- 1Department of Pharmacology, Faculty of Medicine, Universiti Kebangsaan Malaysia, Kuala Lumpur, Malaysia
- 2Department of Biomedical Sciences and Therapeutics, Faculty of Medicine and Health Sciences, Universiti Malaysia Sabah, Kota Kinabalu, Malaysia
Background: Hypertension is a major risk factor for cardiovascular disease (CVD), which is the number one cause of global mortality. The potential use of natural products to alleviate high blood pressure has been demonstrated to exert a cardioprotective effect. Centella asiatica (L.) Urb. belongs to the plant family Apiaceae (Umbelliferae). It contains a high amount of triterpenoid and flavonoid that have antioxidant properties and are involved in the renin-angiotensin-aldosterone system which is an important hormonal system for blood pressure regulation.
Objective: This study aimed to investigate the effects of C. asiatica ethanolic extract on blood pressure and heart in a hypertensive rat model, which was induced using oral N(G)-nitro-l-arginine methyl ester (l-NAME).
Methods: Male Sprague-Dawley rats were divided into five groups and were given different treatments for 8 weeks. Group 1 only received deionized water. Groups 2, 4, and 5 were given l-NAME (40 mg/kg, orally). Groups 4 and 5 concurrently received C. asiatica extract (500 mg/kg, orally) and captopril (5 mg/kg, orally), respectively. Group 3 only received C. asiatica extract (500 mg/kg body weight, orally). Systolic blood pressure (SBP) was measured at weeks 0, 4, and 8, while serum nitric oxide (NO) was measured at weeks 0 and 8. At necropsy, cardiac and aortic malondialdehyde (MDA) contents, cardiac angiotensin-converting enzyme (ACE) activity, and serum level of brain natriuretic peptide (BNP) were measured.
Results: After 8 weeks, the administrations of C. asiatica extract and captopril showed significant (p < 0.05) effects on preventing the elevation of SBP, reducing the serum nitric oxide level, as well as increasing the cardiac and aortic MDA content, cardiac ACE activity, and serum brain natriuretic peptide level.
Conclusion: C. asiatica extract can prevent the development of hypertension and cardiac damage induced by l-NAME, and these effects were comparable to captopril.
Introduction
Cardiovascular disease (CVD) remains the number one cause of global mortality with hypertension contributes to the major risk factor (World Health Organization, 2017). Hypertension promotes mechanical stress that induces cardiomyocyte hypertrophy, apoptosis, and remodeling. Subsequently, the heart can change its size, shape, structure, and function with a consequent cardiac dysfunction (Nadruz, 2015). There is growing evidence showing that the development of hypertension and cardiac damage is associated with elevation of oxidative stress status, suppression of nitric oxide (NO) synthesis in the vasculature, and overactivation of the renin-angiotensin-aldosterone system (RAAS) (Schulz et al., 2011; Münzel et al., 2017; Bakogiannis et al., 2019; Wunpathe et al., 2020).
Elevation of oxidative stress is largely attributable to excessive production of reactive oxygen species (ROS) such as superoxide, hydrogen peroxide, and hydroxyl radicals, which are toxic byproducts of human aerobic metabolism (Sedeek et al., 2009). Importantly, an increase in oxidative stress profile induces endothelial dysfunction, inflammatory processes, and vascular smooth muscle tone (Touyz and Briones, 2011). Meanwhile, NO that is produced in endothelial tissues, activates soluble guanylate cyclase to produce 3′,5′-cyclase guanosine monophosphate (cGMP), which has an important role in vasodilatory processes, inhibition of platelet adhesion, and smooth muscle proliferation (Zhou et al., 2004). This vasodilatory action reduces vascular resistance and blood circulatory pressure, which eventually contributes to a lower risk of hypertension. As expected, vascular NO deficiency is a major finding in dysfunctional endothelium and arterial hypertension (Lüscher and Vanhoutte, 1986; Panza et al., 1990). RAAS is an important neurohormonal system that involves the regulation of blood pressure and tissue perfusion. Angiotensin-converting enzyme (ACE) is one of the RAAS components and it converts angiotensin I (AT-I) into angiotensin II (AT-II). If this hormonal system is overactivated, AT-II will be released excessively and chronically, leading to vasoconstriction, aldosterone secretion, activation of the sympathetic nervous system, anti-natriuretic mechanism, and hypertension. Besides that, AT-II can be produced locally in the heart, primarily induced by an increase in cardiac wall stress. The binding of AT-II to angiotensin II type I receptor (AT1R) stimulates the formation of heart collagen by fibroblast cells, which subsequently increases the risk for cardiac hypertrophy, fibrosis, and cardiomyocyte apoptosis (Singh et al., 2008; Dai et al., 2011; Jia et al., 2012). For instance, AT-II infusion for 2 weeks induced cardiac hypertrophy and elevated oxidative stress markers in rat cardiac tissue (Kakishita et al., 2003).
