- 1Department of Central Laboratory, Xi’an Peihua University, Xi’an, China
- 2Department of Neurosurgery, Bijie Traditional Chinese Medicine Hospital, Bijie, China
- 3Department of Neurosurgery, Xiangya Hospital, Central South University, Changsha, China
- 4The Institute of Skull Base Surgery and Neurooncology at Hunan Province, Changsha, China
Background: Stroke is a major cause of long-term disability and death, but the clinical therapeutic strategy for stroke is limited and more research must be conducted to explore the possible avenues for stroke treatment and recovery. Since ferroptosis is defined, its role in the body has become the focus of attention and discussion, including in stroke.
Methods: In this work, we aim to systematically discuss the “ferroptosis in stroke” research by bibliometric analysis. Documents were retrieved from the Web of Science Core Collection database on October 30, 2021. Statistical analysis and visualization analysis were conducted by the VOSviewer 1.6.15.
Results: Ninety-nine documents were identified for bibliometric analysis. Research on “ferroptosis in stroke” has been rapidly developing and has remained the focus of many scholars and organizations in the last few years, but the Chinese groups in this field still lacked collaboration with others. Documents and citation analysis suggested that Rajiv R. Ratan and Brent R. Stockwell are active researchers, and the research by Qingzhang Tuo, Ishraq Alim, and Qian Li are more important drivers in the development of the field. Keywords associated with lipid peroxidation, ferroptosis, iron, oxidative stress, and cell death had high frequency, but apoptosis, necroptosis, pyroptosis, and autophagy had scant research, and there may be more research ideas in the future by scholars.
Conclusion: Further exploration of the mechanisms of crosstalk between ferroptosis and other programmed cell death may improve clinical applications and therapeutic effects against stroke. Scholars will also continue to pay attention to and be interested in the hot topic “ferroptosis in stroke”, to produce more exciting results and provide new insights into the bottleneck of stroke treatment.
Background
With about 3.0 million new cases occurring every year, stroke is a primary reason of disability and death in China and the United States (Wu et al., 2019; Barthels and Das, 2020). The burden is expected to increase further due to population aging, continued high prevalence of risk factors such as hypertension, and poor management. Although overall access to health services has improved, access to specialist stroke cure and care is patchy across regions and particularly uneven in backward areas (Wu et al., 2019; Zhang et al., 2020). Research has shown that people with hypertension, obesity, and diabetes have a higher incidence of stroke (Barthels and Das, 2020). The vast majority of strokes fall into two categories, hemorrhagic stroke (HS) induced by ruptured brain blood vessels and ischemic stroke (IS) caused by blocked brain arteries, both of which lead to local hypoxia and brain tissue damage (Campbell et al., 2019; Barthels and Das, 2020). Currently, tissue plasminogen activator (tPA), a powerful thrombolysis for the dissolution of acute thromboembolism, is the only ischemic stroke drug approved by the Food and Drug Administration (Kuo et al., 2020). But the tPA has a strict time window for treatment. Stroke patients must receive tPA within 3 h of the onset of stroke symptoms, but no longer than 4.5 h, and if the treatment window is exceeded, it may lead to hemorrhagic transformation, which can cause additional damage to the brain (Hughes et al., 2021). Routine prophylactic drug interventions, such as anticoagulants and blood pressure-lowering and cholesterol-lowering drugs, are necessary for people who have experienced stroke because they are at increased risk for a second stroke occurring immediately after the first stroke (Barthels and Das, 2020). Dismayingly, there is still no effective treatment for HS (Yin et al., 2021). Overall, the clinical therapeutic strategy for stroke is limited, and more research must be conducted to explore possible avenues for stroke treatment and recovery.
Ferroptosis is a programmed cell death (PCD) characterized by iron-dependent lipid peroxidation, including impaired intracellular cysteine uptake, glutathione depletion, membrane damage, and damage-related molecule release (Galluzzi et al., 2018; Tang and Kroemer, 2020; Hassannia et al., 2021; Wu et al., 2021). The term “ferroptosis” was coined by Scott J Dixon in 2012 and used to describe a type of cell death caused by erastin and also suppressed by lipophilic antioxidants or iron chelators such as ferrostatin-1 (Dixon et al., 2012). Recent studies have shown that the cell phenotype and molecular events of ferroptosis are different from apoptosis, autophagy, necroptosis, pyroptosis, and other PCDs (Galluzzi et al., 2018; Zhou et al., 2020). PCD is crucial to the occurrence and development of multiple diseases, including dysgenopathy, immune system diseases, central nervous system (CNS) disease, and cancer (Gibellini and Moro, 2021; Kist and Vucic, 2021; Moujalled et al., 2021). Moreover, common molecular events involved in ferroptosis are logically linked to the occurrence and development of many diseases, such as oxidative stress and abnormal iron, glutamate, and lipid metabolism (Stockwell et al., 2020; Plascencia-Villa and Perry, 2021; Yu et al., 2021). Ferroptosis involves nerve disorders (David et al., 2021), such as Alzheimer’s disease (AD) (Plascencia-Villa and Perry, 2021), Parkinson’s disease (PD) (Mahoney-Sánchez et al., 2021, spinal cord injury (SCI) (Ge et al., 2021), traumatic brain injury (TBI) (Rui et al., 2020), stroke (Alim et al., 2019; Zhou et al., 2020), and depression (Cao et al., 2021). The novel findings provide potential strategies for the clinical treatment and prognosis of stroke, such as targeting ferroptosis. However, to the best of our knowledge, the objective and overall reports on the publishing trend, powerful research, institutions and their collaborations, and the hotspots of “ferroptosis in stroke” are lacking.
In this work, we aim to systematically discuss the “ferroptosis in stroke” research from 2013 to 2021 by bibliometric analysis. The bibliometric analysis combines mathematical and statistical methods with data visualization (Chen G. et al., 2021), to present the annual publications, countries/regions, institutions, journals, authors, and co-citation; to evaluate global patterns of collaboration between authors, institutions, and countries; and to determine the study trend and hotspot in “ferroptosis in stroke”.
Data and Method
Data Collection
The keywords of ferroptosis and stroke were indexed in the Web of Science Core Collection (WOSCC). Articles from 2013 to 2021 (deadline October 30, 2021) were retrieved, and search themes were as follows: “TS=(ferroptosis) OR TS=(ferroptotic)”, AND “TS=(stroke) OR TS=(cerebrovascular accident) OR TS=(cerebrovascular apoplexy)”, AND Language: English, AND Reference Type: Article OR Review”. A total of 99 references were chosen and then used to perform a bibliometric analysis.
Data Analysis
Firstly, through the analysis and retrieval results in WOSCC, the general information of the literature is preliminarily analyzed, including the year of publication, country, organization, journal, and author. Then, the VOSviewer 1.6.15 software was used to conduct bibliometric and visual analysis, including main author, keyword, scientific research partnership, cited analysis, and co-cited analysis. The standard tournament ranking method was used for ranking order, and Linlog/modularization was applied in the VOSviewer software.
Results
Publication Outputs
There were 99 items on “ferroptosis in stroke” in the WOSCC from 2013 to 2021 (October 30, 2021), including 51 articles (51.52%) and 48 reviews (48.48%). The annual publication is exhibited in Figure 1. There was one publication in 2013, which subsequently increased year by year, and it was 28 in 2020 and 42 on October 30, 2021 (Figure 1). The number of publication was small, but it rose steadily rise. A total of 185 sources of funding supported the research on “ferroptosis in stroke”. The top three major sources of funding were the National Natural Science Foundation of China (frequency, 43), National Institutes Of Health (frequency, 22), and United States Department of Health Human Services (frequency, 22) Table 1.
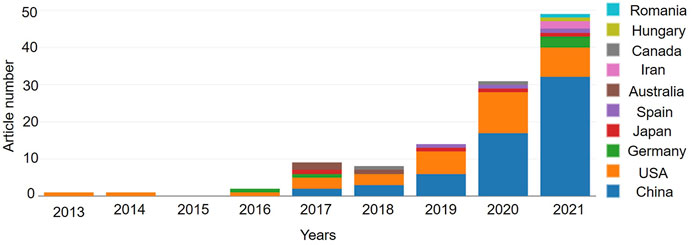
FIGURE 1. The annual publication indexed in the WOSCC from 2013 to 2021 by online bibliometric analysis. And the article number of the top 10 countries/regions with “ferroptosis in stroke” were presented.
