- 1Laboratory of Translational Oncology and Experimental Cancer Therapeutics, The Warren Alpert Medical School, Brown University, Providence, RI, United States
- 2Pediatric Hematology/Oncology, The Warren Alpert Medical School, Brown University, Providence, RI, United States
- 3The Joint Program in Cancer Biology, Brown University and Lifespan Health System, Providence, RI, United States
- 4Pediatric Surgery, The Warren Alpert Medical School, Brown University, Providence, RI, United States
- 5Department of Pathology and Laboratory Medicine, The Warren Alpert Medical School, Brown University, Providence, RI, United States
- 6Cancer Center at Brown University, The Warren Alpert Medical School, Brown University, Providence, RI, United States
- 7Hematology/Oncology Division, Department of Medicine, Lifespan Health System and Brown University, Providence, RI, United States
Chromosomal fusions encoding novel molecular drivers have been identified in several solid tumors, and in recent years the identification of such pathogenetic events in tumor specimens has become clinically actionable. Pediatric sarcomas and other rare tumors that occur in children as well as adults are a group of heterogeneous tumors often with driver gene fusions for which some therapeutics have already been developed and approved, and others where there is opportunity for progress and innovation to impact on patient outcomes. We review the chromosomal rearrangements that represent oncogenic events in pediatric solid tumors outside of the central nervous system (CNS), such as Ewing Sarcoma, Rhabdomyosarcoma, Fibrolamellar Hepatocellular Carcinoma, and Renal Cell Carcinoma, among others. Various therapeutics such as CDK4/6, FGFR, ALK, VEGF, EGFR, PDGFR, NTRK, PARP, mTOR, BRAF, IGF1R, HDAC inhibitors are being explored among other novel therapeutic strategies such as ONC201/TIC10.
Introduction
Pediatric cancer rates have been rising the past few decades, and approximately 400,000 new cases are diagnosed globally each year (Ward et al., 2019a). While the overall 5-year survival rate for pediatric oncology patients in the United States is 84%, outcomes are generally worse in pediatric solid tumors outside of the central nervous system (CNS), especially in cases of up-front metastases, recurrence, or progressive disease (Siegel et al., 2020). Pediatric sarcomas account for approximately 10% of all childhood tumors, and include both soft tissue and bone-related tumors. Pediatric carcinomas, including liver tumors such as fibrolamellar hepatocellular carcinoma, are less common. Of all pediatric sarcoma patients, recurrence occurs in 30–40% of cases, with patients facing poor event-free survival odds after relapse (Stahl et al., 2011; Duchman et al., 2015; Smeland et al., 2019). Similarly, pediatric carcinoma patients who present with up-front metastasis can have an overall survival rate of less than 20% (Schmid and von Schweinitz, 2017).
A subset of extra-cranial pediatric solid tumors are fusion-positive tumors, which are characterized by abnormal chromosomal rearrangements (e.g., translocations, insertions, inversions, and deletions) that result in the fusion of two disparate genes. Fusion genes can lead to constitutively activated oncogenes when a proto-oncogene is upregulated by the promoter and activator sequences of the partner gene in the fusion (Dupain et al., 2017). Additionally, the encoded chimeric oncoprotein can act as an aberrant transcription factor, driving downstream activation of pathways involved in tumorigenesis. Gene fusions may also result in deletion of tumor-suppressor genes, allowing for cancer cell transformation (Dupain et al., 2017).
In the past few decades, detection of these fusions has been of great interest in both basic cancer research and in the clinical setting, as fusions can be used as diagnostic markers, prognostic indicators, and therapeutic targets in the treatment of disease (Table 1). Clinical utility with screening fusion panels in pediatric solid tumors and hematological malignancies has been previously demonstrated, highlighting the need for targeted therapies in fusion-positive pediatric cancers (Chang et al., 2019).
Current therapeutic strategies focus on decreasing long-term treatment toxicity while maintaining excellent outcomes for low-risk patients, while improving outcomes for high-risk patients, often through treatment intensification. In pediatric solid tumors, the combination of cytotoxic chemotherapy, radiation, and surgery has been the standard in pediatric patients for over 2 decades. With the advent of precision medicine, and subsequent increasing understanding of molecular drivers of disease, such as fusion oncogenes, emphasis is currently placed on investigating targeted treatment options.
Ewing Sarcoma
Ewing Sarcoma (ES) is the second most common pediatric bone cancer. ES tumors are thought to arise from mesenchymal progenitor cells in bones and soft tissues in both children and adolescents. ES is most frequently driven by the fusion of a FET/TET family of RNA-binding proteins and an ETS family of transcription factors, with approximately 85–90% of ES cases harboring a common EWSR1-FLI1 fusion (Brohl et al., 2014). These tumors have relatively quiet genome with a low mutational burden, as most of the tumors only have fusion gene rearrangements identified. STAG2 loss is identified in 15% of ES (Brohl et al., 2014). There is inconsistent information about whether mutations in STAG2, CDKN2A and TP53 lead to less favorable prognoses (Honoki et al., 2007; Brohl et al., 2014; Lerman et al., 2015; Brohl et al., 2017).
ES disease progression can be further monitored using a liquid biopsy technique (Hayashi et al., 2016). Liquid biopsies detect circulating tumor DNA (ctDNA) in the peripheral blood. There are promising initial results using capture based next generation sequencing or digital droplet PCR to function as a disease monitoring tool. Circulating tumor DNA has been shown to be effective at detecting disease. One study of ctDNA in the blood found detectable levels in 53% patients tested (Shulman et al., 2018). Detectable ctDNA is associated with inferior outcomes in these patients and can inform how to proceed with management of the disease (Shulman et al., 2018).
Treatment of ES is via a three-pronged approach. Surgery, radiation, and chemotherapy are the mainstays of up-front therapy in ES. Surgery to remove the tumor requires a wide resection (Adzhubei et al., 2010). ES has also expressed sensitivity to radiation therapies in attempts to treat and manage the disease (Ewing, 19211972). It is possible to use radiation therapies as definitive local control for inoperable tumors and radiation may also be used pre- or post-operatively to combat tumor progression or in the setting of marginal or intralesional resection (Zöllner et al., 2021). The optimal utilization of radiation therapy is unclear prior to surgery, but the benefit can be significant (Zöllner et al., 2021). This multimodal treatment strategy of combining cytotoxic chemotherapy with radiation and/or surgery has resulted in a 5-year survival rate of 65–75% in patients with localized disease. In addition, patients with metastatic disease suffer from a survival rate of less than 30% with no appreciable improvements over the past 30 years (Gaspar et al., 2015).
The EWSR1-FLI1 oncoprotein acts as an aberrant transcription factor that binds to the canonical ETS binding site and GGAA microsatellite sequences, thereby deregulating cell cycle genes involved in checkpoint control. Genes regulating cell function, cell migration, signal transduction, and chromatin structure are also affected, leading to malignant transformation.
Several studies have shown the oncogenic effect of the EWSR1-FLI1 fusion, as inhibition of ES cell growth occurs when the EWSR1-FLI1 fusion protein is depleted (Herrero-Martín et al., 2009). This makes for a valuable target for therapies aimed at treating this disease. Despite being recognized as a valuable therapeutic target over 25 years ago, there have been relatively few steps towards inhibiting its tumorigenic characteristics.
Lysine-specific histone demethylase 1 (LSD1) is a part of the nucleosome remodeling and deacetylase (NuRD) co-repressor complex. It is recruited by EWSR1-FLI1 to regulate transcription. In vitro and in vivo experiments with LSD1 inhibition have resulted in reversal of the EWSR1-FLI1 oncogenic activity (Sankar et al., 2013; Sankar et al., 2014). Several of the LSD1 inhibitors have begun to enter clinical trials.
The insulin-like growth factor 1 receptor (IGF-1R) pathway is deregulated by the EWSR1-FLI1 translocation and is an exciting potential target for targeted therapies. There are currently trials ongoing that display at least a partial response in 11.7% of participants, when the IGF-1R inhibitor is administered alone or in combination (van Maldegem et al., 2016). However, a phase II trial combining an IGF-1R inhibitor with an mTOR inhibitor did not show any benefit (Malempati et al., 2012).
The poly (ADP-ribose) polymerase (PARP) pathway is essential for detection of DNA repair and detection of DNA stand breakage in tumor cells. A phase II trial examined 12 patients with ES who were administered 400 mg of oral olaparib, a PARP inhibitor, twice, daily (Choy et al., 2014). While there were no significant toxicities to report, tumor response was not seen in any of the 12 patients, although four of the 12 patients reported stable disease with a median time for progression-free survival (PFS) of 5.7 weeks (Choy et al., 2014). Additionally, there is an open clinical study ongoing testing the PARP inhibitor niraparib to refine dosing and determine dose-limiting toxicities. Similarly, this trial also evaluates escalating doses of temozolomide and/or irinotecan in patients who have recurrent or progressive Ewing Sarcoma that has failed previous treatment (clinicaltrials.gov, NCT 02044120).
There is optimism in a novel ES treatment that focuses on the combination of epigenetic drugs vorinostat and HCI-2509 to inhibit EWSR1-FLI1 and suppress tumor growth. This study analyzed proliferation and cell viability in ES cell lines, showing a synergistic combination of the two epigenetic drugs. These drugs also increased the amount of apoptosis induced in these tumor cells (García-Domínguez et al., 2018). The mechanistic interaction between these epigenetic therapies and EWSR1-FLI1 is not currently well known. However, many of the tumor cells analyzed were stuck in their G1 phase, and apoptosis was induced (García-Domínguez et al., 2018). Treatment with these agents, individually or in combination, resulted in a significant decrease in levels of EWSR1-FLI1 mRNA and protein (García-Domínguez et al., 2018). This result was also confirmed in patient-derived xenograft mice.
Additionally, preclinical work examining the effect of small molecule inhibitors on ETS-transcription factors is ongoing. The molecule YK-4-279 is a small molecule inhibitor that binds to EWSR1-FLI1 and blocks its interaction with RNA helicase A (RHA) (Spriano et al., 2019). RHA is required for efficient EWSR1-FLI1 activity. When the RHA- EWSR1-FLI1 interaction is blocked, ES cellular growth is inhibited, and tumor proliferation is halted. This interaction results in cell cycle inhibition and promotion of apoptosis, resulting in reduced growth in tumor cells (Erkizan et al., 2009). RHA-EWSR1-FLI1 interactions serve as a unique point of optimism in development of ES targeted therapies. The clinical derivative of YK-4-279, known as TK-216, is currently in Phase I clinical trials for patients with refractory or relapsed ES (clinicaltrials.gov, NCT02657005).
Further investigation into the efficacy of targeted therapies and their impact on ES outcomes is needed. There are currently numerous clinical trials underway that are focusing on molecular targets to effectively treat ES and result in better outcomes. Unlike kinase fusions, the EWSR1-FLI1 translocation has yet to be successfully targeted with novel therapies. This represents an area of research that needs further investigation and analysis of in vivo and in vitro studies, as well as clinical trial protocols. Perhaps most intriguing are studies involving small molecule-based therapies. These trials are summarized in Table 2 and are a point of optimism in advancing ES treatment options.
