- 1Department of Radiotherapy, The Second Hospital of Jilin University, Changchun, China
- 2Department of Thyroid Surgery, The Second Hospital of Jilin University, Changchun, China
- 3Department of Neurology, China-Japan Union Hospital of Jilin University, Changchun, China
- 4Department of Breast Surgery, The Second Hospital of Jilin University, Changchun, China
- 5Jilin Ginseng Academy, Changchun University of Chinese Medicine, Changchun, China
- 6Research Center of Traditional Chinese Medicine, The Affiliated Hospital to Changchun University of Chinese Medicine, Changchun, China
The tumor microenvironment (TME) plays a key role in promoting the initiation and progression of tumors, leading to chemoradiotherapy resistance and immunotherapy failure. Targeting of the TME is a novel anti-tumor therapeutic approach and is currently a focus of anti-tumor research. Panax ginseng C. A. Meyer (ginseng), an ingredient of well-known traditional Asia medicines, exerts beneficial anti-tumor effects and can regulate the TME. Here, we present a systematic review that describes the current status of research efforts to elucidate the functions and mechanisms of ginseng active components (including ginsenosides and ginseng polysaccharides) for achieving TME regulation. Ginsenosides have variety effects on TME, such as Rg3, Rd and Rk3 can inhibit tumor angiogenesis; Rg3, Rh2 and M4 can regulate the function of immune cells; Rg3, Rd and Rg5 can restrain the stemness of cancer stem cells. Ginseng polysaccharides (such as red ginseng acidic polysaccharides and polysaccharides extracted from ginseng berry and ginseng leaves) can regulate TME mainly by stimulating immune cells. In addition, we propose a potential mechanistic link between ginseng-associated restoration of gut microbiota and the tumor immune microenvironment. Finally, we describe recent advances for improving ginseng efficacy, including the development of a nano-drug delivery system. Taken together, this review provides novel perspectives on potential applications for ginseng active ingredients as anti-cancer adjuvants that achieve anti-cancer effects by reshaping the tumor microenvironment.
Introduction
Efforts to develop anti-cancer therapies no longer focus specifically on targeting tumor cells themselves, since cancer progression is regulated by the interaction between tumor cells and the tumor-site environment. The tumor microenvironment (TME) is a complex tumor ecosystem that are dominated by tumor and consists of tumor cells, stromal cells, immune cells, and the extracellular matrix (Wang et al., 2021; Zhang et al., 2021). The overall complexity of an anti-tumor treatment depends on interactions between the TME and tumor that support tumor growth and heterogeneity, as well as tumor invasion, metastasis, immune escape, and resistance to radiotherapy and chemotherapy (Cheng et al., 2020; Abou Khouzam et al., 2021). In view of the important role of the TME in tumor development, tumor treatment approaches have evolved from traditional measures aimed at eliminating tumor cells to multi-pronged comprehensive treatment measures focused on eliminating both tumor cells and the TME. Therapeutic strategies targeting the TME include enhancement of anti-tumor immunity, inhibition of tumor angiogenesis, administration of anti-inflammatory agents, and blockage of communication between tumor cells and the extracellular matrix (Pitt et al., 2016). A variety of drugs that target the TME are currently in widespread clinical use. For example, antibody treatments are used to enhance the anti-tumor immune response by blocking negative immunomodulatory effects on “immune checkpoints” that are induced by tumor or immunosuppressive cells within the TME. Such antibodies include anti-CTLA-4 antibodies that restore costimulatory signaling of cytotoxic T lymphocytes (CTLs) and anti-PD-1/PD-L1 antibodies that block PD-1/PD-L1 inhibition of activated CTL function. Meanwhile, drugs targeting tumor angiogenesis are also available that include antibodies targeting the VEGF/VEGFR axis, which block tumor-site endothelial cell angiogenesis and promote vascular normalization. Therefore, targeting of the TME is a novel and promising strategy for the development of anti-tumor drugs.
Panax ginseng C.A. Meyer, known as “the king of herbs,” has been used in Asian medicine for thousands of years to treat illness and was also used primarily as an energy and body balance tonic in ancient times. Nowadays, ginseng has been shown to have benefits for relieving a variety of disorders, such as inflammation, infection, fatigue, effects of aging, and cancer. Active ingredients of ginseng, such as ginsenosides and polysaccharides, have been shown to possess significant anti-tumor activities (Sun et al., 2017; Ahuja et al., 2018; Li X. et al., 2020; Guo et al., 2021). Ginsenosides induce tumor cell death by inducing initiation of programmed death pathways or inhibiting tumor proliferation by interfering with tumor cell cycle and metabolic pathways. In addition, ginsenosides effectively inhibit tumor cell invasion and metastasis, while ginseng polysaccharides have been shown to induce tumor cell apoptosis and inhibit tumor cell metastasis. Furthermore, ginseng may counter immunosuppressive TME effects by modulating immune cell differentiation and functions and regulating immune checkpoints to restore anti-tumor immune functions. Collectively, these results indicate that ginseng anti-tumor efficacy may depend on abilities of its constituents to regulate multiple targets within the TME. In this review, molecular mechanisms underlying TME regulation by ginseng active ingredients are reviewed.
The Role of Ginsenosides in Inhibiting Tumor Angiogenesis
Under normal physiological conditions, blood vessel formation is a tightly controlled process. However, during the process of tumor proliferation, the tumor-dominated microenvironment promotes aberrant angiogenesis by breaking the vascular homeostatic balance (Weis and Cheresh, 2011). A large number of pro-angiogenic and anti-angiogenic factors participate in vascular homeostasis. When these factors are in equilibrium, the vascular system remains stable and endothelial cells do not proliferate. By contrast, when the “angiogenesis switch” is turned on, the vascular homeostatic balance becomes disrupted and tips in favor of pro-angiogenesis (Hanahan and Folkman, 1996; Bergers and Benjamin, 2003; Lugano et al., 2020). Bone marrow-derived endothelial progenitor cells (EPCs) recruited by tumors participate in the pathological neovascularization and growth of early tumors by modulating the angiogenic switch (Nolan et al., 2007; Oh et al., 2007; Plummer et al., 2013; Bonfim-Silva et al., 2017; Xu et al., 2017). Moreover, hypoxic conditions, a hallmark of the TME, are closely related to initiation of tumor angiogenesis, whereby the ubiquitin-proteasome pathway of hypoxia inducible factor-1 (HIF-1) is inhibited under hypoxic conditions, which leads to intracellular HIF-1 complex accumulation that promotes initiation of transcription of pro-angiogenic genes. Pro-angiogenic factors and their homologous receptors effectively co-operate to promote angiogenesis within tumors during which vascular endothelial growth factor and its receptor (VEGF/VEGFR) system play a pivotal role (Weis and Cheresh, 2005; Shibuya, 2013; Ribatti and Tamma, 2019). Furthermore, the leakage and collapse of tumor blood vessels can exacerbate hypoperfusion and hypoxia, leading to increased secretion of VEGF. Meanwhile, other angiogenesis-related factors and molecules (e.g., ANG1, FGF2, PDGF, ephrins, MMPs, etc.) may also contribute to the formation of a defective vascular network in tumors (Lugano et al., 2020) that may explain why neo-vessels in the TME possess abnormal morphology and network structure and have increased blood vessel permeability. In turn, aberrant angiogenesis supports tumor growth, invasion, and metastasis and may intensify the hypoxic microenvironment surrounding the tumor to promote tumor proliferation.
Anti-angiogenesis therapies, which mainly target the VEGF signaling pathway, have been approved for treatment of a variety of tumors (Lugano et al., 2020; Shibuya, 2013), although drug development is ongoing due to drug resistance and adverse reactions. Natural herbs such as ginseng are increasingly being viewed as potential anti-angiogenesis drugs, including various ginsenoside monomers that have been found to inhibit angiogenesis in tumors (Table 1). For example, ginsenoside Rg3 was found to inhibit EPCs differentiation, proliferation, and migration by suppressing VEGF-dependent p38/ERK and Akt/eNOS signal pathways in vitro (Kim et al., 2012a; Kim et al., 2012b), while also attenuating neo-vessel formation and mobilization of EPCs in vivo, leading to delayed tumor progression and angiogenesis (Kim et al., 2012a). According to Tang et al., ginsenoside Rg3 appeared to decrease microvessel density levels in colorectal cancer xenografts by downregulating expression of certain angiogenesis-related genes, such as CSF3, FGF2, MMP1, and PGF (Tang et al., 2018). Under TME hypoxic conditions, HIF-1 complex, of which HIF-1α is a key component, could further activate various downstream angiogenic factors in tumor cells, such as VEGF. In other studies, ginsenoside Rg3 has been shown to inhibit angiogenesis in a variety of tumor models and was shown to inhibit hypoxia-induced VEGF expression in tumor cells (Ge et al., 2014; Li and Qu, 2019; Meng et al., 2019) through regulation of various pathways, such as Akt, ERK, JNK, and STAT3 signaling pathways (Chen et al., 2010; Meng et al., 2019). Another ginsenoside, Rd, was reported to exert anti-angiogenic effects by mitigating VEGF-induced migration, invasion, and capillary formation by human umbilical vascular endothelial cells (HUVECs) and by reducing CD31-positive capillary formation in tumors by inhibiting both VEGF/VEGFR2 signaling cascade pathways Akt/mTOR/p70S6K and HIF-1α expression (Zhang et al., 2017). Meanwhile, ginsenosides Rb1 and Ro have been shown to inhibit HUVEC cell formation into tube-like structures (Lu et al., 2017; Zheng et al., 2019), while the end metabolite of 20(S)-protopanaxadiol-type ginsenosides metabolism, PPD, exerted a pro-apoptotic effect on HUVECs (Wang X. et al., 2019) and ginsenoside Rh2 downregulated tumor cell expression of VEGF and MMPs (Li H. et al., 2018; Zhang et al., 2020).
The tumor vascular network is characterized by dilated, twisted, and disordered immature vessels lacking parietal cells (Figure 1) that exhibit hyperpermeability, poor perfusion, and increased hypoxia (Viallard and Larrivée, 2017). Recent data have demonstrated that some ginsenosides, such as F1 and Rh1, act to reduce vascular leakage induced by VEGF by suppressing mRNA transcription and protein expression of NR4A1 (Kang et al., 2019), leading to vascular normalization that prevents tumor cell extravasation and metastasis.
