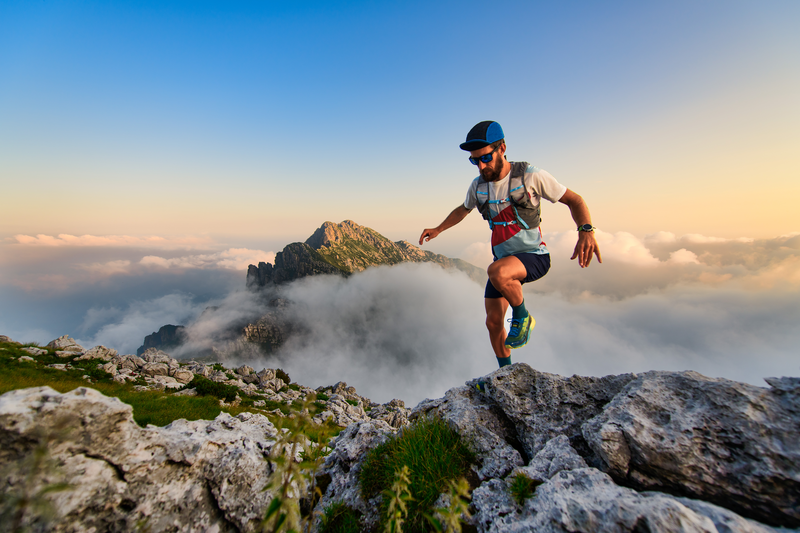
95% of researchers rate our articles as excellent or good
Learn more about the work of our research integrity team to safeguard the quality of each article we publish.
Find out more
ORIGINAL RESEARCH article
Front. Pharmacol. , 19 November 2021
Sec. Ethnopharmacology
Volume 12 - 2021 | https://doi.org/10.3389/fphar.2021.773562
This article is part of the Research Topic Network Pharmacology and Traditional Medicine: Setting the New Standards by Combining In silico and Experimental Work View all 40 articles
Background: Depression is a stress-related disorder that seriously threatens people’s physical and mental health. Xiaoyaosan is a classical traditional Chinese medicine formula, which has been used to treat mental depression since ancient times. More and more notice has been given to the relationship between the occurrence of necroptosis and the pathogenesis of mental disorders.
Objective: The purpose of present study is to explore the potential mechanism of Xiaoyaosan for the treatment of depression using network pharmacology and experimental research, and identify the potential targets of necroptosis underlying the antidepressant mechanism of Xiaoyaosan.
Methods: The mice model of depression was induced by chronic unpredictable mild stress (CUMS) for 6 weeks. Adult C57BL/6 mice were randomly divided into five groups, including control group, chronic unpredictable mild stress group, Xiaoyaosan treatment group, necrostatin-1 (Nec-1) group and solvent group. Drug intervention performed from 4th to 6th week of modeling. The mice in Xiaoyaosan treatment group received Xiaoyaosan by intragastric administration (0.254 g/kg/d), and mice in CUMS group received 0.5 ml physiological saline. Meanwhile, the mice in Nec-1 group were injected intraperitoneally (i.p.) with Nec-1 (10 mg/kg/d), and the equivalent volume of DMSO/PBS (8.3%) was injected into solvent group mice. The behavior tests such as sucrose preference test, forced swimming test and novelty-suppressed feeding test were measured to evaluate depressive-like behaviors of model mice. Then, the active ingredients in Xiaoyaosan and the related targets of depression and necroptosis were compiled through appropriate databases, while the “botanical drugs-active ingredients-target genes” network was constructed by network pharmacology analysis. The expressions of RIPK1, RIPK3, MLKL, p-MLKL were detected as critical target genes of necroptosis and the potential therapeutic target compounds of Xiaoyaosan. Furthermore, the levels of neuroinflammation and microglial activation of hippocampus were measured by detecting the expressions of IL-1β, Lipocalin-2 and IBA1, and the hematoxylin and eosin (H&E) stained was used to observe the morphology in hippocampus sections.
Results: After 6-weeks of modeling, the behavioral data showed that mice in CUMS group and solvent group had obvious depressive-like behaviors, and the medication of Xiaoyaosan or Nec-1 could improve these behavioral changes. A total of 96 active ingredients in Xiaoyaosan which could regulate the 23 key target genes were selected from databases. Xiaoyaosan could alleviate the core target genes in necroptosis and improve the hippocampal function and neuroinflammation in depressed mice.
Conclusion: The activation of necroptosis existed in the hippocampus of CUMS-induced mice, which was closely related to the pathogenesis of depression. The antidepressant mechanism of Xiaoyaosan included the regulation of multiple targets in necroptosis. It also suggested that necroptosis could be a new potential target for the treatment of depression.
Depression is a common clinical mental disorder characterized by constant down in spirits and cognitive impairment, and the prevalence of which is increasing year by year with the lifetime prevalence as high as 10–15% (Moussavi et al., 2007; Chiriţă et al., 2015). According to the latest statistics of the World Health Organization (WHO) in 2019, more than 350 million people worldwide suffer from depression, which has covered all age groups and increased by more than 18% from 2005 to 2015. It is also a major factor contributing to the increase in global burden of disease and medical expenses.
The classic Chinese formula Xiaoyaosan, originated from the book Prescriptions of the Bureau of Taiping People’s Welfare Pharmacy in Song Dynasty of China, is one of the effective traditional Chinese compound recipes in treating psycho-emotional related symptoms and diseases (Zhang et al., 2019). According to the recent clinical and basic studies, Xiaoyaosan has a multi-target regulating effect which is reflected in the treatment of depression-related diseases (Li et al., 2015; Jiao et al., 2019; Xiong et al., 2019; Liu et al., 2020; Zhou et al., 2021). In the pathogenesis study of depression, it is found that psychological and physical stressors can activate the immune system, lead to inflammation, and then participate in the development of depression (Iwata et al., 2013). Overproduction of pro-inflammatory cytokines is a key factor in the interaction between immune system and central nervous system (CNS) (Dowlati et al., 2010). Microglia is innate immune-related glial cell in CNS, which is the most widely-investigated in stress-related neuroinflammation (Sawada et al., 2014). Meanwhile, many studies have confirmed that inhibition of necroptosis can provide neuroprotective effect, so necroptosis is also a potential target for the treatment of CNS-related diseases (Liu et al., 2015). Xiaoyaosan has also been shown to play a therapeutic role by improving inflammation and regulating the function of hippocampal microglia (Li et al., 2017; Jiao et al., 2021).
Necroptosis is an active cell death process characterized by cell necrosis and inflammation. Studies have confirmed that necroptosis has a unique signal transduction pathway, which is closely related to the function of receptor interacting protein kinase 1 (RIPK1) and RIPK3(Galluzzi et al., 2009). Necroptosis is often accompanied by significant inflammatory response, characterized by a large number of inflammatory cell infiltration and activation of inflammatory pathways (Newton and Manning, 2016). The formation of a RIPK1–RIPK3–mixed lineage kinase domain-like (MLKL) complex leads to the initiation of necroptosis, while the phosphorylation of MLKL protein by RIPK3 eventually leads to necroptosis by destroying plasma membrane and cytolysis (Cho et al., 2009; Shan et al., 2018). Moreover, inflammatory response is triggered by the release of intracellular contents from necrotic cells, and inflammatory factors further promote cell death, which forms a circulatory circuit (Pasparakis et al., 2015). Here, Necrostatin-1 (Nec-1) as an effective, selective and permeable necroptosis inhibitor, can effectively block the active sites of RIPK1 and RIPK3, thus inhibit the necroptosis pathway by controlling the phosphorylation (Degterev et al., 2008). Therefore, the detection of RIPK1, RIPK3 and MLKL can verify the occurrence of necroptosis, and the effective measurement to regulate necroptosis may alleviate the inflammatory response of the lesion regions, thereby slowing down the pathological changes of the corresponding diseases (Schwabe and Luedde, 2018).
Under the guidance of the overall concept of traditional Chinese medicine (TCM) and the thought of syndrome differentiation and treatment, TCM formula is a treatment method with specific therapeutic efficacy based on the principles of drug properties, drug properties and compatibility, which contains profound and complex scientific connotation (Wang et al., 2008). However, due to the lack of quantifiable and objective data support, it is very difficult to clarify the mechanism of TCM formula, which has become an important reason why TCM formula is difficult to be accepted by the international community (Luo et al., 2020). Network pharmacology, a new discipline to reveal the mechanism of diseases and the pharmacodynamic mechanism of drugs from the perspective of biological network, is well meet the concept of holism in TCM, while the core element of network pharmacology –“network target, multi-components” theory was also proposed by TCM researchers (Li and Zhang, 2013). Nowadays, network pharmacology has become an important breakthrough in the cross-innovation of information science and medical science, and has been widely used in the research of traditional Chinese medicine (Li et al., 2014; Guo et al., 2021; Sheng et al., 2021). Thus far, studies have shown that necroptosis plays an important role in neuroinflammation and central nervous system related diseases (Chen et al., 2021; Thadathil et al., 2021), and the role of necroptosis remains largely unexamined in the depression-related CNS mechanism. Also, the antidepressant effect of Xiaoyaosan has still not yet been comprehensively studied. Therefore, this study utilized network pharmacology approach to explore the therapeutic effects of Xiaoyaosan on depression via necroptosis pathway, then the antidepressant mechanism was observed in vivo using CUMS mice. Figure 1 showed the flow chart of the study.
FIGURE 1. Flow chart of network pharmacological analysis and experimental study of Xiaoyaosan for depression treatment through necroptosis pathway.
TCM formula Xiaoyaosan consists of the following eight Chinese botanical drugs: Bupleurum chinense DC (Description: Apiaceae), Angelica sinensis (Oliv.) Diels (Description: Apiaceae), Paeonia lactiflora Pall (Description: Paeoniaceae), Poria cocos (Schw.) Wolf (Description: Polyporaceae), Atractylodes macrocephala Koidz (Description: Compositae), Glycyrrhiza uralensis Fisch (Description: Leguminosea), Zingiber officinale Rosc (Description: Zingiberaceae), and Mentha haplocalyx Briq (Description: Lamiaceae) at a ratio of 5:5:5:5:5:4:5:1. The prepared drug in pieces of Xiaoyaosan were purchased from Beijing Tongrentang (Bozhou) Pieces Co. Ltd., Bozhou, China, while the ultra-performance chromatography-electrospray tandem mass spectrometry (UPLC-MS/MS) was used to identify the samples of these decoction pieces (Ding et al., 2017; Yuan et al., 2020). The Xiaoyaosan dry powder (J2447) used in experimental studies was prepared by Jiuzhitang Co. Ltd, Changsha, China, in accord with the process recorded in the Chinese Pharmacopoeia 2015 Edition (Pan et al., 2019; Zhu et al., 2019). 2.36 g of crude drug yielded One Gram the finished dry powder administered experimentally.
