- 1School of Pharmaceutical Sciences, Guangzhou University of Chinese Medicine, Guangzhou, China
- 2School of Chemistry and Molecular Engineering, East China Normal University, Shanghai, China
- 3The Second Clinical College, Guangzhou University of Chinese Medicine, Guangzhou, China
- 4School of Chinese Materia Medica, Guangdong Pharmaceutical University, Guangzhou, China
Vulvovaginal candidiasis (VVC) is an infectious disease caused by Candida species, which affects millions of women worldwide every year. The resistance to available antifungal drugs for clinical treatment is a growing problem. The treatment of refractory VVC caused by azole-resistant Candida is still facing challenges. However, research on new antifungal drugs is progressing slowly. Although a lot of reports on new antifungal drugs, only three new antifungal drugs (Isavuconazole, ibrexafungerp, and rezafungin) and two new formulations of posaconazole were marketed over the last decade. Chinese botanical medicine has advantages in the treatment of drug-resistant VVC, such as outstanding curative effects and low adverse reactions, which can improve patients’ comfort and adherence to therapy. Kangbainian lotion (KBN), a Chinese botanical formulation, has achieved very good clinical effects in the treatment of VVC. In this study, we investigated the antifungal and anti-inflammatory effects of KBN at different doses in fluconazole-resistant (FLC-resistant) VVC model mice. We further studied the antifungal mechanism of KBN against FLC-resistant Candida albicans (C. albicans) and the anti-inflammatory mechanism correlated with the Dectin-1 signaling pathway. In vivo and in vitro results showed that KBN had strong antifungal and anti-inflammatory effects in FLC-resistant VVC, such as inhibiting the growth of C. albicans and vaginal inflammation. Further studies showed that KBN inhibited the biofilm and hypha formation, reduced adhesion, inhibited ergosterol synthesis and the expression of ergosterol synthesis-related genes ERG11, and reduced the expression of drug-resistant efflux pump genes MDR1 and CDR2 of FLC-resistant C. albicans in vitro. In addition, in vivo results showed that KBN reduced the expression of inflammatory factor proteins TNF-α, IL-1β, and IL-6 in vaginal tissues, and inhibited the expression of proteins related to the Dectin-1 signaling pathway. In conclusion, our study revealed that KBN could ameliorate vaginal inflammation in VVC mice caused by FLC-resistance C. albicans. This effect may be related to inhibiting the growth of FLC-resistance C. albicans and Dectin-1 signaling pathway activation.
Introduction
Vulvovaginal candidiasis (VVC) is a common gynecological disease in clinical, and surveys reported that 70–75% of women will suffer from VVC at least once during their lifetime (Farr et al., 2021). Even worse, up to 140 million of these women will develop recurrent VVC (RVVC) defined as ≥4 cases within 1 year (Mckloud et al., 2021). VVC is characterized by redness, burning, and itching of the vulva and vaginal mucosa, frequently accompanied by thick white vaginal secretions (Peters et al., 2014). It even causes dysuria and dyspareunia (Faria et al., 2017), which has a great impact on the quality of life of women in general.
Candida albicans (C. albicans) is the most common species identified in patients with VVC (76–89%), followed by C. glabrata, C. tropicalis, C. parapsilosis, etc (Achkar and Fries, 2010). A variety of azoles are available for the treatment of VVC caused by C. albicans both as topical and systemic agents (Sobel and Sobel, 2018). However, antifungal drugs can have adverse effects such as headaches and gastrointestinal disorders (Felix et al., 2019). Currently, the resistance to available antifungal drugs is a growing problem. In general, widespread use of azoles has significantly increased azoles exposure and resulted in acquired azoles resistance (Pristov and Ghannoum, 2019). Even in drug-naive patients, an increase in resistant Candida species has been observed (Arendrup, 2014). Therefore, there is an urgent need to develop new antifungals. However, research on new antifungal drugs is progressing slowly. Although a lot of reports on new antifungal drugs, only three new antifungal drugs (Isavuconazole, ibrexafungerp, and rezafungin) and two new formulations of posaconazole were marketed over the last decade (Van Daele et al., 2019).
The resistance mechanism of C. albicans has the characteristics of multi-factor and multi-level regulation, mainly concentrated in the following three aspects: mutation or overexpression of ERG11 gene related to ergosterol synthesis (Flowers et al., 2015; Teymuri et al., 2015), increased expression of genes encoding drug efflux pumps (such as MDR1, CDR1, CDR2) (Mukherjee et al., 2003), and biofilm formation (Van Acker et al., 2014). Overexpression of ERG11 increases the amount of Erg11p enzyme and results in elevating ergosterol synthesis, which leads to resistance to the azole drugs (Alizadeh et al., 2017). Erg11p is a target enzyme of azole drugs, and the high concentrations of target enzyme create the need for higher intracellular fluconazole (FLC) concentrations to inhibit all of the enzyme molecules in the cell (Pfaller et al., 2006). The multidrug resistance phenotype in C. albicans has previously been demonstrated to be linked to proteins encoded by MDR1, CDR1, and CDR2 genes, which pump drugs from the fungal cells (Sanglard et al., 1996; Mukherjee et al., 2003).
A large amount of evidence indicates that Dectin-1 regarded as a pattern recognition receptor (PRR) and plays an important role in defense against fungal infection (Drummond and Brown, 2011). Dectin-1, a transmembrane protein, is a member of the C-type lectin receptor (CLR) family. The Dectin-1 receptor is served as the PRR for β-glucan in the fungal cell wall, and it triggers a signaling cascade that can activate NF-κB, which leads to the production of TNF-α、IL-1β、IL-6 (Falsetta et al., 2015; Yang et al., 2018; Tam et al., 2019).
Chinese botanical medicine has advantages in the treatment of drug-resistant VVC, such as outstanding curative effects and low adverse reactions, which can improve patients’ comfort and adherence to therapy. According to traditional Chinese medicine (TCM) theories, excess damp-heat is considered to be the key pathological factor in the pathogenesis of VVC (Yang et al., 2018). Kangbainian lotion (KBN) has been clinically used for VVC treatment for years with the effects of clearing heat and removing dampness (Huang et al., 2019). KBN is composed of Coptis chinensis Franch (Huanglian), Saururus chinensis (Lour.) Baill (Sanbaicao), Isatidis folium (Daqingye), Celosia cristata L. (Amaranthaceae) (Jiguanhua), Elsholtzia ciliata (Thunb.) Hyland (Xiangru), Sophora flavescens Alt. (Kushen), Stemona tuberosa Lour. (Baibu), Gentiana scabra Bunge (Longdan), Eugenia caryophyllata Thunb. (Dingxiang), Cinnamomum camphora (L.) J.Presl (Borneol, Bingpian). These botanical drugs or extracts are considered potential candidates for treating VVC. Their bioactive properties include anti-fungal (Liu et al., 2012; Yang et al., 2015; Niu et al., 2019) and anti-inflammatory (Lee et al., 2014; Yang et al., 2019; Ji et al., 2020; Pudziuvelyte et al., 2020). The previous research found that KBN significantly alleviated the vaginal inflammation in VVC model mice. However, the mechanism of KBN in the treatment of fluconazole-resistant (FLC-resistant) VVC needs further study.
In this study, we investigated the antifungal and anti-inflammatory effects of KBN at different doses in FLC-resistant VVC model mice. We further studied the antifungal mechanism of KBN against FLC-resistant C. albicans and the anti-inflammatory mechanism correlated with the Dectin-1 signaling pathway. Our findings provide better insight into the molecular mechanism of KBN as a treatment for VVC.
Materials and Methods
Preparation and HPLC Analysis of KBN
Preparation of KBN
The composition of the Chinese botanical formulation KBN, plant parts used, and botanical drug dose are presented in table 1. The total dosage of KBN is 206 g. Borneol was purchased from Yunnan Linyuan Spice Co., Ltd. (Yunnan, China), the rest of the botanical drugs were purchased from Guangzhou Zhixin Chinese Medicine Pieces Co., Ltd. (Guangzhou, China). Except for borneol, the rest of the botanical drugs were extracted in a 6-and 4-fold volume of 80% ethanol at 100°C for 1.0 h. After filtrating, both filtrates were mixed and concentrated to 1 g/mL raw botanical drugs. Then the concentrated decoction was added 1 g of borneol dissolved in little ethanol to obtain KBN for in vitro experiments. To obtain 0.4, 0.2, and 0.1 g/mL KBN preparation containing 4% Avicel (Dupont, USA) for in vivo experiments, 200, 100, and 50 mL of KBN were respectively added 20 g of Avicel CL-611with stirring (12,000 r/min) and ultrapure water to 500 mL.
Component Analysis of KBN by HPLC
KBN was determined by the Agilent 1260 high-performance liquid chromatography (HPLC) system (Agilent Technologies, United States) equipped with a VWD detector and a Kromasil 100-5-C18 column (250 × 4.6 mm, 5 μm). The mobile phase consisted of A and B with a gradient elution as follows: 0–10 min for 2–5% B, 10–20 min for 5–10% B, 20–25 min for 10–15% B, 25–55 min for 15% B, 55–60 min for 15–30% B, 65–70 min for 40–50% B, 70–85 min for 50–70% B. A was composed of 0.057 mol/L of sodium dihydrogen phosphate and 0.014 mol/L of sodium dodecyl sulfate and then adjusted pH to 4 with phosphoric acid. B was acetonitrile. The detection wavelength was set at 230 nm, the column temperature was maintained at 40°C, the loading volume was 5 μL and the flow rate was 1.0 mL/min. According to the retention time and peak area of the reference standard, a total of 7 components of KBN was detected, namely, gentiopicrin, eugenol, indirubin, epiberberine, coptisine, palmatine, and berberine (Figure 1).
