- 1Faculty of Pharmacy, Department of Clinical Pharmacy and Pharmacology, University of Dhaka, Dhaka, Bangladesh
- 2Department of Pharmacy, Noakhali Science and Technology University, Noakhali, Bangladesh
- 3Medicines Research Group, School of Health, Sports and Bioscience, University of East London, London, United Kingdom
Antimicrobial resistance has been posing an alarming threat to the treatment of infectious diseases over the years. Ineffectiveness of the currently available synthetic and semisynthetic antibiotics has led the researchers to discover new molecules with potent antimicrobial activities. To overcome the emerging antimicrobial resistance, new antimicrobial compounds from natural sources might be appropriate. Secondary metabolites from natural sources could be prospective candidates in the development of new antimicrobial agents with high efficacy and less side effects. Among the natural secondary metabolites, diterpenoids are of crucial importance because of their broad spectrum of antimicrobial activity, which has put it in the center of research interest in recent years. The present work is aimed at reviewing recent literature regarding different classes of natural diterpenes and diterpenoids with significant antibacterial, antifungal, antiviral, and antiprotozoal activities along with their reported structure–activity relationships. This review has been carried out with a focus on relevant literature published in the last 5 years following the Preferred Reporting Items for Systematic Reviews and Meta-Analyses (PRISMA) guidelines. A total of 229 diterpenoids from various sources like plants, marine species, and fungi are summarized in this systematic review, including their chemical structures, classification, and significant antimicrobial activities together with their reported mechanism of action and structure–activity relationships. The outcomes herein would provide researchers with new insights to find new credible leads and to work on their synthetic and semisynthetic derivatives to develop new antimicrobial agents.
1 Introduction
Over the past few decades, the world population has witnessed an alarming surge of antimicrobial resistance (AMR)—the tip of the iceberg being witnessed during the ongoing coronavirus pandemic. Newer and more lethal pathogens seem to be surfacing, while existing bacteria keep developing newer strategies to resist the action of antibiotics, some even evolving to the “superbug” status. According to a recently published report on tackling drug-resistance infections globally by the United Kingdom government, the global death toll due to drug-resistant infections has been estimated to reach 10 million by 2050 if new antimicrobial strategies are not discovered (O’Neill, 2016). Continued emergence of antibiotic resistance has posed a big risk for health, which increases the mortality and economic burden worldwide (Ciorba et al., 2015). Innovative targeted therapeutic strategies involving newer technology are being considered to deal with these multi-drug-resistant pathogenic bacteria (Yang et al., 2021). New strategies are being developed for sustainable discovery of antibiotics in order to keep up with the ever-increasing demand of novel antimicrobials and reduce the lack of investment in their development (Miethke et al., 2021). Particularly, antimicrobial agents derived from natural sources could be a great tool to deal with these multi-drug-resistant pathogens.
Natural products are a rich source of bioactive compounds and are continuously being investigated to discover new compounds with therapeutic potential to act as lead compounds for drug development (Porras et al., 2021). Several natural products have been adopted as conventional drugs or have been valuable lead compounds for new drug discovery (Newman and Cragg, 2016; Rodrigues et al., 2016). Between 1881 and 2002, about 877 small molecules have been introduced as drugs, among which 49% were natural products, semisynthetic natural products, or synthetic compounds inspired from natural product pharmacophores (Koehn and Carter, 2005). Several important drugs such as morphine, tubocurarine, reserpine, cocaine, vincristine, vinblastine, lovastatin, and paclitaxel originated from natural sources. Natural products also possess potential antimicrobial activity via various mechanisms (Khameneh et al., 2019; Chassagne et al., 2021).
Among natural products, diterpenes and diterpenoids are widely prevalent secondary metabolites, with various significant pharmacological effects, which include antitumor, anti-inflammatory, immune modulation, and so on (Mantaj et al., 2015; Ding et al., 2016; Lin et al., 2016). Diterpenes and diterpenoids are isoprene (C5)-derived chemical compounds consisting of four isoprene units joined head to tail, with the basic molecular formula C20H32, and have diverse possibilities of structure subtypes (Eksi et al., 2020). They are mainly classified based on the number of rings present on their chemical structure. Major classes include acyclic diterpenes (phytane), monocyclic diterpenes (retinol—vitamin A), bicyclic diterpenes (clerodane, halimane, and labdane), tricyclic diterpenes (abietane, rosane, pimarane, podocarpane, cassane, vouacapane, and chinane), tetracyclic diterpenes (kaurene, gibberellane, trachylobane, scopadulane, aphidicolane, atisane, stemodane, beyerene, stemarane), macrocyclic diterpenes (polycyclic—cembrane, jatrophane, taxane, ingenane, daphnane, and tigliane), and miscellaneous structures (Eksi et al., 2020). Although the terms diterpenes and diterpenoids are often used interchangeably in scientific literature, diterpenes are strictly hydrocarbons and have no heteroatoms in their structure, whereas functionalized structures produced by oxidation, substitution, and a wide range of skeletal rearrangements are termed as diterpenoids. They are produced via the mevalonic acid biosynthetic pathway, by condensation reaction of isopentenyl pyrophosphate (IPP) with farnesyl pyrophosphate (FPP), which yields geranylgeranyl pyrophosphate (GGPP) in a few consecutive reactions (Singh and Sharma, 2015). As secondary metabolites, diterpenes and diterpenoids are biosynthesized in various plant, marine, sponge, insect, and fungal species in response to biotic and abiotic stresses (Zi et al., 2014). Several diterpenes have been synthetically produced as well, which possess various potent biological activities (Rahman et al., 2001a; Tanaka et al., 2001, Rahman et al., 2001b; Rahman et al., 2001c; Hayashi et al., 2004; Banerjee et al., 2008).
Medicinal plants that have been widely used in traditional medicines for the treatment of various types of infections are rich in terpenoids—monoterpenes, diterpenes, triterpenes, and tetraterpenes. Diterpene- and diterpenoid-rich herbal plants are traditionally used for the treatment of various diseases. Andrographis paniculata (Burm.f.) Nees, a medicinal herb with the labdane diterpenoid andrographolide as its major constituent, is widely used in Ayurveda and traditional Chinese medicine (TCM) for the treatment of cold, fever, sore throat, swollen and painful gums, and inflammation caused by virus-related diseases (Jiang et al., 2021). Plectranthus madagascariensis (Pers.) Benth., an indigenous South African plant that contains abietane diterpenes in its extracts and oils, has been traditionally used to treat various dermatological and respiratory ailments (Lambrechts and Lall, 2021). Isodon herbs, which are native to Japan and used traditionally as bitter stomachic, contains ent-kaurane diterpenoids as their major phytoconstituent (Tanaka and Ito, 2021). Plants from the Daphne genus contain an abundance of natural diterpenoids and have a long history of traditional use as treatments for acne, rheumatism, and inflammation (Nie et al., 2021). There are numerous similar examples of medicinal plants which contain diterpenes and diterpenoids and are being traditionally used as ailments for various diseases throughout the world.
Remarkable biological activities have been reported by natural diterpenes and diterpenoids, making them potential candidates for lead development (Mafu and Zerbe, 2017). Many existing drugs and herbal medicines, such as paclitaxel (Bernabeu et al., 2017), andrographolide (Kishore et al., 2016; Banerjee et al., 2017), and ginkgolides (Nabavi et al., 2015) are diterpenoids with unique structural scaffolds and potent pharmacological properties. However, due to their vast biodiversity, and even newer ones being discovered each year, few papers have reviewed diterpenes in general, focusing on their antimicrobial potency. Instead, most literature have focused on structure identification and bioactive evaluation of diterpenes or diterpenoids belonging to individual classes. In this present work, we aimed to extensively cover diterpenes and diterpenoids possessing antibacterial, antiviral, antifungal, and antiprotozoal activities, by screening recent studies that have reported isolation of such potent antimicrobials from natural sources. We have systematically summarized these activities, with an emphasis on recent studies that have been reported since 2017, with more than 100 references cited. These insights into the antimicrobial potency of diterpene and diterpenoids will help to identify potential candidates for lead development that would contribute towards the development of more effective clinical antimicrobial drugs in the future.
2 Methods
The study was designed as a systematic review of recent literature for investigation into diterpenes and diterpenoids with potent antimicrobial activity isolated from natural sources. This review was conducted following the protocols of the Preferred Reporting Items for Systematic Reviews and Meta-Analyses (PRISMA). Chemical structures used in this manuscript have been drawn using ChemDraw 16.0 (PerkinElmer, United States).
2.1 Search Strategy
The extensive literature search for relevant articles, papers, and books was conducted systematically using three databases: PubMed, Scopus, and Google Scholar. Literature search included publications from the last 5 years, from 2017 to 2021, to ensure systematic analysis and presentation of recently isolated antimicrobial diterpenes and diterpenoids. Articles pooled from the databases were manually checked, and duplicates were removed. Search terms used for extracting literature included “Diterpenes,” “Diterpenoids,” or their MeSH term “Diterpenes [Mesh]” and were connected with terms such as “Antimicrobial” and “Anti-Infective Agents [Mesh]” using the Boolean operator “AND”. Database-specific filters such as full text, English language, and publication year were applied to specify the search pool. Retrieved articles were initially screened by reading the title, keywords, and abstract to assess the article’s relevance to our research aim. Articles that did not align with the selection criteria were excluded. All relevant articles were downloaded, and the full text was assessed according to the inclusion and exclusion criteria.
2.2 Selection Criteria
Certain inclusion and exclusion criteria were predetermined in order to determine eligibility of relevant literatures for this present review. Inclusion criteria for article selection included journal articles, conference papers, and book chapters that have been published between January 2017 and August 2021, articles published in English language and available as full text, studies which isolated diterpenes and diterpenoids from natural sources (plant, marine species, fungi, etc.), and articles where at least one isolated diterpene or diterpenoid possessed antimicrobial properties (antibacterial, antiviral, antifungal, or antiprotozoal). Exclusion criteria for primarily screened articles included reviews or systematic reviews, articles preceding 2017, papers written in languages other than English or with no full text available, studies where diterpenoids were synthetically produced, and articles where none of the isolated diterpenoids exhibited any sort of antimicrobial characteristics.
3 Results
Based on the selection criteria and search strategy implemented, a total of 170 articles were identified and accumulated from PubMed, Scopus, and Google Scholar. After data cleaning for duplicates, 155 articles were pooled for screening. As shown in the PRISMA flow diagram (Figure 1), screening of titles and abstracts yielded 132 articles. The total number of articles that met the eligibility criteria was 95, and they were critically analyzed to give a comprehensive overview of the isolated diterpenoids, their chemical structures, and the reported antimicrobial activity against different microorganisms.
3.1 Antibacterial Activity
Natural diterpenoids can be potential candidates for designing new antibiotics against the emerging bacterial resistance. A number of classes of natural diterpenoids have been found to have broad-spectrum antibacterial activity (Table 1).
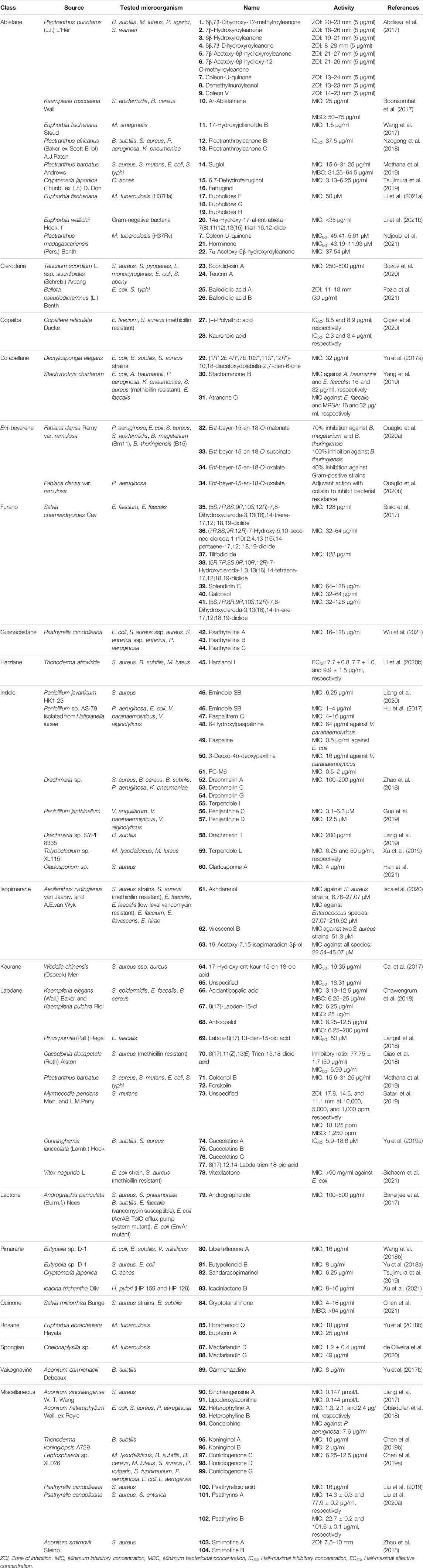
TABLE 1. Different classes of diterpenoids isolated from natural sources with significant antibacterial activity.
3.1.1 Abietane Diterpenoids
Abdissa et al. isolated four novel and thirteen known abietane-type diterpenoids from the roots of the herbaceous perennial plant Plectranthus punctatus (L.f.) L'Hér [Lamiaceae], among which compounds 1–9 were active against the tested microorganisms. The compounds exhibited significant inhibitory activity at 5 μg/ml against a number of Gram-positive and Gram-negative microorganisms, some exhibiting a zone of inhibition (ZOI) superior to that of the reference gentamycin. Compounds 1, 2, 4, 5, and 6 (Figure 2) demonstrated a ZOI in the range 22–28 mm against Staphylococcus warneri, and compounds 5, 6, and 7 showed a ZOI of 24–25 against Micrococcus luteus (Table 1), which were higher than that of gentamycin (ZOI = 21 and 23 mm, respectively). Compounds 3, 8, and 9 exhibited a significant ZOI in the range of 13–23 mm against M. luteus and Bacillus subtilis (Abdissa et al., 2017).
Boonsombat et al. isolated 20 diterpenoids from the whole plant of Kaempferia roscoeana Wall [Zingiberaceae], a popularly used spice in Thai cuisine. The compounds were tested for antibacterial activity against several Gram-positive and Gram-negative organisms along with fungal and malarial species. Only compound 10 exhibited activity against Gram-positive bacteria strains Staphylococcus epidermidis and Bacillus cereus with minimum inhibitory concentration (MIC) values of 25 μg/ml against both species and minimum bactericidal concentration (MBC) values of 75 and 50 μg/ml, respectively. No activity was reported against any of the Gram-negative, fungal, and malarial strains (Boonsombat et al., 2017).
Wang et al. isolated four new ent-abietane types, four new tigliane types, and thirteen other known diterpenoid compounds from the roots of Euphorbia fischeriana Steud [Euphorbiaceae] and investigated their antitubercular activity against Mycobacterium smegmatis by alamarBlue cell viability assay (Thermo Fisher Scientific Inc.) using kanamycin as positive control. Among all the compounds, ent-abietane-type diterpenoids exhibited overall more potent antitubercular activity than the tigliane-type diterpenoids, and compound 11 exhibited the most potent antitubercular activity with an MIC value of 1.5 μg/ml while the MIC values of the other compounds ranged from 100 to 400 μg/ml (Wang et al., 2017).
Nzogong et al. isolated three new and five known abietane diterpenoids from Plectranthus africanus (Baker ex Scott-Elliot) A. J. Paton [Lamiaceae] and examined their antibacterial activity against Gram-positive bacteria (B. subtilis and Staphylococcus aureus) and Gram-negative bacteria (Pseudomonas aeruginosa and Klebsiella pneumoniae). Among the isolated diterpenoids, compounds 12 and 13 showed broad-spectrum antibacterial activity against both Gram-positive and Gram-negative bacteria with a 50% inhibitory concentration (IC50) of 37.5 μg/ml (Nzogong et al., 2018).
Three diterpenoids were isolated from Plectranthus barbatus Andrews [Lamiaceae] by Mothana et al., and their antibacterial activity against S. aureus, Streptococcus mutans, Escherichia coli, and Salmonella typhi wild strain was evaluated using the Mueller Hinton broth (MHB) or Sabouraud dextrose broth micro-well dilution method. Among all the abietanes, compound 14 was found to be the most potent antibacterial agent with an MIC of 15.6–31.25 μg/ml and an MBC of 31.25–64.5 μg/ml (Mothana et al., 2019).
Tsujimaru and coworkers isolated four abietane diterpenoids from the wood drying product of Cryptomeria japonica (Thunb. ex L.f.) D.Don [Cupressaceae] (sugi) and investigated their antibacterial activities against anaerobic Gram-positive bacteria Cutibacterium acnes. Among all the diterpenoids, 15 and 16 showed the most potent antibacterial activity with MIC values ranging from 3.13 to 6.25 μg/ml (Tsujimura et al., 2019).
Li et. al. (2021) isolated 15 ent-abietane diterpenoids including eight unknown eupholides A–H and seven known diterpenoids from the roots of E. fischeriana Steud [Euphorbiaceae], which is traditionally used in Chinese medicine, named as “Langdu,” for the treatment of tuberculosis. All these diterpenoids were subjected to antituberculosis bioassay by co-incubation with Mycobacterium tuberculosis H37Ra by alamarBlue cell viability assay (Thermo Fisher Scientific Inc.) in 96-well microplates using resazurin as a staining agent. Among the isolated diterpenoids, compounds 17–19 showed moderate inhibition of the proliferation of M. tuberculosis with an MIC value of 50 μM (Li DW. et al., 2021).
Three new ent-abietane diterpenoids isolated from Euphorbia wallichii Hook. f [Euphorbiaceae] were tested against several Gram-positive and Gram-negative bacterial strains. The diterpenoids showed significant activity against Gram-negative bacterial strains only (MIC < 60 μg/ml); compound 20 especially showed strong activity (MIC < 35 μg/ml) (Li H. et al., 2021).
Ndjoudi et al. reported five royleanone diterpenoids from P. madagascariensis (Pers.) Benth [Lamiaceae] and tested for their antitubercular activity against the M. tuberculosis H37Rv strain using the standard broth microdilution method. Compounds 21 and 7 showed significant antimycobacterial activity (MIC90 = 43.19–11.93 and 45.41–5.61 μM, respectively, after 14 days), and 22 showed moderate activity (MIC = 37.54 μM after 14 days). The results implied that the activity of the compounds could be affected by the protein binding capacity of the compounds, and a structure–activity relationship (SAR) could be inferred from the activity pattern of the diterpenoids. The presence of a 6β-hydroxy group could be responsible for the reduction of the antimycobacterial potency, and the presence of p-benzoquinone ring C may have a role in the antimycobacterial activities and that the substituents at C-6 and C-7 in ring B could considerably influence the antitubercular activity (Ndjoubi et al., 2021).
3.1.2 Clerodane Diterpenes
Bozov et al. isolated one new neo-clerodane diterpenoid and two known furo-clerodane diterpenoids from the aerial parts of Teucrium scordium L. ssp. scordioides (Schreb.) Arcang [Lamiaceae]. The novel compound scordidesin (23) (Figure 3) and previously identified compound teucrin A (24) were tested for both antibacterial and antifungal activities against several bacterial and fungal species. Both compounds showed similar activities against the bacterial species only, with MIC values ranging between 250 and 500 μg/ml (Bozov et al., 2020).
