- 1CAS Key Laboratory of Tropical Marine Bio-resources and Ecology, Guangdong Key Laboratory of Marine Materia Medica, South China Sea Institute of Oceanology, Chinese Academy of Sciences, Guangzhou, China
- 2College of Earth and Planetary Sciences, University of Chinese Academy of Sciences, Beijing, China
- 3NMPA Key Laboratory for Research and Evaluation of Drug Metabolism, Guangdong Provincial Key Laboratory of New Drug Screening, School of Pharmaceutical Sciences, Southern Medical University, Guangzhou, China
- 4Southern Marine Science and Engineering Guangdong Laboratory (Guangzhou), Guangzhou, China
Nuclear receptors (NRs) are a superfamily of transcription factors induced by ligands and also function as integrators of hormonal and nutritional signals. Among NRs, the liver X receptors (LXRs) and farnesoid X receptor (FXR) have been of significance as targets for the treatment of metabolic syndrome-related diseases. In recent years, natural products targeting LXRs and FXR have received remarkable interests as a valuable source of novel ligands encompassing diverse chemical structures and bioactive properties. This review aims to survey natural products, originating from terrestrial plants and microorganisms, marine organisms, and marine-derived microorganisms, which could influence LXRs and FXR. In the recent two decades (2000–2020), 261 natural products were discovered from natural resources such as LXRs/FXR modulators, 109 agonists and 38 antagonists targeting LXRs, and 72 agonists and 55 antagonists targeting FXR. The docking evaluation of desired natural products targeted LXRs/FXR is finally discussed. This comprehensive overview will provide a reference for future study of novel LXRs and FXR agonists and antagonists to target human diseases, and attract an increasing number of professional scholars majoring in pharmacy and biology with more in-depth discussion.
1 Introduction
Nuclear receptors (NRs), a superfamily of transcription factors incorporating a group of 48 members in humans and 49 in mice (Gronemeyer et al., 2004; Zhang et al., 2004), are integrators of hormonal and nutritional signals, mediating changes to metabolic pathways within the body (Calkin and Tontonoz, 2012). NRs comprise seven subfamilies, namely, thyroid hormone receptor-like (NR1), retinoid X receptor-like (NR2), estrogen receptor-like (NR3), nerve growth factor IB-like (NR4), steroidogenic factor-like (NR5), germ cell nuclear factor-like (NR6), miscellaneous (NR0), etc. (Zhang et al., 2020c). From N-terminus to C-terminus, NRs share common structure features and are composed of an activation function-1 (AF-1) domain, a DNA-binding domain (DBD), a ligand-binding domain (LBD), a ligand-dependent activation function-2 (AF-2) domain, as well as a hinge region linking the DBD and the LBD (Figure 1) (El-Gendy et al., 2018). NRs activate or repress genes expression required for virtually all aspects of development, reproduction, cell growth, metabolism, immunity, and inflammation by binding with regulatory regions of target genes and acting in concert with coactivators and corepressors. The activity of a large subgroup of NRs depends on small lipophilic, membrane-permeable ligands binding to the LBD, such as fatty acids, oxysterols, and bile acids, functioning as important regulators and ideal drug targets in metabolic syndrome-related diseases for drug discovery and development (Lonard and O’Malley, 2007; McKenna et al., 2009; Hollman et al., 2012).
Among the representative NRs, the liver X receptors (LXRs) were identified as an orphan nuclear receptor in the mid-1990s (Willy et al., 1995; Niesor et al., 2001) and have two isoforms: LXRα (NR1H3, initially named OR-1) and LXRβ (NR1H2, initially named NER and UR) (Shinar et al., 1994; El-Gendy et al., 2018). In humans, LXRα consists of 447 amino acids and is highly expressed in the liver, whereas LXRβ consists of 460 amino acids and is expressed ubiquitously in most tissues and organs (Apfel et al., 1994; Shinar et al., 1994; Willy et al., 1995; Lu et al., 2001; Chinetti-Gbaguidi and Staels, 2009). Both LXRα and LXRβ form obligate heterodimers with 9-cis retinoic acid receptor RXR (retinoid X receptor, NR2B1), and thus, LXRs/RXR might be activated by ligands for either LXRs or RXR, such as endogenous oxysterols for LXRs, which are considered as “permissive” (Chinetti-Gbaguidi et al., El-Gendy et al., 2018). As cholesterol sensors, LXRs have attracted sustaining attention in participating in the regulation of cholesterol, fatty acid, and glucose metabolism, inflammation, and immunity (Janowski et al., 1996; Jakobsson et al., 2012).
The farnesoid X receptor (FXR, NR1H4) identified in 1995 has been known as a member of NRs and a xenobiotic receptor (Niesor et al., 2001; Wang D. et al., 2018). FXR was originally named on the basis of the observation that farnesol and related derivatives possessed the potency of activating FXR (Calkin and Tontonoz, 2012; Zhang J. et al., 2020). In the subsequent years, a series of natural cholesterol metabolites including bile acids were identified as endogenous ligands, meaning that FXR is more appropriately classified as a nuclear bile acid (BA) receptor (Parks et al., 1999; Zhang T. et al., 2020). In accordance with its function as chief sensor of BA, FXR is most abundantly expressed in the liver, intestine, kidneys, and adrenal glands, thus, playing a pivotal role in the regulation of BA metabolism (Lefebvre et al., 2009; Chen et al., 2011). Furthermore, FXR has also been reported to exhibit the regulatory potential of several other physiological processes such as glucose and lipid metabolism (Forman et al., 1995; Parks et al., 1999). Similarly, FXR also forms obligate heterodimers with 9-cis retinoic acid receptor RXR like LXRs. FXR exists as two types of encoded genes, namely, FXRα and FXRβ, although the latter is a pseudogene. Hence, for the purpose of this review, FXRα will be simplified as FXR (Modica et al., 2010).
Natural products are a promising source for bioactive agents and lead compounds for new drug research (Hiebl et al., 2018; Wang D. et al., 2020), which not only include ethnopharmacologically used compounds from plants or herbals but also terrestrial or marine-derived microbial metabolites (Yang et al., 2014). There are plenty of bioactive components in traditional Chinese medicines (TCMs) that might serve as selective ligands for their respective receptors (Qiu, 2007; Cragg and Newman, 2013; Li et al., 2015). Furthermore, the oceans, with their unique environment and huge biodiversity, have the potential as a plentiful source of diverse natural products accompanying pharmacological activities (Carazo et al., 2019). In comparison with synthetic and combinatorial compounds, natural products display a great structural and chemical diversity (Jones et al., 2006; Shen 2015). Beyond that, they cover a large range of biodiversity and profuse functionality because of the capacity of interacting with multiple proteins or other biological targets (Wink, 2003; Jones et al., 2006; Hiebl et al., 2018).
However, there are two main drawbacks to the development of natural products, one of which is that natural products are often in limited supply. Another limitation is the difficulty of separation of trace active compounds and elucidation of unknown compounds (Yang et al., 2014). To date, several technological advances have helped to overcome the above drawbacks, particularly in the developments of high-performance liquid chromatography–electrospray ionization mass spectrometry (HPLC-ESI-MS) and high-resolution nuclear magnetic resonance (NMR) technologies, facilitating the identification and structure elucidation of unknown compounds (Strege, 1999; Koehn and Carter, 2005). Additionally, advances in other subjects, such as metabolic engineering, microbial cultivation, as well as genetic methods, might solve the supply of natural products and contribute to the development of natural product-derived drugs (Ling et al., 2015; Shen, 2015).
This review focuses on natural products targeting LXRs/FXR in the recent two decades (2000–2020). For a brief background of LXRs/FXR, we refer to several recent reviews and give comprehensive overviews regarding this topic, and the docking evaluation of desired natural products targeting LXRs/FXR is finally discussed.
2 Physiological functions of liver X receptors and farnesoid X receptor
2.1 The role of liver X receptors and farnesoid X receptor in metabolic processes
2.1.1 Cholesterol and lipid metabolism
A stringent control of systemic and cellular cholesterol levels is essential to physiological homeostasis (Bonamassa and Moschetta, 2013; Hong and Tontonoz, 2014). LXRs sense excess cholesterol and trigger various adaptive mechanisms protecting the cells from cholesterol overload. Activation of LXRs results in reverse cholesterol transport (RCT), inhibition of intestinal cholesterol absorption, and suppression of cholesterol synthesis and uptake by the cells (Beltowski, 2008).
ATP-binding cassette transporters A1 (ABCA1) is one of the earliest identified LXR target genes and one of the most highly regulated LXRs targets, required for the ability of LXRs agonists to stimulate cholesterol efflux to apolipoprotein AI (APOAI) (Ishibashi et al., 2013) acceptors. Another transporter ATP-binding cassette transporters G1 (ABCG1) that promotes cholesterol efflux from macrophages is also an LXR target gene (Tarling and Edwards, 2011). RCT is the stimulation of cholesterol removal from the cell, along with transport to the liver, and biliary excretion (Beltowski, 2008). In this process, LXRs upregulate the expression of transporters involved in cholesterol removal from plasma membrane to extracellular acceptors, namely the ABCA1 and ABCG1 (Cavelier et al., 2006), thus, induce cholesterol mobilization from the plasma membrane of nonhepatocyte cell types, cause the formation of high density lipoprotein (HDL) or apolipoproteins, reduce cholesterol expression in the cell membrane, and disrupt lipid rafts formation (Repa et al., 2000; Bradley et al., 2007). LXRs are also involved in the regulation of intracellular cholesterol traffic. LXRs agonists increase the expression of Niemann–Pick C1 (NPC1) and C2 (NPC2) proteins, two carriers mediating transportation from the endosomal compartment to the plasma membrane before efflux, which result in stimulating redistribution of cholesterol from the endosomal compartment to the plasma membrane where it becomes available for efflux to extracellular acceptors (Castrillo et al., 2003; Duval et al., 2006).
In terms of the indispensable interrelation between the intestinal absorption and the regulation of cholesterol levels within the body, LXRs agonists have been verified to stimulate cholesterol recycling from the enterocyte to the intestinal lumen by upregulating ATP-binding cassette transporters G5 (ABCG5) and ATP-binding cassette transporters G8 (ABCG8) (Yu et al., 2003). Additionally, Niemann–Pick C1-like 1 (NPC1L1) protein contained in the apical membranes of enterocytes and attenuated by LXR activation endows a paramount role in intestinal cholesterol absorption (Wang, 2007).
Collectively, cellular and systemic cholesterol homeostasis are maintained by the coordinated actions of sterol-regulatory element-binding proteins (SREBPs) and LXRs. SREBPs are activated in response to low cellular cholesterol levels, whereas LXRs are activated by elevated cholesterol levels (Calkin and Tontonoz, 2012).
FXR activation not only inhibits the uptake and conversion to bile acids of cholesterol, as well as impact the synthesis and excretion of cholesterol, but also promote the expression of hepatic scavenger receptors leading to the enhanced RCT (Neuschwander-Tetri et al., 2015). Growing lines of evidence indicate that FXR activation is an attractive approach for the regulation of lipid homeostasis. FXR-null mice are featured with hypertriglyceridemia, hypercholesterolemia, and growing intestinal cholesterol absorption, in close association with increase in HDL cholesterol and lipoprotein lipase activity, the generation and characterization of which are a breakthrough for uncovering and verifying the importance of FXR action for lipid metabolism (Sinal et al., 2000; Lambert et al., 2003). LXRs regulate the expression of proteins involved in lipid remodeling. The gene cluster of apolipoproteins E, C1, C2, and C4 (APOE, APOC1, APOC2, and APOC4), belonging to LXRs target genes, are implicated in lipid transport and catabolism (Hiebl et al., 2018). Also, FXR has been demonstrated to possess the ability of regulating LXRs-mediated lipogenesis (Han et al., 2016).
2.1.2 Bile acid metabolism
BAs, the end products of cholesterol catabolism in the liver (Fiorucci et al., 2012a), are amphipathic molecules with a steroidal moiety derived from cholesterol (Han et al., 2016). FXR, widely expressing in nonclassical BA target tissues (Wang et al., 2018b), plays a critical role in maintaining BAs homeostasis by controlling their synthesis, transport, and metabolism (Sinal et al., 2000). Take for instance FXR. It protects the liver from the excess BAs by promoting excretion and preventing synthesis and uptake (Jian et al., 2014). Recent researches have demonstrated that BAs-activated FXR decreases BAs de novo synthesis in the liver, increases BAs secretion into the small intestine, promotes BA intestinal reabsorption, and inhibits hepatic basolateral BAs reuptake (Gadaleta et al., 2015).
The classic BA synthetic pathway, initiated by the first and rate-limiting enzyme converting cholesterol into BA, cholesterol 7α-hydroxylase (CYP7A1), is considered as the major BA biosynthetic pathway in humans (Russell, 2003). Likewise, FXR activation induces intestinal epithelial expression of fibroblast growth factor 19 (FGF19, also known as FGF15 in rodents), which suppresses BA synthesis by inhibiting CYP7A1 (Inagaki et al., 2005). FXR primarily controls BA synthesis via activating small heterodimer partner (SHP) to inhibit the expression of LXRs, which further suppresses the transcription of CYP7A1 (De Fabiani et al., 2001). As has been stated, CYP7A1 contains a responsive element for the LXRs/RXR heterodimer, proposing that LXRs also might regulate the BA synthetic pathways to a certain degree (Li et al., 2019).
2.1.3 Glucose metabolism
Given the interdependence of lipid and carbohydrate metabolism, it is not surprising that the LXR signaling has affected glucose homeostasis and insulin sensitivity (Goodwin et al., 2008). Glucose transporter type 4 (GLUT4), an insulin-responsive glucose transporter expressed primarily in adipose tissues and skeletal muscle, is usually inhibited in diabetic patients and diabetic mice models. Several studies have illuminated that the expression of GLUT4 in some mice models could be regulated throughout direct interaction with LXR response elements (LXREs) (Mi et al., 2003; Goodwin et al., 2008). Indeed, phosphoenolpyruvate carboxy kinase (PEPCK) and glucose-6-phosphatase (G6P), involved in hepatic gluconeogenesis, are dramatically inhibited in insulin-resistant rats administrated with LXR agonists, resulting in decreasing hepatic glucose, insulin sensitivity, and plasma glucose (Cao et al., 2003).
Another unanticipated area of intense study that recently arose is that FXR are currently under clinical investigation for the pleiotropic role to manage glucose homeostasis. Activation of hepatic FXR decreases plasma glucose levels, downregulates the gluconeogenic pathway, and alters the transcription, either indirectly or directly, of several genes that govern gluconeogenesis and glycolysis. Nevertheless, the underlying mechanisms involved in glycemic response remain controversial.
2.2 Liver X receptors and farnesoid X receptor as therapeutic target
The fact that LXRs and FXR play a pivotal role in metabolism homeostasis is of relevance for drug research, the functions of which are evidenced in many pathological conditions as illustrated in Figures 2 and 3.
2.2.1 Diabetic mellitus and obesity
It has been covered that human LXRs genes possess potential connections between obesity and diabetes with the advent of genome-wide association studies (Calkin and Tontonoz, 2012). LXR activation promotes triglyceride (TG) accumulation in skeletal muscle cells, probably through the induction of the expression of lipogenic enzymes. Furthermore, LXR agonist treatment improves glucose tolerance in diet-induced diabetic models, involved in glucose metabolism as a potential approach for the treatment of type 2 diabetes (T2D) characterized by high-blood glucose and insulin resistance (Huang, 2014).
The observation that FXR activation results in insulin substrate receptor 1 (IRS-1) phosphorylation on the tyrosine residues in the liver and adipose tissue manifests a prospect application of FXR agonists for the management of patients with insulin resistance to improve insulin sensitivity, indicating that FXR agonists are suitable for T2D (Gadaleta et al., 2015; Han et al., 2016). Intestinal activation of FXR reduces diet-induced weight gain, hepatic glucose production, and steatosis. Meanwhile, it strongly stimulates FGF19 expression in the intestine, reverses high fat-induced diabetes, and enhances the metabolic rate while decreasing adiposity (Cave et al., 2016).
2.2.2 Cancer
LXRs inhibit the proliferation of multiple cancer cell lines from the liver, lung, skin melanoma, prostate, breast, cervical, epidermis, bone, and squamous carcinoma, as well as leukemia T cells (El-Gendy et al., 2018). LXR ligands have shown antiproliferative effects on different cancer cell types, altogether suggesting a ubiquitous and global effect of LXRs on proliferation and apoptosis, not only on cells with a tumor origin (Jakobsson et al., 2012; Boussac et al., 2013).
According to accumulating bibliographic data, FXR may prevent intestinal and hepatocellular tumorigenesis (Gadaleta et al., 2015; Masaoutis and Theocharis, 2018). Emerging evidence supports that FXR seems to possess various antioncogenic and less common prooncogenic attributes. In the case of the pancreas, elevated FXR expression seems to impart better prognosis to adenocarcinoma. In the case of breast cancer, immunohistochemical FXR positivity is also an independent favorable prognostic factor (Masaoutis and Theocharis, 2018).
2.2.3 Inflammation and immunity
Both LXRα and LXRβ are involved in regulating inflammatory responses since they are expressed in a variety of immune cells, and are involved in innate and adaptive immunity and inflammatory responses (Hong and Tontonoz 2008), the activation of which inhibit proinflammatory cytokine production in macrophages derived from wild-type mice rather than from LXRα- and LXRβ-knockout mice (Cave et al., 2016). Moreover, FXR is downregulated in a variety of disease states accompanying inflammation, such as fibrosis, cirrhosis, cardiovascular inflammatory, and cancer (Han et al., 2016). For the therapy of nonalcoholic steatohepatitis (NASH), chronic hepatitis B virus (HBV) infection (Wang et al., 2018c), and inflammatory bowel disease (IBD), FXR has provided a novel target.
Most of the studies conducted to find LXR modulators possessing therapeutic utility have been directed toward LXR agonists. However, the elevation of plasma TG and hepatic steatosis caused by LXRs in the liver, as a major drawback, has impeded its development into commercial drugs. To overcome this undesirable effect, new strategies have been put forward such as developing inverse agonists, LXRβ-selective agonists, and tissue-selective agonists (El-Gendy et al., 2018).
Based on emerging evidence that FXR activation by agonists treatment has a beneficial effect on the treatment of various diseases in animal models, some FXR agonists have been imported into clinical studies for its value as medicinal drugs (Han et al., 2016). At present, some FXR ligands impacted in cholestasis, T2D and metabolic syndrome, nonalcoholic fatty liver disease (NAFLD) or NASH, and primary BA diarrhea are measured in phases I and II clinical trials. Although there is therapeutic benefit of FXR ligands, the risks of various ligands, particularly on the intestines or kidneys, require profound investigation and cautious consideration (Hollman et al., 2012).
3 Natural products targeting liver X receptors
In this section, natural products regulating LXRs expression in different models are outlined. We categorized and summarized these natural products in Supplementary Tables S1 and S2.
3.1 Natural agonists targeting liver X receptors
Oxysterols, oxygenated derivatives of cholesterol, have been identified as the endogenous agonists of LXRs, including 22R-hydroxycholesterol, 24S,25-epoxycholesterol, 24S-hydroxycholesterol (Janowski et al., 1996; Forman et al., 1997; Lehmann et al., 1997; Huang, 2014), 20S-hydroxycholesterol, 25-hydroxycholesterol, and 27-hydroxycholesterol (Huang, 2014; Schroepfer et al., 2000). These oxysterols possess the ability of activating both LXRα and LXRβ. Interestingly, an endogenous ligand, 5α,6α-epoxycholesterol (Janowski et al., 1996, Lehmann et al., 1997; Hiebl et al., 2018) could act as either agonist, inverse agonist, or antagonist depending on the setting. In this review, these endogenous active ingredients are not listed as natural products targeting LXRs.
3.1.1 Terpenes
Geraniol (1), a major constituent of essential oils from aromatic plants, including Cinnamomum tenuipilum Kosterm (Lauraceae), Valeriana officinalis L. (Caprifoliaceae), and Panax notoginseng (Burk.) F.H. Chen, leads to the activation of LXRα and FXR, as assessed in the hyperlipidemic rats, which induced significant and dose-dependent decrease of serum total cholesterol (TC), TG, and low-density lipoprotein cholesterol in hyperlipidemic rats (Ji and Gong, 2007; Galle et al., 2015; Lei et al., 2019). Cineole (2), also named cajeputol, is a monoterpene and a principal constituent of Mentha longifolia (L.) L., most eucalyptus oils, teas, rosemary, Psidium, and many other essential oils. Treatment with 2 induced remarkable increase in target genes associated with LXRs. It displayed decreased cellular lipid accumulation and reduced cholesterol levels, as evaluated via Oil Red O lipid staining and cholesterol quantification, suggesting practical implications for the development of hypercholesterolemia and atherosclerosis because it not only reduced cholesterol accumulation but also prevented the potential side-effect hepatic steatosis (Jun et al., 2013). Triterpene squalene (3), obtained from dried biomass of Schizochytrium mangrovei PQ6, was presented in nutrition, health care, cosmetics, and medicine. A recent study revealed that 3 significantly regulated target genes associated with RCT via stimulating the transactivation of LXRα/β, further confirmed as a potent pharmaceutical agent for the treatment of atherosclerosis and hypercholesterolemia, and the prevention of the potential side effect of hepatic steatosis as well (Hien et al., 2017).
A pterosin sesquiterpenoid named (2R,3S)-5-hydroxymethylpterosin C (4), isolated from the traditional medicinal plant Pteris cretica L., activated LXRα/β in murine 3T3-L1 adipocytes, and exhibited lipid-lowering effect (Luo et al., 2016). Three diterpenes DTP 1 (5), DTP 3 (6), and DTP 5 (7), widespread in plants and insects, are active compounds from traditional folk medicine Scoparia dulcis L. for the treatment of inflammation and cancer (Cuadrado et al., 2011; Hu et al., 2018). In RAW cells, gene expression analysis demonstrated that 5–7 could activate either LXRα or LXRβ. Moreover, 5–7 strongly induced the expression of established LXR target genes in macrophages and promoted macrophage cholesterol efflux (Traves et al., 2007).
Two new lanostanoid triterpenes, (3β, 24Z)-3,27-dihydroxy-lanosta-8, 24-dien-1-one (8) and (3β, 23S)-3,23-dihydroxy-7,9(11),24-lanostane-triene (9), from the fungus Rigidoporus microporus collected in the rubber tree, activated both LXRα and LXRβ. More detailed data revealed that 8 and 9 were dual LXRα/β agonists at 10 µM using a luciferase reporter assay in HEK293T cells (Rincón et al., 2020). TCM plant Gynostemma pentaphyllum (Thunb.) Makino has been consumed as tea for numerous beneficial effects, for instance, to treat cough and chronic bronchitis. Gynosaponin TR1 (10), from the aerial parts of G. pentaphyllum (Thunb.) Makino, selectively enhanced LXR-mediated transcription activation in HEK293 cells as an LXRα agonist. Notably, 10 possessed higher selectivity for LXRα than LXRβ (Ky et al., 2010; Zhang Y. et al., 2020). Two terpenes, (−)-acanthoic acid (11) isolated from Rollinia pittieri Saff. and R. exsucca (DC.) A.DC., along with polycarpol (12) isolated from Unonopsis glaucopetala R.E.Fr. and Minquartia guianensis Aubl., showed potent binding affinity for LXRα with IC50 of 0.25 and 0.12 µM, respectively. Amazingly, 11 and 12 seemed to be the better LXRα agonists than natural oxysterols, such as 22R-hydroxycholesterol and 24S,25-epoxycholesterol (Jayasuriya et al., 2005). Paeonia lactiflora Pall. is mainly found in Taiwan and frequently used for the treatment of inflammation, hyperlipidemia, and hyperglycemia, a vital natural monoterpene, which is paeoniflorin (13). In a study using mammalian one-hybrid assay with the specificity for LXRα transactivation, 13 transactivated LXRα at 10 mM and was further confirmed as a LXRα agonist (Lin H.-R., 2013).
Ganoderma capense has been documented to possess various pharmacological activities, including defending malignant tumors and hepatitis. Ganocalidin A (14), from the fruiting bodies of G. capense, could increase LXRα and reduced lipid accumulation as an LXRα agonist (Liao et al., 2019). Ganoboninone E (15) and ganoboninketals A-C (16–18), dominating compounds of the fruiting bodies of G. boninense, exhibited remarkable agonistic activity to the transactivation of LXRβ (Ma et al., 2015; Abdullah et al., 2020). Tripterygium wilfordii Hook. f. has been used as a traditional medicine for multiple pharmacological activities, including anticancer and anti-inflammatory effects. A major ingredient triptolide (19) from T. wilfordii Hook. f., influenced either LXRα or FXR through increasing the LXRα protein expression and suppressing FXR protein expression simultaneously, while the clinical application of 19 is greatly constrained by hepatotoxicity (Jiang et al., 2016; Zou et al., 2020).
Tirotundin (20) and tagitinin A (21) from Tithonia diversifolia (Hemsl.) A. Gray, which are used to treat diabetes and hepatoprotection, also act as LXRα/β and FXR agonists (Lin HR., 2013). Platycodin D (22), a triterpene saponin from Platycodon grandiflorum (Jacq.) A.DC., suppressed the generation of proinflammatory cytokine and subsequently inhibited LPS-induced inflammatory response, due to the upregulation of LXRα expression, which is considered as a potential therapeutic drug for mastitis. It exhibited that 22 was capable of attenuating NL-induced inflammatory response in osteoarthritis chondrocyte via LXRα activation (Qu et al., 2016; Wang L. et al., 2017). Saikosaponin A (23), a well-known bioactive component of Radix bupleuri, induced cholesterol efflux from lipid rafts through LXRα–ABCA1 signaling pathway, and knockdown of LXRα led to the abrogation of the antiinflammatory effect of 23, suggesting a potency of attenuating oxidative and inflammatory responses (Fu et al., 2015). Viperidone (24), a major ingredient of Leptocereus quadricostatus (Bello) Britton & Rose, selectively activated LXRα, and its derivatives also owned the same activity (Jayasuriya et al., 2005; Komati et al., 2017).
A highly valued TCM Panax ginseng C.A.Mey. possesses the efficacy of promoting blood circulation and accelerating metabolism and digestion. A ginsenoside, 25-OCH3-PPD (25) from P. ginseng C.A.Mey., proved to ameliorate P2X7 receptor-mediated NLRP3 (nucleotide-binding oligomerization domain, leucine-rich repeat, and pyrin domain-containing 3) inflammasome and hepatic fibrosis through inducing LXRα/β pathway in the development of thioacetamide-stimulated hepatic fibrosis mice (Han et al., 2018). Another study indicated that ginsenosides play an important role in cholesterol metabolism since ginsenosides Ro (26), Rg3 (27), Re (28), Rg1 (29), and Rg2 (30) increased LXRα mRNA levels, followed by the upregulation of CYP7A (Kawase et al., 2012). P. notoginseng (Burk.) F.H. Chen has been used as a natural remedy in traditional medicine for the treatment of cardiovascular diseases. Terpenes from P. notoginseng (Burk.) F.H. Chen, notoginsenoside R1 (31) (Jia et al., 2010; Fan et al., 2012) and ginsenoside CK (32) (Zhang et al., 2020c), could significantly upregulate LXRα functioning as LXRα agonists.
