- 1National Institutes of Nursing Research, NIH, Bethesda, MD, United States
- 2Henry M. Jackson Foundation, Bethesda, MD, United States
- 3Department of Biological Sciences, Notre Dame, IN, United States
- 4Center for Stem Cells and Regenerative Medicine, Galvin Life Sciences, University of Notre Dame, Notre Dame, IN, United States
- 5Center for Neuroscience and Regenerative Medicine, Rockville, MD, United States
- 6Department of Medicine, Uniformed Services University of the Health Sciences, Bethesda, MD, United States
Traumatic brain injury (TBI) affects millions of Americans each year and has been shown to disproportionately impact those subject to greater disparities in health. Female sex is one factor that has been associated with disparities in health outcomes, including in TBI, but sex differences in biomarker levels and behavioral outcomes after TBI are underexplored. This study included participants with both blunt and blast TBI with majority rating their TBI as mild. Time since injury was 5.4 (2.0, 15.5) years for females and 6.8 (2.4, 11.3) years for males. The aim of this cross sectional study is to investigate the relationship between postconcussive, depression, and post-traumatic stress disorder (PTSD) symptoms, as well as health related quality of life (HRQOL), and the levels of glial fibrillary acidic protein (GFAP), total tau (t-tau), neurofilament light chain (NfL), and ubiquitin C-terminal hydrolase-L1 (UCH-L1). Behavioral outcomes were evaluated with the Neurobehavioral Symptom Inventory (NSI), Patient Health Questionnaire-9 (PHQ-9), PTSD Checklist- Civilian Version (PCL-C), short form (SF)-36, and plasma levels of total tau, GFAP, NfL, and UCHL-1 measured with the Simoa-HDX. We observed that females had significantly higher levels of GFAP and tau (ps < 0.05), and higher PHQ-9 scores, NSI total scores, NSI- vestibular, NSI-somatosensory, NSI-affective sub-scale scores (ps < 0.05)), than males. In addition, females had lower scores in HRQOL outcomes of role limitations due to emotional problems, vitality, emotional well-being, social functioning, and pain compared to males (ps < 0.05). Correlation analysis showed positive associations between levels of tau and the NSI-total and NSI-cognitive sub-scale scores (ps < 0.05) in females. No significant associations were found for NfL or GFAP with NSI scores. For female participants, negative correlations were observed between tau and NfL concentrations and the SF-36 physical function subscale (ps < 0.05), as well as tau and the social function subscale (p < 0.001), while GFAP levels positively correlated with role limitations due to emotional problems (p = 0.004). No significant associations were observed in males. Our findings suggest that sex differences exist in TBI-related behavioral outcomes, as well as levels of biomarkers associated with brain injury, and that the relationship between biomarker levels and behavioral outcomes is more evident in females than males. Future studies are warranted to corroborate these results, and to determine the implications for prognosis and treatment. The identification of candidate TBI biomarkers may lead to development of individualized treatment guidelines.
Introduction
Traumatic brain injury (TBI) affects over one million Americans each year and may lead to long-term debilitating behavioral symptoms particularly in groups with greater health disparities (Center for Disease Control and Prevention, National Center for Injury Prevention and Control CDC, 2021). Sex differences in TBI behavioral outcomes have been suggested, but possible molecular underpinnings are unclear. Current TBI treatment guidelines remain largely based on the studies with predominantly male participants (Biegon 2021). Exploring TBI outcomes and potential candidate TBI biomarkers based on sex differences might lead to development of precision medicine therapies.
In a recent review, studies with mild to moderate TBI were more likely to use social-behavioral measures and women reported worse behavioral outcomes than men; however, for severe TBI, studies were more likely to use survival and community integration as outcome measures and women fared better than men (Gupte et al., 2019). In a review of sex differences in mild TBI, researchers found that females have a proportionally higher incidence of concussions in sports; however, comparisons of symptom prevalence between the sexes have had mixed results (Merritt et al., 2019). Other reviews highlight the paucity of studies evaluating sex differences in blood-based biomarkers in TBI (Strathmann et al., 2014; Gupte et al., 2019).
Commonly measured biomarkers of TBI include total tau, neurofilament light chain (NfL), glial fibrillary acidic protein (GFAP), and ubiquitin C-terminal hydrolase-L1 (UCH-L1) (Kawata et al., 2016; Shahim et al., 2020a). In brain tissue of rats, researchers reported that both sexes had upregulation in gene expressions of GFAP, myelin basic protein, and tau following TBI, and while higher levels of GFAP was observed in female rats, in male rats it was significantly decreased (Wright et al., 2017). No sex differences in the biomarkers were noted in separate murine study post severe closed head injury (Rubenstein et al., 2017a). A recent study that examined 20 female and 20 male athletes found that plasma tau levels were significantly higher in females 6 h and 3 days after a concussive event than in males (Mondello et al., 2020). Accumulating evidence suggests that, not only may sex differences exist, but an exploration of outcomes and associations with biomarkers are paramount to furthering the current state of the science.
The aims of this project are to examine sex differences in postconcussive, depressive, and post-traumatic stress disorder (PTSD) symptoms, levels of circulating GFAP, tau, NfL, and the relationship between symptoms and biomarkers as well as health-related quality of life (HRQOL).
Materials and Methods
Study Design
The study used a cross-sectional design. Participants (n = 289) were enrolled in a larger ongoing study at two locations: Fort Belvoir Community Hospital (FBCH, Virginia) and Walter Reed National Military Medical Center (WRNMMC, Bethesda, Maryland). The participants include either active-duty or retired military service members as well as civilians without prior military experience. The participants underwent a medical history and physical exam and had blood samples collected. They also completed a series of behavioral symptom questionnaires. The exclusion criteria in this study included prior diagnosis of psychosis, schizophrenia, schizoaffective disorder, bipolar disorder, conversion disorder, or personality disorder. Institutional review board (IRB) approvals were obtained from the Uniformed Services University of the Health Sciences (USUHS), FBCH, and WRNMMC. Prior to data and sample collection, a written witnessed informed consent was collected from each research participant. Participants with complete blood, clinical, and behavioral outcomes were included in a convenience sample for this analysis.
