- Laboratorio de Etnofarmacología, Departamento de Biología Celular, Facultad de Ciencias, Universidad Nacional Autónoma de México, Mexico City, Mexico
Liver plays a pivotal role in maintaining blood glucose levels through complex processes which involve the disposal, storage, and endogenous production of this carbohydrate. Insulin is the hormone responsible for regulating hepatic glucose production and glucose storage as glycogen, thus abnormalities in its function lead to hyperglycemia in obese or diabetic patients because of higher production rates and lower capacity to store glucose. In this context, two different but complementary therapeutic approaches can be highlighted to avoid the hyperglycemia generated by the hepatic insulin resistance: 1) enhancing insulin function by inhibiting the protein tyrosine phosphatase 1B, one of the main enzymes that disrupt the insulin signal, and 2) direct regulation of key enzymes involved in hepatic glucose production and glycogen synthesis/breakdown. It is recognized that medicinal plants are a valuable source of molecules with special properties and a wide range of scaffolds that can improve hepatic glucose metabolism. Some molecules, especially phenolic compounds and terpenoids, exhibit a powerful inhibitory capacity on protein tyrosine phosphatase 1B and decrease the expression or activity of the key enzymes involved in the gluconeogenic pathway, such as phosphoenolpyruvate carboxykinase or glucose 6-phosphatase. This review shed light on the progress made in the past 7 years in medicinal plants capable of improving hepatic glucose homeostasis through the two proposed approaches. We suggest that Coreopsis tinctoria, Lithocarpus polystachyus, and Panax ginseng can be good candidates for developing herbal medicines or phytomedicines that target inhibition of hepatic glucose output as they can modulate the activity of PTP-1B, the expression of gluconeogenic enzymes, and the glycogen content.
Introduction
Diabetes mellitus (DM) is a chronic metabolic disease characterized by high blood sugar levels (hyperglycemia), caused by insulin malfunctioning, deficient insulin secretion, or both (Liu et al., 2019). Type 2 diabetes (T2D) is the most important type of DM due to its high worldwide prevalence (American Diabetes Association, 2021). It is characterized by insulin resistance, which is defined as a poor response of insulin-sensitive tissues to normal insulin concentration (Mlinar et al., 2007). The main cause of insulin resistance has been associated to an obesogenic environment in which large amounts of free fatty acids and adipokines are responsible for impairing insulin signaling by increasing serine phosphorylation that inhibits tyrosine phosphorylation of insulin receptor (IR) and insulin receptor substrates (IRSs) (DeFronzo et al., 2015). However, it has also been reported that protein tyrosine phosphatases (PTPs) could have a more important role since they are upregulated in insulin resistant states. Insulin action is negative regulated by PTPs, particularly the PTP-1B, because they promote the dephosphorylation of tyrosine residues of IR and IRSs (Saltiel and Kahn, 2001). When insulin signaling is impaired in liver by either insulin resistance or low insulin levels, the glucose storage and production is dysregulated, increasing the hepatic glucose output rates yielding hyperglycemia in diabetic patients.
Liver represents a crucial therapeutic target for treating hyperglycemia in T2D because hepatic glucose output is the pathophysiological abnormality that contributes the most to the hyperglycemic state in fasting and postprandial state as a consequence of hepatic insulin resistance (Sharabi et al., 2015). During the overnight fast (postabsorptive state), the liver of a normal person produces glucose at a rate of approximately 1.8–2 mg/kg. min. However, this rate increases around 0.5 mg/kg min in a patient with T2D, promoting a significant rise in the basal state of glucose production (Cersosimo et al., 2018). After food ingestion and the subsequent increase in insulin levels, the suppression of glucose production is slower in a diabetic patient, promoting an evident postprandial hyperglycemia due to the excess of glucose produced in addition to that from the exogenous source (Rizza, 2010).
Medicinal plants and natural products have shown to have numerous benefits on processes involved in glucose and lipid metabolism, leading to correct homeostasis imbalances that promote metabolic diseases such as T2D (Li J. et al., 2018; Xu L. et al., 2018; Saadeldeen et al., 2020). Unlike the classic “on-target” paradigm in pharmacology, namely a drug with a specific target, the polypharmacology approach, or the binding of a drug to more than one target, could be more effective against a disease as complex as T2D due to its multiple pathophysiological abnormalities (Reddy and Zhang, 2013). In this context, extract plants and phytochemicals isolated from medicinal plants exhibit multiple mechanisms of action on assorted metabolic targets that are involved in glucose homeostasis. Therefore, efforts have been made to describe all the beneficial effects on metabolism of these extracts and molecules in recent years.
The current review summarizes the medicinal plants reported from 2015 that can potentially decrease hyperglycemia resulting from imbalance in hepatic glucose metabolism by two different approaches: improving hepatic insulin resistance by inhibiting PTP-1B and decreasing hepatic glucose output by inhibiting rate-limiting enzymes involved in the storage and production of glucose.
Methodology
Two separate searches were performed based on the Preferred Reporting Items for Systematic Review and Meta-Analysis (PRISMA) (Page et al., 2021) in the following databases: Scopus, Clarivate and PubMed (Figure 1). The first involved studies related to extracts or phytochemicals tested against the activity or expression of PTP-1B enzyme, while in the second, studies with extracts or phytochemicals with an effect on the glucose-producing pathways were sought. Only records related to the study of medicinal plants and their isolated compounds were considered.
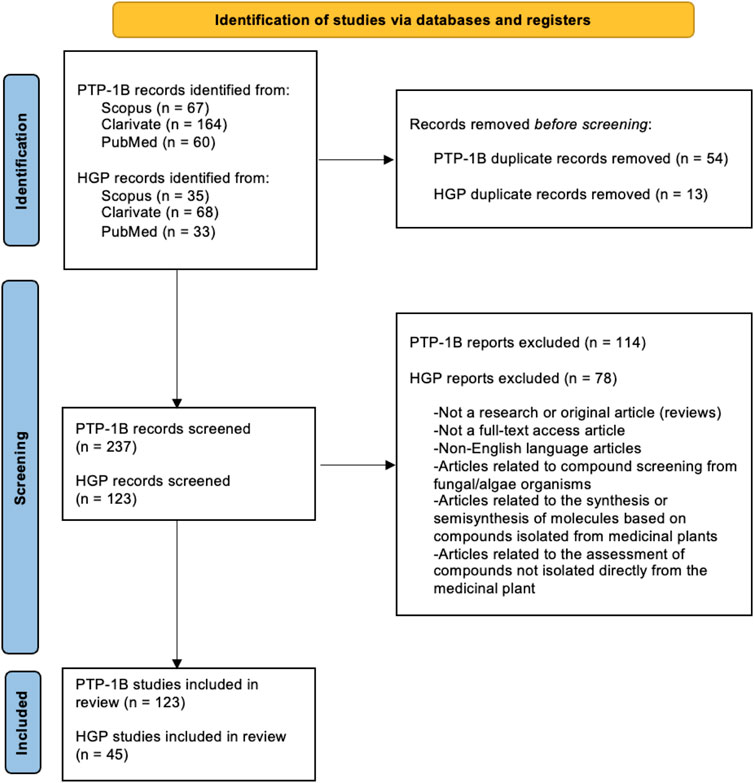
FIGURE 1. PRISMA flowchart. PTP-1B: protein tyrosine phosphatase 1B; HGP: hepatic glucose production.
Therapeutic Approaches to Reduce Hyperglycemia Resulting From Impaired Hepatic Glucose Homeostasis
Each insulin-sensitive tissue presents abnormal characteristics that contribute to hyperglycemia in an insulin-resistant state. The underlying mechanisms that give rise to insulin resistance converge on deficient insulin signalling that limits the activation of factors involved in energy metabolism. In obesity and T2D, insulin resistance has been linked mainly to defects in the signalling pathway of phosphatidylinositol 3-kinase and protein kinase B (PI3K/Akt), particularly to the Akt2 isoform (Cusi et al., 2000; Krook et al., 2000).
In normal conditions, the insulin secreted by pancreatic β cell binds to its receptor in the target cell, activating the tyrosine kinase activity, which promotes the receptor autophosphorylation and the subsequent phosphorylation of IRSs, mainly IRS-1 and IRS-2, in tyrosine residues. Afterwards, the enzyme P13K is recruited and activated by IRS to convert phosphatidylinositol 4,5-bisphosphate (PIP2) from the plasma membrane to phosphatidylinositol 3,4,5-triphosphate (PIP3), which facilitates the phosphorylation and activation of Akt at two important sites: by phosphoinositide-dependent kinase 1 (PDK1) at residue Thr308 of the catalytic domain, and by mammalian target rapamycin complex 2 (mTORC2) at residue Ser473 of the regulatory domain (Schultze et al., 2012). Specifically in liver, the activated Akt enzyme is responsible for phosphorylating different factors that are involved in the regulation of processes such as glycogen synthesis, gluconeogenesis, and glycogenolysis, which are activated or inhibited under different nutritional circumstances (Dimitriadis et al., 2021).
Due to hepatic insulin resistance, this hormone losses its ability to regulate glucose metabolism in liver, resulting in enhanced glucose output that contributes greatly to fasting and postprandial hyperglycemia, namely glycogen synthesis is reduced, and production of glucose is increased (Figure 2). Therefore, we proposed two approaches by which medicinal plants could ameliorated hyperglycemia through enhancing hepatic glucose metabolism: improving the function of insulin in the liver by inhibiting the enzyme PTP-1B and modulating the hepatic production/storage of glucose by regulating the enzymes involved in gluconeogenesis, glycogenolysis, and glycogenesis.
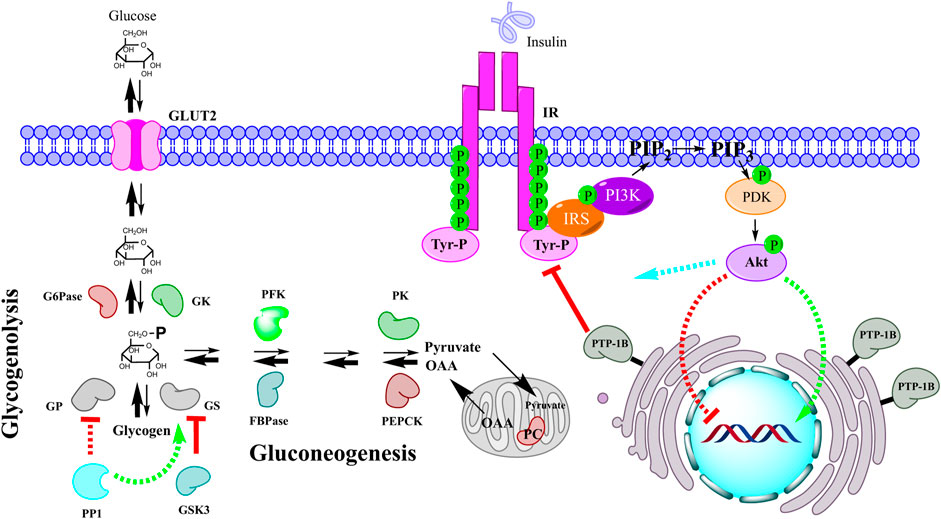
FIGURE 2. Impaired hepatic glucose homeostasis by insulin resistance. When insulin does not work properly either due to overexpression of PTP-1B or other factors, glucose production in liver is upregulated generating a hyperglycemic state. Both gluconeogenesis and glycogenolysis are enhanced due to poor insulin signaling, namely genetic expression of gluconeogenic enzymes is not repressed and enzymes related to glycogen metabolism are not adequately regulated. Akt functions: green color indicates positive regulation, red color indicates negative regulation, and blue color represents direct or indirect regulation by phosphorylation or allosterism. IR: insulin receptor; IRS: insulin receptor substrate; PI3K: phosphoinositide 3-kinase; PIP2: phosphatidylinositol 4,5-bisphosphate; PIP3: phosphatidylinositol 3,4,5-triphosphate; PDK: phosphoinositide-dependent kinase; Akt: protein kinase B; PTP-1B: protein tyrosine phosphatase 1B; PC: pyruvate carboxylase; OAA: oxalacetate; PEPCK: phosphoenolpyruvate carboxykinase; PK: pyruvate kinase; FBPase: fructose 1,6-bisphosphatase; PFK: phosphofructokinase; GS: glycogen synthase; GP: glycogen phosphorylase; PP1: protein phosphatase 1; GSK3: glycogen synthase kinase-3; GK: glucokinase; G6Pase: glucose 6-phosphatase; GLUT2: glucose transporter 2.
