- 1Department of Systematic and Evolutionary Botany, University of Zurich, Zurich, Switzerland
- 2Research Group ‘Pharmacognosy and Phytotherapy’, UCL School of Pharmacy, University of London, London, United Kingdom
The closely related genera Liriope and Ophiopogon (Asparagaceae), collectively known in English as liriopogons, have similar therapeutic uses in treating cough, rheumatoid arthritis, and cleaning heat. The main aim of this review is to understand the current phytochemical and pharmacological knowledge including an assessment of the quality of the scientific evidence. A literature search was conducted in line with PRISMA guidelines, by retrieving available information up to 2020 from five online resources. The bioactive metabolites of liriopogons include steroidal saponins, flavonoids, polysaccharides, organic acids, phenols. Cardiovascular protective, anti-inflammatory, anti-diabetic, anti-oxidant, anti-cancer, neuroprotective, anti-viral, anti-acute myeloid leukemia and hepatoprotective effects have been at the center of attention. From a toxicological perspective Ophiopogon japonicus seems to be safe. Some problems with the quality of the pharmacological evidence stand out including the application of excessive dose level and methodological problems in the design. Additionally, a reasonable link between local/traditional uses and pharmacological assessment is often vague or not reflected in the text. Future researches on liriopogons are required to use rigorous scientific approaches in research on evidence-based natural products for the future benefits of patients.
Introduction
Liriope Lour. and Ophiopogon Ker Gawl. are two closely related genera, collectively known as liriopogons (Fantz, 1993). They comprise a total of some 84 species and are indigenous to Asia, with many species having been traditionally used as medicines in China, with the common label ‘maidong’ or ‘mai men dong’ (for the tuberous roots)- including Ophiopogon japonicus, together with Liriope spicata and L. muscari as alternative sources, is an example of what Linares and Bye (Linares and Bye, 1987) called plant complexes, i.e., different (and not necessarily related) species being classed under the same common name. Interestingly in this case, there is a double labelling one in Chinese but also in popular botanical nomenclature – liriopogons. According to Shenong’s Canon on Materia Medica (ca. 200–250 CE), maidong is categorized as upper herb to extend longevity by ameliorating heart-qi stagnation, vacuity-taxatio, and suppressing vomiting and retching. Furthermore, they are also locally used among China in treating cough, rheumatoid arthritis and cleaning heat (Huang, 1982; Zheng and Xing, 2009; CP Commission, 2020).
Different species of liriopogons exhibit similar phytopharmacological properties; they are rich in saponins, flavonoids and polysaccharides, which have been linked to relevant pharmacological activities, such as cardiovascular protective, anti-inflammatory, immunomodulatory, anti-cancer and anti-diabetic effects (Li et al., 2006; Zheng and Xing, 2009; Chen MH. et al., 2016). Recently, Ophiopogon japonicus (Thunb.) Ker Gawl. has been extensively used in treating COVID-19. Since the start of the COVID-19 pandemic, 31 prescriptions (including a total of 72 medicinal plants) have been recommended by the Chinese authorities, O. japonicus ranks as the fourth frequently used in these 31 prescriptions (Zhang and Li, 2020). Obviously, O. japonicus, plays a predominant role as medicinal plant among liriopogons and its phytopharmacological properties have been investigated without observing significant toxicity (Chen MH. et al., 2016). However, other species of liriopogons have received more limited scientific attention.
The combined complexity of local/traditional phytotherapeutic uses and the resulting biochemical and biomedical investigations makes this group of plants an interesting case study for a review focusing on current approaches in phytopharmacological research and to develop strategies for more robust approaches. Phytopharmacological research, as a flourishing field focusing on complex mixtures, requires as all fields of research, robust and reproducible research. Recently, editors of leading journals called for better designed and reported research, i.e., to consider and cover appropriate models, controls, dosage, reasonable link between local/traditional uses and the assay (Heinrich et al., 2020). Core to this is a greater emphasis on the characterisation of the material under study. This includes botanical, pharmacognositc, chemical as well as other methodological details. Accordingly, in this review, the core aims are to assess:
1) The species most commonly used with regards to the level of information is available on their pharmacological and chemical characteristics,
2) The chemical metabolites or extracts isolated from liriopogons,
3) The corresponding pharmacological effects of the bioactive metabolites, and
4) The rigorousness of these pharmacological studies according to good practice standards.
Methodology
Search Strategy
A literature search was conducted in line with PRISMA guidelines (Moher et al., 2009). Predominantly, four databases, Web of Science (core collection), PubMed, Scopus and SciFinder were consulted from inception until 2020. MeSH terms were used to identify search terms. Ophiopogon and Liriope were searched separately, for each using Boolean operators: Pharmaceutical OR Biological Activity OR Phytochemistry OR Chemical Constituent OR Pharmacology OR Phytopharmacology, respectively. Additional information was retrieved by manual searching through Google scholar. Since liriopogons have been traditionally and widely used in Chinese Medicine, publications in Chinese were considered using the database China National Knowledge Infrastructure (CNKI). Here, 麦冬 (maidong) was jointly searched with 化学成分 (phytochemistry and chemical constituent) OR 活性 (pharmaceutical and biological activity) OR药理 (pharmacology). The application of scientific names was in accordance with the World Flora Online (WFO, 2021). The workflow of our search strategy is shown in Figure 1.
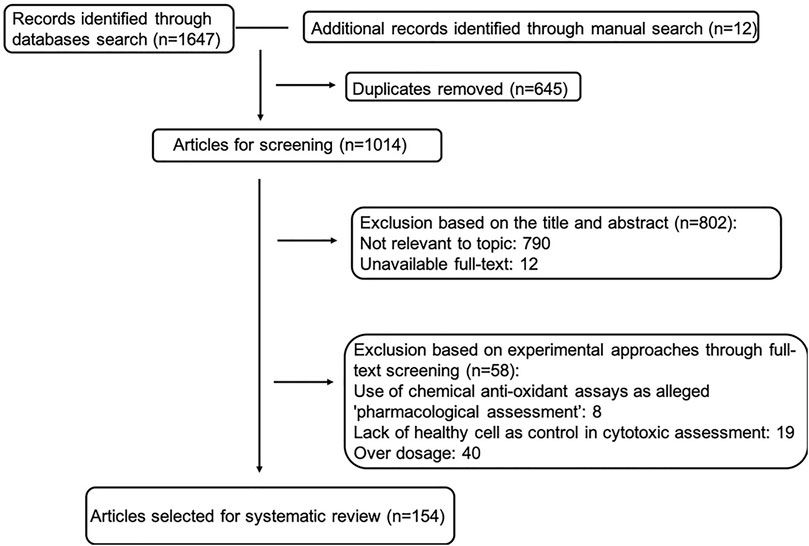
FIGURE 1. Workflow chart of inclusion and exclusion of studies in the systematic review (exclusion based on experimental approaches may overlap, therefore selected article number is slightly different from simple subtraction).
Primary Selection Criteria
Publications were included for further critical assessment if one of these two criteria was met: 1) Isolation of pure metabolites of liriopogons was reported (phytochemical publications, n = 106); 2) Pharmacological effects of extracts, pure metabolites (not including derivatives) were evaluated (pharmacological publications, n = 113). The publications retrieved are summarized in Supplementary Table S6.
Terminology
Since the terminology especially as it relates to the botanical drugs is often not precise or misleading, we standardized the terms used for plant parts as follows- standardized terminology (original sources):
- Tuberous root (tuber)
- Tuberous root (rhizome)
- Tuberous root (tuberous root)
- Fibrous root (fibrous root)
- Subterranean part (underground part)
- Subterranean part (subterranean part)
- Subterranean part (root)
- Aerial part (aerial part)
- Whole plant (whole plant)
- Fruit (fruit, seed)
- Stalk (stalk)
Critical Review of Pharmacological Publications
For the literature analysis we critically assessed the experimental approaches used, following Heinrich et al. (2020). Specifically, we looked at the dosage, antioxidant models, controls (esp. cytotoxic findings); additionally, methodological details were taken into consideration (Table 1). Table 3 (derived from Supplementary Table S6 with all publications before assessment) includes the pharmacological publications included in the analysis after assessment.