Countries and Organization
There were 15 countries and 193 organizations in the 99 documents of “ferroptosis in stroke”, which had been published in the past 8 years. China (63), the United States (38), and Germany (5) were the top three countries/regions, and early research began in the United States and China, which have published documents mainly since 2017 (Figure 1). The United States had the most citation with 2,834, but the citation of publication in China was 2,262. Cooperation on this subject has been concentrated between China and the United States, with weak cooperation between other countries (Figure 2). As shown in Table2, the top 10 institutions in terms of publications were from China (50%) and the United States (50%). The top three institutions in terms of publications were Columbia University (United States, six documents), Weill Cornell Medicine (United States, six documents), and Zhejiang University (China, six documents), but the top three institutions ranked by citations were Columbia University (1,819), Yale University (1,731), and Memorial Sloan Kettering Cancer Center (1,596) (Table 2). Cooperation between institutions is presented in Figure 3. The top organizations showed extensive relationships with others (Figure 3), but some gray circles indicated that the institutions were isolated. The data suggest that the top institutions with “ferroptosis in stroke” research lack cooperation with each other; in particular, the Chinese institutions should improve cooperation with their counterparts.
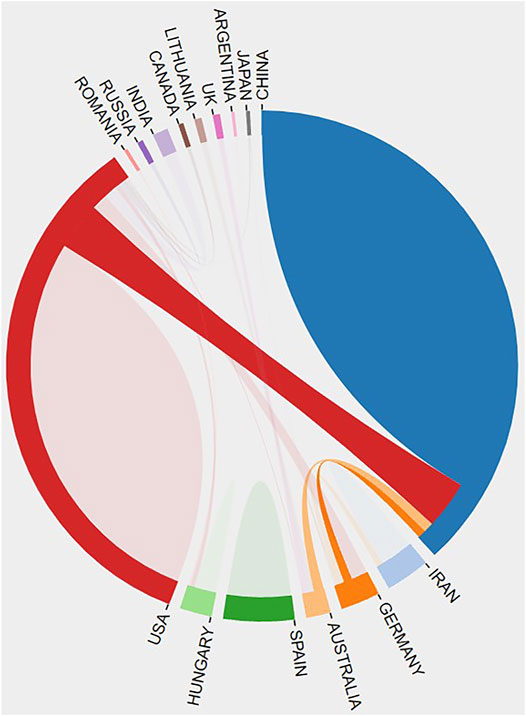
FIGURE 2. The cooperative relationship between countries was generated by the online bibliometric analysis.
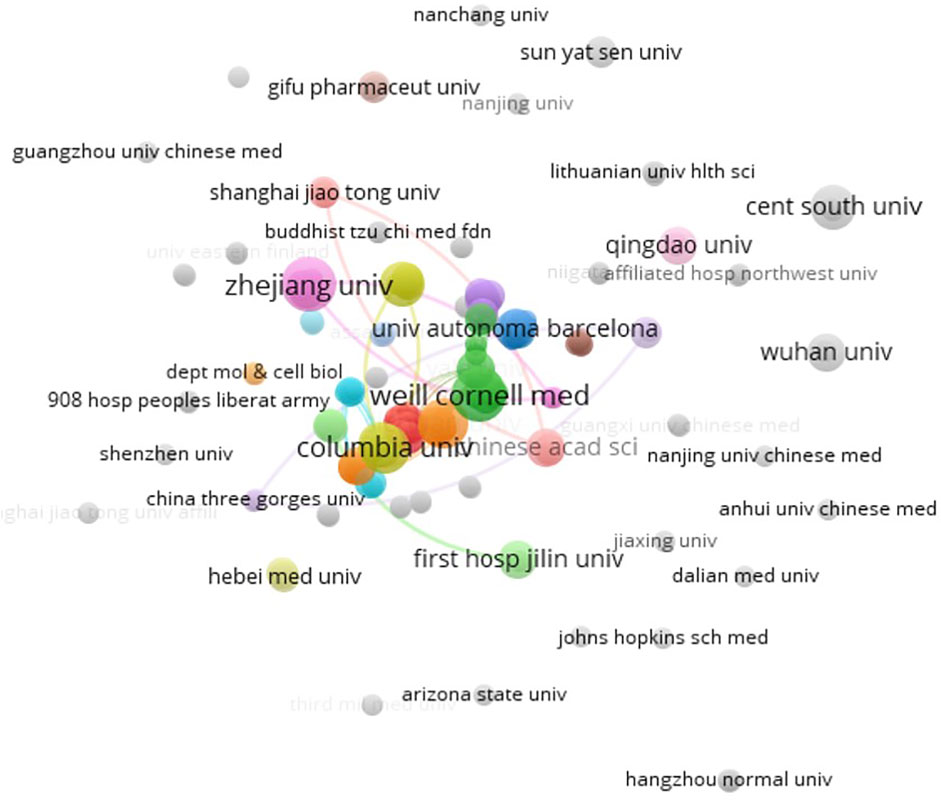
FIGURE 3. Co-author analysis of organizations with network visualization by the VOSviewer 1.6.14. Some of the 190 items in the network. The thickness of the lines indicates the strength of the relationship.
Journals
There were 72 journals with documents on “ferroptosis in stroke”. In Table 4, the top 10 journals were shown to have published about 34.34% of documents (34/99). Frontiers in Cellular Neuroscience was the most active journal, followed by Frontiers in Neuroscience, Frontiers in Cell and Developmental Biology, Frontiers in Pharmacology, Life Sciences, Frontiers in Neurology, Cell, Cellular and Molecular Life Sciences, Pharmacology and Pharmacy, and Cell Chemical Biology. All of the journals had a higher level of JCR partition (70% Q1 and 30% Q2), and Cell Chemical Biology had a minimum IF of 4.003 (Q2) (Table 3). Encouragingly, one article and one review were published in the Cell journal (Q1, 41.584). The publication of these articles in the corresponding high-level journals is enough to indicate that “ferroptosis in stroke” is exciting and interesting.
Authors
A total of 656 authors drafted the 99 documents in “ferroptosis in stroke”. Rajiv R. Ratan (Weill Cornell Medicine) contributed seven documents and ranked first (531 citations), followed by Saravanan S. Karuppagounder (Weill Cornell Medicine), Jian Wang (Johns Hopkins University), Brent R. Stockwell (Columbia University), and Zhenni Guo (Jilin University). Seventy percent of authors were from China (Table 4). In a co-authorship map, yellow indicated that many scholars have only recently begun to work on this topic (Figure 4). Some researchers were also scattered independently with other active scholars, and Rajiv R. Ratan, Jian Wang, and Brent R. Stockwell were the center, but they had not been able to reach all groups (Figure 4). The data suggest that the active authors on “ferroptosis in stroke” still lack collaboration with other scholars.
