- 1Faculty of Medicine, University of Bern, Bern, Switzerland
- 2Institute of Social and Preventive Medicine, University of Bern, Bern, Switzerland
- 3Department of Diabetes, Endocrinology, Nutritional Medicine, and Metabolism, Inselspital, Bern University Hospital, University of Bern, Bern, Switzerland
- 4Department of Cardiology, Inselspital, Bern University Hospital, University of Bern, Bern, Switzerland
Objective: Sodium glucose cotransporter 2 inhibitors (SGLT2-is) are antidiabetic drugs that improve glycemic control by limiting urinary glucose reuptake in the proximal tubule. SGLT2-is might suppress atherosclerotic processes and ameliorate the prognosis of patients with diabetes mellitus diagnosed with or at high risk of atherosclerotic cardiovascular disease (ASCVD). In this mini review, we examine the role of SGLT2-is in the development and progression of atherosclerosis throughout its spectrum, from subclinical atherosclerosis to ASCVD.
Data Sources—PubMed and Google Scholar were searched for publications related to SGLT2-is and atherosclerosis. All types of articles were considered, including clinical trials, animal studies, in vitro observations, and reviews and meta-analyses. Data were examined according to their impact and clinical relevance.
Synopsis of Content—We first review the underlying mechanisms of SGLT2-is on the development and progression of atherosclerosis, including favorable effects on lipid metabolism, reduction of systemic inflammation, and improvement of endothelial function. We then discuss the putative impact of SGLT2-is on the formation, composition, and stability of atherosclerotic plaque. Furthermore, we evaluate the effects of SGLT2-is in subclinical atherosclerosis assessed by carotid intima media thickness and pulse wave velocity. Subsequently, we summarize the effects of SGLT2-is in ASCVD events, including ischemic stroke, angina pectoris, myocardial infarction, revascularization, and peripheral artery disease, as well as major adverse cardiovascular events, cardiovascular mortality, heart failure, and chronic kidney disease. Moreover, we examine factors that could modify the role of SGLT2-is in atherosclerosis, including sex, age, diabetes, glycemic control, ASCVD, and SGLT2-i compounds. Additionally, we propose future directions that can improve our understanding of SGLT2-is and atherosclerosis.
Introduction
Atherosclerosis is a progressive disease process, characterized by focal accumulations of lipids, complex carbohydrates, blood products, fibrous elements, and calcium deposits in the intima of arteries, which are also associated with medial changes (Lusis, 2000; Maurice et al., 2007). In its early stages, atherosclerosis remains clinically silent (i.e., subclinical atherosclerosis) and may further progress to atherosclerotic cardiovascular disease (ASCVD) and death (Ahmadi et al., 2019). In order to stop the progression of atherosclerosis and prevent cardiovascular (CV) events, it is essential to detect and manage it early on (Ahmadi et al., 2019). Besides lifestyle modifications, medications can help reduce progression of atherosclerosis. In addition to lipid- or blood pressure–lowering agents, the novel antidiabetic drugs sodium glucose cotransporter 2 inhibitors (SGLT2-is) might also suppress atherosclerotic processes and ameliorate the patients’ prognosis. SGLT2-is, including canagliflozin, dapagliflozin, and empagliflozin, improve glycemic control by inhibiting glucose reuptake in the proximal tubule and increasing renal glucose excretion (Clar et al., 2012). This narrative mini-review provides an overview on the role of SGLT2-is in the development and progression of atherosclerotic lesions and their possible effects on subclinical and clinical atherosclerosis.
Methods
PubMed and Google Scholar were searched to identify relevant publications on SGLT2-is and atherosclerosis. Key words included the following: “SGLT2 inhibitor, canagliflozin, dapagliflozin, empagliflozin, ertugliflozin, ipragliflozin, luseogliflozin, remogliflozin, sotagliflozin, tofogliflozin, atherosclerosis, carotid intima media thickness, plaque, ankle-brachial index, coronary artery calcification score, pulse wave velocity, transient ischemic attack, ischemic stroke, coronary heart disease, angina pectoris, acute coronary syndrome, myocardial infarction, coronary revascularization, renal artery stenosis, and peripheral artery disease.” All types of articles were considered, including clinical trials, animal studies, in vitro observations, reviews, and meta-analyses. Since this is a narrative mini-review, we prioritized the most clinically relevant and up-to-date articles in the current literature.
SGLT2 Inhibitors and the Pathogenesis of Atherosclerosis
Several underlying mechanisms can explain the role of SLGT2-is in the pathogenesis of atherosclerosis (Figure 1; Table 1). SGLT2-is may prevent the development of atheroma by adjusting dyslipidemia, restoring normal endothelial function, reducing oxidative stress, decreasing inflammation, and inhibiting monocyte-macrophage-foam cell evolution (Terasaki et al., 2015; Leng et al., 2016; Han et al., 2017; Al-Sharea et al., 2018; Dimitriadis et al., 2019; Ganbaatar et al., 2020; Lee et al., 2020; Liu et al., 2021; Park et al., 2021). Furthermore, SGLT2-is may prevent the progression of atherosclerosis by reducing plaque size and burden, altering plaque composition, and improving plaque stability (Terasaki et al., 2015; Leng et al., 2016; Al-Sharea et al., 2018; Lee et al., 2020).
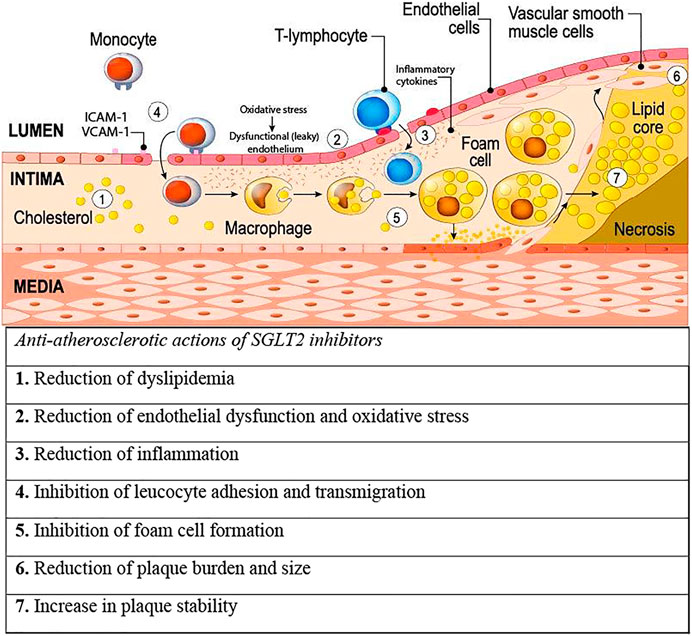
FIGURE 1. Putative mechanisms of action of SGLT2 inhibitors on pathways leading to atherosclerosis. 1–5 summarize effects of SGLT2 inhibitors against pathogenic processes that lead to development of atherosclerosis, while 6 and 7 summarize SGLT2 inhibitor effects against the progression and instability of atherosclerosis. Abbreviations: ICAM-1, intracellular adhesion molecule 1; SGLT2, sodium glucose cotransporter 2; VCAM-1, vascular cell adhesion molecule. Illustration adapted under standard license: designua/stock.adobe.com.
