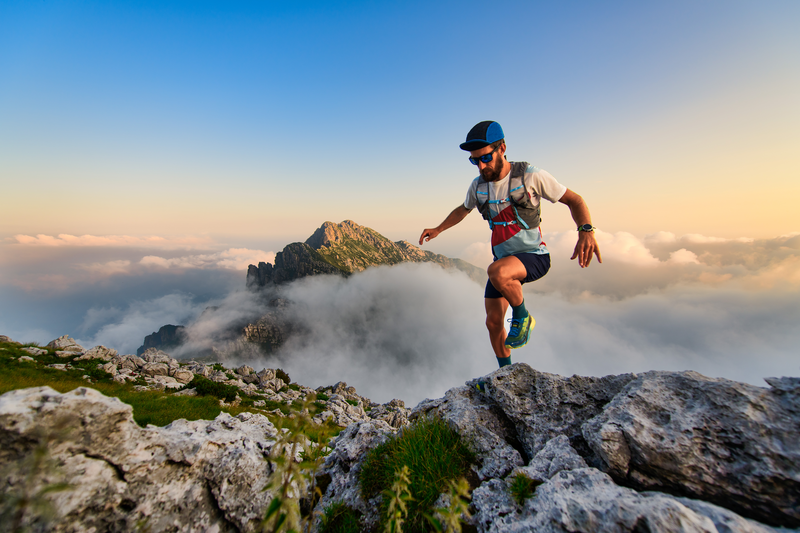
94% of researchers rate our articles as excellent or good
Learn more about the work of our research integrity team to safeguard the quality of each article we publish.
Find out more
ORIGINAL RESEARCH article
Front. Pharmacol. , 29 November 2021
Sec. Ethnopharmacology
Volume 12 - 2021 | https://doi.org/10.3389/fphar.2021.787633
This article is part of the Research Topic Natural Products from the Beehive: Recent Advances in Pharmacology and Therapeutic Applications View all 5 articles
The aim of this study was to obtain a Brazilian red propolis (BRP) enriched composite resin and to perform the characterization of its antibacterial activity, mechanical, and physical-chemical properties. Brazilian red propolis ethyl acetate extract (EABRP) was characterized by LC-ESI-Orbitrap-FTMS, UPLC-DAD, antibacterial activity, total flavonoids content, and radical scavenging capacity. BRP was incorporated to a commercial composite resin (RC) to obtain BRP enriched composite at 0.1, 0.15 and 0.25% (RP10, RP15 and RP25, respectively). The antibacterial activity RPs was evaluated against Streptococcus mutans by contact direct test and expressed by antibacterial ratio. The RPs were characterized as its cytotoxicity against 3T3 fibroblasts, flexural strength (FS), Knoop microhardness (KHN), post-cure depth (CD), degree of conversion (DC%), water sorption (Wsp), water solubility (Wsl), average roughness (Ra), and thermal analysis. Were identified 50 chemical compounds from BRP extract by LC-ESI-Orbitrap-FTMS. EABRP was bacteriostatic and bactericide at 125 and 500 μg/ml, respectively. The RP25 exhibited antibacterial ratio of 90.76% after 1 h of direct contact with S. mutans (p < 0.0001) while RC no showed significative antibacterial activity (p = 0.1865), both compared with cell control group. RPs and RC no showed cytotoxicity. RPs exhibited CD from 2.74 to 4.48 mm, DC% from 80.70 to 83.96%, Wsp from 17.15 to 21.67 μg/mm3, Wsl from 3.66 to 4.20 μg/mm3, Ra from 14.48 to 20.76 nm. RPs showed thermal resistance between 448–455°C. The results support that propolis can be used on development of modified composite resins that show antibacterial activity and that have compatible mechanical and physical-chemical properties to the indicate for composite resins.
Propolis is a natural, nontoxic, raw material collected by Apis mellifera bee from plants sources as exudates, tree barks and leaf buds from various plant sources (Koo et al., 2002; da silva Barboza et al., 2021). Diverse types of propolis are known around the world and each type differs in its chemical composition, this variation occurs in function of its botanic origin, a local main plant source used by bee for propolis obtention (Teixeira et al., 2005; Daugsch et al., 2008; Ristivojević et al., 2015). The botanical origin of red propolis collected in northeastern Brazil is the Dalbergia ecastaphyllum (L.) Taub. (Fabaceae), that is known by has a red resinous exudate from holes in its branches (Daugsch et al., 2008; Piccinelli et al., 2011; Freires et al., 2016; Dantas Silva et al., 2017). The Brazilian red propolis (BRP) can be found in beehives located on the coast of northeastern between states of Bahia, Sergipe, Alagoas, Pernambuco, Paraíba, Rio Grande do Norte, Ceará and Maranhão. The Red propolis from Alagoas state obtained the Geographic Indication (GI) by the Brazilian National Institute of Industrial Property (INPI) (Freires et al., 2016; Dantas Silva et al., 2017). The chemical composition of BRP are rich mainly in isoflavonoids, flavonoids, benzophenones, phenolic acids and terpenes, and has more than 200 compounds as constituents and/or markers (de Mendonça et al., 2015; Freires et al., 2016; do Nascimento et al., 2019). Some pharmacologic activities are attribute to BRP as antibacterial (Sforcin and Bankova, 2011; Bueno-Silva et al., 2013; Dantas Silva et al., 2017; da silva Barboza et al., 2021), anti-inflammatory (Lima Cavendish et al., 2015; Franchin et al., 2016; Corrêa et al., 2017) and wound healing (Jacob et al., 2015; Corrêa et al., 2017; Oryan et al., 2018).
Propolis has been used in dentistry and oral healthcare due to its pharmacological properties and absence of toxicity ensured even by its use in traditional medicine (Sforcin and Bankova, 2011; da silva Barboza et al., 2021; Zulhendri et al., 2021). Among the possibilities of propolis use in dentistry and oral healthcare are: in inhibition of adhesion and biofilm formation of Candida species in dentistry materials (Bezerra et al., 2020), on development of endodontic irrigants (Parolia et al., 2021), on development of varnishes (De Luca et al., 2014), on development of total-etching adhesive system (Porto et al., 2021), as cavity cleaning agent (Celerino de Moraes Porto et al., 2018), on mouthwash development (Santiago et al., 2018), on gel formulation development (González-Serrano et al., 2021) and on oromuco-adhesive films development (Arafa et al., 2018).
Composite resins are dental restorative materials that contain an organic matrix, inorganic fillers, bonding agents and a photoinitiator system (Ferracane, 2011). The organic matrix of composite resin content mainly dimethacrylate monomers as bisphenylglycidyl BisGMA, triethylene glycol dimethacrylate (TEGDMA), ethoxylated bisphenol-A dimethacrylate (BisEMA) and Urethane dimethacrylate (UDMA) (Floyd and Dickens, 2006; Goņçalves et al., 2009). The proportion of each dimethacrylate monomers used influences on formation of cross-links in the 3D structure of the polymer and, consequently, in the mechanical properties of composite (Achilias et al., 2010; Vouvoudi et al., 2015). The viscosity of these composites is established by filler content, and this component is a main responsible for the mechanical strength of composites (Liu et al., 2015). The main filler components used in composite resin are titanium dioxide (TiO2), ceramic, and zirconia (ZrO2) ceramic in form of micro/nanoparticles (Makvandi et al., 2018). Currently, the development of modified composite resins that have antibacterial activity and compatible mechanic and physical-chemical properties with use has been sought (Makvandi et al., 2016; Wang et al., 2017; Liang et al., 2018; Dias et al., 2019).
In this context, the purpose of this study was to modify a commercial composite resin with Brazilian red propolis to obtaining a BRP enriched composite resin that shows antibacterial activity and compatible mechanic and physical-chemical properties with intend use. The null hypothesis tested in this study is that the BRP addiction into a commercial composite resin no provide antibacterial activity, nor compatible mechanic and physical-chemical properties with intend use.
Brazilian red propolis (BRP) raw material was collected in the city of Marechal Deodoro, Alagoas, Brazil in July 2013 (geographic coordinates south latitude: 9° 42.258′, west latitude: 35° 54.391′ and height of 35.5 m above sea level). The access and transportation of Brazilian red propolis was previously authorized by regulatory agencies for control of Brazilian Genetic Heritage and Biodiversity Conservation with protocol number of acceptance 010124/2012-8. The Brazilian red propolis ethanol extract (EBRP) was obtained by extraction of BRP (250 g) through maceration method where raw propolis was manually ground and placed in glass flask with 600 ml of 80% ethanol (v/v) under agitation for 48 h. After this time, the macerate (liquid portion) was removed using a pipette, placed in another glass flask and the resinous mass deposited at the deep of firth glass flask was submitted to new extraction with more 600 ml of 80% ethanol at the same conditions. After the end of 2 cycles of extraction, the total macerate was joined and concentrated in a rotary evaporator (Fisatom, Brazil) in a vacuum at 40°C. The EBRP was then placed in a glass container and left for approximately 3 days for the residual solvent to evaporate. As a result, a solid mass (162 g) with viscous appearance was obtained.
A liquid-liquid extraction of EBRP was carried with hexane and ethyl acetate to eliminate grease and waxes and to concentrate flavonoids, respectively. EBRP (20 g) was solubilized with 60 ml of 70% ethanol (v/v) and successively fractioned with hexane (100 ml, twice) and right after with ethyl acetate (100 ml, twice) giving rise to the Brazilian red propolis hexane extract (HBRP) and the Brazilian red propolis ethyl acetate extract (EABRP), respectively. The EABRP was concentrated in a rotary evaporator to obtain a solid mass (4.0 g) and stored in a freezer at −20°C until further analysis.
The LC-MS analysis was performed using a LC-Orbitrap-FTMS system consisted of an Accela 600 HPLC system combined with an Exactive (Orbitrap) mass spectrometer (Thermo Fisher Scientific, Bremen, Germany) including on-line DAD (200–600 nm) and UV at 280 nm analysis. The chromatographic separation of the compounds from red propolis was performed using an ACE® C18 columns (100 × 4.6 mm; 3 µm) from (Hichrom, Reading United Kingdom) and a mobile phase (0.1% of formic acid in H2O: 0.1% of formic acid in Acetonitrile; A:B) in flow rate of 300 μl/min. The gradient elution was programmed as follows: 0–6 min linear gradient 30–45% of B, 6–14 min linear gradient 45–75% of B, 14–20 min linear gradient 75–100% of B, 20–51 min at 100% of B for elution of the guttiferones and cleaning of the column, 51–54 min decreasing in B to 45%, 54–55 linear gradient 30% of B, 55–60 min isocratic condition with 30% of B to re-equilibration of the column for next run. The injection volume was 10 µl. The MS detection range was from 100 to 1200 m/z set at 30,000 resolutions and the scanning was performed under ESI negative polarity mode with capillary temperature was 250°C.