Inhibition of Protein Tyrosine Phosphatase 1B
The modification of proteins through phosphorylation and dephosphorylation of tyrosine residues represents one of the main mechanisms of cell signaling regulation (Alonso et al., 2016), which is carried out by two superfamilies of enzymes: protein tyrosine kinases (PTKs), and PTPs. In this regard, the classical PTP subfamily possess a domain of 240–250 amino acids characterized by a conserved site that exhibits a catalytic mechanism based on cysteine (Denu and Dixon, 1998). Specifically, the enzyme PTP-1B is a classic intracellular PTP widely distributed in mammalian tissues that is anchored on the cytoplasmic side of the endoplasmic reticulum membrane. Despite its localization, the PTP-1B enzyme can access its substrates located on the surface of the plasma membrane during endocytosis, biosynthesis, and by the movement of the endoplasmic reticulum towards the plasma membrane in specific regions (Bakke and Haj, 2015).
Since its first isolation from the human placenta in 1988 by Tonks et al., 1988 PTP-1B has become an attractive research object due to its direct link with the etiopathogenesis of insulin resistance. In addition to the processes promoted by the obesogenic inflammatory environment, such as the serine/threonine phosphorylation of IR and IRS, and their proteasomal degradation (Mlinar et al., 2007; Ahmed et al., 2021), the dephosphorylation of these components by PTP-1B has also been implied to the termination of the insulin signal (Ahmad et al., 1995; Kenner et al., 1996; Chen et al., 1997).
Experimental data obtained from various studies have shown that the PTP-1B enzyme is one of the main negative regulators of the insulin signaling pathway. For instance, studies performed in PTP-1B knock-out mice have been shown that the absence of this enzyme produces healthy organisms that exhibit enhanced insulin sensitivity, protection against the weight gain generated by high-fat diet, and increased hepatic phosphorylation of IR and IRS after an intraperitoneal insulin injection (Elchebly et al., 1999; Klaman et al., 2000). On the other hand, it has been reported an increased PTP-1B activity in hepatic cytosolic fractions isolated from streptozotocin (STZ)-hyperglycemic rats (Meyerovitch et al., 1989), while augmented hepatic microsomal enzyme activity, content of protein, and mRNA levels have only been observed after 2 weeks of insulin treatment in these insulinopenic organisms, suggesting that elevated insulin levels are necessary to modify PTP-1B content and activity, namely hyperinsulinemia caused by insulin resistance may lead to altered PTP-1B expression and activity (Ahmad and Goldstein, 1995). Additionally, it has also been shown that insulin rises hepatic microsomal PTP-1B activity in rat hepatoma cells (Hashimoto and Goldstein, 1992). Likewise, abnormal expression and activity of PTP-1B have been reported in skeletal muscle of insulin-resistant obese people (Ahmad et al., 1997), as well as in non-obese Goto-Kakizaki rats with spontaneously generated insulin resistance (Dadke et al., 2000), and in STZ-hyperglycemic rats fed with high-fat diet (Wu et al., 2005).
Based on the aforementioned, the PTP-1B inhibition represents a good therapeutic target for the treatment of insulin resistance-related diseases, such as DM2 (Zhang et al., 2006). Hence, an arsenal of molecules with inhibitory capacity of PTP-1B activity has been generated in recent years. The methodological approaches that have been applied are the rational design of synthetic phospho-(tyrosine)-mimetic molecules to be used as competitive inhibitors, considering the structural characteristics of the protein, and the search for molecules from natural sources (Sun et al., 2018). The latter is based on the statement that nature has a great variety of structures that present diverse pharmacological effects (Atanasov et al., 2021), so natural products can be used as a starting point for the creation of powerful inhibitors.
Table 1 summarizes all medicinal plants and their identified compounds that have proved to inhibit the activity or expression of PTP-1B since 2015. It was obtained a total of 125 medicinal plants used in various traditional medicine systems around the world, mainly represented in eastern folk, such as Chinese and Vietnamese. Morus alba L. (Moraceae), a plant used in the traditional Chinese system, has been the most evaluated for this purpose. In addition to direct PTP-1B activity inhibition and molecular docking studies, some extracts and compounds were assessed to improve glucose and lipid metabolism in vivo, such as lowering blood glucose levels, improved insulin resistance and glucose intolerance, and improved lipid profile. Furthermore, their effect on glucose uptake and phosphorylation of some components of insulin signaling, such as IR, IRS, and Akt, was evaluated in cell cultures under insulin-resistant conditions.
Inhibition of Hepatic Glucose Output by Modulating Glucose Metabolism in Liver
The liver is a key organ that plays a crucial role in the regulation of blood glucose because it manages both storage and synthesis of glucose. The latter involves two metabolic pathways: glycogenolysis and gluconeogenesis, which constitute total hepatic glucose production (HGP) (Lee et al., 2015). Glycogenolysis consists of glycogen breakdown into glucose, being half of the basal HGP in fasting and decreasing the glycogen concentration at an almost linear rate during the first 22 h (Rothman et al., 1991; Cersosimo et al., 2018). In fasting, it is controlled by glucagon and epinephrine that activate glycogen phosphorylase (GP), the major enzyme responsible for digesting glycogen by releasing glucose 1-phosphate. In feeding condition, insulin inhibits glycogen breakdown and promotes glycogen synthesis through the activation of Akt and protein phosphatase 1 (PP1), leading the deactivation of both GP and glycogen synthase kinase-3 (GSK3), which in its active form (dephosphorylated), inactivates glycogen synthase (GS) (Han et al., 2016).
Gluconeogenesis, on the other hand, is defined as the production of glucose from a molecule that is not a carbohydrate. Its main substrates are pyruvate, glycerol, and amino acids such as alanine (Hanson and Owen, 2013). Another way to denote gluconeogenesis is as “reverse glycolysis” since both share not only substrates and final products, but also many enzymes. However, the direction of the reactions catalyzed in gluconeogenesis goes in the opposite direction, so the steps that are not shared with glycolysis can be determined as regulatory steps. These reactions are catalyzed by four rate-limiting enzymes: pyruvate carboxylase (PC), which is responsible for converting pyruvate into oxaloacetate; phosphoenolpyruvate carboxykinase (PEPCK), that converts oxaloacetate to phosphoenolpyruvate; fructose 1,6-bisphosphatase (FBPase), that dephosphorylates fructose 1,6-bisphosphate obtaining fructose 6-phosphate; and glucose 6-phosphatase (G6Pase), which is responsible for removing the phosphate group from glucose 6-phosphate, yielding novo synthesized glucose (Postic et al., 2004).
In the diabetic state, increased rates of HGP are observed as a result of an imbalance of various factors, such as the augmented availability of gluconeogenic substrates, the resistance of the liver to the action of insulin, and elevated levels of glucagon that activate HGP (Sharabi et al., 2015). Due to all these factors, the inhibition of HGP turns out to be an important therapeutic target for the reduction of hyperglycemia observed in T2D patients. In this regard, Table 2 summarizes the works made between 2015 and 2021 with extracts or natural products from 47 medicinal plants that showed to modulate hepatic glucose metabolism by inhibiting glucose production or promoting glycogen synthesis. As it can be observed, decreasing the expression of PEPCK and G6Pase is the principal mechanism related to gluconeogenesis inhibition, while phosphorylation of GSK3, promotion of GS activity, and inhibition of GP are the main mechanisms involved in glycogen breakdown and synthesis. Furthermore, although PI3K/Akt pathway stands out as a good pharmacological target to reduce insulin resistance, medicinal plants and their phytochemicals can also decrease HGP through AMP-activated protein kinase (AMPK).
Discussion
Insulin resistance in liver leads to the release of large amounts of glucose into the bloodstream that affects long-term homeostasis. The regulation of hepatic glucose output represents a good pharmacological target for the control of metabolic diseases such as T2D, which are characterized by the presence of this pathophysiological phenomenon. The search for new molecules capable of regulating hepatic glucose metabolism from medicinal plants has focused on screening for phytochemicals that can directly inhibit key enzymes in glucose-producing pathways. However, considering compounds with the ability to also decrease the activity of the enzymes involved in terminating the insulin signal could result in more effective glycemic control.
According to the bibliographic search, plants used in different systems of traditional medicine have shown the ability to inhibit the activity or expression of PTP-1B, which could indicate that they have a potential inhibitory effect on HGP. The determination of biological activity of full extracts and compounds isolated from medicinal plants has been approached through different perspectives. Generally, the medicinal plant is first identified using an ethnopharmacological approach. Afterwards, different types of extracts are elaborated (aqueous, ethanolic, methanolic, etc.) and then tested on the biological activity to be evaluated following several paths: 1) direct inhibition enzymatic assays, which can be complemented with structure-activity relationship (SAR) studies and molecular docking analysis to find the possible structures responsible for the bioactivity, relating them with the binding of amino acid residues present at the catalytic or regulatory sites (regarding isolated compounds); 2) the use of cell cultures to evaluate the effect of the extract or compound on the expression and protein levels of key enzymes; and 3) in vivo studies, where diabetic (hyperglycemic) animals induced with STZ or alloxan, or insulin-resistant animals generated by the consumption of high-fat diet are used.
Regarding PTP-1B, most of the studies published between 2015 and 2021 focused on conducting enzyme activity assays, and few of them had a multidisciplinary approach that encompassed enzyme assays and in vitro or in vivo studies. The main problem with the first type of studies is that, although the inhibition potency and selectivity of the molecule over the enzyme are directly evaluated, the pharmacokinetic properties of the compound are omitted. This particularity stands out since it has been reported that, despite having excellent inhibitory activity, many compounds lack adequate cellular permeability, namely they present poor absorption and low bioavailability (Zhang et al., 2017). Another aspect to highlight is that PTP-1B is almost identical to TC-PTP, another member of the PTP family with 74% identity at the catalytic site, so it is important that the identified inhibitors have a high selectivity towards PTP-1B to avoid unwanted effects (Dewang et al., 2005). Considering these facts, it would be necessary in the future to carry out more studies involving as many approaches as possible to obtain a more integrative panorama and to be able to evaluate potential inhibitors considering their pharmacokinetic properties and selectivity. Also, it is encouraged to directly evaluate the effect of medicinal plants and their compounds with reported PTP-1B inhibitory capacity on hepatic glucose metabolism.
In addition to exhibiting PTP-1B inhibitory capacity, some of the medicinal plants reported in Table 1 also improved hepatic glucose metabolism by promoting glucose consumption and glycogen synthesis, upregulating activity or expression of GS, decreasing activity or expression of key enzymes involved in glycogenolysis and gluconeogenesis such as GSK3, GP, PEPCK, FBPase, and G6Pase, and by modulating insulin signaling. The compounds isolated from these plants could have a greater modulatory capacity of hepatic glucose metabolism because they are capable of directly reducing both insulin resistance and glucose production. These species were Astragalus mongholicus (astragaloside IV), Chaenomeles japonica, Duranta erecta, Eriobotrya japonica (maslinic acid, corosolic acid, oleanolic acid, and ursolic acid), Symplocos cochinchinensis, Thonningia sanguinea (2′-O- (3-O-galloyl-4,6-O-Sa-hexahydroxydiphenoyl-β-d-glucopyranosyl)-3-hydroxyphloretin, 4′-O-(4,6-O-Sa-hexahydroxydiphenoyl-β-d-glucopyranosyl)- phloretin, 2′-O-(3-O-galloyl-4,6-O-Sa-hexahydroxydiphenoyl-β-d-glucopyranosyl)phloretin, thonningianin A, and thonningianin B), Vaccinium uliginosum (cyanidin-3-arabinoside, delphinidin-3-glucoside, cyanidin-3-galactoside, cyanidin-3-glucoside, malvidin-3-galactoside, petunidin-3-glucoside, procyanidin B1, and procyanidin B2), and Vigna radiata. On the other hand, since Coreopsis tinctoria, Lithocarpus polystachyus, and Panax ginseng were documented in both Tables 1, 2, their isolated compounds may have better glycemic control.
This work focused on summarizing the medicinal plants with the potential capacity to reduce hyperglycemia resulting from an imbalance in the hepatic metabolism of glucose, encompassing two different approaches: the inhibition of PTP-1B (improvement of hepatic insulin resistance), and the modulation of enzymes involved in gluconeogenesis and glycogenolysis/glycogenesis (decreased hepatic glucose output). In recent years, PTP-1B research has focused on the characterization of different phytochemicals from medicinal plants, such as phenolic compounds, terpenes, and alkaloids. The main methodology used was to carry out direct enzyme inhibition tests to evaluate the potency of these molecules, omitting important aspects such as selectivity or pharmacokinetics. Therefore, it is proposed to use of multidisciplinary approaches that involve in vitro studies, such as the use of cell lines or primary culture to evaluate the effect of the extracts and compounds on expression and protein levels, and in vivo studies, where the concentration of the compound in systemic circulation and its duration is determined, as well as the transformation processes involved. In this regard, not only the inhibitory activity of the compounds is evaluated, but also the impact on other pharmacological aspects that can only be observed using animal models.