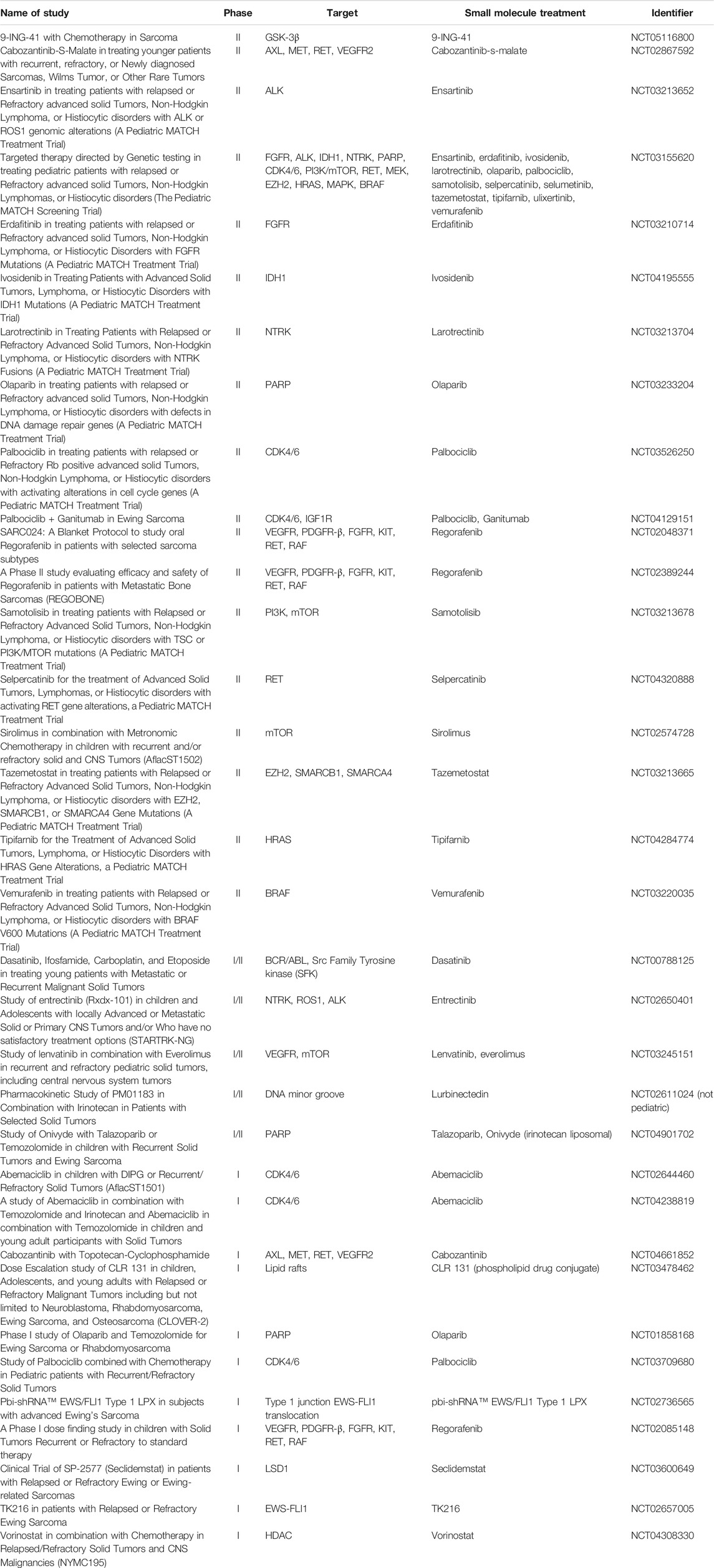
TABLE 2. Small Molecule Clinical Trials that are Recruiting or Active for Adolescent or Pediatric Ewing Sarcoma Patients (All Data from ClinicalTrials.gov).
Abemaciclib is a small molecule inhibitor of CDK4 and CDK6, and it is currently FDA approved for HR+/HER2− advanced breast cancer (Dowless et al., 2018). Additionally, it is available in lung cancer and other solid tumors. In the laboratory, abemaciclib showed tumor suppression in ES cell lines by blocking the G1 phase of the cell cycle (Dowless et al., 2018). Additionally, when cytokine secretion, antigen presentation, and interferon pathway upregulation were measured, abemaciclib was shown to have anti-inflammatory effects (Dowless et al., 2018).
This small molecule is currently being evaluated in two phase I clinical trials in pediatric solid tumors, including ES, either as monotherapy, or in combination with temozolomide and irinotecan, or with temozolomide alone.
Another therapy, recently approved by the FDA in 2020, is lurbinectedin. Lurbinectedin is an inhibitor of RNA polymerase II and induces DNA breaks in cells that result in apoptosis (Markham, 2020). This small molecule therapy covalently binds to guanine, located centrally in the minor groove of DNA, forming adducts capable of inducing DNA double-strand breaks (Markham, 2020). Lurbinectedin is also being investigated as to whether it may induce immunogenic cell death and increase anti-tumor immunity. This is potentially due to the fact that lurbinectedin has been associated with a reduction in tumor associated macrophages and monocytes in pre-clinical in vitro and in vivo models (Markham, 2020). Lurbinectedin has been shown to be an effective anti-tumor treatment in solid tumors, and it is well tolerated by patients. Currently there is one current trial testing lurbinectedin in combination with irinotecan against solid tumors in adults but not in the pediatric population (clinicaltrials.gov, NCT02611024). The trial is based on 2016 laboratory studies where lurbinectedin was shown to cause nuclear redistribution of the EWSR1-FLI1 translocation, resulting in less activity at the promoter and lower levels of mRNA and protein (Harlow et al., 2016). This effect was confirmed in xenograft studies, and when lurbinectedin was combined with irinotecan, there was a complete reversal of EWSR1-FLI1 tumorigenic activity, as ES cells were replaced with benign fat cells. The elimination of tumors in 30–70% of mice in only 11 days from the inception of treatment was observed (Harlow et al., 2016). The targeted therapies discussed above for ES are summarized in Figure 1.
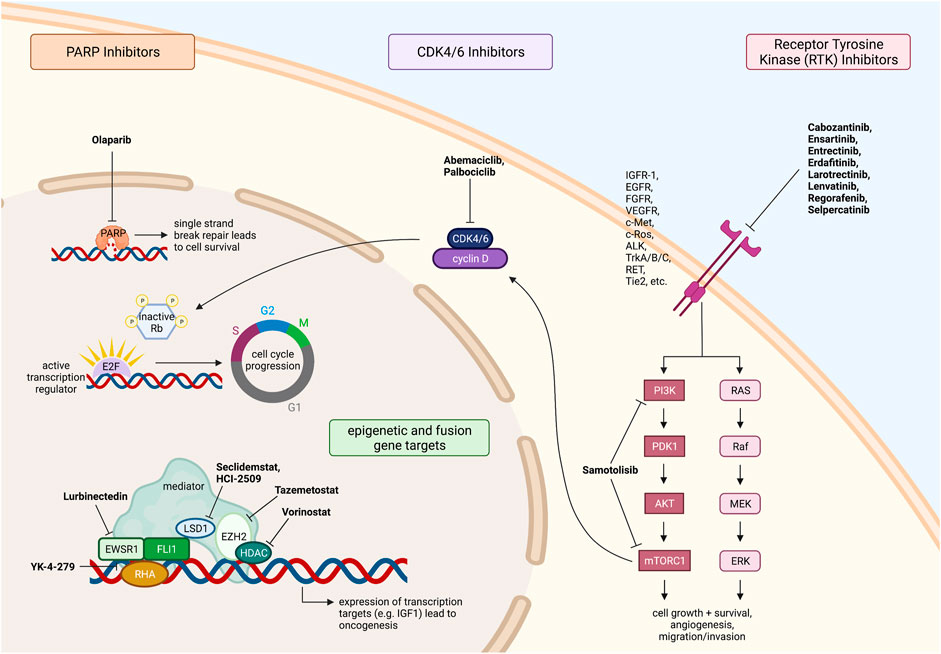
FIGURE 1. Fusion driven pediatric solid tumors with clinically actionable targets. Ewing sarcoma therapeutic targets and drugs under investigation. All figures were created with BioRender.com.
Rhabdomyosarcoma
Rhabdomyosarcoma (RMS), derived from primitive mesenchymal cells in the striated skeletal muscle lineage and myogenic progenitors, is the most common soft tissue sarcoma in children.
For localized RMS cases, gross surgical resection of the primary tumor plus radiation therapy and chemotherapy is considered standard-of-care, although long-term toxicities from RT is a concern in younger patients. Over 90% of low-risk localized RMS patients have relapse-free survival with cytotoxic multi-agent chemotherapy. Most recently vinorelbine and low-dose cyclophosphamide maintenance therapy was trialed in high-risk localized RMS patients and demonstrated improvement in overall survival (Chen et al., 2019). However, overall survival for metastatic and recurrent RMS remains low at 21 and 30%, respectively, indicating the need for novel targeted therapies to improve survival outcomes (Chen et al., 2019).
Alveolar RMS (ARMS), harboring an oncofusion, is the most aggressive subtype due to its high metastasis and recurrence rates (van Erp et al., 2018). ARMS tumors are characterized by the presence of a chromosomal translocation, either the PAX3-FOXO1 fusion gene or the PAX7-FOXO1 fusion gene. The PAX3-FOXO1 rearrangement fuses the DNA-binding domain of paired box gene 3 (PAX3) on chromosome 2 to the transactivation domain of forkhead box protein O1 (FOXO1) on chromosome 13 (van Erp et al., 2018). The PAX-FOXO1 fusion protein is an aberrant oncogenic transcription factor, resulting in downstream transcription and translation of proteins involved in oncogenic transformation (Chen et al., 2019).
The PAX-FOXO1 transcription factor, which is both upstream to many of the signal cascades that promote RMS tumorigenesis, is a direct and promising target, although inhibitors that bind to PAX-FOXO1 with good specificity and affinity have yet to be designed. Another way to suppress PAX-FOXO1 transcription factor activity is through epigenetic targets, with therapy aimed at inhibiting the co-regulators and chromatin-remodeling complexes involved in transcription. Small molecule inhibitors such as JQ1 that target the BET bromodomain-containing protein (BRD4), an epigenetic reader that mediates transcription, can disrupt BRD4 and PAX3-FOXO1 interaction, leading to degradation of PAX3-FOXO1 and reduced transcription of the oncogenic fusion protein (Gryder et al., 2017). Histone deacetylase (HDAC) inhibitors such as entinostat, panobinostat, and vorinostat have also been shown to delay tumor growth in xenograft RMS models (Hedrick et al., 2015).
Receptor tyrosine kinases (RTKs), such as IGFR1, VEGFR, EGFR, FGFR4, and PDGFRα become constitutively activated and cause downstream tumorigenic effects in the presence of PAX-FOXO1 gene fusions and have also been identified as potential targets in RMS. Recently completed and currently ongoing clinical trials that target these RTKS are summarized in Table 3.
