- 1Núcleo de Pesquisa em Morfologia e Imunologia Aplicada, NuPMIA, Faculdade de Medicina, Universidade de Brasília, Brasília, Brazil
- 2Programa de Pós-graduação em Medicina Tropical, PPGMT, Núcleo de Medicina Tropical, NMT, Faculdade de Medicina, UnB, Brasília, Brazil
- 3Instituto de Química, IQ, Universidade de São Paulo, São Paulo, Brazil
- 4Chemistry Department, Faculty of Science, Al-Azhar University, Nasr City-Cairo, Egypt
- 5IPEEC-CONICET, Consejo Nacional de Investigaciones Científicas y Técnicas, Puerto Madryn, Argentina
- 6LAQV/REQUIMTE, Departamento de Química e Bioquímica, Faculdade de Ciências da Universidade do Porto, Porto, Portugal
- 7Joseph Banks Laboratories, The Bridge, School of Chemistry, University of Lincoln, Lincoln, United Kingdom
- 8Bioprospectum, Lda, UPTEC, Porto, Portugal
Urodele amphibians (∼768 spp.), salamanders and newts, are a rich source of molecules with bioactive properties, especially those isolated from their skin secretions. These include pharmacological attributes, such as antimicrobial, antioxidant, vasoactive, immune system modulation, and dermal wound healing activities. Considering the high demand for new compounds to guide the discovery of new drugs to treat conventional and novel diseases, this review summarizes the characteristics of molecules identified in the skin of urodele amphibians. We describe urodele-derived peptides and alkaloids, with emphasis on their biological activities, which can be considered new scaffolds for the pharmaceutical industry. Although much more attention has been given to anurans, bioactive molecules produced by urodeles have the potential to be used for biotechnological purposes and stand as viable alternatives for the development of therapeutic agents.
Introduction
The skin of amphibians exerts a broad spectrum of functions, which are fundamental for their homeostasis and interaction with the environment. Different multicellular skin glands produce compounds involved in both vital processes and defense strategies (Clarke, 1997; Heiss et al., 2009). Mucous glands produce a slippery mucus film, made basically of mucopolysaccharides and mucoproteoglycans, which minimize underwater friction and also make the animal slippery to predators. Other functions of the mucus include the prevention of water loss and the maintenance of a moist surface for skin gas exchange when the animal leaves the water. Granular glands are classified into different types depending on their histological characteristics and, on the other hand, synthesize and release compounds (e.g., amines, peptides and alkaloids) that participate in several defense mechanisms against potential aggressors, which can be either large predators or pathogenic microorganisms (Bucciarelli et al., 2016; Demori et al., 2019). In the first case, the compounds might irritate mucous membranes, cause an unpleasant sensation (e.g., pain or distastefulness), or even be highly toxic and lethal for their aggressors. In the second case, antimicrobial substances, in association with the commensal microbiota, prevent the colonization or infection by fungi, bacteria, and viruses (Brunetti et al., 2021).
The skin secretions of amphibians have been historically used as ethno-pharmaceutical drugs in a variety of cultures. Extensive research on this topic has accumulated over the years and many of the molecules secreted by amphibians have been isolated and characterized to date. These were found to influence different processes in living systems, acting, for example, as myotropic, immunomodulatory, antibiotic, anti-inflammatory, and antioxidant compounds. For this reason, the skin of amphibians has been recognized as a storehouse of bioactive molecules with pharmacological potential. In this context, “bioactivity” refers to the ability of a molecule to exert a given effect in an organism that can be explored for biotechnological applications (e.g., food or pharmaceutical industries), regardless of its original (evolutionary) function in its source. Indeed, amphibian-derived bioactive molecules currently stand as possible substitutes for conventional drugs or as drug leads for the development of therapeutic agents. Among amphibian orders, much more attention has been given to anurans than to that given to caecilians and urodeles in the research of bioactive molecules.
The Urodela order comprises salamanders and newts currently distributed in North and Central America, Europe, Asia, and North Africa, with some species occurring in South America (Baitchman and Herman, 2015). The order is divided into Salamandroidea and Cryptobranchoidea suborders (Jia and Gao, 2016). Salamandroidea differs from its sister clade Cryptobranchoidea regarding anatomic characteristics as bones jaw and ribs, and internal fertilization, and includes the genus Amphiuma, Ambystoma, Plethodon, Salamandra, Tylotriton, and Taricha (Anderson, 2012; Jia and Gao, 2016). Like anurans, bioactive molecules produced by urodeles have the potential to be used for biotechnological purposes, which make this group of amphibians a promising alternative to develop products with application in human health (Afolabi et al., 2018). In this mini review, we provide a brief appraisal of molecules isolated from the skin of urodele amphibians with an overview of their biological activities, with emphasis on peptides and alkaloids.
Alkaloids
Alkaloids have been isolated from phylogenetically diverse organisms, including microorganisms (Zhang et al., 2012; Casciaro et al., 2019), plants (Lelario et al., 2019; Petruczynik et al., 2019), and animals (Dumbacher et al., 2000; Jones et al., 2012; Santos et al., 2012; Saporito et al., 2012; Cushnie et al., 2014; Jeckel et al., 2015; Ligabue-Braun and Carlini, 2015; Knepper et al., 2019). Alkaloids have been shown to possess important biological activities as a defense mechanism against microorganisms and predators (De Luca and Laflamme, 2001; Zhang et al., 2021). They are generally derived from dietary sources of these animals (Macfoy et al., 2005), but certain alkaloids, such as samandarins of salamanders, are synthesized de novo from cholesterol (Habermehl and Haaf, 1968; Daly et al., 2005).