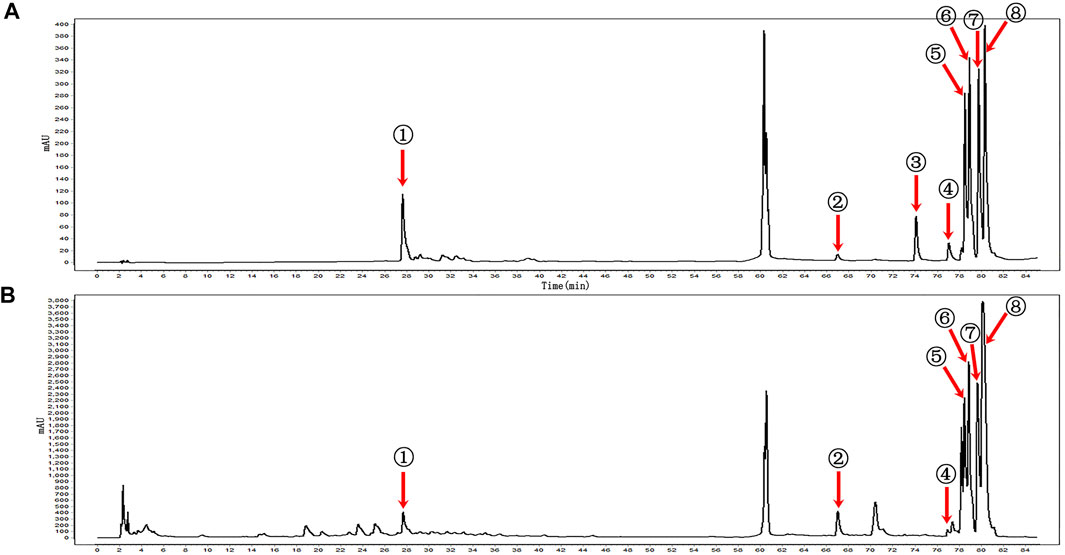
FIGURE 1. HPLC profiles of the main components of KBN. (A) Mixed reference; (B) KBN; ① Gentiopicrin; ② Eugenol; ③ Carvesol; ④ Indirubin; ⑤ Epiberberine; ⑥ Coptisine; ⑦ Palmatine; ⑧ Berberine.
C. albicans Strains and Culture
Four C. albicans strains were tested in this study. The quality control sensitive strain CMSS(F) 98001 was provided by the China National Institute for the Control of Pharmaceutical and Biological Products. The clinically FLC-resistant C. albicans isolates (901, 904, and 311) were donated by Dr. Jiang from the Second Military Medical University. Two distinct colonies of approximately 1 mm diameter were picked and inoculated in 1 mL of yeast extract-peptone-dextrose (YPD) medium (1% yeast extract, 2% peptone, 2% dextrose) at 35°C with constant shaking (200 rpm) for 16 h. Then, the cells were harvested by centrifugation, re-suspended in Roswell Park Memorial Institute (RPMI) 1640 medium with 0.165 M 3-(N-Morpholino) propane sulfonic acid (MOPS) (pH7.0), and counted after serial dilution by a hemocytometer.
Antifungal Susceptibility Test and Time-Kill Curve Analysis of KBN on C. albicans
Antifungal Susceptibility Test of KBN on C. albicans
Using a broth microdilution protocol of the Clinical and Laboratory Standards Institute (CLSI) M27-A3 method, an antifungal susceptibility test was carried out in 96-well tissue culture plates (Corning, USA) with a few modifications (Yan et al., 2019). In brief, the initial concentration of C. albicans in the RPMI 1640 medium was 2.0 × 103 colony-forming units (CFU)/mL, the final concentration ranged from 0.039 to 20 mg/mL for KBN, 0.125–64 μg/mL for FLC, and 0.015–8 μg/mL for ketoconazole. To further test the minimal inhibitory concentration (MIC) of FLC-resistant C. albicans, the final concentration ranged from 4 to 2,048 μg/mL for FLC. Plates were incubated at 35°C for 48 h. MIC endpoints were read as previously described (Li et al., 2019; Yan et al., 2019; Yong et al., 2020). In brief, the MIC was determined as the lowest concentration of the drug that inhibited planktonic C. albicans growth by 80% compared with that of the drug-free C. albicans. The MIC of fluconazole against C. albicans was also tested with the same criteria. Experiments were performed in triplicate.
Time-Kill Curve Assay of KBN on C. albicans
To further study the antifungal activity of KBN, a time-kill curve assay was performed as described previously (Yong et al., 2020). Time-kill experiments were conducted in RPMI 1640 medium with MOPS. The final concentrations were 12.5, 6.25 and 3.125 μg/mL for KBN, 2 μg/mL for FLC, and 4.0 × 104 CFU/mL for C. albicans. A drug-free group served as the negative control. Cultures were incubated at 35°C with constant shaking (200 rpm) after different treatments. 50 μL of aliquots were obtained from each suspension at 0, 3, 6, 12, 24, 36, and 48 h, and serially diluted in sterile phosphate balanced solution (PBS). 50 μL of the diluted fungal suspension was plated on sabouraud dextrose agar (SDA) plates, and colonies were counted following incubation at 35°C for 36 h.
Effect of KBN on the VVC Model Mice Caused by FLC-Resistant C. albicans 901
As previously described (Yano and Fidel, 2011), the VVC model mice were used to demonstrate the antifungal effect of KBN in vivo. Briefly, 6 days before inoculation, SPF KM female mice (Guangdong Medical Laboratory Animal Center) were injected with 0.1 mL of β-estradiol (0.2 mg/mL, Hangzhou Animal Medicine Factory, China) subcutaneously in the lower abdominal. Estrogens were injected every other day, three times in 6 days. After estradiol treatments, infection of the vagina was performed by inoculating the mice intravaginally with 20 μL of FLC-resistant C. albicans 901 suspensions with a concentration of 1.0 × 108 CFU/mL every day, seven times a week. After the establishment of VVC model mice, KBN (0.4, 0.2, and 0.1 g/mL) and FLC (2 mg/mL) were separately delivered into the vagina once a day for 3 weeks, 30 μL per day. Drug-free groups were used as negative control and positive control. On day 1 before administration, day 3, 7, and 14 after treatment, 100 μL of vaginal lavage was collected and cultured on the SDA plates for colony counts. The CFU was counted to analyze the infection burdens. 10 μL of lavage fluid was observed by Gram staining. All mice were killed with anesthetics and the vaginal tissues were observed by hematoxylin and eosin (H&E) staining, periodic acid schiff (PAS) staining, and scanning electron microscopy (SEM). The study was approved by the Animal Ethics Committee of the Institute of Science and Technology at Guangzhou University of Chinese Medicine (Guangzhou, China).
The Mechanism of KBN Inhibiting FLC-Resistant C. albicans
The Effect of KBN on the Biofilm Formation by XTT Reduction Assay
The 2,3-bis-(2-methoxy-4-nitro-5-sulfophenyl)-2H-tetrazolium-5-carboxanilide (XTT) reduction assay was conducted as described previously (Ramage et al., 2001) with slight modifications. Briefly, 100 µL of C. albicans with an initial concentration of 1.0 × 106 CFU/mL was cultured in a sterile 96-well plate at 37°C for 1.5 h and discarded. Biofilm cells were washed with PBS, cultured in 1640 medium with or without drugs at 37°C for 24 h, and discarded. Biofilm cells were washed with PBS and then incubated in 200 μL PBS with 0.5 mg/mL of XTT and 1 µM of menadione at 37°C for 1 h. 100 μL of supernatant was transferred to new microtiter plates, and optical densities were measured at 492 nm.
The Effect of KBN on the Hyphae Growth
The hypha-inducing media (RPMI 1640 medium with MOPS) was used to test the effect of KBN on the hyphal formation. C. albicans cells (1.0 × 105 CFU/mL) were suspended in the hypha-inducing media containing 12.5, 6.25, and 3.125 mg/mL of KBN and added to 12-well tissue culture plates. The plates were incubated at 37°C for 3 h. The morphology of C. albicans was observed and photographed under an inverted microscope.
The Effect of KBN on the Adhesion to VK2/E6E7
VK2/E6E7 kindly gifted from Dr. Chen (Tongji Medical College) is a vaginal epithelial cell. The cells were maintained and passaged in CnT-Prime epithelial culture medium (CELLnTEC, Switzerland) at 37°C with 5% CO2. The adhesion test was conducted as described previously (Gao et al., 2019), with slight modifications. 1.0 × 105 cells/well of C. albicans were inoculated into VK2/E6E7 monolayer cultures in a 24-well plate with or without KBN at 37°Cwith 5% CO2 for 1.5 h. All the mixtures of cell monolayer and fungal cells were fixed with 4% paraformaldehyde for 15 min, stained with 30 μg/mL CFW (Sigma-Aldrich, United States) for 15 min, and then photographed under a fluorescent microscope.
The Effect of KBN on the Ergosterol by HPLC
Total sterols were extracted from whole cells according to a previous report with slight modifications (Xu et al., 2017). Briefly, C. albicans with an initial concentration of 2.0 × 106 CFU/mL were cultured in RPMI 1640 medium with or without KBN at 35°C for 48 h. 6.0 mL of each sample were collected, added 15 mL of saponifier (methanol solution with 10% KOH), and then incubated in an 80°C water bath for 90 min. The total sterols were extracted thrice with 15 mL of petroleum ether (boiling range 30°C-60°C) and incubated in a 60°C water bath until petroleum ether layer volatilized completely. The resulting samples were dissolved in 6 mL of methanol, filtered with a 0.45 μm microporous membrane, and used for HPLC quantification. The ergosterol standard (0.125–64 μg/mL) dissolved in methanol and the sample-derivatized sterols were determined by the Agilent 1260 HPLC system equipped with a VWD detector and a Kromasil 100-5-C18 column (250 × 4.6 mm, 5 μm). The mobile phase consisted of 95% methanol and 5% water. The detection wavelength was set at 282 nm, the column temperature was maintained at 30°C, the flow rate was 1.0 mL/min. The ergosterol standard loading volume was 10 μL, and the sample-derivatized sterols loading volume was 100 μL.