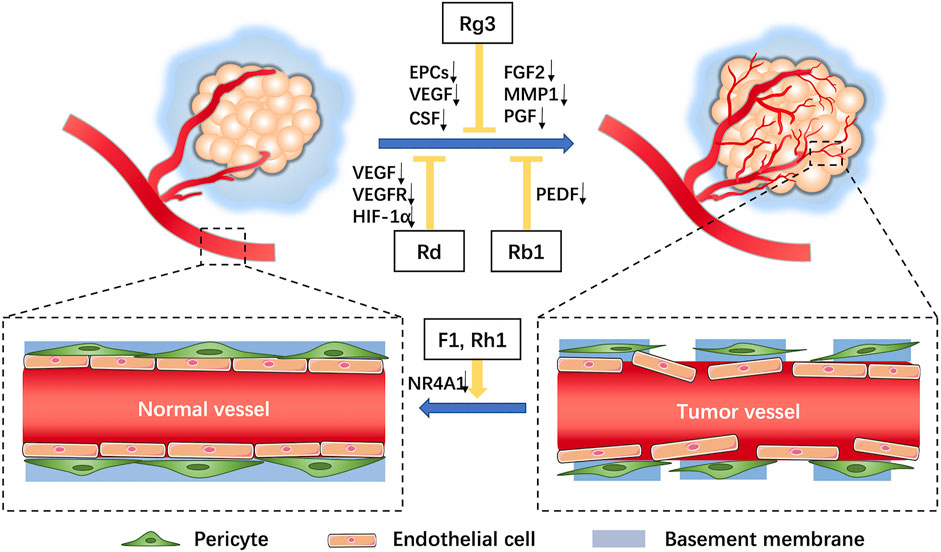
FIGURE 1. Characteristics of tumor vessels and roles of ginsenosides on tumor angiogenesis. In the tumor microenvironment, tumor blood vessels comprise a tortuous, over-branched, and disordered vascular network structure with increased vascular permeability (increased endothelial cell gaps, loss of pericyte coverage, and incomplete basement membrane). Ginsenosides can inhibit effects of angiogenic factors on tumor angiogenesis and facilitate vascular normalization.
Ginseng May Regulate the Tumor Immunosuppressive Microenvironment
An important component of the TME, infiltrating immune cells, can be classified as tumor-suppressors and tumor-promotors based on functions of these tumor-associated cells (Lei et al., 2020). In general, although immune cells within the body are capable of recognizing and killing tumor cells, anti-tumor immune responses in the TME are suppressed due to direct or indirect tumor interference with, and/or inhibition of, functions of tumor-antagonizing immune cells acting via many mechanisms. Ultimately, dysfunctional immunoregulation within the TME promotes proliferation and differentiation of tumor-promoting immune cells that eventually lead to abnormal immunosurveillance and tumor cell immune escape (Li W. et al., 2020; Lei et al., 2020; Liskova et al., 2020). Several active components of ginseng may exert anti-tumor effects by interfering with the differentiation and maturation of tumor-promoting immune cells, leading to reversal of the inhibitory phenotype of tumor-antagonizing immune cells and restoration of anti-tumor functions of innate (Table 2) and acquired immune cells.
Ginseng Enhancement of the Innate Immune Response
Macrophages
Macrophages exhibit developmental plasticity and can differentiate into pro-inflammatory (M1) and anti-inflammatory (M2) phenotypes according to different pathological environments (Wang Y. et al., 2019). Available studies indicate that tumor-associated macrophages (TAMs) derived from circulating monocytes and myeloid-derived suppressor cells (MDSCs) mainly possess characteristics and phenotypes of pro-tumorigenic M2-polarized macrophages that promote tumor angiogenesis, enhance tumor metastasis, and inhibit anti-tumor T cell immunity (Kim and Bae, 2016). Thus, strategies that Increase the M1/M2 ratio or inhibit effects of M2-polarized cells are promising anti-tumor therapeutic approaches that work by targeting the TME.
Recent studies have shown that ginsenosides may help to regulate the two subpopulations of TAMs. One such study declared that ginsenoside Rg3 treatment led to an improved anti-tumor effect based on the re-education and conversion of TAMs from an M2 phenotype to an M1 phenotype when Rg3 was delivered to cells within liposomes that were also loaded with paclitaxel (Rg3-PTX-LPs) (Zhu et al., 2021). Another ginsenoside, Rh2, has also been shown to alter the TME by inducing conversion of TAMs from an M2 to an M1 phenotype that, in turn, prevented tumor cell migration and secretion of tumor angiogenetic factors (Li H. et al., 2018). Meanwhile, ginsenoside Rp1 was shown to inhibit murine macrophage radiation-induced DNA damage. After colon cancer cells were exposed to conditioned medium from radiation-potentiated macrophages, it was found that culture supernatant of Rp1-treated macrophages inhibited growth and metastasis of tumor cells and prolonged survival of tumor-bearing mice in vivo (Baik et al., 2020). In yet another study, treatment of RAW264.7 cells, a macrophage-derived cell line, with heat-processed ginseng (HPG) containing Rg3, Rk1, and Rg5 that enhanced macrophage cell functions that included cytokines production, MHC class I and II expression, and NF-κB transcriptional activity (Shin et al., 2018).
Another component of ginseng, polysaccharides, are recognized as immunomodulators (Zeng et al., 2019; Qi et al., 2021). It has shown that the addition of red ginseng acidic polysaccharide (RGAP) or IFN-γ alone to murine melanoma B16 cells exerted no cytotoxic effect, while each weakly activated macrophages. It is worth noting that RGAP administered with IFN-γ markedly stimulated macrophages to secrete pro-inflammatory cytokines resembling those secreted by M1 macrophages (e.g., IL-1, IL-6, TNF-α) due to triggering of the NF-κB pathway, leading to dramatic enhancement of MHC-unrestricted macrophage-mediated cytotoxicity (Choi et al., 2008). The non-selective cytotoxicity of most chemotherapeutic drugs, while achieving desired anti-tumor effects, can lead to collateral damage of immune cells, prompting researchers to investigate ginseng effects for alleviating immune cell damage. In one such study, ginseng neutral polysaccharides were shown to reverse 5-fluorouracil-induced splenic weight decreases and inhibition of macrophage phagocytosis to restore macrophage production of NO and TNF-α (Ni et al., 2010). Therefore, ginseng active ingredients appear to have the potential to induce macrophages to transform into pro-inflammatory cells with heightened ability to kill tumor cells, while also potentially alleviating macrophage damage caused by effects of radiotherapy and chemotherapy.
Dendritic Cells
Dendritic cells (DCs), which function as professional antigen presenting cells (APCs), play pivotal roles in initiating innate and adaptive immunity, with the latter role associated with DCs presentation of exogenous tumor-associated antigens on MHC I molecules to naive CD8+ T cells to initiate anti-tumor immunity. However, the activity of DCs to induce anti-tumor responses is suppressed by TME dampening of DC maturation, differentiation, or cell migration, with numerous TME effector molecules (e.g., IL-6, IL-10, VEGF, TGF-β, CSF-1) involved in suppression of DC activities (Fu and Jiang, 2018; Bandola-Simon and Roche, 2019; Del Prete et al., 2020). Defective DCs that cannot properly perform their sentinel function have been detected in various cancers, such as breast cancer (Gervais et al., 2005), colorectal cancer (Legitimo et al., 2014), and ovarian cancer (Jiang et al., 2018). Increased immunogenic tumor cell death may contribute to maturation and tumor antigen-presentation activity of DCs resulting from release of damage-associated molecular patterns (DAMPs) molecules from tumor cells, such as chaperone protein calreticulin (CRT), high mobility group box-1 protein (HMGB1), and heat shock proteins (HSPs). Keum-joo Son et al. (Son et al., 2016) reported that ginsenoside Rg3 was able to act as an immunomodulator to increase DC uptake of tumor cells by inducing immunogenic tumor cell death and enhancing immunogenicity of cancer cells. Furthermore, ginsenoside Rg3 could stimulate tumor cells to produce IFN-γ, an anti-tumor cytokine secreted by T cells, while suppressing tumor cell secretion of TGF-β and IL-6 (Son et al., 2016). In other work, Takei et al. (Takei et al., 2004) demonstrated that M1 and M4, end products of steroidal ginseng saponins metabolized within the digestive tract, exerted immunomodulatory effects on DCs by inducing DCs maturation, as reflected by upregulation of DC surface expression of maturation marker molecules CD80, CD83, CD86, and HLA-DR. In turn, mature DCs enhanced the polarization of Th1 cells that increased anti-tumor immunity. In addition, ginseng polysaccharides have been shown to stimulate maturation of murine bone marrow dendritic cells (BMDCs), as revealed by changes in cell morphology, upregulation of membrane phenotypic markers (e.g., CD40, CD80, CD83, CD86, MHC II), and increased pro-inflammatory cytokine production (Meng et al., 2013; Wang et al., 2013).
Natural Killer Cells
As for DCs, natural killer (NK) cells in the TME are also dysfunctional (Round and Mazmanian, 2010). Results of studies based on mouse models of lymphoma clearance and metastatic melanoma demonstrated that ginsenoside F1 could enhance NK cell cytotoxicity against diverse types of cancer cells, while also improving NK cell cancer surveillance ability by upregulating NK cell secretion of cytotoxic mediators and NK activation signal molecules (Kwon et al., 2018). Meanwhile, oral administration of 20(S)-protopanaxatriol (M4), an intestinal bacterial metabolite of ginsenosides, led to complete absorption of M4 by the small intestine followed by transfer of most of the substance to the mesenteric lymphatics, where it was esterified to form EM4 that then spread to other organs in the body. Notably, EM4 was shown to stimulate tumor lysis mediated by NK cells in a B16-BL6 melanoma metastasis mouse model (Hasegawa et al., 2002). In another study, pectin polysaccharide fraction (GS-P) purified from ginseng leaves was shown to inhibit proliferation and metastasis of colon cancer cells; these effects were not based on direct cytotoxicity, but were instead based on stimulation of macrophage and NK cell activities (Shin et al., 2017). A similar conclusion was obtained in a study of ginseng berry polysaccharide portion (GBPP), which stimulated macrophages to secrete anti-tumorigenic cytokines (e.g., IL-6, IL-12, TNF-α) while also promoting NK cells to release IFN-γ and granzyme B that inhibited tumor cells activities (Lee et al., 2019a; Lee et al., 2019b). Moreover, another study of polysaccharides purified from ginseng fruits demonstrated that ginseng polysaccharides could significantly enhance NK cell-mediated cytotoxicity in tumor-bearing mice (Wang et al., 2015). Taken together, these results indicate that active components of ginseng exert anti-tumor effects by correcting impaired NK cell killing of tumor cells that, in turn, effectively inhibit tumor metastasis.
Ginseng Enhancement of the Adaptive Immune Response
Adaptive Immune Cells
In addition to ginseng effects for reversing tumor inhibition of innate immune cell activities, ginseng has also been shown to reverse tumor-inhibited adaptive immune cell activities. For example, regulatory T cells (Tregs) (identified based on the presence of foxp3+CD25+CD4+ cell surface markers) actively engage in maintenance of immunological self-tolerance, as well as in inhibition of immune responses within the TME (Dong et al., 2020). In fact, Tregs have been found to infiltrate the tumor site, where they suppress the anti-tumor response, with suppression reversed by depletion of Tregs. Using chronic intestinal inflammation as a model system of colorectal cancer (due to the close clinical relationship between the two diseases), ginseng berry polysaccharide extract (GBPE) and GBPP obtained from Asian ginseng berries were shown to exert anti-inflammatory effects in vitro that were linked to inhibition of secretion of IL-8, a proinflammatory factor closely tied to intestinal inflammation. Moreover, by inhibiting T cell differentiation into Th1 cells (which promote intestinal inflammation) and Treg cells (which weaken the body’s anti-tumor immunity), intestinal inflammation may be reduced and anti-tumor effects of chemotherapy drugs synergistically enhanced so as to effectively inhibit proliferation of colorectal cancer cells (Wang et al., 2020).