3.1.2 Flavonoids
Cyanidin (33), a very widely distributed flavonoid present in fruits and vegetables, possesses the function of regulating cellular lipid metabolism. It was reported that 33 accelerated LXRα and LXRβ transactivation and induced the recruitment of coactivator peptide with EC50 3.5 and 125.2 μM, respectively. Meanwhile, surface plasmon resonance (SPR) assay demonstrated that 33 directly associated with both LXRα/β with the dissociation equilibrium constant (KD) of 33 to LXRα at 2.16 μM and LXRβ at 73.2 μM, respectively (Jia et al., 2013). Another common flavonoid, hesperetin (34), increased ABCA1 promoter and LXRα enhancer activities, indicating the function of promoting cholesterol efflux (Iio et al., 2012a). Chrysin (35), present in honey, propolis, and plant extracts, significantly enhanced cholesterol efflux and promoted the mRNA level of a set of nuclear receptors including PPAR (peroxisome proliferator-activated receptor) γ, LXRα, ABCA1, and ABCG1 via upregulating the classical PPARγ–LXRα–ABCA1/ABCG1 pathway (Wang et al., 2015). Daidzein (36), widely existing in soybeans, is an indirect modifier of LXRs as it reduced the expression of SREBP-1c through suppressing LXRα, whereas it increased the expression of ABCG8 via activating LXRβ indirectly. Another bioactive flavonoid genistein (37) present in soybeans also was reported to inhibit LXRα activation induced by a synthetic agonist T0901317 while stimulating LXRβ activation. Besides, 37 might prevent NAFLD via the regulation of visceral adipocyte metabolism and adipocytokines (Kim et al., 2010; Gonzalez-Granillo et al., 2012). Naringenin (38), widespread in grapefruits, oranges, and tomatoes, acts as a modulator of LXRα activity. It activated and upregulated LXRα and associated target genes in an AMPK (adenosine 5′-monophosphate-activated protein kinase)-dependent manner, further preventing the deterioration of atherosclerosis and foam cell progression (Wolkow et al., 2010; Saenz et al., 2018). Persimmon tannin is isolated from Diospyros kaki L., also known as proanthocyanidins. In a recent study using high-cholesterol diet mice model, persimmon tannin stimulated the expressions of LXRα, PPARα, and PPARγ, and simultaneously, it promoted their associated downstream gene expressions, further accelerating macrophage reverse cholesterol transport (Ge et al., 2017).
Quercetin (39), the pivotal aglycone in Medicago sativa L., elevated protein levels of LXRα and PPARγ in THP-1cells. Additionally, it increased cholesterol efflux from THP-1 macrophages and lowered the risk of atherosclerosis. A marked antihepatitis C virus (HCV) activity was exhibited by 39 in replicon-containing cells when combined with interferon α, appearing to be an effective inhibitor of HCV replication (Lee et al., 2013; Pisonero-Vaquero et al., 2014; Ren et al., 2018). Iristectorigenin B (40) derived from Belamcanda chinensis, used as a traditional medicine in East Asia for its inflammation property, increased ABCA1 and ABCG1 gene expression function as an agonist for both LXRα and LXRβ significantly. It also stimulated cholesterol efflux in macrophages without inducing hepatic steatosis, providing insights for treating hypercholesterolemia and atherosclerosis (Jun et al., 2012). A flavonoid that is very widespread in food plants like Phaseolus vulgaris L. and in medicinal plants like Cornus alternifolia L. f. (Cornaceae) is kaempferol-3-O-β-D-glucopyranoside (41), as an LXRα/β dual agonist. It exhibited potent LXRα/β agonistic activity with EC50 values of 1.8 μM (Dong et al., 2007; He et al., 2012). Interestingly, its aglycone kaempferol (42) selectively activated LXRβ, further suppressing SREBP-1 to regulate metabolic syndrome (Hoang et al., 2015). Formononetin (43), an isoflavone constituent from Brazilian red propolis, enhanced LXR transcription and promoted ABCG1 activity in THP-1 macrophages, indicating agonistic function of both LXRα and LXRβ (Daugsch et al., 2008; Iio et al., 2012b). Nelumbo nucifera Gaertn., usually regarded as tea in Japan, has been used in traditional medicine; the leaves of Nelumbo nucifera Gaertn. contains quercetin glycosides, especially quercetin-3-O-glucuronide (44). It was proven to increase ABCA1 expression via activating LXRα, providing a potential direction on arteriosclerosis prevention (Ohara et al., 2013). Rhus verniciflua Stokes is used in TCM for the therapy of gastritis, stomach cancer, and atherosclerosis. One of the effective compounds, butein (45), increased CYP7A1 luciferase activity in AML 12 cells via activating LXRα dependently and upregulating the transcriptional expression level of LXRα, which was borne out using the knockdown of LXRα (Jeong et al., 2015). Scutellarein (46), widely found in Erigeron breviscapus (vant.), is a traditional agent for the treatment of inflammation and obesity. Cholesterol output was reduced by 46 by activating the PPARγ–LXRα–ABCA1 pathway, suggesting a potential function in the cholesterol metabolism. Beyond that, treatment with 46 attenuated high-fat diet (HFD)-induced obesity and the associated diseases as it reduced the body weight, inflammatory state, and visceral index as well as improved hyperlipidemia and hepatic dysfunction in C57 mice fed on the HFD-developed obesity (Lin et al., 2019). Puerarin (47), obtained from Radix Pueraria or Pueraria lobate, attenuated acute lung injury via activating LXRα and suppressed LPS-induced inflammatory response (Li et al., 2017; Wang et al., 2018d). Isoliquiritigenin (48), isolated from Glycyrrhizae species, could promote the activation of LXRα and repressed LXRα-dependent hepatic steatosis, further protecting hepatocytes against oxidative injury inflicted caused by fat accumulation (Kim YM. et al., 2010).
The n-butanol fraction isolated from Zanthoxylum bungeanum Maxim., widespread in China as an edible aroma and a traditional medicine, mainly including rutin (49) and hyperin (50), was demonstrated to regulate the lipid metabolism in apolipoprotein E knockout mice via promoting the expression of genes involved in RCT, such as CYP27A1, LXRα, and ABCG1 (Wu et al., 2015). SPF1 (51) and SPF2 (52), two flavonoids derived from the root of Sophora tonkinensis Gagnep. induced the ABCA1 protein in RAW264.7 cells. A more detailed investigation revealed their neuroprotective effect by activating RXR/LXRs heterodimers as a novel approach to the treatment or prevention of Alzheimer’s disease (Inoue et al., 2014; Wang et al., 2019). A recent study using APOE−/− mice model, kuwanon G (53) isolated from the root bark of Morus alba L., significantly decreased intracellular lipid accumulation as well as inflammatory cytokines through activating the LXRα–ABCA1/ABCG1 pathway, which upregulated cholesterol efflux-related proteins, ABCA1 and ABCG1, in an LXRα-dependent way (Liu et al., 2018).
3.1.3 Alkaloids
Tetramethylpyrazine (54), an alkaloid diffusely widespread in foods like fermented Japanese food natto and Chinese black vinegar, also present in medicinal plant like Ligusticum chuanxiong, could directly regulate the expression of PPAR and LXRα gene by elevating the PPARγ–LXRα–ABCA1 pathway, indicating its potency of improving lipid profiles. In a small cohort of patients with pulmonary arterial hypertension or chronic thromboembolic pulmonary, the therapeutic effects of 54 were accompanied by inhibition of intracellular calcium homeostasis in rat distal pulmonary arterial smooth muscle cells as a novel and inexpensive medication for the treatment of pulmonary hypertension (Chen J. et al., 2017; Chen et al., 2020). Leonurine (55) present in Herba leonuri is traditionally used for therapy of gynecological disorders, dysmenorrhea, and menstrual disorders. In a study using Oil Red O staining and liquid scintillation counting assay, 55 mediated cholesterol efflux by promoting ABCA1/G1 induced by PPAR and LXRα, which inhibited lipid accumulation in THP-1 macrophage-derived foam cells via the PPAR and LXRα pathway (Jiang et al., 2017).
A fungus, Penicillium paxilli metabolite paxilline (56), was reported as an LXRα/β dual agonist. It activated LXRα and LXRβ with equivalent potency in HEK293 cells, subsequently leading to the expression of ABCA1 and SREBP (Bramlett et al., 2003). Oxepinamides D–G (57–60), four novel oxepin-containing pyrimidines derived from Aspergillus puniceus F02Z-1744, were revealed as LXRα selective agonists for their agonistic function of LXRα in chimeric receptor reporter gene assay with the EC50 values of 10.6, 12.8, 13.6, and 12.1 μM, respectively (Lu et al., 2011). Oxepinamides H–K (61–64) and four 4-quinazolinone alkaloids, puniceloids A–D (65–68) were obtained from the deep-sea fungus A. puniceus SCSIO z021. Compounds 61–68 remarkably activated LXRα with EC50 values of 1.7–50 μM, with 67 and 68 exhibiting the most potency (Liang et al., 2019). Scequinadoline D (69), an alkaloid derived from the marine fungus Scedosporium apiospermum F41-1, stimulated the mRNA expression of a series target genes, such as LXRα and PPARγ. It was found to facilitate TG accumulation with EC50 values of 0.27 ± 0.03 μM as a potent insulin sensitizer targeting adipocytes and a promising potential for the treatment of T2D (Li CJ. et al., 2020).
3.1.4 Steroids
Phytosterols, including β-sitosterol (70), campesterol (71), sitostanol (72), and YT-32 (73), are equivalent of mammalian cholesterols. Treatment with 70–73 increased the expression of LXRα/β target genes, further supporting subsequent research in intestinal cells (Komati et al., 2017). Two steroids, 24S-stigmast-5-ene-3β,24-ols (74) derived from Ficus pumila L. and 24S-stigmast-5,28-diene-3β,24-ols (75) isolated from genus Sargassum were proved to be selectively LXRβ agonists via luciferase assay with GAL-4 chimeric receptors. Meaningfully, their isomers also promoted the expression of LXR target genes, such as ABCA1 and SREBP1c, serving as LXR positive modulators (Castro Navas et al., 2018).
There have been several marine-derived steroids reported as ligands of LXRs. Fucosterol (76), a steroid widespread in marine algae, could promote the transactivation of both LXRα (+155% at 200 μM) and LXRβ (+83% at 200 μM) serving as an LXRα/β dual agonist, further indicating nutritional implications in hypercholesterolemia and atherosclerosis (Hoang et al., 2012a). 24S-Saringosterol (77) (maybe same as 75) from an edible seaweed Sargassum fusiforme, promoted the transactivation of LXRα/β, and stimulated LXRβ by 14.4-fold, higher than LXRα by 3.8-fold, acting as a selective LXRβ agonist, the discovery of which also further confirmed that phytosterols in Sargassum fusiforme contributed to the well-known antiarteriosclerosis. Beyond that, 77 was in the observed effects on cognition and Aβ plaque load as an attractive option for the treatment of neurodegenerative disorders such as AD (Chen P. et al., 2014; Bogie et al., 2019; Hannan et al., 2020). Present in marine fish and plant roots is 4-Cholesten-3-one (78), but it is also found in a red marine alga Laurencia papillosa. Compound 78 at 12.5 μM resulted in a remarkable enhancement of the mRNA expression of LXRβ as well as its associated target genes compared with untreated cells in monocytic cell line THP-1. To confirm this effect, LXRs inverse agonist and LXRβ knockdown were employed, which resulted in the neutralization. Further study showed that 78 decreased the viability of two breast cancer cell lines, namely MCF-7 and MDA-MB-231, exerting promising antibreast cancer activity by altering LXRs-dependent lipid metabolism in breast cancer cells without increasing lipogenesis (Elia et al., 2019). Gorgostane-3β,9α,5α,6β,11α-tetrol (79) and gorgost-5-ene-3β,9α,11α-triol (80) isolated from the Plexaura species possessed a better affinity with LXRα than LXRβ with EC50 values of 0.45 and 0.05 μM (Jayasuriya et al., 2005).
3.1.5 Phenol derivatives
Ethyl 2,4,6-trihydroxybenzoate (81, ETB) was derived from Celtis biondii Pamp., which is traditionally used in the therapy of cardiovascular disease. In a reporter gene assay by time-resolved fluorescence resonance energy transfer as well as SPR analysis, ETB (81) suppressed cellular cholesterol accumulation via activating LXRα/β-responsive transcriptional genes as a LXRα/β dual agonist (Hoang et al., 2012b). Paeonol (82), a phenol present in Paeonia suffruticosa, is significantly beneficial for various inflammatory diseases, especially atherosclerosis. Treatment with 82 markedly attenuated cholesterol accumulation and suppressed the formation of foam cells in macrophages and APOE−/− mice as it activated LXRα to promote LXRα-ABCA1-dependent cholesterol efflux, providing a novel explanation for the antiatherogenic action of 82 (Zhao et al., 2013). Resveratrol (83), widespread in red wine, berries, and peanuts, elevated LXRα together with the mRNA levels of associated target genes such as ABCA1 and ABCG1. A more detailed investigation revealed that ABCA1-mediated cholesterol efflux and intracellular cholesterol could be mediated by 83 via the PPARγ/LXRα pathway. Pathologic analysis of treatment with 83 (30 mg/kg/day) in HBVX protein transgenic mice showed a therapeutic effect on HBVX protein-induced fatty liver and the early stages of liver damage, considering it as a potential preventive agent for HBV-associated hepatocellular carcinoma (Sevov et al., 2006; Voloshyna et al., 2013). Danshensu (84), rosmarinic acid (85), salvianolic acid A (86), and salvianolic acid B (87), bioactive compounds present in Salvia miltiorrhiza Bunge used for the therapy of angina pectoris, myocardial infarction, and stroke, functioned as LXRα/FXR dual agonists via the transactivation assays and improved the lipid profiles in hyperlipidemic rats (Ji and Gong, 2008; Li et al., 2015). Methyl gallate (88) and ethyl gallate (89) were isolated from Talisia nervosa Radlk, widespread in the tropical moist and wet forest. The evaluation of LXRα transcriptional activity showed that 88 and 89 were 3.16- and 2.62-fold activation of LXRα at a concentration of 100 µg/ml, indicating their potential utility against metabolic syndrome (Vásqueza et al., 2019).
Riccardin C (90), a natural cyclic bibenzyl derivative found in Blasia pusilla L. and Reboulia hemisphaerica, interacts with LXRα as an agonist. 90 resulted in a 15-fold increase of the transactivation of the reporter gene at 30 μM. Moreover, the coactivator association and receptor-mediated transactivation assay demonstrated 90 as an LXRβ antagonist through its competition with the synthetic agonist TO-1317 (Tamehiro et al., 2005; Asakawa, 2008; Harada et al., 2013). Several natural ligands isolated from plant resin have also shown activities towards LXRs. For an instance, podocarpic acid (91), and its derivatives podocarpic acid imide (92), podocarpic acid anhydride (93), and podocarpic acid anhydride acetate (94), bound to both LXRα and LXRβ at 1–2 nM serving as LXRα/β dual agonists. Especially for 91, it was over eight-to 10-fold better at activation of LXRs compared with a natural LXRs agonist 22(R)-hydroxycholesterol (Singh et al., 2005; Komati et al., 2017).
The described biological activities of sesamol (95) found in sesame oil of Sesamum indicum L. mainly include antiinflammatory and antioxidative effects, protecting against hypertension, atherosclerosis, and aging. In a study using pGL3-TK-PPRE-X3-luciferase reporter assay, 95 prominently enhanced LXRα transcriptional activity for 2.6-fold at 100 μM (Periasamy et al., 2013; Majdalawieh and Ro, 2014). Magnolol (96), a natural lignin isolated from Magnolia officinalis Rehder & E.H.Wilson, has proved to activate LXRs and PPARγ target genes and inhibit the NF-κB (nuclear factor kappa-B) and mRNA expression of inflammatory cytokines under Aβ incubation in vitro studies to attenuate Aβ-induced AD (Xie et al., 2020). Honokiol (97), another lignin from M. officinalis Rehder & E.H.Wilson, activated LXRs transcriptional activity and increased its downstream gene ABCA1 expression. Interestingly, it also functions as a dual activator of LXRs/RXR heterodimer (Jung et al., 2010; Xie et al., 2020). Herniarin (98) is a 7-methoxycoumarin derivative found in the aerial part of Artemisia dracunculus L., which is traditionally used to alleviate the symptomatic pain of spasmodic colitis. A detailed research indicated that 98 could upregulate LXRα/β to inhibit the development of breast cancer in Sprague–Dawley rats (Talbi et al., 2016; Pattanayak and Bose, 2019).
Emodin (99) is widespread in the TCM such as Rheum officinale Baill., R. palmatum L., and Polygonam cuspidatum, with its biological activities that range from cardioprotective to antioxidant, anticancer, antibacterial, antifibrotic, and anti-inflammatory effects. Emodin (99) promoted cholesterol efflux from differentiated THP1 macrophages through activating the PPARγ/LXRs/ABCA1/ABCG1 signaling pathway. It prevented atherosclerosis in ApoE−/− mouse model, and in vitro evidence supported this effect commendably, highlighting the therapeutic potential in atherosclerosis (Luo et al., 2021). Six new octulosonic acid derivatives were obtained from the flower heads of Chamaemelum nobile L., while 100 and 101 among them exhibited an increase in LXRα activity at 30 μM (Zhao et al., 2014).
Our previous study revealed eight new ene-yne hydroquinones derived from marine fungus Pestalotiopsis neglecta SCSIO41403, one of them, pestalotioquinoside C (102), functioning as a potential LXRα agonist via upregulating the mRNA level of the target gene ABCA1. Using SPR assay, 102 interacted with LXRα dose-dependently with the KD of 50.0 μM (Wang F. et al., 2020). Further study discovered pestalotiochromones A (103) as a potential LXRs agonist, remarkably upregulated LXRβ and downstream gene ABCA1. Moreover, 103 combined well with LXRα in a dose-dependent manner, the kinetic curves of which resembled those of the potent LXRs agonist GW3965 for binding with LXRα, with KD of 6.2 μM (Liang et al., 2021).
3.1.6 Others
Betaine (104), a natural trimethyl glycine in common foods, including wheat products, spinach, pretzels, and shrimp, has been used for the therapy of NAFLD via upregulating hepatic expression of LXRα and PPARα, along with attenuating the changes in their associated target genes in fructose-induced rat models. 101 also ameliorated hepatic lipid accumulation, gluconeogenesis, and inflammation through a battery of determinations, further confirming potential mechanisms involved in the treatment of NAFLD (Ge et al., 2016; Chen Q. et al., 2017). Allicin (105) is an essential ingredient of garlic, responsible for its favor, and its pharmacological activities range from anti-inflammatory to antioxidative stress and antihypertensive activities. 105 has also been confirmed to attenuate inflammation via increasing the expression of LXRα in a dose-dependent manner (Zhang et al., 2017). Taurine (106), known as 2-aminoethanesulfonic acid is synthesized in the liver to a small extent, which is also isolated from seafood. Macrophage cells incubated with 106 inhibited cholesterol accumulation and regulated genes expression involved in RCT as an LXRα agonist (Hoang et al., 2012c).
5-Hydroxy-3-methoxy-5-methyl-4-bu-tylfuran-2(5H)-one (107), obtained from marine-derived fungus Setosphaeria sp. SCSIO41009, was reported as an LXRα agonist. It decreased ox-low-density lipoprotein-induced lipid accumulation and possessed a greater TG-lowering effect than the positive control T0901317 through targeting LXRα. Taken together, 107 displayed a weak cytotoxicity but had a powerful TC-lowering effect most likely through targeting PPARα, and it exhibited a potential application for the treatment of dyslipidemia (Li et al., 2020b). Setosphapyrone C (108) and D (109) derived from the same fungus, enhanced LXRα/ABC pathways to achieve a lipid-lowering effect, possessing potential application for the treatment of hyperlipidemia (Li et al., 2020c).
In addition, total jiaogulan saponins isolated from Gynostemma pentaphyllum were proven to upregulate LXRα and a series of target genes to increase BA and cholesterol excretions (Liu et al., 2016).
3.2 Natural antagonists targeting liver X receptors
Polyunsaturated fatty acids (PUFAs) are the dominating source of endogenous LXR antagonists, especially for arachidonic acid identified as a dual antagonist as interacting directly with LXRα and LXRβ, which also could be isolated from traditional medicine Acanthopanax koreanum Nakai. (Yoshikawa et al., 2002; Kuang et al., 2012). Prostaglandin F2α, one of the essential metabolites of compound arachidonic acid, has also been verified as a dual antagonist. Notably, prostaglandin F2α could function as an LXRs/RXR heterodimer antagonist (Zhuang et al., 2013). In addition, endogenous antagonist ursodeoxycholic acid, a key secondary BA, has been substantiated to inhibit LXRα-induced lipogenic gene expression as a negative modulator of LXRα signaling (Lee et al., 2014).
3.2.1 Terpenes
Iridoid (110) functions as a predominant compound of Valeriana jatamansi Jones. It has been shown that treatment with 110 resulted in the decrease of lipid biochemical indexes in hyperlipidemic rats by decreasing the expressions of SREBP-1c and LXRα (Zhu et al., 2016). Lucidone (111), a natural occurring terpene derivative present in a folk medicine Lindera erythrocarpa Makino, has been found to inhibit adipogenesis in 3T3-L1 cells by decreasing adipogenic genes transcription levels, including LXRα, indicating that it is a nutraceutical to guard against obesity and subsequent metabolic disorders (Hsieh and Wang 2013; Wong et al., 2014). Two sesquiterpenoids named paraconiothins C (112) and I (113), isolated from the endophytic fungus Paraconiothyrium brasiliense ECN258, were proven to inhibit LXRα at 50 μM (Nakashima et al., 2019).
Ursolic acid (114) is a common pentacyclic triterpenoid in many plants, such as Cornus officinalis Siebold & Zucc., and the described pharmacological activities range from anticancer to antioxidant, antiangiogenic, anti-inflammatory, immunoregulatory, hypolipidemic, and hepatoprotective effects. It was demonstrated to be an LXRα antagonist and displays efficacy in treating NAFLD as it significantly decreased TC accumulation and induced steatosis at 20 μM through modulation of LXRα, transcription factor SREBP-1c, and a battery of downstream target genes (Lin et al., 2018). Potentilla chinensis Ser. found in oriental countries, has been traditionally used in the therapy of immune disorders and liver diseases. Asiatic acid (115), a pivotal constituent isolated from P. chinensis Ser., significantly regulated the key factors associated with lipid metabolism including SREBP-1c and LXRα to restrain the production of hepatocyte lipogenesis. A further study elucidated that 115 effectively alleviated hepatic steatosis and hepatocyte damage, attributed to its ability to alleviate oxidative stress, recruit the antioxidative defense system, inhibit the NF-κB pathway, alleviate hepatocyte apoptosis, and lipid metabolism disorder and, thereby, for the treatment of NAFLD (Wang et al., 2018a). A clerodane diterpenoid borapetoside E (116), derived from Tinospora crispa (L.) Hook. f. & Thomson, which is used in traditional medicine for the therapy of diabetes and other diseases, suppressed the mRNA expressions of SREBP and LXRα in the liver, serving a potential therapy for diet-induced T2D. It improved hyperglycemia and oral glucose tolerance, promoted insulin signaling, improved insulin resistance, and improved lipid levels, etc., in high-fat diet-induced obese mice, these beneficial effects in vivo further demonstrated the promising therapy (Xu W. et al., 2017). A TCM Panax ginseng C. A. Meyer has been regarded as a panacea for centuries in the treatment of various diseases such as metabolic disorders. 20S-Protopanaxatriol (117) is present in the root of this plant encompassing antiinflammatory and antioxidative stress bioactive activities. A synthetic agonist T0901317-dependent LXRα target genes and T0901317-induced TG accumulation were inhibited by 117 in primary hepatocytes, further suggesting it as a potential antilipogenic agent to treat NAFLD (Oh et al., 2015a).
3.2.2 Flavonoids
Luteolin (118), occurring in a broad range of vegetables, fruits, and grains like carrots, peppers, celery, parsley, and spinach, is a common dietary flavonoid exerting numerous biological activities including antioxidant, anticancer, antimicrobial, antiallergic, and antiinflammatory effects. It abrogated agonist-induced LXRα/β transcriptional activity and suppressed the expression of related target genes serving as an LXRα/β antagonist (Francisco et al., 2016). Treatment with 118 inhibited LXR activation in HepG2 cells and eliminated lipid accumulation induced by LXR-SREBP-1c activation, thereby decreasing TG accumulation and primary hepatocytes. Overall, lipid accumulation induced by LXRs-SREBP-1c activation was abolished both in vivo and in vitro after treatment with 118, indicating the potential as a therapeutic agent for treating NAFLD (Yin et al., 2017). Besides, 118 in combination with cisplatin could potentially be used as a new regimen for the treatment of ovarian cancer (Wang et al., 2018c). It exhibited that 118 could upregulate LXRα and downstream target gene expression to control cholesterol metabolism (Park et al., 2020). Morin (119) present in many plants like mulberry, jackfruit, green tea, also in TCM like Tartary buckwheat, could reduce LXRα/β agonism induced by a synthetic agonist GW3965 (Gu et al., 2017a). Licochalcone A (120), present in the licorice root of Glycyrrhiza plant, such as Glycyrrhiza glabra L. and G. inflata Batalin, has been known as “Guolao” in China for thousands of years. The described bioactive properties range from antiparasitic to anticancer, antifungal, antiinflammatory, and osteogenic activities. As an LXRα antagonist, 120 restrained the transcription of lipogenic LXRα and resulted in the diminishment of accumulating TG in primary hepatocytes treated with a synthetic agonist T0901317 (Oh et al., 2015b). Isorhamnetin (121), an active ingredient of Hippophae rhamnoides L., downregulated the mRNA levels of LXRα and PPARγ (Lee et al., 2009). It also promoted the protein expression of PPARγ, LXRα, and CYP7A1 in qPCR analysis, indicating it as a potential agent in lipid homeostasis (Xiao et al., 2021). In HFD mice model, administration of alpinetin (122), derived from Alpinia katsumadai Hayata, efficiently suppressed LXRα and a series of receptors associated with lipid metabolism. Oral glucose and insulin tolerance tests, immunohistochemical and immunofluorescent analyses manifested that 122 attenuated insulin resistance and alleviated liver injury in HFD-induced mice as an ideal natural product in NAFLD (Zhou et al., 2018).