Despite a variety of anti-hypertensive agents such as ACE inhibitors, angiotensin II receptor blockers (ARB), β-adrenergic receptor blocker, and calcium channel antagonists are being used in the treatment of hypertension and cardiac hypertrophy, the progression of myocardial injury and subsequent cardiac dysfunction remains a major problem in chronic hypertensive subjects (Eirin et al., 2014). There has been considerable interest in the potential use of natural products to alleviate blood pressure, which has been demonstrated to exert a cardioprotective effect. One of them is Centella asiatica (L.) Urb., which is also known as Indian pennywort, abundantly found in tropical countries including China, India, and the South-East Asian countries (Brinkhaus et al., 2000). Apart from fever and wound healing, this medicinal plant was used traditionally to treat stomach upset, leprosy, bladder inactivity, and urinary tract infection (Brinkhaus et al., 2000). Triterpenoid compounds such as asiatic acid, madecassic acid, asiaticoside, and madecassoside are the major biologically active compounds in C. asiatica (James and Dubery, 2009). Other compounds isolated from this plant are flavonoids, brahmoside, brahminoside, glycosides isothankuniside, and thankuniside (Azerad, 2016). In modern medicine, C. asiatica has been reported to have high antioxidant property due to its abundant triterpenoid and flavonoid contents (Pittella et al., 2009). The herb and its bioactive compounds may have a role in therapeutic application in diseases associated with oxidative stress, such as hypertension and cardiac failure. Studies using triterpenoid- and flavonoid-rich chloroform fractions of C. asiatica in a hypertensive rat model showed the effectiveness of the extract in reducing the acute rise in blood pressure with a single dose (Harwoko and Nugroho, 2014; Nansy et al., 2015). In addition, asiatic acid, one of the main triterpenoid compounds in C. asiatica, was reported to prevent RAAS activation in metabolic syndrome rats (Maneesai et al., 2016a). Furthermore, flavonoid-rich fruits such as apple and kiwi inhibited ACE activity in some in vitro studies (24, 25), suggesting similar potential cardiovascular benefits offered by C. asiatica.
Although previous in vivo studies have reported the anti-hypertensive effect of C. asiatica (Harwoko and Nugroho, 2014; Nansy et al., 2015), those studies were carried out either in a single dosage of C. asiatica extract or by bioactive compounds isolated from the plant. To date, there has been no studies that investigate the effects of C. asiatica extract on chronic hypertension and cardiac damage. Therefore, to bridge the research gap, this study was conducted to explore the blood pressure-lowering and cardioprotective effects of C. asiatica in chronic hypertensive rats. N(G)-nitro-l-arginine methyl (l-NAME) was administered to induce systemic hypertension and cardiac damage in rats.
Materials and Methods
Drugs, Chemicals and Kits
l-NAME and other chemicals were obtained from Sigma-Aldrich (St. Louis, MO, United States) unless stated otherwise. Captopril tablet was purchased from Y.S.P Industries (Selangor, Malaysia). The kits for cardiac ACE activity and serum brain natriuretic peptide (BNP) level measurements were purchased from Elabscience (Wuhan, China).
Plant Material and Extraction
The fresh leaves of C. asiatica were collected in February 2020 from Kepala Batas, Pulau Pinang, Malaysia. The specimen was identified by a botanist in Universiti Kebangsaan Malaysia Herbarium and a voucher specimen of the plant (UKMB 40434) was deposited at that institute. The cold extraction method was employed using the maceration technique. A 1 kg of the dried leaves was soaked in 4 L of 80% ethanol and agitated on a shaker at 16 rpm for 24 h. The extracts were filtered and the residual sample was soaked again two more times using fresh ethanol, making a total of 12 L. The combined extracts were concentrated and freed of solvent using a rotary evaporator, then dried in a freeze-dryer. The yield percentage of the ethanolic extract was 16.6%.
Animals
Two-month-old male Sprague-Dawley rats (200–250 g) were obtained from Laboratory Animal Resources Unit, Universiti Kebangsaan Malaysia. They were maintained at room temperature (27 ± 2°C) with natural light at the Laboratory Animal Center, Department of Pharmacology, Universiti Kebangsaan Malaysia. All procedures were complied with the standards for the care and use of experimental animals and were approved by the Animal Ethics of Universiti Kebangsaan Malaysia (FAR/PP/2019/NORLIZA/30-OCT./1048-OCT.2019-AUG.-2020). Rats were fed with a commercial diet (Gold Coin Feed-mills (M) Sdn Bhd, Selangor, Malaysia) and tap water ad libitum.