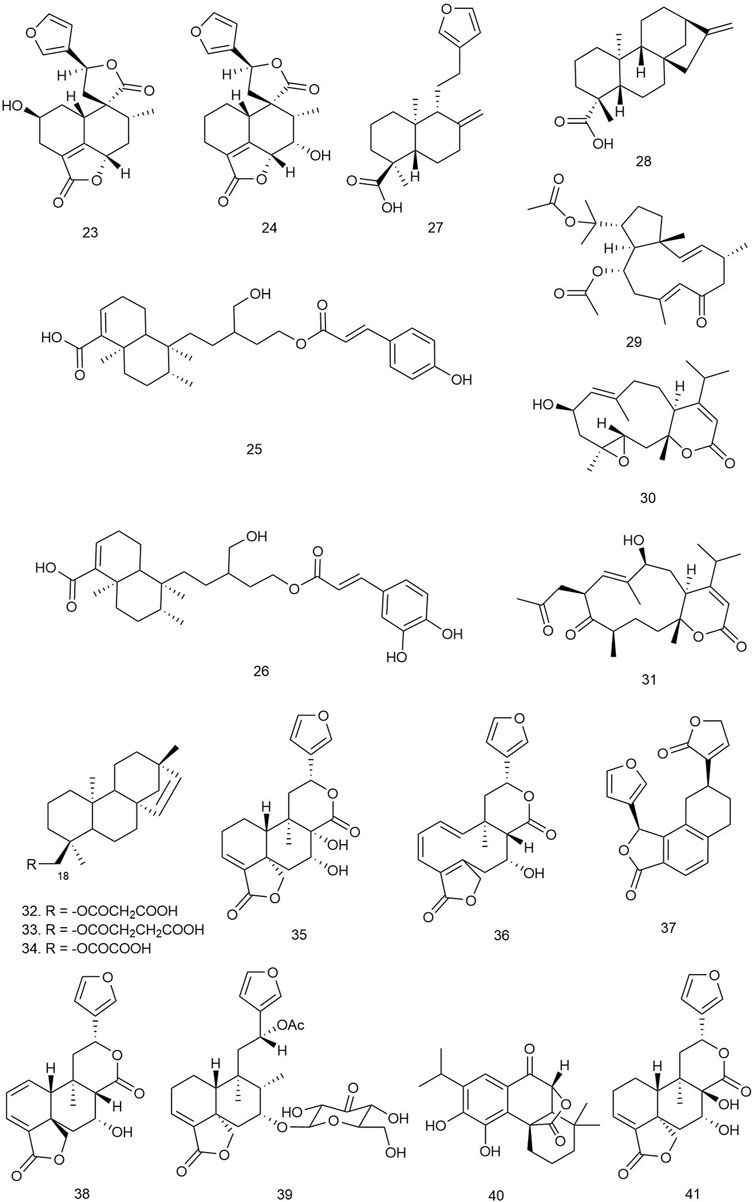
FIGURE 3. Structures of clerodane, copaiba, dolabellane, ent-beyerene, and furano diterpenoids with significant antibacterial activity.
Fozia et al. isolated two novel diterpenes, ballodiolic acids A (25) and B (26), and two other known diterpenes from the root material of Ballota pseudodictamnus (L.) Benth [Lamiaceae]. The compounds were tested for antibacterial activity against a number of bacterial species. At 30 μg/ml concentration, 25 and 26 exhibited the most potent antibacterial properties with ZOIs ranging between 11–13 and 11–12 mm, respectively, against E. coli and S. typhi strains (Fozia et al., 2021).
3.1.3 Copaiba Diterpenoids
Two major copaiba diterpenoids, 27 and 28, were isolated from the oleoresin of Copaifera reticulata Ducke [Fabaceae]. These two compounds and eight of their synthetic derivatives were initially tested for growth inhibition against several bacterial and fungal strains at 100 μg/ml concentration. The natural compounds seemed to be effective only against Enterococcus faecium and methicillin-resistant S. aureus (MRSA) species. Further testing revealed that 27 and 28 were much more potent than their semisynthetic derivatives with IC50 values of 8.5 and 8.9 μg/ml against E. faecium and 2.3 and 3.4 μg/ml against MRSA, respectively (Çiçek et al., 2020).
3.1.4 Dolabellane Diterpenoids
Yu and coworkers isolated two new and two known dolabellane diterpenes from marine sponge Dactylospongia elegans (Thiele) [Thorectidae] and evaluated their antibacterial effects using the broth microdilution assay method against E. coli, B. subtilis, and S. aureus strains. Among the diterpenes, only compound 29 showed potent antibacterial activity (MIC = 32 μg/ml), and other compounds showed mild antibacterial activity against the tested strains (MIC = 32–64 μg/ml) (Yu H.-B. et al., 2017).
Yang et al. isolated three new dolabellane diterpenoids and three new C alkylated dolabellanes, atranones, from a fungus Stachybotrys chartarum [Stachybotryaceae] and evaluated their antibacterial properties against some bacterial strains including extended-spectrum beta-lactamases (ESBL)-producing E. coli, Acinetobacter baumannii, P. aeruginosa, K. pneumoniae, MRSA, and Enterococcus faecalis. Only compound 30 was found to be active against A. baumannii and E. faecalis (MIC = 16 and 32 μg/ml, respectively), and 31 exhibited significant growth inhibition against E. faecalis and MRSA (MIC = 16 and 32 μg/ml, respectively) (Yang et al., 2019).
3.1.5 Ent-Beyerene Diterpenoids
Quaglio et al. reported seven tetracyclic ent-beyerene-type diterpenes from the aerial parts of Fabiana densa Remy var. ramulosa [Solanaceae], which were subjected to antibacterial assay by the broth microdilution method against Gram-negative P. aeruginosa and E. coli and Gram-positive S. aureus, S. epidermidis, Bacillus megaterium Bm11, and Bacillus thuringiensis B15. Among the isolated diterpenes, compounds 32–34 were found to be active against the tested microorganisms. Compound 32 showed significant activity against Gram-positive bacterial strains, especially against Bacillus spp. (70% inhibition), and 33 inhibited 100% growth of B. thuringiensis and 80% growth of S. epidermidis and B. megaterium. Compound 34 showed moderate growth inhibition against other Gram-positive strains (40% growth inhibition). The inactivity of the dimeric diterpenes towards any of the tested microorganisms could indicate that the acidic group at C18 of the tetracyclic ent-beyerene scaffold for the antibacterial effects and the length and flexibility of the alkyl chain between the two carbonyl groups are important factors for the antibacterial activity of the molecule (Quaglio et al., 2020a).
Four ent-beyerene diterpenoids from F. densa var. ramulosa Wedd [Solanaceae] were tested against resistant P. aeruginosa strains to evaluate their adjuvant activity with the antibiotic colistin. Among them, compound 34 was recently patented for its novel colistin adjuvant activity by inhibiting the undecaprenyl phosphate-alpha-4-amino-4-deoxy-L-arabinose arabinosyl transferase (ArnT) enzyme, which is responsible for resistance. The ent-beyerene skeleton was found to be a privileged scaffold for further development of colistin resistance inhibitors (Quaglio et al., 2020b).
3.1.6 Furano Diterpenes
Bisio et al. isolated 13 diterpenes, including seven new furano-diterpene (Figure 3) from the surface extract of the aerial parts of Salvia chamaedryoides Cav [Lamiaceae], a Mexican perennial species of the Flocculosae section. The compounds were tested for antimicrobial activity on 26 clinical strains, which included several multidrug-resistant strains. Although the compounds did not show any growth inhibition of the Gram-negative species, compounds 35–41 inhibited the growth of several E. faecalis and E. faecium strains with MIC values ranging between 32 and 128 μg/ml (Bisio et al., 2017).
3.1.7 Guanacastane Diterpenoids
Wu et al. isolated five new guanacastane diterpenoids from the mushroom Psathyrella candolleana (Fr.) Maire [Psathyrellaceae], and the compounds were subjected to antibacterial assay against E. coli, S. aureus ssp. aureus, Salmonella enterica ssp. enterica, and P. aeruginosa using chloramphenicol as positive control. Among them, compounds 42–44 (Figure 4) were found to be the most potent antibacterial agents with MIC values ranging from 16 to 128 μg/ml (Wu et al., 2021).
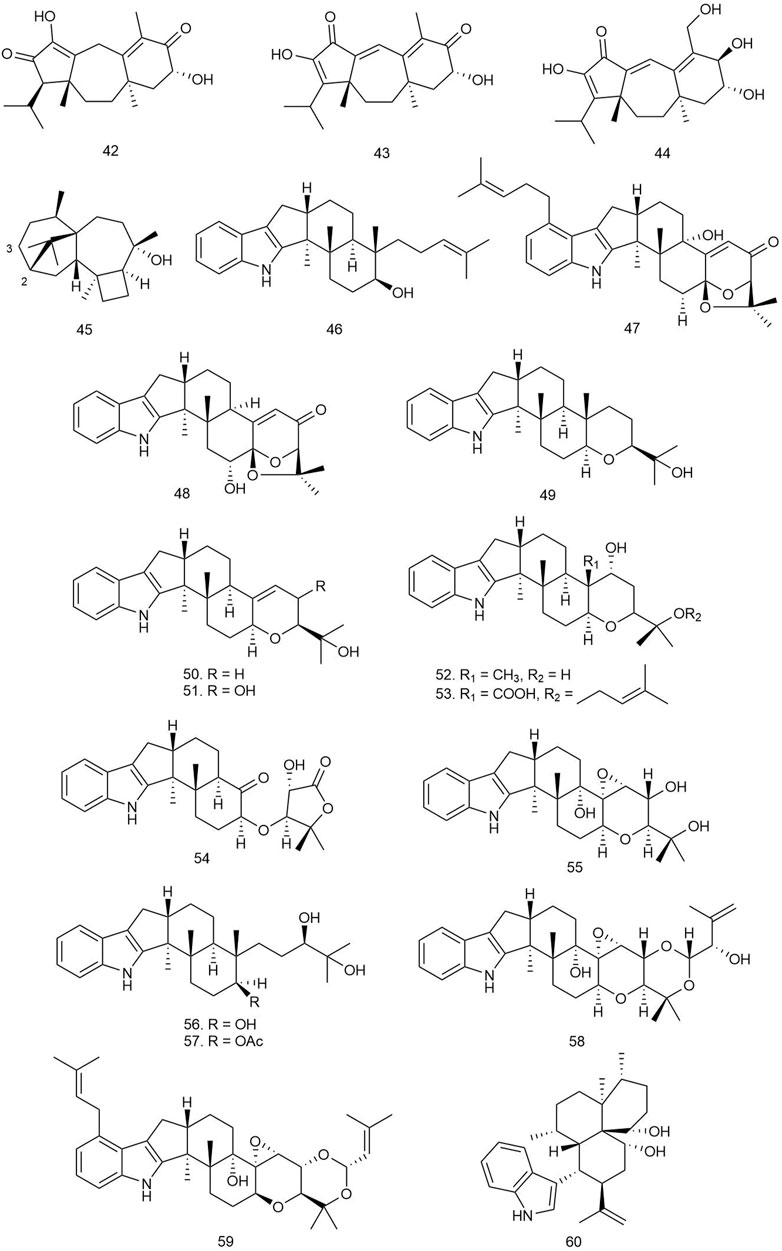
FIGURE 4. Structures of guanacastane, harziane, and indole diterpenoids with significant antibacterial activity.
3.1.8 Harziane Diterpenoids
Four harziane-type diterpenoids isolated from the endophytic fungus Trichoderma atroviride from the healthy flower of a Lamiaceae plant Colquhounia coccinea var. mollis were tested against S. aureus, B. subtilis, and M. luteus by the broth dilution method using ampicillin as positive control. Among all the harzianes, only compound 45 exhibited significant antibacterial activity against S. aureus, B. subtilis, and M. luteus with EC50 values of 7.7 ± 0.8, 7.7 ± 1.0, and 9.9 ± 1.5 μg/ml, respectively. The probable reason behind the inactivity of other harziane diterpenoids may be the functionality at C-2 or C-3 of the compounds, which might be responsible for diminishing their antibacterial activities (Li WY. et al., 2020).
3.1.9 Indole Diterpenoids
Hu et al. isolated 11 indole diterpenoids, including three novel ones, from Penicillium sp. AS-79, a fungal strain isolated from the fresh tissue of the sea anemone Haliplanella luciae. The isolated compounds were tested against several human, aqua, and plant pathogenic microbes. Compounds 46–48 were found to be the most potent ones, exhibiting MIC values of 1–4, 4–16, and 64 μg/ml, respectively, against P. aeruginosa, E. coli, Vibrio parahaemolyticus, and Vibrio alginolyticus species. Compound 46 was also isolated from Penicillium javanicum HK1-23 obtained from mangrove rhizosphere soil, and it exhibited selectivity towards S. aureus ATCC 33591 (MIC = 6.25 μg/ml) (Liang et al., 2020). Compound 49 was bioactive against E. coli with an MIC value of 0.5 μg/ml, while 50 and 51 were active against V. parahaemolyticus with MIC values of 16 and 0.5–2 μg/ml, respectively (Hu et al., 2017).
Zhao and coworkers isolated seven new and four known diterpenoids from Drechmeria sp., isolated from the root of Panax notoginseng and evaluated for their antibacterial activity against S. aureus, B. cereus, B. subtilis, P. aeruginosa, and K. pneumoniae by the broth microdilution method. Among the indole diterpenoids, 52–55 showed weak antibacterial activity with MIC values ranging from 100 to 200 μg/ml. For getting insight into the mechanism of antimicrobial activity of the diterpenoids, molecular docking was performed, targeting peptide deformylase (PDF), which plays an important role in bacterial protein maturation, growth, and survival, and so it has become a pivotal target for designing antimicrobial drugs. Compounds 52–55 along with drechmerin B showed significant accessibility to the ligand-binding domain of the PDF protein (binding energy ranging from –3.16 to –7.08 kcal/mol) interacting with Gln65, Gly60, Cys111, Leu112, Glu155, and Zn2202 (Zhao et al., 2018).
Two new indole diterpenoids, 56 and 57, as well as two other previously identified diterpenoids were isolated from the Bohai Sea fungus Penicillium janthinellum in an effort to discover anti-Vibrio natural products. Conventional broth dilution assay was utilized to measure their antimicrobial activity. Compound 56 demonstrated strong anti-Vibrio activity against Vibrio anguillarum, V. parahaemolyticus, and V. alginolyticus with MIC values of 3.1, 6.3, and 3.1 µM, respectively, and 57 showed moderate activity against the three Vibrio species with the same MIC values of 12.5 µM (Guo et al., 2019).
Liang et al. isolated one new indole diterpenoid, 58, from the fermentation broth of Drechmeria sp. SYPF 8335 strain isolated from the root of P. notoginseng (Burkill) F. H. Chen ex C. Y. Wu & K. M. Feng [Araliaceae] and assayed for antimicrobial effects against Candida albicans, S. aureus, B. cereus, B. subtilis, P. aeruginosa, and K. pneumoniae using the broth microdilution method (NCCL 2202). The compound showed an inhibitory effect against B. subtilis with an MIC of 200 μg/ml and against other microorganisms whose MIC value was more than 400 μg/ml compared to the standard ampicillin and geneticin used as positive control. For predicting the probable mechanism of antimicrobial activity, PDF was used for molecular docking target because of its important role in bacterial protein maturation, growth, and survival by N-formyl group degradation for the polypeptide. The compound was found to be well docked in the catalytic site of PDF with −9.8 kcal/mol binding free energy through hydrogen bonds with Try-88 and Arg-143, which suggested its probable mechanism of antimicrobial action (Liang et al., 2019).
Xu with coworkers reported two new and one known prenylated indole diterpenoids from a mine soil-derived fungus Tolypocladium sp. XL115 and investigated their antibacterial activity against eight human pathological bacterial strains, including Micrococcus lysodeikticus, M. luteus, B. megaterium, Salmonella paratyphi B, S. typhi, P. aeruginosa, E. coli, and Enterobacter aerogenes and using ciprofloxacin as positive controls. Among all three diterpenoids, only compound 59 exhibited remarkable antibacterial activity against M. luteus and M. lysodeikticus with MIC values of 6.25 and 50 μg/ml, respectively (Xu et al., 2019).
Compound 60, a new sterically congested indole diterpenoid alkaloid, was isolated from the fungi Cladosporium sp. Its antimicrobial activity was evaluated against S. aureus and E. coli. Strong antibacterial activity was reported against S. aureus with an MIC value of 4 μg/ml (Han et al., 2021).
3.1.10 Isopimarane Diterpenes
Isca et al. isolated six diterpenoids from the plant Aeollanthus rydingianus van Jaarsv. and A.E.van Wyk belonging to the Lamiaceae family. The compounds were tested for antibacterial activity against several clinical strains of S. aureus and several species of Enterococcus, including two methicillin-resistant strains of S. aureus and one vancomycin-resistant E. faecalis strain. Among all the diterpenes, compounds 61–63 were found to be bioactive against the tested strains. Compound 61 (Figure 5) showed the most potent activity, with an MIC value ranging between 6.76 and 27.07 µM against S. aureus strains and 27.07–216.62 µM against different Enterococcus species. Compound 62 was bioactive only against two strains of S. aureus with an MIC value of 51.3 µM. The diterpene 63 also exhibited potent antibacterial activity with MIC values ranging between 22.54 and 45.07 µM against all tested strains of S. aureus and all species of Enterococcus. The other diterpenes did not show any antimicrobial potency (Isca et al., 2020).
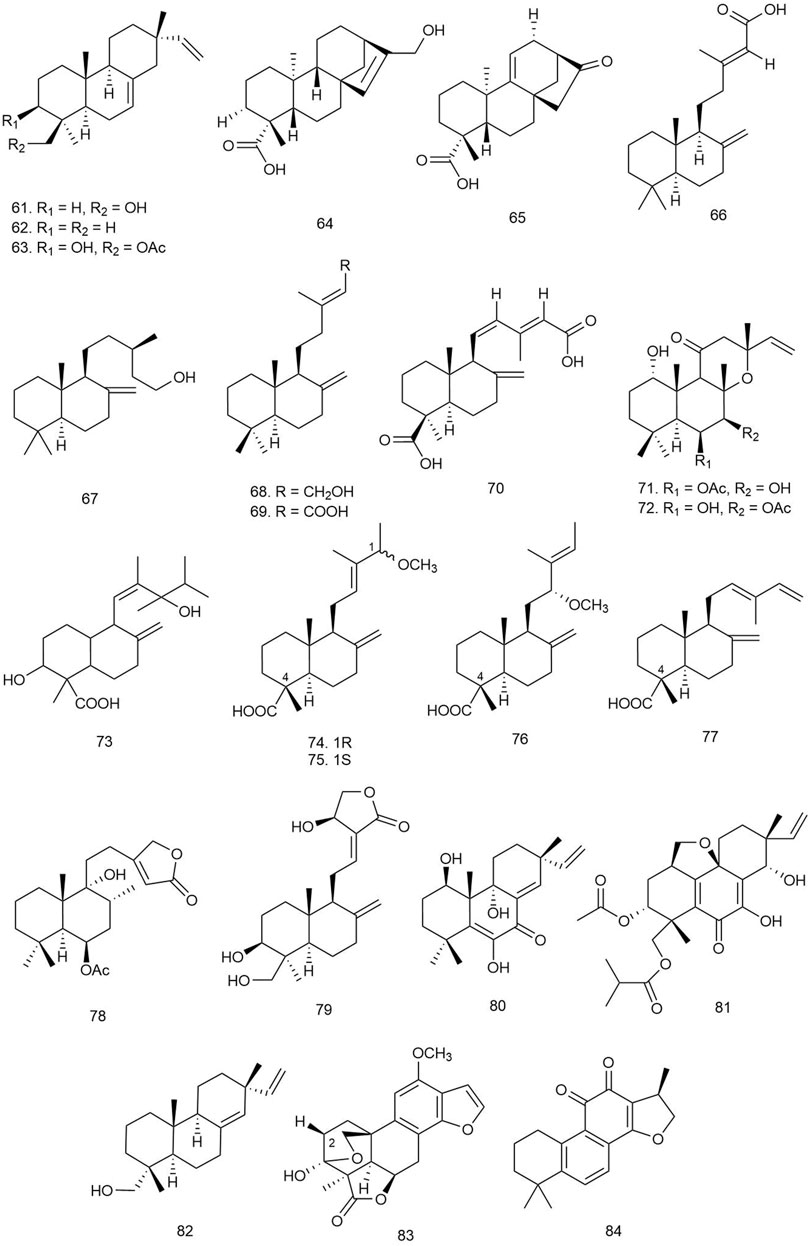
FIGURE 5. Structures of isopimarane, kaurene, labdane, lactone, pimarane, and quinone diterpenoids with significant antibacterial activity.
3.1.11 Kaurane Diterpenoids
Two novel kaurane-type diterpenoids along with 10 other known ones were isolated from the whole plant Wedelia chinensis (Osbeck.) Merr [Compositae]. Some of these compounds were evaluated for their antibacterial activities, and only compounds 64 and 65 exhibited moderate inhibitory activity against S. aureus ssp. aureus with MIC50 values of 19.35 and 18.31 μg/ml, respectively (Cai et al., 2017).
3.1.12 Labdane Diterpenoids
A total of 19 diterpenoids were isolated from the rhizomes of Kaempferia elegans (Wall.) Baker and Kaempferia pulchra Ridl [Zingiberaceae]. The compounds were tested for antimicrobial activities against several Gram-positive and Gram-negative bacterial strains as well as antifungal activity against several yeast and fungal strains. Among the labdanes, compounds 66 and 68 showed antimicrobial activity with MIC/MBC values of 12.5/18.75 and 12.5/200 μg/ml against S. epidermidis; 12.50/25 and 6.25/200 μg/ml against E. faecalis; and 3.13/6.25 and 6.25/6.25 μg/ml against B. cereus, respectively. Compound 67 exhibited activity only against B. cereus with the MIC/MBC values of 6.25/25 μg/ml (Chawengrum et al., 2018).
