- The MOE Key Laboratory for Standardization of Chinese Medicines, Shanghai Key Laboratory of Compound Chinese Medicines and The SATCM Key Laboratory for New Resources and Quality Evaluation of Chinese Medicines, Institute of Chinese Materia Medica, Shanghai University of Traditional Chinese Medicine, Shanghai, China
Si-Miao-Yong-An decoction (SMYAD), a traditional Chinese medicine formula, is mainly used to clear away heat and detoxify and to promote blood circulation and relieve pain. Diabetic retinopathy (DR) is the most common type of microvascular complication caused by diabetes. This study is designed to examine the protective effect of SMYAD against DR and further to reveal the engaged mechanism via integrating network pharmacology and in vivo experimental evidence. Streptozotocin (STZ) was intraperitoneally injected into mice to induce diabetes. The dysfunction of the blood retina barrier (BRB) was observed by conducting Evan’s blue leakage assay, detecting tight junction (TJ) protein expression and counting the number of acellular capillaries in retinas. Our results showed that SMYAD alleviated BRB breakdown in vivo. Network pharmacology results demonstrated that regulating inflammation, immune responses, and angiogenesis might be associated with the efficacy of SMYAD in alleviating DR, in which the tumor necrosis factor (TNF) and hypoxia inducible factor 1 (HIF1) signal pathways were involved. Next, immunofluorescence staining results showed that SMYAD decreased microglia activation in retinas and reduced the enhanced adhesion of leukocytes into retinal vessels. SMYAD reduced the elevated serum TNFα content and retinal TNFα expression. SMYAD abrogated the activation of nuclear factor κB (NFκB) and HIF1α and consequently decreased the enhanced expression of some pro-inflammatory molecules and vascular endothelial growth factor (VEGF) in retinas. These results indicate that SMYAD attenuated DR development through suppressing retinal inflammation and angiogenesis via abrogating NFκB-TNFα and HIF1α-VEGF signal pathways.
Introduction
Diabetic retinopathy (DR), a common microvascular complication caused by diabetes, has become the most serious eye disease leading to the adult blindness in the world (Antonetti et al., 2012; Gardlik and Fusekova, 2015). From 2015 to 2019, the prevalence rate of diabetic macular edema (DME) and DR was 27.0% in the world, among which the early period non-proliferative DR (NPDR) accounted for 25.2%, the later period proliferative DR (PDR) was 1.4%, and DME was 4.6% (Thomas et al., 2019). In China, the pooled prevalence rates in people with diabetes between 1990 and 2007 were 18.45, 15.06, and 0.99% for DR, NPDR, and PDR, respectively (Song et al., 2018). Therefore, more attention shall be paid to DR prevention.
At present, DR therapy is mainly based on drug treatment, laser photocoagulation treatment, and pars plana vitrectomy. Laser photocoagulation is an invasive treatment, which can cause postoperative visual field defects, decreased contrast sensitivity, and some other adverse reactions including central dark spots or para-central dark spots (Oellers and Mahmoud, 2016). These side effects greatly limited the clinical application of laser photocoagulation. The vascular endothelial growth factor (VEGF) antagonist, intravitreally injected when applied, is the most common drug used for DR treatment (Bandello et al., 2013). However, the application of the VEGF antagonist only is incapable of curing DR completely. Moreover, it has some side effects such as causing fibrovascular constriction and the sustained elevation of intraocular pressure (Singh and Kim, 2012; Bandello et al., 2013). Therefore, more drugs with better efficacy and fewer side effects need to be found in the future.
According to the theory of traditional Chinese medicine, the pathogenesis of DR was mainly due to the poor blood circulation and stasis caused by Yin deficiency and fire vigour that burned blood vessels. Recently, many traditional Chinese medicines (TCMs) and their active ingredients have been reported to improve DR. These TCMs include Lonicera japonica Thunb., Salvia miltiorrhiza Bge., Lycium barbarum L., Andrographis paniculata (Burm.f.) Nees, Panax notoginseng (Burkill) F.H.Chen, Dendrobium officinale Kimura and Migo, etc., and they all have functions like eliminating heat, improving blood circulation, nourishing Yin, and dissolving blood stasis (Behl and Kotwani, 2017; Vasant More et al., 2017; Xu et al., 2018).
Si-Miao-Yong-An decoction (SMYAD), first recorded in “YanFangXinBian” (Qing Dynasty), is composed of Scrophularia ningpoensis Hemsl. (Chinese name Xuan-Shen), Lonicera japonica Thunb (Chinese name Jin-Yin-Hua), Angelica sinensis (Oliv.), Diels (Chinese name Dang-Gui), and Glycyrrhiza uralensis Fisch. ex DC. (Chinese name Gan-Cao). It is traditionally applied externally for treating sore, carbuncle, and ulcer. Recently, SMYAD is generally used for the treatment of peripheral vascular diseases such as thromboangiitis obliterans. Among these four TCMs included in SMYAD, Jin-Yin-hua is good at clearing away heat and detoxifying, Xuan-Shen nourishes Yin and relieves fire, Dang-Gui is good at activating blood and dissipating blood stasis, and Gan-Cao reconciles various drugs. Therefore, SMYAD can be used to clear away heat and detoxify, to improve blood circulation, and to relieve pain, which implies its huge potential in DR treatment. Actually, a previous clinical study has demonstrated that SMYAD improved DR and some other diabetic vascular complications in diabetic patients (Zhang et al., 2012). However, the mechanism participating in the SMYAD-provided protection against DR is still not clear.
The pathogenesis of DR is very complex, which implies that modulating multiple targets is required for its treatment. Network pharmacology is generally used to clarify the relationship between the drug’s action and molecular targets (Hopkins, 2008). The therapeutic action of the Chinese medicinal formula may be obtained from the joint action of all compounds in the formula, so its pharmacological activity has multi-effects, multi-pathways, and multi-targets, which is consistent with the core theory and method of network pharmacology (Wang et al., 2021). Recently, network pharmacology is commonly used for the prediction of bioactive ingredients and the clarification of molecular targets in TCM’s research work (Li et al., 2014; Jiao et al., 2021; Wang et al., 2021).
The alleviation of SMYAD on DR was evaluated in vivo, and then, the potential engaged mechanism was investigated by integrating network pharmacology prediction and further in vivo experimental validation in this study.
Materials and Methods
Reagents
Scrophularia ningpoensis Hemsl., Lonicera japonica Thunb., Angelica sinensis (Oliv.) Diels, and Glycyrrhiza uralensis Fisch. ex DC. were purchased from Kangqiao Co., Ltd. (Shanghai, China). These four TCMs were authenticated by Professor Hong Xu, and voucher samples were kept in the Institute of Chinese Materia Medica (Shanghai University of Traditional Chinese Medicine). Chlorogenic acid (Jin-Yin-Hua), luteoloside (Jin-Yin-Hua), harpagide (Xuan-Shen), harpagoside (Xuan-Shen), ferulic acid (Dang-Gui), glycyrrhizinic acid (Gan-Cao), and liquiritin (Gan-Cao) (purity≥98%) were bought from Dalian Meilun Biotechnology Co., Ltd. (Dalian, China). The intercellular adhesion molecule 1 (ICAM1) antibody (ABclonal, Shanghai, China), the ionized calcium-binding adapter molecule 1 (Iba1) antibody (GeneTax Inc., Alton Parkway Irvine, CA), nuclear factor κB (NFκB) p65, ß-actin, Lamin B1, phosphorylated IκB kinase (p-IKK), the phosphorylated NFκ-B inhibitor (p-IκB), VEGF receptor 2 (VEGFR2), and the phosphorylated VEGFR2 antibodies (Cell Signaling Technology, Danvers, MA) were obtained. Tumor necrosis factor-α (TNFα), claudin1, claudin19, hypoxia inducible factor 1α (HIF1α), and vascular cell adhesion protein 1 (VCAM1) antibodies (Santa Cruz, Santa Cruz, CA), Alexa Fluor 568 goat anti-rabbit IgG (BD Biosciences, Franklin Lakes, NJ), and peroxidase-conjugated goat anti-rabbit immunoglobulin G (IgG) (H + L) and anti-mouse IgG (H + L) (Jackson ImmunoResearch, West Grove, PA) were obtained. BCA Protein Assay Kits and NE-PER nuclear and cytoplasmic extraction reagents were bought from Thermo Fisher Scientific (Waltham, MA). Enzyme-linked immunosorbent assay (ELISA) kits were from R and D (Minneapolis, MN). PrimeScript RT Master Mix and SYBR Premix Ex Taq kits (Takara, Shiga, Japan) were obtained. Fluorescein isothiocyanate-coupled concanavalin A (FITC-ConA) was bought from Vector Laboratories (Burlingame, CA). 4’,6-Diamidino-2-phenylindole (DAPI) and Trizol were purchased from Life Technology (Carlsbad, CA). Other reagents were bought from Sigma Chemical Co. (St. Louis, MO).