To characterize the lifetime TBI history of each participant, we used the Ohio State University Traumatic Brain Injury Identification Method (OSU TBI-ID), which is a structured interview carried out by research staff. This interview assesses the number of TBIs that each participant sustained in their lifetime. For each TBI, the OSU TBI-ID reports on age at injury, cause of injury, incidence of loss of consciousness (LOC), and whether the participant was dazed and/or experienced a memory gap. A positive TBI was defined as an insult to the head that resulted in LOC and/or absence of concussion. Participants also reported length of the time they lost consciousness as part of OSU assessment. Because participants had multiple TBIs, the most recent TBI and most significant (higher severity on LOC) were used for the classification of self-reported severity. Self-reported LOC and AOC were used to classify the severity into mild (AOC and/or LOC of zero to 30 min), moderate (LOC greater than 30 min and less than 24 h), and severe (LOC greater than 24 h) (The Management of Concussion-Mild TBI Working Group, 2009). Additional neuroimaging or post-traumatic amnesia were not available for classification at the time of data collection. Time since last injury (TSI) was defined as the difference between the date of last known TBI and the date of blood collection.
Blood-Based Biomarkers
Plasma were separated from peripheral blood collected from study participants following laboratory standard operating procedures and stored at −80°C until further analysis. Protein biomarker measurement was performed on the Simoa HD-x Analyzer (Quanterix Corperation, Lexington, MA), which allows for multiplex detection at single protein molecule level. Single protein molecules are captured and labeled on beads using standard ELISA reagents. After fluorescent labeling of the proteins, the Simoa HD-x Analyzer enables protein concentrations to be determined digitally. By isolating and detecting single immunocomplexes in arrays of femtoliter-volume wells, digital ELISA enables clinically important proteins in serum to be measured at sub-femtomolar concentrations that allows peripheral samples of blood to approximate central activity of neuropeptides. The Simoa Human Neurology 4-Plex Assay (N4PA, Cat# 102153, Quanterix Corperation, MA) measures four important neurology biomarkers detected in cerebrospinal fluid (CSF) and blood. The four targets are total tau, NfL, GFAP, and UCH-L1. The recommended low limit of quantifications (LLOQs) for tau, NfL, and GFAP in plasma are 0.212, 0.964, and 1.868 pg/ml, respectively. All measurements obtained in this study were within the assay quantification range. UCH-L1™ and Banyan GFAP™ are registered trademarks of Banyan Biomarkers. In total 29 samples (23 samples for tau, six samples for NfL) with intra-sample variability of more than 20.4% were excluded from the further analysis (Supplementary Table S1).
UCHL-1 results had CVs greater than 20% in about 59% of total samples and this biomarker was excluded from statistical analysis. The half-life of UCH-L1 is <12 h and it declines after a day to several days thereafter depending on the severity of the injury (Diaz-Arrastia et al., 2014). Because our samples are chronic, it is likely that high CVs in the UCH-L1 dataset were due to having many samples at the LLOQ of this assay. Research staff processing blood-based biomarkers were blind to the male and female groups.
Behavioral Outcomes
Postconcussive symptoms were measured using the Neurobehavioral Symptom Inventory (NSI), a 22-item questionnaire that includes somatosensory, affective, cognitive, and vestibular sub-scales. The questionnaire has been validated in the TBI population with an acceptable test-retest indices (r ranging 0.78–0.94) and good internal consistency (α ≥ 0.80) for NSI total, and test-retest reliability with r ranging 0.52 to 0.91 and α ≥ 0.80 for its subscales (Silva 2020). The items on the questionnaire are rated from 0 (none) to 4 (severe) with higher scores indicating worse symptoms.
Depression symptom severity was measured using a 9-item Patient Health Questionnaire (PHQ-9) that has excellent sensitivity (0.93) and specificity (0.89) and test-retest reliability (r = 0.76, ĸ = 0.46) (Fann et al., 2005). It uses a Likert scale rated from “not at all” to “nearly every day” for each of the 9 items, so that the total score can range from 0 to 27, with higher scores constituting greater severity of depression (Kroenke et al., 2001).
Posttraumatic stress symptom severity was measured with the PTSD Checklist- Civilian Version (PCL-C), a 17-item self-reported questionnaire featuring a Likert scale rated from “not at all” to “extremely” (Ruggiero et al., 2003) on each item. The questionnaire has been previously validated and shown to have high internal consistency for PCL total (α = 0.94) as well as convergent validity (r > 0.75) (Ruggiero et al., 2003). The total score ranges from 17 to 80, where higher scores indicate greater symptom burden.
Health-related quality of life (HRQOL) was measured using the short form (SF)-36 with previously established excellent reliability (≥0.76) and acceptable validity (r ≥ 0.40) (Ware and John 2000). The questionnaire evaluates physical and mental HRQOL and is divided into eight subscales: physical functioning, role-physical, bodily pain, general health, vitality, social functioning, role-emotional, and mental health. The lower scores indicate lower HRQOL and higher scores indicate better HRQOL (Ware et al., 2001).
Statistical Methods
All statistical analyses were performed using SPSS Grad Pack 24.0 and figures were created in GraphPad Prism (version 9.0.0). Demographic, clinical, and biomarker variables were non normally distributed, thus nonparametric test statistics of Mann Whitney U test for continuous variables and Pearson chi -square test or Fisher’s exact test for categorical variables were run to assess group differences between females and males. Binominal logistic regression models were run to control for significant demographic covariates. We performed log base two transformations to decrease skewness of the blood-based biomarkers to improve fit of the logistic regression models. We also ran spearman correlation analyses between biomarker levels and behavioral and HRQOL scores. A simple linear regression line was fit to the correlation data in GraphPad Prism.