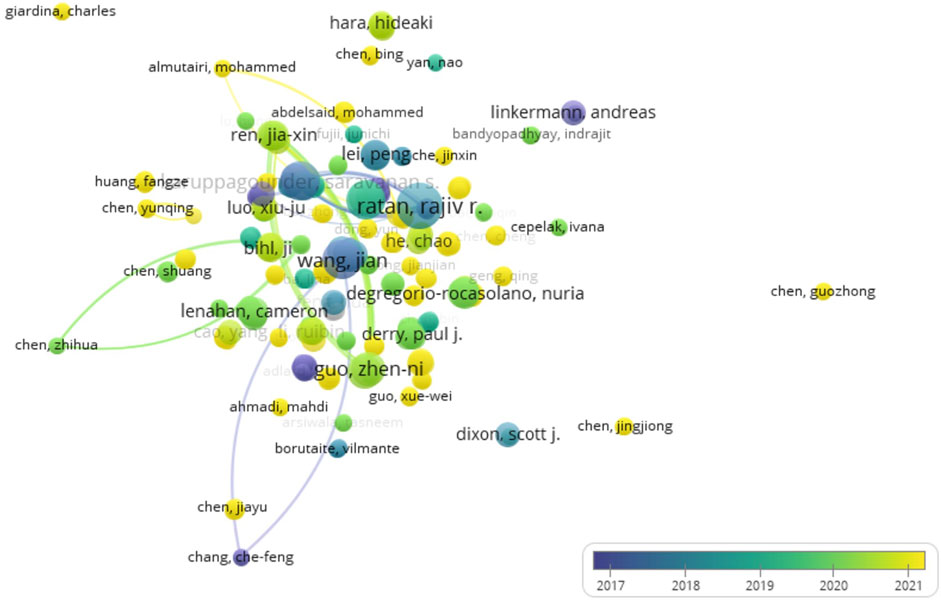
FIGURE 4. Co-occurrence analysis of authors. The author–date (656 items) was generated, and the color means the average published year.
Citations
The top 10 highly cited references and the citation analysis of documents on “ferroptosis in stroke” are shown in Tables 5, 6. “Ferroptosis: an iron-dependent form of nonapoptotic cell death” was the most cited reference in “ferroptosis in stroke” (Table 5), as Dixon et al. (2012) firstly came up with the concept of ferroptosis. Two reviews of Brent R. Stockwell were included in the highly cited references: “Ferroptosis: a regulated cell death nexus linking metabolism, redox biology, and disease” with 1,378 citations and “Emerging mechanisms and disease relevance of ferroptosis” with 84 citations (Table 6). Four of the documents appeared on both lists: “Ferroptosis: a regulated cell death nexus linking metabolism, redox biology, and disease” (review), “Tau-mediated iron export prevents ferroptotic damage after ischemic stroke” (article), “Selenium drives a transcriptional adaptive program to block ferroptosis and treat stroke” (article), and “Inhibition of neuronal ferroptosis protects hemorrhagic brain” (article), which were respectively completed by the team of Brent R. Stockwell, Qingzhang Tuo, Ishraq Alim, and Qian Li. The data suggest that Brent R. Stockwell is very interested in the research on “ferroptosis in stroke”, but the research of Qingzhang Tuo, Ishraq Alim, and Qian Li are more important drivers in the development of the field, and articles of “ferroptosis in stroke” with a high number of citations have been widely accepted and have inspired recent researches, and recent studies should improve scale and breakthrough.
Figure 5 showed the keyword subnetwork and its clustering graph, and the circle size represented the number of occurrences of keywords. The top six keywords in terms of occurrence were “ferroptosis”, “oxidative stress”, “iron”, “cell death”, “lipid peroxidation”, and “intracerebral hemorrhage” (Figure 5). In the overlay visualization (Figure 6), the keywords apoptosis, necroptosis, pyroptosis, autophagy, NLRP3 inflammasome activation, and Chinese herbal medicine were are also related to ferroptosis but had few research.
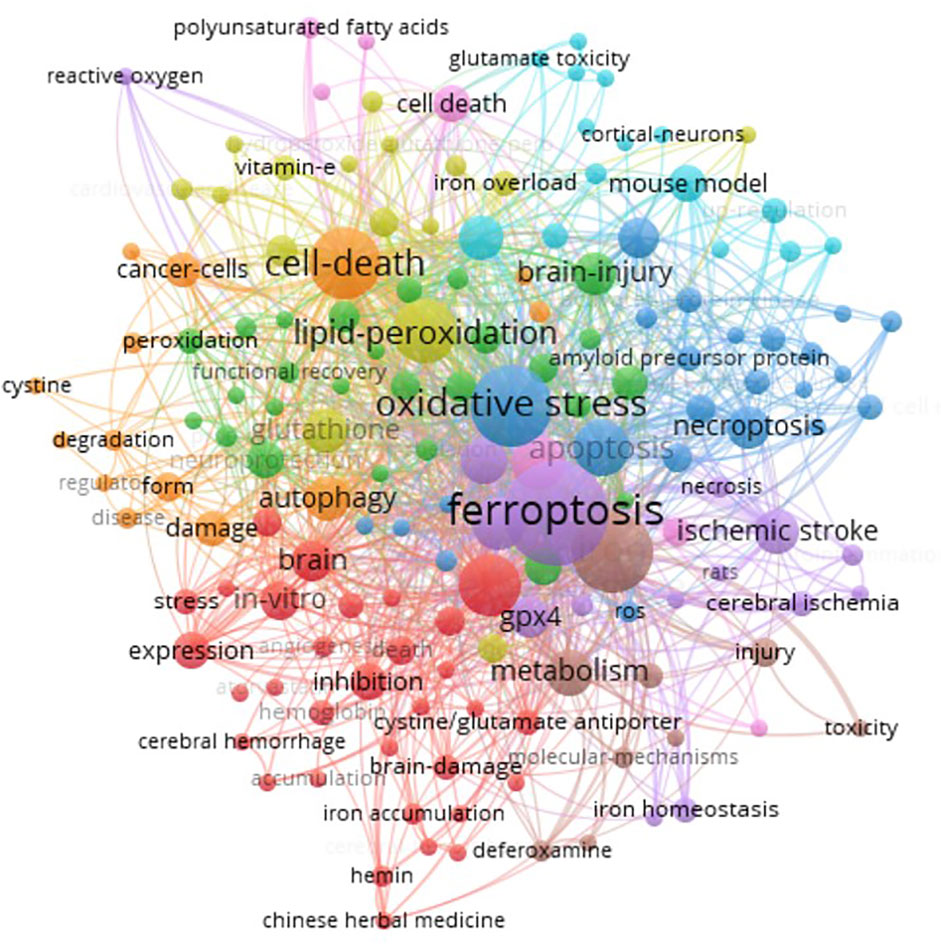
FIGURE 5. Co-occurrence analysis of author keywords. Two minimum number of occurrences of a keyword, and 158 meet the threshold of the 554 keywords. The network visualization of the keywords (158 items) was conducted.
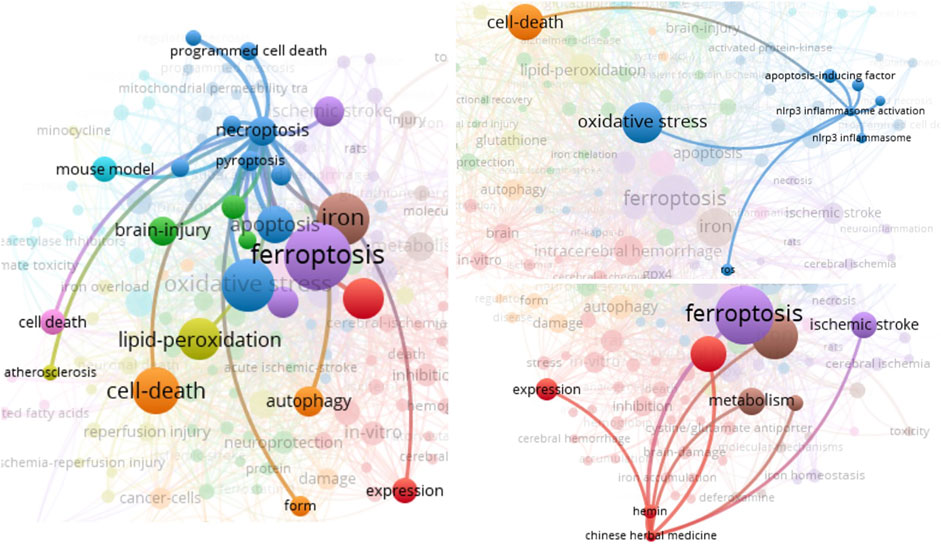
FIGURE 6. The crosstalk of author keywords necroptosis, NLRP3 inflammasome activation, and Chinese herbal medicine was presented.