The Effect of KBN on the Gene Expression by RT-PCR
Reverse transcription-polymerase chain reaction (RT-PCR) was conducted as described previously (Zhong et al., 2017) with slight modifications. Briefly, C. albicans cells with an initial concentration of 3.0 × 106 CFU/mL were cultured in YPD medium at 35°C for 6 h. C. albicans cells were collected and washed, and total RNA was isolated by an EASYspin yeast RNA rapid extraction kit (RN10, Aidlab Biotechnologies, China). cDNA was obtained by a reverse transcription reaction performed with a reverse transcription kit (AG11728, Accurate Biotechnology, China). RT-PCR was performed with a 7500 real-time PCR system (Thermo Fisher, United States), and specific primers were synthesized by Sangon Biotech (Table 2). SYBR green (AG11718, Accurate Biotechnology, China) was used to monitor the amplified products. The expression of each gene was normalized to that of 18S rRNA. The relative genes expression were quantified by the 2−△△Ct method and triplicate independent experiments were performed to obtain a mean value.
Anti-Inflammatory Mechanism of KBN on VVC Model Mice Caused by FLC-Resistant C. albicans 901 by Western Blot
The vaginal tissues were lysed and homogenized in RIPA buffer with phenylmethylsulfonyl fluoride (PMSF) and quantified with a BCA kit (Keygen Biotech, KGP902, China). The protein samples (30 μg/lane) were separated using SDS-PAGE (Beyotim, P0012A, China) and transferred to polyvinylidene difluoride membranes (PVDF) (Merck, IPVH00010, United States) after electrophoresis. The membranes were blocked with 5% skimmed milk for 3 h, followed by incubation with TNF-α (1:1,000, Abcam, ab205587, United States), IL-1β (1:1,000, Abcam, ab234437, United States), IL-6 (1:1,000, Abcam, ab229381, United States), Dectin-1 (1:1,000, Abcam, ab140039, United States), Syk (1:1,000, Cell Signaling Technology, 13198T, United States), PLCγ-2 (1:1,000, Cell Signaling Technology, 3872T, United States), CARD9 (1:500, Affinity, DF8387, China), NF-κB (1:1,000, Cell Signaling Technology, 8242T, USA) and β-actin (1:2,000, Affinity, AF7018, China) antibodies overnight at 4°C. Then, the membranes were incubated with an HRP-conjugated antibody (1:5,000, Affinity, S0001, China) at room temperature for 1 h. The membranes were visualized with a chemiluminescence (ECL) kit by a Tanon 5200 system (Shanghai, China). ImageJ software (Version 1.52a) was used to measure the protein bands based on that of β-actin.
Statistical Analysis
SPSS 20.0 software was used for statistical analysis. The results were expressed as the mean ± standard deviation (SD). One-way ANOVA was utilized for more than two groups. The Levene was used to test the homogeneity of variance, the least significant difference test (LSD-t) was used for inter-group comparisons when the variance was homogeneous, and Dunnett’s T3 was used for inter-group comparisons when the variance was uneven. p < 0.05 was recognized as statistically significant.
Results
KBN Inhibits C. albicans Growth
We first determined the MIC values of KBN on C. albicans strains, including the quality control sensitive CMSS(F) 98001 and the clinically FLC-resistant isolates (901, 904, and 311). The MIC of KBN against planktonic C. albicans was 6.25 mg/mL, for all the tested strains (Table 3). The dynamic antifungal effect of the drug could be monitored by the time-kill curve assays for CMSS(F) 98001 and 901 strains (Yong et al., 2020). The results showed that KBN appeared to exhibit strong antifungal activity against C. albicans. Especially, 12.5 mg/mL of KBN resulted in 99.9% killing within 36 h against C. albicans (Figure 2). It should be noted that KBN exhibited similar antifungal activity on the FLC-sensitive strain and resistant strains.
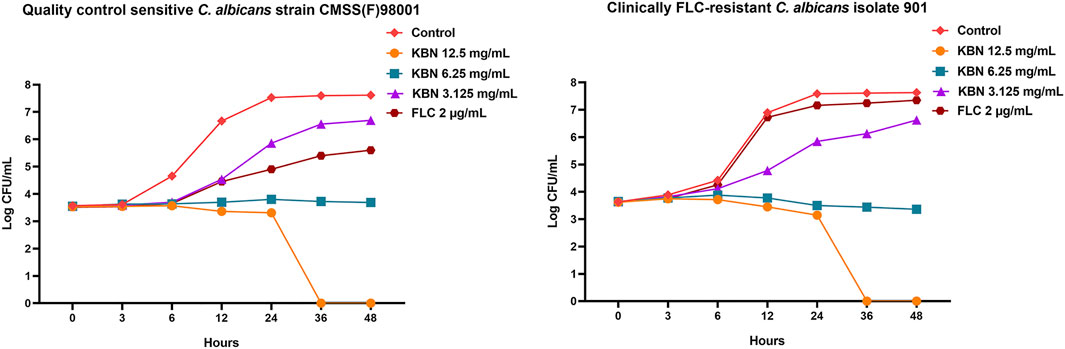
FIGURE 2. Effects of KBN on the time-killing curve of C. albicans. The quality control sensitive C. albicans strain CMSS(F) 98001 and the clinically FLC-resistant C. albicans isolate 901 were used.
KBN Inhibits the Proliferation of C. albicans and Vaginal Inflammation in VVC Model Mice Caused by FLC-Resistant C. albicans
VVC is mainly caused by C. albicans and infections rely on fungal morphogenesis and biofilm formation (Fazly et al., 2013). This mouse vaginal infection model is ideal for evaluating in vivo antifungal potency of KBN. We assessed the antifungal effects of KBN in vaginal infection by colony counts, Gram stain, H&E stain, PAS stain, and SEM (Figure 3). All three KBN groups exhibited markedly reduced fungal burden from day 3 onwards compared with the model group (p < 0.01), and KBN treatment reduced the fungal loads in a dose-dependent manner (Figure 3A). From the images of lavage fluid Gram staining, vaginal PAS staining, and scanning electron, we observed a lot of criss-cross filamentous cells in the model (Figures 3B,D,E). After H&E staining, we observed that the vaginal mucosa was seriously injured in the model, included cellular edema, inflammatory cell infiltration (Figure 3C). By the management of KBN, the cellular symptoms were relieved to different degrees. Noticeably, when exposed to 0.4 or 0.2 g/mL of KBN, the vaginal mucosa was largely recovered, without cell edema and inflammatory cell infiltration. Interestingly, KBN restrained the formation of mycelium in vivo, which was consistent with its hyphal inhibition activity in vitro (Figures 5, 6).
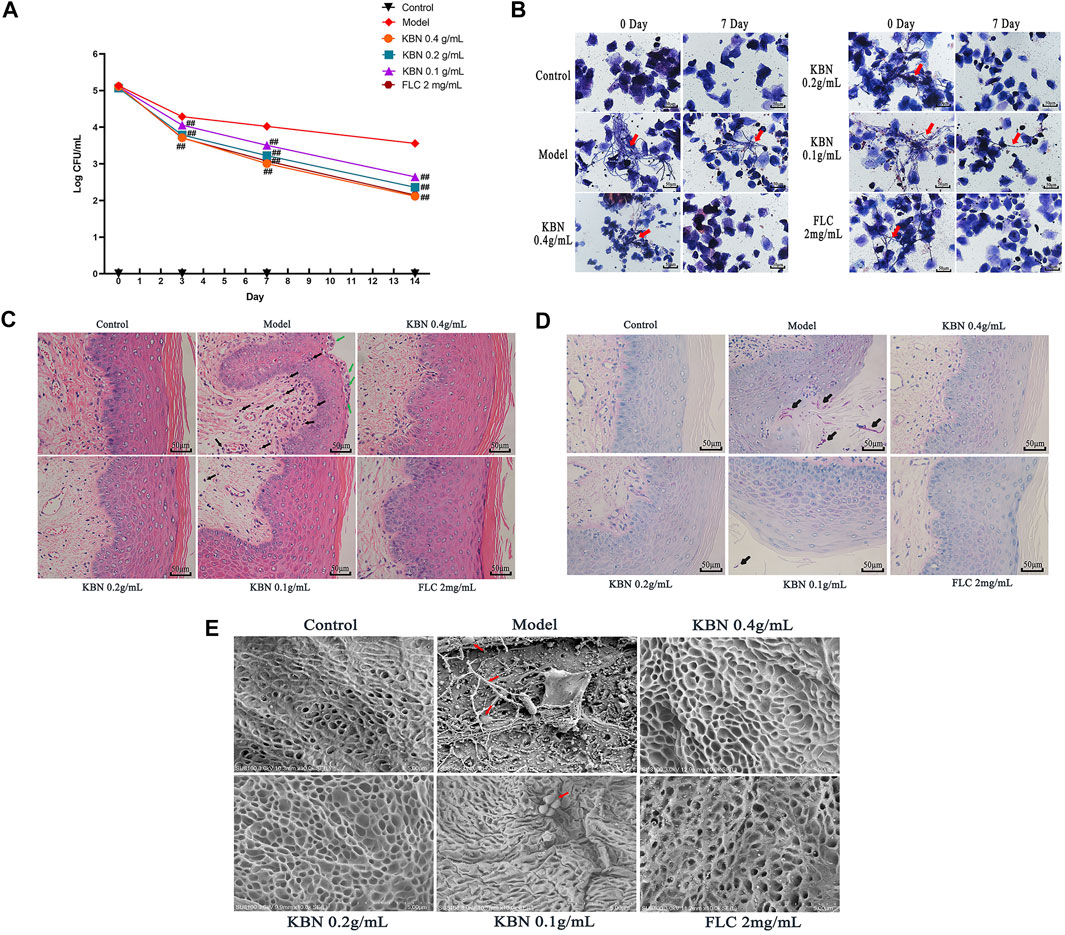
FIGURE 3. The vaginal fungal burden and the intensity of vaginal tissue inflammation in mice infected with FLC-resistant C. albicans 901 after the treatment of KBN. (A) Vaginal fungal burden analyses by colony counting in infected mice. Each value is presented as the mean ± SD, N = 10. ##p < 0.01 as compared to the values from the model group. (B) The formation of hyphae in vaginal excretion after KBN treatment was indicated by Gram staining (400 ×). The red arrows represent visible hyphae in vaginal excretions. (C) Histopathological analysis of vaginal tissue in infected mice by H&E stain (400 ×). The green arrow shows microabscesses and the black arrow shows neutrophils, indicating the presence of inflammation. (D) Histopathological analysis of vaginal tissue in infected animals by PAS (400 ×). The black arrow shows C. albicans. (E) Morphology of the vaginal epithelium by SEM after infection and KBN treatment (10 K ×). The red arrows represent C. albicans.