Results
Demographic and Clinical Characteristics
Demographic, behavioral, and clinical characteristics of 289 TBI participants are presented in Table 1. The sample included 61 female and 228 male participants. The significantly different variables between the groups were age, BMI, race, military status, and highest education completed. Female participants were older (median = 42.0 years, IQR = 33.0–54.5) compared to male participants (median = 38.0, IQR = 32.0–47.0) (p = 0.030). Female participants had lower BMI (median = 27.0, IQR 24.0–29.5) compared to male participants (median = 28.0, IQR 26.0–31.0) (p = 0.008). Over half, 36 (59.0%) of female participants were White and 13 (21.3%) were Black or African American, while male participants were predominantly White 183 (80.3%) (p = 0.003). Twenty-three female participants (37.7%) were active duty service members compared to 169 (74.1%) male; 12 (19.7%) female participants reported no prior or current military service compared to 2 (0.9%) male (p < 0.001). Over a third, 24 (39.3%) female participants had graduate degree compared to 63 (27.6%) male participants, while 14 (22.9%) females obtained high school diploma compared to 81 (35.5%) male (p = 0.004). Majority of participants in both groups reported mild TBI (mTBI) using both most recent and most significant events. While most of the females (60.7%) had blunt force TBI, males had reported both blunt and blast TBI (68.4%) (Table 1).
Biomarker Analysis
Female participants had higher levels of GFAP (p = 0.002) and tau (p = 0.013) compared to male participants (Figure 1). After controlling for age, BMI, race, education, and military status in logistic regression models, t-tau (p = 0.018) and GFAP (p = 0.018) remained significantly different between groups (Table 2). NfL did not differ between female and male participants (p = 0.13). Hindered by the small sample size, biomarker data for controls is available in Supplementary Table S2.
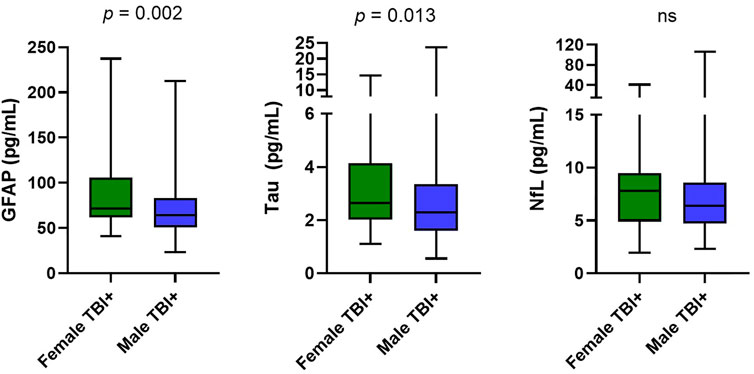
FIGURE 1. Group Differences in biomarkers. Distributions are represented via boxplots, showing median, IQR, and maximum and minimum. Mann-Whitney U results comparing two groups showed significantly higher GFAP (p = 0.002) levels in the female group (median = 71.7, IQR 62.0–106.0) compared to the male group (median = 64.2, IQR 50.7–83.0). Concentrations of t-tau (p = 0.013) were significantly elevated in the female group (median = 2.6, IQR 2.1–4.1) compared to the male group (median = 2.3, IQR 1.6–3.4). NfL in the female group (median = 7.8, IQR 4.9–9.4) did not differ significantly (p = 0.132) from the male group (median = 6.4, IQR 4.7–8.6). Abbreviations: GFAP, Glial fibrillary acidic protein; NfL, Neurofilament light chain; t-tau total tau.
Behavioral Symptoms
We compared behavioral symptoms between female and male participants. PHQ-9 scores were significantly higher in female compared to male participants (p = 0.01). NSI total (p < 0.001), NSI- vestibular (p = 0.002), NSI-somatosensory (p < 0.001), and NSI-affective scores (p = 0.006) were also higher in females. No significant differences were found in NSI-cognitive subscale (Table 1). We also compared HRQOL between the female and male groups. Female participants had lower scores (lower HRQOL) in role limitations due to emotional problems (p = 0.025), vitality (p = 0.024), emotional well-being (p = 0.019), social functioning (p = 0.002), and pain (p = 0.004) compared to male participants (Table 1). There were no differences in SF-36 subscales of physical functioning, role limitations due to physical problems, and general health. We observed no group differences in PCL-C scores. Levels of biomarkers and behavioral symptoms remained significant after removing individuals without military status (Supplementary Table S3). The behavior data for control groups was limited by small sample size in females (n = 22) and is included in Supplementary Table S2.
Correlations Between Biomarkers Levels and Behavioral Symptoms
In females, we observed positive correlations between levels of tau and NSI total (ρ = 0.255, p = 0.047) and NSI-cognitive subscale scores (ρ = 0.263, p = 0.041). Tau was also marginally significantly correlated with NSI somatosensory scores (ρ = 252, p = 0.050). No significant associations were found for NfL/GFAP and the NSI scores. For measures of HRQOL, in the female group, tau (ρ = −0.297, p = 0.020) and NfL (ρ = −0.325, p = 0.011) concentrations negatively correlated with SF-36 subscale of physical functioning. Tau was also inversely correlated with SF-36 subscale of social functioning (ρ = −0.449, p < 0.001) and GFAP had positive association with SF-36 subscale of role limitations due to emotional problems (ρ = 0.365, p = 0.004). No significant associations were observed for measures of behavioral symptoms and HRQOL in the male group (Figure 2, Supplementary Table S4).