Indoleamine-2,3-dioxygenase (IDO) is an intracellular heme-containing enzyme within the kynurenine (Kyn) pathway that catabolizes tryptophan (Trp). Due to its role in tumor immune escape mechanisms, IDO expression has been found in tumor cells, DC cells, endothelial cells and even stromal cells in the TME, where it inhibits CTL infiltration and cell functions while inducing Treg recruitment (Uyttenhove et al., 2003; Löb et al., 2009; Sharma et al., 2010; Munn and Mellor, 2016). Using the ratio of Kyn to Trp as a marker of IDO enzymatic activity, results of a study based on a mouse model of inflammation indicated that ginseng total saponins could decrease the plasma Kyn/Trp ratio (Kang et al., 2011). Since then, researchers have also found that ginsenoside Rg3 treatment similarly reduced IDO activity in the periphery and brain (Kang et al., 2017). In an in vivo liver fibrosis mouse model and in in vitro experiments, ginsenoside Rg1 was also observed to inhibit IDO1 protein expression and enhance DCs activities and T cell infiltration, thereby enhancing the immune response (Mo et al., 2021). Recent studies have shown that ginseng polysaccharides enhanced the anti-tumor response triggered by anti-PD-1 mAb by increasing CD8+ T cell function and decreasing Treg inhibition. These effects may have been due to effects of ginseng polysaccharides on tryptophan metabolism that acted to reshape the gut microbiota that resulted in significantly increased production of L-tryptophan and decreased production of L-kynurenine and IDO expression in tumor cells (Huang et al., 2021). In conclusion, ginsenosides and ginseng polysaccharides appear to enhance the adaptive immune response against tumor cells, warranting further study (Figure 2).
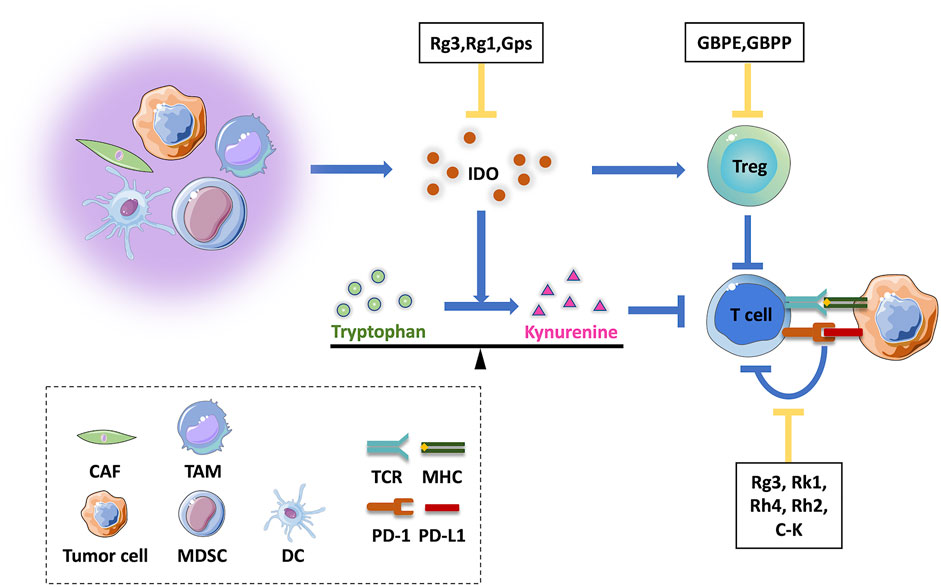
FIGURE 2. Effects of active ingredients of ginseng on acquired immune responses in the tumor microenvironment. A variety of cells in the tumor microenvironment may inhibit T cell functions, either through direct modulation of the IDO molecular switch or indirect effects that maintain immunosuppressive Treg cells within the tumor microenvironment. Tumor cells inhibit T cell functions through the PD-1/PD-L1 axis, resulting in immune escape. Active ingredients of ginseng act to inhibit these processes. CAF, cancer-associated fibroblast; TAM, tumor-associated macrophage; MDSC, myeloid-derived suppressor cell; DC, dendritic cell; GBPE, ginseng berry polysaccharide extract; GBPP, ginseng berry polysaccharide portion; IDO, indoleamine 2,3-dioxygenase.
Enhancement of Immunogenicity of Tumor Cells
Immunogenicity of tumor cells is key to inducing an anti-tumor immune response. Generally, cancer cells could evade anti-tumor immunity by adopting active immunogenicity reduction strategies, including reduced expression of tumor antigens, diminished MHC-I expression for reduced antigenic recognition by T cells, and aberrant expression of immune checkpoint proteins, such as programmed death ligand-1 (PD-L1), which inhibits existing host anti-tumor immunity (Kim and Seo, 2018; Looi et al., 2019; Tang et al., 2014). Furthermore, PD-L1 expression can be up-regulated in tumor cells that are resistant to chemotherapeutic agents (Shen et al., 2019; Wu et al., 2021), including tumor-targeting drugs such as EGFR-TKI (Peng et al., 2019), thus promoting immune escape of tumor cells. Although inhibitors targeting immune checkpoints have been developed and have become a focal point of clinical cancer therapy, current low treatment response rates, high incidence of side-effects, and acquired resistance are still unavoidable challenges. Nevertheless, effective reduction of PD-L1 expression in tumor cells appears to anti-tumor immunity and reduce tumor drug resistance, as shown in a study whereby PD-L1 protein was overexpressed in cisplatin-resistant human non-small cell lung cancer (NSCLC) cell line A549/DDP. In these experiments, ginsenoside Rg3 was shown to significantly block PD-L1 overexpression in A549/DDP cells, an effect that was associated with inhibition of activation of Akt and NK-κB pathways. Additional in vitro experiments confirmed that when A549/DDP cells were co-cultured with CD8+ T cells, Rg3 could target chemotherapy-induced PD-L1 and enhance the immunocytotoxicity of CD8+ T cells (Jiang et al., 2017). Moreover, when loaded into carbon nanotubes (CNT) (Luo et al., 2021), Rg3 inhibited expression of PD-L1 by triple-negative breast cancer cells. Remarkably, CNTs-loaded with Rg3 could attenuate PD-1 expression in activated T cells and reduce PD-1/PD-L1 axis activity in vitro. Meanwhile, other ginsenosides such as Rk1 (Hu et al., 2020), Rh4 (Deng et al., 2020), and Rh2 (Chen Y. et al., 2020) have also been reported to downregulate PD-L1 expression in tumor cells (Figure 2). In addition, ginsenosides have been shown to exert a competitive inhibitory effect on the PD-1/PD-L1 interaction. Specifically, eight ginsenosides (Rd, F2, Rg3, C-K, Rh2, PPD, Rg1, Rh1) were confirmed to block the PD-1/PD-L1 binding interaction, whereby a blocking ability of 35% was observed at the maximum ginsenoside concentration (1 µM), with Rg3 and C-K exerting the most significant effects. Further analyses based on molecular docking and pharmacophore analysis suggested that Rg3 and C-K may achieve an immune checkpoint blockade through multiple hydrophobic and hydrogen bonds with PD-1/PD-L1 molecules (Yim et al., 2020).
Ginseng Could Inhibit Stemness of Cancer Stem Cells
In recent decades, rare stem-like cells known as cancer stem cells (CSCs) have been detected in tumors. CSCs possess characteristics of self-renewal, strong xenograft tumorigenesis properties, and resistance to conventional therapy (radiation and chemotherapy) and resemble bone marrow hematopoietic stem cells. In general, CSCs are dormant except during long-term tumor growth, where they can become activated to engage in self-renewal and differentiation to generate heterogeneous tumor cells with epithelial-mesenchymal transition (EMT) characteristics (López de Andrés et al., 2020). CSCs have been identified using various identification methods, including in vivo limiting-dilution tumorigenicity assays in immunodeficient mice, in vitro tumorsphere formation assays, and assays to detect particular surface markers (Walcher et al., 2020). In multiple types of solid tumors, such as breast cancer (Bai et al., 2018), lung cancer (Heng et al., 2019), brain cancer (Singh et al., 2003), and colon cancer (Gupta et al., 2019), CSCs have been identified and found to play crucial roles in tumor proliferation, metastasis, relapse, and chemotherapy/radiation resistance resulting in failures of anti-cancer therapies. Maintenance of CSCs is dependent on TME characteristics such that the acidic and hypoxic environment of the TME may support the establishment and maintenance of CSCs stemness properties, while initiating and regulating stem cell-like programs through various developmental signaling pathways. Such pathways are crucial for maintenance of stem and progenitor cell homeostasis and functions and include Norch, WNT, Hedgehog, and Hippo pathways (Meurette and Mehlen, 2018; Clara et al., 2020). Recent studies have found that endothelial cells/pericytes within some tumor vessels may be generated via differentiation from CSCs (Krishna Priya et al., 2016), thus highlighting CSCs as a novel promising target for use in regulating the TME.
Ginseng has been reported to decrease the size of the CSCs population (Table 3). A Korean research team fermented red ginseng with Lactobacillus rhamnosus KCTC 5033 (f-RGE) to increase Rg3 content level and found that f-RGE had the potential to inhibit differentiation of breast cancer stem cell-like cells in the presence of carcinogens (Oh et al., 2015). It was further demonstrated that in vitro, Rg3 treatment could reduce the size of the population of CD24+/CD44+/EpCAM+ colon cancer stem cells and inhibit their clone-forming ability, with similar results obtained from an in vivo orthotopic xenograft model study (Tang et al., 2018) and a breast cancer study (Oh et al., 2019). In addition, ginseng had the potential to modulate the CSC phenotype. For example, BST204, a fermented ginseng extract containing high quantities of Rh2 and Rg3, strongly suppressed cancer stemness of embryonic carcinoma cells by downregulating stemness markers and transcription factors at both transcriptional and protein expression levels (Park et al., 2020). Meanwhile, ginsenoside Rg3 was also shown to decrease tumor CSC sphere-forming capacity that led to deregulated expression of stemness-related markers and attenuated CSC tumorigenic activities in several types of cancer (Wang et al., 2018; Phi et al., 2019a; Oh et al., 2019; Tan et al., 2020). Moreover, it has been reported that ginsenoside Rd may downregulate levels of genes related to stemness and EMT by binding to epidermal growth factor receptor (EGFR) (Phi et al., 2019b). Additionally, ginsenosides Rk1 and Rg5 have also been reported to suppress expression levels of lung CSC surface markers CD44 and CD133 and transcriptional regulators Nanog, Oct4, and Sox2 (Kim et al., 2021). Furthermore, the inhibitory effect of ginsenosides on stemness of CSCs has been shown to increase cell sensitivity to chemotherapeutic treatments while also reducing resistance to chemotherapy (Tang et al., 2018; Wang et al., 2018; Tan et al., 2020). At present, mechanisms whereby ginsenosides regulate CSCs are still unclear, while results regarding effects of ginsenosides on CSCs in the TME and effects of other ginseng active ingredients on CSCs await future verification. However, from the body of accumulated data, it is apparent that ginseng holds great promise as a drug for targeting CSCs.