On the other hand, research on medicinal plants that modulate hepatic glucose metabolism has primary focused on testing full extracts rather than compounds. However, it is worth mentioning that mixtures could have synergistic effects capable of regulating multiple targets (Caesar and Cech, 2019) and therefore compound fractions may exhibit more bioactivity than isolated molecules. Further studies are needed to identify potential multi-target phytochemicals in plants listed in Table 2. Finally, it is expected that this review will provide greater knowledge of medicinal plants and compounds for the development of drugs that improving hepatic glucose metabolism as a therapeutic target for the treatment of T2D.
We suggest that Coreopsis tinctoria, Lithocarpus polystachyus, and Panax ginseng can be good candidates for developing herbal medicines or phytomedicines that target inhibition of hepatic glucose output as they can modulate the activity of PTP-1B, the expression of gluconeogenic enzymes, and the glycogen content. However, only their full extracts are tested until now. Therefore, compounds responsible for the effects mentioned above have not been identified, and pharmacological and toxicological tests in animal models are required to assess their efficacy and safety, with the aim of moving forward to carry out clinical studies.
Author Contributions
GM-T and FE-H performed the bibliographical research summarized in tables and wrote the first version of the manuscript. AA-C reviewed and edited the manuscript. All authors have read and agreed to the published version of the manuscript.
Funding
This project was partially sponsored by DGAPA PAPIIT IN226719 and IN213222.
Conflict of Interest
The authors declare that the research was conducted in the absence of any commercial or financial relationships that could be construed as a potential conflict of interest.
Publisher’s Note
All claims expressed in this article are solely those of the authors and do not necessarily represent those of their affiliated organizations, or those of the publisher, the editors and the reviewers. Any product that may be evaluated in this article, or claim that may be made by its manufacturer, is not guaranteed or endorsed by the publisher.
Acknowledgments
Authors acknowledge “Consejo Nacional de Ciencia y Tecnología (CONACyT)” for the doctoral scholarship of GM-T and FE-H.
References
Abdel-Rahman, R. F., Ezzat, S. M., Ogaly, H. A., Abd-Elsalam, R. M., Hessin, A. F., Fekry, M. I., et al. (2020). Ficus Deltoidea Extract Down-Regulates Protein Tyrosine Phosphatase 1B Expression in a Rat Model of Type 2 Diabetes Mellitus: a New Insight into its Antidiabetic Mechanism. J. Nutr. Sci. 9, e2. doi:10.1017/jns.2019.40
Abdel-Sattar, E., El-Maraghy, S. A., El-Dine, R. S., and Rizk, S. M. (2016). Russelioside B, a Pregnane Glycoside Ameliorates Hyperglycemia in Streptozotocin Induced Diabetic Rats by Regulating Key Enzymes of Glucose Metabolism. Chem. Biol. Interact. 252, 47–53. doi:10.1016/j.cbi.2016.03.033
Abdjul, D. B., Yamazaki, H., Maarisit, W., Rotinsulu, H., Wewengkang, D. S., Sumilat, D. A., et al. (2017). Oleanane Triterpenes with Protein Tyrosine Phosphatase 1B Inhibitory Activity from Aerial Parts of Lantana Camara Collected in Indonesia and Japan. Phytochemistry 144, 106–112. doi:10.1016/j.phytochem.2017.08.020
Abdjul, D. B., Yamazaki, H., Maarisit, W., Kirikoshi, R., Takahashi, O., Losung, F., et al. (2018). Protein Tyrosine Phosphatase 1B Inhibitory Components and a New Unique N -alkylamide Derivative with an Endoperoxide Bridge from Aerial Parts of Indonesian Spilanthes Paniculata. Phytochemistry Lett. 24, 71–74. doi:10.1016/j.phytol.2018.01.013
Ahmad, F., Azevedo, J. L., Cortright, R., Dohm, G. L., and Goldstein, B. J. (1997). Alterations in Skeletal Muscle Protein-Tyrosine Phosphatase Activity and Expression in Insulin-Resistant Human Obesity and Diabetes. J. Clin. Invest. 100, 449–458. doi:10.1172/JCI119552
Ahmad, F., and Goldstein, B. J. (1995). Alterations in Specific Protein-Tyrosine Phosphatases Accompany Insulin Resistance of Streptozotocin Diabetes. Am. J. Physiol. 268, E932–E940. doi:10.1152/ajpendo.1995.268.5.e932
Ahmad, F., Li, P. M., Meyerovitch, J., and Goldstein, B. J. (1995). Osmotic Loading of Neutralizing Antibodies Demonstrates a Role for Protein-Tyrosine Phosphatase 1B in Negative Regulation of the Insulin Action Pathway. J. Biol. Chem. 270, 20503–20508. doi:10.1074/jbc.270.35.20503
Ahmed, B., Sultana, R., and Greene, M. W. (2021). Adipose Tissue and Insulin Resistance in Obese. Biomed. Pharmacother. 137, 111315. doi:10.1016/j.biopha.2021.111315
Alonso, A., Nunes-Xavier, C. E., Bayón, Y., and Pulido, R. (2016). “The Extended Family of Protein Tyrosine Phosphatases,” in Methods in Molecular Biology. Editor R. Pulido (New York: Humana Press), 1–23. doi:10.1007/978-1-4939-3746-2_1Protein Tyrosine Phosphatases
American Diabetes Association (2021). 2. Classification and Diagnosis of Diabetes: Standards of Medical Care in Diabetes-2021. Diabetes Care 44, S15–S33. doi:10.2337/dc21-S002
An, J. P., Ha, T. K., Kim, J., Cho, T. O., and Oh, W. K. (2016). Protein Tyrosine Phosphatase 1B Inhibitors from the Stems of Akebia Quinata. Molecules 21. doi:10.3390/molecules21081091
Andrade-Cetto, A., Espinoza-Hernández, F., Mata-Torres, G., and Escandón-Rivera, S. (2021b). Hypoglycemic Effect of Two Mexican Medicinal Plants. Plants (Basel) 10, 2060. doi:10.3390/plants10102060
Andrade-Cetto, A., Espinoza-Hernández, F., and Mata-Torres, G. (2021a). Hypoglycemic Effect of Calea Urticifolia (Mill.) DC. Evidence-Based Complement. Altern. Med. 2021, 1–10. doi:10.1155/2021/6625009
Antu, K. A., Riya, M. P., Nair, A., Mishra, A., Srivastava, A. K., and Raghu, K. G. (2016). Symplocos Cochinchinensis Enhances Insulin Sensitivity via the Down Regulation of Lipogenesis and Insulin Resistance in High Energy Diet Rat Model. J. Ethnopharmacol. 193, 500–509. doi:10.1016/j.jep.2016.09.050
Arancibia-Radich, J., González-Blázquez, R., Alcalá, M., Martín-Ramos, M., Viana, M., Arribas, S., et al. (2019). Beneficial Effects of Murtilla Extract and Madecassic Acid on Insulin Sensitivity and Endothelial Function in a Model of Diet-Induced Obesity. Sci. Rep. 9, 599. doi:10.1038/s41598-018-36555-1
Atanasov, A. G., Zotchev, S., Zotchev, S. B., Dirsch, V. M., and Supuran, C. T. (2021). The International Natural Product Sciences Taskforce, and Supuran, CNatural Products in Drug Discovery: Advances and Opportunities. Nat. Rev. Drug Discov. 20, 200–216. doi:10.1038/s41573-020-00114-z
Bakke, J., and Haj, F. G. (2015). Protein-tyrosine Phosphatase 1B Substrates and Metabolic Regulation. Semin. Cel Dev Biol 37, 58–65. doi:10.1016/j.semcdb.2014.09.020
Bao, S., Wu, Y. L., Wang, X., Han, S., Cho, S., Ao, W., et al. (2020). Agriophyllum Oligosaccharides Ameliorate Hepatic Injury in Type 2 Diabetic Db/db Mice Targeting INS-R/IRS-2/PI3K/AKT/PPAR-γ/Glut4 Signal Pathway. J. Ethnopharmacol. 257, 112863. doi:10.1016/j.jep.2020.112863
Begmatov, N., Li, J., Bobakulov, K., Numonov, S., and Aisa, H. A. (2020). The Chemical Components of Coreopsis Tinctoria Nutt. And Their Antioxidant, Antidiabetic and Antibacterial Activities. Nat. Prod. Res. 34, 1772–1776. doi:10.1080/14786419.2018.1525377
Caesar, L. K., and Cech, N. B. (2019). Synergy and Antagonism in Natural Product Extracts: When 1 + 1 Does Not Equal 2. Nat. Prod. Rep. 36, 869–888. doi:10.1039/c9np00011a
Cai, J., Zhao, L., and Tao, W. (2015). Potent Protein Tyrosine Phosphatase 1B (PTP1B) Inhibiting Constituents from Anoectochilus Chapaensis and Molecular Docking Studies. Pharm. Biol. 53, 1030–1034. doi:10.3109/13880209.2014.957781
Cao, X., Yang, X., Wang, P., Liang, Y., Liu, F., Tuerhong, M., et al. (2017). Polycyclic Phloroglucinols as PTP1B Inhibitors from Hypericum Longistylum: Structures, PTP1B Inhibitory Activities, and Interactions with PTP1B. Bioorg. Chem. 75, 139–148. doi:10.1016/j.bioorg.2017.09.001
Cersosimo, E., Triplitt, C., Solis-Herrera, C., Mandarino, L. J., and DeFronzo, R. A. (2018). “Pathogenesis of Type 2 Diabetes Mellitus,” in ” in Endotext. Editor K. R. Feingold (South Dartmouth: MDText.com, Inc.). . http://www.ncbi.nlm.nih.gov/pubmed/25905339 (Accessed September 1, 2021).