Discussion
In the last few years, research on “ferroptosis in stroke” has been rapidly developing and remained the focus of scholars and organizations. Some countries, organizations, and scholars collaborated on research on “ferroptosis in stroke”, but the Chinese groups in this field still lacked collaboration with others. The documents and citation analysis suggested that Rajiv R. Ratan and Brent R. Stockwell were active researchers, and the research of Qingzhang Tuo, Ishraq Alim, and Qian Li are more important drivers in the development of the field. Keywords associated with lipid peroxidation, ferroptosis, iron, oxidative stress, and cell death were highly frequent, but apoptosis, necroptosis, pyroptosis, and autophagy were used in few research, so there may be more research ideas in the future by scholars. “Ferroptosis in stroke” is an emerging research topic that will continue to produce more exciting results and provide new insights into the bottleneck of stroke treatment.
***Ferroptosis, a newly defined class of PCD in 2012 (Dixon et al., 2012), quickly became the focus of attention and discussion because of its important function in the body, and publication on this topic has increased year by year. In 2013, Louandre et al. demonstrated that sorafenib induces ferroptosis in hepatocellular carcinoma (HCC) cells, and triggering ferroptosis may improve the antitumor effect of sorafenib in HCC (Louandre et al., 2013); Henke et al. (2013) found that dysregulated ORAI1-mediated Ca2+ influx contributes to ferroptosis in HT22 cells; based on their previous research, Speer et al. (2013) suggested that iron chelators inhibit ferroptosis in primary neurons by targeting the HIF prolyl hydroxylases. Since then, research on “ferroptosis” and “ferroptosis in stroke” has been gradually reported.
Studies have confirmed that ferroptosis involves the failure of GPX4 activity and the metabolic processes of iron, amino acids, and lipid peroxide, which results in the accumulation of intracellular reactive oxygen species (ROS) and ferroptotic death (Stockwell et al., 2017; Wan et al., 2019; Cheng et al., 2021). Ferroptosis is mediated by nuclear factor E2-related factor 2 (NRF2) and Hippo pathways by regulating GPX4 activity (Liu et al., 2021). There are still polymolecules involved in ferroptosis, such as acyl-CoA synthetase long-chain family member 4 deferoxamine (ACSL4), ferritin, divalent metal transporter 1 (DMT1), glutathione (GSH), imidazole ketone erastin, lysophosphatidylcholine acyltransferase 3 (LPCAT3), nicotinamide adenine dinucleotide phosphate (NADPH), oxidized glutathione (GSSG), solute carrier family three member 2 (SLC3A2), transferrin, and transferrin receptor 1 (TFR1) (Liu et al., 2021; Zhang et al., 2021). With the deepening of research, the regulatory network of ferroptosis in stroke is gradually revealed (Bai et al., 2020; DeGregorio-Rocasolano, et al., 2019; Zille et al., 2017). Ferritin reduces robust ROS production and GSH consumption; its decrease is necessary for cerebral ischemia-induced hippocampal neuronal ferroptosis through p53 and SLC7A11 in middle cerebral artery occlusion (MCAO) rats (Chen W. et al., 2021), and mice lacking mitochondrial ferritin show graver brain injury and neurological deficits, accompanied by typical ferroptotic event after cerebral ischemia/reperfusion (I/R) (Wang et al., 2021). ACSL4 enhances ischemic stroke by increasing ferroptosis-induced brain damage and neuroinflammation while inhibiting ACSL4, which promotes the recovery of neurological function following stroke (Li et al., 2019; Chen J. et al., 2021; Cui et al., 2021). Tuo et al. (2017) demonstrated that tau suppression reduces MCAO-induced ferroptosis and influences ischemic stroke outcome. Nuclear receptor coactivator 4 (NCOA4) facilitates ferritinophagy-mediated ferroptosis, and NCOA4 deletion protects neurons from ferritinophagy-mediated ferroptosis after ischemic stroke (Li C. et al., 2021). Guo et al. (2021) hypothesized that PIEZO1 involves cerebral I/R injury via ferroptosis regulation. In addition, noncoding RNAs are also involved in ferroptosis-mediated stroke. The lncRNA-MEG3/p53 signaling pathway mediates ferroptosis of rat brain microvascular endothelial cells via regulation of the GPX4 transcription and expression (Chen C. et al., 2021). The level of lncRNA-PVT1 is upregulated and the miR-214 level is downregulated in the plasma of acute ischemic stroke patients, and PVT1 involves ferroptosis via miR-214-mediated TFR1 and TP53 levels in brain I/R (Lu et al., 2020). By the bioinformatic analysis, three ferroptosis-related biomarkers are found as potential diagnostic biomarkers for ischemic stroke, namely, PTGS2, MAP1LC3B, and TLR4, which are upregulated in ischemic stroke and provide more evidence about the important role of ferroptosis (Chen G. et al., 2021). Therefore, extensive evidence suggests that ferroptosis is one of the key pathological mechanisms of nerve injury and neurological dysfunction after stroke, which is a potentially promising therapeutic target (Jin Y. et al., 2021; Li Y. et al., 2021).
Previous studies show that ferroptosis is reversed by iron chelators (deferoxamine and ceruloplasmin), lipophilic antioxidants (ferrostatin-1, trolox, liproxstatin-1, and N-acetylcysteine (NAC)), and selenoprotein activator (selenium) (Figure 7) (Liu et al., 2021; Zhang et al., 2021). Then, significant progress has been made in preclinical studies targeting ferroptosis in the treatment of stroke. Intracerebral hemorrhage-induced neurological defects, dysmnesia, and brain atrophy were decreased by the ferroptosis inhibitor ferrostatin-1 (Chen et al., 2019). NAC, a clinically approved redox regulatory compound containing mercaptan, prevents hemin-induced ferroptosis by inhibiting toxic lipid generated by arachidonic acid-dependent ALOX5 activity (Karuppagounder et al., 2018). Deferoxamine treatment prevents post-stroke cognitive impairment in diabetes while increasing AQP4 polarity and blood–brain barrier permeability (Abdul et al., 2021). Pyridoxal isonicotinoyl hydrazine protects mice against hemorrhage stroke, by reducing ferroptosis, including ROS production, iron accumulation, and lipid peroxidation in perihematoma (Zhang et al., 2021). Pharmacological selenium inhibits GPX4-dependent ferroptotic death, and selenome protects neurons and improves behavior following hemorrhagic stroke through regulating TFAP2c and Sp1 (Alim et al., 2019). And pretreatment with selenium compounds, such as methylselenocysteine or selenocystamine, also protects I/R neuronal ferroptosis in vivo (Tuo et al., 2021). Some natural products and traditional Chinese medicine-related content have also been confirmed to improve the prognosis of stroke by regulating ferroptosis. The baicalin, carthamin yellow, dauricine, (−)-epicatechin, kaempferol, and paeonol ameliorate neuronal ferroptosis in vitro or in vivo stroke models via regulating ACSL4, GPX4, TFR1, Fe2+, and NRF2 pathways (Chang et al., 2014; Duan et al., 2021; Guo et al., 2021; Jin Z. L. et al., 2021; Peng et al., 2021; Yuan et al., 2021), which show great potential in the treatment of stroke. Treating MCAO rats with Naotaifang (a traditional Chinese herbal medicine compound) extract involves inhibition of acute cerebral ischemia-induced neuronal ferroptosis and neurobehavioral disorder through TFR1/DMT1 and SCL7A11/GPX4 pathways (Lan et al., 2020). Electroacupuncture inhibits ferroptosis to protect against MCAO via regulating iron and oxidative stress-related protein (Li G. et al., 2021). Furthermore, the new inhibitors of ferroptosis are also being explored. Yang et al. conducted promethazine derivatives and screened a promising lead compound, 2-(1-(4-(4-methylpiperazin-1-yl)phenyl)ethyl)-10H-phenothiazine, as a new type of ferroptosis inhibitor, which displays favorable pharmacokinetic properties, good ability to permeate the blood–brain barrier, and excellent therapeutic effect in the ischemic stroke model (Yang et al., 2021). Keuters et al. (2021) demonstrated that the benzo[b]thiazine derivative efficiently suppresses GSH or GPX4 inhibition-induced ferroptosis in neuronal cell lines and decreases infarction volume, edema, and pro-inflammatory levels after stroke. Thus, ferroptosis inhibitors and targeting them are potential treatment options for stroke, but more direct clinical evidence remains to be explored.