An ultra-performance liquid chromatography coupled with a photodiode array (UPLC-PDA) analysis was performed using an UPLC-PDA system from Shimadzu (Tokyo, Japan). The UPLC-PDA equipment consisted of the modules: degasser (model DGU-20A3R), two high-pressure pumps (model LC-20ADXR), auto-injector (model SIL-20AXR), oven chromatographic column (Prominence® CTO-20A), photodiode array detector (model SPD-M20A), a controller (model CBM-20A), and Shimadzu Labsolution software. The chromatographic separation of flavonoids from red propolis was performed using a Kinetex® C18- column (150 mm × 4.6 mm; 5 µm) and a mobile phase that consisted of solvent A (Milli-Q water) and solvent B (acetonitrile) pumped at flow rate of 0.3 ml/min. The initial elution gradient consisted of 70% water (A) and 30% acetonitrile (B) (v/v). The column was eluted by varying the percentage of (B) as follows: 0–2 min 30% B, 2–5 min 36% B, 5–8 min 46% B, 8–11 min 52% B, 11–14 min 52% B, 14–17 min 57% B, 17–20 min 62% B, 20–24 min 62% B, 24–28 min 68% B, 28–32 min 72% B, 32–36 min 90% B, 36–42 min 97% B, 42–50 min 100% B, 50–55 min 100% B, 55–57 min acetonitrile was reduced to 30% and this condition was maintained up to 60 min. Volumetric working solutions of analytical standards of the daidzein, liquiritigenin, pinobanksin, isoliquiritigenin, formononetin, pinocembrin, and biochanin A from Sigma-Aldrich (St. Louis, MO, United States) was prepared at 200 μg/ml (ethanolic solutions). After that, the working solutions were diluted to obtain a calibration curve at the concentrations: 7.50, 5.00, 2.50, 1.00, 0.50 and 0.15 μg/ml (n = 3). This calibration curve was used for the quantification of the listed flavonoids in the EABRP dried extract. The EABRP lyophilized extract (EABRP dried extract) were solubilized in ethanol (HPLC grade) to obtain stock solution at 10 mg/ml. Then, 250 µl of EABRP stock solution was pipetted to 5 ml volumetric flasks (n = 3) to obtain work solutions at 500 μg/ml. The injection volume of each sample was 2.0 µl. The UPLC-PDA method was previously validated to the quantification of markers from Brazilian red propolis (do Nascimento et al., 2016). The analysis was carried out at 280 nm.
The EBRP and EABRP were tested against Streptococcus mutans CCT 3440 from Tropical Cultures Collection (CCT). The strain was provided by André Tosello Foundation (Campinas, São Paulo, Brazil). The stock solutions of EBRP and EABRP were prepared at 30 mg/ml in acetone and diluted, first to 10 mg/ml in 1.0% dimethyl sulfoxide (DMSO) and after to 2500 μg/ml (1.0% DMSO). This dilution process was performed to minimize the acetone’s antibacterial action in the assay. The final solutions of EBRP and EABRP (2500 μg/ml in 1.0% DMSO) was made in the same day of the broth microdilution assay. The S. mutans CCT 3440 strain was activated in BHA (brain heart infusion agar) for 18–20 h at 37 ± 1°C in a microaerobic environment. The broth microdilution assay was performed to determination the minimal inhibitory concentration (MIC) of BRP extracts. The broth microdilution assay was carried out in microplates (96 wells) and the procedure according to the Clinical and Laboratory Standards Institute (CLSI, 2012) and Rufatto et al. (Celerino de Moraes Porto et al., 2018). The microplates were prepared with 80 µl of brain heart infusion broth (BHI) and 80 µl of EBRP and EABRP at 2500 μg/ml in each well groups from line A of the microplates, respectively. Serial two-fold dilutions was carried out to obtaining ethanol Brazilian red propolis and ethyl acetate Brazilian red propolis extracts at range from 1000 μg/ml to 7.81 μg/ml, respectively (n = 6). The inoculum was prepared by direct colonies suspension in sterile saline after strain activation period. A bacterial suspension was standardized equivalent to 0.5 McFarland standard suspension by turbidity comparison, resulting in a bacterial suspension containing approximately 1 × 108 colony-forming units (CFU)/ml. The standardized inoculum suspension containing about 1 × 106 CFU/ml was performed by dilution (1:20) of the 1 × 108 CFU/ml bacterial suspension in sterile saline. Exactly 20 µl of the standardized inoculum suspension were added in each well, resulting a final bacterial concentration of 1 × 105 CFU/ml, according CLSI (CLSI, 2012). The negative controls were A (inoculum + medium) and B (inoculum + medium + 1.0% DMSO). The positive control was inoculum + medium + 0.12% chlorhexidine gluconate. The sterility control test was performed for BHI medium. The microplates were incubated in ambient air at 35 ± 2°C for 20 h. After microplates’ incubation, 5.0 µl of each well was pipetted and plated in Petri dishes containing sterile BHA for minimal bactericidal concentration (MBC) determination after new incubation period of 24 h at 35 ± 2°C. Exactly 20 µl of resazurin (7-hydroxy-10-oxidophenoxazin-10-ium-3-one) solution at 0.15 mg/ml and pH 7.4 (50 mM) were added in each well of the microplates that were incubated for more 2 h at 35 ± 2°C for observation of the bacterial growth. MIC values were defined as the lowest concentration where the well’s color remained purple. The MBC values were defined as the lowest concentration where it did not colony growth visually.
The radical scavenging capacity of the extracts were mensurated by DPPH• method following the procedure described by Oliveira et al. (2020), with some modifications. Stock solutions of EBRP and EABRP were performed at 1.0 mg/ml in absolute ethanol. Aliquots of EBRP were transferred to volumetric flask of 5.0 ml, and then 2.0 ml of DPPH solution (39.432 μg/ml; in ethanol) was added obtaining final concentrations of 0.5, 1.0, 1.5, 2.0, 5.0, 10.0, 15.0, 20.0 and 25.0 μg/ml, respectively (n = 3). The EABRP samples were obtained in the same way, but to final concentrations of 0.5, 1.0, 1.5, 2.0, 3.0 and 4.0 μg/ml (n = 3). The scavenging reaction was performed in dark at 25°C for 30 min. After reaction time, the absorbance readings were performed with a spectrophotometer (Model UV-1240, Shimadzu, Kyoto, Japan) at 518 nm and the solvent ethanol was used as blank. The percentage of radical-scavenging capacity (%RSC—DPPH•) of samples was calculated as follows:
where ADPPH is the absorbance of the diluted DPPH solution (2.0 ml of DPPH at 39.432 μg/ml to 5 ml of ethanol); Asample is the absorbance of the tested sample at specific concentration. The IC50 was determined for EBRP and EABRP through of linear equations from its calibration curves y = ax + b, plotted by extract concentration vs. %RSC; where: x is the equivalent concentration to IC50 of %RSC; y is the numeric value 50; a is the angular coefficient (slope) of specific line; b is the linear coefficient of specific line. The positive control was trolox (6-hydroxy-2,5,7,8-tetramethyl-3,4-dihydrochromene-2-carboxylic acid) (Sigma-Aldrich) at final concentration range from 0.25 to 4.0 μg/ml in ethanol.
The total flavonoid content (TFC) was determined by AlCl3 method (Woisky and Salatino, 1998), with some modifications. Therefore, were performed methanolic stock solutions of 5% AlCl3 (w/v), EABRP at 10 mg/ml and quercetin (Sigma-Aldrich) at 1.0 mg/ml, respectively. The assay was performed by add of aliquots of 10 mg/ml EABRP and 100 µl of 5% AlCl3 in a 5 ml volumetric flask (methanol). The final concentration range of EABRP was from 50 to 200 μg/ml (n = 3). The solutions absorbances were measured in a UV-Vis spectrophotometer set at the 425 nm wavelength after 30 min of incubation in dark room at 25°C. Total flavonoids content was calculated using the quercetin calibration plot (Y = 0.0711x + 0.0027, R2 = 0.999) and expressed as mg quercetin equivalent (QE) g−1 of dried EABRP extract.
The composite resin Filtek™ Bulk Fill Flow (3M/ESPE, St. Paul, MN, United States) color A2 was modified by addition of ethyl acetate Brazilian red propolis (EABRP) in 10 µl of 2-hydroxyethyl methacrylate (HEMA solvent) from Sigma-Aldrich (St. Louis. MO, United States) for development of experimental groups. The composition of the commercial composite is described in Table 1. The commercial composite was used as a control group (RC). To test the HEMA solvent effect alone, 10 μl of pure HEMA was added to commercial composite to obtaining the solvent control group (RS). The two controls were used at all characterization assays of enriched composites with Brazilian red propolis. The final concentrations of EABRP in the experimental composites were 0.10, 0.15, and 0.25% (w/v) to obtaining the experimental groups RP10, RP15 and RP25, respectively.
Three EABRP stock solutions were prepared at concentrations of 10.0, 15.0 and 25.0 mg/ml in HEMA solvent, and the mixture was homogenized by vortex in a dark room. The enriched composites resins groups were obtained by the addition of 10 μl of the EABRP-HEMA solution in 100 mg of the commercial composite resin and photoactivated for 20 s using a curing light (LED Emitter A FIT, 1250 mW/cm2, λmax 455 nm, light tip 8 mm, Schuster, Santa Maria, BRA).
The samples of experimental composites groups were prepared in molds with specific dimensions for each type of biological, mechanical, or physicochemical assays and positioned between two polyester strips. Before photoactivation, the upper surface of samples was covered with a glass blade and subjected to a load of 500 g for 1 min to remove excess material and ensure standardization of samples. The glass blade was removed to carry out the photoactivation. The Schematic representation of the process of obtaining and characterization of composite resin enriched with Brazilian red propolis is show in Supplementary Figure S2.
Five disc-shaped samples to each experimental group were prepared with the aid of a stainless metallic matrix (h = 1.0 mm, Ø = 5.0 mm) and then were photoactivated for 20 s, as describe previously. The antibacterial activity of experimental composites was determined in relation to the Streptococcus mutans CCT 3440 strain. The bacterial strain was activated in sterile BHI medium at 37°C for 24 h, and then sowed in BHA medium and incubated at 37°C for 18 h. The inoculum concentration was adjusted in sterile physiologic solution from colonies isolated in BHA. The inoculum was adjusted to 106 UFC/ml for the determination of antibacterial activity by direct contact test.