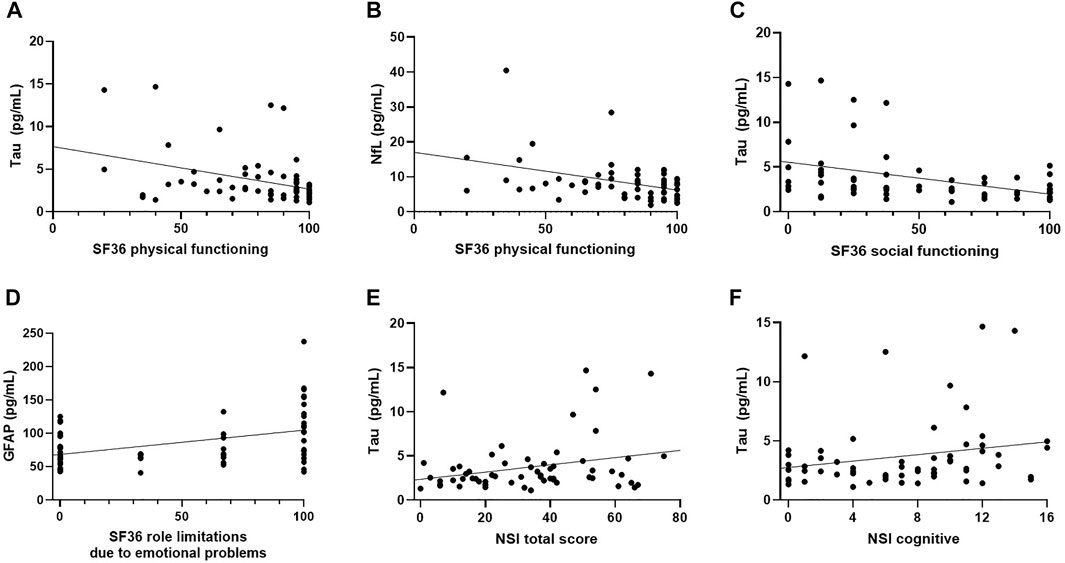
FIGURE 2. Scatter Plots of Significant Correlations in Female TBI Group. (A) T-tau negatively correlated with SF-36 physical functioning (ρ = −0.297, p = 0.020). (B) t-tau negatively correlated with SF-36 social functioning (ρ = −0.449, p < 0.001), (C) NfL negatively correlated with SF-36 physical functioning (ρ = −0.325, p = 0.011), (D) GFAP positively correlated with SF-36 subscale of role limitations due to emotional problems (ρ = 0.365, p = 0.004), (E) t-tau positively correlated with NSI-total (ρ = 0.255, p = 0.047), (F) t-tau had positive association with NSI-cognitive subscale (ρ = 0.263, p = 0.041).
Discussion
To our knowledge, this study is the first to examine levels of circulating biomarkers and symptom scores in the context of sex differences in chronic TBI. In this cross-sectional convenience sample analysis, we found that levels of circulating plasma GFAP and tau were significantly higher in the female group compared to the male group. NfL levels did not differ significantly between groups. Behavioral symptoms of depression, NSI total, NSI-vestibular, NSI-somatosensory, and NSI- affective scores were higher in the female group as well. However, HRQOL of role limitations due to emotional problems, vitality, emotional well-being, social functioning, and pain were higher in the male group indicating better HRQOL. In addition, bivariate correlation results showed positive associations between symptoms, HRQOL scores and levels of GFAP, NfL, and tau.
We observed that levels of GFAP and tau were significantly higher in females. GFAP is an intermediate filament protein found in astrocytes of central nervous system and a marker of reactive astrocytes seen in neurodegenerative disease (Hol and Pekny 2015) and in TBI (Huang et al., 2015; McMahon et al., 2015). Reactive astrocytes can initiate pro-and anti-inflammatory immune mediators, communicate with innate and adaptive immune systems as well as neurons, glia, and vascular cells, and regulate blood-brain barrier in response to TBI (Burda et al., 2016). A recent study suggested that GFAP is a promising biomarker in distinguishing patients with acute mTBI from controls especially when combined with tau and NfL (Gill et al., 2018a). Another study reported that GFAP combined with UCHL-1 has excellent negative predictive value and sensitivity in predicting acute intracranial injuries detected by head computed tomography scans (Bazarian et al., 2018). In subacute and chronic TBI, serum GFAP levels have been shown to be elevated; however in contrast to acute mTBI, these levels were not sensitive in determining injury severity or outcomes (Shahim et al., 2020b). Few clinical studies have examined circulating biomarkers following TBI in relation to sex differences; however, several preclinical investigations have identified sexual dimorphic outcomes. Similar to our study, in mTBI, brain expression of GFAP was found to be elevated in female rats while being significantly lower in male rats (Wright et al., 2017). However, there were no sex differences in repetitive mTBI (Wright et al., 2017) or severe TBI in preclinical models (Rubenstein et al., 2017b).
In addition to GFAP, we also observed sex differences in tau following TBI. Tau is a microtubule-associated protein located on neuronal axons and its dysmetabolism plays a role in a number of neurodegenerative conditions such as Alzheimer’s disease (AD), corticobasal degeneration, as well as TBI (Weingarten et al., 1975; Olivera et al., 2015; Gill et al., 2018b; Didonna 2020). Plasma phosphorylated tau (p-tau) and p-tau-t-tau ratios have been demonstrated to distinguish patients with acute and chronic TBI from healthy controls (Rubenstein et al., 2017b). In chronic mTBI, exosomal tau and p-tau have been shown to be elevated in repetitive mTBI (Kenney et al., 2018). Our findings show that tau is significantly elevated in the female group compared to the male group after controlling for significant covariates. This is similar to findings in sports related concussions, where plasma tau was significantly higher in female athletes up to 3 days after initial injury (Mondello et al., 2020). Early tau depositions in the brain were higher in women measured by positron emission tomography in AD trajectory (Buckley et al., 2019). Higher levels of p-tau were also observed in women versus men among apolipoprotein E e4 (APOE4) carriers (Sundermann et al., 2020). Similarly, in a separate cohort, women had higher tau and p-tau concentrations in early stages of mild cognitive impairment (MCI) and subjective cognitive decline, while among non APOE e4 carriers, women had higher concentrations at the later stages of AD, dementia, and MCI (BabapourMofrad et al., 2020).
While we observed significant changes in GFAP and tau, NfL was not statistically significant between sexes in this report. NfL is 68 kDa subunit of the neurofilaments located on the neuronal cytoplasm which is released in response to CNS axonal damage due to neuroinflammation, neurodegeneration, and/or traumatic or vascular injury (Zetterberg 2016; Gaetani et al., 2019). Serum NfL has been shown to distinguish patients with mTBI, moderate or severe TBI for months and even years after injury (Shahim et al., 2020b). Cerebrospinal fluid NfL and CSF/serum albumin ratio were found to be significantly higher in male aging cohorts of AD (Skillbäck et al., 2021). These studies indicate there are sex differences in the levels of circulating biomarkers and additional studies are needed to corroborate these results.
