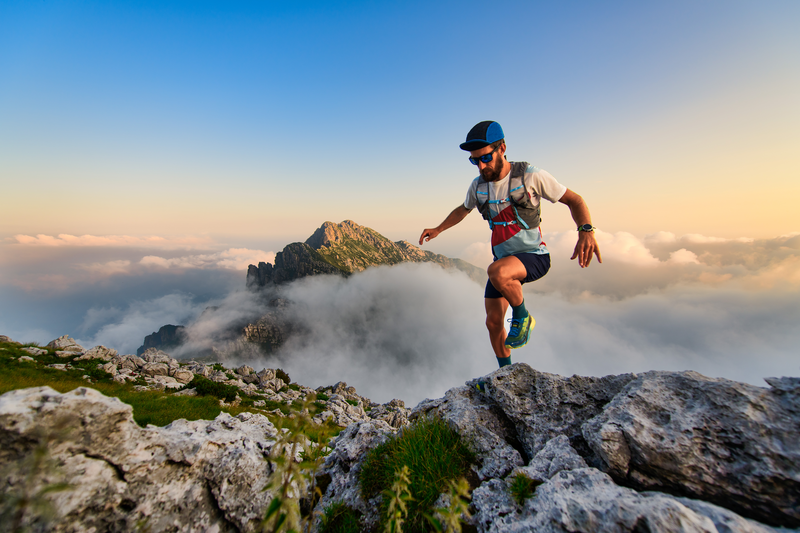
94% of researchers rate our articles as excellent or good
Learn more about the work of our research integrity team to safeguard the quality of each article we publish.
Find out more
REVIEW article
Front. Pharmacol. , 03 January 2022
Sec. Ethnopharmacology
Volume 12 - 2021 | https://doi.org/10.3389/fphar.2021.775084
This article is part of the Research Topic Medicinal Plants in the Treatment of Gastrointestinal Cancers: How can OMICS and other advanced approaches help in understanding their mechanisms of action? View all 8 articles
Parts of this article's content have been modified or rectified in:
Erratum: Current Advances in Coptidis Rhizoma for Gastrointestinal and Other Cancers
Cancer is a serious disease with an increasing number of reported cases and high mortality worldwide. Gastrointestinal cancer defines a group of cancers in the digestive system, e.g., liver cancer, colorectal cancer, and gastric cancer. Coptidis Rhizoma (C. Rhizoma; Huanglian, in Chinese) is a classical Chinese medicinal botanical drug for the treatment of gastrointestinal disorders and has been shown to have a wide variety of pharmacological activity, including antifungal, antivirus, anticancer, antidiabetic, hypoglycemic, and cardioprotective effects. Recent studies on C. Rhizoma present significant progress on its anticancer effects and the corresponding mechanisms as well as its clinical applications. Herein, keywords related to C. Rhizoma, cancer, gastrointestinal cancer, and omics were searched in PubMed and the Web of Science databases, and more than three hundred recent publications were reviewed and discussed. C. Rhizoma extract along with its main components, berberine, palmatine, coptisine, magnoflorine, jatrorrhizine, epiberberine, oxyepiberberine, oxyberberine, dihydroberberine, columbamine, limonin, and derivatives, are reviewed. We describe novel and classic anticancer mechanisms from various perspectives of pharmacology, pharmaceutical chemistry, and pharmaceutics. Researchers have transformed the chemical structures and drug delivery systems of these components to obtain better efficacy and bioavailability of C. Rhizoma. Furthermore, C. Rhizoma in combination with other drugs and their clinical application are also summarized. Taken together, C. Rhizoma has broad prospects as a potential adjuvant candidate against cancers, making it reasonable to conduct additional preclinical studies and clinical trials in gastrointestinal cancer in the future.
Coptidis Rhizoma (C. Rhizoma; Huanglian, in Chinese) is a common botanical drug which has a long history in Asia, especially in China. It is constituted by the dried root of three Coptis species, namely, Coptis chinensis Franch. (Weilian in Chinese), Coptis deltoidea C.Y. Cheng et Hsiao (Yalian), and Coptis teeta Wall. (Yunlian), in the Chinese Pharmacopoeia Edition 2020. Other native species of Coptis, such as Coptis trifolia Salisb and Coptis japonica Makino, are distributed in other regions of the world (Wang et al., 2014a). As recorded in Shennong’s Materia Medica, C. Rhizoma is used to treat high fever, vomiting, diarrhea, abdominal fullness, jaundice, toothache, and eczema, in the traditional dosage form, i.e., powder, pill, decoction, or tablet (Wang et al., 2019). In the past decade, various studies have been conducted to unravel its pharmacological activities and the possible underlying mechanisms of its action. Its wide pharmacological activities include antiviral, antibacterial, antifungal, antihepatic steatosis, antiatherosclerotic, antiarrhythmic, antihypertensive, cardioprotective, antidiabetic, anti-inflammatory, antioxidative, neuroprotective, and anticancer effects (Meng et al., 2018; Lyu et al., 2021).
Cancer is a leading cause of death around the world. Urgent strategies are required to overcome this disease given the population growth and ageing (Omran, 1971; Bray et al., 2018). Gastrointestinal cancer defines a group of cancers that affect the digestive system, including gastric cancer, colorectal cancer (CRC), liver cancer, esophageal cancer, pancreatic cancer, anal cancer, bile duct cancer, gastrointestinal stromal tumor, gallbladder cancer, and small intestine cancer. In particular, digestive system cancers are the first among estimated cancer-related deaths (27.82%, 169,280/608,570) and the second among estimated new cases (17.81%, 338,090/1,898,160) diagnosed at all cancer sites according to the Cancer Statistics 2021, United States (Siegel et al., 2021). As a main treatment for cancer, chemotherapy often indistinguishably kills healthy cells and exerts toxic effects on patients (Zaimy et al., 2017). In addition, cancer metastasis and the development of multidrug resistance (MDR) might occur. Despite the enormous amount of research performed to identify the complex causes of these obstacles and various treatment options, there is still a considerable amount research needed on natural products (Hausman, 2019), especially the conventional botanical drug C. Rhizoma for the treatment of gastrointestinal disorders. The antitumor effects of C. Rhizoma have been known for many years. The corresponding mechanisms involve the exacts as well as the main compounds, such as berberine, palmatine, coptisine, magnoflorine, jatrorrhizine, epiberberine, oxyepiberberine, oxyberberine, columbamine, and limonin. Moreover, the benefits of C. Rhizoma in antiviral and anti-inflammatory effects is closely associated with its anticancer effects, when administered as its traditional prescription (Tang et al., 2009). After all, inflammatory cells and functional polymorphisms of genes encoding inflammatory cytokines are associated with tumor growth and progression and determine whether or not an effective host antitumor response could be achieved in the susceptibility and severity state of cancer (Balkwill and Mantovani, 2001). Given the great potential of C. Rhizoma in cancer treatment, researchers have systematically discussed its druggability in 2015 (Wang et al., 2015). Recent studies have focused on the application of C. Rhizoma in gastrointestinal cancer.
Various typical constituents and abundant secondary metabolites were found in C. Rhizoma, including alkaloids, flavonoids, lignans, phenolic acids, phenylpropanoids, saccharides, and steroids (Meng et al., 2018). Recent studies have improved our understanding of the antitumor activities of C. Rhizoma. Alkaloids are the main components of C. Rhizoma, and the anticancer studies involving C. Rhizoma have mostly concerned on the berberine-type and oxyberberine-type components (Meng et al., 2018). According to their chemical structures, alkaloids can be divided into different subtypes: berberine-type alkaloids, oxyberberine-type alkaloids, methyl-berberine type alkaloid, benzylisoquinolines, benzophenanthridines, protoberberine-type alkaloids, phenethylamines, aporphine, isoquinolines, and other nitrogen-containing molecules (Chen et al., 2008; Li et al., 2012; Yang et al., 2014). Other constituents include lignans with numerous subclasses (Meng et al., 2018), abundant flavonoids (Chen et al., 2012), and phenylpropanoids with various molecular weight and structures. However, there are few published studies investigating the anticancer effects of the latter three compounds isolated from C. Rhizoma. Limonin, another compound isolated from C. Rhizoma, has been indicated to exert broad anticancer effects in a variety of human cancer cells (Fan S. et al., 2019). The chemical structure of the compounds together with their anticancer activities described in this review is shown in Figure 1.
FIGURE 1. Chemical structures of the constitutes derived from Coptidis Rhizoma. The chemical structures of the constitutes derived from Coptidis Rhizoma are shown, including Berberine, Coptisine, Columbamine, Palmatine, Jatrorrhizine, Magnoflorine, Oxyberberine, Epiberberine, Oxoepiberberine, Dihydroberberine, Berberrubine, Worenine, 8-phenyl-berberine, 8-n-butyl-berberine, 13-n-butyl-berberine, 13-n-hepta-berberine, 8-cetylcoptisine, Demethylenetetrahydroberberine, Rhamnetin, Wogonin, Pinoresinol, Secoisolariciresinol, Limonin, and Vanillic acid.
There are many studies describing the antitumor effects and the corresponding mechanisms of C. Rhizoma extract and those of berberine. Common mechanisms include induction of apoptosis, regulation of signal transduction, arresting of the tumor cell cycle, inhibition of tumor migration and invasion, and epigenetic regulation. In recent years, novel mechanisms have emerged, such as regulating autophagy, immunity, inflammation, gut microbiota, and microRNA (miRNA). The current research hotspot is the relationship of tumor, immunity, and inflammation.
Novel mechanisms of action of berberine, including autophagy, immunity, inflammation, modification of the gut microbiota and miRNA, have been the areas of research in recent studies of C. Rhizoma. These mechanisms are briefly elaborated below.
Autophagy is a multistep process, involving the cytoplasmatic autophagosome (Lee et al., 2019). The anticancer mechanisms related to autophagy of berberine are found in a variety of cancer types. mTOR is a major regulator of cell metabolism and are closely associated with cancers. It consists of mTORC1 and mTORC2 (Kim and Guan, 2015). Increased mTORC1 activity contributes to the survival of cancer cells (Martínez-Carreres et al., 2019) and overactivation of protein kinase B (AKT)/mTORC1 signaling leads to excessive proliferation of cancer cells and impairs autophagy-mediated cell death (Erazo et al., 2016). Berberine activates cytostatic autophagy by upregulating the expression of autophagy-related proteins, such as LC3-II, p-ULK1, especially Beclin-1 and suppressing the phosphorylation of AKT, extracellular signal-regulated kinase (ERK), c-Jun N-terminal kinase (JNK), p38, mTOR and p70S6K in gastric cancer (BGC-823) cells. In vivo model, Beclin-1 and LC3-II were increased in tumor tissue, the same inhibitions of phosphorylation were as the above (Zhang Q. et al., 2020). Acute lymphoblastic leukemia (ALL) is a common type of leukemia (Khwaja et al., 2016; Papaemmanuil et al., 2016). In ALL, berberine promotes autophagic cell death and significantly ameliorates the conditions of ALL by increasing LC3-II and Beclin-1 and inactivating the AKT/mTORC1 signaling pathway (Liu et al., 2020a). Murine double minute 2 (MDM2), a proto-oncogene, has been found to be upregulated in a variety of cancers and interacts with p53 (Oliner et al., 1992; Bueso-Ramos et al., 1993), thereby promoting degradation of p53 and inhibiting p53-mediated transactivation (Momand et al., 1992; Haupt et al., 1997). At the transcriptional and post-transcriptional levels, berberine was found to induce autophagy by downregulating MDM2 expression in p53-deficient leukemic cells and contributed to the apoptosis-promoting effect in p53-deficient leukemic cells (Liu et al., 2020c). Temozolomide is often used to treat glioblastoma (Delgado-López and Corrales-García, 2016; Nørøxe et al., 2016; Paolillo et al., 2018), and berberine reduces drug resistance of temozolomide by augmenting autophagy via downregulating the activation of the ERK1/2 signaling pathway. In vivo model, berberine increases glioblastoma sensitivity to temozolomide through the same signaling pathways (Qu et al., 2020). The emerging photodynamic therapy (PDT), which is based on photosensitizer-mediated oxidative cytotoxicity, is regarded as a promising approach in the treatment of cancers. Berberine-associated PDT exerts antiproliferative effects on human malignant melanoma cells, by inducing cleaved Caspase-3-mediated apoptosis, increasing LC3-related autophagy, activating endoplasmic reticulum stress, and promoting a dramatic increase in Reactive Oxygen Species (ROS) (Fang et al., 2021). However, autophagy also plays a negative role in cancer cells. Cancer cells can avoid apoptosis through regulation of autophagy, consequently causing drug resistance and enhancing tumor cell viability (Sui et al., 2014). Hepatocellular carcinoma (HCC) caused by the hepatitis C virus is a deadly disease that induces autophagy and is highly refractory to chemotherapy. Berberine can augment cell apoptosis and necrosis by inhibiting autophagy via targeting ROS and LC3-II in HCC (Huh-7) cells infected with hepatitis C virus RNA (Tai et al., 2020). In addition, berberine inhibits autophagy by downregulating the expression of phosphatase and tensin homolog (PTEN) to increase the phosphorylation of Akt and mTOR. In the case of breast cancer, berberine acts as an autophagy inhibitor to inhibit autophagosome formation in doxorubicin (DOX)-resistant breast cancer (MCF-7) cells and blocks accumulation of LC3-II, and increases expression of the p62 protein, finally reducing cell proliferation and reversing DOX resistance both in vitro and in vivo (Wang et al., 2020). Autophagy is an attractive mechanism that has been identified in recent years. Many unknown areas and details, including a description of the definitive effects and mechanisms of C. Rhizoma on cancer treatment, still need to be resolved.
Immune escape is a major feature of a variety of cancers (Dunn et al., 2004). Berberine and some components of C. Rhizoma have immunomodulatory effects (Li H. et al., 2014). The levels of granulocytic myeloid-derived suppressor cell (G-MDSC), a subset of suppressive myeloid cells, consistently increase in tumor-bearing mice and are closely associated with tumor-induced granulocytic hyperplasia, a process related to tumor vasculogenesis and immune escape (Talmadge and Gabrilovich, 2013). Berberine significantly suppresses acute/chronic hepatic damage in mice by regulating the G-MDSC-like population mediated in part, by the interleukin (IL)-6/STAT3 signaling pathway. This is also involved in the regulation of the gut microbial community represented by the increased Akkermanisa muciniphila (Li S. et al., 2020). Berberine exerts antitumor activity in diffuse large B-cell lymphoma, a subtype of non-Hodgkin lymphoma by modulating the c-myc/CD47 axis. CD47 is a target for improving treatment efficacy, and the overexpression of CD47 is related to immune escape (Casey et al., 2016; Takimoto et al., 2019; Eladl et al., 2020). Berberine, a suppressor of CD47, downregulates CD47 mRNA by suppressing c-Myc expression (Ren et al., 2021). The suppression of CD47 enhances the phagocytosis of macrophages, which contributes to eliminate diffuse large B-cell lymphoma cells both in vitro and in vivo. In addition, berberine can improve the efficiency of the anti-CD47 antibody and rituximab on cell phagocytosis (Chao et al., 2010; Weiskopf et al., 2016; Schürch et al., 2019). Berberine is beneficial in immunochemotherapy based on rituximab in combination with anti-CD47 in diffuse large B-cell lymphoma (Harris et al., 2020; Ren et al., 2021).
Blocking the programmed cell death-1 (PD-1)/programmed cell death ligand-1 (PD-L1) has become the main strategy in cancer immunotherapy (Iwai et al., 2017). In non-small cell lung cancer (NSCLC), berberine reduces the expression of PD-L1 and promotes antitumor immunity by inhibiting the activity of COP9 signalosome 5, along with activation of tumor-infiltrating T cells (Liu Y. et al., 2020). Furthermore, berberine shows a synergistic effect against breast cancer both in vitro and in vivo. This combination activates the immune system, regulates levels of intestinal microbial metabolites, activates the mitochondrial apoptotic signaling pathway, and the Fas death receptor pathway (Ma et al., 2020). Berberine also functions as a potent antioxidant and immunomodulatory agent, having a protective function on the liver. Berberine reverses the elevation of serum and tissue stress induced by DOX, as well as in tissue inflammatory mediators and serum cytokine levels. Some cytochrome P450s (CYP), including 2B1, 2B2 and 2E1, also show significantly reduced expression upon berberine treatment (Sun B. et al., 2020). Additionally, the immune modulation of berberine on gastrointestinal cancer is evident in inflammatory bowel disease (IBD) and in the associated CRC, which is described in the following paragraph.
Chronic inflammation is known to promote carcinogenesis. It was estimated approximately 15–20% of all cancer-related deaths are related to inflammatory processes and the underlying infections (Eiró and Vizoso, 2012). C. Rhizoma and its active component berberine suppress inflammatory processes to ameliorate development of IBD associated with CRC. The anti-inflammatory effects of berberine include the regulation of cell cycle, induction of apoptosis, and prevention of leukocyte migration by inhibiting classic inflammatory pathways and the expression of various chemokines (Xu et al., 2020). To attenuate inflammation in the early phase, berberine interacts with TLR4 and interferes with the TLR4/MyD88/NFκB signaling pathway (Gong et al., 2019a). Researchers identified NIMA-related kinase 7 (NEK7) as a new target of inflammatory diseases related to the nucleotide binding domain (NOD)-like receptor protein 3 (NLRP3) (He et al., 2016; Xu et al., 2016). Berberine acts on NEK7 protein through the hydrogen bond to affect the NEK7−NLRP3 interaction and prevents IL-1β release to exert anti-inflammatory effect both in vitro and in vivo (Zeng et al., 2021). At the same time, berberine is able to directly inhibit the functions of pro-inflammatory Th1 and Th17 cells and their differentiation, which together indirectly reducing Th cell-mediated inflammation by regulating other cells, such as Tregs, that contribute to autoreactive inflammation (Ehteshamfar et al., 2020).
IBD is a recurrent gastrointestinal inflammatory disease that includes two different conditions: ulcerative colitis (UC) and Crohn’s disease (CD) (Gunnarsson et al., 2012). They are associated with a higher risk of colitis-associated CRC with poor prognoses (Watanabe et al., 2011; Zhen et al., 2018). CRC ranks the top three in cancers around the world (Mármol et al., 2017). The highly conserved RNase III enzyme Dicer, an essential component of the RNA interference pathway (Foulkes et al., 2014), is decreased and promotes carcinogenesis (Kumar et al., 2007). The decrease in Dicer expression could increase the levels of cytosolic DNA and IL-6 mRNA under oxidative stress in inflammatory bowel tissues, while berberine alleviates colitis by recovering Dicer expression to exert a preventive effect on colitis-associated tumorigenesis (Wu et al., 2020). In the dextran sulfate sodium (DSS)-induced colitis model, berberine exerts its therapeutic effects by activating the mTORC1 signaling pathway to enhance the number of Treg cells and regulate the levels of intestinal microbiota-associated tryptophan metabolites and the activation of the aryl hydrocarbon receptor (Li Q. et al., 2019; Jing et al., 2021). Similarly, berberine downregulates the levels of COX-2 mRNA, protein, as well as the concentration of prostaglandin E2 in the CRC (HT-29) cells (Tai and Luo, 2003). It attenuates the expression of COX-2 and PGE2 to decrease JAK2 and STAT3 phosphorylation as well as matrix metalloproteinase (MMP)-2 and MMP-9 expression (Liu X. et al., 2015). Berberine also exerts antiproliferative activity by participating in inflammatory response-driven epidermal growth factor receptor (EGFR) signaling pathway, thereby preventing the progression of colitis-associated CRC (Li D. et al., 2017). Cytosolic phospholipase A2a (PLA2G4A), an enzyme that hydrolyzes phosphatidylcholine to lysophosphatidylcholine, decreases in a colitis mouse model and in the inflammatory response of RAW 264.7 macrophages. Berberine binds to PLA2G4A directly and inhibits PLA2G4A activity by suppressing the MAPK/JNK signaling pathway to ameliorate colon inflammation (Zhai et al., 2020).
Berberine could also improve inflammatory status by downregulating cytokines IL-1β and tumor necrosis factor (TNF)-α and upregulating IL-4 in the serum and colon tissue of UC rats through related signaling pathways (Jiang et al., 2021). Berberine is capable of preventing the proinflammatory cytokines producing in colitis (Zhou and Mineshita, 2000; Kawashima et al., 2004; Lee et al., 2010; Zhang et al., 2011; Yan et al., 2012). The relevant mechanism might be the inhibition on proinflammatory cytokines production through the activation of MAPK and NF-κB in lipopolysaccharides (LPS) -induced macrophages (Lee et al., 2010). In addition, berberine exerts a protective effect on UC by regulating the interaction between enteric glial cells (EGCs) and intestinal epithelial cells-immune cells by exerting modulatory effects on the apoptosis of EGCs and the expression of substance p and glial-derived neurotrophic factor (Li et al., 2020b). Furthermore, berberine can also suppress mucosal inflammation by decreasing oncostatin M (OSM) to treat chronic UC. The effects include attenuating intestinal inflammation, modulating pathological changes and interaction between intestinal stromal cells and immune cells. It is closely associated with the inhibition of phosphorylated OSM-mediated signal pathway, such as JAK-STAT, AKT, and MAPK (Li et al., 2020c). Furthermore, inhibition of cytokines expression, like TNF-α、transforming growth factor-β (TGF-β), IL-1, IL-1β, IL-6, IL-12, and interferon (IFN)-γ, decrease the expression of IL-4 and IL-10 at mRNA levels and the inhibition of phosphorylation of STAT3 and NF-κB p65 are implicated in the anti-inflammatory activity of berberine (Zhu L. et al., 2019). Berberine combined with carboxylmethyl chitosan by arylboronic ester can significantly improve the symptoms of colitis and colon damage by regulating IL-6 expression and remodeling the intestinal microbiota (Zhao L. et al., 2021).
The occurrence of breast cancer is closely related to cytokines that alter the microenvironment of inflammatory tumors. Berberine can reduce the migratory ability of breast cancer (MDA-MB-231) cells in an in vitro scratch model, thereby prolonging the wound healing time. In addition, berberine inhibits the phosphorylation of c-Jun and c-Fos. LPS treatment can increase the expression of cytokines, and berberine effectively reduces the expression of IL-6 and TNF-α. Berberine also suppresses the activation of NF-κB by preventing IκBα from degradation (Zhao and Zhang, 2020). In addition to inflammation, the gut microbiota is another aspect of berberine regulation on gastrointestinal cancer, which has been addressed in extensive studies in recent years.
The study of gut microbiota has suggested the inhibitory effects of berberine on CRC. A disruption of the balance of the intestinal flora is characterized by decreased community diversity and decreased abundance of beneficial bacteria, and is a cause of IBD. The mycobiota dysbiosis signature plays an important role in the pathogenesis of CRC (Gao et al., 2017). It is known that one of the mechanisms of berberine treatment of intestinal inflammation is associated with its antibacterial activity (Habtemariam, 2016). A study showed that berberine altered the metabolic and composition of the gut microbiota in azoxymethane/DSS treated mice (Ren et al.). Actinobacteria and Verrucomicrobia at the phylum level, and some pathogenic species, including f_Erysipelotrichaceae and Alistipes at the genus level, are obviously decreased. Meanwhile, some short-chain fatty acid producing bacteria, such as Alloprevotella, Flavonifractor, and Oscillibacter, are increased (Chen YX. et al., 2020). In the case of gut inflammation-associated disease, berberine can decrease harmful bacteria, such as Escherichia coli and enterococci bacteria, and increase Lactobacilli and Bifidobacteria (Habtemariam, 2020). For example, berberine elevates lactic acid-producing bacteria and carbohydrate hydrolysis bacteria, and reduces conditional pathogenic bacteria to treat colonic damage in DSS-induced UC mice (Liao et al., 2020). Furthermore, berberine alleviates DSS-induced colitis by activating AhR, which adjusts the tryptophan metabolite levels associated with the gut microbiota (Jing et al., 2021). Meanwhile, berberine mediates the number of Treg and Th17, two inflammation-related cells, by regulating the intestinal flora in the colon, which works as a therapy for UC (Cui et al., 2018). Another anticancer mechanism of berberine is to promote butyrate production in the gut microbiota. Oral berberine increases the abundance of butyrate-producing bacteria, indirectly changing the composition of intestinal bacterial in mice. Berberine indirectly increases butyrate by inhibiting NADH and bacterial ATP production, hence the levels of phosphotransbutyrylase/butyrate kinase and butyryl-CoA (acetate-CoA transferase) increase (Wang et al., 2017). Furthermore, berberine could alter fecal metabolites to modify the gut microbiota in intestinal mucositis induced by 5-fluorouracil. In addition, berberine significantly increases butyrate and glutamine levels in feces. Meanwhile, berberine decreases Proteobacteria and enriches the abundance of Firmicutes at the phylum level, along with increasing the proportion of unclassified_f_Lachnospiraceae, unclassified_f_Porphyromonadaceae, Lactobacillus, and unclassified_o_Clostridiales at the genus level (Chen et al., 2020a). Given that botanical drugs are characterized by multiple components with multiple effects from a holistic point of view, modulation of the gut microbiota foresees a much larger role for the effects of C. Rhizoma on carcinogenesis.
miRNAs are small non-coding RNA molecules of around 20 nucleotides in length (Ambros, 2004; Bartel, 2004; Gu and Zhou, 2021). miRNAs are capable of suppressing target gene expression via binding to its target mRNA, causing mRNA cleavage or translation inhibition (Wightman et al., 1993; O’carroll and Schaefer, 2013). miRNAs act as essential regulatory elements in many aspects of biological processes and various human diseases, and most miRNAs are dysregulated in cancers (Filipowicz et al., 2008). Dysregulation of miRNAs is usually caused by defects in the miRNA biogenesis pathway (Gurtner et al., 2016). However, some miRNA are reported to directly participate in the formation of cancers and might act as tumor suppressors (e.g. let-7, miR-15a and miR-16–1) or as oncogenes (e.g. the miR-17∼92 cluster, miR-155, and miR-21) (Calin and Croce, 2006; Zhang et al., 2007; Pereira et al., 2013). Berberine was shown to modulate miRNA to affect cell cytotoxicity, apoptosis, and invasion in a variety of multiple cancers (Filipowicz et al., 2008). In multiple myeloma, berberine increases Set9, which damages and suppresses NF-κB, resulting in the decline of miR-21 and Bcl-2 levels, and stimulating ROS generation and apoptosis (Hu et al., 2013). Berberine decreases IL-6 and STAT3 to suppress miR-21 levels, resulting in the upregulation of programmed cell death 4 (PDCD4) (Luo et al., 2014; Jiang et al., 2015; Ayati et al., 2017). The miR-106b/25 cluster participates in numerous cancer-associated signaling pathways and tumorigenesis as an oncogene. Berberine downregulates miR-106b/25 in multiple myeloma cells (Gu et al., 2017).