Conclusion
The TME, a complex ecological system composed of a variety of cells and stroma, is a hotbed of tumor development. Tumor cell interactions with the TME lead to greater tumor cell aggressiveness and resistance to conventional drugs and radiation. Therefore, anti-tumor therapies should both eliminate tumor cells and interfere with communication between tumor cells and the TME.
Due to the fact that targeting of the TME is a promising anti-cancer strategy, agents that target TME inputs hold great promise as future anti-cancer drug treatments. Such agents in current use include monoclonal antibodies against three key targets: immune checkpoints PD-1 and CTLA-4, which enhance tumor-killing T cell immune functions; pro-angiogenic factor VEGF; and integrin adhesion molecules that mediate interactions between tumor cells and the TME matrix. However, anti-cancer drugs inevitably have side effects and development of new anti-cancer compounds and antibodies is extremely expensive, although screening of databases containing information for a huge array of natural compounds is a relatively inexpensive and effective approach for identifying potential anti-cancer drugs.
Ginseng is a versatile natural herbal medicine that has been shown to exert good anti-inflammatory, antioxidant, and anti-aging therapeutic effects. In fact, ginsenosides, which are mainly found in ginseng root, are considered to be the most important biologically active components of ginseng preparations. So far, more than 180 types of ginsenosides have been isolated from ginseng (Xu et al., 2021). In addition, another active ingredient of ginseng, polysaccharides (the structure and extraction method of polysaccharides are listed in Table 4), also possess beneficial activities for modulating immune regulatory functions. Inhibitory effects of some ginsenoside monomers and ginseng polysaccharides on cancer cell activities have been studied, with regulatory effects of ginseng on TME under increasing scrutiny. In this review, ginseng effects on tumor angiogenesis, the tumor immunosuppressive microenvironment, and tumor stem cells are summarized.
The gut microbiota maintains a symbiotic relationship with intestinal mucosa, the largest immune organ of the human body. The importance of this symbiotic interaction to host well-being is based on its ability to shape the host immune system by regulating local and systemic immune responses. For example, gut microbiota and metabolites may activate DCs in the local intestine, thereby activating the transformation of primitive T cells into effector T cells in mesenteric lymph nodes, with special importance for development of Treg and Th17 cells (Round and Mazmanian, 2010; Cheng et al., 2019). Alternatively, metabolites of the gut microbiota could also enter the blood circulation, thus affecting the immune function of the entire body (Rooks and Garrett, 2016; Ohno, 2020). A large body of experimental evidence suggests that gut microbes influence tumorigenesis and functions of immune cells in the TME by regulating production of cytokines (De Almeida et al., 2019; Untersmayr et al., 2019; Li Q. et al., 2020; Chen et al., 2021). In addition, gut microbes are closely associated with the efficacy and toxicity of anti-tumor immunotherapies, such as adoptive cell transfer therapy (Viaud et al., 2013; Nelson et al., 2015; Uribe-Herranz et al., 2018) and immune checkpoint inhibitors (Vétizou et al., 2015; Gopalakrishnan et al., 2018; Routy et al., 2018; Elkrief et al., 2019). Therefore, the gut microbiota not only serves as a new observational index of tumor immunotherapy, but also holds promise as an anti-tumor therapeutic target. In accordance with these concepts, previous studies indicated that the efficacy of oral ginseng was related to gut microbiome-mediated metabolic transformation involving two types of ginsenoside biotransformation: conversion of protopanaxadiol-type ginsenosides to form compound K and ginsenoside Rh2; conversion of protopanaxatriol-type ginsenosides to form ginsenosides Rh1 and protopanaxatriol (Kim, 2018). Interestingly, ginsenosides and ginseng polysaccharides have also been used to regulate the structure of the gut microbiome for treating a variety of diseases, such as obesity (Song et al., 2014; Lee et al., 2021), diabetes (Li J. et al., 2018; Xu et al., 2020), colitis (Wang et al., 2016; Chen L. et al., 2020), and antibiotic-related diarrhea (Qu et al., 2021). With regard to cancer, a recent study showed that oral ginseng polysaccharides combined with anti-PD-1-mAb could improve therapeutic sensitivity of anti-PD-1-mAb in patients with NSCLC. This effect may have been related to ginseng polysaccharides-induced remodeling of gut microbiota structure in chemotherapeutic non-responders that led to increased abundance of metabolites, such as short-chain fatty acids (SCFAs), while also down-regulating IDO activity (Huang et al., 2021). Thus, the immunosuppressive TME associated with NSCLC was altered, leading to heightened immunotherapeutic sensitivity induced by ginseng polysaccharides administration. Consequently, we hypothesized that the regulatory effects of active ingredients of ginseng on anti-tumor immunity and on the tumor immunosuppressive microenvironment may be partly related to the gut microbiome, although we have found only a few published reports describing such an association. Nevertheless, interrelationships between the regulation of gut microbiome by active ginseng ingredients, the tumor immune microenvironment, and tumor immunotherapeutic effects are unknown and require additional evidence, warranting further research.
In order to improve organ/tumor-site targeting, increase solubility of ginseng active ingredients, and reduce drug toxicity toward non-cancerous cells, researchers have combined nanoscale drug delivery systems with ginseng-derived drugs to improve biological activities of ginseng active ingredients for enhanced therapeutic effect. For example, a folic acid-modified targeting-drug delivery system based on bovine serum albumin nanoparticles achieved targeted accumulation of drugs at the cancer focus that significantly increased anti-cancer effectiveness of Rg5 in breast cancer (Dong et al., 2019). Meanwhile, use of multiple nanoparticle-loaded Rg3 was shown to achieve good organ targeting, possess sustained release properties, and exert superior anti-cancer activities, while also facilitating transport of drugs across the blood-brain barrier (Qiu et al., 2019; Ren et al., 2020; Su et al., 2020). These results support potential benefits of ginseng ingredients for use in numerous clinical applications.
Although targets of ginseng anti-cancer effects are unknown, it is undeniable that active ingredients of ginseng influence the interaction between the tumor and the TME through several mechanisms: by inhibiting tumor angiogenesis, regulating the immunosuppressive TME, and by inhibiting stemness of cancer stem cells (Figure 3). Therefore, use of a combination of ginsenosides and/or polysaccharides as cancer adjuvant therapies to target the TME may be a useful anti-tumor therapeutic strategy that may also reduce side effects of chemotherapy or immunotherapy. In addition, the application of red ginseng and white ginseng roots for adjuvant treatment of tumor patients is practical, but the dose must be further standardized and validated using clinical data and the ginseng target network must be further elucidated. In conclusion, this review provides new insights into possible applications of active ingredients of ginseng for achieving TME remodeling.
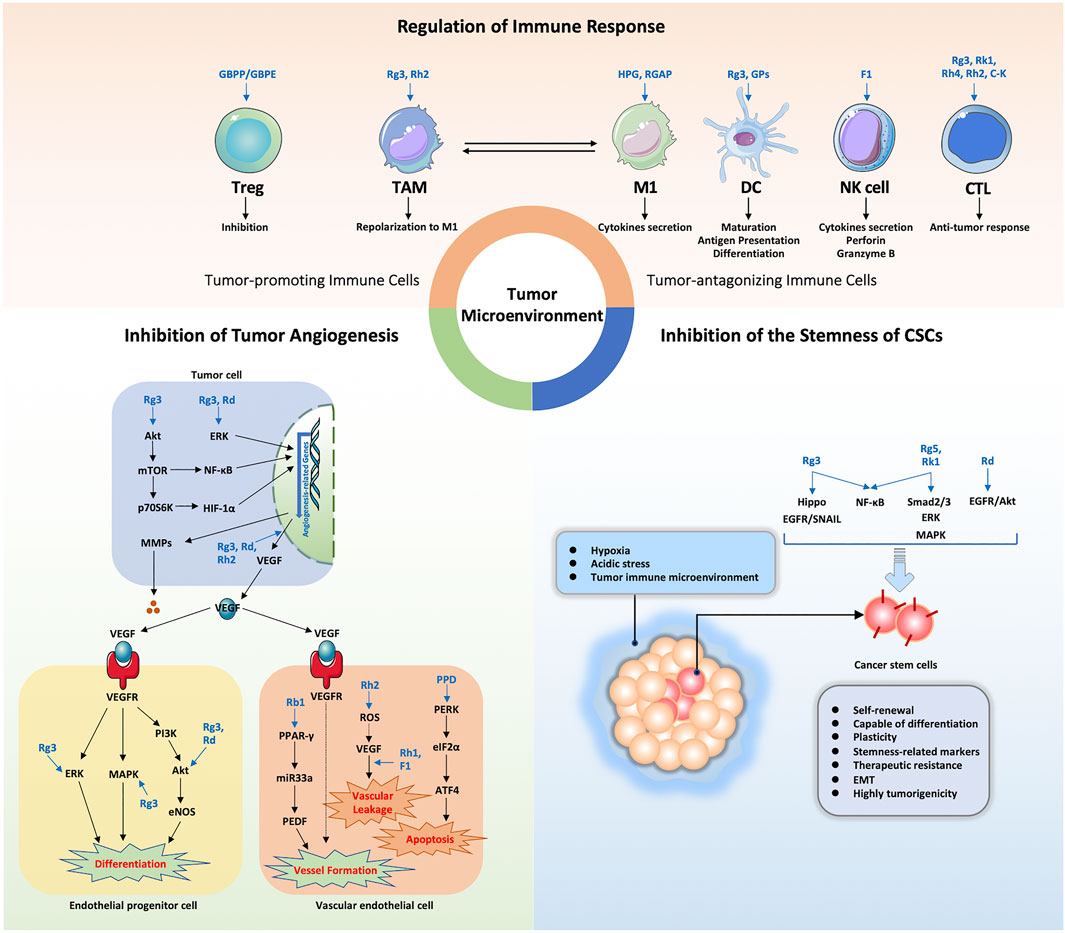
FIGURE 3. Summary of the functional effects and mechanisms underlying TME regulation by ginseng active ingredients via multiple targets. HPG, heat-processed ginseng; RGAP, red ginseng acidic polysaccharide; GPs, ginseng polysaccharides; NK cell, natural killer cell; CTL, cytotoxic T lymphocyte.
Author Contributions
ML: Conceptualization, Writing—original draft, Writing—review and editing. LL and SZ: Conceptualization, Supervision, Funding acquisition, Writing—review and editing. LS: Conceptualization, Supervision, Writing—review and editing. XW, YW, SB, and QC: Writing—review and editing. All authors contributed to the article and approved it for publication.
Funding
This work was supported by the National Natural Science Foundation of China (82104428), Department of Finance of Jilin Province (2020SCZT035) and the Administration of Traditional Chinese Medicine of Jilin Province (No. 2020170).