Dyslipidemia
Excess cholesterol substrates can increase the susceptibility of arterial walls to atherosclerosis. Several studies in animals and humans have shown that SGLT2-is reduce serum total cholesterol and triglyceride levels (Calapkulu et al., 2019; Ganbaatar et al., 2020; Liu et al., 2021). In patients with diabetes, dapagliflozin reduced the potent atherogenic particles of low-density lipoprotein (LDL) (Hayashi et al., 2017), while canagliflozin increased high-density lipoprotein (HDL) levels (Kamijo et al., 2019). In normoglycemic mice models, empagliflozin reduced LDL, but no changes in HDL levels were observed (Liu et al., 2021).
Endothelial Dysfunction and Oxidative Stress
SLGT2-is can reduce endothelial dysfunction directly by affecting endothelial cells or indirectly by reducing oxidative stress and sympathetic activation. Hence, SGLT2-is reverse the upregulation of endothelial cell senescence genes, further reducing the predisposition to endothelial dysfunction (Park et al., 2021). In diabetic mice, SGLT2-is manifested antioxidant effects by reducing reactive oxygen species (ROS) production and by reversing the increased NADPH activity (Leng et al., 2016; Ganbaatar et al., 2020). Empagliflozin also restored and preserved the glycocalyx of human abdominal aortic endothelial cells, resulting in maintained vascular health (Cooper et al., 2019). Oxidative stress was also reversed by empagliflozin in porcine endothelial cells, through inhibition of nitric oxide formation (Park et al., 2021). Additionally, the administration of empagliflozin in normoglycemic mice reduced renin, aldosterone, norepinephrine, and neuropeptide Y (Liu et al., 2021).
Inflammation
SGLT2-is can affect inflammatory cytokines that promote activation and migration of monocytes into the tunica intima in both diabetic and normoglycemic models (Leng et al., 2016; Han et al., 2017; Dimitriadis et al., 2019; Ganbaatar et al., 2020; Lee et al., 2020; Liu et al., 2021). Empagliflozin reduced monocyte chemoattractant protein (MCP)-1, interleukin (IL)-6, IL-10, and tumor necrosis factor (TNF)-α in both normoglycemic and diabetic mice (Han et al., 2017; Dimitriadis et al., 2019; Ganbaatar et al., 2020; Liu et al., 2021). Dapagliflozin reduced IL-1β, IL-18, and NLRP3 inflammasome activation in diabetic mice (Leng et al., 2016). Canagliflozin reduced IL-1β, IL-6, and TNF-α expression in normoglycemic rabbits (Lee et al., 2020). An in vitro study on human plasma samples also suggested that canagliflozin contributes to the reduction of several inflammatory biomarkers (Heerspink et al., 2019).
Leukocyte Adhesion and Transmigration
SGLT2-is can reduce leukocyte adhesion to endothelial cells and transmigration into the intra-intimal space. The adhesion of leukocytes on endothelial surfaces is facilitated by the endothelial adhesion molecules such as vascular cell adhesion molecule (VCAM)-1 and intracellular adhesion molecule (ICAM)-1, with ICAM-1 also facilitating monocyte transmigration (Oppenheimer-Marks et al., 1991; Ley and Huo, 2001; Muller, 2002). In diabetic mice, the upregulation of ICAM-1 was reversed by luseogliflozin (Nakatsu et al., 2017), whereas empagliflozin reduced VCAM-1 (Ganbaatar et al., 2020) and additionally decreased adhesion of proinflammatory monocytes on the endothelial wall (Pennig et al., 2019). These results are also in line with those of a study in an atherosclerotic mouse model, showing a reduction in VCAM-1 by canagliflozin (Nasiri-Ansari et al., 2018).
Foam Cell Formation
The excessive lipid accumulation triggers the transition of macrophages into foam cells, which are key cellular precursors of atheromas (Kang et al., 2020). In diabetic mice models, dapagliflozin, empagliflozin, and ipragliflozin reduced macrophage proliferation, infiltration, and formation of cholesterol esters that are associated with the degree of foam cell formation (Terasaki et al., 2015; Ganbaatar et al., 2020). Additionally, dapagliflozin reduced macrophage infiltration and polarization in a normoglycemic rabbit model (Lee et al., 2020).
Plaque Burden and Size
SGLT2-is can reduce atheroma burden and plaque size (Terasaki et al., 2015; Leng et al., 2016; Al-Sharea et al., 2018). In normoglycemic and diabetic animal models of atherosclerosis, dapagliflozin, empagliflozin, and luseogliflozin reduced the number of atheroma plaques, atherosclerotic lesion size and surface area (Terasaki et al., 2015; Leng et al., 2016; Han et al., 2017; Nakatsu et al., 2017; Dimitriadis et al., 2019; Pennig et al., 2019; Ganbaatar et al., 2020; Lee et al., 2020; Liu et al., 2021). On the other hand, canagliflozin did not reduce plaque size in normoglycemic mice, suggesting that it may abate atherosclerosis progression only in the presence of pronounced hyperglycaemia (Day et al., 2020).
Plaque Composition and Stability
Atherosclerotic plaques cause arterial stenosis and may eventually rupture. The stability of atherosclerotic plaques decreases when plaques have an increased lipid content, increased quantity of foam cells, and increased amount of matrix metalloproteinases (Johnson et al., 2006; Bentzon et al., 2014). Dapagliflozin reduced macrophages and cholesterol crystal content in the atherosclerotic plaques of diabetic mice (Terasaki et al., 2015; Leng et al., 2016). In human myeloid angiogenic cells, dapagliflozin and empagliflozin reduced lipotoxic damage and platelet activation, which may contribute to plaque stabilization and thrombosis inhibition (Spigoni et al., 2020). Moreover, luseogliflozin decreased the amount of matrix metalloproteinases in diabetic mice (Nakatsu et al., 2017). In normoglycemic rabbits, dapagliflozin reduced lipid accumulation within atherosclerotic plaques (Lee et al., 2020).