Another interesting finding is that the female group had higher neurobehavioral and depressive symptom scores and significantly lower scores of HRQOL in role limitations due to emotional problems, vitality, emotional well-being, social functioning, and pain. We did not observe these trends in the male group. These results are consistent with previous reports of women having higher depressive symptoms and stress after mid-to moderate TBI (Bay et al., 2009) and higher likelihood of poor postconcussive symptom scores in mTBI (Bazarian et al., 2010). Our bivariate correlation analysis also showed that tau was positively associated with NSI total, NSI-cognitive, and marginally with NSI-somatosensory, while being negatively correlated with physical and social functioning of HRQOL. NfL also negatively correlated with physical functioning in the female group, indicating that as the concentrations of both tau and NfL increased the score for physical HRQOL functioning decreased. In our previous studies, we found that tau concentrations correlate significantly with postconcussive, post-traumatic, and depressive symptoms (Kenney et al., 2018; Pattinson et al., 2020) and exosomal NfL positively correlated with chronic postconcussive symptoms, PTSD, and depressive symptoms in predominantly male TBI groups (Guedes et al., 2020). In the current study, we also observed higher concentrations of GFAP to be associated with better emotional well-being HRQOL in the female group. While this result reached statistical significance, it is contradictory to the hypothesis that higher GFAP would be associated with lower HRQOL.
A possible mechanism underlying sex differences in TBI might include hormone levels. Several studies implicate the role of hormonal fluctuations during menstrual cycle in symptomatic presentations between sexes (Wunderle et al., 2014; Brown et al., 2015; Sundermann et al., 2020). For example, a study looking at the menstrual cycle phase and levels of progesterone concluded that women during the luteal phase of menstruation have significantly lower QOL metrics compared to women injured during the follicular phase (Wunderle et al., 2014). Lower testosterone levels resulted in higher p-tau concentrations in female APOE4 carriers, suggesting higher testosterone may be inversely related to p-tau levels in AD disease (Sundermann et al., 2020). Although we controlled for age (median age was 42 for women and 38 for men) in our analysis, hormonal fluctuations related to menopause have been documented to affect TBI outcomes. In large retrospective studies, peri- and post-menopausal women (age >50 years of age) had better outcomes and survival rate than men (Davis et al., 2006; Berry et al., 2009).
Another important factor to be considered is the role of gender as a fluid non-binary variable that may impact TBI experiences (Giordano et al., 2020). Societal gender expectations and individual vulnerabilities may affect symptoms and outcomes, coping skills, resources, and ultimately reintegration to society after TBI (Mollayeva et al., 2018). Currently, evidence is lacking on the effects of sex and gender in TBI research (Mollayeva et al., 2018) and often transgender groups are too small to be included in analysis (Giordano et al., 2020). Future clinical studies need to incorporate objective measures to further understand the impact of gender within sex on TBI outcomes.
This study has several limitations. First, our female participants in particular are of limited number. Future studies with larger sample sizes are warranted to corroborate our findings. Second, the injury mechanism of TBI (i.e., blast vs. non-blast TBI) may show a different effect on GFAP and total tau concentration. Future studies should investigate these biomarkers in relation to each injury type. Third, a cross-sectional design with self-reported TBI and symptoms makes it challenging to infer a causal relationship between the biomarkers and behavioral outcomes. Fourth, we did not measure hormonal differences or evaluated menstrual cycle phases. These may be important factors to include in future explorations on sex differences in TBI.
Lastly, the significance of the findings observed in GFAP and Tau between female and male participants in this study are affected by the lack of current clinical reference values for these biomarkers. Though GFAP and UCHL-1 have been shown to have high sensitivity for detection of acute TBI (Bazarian et al., 2018), the establishment of reference values in sex differences in TBI is still novel and clinical reference values are needed in the field. Reference ranges have been established for other biomarkers such as S100 calcium binding protein B (S100B) and neuron-specific enolase (NSE) and have been found to have sex-dependent differences. Specifically, higher s100B was observed in females and higher NSE in males (Hajduková et al., 2015). Several studies published blood-based reference ranges for GFAP and NfL; however, these ranges appear to differ between the studies (van Geel et al., 2002; Mayer et al., 2013). The utility of biomarkers, though promising in diagnostic and prognostic applications is limited by the lack of sex-based reference values. Additional studies are warranted to compare or refute clinical significance.
Despite these study limitations, this analysis shows robust sex differences in the levels of biomarkers and symptom burden in TBI after controlling for significant covariates (age, race, military status, BMI, and education). Our findings indicate that elevated biomarkers may be related to poor behavioral TBI outcomes and may impact overall HRQOL. Future explorations stratified by sex, gender, and age are paramount in delineating individual factors and identifying potential target biomarkers in treatment guidelines.
Data Availability Statement
The original contributions presented in the study are included in the article/Supplementary Files, further inquiries can be directed to the corresponding author.
Ethics Statement
The studies involving human participants were reviewed and approved by the Uniformed Services University of the Health Sciences (USUHS), Fort Belvoir Community Hospital (FBCH), and Walter Reed National Military Medical Center (WRNMMC). The patients/participants provided their written informed consent to participate in this study.
Author Contributions
Original idea, planning and input: DS, VG, and JG; supervision of the project: VG, JG, LS, and MR; Resources: MR and JG; Original Experimental Work: CL, CW, and RV; Technical Experimental Work: CL, CW, and RV; Data Analysis: DS, ES, and VG; Data Curation: KD, CD, JH, and KE; Original Drafting of article: DS, VG, ES, and JG; Review and Editing: DS, ES, VG, RV, SM, KE, JH, KD, CD, CL, CW, DH, LS, MR, and JG.