Preparation of SMYAD Powder
The SMYAD powder was prepared as recorded in classic literature reports. The mixed Scrophularia ningpoensis Hemsl., Lonicera japonica Thunb., Angelica sinensis (Oliv.) Diels, and Glycyrrhiza uralensis Fisch. ex DC. (The ratio of weight is 3:3:2:1) were heated under reflux for 2 h with 10 times the amount of distilled water. The residue was heated under reflux again under the same condition after the extracts were filtered, and this procedure was repeated three times. The filtered extracts were concentrated and further freeze-dried into fine powder with the final yield of 36.5%.
High-Performance Liquid Chromatography Analysis
High-performance liquid chromatography (HPLC) was performed on a Shiseido C18 column (250 nm × 4.6 mm, 5 μm, 30°C). The wavelength was set at 260 nm. The mobile phase consisted of a mixture of acetonitrile–0.05%H3PO4–Et3N (at 0–5 min, 0.2:99.8, v/v; at 5–15 min, increased from 0.2:99.8 to 3:97, v/v; at 15–30 min, 7:93, v/v; at 30–45 min, 8:92, v/v; at 45–65 min, 6:94, v/v; at 65–67 min, 10:90, v/v; at 67–70 min, 11:89, v/v; at 75–90 min, 12:88, v/v; at 90–115 min, 17:83, v/v; at 115–130 min, 20:80, v/v; at 130–135 min, 23:77, v/v; at 135–145 min, 30:70, v/v; at 145–175 min, 50:50, v/v) with a flow rate of 1.0 ml/min. Seven reference compounds including chlorogenic acid, luteoloside, harpagide, harpagoside, ferulic acid, glycyrrhizinic acid, and liquiritin were dissolved in methanol. The peak of seven main compounds in SMYAD was identified by comparing the retention time with that of reference compounds. Additionally, the contents of those above seven compounds in SMYAD were detected by using the external standard method.
Experimental Animals
Specific-pathogen-free male C57BL/6 mice (20 ± 2 g) were obtained from the Shanghai Laboratory Animal Center of Chinese Academy of Science (Shanghai, China) and fed under a controlled room temperature (22°C ± 1 °C) and humidity (65 ± 5%) with a 12:12 h light/dark cycle. Mice have free access to food and water and have received humane care according to the institutional animal care guidelines approved by the Experimental Animal Ethical Committee of Shanghai University of Traditional Chinese Medicine (approved number: PZSHUTCM200724021).
Animal Treatment
A total of 45 mice were intraperitoneally injected (ip) with 55 mg/kg BW of streptozotocin (STZ) (Sigma No. S0130) in citric acid solution and 0.1 M trisodium citrate solution pH = 4.4 (vehicle) for 5 consecutive days. Also, other 15 mice in the control group were injected with physiological saline. Seven days later, mice with a blood glucose concentration over 16.7 mmol/L were considered as diabetic mice and included in this study. These diabetic mice were randomly divided as follows: DM, DM + SMYAD (1.5 g/kg), and DM + SMYAD (4.5 g/kg). Each group has 15 mice. One month later, SMYAD (1.5, 4.5 g/kg, intragastric administration) was orally given to mice for 1 consecutive month, and then, mice were sacrificed to collect blood samples and eyes. The body weight was monitored and the blood glucose concentration was determined with a Accu-Check glucometer (Roche Diagnostics, Germany) every 7 days during the whole experimental process.
Evan’s Blue Leakage Assay
Blood-retina-barrier (BRB) breakdown was detected according to the published method (Mei et al., 2019; Zhang et al., 2019). Briefly, mice were injected with 2% Evan’s blue (10 μl/g, i. p.). Two hours later, retinas were dissected after the Evan’s blue dye was completely removed from retinal vessels. Formamide was used to dissolve the retinas, and the absorbance in the extracts was determined at 620 nm. The amount of Evan’s blue dye was calculated, and the results were normalized to the dried weight of the retina.
Leukostasis Assay
Leukostasis was detected as previously described (Chen et al., 2019). Briefly, FITC-ConA (120 μl) was intravenously injected into mice. Twenty minutes later, the isolated eyes were fixed in a 4% paraformaldehyde (PFA) solution. Retinas were dissected and flat-mounted for the observation under an inverted microscope (IX81, Olympus, Japan). FITC-ConA + cells adhered to retinal blood vessels were counted.
Hematoxylin-Eosin Staining of Acellular Capillaries in Retinas
The retina was fixed in a 4% PFA solution for 24 h and gently rocked overnight after four times of cleaning with ultrapure water and then digested with 3% trypsin and stained with hematoxylin-eosin (H and E) the next day. The morphology of retinal vessels was pictured by using an inverted microscope.
Network Pharmacology Analysis
Network pharmacology analysis was conducted as described in the guidance (Li et al., 2021).
Target Collection: Active targets of SMYAD were acquired from BATMAN-TCM (http://bionet.ncpsb.org.cn/batman-tcm/) and TCMSP (http://tcmspnw.com/) databases. According to the suggested criterion, compounds were selected if the drug-likeness was ≥0.18 and the oral bioavailability was ≥30%. Additionally, the indicator chemical compounds recorded in Chinese Pharmacopoeia from four Chinese botanical drugs included in SMYAD (Chinese Pharmacopoeia Commission, 2020) with low drug-likeness and oral bioavailability values were also retained in this study.
Protein–Protein Interaction (PPI) Network Construction: Diabetic Retinopathy (ICD-11, 9B71.0Z) therapeutic targets were collected by using GeneCards (www.genecards.org/). Cytoscape 3.6.1 software was used to construct a drugs–ingredients–targets network. A target-to-target and function-related PPI network was established via the STRING database.
KEGG and GO Analysis: DAVID 6.8 was used to analyze the KEGG pathway enrichment and GO function of the proteins involved in the PPI network. The R package of ggplot2 was used for enrichment analysis, and the cutoff value was set as p < 0.05.
Immunofluorescence Staining
Retinal Iba1 immunofluorescence staining was conducted according to the published method (Mei et al., 2019; Zhang et al., 2019), but in this study, retinas were incubated with the Alexa fluor 568 goat anti-rabbit IgG (H + L) antibody after incubating with the Iba1 antibody overnight.
qPCR Analysis
Retinal RNA was extracted, cDNA was synthesized, and real-time RT-PCR was conducted as described in kits. The relative expression of target genes was normalized to actin, analyzed by the 2−ΔΔCt method, and calculated as the ratio compared with the control. The primer sequences are shown in Table 1.
Western-Blot Analysis
Proteins were extracted, and the protein concentration was determined. The denatured samples were run on SDS-PAGE gels and electrophoretically transferred to PVDF membranes that were further incubated with primary and secondary antibodies. The protein expression was visualized by using a chemiluminescent reagent and quantified by calculating gray densities of the target protein blots with internal controls.
ELISA
Serum contents of TNFα, monocyte chemoattractant protein-1 (MCP-1), interleukin-6 (IL-6), and VEGF were detected by using commercial ELISA kits.
Statistical Analysis
Data are shown as the mean ± standard error of the mean (SEM). The statistical significance of differences between groups is obtained through one-way ANOVA with the LSD post hoc test. p < 0.05 is considered to be the significant difference.
Results
Chemical Characterization of SMYAD
Data in Figures 1A–G demonstrated the HPLC chromatogram of chlorogenic acid, luteoloside, harpagide, harpagoside, ferulic acid, glycyrrhizinic acid, and liquiritin. Figures 1H–J demonstrate the HPLC chromatogram of SMYAD, and the above seven compounds were pointed out in SMYAD. Next, the content of these above seven compounds in SMYAD was determined. After calculations, the content of chlorogenic acid, luteoloside, harpagide, harpagoside, ferulic acid, glycyrrhizinic acid, and liquiritin in SMYAD was 0.882, 0.034, 0.284, 0.103, 0.034, 0.258, and 0.201%, respectively.