The antibacterial activity of experimental composite RP25 was also determined by direct contact test (Tavassoli Hojati et al., 2013; Zhang et al., 2017). Therefore, 10 μl of suspended bacteria (106 UFC/ml) were placed on each disc-shaped sample (ø = 5 mm; thickness = 1.0 mm) individually positioned in sterile microtubes (n = 5). The disc-shaped sample in contact with inoculum were maintained laminar flow, for 1 h, until the entire liquid suspension evaporated to obtain a thin layer of bacteria. After this period, 200 μl of sterile physiologic solution was added to the each microtubes and the bacterial suspension was homogenized in vortex. To estimate colony formation, bacterial suspension from each specimen was diluted in sterile physiologic solution at 1:10 (10−1) proportion, then 25 μl of this dilution was inoculated in BHA plates for 24 h at 37 ± 1°C in a microaerobic environment. After incubation, the total colonies were counted (n = 5) and the determination of UFC/ml were performed to each group, using the equation:
Where: Nº of colonies is the total colonies present the plate after direct contact test; 40 is the correction factor to 1 ml (25 μl × 40 = 1 ml); 10 is the modulus of dilution used (10−1) to UFC/ml count. The group RC (commercial composite) was used as negative control. The group RS (commercial composite with the solvent HEMA) was used to evaluate the solvent effect in enriched composite resins formulations. A microtube with inoculum without composites was used as cell control. Antibacterial activity was expressed by antibacterial ratio, where:
Where r (%) is the antibacterial ratio; b is the average UFC/ml recovered for bacterial control; c is the average of recovered UFC/ml for the experimental group.
The in vitro cytotoxicity assay was performed for determined the cytotoxicity of BRP enriched composite resin against 3T3 fibroblasts. The composite samples were prepared as describe previously, and 24 h later the samples were immersed in pure Dulbecco’s Modified Eagle Medium (DMEM) medium, incubated for 24 h at 37°C, 5% CO2 and 95% relative humidity to obtain the extracts (composite resins extracts in DMEM). The extracts were prepared at the ratio of 1.0, 2.0, 3.0, 5.0 and 6.0 cm2 of composite/mL of medium to the experimental groups analyzed RC, RS and RP25 (n = 5), according to ISO 10993-12 (International Organization for Standarization, 2006). The samples were taken from the culture medium after the specified time and the obtained extracts were filtered in 0.22 μm membranes (Millipore, Molsheim, France) to ensure sterile conditions.
Fibroblasts of the 3T3 strain (embryonic fibroblasts of mice) were sown in DMEM medium supplemented with 10% bovine fetal serum (SBF), L-glutamine (2.0 μM) and penicillin/streptomycin (0.02 μg/ml) and were kept at 37°C, 5% CO2 and 95% relative humidity. The cells were transferred to 96-well plates (200 μl/well—7.0 × 103 cells/ml of complete culture medium) and incubated for 24 h in the same conditions as described previously. After this period, the supernatant was removed and each well with cells received 180 μl of fresh complete DMEM and 20.0 μl of the extract, and the cells were incubated for 24 h under the same conditions of temperature, CO2 and humidity. The negative control group (cell control) received only fresh complete DMEM. Cell viability was determined by the MTT assay [3-bromide (3,5-dimetiltiazole-2-yl)-2,5-diphenyltrazzole] 24 h after exposure of cells to composite resins extracts in DMEM. The cells were washed with phosphate buffered saline (PBS) and 23 μl of an MTT solution (5.0 mg/ml in PBS) were added to each well as well as 23 μl of an MTT solution (5.0 mg/ml in PBS), then the cells were incubated again for 4 h under the same conditions. The supernatant was discarded and 150 μl dimethyl sulfoxide (DMSO) was added to each well to dissolve the formazan crystals. After 10 min the optical density of the resulting solution was read at 540 nm in a spectrophotometer (Model UV-1240, Shimadzu, Kyoto, Japan). The mean values of optical density obtained from cells exposed to DMEM were used as a negative control reference (100% cell survival). The cytotoxicity of the tested samples was expressed as the percentage of cell viability in relation to the negative control (100%), (n = 5). The in vitro cytotoxicity was determined by decrease of the cell viability as the equation:
where OD540sample is the optical density of cells after sample treatment and OD540 cell control is the optical density of cells control group.
Rectangular samples were made for each experimental group (n = 10) in a stainless-steel matrix (2 mm × 2 mm x 25 mm) according to the specifications of ISO 4049 (International Organization for Standarization, 2000) and then were photoactivated for 20 s, as describe previously. The photoactivation process was carryout according to the ISO 4049 specifications: one moment of photoactivation for each of the three points on top and bottom samples surfaces. After cure, the samples were kept in distilled water at 37°C for 24 h before test. The test was performed in a Microtensile/Semi-universal OM100 test machine (Odeme Dental Research, SC, BRA) with two rods (2 mm in diameter), mounted parallel with 20 mm between its centres, and a third rod (2 mm in diameter) centred between, and parallel to, the other two. The loading ratio and speed applied on samples was of 50 N/min and 0.75 ± 0.25 mm/min, respectively, according to ISO 4049 until the sample fracture. Flexural strength (FS) was expressed in MPa by equation:
where F is the maximum load, in newtons, exerted on the sample; l is the distance, in millimeters, between the supports; b is the width, in millimeters, of the specimen measured immediately prior to testing and h is the height, in millimeters, of the specimen measured immediately prior to testing.
Five disc-shaped samples were made for each group, with the aid of a stainless metallic matrix (h = 1.0 mm, Ø = 5.0 mm) and then were photoactivated for 20 s, as describe previously. The samples were stored in distilled water at 37°C for 24 h before test. Five indentations were performed on the irradiated surface of each sample at the center and more four regions with a load of 50 g for 5 s using an HMV-2000 microhardness (Shimadzu, Tokyo, Japan). The average of five indentations was taken as KHN to each of four samples on each experimental group and the average of four KHN in each experimental group was taken as the KHN to the group. The Knoop microhardness (KHN) was determined by equation:
where P is the load applied on the sample (Kgf); L is the length of the longest diagonal of the indentation (mm2) and C is the indentation constant (0.07028).
The post-cure depth was determined according to ISO 4049 procedure specifications (International Organization for Standarization, 2000). Five cylindric-shaped samples per group were produced with the aid of a cylindrical stainless metallic matrix (h = 6.0 mm, Ø = 2.0 mm) and then were photoactivated for 20 s, as describe previously. Then, the samples were immediately removed from the matrix and the uncured material at the bottom surface was scraped with a plastic spatula. The height of the cylindric-shaped samples was measured with a micrometer (with an accuracy of 0.01 mm) after scraping. The post-cure depth to each experimental groups was determined as the mean of each group samples.
The degree of conversion of the experimental groups was analyzed by Fourier transform infrared spectroscopy (FTIR; IRAffinity-1 Model, Shimadzu, Kyoto, Japan) in attenuated total reflection (ATR) mode. The DC% was measuring by change of height of the band at 1638 cm−1 (corresponding with the aliphatic carbon double bond absorbance). The aromatic carbon double bond absorbance at 1608 cm−1 was used as standard control. Each sample spectrum was acquired with 64 scans, resolution of 4.0 cm−1 and using the absorbance mode. Disc-shaped samples were made with the aid of a stainless metallic matrix (h = 1.0 mm, Ø = 5.0 mm) and then were photoactivated for 20 s, as describe previously. Were analyzed non-polymerized samples (n = 3), immediately polymerized samples (n = 3) and samples with 24 h after polymerization (n = 3) to each experimental group and theirs respective FTIR spectra were obtained. The degree of conversion was calculated as follows:
where: R = (band at 1638 cm−1)/(band at 1608 cm−1).
The water sorption (Wsp) and water solubility (Wsl) in water of the experimental resins were determined according to ISO 4049 specifications (International Organization for Standarization, 2000). Five samples (h = 1.0 mm, Ø = 15.0 mm) per experimental group were prepared in a stainless metallic matrix and were photoactivated at nine points on the upper surface, each point for 20 s. After photoactivation, the samples were arranged in a light-proof container for 24 h and transferred to a desiccator containing silica gel dehydrated (Fischer Scientific®, Leicester, United Kingdom), this set was kept at 37°C in an oven. After 22 h, all groups were transferred to a second desiccator, where they were kept for 2 h at 37°C. At the end of the cycle, each sample was weighed on an analytical balance with precision of 0.01 mg (Ohaus® DV314C Discovery, Pine Brook, United States) and replaced in the initial desiccator. The cycles were repeated until a constant mass was reached M1 (μg), so that the weight variability was not greater than 0.1 mg in 24 h period. The samples were measured with digital micrometer (accurate to 0.01 mm, DIGIMESS-110-250, Digimess® Precision Instruments Ltda, SP, BR) in order to determine the area (mm2) and volume (mm3). Then, the samples were immersed in water at 37 ± 1°C (Fanem® Water Bath 1100, SP, BR), for 7 days. After this period, excess water was removed, and each sample again weighed. The wet mass was recorded as M2 (μg). The design cycles were repeated, as previously described, until the stable weight was reached, corresponding to M3 (μg). The mean values of Wsp and Wsl of each sample were calculated in micrograms per cubic millimeter using equations, respectively:
Disc-shaped samples were made with the aid of a stainless metallic matrix (h = 1.0 mm, Ø = 5.0 mm) and then were photoactivated for 20 s, as describe previously. The average surface roughness (Ra) was determined by an atomic force microscopy (AFM) (Model Multiview 4000TM, Nanonics, Jerusalem, Israel) combined with optical microscope (Model BXFM Olympus, Tokyo, Japan). The AFM system was acoustically isolated, and the instrument was stabilized on an active table of movements. The topography of the samples was determined (256 × 256 pixels) in tapping mode with a scan ratio of 0.3–1.0 Hz in an area of 20 × 20 μm. The probe has a diameter <10 nm, 300 µm cantilever, 30° bend angle, 32 kHz resonance frequency and a Cr coating. Twelve regions per experimental group were analyzed and the average roughness (Ra) was determined using the Software WSxM (Nanotec, Madrid, Spain). The roughness average was calculated as the absolute mean of the heights of the irregularities along the profile as the equation:
Where: N is the number of sample points, zi is the height of each sample point and
Five disc-shaped samples were prepared in a stainless metallic matrix (h = 1.0 mm, Ø = 5.0 mm) and powdered to obtain uniform powder. Thermogravimetry analysis (TGA) was performed in a TGA-51H model equipment (Shimadzu, Kyoto, Japan), from 4.5 mg ± 6.6% of each sample, packed in alumina crucible. The heating ratio was 10°C min−1 in the range of 25–900°C in nitrogen atmosphere with flow of 50 cm3 min−1. The equipment was calibrated under the same conditions with calcium oxalate monohydrate pattern. Differential scanning calorimetry analysis (DSC) was performed in DSC model 60 plus (Shimadzu, Kyoto, Japan) from 2.5 mg ± 4.0% of each sample packed in hermetically sealed alumina crucible. The heating ratio was 5.0°C min−1 in the range of 30–600°C in nitrogen atmosphere and flow of 50 cm3 min−1. The equipment was calibrated under the same conditions with indium and zinc.
The statistical analysis was performed using the software GraphPad prism 9.2.0. The Shapiro-Wilk test was performed to determine the normality distribution of results data, at the level of significance of 95%. The parametric data were analyzed by one-way analysis of variance ANOVA followed by Tukey’s comparisons test (p < 0.05). The data of cytotoxicity assay of were analyzed by two-way ANOVA followed by Tukey’s comparisons test (p < 0.05). The non-parametric data were analyzed by Kruskal-Wallis test followed by Dunn’s comparisons post-test at the level of significance of 95% (p = 0.05).
