- 1School of Pharmaceutical Sciences, State Key Laboratory of Natural and Biomimetic Drugs, Peking University, Beijing, China
- 2Stem Cell Research Center, School of Basic Medical Sciences, Peking University Health Science Center, Beijing, China
Cytochrome P450 (CYP) is the most important phase I drug-metabolizing enzyme, and the effect of drugs on CYP enzymes can lead to decreased pharmacological efficacy or enhanced toxicity of drugs, but there are many deficiencies in the evaluation models of CYP enzymes in vitro. Human-induced hepatocytes (hiHeps) derived from human fibroblasts by transdifferentiation have mature hepatocyte characteristics. The aim was to establish a novel evaluation system for the effect of drugs on CYP3A4, 1A2, 2B6, 2C9, and 2C19 in vitro based on hiHeps. Curcumin can inhibit many CYP enzymes in vitro, and so the inhibition of curcumin on CYP enzymes was compared by human liver microsomes, human hepatocytes, and hiHeps using UPLC-MS and the cocktail method. The results showed that the IC50 values of CYP enzymes in the hiHeps group were similar to those in the hepatocytes group, which proved the effectiveness and stability of the novel evaluation system in vitro. Subsequently, the evaluation system was applied to study the inhibitory activity of notoginseng total saponins (NS), safflower total flavonoids (SF), and the herb pair of NS–SF on five CYP enzymes. The mechanism of improving efficacy after NS and SF combined based on CYP enzymes was elucidated in vitro. The established evaluation system will become a powerful tool for the research of the effect of drugs on the activity of CYP enzymes in vitro, which has broad application prospects in drug research.
Introduction
Drug-metabolizing enzymes play a key role in the process of drug metabolism (Underhill and Khetani, 2018), especially drug-drug interaction (DDI) in which drug-metabolizing enzymes have a significant impact on the safety of clinical drugs (Akamine et al., 2019). At present, the effect of drugs on metabolic enzymes, especially on the activity of cytochrome P450 (CYP) in phase I metabolism, has been listed as an important part of preclinical research of new drugs by the Food and Drug Administration in many countries (Yu et al., 2019; Sudsakorn et al., 2020). With the increasing development of new drugs, a rapid, accurate, stable, and low-cost evaluation system for the effect of drugs on CYP enzymes has become particularly important.
Liver microsomes are the most widely used in drug metabolism research because of their low cost, simple operation, and rapid detection (Williams et al., 2004; Cheng et al., 2018; Lu et al., 2018). However, liver microsomes are difficult to use when simulating the complete metabolic environment in vivo and evaluating the induction ability of drugs on CYP enzymes, so it is hard to accurately and comprehensively reflect the effect of drugs on the activity of CYP enzymes (Diao and Huestis, 2019; Thiengsusuk et al., 2020). Human hepatocytes are the closest model to human liver tissue, which can be used to evaluate the induction and inhibition effect of drugs on CYP enzymes relatively accurately, but human hepatocytes are difficult to obtain, unable to subculture, expensive, and have a short duration for the activity of metabolic enzymes, which is not conducive to extensive application for evaluation of the effect of drugs on CYP enzymes in vitro (Zeilinger et al., 2016; Schink and Dehus, 2017; Inoue et al., 2020). In recent years, some evaluation systems of the effect of drugs on CYP enzymes in vitro were established based on HepG2, HepRG, and other cells (Darnell et al., 2011; Cui et al., 2014), however, the expression of CYP enzyme activity was not stable in these cells, resulting in poor repeatability and accuracy for evaluation of the effect of drugs on CYP enzymes in vitro.
Human-induced hepatocytes (hiHeps), a kind of human hepatocyte model with stable metabolism function, were established through inducing human skin fibroblasts using HNF1A, HNF4A, HNF6, FOXA3, and other cytokines (Du et al., 2014; Huang et al., 2014). A series of phase I and phase II drug-metabolizing enzymes and drug transporters could be expressed in hiHeps, and the metabolic activities of CYP3A4, CYP1A2, CYP2B6, CYP2C9, and CYP2C19 were similar to those of freshly isolated human hepatocytes (Du et al., 2014). The metabolic enzyme activity could be maintained for about 2 weeks, meanwhile, the rapid cell proliferation rate was beneficial to expand the culture. Therefore, hiHeps have an obvious advantage as an evaluation model of related research dependent on CYP enzymes in vitro (Du et al., 2014). However, at present, there is no evaluation system of the effect of drugs on CYP enzymes in vitro based on hiHeps.
The research used hiHeps as a model to establish a new evaluation system for the effect of drugs on CYP enzymes in vitro, which was carried out by determining the Michaelis constant of specific probe substrates of CYP1A2, CYP2B6, CYP2C9, CYP2C19, and CYP3A4, respectively, and investigating the interaction between the probe substrates in the cocktail method. After the establishment of the system, it was compared with the systems of human liver microsomes (HLM) and human hepatocytes to check its validity and stability. Notoginseng and safflower display a synergistic effect in the treatment of cardiovascular disease in clinic and are necessary to explore the mechanism of the synergistic effect of NS and SF through the aspect of metabolism (Meng et al., 2018). Therefore, the system was applied to evaluate the inhibition influences of notoginseng total saponins (NS), safflower total flavonoids (SF), and the herb pair of notoginseng–safflower (namely CNS) on the activity of CYP enzymes. This study aims to set up a novel system based on hiHeps to evaluate the effect of drugs on CYP enzymes in vitro, and provide new methods and ideas for drug safety/toxicity research in preclinical and clinical stages.