The active ingredients and potential targets of TCM formula Xiaoyaosan for application to depression were investigated based on network pharmacology. The active ingredients in Xiaoyaosan were screened according to absorption, distribution, metabolism and enhancement (ADME) of drugs, while the bioavailability (OB) and drug likeness (DL) were used as screening parameters (Vugmeyster et al., 2012). According to ADME (OB ≥ 30% and DL ≥ 0.18), the active ingredients of Xiaoyaosan were collected from traditional Chinese medicine databases including the TCM Systems Pharmacology database and Analysis Platform (TCMSP, https://tcmsp-e.com/) (Ru et al., 2014), the Encyclopedia of Traditional Chinese Medicine (ETCM, http://www.tcmip.cn/ETCM/) (Zhang et al., 2019) and the High-throughput Experiment- and Reference-guided database of Traditional Chinese Medicine (HERB, http://herb.ac.cn/) (Fang et al., 2021), then SwissTargetPrediction database (http://www.swisstargetprediction.ch/) (Daina et al., 2019) was used to predict the targets of active ingredients in Xiaoyaosan, the reliability screening of predicted targets was also carried out (Probability ≥30%).
The related genes with depression and necroptosis pathway were collected from GeneCards database (https://www.genecards.org/) (Stelzer et al., 2016), Online Mendelian Inheritance in Man (OMIM, https://omim.org/) (Amberger et al., 2015), Comparative Toxicogenomic database (CTD, http://ctdbase.org/) (Davis et al., 2020), Therapeutic Target database (TTD, http://db.idrblab.net/ttd/) (Wang et al., 2020), and DrugBank database (http://www.drugbank.ca/) (Wishart et al., 2018), using “Depression”, “Depressive disorder” or “Necroptosis” as the search term. Then, the gene symbols of the ingredients in Xiaoyaosan, depression and necroptosis pathway were searched in the universal Protein Resource (UniProt, https://www.UniProt.org/) to get the unified UniProt gene ID.
The potential therapeutic targets of Xiaoyaosan in depression treatment via necroptosis pathway were obtained by overlapping the predicted targets of the ingredients in Xiaoyaosan and the target genes of depression and necroptosis pathway. Furthermore, the STRING database (https://string-db.org/) (Szklarczyk et al., 2019) was used to generate the protein–protein interaction (PPI) network of potential target genes of Xiaoyaosan with the species limited to human (Homo sapiens) and the interaction score ≥0.4. Then, the Cytoscape software (version 3.7.2, http://www.cytoscape.org/, Boston, MA, United States) was used to construct the “botanical drugs-active ingredients-potential targets” network, and identify the core target genes from the results of PPI network with the cytoHubba plugin (Guo et al., 2021).
The study was approved by the Institutional Animal Care and Use Committee of Beijing University of Chinese Medicine (BUCM-4-2018120401-4053). Experimental protocols applied in our study were performed in agreement with the existing current animal welfare guidelines. Specific-pathogen free (SPF) male C57BL/6 mice aged 8-week-old (SYXK (Jing) 2016-0006, Beijing Vital River Laboratory Animal Technology Limited Company, Beijing, China) were housed separately in a standard animal feeding room (room temperature: 22 ± 2°C; relative humidity: 30–40%; light condition: a 12 h/12 h dark/light cycle) and fed standard rodent diet. Animals were adapted to their new environment for 7 days before CUMS procedure.
A total of 75 mice were randomly assigned to five groups (n = 15), including control group (no stress + physiological saline), CUMS group (CUMS + physiological saline), solvent group [CUMS + dimethyl sulphoxide (DMSO)/phosphate buffered saline (PBS)], Nec-1 group (CUMS + Nec-1), and Xiaoyaosan treatment group (CUMS + Xiaoyaosan). The CUMS modeling was performed as previously reported (Yan et al., 2019). Briefly, animals except the control group mice were subjected to the following stressors for six consecutive weeks: food deprivation for 24 h; water deprivation for 24 h; empty cages for 11 h; crowded cages for 24 h; restraint stress for 3 h; exposed to wet and soiled cages for 24 h; and 4°C cold water swimming for 5 min. The schedule of the study was showed in Figure 2.
Drug intervention lasted for 3 weeks, starting from the 4th week of modeling. The dosage of Xiaoyaosan and the duration of administration were confirmed according to the previous publications (Ding et al., 2017; Liu Y. et al., 2019). The Xiaoyaosan dry powder was dissolved in distilled water, then the mice in Xiaoyaosan treatment group received Xiaoyaosan by intragastric administration (0.254 g/kg/d), meanwhile mice in the CUMS group received 0.5 ml physiological saline (Yan et al., 2018). The concentration and volume of the gavage administration were adjusted once a week according to the changes in the body weight of the mice. Nec-1 (product number: RH82010-10mg, CAS number: 4311-88-0, BIORuler, Danbury, CT, United States) was dissolved in DMSO/PBS (1 mg in 125 μL of DMSO diluted with 1,375 μL of 0.1 M PBS). The Nec-1 group were injected intraperitoneally (i.p.) with Nec-1 (10 mg/kg) daily for 3 weeks, and the equivalent volume of DMSO/PBS (8.3%) was injected into solvent group mice (Dara et al., 2016).
The SP test was performed every week during the modeling process. The scheme lasted for successive 6 weeks, and the 3-weeks’s daily medication of Xiaoyaosan or Nec-1 was conducted. After the modeling, the FS test, and NSF test were carried out, then the mice were sacrificed for further detection.
To evaluate the depressive behaviors of model animals, the sucrose preference (SP) test, forced swimming (FS) test and novelty-suppressed feeding (NSF) test were conducted as previous described with minor modifications (Barfield et al., 2013; Liao et al., 2018).
The SP test was performed every week during the modeling process. In brief, each mouse in this study was given two bottles filled with deionized water and 1% sucrose solution after 24-h fasting and water deprivation, then the consumption of sucrose solution and deionized water in 1 h was recorded, respectively. The sucrose preference rate was presented as the percentage of the 1% sucrose solution consumed out of the total amount of liquid consumed, which represented the parameter of hedonic behavior.
The FS test and NSF test were conducted after the 6-weeks modeling to further assess the depressed behaviors in mice. In the FS test, each mouse was placed in a clear glass aquarium (24 cm height and 19 cm diameter) containing approximately 6 cm of water (24 ± 1°C) for 5 min, and the whole time was recorded using a high definition camera. The mouse was accepted and considered to be immobile when it stopped swimming and kept afloat on the water which reflected the despair of animals after they failed to cope with the attempt, the immobility time was recorded in our study. The NSF test was performed in a white plastic box (25 × 25 × 20 cm) with a single food pellet of regular chow placed in the center. After 24 h of food deprivation, the mouse was placed in a corner of the box, the latency to chew the food pellet was recorded, followed by the measurement of the food consumption in the subsequent 5 min.
After 6 weeks of modeling, mice sacrificed by decapitation after behavioral tests. The hippocampal tissues of five mice in each group were collected for western blot analysis, the RNA preservation solution (Biotech, #2714) was used to preserve the hippocampi of five mice in each group for quantitative RT-PCR assay. The whole brains of the remaining mice in each group were fixed in 4% paraformaldehyde (PFA) solution (4% PFA, 2.5% glutaraldehyde, and 0.1 M PBS) and prepared for tissue slicing.
Total RNA of hippocampus was extracted using Trizol® reagent (Applied Biosystems, Waltham, MA, United States), then the quality and concentration of total RNA were determined by 1% agarose gel electrophoresis and Q3000 micro-volume spectrophotometer (Quawell Technology, San Jose, CA, United States). The RevertAid First Strand cDNA Synthesis Kit (Termo Fisher Scientifc, Waltham, MA, United States) was used to transfer total RNA into cDNA. Table 1 showed the sequences for primers used in our study which were designed based on published mRNA sequences in NCBI and synthesized by Sangon Biotech Co., Ltd, Shanghai, China. HPRT1 (BBI LifeScience, Amherst, MA, United States) was selected as the housekeeping gene in the experiment through reference genes selection method by geNorm, NormFinder, and BestKeeper software (Dongliang et al., 2011). The quantitative RT-PCR (qRT-PCR) reaction system was prepared by SYBR ® Green PCR Master Mix (Applied Biosystems) in a final volume of 25 μL, then performed on Multicolor Real-time PCR Detection System (Bio-Rad, Hercules, CA, United States) with the following thermal cycling conditions: preincubation at 94 °C for 3 min, followed by 40 cycles of denaturation at 94°C for 30 s, annealing at 55°C for 30 s, and extending at 72°C for 30 s. The signals were normalized to HPRT1 and the relative expression of mRNA in each sample was calculated by 2−∆∆Ct method.
Protein levels of IBA1, IL-1β, Lipocalin-2, RIPK1, RIPK3, MLKL, and p-MLKL were measured by western blot analysis. The total protein of hippocampal tissues was extracted using RIPA Lysis buffer (Biomiga, Santiago, CA, United States). The proteins were loaded onto 12% SDS-PAGE gels at 30 µg per lane, and then transferred onto polyvinylidene fluoride (PVDF) membranes. The 5% non-fat dry milk in 0.05% tris buffered saline tween (TBST) was used to block the membranes, and the membranes were incubated with primary antibodies at 4 °C overnight (IBA1, 1:1,000 dilution, ab178847, Abcam, San Francisco, CA, United States; IL-1β, ab9722, 1:1,000 dilution, Abcam; Lipocalin-2, ab216462, 1:100 dilution, Abcam; RIPK1, 1:1,000 dilution, ab72139, Abcam; RIPK3, 1:1,000 dilution, ab56164, Abcam; phosphorylated MLKL (p-MLKL), 1:1,000 dilution, ab196436, Abcam; MLKL, 1:2000 dilution, orb32399, Biorbyt, Cambridge, UK; β-actin, 1:5,000 dilution, #5125, Cell Signal, Danvers, MA, United States). Then, the membranes were incubated with horseradish peroxidase- (HRP-) conjugated secondary antibody [Goat Anti-Rabbit IgG H&L (HRP), 1:10,000 dilution, ab205718, Abcam; Goat Anti-Mouse IgG H&L (HRP), 1:10,000 dilution, ab205719, Abcam] for 1 h at room temperature. The enhanced chemiluminescence (ECL) detection reagent (Thermo Fisher Scientific) was used to develop the membranes for 1 min, and the Tanon-5200 system (Tanon, Shanghai, China) was used to visualize the protein bands. The optical density of protein bands was quantified by Tanon Gis software (Tanon).