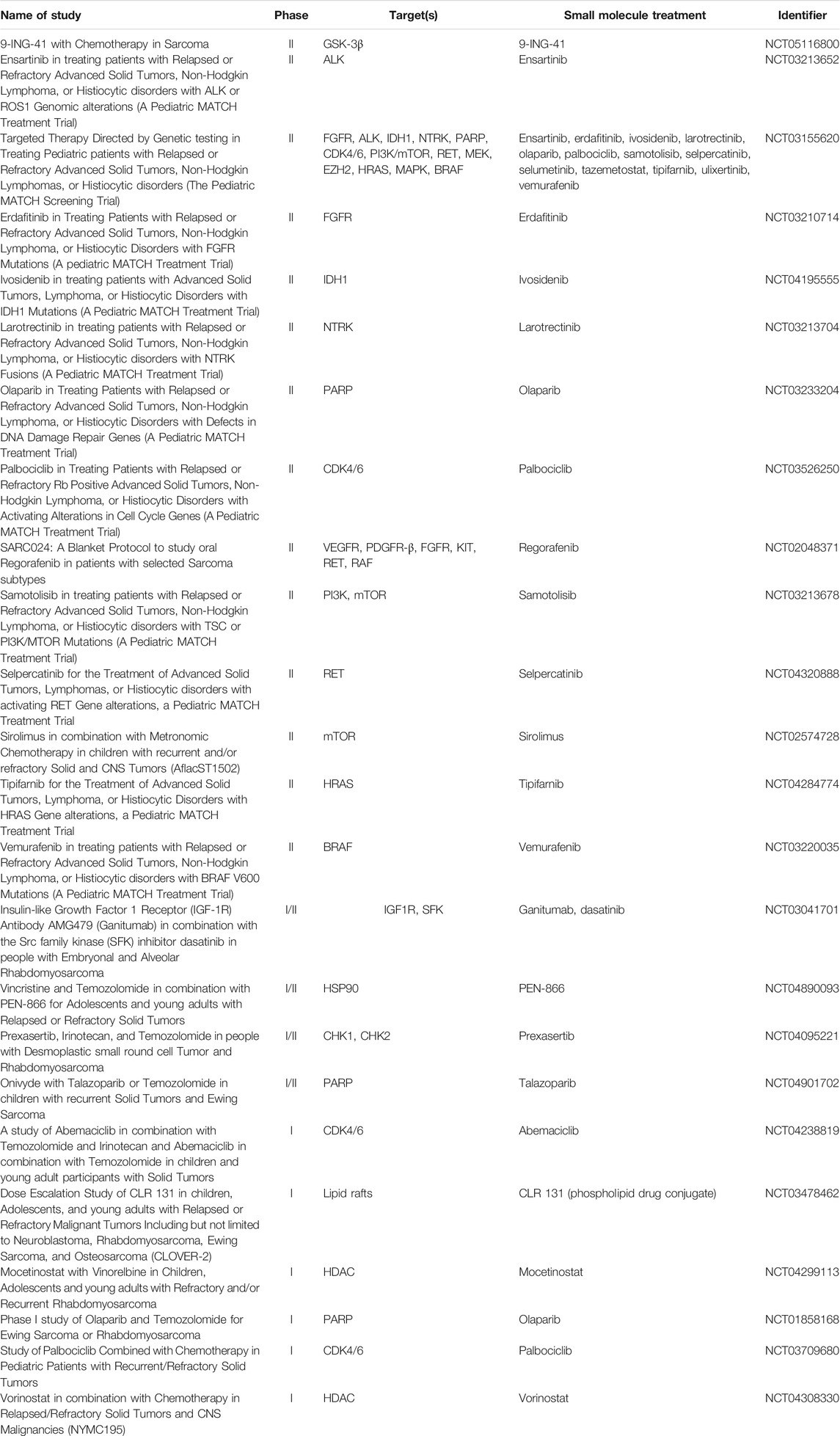
TABLE 3. Small Molecule Clinical Trials that are Recruiting or Active for Pediatric and Adolescent Rhabdomyosarcoma Patients (All Data from ClinicalTrials.gov).
A phase II clinical trial compared the IGF-1R inhibitor cixutumumab to conventional multi-agent chemotherapy in pediatric and adult patients with metastatic alveolar and embryonal RMS. Event-free survival (EFS) at 18 months was initially higher in patients receiving cixutumumab treatment (68%) compared to combinational chemotherapy (39%), but at 3-year follow-up, cixutumumab therapy had an event-free survival rate of 16% (Malempati et al., 2015; van Erp et al., 2018; Malempati et al., 2019). A phase II trial of the IGF-1R inhibitor ganitumab in combination with the Src Family Kinase (SFK) inhibitor dasatinib is ongoing in patients with relapsed or refractory RMS (clinicaltrials.gov, NCT03041701).
In a phase II clinical trial comparing conventional chemotherapy against an experimental arm adding the VEGFR inhibitor bevacizumab to cytotoxic chemotherapy in pediatric and adolescent patients with metastatic RMS and soft-tissue sarcomas, median EFS was higher in patients treated with bevacizumab (20.6 months, compared to 14.9 months for chemotherapy only). Higher objective response with bevacizumab was also observed at 54%, compared to 36% in the chemotherapy cohort, but findings were not statistically significant (Chisholm et al., 2017). In another phase I clinical trial evaluating the efficacy of bevacizumab with sorafenib and low-dose cyclophosphamide, 1 of 2 patients with refractory/recurrent RMS experienced a partial response (Navid et al., 2013).
EGFR is over-expressed in about 15–20% of ARMS tumors. Although no in vivo experiments targeting EGFR have been conducted yet, in vitro experiments in ARMS cell lines found that combination therapy of the anti-EGFR antibody cetuximab and the standard chemotherapy dactinomycin had synergistic effects in inhibiting cell growth and inducing apoptosis, with greater anticancer toxicity than dactinomycin alone (Yamamoto et al., 2013).
PAX3-FOXO1 also amplifies FGFR4 expression in ARMS, and FGFR4 has been implicated in resistance to apoptosis in tumors treated with therapeutics targeting the IGF1R-PI3K-mTOR pathway. However, the FGFR4 inhibitor BGJ398 showed synergy with IGF-1R inhibitor AEW54 in an ARMS cell line, suggesting that FGFR4 may be a promising target in treatment resistance prevention (Wachtel et al., 2014). Relapsed ARMS has also been shown to be responsive to pazopanib, a multi-kinase inhibitor currently in use for soft tissue sarcomas (Heske and Mascarenhas, 2021).
In the Children’s Oncology Group phase II clinical trial ARST0921, treatment of first-relapse RMS patients with a vinorelbine and cyclophosphamide chemotherapy backbone in combination with either the mTOR inhibitor temsirolimus or the VEGFR inhibitor bevacizumab resulted in 6-month event-free survival rates of 69 and 55%, respectively, with a greater proportion of partial responses in patients treated with temsirolimus (Heske and Mascarenhas, 2021). This data was promising enough to lead to further investigation of temsirolimus in the upfront setting for patients with newly diagnosed intermediate-risk RMS. This randomized phase III trial compares conventional cytotoxic chemotherapy with VAC/VI (alternating vincristine, dactinomycin, cyclophosphamide, and vincristine, irinotecan), with the addition of temsirolimus to the conventional VAC/VI chemotherapy backbone (Heske and Mascarenhas, 2021).
Developmental pathways may also provide therapeutic targets in RMS. GLI transcription factor activation through the constitutively active Hedgehog signaling pathway in RMS is involved in tumorigenesis. ERMS and ARMS xenograft models treated with GLI1/2 inhibitor GANT-61 combined with either temsirolimus or vincristine showed inhibition of proliferation via cell cycle arrest at the G0/G1 phase and significant reductions in tumor growth (Srivastava et al., 2014). Further research is needed to establish the potential of GLI inhibitors in RMS treatment.
Therapeutic targets may also lie in the apoptosis pathway. The Bcl-2 family of apoptotic proteins is involved in cancer cell survival and proliferation, and combination of Bcl-2 inhibitor ABT-737 and mTOR inhibitor AZD8055 was highly synergistic in inducing caspase-dependent apoptosis in ARMS and ERMS cells (Preuss et al., 2013).
Numerous targets for RMS are being investigated, yet there are currently no clinically impactful novel agents for the treatment of RMS. Inhibitors of the PAX-FOXO1 fusion protein have yet to be developed as well, despite the discovery of this oncogenic driver since 1993 (Galili et al., 1993). As prognosis for recurrent and metastatic RMS remains poor, this is an area of research that greatly needs further investigation and analysis of both in vivo and in vitro studies and clinical trials. The targeted therapies discussed above for RMS are summarized in Figure 2.
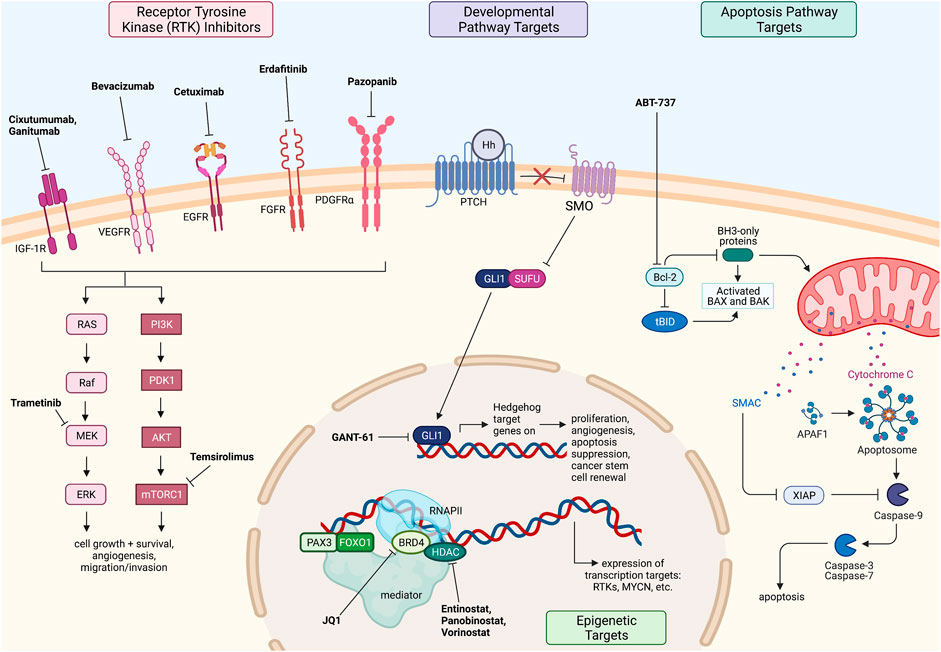
FIGURE 2. Fusion driven pediatric solid tumors with clinically actionable targets. Rhabdomyosarcoma therapeutic targets and drugs under investigation. All figures were created with BioRender.com.
NTRK-Fused Infantile Fibrosarcoma
Infantile fibrosarcoma (IFS) is a soft tissue sarcoma that presents as a localized, but large and rapidly growing tumor in the neonatal setting. IFS accounts for 24.5% of all soft tissue sarcomas found in children under the age of one. IFS has a very good overall prognosis, with studies demonstrating a 5-year survival rate of approximately 90%.
The optimal form of treatment for IFS is surgical resection, but wide local excision often results in mutilating surgery. In particular cases, neoadjuvant chemotherapy may be used to improve the probability of achieving a complete surgical resection; radiotherapy is used when surgical resection is deemed impossible (Parida et al., 2013). Thus there is a need for novel therapies in those IFS patients with extensive or unresectable disease, as well as those with metastatic IFS.
Nearly all IFS tumors harbor a neurotrophic tyrosine receptor kinase (NTRK) fusion, with 70% of IFS cases containing the ETS Variant Transcription Factor 6 (ETV6)-NTRK3 gene rearrangement (Knezevich et al., 1998). In NTRK rearrangements, the 5’ fusion partner induces ligand-independent constitutive activation of the tropomyosin receptor kinase (TRK). This leads to uninterrupted downstream signaling of the RAS/RAF/MEK/ERK and PI3K/AKT pathways, which promote cancer cell survival, invasion, and proliferation (Amatu et al., 2019).
Larotrectinib is a TRKA, TRKB, and TRKC inhibitor that prevents neurotrophin-TRK interaction and activation, inducing apoptosis and inhibition of tumor growth. Larotrectinib has been approved by the FDA for use in children and adults with NTRK-fusion-positive tumors that are metastatic and/or will result in severe morbidity upon surgical removal and do not have any resistance mutations (Dunn, 2020).
Various clinical trials have shown efficacy of larotrectinib against ETV6-NTRK3 fusion-positive IFS with negligible toxicity. In a phase I clinical trial, a 16-month-old female patient with refractory IFS was treated with larotrectinib. One month later, MRI scans revealed a reduction in tumor size by over 90%, and continued clinical response was also observed in later cycles of therapy (Nagasubramanian et al., 2016). Multiple phase 1 studies and case reports show reduction in tumor size, facilitating complete surgical resection, and remarkable reduction in size in other cases without surgical intervention (Caldwell et al., 2020).