Conflict of Interest
The authors declare that the research was conducted in the absence of any commercial or financial relationships that could be construed as a potential conflict of interest.
Publisher’s Note
All claims expressed in this article are solely those of the authors and do not necessarily represent those of their affiliated organizations, or those of the publisher, the editors and the reviewers. Any product that may be evaluated in this article, or claim that may be made by its manufacturer, is not guaranteed or endorsed by the publisher.
References
Abou Khouzam, R., Rao, S. P., Venkatesh, G. H., Zeinelabdin, N. A., Buart, S., Meylan, M., et al. (2021). An Eight-Gene Hypoxia Signature Predicts Survival in Pancreatic Cancer and Is Associated with an Immunosuppressed Tumor Microenvironment. Front. Immunol. 12, 680435. doi:10.3389/fimmu.2021.680435
Ahuja, A., Kim, J. H., Kim, J. H., Yi, Y. S., and Cho, J. Y. (2018). Functional Role of Ginseng-Derived Compounds in Cancer. J. Ginseng Res. 42 (3), 248–254. doi:10.1016/j.jgr.2017.04.009
Bai, X., Ni, J., Beretov, J., Graham, P., and Li, Y. (2018). Cancer Stem Cell in Breast Cancer Therapeutic Resistance. Cancer Treat. Rev. 69, 152–163. doi:10.1016/j.ctrv.2018.07.004
Baik, J. S., Seo, Y. N., Yi, J. M., Rhee, M. H., Park, M. T., and Kim, S. D. (2020). Ginsenoside-Rp1 Inhibits Radiation-Induced Effects in Lipopolysaccharide-Stimulated J774A.1 Macrophages and Suppresses Phenotypic Variation in CT26 colon Cancer Cells. J. Ginseng Res. 44 (6), 843–848. doi:10.1016/j.jgr.2020.01.006
Bandola-Simon, J., and Roche, P. A. (2019). Dysfunction of Antigen Processing and Presentation by Dendritic Cells in Cancer. Mol. Immunol. 113, 31–37. doi:10.1016/j.molimm.2018.03.025
Bergers, G., and Benjamin, L. E. (2003). Tumorigenesis and the Angiogenic Switch. Nat. Rev. Cancer 3 (6), 401–410. doi:10.1038/nrc1093
Bonfim-Silva, R., Souza, L. E., Melo, F. U., Oliveira, V. C., Magalhães, D. A., Oliveira, H. F., et al. (2017). Bone Marrow-Derived Cells Are Recruited by the Melanoma Tumor with Endothelial Cells Contributing to Tumor Vasculature. Clin. Transl Oncol. 19 (1), 125–133. doi:10.1007/s12094-016-1515-z
Chen, Q. J., Zhang, M. Z., and Wang, L. X. (2010). Gensenoside Rg3 Inhibits Hypoxia-Induced VEGF Expression in Human Cancer Cells. Cell Physiol Biochem 26 (6), 849–858. doi:10.1159/000323994
Chen, Y., Liu, B., Wei, Y., and Kuang, D. M. (2021). Influence of Gut and Intratumoral Microbiota on the Immune Microenvironment and Anti-cancer Therapy. Pharmacol. Res. 174, 105966. doi:10.1016/j.phrs.2021.105966
Chen, Y., Zhang, Y., Song, W., Zhang, Y., Dong, X., and Tan, M. (2020). Ginsenoside Rh2 Improves the Cisplatin Anti-tumor Effect in Lung Adenocarcinoma A549 Cells via Superoxide and PD-L1. Anticancer Agents Med. Chem. 20 (4), 495–503. doi:10.2174/1871520619666191209091230
Chen, L., Chen, M. Y., Shao, L., Zhang, W., Rao, T., Zhou, H. H., et al. (2020). Panax Notoginseng Saponins Prevent Colitis-Associated Colorectal Cancer Development: the Role of Gut Microbiota. Chin. J. Nat. Med. 18 (7), 500–507. doi:10.1016/S1875-5364(20)30060-1
Cheng, H., Guan, X., Chen, D., and Ma, W. (2019). The Th17/Treg Cell Balance: A Gut Microbiota-Modulated Story. Microorganisms 7 (12), 583. doi:10.3390/microorganisms7120583
Cheng, J., Meng, J., Zhu, L., and Peng, Y. (2020). Exosomal Noncoding RNAs in Glioma: Biological Functions and Potential Clinical Applications. Mol. Cancer 19 (1), 66. doi:10.1186/s12943-020-01189-3
Choi, H. S., Kim, K. H., Sohn, E., Park, J. D., Kim, B. O., Moon, E. Y., et al. (2008). Red Ginseng Acidic Polysaccharide (RGAP) in Combination with IFN-Gamma Results in Enhanced Macrophage Function through Activation of the NF-kappaB Pathway. Biosci. Biotechnol. Biochem. 72 (7), 1817–1825. doi:10.1271/bbb.80085
Clara, J. A., Monge, C., Yang, Y., and Takebe, N. (2020). Targeting Signalling Pathways and the Immune Microenvironment of Cancer Stem Cells - a Clinical Update. Nat. Rev. Clin. Oncol. 17 (4), 204–232. doi:10.1038/s41571-019-0293-2
De Almeida, C. V., de Camargo, M. R., Russo, E., and Amedei, A. (2019). Role of Diet and Gut Microbiota on Colorectal Cancer Immunomodulation. World J. Gastroenterol. 25 (2), 151–162. doi:10.3748/wjg.v25.i2.151
Del Prete, A., Sozio, F., Barbazza, I., Salvi, V., Tiberio, L., Laffranchi, M., et al. (2020). Functional Role of Dendritic Cell Subsets in Cancer Progression and Clinical Implications. Int. J. Mol. Sci. 21 (11), 3930. doi:10.3390/ijms21113930
Deng, X., Zhao, J., Qu, L., Duan, Z., Fu, R., Zhu, C., et al. (2020). Ginsenoside Rh4 Suppresses Aerobic Glycolysis and the Expression of PD-L1 via Targeting AKT in Esophageal Cancer. Biochem. Pharmacol. 178, 114038. doi:10.1016/j.bcp.2020.114038
Dong, Y., Fu, R., Yang, J., Ma, P., Liang, L., Mi, Y., et al. (2019). Folic Acid-Modified Ginsenoside Rg5-Loaded Bovine Serum Albumin Nanoparticles for Targeted Cancer Therapy In Vitro and In Vivo. Int. J. Nanomedicine 14, 6971–6988. doi:10.2147/IJN.S210882
Dong, Y., Han, Y., Huang, Y., Jiang, S., Huang, Z., Chen, R., et al. (2020). PD-L1 Is Expressed and Promotes the Expansion of Regulatory T Cells in Acute Myeloid Leukemia. Front. Immunol. 11, 1710. doi:10.3389/fimmu.2020.01710
Duan, Z., Deng, J., Dong, Y., Zhu, C., Li, W., and Fan, D. (2017). Anticancer Effects of Ginsenoside Rk3 on Non-small Cell Lung Cancer Cells: In Vitro and In Vivo. Food Funct. 8 (10), 3723–3736. doi:10.1039/c7fo00385d
Elkrief, A., Derosa, L., Zitvogel, L., Kroemer, G., and Routy, B. (2019). The Intimate Relationship between Gut Microbiota and Cancer Immunotherapy. Gut Microbes 10 (3), 424–428. doi:10.1080/19490976.2018.1527167
Fu, C., and Jiang, A. (2018). Dendritic Cells and CD8 T Cell Immunity in Tumor Microenvironment. Front. Immunol. 9, 3059. doi:10.3389/fimmu.2018.03059
Ge, X., Zhen, F., Yang, B., Yang, X., Cai, J., Zhang, C., et al. (2014). Ginsenoside Rg3 Enhances Radiosensitization of Hypoxic Oesophageal Cancer Cell Lines through Vascular Endothelial Growth Factor and Hypoxia Inducible Factor 1α. J. Int. Med. Res. 42 (3), 628–640. doi:10.1177/0300060513505491
Gervais, A., Levêque, J., Bouet-Toussaint, F., Burtin, F., Lesimple, T., Sulpice, L., et al. (2005). Dendritic Cells Are Defective in Breast Cancer Patients: a Potential Role for Polyamine in This Immunodeficiency. Breast Cancer Res. 7 (3), R326–R335. doi:10.1186/bcr1001
Gopalakrishnan, V., Spencer, C. N., Nezi, L., Reuben, A., Andrews, M. C., Karpinets, T. V., et al. (2018). Gut Microbiome Modulates Response to Anti-PD-1 Immunotherapy in Melanoma Patients. Science 359 (6371), 97–103. doi:10.1126/science.aan4236
Guo, M., Shao, S., Wang, D., Zhao, D., and Wang, M. (2021). Recent Progress in Polysaccharides from Panax Ginseng C. A. Meyer. Food Funct. 12 (2), 494–518. doi:10.1039/d0fo01896a
Gupta, R., Bhatt, L. K., Johnston, T. P., and Prabhavalkar, K. S. (2019). Colon Cancer Stem Cells: Potential Target for the Treatment of Colorectal Cancer. Cancer Biol. Ther. 20 (8), 1068–1082. doi:10.1080/15384047.2019.1599660
Hanahan, D., and Folkman, J. (1996). Patterns and Emerging Mechanisms of the Angiogenic Switch during Tumorigenesis. Cell 86 (3), 353–364. doi:10.1016/s0092-8674(00)80108-7
Hasegawa, H., Suzuki, R., Nagaoka, T., Tezuka, Y., Kadota, S., and Saiki, I. (2002). Prevention of Growth and Metastasis of Murine Melanoma through Enhanced Natural-Killer Cytotoxicity by Fatty Acid-Conjugate of Protopanaxatriol. Biol. Pharm. Bull. 25 (7), 861–866. doi:10.1248/bpb.25.861
Heng, W. S., Gosens, R., and Kruyt, F. A. E. (2019). Lung Cancer Stem Cells: Origin, Features, Maintenance Mechanisms and Therapeutic Targeting. Biochem. Pharmacol. 160, 121–133. doi:10.1016/j.bcp.2018.12.010