Langat et al. isolated pumilol-a rare strobane-type diterpenoid from the bark of Pinus pumila (Pall.) Regel [Pinaceae], more commonly known as Siberian dwarf pine or Japanese stone pine, along with nine other previously identified labdane- and abietane-type diterpenoids. The compounds were primarily screened for antimicrobial activity by administering 50 µM on five bacterial and one fungal strain, and only 69 showed more than 90% growth inhibition of E. faecalis (Langat et al., 2018).
Qiao et al. reported two C20 epimeric diterpenoids and one new labdane diterpenoid from the leaves of the medicinal plant Caesalpinia decapetala (Roth) Alston [Fabaceae]. These compounds were subjected to an antibacterial test by a microdilution assay in sterile 96-well microtiter plates using standard penicillin G and ceftazidime against E. coli, S. aureus, S. enterica, and P. aeruginosa. Among the isolated diterpenoids, compound 70 inhibited the growth of MRSA at 50 μg/ml (inhibitory ratio value 77.745 ± 1.704), and the MIC50 of the compound was found to be 5.99 μg/ml (Qiao et al., 2018).
Mothana et al. isolated two labdane diterpenes from P. barbatus Andrews [Lamiaceae], which were subjected to the MHB or Sabouraud dextrose broth micro-well dilution method to evaluate antibacterial activity against S. aureus, S. mutans, E. coli, and S. typhi wild-type strains. Among the compounds, 71 and 72 were found to exhibit the most potent antimicrobial activity with MIC values between 15.6 and 31.25 μg/ml. The lipophilic nature of the diterpenoids could be an attributing factor for easy transport through the cell membrane and accumulation inside the cell to affect the cells (Mothana et al., 2019).
Satari et al. reported a labdane diterpene from Myrmecodia pendens Merr. and L.M.Perry [Rubiaceae] and tested it against S. mutans by the Kirby–Bauer method for zone inhibition using chlorhexidine as positive control. The diterpene 73 was found to possess significant antibacterial activity against the tested strain with ZOIs of 17.8, 14.5, and 11.1 mm at doses of 10,000, 5,000, and 1,000 ppm and MIC and MBC values of 18.125 and 1,250 ppm, respectively (Satari et al., 2019).
Yu et al. isolated four new (three labdane one abietane type) and three known labdane-type diterpenoids from the leaves of Cunninghamia lanceolata (Lamb.) Hook [Taxodiaceae] and evaluated their antibacterial activity against B. subtilis and S. aureus by the liquid growth inhibition method using penicillin as positive control. Among these compounds, four labdane derivatives, 74–77, showed significant antibacterial activity (IC50 values ranging from 5.9 to 18.6 μM), which indicates that the presence of the 4α-carboxyl group in the labdane-type diterpenoids could be of crucial importance for their significant antibacterial activities (Yu JH. et al., 2019).
Four labdane and one halimane diterpenoid from the leaves of Vitex negundo L [Verbenaceae] were isolated and tested against an ESBL-producing E. coli strain and MRSA using spiramycin as positive control. Only 78 showed significant growth inhibition against the E. coli strain (MIC > 90 mg/ml) (Sichaem et al., 2021).
3.1.13 Lactone Diterpenoids
Andrographolide (79), a diterpenoid lactone found in traditional medicinal herb A. paniculata (Burm.f) Nees [Acanthaceae], was tested for its antimicrobial property against 14 Gram-negative strains and 7 Gram-positive strains. Among the Gram-positive strains, methicillin-susceptible S. aureus (MSSA) was the most susceptible strain to the diterpenoid with an MIC value of 100 μg/ml. S. pneumoniae and B. subtilis both required an MIC of 250 μg/ml while vancomycin-susceptible E. faecalis (VSE) required 500 μg/ml. Among the Gram-negative microorganisms, both the AcrAB-TolC efflux pump system mutant E. coli and EnvA1 mutant E. coli were susceptible to 79, with MIC values of 125 and 250 μg/ml, respectively. Further investigation to determine the mechanism of action of the lactone diterpenoid revealed that it interferes with RNA and protein synthesis of microbes by impairing their DNA synthesis. This results in the inhibition of the downstream biosynthetic pathway. The compound is also thought to prevent biofilm formation as it efficiently inhibited biofilm formation of S. aureus (Banerjee et al., 2017).
3.1.14 Pimarane Diterpenoids
Two new and five known pimarane diterpenoids were isolated from an arctic fungus Eutypella sp. D-1, and their antibacterial potential was checked against E. coli, S. aureus, B. subtilis, Vibrio vulnificus, V. alginolyticus, Aeromonas hydrophila, and Streptococcus agalactiae. Only compound 80 was found to show weak antibacterial activity against E. coli, B. subtilis, and V. vulnificus with an MIC value of 16 μg/ml (Wang X. et al., 2018).
Three new and one known diterpenoids were isolated from Eutypella sp. D-1 and tested against S. aureus, E. coli, B. subtilis, V. alginolyticus, V. vulnificus, S. agalactiae, and A. hydrophila. Among the compounds, only 81 showed antibacterial activity against S. aureus and E. coli with an MIC value of 8 μg/ml (Yu H.-B. et al., 2018).
Among the isolated pimarane-type diterpenoids from the wood drying product of C. japonica (Thunb. ex L. f.) D. Don [Cupressaceae] (sugi), 82 showed significant activity against C. acnes with an MIC value of 6.25 μg/ml (Tsujimura et al., 2019).
Xu et al. reported the presence of two new and ten known pimarane-derived diterpenoids from the tuber of Icacina trichantha Oliv [Icacinaceae] and their antimicrobial activity against both standard and multidrug-resistant strains of Helicobacter pylori (HP 159 and HP 129) by the broth microdilution method using metronidazole as positive control. All diterpenoids were found to be potent antibacterial agents against both strains (MIC = 8–64 μg/ml); 83 especially showed the most prominent activity (MIC = 8–16 μg/ml). Drug interactions between the compound and antibiotics like metronidazole and clarithromycin were tested by the checker-board assay method, which exhibited an additive effect in combination with both metronidazole and clarithromycin, each against G27 strains. The most effective additive action was found in combination with metronidazole or amoxicillin against the clinical strain HP 159 with a fractional inhibitory concentration index (FICI) value of 0.56. The more potent antimicrobial effect of 83 implies the greater contribution of CH3O-12 than 12-OH moiety in the Structure Activity Relationship (SAR) study of the isolated diterpenoids (Xu et al., 2021).
3.1.15 Quinone Diterpenoids
Cryptotanshinone (84), a potential diterpenoid quinone with antimicrobial properties, is found in the root of Salvia miltiorrhiza Bunge [Lamiaceae]. It is used as the major active ingredient in several Chinese patent medicines used for the treatment of acne vulgaris and other skin infections. Chen et al. attempted to investigate the molecular mechanism behind its antimicrobial activity. The diterpenoid was tested against several clinical strains of S. aureus and a single strain of B. subtilis. The compound exhibited significant antimicrobial activity, with MIC/MBC values ranging between 4 and 16/>64 μg/ml. The compound is thought to be a bacteriostatic agent, as it demonstrated MBC/MIC ratios higher than 4 against all strains tested. Test results suggest that 84 works as a respiratory chain inhibitor by targeting NDH-2 (type-II NADH dehydrogenase). It disrupts the NAD+/NADH balance of the bacterial membrane without causing significant membrane damage. It also rapidly dissipates bacterial membrane potential (Chen et al., 2021).
3.1.16 Rosane Diterpenoids
Yu et al. isolated 15 rosane-, abietane-, isopimarane-, and lathyrane-type diterpenoids from the roots of Euphorbia ebracteolata Hayata [Euphorbiaceae] and tested them against M. tuberculosis by an alamarBlue cell viability assay (Thermo Fisher Scientific Inc.) using kanamycin as positive control. Among all the isolated diterpenoids, compounds 85 and 86 (both rosane analogues possessing α,β-unsaturated-ketone and terminal olefinic bonds; Figure 6) showed significant inhibitory activity with MIC values of 18 and 25 μg/ml, respectively. This implies that the unsaturated ketone (C-1/C-2/C-3) could act as a key moiety for the activity. The compounds were also tested for their N-acetylglucosamine-1-phosphate uridyltransferase (GlmU) inhibitory activity by a GlmU acetyltransferase assay, and 85 inhibited GlmU activity moderately (IC50 value 12.5 μg/ml). GlmU protein is a bifunctional enzyme with both acetyltransferase and uridylyltransferase (pyrophosphorylase) activities, which are mainly responsible for catalyzing the formation of UDP-N-acetylglucosamine (UDP-GlcNAc) from glucosamine-1-P (GlcN-1-P), UTP, and acetyl-CoA (Ac-CoA), and the final product UDP-GlcNAc is essential for two important biosynthetic pathways of the cell wall, lipopolysaccharide and peptidoglycan synthesis, which makes GlmU protein a universal target of antibacterial therapy. In consideration of the inhibitory effect of 85 on both M. tuberculosis and GlmU protein, the mechanism of antitubercular activity of the compound could be suggested by this pathway (Yu Z. et al., 2018).
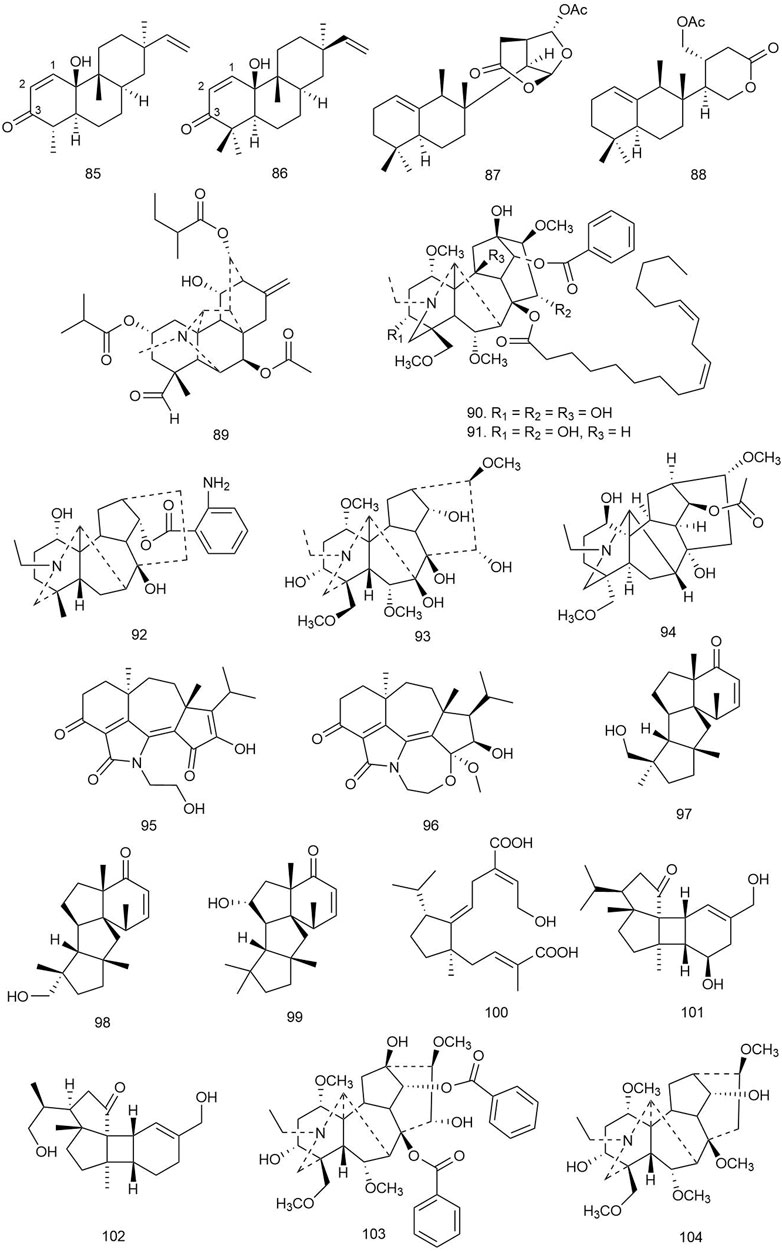
FIGURE 6. Structures of rosane, spongian, vakognavine, and miscellaneous diterpenoids with significant antibacterial activity.
3.1.17 Spongian Diterpenoids
Four diterpenoids were isolated from crude extracts of the marine sponge Chelonaplysilla sp. and tested for antitubercular activity against M. tuberculosis where 87 exhibited potent activity with an MIC of 1.2 ± 0.4 μg/ml, which is ideal for a hit-to-lead antitubercular drug development project as the MIC is lesser than even 5 μg/ml. Compound 88 also inhibited the growth of M. tuberculosis with an MIC value of 49 μg/ml (de Oliveira et al., 2020).
3.1.18 Vakognavine Diterpenoids
Yu et al. isolated one new C20 diterpenoid alkaloid and six known diterpenoids from the roots of Aconitum carmichaelii Debeaux [Ranunculaceae]. The compounds were tested for antibacterial activity against B. subtilis, and only 89 was found to be a potent antibacterial agent like the standard kanamycin used in this study (MIC = 8 μg/ml) (Yu J. et al., 2017).
3.1.19 Miscellaneous Diterpenoids
Liang et al. isolated 15 diterpenoid alkaloids including one novel C19 diterpenoid alkaloid, 90, and 14 known diterpenoids from the root barks of Aconitum sinchiangense W. T. Wang [Ranunculaceae], a traditional Chinese herb. The diterpenoids were tested against Gram-positive S. aureus and Gram-negative E. coli strains by the microdilution method. Among the compounds, 90 and 91 showed more potent antibacterial activity (MIC = 0.147 and 0.144 μmol/L, respectively) against S. aureus compared to the standard berberine hydrochloride (Liang et al., 2017).
Two new and one known C19 diterpenoid alkaloids were isolated from the roots of Aconitum heterophyllum Wall. ex Royle [Ranunculaceae] and tested against E. coli, B. subtilis, Shigella flexneri (clinical isolate), S. aureus, P. aeruginosa, and S. typhi by hole diffusion and broth microdilution methods using imipenem as standard, and the new compounds 92 and 93 showed significant antibacterial activity (MIC = 1.3, 2.1, and 2.4 μg/ml, respectively) against E. coli, S. aureus, and P. aeruginosa, and 94 showed moderate antibacterial activity only against P. aeruginosa (MIC = 7.6 μg/ml) (Obaidullah et al., 2018).
Three new diterpenes were reported by Chen and coworkers from the endophytic fungus Trichoderma koningiopsis A729 and tested for their antibacterial activity against S. aureus, B. subtilis, and E. coli by the resazurin staining method. Compounds 95 and 96 exhibited the most potent antibacterial activities against B. subtilis with MIC values of 10 and 2 μg/ml, respectively (Chen S. et al., 2019).
Five diterpenes were reported from Leptosphaeria sp. XL026 isolated from P. notoginseng (Burkill) F. H. Chen [Araliaceae] and investigated against 10 bacterial strains, namely, M. lysodeikticus, B. subtilis, B. cereus, M. luteus, S. aureus, Proteus vulgaris, Salmonella typhimurium, P. aeruginosa, E. coli, and E. aerogenes. Among the isolated diterpenoids, compounds 97–99 showed moderate antibacterial activity against the selected strains with MIC values ranging from 12.5 to 6.25 μg/ml (Chen HY. et al., 2019).
Liu et al. isolated a novel monocyclic diterpenoid, psathyrelloic acid (100), from the cultures of the edible mushroom basidiomycete P. candolleana and evaluated for its antibacterial activity using the MHB dilution method against E. coli, S. aureus ssp. aureus, S. enterica ssp. enterica, and P. aeruginosa using penicillin G sodium salt and ceftazidime as positive controls. The novel diterpenoid showed significant antibacterial activity against S. aureus with an MIC of 16 μg/ml (Liu et al., 2019).
Two skeletally novel tetracyclic diterpenoids were characterized by Liu et al. from the cultures of the basidiomycete P. candolleana. They were screened for antibacterial activities using the MHB dilution method against S. aureus ssp. aureus, S. enterica ssp. enterica, and P. aeruginosa where penicillin G sodium salt and ceftazidime were used as positive inhibitor controls. Compounds 101 and 102 exhibited weak activities against S. aureus (MIC = 14.3 ± 0.3 and 22.7 ± 0.2 μg/ml, respectively) and S. enterica (MIC = 77.9 ± 0.2 and 101.6 ± 0.1 μg/ml, respectively), but not against P. aeruginosa (MIC > 128 μg/ml) (Liu YP. et al., 2020).
Zhao et al. isolated seven diterpenoids including two C19-diterpenoids from Aconitum smirnovii Steinb [Ranunculaceae]. Antibacterial activity of these compounds was tested against S. aureus and E. coli by the disc diffusion method using ampicillin as positive control, and only compounds 103 and 104 exhibited mild antibacterial effects against S. aureus with a ZOI of 7.5–10 mm (Zhao B. et al., 2020).
3.2 Antiviral Activity
A considerable number of diterpenoids isolated from natural sources have been identified with their significant antiviral activity against several viruses (Table 2).
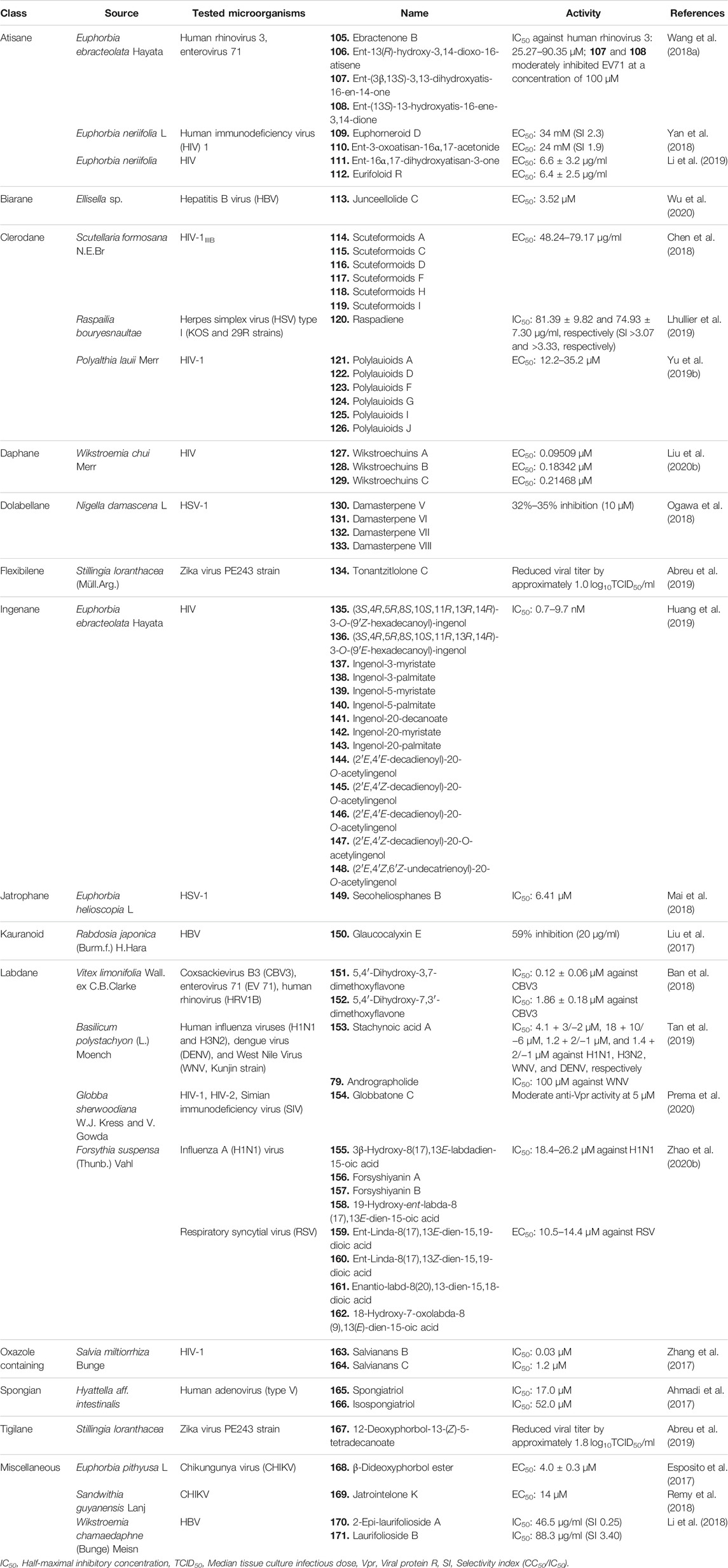
TABLE 2. Different classes of diterpenoids isolated from natural sources with significant antiviral activity.