Sophoricoside (123), an LXRβ antagonist, present in the dried fruit of Styphnolobium japonicum (L.) Schott, has been reported to possess an antioxidant property. It could decrease the transcriptional activity of LXRβ concentration dependently (Zhang et al., 2020d). Xanthohumol (124), a critical flavonoid present in the female inflorescences of Humulus lupulus L., remarkably attenuated the mRNA expression of a direct target for LXRα transcriptional activation, inducible degrader of the low-density lipoprotein receptor (LDLR) in hepatic cells, which further inferenced a potential role of counteracting LXRs activation (Chen SF. et al., 2017). A flavonolignan silymarin (125), obtained from Silybum marianum (L.) Gaertn., has beneficial effects on liver diseases. It has been shown to reduce de novo hepatic lipogenesis, recover in insulin sensitivity, and protect against exacerbated myocardial ischemia reperfusion injury. Administration of 125 at 100 and 300 mg/kg depressed the upregulation of LXRβ and related genes expression in the liver of high fructose diet mice (Prakash et al., 2014; Chen et al., 2017b). Citrus fruits contain ample bioactive compounds including flavonoids, carotenoids, and coumarins. Citrange peel extract and citrange flesh and seed extract were proved to decrease LXRα/β and PPARγ level, emerging as a potential candidate for the treatment of obesity and related metabolic disorders (Lu et al., 2013).
3.2.3 Steroids
Natural steroids antagonists for LXRα principally include ergosterol (126), ergostan-6,8,22-trien-3-ol (127), and ergostan-4,6,8,22-tetraen-3-one (128) from Tolypocladium niveum, 129 from Colletotrichum dematium, 130 from Acremonium sordidulum, and cycloeucalenone (131) from an unidentified fungus. These compounds suppressed LXRα to varying degrees with binding IC50 ranging from 0.5 to 6.5 μM (Ondeyka et al., 2005). Diosgenin (132), obtained from Dioscorea villosa L., Rhizoma Dioscorea Nipponicae, and Trigonella foenum-graecum L., has been reported to repress the accumulation of TG and lipogenic genes expression in HepG2 cells at 5 and 10 μmol/L via suppressing LXRα. Compound 132 inhibited an increase in LXRα mRNA in HepG2 cells, which were increased upon high glucose or a synthetic agonist T0901317 treatment. As determined by hematoxylin and eosin and Oil red O staining, along with detection of serum AST and ALT activity, 132 administration obviously ameliorated lipid accumulation in the liver and reduced the elevated serum ALT level in NAFLD rats, suggesting it as a potential agent for preventing NAFLD (Uemura et al., 2011; Cheng et al., 2018).
3.2.4 Phenol derivatives
Curcumin (133), an active phenol derivative obtained from Curcuma longa L., could suppress the hepatic overexpression of LXRα, PPARγ, and fatty acid synthase. An immunoblot analysis also verified that 133 decreased the protein expression of LXRα and SREBP1c in the liver. Moreover, histological and serum biochemical analyses indicated that 133 apparently attenuated the hepatic lipid accumulation and decreased TG, TC, and nonesterified fatty acid levels in NAFLD mice model on account of the role for the prevention and treatment of NAFLD (Maithilikarpagaselvi et al., 2016; Chen et al., 2017b; Auger et al., 2018).
Meso-dihydroguaiaretic acid (134, MDGA), active dibenzylbutane lignan in Machilus thunbergia Siebold & Zucc., appears helpful in inflammation and neurovirulence. In HFD mice model, treatment with 134 reduced LXRα and associated target gene product expression, implying the purpose of attenuating NASH as a selective LXRα antagonist (Sim et al., 2014; Zanellaa et al., 2017). Sesamin (135), a major constituent in Sesamum indicum L., exerted antihyperlipidemic effects via decreasing LXRα expression and its downstream target genes, which were induced upon T0901317 treatment (Tai et al., 2019; Majdalawieh et al., 2020). Sauchinone (136), obtained from Saururus chinensis (Lour.) Baill., significantly decreased luciferase activity of LXRα activated by T0901317 and further inhibited LXRα-mediated SREBP-1c induction in HepG2 cells, preventing fat accumulation of hepatocytes (Kim YW. et al., 2010). A potential cholesterol-lowering agent alkaloid present in Piper longum L. is piperine (137), for which various biological activities, including anti-inflammatory, antiasthmatic and antitumor effects have been described. It inhibited the expression of ABCG5/8 and LXRα in the liver as a potential agent in preventing cholesterol gallstone formation and reduced biliary cholesterol secretion induced by lithogenic diet in C57BL/6 mice (Song et al., 2015).
Cyclic bibenzyl derivative riccardin F (138), present in liverworts, such as Blasia pusilla L., suppressed LXRE-dependent luciferase transcription in HepG2 cells functioning as an LXRα antagonist as well, and its homologous compound 90 has been described above as an LXRβ antagonist (Tamehiro et al., 2005; Asakawa, 2008). An LXRα antagonist biacetophenone cynandione A (139) found in the dried roots of Cynanchum wilfordi is both an endemic tonic and a traditional herbal medicine to promote liver and renal function. In HepG2 cells, treatment with 139 inhibited the mRNA levels of SREBP-1c as well as the expression of each enzyme upregulation promoted by two extrinsic LXRα agonists, GW3954 and T0901317 (Kim et al., 2020). Guttiferone I (140) is a naturally occurring polyisoprenylated benzophenone found in Garcinia humilis (Vahl) C.D.Adams. It has been found to bind with LXRα as an LXRα antagonist earlier on (Herath et al., 2005).
Rhein (141) is a key phytochemical of Rheum palmatum L., which is used as a TCM for thousands of years to treat obesity, constipation, ulcers, and gastrointestinal hemorrhage. It resulted into LXRs antagonism, dose-dependently interacted with LXRα and LXRβ with KD values of 46.7 and 31.97 μM in SPR assay, respectively. Moreover, 141 suppressed the transcriptional activity of SREBP-1c and further dose-dependently reduced the transaction of LXRs induced by GW3965. A further detailed study showed that 141 suppressed the expression levels of LXRs target genes. According to a series pharmacological experiment in an HFD-induced obese mouse model such as intraperitoneal glucose tolerance test and serum, fecal, and liver lipid content analysis, 141 showed several beneficial treatment effects on NAFLD in mice model, including decreased body and fat weight, lowered serum and hepatic lipid levels, improved insulin resistance, and reversed hepatic steatosis (Sheng et al., 2011a; Sheng et al., 2011b; Xue et al., 2020).
3.2.5 Others
Our recent study revealed that piericidin A (142, PA) and its glycoside glucopiericidin A (143, GPA), isolated from a marine-derived Streptomyces strain, could decrease the protein level of LXRα rather than mRNA expression. SPR assay was further employed to explore, along with several other piericidin analogs. As expected, PA presented a similar LXRα protein affinity (KD 3.24 × 10−5 M) compared with GW3965 (KD 6.97 × 10−5 M). Another piericidin aglycone, 10-ketone PA (144), could also serve as an LXRα ligand with a KD value of 7.22 × 10−5 (M). However, the affinity for GPA–LXRα binding was much weaker in nonkinetic simulation binding mode, with the KD value of 3.492 (M). Other piericidin glycosides, such as 13-OH GPA and 6″-Gal GPA, also exhibited no binding activity. The molecular docking analysis also showed the significant differences in the binding effects of glycosides and glycosides with LXRα protein. Intriguingly, PA (142) and GPA (143) have been considered as antirenal cell carcinoma (RCC) drug candidates, whereas on account of LXRα, PA/GPA caused specific hepatotoxicity in RCC xenograft mice-induced ALT to 2.6- and 2.3-fold, as well as the AST to 1.6- and 2.2-fold higher than that of the control group, respectively. Further study demonstrated that PA/GPA aggravated hepatotoxicity in high cholesterol diet-fed LXRα-activated mice while exhibiting no toxicity in chow diet-fed mice, indicating that the accumulation as well as the delay metabolism process of cholesterol resulted in hepatotoxicity and cholestasis. Pharmacokinetic study discovered that GPA existed as a prodrug in the liver and exerted toxic effect due to transforming into PA, different from PA directly combined with LXRα as an inhibitor (Zhou et al., 2019; Liang et al., 2020).
3R-1,7-Diphenyl-4E,6E-4,6-heptadien-3-ol (145), namely DPHD, is present in an indigenous medicinal herb Curcuma comosa Roxb. 145 upregulated mRNA and protein expressions of LXRα, SREBP1c, and their downstream targets in bilateral ovariectomy rats, exerting a lipid-lowering effect (Sutjarit et al., 2017). An endogenous LXR antagonist, arachidonic acid (146) (Ou et al., 2001) was also reported as a natural product from traditional medicine Acanthopanax koreanum Nakai. (Yoshikawa et al., 2002; Kuang et al., 2012). Another LXRα antagonist siphonaxanthin (147), a carotenoid present in green algae, such as Codium fragile, Umbraulva japonica, and Caulerpa lentillifera, repressed the excess accumulation of triacylglycerol induced by LXRα agonists, this effect was due to the downregulation of SREBP-1C and a series of associated target genes (Zheng J. et al., 2018).
It exhibited that schisandra polysaccharides, isolated from Schisandra chinensis (Turcz.) Baill (Wang et al., 2016), phenol, flavonoid, and sugar collected in the fruit of Abelmoschus esculentus (Zhang et al., 2020b), and total glycosides, isolated from Ligustrum lucidum L. Moench (Zhao and Liu, 2020), have the potency to downregulate LXRα and a set of associated genes, indicating both preventive and therapeutic role in regard to metabolic disorders.
4 Natural products targeting farnesoid X receptor
In this section, we gather natural products regulating FXR expression in different models and categories. These are illustrated in Supplementary Tables S3 and S4.
4.1 Natural agonists targeting farnesoid X receptor
BAs are endogenous agonists targeting FXR/PXR, with chenodeoxycholic acid (CDCA) being most effective. The activation of BAs targeting FXR follows a rank order of potency as CDCA > deoxycholic acid (DCA) > lithocholic acid (LCA) > cholic acid (Parks et al., 1999; Carottiet al., 2014).
4.1.1 Terpenes and steroids
Forman et al. identified and described an orphan nuclear receptor, farnesoid X-activated receptor, which is activated by a naturally occurring isoprenoid farnesol (148) and related derivatives. Farnesol (148), present in many essential oils, activated FXR-RXR at the concentrations ranging between 5 and 50 μM as an FXR agonist, further inhibiting hepatic TG synthesis via regulating the mRNA expressions of related molecules involved in lipid metabolism (Forman et al., 1995). Further study indicated that 148 activated both PPARα and FXR at similar concentrations (30 or 50 μM) in CV-1 cells (Goto et al., 2011).
Lepidozenolide (149), a sesquiterpenoid isolated from the liverwort Lepidozia fauriana, could significantly activate FXR in a dose-dependent manner (Lin, 2015). Cafestol (150), a well-known ingredient of coffee, has been identified as a dual FXR/PXR agonist, as assessed by a double FXR/PXR KO mice model. It increased the expression of FXR-target genes in pathways of BA biosynthesis and intestinal FGF15 via the contribution of FXR/PXR activation (Ricketts et al., 2007). A naturally occurring terpenoid found in Ferula ovina Boiss. is tschimgine (151), capable of activating FXR and bind ideally with the LBD of FXR in molecular docking. Interestingly, 151 inhibited the induction of inflammation genes in an FXR-dependent manner (Zheng W. et al., 2017). Dihydroartemisinin (152), the derivative of artemisinin, is present in Artemisia carvifolia Buch.-Ham. ex Roxb. and emerged as a potential candidate for the treatment of liver cancer and fibrogenesis. Compound 152 activated FXR to inhibit contractility of cultured hepatic stellate cells as a potential natural product to attenuate portal hypertension in fibrotic rodents (Xu J. et al., 2016; Xu Y. et al., 2017; Ma et al., 2020). Scrophularia dentatais, a folk herbal medicine found in Tibet, is emerging as a potential candidate for the treatment of smallpox, measles, high-heat plague, and poisoning. Zhang et al. isolated four compounds from the whole plant of Scrophularia dentatais Royle ex Benth., all of them being C19-diterpenoids. One is scrodentoids F (153) that dose-dependently activated FXR in dual-luciferase reporter assay system in 293T cells (Zhang et al., 2016). Picroside II (154), the major terpene in Picrorhiza scrophulariiflora Pennell, activated FXR via transient transfection in a dual-luciferase reporter assay. It decreased CYP7A1 and increased bile salt export pump and UDP-glucuronosyltransferase 1a1 mRNA expression, the latter two of which were inhibited upon knockdown of FXR. Meanwhile, 154 dose-dependently reversed α-naphthylisothiocyanate (ANIT)-induced alteration in serum markers and thereby exerted protective effect on ANIT-induced cholestasis, possibly through FXR activation (Li et al., 2020d; Li et al., 2020e). Geniposide (155), a pivotal constituent in Gardenia jasminoides Ellis, activated FXR, PXR, and SHP. Blood biochemical determination discovered that 155 at 25, 50, or 100 mg/kg increased bile flow rate and reversed the ANIT-induced increases in serum markers for hepatotoxicity and cholestasis in a dose-dependent manner (Wang Y. et al., 2017).
Genet et al. screened betulinic acid (156) from the Betula species, oleanolic acid (157) from Olea europaea L., and ursolic acid (114) from Cornus officinalis Siebold & Zucc., and tested them regarding their ability to activate FXR. In the results, 156, 157, and 114 activated FXR along with potential impact in diabetes (Genet et al., 2010), and 157 also generated frail FXR agonism, along with moderate PXR agonism, which protected against LCA-induced hepatotoxicity and cholestasis (Chen Z. et al., 2014). Hedragonic acid (158), from the stem and root of Celastrus orbicalatus Thunb., remarkably activated FXR and possessed therapeutic effects on liver injury and inflammation in acetyl-para-aminophenol-induced mice model as an FXR agonist (Lu et al., 2018). Ergosterol peroxide (159) and four triterpenoids, ganoderiol F (160), ganodermanontriol (161), lucidumol A (162), and ganoderic acid TR (163), are bioactive lanostane metabolites of Ganoderma lucidum Karst., which has been used in Asian medicines since 2,000 years against a variety of diseases including tumor, inflammation, obesity, and diabetes. 159–163 were verified as FXR agonists as inducing FXR with the low micromolar range in HEK293 cells (Grienke et al., 2011). Ginsenoside Rg1 (29) from Panax ginseng C.A.Mey. is both FXR and LXRs agonist, the function of 29 on LXRs has been described above. Herein, 29 also was reported to activate FXR to promote bile secretion and normalize enzyme activity in the serum in intrahepatic cholestasis mice model (Xiao et al., 2020).
Astragalus membranaceus (Fisch.) Bunge, widespread in Europe and Asia, is beneficial to the treatment of diabetes, hyperlipidemia, atherosclerosis, and cancers, with its key constituents like astragaloside IV and cycloastragenol (164). Compound 164 stimulated FXR transcription activities and regulated the expression of FXR target gene in HepG2 cells as a potential candidate for NAFLD. Meanwhile, it improved metabolic profiles, ameliorated hepatic steatosis, altered BA composition, and activated FXR signaling and feedback loops in diet-induced obesity mice, further confirming the promise in ameliorating NAFLD. Besides, 164 also alleviated hepatic steatosis in methionine and choline-deficient L-amino acid diet-induced NASH mice (Gu et al., 2017b). Alisol M 23-acetate (165) and alisol A 23-acetate (166) present in Alisma orientalis (Sam.) Juzep. have been described as FXR agonists by transactivating FXR to modulate promoter action (Lin 2012). Another FXR agonist present in A. orientale (Sam.) Juzep and Rhizoma alismatis is alisol B 23-acetate (167), protecting against NASH and CCl4-induced hepatotoxicity via FXR activation (Meng et al., 2015; Meng et al., 2017; Huo et al., 2018). In addition, 167, aisol F (168), aisol A (169), and 25-anhydro alisol A (170) found in A. orientale (Sam.) Juzep. activated FXR in a dose-dependent manner (Huo et al., 2018).
Recently, another protostane-type triterpenoid 23S-11β,23-dihydroxy-8α, 9β,14β-dammar-13(17)-ene-3,24-dione (171) from A. orientale (Sam.) Juzep. was confirmed with the activities of activating FXR with an EC50 value of 90 nM (Luan et al., 2019). An oleanane triterpenoid arjunolic acid (172) is present in the heartwood of Terminalia arjuna W. & Arn., a common traditional medicine in India. Compound 172 was reported to upregulate the FXR and PPARα expression, as well as downregulated PPARγ expression in HFD-fed rats as a lead molecule for the therapy of NAFLD (Toppo et al., 2018).
Four tirucallanes xylocarpols E (173), agallochols A (174), B (175), and D (176) isolated from mangrove plants, activated FXR at the concentration of 10.0 µM in a luciferase reporter assay in HepG2 cells (Jiang et al., 2018). Dioscoreae rhizome, also known as “Shaoyao,” acts as either a food or a traditional medicine because of its protectivity of the kidneys and liver. Dioscin (177), a steroid saponin from D. rhizome, attenuated oxidative stress and inhibited inflammation to improve thioacetamide-induced acute liver injury in Sprague–Dawley rats as upregulating the expression levels of FXR and AMPKα via FXR/AMPK signal pathway, providing a new insight in the treatment of acute liver injury (Zheng L. et al., 2018).
4.1.2 Flavonoids
Calycosin (178), present in the dry roots of Radix Astragali, has been demonstrated to improve TG metabolism, mitigate obesity, and hyperlipidemia. It played an emerging role in protecting against liver injury induced by CCl4 on account of activating FXR and its target genes as an FXR agonist (Chen et al., 2005b). A more detailed study showed that 178 attenuated TG accumulation and hepatic fibrosis to protect against NASH through FXR activation (Duan et al., 2017). Cryptocarya chinensis (Hance) Hemsl. is an endemic famous for its antioxidant, immunological, and antitumor effects in southern China, Japan, and Taiwan. Four naturally occurring tetrahydroflavanone cryptochinones A–D (179–182), obtained from this plant, transactivated FXR dose dependently in a transient transfection reporter assay, semblable to CDCA (Lin et al., 2014). Xanthohumol (124) is not only a counteractor of LXRs activation, but also an FXR modulator. It activated FXR dose-dependently in the transient transfection assay. Furthermore, 124-fed mice possessed lowered levels of SREBP-1c, gluconeogenetic genes, as well as targets involved in fatty acid synthesis in KKA-(y) mice, indicating a promising role in attenuating diabetes (Nozawa, 2005).
A flavanone glycoside abundantly found in lemons and oranges is hesperidin (183), treatment with which prevented cholestatic liver injury and reduced BA toxicity in HepaRG cells via activating FXR. Compound 183 dose-dependently protected against 75 mg/kg dose of ANIT-induced cholestasis and liver injury as reversing increases in the liver index, biliary index, serum AST, ALT, alkaline phosphatase, and total bilirubin, functioning as an effective agent for the prevention and therapy of cholestatic liver disease (Zhang et al., 2020a). Desmodium styracifolium has extensive biological activities, ranging from cholesterol level-lowering to cancer prevention and inflammatory inhibition. Schaftoside (184), a flavanone glycoside found in D. styracifolium, ameliorated oxidative injury and inflammation as an FXR agonist. Particularly, 184 attenuated acetaminophen-induced hepatotoxicity via restraining NF-κB signaling and fine-tuning the generation of pro- and antiinflammatory eicosanoids, providing a promising agent to alleviate liver injury induced by acetaminophen overdose (Liu et al., 2020a). In another study, HFD-induced lipid accumulation in the liver was decreased after treatment with 184, as indicated by reduced AST and cholesterol in serum, along with intracellular TG levels in the serum and liver tissue, subsequently resulting in attenuation of liver histopathological injury. As assessed by RT-PCR and Western blotting, 184 ameliorated HFD-induced NAFLD probably via FXR–SREBP1 signaling (Liu et al., 2020b). A crucial tea catechin, epigallocatechin-3-gallate (185, EGCG), is well known as a potential tonic for cardiovascular diseases and a variety of cancers. EGCG activated FXR with the EC50 value of 2.99 μM, nevertheless, which seemed to be specific because of the nonactivation of other nuclear receptors. It was regarded as a selective SBARM (a selective bile acid receptor modulator) (Li et al., 2012). EGCG possessed the strongest anti-HBV activity toward HBsAg and HBeAg among the crude green tea catechin tests. Meanwhile, the interaction between 185 and FXR has been confirmed to decrease the transcriptional activation of HBV, serving as an FXR antagonist and anti-HBV agent (Xu W. et al., 2016).
Penthorum chinense Pursh, also known as “GanHuang-Cao,” has been used as food and Chinese tea for thousands of years, with various biological activities, including antioxidation and antihepatitis virus effects. Notably, Gansu granule made up of the extracts of P. chinense has been used in clinics for the treatment of acute hepatitis. Besides, pinocembrin-7-O-[2″-O-galloyl-4″,6″-hexahydroxydiphenoyl]-β-D-glucose (186) and 2′,6-dihydroxydihydrochalcone-4′-O-[2″-O-galloyl-4″, 6″-hexahydroxydiphenoyl]-β-D-glucopyranoside (187) present in P. chinense activated hepatic FXR to BA homeostasis and regulated lipid metabolism (Zhao et al., 2021).
Total flavonoids of Astmgali Radix (TFA) are the essential ingredients of A. Radix, which contributes to the pharmacological efficacy including antiinjury, antimutation, and antitumor effects of this herb. TFA reversed the decrease in mRNA and protein levels of FXR induced by dimethylnitrosamine in rats and mitigated liver function in rats with liver cirrhosis (Cheng et al., 2017). Procyanidins is abundant in grapes, apples, red grape juice, and red wine, whose folkloric applications embody in preventing and ameliorating atherosclerosis and other cardiovascular disease. A SBARM grape seed procyanidin extract promoted FXR transcriptional activity induced by BA and FXR dependently resulted in a reduction in TG in vivo (Del Bas et al., 2009).
4.1.3 Phenol derivatives
A farnesyl phenolic compound grifolin (188) and ginkgolic acid 15:1 (189) from mushroom or Ginkgo biloba L., and geranyl caffeate (190) from Himalayan poplar, activated FXR ranging from 20 to 30 μM in monkey kidney cell line (Suzuki et al., 2006). Marchantins A (191) and E (192), macrocyclic bis-bibenzyls present in the liverworts of Marchantia paleacea Bertol., activated FXR at 10 μM (Suzuki et al., 2008; Asakawa and Ludwiczuk, 2018). A fungal metabolite altenusin (193), isolated from Alternaria sp. present in the leaves of polygonumse negalense, functioned as an FXR agonist with an EC50 value of 3.4 μM. Additionally, weight and fat mass, along with blood and glucose serum insulin level were reduced after treatment with 193 in HFD-fed mice. It reversed hepatic lipid droplet accumulation and macrovesicular steatosis, which were abolished in FXR-knockout mice (Zheng Z. et al., 2017).
Papaverine (194) is a bioactive constituent present in Papaver somniferum L. and contributes to the pharmacological efficacy of this herb, appearing to be an FXR agonist in a full length FXR transactivation assay (Steri et al., 2012). Another famous natural product berberine (195), beneficial for diarrhea, isolated from the roots of Coptis chinensi, regulated BA metabolism and, thus, exerted a lipid-lowering effect, functioning as an intestine-restricted FXR agonist. It prevented diet-induced obesity and TG accumulation in the liver with a significant decrease in serum and hepatic TG by 19% and 47%, respectively. A further mechanism study discovered that the antiobesity and lipid-lowering effects of 195 are primarily due to the activation of intestinal FXR and thereby result into a reduction in the CD36 expression (Sun et al., 2017; Tian et al., 2019).
Three naturally occurring phenylpropanoids, nelumol A (196) and nelumal A (197), from Ligularia nelumbifolia Hand. Mazz. (Bruyere et al., 2011), and auraptene (198), from Citrus aurantium, exhibited significant agonistic activity of FXR as FXR agonists (Epifano et al., 2012). The activation of FXR via 198 also resulted in hepatoprotective effect against cholestatic liver injury and increasing effect of BA efflux from the liver into the intestine (Gao et al., 2017). Moreover, it showed that 198 significantly attenuated apparent collagen deposition between liver lobules in thioacetamide-induced liver fibrosis mice. Treatment with 198 at 30 mg kg−1 significantly lowered the grade of fibrosis compared with the thioacetamide group. Further gene expression involved in hepatic stellate cell activation and fibrosis also confirmed its efficacious potency. Overall, 198 seemed to be beneficial to hepatic fibrosis as reducing toxic BAs and inhibiting hepatic stellate cell activation and inflammation due to FXR activation (Gao et al., 2018). Another phenylpropanoid present in the rhizomes of the Podophyllum species, podophyllotoxin (199), could transactivate FXR at a concentration of 10 μM in HeLa cells (Hiebl et al., 2018).
“Se-Ji-Mei-Duo,” recognized as having auspicious and beautiful flowers, is a traditional medicine, Herpetospermum pedunculosum (Ser.) C.B. Clarke, for which various biological properties including anti-HBV, anti-inflammatory, and antioxidant effects have been described. Five lignans, herpetotriol (200), spathulatd (201), lariciresinol (202), dehydrodiconiferyl alcohol (203), and herpetrione (204), have emerged with significant FXR agonistic activity in vitro and, thus, were identified as the main bioactive constituents in the seeds of H. pedunculosum (Ser.) C.B. Clarke (Wei et al., 2020). Furthermore, dehydrodiconiferyl alcohol (205) is also an FXR agonist found in the seeds of H. dunculosum (Ser.) C.B. Clarke. To confirm its agonistic activity, knockdown of the expression of FXR in L-02 cells was performed. The mRNA and protein levels of FXR as well as a range of keys downstream has been remarkably upregulated after treatment with 205, which were decreased in Si-FXR cells (Wei et al., 2021).