The Mechanism of KBN Inhibiting FLC-Resistant C. albicans 901
KBN Inhibits the Biofilms Formation
KBN significantly inhibited biofilm formation of FLC-resistant C. albicans 901 in a dose-dependent manner (Figure 4). More specifically, 3.125 mg/mL of KBN inhibited biofilm formation by 40.15%, and the inhibitory effect on biofilm formation was enhanced when the concentration of KBN was increased. 6.25 mg/mL of KBN inhibited biofilm formation by 56.86%, and 12.5 mg/mL of KBN inhibited biofilm formation by 75.54%. 64 μg/mL of FLC only inhibited biofilm formation by 22.23%.
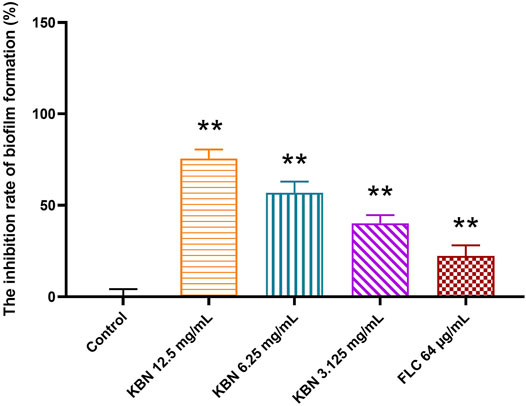
FIGURE 4. Effects of different concentrations of KBN on FLC-resistant C. albicans 901 biofilm formation. Data are expressed as the mean ± SD of the independent assays in sextuplicate. ∗∗p < 0.05 as compared to the control group.
KBN Inhibits the Hyphae Growth
Since hypha growth is a key factor in C. albicans biofilm formation and virulence, we tested the effect of KBN on hypha growth. In the absence of KBN, FLC-resistant C. albicans 901 underwent hyphal initiation, produced elongated and regular hyphal cells (Figure 5). When 12.5 or 6.25 mg/mL of KBN was added, the hypha growth was remarkably inhibited. Specifically, the hypha growth was completely inhibited by 12.5 mg/mL of KBN as only yeast cells were observed.
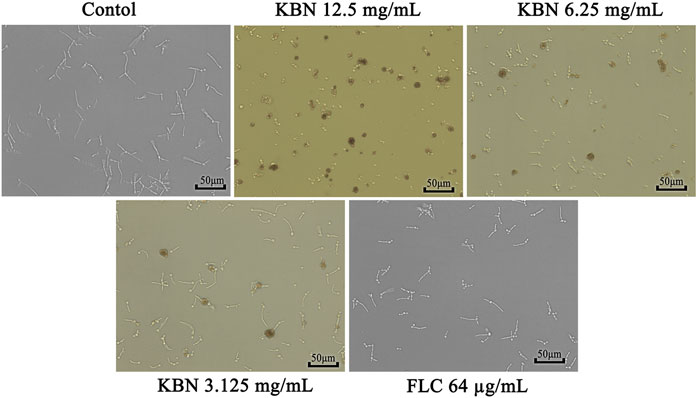
FIGURE 5. Effects of KBN on hyphae growth of FLC-resistant C. albicans 901. The cellular morphology was photographed after incubating at 37°C for 3 h.
KBN Inhibits the Adhesion to VK2/E6E7
We further analyzed the effect of KBN on FLC-resistant C. albicans 901 interacting with VK2/E6E7 by analyzing adhesion. 12.5 or 6.25 mg/mL of KBN significantly inhibited the adhesion of FLC-resistant C. albicans 901 to the surface of VK2/E6E7 cells and reduced the damage of C. albicans to VK2/E6E7 cells (Figure 6). In addition, 12.5 or 6.25 mg/mL of KBN significantly inhibited the hyphae growth of FLC-resistant C. albicans 901.
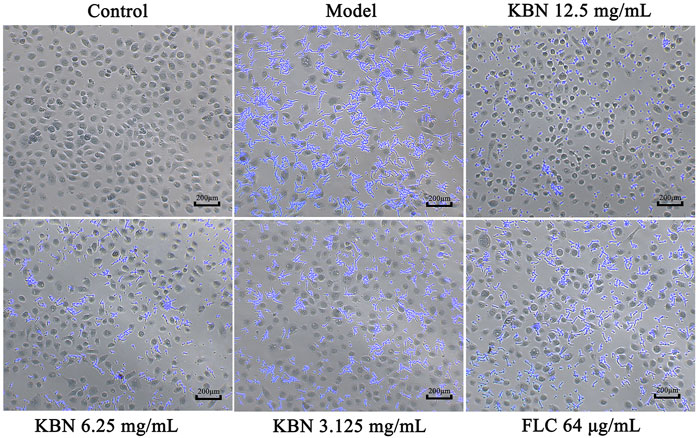
FIGURE 6. KBN inhibited the adhesion of FLC-resistant C. albicans 901 to VK2/E6E7 cells. The treatment concentration for KBN were 12.5, 6.25, and 3.125 mg/mL.
KBN Reduces the Content of Ergosterol and Overexpression of Related Gene ERG11
In this study, we investigated the resistance mechanism of ergosterol in FLC-resistant C. albicans 901 and the effect of KBN on it. First, the cellular ergosterol levels in FLC-resistant C. albicans 901 were measured after KBN or FLC treatment by HPLC analysis. The ergosterol content significantly treated with 6.25 or 3.125 mg/mL of KBN decreased in the cells compared with the control group (p < 0.01) (Figures 7A,B). Changes in the expression of ergosterol synthesis-related gene ERG11 in FLC-resistant C. albicans 901 and sensitive C. albicans 98001 were investigated by RT-PCR analysis. The expression of the ERG11 gene in FLC-resistant C. albicans 901 was 1.2 times that of sensitive C. albicans 98001 (Figure 7C). The effect of KBN on the overexpression of ERG11 in C. albicans 901 was further investigated. 6.25 or 3.125 mg/mL of KBN significantly reduced the expression level of ERG11 (p < 0.05) (Figure 7D). Interestingly, FLC significantly increased the expression of ERG11.
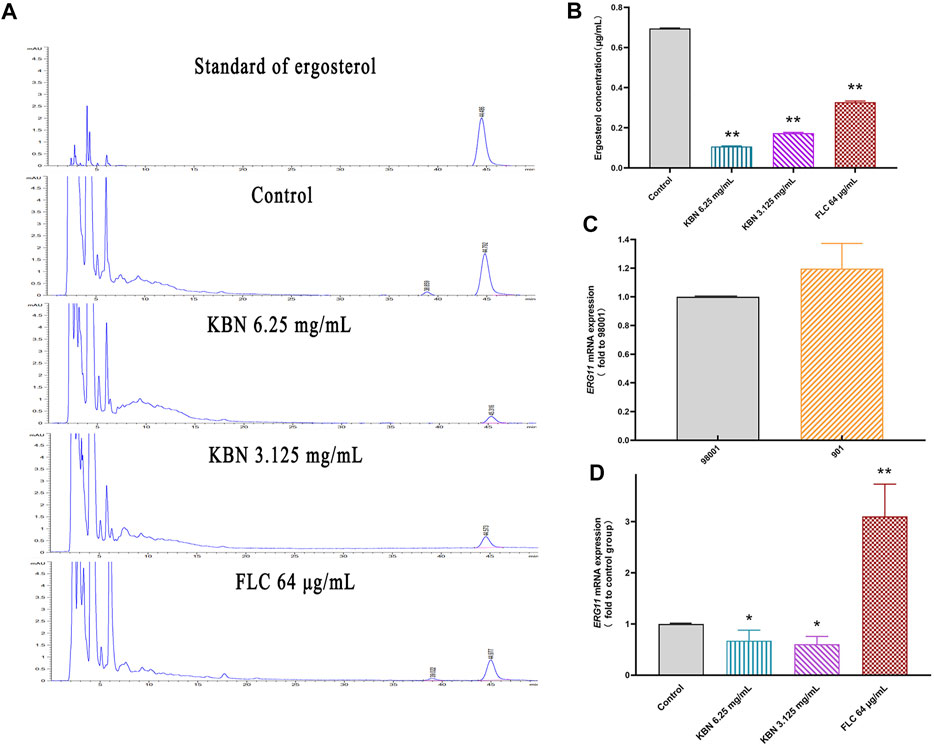
FIGURE 7. Effect of KBN on ergosterol content of FLC-resistant C. albicans 901 and expression of related gene ERG11. (A) Quantitative analysis of cellular ergosterol in FLC-resistant C. albicans 901 treated with KBN or FLC for 48 h by HPLC. (B) Ergosterol content of cells treated with KBN. Values were described as ergosterol (μg/mL). (C) The expression of ergosterol synthesis gene ERG11 in FLC-resistant C. albicans 901 and sensitive C. albicans 98001. (D) Changes in the expression of ergosterol synthesis gene ERG11 in FLC-resistant C. albicans 901 treated with KBN or FLC for 6 h. The gene was examined by RT-PCR with gene-specific primers. All values are expressed as the mean ± SD of triplicate assays. *p < 0.05 versus control group, **p < 0.01 versus control group.