Materials and Methods
Chemicals and Reagents
Nicotinamide adenine dinucleotide phosphate (NADPH), MgCl2, phenacetin (PHE), diclofenac (DIC), acetaminophen (ACT), ticlopidine (TIC), thiotepa (THI), sulfaphenazole (SUL), α-naphthoflavone (NAP), alamethicin, and DMSO were purchased from the Sigma–Aldrich Company (St. Louis, United States). Bupropion (BUP), carbamazepine (CAR, internal standard), ketoconazole (KET), and midazolam (MID) were obtained from National Institutes for Food and Drug Control (Beijing, China). Mephenytoin (MEP), hydroxybupropion, 4′-hydroxydiclofenac, 4′-hydroxymephenytoin, and 1′-hydroxymidazolam were gained from the Toronto Research Chemicals Company (Toronto, Canada). Curcumin was obtained from Chengdu Manster Biotechnology Co., Ltd. (Chengdu, China). The purities of all standards were more than 98%. InVitro GRO CP culture medium and fetal bovine serum (FBS) were purchased from Celsis In Vitro Technologies Inc (Maryland, United States). HLM (batch number: X008067) and human hepatocytes (batch number: X008000) were purchased from Research Institute for Liver disease Co., Ltd (Shanghai, China). Methanol, acetonitrile, and formic acid of LC-MS grade were obtained from Merck (Darmstadt, Germany). Ultrapure water was produced in the Millipore-Q system (Milford, MA, United States). All the reagents were at least of analytical grade.
NS, consisting of notoginsenoside R1 (6.2%), ginsenoside Rb1 (32.5%), Rd (6.6%), Re (4.1%), and Rg1 (26.6%), was collected by Yunnan Plant Pharmaceutical Co., Ltd (Kunming, China). Safflower, which was provided from Xinjiang Uygur Autonomous Region in China, was authenticated by PFT. The voucher specimen (No. 20110301) was deposited in the Modern Research Center for Traditional Chinese Medicine, Peking University (Beijing, China). The materials of safflower were refluxed with deionized water at 80°C three times (120 L for 1 h, 100 L for 0.5 h, and 100 L for 0.5 h), and the extract was filtered and concentrated in a vacuum. The concentrated solution was subjected to a D101 macroporous resin column eluted with deionized water and 50% aqueous ethanol. The 50% aqueous ethanol eluate was dried by spray drying to obtain SF and the amount of HSYA in SF was more than 8.0% verified by high performance liquid chromatography. CNS was composed of NS and SF with the ratio of 6:5.
Sample Pretreatment
A total of 250 μL of incubation solution was added to the corresponding 1.5 ml EP tube containing 750 μL of methanol consisting of internal standard (IS). The ice bath was maintained for 60 min after vortex-mixing, and then the solution was centrifuged at 14,000 rpm for 15 min at 4°C. The supernatant was centrifuged twice and 400 μL of the supernatant was evaporated to dryness under reduced pressure at 25°C. The residue was dissolved in 100 μL of 50% methanol (methanol: water, v/v), centrifuged at 14,000 rpm for 15 min, and then the supernatant was injected into the UPLC-MS/MS system for analysis.
UPLC-MS/MS Conditions
The analysis was conducted on a UPLC ACQUITY H-Class system (Waters, Milford, MA, United States) coupled with an AB SCIEX QTRAP 4500 mass spectrometer (SCIEX, Foster, United States) via an ESI interface. Sample separations were performed on an Acquity UPLC Xbridge BEH C18 column (2.1 mm × 50 mm, 2.5 μm, Waters, Milford, United States) and column temperature was set at 40°C. The mobile phase with the flow rate of 0.6 ml/min consisted of (A) 0.1% formic acid in water and (B) 0.1% formic acid in acetonitrile. Gradient elution condition was as follows: 0–1.5 min, 5% B; 1.5–3.5 min, 5%–45% B; 3.5–8.0 min, 45%–100% B; 8.0–11.0 min, 100% B. The injection volume was 1.0 μL. The ion source parameters of MS were kept as follows: MRM mode; positive ion mode; collisional activated dissociation (CAD) gas, medium level; ion spray needle voltage, 5500 V/−4500 V; GS1, 45 psi; GS2, 45 psi; CUR, 35 psi; and turbo gas temperature, 550°C. Analyst 1.6.2 was used to analyze data in this experiment. The MS parameters of probe drugs and corresponding metabolites are shown in Supplementary Table S1.
The quantitative determination method of probe drug metabolites (acetaminophen, hydroxybupropion, 4′-hydroxydiclofenac, 4′-hydroxymephenytoin, and 1′-hydroxymidazolam) was verified by specificity, linearity, precision, accuracy, recovery, and matrix effects. Calibration curves had to have correlation coefficients (r) of 0.99 or better. The lower limit of quantification (S/N ≥ 10) was expressed as the lowest concentration of the standard curve. Relative standard deviation (RSD) and relative error (RE) were defined as precision and accuracy, which were set to be less than ±15% (for the low concentration, the acceptance criterion was less than 20%). The extraction recoveries of the analytes were calculated by determining the peak area ratios of the analytes in the post-treatment spiked samples to that acquired from pre-treatment spiked samples. The matrix effect was investigated by comparing the peak areas of the analytes dissolved in the pre-treated blank incubation solution with that of the pure standard solutions containing equivalent amounts of the analytes.
Establishment of an Evaluation System for the Effect of Drugs on the Activity of Cytochrome P450 Enzymes in vitro Based on Human-Induced Hepatocytes
Determination of Michaelis Constant of Five Cytochrome P450 Enzymes in Human-Induced Hepatocytes
The hiHeps were digested with accutase; the cell-survival rate was detected with trypan blue staining, and counted with hemocytometer measurement. In total, 2×105 cells per milliliter of culture medium was used as the cell suspension. The standard working solutions of probe drugs were prepared by a spiking incubation solution to give the nominal concentrations of 20, 40, 80, 120, 160, and 200 μM for PHE (CYP1A2, substrate), 50, 100, 200, 400, 600, and 1000 μM for BUP (CYP2B6, substrate), 12.5, 25, 50, 100, 200, and 400 μM for DIC (CYP2C9, substrate), and 5, 10, 20, 50, 100, and 200 μM for MEP (CYP2C19, substrate) and MID (CYP3A4, substrate). A 250 μL cell suspension and 250 μL probe incubation solution were mixed in a 5 ml flow tube, which was placed in a 37°C shaker and incubated for 60 min. The samples were pretreated according to “Sample Pretreatment.”