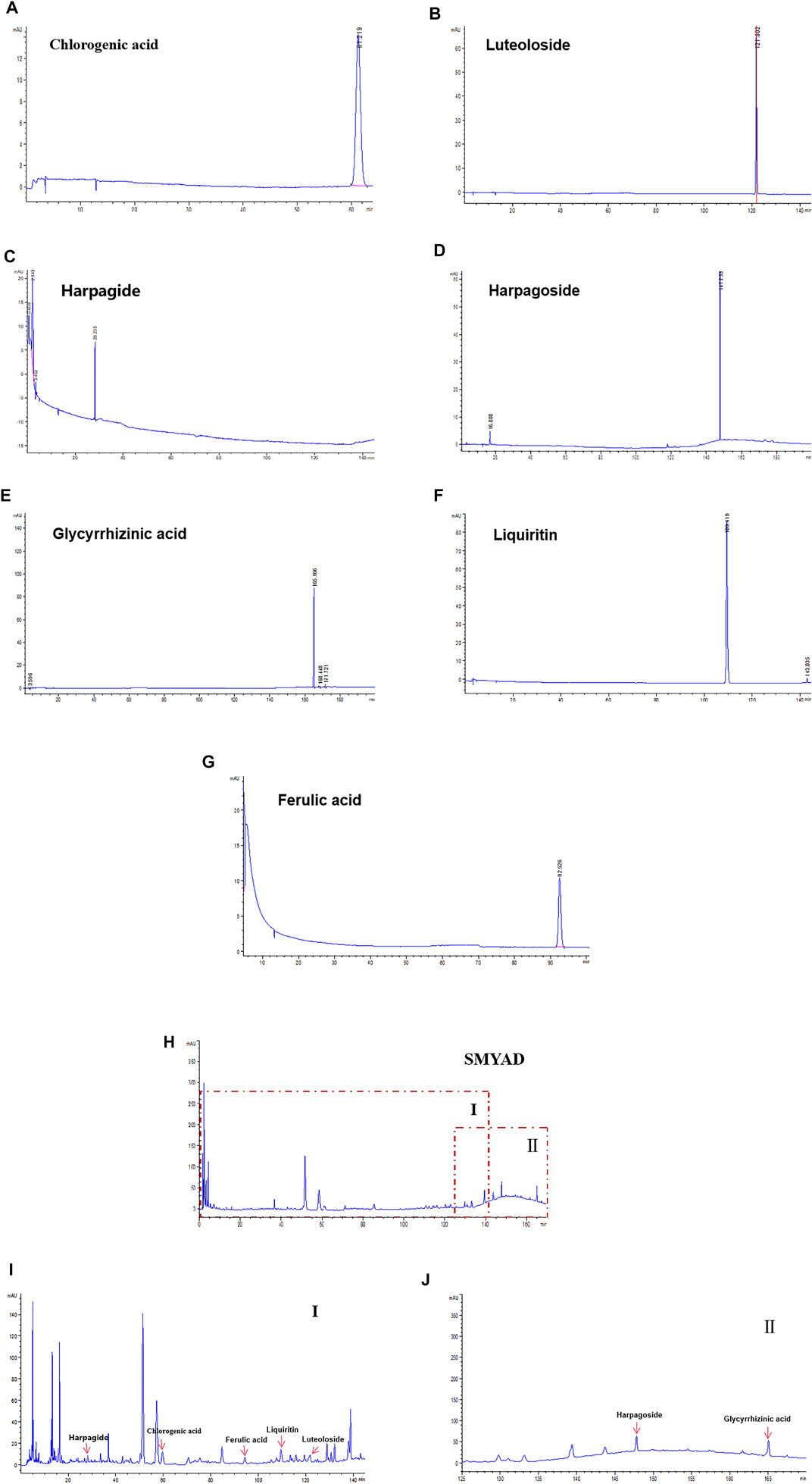
FIGURE 1. HPLC analysis. HPLC chromatogram of reference compounds including (A) chlorogenic acid, (B) luteoloside, (C) harpagide, (D) harpagoside, (E) glycyrrhizinic acid, (F) liquiritin, and (G) ferulic acid. HPLC chromatogram of (H) SMYAD and (I) SMYAD in areaⅠand (J) SMYAD in areaⅡ.
SMYAD Alleviated BRB Dysfunction in vivo
The increased blood glucose concentration and the reduced body weight of diabetic mice did not change after the administration of SMYAD (1.5, 4.5 g/kg) (data not shown). The elevated Evan’s blue dye leakage in retinas from diabetic mice was reduced after the administration of SMYAD (1.5, 4.5 g/kg) (Figure 2A). As shown in Figure 2B, the decreased expression of retinal claudin1 and claudin19 (two typical tight junctions, TJs) in diabetic mice was enhanced when diabetic mice received SMYAD (4.5 g/kg). Moreover, the decreased number of acellular capillaries marked with black arrows in retinas from diabetic mice was enhanced after the administration of SMYAD (1.5, 4.5 g/kg) (Figure 2C).
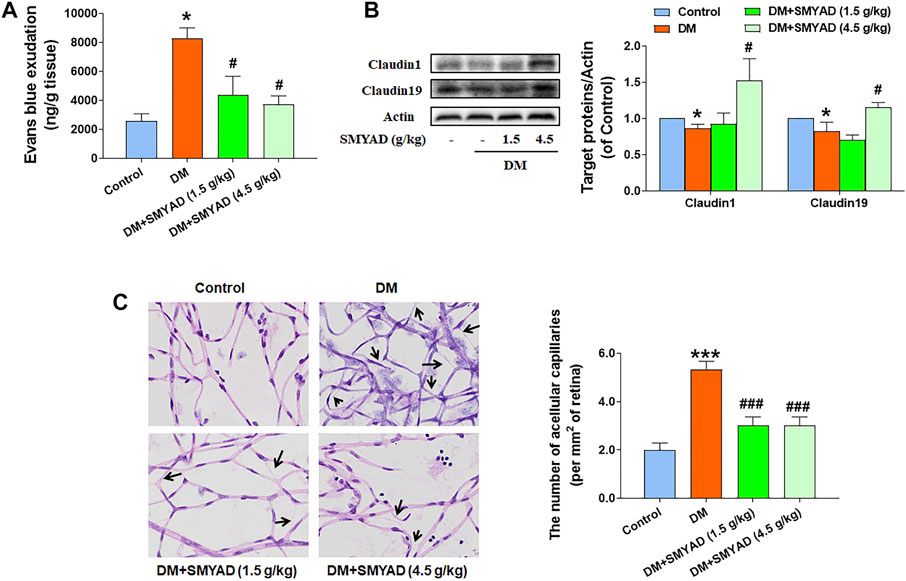
FIGURE 2. SMYAD alleviated BRB dysfunction in vivo. (A) Evan’s blue leakage assay (n = 4). (B) Claudin1 and claudin19 expression in retinas. The quantitative result is shown at right (n = 3). (C) Retinal H and E staining. The number of acellular capillaries per field in retinas is shown at right (n = 3). Black arrows indicate acellular capillaries. Data = mean ± SEM. *p < 0.05, ***p < 0.001 vs. control; #p < 0.05, ###p < 0.001 vs. DM.
Network Pharmacology Analysis
A total of 109 active compounds and 398 compound-related targets were acquired from TCMSP and BATMAN-TCM. A DR target network was established to find the relationship between compounds in SMYAD and common targets of DR. As shown in Figure 3A, the yellow diamond-shape node in the central area represents the total 398 compounds-related targets, the green circular-shape node on the upper-left corner represents 14 active compounds from Jin-Yin-Hua, the red circular-shape node on the upper-right corner represents five compounds from Xuan-Shen, the purple circular-shape node on the bottom-left corner represents one compound from Dang-Gui, the pink circular-shape node on the bottom-right corner represents 84 compounds from Gan-Cao, and the blue circular-shape node represents five co-owned compounds from Jin-Yin-Hua, Xuan-Shen, Dang-Gui, and Gan-Cao. A total of 3,497 targets involved in DR development were obtained from the GeneCards database. After integrating the targets of compounds from SMYAD and the targets involved in DR development, 198 common matched-targets were found, and the Venn diagram is shown in Figure 3B. Next, those above 198 common matched-targets were imported into the STRING database to construct a PPI network. After removing 60 disconnected nodes, there were a total of 138 nodes (representing 138 common matched targets) and 817 edges (representing the interaction between two targets) (Figure 3C). After screening according to the degree of these nodes, the core targets are shown in Figure 3D. The node became larger and its color changed from yellow to red with the increased degree of the targets. Among these core targets, the first six molecular targets with higher degrees were protein kinase B (AKT1), IL-6, tumor protein p53 (TP53), TNF, mitogen-activated protein kinase 8 (MAPK8), and VEGFA.