The BRP chemical composition was identified by LC-ESI-Orbitrap-FTMS, where was possible to identify fifty compounds from BRP belonging to the class of phenolic acids, flavonoids (isoflavones, isoflavans, flavones, flavanonols, flavonols, flavanols, flavanones, C30 isoflavones, pterocarpan), chalcones, triterpenes and prenylated benzophenones. The EABRP LC-ESI-Orbitrap-FTMS chromatogram shows in Figure 1. Furthermore, is observed that the compounds 4,4′-dihydroxy-2-methoxychalcone (26), formononetin (23), formononetin (22), vestitol (28), liquiritigenin (13), and isoliquiritigenin (21) are the major constituents of this EABRP extract. The identified compounds in the EABRP extract are listed in Table 2. The chemical structures of some of compounds identified from EABRP are show in the Supplementary Figure S1.
The quantification of seven markers from EABRP was performed by UPLC-DAD. The flavonoids daidzein, liquiritigenin, pinobanksin, formononetin, pinocembrin, biochanin A and the chalcone isoliquiritigenin were identified and quantified from EABRP dried extract. Its respective concentrations are showed in Table 3. The EABRP UPLC-DAD chromatogram too shows formononetin, liquiritigenin and isoliquiritigenin as majority compounds of EABRP dried extract Figure 2.
FIGURE 2. EABRP UPLC-DAD chromatogram at 280 nm. 1 (daidzein), 2 (liquiritigenin), 3 (pinobanksin), 4 (isoliquiritigenin), 5 (formononetin), 6 (pinocembrin) and 7 (biochanin A).
In the present work, the total flavonoids content (TFC) was determined by a colorimetric method using AlCl3 reagent. The TFC were expressed as mg quercetin equivalent (mg QE g−1) of dried EABRP extract. The EABRP extract contain 54.56 ± 0.25 mg QE g−1 of dried EABRP extract Table 4. The radical scavenging capacity (RSC) of EBRP and EABRP were determined by DPPH• method, the EABRP showed RSC fifteen times bigger than EBRP (p < 0.05) Table 4. The more capacity radical scavenge verified to EABRP was expected because the liquid-liquid extraction leads to it obtain as a more flavonoids enriched extract. The RSC to EABRP not showed statistical difference in comparation with the RSC to the trolox standard (p > 0.05). One of characteristics of a flavonoid enriched extract is precisely its radical scavenging capacity.
TABLE 4. Radical scavenge capacity, total flavonoids content, minimal inhibitory concentration and minimal bactericidal concentration of BRP extracts.
The antibacterial activity of EBRP and EARP against Streptococcus mutans CCT 3440 was determined by broth microdilution assay where the minimal inhibitory concentration (MIC) and the minimal bactericidal concentration (MBC), were determined to each extract. The MIC values to EBRP and EABRP were of 125 μg/ml, being both characterized as bacteriostatic extracts at this concentration for this bacterial strain. The EABRP extract showed MBC value of 500 μg/ml, being twice more active than EBRP extract that showed MBC value of 1000 μg/ml, Table 4.
Brazilian red propolis enriched composite resins were developed by the addiction of EABRP extract at the concentrations of 0.10%, 0.15%, and 0.25% (w/w) in a commercial composite resin to obtaining the formulations RP10, RP15 and RP25, respectively. The biologic characterization of enriched composites was carried out for determination of the antibacterial activity against Streptococcus mutans CCT 3440 and the cytotoxicity to 3T3 fibroblasts, respectively.
The antibacterial activity of Brazilian red propolis enriched composite resins was performed by direct contact test and the antibacterial ratio (r %) was determined Figure 3. The statistical results shows that the antibacterial ratio of RP25 was 90.76 ± 6.43 (p < 0.0001) and that negative control (RC) did not exhibit significant antibacterial ratio (p = 0.1865) after 1 h of direct contact, both in comparison with cell control. The addition of EABRP to the commercial composite resulted in an enriched composite and with antibacterial activity against S. mutans CCT 3440 after 1 h of direct contact. The use of methacrylate’s monomer hema as solvent for incorporate propolis to commercial composite in the development phase contributed to the antibacterial activity verified to RP25. This fact is confirmed by antibacterial activity exhibit for RS with an antibacterial ratio of 69.23 ± 12.16 (p = 0.0006) when compared with cell control. When comparing RP25 and RS with RC, separately, both shows statistical difference in relation of this commercial composite resin (p = 0.0019 and p = 0.0454, respectively) Table 5. Thereby, the null hypothesis was discarded and considered the test hypothesis that the addition of EABRP in hema in commercial composite resin led to obtainment of a EABRP enriched composite resin with antibacterial activity.
FIGURE 3. Antibacterial ratio (r %) of EABRP enriched composite resin against S. mutans after 1h of direct contact test. CC, cell control; RC, commercial resin; RS, commercial resin + HEMA solvent. RP25, enriched EABRP composite resin at 0.25% (w/w). ***: p = 0.002 ****: p < 0.0001.
The cytotoxicity of BRP enriched composite resins was determined by MTT assay against fibroblasts 3T3. In this assay were observed the effect of addition of BRP in the commercial composite resins and the effect of composite resins dose on the cytotoxicity. The usual dose of composite resin for this assay was defined as ratio of 3.0 cm2 of composite resin/mL (surface area/volume), conforming ISO 10993-12 (International Organization for Standarization, 2006). The result shows that the groups RP25, RC and RS no presented cytotoxicity to 3T3 fibroblasts, once that after 24 h of exposition to composite resins extracts the cell viability were bigger than 70% Figure 4. Nevertheless, was observed statistically significant difference on the cell viability between experimental groups when analyzed the addition of BRP to the composite resin (p < 0.0001). The dose effect was not statistically significant (p = 0.0611). Comparing the results for groups RP25, RC and RS at ratio of 3.0 cm2 is possible observed that the cells show bigger viability after exposition to RP25 than after to RC and to RS exposition (p < 0.05), respectively, as shows Table 5.
FIGURE 4. Cytotoxicity of EABRP enriched composite resin against 3T3 fibroblast. Dot line: cell control (100% viability); RC, commercial resin; RS, commercial resin + HEMA solvent; RP25, enriched EABRP composite resin at 0.25% (w/w). *: p < 0.05 (comparison between RP and RC).
BRP enriched composite resins exhibit flexural strength above of 100 MPa, according to the ISO 4049 specifications (minimal FS of 80 MPa). Formulations RP10, RP15, RP25 and RS are statistically similar (p > 0.05) and RC shows the biggest FS 125.57 MPa ± 11.49 (p < 0.05) Figure 5A. KHN for RS, RP10, RP15 and RP25 were 19.4 ± 1.5, 18.6 ± 1.3, 18.2 ± 1.3 and 18.8 ± 1.1, respectively Figure 5B. The KHN for commercial composite resin (RC) was 24.8 ± 1.0. The addiction of HEMA, a methacrylate monomer solvent used as diluent in composite resin development was responsible by decrease of the mechanical properties of BRP enriched composite resins compared with RC (p < 0.05) once that promotes the decrease of viscosity in comparation. Decrease of viscosity in composite resins is caused by decreased of filler proportion in the composite.
FIGURE 5. Mechanical characterization of EABRP enriched composite resin. (A): Flexural strength determination (MPa). Dot line: minimal limit of 80 MPa (ISO 4049). (B): Knoop microhardness. RC, commercial resin; RS, commercial resin + HEMA solvent; RP10, RP15 and RP25, enriched EABRP composite resins at 0.10, 0.15 and 0.25% (w/w).
Composites enriched with red propolis showed a cure depth compatible with the commercial composites used as a basis in this study, the bulk composites (with increment of ≥ 4.0 ± 0.1 mm), except RP25. The post-cure depth of RP25 and RP15 was 2.74 ± 0.06 mm and 3.80 ± 0.30 mm, respectively, both smaller than RC (5.86 ± 0.03 mm), RS (5.69 ± 0.05 mm) and RP10 (4.48 ± 0.34 mm) composites Figure 6A. The post-cure depth among the enriched composites was inversely proportional to the red propolis concentration: RP10 > RP15 > RP25. This phenomenon can be explained because the increase of red propolis concentration at composites led to decrease of its translucency, decreasing the capacity of irradiation of the curing light through sample. The Shapiro-Wilk normality test exhibit a non-normal distribution of post-cure depth results; therefore, the Kruskal-Wallis nonparametric test was performed. There was not statistically significant difference between three formulations of enriched composite resins (p > 0.05), but there was statistically significant difference between: RP25 and RC (p = 0.0002); RC and RP15 (p = 0.0171). The statistical analysis demonstrated that there was not significant difference between RC and RP10 (p = 0.2546). In this way, RP10 can be considered a bulk enriched composite resin.
FIGURE 6. Physical-chemical characterization of EABRP enriched composite resins. (A): Depth of cure. (B): Degree of conversion. (C): Water sorption. (D): Water solubility. RC, commercial resin; RS, commercial resin + HEMA solvent; RP10, RP15 and RP25, enriched EABRP composite resins at 0.10, 0.15 and 0.25% (w/w).
This study analyzed the degree of conversion of BRP enriched composite resins by FTIR spectroscopy in twice moments: immediately after light curing photoactivation and 24 h after photoactivation. Was observed that the addition of EARP-HEMA to the commercial composite promoted a significant increase in the DC% of enriched composites when compared to the RC Figure 6B. When analyzing each moment separately, is possible verify that at first moment RS, RP10, RP15 and RP25 are statistically similar each other (p > 0.05) showing DC% among 76.95 ± 2.24% to 79.81 ± 0.39% and have bigger DC% than RC with 60.17 ± 3.09% (p < 0.0001). At the second moment this result profile remains: RS, RP10, RP15 and RP25 are statistically similar each other (p > 0.05) showing DC% from 80.70 ± 4.07% to 83.96 ± 2.87% and have bigger DC% than RC with 69.58 ± 4.51% (p < 0.029).
The BRP enriched composite resins, RC and RS controls exhibited values conforming ISO 4049 specification for Wsp (≤40.0 μg/mm3) Figure 6C. RS, RP10, RP15 and RP25 were statistically like each other (p > 0.05), but smaller than of RC (p < 0.028). The Wsl for BRP enriched composite resins was conforming ISO 4049 (≤7.5 μg/mm3) Figure 6D. The Shapiro-Wilk test demonstrated that Wsl data have non-normal distribution, therefore was conducted the Kruskal-Wallis test (p < 0.05) for this assay. Was observed that RP25 showed a median of 3.9 μg/mm3, exhibiting a bigger Wsl than RC with 0.68 μg/mm3 (p = 0.0109). The groups RC, RS, RP10 and RP15 no showed statistically significant difference (p > 0.05) each other.
