- 1School of Veterinary Medicine and Animal Resources, Makerere University, Kampala, Uganda
- 2Infection Medicine, Deanery of Biomedical Sciences, College of Medicine and Veterinary Medicine, University of Edinburgh, Scotland, United Kingdom
- 3School of Medicine, Kabale University, Kabale, Uganda
- 4Department of Pharmacology and Therapeutics, Faculty of Veterinary Medicine, Damanhour University, Albeheira, Egypt
- 5Department of Animal Production and Management, Faculty of Agriculture and Animal Sciences, Busitema University, Soroti, Uganda
- 6School of Pharmacy, Kampala International University Western Campus, Bushenyi, Uganda
- 7Biology Department, Faculty of Applied Sciences, Umm Al-Qura University, Makkah, Saudi Arabia
- 8Department of Parasitology, Faculty of Veterinary Medicine, Aswan University, Aswan, Egypt
- 9Pharmacology and Toxicology Department, Faculty of Pharmacy, Modern University for Technology and Information, Cairo, Egypt
- 10Department of Pharmaceutics, College of Pharmacy, King Saud University, Riyadh, Saudi Arabia
- 11Department of Pharmaceutical Chemistry, College of Pharmacy, Jouf University, Al Jouf, Saudi Arabia
Limited pharmacological studies have been conducted on plant species used against poultry helminths. The objective of this study was to provide a basis for plant based anthelmintics as possible alternatives against poultry anthelmintic resistance. The study justified the need for alternative anthelmintics. The study places emphasis on the increasing anthelmintic resistance, mechanism of resistance, and preparational protocols for plant anthelmintics and their associated mechanism of action. Pharmaceutical studies on plants as alternative therapies for the control of helminth parasites have not been fully explored especially in several developing countries. Plants from a broad range of species produce a wide variety of compounds that are potential anthelmintics candidates. Important phenolic acids have been found in Brassica rapa L. and Terminalia avicenniodes Guill. and Perri that affect the cell signaling pathways and gene expression. Benzo (c) phenanthridine and isoquinoline alkaloids are neurotoxic to helminths. Steroidal saponins (polyphyllin D and dioscin) interact with helminthic mitochondrial activity, alter cell membrane permeability, vacuolation and membrane damage. Benzyl isothiocyanate glucosinolates interfere with DNA replication and protein expression, while isoflavones from Acacia oxyphylla cause helminth flaccid paralysis, inhibit energy generation, and affect calcium utilization. Condensed tannins have been shown to cause the death of nematodes and paralysis leading to expulsion from the gastro-intestinal tract. Flavonoids from Chenopodium album L and Mangifera indica L act through the action of phosphodiesterase and Ca2+-ATPase, and flavonoids and tannins have been shown to act synergistically and are complementary to praziquantel. Artemisinins from Artemisia cina O. Berg are known to disrupt mitochondrial ATP production. Terpenoids from Cucurbita moschata L disrupt neurotransmission leading to paralysis as well as disruption of egg hatching. Yeast particle encapsulated terpenes are effective for the control of albendazole-resistant helminths.
1 Introduction
Ethnoveterinary medicine is an established practice, however, information on the pharmacology of plant anthelmintics for use in poultry is scarce. In Africa, the absence of pharmacovigilance policies and a lack of research on the pharmacology of plants for use in the control of helminths continues to impede innovation in this field.
Domesticated birds including turkeys, chickens, geese, and ratites are generally referred to as poultry in the United States while in Europe, poultry also includes domesticated birds kept for the benefit of humans in production (Patel et al., 2018). Productivity in poultry is compromised by an emerging helminthic burden with birds being affected by a variety of nematodes, cestodes, and trematodes (Table 1) (Ola-Fadunsin et al., 2019). Many drugs are available for the helminth control including benzimidazoles, macrocyclic lactones, and imidazothiazoles (Patel et al., 2018). Most anthelmintics exert their effects by either stunting or killing helminths. The large diversity of helminths causing parasitic infections in poultry is a challenge. There is a growing interest in the application of plant-based anthelmintics in poultry, as they are considered safer than synthetic compounds. Plant alternatives offer a cheap natural resource and some plant-based anthelmintics are more effective than synthetic anthelmintics (Karumari et al., 2014). Plant-based medicinal compounds however have unknown safety profiles and plant phytochemical composition is highly variable. There is a need to identify the bioactive compounds in ethnoveterinary products used against helminths to guide pharmacognosy and policy development.
2 Methods
A scoping review approach was used to get evidence on use of plants to control helminths (Sucharew and Macaluso, 2019). Structured searches were done to get information on the use of plant medicines to treat helminths in poultry. The scope of the literature included opportunities of plants used in livestock and not documented as already used in poultry against helminths. Electronic databases of Medline, PubMed, Embase, CABI Abstracts using the ovid interface, Web of Science, Scorpus were used to access published articles while google scholar was used on grey literature. The searches were done using keywords i.e., Ethnobotany, ethnopharmacology, plant anthelmintics, poultry helminths, poultry anthelmintic resistance, poultry anthelmintics, ethnoveterinary medicine. The research questions were; what plant anthelmintics are available against poultry helminths? Can plant anthelmintics be an alternative to synthetic anthelmintics? Are plant anthelmintics an answer to synthetic anthelmintic drug resistance? Literature on use of plant anthelmintics in poultry were included. Data were extracted into tables and then discussed as narrative sections in an effort to address the research questions.
3 Results
3.1 Ethnobotany in Anthelmintic Control
Plant alternatives offer an attractive option in organic farming of poultry products, lowering the poultry production costs. Many plant species have been tested for their efficacy against helminths (Table 2). For example, ginger and curcumin extracts were fairly effective by paralyzing Ascaridia galli after 48 h of exposure (Bazh and El-bahy, 2013). Garlic (Allium sativum L.) also showed anthelmintic activity (Landman, 2005). Aqueous and ethanolic extracts of Areca catechu reduced parasitic infestation by Ascaridia galli in poultry (Mubarokah et al., 2019). The latex from papaya fruits and sap also showed anthelmintic activity in chickens against Ascaridia galli and Heterakis gallinae infections (Bilandz et al., 2018).

TABLE 2. Major ethnoveterinary options for control of helminths, preparation, composition and target vector species. Leaves, roots and stems have been used on limited helmintic species, however studies on their pharmacology remain scarce. Information on effective dosage, effective concentration and safety are still unknown.
Synthetic anthelmintics leave residue in poultry products, residues which have been associated with carcinogenesis and anthelmintic resistance (Patel et al., 2018). The synthetic anthelmintics are not only expensive but also reduce the acceptability of poultry products (Hammond et al., 1997). These issues are of particular concern especially in low and middle-income countries where weak legislation and infrastructure for monitoring anthelmintics in animal products continues to prevail. Investing capital to promote research towards the promotion of reliable and tested plant based products would be of benefit to farmers and could provide novel alternatives for the treatment of emerging anthelmintic resistant strains in poultry. This is important since such plants can easily be grown for farm (plant medicines are acceptable in organic farming) or industrial use. However, the concentration of the phyto-compounds varies with the seasons and locations. Some plants are only found in particular parts of the world and may not even grow in others. The farm methods of using plant anthelmintics are more likely to cause toxic effects than the industrial laboratory methods in the absence of clear clinical data to guide their adoption (Hammond et al., 1997).
3.2 The Demand for Alternative Anthelmintic Options for Poultry Production
Intestinal helminths infections are a major cause for concern (Bazh and El-bahy, 2013). While helminths have many different predilection sites (Table 1), helminth species in poultry are characterized by their hepato-pulmonary migration with an escape to the peritoneum which results in abdominal peritonitis and intestinal perforation (Bazh and El-bahy, 2013). Ascaridia galli, Heterakis gallinarum, and Capillaria spp. penetrate the mucosa causing hemorrhage and subsequently return to the lumen to reach maturity and this infection has been associated with reduced weight gain and productivity losses (Bessell et al., 2012; Collins et al., 2015). The lack of a humoral immune response (Thapa et al., 2018) against helminths has led to dependence on anthelmintics and the search for more potent chemotherapeutical agents.
Benzimidazoles (BMZ) are commonly used in poultry including flubendazole, fenbendazole, and albendazole. Flimabend® (flubendazole) is used to treat Ascaridia galli, Heterakis gallinarum, and Capillaria spp. Flubendazole ([5-(4-fluorobenzoyl)-1H-benzimidazole-2-y1]-carbamic acid methyl ester) a benzimidazole carbamate (Levkut et al., 2017) impairs tubulin polymerization into microtubules, contributing to death of parasite (Zamanian et al., 2018). These drugs are applied daily to achieve therapeutical effects but increasing drug resistance leads to rapid reinfection, inadequate drug delivery, and increased farm losses through increased production costs (Collins et al., 2015). Overuse of anthelmintics including levamisole subsequently leads to drug residues in poultry products (Bilandz et al., 2018). In humans, consuming such products leads to hypersensitivity reactions including nausea, gastrointestinal manifestations, fever, and neurological effects (Levkut et al., 2017).
The lack of scientific studies on alternative anthelmintic options undermines efforts for alternative medicinal options (Bazh & El-bahy, 2013; Rana & Misra-Bhattacharya, 2013). Limited availability and high cost of synthetic anthelmintics have generated an increased interest in ethnoveterinary medicines and the search for new plant compounds for helminth control (Rana & Misra-Bhattacharya, 2013; Scantlebury et al., 2013). There is a growing movement in some low and middle-income countries to recognize the value of traditional medicinal approaches. There is a need to document the use of anthelmintic agents in communities as oral traditions in local medicine systems are fragile and there is a real risk of loss (Mcgaw et al., 2020).
Plant products are used in various regions for the treatment of poultry diseases. Farmatan® is a natural extract derived from chestnut wood (Castanea sativa miller), from the Fabaceae family. The primary component is water-soluble vegetable polyphenols—tannins that impairs nematode larval development and viability (Levkut et al., 2017). Ethnoveterinary medicine offers a cheaper and accessible option because products are locally available (Nghonjuyi et al., 2016). Plant compounds vary in quality and quantity with the different geographical zones; season of the year, nature of the solvent used, and particular edaphic factors. Not all compounds have been tested on synthetic anthelmintic resistant helminths but some terpenoids were effective against albendazole resistant helminths (Mirza et al., 2020). The details that make particular plant compounds effective against helminths resistant to synthetic anthelmintics are not yet known. There are several plant candidates whose compounds have been proven to be effective against helminths in animal species other than poultry, those have been listed in Table 2 as possible opportunities. However, the lack of information on efficacy, standardizations, and toxicity of the plant compounds in animals like chickens continues to challenge possible ratification (Mcgaw et al., 2020).
3.3 Ethnoveterinary Medicine in Veterinary Practice
Ethnoveterinary practices are commonly used to target poultry helminths. A pool of plants and practices have passed through generations that are used to manage poultry diseases (Gueye, 1999; Mwale et al., 2005). Usually, one herb is said to be effective on a variety of diseases including helminthiasis (Dhama et al., 2015). The value of such products is sometimes doubted because of a lack of scientific proof (Toyang et al., 1995). Practices are passed from one generation to the next through informal classes and storytelling. This inadvertent research on ethnoveterinary medicine has acceptance because it is tailored to the cultures and traditions of various communities. While plants used tend to be available locally, extraction is challenging and can affect the result. Polyphenolic compounds of Rubus ulmifolius schott varied with the varying polarity and quality of solvents (Dev et al., 2015; Akkari et al., 2016) Even the efficacy of extracts depends on the solvent used (Wang, 2011; Akkari et al., 2016). Helminths are possibly the most important poultry parasites (Ruff, 1999) and ethnoveterinary practices may offer alternative products to combat anthelmintic resistance.
3.4 Plant Metabolites
3.4.1 Phenolics
The phenolics include tannins, flavonoids, and phenolic acids (example of structures shown as Figure 1A) with catechins, anthocyanins, and coumarins being derivatives of phenolics (Dai and Mumper, 2010; Shen et al., 2017). Tannins are plant polyphenols (Salminen et al., 2011) with a similar structure to that of synthetic phenols. Tannins are found in many families of plants including Asteraceae, Anacardiaceae, Leguminoseae, Lumiaceae, Apiaceae and Cucurbitaceae (Table 2). Catechins and epicatechins are monomers that make the tannins (Duval and Avérous, 2016). Tannins can only be degraded at very high temperatures (190°C) and can be processed at high temperatures (García et al., 2014). Condensed tannins (CT) from dicotyledonous plants (Kahn and Diaz-Hernandez, 1999) are the major metabolites that cause the plant anthelmintic properties (Anthanasiadou et al., 2001; Patilaya et al., 2017). Although tannins have beneficial effects they require to be administered with care (Marzoni et al., 2020) due to their anti-nutritional character (Mansoori and Modirsanei, 2012). They are effective anthelmintic agents (Cenci et al., 2007; Sandoval-Castro et al., 2012) and cause nematode paralysis and death (Figure 1B), leading to expulsion from the gastro-intestinal tract (Kane et al., 2009). Controlled feeding of animals on tannins has many advantages (Kabasa et al., 2000; Alonso-Díaz et al., 2010).
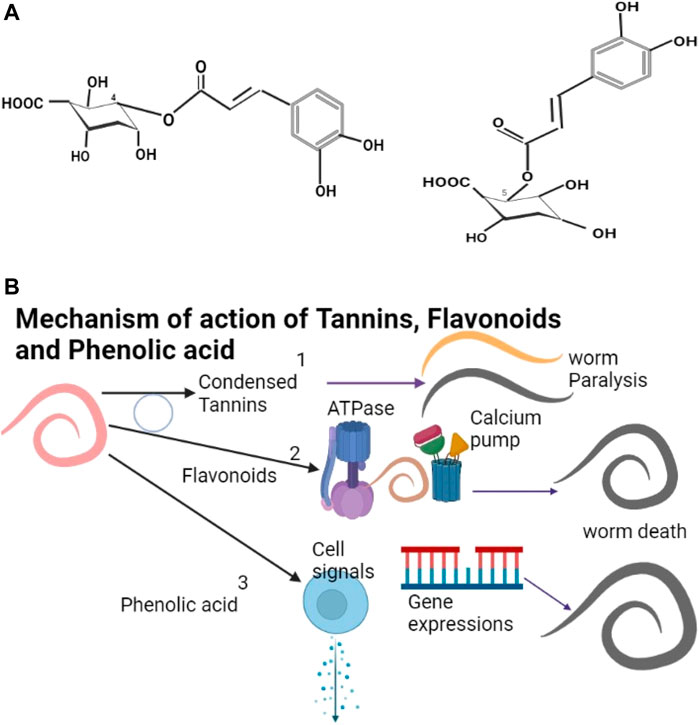
FIGURE 1. (A) 4-O-Caffeoylquinic acid and 5-O-caffeoylquinic acids are examples of phenolic acids in green coffee beans (Wei and Tanokura, 2015). (B) (1) Condensed Tannins cause paralysis and death of helminths. (2) Flavonoids affect the calcium pump and ATPase leading to the death of the helminth. (3) Phenolic acids affect cell signaling pathways and gene expressions leading to the death of the helminth. The blue circle shows possible synergistic actions between Condensed Tannins and Flavonoids.
Flavonoids are polyphenolic compounds (Cook and Samman, 1996; Croft, 1998; Madhusudhana et al., 2010) for which there is no database on the physicochemical properties (Kinoshita et al., 2005). Flavonoids may act by the various enzymes (Figure 1B) like phosphodiesterase and Ca2+-ATPase (Cazarolli et al., 2008; Rathee et al., 2009) but little is known as to their mode of action (Kerboeuf et al., 2008). Flavonoids have been identified in the families Rosaceae, Solanaceae, Fabaceae, Anacardiaceae, Euphorbiaceae, and Brassicaceae (Table 2). Flavonoids are effective against helminths (Pereira et al., 2016; Fomum and Nsahlai, 2017) and act synergistically with tannins (Klongsiriwet et al., 2015); they also potentiate the action of praziquantel against helminths (Hrckova & Velebny, 2010). Catechins can form tannin substances (Smeriglio, Barreca, Bellocco, and Trombetta, 2016) but are unstable and have limited application (Castañeda-Ovando et al., 2009; Dube et al., 2010). Catechins can be stabilized by encapsulation in B-cyclodextrin (Ho et al., 2017) or chitosan-tripolyphosphate (Dube et al., 2010) and their release from complexes increases with an increase in temperature (Bian et al., 2019). Coumarins also have anthelmintic effects (Kamble et al., 2013; Torres et al., 2014) and can be easily transformed into various useful derivatives (Katsori and Hadjipavlou-Litina, 2014; Stefanachi et al., 2018). Phenolic acids have been found in some members of the plant families of Colchicaceae, Solanaceae, Cucurbitaceae, Euphorbiaceae, Malyaceae and Brassicaceae and show anthelmintic properties (Akter et al., 2014; Ndhlala et al., 2015) (Table 2). Phenolics affect cell signaling pathways and gene expression (Figure 1B).
3.4.2 Alkaloids
Alkaloids are secondary metabolites, this group includes morphine, quinine, strychnine, atropine, colchine, and nicotine (Gutiérrez-Grijalva et al., 2020). An example of an alkaloid structure is shown as Figure 2A. They are most common in herbaceous plants (Jirschitzka et al., 2013; Fester, 2018). Alkaloids are found in abundance the Solanaceae and Erythroxylaceae plant families (Kohnen-Johannsen and Kayser, 2019) but are also found in some plants in the families of Leguminoseae, Meliaceae, Caprifoliaceae, and Euphorbiaceae. They are effective against helminths (Perrett and Whitefield, 1995; Ayers et al., 2007; Rocha et al., 2017) though toxic (Moreira et al., 2018); they show promising neurotoxic pharmacological properties in helminths (Terada et al., 1982; Athanasiadou et al., 2007) (Figure 2B) (Table 2).