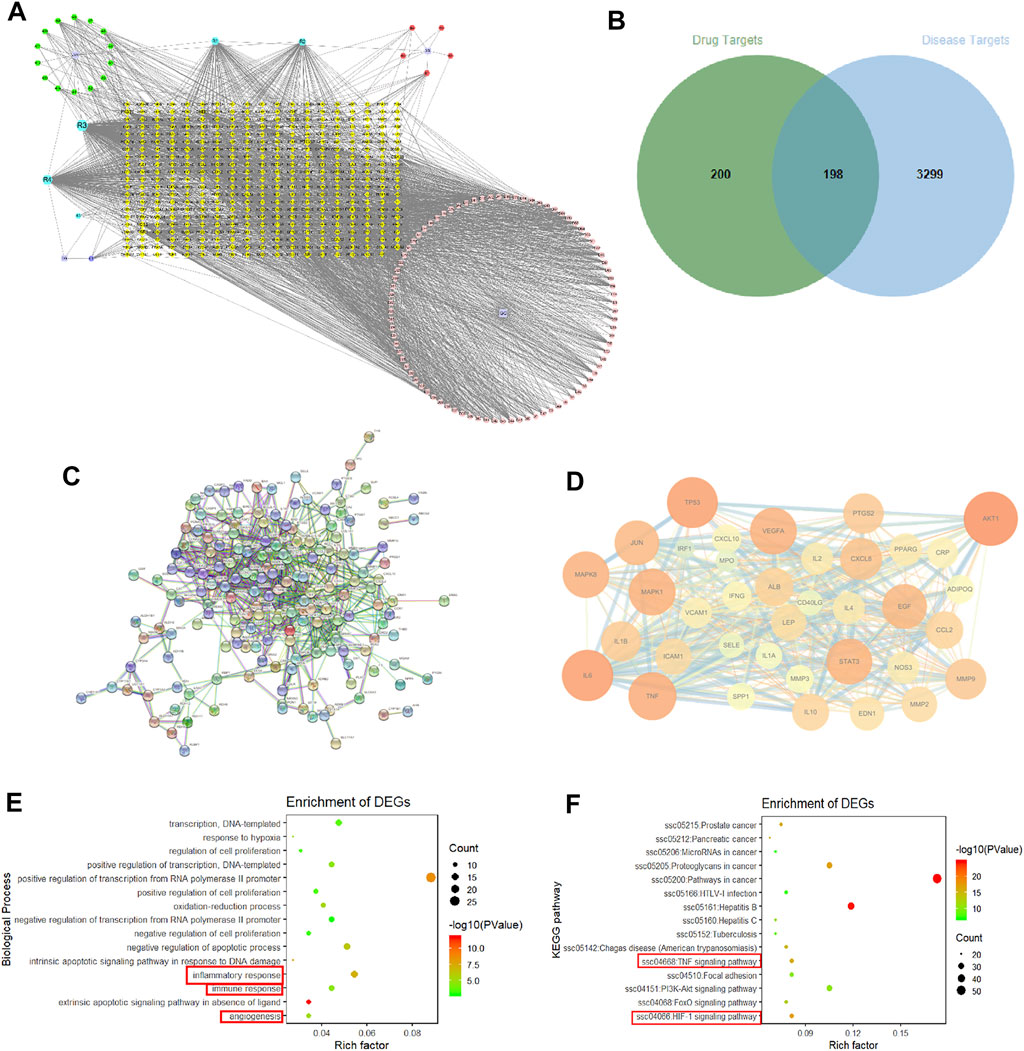
FIGURE 3. Network pharmacology. (A) Drugs–compounds–targets network. The node representing the compound is represented by a multi-color hexagon, and the target point is represented by a yellow diamond. (B) Venn diagram. (C) PPI network. (D) PPI network of core targets extracted from degree centrality. (E) GO enrichment analyses. A total of 15 biological process items in descending order of p-values in the DAVID database. (F) KEGG enrichment analyses.
These above 198 common matched-targets were further imported into the DAVID database, and a total of 960 GO items and 133 KEGG pathways were obtained. As shown in Figure 3E, inflammation (GO: 0006954), immune responses (GO: 0006955), and angiogenesis (GO: 0001525) marked with a red square accounted for a large proportion of biological processes. Moreover, the results of KEGG pathways showed that the TNF signaling pathway (hsa04668) (critically involved in inflammation and immune responses) and the HIF1 signaling pathway (hsa04066) (critically involved in angiogenesis) marked with a red square were the key signal pathways involved in the SMYAD-provided protection against DR.
Next, we consulted some transcriptome chips conducted in DR research included in GEO Databases (http://www.ncbi.nlm.nih.gov/gds/). The transcriptome chip results from mice at about 6 weeks after STZ injection (GSE111465) showed that the TNF signaling pathway marked with a red square was the most important signaling pathway involved in DR development in mice at 6 weeks after STZ treatment (Supplementary Figure S1A). Additionally, the transcriptome chip results from mice at about 12 weeks after STZ injection (GSE19122) showed that the VEGF signaling pathway marked with a red square was the most important signaling pathway involved in DR development in mice at 12 weeks after STZ treatment (Supplementary Figure S1B).
SMYAD Alleviated Retinal Inflammation in vivo
Studies have reported that Iba1 is a microglia/macrophage specific marker, which is widely used to label microglia (Kanazawa et al., 2002; Ibrahim et al., 2011). The enhanced number of Iba1-staining microglia (marked with white arrows) in retinas from diabetic mice was decreased after the administration of SMYAD (Figure 4A). The elevated retinal Iba1 expression in diabetic mice was decreased when diabetic mice received SMYAD (4.5 g/kg) (Figure 4B). The increased number of leukocytes adhered to the retinal capillary (marked with white arrows) from diabetic mice was also decreased after the administration of SMYAD (4.5 g/kg) (Figure 4C). Next results showed that the enhanced serum IL-6 and MCP-1 contents in diabetic mice were decreased when diabetic mice received SMYAD (1.5, 4.5 g/kg) (Figures 4D,E). The elevated ICAM1 mRNA expression in retinas from diabetic mice was decreased after the administration of SMYAD (4.5 g/kg), and the elevated retinal MCP-1 mRNA expression in diabetic mice was decreased after the administration of SMYAD (1.5, 4.5 g/kg) (Figure 4F). Moreover, the elevated protein expression of both ICAM1 and VCAM1 in retinas from diabetic mice was decreased when diabetic mice received SMYAD (4.5 g/kg) (Figure 4G).