KBN Reduces the Overexpression Levels of Efflux Pump Gene
First, the sensitive C. albicans 98001 was used as the quality control fungus to compare the expression of different efflux pump genes in FLC-resistant C. albicans 901. 18S rRNA was used as an internal reference for each gene. There were no primer-dimers or nonspecific amplification products according to the melting curves and melting peaks of all the tested genes. The expression of MDR1, CDR1, and CDR2 genes in FLC-resistant C. albicans 901 was higher than that of sensitive C. albicans 98001, which were 5.67, 1.68, and 3.00 times of those in FLC-sensitive C. albicans 98001, respectively (Figure 8A). For FLC-resistant C. albicans 901 strain, the MDR1 and CDR2 expression levels among treatments with 6.25 and 12.5 mg/mL of KBN significantly decreased (p < 0.01). However, there was no significant difference in the CDR1 expression level among treatments (p > 0.05) (Figure 8B).
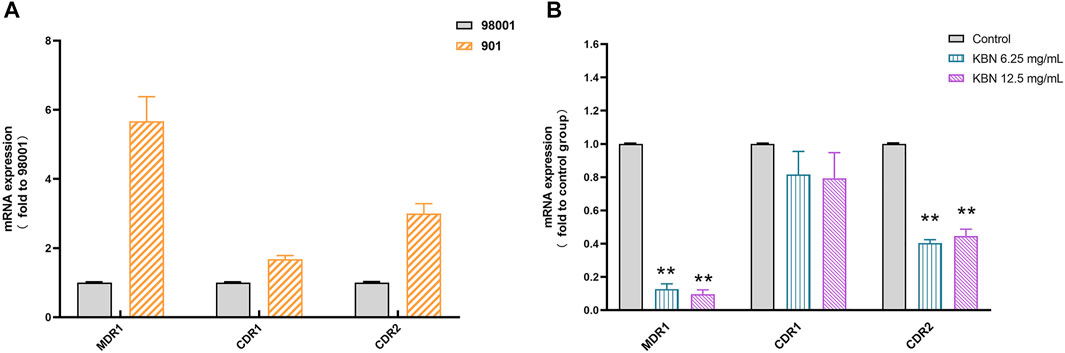
FIGURE 8. Relative expression of the efflux-pump genes in C. albicans strains. (A) The expression levels of efflux pump genes MDR1, CDR1, and CDR2 in sensitive C. albicans 98001 and FLC-resistant C. albicans 901. (B) The expression levels of the efflux-pump genes MDR1, CDR1, and CDR2 in FLC-resistant C. albicans 901 cells that were treated with KBN relative to those in untreated cells. Values represent the mean ± SD for three independent experiments. ∗∗p < 0.01 versus control group.
Anti-Inflammatory Mechanism of KBN on VVC Model Mice Caused by FLC-Resistant C. albicans 901
KBN Decreased Inflammation Levels of Vaginal Tissue in VVC Model Mice
To assess the level of inflammation induced by infection, vaginal tissues were collected and the protein levels of TNF-α, IL-1β, and IL-6 were analyzed by Western Blot. The protein expression levels of TNF-α、IL-1β, and IL-6 were significantly upregulated in the model group compared with that in the control group (p < 0.05), the expression of these proteins was significantly downregulated while KBN and FLC treatments (p < 0.05) (Figure 9).
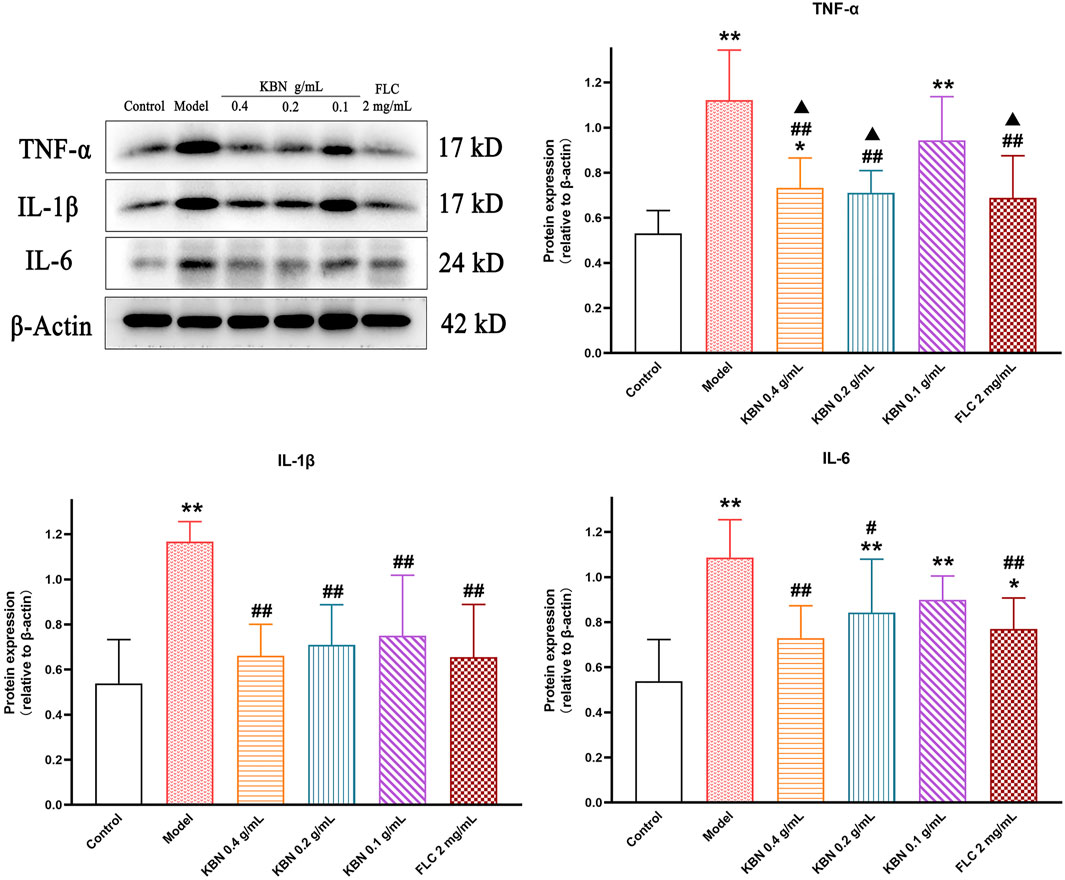
FIGURE 9. Effect of KBN on the protein expression of TNF-α, IL-1β, and IL-6 in the vaginal tissues. N = 6. Each value is presented as the mean ± SD. *p < 0.05, **p < 0.01 versus the control group; #p < 0.05, ##p < 0.01 versus the model group; ▲p < 0.05 versus the KBN 0.1 g/mL group.
KBN Regulated the Expression of Proteins Related to the Dectin-1 Signaling Pathway
To determine the potential molecular mechanism for the antifungal and anti-inflammatory effects of KBN, we studied the protein expression levels related to the Dectin-1 signaling pathway in vaginal tissues. The protein expression levels of Dectin-1, Syk, PLCγ-2, CARD9, and NF-κB showed a significant reduction in the model group (p < 0.05), and this trend was reversed after KBN treatment (p < 0.05) (Figure 10).
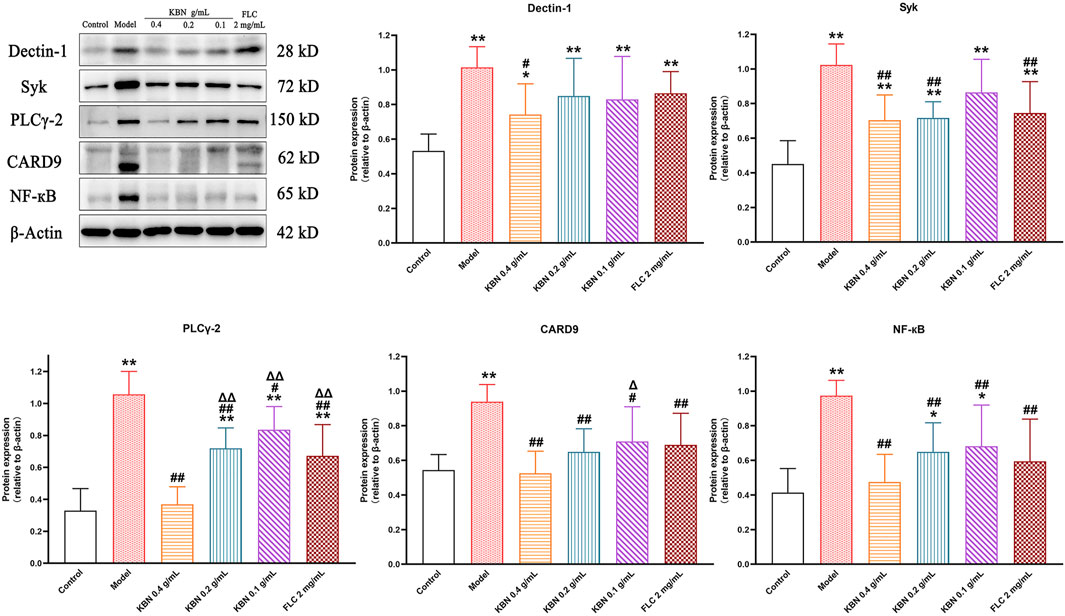
FIGURE 10. Effect of KBN on the protein expression of Dectin-1, Syk, PLCγ-2, CARD9, and NF-κB in the vaginal tissues. N = 6. Each value is presented as the mean ± SD. *p < 0.05, **p < 0.01 as compared to the control group; #p < 0.05, ##p < 0.01 as compared to the model group; △p < 0.05, △△p < 0.01 as compared to the KBN 0.4 g/mL group.
Discussion
VVC is the second most common inflammatory disease in the female vagina, which has a serious impact on their quality of life (Dovnik et al., 2015). At present, an important factor in the refractory treatment of VVC is that fungus-inducing VVC has drug-resistance. Thus, there is an urgent need for new antifungal drugs that can efficiently treat VVC caused by drug-resistant C. albicans. In this study, we found that KBN had strong antifungal and anti-inflammatory effects in FLC-resistant VVC, such as inhibiting the growth of C. albicans and vaginal inflammation. Further studies showed that KBN inhibited the biofilm and hypha formation, reduced adhesion, inhibited ergosterol synthesis and the expression of ergosterol synthesis-related genes ERG11, and reduced the expression of drug-resistant efflux pump genes MDR1 and CDR2 of FLC-resistant C. albicans in vitro. In addition, in vivo results showed that KBN reduced the expression of the proteins of inflammatory factors TNF-α, IL-1β, and IL-6 in vaginal tissues, and inhibited the expression of the proteins related to the Dectin-1 signaling pathway.