Topographic images obtained in AFM, representative of the average surface roughness (Ra) of the experimental groups are presented in Figure 7A. The values of Ra for BRP enriched composite resins are presented in Figure 7B and show that all groups presented average surface roughness below 21 nm. RS, RP10, RP15 and RP25 exhibited small Ra than RC (p < 0.0001), being RC presented the lowest Ra (6.59 ± 1.94 nm), followed for RP15 (14.48 ± 1.63 nm). There was no significant difference (p > 0.05) between the Ra values of groups RS (19.24 ± 1.87 nm), RP10 (20.58 ± 3.86 nm) and RP25 (20.76 ± 2.31 nm).
FIGURE 7. Average roughness (Ra) of EABRP enriched composite resins. (A): Topographic images obtained by AFM for experimental groups. (B): Average roughness values (Ra) for all resins analyzed. RC, commercial resin; RS, commercial resin + HEMA solvent; RP10, RP15 and RP25, enriched EABRP composite resins at 0.10, 0.15 and 0.25% (w/w).
Was performed the thermal analysis for BRP enriched composite resins for determination of its thermal events of degradation that explain the degree of formation of covalent bonds in the composite polymeric chains. The more resistant to thermal degradation below 600°C, the greater the number of covalent bonds existing in the three-dimensional structure of the composite. The TGA, DTG and DSC thermograms are shown in Figure 8. The thermogravimetric profiles of the studied groups were similar, where all groups presented two events of mass loss, one between 334–379°C and the other between 448–455°C. All composite resins formulations analyzed presented thermal resistance between 300–350°C, since no group had mass loss of up to 5% below these temperatures Figure 8A. The values of thermogravimetric events can be observed in Table 6, where it is observed that the addition of EARP-HEMA led to increased thermal resistance of composite resins.
FIGURE 8. Overlapping thermograms of experimental and control resins. (A): Overlapping TGA and DTG thermograms. (B): Overlapping DSC thermograms.
TABLE 6. Thermal resistance, T0, T1, T2 and residual mass at 600°C of EABRP enriched composite resins.
All samples presented similar DSC profile between each other, with two events of thermal decomposition of the polymer matrix in 368°C and 407°C, and the beginning of thermal degradation of the composite stars at ∼ 560°C Figure 8B. It was observed that the addition of EARP-HEMA led to a increase in the heat variation involved in the thermal decomposition of the polymer matrix of enriched composite resins. The values of DSC events can be observed in Table 7.
The Ethyl acetate Brazilian red propolis extract (EABRP) was chose for enriched composite resin development because its antibacterial activity against Streptococcus mutans CCT 3440. Main idea in development of BRP enriched composite resin was the obtain of a antibacterial composite resin against Streptococcus mutans, the primary etiologic agent of caries (Koo et al., 2003). Phenolic acids, flavonoids, chalcones, triterpenes and prenylated benzophenones detected by LC-ESI-Orbitrap-FTMS in BRP extracts are characteristic for Brazilian red propolis collected in the northeast Brazil region (Frozzada et al., 2013; López et al., 2014; de Mendonça et al., 2015; Freires et al., 2016; Rufatto et al., 2018). The flavonoids formononetin, biochanin A, daidzein, liquiritigenin and the chalcone isoliquiritigenin, quantify on this study, are some of markers describes for BRP (Andrade et al., 2017; Picolotto et al., 2019). The total flavonoid content for EABRP observed in this work is bigger than ethanol BRP extract describes in another work (31.48 mg QE g−1) because the liquid-liquid extraction carried out concentrated the flavonoids in the acetate phase (Andrade et al., 2017).
The antibacterial results observed for EABRP against S. mutans was compatible with results in another studies where ethanol extract of BRP was tested against S. mutans UA159 and exhibited MIC and MBC values of 200.0 μg/ml for both assay (Bueno-Silva et al., 2013). In another study chloroform extract of BRP exhibited MIC and MBC against S mutans of 125 and 500 μg/ml, respectively (Oldoni et al., 2011). In a study, the antibacterial activity of eight ethanolic Brazilian propolis extracts from 3 types (red, green, and brown) was analyzed against Enterococcus sp. ATCC 2912, Staphylococcus aureus ATCC 25923 and Klebsiella sp. ATCC 1706/700603, and red propolis showed the highest antibacterial activity against this bacteria with MIC values of 31.3, 62.5, and 31.3 μg/ml, respectively (Dantas Silva et al., 2017). These variations in antibacterial results for Brazilian red propolis from the same origin can be explained by seasonality. It is known that the antibacterial activity of propolis may vary depending on seasonality because the presence and concentration of its markers are related with the season, which in turn influences the production of secondary metabolites by the botanical origin of propolis (Regueiro et al., 2017; do Nascimento et al., 2019).
The use of propolis on technological development in dentistry and oral health has been frequent because it is a nontoxic raw material, with traditional use in natural medicine and that shows some known pharmacological activities as antimicrobial, anti-inflammatory and wound healing (Koo et al., 2002; Freires et al., 2016; Oliveirados et al., 2017; de Carvalho Furtado et al., 2018; Bezerra et al., 2020; da silva Barboza et al., 2021). It has been reported in scientific literature the use of propolis on development of varnish (De Luca et al., 2014; Neto et al., 2020), toothpaste (Wiatrak et al., 2017; Peycheva et al., 2019), total-etching adhesive system (Porto et al., 2021), cavity cleaning agent (Celerino de Moraes Porto et al., 2018) and endodontic irrigant (Parolia et al., 2021). The use of propolis was not found in the development of composite resins in scientific literature and in technological literature.
The Brazilian red propolis enriched composite resin developed in this study showed antibacterial activity against S. mutans CCT 3440 after 1 h of direct contact and biocompatibility with 3T3 fibroblasts. Direct contact test has been used for determination of antibacterial and antifungal activity of enriched dental materials (Tavassoli Hojati et al., 2013; Zhang et al., 2017). The antibacterial activity of Brazilian red propolis enriched composite resins is a result of synergism of several markers present in BRP (Xie et al., 2014). Some examples of antimicrobial action mechanism for flavonoids presents in BRP are listed in the scientific literature as inhibition of nuclei acids synthesis (quercetin, chrysin, kaempferol), inhibition of quorum sensing (naringenin, kaempferol) and membrane disruption (naringenin, catechin) (Silva et al., 2016; Wang et al., 2018; Biharee et al., 2020).
In a study, the isolated flavanone naringenin had a MIC value of 500 μg/ml (1.84 mM) against S. aureus, a Gram positive bacteria (Wang et al., 2018). The same study showed that at 400 μg/ml (1.47 mM), naringenin down-regulated expression levels of fabD, fabF, fabG, fabH and fabI, genes associated with biosynthesis of fatty acid from cell membrane of S. aureus. The authors report that this down-regulating leading to modification of cell membrane fatty acid content, increasing the membrane fluidity and resulting in decrease of bacterial cell viability (Wang et al., 2018). Naringenin and other flavanones as liquiritigenin, 6-methoxyflavanone, 6-hydroxynaringenin, pinostrobin and pinocembrin was identified in EABRP used in this study. In the scientific literature, the structure activity relationship of flavanones was evaluated for the against Gram positive and was observed that two hydroxyl groups in the ring A at the C-5 and C-7 positions and none hydroxyl group in the ring B is a major contributing factor towards antibacterial activity, as pinocembrin (Echeverr et al., 2017). Studies of Chalcones structure activity relationship highlight the importance of hydroxyl group at C2′, C4′ and C4 for antibacterial activity (Ávila et al., 2008; Xie et al., 2014). The chalcone isoliquiritigenin, quantified in EABRP at concentration of 4.53 μg/ml, has this chemical arrangement.
New dental composites have been developed from the incorporation of antibacterial agents (Beyth et al., 2014). These agents can be inorganic particles, modified monomers or additives incorporated into both the polymer matrix and the charge particles, such as zinc oxide nanoparticles (Beyth et al., 2013, 2014; Rüttermann et al., 2013; Tavassoli Hojati et al., 2013; Inagaki et al., 2016), silica nanoparticles functionalized with amphotericin B (Lino et al., 2013), silver nanoparticles (Fan et al., 2011), modified monomers containing quaternary ammonium (Imazato et al., 2003; Makvandi et al., 2016, 2018) and antibacterial agents immobilized in composite (Namba et al., 2009; Mankovskaia et al., 2013). The concept of modified surfaces is reported in the scientific literature mainly for the purpose of developing antimicrobial and bioequivalent health materials (Busscher et al., 2012; Silva et al., 2016). Thus, surface-modified materials can be classified in five different ways: nonadhesive, tissue-integrating, contact-killing, antimicrobial-releasing coatings and multifunction coatings (Busscher et al., 2012).
The evidence that composite resins normally release some free monomers both in the oral cavity and in the dental pulp and that this release can cause toxicity is already well established in the literature (Schweikl et al., 2006; Nocca et al., 2011). In addition, there is a positive correlation between the toxicity of these composites and their monomers with oxidative stress that they can cause to normal cells in the oral cavity (Krifka et al., 2012, 2013; Perduns et al., 2019). The enzyme glutathione peroxidase (GPx1) it is present in both cell cytoplasm and mitochondrial matrix and acts reducing H2O2 to H2O when reduced glutathione (GSH), an tripeptide and endogenous antioxidant, levels are high (Krifka et al., 2012; Sies et al., 2017). The mechanism of oxidative stress in oral cell caused by methacrylate monomers involves the decrease in cellular levels of GSH due to the formation of the GSH-methacrylate monomer adduct that leads to glutathione peroxidase (GPx1) inhibition with a consequent increase in H2O2 levels in cell. Accumulation of H2O2 into oral cells leads to oxidative stress, in this process started by exposure to methacrylate monomers (Krifka et al., 2012). To reduce this GSH-methacrylate monomer adduct formation, studies have developed composite resins containing antioxidants species as ascorbic acid and N-acetylcysteine (Yang et al., 2019). The Brazilian red propolis DPPH radical scavenging capacity suggest a possible explanation for the statistical difference found between enriched and commercial composite resin in the cytotoxicity assay in this study, when the phenolic compounds of BRP can be inhibition the GSH-methacrylate monomer. More studies are necessary to confirm this supposition.
The enriched Brazilian red propolis composite resin exhibited flexural resistance higher than 100 MPa, exceeding the 80 MPa values indicated for polymer-based restorative materials (International Organization for Standarization, 2000), In the mechanical tests of flexural resistance and Knoop microhardness, the insertion of the HEMA diluent was determinant for the reduction of the properties observed between the RC group and the other groups. The DC% of 60% for filtek bulk fill flow composite resin is according with scientific literature data (Pongprueksa et al., 2015). The increase of DG% exhibited by modified composite resin (RS and RPs) was provided by EABRP-HEMA incorporation. This fact is confirmed by thermal analysis data, where RP15 and RP25 showed increase in thermal resistance when compared to RC. This increase on thermal resistance happens by EABRP-HEMA addition that promoted formation of cross-links in the 3D structure of the polymer (Achilias et al., 2010; Vouvoudi et al., 2015). The main monomer at the RPs composition, the urethane dimethacrylate, is able to form hydrogen bonds with the phenolic compounds present in the red propolis, making the polymeric network more cross-linked and consequently more thermal resistant (Achilias et al., 2008). The bigger DC% for RP25 in comparation with DC% for RC mean a smaller amount of free monomers in enriched composites that contributes for the smaller cytotoxicity exhibited by RP25.