Chen, H., Wertheimer, S. J., Lin, C. H., Katz, S. L., Amrein, K. E., Burn, P., et al. (1997). Protein-tyrosine Phosphatases PTP1B and Syp Are Modulators of Insulin-Stimulated Translocation of GLUT4 in Transfected Rat Adipose Cells. J. Biol. Chem. 272, 8026–8031. doi:10.1074/jbc.272.12.8026
Choi, J. S., Ali, M. Y., Jung, H. A., Oh, S. H., Choi, R. J., and Kim, E. J. (2015). Protein Tyrosine Phosphatase 1B Inhibitory Activity of Alkaloids from Rhizoma Coptidis and Their Molecular Docking Studies. J. Ethnopharmacol. 171, 28–36. doi:10.1016/j.jep.2015.05.020
Cusi, K., Maezono, K., Osman, A., Pendergrass, M., Patti, M. E., Pratipanawatr, T., et al. (2000). Insulin Resistance Differentially Affects the PI 3-kinase- and MAP Kinase-Mediated Signaling in Human Muscle. J. Clin. Invest. 105, 311–320. doi:10.1172/JCI7535
Dadke, S. S., Li, H. C., Kusari, A. B., Begum, N., and Kusari, J. (2000). Elevated Expression and Activity of Protein-Tyrosine Phosphatase 1B in Skeletal Muscle of Insulin-Resistant Type II Diabetic Goto-Kakizaki Rats. Biochem. Biophys. Res. Commun. 274, 583–589. doi:10.1006/bbrc.2000.3188
de Oliveira, K. A., Moreira Gomes, M. D., Vasconcelos, R. P., de Abreu, E. S., Fortunato, R. S., Carneiro Loureiro, A. C., et al. (2019). Phytomodulatory Proteins Promote Inhibition of Hepatic Glucose Production and Favor Glycemic Control via the AMPK Pathway. Biomed. Pharmacother. 109, 2342–2347. doi:10.1016/j.biopha.2018.11.139
DeFronzo, R. A., Ferrannini, E., Groop, L., Henry, R. R., Herman, W. H., Holst, J. J., et al. (2015). Type 2 Diabetes Mellitus. Nat. Rev. Dis. Primers 1, 15019–15022. doi:10.1038/nrdp.2015.19
Denu, J. M., and Dixon, J. E. (1998). Protein Tyrosine Phosphatases: Mechanisms of Catalysis and Regulation. Curr. Opin. Chem. Biol. 2, 633–641. doi:10.1016/S1367-5931(98)80095-1
Dewang, P. M., Hsu, N. M., Peng, S. Z., and Li, W. R. (2005). Protein Tyrosine Phosphatases and Their Inhibitors. Curr. Med. Chem. 12, 1–22. doi:10.2174/97816080520731090401037110.2174/0929867053363504
Dimitriadis, G. D., Maratou, E., Kountouri, A., Board, M., and Lambadiari, V. (2021). Regulation of Postabsorptive and Postprandial Glucose Metabolism by Insulin-dependent and Insulin-independent Mechanisms: An Integrative Approach. Nutrients 13, 1–33. doi:10.3390/nu13010159
Divya, N., Rengarajan, R. L., Radhakrishnan, R., Fathi Abd Allah, E., Alqarawi, A. A., Hashem, A., et al. (2019). Phytotherapeutic Efficacy of the Medicinal Plant Terminalia catappa L. Saudi J. Biol. Sci. 26, 985–988. doi:10.1016/j.sjbs.2018.12.010
Edirs, S., Jiang, L., Xin, X., and Aisa, H. A. (2018). Anti-diabetic Effect and Mechanism of Kursi Wufarikun Ziyabit in L6 Rat Skeletal Muscle Cells. J. Pharmacol. Sci. 137, 212–219. doi:10.1016/j.jphs.2018.06.011
Ekakitie, L. I., Oyinloye, B. E., and Ajiboye, B. O. (2021). The Ameliorative Activity of Chrysobalanus Orbicularis in Streptozotocin-Induced Type II Diabetes Mellitus Rat Model. Heliyon 7, e06596. doi:10.1016/j.heliyon.2021.e06596
Elchebly, M., Payette, P., Michaliszyn, E., Cromlish, W., Collins, S., Loy, A. L., et al. (1999). Increased Insulin Sensitivity and Obesity Resistance in Mice Lacking the Protein Tyrosine phosphatase-1B Gene. Science 283, 1544–1548. doi:10.1126/science.283.5407.1544
Erukainure, O. L., Ijomone, O. M., Chukwuma, C. I., Xiao, X., Salau, V. F., and Islam, M. S. (2020). Dacryodes Edulis (G. Don) H.J. Lam Modulates Glucose Metabolism, Cholinergic Activities and Nrf2 Expression, while Suppressing Oxidative Stress and Dyslipidemia in Diabetic Rats. J. Ethnopharmacol. 255, 112744. doi:10.1016/j.jep.2020.112744
Erukainure, O. L., Mopuri, R., Oyebode, O. A., Koorbanally, N. A., and Islam, M. S. (2017). Dacryodes Edulis Enhances Antioxidant Activities, Suppresses DNA Fragmentation in Oxidative Pancreatic and Hepatic Injuries; and Inhibits Carbohydrate Digestive Enzymes Linked to Type 2 Diabetes. Biomed. Pharmacother. 96, 37–47. doi:10.1016/j.biopha.2017.09.106
Erukainure, O. L., Oyebode, O. A., Ijomone, O. M., Chukwuma, C. I., Koorbanally, N. A., and Islam, M. S. (2019a). Raffia palm (Raphia Hookeri G. Mann & H. Wendl) Wine Modulates Glucose Homeostasis by Enhancing Insulin Secretion and Inhibiting Redox Imbalance in a Rat Model of Diabetes Induced by High Fructose Diet and Streptozotocin. J. Ethnopharmacol. 237, 159–170. doi:10.1016/j.jep.2019.03.039
Erukainure, O. L., Sanni, O., Ijomone, O. M., Ibeji, C. U., Chukwuma, C. I., and Islam, M. S. (2019b). The Antidiabetic Properties of the Hot Water Extract of Kola Nut (Cola Nitida (Vent.) Schott & Endl.) in Type 2 Diabetic Rats. J. Ethnopharmacol. 242, 112033. doi:10.1016/j.jep.2019.112033
Espinoza-Hernández, F., Andrade-Cetto, A., Escandón-Rivera, S., Mata-Torres, G., and Mata, R. (2021). Contribution of Fasting and Postprandial Glucose-Lowering Mechanisms to the Acute Hypoglycemic Effect of Traditionally Used Eryngium Cymosum F.Delaroche. J. Ethnopharmacology 279, 114339. doi:10.1016/j.jep.2021.114339
Govindarajan, S., Babu, S. N., Vijayalakshmi, M. A., Manohar, P., and Noor, A. (2021). Aloe Vera Carbohydrates Regulate Glucose Metabolism through Improved Glycogen Synthesis and Downregulation of Hepatic Gluconeogenesis in Diabetic Rats. J. Ethnopharmacol. 281, 114556. doi:10.1016/j.jep.2021.114556
Gujjala, S., Putakala, M., Nukala, S., Bangeppagari, M., Rajendran, R., and Desireddy, S. (2017). Modulatory Effects of Caralluma Fimbriata Extract against High-Fat Diet Induced Abnormalities in Carbohydrate Metabolism in Wistar Rats. Biomed. Pharmacother. 92, 1062–1072. doi:10.1016/j.biopha.2017.06.016
Guo, Y., Dai, R., Deng, Y., Sun, L., Meng, S., and Xin, N. (2019). Hypoglycemic Activity of the Extracts of Belamcanda Chinensis Leaves (BCLE) on KK-Ay Mice. Biomed. Pharmacother. 110, 449–455. doi:10.1016/j.biopha.2018.11.094
Guo, Z., Niu, X., Xiao, T., Lu, J., Li, W., and Zhao, Y. (2015). Chemical Profile and Inhibition of α-glycosidase and Protein Tyrosine Phosphatase 1B (PTP1B) Activities by Flavonoids from Licorice (Glycyrrhiza Uralensis Fisch). J. Funct. Foods 14, 324–336. doi:10.1016/j.jff.2014.12.003
Ha, M. T., Shrestha, S., Tran, T. H., Kim, J. A., Woo, M. H., Choi, J. S., et al. (2020). Inhibition of PTP1B by Farnesylated 2-arylbenzofurans Isolated from Morus alba Root Bark: Unraveling the Mechanism of Inhibition Based on In Vitro and In Silico Studies. Arch. Pharm. Res. 43, 961–975. doi:10.1007/s12272-020-01269-4
Han, H. S., Kang, G., Kim, J. S., Choi, B. H., and Koo, S. H. (2016). Regulation of Glucose Metabolism from a Liver-Centric Perspective. Exp. Mol. Med. 48, e218–10. doi:10.1038/emm.2015.122
Han, S.-w., Shi, S.-m., Zou, Y.-x., Wang, Z.-c., Wang, Y.-q., Shi, L., et al. (2020). Chemical Constituents from Acid Hydrolyzates of Panax Quinquefolius Total Saponins and Their Inhibition Activity to α-glycosidase and Protein Tyrosine Phosphatase 1B. Chin. Herbal Medicines 12, 195–199. doi:10.1016/j.chmed.2020.03.003
Hanson, R. W., and Owen, O. E. (2013). “Gluconeogenesis,” in Metabolism Vitamins And Hormones. Elsevier, 381–386. doi:10.1016/B978-0-12-378630-2.00040-2
Hao, J., Han, L., Zhang, Y., and Wang, T. (2020). Docking Studies on Potential Mechanisms for Decreasing Insulin Resistance by the Tangzhiqing Herbal Formula. Evidence-Based Complement. Altern. Med. 2020, 1–11. doi:10.1155/2020/1057648
Hashimoto, N., and Goldstein, B. J. (1992). Differential Regulation of mRNAs Encoding Three Protein-Tyrosine Phosphatases by Insulin and Activation of Protein Kinase C. Biochem. Biophys. Res. Commun. 188, 1305–1311. doi:10.1016/0006-291X(92)91373-X
He, X. F., Chen, J. J., Li, T. Z., Zhang, X. K., Guo, Y. Q., Zhang, X. M., et al. (2020). Nineteen New Flavanol-Fatty Alcohol Hybrids with α-Glucosidase and PTP1B Dual Inhibition: One Unusual Type of Antidiabetic Constituent from Amomum Tsao-Ko. J. Agric. Food Chem. 68, 11434–11448. doi:10.1021/acs.jafc.0c04615
Hou, J. Q., Fan, C. L., Pei, X., Zhang, P. L., Deng, F., Jiang, W. Q., et al. (2019). Psiguadiols A-J, Rearranged Meroterpenoids as Potent PTP1B Inhibitors from Psidium Guajava. J. Nat. Prod. 82, 3267–3278. doi:10.1021/acs.jnatprod.9b00333
Hu, Y., Li, J., Chang, A. K., Li, Y., Tao, X., Liu, W., et al. (2021). Screening and Tissue Distribution of Protein Tyrosine Phosphatase 1B Inhibitors in Mice Following Oral Administration of Garcinia Mangostana L. Ethanolic Extract. Food Chem. 357, 129759–129768. doi:10.1016/j.foodchem.2021.129759
Huang, G. H., Lei, C., Zhu, K. X., Li, J. Y., Li, J., and Hou, A. J. (2019). Enantiomeric Pairs of Meroterpenoids from Rhododendron Fastigiatum. Chin. J. Nat. Med. 17, 963–969. doi:10.1016/S1875-5364(19)30119-0
Ighodaro, O. M., Akinloye, O. A., Ugbaja, R. N., and Omotainse, S. O. (2017). Sapium Ellipticum (Hochst) Pax Ethanol Leaf Extract Modulates Glucokinase and Glucose-6-Phosphatase Activities in Streptozotocin Induced Diabetic Rats. Asian Pac. J. Trop. Biomed. 7, 544–548. doi:10.1016/j.apjtb.2017.05.009
Jhuang, H. J., Hsu, W. H., Lin, K. T., Hsu, S. L., Wang, F. S., Chou, C. K., et al. (2015). Gluconeogenesis, Lipogenesis, and HBV Replication Are Commonly Regulated by PGC-1α-dependent Pathway. Oncotarget 6, 7788–7803. doi:10.18632/oncotarget.3050
Ji, S., Li, Z., Song, W., Wang, Y., Liang, W., Li, K., et al. (2016). Bioactive Constituents of Glycyrrhiza Uralensis (Licorice): Discovery of the Effective Components of a Traditional Herbal Medicine. J. Nat. Prod. 79, 281–292. doi:10.1021/acs.jnatprod.5b00877
Jiang, B.-p., Lv, Q.-y., Xiang, J.-m., Le, L., Hu, K.-p., Xu, L.-j., et al. (2018a). Inhibition of Metabolic Disorders In Vivo and In Vitro by Main Constituent of Coreopsis Tinctoria. Chin. Herbal Medicines 10, 157–168. doi:10.1016/j.chmed.2018.03.004
Jiang, L., Numonov, S., Bobakulov, K., Qureshi, M. N., Zhao, H., and Aisa, H. A. (2015). Phytochemical Profiling and Evaluation of Pharmacological Activities of Hypericum Scabrum L. Molecules 20, 11257–11271. doi:10.3390/molecules200611257
Jiang, W., Si, L., Li, P., Bai, B., Qu, J., Hou, B., et al. (2018b). Serum Metabonomics Study on Antidiabetic Effects of Fenugreek Flavonoids in Streptozotocin-Induced Rats. J. Chromatogr. B Analyt Technol. Biomed. Life Sci. 1092, 466–472. doi:10.1016/j.jchromb.2018.06.041