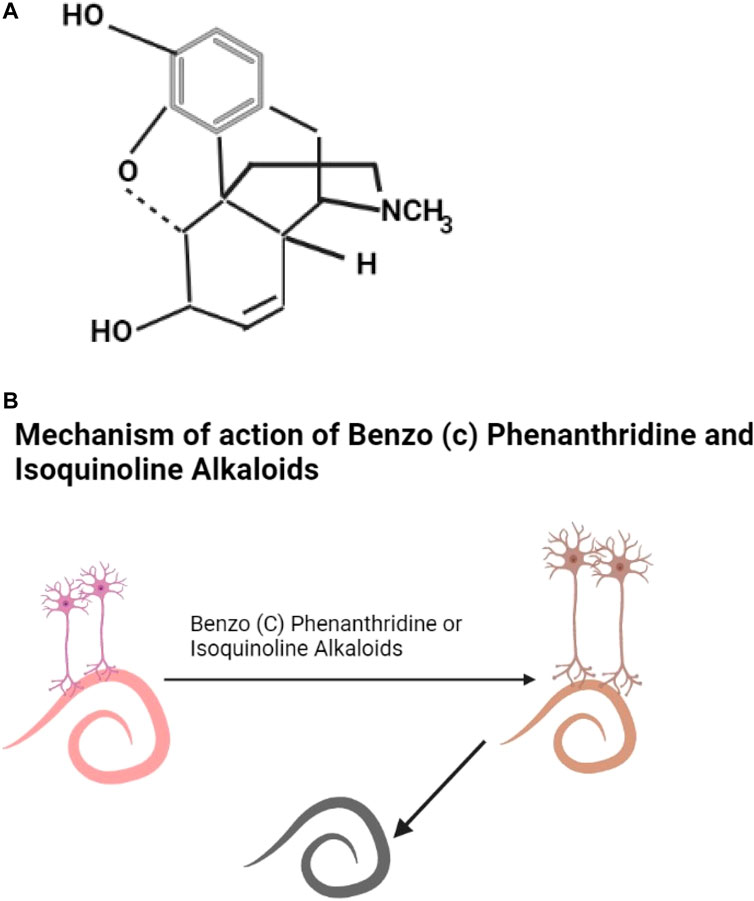
FIGURE 2. (A) The structure of morphine (Verpoorte, 2005). (B) Benzo (c) phenanthridine or isoquinoline alkaloids damage helminth neurons leading to the death of the helminth.
3.4.3 Saponins
Saponins include triterpenoids, steroids, gensenosides, allium saponins, glycoalkaloid saponins (Savage et al., 2003; Sobolewska et al., 2016). The structure of digitalin which is a steroidal saponin is shown as Figure 3A. Plant saponins are found in plants of the families of Fabaceae, Zygophyllaceae, Rosaceae, Apiaceae, and Verbenaceae (Table 2). They contain triterpene and sugar chains of varying lengths (Guclu-Ustundag and Mazza, 2007) and have the properties of foaming, solubilization, and emulsification (Ribeiro et al., 2013). Saponins are amphiphilic (Singh and Chaudhuri, 2018) and interact with sterols to form a variety of biological compounds of various categories of pharmaceutical values (Ribeiro et al., 2013). Ginseng plants are rich sources of saponins (Shi et al., 2019; Wang et al., 2020). Saponins have anthelmintic properties (Wang et al., 2010; Idris et al., 2017) affecting mitochondrial action (Santos et al., 2018) and altering the permeability of the helminth cell membrane, leading to damage of the helminth (Melzig et al., 2001) (Figure 3B).
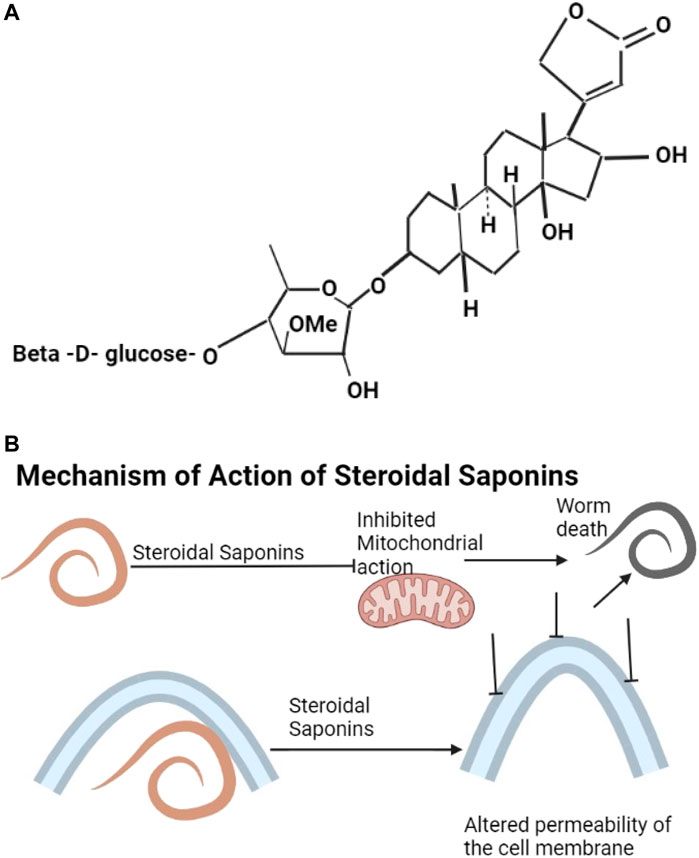
FIGURE 3. (A) The structure of digitalin is an example of steroidal saponins (Morgan and Wilson, 1999). (B) Saponins affect mitochondrial action and also alter the permeability of the cell membrane leading to death of the helminth.
3.4.4 Terpenoids
Digoxin, cicutoxin, atractyloside, daphetoxin, gibberellic acid (The example given as Figure 4A), betulinic acid, lupeol, ursolic acid, and oleanolic acid are the different types of terpenoids. Digoxin is from plants like Digitalis lanata, it affects muscle contraction through increasing calcium ions (Joel Edwards, 2009). Atractyloside is found in Callilepsis laureola and Atractylis glummifera, it blocks oxidative phosphorylation (Mbaveng et al., 2014). Gibberellic acids are found in Capsicum annuum, they decrease anti-oxidant enzymes (Mbaveng et al., 2014). Diphnetoxins are found in plants of the family Thymelaeaceae, they inhibit ATP synthase and the mitochondrial respiratory chain (Diogo et al., 2009). Betulinic acids are found in the bark of various plants, information about their actions is not available, they are thought to increase cytochrome C release by acting on the mitochondria (Varsha et al., 2017). Lupeol is from the bark of Bombax ceiba and Albizia adianthifolia (Fabaceae), lupeol suppresses the cells of inflammation (Dev et al., 2017; Saleem, 2009). Oleanolic acids are from fruits and vegetables especially Olea europaea, they lower glucose levels by mechanisms that are not well understood (Rodriguez-Rodriguez and Ruiz-Gutierrez, 2010; Yagishita et al., 2016). Ursolic acids are found in bilberries, apple peels, and peppermint, they cause increased Akt activity and increased energy utilization (Baliga et al., 2019; Wicks et al., 2018). Terpenoids with known anthelmintic properties include menthol and camphor (Mukherjee et al., 2016). Terpenoids hinder neurotransmission leading to helminth paralysis and inhibition of worm egg hatching (Mukherjee et al., 2016; Pillai & Nair, 2011) (Figure 4B). Terpenes have been shown to control albendazole-resistant helminths (Mirza et al., 2020). The specific properties that make particular terpenes effective against albendazole-resistant helminths are not known.
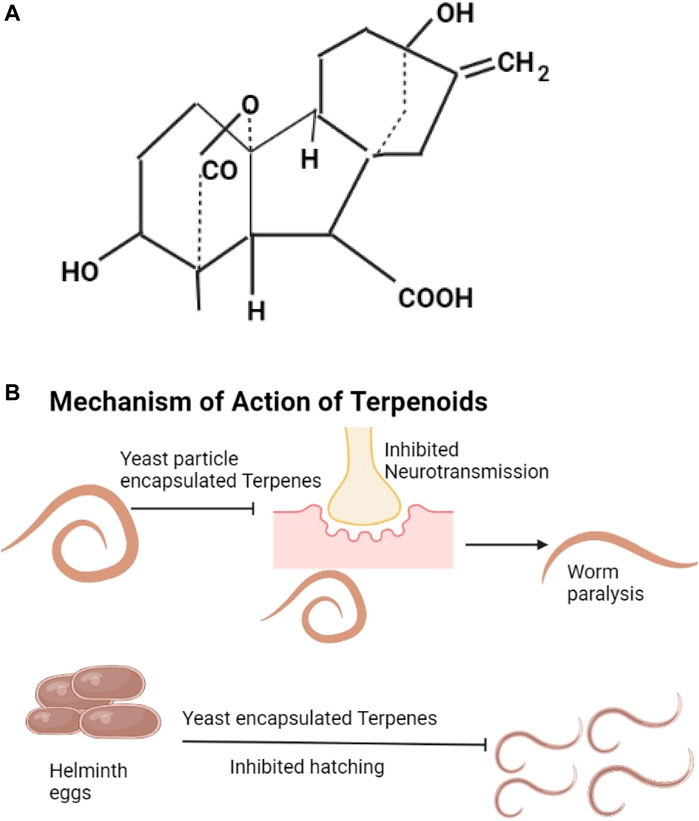
FIGURE 4. (A) The structure of gibberellic acid (Sponsel, 2003). (B) Yeast encapsulated terpenes inhibit neurotransmission and lead to helminth paralysis, they also inhibit hatching of helminths eggs.
3.4.5 Glucosinolates
An example of glucosinolate is shown (Figure 5A), they are natural glucosides that can be aliphatic, indole, and aromatic (Ishida et al., 2014). Annonaceae (Papaya) has anthelmintic properties; the papaya seeds have benzyl glucosinolate that is hydrolyzed by the enzyme myrosinase to benzyl Isothiocyanate (BITC) (Wilson et al., 2002) known to be effective against helminths (Kermanshai et al., 2001). BITC is thought to act by protein modification (Goosen et al., 2000) or by causing DNA damage (Kassie et al., 1999) (Figure 5B). Cysteine proteinases from papaya include Papain and chymopapain which digest the helminth cuticle (Stepek et al., 2004; Behnke et al., 2008).
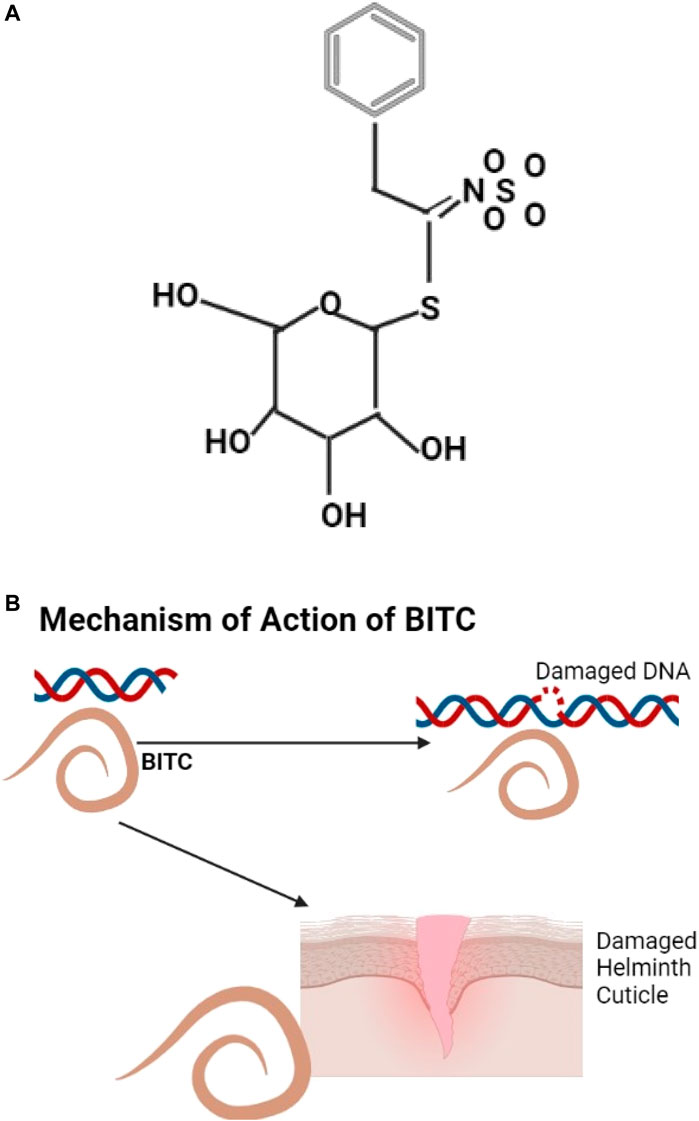
FIGURE 5. (A) The structure of Benzyl glucosinolate, metabolites of glucosinolates (Akram et al., 2021). (B) BITC causes helminth DNA and cuticle damage.
3.4.6 Isoflavones
Daidzein shown is an example of an isoflavone (Figure 6A). They are found in plants like Trifolium subterraneum, Medicago spp. (Barreira et al., 2015) and in soya agricultural waste (Carneiro et al., 2020). Known isoflavones include genistein, formononetin, pseudobaptigenin, and daidzein that cause helminth flaccid paralysis, inhibit energy generation, and affect calcium utilization (Das et al., 2006; Nirala et al., 2019) (Figure 6B).
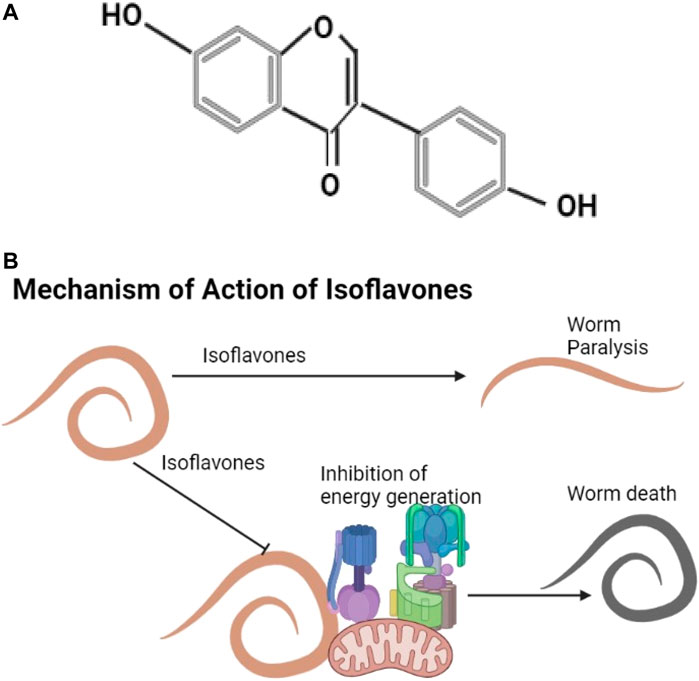
FIGURE 6. (A) The structure of daidzein (Hampl et al., 2009). (B) Isoflavones cause helminth paralysis and inhibit energy utilization.
3.4.7 Artemisinin and Its Derivatives
The structure of artemisinin is shown below (Figure 7A). Artemisinin and its derivatives are found in Artemisia annua. They produce oxygen radicals and can influence inhibitory neurotransmission (Pacios-Michelena et al., 2021). These cause oxidative stress through the effects on mitochondrial action and electron transfer in the parasite (Cumming et al., 1998; Beshay, 2018) and kill helminths (Fathy, 2011; Cala et al., 2014) (Figure 7B).
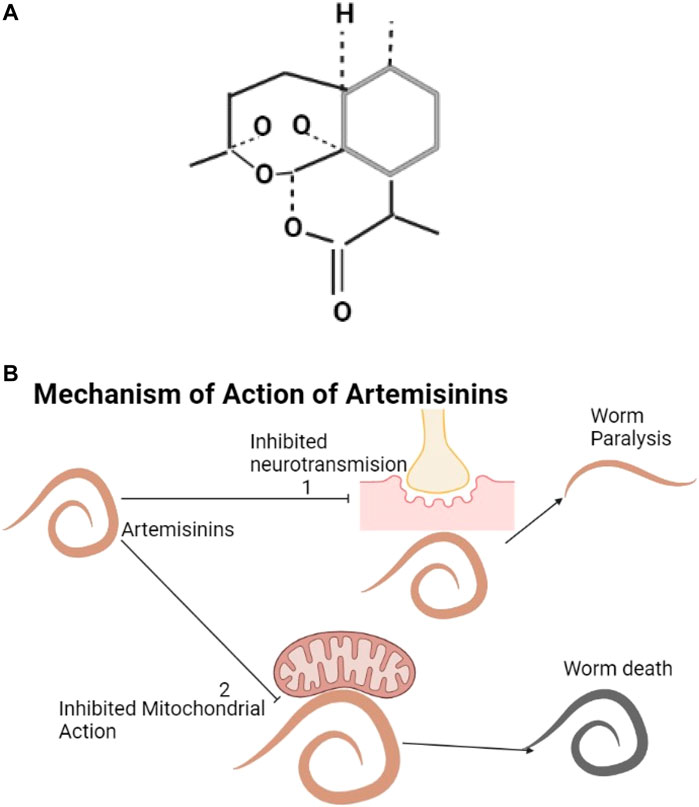
FIGURE 7. (A) The structure of artemisinin (Zeyuan, Yulin, and Meiyi, 2018). (B) Artemisinin and its derivatives inhibit neurotransmission resulting into worm paralysis (1) and can affect mitochondrial action resulting into worm death (2).
3.5 Relevance of the Review to Drug Policy and Planning
The efficacy of synthetic chemical anthelmintics is challenged by the escalating anthelmintic resistance and ethno-compounds are a growing resilient substitute due to their ecological stability. Resource-poor farmers can be helped to develop cheap plant resources which are sustainable and easy to process. Anthelmintic ethno-compounds can be a basis for nano-anthelmintics or green synthesis for possibly better-performing anthelmintics. Silver nanoparticles have been shown to potentiate the action of Momordica charantia L. fruit extract against helminths (Rashid et al., 2016) and gold nanoparticles make the fungus Nigrospora oryzae more effective against poultry tapeworms (Raillietina spp) (Kar et al., 2014).
While in China and the Himalayan regions ethnobotany has guided the new drug development (Sheng-Ji, 2001) this has not occurred in many low-income countries. The People’s Republic of China has developed ethnopharmacology alongside Western medicine by the establishment of a robust ethnomedicinal databank and funding research in this field. In comparison, developing countries (especially in Africa) lack clear policies on community-based research and sustainable ethnoveterinary resource management since legislation is crucial for national and international adoption of ethnomedicines.