Borneol, including natural borneol (D-borneol) and synthetic borneol (Borneol, isoborneol, and camphor) (Chinese Pharmacopoeia Commission, 2020), has been used in TCM clinical applications for more than 2,000 years (Wang et al., 2017). Our previous study showed that the MIC of borneol against sensitive or FLC-resistant C. albicans was >1.28 mg/mL, which was similar to the previous report (E Silva et al., 2018). The MIC of KBN against C. albicans was 6.25 mg/mL, and the concentration of borneol in it was only 0.03 mg/mL. It was speculated that borneol had no obvious inhibitory effect on C. albicans at this concentration. Therefore, the borneol control group was not established in the series of C. albicans trials.
To explore the effect of KBN treating VVC, we used a VVC model mouse induced by FLC-resistant C. albicans 901. The estrogen required by this model can promote the adhesion of C. albicans in the vagina by increasing the proliferation and thickening of epithelial cells, and also accelerating the growth of C. albicans by enhancing glycogen levels in vaginal tissues (Yang et al., 2018). One important indicator for determining antifungal effects is the effects on inhibiting proliferation and adhesion of fungus. KBN significantly reduced the C. albicans burden from day 3 onwards. We found that KBN inhibited the growth of C. albicans hyphae by Gram stain, and reduced the adhesion of C. albicans to the vagina by PAS staining and SEM. The above results support that KBN effectively reduces the growth and adhesion of C. albicans in the vagina.
The hyphal formation is an important virulence factor of C. albicans associated with biofilm (Shin and Eom, 2019). Hyphae can attach to host cells, damage host tissues, and evade host immune defenses (Rossoni et al., 2018). When the body’s microecological imbalance or immune function is inhibited, C. albicans can transform from a yeast state to a hyphae state, and its invasiveness is enhanced (Thomson et al., 2015). During C. albicans infecting epithelial cells, adhesion and hyphal formation are linked: adhesion promotes the hyphal formation and hyphal formation stimulates adhesion (Wächtler et al., 2011). In this study, we found that KBN reduced the adhesion of drug-resistant C. albicans 901 to abiotic surfaces (Polystyrene) and VK2/E6E7 cells, inhibited the formation of hyphae, and has a protective effect on VK2/E6E7 cells.
The antifungal drugs used in the treatment of VVC mainly include azoles. After azole treatment of VVC, C. albicans may become highly drug-resistant, especially to FLC (Marchaim et al., 2012). Multiple molecular mechanisms may operate in FLC-resistance C. albicans at the same time. These mechanisms include decreased overexpression and mutations of the ERG11 gene, alterations in the sterol biosynthesis pathway, and intracellular drug accumulation (Siikala et al., 2010). One of the biofilm resistance mechanisms of C. albicans is the slow penetration of the antimicrobial agent through the biofilm (Van Acker et al., 2014). The resistance of biofilm is also closely related to the increase of extracellular matrix (Desai and Mitchell, 2015). In this study, the antifungal effect of KBN was stronger as compared to FLC. KBN inhibited FLC-resistance C. albicans 901 biofilm and 12.5 mg/mL of KBN inhibited 75.54% of biofilm formation, while 64 μg/mL of FLC only inhibited 22.23%. KBN significantly reduced the synthesis of ergosterol of FLC-resistant C. albicans 901. The expression of ergosterol synthesis-related gene ERG11 in FLC-resistant C. albicans 901 was 1.2 times that of sensitive C. albicans 98001, indicating that the overexpression of ERG11 is one of the FLC-resistance mechanisms of C. albicans 901, while KBN down-regulated its overexpression. Interestingly, 64 μg/mL of FLC up-regulated the expression of the FLC-resistant C. albicans 901 ERG11 gene, which was consistent with previous reports (Morais et al., 2021). These results as a whole suggest that the antifungal effect of KBN may reduce the synthesis of ergosterol by inhibiting the gene expression of ERG11.
The multidrug resistance in C. albicans has previously been proved to be linked to proteins encoded by efflux pump genes MDR1, CDR1, and CDR2 (Mukherjee et al., 2003). To further reveal the potential molecular basis of KBN against drug-resistant fungus, we tested the transcription levels of drug resistance genes MDR1, CDR1, and CDR2. In our study, the overexpression of efflux pump genes MDR1, CDR1, and CDR2 in FLC-resistant C. albicans strain are consistent with previous reports (White et al., 2002; Park and Perlin, 2005; Feng et al., 2018). The results of this experiment showed that KBN significantly down-regulated the expression levels of efflux pump genes MDR1 and CDR2 in drug-resistant C. albicans 901. The results of this experiment suggested the same antifungal effect of KBN on FLC-sensitive and resistant C. albicans might be related to the inhibition of the expression of drug-resistant efflux pump genes MDR1 and CDR2.
A protective immune response is caused by VVC, accompanied by tissue inflammation. Dectin-1 is a type of PRR, which recognizes β-glucan in the fungal cell wall (Hardison and Brown, 2012). Recognition of β-glucans by Dectin-1 leads to the induction of numerous cellular responses, including respiratory burst, formation of phagosomes, the production of arachidonic acid metabolites, the induction of multiple cytokines, and chemokines (Huysamen and Brown, 2009). After Dectin-1 binds to β-glucan, Src family kinases are phosphorylated and the immunoreceptor tyrosine-based activation motif (ITAM)-like motif is activated, which activates Syk; the activated Syk activates CARD9 by the downstream products produced by PLCγ2, forming the CARD9-BCL10-MALT1 complex (Strasser et al., 2012). The CARD9 complex promotes the formation of the IKK complex to enable IκB protein phosphate and ultimately activate NF-κB (Gross et al., 2006), which produces inflammatory cytokines and chemokines (Li et al., 2009). It was previously reported that the levels of pro-inflammatory factors TNF-α, IL-1β, and IL-6 in VVC model mice were elevated (Trinh et al., 2011; Joo et al., 2012). In this study, KBN inhibited the expression of TNF-α, IL-1β, and IL-6 in vaginal tissues, similar to previous reports in vaginal epithelial cells A431 and VK2 (E6/E7) cells (Trinh et al., 2011; Wagner and Johnson, 2012). The Dectin-1/Syk/PLCγ2/Card9/NF-κB signaling pathway was activated in the vaginal tissues in the model group, and KBN significantly inhibited its activation. The above results suggest that KBN can alleviate vaginal inflammation in FLC-resistant VVC model mice by inhibiting the Dectin-1 signaling pathway.
The current study has several limitations. Firstly, KBN is a prescription in TCM with multiple components, and the bioactive components from KBN that relieve VVC remain unclear. Secondly, VVC is also caused by C. glabrata, C. krusei, C. tropicalis, C. parapsilosis, etc, and the effect of KBN on FLC-resistant VVC caused by these fungi needs to be further evaluated. Thirdly, the downstream targets of the Dectin-1 signaling pathway are also regulated by other factors, and the in-depth study of downstream mechanisms is crucial for future research. Finally, this study did not assess the change of vaginal microbiota and the effect of KBN on vaginal beneficial bacteria (such as Lactobacilli) of VVC mice is unknown. Therefore, we will further research the bioactive components of KBN treating VVC and their interactions, the effect of KBN treating VVC caused by other FLC-resistant fungi, the in-depth downstream mechanisms of the Dectin-1 signaling pathway, and the effect of KBN on vaginal beneficial bacteria in VVC mice in the future.
In conclusion, our study reveals that KBN can ameliorate vaginal inflammation in VVC mice caused by FLC-resistance C. albicans. This effect may be related to inhibiting the growth of FLC-resistance C. albicans and Dectin-1 activation. Furthermore, our study revealed the potential mechanism of KBN against FLC-resistance C. albicans. As the development of new antifungal drugs is progressing slowly and the azole drugs on the market are facing the challenge of resistant fungi, the development of KBN may provide a new treatment option for superficial drug-resistant fungal infections.
Data Availability Statement
The raw data supporting the conclusions of this article will be made available by the authors, without undue reservation.
Ethics Statement
The animal study was approved by the Animal Ethics Committee of the Institute of Science and Technology at Guangzhou University of Chinese Medicine (Guangzhou, China).
Author Contributions
JZ, FW, and ZC designed the experiments. ZC, WL, and TL performed animal experiments. ZC, FY, GC, and FH conducted the cell experiments. ZC, WL, and MC analyzed the data. ZC and TL wrote the manuscript. All authors have read and agreed to the published version of the manuscript.
Funding
The Science and Technology Planning Project of Guangdong Province (2016B020239005) supported this study.
Conflict of Interest
The authors declare that the research was conducted in the absence of any commercial or financial relationships that could be construed as a potential conflict of interest.
Publisher’s Note
All claims expressed in this article are solely those of the authors and do not necessarily represent those of their affiliated organizations, or those of the publisher, the editors and the reviewers. Any product that may be evaluated in this article, or claim that may be made by its manufacturer, is not guaranteed or endorsed by the publisher.
Acknowledgments
We thank Yuanying Jiang (the Second Military Medical University, China) for providing clinical drug-resistance C. albicans isolates. We also thank Zhuo Chen (Tongji Hospital, Tongji Medical College, China) for providing VK2/E6E7 cells.