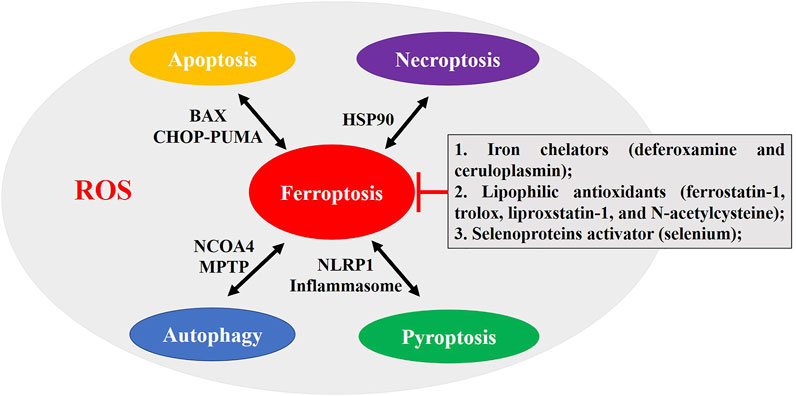
FIGURE 7. The crosstalk between ferroptosis and autophagy, apoptosis, necroptosis, and pyroptosis. The iron chelators (deferoxamine and ceruloplasmin), lipophilic antioxidants (ferrostatin-1, trolox, liproxstatin-1, and N-acetylcysteine), and selenoprotein activator (selenium) can reverse ferroptosis. Mitochondrial function and ROS production may be key to the crosstalk. Some molecules participate in the crosstalk between them, including ROS; BAX; HSP90; mitochondrial permeability transition pore (MPTP); NCOA4; NACHT, LRR, and PYD domains-containing protein 1 (NLRP1) inflammasome; C/EBP-homologous protein (CHOP); and p53 upregulated modulator of apoptosis (PUMA).
The keyword analysis revealed that multiple PCDs were involved in the progression of stroke pathology, and apoptosis, necroptosis, pyroptosis, and autophagy were related to ferroptosis. Some reports show that apoptosis, autophagy, pyroptosis, and ferroptosis are present together in stroke-induced neuronal damage (Guo et al., 2021; Li et al., 2018). The crosstalk between ferroptosis and other PCDs is fascinating (Figure 7). Lee et al. (2018, 2020) showed ferroptotic and apoptotic agent interactions via the B-cell lymphoma-2 associated X (BAX)-dependent mitochondrial pathway and ER stress-mediated PERK-eIF2α-ATF4-CHOP-PUMA pathway. Studies have found that ferritinophagy-mediated ROS production contributes to ferroptosis and apoptosis (Li L. et al., 2021). Ferroptosis, an autophagic cell death process, leads to autophagy activation and consequent ferritin and NCOA4 degradation (Gao et al., 2016), and NCOA4-regulated ferritinophagy maintains ferroptosis via mediating cellular iron homeostasis, which is also present in stroke (Li C. et al., 2021). Mitochondrial permeability transition pore opening is a common event of ferroptosis and necroptosis, which stimulates formation of autophagosome, mitophagy, and ROS production, causing necroptosis/ferroptosis (Basit et al., 2017). Heat shock protein 90 (HSP90) is defined as a common regulatory nodal between ferroptosis and necroptosis (Wu et al., 2019). Friedmann Angeli et al. demonstrated that necrostatin-1 (RIP1 inhibitor) inhibits ferroptosis through a necroptosis/RIP1-independent manner, but ferroptosis inhibitors cannot inhibit necroptosis (Friedmann Angeli et al., 2014) and the specific link between ferroptosis and necroptosis remains to be clarified after stroke (Zille et al., 2017; Zhou et al., 2021). Carthamin yellow attenuates cerebral I/R injury via suppressing NF-κB/NLRP3-mediated pyroptosis and ACSL4-mediated ferroptosis in rats (Guo et al., 2021b). The regulatory relationship between ferroptosis and pyroptosis has been proved. Meihe et al. found that silenced or upregulated NLRP1 inflammasome positively affects ferroptosis in the oxidative stress model, and NLRP1, NLRP3, IL-1β, and caspase-1 levels are positively correlated with ferroptosis following ferroptosis inhibition or ferroptosis activation (Meihe et al., 2021). However, whether ferroptosis facilitates apoptosis, autophagy, necroptosis, pyroptosis, or other PCDs or their mutual regulation following stroke requires the further studies.
Limitations
This is the first bibliometric analysis of “ferroptosis in stroke”, but some limitations are presented. Firstly, the retrieval time is October 30, 2021, but the database continues to be updated. Secondly, the search terms “TS=(Ferroptosis) OR TS=(Ferroptotic)”, AND “TS=(stroke) OR TS=(cerebrovascular accident) OR TS=(cerebrovascular apoplexy)”, AND Language: English, AND Reference Type: Article OR Review” are used to define the topic of this studies in the WOSCC database; some productions may not be contained. Thirdly, there are still some articles that are not included in the WOSCC database, so they are left out. However, WOSCC is the dominant database for bibliometric analysis, and we believe this work could represent the overall situation and general trend for “ferroptosis in stroke”.
Conclusion
Our study discussed the research status of “ferroptosis in stroke” by bibliometric analysis, which is an increasingly hot research topic, with more and more scholars, institutions, and countries pouring in and publishing a lot of high-quality productions. Most of them are Chinese scholars, but most of them are isolated and lack communication and cooperation. Researchers need to strengthen the sharing of results on this topic and promote the exploration of relevant hotspots. The mechanisms of apoptosis, necroptosis, pyroptosis, and autophagy engage crosstalk with ferroptosis in the pathological processes of stroke, and the different types of PCD is as a single, but in which the individual pathway is highly interconnected and concertedly compensated for others (Bedoui et al., 2020). The ferroptosis inhibitors and targeting them are potential treatment options for stroke, but more direct clinical evidence remains to be explored. Further exploring the mechanisms of crosstalk between ferroptosis and other PCDs improves clinical applications and therapeutic effects against stroke, and a combination of ferroptosis with other harmful pathway suppression may provide valid therapies for stroke and brain disorder. The scholars will also continue to pay attention to and be interested in the hot topic of “ferroptosis in stroke”.
Author Contributions
Conceptualization: YC, TL, and CZ. Data collection and analysis: QX and TL. Writing—original draft: YC. Writing—review and editing: CZ.
Funding
This study was supported by the National Natural Science Foundation of China (82072229 and 81901270), Guizhou Traditional Chinese Medicine Administration (201815797), and Guizhou Provincial Health and Family Planning Commission (2019XMSB00022878).
Conflict of Interest
The authors declare that the research was conducted in the absence of any commercial or financial relationships that could be construed as a potential conflict of interest.
Publisher’s Note
All claims expressed in this article are solely those of the authors and do not necessarily represent those of their affiliated organizations, or those of the publisher, the editors and the reviewers. Any product that may be evaluated in this article, or claim that may be made by its manufacturer, is not guaranteed or endorsed by the publisher.