Soybean coumestrol (206) with 50 μM stimulated FXR transcriptional activity to 2.0-fold over the control group in HEK293T cells. ORO staining was employed to investigate the effect of 206 on differentiation of 3T3-L1 preadipocytes into mature adipocytes. It was observed that 206 dose-dependently suppressed MDI (mixture of 3-isobutyl-1-methylxanthine, dexamethasone, and insulin)-induced lipid accumulation in a manner, 206 at 20 or 40 µM decreased MDI-induced lipid accumulation by 38.8% and 77.4%, respectively. Together with RT-PCR analysis, 206 could be considered as an agent to prevent adipogenesis (Takahashi et al., 2008; Jang et al., 2016). Yangonin (207), a kavalactone found in Kava Piper methysticum, has been demonstrated to bind with the LBD of FXR and activate FXR to alleviate estrogen-caused cholestasis. Moreover, 207 caused an increase in BA efflux and detoxification as well as a decrease in BA uptake and synthesis via activating FXR, thereby exerting potential hepatoprotective effect. Moreover, in estrogen-induced cholestasis rat model, 17α-ethinylestradiol-induced growth retardation was reduced in a dose-dependent manner by 207, and relative liver weight was significantly decreased after treatment with 207, thereby alleviating estrogen-induced cholestasis (Dong et al., 2019).
4.1.4 Others
Two secondary metabolites collected in Monascus-fermented rice, monascin (208) and ankaflavin (209), have been confirmed to upregulate FXR and PPARα expressions and, thus, result in fatty acid oxidation as food supplements to prevent against diabetes and obesity (Hsu et al., 2014).
4.2 Natural antagonists targeting farnesoid X receptor
4.2.1 terpenes
Atractylenolides II (210) and III (211) are two naturally occurring sesquiterpenoids of Atractylodes macrocephala Koidz. responsible for treating excessive vaginal bleeding and exerting antihyperlipidemic effect. Compounds 210 and 211 at 50 µM exerted approximately 50% antagonistic effect against SHP gene promoter transactivation activity induced by 10 µM CDCA. Intriguingly, 100 µM of 210 and 211 entirely antagonized the transactivation activity of SHP in HepG2 cells at 0.9 and 0.85, respectively, induced by 10 µM of CDCA, which confirmed the function as FXR antagonists (Tsai et al., 2012). Andrographolide (212), a major constituent of “King of Bitte” Andrographis panniculata (Burm.f.) Nees, contributes to the pharmacological efficacy of this herb, owning medicinal potencies including anti-inflammation, antidiabetic, and anticancer (Kandanur et al., 2019; Kumar et al., 2020). In a study using transient transfection and FXR luciferase reporter assay in 293T cells, 212 was proven to be an FXR antagonist with the IC50 value of 9.7 µM (Liu et al., 2014).
Oleanolic acid (157) not only activates LXRs as a promising treatment for NAFLD but also functions as an antagonist of FXR. It could bind with the LBD of FXR and inhibit the activity of FXR–LBD induced upon an endogenous ligand CDCA (Liu and Wong 2010). It also ameliorated obstructive cholestasis in mice after treatment with bile duct ligation due to FXR antagonism. Further histological and biochemical assessment showed that pretreatment with 20 mg/kg of 157 significantly ameliorated pathological alteration, such as extrahepatic cholestatic liver injury, bile duct hyperplasia, and portal infiltrates along with extensive foci of liver necrosis in bile duct-ligation mice (Chen et al., 2015a). Schisandra glaucescens Diels is both a traditional medicine and a food locally, as a potential candidate for the therapy of spontaneous sweat, night sweat, chronic diarrhea, along with neurasthenia in Hubei and Sichuan provinces of China. Cycloartane triterpenoids 6β-hydroxynigranoic acid (213) and schiglausin N (214), from the stems of S. glaucescens Diels, exhibited significant inhibitory effect on FXR as antagonists. Compound 213 restrained FXR with an IC50 of 1.50 μM, and simultaneously 214 emerged with the inhibitory rate of FXR of more than 20% at 25 μM (Zou et al., 2012a). Other six triterpenoids from S. glaucescens Diels, schiglausins D–G (215–218), kadsuric acid (219), and 3β-hydroxy-lanost-9(11),24-dien-26-oic acid (220), revealed antagonistic activity against FXR together with the inhibitory rate higher than 20% at 25 µM (Zou et al., 2012b). An FXR antagonist, platycodin D (22), from Platycodon grandiflorum (Jacq.) A.DC., is capable of modulating hepatic lipogenesis in high glucose-exposed HepG2 cells and in HFD-fed rats (Hwang et al., 2013). Treatment with 22 exhibited an antagonistic ratio at 40%–50%, compared with the control value of CDCA in a coactivator recruitment assay for FXR as a novel cholesterol-lowering and anti-atherogenic candidate (Zhao et al., 2006).
Marine organisms, such as sponges and tunicates, have received remarkable interests as a valuable source of novel bioactive natural products encompassing diverse chemical structures and pharmacological activities. Suvanine (221), a sesterterpene present in sponge Coscinoder mamathewsi, has been confirmed to be an FXR antagonist, effectively antagonizing FXR transactivation (Di Leva et al., 2013). In addition, 12-O-deacetyl-12-epi-19-deoxy-21-hydroxyscalarin (222), 12-O-deacetyl-12-epi-19-deoxy-22-hydroxyscalarin (223), 12-O-deacetyl- 12-epi-19-O-methylscalarin (224), 12-O-deacetyl-12-epi-scalarin (225), 12-epi-scalarin (226), and 12-O-deacetyl-12-epi-19-deoxyscalarin (227) found in the genus of Spongia also antagonized FXR transactivation. They decreased the affinity of FXR LBD for a coactivator peptide facilitated by CDCA in SPR assay, especially for 224–227, disrupted very strong interaction between FXR and a coactivator peptide (Nam et al., 2006; Nam et al., 2007). An isoprenoid tuberatolide A (228), and five meroterpenoids tuberatolide B (229), 2′-epi-tuberatolide B (230), yezoquinolide (231), R-sargachromenol (232), and S-sargachromenol (233), were isolated from Korean marine tunicate Botryllus tuberatus, and all of them exhibited significant antagonism on FXR with IC50 values as low as 1.5 µM. Compounds 228–233 released the coactivator peptide from the CDCA-bound hFXR LBD in cell-free SPR experiments, indicating the characterization of their potent FXR antagonists (Choi et al., 2011).
4.2.2 Steroids
Guggulsterone, existing in two isomeric forms, namely, E-guggulsterone (234) and Z-guggulsterone (235), is the active agent isolated from the resin of Commiphora mukul (Arn.) Bhandari, responsible for antihyperlipidemic effect. E/Z-guggulsterones 234 and 235 have been identified as FXR antagonists directly, to decrease hepatic cholesterol levels in rodent models. Moreover, Z-guggulsterone (235) exhibited remarkable FXR antagonism with an IC50 of 1–5 μM in HepG2 cells, increased the cholesterol CYP7A1, and further decreased the circulating cholesterol level (Urizar et al., 2002; Urizar and Moore, 2003; Bhutani et al., 2007; Yu et al., 2009; Singh and Sashidhara, 2017). A very well established FXR antagonist from a natural source is stigmasterol acetate (226), a principal phytosterol extracted from soybeans. Compound 236 inhibited the expression of FXR target genes induced by CDCA in a Gal4-responsive luciferase reporter gene assay in HepG2 cells. Intriguingly, except for FXR, 236 also antagonized the LBD of PXR (Carter et al., 2007).
Ergostane-3,4,21,26-tetrol 3,21-bis (hydrogensulfate) (237) is a sulfated polyhydroxy sterol present in the marine invertebrate Ophiolepis superba. It resulted in a potent antagonism of FXR transactivation concentration dependently, and treatment with 237 at 100 μM completely reversed the effect exerted by CDCA on FXR expression. Taken together, 237 might be a potential candidate in regulating BA metabolism (Sepe et al., 2011; Fiorucci et al., 2012b). Some of polyhydroxylated steroids isolated from marine sponge Theonella swinhoei have been identified as FXR antagonists.
Swinhosterol B (238), conicasterols I (239), J (240), and conicasterol (241) are polyhydroxylated steroids identified from Theonella swinhoei. In a luciferase reporter assay transfected with FXR and RXR, 238 at 50 μM showed the most potential antagonism of 10 μM of CDCA-induced FXR, followed by 239–241. Simultaneously, 238–241 were also endowed with a potent PXR agonistic activity (De Marino et al., 2012; Fiorucci et al., 2012a). 4-Methylene-24-ethylsteroid, also called theonellasterol (242), also isolated from T. swinhoei, directly antagonized the activation of FXR at 50 μM and activated PXR as well (Fiorucci et al., 2012a; Renga et al., 2012; Sepe et al., 2014). Other eight polyhydroxylated steroids from T. swinhoei, conicasterols B–D (243–245), theonellasterols B, C, and E–G (246–250), functioned as FXR antagonists in the presence of CDCA with 10 μM, but also behaved as PXR agonists (De Marino et al., 2011, Fiorucci et al., 2012). Moreover, conicasterol E (251), from the same sponge, also has been demonstrated as an SBARM endowed with PXR agonistic activity (Sepe et al., 2012). 3-Oxocholest-1, 22-dien-12β-ol (252) and 3-oxocholest-1, 4-dien-20β-ol (253), two sterols from the soft coral Dendronephthya gigantea, have been verified as FXR antagonists, as assessed by a cotransfection assay in CV-1 cells, which exhibited notable antagonistic activity against FXR with IC50 values of 14 and 15 μM (Shin et al., 2012).
4.2.3 Others
Three PUFAs FXR antagonists, arachidonic acid (146), docosahexaenoic acid (254) from Crocodylus siamensis, and linolenic acid (255) from Perilla frutesccns, exhibited antagonistic activity of CDCA-activated FXR with IC50 ranging from 0.9 to 4.7 μM (Zhao et al., 2004). Naringin (256), one of the most abundant and common flavonoids in citrus fruits and grapefruits, has been reported to relieve atherosclerosis. In ApoE−/−mice fed with an HFD, 256 ameliorated atherosclerosis, lowered the serum and liver cholesterol levels by 24.04% and 28.37%, increased lipid and BA excretion in feces, and regulated the gut microbiota associated with cholesterol metabolism. Moreover, 256 upregulated CYP7A1 via suppressing FXR/FGF15 pathway, and facilitated BA synthesis, providing insight into the atherosclerosis-amelioration mechanisms (Wang J. et al., 2020). In addition, 256 also could exert hepato- and nephroprotective effect in rats via the role of FXR and renal injury molecule-1 (Adil et al., 2016).
Maninsigins A (257), isolated from Magnolia officinalis as a TCM for the therapy of asthma, dyspepsia, and asthmatic cough, suppressed FXR activation induced by CDCA with an IC50 value of 55.6 μM as an FXR antagonist (Shang et al., 2013). An isoquinoline alkaloid palmatine (258, PAL) from Rhizoma coptidis contributes to the pharmacological efficacy of the herb such as obesity, diabetes mellitus, hyperlipidemia, and hyperglycemia–cardiovascular diseases. PAL-treated rats were characterized by decreased TC, TG, low-density lipoprotein cholesterol levels, serum total bile acid (TBA) levels, and increased fecal TBA and TC levels. It showed that 258 reduced HFD-induced lipid accumulation in the liver. So, it indicated the curative effect of 258 for hyperlipidemia (Ning et al., 2020).
Halicylindramides F (259), A (260), and C (261) are three naturally occurring depsipeptides from a Petrosia species marine sponge collected in Korean waters. In a cotransfection assay in CV-1 cells, 259–261 possessed potent inhibitory effects of CDCA-induced hFXR transactivation with IC50 values of 6.0, 0.5, and 5.0 μM, respectively. Nevertheless, the function of hFXR antagonists they exerted did not bind directly to hFXR. However, SPR assay showed that 259–261 did not appear to have any significant inhibition of the recruitment of cofactor coactivator peptide to hFXR at concentrations higher than their IC50 values determined in a cell-based cotransfection assay. Thereby, the antagonism between FXR and 259–261 might be achieved by an indirect manner, not by a direct binding to LBD of FXR (Hahn et al., 2016).
5 Molecular docking analysis of liver X receptors and farnesoid X receptor
In silico molecular docking is an important virtual screening method in the drug discovery process, which mainly focuses on structures of targets and ligands. It predicts the binding geometries as well as the binding energy of the ligand–target complex. To better understand the above-reported active natural products targeting LXR/FXR, most of them were performed together with molecular docking analyses, using the same method and parameters, although similar work has been done on some compounds in the literatures. As results, some natural compounds, like 7, 47, 54, 58, 60, 76, 77, 92, 93, 94, 136, 145, 156, 170, 189, 223, 226, 230, 232, 236, 238, 240, 244, 249, 250, 257, and 258, exhibited optimal property for targeting LXRs or FXR in molecular docking evaluation, suggesting that these compounds are even more promising.
5.1 Molecular docking analysis of liver X receptors
Based on the abovementioned LXR ligands, we have then analyzed, by means of molecular docking calculations, the interactions between LXRs ligands and LXRs proteins to understand and shed light on the binding mechanism of LXRs ligands. The crystal structures of the LXRα LBD in a complex with T0901317 (PDB ID: 1UHL) (Svensson et al., 2014) and GW3965 (PDB ID: 3IPQ) (Fradera et al., 2010), along with the LXRβ LBD in a complex with 2 (PDB ID: 1P8D) (Williams et al., 2003), were selected and subjected to an in silico molecular docking analysis, using the induced-fit module in the Schrödinger software suite. Molecular docking results (S values listed in Supplementary Tables S1 and S2) indicated that majority of the compounds fitted comfortably into the binding pocket, and the most potential 2D and 3D binding models of each protein are displayed in Supplementary Figures S1–S3, respectively.
As the docking results shown in Supplementary Figure 1A, the hydroxy in the saccharide moiety of 27 formed hydrogen bonds with the active-site residue SER 228 of 1UHL, the S value of which was −8.779, followed by 130 (−8.770), and 47 (−8.732), comparative with the ligand T0901317 (−8.931). In line with the abovementioned SPR assay of 33, 81, 102, 103, and 141, they could directly combine with LXRα protein 1UHL with S values from −5.763 to −9.187 (Supplementary Figures S1B–F). In the 2D binding models, the hydroxy or carbonyl groups in the hexatomic ring formed hydrogen bonds with the active site residue THR258, HID421, LYS434, THR302, TRP443, SRE264, or ARG305 of LXRα.
The docking model between LXRα 3IPQ and 58 along with 60 (Supplementary Figures S2A,B) exhibited that 58 and 60 formed hydrogen bonds with the active-site residue PHE 257, and the hexatomic ring plays a key role to form a π–π stacking interaction with PHE 315 with the docking score of −10.776 and −10.567 individually. Compounds 33, 81, 102, 103, 141, 142, and 144 also appeared to interact with LXRα protein 3IPQ perfectly with the S values from −5.763 to −9.187 (Supplementary Figures S2C–I), consistent with the corresponding SPR assay. 2D binding models showed that the phenolic hydroxy groups in the hexatomic ring formed hydrogen bonds with the active site residue ARG305, GLU267, MET298, THR302, PHE257, or SER264 of LXRα. Additionally, the aromatic hexatomic ring of 33, 103, and 141 formed a π–π stacking interaction with PHE 315 or PHE 326 of LXRα.
As depicted in Supplementary Figures S3A–D, 94, 76, 27-hydroxycholesterol, and its own ligand 24(S),25-epoxycholesterol all fitted well into LXRβ 1P8D and formed a hydrogen bond with ASN 239. Moreover, 27-hydroxycholesterol contained an additional hydrogen bond with HID 435. In fact, with respect to compounds 76, 77, 92–94, and 125, the S values of which are from −10.056 to −11.457, were better than or comparative to 24(S),25-epoxycholesterol (−9.941). Overall, ASN 239, GLU 281, and HID 435 might be regarded as key residues for the interactions and activity of 1P8D. In accordance with the SPR assay of 33, 81, and 141, they could directly combine with LXRβ protein 1P8D with the S values from −5.578 to −8.715 (Supplementary Figures S3E–G). The phenolic hydroxy groups in the hexatomic ring formed hydrogen bonds with the active site residues ASN239, GLU281, THR316, or MET312 of LXRβ, and there exist the formation of π–π stacking and π cation with PHE239 or PHE 243.
5.2 Molecular docking analysis of farnesoid X receptor
To gain an insight into the molecular interactions between FXR ligands and FXR proteins as well as generate a structure and activity relationship at the atomic level, molecular docking calculations were carried out using the crystal structures of the FXR LBD in a complex with obeticholic acid (PDB ID: 1OSV and 1OT7) (Mi et al., 2003) and DB08220 (PDB ID: 3BEJ) (Soisson et al., 2008) to explore the molecular interactions (S values listed in Supplementary Tables S3, S4). The most potential 2D and 3D binding models of each protein are shown in Supplementary Figures S4–S6, respectively.
As illustrated in Supplementary Figures S4A, B, 239 and 250 occupied the LBD similar to obeticholic acid (the S value −11.769) with similar interaction (Supplementary Figure S4B3), which bound to 1OSV with the S values of −10.917 and −10.415, respectively. The hydroxy groups in the steroidal ring moiety of these molecules formed hydrogen bonds with the active-site residue LEU 284 and SER 329. Overall, the docking models 235 also showed the most potency with a docking score over −10. Compounds 222–233 were a series of steroids isolated from the genus of Spongia. SPR assay indicated that they all decreased the affinity of FXR LBD for a coactivator peptide facilitated by CDCA. In line with SPR, docking indicated that they fitted comfortably into the binding pocket for the agonist obeticholic acid with the similar binding positions, with the S values from −8.941 to −9.179, except for 225 with the S value of −5.849 (Supplementary Figures S4C–N). The ester and phenolic hydroxyl groups formed hydrogen bonds with the active-site residue ARG 328 or TYR358 of FXR. Furthermore, both 226 and 227 formed π–π stacking interaction with TRP451 and TYR328.
Compound 168 interacted with FXR 1OT7 with the highest S value of −10.884, comparative to the ligand obeticholic acid (docking score −11.569) and CDCA (docking score −10.610). The hydroxy groups formed hydrogen bonds with the active-site residue TYR 358, HID 444, LEU 284, and ARG 328 (Supplementary Figure 5A). Meanwhile, 163, 218, and 168 also ideally fitted into the LBD of 1OT7 along with a docking score over −10. 2D binding models of 222–233 with 1OT7 supporting the corresponding SPR results excellently with the S values from −7.906 to −9.352, except for 225 with the S value of −5.899 (Supplementary Figures S5B–M). The ester and phenolic hydroxyl groups of 226, 232, and 236 formed hydrogen bonds with the active-site residue ARG 328 or TYR358 of FXR.
Compound 233 docked into FXR 3BEJ with the highest score of −11.025, though only inferior to DB08220 (the S value of −12.625), both formed hydrogen bonds with the active-site residue THR 288 and ARG 331. Differently, there is a salt bridge between DB08220 and ARG 331 of 3BEJ. The docking models of 233, 204, 244, 252, 228, 230, 253, and 255 predicted the significant role of the active-site residue THR 288 and ARG 331. Interestingly, direct binding of 233, along with 222–232 to the LBD of FXR, was monitored by SPR assay. In molecular docking, they also fitted comfortably into the binding pocket for the agonist obeticholic acid with the similar binding positions as well, with the S values from −8.894 to −10.113, except for 228 with the S value of −5.861 (Supplementary Figures S6A–K). In the 2D binding models, the ester and hydroxyl groups formed hydrogen bonds with the active-site residue THR288, ARG331 or HID447 of FXR.
6 Conclusion and perspective
Concurrent with our increasing knowledge of the roles of LXRs/FXR in cholesterol, lipid, bile acid, and glucose metabolism, selective and potent LXRs/FXR ligands have gained significant ground for shedding light on the functions of LXRs and FXR in metabolic syndrome-related diseases as promising chemical biology tools (Jian et al., 2014; Pu et al., 2019).
In the recent two decades (2000–2020), 261 natural products were discovered from natural resources, such as LXRs/FXR modulators, 109 agonists and 38 antagonists targeting LXRs, while 72 agonists and 55 antagonists are targeting FXR. As shown in Figure 4 and Figure 5, LXR ligands exhibited higher chemical diversity than FXR ligands, terpenes, flavonoids, phenol derivatives, and steroids constituted 29%, 19%, 21%, and 10% of the reported LXR agonists, constituted 21%, 21%, 24%, and 18% of the reported LXR antagonists, respectively, while for FXR ligands, terpenoids accounted for a large proportion, close to half, for 49% and 47% of the reported FXR agonists and antagonists, respectively. Sterols, primary type of FXR antagonists, the reason of which is a series of endogenous BAs that possess distinguishing potency of targeting BA-receptor FXR. It is a remarkable fact that several of the mentioned natural products are food constituents, such as compounds 41, 42, 54, 95, 96, 104, 106, 125, 127, 208, 210–212, and 214–220, thus, potential candidates for dietary interventions for the prevention and treatment of corresponding diseases. Terrestrial plants are a promising source for the discovery of new drug leads, especially for TCMs having a history of more than 2,000 years. In recent years, marine organisms and microorganisms have gradually become a vital source of LXRs/FXR modulators with the development of marine natural product research. For instance, 38% of FXR antagonists originated from marine organisms. Especially for 222–227 and 228–233, a series of steroids isolated from the genus of Spongia have been verified as FXR antagonists via various representative assays, including SPR, indicating the significance of discovering marine natural steroids associated with FXR in the genus of Spongia. Overall, it is believed and prospective that LXR/FXR modulators from marine sources, especially marine microbial sources, will take a greater proportion in the future.
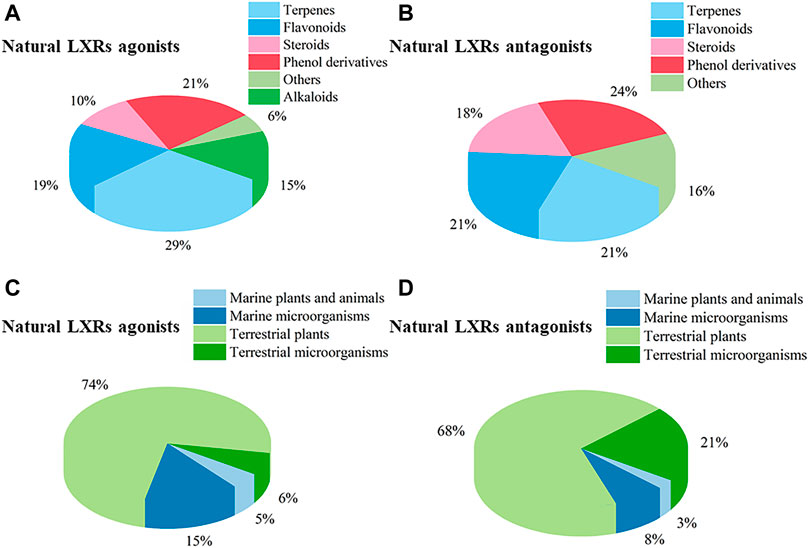
FIGURE 4. Chemical types (A, B) and resources (C, D) of natural-derived LXRs agonists and antagonists.
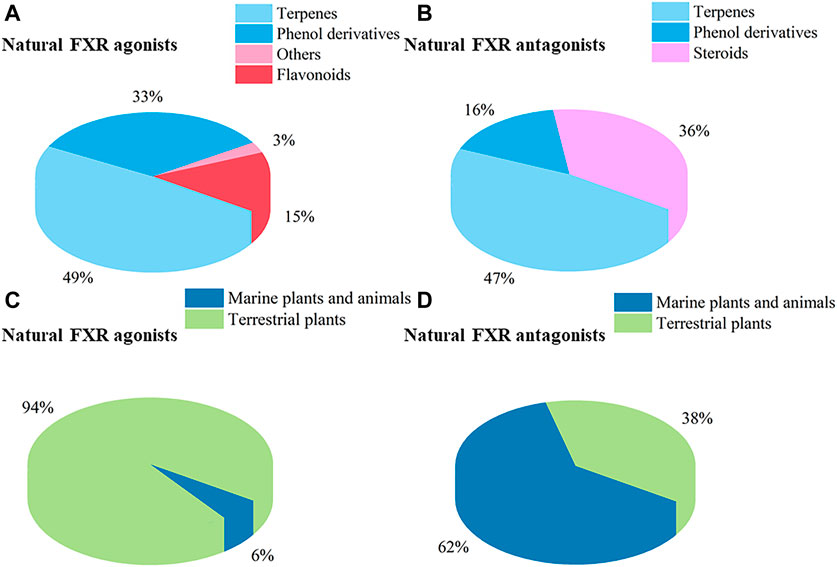
FIGURE 5. Chemical types (A, B) and resources (C, D) of natural-derived FXR agonists and antagonists.
LXRs and FXR are highly expressed in the livers of patients with NAFLD, increasing with the severity of NASH (Zheng Z. et al., 2017, Mi et al., 2019). Some natural compounds especially for 2, 3, 37, 42, 83, 104, 106, 114, 115, 117, 118, 122, 132–134, 141, 157, 164, 167, 178, and 193, proved effective in ameliorating NAFLD and NASH by targeting LXR/FXR or through the LXR/FXR pathway, presenting a promising therapeutic prospect. Risk of cardiovascular diseases has been reported to be associated with cellular cholesterol levels, that is, with excess cellular cholesterol often increasing the risk of cardiovascular diseases (Jun et al., 2013). Our review discovered that 3, 22, 39, 40, 44, 54, 76, 77, 82, 83, 99, 107–109, 152, 210, 211, 256, and 258 played a vital role in hypercholesterolemia, hypertension, or atherosclerosis, indicating the prospect of further exploration in cardiovascular diseases focusing on LXR/FXR ligands. Additionally, 25, 38, 47, 48, 83, 152, 154, 155, 157, 177, and 183–185 have represented tremendous potential for the treatment of various liver diseases including HBV, HCV, hepatic fibrosis, acute lung injury, cholestatic liver injury, liver cancer, and cholestasis. Previous reports have elicited that the ligands targeting the LXR/FXR might be one of the most effective ways to intervene with diabetes and obesity (Pu et al., 2019). It is suggested that 46, 114, 116, 124, 156, 157, 195, 208, and 209 could be developed as prospective candidates to reduce the risk of diabetes and obesity. Intriguingly, 51, 52, 77, and 96 have proved to be beneficial for the treatment of AD; meanwhile, 22, 78, 98, and 99 have contributed to alleviate the development of mastitis and breast cancer. Overall, the abovementioned provides multiple alternative approaches to LXR/FXR-related drug development.
At this point it needs, nevertheless, to be mentioned that most lead compounds failed in preclinical and clinical developments mostly due to toxicological and/or pharmacokinetic issues. For instance, the clinical application of LXRα agonist triptolide (19) is greatly constrained by hepatotoxicity, suggesting the risk of LXRs as toxicity targets. Our recent study revealed that, different from PA (142) directly combined with LXRα as an inhibitor, another anti-RCC drug candidate GPA (143) exists as a prodrug in the liver and exerts a toxic effect due to transformation into PA. Longer survival time of GPA-treated mice indicates that further exploration in anti-RCC drug research should focus on reducing glycosides transformed into PA and concentrating in kidney tumor rather than the liver for lowering hepatotoxicity risk.