The search for alkaloids in amphibians of the Urodela order was pioneered by Zalesky in 1866, who isolated an alkaloid named samandarine from fire salamander (Salamandra salamandra) secretion. Then, Schöpf and Habermehl described a series of steroidal alkaloids and elucidated their absolute configurations (Weitz and Wolfel, 1962; Habermehl, 1967; Knepper et al., 2019). To date, the alkaloids characterized from the skin secretion of fire salamanders include samanine (1), samandinine (2), samandenone (3), samandarone (4), samandarine (5), samandaridine (6), cycloneosamandione (7), O-Acetyl-samandarine (8) and isocycloneosamandaridine (9) (Habermehl, 1964; Habermehl, 1967; Habermehl and Vogel, 1969; Habermehl, 1971; Daly et al., 2005), samanone (10) and O-(S)-3-hydroxybutanoylsamandarine (11) (Knepper et al., 2019) (Figure 1). A study of toxins using gas chromatography/mass spectrometry confirmed the presence of samandarine and/or samandarone steroidal alkaloids in all species of Salamandra as well as in representatives of Lyciasalamandra group. Other salamandrids, such as Calotriton, Euproctus, Lissotriton, and Triturus, also present low concentrations of samandarone (Vences et al., 2014), evidencing the widespread of alkaloids in the skin secretion of this group of animals.
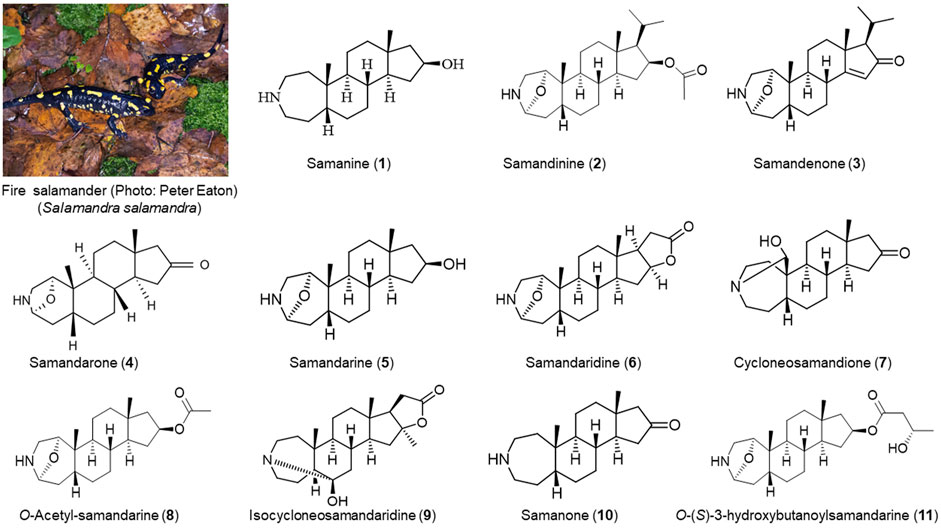
FIGURE 1. Alkaloids identified in the skin secretion of fire salamander (Salamandra salamandra). (1): (Habermehl, 1967; Habermehl and Haaf, 1968; Habermehl and Preusser, 1969; Habermehl, 1971); (2) (Habermehl and Vogel, 1969); (3,4,5,6, and 7): (Habermehl, 1964, Habermehl, 1967; Habermehl and Haaf, 1968; Habermehl and Preusser, 1969; Habermehl, 1971); (8) (Habermehl, 1964); (9) (Daly et al., 2005); (10,11) (Knepper et al., 2019).
Antibiotic resistance has become one of the major concerns for global health systems and the worldwide emergence of microbial resistance to available antibiotic drugs has led to an exhaustive search for new molecules with antimicrobial properties, and those from urodeles are valid alternatives. The alkaloids from the secretion of S. salamandra were able to inhibit the growth of fungi (Geotrichum candidum) and bacteria (Bacillus subtilis) (Habermehl and Preusser, 1969). Samandarone completely inhibited the growth of fungi (Phycomyces blakesleeanus) at a minimum effective concentration of 3 × 10−6 M. Samandarone and salamandarine from S. maculosa inhibit the growth of Escherichia coli, Proteus mirabilis, Bacillus subtilis, Phycomyces blakesleeanus, Saccharomyces cerevisiae, Geotrichum candidum, and Trichoderma viride in agar-diffusion tests. Samandarone presented the widest zones of inhibition for all the strains and also inhibited the growth of Penicillium expansum. Samandarone, samandarine, and samandaridine completely inhibited the growth of Saccharomyces cerevisiae at a minimum effective concentration of 1.5 × 10−6 M (Preusser et al., 1975). Although the performance of alkaloids as antibiotics against a variety of microorganisms stand out, their toxicity in major groups of vertebrates have also been documented discouraging its pharmacological use (von Byern et al., 2017). Indeed, alkaloids from urodele amphibians are known to be neurotoxins towards vertebrates (Phisalix, 1900; Mebs and Pogoda, 2005; Erjavec et al., 2017; Preißler et al., 2019).
Peptides
Peptides form another group of molecules with antibiotic activity produced by the skin of salamanders and newts, but with presumed (and in some cases, proven) low toxicity (Peters et al., 2010). Among these, antimicrobial peptides (AMPs) are found in several species (Jenssen et al., 2006). In general, antimicrobial peptides have between 12 and 100 amino acid residues, are cationic, amphipathic and have a range of typical secondary structures, as α-helices, β-sheets, and extended and flexible loops (Nguyen et al., 2011; Gao et al., 2017). The development of resistance by microorganisms to AMPs is considered relatively unlikely, mainly because they target the cell membrane directly. It has also been claimed that they have a poorly mutable structure, and present a wide range of epitopes, which makes their recognition difficult (Yeaman and Yount, 2003; Shen et al., 2018). Therefore, AMPs have been considered as alternative candidates for the development of new antimicrobial agents either alone (Nicola et al., 2019) or in combination with conventional antibiotics (Choi and Lee, 2012; Nuding et al., 2014).