References
Abdul, Y., Li, W., Ward, R., Abdelsaid, M., Hafez, S., Dong, G., et al. (2021). Deferoxamine Treatment Prevents Post-Stroke Vasoregression and Neurovascular Unit Remodeling Leading to Improved Functional Outcomes in Type 2 Male Diabetic Rats: Role of Endothelial Ferroptosis. Transl Stroke Res. 12 (4), 615–630. doi:10.1007/s12975-020-00844-7
Alim, I., Caulfield, J. T., Chen, Y., Swarup, V., Geschwind, D. H., Ivanova, E., et al. (2019). Selenium Drives a Transcriptional Adaptive Program to Block Ferroptosis and Treat Stroke. Cell 177 (5), 1262–e25. doi:10.1016/j.cell.2019.03.032
Bai, Q., Liu, J., and Wang, G. (2020). Ferroptosis, a Regulated Neuronal Cell Death Type after Intracerebral Hemorrhage. Front Cel Neurosci 14, 591874. doi:10.3389/fncel.2020.591874
Barthels, D., and Das, H. (2020). Current Advances in Ischemic Stroke Research and Therapies. Biochim. Biophys. Acta Mol. Basis Dis. 1866 (4), 165260. doi:10.1016/j.bbadis.2018.09.012
Basit, F., van Oppen, L. M., Schöckel, L., Bossenbroek, H. M., van Emst-de Vries, S. E., Hermeling, J. C., et al. (2017). Mitochondrial Complex I Inhibition Triggers a Mitophagy-dependent ROS Increase Leading to Necroptosis and Ferroptosis in Melanoma Cells. Cell Death Dis 8 (3), e2716. doi:10.1038/cddis.2017.133
Bedoui, S., Herold, M. J., and Strasser, A. (2020). Emerging Connectivity of Programmed Cell Death Pathways and its Physiological Implications. Nat. Rev. Mol. Cel Biol 21 (11), 678–695. doi:10.1038/s41580-020-0270-8
Campbell, B. C. V., De Silva, D. A., Macleod, M. R., Coutts, S. B., Schwamm, L. H., Davis, S. M., et al. (2019). Ischaemic Stroke. Nat. Rev. Dis. Primers 5 (1), 70. doi:10.1038/s41572-019-0118-8
Cao, H., Zuo, C., Huang, Y., Zhu, L., Zhao, J., Yang, Y., et al. (2021). Hippocampal Proteomic Analysis Reveals Activation of Necroptosis and Ferroptosis in a Mouse Model of Chronic Unpredictable Mild Stress-Induced Depression. Behav. Brain Res. 407, 113261. doi:10.1016/j.bbr.2021.113261
Chang, C. F., Cho, S., and Wang, J. (2014). (-)-Epicatechin Protects Hemorrhagic Brain via Synergistic Nrf2 Pathways. Ann. Clin. Transl Neurol. 1 (4), 258–271. doi:10.1002/acn3.54
Chen, B., Chen, Z., Liu, M., Gao, X., Cheng, Y., Wei, Y., et al. (2019). Inhibition of Neuronal Ferroptosis in the Acute Phase of Intracerebral Hemorrhage Shows Long-Term Cerebroprotective Effects. Brain Res. Bull. 153, 122–132. doi:10.1016/j.brainresbull.2019.08.013
Chen, C., Huang, Y., Xia, P., Zhang, F., Li, L., Wang, E., et al. (2021). Long Noncoding RNA Meg3 Mediates Ferroptosis Induced by Oxygen and Glucose Deprivation Combined with Hyperglycemia in Rat Brain Microvascular Endothelial Cells, through Modulating the p53/GPX4 axis. Eur. J. Histochem. 65 (3), 3224. doi:10.4081/ejh.2021.3224
Chen, G., Li, L., and Tao, H. (2021). Bioinformatics Identification of Ferroptosis-Related Biomarkers and Therapeutic Compounds in Ischemic Stroke. Front. Neurol. 12, 745240. doi:10.3389/fneur.2021.745240
Chen, J., Yang, L., Geng, L., He, J., Chen, L., Sun, Q., et al. (2021). Inhibition of Acyl-CoA Synthetase Long-Chain Family Member 4 Facilitates Neurological Recovery after Stroke by Regulation Ferroptosis. Front. Cel Neurosci 15, 632354. doi:10.3389/fncel.2021.632354
Chen, W., Jiang, L., Hu, Y., Tang, N., Liang, N., Li, X. F., et al. (2021). Ferritin Reduction Is Essential for Cerebral Ischemia-Induced Hippocampal Neuronal Death through p53/SLC7A11-Mediated Ferroptosis. Brain Res. 1752, 147216. doi:10.1016/j.brainres.2020.147216
Cheng, Y., Song, Y., Chen, H., Li, Q., Gao, Y., Lu, G., et al. (2021). Ferroptosis Mediated by Lipid Reactive Oxygen Species: A Possible Causal Link of Neuroinflammation to Neurological Disorders. Oxid Med. Cel Longev 2021, 20215005136. doi:10.1155/2021/5005136
Cui, Y., Zhang, Y., Zhao, X., Shao, L., Liu, G., Sun, C., et al. (2021). ACSL4 Exacerbates Ischemic Stroke by Promoting Ferroptosis-Induced Brain Injury and Neuroinflammation. Brain Behav. Immun. 93, 312–321. doi:10.1016/j.bbi.2021.01.003
David, S., Jhelum, P., Ryan, F., Jeong, S. Y., and Kroner, A. (2021). Dysregulation of Iron Homeostasis in the CNS and the Role of Ferroptosis in Neurodegenerative Disorders. Antioxid. Redox Signaling. doi:10.1089/ars.2021.0218
DeGregorio-Rocasolano, N., Martí-Sistac, O., and Gasull, T. (2019). Deciphering the Iron Side of Stroke: Neurodegeneration at the Crossroads between Iron Dyshomeostasis, Excitotoxicity, and Ferroptosis. Front. Neurosci. 13, 85. doi:10.3389/fnins.2019.00085
Dixon, S. J., Lemberg, K. M., Lamprecht, M. R., Skouta, R., Zaitsev, E. M., Gleason, C. E., et al. (2012). Ferroptosis: an Iron-dependent Form of Nonapoptotic Cell Death. Cell 149 (5), 1060–1072. doi:10.1016/j.cell.2012.03.042
Duan, L., Zhang, Y., Yang, Y., Su, S., Zhou, L., Lo, P. C., et al. (2021). Baicalin Inhibits Ferroptosis in Intracerebral Hemorrhage. Front. Pharmacol. 12, 62937. doi:10.3389/fphar.2021.629379
Friedmann Angeli, J. P., Schneider, M., Proneth, B., Tyurina, Y. Y., Tyurin, V. A., Hammond, V. J., et al. (2014). Inactivation of the Ferroptosis Regulator Gpx4 Triggers Acute Renal Failure in Mice. Nat. Cel Biol 16 (12), 1180–1191. doi:10.1038/ncb3064
Galluzzi, L., Vitale, I., Aaronson, S. A., Abrams, J. M., Adam, D., Agostinis, P., et al. (2018). Molecular Mechanisms of Cell Death: Recommendations of the Nomenclature Committee on Cell Death 2018. Cell Death Differ 25 (3), 486–541. doi:10.1038/s41418-017-0012-4
Gao, M., Monian, P., Pan, Q., Zhang, W., Xiang, J., and Jiang, X. (2016). Ferroptosis Is an Autophagic Cell Death Process. Cell Res 26 (9), 1021–1032. doi:10.1038/cr.2016.95
Ge, H., Xue, X., Xian, J., Yuan, L., Wang, L., Zou, Y., et al. (2021). Ferrostatin-1 Alleviates White Matter Injury via Decreasing Ferroptosis Following Spinal Cord Injury. Mol. Neurobiol. doi:10.1007/s12035-021-02571-y
Gibellini, L., and Moro, L. (2021). Programmed Cell Death in Health and Disease. Cells 10 (7), 1765. doi:10.3390/cells10071765
Guo, H., Zhu, L., Tang, P., Chen, D., Li, Y., Li, J., et al. (2021b). Carthamin Yellow Improves Cerebral Ischemia-reperfusion I-njury by A-ttenuating I-nflammation and F-erroptosis in R-ats. Int. J. Mol. Med. 47 (4), 52. doi:10.3892/ijmm.2021.4885
Guo, X. W., Lu, Y., Zhang, H., Huang, J. Q., and Li, Y. W. (2021). PIEZO1 Might Be Involved in Cerebral Ischemia-Reperfusion Injury through Ferroptosis Regulation: a Hypothesis. Med. Hypotheses 146, 110327. doi:10.1016/j.mehy.2020.110327
Hassannia, B., Van Coillie, S., and Vanden Berghe, T. (2021). Ferroptosis: Biological Rust of Lipid Membranes. Antioxid. Redox Signal. 35 (6), 487–509. doi:10.1089/ars.2020.8175
Henke, N., Albrecht, P., Bouchachia, I., Ryazantseva, M., Knoll, K., Lewerenz, J., et al. (2013). The Plasma Membrane Channel ORAI1 Mediates Detrimental Calcium Influx Caused by Endogenous Oxidative Stress. Cel Death Dis 4 (1), e470. doi:10.1038/cddis.2012.216
Hughes, R. E., Tadi, P., and Bollu, P. C. (2021). “TPA Therapy,” in StatPearls. Treasure Island, Florida: StatPearls Publishing.