Thereby, the therapeutic effects of these on diseases associated with LXRs and FXR expression remain to be further investigated in preclinical or clinical studies. Even if some compounds possessed grand effects on LXRs or FXR at a high concentration without toxicity in cell models, there exists a difference in vivo. Besides, overactivation or repression of LXRs and FXR is likely to cause other diseases, the discovery of the right balance between activating and inhibiting LXRs and/or FXR effects will be crucial for the development of targeting agents.
Author Contributions
JS searched the literature and wrote the draft of the manuscript. TG and XP improved the manuscript. YL and LT revised the manuscript and discussed the conclusion. XZ established the concept and approved the final manuscript.
Funding
This work was supported by grants from the Finance Science and Technology Project of Hainan Province (ZDKJ202018), Marine Economy Development Project of Guangdong Province (GDNRC2021052), Key Special Project for Introduced Talents Team of Southern Marine Science and Engineering Guangdong Laboratory (Guangzhou) (GML2019ZD0406), National Natural Science Foundation of China (U20A20101, 81973235, 81973388, 81673677), Guangdong Local Innovation Team Program (2019BT02Y262), and K. C. Wong Education Foundation (GJTD-2020-12).
Conflict of Interest
The authors declare that the research was conducted in the absence of any commercial or financial relationships that could be construed as a potential conflict of interest.
Publisher’s Note
All claims expressed in this article are solely those of the authors and do not necessarily represent those of their affiliated organizations, or those of the publisher, the editors, and the reviewers. Any product that may be evaluated in this article, or claim that may be made by its manufacturer, is not guaranteed or endorsed by the publisher.
Supplementary Material
The Supplementary Material for this article can be found online at: https://www.frontiersin.org/articles/10.3389/fphar.2021.772435/full#supplementary-material
References
Abdullah, S., Jang, S. E., Kwak, M. K., and Chong, K. (2020). Ganoderma Boninense Mycelia for Phytochemicals and Secondary Metabolites with Antibacterial Activity. J. Microbiol. 58, 1054–1064. doi:10.1007/s12275-020-0208-z
Adil, M., Kandhare, A. D., Ghosh, P., Venkata, S., Raygude, K. S., and Bodhankar, S. L. (2016). Ameliorative Effect of Naringin in Acetaminophen-Induced Hepatic and Renal Toxicity in Laboratory Rats: Role of FXR and KIM-1. Ren. Fail. 38, 1007–1020. doi:10.3109/0886022X.2016.1163998
Apfel, R., Benbrook, D., Lernhardt, E., Ortiz, M. A., Salbert, G., and Pfahl, M. (1994). A Novel Orphan Receptor Specific for a Subset of Thyroid Hormone-Responsive Elements and its Interaction with the Retinoid/thyroid Hormone Receptor Subfamily. Mol. Cel. Biol. 14, 7025–7035. doi:10.1128/mcb.14.10.7025
Asakawa, Y., and Ludwiczuk, A. (2018). Chemical Constituents of Bryophytes: Structures and Biological Activity. J. Nat. Prod. 81, 641–660. doi:10.1021/acs.jnatprod.6b01046
Asakawa, Y. (2008). Recent Advances of Biologically Active Substances from the Marchantiophyta. Nat. Product. Commun. 3, 1934578X0800300–92. doi:10.1177/1934578X0800300116
Auger, F., Martin, F., Pétrault, O., Samaillie, J., Hennebelle, T., Trabelsi, M. S., et al. (2018). Risperidone-induced Metabolic Dysfunction Is Attenuated by Curcuma Longa Extract Administration in Mice. Metab. Brain Dis. 33, 63–77. doi:10.1007/s11011-017-0133-y
Bełtowski, J. (2008). Liver X Receptors (LXR) as Therapeutic Targets in Dyslipidemia. Cardiovasc. Ther. 26, 297–316. doi:10.1111/j.1755-5922.2008.00062.x
Bhutani, K. K., Birari, R., and Kapat, K. (2007). Potential Anti-obesity and Lipid Lowering Natural Products: A Review. Nat. Product. Commun. 2, 1934578X0700200–348. doi:10.1177/1934578X0700200316
Bogie, J., Hoeks, C., Schepers, M., Tiane, A., Cuypers, A., Leijten, F., et al. (2019). Dietary Sargassum Fusiforme Improves Memory and Reduces Amyloid Plaque Load in an Alzheimer’s Disease Mouse Model. Sci. Rep. 9, 4908. doi:10.1038/s41598-019-41399-4
Bonamassa, B., and Moschetta, A. (2013). Atherosclerosis: Lessons from LXR and the Intestine. Trends Endocrinol. Metab. 24, 120–128. doi:10.1016/j.tem.2012.10.004
Bradley, M. N., Hong, C., Chen, M., Joseph, S. B., Wilpitz, D. C., Wang, X., et al. (2007). Ligand Activation of LXR Beta Reverses Atherosclerosis and Cellular Cholesterol Overload in Mice Lacking LXR Alpha and apoE. J. Clin. Invest. 117, 2337–2346. doi:10.1172/JCI31909
Bramlett, K. S., Houck, K. A., Borchert, K. M., Dowless, M. S., Kulanthaivel, P., Zhang, Y., et al. (2003). A Natural Product Ligand of the Oxysterol Receptor, Liver X Receptor. J. Pharmacol. Exp. Ther. 307, 291–296. doi:10.1124/jpet.103.052852
Bruyère, C., Genovese, S., Lallemand, B., Ionescu-Motatu, A., Curini, M., Kiss, R., et al. (2011). Growth Inhibitory Activities of Oxyprenylated and Non-prenylated Naturally Occurring Phenylpropanoids in Cancer Cell Lines. Bioorg. Med. Chem. Lett. 21, 4174–4179. doi:10.1016/j.bmcl.2011.05.089
Calkin, A. C., and Tontonoz, P. (2012). Transcriptional Integration of Metabolism by the Nuclear Sterol-Activated Receptors LXR and FXR. Nat. Rev. Mol. Cel Biol. 13, 213–224. doi:10.1038/nrm3312
Cao, G., Liang, Y., Broderick, C. L., Oldham, B. A., Beyer, T. P., Schmidt, R. J., et al. (2003). Antidiabetic Action of a Liver X Receptor Agonist Mediated by Inhibition of Hepatic Gluconeogenesis. J. Biol. Chem. 278, 1131–1136. doi:10.1074/jbc.M210208200
Carazo, A., Mladěnka, P., and Pávek, P. (2019). Marine Ligands of the Pregnane X Receptor (PXR): An Overview. Mar. Drugs 17, 554. doi:10.3390/md17100554
Carotti, A., Marinozzi, M., Custodi, C., Cerra, B., Pellicciari, R., Gioiello, A., et al. (2014). Beyond Bile Acids: Targeting Farnesoid X Receptor (FXR) with Natural and Synthetic Ligands. Curr. Top. Med. Chem. 14, 2129–2142. doi:10.2174/1568026614666141112094058
Carter, B. A., Taylor, O. A., Prendergast, D. R., Zimmerman, T. L., Von Furstenberg, R., Moore, D. D., et al. (2007). Stigmasterol, a Soy Lipid-Derived Phytosterol, Is an Antagonist of the Bile Acid Nuclear Receptor FXR. Pediatr. Res. 62, 301–306. doi:10.1203/PDR.0b013e3181256492
Castrillo, A., Joseph, S. B., Marathe, C., Mangelsdorf, D. J., and Tontonoz, P. (2003). Liver X Receptor-dependent Repression of Matrix Metalloproteinase-9 Expression in Macrophages. J. Biol. Chem. 278, 10443–10449. doi:10.1074/jbc.M213071200
Castro Navas, F. F., Giorgi, G., Maggioni, D., Pacciarini, M., Russo, V., and Marinozzi, M. (2018). C24-hydroxylated Stigmastane Derivatives as Liver X Receptor Agonists. Chem. Phys. Lipids 212, 44–50. doi:10.1016/j.chemphyslip.2018.01.005
Cave, M. C., Clair, H. B., Hardesty, J. E., Falkner, K. C., Feng, W., Clark, B. J., et al. (2016). Nuclear Receptors and Nonalcoholic Fatty Liver Disease. Biochim. Biophys. Acta 1859, 1083–1099. doi:10.1016/j.bbagrm.2016.03.002
Cavelier, C., Lorenzi, I., Rohrer, L., and von Eckardstein, A. (2006). Lipid Efflux by the ATP-Binding Cassette Transporters ABCA1 and ABCG1. Biochim. Biophys. Acta 1761, 655–666. doi:10.1016/j.bbalip.2006.04.012
Chen, J., Tian, J., Ge, H., Liu, R., and Xiao, J. (2017a). Effects of Tetramethylpyrazine from Chinese Black Vinegar on Antioxidant and Hypolipidemia Activities in HepG2 Cells. Food Chem. Toxicol. 109, 930–940. doi:10.1016/j.fct.2016.12.017
Chen, P., Li, J., Fan, X., Zeng, H., Deng, R., Li, D., et al. (2015a). Oleanolic Acid Attenuates Obstructive Cholestasis in Bile Duct-Ligated Mice, Possibly via Activation of NRF2-MRPs and FXR Antagonism. Eur. J. Pharmacol. 765, 131–139. doi:10.1016/j.ejphar.2015.08.029
Chen, P., Zeng, H., Wang, Y., Fan, X., Xu, C., Deng, R., et al. (2014a). Low Dose of Oleanolic Acid Protects against Lithocholic Acid-Induced Cholestasis in Mice: Potential Involvement of Nuclear Factor-E2-Related Factor 2-mediated Upregulation of Multidrug Resistance-Associated Proteins. Drug Metab. Dispos. 42, 844–852. doi:10.1124/dmd.113.056549
Chen, Q., Wang, T., Li, J., Wang, S., Qiu, F., Yu, H., et al. (2017b). Effects of Natural Products on Fructose-Induced Nonalcoholic Fatty Liver Disease (NAFLD). Nutrients 9, 96. doi:10.3390/nu9020096
Chen, S. F., Chen, P. Y., Hsu, H. J., Wu, M. J., and Yen, J. H. (2017c). Xanthohumol Suppresses Mylip/Idol Gene Expression and Modulates Ldlr Abundance and Activity in HepG2 Cells. J. Agric. Food Chem. 65, 7908–7918. doi:10.1021/acs.jafc.7b02282
Chen, W. D., Wang, Y. D., Meng, Z., Zhang, L., and Huang, W. (2011). Nuclear Bile Acid Receptor FXR in the Hepatic Regeneration. Biochim. Biophys. Acta 1812, 888–892. doi:10.1016/j.bbadis.2010.12.006
Chen, X. L., Meng, Q., Wang, C. Y., Liu, Q., Sun, H. J., Huo, X. K., et al. (2015b). Protective Effects of Calycosin Against ccl4-Induced Liver Injury With Activation of fxr and Stat3 in Mice. Pharm. Res. 32, 538–548. doi:10.1007/s11095-014-1483-3
Chen, Y., Lu, W., Yang, K., Duan, X., Li, M., Chen, X., et al. (2020). Tetramethylpyrazine: A Promising Drug for the Treatment of Pulmonary Hypertension. Br. J. Pharmacol. 177, 2743–2764. doi:10.1111/bph.15000
Chen, Z., Liu, J., Fu, Z., Ye, C., Zhang, R., Song, Y., et al. (2014b). 24(S)-Saringosterol from Edible marine Seaweed Sargassum Fusiforme Is a Novel Selective LXRβ Agonist. J. Agric. Food Chem. 62, 6130–6137. doi:10.1021/jf500083r
Cheng, S., Liang, S., Liu, Q., Deng, Z., Zhang, Y., Du, J., et al. (2018). Diosgenin Prevents High-Fat Diet-Induced Rat Non-alcoholic Fatty Liver Disease through the AMPK and LXR Signaling Pathways. Int. J. Mol. Med. 41, 1089–1095. doi:10.3892/ijmm.2017.3291
Cheng, Y., Mai, J. Y., Wang, M. F., Chen, G. F., and Ping, J. (2017). Antifibrotic Effect of Total Flavonoids of Astmgali Radix on Dimethylnitrosamine-Induced Liver Cirrhosis in Rats. Chin. J. Integr. Med. 23, 48–54. doi:10.1007/s11655-016-2627-6
Chinetti-Gbaguidi, G., and Staels, B. (2009). Lipid Ligand-Activated Transcription Factors Regulating Lipid Storage and Release in Human Macrophages. Biochim. Biophys. Acta 1791, 486–493. doi:10.1016/j.bbalip.2009.01.009
Choi, H., Hwang, H., Chin, J., Kim, E., Lee, J., Nam, S. J., et al. (2011). Tuberatolides, Potent FXR Antagonists from the Korean marine Tunicate Botryllus Tuberatus. J. Nat. Prod. 74, 90–94. doi:10.1021/np100489u
Cragg, G. M., and Newman, D. J. (2013). Natural Products: a Continuing Source of Novel Drug Leads. Biochim. Biophys. Acta 1830, 3670–3695. doi:10.1016/j.bbagen.2013.02.008
Cuadrado, I., Fernández-Velasco, M., Boscá, L., and de Las Heras, B. (2011). Labdane Diterpenes Protect against Anoxia/reperfusion Injury in Cardiomyocytes: Involvement of Akt Activation. Cell Death Dis 2, e229. doi:10.1038/cddis.2011.113
Daugsch, A., Moraes, C. S., Fort, P., and Park, Y. K. (2008). Brazilian Red Propolis-Cchemical Composition and Botanical Origin. Evid. Based Complement. Alternat Med. 5, 435–441. doi:10.1093/ecam/nem057
De Boussac, H., Alioui, A., Viennois, E., Dufour, J., Trousson, A., Vega, A., et al. (2013). Oxysterol Receptors and Their Therapeutic Applications in Cancer Conditions. Expert Opin. Ther. Targets 17, 1029–1038. doi:10.1517/14728222.2013.820708
De Fabiani, E., Mitro, N., Anzulovich, A. C., Pinelli, A., Galli, G., and Crestani, M. (2001). The Negative Effects of Bile Acids and Tumor Necrosis Factor-Alpha on the Transcription of Cholesterol 7alpha-Hydroxylase Gene (CYP7A1) Converge to Hepatic Nuclear Factor-4: a Novel Mechanism of Feedback Regulation of Bile Acid Synthesis Mediated by Nuclear Receptors. J. Biol. Chem. 276, 30708–30716. doi:10.1074/jbc.M103270200
De Marino, S., Ummarino, R., D’Auria, M. V., Chini, M. G., Bifulco, G., D’Amore, C., et al. (2012). 4-Methylenesterols from Theonella Swinhoei Sponge Are Natural Pregnane-X-Receptor Agonists and Farnesoid-X-Receptor Antagonists that Modulate Innate Immunity. Steroids 77, 484–495. doi:10.1016/j.steroids.2012.01.006
De Marino, S., Ummarino, R., D’Auria, M. V., Chini, M. G., Bifulco, G., Renga, B., et al. (2011). Theonellasterols and Conicasterols from Theonella Swinhoei. Novel marine Natural Ligands for Human Nuclear Receptors. J. Med. Chem. 54, 3065–3075. doi:10.1021/jm200169t
Del Bas, J. M., Ricketts, M. L., Vaqué, M., Sala, E., Quesada, H., Ardevol, A., et al. (2009). Dietary Procyanidins Enhance Transcriptional Activity of Bile Acid-Activated FXR In Vitro and Reduce Triglyceridemia In Vivo in a FXR-dependent Manner. Mol. Nutr. Food Res. 53, 805–814. doi:10.1002/mnfr.200800364
Di Leva, F. S., Festa, C., D’Amore, C., De Marino, S., Renga, B., D’Auria, M. V., et al. (2013). Binding Mechanism of the Farnesoid X Receptor marine Antagonist Suvanine Reveals a Strategy to Forestall Drug Modulation on Nuclear Receptors. Design, Synthesis, and Biological Evaluation of Novel Ligands. J. Med. Chem. 56, 4701–4717. doi:10.1021/jm400419e
Dong, M., He, X., and Liu, R. H. (2007). Phytochemicals of Black Bean Seed coats: Isolation, Structure Elucidation, and Their Antiproliferative and Antioxidative Activities. J. Agric. Food Chem. 55, 6044–6051. doi:10.1021/jf070706d
Dong, R., Wang, J., Gao, X., Wang, C., Liu, K., Wu, J., et al. (2019). Yangonin Protects against Estrogen-Induced Cholestasis in a Farnesoid X Receptor-dependent Manner. Eur. J. Pharmacol. 857, 172461. doi:10.1016/j.ejphar.2019.172461
Duan, X., Meng, Q., Wang, C., Liu, Z., Liu, Q., Sun, H., et al. (2017). Calycosin Attenuates Triglyceride Accumulation and Hepatic Fibrosis in Murine Model of Non-alcoholic Steatohepatitis via Activating Farnesoid X Receptor. Phytomedicine 25, 83–92. doi:10.1016/j.phymed.2016.12.006
Duval, C., Touche, V., Tailleux, A., Fruchart, J. C., Fievet, C., Clavey, V., et al. (2006). Niemann-Pick C1 like 1 Gene Expression Is Down-Regulated by LXR Activators in the Intestine. Biochem. Biophys. Res. Commun. 340, 1259–1263. doi:10.1016/j.bbrc.2005.12.137
El-Gendy, B. E. M., Goher, S. S., Hegazy, L. S., Arief, M. M. H., and Burris, T. P. (2018). Recent Advances in the Medicinal Chemistry of Liver X Receptors. J. Med. Chem. 61, 10935–10956. doi:10.1021/acs.jmedchem.8b00045
Elia, J., Carbonnelle, D., Logé, C., Ory, L., Huvelin, J. M., Tannoury, M., et al. (2019). 4-cholesten-3-one Decreases Breast Cancer Cell Viability and Alters Membrane Raft-Localized EGFR Expression by Reducing Lipogenesis and Enhancing LXR-dependent Cholesterol Transporters. Lipids Health Dis. 18, 168. doi:10.1186/s12944-019-1103-7
Epifano, F., Genovese, S., James Squires, E., and Gray, M. A. (2012). Nelumal A, the Active Principle from Ligularia Nelumbifolia, Is a Novel Farnesoid X Receptor Agonist. Bioorg. Med. Chem. Lett. 22, 3130–3135. doi:10.1016/j.bmcl.2012.03.057
Fan, J. S., Liu, D. N., Huang, G., Xu, Z. Z., Jia, Y., Zhang, H. G., et al. (2012). Panax Notoginseng Saponins Attenuate Atherosclerosis via Reciprocal Regulation of Lipid Metabolism and Inflammation by Inducing Liver X Receptor Alpha Expression. J. Ethnopharmacol. 142, 732–738. doi:10.1016/j.jep.2012.05.053
Fiorucci, S., Distrutti, E., Bifulco, G., D’Auria, M. V., and Zampella, A. (2012a). Marine Sponge Steroids as Nuclear Receptor Ligands. Trends Pharmacol. Sci. 33, 591–601. doi:10.1016/j.tips.2012.08.004
Fiorucci, S., Zampella, A., and Distrutti, E. (2012b). Development of FXR, PXR and CAR Agonists and Antagonists for Treatment of Liver Disorders. Curr. Top. Med. Chem. 12, 605–624. doi:10.2174/156802612799436678
Forman, B. M., Goode, E., Chen, J., Oro, A. E., Bradley, D. J., Perlmann, T., et al. (1995). Identification of a Nuclear Receptor that Is Activated by Farnesol Metabolites. Cell 81, 687–693. doi:10.1016/0092-8674(95)90530-8
Forman, B. M., Ruan, B., Chen, J., Schroepfer, G. J., and Evans, R. M. (1997). The Orphan Nuclear Receptor LXRalpha Is Positively and Negatively Regulated by Distinct Products of Mevalonate Metabolism. Proc. Natl. Acad. Sci. U S A. 94, 10588–10593. doi:10.1073/pnas.94.20.10588
Fradera, X., Vu, D., Nimz, O., Skene, R., Hosfield, D., Wynands, R., et al. (2010). X-ray Structures of the LXRalpha LBD in its Homodimeric Form and Implications for Heterodimer Signaling. J. Mol. Biol. 399, 120–132. doi:10.1016/j.jmb.2010.04.005
Francisco, V., Figueirinha, A., Costa, G., Liberal, J., Ferreira, I., Lopes, M. C., et al. (2016). The Flavone Luteolin Inhibits Liver X Receptor Activation. J. Nat. Prod. 79, 1423–1428. doi:10.1021/acs.jnatprod.6b00146
Fu, Y., Hu, X., Cao, Y., Zhang, Z., and Zhang, N. (2015). Saikosaponin a Inhibits Lipopolysaccharide-Oxidative Stress and Inflammation in Human Umbilical Vein Endothelial Cells via Preventing TLR4 Translocation into Lipid Rafts. Free Radic. Biol. Med. 89, 777–785. doi:10.1016/j.freeradbiomed.2015.10.407
Gadaleta, R. M., Cariello, M., Sabbà, C., and Moschetta, A. (2015). Tissue-specific Actions of FXR in Metabolism and Cancer. Biochim. Biophys. Acta 1851, 30–39. doi:10.1016/j.bbalip.2014.08.005
Galle, M., Kladniew, B. R., Castro, M. A., Villegas, S. M., Lacunza, E., Polo, M., et al. (2015). Modulation by Geraniol of Gene Expression Involved in Lipid Metabolism Leading to a Reduction of Serum-Cholesterol and Triglyceride Levels. Phytomedicine 22, 696–704. doi:10.1016/j.phymed.2015.04.005
Gao, X., Fu, T., Wang, C., Ning, C., Kong, Y., Liu, Z., et al. (2017). Computational Discovery and Experimental Verification of Farnesoid X Receptor Agonist Auraptene to Protect against Cholestatic Liver Injury. Biochem. Pharmacol. 146, 127–138. doi:10.1016/j.bcp.2017.09.016
Gao, X., Wang, C., Ning, C., Liu, K., Wang, X., Liu, Z., et al. (2018). Hepatoprotection of Auraptene from Peels of Citrus Fruits against Thioacetamide-Induced Hepatic Fibrosis in Mice by Activating Farnesoid X Receptor. Food Funct. 9, 2684–2694. doi:10.1039/c8fo00107c
Ge, C. X., Yu, R., Xu, M. X., Li, P. Q., Fan, C. Y., Li, J. M., et al. (2016). Betaine Prevented Fructose-Induced NAFLD by Regulating LXRα/PPARα Pathway and Alleviating ER Stress in Rats. Eur. J. Pharmacol. 770, 154–164. doi:10.1016/j.ejphar.2015.11.043
Ge, Z., Zhang, M., Deng, X., Zhu, W., Li, K., and Li, C. (2017). Persimmon Tannin Promoted Macrophage Reverse Cholesterol Transport through Inhibiting ERK1/2 and Activating PPARγ Both In Vitro and In Vivo. J. Funct. Foods 38, 338–348. doi:10.1016/j.jff.2017.09.023
Genet, C., Strehle, A., Schmidt, C., Boudjelal, G., Lobstein, A., Schoonjans, K., et al. (2010). Structure-activity Relationship Study of Betulinic Acid, a Novel and Selective TGR5 Agonist, and its Synthetic Derivatives: Potential Impact in Diabetes. J. Med. Chem. 53, 178–190. doi:10.1021/jm900872z
Goldwasser, J., Cohen, P. Y., Yang, E., Balaguer, P., Yarmush, M. L., Nahmias, Y., et al. (2010). Transcriptional Regulation of Human and Rat Hepatic Lipid Metabolism by the Grapefruit Flavonoid Naringenin: Role of PPARα, PPARγ and LXRα. Plos One 5, e12399. doi:10.1371/journal.pone.0012399
González-Granillo, M., Steffensen, K. R., Granados, O., Torres, N., Korach-André, M., Ortíz, V., et al. (2012). Soy Protein Isoflavones Differentially Regulate Liver X Receptor Isoforms to Modulate Lipid Metabolism and Cholesterol Transport in the Liver and Intestine in Mice. Diabetologia 55, 2469–2478. doi:10.1007/s00125-012-2599-9
Goodwin, B. J., Zuercher, W. J., and Collins, J. L. (2008). Recent Advances in Liver X Receptor Biology and Chemistry. Curr. Top. Med. Chem. 8, 781–791. doi:10.2174/156802608784535075
Goto, T., Kim, Y. I., Funakoshi, K., Teraminami, A., Uemura, T., Hirai, S., et al. (2011). Farnesol, an Isoprenoid, Improves Metabolic Abnormalities in Mice via Both PPARα-dependent and -independent Pathways. Am. J. Physiol. Endocrinol. Metab. 301, E1022–E1032. doi:10.1152/ajpendo.00061.2011
Grienke, U., Mihály-Bison, J., Schuster, D., Afonyushkin, T., Binder, M., Guan, S. H., et al. (2011). Pharmacophore-based Discovery of FXR-Agonists. Part II: Identification of Bioactive Triterpenes from Ganoderma Lucidum. Bioorg. Med. Chem. 19, 6779–6791. doi:10.1016/j.bmc.2011.09.039
Gronemeyer, H., Gustafsson, J. A., and Laudet, V. (2004). Principles for Modulation of the Nuclear Receptor Superfamily. Nat. Rev. Drug Discov. 3, 950–964. doi:10.1038/nrd1551
Gu, M., Zhang, S., Zhao, Y., Huang, J., Wang, Y., Li, Y., et al. (2017a). Cycloastragenol Improves Hepatic Steatosis by Activating Farnesoid X Receptor Signalling. Pharmacol. Res. 121, 22–32. doi:10.1016/j.phrs.2017.04.021