Hu, M., Yang, J., Qu, L., Deng, X., Duan, Z., Fu, R., et al. (2020). Ginsenoside Rk1 Induces Apoptosis and Downregulates the Expression of PD-L1 by Targeting the NF-Κb Pathway in Lung Adenocarcinoma. Food Funct. 11 (1), 456–471. doi:10.1039/c9fo02166c
Huang, J., Liu, D., Wang, Y., Liu, L., Li, J., Yuan, J., et al. (2021). Ginseng Polysaccharides Alter the Gut Microbiota and Kynurenine/tryptophan Ratio, Potentiating the Antitumour Effect of Antiprogrammed Cell Death 1/programmed Cell Death Ligand 1 (Anti-PD-1/PD-L1) Immunotherapy. Gut. doi:10.1136/gutjnl-2020-321031
Jiang, L., Fang, X., Wang, H., Li, D., and Wang, X. (2018). Ovarian Cancer-Intrinsic Fatty Acid Synthase Prevents Anti-tumor Immunity by Disrupting Tumor-Infiltrating Dendritic Cells. Front. Immunol. 9, 2927. doi:10.3389/fimmu.2018.02927
Jiang, Z., Yang, Y., Yang, Y., Zhang, Y., Yue, Z., Pan, Z., et al. (2017). Ginsenoside Rg3 Attenuates Cisplatin Resistance in Lung Cancer by Downregulating PD-L1 and Resuming Immune. Biomed. Pharmacother. 96, 378–383. doi:10.1016/j.biopha.2017.09.129
Kang, A., Hao, H., Zheng, X., Liang, Y., Xie, Y., Xie, T., et al. (2011). Peripheral Anti-inflammatory Effects Explain the Ginsenosides Paradox between Poor Brain Distribution and Anti-depression Efficacy. J. Neuroinflammation 8, 100. doi:10.1186/1742-2094-8-100
Kang, A., Xie, T., Zhu, D., Shan, J., Di, L., and Zheng, X. (2017). Suppressive Effect of Ginsenoside Rg3 against Lipopolysaccharide-Induced Depression-like Behavior and Neuroinflammation in Mice. J. Agric. Food Chem. 65 (32), 6861–6869. doi:10.1021/acs.jafc.7b02386
Kang, J. I., Choi, Y., Cui, C. H., Lee, D., Kim, S. C., and Kim, H. M. (2019). Pro-angiogenic Ginsenosides F1 and Rh1 Inhibit Vascular Leakage by Modulating NR4A1. Sci. Rep. 9 (1), 4502. doi:10.1038/s41598-019-41115-2
Kim, D. H. (2018). Gut Microbiota-Mediated Pharmacokinetics of Ginseng Saponins. J. Ginseng Res. 42 (3), 255–263. doi:10.1016/j.jgr.2017.04.011
Kim, H., Choi, P., Kim, T., Kim, Y., Song, B. G., Park, Y. T., et al. (2021). Ginsenosides Rk1 and Rg5 Inhibit Transforming Growth Factor-Β1-Induced Epithelial-Mesenchymal Transition and Suppress Migration, Invasion, Anoikis Resistance, and Development of Stem-like Features in Lung Cancer. J. Ginseng Res. 45 (1), 134–148. doi:10.1016/j.jgr.2020.02.005
Kim, H. S., and Seo, H. K. (2018). Immune Checkpoint Inhibitors for Urothelial Carcinoma. Investig. Clin. Urol. 59 (5), 285–296. doi:10.4111/icu.2018.59.5.285
Kim, J., and Bae, J. S. (2016). Tumor-Associated Macrophages and Neutrophils in Tumor Microenvironment. Mediators Inflamm. 2016, 6058147. doi:10.1155/2016/6058147
Kim, J. W., Jung, S. Y., Kwon, Y. H., Lee, J. H., Lee, Y. M., Lee, B. Y., et al. (2012a). Ginsenoside Rg3 Attenuates Tumor Angiogenesis via Inhibiting Bioactivities of Endothelial Progenitor Cells. Cancer Biol. Ther. 13 (7), 504–515. doi:10.4161/cbt.19599
Kim, J. W., Jung, S. Y., Kwon, Y. H., Lee, S. H., Lee, J. H., Lee, B. Y., et al. (2012b). Ginsenoside Rg3 Inhibits Endothelial Progenitor Cell Differentiation through Attenuation of VEGF-dependent Akt/eNOS Signaling. Phytother Res. 26 (9), 1286–1293. doi:10.1002/ptr.3722
Krishna Priya, S., Nagare, R. P., Sneha, V. S., Sidhanth, C., Bindhya, S., Manasa, P., et al. (2016). Tumour Angiogenesis-Origin of Blood Vessels. Int. J. Cancer 139 (4), 729–735. doi:10.1002/ijc.30067
Kwon, H. J., Lee, H., Choi, G. E., Kwon, S. J., Song, A. Y., Kim, S. J., et al. (2018). Ginsenoside F1 Promotes Cytotoxic Activity of NK Cells via Insulin-like Growth Factor-1-dependent Mechanism. Front. Immunol. 9, 2785. doi:10.3389/fimmu.2018.02785
Lee, D. Y., Park, C. W., Lee, S. J., Park, H. R., Kim, S. H., Son, S. U., et al. (2019a). Anti-Cancer Effects of Panax Ginseng Berry Polysaccharides via Activation of Immune-Related Cells. Front. Pharmacol. 10, 1411. doi:10.3389/fphar.2019.01411
Lee, D. Y., Park, C. W., Lee, S. J., Park, H. R., Seo, D. B., Park, J. Y., et al. (2019b). Immunostimulating and Antimetastatic Effects of Polysaccharides Purified from Ginseng Berry. Am. J. Chin. Med. 47 (4), 823–839. doi:10.1142/S0192415X19500435
Lee, S. Y., Yuk, H. G., Ko, S. G., Cho, S.-G., and Moon, G.-S. (2021). Gut Microbiome Prolongs an Inhibitory Effect of Korean Red Ginseng on High-Fat-Diet-Induced Mouse Obesity. Nutrients 13 (3), 926. doi:10.3390/nu13030926
Legitimo, A., Consolini, R., Failli, A., Orsini, G., and Spisni, R. (2014). Dendritic Cell Defects in the Colorectal Cancer. Hum. Vaccin. Immunother. 10 (11), 3224–3235. doi:10.4161/hv.29857
Lei, X., Lei, Y., Li, J. K., Du, W. X., Li, R. G., Yang, J., et al. (2020). Immune Cells within the Tumor Microenvironment: Biological Functions and Roles in Cancer Immunotherapy. Cancer Lett. 470, 126–133. doi:10.1016/j.canlet.2019.11.009
Li, B., and Qu, G. (2019). Inhibition of the Hypoxia-Induced Factor-1α and Vascular Endothelial Growth Factor Expression through Ginsenoside Rg3 in Human Gastric Cancer Cells. J. Cancer Res. Ther. 15 (7), 1642–1646. doi:10.4103/jcrt.JCRT_77_17
Li, H., Huang, N., Zhu, W., Wu, J., Yang, X., Teng, W., et al. (2018). Modulation the Crosstalk between Tumor-Associated Macrophages and Non-small Cell Lung Cancer to Inhibit Tumor Migration and Invasion by Ginsenoside Rh2. BMC cancer 18 (1), 579. doi:10.1186/s12885-018-4299-4
Li, J., Li, R., Li, N., Zheng, F., Dai, Y., Ge, Y., et al. (2018). Mechanism of Antidiabetic and Synergistic Effects of Ginseng Polysaccharide and Ginsenoside Rb1 on Diabetic Rat Model. J. Pharm. Biomed. Anal. 158, 451–460. doi:10.1016/j.jpba.2018.06.024
Li, X., Chu, S., Lin, M., Gao, Y., Liu, Y., Yang, S., et al. (2020). Anticancer Property of Ginsenoside Rh2 from Ginseng. Eur. J. Med. Chem. 203, 112627. doi:10.1016/j.ejmech.2020.112627
Li, W., Peng, A., Wu, H., Quan, Y., Li, Y., Lu, L., et al. (2020). Anti-Cancer Nanomedicines: A Revolution of Tumor Immunotherapy. Front. Immunol. 11, 601497. doi:10.3389/fimmu.2020.601497
Li, Q., Jin, M., Liu, Y., and Jin, L. (2020). Gut Microbiota: Its Potential Roles in Pancreatic Cancer. Front Cel Infect Microbiol 10, 572492. doi:10.3389/fcimb.2020.572492
Liskova, A., Koklesova, L., Samec, M., Smejkal, K., Samuel, S. M., Varghese, E., et al. (2020). Flavonoids in Cancer Metastasis. Cancers (Basel) 12 (6), 1498. doi:10.3390/cancers12061498
Löb, S., Königsrainer, A., Rammensee, H.-G., Opelz, G., and Terness, P. (2009). Inhibitors of Indoleamine-2,3-Dioxygenase for Cancer Therapy: Can We See the wood for the Trees. Nat. Rev. Cancer 9 (6), 445–452. doi:10.1038/nrc2639
Looi, C. K., Chung, F. F., Leong, C. O., Wong, S. F., Rosli, R., and Mai, C. W. (2019). Therapeutic Challenges and Current Immunomodulatory Strategies in Targeting the Immunosuppressive Pancreatic Tumor Microenvironment. J. Exp. Clin. Cancer Res. 38 (1), 162. doi:10.1186/s13046-019-1153-8
López de Andrés, J., Griñán-Lisón, C., Jiménez, G., and Marchal, J. A. (2020). Cancer Stem Cell Secretome in the Tumor Microenvironment: a Key point for an Effective Personalized Cancer Treatment. J. Hematol. Oncol. 13 (1), 136. doi:10.1186/s13045-020-00966-3
Lu, H., Zhou, X., Kwok, H. H., Dong, M., Liu, Z., Poon, P. Y., et al. (2017). Ginsenoside-Rb1-Mediated Anti-angiogenesis via Regulating PEDF and miR-33a through the Activation of PPAR-γ Pathway. Front. Pharmacol. 8, 783. doi:10.3389/fphar.2017.00783
Lugano, R., Ramachandran, M., and Dimberg, A. (2020). Tumor Angiogenesis: Causes, Consequences, Challenges and Opportunities. Cell Mol Life Sci 77 (9), 1745–1770. doi:10.1007/s00018-019-03351-7
Luo, X., Wang, H., and Ji, D. (2021). Carbon Nanotubes (CNT)-loaded Ginsenosides Rb3 Suppresses the PD-1/pd-L1 Pathway in Triple-Negative Breast Cancer. Aging 13, 17177–17189. doi:10.18632/aging.203131