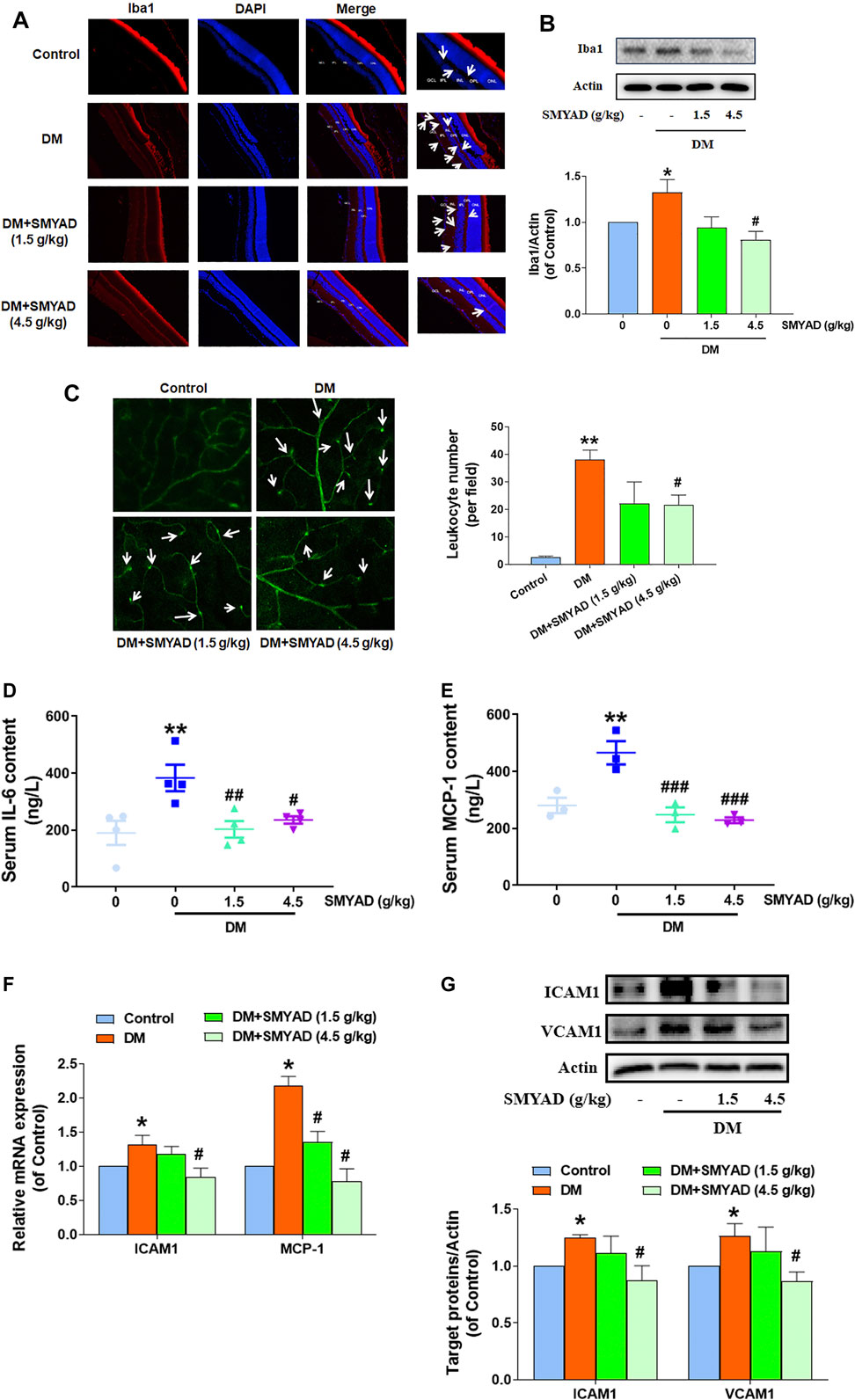
FIGURE 4. SMYAD alleviated retinal inflammation in vivo. (A) Representative images of retinal Iba1 immunofluorescence staining (scale bar: 20 mm). Enlarged images are shown at right (scale bar: 5 mm). White arrows indicate microglia. (B) SMYAD reduced the enhanced Iba1 expression in retinas. The quantitative result is shown below (n = 3). (C) Representative images of retinal FITC-ConA staining. The number of entrapped leukocytes in retinal vessels was calculated and is shown at right (n = 3). (D) Serum IL-6 amount (n = 4). (E) Serum MCP-1 amount (n = 3). (F) Retinal mRNA expression of ICAM1 and MCP-1 (n = 3). (G) SMYAD decreased the increased retinal expression of ICAM1 and VCAM1. The quantitative result is shown below (n = 3). Data = mean ± SEM. *p < 0.05, **p < 0.01 vs. control; #p < 0.05, ##p < 0.01, ###p < 0.001 vs. DM.
SMYAD Reduced TNFα Expression and Inhibited NFκB Activation in vivo
Data in Figure 5A showed that the enhanced serum TNF-α content in diabetic mice was obviously decreased when diabetic mice received SMYAD (1.5, 4.5 g/kg). Meanwhile, the enhanced retinal TNF-α mRNA and protein expression in diabetic mice was also decreased when diabetic mice received SMYAD (4.5 g/kg) (Figures 5B,C). Next results showed that SMYAD (4.5 g/kg) abrogated the nuclear accumulation of NFκ-B subunit p65 in retinas from diabetic mice (Figure 5D). Moreover, the elevated phosphorylation of IκB and IKK in retinas from diabetic mice was decreased when diabetic mice received SMYAD (1.5, 4.5 g/kg) (Figure 5E).
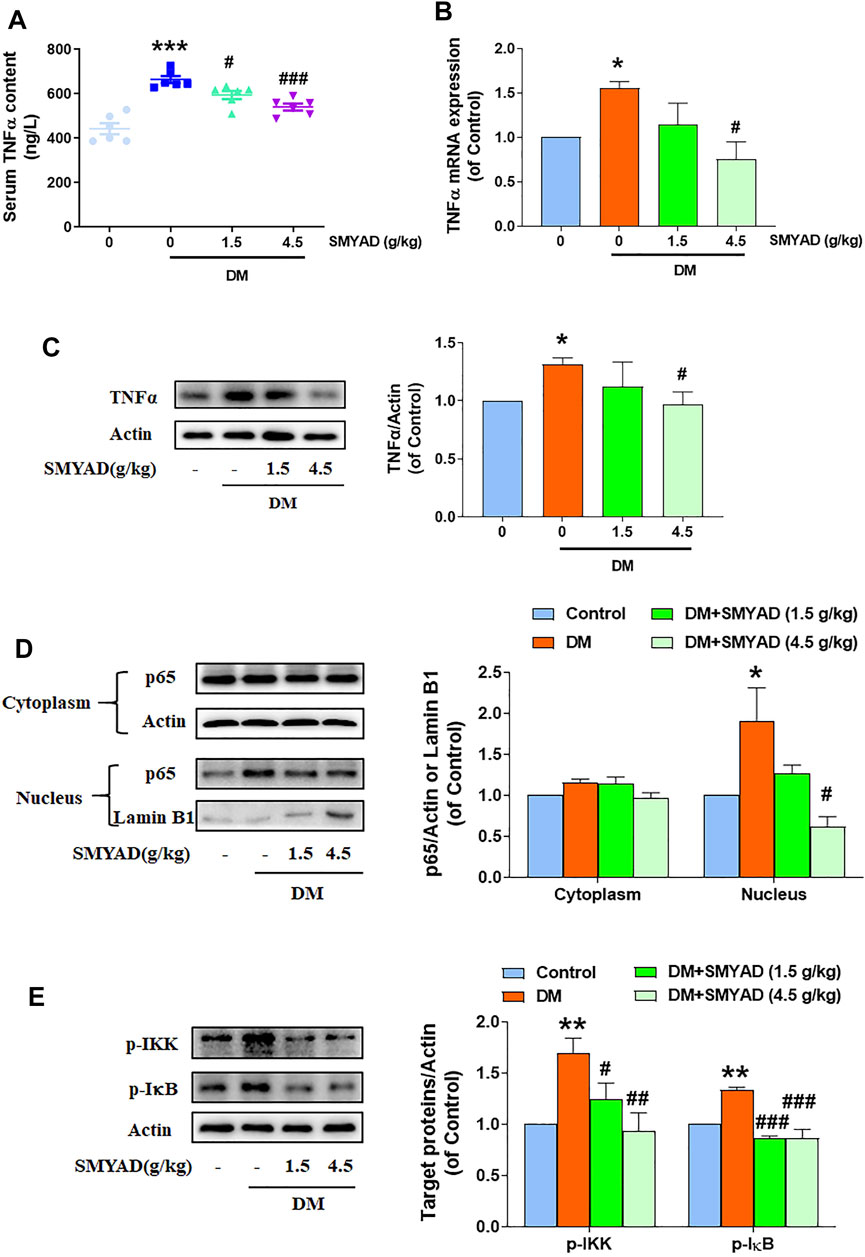
FIGURE 5. SMYAD reduced TNFα expression and blocked NFκB activation in vivo. (A) Serum TNFα content (n = 6). (B) Retinal mRNA expression of TNFα (n = 3). (C) SMYAD reduced the enhanced TNFα expression in retinas. The quantitative result is shown below (n = 3). (D) SMYAD abrogated the nuclear accumulation of NFκBp65 in retinas. The quantitative result is shown at right (n = 3). (E) SMYAD decreased the enhanced phosphorylation of IκB and IKK. The quantitative result is shown at right (n = 3). Data = mean ± SEM. *p < 0.05, **p < 0.01, ***p < 0.001 vs. control; #p < 0.05, ##p < 0.01, ###p < 0.001 vs. DM.