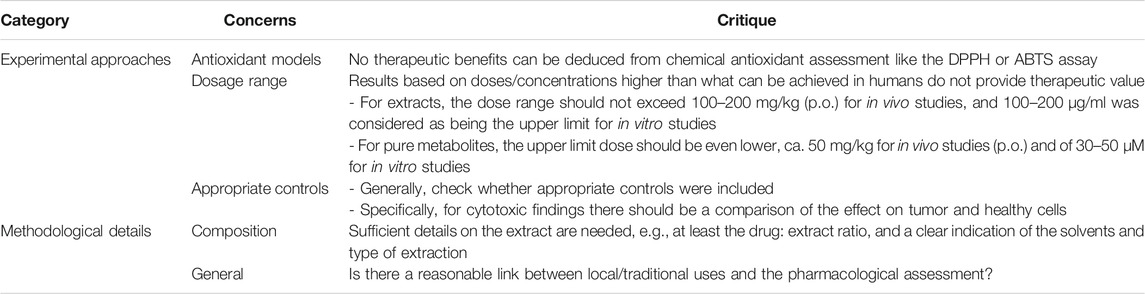
TABLE 1. Criteria for the exclusion of studies which are considered to be of limited relevance in a pharmacological context (based on Heinrich et al., 2020).
Phytochemistry
Various metabolites have been isolated and characterized from different parts (tuberous roots, fibrous roots and aerial parts) of liriopogons, including steroidal saponins (Supplementary Table S1), flavonoids (Supplementary Table S2), polysaccharides (Supplementary Table S3), phenols and organic acids (Supplementary Table S4) and other types of metabolites (Supplementary Table S5). Steroidal saponins are a core group of secondary metabolites of liriopogons, followed by flavonoids (Figure 2). Chemical structures of pharmacologically active metabolites are shown in Table 2. As main bioactive metabolites, the chemical structures of steroidal saponins and flavonoids are shown in Supplementary Tables S1, S2 and Supplementary Figure S2.
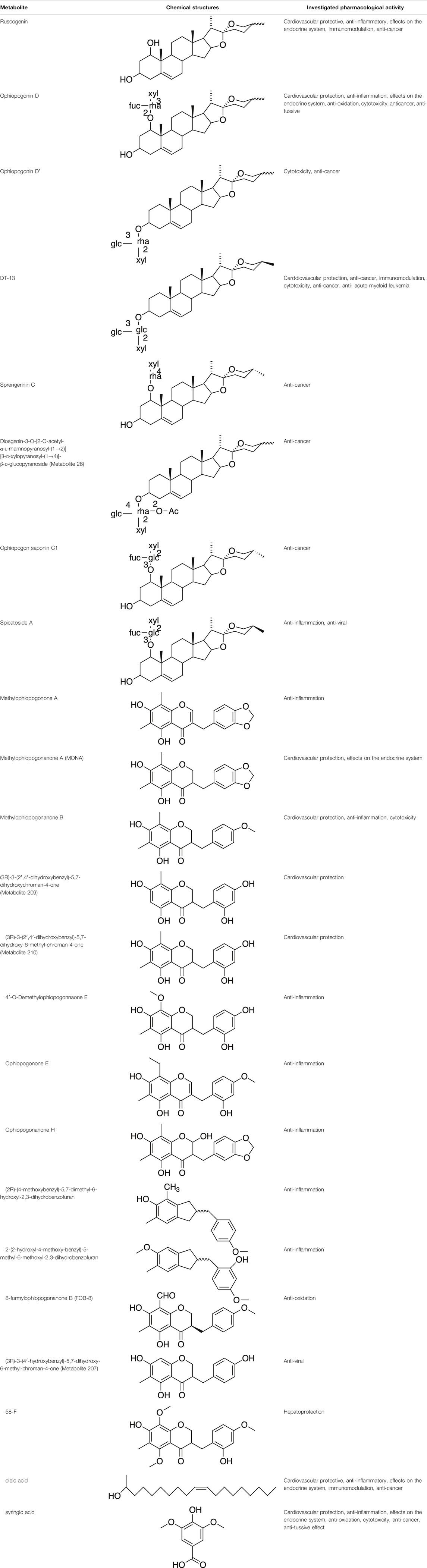
TABLE 2. Chemical structures of pharmacologically investigated metabolites with corresponding activities of liriopogons (details of activities can be found in Table 3).
Ophiopogon japonicus, Liriope muscari and L. spicata have been well studied phytochemically, while less attention paid to other species. Overall, 337 metabolites have been isolated from liriopogons, only three metabolites have been reported each from Ophiopogon planiscapus Nakai and Ophiopogon jaburan (Siebold) Lodd., one from Ophiopogon intermedius D. Don and 15 from Lirioipe graminifolia (Drude) Becc., the rest of them are found in O. japonicus, L. muscari and L. spicata.
Steroidal Saponins
The absolute configurations of many steroidal saponins have been comprehensively determined by 1D, 2D, NMR, CD and MS spectral data analysis. Overall, so far, 156 steroidal saponins have been isolated and characterized from liriopogons from tuberous and fibrous roots, including 88 from O. japonicus; 37 from L. muscari; 31 from L. spicata; nine from L. graminifolia; two from O. jaburan; five from O. planiscapus; one from O. intermedius (Rawat et al., 1988). In addition, metabolites 53, 57, 58 (DT-13),71 (Ophiopogon A), 72 (Ophiopogon B) and 76 (Ophiopogon D) have been isolated both from Ophiopogon and Liriope spp., and most of them have been further evaluated focusing on a broad spectrum of bioactivities.
Flavonoids
Generally, flavonoids including flavanones, isoflavanones and homoisoflavanones have been reported mainly from tuberous roots and fibrous roots of liriopogons, a few are from the aerial parts and fruits (Lee and Choung, 2011; Tsai et al., 2015). Flavonoids isolated from Ophiopogon spp. are mainly homoisoflavones; whereas, the diversity of flavones and isoflavones is higher in Liriope spp., together with several homoisoflavones.
In total, 84 flavonoids have been isolated from liriopogons, including 47 from O. japonicus; 29 from L. muscari; six from L. graminifolia; one from O. jaburan. Only, methylophiopogonanone B was isolated both from O. japonicus and L. graminfolia.
Polysaccharides
Polysaccharides in the tuberous roots of L. spicata, L. muscari and O. japonicus have been evaluated reaching 53.2%, 54.7 and 55.2%, respectively, and their structures were distinctively different kim (Gong et al., 2017). In total, 18 polysaccharides have been isolated and identified from liriopogons.
Others
Other metabolites have also been isolated from the tuberous roots, fibrous roots and aerial part of liriopogons, including 19 organic acids (metabolite 260–278, 26 phenols (metabolite 279–304), 13 glycosides (metabolite 305–317) and 20 other types of metabolites (metabolite 318–337). Among them, three organic acids existed both in Ophiopogon and Liriope spp. Vanillic acid were found existing in O. japonicus, L. spicata and L. muscari. Oleanolic acid and palmitic acid have been both isolated from O. japonicus and L. muscari.
Pharmacological Properties of Liriopogons
Several species of liriopogons have been used in local/traditional medicines in South-East Asia, and are especially popular within China. Although only four species of liriopogons have been pharmacologically investigated in vitro or in vivo, a variety of pharmacological properties have been reported including anti-inflammatory, immunomodulatory, antioxidant, anti-cancer, anti-tussive, neuroprotective, anti-viral activities, and the effects on the cardiovascular and endocrine system. All pharmacological findings performed with extracts and pure metabolites isolated from liriopogons together with an overview of tested species (with main focus on O. japonicus, L. muscari and L. spicata) for each pharmacological effect are summarized in Table 3. Generally, O. japonicus represents the most important medicinal species of liriopogons and has been widely studied both in vitro and in vivo, exhibiting anti-inflammatory, immunomodulatory, antioxidant, anti-cancer, and anti-tussive activities, as well as the effects on the cardiovascular and endocrine system. In addition to neuroprotective, anti-infective and hepatoprotective effects, Liriope muscari possesses similar pharmacological activities as O. japonicus does (except anti-oxidative, anti-tussive and immunomodulatory activities). Liriope spicata exhibits the effects on the endocrine system and inflammatory diseases. Meanwhile, the cytotoxic effects of liriopogons on various tumor cells and traditional therapeutic effects of L. muscari on dry eye syndrome, gastrointestinal motility and bronchial asthma have also been investigated (Kim et al., 2016; Lee et al., 2019; Song et al., 2019). During the search we did not identify any clinical studies of relevance.