A lack of poultry drug use policy in developing countries makes farmers vulnerable to unscrupulous dealers (unlicensed drug dealers) who exploit farmers with fake products for the sake of money. Farmers may buy useless products with the hope of treating their flocks and end up losing their flocks. For example, the use of opium poppy (Papaver somniferum L.) in control of poultry helminths is very common (Lans and Turner, 2011) but some low-income countries lack clear regulations and policies on the medicinal use of narcotic plants. There are no frameworks of access and benefit-sharing (ABS) protocols for intellectual property in most African countries including Uganda. Even in countries with ABS protocols, there are challenges to equitable sharing of indigenous knowledge (Van Overwalle, 2005; Chikombero and Luseba, 2010). ABS’s objective is to make biodiversity a private good with market opportunities. However, ABS legislation is expensive, unnecessarily bureaucratic, and usually at risk of biopiracy (Richerzhagen, 2007). Traditional knowledge doesn’t fit in the jurisdictions of the patent protection laws (Richerzhagen, 2007). Patent restrictions only apply to processed materials and not to raw materials (Van Overwalle, 2005). Even with set biodiversity authorities, the concept and scope of biodiversity is not clear cut since it is a multi-agency structure (Siebenhüner et al., 2005). The Convention on Biological Diversity (CBD) is non-specific (Siebenhüner and Suplie, 2005). The International Treaty on Plant Genetic resources for food and agriculture does not cover all crops complicating ABS design for some plants (Richerzhagen and Virchow, 2007). There is a need for a government agency to be responsible for granting access permits to companies and international organizations because the local communities are not able to trade this resource (Chikombero and Luseba, 2010). Communities ought to benefit from their ideas but the challenge is the long time lag to confirm research (Richerzhagen and Holm-Mueller, 2005).
4 Discussion
4.1 Emerging Poultry Anthelmintic Resistance and Plant-Anthelmintics
Anthelmintic resistance emerges when worms lose sensitivity to a chemical that is known to be lethal to them at the recommended concentration (Beech et al., 2011; Von Samson-Himmelstjerna and Blackhall et al., 2005). There are multiple reports of emerging anthelmintic resistance (Giri and Roy, 2015; Tarbiat, 2018) but studies in low and middle-income countries are scarce. Anthelmintic resistance is a common phenomenon (Kaplan and Vidyashankar, 2012; Whittaker et al., 2017) as a result of evolutionary modifications which take place following chronic exposure to an agent (Figure 8). Because of frequent and/or unlimited use of anthelmintics, resistance is increasing in several countries (Lalthanpuii and Lalchhandama, 2020), although this continues to be under-reported in resource-limited countries probably due to a lack of clear policies for the promotion of biomedical research. Nicotinic agonists such as levamisole become ineffective due to altered drug targets but the resistance mechanism is not fully understood (Köhler, 2001). Levamisole resistance may be linked to change in the nicotinic cholinergic receptor channels among resistant nematodes (Prichard, 1994). Changes in the nicotinic Acetylcholine Receptor (nAchR) subunit encoding genes are associated with Levamisole resistance (Köhler, 2001). Studies of various nAchRs by patch-clamp technique showed variations in Levamisole activated receptor channel currents for levamisole sensitive and levamisole resistant isolates of nematodes (Robertson et al., 1999; Martin et al., 2012). Resistant groups showed a lower number of activated receptor channels (Robertson et al., 1999). Levamisole receptors were observed to be deactivated in resistant isolates (Qian et al., 2008) resulting in a shift in the proportion of nAchR subtypes towards the less sensitive to levamisole (Köhler, 2001).
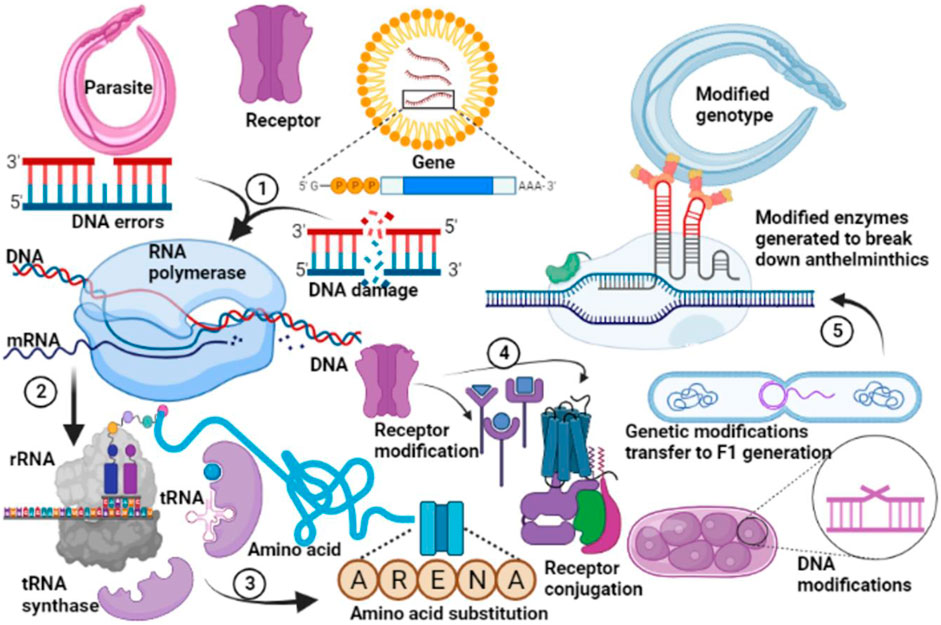
FIGURE 8. General mechanism of resistance to anthelmintic drugs. Genetic modifications in the parasite occur following decades of application of allopathic anthelmintics. DNA replication errors subsequently promote evolutionary changes in the gene of the parasites to form stable DNA (1). This undergoes transcription (2), and translation (3) with substitution of primary amino acids associated with susceptibility with those which favour resistances against anthelmintics. Subsequent protein modifications (4) favour expression of receptors which inhibit or reduce anthelmintic binding thus protecting the parasite from anthelmintic action. These genetic modifications are transferred to the offspring, favouring selective evolutionary changes which produce metabolic enzymes which degrade anthelmintics (5). A modified genotype is subsequently created which produces subsequent offsprings which are completely resistant to anthelmintics.
Macrocyclic lactones such as ivermectin are agonists of inhibitory chloride channels activated by glutamic acid (Geary and Moreno, 2012; Wolstenholme and Kaplan, 2012). Resistance to Macrocyclic lactones is not fully understood although various studies have inferred that it is likely genetically controlled (Gill and Lacey, 1998; Jambre et al., 2000). Resistance develops when mutations take place concurrently in not less than three genes that encode GluCl alpha-type subunits (Wang et al., 2010). It is possible mutations in genes other than those that encode GluCl that lead to resistance (Holden-Dye and Walker, 2006). The mutations lead to physiological changes in the drug target which is the pharyngeal muscle (Keane and Avery, 2003). Ivermectin resistance is also linked to the alteration of the ivermectin receptors (Prichard, 1994).
Benzimidazole resistance is associated with a change in β-tubulin genes (Roos, 1997); precisely substitution of specific amino acids in β -tubulin (Beech et al., 2011). Unregulated use of anthelmintics increases selection for resistance (Van Wyk, 2001; Coles, 2005). Resistant isolates emerge when the administration of benzimidazoles is done when the number of worm eggs and larval stages in the environment is low (Köhler, 2001). This leads to a change in gene expression and subsequent cross anthelmintic resistance (Von Samson-Himmelstjerna and Blackhall, 2005). Ascaridia galli is completely susceptible to benzimidazoles (Tarbiat, 2018), demonstrating selective species resistance in the helminths. However many helminths have genetic advantages that leverage their anthelmintic resistance (Shalaby, 2013). Changes in the β -tubulin genes leads to receptor loss or decreased affinity of the binding site for benzimidazole (Lubega and Prichard, 1990).
The poultry industry suffers direct and indirect losses due to anthelmintic resistant varieties of worms (Raza et al., 2016). It is reported that the effects of the increasing global warming are worsening the development of resistance to various anthelmintics (Yazwinski et al., 2013). Medicinal plants are becoming realistic alternatives against helminths that have proven resistant to synthetic anthelmintics (Mahdi et al., 2019). For example, yeast particle encapsulated terpenoids are effective against albendazole-resistant helminths (Mirza et al., 2020). However, the reasons why certain ethnomedicines may be effective against helminths resistant to synthetic anthelmintics are not fully known. The mechanism of action of plant phytochemicals may sometimes be similar to that of synthetic anthelmintics. The action of some saponins is said to be similar to praziquantel (Wang et al., 2010). The plant phytochemicals and their metabolites are diverse with many that need to be tested against the resistant helminths.
4.2 Preparation Methods of Ethnoveterinary Anthelmintics
Plant parts commonly used include leaves, barks, and roots (Yirga, 2012; Ritter et al., 2012). Freshly prepared plant parts are more frequently used than dried preparations (Eshetu et al., 2015). Preparation methods are either the traditional on farm practices or the industrial laboratory for plant-based medicines. Traditional on farm methods of preparation can be infusion, decoction and ground fresh materials (McGaw et al., 2007) and macerations (Table 2). Decoctions of barks of Anogeissus leiocarpus and Khaya senegalensis had anthelmintic potential (Hammond et al., 1997). Traditional maceration methods involve dipping the plant parts in a solvent; the harvested ethno-compound depends on the nature of the solvent and the solid to a solvent ratio (Ćujić et al., 2016).
Industrial laboratory methods can be simulated macerations (Khafagi and Dewedar, 2000; González-Manzano et al., 2004), by use of maceration enzymes (Bautista-Ortín et al., 2005). Extraction time, temperature, and concentration of extraction solvent determine the content of the extract (Chew et al., 2011). Multiple solvents can be used sequentially from the least polar to the most polar. When the polarity of the solvent is almost equal to that of the solute there is a better yield of the extract because the solute dissolves well (Altemimi et al., 2017).
Ethnoveterinary compounds can also be harvested by microwave-assisted extraction (Rafiee et al., 2011). This involves the use of a magnetic field and an electric field to cause extraction. The method is fast and uses less volume of the solvent (Altemimi et al., 2017). Ultrasonic-assisted extraction methods use a particular wavelength that distorts the plant cell wall and enables the solvent to penetrate plant cells (Lianfu and Zelong, 2008; Tian et al., 2013). Smashed samples which are well mixed with a solvent are monitored in an ultrasonic bath.
Phenolics are best harvested by mixing air-dried plant parts with the solvents (Djeridane et al., 2006). Alkaloids are harvested using gold seal-based pressurized hot water, reflux, or ultrasonic methods (Mokgadi et al., 2013). Saponins are harvested by accelerated solvent extraction, ultrasonic-assisted extractions, or pressurized low polarity water methods (Guclu-Ustundag and Mazza, 2007). To harvest terpenoids, the supercritical fluid extraction method was better than the soxhlet extraction methods when n-hexane or ethyl alcohol solvent were used (Kristo et al., 2001). Papaya metabolomes are harvested using the soxhlet extraction methods (Bah et al., 2006; Nakamura et al., 2019). Isoflavones could be harvested by soxhlet, shaking, vortexing, sonication, pressurized liquid extraction, or stirring all with a solvent (Luthria et al., 2007). Artemisinins are harvested by microwave-assisted extraction with the diameter of materials at most 0.125 mm (Hao et al., 2002). Modern preparations of the plant products ought to simulate the traditional preparation which initiated the claim, however, this is not always practical. The solvents and purification equipment are very expensive for low resource country early-career scientists interested in ethnoveterinary anthelmintics.
4.3 Traditional Use of Plants in Controlling Poultry Helminths
Use of plant-based ethnomedicines in managing poultry helminth infections has been well documented for other parts of the world but is scanty for sub-Saharan Africa. However their use is widespread as alternative to conventional medicines or when it’s the only accessible option for attempting treatment (Nyamanga, Suda, and Aagaard-Hansen, 2008).
Decoctions of Cannabis sativa L leaves (Iqbal et al., 2006; Hartady et al., 2021), Sanseveria nilotica Baker leaves (Nabukenya et al., 2014), Carica papaya L roots, cassia occidentalis L either roots or leaves and Boerhivia diffusa L leaves are used against poultry helminths. These decoctions are prepared by boiling plant parts in water and left to simmer, cooled and orally administered to poultry. Roots or barks of Aloe vera L are used in making decoctions for use against poultry helminths (Iqbal et al., 2006; Hartady et al., 2021).
Infusions of Cannabis sativa L leaves and Carica papaya L roots are also used against poultry helminths (Nabukenya et al., 2014), infusions of Nicotiana tabacum L leaves are also used for the same purpose (Iqbal et al., 2006; Hartady et al., 2021). The infusions are made by soaking plant parts in water which may be hot or cold. The plant parts are left to stand in the water to allow the plant active compounds to move into the water. The use of plant infusions in traditional poultry medicine is highly acceptable by communities although such practices need substantiation (Gueye, 1999).
Concoctions of various plants can be used to generate a blanket treatment against gastro-intestinal disorders (Nalubega, 2010). The use of plant combinations is the most used in poultry plant based treatments because they target many disease causing agents (Haniarti et al., 2019; Moreki, 2012). Various plants are combined together and crushed to generate juice for treatment or crushed and preserved for making infusions in the future.
In Uganda (Africa), traditionally prepared materials are also available as dried plant parts for infusions which are sold in packets as general poultry care products against gastro-intestinal disorders. The details of the composition and method of preparation usually remain secretive as a source of livelihood for the herbalists in the communities. However it is thought that some other non-plant materials are added to the preparations as a custom or procedure of getting the most desired outcomes or preventing hazardous outcomes. Materials like unspecified wood ash, common salt, cooking oils and alcohol are said to be sometimes added to the plant materials. Traditional use of plants in poultry medicine is challenged by lack of clear policies on use and protection of intellectual property. Plant compositions are affected by many factors like season of year and edaphic factors, which affect standardization of use. However industrial research on the traditionally used plants may provide links to developing new anthelmintics.
4.4 Future Research
Studies on the pharmacokinetics and dynamics of various ethno-anthelmintics are needed. Profiling of ethno-anthelmintics and susceptibility patterns of thelmintic species would help guide policy for the promotion of these products. Pharmacological experiments would help improve vigilance on the use of ethnomedicines for animal production and help reverse the current trend of drug abuse by ethnomedicinal practitioners (for example in Uganda (Schillhorn and Van Veen, 1997). Phytochemical analyses have been developed (Heinrich, 2008) but need to be applied to all-natural medicines. The metabolomics of several plant candidates need to be undertaken and a systematic ethnoveterinary anthelmintic database created. There is a need for collaborations between biomedical scientists to move study findings from the laboratory to the field. Studies on the anthelmintic synergy of different plant species combinations or ethnomedicine and synthetic combinations need to be considered.
5 Conclusion
Ethnoveterinary anthelmintics are an inadequately explored alternative to synthetic anthelmintics. They are an option in the era of organic farming and reducing synthetic drug residues in poultry products. In some cases, they have been claimed to be effective against helminths resistant to synthetic anthelmintics. Though these plant alternatives may be cheap and accessible, they have limitations. The efficacy and toxicity of most of the plant alternatives are usually controversial or completely unknown. Most inferences have been based only on in vitro assays and very limited in vivo assays have been reported in poultry. Very few species of helminths have been used as models (usually Ascaridia galli), it’s not known whether all other helminths would respond like the selected models. Licensing and regulating of ethnoveterinary anthelmintics can be leveraged by robust studies of these plant alternatives in poultry medicine. Ethnoveterinary anthelmintics also offer the basis for the new generation of anthelmintics made as a combination with nanoparticles. The identified bio-active molecules effective against poultry helminths could be studied to guide the development of new generations of anthelmintics. Several opportunities could be achieved from ethnomedicinal alternatives by exploring synergistic possibilities with conventional anthelmintics.
Author Contributions
GZ conceptualized the study; GZ, SB, JK, and JAO designed the study; GZ and KIK collected the data. GZ, SB, KIK, GES, JK, PV, SN, DO, MK, KM, MRK, MMG, MA, SSZ, SA, MAA and JAO interpreted the data. GZ wrote the initial draft, while all authors reviewed the manuscript for intellectual content, approved the manuscript for submission for potential publication and all authors remain in agreement on all aspects of the work.
Conflict of Interest
The authors declare that the research was conducted in the absence of any commercial or financial relationships that could be construed as a potential conflict of interest.
Publisher’s Note
All claims expressed in this article are solely those of the authors and do not necessarily represent those of their affiliated organizations, or those of the publisher, the editors, and the reviewers. Any product that may be evaluated in this article, or claim that may be made by its manufacturer, is not guaranteed or endorsed by the publisher.
Acknowledgments
The authors are grateful for the guidance from Professor Susan Christiana Welburn at the University of Edinburgh, United Kingdom. The authors deeply acknowledge the Researchers Supporting Program (TUMA-Project-2021-6), Al Maarefa University, Riyadh, Saudi Arabia for supporting steps of this work.
Abbreviations
HPLC, High Performance Liquid Chromatography; GluCl, Glutamate-gated Chloride; CBD, Convention on Biological Diversity; ABS, Access and Benefit Sharing; IP, Intellectual Property; h, hour; Spp, Species; BITC, Benzyl Isothiocyanate; DNA, Deoxyribonucleic acid; CT, Condensed Tannins; EVM, Ethnoveterinary Medicine; ABZ, Albendazole; ABZSO, Albendazole sulfoxide; ABZSO2, Albendazole sulphone; T1/2, Half life; µg, Micro-grams; Kg, Kilograms; ADI, Acceptable Daily intake; EC, European Commission; BW, Body weight; SDG, Sustainable Development Goal.
References
Abdel Aziz, A. R., Aziz, M., Omar, M. A., Sultan, K., and Sultan, K. (2018). In Vitro and In Vivo Anthelmintic Activity of Pumpkin Seeds and Pomegranate Peels Extracts against Ascaridia Galli. Beni-Suef Univ. J. Basic Appl. Sci. 7 (2), 231–234. doi:10.1016/j.bjbas.2018.02.003
Adate, P. S., Parmesawaran, S., and Chauhan, Y. (2012). In Vitro anthelmintic Activity of Stem Extracts of Piper Betle linn against Pheritima Posthuma. Pharmacognosy J. 4 (29), 61–65. doi:10.5530/pj.2012.29.10
Ade-Ademilua, O. E., and Okpoma, M. O. (2018). Gossypium Hirsutum L. And Gossypium barbadense L.: Differences in Phytochemical Contents, Antioxidant and Antimicrobial Properties. Ife J. Sci. 20 (1), 77. doi:10.4314/ijs.v20i1.8
Ade-Ajayi, A., Ogabiela, E., and Udiba, U. (2011). Preliminary Phytochemical and Antimicrobial Screening of Agave Sisalana Perrine Juice (Waste). J. Environ. Chem. Ecotoxicology 3, 180. doi:10.5897/JECE.9000051
Adu, O., Akingboye, K. A., and Akinfemi, A. (2009). Potency of Pawpaw (Carica Papaya) Latex as an Anthelmintic in Poultry Production. Bot. Res. Int. 2 (3), 139–142.