Sorption has an important negative correction with the amount of composite filler. When the percentage filler increases, the polymer matrix decreases and consequently decreases water sorption, which is a phenomenon associated mainly with the polymer matrix (Alshali et al., 2015). In this study, all BRP enriched resins showed a significant increase in water sorption. Even so, the values were lower than the maximum recommended value of 40 μg/mm3 according to the ISO standard for restorative resins (International Organization for Standarization, 2000). Finally, the roughness of composite resins can be classify as mild (Ra ≤ 25 nm), moderately rough (25 mn > Ra ≤ 150 nm) and rough (>150 nm) (Mei et al., 2011). According to this classification, all composites tested RC, RS, RP10, RP15 and RP25 are considered as mildly roughness.
Up to our knowledge, this is a first study which describes the use of propolis on composite resins development. The phytochemical profile of Brazilian red propolis was characterized and used BRP extract showed up bacteriostatic and bactericide against Streptococcus mutans. A BRP enriched composite resin (RP25) was obtained by commercial composite resin modification. RP25 exhibited antibacterial activity against S. mutans by direct contact test and no showed cytotoxicity against 3T3 fibroblasts. The RP25 exhibited compatible mechanical and physical-chemical properties to the indicate for composite resins. Finally, more one application of propolis on development of materials for healthcare was carried, obtaining a BRP enriched composite resin characterized as an unprecedented, biocompatible, and antibacterial dental material.
The raw data supporting the conclusions of this article will be made available by the corresponding author on request.
Conceived and designed the experiments: JO, IP, TN, and JT. Conducted the experiment(s): JO, IP, TC, FA, AS, and RL. Analyzed and interpreted the data: JO, DS, IP, RV, JF, EB, SS, RK, TN, and JT. Contributed reagents, materials, analysis tools or data: IP, RV, JF, EB, SS, RK, TN, and JT. Gathered the literature data: JO, IP, TC, DS, FA, IL, TN, and JT. Wrote the paper: JO, IP, TC, DS, FA, AS, IL, RL, RV, JF, EB, SS, RK, TN, and JT. All authors reviewed the manuscript.
This work was supported by the Brazilian National Council for Scientific and Technological Development (CNPq), project 870220/2000-4, process 140765/2016-6. The funding received was from a doctoral grant.
The authors declare that the research was conducted in the absence of any commercial or financial relationships that could be construed as a potential conflict of interest.
All claims expressed in this article are solely those of the authors and do not necessarily represent those of their affiliated organizations, or those of the publisher, the editors and the reviewers. Any product that may be evaluated in this article, or claim that may be made by its manufacturer, is not guaranteed or endorsed by the publisher.
The authors acknowledge the CNPq (Brazilian National Council for Scientific and Technological Development) for funding the project. The authors would like to acknowledge FAPEAL (Foundation for Sponsoring Research in the State of Alagoas) and CAPES (The Brazilian Coordination for the Personal Improvement of Superior Education) for supporting the groups infrastructure.
The Supplementary Material for this article can be found online at: https://www.frontiersin.org/articles/10.3389/fphar.2021.787633/full#supplementary-material
Achilias, D. S., Karabela, M. M., and Sideridou, I. D. (2010). Thermal Degradation and Isoconversional Kinetic Analysis of Light-Cured Dimethacrylate Copolymers. J. Therm. Anal. Calorim. 99, 917–923. doi:10.1007/s10973-009-0526-1
Achilias, D. S., Karabela, M. M., and Sideridou, I. D. (2008). Thermal Degradation of Light-Cured Dimethacrylate Resins. Thermochim. Acta. 472, 74–83. doi:10.1016/j.tca.2008.02.004
Alshali, R. Z., Salim, N. A., Satterthwaite, J. D., and Silikas, N. (2015). Post-irradiation Hardness Development, Chemical Softening, and thermal Stability of Bulk-Fill and Conventional Resin-Composites. J. Dent. 43, 209–218. doi:10.1016/j.jdent.2014.12.004
Andrade, J. K. S., Denadai, M., de Oliveira, C. S., Nunes, M. L., and Narain, N. (2017). Evaluation of Bioactive Compounds Potential and Antioxidant Activity of Brown, green and Red Propolis from Brazilian Northeast Region. Food Res. Int. 101, 129–138. doi:10.1016/j.foodres.2017.08.066
Arafa, M. G., Ghalwash, D., El-Kersh, D. M., and Elmazar, M. M. (2018). Propolis-based Niosomes as Oromuco-Adhesive Films: A Randomized Clinical Trial of a Therapeutic Drug Delivery Platform for the Treatment of Oral Recurrent Aphthous Ulcers. Sci. Rep. 8, 18056. doi:10.1038/s41598-018-37157-7
Ávila, H. P., Smânia, E. F., Monache, F. D., and Smânia, A. (2008). Structure-activity Relationship of Antibacterial Chalcones. Bioorg. Med. Chem. 16, 9790–9794. doi:10.1016/j.bmc.2008.09.064
Beyth, N., Farah, S., Domb, A. J., and Weiss, E. I. (2013). Antibacterial Dental Resin Composites. Reactive Funct. Polym. 75, 81–88. doi:10.1016/j.reactfunctpolym.2013.11.011
Beyth, N., Farah, S., Domb, A. J., and Weiss, E. I. (2014). Reactive & Functional Polymers Antibacterial Dental Resin Composites. React. Funct. Polym. 75, 81–88. doi:10.1016/j.reactfunctpolym.2013.11.011
Bezerra, C. R. F., Assunção Borges, K. R., Alves, R. d. N. S., Teles, A. M., Pimentel Rodrigues, I. V., Da Silva, M. A. C. N., et al. (2020). Highly Efficient Antibiofilm and Antifungal Activity of green Propolis against Candida Species in Dentistry Materials. PLoS One 15, e0228828. doi:10.1371/journal.pone.0228828
Biharee, A., Sharma, A., Kumar, A., and Jaitak, V. (2020). Antimicrobial Flavonoids as a Potential Substitute for Overcoming Antimicrobial Resistance. Fitoterapia 146, 104720. doi:10.1016/j.fitote.2020.104720
Bueno-Silva, B., Alencar, S. M., Koo, H., Ikegaki, M., Silva, G. V., Napimoga, M. H., et al. (2013). Anti-inflammatory and Antimicrobial Evaluation of Neovestitol and Vestitol Isolated from Brazilian Red Propolis. J. Agric. Food Chem. 61, 4546–4550. doi:10.1021/jf305468f
Busscher, H. J., Van Der Mei, H. C., Subbiahdoss, G., Jutte, P. C., Van Den Dungen, J. J., Zaat, S. A., et al. (2012). Biomaterial-associated Infection: Locating the Finish Line in the Race for the Surface. Sci. Transl. Med. 4, 153rv10. doi:10.1126/scitranslmed.3004528
Celerino de Moraes Porto, I. C., Chaves Cardoso de Almeida, D., Vasconcelos Calheiros de Oliveira Costa, G., Sampaio Donato, T. S., Moreira Nunes, L., Gomes do Nascimento, T., et al. (2018). Mechanical and Aesthetics Compatibility of Brazilian Red Propolis Micellar Nanocomposite as a Cavity Cleaning Agent. BMC Complement. Altern. Med. 18, 219. doi:10.1186/s12906-018-2281-y
CLSI (2012). Methods for Dilution Antimicrobial Susceptibility Tests for Bacteria that Grow Aerobically ;Approved Standard. Ninth Edition. Wayne (Pennsylvania): Clinical and Laboratory Standards Institute.