In amphibians of the Urodela order, peptides with potential biological activities were isolated particularly from the skin secretion, although also from different parts of the animals (Table 1) (Pei et al., 2018). The peptide andricin 01 was isolated from the skin secretion of giant Chinese salamander Andrias davidianus. Andricin 01 showed antimicrobial activity against Gram-negative and Gram-positive bacteria however, it did not show cytotoxic activity to human hepatocytes or renal cells, and no hemolytic activity was observed (Pei and Jiang, 2017).
The 13.75 kDa peptide F15 was isolated from the skin of the red-backed salamander Plethodon cinereus. In a colony counting assay, F15 reduced S. aureus by 90% in 2 h, thus showing strong antibacterial activity against Gram-positive bacteria (Fredericks and Dankert, 2000). Another work identified CFBD-1 in the skin of the newt Cynops fudingens, a peptide of the β-defensin class, consisting of 41 amino acids, with a molecular mass of 4251.37 Da. This defensin was shown to be active mainly against the Gram-positive bacteria S. aureus, similarly to the previously described F15 peptide, with a minimum inhibitory concentration (MIC) of 65 μg/ml (Meng et al., 2013).
As a first approximation, numerous colleagues determined the antimicrobial activity of the total crude extract or the protein fraction of the skin secretion of several urodels. A study performed with the analysis of the total number of peptides isolated from the skin of salamander larvae and adults of the Ambystoma tigrinum species revealed antibacterial activity against Bacillus dendrobatidis, Staphylococcus aureus, and Klebsiella sp. (Sheafor et al., 2008). Studies with the crude extract of the cutaneous secretions of the species Lissotriton vulgaris and Triturus ivanbureschi showed that these secretions present a total of 18 and 20 protein fractions, respectively. These extracts showed antimicrobial activity for several bacteria, Escherichia coli and Enterococcus faecalis, and fungi, Candida albicans, strains, and hemolytic activity for human and rabbit red blood cells (Mert et al., 2018). The antimicrobial activity may be is a result of the action of active peptides (Pukala et al., 2006). In addition, they also showed high cytotoxic effects for cancer cell lines, with the highest anti-cancer activity of both secretion samples (L.vulgaris and T. ivanbureschi) being for the MDA-MB-231 breast cancer cell line (Mert et al., 2018). These preliminary findings highlight the importance of further research on the skin secretions of these animals, to characterize the molecules responsible for the antibiotic activities.
In addition to AMPs, other peptides isolated from urodeles can also have diverse pharmacological properties, such as antioxidant, and immunomodulatory activities (Coorens et al., 2017; Holthausen et al., 2017; Lee et al., 2019; Woodhams et al., 2020). Some examples of the last one is the neutralization of endotoxins, chemotaxis, and wound healing activities (Mangoni and Casciaro, 2020).
Reactive oxygen species (ROS) are necessary for the normal biochemical processes of cells (Litescu et al., 2011). However, in excessive amounts, when oxidative stress occurs, they can damage some structures, like DNA, proteins, and lipids (Duarte and Lunec, 2005). This process is related to cardiovascular disorders, diabetes, neurodegenerative diseases, cancer, chronic inflammatory diseases (Llesuy et al., 2001).
Synthetic chemical antioxidants often have low stability, cytotoxic, and carcinogenic effects, which led to search for natural antioxidants with low cytotoxicity (Gürlek et al., 2020). Salamandrin-I is a recently described molecule that showed relevant antioxidant activity. This molecule was the first peptide identified in the skin of the European fire salamander (S. salamandra). The peptide neutralizes the free radicals DPPH and ABTS at nontoxic concentrations for microglial cells and human red blood cells (Plácido et al., 2020). The discovery of this peptide paves the way for further investigation of other antioxidant peptides in the skin secretion of urodeles.
The medication currently available for wound healing is costly, has low activity, and produces hyperplastic scars necessitating the search for new drugs (Hardwicke et al., 2008). The amphibian peptides have shown potential as alternative treatment (Cao et al., 2018). The peptide tylotoin identified from the salamander Tylototriton verrucosus skin has shown to be effective in assay with dermal wounds in mice. It showed a similar wound healing capacity as the epidermal growth factor. The peptide has been shown to promote increased motility and proliferation of keratinocytes, vascular endothelial cells, and fibroblasts, resulting in accelerated re-epithelialization and formation of granulation tissue at the wound site. Tylotoin promotes the release of transforming growth factor β1 (TGF-β1) and Interleukin 6 (IL-6), which are essential in the wound healing response (Mu et al., 2014).
In general, due to its distinct biochemical and therapeutic properties, peptides are under development to disrupt protein-protein interactions and target or inhibit intracellular molecules such as proteinaceous receptors (Lee et al., 2019). Moreover, numerous peptide molecules were described to interact with microbial membranes through different mechanisms, destabilizing them, making peptides a unique class of pharmaceutical compounds (Vineeth Kumar and Sanil, 2017). The field of peptide drug discovery has evolved from both computational-aided rational design and combinatorial chemistry to the discovery of new molecules as stepping stones to new drug design, involving academic groups and private companies (de la Torre and Albericio, 2020; Al Shaer et al., 2020; Sampaio de Oliveira et al., 2020). With a high level of dynamism several peptide drugs are approved for clinical use in the United States, Europe, and Japan, and near 400 peptides are already in clinical development (Lee et al., 2019). The urodel peptides deserve more attention to clarify their structural features and mechanism of action.