Study on the Interaction Between Probe Drugs
The standard working solutions of probe drugs were prepared by diluting the stock solution to obtain the nominal concentrations of 125 μM for PHE and BUP, 50 μM for DIC and MEP, and 25 μM for MID. Serial dilutions of NAP (CYP1A2 inhibitor, 0, 0.001, 0.005, 0.01, 0.05, and 0.1 μM), THI (CYP2B6 inhibitor, 0, 0.1, 1, 10, 50, and 100 μM), SUL (CYP2C9 inhibitor, 0, 0.05, 0.5, 5, 50, and 500 μM), TIC (CYP2C19 inhibitor, 0, 0.05, 0.5, 5, 50, and 500 μM), KET (CYP3A4 inhibitor, 0, 0.005, 0.01, 0.05, 0.1, and 1 μM), and cocktail solution that included the above drugs were prepared for use. A 250 μL cell suspension, 200 μL probe incubation solution, and 50 μL inhibitor incubation solution were mixed in a 5 ml flow tube, which was placed in a 37°C shaker and incubated for 60 min.
Validation of System for the Effect of Drugs on the Activity of Cytochrome P450 Enzymes in vitro Based on Human-Induced Hepatocytes
Inhibitory activity of Curcumin on Five Cytochrome P450 Enzymes in Human Liver Microsomes
Human liver microsomes (HLM, 20 mg/ml) were added to an equal volume of Tris-HCl and double volume of 1 mg/ml of macromolecular surfactant (Brij), which had no effect on the activity of CYP enzymes, and were activated by ice bath for 5 min. The activated HLM were added into the corresponding channel of 96-well plates, then MgCl2 and alamethicin were added, and an ice bath was used for 20 min for reactivation. The mixed solution of probe drugs consisting of 50 μM PHE, 50 μM BUP, 20 μM DIC, 20 μM MEP, and 10 μM MID and serial dilutions of curcumin (0, 1, 10, 50, 100, and 500 μM) were added to the reaction system. The reaction system was pre-incubated at 37°C for 20 min, then NADPH was added, and incubated at 37°C for 60 min. The samples were pretreated according to “Sample Pretreatment.”
Inhibitory activity of Curcumin on Five Cytochrome P450 Enzymes in Human Hepatocytes
In total, 45 ml of InVitroGro CP culture medium (without FBS) was preheated to 37°C, and then 5 ml of FBS and 1 ml of Torpedo Antibiotic Mix was added. Then, 5 ml of InVitroGro CP culture medium was transferred to a 50 ml sterile conical tube. Human hepatocytes were transferred into the preheated InVitroGro CP culture medium and the tube was gently reversed three times to suspend hepatocytes again. The cell-survival rate was detected by trypan blue staining and counted by hemocytometer measurement. Overall, 2 × 105 cells per milliliter of culture medium was used as the cell suspension. The remaining treatments were the same as the method described in the “Inhibitory activity of curcumin on five CYP enzymes in human liver microsomes” section.
Inhibitory activity of Curcumin on Five Cytochrome P450 Enzymes in Human-Induced Hepatocytes
The hiHeps to be detected were digested with accutase, the cell-survival rate was detected by trypan blue staining, and counted with hemocytometer measurement. A total of 2 × 105 cells per milliliter of culture medium was used as the cell suspension. The remaining treatments were the same as the method described in the “Inhibitory activity of curcumin on five CYP enzymes in human hepatocytes” section.
Application of System for Cytochrome P450 Enzyme Activity in vitro Based on Human-Induced Hepatocytes
Inhibitory activity of CNS, NS, and SF on Five Cytochrome P450 Isoenzymes in Human-Induced Hepatocytes
The treatment of hiHeps was the same as the method described in the section of “Inhibitory activity of curcumin on five CYP enzymes in hiHeps.” The stock solutions of SF, NS, and CNS were diluted to provide incubation solutions (5 mg/ml, 6 mg/ml, and 11 mg/ml), respectively. A 250 μL cell suspension, 200 μL incubation solution of probe drugs, and 50 μL incubation solution of SF, NS, or CNS were mixed in a 5 ml flow tube, placed in a 37°C shaker, and incubated for 60 min. The samples were pretreated according to “Sample Pretreatment.”
Enzyme Kinetic Parameters and Statistical Analysis
The kinetic parameter Michaelis constant (Km) of the five probe drugs was estimated by nonlinear regression from a Lineweaver–Burk plot on the basis of the Michaelis–Menten equation, 1/v = 1/Vmax+(Km/Vmax)×1/[S], where v is the rate of reaction and [S] is the substrate concentration. Drug inhibition was calculated on the basis of the metabolite response observed in the presence of a test drug to the vehicle control. Non-linear fitting and determination of IC50 values were calculated using IBM SPSS Statistics 20.0. The data were expressed as the mean ± standard deviation (SD). Statistical analysis was determined by Student’s t-test with a two-tailed distribution and one-way analysis of variance (ANOVA), and a p value below 0.05 was considered statistically significant.