Indirect Mechanisms
SGLT2-is may indirectly reduce the risk of atherosclerotic processes via several beneficial CV effects, including reduction in blood pressure, body weight, and epicardial fat volume (Storgaard et al., 2016; Verma and McMurray, 2018). Via natriuretic and osmotic diuretic properties, SGLT2-is reduce the preload and afterload, which further results in decreased blood pressure and reduction of atherosclerosis (Verma and McMurray, 2018). SGLT2-is also reduce body weight through renal excretion of glucose and corresponding calories (Storgaard et al., 2016; Pereira and Eriksson, 2019). Moreover, the administration of SGLT2-is in patients with diabetes and coronary artery disease can decrease epicardial fat volume, most likely by reductions in body weight and inflammation markers (Sato et al., 2018; Verma and McMurray, 2018).
SGLT2 Inhibitors and Subclinical Atherosclerosis
Several noninvasive measures of subclinical atherosclerosis, including carotid intima media thickness (cIMT) and pulse wave velocity (PWV), are used to quantify the atherosclerotic burden in asymptomatic individuals and are predictive for future CV events (Folsom et al., 2008; Van Bortel et al., 2012; Zhong et al., 2018; Ahmadi et al., 2019). The UTOPIA study, a prospective, randomized, open-label, parallel-design trial performed in 340 subjects with type 2 diabetes mellitus (T2DM) and no history of CV disease, investigated whether tofogliflozin has favorable effects on cIMT compared to conventional treatment using drugs other than SGLT2-is (Katakami et al., 2020). After 104 weeks, tofogliflozin reduced the common mean cIMT compared to the baseline (mean change ±standard error, −0.132 ± 0.007, p < 0.001), but there was no significant difference between the tofogliflozin and the conventional treatment group. In a prespecified subanalysis of the UTOPIA trial, a significant attenuation of PWV was found in the tofogliflozin group compared to the conventional treatment group (mean change,-104.7 cm/s; 95% confidence interval [95%CI],−177 to −32.4) (Katakami et al., 2021). Another prospective, randomized study in 160 patients with T2DM also found significantly improved PWV in patients treated with SGLT2-is compared to those treated with basal insulin after a 12-month treatment period (Ikonomidis et al., 2020). Overall, the current evidence regarding the potential influence of SGLT2-is on surrogate measures of subclinical atherosclerosis is limited, due to the restricted number of existing trials, relatively small sample sizes of previous studies, the relatively short follow-up time, potential differences in baseline characteristics between the treatment and control groups, and inter-sonographer variability of measurements. Further studies may also consider assessing the coronary artery calcification score (CAC), which is superior to other measures of subclinical atherosclerosis in the prediction of future CV events (Folsom et al., 2008).
SGLT2 Inhibitors and Clinical Atherosclerosis
ASCVD comprises acute coronary syndromes (myocardial infarction [MI] or unstable angina), stable angina, stroke, transient ischemic attack (TIA), arterial revascularization, and peripheral artery disease (PAD) (Grundy et al.; ADA, 2016; Mach et al., 2020). Major adverse cardiovascular events (MACEs), CV mortality, heart failure (HF), and chronic kidney disease (CKD) are greatly driven by atherosclerosis and therefore will also be discussed below. Given the large body of evidence on SGLT2-is and clinical atherosclerosis, this section mainly reports the results of previous systematic reviews and meta-analyses.
Major Adverse Cardiovascular Events, Cardiovascular Mortality, Heart Failure, and Chronic Kidney Disease
Previous studies have consistently shown beneficial effects of SGLT2-is in reducing the risk of MACEs, CV mortality, HF, and CKD (Table 2). Although these diseases are commonly of atherosclerotic origin, we acknowledge that they can also be influenced by mechanisms other than atherosclerosis (Hupfeld and Mudaliar, 2019). In particular, HF may occur in the form of “diabetic cardiomyopathy” that is characterized by ventricular dysfunction in a patient with diabetes in the absence of coronary artery disease or hypertension (Hupfeld and Mudaliar, 2019).
MACE. Although its definitions vary across studies, MACE is commonly defined as a three-point composite of CV mortality, nonfatal MI, and nonfatal stroke. An umbrella review of meta-analyses of 32 trials including a total of 55,283 participants with T2DM, pre-diabetes, or high risk of diabetes indicated that SGLT2-is are associated with a 13% risk reduction in MACE (relative risk [RR], 0.87; 95%CI, 0.82–0.93) (Zhu et al., 2020). In line, another meta-analysis with a total of 38,723 participants, including four cardiovascular outcome trials (CVOTs), namely, EMPA-REG OUTCOME, CANVAS, DECLARE-TIMI 58, and CREDENCE, showed a 12% lower risk of atherosclerotic MACE in the SGLT2-i group compared with the standard of care or placebo [hazard ratio (HR), 0.88; 95%CI, 0.82–0.94] (Ghosh-Swaby et al., 2020).
CV mortality. Two meta-analyses revealed an 18% (RR, 0.82; 95%CI, 0.75–0.90) and 21% risk reduction (HR, 0.79; 95%CI, 0.69–0.91) of CV mortality in SGLT2-i users compared to controls, respectively (Zheng et al., 2018; Zhu et al., 2020).
HF. A meta-analysis including 17 studies with a total of 51,348 participants with T2DM, pre-diabetes, or high risk of diabetes reported a 32% reduction of HF risk (RR, 0.68; 95%CI, 0.63–0.73) in SGLT2-i users (Zhu et al., 2020). Another meta-analysis of randomized controlled trials (RCTs) including 64,351 patients with diabetes found a 38% risk reduction for HF (HR, 0.62; 95%CI, 0.54–0.72) (Zheng et al., 2018).
CKD. A meta-analysis of four CVOTs (EMPA-REG OUTCOME, CANVAS, CREDENCE, and DECLARE-TIMI 58) including a total of 38,723 participants with diabetes found a 35% risk reduction of end-stage kidney disease (RR, 0.65; 95%CI, 0.53–0.81) in SGLT2-i users (Neuen et al., 2019).
Distinct Atherosclerotic Cardiovascular Disease Events
Current evidence suggests that SGLT2-is reduce the risk of MI but do not reduce the risk of unstable angina, stroke, TIA, arterial revascularization, and PAD (Table 2).
MI and angina pectoris. Analyzing 40 trials with approximately 60,000 participants, an umbrella review showed that SGLT2-i users have a 14% lower risk of incident MI compared to controls (HR, 0.86; 95%CI, 0.78–0.94) (Zhu et al., 2020). These results were consistent with another meta-analysis of RCTs in patients with diabetes (Zheng et al., 2018). However, the aforementioned meta-analyses did not show differences in the risk of unstable angina between SGLT2-i users and controls (Zheng et al., 2018; Zhu et al., 2020).