Other Molecules
In addition to the alkaloids and peptides, other compounds have been described from salamanders and newts. CCK-TV is a molecule of the cholecystokinin class, a gastrointestinal hormone, identified from salamander skin Tylototriton verrucosus. CCK-TV showed potential for inducing muscle contraction isolated smooth striatum from the porcine gallbladder in concentrations range of 5.0 × 10−11 to 2.0 × 10−6 M (Jiang et al., 2015).
Dermal secretion analysis of salamander Plethodon cinereus, shows the presence of three fatty acids with antibacterial activity. The inhibition test showed that myristoleic acid, linolenic acid, and palmitoleic acid, inhibited the growth of Bacillus cereus in 24 h, at concentrations of 27, 7, and 6.9 µg, respectively, with halos of inhibition around 8.0–6.5 mm in diameter (Rickrode et al., 1986).
Another class of bioactive molecules found in amphibian skin are biogenic amines. Amines, such as putrescine, histamine, tryptamine, and phenylethylamine, are low molecular weight bases found in living organisms or as breakdown products of the fermentation process (Erspamer, 1971; Jovaisiene et al., 2017). Studies reported the presence of high concentrations of tryptamine and serotonin in the skin of salamander species (Erspamer, 1971; Roseghini et al., 1976; Luddecke et al., 2018).
Conclusion
Understanding the Earth’s biodiversity is important for describing genetic diversity and prospecting for bioactive molecules from diverse organisms. Skin secretion of amphibians of order Urodela are a unique source of alkaloids, peptides, biogenic amines and other compounds with diverse structures and functions to be considered as bioactive compounds. The studies compile in this review shows that steroidal alkaloids and peptides from Urodela skin secretions are considered the first line of defense against pathogens, however diverse limitations of the studies performed as difficulties to obtain toxins or to synthetize it by artificial methods made that the full capacity of these unique molecules as antimicrobials has not been properly studied and most of the studies described are still preliminary. In the case of peptides, the availability of synthetic methodology has provided access to explore the structures of analogues while in case of complex molecules, the partial or total synthesis can be more challenging specially when only putative compounds are inferred from the mass spectrometric data and the number of chiral centers and the number of possible diastereoisomers makes the task even more complex.
Despite considerable knowledge on alkaloids from urodeles, studies of secretions in terms as-of-yet unidentified antimicrobial components are needed to improve the current understanding of the complex toxin system of their skin (Luddecke et al., 2018). Urodele-derived molecules are underexplored therapeutic alternatives to conventional antibiotics used to treat fungal and bacterial infections.
Author Contributions
ALANB: Data curation; Methodology; Writing-original draft; Conceptualization. AH: Data curation; Methodology; Writing-original draft; Conceptualization. MM: Data curation; Methodology; Writing-original draft; Conceptualization. DCM: Data curation; Methodology; Writing-original draft; Conceptualization. PE: Data curation; Methodology; Writing-original draft; Funding acquisition; Conceptualization. AP: Data curation; Methodology; Writing-original draft; Funding acquisition; Conceptualization. MJK: Data curation; Methodology; Writing-original draft; Funding acquisition; Conceptualization. JRSAL: Data curation; Methodology; Writing-original draft; Funding acquisition; Conceptualization.
Funding
This study is part of the project VIDA-FROG, which is funded by Fundação para a Ciência e a Tecnologia (FCT, Portugal), grant number PTDC/BII-BIO/31158/2017, and Fundação de Amparo à Pesquisa do Estado de São Paulo (FAPESP, Brazil), grant number 2018/07999-7. A.P. is a recipient of a post-doctoral grant from the same project (FCT, PTDC/BII-BIO/31158/2017). This study was financed in part by the Coordenação de Aperfeiçoamento de Pessoal de Nível Superior (CAPES, Brazil) - Finance Code 001.
Conflict of Interest
The authors declare that the research was conducted in the absence of any commercial or financial relationships that could be construed as a potential conflict of interest.
Publisher’s Note
All claims expressed in this article are solely those of the authors and do not necessarily represent those of their affiliated organizations, or those of the publisher, the editors and the reviewers. Any product that may be evaluated in this article, or claim that may be made by its manufacturer, is not guaranteed or endorsed by the publisher.
Acknowledgments
MM thanks the support of Consejo Nacional de Investigaciones Científicas y Técnicas (Puerto Madryn, Argentina).