3.2.1 Atisane Diterpenoids
Wang et al. isolated two novel and four known ent-atisane-type diterpenoids from E. ebracteolata Hayata [Euphorbiaceae] and tested for their antiviral activity against human rhinovirus 3 and enterovirus 71 (EV71). Among them, compounds 105–108 (Figure 7) displayed significant antiviral activities against human rhinovirus 3, with IC50 values of 25.27–90.35 μM. Compounds 107 and 108 showed moderate antiviral activities against EV71 at a concentration of 100 μM (Table 2) (Wang B. et al., 2018).
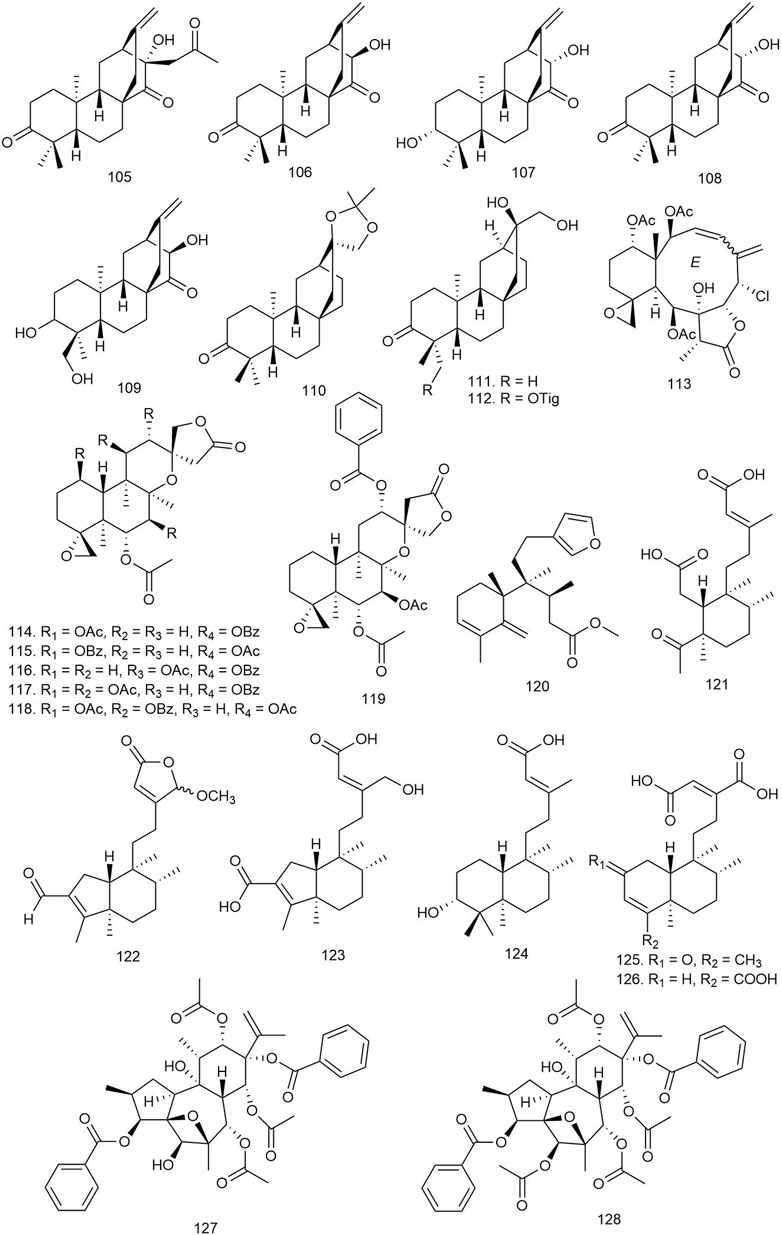
FIGURE 7. Structures of atisane, biarane, and clerodane diterpenoids with significant antiviral activity.
Among the 17 ent-atisane diterpenoids including six new and eleven known molecules isolated from Euphorbia neriifolia L [Euphorbiaceae], only 109 and 110 showed moderate anti-human immunodeficiency virus (HIV)-1 activities, with EC50 values of 34 mM (selectivity index, SI = 2.3) and 24 mM (SI = 1.9), respectively, when tested against HIV-1 at the dose of 50 median tissue culture infectious dose (TCID50 per well) (Yan et al., 2018).
Fifteen diterpenoids including three new ones were isolated from E. neriifolia L [Euphorbiaceae], and their antiviral potential was tested against HIV. Among all these diterpenes, only 111 and 112 showed potential anti-HIV-1 activities with EC50 values of 6.6 ± 3.2 and 6.4 ± 2.5 μg/ml, respectively (Li et al., 2019).
3.2.2 Biarane Diterpenoids
Twelve biarane diterpenoids including eight new congeners isolated from Ellisella sp. were tested against hepatitis B virus (HBV) at a dose of 10 μl. Among the biaranes, 113 reduced the HBV DNA, HBV RNA, and hepatitis B e-antigen (HBeAg) production in a dose-dependent manner (EC50 = 3.52 μM). It also significantly reduced the HBV covalently closed circular DNA (cccDNA) replenishment and enhanced the existing HBV cccDNA degradation. These outcomes suggest that 113 acts as a transcription inhibitor of cccDNA and is a promising lead for new anti-HBV agent development (Wu et al., 2020).
3.2.3 Clerodane Diterpenoids
Chen et al. described ten new and one known neo-clerodane-type diterpenoids from Scutellaria formosana N.E.Br [Lamiaceae] and tested for antiviral activity against HIV-1IIIB by the inhibition assay for the cytopathic effects of HIV-1 (EC50) using zidovudine as standard. Compounds 114–119 showed weak anti-HIV activities, with EC50 values ranging from 48.24 to 79.17 μg/ml (Chen et al., 2018).
A total of six clerodane diterpenoids were isolated from marine sponge Raspailia bouryesnaultae, and their anti-herpes activity was tested against herpes simplex virus type 1 (HSV-1). All of the diterpenoids displayed potential antiviral activity with an IC50 lower than 25 μM, especially the new compound 120, which inhibited HSV-1 (KOS and 29R strains) replication by 83% and 74% (IC50 = 81.39 ± 9.82 and 74.93 ± 7.30 μg/ml) respectively (Lhullier et al., 2019).
Fifteen clerodanes including ten new diterpenoids were isolated from Polyalthia lauii Merr [Annonaceae] and tested against HIV-1. The new compounds 121–126 exhibited anti-HIV activities with EC50 ranging from 12.2 to 35.2 μM (Yu ZX. et al., 2019).
3.2.4 Daphane Diterpenoids
Three new and eight known daphnane diterpenes isolated from Wikstroemia chuii Merr [Thymelaeaceae] showed potent anti-HIV reverse transcriptase (RT) effects with EC50 values ranging from 0.09509 to 8.62356 μM. The new daphanes, 127–129 (Figures 7, 8), were found to be the most potent antiviral agents (EC50 = 0.09509, 0.18432, and 0.21468 μM, respectively) (Liu YY. et al., 2020).
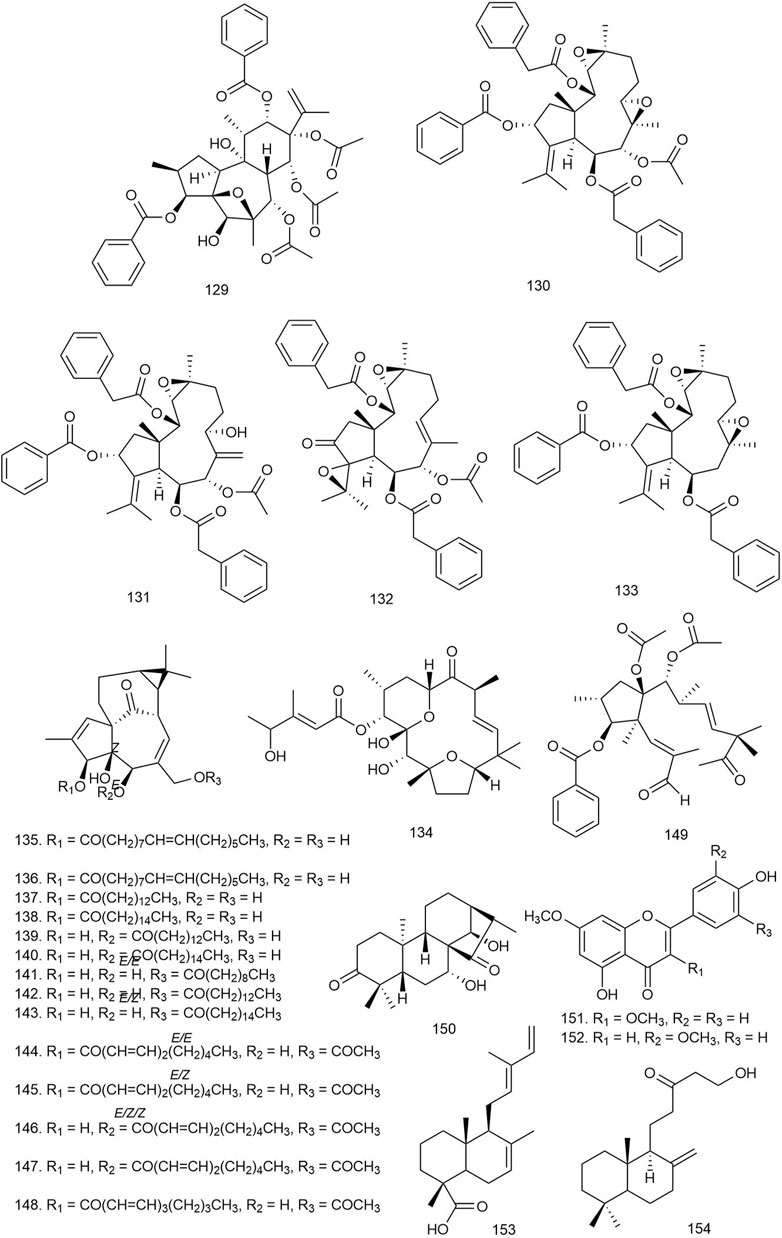
FIGURE 8. Structures of daphane, dolabellane, and flexibilene diterpenoids with significant antiviral activity.
3.2.5 Dolabellane Diterpenoids
Eighteen dolabellane diterpenoids including five new dolabellanes were isolated from Nigella damascena L [Ranunculaceae] and tested against HSV-1. Four new compounds, 130–133, showed significant anti-HSV-1 activity at 10 μM concentration with 32%–35% inhibition. The results indicated that the diacylated diterpenes and diterpenes with a nicotinoyl group displayed better antiviral activity (Ogawa et al., 2018).
3.2.6 Flexibilene Diterpenes
Three known flexibilene diterpenes, tonantzitlolones A–C, were isolated from the root bark of Stillingia loranthacea (Müll.Arg.) [Euphorbiaceae]. The compounds were tested for antiviral activity against the Zika virus PE243 strain where only 134 exhibited significant inhibition of Zika virus replication by reducing viral titer by approximately 1.0 log10TCID50/ml (Abreu et al., 2019).
3.2.7 Ingenane Diterpenoids
Huang et al. isolated two new and fourteen known ingenane diterpenoids (135–148) from E. ebracteolata Hayata [Euphorbiaceae] and tested their anti-HIV activity. All of the aliphatic diterpenoids with aliphatic side chains (135–148) displayed potent activity against HIV-1, with IC50 values of 0.7–9.7 nM. These results imply that aliphatic side chain substituents are crucial factors for the antiviral activity of the ingenane diterpenoids (Huang et al., 2019).
3.2.8 Jatrophane Diterpenoids
Five diterpenoids isolated from Euphorbia helioscopia L [Euphorbiaceae] were tested for their antiviral potential against HSV-1, and among them, compound 149 showed moderate activity against HSV-1 with an IC50 value of 6.41 μM. The SAR study of the diterpenes revealed that seco-jatrophane skeleton exhibited more potent antiviral activity against HSV-1 than the jatrophane skeleton (Mai et al., 2018).
3.2.9 Kauranoid Diterpenoids
Three new and nine known ent-kauranoid diterpenoids isolated from Rabdosia japonica (Burm.f.) H. Hara [Lamiaceae] were tested against HBV, and only compound 150 exhibited the most potent antiviral action by inhibiting the HBV surface antigen (HBsAg) with a 59% inhibition ratio at a concentration of 20 μg/ml, which is more potent than the standard adefovir. The results imply that diterpenoids without the moiety of α-methylene cyclopentanone possess more potent antiviral activity (Liu et al., 2017).
3.2.10 Labdane Diterpenoids
Ban et al. reported three new and eight known labdane diterpenoids from Vitex limonifolia Wall. ex C.B.Clarke [Lamiaceae], and their antiviral properties were evaluated against Coxsackievirus B3 (CBV3), enterovirus 71 (EV 71), and human rhinovirus (HRV1B). Among the compounds, 151 and 152 exhibited potent antiviral activity against CBV3 infection with IC50 values of 0.12 ± 0.06 and 1.86 ± 0.18 μM, respectively. Compound 151 also showed antiviral activity against the EV71 virus, indicating broad-spectrum antiviral activity of the compound (Ban et al., 2018).
Tan et al. isolated two labdane-type and three pimarane-type diterpenoids from Basilicum polystachyon (L.) Moench [Lamiaceae] and tested for antiviral activity against Madin-Darby Canine Kidney (MDCK) cells (for human influenza viruses H1N1 and H3N2) and Vero cells (African green monkey kidney) (for flaviviruses dengue virus (DENV) and West Nile virus (WNV) Kunjin strain) by plaque reduction neutralization (PRNT) assays. The labdane diterpenoids showed more potent antiviral activity than the pimarane ones. Compound 153 showed broad-spectrum antiviral activity against the tested viruses with IC50 values of 1.2 + 2/−1 μM against WNV, 4.1 + 3/−2 μM against H1N1, 18 + 10/−6 μM against H3N2, 1.2 + 2/−1 μM against WNVKun, and 1.4 + 2/−1 μM against DENV, with low toxicity. Compound 79 showed mild antiviral activity against WNVKun (IC50 = 100 μM) only. The mechanism of broad-spectrum antiviral activity of 153 can be suggested as its capability of blocking viral RNA replication either through direct or indirect means. It could act on the cellular pathways broadly utilized by the enveloped viruses or could activate innate antiviral responses. Alternatively, 79 can act in different mechanisms as it showed narrow-spectrum antiviral activity (Tan et al., 2019).
One new and nine previously reported labdane diterpenoids isolated from Globba sherwoodiana W.J. Kress and V. Gowda [Zingiberaceae] were tested for their anti-viral protein R (anti-Vpr) activity against HIV-1, HIV-2, and simian immunodeficiency virus (SIV). All labdanes showed weak anti-Vpr activity at 10 μM concentration except 154, which showed more potency at the 5 μM dose. This suggests that the lactone ring and carbonyl group could be important functionalities to increase the anti-Vpr activity of labdane diterpenoids (Prema et al., 2020).
Eight labdane-type diterpenoids isolated from Forsythia suspensa (Thunb.) Vahl [Oleaceae] were tested for antiviral activity against influenza A (H1N1) virus and respiratory syncytial virus (RSV). All of the isolated labdane diterpenoids (155–162; Figure 9) displayed moderate antiviral activities against H1N1 virus and RSV, with IC50 values ranging from 18.4 to 26.2 μM and EC50 values ranging from 10.5 to 14.4 μM, respectively (Zhao L. et al., 2020).
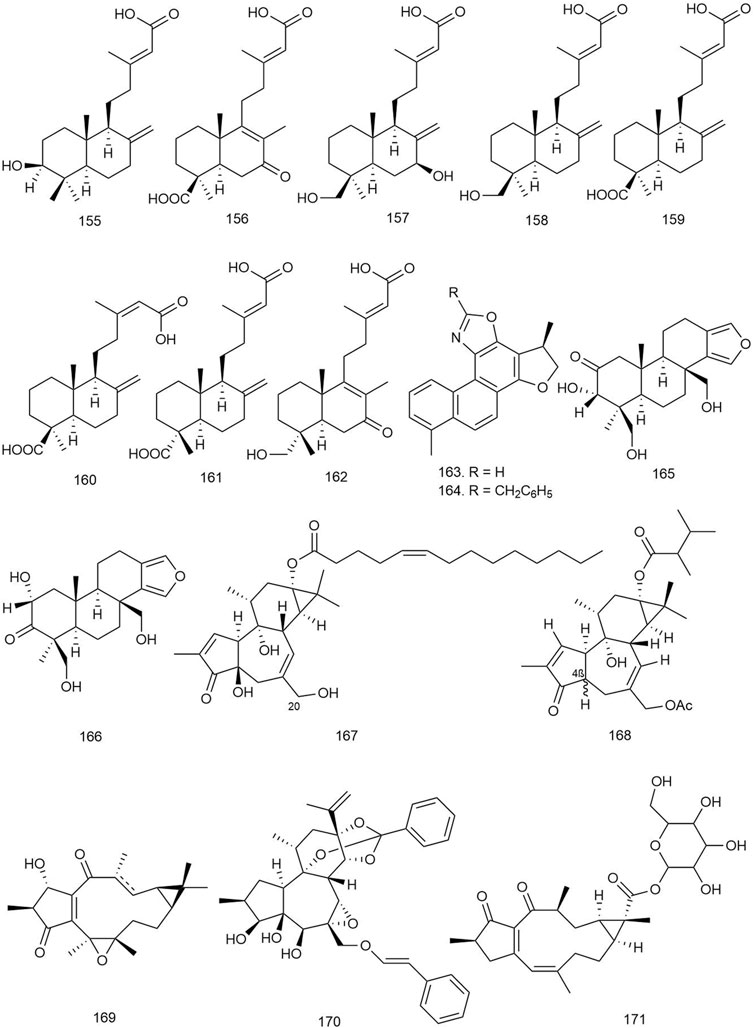
FIGURE 9. Structures of ingenane, jatrophane, kauranoid, labdane, oxazole-containing, spongian, tigilane, and miscellaneous diterpenoids with significant antiviral activity.
3.2.11 Oxazole-Containing Diterpenoids
Zhang et al. isolated four new and three known diterpenoids from S. miltiorrhiza Bunge [Lamiaceae] and tested for their anti-HIV-1 activity. Among them, compounds 163 and 164 showed inhibition against HIV-1 with IC50 values of 0.03 and 1.2 μM, respectively. The time-of-addition (TOA) assay and long terminal repeat (LTR) luciferase reporter assay results suggested that compound 163 might inhibit the wild-type HIV-1 transcription, leading to the blocking of HIV-1 replication at the submicromolar level (Zhang et al., 2017).
3.2.12 Spongian Diterpenoids
Ahmadi et al. isolated three novel and six known spongian diterpenoids from the sponge Hyattella aff. intestinalis and tested for their antiviral activity against human adenovirus (type V) at 100 μl of twofold serially diluted dose. Among the diterpenoids, 165 and 166 showed significant antiviral activity with IC50 values of 17.0 and 52.0 μM, respectively (Ahmadi et al., 2017).
3.2.13 Tigilane Diterpenes
Abreu et al. isolated four new and three known tigliane-type diterpenes from the root bark of S. loranthacea (Müll.Arg.) Pax [Euphorbiaceae]. The compounds were significant inhibitors of Zika virus replication. Compound 167 significantly reduced Zika virus replication. Compared to the titer of 6.7 log10TCID50/ml seen in untreated cells, compound 167 had the highest anti-Zika viral activity by reducing viral titer by 1.8 log10TCID50/ml. It was also reported that the presence of a hydroxyl group in the C20 position was necessary for activity while the presence of a formyl group seemed to decrease activity. These compounds are definitely potential antiviral candidates against the Zika virus and require further testing in animal models (Abreu et al., 2019).
3.2.14 Miscellaneous Diterpenoids
Esposito et al. isolated six new premyrsinol esters and one new myrsinol ester from Euphorbia pithyusa L [Euphorbiaceae] and tested for their antiviral activity against Chikungunya virus (CHIKV) viral strain on Vero cells. Among the isolated compounds, 168 was the most active one, with an EC50 value of 4.0 ± 0.3 μM and an SI of 10.6. There could be an important role of the spatial configuration of H-4 in causing the anti-CHIKV activity of 168 (Esposito et al., 2017).
Seventeen new and two known diterpenoids isolated from Sandwithia guyanensis Lanj [Euphorbiaceae] were tested against CHIKV, and only 169 displayed moderate anti-CHIKV activity with an EC50 value of 14 μM (Remy et al., 2018).