Stroke and TIA. Several meta-analyses and reviews reported that SGLT2-is, including canagliflozin, dapagliflozin, and empagliflozin, do not affect the risk of stroke (Milonas and Tziomalos, 2018; Zheng et al., 2018; Sinha and Ghosal, 2019b; Sinha and Ghosal, 2019a; Zhu et al., 2020). In line, the empagliflozin and placebo arms in the EMPA-REG OUTCOME trial did not differ in the risk of TIA (Zinman et al., 2015). Further investigations evaluating the role of SGLT2-is in ischemic stroke are needed.
Arterial revascularization. A limited number of studies have investigated the likelihood of arterial revascularization among SGLT2-i users. In the EMPA-REG OUTCOME trial on empagliflozin and in the DECLARE-TIMI 58 trial on dapagliflozin, the administration of SGLT2-is did not affect the risks of coronary revascularization or lower extremity revascularization, respectively (Zinman et al., 2015; Bonaca et al., 2020).
PAD. There is inconsistent evidence regarding the role of SGLT2-is on PAD risk and subsequent lower limb amputations. Patients with diabetes are already at high risk of PAD, and SGLT2-is can presumably increase the risk of PAD even further via promoting glucosuria, volume depletion, and haemoconcentration (Shah et al., 2012). The CANVAS Program found increased risk of lower extremity amputations in the canagliflozin group compared to the placebo (HR, 1.97; 95%CI, 1.41–2.75) (Neal et al., 2017). However, the effects of canagliflozin on PAD may not be generalizable to other SGLT2-i compounds. In meta-analyses, dapagliflozin and empagliflozin were not associated with increased risk of amputations (Dicembrini et al., 2019; Heyward et al., 2020; Huang and Lee, 2020). Further studies are warranted to compare the risk of PAD between users of canagliflozin and users of other SGLT2-i compounds.
SGLT2 Inhibitors and Atherosclerosis: Potential Effect Modifiers
Various factors including sex, age, diabetes, glycemic control, ASCVD, and SGLT2-i compounds can modify the association of SGLT2-is with atherosclerosis. The identification of effect modifiers is important, as it can help explain heterogeneity, improve current recommendations, and foster personalized treatment.
Sex. Men and women might have different responses to SGLT2-is, possibly due to differences in drug pharmacokinetics, pharmacodynamics, and adherence. However, a meta-analysis of four RCTs in patients with T2DM (EMPA-REG OUTCOME, CANVAS, DECLARE-TIMI 58, and CREDENCE) suggested that SGLT2-is may have comparable effects in men and women (Rådholm et al., 2020). Overall, evidence on sex differences remains inconclusive, given that women are often underrepresented in RCTs of SGLT2-is (O’Donoghue et al., 2021). In the CANVAS study on canagliflozin and the DECLARE-TIMI 58 study on dapagliflozin, less than 38% of participants were women (Neal et al., 2017; Wiviott et al., 2019). Future studies should include comparable proportions of men and women, which can allow an adequate assessment of possible sex differences in the safety and efficacy of SGLT2-is.
Age. Aging can alter the effects of SGLT2-is via affecting drug metabolism. Increasing age is associated with a gradual reduction in the glomerular filtration rate, which may downregulate the tubular expression of SGLT2 and further decline the glucose lowering effect of SGLT2-is (Cintra et al., 2019). Aging is also associated with decreased insulin sensitivity, sarcopenia, weight gain, and elevated adiposity, which influence the risk of hypoglycemia and could further affect the effectiveness of SGLT2-is (Cintra et al., 2019). Therefore, it would be of major importance to assess whether the potential anti-atherosclerotic properties of SGLT2-is differ by age. However, the recruitment of older participants is usually challenging. Further studies need to perform predefined analyses accounting for age.
Diabetes and glycemic control. Given that the main indication for SGLT2-is is the reduction of blood glucose, most clinical studies evaluating the role of SGLT2-is in ASCVD are conducted in patients with T2DM. However, SGLT2-is can also have beneficial effects in normoglycemic individuals. This assumption is supported by a meta-analysis of two RCTs (DAPA-HF for dapagliflozin and EMPEROR-Reduced for empagliflozin) in patients with HF, which showed that SGLT2-i users with and without diabetes have a similar reduction in the risk of a composite endpoint consisting of hospitalization for HF (HHF) and CV death (Zannad et al., 2020). Furthermore, the EMPA-REG OUTCOME trial including patients with T2DM and cardiovascular disease suggested that the benefits of empagliflozin in reducing the risk of HHF and CV death are independent of glycemic control (Inzucchi et al., 2018). Similarly, the DAPA-CKD study performed in patients with CKD suggested protective effects of SGLT2-is on the kidney, regardless of the presence or absence of diabetes (Heerspink et al., 2020). Future studies are needed to evaluate whether diabetes status, duration, and glycemic control modify the effects of SGLT2-is on distinct ASCVD events.
ASCVD. In RCTs of SGLT2-is, the cardiovascular risk profile of eligible participants varies according to the study inclusion criteria. Some RCTs (EMPA-REG-OUTCOME and VERTIS) included only patients with pre-existent ASCVD, whereas other RCTs (CANVAS, DECLARE-TIMI 58, and CREDENCE) included participants with and without ASCVD (Zinman et al., 2015; Neal et al., 2017; Perkovic et al., 2019; Wiviott et al., 2019; Cannon et al., 2020). A recent meta-analysis of RCTs in patients with diabetes concluded that the presence or absence of ASCVD does not modify the association of SGLT2-is with MACEs, CV deaths, and HHF, respectively (McGuire et al., 2021). Further stratified analyses by CV risk factors (e.g., hypertension and obesity) are warranted.
SGLT2-i compounds. Various SGLT2-i compounds have different selectivities for the SGLT2 receptor; thereby, effect differences may exist across compounds even within the SGLT2-i class. A meta-analysis of RCTs in patients with diabetes suggested that empagliflozin is associated with reduced risks of CV death and MACE and canagliflozin is associated with reduced risk of MACEs, while all analyzed SGLT2-is, including empagliflozin, canagliflozin, dapagliflozin, and ertugliflozin, are associated with reduced risk of HHF (McGuire et al., 2021).