References
Afolabi, L. T., Saeed, F., Hashim, H., and Petinrin, O. O. (2018). Ensemble Learning Method for the Prediction of New Bioactive Molecules. PLoS One 13 (1), e0189538. doi:10.1371/journal.pone.0189538
Al Shaer, D., Al Musaimi, O., Albericio, F., and de la Torre, B. G. (2020). 2019 FDA TIDES (Peptides and Oligonucleotides) Harvest. Pharmaceuticals (Basel) 13 (3). doi:10.3390/ph13030040
Anderson, J. S. (2012). Fossils, Molecules, Divergence Times, and the Origin of Salamandroidea. Proc. Natl. Acad. Sci. U S A. 109 (15), 5557–5558. doi:10.1073/pnas.1202491109
Baitchman, E. J., and Herman, T. A. (2015). “Caudata (Urodela),” in Fowler's Zoo and Wild Animal Medicine. Editors R. E. Miller, and M. E. Fowler (St. Louis: W.B. Saunders), 8, 13–20. doi:10.1016/b978-1-4557-7397-8.00002-5
Brunetti, A. E., Bunk, B., Lyra, M. L., Fuzo, C. A., Marani, M. M., Spröer, C., et al. (2021). Molecular Basis of a Bacterial-Amphibian Symbiosis Revealed by Comparative Genomics, Modeling, and Functional Testing. ISME J. doi:10.1038/s41396-021-01121-7
Bucciarelli, G. M., Green, D. B., Shaffer, H. B., and Kats, L. B. (2016). Individual Fluctuations in Toxin Levels Affect Breeding Site Fidelity in a Chemically Defended Amphibian. Proc. Biol. Sci. 283 (1831). doi:10.1098/rspb.2016.0468
Cao, X., Wang, Y., Wu, C., Li, X., Fu, Z., Yang, M., et al. (2018). Cathelicidin-OA1, a Novel Antioxidant Peptide Identified from an Amphibian, Accelerates Skin Wound Healing. Sci. Rep. 8 (1), 943. doi:10.1038/s41598-018-19486-9
Casciaro, B., Calcaterra, A., Cappiello, F., Mori, M., Loffredo, M. R., Ghirga, F., et al. (2019). Nigritanine as a New Potential Antimicrobial Alkaloid for the Treatment of Staphylococcus Aureus-Induced Infections. Toxins (Basel) 11 (9). doi:10.3390/toxins11090511
Choi, H., and Lee, D. G. (2012). Synergistic Effect of Antimicrobial Peptide Arenicin-1 in Combination with Antibiotics against Pathogenic Bacteria. Res. Microbiol. 163 (6-7), 479–486. doi:10.1016/j.resmic.2012.06.001
Clarke, B. T. (1997). The Natural History of Amphibian Skin Secretions, Their normal Functioning and Potential Medical Applications. Biol. Rev. Camb Philos. Soc. 72 (3), 365–379. doi:10.1017/s0006323197005045
Coorens, M., Scheenstra, M. R., Veldhuizen, E. J., and Haagsman, H. P. (2017). Interspecies Cathelicidin Comparison Reveals Divergence in Antimicrobial Activity, TLR Modulation, Chemokine Induction and Regulation of Phagocytosis. Sci. Rep. 7, 40874. doi:10.1038/srep40874
Cushnie, T. P., Cushnie, B., and Lamb, A. J. (2014). Alkaloids: an Overview of Their Antibacterial, Antibiotic-Enhancing and Antivirulence Activities. Int. J. Antimicrob. Agents 44 (5), 377–386. doi:10.1016/j.ijantimicag.2014.06.001
Daly, J. W., Spande, T. F., and Garraffo, H. M. (2005). Alkaloids from Amphibian Skin: a Tabulation of over Eight-Hundred Compounds. J. Nat. Prod. 68 (10), 1556–1575. doi:10.1021/np0580560
De Luca, V., and Laflamme, P. (2001). The Expanding Universe of Alkaloid Biosynthesis. Curr. Opin. Plant Biol. 4 (3), 225–233. doi:10.1016/s1369-5266(00)00165-5
Demori, I., Rashed, Z. E., Corradino, V., Catalano, A., Rovegno, L., Queirolo, L., et al. (2019). Peptides for Skin Protection and Healing in Amphibians. Molecules 24 (2). doi:10.3390/molecules24020347
Duarte, T. L., and Lunec, J. (2005). Review: When Is an Antioxidant Not an Antioxidant? A Review of Novel Actions and Reactions of Vitamin C. Free Radic. Res. 39 (7), 671–686. doi:10.1080/10715760500104025
Dumbacher, J. P., Spande, T. F., and Daly, J. W. (2000). Batrachotoxin Alkaloids from Passerine Birds: A Second Toxic Bird Genus (Ifrita Kowaldi) from New Guinea. Proc. Natl. Acad. Sci. U S A. 97 (24), 12970–12975. doi:10.1073/pnas.200346897
Erjavec, V., Lukanc, B., and Žel, J. (2017). Intoxication of a Dog with Alkaloids of the Fire Salamander. Medycyna Weterynaryjna 73, 186–188. doi:10.21521/mw.5648
Erspamer, V. (1971). Biogenic Amines and Active Polypeptides of the Amphibian Skin. Annu. Rev. Pharmacol. 11, 327–350. doi:10.1146/annurev.pa.11.040171.001551
Fredericks, L. P., and Dankert, J. R. (2000). Antibacterial and Hemolytic Activity of the Skin of the Terrestrial Salamander, Plethodon cinereus. J. Exp. Zool 287, 3402–3459. doi:10.1002/1097-010X(20001001)287:53.0.COdoi:10.1002/1097-010x(20001001)287:5<340:aid-jez2>3.0.co;2-9
Gao, Y., Wu, D., Wang, L., Lin, C., Ma, C., Xi, X., et al. (2017). Targeted Modification of a Novel Amphibian Antimicrobial Peptide from Phyllomedusa tarsius to Enhance its Activity against MRSA and Microbial Biofilm. Front. Microbiol. 8, 628. doi:10.3389/fmicb.2017.00628