Jin, Y., Zhuang, Y., Liu, M., Che, J., and Dong, X. (2021). Inhibiting Ferroptosis: A Novel Approach for Stroke Therapeutics. Drug Discov. Today 26 (4), 916–930. doi:10.1016/j.drudis.2020.12.020
Jin, Z. L., Gao, W. Y., Liao, S. J., Yu, T., Shi, Q., Yu, S. Z., et al. (2021). Paeonol Inhibits the Progression of Intracerebral Haemorrhage by Mediating the HOTAIR/UPF1/ACSL4 axis. ASN Neuro 13, 17590914211010647. doi:10.1177/17590914211010647
Karuppagounder, S. S., Alin, L., Chen, Y., Brand, D., Bourassa, M. W., Dietrich, K., et al. (2018). N-acetylcysteine Targets 5 Lipoxygenase-Derived, Toxic Lipids and Can Synergize with Prostaglandin E2 to Inhibit Ferroptosis and Improve Outcomes Following Hemorrhagic Stroke in Mice. Ann. Neurol. 84 (6), 854–872. doi:10.1002/ana.25356
Keuters, M. H., Keksa-Goldsteine, V., Dhungana, H., Huuskonen, M. T., Pomeshchik, Y., Savchenko, E., et al. (2021). An Arylthiazyne Derivative Is a Potent Inhibitor of Lipid Peroxidation and Ferroptosis Providing Neuroprotection In Vitro and In Vivo. Sci. Rep. 11 (1), 3518. doi:10.1038/s41598-021-81741-3
Kist, M., and Vucic, D. (2021). Cell Death Pathways: Intricate Connections and Disease Implications. EMBO J. 40 (5), e106700. doi:10.15252/embj.2020106700
Kuo, P. C., Weng, W. T., Scofield, B. A., Furnas, D., Paraiso, H. C., Intriago, A. J., et al. (2020). Interferon-β Alleviates Delayed tPA-Induced Adverse Effects via Modulation of MMP3/9 Production in Ischemic Stroke. Blood Adv. 4 (18), 4366–4381. doi:10.1182/bloodadvances.2020001443
Lan, B., Ge, J. W., Cheng, S. W., Zheng, X. L., Liao, J., He, C., et al. (2020). Extract of Naotaifang, a Compound Chinese Herbal Medicine, Protects Neuron Ferroptosis Induced by Acute Cerebral Ischemia in Rats. J. Integr. Med. 18 (4), 344–350. doi:10.1016/j.joim.2020.01.008
Lee, Y. S., Kalimuthu, K., Park, Y. S., Luo, X., Choudry, M. H. A., Bartlett, D. L., et al. (2020). BAX-dependent Mitochondrial Pathway Mediates the Crosstalk between Ferroptosis and Apoptosis. Apoptosis 25 (9-10), 625–631. doi:10.1007/s10495-020-01627-z
Lee, Y. S., Lee, D. H., Choudry, H. A., Bartlett, D. L., and Lee, Y. J. (2018). Ferroptosis-Induced Endoplasmic Reticulum Stress: Cross-Talk between Ferroptosis and Apoptosis. Mol. Cancer Res. 16 (7), 1073–1076. doi:10.1158/1541-7786.MCR-18-0055
Li, C., Sun, G., Chen, B., Xu, L., Ye, Y., He, J., et al. (2021). Nuclear Receptor Coactivator 4-mediated Ferritinophagy Contributes to Cerebral Ischemia-Induced Ferroptosis in Ischemic Stroke. Pharmacol. Res. 174, 105933. doi:10.1016/j.phrs.2021.105933
Li, G., Li, X., Dong, J., and Han, Y. (2021). Electroacupuncture Ameliorates Cerebral Ischemic Injury by Inhibiting Ferroptosis. Front. Neurol. 12, 619043. doi:10.3389/fneur.2021.619043
Li, L., Li, H., Li, Y., Feng, J., Guan, D., Zhang, Y., et al. (2021). Ferritinophagy-Mediated ROS Production Contributed to Proliferation Inhibition, Apoptosis, and Ferroptosis Induction in Action of Mechanism of 2-Pyridylhydrazone Dithiocarbamate Acetate. Oxid Med. Cel Longev 2021, 5594059. doi:10.1155/2021/5594059
Li, Q., Weiland, A., Chen, X., Lan, X., Han, X., Durham, F., et al. (2018). Ultrastructural Characteristics of Neuronal Death and White Matter Injury in Mouse Brain Tissues after Intracerebral Hemorrhage: Coexistence of Ferroptosis, Autophagy, and Necrosis. Front. Neurol. 9, 581. doi:10.3389/fneur.2018.00581
Li, Y., Feng, D., Wang, Z., Zhao, Y., Sun, R., Tian, D., et al. (2019). Ischemia-induced ACSL4 Activation Contributes to Ferroptosis-Mediated Tissue Injury in Intestinal Ischemia/reperfusion. Cel Death Differ 26 (11), 2284–2299. doi:10.1038/s41418-019-0299-4
Li, Y., Liu, Y., Wu, P., Tian, Y., Liu, B., Wang, J., et al. (2021). Inhibition of Ferroptosis Alleviates Early Brain Injury after Subarachnoid Hemorrhage In Vitro and In Vivo via Reduction of Lipid Peroxidation. Cell Mol Neurobiol 41 (2), 263–278. doi:10.1007/s10571-020-00850-1
Liu, Y., Fang, Y., Zhang, Z., Luo, Y., Zhang, A., Lenahan, C., et al. (2021). Ferroptosis: An Emerging Therapeutic Target in Stroke. J. Neurochem. 160, 64–73. doi:10.1111/jnc.15351
Louandre, C., Ezzoukhry, Z., Godin, C., Barbare, J. C., Mazière, J. C., Chauffert, B., et al. (2013). Iron-dependent Cell Death of Hepatocellular Carcinoma Cells Exposed to Sorafenib. Int. J. Cancer 133 (7), 1732–1742. doi:10.1002/ijc.28159
Lu, J., Xu, F., and Lu, H. (2020). LncRNA PVT1 Regulates Ferroptosis through miR-214-Mediated TFR1 and P53. Life Sci. 260, 118305. doi:10.1016/j.lfs.2020.118305
Mahoney-Sánchez, L., Bouchaoui, H., Ayton, S., Devos, D., Duce, J. A., and Devedjian, J. C. (2021). Ferroptosis and its Potential Role in the Physiopathology of Parkinson's Disease. Prog. Neurobiol. 196, 101890. doi:10.1016/j.pneurobio.2020.101890
Meihe, L., Shan, G., Minchao, K., Xiaoling, W., Peng, A., Xili, W., et al. (2021). The Ferroptosis-NLRP1 Inflammasome: The Vicious Cycle of an Adverse Pregnancy. Front Cel Dev Biol 9, 707959. doi:10.3389/fcell.2021.707959
Moujalled, D., Strasser, A., and Liddell, J. R. (2021). Molecular Mechanisms of Cell Death in Neurological Diseases. Cel Death Differ 28 (7), 2029–2044. doi:10.1038/s41418-021-00814-y
Peng, C., Fu, X., Wang, K., Chen, L., Luo, B., Huang, N., et al. (2022). Dauricine Alleviated Secondary Brain Injury after Intracerebral Hemorrhage by Upregulating GPX4 Expression and Inhibiting Ferroptosis of Nerve Cells. Eur. J. Pharmacol. 914, 174461. doi:10.1016/j.ejphar.2021.174461
Plascencia-Villa, G., and Perry, G. (2021). Preventive and Therapeutic Strategies in Alzheimer's Disease: Focus on Oxidative Stress, Redox Metals, and Ferroptosis. Antioxid. Redox Signal. 34 (8), 591–610. doi:10.1089/ars.2020.8134
Rui, T., Li, Q., Song, S., Gao, Y., and Luo, C. (2020). Ferroptosis-relevant Mechanisms and Biomarkers for Therapeutic Interventions in Traumatic Brain Injury. Histol. Histopathol 35 (10), 1105–1113. doi:10.14670/HH-18-229