Gu, M., Zhang, Y., Liu, C., Wang, D., Feng, L., Fan, S., et al. (2017b). Morin, a Novel Liver X Receptor α/β Dual Antagonist, Has Potent Therapeutic Efficacy for Nonalcoholic Fatty Liver Diseases. Br. J. Pharmacol. 174 (18), 3032–3044. doi:10.1111/bph.13933
Hahn, D., Kim, H., Yang, I., Chin, J., Hwang, H., Won, D. H., et al. (2016). The Halicylindramides, Farnesoid X Receptor Antagonizing Depsipeptides from a Petrosia Sp. Marine Sponge Collected in Korea. J. Nat. Prod. 79, 499–506. doi:10.1021/acs.jnatprod.5b00871
Han, C. Y., Kim, T. H., Koo, J. H., and Kim, S. G. (2016). Farnesoid X Receptor as a Regulator of Fuel Consumption and Mitochondrial Function. Arch. Pharm. Res. 39, 1062–1074. doi:10.1007/s12272-016-0812-y
Han, X., Song, J., Lian, L. H., Yao, Y. L., Shao, D. Y., Fan, Y., et al. (2018). Ginsenoside 25-OCH3-PPD Promotes Activity of LXRs to Ameliorate P2X7R-Mediated NLRP3 Inflammasome in the Development of Hepatic Fibrosis. J. Agric. Food Chem. 66, 7023–7035. doi:10.1021/acs.jafc.8b01982
Hannan, M. A., Sohag, A. A. M., Dash, R., Haque, M. N., Mohibbullah, M., Oktaviani, D. F., et al. (2020). Phytosterols of marine Algae: Insights into the Potential Health Benefits and Molecular Pharmacology. Phytomedicine 69, 153201. doi:10.1016/j.phymed.2020.153201
Harada, K., Makino, K., Shima, N., Okuyama, H., Esumi, T., Kubo, M., et al. (2013). Total Synthesis of Riccardin C and (±)-cavicularin via Pd-Catalyzed Ar-Ar Cross Couplings. Tetrahedron 69, 6959–6968. doi:10.1016/j.tet.2013.06.064
He, Y. Q., Ma, G. Y., Peng, J. N., Ma, Z. Y., and Hamann, M. T. (2012). Liver X Receptor and Peroxisome Proliferator-Activated Receptor Agonist from Cornus Alternifolia. Biochim. Biophys. Acta 1820, 1021–1026. doi:10.1016/j.bbagen.2012.02.004
Herath, K. B., Jayasuriya, H., Guan, Z., Schulman, M., Ruby, C., Sharma, N., et al. (2005). Anthrabenzoxocinones from Streptomyces Sp. As Liver X Receptor Ligands and Antibacterial Agents. J. Nat. Prod. 68, 1437–1440. doi:10.1021/np050176k
Hiebl, V., Ladurner, A., Latkolik, S., and Dirsch, V. M. (2018). Natural Products as Modulators of the Nuclear Receptors and Metabolic Sensors LXR, FXR and RXR. Biotechnol. Adv. 36, 1657–1698. doi:10.1016/j.biotechadv.2018.03.003
Hien, H. T. M., Ha, N. C., Thom, L. T., and Hong, D. D. (2017). Squalene Promotes Cholesterol Homeostasis in Macrophage and Hepatocyte Cells via Activation of Liver X Receptor (LXR) α and β. Biotechnol. Lett. 39, 1101–1107. doi:10.1002/asna.20081115010.1007/s10529-017-2345-y
Hoang, M. H., Jia, Y., Jun, H. J., Lee, J. H., Hwang, K. Y., Choi, D. W., et al. (2012b). Taurine Is a Liver X Receptor-α Ligand and Activates Transcription of Key Genes in the Reverse Cholesterol Transport without Inducing Hepatic Lipogenesis. Mol. Nutr. Food Res. 56, 900–911. doi:10.1002/mnfr.201100611
Hoang, M. H., Jia, Y., Jun, H. J., Lee, J. H., Lee, B. Y., and Lee, S. J. (2012c). Fucosterol Is a Selective Liver X Receptor Modulator that Regulates the Expression of Key Genes in Cholesterol Homeostasis in Macrophages, Hepatocytes, and Intestinal Cells. J. Agric. Food Chem. 60, 11567–11575. doi:10.1021/jf3019084
Hoang, M. H., Jia, Y., Jun, H. J., Lee, J. H., Lee, D. H., Hwang, B. Y., et al. (2012a). Ethyl 2,4,6-trihydroxybenzoate Is an Agonistic Ligand for Liver X Receptor that Induces Cholesterol Efflux from Macrophages without Affecting Lipid Accumulation in HepG2 Cells. Bioorg. Med. Chem. Lett. 22, 4094–4099. doi:10.1016/j.bmcl.2012.04.071
Hoang, M. H., Jia, Y., Mok, B., Jun, H. J., Hwang, K. Y., and Lee, S. J. (2015). Kaempferol Ameliorates Symptoms of Metabolic Syndrome by Regulating Activities of Liver X Receptor-β. J. Nutr. Biochem. 26, 868–875. doi:10.1016/j.jnutbio.2015.03.005
Hollman, D. A., Milona, A., van Erpecum, K. J., and van Mil, S. W. (2012). Anti-inflammatory and Metabolic Actions of FXR: Insights into Molecular Mechanisms. Biochim. Biophys. Acta 1821, 1443–1452. doi:10.1016/j.bbalip.2012.07.004
Hong, C., and Tontonoz, P. (2008). Coordination of Inflammation and Metabolism by PPAR and LXR Nuclear Receptors. Curr. Opin. Genet. Dev. 18, 461–467. doi:10.1016/j.gde.2008.07.016
Hong, C., and Tontonoz, P. (2014). Liver X Receptors in Lipid Metabolism: Opportunities for Drug Discovery. Nat. Rev. Drug Discov. 13, 433–444. doi:10.1038/nrd4280
Hsieh, Y. H., and Wang, S. Y. (2013). Lucidone from Lindera Erythrocarpa Makino Fruits Suppresses Adipogenesis in 3T3-L1 Cells and Attenuates Obesity and Consequent Metabolic Disorders in High-Fat Diet C57BL/6 Mice. Phytomedicine 20, 394–400. doi:10.1016/j.phymed.2012.11.007
Hsu, W. H., Chen, T. H., Lee, B. H., Hsu, Y. W., and Pan, T. M. (2014). Monascin and Ankaflavin Act as Natural AMPK Activators with PPARα Agonist Activity to Down-Regulate Nonalcoholic Steatohepatitis in High-Fat Diet-Fed C57BL/6 Mice. Food Chem. Toxicol. 64, 94–103. doi:10.1016/j.fct.2013.11.015
Hu, X., Zhang, N., and Fu, Y. (2018). Role of Liver X Receptor in Mastitis Therapy and Regulation of Milk Fat Synthesis. J. Mammary Gland Biol. Neoplasia 24, 73–83. doi:10.1007/s10911-018-9403-5
Huang, C. (2014). Natural Modulators of Liver X Receptors. J. Integr. Med. 12, 76–85. doi:10.1016/S2095-4964(14)60013-3
Huang, H., Xu, Y., Zhu, J., and Li, J. (2014). Recent Advances in Non-steroidal FXR Antagonists Development for Therapeutic Applications. Curr. Top. Med. Chem. 14, 2175–2187. doi:10.2174/1568026614666141112101840
Huo, X. K., Liu, J., Yu, Z. L., Wang, Y. F., Wang, C., Tian, X. G., et al. (2018). Alisma Orientale Extract Exerts the Reversing Cholestasis Effect by Activation of Farnesoid X Receptor. Phytomedicine 42, 34–42. doi:10.1016/j.phymed.2018.03.017
Hwang, Y. P., Choi, J. H., Kim, H. G., Khanal, T., Song, G. Y., Nam, M. S., et al. (2013). Saponins, Especially Platycodin D, from Platycodon Grandiflorum Modulate Hepatic Lipogenesis in High-Fat Diet-Fed Rats and High Glucose-Exposed HepG2 Cells. Toxicol. Appl. Pharmacol. 267, 174–183. doi:10.1016/j.taap.2013.01.001
Iio, A., Ohguchi, K., Iinuma, M., Nozawa, Y., and Ito, M. (2012a). Hesperetin Upregulates ABCA1 Expression and Promotes Cholesterol Efflux from THP-1 Macrophages. J. Nat. Prod. 75 (4), 563–566. doi:10.1021/np200696r
Iio, A., Ohguchi, K., Maruyama, H., Tazawa, S., Araki, Y., Ichihara, K., et al. (2012b). Ethanolic Extracts of Brazilian Red Propolis Increase ABCA1 Expression and Promote Cholesterol Efflux from THP-1 Macrophages. Phytomedicine 19, 383–388. doi:10.1016/j.phymed.2011.10.007
Inagaki, T., Choi, M., Moschetta, A., Peng, L., Cummins, C. L., McDonald, J. G., et al. (2005). Fibroblast Growth Factor 15 Functions as an Enterohepatic Signal to Regulate Bile Acid Homeostasis. Cell Metab 2, 217–225. doi:10.1016/j.cmet.2005.09.001
Inoue, M., Tanabe, H., Nakashima, K., Ishida, Y., and Kotani, H. (2014). Rexinoids Isolated from Sophora Tonkinensis with a Gene Expression Profile Distinct from the Synthetic Rexinoid Bexarotene. J. Nat. Prod. 77, 1670–1677. doi:10.1021/np5002016
Ishibashi, M., Filomenko, R., Rébé, C., Chevriaux, A., Varin, A., Derangère, V., et al. (2013). Knock-down of the Oxysterol Receptor LXRα Impairs Cholesterol Efflux in Human Primary Macrophages: Lack of Compensation by LXRβ Activation. Biochem. Pharmacol. 86, 122–129. doi:10.1016/j.bcp.2012.12.024
Jakobsson, T., Treuter, E., Gustafsson, J. Å., and Steffensen, K. R. (2012). Liver X Receptor Biology and Pharmacology: New Pathways, Challenges and Opportunities. Trends Pharmacol. Sci. 33, 394–404. doi:10.1016/j.tips.2012.03.013
Jang, Y. J., Son, H. J., Ahn, J., Jung, C. H., and Ha, T. (2016). Coumestrol Modulates Akt and Wnt/β-Catenin Signaling during the Attenuation of Adipogenesis. Food Funct. 7, 4984–4991. doi:10.1039/c6fo01127f
Janowski, B. A., Willy, P. J., Devi, T. R., Falck, J. R., and Mangelsdorf, D. J. (1996). An Oxysterol Signalling Pathway Mediated by the Nuclear Receptor LXR Alpha. Nature 383, 728–731. doi:10.1038/383728a0
Jayasuriya, H., Herath, K. B., Ondeyka, J. G., Guan, Z., Borris, R. P., Tiwari, S., et al. (2005). Diterpenoid, Steroid, and Triterpenoid Agonists of Liver X Receptors from Diversified Terrestrial Plants and marine Sources. J. Nat. Prod. 68, 1247–1252. doi:10.1002/ibd.2045710.1021/np050182g
Jeong, S. J., Park, J. G., Kim, S., Kweon, H. Y., Seo, S., Na, D. S., et al. (2015). Extract of Rhus Verniciflua Stokes Protects the Diet-Induced Hyperlipidemia in Mice. Arch. Pharm. Res. 38, 2049–2058. doi:10.1007/s12272-015-0579-6
Ji, W., and Gong, B. Q. (2008). Hypolipidemic Activity and Mechanism of Purified Herbal Extract of Salvia Miltiorrhiza in Hyperlipidemic Rats. J. Ethnopharmacol. 119, 291–298. doi:10.1016/j.jep.2008.07.013
Ji, W., and Gong, B. Q. (2007). Hypolipidemic Effects and Mechanisms of Panax Notoginseng on Lipid Profile in Hyperlipidemic Rats. J. Ethnopharmacol. 113, 318–324. doi:10.1016/j.jep.2007.06.022
Jia, Y., Hoang, M. H., Jun, H. J., Lee, J. H., and Lee, S. J. (2013). Cyanidin, a Natural Flavonoid, Is an Agonistic Ligand for Liver X Receptor Alpha and Beta and Reduces Cellular Lipid Accumulation in Macrophages and Hepatocytes. Bioorg. Med. Chem. Lett. 23, 4185–4190. doi:10.1016/j.bmcl.2013.05.030
Jia, Y., Li, Z. Y., Zhang, H. G., Li, H. B., Liu, Y., and Li, X. H. (2010). Panax Notoginseng Saponins Decrease Cholesterol Ester via Up-Regulating ATP-Binding Cassette Transporter A1 in Foam Cells. J. Ethnopharmacol. 132, 297–302. doi:10.1016/j.jep.2010.08.033
Jiang, T., Ren, K., Chen, Q., Li, H., Yao, R., Hu, H., et al. (2017). Leonurine Prevents Atherosclerosis via Promoting the Expression of ABCA1 and ABCG1 in a Pparγ/Lxrα Signaling Pathway-dependent Manner. Cell Physiol. Biochem. 43, 1703–1717. doi:10.1159/000484031
Jiang, Z., Huang, X., Huang, S., Guo, H., Wang, L., Li, X., et al. (2016). Sex-Related Differences of Lipid Metabolism Induced by Triptolide: The Possible Role of the LXRα/SREBP-1 Signaling Pathway. Front. Pharmacol. 7, 87. doi:10.3389/fphar.2016.00087
Jiang, Z. P., Luan, Z. L., Liu, R. X., Zhang, Q., Ma, X. C., Shen, L., et al. (2018). Mangrove Tirucallane- and Apotirucallane-type Triterpenoids: Structure Diversity of the C-17 Side-Chain and Natural Agonists of Human Farnesoid/Pregnane⁻X⁻Receptor. Mar. Drugs 16, 488. doi:10.3390/md16120488
Jones, W. P., Chin, Y. W., and Kinghorn, A. D. (2006). The Role of Pharmacognosy in Modern Medicine and Pharmacy. Curr. Drug Targets 7, 247–264. doi:10.2174/138945006776054915
Jun, H. J., Hoang, M. H., Lee, J. W., Yaoyao, J., Lee, J. H., Lee, D. H., et al. (2012). Iristectorigenin B Isolated from Belamcanda Chinensis Is a Liver X Receptor Modulator that Increases ABCA1 and ABCG1 Expression in Macrophage RAW 264.7 Cells. Biotechnol. Lett. 34, 2213–2221. doi:10.1007/s10529-012-1036-y
Jun, H. J., Hoang, M. H., Yeo, S. K., Jia, Y., and Lee, S. J. (2013). Induction of ABCA1 and ABCG1 Expression by the Liver X Receptor Modulator Cineole in Macrophages. Bioorg. Med. Chem. Lett. 23, 579–583. doi:10.1016/j.bmcl.2012.11.012
Jung, C. G., Horike, H., Cha, B. Y., Uhm, K. O., Yamauchi, R., Yamaguchi, T., et al. (2010). Honokiol Increases ABCA1 Expression Level by Activating Retinoid X Receptor Beta. Biol. Pharm. Bull. 33, 1105–1111. doi:10.1248/bpb.33.1105
Kandanur, S. G. S., Tamang, N., Golakoti, N. R., and Nanduri, S. (2019). Andrographolide: A Natural Product Template for the Generation of Structurally and Biologically Diverse Diterpenes. Eur. J. Med. Chem. 176, 513–533. doi:10.1016/j.ejmech.2019.05.022
Kawase, A., Yamada, A., Gamou, Y., Tahara, C., Takeshita, F., Murata, K., et al. (2012). Increased Effects of Ginsenosides on the Expression of Cholesterol 7α-Hydroxylase but Not the Bile Salt export Pump Are Involved in Cholesterol Metabolism. J. Nat. Med. 67, 545–553. doi:10.1007/s11418-012-0713-4
Kim, M. H., Kang, K. S., and Lee, Y. S. (2010a). The Inhibitory Effect of Genistein on Hepatic Steatosis Is Linked to Visceral Adipocyte Metabolism in Mice with Diet-Induced Non-alcoholic Fatty Liver Disease. Br. J. Nutr. 104, 1333–1342. doi:10.1017/s0007114510002266
Kim, S., Yoon, Y. Y., Park, Y. W., Whang, W. K., Park, S. Y., and Hwang, K. W. (2020). Cynandione A from Cynanchum Wilfordii Inhibits Hepatic De Novo Lipogenesis by Activating the LKB1/AMPK Pathway in HepG2 Cells. J. Nat. Med. 74, 142–152. doi:10.1007/s11418-019-01356-x
Kim, Y. M., Kim, T. H., Kim, Y. W., Yang, Y. M., Ryu, D. H., Hwang, S. J., et al. (2010b). Inhibition of Liver X Receptor-α-dependent Hepatic Steatosis by Isoliquiritigenin, a Licorice Antioxidant Flavonoid, as Mediated by JNK1 Inhibition. Free Radic. Biol. Med. 49, 1722–1734. doi:10.1016/j.freeradbiomed.2010.09.001
Kim, Y. W., Kim, Y. M., Yang, Y. M., Kim, T. H., Hwang, S. J., Lee, J. R., et al. (2010c). Inhibition of SREBP-1c-Mediated Hepatic Steatosis and Oxidative Stress by Sauchinone, an AMPK-Activating Lignan in Saururus Chinensis. Free Radic. Biol. Med. 48, 567–578. doi:10.1016/j.freeradbiomed.2009.12.006
Koehn, F. E., and Carter, G. T. (2005). The Evolving Role of Natural Products in Drug Discovery. Nat. Rev. Drug Discov. 4, 206–220. doi:10.1038/nrd1657
Komati, R., Spadoni, D., Zheng, S., Sridhar, J., Riley, K. E., and Wang, G. (2017). Ligands of Therapeutic Utility for the Liver X Receptors. Molecules 22, 88. doi:10.3390/molecules22010088
Kuang, Y. L., Paulson, K. E., Lichtenstein, A. H., and Lamon-Fava, S. (2012). Regulation of the Expression of Key Genes Involved in HDL Metabolism by Unsaturated Fatty Acids. Br. J. Nutr. 108, 1351–1359. doi:10.1017/s0007114511006854
Kumar, G., Singh, D., Tali, J. A., Dheer, D., and Shankar, R. (2020). Andrographolide: Chemical Modification and its Effect on Biological Activities. Bioorg. Chem. 95, 103511. doi:10.1016/j.bioorg.2019.103511
Ky, P. T., Huong, P. T., My, T. K., Anh, P. T., Kiem, P. V., Minh, C. V., et al. (2010). Dammarane-type Saponins from Gynostemma Pentaphyllum. Phytochemistry 71, 994–1001. doi:10.1016/j.phytochem.2010.03.009
Lambert, G., Amar, M. J., Guo, G., Brewer, H. B., Gonzalez, F. J., and Sinal, C. J. (2003). The Farnesoid X-Receptor Is an Essential Regulator of Cholesterol Homeostasis. J. Biol. Chem. 278, 2563–2570. doi:10.1074/jbc.M209525200
Lee, J., Jung, E., Lee, J., Kim, S., Huh, S., Kim, Y., et al. (2009). Isorhamnetin Represses Adipogenesis in 3T3-L1 Cells. Obesity (Silver Spring) 17, 226–232. doi:10.1038/oby.2008.472
Lee, J. M., Gang, G. T., Kim, D. K., Kim, Y. D., Koo, S. H., Lee, C. H., et al. (2014). Ursodeoxycholic Acid Inhibits Liver X Receptor α-mediated Hepatic Lipogenesis via Induction of the Nuclear Corepressor SMILE. J. Biol. Chem. 289, 1079–1091. doi:10.1074/jbc.M113.491522
Lee, S. M., Moon, J., Cho, Y., Chung, J. H., and Shin, M. J. (2013). Quercetin Up-Regulates Expressions of Peroxisome Proliferator-Activated Receptor γ, Liver X Receptor α, and ATP Binding Cassette Transporter A1 Genes and Increases Cholesterol Efflux in Human Macrophage Cell Line. Nutr. Res. 33, 136–143. doi:10.1016/j.nutres.2012.11.010
Lefebvre, P., Cariou, B., Lien, F., Kuipers, F., and Staels, B. (2009). Role of Bile Acids and Bile Acid Receptors in Metabolic Regulation. Physiol. Rev. 89, 147–191. doi:10.1152/physrev.00010.2008
Lehmann, J. M., Kliewer, S. A., Moore, L. B., Smith-Oliver, T. A., Oliver, B. B., Su, J. L., et al. (1997). Activation of the Nuclear Receptor Lxr by Oxysterols Defines a New Hormone Response Pathway. J. Biol. Chem. 272, 3137–3140. doi:10.1074/jbc.272.6.3137
Lei, Y., Fu, P., Jun, X., and Cheng, P. (2019). Pharmacological Properties of Geraniol – A Review. Planta. Med. 85, 48–55. doi:10.1055/a-0750-6907
Li, C., Li, Y., and Gai, Z. (2019). Bile Acids and Farnesoid X Receptor: Novel Target for the Treatment of Diabetic Cardiomyopathy. Curr. Protein Pept. Sci. 20, 976–983. doi:10.2174/1389203720666190726152847
Li, C. H., Gong, D., Chen, L. Y., Zhang, M., Xia, X. D., Cheng, H. P., et al. (2017). Puerarin Promotes ABCA1-Mediated Cholesterol Efflux and Decreases Cellular Lipid Accumulation in THP-1 Macrophages. Eur. J. Pharmacol. 811, 74–86. doi:10.1016/j.ejphar.2017.05.055
Li, C. J., Chen, P. N., Li, H. J., Mahmud, T., Wu, D. L., Xu, J., et al. (2020a). Potential Antidiabetic Fumiquinazoline Alkaloids from the marine-derived Fungus Scedosporium Apiospermum F41-1. J. Nat. Prod. 83, 1082–1091. doi:10.1021/acs.jnatprod.9b01096
Li, G., Lin, W., Araya, J. J., Chen, T., Timmermann, B. N., and Guo, G. L. (2012). A tea Catechin, Epigallocatechin-3-Gallate, Is a Unique Modulator of the Farnesoid X Receptor. Toxicol. Appl. Pharmacol. 258, 268–274. doi:10.1016/j.taap.2011.11.006
Li, L., Bonneton, F., Chen, X. Y., and Laudet, V. (2015). Botanical Compounds and Their Regulation of Nuclear Receptor Action: the Case of Traditional Chinese Medicine. Mol. Cel Endocrinol. 401, 221–237. doi:10.1016/j.mce.2014.10.028
Li, T., Hu, S. M., Pang, X. Y., Wang, J. F., Yin, J. Y., Li, F. H., et al. (2020b). The marine-derived Furanone Reduces Intracellular Lipid Accumulation In Vitro by Targeting LXRα and PPARα. J. Cel Mol. Med. 24, 3384–3398. doi:10.1111/jcmm.15012
Li, T., Xu, L., Zheng, R., Wang, X., Li, L., Ji, H., et al. (2020c). Picroside II Protects against Cholestatic Liver Injury Possibly through Activation of Farnesoid X Receptor. Phytomedicine 68, 153153. doi:10.1016/j.phymed.2019.153153
Li, T., Yin, J., Ji, Y., Lin, P., Li, Y., Yang, Z., et al. (2020d). Setosphapyrone C and D Accelerate Macrophages Cholesterol Efflux by Promoting LXRα/ABCA1 Pathway. Arch. Pharm. Res. 43, 788–797. doi:10.1007/s12272-020-01255-w
Li, T., Zheng, R., Xu, L., Zhou, M., Wang, X., Guo, Q., et al. (2020e). Picroside II Alleviates Liver Injury Induced by Alpha-Naphthylisothiocyanate through AMPK-FXR Pathway. Toxicol. Appl. Pharmacol. 408, 115248. doi:10.1016/j.taap.2020.115248
Liang, X., Zhang, X., Lu, X., Zheng, Z., Ma, X., and Qi, S. (2019). Diketopiperazine-Type Alkaloids from a Deep-Sea-Derived Aspergillus puniceus Fungus and Their Effects on Liver X Receptor α. J. Nat. Prod. 82, 1558–1564. doi:10.1021/acs.jnatprod.9b00055
Liang, Z., Gu, T., Wang, J., She, J., Ye, Y., Cao, W., et al. (2021). Chromene and Chromone Derivatives as Liver X Receptors Modulators from a marine-derived Pestalotiopsis Neglecta Fungus. Bioorg. Chem. 112, 104927. doi:10.1016/j.bioorg.2021.104927
Liang, Z., Chen, Y., Gu, T., She, J., Dai, F., Jiang, H., et al. (2021). LXR-mediated Regulation of Marine-Derived Piericidins Aggravates High-Cholesterol Diet-Induced Cholesterol Metabolism Disorder in Mice. J. Med. Chem. 64, 9943–9959. doi:10.1021/acs.jmedchem.1c00175
Liao, G. F., Wu, Z. H., Liu, Y., Yan, Y. M., Lu, R. M., and Cheng, Y. X. (2019). Ganocapenoids A-D: Four New Aromatic Meroterpenoids from Ganoderma Capense. Bioorg. Med. Chem. Lett. 29, 143–147. doi:10.1016/j.bmcl.2018.12.011
Lin, H.-R. (2013a). Identification of Liver X Receptor and Farnesoid X Receptor Dual Agonists from Tithonia Diversifolia. Med. Chem. Res. 22, 3270–3281. doi:10.1007/s00044-012-0359-5
Lin, H. R., Chou, T. H., Huang, D. W., and Chen, I. S. (2014). Cryptochinones from Cryptocarya Chinensis Act as Farnesoid X Receptor Agonists. Bioorg. Med. Chem. Lett. 24, 4181–4186. doi:10.1016/j.bmcl.2014.07.045
Lin, H. R. (2015). Lepidozenolide from the Liverwort Lepidozia Fauriana Acts as a Farnesoid X Receptor Agonist. J. Asian Nat. Prod. Res. 17, 149–158. doi:10.1080/10286020.2014.964689
Lin, H. R. (2013b). Paeoniflorin Acts as a Liver X Receptor Agonist. J. Asian Nat. Prod. Res. 15, 35–45. doi:10.1080/10286020.2012.742510
Lin, H. R. (2012). Triterpenes from Alisma Orientalis Act as Farnesoid X Receptor Agonists. Bioorg. Med. Chem. Lett. 22, 4787–4792. doi:10.1016/j.bmcl.2012.05.057
Lin, Y., Ren, N., Li, S., Chen, M., and Pu, P. (2019). Novel Anti-obesity Effect of Scutellarein and Potential Underlying Mechanism of Actions. Biomed. Pharmacother. 117, 109042. doi:10.1016/j.biopha.2019.109042
Lin, Y. N., Wang, C. C. N., Chang, H. Y., Chu, F. Y., Hsu, Y. A., Cheng, W. K., et al. (2018). Ursolic Acid, a Novel Liver X Receptor α (LXRα) Antagonist Inhibiting Ligand-Induced Nonalcoholic Fatty Liver and Drug-Induced Lipogenesis. J. Agric. Food Chem. 66, 11647–11662. doi:10.1021/acs.jafc.8b04116
Ling, L. L., Schneider, T., Peoples, A. J., Spoering, A. L., Engels, I., Conlon, B. P., et al. (2015). Erratum: A New Antibiotic Kills Pathogens without Detectable Resistance. Nature 520, 388–459. doi:10.1038/nature1409810.1038/nature14303
Liu, J., Li, Y., Shi, H., Wang, T., Wu, X., Sun, X., et al. (2016). Components Characterization of Total Tetraploid Jiaogulan ( Gynostemma Pentaphyllum ) Saponin and its Cholesterol-Lowering Properties. J. Funct. Foods 23, 542–555. doi:10.1016/j.jff.2016.03.013