Gürlek, C., Yarkent, Ç., Köse, A., Tuğcu, B., Gebeloğlu, I. K., Öncel, S. Ş., et al. (2020). Screening of Antioxidant and Cytotoxic Activities of Several Microalgal Extracts with Pharmaceutical Potential. Health Technol. 10 (1), 111–117. doi:10.1007/s12553-019-00388-3
Habermehl, G., and Haaf, A. (1968). Cholesterol as the First Step in the Biosynthesis of the Salamander Alkaloids. Chem. Ber 101 (1), 198–200. doi:10.1002/cber.19681010126
Habermehl, G., and Vogel, G. (1969). Samandinine, a Minor Alkaloid from Salamandra Maculosa Laur. Toxicon 7 (2), 163–164. doi:10.1016/0041-0101(69)90080-4
Habermehl, G. (1967). “Chapter 9 the Steroid Alkaloids: The Salamandra Group,” in The Alkaloids: Chemistry and Physiology. Editor R. H. F. Manske (Academic Press), 427–439. doi:10.1016/s1876-0813(08)60205-5
Habermehl, G. (1964). O-Acetyl-samandarin im Gift von Salamandra Maculosa. Justus Liebigs Ann. Chem. 679 (1), 164–167. doi:10.1002/jlac.19646790123
Habermehl, G., and Preusser, H.-J. (1969). Hemmung des Wachstums von Pilzen und Bakterien durch das Hautdrüsensekret von Salamandra maculosa. Z. für Naturforschung B 24(12), 1599–1601. doi: doi:doi:10.1515/znb-1969-1220
Habermehl, G. (1971). “Toxicology, Pharmacology, Chemistry, and Biochemistry of Salamander Venom,” in Venomous Animals and Their Venoms. Editors W. BÜCherl, and E. E. Buckley (Academic Press), 569–584. doi:10.1016/b978-0-12-138902-4.50029-5
Hardwicke, J., Schmaljohann, D., Boyce, D., and Thomas, D. (2008). Epidermal Growth Factor Therapy and Wound Healing-Ppast, Present and Future Perspectives. Surgeon 6 (3), 172–177. doi:10.1016/S1479-666X(08)80114-X
Heiss, E., Natchev, N., Rabanser, A., Weisgram, J., and Hilgers, H. (2009). Three Types of Cutaneous Glands in the Skin of the Salamandrid Pleurodeles waltl. A Histological and Ultrastructural Study. J. Morphol. 270 (7), 892–902. doi:10.1002/jmor.10728
Holthausen, D. J., Lee, S. H., Kumar, V. T., Bouvier, N. M., Krammer, F., Ellebedy, A. H., et al. (2017). An Amphibian Host Defense Peptide Is Virucidal for Human H1 Hemagglutinin-Bearing Influenza Viruses. Immunity 46 (4), 587–595. doi:10.1016/j.immuni.2017.03.018
Jeckel, A. M., Grant, T., and Saporito, R. A. (2015). Sequestered and Synthesized Chemical Defenses in the Poison Frog Melanophryniscus moreirae. J. Chem. Ecol. 41 (5), 505–512. doi:10.1007/s10886-015-0578-6
Jenssen, H., Hamill, P., and Hancock, R. E. (2006). Peptide Antimicrobial Agents. Clin. Microbiol. Rev. 19 (3), 491–511. doi:10.1128/CMR.00056-05
Jia, J., and Gao, K. Q. (2016). A New Basal Salamandroid (Amphibia, Urodela) from the Late Jurassic of Qinglong, Hebei Province, China. PLoS One 11 (5), e0153834. doi:10.1371/journal.pone.0153834
Jiang, W. B., Hakim, M., Luo, L., Li, B. W., Yang, S. L., Song, Y. Z., et al. (2015). Purification and Characterization of Cholecystokinin from the Skin of Salamander Tylototriton verrucosus. Dongwuxue Yanjiu 36 (3), 174–177.
Jones, T. H., Adams, R. M., Spande, T. F., Garraffo, H. M., Kaneko, T., and Schultz, T. R. (2012). Histrionicotoxin Alkaloids Finally Detected in an Ant. J. Nat. Prod. 75 (11), 1930–1936. doi:10.1021/np300485v
Jovaišienė, J., Bakutis, B., Baliukonienė, V., Matusevičius, P., Lipiński, K., Antoszkiewicz, Z., et al. (2017). Biogenic Amines and Mycotoxins Concentrations in Baled Silage from Organic and Conventional Farms. Arq. Bras. Med. Vet. Zootec. 69, 269–277. doi:10.1590/1678-4162-9130
Karış, M., Şener, D., Yalçın, H. T., Nalbantsoy, A., and Göçmen, B. (2018). Major Biological Activities and Protein Profiles of Skin Secretions of Lissotriton vulgaris and Triturus Ivanbureschi. Turkish J. Biochem. 43 (6), 605–612. doi:10.1515/tjb-2017-0306
Knepper, J., Lüddecke, T., Preissler, K., Vences, M., and Schulz, S. (2019). Isolation and Identification of Alkaloids from Poisons of Fire Salamanders ( Salamandra salamandra). J. Nat. Prod. 82 (5), 1319–1324. doi:10.1021/acs.jnatprod.9b00065
Lee, A. C., Harris, J. L., Khanna, K. K., and Hong, J. H. (2019). A Comprehensive Review on Current Advances in Peptide Drug Development and Design. Int. J. Mol. Sci. 20 (10). doi:10.3390/ijms20102383
Lelario, F., De Maria, S., Rivelli, A. R., Russo, D., Milella, L., Bufo, S. A., et al. (2019). A Complete Survey of Glycoalkaloids Using LC-FTICR-MS and IRMPD in a Commercial Variety and a Local Landrace of Eggplant (Solanum Melongena L.) and Their Anticholinesterase and Antioxidant Activities. Toxins (Basel) 11 (4). doi:10.3390/toxins11040230
Ligabue-Braun, R., and Carlini, C. R. (2015). Poisonous Birds: A Timely Review. Toxicon 99, 102–108. doi:10.1016/j.toxicon.2015.03.020
Litescu, S. C., Eremia, S., Diaconu, M., Tache, A., and Radu, G. (2011). Biosensors Applications on Assessment of Reactive Oxygen Species and Antioxidants.