Larotrectinib can also be used as a pre-surgical therapy to reduce tumor size, preventing radical and disfiguring surgeries. Larotrectinib treatment resulted in rapid and durable tumor regression and allowed for limb-sparing surgeries in patients who otherwise would have undergone amputations, confirming larotrectinib as a promising therapy for ETV6-NTRK3 fusion-positive IFS (DuBois et al., 2018). In a multi-center, phase I clinical study enrolling eight NTRK-fusion-positive IFS patients between 1 month and 21 years of age, larotrectinib was well-tolerated and demonstrated antitumor activity in all eight patients. Four patients avoided disfiguring surgery and instead underwent R0 (negative resection margins with no tumor at marked resection region) and R1 (microscopic residual tumor at resection margin) surgical resection following larotrectinib treatment, indicating that larotrectinib may represent a new standard of care in controlling disease without morbid surgery (Laetsch et al., 2018).
Another gene fusion identified in IFS is the non-classical LMNA-NTRK1 fusion. Crizotinib, an ALK inhibitor, has been shown to be effective in treating these tumors. Case reports show patients with refractory and metastatic IFS on crizotinib therapy had responses ranging from stable disease to complete and durable responses (Wong et al., 2016; Bender et al., 2019). The targeted therapies discussed above for IFS are summarized in Figure 3.
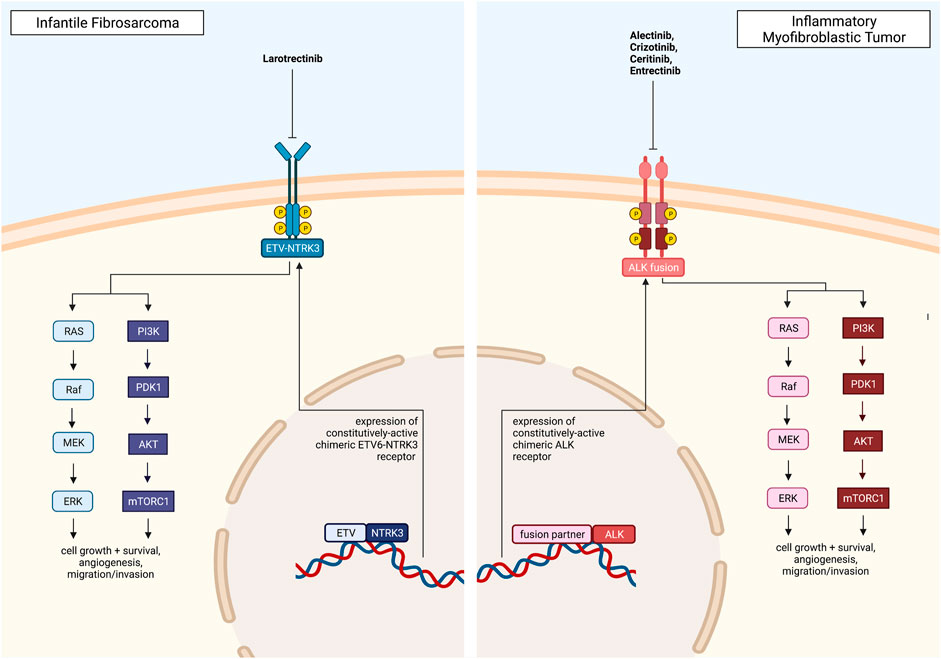
FIGURE 3. Pediatric solid tumors with a direct, targetable fusion kinase, including Infantile Fibrosarcoma and Inflammatory Myofibroblastic Tumor, and clinically approved drugs. All figures were created with BioRender.com.
Alveolar Soft Part Sarcoma
Alveolar soft part sarcoma (ASPS) is a rare, slow-growing but highly-angiogenic soft tissue sarcoma that often remains undetected until metastasis. ASPS is characterized by the TFE3-ASPSCR1 fusion gene, which induces overexpression of the MET receptor tyrosine kinase. This leads to downstream activation of MEK1/2 and AKT, which promote tumor cell proliferation and angiogenesis (Mitton and Federman, 2012).
The 5-year survival rates of localized and metastasized ASPS are 80% and 10–40%, respectively. The main form of treatment for ASPS is surgical removal; radiation therapy may also be used to prevent regrowth following surgery. For unresectable tumors, however, ASPS is resistant to conventional chemotherapies, indicating the need for novel therapeutic targets such as receptor tyrosine kinase inhibitors and other anti-angiogenic agents in the treatment of ASPS (Mitton and Federman, 2012).
Tivantinib (ARQ 197), a selective inhibitor of MET receptor tyrosine kinase, has been investigated in a Phase II clinical trial involving 27 patients ≥13 years with metastatic and/or surgically unresectable ASPS. After 10 cycles of tivantinib, 78% of ASPS patients had stable disease for at least 4 months, and median PFS was 6 months (Wagner et al., 2012).
Sunitinib, a PDGFR and VEGFR tyrosine kinase inhibitor that reduces tumor angiogenesis and triggers cancer cell apoptosis, has demonstrated efficacy in controlling progressive metastatic ASPS in adults. In a case series of 10 adult patients, with a median age of 24, treated with daily sunitinib, treatment was well-tolerated and induced long-lasting responses. Partial response and stable disease were confirmed in 9 cases after 6 months, and median PFS was 17 months (Stacchiotti et al., 2011). A further case study reporting a patient with refractory metastatic ASPS treated with sunitinib described complete primary tumor regression and stabilization of the metastases (Ghose et al., 2012). These anecdotal reports of sunitinib efficacy in adult ASPS tumors suggest a potential therapeutic role of sunitinib in pediatric ASPS patients as well.
Cediranib, a potent inhibitor of VEGFR tyrosine kinase, has also been evaluated in treating adult ASPS. In a phase II clinical trial involving 46 patients, with a median age of 27, with unresectable and metastatic ASPS, 35% had partial response and 60% had stable disease after 24 weeks of daily cediranib treatment (Kummar et al., 2013).
Inflammatory Myofibroblastic Tumor
Inflammatory myofibroblastic tumors (IMT) are rare soft tissue neoplasms made up of myofibroblastic spindle cells, typically characterized by benign local recurrence and rare metastasis. The standard treatment for IMT is complete surgical resection, which has favorable prognosis with most patients surviving past 10 years after complete tumor removal. However, therapy remains lacking for malignant unresectable IMT, especially since chemotherapy and radiation are not particularly effective (Maruyama et al., 2017).
About half of all IMTs are anaplastic lymphoma kinase (ALK) fusion-positive, consisting of cells that express an ALK gene rearranged with over 30 different identified 5’ fusion partners (Coffin et al., 2001). Rearrangement of ALK over-activates ALK receptor tyrosine kinase, which leads to downstream activation of the PI3K/AKT, JAK/STAT, and Ras/ERK pathways, increasing cancer cell proliferation and survival.
Crizotinib, a receptor tyrosine kinase inhibitor, inhibits ALK phosphorylation and its downstream signaling pathways, leading to G1/S cell cycle arrest and apoptosis. Crizotinib is currently used to treat ALK-rearranged cancers, such as anaplastic large-cell lymphoma (ALCL) and non-small cell lung cancer (NSCLC), and crizotinib has demonstrated efficacy in treating ALK-positive IMT as well.
In a 2017 study involving 14 unresectable, ALK-positive IMT pediatric patients who were given two doses of oral crizotinib daily, complete and partial responses were observed in five and seven patients, respectively (Mossé et al., 2017). In a cohort of eight ALK-positive IMT patients diagnosed with IMT between 2009-2016 and treated with crizotinib, four achieved a complete response, 3 a partial response, and one patient had stable disease. When preoperative crizotinib was used to decrease tumor size prior to surgical resection, 2 of the 3 partial responses achieved complete response. Furthermore, seven of eight patients were still alive at long-term follow-up, with no evidence of disease in six patients. Crizotinib was also found to be well-tolerated. As such, crizotinib combined with surgical resection appears to be effective in long-term disease control of ALK-positive IMT (Trahair et al., 2019).
For patients with RANBP2-ALK fusion positive epithelioid inflammatory myofibroblastic sarcoma (eIMS), a malignant variant of ALK-fusion-positive IMT, CD30 and ALK combination therapy may have high therapeutic potency. RANBP2-ALK eIMS xenografts treated with brentuximab-vedotin, targeting CD30 + tumor cells, and crizotinib resulted in tumor shrinkage and prolonged disease-free survival. In the diagnosis eIMS model, the majority of mice were confirmed as tumor-free 180 days past the study end date. However, disease recurrence was observed in all mice in the relapsed eIMS model, indicating that CD30 and ALK combination therapy may be most effective as early treatment for eIMS (Fordham et al., 2020).
Ceritinib, another ALK receptor tyrosine kinase inhibitor, has demonstrated efficacy against crizotinib-resistant tumors, with stronger potency in both crizotinib-naïve and crizotinib-refractory patients. Ceritinib is currently approved for use in ALK-positive NSCLC, but it has also shown excellent disease control in various ALK-positive IMT case studies (Santarpia et al., 2017). Recent case reports also show response in stage IV IMT or unresectable IMTs with ceritinib therapy and tumor response, allowing for surgical resection or complete response with ceritinib therapy alone (Mittal et al., 2021).
Alectinib is another ALK-inhibitor that has demonstrated efficacy against ALK-fusion-positive IMT. A case study in which a 26-year-old male with hyper-progressive ALK-fusion-positive IMT experienced a significant and durable response to treatment with alectinib was reported in 2017 (Saiki et al., 2017).
Entrectinib is an inhibitor of TRK, ROS1, and ALK, and has been shown to induce rapid and durable anti-cancer responses in NTRK, ROS1, and ALK-fusion tumors. A 16-year-old female patient with unresectable DCTN1-ALK-fusion-positive IMT had a complete response to entrectinib therapy, which controlled disease with low toxicity (Ambati et al., 2018).
Other gene translocations observed in IMT include proto-oncogene tyrosine-protein kinase (ROS1) fusions, which lead to tumorigenesis in mechanisms similar to ALK fusions. In a case study reported in 2021, the ALK- and ROS1-inhibitor lorlatinib was reported successful in treating a patient with refractory TFG-ROS1 fusion-positive IMT (Carcamo et al., 2021).
Fibrolamellar Hepatocellular Carcinoma
Fibrolamellar hepatocellular carcinoma (FL-HCC) is a rare form of hepatocellular carcinoma (HCC), affecting adolescents and young adults with no prior history of liver disease or risk factors for live cancer. In FL-HCC, a ∼400 kilobase deletion on chromosome 19 gives rise to the DnaJ Heat Shock Protein Family (HSP40) Member B1 (DNAJB1)-Protein Kinase CAMP-Activated Catalytic Subunit Alpha (PRKACA) fusion gene, and the corresponding DNAJB1-PRKACA-fusion protein has upregulated protein kinase activity to promote tumorigenesis (Honeyman et al., 2014).
Current treatments for FL-HCC remain in development. Chemotherapy for unresectable hepatocellular carcinoma (HCC) is a combination of atezolizumab and bevacizumab, but this therapy showed no clinical benefits in case studies in two patients with advanced FL-HCC (Al Zahrani and Alfakeeh, 2021). Sorafenib is another multi-tyrosine kinase inhibitor in use against advanced HCC; however, sorafenib has limited efficacy against FL-HCC, with only delayed progression of disease as the best response (Ang et al., 2013).
The only potentially curative treatment for FL-HCC is liver resection or liver transplantation; if the tumor is not completely removed, likelihood of recurrence is high. For patients with metastatic and/or unresectable disease, there are no effective treatments available and FL-HCC is progressive and fatal; median survival time is less than 12 months (Kassahun, 2016).
However, novel combination and targeted therapies are being investigated in the treatment of FL-HCC. In a phase II trial of continuous IV fluorouracil and thrice weekly recombinant interferon alfa-2b administered to eight FL-HCC patients, one patient had complete response while four had partial responses, and overall median survival was 23.1 months (Patt et al., 2003). In a case study involving a 27-year-old woman with FL-HCC, 10 cycles of gemcitabine and oxaliplatin were administered, and 5 years after the regimen had been completed, the woman remained in complete remission (Gras et al., 2012).