Liu, M., Zhang, G., Song, M., Wang, J., Shen, C., Chen, Z., et al. (2020a). Activation of Farnesoid X Receptor by Schaftoside Ameliorates Acetaminophen-Induced Hepatotoxicity by Modulating Oxidative Stress and Inflammation. Antioxid. Redox Signal. 33, 87–116. doi:10.1089/ars.2019.7791
Liu, M., Zhang, G., Wu, S., Song, M., Wang, J., Cai, W., et al. (2020b). Schaftoside Alleviates HFD-Induced Hepatic Lipid Accumulation in Mice via Upregulating Farnesoid X Receptor. J. Ethnopharmacol. 255, 112776. doi:10.1016/j.jep.2020.112776
Liu, W., and Wong, C. (2010). Oleanolic Acid Is a Selective Farnesoid X Receptor Modulator. Phytother. Res. 24, 369–373. doi:10.1002/ptr.2948
Liu, X. X., Zhang, X. W., Wang, K., Wang, X. Y., Ma, W. L., Cao, W., et al. (2018). Kuwanon G Attenuates Atherosclerosis by Upregulation of LXRα-Abca1/abcg1 and Inhibition of NFκB Activity in Macrophages. Toxicol. Appl. Pharmacol. 341, 56–63. doi:10.1016/j.taap.2018.01.007
Liu, Z., Law, W.-K., Wang, D., Nie, X., Sheng, D., Song, G., et al. (2014). Synthesis and Discovery of Andrographolide Derivatives as Non-steroidal Farnesoid X Receptor (FXR) Antagonists. RSC Adv. 4, 13533–13545. doi:10.1039/c3ra46715e
Lonard, D. M., and O’Malley, B. W. (2007). Nuclear Receptor Coregulators: Judges, Juries, and Executioners of Cellular Regulation. Mol. Cel. 27, 691–700. doi:10.1016/j.molcel.2007.08.012
Lu, T. T., Repa, J. J., and Mangelsdorf, D. J. (2001). Orphan Nuclear Receptors as eLiXiRs and FiXeRs of Sterol Metabolism. J. Biol. Chem. 276, 37735–37738. doi:10.1074/jbc.R100035200
Lu, X.-H., Shi, Q.-W., Zheng, Z.-H., Ke, A.-B., Zhang, H., Huo, C.-H., et al. (2011). Oxepinamides: Novel Liver X Receptor Agonists from Aspergillus puniceus. Eur. J. Org. Chem. 2011 (4), 802–807. doi:10.1002/ejoc.201000812
Lu, Y., Xi, W., Ding, X., Fan, S., Zhang, Y., Jiang, D., et al. (2013). Citrange Fruit Extracts Alleviate Obesity-Associated Metabolic Disorder in High-Fat Diet-Induced Obese C57BL/6 Mouse. Int. J. Mol. Sci. 14, 23736–23750. doi:10.3390/ijms141223736
Lu, Y., Zheng, W., Lin, S., Guo, F., Zhu, Y., Wei, Y., et al. (2018). Identification of an Oleanane-type Triterpene Hedragonic Acid as a Novel Farnesoid X Receptor Ligand with Liver Protective Effects and Anti-inflammatory Activity. Mol. Pharmacol. 93, 63–72. doi:10.1124/mol.117.109900
Luan, Z. L., Huo, X. K., Dong, P. P., Tian, X. G., Sun, C. P., Lv, X., et al. (2019). Highly Potent Non-steroidal FXR Agonists Protostane-type Triterpenoids: Structure-Activity Relationship and Mechanism. Eur. J. Med. Chem. 182, 111652. doi:10.1016/j.ejmech.2019.111652
Luo, N., Fang, J., Wei, L., Sahebkar, A., Little, P. J., Xu, S., et al. (2021). Emodin in Atherosclerosis Prevention: Pharmacological Actions and Therapeutic Potential. Eur. J. Pharmacol. 890, 173617. doi:10.1016/j.ejphar.2020.173617
Luo, X., Li, C., Luo, P., Lin, X., Ma, H., Seeram, N. P., et al. (2016). Pterosin Sesquiterpenoids from Pteris Cretica as Hypolipidemic Agents via Activating Liver X Receptors. J. Nat. Prod. 79, 3014–3021. doi:10.1021/acs.jnatprod.6b00558
Ma, K., Li, L., Bao, L., He, L., Sun, C., Zhou, B., et al. (2015). Six New 3,4-Seco-27-Norlanostane Triterpenes from the Medicinal Mushroom Ganoderma Boninense and Their Antiplasmodial Activity and Agonistic Activity to LXRβ. Tetrahedron 71, 1808–1814. doi:10.1016/j.tet.2015.02.002
Ma, X., Jiang, Y., Wen, J., Zhao, Y., Zeng, J., and Guo, Y. (2020). A Comprehensive Review of Natural Products to Fight Liver Fibrosis: Alkaloids, Terpenoids, Glycosides, Coumarins and Other Compounds. Eur. J. Pharmacol. 888, 173578. doi:10.1016/j.ejphar.2020.173578
Maithilikarpagaselvi, N., Sridhar, M. G., Swaminathan, R. P., Sripradha, R., and Badhe, B. (2016). Curcumin Inhibits Hyperlipidemia and Hepatic Fat Accumulation in High-Fructose-Fed Male Wistar Rats. Pharm. Biol. 54, 2857–2863. doi:10.1080/13880209.2016.1187179
Majdalawieh, A. F., Dalibalta, S., and Yousef, S. M. (2020). Effects of Sesamin on Fatty Acid and Cholesterol Metabolism, Macrophage Cholesterol Homeostasis and Serum Lipid Profile: A Comprehensive Review. Eur. J. Pharmacol. 885, 173417. doi:10.1016/j.ejphar.2020.173417
Majdalawieh, A. F., and Ro, H. S. (2014). Sesamol and Sesame (Sesamum indicum) Oil Enhance Macrophage Cholesterol Efflux via Up-Regulation of PPARγ1 and LXRα Transcriptional Activity in a MAPK-dependent Manner. Eur. J. Nutr. 54, 691–700. doi:10.1007/s00394-014-0747-3
Masaoutis, C., and Theocharis, S. (2018). The Farnesoid X Receptor: a Potential Target for Expanding the Therapeutic Arsenal against Kidney Disease. Expert Opin. Ther. Targets 23, 107–116. doi:10.1080/14728222.2019.1559825
McKenna, N. J., Cooney, A. J., DeMayo, F. J., Downes, M., Glass, C. K., Lanz, R. B., et al. (2009). Minireview: Evolution of NURSA, the Nuclear Receptor Signaling Atlas. Mol. Endocrinol. 23, 740–746. doi:10.1210/me.2009-0135
Meng, Q., Chen, X., Wang, C., Liu, Q., Sun, H., Sun, P., et al. (2015). Protective Effects of Alisol B 23-acetate from Edible Botanical Rhizoma Alismatis against Carbon Tetrachloride-Induced Hepatotoxicity in Mice. Food Funct. 6, 1241–1250. doi:10.1039/c5fo00082c
Meng, Q., Duan, X. P., Wang, C. Y., Liu, Z. H., Sun, P. Y., Huo, X. K., et al. (2017). Alisol B 23-acetate Protects against Non-alcoholic Steatohepatitis in Mice via Farnesoid X Receptor Activation. Acta Pharmacol. Sin. 38, 69–79. doi:10.1038/aps.2016.119
Mi, L. Z., Devarakonda, S., Harp, J. M., Han, Q., Pellicciari, R., Willson, T. M., et al. (2003). Structural Basis for Bile Acid Binding and Activation of the Nuclear Receptor FXR. Mol. Cel. 11, 1093–1100. doi:10.1016/S1097-2765(03)00112-6
Modica, S., Gadaleta, R. M., and Moschetta, A. (2010). Deciphering the Nuclear Bile Acid Receptor FXR Paradigm. Nucl. Recept. Signal. 8, e005. doi:10.1621/nrs.08005
Nakashima, K. I., Tomida, J., Hirai, T., Kawamura, Y., and Inoue, M. (2019). Paraconiothins A-J: Sesquiterpenoids from the Endophytic Fungus Paraconiothyrium Brasiliense ECN258. J. Nat. Prod. 82, 3347–3356. doi:10.1021/acs.jnatprod.9b00638
Nam, S. J., Ko, H., Ju, M. K., Hwang, H., Chin, J., Ham, J., et al. (2007). Scalarane Sesterterpenes from a marine Sponge of the Genus Spongia and Their Fxr Antagonistic Activity. J. Nat. Prod. 70, 1691–1695. doi:10.1021/np070024k
Nam, S. J., Ko, H., Shin, M., Ham, J., Chin, J., Kim, Y., et al. (2006). Farnesoid X-Activated Receptor Antagonists from a marine Sponge Spongia Sp. Bioorg. Med. Chem. Lett. 16, 5398–5402. doi:10.1016/j.bmcl.2006.07.079
Neuschwander-Tetri, B. A., Loomba, R., Sanyal, A. J., Lavine, J. E., Van Natta, M. L., Abdelmalek, M. F., et al. (2015). Farnesoid X Nuclear Receptor Ligand Obeticholic Acid for Non-cirrhotic, Non-alcoholic Steatohepatitis (FLINT): a Multicentre, Randomised, Placebo-Controlled Trial. Lancet 385, 956–965. doi:10.1016/s0140-6736(14)61933-4
Niesor, E. J., Flach, J., Lopes-Antoni, I., Perez, A., and Bentzen, C. L. (2001). The Nuclear Receptors FXR and LXRalpha: Potential Targets for the Development of Drugs Affecting Lipid Metabolism and Neoplastic Diseases. Curr. Pharm. Des. 7, 231–259. doi:10.2174/1381612013398185
Ning, Y., Xu, F., Xin, R., and Yao, F. (2020). Palmatine Regulates Bile Acid Cycle Metabolism and Maintains Intestinal flora Balance to Maintain Stable Intestinal Barrier. Life Sci. 262, 118405. doi:10.1016/j.lfs.2020.118405
Nozawa, H. (2005). Xanthohumol, the Chalcone from Beer Hops (Humulus Lupulus L.), Is the Ligand for Farnesoid X Receptor and Ameliorates Lipid and Glucose Metabolism in KK-A(y) Mice. Biochem. Biophys. Res. Commun. 336, 754–761. doi:10.1016/j.bbrc.2005.08.159
Oh, G. S., Lee, G. G., Yoon, J., Oh, W. K., and Kim, S. W. (2015b). Selective Inhibition of Liver X Receptor α-mediated Lipogenesis in Primary Hepatocytes by Licochalcone A. Chin. Med. 10, 8. doi:10.1186/s13020-015-0037-x
Oh, G. S., Yoon, J., Lee, G. G., Oh, W. K., and Kim, S. W. (2015a). 20(S)-protopanaxatriol Inhibits Liver X Receptor α-mediated Expression of Lipogenic Genes in Hepatocytes. J. Pharmacol. Sci. 128, 71–77. doi:10.1016/j.jphs.2015.05.007
Ohara, K., Wakabayashi, H., Taniguchi, Y., Shindo, K., Yajima, H., and Yoshida, A. (2013). Quercetin-3-O-glucuronide Induces ABCA1 Expression by LXRα Activation in Murine Macrophages. Biochem. Biophys. Res. Commun. 441, 929–934. doi:10.1016/j.bbrc.2013.10.168
Ondeyka, J. G., Jayasuriya, H., Herath, K. B., Guan, Z., Schulman, M., Collado, J., et al. (2005). Steroidal and Triterpenoidal Fungal Metabolites as Ligands of Liver X Receptors. J. Antibiot. (Tokyo) 58, 559–565. doi:10.1038/ja.2005.76
Ou, J., Tu, H., Shan, B., Luk, A., DeBose-Boyd, R. A., Bashmakov, Y., et al. (2001). Unsaturated Fatty Acids Inhibit Transcription of the Sterol Regulatory Element-Binding Protein-1c (SREBP-1c) Gene by Antagonizing Ligand-dependent Activation of the LXR. Proc. Natl. Acad. Sci. U S A. 98, 6027–6032. doi:10.1073/pnas.111138698
Park, H. S., Lee, K., Kim, S. H., Hong, M. J., Jeong, N. J., and Kim, M. S. (2020). Luteolin Improves Hypercholesterolemia and Glucose Intolerance through LXRα-dependent Pathway in Diet-Induced Obese Mice. J. Food Biochem. 44, e13358. doi:10.1111/jfbc.13358
Parks, D. J., Blanchard, S. G., Bledsoe, R. K., Chandra, G., Consler, T. G., Kliewer, S. A., et al. (1999). Bile Acids: Natural Ligands for an Orphan Nuclear Receptor. Science 284, 1365–1368. doi:10.1126/science.284.5418.1365
Pattanayak, S., and Bose, P. (2019). Herniarin, a Natural Coumarin, Inhibits Mammary Carcinogenesis by Modulating Liver X Receptor-Α/β-Pi3k-Akt-Maf1 Pathway in prague-dawley Rats. Phcog Mag. 15, 510. doi:10.4103/pm.pm_264_19
Periasamy, S., Hsu, D. Z., Chandrasekaran, V. R., and Liu, M. Y. (2013). Sesame Oil Accelerates Healing of 2,4,6-trinitrobenzenesulfonic Acid-Induced Acute Colitis by Attenuating Inflammation and Fibrosis. JPEN J. Parenter. Enteral Nutr. 37, 674–682. doi:10.1177/0148607112468768
Pisonero-Vaquero, S., García-Mediavilla, M. V., Jorquera, F., Majano, P. L., Benet, M., Jover, R., et al. (2014). Modulation of PI3K-lxrα-dependent Lipogenesis Mediated by Oxidative/nitrosative Stress Contributes to Inhibition of HCV Replication by Quercetin. Lab. Invest. 94, 262–274. doi:10.1038/labinvest.2013.156
Prakash, P., Singh, V., Jain, M., Rana, M., Khanna, V., Barthwal, M. K., et al. (2014). Silymarin Ameliorates Fructose Induced Insulin Resistance Syndrome by Reducing De Novo Hepatic Lipogenesis in the Rat. Eur. J. Pharmacol. 727, 15–28. doi:10.1016/j.ejphar.2014.01.038
Pu, S., Wu, X., Yang, X., Zhang, Y., Dai, Y., Zhang, Y., et al. (2019). The Therapeutic Role of Xenobiotic Nuclear Receptors against Metabolic Syndrome. Curr. Drug Metab. 20, 15–22. doi:10.2174/1389200219666180611083155
Qiu, J. (2007). Traditional Chinese Medicine and Western Science Face Almost Irreconcilable differences.Can Systems Biology Bring Them Together? Nature 448, 126–128. doi:10.1038/448126a
Qu, Y., Zhou, L., and Wang, C. (2016). Effects of Platycodin D on IL-1β-induced Inflammatory Response in Human Osteoarthritis Chondrocytes. Int. Immunopharmacol. 40, 474–479. doi:10.1016/j.intimp.2016.09.025
Ren, K., Jiang, T., and Zhao, G. J. (2018). Quercetin Induces the Selective Uptake of HDL-Cholesterol via Promoting SR-BI Expression and the Activation of the PPARγ/LXRα Pathway. Food Funct. 9, 624–635. doi:10.1039/c7fo01107e
Renga, B., Mencarelli, A., D’Amore, C., Cipriani, S., D’Auria, M. V., Sepe, V., et al. (2012). Discovery that Theonellasterol a marine Sponge Sterol Is a Highly Selective FXR Antagonist that Protects against Liver Injury in Cholestasis. Plos One 7, e30443. doi:10.1371/journal.pone.0030443
Repa, J. J., Turley, S. D., Lobaccaro, J. A., Medina, J., Li, L., Lustig, K., et al. (2000). Regulation of Absorption and ABC1-Mediated Efflux of Cholesterol by RXR Heterodimers. Science 289, 1524–1529. doi:10.1126/science.289.5484.1524
Ricketts, M. L., Boekschoten, M. V., Kreeft, A. J., Hooiveld, G. J., Moen, C. J., Müller, M., et al. (2007). The Cholesterol-Raising Factor from Coffee Beans, Cafestol, as an Agonist Ligand for the Farnesoid and Pregnane X Receptors. Mol. Endocrinol. 21, 1603–1616. doi:10.1210/me.2007-0133
Rincón, Y. A., Siless, G. E., Amado, L. D., Dansey, M. V., Grassi, E., Schenone, N., et al. (2020). Lanostanoid Triterpenes from the Fungus Rigidoporus Microporus. Nat. Prod. Res. 35, 1–10. doi:10.1080/14786419.2020.1752205
Russell, D. W. (2003). The Enzymes, Regulation, and Genetics of Bile Acid Synthesis. Annu. Rev. Biochem. 72, 137–174. doi:10.1146/annurev.biochem.72.121801.161712
Saenz, J., Santa-María, C., Reyes-Quiroz, M. E., Geniz, I., Jiménez, J., Sobrino, F., et al. (2018). Grapefruit Flavonoid Naringenin Regulates the Expression of LXRα in THP-1 Macrophages by Modulating AMP-Activated Protein Kinase. Mol. Pharm. 15, 1735–1745. doi:10.1021/acs.molpharmaceut.7b00797
Schroepfer, G. J. (2000). Oxysterols: Modulators of Cholesterol Metabolism and Other Processes. Physiol. Rev. 80, 361–554. doi:10.1152/physrev.2000.80.1.361
Sepe, V., Bifulco, G., Renga, B., D’Amore, C., Fiorucci, S., and Zampella, A. (2011). Discovery of Sulfated Sterols from marine Invertebrates as a New Class of marine Natural Antagonists of Farnesoid-X-Receptor. J. Med. Chem. 54, 1314–1320. doi:10.1021/jm101336m
Sepe, V., D’Amore, C., Ummarino, R., Renga, B., D’Auria, M. V., Novellino, E., et al. (2014). Insights on Pregnane-X-Receptor Modulation. Natural and Semisynthetic Steroids from Theonella marine Sponges. Eur. J. Med. Chem. 73, 126–134. doi:10.1016/j.ejmech.2013.12.005
Sepe, V., Ummarino, R., D’Auria, M. V., Chini, M. G., Bifulco, G., Renga, B., et al. (2012). Conicasterol E, a Small Heterodimer Partner Sparing Farnesoid X Receptor Modulator Endowed with a Pregnane X Receptor Agonistic Activity, from the marine Sponge Theonella Swinhoei. J. Med. Chem. 55, 84–93. doi:10.1021/jm201004p
Sevov, M., Elfineh, L., and Cavelier, L. B. (2006). Resveratrol Regulates the Expression of LXR-Alpha in Human Macrophages. Biochem. Biophys. Res. Commun. 348, 1047–1054. doi:10.1016/j.bbrc.2006.07.155
Shang, S. Z., Kong, L. M., Yang, L. P., Jiang, J., Huang, J., Zhang, H. B., et al. (2013). Bioactive Phenolics and Terpenoids from Manglietia Insignis. Fitoterapia 84, 58–63. doi:10.1016/j.fitote.2012.10.010
Shen, B. (2015). A New golden Age of Natural Products Drug Discovery. Cell 163, 1297–1300. doi:10.1016/j.cell.2015.11.031
Sheng, X., Wang, M., Lu, M., Xi, B., Sheng, H., and Zang, Y. Q. (2011a). Rhein Ameliorates Fatty Liver Disease through Negative Energy Balance, Hepatic Lipogenic Regulation, and Immunomodulation in Diet-Induced Obese Mice. Am. J. Physiol. Endocrinol. Metab. 300, E886–E893. doi:10.1152/ajpendo.00332.2010
Sheng, X., Zhu, X., Zhang, Y., Cui, G., Peng, L., Lu, X., et al. (2011b). Rhein Protects against Obesity and Related Metabolic Disorders through Liver X Receptor-Mediated Uncoupling Protein 1 Upregulation in Brown Adipose Tissue. Int. J. Biol. Sci. 8, 1375–1384. doi:10.7150/ijbs.4575
Shin, K., Chin, J., Hahn, D., Lee, J., Hwang, H., Won, D. H., et al. (2012). Sterols from a Soft Coral, Dendronephthya Gigantea as Farnesoid X-Activated Receptor Antagonists. Steroids 77 (5), 355–359. doi:10.1016/j.steroids.2011.12.027
Shinar, D. M., Endo, N., Rutledge, S. J., Vogel, R., Rodan, G. A., and Schmidt, A. (1994). Ner, a New Member of the Gene Family Encoding the Human Steroid Hormone Nuclear Receptor. Gene 147, 273–276. doi:10.1016/0378-1119(94)90080-9
Sim, W. C., Park, S., Lee, K. Y., Je, Y. T., Yin, H. Q., Choi, Y. J., et al. (2014). LXR-α Antagonist Meso-Dihydroguaiaretic Acid Attenuates High-Fat Diet-Induced Nonalcoholic Fatty Liver. Biochem. Pharmacol. 90, 414–424. doi:10.1016/j.bcp.2014.06.013
Sinal, C. J., Tohkin, M., Miyata, M., Ward, J. M., Lambert, G., and Gonzalez, F. J. (2000). Targeted Disruption of the Nuclear Receptor FXR/BAR Impairs Bile Acid and Lipid Homeostasis. Cell 102, 731–744. doi:10.1016/S0092-8674(00)00062-3
Singh, S. B., Ondeyka, J. G., Liu, W., Chen, S., Chen, T. S., Li, X., et al. (2005). Discovery and Development of Dimeric Podocarpic Acid Leads as Potent Agonists of Liver X Receptor with HDL Cholesterol Raising Activity in Mice and Hamsters. Bioorg. Med. Chem. Lett. 15, 2824–2828. doi:10.1016/j.bmcl.2005.03.100
Singh, S. P., and Sashidhara, K. V. (2017). Lipid Lowering Agents of Natural Origin: An Account of Some Promising Chemotypes. Eur. J. Med. Chem. 140, 331–348. doi:10.1016/j.ejmech.2017.09.020
Soisson, S. M., Parthasarathy, G., Adams, A. D., Sahoo, S., Sitlani, A., Sparrow, C., et al. (2008). Identification of a Potent Synthetic Fxr Agonist with an Unexpected Mode of Binding and Activation. Proc. Natl. Acad. Sci. U S A. 105, 5337–5342. doi:10.1073/pnas.0710981105
Song, X. Y., Xu, S., Hu, J. F., Tang, J., Chu, S. F., Liu, H., et al. (2015). Piperine Prevents Cholesterol Gallstones Formation in Mice. Eur. J. Pharmacol. 751, 112–117. doi:10.1016/j.ejphar.2015.01.038
Steri, R., Achenbach, J., Steinhilber, D., Schubert-Zsilavecz, M., and Proschak, E. (2012). Investigation of Imatinib and Other Approved Drugs as Starting Points for Antidiabetic Drug Discovery with FXR Modulating Activity. Biochem. Pharmacol. 83, 1674–1681. doi:10.1016/j.bcp.2012.02.027
Strege, M. A. (1999). High-performance Liquid Chromatographic-Electrospray Ionization Mass Spectrometric Analyses for the Integration of Natural Products with Modern High-Throughput Screening. J. Chromatogr. B. Biomed. Sci. Appl. 725, 67–78. doi:10.1016/S0378-4347(98)00553-2
Sun, R., Yang, N., Kong, B., Cao, B., Feng, D., Yu, X., et al. (2017). Orally Administered Berberine Modulates Hepatic Lipid Metabolism by Altering Microbial Bile Acid Metabolism and the Intestinal FXR Signaling Pathway. Mol. Pharmacol. 91, 110–122. doi:10.1124/mol.116.106617
Sutjarit, N., Sueajai, J., Boonmuen, N., Sornkaew, N., Suksamrarn, A., Tuchinda, P., et al. (2017). Curcuma Comosa Reduces Visceral Adipose Tissue and Improves Dyslipidemia in Ovariectomized Rats. J. Ethnopharmacol. 215, 167–175. doi:10.1016/j.jep.2017.12.027
Suzuki, T., Nishimaki-Mogami, T., Kawai, H., Kobayashi, T., Shinozaki, Y., Sato, Y., et al. (2006). Screening of Novel Nuclear Receptor Agonists by a Convenient Reporter Gene Assay System Using green Fluorescent Protein Derivatives. Phytomedicine 13, 401–411. doi:10.1016/j.phymed.2005.09.003
Suzuki, T., Tamehiro, N., Sato, Y., Kobayashi, T., Ishii-Watabe, A., Shinozaki, Y., et al. (2008). The Novel Compounds that Activate Farnesoid X Receptor: the Diversity of Their Effects on Gene Expression. J. Pharmacol. Sci. 107, 285–294. doi:10.1254/jphs.08006fp
Svensson, S., Ostberg, T., Jacobsson, M., Norström, C., Stefansson, K., Hallén, D., et al. (2014). Crystal Structure of the Heterodimeric Complex of LXRalpha and RXRbeta Ligand-Binding Domains in a Fully Agonistic Conformation. EMBO J. 22, 4625–4633. doi:10.1093/emboj/cdg456
Tai, T. S., Tien, N., Shen, H. Y., Chu, F. Y., Wang, C. C. N., Lu, C. H., et al. (2019). Sesamin, a Naturally Occurring Lignan, Inhibits Ligand-Induced Lipogenesis through Interaction with Liver X Receptor Alpha (LXRα) and Pregnane X Receptor (PXR). Evid. Based. Complement. Alternat. Med. 2019, 9401648. doi:10.1155/2019/9401648
Takahashi, M., Kanayama, T., Yashiro, T., Kondo, H., Murase, T., Hase, T., et al. (2008). Effects of Coumestrol on Lipid and Glucose Metabolism as a Farnesoid X Receptor Ligand. Biochem. Biophys. Res. Commun. 372, 395–399. doi:10.1016/j.bbrc.2008.04.136
Talbi, M., Saadali, B., Boriky, D., Bennani, L., Elkouali, M., and Ainane, T. (2016). Two Natural Compounds – a Benzofuran and a Phenylpropane – from Artemisia Dracunculus. J. Asian Nat. Prod. Res. 18, 724–729. doi:10.1080/10286020.2016.1158708
Tamehiro, N., Sato, Y., Suzuki, T., Hashimoto, T., Asakawa, Y., Yokoyama, S., et al. (2005). Riccardin C: a Natural Product that Functions as a Liver X Receptor (LXR)alpha Agonist and an LXRbeta Antagonist. FEBS Lett. 579, 5299–5304. doi:10.1016/j.febslet.2005.08.054
Tarling, E. J., and Edwards, P. A. (2011). ATP Binding Cassette Transporter G1 (ABCG1) Is an Intracellular Sterol Transporter. Proc. Natl. Acad. Sci. U S A. 108, 19719–19724. doi:10.1073/pnas.1113021108
Tian, Y., Cai, J., Gui, W., Nichols, R. G., Koo, I., Zhang, J., et al. (2019). Berberine Directly Affects the Gut Microbiota to Promote Intestinal Farnesoid X Receptor Activation. Drug Metab. Dispos. 47, 86–93. doi:10.1124/dmd.118.083691