SMYAD Inhibited the HIF1α-VEGF/VEGFR2 Signal Pathway in vivo
SMYAD (1.5, 4.5 g/kg) abrogated the nuclear accumulation of HIF1α in retinas from diabetic mice (Figure 6A). Data in Figure 6B showed that the elevated serum VEGF content in diabetic mice was decreased after the administration of SMYAD (1.5, 4.5 g/kg). The increased retinal VEGF mRNA expression in diabetic mice was decreased when diabetic mice received SMYAD (4.5 g/kg) (Figure 6C). The enhanced retinal HIF1α-mRNA expression in diabetic mice was also obviously decreased when diabetic mice received SMYAD (1.5, 4.5 g/kg) (Figure 6C). Moreover, the enhanced VEGFR2 phosphorylation in retinas from diabetic mice was decreased when diabetic mice received SMYAD (4.5 g/kg) (Figure 6D).
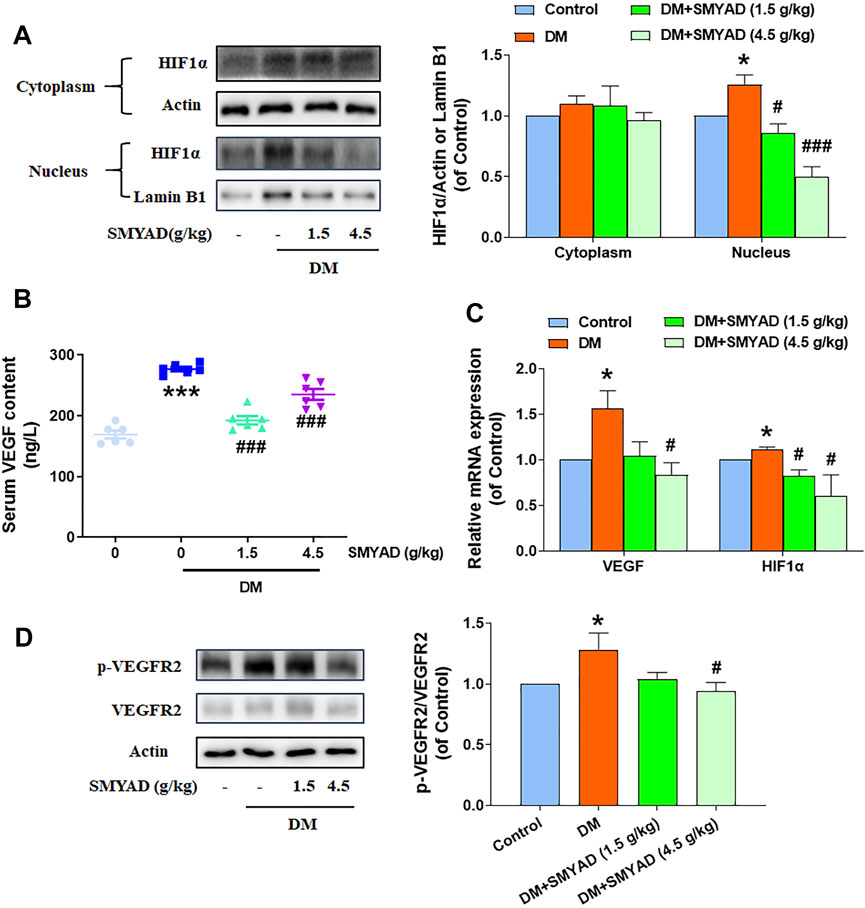
FIGURE 6. SMYAD inhibited the HIF1α-VEGF/VEGFR2 signal pathway in vivo. (A) SMYAD reduced nuclear accumulation of HIF1α in retinas. The quantitative result is shown at right (n = 3). (B) Serum VEGF content (n = 6). (C) HIF1α and VEGF mRNA expression in retinas (n = 3). (D) SMYAD decreased the elevated phosphorylation of VEGFR2. The quantitative result is shown at right (n = 3). Data = mean ± SEM. *p < 0.05, ***p < 0.001 vs. control; #p < 0.05, ###p < 0.001 vs. DM.
DISCUSSION
The dysfunction of BRB is commonly recognized as the earliest and typical event during DR progression (Zhang et al., 2014). BRB dysfunction is a crucial indicator for reflecting retinal microvascular injury in diabetic patients, which eventually causes severe visual impairment when no prompt intervention is applied (Rudraraju et al., 2020). Claudin1 and claudin19, two important TJs, greatly contributed to maintain the BRB integrity (Erickson et al., 2007). In this study, SMYAD reduced the leakage of Evan’s blue dye in retinas. SMYAD also restored the decreased retinal claudin1 and claudin19 expression in vivo. These above results indicate that SMYAD ameliorated BRB dysfunction in vivo. Acellular capillaries are a kind of microvessels with the blood vessel basal membrane and without endothelial cells. The occurrence of acellular capillaries was commonly used for reflecting the severity of retinopathy (Douglas et al., 2012; Chen et al., 2019). SMYAD decreased the enhanced number of acellular capillaries in retinas from diabetic mice. All these above results clearly demonstrated that SMYAD alleviated BRB dysfunction during DR progression.
Next, network pharmacology was performed to find the potential molecular targets involved in the SMYAD-provided alleviation on DR. Among those core molecular targets obtained from network pharmacology, the first six targets with higher degrees were AKT1, IL-6, TP53, TNF, MAPK8, and VEGFA, and they are all reported to regulate inflammation and angiogenesis (Kaštelan et al., 2020; Zhang et al., 2021; Zhou et al., 2021). Moreover, the enrichment results imply the crucial involvement of inflammation, immune responses, and angiogenesis in the SMYAD-provided protection against DR, as well as the potential participation of the TNF signaling pathway and HIF1 signaling pathway. Next, the results from two transcriptome chips conducted in DR research from GEO Databases further validated the previous experimental hints from network pharmacology that TNFα and HIF1α-VEGF signaling pathways participated in the progression of DR. The persistent low-grade inflammation caused by the elevated pro-inflammatory cytokines and other mediators was reported to be closely associated with the injury of retinal vasculature, which will cause BRB breakdown and induce retinal neovascularization during DR progression (Adamis, 2002; Aveleira, et al., 2010; Mesquida, et al., 2019). Therefore, retinal inflammation and neoangiogenesis are crucially involved in the whole course of DR. Our results from network pharmacology indicate that SMYAD may alleviate DR via inhibiting TNFα-mediated inflammation and HIF1α-initiated neoangiogenesis in retinas. Meanwhile, these prediction results from network pharmacology were further validated in the next following experiments.
Retinal inflammation initiated by the activated microglia is reported to be associated with DR progression (Baptista et al., 2017; Xu and Chen, 2017). This study showed that SMYAD reduced the enhanced Iba1 expression in retinas, suggesting that SMYAD inhibited retinal microglia activation in vivo. Leukostasis is a mild intra-vascular inflammatory response, and it is also an early event during DR progression reflecting retinal ischemia in diabetic patients (Adamis, 2002; Joussen et al., 2004). MCP-1 can recruit monocytes and macrophages, and its expression was elevated during DR development (Taghavi et al., 2019). ICAM1 and VCAM1 can recruit leukocytes to adhere to the endothelium and thus regulate leukostasis (Barouch et al., 2000). We found that SMYAD down-regulated MCP-1, ICAM1, VCAM1, and IL-6 expression and reduced leukostasis in retinas. These results suggest that SMYAD suppressed microglia activation and leukostasis in retina and thus alleviated retinal inflammation during DR development. Moreover, the results further validated the previous network pharmacology prediction that SMYAD alleviated BRB dysfunction during DR progression via inhibiting retinal inflammation.
As a main pro-inflammatory cytokine, TNFα was reported to regulate intra-ocular inflammatory responses and DR pathogenesis (Joussen, et al., 2002; Aveleira et al., 2010). TNFα was found to promote the adhesion of leukocytes to the endothelium in the retina and thus caused BRB damage (Joussen et al., 2002; Behl et al., 2008; Aveleira et al., 2010). As predicted in network pharmacology, the TNF signaling pathway participated in the SMYAD-provided amelioration on DR. Next experimental results further proved that SMYAD actually lowered the elevated retinal and serum TNFα contents. Transcription factor NFκB regulates the expression of many pro-inflammatory molecules including TNFα, IL-6, MCP-1, ICAM1, and VCAM1 (Shih et al., 2015). The NFκB signaling pathway is crucial for regulating retinal inflammation during DR progression (Kern, 2007). Our results found that SMYAD suppressed NFκB transcriptional activation and thus contributed to the reduced TNFα amount. All these will contribute to its inhibition on retinal inflammation, which shall be helpful for the alleviation of DR.