Li et al. described the isolation and antiviral activity of seven new and four known diterpenes from Wikstroemia chamaedaphne (Bunge) Meisn [Thymelaeaceae]. The compounds were tested against HBV at below 50% cytotoxic concentration (CC50) (50 μl per well) dose, and 170 and 171 displayed the most potent anti-HBV activities (IC50 = 46.5 and 88.3 μg/ml, SI = 0.25 and 3.40, respectively) by inhibiting HBsAg. Other compounds may possess some inhibitory effects on the replication of HBV-DNA (Li et al., 2018).
3.3 Antifungal Activity
Several classes of natural diterpenoids have been recognized for their potential antifungal activity against a number of human and plant pathogens (Table 3).
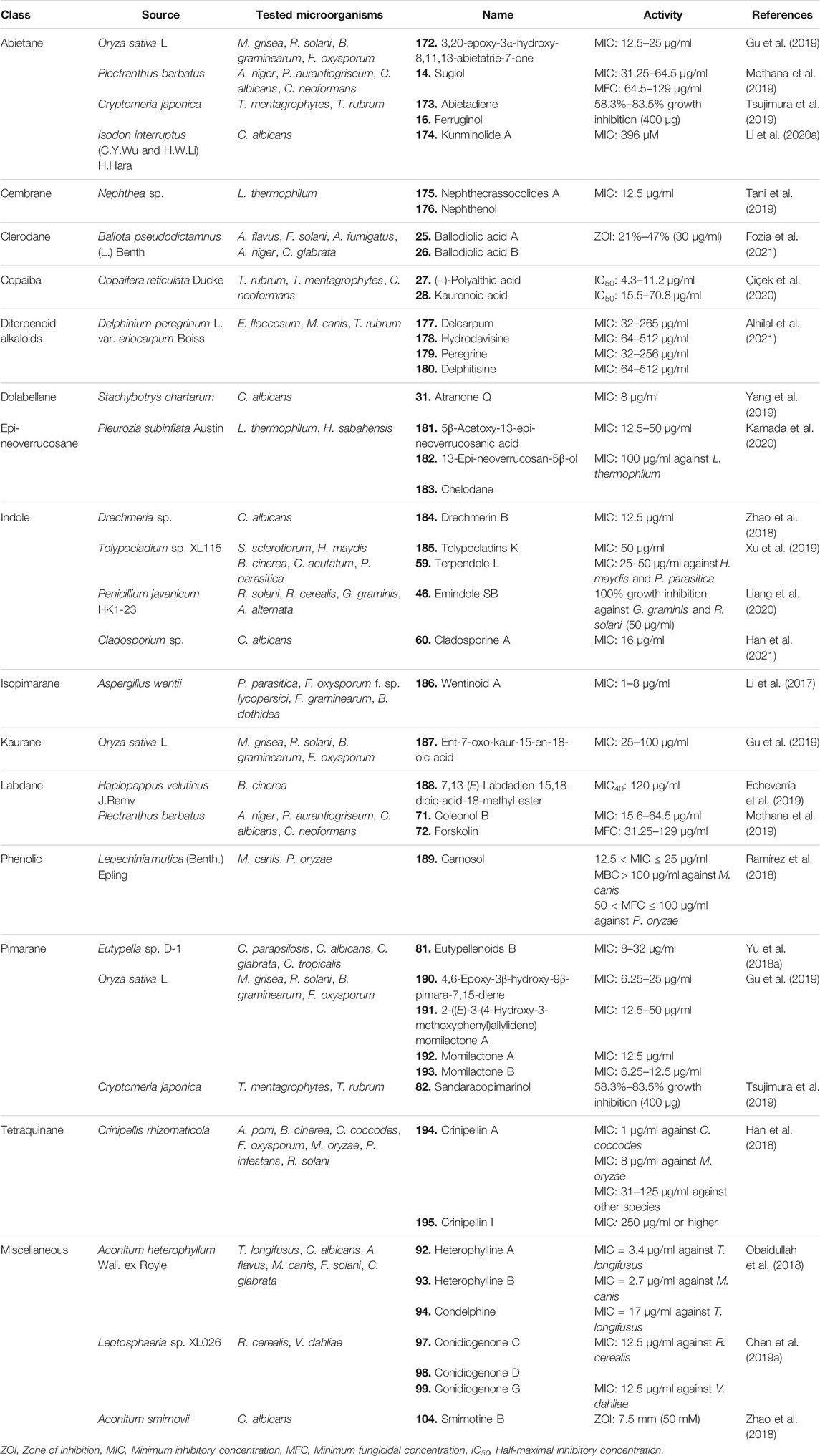
TABLE 3. Different classes of diterpenoids isolated from natural sources with significant antifungal activity.
3.3.1 Abietane Diterpenoids
A novel abietane diterpenoid (172) (Figure 10) was isolated from the hulls of rice Oryza sativa L [Poaceae]. The compound was tested for antifungal activity against four crop pathogenic fungal strains. It exhibited potent antifungal properties with MIC values ranging from 12.5 to 25 μg/ml (Table 3) (Gu et al., 2019).
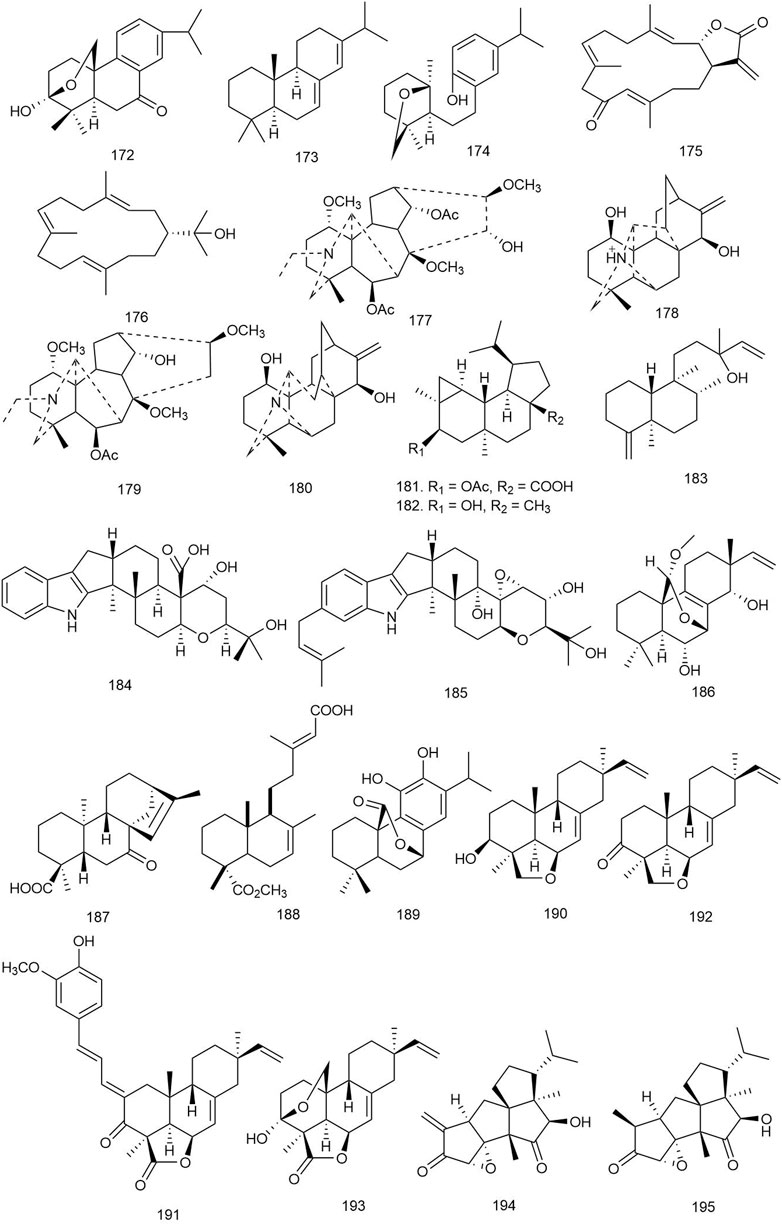
FIGURE 10. Structures of abietane, cembrane, diterpenoid alkaloids, epi-neoverrucosane, indole, isopimarane, kaurene, labdane, phenolic, pimarane, tetraquinane, and miscellaneous diterpenoids with significant antifungal activity.
Mothana et al. reported three abietane diterpenoids with their antifungal activity tested against Aspergillus niger, Penicillium aurantiogriseum, C. albicans, and Cryptococcus neoformans wild strains. Among them, 14 (Figure 2) exhibited moderate antifungal activity with MIC and minimum fungicidal concentration (MFC) values of 31.25–64.5 and 64.5–129 μg/ml, respectively (Mothana et al., 2019).
Tsujimaru et al. isolated four abietanes from the wood drying product of C. japonica (Thunb. ex L. f.) D. Don [Cupressaceae] (sugi) and investigated their antifungal activities against two fungal strains Trichophyton mentagrophytes and Trichophyton rubrum. Compounds 173 and 16 showed the maximum fungal growth inhibition against the selected fungal strains ranging from 58.3% to 83.5% growth inhibition at a dose of 400 μg in the Tukey–Kramer test, p < 0.05 (Tsujimura et al., 2019).
A new abietane diterpenoid (174) was isolated from Isodon interruptus (C.Y. Wu and H.W. Li) H. Hara [Lamiaceae], and its potential against the growth inhibition of C. albicans was observed. The abietane showed antifungal activity at a dose of 396 μM by breaking down the biofilm of the pathogen (Li QJ. et al., 2020).
3.3.2 Cembrane Diterpenoids
Tani et al. isolated three new and three known cembrane diterpenes from Nephthea sp., which were tested against Exophiala sp., Fusarium moniliforme, Fusarium oxysporum, Fusarium solani, Haliphthoros milfordensis, Haliphthoros sabahensis, and Lagenidium thermophilum by evaluating the MIC of the fungistatic effect on hyphae using itraconazole as positive control. Among all the compounds, 175 and 176 exhibited significant antifungal activity against L. thermophilum with an MIC value of 12.5 μg/ml (Tani et al., 2019).
3.3.3 Clerodane Diterpenes
Compounds 25 and 26 extracted from the roots of B. pseudodictamnus (L.) Benth [Lamiaceae] exhibited strong antifungal property. At 30 μg/ml concentration, 25 and 26 gave prominent antifungal activity, with % ZOIs ranging between 21% and 45% and between 25% and 47%, respectively, against different fungal strains compared to the standard drug miconazole (100%) (Fozia et al., 2021).
3.3.4 Copaiba Diterpenoids
Copaiba-type diterpenoids (27 and 28) (Figure 2) demonstrated strong antifungal properties. Compound 27 was active against T. rubrum, T. mentagrophytes, and C. neoformans fungal species with IC50 values of 6.8, 4.3, and 11.2 μg/ml, respectively. Compound 28 was active against T. rubrum and T. mentagrophytes only with IC50 values of 70.8 and 15.5 μg/ml, respectively (Çiçek et al., 2020).
3.3.5 Diterpenoid Alkaloids
Alhilal et al. isolated four diterpenoid alkaloids, including two novel alkaloids delcarpum (177) and hydrodavisine (178) from the aerial parts of Delphinium peregrinum L. var. eriocarpum Boiss [Ranunculaceae], a plant commonly grown in Syria. The compounds were tested for antifungal properties using MIC and MFC assays. Compound 179 was the most effective among the four diterpenoids with MIC levels ranging between 128 and 256, 32–64, and 32 g/ml for Epidermophyton floccosum, Microsporum canis, and T. rubrum, respectively, compared to 32–64, 16, and 32 μg/ml in the case of standard fluconazole. Interestingly, a mixture of the four alkaloids had significantly lesser MIC (16–64 μg/ml) and MFC (64–258 μg/ml) levels compared to each individual alkaloid, which hints at the synergistic activity of these plant compounds against microorganisms (Alhilal et al., 2021).
3.3.6 Dolabellane Diterpenoids
Six new dolabellane-type diterpenoids were isolated from a fungal strain Stachybotrys chartarum and evaluated for their antifungal activity against C. albicans. Among them, compound 31 significantly inhibited the growth of C. albicans (MIC = 8 μg/ml). Transmission electron microscopy (TEM) was employed for visualizing the morphological changes of C. albicans caused by 31, and it showed that at 8 μg/ml dose, the compound caused agglutination in the cytoplasm and thinning of the cells, leading to deformity, wrinkles, and irregularity in shape. At a higher dose, i.e., 16 μg/ml, the cell wall and cell membrane were deformed, vacuoles appeared in the cytoplasm, and the cell contents were partially or completely leaked, which implies a dose-dependent destructive effect on the cell wall and cell membrane by the compound on C. albicans (Yang et al., 2019).
3.3.7 Epi-Neoverrucosane Diterpenoid
Kamada et al. isolated a novel epi-neoverrucosane-type diterpenoid and three other known secondary metabolites from the methanolic extract of east Malaysia’s liverwort Pleurozia subinflata Austin [Pleuroziaceae]. The compounds were tested against six fungal strains isolated from the Bornean ocean. The newly identified compound 181 showed the strongest activity, with MIC values of 12.5 and 50 μg/ml against L. thermophilum and H. sabahensis, respectively. Among the other identified diterpenoids, 182 and 183 were both moderately active with an MIC value of 100 μg/ml against L. thermophilum (Kamada et al., 2020).
3.3.8 Indole Diterpenoids
Zhao et al. reported 11 indole diterpenoids from Drechmeria sp., isolated from the root of P. notoginseng, and tested against C. albicans using geneticin as positive control. Among all the indole diterpenoids, only 184 showed a significant inhibitory effect against C. candida with an MIC value of 12.5 μg/ml. It also showed significant binding affinity in the ligand-binding site of the PDF enzyme, implying a probable mechanism of its antifungal activity (Zhao et al., 2018).
Three prenylated indole diterpenoids from a mine soil-derived fungus Tolypocladium sp. XL115 were investigated against seven agricultural pathological fungal strains, Sclerotinia sclerotiorum, Helminthosporium maydis, Verticillium dahliae Kleb, Phytophthora parasitica, Gibberella saubinetii, Botrytis cinerea Pers., and Colletotrichum acutatum Simmonds, using ketoconazole as positive control. Compound 185 displayed moderate antifungal activity against S. sclerotiorum, H. maydis, B. cinerea Pers., and C. acutatum Simmonds with an MIC value of 50 μg/ml, and 59 also showed significant antifungal activity against H. maydis and P. parasitica with MIC values ranging from 25 to 50 μg/ml (Xu et al., 2019).
Seven indole diterpenes were isolated from the fungus P. javanicum HK1-23 by Liang and coworkers and were screened for antifungal activities against crop pathogens such as Rhizoctonia solani, Rhizoctonia cerealis, Gaeumannomyces graminis, and Alternaria alternata on the basis of the hyphal radial growth rate of filamentous fungi, and 46 (Figure 4) showed the most potent antifungal activity, especially against G. graminis and R. solani with 100% growth inhibition at 50 μg/ml (Liang et al., 2020).
Han et al. isolated cladosporine A (60) and tested it for antifungal activity against A. niger and C. albicans. Potent antifungal activity was observed against C. albicans with an MIC value of 16 μg/ml (Han et al., 2021).
3.3.9 Isopimarane Diterpenoids
Li et al. isolated six new isopimarane-type diterpenoids, named as wentinoids A–F, from Aspergillus wentii—a sediment-derived fungus found in the deep seas. The diterpenoids were tested for antimicrobial activity against 11 human and aqua-pathogenic bacterial strains and also for antifungal activity against seven plant pathogenic fungi. Only 186 exhibited antifungal activity against four fungal species—P. parasitica, F. oxysporum f. sp. lycopersici, Fusarium graminearum, and Botryosphaeria dothidea with MIC values of 8.0, 4.0, 1.0, and 4.0 μg/ml respectively (Li et al., 2017).
3.3.10 Kaurane Diterpenoids
A kaurene-type diterpenoid (187) was isolated from the hulls of rice O. sativa L [Poaceae]. It exhibited potent antifungal properties against four plant pathogenic fungi with MIC values ranging from 25 to 100 μg/ml (Gu et al., 2019).
3.3.11 Labdane Diterpenoids
Two labdane diterpenoids, including the novel 7,13-(E)-labdadien-15,18-dioic-acid-18-methyl ester (188), were isolated from the resinous exudate of Haplopappus velutinus J. Remy [Asteraceae], an herbaceous shrub. The compounds were tested against the phytopathogen B. cinerea, and the novel compound 188 significantly inhibited the mycelial growth of B. cinerea by approximately 40% at 120 μg/ml concentration (Echeverría et al., 2019).
Two diterpenoids (71 and 72) isolated by Mothana et al. were tested for their antifungal activity against A. niger, P. aurantiogriseum, C. albicans, and C. neoformans wild-type strains using nystatin as standard, and 71 and 72 showed potent antifungal activity against the selected strains (MIC = 15.6–64.5 μg/ml, MFC = 31.25–129 μg/ml) (Mothana et al., 2019).
3.3.12 Phenolic Diterpenoids
Ramirez et al. isolated a new phenolic-type diterpenoid from the leaves of Lepechinia mutica (Benth.) Epling [Lamiaceae], which was testes against M. canis (a human dermatophyte fungus) and Pyricularia oryzae (a plant pathogenic fungus, LM120 strain) using itraconazole and flutriafol as standard. Only compound 189 showed significant antifungal activity against M. canis (0.0250 < MIC ≤ 0.0500, MBC > 0.1 mg/ml) and P. oryzae (0.0125 < MIC ≤ 0.025, 0.0500 < MFC ≤0.1000 mg/ml) (Ramírez et al., 2018).
3.3.13 Pimarane Diterpenoids
Among the pimarane diterpenoids isolated from Eutypella sp. D-1, 81 showed broad-spectrum antifungal activity against Candida parapsilosis, C. albicans, C. glabrata, and Candida tropicalis with MIC values of 8, 8, 16, and 32 μg/ml, respectively (Yu H.-B. et al., 2018).
Four pimaranes, including two new ones, were isolated from the hulls of rice O. sativa L [Poaceae]. The diterpenes (190–193; Figure 10) were tested for antifungal activity against four crop pathogenic fungal species where all of them exhibited potent antifungal properties with MIC values ranging from 6.25 to 50 μg/ml. The result indicates that rice can produce secondary metabolites that are capable of preventing fungal growth and can also be used as potential natural leads for development of future fungicidal drugs (Gu et al., 2019).
The pimarane diterpenoids isolated from C. japonica (Thunb. ex L.f.) D.Don [Cupressaceae] (sugi) were tested against two fungal strains, namely, T. mentagrophytes and T. rubrum, using miconazole nitrate as positive control, and 82 showed significant antifungal activity at 400 μg dose with 58.3%–83.5% growth inhibition (Tsujimura et al., 2019).
3.3.14 Tetraquinane Diterpenoids
Two diterpenoids, crinipellin A (194) and crinipellin I (195), were isolated from the culture filtrate of the basidiomycete fungus Crinipellis rhizomaticola. The compounds were tested for antifungal activity against seven plant pathogenic fungi and antibacterial activity against nine plant pathogenic bacteria. Compound 194 demonstrated strong antifungal activity against Colletotrichum coccodes with an MIC of only 1 μg/ml. It also strongly inhibited the growth of other fungal strains with MIC levels ranging from 8 to 125 μg/ml. Compound 195 had weak (MIC = 250 μg/ml) or no antifungal activity against the tested strains. Compound 194 also exclusively inhibited the growth of leaf blight-causing bacteria Acidovorax avenae ssp. cattleyae (MIC = 31 μg/ml) (Han et al., 2018).
3.3.15 Miscellaneous Diterpenoids
Compounds 92 and 93 were tested against Trichophyton longifusus (clinical isolate), C. albicans, Aspergillus flavus, M. canis (ATCC 11622), F. solani, and C. glabrata by the agar tube dilution method using amphotericin B as positive control, and they were found to exhibit significant antifungal activity against T. longifusus (MIC = 3.4 μg/ml) and M. canis (MIC = 2.7 μg/ml), respectively. Compound 94 showed moderate antifungal activity against T. longifusus (MIC = 17 μg/ml) (Obaidullah et al., 2018).
Compounds 97–99 were tested against 10 fungal strains, namely, B. dothidea, A. alternata f. sp. mali, F. graminearum, S. sclerotiorum, V. dahliae Kleb, Bipolaris carbonum Wilson, P. parasitica, A. alternata, R. cerealis, and B. cinerea Pers. Among the diterpenes, 97 and 99 showed moderate antifungal activity against R. cerealis, and 98 showed activity against V. dahliae Kleb with an MIC value of 12.5 μg/ml (Chen HY. et al., 2019).