Experimental Protocol
Thirty rats were randomly divided into five groups (n = six rats/group). At a baseline, rat blood was taken via cardiac puncture after being given ketamine (0.1 ml/100 g body weight) and xylazine (0.01 ml/100 g body weight) via intraperitoneal injection as anesthesia. Groups 2, 4, and 5 were given l-NAME (40 mg/kg body weight, orally) for 8 weeks. Groups 4 and 5 concurrently received C. asiatica extract (500 mg/kg body weight, orally) and captopril (5 mg/kg, orally), respectively. Groups 1 and 3 only received deionized water and C. asiatica extract (500 mg/kg, orally body weight) respectively. The dose of the extract was selected based on a pilot study (Supplementary Material). All treatments were given every day for 8 weeks via oral gavage. Body weight was measured at the baseline and after the study ended. After 8 weeks of study, blood was taken via cardiac puncture, and all animals were sacrificed. Heart and aortic tissues were harvested after completing the study for biochemical analysis.
Indirect Measurement of Blood Pressure in Conscious Rats
Animal systolic blood pressure (SBP) was measured at baseline, fourth week and eighth week of study using non-invasive tail-cuff plethysmography (Kent Scientific Cooperation, Torrington, US). In brief, conscious rats were placed in a restrainer and allowed to be calm before SBP measurement. The rat tail was placed inside the tail-cuff, and the cuff was automatically inflated and released. For each rat, five stable SBP readings were chosen and averaged from each cycle.
Biochemical Analysis
Serum nitric oxide (NO) level was measured using Griess reagent against sodium nitrite standard curve. It was expressed as the percentage difference between weeks 0 and 8. Cardiac and aortic malondialdehyde (MDA) contents were measured as thiobarbituric acid reactive substance (TBARS) according to the method of Ledwozyw et al. (1986). Malondialdehyde tetraethyl acetal was used as the standard and the TBARS content was expressed as MDA per protein (μmol/mg). The protein content was determined based on the method described by Bradford (1976). Cardiac ACE activity and serum BNP level were determined using a commercial kit (Elabscience, Wuhan, China) based on the sandwich enzyme immunoassay principle.
Statistical Analysis
All results were expressed as mean ± standard error of the mean (SEM). The data were analyzed for normality test using the Shapiro-Wilk test. Analysis of variance (ANOVA) with Bonferroni posthoc test was used for the SBP parameter. The differences between the groups for body weight, NO, MDA, BNP, and ACE parameters were compared using one-way ANOVA with Tukey’s Honestly Significant Differences posthoc test. Statistical significance was defined as p < 0.05. Statistical analyses were conducted using the Statistical Product for Social Science 23 software (SPSS Inc., Chicago, IL).
Results
Body Weight
There was no significant difference (p > 0.05) in body weight among the rat groups at the baseline (Figure 1). After 8 weeks of treatment, there was a significant increase (p < 0.05) in body weight seen in all groups compared to the baseline. However, group 2 (366.17 ± 5.04 g) demonstrated a significantly lower (p < 0.05) body weight when compared to the control group (391.17 ± 2.09 g).
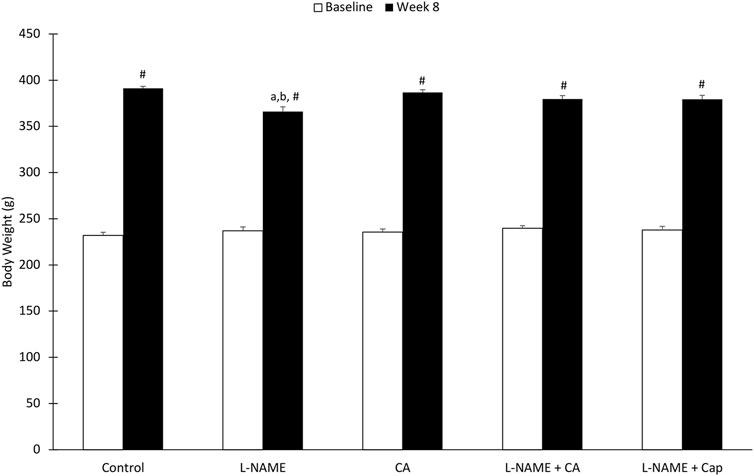
FIGURE 1. Rat body weight before and after administration of l-NAME (40 mg/kg orally) and C. asiatica extract (500 mg/kg orally) or captopril (5 mg/kg orally) during the 8-weeks of study. The values represent mean ± SEM (n = 6). #versus baseline (p < 0.05), aversus control (p < 0.05), bversus C. asiatica (p < 0.05).