The PRKACA kinase domain was also found to play an essential role in FL-HCC tumor formation, suggesting the potential for small molecule PRKACA-inhibitors as a therapy for FL-HCC (Kastenhuber et al., 2017). However, further research is needed to investigate the efficacy and viability of PRKACA inhibitors against FL-HCC.
Renal Cell Carcinoma
Pediatric translocation renal cell carcinoma (tRCC) is a rare kidney cancer found in children, accounting for 2–5% of all childhood renal neoplasms. Less-advanced tRCC tumors can be treated via surgical resection, whereas more-advanced tRCC is treated with targeted therapies.
The most common mutation associated with RCC is the TFE3-fusion gene, where the TFE3 gene on chromosome Xp11.12 is rearranged with a fusion partner (i.e., PRCC, ASPSCR1, NONO, CLTC, SFPQ, etc.) from a different chromosome. The TFE3-fusion gene is a constitutively active promoter, causing dysregulated transcriptional TFE3 activity that leads to tumorigenesis through downstream upregulation of the PI3K/AKT/mTOR signaling pathway (Damayanti et al., 2018).
Cabozantinib is a small-molecule VEGFR2 and MET tyrosine kinase inhibitor that has already been approved for use in medullary thyroid cancer and renal cell carcinoma. For tRCC tumors that express MET, cabozantinib has been shown to regress disease with tolerable toxicity. In a 16-year-old female patient presenting with refractory, locally recurrent TFE3-fusion-positive tRCC with lung metastases, treatment with cabozantinib led to prompt and durable disease control. The patient was also noted to have reduction in pain, weight gain of 90% of weight previously lost on chemotherapy, and improved quality of life. In a 12-year-old male patient with stage IV TFE3-fusion-positive tRCC, treatment with cabozantinib demonstrated excellent disease control, including reduced pain just after 3 months and halted disease progression even 18 months later (Wedekind et al., 2017).
VEGFR tyrosine kinase and mTOR inhibitors, which are used in metastatic RCC therapies, have also demonstrated efficacy in disease control of tRCC. In a case study of 53 patients with tRCC, 21 patients who were TFE3-fusion-positive received targeted therapy. Fourteen patients treated with sunitinib had a median PFS of 8.2 months, with seven patients achieving either a partial or complete response. Eight patients treated with sorafenib had a median PFS of more than 6 months. Two pediatric patients had partial responses with single agent therapy of either sunitinib or sorafenib, and both patients continued with stable disease after 29 months. For patients that experienced disease progression while being treated with VEGFR inhibitors, switching to mTOR inhibitors temsirolimus and everolimus resulted in stable disease (Malouf et al., 2010).
Synovial Sarcoma
Synovial sarcoma (SS) is a rare soft tissue sarcoma that occurs in adolescents and young adults, with a pathognomonic t (X; 18) (p11.2; q11.2) chromosomal translocation, fusing the SS18 (formerly known as SYT) gene with the SSX1, SSX2, or SSX4 gene (Clark et al., 1994; Shipley et al., 1994). Therapeutic approaches involve systemic chemotherapy with doxorubicin and ifosfamide combined with radiation therapy and/or surgery for local control.
The SS18-SSX fusion protein acts as an epigenetic modifier, driving tumorigenesis in SS cells (Kadoch and Crabtree, 2013). SWItch Sucrose Non-Fermentable (SWI/SNF) chromatin remodeling complexes, containing the BAF (BRG1 or BRM associated factors) complex, are disrupted in SS cells when the SS18-SSX fusion protein competitively replaces the wild-type SS18 in the BAF complex (Kadoch and Crabtree, 2013). The fusion SS18-SSX protein is found to co-localize with Polycomb Repressive Complexes 1 and 2 (PRC1, PRC2) (Soulez et al., 1999; Lubieniecka et al., 2008). PRC2 silences chromatin with its catalytic subunit, the histone methyltransferase Enhancer of Zeste 2 (EZH2) (Wilson et al., 2010). The relocalization of oncogenic BAF complexes to PRC repressed domains, recruitment of RNA Polymerase II, and initiation of transcription leads to epigenetic reprogramming and drives tumorigenesis (McBride et al., 2018).
Preclinical studies using EZH2 inhibitors in SS cell lines were promising, showing a decrease in cellular proliferation and migration in vitro, and with decreased tumor burden in xenograft models (Kawano et al., 2016; Shen et al., 2016). However, in a phase II clinical trial in adult patients with synovial sarcoma, the selective EZH2 inhibitor tazemetostat exhibited less promising preliminary results. Tazemetostat was well tolerated by patients with few adverse events, but the best response observed was stable disease, which occurred in 33% of patients (Schoffski P et al., 2017).
Other Novel Approaches Such as Immunotherapy and ONC201/TIC10
Various strategies have been employed to promote the immune response against pediatric solid tumors. These include oncolytic virus-based therapy, antibody-dependent cellular cytotoxicity (ADCC), bispecific antibodies, immune checkpoint inhibitors, tumor microenvironment targeted therapies (cancer-associated fibroblasts, macrophages), cytokines, growth factors and CAR-T cells (Marayati et al., 2019).
TRAIL-Inducing Compound #10 (TIC10)/ONC201 is a novel agent that activates a potent innate immune pro-apoptotic anti-cancer response through the integrated stress response (Allen et al., 2013; Kline et al., 2016; Wagner et al., 2018; Prabhu et al., 2020). ONC201 has potential for further development in pediatric solid tumors including in combination with epigenetic modulators (Chang et al., 2020; Chang et al., 2021; Honeyman et al., 2021; Zhang et al., 2021).
Conclusion
Pediatric non-CNS solid tumors are a diverse group of tumors that are best managed with a multi-disciplinary approach. Combinations of chemotherapy, surgery and radiation have improved patient outcomes (Ward et al., 2019b), but these approaches have changed little in the previous 2 decades. In addition, patients with unresectable, metastatic, and recurrent disease typically face dismal outcomes with current multimodal therapy. With the ability to analyze the genomes of individual patients and identify molecular drivers specific to an individual cancer, opportunities for clinical trials, such as the Pediatric MATCH trial run by the Children’s Oncology group, are now available. Currently, there exists promising novel therapies in preclinical investigation as well as ongoing clinical trials. Investigation continues in these targeted therapies, their toxicities, and administration in the pediatric population. Novel therapies, including small molecule agents, targeted therapies, and other precision medicine-based treatments, can offer an opportunity to examine unique mechanisms in treating pediatric sarcomas and other solid tumors.
Preclinical strategies to target gene fusions include both the utilization of existing inhibitors as well as the development of novel drugs through rational design approaches. The development of novel therapies requires a significant development time and multidisciplinary effort that includes expertise in genomics, molecular biology, pharmacology, clinical trials, and clinical oncology. Using these resources more effectively will not only help drive the development of novel therapies, but also better inform individual patient treatment decisions. As our understanding of the molecular drivers of pediatric fusion-positive cancers increases, so do our abilities to tailor therapies toward better outcomes for this patient population.
Data Availability Statement
The original contributions presented in the study are included in the article/Supplementary Material, further inquiries can be directed to the corresponding authors.
Author Contributions
All authors listed have made a substantial, direct, and intellectual contribution to the work and approved it for publication.
Funding
This work was supported by a grant from the Rhode Island Foundation (W-IC) and an NIH grant (CA173453) to WE-D. WE-D is an American Cancer Society Research Professor and is supported by the Mencoff Family University Professorship at Brown University.
Conflict of Interest
WE-D is a co-founder of Oncoceutics, Inc., a subsidiary of Chimerix. WE-D has disclosed his relationship with Oncoceutics and potential conflict of interest to his academic institution/employer and is fully compliant with NIH and institutional policy that is managing this potential conflict of interest.
The remaining authors declare that the research was conducted in the absence of any commercial or financial relationships that could be construed as a potential conflict of interest
Publisher’s Note
All claims expressed in this article are solely those of the authors and do not necessarily represent those of their affiliated organizations, or those of the publisher, the editors and the reviewers. Any product that may be evaluated in this article, or claim that may be made by its manufacturer, is not guaranteed or endorsed by the publisher.
Acknowledgments
All figures were created with BioRender.com.
References
Adzhubei, I. A., Schmidt, S., Peshkin, L., Ramensky, V. E., Gerasimova, A., Bork, P., et al. (2010). A Method and Server for Predicting Damaging Missense Mutations. Nat. Methods 7 (4), 248–249. doi:10.1038/nmeth0410-248
Akgul, M., Williamson, S. R., Ertoy, D., Argani, P., Gupta, S., Caliò, A., et al. (2021). Diagnostic Approach in TFE3-Rearranged Renal Cell Carcinoma: a Multi-Institutional International Survey. J. Clin. Pathol. 74 (5), 291–299. doi:10.1136/jclinpath-2020-207372
Al Zahrani, A., and Alfakeeh, A. (2021). Fibrolamellar Hepatocellular Carcinoma Treated with Atezolizumab and Bevacizumab: Two Case Reports. J. Med. Case Rep. 15 (1), 132. doi:10.1186/s13256-021-02695-8
Allen, J. E., Krigsfeld, G., Mayes, P. A., Patel, L., Dicker, D. T., Patel, A. S., et al. (2013). Dual Inactivation of Akt and ERK by TIC10 Signals Foxo3a Nuclear Translocation, TRAIL Gene Induction, and Potent Antitumor Effects. Sci. Transl Med. 5 (171), 171ra17. doi:10.1126/scitranslmed.3004828
Amatu, A., Sartore-Bianchi, A., Bencardino, K., Pizzutilo, E. G., Tosi, F., and Siena, S. (2019). Tropomyosin Receptor Kinase (TRK) Biology and the Role of NTRK Gene Fusions in Cancer. Ann. Oncol. 30 (Suppl. l_8), viii5–viii15. doi:10.1093/annonc/mdz383
Ambati, S. R., Slotkin, E. K., Chow-Maneval, E., and Basu, E. M. (2018). Entrectinib in Two Pediatric Patients with Inflammatory Myofibroblastic Tumors Harboring ROS1 or ALK Gene Fusions. JCO Precis Oncol. 2018, 1–6. doi:10.1200/PO.18.00095
Ang, C. S., Kelley, R. K., Choti, M. A., Cosgrove, D. P., Chou, J. F., Klimstra, D., et al. (2013). Clinicopathologic Characteristics and Survival Outcomes of Patients with Fibrolamellar Carcinoma: Data from the Fibrolamellar Carcinoma Consortium. Gastrointest. Cancer Res. 6 (1), 3–9.