Conclusion and Future Directions
SGLT2-is can exert anti-atherosclerotic properties via affecting various pathways of atherogenesis, including dyslipidemia, endothelial dysfunction, oxidative stress, inflammation, leucocyte adhesion and transmigration, plaque composition and instability. Still, the exact underlying mechanisms linking SGLT2-is to atherosclerotic processes are yet to be fully elucidated. Furthermore, SGLT2-is have manifested beneficial effects in reducing the risk of MACEs, CV mortality, HF, and CKD, which are largely of atherosclerotic origin. However, the role of SGLT2-is in distinct ASCVD events remains to be explored more extensively. Current evidence supports that SGLT2-is can reduce the risk of MI, while the risks of unstable angina, stroke, TIA, arterial revascularization, and PAD seem to be unaffected by SGLT2-is. Further experimental and observational studies of high quality, with adequate number of events and follow-up time, need to examine the potential role of SGLT2-is in subclinical atherosclerosis and ASCVD events, not only in those with diabetes and pre-diabetes but also in normoglycemic individuals. The effects of SGLT2-i compounds need to be compared with one another and with those of other antidiabetic medications. Potential effect modification by age, sex, and comorbidities needs to be extensively explored.
Author Contributions
Conceptualization: AB; methodology: AP and AB; writing—original draft preparation: AP, DS, and AB; writing—reviewing and editing: AP, DS, ML, TM, MW, and AB.
Conflict of Interest
The authors declare that the research was conducted in the absence of any commercial or financial relationships that could be construed as a potential conflict of interest.
Publisher’s Note
All claims expressed in this article are solely those of the authors and do not necessarily represent those of their affiliated organizations, or those of the publisher, the editors, and the reviewers. Any product that may be evaluated in this article, or claim that may be made by its manufacturer, is not guaranteed or endorsed by the publisher.
References
Ada (2016). 8. Cardiovascular Disease and Risk Management. Dia Care 39, S60–S71. doi:10.2337/dc16-s011
Ahmadi, A., Argulian, E., Leipsic, J., Newby, D. E., and Narula, J. (2019). From Subclinical Atherosclerosis to Plaque Progression and Acute Coronary Events: JACC State-Of-The-Art Review. J. Am. Coll. Cardiol. 74(12):1608–1617. doi:10.1016/j.jacc.2019.08.012
Al-Sharea, A., Murphy, A. J., Huggins, L. A., Hu, Y., Goldberg, I. J., and Nagareddy, P. R. (2018). SGLT2 Inhibition Reduces Atherosclerosis by Enhancing Lipoprotein Clearance in Ldlr-/- Type 1 Diabetic Mice. Atherosclerosis 271, 166–176. doi:10.1016/j.atherosclerosis.2018.02.028
Bentzon, J. F., Otsuka, F., Virmani, R., and Falk, E. (2014). Mechanisms of Plaque Formation and Rupture. Circ. Res. 114, 1852–1866. doi:10.1161/CIRCRESAHA.114.302721
Bonaca, M. P., Wiviott, S. D., Zelniker, T. A., Mosenzon, O., Bhatt, D. L., Leiter, L. A., et al. (2020). Dapagliflozin and Cardiac, Kidney, and Limb Outcomes in Patients with and without Peripheral Artery Disease in DECLARE-TIMI 58. Circulation 142, 734–747. doi:10.1161/CIRCULATIONAHA.119.044775
Calapkulu, M., Cander, S., Gul, O. O., and Ersoy, C. (2019). Lipid Profile in Type 2 Diabetic Patients with New Dapagliflozin Treatment; Actual Clinical Experience Data of Six Months Retrospective Lipid Profile from Single center. Diabetes Metab. Syndr. 13, 1031–1034. doi:10.1016/j.dsx.2019.01.016
Cannon, C. P., Pratley, R., Dagogo-Jack, S., Mancuso, J., Huyck, S., Masiukiewicz, U., et al. (2020). Cardiovascular Outcomes with Ertugliflozin in Type 2 Diabetes. N. Engl. J. Med. 383, 1425–1435. doi:10.1056/NEJMoa2004967
Cintra, R., Moura, F. A., De Carvalho, L. S. F., Barreto, J., Tambascia, M., Pecoits-Filho, R., et al. (2019). Inhibition of the Sodium-Glucose Co-transporter 2 in the Elderly: Clinical and Mechanistic Insights into Safety and Efficacy. Revista da Associacao Medica Brasileira. (São Paulo, Brazil: Associacao Medica Brasileira). doi:10.1590/1806-9282.65.1.70
Clar, C., Gill, J. A., Court, R., and Waugh, N. (2012). Systematic Review of SGLT2 Receptor Inhibitors in Dual or Triple Therapy in Type 2 Diabetes. BMJ Open 2, 1007. doi:10.1136/bmjopen-2012-001007
Cooper, S., Teoh, H., Campeau, M. A., Verma, S., and Leask, R. L. (2019). Empagliflozin Restores the Integrity of the Endothelial Glycocalyx In Vitro. Mol. Cel Biochem 459, 121–130. doi:10.1007/s11010-019-03555-2
Day, E. A., Ford, R. J., Lu, J. H., Lu, R., Lundenberg, L., Desjardins, E. M., et al. (2020). The SGLT2 Inhibitor Canagliflozin Suppresses Lipid Synthesis and Interleukin-1 Beta in ApoE Deficient Mice. Biochem. J. 477, 2347–2361. doi:10.1042/BCJ20200278
Dicembrini, I., Tomberli, B., Nreu, B., Baldereschi, G. I., Fanelli, F., Mannucci, E., et al. (2019). Peripheral Artery Disease and Amputations with Sodium-Glucose Co-transporter-2 (SGLT-2) Inhibitors: A Meta-Analysis of Randomized Controlled Trials. Diabetes Res. Clin. Pract. 153, 138–144. doi:10.1016/j.diabres.2019.05.028
Dimitriadis, G. K., Nasiri-Ansari, N., Agrogiannis, G., Kostakis, I. D., Randeva, M. S., Nikiteas, N., et al. (2019). Empagliflozin Improves Primary Haemodynamic Parameters and Attenuates the Development of Atherosclerosis in High Fat Diet Fed APOE Knockout Mice. Mol. Cel Endocrinol 494, 110487. doi:10.1016/j.mce.2019.110487
Folsom, A. R., Kronmal, R. A., Detrano, R. C., O'leary, D. H., Bild, D. E., Bluemke, D. A., et al. (2008). Coronary Artery Calcification Compared with Carotid Intima-media Thickness in the Prediction of Cardiovascular Disease Incidence: The Multi-Ethnic Study of Atherosclerosis (MESA). Arch. Intern. Med. 168, 1333–1339. doi:10.1001/archinte.168.12.1333
Ganbaatar, B., Fukuda, D., Shinohara, M., Yagi, S., Kusunose, K., Yamada, H., et al. (2020). Empagliflozin Ameliorates Endothelial Dysfunction and Suppresses Atherogenesis in Diabetic Apolipoprotein E-Deficient Mice. Eur. J. Pharmacol. 875, 173040. doi:10.1016/j.ejphar.2020.173040
Ghosh-Swaby, O. R., Goodman, S. G., Leiter, L. A., Cheng, A., Connelly, K. A., Fitchett, D., et al. (2020). Glucose-lowering Drugs or Strategies, Atherosclerotic Cardiovascular Events, and Heart Failure in People with or at Risk of Type 2 Diabetes: an Updated Systematic Review and Meta-Analysis of Randomised Cardiovascular Outcome Trials. Lancet Diabetes Endocrinol. 8, 418–435. doi:10.1016/S2213-8587(20)30038-3
Grundy, S. M., Stone, N. J., Chair, V., Bailey, A. L., Beam, C., Birtcher, K. K., et al. (2019). Writing Committee Members Cholesterol Clinical Practice Guidelines 2018 AHA/ACC/AACVPR/AAPA/ABC/ACPM/ADA/AGS/ APhA/ASPC/NLA/PCNA Guideline on the Management of Blood Cholesterol: Executive Summary Circulation. Circulation 139, 1046–1081.