Speer, R. E., Karuppagounder, S. S., Basso, M., Sleiman, S. F., Kumar, A., Brand, D., et al. (2013). Hypoxia-inducible Factor Prolyl Hydroxylases as Targets for Neuroprotection by "antioxidant" Metal Chelators: From Ferroptosis to Stroke. Free Radic. Biol. Med. 62, 26–36. doi:10.1016/j.freeradbiomed.2013.01.026
Stockwell, B. R., Friedmann Angeli, J. P., Bayir, H., Bush, A. I., Conrad, M., Dixon, S. J., et al. (2017). Ferroptosis: A Regulated Cell Death Nexus Linking Metabolism, Redox Biology, and Disease. Cell 171 (2), 273–285. doi:10.1016/j.cell.2017.09.021
Stockwell, B. R., Jiang, X., and Gu, W. (2020). Emerging Mechanisms and Disease Relevance of Ferroptosis. Trends Cel Biol 30 (6), 478–490. doi:10.1016/j.tcb.2020.02.009
Tang, D., and Kroemer, G. (2020). Ferroptosis. Curr. Biol. 30 (21), R1292–R1297. doi:10.1016/j.cub.2020.09.068
Tuo, Q.-Z., Masaldan, S., Southon, A., Mawal, C., Ayton, S., Bush, A. I., et al. (2021). Characterization of Selenium Compounds for Anti-ferroptotic Activity in Neuronal Cells and after Cerebral Ischemia-Reperfusion Injury. Neurotherapeutics. doi:10.1007/s13311-021-01111-9
Tuo, Q. Z., Lei, P., Jackman, K. A., Li, X. L., Xiong, H., Li, X. L., et al. (2017). Tau-mediated Iron export Prevents Ferroptotic Damage after Ischemic Stroke. Mol. Psychiatry 22 (11), 1520–1530. doi:10.1038/mp.2017.171
Wan, J., Ren, H., and Wang, J. (2019). Iron Toxicity, Lipid Peroxidation and Ferroptosis after Intracerebral Haemorrhage. Stroke Vasc. Neurol. 4 (2), 93–95. doi:10.1136/svn-2018-000205
Wang, P., Cui, Y., Ren, Q., Yan, B., Zhao, Y., Yu, P., et al. (2021). Mitochondrial Ferritin Attenuates Cerebral Ischaemia/reperfusion Injury by Inhibiting Ferroptosis. Cel Death Dis 12 (5), 447. doi:10.1038/s41419-021-03725-5
Wu, H., Wang, Y., Tong, L., Yan, H., and Sun, Z. (2021). Global Research Trends of Ferroptosis: A Rapidly Evolving Field with Enormous Potential. Front. Cel Dev Biol 9, 646311. doi:10.3389/fcell.2021.646311
Wu, S., Wu, B., Liu, M., Chen, Z., Wang, W., Anderson, C. S., et al. (2019). Stroke in China: Advances and Challenges in Epidemiology, Prevention, and Management. Lancet Neurol. 18 (4), 394–405. doi:10.1016/S1474-4422(18)30500-3
Wu, Z., Geng, Y., Lu, X., Shi, Y., Wu, G., Zhang, M., et al. (2019). Chaperone-mediated Autophagy Is Involved in the Execution of Ferroptosis. Proc. Natl. Acad. Sci. U S A. 116 (8), 2996–3005. doi:10.1073/pnas.1819728116
Yang, W., Liu, X., Song, C., Ji, S., Yang, J., Liu, Y., et al. (2021). Structure-activity Relationship Studies of Phenothiazine Derivatives as a New Class of Ferroptosis Inhibitors Together with the Therapeutic Effect in an Ischemic Stroke Model. Eur. J. Med. Chem. 209, 112842. doi:10.1016/j.ejmech.2020.112842
Yin, J., Wan, J., Zhu, J., Zhou, G., Pan, Y., and Zhou, H. (2021). Global Trends and Prospects about Inflammasomes in Stroke: a Bibliometric Analysis. Chin. Med. 16 (1), 53. doi:10.1186/s13020-021-00464-9
Yu, Y., Yan, Y., Niu, F., Wang, Y., Chen, X., Su, G., et al. (2021). Ferroptosis: a Cell Death Connecting Oxidative Stress, Inflammation and Cardiovascular Diseases. Cell Death Discov 7 (1), 193. doi:10.1038/s41420-021-00579-w
Yuan, Y., Zhai, Y., Chen, J., Xu, X., and Wang, H. (2021). Kaempferol Ameliorates Oxygen-Glucose Deprivation/Reoxygenation-Induced Neuronal Ferroptosis by Activating Nrf2/SLC7A11/GPX4 Axis. Biomolecules 11 (7), 923. doi:10.3390/biom11070923
Zhang, H., Wen, M., Chen, J., Yao, C., Lin, X., Lin, Z., et al. (2021). Pyridoxal Isonicotinoyl Hydrazone Improves Neurological Recovery by Attenuating Ferroptosis and Inflammation in Cerebral Hemorrhagic Mice. Biomed. Res. Int., 20219916328. doi:10.1155/2021/9916328
Zhang, T., Zhao, J., Li, X., Bai, Y., Wang, B., Qu, Y., et al. (2020). Chinese Stroke Association Guidelines for Clinical Management of Cerebrovascular Disorders: Executive Summary and 2019 Update of Clinical Management of Stroke Rehabilitation. Stroke Vasc. Neurol. 5 (3), 250–259. doi:10.1136/svn-2019-000321
Zhang, Y., Lu, X., Tai, B., Li, W., and Li, T. (2021). Ferroptosis and its Multifaceted Roles in Cerebral Stroke. Front. Cel Neurosci 15, 615372. doi:10.3389/fncel.2021.615372
Zhou, S. Y., Cui, G. Z., Yan, X. L., Wang, X., Qu, Y., Guo, Z. N., et al. (2020). Mechanism of Ferroptosis and its Relationships with Other Types of Programmed Cell Death: Insights for Potential Interventions after Intracerebral Hemorrhage. Front. Neurosci. 14, 589042. doi:10.3389/fnins.2020.589042
Zhou, Y., Liao, J., Mei, Z., Liu, X., and Ge, J. (2021). Insight into Crosstalk between Ferroptosis and Necroptosis: Novel Therapeutics in Ischemic Stroke. Oxid Med. Cel Longev, 20219991001. doi:10.1155/2021/9991001
Keywords: bibliometric analysis, Web of Science, stroke, ferroptosis, programmed cell death
Citation: Chen Y, Long T, Xu Q and Zhang C (2022) Bibliometric Analysis of Ferroptosis in Stroke From 2013 to 2021. Front. Pharmacol. 12:817364. doi: 10.3389/fphar.2021.817364
Received: 18 November 2021; Accepted: 29 December 2021;
Published: 21 February 2022.
Edited by:
Toshiko Yamazawa, Jikei University School of Medicine, JapanReviewed by:
Hartmut Kuhn, Charité University Medicine Berlin, GermanyCharareh Pourzand, University of Bath, United Kingdom
Copyright © 2022 Chen, Long, Xu and Zhang. This is an open-access article distributed under the terms of the Creative Commons Attribution License (CC BY). The use, distribution or reproduction in other forums is permitted, provided the original author(s) and the copyright owner(s) are credited and that the original publication in this journal is cited, in accordance with accepted academic practice. No use, distribution or reproduction is permitted which does not comply with these terms.
*Correspondence: Chi Zhang, chizhang25@163.com
†These authors have contributed equally to this work and share first authorship