Funding
This work was supported by the National Institute of Nursing Research Intramural Research Program, National Institutes of Health, Center for Neuroscience and Regenerative Medicine, the Uniformed Services University of the Health Sciences and the Henry M. Jackson Foundation.
Author Disclaimer
Any views or opinions expressed herein are solely those of the authors and are not to be construed as representing official opinions of the U.S. government, the Department of Defense, Uniformed Services University, or the National Institutes of Health.
Conflict of Interest
The authors declare that the research was conducted in the absence of any commercial or financial relationships that could be construed as a potential conflict of interest.
The handling editor declared a past collaboration with several of the authors VG, CL, and JG.
Publisher’s Note
All claims expressed in this article are solely those of the authors and do not necessarily represent those of their affiliated organizations, or those of the publisher, the editors and the reviewers. Any product that may be evaluated in this article, or claim that may be made by its manufacturer, is not guaranteed or endorsed by the publisher.
Acknowledgments
We thank all military and non-military members who participated in the study.
Supplementary Material
The Supplementary Material for this article can be found online at: https://www.frontiersin.org/articles/10.3389/fphar.2021.746491/full#supplementary-material
References
Babapour Mofrad, R., Tijms, B. M., Scheltens, P., Barkhof, F., van der Flier, W. M., SietskeSikkes, A. M., et al. (2020). Sex Differences in CSF Biomarkers Vary by Alzheimer Disease Stage and APOE ε4 Genotype. Neurology 95 (17), e2378–e2388. doi:10.1212/WNL.0000000000010629
Bay, E., Sikorskii, A., and Saint-Arnault, D. (2009). Sex Differences in Depressive Symptoms and Their Correlates after Mild-To-Moderate Traumatic Brain Injury. J. Neurosci. Nurs. 41 (6), 298. doi:10.1097/jnn.0b013e3181b6be81
Bazarian, J. J., Biberthaler, P., Welch, R. D., Lewis, L. M., Barzo, P., Bogner-Flatz, V., et al. (2018). Serum GFAP and UCH-L1 for Prediction of Absence of Intracranial Injuries on Head CT (ALERT-TBI): a Multicentre Observational Study. Lancet Neurol. 17 (9), 782–789. doi:10.1016/S1474-4422(18)30231-X
Bazarian, J. J., Blyth, B., Mookerjee, S., He, H., and McDermott, M. P. (2010). Sex Differences in Outcome after Mild Traumatic Brain Injury. J. Neurotrauma. 27 (3), 527–539. doi:10.1089/neu.2009.1068
Berry, C., LeyLey, E. J., Tillou, A., Cryer, G., Margulies, D. R., and Salim, A. (2009). The Effect of Gender on Patients with Moderate to Severe Head Injuries. J. Trauma 67 (5), 950–953. doi:10.1097/TA.0b013e3181ba3354
Biegon, A. (2021). Considering Biological Sex in Traumatic Brain Injury. Front. Neurol. 12, 1–12. doi:10.3389/fneur.2021.576366
Brown, D. A., Elsass, J. A., Miller, A. J., Reed, L. E., and Reneker, J. C. (2015). Differences in Symptom Reporting between Males and Females at Baseline and after a Sports-Related Concussion: a Systematic Review and Meta-Analysis. Sports Med. 45 (7), 1027–1040. doi:10.1007/s40279-015-0335-6
Buckley, R. F., Mormino, E. C., RabinRabin, J. S., Hohman, T. J., Landau, S., Hanseeuw, B. J., et al. (2019). Sex Differences in the Association of Global Amyloid and Regional Tau Deposition Measured by Positron Emission Tomography in Clinically normal Older Adults. JAMA Neurol. 76 (5), 542–551. doi:10.1001/jamaneurol.2018.4693
Burda, J. E., Bernstein, A. M., and Sofroniew, M. V. (2016). Astrocyte Roles in Traumatic Brain Injury. Exp. Neurol. 275, 305–315. doi:10.1016/j.expneurol.2015.03.020
Center for Disease Control and Prevention, National Center for Injury Prevention and Control Cdc (2021). Health Disparities and TBI. Last Modified May 12, 2021. Available at: https://www.cdc.gov/traumaticbraininjury/health-disparities-tbi.html (Accessed July 7th, 2021).
Davis, D. P., DouglasDouglas, D. J., Smith, W., Sise, M. J., Vilke, G. M., Holbrook, T. L., et al. (2006). Traumatic Brain Injury Outcomes in Pre- and post- Menopausal Females versus Age-Matched Males. J. Neurotrauma 23 (2), 140–148. doi:10.1089/neu.2006.23.140
Diaz-Arrastia, R., Wang, K. K., Papa, L., Sorani, M. D., YuePuccio, J. K., Puccio, A. M., et al. (2014). Acute Biomarkers of Traumatic Brain Injury: Relationship between Plasma Levels of Ubiquitin C-Terminal Hydrolase-L1 and Glial Fibrillary Acidic Protein. J. Neurotrauma 31 (1), 19–25. doi:10.1089/neu.2013.3040
Didonna, A. (2020). Tau at the Interface between Neurodegeneration and Neuroinflammation. Genes Immun. 21 (5), 288–300. doi:10.1038/s41435-020-00113-5
Fann, J. R., Bombardier, C. H., Dikmen, S., Esselman, P., Warms, C. A., Pelzer, E., et al. (2005). Validity of the Patient Health Questionnaire-9 in Assessing Depression Following Traumatic Brain Injury. J. Head Trauma Rehabil. 20 (6), 501–511. doi:10.1097/00001199-200511000-00003