Among the seven diterpenoids isolated from A. smirnovii Steinb [Ranunculaceae] by Zhao et al., only 104 showed moderate antifungal activity (7.5 mm inhibitory zone) against C. albicans evaluated by the disc diffusion method using amphotericin B as positive control (Zhao B. et al., 2020).
3.4 Antiprotozoal Activity
A number of diterpenoids from natural sources have been found to be active against several parasites responsible for causing different parasitic diseases like malaria, leishmaniasis, giardiasis, Chagas disease, and trichomoniasis (Table 4).
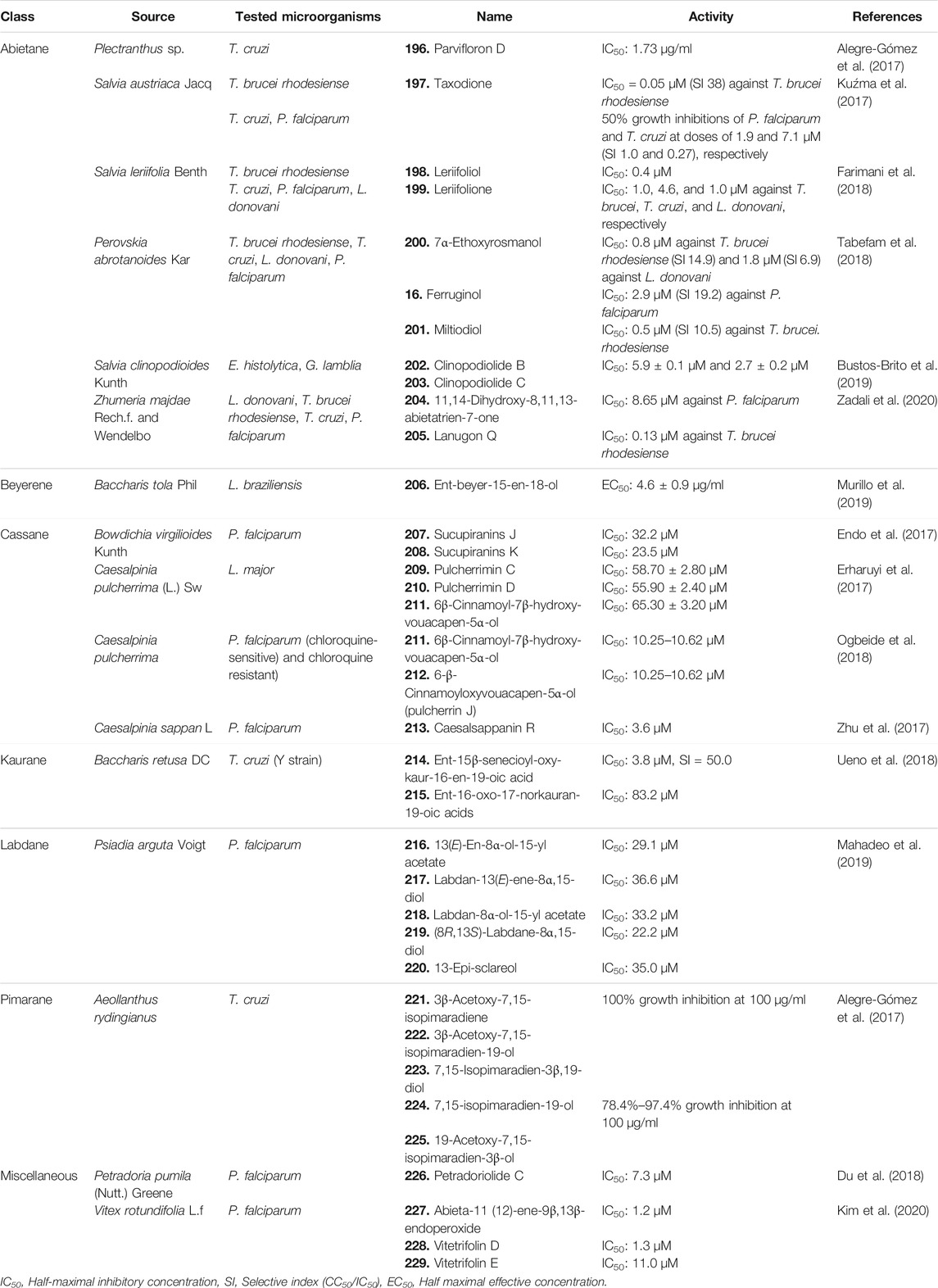
TABLE 4. Different classes of diterpenoids isolated from natural sources with significant antiprotozoal activity.
3.4.1 Abietane Diterpenoids
Ten diterpenoids, including abietanes, labdanes, and halimane, were isolated from Plectranthus spp. [Lamiaceae]. The compounds were tested for their antiparasitic effect against Trypanosoma cruzi, a protozoal parasite responsible for the fatal Chagas disease, which affects the heart and gastrointestinal system. Several of the isolated abietanes completely inhibited the growth of epimastigote forms of T. cruzi at 100 μg/ml concentration. However, at much lesser concentrations of 10 and 1 μg/ml, only 196 (Figure 11) seemed to significantly inhibit the growth of T. cruzi having an IC50 concentration of 1.73 μg/ml. The activity of 196 could be attributed to its increased lipophilicity and membrane interactions due to the presence of a C-2 para-substituted aromatic ester with a hydroxyl group (Alegre-Gómez et al., 2017).
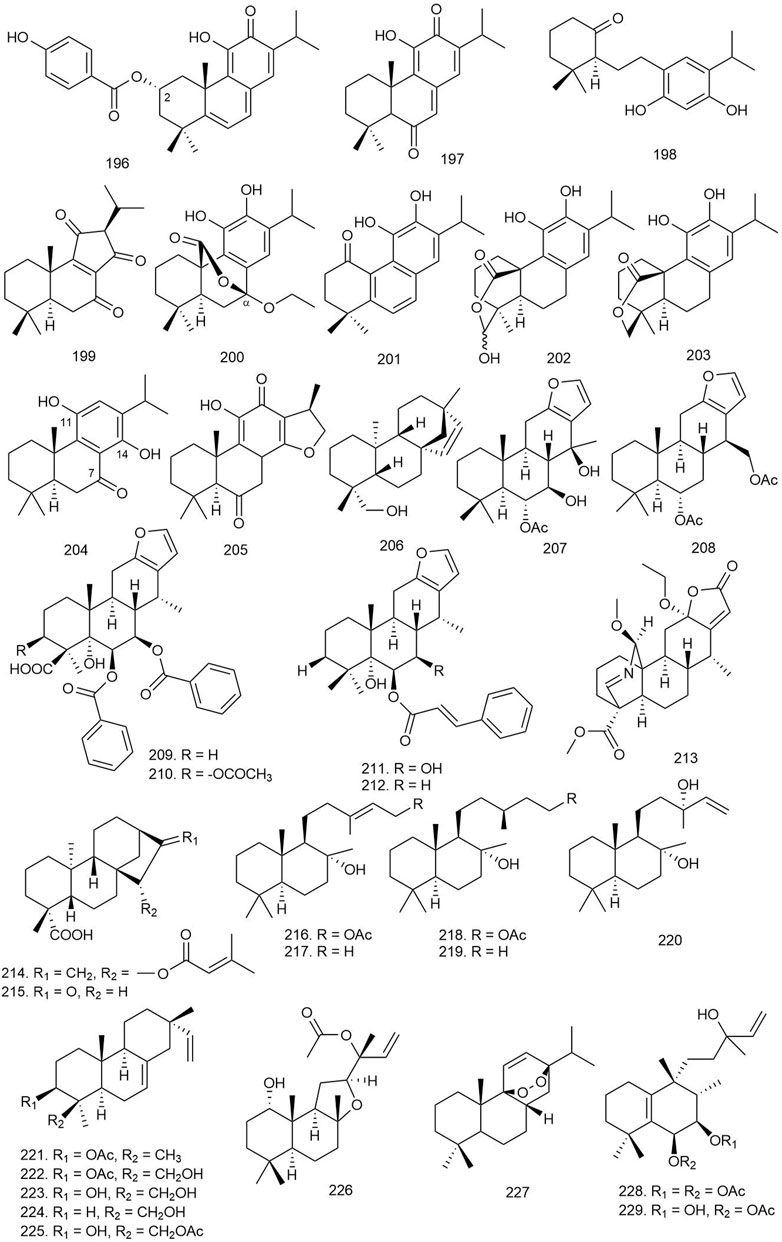
FIGURE 11. Structures of abietane, beyerene, cassane, kaurene, labdane, pimarane, and miscellaneous diterpenoids with significant antiprotozoal activity.
Four abietane diterpenoids were isolated from Salvia austriaca Jacq [Lamiaceae], and their antiprotozoal activity was tested against several parasites including Trypanosoma brucei rhodesiense, T. cruzi, and Plasmodium falciparum. Among them, compound 197 was found to be the most active antiprotozoal agent against T. brucei rhodesiense (IC50 = 0.05 μM with high selectivity, SI = 38), and the growth inhibition of P. falciparum and T. cruzi was 50% at doses of 1.9 and 7.1 μM respectively (SI = 1.0 and 0.27). Other diterpenoids exhibited weaker activity against the tested parasites (IC50 = 0.62–194.7 μM with lower selectivity, SI = 0.4–5.0) (Kuźma et al., 2017).
Farimani et al. isolated two new and ten known abietanes from Salvia leriifolia Benth [Lamiaceae], and their antiprotozoal activity was examined against T. brucei rhodesiense, T. cruzi, P. falciparum, and Leishmania donovani. Among all the diterpenoids, compound 198 displayed antimalarial activity with an IC50 value of 0.4 μM, and 199 displayed activity against T. brucei, T. cruzi, and L. donovani, with IC50 values of 1.0, 4.6, and 1.0 μM, respectively; however, SI values <10 indicated the general toxicity of the compound (Farimani et al., 2018).
Seventeen diterpenoids isolated from Perovskia abrotanoides Kar [Lamiaceae] were evaluated against T. brucei rhodesiense, T. cruzi, L. donovani, and P. falciparum to examine their antiparasitic activity. Among the diterpenoids, 200, 201, and 16 showed antiprotozoal activity against the tested microorganisms. Compound 200 with an IC50 of 0.8 μM against T. brucei rhodesiense (SI = 14.9) and an IC50 of 1.8 μM (SI = 6.9) against L. donovani, compound 16 (Figure 2) with an IC50 of 2.9 μM (SI = 19.2) against P. falciparum, and compound 201 with an IC50 of 0.5 μM (SI = 10.5) against T. brucei rhodesiense showed significant antiprotozoal activity. None of the compounds showed any selective activity against T. cruzi (SI ≤ 1.6) (Tabefam et al., 2018).
Four new diterpenoids including three new abietanes and one new icetexane were reported from the aerial parts of Salvia clinopodioides Kunth [Lamiaceae] and tested against Entamoeba histolytica and Giardia lamblia. Compounds 202 and 203 showed better effects in the inhibition of lipid peroxidation with IC50 values of 5.9 ± 0.1 and 2.7 ± 0.2 μM, respectively (Bustos-Brito et al., 2019).
Eight abietane diterpenoids were isolated from the roots of Zhumeria majdae Rech. f. and Wendelbo [Lamiaceae], and their antiprotozoal activity was tested against L. donovani, T. brucei rhodesiense, T. cruzi, and P. falciparum. Among all diterpenoids, 204 showed significant inhibition against P. falciparum (IC50 = 8.65 μM), with an SI of 4.6, and 205 inhibited T. brucei rhodesiense with an IC50 value of 0.13 μM and an SI of 15.4. The presence of a keto group at C-7 and OH group at C-11 and C-14 could be a crucial factor of the reported antiprotozoal activity of 204 (Zadali et al., 2020).
3.4.2 Beyerene Diterpenoids
Two natural ent-beyerene-type diterpenoids isolated from Baccharis tola Phil [Compositae] were tested for their antileishmanial potential against Leishmania braziliensis intracellular amastigotes, and 206 was found to be most potent against the pathogen with an EC50 of 4.6 ± 0.9 μg/ml (Murillo et al., 2019).
3.4.3 Cassane Diterpenoids
Twelve new and three known furanocassane-type diterpenoids were isolated from the seeds of Bowdichia virgilioides Kunth [Fabaceae] and investigated for their antiplasmodic activity against P. falciparum. Among the diterpenoids, 207 and 208 exhibited weak antimalarial activity with IC50 values of 32.2 and 23.5 μM and selectivity indices of 4.3 and 1.9, respectively, in Medical Research Council Cell line 5, K1 strain (MRC-5/K1) (Endo et al., 2017).
Erharuyi et al. isolated 13 known furanocassane diterpenoids from Caesalpinia pulcherrima (L.) Sw [Fabaceae], and their leishmanicidal potential was evaluated against the promastigotes of Leishmania major. Compounds 209–211 showed significant activity against promastigotes of L. major (IC50 = 58.70 ± 2.80, 55.90 ± 2.40, and 65.30 ± 3.20 μM, respectively) (Erharuyi et al., 2017). Compounds 211 and 212 were also found to exhibit moderate antimalarial activity by inhibiting two strains of P. falciparum, i.e., Sierra Leone D6 (chloroquine sensitive) and Indochina W2 (chloroquine resistant) (IC50 = 10.25–10.62 µM) (Ogbeide et al., 2018).
Two diterpenoids including one new cassane-type diterpenoid were isolated from Caesalpinia sappan L [Fabaceae] and tested for their antimalarial activity against P. falciparum. Compound 213 exhibited relatively good antiplasmodial activity in vitro with an IC50 value of 3.6 μM, compared with chloroquine and caesalsappanin S, but showed only weak activity against the chloroquine-resistant K1 strain of P. falciparum. It indicates that the presence of the N-bridge in cassane-type diterpenoids can be responsible for increasing activity against the chloroquine-resistant K1 strain of P. falciparum (Zhu et al., 2017).
3.4.4 Kaurane Diterpenoids
Three new kaurane diterpenoids were isolated from Baccharis retusa DC [Asteraceae] and their antitrypanosomal activity was evaluated against T. cruzi (Y strain). Among them, compound 214 showed enhanced activity against trypomastigotes of T. cruzi (IC50 = 3.8 μM, SI = 50.0), while 215 displayed better activity against intracellular amastigotes of the parasite (IC50 = 83.2 μM). The results implied an expressive interference with the plasma membrane permeability in the parasites which were treated with the diterpenes (Ueno et al., 2018).
3.4.5 Labdane Diterpenoids
Four new and five known labdane diterpenoids were isolated from Psiadia arguta (Pers.) Voigt [Asteraceae], and their antimalarial property was evaluated against P. falciparum. The known labdanes (216–220) showed significant antimalarial activity with IC50 values of 29.1, 36.6, 33.2, 22.2, and 35.0 μM, respectively. A lactone or an endoperoxide group in their structure might lead to their interference with parasite development (Mahadeo et al., 2019).
3.4.6 Pimarane Diterpenoids
Seven natural pimaranes were isolated from Aeollanthus rydingianus van Jaarsv. and A.E.van Wyk [Lamiaceae]. The compounds were tested for their antiparasitic effect against T. cruzi, where compounds 221–223 completely inhibited the growth of the epimastigote forms of T. cruzi at 100 μg/ml concentration, while 224 and 225 inhibited growth ranging from 78.4% to 97.4% at 100 μg/ml (Alegre-Gómez et al., 2017).
3.4.7 Miscellaneous Diterpenoids
Du et al. isolated five new diterpenoids from Petradoria pumila (Nutt.) Greene [Asteraceae] and investigated against P. falciparum to identify their antimalarial activity. Among the diterpenes, 226 showed moderate antiplasmodial activity, with an IC50 value of 7.3 μM (Du et al., 2018).
Three new and five known diterpenoids were isolated from Vitex rotundifolia L. f [Verbenaceae] and tested against P. falciparum to evaluate their antimalarial property. Among the isolated diterpenoids, 227–229 showed significant antimalarial activity with IC50 values of 1.2, 1.3, and 11.0 μM, respectively (Kim et al., 2020).
4 Discussion
The natural diterpenoids could be potential compounds to confront the continuous outbreaks of new viruses and viral strains. In the current review work, a total of 229 natural diterpenoids of different chemical classes have been summarized along with their sources (plants, fungi, marine species, etc.); antimicrobial activities against several bacterial, viral, fungal, protozoal species with their reported testing methods; and MIC and IC50 values. Among them, there have been some promising molecules like andrographolide, koninginol A and B, and psathyrins A and B with significant MIC, which could be further investigated for discovering new antimicrobial agents. For example, some daphane diterpenoids, wikstroechuins A–C (127–129), were found to exhibit potent anti-HIV activity with very low EC50 values (Table 2). Some other classes like labdanes, ingenane, and jatrophanes were found to be active against CBV3, HSV-1, CHIKV, HBV, etc. in different in vitro tests, which should be further substantiated with proper in vivo studies. Abietane- and cassane-type diterpenoids were found to be most active against parasites causing trypanosomal disease and malaria (Table 4). Several diterpenoids were found to have a synergistic antibacterial action which could improve the activity of available antibiotics. For example, ent-beyer-15-en-18-O-oxalate has been patented as an adjuvant therapy with the available antibiotic colistin because of its ability to block the ArnT enzyme responsible for causing resistance. Moreover, in several cases, a combination of diterpenoids with standard antibiotics and combination of different diterpenoids reduced the MIC values for the tested strains. Therefore, the role of diterpenoids as synergists and adjuvant therapies should be explored extensively to potentiate the available antibiotics.
In numerous in vitro studies in recent years, it has been demonstrated that the diterpenoid compounds are capable of inhibiting the growth of different strains of resistant bacteria emerging from irrational use of antibiotics. However, clinical trials of diterpenoids as antimicrobial agents are yet to be explored because of lack of sufficient in vivo data, unclear mode of action, lack of selectivity, etc. Most of the diterpenoids consist of diverse lipophilic compounds, which poses a new challenge in developing drug delivery systems to improve bioavailability of the compounds. Another challenge in the path of new drug development from natural diterpenoids is toxicity and lack of selectivity. Most of the diterpenoids in their pure form have the capacity to be absorbed in the epithelial cells before reaching the site of infection because of their highly lipophilic nature. So an exquisite delivery system is necessary for in vivo studies, leading to clinical trials of these compounds which will help to identify the mode of action of these compounds. Several in silico studies have been conducted targeting some bacterial proteins and enzymes like PDF, GlmU, and NADH-2 to evaluate the binding pattern of diterpenoids in the active sites of these proteins, which have shed some light on their mechanisms of actions. More extensive research on their SAR is required by synthesizing new synthetic and semisynthetic derivatives from the natural diterpenoids to develop new antimicrobial agents to combat the upcoming pre-antibiotic era.
5 Conclusion
AMR has created a global challenge for effective treatment of infectious diseases. Although most of the commonly used antimicrobial drugs have gradually become ineffective or less effective, inclusion of new drugs to overcome the situation is not satisfactory. Natural sources, especially plants and microorganisms, contain several secondary metabolites that have potential antimicrobial properties. In this review, we have concentrated on searching for natural diterpenoids possessing potential antimicrobial properties. This review summarizes 229 prospective diterpenoids for the last 5 years with their promising antimicrobial properties such as antibacterial, antiviral, antifungal, and antiprotozoal properties. Additionally, SAR data have been presented in different sections where the SAR studies were accomplished by the authors. This review will enable the potential researchers identifying credible lead compounds for antimicrobial drug development to combat AMR. Chemical synthesis of the potential leads along with their derivatization followed by further SAR studies might be useful for effective drug discovery for infectious diseases.
Data Availability Statement
The original contributions presented in the study are included in the article/Supplementary Material, further inquiries can be directed to the corresponding authors.
Author Contributions
SMAR and MMR developed the idea of the article. PS, FIR, and FH performed the literature search and data analysis. PS, FIR, and SMAR wrote the original draft of the manuscript. All authors reviewed and approved the final manuscript.
Conflict of Interest
The authors declare that the research was conducted in the absence of any commercial or financial relationships that could be construed as a potential conflict of interest.
Publisher’s Note
All claims expressed in this article are solely those of the authors and do not necessarily represent those of their affiliated organizations, or those of the publisher, the editors and the reviewers. Any product that may be evaluated in this article, or claim that may be made by its manufacturer, is not guaranteed or endorsed by the publisher.