Corrêa, F. R., Schanuel, F. S., Moura-Nunes, N., Monte-Alto-Costa, A., and Daleprane, J. B. (2017). Brazilian Red Propolis Improves Cutaneous Wound Healing Suppressing Inflammation-Associated Transcription Factor NFκB. Biomed. Pharmacother. 86, 162–171. doi:10.1016/j.biopha.2016.12.018
da Silva Barboza, A., Aitken-Saavedra, J. P., Ferreira, M. L., Fábio Aranha, A. M., and Lund, R. G. (2021). Are Propolis Extracts Potential Pharmacological Agents in Human Oral Health? - A Scoping Review and Technology Prospecting. J. Ethnopharmacology 271, 113846. doi:10.1016/j.jep.2021.113846
Dantas Silva, R. P., Machado, B. A., Barreto, G. A., Costa, S. S., Andrade, L. N., Amaral, R. G., et al. (2017). Antioxidant, Antimicrobial, Antiparasitic, and Cytotoxic Properties of Various Brazilian Propolis Extracts. PLoS One 12, e0172585. doi:10.1371/journal.pone.0172585
Daugsch, A., Moraes, C. S., Fort, P., and Park, Y. K. (2008). Brazilian Red Propolis-Cchemical Composition and Botanical Origin. Evid. Based Complement. Alternat Med. 5, 435–441. doi:10.1093/ecam/nem057
de Carvalho Furtado, J. H., Rocha Valadas, L. A., Mendonça, K. S., de Oliveira Filho, R. D., Gadelha, L. M. U., de Mello Fiallos, N., et al. (2018). Propolis and its Dental Applications: A Technological Prospection. Biot 12, 288–296. doi:10.2174/2211550107666180815114855
De Luca, M. P., Franca, J. R., Macedo, F. A. F. F., Grenho, L., Cortes, M. E., Faraco, A. A. G., et al. (2014). Propolis Varnish: Antimicrobial Properties against Cariogenic Bacteria, Cytotoxicity, and Sustained-Release Profile. Biomed. Res. Int. 2014, 1–6. doi:10.1155/2014/348647
de Mendonça, I. C., Porto, I. C., do Nascimento, T. G., de Souza, N. S., Oliveirados, J. M. S., Arrudados, R. E. S., et al. (2015). Brazilian Red Propolis: Phytochemical Screening, Antioxidant Activity and Effect against Cancer Cells. BMC Complement. Altern. Med. 15, 357. doi:10.1186/s12906-015-0888-9
Dias, H. B., Bernardi, M. I. B., Bauab, T. M., Hernandes, A. C., and de Souza Rastelli, A. N. (2019). Titanium Dioxide and Modified Titanium Dioxide by Silver Nanoparticles as an Anti Biofilm Filler Content for Composite Resins. Dent. Mater. 35, e36–e46. doi:10.1016/j.dental.2018.11.002
do Nascimento, T. G., da Silva, P. F., Azevedo, L. F., da Rocha, L. G., de Moraes Porto, I. C., Lima E Moura, T. F., et al. (2016). Polymeric Nanoparticles of Brazilian Red Propolis Extract: Preparation, Characterization, Antioxidant and Leishmanicidal Activity. Nanoscale Res. Lett. 11, 301. doi:10.1186/s11671-016-1517-3
do Nascimento, T. G., Dos Santos Arruda, R. E., da Cruz Almeida, E. T., Dos Santos Oliveirados, J. M. S., Basílio-Júnior, I. D., Celerino de Moraes Porto, I. C., et al. (2019). Comprehensive Multivariate Correlations between Climatic Effect, Metabolite-Profile, Antioxidant Capacity and Antibacterial Activity of Brazilian Red Propolis Metabolites during Seasonal Study. Sci. Rep. 9, 18293. doi:10.1038/s41598-019-54591-3
Echeverría, J., Opazo, J., Mendoza, L., Urzúa, A., and Wilkens, M. (2017). Structure-Activity and Lipophilicity Relationships of Selected Antibacterial Natural Flavones and Flavanones of Chilean Flora. Molecules 22, 608. doi:10.3390/molecules22040608
Fan, C., Chu, L., Rawls, H. R., Norling, B. K., Cardenas, H. L., and Whang, K. (2011). Development of an Antimicrobial Resin-Aa Pilot Study. Dent. Mater. 27, 322–328. doi:10.1016/j.dental.2010.11.008
Ferracane, J. L. (2011). Resin Composite-Sstate of the Art. Dent. Mater. 27, 29–38. doi:10.1016/j.dental.2010.10.020
Floyd, C. J., and Dickens, S. H. (2006). Network Structure of Bis-GMA- and UDMA-Based Resin Systems. Dent. Mater. 22, 1143–1149. doi:10.1016/j.dental.2005.10.009
Franchin, M., Colón, D. F., Da Cunha, M. G., Castanheira, F. V., Saraiva, A. L., Bueno-Silva, B., et al. (2016). Neovestitol, an Isoflavonoid Isolated from Brazilian Red Propolis, Reduces Acute and Chronic Inflammation: Involvement of Nitric Oxide and IL-6. Sci. Rep. 6, 36401–36412. doi:10.1038/srep36401
Freires, I. A., de Alencar, S. M., and Rosalen, P. L. (2016). A Pharmacological Perspective on the Use of Brazilian Red Propolis and its Isolated Compounds against Human Diseases. Eur. J. Med. Chem. 110, 267–279. doi:10.1016/j.ejmech.2016.01.033
Frozzada, C. O. S., Garcia, C. S., Gambato, G., de Souza, M. D., Salvador, M., Moura, S., et al. (2013). Chemical Characterization, Antioxidant and Cytotoxic Activities of Brazilian Red Propolis. Food Chem. Toxicol. 52, 137–142. doi:10.1016/j.fct.2012.11.013
González-Serrano, J., López-Pintor, R. M., Serrano, J., Torres, J., Hernández, G., and Sanz, M. (2021). Short-term Efficacy of a Gel Containing Propolis Extract, Nanovitamin C and Nanovitamin E on Peri-Implant Mucositis: A Double-Blind, Randomized, Clinical Trial. J. Periodont Res. 56, 897–906. doi:10.1111/jre.12886
Gonçalves, F., Kawano, Y., Pfeifer, C., Stansbury, J. W., and Braga, R. R. (2009). Influence of BisGMA, TEGDMA, and BisEMA Contents on Viscosity, Conversion, and Flexural Strength of Experimental Resins and Composites. Eur. J. Oral Sci. 117, 442–446. doi:10.1111/j.1600-0722.2009.00636.x
Imazato, S., Ebi, N., Takahashi, Y., Kaneko, T., Ebisu, S., and Russell, R. R. (2003). Antibacterial Activity of Bactericide-Immobilized Filler for Resin-Based Restoratives. Biomaterials 24, 3605–3609. doi:10.1016/s0142-9612(03)00217-5
Inagaki, L. T., Alonso, R. C., Araújo, G. A., de Souza-Junior, E. J., Anibal, P. C., Höfling, J. F., et al. (2016). Effect of Monomer Blend and Chlorhexidine-Adding on Physical, Mechanical and Biological Properties of Experimental Infiltrants. Dent. Mater. 32, e307–e313. doi:10.1016/j.dental.2016.09.028
International Organization for Standarization (2006). International Organization for Standarization ISO 10993-12 Biological Evaluation of Medical Devices — Part 12: Sample Preparation and Reference Materials. Brussels: European Committee For Standardization.
International Organization for Standarization (2000). ISO 4049:2000 Dentistry Polymer Based Restorative Materials. Switzerland: International Organization.
Jacob, A., Parolia, A., Pau, A., and Davamani Amalraj, F. (2015). The Effects of Malaysian Propolis and Brazilian Red Propolis on Connective Tissue Fibroblasts in the Wound Healing Process. BMC Complement. Altern. Med. 15, 294. doi:10.1186/s12906-015-0814-1
Koo, H., Hayacibara, M. F., Schobel, B. D., Cury, J. A., Rosalen, P. L., Park, Y. K., et al. (2003). Inhibition of Streptococcus Mutans Biofilm Accumulation and Polysaccharide Production by Apigenin and Tt-Farnesol. J. Antimicrob. Chemother. 52, 782–789. doi:10.1093/jac/dkg449
Koo, H., Rosalen, P. L., Cury, J. A., Park, Y. K., and Bowen, W. H. (2002). Effects of Compounds Found in Propolis on Streptococcus Mutans Growth and on Glucosyltransferase Activity. Antimicrob. Agents Chemother. 46, 1302–1309. doi:10.1128/AAC.46.5.130210.1128/aac.46.5.1302-1309.2002
Krifka, S., Hiller, K. A., Spagnuolo, G., Jewett, A., Schmalz, G., and Schweikl, H. (2012). The Influence of Glutathione on Redox Regulation by Antioxidant Proteins and Apoptosis in Macrophages Exposed to 2-hydroxyethyl Methacrylate (HEMA). Biomaterials 33, 5177–5186. doi:10.1016/j.biomaterials.2012.04.013
Krifka, S., Spagnuolo, G., Schmalz, G., and Schweikl, H. (2013). A Review of Adaptive Mechanisms in Cell Responses towards Oxidative Stress Caused by Dental Resin Monomers. Biomaterials 34, 4555–4563. doi:10.1016/j.biomaterials.2013.03.019
Liang, J., Li, M., Ren, B., Wu, T., Xu, H. H. K., Liu, Y., et al. (2018). The Anti-caries Effects of Dental Adhesive Resin Influenced by the Position of Functional Groups in Quaternary Ammonium Monomers. Dent. Mater. 34, 400–411. doi:10.1016/j.dental.2017.11.021
Lima Cavendish, R., de Souza Santos, J., Belo Neto, R., Oliveira Paixão, A., Valéria Oliveira, J., Divino de Araujo, E., et al. (2015). Antinociceptive and Anti-inflammatory Effects of Brazilian Red Propolis Extract and Formononetin in Rodents. J. Ethnopharmacol. 173, 127–133. doi:10.1016/j.jep.2015.07.022
Lino, M. M., Paulo, C. S., Vale, A. C., Vaz, M. F., and Ferreira, L. S. (2013). Antifungal Activity of Dental Resins Containing Amphotericin B-Conjugated Nanoparticles. Dent. Mater. 29, e252–62. doi:10.1016/j.dental.2013.07.023
Liu, F., Jiang, X., Bao, S., Wang, R., Sun, B., and Zhu, M. (2015). Effect of Hydroxyapatite Whisker Surface Graft Polymerization on Water Sorption, Solubility and Bioactivity of the Dental Resin Composite. Mater. Sci. Eng. C Mater. Biol. Appl. 53, 150–155. doi:10.1016/j.msec.2015.04.043
López, B. G., Schmidt, E. M., Eberlin, M. N., and Sawaya, A. C. (2014). Phytochemical Markers of Different Types of Red Propolis. Food Chem. 146, 174–180. doi:10.1016/j.foodchem.2013.09.063
Makvandi, P., Jamaledin, R., Jabbari, M., Nikfarjam, N., and Borzacchiello, A. (2018). Antibacterial Quaternary Ammonium Compounds in Dental Materials: A Systematic Review. Dent. Mater. 34, 851–867. doi:10.1016/j.dental.2018.03.014
Makvandi, P., Ghaemy, M., and Mohseni, M. (2016). Synthesis and Characterization of Photo-Curable Bis-Quaternary Ammonium Dimethacrylate with Antimicrobial Activity for Dental Restoration Materials. Eur. Polym. J. 74, 81–90. doi:10.1016/j.eurpolymj.2015.11.011
Mankovskaia, A., Lévesque, C. M., and Prakki, A. (2013). Catechin-incorporated Dental Copolymers Inhibit Growth of Streptococcus Mutans. J. Appl. Oral Sci. 21, 203–207. doi:10.1590/1678-7757201302430
Mei, L., Busscher, H. J., van der Mei, H. C., and Ren, Y. (2011). Influence of Surface Roughness on Streptococcal Adhesion Forces to Composite Resins. Dent. Mater. 27, 770–778. doi:10.1016/j.dental.2011.03.017
Namba, N., Yoshida, Y., Nagaoka, N., Takashima, S., Matsuura-Yoshimoto, K., Maeda, H., et al. (2009). Antibacterial Effect of Bactericide Immobilized in Resin Matrix. Dent. Mater. 25, 424–430. doi:10.1016/j.dental.2008.08.012
Neto, E. M. R., Valadas, L. A. R., Lobo, P. L. D., Fernandes, A. M. B., da Cruz Fonseca, S. G., Fechine, F. V., et al. (2020). Dose-response Evaluation of Propolis Dental Varnish in Children: A Randomized Control Study. Recent Pat. Biotechnol. 14, 41–48. doi:10.2174/1872208313666190826145453