Jin, D.-X., He, J.-F., Luo, X.-G., and Zhang, T.-C. (2019). Hypoglycemic Effect of Hypericum Attenuatum Choisy Extracts on Type 2 Diabetes by Regulating Glucolipid Metabolism and Modulating Gut Microbiota. J. Funct. Foods 52, 479–491. doi:10.1016/j.jff.2018.11.031
Jung, H. A., Ali, M. Y., and Choi, J. S. (2016). Promising Inhibitory Effects of Anthraquinones, Naphthopyrone, and Naphthalene Glycosides, from Cassia Obtusifolia on α-Glucosidase and Human Protein Tyrosine Phosphatases 1B. Molecules 22, 28. doi:10.3390/molecules22010028
Jung, H. J., Seong, S. H., Ali, M. Y., Min, B. S., Jung, H. A., and Choi, J. S. (2017). α-Methyl Artoflavanocoumarin from Juniperus Chinensis Exerts Anti-diabetic Effects by Inhibiting PTP1B and Activating the PI3K/Akt Signaling Pathway in Insulin-Resistant HepG2 Cells. Arch. Pharm. Res. 40, 1403–1413. doi:10.1007/s12272-017-0992-0
Kasangana, P. B., Eid, H. M., Nachar, A., Stevanovic, T., and Haddad, P. S. (2019). Further Isolation and Identification of Anti-diabetic Principles from Root Bark of Myrianthus Arboreus P. Beauv.: The Ethyl Acetate Fraction Contains Bioactive Phenolic Compounds that Improve Liver Cell Glucose Homeostasis. J. Ethnopharmacol. 245, 112167. doi:10.1016/j.jep.2019.112167
Kasangana, P. B., Nachar, A., Eid, H. M., Stevanovic, T., and Haddad, P. S. (2018). Root Bark Extracts of Myrianthus Arboreus P. Beauv. (Cecropiaceae) Exhibit Anti-diabetic Potential by Modulating Hepatocyte Glucose Homeostasis. J. Ethnopharmacol. 211, 117–125. doi:10.1016/j.jep.2017.09.017
Kenner, K. A., Anyanwu, E., Olefsky, J. M., and Kusari, J. (1996). Protein-tyrosine Phosphatase 1B Is a Negative Regulator of Insulin- and Insulin-like Growth Factor-I-Stimulated Signaling. J. Biol. Chem. 271, 19810–19816. doi:10.1074/jbc.271.33.19810
Khanal, P., and Patil, B. M. (2021). Integration of Network and Experimental Pharmacology to Decipher the Antidiabetic Action of Duranta Repens L. J. Integr. Med. 19, 66–77. doi:10.1016/j.joim.2020.10.003
Kim, D. H., Jung, H. A., Sohn, H. S., Kim, J. W., and Choi, J. S. (2017a). Potential of Icariin Metabolites from Epimedium Koreanum Nakai as Antidiabetic Therapeutic Agents. Molecules 22. doi:10.3390/molecules22060986
Kim, D. H., Lee, S., Chung, Y. W., Kim, B. M., Kim, H., Kim, K., et al. (2016). Antiobesity and Antidiabetes Effects of a Cudrania Tricuspidata Hydrophilic Extract Presenting PTP1B Inhibitory Potential. Biomed. Res. Int. 2016, 8432759. doi:10.1155/2016/8432759
Kim, D. H., Paudel, P., Yu, T., Ngo, T. M., Kim, J. A., Jung, H. A., et al. (2017b). Characterization of the Inhibitory Activity of Natural Tanshinones from Salvia Miltiorrhiza Roots on Protein Tyrosine Phosphatase 1B. Chem. Biol. Interact. 278, 65–73. doi:10.1016/j.cbi.2017.10.013
Klaman, L. D., Boss, O., Peroni, O. D., Kim, J. K., Martino, J. L., Zabolotny, J. M., et al. (2000). Increased Energy Expenditure, Decreased Adiposity, and Tissue-specific Insulin Sensitivity in Protein-Tyrosine Phosphatase 1B-Deficient Mice. Mol. Cel. Biol. 20, 5479–5489. doi:10.1128/mcb.20.15.5479-5489.2000
Kowalczuk, A., Bourebaba, N., Kornicka-Garbowska, K., Turlej, E., Marycz, K., and Bourebaba, L. (2021). Hyoscyamus Albus Nortropane Alkaloids Reduce Hyperglycemia and Hyperinsulinemia Induced in HepG2 Cells through the Regulation of SIRT1/NF-kB/JNK Pathway. Cell Commun. Signal. 19, 61. doi:10.1186/s12964-021-00735-w
Krishnasamy, R., and Periyasamy, S. (2019). Regulating Role of Ethyl Acetate Fraction of Tephrosia Tinctoria Pers. In Carbohydrate Metabolism and Oxidative Stress in Diabetic Rats. Biomed. Pharmacother. 114, 108842. doi:10.1016/j.biopha.2019.108842
Krook, A., Björnholm, M., Galuska, D., Jiang, X. J., Fahlman, R., Myers, M. G., et al. (2000). Characterization of Signal Transduction and Glucose Transport in Skeletal Muscle from Type 2 Diabetic Patients. Diabetes 49, 284–292. doi:10.2337/diabetes.49.2.284
Kurup, S. B., and Mini, S. (2017). Protective Potential of Averrhoa Bilimbi Fruits in Ameliorating the Hepatic Key Enzymes in Streptozotocin-Induced Diabetic Rats. Biomed. Pharmacother. 85, 725–732. doi:10.1016/j.biopha.2016.11.088
Lang, G. Z., Li, C. J., Gaohu, T. Y., Li, C., Ma, J., Yang, J. Z., et al. (2020). Bioactive Flavonoid Dimers from Chinese Dragon's Blood, the Red Resin of Dracaena Cochinchinensis. Bioorg. Chem. 97, 103659. doi:10.1016/j.bioorg.2020.103659
Le, D. D., Nguyen, D. H., Zhao, B. T., Seong, S. H., Choi, J. S., Kim, S. K., et al. (2017). PTP1B Inhibitors from Selaginella Tamariscina (Beauv.) Spring and Their Kinetic Properties and Molecular Docking Simulation. Bioorg. Chem. 72, 273–281. doi:10.1016/j.bioorg.2017.05.001
Lee, S. Y., Lai, F. Y., Shi, L. S., Chou, Y. C., Yen, I. C., and Chang, T. C. (2015). Rhodiola Crenulata Extract Suppresses Hepatic Gluconeogenesis via Activation of the AMPK Pathway. Phytomedicine 22, 477–486. doi:10.1016/j.phymed.2015.01.016
Lei, C., Zhang, L.-B., Yang, J., Gao, L.-X., Li, J.-Y., Li, J., et al. (2016). Macdentichalcone, a Unique Polycyclic Dimeric Chalcone from Macaranga Denticulata. Tetrahedron Lett. 57, 5475–5478. doi:10.1016/j.tetlet.2016.10.090
Li, B., Fu, R., Tan, H., Zhang, Y., Teng, W., Li, Z., et al. (2021). Characteristics of the Interaction Mechanisms of Procyanidin B1 and Procyanidin B2 with Protein Tyrosine phosphatase-1B: Analysis by Kinetics, Spectroscopy Methods and Molecular Docking. Spectrochimica Acta A: Mol. Biomol. Spectrosc. 259, 119910. doi:10.1016/j.saa.2021.119910
Li, C., Li, C. J., Ma, J., Chen, F. Y., Li, L., Wang, X. L., et al. (2018a). Magterpenoids A-C, Three Polycyclic Meroterpenoids with PTP1B Inhibitory Activity from the Bark of Magnolia Officinalis Var. Biloba. Org. Lett. 20, 3682–3686. doi:10.1021/acs.orglett.8b01476
Li, C., Li, C. J., Ma, J., Huang, J. W., Wang, X. Y., Wang, X. L., et al. (2019a). Magmenthanes A-H: Eight New Meroterpenoids from the Bark of Magnolia Officinalis Var. Biloba. Bioorg. Chem. 88, 102948. doi:10.1016/j.bioorg.2019.102948
Li, C., Li, C. J., Xu, K. L., Ma, J., Huang, J. W., Ye, F., et al. (2020a). Novel Oligomeric Neolignans with PTP1B Inhibitory Activity from the Bark of Magnolia Officinalis Var. Biloba. Bioorg. Chem. 104, 104319. doi:10.1016/j.bioorg.2020.104319
Li, F., Li, Y., Li, Q., and Shi, X. (2020b). Eriobotrya Japonica Leaf Triterpenoid Acids Ameliorate Metabolic Syndrome in C57BL/6J Mice Fed with High-Fat Diet. Biomed. Pharmacother. 132, 110866. doi:10.1016/j.biopha.2020.110866
Li, J., Yu, H., Wang, S., Wang, W., Chen, Q., Ma, Y., et al. (2018b). Natural Products, an Important Resource for Discovery of Multitarget Drugs and Functional Food for Regulation of Hepatic Glucose Metabolism. Drug Des. Devel. Ther. 12, 121–135. doi:10.2147/DDDT.S151860
Li, J. L., Li, N., Xing, S. S., Zhang, N., Li, B. B., Chen, J. G., et al. (2017). New Neo-Lignan from Acanthopanax Senticosus with Protein Tyrosine Phosphatase 1B Inhibitory Activity. Arch. Pharm. Res. 40, 1265–1270. doi:10.1007/s12272-015-0659-7
Li, W., Pu, Z., Yi, W., Ma, Q., Lin, Q., Zhong, G., et al. (2019b). Unusual Prenylated Stilbene Derivatives with PTP1B Inhibitory Activity from Artocarpus Styracifolius. Planta Med. 85, 1263–1274. doi:10.1055/a-1013-1417
Li, X., Xia, H., Wang, L., Xia, G., Qu, Y., Shang, X., et al. (2019c). Lignans from the Twigs of Litsea Cubeba and Their Bioactivities. Molecules 24. doi:10.3390/molecules24020306
Li, Z. H., Guo, H., Xu, W. B., Ge, J., Li, X., Alimu, M., et al. (2016). Rapid Identification of Flavonoid Constituents Directly from PTP1B Inhibitive Extract of Raspberry (Rubus Idaeus L.) Leaves by HPLC-ESI-QTOF-MS-MS. J. Chromatogr. Sci. 54, 805–810. doi:10.1093/chromsci/bmw016
Liang, C., Staerk, D., and Kongstad, K. T. (2020). Potential of Myrtus Communis Linn. As a Bifunctional Food: Dual High-Resolution PTP1B and α-glucosidase Inhibition Profiling Combined with HPLC-HRMS and NMR for Identification of Antidiabetic Triterpenoids and Phloroglucinol Derivatives. J. Funct. Foods 64, 103623. doi:10.1016/j.jff.2019.103623
Liao, Z., Zhang, J., Liu, B., Yan, T., Xu, F., Xiao, F., et al. (2019). Polysaccharide from Okra (Abelmoschus Esculentus (L.) Moench) Improves Antioxidant Capacity via PI3K/AKT Pathways and Nrf2 Translocation in a Type 2 Diabetes Model. Molecules 24, 1906. doi:10.3390/molecules24101906
Lin, G. M., Chen, Y. H., Yen, P. L., and Chang, S. T. (2016). Antihyperglycemic and Antioxidant Activities of Twig Extract from Cinnamomum Osmophloeum. J. Tradit. Complement. Med. 6, 281–288. doi:10.1016/j.jtcme.2015.08.005
Lin, Y., Kuang, Y., Li, K., Wang, S., Song, W., Qiao, X., et al. (2017). Screening for Bioactive Natural Products from a 67-compound Library of Glycyrrhiza Inflata. Bioorg. Med. Chem. 25, 3706–3713. doi:10.1016/j.bmc.2017.05.009
Liu, Y., Wang, C., Li, J., Mei, Y., and Liang, Y. (2019). Hypoglycemic and Hypolipidemic Effects of Phellinus Linteus Mycelial Extract from Solid-State Culture in A Rat Model of Type 2 Diabetes. Nutrients 11, 296. doi:10.3390/nu11020296
Liu, Y. P., Li, Y. J., Zhao, Y. Y., Guo, J. M., Liu, Y. Y., Wang, X. P., et al. (2021). Carbazole Alkaloids from the Fruits of Clausena Anisum-Olens with Potential PTP1B and α-glucosidase Inhibitory Activities. Bioorg. Chem. 110, 104775. doi:10.1016/j.bioorg.2021.104775
Liu, Z., Cheng, Z., He, Q., Lin, B., Gao, P., Li, L., et al. (2016). Secondary Metabolites from the Flower Buds of Lonicera japonica and Their In Vitro Anti-diabetic Activities. Fitoterapia 110, 44–51. doi:10.1016/j.fitote.2016.02.011
Ma, Q., Wei, R., Wang, Z., Liu, W., Sang, Z., Li, Y., et al. (2017). Bioactive Alkaloids from the Aerial Parts of Houttuynia Cordata. J. Ethnopharmacol. 195, 166–172. doi:10.1016/j.jep.2016.11.013
Manimegalai, S., Mahboob, S., Al-Ghanim, K. A., Al-Misned, F., Govindarajan, M., Anbarasu, K., et al. (2020). Down-regulation of Hepatic G-6-Pase Expression in Hyperglycemic Rats: Intervention with Biogenic Gold Nanoconjugate. Saudi J. Biol. Sci. 27, 3334–3341. doi:10.1016/j.sjbs.2020.09.026