The brain samples fixed in 4% PFA solution were used to prepared paraffin-embedded tissue sections which included hippocampal CA1 and CA3 regions based on the stereotaxic atlases of the mouse brain (Konsman, 2003). The sections were stained with hematoxylin-eosin (H&E) to observe the cellular morphology and infiltration of inflammatory cells. A four-point severity scale (0, normal; 1, mild; 2, moderate; 3, severe) was used to score the H&E stained sections by an experienced pathologist in a blinded manner.
Immunohistochemical (IHC) staining was used to observe the levels of IBA1 and p-MLKL in hippocampus followed the previous described procedures (Yan et al., 2018). After dewaxing, dehydration and antigen retrieval, the brain sections were incubated with 3% H2O2 solution at room temperature for 10 min. Next, sections were incubated with primary antibodies (IBA1, 1:8,000 dilution, ab178847, Abcam; p-MLKL, 1:250 dilution, ab196436, Abcam) overnight at 4 °C. Then sections were incubated with secondary antibodies, and the diaminobezidin 3, 3 (DAB) solution (Invitrogen, Carlsbad, CA, United States) was used for coloration. For quantitative analysis, the original immunohistochemical images were collected with an image analyzer (MIAS99, Fubo-Tech Co., Beijing, China) with a color video camera (TK-C1381, JVC, Beijing, China) and BX50 microscope (Olympus Co., Tokyo, Japan), and the positive staining of hippocampal CA1 and CA3 regions at high power magnification (×400) was assessed by mean optical density (MOD) using Image-pro Plus software (version 6.0, Rockville, MD, United States).
Immunofluorescence (IF) staining was used to observe the expression of p-MLKL as previous described (Qu et al., 2017). The brain sections were incubated with 0.5% TritonX-100 solution at room temperature for 10 min. Next, sections were incubated with primary antibody (p-MLKL, 1:100 dilution, ab196436, Abcam) overnight at 4°C. Then sections were incubated with fluorescent-conjugated goat anti-rabbit IgG, follow by the Thioflavin T and DAPI staining. The fluorescence signal of sections was observed and photographed under an Olympus BX53 fluorescence microscope (Olympus Co., Tokyo, Japan), and the mean fluorescence intensity (MFI) was analyzed using Image-pro Plus software.
In this study, all data were analyzed using SPSS software (version 20.0, Chicago, IL, United States) and expressed as means ± SEM. The statistical analyses were performed by one-way analysis of variance (ANOVA) with least significant difference (LSD) post hoc multiple comparisons when equal variances were assumed, Dunnett’s T3 test was used when the data had a normal distribution but the variances are not homogeneous. A p-value of 0.05 was considered statistically significant.
The network pharmacology method was used to initial investigate whether the active components of Xiaoyaosan could improve necroptosis pathway in depression.
As showed in Figure 3, a total of 148 active ingredients in the eight botanical drugs of Xiaoyaosan were collected based on the ADME parameters (OB ≥ 30% and DL ≥ 0.18), and we obtained 23 potential treatment targets (BAX, BCL2, CAPN1, CAPN2, CASP1, CASP8, EIF2AK2, IL1B, JAK1, JAK2, JAK3, MLKL, NLRP3, PARP1, PPIA, RIPK1, RIPK3, STAT1, STAT3, STAT6, TLR4, TNF, XIAP) that might be associated with the antidepressant mechanism of Xiaoyaosan through necroptosis pathway by taking the intersection of 1708 putative targets of the active ingredients in Xiaoyaosan, 12,608 therapeutic targets for depression and 97 necroptosis related targets.
FIGURE 3. Wynn maps for the active ingredients of Xiaoyaosan and potential treatment targets (A) Based on the ADME parameters (OB ≥ 30% and DL ≥ 0.18), there were 17 ingredients in Radix Bupleuri, two ingredients in Radix Angelicae Sinensis, 13 ingredients in Radix Paeoniae Alba, 15 ingredients in Poria, seven ingredients in Rhizoma Atractylodis Macrocephalae, 91 ingredients in Radix Glycyrrhizae, five ingredients in Rhizoma Zingiberis Recens and 10 ingredients in Herba Menthae (B) A total of 1708 predicted targets of TCM formula Xiaoyaosan, 12,608 therapeutic targets for depression and 97 necroptosis related targets were intersected, and there were 23 potential treatment target genes.
Figure 4A showed the “botanical drugs-active ingredients-potential targets” network, eight botanical drugs of TCM formula Xiaoyaosan had 96 active ingredients which could regulate the 23 key target genes. Therefore, based on the network pharmacological analysis we can speculate that traditional Chinese formula Xiaoyaosan could regulate the necroptosis pathway in depression. Figure 4B showed the PPI network of potential target genes of Xiaoyaosan and the sub-network of the top 10 core targets which was picked up using the cytoHubba plugin in Cytoscape software. As showed in Table 2, the hub nodes of sub-network were ranked by maximal clique centrality (MCC) method, and MLKL, IL1B, CASP8, CASP1, RIPK1, TLR4, NLRP3, RIPK3, TNF, STAT3 were the top 10 potential treatment targets of Xiaoyaosan.
FIGURE 4. “Botanical drugs-active ingredients-potential targets” network and PPI network of potential target genes of Xiaoyaosan (A) The “Botanical drugs-active ingredients-potential targets” network: These eight botanical drugs of TCM formula Xiaoyaosan had 96 active ingredients which could regulate the 23 key target genes according to the network pharmacological analysis (B) PPI network of the 23 key target genes: The sub-network of the top 10 core targets was picked up from the PPI network of potential target genes of Xiaoyaosan using the cytoHubba plugin in Cytoscape software.
We performed the sucrose preference (SP) test, forced swimming (FS) test and novelty-suppressed feeding (NSF) test to observe the effects of Xiaoyaosan on depressive-like behaviors. As shown in Figure 5A, the baseline sucrose preference rate of control group, CUMS group, solvent group, Nec-1 group and Xiaoyaosan group had no significant differences. However, after CUMS modeling, the mice in CUMS group had a significant drop in sucrose preference rate compared with the control group mice (p < 0.01), while the Nec-1 group and Xiaoyaosan treatment group had no differences with the stress groups. Then, the CUMS progress continued for another 3 weeks with medication procedure, the mice in CUMS group and solvent group still had a low sucrose preference rate (p < 0.01), while the sucrose preference rate of Nec-1 group and Xiaoyaosan treatment group mice was significant increased (both p < 0.01). The CUMS group and solvent group mice exhibited significantly longer immobility time in the FS test (p < 0.01), and Xiaoyaosan or Nec-1 ameliorated this depressive-like behavior in comparison with the CUMS group and solvent group (both p < 0.01) (Figure 5B). For the NSF test, it was shown that mice in CUMS group had a significant longer latency to chew the food pellet compared with the control mice (p < 0.01) (Figure 5C), and the food consumption significantly decreased (p < 0.01) (Figure 5D). There was no significant difference between the solvent group and the CUMS group. Xiaoyaosan or Nec-1 could reverse these changes in the NSF test (both p < 0.05).
FIGURE 5. Changes in the Depressive-like behaviors of CUMS mice (A) Changes of sucrose preference rate during the CUMS modeling period (n = 15) (B) FS test results after the 6-weeks modeling (n = 15) (C) The results of latency to feed in NSF test after the 6-weeks modeling (n = 15) (D) The results of food consumption in NSF test after the 6-weeks modeling (n = 15). Data were expressed as means ± SEM, **p < 0.01 versus control group; Δ p < 0.05, ΔΔ p < 0.01 versus CUMS group; #p < 0.05, ##p < 0.01 versus solvent group.
In order to explore the possible mechanism of necroptosis in the mouse model of depression, the expressions of RIPK1, RIPK3, MLKL, p-MLKL were detected by western blot assay. As shown in Figure 6, the levels of RIPK1, RIPK3, MLKL and p-MLKL significant up-regulated in the hippocampi of CUMS-exposed mice compared to the control group (p < 0.01). Compared with the solvent group, these necroptosis biomarkers in Nec-1 group were significant down-regulated (both p < 0.01). Also, the effects of Xiaoyaosan were similar to Nec-1, which could mitigate the stress-induced elevation of RIPK1, RIPK3, MLKL and p-MLKL levels, as shown in the comparison between Xiaoyaosan treatment group and CUMS group (p < 0.05 or p < 0.01).
FIGURE 6. Changes of necroptosis biomarkers in the hippocampi of CUMS mice (A) Representative micrographs of WB for necroptosis biomarkers (B) The expression of RIPK1 in the hippocampus of experimental mice (n = 5) (C) The expression of RIPK3 in the hippocampus of experimental mice (n = 5) (D) The expression of MLKL in the hippocampus of experimental mice (n = 5) (E) The expression of p-MLKL in the hippocampus of experimental mice (n = 5). Data were expressed as means ± SEM, **p < 0.01 versus control group; Δ p < 0.05, ΔΔ p < 0.01 versus CUMS group; ##p < 0.01 versus solvent group.
To further verified the process of necroptosis in CUMS-induced mice, the IHC and IF staining methods were used to observe the distribution of p-MLKL in hippocampus. Figure 7 exhibited the representative micrographs of IHC and IF staining for p-MLKL in the CA1 and CA3 regions of hippocampus, and the results were set out in Figure 8. The MOD of p-MLKL in both CA1 and CA3 regions of CUMS group mice was significantly increased when compared with the control group (p < 0.05, p < 0.01, respectively), and there was no significant difference between solvent group and CUMS group. Then the treatment of Nec-1 or Xiaoyaosan reversed the CUMS-induced p-MLKL changes in the CA1 and CA3 (p < 0.05 or p < 0.01, Figures 8A,B). Compared with the control group, the MFI of p-MLKL of CUMS group mice was significantly increased, and CUMS group had no significant difference with solvent group. Similarly to IHC staining results, the p-MLKL MFI was decreased in Nec-1 and Xiaoyaosan group mice (p < 0.05, p < 0.01, Figures 8C,D). The outcomes of thioflavine T staining showed that the MFI of thioflavine T labelled amyloid-like polymers was also increased in the CA1 and CA3 regions of CUMS group mice compared to the control group (both p < 0.01, Figures 8E,F). In the end, the IF staining results revealed that high levels of the p-MLKL colocalized with thioflavin T staining was detected in the CA1 and CA3 regions of CUMS group mice, in contrast with the low colocalization in control group (p < 0.01, Figures 8G,H). The low levels of colocalization were observed in the CA1 and CA3 regions of mice in Xiaoyaosan group and Nec-1 group as well (p < 0.05, p < 0.01).