Han, J. H., Oh, T. J., Lee, G., Maeng, H. J., Lee, D. H., Kim, K. M., et al. (2017). The Beneficial Effects of Empagliflozin, an SGLT2 Inhibitor, on Atherosclerosis in ApoE -/- Mice Fed a Western Diet. Diabetologia 60, 364–376. doi:10.1007/s00125-016-4158-2
Hayashi, T., Fukui, T., Nakanishi, N., Yamamoto, S., Tomoyasu, M., Osamura, A., et al. (2017). Correction to: Dapagliflozin Decreases Small Dense Low-Density Lipoprotein-Cholesterol and Increases High-Density Lipoprotein 2-cholesterol in Patients with Type 2 Diabetes: Comparison with Sitagliptin. Cardiovasc. Diabetol. 16, 149. doi:10.1186/s12933-017-0608-5
Heerspink, H. J. L., Perco, P., Mulder, S., Leierer, J., Hansen, M. K., Heinzel, A., et al. (2019). Canagliflozin Reduces Inflammation and Fibrosis Biomarkers: a Potential Mechanism of Action for Beneficial Effects of SGLT2 Inhibitors in Diabetic Kidney Disease. Diabetologia 62, 1154–1166. doi:10.1007/s00125-019-4859-4
Heerspink, H. J. L., Stefánsson, B. V., Correa-Rotter, R., Chertow, G. M., Greene, T., Hou, F. F., et al. (2020). Dapagliflozin in Patients with Chronic Kidney Disease. N. Engl. J. Med. 383, 1436–1446. doi:10.1056/NEJMoa2024816
Heyward, J., Mansour, O., Olson, L., Singh, S., and Alexander, G. C. (2020). Association between Sodium-Glucose Cotransporter 2 (SGLT2) Inhibitors and Lower Extremity Amputation: A Systematic Review and Meta-Analysis. PLOS ONE 15, e0234065. doi:10.1371/journal.pone.0234065
Huang, C. Y., and Lee, J. K. (2020). Sodium-glucose Co-transporter-2 Inhibitors and Major Adverse Limb Events: A Trial-Level Meta-Analysis Including 51 713 Individuals. Diabetes Obes. Metab. 22, 2348–2355. doi:10.1111/dom.14159
Hupfeld, C., and Mudaliar, S. (2019). Navigating the "MACE" in Cardiovascular Outcomes Trials and Decoding the Relevance of Atherosclerotic Cardiovascular Disease Benefits versus Heart Failure Benefits. Diabetes Obes. Metab. 21, 1780–1789. doi:10.1111/dom.13740
Ikonomidis, I., Pavlidis, G., Thymis, J., Birba, D., Kalogeris, A., Kousathana, F., et al. (2020). Effects of Glucagon-like Peptide-1 Receptor Agonists, Sodium-Glucose Cotransporter-2 Inhibitors, and Their Combination on Endothelial Glycocalyx, Arterial Function, and Myocardial Work Index in Patients with Type 2 Diabetes Mellitus after 12-Month Treatment. J. Am. Heart Assoc. 9, e015716. doi:10.1161/JAHA.119.015716
Inzucchi, S. E., Kosiborod, M., Fitchett, D., Wanner, C., Hehnke, U., Kaspers, S., et al. (2018). Improvement in Cardiovascular Outcomes with Empagliflozin Is Independent of Glycemic Control. Circulation 138, 1904–1907. doi:10.1161/CIRCULATIONAHA.118.035759
Johnson, J. L., Baker, A. H., Oka, K., Chan, L., Newby, A. C., Jackson, C. L., et al. (2006). Suppression of Atherosclerotic Plaque Progression and Instability by Tissue Inhibitor of Metalloproteinase-2: Involvement of Macrophage Migration and Apoptosis. Circulation 113, 2435–2444. doi:10.1161/CIRCULATIONAHA.106.613281
Kamijo, Y., Ishii, H., Yamamoto, T., Kobayashi, K., Asano, H., Miake, S., et al. (2019). Potential Impact on Lipoprotein Subfractions in Type 2 Diabetes. Clin. Med. Insights Endocrinol. Diabetes 12, 1179551419866811. doi:10.1177/1179551419866811
Kang, Y., Zhan, F., He, M., Liu, Z., and Song, X. (2020). Anti-inflammatory Effects of Sodium-Glucose Co-transporter 2 Inhibitors on Atherosclerosis. Vasc. Pharmacol. 133-134:106779. doi:10.1016/j.vph.2020.106779
Katakami, N., Mita, T., Yoshii, H., Shiraiwa, T., Yasuda, T., Okada, Y., et al. (2020). Tofogliflozin Does Not Delay Progression of Carotid Atherosclerosis in Patients with Type 2 Diabetes: A Prospective, Randomized, Open-Label, Parallel-Group Comparative Study. Cardiovasc. Diabetol. 19, 110. doi:10.1186/s12933-020-01079-4