Systolic Blood Pressure
SBP was significantly elevated (p < 0.05) in group 2 (148.50 ± 0.64 mmHg) with chronic l-NAME exposure when compared with the control group (group 1) after 8 weeks (127.22 ± 0.99 mmHg) (Figure 2). The increase in SBP induced by l-NAME was attenuated in groups 4 and 5 given C. asiatica (131.78 ± 1.60 mmHg) and captopril (130.72 ± 0.83 mmHg), respectively. No significant difference (p > 0.05) was noted between these two groups. C. asiatica had no effect (p > 0.05) on SBP in C. asiatica control rats of group 3 (127.83 ± 1.46 mmHg).
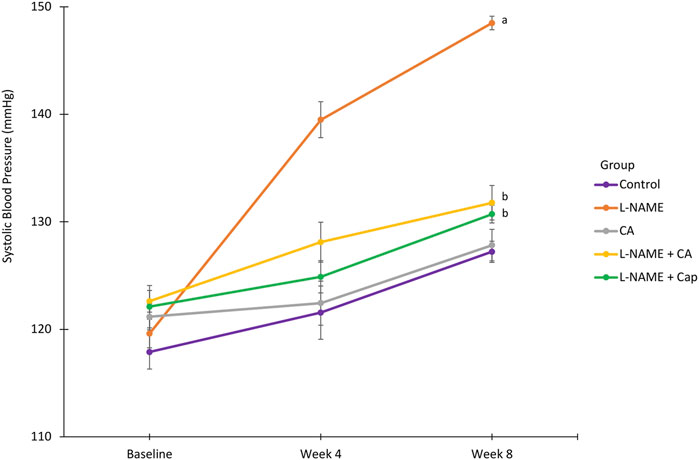
FIGURE 2. Systolic blood pressure in rats treated with concurrent l-NAME (40 mg/kg orally) and C. asiatica (500 mg/kg orally) or captopril (5 mg/kg orally). Line graph represent mean ± SEM (n = 6). aversus control (p < 0.05), bversus l-NAME (p < 0.05).
Serum Nitric Oxide Level
A significant (p < 0.05) reduction in serum NO was seen in the group 2 (−18.68 ± 1.81% mmHg) after 8 weeks compared to the control of group 1 (−1.94 ± 1.54% mmHg) (Figure 3). Concurrent treatment of l-NAME administered in groups 4 and 5 with C. asiatica (−6.65 ± 0.92% mmHg) and captopril (−7.33 ± 0.69% mmHg), respectively had significantly (p < 0.05) prevented the NO reduction induced by l-NAME.
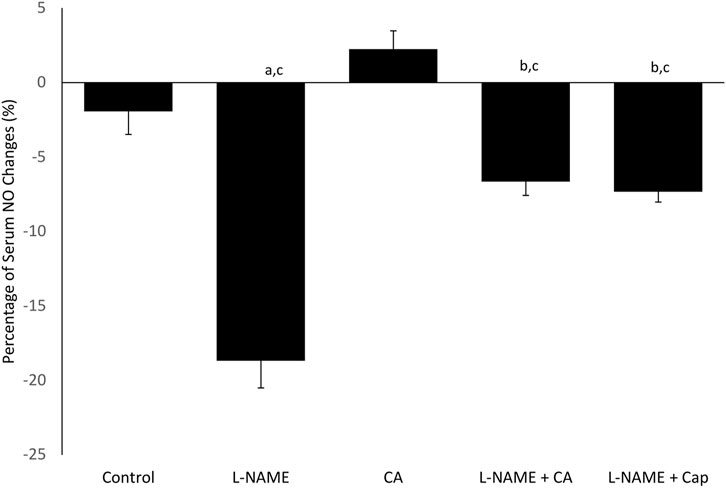
FIGURE 3. Percentage of plasma nitric oxide (NO) change in rats given C. asiatica extract (500 mg/kg orally) and captopril (5 mg/kg orally) together with l-NAME (40 mg/kg orally) for 8 weeks. Bars represent mean ± SEM (n = 6). aversus control (p < 0.05), bversus l-NAME (p < 0.05), cversus C. asiatica (p < 0.05).
Cardiac Angiotensin-Converting Enzyme Activity
The ACE activity in the heart was significantly (p < 0.05) elevated in the group 2 (7.26 ± 0.25 μg/mg) as compared to the control of group 1 (4.11 ± 0.23 μg/mg). The elevation was inhibited by the concurrent treatment of C. asiatica (4.90 ± 0.47 μg/mg) and captopril (3.58 ± 0.30 μg/mg) in groups 4 and 5, respectively (Figure 4). No significant (p > 0.05) difference in cardiac ACE activity was observed among groups 1, 2, and 5.