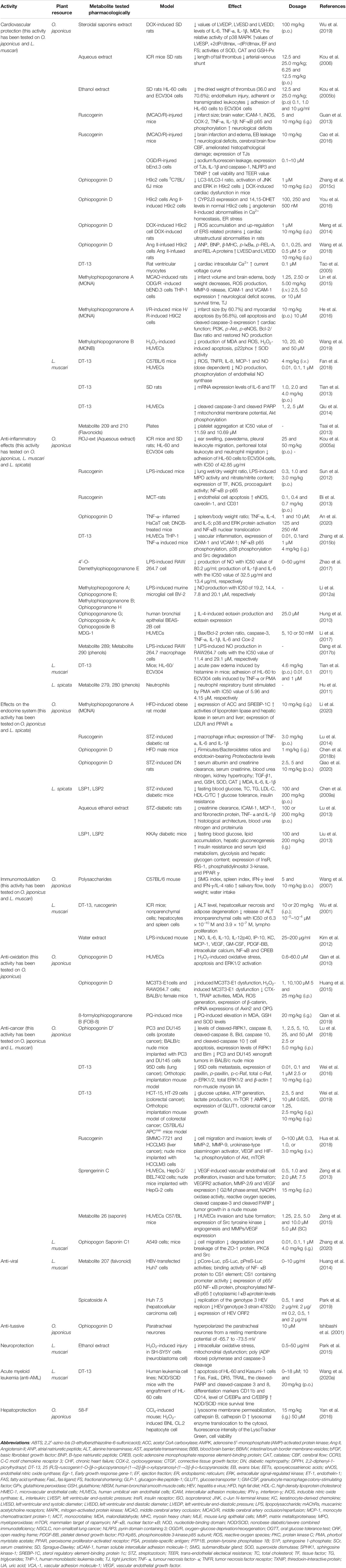
TABLE 3. Summary of pharmacological studies on extracts/metabolites isolated from liriopogons included in this review.
A critical assessment of pharmacological findings retrieved was conducted (Table 1). The application of excessively high dose, with over 1/3 publications, is seen as the most comm problem among the studies on liriopogons. Additionally, most of the high dose studies are on the pharmacological investigation of polysaccharides. The lack of controls using healthy cells in the evaluation of cytotoxic effects limits the scientific conclusion that can be drawn. Therefore, such studies were excluded. Similarly, eight out of eleven studies on ‘antioxidant’ effects included in the initial list of sources merely rely on chemical assays, which are of no therapeutic relevance and, therefore, were excluded. Methodological details are also assessed but not considered as exclusion criteria.
Cardiovascular Protection
Ophiopogon japonicus
Protective effects on the cardiovascular system have been a core focus of research using both extracts and many metabolites isolated from Ophiopogon japonicus, including steroidal saponins and flavonoids. In essence, the level of cytokines such as interleukin (IL)-6, tumor necrosis factor (TNF)-α and IL-1β were reduced which inhibited the activation of NF-κB and MAPK pathway. Additionally, this protective effect is associated with antioxidant effect through improving antioxidant enzymes (superoxide dismutase (SOD), glutathione peroxidase (GSH-Px), catalase (CAT)).
Steroidal saponins from Ophiopogon japonicus mainly consisting of ophiopogonin B, ophiopogonin D (OPD) and ophiopogonin D’, significantly improved cardiac function of doxorubicin (DOX)-induced chronic heart failure (CHF) in rats linked to the increased blood pressure values of markers for left ventricular function, and the decreased value of left ventricular end-diastolic pressure (LVEDP), left ventricular end systolic diameter (LVESD) and left ventricular end diastolic diameter (LVEDD). This protective effect was achieved through suppressing oxidative stress and inflammatory response by improving SOD, GSH-Px, CAT and reducing inflammatory cytokine levels including IL-6, TNF-α and IL-1β (Wu et al., 2019). Methylophiopogonanone (MONB) exerted protective effects by increasing antioxidant potential in human umbilical vein endothelial cells (HUVECs), which is evidenced by the decreased production of malondialdehyde (MDA), ROS and increased SOD activity. Moreover, this effect might be associated to NADPH-related signaling by suppressing the expression of p22phox (an important component of NADPH oxidase) (Wang et al., 2019).
Liriope muscari
DT-13, a key biologically active steroidal saponin isolated from L. muscari has been investigated for the protective effects on the cardiovascular system through diverse ways. It protected C57BL/6 mice endothelium through inhibiting endothelium vascular inflammation by regulating nitric oxide (NO) production and the expression of ROS, tumor necrosis factor receptor (TNFR), IL-8, monocyte chemoattractant protein (MCP)-1 (Fan et al., 2018). The anti-thrombotic activity of DT-13 was observed in SD (Sprague-Dawley) rats by inhibiting thrombosis and down-regulating mRNA expression levels of IL-6 and tissue factor (TF) (Tian et al., 2013). Additionally, DT-13 showed anti-apoptosis activity on HUVECs by decreasing the expression of cleaved caspase-3 and cleaved poly (ADP ribose) polymerase (PARP) through regulating PI3 K/Akt signaling pathway (Qiu et al., 2014). Two homoisoflavonoids (metabolite 209 and 210) (Supplementary Table S2) exhibited anti-platelet activity at IC50 value of 11.59 and 10.69 μM (Tsai et al., 2013).
Anti-Inflammatory Effects
Steroidal saponins, flavonoids and polysaccharide-rich fraction have been broadly studied for anti-inflammatory activities both in vitro and in vivo. In addition, a few phenols have also been assessed for anti-inflammatory activity. Lipopolysaccharides (LPS), monocrotaline (MCT), DNCB, IL-4 are applied for establishing the inflammatory models. In general, the bioactive metabolites inhibit the production of inflammatory cytokines, e.g., NO, IL-Iβ, IL-6, TNF-α etc., and suppress the phosphorylation of MAPK and NF-κB signaling pathways. Moreover, the anti-inflammatory effect may be achieved through reducing cell adhesion.
Ophiopogon japonicus
Sun et al. (2012) looked at the inhibitory effect of ruscogenin on LPS-induced mice with acute lung injury. Ruscogenin remarkably alleviated lung injury by attenauting LPS- induced myeloperoxidase (MPO) activity and nitrate/nitrite content, downregulating the expression of TF, iNOS, and regulating NF-κB pathway and NF-κB p-p65. The inhibitory effects of DT-13 on TNF-α-induced vascular inflammation and the potential molecular mechanisms were investigated as well. It diminished vascular inflammation through reducing adhesion molecules tandemed with regulating the Src/NF-kappa B/MAPK pathway by suppressing NF-кB p65 phosphorylation, TNF-α induced luciferase activities of ICAM-1 and VCAM (vascular adhesion molecule)-1, and p38 phosphorylation and Src degradation (Zhang et al., 2015b).
A range of homoisoflavonoids have been assessed for anti-inflammatory activity in vitro. 4′-O-Demethylophiopogonanone E, and MONA, ophiopogonone E, MONB and ophiopogonanone H were observed with signidicant anti-inflammatory activity. In LPS-induced RAW 264.7 cell and LPS-induced murine microglial cell BV-2, the production of NO was significantly suppressed, along with the decreased level of IL-1β and IL-6 (Li N. et al., 2012; Zhao et al., 2017).
Liriope muscari
The anti-inflammatory effect of DT-13 isolated from L. muscari was reported both in vitro and in vivo. Acute paw edema induced by histamine was reduced up to 17.2% by DT-13, in vitro assay indicated it also significantly suppressed the adhesion of HL-60 to ECV304 cells induced by TNF-α or 12-myristate 13-acetate (PMA) (Tian et al., 2011).