Gaetani, L., Blennow, K., Calabresi, P., Di Filippo, M., Parnetti, L., and Zetterberg, H. (2019). Neurofilament Light Chain as a Biomarker in Neurological Disorders. J. Neurol. Neurosurg. Psychiatry 90 (8), 870–881. doi:10.1136/jnnp-2018-320106
Gill, J., Latour, L., Diaz-Arrastia, R., Motamedi, V., Turtzo, C., Shahim, P., et al. (2018a). Glial Fibrillary Acidic Protein Elevations Relate to Neuroimaging Abnormalities after Mild TBI. Neurology 91 (15), e1385–e1389. doi:10.1212/WNL.0000000000006321
Gill, J., Mustapic, M., Diaz-Arrastia, R., Lange, R., Gulyani, S., Diehl, T., et al. (2018b). Higher Exosomal Tau, Amyloid-Beta 42 and IL-10 Are Associated with Mild TBIs and Chronic Symptoms in Military Personnel. Brain Inj. 32 (11), 1277–1284. doi:10.1080/02699052.2018.1471738
Giordano, K. R., Rojas-Valencia, L. M., Bhargava, V., and Lifshitz, J. (2020). Beyond Binary: Influence of Sex and Gender on Outcome after Traumatic Brain Injury. J. Neurotrauma 37 (23), 2454–2459. doi:10.1089/neu.2020.7230
Guedes, V. A., Kenney, K., Shahim, P., Qu, B. X., Lai, C., Devoto, C., et al. (2020). Exosomal Neurofilament Light: A Prognostic Biomarker for Remote Symptoms after Mild Traumatic Brain Injury? Neurology 94 (23), e2412–e2423. doi:10.1212/WNL.0000000000009577
Gupte, R., Brooks, W., Vukas, R., Pierce, J., and Harris, J. (2019). Sex Differences in Traumatic Brain Injury: what We Know and what We Should Know. J. Neurotrauma 36 (22), 3063–3091. doi:10.1089/neu.2018.6171
Hajduková, L., Sobek, O., Prchalová, D., Bílková, Z., Koudelková, M., Lukášková, J., et al. (2015). Biomarkers of Brain Damage: S100B and NSE Concentrations in Cerebrospinal Fluid—A Normative Study. Biomed. Research International 2015, 1–7. doi:10.1155/2015/379071
Hol, E. M., and Pekny, M. (2015). Glial Fibrillary Acidic Protein (GFAP) and the Astrocyte Intermediate Filament System in Diseases of the central Nervous System. Curr. Opin. Cel Biol. 32, 121–130. doi:10.1016/j.ceb.2015.02.004
Huang, X. J., Glushakova, O., Mondello, S., Van, K., Hayes, R. L., and Lyeth, B. G. (2015). Acute Temporal Profiles of Serum Levels of UCH-L1 and GFAP and Relationships to Neuronal and Astroglial Pathology Following Traumatic Brain Injury in Rats. J. Neurotrauma 32 (16), 1179–1189. doi:10.1089/neu.2015.3873
Kawata, K., Liu, C. Y., Merkel, S. F., Ramirez, S. H., Tierney, R. T., and Langford, D. (2016). Blood Biomarkers for Brain Injury: What Are We Measuring? Neurosci. Biobehav Rev. 68, 460–473. doi:10.1016/j.neubiorev.2016.05.009
Kenney, K., Qu, B. X., Lai, C., Devoto, C., Motamedi, V., Walker, W. C., et al. (2018). Higher Exosomal Phosphorylated Tau and Total Tau Among Veterans with Combat-Related Repetitive Chronic Mild Traumatic Brain Injury. Brain Inj. 32 (10), 1276–1284. doi:10.1080/02699052.2018.1483530
Kroenke, K., Spitzer, R. L., and Williams, J. B. (2001). The PHQ-9: Validity of a Brief Depression Severity Measure. J. Gen. Intern. Med. 16 (9), 606–613. doi:10.1046/j.1525-1497.2001.016009606.x
Mayer, C. A., Brunkhorst, R., Niessner, M., Pfeilschifter, W., Steinmetz, H., and Foerch, C. (2013). Blood Levels of Glial Fibrillary Acidic Protein (GFAP) in Patients with Neurological Diseases. PloS one 8 (4), e62101. doi:10.1371/journal.pone.0062101
McMahon, P. J., Panczykowski, D. M., Yue, J. K., PuccioPuccio, A. M., Inoue, T., Sorani, M. D., et al. (2015). Measurement of the Glial Fibrillary Acidic Protein and its Breakdown Products GFAP-BDP Biomarker for the Detection of Traumatic Brain Injury Compared to Computed Tomography and Magnetic Resonance Imaging. J. Neurotrauma 32 (8), 527–533. doi:10.1089/neu.2014.3635
Merritt, V. C., Padgett, C. R., and JakJak, A. J. (2019). A Systematic Review of Sex Differences in Concussion Outcome: what Do We Know? Clin. Neuropsychol. 33 (6), 1016–1043. doi:10.1080/13854046.2018.1508616
Mollayeva, T., Mollayeva, S., and Colantonio, A. (2018). Traumatic Brain Injury: Sex, Gender and Intersecting Vulnerabilities. Nat. Rev. Neurol. 14 (12), 711–722. doi:10.1038/s41582-018-0091-y
Mondello, S., Guedes, V. A., Lai, C., Jeromin, A., Bazarian, J. J., and Gill, J. M. (2020). Sex Differences in Circulating T-Tau Trajectories after Sports-Concussion and Correlation with Outcome. Front. Neurol. 11, 651. doi:10.3389/fneur.2020.00651
Olivera, A., Lejbman, N., Jeromin, A., French, L. M., Kim, H. S., Cashion, A., et al. (2015). Peripheral Total Tau in Military Personnel Who Sustain Traumatic Brain Injuries during Deployment. JAMA Neurol. 72 (10), 1109–1116. doi:10.1001/jamaneurol.2015.1383
Pattinson, C. L., Shahim, P., Taylor, P., Dunbar, K., Guedes, V. A., Motamedi, V., et al. (2020). Elevated Tau in Military Personnel Relates to Chronic Symptoms Following Traumatic Brain Injury. J. Head Trauma Rehabil. 35 (1), 66–73. doi:10.1097/HTR.0000000000000485