Ma, J., Liu, H., and Wang, X. (2014). Effect of Ginseng Polysaccharides and Dendritic Cells on the Balance of Th1/Th2 T Helper Cells in Patients with Non-small Cell Lung Cancer. J. Tradit Chin. Med. 34 (6), 641–645. doi:10.1016/s0254-6272(15)30076-5
Meng, J., Meng, Y., Liang, Z., Du, L., Zhang, Z., Hu, X., et al. (2013). Phenotypic and Functional Analysis of the Modification of Murine Bone Marrow Dendritic Cells (BMDCs) Induced by Neutral Ginseng Polysaccharides (NGP). Hum. Vaccin. Immunother. 9 (2), 233–241. doi:10.4161/hv.22612
Meng, L., Ji, R., Dong, X., Xu, X., Xin, Y., and Jiang, X. (2019). Antitumor Activity of Ginsenoside Rg3 in Melanoma through Downregulation of the ERK and Akt Pathways. Int. J. Oncol. 54 (6), 2069–2079. doi:10.3892/ijo.2019.4787
Meurette, O., and Mehlen, P. (2018). Notch Signaling in the Tumor Microenvironment. Cancer Cell 34 (4), 536–548. doi:10.1016/j.ccell.2018.07.009
Mo, C., Xie, S., Zeng, T., Lai, Y., Huang, S., Zhou, C., et al. (2021). Ginsenoside-Rg1 Acts as an Ido1 Inhibitor, Protects against Liver Fibrosis via Alleviating Ido1-mediated the Inhibition of DCs Maturation. Phytomedicine 84, 153524. doi:10.1016/j.phymed.2021.153524
Munn, D. H., and Mellor, A. L. (2016). Ido in the Tumor Microenvironment: Inflammation, Counter-regulation, and Tolerance. Trends Immunol. 37 (3), 193–207. doi:10.1016/j.it.2016.01.002
Nelson, M. H., Diven, M. A., Huff, L. W., and Paulos, C. M. (2015). Harnessing the Microbiome to Enhance Cancer Immunotherapy. J. Immunol. Res. 2015, 368736. doi:10.1155/2015/368736
Ni, W., Zhang, X., Wang, B., Chen, Y., Han, H., Fan, Y., et al. (2010). Antitumor Activities and Immunomodulatory Effects of Ginseng Neutral Polysaccharides in Combination with 5-fluorouracil. J. Med. Food 13 (2), 270–277. doi:10.1089/jmf.2009.1119
Nolan, D. J., Ciarrocchi, A., Mellick, A. S., Jaggi, J. S., Bambino, K., Gupta, S., et al. (2007). Bone Marrow-Derived Endothelial Progenitor Cells Are a Major Determinant of Nascent Tumor Neovascularization. Genes Dev. 21 (12), 1546–1558. doi:10.1101/gad.436307
Oh, H. K., Ha, J. M., Eunju, O., Lee, S. K., Shim, B. S., Hong, Y. K., et al. (2007). Tumor Angiogenesis Promoted by Ex Vivo Differentiated Endothelial Progenitor Cells Is Effectively Inhibited by an Angiogenesis Inhibitor, TK1-2. Cancer Res. 67 (10), 4851–4859. doi:10.1158/0008-5472.Can-06-2979
Oh, J., Jeon, S. B., Lee, Y., Lee, H., Kim, J., Kwon, B. R., et al. (2015). Fermented Red Ginseng Extract Inhibits Cancer Cell Proliferation and Viability. J. Med. Food 18 (4), 421–428. doi:10.1089/jmf.2014.3248
Oh, J., Yoon, H. J., Jang, J. H., Kim, D. H., and Surh, Y. J. (2019). The Standardized Korean Red Ginseng Extract and its Ingredient Ginsenoside Rg3 Inhibit Manifestation of Breast Cancer Stem Cell-like Properties through Modulation of Self-Renewal Signaling. J. Ginseng Res. 43 (3), 421–430. doi:10.1016/j.jgr.2018.05.004
Ohno, H. (2020). The Impact of Metabolites Derived from the Gut Microbiota on Immune Regulation and Diseases. Int. Immunol. 32 (10), 629–636. doi:10.1093/intimm/dxaa041
Park, J. W., Park, J. H., and Han, J. W. (2020). Fermented Ginseng Extract, BST204, Suppresses Tumorigenesis and Migration of Embryonic Carcinoma through Inhibition of Cancer Stem Cell Properties. Molecules 25 (14), 3128. doi:10.3390/molecules25143128
Peng, S., Wang, R., Zhang, X., Ma, Y., Zhong, L., Li, K., et al. (2019). EGFR-TKI Resistance Promotes Immune Escape in Lung Cancer via Increased PD-L1 Expression. Mol. Cancer 18 (1), 165. doi:10.1186/s12943-019-1073-4
Phi, L. T. H., Wijaya, Y. T., Sari, I. N., Kim, K. S., Yang, Y. G., Lee, M. W., et al. (2019a). 20(R)-Ginsenoside Rg3 Influences Cancer Stem Cell Properties and the Epithelial-Mesenchymal Transition in Colorectal Cancer via the SNAIL Signaling Axis. Onco Targets Ther. 12, 10885–10895. doi:10.2147/OTT.S219063
Phi, L. T. H., Sari, I. N., Wijaya, Y. T., Kim, K. S., Park, K., Cho, A. E., et al. (2019b). Ginsenoside Rd Inhibits the Metastasis of Colorectal Cancer via Epidermal Growth Factor Receptor Signaling Axis. IUBMB Life 71 (5), 601–610. doi:10.1002/iub.1984
Pitt, J. M., Marabelle, A., Eggermont, A., Soria, J. C., Kroemer, G., and Zitvogel, L. (2016). Targeting the Tumor Microenvironment: Removing Obstruction to Anticancer Immune Responses and Immunotherapy. Ann. Oncol. 27 (8), 1482–1492. doi:10.1093/annonc/mdw168
Plummer, P. N., Freeman, R., Taft, R. J., Vider, J., Sax, M., Umer, B. A., et al. (2013). MicroRNAs Regulate Tumor Angiogenesis Modulated by Endothelial Progenitor Cells. Cancer Res. 73 (1), 341–352. doi:10.1158/0008-5472.Can-12-0271
Qi, H., Zhang, Z., Liu, J., Chen, Z., Huang, Q., Li, J., et al. (2021). Comparisons of Isolation Methods, Structural Features, and Bioactivities of the Polysaccharides from Three Common Panax Species: A Review of Recent Progress. Molecules 26 (16), 4997. doi:10.3390/molecules26164997
Qiu, R., Qian, F., Wang, X., Li, H., and Wang, L. (2019). Targeted Delivery of 20(S)-ginsenoside Rg3-Based Polypeptide Nanoparticles to Treat colon Cancer. Biomed. Microdevices 21 (1), 18. doi:10.1007/s10544-019-0374-0
Qu, Q., Yang, F., Zhao, C., Liu, X., Yang, P., Li, Z., et al. (2021). Effects of Fermented Ginseng on the Gut Microbiota and Immunity of Rats with Antibiotic-Associated Diarrhea. J. Ethnopharmacol 267, 113594. doi:10.1016/j.jep.2020.113594
Ren, Z., Chen, X., Hong, L., Zhao, X., Cui, G., Li, A., et al. (2020). Nanoparticle Conjugation of Ginsenoside Rg3 Inhibits Hepatocellular Carcinoma Development and Metastasis. Small 16 (2), e1905233. doi:10.1002/smll.201905233
Ribatti, D., and Tamma, R. (2019). Hematopoietic Growth Factors and Tumor Angiogenesis. Cancer Lett. 440-441, 47–53. doi:10.1016/j.canlet.2018.10.008
Rooks, M. G., and Garrett, W. S. (2016). Gut Microbiota, Metabolites and Host Immunity. Nat. Rev. Immunol. 16 (6), 341–352. doi:10.1038/nri.2016.42
Round, J. L., and Mazmanian, S. K. (2010). Inducible Foxp3+ Regulatory T-Cell Development by a Commensal Bacterium of the Intestinal Microbiota. Proc. Natl. Acad. Sci. U S A. 107 (27), 12204–12209. doi:10.1073/pnas.0909122107
Routy, B., Le Chatelier, E., Derosa, L., Duong, C. P. M., Alou, M. T., Daillère, R., et al. (2018). Gut Microbiome Influences Efficacy of PD-1-Based Immunotherapy against Epithelial Tumors. Science 359 (6371), 91–97. doi:10.1126/science.aan3706
Sharma, M. D., Hou, D. Y., Baban, B., Koni, P. A., He, Y., Chandler, P. R., et al. (2010). Reprogrammed Foxp3(+) Regulatory T Cells Provide Essential Help to Support Cross-Presentation and CD8(+) T Cell Priming in Naive Mice. Immunity 33 (6), 942–954. doi:10.1016/j.immuni.2010.11.022
Shen, M., Xu, Z., Xu, W., Jiang, K., Zhang, F., Ding, Q., et al. (2019). Inhibition of ATM Reverses EMT and Decreases Metastatic Potential of Cisplatin-Resistant Lung Cancer Cells through JAK/STAT3/PD-L1 Pathway. J. Exp. Clin. Cancer Res. 38 (1), 149. doi:10.1186/s13046-019-1161-8
Shibuya, M. (2013). Vascular Endothelial Growth Factor and its Receptor System: Physiological Functions in Angiogenesis and Pathological Roles in Various Diseases. J. Biochem. 153 (1), 13–19. doi:10.1093/jb/mvs136
Shin, M. S., Hwang, S. H., Yoon, T. J., Kim, S. H., and Shin, K. S. (2017). Polysaccharides from Ginseng Leaves Inhibit Tumor Metastasis via Macrophage and NK Cell Activation. Int. J. Biol. Macromol 103, 1327–1333. doi:10.1016/j.ijbiomac.2017.05.055
Shin, M. S., Song, J. H., Choi, P., Lee, J. H., Kim, S. Y., Shin, K. S., et al. (2018). Stimulation of Innate Immune Function by Panax Ginseng after Heat Processing. J. Agric. Food Chem. 66 (18), 4652–4659. doi:10.1021/acs.jafc.8b00152
Singh, S. K., Clarke, I. D., Terasaki, M., Bonn, V. E., Hawkins, C., Squire, J., et al. (2003). Identification of a Cancer Stem Cell in Human Brain Tumors. Cancer Res. 63 (18), 5821–5828.