References
Abdissa, N., Frese, M., and Sewald, N. (2017). Antimicrobial Abietane-type Diterpenoids from Plectranthus Punctatus. Molecules 22, 1919. doi:10.3390/molecules22111919
Abreu, L. S., Do Nascimento, Y. M., Costa, R. D. S., Guedes, M. L. S., Souza, B. N. R. F., Pena, L. J., et al. (2019). Tri- and Diterpenoids from Stillingia Loranthacea as Inhibitors of Zika Virus Replication. J. Nat. Prod. 82, 2721–2730. doi:10.1021/acs.jnatprod.9b00251
Ahmadi, P., Haruyama, T., Kobayashi, N., de Voogd, N. J., and Tanaka, J. (2017). Spongian Diterpenes from the Sponge Hyattella Aff. Intestinalis. Chem. Pharm. Bull. (Tokyo) 65, 874–877. doi:10.1248/cpb.c17-00297
Alegre-Gómez, S., Sainz, P., Simões, M. F., Rijo, P., Moiteiro, C., González-Coloma, A., et al. (2017). Antiparasitic Activity of Diterpenoids against Trypanosoma Cruzi. Planta Med. 83, 306–311. doi:10.1055/s-0042-115646
Alhilal, M., Sulaiman, Y. A. M., Alhilal, S., Gomha, S. M., and Ouf, S. A. (2021). Antifungal Activity of New Diterpenoid Alkaloids Isolated by Different Chromatographic Methods from Delphinium Peregrinum L. Var. Eriocarpum Boiss. Molecules 26, 1375. doi:10.3390/molecules26051375
Ban, N. K., Thoa, N. T. K., Linh, T. M., Trang, D. T., Van Kiem, P., Nhiem, N. X., et al. (2018). Labdane-type Diterpenoids from Vitex Limonifolia and Their Antivirus Activities. J. Nat. Med. 72, 290–297. doi:10.1007/s11418-017-1125-2
Banerjee, A., Laya, M., Mora, H., and Cabrera, E. (2008). The Chemistry of Bioactive Diterpenes. Coc 12, 1050–1070. doi:10.2174/138527208785740292
Banerjee, M., Parai, D., Chattopadhyay, S., and Mukherjee, S. K. (2017). Andrographolide: Antibacterial Activity against Common Bacteria of Human Health Concern and Possible Mechanism of Action. Folia Microbiol. (Praha) 62, 237–244. doi:10.1007/s12223-017-0496-9
Bernabeu, E., Cagel, M., Lagomarsino, E., Moretton, M., and Chiappetta, D. A. (2017). Paclitaxel: What Has Been Done and the Challenges Remain Ahead. Int. J. Pharm. 526, 474–495. doi:10.1016/J.IJPHARM.2017.05.016
Bisio, A., De Mieri, M., Milella, L., Schito, A. M., Parricchi, A., Russo, D., et al. (2017). Antibacterial and Hypoglycemic Diterpenoids from Salvia Chamaedryoides. J. Nat. Prod. 80, 503–514. doi:10.1021/acs.jnatprod.6b01053
Boonsombat, J., Mahidol, C., Chawengrum, P., Reuk-Ngam, N., Chimnoi, N., Techasakul, S., et al. (2017). Roscotanes and Roscoranes: Oxygenated Abietane and Pimarane Diterpenoids from Kaempferia Roscoeana. Phytochemistry 143, 36–44. doi:10.1016/j.phytochem.2017.07.008
Bozov, P. I., Penchev, P. N., Girova, T. D., and Gochev, V. K. (2020). Diterpenoid Constituents of Teucrium Scordium L. Subsp. Scordioides (Shreb.) Maire Et Petitmengin. Nat. Product. Commun. 15, 1934578X2095952–6. doi:10.1177/1934578X20959525
Bustos-Brito, C., Joseph-Nathan, P., Burgueño-Tapia, E., Martínez-Otero, D., Nieto-Camacho, A., Calzada, F., et al. (2019). Structure and Absolute Configuration of Abietane Diterpenoids from Salvia Clinopodioides: Antioxidant, Antiprotozoal, and Antipropulsive Activities. J. Nat. Prod. 82, 1207–1216. doi:10.1021/acs.jnatprod.8b00952
Cai, C., Zhang, Y., Yang, D., Hao, X., and Li, S. (2017). Two New Kaurane-type Diterpenoids from Wedelia Chinensis (Osbeck.) Merr. Nat. Prod. Res. 31, 2531–2536. doi:10.1080/14786419.2017.1317775
Chassagne, F., Samarakoon, T., Porras, G., Lyles, J. T., Dettweiler, M., Marquez, L., et al. (2021). A Systematic Review of Plants With Antibacterial Activities: A Taxonomic and Phylogenetic Perspective. Front. pharmacol. 11, 2069. doi:10.3389/fphar.2020.586548
Chawengrum, P., Boonsombat, J., Kittakoop, P., Mahidol, C., Ruchirawat, S., and Thongnest, S. (2018). Cytotoxic and Antimicrobial Labdane and Clerodane Diterpenoids from Kaempferia Elegans and Kaempferia Pulchra. Phytochemistry Lett. 24, 140–144. doi:10.1016/j.phytol.2018.02.009
Chen, B.-C., Ding, Z.-S., Dai, J.-S., Chen, N.-P., Gong, X.-W., Ma, L.-F., et al. (2021). New Insights into the Antibacterial Mechanism of Cryptotanshinone, a Representative Diterpenoid Quinone from Salvia Miltiorrhiza Bunge. Front. Microbiol. 12, 372. doi:10.3389/fmicb.2021.647289
Chen, H. Y., Liu, T. K., Shi, Q., and Yang, X. L. (2019a). Sesquiterpenoids and Diterpenes with Antimicrobial Activity from Leptosphaeria Sp. XL026, an Endophytic Fungus in Panax Notoginseng. Fitoterapia 137, 104243. doi:10.1016/j.fitote.2019.104243
Chen, S., Li, H., Chen, Y., Li, S., Xu, J., Guo, H., et al. (2019b). Three New Diterpenes and Two New Sesquiterpenoids from the Endophytic Fungus Trichoderma Koningiopsis A729. Bioorg. Chem. 86, 368–374. doi:10.1016/j.bioorg.2019.02.005
Chen, X., Chen, W., Chen, G., Han, C., He, J. J., Zhou, X., et al. (2018). Neo-clerodane Diterpenoids from the Whole Plants of Scutellaria Formosana. Phytochemistry 145, 1–9. doi:10.1016/j.phytochem.2017.09.002
Ciorba, V., Odone, A., Veronesi, L., Pasquarella, C., and Signorelli, C. (2015). Antibiotic Resistance as a Major Public Health Concern: Epidemiology and Economic Impact. Ann. Ig. 27, 562–579. doi:10.7416/AI.2015.2048
Çiçek, S. S., Wenzel-Storjohann, A., Girreser, U., and Tasdemir, D. (2020). Biological Activities of Two Major Copaiba Diterpenoids and Their Semi-synthetic Derivatives. Rev. Bras. Farmacogn. 30, 18–27. doi:10.1007/s43450-020-00002-y
de Oliveira, J. A. M., Williams, D. E., Bonnett, S., Johnson, J., Parish, T., and Andersen, R. J. (2020). Diterpenoids Isolated from the Samoan marine Sponge Chelonaplysilla Sp. Inhibit Mycobacterium tuberculosis Growth. J. Antibiot. (Tokyo) 73, 568–573. doi:10.1038/s41429-020-0315-4
Ding, Y., Ding, C., Ye, N., Liu, Z., Wold, E. A., Chen, H., et al. (2016). Discovery and Development of Natural Product Oridonin-Inspired Anticancer Agents. Eur. J. Med. Chem. 122, 102–117. doi:10.1016/J.EJMECH.2016.06.015
Du, Y., Valenciano, A. L., Goetz, M., Cassera, M. B., and Kingston, D. G. I. (2018). Antiplasmodial Diterpenoids and a Benzotropolone from Petradoria Pumila. J. Nat. Prod. 81, 1260–1265. doi:10.1021/acs.jnatprod.8b00135
Echeverría, J., González-Teuber, M., and Urzúa, A. (2019). Antifungal Activity against Botrytis Cinerea of Labdane-type Diterpenoids Isolated from the Resinous Exudate of Haplopappus Velutinus Remy (Asteraceae). Nat. Prod. Res. 33, 2408–2412. doi:10.1080/14786419.2018.1443093
Eksi, G., Kurbanoglu, S., and Erdem, S. A. (2020). “Analysis of Diterpenes and Diterpenoids,” in Recent Advances In Natural Products Analysis. Editors S. M. Nabavi, M. Saeedi, S. F. Nabavi, and A. S. Silva. 1st ed. (Amsterdam: Elsevier), 313–345. doi:10.1016/b978-0-12-816455-6.00009-3
Endo, Y., Kasahara, T., Harada, K., Kubo, M., Etoh, T., Ishibashi, M., et al. (2017). Sucupiranins A-L, Furanocassane Diterpenoids from the Seeds of Bowdichia Virgilioides. J. Nat. Prod. 80, 3120–3127. doi:10.1021/acs.jnatprod.7b00249
Erharuyi, O., Adhikari, A., FalodunJabeen, A., Jabeen, A., Imad, R., Ammad, M., et al. (2017). Cytotoxic, Anti-inflammatory, and Leishmanicidal Activities of Diterpenes Isolated from the Roots of Caesalpinia Pulcherrima. Planta Med. 83, 104–110. doi:10.1055/s-0042-110407
Esposito, M., Nothias, L. F., Retailleau, P., Costa, J., Roussi, F., Neyts, J., et al. (2017). Isolation of Premyrsinane, Myrsinane, and Tigliane Diterpenoids from Euphorbia Pithyusa Using a Chikungunya Virus Cell-Based Assay and Analogue Annotation by Molecular Networking. J. Nat. Prod. 80, 2051–2059. doi:10.1021/acs.jnatprod.7b00233
Farimani, M. M., Khodaei, B., Moradi, H., Aliabadi, A., Ebrahimi, S. N., De Mieri, M., et al. (2018). Phytochemical Study of Salvia Leriifolia Roots: Rearranged Abietane Diterpenoids with Antiprotozoal Activity. J. Nat. Prod. 81, 1384–1390. doi:10.1021/acs.jnatprod.7b01019
Fozia, A., Shaheen, A., Ahmad, I., Amin, S., Ahmad, N., Ullah, R., et al. (2021). Ballodiolic Acid A and B: Two New ROS, (OH), (ONOO−) Scavenging and Potent Antimicrobial Constituents Isolated from Ballota Pseudodictamnus (L.) Benth. Pharmaceutics 13, 402. doi:10.3390/pharmaceutics13030402
Gu, C. Z., Xia, X. M., Lv, J., Tan, J. W., Baerson, S. R., Pan, Z. Q., et al. (2019). Diterpenoids with Herbicidal and Antifungal Activities from Hulls of rice (Oryza Sativa). Fitoterapia 136, 104183. doi:10.1016/j.fitote.2019.104183
Guo, X. C., Xu, L. L., Yang, R. Y., Yang, M. Y., Hu, L. D., Zhu, H. J., et al. (2019). Anti-vibrio Indole-Diterpenoids and C-25 Epimeric Steroids from the marine-derived Fungus Penicillium janthinellum. Front. Chem. 7, 80. doi:10.3389/fchem.2019.00080
Han, J. W., Oh, M., Lee, Y. J., Choi, J., Choi, G. J., and Kim, H. (2018). Crinipellins A and I, Two Diterpenoids from the Basidiomycete Fungus Crinipellis Rhizomaticola, as Potential Natural Fungicides. Molecules 23, 2377. doi:10.3390/molecules23092377
Han, X., Bao, X. F., Wang, C. X., Xie, J., Song, X. J., Dai, P., et al. (2021). Cladosporine A, a New Indole Diterpenoid Alkaloid with Antimicrobial Activities from Cladosporium Sp. Nat. Prod. Res. 35, 1115–1121. doi:10.1080/14786419.2019.1641807
Hayashi, K., Ohno, H., Tanaka, T., Toyoka, N., Nemoto, H., and Hayashi, T. (2004). Evaluation of Scopadulciol-Related Molecules for Their Stimulatory Effect on the Cytotoxicity of Acyclovir and Ganciclovir Against Herpes Simplex Virus Type 1 Thymidine Kinase Gene-Transfected HeLa cells. Chem. Pharm. Bull. 52 (8), 1015–1017.
Hu, X. Y., Meng, L. H., Li, X., Yang, S. Q., Li, X. M., and Wang, B. G. (2017). Three New Indole Diterpenoids from the Sea-Anemone-Derived Fungus Penicillium Sp. AS-79. Mar. Drugs 15, 137. doi:10.3390/md15050137
Huang, Y. S., Lu, Y., Chen, C. H., Lee, K. H., and Chen, D. F. (2019). Potent Anti-HIV Ingenane Diterpenoids from Euphorbia Ebracteolata. J. Nat. Prod. 82, 1587–1592. doi:10.1021/acs.jnatprod.9b00088
Isca, V. M. S., Andrade, J., Fernandes, A. S., Paixão, P., Uriel, C., Gómez, A. M., et al. (2020). In Vitro Antimicrobial Activity of Isopimarane-type Diterpenoids. Molecules 25, 4250. doi:10.3390/molecules25184250
Jiang, M., Sheng, F., Zhang, Z., Ma, X., Gao, T., Fu, C., et al. (2021). Andrographis Paniculata (Burm.f.) Nees and its Major Constituent Andrographolide as Potential Antiviral Agents. J. Ethnopharmacol. 272, 113954. doi:10.1016/j.jep.2021.113954
Kamada, T., Johanis, M. L., Ng, S. Y., Phan, C. S., Suleiman, M., and Vairappan, C. S. (2020). A New Epi-neoverrucosane-type Diterpenoid from the Liverwort Pleurozia Subinflata in Borneo. Nat. Prod. Bioprospect 10, 51–56. doi:10.1007/s13659-020-00232-6
Khameneh, B., Iranshahy, M., Soheili, V., Fazly Bazzaz, B. S., and Bazzaz, F. (2019). Review on Plant Antimicrobials: a Mechanistic Viewpoint. Antimicrob. Resist. Infect. Control. 8, 118. doi:10.1186/s13756-019-0559-6
Kim, Y. A., Latif, A., Kong, C. S., Seo, Y., Dalal, S. R., Cassera, M. B., et al. (2020). Antimalarial Diterpenoids from Vitex Rotundifolia: Isolation, Structure Elucidation, and In Vitro Antiplasmodial Activity. Bioorg. Chem. 100, 103925. doi:10.1016/j.bioorg.2020.103925
Kishore, V., Yarla, N. S., Bishayee, A., Putta, S., Malla, R., Neelapu, N. R., et al. (2016). Multi-targeting Andrographolide and its Natural Analogs as Potential Therapeutic Agents. Curr. Top. Med. Chem. 17, 845–857. doi:10.2174/1568026616666160927150452
Koehn, F. E., and Carter, G. T. (2005). The Evolving Role of Natural Products in Drug Discovery. Nat. Rev. Drug Discov. 4, 206–220. doi:10.1038/nrd1657
Kuźma, Ł., Kaiser, M., and Wysokińska, H. (2017). The Production and Antiprotozoal Activity of Abietane Diterpenes in Salvia Austriaca Hairy Roots Grown in Shake Flasks and Bioreactor. Prep. Biochem. Biotechnol. 47, 58–66. doi:10.1080/10826068.2016.1168745
Lambrechts, I. A., and Lall, N. (2021). Traditional Usage and Biological Activity of Plectranthus Madagascariensis and its Varieties: a Review. J. Ethnopharmacol. 269, 113663. doi:10.1016/j.jep.2020.113663
Langat, M. K., Helfenstein, A., Horner, C., Tammela, P., Hokkanen, H., Izotov, D., et al. (2018). Pumilol, a Diterpenoid with a Rare Strobane Skeleton from Pinus Pumila (Pinaceae). Chem. Biodivers. 15, e1800056. doi:10.1002/cbdv.201800056
Lhullier, C., De Oliveira Tabalipa, E., Nienkötter Sardá, F., Sandjo, L. P., Zanchett Schneider, N. F., Carraro, J. L., et al. (2019). Clerodane Diterpenes from the marine Sponge Raspailia Bouryesnaultae Collected in South Brazil. Mar. Drugs 17, 57. doi:10.3390/md17010057
Li, D. W., Deng, X. P., He, X., Han, X. Y., Ma, Y. F., Huang, H. L., et al. (2021a). Eupholides A-H, Abietane Diterpenoids from the Roots of Euphorbia Fischeriana, and Their Bioactivities. Phytochemistry 183, 112593. doi:10.1016/j.phytochem.2020.112593
Li, H., Yang, P., Zhang, E. H., Kong, L. M., and Meng, C. Y. (2021b). Antimicrobial Ent-abietane-type Diterpenoids from the Roots of Euphorbia Wallichii. J. Asian Nat. Prod. Res. 23, 652–659. doi:10.1080/10286020.2020.1758931
Li, J. C., Feng, X. Y., Liu, D., Zhang, Z. J., Chen, X. Q., Li, R. T., et al. (2019). Diterpenoids from Euphorbia Neriifolia and Their Related Anti-HIV and Cytotoxic Activity. Chem. Biodivers. 16, e1900495. doi:10.1002/cbdv.201900495
Li, Q. J., Zhao, C. L., Ku, C. F., Zhu, Y., Zhu, X. J., Zhang, J. J., et al. (2020a). Two New Bioactive Diterpenes Identified from Isodon Interruptus. Bioorg. Chem. 95, 103512. doi:10.1016/j.bioorg.2019.103512
Li, S. F., Jiao, Y. Y., Zhang, Z. Q., Chao, J. B., Jia, J., Shi, X. L., et al. (2018). Diterpenes from Buds of Wikstroemia Chamaedaphne Showing Anti-hepatitis B Virus Activities. Phytochemistry 151, 17–25. doi:10.1016/j.phytochem.2018.01.021
Li, W. Y., Liu, Y., Lin, Y. T., Liu, Y. C., Guo, K., Li, X. N., et al. (2020b). Antibacterial Harziane Diterpenoids from a Fungal Symbiont Trichoderma Atroviride Isolated from Colquhounia Coccinea Var. Mollis. Phytochemistry 170, 112198. doi:10.1016/j.phytochem.2019.112198
Li, X., Li, X.-D., Li, X.-M., Xu, G.-M., Liu, Y., and Wang, B.-G. (2017). Wentinoids A-F, Six New Isopimarane Diterpenoids from Aspergillus wentii SD-310, a Deep-Sea Sediment Derived Fungus. RSC Adv. 7, 4387–4394. doi:10.1039/C6RA27209F
Liang, J. H., Huo, X. K., Cheng, Z. B., Sun, C. P., Zhao, J. C., Kang, X. H., et al. (2019). An Indole Diterpenoid Isolated from the Fungus Drechmeria Sp. And its Antimicrobial Activity. Nat. Prod. Res. 33, 2770–2776. doi:10.1080/14786419.2018.1501050
Liang, X., Chen, L., Song, L., Fei, W., He, M., He, C., et al. (2017). Diterpenoid Alkaloids from the Root of Aconitum Sinchiangense W. T. Wang with Their Antitumor and Antibacterial Activities. Nat. Prod. Res. 31, 2016–2023. doi:10.1080/14786419.2016.1272113
Liang, Z.-Y., Shen, N.-X., Zhou, X.-J., Zheng, Y.-Y., Chen, M., and Wang, C.-Y. (2020). Bioactive Indole Diterpenoids and Polyketides from the Marine-Derived Fungus Penicillium javanicum. Chem. Nat. Compd. 56, 379–382. doi:10.1007/s10600-020-03039-6
Lin, L. G., Ung, C. O., Feng, Z. L., Huang, L., and Hu, H. (2016). Naturally Occurring Diterpenoid Dimers: Source, Biosynthesis, Chemistry and Bioactivities. Planta Med. 82, 1309–1328. doi:10.1055/S-0042-114573
Liu, H. C., Xiang, Z. B., Wang, Q., Li, B. Y., Jin, Y. S., and Chen, H. S. (2017). Monomeric and Dimeric Ent-kauranoid-type Diterpenoids from Rabdosia Japonica and Their Cytotoxicity and Anti-HBV Activities. Fitoterapia 118, 94–100. doi:10.1016/j.fitote.2017.03.006
Liu, Y.-P., Dai, Q., Pu, C.-J., Wang, M., Li, Z.-H., Liu, J.-K., et al. (2019). Psathyrellanic Acid, a Monocyclic Diterpenoid from the Basidiomycete Psathyrella Candolleana. Nat. Product. Commun. 14, 1934578X1985095–4. doi:10.1177/1934578X19850958
Liu, Y. P., Dai, Q., Wang, W. X., He, J., Li, Z. H., Feng, T., et al. (2020a). Psathyrins: Antibacterial Diterpenoids from Psathyrella Candolleana. J. Nat. Prod. 83, 1725–1729. doi:10.1021/acs.jnatprod.0c00269
Liu, Y. Y., Liu, Y. P., Wang, X. P., Qiao, Z. H., Yu, X. M., Zhu, Y. Z., et al. (2020b). Bioactive Daphnane Diterpenes from Wikstroemia Chuii with Their Potential Anti-inflammatory Effects and Anti-HIV Activities. Bioorg. Chem. 105, 104388. doi:10.1016/j.bioorg.2020.104388
Mafu, S., and Zerbe, P. (2017). Plant Diterpenoid Metabolism for Manufacturing the Biopharmaceuticals of Tomorrow: Prospects and Challenges. Phytochem. Rev. 17, 113–130. doi:10.1007/s11101-017-9513-5
Mahadeo, K., Herbette, G., Grondin, I., Jansen, O., Kodja, H., Soulange, J., et al. (2019). Antiplasmodial Diterpenoids from Psiadia Arguta. J. Nat. Prod. 82, 1361–1366. doi:10.1021/acs.jnatprod.8b00698
Mai, Z. P., Ni, G., Liu, Y. F., Li, Y. H., Li, L., Li, J. Y., et al. (2018). Secoheliosphanes A and B and Secoheliospholane A, Three Diterpenoids with Unusual Seco-Jatrophane and Seco-Jatropholane Skeletons from Euphorbia Helioscopia. J. Org. Chem. 83, 167–173. doi:10.1021/acs.joc.7b02558
Mantaj, J., Rahman, S. M. A., Bokshi, B., Hasan, C. M., Jackson, P. J., Parsons, R. B., et al. (2015). Crispene E, a Cis-Clerodane Diterpene Inhibits STAT3 Dimerization in Breast Cancer Cells. Org. Biomol. Chem. 13, 3882–3886. doi:10.1039/C5OB00052A
Miethke, M., Pieroni, M., Weber, T., Brönstrup, M., Hammann, P., Halby, L., et al. (2021). Towards the Sustainable Discovery and Development of New Antibiotics. Nat. Rev. Chem. 5, 726–749. doi:10.1038/s41570-021-00313-1
Mothana, R. A., Khaled, J. M., El-Gamal, A. A., Noman, O. M., Kumar, A., Alajmi, M. F., et al. (2019). Comparative Evaluation of Cytotoxic, Antimicrobial and Antioxidant Activities of the Crude Extracts of Three Plectranthus Species Grown in Saudi Arabia. Saudi Pharm. J. 27, 162–170. doi:10.1016/j.jsps.2018.09.010
Murillo, J. A., Gil, J. F., Upegui, Y. A., Restrepo, A. M., Robledo, S. M., Quiñones, W., et al. (2019). Antileishmanial Activity and Cytotoxicity of Ent-Beyerene Diterpenoids. Bioorg. Med. Chem. 27, 153–160. doi:10.1016/j.bmc.2018.11.030
Nabavi, S. M., Habtemariam, S., Daglia, M., Braidy, N., Loizzo, M. R., Tundis, R., et al. (2015). Neuroprotective Effects of Ginkgolide B against Ischemic Stroke: A Review of Current Literature. Curr. Top. Med. Chem. 15, 2222–2232. doi:10.2174/1568026615666150610142647
Ndjoubi, K. O., Sharma, R., Badmus, J. A., Jacobs, A., Jordaan, A., Marnewick, J., et al. (2021). Antimycobacterial, Cytotoxic, and Antioxidant Activities of Abietane Diterpenoids Isolated from Plectranthus Madagascariensis. Plants 10, 175. doi:10.3390/plants10010175
Newman, D. J., and Cragg, G. M. (2016). Natural Products as Sources of New Drugs from 1981 to 2014. J. Nat. Prod. 79, 629–661. doi:10.1021/ACS.JNATPROD.5B01055
Nie, Y. W., Li, Y., Luo, L., Zhang, C. Y., Fan, W., Gu, W. Y., et al. (2021). Phytochemistry and Pharmacological Activities of the Diterpenoids from the Genus Daphne. Molecules 26, 6598. doi:10.3390/molecules26216598
Nzogong, R. T., Nganou, B. K., Tedonkeu, A. T., Awouafack, M. D., Tene, M., Ito, T., et al. (2018). Three New Abietane-type Diterpenoids from Plectranthus Africanus and Their Antibacterial Activities. Planta Med. 84, 59–64. doi:10.1055/s-0043-114426
Obaidullah, M. N. A., Ahmad, M. N., Ahmad, W., Tariq, S. A., Rahman, N., Ahmad, S., et al. (2018). Isolation and Characterization of C19-Diterpenoid Alkaloids from the Roots of Aconitum Heterophyllum Wall. J. Med. Chem. Drug Des. 1, 101.