In HCC, berberine upregulates miR-21-3p to modulate the expression of methionine adenosyltransferase 2A and methionine adenosyltransferase 2B, leading to cell apoptosis and viability and reduction of proliferation (Lo et al., 2013). miR-22-3p is decreased in HCC and berberine increases its expression to suppress cell proliferation by targeting Sp1 (Chen J. et al., 2016). Berberine also induces miR-23a expression and suppresses NEK6, which is a negative regulator of p53 in human cancers (Wang et al., 2014b). The combination of berberine and heat shock protein 90 (Hsp90) inhibitors has synergistic antiproliferative effects on cell growth arrest by suppressing the overexpression of CDK4 as that of miRNA-296-5p, which leads to the activation of the Pin1-β-catenin-cyclin D1 signaling pathway in CRC (Su et al., 2015). Berberine also inhibits miR-21 expression by mediating the miR-21-integrin β4-PDCD4 pathway in CRC (HCT 116) cells (Lü et al., 2018). As for gastric cancer, berberine suppresses cell invasion by upregulating miR-203 which targets Bcl-w, resulting in sensitizing cisplatin-resistant cells to initiate a caspase-dependent apoptosis (You et al., 2016). Some studies have also reported that miRNA target genes are associated with the regulation of the cell cycle, the Ras signaling pathway, and the JAK-STAT signaling pathway in gastric cancer (SGC-7901) cells (Yang et al., 2018). miR-212 is a cancer-related miRNA with dual functions, acting as a tumor suppressor or an oncogene. Overexpression of miR-212 is associated with poor outcomes in patients with esophageal squamous cell carcinoma (ESCC). By activating epithelial-mesenchymal transition and degrading the extracellular matrix (ECM), miR-212 promotes multiple signaling cascades, cell motility and invasion in ESCC. Berberine may downregulate miR-212 to eventually inhibit cell migration (Chen et al., 2019). In ovarian cancer, miR-21 enhances tumor resistance to chemotherapy. Berberine increases cell sensitivity to cisplatin through the miR-21/PDCD4 axis, by decreasing miR-21 expression and function by enhancing the levels of its target PDCD4, an important tumor suppressor of ovarian cancer (Liu S. et al., 2013). Studies on miRNAs have attracted great attention and contributed to the understanding of the relationship between miRNA and the active components derived from C. Rhizoma.
The C. Rhizoma extract plays its antitumor role in various cancers through induction of apoptosis. In two different human squamous cell carcinoma (SCC-25, KB) cells:, C. Rhizoma extract influenced cell differentiation and apoptosis by targeting STAT3, p53, and BRCA1 (Wang et al., 2011). The Bcl-2 family members induce apoptosis (Korsmeyer, 1999). There are two functionally distinct groups of the Bcl-2 family members: anti-apoptotic and pro-apoptotic factors. Bcl-2, an anti-apoptotic protein, mediates the apoptotic pathways and protects cell viability, while Bax, a pro-apoptotic protein, is abundantly and selectively expressed during cell apoptosis, promoting cell death (Oltvai et al., 1993). The pro-apoptotic effects induced by the extracts of C. Rhizoma in HCC (HepG2) cells are expressed by downregulating Bcl-2, activating procaspase-3 and procaspase-9, as well as cleaving poly (ADP-ribose) polymerase (PARP) (Auyeung and Ko, 2009). The activity of caspases, particularly Caspase-3, led to a series of morphological and biochemical changes in the apoptosis execution phase (Thornberry and Lazebnik, 1998). As for CRC, the methanol extract of C. Rhizoma exerts its anticancer role by activating intracellular death-related pathways, resulting in Caspase-3 activation in human CRC (SNU-C4) cells (Kim et al., 2004). The water extract C. Rhizoma influences the viability of melanoma (A2058, UACC257, UACC62, SK-Mel-2, MeWo, M14, Malme-3M) cells, and mouse fibroblast cells. These effects might be partly attributed to apoptosis induction, which may involve the suppression of anti-apoptotic proteins, including BCL2A1, Mcl-1 and Bcl-w, and the activation on multidomain pro-apoptotic proteins, such as Bax and Bak (Xu et al., 2017). C. Rhizoma extract induces glioma cell apoptosis. Under treatment with C. Rhizoma extract, the expression of proteins associated with apoptosis was altered and involved the reduction of total Caspase-3 and induced cleavage Caspase-3 (Li J. et al., 2017). The water extracts of C. Rhizoma exerted apoptotic effects in immortalized human oral keratinocytes through the mitochondrial signaling pathway, represented by mitochondrial cytochrome-C release and Caspase-3 activation (Lee et al., 2006). The root extract of Coptis japonica var. dissecta (another species of C. Rhizoma), functions as an apoptosis inducer by activating Bax-dependent Caspase-3 in human gastric cancer (SNU-668) cells (Park et al., 2005).
Berberine was found to induce apoptosis in many cancer cell lines, including HCC (HepG2) cells (Hyun et al., 2010) and other liver cancer cells (Wang et al., 2010b; Yip and Ho, 2013), human CRC cancer (SW620) cells (Hsu et al., 2007), human gastric cancer (BGC-823) cells (Yi et al., 2015), human cholangiocarcinoma (QBC939) cells (He W. et al., 2012), human pancreatic cancer (MIA-PaCa2, PANC-1) cells (Park et al., 2015), human glioblastoma (87 MG) cells (Palma et al., 2020), and human osteosarcoma (MG-63) cells (Zhu Y. et al., 2014). Apoptosis was mediated by the typical mitochondria-dependent apoptotic signaling pathway, although the activation of the JNK/p38 pathway (Hsu et al., 2007), caspase-independent cell death (Wang et al., 2012), nonsteroidal anti-inflammatory drug (NSAID)-activated gene-1 (NAG-1) activation (Auyeung and Ko, 2009), AKT/mTOR/p70S6/S6 pathway suppression (Yi et al., 2015), ROS generation and cytochrome-C release were also involved in berberine-induced apoptosis (Tillhon et al., 2012). Furthermore, berberine could modulate the MMP-2 and the Bcl-2/Bax signaling pathway to induce cell apoptosis in NSCLC (Li et al., 2018). Meanwhile, berberine induced cell apoptosis in HCC by decreasing phosphorylated AKT and phosphatidylinositol 3-kinase (PI3K) levels in HCC (MHCC97-H and HepG2) cells (Song et al., 2019).
The STAT protein family is an important transcription factor. STATs regulate the active sites of many proteins through phosphorylation and acetylation (Yang and Seto, 2007). The body adapts to the environment and maintains homeostasis through the precise control of the STAT signaling pathway (Yang and Seto, 2007). STAT3 has proven to be an ideal drug target, as sustained activation of STAT3 and strong dependence on STAT3 activity has been identified in approximately 70% of blood and solid tumors, and is involved in tumor proliferation, survival, self-renewal, invasion, and angiogenesis (Calò et al., 2003; Frank, 2007). Aberrant expression or mutation of genes encoding histone acetyltransferase or histone deacetylase (HDAC) enzymes is associated with tumorigenesis (Mai et al., 2005; Leng et al., 2006; Yang and Seto, 2007; Nguyen et al., 2010). C. Rhizoma extract acts as an HDAC inhibitor and can inhibit STAT3 phosphorylation by lowering the expression of histone acetyltransferase 3, thus affecting the function and biological characteristics of glioma cells (Li J. et al., 2017). MAPK and PI3K signaling pathways are also involved. C. Rhizoma extract regulates MAPK signaling by targeting Raf-1, ERK1/2, p38 and ERK, along with the PI3K signaling pathway by targeting AKT and PTEN in squamous cell carcinoma (KB and SCC-25)cells (Wang et al., 2011).
Adenosine monophosphate-activated protein kinase (AMPK) and PI3K/AKT signaling exert antagonistic activity in cancer development (Tan et al., 2019). Berberine exerts anticancer activity by modulating the AMPK and PI3K/AKT signaling pathways (Huang et al., 2021). For example, berberine exerts anticancer activity on CRC both in vivo and in vitro by inhibiting PI3K/AKT signaling and by downregulating insulin-like growth factor 2 (IGF2) mRNA-binding protein 3 (IGF2BP3) (Zhang Y. et al., 2020), a member of the oncofetal RNA-binding protein family, and is highly expressed in many different types of cancers (Mancarella et al., 2018). IGF2BP3 activates IGF2 translation, which leads to activate the direct downstream effector PI3K/AKT pathway (Suvasini et al., 2011; Lederer et al., 2014; Zhang Y. et al., 2020). Overexpression of COX-2 correlates with CRC tumorigenesis and leads to cell proliferation and apoptosis inhibition. Furthermore, COX-2 enhances tumor angiogenesis, cell attachment as well as migration and invasion (Wang and Dubois, 2010). In CRC, the JAK2/STAT3 signaling pathway is persistently activated and therefore, resulting in upregulation of the expression of downstream genes, e.g. MMP-2 and MMP-9 (Yang J. et al., 2013; Slattery et al., 2013). Berberine also suppresses CRC cells invasion and metastasis via mediating the expression of COX-2 and PGE2 (Prostaglandin E2, the main product of COX-2 (Wang and Dubois, 2006) to decrease p-JAK2/STAT3 signaling and downstream genes MMP-2 and MMP-9 expression (Liu X. et al., 2015). In addition, berberine has a promising antitumor effect in HCC by inhibiting phosphorylation of AKT and PI3K to suppress cell growth, migration, invasion and induce cell apoptosis in HCC (MHCC97-H and HepG2) cells (Song et al., 2019).
The erythropoietin-producing hepatoma (Eph) receptors family is the largest type of receptor tyrosine kinases (RTKs) (Salgia et al., 2018). Two groups exist: Eph A and B. Eph tyrosine kinase receptors and the ephrin ligands are expressed in many types of tumors (Genander and Frisén, 2010). For example, EphB4 is overexpressed in many cancers such as colon, lung, ovarian, prostate, breast, melanoma, endometrial, and pancreatic cancers which is associated with tumor development. (Huang and Li, 2015; Stephenson et al., 2015) (Mertens-Walker et al., 2015; Lv et al., 2016; Merchant et al., 2017). Stimulating Ephrin induces Eph receptor dimerization and autophosphorylation, resulting in activating downstream signaling molecules including PI3K and MAPK (Germain and Eichmann, 2010). The downstream signaling pathways of PI3K and MAPK contribute to cell growth, survival, and migration of different types of cancers (Hu et al., 2014). Therefore, EphB4 is a potential therapeutic target for malignancies (Salgia et al., 2018). Some reports suggested that berberine drives EphB4 inhibitory activity on cancer cell growth (Zhu M. et al., 2019).
The C. Rhizoma extract inhibits cell proliferation and causes G2/M phase arrest in HCC (HepG2) cells to arrest the cell cycle (Auyeung and Ko, 2009). In addition to glioma (BV2, H4, LN299, U251, U87) cells C. Rhizoma induces G2/M arrest and markedly suppresses cell proliferation, tumor formation, and migration, and prolongs the survival time of glioma cell-bearing mice in vivo (Li J. et al., 2017). Cell cycle progression is regulated by cyclins and cyclin-dependent kinases (CDKs) complexes (Vidal and Koff, 2000). Coptis japonica Makino (another species of C. Rhizoma) extract was also reported to block vascular endothelial growth factor (VEGF)-induced G0/G1 phase transition by downregulating the expression of cell cycle-regulated proteins, including cyclin D, cyclin E, CDK2, and CDK4 (Kim SH. et al., 2016). C. Rhizoma extract regulates the cell cycle in squamous cell carcinoma (KB, SCC-25) cells mediated by CDK4, CDK6, cyclin B1, cyclin E, cyclin D1, and p27 (Wang et al., 2011). Berberine induces G0/G1 phase arrest in human cholangiocarcinoma (QBC939) cells (He W. et al., 2012), and G0/G1 phase arrest in CRC and rectal cancer cells (Zhang Y. et al., 2020). Moreover, berberine induces G2/M arrest in leukemia (HL-60, WEHI-3) cells by inhibiting cyclin B1 and promoting Wee1 expression (Lin et al., 2006).
Angiogenesis is a significant part in tumor growth and metastasis (Folkman, 1990; Folkman and Shing, 1992), and endothelial migration is an important step in the process of angiogenesis. Cell invasion is another important characteristic of cancer cells. It is initiated by ECM breakdown by MMPs (Nabeshima et al., 2002). C. Rhizoma extract significantly suppresses proliferation, migration, and invasion of human umbilical vein endothelial cells (HUVECs) stimulated by VEGF in vitro and inhibits VEGF-induced tube formation in vitro and micro-vessel sprouting ex vivo (Kim SH. et al., 2016). Furthermore, The Coptis japonica Makino extract inhibits the expression of MMP-2 and MMP-9.
F-actin, a type of stress fiber, regulates cell motility and polarization. Its reduction inhibits the migration of cancer cells (Chen et al., 2007; Havaki et al., 2007; Smerling et al., 2007; Shao et al., 2018). The Rho/ROCK signaling pathway plays a pivotal role in cancer metastasis (Itoh et al., 1999; Takamura et al., 2001; Leung et al., 2005; Wong et al., 2008). C. Rhizoma aqueous extract suppresses the migration and invasion of a highly metastatic HCC (MHCC97-L) cells. It reduces F-actin polymerization and damages to cytoskeleton network (Wang et al., 2010a). Furthermore, C. Rhizoma extract affects cell adhesion mediated by E-cadherin and osteopontin in human squamous cell carcinoma cell lines (KB and SCC-25) (Wang et al., 2011). Metadherin (MTDH) functions as an oncogene that facilitates tumor cell invasion and migration, resulting in poor prognosis (Liu Y. et al., 2015). MTDH is highly expressed in many types of cancers, and involved in tumorigenesis and tumor progression in multiple aspects (Liang et al., 2011). In breast cancer, the overexpression of MTDH is closely associated with carcinogenesis, development, metastasis, and chemoresistance (Su et al., 2010). Berberine exerts anticancer activity partially by regulating MTDH expression in breast cancer (Sun et al., 2019b).
miRNAs suppress their target genes and inhibit protein translation, or cause mRNA cleavage (Lu et al., 2005; Volinia et al., 2006; Fabbri et al., 2008; Krol et al., 2010). miR-145, a tumor suppressor gene (Du and Pertsemlidis, 2010; Huang et al., 2012; Dip et al., 2013), is generally downregulated in various types of cancers (Xu et al., 2012; Yang D. et al., 2013). MMP-16 is a target gene of miR-145. Berberine inhibits migration and invasion by promoting miR-145 expression and decreasing MMP-16 expression in the human ovarian cancer (SK-OV-3, 3AO) cells (Li P. et al., 2021). Furthermore, berberine inhibits migration in breast cancer (ZR-75–30) cells by targeting ephrin-B2 (Ma et al., 2017). Ephrin-B2 is a cell-surface protein that contributes to cancer cell survival, invasion, and migration (Lisle et al., 2015; Chen II. et al., 2016; Yang et al., 2016). Some studies have shown that berberine is sensitive to various growth factors (Jabbarzadeh Kaboli et al., 2014). Ephrin-B2 mediates VEGFR2 internalization to inhibit activating the downstream signaling (Sawamiphak et al., 2010; Sentürk et al., 2011). Berberine exerts inhibitory activity in cells with high expression of Ephrin-B2 by downregulating the expression of Ephrin-B2, Syntenin 1, PICK1, MMP-2 and MMP-9, as well as inhibiting the phosphorylation of VEGFR2 and AKT (Ma et al., 2017). Berberine functions through attenuating the expression of COX-2 and PGE2 to decrease JAK2 and STAT3 phosphorylation as well as downstream genes MMP-2 and MMP-9 expression of CRC cells both in vitro and in vivo (Liu X. et al., 2015). In human A549 lung carcinoma cells, berberine hydrochloride inhibits cell proliferation and promotes cell apoptosis via regulating the MMP-2 and the Bcl-2/Bax signaling pathways (Li et al., 2018).
Various carcinogenesis-related genetic and epigenetic events have been discovered. An epigenetic modification is a transient, reversible, and heritable change in gene expression with no modification in the DNA sequence and is associated with gene silencing of tumor inhibitors and oncogene activation. Aberrant expression of miRNA is associated with the growth and development of cancers (Calin and Croce, 2006; Pereira et al., 2013). C. Rhizoma extract alters miRNA expression profiles and, consequently, hinders cancer development, induces apoptosis, and improves drug sensitivity (Mohammadi et al., 2017). For example, in human liver cancer (MHCC97-L) cells, the aqueous extract of C. Rhizoma upregulates miR-21 and miR-23a to exert its anticancer role (Zhu et al., 2011).
DNA methylation most commonly occurs at the cytosine moiety of the CpG dinucleotide and histone, thus affecting the interaction with DNA and chromatin modifying protein. Studies have focused on the role of hypermethylation of tumor suppressor genes and global hypomethylation of oncogenes (Puneet et al., 2018). There are two forms of human CpG: one is dispersed in genomic DNA and the other is highly clustered to form CpG islands. When a tumor occurs, the degree of CpG unmethylation increases outside CpG islands, while those located within CpG islands are highly methylated, causing the overall decrease of methylation level of the genome and CpG islands (Crawford et al., 2018). The DNMT family related to DNA methylation includes DNMT1, DNMT3A, and DNMT3B (Jackson et al., 2004; Smith and Meissner, 2013). In multiple myeloma (U266) cells, berberine inhibits the expression of DNMT1 and DNMT3B to alter the CpG methylation of p53, which affects the mRNA levels of apoptosis-related proteins and thus, induces cell apoptosis and cell cycle arrest (Qing et al., 2014; Liu et al., 2019). In CRC, berberine increases the expression of DNMT1, DNMT3A, DNMT3B and miR-152, miR-429, miR-29a (Huang C. et al., 2017). Additionally, histones are associated with tumorigenesis and development. Histones protect DNA structure and genetic information and regulate gene expression. Berberine downregulates histone deacetylases in lung cancer (A549) cells, resulting in a decrease in the expression of mRNA and protein of the MMP-2 and MMP-9, inhibiting cell migration and invasion (Kalaiarasi et al., 2016).
In recent years, the pharmacological effects of other alkaloids derived from C. Rhizoma have been explored gradually, including coptisine, columbamine, palmatine, jatrorrhizine, magnoflorine, oxyberberine, epiberberine, oxyepiberberine, dihydroberberine, berberrubine, and worenine.
Coptisine exerts strong antiproliferative activities in pancreatic cancer (MiaPaCa-2, Panc-1) cells (Hara et al., 2005). It also exerts active anticancer effect on hepatoma (HepG2, Hep3B, SK-Hep1, and PLC/PRF/5), leukemia (K562, U937, P3H1, and Raji) and osteosarcoma (MG63) cells (Lin et al., 2004; Yu et al., 2014). An in vivo study using HCT-116 xenograft mouse mode shows its anticancer effects in breast cancer therapy (Luo et al., 2014; Huang T. et al., 2017). Briefly, the corresponding mechanisms of action involve cell cycles arrest, apoptosis induction, and metastasis inhibition. Coptisine effectively induces G0/G1 phase arrest in CRC (HCT-116 and FHC) cells and pancreatic carcinoma (PANC-1) cells (Huang T. et al., 2017; Zhang Y. et al., 2020). It also causes G0/G1 phase arrest by downregulating the expression of CDK4 and cyclin D1 in a xenografted mouse model (Yu et al., 2014). Furthermore, coptisine can also induce cell apoptosis. In HCT-116 cells, coptisine induces G1 phase arrest and caspase-dependent/independent apoptosis through suppressing the levels of PI3K and AKT, mediating Bcl-2 family to the mitochondria-associated apoptotic pathways (Huang T. et al., 2017; Han et al., 2018). Finally, coptisine plays an important role in inhibiting tumor migration and invasion. In osteosarcoma, it decreases the mRNA levels of some tumor angiogenic genes, such as VE-cadherin and integrin β3 and suppresses STAT3 phosphorylation so as to potently impede cell migration and invasion (Yu et al., 2014). In human breast cancer cells with high metastasis potential, coptisine exerts antimetastatic function by downregulation of MMP-9 in combination with increases in tissue inhibitor of metalloproteinase 1 (TIMP-1) (Luo et al., 2014). Furthermore, coptisine also triggers autophagy in Hep3B cells via downregulating the phosphorylation of mTOR and ULK-1 as well as its upstream pathway PI3K/AKT and upregulation of ROS-mediated mitochondrial dysfunction (Kim et al., 2021).
Columbamine exerts antiproliferative, anti-metastasis, anti-vasculogenic, and cytotoxic effects in various cancer types, including human CRC, HCC, and metastatic osteosarcoma (Zhang et al., 2014; Lei et al., 2019). Columbamine significantly decreases tumor volumes in HCT116 or SMMC7721 xenograft mouse model, as well as induces cell apoptosis and G2/M phase arrest via Wnt/β-catenin, MAPK, CDK6, STAT3 signaling pathways in in vitro models (Lin et al., 2019). Simultaneously, it downregulates MMP-2 expression and reduces cell migration and invasion in HCT116, LoVo, and U2OS cells (Wang et al., 2012).
Palmatine has been reported to have anticancer effects in CRC, HCC, pancreatic cancer, oral squamous cell carcinoma, breast cancer, ovarian cancer, and prostate cancer both in vitro and in vivo (Long et al., 2019; Qi et al., 2019). It induces antiproliferative effects, anti-inflammatory effects, G2/M phase arrest, and cell apoptosis via mitochondrial pathways by targeting cytokines and aurora kinase A (AURKA) in CRC cells (Ma et al., 2016; Liu et al., 2020e). Particularly, it is identified to exert anti-Helicobacter pylori activity, which is a trigger for gastritis and gastric cancer, showing a great potential in prevention and therapy of Helicobacter pylori-induced gastric cancer (Jung et al., 2014).
Jatrorrhizine has been reported to have an inhibitory effect on HCC, breast cancer, and melanoma. For breast cancer, it induces apoptosis through caspase-mediated mitochondrial pathway and potentially inhibits cell proliferation and metastasis by repressing Traf2 and Nck interacting serine protein kinase (TNIK) and epithelial-mesenchymal transition (EMT) via Wnt/β-catenin signaling in vitro and in 4T1 tumor-bearing mice (Sun et al., 2019a). Similarly, jatrorrhizine suppresses proliferation of metastatic melanoma cells by inducing G0/G1 phase arrest by enhancing the expression of p21 and p27 genes, and hinders human melanoma C8161 cell-mediated neovascularization in vitro and in vivo, which is accompanied by downregulation of VE-cadherin (Liu R. et al., 2013).
Magnoflorine suppresses cell proliferation of human gastric cancer by generating ROS, especially in SGC7901 cells and the xenograft tumor, by inducing autophagy via LC3B-II upregulation, G2/M phase arrest via p27 and p21 upregulation, and cell death via caspase activation (Sun XL. et al., 2020). Moreover, for breast cancer, it improves cell sensitivity to doxorubicin through inducing autophagy and apoptosis by elevating LC3-II and activating Caspase-3 via MAPK signaling pathway (Wei T. et al., 2020).
Oxyberberine can significantly alleviate DSS-induced colitis in mice and the effect is superior to berberine. Furthermore, oxyberberine can improve the colonic inflammatory reaction and intestinal epithelial barrier function. It inhibits the inflammatory signaling pathway via downregulation of inflammatory cytokines and the expression of TLR4 and MyD88, which inhibits the phosphorylated IκBα, and the translocation of NF-κB-p65 from the cytoplasm to the nucleus. In addition, oxyberberine markedly modulates DSS-induced intestinal dysbiosis and restores the balance of intestinal microbiota (Li C. et al., 2020).
In MKN-45-related gastric cancer, which harbors wild type p53, epiberberine shows great potential in inhibiting cell growth by inducing ROS, mitochondrial apoptosis and cell cycle arrest in vitro and inhibiting tumor growth in vivo via the p53/Bax pathway, and also inhibiting cell growth in the human gastric cancer (HGC-27) cells, which harbors mutated p53 (Zhai et al., 2020). Lysine-specific demethylase 1 (LSD1) is considered a critical target in many cancer treatments (Fiskus et al., 2014; Hong et al., 2018; Hu et al., 2019). Epiberberine acts as a LSD1 inhibitor in THP-1 and HL-60 cells (Li ZR. et al., 2020). Meanwhile, epiberberine also inhibits telomerase by disrupting telomere maintenance and capping to induce cell apoptosis (Liu L. et al., 2020). Furthermore, epiberberine induces low expression of Bcl-2 and the X-linked inhibitor of apoptosis protein (XIAP), the high expression of Bax, p53 and release of cytochrome C, and the activation of Caspase-3 to promote gastric cancer cell apoptosis (Liu L. et al., 2020). Oxyepiberberine has been reported to inhibit proliferation of CRC LS-1034 cells both in vitro and in vivo, accompanied by inducing apoptosis and inhibiting migration and tubulin polymerization (Ning et al., 2021). In addition, it inhibits cancer lung metastasis, impeding TGF-β1-induced EMT in cancer cells, and preferentially interfering with the Smad3 promoter activity in EMT (Liu et al., 2020f).
Dihydroberberine, an alkaloid of isoquinoline with various bioactivities, was identified in many plants including C. Rhizoma. Dihydroberberine has a therapeutic effect on UC induced by DSS in vivo by suppressing the immune-inflammatory response. It may decrease IL-6, IL-1β, IL-17, IFN-γ, TNF-α, and IgA by suppressing TLR4/MyD88/NF-κB signaling pathway to alleviate colonic immune-inflammation (Li C. et al., 2021). Meanwhile, it ameliorates inflammation by improving gut barrier function by upregulating the levels of claudin-1, occludin, junctional adhesion molecules A (JAM-A), and mucins (Li G. et al., 2021).