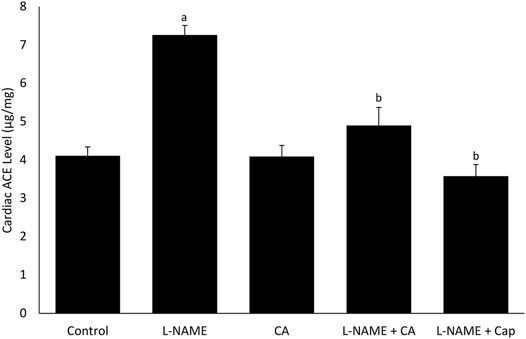
FIGURE 4. Cardiac angiotensin-converting enzyme (ACE) activity in rats treated with C. asiatica extract (500 mg/kg orally) or captopril (5 mg/kg orally) together with l-NAME (40 mg/kg orally) for 8 weeks. Bars represent mean ± SEM (n = 6). aversus control (p < 0.05), bversus l-NAME (p < 0.05).
Cardiac and Aortic Thiobarbituric Acid Reactive Substance Content
l-NAME administration in group 2 for 8 weeks significantly (p < 0.05) increased cardiac and aortic TBARS content when compared to the control group (group 1) (Figures 5A,B). Treatments with C. asiatica and captopril in groups 4 and 5, respectively had similarly prevented the elevation of TBARS content induced by l-NAME in both heart and aorta.
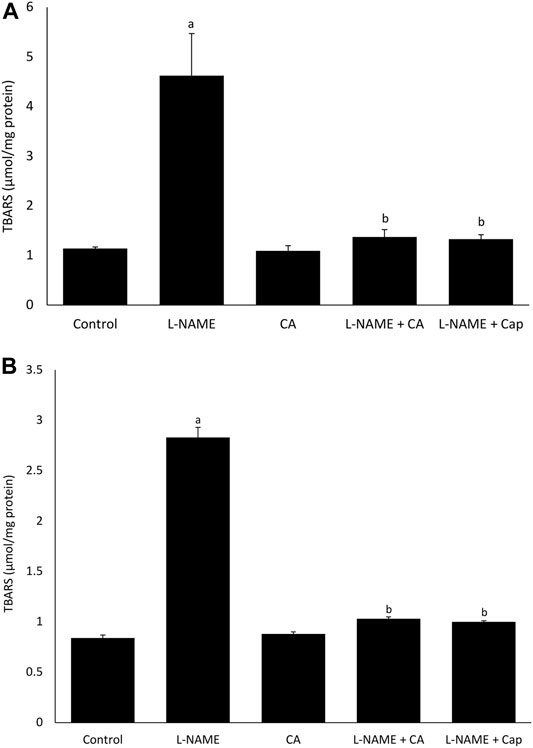
FIGURE 5. The effects of C. asiatica (500 mg/kg orally) and captopril (5 mg/kg orally) on (A) aortic and (B) cardiac TBARS content in l-NAME-administered rats (40 mg/kg orally) after 8 weeks. Bars represent mean ± SEM (n = 6). aversus control (p < 0.05), bversus l-NAME (p < 0.05).
Serum Brain Natriuretic Peptide Level
Serum BNP level was significantly (p < 0.05) higher in group 2 (652.42 ± 20.79 pg/ml) receiving l-NAME for 8 weeks than the control group 1 (373.56 ± 30.59 pg/ml) (Figure 6). Treatments with C. asiatica (398.88 ± 28.17 pg/ml) and captopril (353.27 ± 21.18 pg/ml) in groups 4 and 5, respectively had similarly prevented the rise in the serum BNP level.
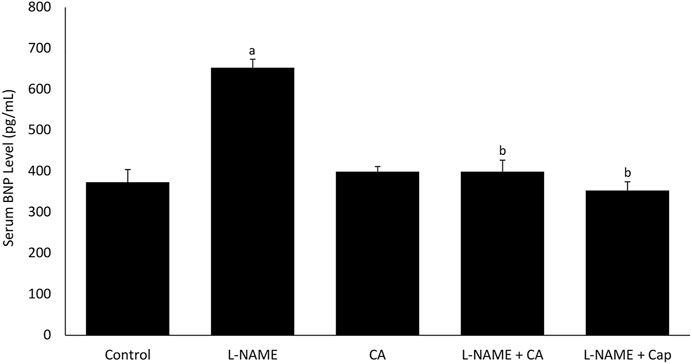
FIGURE 6. Serum B-natriuretic peptide (BNP) level in rats co-treated with l-NAME (40 mg/kg orally) and C. asiatica extract (500 mg/kg orally) or captopril (5 mg/kg orally) for 8 weeks. Bars represent mean ± SEM (n = 6). aversus control (p < 0.05), bversus l-NAME (p < 0.05).