Llesuy, S., Evelson, P., Campos, A. M., and Lissi, E. (2001). Methodologies for Evaluation of Total Antioxidant Activities in Complex Mixtures. A Critical Review. Biol. Res. 34, 51–73. doi:10.4067/s0716-97602001000200009
Lüddecke, T., Schulz, S., Steinfartz, S., and Vences, M. (2018). A Salamander's Toxic Arsenal: Review of Skin Poison Diversity and Function in True Salamanders, Genus Salamandra. Naturwissenschaften 105 (9-10), 56. doi:10.1007/s00114-018-1579-4
Macfoy, C., Danosus, D., Sandit, R., Jones, T. H., Garraffo, H. M., Spande, T. F., et al. (2005). Alkaloids of Anuran Skin: Antimicrobial Function. Z. Naturforsch C J. Biosci. 60 (11-12), 932–937. doi:10.1515/znc-2005-11-1218
Makarova, M., Rycek, L., Hajicek, J., Baidilov, D., and Hudlicky, T. (2019). Tetrodotoxin: History, Biology, and Synthesis. Angew. Chem. Int. Ed. Engl. 58 (51), 18338–18387. doi:10.1002/anie.201901564
Mangoni, M. L., and Casciaro, B. (2020). Development of Antimicrobial Peptides from Amphibians. Antibiotics (Basel) 9 (11). doi:10.3390/antibiotics9110772
Mebs, D., and Pogoda, W. (2005). Variability of Alkaloids in the Skin Secretion of the European Fire Salamander (Salamandra Salamadra Terrestris). Toxicon 45 (5), 603–606. doi:10.1016/j.toxicon.2005.01.001
Meng, P., Yang, S., Shen, C., Jiang, K., Rong, M., and Lai, R. (2013). The First Salamander Defensin Antimicrobial Peptide. PLoS One 8 (12), e83044. doi:10.1371/journal.pone.0083044
Mookherjee, N., and Hancock, R. E. (2007). Cationic Host Defence Peptides: Innate Immune Regulatory Peptides as a Novel Approach for Treating Infections. Cell Mol Life Sci 64 (7-8), 922–933. doi:10.1007/s00018-007-6475-6
Mu, L., Tang, J., Liu, H., Shen, C., Rong, M., Zhang, Z., et al. (2014). A Potential Wound-Healing-Promoting Peptide from Salamander Skin. FASEB J. 28 (9), 3919–3929. doi:10.1096/fj.13-248476
Nguyen, L. T., Haney, E. F., and Vogel, H. J. (2011). The Expanding Scope of Antimicrobial Peptide Structures and Their Modes of Action. Trends Biotechnol. 29 (9), 464–472. doi:10.1016/j.tibtech.2011.05.001
Nicola, A. M., Albuquerque, P., Paes, H. C., Fernandes, L., Costa, F. F., Kioshima, E. S., et al. (2019). Antifungal Drugs: New Insights in Research & Development. Pharmacol. Ther. 195, 21–38. doi:10.1016/j.pharmthera.2018.10.008
Nuding, S., Frasch, T., Schaller, M., Stange, E. F., and Zabel, L. T. (2014). Synergistic Effects of Antimicrobial Peptides and Antibiotics against Clostridium difficile. Antimicrob. Agents Chemother. 58 (10), 5719–5725. doi:10.1128/AAC.02542-14
Pei, J., and Jiang, L. (2017). Antimicrobial Peptide from Mucus of Andrias davidianus: Screening and Purification by Magnetic Cell Membrane Separation Technique. Int. J. Antimicrob. Agents 50 (1), 41–46. doi:10.1016/j.ijantimicag.2017.02.013
Pei, J., Feng, Z., Ren, T., Sun, H., Han, H., Jin, W., et al. (2018). Purification, Characterization and Application of a Novel Antimicrobial Peptide from Andrias davidianus Blood, 1472–765X. (Electronic)).
Peters, B. M., Shirtliff, M. E., and Jabra-Rizk, M. A. (2010). Antimicrobial Peptides: Primeval Molecules or Future Drugs. Plos Pathog. 6 (10), e1001067. doi:10.1371/journal.ppat.1001067
Petruczynik, A., Plech, T., Tuzimski, T., Misiurek, J., Kaproń, B., Misiurek, D., et al. (2019). Determination of Selected Isoquinoline Alkaloids from Mahonia Aquifolia; Meconopsis Cambrica; Corydalis Lutea; Dicentra Spectabilis; Fumaria Officinalis; Macleaya Cordata Extracts by HPLC-DAD and Comparison of Their Cytotoxic Activity. Toxins 11 (10), 575. doi:10.3390/toxins11100575
Phisalix, M. (1900). Recherches embryologiques, histologiques et physiologiques sur les glandes à venin de la salamandre terrestre. Pariséditeurs: Librairie C. Reinwald, Schleicher frères.