Berberrubine, a specific poison of topoisomerase II, has a potent antitumor activity by inducing DNA cleavage in vitro and exerts remarkable upgradation resistance to CRC associated with downregulation of topoisomerase IIα in vivo (Kang and Chung, 2002).
Worenine inhibits cell viability and proliferation, as well as induces G2/M phase arrest by balancing the Warburg effect via HIF-1α signaling in CRC cells (Ji L. J. et al., 2021).
The chemical structures of compounds of C. Rhizoma and some active derivatives have been identified, as shown in Figure 1. A series of compounds have been synthesized that exhibit differences relative lipophilicity due to the size of the substituent achieved by extending the alkyl side chain. This structure modification increases cytotoxic activity, and some derivatives (8-phenyl-berberine and 8-n-butyl-berberine) show better selectivity for breast cancer cells as well. 13-n-butyl-berberine and 13-n-hepta-berberine increase cytotoxic activity and are very effective against lung cancer (Iwasa et al., 2001). A study showed that 13-methylberberine and 13-ethylberberine could be used as potential immunotherapeutic compounds to induce IL-12, and combined with an iNOS inhibitor has potential value in cancer treatment (Lee et al., 2003). 8-cetylcoptisine, a new derivative of coptisine, exerts anticancer activity by inducing mitochondria-related apoptosis and G0/G1 phase arrest. 8-cetylcoptisine significantly delays tumor development in NSCLC (A549) cells-bearing mice, which is much stronger than coptisine. In addition to A549 cells, 8-cetylcoptisine also inhibits cell viability of BGC-823, HepG2, HCT-116 and MDA-MB-231 cells (Han et al., 2019).
Demethylenetetrahydroberberine (DMTHB) is a new derivative of berberine that can improve the symptoms of nonalcoholic fatty liver disease (NAFLD) by repressing the NLRP3 inflammasome and oxidative stress in mice. DMTHB targets NLRP3 inflammasome and TLR4/NF-κB signaling to suppress inflammatory response and inhibits CYP2E1 and C/EBP homologous protein (Kumar et al.), therefore activating transcription factor 4 (ATF4) to repress the over-expression of ROS and endoplasmic reticulum stress (Zhang Y. et al., 2021).
Some flavonoids, lignans, and other natural compounds are derived from C. Rhizoma and exists in many other botanical drugs. Limonin has potential effects against CRC, HCC, breast cancer, panchromatic islet cancer, meningioma, lung cancer, leukemia, and cervical carcinoma (Shimizu et al., 2015; Tang et al., 2019; Chen et al., 2021). The critical targets of action of limonin were described in detail in a recent review, including NAD(p)H quinone oxidoreductase 1 (NQO1), Yes-associated protein 1 (YAP1), NF-κB, p53, Wnt, and STAT3 (Fan S. et al., 2019). Limonin also overcomes MDR of antitumor agents (e.g., DOX) by inhibiting the activity of P-glycoprotein (P-gp) (El-Readi et al., 2010; Fan S. et al., 2019).
Rhamnetin induces cell death by provoking apoptosis, suppresses cell migration by inhibiting EMT, reverses MDR by reducing P-gp and breast cancer resistance protein (BCRP) expression, and enhances the antitumor effects of sorafenib, etoposide, paclitaxel, irradiation via the miR-34a/Notch-1 and miR-148a/PXR axis in HCC (Jia et al., 2016; Li B. et al., 2021), breast cancer (Lan et al., 2019), and NSCLC (Kang et al., 2013).
Growing evidence proved that Wogonin (Ming et al., 2020), Pinoresinol (Sain et al., 2021), Secoisolariciresionol (Ozgocmen et al., 2021), and Vanillic acid (Gong et al., 2019b) have a high potential to tackle gastrointestinal cancer through regulating cell cycle arrest, cellular senescence, autophagy, as well as inhibiting angiogenesis via multiple mechanisms.
Undoubtedly, traditional approaches for biological analysis require extensive resources to evaluate the increasing information derived from scale data sets in cancer research and drug discovery. With the rapid development of science and technology, omics offers a multidimensional perspective in a convenient approach to anticancer drug discovery, and includes genomics, proteomics, metabolomics, and microbiomics.
Genome-wide expression profiling of cancer cells treated with C. Rhizoma and its components have been investigated using cDNA microarray, oligonucleotide microarray, and RNA sequencing. These studies reveal the anticancer effects of C. Rhizoma by the regulation of altered gene expression profiles. A genome-wide biological response fingerprinting (BioReF) study showed that most genes are enriched in the terpenoid backbone biosynthesis pathway in HCC (HepG2) cells, which is remarkably influenced by treatment with C. Rhizoma originating from specific growing regions, along with six downregulated gene sets involved in the mevalonate pathway (Feng et al., 2018). To further explore this relationship, a total of 27 differentially expressed genes (DEGs) selected among 12,600 genes were identified to be involved in the regulation of signal transduction, cell metabolism, and cell invasion (MMP-14 and PAK1), that contribute to the anticancer effects of C. Rhizoma, as well as 3,726 genes that were downregulated and 3,642 genes upregulated by berberine in pancreatic cancer cells (Hara et al., 2005; Liu et al., 2020b). In addition, bioinformatics is an indispensable tool in omics analysis, which reveal the underlying mechanisms of the anticancer activity of C. Rhizoma. For example, the comparison of gene expression and survival analysis demonstrates that MYC is overexpressed in colorectal tumors and is highly associated with poor overall survival. C. Rhizoma was identified to target MYC using the TCMSP (https://tcmsp-e.com/) (Ru et al., 2014) and TCM-MESH (http://mesh.tcm.microbioinformatics.org/) (Zhang et al., 2017) databases, respectively (Dong et al., 2019). Moreover, 56 upregulated and 8 downregulated genes of berberine-treated HCC (HepG2) cells were identified and enriched in cell cycle, cell apoptosis, and transcription (Hu et al., 2018). Berberine could suppress cell proliferation and induce apoptosis by upregulating 1,960 genes and downregulating 4,837 genes, that are involved in cellular, metabolic, and single-organism processes in gastric cancer (SGC-7901) cells (Yang et al., 2018). A total of 2,706 and 3,397 DEGs involved in the regulation of cell apoptosis, cell cycle, and cell migration are regulated by berberine in breast cancer (MDA-MB-231 and MCF-7) cells. (Wen et al., 2013). Collectively, the application of genomics in anticancer research on C. Rhizoma is still in its infancy. In particular, genomic approaches help to accelerate high-throughput screening to identify the target genes of C. Rhizoma. However, a transcriptomic analysis has rarely been employed to reveal the anticancer action of C. Rhizoma and its components.
Proteomics analysis, the application of gel electrophoresis combined with mass spectrometry (Chou et al.), has been applied to reveal the potential therapeutic targets of berberine in CRC, HCC, breast cancer, cervix adenocarcinoma, and melanoma. Proteomic data demonstrated differentially expressed proteins (DEPs) among 5,130 or 8,051 identified proteins on berberine-treated CRC DLD-1 (675 proteins), HCT-116 (865 proteins), Caco-2 (503 proteins), and LOVO (277 proteins) cells, respectively. Among these, a total of 54 DEPs (22 upregulated and 32 downregulated proteins) overlapped in DLD1 and HCT116 cells, as well as 83 DEPs (the most downregulated proteins) overlapped in Caco-2 and LOVO cells were mainly involved in specific pathways, including calcium mobilization (LAT2/NTAL/LAB), metabolism of fat-soluble vitamins (LDLR and VKORC1), mitochondrial protein synthesis (GTPase ERAL1, MRPL11, and MRPL 48), tricarboxylic acid cycle (TCA) (citrate synthase, CS), and respiratory electron transport pathway (NDUFS2 and COX7A2L) (Tong et al., 2020; Li P. et al., 2021). Moreover, a total of 96 and 22 DEPs screened from 1,800 identified proteins showed altered protein expression and thiol reactivity on berberine-treated breast cancer (MCF-7) cells, respectively, which are attributable to gene regulation (HDAC1), protein folding (HSP27 and PPIA), signal transduction (KCIP-1 and NRG2), and metabolism (ENO1 and TPI1) (Chou et al., 2012). In berberine-treated cervix adenocarcinoma HeLa cells, a total of 51 DEPs screened among more than 700 proteins were strongly associated with cellular metabolic process (PDHB and MAPK13), cellular component organization (HSPA8 and VIM), and cell apoptosis and proliferation (ANXA5 and PHB) (Lu et al., 2012). A total of 23 DEPs (3 upregulated and 20 downregulated proteins) identified from approximately 806 proteins were associated with cell death (Annexin A1, Ezrin, and Septin-8), molecular chaperone (TCP-1 and Ferritin), and metabolism (Elongation Factor Tu and UQC. RHIZOMAC1) in melanoma (B16F10) cells treated with berberine (Kim J.-H. et al., 2016). In addition, a total of 8 DEPs (3 upregulated and 5 downregulated proteins) from berberine-treated HCC (HepG2) cells were associated with cell proliferation (MAPK4), cell metabolism, cell cycle, and DNA damage response (Tan et al., 2006). Accumulating evidence has shown that proteomic approaches could be helpful to investigate the mechanism of anticancer activity of berberine, but few analyses of proteomics, metabolomics, and microbiomics have been performed to identify the therapeutic targets of C. Rhizoma extract and other bioactive components in cancer cells. Further experimental verification, such as Western blotting, immunoprecipitation, and immunofluorescence, should be performed to confirm the potential targets identified from high-throughput proteomic data.
With the advancement of technologies, newly developed targeted metabolomics approaches combined with MS have been applied to accurately identify berberine-induced changes in metabolism in CRC, HCC, pancreatic cancer, and prostate cancer. In berberine-treated pancreatic cancer cells, the metabolism of 78 differential metabolites (DMs) was significantly changed at the phenotype level. These DMs included metabolism of some amino acids and nucleotides, upregulated energetic metabolism (glycolysis and glutamine)-associated metabolites and downregulated TCA cycle-associated metabolites (citrate), which interfere with mitochondrial structure and dysregulate energy metabolism (Eladl et al., 2020). Moreover, a total of 14 DMs (3 upregulated metabolites and 11 downregulated metabolites) in prostate cancer cells and 30 DMs (16 upregulated metabolites and 14 downregulated metabolites) in blood are closely associated with the metabolism of phenylalanine, d-arginine, d-ornithine, tyrosine, and the metabolism of arachidonic acid, glycerophospholipid, linoleic acid, purine, sphingolipid, retinol, the TCA cycle, arginine and proline (e.g., arachidonic acid, cholines, citric acid, eicosapentaenoic acid, prostaglandin A1/A2, thromboxane, and uric acid) (Li X. et al., 2017). Recently, Feng et al. from the University of Hong Kong reported that a total of 53 metabolites from tumors and 25 metabolites from HCC (MHCC97L) cells have been identified that could mediate berberine in the regulation of the glucose–alanine cycle (e.g., sugar, fatty acid, amino acid, and organic acid) (Guo et al., 2020).
Phylogenetic Investigation of Communities by Reconstruction of Unobserved States (PICRSt) analysis was used to predict the metabolic functions for berberine treatment based on 16S rRNA. The results indicated that berberine intervention along with the reduction of intestinal tumor development could improve metabolic abnormalities by reversing glucose metabolism (glycolysis/gluconeogenesis, fructose and mannose, and galactose), amino acid-related metabolism (lysine degradation), and lipid metabolism (fatty acid biosynthesis and arachidonic acid). In vivo animal models confirmed the above mechanisms by analyzing a total of 8 DMs from the detected 44 of fecal metabolites regulated by berberine in the azoxymethane/DSS-induced CRC mouse model (Chen et al., 2020b). To uncover the interactions of DMs and further investigate the underlying mechanisms of action of berberine against prostate cancer, bioinformatics analysis was performed to identify the functions of metabolites using Ingenuity Pathway Analysis (IPA) software (Li X. et al., 2017). Hence, further in-depth study on large-scale biological data sets is required using multiple bioinformatics approaches to provide a more holistic view, which also include the crosstalk between metabolites and microbiota.
The microbiota exists in all multicellular organisms and plays an important role in carcinogenesis. Growing attention has been focused on the gut microbiota and its impact on cancer progression (Elinav et al., 2019; Jaye et al., 2021; Matson et al., 2021). Microbiomics is a feasible approach to detect alterations in the gut microbiota including bacteria, fungi, archaea, and viruses using 16S rRNA gene sequencing, whole metagenome sequencing (WMS), pyrosequencing sequencing, gene chip, and fluorescence in situ hybridization (Petrosino et al., 2009). Accumulating evidence suggests that berberine exerts anticancer effects by improving intestinal dysbacteriosis in CRC and breast cancer. Below we will examine the so-called hidden organ (gut microbiota) effects induced by berberine.
In fecal or mucosal samples, berberine treatment greatly rescues colorectal tumorigenesis by improving the imbalance of the gut microbiota and modulating the tumor microenvironment by decreasing the richness of microbiota community and relative abundance of Actinobacteria and Verrucomicrbia at the phylum level, as well as suppressing pathogenic species (e.g., F. nucleatum, f_Erysipelotrichaceae and Alistipes) and elevating some bacteria producing short-chain fatty acid (SCFA) (Alloprevotella, Flavonifractor and Oscillibacter) at the genus level in AOM/DSS-induced CRC mouse model (Yu et al., 2015; Chen et al., 2020b). Interestingly, exercise therapy combined with berberine treatment produces the lowest effects on species richness and the highest relative abundance of bacteroidaceae to achieve a synergistic anticancer effect, compared with negative control and single administration in 4T1 breast cancer-bearing mice (Ma et al., 2020). Strong evidence supports that dysbiosis of gut microbiota involved in inflammatory and immune response is directly correlated with the development and concurrence of CRC (Alexander et al., 2017), thus, most studies for the anticancer effects of berberine have concentrated on the gut microbiota and inflammation in CRC. Further studies should also be performed to explore microbiota in the other cancer models for anticancer effects of C. Rhizoma and its components.
By changing drug delivery systems, a novel mode of drug administration with improved physical and/or chemical properties and bioavailability could be obtained, and the expected activity or efficacy could also be improved. Systemic/targeted drug delivery systems, including liposomes, nanoparticles, and hydrogels, could decrease side effects, toxicity, and the frequency of administration, also overcome MDR, improve symptoms and the survival rates in cancer treatment.
To improve bioavailability and optimize berberine immunoregulatory effects in a specific part of the gastrointestinal tract, a micro- and nanoencapsulated hybrid delivery system, targeted colon by oral administration, was established by encapsulation of poly nanoparticles loaded with berberine into a pH-sensitive Eudragit FS30D matrix pre-entrapped with berberine to form a hybrid microparticle. The nanoparticles are able to improve release of berberine subsequent to their intestinal absorption. Treatment with this novel system results in a more effective improvement than free berberine in acutely and chronically induced colitis by DSS. The improved efficacy is accompanied by a decrease in colon inflammation (Zhang L. et al., 2021).
Berberine combined with carboxylmethyl chitosan by arylboronic ester becomes a new oxidation-responsive nanoprodrug. It is responsive ROS and effectively delivers drugs to inflamed tissues (Lamprecht et al., 2001; Wilson et al., 2010; Zhang et al., 2016). The combination of carboxylmethyl chitosan modified with phenylboronic esters and berberine can significantly improve the symptoms of colitis and colon damage by regulating IL-6 expression and remodeling the intestinal microbiota (Zhao L. et al., 2021).
Synthesized silver nanoparticles (AgNPs) have attracted great attention because of their excellent characteristics, such as easy surface modification and synthesis, and superior biocompatibility (Gupta and Gupta, 2017). In a study, researchers found that AgNPs in combination with an aqueous extract of C. Rhizoma inhibited proliferation, migration, and invasion in NSCLC (A549) cells, and induced apoptosis likely involving the mitochondria-mediated pathway (Pei et al., 2019). To improve the poor oral bioavailability of berberine, new formulations of nano emulsion protocols have been explored and have achieved better bioavailability, efficacy, and pharmacological activity via the P-gp efflux system (Kumar et al., 2015; Liu et al., 2016). When berberine nanoemulsions are combined with photodynamic therapy, this novel treatment modality induced significant phototoxicity in the cervical carcinoma (Caski) cells and spontaneously immortalized non-tumorigenic human skin HaCaT keratinocytes, exhibiting a great potential for working as photosensitizing agents in cervical carcinoma treatment (Floriano et al., 2021).
Folate acid-modified chitosan nanoparticles loaded berberine hydrochloride are effective in regulating cell proliferation, migration, and apoptosis by improving its hydrophobic properties, poor stability, and bioavailability in nasopharyngeal carcinoma (CNE-1) cells both in vivo and in vitro (Wang et al., 2018). Although coptisine was reported to show positive anticancer effects in some studies (Lin et al., 2004; Hara et al., 2005; Luo et al., 2014; Yu et al., 2014; Huang T. et al., 2017; Han et al., 2018; Zhang YL. et al., 2020; Kim et al., 2021), its poor absorption and low bioavailability were still obstacles for its application. Therefore, nano strategies, microrods and salt formulation could also be employed to promote the intestinal dissolution (Wu et al., 2019).
In most cases, monotherapy is not as effective as expected, and is always required excessive dosages and accompanied with significant side effects. Combination of anticancer drugs with C. Rhizoma extract or active ingredients could improve MDR, exert synergistic effects, and reduce side effects, which contributes to improving clinical outcomes in chemotherapy and is regarded as a beneficial treatment for cancers. There are many studies that support the potential of C. Rhizoma as an adjuvant agent in chemotherapy.
MDR is a critical limitation of chemotherapy, and its corresponding mechanisms have been extensively explored. The potential mechanisms of MDR include alterations in the expression of the ABC transporter family members, apoptosis induction, autophagy induction, cancer stem cell regulation, miRNA regulation, hypoxia induction, DNA damage and repair, and epigenetic regulation (Wu et al., 2014). The mechanisms involving C. Rhizoma that improve MDR are described below.
P-gp transporter and multidrug resistance protein 1 (MRP1) are active members of ABC transporters in the gastrointestinal system. Overexpression of P-gp in CRC patients leads to drug efflux and drug resistance (Lee et al., 2018). In the human CRC (HCT15) cells and the human MDR uterine sarcoma (MES-SA/DX5) cells, 8-oxocoptisine, which is obtained from the rhizome of Coptis japonica Makino, shows significant efficacy as a P-gp inhibitor. Its inhibitory activity on P-gp is similar to that of verapamil (Min et al., 2006). Thymidylate synthase (TS) is reported highly expressed in CRC patients and is associated with overall survival (Popat et al., 2004). C. Rhizoma extract significantly reduced the IC50 value of fluorouracil (5-FU) in human CRC (HCT116) cells, and could act as a potential adjuvant agent against 5-FU-resistant CRCs by attenuating the expression of TS gene (Kang et al., 2021). In addition, C. Rhizoma extract improves the sensitivity of HeLa cells to paclitaxel or 5-FU, presumably via the inhibition of P-gp function (Takara et al., 2005). TNF-related apoptosis inducing ligand (TRAIL) is considered a potential adjuvant in cancer treatment (Johnstone et al., 2008). However, some cancer cells appear to resistant to TRAIL-induced apoptosis (Stegehuis et al., 2010). TRAIL resistance was reported to be reduced by the combination of TRAIL and C. Rhizoma aqueous extract in TRAIL-resistant A549 cells (Chiang et al., 2018). As a potential anticancer agent for NSCLC, C. Rhizoma extract and its major constituent berberine repress ROS production, ameliorate MDR, and enhance the inhibitory effects of chemotherapeutic agents in A549 cells (He C. et al., 2012). The EGFR tyrosine kinase inhibitor (TKI), gefitinib, is used in the treatment of NSCLC with EGFR mutations (Rawluk and Waller, 2018). C. Rhizoma extracts suppress EGFR/AKT signaling and the expression of overexpressed antiapoptotic proteins, Mcl-1 and Bcl-2 in gefitinib-resistant NSCLC cells (Kim et al., 2020).
Long term treatment of DOX can induce MDR by transforming N1 into N2 neutrophil phenotypes via upregulation of CD133, CD309, and PD-L1 expression in HL-60 cells. Treatment using the combination of berberine and DOX can maintain the N1 neutrophil polarization and stimulate immune checkpoints to reverse MDR by downregulating CD133, CD309, PD-1, and PD-L1 expression in HCC allograft model (Zhang et al., 2019).
Drug combinations often exhibit synergistic effects, which can be achieved by a combination of two or more drugs, making combined treatment much more effective than monotherapy. Aiming at the same target, increasing the effect of the former drug or improving the microenvironment in which the drug acts, all may effectively increase clinical efficacy. The primary metabolites of the C. Rhizoma extract, known as natural deep eutectic solvents, improves the pharmacokinetics of orally administered berberine (Zhao J. et al., 2021).
C. Rhizoma extract and 5-FU act on the same target. Cotreatment with C. Rhizoma extract and 5-FU significantly augments G0/G1 phase arrest, especially in 5-FU-resistant CRC. The potential mechanisms might be associated with modulation of TS expression (Kang et al., 2021). Additionally, cotreatment with C. Rhizoma extract and estrogen receptor (ER) antagonists increase the anticancer effect. A majority of breast cancer patients are ER positive and the disease progresses in the presence of high levels of estrogen (Jordan, 2008). ER antagonists, such as tamoxifen and fulvestrant, have been widely used in the treatment of ER-positive breast cancer, but the drug efficacy and resistance remain a concern (Shou et al., 2004; Abukhdeir et al., 2008; Jordan, 2008; Lee et al., 2008). Combined treatment of C. Rhizoma extracts or berberine with tamoxifen were found to enhance the inhibitory effects on ER-positive breast cancer (MCF-7) cells via downregulating the expression of EGFR, HER2, bcl-2 and COX-2 and upregulating IFN-β and p21 (Liu et al., 2009).
The ErbB family is a type of TK receptors, with high expression in breast cancer patients (Scott et al., 1991; Witters et al., 2007). EGFR and HER2, two members of the ErbB family, antagonize the anticancer effects of tamoxifen and induce drug resistance via activating ER and co-regulatory proteins. Bcl-2 inhibitors induce apoptosis and improve cell sensitivity to other therapies. It would be beneficial to block the ErbB family and the Bcl-2 family simultaneously (Witters et al., 2007). High expression level of COX-2 also reduces the inhibitory effects of tamoxifen on breast cancer cell growth (Tari et al., 2005). The upregulation of IFN-
The combination of berberine and PEGylated liposomal doxorubicin (PEG-lip-DOX) suppresses tumor growth, which is much more effective than that achieved by monotherapy with berberine or PEG-lip-DOX treatment alone. This combination also exhibits antiproliferative activity on Meth A murine sarcoma cells (Yahuafai et al., 2018). Berberine improves the sensitivity to cisplatin of breast cancer (MCF-7) cells. Pro-apoptotic Capase-3 and cleaved Caspase-3 and Caspase-9 were induced and Bcl-2 was downregulated after cotreatment of berberine and cisplatin (Zhao et al., 2016). Proliferating cell nuclear antigen (PCNA) is a DNA sliding clamp required for DNA pol δ to replicate DNA and is crucial in DNA repair (Zhu Q. et al., 2014). Berberine restrains the expression of cellular PCNA and increases DNA damage induced by cisplatin. A decrease in the cellular DNA repair ability may result in cell sensitizing to genotoxic cisplatin (Zhao et al., 2016).
Berberine enhances the expected maximum antitumor activity of 17-AAG (the Hsp90 inhibitor) and SAHA (the HDAC inhibitor) alone in CRC (SW480) cells. The combination of the three appear the most effect (Li J. et al., 2021). Magnoflorine improves the cell sensitivity to doxorubicin through inducing autophagy and apoptosis by elevating LC3-II and activating Caspase-3 via MAPK pathway in breast cancer. This combination suppresses migration and invasion, induces G2/M arrest, apoptosis and autophagy (Wei T. et al., 2020).
Cachexia is a complex syndrome that often occurs in advanced cancer patients, with the main symptoms including anorexia, weight loss, and loss of adipose tissue and skeletal muscle (Evans et al., 2008). IL-6 plays an important role in cancer-induced cachexia. It has been demonstrated that downregulation of IL-6 levels would improve cachexia or malnutrition in patients (Strassmann et al., 1992; Fujita et al., 1996; Oka et al., 1996; Cahlin et al., 2000). Botanical drugs might represent an effective approach to cancer-related cachexia as well as in adjuvant therapy (Park et al., 2019). C. Rhizoma water extract has anti-inflammatory effects, exerting an anticachectic effect in esophageal cancer (YES-2) cells-bearing mice (Iizuka et al., 2000). The inhibitory effects on the production of pro-inflammatory cytokines by C. Rhizoma water extract and berberine were also found to be syngeneic in CRC cells-bearing mice (Iizuka et al., 2002). Furthermore, some adverse effects can also be alleviated by C. Rhizoma. Oral mucositis (OM) is a common complication of radiotherapy and chemotherapy which brings pain to head and neck cancer patients due to ROS generation. Hangeshashinto (HST), a Japanese traditional medicine, contains C. Rhizoma as one of its components. C. Rhizoma and berberine alleviate OM by reducing ROS levels (Matsumoto et al., 2015).
C. Rhizoma is often formulated in combination with other botanical drugs. These types of herbal formulas play an important role in cancer treatment. They exert special synergistic mechanisms and therapeutic characteristics. There are some common classical prescriptions, such as Zuojinwan, San-Huang-Xie-Xin Decoction, Chingwaysan, and Gegen Qinlian Decoction.