FIGURE 7. Representative micrographs of IHC and IF staining for p-MLKL (scale bar = 200 μm, ×400 magnification) in the CA1 and CA3 regions.
FIGURE 8. Expression of p-MLKL in CA1 and CA3 regions of the hippocampus (A) The MOD level of p-MLKL in the CA1 region (n = 5) (B) The MOD level of p-MLKL in the CA3 region (n = 5) (C) The MFI level of p-MLKL in the CA1 region (n = 5) (D) The MFI level of p-MLKL in the CA3 region (n = 5) (E) The MFI level of thioflavine T staining in the CA1 region (n = 5) (F) The MFI level of thioflavine T staining in the CA3 region (n = 5) (G) The MFI level of p-MLKL colocalized with thioflavin T staining in the CA1 region (n = 5) (H) The MFI level of p-MLKL colocalized with thioflavin T staining in the CA3 region (n = 5). Data were expressed as means ± SEM, *p < 0.05, **p < 0.01 versus control group; Δ p < 0.05, ΔΔ p < 0.01 versus CUMS group; #p < 0.05, ##p < 0.01 versus solvent group.
The H&E staining was performed in order to evaluate the inflammatory infiltrates in mice hippocampal CA1 and CA3 regions. As exhibited in Figure 9A, a large number of inflammatory cell infiltration was observed in the hippocampus of the mice in chronically stressed groups treated with vehicle (physiological saline and solvent group). The histological scores of the hippocampus in each group were judged, it was found that the scores of CUMS group and solvent group in CA1 and CA3 regions were significantly higher than that of control group (both p < 0.01), Xiaoyaosan or Nec-1 treatment notably mitigated CUMS-induced inflammatory infiltrates (both p < 0.05) (Figures 9B,C). The expression levels of IL-1β and Lipocalin-2 were measured by qRT-PCR and western blot. The results indicated that the 6-weeks CUMS significant up-regulated the mRNA and protein levels of IL-1β in the hippocampus of CUMS-exposed mice (both p < 0.01), and the mice in the Xiaoyaosan treatment group and Nec-1 group showed a significant increase in IL-1β expression compared with the CUMS group mice (both p < 0.01) (Figures 10A,C). The CUMS modeling also increased the Lipocalin-2 level (p < 0.01), whereas the administration of Xiaoyaosan and Nec-1 noticeably inhibited the expressions of Lipocalin-2 (both p < 0.01) (Figures 10B,D).
FIGURE 9. The histological changes in CA1 and CA3 regions of the hippocampi in CUMS mice (A) Representative micrographs of H&E staining (scale bar = 200 μm, ×400 magnification) in the CA1 and CA3 regions (B) The histological scores in the CA1 region (n = 5) (C) The histological scores in the CA1 region (n = 5). Data were expressed as means ± SEM, **p < 0.01 versus control group; Δ p < 0.05 versus CUMS group; #p < 0.05 versus Solvent group.
FIGURE 10. The levels of IL-1β and Lipocalin-2 in the hippocampus of CUMS mice (A) The mRNA results of IL-1β (n = 5) (B) The mRNA results of Lipocalin-2 (n = 5) (C) The protein results of IL-1β (n = 5) (D) The protein results of Lipocalin-2 (n = 5) (E) Representative micrographs of WB for IL-1β and Lipocalin-2. Data were expressed as means ± SEM, **p < 0.01 versus control group; ΔΔ p < 0.01 versus CUMS group; ##p < 0.01 versus Solvent group.
To initial test the level of microglial activation in the hippocampi of CUMS-exposed mice, the expression of IBA1 was detected. Figure 11A presented the representative micrographs of IHC staining for IBA1 in the CA1 and CA3 regions of hippocampus. As showed in Figures 11B,C, the MOD of IBA1 in CA1 and CA3 of CUMS mice was significantly increased when compared to the control group (both p < 0.05), whereas the expression of IBA1 in CA1 and CA3 was noticeably reduced by the interference of Nec-1 and Xiaoyaosan (p < 0.05, p < 0.01). As showed in Figures 11D,E, the qRT-PCR and western blot data also demonstrated the high level of IBA1 in the hippocampus of the chronically stressed mice (both p < 0.01), and the treatment with Nec-1 or Xiaoyaosan significantly reduced the expression of IBA1 as compared to stressed groups (both p < 0.01).
FIGURE 11. Expression of IBA1 in CA1 and CA3 regions of the hippocampi in CUMS mice (A) Representative micrographs of immunohistochemical staining for IBA1 (scale bar = 200 μm, ×400 magnification) in the CA1 and CA3 regions (B) The MOD level of IBA1 in the CA1 region (n = 5) (C) The MOD level of IBA1 in the CA3 region (n = 5) (D) The mRNA results of IBA1 (n = 5) (E) The protein results of IBA1 (n = 5). Data were expressed as means ± SEM, *p < 0.05, **p < 0.01 versus control group; Δ p < 0.05, ΔΔ p < 0.01 versus CUMS group; #p < 0.05, ##p < 0.01 versus Solvent group.
Our study proposed that necroptosis was involved in the antidepressant mechanism of Xiaoyaosan. A total of 23 potential treatment targets were screened out through network pharmacological analysis that might be related to the active ingredients of Xiaoyaosan intervened necroptosis pathway in depression. In the meantime, the animal experiment revealed that Xiaoyaosan displayed antidepressant-like effects via regulating the expression of RIPK1, RIPK3, MLKL, and the phosphorylation level of MLKL of depressed mice, and then improving the neuroinflammation and microglia function in the hippocampus.
Depression is a prevalent and persistent psychiatric illness affecting millions of people worldwide. Many hypotheses such as inflammatory cytokines, hypothalamic pituitary adrenal (HPA) axis, neurotransmitter system, brain-derived neurotrophic factor (BDNF) in the brain and endogenous metabolites are involved in depression pathogenesis, but it is still obscure (Peng et al., 2015). Today, there is still lack of precise and effective diagnostic or therapeutic methods for depression. Xiaoyaosan is a common TCM formula for treating syndrome of stagnation of liver qi and spleen deficiency with the characteristics of multiple ingredients, multiple targets and multiple pathways, and it also has a good therapeutic effect on depressive diseases (Chen et al., 2020). Although previous studies have revealed some antidepressant mechanisms of the active ingredients of Xiaoyaosan (Liu X. et al., 2021; Liu XJ. et al., 2021), while whether the components of Xiaoyaosan could improve necroptosis pathway in depression remains to be discussed. The present study preliminary disclosure that Xiaoyaosan could regulate the necroptosis pathway in depression via its 96 active ingredients that could regulate the 23 key target genes based on the network pharmacological analysis. Through PPI network analysis of these 23 target genes, we confirmed that MLKL, IL1B, CASP8, CASP1, RIPK1, TLR4, NLRP3, RIPK3, TNF, STAT3 as the potential target genes of Xiaoyaosan in the treatment of depression, among which the MCC score of MLKL was the highest. Mounting evidence suggested that necroptosis depended on RIPK1/RIPK3/MLKL activities, RIPK1 and RIPK3 upregulated upon activation of death receptors, triggered a signaling cascade involving phosphorylation of MLKL which contributes to the change of plasm membrane permeability, then resulting in cellular necrosis (Liu C. et al., 2021; Marunouchi et al., 2021; Yu et al., 2021). Therefore, the expressions of RIPK1, RIPK3, MLKL were evaluated as biomarkers of necroptosis in the study.
Stress was closely related to neurological disorders, and the sustained psychosocial stress is one of the main causes of depression. Chronic or repeated stress can cause neuronal disturbances, which was similar to changes observed in the brain during depression (de Kloet and Joëls, 2005). Animal model of depression was an important method to explore the dysregulatory mechanisms of depression and the regulatory mechanisms by which antidepressant treatments alleviate the various depressive symptoms (Frazer and Morilak, 2005). The depression model by CUMS method was widely recognized and applied for mechanism research and drug selection. Also, some studies showed that the depressive behaviors induced by CUMS was similar to the clinical manifestations of depression induced by long-term exposure to multiple stresses (D'Aquila et al., 1994; Papp et al., 1996). Here, we found that after 6 weeks of CUMS, mice were observed to exhibit significant depressive symptoms through behavioral evaluation, which was consistent with other studies (Cao et al., 2013). This study used classical evaluation methods to observe depressive behavior in CUMS-induced mice, including SP test, FS test and NSF test. The performance of anhedonia was manifested by lack of interest in reward stimulus, which was a manifestation of affective disorders including depression, while SP test was a method to evaluate the degree of pleasure loss in rodents (Weiss, 1997). FS test was a kind of negative stress experiment that animals could not escape from hostile environment and produce hopeless behaviors, the immobility time was used as an important index to evaluate depressive behaviors (Bächli et al., 2008). So, it was widely used in the exploration of the pathogenesis of stress and the pharmacological study of potential antidepressants. The NSF test observed the contradiction between the fear of new environment and the great need for things, the food consumption and latency of feed could represent the degree of depression and anxiety in animals (Shalom et al., 2006). The results showed that there were obvious behavioral changes in the CUMS model group, including decreased sucrose preference rate, prolonged immobility time, longer latency of feed and decreased appetite. Thus, it could be proved that the 6-weeks modeling successfully established depressive-like changes in mice. In the meantime, we found that the treatment of Xiaoyaosan could significantly improve the depressive-like behavioral changes in mice which was consistent with the results of previous studies, indicating that Xiaoyaosan exhibited good antidepressant performances (Wang et al., 2018; Liu X. et al., 2019).