Plácido, A., Bueno, J., Barbosa, E. A., Moreira, D. C., Dias, J. D. N., Cabral, W. F., et al. (2020). The Antioxidant Peptide Salamandrin-I: First Bioactive Peptide Identified from Skin Secretion of Salamandra Genus (Salamandra salamandra). Biomolecules 10 (4). doi:10.3390/biom10040512
Preißler, K., Gippner, S., Lüddecke, T., Krause, E. T., Schulz, S., Vences, M., et al. (2019). More Yellow More Toxic? Sex rather Than Alkaloid Content Is Correlated with Yellow Coloration in the Fire Salamander. J. Zool 308 (4), 293–300. doi:10.1111/jzo.12676
Preusser, H. J., Habermehl, G., Sablofski, M., and Schmall-Haury, D. (1975). Antimicrobial Activity of Alkaloids from Amphibian Venoms and Effects on the Ultrastructure of Yeast Cells. Toxicon 13 (4), 285–289. doi:10.1016/0041-0101(75)90135-X
Pukala, T. L., Bowie, J. H., Maselli, V. M., Musgrave, I. F., and Tyler, M. J. (2006). Host-defence Peptides from the Glandular Secretions of Amphibians: Structure and Activity. Nat. Prod. Rep. 23 (3), 368–393. doi:10.1039/b512118n
Rickrode, T. E., Mueller, C. F., and Taylor, D. (1986). Identification and Antibiotic Activity of Fatty Acids in Dermal Secretions of Plethodon cinereus. Am. Midland Naturalist 115 (1), 198–200. doi:10.2307/2425850
Roseghini, M., Erspamer, V., and Endean, R. (1976). Indole-, Imidazole- and Phenyl-Alkylamines in the Skin of One Hundred Amphibian Species from Australia and Papua New Guinea. Comp. Biochem. Physiol. C Comp. Pharmacol. 54 (1), 31–43. doi:10.1016/0306-4492(76)90022-8
Sampaio de Oliveira, K. B., Leite, M. L., Rodrigues, G. R., Duque, H. M., da Costa, R. A., Cunha, V. A., et al. (2020). Strategies for Recombinant Production of Antimicrobial Peptides with Pharmacological Potential. Expert Rev. Clin. Pharmacol. 13 (4), 367–390. doi:10.1080/17512433.2020.1764347
Santos, I. J. M., Melo Coutinho, H. D., Ferreira Matias, E. F., Martins da Costa, J. G., Nóbrega Alves, R. R., and de Oliveira Almeida, W. (2012). Antimicrobial Activity of Natural Products from the Skins of the Semiarid Living Lizards Ameiva ameiva (Linnaeus, 1758) and Tropidurus Hispidus (Spix, 1825). J. Arid Environments 76, 138–141. doi:10.1016/j.jaridenv.2011.08.005
Saporito, R. A., Donnelly, M. A., Spande, T. F., and Garraffo, H. M. (2012). A Review of Chemical Ecology in Poison Frogs. Chemoecology 22 (3), 159–168. doi:10.1007/s00049-011-0088-0
Sheafor, B., Davidson, E. W., Parr, L., and Rollins-Smith, L. (2008). Antimicrobial Peptide Defenses in the Salamander, Ambystoma tigrinum, against Emerging Amphibian Pathogens. J. Wildl. Dis. 44 (2), 226–236. doi:10.7589/0090-3558-44.2.226
Shen, W., He, P., Xiao, C., and Chen, X. (2018). From Antimicrobial Peptides to Antimicrobial Poly(α-Amino Acid)s. Adv. Healthc. Mater. 7 (20), e1800354. doi:10.1002/adhm.201800354
Teranishi, H., Muneoka, Y., Takao, T., Shimonishi, Y., and Kojima, M. (2004). Isolation and Characterization of Four VIP-Related Peptides from Red-Bellied Newt, Cynops pyrrhogaster. Regul. Pept. 123 (1-3), 173–179. doi:10.1016/j.regpep.2004.04.024
Vences, M., Sanchez, E., Hauswaldt, J. S., Eikelmann, D., Rodríguez, A., Carranza, S., et al. (2014). Nuclear and Mitochondrial Multilocus Phylogeny and Survey of Alkaloid Content in True Salamanders of the Genus Salamandra (Salamandridae). Mol. Phylogenet. Evol. 73, 208–216. doi:10.1016/j.ympev.2013.12.009
Vineeth Kumar, T. V., and Sanil, G. (2017). A Review of the Mechanism of Action of Amphibian Antimicrobial Peptides Focusing on Peptide-Membrane Interaction and Membrane Curvature. Curr. Protein Pept. Sci. 18 (12), 1263–1272. doi:10.2174/1389203718666170710114932
von Byern, J., Mebs, D., Heiss, E., Dicke, U., Wetjen, O., Bakkegard, K., et al. (2017). Salamanders on the Bench - A Biocompatibility Study of Salamander Skin Secretions in Cell Cultures. Toxicon 135, 24–32. doi:10.1016/j.toxicon.2017.05.021
Weitz, G., and Wölfel, E. (1962). The Structure of Salamander Alkaloids. I. On the Structure of Samandarine-Hydrobromide. Acta Cryst. 15(5), 484–491. doi:10.1107/S0365110X6200119X
Woodhams, D. C., Rollins-Smith, L. A., Reinert, L. K., Lam, B. A., Harris, R. N., Briggs, C. J., et al. (2020). Probiotics Modulate a Novel Amphibian Skin Defense Peptide that Is Antifungal and Facilitates Growth of Antifungal Bacteria. Microb. Ecol. 79 (1), 192–202. doi:10.1007/s00248-019-01385-9
Yeaman, M. R., and Yount, N. Y. (2003). Mechanisms of Antimicrobial Peptide Action and Resistance. Pharmacol. Rev. 55 (1), 27–55. doi:10.1124/pr.55.1.2
Zhang, J., Morris-Natschke, S. L., Ma, D., Shang, X. F., Yang, C. J., Liu, Y. Q., et al. (2021). Biologically Active Indolizidine Alkaloids. Med. Res. Rev. 41 (2), 928–960. doi:10.1002/med.21747
Keywords: amphibians, urodela, bioactive molecules, peptides, alkaloids
Citation: Barros ALAN, Hamed A, Marani M, Moreira DC, Eaton P, Plácido A, Kato MJ and Leite JRSA (2022) The Arsenal of Bioactive Molecules in the Skin Secretion of Urodele Amphibians. Front. Pharmacol. 12:810821. doi: 10.3389/fphar.2021.810821
Received: 08 November 2021; Accepted: 24 December 2021;
Published: 14 January 2022.
Edited by:
Jean-Marc Sabatier, Aix-Marseille Université, FranceReviewed by:
Sarah Woodley, Duquesne University, United StatesElvira Brunelli, University of Calabria, Italy
Copyright © 2022 Barros, Hamed, Marani, Moreira, Eaton, Plácido, Kato and Leite. This is an open-access article distributed under the terms of the Creative Commons Attribution License (CC BY). The use, distribution or reproduction in other forums is permitted, provided the original author(s) and the copyright owner(s) are credited and that the original publication in this journal is cited, in accordance with accepted academic practice. No use, distribution or reproduction is permitted which does not comply with these terms.
*Correspondence: José Roberto S. A. Leite, jrsaleite@gmail.com, jrleite@pq.cnpq.br; Massuo J. Kato, majokato@iq.usp.br