Aftab, A., Khan, Z. D., Yousaf, Z., Aftab, Z.-e.--H., Javad, S., Shamsheer, B., et al. (2017). Exploration of Ethnopharmacological Potential of Antimicrobial, Antioxidant, Anthelmintic and Phytochemical Analysis of Medicinally Important Plant Centella Asiatica (L.) Urban in Mart. Eichl. Am. J. Plant Sci. 08 (02), 201–211. doi:10.4236/ajps.2017.82016
Ahmad, S. U. F. I. Y. A. N., and khan, M. O. H. I. (2013). Pharmacognostical and Preliminary Phytochemical Investigation on Stems of Tephrosia Villosa Pers. Int. J. Pharm. Res. 5 (2), 131–134.
Akaberi, M., Sahebkar, A., Azizi, N., and Emami, S. A. (2019). Everlasting Flowers: Phytochemistry and Pharmacology of the Genus Helichrysum. Ind. Crops Prod. 138 (March), 111471. doi:10.1016/j.indcrop.2019.111471
Akbar, S. (2020). “Juniperus Communis L. (Cupressaceae),” in Handbook of 200 Medicinal Plants (Berlin, Germany: Springer International Publishing), 1049–1057. doi:10.1007/978-3-030-16807-0_113
Akhtar, M. S., and Ahmad, I. (1992). Comparative Efficacy of Mallotus Philippinensis Fruit (Kamala) or Nilzan® Drug against Gastrointestinal Cestodes in Beetal Goats. Small Ruminant Res. 8 (1–2), 121–128. doi:10.1016/0921-4488(92)90013-T
Akkari, H., Hajaji, S., B’chir, F., Rekik, M., and Gharbi, M. (2016). Correlation of Polyphenolic Content with Radical-Scavenging Capacity and Anthelmintic Effects of Rubus Ulmifolius (Rosaceae) against Haemonchus contortus. Vet. Parasitol. 221, 46–53. doi:10.1016/j.vetpar.2016.03.007
Akram, M., Jabeen, F., Riaz, M., Khan, F. S., Okushanova, E., Imran, M., et al. (2021). Health Benefits of Glucosinolate Isolated from Cruciferous and Other Vegetables. Preparation Phytopharmaceuticals Manage. Disord. 2021, 361–371. doi:10.1016/B978-0-12-820284-5.00006-X
Akter, K. N., Karmakar, P., Das, A., Anonna, S. N., Shoma, S. A., and Sattar, M. M. (2014). Evaluation of Antibacterial and Anthelmintic Activities with Total Phenolic Contents of Piper Betel Leaves. Avicenna J. Phytomedicine 4 (5), 320–329. Available at: http://www.ncbi.nlm.nih.gov/pubmed/25386394.
Alam, M., Alam, K., Begum, N., and Amin, M. (2014). Comparative Efficacy of Different Herbal and Modern Anthelmintics against Gastrointestinal Nematodiasis in Fowl. Int. J. Biol. Res. 2 (2), 145. doi:10.14419/ijbr.v2i2.3584
Alawa Ab, C. B. I., Adamu, A. M., Gefu, J. O., Ajanusi, O. J., Abdu, P. A., and Chiezey, N. P. (2010). Pakistan Veterinary Journal In Vivo Efficacy of Vernonia Amygdalina (Compositae) against Natural Helminth Infection in Bunaji (Bos indicus) Calves. Available at: www.pvj.com.pk.
Alawa, J., Jokthan, G., and Akut, K. (2002). Ethnoveterinary Medical Practice for Ruminants in the Subhumid Zone of Northern Nigeria. Prev. Vet. Med. 54 (1), 79–90. doi:10.1016/S0167-5877(01)00273-2
Ali, N., Aleem, U., Shah, S. W. A., Shah, I., Junaid, M., Ahmed, G., et al. (2013). Acute Toxicity, Brine Shrimp Cytotoxicity, Anthelmintic and Relaxant Potentials of Fruits of Rubus Fruticosus Agg. BMC Complement. Altern. Med. 13, 138. doi:10.1186/1472-6882-13-138
Alonso-Díaz, M. A., Torres-Acosta, J. F. J., Sandoval-Castro, C. A., and Hoste, H. (2010). Tannins in Tropical Tree Fodders Fed to Small Ruminants: A Friendly Foe? Small Ruminant Res. 89 (2–3), 164–173. doi:10.1016/j.smallrumres.2009.12.040
Altemimi, A., Lakhssassi, N., Baharlouei, A., and Watson, D. G. (2017). Phytochemicals: Extraction, Isolation, and Identification of Bioactive Compounds from Plant Extracts. Plants 6 (42), 23. doi:10.3390/plants6040042
Ameen, S. A., Azeez, O. M., Baba, Y. A., Raji, L. O., Basiru, A., Biobaku, K. T., et al. (2018). Anthelmintic Potency of Carica Papaya Seeds against Gastro-Intestinal Helminths in Red Sokoto Goat. Ceylon J. Sci. 47 (2), 137. doi:10.4038/cjs.v47i2.7509
Amin Mir, M., Sawhney, S., and Jassal, M. (2013). 2013 Wudpecker Journals Qualitative and Quantitative Analysis of Phytochemicals of Taraxacum officinale. Wudpecker J. Pharm. Pharmocology 2 (1), 1–005. Available at: https://pdfs.semanticscholar.org/fb65/97543d933256cbe8db76f99cef94e426860c.pdf.
Anthanasiadou, S., Kyriazakis, I., Jackson, F., and Coop, R. (2001). Anthelmintic Effects of Condensed Tannins toward Different Gastrointestinal Nematodes of Sheep. In Vivo and In Vitro Studies. Vet. Parasitol. 99, 205–219.
Asadullar, M., and Sabir, M. (1980). In Vitro anthelmintic Action of Bassia Latifolia against Ascaridia Galli Worms. Vet. Res. J. 3 (2), 131–133.
Ashokkumar, K. (2015). Gloriosa Superba (L.): A Brief Review of its Phytochemical Properties and Pharmacology. Int. J. Pharmacognosy Phytochem. Res. 7 (6), 1190–1193.
Athanasiadou, S., Githiori, J., and Kyriazakis, I. (2007). Medicinal Plants for Helminth Parasite Control: Facts and Fiction. Animal 1 (9), 1392–1400. doi:10.1017/S1751731107000730
Ayers, S., Zink, D. L., Mohn, K., Powell, J. S., Brown, C. M., Murphy, T., et al. (2007). Anthelmintic Activity of Aporphine Alkaloids from Cissampelos Capensis. Planta Med. 73 (3), 296–297. doi:10.1055/s-2007-967124
Bachaya, H. A., Iqbal, Z., Khan, M. N., Sindhu, Z. ud. D., and Jabbar, A. (2009). Anthelmintic Activity of Ziziphus Nummularia (Bark) and Acacia Nilotica (Fruit) against Trichostrongylid Nematodes of Sheep. J. Ethnopharmacology 123 (2), 325–329. doi:10.1016/j.jep.2009.02.043
Bah, S., Paulsen, B. S., Diallo, D., and Johansen, H. T. (2006). Characterization of Cysteine Proteases in Malian Medicinal Plants. J. Ethnopharmacology 107 (2), 189–198. doi:10.1016/j.jep.2006.03.008
Baliga, M. S., Shivashankara, A. R., Venkatesh, S., Bhat, H. P., Palatty, P. L., Bhandari, G., et al. (2019). Phytochemicals in the Prevention of Ethanol-Induced Hepatotoxicity: A Revisit. Dietary Interventions Liver Dis. Foods Nutrients, Dietary Supplements 2019, 79–89. doi:10.1016/B978-0-12-814466-4.00007-0
Banaras, S., Javaid, A., Shoaib, A., and Ahmed, E. (2017). Atividade antifúngica dos extratos de cirsium arvense contra o fungo fitopatogênico macrophomina phaseolina. Planta Daninha 35, e017162738. doi:10.1590/S0100-83582017350100014
Banso, A. (2009). Phytochemical and Antibacterial Investigation of Bark Extracts of Acacia Nilotica. J. Med. Plants Res. 3 (2), 082–085.
Barreira, J. C. M., Visnevschi-Necrasov, T., Nunes, E., Cunha, S. C., Pereira, G., and Oliveira, M. B. P. P. (2015). Medicago Spp. As Potential Sources of Bioactive Isoflavones: Characterization According to Phylogenetic and Phenologic Factors. Phytochemistry 116 (1), 230–238. doi:10.1016/J.PHYTOCHEM.2015.04.011
Bartolome, A. P., Villaseñor, I. M., and Yang, W. (2013). Bidens Pilosa L. (Asteraceae): Botanical Properties, Traditional Uses, Phytochemistry, and Pharmacology. Evidence-Based Complement. Altern. Med. 2013, 340215. doi:10.1155/2013/340215
Bautista-Ortín, A. B., Martínez-Cutillas, A., Ros-García, J. M., López-Roca, J. M., and Gómez-Plaza, E. (2005). Improving Colour Extraction and Stability in Red Wines: The Use of Maceration Enzymes and Enological Tannins. Int. J. Food Sci. Technol. 40 (8), 867–878. doi:10.1111/j.1365-2621.2005.01014.x
Bazh, E. K. A., and El-bahy, N. M. (2013). In Vitro and In Vivo Screening of Anthelmintic Activity of Ginger and Curcumin on Ascaridia Galli. Parasitol. Res. 112, 3679–3686. doi:10.1007/s00436-013-3541-x
Beech, R. N., Skuce, P., Bartley, D. J., Martin, R. J., Prichard, R. K., and Gilleard, J. S. (2011). Anthelmintic Resistance: Markers for Resistance, or Susceptibility? Parasitology 138, 160. doi:10.1017/S0031182010001198
Behnke, J. M., Buttle, D. J., Stepek, G., Lowe, A., and Duce, I. R. (2008). Developing Novel Anthelmintics from Plant Cysteine Proteinases. Parasites and Vectors 1 (1), 1–18. doi:10.1186/1756-3305-1-29
Beshay, E. V. N. (2018). Therapeutic Efficacy of Artemisia Absinthium against Hymenolepis Nana: In Vitro and In Vivo Studies in Comparison with the Anthelmintic Praziquantel. J. Helminthol. 92 (3), 298–308. doi:10.1017/S0022149X17000529
Bessell, P. R., Dash, R., Prasad, S., Al-riyami, L., Gammon, N., Stuke, K., et al. (2012). Estimating the Impact of Administration of Dewormers on Smallholder Chickens in Odisha State, India. Poult. Sci. 98, 1692. doi:10.3382/ps/pey526
Bian, J., Shi, X., Li, Q., Zhao, M., Wang, L., and Lee, J. (2019). Journal of Trace Elements in Medicine and Biology A Novel Functional Role of Nickel in Sperm Motility and Eukaryotic Cell Growth. J. Trace Elem. Med. Biol. 54 (January), 142–149. doi:10.1016/j.jtemb.2019.04.017
Bilandz, N., Varga, I., Cvetnic, L., and Su, J. (2018). Estimation of the Withdrawal Time of Levamisole in Eggs after Oral Administration to Laying Hens. J. Food Prot. 81 (10), 1627–1634. doi:10.4315/0362-028X.JFP-18-194
Blakemore, R. C., Bowden, K., Broadbent, J. L., and Drysdale, A. C. (1964). Anthelmintic Constituents of Ferns. J. Pharm. Pharmacol. 16 (7), 464–471. doi:10.1111/j.2042-7158.1964.tb07495.x
Blancad, D., Lecoq, H., and Pitrat, M. (1991). Diseases of Cucurbitaceae: Observation, Identification and Control. Paris. Available at: https://www.cabdirect.org/cabdirect/search/?q=do%3A%22Maladies+des+Cucurbitacees%3A+observer%2C+identifier%2C+lutter%22.
Botura, M. B., Silva, G. D., Lima, H. G., Oliveira, J. V. A., Souza, T. S., Santos, J. D. G., et al. (2011). In Vivo anthelmintic Activity of an Aqueous Extract from Sisal Waste (Agave Sisalana Perr.) against Gastrointestinal Nematodes in Goats. Vet. Parasitol. 177 (1–2), 104–110. doi:10.1016/j.vetpar.2010.11.039
Cala, A. C., Ferreira, J. F. S., Chagas, A. C. S., Gonzalez, J. M., Rodrigues, R. A. F., Foglio, M. A., et al. (2014). Anthelmintic Activity of Artemisia Annua L. Extracts In Vitro and the Effect of an Aqueous Extract and Artemisinin in Sheep Naturally Infected with Gastrointestinal Nematodes. Parasitol. Res. 113 (6), 2345–2353. doi:10.1007/s00436-014-3891-z
Calzetta, L., Pistocchini, E., Leo, A., Roncada, P., Ritondo, B. L., Palma, E., et al. (2020). Anthelminthic Medicinal Plants in Veterinary Ethnopharmacology: A Network Meta-Analysis Following the PRISMA-P and PROSPERO Recommendations. Heliyon 6 (2), e03256. doi:10.1016/j.heliyon.2020.e03256
Carneiro, A. M., Moreira, E. A., Bragagnolo, F. S., Borges, M. S., Pilon, A. C., Rinaldo, D., et al. (2020). Soya Agricultural Waste as a Rich Source of Isoflavones. Food Res. Int. 130, 108949. doi:10.1016/J.FOODRES.2019.108949
Castañeda-Ovando, A., Pacheco-Hernández, M. de. L., Páez-Hernández, M. E., Rodríguez, J. A., and Galán-Vidal, C. A. (2009). Chemical Studies of Anthocyanins: A Review. Food Chemistry. Amsterdam: Elsevier. doi:10.1016/j.foodchem.2008.09.001
Cazarolli, L., Zanatta, L., and Alberton, E. (2008). Flavonoids: Cellular and Molecular Mechanism of Action in Glucose Homeostasis. Mini-Reviews Med. Chem. 8 (10), 1032–1038. doi:10.2174/138955708785740580
Cenci, F. B., Louvandini, H., McManus, C. M., DelľPorto, A., Costa, D. M., Araújo, S. C., et al. (2007). Effects of Condensed Tannin from Acacia Mearnsii on Sheep Infected Naturally with Gastrointestinal Helminthes. Vet. Parasitol. 144 (1–2), 132–137. doi:10.1016/j.vetpar.2006.09.021
Chand, J., Naaz, Y., and Nainwal, P. (2019). In Vitro Anthelmintic Activity of Peel Extracts of Cucurbita Maxima. Int. J. Pharm. Res. 10 (1), 22–25.
Chew, K. K., Khoo, M. Z., Ng, S. Y., Thoo, Y. Y., Aida, W. W. M., and Ho, C. W. (2011). Effect of Ethanol Concentration, Extraction Time and Extraction Temperature on the Recovery of Phenolic Compounds and Antioxidant Capacity of Orthosiphon Stamineus Extracts. Int. Food Res. J. 18 (4), 1427–1435.
Chhatre, S., Nesari, T., Somani, G., Kanchan, D., and Sathaye, S. (2014). Phytopharmacological Overview of Tribulus Terrestris. Pharmacognosy Rev. 8, 45. doi:10.4103/0973-7847.125530
Chikombero, M., and Luseba, D. (2010). “2 Logistical and Legal Considerations in Ethnoveterinary Botanical Medicine,” in Herbal Medicines for Animal Health, 25.
Chota, A., Sikasunge, C. S., Phiri, A. M., Musukwa, M. N., Haazele, F., and Phiri, I. K. (2010). A Comparative Study of the Efficacy of Piperazine and Carica Papaya for the Control of Helminth Parasites in Village Chickens in Zambia. Trop. Anim. Health Prod. 42 (3), 315–318. doi:10.1007/s11250-009-9432-6
Coles, G. C. (2005). Anthelmintic Resistance - Looking to the Future: A UK Perspective. Research in Veterinary Science. Amsterdam: Elsevier. doi:10.1016/j.rvsc.2004.09.001
Collins, J. B., Jordan, B., Baldwin, L., Hebron, C., Paras, K., Vidyashankar, A. N., et al. (2015). Resistance to Fenbendazole in Ascaridia Dissimilis, an Important Nematode Parasite of Turkeys. Poult. Sci. 98, 5412. doi:10.3382/ps/pez379
Cook, N. C., and Samman, S. (1996). Flavonoids—Chemistry, Metabolism, Cardioprotective Effects, and Dietary Sources. J. Nutr. Biochem. 7 (2), 66–76. doi:10.1016/0955-2863(95)00168-9
Couet, C. E., Crews, C., and Hanley, A. B. (1996). Analysis, Separation, and Bioassay of Pyrrolizidine Alkaloids from comfrey (Symphytum Officinale). Nat. Toxins 4 (4), 163–167. doi:10.1002/19960404NT3
Croft, K. D. (1998). The Chemistry and Biological Effects of Flavonoids and Phenolic Acids. Ann. N Y Acad. Sci. 854, 435–442. doi:10.1111/j.1749-6632.1998.tb09922.x
Ćujić, N., Šavikin, K., Janković, T., Pljevljakušić, D., Zdunić, G., and Ibrić, S. (2016). Optimization of Polyphenols Extraction from Dried Chokeberry Using Maceration as Traditional Technique. Food Chem. 194, 135–142. doi:10.1016/j.foodchem.2015.08.008
Cumming, J. N., Wang, D., Park, S. B., Shapiro,Ta, , and Posner, G. (1998). Design, Synthesis, Derivatization, and Structure−Activity Relationships of Simplified, Tricyclic, 1,2,4-Trioxane Alcohol Analogues of the Antimalarial Artemisinin. J. Med. Chem. 41 (6), 952–964. doi:10.1021/jm970711g
Dai, D. N., Thang, T. D., Ogunmoye, A., Eresanya, O. I., and Ogunwande, I. A. (2015). Chemical Constituents of Essential Oils from the Leaves of Tithonia Diversifolia, Houttuynia Cordata and Asarum Glabrum Grown in Vietnam. Am. J. Essent. Oils Naturals Prod. 2 (4), 17–21.