The mechanism of cell death had always been one of the focuses of biomedical research, and it was common believed that cell death patterns included apoptosis, necrosis and autophagy at present. Apoptosis and autophagy required the synthesis of new proteins and energy supplies, which was an active process of cell self-regulation, also known as programmed death (M Stacey and Wei-Xing, 2006). Cell necrosis was previously considered to be a passive disordered process that occurred under overwhelming physical and chemical damage from the outside world, and therefore could not be regulated (Vandenabeele et al., 2008). With the in-depth study of the mechanism of cell death, it was found that cell necrosis was also a programmed death process with notable necrosis features, then it was redefined as necroptosis (Degterev et al., 2005). Necroptosis was mainly initiated by the members of tumor necrosis factor (TNF) receptor family and toll-like receptor (TLR) family, which phosphorylated MLKL by transmitting death signals through RIPK1 and RIPK3(Galluzzi and Kroemer, 2008). Thus, as the executor of cell death, phosphorylation of MLKL eventually led to cell necrosis. The necrotic cells released their contents as damage-associated molecular pattern molecules (DAMPs) to stimulate inflammation in peripheral cells, then activated immune responses of the body (Sonenshine and Macaluso, 2017). Therefore, the role of necroptosis in the neurological diseases involved a variety of biological mechanisms related to neuroinflammation (Liu et al., 2014; Bian et al., 2017; Shao et al., 2018). Nec-1 could inhibit necroptosis and reduce inflammation, which was beneficial to the improvement of pathological damage (Takahashi et al., 2012). The increased inflammation was associated with stress-related affective disorders (Stein et al., 2018), while the activations of immune system and inflammatory cytokines might be involved in the pathogenesis of some patients with depression (Haapakoski et al., 2016). The involvement of chronic low-grade inflammatory response, compensatory anti-inflammatory reflex system, and cellular mediated immunity might be the key factors leading to depression (Maes, 1995). Also, higher level of inflammation was associated with increased risk of depression (Pasco et al., 2010). The treatment of antidepressants, especially selective serotonin reuptake inhibitors (SSRIs), could significantly reduce the production of pro-inflammatory cytokines and inflammatory markers, such as IL-1β, IL-6 and TNF-α, and increase the production of anti-inflammatory cytokines, such as IL-10 (Berk et al., 2013). Accordingly, anti-inflammatory could be an effective strategy for the treatment of depression and had great research value (Müller and Schwarz, 2010; Hayley, 2011).
Our results explained that the necroptosis inhibitor Nec-1 could improve depressive-like behaviors in mice, which suggested that the necroptosis pathway might be associated with the pathogenesis of depression. Hippocampus, as the most learning and memory function-related brain area, was also the main target of stress injury (Christie, 2015). Studies have found that inflammation of hippocampus and overactivation of microglia were important central mechanisms of depression (Streit et al., 2004; Xu et al., 2015), and the regulation of necroptosis on CNS function was also closely related to the occurrence of neuroinflammation (Antunes et al., 2019). Therefore, in order to further investigate the results of network pharmacology, and further explore the relationship between the pathogenesis of depression and necroptosis, we detected the expressions of RIPK1, RIPK3, MLKL and p-MLKL in hippocampus of CUMS-induced mice to confirm the level of necroptosis. It was observed that RIPK1, RIPK3 and MLKL in hippocampus of model mice were significantly up-regulated, and the phosphorylation level of MLKL was also increased, meanwhile, Nec-1 played a significant role in regulating the expressions of the above indicators. Phosphorylated MLKL was the most precise protein, which could definitely indicate the necroptosis activated via RIPK-mediated MLKL signaling (Rodriguez et al., 2016). In other words, the phosphorylation of MLKL at ser345 was crucial for the recruitment of MLKL and the activation of necroptosis pathway (Rodriguez et al., 2016). We also found high level of the p-MLKL colocalized with thioflavin T staining in the hippocampus of CUMS group mice, indicating that MLKL was phosphorylated in the presence of amyloid-like polymers, characteristic of necrosome complexes (Li et al., 2012; Liu et al., 2017).
Finally, our results displayed a large number of inflammatory cells infiltrated into the hippocampus of CUMS-exposed mice and revealed that the expressions of IL-1β, Lipocalin-2 and IBA1 were significantly increased, which proved that the existence of neuroinflammation and excessive activation of microglia were associated with the necroptosis pathway. Necroptosis can be specifically blocked by small molecule compound Nec-1, but not by specific inhibitors of apoptosis and autophagy, such as z-vad. fmk (N-benzyloxycarbonyl Val ala ASP (o-me) fluoromethylketone) and 3-methyladenine (3 mA). Many studies on different perspectives have revealed that the inhibition of necroptosis mediated by RIPK1/RIPK3/MLKL provides a protective effect against inflammation (Moriwaki and Chan, 2016; Duan et al., 2020; Kumari et al., 2021). Therefore, it was found that Nec-1 could also suppress neuroinflammation and activation of microglia in hippocampus. It was reported that Lipocalin-2 was an inflammatory-related protein closely correlated to hippocampal structure and function, and it might be a key regulator of emotional behaviors and cognitive function (Ferreira et al., 2013). Lipocalin-2 secreted by microglia and astrocytes participated in the activation of microglia, then excessive activation of microglia caused neuroinflammation and injured the structure and function of neurons (Kim et al., 2017). Normally, there was low expression of Lipocalin-2 in hippocampus, but it might continuously increase under stress stimulation, which was related to its ability to regulate the formation and maturation of dendritic spines of neurons in hippocampal CA1-CA3 regions (Mucha et al., 2011). Moreover, the activation of MLKL during necroptosis triggered the release of IL-1β, IL-6 and TNF(Wu et al., 2013). The inflammatory cytokines such as IL-1β might be the key substance regulating the expression of Lipocalin-2 (Hu et al., 2015). At this point we could conclude that necroptosis might activate the Lipocalin-2 involved inflammatory pathway, induce the neuroinflammation and microglial over-activation, and then participate in the functional changes of hippocampus leading to depressive-like behaviors in depressed mice. Grounded on rigorous science, the TCM formula Xiaoyaosan was an antidepressant therapy conceptually based on a multiple component, multiple targeting principle. As showed in this study, Xiaoyaosan might mediate the RIPK1-RIPK3-MLKL signaling and its subsequent inflammatory pathway, regulate the inflammatory-related mediator Lipocalin-2, and then improve the function of hippocampal microglia to exert antidepressant and anti-inflammatory effect.
According to the network pharmacological study, the active ingredients of Xiaoyaosan may exert the antidepressant effect via 23 necroptosis related potential targets. Then, the animal experiment found that the RIPK1-RIPK3-MLKL signaling was triggered in the stress-induced depression in mice. These findings were consistent with the cytokine hypothesis of depression that postulated stress may activate the necroptosis pathway in hippocampus, leading to neuroinflammation and microglial activation, then contribute to the development of depressive-like behaviors. Moreover, our findings further illustrated the possible mechanism of Xiaoyaosan with explicit experiment results that it could regulate the necroptosis mediated inflammatory signaling pathway to alleviated the depressive symptoms of mice, which provided an experimental basis for the clinical application of Xiaoyaosan in the treatment of depression. For future research, whether necroptosis can be used as a new potential pathway for the treatment of depression still needs to be confirmed with RIPK or MLKL knockout mice, and the functional impact of necroptosis in depression with the specific role of the active components of Xiaoyaosan on neuroinflammation could be further explored by more novel and accurate network pharmacological analysis.
The original contributions presented in the study are included in the article, further inquiries can be directed to the corresponding authors.
The animal study was reviewed and approved by The study was approved by the Institutional Animal Care and Use Committee of Beijing University of Chinese Medicine (BUCM-4-2018120401-4053).
Conceptualization, ZY, HJ and JC; Data curation, ZY and HJ; Formal analysis, ZY and HJ; Funding acquisition, JC; Investigation, ZY, JC, KZ and XW; Methodology, HJ, JC and XW; Project administration, ZY and KZ; Visualization, ZY and HJ; Writing — original draft, ZY and HJ; Writing — review andamp; editing, YJ, YL, ZX, QM, XL and JC.
This research was supported by grants from National Natural Science Foundation of China (No. 81630104, 81973748), and Huang Zhendong Research Fund for Traditional Chinese Medicine of Jinan University (No. 201911).
The authors declare that the research was conducted in the absence of any commercial or financial relationships that could be construed as a potential conflict of interest.
All claims expressed in this article are solely those of the authors and do not necessarily represent those of their affiliated organizations, or those of the publisher, the editors and the reviewers. Any product that may be evaluated in this article, or claim that may be made by its manufacturer, is not guaranteed or endorsed by the publisher.
The Supplementary Material for this article can be found online at: https://www.frontiersin.org/articles/10.3389/fphar.2021.773562/full#supplementary-material
ADME, absorption, distribution, metabolism and enhancement; ANOVA, one-way analysis of variance; BDNF, brain-derived neurotrophic factor; CTD, Comparative Toxicogenomic database; CUMS, chronic unpredictable mild stress; CNS, central nervous system; DL, drug likeness; DMSO, dimethyl sulphoxide; DAMPs, damage-associated molecular pattern molecules; ECL, enhanced chemiluminescence; ETCM, Encyclopedia of Traditional Chinese Medicine; FS, forced swimming; H&E, hematoxylin and eosin; HPA, hypothalamic pituitary adrenal; HRP, horseradish peroxidase; HERB, High-throughput Experiment- and Reference-guided database of Traditional Chinese Medicine; IF, immunofluorescence; IHC, immunohistochemical; LSD, least significant difference; MOD, mean optical density; MFI, mean fluorescence intensity; MLKL, mixed lineage kinase domain-like; Nec-1, necrostatin-1; NSF, novelty-suppressed feeding; OB, bioavailability; OMIM, Online Mendelian Inheritance in Man; PFA, paraformaldehyde; PPI, protein–protein interaction; PVDF, polyvinylidene fluoride; RIPK, receptor interacting protein kinas; SAC, sacrificed; SP, sucrose preference; SPF, specific-pathogen free; SEM, standard error of mean; SSRIs, selective serotonin reuptake inhibitors; TCM, traditional Chinese medicine; TCMSP, TCM Systems Pharmacology database and Analysis Platform; TBST, tris buffered saline tween; TLR, toll-like receptor; TNF, tumor necrosis factor; TTD, Therapeutic Target database; UPLC-MS/MS, ultra-performance chromatography-electrospray tandem mass spectrometr; UniProt, universal Protein Resource; WB, western blot; WHO, World Health Organization.