Katakami, N., Mita, T., Yoshii, H., Shiraiwa, T., Yasuda, T., Okada, Y., et al. (2021). Effect of Tofogliflozin on Arterial Stiffness in Patients with Type 2 Diabetes: Prespecified Sub-analysis of the Prospective, Randomized, Open-Label, Parallel-Group Comparative UTOPIA Trial. Cardiovasc. Diabetology 20, 4. doi:10.1186/s12933-020-01206-1
Lee, S. G., Lee, S. J., Lee, J. J., Kim, J. S., Lee, O. H., Kim, C. K., et al. (2020). Anti-Inflammatory Effect for Atherosclerosis Progression by Sodium-Glucose Cotransporter 2 (SGLT-2) Inhibitor in a Normoglycemic Rabbit Model. Korean Circ. J. 50, 443–457. doi:10.4070/kcj.2019.0296
Leng, W., Ouyang, X., Lei, X., Wu, M., Chen, L., Wu, Q., et al. (2016). The SGLT-2 Inhibitor Dapagliflozin Has a Therapeutic Effect on Atherosclerosis in Diabetic ApoE-/- Mice. Mediators Inflamm. 2016, 6305735. doi:10.1155/2016/6305735
Ley, K., and Huo, Y. (2001). VCAM-1 Is Critical in Atherosclerosis. J. Clin. Invest. 107, 1209–1210. doi:10.1172/JCI13005
Liu, Y., Xu, J., Wu, M., Xu, B., and Kang, L. (2021). Empagliflozin Protects against Atherosclerosis Progression by Modulating Lipid Profiles and Sympathetic Activity. Lipids Health Dis. 20, 5. doi:10.1186/s12944-021-01430-y
Mach, F., Baigent, C., Catapano, A. L., Koskinas, K. C., Casula, M., Badimon, L., et al. (2020). 2019 ESC/EAS Guidelines for the Management of Dyslipidaemias: Lipid Modification to Reduce Cardiovascular Risk. Eur. Heart J. 41, 111–188. doi:10.1093/eurheartj/ehz455
Maurice, R. L., Fromageau, J., Brusseau, E., Finet, G., Rioufol, G., and Cloutier, G. (2007). On the Potential of the Lagrangian Estimator for Endovascular Ultrasound Elastography: In Vivo Human Coronary Artery Study. Ultrasound Med. Biol. 33, 1199–1205. doi:10.1016/j.ultrasmedbio.2007.01.018
McGuire, D. K., Shih, W. J., Cosentino, F., Charbonnel, B., Cherney, D. Z. I., Dagogo-Jack, S., et al. (2021). Association of SGLT2 Inhibitors with Cardiovascular and Kidney Outcomes in Patients with Type 2 Diabetes: A Meta-Analysis. JAMA Cardiol. 6, 148–158. doi:10.1001/jamacardio.2020.4511
Milonas, D., and Tziomalos, K. (2018). Sodium-glucose Cotransporter 2 Inhibitors and Ischemic Stroke. Cardiovasc. Hematol. Disord. Drug Targets 18, 134–138. doi:10.2174/1871529X18666180206120444
Muller, W. A. (2002). Leukocyte-Endothelial Cell Interactions in the Inflammatory Response. Lab. Invest. 82, 521–533. doi:10.1038/labinvest.3780446
Nakatsu, Y., Kokubo, H., Bumdelger, B., Yoshizumi, M., Yamamotoya, T., Matsunaga, Y., et al. (2017). The SGLT2 Inhibitor Luseogliflozin Rapidly Normalizes Aortic mRNA Levels of Inflammation-Related but Not Lipid-Metabolism-Related Genes and Suppresses Atherosclerosis in Diabetic ApoE KO Mice. Int. J. Mol. Sci. 18, 1704. doi:10.3390/ijms18081704
Nasiri-Ansari, Ν., Dimitriadis, G. K., Agrogiannis, G., Perrea, D., Kostakis, I. D., Kaltsas, G., et al. (2018). Canagliflozin Attenuates the Progression of Atherosclerosis and Inflammation Process in APOE Knockout Mice. Cardiovasc. Diabetol. 17, 106. doi:10.1186/s12933-018-0749-1
Neal, B., Perkovic, V., Mahaffey, K. W., De Zeeuw, D., Fulcher, G., Erondu, N., et al. (2017). Canagliflozin and Cardiovascular and Renal Events in Type 2 Diabetes. N. Engl. J. Med. 377, 644–657. doi:10.1056/NEJMoa1611925
Neuen, B. L., Young, T., Heerspink, H. J. L., Neal, B., Perkovic, V., Billot, L., et al. (2019). SGLT2 Inhibitors for the Prevention of Kidney Failure in Patients with Type 2 Diabetes: a Systematic Review and Meta-Analysis. Lancet Diabetes Endocrinol. 7, 845–854. doi:10.1016/S2213-8587(19)30256-6
O’Donoghue, M. L., Kato, E. T., Mosenzon, O., Murphy, S. A., Cahn, A., Herrera, M., et al. (2021). The Efficacy and Safety of Dapagliflozin in Women and Men with Type 2 Diabetes Mellitus. Diabetologia 64 (6), 1226–1234. doi:10.1007/s00125-021-05399-2
Oppenheimer-Marks, N., Davis, L. S., Bogue, D. T., Ramberg, J., and Lipsky, P. E. (1991). Differential Utilization of ICAM-1 and VCAM-1 during the Adhesion and Transendothelial Migration of Human T Lymphocytes. J. Immunol. 147, 2913–2921.