Dai, J., and Mumper, R. J. (2010). Plant Phenolics: Extraction, Analysis and Their Antioxidant and Anticancer Properties. Molecules 15 (10), 7313–7352. doi:10.3390/molecules15107313
Das, A., Chaudhuri, D., Ghate, N. B., Chatterjee, A., and Mandal, N. (2013). Comparative Assessment of Phytochemicals and Antioxidant Potential of Methanolic and Aqueous Extracts of Clerodendrum Colebrookianum Walp. Leaf from north-east India. Int. J. Pharm. Pharm. Sci. 5 (4), 420–427.
Das, A., Shakya, A., Ghosh, S. K., Singh, U. P., and Bhat, H. R. (2020). A Review of Phytochemical and Pharmacological Studies of Inula Species. Curr. Bioactive Compd. 16 (5), 557–567. doi:10.2174/1573407215666190207093538
Das, B., Tandon, V., and Saha, N. (2006). Effect of Isoflavone from Flemingia Vestita (Fabaceae) on the Ca 2+ Homeostasis in Raillietina Echinobothrida, the Cestode of Domestic Fowl. Parasitol. Int. 55 (1), 17–21. doi:10.1016/j.parint.2005.08.002
Deepak, M., Dipankar, G., Prashanth, D., Asha, M. K., Amit, A., and Venkataraman, B. V. (2010). Tribulosin and β-sitosterol-D-glucoside, the Anthelmintic Principles of Tribulus Terrestris. Phytomedicine 9 (8), 753–756. doi:10.1078/094471102321621395
Dev, K., Ramakrishna, E., and Maurya, R. (2017). Glucose Transporter 4 Translocation Activators from Nature. Discov. Develop. Antidiabetic Agents Nat. Prod. Nat. Product. Drug Discov. 2017, 113–145. doi:10.1016/B978-0-12-809450-1.00004-1
Dev, U. K., Hossain, M. T., and Islam, M. Z. (2015). Phytochemical Investigation, Antioxidant Activity and Anthelmintic Activity of Mikania Micrantha Leaves. World J. Pharm. Res. 4 (5), 121–133. Available at: http://www.wjpr.net/download/article/1430372421.pdf.
Dhama, K., Latheef, S. K., Mani, S., Samad, H. A., Karthik, K., Tiwari, R., et al. (2015). Multiple Beneficial Applications and Modes of Action of Herbs in Poultry Health and Production-A Review. Int. J. Pharmacol. 11, 152. doi:10.3923/ijp.2015.152.176
Diogo, C. V., Félix, L., Vilela, S., Burgeiro, A., Barbosa, I. A., Carvalho, M. J. M., et al. (2009). Mitochondrial Toxicity of the Phyotochemicals Daphnetoxin and Daphnoretin – Relevance for Possible Anti-cancer Application. Toxicol. Vitro 23 (5), 772–779. doi:10.1016/j.tiv.2009.04.002
Djeridane, A., Yousfi, M., Nadjemi, B., Boutassouna, D., Stocker, P., and Vidal, N. (2006). Antioxidant Activity of Some Algerian Medicinal Plants Extracts Containing Phenolic Compounds. Food Chem. 97 (4), 654–660. doi:10.1016/j.foodchem.2005.04.028
Dube, A., Ng, K., Nicolazzo, J. A., and Larson, I. (2010). Effective Use of Reducing Agents and Nanoparticle Encapsulation in Stabilizing Catechins in Alkaline Solution. Food Chem. 122 (3), 662–667. doi:10.1016/j.foodchem.2010.03.027
Dube, A., Nicolazzo, J. A., and Larson, I. (2010). Chitosan Nanoparticles Enhance the Intestinal Absorption of the green tea Catechins (+)-catechin and (-)-epigallocatechin Gallate. Eur. J. Pharm. Sci. 41 (2), 219–225. doi:10.1016/j.ejps.2010.06.010
Duval, A., and Avérous, L. (2016). Characterization and Physicochemical Properties of Condensed Tannins from Acacia Catechu. J. Agric. Food Chem. 64 (8), 1751–1760. doi:10.1021/acs.jafc.5b05671
Eshetu, G. R., Dejene, T. A., Telila, L. B., and Bekele, D. F. (2015). Ethnoveterinary Medicinal Plants: Preparation and Application Methods by Traditional Healers in Selected Districts of Southern Ethiopia. Vet. World 8 (5), 674–684. doi:10.14202/vetworld.2015.674-684
Fathy, F. (2011). Anthelmintic Effect of Artesunate in Experimental Heterophyid Infection. J. Egypt. Soc. Parasitol. 41 (2), 469–483.
Fester, R. (2018). Plant Alkaloids in: Encyclopedia of Life Sciences. Plant Alkaloids. doi:10.1201/9780203743218
Fomum, S. W., and Nsahlai, I. V. (2017). In Vitro control of Parasitic Nematodes of Small Ruminants Using Some Plant Species Containing Flavonoids. Trop. Anim. Health Prod. 49 (2), 375–382. doi:10.1007/s11250-016-1203-6
Freire, M. M., Jham, G. N., Dhingra, O. D., Jardim, C. M., Barcelos, R. C., and Valente, V. M. M. (2012). Composition, Antifungal Activity and Main Fungitoxic Components of the Essential Oil of Mentha Piperita L. J. Food Saf. 32 (1), 29–36. doi:10.1111/j.1745-4565.2011.00341.x
García, D. E., Glasser, W. G., Pizzi, T. A., Osorio-Madrazo, A., and Laborie, M. P. G. (2014). Synthesis and Physicochemical Properties of Hydroxypropyl Tannins from Maritime pine Bark (Pinus pinaster Ait.). Holzforschung 68 (4), 411–418. doi:10.1515/hf-2013-0145
Geary, G. T., and Moreno, Y. (2012). Macrocyclic Lactone Anthelmintics: Spectrum of Activity and Mechanism of Action. Curr. Pharm. Biotechnol. 13 (6), 866–872. doi:10.2174/138920112800399077
Ghoulami, S., Il Idrissi, A., and Fkih-Tetouani, S. (2001). Phytochemical Study of Mentha Longifolia of Morocco. Fitoterapia 72 (5), 596–598. doi:10.1016/S0367-326X(01)00279-9
Gill, J. H., and Lacey, E. (1998). Avermectin/milbemycin Resistance in Trichostrongyloid Nematodes. Int. J. Parasitol. 28, 863–877. doi:10.1016/S0020-7519(98)00068-X
Giri, B. R., and Roy, B. (2015). Resveratrol- and α-viniferin-induced Alterations of Acetylcholinesterase and Nitric Oxide Synthase in Raillietina Echinobothrida. Parasitol. Res. 114 (10), 3775–3781. doi:10.1007/s00436-015-4607-8
Girme, A., Bhalke, R., Ghogare, P., Tambe, V., Jadhav, R., and Nirmal, S. (1970). Comparative In Vitro Anthelmintic Activity of Mentha Piperita and Lantana Camara from Western India. Dhaka Univ. J. Pharm. Sci. 5 (1), 5–7. doi:10.3329/dujps.v5i1.220
Githiori, J. B., Höglund, J., and Waller, P. J. (2005). Ethnoveterinary Plant Preparations as Livestock Dewormers: Practices, Popular Beliefs, Pitfalls and Prospects for the Future. Anim. Health Res. Rev. 6 (1), 91–103. doi:10.1079/ahr2005099
Githiori, J. B., Höglund, J., Waller, P. J., and Baker, R. L. (2004). Evaluation of Anthelmintic Properties of Some Plants Used as Livestock Dewormers against Haemonchus contortus Infections in Sheep. Parasitology 129 (2), 245–253. doi:10.1017/S0031182004005566
González-Manzano, S., Rivas-Gonzalo, J. C., and Santos-Buelga, C. (2004). “Extraction of Flavan-3-Ols from Grape Seed and Skin into Wine Using Simulated Maceration,” in Analytica Chimica Acta (Amsterdam: Elsevier), Vol. 513, 283–289. doi:10.1016/j.aca.2003.10.019
Goosen, T. C., Kent, U. M., Brand, L., and Hollenberg, P. F. (2000). Inactivation of Cytochrome P450 2B1 by Benzyl Isothiocyanate, a Chemopreventative Agent from Cruciferous Vegetables. Chem. Res. Toxicol. 13 (12), 1349–1359. doi:10.1021/tx000133y
Gradé, J. T., Arble, B. L., Weladji, R. B., and Van Damme, P. (2008). Anthelmintic Efficacy and Dose Determination of Albizia Anthelmintica against Gastrointestinal Nematodes in Naturally Infected Ugandan Sheep. Vet. Parasitol. 157 (3–4), 267–274. doi:10.1016/j.vetpar.2008.07.021
Grover, J. K., and Yadav, S. P. (2004). Pharmacological Actions and Potential Uses of Momordica Charantia: A Review. J. Ethnopharmacology 93 (1), 123–132. doi:10.1016/j.jep.2004.03.035
Grzybek, M., Kukula-Koch, W., Strachecka, A., Jaworska, A., Phiri, A. M., Paleolog, J., et al. (2016). Evaluation of Anthelmintic Activity and Composition of Pumpkin (Cucurbita Pepo L.) Seed Extracts—In Vitro and In Vivo Studies. Int. J. Mol. Sci. 17 (9), 1–21. doi:10.3390/ijms17091456
Guclu-Ustundag, Ö., and Mazza, G. (2007). Saponins: Properties, Applications and Processing. Crit. Rev. Food Sci. Nutr. 47 (3), 231–258. doi:10.1080/10408390600698197
Gueye, E. F. (1999). Ethnoveterinary Medicine against Poultry Diseases in African Villages. World’s Poult. Sci. J. 55 (2), 187–198. doi:10.1079/WPS19990013
Gutiérrez-Grijalva, E. P., López-Martínez, L. X., Contreras-Angulo, L. A., Elizalde-Romero, C. A., and Heredia, J. B. (2020). Plant Alkaloids: Structures and Bioactive Properties. In Plant-Derived Bioactives: Chemistry and Mode of Action. Singapore: Springer, 85–117. doi:10.1007/978-981-15-2361-8_5
Hajaji, S., Alimi, D., Jabri, M. A., Abuseir, S., Gharbi, M., and Akkari, H. (2018). Anthelmintic Activity of Tunisian Chamomile (Matricaria Recutita L.) against Haemonchus contortus. J. Helminthol. 92 (2), 168–177. doi:10.1017/S0022149X17000396
Hammond, J. A., Fielding, D., and Bishop, S. C. (1997). Prospects for Plant Anthelmintics in Tropical Veterinary Medicine. Vet. Res. Commun. 21 (3), 213–228. doi:10.1023/A:1005884429253
Hampl, R., Ostatníková, D., Celec, P., Putz, Z., Bílek, R., Lapčík, O., et al. (2009). Correlation between Soy Phytoestrogens and Thyroid Laboratory Parameters: Implications for Iodine Nutrition. Compr. Handbook Iodine, 353–363. doi:10.1016/B978-0-12-374135-6.00038-8
Hao, J-Y., Han, W., and Huang, S. de. (2002). Microwave-assisted Extraction of Artemisinin from Artemisia Annua L. Separat. Purif. Technol. 28 (3), 191–196. doi:10.1016/S1383-5866(02)00043-6
Hartady, T., Syamsunarno, M., Priosoeryanto, B. P., Jasni, S., and Balia, R. L. (2021). Review of Herbal Medicine Works in the Avian Species. Veterinary world 14 (11), 2889–2906. doi:10.14202/vetworld.2021.2889-2906
Heinrich, M. (2008). Ethnopharmacy and Natural Product Research-Multidisciplinary Opportunities for Research in the Metabolomic Age. Phytochemistry Letters. Amsterdam: Elsevier. doi:10.1016/j.phytol.2007.11.003
Hirazumi, A., and Furusawa, E. (1999). An Immunomodulatory Polysaccharide-Rich Substance from the Fruit Juice of Morinda citrifolia (Noni) with Antitumour Activity. Phytotherapy Res. 13 (5), 380–387. doi:10.1002/(SICI)1099-1573(199908/09)13:5<380:AID-PTR463>3.0.CO;2-M
Ho, S., Thoo, Y. Y., Young, D. J., and Siow, L. F. (2017). Inclusion Complexation of Catechin by β-cyclodextrins: Characterization and Storage Stability. LWT 86, 555–565. doi:10.1016/j.lwt.2017.08.041
Holden-Dye, L., and Walker, R. J. (2006). Actions of Glutamate and Ivermectin on the Pharyngeal Muscle of Ascaridia Galli: A Comparative Study with Caenorhabditis elegans. Int. J. Parasitol. 36 (4), 395–402. doi:10.1016/j.ijpara.2005.11.006
Hrckova, G., and Velebny, S. (2010). Flavonoid Silymarin Potentiates Antiheimintic Effect of Praziquantel via Down-Regulation of Oxidative Stress and Fibrogenesis in the Liver. Proc. World Med. Conf. 2010, 250–257.
Hussain, A., Khan, M. N., Iqbal, Z., and Sajid, M. S. (2008). An Account of the Botanical Anthelmintics Used in Traditional Veterinary Practices in Sahiwal District of Punjab, Pakistan. J. Ethnopharmacology 119 (1), 185–190. doi:10.1016/j.jep.2008.06.034
I, O. A., and J, N. E. (2011). Anthelmintic Efficacy of Cashew (Anarcadium Occidentale L.) on In Vitro Susceptibility of the Ova and Larvae of Haemonchus contortus. Afr. J. Biotechnol. 10 (47), 9700–9705. doi:10.5897/ajb09.1706
Ibrahim, M., Nwude, N., Ogunsusi, R., and Aliu, Y. (1984). Screening of West African Plants for Anthelmintic Activity. ILCA Bull. 17 (1964), 19–23. Available at: http://www.ilri.cgiar.org/InfoServ/Webpub/Fulldocs/Bulletin17/Screening.htm.
Ibrahim, T. A., and Fagbohun, E. (2013). Phytochemical and Mineral Quality of Dried Seeds of Buchholzia Coriacea. J. Appl. Phytotechnology Environ. Sanitation 2 (4), 121–126.
Idris, O. A., Wintola, O. A., and Afolayan, A. J. (2017). Phytochemical and Antioxidant Activities of Rumex Crispus L. In Treatment of Gastrointestinal Helminths in Eastern Cape Province, South Africa. Asian Pac. J. Trop. Biomed. 7 (12), 1071–1078. doi:10.1016/j.apjtb.2017.10.008
Iqbal, Z., Lateef, M., Jabbar, A., Ghayur, M. N., and Gilani, A. H. (2006). In vitro and In vivo Anthelmintic Activity of Nicotiana tabacum L. Leaves Against Gastrointestinal Nematodes of sheep. Phytotherapy research: PTR. 20 (1), 46–48. doi:10.1002/ptr.1800
Ishida, M., Hara, M., Fukino, N., Kakizaki, T., and Morimitsu, Y. (2014). Glucosinolate Metabolism, Functionality and Breeding for the Improvement of Brassicaceae Vegetables. Breed. Sci. 64, 48. doi:10.1270/jsbbs.64.48
Jabbar, A., Zaman, M. A., Iqbal, Z., Yaseen, M., and Shamim, A. (2007). Anthelmintic Activity of Chenopodium album (L.) and Caesalpinia Crista (L.) against Trichostrongylid Nematodes of Sheep. J. Ethnopharmacology 114 (1), 86–91. doi:10.1016/j.jep.2007.07.027
Jaiarj, P., Khoohaswan, P., Wongkrajang, Y., Peungvicha, P., Suriyawong, P., Saraya, M. L. S., et al. (1999). Anticough and Antimicrobial Activities of Psidium Guajava Linn. Leaf Extract. J. Ethnopharmacology 67 (2), 203–212. doi:10.1016/S0378-8741(99)00022-7
Jambre, L. F. L., Gill, J. H., Lenane, I. J., and Baker, P. (2000). Inheritance of Avermectin Resistance in Haemonchus contortus. Int. J. Parasitol. 30 (1), 105–111. doi:10.1016/S0020-7519(99)00172-1
Jirschitzka, J., Dolke, F., and D’Auria, J. C. (2013). “Increasing the Pace of New Discoveries in Tropane Alkaloid Biosynthesis,” in Advances in Botanical Research (Cambridge: Academic Press), Vol. 68, 39–72. doi:10.1016/B978-0-12-408061-4.00002-X
Jisaka, M., Kawanaka, M., Sugiyama, H., Takegawa, K., Huffman, M. A., Ohigashi, H., et al. (1992). NII-electronic Library Service. Chem. Pharm. Bull. 56 (5), 845–846. doi:10.1271/bbb.56.845
Joel Edwards, N. (2009). Digoxin. Small Anim. Crit. Care Med. 2009, 805–806. doi:10.1016/B978-1-4160-2591-7.10189-4
Juma Kk, A. Y., Njagi Jm, N. D., Ngugi Mp, A. D., Njagi, J., Agyirifo, D. S., Ngugi, M. P., et al. (2015). In-Vivo Anti-hyperglycemic Activity and Safety of the Aqueous Stem Bark Extracts of Aloe Secundiflora. Med. Aromat Plants s1, 1–8. doi:10.4172/2167-0412.s1-003
Kabasa, J. D., Opuda-Asibo, J., and Ter Meulen, U. (2000). The Effect of Oral Administration of Polyethylene Glycol on Faecal Helminth Egg Counts in Pregnant Goats Grazed on Browse Containing Condensed Tannins. Trop. Anim. Health Prod. 32 (2), 73–86. doi:10.1023/A:1005274502184
Kabasa, J., Tuyisenge, R., and Ugirinshuti, V. (2014). Preliminary Investigation on Anthelmintic Activity and Phytochemical Screening of Leaf Crude Extracts of Tithonia Diversifolia and Tephrosia Vogelii. Afr. J. Microbiol. Res. 8 (25), 2449–2457. doi:10.5897/ajmr2013.6525
Kahn, L., and Diaz-Hernandez, A. (1999). “Tannins and Anthelmintic Properties,” in 070708 Veterinary Parasitology. Editor J. D. Brooker (Adelaide: Brown Prior Anderson), 130–139. Available at: https://hdl.handle.net/1959.11/2594.