Liriope spicata
Two phenols (metabolite 279, 280) (Supplementary Table S4) from L. spicata were investigated for anti-inflammatory activities against neutrophil respiratory burst stimulated by PMA with IC50 value of 4.15 and 5.96 μM, respectively (Hu et al., 2011).
Effects on the Endocrine System
A small number of studies have revealed the effects of metabolites isolated from liriopogons on the endocrine system, such as anti-obesity, anti-hyperlipidemic and anti-diabetic activities. Generally, KKay mice, rats (mice) fed with high fat diet (HFD) or induced by streptozotocin (STZ), and diabetic rats/mice models are normally applied to study the regulatory potential on the endocrine system.
Ophiopogon japonicus
Ruscogenin and OPD isolated from O. japonicus act on the endocrine system. OPD exerted anti-obesity effect on HFD-induced metabolic syndrome mice through regulating gut microbiota, precisely, by reducing Firmicutes/Bacteroidetes ratios and endotoxin-bearing Proteobacteria levels (Chen S. et al., 2018). In addition, OPD played a protective role against renal damage in STZ-induced diabetic nephropathy through suppressing oxidative damage as evidence by the decreased level of MDA and increased activity of SOD, GSH, CAT, along with inflammatory response by reducing pro-inflammatory cytokines (IL-6, IL-1β) (Qiao et al., 2020). Ruscogenin was also reported can improve diabetic nephropathy (Lu et al., 2014).
Pretreatment with MO-A (10 mg/kg), a homoisoflavonoid, significantly ameliorated the hyperlipidemia in rats induced by high fat diet HFD through regulating the serum lipid profile by modulating the expression levels involved in lipogenesis and lipid oxidation, i.e., inducing the expression levels of both low-density lipoprotein receptor (LDLR) and peroxisome proliferators-activated receptors (PPAR) α, and suppressing the expression levels of both acetyl CoA carboxylase (ACC) and sterol regulatory element-binding protein 1c (SREBP-1C) (Li et al., 2020).
Liriope spicata
Two polysaccharides (LSP1, LSP2) obtained from L. spicata have been investigated for anti-diabetic activity. Chen et al. (2009a) evaluated their effects on STZ-induced diabetic mice and observed a remarkable reduction of fasting blood glucose, improvement of glucose tolerance and insulin resistance, as well as the decreased level of cholesterol. Moreover, they also exerted anti-diabetic effects on insulin-resistant diabetic KKAy mice through up-regulating the expression of insulin-receptor (InsR), insulin-receptor substrate-1 (IRS-1), phosphatidylinositol 3-kinase, and PPAR γ (Liu et al., 2013). The anti-diabetic activity of aqueous-ethanol extract of L. spicata was observed through inhibiting the expression of ICAM-1, MCP-1, and fibronectin protein and inflammatory cytokines (Lu et al., 2013).
Immunomodulation
Th1/Th2 cytokine imbalance may play a role in the pathogenesis of Sjogren’s syndrome SS (Price and Venables, 1995). O. japonicus polysaccharides (OJP) was found to significantly improve the SS in mouse via the regulation of Th1/Th2 cytokine imbalance by reducing IFN-γ level and IFN-γ/IL-4 ratio (Wang et al., 2007).
Antioxidative Effects
Up to now, OPD and FOB-8, the bioactive metabolites from O. japonicus have been reported for significant antioxidative activities both in vitro and in vivo.
OPD played a protective role as an effective antioxidant agent in H2O2-induced endothelial injury by decreasing H2O2-induced oxidative stress through inhibiting the activation of ERK1/2 (Qian et al., 2010). Moreover, it demonstrated anti-osteoporosis activity both in vitro and in vivo, through decreasing oxidative stress which was related to FoxO3a-β-catenin signaling pathway by down-regulating the protein expression of β-catenin, mRNA expressions of Axin2 and OPG (Huang et al., 2015).
8-FOB showed protective effect against paraquat-induced hepatotoxicity through suppressing oxidative stress by attenuating MDA levels and GSH and SOD levels (Qian et al., 2019).
Cytotoxic and Anti-Cancer Activity
The anti-cytotoxic and cancer activities of steroidal saponins isolated from liriopogons have been investigated, including the effects on prostate cancer, lung cancer, colorectal cancer, liver cancer, and the possible mechanisms were also studied. Generally, the anti-cancer effects of liriopogons are achieved by inducing apoptosis, suppressing glucose transporter 1 (GLUT1) transmembrane glucose pathway, and inhibiting proliferation and angiogenesis.
Ophiopogon Japonicus
Ophiopogonin D′, an active metabolite from O. japonicus, suppressed the growth of PC3 and DU145 xenograft tumors (prostate cancer) in BALB/c nude mice through inducing apoptosis. The cellular mechanism of this activity might be through modulating RIPK1-related pathway as evidenced by the increased protein expression of RIPK1 and Bcl-2-like protein 11, and the decreased levels of cleaved-RIPK1, caspase 8, cleaved-caspase 8, Bid, caspase 10, and cleaved-caspase 10 (Lu et al., 2018). DT-13 inhibited the proliferation of colorectal cancer in orthotopic implantation mouse model of colorectal cancer model and C57BL/6J APCmin mice model were reported, which is associated with GLUT1 transmembrane glucose pathway. The results indicated that DT-13 significantly suppressed GLUT1 and activating AMPK/mTOR pathway (Wei et al., 2019). Sprengerinin C inhibited the angiogenesis in HUVECs through repressing the activation of VEGFR2-dependent PI3K/Akt/mTOR and p38 MAPK signaling pathways by down-regulating the expression of MMP-2/9 and VEGF. Meanwhile, a significant improvement of reactive oxygen species, cleaved caspase-3 and cleaved PARP was detected after DT-13 treatment on HepG-2 and BEL7402 cells, which induced the apoptosis (Zeng et al., 2013).
Liriope muscari
Ophiopogon Saponin C1, the bioactive metabolite of L. muscari against lung tumor through stablizing endothelium permeability by inhibiting the disassembly of ZO-1 protein, TNF-α and repressing PKCδ and Src kinase (Zhang et al., 2020).
Anti-Viral Activity
Only two steroidal saponins isolated from L. muscari have been investigated for anti-viral activity in vitro.
The effect of metabolite 207 (Supplementary Table S2) against hepatitis B virus was reported (Huang et al., 2014) as shown by the decreased level of viral gene expression and viral DNA replication. This was possibly regulated through NF-κB signaling pathway by decreasing the expression of p65/p50 NF- κB protein and phosphorylated NF-κB p65, simultaneously elevating cytoplasmic IκBα protein levels.
Park et al. (2019) looked at the anti-viral activity of the steroidal saponin spicatoside A on hepatitis E virus (HEV). It inhibited the replication of HEV genotype 3 strain 47832c replicon in a concentration-dependent manner, and down-regulating the expression of HEV open reading frame 2 (ORF2).
Others
Other pharmacological benefits such as anti-tussive and neuroprotective effects and the therapeutic effects on acute myeloid leukemia (AML) have also been evaluated, but received far less attention.
Ophiopogonin D isolated from O. japonicus exerted anti-tussive activity by hyperpolarizing the paratracheal neurones from a resting membrane potential of -65.7 to -73.5 mV (Ishibashi et al., 2001). Ethanol extract of L. muscari was reported for neuroprotective effect by attenuating intracellular oxidative stress and mitochondrial dysfunction, where PARP and caspase-3 cleavage was suppressed (Park et al., 2015).
The anti-AML activity of DT-13 was investigated in vitro and in vivo. It induced the apoptosis AME cells, especially HL-60 and Kasumi-1 cells through modulating death receptor pathway by enhancing the expression of cleaved-PARP and cleaved-caspase 3 and 8. Moreover, the differentiation of AML cells was promoted by DT-13 as shown by the increased level of differentiation markers CD11b and CD14, as well as transcription factor C/EBPα and C/EBPβ. In vivo evaluation was carried out on NOD/SCID mice with the engraftment of HL-60 cells revealing the anti-leukemia activity of DT-13 (Wang C. et al., 2020).