Zuojinwan is a representative Chinese herbal formula, consisting of C. Rhizoma and Euodiae Fructus at a ratio of 6:1 (w/w). In human gastric carcinoma (SGC-7901) cells, water extracts of C. Rhizoma and Evodiae Fructus inhibit proliferation and induce apoptosis. The optimal proportion is also 6:1 (w/w) in treating cancer (Peng et al., 2011). Meanwhile, water extracts of C. Rhizoma and Evodiae Fructus significantly inhibit aberrant crypt foci formation in model animals. A clinical therapeutic effect is observed in CRC, in part due to inhibition of proliferation of the middle and distal crypts and the promotion of apoptosis (Dong et al., 2010). The anticancer activity of Zuojinwan is superior to that of C. Rhizoma or Euodiae Fructus used alone. An obvious synergistic effect could be associated with gene expression and the activities of tumor markers in the serum (Wang et al., 2009). Berberine and evodiamine are the two main bioactive compounds in Zuojinwan, both inhibit AP-1 and/or NF-κB activities and suppress anchorage-independent growth of HCC (HepG2) cells (Chao et al., 2011). Another study showed that c-myc plays a critical role in its cytotoxic effect (Chou et al., 2017). The combination of berberine and evodiamine in human HCC (SMMC-7721) cells significantly enhanced apoptosis and showed the highest inhibitory effect when compared with either used individually (Wang et al., 2008). Furthermore, the combination of berberine and evodiamine also exerts synergistic anticancer effect on P-gp positive CRC cells by decreasing the overexpression of P-gp. Moreover, in other types of cancer cells that are P-gp-positive, namely breast cancer or ovarian cancer cells, this combination also shows synergistic inhibitory effect. Berberine did not enhance the cytotoxicity of evodiamine in normal cells (Guan et al., 2020). In a different study, although evodiamine displayed antiproliferative and antimigratory activities in human gastric cancer (AGS) cells, it promoted metastasis by the concomitant increase in IL-8 secretion and mRNA expression of vascular cell adhesion protein. Further, berberine was shown to counteract this side-effect and maintain the antiproliferative and antimigratory properties of evodiamine to improve its therapeutic effects (Shi et al., 2013).
San-Huang-Xie-Xin Decoction, containing Rhei Rhizoma, C. Rhizoma, and Radix Scutellariae at a ratio of 2:1:1 (w/w/w), was reported to regulate p53 signaling pathway related genes and caused apoptosis and DNA damage in the HCC (HepG2) cells. The main pharmacological effect might be due to the presence of C. Rhizoma (Cheng et al., 2008).
Chingwaysan (Qingweisan), a well-known Chinese herbal formula, consists of Angelicae Sinensis Radix, Rehmanniae Radixet Rhizoma, Moutan Radicis Cortex, C. Rhizoma, and Cimicfugae Rhizoma at a ratio of 3:3:5:5:10 (w/w/w/w/w). Chingwaysan inhibits cell viability by inducing cell apoptosis via the Bax signaling pathway in human oral cancer (OC2 and TSCCa) cells (Liao et al., 2005).
Gegen Qinlian Decoction, containing Radix Puerariae, Radix Scutellariae, C. Rhizoma and Glycyrrhiza at a rate of 5:3:3:2 (w/w/w/w), is frequently used to treat UC by means of its anti-inflammatory and antioxidative properties and inhibition of the transcription of oncogenes (Wei M. et al., 2020). It not only exerts antitumor effects when used alone, as described in a recent review (Lu et al., 2021), but also improves clinical symptoms and reduces adverse events in combination with western medicines (Fan Y. et al., 2019).
Xiao-Xian-Xiong Decoction contains C. Rhizoma, Pinellia ternate, and Fructus trichosanthis. It induces proliferative switching by inducing G0/G1 phase arrest via the inhibition of Facilitates chromatin transcription (FACT) and c-MYC transcription in quiescent lung cancer in vitro and in vivo (Bi et al., 2020).
Prostacaid is a 33-ingredient dietary supplement with a mixture of vitamins, minerals, multi-botanical extracts, and derivatives, which hinders abnormal cell proliferation and promotes cell apoptosis in androgen-independent or dependent prostate cancer of mouse and human. C. Rhizoma is one of the ingredients (Yan and Katz, 2010). Prostacaid suppresses cell proliferation by inducing G2/M phase arrest, induction of apoptosis and regulating the expression of CCND1, CDK4, CDKN1A, E2F1, MAPK6, and PCNA genes (Yan and Katz, 2010; Jiang et al., 2011). Furthermore, it also inhibits the metastatic behavior of the human prostate cancer cells by inhibiting cell adhesion, invasion, and invasion, by downregulating of the expression of the CAV1, NR2F1, PLAU, and IGF2 genes and suppressing the secretion of urokinase plasminogen activator (Jiang et al., 2011).
Besides being used in combination with chemotherapeutic drugs and botanical drugs, berberine is also found to have protective effects against radiotherapy. Berberine can simultaneously act as a radiosensitizer and a photothermal agent to supplement effects of chemo-radiotherapy of liver cancer when it was loaded into folic acid with Janus gold mesoporous silica nanocarrier in advance (Li XD. et al., 2019). In addition, berberine can sensitize human HCC cells to ionizing radiation therapy by blocking autophagy at LC3-II manner and inducing G2/M phase arrest via the upregulation of mitochondrial oxidative stress and the inhibition of ATP levels, resulting in senescence (Ramesh et al., 2020). Additionally, berberine induces DNA repair by affecting DNA repair protein XRCC1-mediated base excision repair to enhance the sensitization of breast cancer cells to cisplatin, camptothecin, and methyl methanesulfonate (Gao et al., 2019). Other sensitization enhancements were also found in human ovarian cancer cells to cisplatin and the radiosensitivity of esophageal squamous cancer after berberine treatment (Yang X. et al., 2013; Chen et al., 2015).
Taken together, C. Rhizoma have potent anticancer effects against gastrointestinal and other cancers, as shown in Table 1.
Clinical research on C. Rhizoma has mostly focused on berberine in colorectal adenomas and NSCLC. Since 2002, over 2000 participants have taken part in the clinical trials for cancer therapy with berberine. Published results show that berberine exerts protective effects on lung damage caused by radiation via intercellular adhesion molecular-1 (ICAM-1) and TGF-β1 in patients with lung cancer. In a randomized, double-blind study, 90 sampled patients with NSCLC were divided into two groups. Berberine significantly decreased the incidence of radiation-induced lung damage as well as the levels of soluble ICAM-1 and TGF-β1, and improved pulmonary function (Liu et al., 2008a). Radiation-induced acute intestinal symptoms (RIAISs) are the most relevant complication that happened in patients of abdominal or pelvic radiotherapy. Berberine exerts preventive effects on acute RIAIS. In one study, 42 randomly sampled patients with cervical cancer were divided into two groups. Patients who had more severe symptoms were in the control group. The study illustrated that berberine could reduce the incidence and severity of RIAISs (Li et al., 2010).
Collectively, there are few clinical studies evaluating C. Rhizoma and its active ingredients, most of which are concentrated on berberine, as shown in Table 2. In clinical trials, berberine is generally used as an anticancer adjuvant in NSCLC rather than a major chemotherapy agent as in colorectal adenomas. We look forward to many more studies evaluating the application of C. Rhizoma in gastrointestinal cancer in the future.
Cancer is a complex systemic chronic disease threatening human health and quality of life. As a traditional Chinese medicine for the treatment of gastrointestinal disorders, C. Rhizoma has the unparalleled advantage in treating digestive system cancers. The percentage of estimated deaths of digestive system cancers is increased in recent years (25.69%, 153,030/595,690 for year 2016; 26.24%, 157,700/600,920 for year 2017; 26.38%, 160,820/609,640 for year 2018; 27.26%, 165,460/606,880 for year 2019; 27.66%, 167,790/606,520 for year 2020; 27.82%, 169,280/608,570 for year 2021) according to the cancer statistics over the past 5 years in the United States. The anticancer research advance of C. Rhizoma highlights the need for a systematic review.
From the current advances of using C. Rhizoma for the treatment of gastrointestinal and other cancers, some achievements and limitations could be appreciated, as well as the most promising direction for future research. Most of the studies are mainly in vitro, and there are a few in vivo investigations, especially in human clinical trials. In the limited clinical studies on C. Rhizoma and its active ingredients, berberine has been used as an anticancer adjuvant. In fact, several types of secondary metabolites of C. Rhizoma are in need of in-depth study for their properties, that would be clinically valuable and might represent a research hotspot in the future. The chemical structures of the active ingredients from C. Rhizoma have been investigated to improve their pharmacological activities, which commonly results in modification of the molecular structure and OMICS approaches in natural product research. In order to obtain superior physical and/or chemical properties and bioavailability, novel drug delivery systems have been a focus of active research and shown great promise, such as hybrid microparticle of berberine, synthesized silver nanoparticles combined with aqueous extract of C. Rhizoma, berberine nanoemulsion and more. Moreover, combinations with other drugs or therapies aiming at enhancing efficacy and reducing toxicity is also a significant way to improve clinical outcomes.
Taken together, Figure 2 summarizes the broad prospects of C. Rhizoma as an adjuvant candidate against cancers in the present review, and illustrates the rational for pre-clinical studies and clinical trials for this promising preparation.
FIGURE 2. A scheme for current advance in Coptidis Rhizoma for gastrointestinal and other cancers. A brief scheme showing main features of Coptidis Rhizoma and key roles and relationship with cancers.
LH and ZZ wrote and revised this manuscript. MC and QL helped to revise the manuscript and provided valuable feedback to this conception. ZZ and WT revised the entire manuscript and edited the language for scientific presentation. The corresponding authors, YW and WT conceived and organized this study.
This study was supported by the Young Scientists Fund of National Natural Science Fund (81903844), the Gansu Province Science Fund for Distinguished Young Scholars (20JR10RA650), and supported by Outstanding Youth of the Fundamental Research Funds for the Central Universities (lzujbky-2021-ey21). Meanwhile, it was also supported by the Macao Science and Technology Development Fund (FDCT 007/2020/ALC), the Research Fund of University of Macau (CPG 2021–00022-ICMS). Hong Kong, Macao and Mainland University Teacher and Student Exchange Program for year 2020 and 2021 (201910280036, 202010270012), support the design of the study and collection and analysis and interpretation of data. When in writing manuscript, the Natural Science Foundation of Guangdong Province (2018A030310226, 2020A1515010922) and Traditional Chinese Medicine Bureau of Guangdong Province, China (20201183) also support the present study.
The authors declare that the research was conducted in the absence of any commercial or financial relationships that could be construed as a potential conflict of interest.
All claims expressed in this article are solely those of the authors and do not necessarily represent those of their affiliated organizations, or those of the publisher, the editors and the reviewers. Any product that may be evaluated in this article, or claim that may be made by its manufacturer, is not guaranteed or endorsed by the publisher.
We want to thank all the colleagues and friends for their sincere and generous help.
Abukhdeir, A. M., Vitolo, M. I., Argani, P., De Marzo, A. M., Karakas, B., Konishi, H., et al. (2008). Tamoxifen-stimulated Growth of Breast Cancer Due to P21 Loss. Proc. Natl. Acad. Sci. U S A. 105, 288–293. doi:10.1073/pnas.0710887105
Alexander, J. L., Wilson, I. D., Teare, J., Marchesi, J. R., Nicholson, J. K., and Kinross, J. M. (2017). Gut Microbiota Modulation of Chemotherapy Efficacy and Toxicity. Nat. Rev. Gastroenterol. Hepatol. 14, 356–365. doi:10.1038/nrgastro.2017.20
Auyeung, K. K., and Ko, J. K. (2009). Coptis Chinensis Inhibits Hepatocellular Carcinoma Cell Growth through Nonsteroidal Anti-inflammatory Drug-Activated Gene Activation. Int. J. Mol. Med. 24, 571–577. doi:10.3892/ijmm_00000267
Ayati, S. H., Fazeli, B., Momtazi-Borojeni, A. A., Cicero, A. F. G., Pirro, M., and Sahebkar, A. (2017). Regulatory Effects of Berberine on microRNome in Cancer and Other Conditions. Crit. Rev. Oncol. Hematol. 116, 147–158. doi:10.1016/j.critrevonc.2017.05.008
Balkwill, F., and Mantovani, A. (2001). Inflammation and Cancer: Back to Virchow? Lancet 357, 539–545. doi:10.1016/S0140-6736(00)04046-0
Bao, M., Cao, Z., Yu, D., Fu, S., Zhang, G., Yang, P., et al. (2012). Columbamine Suppresses the Proliferation and Neovascularization of Metastatic Osteosarcoma U2OS Cells with Low Cytotoxicity. Toxicol. Lett. 215, 174–180. doi:10.1016/j.toxlet.2012.10.015
Bartel, D. P. (2004). MicroRNAs: Genomics, Biogenesis, Mechanism, and Function. Cell 116, 281–297. doi:10.1016/s0092-8674(04)00045-5
Bi, L., Xie, C., Jiao, L., Jin, S., Hnit, S. S. T., Mu, Y., et al. (2020). CPF Impedes Cell Cycle Re-entry of Quiescent Lung Cancer Cells through Transcriptional Suppression of FACT and C-MYC. J. Cel Mol Med 24, 2229–2239. doi:10.1111/jcmm.14897
Bray, F., Ferlay, J., Soerjomataram, I., Siegel, R. L., Torre, L. A., and Jemal, A. (2018). Global Cancer Statistics 2018: GLOBOCAN Estimates of Incidence and Mortality Worldwide for 36 Cancers in 185 Countries. CA Cancer J. Clin. 68, 394–424. doi:10.3322/caac.21492
Bueso-Ramos, C. E., Yang, Y., Deleon, E., Mccown, P., Stass, S. A., and Albitar, M. (1993). The Human MDM-2 Oncogene Is Overexpressed in Leukemias. Blood 82, 2617–2623. doi:10.1182/blood.v82.9.2617.2617
Buzzi, F., Brugia, M., Rossi, G., Giustini, L., Scoponi, C., and Sica, G. (1992). Combination of Beta-Interferon and Tamoxifen as a New Way to Overcome Clinical Resistance to Tamoxifen in Advanced Breast Cancer. Anticancer Res. 12, 869–871.
Cahlin, C., Körner, A., Axelsson, H., Wang, W., Lundholm, K., and Svanberg, E. (2000). Experimental Cancer Cachexia: the Role of Host-Derived Cytokines Interleukin (IL)-6, IL-12, Interferon-Gamma, and Tumor Necrosis Factor Alpha Evaluated in Gene Knockout, Tumor-Bearing Mice on C57 Bl Background and Eicosanoid-dependent Cachexia. Cancer Res. 60, 5488–5493.
Calin, G. A., and Croce, C. M. (2006). MicroRNA-cancer Connection: the Beginning of a New Tale. Cancer Res. 66, 7390–7394. doi:10.1158/0008-5472.CAN-06-0800
Calò, V., Migliavacca, M., Bazan, V., Macaluso, M., Buscemi, M., Gebbia, N., et al. (2003). STAT Proteins: from normal Control of Cellular Events to Tumorigenesis. J. Cel Physiol 197, 157–168. doi:10.1002/jcp.10364
Casey, S. C., Tong, L., Li, Y., Do, R., Walz, S., Fitzgerald, K. N., et al. (2016). Erratum for the Report "MYC Regulates the Antitumor Immune Response through CD47 and PD-L1" by S. C. Casey, L. Tong, Y. Li, R. Do, S. Walz, K. N. Fitzgerald, A. M. Gouw, V. Baylot, I. Gütgemann, M. Eilers, D. W. Felsher. Science 352, 227–231. doi:10.1126/science.aaf7984
Chao, D. C., Lin, L. J., Kao, S. T., Huang, H. C., Chang, C. S., Liang, J. A., et al. (2011). Inhibitory Effects of Zuo-Jin-Wan and its Alkaloidal Ingredients on Activator Protein 1, Nuclear Factor-Κb, and Cellular Transformation in HepG2 Cells. Fitoterapia 82, 696–703. doi:10.1016/j.fitote.2011.02.009
Chao, M. P., Alizadeh, A. A., Tang, C., Myklebust, J. H., Varghese, B., Gill, S., et al. (2010). Anti-CD47 Antibody Synergizes with Rituximab to Promote Phagocytosis and Eradicate Non-hodgkin Lymphoma. Cell 142, 699–713. doi:10.1016/j.cell.2010.07.044
Chen, H., Zhang, F., Li, R., Liu, Y., Wang, X., Zhang, X., et al. (2020a). Berberine Regulates Fecal Metabolites to Ameliorate 5-fluorouracil Induced Intestinal Mucositis through Modulating Gut Microbiota. Biomed. Pharmacother. 124, 109829. doi:10.1016/j.biopha.2020.109829
Chen, H., Zhang, F., Zhang, J., Zhang, X., Guo, Y., and Yao, Q. (2020b). A Holistic View of Berberine Inhibiting Intestinal Carcinogenesis in Conventional Mice Based on Microbiome-Metabolomics Analysis. Front. Immunol. 11, 588079. doi:10.3389/fimmu.2020.588079
Chen, I. I., Caprioli, A., Ohnuki, H., Kwak, H., Porcher, C., and Tosato, G. (2016a). EphrinB2 Regulates the Emergence of a Hemogenic Endothelium from the Aorta. Sci. Rep. 6, 27195. doi:10.1038/srep27195
Chen, J., Wang, F., Liu, J., Lee, F. S., Wang, X., and Yang, H. (2008). Analysis of Alkaloids in Coptis Chinensis Franch by Accelerated Solvent Extraction Combined with Ultra Performance Liquid Chromatographic Analysis with Photodiode Array and Tandem Mass Spectrometry Detections. Anal. Chim. Acta 613, 184–195. doi:10.1016/j.aca.2008.02.060
Chen, J., Wu, F. X., Luo, H. L., Liu, J. J., Luo, T., Bai, T., et al. (2016b). Berberine Upregulates miR-22-3p to Suppress Hepatocellular Carcinoma Cell Proliferation by Targeting Sp1. Am. J. Transl Res. 8, 4932–4941.
Chen, L., Wang, L., Zhang, Q., Zhang, S., and Ye, W. (2012). [Non-alkaloid Chemical Constituents from Coptis Chinensis]. Zhongguo Zhong Yao Za Zhi 37, 1241–1244. doi:10.4268/cjcmm20120915
Chen, P. S., Wang, M. Y., Wu, S. N., Su, J. L., Hong, C. C., Chuang, S. E., et al. (2007). CTGF Enhances the Motility of Breast Cancer Cells via an Integrin-alphavbeta3-erk1/2-dependent S100A4-Upregulated Pathway. J. Cel Sci 120, 2053–2065. doi:10.1242/jcs.03460
Chen, Q., Qin, R., Fang, Y., and Li, H. (2015). Berberine Sensitizes Human Ovarian Cancer Cells to Cisplatin through miR-93/PTEN/Akt Signaling Pathway. Cell Physiol Biochem 36, 956–965. doi:10.1159/000430270
Chen, Y., Liang, J., Liang, X., Chen, J., Wang, Y., Cao, J., et al. (2021). Limonin Induces Apoptosis of HL-60 Cells by Inhibiting NQO1 Activity. Food Sci. Nutr. 9, 1860–1869. doi:10.1002/fsn3.2109
Chen, Y. X., Gao, Q. Y., Zou, T. H., Wang, B. M., Liu, S. D., Sheng, J. Q., et al. (2020c). Berberine versus Placebo for the Prevention of Recurrence of Colorectal Adenoma: a Multicentre, Double-Blinded, Randomised Controlled Study. Lancet Gastroenterol. Hepatol. 5, 267–275. doi:10.1016/S2468-1253(19)30409-1
Chen, Z., Liu, Y., Qi, B., Gu, C., Wei, X., Guo, L., et al. (2019). MicroRNA-212 F-acilitates the M-otility and I-nvasiveness of E-sophageal S-quamous C-arcinoma C-ells. Mol. Med. Rep. 20, 3633–3641. doi:10.3892/mmr.2019.10647
Cheng, W. Y., Wu, S. L., Hsiang, C. Y., Li, C. C., Lai, T. Y., Lo, H. Y., et al. (2008). Relationship between San-Huang-Xie-Xin-Tang and its Herbal Components on the Gene Expression Profiles in HepG2 Cells. Am. J. Chin. Med. 36, 783–797. doi:10.1142/S0192415X08006235
Chiang, S. C., Choi, Y. J., Kang, S. E., Yun, M., and Lee, B. J. (2018). Herbal Medicines Showing Synergistic Effects with Tumor Necrosis Factor-Related Apoptosis-Inducing Ligand (TRAIL) against A549 TRAIL-Resistant Lung Cancer Cells: A Screening Study. Pharmacogn Mag. 14, 145–148. doi:10.4103/pm.pm_270_17
Chidambara Murthy, K. N., Jayaprakasha, G. K., Kumar, V., Rathore, K. S., and Patil, B. S. (2011). Citrus Limonin and its Glucoside Inhibit colon Adenocarcinoma Cell Proliferation through Apoptosis. J. Agric. Food Chem. 59, 2314–2323. doi:10.1021/jf104498p
Chou, H. C., Lu, Y. C., Cheng, C. S., Chen, Y. W., Lyu, P. C., Lin, C. W., et al. (2012). Proteomic and Redox-Proteomic Analysis of Berberine-Induced Cytotoxicity in Breast Cancer Cells. J. Proteomics 75, 3158–3176. doi:10.1016/j.jprot.2012.03.010
Chou, S. T., Hsiang, C. Y., Lo, H. Y., Huang, H. F., Lai, M. T., Hsieh, C. L., et al. (2017). Exploration of Anti-cancer Effects and Mechanisms of Zuo-Jin-Wan and its Alkaloid Components In Vitro and in Orthotopic HepG2 Xenograft Immunocompetent Mice. BMC Complement. Altern. Med. 17, 121. doi:10.1186/s12906-017-1586-6
Crawford, B., Craig, Z., Mansell, G., White, I., Smith, A., Spaull, S., et al. (2018). DNA Methylation and Inflammation Marker Profiles Associated with a History of Depression. Hum. Mol. Genet. 27, 2840–2850. doi:10.1093/hmg/ddy199
Cui, H., Cai, Y., Wang, L., Jia, B., Li, J., Zhao, S., et al. (2018). Berberine Regulates Treg/Th17 Balance to Treat Ulcerative Colitis through Modulating the Gut Microbiota in the Colon. Front. Pharmacol. 9, 571. doi:10.3389/fphar.2018.00571
Delgado-López, P. D., and Corrales-García, E. M. (2016). Survival in Glioblastoma: a Review on the Impact of Treatment Modalities. Clin. Transl Oncol. 18, 1062–1071. doi:10.1007/s12094-016-1497-x
Dip, N., Reis, S. T., Srougi, M., Dall'oglio, M. F., and Leite, K. R. (2013). Expression Profile of Microrna-145 in Urothelial Bladder Cancer. Int. Braz. J. Urol. 39, 95–102. doi:10.1590/S1677-5538.IBJU.2013.01.12