Results and Discussion
Method Validation
There was no interference observed from endogenous substances in the samples at the retention time of acetaminophen, hydroxybupropion, 4′-hydroxydiclofenac, 4′-hydroxymephenytoin, 1′-hydroxymidazolam, and IS (Supplementary Figure S1). We detected the standard curve, linear range, correlation coefficient, and lower limit of quantitation (LLOQ) for each metabolite of probe substrate by UPLC-MS (Supplementary Table S2). The intra-day and inter-day precisions were less than 5.21% and accuracies changed from 98.55 to 102.54% (Supplementary Table S3). The matrix effects and extraction recoveries were more than 78.59 and 86.36%, respectively (Supplementary Table S4).
Determination of Michaelis Constant for the Five Probe Drugs
The Km could reflect the catalytic ability of the metabolic enzyme, and also provide the necessary reference value of the probe drug for the evaluation system of CYP enzyme activity in vitro (Hakkola et al., 2020; Kong et al., 2020). We used the Lineweaver-Burk plot of CYP1A2 1), CYP2B6 2), CYP2C9 3), CYP2C19 4), and CYP3A4 5) in a hiHeps model to reveal the enzyme kinetics (Figure 1). The Km values of the five probe drugs were calculated as follows: 115.9 μM for PHE, 150.8 μM for BUP, 41.9 μM for DIC, 33.6 μM for MEP, and 13.9 μM for MID. According to the references, the Km values of PHE, BUP, DIC, MEP, and MID were as follows: 1.7–152.0 μM, 67.0–168.0 μM, 3.4–52.0 μM, 13.0–35.0 μM, and 1.0–14.0 μM, respectively. All Km values of probe drugs in the hiHeps model were within the scope of literature, which indicates that the activity of CYP1A2, CYP2B6, CYP2C9, CYP2C19, and CYP3A4 in this hiHeps model met the requirements of an evaluation system for the effect of drugs on the activity of CYP enzymes in vitro. Secondly, according to the measured Km in this experiment and the requirements of the cocktail method for the evaluation system, the concentration near the Km and as low as possible was selected as the final concentration of the probe drugs (Chen et al., 2020). Therefore, the concentrations of the five probe drugs were selected in this system as follows: 50 μM for PHE, 50 μM for BUP, 20 μM for DIC, 20 μM for MEP, and 10 μM for MID. Moreover, when the incubation time was less than 60 min, the consumption curves of each probe drug had good linearity (r > 0.95). Therefore, 60 min was chosen as the final incubation time for the evaluation system.
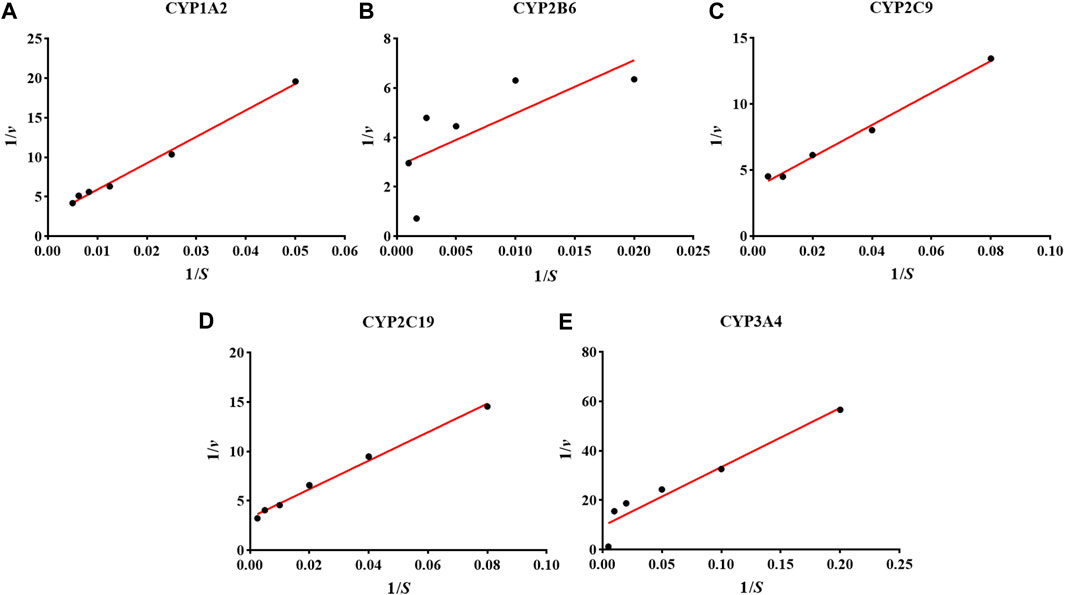
FIGURE 1. The results of the Lineweaver-Burk plot of CYP1A2 (A), CYP2B6 (B), CYP2C9 (C), CYP2C19 (D), and CYP3A4 (E) in the hiHeps model.
Study on the Interaction of Probe Substrates
There was no significant difference in the activities of the five CYP enzymes in the hiHeps model between single probe substrates and cocktail probe substrates (p > 0.05) (Figure 2). The interaction between the probe substrates was not obvious under the conditions of substrate concentration and incubation time. Therefore, CYP enzyme activity in the evaluation system based on the hiHeps model could be rapid and detected by the cocktail method. A novel evaluation system for the effect of drugs on the activity of CYP enzymes in vitro based on hiHeps was successfully established.