Previous network pharmacology results predicted the participation of angiogenesis and the important involvement of the HIF1 signaling pathway in the SMYAD-provided alleviation on DR. The dysfunction of the HIF1α-signaling pathway has already been found to be related with DR progression (Catrina and Zheng, 2021). Our experimental results further evidenced the inhibition of SMYAD on HIF1α activation in retinas in vivo. HIF1α can regulate the expression of VEGF, which drives neoangiogenesis via binding to its receptors, including VEGFR1, VEGFR2, and VEGFR3, and thus regulates pathological angiogenesis and the elevated vascular permeability during DR development (Witmer et al., 2003; Vriend and Reiter, 2016). SMYAD decreased the enhanced expression of VEGF and phosphorylated VEGFR2 in retinas. These above results suggest that SMYAD reduced retinal angiogenesis through suppressing the HIF1α-VEGF/VEGFR2 signaling cascade.
Our results demonstrated the alleviation of SMYAD on DR in vivo for the first time. After integrating the results from network pharmacology and the in vivo experimental validation, we found that SMYAD improved DR by inhibiting inflammation and angiogenesis through abrogating the NFκB and HIF1α-VEGF/VEGFR2 signal pathways. However, we must also recognize that the current network pharmacology database cannot yet contain all the signaling molecules involved in DR development, so there may be some other important molecules that have not been found in our study, which needs further deeper investigation in the future. This study provides an experimental basis for the clinical application of SMYAD in patients with DR in the future.
Data Availability Statement
The original contributions presented in the study are included in the article/Supplementary Material, and further inquiries can be directed to the corresponding authors.
Ethics Statement
The animal study was reviewed and approved by the Experimental Animal Ethical Committee of Shanghai University of Traditional Chinese Medicine.
Author Contributions
AD, YX, HO, BL, and WJ performed the experiments and analyzed the data. AD drafted the manuscript. HX contributed to the discussion of this study and the revision of this manuscript. LJ conceived and designed this study, wrote and revised the manuscript, conducted the project administration, and acquired the funding grants.
Funding
This work was financially supported by the National Natural Science Foundation of China (82074084 and U19A2009) and the leadership in Science and Technology innovation of the third batch of national “Ten Thousand People Plan” for LJ.
Conflict of Interest
The authors declare that the research was conducted in the absence of any commercial or financial relationships that could be construed as a potential conflict of interest.
Publisher’s Note
All claims expressed in this article are solely those of the authors and do not necessarily represent those of their affiliated organizations or those of the publisher, the editors, and the reviewers. Any product that may be evaluated in this article or claim that may be made by its manufacturer is not guaranteed or endorsed by the publisher.
Supplementary Material
The Supplementary Material for this article can be found online at: https://www.frontiersin.org/articles/10.3389/fphar.2021.763163/full#supplementary-material
References
Adamis, A. P. (2002). Is Diabetic Retinopathy an Inflammatory Disease? Br. J. Ophthalmol. 86, 363–365. doi:10.1136/bjo.86.4.363
Antonetti, D. A., Klein, R., and Gardner, T. W. (2012). Diabetic Retinopathy. N. Engl. J. Med. 366, 1227–1239. doi:10.1056/NEJMra1005073
Aveleira, C. A., Lin, C. M., Abcouwer, S. F., Ambrósio, A. F., and Antonetti, D. A. (2010). TNF-α Signals through PKCζ/NF-Κb to Alter the Tight junction Complex and Increase Retinal Endothelial Cell Permeability. Diabetes 59, 2872–2882. doi:10.2337/db09-1606
Bandello, F., Lattanzio, R., Zucchiatti, I., and Del Turco, C. (2013). Pathophysiology and Treatment of Diabetic Retinopathy. Acta Diabetol. 50, 1–20. doi:10.1007/s00592-012-0449-3
Baptista, F. I., Aveleira, C. A., Castilho, Á. F., and Ambrósio, A. F. (2017). Elevated Glucose and Interleukin-1β Differentially Affect Retinal Microglial Cell Proliferation. Mediators Inflamm. 2017, 4316316. doi:10.1155/2017/4316316
Barouch, F. C., Miyamoto, K., Allport, J. R., Fujita, K., Bursell, S. E., Aiello, L. P., et al. (2000). Integrin-mediated Neutrophil Adhesion and Retinal Leukostasis in Diabetes. Invest. Ophthalmol. Vis. Sci. 41, 1153–1158.
Behl, T., and Kotwani, A. (2017). Chinese Herbal Drugs for the Treatment of Diabetic Retinopathy. J. Pharm. Pharmacol. 69, 223–235. doi:10.1111/jphp.12683
Behl, Y., Krothapalli, P., Desta, T., DiPiazza, A., Roy, S., and Graves, D. T. (2008). Diabetes-enhanced Tumor Necrosis Factor-Alpha Production Promotes Apoptosis and the Loss of Retinal Microvascular Cells in Type 1 and Type 2 Models of Diabetic Retinopathy. Am. J. Pathol. 172, 1411–1418. doi:10.2353/ajpath.2008.071070
Catrina, S. B., and Zheng, X. (2021). Hypoxia and Hypoxia-Inducible Factors in Diabetes and its Complications. Diabetologia 64 (4), 709–716. doi:10.1007/s00125-021-05380-z
Chen, M., Obasanmi, G., Armstrong, D., Lavery, N. J., Kissenpfennig, A., Lois, N., et al. (2019). STAT3 Activation in Circulating Myeloid-Derived Cells Contributes to Retinal Microvascular Dysfunction in Diabetes. J. Neuroinflammation. 16, 138. doi:10.1186/s12974-019-1533-1
Chinese Pharmacopoeia Commission (2020). Pharmacopeia of the People’s republic of China. Beijing: The Medicine Science and Technology Press of China. p230, p121, p139, p88.