Discussions
The present study exhibited the effects of ethanolic extract of Centella asiatica (L.) Urb. on the cardiovascular system in l-NAME-induced hypertensive rats as summarized in Table 1. Ethanol extraction was chosen because it was proven to produce a high yield of flavonoid (Andarwulan et al., 2010; Nansy et al., 2015) and triterpenoids such as asiatic acid, asiaticoside, madecassic acid, and madecacosside (Hashim et al., 2011). Nansy et al. (2015) and Harwoko and Nugroho (2014) showed that triterpenoid and flavonoid-rich fractions isolated from ethanolic extract of C. asiatica and administered at bolus dose, respectively, reduced blood pressure in phenylephrine-induced hypertensive rats. Asiatic acid, another bioactive compound in C. asiatica, had been shown to exert significant anti-hypertensive effect in numerous types of hypertensive rat model (Bunbupha et al., 2014; Maneesai et al., 2016a; Maneesai et al., 2017). The ethanolic extract was also reported to possess cardioprotective benefit in myocardial infarction (Pragada et al., 2004) in addition to its high antioxidant activities (Muchtaromah et al., 2016). Nevertheless, we could not find any literature on the cardioprotective ability of C. asiatica extracted using other methods.
In the current study, we used l-NAME to induce chronic hypertension in our rats. It appeared that this compound had a detrimental effect on the rat’s growth as evidence by the reduction in body weight. Chronic l-NAME administration was shown to attenuate weight gain in normal and high-fat diet-fed rats, possibly due to decreased food intake in the l-NAME group (Tsuchiya et al., 2007). Nonetheless, concomitant treatment with C. asiatica reversed the effect in the present study. This herbal extract was capable of overcoming this noxious effect on weight gain, conceivably due to its high flavonoid content, which contributed to lower energy consumption and a more efficient digestive system (Ke et al., 2015). The safety of C. asiatica consumption was proven in an acute toxicity testing, in which C. asiatica extract at the maximum dose of 2000 mg/kg body weight showed no toxic signs and mortality in rats. In a subacute toxicity testing, there was no significant alteration in body weight, general health, and food intake in rats after receiving C. asiatica extract ranged from 10 to 1,000 mg/kg/day for 90 days in comparison to the control group (Kumari et al., 2016).
The paradigm of using l-NAME to establish experimental hypertension has become a widely accepted method for testing anti-hypertensive drugs. Our observation that chronic eNOS inhibition is responsible for the development of hypertension, which is also supported by other studies (Sung et al., 2013; Bunbupha et al., 2014). l-NAME is a potent inhibitor of eNOS that depreciates NO synthesis in cells. In blood vessels, it diminishes vascular relaxation due to a reduction in the availability of NO, a potent vasodilator. As expected, group 2 administered with l-NAME alone demonstrated a greater reduction in NO at the end of the study. This led to an increase in blood pressure, as similarly reported by Aluko et al. (2019) and Berkban et al. (2015). However, concomitant treatment of C. asiatica for 8 weeks managed to restore the serum NO level and eventually prevented the elevation of blood pressure, despite the chronic l-NAME exposure (Figure 7). NO promotes vasodilation via induction of soluble guanylate cyclase and increases cyclic guanosine monophosphate (cGMP) in smooth muscle cells (Capettini et al., 2010; Förstermann and Sessa, 2012). Previous evidence suggested that a high content of asiatic acid in C. asiatica might contribute to the normalization of serum NO level and blood pressure, as reported in a range of different experimental models of hypertension including metabolic syndrome, renovascular, and l-NAME-induced hypertension (Bunbupha et al., 2014; Maneesai et al., 2016a; Maneesai et al., 2017). Asiatic acid also upregulated eNOS protein expression and ameliorated systemic vasodilation in these studies, further explaining the anti-hypertensive effects of C. asiatica on chronic NO deficiency induced by l-NAME.
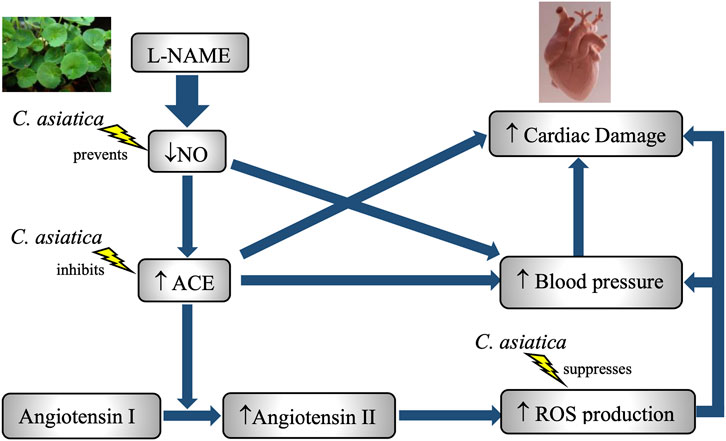
FIGURE 7. A schematic diagram on the role of NO deficiency in l-NAME-induced hypertension and cardiotoxicity in rats. Treatment with C. asiatica extract exhibited anti-hypertensive and cardioprotective effects via the enhancement of NO bioavailability, the suppression of RAAS and amelioration of oxidative stress status in l-NAME-treated group.