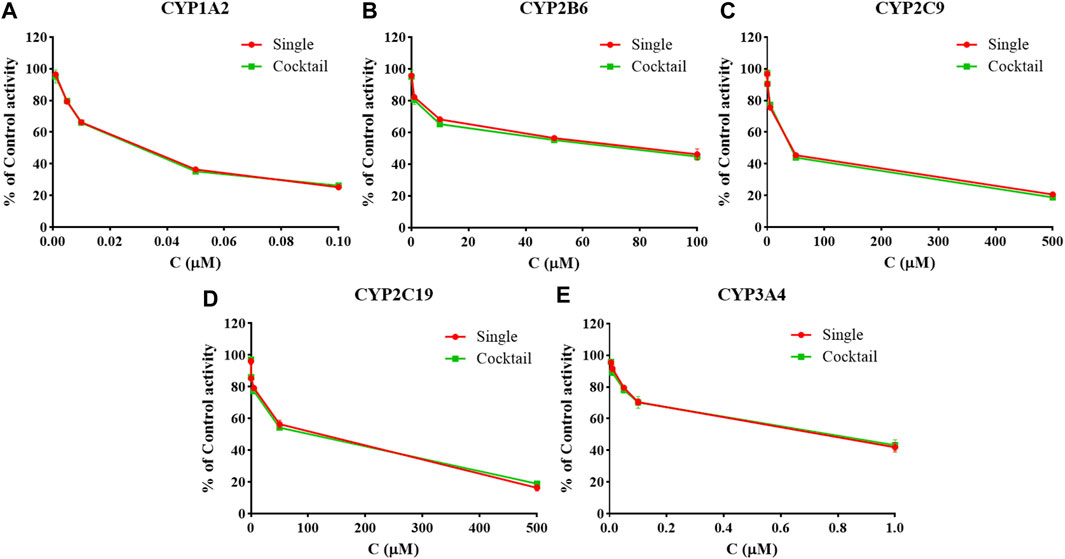
FIGURE 2. The activities of CYP1A2 (A), CYP2B6 (B), CYP2C9 (C), CYP2C19 (D), and CYP3A4 (E) in the hiHeps model between single probe substrates and cocktail probe substrates (mean ± SD, n = 3).
Comparison of Inhibitory Activity of Curcumin on Cytochrome P450 Enzymes in Three Models
The inhibition curves of curcumin on the five CYP enzymes in HLM, hepatocytes, and hiHeps groups are shown in Figure 3, and the IC50 values of curcumin on the five CYP enzymes were compared among HLM, hepatocytes, and hiHeps groups (Table 1). In the hiHeps group, the IC50 value of curcumin on the CYP1A2 isoenzyme was 68.55 ± 4.89 μM, which indicated that curcumin had a weak inhibitory effect on the CYP1A2 isoenzyme. The IC50 value of curcumin on CYP2B6 and CYP3A4 was 35.96 ± 1.57 μM and 21.91 ± 1.76 μM, both were less than 50 μM, which belonged to the range of moderate inhibitory effect. The IC50 value of curcumin on CYP2C9 was 9.91 ± 1.12 μM suggesting that curcumin had a strong inhibitory effect on CYP2C9, and curcumin had no significant inhibitory effect on CYP2C19 (IC50 > 500 μM). In the HLM group, the IC50 values of curcumin on CYP1A2, CYP2B6, CYP2C9, CYP2C19, and CYP3A4 were 50.10 ± 2.71 μM, 30.00 ± 2.09 μM, 8.70 ± 1.36 μM, >500 μM, and 21.00 ± 1.27 μM, respectively. In the hepatocytes group, the IC50 values of curcumin on CYP1A2, CYP2B6, CYP2C9, CYP2C19, and CYP3A4 were 67.13 ± 3.81 μM, 37.72 ± 2.97 μM, 10.77 ± 1.43 μM, >500 μM, and 22.09 ± 1.63 μM, respectively.
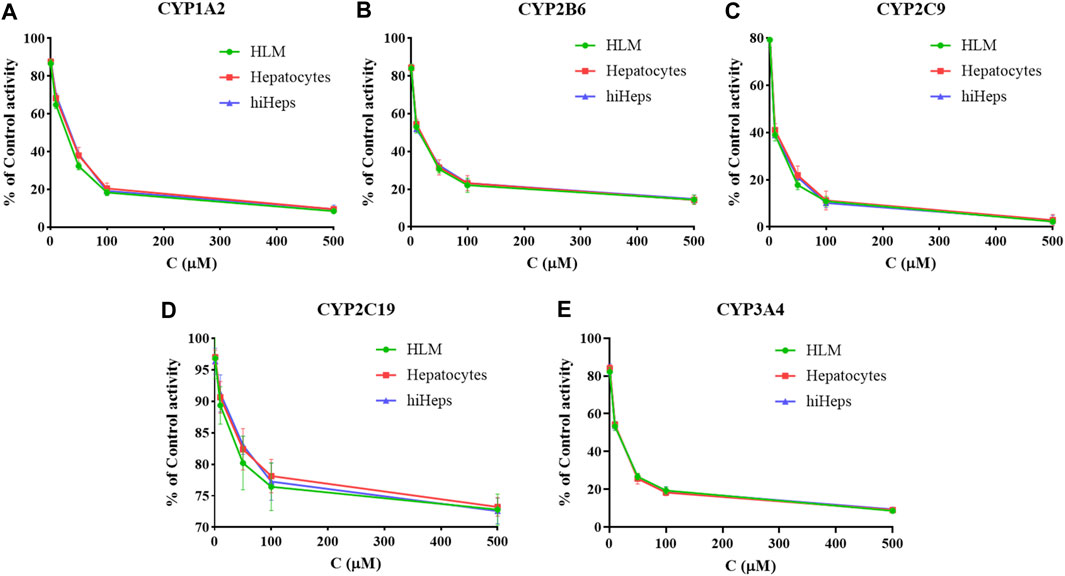
FIGURE 3. The inhibition curves of curcumin on CYP1A2 (A), CYP2B6 (B), CYP2C9 (C), CYP2C19 (D), and CYP3A4 (E) in HLM, hepatocytes, and hiHeps groups (mean ± SD, n = 3).