Kaingu, F., Kibor, A., Waihenya, R., Shivairo, R., and Mungai, L. (2013). Efficacy of Aloe Secundiflora Crude Extracts on Ascaridia Galli In Vitro. Sustain. Agric. Res. 2 (2), 49. doi:10.5539/sar.v2n2p49
Kamble, R. D., Jawadwar, G. V., Patil, S. D., Hese, S. V., Acharya, A. P., Dawane, B. S., et al. (2013). Bleaching Earth clay (Ph 12.5): A Novel and Reusable Catalyst for Rapid Synthesis of 7-hydroxy 4-styryl Coumarin Derivatives and Their Antihelmintic Activity. Org. Commun. 6 (2), 95–101.
Kane, S. R., Mohite, S. K., and Shete, J. S. (2009). Antihelmintic Activity of Aqueous and Methanolic Extracts of Euphorbia Thymifolia Linn. Int. J. PharmTech Res. 1 (3), 666–669.
Kaplan, R. M., and Vidyashankar, A. N. (2012). An Inconvenient Truth: Global Worming and Anthelmintic Resistance. Vet. Parasitol. 186 (1–2), 70–78. doi:10.1016/j.vetpar.2011.11.048
Kar, P. K., Murmu, S., Saha, S., Tandon, V., and Acharya, K. (2014). Anthelmintic Efficacy of Gold Nanoparticles Derived from a Phytopathogenic Fungus, Nigrospora Oryzae. PLoS ONE 9 (1), 1–9. doi:10.1371/journal.pone.0084693
Karumari, R. J., Sumathi, S., Vijayalakshmi, K., and Ezhilarasi, S. (2014). Anthelmintic Efficacy of Sesbania Grandiflora Leaves and Solanum Torvum Fruits against the Nematode Parasite Ascaridia Galli. Am. J. Ethnomedicine 1 (5), 326–333.
Kassie, F., Pool-Zobel, B., Parzefall, W., and Knasmüller, S. (1999). Genotoxic Effects of Benzyl Isothiocyanate, a Natural Chemopreventive Agent. Mutagenesis 14 (6), 595–603. doi:10.1093/mutage/14.6.595
Kateregga, J. N., Nabayunga, M., Vudriko, P., and Ndukui, J. (2014). Anthelmintic Activity of Cassia Occidentalis L. Methanolic Leaf Extract on Ascaridia Galli and Heterakis Gallinarum and its Acute Toxicity. Int. J. Basic Clin. Pharmacol. 3 (1), 114. doi:10.5455/2319-2003.ijbcp20140213
Katsori, A. M., and Hadjipavlou-Litina, D. (2014). Coumarin Derivatives: An Updated Patent Review (2012-2014). Expert Opin. Ther. Pat 24, 1323. doi:10.1517/13543776.2014.972368
Keane, J., and Avery, L. (2003). Mechanosensory Inputs Influence Caenorhabditis elegans Pharyngeal Activity via Ivermectin Sensitivity Genes. Genetics 164 (1), 153. doi:10.1093/genetics/164.1.153
Kerboeuf, D., Riou, M., and Guegnard, F. (2008). Flavonoids and Related Compounds in Parasitic Disease Control. Mini-Reviews Med. Chem. 8 (2), 116–128. doi:10.2174/138955708783498168
Kermanshai, R., McCarry, B. E., Rosenfeld, J., Summers, P. S., Weretilnyk, E. A., and Sorger, G. J. (2001). Benzyl Isothiocyanate Is the Chief or Sole Anthelmintic in Papaya Seed Extracts. Phytochemistry 57 (3), 427–435. doi:10.1016/S0031-9422(01)00077-2
Khafagi, I. K., and Dewedar, A. (2000). The Efficiency of Random versus Ethno-Directed Research in the Evaluation of Sinai Medicinal Plants for Bioactive Compounds. J. Ethnopharmacology 71 (3), 365–376. doi:10.1016/S0378-8741(00)00164-1
Kinoshita, T., Lepp, Z., and Chuman, H. (2005). Construction of a Novel Database for Flavonoids. J. Med. Invest. 52 (Suppl. ment), 291–292. doi:10.2152/jmi.52.291
Klongsiriwet, C., Quijada, J., Williams, A. R., Mueller-Harvey, I., Williamson, E. M., and Hoste, H. (2015). Synergistic Inhibition of haemonchus Contortus Exsheathment by Flavonoid Monomers and Condensed Tannins. Int. J. Parasitol. Drugs Drug Resist. 5 (3), 127–134. doi:10.1016/j.ijpddr.2015.06.001
Köhler, P. (2001). The Biochemical Basis of Anthelmintic Action and Resistance. International Journal for Parasitology. Amsterdam: Elsevier. doi:10.1016/S0020–7519(01)00131-X
Kohnen-Johannsen, K., and Kayser, O. (2019). Tropane Alkaloids: Chemistry, Pharmacology, Biosynthesis and Production. Molecules 24 (4), 796. doi:10.3390/molecules24040796
Kristo, T., Terdy, P. P., Simandi, B., Szoke, E., Lemberkovics, E., and Kery, A. (2001). Efficiency of Supercritical Fluid Extraction for the Production of Non-volatile Terpenoids from Taraxaci Radix. Acta Pharm. Hung 71 (3), 318–324.
Lalthanpuii, P. B., and Lalchhandama, K. (2020). Scanning Electron Microscopic Study of the Anthelmintic Effects of Some Anthelmintic Drugs on Poultry Nematode, Ascaridia Galli. Adv. Anim. Vet. Sci. 8 (8), 793. doi:10.17582/journal.aavs/2020/8.8.788.793
Landman, W. J. M. (2005). Efficacy of Allicin from Garlic against Ascaridia Galli Infection in Chickens. Poult. Sci. 90, 364–368. doi:10.3382/ps.2010-01090
Lans, C., and Turner, N. (2011). Organic Parasite Control for Poultry and Rabbits in British Columbia, Canada. J. Ethnobiol. Ethnomedicine 7 (1), 1–10. doi:10.1186/1746-4269-7-21
Levkut, M., Revajov, V., and Sevˇ, Z. (2017). The Influence of Farmatan R and Flimabend R. on mucosal Immun. broiler chicken 1, 1–6.
Lian, K., Zhang, M., Zhou, L., Song, Y., and Guan, X. (2020). Screening of Chinese Medicinal Herbs for Anthelmintic Efficacy against Gyrodactylus Kobayashii (Monogenea) in Goldfish (carassius Auratus). Kafkas Universitesi Veteriner Fakultesi Dergisi 26 (3), 357–363. doi:10.9775/kvfd.2019.23196
Lianfu, Z., and Zelong, L. (2008). Optimization and Comparison of Ultrasound/microwave Assisted Extraction (UMAE) and Ultrasonic Assisted Extraction (UAE) of Lycopene from Tomatoes. Ultrason. Sonochem. 15 (5), 731–737. doi:10.1016/j.ultsonch.2007.12.001
Lone, B. A., Bandh, S. A., Chishti, M. Z., Bhat, F. A., Tak, H., and Nisa, H. (2013). Anthelmintic and Antimicrobial Activity of Methanolic and Aqueous Extracts of Euphorbia Helioscopia L. Trop. Anim. Health Prod. 45 (3), 743–749. doi:10.1007/s11250-012-0283-1
Lubega, G. W., and Prichard, R. K. (1990). Specific Interaction of Benzimidazole Anthelmintics with Tubulin: High-Affinity Binding and Benzimidazole Resistance in Haemonchus contortus. Mol. Biochem. Parasitol. 38 (2), 221–232. doi:10.1016/0166-6851(90)90025-H
Luthria, D. L., Biswas, R., and Natarajan, S. (2007). Comparison of Extraction Solvents and Techniques Used for the Assay of Isoflavones from Soybean. Food Chem. 105 (1), 325–333. doi:10.1016/j.foodchem.2006.11.047
MacDonald, D., VanCrey, K., Harrison, P., Rangachari, P. K., Rosenfeld, J., Warren, C., et al. (2004). Ascaridole-less Infusions of Chenopodium Ambrosioides Contain a Nematocide(s) that Is(are) Not Toxic to Mammalian Smooth Muscle. J. Ethnopharmacology 92 (2–3), 215–221. doi:10.1016/j.jep.2004.02.018
Madhusudhana, J., Reddy, R. V. N., Reddy, B. A. K., Reddy, M. V. B., Gunasekar, D., Deville, A., et al. (2010). Two New Geranyl Flavanones from Tephrosia Villosa. Nat. Product. Res. 24 (8), 743–749. doi:10.1080/14786410903020560
Madrigal-Carballo, S., Rodriguez, G., Krueger, C. G., Dreher, M., and Reed, J. D. (2009). Pomegranate (Punica Granatum) Supplements: Authenticity, Antioxidant and Polyphenol Composition. J. Funct. Foods 1 (3), 324–329. doi:10.1016/j.jff.2009.02.005
Mahajan, A., and Dua, S. (1998). Role of Enzymatic Treatments in Modifying the Functional Properties of Rapeseed (Brassica Campestris Var. Toria) Meal. Int. J. Food Sci. Nutr. 49 (6), 435–440. doi:10.3109/09637489809086422
Mahdi, A. A., Rashed, M. M. A., Al-Ansi, W., Ahmed, M. I., Obadi, M., Jiang, Q., et al. (2019). Enhancing Bio-Recovery of Bioactive Compounds Extracted from Citrus Medica L. Var. Sarcodactylis: Optimization Performance of Integrated of Pulsed-Ultrasonic/microwave Technique. J. Food Meas. Characterization 13 (3), 1661–1673. doi:10.1007/s11694-019-00083-x
Mammam, A. A. E. (2014). Preliminary Phytochemical Screening of Diospyros Mespiliformis. Anat. Physiol. 04 (04), 5–7. doi:10.4172/2161-0940.1000156
Mann, A., Yahaya, Y., Banso, A., and Ajayi, G. O. (2008). Phytochemical and Antibacterial Screening of Anogeissus Leiocarpus against Some Microorganisms Associated with Infectious Wounds. Afr. J. Microbiol. Res. 2 (3), 60–62.
Mansoori, B., and Modirsanei, M. (2012). Effects of Dietary Tannic Acid and Vaccination on the Course of Coccidiosis in Experimentally Challenged Broiler Chicken. Vet. Parasitol. 187 (1–2), 119–122. doi:10.1016/j.vetpar.2011.12.016
Maphosa, V., Masika, P. J., Bizimenyera, E. S., and Eloff, J. N. (2010). In-vitro Anthelminthic Activity of Crude Aqueous Extracts of Aloe Ferox, Leonotis Leonurus and Elephantorrhiza Elephantina against Haemonchus contortus. Trop. Anim. Health Prod. 42 (2), 301–307. doi:10.1007/s11250-009-9421-9
Marie-magdeleine, C., Mahieu, M., Archimède, H., Marie-magdeleine, C., Mahieu, M., Archimède, H., et al. (2011). Pumpkin (Cucurbita Moschata Duchesne Ex Poir.) Seeds as an Anthelmintic Agent ? to Cite This Version : HAL Id : Hal-02810473 Author ’ S Personal Copy.
Maroyi, A. (2016). From Traditional Usage to Pharmacological Evidence: Systematic Review of Gunnera Perpensa L. Evidence-Based Complement. Altern. Med. 2016, 1720123. doi:10.1155/2016/1720123
Martin, R. J., Robertson, A. P., Buxton, S. K., Beech, R. N., Charvet, C. L., and Neveu, C. (2012). “Levamisole Receptors: A Second Awakening,” in Trends in Parasitology (Amsterdam: Elsevier Current Trends). doi:10.1016/j.pt.2012.04.003
Martins, N., Petropoulos, S., and Ferreira, I. C. F. R. (2016). Chemical Composition and Bioactive Compounds of Garlic (Allium Sativum L.) as Affected by Pre- and post-harvest Conditions: A Review. Food Chem. 211, 41–50. doi:10.1016/j.foodchem.2016.05.029
Marzoni, M., Castillo, A., Franzoni, A., Nery, J., Fortina, R., Romboli, I., et al. (2020). Effects of Dietary Quebracho Tannin on Performance Traits and Parasite Load in an Italian Slow-Growing Chicken (White Livorno Breed). Animals 10 (4), 684. doi:10.3390/ani10040684
Masihuddin, M., Jafri, M., Siddiqui, A., and Chaudhary, S. (2018). Traditional Uses, Phytochemistry and Pharmacological Activities of Papaver Somniferum with Special Reference of Unani Medicine an Updated Review. J. Drug Deliv. Ther. 8 (5-s), 110–114. doi:10.22270/jddt.v8i5-s.2069
Matsumoto, T., Hosono-Nishiyama, K., and Yamada, H. (2006). Antiproliferative and Apoptotic Effects of Butyrolactone Lignans from Arctium Lappa on Leukemic Cells. Planta Med. 72 (3), 276–278. doi:10.1055/s-2005-916174
Mbaveng, A. T., Hamm, R., and Kuete, V. (2014). Harmful and Protective Effects of Terpenoids from African Medicinal Plants. Toxicol. Surv. Afr. Med. Plants, 557–576. doi:10.1016/B978-0-12-800018-2.00019-4
Mcgaw, L. J., Famuyide, I. M., Khunoana, E. T., and Aremu, A. O. (2020). Ethnoveterinary Botanical Medicine in South Africa : A Review of Research from the Last Decade ( 2009 to 2019 ). J. Ethnopharmacology 257 (July 2019), 112864. doi:10.1016/j.jep.2020.112864
McGaw, L. J., Van der Merwe, D., and Eloff, J. N. (2007). In Vitro anthelmintic, Antibacterial and Cytotoxic Effects of Extracts from Plants Used in South African Ethnoveterinary Medicine. Vet. J. 173 (2), 366–372. doi:10.1016/j.tvjl.2005.09.004
Melzig, M. F., Bader, G., and Loose, R. (2001). Investigations of the Mechanism of Membrane Activity of Selected Triterpenoid Saponins. Planta Med. 67 (1), 43–48. doi:10.1055/s-2001-10632
Mirza, Z., Soto, E. R., Hu, Y., Nguyen, T.-T., Koch, D., Aroian, R. V., et al. (2020). Anthelmintic Activity of Yeast Particle-Encapsulated Terpenes. Molecules 25 (13), 2958. doi:10.3390/molecules25132958
Mohamed, N., El-Masry, R., Awad, A., and Badr, H. (2019). Chemical Composition and Antibacterial Activity of Codiaeum Variegatum Leaves. Zagazig J. Agric. Res. 46 (4), 1133–1140. doi:10.21608/zjar.2019.47093
Mokgadi, J., Turner, C., and Torto, N. (2013). Pressurized Hot Water Extraction of Alkaloids in Goldenseal. Am. J. Anal. Chem. 04 (08), 398–403. doi:10.4236/ajac.2013.48050
Moreira, R., Pereira, D., Valentão, P., and Andrade, P. (2018). Pyrrolizidine Alkaloids: Chemistry, Pharmacology, Toxicology and Food Safety. Int. J. Mol. Sci. 19 (6), 1668. doi:10.3390/ijms19061668
Morgan, E. D., and Wilson, I. D. (1999). Insect Hormones and Insect Chemical Ecology. Compr. Nat. Prod. Chem., 263–375. doi:10.1016/B978-0-08-091283-7.00053-9
Mubarokah, W. W., Nurcahyo, W., Prastowo, J., and Kurniasih, K. (2019). In Vitro and In Vivo Areca Catechu Crude Aqueous Extract as an Anthelmintic against Ascaridia Galli Infection in Chickens. Vet. World 12 (6), 877–882. doi:10.14202/vetworld.2019.877-882
Mukherjee, N., Mukherjee, S., Saini, P., Roy, P., and Sinha Babu, P. S. (2016). Phenolics and Terpenoids; the Promising New Search for Anthelmintics: A Critical Review. Mini-Reviews Med. Chem. 16 (17), 1415–1441. doi:10.2174/1389557516666151120121036
Mursof, E. P., and Simon, H. (1991). A Potential Role of Papaya Latex as an Anthelmintic against Patent Ascaridia Galli Infection in Chicken. Hemera Zoa 74 (3). Available at: http://journal.ipb.ac.id/index.php/hemera/article/view/4842.
Mustapha, A. (2013). Annona Senegalensis Persoon: a Multipurpose Shrub, its Phytotherapic, Phytopharmacological and Phytomedicinal Uses. Int. J. Sci. Technol. 2 (12), 862–865.
Mutai, C., Abatis, D., Vagias, C., Moreau, D., Roussakis, C., and Roussis, V. (2004). Cytotoxic Lupane-type Triterpenoids from Acacia Mellifera. Phytochemistry 65 (8), 1159–1164. doi:10.1016/j.phytochem.2004.03.002
Mwale, M., Bhebhe, E., Chimonyo, M., and Halimani, T. E. (2005). Use of Herbal Plants in Poultry Health Management in the Mushagashe Small-Scale Commercial Farming Area in Zimbabwe. Zimbabwe Intern. J. Appl. Res. Vet. Med. 3.
Mwale, M., and Masika, P. J. (2015). In Vitro Anthelmintic Efficacy of Medicinal Plants against Heterakis Gallinarum in Village Chickens. J. Agric. Sci. 7 (12), 247. doi:10.5539/jas.v7n12p247
Mwale, M., Masika, P. J., and Masika, P. J. (2015). In Vitro Anthelmintic Efficacy of Medicinal Plants against Heterakis Gallinarum in Village Chickens Contract Farming Approach to Essential Oil Production in the Eastern Cape Province of South Africa View Project Eisenia Foetida as Feed Ingredient for Broilers View Project In Vitro Anthelmintic Efficacy of Medicinal Plants against Heterakis Gallinarum in Village Chickens. J. Agric. Sci. 7 (12), 247. doi:10.5539/jas.v7n12p247
Mwale, M., and Masika, P. J. (2009). Ethno-veterinary Control of Parasites, Management and Role of Village Chickens in Rural Households of Centane District in the Eastern Cape, South Africa. Trop. Anim. Health Prod. 41 (8), 1685–1693. doi:10.1007/s11250-009-9366-z
Nabukenya, I., Rubaire-Akiiki, C., Olila, O., Ikwap, K., and Höglund, J. (2014). Ethnopharmacological Practices by Livestock Farmers in Uganda: Survey Experiences from Mpigi and Gulu Districts. J. Ethnobiol. Ethnomedicine. 10, 9. doi:10.1186/1746-4269-10-9
Nakamura, T., Murata, Y., and Nakamura, Y. (2019). Characterization of Benzyl Isothiocyanate Extracted from Mashed green Papaya by Distillation. Food Chem. 299, 125118. doi:10.1016/j.foodchem.2019.125118
Nalule, A., and Mbaria, J. (2013). In Vitro anthelmintic Potential of Vernonia Amygdalina and Secamone Africana on Gastrointestinal Nematodes. Agric. Biol. J. North America 4 (1), 54–66. doi:10.5251/abjna.2013.4.1.54.66
Nandeeshwar, P., Kumar, V. K., Krishnasai, K., and Ramanjaneyulu, K. (2019). In Vitro anthelmintic Activity of Gossypium Herbaceum. Int. Res. J. Pharm. 8 (2), 1624–1626.