Dong, L., Shi, H., Ji, G., and Wu, D. (2010). [Effects of Coptis Chinensis and Evodia Rutaecarpa Water Extract on DMH-Induced Precancerous Lesion of colon]. Zhongguo Zhong Yao Za Zhi 35, 1185–1188. doi:10.4268/cjcmm20100923
Dong, S., Ding, Z., Zhang, H., and Chen, Q. (2019). Identification of Prognostic Biomarkers and Drugs Targeting Them in Colon Adenocarcinoma: A Bioinformatic Analysis. Integr. Cancer Ther. 18, 1534735419864434. doi:10.1177/1534735419864434
Du, L., and Pertsemlidis, A. (2010). microRNAs and Lung Cancer: Tumors and 22-mers. Cancer Metastasis Rev. 29, 109–122. doi:10.1007/s10555-010-9204-9
Dunn, G. P., Old, L. J., and Schreiber, R. D. (2004). The Immunobiology of Cancer Immunosurveillance and Immunoediting. Immunity 21, 137–148. doi:10.1016/j.immuni.2004.07.017
Ehteshamfar, S. M., Akhbari, M., Afshari, J. T., Seyedi, M., Nikfar, B., Shapouri-Moghaddam, A., et al. (2020). Anti-inflammatory and Immune-Modulatory Impacts of Berberine on Activation of Autoreactive T Cells in Autoimmune Inflammation. J. Cel Mol Med 24, 13573–13588. doi:10.1111/jcmm.16049
Eiró, N., and Vizoso, F. J. (2012). Inflammation and Cancer. World J. Gastrointest. Surg. 4, 62–72. doi:10.4240/wjgs.v4.i3.62
El-Readi, M. Z., Hamdan, D., Farrag, N., El-Shazly, A., and Wink, M. (2010). Inhibition of P-Glycoprotein Activity by Limonin and Other Secondary Metabolites from Citrus Species in Human colon and Leukaemia Cell Lines. Eur. J. Pharmacol. 626, 139–145. doi:10.1016/j.ejphar.2009.09.040
Eladl, E., Tremblay-Lemay, R., Rastgoo, N., Musani, R., Chen, W., Liu, A., et al. (2020). Role of CD47 in Hematological Malignancies. J. Hematol. Oncol. 13, 96. doi:10.1186/s13045-020-00930-1
Elinav, E., Garrett, W. S., Trinchieri, G., and Wargo, J. (2019). The Cancer Microbiome. Nat. Rev. Cancer 19, 371–376. doi:10.1038/s41568-019-0155-3
Erazo, T., Lorente, M., López-Plana, A., Muñoz-Guardiola, P., Fernández-Nogueira, P., García-Martínez, J. A., et al. (2016). The New Antitumor Drug ABTL0812 Inhibits the Akt/mTORC1 Axis by Upregulating Tribbles-3 Pseudokinase. Clin. Cancer Res. 22, 2508–2519. doi:10.1158/1078-0432.CCR-15-1808
Evans, W. J., Morley, J. E., Argilés, J., Bales, C., Baracos, V., Guttridge, D., et al. (2008). Cachexia: a New Definition. Clin. Nutr. 27, 793–799. doi:10.1016/j.clnu.2008.06.013
Fabbri, M., Croce, C. M., and Calin, G. A. (2008). MicroRNAs. Cancer J. 14, 1–6. doi:10.1097/PPO.0b013e318164145e
Fan, S., Zhang, C., Luo, T., Wang, J., Tang, Y., Chen, Z., et al. (2019a). Limonin: A Review of its Pharmacology, Toxicity, and Pharmacokinetics. Basel, Switzerland: Molecules, 24. doi:10.3390/molecules24203679
Fan, Y., Yi, W., Huang, H., Mei, Z., and Feng, Z. (2019b). Efficacy of Herbal Medicine (Gegen Qinlian Decoction) on Ulcerative Colitis: A Systematic Review of Randomized Controlled Trials. Medicine (Baltimore) 98, e18512. doi:10.1097/MD.0000000000018512
Fang, J., Huang, X., Yang, Y., Wang, X., Liang, X., and Liu, J. (2021). Berberine-photodynamic Induced Apoptosis by Activating Endoplasmic Reticulum Stress-Autophagy Pathway Involving CHOP in Human Malignant Melanoma Cells. Biochem. Biophys. Res. Commun. 552, 183–190. doi:10.1016/j.bbrc.2021.02.147
Feng, J., Li, H., Zhao, W., Dang, H., Wang, R., Luo, K., et al. (2018). Biological-Profiling-Based Systematic Analysis of Rhizoma Coptidis from Different Growing Regions and its Anticholesterol Biosynthesis Activity on HepG2 Cells. Mol. Pharm. 15, 2234–2245. doi:10.1021/acs.molpharmaceut.8b00078
Filipowicz, W., Bhattacharyya, S. N., and Sonenberg, N. (2008). Mechanisms of post-transcriptional Regulation by microRNAs: Are the Answers in Sight? Nat. Rev. Genet. 9, 102–114. doi:10.1038/nrg2290
Fiskus, W., Sharma, S., Shah, B., Portier, B. P., Devaraj, S. G., Liu, K., et al. (2014). Highly Effective Combination of LSD1 (KDM1A) Antagonist and Pan-Histone Deacetylase Inhibitor against Human AML Cells. Leukemia 28, 2155–2164. doi:10.1038/leu.2014.119
Floriano, B. F., Carvalho, T., Lopes, T. Z., Takahashi, L. A. U., Rahal, P., Tedesco, A. C., et al. (2021). Effect of Berberine Nanoemulsion Photodynamic Therapy on Cervical Carcinoma Cell Line. Photodiagnosis Photodyn Ther. 33, 102174. doi:10.1016/j.pdpdt.2020.102174
Folkman, J., and Shing, Y. (1992). Angiogenesis. J. Biol. Chem. 267, 10931–10934. doi:10.1016/s0021-9258(19)49853-0
Folkman, J. (1990). What Is the Evidence that Tumors Are Angiogenesis Dependent? J. Natl. Cancer Inst. 82, 4–6. doi:10.1093/jnci/82.1.4
Foulkes, W. D., Priest, J. R., and Duchaine, T. F. (2014). DICER1: Mutations, microRNAs and Mechanisms. Nat. Rev. Cancer 14, 662–672. doi:10.1038/nrc3802
Frank, D. A. (2007). STAT3 as a central Mediator of Neoplastic Cellular Transformation. Cancer Lett. 251, 199–210. doi:10.1016/j.canlet.2006.10.017
Fujita, J., Tsujinaka, T., Yano, M., Ebisui, C., Saito, H., Katsume, A., et al. (1996). Anti-interleukin-6 Receptor Antibody Prevents Muscle Atrophy in colon-26 Adenocarcinoma-Bearing Mice with Modulation of Lysosomal and ATP-ubiquitin-dependent Proteolytic Pathways. Int. J. Cancer 68, 637–643. doi:10.1002/(SICI)1097-0215(19961127)68:5<637::AID-IJC14>3.0.CO;2-Z
Gao, R., Kong, C., Li, H., Huang, L., Qu, X., Qin, N., et al. (2017). Dysbiosis Signature of Mycobiota in colon Polyp and Colorectal Cancer. Eur. J. Clin. Microbiol. Infect. Dis. 36, 2457–2468. doi:10.1007/s10096-017-3085-6
Gao, X., Wang, J., Li, M., Wang, J., Lv, J., Zhang, L., et al. (2019). Berberine Attenuates XRCC1-Mediated Base Excision Repair and Sensitizes Breast Cancer Cells to the Chemotherapeutic Drugs. J. Cel Mol Med 23, 6797–6804. doi:10.1111/jcmm.14560
Genander, M., and Frisén, J. (2010). Ephrins and Eph Receptors in Stem Cells and Cancer. Curr. Opin. Cel Biol 22, 611–616. doi:10.1016/j.ceb.2010.08.005
Germain, S., and Eichmann, A. (2010). VEGF and Ephrin-B2: a Bloody Duo. Nat. Med. 16, 752–754. doi:10.1038/nm0710-752
Gong, J., Li, J., Dong, H., Chen, G., Qin, X., Hu, M., et al. (2019a). Inhibitory Effects of Berberine on Proinflammatory M1 Macrophage Polarization through Interfering with the Interaction between TLR4 and MyD88. BMC Complement. Altern. Med. 19, 314. doi:10.1186/s12906-019-2710-6
Gong, J., Zhou, S., and Yang, S. (2019b). Vanillic Acid Suppresses HIF-1α Expression via Inhibition of mTOR/p70S6K/4E-BP1 and Raf/MEK/ERK Pathways in Human Colon Cancer HCT116 Cells. Int. J. Mol. Sci. 20, 465. doi:10.3390/ijms20030465
Gu, C., Li, T., Yin, Z., Chen, S., Fei, J., Shen, J., et al. (2017). Integrative Analysis of Signaling Pathways and Diseases Associated with the miR-106b/25 Cluster and Their Function Study in Berberine-Induced Multiple Myeloma Cells. Funct. Integr. Genomics 17, 253–262. doi:10.1007/s10142-016-0519-7
Gu, Y., and Zhou, Z. (2021). Berberine Inhibits the Proliferation, Invasion and Migration of Endometrial Stromal Cells by Downregulating miR-429. Mol. Med. Rep. 23, 416. doi:10.3892/mmr.2021.12055
Guan, X., Zheng, X., Vong, C. T., Zhao, J., Xiao, J., Wang, Y., et al. (2020). Combined Effects of Berberine and Evodiamine on Colorectal Cancer Cells and Cardiomyocytes In Vitro. Eur. J. Pharmacol. 875, 173031. doi:10.1016/j.ejphar.2020.173031
Gunnarsson, C., Chen, J., Rizzo, J. A., Ladapo, J. A., and Lofland, J. H. (2012). Direct Health Care Insurer and Out-Of-Pocket Expenditures of Inflammatory Bowel Disease: Evidence from a US National Survey. Dig. Dis. Sci. 57, 3080–3091. doi:10.1007/s10620-012-2289-y
Guo, W., Tan, H. Y., Li, S., Wang, N., and Feng, Y. (2020). Glutamic-Pyruvic Transaminase 1 Facilitates Alternative Fuels for Hepatocellular Carcinoma Growth-A Small Molecule Inhibitor, Berberine. Cancers (Basel) 12, 1854. doi:10.3390/cancers12071854
Gupta, S., and Gupta, M. K. (2017). Possible Role of Nanocarriers in Drug Delivery against Cervical Cancer. Nano Rev. Exp. 8, 1335567. doi:10.1080/20022727.2017.1335567
Gurtner, A., Falcone, E., Garibaldi, F., and Piaggio, G. (2016). Dysregulation of microRNA Biogenesis in Cancer: the Impact of Mutant P53 on Drosha Complex Activity. J. Exp. Clin. Cancer Res. 35, 45. doi:10.1186/s13046-016-0319-x
Habtemariam, S. (2016). Berberine and Inflammatory Bowel Disease: A Concise Review. Pharmacol. Res. 113, 592–599. doi:10.1016/j.phrs.2016.09.041
Habtemariam, S. (2020). Berberine Pharmacology and the Gut Microbiota: A Hidden Therapeutic Link. Pharmacol. Res. 155, 104722. doi:10.1016/j.phrs.2020.104722
Han, B., Jiang, P., Li, Z., Yu, Y., Huang, T., Ye, X., et al. (2018). Coptisine-induced Apoptosis in Human colon Cancer Cells (HCT-116) Is Mediated by PI3K/Akt and Mitochondrial-Associated Apoptotic Pathway. Phytomedicine 48, 152–160. doi:10.1016/j.phymed.2017.12.027
Han, B., Jiang, P., Xu, H., Liu, W., Zhang, J., Wu, S., et al. (2019). 8-Cetylcoptisine, a New Coptisine Derivative, Induces Mitochondria-dependent Apoptosis and G0/G1 Cell Cycle Arrest in Human A549 Cells. Chem. Biol. Interact 299, 27–36. doi:10.1016/j.cbi.2018.11.005
Hara, A., Iizuka, N., Hamamoto, Y., Uchimura, S., Miyamoto, T., Tsunedomi, R., et al. (2005). Molecular Dissection of a Medicinal Herb with Anti-tumor Activity by Oligonucleotide Microarray. Life Sci. 77, 991–1002. doi:10.1016/j.lfs.2005.01.018
Harris, L. J., Patel, K., and Martin, M. (2020). Novel Therapies for Relapsed or Refractory Diffuse Large B-Cell Lymphoma. Int. J. Mol. Sci. 21, 8553. doi:10.3390/ijms21228553
Haupt, Y., Maya, R., Kazaz, A., and Oren, M. (1997). Mdm2 Promotes the Rapid Degradation of P53. Nature 387, 296–299. doi:10.1038/387296a0
Havaki, S., Kouloukoussa, M., Amawi, K., Drosos, Y., Arvanitis, L. D., Goutas, N., et al. (2007). Altered Expression Pattern of Integrin Alphavbeta3 Correlates with Actin Cytoskeleton in Primary Cultures of Human Breast Cancer. Cancer Cel Int 7, 16. doi:10.1186/1475-2867-7-16
He, C., Rong, R., Liu, J., Wan, J., Zhou, K., and Kang, J. X. (2012a). Effects of Coptis Extract Combined with Chemotherapeutic Agents on ROS Production, Multidrug Resistance, and Cell Growth in A549 Human Lung Cancer Cells. Chin. Med. 7, 11. doi:10.1186/1749-8546-7-11
He, W., Wang, B., Zhuang, Y., Shao, D., Sun, K., and Chen, J. (2012b). Berberine Inhibits Growth and Induces G1 Arrest and Apoptosis in Human Cholangiocarcinoma QBC939 Cells. J. Pharmacol. Sci. 119, 341–348. doi:10.1254/jphs.12052fp
He, Y., Zeng, M. Y., Yang, D., Motro, B., and Núñez, G. (2016). NEK7 Is an Essential Mediator of NLRP3 Activation Downstream of Potassium Efflux. Nature 530, 354–357. doi:10.1038/nature16959
Hong, M., Almutairi, M. M., Li, S., and Li, J. (2020). Wogonin Inhibits Cell Cycle Progression by Activating the Glycogen Synthase Kinase-3 Beta in Hepatocellular Carcinoma. Phytomedicine 68, 153174. doi:10.1016/j.phymed.2020.153174
Hong, X., Huang, H., Qiu, X., Ding, Z., Feng, X., Zhu, Y., et al. (2018). Targeting Posttranslational Modifications of RIOK1 Inhibits the Progression of Colorectal and Gastric Cancers. eLife 7, e29511. doi:10.7554/eLife.29511
Hsu, W. H., Hsieh, Y. S., Kuo, H. C., Teng, C. Y., Huang, H. I., Wang, C. J., et al. (2007). Berberine Induces Apoptosis in SW620 Human Colonic Carcinoma Cells through Generation of Reactive Oxygen Species and Activation of JNK/p38 MAPK and FasL. Arch. Toxicol. 81, 719–728. doi:10.1007/s00204-006-0169-y
Hu, F., Tao, Z., Shen, Z., Wang, X., and Hua, F. (2014). Down-regulation of EphB4 Phosphorylation Is Necessary for Esophageal Squamous Cell Carcinoma Tumorigenecity. Tumour Biol. 35, 7225–7232. doi:10.1007/s13277-014-1955-4
Hu, H. Y., Li, K. P., Wang, X. J., Liu, Y., Lu, Z. G., Dong, R. H., et al. (2013). Set9, NF-Κb, and microRNA-21 Mediate Berberine-Induced Apoptosis of Human Multiple Myeloma Cells. Acta Pharmacol. Sin 34, 157–166. doi:10.1038/aps.2012.161
Hu, W. Q., Wang, W., Fang, D. L., and Yin, X. F. (2018). Identification of Biological Targets of Therapeutic Intervention for Hepatocellular Carcinoma by Integrated Bioinformatical Analysis. Med. Sci. Monit. 24, 3450–3461. doi:10.12659/MSM.909290
Hu, X., Xiang, D., Xie, Y., Tao, L., Zhang, Y., Jin, Y., et al. (2019). LSD1 Suppresses Invasion, Migration and Metastasis of Luminal Breast Cancer Cells via Activation of GATA3 and Repression of TRIM37 Expression. Oncogene 38, 7017–7034. doi:10.1038/s41388-019-0923-2
Huang, C., Liu, H., Gong, X. L., Wu, L. Y., and Wen, B. (2017a). Effect of Evodiamine and Berberine on the Interaction between DNMTs and Target microRNAs during Malignant Transformation of the colon by TGF-Β1. Oncol. Rep. 37, 1637–1645. doi:10.3892/or.2017.5379
Huang, G., and Li, M. (2015). The Role of EphB4 and IGF-IR Expression in Breast Cancer Cells. Int. J. Clin. Exp. Pathol. 8, 5997–6004.
Huang, J., Feng, W., Li, S., Tang, H., Qin, S., Li, W., et al. (2021). Berberine Exerts Anti-cancer Activity by Modulating Adenosine Monophosphate- Activated Protein Kinase (AMPK) and the Phosphatidylinositol 3-Kinase/Protein Kinase B (PI3K/AKT) Signaling Pathways. Curr. Pharm. Des. 27, 565–574. doi:10.2174/1381612826666200928155728
Huang, L., Lin, J. X., Yu, Y. H., Zhang, M. Y., Wang, H. Y., and Zheng, M. (2012). Downregulation of Six microRNAs Is Associated with Advanced Stage, Lymph Node Metastasis and Poor Prognosis in Small Cell Carcinoma of the Cervix. PloS one 7, e33762. doi:10.1371/journal.pone.0033762
Huang, T., Xiao, Y., Yi, L., Li, L., Wang, M., Tian, C., et al. (2017b). Coptisine from Rhizoma Coptidis Suppresses HCT-116 Cells-Related Tumor Growth In Vitro and In Vivo. Sci. Rep. 7, 38524. doi:10.1038/srep38524
Hyun, M. S., Hur, J. M., Mun, Y. J., Kim, D., and Woo, W. H. (2010). BBR Induces Apoptosis in HepG2 Cell through an Akt-ASK1-ROS-p38MAPKs-Linked cascade. J. Cel Biochem 109, 329–338. doi:10.1002/jcb.22384
Iizuka, N., Hazama, S., Yoshimura, K., Yoshino, S., Tangoku, A., Miyamoto, K., et al. (2002). Anticachectic Effects of the Natural Herb Coptidis Rhizoma and Berberine on Mice Bearing colon 26/clone 20 Adenocarcinoma. Int. J. Cancer 99, 286–291. doi:10.1002/ijc.10338
Iizuka, N., Miyamoto, K., Hazama, S., Yoshino, S., Yoshimura, K., Okita, K., et al. (2000). Anticachectic Effects of Coptidis Rhizoma, an Anti-inflammatory Herb, on Esophageal Cancer Cells that Produce Interleukin 6. Cancer Lett. 158, 35–41. doi:10.1016/s0304-3835(00)00496-1
Itoh, K., Yoshioka, K., Akedo, H., Uehata, M., Ishizaki, T., and Narumiya, S. (1999). An Essential Part for Rho-Associated Kinase in the Transcellular Invasion of Tumor Cells. Nat. Med. 5, 221–225. doi:10.1038/5587
Iwai, Y., Hamanishi, J., Chamoto, K., and Honjo, T. (2017). Cancer Immunotherapies Targeting the PD-1 Signaling Pathway. J. Biomed. Sci. 24, 26. doi:10.1186/s12929-017-0329-9
Iwasa, K., Moriyasu, M., Yamori, T., Turuo, T., Lee, D. U., and Wiegrebe, W. (2001). In Vitro cytotoxicity of the Protoberberine-type Alkaloids. J. Nat. Prod. 64, 896–898. doi:10.1021/np000554f
Jabbarzadeh Kaboli, P., Rahmat, A., Ismail, P., and Ling, K. H. (2014). Targets and Mechanisms of Berberine, a Natural Drug with Potential to Treat Cancer with Special Focus on Breast Cancer. Eur. J. Pharmacol. 740, 584–595. doi:10.1016/j.ejphar.2014.06.025
Jackson, M., Krassowska, A., Gilbert, N., Chevassut, T., Forrester, L., Ansell, J., et al. (2004). Severe Global DNA Hypomethylation Blocks Differentiation and Induces Histone Hyperacetylation in Embryonic Stem Cells. Mol. Cel Biol 24, 8862–8871. doi:10.1128/MCB.24.20.8862-8871.2004
Jaye, K., Li, C. G., and Bhuyan, D. J. (2021). The Complex Interplay of Gut Microbiota with the Five Most Common Cancer Types: From Carcinogenesis to Therapeutics to Prognoses. Crit. Rev. Oncol. Hematol. 165, 103429. doi:10.1016/j.critrevonc.2021.103429
Ji, L. J., Shen, W. X., Zhang, F., Qian, J., Jiang, J., Weng, L. P., et al. (2021b). Worenine Reverses the Warburg Effect and Inhibits colon Cancer Cell Growth by Negatively Regulating HIF-1 Alpha. Cell Mol. Biol. Lett. 26, 19. doi:10.1186/s11658-021-00263-y
Ji, L., Shen, W., Zhang, F., Qian, J., Jiang, J., Weng, L., et al. (2021a). Worenine Reverses the Warburg Effect and Inhibits colon Cancer Cell Growth by Negatively Regulating HIF-1α. Cell Mol Biol Lett 26, 19. doi:10.1186/s11658-021-00263-y
Jia, H., Yang, Q., Wang, T., Cao, Y., Jiang, Q. Y., Ma, H. D., et al. (2016). Rhamnetin Induces Sensitization of Hepatocellular Carcinoma Cells to a Small Molecular Kinase Inhibitor or Chemotherapeutic Agents. Biochim. Biophys. Acta 1860, 1417–1430. doi:10.1016/j.bbagen.2016.04.007
Jiang, J., Eliaz, I., and Sliva, D. (2011). Suppression of Growth and Invasive Behavior of Human Prostate Cancer Cells by ProstaCaid™: Mechanism of Activity. Int. J. Oncol. 38, 1675–1682. doi:10.3892/ijo.2011.996
Jiang, L. H., Ge, M. H., Hou, X. X., Cao, J., Hu, S. S., Lu, X. X., et al. (2015). miR-21 Regulates Tumor Progression through the miR-21-PDCD4-Stat3 Pathway in Human Salivary Adenoid Cystic Carcinoma. Lab. Invest. 95, 1398–1408. doi:10.1038/labinvest.2015.105
Jiang, Y., Zhao, L., Chen, Q., and Zhou, L. (2021). Exploring the Mechanism of Berberine Intervention in Ulcerative Colitis from the Perspective of Inflammation and Immunity Based on Systemic Pharmacology. Evid. Based Complement. Alternat Med. 2021, 9970240. doi:10.1155/2021/9970240
Jing, W., Dong, S., Luo, X., Liu, J., Wei, B., Du, W., et al. (2021). Berberine Improves Colitis by Triggering AhR Activation by Microbial Tryptophan Catabolites. Pharmacol. Res. 164, 105358. doi:10.1016/j.phrs.2020.105358
Johnstone, R. W., Frew, A. J., and Smyth, M. J. (2008). The TRAIL Apoptotic Pathway in Cancer Onset, Progression and Therapy. Nat. Rev. Cancer 8, 782–798. doi:10.1038/nrc2465
Jordan, V. C. (2008). Tamoxifen: Catalyst for the Change to Targeted Therapy. Eur. J. Cancer 44, 30–38. doi:10.1016/j.ejca.2007.11.002
Jung, J., Choi, J. S., and Jeong, C. S. (2014). Inhibitory Activities of Palmatine from Coptis Chinensis against Helicobactor Pylori and Gastric Damage. Toxicol. Res. 30, 45–48. doi:10.5487/TR.2014.30.1.045
Kalaiarasi, A., Anusha, C., Sankar, R., Rajasekaran, S., John Marshal, J., Muthusamy, K., et al. (2016). Plant Isoquinoline Alkaloid Berberine Exhibits Chromatin Remodeling by Modulation of Histone Deacetylase to Induce Growth Arrest and Apoptosis in the A549 Cell Line. J. Agric. Food Chem. 64, 9542–9550. doi:10.1021/acs.jafc.6b04453
Kang, J., Kim, E., Kim, W., Seong, K. M., Youn, H., Kim, J. W., et al. (2013). Rhamnetin and Cirsiliol Induce Radiosensitization and Inhibition of Epithelial-Mesenchymal Transition (EMT) by miR-34a-Mediated Suppression of Notch-1 Expression in Non-small Cell Lung Cancer Cell Lines. J. Biol. Chem. 288, 27343–27357. doi:10.1074/jbc.M113.490482
Kang, M. R., and Chung, I. K. (2002). Down-regulation of DNA Topoisomerase IIalpha in Human Colorectal Carcinoma Cells Resistant to a Protoberberine Alkaloid, Berberrubine. Mol. Pharmacol. 61, 879–884. doi:10.1124/mol.61.4.879
Kang, Y.-H., Lee, J.-S., Lee, N.-H., Kim, S.-H., Seo, C.-S., and Son, C.-G. (2021). Extract Reverses 5-Fluorouracil Resistance in HCT116 Human Colorectal Cancer Cells via Modulation of Thymidylate Synthase. Basel, Switzerland: Molecules, 26. doi:10.3390/molecules26071856
Kawashima, K., Nomura, A., Makino, T., Saito, K., and Kano, Y. (2004). Pharmacological Properties of Traditional Medicine (XXIX): Effect of Hange-Shashin-To and the Combinations of its Herbal Constituents on Rat Experimental Colitis. Biol. Pharm. Bull. 27, 1599–1603. doi:10.1248/bpb.27.1599
Khwaja, A., Bjorkholm, M., Gale, R. E., Levine, R. L., Jordan, C. T., Ehninger, G., et al. (2016). Acute Myeloid Leukaemia. Nat. Rev. Dis. Primers 2, 16010. doi:10.1038/nrdp.2016.10
Kim, J.-H., Ryu, A.-R., Kang, M.-J., and Lee, M.-Y. (2016a). Berberine-induced Changes in Protein Expression and Antioxidant Enzymes in Melanoma Cells. Mol. Cel. Toxicol. 12, 53–61. doi:10.1007/s13273-016-0008-z
Kim, J. H., Ko, E. S., Kim, D., Park, S. H., Kim, E. J., Rho, J., et al. (2020). Cancer Cell-specific A-nticancer E-ffects of Coptis chinensis on G-efitinib-resistant L-ung C-ancer C-ells A-re M-ediated through the S-uppression of Mcl-1 and Bcl-2. Int. J. Oncol. 56, 1540–1550. doi:10.3892/ijo.2020.5025
Kim, S. H., Kim, E. C., Kim, W. J., Lee, M. H., Kim, S. Y., and Kim, T. J. (2016b). Coptis Japonica Makino Extract Suppresses Angiogenesis through Regulation of Cell Cycle-Related Proteins. Biosci. Biotechnol. Biochem. 80, 1095–1106. doi:10.1080/09168451.2016.1148574
Kim, S. Y., Hwangbo, H., Kim, M. Y., Ji, S. Y., Lee, H., Kim, G. Y., et al. (2021). Coptisine Induces Autophagic Cell Death through Down-Regulation of PI3K/Akt/mTOR Signaling Pathway and Up-Regulation of ROS-Mediated Mitochondrial Dysfunction in Hepatocellular Carcinoma Hep3B Cells. Arch. Biochem. Biophys. 697, 108688. doi:10.1016/j.abb.2020.108688
Kim, Y. C., and Guan, K. L. (2015). mTOR: a Pharmacologic Target for Autophagy Regulation. J. Clin. Invest. 125, 25–32. doi:10.1172/JCI73939
Kim, Y. J., Kang, S. A., Hong, M. S., Park, H. J., Kim, M. J., Park, H. J., et al. (2004). Coptidis Rhizoma Induces Apoptosis in Human Colorectal Cancer Cells SNU-C4. Am. J. Chin. Med. 32, 873–882. doi:10.1142/S0192415X0400248X
Korsmeyer, S. J. (1999). BCL-2 Gene Family and the Regulation of Programmed Cell Death. Cancer Res. 59, 1693s–1700s.