The IC50 values in the HLM group were generally lower than that in the hepatocytes group and hiHeps group. This might be due to the direct exposure of enzyme proteins in HLM to inhibitors, while most enzyme proteins in the cell model were in the endoplasmic reticulum, and the inhibitors needed transmembrane transport to make contact with enzyme proteins. It suggested that there were differences in function of CYP enzymes between HLM and human liver tissue. The results showed that the activities of CYP1A2, CYP2B6, CYP2C9, CYP2C19, and CYP3A4 in the hiHeps group were similar to that in the hepatocytes group. Detection results of the hiHeps model were stable and reliable, which could be used to evaluate the effect of drugs on the activity of CYP enzymes in vitro. Due to rapid cell passage and good stability of CYP enzymes in hiHeps, the established evaluation system will become a powerful tool for the research of the effect of drugs on the activity of CYP enzymes in vitro, which has broad application prospects in drug research.
Effect of NS, SF, and CNS on the Activity of Cytochrome P450 Enzymes in vitro
We assessed the effects of CNS, NS, and SF on the activity of CYP1A2, CYP2B6, CYP2C9, CYP2C19, and CYP3A4 (Figure 4 and Table 2). The IC50 values of NS on CYP1A2, CYP2B6, CYP2C9, CYP2C19, and CYP3A4 were 103.33 ± 1.53 μM, 101.67 ± 3.05 μM, 104.33 ± 1.15 μM, 102.83 ± 2.02 μM, and 96.21 ± 3.51 μM, respectively. The results suggested that NS had no significant effects on CYP1A2, CYP2B6, CYP2C9, CYP2C19, and CYP3A4 (p > 0.05). The IC50 values of SF on CYP1A2, CYP2B6, CYP2C9, CYP2C19, and CYP3A4 were 55.67 ± 2.52 μM, 72.00 ± 2.65 μM, 79.67 ± 2.52 μM, 102.67 ± 1.53 μM, and 91.82 ± 1.50 μM, respectively, which indicated that SF had significant inhibitory effects on CYP1A2, CYP2B6, and CYP2C9 enzymes (p < 0.05), but had no significant effects on CYP2C19 and CYP3A4 enzymes (p > 0.05). CNS had significant inhibitory effects on CYP1A2 (IC50 = 68.55 ± 4.89 μM), CYP2B6 (IC50 = 87.33 ± 1.53 μM), CYP2C9 (IC50 = 91.33 ± 2.08 μM), and CYP3A4 (IC50 = 80.11 ± 3.54 μM) (p < 0.05), but had no significant effect on the activity of CYP2C19 (IC50 = 95.67 ± 1.53 μM) (p > 0.05).
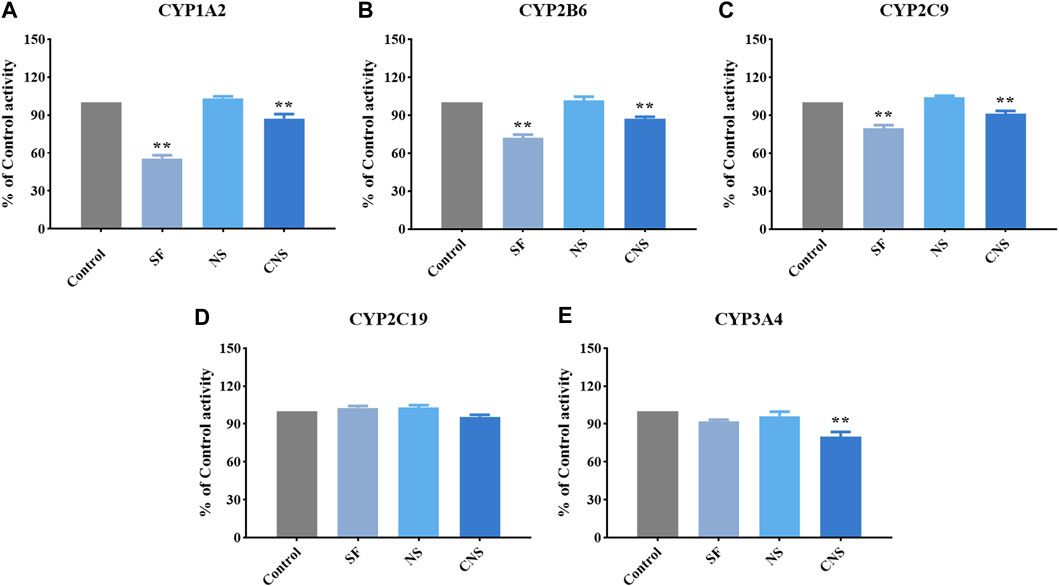
FIGURE 4. Effects of CNS, NS, and SF on the activity of CYP1A2 (A), CYP2B6 (B), CYP2C9 (C), CYP2C19 (D), and CYP3A4 (E) (mean ± SD, n = 3, **p < 0.01).
CYP3A4 is the most predominant CYP enzyme and metabolizes approximately 50% of market drugs (Mann, 2006). Human CYP3A4 and CYP3A5 were the major enzymes in charge of the metabolism of 20(S)-protopanaxadiol or 20(S)-protopanaxatriol in NS (Hu et al., 2013). SF and NS alone had no significant effect on the activity of CYP3A4, but CNS (the compatibility form of SF and NS) significantly reduced the percentage of CYP3A4 enzyme activity, which showed an obvious inhibitory effect (p < 0.05). Therefore, the combined administration of SF and NS was beneficial to reduce the phase I metabolism of ginsenosides mediated by CYP3A4, and increase the exposure levels of the main bioactive components of NS in vivo. Compared with NS, the activity percentages of CYP1A2, CYP2B6, CYP2C9, and CYP3A4 were significantly decreased by CNS, which was of benefit for reducing the metabolism and enhancing the exposure levels of the main bioactive components in NS by CYP isozymes, and improved the exposure levels in vivo. The CYP2C subfamily make up about 20% of the total content of hepatic CYPs and metabolize about 20% of market drugs (Isvoran et al., 2017). SF, NS, and CNS had no significant effect on the activity of CYP2C19, however, CNS significantly reduced the activity percentage of CYP2C19 compared with SF and NS (p < 0.05). The results suggested that CNS reduced the phase I metabolism of saponins and flavonoids mediated by CYP2C19, and thus increased the exposure levels of the main bioactive ingredients of SF and NS in vivo.