References
Achkar, J. M., and Fries, B. C. (2010). Candida Infections of the Genitourinary Tract. Clin. Microbiol. Rev. 23 (2), 253–273. doi:10.1128/CMR.00076-09
Alizadeh, F., Khodavandi, A., and Zalakian, S. (2017). Quantitation of Ergosterol Content and Gene Expression Profile of ERG11 Gene in Fluconazole-Resistant Candida Albicans. Curr. Med. Mycol. 3 (1), 13–19. doi:10.29252/cmm.3.1.13
Arendrup, M. C. (2014). Update on Antifungal Resistance in Aspergillus and Candida. Clin. Microbiol. Infect. 20 (Suppl. 6), 42–48. doi:10.1111/1469-0691.12513
Chinese Pharmacopoeia Commission (2020). Chinese Pharmacopoeia. Beijing: China Medical Science Press.
Desai, J. V., and Mitchell, A. P. (2015). Candida Albicans Biofilm Development and its Genetic Control. Microbiol. Spectr. 3 (3), 1–19. doi:10.1128/microbiolspec.MB-0005-2014
Dovnik, A., Golle, A., Novak, D., Arko, D., and Takač, I. (2015). Treatment of Vulvovaginal Candidiasis: a Review of the Literature. Acta Dermatovenerol Alp Pannonica Adriat. 24 (1), 5–7. doi:10.15570/actaapa.2015.2
Drummond, R. A., and Brown, G. D. (2011). The Role of Dectin-1 in the Host Defence against Fungal Infections. Curr. Opin. Microbiol. 14 (4), 392–399. doi:10.1016/j.mib.2011.07.001
E Silva, A. T. M., Pereira, V. V., Takahashi, J. A., Silva, R. R., and Duarte, L. P. (2018). Microwave-assisted Synthesis of Borneol Esters and Their Antimicrobial Activity. Nat. Prod. Res. 32 (14), 1714–1720. doi:10.1080/14786419.2017.1399380
Falsetta, M. L., Foster, D. C., Woeller, C. F., Pollock, S. J., Bonham, A. D., Haidaris, C. G., et al. (2015). Identification of Novel Mechanisms Involved in Generating Localized Vulvodynia Pain. Am. J. Obstet. Gynecol. 213 (1), 38.e12. doi:10.1016/j.ajog.2015.02.002
Faria, D. R., Sakita, K. M., Akimoto-Gunther, L. S., Kioshima, É. S., Svidzinski, T. I. E., and Bonfim-Mendonça, P. S. (2017). Cell Damage Caused by Vaginal Candida Albicans Isolates from Women with Different Symptomatologies. J. Med. Microbiol. 66 (8), 1225–1228. doi:10.1099/jmm.0.000547
Farr, A., Effendy, I., Frey Tirri, B., Hof, H., Mayser, P., Petricevic, L., et al. (2021). Guideline: Vulvovaginal Candidosis (AWMF 015/072, Level S2k). Mycoses. 64 (6), 583–602. doi:10.1111/myc.13248
Fazly, A., Jain, C., Dehner, A. C., Issi, L., Lilly, E. A., Ali, A., et al. (2013). Chemical Screening Identifies Filastatin, a Small Molecule Inhibitor of Candida Albicans Adhesion, Morphogenesis, and Pathogenesis. Proc. Natl. Acad. Sci. U S A. 110 (33), 13594–13599. doi:10.1073/pnas.1305982110
Felix, T. C., de Brito Röder, D. V. D., and Dos Santos Pedroso, R. (2019). Alternative and Complementary Therapies for Vulvovaginal Candidiasis. Folia Microbiol (Praha). 64 (2), 133–141. doi:10.1007/s12223-018-0652-x
Feng, W., Yang, J., Yang, L., Li, Q., Zhu, X., Xi, Z., et al. (2018). Research of Mrr1, Cap1 and MDR1 in Candida Albicans Resistant to Azole Medications. Exp. Ther. Med. 15 (2), 1217–1224. doi:10.3892/etm.2017.5518
Flowers, S. A., Colón, B., Whaley, S. G., Schuler, M. A., and Rogers, P. D. (2015). Contribution of Clinically Derived Mutations in ERG11 to Azole Resistance in Candida Albicans. Antimicrob. Agents Chemother. 59 (1), 450–460. doi:10.1128/AAC.03470-14
Gao, Y., Liang, G., Wang, Q., She, X., Shi, D., Shen, Y., et al. (2019). Different Host Immunological Response to C. Albicans by Human Oral and Vaginal Epithelial Cells. Mycopathologia. 184 (1), 1–12. doi:10.1007/s11046-018-0301-6
Gross, O., Gewies, A., Finger, K., Schäfer, M., Sparwasser, T., Peschel, C., et al. (2006). Card9 Controls a Non-TLR Signalling Pathway for Innate Anti-fungal Immunity. Nature. 442 (7103), 651–656. doi:10.1038/nature04926
Hardison, S. E., and Brown, G. D. (2012). C-type Lectin Receptors Orchestrate Antifungal Immunity. Nat. Immunol. 13 (9), 817–822. doi:10.1038/ni.2369
Huang, Z., Shen, C., Feng, H., Hou, X., Wu, W., and Zhang, J. (2019). Anti-inflammatory Effects of KBN Thixotropic Gel on Animal Models with Non-infective Inflammation and its Mechanism. J. Guangzhou Univ. Traditional Chin. Med. 36 (8), 1225–1231. doi:10.13359/j.cnki.gzxbtcm.2019.08.022
Huysamen, C., and Brown, G. D. (2009). The Fungal Pattern Recognition Receptor, Dectin-1, and the Associated Cluster of C-type Lectin-like Receptors. FEMS Microbiol. Lett. 290 (2), 121–128. doi:10.1111/j.1574-6968.2008.01418.x
Ji, J., Zhang, R., Li, H., Zhu, J., Pan, Y., and Guo, Q. (2020). Analgesic and Anti-inflammatory Effects and Mechanism of Action of Borneol on Photodynamic Therapy of Acne. Environ. Toxicol. Pharmacol. 75, 103329. doi:10.1016/j.etap.2020.103329
Joo, H. M., Kim, K. A., Myoung, K. S., Ahn, Y. T., Lee, J. H., Huh, C. S., et al. (2012). Lactobacillus Helveticus HY7801 Ameliorates Vulvovaginal Candidiasis in Mice by Inhibiting Fungal Growth and NF-Κb Activation. Int. Immunopharmacol. 14 (1), 39–46. doi:10.1016/j.intimp.2012.05.023
Lee, H., Jung, K. H., Park, S., Kil, Y. S., Chung, E. Y., Jang, Y. P., et al. (2014). Inhibitory Effects of Stemona Tuberosa on Lung Inflammation in a Subacute Cigarette Smoke-Induced Mouse Model. BMC Complement. Altern. Med. 14, 513. doi:10.1186/1472-6882-14-513
Li, M., Chen, Q., Shen, Y., and Liu, W. (2009). Candida Albicans Phospholipomannan Triggers Inflammatory Responses of Human Keratinocytes through Toll-like Receptor 2. Exp. Dermatol. 18 (7), 603–610. doi:10.1111/j.1600-0625.2008.00832.x
Li, X., Wu, X., Gao, Y., and Hao, L. (2019). Synergistic Effects and Mechanisms of Combined Treatment with Harmine Hydrochloride and Azoles for Resistant Candida Albicans. Front. Microbiol. 10, 2295. doi:10.3389/fmicb.2019.02295
Liu, Q., Luyten, W., Pellens, K., Wang, Y., Wang, W., Thevissen, K., et al. (2012). Antifungal Activity in Plants from Chinese Traditional and Folk Medicine. J. Ethnopharmacol. 143 (3), 772–778. doi:10.1016/j.jep.2012.06.019
Marchaim, D., Lemanek, L., Bheemreddy, S., Kaye, K. S., and Sobel, J. D. (2012). Fluconazole-resistant Candida Albicans Vulvovaginitis. Obstet. Gynecol. 120 (6), 1407–1414. doi:10.1097/aog.0b013e31827307b2
Mckloud, E., Delaney, C., Sherry, L., Kean, R., Williams, S., Metcalfe, R., et al. (2021). Recurrent Vulvovaginal Candidiasis: a Dynamic Interkingdom Biofilm Disease of Candida and Lactobacillus. mSystems. 6 (4), e0062221. doi:10.1128/mSystems.00622-21
Morais, V. O. J., Conceição Oliver, J., Latércia, T. D. A., Barbosa Padovan, A. C., Siqueira, C. E., and Caixeta, F. A. M. (2021). Detection of ERG11 Overexpression in Candida Albicans Isolates from Environmental Sources and Clinical Isolates Treated with Inhibitory and Subinhibitory Concentrations of Fluconazole. Mycoses. 64 (2), 220–227. doi:10.1111/myc.13208
Mukherjee, P. K., Chandra, J., Kuhn, D. M., and Ghannoum, M. A. (2003). Mechanism of Fluconazole Resistance in Candida Albicans Biofilms: Phase-specific Role of Efflux Pumps and Membrane Sterols. Infect. Immun. 71 (8), 4333–4340. doi:10.1128/iai.71.8.4333-4340.2003
Niu, H. X., Duan, Q. J., Shi, G. X., Wu, D. Q., Shao, J., Wang, T. M., et al. (2019). Inhibitory Effect of Extract of Coptidis Rhizoma on Invasion of Candida Albicans Hyphae In Vitro. Zhongguo Zhong Yao Za Zhi. 44 (1), 125–130. doi:10.19540/j.cnki.cjcmm.20180912.004
Park, S., and Perlin, D. S. (2005). Establishing Surrogate Markers for Fluconazole Resistance in Candida Albicans. Microb. Drug Resist. 11 (3), 232–238. doi:10.1089/mdr.2005.11.232
Peters, B. M., Palmer, G. E., Nash, A. K., Lilly, E. A., Fidel, P. L., and Noverr, M. C. (2014). Fungal Morphogenetic Pathways Are Required for the Hallmark Inflammatory Response during Candida Albicans Vaginitis. Infect. Immun. 82 (2), 532–543. doi:10.1128/IAI.01417-13
Pfaller, M. A., Diekema, D. J., and Sheehan, D. J. (2006). Interpretive Breakpoints for Fluconazole and Candida Revisited: a Blueprint for the Future of Antifungal Susceptibility Testing. Clin. Microbiol. Rev. 19 (2), 435–447. doi:10.1128/CMR.19.2.435-447.2006