Park, S. H., Belcastro, E., Hasan, H., Matsushita, K., Marchandot, B., Abbas, M., et al. (2021). Angiotensin II-Induced Upregulation of SGLT1 and 2 Contributes to Human Microparticle-Stimulated Endothelial Senescence and Dysfunction: Protective Effect of Gliflozins. Cardiovasc. Diabetol. 20, 65. doi:10.1186/s12933-021-01252-3
Pennig, J., Scherrer, P., Gissler, M. C., Anto-Michel, N., Hoppe, N., Füner, L., et al. (2019). Glucose Lowering by SGLT2-Inhibitor Empagliflozin Accelerates Atherosclerosis Regression in Hyperglycemic STZ-Diabetic Mice. Sci. Rep. 9, 17937. doi:10.1038/s41598-019-54224-9
Pereira, M. J., and Eriksson, J. W. (2019). Emerging Role of SGLT-2 Inhibitors for the Treatment of Obesity. Drugs 79, 219–230. doi:10.1007/s40265-019-1057-0
Perkovic, V., Jardine, M. J., Neal, B., Bompoint, S., Heerspink, H. J. L., Charytan, D. M., et al. (2019). Canagliflozin and Renal Outcomes in Type 2 Diabetes and Nephropathy. N. Engl. J. Med. 380, 2295–2306. doi:10.1056/NEJMoa1811744
Rådholm, K., Zhou, Z., Clemens, K., Neal, B., and Woodward, M. (2020). Effects of Sodium-Glucose Co-transporter-2 Inhibitors in Type 2 Diabetes in Women versus Men. Diabetes Obes. Metab. 22, 263–266. doi:10.1111/dom.13876
Sato, T., Aizawa, Y., Yuasa, S., Kishi, S., Fuse, K., Fujita, S., et al. (2018). The Effect of Dapagliflozin Treatment on Epicardial Adipose Tissue Volume. Cardiovasc. Diabetol. 17, 6. doi:10.1186/s12933-017-0658-8
Shah, N. K., Deeb, W. E., Choksi, R., and Epstein, B. J. (2012). Dapagliflozin: a Novel Sodium-Glucose Cotransporter Type 2 Inhibitor for the Treatment of Type 2 Diabetes Mellitus. Pharmacotherapy 32, 80–94. doi:10.1002/PHAR.1010
Sinha, B., and Ghosal, S. (2019a). Meta-analyses of the Effects of DPP-4 Inhibitors, SGLT2 Inhibitors and GLP1 Receptor Analogues on Cardiovascular Death, Myocardial Infarction, Stroke and Hospitalization for Heart Failure. Diabetes Res. Clin. Pract. 150, 8–16. doi:10.1016/j.diabres.2019.02.014
Sinha, B., and Ghosal, S. (2019b). Sodium-Glucose Cotransporter-2 Inhibitors (SGLT-2i) Reduce Hospitalization for Heart Failure Only and Have No Effect on Atherosclerotic Cardiovascular Events: A Meta-Analysis. Diabetes Ther. 10, 891–899. doi:10.1007/s13300-019-0597-3
Spigoni, V., Fantuzzi, F., Carubbi, C., Pozzi, G., Masselli, E., Gobbi, G., et al. (2020). Sodium-glucose Cotransporter 2 Inhibitors Antagonize Lipotoxicity in Human Myeloid Angiogenic Cells and ADP-dependent Activation in Human Platelets: Potential Relevance to Prevention of Cardiovascular Events. Cardiovasc. Diabetol. 19, 46. doi:10.1186/s12933-020-01016-5
Storgaard, H., Gluud, L. L., Bennett, C., Grøndahl, M. F., Christensen, M. B., Knop, F. K., et al. (2016). Benefits and Harms of Sodium-Glucose Co-transporter 2 Inhibitors in Patients with Type 2 Diabetes: A Systematic Review and Meta-Analysis. PLoS One 11, e0166125. doi:10.1371/journal.pone.0166125
Terasaki, M., Hiromura, M., Mori, Y., Kohashi, K., Nagashima, M., Kushima, H., et al. (2015). Amelioration of Hyperglycemia with a Sodium-Glucose Cotransporter 2 Inhibitor Prevents Macrophage-Driven Atherosclerosis through Macrophage Foam Cell Formation Suppression in Type 1 and Type 2 Diabetic Mice. PLoS One 10, e0143396. doi:10.1371/journal.pone.0143396
Van Bortel, L. M., Laurent, S., Boutouyrie, P., Chowienczyk, P., Cruickshank, J. K., De Backer, T., et al. (2012). Expert Consensus Document on the Measurement of Aortic Stiffness in Daily Practice Using Carotid-Femoral Pulse Wave Velocity. J. Hypertens. 30(3):445–448. doi:10.1097/hjh.0b013e32834fa8b0
Verma, S., and Mcmurray, J. J. V. (2018). SGLT2 Inhibitors and Mechanisms of Cardiovascular Benefit: a State-Of-The-Art Review. Diabetologia 61, 2108–2117. doi:10.1007/s00125-018-4670-7
Wiviott, S. D., Raz, I., Bonaca, M. P., Mosenzon, O., Kato, E. T., Cahn, A., et al. (2019). Dapagliflozin and Cardiovascular Outcomes in Type 2 Diabetes. N. Engl. J. Med. 380, 347–357. doi:10.1056/NEJMoa1812389
Zannad, F., Ferreira, J. P., Pocock, S. J., Anker, S. D., Butler, J., Filippatos, G., et al. (2020). SGLT2 Inhibitors in Patients with Heart Failure with Reduced Ejection Fraction: a Meta-Analysis of the EMPEROR-Reduced and DAPA-HF Trials. Lancet 396, 819–829. doi:10.1016/S0140-6736(20)31824-9
Zheng, S. L., Roddick, A. J., Aghar-Jaffar, R., Shun-Shin, M. J., Francis, D., Oliver, N., et al. (2018). Association between Use of Sodium-Glucose Cotransporter 2 Inhibitors, Glucagon-like Peptide 1 Agonists, and Dipeptidyl Peptidase 4 Inhibitors with All-Cause Mortality in Patients with Type 2 Diabetes: A Systematic Review and Meta-Analysis. JAMA 319, 1580–1591. doi:10.1001/jama.2018.3024
Zhong, Q., Hu, M. J., Cui, Y. J., Liang, L., Zhou, M. M., Yang, Y. W., et al. (2018). Carotid-Femoral Pulse Wave Velocity in the Prediction of Cardiovascular Events and Mortality: An Updated Systematic Review and Meta-Analysis. Angiology 69, 617–629. doi:10.1177/0003319717742544
Zhu, J., Yu, X., Zheng, Y., Li, J., Wang, Y., Lin, Y., et al. (2020). Association of Glucose-Lowering Medications with Cardiovascular Outcomes: an Umbrella Review and Evidence Map. Lancet Diabetes Endocrinol. 8, 192–205. doi:10.1016/S2213-8587(19)30422-X
Keywords: atherosclerotic cardiovascular disease, SGLT2-inhibitors, subclinical atherosclerosis, diabetes, review
Citation: Pahud de Mortanges A, Salvador Jr. D, Laimer M, Muka T, Wilhelm M and Bano A (2021) The Role of SGLT2 Inhibitors in Atherosclerosis: A Narrative Mini-Review. Front. Pharmacol. 12:751214. doi: 10.3389/fphar.2021.751214
Received: 31 July 2021; Accepted: 01 October 2021;
Published: 05 November 2021.
Edited by:
Takuya Miyawaki, Okayama University, JapanReviewed by:
Didac Mauricio, Hospital de la Santa Creu i Sant Pau, SpainKarin Bartel, Ludwig-Maximilians-University Munich, Germany
Copyright © 2021 Pahud de Mortanges, Salvador Jr., Laimer, Muka, Wilhelm and Bano. This is an open-access article distributed under the terms of the Creative Commons Attribution License (CC BY). The use, distribution or reproduction in other forums is permitted, provided the original author(s) and the copyright owner(s) are credited and that the original publication in this journal is cited, in accordance with accepted academic practice. No use, distribution or reproduction is permitted which does not comply with these terms.
*Correspondence: Arjola Bano, YXJqb2xhLmJhbm9AaXNwbS51bmliZS5jaA==