Amberger, J. S., Bocchini, C. A., Schiettecatte, F., Scott, A. F., and Hamosh, A. (2015). OMIM.org: Online Mendelian Inheritance in Man (OMIM®), an Online Catalog of Human Genes and Genetic Disorders. Nucleic Acids Res. 43, D789–D798. doi:10.1093/nar/gku1205
Antunes, D. P. E., Rodrigues, O. S., Dias, A. J. S. o. J., and Pereira, R. C. M. (2019). Loss of Microglial Parkin Inhibits Necroptosis and Contributes to Neuroinflammation. Mol. Neurobiol., 1–15. doi:10.1007/s12035-018-1264-9
Bächli, H., Steiner, M. A., Habersetzer, U., and Wotjak, C. T. (2008). Increased Water Temperature Renders Single-Housed C57BL/6J Mice Susceptible to Antidepressant Treatment in the Forced Swim Test. Behav. Brain Res. 187 (1), 67–71. doi:10.1016/j.bbr.2007.08.029
Barfield, E. T., Moser, V. A., Hand, A., and Grisel, J. E. (2013). β-Endorphin Modulates the Effect of Stress on Novelty-Suppressed Feeding. Front. Behav. Neurosci. 7 (6), 19. doi:10.3389/fnbeh.2013.00019
Berk, M., Williams, L. J., Jacka, F. N., O'Neil, A., Pasco, J. A., Moylan, S., et al. (2013). So Depression Is an Inflammatory Disease, but Where Does the Inflammation Come From? BMC Med. 11 (1), 200. doi:10.1186/1741-7015-11-200
Bian, P., Zheng, X., Wei, L., Ye, C., Fan, H., Cai, Y., et al. (2017). MLKL Mediated Necroptosis Accelerates JEV-Induced Neuroinflammation in Mice. Front. Microbiol. 8, 303. doi:10.3389/fmicb.2017.00303
Cao, X., Li, L. P., Wang, Q., Wu, Q., Hu, H. H., Zhang, M., et al. (2013). Astrocyte-Derived ATP Modulates Depressive-Like Behaviors. Nat. Med. 19 (1), 773–777. doi:10.1038/nm.3162
Chen, C., Yin, Q., Tian, J., Gao, X., Qin, X., Du, G., et al. (2020). Studies on the Potential Link Between Antidepressant Effect of Xiaoyao San and its Pharmacological Activity of Hepatoprotection Based on Multi-Platform Metabolomics. J. Ethnopharmacol. 249, 112432. doi:10.1016/j.jep.2019.112432
Chen, Y., Gong, K., Guo, L., Zhang, B., Chen, S., Li, Z., et al. (2021). Downregulation of Phosphoglycerate Mutase 5 Improves Microglial Inflammasome Activation after Traumatic Brain Injury. Cell Death Discov. 7 (1), 290. doi:10.1038/s41420-021-00686-8
Chiriţă, A. L., Gheorman, V., Bondari, D., and Rogoveanu, I. (2015). Current Understanding of the Neurobiology of Major Depressive Disorder. Rom. J. Morphol. Embryol. 56 (2 Suppl. l), 651–658.
Cho, Y. S., Challa, S., Moquin, D., Genga, R., Ray, T. D., Guildford, M., et al. (2009). Phosphorylation-Driven Assembly of the RIP1-RIP3 Complex Regulates Programmed Necrosis and Virus-Induced Inflammation. Cell. 137 (6), 1112–1123. doi:10.1016/j.cell.2009.05.037
D'Aquila, P. S., Brain, P., and Willner, P. (1994). Effects of Chronic Mild Stress on Performance in Behavioural Tests Relevant to Anxiety and Depression. Physiol. Behav. 56 (5), 861–867. doi:10.1016/0031-9384(94)90316-6
Daina, A., Michielin, O., and Zoete, V. (2019). SwissTargetPrediction: Updated Data and New Features for Efficient Prediction of Protein Targets of Small Molecules. Nucleic Acids Res. 47 (W1), W357–w364. doi:10.1093/nar/gkz382
Dara, L., Johnson, H., Suda, J., Win, S., Gaarde, W., Han, D., et al. (2016). Receptor Interacting Protein Kinase 1 Mediates Murine Acetaminophen Toxicity Independent of the Necrosome and Not Through Necroptosis. Hepatology. 62 (6), 1847–1857. doi:10.1002/hep.27939
Davis, A. P., Grondin, C. J., Johnson, R. J., Sciaky, D., Wiegers, J., Wiegers, T. C., et al. (2020). Comparative Toxicogenomics Database (CTD): Update 2021. Nucleic Acids Res. 49, D1138–D1143. doi:10.1093/nar/gkaa891
de Kloet, E. R., and Joëls, F. (2005). Jo?lsStress and the Brain: From Adaptation to Disease. Nat. Rev. Neurosci. 6 (6), 463–475. doi:10.1038/nrn1683
Degterev, A., Hitomi, J., Germscheid, M., Ch'En, I. L., Korkina, O., Teng, X., et al. (2008). Identification of RIP1 Kinase as a Specific Cellular Target of Necrostatins. Nat. Chem. Biol. 4 (5), 313–321. doi:10.1038/nchembio.83
Degterev, A., Huang, Z., Boyce, M., Li, Y., Jagtap, P., Mizushima, N., et al. (2005). Chemical Inhibitor of Nonapoptotic Cell Death With Therapeutic Potential for Ischemic Brain Injury. Nat. Chem. Biol. 1 (2), 112–119. doi:10.1038/nchembio711
Ding, X. F., Li, Y. H., Chen, J. X., Sun, L. J., Jiao, H. Y., Wang, X. X., et al. (2017). Involvement of the Glutamate/Glutamine Cycle and Glutamate Transporter GLT-1 in Antidepressant-like Effects of Xiao Yao San on Chronically Stressed Mice. BMC Complement. Altern. Med. 17 (1), 326. doi:10.1186/s12906-017-1830-0
Dongliang, C., Xiaoping, P., Peng, X., Farwell, M. A., and Baohong, Z. (2011). Evaluation and Identification of Reliable Reference Genes for Pharmacogenomics, Toxicogenomics, and Small RNA Expression Analysis. J. Cell Physiol. 226 (10), 2469–2477. doi:10.1002/jcp.22725
Dowlati, Y., Herrmann, N., Swardfager, W., Liu, H., Sham, L., Reim, E. K., et al. (2010). A Meta-Analysis of Cytokines in Major Depression. Biol. Psychiatry. 67 (5), 446–457. doi:10.1016/j.biopsych.2009.09.033
Duan, X., Liu, X., Liu, N., Huang, Y., Jin, Z., Zhang, S., et al. (2020). Inhibition of Keratinocyte Necroptosis Mediated by RIPK1/RIPK3/MLKL Provides a Protective Effect against Psoriatic Inflammation. Cell Death Dis. 11 (2), 134. doi:10.1038/s41419-020-2328-0
Fang, S., Dong, L., Liu, L., Guo, J., Zhao, L., Zhang, J., et al. (2021). HERB: a High-Throughput Experiment- and Reference-Guided Database of Traditional Chinese Medicine. Nucleic Acids Res. 49 (D1), D1197–d1206. doi:10.1093/nar/gkaa1063
Ferreira, A. C., Pinto, V., Dá Mesquita, S., Novais, A., Sousa, J. C., Correia-Neves, M., et al. (2013). Lipocalin-2 Is Involved in Emotional Behaviors and Cognitive Function. Front Cell Neurosci. 7 (31), 122. doi:10.3389/fncel.2013.00122
Frazer, A., and Morilak, D. A. (2005). What Should Animal Models of Depression Model? Neurosci. Biobehav Rev. 29 (4), 515–523. doi:10.1016/j.neubiorev.2005.03.006
Galluzzi, L., Kepp, O., and Kroemer, G. (2009). RIP Kinases Initiate Programmed Necrosis. J. Mol. Cell Biol. 1 (1), 8–10. doi:10.1093/jmcb/mjp007
Galluzzi, L., and Kroemer, G. (2008). Necroptosis: A Specialized Pathway of Programmed Necrosis. Cell. 135 (7), 1161–1163. doi:10.1016/j.cell.2008.12.004
Guo, X., Wu, Y., Zhang, C., Wu, L., Qin, L., and Liu, T. (2021). Network Pharmacology Analysis of ZiShenWan for Diabetic Nephropathy and Experimental Verification of its Anti-Inflammatory Mechanism. Drug Des. Devel Ther. 15, 1577–1594. doi:10.2147/dddt.s297683
Haapakoski, R., Ebmeier, K. P., Alenius, H., and Kivimäki, M. (2016). Innate and Adaptive Immunity in the Development of Depression: An Update on Current Knowledge and Technological Advances. Prog. Neuropsychopharmacol. Biol. Psychiatry. 66, 63–72. doi:10.1016/j.pnpbp.2015.11.012
Hayley, S. (2011). Toward an Anti-inflammatory Strategy for Depression. Front. Behav. Neurosci. 5 (19), 19. doi:10.3389/fnbeh.2011.00019
Hu, Y., Xue, J., Yang, Y., Zhou, X., Qin, C., Zheng, M., et al. (2015). Lipocalin 2 Upregulation Protects Hepatocytes from IL1-β-Induced Stress. Cell Physiol Biochem. 36 (2), 753–762. doi:10.1159/000430135
Iwata, M., Ota, K. T., and Duman, R. S. (2013). The Inflammasome: Pathways Linking Psychological Stress, Depression, and Systemic Illnesses. Brain Behav. Immun. 31 (7), 105–114. doi:10.1016/j.bbi.2012.12.008
Jiao, H., Yan, Z., Ma, Q., Li, X., Jiang, Y., Liu, Y., et al. (2019). Influence of Xiaoyaosan on Depressive-Like Behaviors in Chronic Stress-Depressed Rats Through Regulating Tryptophan Metabolism in Hippocampus. Neuropsychiatr. Dis. Treat. 15, 21–31. doi:10.2147/ndt.S185295
Jiao, H., Yang, H., Yan, Z., Chen, J., Xu, M., Jiang, Y., et al. (2021). Traditional Chinese Formula Xiaoyaosan Alleviates Depressive-Like Behavior in CUMS Mice by Regulating PEBP1-GPX4-Mediated Ferroptosis in the Hippocampus. Neuropsychiatr. Dis. Treat. 17, 1001–1019. doi:10.2147/ndt.s302443
Kim, J. H., Ko, P. W., Lee, H. W., Jeong, J. Y., Lee, M. G., Kim, J. H., et al. (2017). Astrocyte-Derived Lipocalin-2 Mediates Hippocampal Damage and Cognitive Deficits in Experimental Models of Vascular Dementia. Glia. 65 (9), 1471–1490. doi:10.1002/glia.23174
Konsman, J.-P. (2003). The Mouse Brain in Stereotaxic Coordinates. Psychoneuroendocrinology. 28 (6), 827–828. doi:10.1016/s0306-4530(03)00088-x