Marvibaigi, M., Hosseini, S. M., and Amini, N. (2021). Launaea Acanthodes (Boiss) O. Kuntze Mediates Hepatic Glucose Metabolism and Ameliorates Impaired Pancreatic Function in Streptozotocin-Induced Diabetic Rats. J. Ethnopharmacol. 268, 113577. doi:10.1016/j.jep.2020.113577
Mata-Torres, G., Andrade-Cetto, A., Espinoza-Hernández, F. A., and Cárdenas-Vázquez, R. (2020). Hepatic Glucose Output Inhibition by Mexican Plants Used in the Treatment of Type 2 Diabetes. Front. Pharmacol. 11, 215–219. doi:10.3389/fphar.2020.00215
Meng, Y., Ding, L., Wang, Y., Nie, Q. T., Xing, Y. Y., and Ren, Q. (2020). Phytochemical Identification of Lithocarpus Polystachyus Extracts by Ultra-high-performance Liquid Chromatography-Quadrupole Time-Of-Flight-MS and Their Protein Tyrosine Phosphatase 1B and α-glucosidase Activities. Biomed. Chromatogr. 34, e4705. doi:10.1002/bmc.4705
Meyerovitch, J., Backer, J. M., and Kahn, C. R. (1989). Hepatic Phosphotyrosine Phosphatase Activity and its Alterations in Diabetic Rats. J. Clin. Invest. 84, 976–983. doi:10.1172/JCI114261
Mlinar, B., Marc, J., Janez, A., and Pfeifer, M. (2007). Molecular Mechanisms of Insulin Resistance and Associated Diseases. Clin. Chim. Acta 375, 20–35. doi:10.1016/j.cca.2006.07.005
Mohanasundaram, S., Rangarajan, N., Sampath, V., Porkodi, K., and Pennarasi, M. (2021). GC-MS and HPLC Analysis of Antiglycogenolytic and Glycogenic Compounds in Kaempferol 3-O-Gentiobioside Containing Senna alata L Leaves in Experimental Rats. Translational Metab. Syndr. Res. 4, 10–17. doi:10.1016/j.tmsr.2021.07.001
Muñoz, E. B., Luna-Vital, D. A., Fornasini, M., Baldeón, M. E., and Gonzalez de Mejia, E. (2018). Gamma-conglutin Peptides from Andean Lupin Legume (Lupinus Mutabilis Sweet) Enhanced Glucose Uptake and Reduced Gluconeogenesis In Vitro. J. Funct. Foods 45, 339–347. doi:10.1016/j.jff.2018.04.021
Na, B., Nguyen, P. H., Zhao, B. T., Vo, Q. H., Min, B. S., and Woo, M. H. (2016). Protein Tyrosine Phosphatase 1B (PTP1B) Inhibitory Activity and Glucosidase Inhibitory Activity of Compounds Isolated from Agrimonia Pilosa. Pharm. Biol. 54, 474–480. doi:10.3109/13880209.2015.1048372
Nguyen, D. H., Seo, U. M., Zhao, B. T., Le, D. D., Seong, S. H., Choi, J. S., et al. (2017). Ellagitannin and Flavonoid Constituents from Agrimonia Pilosa Ledeb. With Their Protein Tyrosine Phosphatase and Acetylcholinesterase Inhibitory Activities. Bioorg. Chem. 72, 293–300. doi:10.1016/j.bioorg.2017.04.017
Nguyen, D. T., To, D. C., Tran, T. T., Tran, M. H., and Nguyen, P. H. (2021). PTP1B and α-glucosidase Inhibitors from Selaginella Rolandi-Principis and Their Glucose Uptake Stimulation. J. Nat. Med. 75, 186–193. doi:10.1007/s11418-020-01448-z
Nguyen, P. H., Ji, D. J., Han, Y. R., Choi, J. S., Rhyu, D. Y., Min, B. S., et al. (2015). Selaginellin and Biflavonoids as Protein Tyrosine Phosphatase 1B Inhibitors from Selaginella Tamariscina and Their Glucose Uptake Stimulatory Effects. Bioorg. Med. Chem. 23, 3730–3737. doi:10.1016/j.bmc.2015.04.007
Nguyen, P. H., Tuan, H. N., Hoang, D. T., Vu, Q. T., Pham, M. Q., Tran, M. H., et al. (2019). Glucose Uptake Stimulatory and PTP1B Inhibitory Activities of Pimarane Diterpenes from Orthosiphon Stamineus Benth. Biomolecules 9, 859. doi:10.3390/biom9120859
Niu, S. L., Tong, Z. F., Zhang, Y., Liu, T. L., Tian, C. L., Zhang, D. X., et al. (2020). Novel Protein Tyrosine Phosphatase 1B Inhibitor-Geranylated Flavonoid from Mulberry Leaves Ameliorates Insulin Resistance. J. Agric. Food Chem. 68, 8223–8231. doi:10.1021/acs.jafc.0c02720
Numonov, S., Edirs, S., Bobakulov, K., Qureshi, M. N., Bozorov, K., Sharopov, F., et al. (2017). Evaluation of the Antidiabetic Activity and Chemical Composition of Geranium Collinum Root Extracts-Computational and Experimental Investigations. Molecules 22. doi:10.3390/molecules22060983
Ojo, O. A. (2021). Puerarin as a Potential Drug Candidate for the Management of Type-2 Diabetes: Molecular Docking and Pharmacophore Modeling Studies. Biointerface Res. Appl. Chem. 11, 8751–8759. doi:10.33263/BRIAC112.87518759
Ovalle-Magallanes, B., Navarrete, A., Haddad, P. S., Tovar, A. R., Noriega, L. G., Tovar-Palacio, C., et al. (2019). Multi-target Antidiabetic Mechanisms of Mexicanolides from Swietenia Humilis. Phytomedicine 58, 152891. doi:10.1016/j.phymed.2019.152891
Page, M. J., Moher, D., Bossuyt, P. M., Boutron, I., Hoffmann, T. C., Mulrow, C. D., et al. (2021). PRISMA 2020 Explanation and Elaboration: Updated Guidance and Exemplars for Reporting Systematic Reviews. BMJ 372, n160. doi:10.1136/bmj.n160
Parveen, A., Farooq, M. A., and Kyunn, W. W. (2020). A New Oleanane Type Saponin from the Aerial Parts of Nigella Sativa with Anti-oxidant and Anti-diabetic Potential. Molecules 25, 1–13. doi:10.3390/molecules25092171
Pedersen, H. A., Ndi, C., Semple, S. J., Buirchell, B., Møller, B. L., and Staerk, D. (2020). PTP1B-Inhibiting Branched-Chain Fatty Acid Dimers from Eremophila Oppositifolia Subsp. Angustifolia Identified by High-Resolution PTP1B Inhibition Profiling and HPLC-PDA-HRMS-SPE-NMR Analysis. J. Nat. Prod. 83, 1598–1610. doi:10.1021/acs.jnatprod.0c00070
Pham, H. T. T., Ryu, B., Cho, H. M., Lee, B. W., Yang, W. Y., Park, E. J., et al. (2020). Oleanane Hemiacetal Glycosides from Gymnema Latifolium and Their Inhibitory Effects on Protein Tyrosine Phosphatase 1B. Phytochemistry 170, 112181. doi:10.1016/j.phytochem.2019.112181
Pitschmann, A., Zehl, M., Heiss, E., Purevsuren, S., Urban, E., Dirsch, V. M., et al. (2016). Quantitation of Phenylpropanoids and Iridoids in Insulin-Sensitising Extracts of Leonurus Sibiricus L. (Lamiaceae). Phytochem. Anal. 27, 23–31. doi:10.1002/pca.2583
Pompermaier, L., Heiss, E. H., Alilou, M., Mayr, F., Monizi, M., Lautenschlaeger, T., et al. (2018). Dihydrochalcone Glucosides from the Subaerial Parts of Thonningia Sanguinea and Their In Vitro PTP1B Inhibitory Activities. J. Nat. Prod. 81, 2091–2100. doi:10.1021/acs.jnatprod.8b00450
Postic, C., Dentin, R., and Girard, J. (2004). Role of the Liver in the Control of Carbohydrate and Lipid Homeostasis. Diabetes Metab. 30, 398–408. doi:10.1016/S1262-3636(07)70133-7
Qin, N. B., Jia, C. C., Xu, J., Li, D. H., Xu, F. X., Bai, J., et al. (2017). New Amides from Seeds of Silybum marianum with Potential Antioxidant and Antidiabetic Activities. Fitoterapia 119, 83–89. doi:10.1016/j.fitote.2017.04.008
Quang, T. H., Ngan, N. T., Yoon, C. S., Cho, K. H., Kang, D. G., Lee, H. S., et al. (2015). Protein Tyrosine Phosphatase 1B Inhibitors from the Roots of Cudrania Tricuspidata. Molecules 20, 11173–11183. doi:10.3390/molecules200611173
Qureshi, M. N., Numonov, S., and Aisa, H. A. (2019). Chemical and Pharmacological Evaluation of Hulls of Prunus Dulcis Nuts. Int. J. Anal. Chem. 2019, 5861692. doi:10.1155/2019/5861692
Reddy, A. S., and Zhang, S. (2013). Polypharmacology: Drug Discovery for the Future. Expert Rev. Clin. Pharmacol. 6, 41–47. doi:10.1586/ECP.12.74
Ren, C., Zhang, Y., Cui, W., Lu, G., Wang, Y., Gao, H., et al. (2015). A Polysaccharide Extract of mulberry Leaf Ameliorates Hepatic Glucose Metabolism and Insulin Signaling in Rats with Type 2 Diabetes Induced by High Fat-Diet and Streptozotocin. Int. J. Biol. Macromol. 72, 951–959. doi:10.1016/j.ijbiomac.2014.09.060
Riya, M. P., Antu, K. A., Pal, S., Chandrakanth, K. C., Anilkumar, K. S., Tamrakar, A. K., et al. (2015). Antidiabetic Property of Aerva Lanata (L.) Juss. Ex Schult. Is Mediated by Inhibition of Alpha Glucosidase, Protein Glycation and Stimulation of Adipogenesis. J. Diabetes 7, 548–561. doi:10.1111/1753-0407.12216
Rizza, R. A. (2010). Pathogenesis of Fasting and Postprandial Hyperglycemia in Type 2 Diabetes: Implications for Therapy. Diabetes 59, 2697–2707. doi:10.2337/db10-1032
Rothman, D. L., Magnusson, I., Katz, L. D., Shulman, R. G., and Shulman, G. I. (1991). Quantitation of Hepatic Glycogenolysis and Gluconeogenesis in Fasting Humans with 13C NMR. Science 254, 573–576. doi:10.1126/science.1948033
Rozenberg, K., and Rosenzweig, T. (2018). Sarcopoterium Spinosum Extract Improved Insulin Sensitivity in Mice Models of Glucose Intolerance and Diabetes. PLoS One 13, e0196736. doi:10.1371/journal.pone.0196736
Ryu, B., Cho, H. M., Zhang, M., Lee, B. W., Doan, T. P., Park, E. J., et al. (2021). Meroterpenoids from the Leaves of Psidium Guajava (Guava) Cultivated in Korea Using MS/MS-based Molecular Networking. Phytochemistry 186, 112723. doi:10.1016/j.phytochem.2021.112723
Saadeldeen, F. S. A., Niu, Y., Wang, H., Zhou, L., Meng, L., Chen, S., et al. (2020). Natural Products: Regulating Glucose Metabolism and Improving Insulin Resistance. Food Sci. Hum. Wellness 9, 214–228. doi:10.1016/j.fshw.2020.04.005
Saeting, O., Chandarajoti, K., Phongphisutthinan, A., Hongsprabhas, P., and Sae-Tan, S. (2021). Water Extract of Mungbean (Vigna Radiata L.) Inhibits Protein Tyrosine Phosphatase-1B in Insulin-Resistant HepG2 Cells. Molecules 26, 1452. doi:10.3390/molecules26051452
Sai, C.-M., Qin, N.-B., Jia, C.-C., Li, D.-H., Wang, K.-B., Pei, Y.-H., et al. (2016). Macleayine, a New Alkaloid from Macleaya Cordata. Chin. Chem. Lett. 27, 1717–1720. doi:10.1016/j.cclet.2016.06.017
Saifudin, A., Lallo, S. A., and Tezuka, Y. (2016a). The Potent Inhibitors of Protein Tyrosine Phosphatase 1B from the Fruits of Melaleuca Leucadendron. Pharmacognosy Res. 8, S38–S41. doi:10.4103/0974-8490.178644
Saifudin, A., Usia, T., AbLallo, S., Morita, H., Tanaka, K., and Tezuka, Y. (2016b). Potent Water Extracts of Indonesian Medicinal Plants against PTP1B. Asian Pac. J. Trop. Biomed. 6, 38–43. doi:10.1016/j.apjtb.2015.09.021
Salinas-Arellano, E., Pérez-Vásquez, A., Rivero-cruz, I., Torres-Colin, R., González-Andrade, M., Rangel-Grimaldo, M., et al. (2020). Flavonoids and Terpenoids with PTP-1B Inhibitory Properties from the Infusion of Salvia Amarissima Ortega. Molecules 25, 1–18. doi:10.3390/molecules25153530
Saltiel, A. R., and Kahn, C. R. (2001). Insulin Signalling and the Regulation of Glucose and Lipid Metabolism. Nature 414, 799–806. doi:10.1038/414799a