Antonescu, C. R., Tschernyavsky, S. J., Woodruff, J. M., Jungbluth, A. A., Brennan, M. F., and Ladanyi, M. (2002). Molecular Diagnosis of clear Cell Sarcoma: Detection of EWS-ATF1 and MITF-M Transcripts and Histopathological and Ultrastructural Analysis of 12 Cases. J. Mol. Diagn. 4 (1), 44–52. doi:10.1016/S1525-1578(10)60679-4
Arnold, M. A., and Barr, F. G. (2017). Molecular Diagnostics in the Management of Rhabdomyosarcoma. Expert Rev. Mol. Diagn. 17 (2), 189–194. doi:10.1080/14737159.2017.1275965
Aulmann, S., Longerich, T., Schirmacher, P., Mechtersheimer, G., and Penzel, R. (2007). Detection of the ASPSCR1-TFE3 Gene Fusion in Paraffin-Embedded Alveolar Soft Part Sarcomas. Histopathology 50 (7), 881–886. doi:10.1111/j.1365-2559.2007.02693.x
Bender, J., Anderson, B., Bloom, D. A., Rabah, R., McDougall, R., Vats, P., et al. (2019). Refractory and Metastatic Infantile Fibrosarcoma Harboring LMNA-NTRK1 Fusion Shows Complete and Durable Response to Crizotinib. Cold Spring Harb Mol. Case Stud. 5 (1). doi:10.1101/mcs.a003376
Bourgeois, J. M., Knezevich, S. R., Mathers, J. A., and Sorensen, P. H. (2000). Molecular Detection of the ETV6-NTRK3 Gene Fusion Differentiates Congenital Fibrosarcoma from Other Childhood Spindle Cell Tumors. Am. J. Surg. Pathol. 24 (7), 937–946. doi:10.1097/00000478-200007000-00005
Brohl, A. S., Patidar, R., Turner, C. E., Wen, X., Song, Y. K., Wei, J. S., et al. (2017). Frequent Inactivating Germline Mutations in DNA Repair Genes in Patients with Ewing Sarcoma. Genet. Med. 19 (8), 955–958. doi:10.1038/gim.2016.206
Brohl, A. S., Solomon, D. A., Chang, W., Wang, J., Song, Y., Sindiri, S., et al. (2014). The Genomic Landscape of the Ewing Sarcoma Family of Tumors Reveals Recurrent STAG2 Mutation. Plos Genet. 10 (7), e1004475. doi:10.1371/journal.pgen.1004475
Caldwell, K. J., De La Cuesta, E., Morin, C., Pappo, A., and Helmig, S. (2020). A Newborn with a Large NTRK Fusion Positive Infantile Fibrosarcoma Successfully Treated with Larotrectinib. Pediatr. Blood Cancer 67 (9), e28330. doi:10.1002/pbc.28330
Carcamo, B., Bista, R., Wilson, H., Reddy, P., and Pacheco, J. (2021). Rapid Response to Lorlatinib in a Patient with TFG-ROS1 Fusion Positive Inflammatory Myofibroblastic Tumor of the Chest Wall Metastatic to the Brain and Refractory to First and Second Generation ROS1 Inhibitors. J. Pediatr. Hematol. Oncol. 43 (5), e718–e722. doi:10.1097/MPH.0000000000002185
Chang, F., Lin, F., Cao, K., Surrey, L. F., Aplenc, R., Bagatell, R., et al. (2019). Development and Clinical Validation of a Large Fusion Gene Panel for Pediatric Cancers. J. Mol. Diagn. 21 (5), 873–883. doi:10.1016/j.jmoldx.2019.05.006
Chang, W.-i., Zhou, L., Seyhan, A. A., Prabhu, V. V., and El-Deiry, W. S. (2021). Abstract 1060: Combinatorial Therapy of Imipridones and Histone Deacetylase Inhibitors in Ewing Sarcoma Cell Lines Demonstrates Synergistic Cell Death. Cancer Res. 81 (13 Suppl. ment), 1060. doi:10.1158/1538-7445.am2021-1060
Chang, W.-I., Zhou, L., Seyhan, A. A., Zhang, Y., and El-Deiry, W. S. (2020). Abstract 3902: Novel Therapeutic Targeting of Epigenetic Aberrations in Pediatric Sarcomas through Combination of ONC201 and HDAC Inhibitors. Cancer Res. 80 (16 Suppl. ment), 3902. doi:10.1158/1538-7445.am2020-3902
Chen, C., Dorado Garcia, H., Scheer, M., and Henssen, A. G. (2019). Current and Future Treatment Strategies for Rhabdomyosarcoma. Front. Oncol. 9, 1458. doi:10.3389/fonc.2019.01458
Chisholm, J. C., Merks, J. H. M., Casanova, M., Bisogno, G., Orbach, D., Gentet, J. C., et al. (2017). Open-label, Multicentre, Randomised, Phase II Study of the EpSSG and the ITCC Evaluating the Addition of Bevacizumab to Chemotherapy in Childhood and Adolescent Patients with Metastatic Soft Tissue Sarcoma (The BERNIE Study). Eur. J. Cancer 83, 177–184. doi:10.1016/j.ejca.2017.06.015
Choy, E., Butrynski, J. E., Harmon, D. C., Morgan, J. A., George, S., Wagner, A. J., et al. (2014). Phase II Study of Olaparib in Patients with Refractory Ewing Sarcoma Following Failure of Standard Chemotherapy. BMC Cancer 14, 813. doi:10.1186/1471-2407-14-813
Clark, J., Rocques, P. J., Crew, A. J., Gill, S., Shipley, J., Chan, A. M., et al. (1994). Identification of Novel Genes, SYT and SSX, Involved in the t(X;18)(p11.2;q11.2) Translocation Found in Human Synovial Sarcoma. Nat. Genet. 7 (4), 502–508. doi:10.1038/ng0894-502
Coffin, C. M., Patel, A., Perkins, S., Elenitoba-Johnson, K. S., Perlman, E., and Griffin, C. A. (2001). ALK1 and P80 Expression and Chromosomal Rearrangements Involving 2p23 in Inflammatory Myofibroblastic Tumor. Mod. Pathol. 14 (6), 569–576. doi:10.1038/modpathol.3880352
Cook, J. R., Dehner, L. P., Collins, M. H., Ma, Z., Morris, S. W., Coffin, C. M., et al. (2001). Anaplastic Lymphoma Kinase (ALK) Expression in the Inflammatory Myofibroblastic Tumor: a Comparative Immunohistochemical Study. Am. J. Surg. Pathol. 25 (11), 1364–1371. doi:10.1097/00000478-200111000-00003
Damayanti, N. P., Budka, J. A., Khella, H. W. Z., Ferris, M. W., Ku, S. Y., Kauffman, E., et al. (2018). Therapeutic Targeting of TFE3/IRS-1/PI3K/mTOR Axis in Translocation Renal Cell Carcinoma. Clin. Cancer Res. 24 (23), 5977–5989. doi:10.1158/1078-0432.CCR-18-0269
de Alava, E., Panizo, A., Antonescu, C. R., Huvos, A. G., Pardo-Mindán, F. J., Barr, F. G., et al. (2000). Association of EWS-FLI1 Type 1 Fusion with Lower Proliferative Rate in Ewing's Sarcoma. Am. J. Pathol. 156 (3), 849–855. doi:10.1016/S0002-9440(10)64953-X
Dowless, M., Lowery, C. D., Shackleford, T., Renschler, M., Stephens, J., Flack, R., et al. (2018). Abemaciclib Is Active in Preclinical Models of Ewing Sarcoma via Multipronged Regulation of Cell Cycle, DNA Methylation, and Interferon Pathway Signaling. Clin. Cancer Res. 24 (23), 6028–6039. doi:10.1158/1078-0432.CCR-18-1256
DuBois, S. G., Laetsch, T. W., Federman, N., Turpin, B. K., Albert, C. M., Nagasubramanian, R., et al. (2018). The Use of Neoadjuvant Larotrectinib in the Management of Children with Locally Advanced TRK Fusion Sarcomas. Cancer 124 (21), 4241–4247. doi:10.1002/cncr.31701
Duchman, K. R., Gao, Y., and Miller, B. J. (2015). Prognostic Factors for Survival in Patients with Ewing's Sarcoma Using the Surveillance, Epidemiology, and End Results (SEER) Program Database. Cancer Epidemiol. 39 (2), 189–195. doi:10.1016/j.canep.2014.12.012
Dunn, D. B. (2020). Larotrectinib and Entrectinib: TRK Inhibitors for the Treatment of Pediatric and Adult Patients with NTRK Gene Fusion. J. Adv. Pract. Oncol. 11 (4), 418–423. doi:10.6004/jadpro.2020.11.4.9
Dupain, C., Harttrampf, A. C., Urbinati, G., Geoerger, B., and Massaad-Massade, L. (2017). Relevance of Fusion Genes in Pediatric Cancers: Toward Precision Medicine. Mol. Ther. Nucleic Acids 6, 315–326. doi:10.1016/j.omtn.2017.01.005
Erkizan, H. V., Kong, Y., Merchant, M., Schlottmann, S., Barber-Rotenberg, J. S., Yuan, L., et al. (2009). A Small Molecule Blocking Oncogenic Protein EWS-FLI1 Interaction with RNA Helicase A Inhibits Growth of Ewing's Sarcoma. Nat. Med. 15 (7), 750–756. doi:10.1038/nm.1983
Ewing, J. (19211972). Classics in Oncology. Diffuse Endothelioma of Bone. James Ewing. Proceedings of the New York Pathological Society, 1921. CA Cancer J. Clin. 22 (2), 95–98. doi:10.3322/canjclin.22.2.95
Fordham, A. M., Xie, J., Gifford, A. J., Wadham, C., Morgan, L. T., Mould, E. V. A., et al. (2020). CD30 and ALK Combination Therapy Has High Therapeutic Potency in RANBP2-ALK-Rearranged Epithelioid Inflammatory Myofibroblastic Sarcoma. Br. J. Cancer 123 (7), 1101–1113. doi:10.1038/s41416-020-0996-2
Galili, N., Davis, R. J., Fredericks, W. J., Mukhopadhyay, S., Rauscher, F. J., Emanuel, B. S., et al. (1993). Fusion of a fork Head Domain Gene to PAX3 in the Solid Tumour Alveolar Rhabdomyosarcoma. Nat. Genet. 5 (3), 230–235. doi:10.1038/ng1193-230
García-Domínguez, D. J., Hontecillas-Prieto, L., Rodríguez-Núñez, P., Pascual-Pasto, G., Vila-Ubach, M., García-Mejías, R., et al. (2018). The Combination of Epigenetic Drugs SAHA and HCI-2509 Synergistically Inhibits EWS-FLI1 and Tumor Growth in Ewing Sarcoma. Oncotarget 9 (59), 31397–31410. doi:10.18632/oncotarget.25829
Gaspar, N., Hawkins, D. S., Dirksen, U., Lewis, I. J., Ferrari, S., Le Deley, M. C., et al. (2015). Ewing Sarcoma: Current Management and Future Approaches through Collaboration. J. Clin. Oncol. 33 (27), 3036–3046. doi:10.1200/JCO.2014.59.5256
Ghose, A., Tariq, Z., and Veltri, S. (2012). Treatment of Multidrug Resistant Advanced Alveolar Soft Part Sarcoma with Sunitinib. Am. J. Ther. 19 (1), e56–8. doi:10.1097/MJT.0b013e3181e70d20
Gras, P., Truant, S., Boige, V., Ladrat, L., Rougier, P., Pruvot, F. R., et al. (2012). Prolonged Complete Response after GEMOX Chemotherapy in a Patient with Advanced Fibrolamellar Hepatocellular Carcinoma. Case Rep. Oncol. 5 (1), 169–172. doi:10.1159/000338242
Gryder, B. E., Yohe, M. E., Chou, H. C., Zhang, X., Marques, J., Wachtel, M., et al. (2017). PAX3-FOXO1 Establishes Myogenic Super Enhancers and Confers BET Bromodomain Vulnerability. Cancer Discov. 7 (8), 884–899. doi:10.1158/2159-8290.CD-16-1297
Harlow, M. L., Maloney, N., Roland, J., Guillen Navarro, M. J., Easton, M. K., Kitchen-Goosen, S. M., et al. (2016). Lurbinectedin Inactivates the Ewing Sarcoma Oncoprotein EWS-FLI1 by Redistributing it within the Nucleus. Cancer Res. 76 (22), 6657–6668. doi:10.1158/0008-5472.CAN-16-0568
Hayashi, M., Chu, D., Meyer, C. F., Llosa, N. J., McCarty, G., Morris, C. D., et al. (2016). Highly Personalized Detection of Minimal Ewing Sarcoma Disease burden from Plasma Tumor DNA. Cancer 122 (19), 3015–3023. doi:10.1002/cncr.30144
Hedrick, E., Crose, L., Linardic, C. M., and Safe, S. (2015). Histone Deacetylase Inhibitors Inhibit Rhabdomyosarcoma by Reactive Oxygen Species-dependent Targeting of Specificity Protein Transcription Factors. Mol. Cancer Ther. 14 (9), 2143–2153. doi:10.1158/1535-7163.MCT-15-0148
Herrero-Martín, D., Osuna, D., Ordóñez, J. L., Sevillano, V., Martins, A. S., Mackintosh, C., et al. (2009). Stable Interference of EWS-FLI1 in an Ewing Sarcoma Cell Line Impairs IGF-1/IGF-1R Signalling and Reveals TOPK as a New Target. Br. J Cancer 101 (1), 80–90. doi:10.1038/sj.bjc.6605104
Heske, C. M., and Mascarenhas, L. (2021). Relapsed Rhabdomyosarcoma. J. Clin. Med. 10, 804. doi:10.3390/jcm10040804