Pristov, K. E., and Ghannoum, M. A. (2019). Resistance of Candida to Azoles and Echinocandins Worldwide. Clin. Microbiol. Infect. 25 (7), 792–798. doi:10.1016/j.cmi.2019.03.028
Pudziuvelyte, L., Liaudanskas, M., Jekabsone, A., Sadauskiene, I., and Bernatoniene, J. (2020). Elsholtzia Ciliata (Thunb.) Hyl. Extracts from Different Plant Parts: Phenolic Composition, Antioxidant, and Anti-inflammatory Activities. Molecules. 25 (5), 1153. doi:10.3390/molecules25051153
Ramage, G., Vande Walle, K., Wickes, B. L., and López-Ribot, J. L. (2001). Standardized Method for In Vitro Antifungal Susceptibility Testing of Candida Albicans Biofilms. Antimicrob. Agents Chemother. 45 (9), 2475–2479. doi:10.1128/AAC.45.9.2475-2479.2001
Rossoni, R. D., Dos Santos Velloso, M., Figueiredo, L. M. A., Martins, C. P., Jorge, A. O. C., and Junqueira, J. C. (2018). Clinical Strains of Lactobacillus Reduce the Filamentation of Candida Albicans and Protect Galleria Mellonella against Experimental Candidiasis. Folia Microbiol. (Praha). 63 (3), 307–314. doi:10.1007/s12223-017-0569-9
Sanglard, D., Ischer, F., Monod, M., and Bille, J. (1996). Susceptibilities of Candida Albicans Multidrug Transporter Mutants to Various Antifungal Agents and Other Metabolic Inhibitors. Antimicrob. Agents Chemother. 40 (10), 2300–2305. doi:10.1128/AAC.40.10.2300
Shin, D. S., and Eom, Y. B. (2019). Zerumbone Inhibits Candida Albicans Biofilm Formation and Hyphal Growth. Can. J. Microbiol. 65 (10), 713–721. doi:10.1139/cjm-2019-0155
Siikala, E., Rautemaa, R., Richardson, M., Saxen, H., Bowyer, P., and Sanglard, D. (2010). Persistent Candida Albicans Colonization and Molecular Mechanisms of Azole Resistance in Autoimmune Polyendocrinopathy-Candidiasis-Ectodermal Dystrophy (APECED) Patients. J. Antimicrob. Chemother. 65 (12), 2505–2513. doi:10.1093/jac/dkq354
Sobel, J. D., and Sobel, R. (2018). Current Treatment Options for Vulvovaginal Candidiasis Caused by Azole-Resistant Candida Species. Expert Opin. Pharmacother. 19 (9), 971–977. doi:10.1080/14656566.2018.1476490
Strasser, D., Neumann, K., Bergmann, H., Marakalala, M. J., Guler, R., Rojowska, A., et al. (2012). Syk Kinase-Coupled C-type Lectin Receptors Engage Protein Kinase C-δ to Elicit Card9 Adaptor-Mediated Innate Immunity. Immunity. 36 (1), 32–42. doi:10.1016/j.immuni.2011.11.015
Tam, J. M., Reedy, J. L., Lukason, D. P., Kuna, S. G., Acharya, M., Khan, N. S., et al. (2019). Tetraspanin CD82 Organizes Dectin-1 into Signaling Domains to Mediate Cellular Responses to Candida Albicans. J. Immunol. 202 (11), 3256–3266. doi:10.4049/jimmunol.1801384
Teymuri, M., Mamishi, S., Pourakbari, B., Mahmoudi, S., Ashtiani, M. T., Sadeghi, R. H., et al. (2015). Investigation of ERG11 Gene Expression Among Fluconazole-Resistant Candida Albicans: First Report from an Iranian Referral Paediatric Hospital. Br. J. Biomed. Sci. 72 (1), 28–31. doi:10.1080/09674845.2015.11666792
Thomson, D. D., Wehmeier, S., Byfield, F. J., Janmey, P. A., Caballero-Lima, D., Crossley, A., et al. (2015). Contact-induced Apical Asymmetry Drives the Thigmotropic Responses of Candida Albicans Hyphae. Cell. Microbiol. 17 (3), 342–354. doi:10.1111/cmi.12369
Trinh, H. T., Lee, I. A., Hyun, Y. J., and Kim, D. H. (2011). Artemisia Princeps Pamp. Essential Oil and its Constituents Eucalyptol and α-terpineol Ameliorate Bacterial Vaginosis and Vulvovaginal Candidiasis in Mice by Inhibiting Bacterial Growth and NF-Κb Activation. Planta Med. 77 (18), 1996–2002. doi:10.1055/s-0031-1280094
Van Acker, H., Van Dijck, P., and Coenye, T. (2014). Molecular Mechanisms of Antimicrobial Tolerance and Resistance in Bacterial and Fungal Biofilms. Trends Microbiol. 22 (6), 326–333. doi:10.1016/j.tim.2014.02.001
Van Daele, R., Spriet, I., Wauters, J., Maertens, J., Mercier, T., Van Hecke, S., et al. (2019). Antifungal Drugs: What Brings the Future? Med. Mycol. 57 (Suppl. ment_3), S328–S343. doi:10.1093/mmy/myz012
Wächtler, B., Wilson, D., and Hube, B. (2011). Candida Albicans Adhesion to and Invasion and Damage of Vaginal Epithelial Cells: Stage-specific Inhibition by Clotrimazole and Bifonazole. Antimicrob. Agents Chemother. 55 (9), 4436–4439. doi:10.1128/AAC.00144-11
Wagner, R. D., and Johnson, S. J. (2012). Probiotic Lactobacillus and Estrogen Effects on Vaginal Epithelial Gene Expression Responses to Candida Albicans. J. Biomed. Sci. 19 (1), 58. doi:10.1186/1423-0127-19-58
Wang, S., Zhang, D., Hu, J., Jia, Q., Xu, W., Su, D., et al. (2017). A Clinical and Mechanistic Study of Topical Borneol-Induced Analgesia. EMBO Mol. Med. 9 (6), 802–815. doi:10.15252/emmm.201607300
White, T. C., Holleman, S., Dy, F., Mirels, L. F., and Stevens, D. A. (2002). Resistance Mechanisms in Clinical Isolates of Candida Albicans. Antimicrob. Agents Chemother. 46 (6), 1704–1713. doi:10.1128/AAC.46.6.1704-1713.2002
Xu, Y., Quan, H., Wang, Y., Zhong, H., Sun, J., Xu, J., et al. (2017). Requirement for Ergosterol in Berberine Tolerance Underlies Synergism of Fluconazole and Berberine against Fluconazole-Resistant Candida Albicans Isolates. Front Cell Infect Microbiol. 7, 491. doi:10.3389/fcimb.2017.00491
Yan, Y., Tan, F., Miao, H., Wang, H., and Cao, Y. (2019). Effect of Shikonin against Candida Albicans Biofilms. Front. Microbiol. 10, 1085. doi:10.3389/fmicb.2019.01085
Yang, B., Kim, S., Kim, J. H., Lim, C., Kim, H., and Cho, S. (2019). Gentiana Scabra Bunge Roots Alleviates Skin Lesions of Contact Dermatitis in Mice. J. Ethnopharmacol. 233, 141–147. doi:10.1016/j.jep.2018.12.046
Yang, F., Ding, S., Liu, W., Liu, J., Zhang, W., Zhao, Q., et al. (2015). Antifungal Activity of 40 TCMs Used Individually and in Combination for Treatment of Superficial Fungal Infections. J. Ethnopharmacol. 163, 88–93. doi:10.1016/j.jep.2015.01.025
Yang, L., Wu, H., Qiu, W., Guo, L., Du, X., Yu, Q., et al. (2018). Pulsatilla Decoction Inhibits Candida Albicans Proliferation and Adhesion in a Mouse Model of Vulvovaginal Candidiasis via the Dectin-1 Signaling Pathway. J. Ethnopharmacol. 223, 51–62. doi:10.1016/j.jep.2018.05.018
Yano, J., and Fidel, P. L. (2011). Protocols for Vaginal Inoculation and Sample Collection in the Experimental Mouse Model of Candida Vaginitis. J. Vis. Exp. 58, 3382. doi:10.3791/3382
Yong, J., Zu, R., Huang, X., Ge, Y., and Li, Y. (2020). Synergistic Effect of Berberine Hydrochloride and Fluconazole against Candida Albicans Resistant Isolates. Front. Microbiol. 11, 1498. doi:10.3389/fmicb.2020.01498
Keywords: kangbainian lotion, fluconazole-resistant, vulvovaginal candidiasis, Candida albicans, dectin-1 signaling pathway
Citation: Chen Z, Luo T, Huang F, Yang F, Luo W, Chen G, Cao M, Wang F and Zhang J (2022) Kangbainian Lotion Ameliorates Vulvovaginal Candidiasis in Mice by Inhibiting the Growth of Fluconazole-Resistant Candida albicans and the Dectin-1 Signaling Pathway Activation. Front. Pharmacol. 12:816290. doi: 10.3389/fphar.2021.816290
Received: 16 November 2021; Accepted: 27 December 2021;
Published: 20 January 2022.
Edited by:
Priyia Pusparajah, Monash University Malaysia, MalaysiaReviewed by:
Letizia Angiolella, Sapienza University of Rome, ItalyEugénia Pinto, University of Porto, Portugal
Copyright © 2022 Chen, Luo, Huang, Yang, Luo, Chen, Cao, Wang and Zhang. This is an open-access article distributed under the terms of the Creative Commons Attribution License (CC BY). The use, distribution or reproduction in other forums is permitted, provided the original author(s) and the copyright owner(s) are credited and that the original publication in this journal is cited, in accordance with accepted academic practice. No use, distribution or reproduction is permitted which does not comply with these terms.
*Correspondence: Jun Zhang, zhangjun@gzucm.edu.cn; Fengyun Wang, wfycn2000@163.com
†These authors have contributed equally to this work and share first authorship