This result was consistent with the experimental data in vivo, which verified the reliability and stability of the evaluation system. Moreover, the mechanism of effective compatibility of NS and SF based on a phase I metabolic enzyme was elucidated from in vitro experiments on the basis of the hiHeps model. In addition, the ability of hiHeps in evaluating the induced effect of drugs on CYP enzymes was found in this research. The application of hiHeps in metabolism research will continue to be improved in the follow-up study.
Conclusion
This research established a new evaluation system for the effect of drugs on CYP enzymes in vitro based on hiHeps. The validity and stability of the evaluation system were verified by comparing those of HLM and human hepatocytes. Subsequently, the system was applied to evaluate the inhibition effect of NS, SF, and CNS on the activity of CYP enzymes, which explained the mechanism of effective compatibility of NS and SF based on CYP enzymes. This study provided new methods and ideas for drug safety/toxicity research in preclinical and clinical stages.
Data Availability Statement
The original contributions presented in the study are included in the article/Supplementary Material, further inquiries can be directed to the corresponding authors.
Author Contributions
XG, PT, YS, and YJ contributed to the conception and design of the study; YL, JJ, and LZ performed experiments; YL and MF performed the statistical analysis; YL wrote the first draft of the manuscript; XG finalized the writing. All authors read and approved the final manuscript.
Funding
This work was supported by the National Natural Science Foundation of China (grant nos. 81974551 and 81573684), Beijing Municipal Science and Technology Project (grant no. Z181100002218028), and National Key Technology R&D Program “New Drug Innovation” of China (grant no. 2018ZX09711001-008-003).
Conflict of Interest
The authors declare that the research was conducted in the absence of any commercial or financial relationships that could be construed as a potential conflict of interest.
Publisher’s Note
All claims expressed in this article are solely those of the authors and do not necessarily represent those of their affiliated organizations, or those of the publisher, the editors and the reviewers. Any product that may be evaluated in this article, or claim that may be made by its manufacturer, is not guaranteed or endorsed by the publisher.
Supplementary Material
The Supplementary Material for this article can be found online at: https://www.frontiersin.org/articles/10.3389/fphar.2021.748658/full#supplementary-material
References
Akamine, Y., Yasui-Furukori, N., and Uno, T. (2019). Drug-drug Interactions of P-Gp Substrates Unrelated to CYP Metabolism. Curr. Drug Metab. 20, 124–129. doi:10.2174/1389200219666181003142036
Chen, J., Guo, X., Lu, Y., Shi, M., Mu, H., Qian, Y., et al. (2020). Large Volume Direct Injection Ultra-high Performance Liquid Chromatography-Tandem Mass Spectrometry-Based Comparative Pharmacokinetic Study between Single and Combinatory Uses of Carthamus Tinctorius Extract and Notoginseng Total Saponins. Pharmaceutics 12, 180. doi:10.3390/pharmaceutics12020180
Cheng, H.-X., Lu, Y.-Y., Wang, X., Ren, H., Li, Q., Wang, S.-M., et al. (2018). Interaction between 3,4-dichlorophenyl-propenoyl-sec.B-utylamine (3,4-DCPB), an A-ntiepileptic D-rug, and C-ytochrome P450 in R-at L-iver M-icrosomes and R-ecombinant H-uman E-nzymes In V-itro. Eur. J. Pharm. Sci. 123, 241–248. doi:10.1016/j.ejps.2018.07.018
Cui, H. M., Zhang, Q. Y., Wang, J. L., Chen, J. L., Zhang, Y. L., and Tong, X. L. (2014). In Vitro studies of Berberine Metabolism and its Effect of Enzyme Induction on Hepg2 Cells. J. Ethnopharmacol 158 Pt A, 388–396. doi:10.1016/j.jep.2014.10.018
Darnell, M., Schreiter, T., Zeilinger, K., Urbaniak, T., Söderdahl, T., Rossberg, I., et al. (2011). Cytochrome P450-dependent Metabolism in HepaRG Cells Cultured in a Dynamic Three-Dimensional Bioreactor. Drug Metab. Dispos. 39, 1131–1138. doi:10.1124/dmd.110.037721
Diao, X., and Huestis, M. A. (2019). New Synthetic Cannabinoids Metabolism and Strategies to Best Identify Optimal Marker Metabolites. Front. Chem. 7, 109. doi:10.3389/fchem.2019.00109
Du, Y., Wang, J., Jia, J., Song, N., Xiang, C., Xu, J., et al. (2014). Human Hepatocytes with Drug Metabolic Function Induced from Fibroblasts by Lineage Reprogramming. Cell Stem Cell. 14, 394–403. doi:10.1016/j.stem.2014.01.008
Hakkola, J., Hukkanen, J., Turpeinen, M., and Pelkonen, O. (2020). Inhibition and Induction of CYP Enzymes in Humans: an Update. Arch. Toxicol. 94, 3671–3722. doi:10.1007/s00204-020-02936-7