Kumari, S., Van, T. M., Preukschat, D., Schuenke, H., Basic, M., Bleich, A., et al. (2021). NF-κB Inhibition in Keratinocytes Causes RIPK1-Mediated Necroptosis and Skin Inflammation. Life Sci. Alliance. 4 (6). doi:10.26508/lsa.202000956
Li, H., Zhao, L., Zhang, B., Jiang, Y., Wang, X., Guo, Y., et al. (2014). A Network Pharmacology Approach to Determine Active Compounds and Action Mechanisms of Ge-Gen-Qin-Lian Decoction for Treatment of Type 2 Diabetes. Evid. Based Complement. Alternat Med. 2014, 495840. doi:10.1155/2014/495840
Li, J., McQuade, T., Siemer, A. B., Napetschnig, J., Moriwaki, K., Hsiao, Y. S., et al. (2012). The RIP1/RIP3 Necrosome Forms a Functional Amyloid Signaling Complex Required for Programmed Necrosis. Cell. 150 (2), 339–350. doi:10.1016/j.cell.2012.06.019
Li, P., Tang, X. D., Cai, Z. X., Qiu, J. J., Lin, X. L., Zhu, T., et al. (2015). CNP Signal Pathway Up-Regulated in Rectum of Depressed Rats and the Interventional Effect of Xiaoyaosan. World J. Gastroenterol. 21 (5), 1518–1530. doi:10.3748/wjg.v21.i5.1518
Li, S., and Zhang, B. (2013). Traditional Chinese Medicine Network Pharmacology: Theory, Methodology and Application. Chin. J. Nat. Med. 11 (2), 110–120. doi:10.1016/s1875-5364(13)60037-0
Li, X. J., Ma, Q. Y., Jiang, Y. M., Bai, X. H., Yan, Z. Y., Liu, Q., et al. (2017). Xiaoyaosan Exerts Anxiolytic-Like Effects by Down-Regulating the TNF-Α/jak2-STAT3 Pathway in the Rat hippocampus. Sci. Rep. 7 (1), 353. doi:10.1038/s41598-017-00496-y
Liao, D., Xiang, D., Dang, R., Xu, P., Wang, J., Han, W., et al. (2018). Neuroprotective Effects of Dl-3-N-Butylphthalide Against Doxorubicin-Induced Neuroinflammation, Oxidative Stress, Endoplasmic Reticulum Stress, and Behavioral Changes. Oxid Med. Cell Longev. 2018, 9125601–9125613. doi:10.1155/2018/9125601
Liu, C., Chen, Y., Cui, W., Cao, Y., Zhao, L., Wang, H., et al. (2021a). Inhibition of Neuronal Necroptosis Mediated by RIP1/RIP3/MLKL Provides Neuroprotective Effects on Kaolin‐Induced Hydrocephalus in Mice. Cell Prolif. 54, e13108. doi:10.1111/cpr.13108
Liu, X., Wei, F., Liu, H., Zhao, S., Du, G., and Qin, X. (2021b). Integrating Hippocampal Metabolomics and Network Pharmacology Deciphers the Antidepressant Mechanisms of Xiaoyaosan. J. Ethnopharmacol. 268, 113549. doi:10.1016/j.jep.2020.113549
Liu, X. J., Wang, Y. Z., Wei, F. X., Lv, M., Qu, P., Chen, S. J., et al. (2021c). The Synergistic Anti-Depression Effects of Different Efficacy Groups of Xiaoyaosan as Demonstrated by the Integration of Network Pharmacology and Serum Metabolomics. J. Pharm. Biomed. Anal. 197, 113949. doi:10.1016/j.jpba.2021.113949
Liu, S., Liu, H., Johnston, A., Hanna-Addams, S., Reynoso, E., Xiang, Y., et al. (2017). MLKL Forms Disulfide Bond-Dependent Amyloid-like Polymers to Induce Necroptosis. Proc. Natl. Acad. Sci. U S A. 114 (36), E7450–e7459. doi:10.1073/pnas.1707531114
Liu, S., Wang, X., Li, Y., Xu, L., Yu, X., Ge, L., et al. (2014). Necroptosis Mediates TNF-Induced Toxicity of Hippocampal Neurons. Biomed. Res. Int. 2014 (11), 290182. doi:10.1155/2014/290182
Liu, T., Bao, Y. H., Wang, Y., and Jiang, J. Y. (2015). The Role of Necroptosis in Neurosurgical Diseases. Braz. J. Med. Biol. Res. 48 (4), 292–298. doi:10.1590/1414-431X20144310
Liu, X., Liu, C., Tian, J., Gao, X., Li, K., Du, G., et al. (2020). Plasma Metabolomics of Depressed Patients and Treatment With Xiaoyaosan Based on Mass Spectrometry Technique. J. Ethnopharmacol. 246, 112219. doi:10.1016/j.jep.2019.112219
Liu, X., Zheng, X., Du, G., Li, Z., and Qin, X. (2019a). Brain Metabonomics Study of the Antidepressant-Like Effect of Xiaoyaosan on the CUMS-Depression Rats by 1H NMR Analysis. J. Ethnopharmacol 235, 141–154. doi:10.1016/j.jep.2019.01.018
Liu, Y., Ding, X. F., Wang, X. X., Zou, X. J., Li, X. J., Liu, Y. Y., et al. (2019b). Xiaoyaosan Exerts Antidepressant-Like Effects by Regulating the Functions of Astrocytes and EAATs in the Prefrontal Cortex of Mice. BMC Complement. Altern. Med. 19 (1), 215. doi:10.1186/s12906-019-2613-6
Luo, T. T., Lu, Y., Yan, S. K., Xiao, X., Rong, X. L., and Guo, J. (2020). Network Pharmacology in Research of Chinese Medicine Formula: Methodology, Application and Prospective. Chin. J. Integr. Med. 26 (1), 72–80. doi:10.1007/s11655-019-3064-0
Maes, M. (1995). Evidence for an Immune Response in Major Depression: A Review and Hypothesis. Prog. Neuropsychopharmacol. Biol. Psychiatry. 19 (1), 11–38. doi:10.1016/0278-5846(94)00101-m
Marunouchi, T., Ito, T., Onda, S., Kyo, L., Takahashi, K., Uchida, M., et al. (2021). Effects of 17-AAG on the RIP1/RIP3/MLKL Pathway During the Development of Heart Failure Following Myocardial Infarction in Rats. J. Pharmacol. Sci. 147 (2), 192–199. doi:10.1016/j.jphs.2021.06.009
Moriwaki, K., and Chan, F. K. (2016). Necroptosis-Independent Signaling by the RIP Kinases in Inflammation. Cell Mol Life Sci. 73 (11-12), 2325–2334. doi:10.1007/s00018-016-2203-4
Moussavi, S., Chatterji, S., Verdes, E., Tandon, A., Patel, V., and Ustun, B. (2007). Depression, Chronic Diseases, and Decrements in Health: Results From the World Health Surveys. Lancet. 370 (9590), 851–858. doi:10.1016/s0140-6736(07)61415-9
Mucha, M., Skrzypiec, A. E., Schiavon, E., Attwood, B. K., Kucerova, E., and Pawlak, R. (2011). Lipocalin-2 Controls Neuronal Excitability and Anxiety by Regulating Dendritic Spine Formation and Maturation. Proc. Natl. Acad. Sci. U S A. 108 (45), 18436–18441. doi:10.1073/pnas.1107936108
Müller, N., and Schwarz, M. J. (2010). S03-03 - Antiinflammatory Treatment Approaches in Major Depression. Eur. Psychiatry. 25 (10), 120. doi:10.1016/s0924-9338(10)70120-6
Newton, K., and Manning, G. (2016). Necroptosis and Inflammation. Annu. Rev. Biochem. 85 (1), 743–763. doi:10.1146/annurev-biochem-060815-014830
Pan, Q., Wu, J., Liu, Y., Li, X., and Chen, J. (2019). Involvement of Hepatic SHIP2 and PI3K/Akt Signalling in the Regulation of Plasma Insulin by Xiaoyaosan in Chronic Immobilization-Stressed Rats. Molecules. 24 (3), 480. doi:10.3390/molecules24030480
Papp, M., Moryl, E., and Willner, P. (1996). Pharmacological Validation of the Chronic Mild Stress Model of Depression. Eur. J. Pharmacol. 296 (2), 129–136. doi:10.1016/0014-2999(95)00697-4
Pasco, J. A., Nicholson, G. C., Williams, L. J., Jacka, F. N., Henry, M. J., Kotowicz, M. A., et al. (2010). Association of High-Sensitivity C-Reactive Protein With De Novo Major Depression. Br. J. Psychiatry. 197 (5), 372–377. doi:10.1192/bjp.bp.109.076430
Pasparakis, M., and Vandenabeele, P. (2015). Necroptosis and its Role in Inflammation. Nature. 517 (7534), 311–320. doi:10.1038/nature14191
Peng, G. J., Tian, J. S., Gao, X. X., Zhou, Y. Z., and Qin, X. M. (2015). Research on the Pathological Mechanism and Drug Treatment Mechanism of Depression. Curr. Neuropharmacol. 13 (4), 514–523. doi:10.2174/1570159x1304150831120428
Qu, Y., Tang, J., Wang, H., Li, S., Zhao, F., Zhang, L., et al. (2017). RIPK3 Interactions With MLKL and CaMKII Mediate Oligodendrocytes Death in the Developing Brain. Cell Death Dis. 8 (2), e2629. doi:10.1038/cddis.2017.54
Rodriguez, D. A., Weinlich, R., Brown, S., Guy, C., Fitzgerald, P., Dillon, C. P., et al. (2016). Characterization of RIPK3-Mediated Phosphorylation of the Activation Loop of MLKL during Necroptosis. Cell Death Differ. 23 (1), 76–88. doi:10.1038/cdd.2015.70
Ru, J., Li, P., Wang, J., Zhou, W., Li, B., Huang, C., et al. (2014). TCMSP: a Database of Systems Pharmacology for Drug Discovery From Herbal Medicines. J. Cheminform. 6, 13. doi:10.1186/1758-2946-6-13
Sawada, A., Niiyama, Y., Ataka, K., Nagaishi, K., Yamakage, M., and Fujimiya, M. (2014). Suppression of Bone Marrow-Derived Microglia in the Amygdala Improves Anxiety-Like Behavior Induced by Chronic Partial Sciatic Nerve Ligation in Mice. Pain. 155 (9), 1762–1772. doi:10.1016/j.pain.2014.05.031
Schwabe, R. F., and Luedde, T. (2018). Apoptosis and Necroptosis in the Liver: a Matter of Life and Death. Nat. Rev. Gastroenterol. Hepatol. 15 (12), 738–752. doi:10.1038/s41575-018-0065-y