Nocca, G., Ragno, R., Carbone, V., Martorana, G. E., Rossetti, D. V., Gambarini, G., et al. (2011). Identification of Glutathione-Methacrylates Adducts in Gingival Fibroblasts and Erythrocytes by HPLC-MS and Capillary Electrophoresis. Dent. Mater. 27, e87–98. doi:10.1016/j.dental.2011.01.002
Oldoni, T. L. C., Cabral, I. S. R., d’Arce, M. A. B. R., Rosalen, P. L., Ikegaki, M., Nascimento, A. M., et al. (2011). Isolation and Analysis of Bioactive Isoflavonoids and Chalcone from a New Type of Brazilian Propolis. Separat. Purif. Tech. 77, 208–213. doi:10.1016/j.seppur.2010.12.007
Oliveirad, J. M. S., Silveira Cavalcanti, T. F., de Souza, L. C., Celerino de Moraes Porto, I. C., Gomes do Nascimento, T., De Paiva e Silva Zanta, C. L., et al. (2017). Uso De Própolis No Desenvolvimento De Resinas Dentárias: Um Estudo Prospectivo. Cp 10, 285–299. doi:10.9771/cp.v10i2.20531
Oliveira, M. B. S., Valentim, I. B., Rocha, T. S., Santos, J. C., Pires, K. S. N., Tanabe, E. L. L., et al. (2020). Schinus Terebenthifolius Raddi Extracts: From Sunscreen Activity toward protection of the Placenta to Zika Virus Infection, New Uses for a Well-Known Medicinal Plant. Ind. Crops Prod. 152, 112503. doi:10.1016/j.indcrop.2020.112503
Oryan, A., Alemzadeh, E., and Moshiri, A. (2018). Potential Role of Propolis in Wound Healing: Biological Properties and Therapeutic Activities. Biomed. Pharmacother. 98, 469–483. doi:10.1016/j.biopha.2017.12.069
Parolia, A., Kumar, H., Ramamurthy, S., Madheswaran, T., Davamani, F., Pichika, M. R., et al. (2021). Effect of Propolis Nanoparticles against Enterococcus faecalis Biofilm in the Root Canal. Molecules 26, 715. doi:10.3390/molecules26030715
Perduns, R., Volk, J., Schertl, P., Leyhausen, G., and Geurtsen, W. (2019). HEMA Modulates the Transcription of Genes Related to Oxidative Defense, Inflammatory Response and Organization of the ECM in Human Oral Cells. Dent. Mater. 35, 501–510. doi:10.1016/j.dental.2019.01.011
Peycheva, S., Apostolova, E., Gardjeva, P., Peychev, Z., Kokova, V., Angelov, A., et al. (2019). Effect of Bulgarian Propolis on the Oral Microflora in Adolescents with Plaque-Induced Gingivitis. Revista Brasileira de Farmacognosia 29, 271–277. doi:10.1016/j.bjp.2018.11.001
Piccinelli, A. L., Lotti, C., Campone, L., Cuesta-Rubio, O., Campo Fernandez, M., and Rastrelli, L. (2011). Cuban and Brazilian Red Propolis: Botanical Origin and Comparative Analysis by High-Performance Liquid Chromatography-Photodiode Array Detection/electrospray Ionization Tandem Mass Spectrometry. J. Agric. Food Chem. 59, 6484–6491. doi:10.1021/jf201280z
Picolotto, A., Pergher, D., Pereira, G. P., Machado, K. G., da Silva Barud, H., Roesch-Ely, M., et al. (2019). Bacterial Cellulose Membrane Associated with Red Propolis as Phytomodulator: Improved Healing Effects in Experimental Models of Diabetes Mellitus. Biomed. Pharmacother. 112, 108640. doi:10.1016/j.biopha.2019.108640
Pongprueksa, P., De Munck, J., Duca, R. C., Poels, K., Covaci, A., Hoet, P., et al. (2015). Monomer Elution in Relation to Degree of Conversion for Different Types of Composite. J. Dent. 43, 1448–1455. doi:10.1016/j.jdent.2015.10.013
Porto, I. C. C. d. M., Rocha, A. B. d. B., Ferreira, I. I. S., de Barros, B. M., Ávila, E. C., da Silva, M. C., et al. (2021). Polyphenols and Brazilian Red Propolis Incorporated into a Total-Etching Adhesive System Help in Maintaining Bonding Durability. Heliyon 7, e06237. doi:10.1016/j.heliyon.2021.e06237
Regueira, M. S., Tintino, S. R., da Silva, A. R. P., Costa, M. D. S., Boligon, A. A., Matias, E. F. F., et al. (2017). Seasonal Variation of Brazilian Red Propolis: Antibacterial Activity, Synergistic Effect and Phytochemical Screening. Food Chem. Toxicol. 107, 572–580. doi:10.1016/j.fct.2017.03.052
Ristivojević, P., Trifković, J., Andrić, F., and Milojković-Opsenica, D. (2015). Poplar-type Propolis: Chemical Composition, Botanical Origin and Biological Activity. Nat. Prod. Commun. 10, 1869–1876. doi:10.1177/1934578x1501001117
Rufatto, L. C., Luchtenberg, P., Garcia, C., Thomassigny, C., Bouttier, S., Henriques, J. A. P., et al. (2018). Brazilian Red Propolis: Chemical Composition and Antibacterial Activity Determined Using Bioguided fractionationBrazilian Red Propolis: Chemical Composition and Antibacterial Activity Determined Using Bioguided Fractionation. Microbiol. Res.Microbiol. Res. 214, 774–782. doi:10.1016/j.micres.2018.05.003
Rüttermann, S., Trellenkamp, T., Bergmann, N., Beikler, T., Ritter, H., and Janda, R. (2013). Bacterial Viability and Physical Properties of Antibacterially Modified Experimental Dental Resin Composites. PLoS One 8, e79119. doi:10.1371/journal.pone.0079119
Santiago, K. B., Piana, G. M., Conti, B. J., Cardoso, E. O., Murbach Teles Andrade, B. F., Zanutto, M. R., et al. (2018). Microbiological Control and Antibacterial Action of a Propolis-Containing Mouthwash and Control of Dental Plaque in Humans. Nat. Prod. Res. 32, 1441–1445. doi:10.1080/14786419.2017.1344664
Schweikl, H., Spagnuolo, G., and Schmalz, G. (2006). Genetic and Cellular Toxicology of Dental Resin Monomers. J. Dent. Res. 85, 870–877. doi:10.1177/154405910608501001
Sforcin, J. M., and Bankova, V. (2011). Propolis: Is There a Potential for the Development of New Drugs? J. Ethnopharmacol. 133, 253–260. doi:10.1016/j.jep.2010.10.032
Sies, H., Berndt, C., and Jones, D. P. (2017). Oxidative Stress. Annu. Rev. Biochem. 86, 715–748. doi:10.1146/annurev-biochem-061516-045037
Silva, L. N., Zimmer, K. R., Macedo, A. J., and Trentin, D. S. (2016). Plant Natural Products Targeting Bacterial Virulence Factors. Chem. Rev. 116, 9162–9236. doi:10.1021/acs.chemrev.6b00184
Tavassoli Hojati, S., Alaghemand, H., Hamze, F., Ahmadian Babaki, F., Rajab-Nia, R., Rezvani, M. B., et al. (2013). Antibacterial, Physical and Mechanical Properties of Flowable Resin Composites Containing Zinc Oxide Nanoparticles. Dent. Mater. 29, 495–505. doi:10.1016/j.dental.2013.03.011
Teixeira, E. W., Negri, G., Meira, R. M., Message, D., and Salatino, A. (2005). Plant Origin of green Propolis: Bee Behavior, Plant Anatomy and Chemistry. Evid. Based Complement. Alternat Med. 2, 85–92. doi:10.1093/ecam/neh055
Vouvoudi, E. C., Achilias, D. S., and Sideridou, I. D. (2015). Dental Light-Cured Nanocomposites Based on a Dimethacrylate Matrix: Thermal Degradation and Isoconversional Kinetic Analysis in N2 Atmosphere. Thermochim. Acta 599, 63–72. doi:10.1016/j.tca.2014.11.017
Wang, J., Dong, X., Yu, Q., Baker, S. N., Li, H., Larm, N. E., et al. (2017). Incorporation of Antibacterial Agent Derived Deep Eutectic Solvent into an Active Dental Composite. Dent. Mater. 33, 1445–1455. doi:10.1016/j.dental.2017.09.014
Wang, L. H., Zeng, X. A., Wang, M. S., Brennan, C. S., and Gong, D. (2018). Modification of Membrane Properties and Fatty Acids Biosynthesis-Related Genes in Escherichia coli and Staphylococcus aureus: Implications for the Antibacterial Mechanism of Naringenin. Biochim. Biophys. Acta Biomembr 1860, 481–490. doi:10.1016/j.bbamem.2017.11.007
Wiatrak, K., Morawiec, T., Rój, R., Mertas, A., Machorowska-Pieniążek, A., Kownacki, P., et al. (2017). Oral Health of Patients Treated with Acrylic Partial Dentures Using a Toothpaste Containing Bee Product. Evidence-Based Complement. Altern. Med. 2017, 1–12. doi:10.1155/2017/4034179
Woisky, R. G., and Salatino, A. (1998). Analysis of Propolis: Some Parameters and Procedures for Chemical Quality Control. J. Apicultural Res. 37, 99–105. doi:10.1080/00218839.1998.11100961
Xie, Y., Yang, W., Tang, F., Chen, X., and Ren, L. (2014). Antibacterial Activities of Flavonoids: Structure-Activity Relationship and Mechanism. Curr. Med. Chem. 22, 132–149. doi:10.2174/0929867321666140916113443
Yang, Y., Reichl, F. X., Ilie, N., Shi, J., Dhein, J., Hickel, R., et al. (2019). Antioxidants as a Novel Dental Resin-Composite Component: Effect on Elution and Degree of Conversion. Dent. Mater. 35, 650–661. doi:10.1016/j.dental.2019.02.003
Zhang, Y., Chen, Y. Y., Huang, L., Chai, Z. G., Shen, L. J., and Xiao, Y. H. (2017). The Antifungal Effects and Mechanical Properties of Silver Bromide/cationic Polymer Nano-Composite-Modified Poly-Methyl Methacrylate-Based Dental Resin. Sci. Rep. 7, 1547. doi:10.1038/s41598-017-01686-4
Keywords: propolis, antibacterial activity, Streptococcus mutans, dental materials, mechanical properties, fibroblasts, composite resin, direct contact test
Citation: Oliveira JMdS, Cavalcanti TFS, Leite IF, dos Santos DMRC, Porto ICCdM, de Aquino FLT, Sonsin AF, Lins RML, Vitti RP, de Freitas JD, Barreto EdO, de Souza ST, Kamiya RU, do Nascimento TG and Tonholo J (2021) Propolis in Oral Healthcare: Antibacterial Activity of a Composite Resin Enriched With Brazilian Red Propolis. Front. Pharmacol. 12:787633. doi: 10.3389/fphar.2021.787633
Received: 01 October 2021; Accepted: 03 November 2021;
Published: 29 November 2021.
Edited by:
Jose Mauricio Sforcin, São Paulo State University, BrazilReviewed by:
Luis A. Salazar, University of La Frontera, ChileCopyright © 2021 Oliveira, Cavalcanti, Leite, dos Santos, Porto, de Aquino, Sonsin, Lins, Vitti, de Freitas, Barreto, de Souza, Kamiya, do Nascimento and Tonholo. This is an open-access article distributed under the terms of the Creative Commons Attribution License (CC BY). The use, distribution or reproduction in other forums is permitted, provided the original author(s) and the copyright owner(s) are credited and that the original publication in this journal is cited, in accordance with accepted academic practice. No use, distribution or reproduction is permitted which does not comply with these terms.
*Correspondence: José Marcos dos Santos Oliveira, am9zZV9tYXJjb3NfY2JqckBob3RtYWlsLmNvbQ==, b2xpdmVpcmEuam1zOTFAZ21haWwuY29t
Disclaimer: All claims expressed in this article are solely those of the authors and do not necessarily represent those of their affiliated organizations, or those of the publisher, the editors and the reviewers. Any product that may be evaluated in this article or claim that may be made by its manufacturer is not guaranteed or endorsed by the publisher.
Research integrity at Frontiers
Learn more about the work of our research integrity team to safeguard the quality of each article we publish.