Hu, Z., Yang, J., Cheng, C., Huang, Y., Du, F., Wang, F., et al. (2013). Combinatorial Metabolism Notably Affects Human Systemic Exposure to Ginsenosides from Orally Administered Extract of Panax Notoginseng Roots (Sanqi). Drug Metab. Dispos. 41, 1457–1469. doi:10.1124/dmd.113.051391
Huang, P., Zhang, L., Gao, Y., He, Z., Yao, D., Wu, Z., et al. (2014). Direct Reprogramming of Human Fibroblasts to Functional and Expandable Hepatocytes. Cell Stem Cell. 14, 370–384. doi:10.1016/j.stem.2014.01.003
Inoue, T., Iwazaki, N., Araki, T., and Hitotsumachi, H. (2020). Human-induced Pluripotent Stem Cell-Derived Hepatocytes and Their Culturing Methods to Maintain Liver Functions for Pharmacokinetics and Safety Evaluation of Pharmaceuticals. Curr. Pharm. Biotechnol. 21, 773–779. doi:10.2174/1389201021666200131123524
Isvoran, A., Louet, M., Vladoiu, D. L., Craciun, D., Loriot, M. A., Villoutreix, B. O., et al. (2017). Pharmacogenomics of the Cytochrome P450 2c Family: Impacts of Amino Acid Variations on Drug Metabolism. Drug Discov. Today 22, 366–376. doi:10.1016/j.drudis.2016.09.015
Kong, R., Ma, J., Hwang, S., Moon, Y. C., Welch, E. M., Weetall, M., et al. (2020). In Vitro metabolism, Reaction Phenotyping, Enzyme Kinetics, CYP Inhibition and Induction Potential of Ataluren. Pharmacol. Res. Perspect. 8, e00576. doi:10.1002/prp2.576
Lu, Y. Y., Du, Z. Y., Li, Y., Wang, J. L., Zhao, M. B., Jiang, Y., et al. (2018). Effects of Baoyuan Decoction, a Traditional Chinese Medicine Formula, on the Activities and mRNA Expression of Seven CYP Isozymes in Rats. J. Ethnopharmacol 225, 327–335. doi:10.1016/j.jep.2018.07.023
Mann, H. J. (2006). Drug-associated Disease: Cytochrome P450 Interactions. Crit. Care Clin. 22, 329–vii. doi:10.1016/j.ccc.2006.02.004
Meng, Y., Du, Z., Li, Y., Wang, L., Gao, P., Gao, X., et al. (2018). Integration of Metabolomics with Pharmacodynamics to Elucidate the Anti-myocardial Ischemia Effects of Combination of Notoginseng Total Saponins and Safflower Total Flavonoids. Front. Pharmacol. 9, 667. doi:10.3389/fphar.2018.00667
Schink, M., and Dehus, O. (2017). Effects of Mistletoe Products on Pharmacokinetic Drug Turnover by Inhibition and Induction of Cytochrome P450 Activities. BMC Complement. Altern. Med. 17, 521. doi:10.1186/s12906-017-2028-1
Sudsakorn, S., Bahadduri, P., Fretland, J., and Lu, C. (2020). 2020 FDA Drug-Drug Interaction Guidance: a Comparison Analysis and Action Plan by Pharmaceutical Industrial Scientists. Curr. Drug Metab. 21, 403–426. doi:10.2174/1389200221666200620210522
Thiengsusuk, A., Boonprasert, K., and Na-Bangchang, K. (2020). A Systematic Review of Drug Metabolism Studies of Plants with Anticancer Properties: Approaches Applied and Limitations. Eur. J. Drug Metab. Pharmacokinet. 45, 173–225. doi:10.1007/s13318-019-00582-8
Underhill, G. H., and Khetani, S. R. (2018). Advances in Engineered Human Liver Platforms for Drug Metabolism Studies. Drug Metab. Dispos. 46, 1626–1637. doi:10.1124/dmd.118.083295
Williams, J. A., Hyland, R., Jones, B. C., Smith, D. A., Hurst, S., Goosen, T. C., et al. (2004). Drug-drug Interactions for UDP-Glucuronosyltransferase Substrates: a Pharmacokinetic Explanation for Typically Observed Low Exposure (AUCi/AUC) Ratios. Drug Metab. Dispos. 32, 1201–1208. doi:10.1124/dmd.104.000794
Yu, J., Petrie, I. D., Levy, R. H., and Ragueneau-Majlessi, I. (2019). Mechanisms and Clinical Significance of Pharmacokinetic-Based Drug-Drug Interactions with Drugs Approved by the U.S. Food and Drug Administration in 2017. Drug Metab. Dispos. 47, 135–144. doi:10.1124/dmd.118.084905
Keywords: human induced hepatocytes, cytochrome P450, cocktail, notoginseng, safflower, compatibility
Citation: Li Y, Lu Y-Y, Jia J, Fang M, Zhao L, Jiang Y, Shi Y, Tu P-F and Guo X-Y (2021) A Novel System for Evaluating the Inhibition Effect of Drugs on Cytochrome P450 Enzymes in vitro Based on Human-Induced Hepatocytes (hiHeps). Front. Pharmacol. 12:748658. doi: 10.3389/fphar.2021.748658
Received: 28 July 2021; Accepted: 11 October 2021;
Published: 28 October 2021.
Edited by:
Massimo Valoti, University of Siena, ItalyReviewed by:
José V. Castell, University of Valencia, SpainStanislav Yanev, Bulgarian Academy of Sciences (BAS), Bulgaria
Copyright © 2021 Li, Lu, Jia, Fang, Zhao, Jiang, Shi, Tu and Guo. This is an open-access article distributed under the terms of the Creative Commons Attribution License (CC BY). The use, distribution or reproduction in other forums is permitted, provided the original author(s) and the copyright owner(s) are credited and that the original publication in this journal is cited, in accordance with accepted academic practice. No use, distribution or reproduction is permitted which does not comply with these terms.
*Correspondence: Yan Shi, shiyan@bjmu.edu.cn; Peng-Fei Tu, pengfeitu@bjmu.edu.cn; Xiao-Yu Guo, guoxiaoyu@bjmu.edu.cn
†These authors have contributed equally to this work.