Shalom, G., Lifschytz, T., Lerer, B., and Newman, M. E. (2006). Effects of T3 and Fluoxetine in the Novelty-Suppressed Feeding Test Model of Depression and Anxiety: Gender Differences. Eur. Neuropsychopharmacol. 16 (06), S48–S49. doi:10.1016/s0924-977x(06)80058-1
Shan, B., Pan, H., Najafov, A., and Yuan, J. (2018). Necroptosis in Development and Diseases. Genes Dev. 32 (5-6), 327–340. doi:10.1101/gad.312561.118
Shao, L., Liu, X., Zhu, S., Liu, C., Gao, Y., and Xu, X. (2018). The Role of Smurf1 in Neuronal Necroptosis after Lipopolysaccharide-Induced Neuroinflammation. Cell Mol Neurobiol. 38 (4), 809–816. doi:10.1007/s10571-017-0553-6
Sheng, Q., Du, R., Ma, C., Zhou, Y., Shen, X., Hou, X., et al. (2021). NMPA-Approved Traditional Chinese Medicine-Pingwei Pill: New Indication for Colistin Recovery Against MCR-Positive Bacteria Infection. Chin. Med. 16 (1), 106. doi:10.1186/s13020-021-00518-y
Sonenshine, D. E., and Macaluso, R. K. (2017). Microbial Invasion vs. Tick Immune Regulation. Front. Cell Infect. Microbiol. 7, 390. doi:10.3389/fcimb.2017.00390
Stacey, R., and Wei-Xing, Z. (2006). Chemotherapeutic Approaches for Targeting Cell Death Pathways. Oncologist. 11 (4), 342–357. doi:10.1634/theoncologist.11-4-342
Stein, D. J., Naudé, P. J., and Berk, M. (2018). Stress, Depression, and Inflammation: Molecular and Microglial Mechanisms. Biol. Psychiatry. 83 (1), 5. doi:10.1016/j.biopsych.2017.10.025
Stelzer, G., Rosen, N., Plaschkes, I., Zimmerman, S., Twik, M., Fishilevich, S., et al. (2016). The GeneCards Suite: From Gene Data Mining to Disease Genome Sequence Analyses. Curr. Protoc. Bioinformatics. 54, 131–3031. doi:10.1002/cpbi.5
Streit, W. J., Mrak, R. E., and Griffin, W. S. T. (2004). Microglia and Neuroinflammation: a Pathological Perspective. J. Neuroinflammation. 1 (1), 14. doi:10.1186/1742-2094-1-14
Szklarczyk, D., Gable, A. L., Lyon, D., Junge, A., Wyder, S., Huerta-Cepas, J., et al. (2019). STRING V11: Protein-Protein Association Networks With Increased Coverage, Supporting Functional Discovery in Genome-Wide Experimental Datasets. Nucleic Acids Res. 47 (D1), D607–d613. doi:10.1093/nar/gky1131
Takahashi, N., Duprez, L., Grootjans, S., Cauwels, A., Nerinckx, W., DuHadaway, J. B., et al. (2012). Necrostatin-1 Analogues: Critical Issues on the Specificity, Activity and In Vivo Use in Experimental Disease Models. Cell Death Dis. 3 (11), e437. doi:10.1038/cddis.2012.176
Thadathil, N., Nicklas, E. H., Mohammed, S., Lewis, T. L., Richardson, A., and Deepa, S. S. (2021). Necroptosis Increases With Age in the Brain and Contributes to Age-Related Neuroinflammation. Geroscience. doi:10.1007/s11357-021-00448-5
Vandenabeele, P., Declercq, W., and Berghe, T. V. (2008). Necrotic Cell Death and ‘Necrostatins’: Now We Can Control Cellular Explosion. Trends Biochem. Sci. 33 (8), 352–355. doi:10.1016/j.tibs.2008.05.007
Vugmeyster, Y., Harrold, J., and Xu, X. (2012). Absorption, Distribution, Metabolism, and Excretion (ADME) Studies of Biotherapeutics for Autoimmune and Inflammatory Conditions. Aaps J. 14 (4), 714–727. doi:10.1208/s12248-012-9385-y
Wang, L., Zhou, G. B., Liu, P., Song, J. H., Liang, Y., Yan, X. J., et al. (2008). Dissection of Mechanisms of Chinese Medicinal Formula Realgar-Indigo Naturalis as an Effective Treatment for Promyelocytic Leukemia. Proc. Natl. Acad. Sci. U S A. 105 (12), 4826–4831. doi:10.1073/pnas.0712365105
Wang, M., Huang, W., Gao, T., Zhao, X., and Lv, Z. (2018). Effects of Xiao Yao San on Interferon-Alpha-Induced Depression in Mice. Brain Res. Bull. 139, 197–202. doi:10.1016/j.brainresbull.2017.12.001
Wang, Y., Zhang, S., Li, F., Zhou, Y., Zhang, Y., Wang, Z., et al. (2020). Therapeutic Target Database 2020: Enriched Resource for Facilitating Research and Early Development of Targeted Therapeutics. Nucleic Acids Res. 48 (D1), D1031–d1041. doi:10.1093/nar/gkz981
Weiss, J. M. (1997). Does Decreased Sucrose Intake Indicate Loss of Preference in CMS Model? Psychopharmacology. 134 (4), 368–370. doi:10.1007/s002130050472
Wishart, D. S., Feunang, Y. D., Guo, A. C., Lo, E. J., Marcu, A., Grant, J. R., et al. (2018). DrugBank 5.0: a Major Update to the DrugBank Database for 2018. Nucleic Acids Res. 46 (D1), D1074–d1082. doi:10.1093/nar/gkx1037
Wu, J., Huang, Z., Ren, J., Zhang, Z., He, P., Li, Y., et al. (2013). Mlkl Knockout Mice Demonstrate the Indispensable Role of Mlkl in Necroptosis. Cell Res. 23 (8), 994–1006. doi:10.1038/cr.2013.91
Xiong, X., Wang, P., Duan, L., Liu, W., Chu, F., Li, S., et al. (2019). Efficacy and Safety of Chinese Herbal Medicine Xiao Yao San in Hypertension: A Systematic Review and Meta-Analysis. Phytomedicine. 61, 152849. doi:10.1016/j.phymed.2019.152849
Xu, Y., Sheng, H., Tang, Z., Lu, J., and Ni, X. (2015). Inflammation and Increased Ido in hippocampus Contribute to Depression-Like Behavior Induced by Estrogen Deficiency. Behav. Brain Res. 288, 71–78. doi:10.1016/j.bbr.2015.04.017
Yan, Z., Jiao, H., Ding, X., Ma, Q., and Chen, J. (2018). Xiaoyaosan Improves Depressive-Like Behaviors in Mice Through Regulating Apelin-APJ System in Hypothalamus. Molecules. 23 (5), 1073. doi:10.3390/molecules23051073
Yan, Z. Y., Li, X. J., Ding, X. F., Liu, Y. Y., and Chen, J. X. (2019). Evaluating the Anti-sepression Effect of Xiaoyaosan on Chronically-Stressed Mice. J. Vis. Exp. 143. doi:10.3791/58276
Yu, Z., Jiang, N., Su, W., and Zhuo, Y. (2021). Necroptosis: A Novel Pathway in Neuroinflammation. Front. Pharmacol. 12, 701564. doi:10.3389/fphar.2021.701564
Yuan, N., Gong, L., Tang, K., He, L., Hao, W., Li, X., et al. (2020). An Integrated Pharmacology-Based Analysis for Antidepressant Mechanism of Chinese Herbal Formula Xiao-Yao-San. Front. Pharmacol. 11, 284. doi:10.3389/fphar.2020.00284
Zhang, B. B., Liu, Y. M., Hu, A. L., Xu, S. F., Fan, L. D., Cheng, M. L., et al. (2019). HgS and Zuotai Differ From HgCl(2) and Methyl Mercury in Intestinal Hg Absorption, Transporter Expression and Gut Microbiome in Mice. Toxicol. Appl. Pharmacol. 379, 114615. doi:10.1016/j.taap.2019.114615
Zhou, X. M., Liu, C. Y., Liu, Y. Y., Ma, Q. Y., Zhao, X., Jiang, Y. M., et al. (2021). Xiaoyaosan Alleviates Hippocampal Glutamate-Induced Toxicity in the CUMS Rats via NR2B and PI3K/Akt Signaling Pathway. Front. Pharmacol. 12, 586788. doi:10.3389/fphar.2021.586788
Keywords: xiaoyaosan, chronic unpredictable mild stress, depression, necroptosis, neuroinflammation, microglial activation
Citation: Yan Z-Y, Jiao H-Y, Chen J-B, Zhang K-W, Wang X-H, Jiang Y-M, Liu Y-Y, Xue Z, Ma Q-Y, Li X-J and Chen J-X (2021) Antidepressant Mechanism of Traditional Chinese Medicine Formula Xiaoyaosan in CUMS-Induced Depressed Mouse Model via RIPK1-RIPK3-MLKL Mediated Necroptosis Based on Network Pharmacology Analysis. Front. Pharmacol. 12:773562. doi: 10.3389/fphar.2021.773562
Received: 10 September 2021; Accepted: 01 November 2021;
Published: 19 November 2021.
Edited by:
Shao Li, Tsinghua University, ChinaReviewed by:
Wenda Xue, Nanjing University of Chinese Medicine, ChinaCopyright © 2021 Yan, Jiao, Chen, Zhang, Wang, Jiang, Liu, Xue, Ma, Li and Chen. This is an open-access article distributed under the terms of the Creative Commons Attribution License (CC BY). The use, distribution or reproduction in other forums is permitted, provided the original author(s) and the copyright owner(s) are credited and that the original publication in this journal is cited, in accordance with accepted academic practice. No use, distribution or reproduction is permitted which does not comply with these terms.
*Correspondence: Qing-Yu Ma, dG1hcWluZ3l1QGpudS5lZHUuY24=; Xiao-Juan Li, bGl4aWFvanVhbkBqbnUuZWR1LmNu; Jia-Xu Chen, Y2hlbmppYXh1QGhvdG1haWwuY29t
†These authors contributed equally to this work
Disclaimer: All claims expressed in this article are solely those of the authors and do not necessarily represent those of their affiliated organizations, or those of the publisher, the editors and the reviewers. Any product that may be evaluated in this article or claim that may be made by its manufacturer is not guaranteed or endorsed by the publisher.
Research integrity at Frontiers
Learn more about the work of our research integrity team to safeguard the quality of each article we publish.