The hepatoprotective effect has also been reported. 58-F, a flavanone isolated from O. japonicus, protected against hepatocyte from death through lowering lysosomal membrane permeability as shown by the increased the fluorescence intensity of the LysoTracker Green and cell viability, and through elevating lysosomal enzyme translocation to the cytosol as evidenced by the suppressed activity of cathepsin B and cathepsin D (Yan et al., 2016).
Toxicological Assessments of Liriopogons
Liriopogons are noted for their therapeutic benefits with little recorded toxicity since Shenong’s Canon on Materia Medica (ca. 200–250 CE), but also in numerous contemporary medical monographs (Huang, 1982; Li et al., 2011). Only a few scientific studies have been conducted on toxicological properties of liriopogons but confirmed the traditional cognition.
O. japonicus decoction showed no chromosome damage of bone marrow cells in ICR mice, and no genotoxicity in vivo with metabolic activation (Hu et al., 2009). Moreover, a O. japonicus decoction was investigated for the potential development of toxicity in rats by evaluating the maternal body weight, fetus weight and viability, incidences of fetalmal formation and variation, showing no obvious adverse effect (Min et al., 2010).
Conclusion and Perspectives
The metabolites and pharmacological activities of liriopogons are reasonably well understood and this supports the idea of the two genera Ophiopogon and Liriope forming – in ethnopharmacological terms – a plant complex. Some species are also relatively well known pharmacologically. Steroidal saponins, flavonoids and polysaccharides are the major classes of metabolites in both genera. Several organic acids, phenols and other types of metabolites have also been isolated. Crude extracts and isolated pure metabolites from liriopogons exhibit a wide spectrum of reported pharmacological properties. Especially, steroidal saponins and flavonoids have been linked to experimental pharmacological studies focusing on cardiovascular diseases and inflammatory syndromes. However, clinical evidence needs to be developed. Despite the extensive studies on liriopogons, the focus has mainly been on three species - O. japonicus, L. muscari and L. spicata. Less emphasis has been placed on other species, which are also traditionally used as local and traditional medicines, such as L. gramilifolia, O. dracaenoides, O. platyphyllus and O. reversus (Li et al., 2006; Zheng and Xing, 2009), leaving a large open area for future investigations. On the other hand, studies generally have only focused on broad ranging in vivo effects and not on molecular mechanisms, which need to be explored further, e.g., the modulation of pathways, along with related cytokines and genes.
Limited evidence exists with regards to the species’ safety and specifically, there is a lack of assessing potential toxicological effects of liriopogons. In general, the findings indicated that, consistent with traditional perception, decoctions derived from the species have a low toxicity. However, this is clearly insufficient from a clinical perspective.
We also critically assessed the experimental approaches (Table 1) and identified a number of problems, which make an assessment of the species’ potential benefits difficult if not impossible. In experimental terms, the use of excessively high dose levels needs to be addressed. Numerous studies reviewed use high dose levels resulting in these results being of very limited scientific relevance. Especially in case of in vivo studies, the dose per day and kg body weight often seems to be of limited or no therapeutic relevance. The use of such high doses is often justified with the rate of metabolism being higher in rodent models. While the calculations commonly used in drug discovery (where the starting values in humans are nano or microMol) makes sense, this is not meaningful if the starting dose is higher like in traditional (tea) preparations. Moreover, the majority of high dose studies are on pharmacological investigations of polysaccharides. Evaluations of potential cytotoxic effects on liriopogons lack controls using healthy cells, making it impossible to assess the specificity of the effect. Investigations of potential chemical antioxidant effects cannot make pharmacological claims based on such assays.
In the current review, we present the case study of the liriopogons in order to assess how the pharmacological evaluation of extracts needs to be improved in experimental terms. Methodological details provided are also evaluated (Table 1). In general, the extraction process of the crude extracts or pure metabolites and characterization are well described. However, the link between local/traditional uses and the pharmacological assessment is often vague or is not reflected in the publications.
All this is not just specific to studies on liriopogons, but represents a more general situation of the current state of ethnopharmacological research. When conducting pharmacological assays, researchers need to reassess what constitute therapeutically meaningful doses, in particular for the pharmacological assessment of polysaccharides. Moreover, the assessment of anti-oxidant effects need to of pharmacological relevance. Appropriate controls in cytotoxic studies, further investigation on toxicological properties, and molecular mechanism and clinical evidence of diverse pharmacological activities are also required.
Therefore, it is essential, and our responsibility, to use rigorous scientific approaches and to deliver high quality findings for the future benefits of patients, and for the better development of evidence-based natural products.
Author Contributions
FL, MH, and CW developed the concept for the study. FL conducted the literature survey and drafted the paper. MH and CW supervised the project and revised the paper.
Funding
This work was financially supported by the Chinese Government Scholarship (No. 201906910062), the Forschungskredit Candoc (No. FK-20-091) and the Georges und Antoine Claraz Schenkung.
Conflict of Interest
The authors declare that the research was conducted in the absence of any commercial or financial relationships that could be construed as a potential conflict of interest.
Publisher’s Note
All claims expressed in this article are solely those of the authors and do not necessarily represent those of their affiliated organizations, or those of the publisher, the editors and the reviewers. Any product that may be evaluated in this article, or claim that may be made by its manufacturer, is not guaranteed or endorsed by the publisher.
Acknowledgments
We thank Reto Nyffeler (Zuerich) for his input into the project and Yaqin Chen (Chengdu) and Yuping Fu (Oslo) for advice on pharmacological aspects.
Supplementary Material
The Supplementary Material for this article can be found online at: https://www.frontiersin.org/articles/10.3389/fphar.2021.769929/full#supplementary-material
References
An, E. J., Kim, Y., Lee, S. H., Choi, S. H., Chung, W. S., and Jang, H. J. (2020). Ophiopogonin D Ameliorates DNCB-Induced Atopic Dermatitis-like Lesions in BALB/c Mice and TNF-α- Inflamed HaCaT Cell. Biochem. Biophys. Res. Commun. 522 (1), 40–46. doi:10.1016/j.bbrc.2019.10.190