Nandhini, S., Narayanan, K. B., and Ilango, K. (2018). Valeriana Officinalis: A Review of its Traditional Uses, Phytochemistry and Pharmacology. Asian J. Pharm. Clin. Res. 11 (1), 36–41. doi:10.22159/ajpcr.2018.v11i1.22588
Ndhlala, A. R., Ghebrehiwot, H. M., Ncube, B., Aremu, A. O., Gruz, J., Šubrtová, M., et al. (2015). Antimicrobial, Anthelmintic Activities and Characterisation of Functional Phenolic Acids of Achyranthes aspera Linn.: A Medicinal Plant Used for the Treatment of Wounds and Ringworm in East Africa. Front. Pharmacol. 6 (NOV), 274. doi:10.3389/fphar.2015.00274
Nghonjuyi, N. W., Keambou, C. T., Sofeu-Feugaing, D. D., Taiwe, G. S., Aziz, A. R. A., Lisita, F., et al. (2020). Mimosa Pudica and Carica Papaya Extracts on Ascaridia Galli - Experimentally Infected Kabir Chicks in Cameroon: Efficacy, Lipid and Hematological Profile. Vet. Parasitol. Reg. Stud. Rep. 19 (August 2019), 100354. doi:10.1016/j.vprsr.2019.100354
Nghonjuyi, N. W., Tiambo, C. K., Taïwe, G. S., Toukala, J. P., Lisita, F., Juliano, R. S., et al. (2016). Acute and Sub-chronic Toxicity Studies of Three Plants Used in Cameroonian Ethnoveterinary Medicine: Aloe Vera (L.) Burm. F. (Xanthorrhoeaceae) Leaves, Carica Papaya L. (Caricaceae) Seeds or Leaves, and Mimosa Pudica L. (Fabaceae) Leaves in Kabir Chicks. J. Ethnopharmacology 178, 40–49. doi:10.1016/j.jep.2015.11.049
Nirala, R. K., Raj, P., Anjana, K., and Mandal, K. G. (2019). Medicinal Plants and its Activity against Helminth. A Rev. 8 (5), 2348–2355.
Nweze, N. E., and Asuzu, I. (2006). The Anthelmintic Effects of Buchholzia Coriacea Seed. Niger. Vet. J. 27 (2), 60–65.
Odhong, C., Wahome, R., Vaarst, M., Nalubwama, S., Kiggundu, M., Halberg, N., et al. (2014). In Vitro anthelmintic Effects of Crude Aqueous Extracts of Tephrosia Vogelii, Tephrosia Villosa and Carica Papaya Leaves and Seeds. Afr. J. Biotechnol. 13 (52), 4667–4672. doi:10.5897/ajb2014.14048
Ogunwande, I. A., Olawore, N. O., Adeleke, K. A., Ekundayo, O., and Koenig, W. A. (2003). Chemical Composition of the Leaf Volatile Oil of Psidium Guajava L. Growing in Nigeria. Flavour Fragrance J. 18 (2), 136–138. doi:10.1002/ffj.1175
Ola-Fadunsin, S. D., Ganiyu, I. A., Rabiu, M., Hussain, K., Sanda, I. M., Musa, S. A., et al. (2019). Gastrointestinal Parasites of Different Avian Species in Ilorin, North Central Nigeria. J. Adv. Vet. Anim. Res. 6 (1), 108–116. doi:10.5455/javar.2019.f320
Oliveira, A. F., Junior, L. M. C., Lima, A. S., Silva, C. R., Ribeiro, M. N. S., Mesquista, J. W. C., et al. (2017). Anthelmintic Activity of Plant Extracts from Brazilian savanna. Vet. Parasitol. 236, 121–127. doi:10.1016/j.vetpar.2017.02.005
Oyeyemi, I. T., Akinlabi, A. A., Adewumi, A., Aleshinloye, A. O., and Oyeyemi, O. T. (2018). Vernonia Amygdalina : A Folkloric Herb with Anthelminthic Properties. Beni-Suef Univ. J. Basic Appl. Sci. 7 (1), 43–49. doi:10.1016/j.bjbas.2017.07.007
Ozaraga, M. I. S., and Ozaraga, B. P. (2017). EFFICACY of IPIL-IPIL (Leucaena Leucocephala), BETEL NUT (Areca Catechu) and PAPAYA (Carica Papaya) SEEDS against ROUNDWORMS of DARAG NATIVE CHICKEN. Anim. Sci. 43.Available at: http://pjvas.org/index.php/pjvas/article/view/173.
Pacios-Michelena, A., Kasaragod, V. B., and Schindelin, H. (2021). Artemisinins and Their Impact on Inhibitory Neurotransmission. Curr. Opin. Pharmacol. 59, 19–25. doi:10.1016/J.COPH.2021.04.008
Pal Singh, I., and Bharate, S. B. (2006). Phloroglucinol Compounds of Natural Origin. Nat. Product. Rep. 23 (4), 558–591. doi:10.1039/b600518g
Pande, P. C., and Tiwari, L. P. H. (2007). NOPR: Ethnoveterinary Plants of Uttaranchal — A Review. Indian J. Traditional Knowledge 6 (3), 444–458. Available at: http://nopr.niscair.res.in/handle/123456789/977.
Patel, T., Marmulak, T., Gehring, R., Pitesky, M., Clapham, M. O., and Tell, L. A. (2018). Drug Residues in Poultry Meat: A Literature Review of Commonly Used Veterinary Antibacterials and Anthelmintics Used in Poultry. J. Vet. Pharmacol. Ther. 41 (6), 761–789. doi:10.1111/jvp.12700
Patilaya, P., Husori, D. I., and Sumantri, I. B. (2017). The Anthelmintic Effects of Ethanol Extract of Curanga Fel-Terrae Leaves on Ascaridia Galli. Asian J. Pharm. Clin. Res. 10 (3), 117–119. doi:10.22159/ajpcr.2017.v10i3.15504
Patočka, J., and Plucar, B. (2003). Pharmacology and Toxicology of Absinthe. J. Appl. Biomed. 1 (4), 199–205. doi:10.32725/jab.2003.036
Pawar, B. M., Wavhal, V. P., Pawar, N. D., Agarwal, M. R., Shinde, B. P., and Kamble, H. (2010). Anthelmintic Activity of Gloriosa Superba Linn (Liliaceae). Int. J. PharmTech Res. 2 (2), 1483–1487.
Pereira, C. A. J., Oliveira, L. L. S., Coaglio, A. L., Santos, F. S. O., Cezar, R. S. M., Mendes, T., et al. (2016). Anti-helminthic Activity of Momordica Charantia L. Against Fasciola Hepatica Eggs after Twelve Days of Incubation In Vitro. Vet. Parasitol. 228, 160–166. doi:10.1016/j.vetpar.2016.08.025
Perrett, S., and Whitfield, P. (1995). Atanine (3-Dimethylallyl-4-Methoxy-2-Quinolone), an Alkaloid with Anthelmintic Activity from the Chinese Medicinal Plant. Evodia Rutaecarpa. Planta Med. 61 (03), 276–278. doi:10.1055/s-2006-958073
Pillai, L. S., and Nair, B. R. (2011). A Comparative Study of the Anthelmintic Potential of Cleome Viscosa L. And Cleome Burmanni W. and A. Indian J. Pharm. Sci. 73 (1), 98–100. doi:10.4103/0250-474x.89766
Piña-Vázquez, D. M., Mayoral-Peña, Z., Gómez-Sánchez, M., Salazar-Olivo, L. A., and Arellano-Carbajal, F. (2017). Anthelmintic Effect of Psidium Guajava and Tagetes erecta on Wild-type and Levamisole-Resistant Caenorhabditis elegans Strains. J. Ethnopharmacology 202, 92–96. doi:10.1016/j.jep.2017.03.004
Polovetskaya, O. S. T. A. V., Nikishina, M. B., and Ivanova, E. V. Z. K. N. (2017). (Tanaceti Vulgaris Flores) Morpho-Anatomical Diagnosis of the Crushed. Tanaceti vulgaris flores 3, 265–269. doi:10.17117/na.2017.05.03.265
Poolperm, S., and Jiraungkoorskul, W. (2017). An Update Review on the Anthelmintic Activity of Bitter Gourd, Momordica Charantia. Pharmacogn Rev. 11, 31. doi:10.4103/phrev.phrev_52_16
Prichard, R. (1994). Anthelmintic Resistance. Vet. Parasitol. 54 (1–3), 259–268. doi:10.1016/0304-4017(94)90094-9
Qian, H., Robertson, A. P., Powell-Coffman, J. A., and Martin, R. J. (2008). Levamisole Resistance Resolved at the Single-Channel Level in Caenorhabditis elegans. FASEB J. 22 (9), 3247–3254. doi:10.1096/fj.08-110502
Rafiee, Z., Jafari, S. M., Alami, M., and Khomeiri, M. (2011). Microwave-Assisted Extraction of Phenolic Compounds from Olive Leaves; a Comparison with Maceration. J. Anim. Plant Sci. 21, 738–745.
Rana, A. K., and Misra-Bhattacharya, S. (2013). Current Drug Targets for Helminthic Diseases. Parasitol. Res. 112 (5), 1819–1831. doi:10.1007/s00436-013-3383-6
Rashid, M. M. O., Ferdous, J., Banik, S., Islam, M. R., Uddin, A. H. M. M., and Robel, F. N. (2016). Anthelmintic Activity of Silver-Extract Nanoparticles Synthesized from the Combination of Silver Nanoparticles and M. Charantia Fruit Extract. BMC Complement. Altern. Med. 16 (1), 1–6. doi:10.1186/s12906-016-1219-5
Rathee, P., Chaudhary, H., Rathee, S., Rathee, D., Kumar, V., and Kohli, K. (2009). Mechanism of Action of Flavonoids as Anti-inflammatory Agents: A Review. Inflamm. Allergy - Drug Targets 8 (3), 229–235. doi:10.2174/187152809788681029
Raza, A., Muhammad, F., Bashir, S., Aslam, B., Anwar, M. I., and Naseer, M. U. (2016). In-vitro and In-Vivo Anthelmintic Potential of Different Medicinal Plants against Ascaridia Galli Infection in Poultry Birds. World’s Poultry Science Journal. Cambridge: Cambridge University Press. doi:10.1017/S0043933915002615
Ribeiro, B. D., Alviano, D. S., Barreto, D. W., and Coelho, M. A. Z. (2013). Functional Properties of Saponins from Sisal (Agave Sisalana) and Juá (Ziziphus Joazeiro): Critical Micellar Concentration, Antioxidant and Antimicrobial Activities. Colloids and Surfaces A:. Physicochemical Eng. Aspects 436, 736–743. doi:10.1016/j.colsurfa.2013.08.007
Richerzhagen, C. (2007). Effectiveness and Perspectives of Access and Benefit-sharing Regimes in the Convention on Biological Diversity - A Comparative Analysis of Costa Rica, the Philippines, Ethiopia and the European Union. Universitäts- und Landesbibliothek Bonn. Available at: http://hss.ulb.uni-bonn.de/diss_online.
Richerzhagen, C., and Holm-Mueller, K. (2005). The Effectiveness of Access and Benefit Sharing in Costa Rica: Implications for National and International Regimes. Ecol. Econ. 53 (4), 445–460. doi:10.1016/j.ecolecon.2004.06.031
Richerzhagen, C., and Virchow, D. (2007). Sustainable Utilisation of Crop Genetic Diversity through Property Rights Mechanisms: The Case of Coffee Genetic Resources in Ethiopia. Int. J. Biotechnol. 9 (1), 60–86. doi:10.1504/IJBT.2007.012169
Ritter, R. A., Monteiro, M. V. B., Monteiro, F. O. B., Rodrigues, S. T., Soares, M. L., Silva, J. C. R., et al. (2012). Ethnoveterinary Knowledge and Practices at Colares Island, Pará State, Eastern Amazon, Brazil. J. Ethnopharmacology 144 (2), 346–352. doi:10.1016/j.jep.2012.09.018
Robertson, A. P., Bjorn, H. E., and Martin, R. J. (1999). Resistance to Levamisole Resolved at the Single-Channel Level. FASEB J. 13 (6), 749–760. doi:10.1096/fasebj.13.6.749
Rocha, J. A., Andrade, I. M., Véras, L. M. C., Quelemes, P. V., Lima, D. F., Soares, M. J. S., et al. (2017). Anthelmintic, Antibacterial and Cytotoxicity Activity of Imidazole Alkaloids from Pilocarpus Microphyllus Leaves. Phytotherapy Res. 31 (4), 624–630. doi:10.1002/ptr.5771
Rodriguez-Rodriguez, R., and Ruiz-Gutierrez, V. (2010). Functional Properties of Pentacyclic Triterpenes Contained in Pomace Olive Oil. Olives Olive Oil Health Dis. Prev., 1431–1438. doi:10.1016/B978-0-12-374420-3.00159-5
Roos, M. H. (1997). The Role of Drugs in the Control of Parasitic Nematode Infections: Must We Do without? Parasitology 114 (Suppl. 1), S137. doi:10.1017/s0031182097008986
Ruff, M. D. (1999). Important Parasites in Poultry Production Systems. Vet. Parasitol. 84 (3–4), 337–347. doi:10.1016/S0304-4017(99)00076-X
Sable, S. D., and Dhawale, S. C. (2013). Phytochemical Analysis and In-Vitro Anthelmintic Activity of Musa Paradisiaca linn and Sesbania Grandiflora. Int. J. Chem. Pharm. Sci. 4 (2), 69–73.
Sabudak, T., and Guler, N. (2009). Trifolium L. - A Review on its Phytochemical and Pharmacological Profile. Phytotherapy Res. 23 (3), 439–446. doi:10.1002/ptr.2709
Salau, A., Yakubu, M., and Oladiji, A. (2013). Cytotoxic Activity of Aqueous Extracts of Anogeissus Leiocarpus and Terminalia Avicennioides Root Barks against Ehrlich Ascites Carcinoma Cells. Indian J. Pharmacol. 45 (4), 381–385. doi:10.4103/0253-7613.115023
Saleem, M. (2009). Lupeol, a Novel Anti-inflammatory and Anti-cancer Dietary Triterpene. Cancer Lett. 285 (2), 109–115. doi:10.1016/J.CANLET.2009.04.033
Salminen, J.-P., Karonen, M., and Sinkkonen, J. (2011). Chemical Ecology of Tannins: Recent Developments in Tannin Chemistry Reveal New Structures and Structure-Activity Patterns. Chem. - A Eur. J. 17 (10), 2806–2816. doi:10.1002/chem.201002662
Sandabe, U. K., Onyeyili, P. A., and Chibuzo, G. A. (2006). Phytochemical Screening and Effect of Aqueous Extract of Ficus Sycomorus L. (Moraceae) Stembark on Muscular Activity in Laboratory Animals. J. Ethnopharmacology 104 (1–2), 283–285. doi:10.1016/j.jep.2005.08.06610.1016/j.jep.2005.08.025
Sandoval-Castro, C. A., Torres-Acosta, J. F. J., Hoste, H., Salem, A. Z. M., and Chan-Pérez, J. I. (2012). Using Plant Bioactive Materials to Control Gastrointestinal Tract Helminths in Livestock. Anim. Feed Sci. Technol. 176 (1–4), 192–201. doi:10.1016/j.anifeedsci.2012.07.023
Santos, A. C. V., Santos, F. O., Lima, H. G., Silva, G. D. Da., Uzêda, R. S., Dias, Ê. R., et al. (2018). In Vitro ovicidal and Larvicidal Activities of Some Saponins and Flavonoids against Parasitic Nematodes of Goats. Parasitology 145 (14), 1884–1889. doi:10.1017/S0031182018000689
Satrija, F., Retnani, E. B., Ridwan, Y., and Tiuria, R. (2001a). “Potential Use of Herbal Anthelmintics as Alternative Antiparasitic Drugs for Small,” in Proceedings of the 10th Conference of the Association of Institutions for Tropical Veterinary Medicine, Copenhagen, Denmark, 1–10.
Satrija, F., Retnani, E., Ridwan, Y., and Tiuria, R. (2001b). Potential Use of Herbal Anthelmintics as Alternative Antiparasitic Drugs for Small Holder Farms in Developing Countries. Undefined.
Savage, S. R., Joranson, D. E., Covington, E. C., Schnoll, S. H., Heit, H. A., and Gilson, A. M. (2003). Definitions Related to the Medical Use of Opioids: Evolution towards Universal Agreement. J. Pain Symptom Manage. 26 (1), 655–667. doi:10.1016/S0885-3924(03)00219-7
Scantlebury, C. E., Peachey, L., Hodgkinson, J., Matthews, J. B., Trawford, A., Mulugeta, G., et al. (2013). Participatory Study of Medicinal Plants Used in the Control of Gastrointestinal Parasites in Donkeys in Eastern Shewa and Arsi Zones of Oromia Region, Ethiopia. BMC Vet. Res. 9 (1), 1–12. doi:10.1186/1746-6148-9-179
Schillhorn Van Veen, T. W. (1997). Sense or Nonsense? Traditional Methods of Animal Parasitic Disease Control. Vet. Parasitol. 71, 177–194. doi:10.1016/S0304-4017(97)00031-9
Shah, H., and Khan, A. A. (2017). Phytochemical Characterisation of an Important Medicinal Plant, Chenopodium Ambrosioides Linn. Nat. Product. Res. 31 (19), 2321–2324. doi:10.1080/14786419.2017.1299722
Shah, K., Patel, M., Patel, R., and Parmar, P. (2010). Mangifera Indica (Mango). Pharmacognosy Rev. 4, 42. doi:10.4103/0973-7847.65325
Shailajan, S., Kumaria, S., Pednekar, S., Menon, S., Joshi, H., and Matani, A. (2014). Chromatographic Evaluation of a Phytoestrogen Genistein from Flemingia Vestita Benth: an Endemic Plant of Northeast India. Pharmacognosy Commun. 4 (4). doi:10.5530/pc.2014.4.2
Shalaby, H. A. (2013). Anthelmintics Resistance; How to Overcome it? Iranian J. Parasitol. 8 (1), 18–32. Available at: http://ijpa.tums.ac.ir.