Krol, J., Loedige, I., and Filipowicz, W. (2010). The Widespread Regulation of microRNA Biogenesis, Function and Decay. Nat. Rev. Genet. 11, 597–610. doi:10.1038/nrg2843
Kumar, A., EkavaliChopra, K., Chopra, K., Mukherjee, M., Pottabathini, R., and Dhull, D. K. (2015). Current Knowledge and Pharmacological Profile of Berberine: An Update. Eur. J. Pharmacol. 761, 288–297. doi:10.1016/j.ejphar.2015.05.068
Kumar, M. S., Lu, J., Mercer, K. L., Golub, T. R., and Jacks, T. (2007). Impaired microRNA Processing Enhances Cellular Transformation and Tumorigenesis. Nat. Genet. 39, 673–677. doi:10.1038/ng2003
Kumar, R., Awasthi, M., Sharma, A., Padwad, Y., and Sharma, R. (2020). Berberine Induces Dose-dependent Quiescence and Apoptosis in A549 Cancer Cells by Modulating Cell Cyclins and Inflammation Independent of mTOR Pathway. Life Sci. 244, 117346. doi:10.1016/j.lfs.2020.117346
Kuo, H. P., Chuang, T. C., Yeh, M. H., Hsu, S. C., Way, T. D., Chen, P. Y., et al. (2011). Growth Suppression of HER2-Overexpressing Breast Cancer Cells by Berberine via Modulation of the HER2/PI3K/Akt Signaling Pathway. J. Agric. Food Chem. 59, 8216–8224. doi:10.1021/jf2012584
Lamprecht, A., Schäfer, U., and Lehr, C. M. (2001). Size-dependent Bioadhesion of Micro- and Nanoparticulate Carriers to the Inflamed Colonic Mucosa. Pharm. Res. 18, 788–793. doi:10.1023/a:1011032328064
Lan, L., Wang, Y., Pan, Z., Wang, B., Yue, Z., Jiang, Z., et al. (2019). Rhamnetin Induces Apoptosis in Human Breast Cancer Cells via the miR-34a/Notch-1 Signaling Pathway. Oncol. Lett. 17, 676–682. doi:10.3892/ol.2018.9575
Langeswaran, K., Gowthamkumar, S., Vijayaprakash, S., Revathy, R., and Balasubramanian, M. P. (2013). Influence of Limonin on Wnt Signalling Molecule in HepG2 Cell Lines. J. Nat. Sci. Biol. Med. 4, 126–133. doi:10.4103/0976-9668.107276
Lederer, M., Bley, N., Schleifer, C., and Hüttelmaier, S. (2014). The Role of the Oncofetal IGF2 mRNA-Binding Protein 3 (IGF2BP3) in Cancer. Semin. Cancer Biol. 29, 3–12. doi:10.1016/j.semcancer.2014.07.006
Lee, D. U., Kang, Y. J., Park, M. K., Lee, Y. S., Seo, H. G., Kim, T. S., et al. (2003). Effects of 13-Alkyl-Substituted Berberine Alkaloids on the Expression of COX-II, TNF-Alpha, iNOS, and IL-12 Production in LPS-Stimulated Macrophages. Life Sci. 73, 1401–1412. doi:10.1016/s0024-3205(03)00435-1
Lee, G., Joung, J.-Y., Cho, J.-H., Son, C.-G., and Lee, N. (2018). Overcoming P-Glycoprotein-Mediated Multidrug Resistance in Colorectal Cancer: Potential Reversal Agents Among Herbal Medicines. eCAM 2018, 3412074. doi:10.1155/2018/3412074
Lee, H. J., Son, D. H., Lee, S. K., Lee, J., Jun, C. D., Jeon, B. H., et al. (2006). Extract of Coptidis Rhizoma Induces Cytochrome-C Dependent Apoptosis in Immortalized and Malignant Human Oral Keratinocytes. Phytother Res. 20, 773–779. doi:10.1002/ptr.1956
Lee, I. A., Hyun, Y. J., and Kim, D. H. (2010). Berberine Ameliorates TNBS-Induced Colitis by Inhibiting Lipid Peroxidation, Enterobacterial Growth and NF-Κb Activation. Eur. J. Pharmacol. 648, 162–170. doi:10.1016/j.ejphar.2010.08.046
Lee, S., Hallis, S. P., Jung, K. A., Ryu, D., and Kwak, M. K. (2019). Impairment of HIF-1α-Mediated Metabolic Adaption by NRF2-Silencing in Breast Cancer Cells. Redox Biol. 24, 101210. doi:10.1016/j.redox.2019.101210
Lee, W. L., Cheng, M. H., Chao, H. T., and Wang, P. H. (2008). The Role of Selective Estrogen Receptor Modulators on Breast Cancer: from Tamoxifen to Raloxifene. Taiwan J. Obstet. Gynecol. 47, 24–31. doi:10.1016/S1028-4559(08)60051-0
Lei, C., Yao, Y., Shen, B., Liu, J., Pan, Q., Liu, N., et al. (2019). Columbamine Suppresses the Proliferation and Malignization of colon Cancer Cells via Abolishing Wnt/β-Catenin Signaling Pathway. Cancer Manag. Res. 11, 8635–8645. doi:10.2147/CMAR.S209861
Leng, C., Gries, M., Ziegler, J., Lokshin, A., Mascagni, P., Lentzsch, S., et al. (2006). Reduction of Graft-Versus-Host Disease by Histone Deacetylase Inhibitor Suberonylanilide Hydroxamic Acid Is Associated with Modulation of Inflammatory Cytokine Milieu and Involves Inhibition of STAT1. Exp. Hematol. 34, 776–787. doi:10.1016/j.exphem.2006.02.014
Leung, T. H., Ching, Y. P., Yam, J. W., Wong, C. M., Yau, T. O., Jin, D. Y., et al. (2005). Deleted in Liver Cancer 2 (DLC2) Suppresses Cell Transformation by Means of Inhibition of RhoA Activity. Proc. Natl. Acad. Sci. U S A. 102, 15207–15212. doi:10.1073/pnas.0504501102
Li, B., Feng, F., Jia, H., Jiang, Q., Cao, S., Wei, L., et al. (2021a). Rhamnetin Decelerates the Elimination and Enhances the Antitumor Effect of the Molecular-Targeting Agent Sorafenib in Hepatocellular Carcinoma Cells via the miR-148a/PXR axis. Food Funct. 12, 2404–2417. doi:10.1039/d0fo02270e
Li, C., Ai, G., Wang, Y., Lu, Q., Luo, C., Tan, L., et al. (2020a). Oxyberberine, a Novel Gut Microbiota-Mediated Metabolite of Berberine, Possesses superior Anti-colitis Effect: Impact on Intestinal Epithelial Barrier, Gut Microbiota Profile and TLR4-MyD88-NF-Κb Pathway. Pharmacol. Res. 152, 104603. doi:10.1016/j.phrs.2019.104603
Li, C., Dong, N., Wu, B., Mo, Z., Xie, J., and Lu, Q. (2021b). Dihydroberberine, an Isoquinoline Alkaloid, Exhibits Protective Effect against Dextran Sulfate Sodium-Induced Ulcerative Colitis in Mice. Phytomedicine 90, 153631. doi:10.1016/j.phymed.2021.153631
Li, D., Zhang, Y., Liu, K., Zhao, Y., Xu, B., Xu, L., et al. (2017a). Berberine Inhibits Colitis-Associated Tumorigenesis via Suppressing Inflammatory Responses and the Consequent EGFR Signaling-Involved Tumor Cell Growth. Lab. Invest. 97, 1343–1353. doi:10.1038/labinvest.2017.71
Li, G., Zhang, C., Liang, W., Zhang, Y., Shen, Y., and Tian, X. (2021c). Berberine Regulates the Notch1/PTEN/PI3K/AKT/mTOR Pathway and Acts Synergistically with 17-AAG and SAHA in SW480 colon Cancer Cells. Pharm. Biol. 59, 21–30. doi:10.1080/13880209.2020.1865407
Li, G. H., Wang, D. L., Hu, Y. D., Pu, P., Li, D. Z., Wang, W. D., et al. (2010). Berberine Inhibits Acute Radiation Intestinal Syndrome in Human with Abdomen Radiotherapy. Med. Oncol. 27, 919–925. doi:10.1007/s12032-009-9307-8
Li, H., Fan, C., Lu, H., Feng, C., He, P., Yang, X., et al. (2020b). Protective Role of Berberine on Ulcerative Colitis through Modulating Enteric Glial Cells-Intestinal Epithelial Cells-Immune Cells Interactions. Acta Pharm. Sin B 10, 447–461. doi:10.1016/j.apsb.2019.08.006
Li, H., Feng, C., Fan, C., Yang, Y., Yang, X., Lu, H., et al. (2020c). Intervention of Oncostatin M-Driven Mucosal Inflammation by Berberine Exerts Therapeutic Property in Chronic Ulcerative Colitis. Cell Death Dis 11, 271. doi:10.1038/s41419-020-2470-8
Li, H., Li, X. L., Zhang, M., Xu, H., Wang, C. C., Wang, S., et al. (2014a). Berberine Ameliorates Experimental Autoimmune Neuritis by Suppressing Both Cellular and Humoral Immunity. Scand. J. Immunol. 79, 12–19. doi:10.1111/sji.12123
Li, J., Liu, F., Jiang, S., Liu, J., Chen, X., Zhang, S., et al. (2018). Berberine Hydrochloride Inhibits Cell Proliferation and Promotes Apoptosis of Non-small Cell Lung Cancer via the Suppression of the MMP2 and Bcl-2/Bax Signaling Pathways. Oncol. Lett. 15, 7409–7414. doi:10.3892/ol.2018.8249
Li, J., Ni, L., Li, B., Wang, M., Ding, Z., Xiong, C., et al. (2017b). Coptis Chinensis Affects the Function of Glioma Cells through the Down-Regulation of Phosphorylation of STAT3 by Reducing HDAC3. BMC Complement. Altern. Med. 17, 524. doi:10.1186/s12906-017-2029-0
Li, J., Qiu, D. M., Chen, S. H., Cao, S. P., and Xia, X. L. (2014b). Suppression of Human Breast Cancer Cell Metastasis by Coptisine In Vitro. Asian Pac. J. Cancer Prev. 15, 5747–5751. doi:10.7314/apjcp.2014.15.14.5747
Li, J., Zhang, S., Wu, L., Pei, M., and Jiang, Y. (2021d). Berberine Inhibited Metastasis through miR-145/MMP16 axis In Vitro. J. Ovarian Res. 14, 4. doi:10.1186/s13048-020-00752-2
Li, P., Hao, Z., Liu, H., Zhu, B., Dang, L., Ma, C., et al. (2021e). Quantitative Proteomics Analysis of Berberine-Treated Colon Cancer Cells Reveals Potential Therapy Targets. Biology (Basel) 10, 250. doi:10.3390/biology10030250
Li, Q., Qu, X., Pang, X., Song, Y., Chen, L., Xiao, Q., et al. (2019a). Berberine Protects Mice against Dextran Sulfate Sodium-Induced Colitis by Activating mTORC1 Pathway. Front. Pharmacol. 10, 786. doi:10.3389/fphar.2019.00786
Li, S., Wang, N., Tan, H. Y., Chueng, F., Zhang, Z. J., Yuen, M. F., et al. (2020d). Modulation of Gut Microbiota Mediates Berberine-Induced Expansion of Immuno-Suppressive Cells to against Alcoholic Liver Disease. Clin. Transl Med. 10, e112. doi:10.1002/ctm2.112
Li, X., Zhang, A., Sun, H., Liu, Z., Zhang, T., Qiu, S., et al. (2017c). Metabolic Characterization and Pathway Analysis of Berberine Protects against Prostate Cancer. Oncotarget 8, 65022–65041. doi:10.18632/oncotarget.17531
Li, X. D., Wang, Z., Wang, X. R., Shao, D., Zhang, X., Li, L., et al. (2019b). Berberine-loaded Janus Gold Mesoporous Silica Nanocarriers for Chemo/radio/photothermal Therapy of Liver Cancer and Radiation-Induced Injury Inhibition. Int. J. Nanomedicine 14, 3967–3982. doi:10.2147/IJN.S206044
Li, Z. F., Wang, Q., Feng, Y. L., Luo, X. J., Fan, M. M., and Yang, S. L. (2012). [Chemical Constituents from Coptis Chinensis]. Zhong Yao Cai 35, 1438–1441.
Li, Z. R., Suo, F. Z., Guo, Y. J., Cheng, H. F., Niu, S. H., Shen, D. D., et al. (2020e). Natural Protoberberine Alkaloids, Identified as Potent Selective LSD1 Inhibitors, Induce AML Cell Differentiation. Bioorg. Chem. 97, 103648. doi:10.1016/j.bioorg.2020.103648
Liang, Y., Fu, D., and Hu, G. (2011). Metadherin: An Emerging Key Regulator of the Malignant Progression of Multiple Cancers. Thorac. Cancer 2, 143–148. doi:10.1111/j.1759-7714.2011.00064.x
Liao, P. H., Chen, S. L., Shih, H. C., and Chou, M. Y. (2005). Induction of Apoptosis in Human Oral Cancer Cell Lines, OC2 and TSCCa, by Chingwaysan. Am. J. Chin. Med. 33, 21–27. doi:10.1142/S0192415X0500262X
Liao, Z., Xie, Y., Zhou, B., Zou, B., Xiao, D., Liu, W., et al. (2020). Berberine Ameliorates Colonic Damage Accompanied with the Modulation of Dysfunctional Bacteria and Functions in Ulcerative Colitis Rats. Appl. Microbiol. Biotechnol. 104, 1737–1749. doi:10.1007/s00253-019-10307-1
Lin, C. C., Lin, S. Y., Chung, J. G., Lin, J. P., Chen, G. W., and Kao, S. T. (2006). Down-regulation of Cyclin B1 and Up-Regulation of Wee1 by Berberine Promotes Entry of Leukemia Cells into the G2/M-phase of the Cell Cycle. Anticancer Res. 26, 1097–1104.
Lin, C. C., Ng, L. T., Hsu, F. F., Shieh, D. E., and Chiang, L. C. (2004). Cytotoxic Effects of Coptis Chinensis and Epimedium Sagittatum Extracts and Their Major Constituents (Berberine, Coptisine and Icariin) on Hepatoma and Leukaemia Cell Growth. Clin. Exp. Pharmacol. Physiol. 31, 65–69. doi:10.1111/j.1440-1681.2004.03951.x
Lin, Z., Li, S., Guo, P., Wang, L., Zheng, L., Yan, Z., et al. (2019). Columbamine Suppresses Hepatocellular Carcinoma Cells through Down-Regulation of PI3K/AKT, P38 and ERK1/2 MAPK Signaling Pathways. Life Sci. 218, 197–204. doi:10.1016/j.lfs.2018.12.038
Lisle, J. E., Mertens-Walker, I., Stephens, C. R., Stansfield, S. H., Clements, J. A., Herington, A. C., et al. (2015). Murine, but Not Human, Ephrin-B2 Can Be Efficiently Cleaved by the Serine Protease Kallikrein-4: Implications for Xenograft Models of Human Prostate Cancer. Exp. Cel Res 333, 136–146. doi:10.1016/j.yexcr.2015.02.014
Liu, C. S., Zheng, Y. R., Zhang, Y. F., and Long, X. Y. (2016). Research Progress on Berberine with a Special Focus on its Oral Bioavailability. Fitoterapia 109, 274–282. doi:10.1016/j.fitote.2016.02.001
Liu, D., Meng, X., Wu, D., Qiu, Z., and Luo, H. (2019). A Natural Isoquinoline Alkaloid with Antitumor Activity: Studies of the Biological Activities of Berberine. Front. Pharmacol. 10, 9. doi:10.3389/fphar.2019.00009
Liu, J., He, C., Zhou, K., Wang, J., and Kang, J. X. (2009). Coptis Extracts Enhance the Anticancer Effect of Estrogen Receptor Antagonists on Human Breast Cancer Cells. Biochem. Biophys. Res. Commun. 378, 174–178. doi:10.1016/j.bbrc.2008.10.169
Liu, J., Liu, P., Xu, T., Chen, Z., Kong, H., Chu, W., et al. (2020a). Berberine Induces Autophagic Cell Death in Acute Lymphoblastic Leukemia by Inactivating AKT/mTORC1 Signaling. Drug Des. Devel Ther. 14, 1813–1823. doi:10.2147/DDDT.S239247
Liu, J., Luo, X., Guo, R., Jing, W., and Lu, H. (2020b). Cell Metabolomics Reveals Berberine-Inhibited Pancreatic Cancer Cell Viability and Metastasis by Regulating Citrate Metabolism. J. Proteome Res. 19, 3825–3836. doi:10.1021/acs.jproteome.0c00394
Liu, J., Zhu, Z., Liu, Y., Wei, L., Li, B., Mao, F., et al. (2020c). MDM2 Inhibition-Mediated Autophagy Contributes to the Pro-apoptotic Effect of Berberine in P53-Null Leukemic Cells. Life Sci. 242, 117228. doi:10.1016/j.lfs.2019.117228
Liu, L., Li, J., and He, Y. (2020d). Multifunctional Epiberberine Mediates Multi-Therapeutic Effects. Fitoterapia 147, 104771. doi:10.1016/j.fitote.2020.104771
Liu, R., Cao, Z., Pan, Y., Zhang, G., Yang, P., Guo, P., et al. (2013a). Jatrorrhizine Hydrochloride Inhibits the Proliferation and Neovascularization of C8161 Metastatic Melanoma Cells. Anticancer Drugs 24, 667–676. doi:10.1097/CAD.0b013e328361ab28
Liu, S., Fang, Y., Shen, H., Xu, W., and Li, H. (2013b). Berberine Sensitizes Ovarian Cancer Cells to Cisplatin through miR-21/PDCD4 axis. Acta Biochim. Biophys. Sin (Shanghai) 45, 756–762. doi:10.1093/abbs/gmt075
Liu, X., Ji, Q., Ye, N., Sui, H., Zhou, L., Zhu, H., et al. (2015a). Berberine Inhibits Invasion and Metastasis of Colorectal Cancer Cells via COX-2/PGE2 Mediated JAK2/STAT3 Signaling Pathway. PloS one 10, e0123478. doi:10.1371/journal.pone.0123478
Liu, X., Zhang, Y., Wu, S., Xu, M., Shen, Y., Yu, M., et al. (2020e). Palmatine Induces G2/M Phase Arrest and Mitochondrial-Associated Pathway Apoptosis in colon Cancer Cells by Targeting AURKA. Biochem. Pharmacol. 175, 113933. doi:10.1016/j.bcp.2020.113933
Liu, X., Zhang, Y., Zhou, G. J., Hou, Y., Kong, Q., Lu, J. J., et al. (2020f). Natural Alkaloid 8-Oxo-Epiberberine Inhibited TGF-Β1-Triggred Epithelial-Mesenchymal Transition by Interfering Smad3. Toxicol. Appl. Pharmacol. 404, 115179. doi:10.1016/j.taap.2020.115179
Liu, Y., Kong, X., Li, X., Li, B., and Yang, Q. (2015b). Knockdown of Metadherin Inhibits Angiogenesis in Breast Cancer. Int. J. Oncol. 46, 2459–2466. doi:10.3892/ijo.2015.2973
Liu, Y., Liu, X., Zhang, N., Yin, M., Dong, J., Zeng, Q., et al. (2020g). Berberine Diminishes Cancer Cell PD-L1 Expression and Facilitates Antitumor Immunity via Inhibiting the Deubiquitination Activity of CSN5. Acta Pharm. Sin B 10, 2299–2312. doi:10.1016/j.apsb.2020.06.014
Liu, Y., Yu, H., Zhang, C., Cheng, Y., Hu, L., Meng, X., et al. (2008b). Protective Effects of Berberine on Radiation-Induced Lung Injury via Intercellular Adhesion Molecular-1 and Transforming Growth Factor-Beta-1 in Patients with Lung Cancer. Eur. J. Cancer 44, 2425–2432. doi:10.1016/j.ejca.2008.07.040
Liu, Y., Yu, H., Zhang, C., Cheng, Y., Hu, L., Meng, X., et al. (2008a). Protective Effects of Berberine on Radiation-Induced Lung Injury via Intercellular Adhesion Molecular-1 and Transforming Growth Factor-Beta-1 in Patients with Lung Cancer. Eur. J. Cancer 44, 2425–2432. doi:10.1016/j.ejca.2008.07.040
Lo, T. F., Tsai, W. C., and Chen, S. T. (2013). MicroRNA-21-3p, a Berberine-Induced miRNA, Directly Down-Regulates Human Methionine Adenosyltransferases 2A and 2B and Inhibits Hepatoma Cell Growth. PloS one 8, e75628. doi:10.1371/journal.pone.0075628
Long, J., Song, J., Zhong, L., Liao, Y., Liu, L., and Li, X. (2019). Palmatine: A Review of its Pharmacology, Toxicity and Pharmacokinetics. Biochimie 162, 176–184. doi:10.1016/j.biochi.2019.04.008
Lu, B., Zhao, J., Xu, L., Xu, Y., Wang, X., and Peng, J. (2012). Identification of Molecular Target Proteins in Berberine-Treated Cervix Adenocarcinoma HeLa Cells by Proteomic and Bioinformatic Analyses. Phytother Res. 26, 646–656. doi:10.1002/ptr.3615
Lu, J., Getz, G., Miska, E. A., Alvarez-Saavedra, E., Lamb, J., Peck, D., et al. (2005). MicroRNA Expression Profiles Classify Human Cancers. Nature 435, 834–838. doi:10.1038/nature03702
Lu, J. Z., Ye, D., and Ma, B. L. (2021). Constituents, Pharmacokinetics, and Pharmacology of Gegen-Qinlian Decoction. Front. Pharmacol. 12, 668418. doi:10.3389/fphar.2021.668418
Lü, Y., Han, B., Yu, H., Cui, Z., Li, Z., and Wang, J. (2018). Berberine Regulates the microRNA-21-Itgβ4-PDCD4 axis and Inhibits colon Cancer Viability. Oncol. Lett. 15, 5971–5976. doi:10.3892/ol.2018.7997
Luo, X., Gu, J., Zhu, R., Feng, M., Zhu, X., Li, Y., et al. (2014). Integrative Analysis of Differential miRNA and Functional Study of miR-21 by Seed-Targeting Inhibition in Multiple Myeloma Cells in Response to Berberine. BMC Syst. Biol. 8, 82. doi:10.1186/1752-0509-8-82
Lv, J., Xia, Q., Wang, J., Shen, Q., Zhang, J., and Zhou, X. (2016). EphB4 Promotes the Proliferation, Invasion, and Angiogenesis of Human Colorectal Cancer. Exp. Mol. Pathol. 100, 402–408. doi:10.1016/j.yexmp.2016.03.011
Lyu, Y., Lin, L., Xie, Y., Li, D., Xiao, M., Zhang, Y., et al. (2021). Blood-Glucose-Lowering Effect of Coptidis Rhizoma Extracts from Different Origins via Gut Microbiota Modulation in Db/db Mice. Front. Pharmacol. 12, 684358. doi:10.3389/fphar.2021.684358
Ma, W., Zhang, Y., Yu, M., Wang, B., Xu, S., Zhang, J., et al. (2020). In-vitro and In-Vivo Anti-breast Cancer Activity of Synergistic Effect of Berberine and Exercise through Promoting the Apoptosis and Immunomodulatory Effects. Int. Immunopharmacol 87, 106787. doi:10.1016/j.intimp.2020.106787
Ma, W., Zhu, M., Zhang, D., Yang, L., Yang, T., Li, X., et al. (2017). Berberine Inhibits the Proliferation and Migration of Breast Cancer ZR-75-30 Cells by Targeting Ephrin-B2. Phytomedicine 25, 45–51. doi:10.1016/j.phymed.2016.12.013
Ma, W. K., Li, H., Dong, C. L., He, X., Guo, C. R., Zhang, C. F., et al. (2016). Palmatine from Mahonia Bealei Attenuates Gut Tumorigenesis in ApcMin/+ Mice via Inhibition of Inflammatory Cytokines. Mol. Med. Rep. 14, 491–498. doi:10.3892/mmr.2016.5285
Mai, A., Massa, S., Rotili, D., Cerbara, I., Valente, S., Pezzi, R., et al. (2005). Histone Deacetylation in Epigenetics: an Attractive Target for Anticancer Therapy. Med. Res. Rev. 25, 261–309. doi:10.1002/med.20024
Mancarella, C., Pasello, M., Manara, M. C., Toracchio, L., Sciandra, E. F., Picci, P., et al. (2018). Insulin-Like Growth Factor 2 mRNA-Binding Protein 3 Influences Sensitivity to Anti-IGF System Agents through the Translational Regulation of IGF1R. Front. Endocrinol. (Lausanne) 9, 178. doi:10.3389/fendo.2018.00178
Mantena, S. K., Sharma, S. D., and Katiyar, S. K. (2006). Berberine, a Natural Product, Induces G1-phase Cell Cycle Arrest and Caspase-3-dependent Apoptosis in Human Prostate Carcinoma Cells. Mol. Cancer Ther. 5, 296–308. doi:10.1158/1535-7163.MCT-05-0448
Mármol, I., Sánchez-de-Diego, C., Pradilla Dieste, A., Cerrada, E., and Rodriguez Yoldi, M. J. (2017). Colorectal Carcinoma: A General Overview and Future Perspectives in Colorectal Cancer. Int. J. Mol. Sci. 18, 197. doi:10.3390/ijms18010197
Martínez-Carreres, L., Puyal, J., Leal-Esteban, L. C., Orpinell, M., Castillo-Armengol, J., Giralt, A., et al. (2019). CDK4 Regulates Lysosomal Function and mTORC1 Activation to Promote Cancer Cell Survival. Cancer Res. 79, 5245–5259. doi:10.1158/0008-5472.CAN-19-0708
Matson, V., Chervin, C. S., and Gajewski, T. F. (2021). Cancer and the Microbiome-Influence of the Commensal Microbiota on Cancer, Immune Responses, and Immunotherapy. Gastroenterology 160, 600–613. doi:10.1053/j.gastro.2020.11.041
Matsumoto, C., Sekine-Suzuki, E., Nyui, M., Ueno, M., Nakanishi, I., Omiya, Y., et al. (2015). Analysis of the Antioxidative Function of the Radioprotective Japanese Traditional (Kampo) Medicine, Hangeshashinto, in an Aqueous Phase. J. Radiat. Res. 56, 669–677. doi:10.1093/jrr/rrv023
Meeran, S. M., Katiyar, S., and Katiyar, S. K. (2008). Berberine-induced Apoptosis in Human Prostate Cancer Cells Is Initiated by Reactive Oxygen Species Generation. Toxicol. Appl. Pharmacol. 229, 33–43. doi:10.1016/j.taap.2007.12.027
Meng, F. C., Wu, Z. F., Yin, Z. Q., Lin, L. G., Wang, R., and Zhang, Q. W. (2018). Coptidis Rhizoma and its Main Bioactive Components: Recent Advances in Chemical Investigation, Quality Evaluation and Pharmacological Activity. Chin. Med. 13, 13. doi:10.1186/s13020-018-0171-3
Merchant, A. A., Jorapur, A., Mcmanus, A., Liu, R., Krasnoperov, V., Chaudhry, P., et al. (2017). EPHB4 Is a Therapeutic Target in AML and Promotes Leukemia Cell Survival via AKT. Blood Adv. 1, 1635–1644. doi:10.1182/bloodadvances.2017005694
Mertens-Walker, I., Fernandini, B. C., Maharaj, M. S., Rockstroh, A., Nelson, C. C., Herington, A. C., et al. (2015). The Tumour-Promoting Receptor Tyrosine Kinase, EphB4, Regulates Expression of Integrin-Β8 in Prostate Cancer Cells. BMC cancer 15, 164. doi:10.1186/s12885-015-1164-6
Min, Y. D., Yang, M. C., Lee, K. H., Kim, K. R., Choi, S. U., and Lee, K. R. (2006). Protoberberine Alkaloids and Their Reversal Activity of P-Gp Expressed Multidrug Resistance (MDR) from the Rhizome of Coptis Japonica Makino. Arch. Pharm. Res. 29, 757–761. doi:10.1007/BF02974076
Ming, H., Mohammed, M. A., Li, S. Y., and Li, J. K. (2020). Wogonin Inhibits Cell Cycle Progression by Activating the Glycogen Synthase Kinase-3 Beta in Hepatocellular Carcinoma. Phytomedicine 68, 153174. doi:10.1016/j.phymed.2020.153174
Mohammadi, A., Mansoori, B., and Baradaran, B. (2017). Regulation of miRNAs by Herbal Medicine: An Emerging Field in Cancer Therapies. Biomed. Pharmacother. 86, 262–270. doi:10.1016/j.biopha.2016.12.023
Momand, J., Zambetti, G. P., Olson, D. C., George, D., and Levine, A. J. (1992). The Mdm-2 Oncogene Product Forms a Complex with the P53 Protein and Inhibits P53-Mediated Transactivation. Cell 69, 1237–1245. doi:10.1016/0092-8674(92)90644-r
Nabeshima, K., Inoue, T., Shimao, Y., and Sameshima, T. (2002). Matrix Metalloproteinases in Tumor Invasion: Role for Cell Migration. Pathol. Int. 52, 255–264. doi:10.1046/j.1440-1827.2002.01343.x
Nguyen, T. L., Wilson, M. G., and Hiscott, J. (2010). Oncolytic Viruses and Histone Deacetylase Inhibitors-Aa Multi-Pronged Strategy to Target Tumor Cells. Cytokine Growth Factor. Rev. 21, 153–159. doi:10.1016/j.cytogfr.2010.03.002
Ning, H., Lu, W., Jia, Q., Wang, J., Yao, T., Lv, S., et al. (2021). Discovery of Oxyepiberberine as a Novel Tubulin Polymerization Inhibitor and an Anti-colon Cancer Agent against LS-1034 Cells. Invest. New Drugs 39, 386–393. doi:10.1007/s10637-020-01006-0
Nørøxe, D. S., Poulsen, H. S., and Lassen, U. (2016). Hallmarks of Glioblastoma: a Systematic Review. ESMO open 1, e000144. doi:10.1136/esmoopen-2016-000144
O'carroll, D., and Schaefer, A. (2013). General Principals of miRNA Biogenesis and Regulation in the Brain. Neuropsychopharmacology 38, 39–54. doi:10.1038/npp.2012.87
Oka, M., Yamamoto, K., Takahashi, M., Hakozaki, M., Abe, T., Iizuka, N., et al. (1996). Relationship between Serum Levels of Interleukin 6, Various Disease Parameters and Malnutrition in Patients with Esophageal Squamous Cell Carcinoma. Cancer Res. 56, 2776–2780.