Excessive lipid peroxidation alarms a weak antioxidant defense and subsequent oxidative damage that increases the oxidative status, resulting from overproduction of ROS (Yu, 1994). Oxidative stress is a pro-hypertensive factor that aggravates endothelial dysfunction and enhances vasoconstriction, which together contributes to increasing systemic vascular resistance that results in BP elevation (Sorriento et al., 2018). MDA is a product of the reaction between lipid bilayer of membrane plasma with ROS (Samhan-Arias et al., 2011), which can react with thiobarbituric acid to produce TBARS. In the l-NAME-induced hypertensive rat, increased TBARS level in the aorta indicated a worsening oxidative stress profile, which was observed similarly in other studies (Rajeshwari et al., 2014; Kamisah et al., 2017). Endogenous NO suppresses vascular p47phox protein expression and superoxide production, which contribute to the source of vascular oxidative stress that initiates vascular inflammation and dysfunction (Harrison et al., 2010). Reduced bioavailability of NO may underlie the development of oxidative stress-related hypertension in the present study. Besides, a chronic NO inhibition promotes a higher expression of NADPH-dependent oxidase in aortic smooth muscle cells and a subsequent exacerbation in vascular superoxide (O2−) formation via excessive production of AT-II (Touyz and Schiffrin, 1999; Giani et al., 2014).
The augmented generation of ROS by l-NAME was overcome by C. asiatica in this study based on the low content of TBARS in aortic tissue, which was comparable to that of the control group. Our finding is in line with previous literature that demonstrates the antioxidant property of C. asiatica extract (Pittella et al., 2009; Kumari et al., 2016). The astounding ROS scavenging ability of this herb is also apparent in various models of organ injury (Wei et al., 2013; Pakdeechote et al., 2014). In a study by Bunbupha et al. (2014), asiatic acid was demonstrated to inhibit the overproduction of O2− in aortic tissue and plasma MDA via downregulation of p47phox expression, which subsequently restored the vascular function and improved the hemodynamic parameters. A similar finding was also reported by Maneesai et al. (2016a) showing that anti-hypertensive effects of asiatic acid in the metabolic syndrome model were contributed by its antioxidant and anti-inflammatory properties. Besides, protection against oxidative damage in cells is mainly depending on the scavenging enzymes, which break down free radicals including superoxide and hydroxyl, and subsequently improve the oxidative stress status (Robaczewska et al., 2016; Ahmadinejad et al., 2017). C. asiatica extract can ward off the free radical excess via enhancement of free radical scavenging enzymes including catalase, superoxide dismutase (SOD), glutathione peroxidase (GPx), and glutathione-S-transferase (GST), which attenuate the superoxide production, ameliorate the vascular oxidative stress, and reverse the free radical-induced cellular damage (Tabassum et al., 2013). NO can react with O2− to produce peroxynitrite (ONOO−), thus mitigate the biological effect of O2− (Pryor and Squadrito, 1995). Our current work showed an inverse relationship between NO and ROS level in C. asiatica-treated group, suggesting that this herbal extract reduced the ROS level via upregulation of NO bioavailability.