Shang, H., Zhou, H., Duan, M., Li, R., Wu, H., and Lou, Y. (2018). Extraction Condition Optimization and Effects of Drying Methods on Physicochemical Properties and Antioxidant Activities of Polysaccharides from comfrey (Symphytum Officinale L.) Root. Int. J. Biol. Macromolecules 112, 889–899. doi:10.1016/j.ijbiomac.2018.01.198
Sharma, A., Sharma, A. K., Chand, T., Khardiya, M., and Yadav, K. C. (2013). Preliminary Phytochemical Evaluation of Seed Extracts of Cucurbita Maxima Duchense. J. Pharmacol. Phytochemistry 2 (3), 62–65.
Shen, X., Chen, W., Zheng, Y., Lei, X., Tang, M., Wang, H., et al. (2017). Chemical Composition, Antibacterial and Antioxidant Activities of Hydrosols from Different Parts of Areca Catechu L. And Cocos Nucifera L. Ind. Crops Prod. 96, 110–119. doi:10.1016/j.indcrop.2016.11.053
Sheng-Ji, P. (2001). Ethnobotanical Approaches of Traditional Medicine Studies: Some Experiences from Asia. Pharm. Biol. 39 (Suppl. 1), 74–79. doi:10.1076/phbi.39.s1.74.0005
Shi, Z.-Y., Zeng, J.-Z., and Wong, A. S. T. (2019). Chemical Structures and Pharmacological Profiles of Ginseng Saponins. Molecules 24 (13), 2443. doi:10.3390/molecules24132443
Siamba, D. N., Okitoi, L. O., Watai, M. K., Wachira, A. M., Lukibisi, F. B., and Mukisira, E. (2007). Efficacy of Tephrosia Vogelli and Vernonia Amygdalina as Anthelmintics against Ascaridia Galli in Indigenous Chicken. Livestock Res. Rural Develop. 19 (12).
Siebenhüner, B., Dedeurwaerdere, T., and Brousseau, E. (2005). Introduction and Overview to the Special Issue on Biodiversity Conservation, Access and Benefit-Sharing and Traditional Knowledge. Ecological Economics. Amsterdam: Elsevier. doi:10.1016/j.ecolecon.2004.12.012
Siebenhüner, B., and Suplie, J. (2005). Implementing the Access and Benefit-Sharing Provisions of the CBD: A Case for Institutional Learning. Ecol. Econ. 53 (4), 507–522. doi:10.1016/j.ecolecon.2004.10.012
Singh, D., and Chaudhuri, P. K. (2018). Structural Characteristics, Bioavailability and Cardioprotective Potential of Saponins. Integr. Med. Res. 7 (1), 33–43. doi:10.1016/j.imr.2018.01.003
Smeriglio, A., Barreca, D., Bellocco, E., and Trombetta, D. (2016). Chemistry, Pharmacology and Health Benefits of Anthocyanins. Phytotherapy Res. 30, 1265. doi:10.1002/ptr.5642
Sobolewska, D., Michalska, K., Podolak, I., and Grabowska, K. (2016). Steroidal Saponins from the Genus Allium. Phytochemistry Rev. 15 (1), 1–35. doi:10.1007/s11101-014-9381-1
Sponsel, V. M. (2003). Gibberellins. Encyclopedia Horm. 2003, 29–40. doi:10.1016/B0-12-341103-3/00108-X
Stefanachi, A., Leonetti, F., Pisani, L., Catto, M., and Carotti, A. (2018). Coumarin: A Natural, Privileged and Versatile Scaffold for Bioactive Compounds. Molecules 23 (2), 250. doi:10.3390/molecules23020250
Stepek, G., Behnke, J. M., Buttle, D. J., and Duce, I. R. (2004). Natural Plant Cysteine Proteinases as Anthelmintics? Trends Parasitol. 20 (7), 322–327. doi:10.1016/j.pt.2004.05.003
Sucharew, H., and Macaluso, M. (2019). Methods for Research Evidence Synthesis: The Scoping Review Approach. J. Hosp. Med. 14 (7), 416–418. doi:10.12788/JHM.3248
Suleiman, M. M., Mamman, M., Sidiama, A., Ighoja, E. J., Tauheed, M., and Talba, A. M. (2014). Evaluation of Anthelmintic Activity of Nigerian Ethnoveterinary Plants; Cassia Occidentalis and Guiera Senegalensis. Vet. World 7 (7), 536–541. doi:10.14202/vetworld.2014.536-541
Suryavanshi, S., Rai, G., Malviya, S. N., and Suryavanshi, S. (2012). Evaluation of Anti-microbial and Anthelmintic Activity of Gloriosa, 2(March), 45–52.
Susmitha, S., Vidyamol, K. K., Ranganayaki, P., and Vijayaragavan, R. (2013). Phytochemical Extraction and Antimicrobial Properties of Azadirachta Indica (Neem). Glob. J. Pharmacol. 7 (3), 316–320. doi:10.5829/idosi.gjp.2013.7.3.1107
Tamilarasi, T., and Ananthi, T. (2012). Phytochemical Analysis and Anti Microbial Activity of. Res. J. Chem. Sci. 2 (2), 72–74. Available at: www.isca.in.
Tandon, V., Lucknow, B. P., Yadav, A. K., Roy, B., and Das, B. (2011). Worm Infections in Traditional Medicine Systems, (April 2014).
Tandon, V., Yadav, A. K., Roy, B., and Das, B. (2011). Phytochemicals as Cure of Worm Infections in Traditional Medicine Systems Evaluation of Mitochondrial Glycerophosphate Dehydrogenase (mGPDH) for its Role in Type 2 Diabetes Mellitus (T2DM) View Project Nematode Fauna View Project. Available at: https://www.researchgate.net/publication/233951689.
Tandon, V., Pal, P., Roy, B., Rao, H. S. P., and Reddy, K. S. (1997). In Vitro anthelmintic Activity of Root-Tuber Extract of Flemingia Vestita, an Indigenous Plant in Shillong, India. Parasitol. Res. 83 (5), 492–498. doi:10.1007/s004360050286
Tangpu, V., Temjenmongla, K., and Yadav, A. K. (2004). Anticestodal Activity of Trifolium Repens Extract. Pharm. Biol. 42 (8), 656–658. doi:10.1080/13880200490902617
Tarbiat, B. (2018). Ascaridia Galli in Laying Hens: Adaptation of a Targeted Treatment Strategy with Attention to Anti-helmintic Resistance - Ascaridia Galli. Uppsala, Sweden: Swedish University of Agriculture Sciences.
Terada, M., Sano, M., Ishii, A. I., Kino, H., Fukushima, S., and Noro, T. (1982). Studies on Chemotherapy of Parasitic Helminths (IV). Effects of Alkaloids from Sophora Flavescens on the Motility of Parasitic Helminths and Isolated Host Tissues. Folia Pharmacologica Japonica 79 (2), 105–111. doi:10.1254/fpj.79.105
Thapa, S., Thamsborg, S. M., Wang, R., Meyling, N. V., Dalgaard, T. S., Petersen, H. H., et al. (2018). Effect of the Nematophagous Fungus Pochonia Chlamydosporia on Soil Content of Ascarid Eggs and Infection Levels in Exposed Hens. Parasites and Vectors 11 (1), 1–11. doi:10.1186/s13071-018-2898-1
Tian, Y., Xu, Z., Zheng, B., and Martin Lo, Y. (2013). Optimization of Ultrasonic-Assisted Extraction of Pomegranate (Punica Granatum L.) Seed Oil. Ultrason. Sonochem. 20 (1), 202–208. doi:10.1016/j.ultsonch.2012.07.010
Torres, F. C., Brucker, N., Fernandes Andrade, S., Fabio Kawano, D., Cristina Garcia, S., Lino von Poser, G., et al. (2014). New Insights into the Chemistry and Antioxidant Activity of Coumarins. Curr. Top. Med. Chem. 14 (22), 2600. doi:10.2174/1568026614666141203144551
Toyang, N. J., Ndi, C., Django, S., Nuwanyakpa, M., and Kinyuy, W. C. (1995). Ethnoveterinary Medicine Practices in the Northwest Province of Cameroon. Indigenous Knowledge Develop. Monitor 3 (3), 20–22.
Tulloch, A. P., and Hoffman, L. L. (1982). Epicuticular Wax of Cirsium Arvense. Phytochemistry 21 (7), 1639–1642. doi:10.1016/S0031-9422(82)85031-0
Tuwangye, I., and Olila, D. (2006). The Anthelmintic Activity of Selected Indigenous Medicinal Plants Used by the Banyankole of Western Uganda. J. Anim. Vet. Adv. Available at: http://198.170.104.138/medwellonline/java/2006/712-717.pdf.
Udobi, M. I., Nzeakor, T. A., Eke, I. G., Ezeh, I. O., Onyeabor, A., Idika, I. K., et al. (2018). Evaluation of the Anthelminthic Potential of Duranta Erecta L. (Verbenaceae) Fruits Used in Nigerian Ethnomedicine as a Vermifuge. J. Ethnopharmacology 216 (January), 57–62. doi:10.1016/j.jep.2018.01.030
Urban, J., Kokoska, L., Langrova, I., and Matejkova, J. (2008). In Vitro anthelmintic Effects of Medicinal Plants Used in Czech Republic. Pharm. Biol. 46 (10–11), 808–813. doi:10.1080/13880200802315618
Uzma, S., Khalid, H., Mobasher, A., Nadeem, B. I., Arif, M., and Bashir, A. (2014). Physicochemical and Phytochemical Analysis of Euphorbia Helioscopia (L.). Pakistan J. Pharm. Sci. 27 (3), 577–585.
Valentynivna, T. K., and Ivanivna, V. L. (2017). Scientific Justification of Anthelmintic Medicines Based on Medicinal Plant Material. Int. J. Green Pharm. 11 (3), 154–159.
Van Overwalle, G. (2005). Protecting and Sharing Biodiversity and Traditional Knowledge: Holder and User Tools. Ecol. Econ. 53 (4), 585–607. doi:10.1016/j.ecolecon.2004.10.014
Van Wyk, J. A. (2001). Refugia-overlooked as Perhaps the Most Potent Factor Concerning the Development of Anthelmintic Resistance Onderstepoort. J. Vet. Res. 68, 55. Available at: https://repository.up.ac.za/handle/2263/18482.
Varsha, K., Sharma, A., Kaur, A., Madan, J., Pandey, R. S., Jain, U. K., et al. (2017). Natural Plant-Derived Anticancer Drugs Nanotherapeutics: a Review on Preclinical to Clinical success. Nanostructures Cancer Ther. 2017, 775–809. doi:10.1016/B978-0-323-46144-3.00028-3
Velkers, F. C., Dieho, K., Pecher, F. W. M., Vernooij, J. C. M., Van Eck, J. H. H., and Landman, W. J. M. (2011). Efficacy of Allicin from Garlic against Ascaridia Galli Infection in Chickens. Poult. Sci. 90 (2), 364–368. doi:10.3382/ps.2010-01090
Verpoorte, R. (2005). Encyclopedia of Analytical Science. Second. Amsterdam: Elsevier, 56–61. doi:10.1016/b0-12-369397-7/00010-8
Von Samson-Himmelstjerna, G., and Blackhall, W. (2005). Will Technology Provide Solutions for Drug Resistance in Veterinary Helminths? Vet. Parasitol. 132 (3-4 SPEC. ISS.), 223–239. doi:10.1016/j.vetpar.2005.07.014
Wang, C., Liu, J., Deng, J., Wang, J., Weng, W., Chu, H., et al. (2020). Advances in the Chemistry, Pharmacological Diversity, and Metabolism of 20(R)-ginseng Saponins. J. Ginseng Res. 44, 14. doi:10.1016/j.jgr.2019.01.005
Wang, G. X., Han, J., Zhao, L. W., Jiang, D. X., Liu, Y. T., and Liu, X. L. (2010). Anthelmintic Activity of Steroidal Saponins from Paris Polyphylla. Phytomedicine 17 (14), 1102–1105. doi:10.1016/j.phymed.2010.04.012
Wang, Y., Lou, Z., Wu, Q. B., and Guo, M. L. (2010). A Novel Hepatoprotective Saponin from Celosia Cristata L. Fitoterapia 81 (8), 1246–1252. doi:10.1016/j.fitote.2010.08.011
Wang, Z. (2011). Extract of Phenolics from Pomegranate Peels. Open Food Sci. J. 5 (1), 17–25. doi:10.2174/1874256401105010017
Wei, F., and Tanokura, M. (2015). Organic Compounds in Green Coffee Beans. Coffee Health Dis. Prev., 149–162. doi:10.1016/B978-0-12-409517-5.00017-6
Whittaker, J. H., Carlson, S. A., Jones, D. E., and Brewer, M. T. (2017). Molecular Mechanisms for Anthelmintic Resistance in Strongyle Nematode Parasites of Veterinary Importance. J. Vet. Pharmacol. Ther. 40 (2), 105–115. doi:10.1111/jvp.12330
Wicks, S. M., Salamon, I., Calderon, A. I., Carcache de Blanco, E. J., and Mahady, G. B. (2018). Sarcopenia, Diabetes, and Nutritional Intervention. Nutr. Ther. Interventions Diabetes Metab. Syndr. 2018, 279–292. doi:10.1016/B978-0-12-812019-4.00023-4
Wilson, R. K., Kwan, T. K., Kwan, C. Y., and Sorger, G. J. (2002). Effects of Papaya Seed Extract and Benzyl Isothiocyanate on Vascular Contraction. Life Sci. 71 (5), 497–507. doi:10.1016/S0024-3205(02)01708-3
Woerdenbag, H. J., Van Uden, W., and Pras, N. (1997). Artemisia Cina 3 (Dab 6), 15–22. doi:10.1007/978-3-642-60367-9_2
Wolstenholme, J. A., and Kaplan, M. R. (2012). Resistance to Macrocyclic Lactones. Curr. Pharm. Biotechnol. 13 (6), 873–887. doi:10.2174/138920112800399239
Yadav, A. K., and Temjenmongla, T. T. (2012). In Vivo anthelmintic Activity of Clerodendrum Colebrookianum walp., a Traditionally Used Taenicidal Plant in Northeast india. Parasitol. Res. 111 (4), 1841–1846. doi:10.1007/s00436-012-2908-8
Yagishita, Y., Uruno, A., and Yamamoto, M. (2016). NRF2-Mediated Gene Regulation and Glucose Homeostasis. Mol. Nutr. Diabetes A Volume Mol. Nutr. Ser. 2016, 331–348. doi:10.1016/B978-0-12-801585-8.00027-0
Yazwinski, T. A., Tucker, C. A., Wray, E., Jones, L., Reynolds, J., Hornsby, P., et al. (2013). Control Trial and Fecal Egg Count Reduction Test Determinations of Nematocidal Efficacies of Moxidectin and Generic Ivermectin in Recently Weaned, Naturally Infected Calves. Vet. Parasitol. 195 (1–2), 95–101. doi:10.1016/j.vetpar.2012.12.061
Yirga, G. (2012). Plants Used in Ethnoveterinary Practices in Medebay-Zana District, Northern Ethiopia. J. Med. Plants Res. 6 (3), 433–438. doi:10.5897/jmpr11.1133
Yu, Z. M., Tm, H., Ah, K., Tv, I., Ov, K., and Yu, Z. M. (2017). CODEN (USA): PCHHAX Study of Dry Extract of Tansy (Tanacetum Vulgare) Using the Method of High- Performance Liquid Chromatography, 9(11), 1–4. doi:10.1016/j.chroma.2016.11.063
Zamanian, M., Cook, D. E., Zdraljevic, S., Brady, S. C., Lee, D., Lee, J., et al. (2018). Discovery of Genomic Intervals that Underlie Nematode Responses to Benzimidazoles. PLoS Negl. Trop. Dis. 12 (3), 1–20. doi:10.1371/journal.pntd.0006368
Keywords: synthetic, toxicity, safety, medicine, ethnoveterinary, parasites, nematodes, plant
Citation: Zirintunda G, Biryomumaisho S, Kasozi KI, Batiha GE-S, Kateregga J, Vudriko P, Nalule S, Olila D, Kajoba M, Matama K, Kwizera MR, Ghoneim MM, Abdelhamid M, Zaghlool SS, Alshehri S, Abdelgawad MA and Acai-Okwee J (2022) Emerging Anthelmintic Resistance in Poultry: Can Ethnopharmacological Approaches Offer a Solution?. Front. Pharmacol. 12:774896. doi: 10.3389/fphar.2021.774896
Received: 13 September 2021; Accepted: 30 November 2021;
Published: 14 February 2022.
Edited by:
Adolfo Andrade-Cetto, National Autonomous University of Mexico, MexicoReviewed by:
Fabio Boylan, Trinity College Dublin, IrelandEdson Roberto Silva, University of São Paulo, Brazil
Copyright © 2022 Zirintunda, Biryomumaisho, Kasozi, Batiha, Kateregga, Vudriko, Nalule, Olila, Kajoba, Matama, Kwizera, Ghoneim, Abdelhamid, Zaghlool, Alshehri, Abdelgawad and Acai-Okwee. This is an open-access article distributed under the terms of the Creative Commons Attribution License (CC BY). The use, distribution or reproduction in other forums is permitted, provided the original author(s) and the copyright owner(s) are credited and that the original publication in this journal is cited, in accordance with accepted academic practice. No use, distribution or reproduction is permitted which does not comply with these terms.
*Correspondence: Gerald Zirintunda, gzerald777@gmail.com; James Acai-Okwee, jokwee@yahoo.com