Oliner, J. D., Kinzler, K. W., Meltzer, P. S., George, D. L., and Vogelstein, B. (1992). Amplification of a Gene Encoding a P53-Associated Protein in Human Sarcomas. Nature 358, 80–83. doi:10.1038/358080a0
Oltvai, Z. N., Milliman, C. L., and Korsmeyer, S. J. (1993). Bcl-2 Heterodimerizes In Vivo with a Conserved Homolog, Bax, that Accelerates Programmed Cell Death. Cell 74, 609–619. doi:10.1016/0092-8674(93)90509-o
Omran, A. R. (1971). The Epidemiologic Transition. A Theory of the Epidemiology of Population Change. Milbank Mem. Fund Q. 49, 509–538. doi:10.2307/3349375
Özgöçmen, M., Bayram, D., Yavuz Türel, G., Toğay, V. A., and Şahin Calapoğlu, N. (2021). Secoisolariciresinol Diglucoside Induces Caspase-3-Mediated Apoptosis in Monolayer and Spheroid Cultures of Human colon Carcinoma Cells. J. Food Biochem. 45, e13719. doi:10.1111/jfbc.13719
Palma, T. V., Lenz, L. S., Bottari, N. B., Pereira, A., Schetinger, M. R. C., Morsch, V. M., et al. (2020). Berberine Induces Apoptosis in Glioblastoma Multiforme U87MG Cells via Oxidative Stress and Independent of AMPK Activity. Mol. Biol. Rep. 47, 4393–4400. doi:10.1007/s11033-020-05500-9
Paolillo, M., Boselli, C., and Schinelli, S. (2018). Glioblastoma under Siege: An Overview of Current Therapeutic Strategies. Brain Sci. 8, 15. doi:10.3390/brainsci8010015
Papaemmanuil, E., Gerstung, M., Bullinger, L., Gaidzik, V. I., Paschka, P., Roberts, N. D., et al. (2016). Genomic Classification and Prognosis in Acute Myeloid Leukemia. N. Engl. J. Med. 374, 2209–2221. doi:10.1056/NEJMoa1516192
Park, B., You, S., Cho, W. C. S., Choi, J. Y., and Lee, M. S. (2019). A Systematic Review of Herbal Medicines for the Treatment of Cancer Cachexia in Animal Models. J. Zhejiang Univ. Sci. B 20, 9–22. doi:10.1631/jzus.B1800171
Park, H. J., Kim, Y. J., Leem, K., Park, S. J., Seo, J. C., Kim, H. K., et al. (2005). Coptis Japonica Root Extract Induces Apoptosis through Caspase3 Activation in SNU-668 Human Gastric Cancer Cells. Phytother Res. 19, 189–192. doi:10.1002/ptr.1539
Park, S. H., Sung, J. H., Kim, E. J., and Chung, N. (2015). Berberine Induces Apoptosis via ROS Generation in PANC-1 and MIA-PaCa2 Pancreatic Cell Lines. Braz. J. Med. Biol. Res. 48, 111–119. doi:10.1590/1414-431X20144293
Pei, J., Fu, B., Jiang, L., and Sun, T. (2019). Biosynthesis, Characterization, and Anticancer Effect of Plant-Mediated Silver Nanoparticles Using Coptis Chinensis. Int. J. Nanomedicine 14, 1969–1978. doi:10.2147/IJN.S188235
Peng, Q. X., Yang, D. J., Shi, J., Cai, H. B., and Mo, Z. X. (2011). [Proportion of Coptidis Rhizoma and Evodiae Fructus in the Compound Preparation: its Effect in Inducing Apoptosis of SGC-7901 Cells]. Nan Fang Yi Ke Da Xue Xue Bao 31, 226–229. doi:10.12122/j.issn.1673-4254.2011.02.007
Pereira, D. M., Rodrigues, P. M., Borralho, P. M., and Rodrigues, C. M. (2013). Delivering the Promise of miRNA Cancer Therapeutics. Drug Discov. Today 18, 282–289. doi:10.1016/j.drudis.2012.10.002
Petrosino, J. F., Highlander, S., Luna, R. A., Gibbs, R. A., and Versalovic, J. (2009). Metagenomic Pyrosequencing and Microbial Identification. Clin. Chem. 55, 856–866. doi:10.1373/clinchem.2008.107565
Popat, S., Matakidou, A., and Houlston, R. S. (2004). Thymidylate Synthase Expression and Prognosis in Colorectal Cancer: A Systematic Review and Meta-Analysis. J. Clin. Oncol. 22, 529–536. doi:10.1200/JCO.2004.05.064
Puneet, , , Kazmi, H. R., Kumari, S., Tiwari, S., Khanna, A., and Narayan, G. (2018). Epigenetic Mechanisms and Events in Gastric Cancer-Emerging Novel Biomarkers. Pathol. Oncol. Res. 24, 757–770. doi:10.1007/s12253-018-0410-z
Qi, F., Sun, Y., Lv, M., Qin, F., Cao, W., and Bi, L. (2019). Effects of Palmatine Hydrochloride Mediated Photodynamic Therapy on Oral Squamous Cell Carcinoma. Photochem. Photobiol. Sci. 18, 1596–1605. doi:10.1039/c9pp00040b
Qing, Y., Hu, H., Liu, Y., Feng, T., Meng, W., Jiang, L., et al. (2014). Berberine Induces Apoptosis in Human Multiple Myeloma Cell Line U266 through Hypomethylation of P53 Promoter. Cell Biol Int 38, 563–570. doi:10.1002/cbin.10206
Qu, H., Song, X., Song, Z., Jiang, X., Gao, X., Bai, L., et al. (2020). Berberine Reduces Temozolomide Resistance by Inducing Autophagy via the ERK1/2 Signaling Pathway in Glioblastoma. Cancer Cel Int 20, 592. doi:10.1186/s12935-020-01693-y
Ramesh, G., Das, S., and Bola Sadashiva, S. R. (2020). Berberine, a Natural Alkaloid Sensitizes Human Hepatocarcinoma to Ionizing Radiation by Blocking Autophagy and Cell Cycle Arrest Resulting in Senescence. J. Pharm. Pharmacol. 72, 1893–1908. doi:10.1111/jphp.13354
Rawluk, J., and Waller, C. F. (2018). Gefitinib. Recent Results Cancer Res. 211, 235–246. doi:10.1007/978-3-319-91442-8_16
Ren, S., Cai, Y., Hu, S., Liu, J., Zhao, Y., Ding, M., et al. (2021). Berberine Exerts Anti-tumor Activity in Diffuse Large B-Cell Lymphoma by Modulating C-myc/CD47 axis. Biochem. Pharmacol. 188, 114576. doi:10.1016/j.bcp.2021.114576
Ru, J., Li, P., Wang, J., Zhou, W., Li, B., Huang, C., et al. (2014). TCMSP: a Database of Systems Pharmacology for Drug Discovery from Herbal Medicines. J. Cheminform 6, 13. doi:10.1186/1758-2946-6-13
Sain, A., Kandasamy, T., and Naskar, D. (2021). In Silico approach to Target PI3K/Akt/mTOR axis by Selected Olea Europaea Phenols in PIK3CA Mutant Colorectal Cancer. J. Biomol. Struct. Dyn. 23, 1–16. doi:10.1080/07391102.2021.1953603
Salgia, R., Kulkarni, P., and Gill, P. S. (2018). EphB4: A Promising Target for Upper Aerodigestive Malignancies. Biochim. Biophys. Acta Rev. Cancer 1869, 128–137. doi:10.1016/j.bbcan.2018.01.003
Sawamiphak, S., Seidel, S., Essmann, C. L., Wilkinson, G. A., Pitulescu, M. E., Acker, T., et al. (2010). Ephrin-B2 Regulates VEGFR2 Function in Developmental and Tumour Angiogenesis. Nature 465, 487–491. doi:10.1038/nature08995
Schürch, C. M., Roelli, M. A., Forster, S., Wasmer, M. H., Brühl, F., Maire, R. S., et al. (2019). Targeting CD47 in Anaplastic Thyroid Carcinoma Enhances Tumor Phagocytosis by Macrophages and Is a Promising Therapeutic Strategy. Thyroid 29, 979–992. doi:10.1089/thy.2018.0555
Scott, G. K., Dodson, J. M., Montgomery, P. A., Johnson, R. M., Sarup, J. C., Wong, W. L., et al. (1991). p185HER2 Signal Transduction in Breast Cancer Cells. J. Biol. Chem. 266, 14300–14305. doi:10.1016/s0021-9258(18)98683-7
Sentürk, A., Pfennig, S., Weiss, A., Burk, K., and Acker-Palmer, A. (2011). Ephrin Bs Are Essential Components of the Reelin Pathway to Regulate Neuronal Migration. Nature 472, 356–360. doi:10.1038/nature09874
Shao, J., Zhang, H., and Wang, Z. (2018). Coronin 1c and F-Actin Promote Metastasis of Breast Cancer. Med. Sci. Monit. 24, 5980–5987. doi:10.12659/MSM.908929
Shi, H. L., Wu, X. J., Liu, Y., and Xie, J. Q. (2013). Berberine Counteracts Enhanced IL-8 Expression of AGS Cells Induced by Evodiamine. Life Sci. 93, 830–839. doi:10.1016/j.lfs.2013.09.010
Shimizu, S., Miyamoto, S., Fujii, G., Nakanishi, R., Onuma, W., Ozaki, Y., et al. (2015). Suppression of Intestinal Carcinogenesis in Apc-Mutant Mice by Limonin. J. Clin. Biochem. Nutr. 57, 39–43. doi:10.3164/jcbn.15-28
Shou, J., Massarweh, S., Osborne, C. K., Wakeling, A. E., Ali, S., Weiss, H., et al. (2004). Mechanisms of Tamoxifen Resistance: Increased Estrogen Receptor-HER2/neu Cross-Talk in ER/HER2-positive Breast Cancer. J. Natl. Cancer Inst. 96, 926–935. doi:10.1093/jnci/djh166
Siegel, R. L., Miller, K. D., Fuchs, H. E., and Jemal, A. (2021). Cancer Statistics, 2021. CA A. Cancer J. Clin. 71, 7–33. doi:10.3322/caac.21654
Slattery, M. L., Lundgreen, A., Kadlubar, S. A., Bondurant, K. L., and Wolff, R. K. (2013). JAK/STAT/SOCS-signaling Pathway and colon and Rectal Cancer. Mol. Carcinog 52, 155–166. doi:10.1002/mc.21841
Smerling, C., Tang, K., Hofmann, W., and Danker, K. (2007). Role of the Alpha(1) Integrin Cytoplasmic Tail in the Formation of Focal Complexes, Actin Organization, and in the Control of Cell Migration. Exp. Cel Res 313, 3153–3165. doi:10.1016/j.yexcr.2007.06.003
Smith, Z. D., and Meissner, A. (2013). DNA Methylation: Roles in Mammalian Development. Nat. Rev. Genet. 14, 204–220. doi:10.1038/nrg3354
Song, L., Luo, Y., Wang, X., Almutairi, M. M., Pan, H., Li, W., et al. (2019). Exploring the Active Mechanism of Berberine against HCC by Systematic Pharmacology and Experimental Validation. Mol. Med. Rep. 20, 4654–4664. doi:10.3892/mmr.2019.10698
Stegehuis, J. H., De Wilt, L. H. a. M., De Vries, E. G. E., Groen, H. J., De Jong, S., and Kruyt, F. a. E. (2010). TRAIL Receptor Targeting Therapies for Non-small Cell Lung Cancer: Current Status and Perspectives. Drug Resist. Updat. 13, 2–15. doi:10.1016/j.drup.2009.11.001
Stephenson, S. A., Douglas, E. L., Mertens-Walker, I., Lisle, J. E., Maharaj, M. S., and Herington, A. C. (2015). Anti-tumour Effects of Antibodies Targeting the Extracellular Cysteine-Rich Region of the Receptor Tyrosine Kinase EphB4. Oncotarget 6, 7554–7569. doi:10.18632/oncotarget.3199
Strassmann, G., Jacob, C. O., Evans, R., Beall, D., and Fong, M. (1992). Mechanisms of Experimental Cancer Cachexia. Interaction between Mononuclear Phagocytes and colon-26 Carcinoma and its Relevance to IL-6-mediated Cancer Cachexia. J. Immunol. 148, 3674–3678.
Su, P., Zhang, Q., and Yang, Q. (2010). Immunohistochemical Analysis of Metadherin in Proliferative and Cancerous Breast Tissue. Diagn. Pathol. 5, 38. doi:10.1186/1746-1596-5-38
Su, Y. H., Tang, W. C., Cheng, Y. W., Sia, P., Huang, C. C., Lee, Y. C., et al. (2015). Targeting of Multiple Oncogenic Signaling Pathways by Hsp90 Inhibitor Alone or in Combination with Berberine for Treatment of Colorectal Cancer. Biochim. Biophys. Acta 1853, 2261–2272. doi:10.1016/j.bbamcr.2015.05.012
Su, Z., Wang, C., Chang, D., Zhu, X., Sai, C., and Pei, J. (2019). Limonin Attenuates the Stemness of Breast Cancer Cells via Suppressing MIR216A Methylation. Biomed. Pharmacother. 112, 108699. doi:10.1016/j.biopha.2019.108699
Sui, X., Kong, N., Ye, L., Han, W., Zhou, J., Zhang, Q., et al. (2014). p38 and JNK MAPK Pathways Control the Balance of Apoptosis and Autophagy in Response to Chemotherapeutic Agents. Cancer Lett. 344, 174–179. doi:10.1016/j.canlet.2013.11.019
Sun, B., Yang, Y., He, M., Jin, Y., Cao, X., Du, X., et al. (2020a). Hepatoprotective Role of Berberine on Doxorubicin Induced Hepatotoxicity - Involvement of Cyp. Curr. Drug Metab. 21, 541–547. doi:10.2174/1389200221666200620203648
Sun, X. L., Zhang, X. W., Zhai, H. J., Zhang, D., and Ma, S. Y. (2020b). Magnoflorine Inhibits Human Gastric Cancer Progression by Inducing Autophagy, Apoptosis and Cell Cycle Arrest by JNK Activation Regulated by ROS. Biomed. Pharmacother. 125, 109118. doi:10.1016/j.biopha.2019.109118
Sun, Y., Gao, X., Wu, P., Wink, M., Li, J., Dian, L., et al. (2019a). Jatrorrhizine Inhibits Mammary Carcinoma Cells by Targeting TNIK Mediated Wnt/β-Catenin Signalling and Epithelial-Mesenchymal Transition (EMT). Phytomedicine 63, 153015. doi:10.1016/j.phymed.2019.153015
Sun, Y., Wang, W., and Tong, Y. (2019b). Berberine Inhibits Proliferative Ability of Breast Cancer Cells by Reducing Metadherin. Med. Sci. Monit. 25, 9058–9066. doi:10.12659/MSM.914486
Suvasini, R., Shruti, B., Thota, B., Shinde, S. V., Friedmann-Morvinski, D., Nawaz, Z., et al. (2011). Insulin Growth Factor-2 Binding Protein 3 (IGF2BP3) Is a Glioblastoma-specific Marker that Activates Phosphatidylinositol 3-Kinase/mitogen-Activated Protein Kinase (PI3K/MAPK) Pathways by Modulating IGF-2. J. Biol. Chem. 286, 25882–25890. doi:10.1074/jbc.M110.178012
Tai, C.-J., Jassey, A., Liu, C.-H., Tai, C.-J., Richardson, C. D., Wong, S. H., et al. (2020). Targeting Autophagy Augments BBR-Mediated Cell Death in Human Hepatoma Cells Harboring Hepatitis C Virus RNA. Cells 9, 908. doi:10.3390/cells9040908
Tai, W. P., and Luo, H. S. (2003). [The Inhibit Effect of Berberine on Human colon Cell Line Cyclooxygenase-2]. Zhonghua nei ke za zhi 42, 558–560. doi:10.3760/j.issn:0578-1426.2003.08.010
Takamura, M., Sakamoto, M., Genda, T., Ichida, T., Asakura, H., and Hirohashi, S. (2001). Inhibition of Intrahepatic Metastasis of Human Hepatocellular Carcinoma by Rho-Associated Protein Kinase Inhibitor Y-27632. Hepatology 33, 577–581. doi:10.1053/jhep.2001.22652
Takara, K., Horibe, S., Obata, Y., Yoshikawa, E., Ohnishi, N., and Yokoyama, T. (2005). Effects of 19 Herbal Extracts on the Sensitivity to Paclitaxel or 5-fluorouracil in HeLa Cells. Biol. Pharm. Bull. 28, 138–142. doi:10.1248/bpb.28.138
Takimoto, C. H., Chao, M. P., Gibbs, C., Mccamish, M. A., Liu, J., Chen, J. Y., et al. (2019). The Macrophage 'Do Not Eat Me' Signal, CD47, Is a Clinically Validated Cancer Immunotherapy Target. Ann. Oncol. 30, 486–489. doi:10.1093/annonc/mdz006
Talmadge, J. E., and Gabrilovich, D. I. (2013). History of Myeloid-Derived Suppressor Cells. Nat. Rev. Cancer 13, 739–752. doi:10.1038/nrc3581
Tan, W., Zhong, Z., Carney, R. P., Men, Y., Li, J., Pan, T., et al. (2019). Deciphering the Metabolic Role of AMPK in Cancer Multi-Drug Resistance. Semin. Cancer Biol. 56, 56–71. doi:10.1016/j.semcancer.2018.09.005
Tan, Y. L., Goh, D., and Ong, E. S. (2006). Investigation of Differentially Expressed Proteins Due to the Inhibitory Effects of Berberine in Human Liver Cancer Cell Line HepG2. Mol. Biosyst. 2, 250–258. doi:10.1039/b517116d
Tang, J., Feng, Y., Tsao, S., Wang, N., Curtain, R., and Wang, Y. (2009). Berberine and Coptidis Rhizoma as Novel Antineoplastic Agents: a Review of Traditional Use and Biomedical Investigations. J. Ethnopharmacol 126, 5–17. doi:10.1016/j.jep.2009.08.009
Tang, Z., Tang, Y., Li, L., Liu, T., and Yang, J. (2019). Limonin Provokes Hepatocellular Carcinoma Cells with Stemness Entry into Cycle via Activating PI3K/Akt Signaling. Biomed. Pharmacother. 117, 109051. doi:10.1016/j.biopha.2019.109051
Tari, A. M., Simeone, A. M., Li, Y. J., Gutierrez-Puente, Y., Lai, S., and Symmans, W. F. (2005). Cyclooxygenase-2 Protein Reduces Tamoxifen and N-(4-hydroxyphenyl)retinamide Inhibitory Effects in Breast Cancer Cells. Lab. Invest. 85, 1357–1367. doi:10.1038/labinvest.3700339
Thornberry, N. A., and Lazebnik, Y. (1998). Caspases: Enemies within. Science 281, 1312–1316. doi:10.1126/science.281.5381.1312
Tillhon, M., Guamán Ortiz, L. M., Lombardi, P., and Scovassi, A. I. (2012). Berberine: New Perspectives for Old Remedies. Biochem. Pharmacol. 84, 1260–1267. doi:10.1016/j.bcp.2012.07.018
Tong, M., Liu, H., Hao, J., and Fan, D. (2020). Comparative Pharmacoproteomics Reveals Potential Targets for Berberine, a Promising Therapy for Colorectal Cancer. Biochem. Biophys. Res. Commun. S0006-291X (20), 30320–X. doi:10.1016/j.bbrc.2020.02.052
Vidal, A., and Koff, A. (2000). Cell-cycle Inhibitors: Three Families United by a Common Cause. Gene 247, 1–15. doi:10.1016/s0378-1119(00)00092-5
Volinia, S., Calin, G. A., Liu, C. G., Ambs, S., Cimmino, A., Petrocca, F., et al. (2006). A microRNA Expression Signature of Human Solid Tumors Defines Cancer Gene Targets. Proc. Natl. Acad. Sci. U S A. 103, 2257–2261. doi:10.1073/pnas.0510565103
Wang, D., and Dubois, R. N. (2006). Prostaglandins and Cancer. Gut 55, 115–122. doi:10.1136/gut.2004.047100
Wang, D., and Dubois, R. N. (2010). The Role of COX-2 in Intestinal Inflammation and Colorectal Cancer. Oncogene 29, 781–788. doi:10.1038/onc.2009.421
Wang, H., Mu, W., Shang, H., Lin, J., and Lei, X. (2014a2014). The Antihyperglycemic Effects of Rhizoma Coptidis and Mechanism of Actions: a Review of Systematic Reviews and Pharmacological Research. Biomed. Res. Int. 2014, 798093. doi:10.1155/2014/798093
Wang, H., Zhang, F., Ye, F., Ma, Y., and Zhang, D. Y. (2011). The Effect of Coptis Chinensis on the Signaling Network in the Squamous Carcinoma Cells. Front. Biosci. (Elite Ed. 3, 326–340. doi:10.2741/e248
Wang, J., Wang, L., Lou, G. H., Zeng, H. R., Hu, J., Huang, Q. W., et al. (2019). Coptidis Rhizoma: a Comprehensive Review of its Traditional Uses, Botany, Phytochemistry, Pharmacology and Toxicology. Pharm. Biol. 57, 193–225. doi:10.1080/13880209.2019.1577466
Wang, L., Liu, L., Shi, Y., Cao, H., Chaturvedi, R., Calcutt, M. W., et al. (2012). Berberine Induces Caspase-independent Cell Death in colon Tumor Cells through Activation of Apoptosis-Inducing Factor. PloS one 7, e36418. doi:10.1371/journal.pone.0036418
Wang, N., Feng, Y., Lau, E. P., Tsang, C., Ching, Y., Man, K., et al. (2010a). F-actin Reorganization and Inactivation of Rho Signaling Pathway Involved in the Inhibitory Effect of Coptidis Rhizoma on Hepatoma Cell Migration. Integr. Cancer Ther. 9, 354–364. doi:10.1177/1534735410379121
Wang, N., Feng, Y., Zhu, M., Tsang, C. M., Man, K., Tong, Y., et al. (2010b). Berberine Induces Autophagic Cell Death and Mitochondrial Apoptosis in Liver Cancer Cells: the Cellular Mechanism. J. Cel Biochem 111, 1426–1436. doi:10.1002/jcb.22869
Wang, N., Tan, H. Y., Li, L., Yuen, M. F., and Feng, Y. (2015). Berberine and Coptidis Rhizoma as Potential Anticancer Agents: Recent Updates and Future Perspectives. J. Ethnopharmacol 176, 35–48. doi:10.1016/j.jep.2015.10.028
Wang, N., Zhu, M., Wang, X., Tan, H. Y., Tsao, S. W., and Feng, Y. (2014b). Berberine-induced Tumor Suppressor P53 Up-Regulation Gets Involved in the Regulatory Network of MIR-23a in Hepatocellular Carcinoma. Biochim. Biophys. Acta 1839, 849–857. doi:10.1016/j.bbagrm.2014.05.027
Wang, X.-N., Xu, L.-N., Peng, J.-Y., Liu, K.-X., Zhang, L.-H., and Zhang, Y.-K. (2009). In Vivo inhibition of S180 Tumors by the Synergistic Effect of the Chinese Medicinal Herbs Coptis Chinensis and Evodia Rutaecarpa. Planta Med. 75, 1215–1220. doi:10.1055/s-0029-1185538
Wang, X. N., Han, X., Xu, L. N., Yin, L. H., Xu, Y. W., Qi, Y., et al. (2008). Enhancement of Apoptosis of Human Hepatocellular Carcinoma SMMC-7721 Cells through Synergy of Berberine and Evodiamine. Phytomedicine 15, 1062–1068. doi:10.1016/j.phymed.2008.05.002
Wang, Y., Liu, Y., Du, X., Ma, H., and Yao, J. (2020). Berberine Reverses Doxorubicin Resistance by Inhibiting Autophagy through the PTEN/Akt/mTOR Signaling Pathway in Breast Cancer. Onco Targets Ther. 13, 1909–1919. doi:10.2147/OTT.S241632
Wang, Y., Shou, J. W., Li, X. Y., Zhao, Z. X., Fu, J., He, C. Y., et al. (2017). Berberine-induced Bioactive Metabolites of the Gut Microbiota Improve Energy Metabolism. Metabolism 70, 72–84. doi:10.1016/j.metabol.2017.02.003
Wang, Y., Wen, B., Yu, H., Ding, D., Zhang, J., Zhang, Y., et al. (2018). Berberine Hydrochloride-Loaded Chitosan Nanoparticles Effectively Targets and Suppresses Human Nasopharyngeal Carcinoma. J. Biomed. Nanotechnol 14, 1486–1495. doi:10.1166/jbn.2018.2596
Watanabe, T., Konishi, T., Kishimoto, J., Kotake, K., Muto, T., and Sugihara, K. (2011). Ulcerative Colitis-Associated Colorectal Cancer Shows a Poorer Survival Than Sporadic Colorectal Cancer: a Nationwide Japanese Study. Inflamm. Bowel Dis. 17, 802–808. doi:10.1002/ibd.21365