Son, K. J., Choi, K. R., Lee, S. J., and Lee, H. (2016). Immunogenic Cell Death Induced by Ginsenoside Rg3: Significance in Dendritic Cell-Based Anti-tumor Immunotherapy. Immune Netw. 16 (1), 75–84. doi:10.4110/in.2016.16.1.75
Song, M. Y., Kim, B. S., and Kim, H. (2014). Influence of Panax Ginseng on Obesity and Gut Microbiota in Obese Middle-Aged Korean Women. J. Ginseng Res. 38 (2), 106–115. doi:10.1016/j.jgr.2013.12.004
Su, X., Zhang, D., Zhang, H., Zhao, K., and Hou, W. (2020). Preparation and Characterization of Angiopep-2 Functionalized Ginsenoside-Rg3 Loaded Nanoparticles and the Effect on C6 Glioma Cells. Pharm. Dev. Technol. 25 (3), 385–395. doi:10.1080/10837450.2018.1551901
Sun, M., Ye, Y., Xiao, L., Duan, X., Zhang, Y., and Zhang, H. (2017). Anticancer Effects of Ginsenoside Rg3 (Review). Int. J. Mol. Med. 39 (3), 507–518. doi:10.3892/ijmm.2017.2857
Takei, M., Tachikawa, E., Hasegawa, H., and Lee, J. J. (2004). Dendritic Cells Maturation Promoted by M1 and M4, End Products of Steroidal Ginseng Saponins Metabolized in Digestive Tracts, Drive a Potent Th1 Polarization. Biochem. Pharmacol. 68 (3), 441–452. doi:10.1016/j.bcp.2004.04.015
Tan, Q., Lin, S., Zeng, Y., Yao, M., Liu, K., Yuan, H., et al. (2020). Ginsenoside Rg3 Attenuates the Osimertinib Resistance by Reducing the Stemness of Non-small Cell Lung Cancer Cells. Environ. Toxicol. 35 (6), 643–651. doi:10.1002/tox.22899
Tang, C., Wang, X., Soh, H., Seyedin, S., Cortez, M. A., Krishnan, S., et al. (2014). Combining Radiation and Immunotherapy: a New Systemic Therapy for Solid Tumors. Cancer Immunol. Res. 2 (9), 831–838. doi:10.1158/2326-6066.Cir-14-0069
Tang, Y. C., Zhang, Y., Zhou, J., Zhi, Q., Wu, M. Y., Gong, F. R., et al. (2018). Ginsenoside Rg3 Targets Cancer Stem Cells and Tumor Angiogenesis to Inhibit Colorectal Cancer Progression In Vivo. Int. J. Oncol. 52 (1), 127–138. doi:10.3892/ijo.2017.4183
Untersmayr, E., Bax, H. J., Bergmann, C., Bianchini, R., Cozen, W., Gould, H. J., et al. (2019). AllergoOncology: Microbiota in Allergy and Cancer-A European Academy for Allergy and Clinical Immunology Position Paper. Allergy 74 (6), 1037–1051. doi:10.1111/all.13718
Uribe-Herranz, M., Bittinger, K., Rafail, S., Guedan, S., Pierini, S., Tanes, C., et al. (2018). Gut Microbiota Modulates Adoptive Cell Therapy via CD8α Dendritic Cells and IL-12. JCI Insight 3 (4), e94952. doi:10.1172/jci.insight.94952
Uyttenhove, C., Pilotte, L., Théate, I., Stroobant, V., Colau, D., Parmentier, N., et al. (2003). Evidence for a Tumoral Immune Resistance Mechanism Based on Tryptophan Degradation by Indoleamine 2,3-dioxygenase. Nat. Med. 9 (10), 1269–1274. doi:10.1038/nm934
Vétizou, M., Pitt, J. M., Daillère, R., Lepage, P., Waldschmitt, N., Flament, C., et al. (2015). Anticancer Immunotherapy by CTLA-4 Blockade Relies on the Gut Microbiota. Science 350 (6264), 1079–1084. doi:10.1126/science.aad1329
Viallard, C., and Larrivée, B. (2017). Tumor Angiogenesis and Vascular Normalization: Alternative Therapeutic Targets. Angiogenesis 20 (4), 409–426. doi:10.1007/s10456-017-9562-9
Viaud, S., Saccheri, F., Mignot, G., Yamazaki, T., Daillère, R., Hannani, D., et al. (2013). The Intestinal Microbiota Modulates the Anticancer Immune Effects of Cyclophosphamide. Science 342 (6161), 971–976. doi:10.1126/science.1240537
Walcher, L., Kistenmacher, A. K., Suo, H., Kitte, R., Dluczek, S., Strauß, A., et al. (2020). Cancer Stem Cells-Origins and Biomarkers: Perspectives for Targeted Personalized Therapies. Front. Immunol. 11, 1280. doi:10.3389/fimmu.2020.01280
Wang, C. Z., Hou, L., Wan, J. Y., Yao, H., Yuan, J., Zeng, J., et al. (2020). Ginseng berry Polysaccharides on Inflammation-Associated colon Cancer: Inhibiting T-Cell Differentiation, Promoting Apoptosis, and Enhancing the Effects of 5-fluorouracil. J. Ginseng Res. 44 (2), 282–290. doi:10.1016/j.jgr.2018.12.010
Wang, C. Z., Yu, C., Wen, X. D., Chen, L., Zhang, C. F., Calway, T., et al. (2016). American Ginseng Attenuates Colitis-Associated Colon Carcinogenesis in Mice: Impact on Gut Microbiota and Metabolomics. Cancer Prev. Res. (Phila) 9 (10), 803–811. doi:10.1158/1940-6207.CAPR-15-0372
Wang, J., Tian, L., Khan, M. N., Zhang, L., Chen, Q., Zhao, Y., et al. (2018). Ginsenoside Rg3 Sensitizes Hypoxic Lung Cancer Cells to Cisplatin via Blocking of NF-Κb Mediated Epithelial-Mesenchymal Transition and Stemness. Cancer Lett. 415, 73–85. doi:10.1016/j.canlet.2017.11.037
Wang, X., Mao, J., Zhou, T., Chen, X., Tu, H., Ma, J., et al. (2021). Hypoxia-induced Myeloid Derived Growth Factor Promotes Hepatocellular Carcinoma Progression through Remodeling Tumor Microenvironment. Theranostics 11 (1), 209–221. doi:10.7150/thno.49327
Wang, Y., Huang, M., Sun, R., and Pan, L. (2015). Extraction, Characterization of a Ginseng Fruits Polysaccharide and its Immune Modulating Activities in Rats with Lewis Lung Carcinoma. Carbohydr. Polym. 127, 215–221. doi:10.1016/j.carbpol.2015.03.070
Wang, X., Xia, H. Y., Qin, H. Y., Kang, X. P., Hu, H. Y., Zheng, J., et al. (2019). 20(S)-protopanaxadiol Induces Apoptosis in Human Umbilical Vein Endothelial Cells by Activating the PERK-eIF2alpha-ATF4 Signaling Pathway. J. Cel Biochem 120 (4), 5085–5096. doi:10.1002/jcb.27785
Wang, Y., Smith, W., Hao, D., He, B., and Kong, L. (2019). M1 and M2 Macrophage Polarization and Potentially Therapeutic Naturally Occurring Compounds. Int. Immunopharmacol 70, 459–466. doi:10.1016/j.intimp.2019.02.050
Wang, Z., Meng, J., Xia, Y., Meng, Y., Du, L., Zhang, Z., et al. (2013). Maturation of Murine Bone Marrow Dendritic Cells Induced by Acidic Ginseng Polysaccharides. Int. J. Biol. Macromol 53, 93–100. doi:10.1016/j.ijbiomac.2012.11.009
Weis, S. M., and Cheresh, D. A. (2005). Pathophysiological Consequences of VEGF-Induced Vascular Permeability. Nature 437 (7058), 497–504. doi:10.1038/nature03987
Weis, S. M., and Cheresh, D. A. (2011). Tumor Angiogenesis: Molecular Pathways and Therapeutic Targets. Nat. Med. 17 (11), 1359–1370. doi:10.1038/nm.2537
Wu, L., Cai, S., Deng, Y., Zhang, Z., Zhou, X., Su, Y., et al. (2021). PD-1/PD-L1 Enhanced Cisplatin Resistance in Gastric Cancer through PI3K/AKT Mediated P-Gp Expression. Int. Immunopharmacol 94, 107443. doi:10.1016/j.intimp.2021.107443
Xu, H., Wang, E., Chen, F., Xiao, J., and Wang, M. (2021). Neuroprotective Phytochemicals in Experimental Ischemic Stroke: Mechanisms and Potential Clinical Applications. Oxid Med. Cel Longev 2021, 6687386. doi:10.1155/2021/6687386
Xu, J., Li, T., Xia, X., Fu, C., Wang, X., and Zhao, Y. (2020). Dietary Ginsenoside T19 Supplementation Regulates Glucose and Lipid Metabolism via AMPK and PI3K Pathways and its Effect on Intestinal Microbiota. J. Agric. Food Chem. 68 (49), 14452–14462. doi:10.1021/acs.jafc.0c04429
Xu, W. W., Li, B., Guan, X. Y., Chung, S. K., Wang, Y., Yip, Y. L., et al. (2017). Cancer Cell-Secreted IGF2 Instigates Fibroblasts and Bone Marrow-Derived Vascular Progenitor Cells to Promote Cancer Progression. Nat. Commun. 8, 14399. doi:10.1038/ncomms14399
Yim, N. H., Kim, Y. S., and Chung, H. S. (2020). Inhibition of Programmed Death Receptor-1/Programmed Death Ligand-1 Interactions by Ginsenoside Metabolites. Molecules 25 (9), 2068. doi:10.3390/molecules25092068
Zeng, P., Li, J., Chen, Y., and Zhang, L. (2019). The Structures and Biological Functions of Polysaccharides from Traditional Chinese Herbs. Prog. Mol. Biol. Transl Sci. 163, 423–444. doi:10.1016/bs.pmbts.2019.03.003
Zhang, B. P., Li, B., Cheng, J. Y., Cao, R., Gao, S. T., Huang, C. J., et al. (2020). Anti-cancer Effect of 20(S)-Ginsenoside-Rh2 on Oral Squamous Cell Carcinoma Cells via the Decrease in ROS and Downregulation of MMP-2 and VEGF. Biomed. Environ. Sci. 33 (9), 713–717. doi:10.3967/bes2020.093
Zhang, E., Shi, H., Yang, L., Wu, X., and Wang, Z. (2017). Ginsenoside Rd Regulates the Akt/mTOR/p70S6K Signaling cascade and Suppresses Angiogenesis and Breast Tumor Growth. Oncol. Rep. 38 (1), 359–367. doi:10.3892/or.2017.5652
Zhang, W., Cho, S. Y., Xiang, G., Min, K. J., Yu, Q., and Jin, J. O. (2015). Ginseng Berry Extract Promotes Maturation of Mouse Dendritic Cells. PloS one 10 (6), e0130926. doi:10.1371/journal.pone.0130926
Zhang, Z., Zeng, P., Gao, W., Zhou, Q., Feng, T., and Tian, X. (2021). Circadian Clock: a Regulator of the Immunity in Cancer. Cell Commun Signal 19 (1), 37. doi:10.1186/s12964-021-00721-2
Zheng, S. W., Xiao, S. Y., Wang, J., Hou, W., and Wang, Y. P. (2019). Inhibitory Effects of Ginsenoside Ro on the Growth of B16F10 Melanoma via its Metabolites. Molecules 24 (16), 2985. doi:10.3390/molecules24162985
Keywords: tumor microenvironment, tumor angiogenesis, tumor stem cell, immune response, Panax ginseng (C.A. Meyer), cancer therapy
Citation: Li M, Wang X, Wang Y, Bao S, Chang Q, Liu L, Zhang S and Sun L (2021) Strategies for Remodeling the Tumor Microenvironment Using Active Ingredients of Ginseng—A Promising Approach for Cancer Therapy. Front. Pharmacol. 12:797634. doi: 10.3389/fphar.2021.797634
Received: 21 October 2021; Accepted: 06 December 2021;
Published: 22 December 2021.
Edited by:
Ruiwen Zhang, University of Houston, United StatesReviewed by:
Lian Xiang Luo, Guangdong Medical University, ChinaAn-Sik Chung, Korea Advanced Institute of Science and Technology, South Korea
Copyright © 2021 Li, Wang, Wang, Bao, Chang, Liu, Zhang and Sun. This is an open-access article distributed under the terms of the Creative Commons Attribution License (CC BY). The use, distribution or reproduction in other forums is permitted, provided the original author(s) and the copyright owner(s) are credited and that the original publication in this journal is cited, in accordance with accepted academic practice. No use, distribution or reproduction is permitted which does not comply with these terms.
*Correspondence: Linlin Liu, bGl1bGlubEBqbHUuZWR1LmNu; Shuai Zhang, emhhbmdzNTMwQG5lbnUuZWR1LmNu; Liwei Sun, c3VubnlsaWx3ZWlAMTYzLmNvbQ==