Douglas, J. Y., Bhatwadekar, A. D., Li Calzi, S., Shaw, L. C., Carnegie, D., Caballero, S., et al. (2012). Bone Marrow-CNS Connections: Implications in the Pathogenesis of Diabetic Retinopathy. Prog. Retin. Eye Res. 31, 481–494. doi:10.1016/j.preteyeres.2012.04.005
Erickson, K. K., Sundstrom, J. M., and Antonetti, D. A. (2007). Vascular Permeability in Ocular Disease and the Role of Tight Junctions. Angiogenesis 10, 103–117. doi:10.1007/s10456-007-9067-z
Gardlik, R., and Fusekova, I. (2015). Pharmacologic Therapy for Diabetic Retinopathy. Semin. Ophthalmol. 30, 252–263. doi:10.3109/08820538.2013.859280
Hopkins, A. L. (2008). Network Pharmacology: the Next Paradigm in Drug Discovery. Nat. Chem. Biol. 4, 682–690. doi:10.1038/nchembio.118
Ibrahim, A. S., El-Remessy, A. B., Matragoon, S., Zhang, W., Patel, Y., Khan, S., et al. (2011). Retinal Microglial Activation and Inflammation Induced by Amadori-Glycated Albumin in a Rat Model of Diabetes. Diabetes 60, 1122–1133. doi:10.2337/db10-1160
Jiao, X., Jin, X., Ma, Y., Yang, Y., Li, J., Liang, L., et al. (2021). A Comprehensive Application: Molecular Docking and Network Pharmacology for the Prediction of Bioactive Constituents and Elucidation of Mechanisms of Action in Component-Based Chinese Medicine. Comput. Biol. Chem. 90, 107402. doi:10.1016/j.compbiolchem.2020.107402
Joussen, A. M., Poulaki, V., Le, M. L., Koizumi, K., Esser, C., Janicki, H., et al. (2004). A central Role for Inflammation in the Pathogenesis of Diabetic Retinopathy. FASEB J. 18, 1450–1452. doi:10.1096/fj.03-1476fje
Joussen, A. M., Poulaki, V., Mitsiades, N., Kirchhof, B., Koizumi, K., Döhmen, S., et al. (2002). Nonsteroidal Anti-inflammatory Drugs Prevent Early Diabetic Retinopathy via TNF-Alpha Suppression. FASEB J. 16, 438–440. doi:10.1096/fj.01-0707fje
Kanazawa, H., Ohsawa, K., Sasaki, Y., Kohsaka, S., and Imai, Y. (2002). Macrophage/microglia-specific Protein Iba1 Enhances Membrane Ruffling and Rac Activation via Phospholipase C-Gamma -dependent Pathway. J. Biol. Chem. 277, 20026–20032. doi:10.1074/jbc.M109218200
Kaštelan, S., Orešković, I., Bišćan, F., Kaštelan, H., and Gverović Antunica, A. (2020). Inflammatory and Angiogenic Biomarkers in Diabetic Retinopathy. Biochem. Med. (Online) 30 (3), 030502. doi:10.11613/BM.2020.030502
Kern, T. S. (2007). Contributions of Inflammatory Processes to the Development of the Early Stages of Diabetic Retinopathy. Exp. Diabetes Res. 2007, 95103. doi:10.1155/2007/95103
Li, H., Zhao, L., Zhang, B., Jiang, Y., Wang, X., Guo, Y., et al. (2014). A Network Pharmacology Approach to Determine Active Compounds and Action Mechanisms of Ge-Gen-Qin-Lian Decoction for Treatment of Type 2 Diabetes. Evid. Based Complement. Alternat. Med. 2014, 495840. doi:10.1155/2014/495840
Li, S., Chen, Y. T., Ding, Q. Y., Dai, J. Y., Duan, X. C., Hu, Y. J., et al. (2021). Network Pharmacology Evaluation Method Guidance-Draft. World J. Tradit. Chin. Med. 7 (1), 146–154. doi:10.4103/wjtcm.wjtcm_11_21
Mei, X., Zhang, T., Ouyang, H., Lu, B., Wang, Z., and Ji, L. (2019). Scutellarin Alleviates Blood-Retina-Barrier Oxidative Stress Injury Initiated by Activated Microglia Cells during the Development of Diabetic Retinopathy. Biochem. Pharmacol. 159, 82–95. doi:10.1016/j.bcp.2018.11.011
Mesquida, M., Drawnel, F., and Fauser, S. (2019). The Role of Inflammation in Diabetic Eye Disease. Semin. Immunopathol. 41, 427–445. doi:10.1007/s00281-019-00750-7
Oellers, P., and Mahmoud, T. H. (2016). Surgery for Proliferative Diabetic Retinopathy: New Tips and Tricks. J. Ophthalmic Vis. Res. 11, 93–99. doi:10.4103/2008-322X.180697
Rudraraju, M., Narayanan, S. P., and Somanath, P. R. (2020). Regulation of Blood-Retinal Barrier Cell-Junctions in Diabetic Retinopathy. Pharmacol. Res. 161, 105115. doi:10.1016/j.phrs.2020.105115
Shih, R. H., Wang, C. Y., and Yang, C. M. (2015). NF-kappaB Signaling Pathways in Neurological Inflammation: A Mini Review. Front. Mol. Neurosci. 8, 77. doi:10.3389/fnmol.2015.00077
Singh, R. S., and Kim, J. E. (2012). Ocular Hypertension Following Intravitreal Anti-vascular Endothelial Growth Factor Agents. Drugs Aging 29, 949–956. doi:10.1007/s40266-012-0031-2
Song, P., Yu, J., Chan, K. Y., Theodoratou, E., and Rudan, I. (2018). Prevalence, Risk Factors and burden of Diabetic Retinopathy in China: a Systematic Review and Meta-Analysis. J. Glob. Health 8, 010803. doi:10.7189/jogh.08.010803
Taghavi, Y., Hassanshahi, G., Kounis, N. G., Koniari, I., and Khorramdelazad, H. (2019). Monocyte Chemoattractant Protein-1 (MCP-1/CCL2) in Diabetic Retinopathy: Latest Evidence and Clinical Considerations. J. Cel Commun. Signal 13, 451–462. doi:10.1007/s12079-018-00500-8
Thomas, R. L., Halim, S., Gurudas, S., Sivaprasad, S., and Owens, D. R. (2019). IDF Diabetes Atlas: A Review of Studies Utilising Retinal Photography on the Global Prevalence of Diabetes Related Retinopathy between 2015 and 2018. Diabetes Res. Clin. Pract. 157, 107840. doi:10.1016/j.diabres.2019.107840
Vasant More, S., Kim, I. S., and Choi, D. K. (2017). Recent Update on the Role of Chinese Material Medica and Formulations in Diabetic Retinopathy. Molecules 22, 76. doi:10.3390/molecules22010076
Vriend, J., and Reiter, R. J. (2016). Melatonin and the von Hippel-Lindau/HIF-1 oxygen sensing mechanism: A review. Biochim. Biophys. Acta 1865, 176–183. doi:10.1016/j.bbcan.2016.02.004
Wang, X., Wang, Z.-Y., Zheng, J.-H., and Li, S. (2021). TCM Network Pharmacology: A New Trend towards Combining Computational, Experimental and Clinical Approaches. Chin. J. Nat. Med. 19, 1–11. doi:10.1016/S1875-5364(21)60001-8
Witmer, A. N., Vrensen, G. F., Van Noorden, C. J., and Schlingemann, R. O. (2003). Vascular Endothelial Growth Factors and Angiogenesis in Eye Disease. Prog. Retin. Eye Res. 22, 1–29. doi:10.1016/s1350-9462(02)00043-5
Xu, H., and Chen, M. (2017). Diabetic Retinopathy and Dysregulated Innate Immunity. Vis. Res 139, 39–46. doi:10.1016/j.visres.2017.04.013
Xu, Z. H., Gao, Y. Y., Zhang, H. T., Ruan, K. F., and Feng, Y. (2018). Progress in Experimental and Clinical Research of the Diabetic Retinopathy Treatment Using Traditional Chinese Medicine. Am. J. Chin. Med. 46, 1–27. doi:10.1142/S0192415X1850074X
Zhang, C., Liu, J., Wang, J., Zhang, T., Xu, D., Hu, W., et al. (2021). The Interplay between Tumor Suppressor P53 and Hypoxia Signaling Pathways in Cancer. Front. Cel Dev. Biol. 9, 648808. doi:10.3389/fcell.2021.648808
Zhang, C., Wang, H., Nie, J., and Wang, F. (2014). Protective Factors in Diabetic Retinopathy: Focus on Blood-Retinal Barrier. Discov. Med. 18, 105–112.
Zhang, R. X., Chen, G. L., and Fang, C. H. (2012). The Application and Mechanism of Simiao Yongan Decoction in Diabetic Complications. Lishizhen Med. Materia Med. Res. 23, 2739–2740. doi:10.3969/j.issn.1008-0805.2012.11.033
Zhang, T., Ouyang, H., Mei, X., Lu, B., Yu, Z., Chen, K., et al. (2019). Erianin Alleviates Diabetic Retinopathy by Reducing Retinal Inflammation Initiated by Microglial Cells via Inhibiting Hyperglycemia-Mediated ERK1/2-NF-Κb Signaling Pathway. FASEB J. 33, 11776–11790. doi:10.1096/fj.201802614RRR
Keywords: Si-Miao-Yong-An decoction, Diabetic retinopathy, network pharmacology, inflammation, angiogenesis
Citation: Du A, Xie Y, Ouyang H, Lu B, Jia W, Xu H and Ji L (2021) Si-Miao-Yong-An Decoction for Diabetic Retinopathy: A Combined Network Pharmacological and In Vivo Approach. Front. Pharmacol. 12:763163. doi: 10.3389/fphar.2021.763163
Received: 23 August 2021; Accepted: 25 October 2021;
Published: 26 November 2021.
Edited by:
Shao Li, Tsinghua University, ChinaReviewed by:
Cristina Revilla-Monsalve, Mexican Social Security Institute (IMSS), MexicoDeqiang Dou, Liaoning University of Traditional Chinese Medicine, China
Copyright © 2021 Du, Xie, Ouyang, Lu, Jia, Xu and Ji. This is an open-access article distributed under the terms of the Creative Commons Attribution License (CC BY). The use, distribution or reproduction in other forums is permitted, provided the original author(s) and the copyright owner(s) are credited and that the original publication in this journal is cited, in accordance with accepted academic practice. No use, distribution or reproduction is permitted which does not comply with these terms.
*Correspondence: Hong Xu, xuhongtcm@163.com; Lili Ji, lichenyue1307@126.com