Wei, M., Li, H., Li, Q., Qiao, Y., Ma, Q., Xie, R., et al. (2020a). Based on Network Pharmacology to Explore the Molecular Targets and Mechanisms of Gegen Qinlian Decoction for the Treatment of Ulcerative Colitis. Biomed. Res. Int. 2020, 5217405. doi:10.1155/2020/5217405
Wei, T., Xiaojun, X., and Peilong, C. (2020b). Magnoflorine Improves Sensitivity to Doxorubicin (DOX) of Breast Cancer Cells via Inducing Apoptosis and Autophagy through AKT/mTOR and P38 Signaling Pathways. Biomed. Pharmacother. 121, 109139. doi:10.1016/j.biopha.2019.109139
Weiskopf, K., Anderson, K. L., Ito, D., Schnorr, P. J., Tomiyasu, H., Ring, A. M., et al. (2016). Eradication of Canine Diffuse Large B-Cell Lymphoma in a Murine Xenograft Model with CD47 Blockade and Anti-CD20. Cancer Immunol. Res. 4, 1072–1087. doi:10.1158/2326-6066.CIR-16-0105
Wen, C. J., Wu, L. X., Fu, L. J., Yu, J., Zhang, Y. W., Zhang, X., et al. (2013). Genomic Screening for Targets Regulated by Berberine in Breast Cancer Cells. Asian Pac. J. Cancer Prev. 14, 6089–6094. doi:10.7314/apjcp.2013.14.10.6089
Wightman, B., Ha, I., and Ruvkun, G. (1993). Posttranscriptional Regulation of the Heterochronic Gene Lin-14 by Lin-4 Mediates Temporal Pattern Formation in C. elegans. Cell 75, 855–862. doi:10.1016/0092-8674(93)90530-4
Wilson, D. S., Dalmasso, G., Wang, L., Sitaraman, S. V., Merlin, D., and Murthy, N. (2010). Orally Delivered Thioketal Nanoparticles Loaded with TNF-α-siRNA Target Inflammation and Inhibit Gene Expression in the Intestines. Nat. Mater. 9, 923–928. doi:10.1038/nmat2859
Witters, L. M., Witkoski, A., Planas-Silva, M. D., Berger, M., Viallet, J., and Lipton, A. (2007). Synergistic Inhibition of Breast Cancer Cell Lines with a Dual Inhibitor of EGFR-HER-2/neu and a Bcl-2 Inhibitor. Oncol. Rep. 17, 465–469. doi:10.3892/or.17.2.465
Wong, C. C., Wong, C. M., Ko, F. C., Chan, L. K., Ching, Y. P., Yam, J. W., et al. (2008). Deleted in Liver Cancer 1 (DLC1) Negatively Regulates Rho/ROCK/MLC Pathway in Hepatocellular Carcinoma. PloS one 3, e2779. doi:10.1371/journal.pone.0002779
Wu, J., Luo, Y., Deng, D., Su, S., Li, S., Xiang, L., et al. (2019). Coptisine from Coptis Chinensis Exerts Diverse Beneficial Properties: A Concise Review. J. Cel Mol Med 23, 7946–7960. doi:10.1111/jcmm.14725
Wu, Q., Yang, Z., Nie, Y., Shi, Y., and Fan, D. (2014). Multi-drug Resistance in Cancer Chemotherapeutics: Mechanisms and Lab Approaches. Cancer Lett. 347, 159–166. doi:10.1016/j.canlet.2014.03.013
Wu, X., Chen, X., Liu, H., He, Z. W., Wang, Z., Wei, L. J., et al. (2020). Rescuing Dicer Expression in Inflamed colon Tissues Alleviates Colitis and Prevents Colitis-Associated Tumorigenesis. Theranostics 10, 5749–5762. doi:10.7150/thno.41894
Xu, J., Lu, L., and Li, L. (2016). NEK7: a Novel Promising Therapy Target for NLRP3-Related Inflammatory Diseases. Acta Biochim. Biophys. Sin (Shanghai) 48, 966–968. doi:10.1093/abbs/gmw080
Xu, Q., Liu, L. Z., Qian, X., Chen, Q., Jiang, Y., Li, D., et al. (2012). MiR-145 Directly Targets p70S6K1 in Cancer Cells to Inhibit Tumor Growth and Angiogenesis. Nucleic Acids Res. 40, 761–774. doi:10.1093/nar/gkr730
Xu, X., Yokoyama, S., Hayakawa, Y., and Saiki, I. (2017). Coptidis Rhizoma Induces Intrinsic Apoptosis through BAX and BAK Activation in Human Melanoma. Oncol. Rep. 38, 538–544. doi:10.3892/or.2017.5672
Xu, X., Zhang, L., Zhao, Y., Xu, B., Qin, W., Yan, Y., et al. (2020). Anti-inflammatory M-echanism of B-erberine on L-ipopolysaccharide-induced IEC-18 M-odels B-ased on C-omparative T-ranscriptomics. Mol. Med. Rep. 22, 5163–5180. doi:10.3892/mmr.2020.11602
Yahuafai, J., Asai, T., Oku, N., and Siripong, P. (2018). Anticancer Efficacy of the Combination of Berberine and PEGylated Liposomal Doxorubicin in Meth A Sarcoma-Bearing Mice. Biol. Pharm. Bull. 41, 1103–1106. doi:10.1248/bpb.b17-00989
Yan, F., Wang, L., Shi, Y., Cao, H., Liu, L., Washington, M. K., et al. (2012). Berberine Promotes Recovery of Colitis and Inhibits Inflammatory Responses in Colonic Macrophages and Epithelial Cells in DSS-Treated Mice. Am. J. Physiol. Gastrointest. Liver Physiol. 302, G504–G514. doi:10.1152/ajpgi.00312.2011
Yan, J., and Katz, A. E. (2010). ProstaCaid Induces G2/M Cell Cycle Arrest and Apoptosis in Human and Mouse Androgen-dependent And-independent Prostate Cancer Cells. Integr. Cancer Ther. 9, 186–196. doi:10.1177/1534735410371478
Yang, D., Jin, C., Ma, H., Huang, M., Shi, G. P., Wang, J., et al. (2016). EphrinB2/EphB4 Pathway in Postnatal Angiogenesis: a Potential Therapeutic Target for Ischemic Cardiovascular Disease. Angiogenesis 19, 297–309. doi:10.1007/s10456-016-9514-9
Yang, D., Sun, Y., Hu, L., Zheng, H., Ji, P., Pecot, C. V., et al. (2013a). Integrated Analyses Identify a Master microRNA Regulatory Network for the Mesenchymal Subtype in Serous Ovarian Cancer. Cancer cell 23, 186–199. doi:10.1016/j.ccr.2012.12.020
Yang, J., Li, T. Z., Xu, G. H., Luo, B. B., Chen, Y. X., and Zhang, T. (2013b). Low-concentration Capsaicin Promotes Colorectal Cancer Metastasis by Triggering ROS Production and Modulating Akt/mTOR and STAT-3 Pathways. Neoplasma 60, 364–372. doi:10.4149/neo_2013_048
Yang, T. C., Chao, H. F., Shi, L. S., Chang, T. C., Lin, H. C., and Chang, W. L. (2014). Alkaloids from Coptis Chinensis Root Promote Glucose Uptake in C2C12 Myotubes. Fitoterapia 93, 239–244. doi:10.1016/j.fitote.2014.01.008
Yang, X., Yang, B., Cai, J., Zhang, C., Zhang, Q., Xu, L., et al. (2013c). Berberine Enhances Radiosensitivity of Esophageal Squamous Cancer by Targeting HIF-1α In Vitro and In Vivo. Cancer Biol. Ther. 14, 1068–1073. doi:10.4161/cbt.26426
Yang, X. J., and Seto, E. (2007). HATs and HDACs: from Structure, Function and Regulation to Novel Strategies for Therapy and Prevention. Oncogene 26, 5310–5318. doi:10.1038/sj.onc.1210599
Yang, Y., Zhang, N., Li, K., Chen, J., Qiu, L., and Zhang, J. (2018). Integration of microRNA-mRNA Profiles and Pathway Analysis of Plant Isoquinoline Alkaloid Berberine in SGC-7901 Gastric Cancers Cells. Drug Des. Devel Ther. 12, 393–408. doi:10.2147/DDDT.S155993
Yi, T., Zhuang, L., Song, G., Zhang, B., Li, G., and Hu, T. (2015). Akt Signaling Is Associated with the Berberine-Induced Apoptosis of Human Gastric Cancer Cells. Nutr. Cancer 67, 523–531. doi:10.1080/01635581.2015.1004733
Yip, N. K., and Ho, W. S. (2013). Berberine Induces Apoptosis via the Mitochondrial Pathway in Liver Cancer Cells. Oncol. Rep. 30, 1107–1112. doi:10.3892/or.2013.2543
You, H. Y., Xie, X. M., Zhang, W. J., Zhu, H. L., and Jiang, F. Z. (2016). Berberine Modulates Cisplatin Sensitivity of Human Gastric Cancer Cells by Upregulation of miR-203. In Vitro Cel Dev Biol Anim 52, 857–863. doi:10.1007/s11626-016-0044-y
Yu, D., Fu, S., Cao, Z., Bao, M., Zhang, G., Pan, Y., et al. (2014). Unraveling the Novel Anti-osteosarcoma Function of Coptisine and its Mechanisms. Toxicol. Lett. 226, 328–336. doi:10.1016/j.toxlet.2014.02.021
Yu, M., Ren, L., Liang, F., Zhang, Y., Jiang, L., Ma, W., et al. (2020). Effect of Epiberberine from Coptis Chinensis Franch on Inhibition of Tumor Growth in MKN-45 Xenograft Mice. Phytomedicine 76, 153216. doi:10.1016/j.phymed.2020.153216
Yu, Y. N., Yu, T. C., Zhao, H. J., Sun, T. T., Chen, H. M., Chen, H. Y., et al. (2015). Berberine May rescue Fusobacterium Nucleatum-Induced Colorectal Tumorigenesis by Modulating the Tumor Microenvironment. Oncotarget 6, 32013–32026. doi:10.18632/oncotarget.5166
Zaimy, M. A., Saffarzadeh, N., Mohammadi, A., Pourghadamyari, H., Izadi, P., Sarli, A., et al. (2017). New Methods in the Diagnosis of Cancer and Gene Therapy of Cancer Based on Nanoparticles. Cancer Gene Ther. 24, 233–243. doi:10.1038/cgt.2017.16
Zeng, Q., Deng, H., Li, Y., Fan, T., Liu, Y., Tang, S., et al. (2021). Berberine Directly Targets the NEK7 Protein to Block the NEK7-NLRP3 Interaction and Exert Anti-inflammatory Activity. J. Med. Chem. 64, 768–781. doi:10.1021/acs.jmedchem.0c01743
Zhai, L., Huang, T., Xiao, H. T., Wu, P. G., Lin, C. Y., Ning, Z. W., et al. (2020). Berberine Suppresses Colonic Inflammation in Dextran Sulfate Sodium-Induced Murine Colitis through Inhibition of Cytosolic Phospholipase A2 Activity. Front. Pharmacol. 11, 576496. doi:10.3389/fphar.2020.576496
Zhang, B., Pan, X., Cobb, G. P., and Anderson, T. A. (2007). microRNAs as Oncogenes and Tumor Suppressors. Dev. Biol. 302, 1–12. doi:10.1016/j.ydbio.2006.08.028
Zhang, L. L., Ma, L. N., Yan, D., Zhang, C. E., Gao, D., Xiong, Y., et al. (2014). Dynamic Monitoring of the Cytotoxic Effects of Protoberberine Alkaloids from Rhizoma Coptidis on HepG2 Cells Using the xCELLigence System. Chin. J. Nat. Med. 12, 428–435. doi:10.1016/S1875-5364(14)60067-4
Zhang, L., Li, M., Zhang, G., Gao, C., Wang, S., Zhang, T., et al. (2021a). Micro- and Nanoencapsulated Hybrid Delivery System (MNEHDS): A Novel Approach for Colon-Targeted Oral Delivery of Berberine. Mol. Pharmaceutics 18, 1573–1581. doi:10.1021/acs.molpharmaceut.0c00970
Zhang, M., Long, Y., Sun, Y., Wang, Y., Li, Q., Wu, H., et al. (2011). Evidence for the Complementary and Synergistic Effects of the Three-Alkaloid Combination Regimen Containing Berberine, Hypaconitine and Skimmianine on the Ulcerative Colitis Rats Induced by Trinitrobenzene-Sulfonic Acid. Eur. J. Pharmacol. 651, 187–196. doi:10.1016/j.ejphar.2010.10.030
Zhang, Q., Tao, H., Lin, Y., Hu, Y., An, H., Zhang, D., et al. (2016). A Superoxide Dismutase/catalase Mimetic Nanomedicine for Targeted Therapy of Inflammatory Bowel Disease. Biomaterials 105, 206–221. doi:10.1016/j.biomaterials.2016.08.010
Zhang, Q., Wang, X., Cao, S., Sun, Y., He, X., Jiang, B., et al. (2020a). Berberine Represses Human Gastric Cancer Cell Growth In Vitro and In Vivo by Inducing Cytostatic Autophagy via Inhibition of MAPK/mTOR/p70S6K and Akt Signaling Pathways. Biomed. Pharmacother. 128, 110245. doi:10.1016/j.biopha.2020.110245
Zhang, R. Z., Yu, S. J., Bai, H., and Ning, K. (2017). TCM-mesh: The Database and Analytical System for Network Pharmacology Analysis for TCM Preparations. Sci. Rep. 7, 2821. doi:10.1038/s41598-017-03039-7
Zhang, S., Zhou, L., Zhang, M., Wang, Y., Wang, M., Du, J., et al. (2019). Berberine Maintains the Neutrophil N1 Phenotype to Reverse Cancer Cell Resistance to Doxorubicin. Front. Pharmacol. 10, 1658. doi:10.3389/fphar.2019.01658
Zhang, Y., Liu, X., Yu, M., Xu, M., Xiao, Y., Ma, W., et al. (2020c). Berberine Inhibits Proliferation and Induces G0/G1 Phase Arrest in Colorectal Cancer Cells by Downregulating IGF2BP3. Life Sci. 260, 118413. doi:10.1016/j.lfs.2020.118413
Zhang, Y., Wen, J., Liu, D., Qiu, Z., Zhu, Q., Li, R., et al. (2021b). Demethylenetetrahydroberberine Alleviates Nonalcoholic Fatty Liver Disease by Inhibiting the NLRP3 Inflammasome and Oxidative Stress in Mice. Life Sci. 281, 119778. doi:10.1016/j.lfs.2021.119778
Zhang, Y. L., Zhang, X., Miao, X. Z., Yuan, Y. Y., Gao, J., Li, X., et al. (2020b). Coptisine Suppresses Proliferation and Inhibits Metastasis in Human Pancreatic Cancer PANC-1 Cells. J. Asian Nat. Prod. Res. 22, 452–463. doi:10.1080/10286020.2019.1585820
Zhao, J., Zhou, T., Lu, J. Z., Ye, D., Mu, S., Tian, X. H., et al. (2021a). Intra-Herb Interactions: Primary Metabolites in Coptidis Rhizoma Extract Improved the Pharmacokinetics of Oral Berberine Hydrochloride in Mice. Front. Pharmacol. 12, 675368. doi:10.3389/fphar.2021.675368
Zhao, L., Du, X., Tian, J., Kang, X., Li, Y., Dai, W., et al. (2021b). Berberine-Loaded Carboxylmethyl Chitosan Nanoparticles Ameliorate DSS-Induced Colitis and Remodel Gut Microbiota in Mice. Front. Pharmacol. 12, 644387. doi:10.3389/fphar.2021.644387
Zhao, L., and Zhang, C. (2020). Berberine Inhibits MDA-MB-231 Cells by Attenuating Their Inflammatory Responses. Biomed. Res. Int. 2020, 3617514. doi:10.1155/2020/3617514
Zhao, Y., Jing, Z., Li, Y., and Mao, W. (2016). Berberine in Combination with Cisplatin Suppresses Breast Cancer Cell Growth through Induction of DNA Breaks and Caspase-3-dependent Apoptosis. Oncol. Rep. 36, 567–572. doi:10.3892/or.2016.4785
Zhen, Y., Luo, C., and Zhang, H. (2018). Early Detection of Ulcerative Colitis-Associated Colorectal Cancer. Gastroenterol. Rep. (Oxf) 6, 83–92. doi:10.1093/gastro/goy010
Zhou, H., and Mineshita, S. (2000). The Effect of Berberine Chloride on Experimental Colitis in Rats In Vivo and In Vitro. J. Pharmacol. Exp. Ther. 294, 822–829.
Zhou, L., Yang, F., Li, G., Huang, J., Liu, Y., Zhang, Q., et al. (2018). Coptisine Induces Apoptosis in Human Hepatoma Cells through Activating 67-kDa Laminin Receptor/cGMP Signaling. Front. Pharmacol. 9, 517. doi:10.3389/fphar.2018.00517
Zhu, L., Gu, P., and Shen, H. (2019a). Protective Effects of Berberine Hydrochloride on DSS-Induced Ulcerative Colitis in Rats. Int. Immunopharmacol 68, 242–251. doi:10.1016/j.intimp.2018.12.036
Zhu, M., Cui, Y., Yang, L., Yang, T., Wang, H., Zhang, D., et al. (2019b). Ephrin Type-B Receptor 4 Affinity Chromatography: An Effective and Rapid Method Studying the Active Compounds Targeting Ephrin Type-B Receptor 4. J. Chromatogr. A. 1586, 82–90. doi:10.1016/j.chroma.2018.12.005
Zhu, M., Wang, N., Tsao, S. W., Yuen, M. F., Feng, Y., Wan, T. S., et al. (2011). Up-regulation of microRNAs, miR21 and miR23a in Human Liver Cancer Cells Treated with Coptidis Rhizoma Aqueous Extract. Exp. Ther. Med. 2, 27–32. doi:10.3892/etm.2010.164
Zhu, Q., Chang, Y., Yang, J., and Wei, Q. (2014a). Post-translational Modifications of Proliferating Cell Nuclear Antigen: A Key Signal Integrator for DNA Damage Response (Review). Oncol. Lett. 7, 1363–1369. doi:10.3892/ol.2014.1943
Zhu, Y., Ma, N., Li, H. X., Tian, L., Ba, Y. F., and Hao, B. (2014b). Berberine Induces Apoptosis and DNA Damage in MG-63 H-uman O-steosarcoma C-ells. Mol. Med. Rep. 10, 1734–1738. doi:10.3892/mmr.2014.2405
5-FU 5-fluorouracil
AgNPs synthesized silver nanoparticles
Akt protein kinase B
ALL acute lymphoblastic leukemia
AMPK adenosine monophosphate-activated protein kinase
AOM azoxymethane
ATF4 transcription factor 4
AURKA aurora kinase A
BBR berberine
Bcl-2 B-cell lymphoma 2
BCRP breast cancer resistance protein
BioReF biological response fingerprinting
CD Crohn’s disease
CDKs cyclin-dependent kinases
COX-2 cyclooxygenase-2
CRC colorectal cancer
CYP cytochrome P450s
DEGs differentially expressed genes
DMs differential metabolites
DMTHB demethylenetetrahydroberberine
DOX doxorubicin
DSS dextran sulfate sodium
ECM extracellular matrix
EGCs enteric glial cells
EGFR epidermal growth factor receptor
EMT epithelial-mesenchymal transition
Eph erythropoietin-producing hepatoma
EphB4 erythropoietin-producing hepatocyte B4
ER estrogen receptor
ERK extracellular signal-regulated kinase
ESCC esophageal squamous cell carcinoma
FACT facilitates chromatin trancription
FISH fluorescence in situ hybridization
G-MDSC granulocytic-myeloid-derived suppressor cell
HCC hepatocellular Carcinoma
HCV hepatitis C virus
HDAC3 histone acetyltransferase 3
HDCA histone deacetylase
HST Hangeshashinto
HUVECs human umbilical vein endothelial cells
IBD inflammatory bowel disease
ICAM-1 intercellular adhesion molecular-1
IFN interferon
IGF2 insulin-like growth factor 2
IGF2BP3 Insulin-like growth factor 2 mRNA-binding protein 3
IL interleukin
IPA Ingenuity Pathway Analysis
JNK c-Jun N-terminal kinase
LC3 light chain 3
MDM2 murine double minute 2 murine double minute 2
LPS lipopolysaccharide
LSD1 lysine-specific demethylase 1
MAPK mitogen-activated protein kinase
MDM2 murine double minute 2 murine double minute 2
MDR multiple drug resistance
MRP1 multidrug resistance protein 1
miR microRNA
MMP matrix metalloproteinase
MNEHDS micro- and nano-encapsulated hybrid delivery system
MS mass spectrometry
MTDH metadherin
NAFLD nonalcoholic fatty liver disease
NAG-1 nonsteroidal anti-inflammatory drug-activated gene-1
NEK NIMA-related kinase
NF-κB nuclear factor kappa-B
NLRP3 nucleotide-binding domain-like receptor protein 3
NOD nucleotide-binding domain
NQO1 NAD(P)H quinone oxidoreductase 1
NSAID nonsteroidal anti-inflammatory drug
NSCLC non-small cell lung cancer
OBB oxyberberine
OM oral mucositis
OSM oncostatin M
PCNA proliferating cell nuclear antigen
PD-1 programmed cell death-1
PDCD4 programmed cell death 4
PD-L1 programmed cell death ligand-1
PDT photodynamic therapy
PEG-lip-DOX Pegylated liposomal doxorubicin
PGE2 prostaglandin E2
P-gp P-glycoprotein
PI3K phosphatidylinositol 3-kinase
PICRSt Phylogenetic Investigation of Communities by Reconstruction of Unobserved States
PLA2G4A Phospholipase A2a
RBPs RNA-binding proteins
RIAISs radiation-induced acute intestinal symptoms
ROS reactive oxygen species
RTKs receptor tyrosine kinases
SCFA short-chain fatty acids
SPT16 suppressor of Ty homolog-16
SSRP1 structure-specific recognition protein 1
TGF-β1 transforming growth factor-beta-1
Th T helper
TIMP-1 tissue inhibitor of metalloproteinase 1
TKI tyrosine kinase inhibitor
TNF tumor necrosis factor
TNIK Traf2 and Nck interacting serine protein kinase
TRAIL tumor necrosis factor related apoptosis inducing ligand
Treg cell T regulatory cell
TS thymidylate synthase
UC ulcerative colitis
uPA Urokinase plasminogen activator
VEGF vascular endothelial growth factor
VEGFR2 vascular endothelial growth factor receptor 2
WMS whole metagenome sequencing
XIAP X-linked inhibitor of apoptosis protein
Keywords: coptidis rhizoma, medicinal plant, gastrointestinal cancer, omics, clinical research
Citation: He L, Zhong Z, Chen M, Liang Q, Wang Y and Tan W (2022) Current Advances in Coptidis Rhizoma for Gastrointestinal and Other Cancers. Front. Pharmacol. 12:775084. doi: 10.3389/fphar.2021.775084
Received: 13 September 2021; Accepted: 08 November 2021;
Published: 03 January 2022.
Edited by:
Zhe-Sheng Chen, St. John’s University, United StatesReviewed by:
Wang Lingchong, Nanjing University of Chinese Medicine, ChinaCopyright © 2022 He, Zhong, Chen, Liang, Wang and Tan. This is an open-access article distributed under the terms of the Creative Commons Attribution License (CC BY). The use, distribution or reproduction in other forums is permitted, provided the original author(s) and the copyright owner(s) are credited and that the original publication in this journal is cited, in accordance with accepted academic practice. No use, distribution or reproduction is permitted which does not comply with these terms.
*Correspondence: Zhangfeng Zhong, emhhbmdmZW5nemhvbmdAdW0uZWR1Lm1v; Yitao Wang, eXR3YW5nQHVtLmVkdS5tbw==; Wen Tan, dGFud2VuQGx6dS5lZHUuY24=
†These authors contributed equally to this work
Disclaimer: All claims expressed in this article are solely those of the authors and do not necessarily represent those of their affiliated organizations, or those of the publisher, the editors and the reviewers. Any product that may be evaluated in this article or claim that may be made by its manufacturer is not guaranteed or endorsed by the publisher.
Research integrity at Frontiers
Learn more about the work of our research integrity team to safeguard the quality of each article we publish.