Honeyman, J. N., Simon, E. P., Robine, N., Chiaroni-Clarke, R., Darcy, D. G., Lim, I. I., et al. (2014). Detection of a Recurrent DNAJB1-PRKACA Chimeric Transcript in Fibrolamellar Hepatocellular Carcinoma. Science 343 (6174), 1010–1014. doi:10.1126/science.1249484
Honeyman, J. N., Chang, W-I., Prabhu, V. V., Allen, J. E., Zhou, L., and El-Deiry, W. S. (2021). Abstract 1040: Imipridones Exhibit Synergy with Sorafenib, HDAC Inhibition, PARP Inhibition, and Proteasome Inhibition in Liver Cancer Cell Lines. Cancer Res. 81 (13 Suppl. ment), 1040. doi:10.1158/1538-7445.am2021-1040
Honoki, K., Stojanovski, E., McEvoy, M., Fujii, H., Tsujiuchi, T., Kido, A., et al. (2007). Prognostic Significance of P16 INK4a Alteration for Ewing Sarcoma: a Meta-Analysis. Cancer 110 (6), 1351–1360. doi:10.1002/cncr.22908
Kadoch, C., and Crabtree, G. R. (2013). Reversible Disruption of mSWI/SNF (BAF) Complexes by the SS18-SSX Oncogenic Fusion in Synovial Sarcoma. Cell 153 (1), 71–85. doi:10.1016/j.cell.2013.02.036
Kassahun, W. T. (2016). Contemporary Management of Fibrolamellar Hepatocellular Carcinoma: Diagnosis, Treatment, Outcome, Prognostic Factors, and Recent Developments. World J. Surg. Oncol. 14 (1), 151. doi:10.1186/s12957-016-0903-8
Kastenhuber, E. R., Lalazar, G., Houlihan, S. L., Tschaharganeh, D. F., Baslan, T., Chen, C. C., et al. (2017). DNAJB1-PRKACA Fusion Kinase Interacts with β-catenin and the Liver Regenerative Response to Drive Fibrolamellar Hepatocellular Carcinoma. Proc. Natl. Acad. Sci. U S A. 114 (50), 13076–13084. doi:10.1073/pnas.1716483114
Kawano, S., Grassian, A. R., Tsuda, M., Knutson, S. K., Warholic, N. M., Kuznetsov, G., et al. (2016). Preclinical Evidence of Anti-tumor Activity Induced by EZH2 Inhibition in Human Models of Synovial Sarcoma. PLoS One 11 (7), e0158888. doi:10.1371/journal.pone.0158888
Kline, C. L., Van den Heuvel, A. P., Allen, J. E., Prabhu, V. V., Dicker, D. T., and El-Deiry, W. S. (2016). ONC201 Kills Solid Tumor Cells by Triggering an Integrated Stress Response Dependent on ATF4 Activation by Specific eIF2α Kinases. Sci. Signal. 9 (415), ra18. doi:10.1126/scisignal.aac4374
Knezevich, S. R., McFadden, D. E., Tao, W., Lim, J. F., and Sorensen, P. H. (1998). A Novel ETV6-NTRK3 Gene Fusion in Congenital Fibrosarcoma. Nat. Genet. 18 (2), 184–187. doi:10.1038/ng0298-184
Kummar, S., Allen, D., Monks, A., Polley, E. C., Hose, C. D., Ivy, S. P., et al. (2013). Cediranib for Metastatic Alveolar Soft Part Sarcoma. J. Clin. Oncol. 31 (18), 2296–2302. doi:10.1200/JCO.2012.47.4288
Ladanyi, M., and Gerald, W. (1994). Fusion of the EWS and WT1 Genes in the Desmoplastic Small Round Cell Tumor. Cancer Res. 54 (11), 2837–2840.
Laetsch, T. W., DuBois, S. G., Mascarenhas, L., Turpin, B., Federman, N., Albert, C. M., et al. (2018). Larotrectinib for Paediatric Solid Tumours Harbouring NTRK Gene Fusions: Phase 1 Results from a Multicentre, Open-Label, Phase 1/2 Study. Lancet Oncol. 19 (5), 705–714. doi:10.1016/S1470-2045(18)30119-0
Lerman, D. M., Monument, M. J., McIlvaine, E., Liu, X. Q., Huang, D., Monovich, L., et al. (2015). Tumoral TP53 And/or CDKN2A Alterations Are Not Reliable Prognostic Biomarkers in Patients with Localized Ewing Sarcoma: a Report from the Children's Oncology Group. Pediatr. Blood Cancer 62 (5), 759–765. doi:10.1002/pbc.25340
Lubieniecka, J. M., de Bruijn, D. R., Su, L., van Dijk, A. H., Subramanian, S., van de Rijn, M., et al. (2008). Histone Deacetylase Inhibitors Reverse SS18-SSX-Mediated Polycomb Silencing of the Tumor Suppressor Early Growth Response 1 in Synovial Sarcoma. Cancer Res. 68 (11), 4303–4310. doi:10.1158/0008-5472.CAN-08-0092
Malempati, S., Weigel, B., Ingle, A. M., Ahern, C. H., Carroll, J. M., Roberts, C. T., et al. (2012). Phase I/II Trial and Pharmacokinetic Study of Cixutumumab in Pediatric Patients with Refractory Solid Tumors and Ewing Sarcoma: a Report from the Children's Oncology Group. J. Clin. Oncol. 30 (3), 256–262. doi:10.1200/JCO.2011.37.4355
Malempati, S., Weigel, B. J., Chi, Y. Y., Tian, J., Anderson, J. R., Parham, D. M., et al. (2019). The Addition of Cixutumumab or Temozolomide to Intensive Multiagent Chemotherapy Is Feasible but Does Not Improve Outcome for Patients with Metastatic Rhabdomyosarcoma: A Report from the Children's Oncology Group. Cancer 125 (2), 290–297. doi:10.1002/cncr.31770
Malempati, S. W., Anderson, J., Parham, D., Teot, L. A., Rodeberg, D. A., Yock, T. I., et al. (2015). Early Results from Children’s Oncology Group (COG) ARST08P1: Pilot Studies of Cixutumumab or Temozolomide with Intensive Multiagent Chemotherapy for Patients with Metastatic Rhabdomyosarcoma (RMS). J. Clin. Oncol. 33 (15), 10015. doi:10.1200/jco.2015.33.15_suppl.10015
Malouf, G. G., Camparo, P., Oudard, S., Schleiermacher, G., Theodore, C., Rustine, A., et al. (2010). Targeted Agents in Metastatic Xp11 translocation/TFE3 Gene Fusion Renal Cell Carcinoma (RCC): a Report from the Juvenile RCC Network. Ann. Oncol. 21 (9), 1834–1838. doi:10.1093/annonc/mdq029
Marayati, R., Quinn, C. H., and Beierle, E. A. (2019). Immunotherapy in Pediatric Solid Tumors-A Systematic Review. Cancers (Basel) 11 (12), 2022. doi:10.3390/cancers11122022
Markham, A. (2020). Lurbinectedin: First Approval. Drugs 80 (13), 1345–1353. doi:10.1007/s40265-020-01374-0
Maruyama, Y., Fukushima, T., Gomi, D., Kobayashi, T., Sekiguchi, N., Sakamoto, A., et al. (2017). Relapsed and Unresectable Inflammatory Myofibroblastic Tumor Responded to Chemotherapy: A Case Report and Review of the Literature. Mol. Clin. Oncol. 7 (4), 521–524. doi:10.3892/mco.2017.1383
McBride, M. J., Pulice, J. L., Beird, H. C., Ingram, D. R., D'Avino, A. R., Shern, J. F., et al. (2018). The SS18-SSX Fusion Oncoprotein Hijacks BAF Complex Targeting and Function to Drive Synovial Sarcoma. Cancer Cell 33 (6), 1128–e7. doi:10.1016/j.ccell.2018.05.002
Mittal, A., Gupta, A., Rastogi, S., Barwad, A., and Sharma, S. (2021). Near-complete Response to Low-Dose Ceritinib in Recurrent Infantile Inflammatory Myofibroblastic Tumour. Ecancermedicalscience 15, 1215. doi:10.3332/ecancer.2021.1215
Mitton, B., and Federman, N. (2012). Alveolar Soft Part Sarcomas: Molecular Pathogenesis and Implications for Novel Targeted Therapies. Sarcoma 2012, 428789. doi:10.1155/2012/428789
Mossé, Y. P., Voss, S. D., Lim, M. S., Rolland, D., Minard, C. G., Fox, E., et al. (2017). Targeting ALK with Crizotinib in Pediatric Anaplastic Large Cell Lymphoma and Inflammatory Myofibroblastic Tumor: A Children's Oncology Group Study. J. Clin. Oncol. 35 (28), 3215–3221. doi:10.1200/JCO.2017.73.4830
Nagasubramanian, R., Wei, J., Gordon, P., Rastatter, J. C., Cox, M. C., and Pappo, A. (2016). Infantile Fibrosarcoma with NTRK3-ETV6 Fusion Successfully Treated with the Tropomyosin-Related Kinase Inhibitor LOXO-101. Pediatr. Blood Cancer 63 (8), 1468–1470. doi:10.1002/pbc.26026
Navid, F., Baker, S. D., McCarville, M. B., Stewart, C. F., Billups, C. A., Wu, J., et al. (2013). Phase I and Clinical Pharmacology Study of Bevacizumab, Sorafenib, and Low-Dose Cyclophosphamide in Children and Young Adults with Refractory/recurrent Solid Tumors. Clin. Cancer Res. 19 (1), 236–246. doi:10.1158/1078-0432.CCR-12-1897
Parida, L., Fernandez-Pineda, I., Uffman, J. K., Davidoff, A. M., Krasin, M. J., Pappo, A., et al. (2013). Clinical Management of Infantile Fibrosarcoma: a Retrospective Single-Institution Review. Pediatr. Surg. Int. 29 (7), 703–708. doi:10.1007/s00383-013-3326-4
Patt, Y. Z., Hassan, M. M., Lozano, R. D., Brown, T. D., Vauthey, J. N., Curley, S. A., et al. (2003). Phase II Trial of Systemic Continuous Fluorouracil and Subcutaneous Recombinant Interferon Alfa-2b for Treatment of Hepatocellular Carcinoma. J. Clin. Oncol. 21 (3), 421–427. doi:10.1200/JCO.2003.10.103
Prabhu, V. V., Morrow, S., Rahman Kawakibi, A., Zhou, L., Ralff, M., Ray, J., et al. (2020). ONC201 and Imipridones: Anti-cancer Compounds with Clinical Efficacy. Neoplasia 22 (12), 725–744. doi:10.1016/j.neo.2020.09.005
Preuss, E., Hugle, M., Reimann, R., Schlecht, M., and Fulda, S. (2013). Pan-mammalian Target of Rapamycin (mTOR) Inhibitor AZD8055 Primes Rhabdomyosarcoma Cells for ABT-737-Induced Apoptosis by Down-Regulating Mcl-1 Protein. J. Biol. Chem. 288 (49), 35287–35296. doi:10.1074/jbc.M113.495986
Saiki, M., Ohyanagi, F., Ariyasu, R., Koyama, J., Sonoda, T., Nishikawa, S., et al. (2017). Dramatic Response to Alectinib in Inflammatory Myofibroblastic Tumor with Anaplastic Lymphoma Kinase Fusion Gene. Jpn. J. Clin. Oncol. 47 (12), 1189–1192. doi:10.1093/jjco/hyx133
Sankar, S., Bell, R., Stephens, B., Zhuo, R., Sharma, S., Bearss, D. J., et al. (2013). Mechanism and Relevance of EWS/FLI-mediated Transcriptional Repression in Ewing Sarcoma. Oncogene 32 (42), 5089–5100. doi:10.1038/onc.2012.525
Sankar, S., Theisen, E. R., Bearss, J., Mulvihill, T., Hoffman, L. M., Sorna, V., et al. (2014). Reversible LSD1 Inhibition Interferes with Global EWS/ETS Transcriptional Activity and Impedes Ewing Sarcoma Tumor Growth. Clin. Cancer Res. 20 (17), 4584–4597. doi:10.1158/1078-0432.CCR-14-0072
Santarpia, M., Daffinà, M. G., D'Aveni, A., Marabello, G., Liguori, A., Giovannetti, E., et al. (2017). Spotlight on Ceritinib in the Treatment of ALK+ NSCLC: Design, Development and Place in Therapy. Drug Des. Devel Ther. 11, 2047–2063. doi:10.2147/DDDT.S113500
Schmid, I., and von Schweinitz, D. (2017). Pediatric Hepatocellular Carcinoma: Challenges and Solutions. J. Hepatocell Carcinoma 4, 15–21. doi:10.2147/JHC.S94008
Schoffski P, A. M., Stacchiotti, S., Davis, L. E., Villalobos, V. M., Italiano, A., George, S., et al. (2017). Phase 2 Multicenter Study of the EZH2 Inhibitor Tazemetostat in Adults with Synovial Sarcoma (NCT02601950). J. Clin. Oncol. 35 (15), 11057. doi:10.1200/jco.2017.35.15_suppl.11057
Shen, J. K., Cote, G. M., Gao, Y., Choy, E., Mankin, H. J., Hornicek, F. J., et al. (2016). Targeting EZH2-Mediated Methylation of H3K27 Inhibits Proliferation and Migration of Synovial Sarcoma In Vitro. Sci. Rep. 6, 25239. doi:10.1038/srep25239
Shipley, J. M., Clark, J., Crew, A. J., Birdsall, S., Rocques, P. J., Gill, S., et al. (1994). The t(X;18)(p11.2;q11.2) Translocation Found in Human Synovial Sarcomas Involves Two Distinct Loci on the X Chromosome. Oncogene 9 (5), 1447–1453.