Ogawa, K., Nakamura, S., Hosokawa, K., Ishimaru, H., Saito, N., Ryu, K., et al. (2018). New Diterpenes from Nigella Damascena Seeds and Their Antiviral Activities against Herpes Simplex Virus Type-1. J. Nat. Med. 72, 439–447. doi:10.1007/s11418-017-1166-6
Ogbeide, O., Dickson, V. O., Dickson, V., Jebba, R., Owhiroro, D., Olaoluwa, M., et al. (2018). Antiplasmodial and Acute Toxicity Studies of Fractions and Cassane-type Diterpenoids from the Stem Bark of Caesalpinia Pulcherrima (L.) Sw. Tjnpr 2, 179–184. doi:10.26538/tjnpr/v2i4.5
O’Neill, J. (2016). Tackling Drug-resistant Infections Globally: Final Report and Recommendations. Review on Antimicrobial Resistance. Available at: https://amr-review.org/sites/default/files/160518_Final%20paper_with%20cover.pdf (Accessed December 13, 2021).
Porras, G., Chassagne, F., Lyles, J. T., Marquez, L., Dettweiler, M., Salam, A. M., et al. (2021). Ethnobotany and the Role of Plant Natural Products in Antibiotic Drug Discovery. Chem. Rev. 121, 3495–3560. doi:10.1021/acs.chemrev.0c00922
Prema, , Kodama, T., Wong, C. P., El-Desoky, A. H., Nyunt, H. H. W., Ngwe, H., et al. (2020). Anti-Vpr Activities of Homodrimane Sesquiterpenoids and Labdane Diterpenoids from Globba Sherwoodiana Rhizomes. Fitoterapia 146, 104705. doi:10.1016/j.fitote.2020.104705
Qiao, Y., Liu, Y., Duan, X., Chen, C., Liu, J., Zhu, H., et al. (2018). A Pair of Epimeric Cassane-type Diterpenoids and a New Labdane-type Derivative from Caesalpinia Decapetala. Tetrahedron 74, 3852–3857. doi:10.1016/j.tet.2018.05.028
Quaglio, D., Corradi, S., Erazo, S., Vergine, V., Berardozzi, S., Sciubba, F., et al. (2020a). Structural Elucidation and Antimicrobial Characterization of Novel Diterpenoids from Fabiana Densa Var. Ramulosa. ACS Med. Chem. Lett. 11, 760–765. doi:10.1021/acsmedchemlett.9b00605
Quaglio, D., Mangoni, M. L., Stefanelli, R., Corradi, S., Casciaro, B., Vergine, V., et al. (2020b). Ent-Beyerane Diterpenes as a Key Platform for the Development of ArnT-Mediated Colistin Resistance Inhibitors. J. Org. Chem. 85, 10891–10901. doi:10.1021/acs.joc.0c01459
Rahman, S. M. A., Ohno, H., Murata, T., Yoshino, H., Satoh, N., Murakami, K., et al. (2001a). The First Total Synthesis of Scopadulin, An Antiviral Aphidicolane Diterpene. Org. Lett. 3, 619. doi:10.1021/ol007059v
Rahman, S. M. A., Ohno, H., Yoshino, H., Satoh, N., Tsukaguchi, M., Murakami, K., et al. (2001b). A Model Study for the Total Synthesis of (±)-Scopadulin: Stereoselective Construction of the A/B Ring System With Desired Functionalities. Tetrahedron 57, 127–134. doi:10.1016/s0040-4020(00)00995-9
Rahman, S. M. A., Ohno, H., Murata, T., Yoshino, H., Satoh, N., Murakami, K., et al. (2001c). Total Synthesis of (+/-)-scopadulin. J. Org. Chem. 66, 4831–4840. doi:10.1021/OL007059V10.1021/jo015590d
Ramírez, J., Gilardoni, G., Ramón, E., Tosi, S., Picco, A. M., Bicchi, C., et al. (2018). Phytochemical Study of the Ecuadorian Species Lepechinia Mutica (Benth.) Epling and High Antifungal Activity of Carnosol against Pyricularia Oryzae. Pharmaceuticals (Basel) 11, 33. doi:10.3390/ph11020033
Remy, S., Olivon, F., Desrat, S., Blanchard, F., Eparvier, V., Leyssen, P., et al. (2018). Structurally Diverse Diterpenoids from Sandwithia Guyanensis. J. Nat. Prod. 81, 901–912. doi:10.1021/acs.jnatprod.7b01025
Rodrigues, T., Reker, D., Schneider, P., and Schneider, G. (2016). Counting on Natural Products for Drug Design. Nat. Chem. 8, 531–541. doi:10.1038/nchem.2479
Satari, M. H., Situmeang, B., Yudha, I. P., and Kurnia, D. (2019). Antibacterial Diterpenoid against Pathogenic Oral Bacteria of Streptococcus Mutans ATCC 25175 Isolated from Sarang Semut (Myrmecodia Pendans). Valensi 5, 218–223. doi:10.15408/jkv.v5i2.8864
Sichaem, J., Nguyen, H. H., Nguyen, V. H., Mac, D. H., Mai, D. T., Nguyen, H. C., et al. (2021). A New Labdane-type Diterpenoid from the Leaves of Vitex Negundo L. Nat. Prod. Res. 35, 2329–2334. doi:10.1080/14786419.2019.1672687
Singh, B., and Sharma, R. A. (2015). Plant Terpenes: Defense Responses, Phylogenetic Analysis, Regulation and Clinical Applications. 3 Biotech. 5, 129–151. doi:10.1007/S13205-014-0220-2
Tabefam, M., Farimani, M. M., Danton, O., Ramseyer, J., Kaiser, M., Ebrahimi, S. N., et al. (2018). Antiprotozoal Diterpenes from Perovskia Abrotanoides. Planta Med. 84, 913–919. doi:10.1055/a-0608-4946
Tan, Y. P., Xue, Y., Savchenko, A. I., Houston, S. D., Modhiran, N., McMillan, C. L. D., et al. (2019). Basimarols A, B, and C, Highly Oxygenated Pimarane Diterpenoids from Basilicum Polystachyon. J. Nat. Prod. 82, 2828–2834. doi:10.1021/acs.jnatprod.9b00522
Tanaka, S., and Ito, M. (2021). Quantitative Analysis of Ent-Kaurane Diterpenoids in Isodon Herb (Enmei-So) by HPLC-UV. Chem. Pharm. Bull. 69, 246–252. doi:10.1248/cpb.c20-00769
Tanaka, T., Murakami, K., Kanda, A., Patra, D., Yamamoto, S., Satoh, N., et al. (2001). Total Synthesis of Stemodinone via an Efficient Ring Exchange Strategy. J. Org. Chem. 66, 7107. doi:10.1021/jo015808w
Tani, K., Kamada, T., Phan, C. S., and Vairappan, C. S. (2019). New Cembrane-type Diterpenoids from Bornean Soft Coral Nephthea Sp. With Antifungal Activity against Lagenidium Thermophilum. Nat. Prod. Res. 33, 3343–3349. doi:10.1080/14786419.2018.1475387
Tsujimura, M., Goto, M., Tsuji, M., Yamaji, Y., Ashitani, T., Kimura, K.-i., et al. (2019). Isolation of Diterpenoids from Sugi wood-drying Byproducts and Their Bioactivities. J. Wood Sci. 65, 19. doi:10.1186/s10086-019-1787-3
Ueno, A. K., Barcellos, A. F., Costa-Silva, T. A., Mesquita, J. T., Ferreira, D. D., Tempone, A. G., et al. (2018). Antitrypanosomal Activity and Evaluation of the Mechanism of Action of Diterpenes from Aerial Parts of Baccharis Retusa (Asteraceae). Fitoterapia 125, 55–58. doi:10.1016/j.fitote.2017.12.016
Wang, B., Wei, Y., Zhao, X., Tian, X., Ning, J., Zhang, B., et al. (2018a). Unusual Ent-Atisane Type Diterpenoids with 2-oxopropyl Skeleton from the Roots of Euphorbia Ebracteolata and Their Antiviral Activity against Human Rhinovirus 3 and Enterovirus 71. Bioorg. Chem. 81, 234–240. doi:10.1016/j.bioorg.2018.08.029
Wang, C. J., Yan, Q. L., Ma, Y. F., Sun, C. P., Chen, C. M., Tian, X. G., et al. (2017). Ent-Abietane and Tigliane Diterpenoids from the Roots of Euphorbia Fischeriana and Their Inhibitory Effects against Mycobacterium Smegmatis. J. Nat. Prod. 80, 1248–1254. doi:10.1021/acs.jnatprod.6b00786
Wang, X., Sun, K., and Wang, B. (2018b). Bioactive Pimarane Diterpenes from the Arctic Fungus Eutypella Sp. D-1. Chem. Biodivers. 15, e1700501. doi:10.1002/cbdv.201700501
Wu, H., Yang, H.-X., Li, Z.-H., Feng, T., and Liu, J.-K. (2021). Psathyrellins A-E, Antibacterial Guanacastane Diterpenoids from Mushroom Psathyrella Candolleana. Nat. Prod. Bioprospect. 11, 447–452. doi:10.1007/s13659-021-00316-x
Wu, J., Li, X., Guo, X., Cheng, Z., Meng, J., Cheng, W., et al. (2020). Briarane-type Diterpenoids from a Gorgonian Coral Ellisella Sp. With Anti-HBV Activities. Bioorg. Chem. 105, 104423. doi:10.1016/j.bioorg.2020.104423
Xu, L. L., Pang, X. J., Shi, Q., Xian, P. J., Tao, Y. D., and Yang, X. L. (2019). Two New Prenylated Indole Diterpenoids from Tolypocladium Sp. And Their Antimicrobial Activities. Chem. Biodivers. 16, e1900116. doi:10.1002/cbdv.201900116
Xu, M.-M., Zhou, J., Zeng, L., Xu, J., Onakpa, M. M., Duan, J.-A., et al. (2021). Pimarane-derived Diterpenoids with Anti-Helicobacter pylori Activity from the Tuber of Icacina Trichantha. Org. Chem. Front. 8, 3014–3022. doi:10.1039/d1qo00374g
Yan, S. L., Li, Y. H., Chen, X. Q., Liu, D., Chen, C. H., and Li, R. T. (2018). Diterpenes from the Stem Bark of Euphorbia Neriifolia and Their In Vitro Anti-HIV Activity. Phytochemistry 145, 40–47. doi:10.1016/j.phytochem.2017.10.006
Yang, B., He, Y., Lin, S., Zhang, J., Li, H., Wang, J., et al. (2019). Antimicrobial Dolabellanes and Atranones from a Marine-Derived Strain of the Toxigenic Fungus Stachybotrys chartarum. J. Nat. Prod. 82, 1923–1929. doi:10.1021/acs.jnatprod.9b00305
Yang, B., Fang, D., Lv, Q., Wang, Z., and Liu, Y. (2021). Targeted Therapeutic Strategies in the Battle against Pathogenic Bacteria. Front. Pharmacol. 12, 1090. doi:10.3389/FPHAR.2021.673239
Yu, H.-B., Gu, B.-B., Wang, S.-P., Cheng, C.-W., Yang, F., and Lin, H.-W. (2017a). New Diterpenoids from the marine Sponge Dactylospongia Elegans. Tetrahedron 73, 6657–6661. doi:10.1016/j.tet.2017.10.023
Yu, H.-B., Wang, X.-L., Xu, W.-H., Zhang, Y.-X., Qian, Y.-S., Zhang, J.-P., et al. (2018a). Eutypellenoids A-C, New Pimarane Diterpenes from the Arctic Fungus Eutypella Sp. D-1. Mar. Drugs 16, 284. doi:10.3390/md16080284
Yu, J., Yin, T. P., Wang, J. P., Mei, R. F., Cai, L., and Ding, Z. T. (2017b). A New C20-Diterpenoid Alkaloid from the Lateral Roots of Aconitum Carmichaeli. Nat. Prod. Res. 31, 228–232. doi:10.1080/14786419.2016.1219863
Yu, J. H., Yu, Z. P., Wu, D. X., Yan, X., Wang, Y. Y., and Zhang, H. (2019a). Cuceolatins A-D: New Bioactive Diterpenoids from the Leaves of Cunninghamia Lanceolata. Chem. Biodivers. 16, e1900317. doi:10.1002/cbdv.201900317
Yu, Z., Wei, Y., Tian, X., Yan, Q., Yan, Q., Huo, X., et al. (2018b). Diterpenoids from the Roots of Euphorbia Ebracteolata and Their Anti-tuberculosis Effects. Bioorg. Chem. 77, 471–477. doi:10.1016/j.bioorg.2018.02.007
Yu, Z. X., Zheng, C. J., Chen, G. Y., Huang, R. L., Zhou, X. M., Niu, Z. G., et al. (2019b). 3,4- Seco-Norclerodane Diterpenoids from the Roots of Polyalthia Laui. J. Nat. Prod. 82, 27–34. doi:10.1021/acs.jnatprod.8b00243
Zadali, R., Nejad Ebrahimi, S., Tofighi, Z., Es-haghi, A., Hamburger, M., Kaiser, M., et al. (2020). Antiprotozoal Activity of Diterpenoids Isolated from Zhumeria Majdae- Absolute Configuration by Circular Dichroism. Daru 28, 455–462. doi:10.1007/s40199-020-00345-w
Zhang, D., Guo, J., Zhang, M., Liu, X., Ba, M., Tao, X., et al. (2017). Oxazole-Containing Diterpenoids from Cell Cultures of Salvia Miltiorrhiza and Their Anti-HIV-1 Activities. J. Nat. Prod. 80, 3241–3246. doi:10.1021/acs.jnatprod.7b00659
Zhao, B., Ablajan, N., Zhao, J.-Y., Kodirova, D. R., Sagdullaev, S. S., and Aisa, H. A. (2020a). Two New C19-Diterpenoid Alkaloids from Aconitum Smirnovii. Phytochemistry Lett. 38, 96–100. doi:10.1016/j.phytol.2020.05.007
Zhao, J. C., Wang, Y. L., Zhang, T. Y., Chen, Z. J., Yang, T. M., Wu, Y. Y., et al. (2018). Indole Diterpenoids from the Endophytic Fungus Drechmeria Sp. As Natural Antimicrobial Agents. Phytochemistry 148, 21–28. doi:10.1016/j.phytochem.2018.01.010
Zhao, L., Xiang, K. L., Liu, R. X., Xie, Z. P., Zhang, S. M., and Dai, S. J. (2020b). Anti-inflammatory and Anti-viral Labdane Diterpenoids from the Fruits of Forsythia Suspensa. Bioorg. Chem. 96, 103651. doi:10.1016/j.bioorg.2020.103651
Zhu, N. L., Sun, Z. H., Hu, M. G., Wu, T. Y., Yuan, J. Q., Wu, H. F., et al. (2017). New Cassane Diterpenoids from caesalpinia Sappan and Their Antiplasmodial Activity. Molecules 22, 1751. doi:10.3390/molecules22101751
Keywords: diterpenes, diterpenoids, antimicrobial resistance, antibacterial activity, antifungal activity, antiviral activity, antiprotozoal activity
Citation: Saha P, Rahman FI, Hussain F, Rahman SMA and Rahman MM (2022) Antimicrobial Diterpenes: Recent Development From Natural Sources. Front. Pharmacol. 12:820312. doi: 10.3389/fphar.2021.820312
Received: 22 November 2021; Accepted: 30 December 2021;
Published: 28 February 2022.
Edited by:
Michael Heinrich, University College London, United KingdomReviewed by:
François Chassagne, IRD UMR152 Pharmacochimie et Biologie Pour le Développement (PHARMADEV), FranceLouis Pergaud Sandjo, Federal University of Santa Catarina, Brazil
Copyright © 2022 Saha, Rahman, Hussain, Rahman and Rahman. This is an open-access article distributed under the terms of the Creative Commons Attribution License (CC BY). The use, distribution or reproduction in other forums is permitted, provided the original author(s) and the copyright owner(s) are credited and that the original publication in this journal is cited, in accordance with accepted academic practice. No use, distribution or reproduction is permitted which does not comply with these terms.
*Correspondence: S. M. Abdur Rahman, smarahman@du.ac.bd; M. Mukhlesur Rahman, m.rahman@uel.ac.uk
†These authors have contributed equally to this work