Sawant, L., Singh, V. K., Dethe, S., Bhaskar, A., Balachandran, J., Mundkinajeddu, D., et al. (2015). Aldose Reductase and Protein Tyrosine Phosphatase 1B Inhibitory Active Compounds from Syzygium Cumini Seeds. Pharm. Biol. 53, 1176–1182. doi:10.3109/13880209.2014.967784
Schultze, S. M., Hemmings, B. A., Niessen, M., and Tschopp, O. (2012). PI3K/AKT, MAPK and AMPK Signalling: Protein Kinases in Glucose Homeostasis. Expert Rev. Mol. Med. 14, e1–21. doi:10.1017/S1462399411002109
Selvaraj, G., Kaliamurthi, S., and Thirugnasambandan, R. (2016). Effect of Glycosin Alkaloid from Rhizophora apiculata in Non-insulin Dependent Diabetic Rats and its Mechanism of Action: In Vivo and In Silico Studies. Phytomedicine 23, 632–640. doi:10.1016/j.phymed.2016.03.004
Semaan, D. G., Igoli, J. O., Young, L., Gray, A. I., Rowan, E. G., and Marrero, E. (2018). In Vitro anti-diabetic Effect of Flavonoids and Pheophytins from Allophylus Cominia Sw. On the Glucose Uptake Assays by HepG2, L6, 3T3-L1 and Fat Accumulation in 3T3-L1 Adipocytes. J. Ethnopharmacol. 216, 8–17. doi:10.1016/j.jep.2018.01.014
Semaan, D. G., Igoli, J. O., Young, L., Marrero, E., Gray, A. I., and Rowan, E. G. (2017). In Vitro anti-diabetic Activity of Flavonoids and Pheophytins from Allophylus Cominia Sw . On PTP1B, DPPIV, Alpha-Glucosidase and Alpha-Amylase Enzymes. J. Ethnopharmacol. 203, 39–46. doi:10.1016/j.jep.2017.03.023
Seo, Y. S., Shon, M. Y., Kong, R., Kang, O. H., Zhou, T., Kim, D. Y., et al. (2016). Black Ginseng Extract Exerts Anti-hyperglycemic Effect via Modulation of Glucose Metabolism in Liver and Muscle. J. Ethnopharmacol. 190, 231–240. doi:10.1016/j.jep.2016.05.060
Seong, S. H., Roy, A., Jung, H. A., Jung, H. J., and Choi, J. S. (2016). Protein Tyrosine Phosphatase 1B and α-glucosidase Inhibitory Activities of Pueraria Lobata Root and its Constituents. J. Ethnopharmacol. 194, 706–716. doi:10.1016/j.jep.2016.10.007
Sharabi, K., Tavares, C. D., Rines, A. K., and Puigserver, P. (2015). Molecular Pathophysiology of Hepatic Glucose Production. Mol. Aspects Med. 46, 21–33. doi:10.1016/j.mam.2015.09.003
Siqueira, J. T., Batistela, E., Pereira, M. P., da Silva, V. C., de Sousa Junior, P. T., Andrade, C. M., et al. (2016). Combretum Lanceolatum Flowers Ethanol Extract Inhibits Hepatic Gluconeogenesis: an In Vivo Mechanism Study. Pharm. Biol. 54, 1671–1679. doi:10.3109/13880209.2015.1120321
Su, C., Duan, Y., Tian, J., Liu, J., Xie, K., Chen, D., et al. (2020). Morusalisins A-F, Six New Diels-Alder Type Adducts, as Potential PTP1B Inhibitors from Cell Cultures of Morus alba. Fitoterapia 146, 104682. doi:10.1016/j.fitote.2020.104682
Su, C., Tao, X., Yin, Z., Zhang, X., Tian, J., Chen, R., et al. (2019). Morusalones A-D, Diels-Alder Adducts with 6/7/6/6/6/6 Hexacyclic Ring Systems as Potential PTP1B Inhibitors from Cell Cultures of Morus alba. Org. Lett. 21, 9463–9467. doi:10.1021/acs.orglett.9b03664
Sun, J., Wang, Y., Fu, X., Chen, Y., Wang, D., Li, W., et al. (2015). Magnolia Officinalis Extract Contains Potent Inhibitors against PTP1B and Attenuates Hyperglycemia in Db/db Mice. Biomed. Res. Int. 2015, 139451. doi:10.1155/2015/139451
Sun, J., Qu, C., Wang, Y., Huang, H., Zhang, M., Li, H., et al. (2018). PTP1B, A Potential Target of Type 2 Diabetes Mellitus. Mol. Biol. 05. doi:10.4172/2168-9547.1000174
Sun, R., Deng, X., Zhang, D., Xie, F., Wang, D., Wang, J., et al. (2019). Anti-diabetic Potential of Pueraria Lobata Root Extract through Promoting Insulin Signaling by PTP1B Inhibition. Bioorg. Chem. 87, 12–15. doi:10.1016/j.bioorg.2019.02.046
Sureka, C., Elango, V., Al-Ghamdi, S., Aldossari, K. K., Alsaidan, M., Geddawy, A., et al. (2021). Ameliorative Property of Sesbania Grandiflora on Carbohydrate Metabolic Enzymes in the Liver and Kidney of Streptozotocin-Induced Diabetic Rats. Saudi J. Biol. Sci. 28, 3669–3677. doi:10.1016/j.sjbs.2021.05.002
Tahtah, Y., Wubshet, S. G., Kongstad, K. T., Heskes, A. M., Pateraki, I., Møller, B. L., et al. (2016). High-resolution PTP1B Inhibition Profiling Combined with High-Performance Liquid Chromatography-High-Resolution Mass Spectrometry-solid-phase Extraction-Nuclear Magnetic Resonance Spectroscopy: Proof-Of-Concept and Antidiabetic Constituents in Crude Extract of Eremophila Lucida. Fitoterapia 110, 52–58. doi:10.1016/j.fitote.2016.02.008
Tang, D., Chen, Q. B., Xin, X. L., and Aisa, H. A. (2017). Anti-diabetic Effect of Three New Norditerpenoid Alkaloids In Vitro and Potential Mechanism via PI3K/Akt Signaling Pathway. Biomed. Pharmacother. 87, 145–152. doi:10.1016/j.biopha.2016.12.058
Tian, J. L., Liao, X. J., Wang, Y. H., Si, X., Shu, C., Gong, E. S., et al. (2019). Identification of Cyanidin-3-Arabinoside Extracted from Blueberry as a Selective Protein Tyrosine Phosphatase 1B Inhibitor. J. Agric. Food Chem. 67, 13624–13634. doi:10.1021/acs.jafc.9b06155
Tian, J. Y., Tao, R. Y., Zhang, X. L., Liu, Q., He, Y. B., Su, Y. L., et al. (2015). Effect of Hypericum perforatum L. Extract on Insulin Resistance and Lipid Metabolic Disorder in High-Fat-Diet Induced Obese Mice. Phytother. Res. 29, 86–92. doi:10.1002/ptr.5230
Tiong, S. H., Looi, C. Y., Arya, A., Wong, W. F., Hazni, H., Mustafa, M. R., et al. (2015). Vindogentianine, a Hypoglycemic Alkaloid from Catharanthus Roseus (L.) G. Don (Apocynaceae). Fitoterapia 102, 182–188. doi:10.1016/j.fitote.2015.01.019
To, D. C., Bui, T. Q., Nhung, N. T. A., Tran, Q. T., Do, T. T., Tran, M. H., et al. (2021). On the Inhibitability of Natural Products Isolated from Tetradium Ruticarpum towards Tyrosine Phosphatase 1B (PTP1B) and α-Glucosidase (3W37): An In Vitro and In Silico Study. Molecules 26. doi:10.3390/molecules26123691
Tonks, N. K., Diltz, C. D., and Fischer, E. H. (1988). Characterization of the Major Protein-Tyrosine-Phosphatases of Human Placenta. J. Biol. Chem. 263, 6731–6737. doi:10.1016/s0021-9258(18)68703-4
Trinh, B. T., Quach, T. T., Bui, D. N., Staerk, D., Nguyen, L. D., and Jäger, A. K. (2017b). Xanthones from the Twigs of Garcinia Oblongifolia and Their Antidiabetic Activity. Fitoterapia 118, 126–131. doi:10.1016/j.fitote.2017.03.003
Trinh, B. T. D., Jäger, A. K., and Staerk, D. (2017a). High-Resolution Inhibition Profiling Combined with HPLC-HRMS-SPE-NMR for Identification of PTP1B Inhibitors from Vietnamese Plants. Molecules 22. doi:10.3390/molecules22071228
Uwazie, J. N., Yakubu, M. T., Ashafa, A. O. T., and Ajiboye, T. O. (2020). Identification and Characterization of Anti-diabetic Principle in Senna alata (Linn.) Flower Using Alloxan-Induced Diabetic Male Wistar Rats. J. Ethnopharmacol. 261, 112997. doi:10.1016/j.jep.2020.112997
Vo, Q. H., Nguyen, P. H., Zhao, B. T., Ali, M. Y., Choi, J. S., Min, B. S., et al. (2015). Protein Tyrosine Phosphatase 1B (PTP1B) Inhibitory Constituents from the Aerial Parts of Tradescantia Spathacea Sw. Fitoterapia 103, 113–121. doi:10.1016/j.fitote.2015.03.017
Vu, N. K., Kim, C. S., Ha, M. T., Ngo, Q. T., Park, S. E., Kwon, H., et al. (2020). Antioxidant and Antidiabetic Activities of Flavonoid Derivatives from the Outer Skins of Allium cepa L. J. Agric. Food Chem. 68, 8797–8811. doi:10.1021/acs.jafc.0c02122
Wang, H., Gu, D., Wang, M., Guo, H., Wu, H., Tian, G., et al. (2017a). A Strategy Based on Gas Chromatography-Mass Spectrometry and Virtual Molecular Docking for Analysis and Prediction of Bioactive Composition in Natural Product Essential Oil. J. Chromatogr. A. 1501, 128–133. doi:10.1016/j.chroma.2017.04.031
Wang, J., Huang, Y., Li, K., Chen, Y., Vanegas, D., McLamore, E. S., et al. (2016a). Leaf Extract from Lithocarpus Polystachyus Rehd. Promote Glycogen Synthesis in T2DM Mice. PLoS One 11, e0166557. doi:10.1371/journal.pone.0166557
Wang, J., Yang, J. L., Zhou, P. P., Meng, X. H., and Shi, Y. P. (2017b). Further New Gypenosides from Jiaogulan (Gynostemma Pentaphyllum). J. Agric. Food Chem. 65, 5926–5934. doi:10.1021/acs.jafc.7b01477
Wang, L.-J., Xiong, J., Zou, Y., Mei, Q.-B., Wang, W.-X., and Hu, J.-F. (2016b). Sesquiterpenoids from the Chinese Endangered Plant Manglietia Aromatica. Phytochemistry Lett. 18, 202–207. doi:10.1016/j.phytol.2016.10.018
Wang, M., Yu, B. W., Yu, M. H., Gao, L. X., Li, J. Y., Wang, H. Y., et al. (2015). New Isoprenylated Phenolic Compounds from Morus Laevigata. Chem. Biodivers. 12, 937–945. doi:10.1002/cbdv.201400210
Wu, Y., Ouyang, J. P., Wu, K., Wang, S. S., Wen, C. Y., and Xia, Z. Y. (2005). Rosiglitazone Ameliorates Abnormal Expression and Activity of Protein Tyrosine Phosphatase 1B in the Skeletal Muscle of Fat-Fed, Streptozotocin-Treated Diabetic Rats. Br. J. Pharmacol. 146, 234–243. doi:10.1038/sj.bjp.0706306
Xiao, T., Guo, Z., Sun, B., and Zhao, Y. (2017b). Identification of Anthocyanins from Four Kinds of Berries and Their Inhibition Activity to α-Glycosidase and Protein Tyrosine Phosphatase 1B by HPLC-FT-ICR MS/MS. J. Agric. Food Chem. 65, 6211–6221. doi:10.1021/acs.jafc.7b02550
Xiao, T., Guo, Z., Bi, X., and Zhao, Y. (2017a). Polyphenolic Profile as Well as Anti-oxidant and Anti-diabetes Effects of Extracts from Freeze-Dried Black Raspberries. J. Funct. Foods 31, 179–187. doi:10.1016/j.jff.2017.01.038
Xiong, J., Hu, C. L., Wang, P. P., Gao, D. D., Huang, F., Li, J., et al. (2020). Spirobiflavonoid Stereoisomers from the Endangered conifer Glyptostrobus Pensilis and Their Protein Tyrosine Phosphatase 1B Inhibitory Activity. Bioorg. Med. Chem. Lett. 30, 126943. doi:10.1016/j.bmcl.2019.126943
Xiong, J., Wan, J., Ding, J., Wang, P. P., Ma, G. L., Li, J., et al. (2017). Camellianols A-G, Barrigenol-like Triterpenoids with PTP1B Inhibitory Effects from the Endangered Ornamental Plant Camellia Crapnelliana. J. Nat. Prod. 80, 2874–2882. doi:10.1021/acs.jnatprod.7b00241
Xu, J., Wang, X., Yue, J., Sun, Y., Zhang, X., and Zhao, Y. (2018b). Polyphenols from Acorn Leaves (Quercus Liaotungensis) Protect Pancreatic Beta Cells and Their Inhibitory Activity against α-Glucosidase and Protein Tyrosine Phosphatase 1B. Molecules 23. doi:10.3390/molecules23092167
Xu, J., Yang, L., Wang, R., Zeng, K., Fan, B., and Zhao, Z. (2019a). The Biflavonoids as Protein Tyrosine Phosphatase 1B Inhibitors from Selaginella Uncinata and Their Antihyperglycemic Action. Fitoterapia 137, 104255. doi:10.1016/j.fitote.2019.104255