Shulman, D. S., Klega, K., Imamovic-Tuco, A., Clapp, A., Nag, A., Thorner, A. R., et al. (2018). Detection of Circulating Tumour DNA Is Associated with Inferior Outcomes in Ewing Sarcoma and Osteosarcoma: a Report from the Children's Oncology Group. Br. J Cancer 119 (5), 615–621. doi:10.1038/s41416-018-0212-9
Siegel, D. A., Richardson, L. C., Henley, S. J., Wilson, R. J., Dowling, N. F., Weir, H. K., et al. (2020). Pediatric Cancer Mortality and Survival in the United States, 2001-2016. Cancer 126 (19), 4379–4389. doi:10.1002/cncr.33080
Smeland, S., Bielack, S. S., Whelan, J., Bernstein, M., Hogendoorn, P., Krailo, M. D., et al. (2019). Survival and Prognosis with Osteosarcoma: Outcomes in More Than 2000 Patients in the EURAMOS-1 (European and American Osteosarcoma Study) Cohort. Eur. J. Cancer 109, 36–50. doi:10.1016/j.ejca.2018.11.027
Soulez, M., Saurin, A. J., Freemont, P. S., and Knight, J. C. (1999). SSX and the Synovial-sarcoma-specific Chimaeric Protein SYT-SSX Co-localize with the Human Polycomb Group Complex. Oncogene 18 (17), 2739–2746. doi:10.1038/sj.onc.1202613
Spriano, F., Chung, E. Y. L., Gaudio, E., Tarantelli, C., Cascione, L., Napoli, S., et al. (2019). The ETS Inhibitors YK-4-279 and TK-216 Are Novel Antilymphoma Agents. Clin. Cancer Res. 25 (16), 5167–5176. doi:10.1158/1078-0432.CCR-18-2718
Srivastava, R. K., Kaylani, S. Z., Edrees, N., Li, C., Talwelkar, S. S., Xu, J., et al. (2014). GLI Inhibitor GANT-61 Diminishes Embryonal and Alveolar Rhabdomyosarcoma Growth by Inhibiting Shh/AKT-mTOR axis. Oncotarget 5 (23), 12151–12165. doi:10.18632/oncotarget.2569
Stacchiotti, S., Negri, T., Zaffaroni, N., Palassini, E., Morosi, C., Brich, S., et al. (2011). Sunitinib in Advanced Alveolar Soft Part Sarcoma: Evidence of a Direct Antitumor Effect. Ann. Oncol. 22 (7), 1682–1690. doi:10.1093/annonc/mdq644
Stahl, M., Ranft, A., Paulussen, M., Bölling, T., Vieth, V., Bielack, S., et al. (2011). Risk of Recurrence and Survival after Relapse in Patients with Ewing Sarcoma. Pediatr. Blood Cancer 57 (4), 549–553. doi:10.1002/pbc.23040
Trahair, T., Gifford, A. J., Fordham, A., Mayoh, C., Fadia, M., Lukeis, R., et al. (2019). Crizotinib and Surgery for Long-Term Disease Control in Children and Adolescents with ALK-Positive Inflammatory Myofibroblastic Tumors. JCO Precis Oncol. 3 (3), 1–11. doi:10.1200/PO.18.00297
van Erp, A. E. M., Versleijen-Jonkers, Y. M. H., van der Graaf, W. T. A., and Fleuren, E. D. G. (2018). Targeted Therapy-Based Combination Treatment in Rhabdomyosarcoma. Mol. Cancer Ther. 17 (7), 1365–1380. doi:10.1158/1535-7163.MCT-17-1131
van Maldegem, A. M., Bovée, J. V., Peterse, E. F., Hogendoorn, P. C., and Gelderblom, H. (2016). Ewing Sarcoma: The Clinical Relevance of the Insulin-like Growth Factor 1 and the Poly-ADP-Ribose-Polymerase Pathway. Eur. J. Cancer 53, 171–180. doi:10.1016/j.ejca.2015.09.009
Wachtel, M., Rakic, J., Okoniewski, M., Bode, P., Niggli, F., and Schäfer, B. W. (2014). FGFR4 Signaling Couples to Bim and Not Bmf to Discriminate Subsets of Alveolar Rhabdomyosarcoma Cells. Int. J. Cancer 135 (7), 1543–1552. doi:10.1002/ijc.28800
Wagner, A. J., Goldberg, J. M., Dubois, S. G., Choy, E., Rosen, L., Pappo, A., et al. (2012). Tivantinib (ARQ 197), a Selective Inhibitor of MET, in Patients with Microphthalmia Transcription Factor-Associated Tumors: Results of a Multicenter Phase 2 Trial. Cancer 118 (23), 5894–5902. doi:10.1002/cncr.27582
Wagner, J., Kline, C. L., Zhou, L., Campbell, K. S., MacFarlane, A. W., Olszanski, A. J., et al. (2018). Dose Intensification of TRAIL-Inducing ONC201 Inhibits Metastasis and Promotes Intratumoral NK Cell Recruitment. J. Clin. Invest. 128 (6), 2325–2338. doi:10.1172/JCI96711
Ward, Z. J., Yeh, J. M., Bhakta, N., Frazier, A. L., and Atun, R. (2019). Estimating the Total Incidence of Global Childhood Cancer: a Simulation-Based Analysis. Lancet Oncol. 20 (4), 483–493. doi:10.1016/S1470-2045(18)30909-4
Ward, Z. J., Yeh, J. M., Bhakta, N., Frazier, A. L., and Atun, R. (2019). Estimating the Total Incidence of Global Childhood Cancer: a Simulation-Based Analysis. Lancet Oncol. 20 (4), 483–493. doi:10.1016/S1470-2045(18)30909-4
Wedekind, M. F., Ranalli, M., and Shah, N. (2017). Clinical Efficacy of Cabozantinib in Two Pediatric Patients with Recurrent Renal Cell Carcinoma. Pediatr. Blood Cancer 64 (11). doi:10.1002/pbc.26586
Wexler, L. H., and Ladanyi, M. (2010). Diagnosing Alveolar Rhabdomyosarcoma: Morphology Must Be Coupled with Fusion Confirmation. J. Clin. Oncol. 28 (13), 2126–2128. doi:10.1200/JCO.2009.27.5339
Wilson, B. G., Wang, X., Shen, X., McKenna, E. S., Lemieux, M. E., Cho, Y. J., et al. (2010). Epigenetic Antagonism between Polycomb and SWI/SNF Complexes during Oncogenic Transformation. Cancer Cell 18 (4), 316–328. doi:10.1016/j.ccr.2010.09.006
Wong, V., Pavlick, D., Brennan, T., Yelensky, R., Crawford, J., Ross, J. S., et al. (2016). Evaluation of a Congenital Infantile Fibrosarcoma by Comprehensive Genomic Profiling Reveals an LMNA-NTRK1 Gene Fusion Responsive to Crizotinib. J. Natl. Cancer Inst. 108 (1). doi:10.1093/jnci/djv307
Yamamoto, Y., Fukuda, K., Fuchimoto, Y., Matsuzaki, Y., Saikawa, Y., Kitagawa, Y., et al. (2013). Cetuximab Promotes Anticancer Drug Toxicity in Rhabdomyosarcomas with EGFR Amplification In Vitro. Oncol. Rep. 30 (3), 1081–1086. doi:10.3892/or.2013.2588
Zhang, Y., Zhou, L., Safran, H., Borsuk, R., Lulla, R., Tapinos, N., et al. (2021). EZH2i EPZ-6438 and HDACi Vorinostat Synergize with ONC201/TIC10 to Activate Integrated Stress Response, DR5, Reduce H3K27 Methylation, ClpX and Promote Apoptosis of Multiple Tumor Types Including DIPG. Neoplasia 23 (8), 792–810. doi:10.1016/j.neo.2021.06.007
Keywords: sarcoma, pediatric, fusion-positive, molecular targets, solid tumors
Citation: Chang W-I, Lin C, Liguori N, Honeyman JN, DeNardo B and El-Deiry W (2022) Molecular Targets for Novel Therapeutics in Pediatric Fusion-Positive Non-CNS Solid Tumors. Front. Pharmacol. 12:747895. doi: 10.3389/fphar.2021.747895
Received: 27 July 2021; Accepted: 03 December 2021;
Published: 20 January 2022.
Edited by:
Anne Lorant, Laboratoire de Biologie Moléculaire et Cellulaire du Cancer (LBMCC), LuxembourgReviewed by:
Lawrence Panasci, Segal Cancer Centre, CanadaPankaj Pathak, National Institutes of Health (NIH), United States
Copyright © 2022 Chang, Lin, Liguori, Honeyman, DeNardo and El-Deiry. This is an open-access article distributed under the terms of the Creative Commons Attribution License (CC BY). The use, distribution or reproduction in other forums is permitted, provided the original author(s) and the copyright owner(s) are credited and that the original publication in this journal is cited, in accordance with accepted academic practice. No use, distribution or reproduction is permitted which does not comply with these terms.
*Correspondence: Wen-I Chang, d2VuLWlfY2hhbmdAYnJvd24uZWR1; Wafik El-Deiry, d2FmaWtAYnJvd24uZWR1