Bi, L. Q., Zhu, R., Kong, H., Wu, S. L., Li, N., Zuo, X. R., et al. (2013). Ruscogenin Attenuates Monocrotaline-Induced Pulmonary Hypertension in Rats. Int. Immunopharmacol. 16 (1), 7–16. doi:10.1016/j.intimp.2013.03.010
Cao, G., Jiang, N., Hu, Y., Zhang, Y., Wang, G., Yin, M., et al. (2016). Ruscogenin Attenuates Cerebral Ischemia-Induced Blood-Brain Barrier Dysfunction by Suppressing TXNIP/NLRP3 Inflammasome Activation and the MAPK Pathway. Int. J. Mol. Sci. 17 (9), 1–17. doi:10.3390/ijms17091418
Chen, M. H., Chen, X. J., Wang, M., Lin, L. G., and Wang, Y. T. (2016a). Ophiopogon Japonicus--A Phytochemical, Ethnomedicinal and Pharmacological Review. J. Ethnopharmacol. 181, 193–213. doi:10.1016/j.jep.2016.01.037
Chen, S., Li, X., Liu, L., Liu, C., and Han, X. (2018b). Ophiopogonin D Alleviates High-Fat Diet-Induced Metabolic Syndrome and Changes the Structure of Gut Microbiota in Mice. FASEB J. 32 (3), 1139–1153. doi:10.1096/fj.201700741RR
Chen, X., Bai, X., Liu, Y., Tian, L., Zhou, J., Zhou, Q., et al. (2009a). Anti-diabetic Effects of Water Extract and Crude Polysaccharides from Tuberous Root of Liriope Spicata Var. Prolifera in Mice. J. Ethnopharmacol 122, 205–209. doi:10.1016/j.jep.2009.01.016
Dang, N. H., Chung, N. D., Tuan, H. M., Van Thanh, N., Hiep, N. T., Lee, D., et al. (2017b). 2-Benzyl-benzofurans from the Tubers of Ophiopogon Japonicus. Chem. Cent. J. 11 (1), 15–16. doi:10.1186/s13065-017-0242-z
Fan, R., Han, Y., Han, H., Chen, Z., Yu, B., Kou, J., et al. (2018). DT-13 Ameliorates TNF-α-Induced Nitric Oxide Production in the Endothelium In vivo and In vitro. Biochem. Biophys. Res. Commun. 495 (1), 1175–1181. doi:10.1016/j.bbrc.2017.11.009
Fantz, P. R. (1993). Taxonomic Problems in Cultivated Liriopogons. horttech 3 (2), 146–150. doi:10.21273/horttech.3.2.146
Gong, Y., Zhang, J., Gao, F., Zhou, J., Xiang, Z., Zhou, C., et al. (2017). Structure Features and In Vitro Hypoglycemic Activities of Polysaccharides from Different Species of Maidong. Carbohydr. Polym. 173, 215–222. doi:10.1016/j.carbpol.2017.05.076
Guan, T., Liu, Q., Qian, Y., Yang, H., Kong, J., Kou, J., et al. (2013). Ruscogenin Reduces Cerebral Ischemic Injury via NF-Κb-Mediated Inflammatory Pathway in the Mouse Model of Experimental Stroke. Eur. J. Pharmacol. 714, 303–311. doi:10.1016/j.ejphar.2013.07.036
He, F., Xu, B. L., Chen, C., Jia, H. J., Wu, J. X., Wang, X. C., et al. (2016). Methylophiopogonanone A Suppresses Ischemia/reperfusion-Induced Myocardial Apoptosis in Mice via Activating PI3K/Akt/eNOS Signaling Pathway. Acta Pharmacol. Sin. 37, 763–771. doi:10.1038/aps.2016.14
Heinrich, M., Appendino, G., Efferth, T., Fürst, R., Izzo, A. A., Kayser, O., et al. (2020). Best Practice in Research - Overcoming Common Challenges in Phytopharmacological Research. J. Ethnopharmacol. 246, 112230. doi:10.1016/j.jep.2019.112230
Hu, Y. P., Song, J., Wang, X., Zhang, M., Wang, X. W., and Li, B. (2009). Genotoxicity Study of Radix Ophiopogonis Decoction. Chin. J. Inf. TCM 16 (7), 38–40. doi:10.3969/j.issn.1005-5304.2009.07.016
Hu, Z. F., Chen, L. L., Qi, J., Wang, Y. H., Zhang, H., and Yu, B. Y. (2011). Two New Benzofuran Derivatives with Anti-inflammatory Activity from Liriope Spicata Var. Prolifera. Fitoterapia 82 (2), 190–192. doi:10.1016/j.fitote.2010.09.002
Hua, H., Zhu, Y., and Song, Y. H. (2018). Ruscogenin Suppressed the Hepatocellular Carcinoma Metastasis via PI3K/Akt/mTOR Signaling Pathway. Biomed. Pharmacother. 101, 115–122. doi:10.1016/j.biopha.2018.02.031
Huang, Q., Gao, B., Wang, L., Zhang, H. Y., Li, X. J., Shi, J., et al. (2015). Ophiopogonin D: A New Herbal Agent against Osteoporosis. Bone 74, 18–28. doi:10.1016/j.bone.2015.01.002
Huang, T. J., Tsai, Y. C., Chiang, S. Y., Wang, G. J., Kuo, Y. C., Chang, Y. C., et al. (2014). Anti-viral Effect of a Compound Isolated from Liriope Platyphylla against Hepatitis B Virus In Vitro. Virus. Res. 192, 16–24. doi:10.1016/j.virusres.2014.07.015
Hung, T. M., Thu, C. V., Dat, N. T., Dat, S. W., Lee, J. H., Kim, J. C., et al. (2010). Homoisoflavonoid Derivatives from the Roots of Ophiopogon Japonicus and Their In Vitro Anti-inflammation Activity. Bioorg. Med. Chem. Lett. 20 (8), 2412–2416. doi:10.1016/j.bmcl.2010.03.043
Ishibashi, H., Mochidome, T., Okai, J., Ichiki, H., Shimada, H., and Takahama, K. (2001). Activation of Potassium Conductance by Ophiopogonin-D in Acutely Dissociated Rat Paratracheal Neurones. Br. J. Pharmacol. 132 (2), 461–466. doi:10.1038/sj.bjp.0703818
Kim, H. J., Park, S. Y., Kim, D. G., Park, S. H., Lee, H., Hwang, D. Y., et al. (2016). Effects of the roots of Liriope Platyphylla Wang et tang on gastrointestinal motility function. J. Ethnopharmacol. 184, 144–153. doi:10.1016/j.jep.2016.03.012
Kim, H. K., Lee, J. Y., Han, H. S., Kim, Y. J., Kim, H. J., Kim, Y. S., et al. (2012). Immunomodulatory Effects of Liriope Platyphylla Water Extract on Lipopolysaccharide-Activated Mouse Macrophage. Nutrients 4, 1887–1897. doi:10.3390/nu4121887
Kou, J., Sun, Y., Lin, Y., Cheng, Z., Zheng, W., Yu, B., et al. (2005a). Anti-inflammatory Activities of Aqueous Extract from Radix Ophiopogon Japonicus and its Two Constituents. Biol. Pharm. Bull. 28 (7), 1234–1238. doi:10.1248/bpb.28.1234
Kou, J., Tian, Y., Tang, Y., Yan, J., and Yu, B. (2006). Antithrombotic Activities of Aqueous Extract from Radix Ophiopogon Japonicus and its Two Constituents. Biol. Pharm. Bull. 29 (6), 1267–1270. doi:10.1248/bpb.29.1267
Kou, J., Yu, B., and Xu, Q. (2005b). Inhibitory Effects of Ethanol Extract from Radix Ophiopogon Japonicus on Venous Thrombosis Linked with its Endothelium-Protective and Anti-adhesive Activities. Vascul. Pharmacol. 43 (3), 157–163. doi:10.1016/j.vph.2005.06.004
Lee, I. S., Kim, D. H., Kim, K. H., Park, J., Kim, Y., An, E. J., et al. (2019). Prevention and Relaxation Effects of Liriope Platyphylla on Bronchial Asthma In Vitro Model by Suppressing the Activities of MAPK/NF-κB Pathway. Mol. Cel. Toxicol. 15 (3), 325–334. doi:10.1007/s13273-019-0036-6
Lee, J. H., and Choung, M.-G. (2011). Identification and Characterisation of Anthocyanins in the Antioxidant Activity-Containing Fraction of Liriope Platyphylla Fruits. Food Chem. 127, 1686–1693. doi:10.1016/j.foodchem.2011.02.037
Li, L. C., Wang, Z. W., Hu, X. P., Wu, Z. Y., Hu, Z. P., and RuanLe, Y. L. (2017). MDG-1 I-nhibits H2O2-induced A-poptosis and I-nflammation in H-uman U-mbilical V-ein E-ndothelial C-ells. Mol. Med. Rep. 16 (3), 3673–3679. doi:10.3892/mmr.2017.6957
Li, N., Zhang, J. Y., Zeng, K. W., Zhang, L., Che, Y. Y., and Tu, P. F. (2012a). Anti-inflammatory Homoisoflavonoids from the Tuberous Roots of Ophiopogon Japonicus. Fitoterapia 83 (6), 1042–1045. doi:10.1016/j.fitote.2012.05.011
Li, S., Long, C., Liu, F., Lee, S., Guo, Q., Li, R., et al. (2006). Herbs for Medicinal Baths Among the Traditional Yao Communities of China. J. Ethnopharmacol. 108 (1), 59–67. doi:10.1016/j.jep.2006.04.014
Li, S. Z., Liu, H. R., and Liu, S. Y. (2011). Compendium of Materia Medica. Beijing: Huaxia Publishing House.