Xu, J., Cao, J., Yue, J., Zhang, X., and Zhao, Y. (2018a). New Triterpenoids from Acorns of Quercus Liaotungensis and Their Inhibitory Activity against α -glucosidase, α -amylase and Protein-Tyrosine Phosphatase 1B. J. Funct. Foods 41, 232–239. doi:10.1016/j.jff.2017.12.054
Xu, L., Li, Y., Dai, Y., and Peng, J. (2018c). Natural Products for the Treatment of Type 2 Diabetes Mellitus: Pharmacology and Mechanisms. Pharmacol. Res. 130, 451–465. doi:10.1016/j.phrs.2018.01.015
Xu, Q. Q., Chen, X. L., Xu, J. F., Wang, S. B., Luo, J. G., and Kong, L. Y. (2019b). Acetophenone Derivatives from the Roots of Melicope Ptelefolia. Fitoterapia 132, 40–45. doi:10.1016/j.fitote.2018.11.013
Yan, H. W., Zhu, H., Yuan, X., Yang, Y. N., Feng, Z. M., Jiang, J. S., et al. (2019). Eight New Biflavonoids with Lavandulyl Units from the Roots of Sophora Flavescens and Their Inhibitory Effect on PTP1B. Bioorg. Chem. 86, 679–685. doi:10.1016/j.bioorg.2019.01.058
Yang, J. B., Ye, F., Tian, J. Y., Song, Y. F., Gao, H. Y., Liu, Y., et al. (2020). Multiflorumisides HK, Stilbene Glucosides Isolated from Polygonum Multiflorum and Their In Vitro PTP1B Inhibitory Activities. Fitoterapia 146, 104703. doi:10.1016/j.fitote.2020.104703
Yang, J. L., Ha, T. K. Q., Lee, B. W., Kim, J., and Oh, W. K. (2017). PTP1B Inhibitors from the Seeds of Iris Sanguinea and Their Insulin Mimetic Activities via AMPK and ACC Phosphorylation. Bioorg. Med. Chem. Lett. 27, 5076–5081. doi:10.1016/j.bmcl.2017.09.031
Yang, N., Zhang, S., Yang, S., Guo, Z., Zhang, X., and Zhao, Y. (2016). The Inhibition of α-glycosidase and Protein Tyrosine Phosphatase 1B (PTP1B) Activities by Ginsenosides from Panax Ginseng C.A. Meyer and Simultaneous Determination by HPLC-ELSD. J. Funct. Foods 23, 188–197. doi:10.1016/j.jff.2015.12.018
Yin, X., Huang, Y., Jung, D. W., Chung, H. C., Choung, S. Y., Shim, J. H., et al. (2015). Anti-Diabetic Effect of Aster Sphathulifolius in C57BL/KsJ-Db/db Mice. J. Med. Food 18, 987–998. doi:10.1089/jmf.2014.3416
Yousof Ali, M., Jung, H. A., and Choi, J. S. (2015). Anti-diabetic and Anti-alzheimer's Disease Activities of Angelica Decursiva. Arch. Pharm. Res. 38, 2216–2227. doi:10.1007/s12272-015-0629-0
Yu, J. H., Liu, Q. F., Sheng, L., Wang, G. C., Li, J., and Yue, J. M. (2016a). Cipacinoids A-D, Four Limonoids with Spirocyclic Skeletons from Cipadessa Cinerascens. Org. Lett. 18, 444–447. doi:10.1021/acs.orglett.5b03487
Yu, Y., Gan, L. S., Yang, S. P., Sheng, L., Liu, Q. F., Chen, S. N., et al. (2016b). Eucarobustols A-I, Conjugates of Sesquiterpenoids and Acylphloroglucinols from Eucalyptus Robusta. J. Nat. Prod. 79, 1365–1372. doi:10.1021/acs.jnatprod.6b00090
Yuan, Y. C., Bai, X. L., Liu, Y. M., Tang, X. Y., Yuan, H., and Liao, X. (2021). Ligand Fishing Based on Cell Surface Display of Enzymes for Inhibitor Screening. Anal. Chim. Acta 1156, 338359. doi:10.1016/j.aca.2021.338359
Yue, J., Xu, J., Cao, J., Zhang, X., and Zhao, Y. (2017). Cucurbitane Triterpenoids from Momordica Charantia L. And Their Inhibitory Activity against α-glucosidase, α-amylase and Protein Tyrosine Phosphatase 1B (PTP1B). J. Funct. Foods 37, 624–631. doi:10.1016/j.jff.2017.07.041
Zakłos-Szyda, M., and Pawlik, N. (2018). Japanese Quince (Chaenomeles Japonica L.) Fruit Polyphenolic Extract Modulates Carbohydrate Metabolism in HepG2 Cells via AMP-Activated Protein Kinase. Acta Biochim. Pol. 65, 67–78. doi:10.18388/abp.2017_1604
Zhang, C. C., Geng, C. A., Huang, X. Y., Zhang, X. M., and Chen, J. J. (2019a). Antidiabetic Stilbenes from Peony Seeds with PTP1B, α-Glucosidase, and DPPIV Inhibitory Activities. J. Agric. Food Chem. 67, 6765–6772. doi:10.1021/acs.jafc.9b01193
Zhang, P. Z., Gu, J., and Zhang, G. L. (2015). Novel Stilbenes from Artocarpus Nanchuanensis. J. Asian Nat. Prod. Res. 17, 217–223. doi:10.1080/10286020.2015.1006202
Zhang, R., Yu, R., Xu, Q., Li, X., Luo, J., Jiang, B., et al. (2017). Discovery and Evaluation of the Hybrid of Bromophenol and Saccharide as Potent and Selective Protein Tyrosine Phosphatase 1B Inhibitors. Eur. J. Med. Chem. 134, 24–33. doi:10.1016/j.ejmech.2017.04.004
Zhang, S. N., Zeng, J., Tan, Y. N., Ma, R. J., Zhang, G. J., Wang, H. S., et al. (2019b). 3α,19-Dihydroxyl-ent-pimara-8(14),15-diene, a New Diterpenoid from the Rhizomes of Ricinus communis. J. Asian Nat. Prod. Res. 21, 522–527. doi:10.1080/10286020.2018.1461087
Zhang, W., Hong, D., Zhou, Y., Zhang, Y., Shen, Q., Li, J. Y., et al. (2006). Ursolic Acid and its Derivative Inhibit Protein Tyrosine Phosphatase 1B, Enhancing Insulin Receptor Phosphorylation and Stimulating Glucose Uptake. Biochim. Biophys. Acta 1760, 1505–1512. doi:10.1016/j.bbagen.2006.05.009
Zhang, X., Lv, Q., Jia, S., Chen, Y., Sun, C., Li, X., et al. (2016a). Effects of flavonoid-rich Chinese bayberry (Morella rubra Sieb. et Zucc.) fruit extract on regulating glucose and lipid metabolism in diabetic KK-A(y) mice. Food Funct. 7, 3130–3140. doi:10.1039/c6fo00397d
Zhang, X. Y., Li, W., Wang, J., Li, N., Cheng, M. S., and Koike, K. (2019c). Protein Tyrosine Phosphatase 1B Inhibitory Activities of Ursane-type Triterpenes from Chinese Raspberry, Fruits of Rubus Chingii. Chin. J. Nat. Med. 17, 15–21. doi:10.1016/S1875-5364(19)30004-4
Zhang, Y., Chen, J., Zeng, Y., Huang, D., and Xu, Q. (2019d). Involvement of AMPK Activation in the Inhibition of Hepatic Gluconeogenesis by Ficus Carica Leaf Extract in Diabetic Mice and HepG2 Cells. Biomed. Pharmacother. 109, 188–194. doi:10.1016/j.biopha.2018.10.077
Zhang, Y., Feng, F., Chen, T., Li, Z., and Shen, Q. W. (2016b). Antidiabetic and Antihyperlipidemic Activities of Forsythia Suspensa (Thunb.) Vahl (Fruit) in Streptozotocin-Induced Diabetes Mice. J. Ethnopharmacol. 192, 256–263. doi:10.1016/j.jep.2016.07.002
Zhang, Y., Yan, L. S., Ding, Y., Cheng, B. C. Y., Luo, G., Kong, J., et al. (2020). Edgeworthia Gardneri (Wall.) Meisn. Water Extract Ameliorates Palmitate Induced Insulin Resistance by Regulating IRS1/GSK3β/FoxO1 Signaling Pathway in Human HepG2 Hepatocytes. Front. Pharmacol. 10, 1666. doi:10.3389/fphar.2019.01666
Zhao, B. T., Le, D. D., Nguyen, P. H., Ali, M. Y., Choi, J. S., Min, B. S., et al. (2016). PTP1B, α-glucosidase, and DPP-IV Inhibitory Effects for Chromene Derivatives from the Leaves of Smilax china L. Chem. Biol. Interact. 253, 27–37. doi:10.1016/j.cbi.2016.04.012
Zhao, C. C., Chen, J., Shao, J. H., Zhang, X. H., Gu, W. Y., Shen, J., et al. (2020). Lignan Constituents from the Fruits of Viburnum Macrocephalum F. Keteleeri and Their α-Amylase, α-Glucosidase, and Protein Tyrosine Phosphatase 1B Inhibitory Activities. J. Agric. Food Chem. 68, 11151–11160. doi:10.1021/acs.jafc.0c03353
Zhao, Y., Chen, M. X., Kongstad, K. T., Jäger, A. K., and Staerk, D. (2017). Potential of Polygonum Cuspidatum Root as an Antidiabetic Food: Dual High-Resolution α-Glucosidase and PTP1B Inhibition Profiling Combined with HPLC-HRMS and NMR for Identification of Antidiabetic Constituents. J. Agric. Food Chem. 65, 4421–4427. doi:10.1021/acs.jafc.7b01353
Zhao, Y., Kjaerulff, L., Kongstad, K. T., Heskes, A. M., Møller, B. L., and Staerk, D. (2019a). 2(5H)-Furanone Sesquiterpenes from Eremophila bignoniiflora: High-Resolution Inhibition Profiling and PTP1B Inhibitory Activity. Phytochemistry 166, 112054. doi:10.1016/j.phytochem.2019.112054
Zhao, Y., Kongstad, K. T., Liu, Y., He, C., and Staerk, D. (2019b). Unraveling the Complexity of Complex Mixtures by Combining High-Resolution Pharmacological, Analytical and Spectroscopic Techniques: Antidiabetic Constituents in Chinese Medicinal Plants. Faraday Discuss. 218, 202–218. doi:10.1039/c8fd00223a
Zhao, Y., Kongstad, K. T., Jäger, A. K., Nielsen, J., and Staerk, D. (2018). Quadruple High-Resolution α-glucosidase/α-amylase/PTP1B/radical Scavenging Profiling Combined with High-Performance Liquid Chromatography-High-Resolution Mass Spectrometry-solid-phase Extraction-Nuclear Magnetic Resonance Spectroscopy for Identification of Antidiabetic Constituents in Crude Root Bark of Morus alba L. J. Chromatogr. A 1556, 55–63. doi:10.1016/j.chroma.2018.04.041
Zheng, X. M., Meng, F. W., Geng, F., Qi, M., Luo, C., Yang, L., et al. (2015). Plantadeprate A, a Tricyclic Monoterpene Zwitterionic Guanidium, and Related Derivatives from the Seeds of Plantago Depressa. J. Nat. Prod. 78, 2822–2826. doi:10.1021/acs.jnatprod.5b00368
Zhou, J., Wu, Z., Oyawaluja, B. O., Coker, H. A. B., Odukoya, O. A., Yao, G., et al. (2019). Protein Tyrosine Phosphatase 1B Inhibitory Iridoids from Psydrax Subcordata. J. Nat. Prod. 82, 2916–2924. doi:10.1021/acs.jnatprod.9b00770
Zhou, X., Wang, L. L., Tang, W. J., and Tang, B. (2021). Astragaloside IV Inhibits Protein Tyrosine Phosphatase 1B and Improves Insulin Resistance in Insulin-Resistant HepG2 Cells and Triglyceride Accumulation in Oleic Acid (OA)-treated HepG2 Cells. J. Ethnopharmacol. 268, 113556. doi:10.1016/j.jep.2020.113556
Keywords: medicinal plants, hyperglycemia, hepatic glucose output, insulin resistance, PTP-1B inhibitors, natural products
Citation: Mata-Torres G, Andrade-Cetto A and Espinoza-Hernández F (2021) Approaches to Decrease Hyperglycemia by Targeting Impaired Hepatic Glucose Homeostasis Using Medicinal Plants. Front. Pharmacol. 12:809994. doi: 10.3389/fphar.2021.809994
Received: 05 November 2021; Accepted: 30 November 2021;
Published: 23 December 2021.
Edited by:
Valentina Echeverria Moran, Bay Pines VA Healthcare System, United StatesReviewed by:
Luis Goya, Spanish National Research Council (CSIC), SpainSakina Mohamed Yagi, University of Khartoum, Sudan
Copyright © 2021 Mata-Torres, Andrade-Cetto and Espinoza-Hernández. This is an open-access article distributed under the terms of the Creative Commons Attribution License (CC BY). The use, distribution or reproduction in other forums is permitted, provided the original author(s) and the copyright owner(s) are credited and that the original publication in this journal is cited, in accordance with accepted academic practice. No use, distribution or reproduction is permitted which does not comply with these terms.
*Correspondence: Adolfo Andrade-Cetto, YWFjQGNpZW5jaWFzLnVuYW0ubXg=