Toppo, E., Sylvester Darvin, S., Esakkimuthu, S., Buvanesvaragurunathan, K., Ajeesh Krishna, T. P., Antony Caesar, S., et al. (2018). Curative Effect of Arjunolic Acid from Terminalia Arjuna in Non-alcoholic Fatty Liver Disease Models. Biomed. Pharmacother. 107, 979–988. doi:10.1016/j.biopha.2018.08.019
Traves, P. G., Hortelano, S., Zeini, M., Chao, T. H., Lam, T., Neuteboom, S. T., et al. (2007). Selective Activation of Liver X Receptors by Acanthoic Acid-Related Diterpenes. Mol. Pharmacol. 71, 1545–1553. doi:10.1124/mol.106.031906
Tsai, C. J., Liang, J. W., and Lin, H. R. (2012). Sesquiterpenoids from Atractylodes Macrocephala Act as Farnesoid X Receptor and Progesterone Receptor Modulators. Bioorg. Med. Chem. Lett. 22, 2326–2329. doi:10.1016/j.bmcl.2012.01.048
Uemura, T., Goto, T., Kang, M. S., Mizoguchi, N., Hirai, S., Lee, J. Y., et al. (2011). Diosgenin, the Main Aglycon of Fenugreek, Inhibits LXRα Activity in HepG2 Cells and Decreases Plasma and Hepatic Triglycerides in Obese Diabetic Mice. J. Nutr. 141, 17–23. doi:10.3945/jn.110.125591
Urizar, N. L., Liverman, A. B., Dodds, D. T., Silva, F. V., Ordentlich, P., Yan, Y., et al. (2002). A Natural Product that Lowers Cholesterol as an Antagonist Ligand for FXR. Science 296, 1703–1706. doi:10.1126/science.1072891
Urizar, N. L., and Moore, D. D. (2003). GUGULIPID: A Natural Cholesterol-Lowering Agent. Annu. Rev. Nutr. 23, 303–313. doi:10.1146/annurev.nutr.23.011702.073102
Vásquez, Y., Zhao, J., Khan, S. I., Gupta, M. P., and Khan, I. A. (2019). Constituents of Talisia Nervosa with Potential Utility against Metabolic Syndrome. Nat. Product. Commun. 14, 1934578X1901400–54. doi:10.1177/1934578X1901400114
Voloshyna, I., Hai, O., Littlefield, M. J., Carsons, S., and Reiss, A. B. (2013). Resveratrol Mediates Anti-atherogenic Effects on Cholesterol Flux in Human Macrophages and Endothelium via PPARγ and Adenosine. Eur. J. Pharmacol. 698, 299–309. doi:10.1016/j.ejphar.2012.08.024
Wang, C. M., Yuan, R. S., Zhuang, W. Y., Sun, J. H., Wu, J. Y., Li, H., et al. (2016). Schisandra Polysaccharide Inhibits Hepatic Lipid Accumulation by Downregulating Expression of SREBPs in NAFLD Mice. Lipids Health Dis. 15, 195. doi:10.1186/s12944-016-0358-5
Wang, D., Hiebl, V., Xu, T., Ladurner, A., Atanasov, A. G., Heiss, E. H., et al. (2020a). Impact of Natural Products on the Cholesterol Transporter ABCA1. J. Ethnopharmacol. 249, 112444. doi:10.1016/j.jep.2019.112444
Wang, D., Lao, L., Pang, X., Qiao, Q., Pang, L., Feng, Z., et al. (2018a). Asiatic Acid from Potentilla Chinensis Alleviates Non-alcoholic Fatty Liver by Regulating Endoplasmic Reticulum Stress and Lipid Metabolism. Int. Immunopharmacology 65, 256–267. doi:10.1016/j.intimp.2018.10.013
Wang, D. Q. (2007). Regulation of Intestinal Cholesterol Absorption. Annu. Rev. Physiol. 69, 221–248. doi:10.1146/annurev.physiol.69.031905.160725
Wang, F., Zhao, C., Tian, G., Wei, X., Ma, Z., Cui, J., et al. (2020b). Naringin Alleviates Atherosclerosis in ApoE-/- Mice by Regulating Cholesterol Metabolism Involved in Gut Microbiota Remodeling. J. Agric. Food Chem. 68, 12651–12660. doi:10.1021/acs.jafc.0c05800
Wang, H., He, Q., Wang, G., Xu, X., and Hao, H. (2018b). FXR Modulators for Enterohepatic and Metabolic Diseases. Expert Opin. Ther. Pat. 28, 765–782. doi:10.1080/13543776.2018.1527906
Wang, H., Luo, Y., Qiao, T., Wu, Z., and Huang, Z. (2018c). Luteolin Sensitizes the Antitumor Effect of Cisplatin in Drug-Resistant Ovarian Cancer via Induction of Apoptosis and Inhibition of Cell Migration and Invasion. J. Ovarian. Res. 11, 93. doi:10.1021/acs.jafc.0c0580010.1186/s13048-018-0468-y
Wang, J., Liang, Z., Li, K., Yang, B., Liu, Y., Fang, W., et al. (2020c). Ene-yne Hydroquinones from a Marine-derived Strain of the Fungus Pestalotiopsis Neglecta with Effects on Liver X Receptor Alpha. J. Nat. Prod. 83, 1258–1264. doi:10.1021/acs.jnatprod.0c00050
Wang, L., Wu, G., Wu, F., Jiang, N., and Lin, Y. (2017a). Geniposide Attenuates ANIT-Induced Cholestasis through Regulation of Transporters and Enzymes Involved in Bile Acids Homeostasis in Rats. J. Ethnopharmacol. 196, 178–185. doi:10.1016/j.jep.2016.12.022
Wang, S., Zhang, X., Liu, M., Luan, H., Ji, Y., Guo, P., et al. (2015). Chrysin Inhibits Foam Cell Formation through Promoting Cholesterol Efflux from RAW264.7 Macrophages. Pharm. Biol. 53, 1481–1487. doi:10.3109/13880209.2014.986688
Wang, W., Nakashima, K. I., Hirai, T., and Inoue, M. (2019). Correction to: Neuroprotective Effect of Naturally Occurring RXR Agonists Isolated from Sophora Tonkinensis Gagnep. On Amyloid-β-Induced Cytotoxicity in PC12 Cells. J. Nat. Med. 73, 683–684. doi:10.1007/s11418-018-1257-z10.1007/s11418-019-01305-8
Wang, X., Yan, J., Xu, X., Duan, C., Xie, Z., Su, Z., et al. (2018d). Puerarin Prevents LPS-Induced Acute Lung Injury via Inhibiting Inflammatory Response. Microb. Pathog. 118, 170–176. doi:10.1016/j.micpath.2018.03.033
Wang, Y., Zhang, X., Wei, Z., Wang, J., Zhang, Y., Shi, M., et al. (2017b). Platycodin D Suppressed LPS-Induced Inflammatory Response by Activating LXRα in LPS-Stimulated Primary Bovine Mammary Epithelial Cells. Eur. J. Pharmacol. 814, 138–143. doi:10.1016/j.ejphar.2017.07.037
Wei, X., Fan, X., Feng, Z., Ma, Y., Lan, X., and Chen, M. (2020). Ethyl Acetate Extract of Herpetospermum Pedunculosum Alleviates α-naphthylisothiocyanate-induced Cholestasis by Activating the Farnesoid X Receptor and Suppressing Oxidative Stress and Inflammation in Rats. Phytomedicine 76, 153257. doi:10.1016/j.phymed.2020.153257
Wei, X., Ma, Y., Dong, Z., Wang, G., Lan, X., Liao, Z., et al. (2021). Dehydrodiconiferyl Alcohol, a Lignan from Herpetospermum Pedunculosum, Alleviates Cholestasis by Activating Pathways Associated with the Farnesoid X Receptor. Phytomedicine 80, 153378. doi:10.1016/j.phymed.2020.153378
Williams, S., Bledsoe, R. K., Collins, J. L., Boggs, S., Lambert, M. H., Miller, A. B., et al. (20032003). X-ray crystal Structure of the Liver X Receptor Beta Ligand Binding Domain: Regulation by a Histidine-Tryptophan Switch. J. Biol. Chem. 278, 27138–27143. doi:10.1074/jbc.M302260200
Willy, P. J., Umesono, K., Ong, E. S., Evans, R. M., Heyman, R. A., and Mangelsdorf, D. J. (1995). LXR, a Nuclear Receptor that Defines a Distinct Retinoid Response Pathway. Genes Dev. 9, 1033–1045. doi:10.1101/gad.9.9.1033
Wink, M. (2003). Evolution of Secondary Metabolites from an Ecological and Molecular Phylogenetic Perspective. Phytochemistry 64, 3–19. doi:10.1016/S0031-9422(03)00300-5
Wong, C. P., Kaneda, T., and Morita, H. (2014). Plant Natural Products as an Anti-lipid Droplets Accumulation Agent. J. Nat. Med. 68, 253–266. doi:10.1007/s11418-014-0822-3
Wu, T., Zhong, L., Hong, Z., Li, Y., Liu, X., Pan, L., et al. (2015). The Effects of Zanthoxylum Bungeanum Extract on Lipid Metabolism Induced by Sterols. J. Pharmacol. Sci. 127, 251–259. doi:10.1016/j.jphs.2014.12.002
Xiao, P. T., Liu, S. Y., Kuang, Y. J., Jiang, Z. M., Lin, Y., Xie, Z. S., et al. (2021). Network Pharmacology Analysis and Experimental Validation to Explore the Mechanism of Sea Buckthorn Flavonoids on Hyperlipidemia. J. Ethnopharmacol. 264, 113380. doi:10.1016/j.jep.2020.113380
Xiao, Q., Zhang, S., Ren, H., Du, R., Li, J., Zhao, J., et al. (2020). Ginsenoside Rg1 Alleviates ANIT-Induced Intrahepatic Cholestasis in Rats via Activating Farnesoid X Receptor and Regulating Transporters and Metabolic Enzymes. Chem. Biol. Interact. 324, 109062. doi:10.1016/j.cbi.2020.109062
Xie, Z., Zhao, J., Wang, H., Jiang, Y., Yang, Q., Fu, Y., et al. (2020). Magnolol Alleviates Alzheimer’s Disease-like Pathology in Transgenic C. elegans by Promoting Microglia Phagocytosis and the Degradation of Beta-Amyloid through Activation of PPAR-γ. Biomed. Pharmacother. 124, 109886. doi:10.1016/j.biopha.2020.109886
Xu, J., Gu, W., Li, C., Li, X., Xing, G., Li, Y., et al. (2016a). Epigallocatechin Gallate Inhibits Hepatitis B Virus via Farnesoid X Receptor Alpha. J. Nat. Med. 70, 584–591. doi:10.1007/s11418-016-0980-6
Xu, W., Lu, C., Zhang, F., Shao, J., Yao, S., and Zheng, S. (2017a). Dihydroartemisinin Counteracts Fibrotic portal Hypertension via Farnesoid X Receptor-dependent Inhibition of Hepatic Stellate Cell Contraction. FEBS. J. 284, 114–133. doi:10.1111/febs.13956
Xu, W., Lu, C., Zhang, F., Shao, J., and Zheng, S. (2016b). Dihydroartemisinin Restricts Hepatic Stellate Cell Contraction via an FXR-S1PR2-dependent Mechanism. IUBMB Life 68, 376–387. doi:10.1002/iub.1492
Xu, Y., Niu, Y., Gao, Y., Wang, F., Qin, W., Lu, Y., et al. (2017b). Borapetoside E, a Clerodane Diterpenoid Extracted from Tinospora Crispa, Improves Hyperglycemia and Hyperlipidemia in High-Fat-Diet-Induced Type 2 Diabetes Mice. J. Nat. Prod. 80, 2319–2327. doi:10.1021/acs.jnatprod.7b00365
Xue, X., Quan, Y., Gong, L., Gong, X., and Li, Y. (2020). A Review of the Processed Polygonum Multiflorum (Thunb.) for Hepatoprotection: Clinical Use, Pharmacology and Toxicology. J. Ethnopharmacol. 261, 113121. doi:10.1016/j.jep.2020.113121
Yang, C., Li, Q., and Li, Y. (2014). Targeting Nuclear Receptors with marine Natural Products. Mar. Drugs 12, 601–635. doi:10.3390/md12020601
Yin, Y., Gao, L., Lin, H., Wu, Y., Han, X., Zhu, Y., et al. (2017). Luteolin Improves Non-alcoholic Fatty Liver Disease in Db/db Mice by Inhibition of Liver X Receptor Activation to Down-Regulate Expression of Sterol Regulatory Element Binding Protein 1c. Biochem. Biophys. Res. Commun. 482, 720–726. doi:10.1016/j.bbrc.2016.11.101
Yoshikawa, T., Shimano, H., Yahagi, N., Ide, T., Amemiya-Kudo, M., Matsuzaka, T., et al. (2002). Polyunsaturated Fatty Acids Suppress Sterol Regulatory Element-Binding Protein 1c Promoter Activity by Inhibition of Liver X Receptor (LXR) Binding to LXR Response Elements. J. Biol. Chem. 277 (3), 1705–1711. doi:10.1074/jbc.M105711200
Yu, B. Z., Kaimal, R., Bai, S., El Sayed, K. A., Tatulian, S. A., Apitz, R. J., et al. (2009). Effect of Guggulsterone and Cembranoids of Commiphora Mukul on Pancreatic Phospholipase A(2): Role in Hypocholesterolemia. J. Nat. Prod. 72, 24–28. doi:10.1021/np8004453
Yu, L., York, J., von Bergmann, K., Lutjohann, D., Cohen, J. C., and Hobbs, H. H. (2003). Stimulation of Cholesterol Excretion by the Liver X Receptor Agonist Requires ATP-Binding Cassette Transporters G5 and G8. J. Biol. Chem. 278, 15565–15570. doi:10.1074/jbc.M301311200
Zanella, I., Biasiotto, G., Holm, F., and di Lorenzo, D. (2017). Cereal Lignans, Natural Compounds of Interest for Human Health? Nat. Product. Commun. 12, 1934578X1701200–146. doi:10.1177/1934578X1701200139
Zhang, G., Sun, X., Wen, Y., Shi, A., Zhang, J., Wei, Y., et al. (2020a). Hesperidin Alleviates Cholestasis via Activation of the Farnesoid X Receptor In Vitro and In Vivo. Eur. J. Pharmacol. 885, 173498. doi:10.1016/j.ejphar.2020.173498
Zhang, J., Zhao, Y., Ren, D., and Yang, X. (2020b). Effect of Okra Fruit Powder Supplementation on Metabolic Syndrome and Gut Microbiota Diversity in High Fat Diet-Induced Obese Mice. Food Res. Int. 130, 108929. doi:10.1016/j.foodres.2019.108929
Zhang, L.-q., Zhao, Y.-y., Huang, C., Chen, K.-x., and Li, Y.-m. (2016). Scrodentoids F-I, Four C19-Norditerpenoids from Scrophularia Dentata. Tetrahedron 72, 8031–8035. doi:10.1016/j.tet.2016.10.035
Zhang, M., Pan, H., Xu, Y., Wang, X., Qiu, Z., and Jiang, L. (2017). Allicin Decreases Lipopolysaccharide-Induced Oxidative Stress and Inflammation in Human Umbilical Vein Endothelial Cells through Suppression of Mitochondrial Dysfunction and Activation of Nrf2. Cel Physiol. Biochem. 41, 2255–2267. doi:10.1159/000475640
Zhang, T., Zhong, S., Li, T., and Zhang, J. (2020c). Saponins as Modulators of Nuclear Receptors. Crit. Rev. Food Sci. Nutr. 60, 94–107. doi:10.1080/10408398.2018.1514580
Zhang, Y., Li, F., Jiang, X., Jiang, X., Wang, Y., Zhang, H., et al. (2020d). Sophoricoside Is a Selective LXRβ Antagonist with Potent Therapeutic Effects on Hepatic Steatosis of Mice. Phytother. Res. 34, 3168–3179. doi:10.1002/ptr.6747
Zhang, Z., Burch, P. E., Cooney, A. J., Lanz, R. B., Pereira, F. A., Wu, J., et al. (2004). Genomic Analysis of the Nuclear Receptor Family: New Insights into Structure, Regulation, and Evolution from the Rat Genome. Genome Res. 14, 580–590. doi:10.1101/gr.2160004
Zhao, A., Yu, J., Lew., J. L., Huang, L., Wright, S. D., and Cui, J. (2004). Polyunsaturated Fatty Acids Are FXR Ligands and Differentially Regulate Expression of FXR Targets. DNA Cel Biol 23, 519–526. doi:10.1089/1044549041562267
Zhao, H. L., Cho, K. H., Ha, Y. W., Jeong, T. S., Lee, W. S., and Kim, Y. S. (2006). Cholesterol-lowering Effect of Platycodin D in Hypercholesterolemic ICR Mice. Eur. J. Pharmacol. 537, 166–173. doi:10.1016/j.ejphar.2006.03.032
Zhao, J., Khan, S. I., Wang, M., Vasquez, Y., Yang, M. H., Avula, B., et al. (2014). Octulosonic Acid Derivatives from Roman Chamomile (Chamaemelum Nobile) with Activities against Inflammation and Metabolic Disorder. J. Nat. Prod. 77, 509–515. doi:10.1021/np400780n
Zhao, J. F., Jim Leu, S. J., Shyue, S. K., Su, K. H., Wei, J., and Lee, T. S. (2013). Novel Effect of Paeonol on the Formation of Foam Cells: Promotion of LXRα-ABCA1-dependent Cholesterol Efflux in Macrophages. Am. J. Chin. Med. 41, 1079–1096. doi:10.1142/s0192415x13500730
Zhao, W. W., Guo, W. W., Guo, J. F., Wang, X., Chen, X. Q., and Wu, X. (2021). Three New Flavonoids from Penthorum Chinense Pursh and Their Docking Studies. Nat. Prod. Res. 35, 49–56. doi:10.1080/14786419.2019.1613394
Zhao, X., and Liu, J. (2020). Chemical Constituents from the Fruits ofLigustrum Lucidum W.T.Aitonand Their Role on the Medicinal Treatment. Nat. Product. Commun. 15, 1934578X2092233. doi:10.1177/1934578x20922338
Zheng, J., Li, Z., Manabe, Y., Kim, M., Goto, T., Kawada, T., et al. (2018a). Siphonaxanthin, a Carotenoid from Green Algae, Inhibits Lipogenesis in Hepatocytes via the Suppression of Liver X Receptor α Activity. Lipids 53, 41–52. doi:10.1002/lipd.12002
Zheng, L., Yin, L., Xu, L., Qi, Y., Li, H., Xu, Y., et al. (2018b). Protective Effect of Dioscin against Thioacetamide-Induced Acute Liver Injury via FXR/AMPK Signaling Pathway In Vivo. Biomed. Pharmacother. 97, 481–488. doi:10.1016/j.biopha.2017.10.153
Zheng, W., Lu, Y., Lin, S., Wang, R., Qiu, L., Zhu, Y., et al. (2017a). A Novel Class of Natural FXR Modulators with a Unique Mode of Selective Co-regulator Assembly. Chembiochem 18, 721–725. doi:10.1002/cbic.201700059
Zheng, Z., Zhao, Z., Li, S., Lu, X., Jiang, M., Lin, J., et al. (2017b). Altenusin, a Nonsteroidal Microbial Metabolite, Attenuates Nonalcoholic Fatty Liver Disease by Activating the Farnesoid X Receptor. Mol. Pharmacol. 92, 425–436. doi:10.1124/mol.117.108829
Zhou, X., Liang, Z., Li, K., Fang, W., Tian, Y., Luo, X., et al. (2019). Exploring the Natural Piericidins as Anti-renal Cell Carcinoma Agents Targeting Peroxiredoxin 1. J. Med. Chem. 62, 7058–7069. doi:10.1021/acs.jmedchem.9b00598
Zhou, Y., Ding, Y. L., Zhang, J. L., Zhang, P., Wang, J. Q., and Li, Z. H. (2018). Alpinetin Improved High Fat Diet-Induced Non-alcoholic Fatty Liver Disease (NAFLD) through Improving Oxidative Stress, Inflammatory Response and Lipid Metabolism. Biomed. Pharmacother. 97, 1397–1408. doi:10.1016/j.biopha.2017.10.035
Zhu, J., Xu, K., Zhang, X., Cao, J., Jia, Z., Yang, R., et al. (2016). Studies on the Regulation of Lipid Metabolism and its Mechanism of the Iridoids Rich Fraction in Valeriana Jatamansi Jones. Biomed. Pharmacother. 84, 1891–1898. doi:10.1016/j.biopha.2016.10.099
Zhuang, J., Zhang, H., Zhou, R., Chen, L., Chen, J., and Shen, X. (2013). Regulation of Prostaglandin F2α against β Amyloid Clearance and its Inflammation Induction through LXR/RXR Heterodimer Antagonism in Microglia. Prostaglandins Other Lipid Mediat 106, 45–52. doi:10.1016/j.prostaglandins.2013.09.002
Zou, J., Jiang, J., Diao, Y. Y., Yang, L. B., Huang, J., Li, H. L., et al. (2012a). Cycloartane Triterpenoids from the Stems of Schisandra Glaucescens and Their Bioactivity. Fitoterapia 83, 926–931. doi:10.1016/j.fitote.2012.04.007
Zou, J., Yang, L. B., Jiang, J., Diao, Y. Y., Li, X. N., Huang, J., et al. (2012b). Lanostane Triterpenoids from the Stems of Schisandra Glaucescens. Planta. Med. 78, 472–479. doi:10.1055/s-0031-1298214
Zou, M., Nong, C., Yu, Z., Cai, H., Jiang, Z., Xue, R., et al. (2020). The Role of Invariant Natural Killer T Cells and Associated Immunoregulatory Factors in Triptolide-Induced Cholestatic Liver Injury. Food Chem. Toxicol. 146, 111777. doi:10.1016/j.fct.2020.111777
Glossary
ABCA1 ATP-binding cassette transporters A1
ABCG1 ATP-binding cassette transporters G1
ABCG5 ATP-binding cassette transporters G5
ABCG8 ATP-binding cassette transporters G8
AD alzheimer’s disease
AF-1 activation function-1
AF-2 activation function-2
ALT alanine aminotransferase
AMPK adenosine 5′-monophosphate-activated protein kinase
ANIT α-naphthylisothiocyanate
APOAI apolipoprotein AI
APOC1 apolipoproteins C1
APOC2 apolipoproteins C2
APOC4 apolipoproteins C3
APOE apolipoproteins E
AST aspartate aminotransferase
BA bile acid
CDCA chenodeoxycholic acid
CYP7A1 cholesterol 7α-hydroxylase
DBD DNA-binding domain
DCA deoxycholic acid
EC50 half maximal effective concentration
FGF19 fibroblast growth factor 19
FXR farnesoid X receptor
GLUT4 glucose transporter type 4
G6P glucose-6-phosphatase
GPA glucopiericidin A
HBV hepatitis B virus
HCV hepatitis C virus
HDL high-density lipoprotein
HFD high-fat diet
HPLC-ESI-MS high-performance liquid chromatography-electrospray ionization mass spectrometry
IBD inflammatory bowel disease
IC50 half maximal inhibitory concentration
IRS-1 insulin substrate receptor 1
KD dissociation equilibrium constant
LBD ligand-binding domain
LCA lithocholic acid
LCAT cholesterol acyltransferase enzyme
LDL-C low-density lipoprotein-cholesterol
LDLR low-density lipoprotein receptor
LPS lipopolysaccharide
LXRs liver X receptors
LXREs LXRs response elements
MDI mixture of 3-isobutyl-1-methylxanthine, dexamethasone and insulin
NAFLD nonalcoholic fatty liver disease
NASH nonalcoholic steatohepatitis
NLRP3 nucleotide-binding oligomerization domain, leucine-rich repeat and pyrin domain-containing 3
NMR nuclear magnetic resonance
NPC1 Niemann–Pick C1
NPC2 Niemann-Pick C2
NPC1L1 Niemann-Pick C1-like 1
NRs nuclear receptors
NR0 miscellaneous
NR1 thyroid hormone receptor-like
NR2 retinoid X receptor-like
NR3 estrogen receptor-like
NR4 nerve growth factor IB-like
NR5 steroidogenic factor-like
NR6 germ cell nuclear factor-like
NF-κB nuclear factor kappa-B
PA piericidin A
PEPCK phosphoenolpyruvate carboxy kinase
PPAR peroxisome proliferator-activated receptor
PUFAs polyunsaturated fatty acids
qPCR quantitative polymerase chain reaction
RCC renal cell carcinoma
RCT reverse cholesterol transport
RT-qPCR real-time quantitative polymerase chain reaction
RT-PCR real-time polymerase chain reaction
RXR retinoid X receptor
S value negative-binding free energy value
SBARM A selective bile acid receptor modulator
SHP small heterodimer partner
SPR surface plasmon resonance
SREBPs sterol-regulatory element-binding proteins
TBA total bile acid
TC total cholesterol
TCMs traditional Chinese medicines
T2D type 2 diabetes
TG triglyceride
TFA total flavonoids of Astmgali Radix
Keywords: natural products, liver X receptor, farnesoid X receptor, docking, agonists, antagonists
Citation: She J, Gu T, Pang X, Liu Y, Tang L and Zhou X (2022) Natural Products Targeting Liver X Receptors or Farnesoid X Receptor. Front. Pharmacol. 12:772435. doi: 10.3389/fphar.2021.772435
Received: 08 September 2021; Accepted: 22 November 2021;
Published: 05 January 2022.
Edited by:
Lei Chen, Guangdong Ocean University, ChinaReviewed by:
Prasanna K. Santhekadur, JSS Academy of Higher Education and Research, IndiaMaria Costa, Universidade Lusófona Research Center for Biosciences & Health Technologies, Portugal
Sheikh Mansoor, Sher-I-Kashmir Institute of Medical Sciences, India
Copyright © 2022 She, Gu, Pang, Liu, Tang and Zhou. This is an open-access article distributed under the terms of the Creative Commons Attribution License (CC BY). The use, distribution or reproduction in other forums is permitted, provided the original author(s) and the copyright owner(s) are credited and that the original publication in this journal is cited, in accordance with accepted academic practice. No use, distribution or reproduction is permitted which does not comply with these terms.
*Correspondence: Lan Tang, dGw0MDVAc211LmVkdS5jbg==; Xuefeng Zhou, eGZ6aG91QHNjc2lvLmFjLmNu