Li, Z., Wu, Y. Y., and Yu, B. X. (2020). Methylophiopogonanone a, an Ophiopogon Homoisoflavonoid, Alleviates High-Fat Diet-Induced Hyperlipidemia: Assessment of its Potential Mechanism. Braz. J. Med. Biol. Res. 53, e9201–10. doi:10.1590/1414-431x20199201
Lin, M., Sun, W., Gong, W., Zhou, Z., Ding, Y., and Hou, Q. (2015). Methylophiopogonanone A Protects against Cerebral Ischemia/Reperfusion Injury and Attenuates Blood-Brain Barrier Disruption In Vitro. PLoS One 10, e0124558. doi:10.1371/journal.pone.0124558
Linares, E., and Bye, R. A. (1987). A Study of Four Medicinal Plant Complexes of Mexico and Adjacent United States. J. Ethnopharmacol. 19 (2), 153–183. doi:10.1016/0378-8741(87)90039-0
Liu, Y. H., Wan, L., Xiao, Z., Wang, J., Wang, Y., and Chen, J. (2013). Antidiabetic Activity of Polysaccharides from Tuberous Root of Liriope Spicata Var. Prolifera in KKAy Mice. Evidence-based Complement. Altern. Med. 2013, 349790. doi:10.1155/2013/349790
Liu, Y. X. (2013). Study on Steroidal Saponins from Ophiopogon Japonicus. Hengyang: University of South China.
Lu, H. J., Tzeng, T. F., Hsu, J. C., Kuo, S. H., Chang, C. H., Huang, S. Y., et al. (2013). An Aqueous-Ethanol Extract of Liriope Spicata Var. Prolifera Ameliorates Diabetic Nephropathy through Suppression of Renal Inflammation. Evid. Based Complement. Alternat Med. 2013, 201643. doi:10.1155/2013/201643
Lu, H. J., Tzeng, T. F., Liou, S. S., Da Lin, S., Wu, M. C., and Liu, I. M. (2014). Ruscogenin Ameliorates Diabetic Nephropathy by its Anti-inflammatory and Anti-fibrotic Effects in Streptozotocin-Induced Diabetic Rat. BMC Complement. Altern. Med. 14, 110. doi:10.1186/1472-6882-14-110
Lu, Z., Wang, H., Zhu, M., Song, W., Wang, J., Wu, C., et al. (2018). Ophiopogonin D', a Natural Product from Radix Ophiopogonis, Induces In Vitro and In Vivo RIPK1-dependent and Caspase-independent Apoptotic Death in Androgen-independent Human Prostate Cancer Cells. Front. Pharmacol. 9, 432. doi:10.3389/fphar.2018.00432
Meng, C., Yuan, C. H., Zhang, C. C., Wen, M. D., Gao, Y. H., Gao, X. Y., et al. (2014). [Ophiopogonin D Protects Cardiomyocytes against Doxorubicin-Induced Injury through Suppressing Endoplasmic Reticulum Stress]. Yao Xue Xue Bao 49 (08), 1117–1123.
Moher, D., Liberati, A., Tetzlaff, J., and Altman, D. G. (2009). Preferred Reporting Items for Systematic Reviews and Meta-Analyses: The PRISMA Statement. BMJ 339 (7716), b2535–336. doi:10.1136/bmj.b2535
Park, G., Parveen, A., Kim, J. E., Cho, K. H., Kim, S. Y., Park, B. J., et al. (2019). Spicatoside A Derived from Liriope Platyphylla Root Ethanol Extract Inhibits Hepatitis E Virus Genotype 3 Replication In Vitro. Sci. Rep. 9 (1), 4397–4411. doi:10.1038/s41598-019-39488-5
Park, H. R., Lee, H., Park, H., Jeon, J. W., Cho, W. K., and Ma, J. Y. (2015). Neuroprotective Effects of Liriope Platyphylla Extract against Hydrogen Peroxide-Induced Cytotoxicity in Human Neuroblastoma SH-Sy5y Cells. BMC Complement. Altern. Med. 15, 171. doi:10.1186/s12906-015-0679-3
Price, E. J., and Venables, P. J. (1995). The Etiopathogenesis of Sjögren's Syndrome. Semin. Arthritis Rheum. 25, 117–133. doi:10.1016/S0049-0172(95)80025-5
Qian, J., Jiang, F., Wang, B., Yu, Y., Zhang, X., Yin, Z., et al. (2010). Ophiopogonin D Prevents H2O2-Induced Injury in Primary Human Umbilical Vein Endothelial Cells. J. Ethnopharmacol. 128 (2), 438–445. doi:10.1016/j.jep.2010.01.031
Qian, J. Y., Deng, P., Liang, Y. D., Pang, L., Wu, L. C., Yang, L. L., et al. (2019). 8-Formylophiopogonanone B Antagonizes Paraquat-Induced Hepatotoxicity by Suppressing Oxidative Stress. Front. Pharmacol. 10 (OCT), 1–12. doi:10.3389/fphar.2019.01283
Qiao, Y., Jiao, H., Wang, F., and Niu, H. (2020). Ophiopogonin D of Ophiopogon Japonicus Ameliorates Renal Function by Suppressing Oxidative Stress and Inflammatory Response in Streptozotocin-Induced Diabetic Nephropathy Rats. Braz. J. Med. Biol. Res. 53, e9628. doi:10.1590/1414-431x20209628
Qiu, C., Jozsef, L., Yu, B., and Yu, J. (2014). Saponin Monomer 13 of dwarf Lilyturf Tuber (DT-13) Protects Serum Withdrawal-Induced Apoptosis through PI3K/Akt in HUVEC. Biochem. Biophys. Res. Commun. 443 (1), 74–79. doi:10.1016/j.bbrc.2013.11.056
Rawat, M. S., Negi, D., Panwar, M., and Pant, G. (1988). A Spirostanol Glycoside from the Rhizome of Ophiopogon Intermedius. Phytochemistry 27 (1982), 3326–3327. doi:10.1016/0031-9422(88)80058-x
Song, S. J., Hyun, S. W., Lee, T. G., Park, B., Jo, K., Lee, I. S., et al. (2019). Topical Application of Liriope Platyphylla Extract Attenuates Dry Eye Syndrome Induced by Particulate Matter. J. Ophthalmol. 2019, 1429548. doi:10.1155/2019/1429548
Sun, Q., Chen, L., Gao, M., Jiang, W., Shao, F., Li, J., et al. (2012). Ruscogenin Inhibits Lipopolysaccharide-Induced Acute Lung Injury in Mice: Involvement of Tissue Factor, Inducible NO Synthase and Nuclear Factor (NF)-κB. Int. Immunopharmacol. 12 (1), 88–93. doi:10.1016/j.intimp.2011.10.018
Tao, J., Wang, H., Zhou, H., and Li, S. (2005). The Saponin Monomer of dwarf Lilyturf Tuber, DT-13, Reduces L-type Calcium Currents during Hypoxia in Adult Rat Ventricular Myocytes. Life Sci. 77 (24), 3021–3030. doi:10.1016/j.lfs.2005.01.039
Tian, Y., Ma, S., Lin, B., Kou, J., and Yu, B. (2013). Anti-thrombotic Activity of DT-13, a Saponin Isolated from the Root Tuber of Liriope Muscari. Indian J. Pharmacol. 45 (3), 283–285. doi:10.4103/0253-7613.111896
Tian, Y. Q., Kou, J. P., Li, L. Z., and Yu, B. Y. (2011). Anti-inflammatory Effects of Aqueous Extract from Radix Liriope Muscari and its Major Active Fraction and Component. Chin. J. Nat. Med. 9 (3), 222–226. doi:10.3724/SP.J.1009.2011.00222
Tsai, Y. C., Chiang, S. Y., El-Shazly, M., Wu, C. C., Beerhues, L., Lai, W. C., et al. (2013). The Oestrogenic and Anti-platelet Activities of Dihydrobenzofuroisocoumarins and Homoisoflavonoids from Liriope Platyphylla Roots. Food Chem. 140, 305–314. doi:10.1016/j.foodchem.2013.02.069
Tsai, Y. C., Hsu, C. C., El-Shazly, M., Chiang, S. Y., Wu, C. C., Wu, C. C., et al. (2015). Phytochemicals and Estrogen-Receptor Agonists from the Aerial Parts of Liriope Platyphylla. Molecules 20 (4), 6844–6855. doi:10.3390/molecules20046844
Wang, C., He, H., Liu, G., Ma, H., Li, L., Jiang, M., et al. (2020a). DT-13 Induced Apoptosis and Promoted Differentiation of Acute Myeloid Leukemia Cells by Activating AMPK-KLF2 Pathway. Pharmacol. Res. 158, 104864. doi:10.1016/j.phrs.2020.104864
Wang, L., Zhou, Y., Qin, Y., Wang, Y., Liu, B., Fang, R., et al. (2019). Methylophiopogonanone B of Radix Ophiopogonis Protects Cells from H2O2-induced A-poptosis through the NADPH O-xidase P-athway in HUVECs. Mol. Med. Rep. 20 (4), 3691–3700. doi:10.3892/mmr.2019.10625
Wang, Y., Huang, X., Ma, Z., Wang, Y., Chen, X., and Gao, Y. (2018). Ophiopogonin D Alleviates Cardiac Hypertrophy in Rat by Upregulating CYP2J3 In Vitro and Suppressing Inflammation In Vivo. Biochem. Biophys. Res. Commun. 503 (2), 1011–1019. doi:10.1016/j.bbrc.2018.06.110
Wang, Y., Yan, T., Shen, J., Guo, H., and Xiang, X. (2007). Preventive Effect of Ophiopogon Japonicus Polysaccharides on an Autoallergic Mouse Model for Sjogren's Syndrome by Regulating the Th1/Th2 Cytokine Imbalance. J. Ethnopharmacol. 114, 246–253. doi:10.1016/j.jep.2007.08.014
Wei, X., Mao, T., Li, S., He, J., Hou, X., Li, H., et al. (2019). DT-13 Inhibited the Proliferation of Colorectal Cancer via Glycolytic Metabolism and AMPK/mTOR Signaling Pathway. Phytomedicine 54, 120–131. doi:10.1016/j.phymed.2018.09.003
Wei, X. H., Lin, S. S., Liu, Y., Liu, R. P., Khan, G. J., Du, H. Z., et al. (2016). DT-13 Attenuates Human Lung Cancer Metastasis via Regulating NMIIA Activity under Hypoxia Condition. Oncol. Rep. 36 (2), 991–999. doi:10.3892/or.2016.4879
WFO (2021). World Flora Online. Published on the Internet. Available at: http://www.worldfloraonline.org.