Rubenstein, R., Chang, B., Grinkina, N., Drummond, E., Davies, P., Ruditzky, M., et al. (2017a). Tau Phosphorylation Induced by Severe Closed Head Traumatic Brain Injury Is Linked to the Cellular Prion Protein. Acta Neuropathol. Commun. 5 (1), 30–17. doi:10.1186/s40478-017-0435-7
Rubenstein, R., Chang, B., Yue, J. K., Chiu, A., Winkler, E. A., PuccioPuccio, A. M., et al. (2017b). Comparing Plasma Phospho Tau, Total Tau, and Phospho Tau-Total Tau Ratio as Acute and Chronic Traumatic Brain Injury Biomarkers. JAMA Neurol. 74 (9), 1063–1072. doi:10.1001/jamaneurol.2017.0655
Ruggiero, K. J., Del Ben, K., Scotti, J. R., and RabalaisRabalais, A. E. (2003). Psychometric Properties of the PTSD Checklist-Civilian Version. J. Trauma Stress 16 (5), 495–502. doi:10.1023/A:1025714729117
Shahim, P., Politis, A., van der Merwe, A., Moore, B., Chou, Y. Y., and Pham, D. L (2020a). Neurofilament Light as a Biomarker in Traumatic Brain Injury. Neurology 95 (6), e610–e622. doi:10.1212/WNL.0000000000009983
Shahim, P., Politis, A., van der Merwe, A., Moore, B., Ekanayake, V., Lippa, S. M., et al. (2020b). Time Course and Diagnostic Utility of NfL, Tau, GFAP, and UCH-L1 in Subacute and Chronic TBI. Neurology 95 (6), e623–e636. doi:10.1212/WNL.0000000000009985
Silva, M. A. (2020). Review of the Neurobehavioral Symptom Inventory. Rehabil. Psychol. 66, 170–182. doi:10.1037/rep0000367
Skillbäck, T., Blennow, K., Zetterberg, H., Shams, S., Machado, A., Pereira, J., et al. (2021). Sex Differences in CSF Biomarkers for Neurodegeneration and Blood‐brain Barrier Integrity. Alzheimer's Demen. Diagn. Assess. Dis. Monit. 13 (1), e12141. doi:10.1002/dad2.12141
Strathmann, F. G., Schulte, S., Goerl, K., Petron, D. J., and Petron, D. J. (2014). Blood-based Biomarkers for Traumatic Brain Injury: Evaluation of Research Approaches, Available Methods and Potential Utility from the Clinician and Clinical Laboratory Perspectives. Clin. Biochem. 47 (10-11), 876–888. doi:10.1016/j.clinbiochem.2014.01.028
Sundermann, E. E., Panizzon, M. S., Chen, X., Andrews, M., Galasko, D., and Banks, S. J. (2020). Sex Differences in Alzheimer's-Related Tau Biomarkers and a Mediating Effect of Testosterone. Biol. Sex. Differ. 11 (1), 33–10. doi:10.1186/s13293-020-00310-x
The Management of Concussion Mild TBI Working Group (2009). VA/DoD Clinical Practice Guideline for Management of Concussion/mild Traumatic Brain Injury. J. Rehabil. Res. Dev. 46 (6), 1–60.
van Geel, W. J., de Reus, H. P., Nijzing, H., Verbeek, M. M., Vos, P. E., Lamers, K. J., et al. (2002). Measurement of Glial Fibrillary Acidic Protein in Blood: an Analytical Method. Clin. Chim. Acta 326 (1-2), 151–154. doi:10.1016/s0009-8981(02)00330-3
Ware, J. E., Kosinski, M., and Keller, S. (2001). SF-36 Physical and Mental Health Summary Scales: A User's Manual:1994. Boston, MA: Health Assessment Lab, New England Medical Center.
Ware, , and John, E. (2000). SF-36 Health Survey Update. Spine (Phila Pa 1976) 25 (24), 3130–3139. doi:10.1097/00007632-200012150-00008
Weingarten, M. D., Lockwood, A. H., Hwo, S. Y., and Kirschner, M. W. (1975). A Protein Factor Essential for Microtubule Assembly. Proc. Natl. Acad. Sci. U S A. 72 (5), 1858–1862. doi:10.1073/pnas.72.5.1858
Wright, D. K., O'BrienO'Brien, T. J., Shultz, S. R., and Mychasiuk, R. (2017). Sex Matters: Repetitive Mild Traumatic Brain Injury in Adolescent Rats. Ann. Clin. Transl Neurol. 4 (9), 640–654. doi:10.1002/acn3.441
Wunderle, K., Hoeger, K. M., Wasserman, E., and Bazarian, J. J. (2014). Menstrual Phase as Predictor of Outcome after Mild Traumatic Brain Injury in Women. J. Head Trauma Rehabil. 29 (5), E1–E8. doi:10.1097/HTR.0000000000000006
Keywords: biomarkers, traumatic brain injury, sex, behavioral symptoms, gender
Citation: Sass D, Guedes VA, Smith EG, Vorn R, Devoto C, Edwards KA, Mithani S, Hentig J, Lai C, Wagner C, Dunbar K, Hyde DR, Saligan L, Roy MJ and Gill J (2021) Sex Differences in Behavioral Symptoms and the Levels of Circulating GFAP, Tau, and NfL in Patients With Traumatic Brain Injury. Front. Pharmacol. 12:746491. doi: 10.3389/fphar.2021.746491
Received: 23 July 2021; Accepted: 08 November 2021;
Published: 26 November 2021.
Edited by:
Stefania Mondello, University of Messina, ItalyReviewed by:
Damir Janigro, Case Western Reserve University, United StatesAlexander V. Glushakov, University of Florida, United States
Copyright © 2021 Sass, Guedes, Smith, Vorn, Devoto, Edwards, Mithani, Hentig, Lai, Wagner, Dunbar, Hyde, Saligan, Roy and Gill. This is an open-access article distributed under the terms of the Creative Commons Attribution License (CC BY). The use, distribution or reproduction in other forums is permitted, provided the original author(s) and the copyright owner(s) are credited and that the original publication in this journal is cited, in accordance with accepted academic practice. No use, distribution or reproduction is permitted which does not comply with these terms.
*Correspondence: Jessica Gill, jessicagill@jhu.edu