Wu, F., Cao, J., Jiang, J., Yu, B., and Xu, Q. (2001). Ruscogenin Glycoside (Lm-3) Isolated from Liriope Muscari Improves Liver Injury by Dysfunctioning Liver-Infiltrating Lymphocytes. J. Pharm. Pharmacol. 53 (5), 681–688. doi:10.1211/0022357011775802
Wu, Z., Zhao, X., Miyamoto, A., Zhao, S., Liu, C., Zheng, W., et al. (2019). Effects of Steroidal Saponins Extract from Ophiopogon Japonicus Root Ameliorates Doxorubicin-Induced Chronic Heart Failure by Inhibiting Oxidative Stress and Inflammatory Response. Pharm. Biol. 57 (1), 176–183. doi:10.1080/13880209.2019.1577467
Yan, X., Ye, T., Hu, X., Zhao, P., and Wang, X. (2016). 58-F, a Flavanone from Ophiopogon Japonicus, Prevents Hepatocyte Death by Decreasing Lysosomal Membrane Permeability. Sci. Rep. 6, 27875–27915. doi:10.1038/srep27875
You, W. T., Zhou, T., Ma, Z. C., Liang, Q. D., Xiao, C. R., Tang, X. L., et al. (2016). Ophiopogonin D Maintains Ca2+ Homeostasis in Rat Cardiomyocytes In Vitro by Upregulating CYP2J3/EETs and Suppressing ER Stress. Acta Pharmacol. Sin. 37 (3), 368–381. doi:10.1038/aps.2015.146
Zeng, K. W., Li, N., Dong, X., Ma, Z. Z., Jiang, Y., Jin, H. W., et al. (2013). Sprengerinin C Exerts Anti-tumorigenic Effects in Hepatocellular Carcinoma via Inhibition of Proliferation and Angiogenesis and Induction of Apoptosis. Eur. J. Pharmacol. 714 (1–3), 261–273. doi:10.1016/j.ejphar.2013.04.026
Zeng, K. W., Song, F. J., Li, N., Dong, X., Jiang, Y., and Tu, P. F. (2015). ASC, a Bioactive Steroidal Saponin from Ophitopogin Japonicas, Inhibits Angiogenesis through Interruption of Src Tyrosine Kinase-dependent Matrix Metalloproteinase Pathway. Basic Clin. Pharmacol. Toxicol. 116 (2), 115–123. doi:10.1111/bcpt.12305
Zhang, J., and Li, X. D. (2020). Study on the Law of Chinese Medicine Prescription in Convalescence Period of corona Virus Disease-19 (COVID-19) in Various Regions Based on Data Mining. J. Hubei Univ. Chin. Med. 06, 117–121. doi:10.3969/j.issn.1008987x.2020.06.33
Zhang, M., Liu, X., Song, J., Hu, Y., Wang, X., and Li, B. (2010). [Effects of Radix Ophiopogonis Decoction on Embryo-Fetal Development in Rats]. Zhongguo Zhong Yao Za Zhi 35, 2334–2337. doi:10.4268/cjcmm20100821
Zhang, Y., Sun, M., Han, Y., Zhai, K., Tang, Y., Qin, X., et al. (2015b). The Saponin DT-13 Attenuates Tumor Necrosis Factor-α-Induced Vascular Inflammation Associated with Src/NF-Кb/MAPK Pathway Modulation. Int. J. Biol. Sci. 11 (8), 970–981. doi:10.7150/ijbs.11635
Zhang, Y., Zhao, Y., Wu, Y., Qi, J., Li, F., Kou, J., et al. (2020). Ophiopogon Saponin C1 Inhibits Lung Tumors by Stabilizing Endothelium Permeability via Inhibition of PKCδ. Int. J. Biol. Sci. 16 (3), 396–407. doi:10.7150/ijbs.34978
Zhang, Y. Y., Meng, C., Zhang, X. M., Yuan, C. H., Wen, M. D., Chen, Z., et al. (2015c). Ophiopogonin D Attenuates Doxorubicin-Induced Autophagic Cell Death by Relieving Mitochondrial Damage In Vitro and In Vivo. J. Pharmacol. Exp. Ther. 352 (1), 166–174. doi:10.1124/jpet.114.219261
Zhao, J. W., Chen, D. S., Deng, C. S., Wang, Q., Zhu, W., and Lin, L. (2017). Evaluation of Anti-inflammatory Activity of Compounds Isolated from the Rhizome of Ophiopogon Japonicas. BMC Complement. Altern. Med. 17, 7. doi:10.1186/s12906-016-1539-5
Keywords: ophiopogon, liriope, liriopogons, phytochemistry, pharmacology, critical review
Citation: Lei F, Weckerle CS and Heinrich M (2021) Liriopogons (Genera Ophiopogon and Liriope, Asparagaceae): A Critical Review of the Phytochemical and Pharmacological Research. Front. Pharmacol. 12:769929. doi: 10.3389/fphar.2021.769929
Received: 02 September 2021; Accepted: 29 October 2021;
Published: 03 December 2021.
Edited by:
Hung-Rong Yen, China Medical University, TaiwanReviewed by:
Xiaoxiao Huang, Shenyang Pharmaceutical University, ChinaSubhash C. Mandal, Directorate of Drugs Control, India
Copyright © 2021 Lei, Weckerle and Heinrich. This is an open-access article distributed under the terms of the Creative Commons Attribution License (CC BY). The use, distribution or reproduction in other forums is permitted, provided the original author(s) and the copyright owner(s) are credited and that the original publication in this journal is cited, in accordance with accepted academic practice. No use, distribution or reproduction is permitted which does not comply with these terms.
*Correspondence: Feiyi Lei, feiyi.lei@systbot.uzh.ch, l_feiyi@hotmail.com; Michael Heinrich, m.heinrich@ucl.ac.uk