BNP is a biomarker that plays an important role in natriuresis, vasodilatation, RAAS inhibition, and the sympathetic nervous system (Weber and Hamm, 2006). It is released by injured cardiac tissue due to increased mechanical stretch, hypoxia, and tissue ischemia (D'Souza and Baxter, 2003; Kinnunen et al., 1993; Tóth et al., 1994). Its level correlates with mass increase and tissue damage in the cardiac ventricle (Conen et al., 2006). In the current study, administration of l-NAME increased the availability of BNP in plasma indicating that this compound could feasibly induce cardiac injury. Our observation is consistent with other studies which showed that there was an increase in other cardiac biomarkers such as troponin T and creatinine kinase-MB (CKMB) after 4 weeks of l-NAME administration (Adamcova et al., 2013; Aldubayan et al., 2020). These findings suggested the detrimental effects of l-NAME on cardiac tissue. More evidence was observed in our study, in which the deficient state of NO was associated with cardiac damage induced by l-NAME. Significant works had been done to show the role of NO in influencing cardiac performance. Early observation exhibited that NO enhanced Frank-Starling response and cardiac distensibility (Grocott-Mason et al., 1994). A later study by Casadei and Sears (2003) generated similar findings with intracardiac NO improved diastolic relaxation and cardiac stiffness. In addition, a downregulation of eNOS activity followed by reduced bioavailability of NO was reported to deteriorate the ventricular hypertrophy and dysfunction in chronic l-NAME exposure in rats (Kumar et al., 2014; Jin et al., 2017). In a more detailed framework, an interaction between eNOS and cardiac ACE activities was reported via a feedback regulation (Wiemer et al., 1997; Takemoto et al., 1997), which contributed to the pathophysiology of l-NAME-induced cardiac damage. Silambarasan et al. (2014) reported that increased myocardial dysfunction, cardiac hypertrophy, and fibrosis were positively correlated to the oxidative markers and ACE activity in nitric oxide inhibited rats. AT-II is produced during the early stage of heart failure via cardiac ACE action to compensate for the reduced cardiac function during the chronic deficient state of nitric oxide. However, as the disease progresses, RAAS is excessively activated. This results in increased cardiac preload and afterload, promotes cardiac hypertrophy, fibrosis, and cardiomyocyte apoptosis (Singh et al., 2008; Dai et al., 2011; Jia et al., 2012), and subsequently worsens the heart function (Jackson et al., 2000). In addition, cardiac AT-II also enhances the mitochondrial ROS generation in cardiomyocytes including the excessive production of NADPH (De Giusti et al., 2009; De Giusti et al., 2013). The elevation of oxidative stress seems to augment the progression of heart remodeling and the subsequent cardiac damage (Rababa'h et al., 2018).
Systemic administration of C. asiatica extract inhibited an increase in serum BNP level induced by l-NAME in our study. Possible explanations for the beneficial effects of C. asiatica could be the enhancement of NO bioavailability, reduction of cardiac ACE activity, and improvement of oxidative stress status. Pragada et al. (2004) reported that C. asiatica extract possessed a cardio-protective effect that was attributable to its high content of triterpenoid. Asiatic acid was demonstrated to prevent cardiac and aorta remodeling including left ventricular hypertrophy, myocardial fibrosis, collagen deposition, and aortic wall thickening in a chronic NO deficiency state, as reported by Bunbupha et al. (2015). Another triterpenoid compound, asiaticoside was reported to have anti-hypertensive and cardioprotective effects in pulmonary hypertension and right ventricular hypertrophy rat model via induction of PI3K/Akt/eNOS signaling pathway (Wang et al., 2018). Based on the previous literature, we speculate that the triterpenoid in C. asiatica upregulated eNOS activity and increased NO content in l-NAME treated rats, and these mechanisms might play a beneficial role in the amelioration of cardiac damage.
C. asiatica also inhibited the increase in cardiac ACE activity induced by l-NAME. Reports from the previous studies noted that asiatic acid managed to attenuate excessive RAAS activation in several models of hypertensive rats (Maneesai et al., 2016a; Maneesai et al., 2017). Some studies showed that flavonoid compounds isolated from kiwi and apple peel inhibited ACE activities in vitro (Balasuriya and Rupasinghe, 2012; Hettihewa et al., 2018). These findings may suggest the role of flavonoids in C. asiatica might be responsible for the suppression of RAAS activation. In addition, the high antioxidant property of C. asiatica showed a significant role in reducing oxidative stress in cardiomyocytes (Zainol et al., 2003; Pittella et al., 2009; Kumari et al., 2016). Ventricular hypertrophy and myocardial damage are among the complications of hypertension. In the presence of pressure overload due to systemic hypertension, the left ventricular wall thickens to minimize the wall stress (Drazner, 2011). Dysregulation of protein synthesis/processing within the endoplasmic reticulum during the hypertrophic response may be linked to the cardiomyocyte apoptosis process (González et al., 2018). Hence, the present study suggested that the protective effect of C. asiatica against hypertension was significant in the reduction in cardiac tissue damage in l-NAME treated group.
Our study also demonstrated the effects of captopril on SBP, serum NO, oxidative stress profile as well as a cardiac marker, and ACE activities. Captopril is one of anti-hypertensive drugs that belong to the ACE inhibitor group. This drug was chosen as a positive control since ACE inhibitors are the first line of treatment in hypertension and cardiac failure (Kementerian Kesihatan Malaysia, 2019) and it also possesses antioxidant activity (Bartosz et al., 1997; Benzie and Tomlinson, 1998). In the current study, captopril prevented a reduction in serum NO level, as well as an increase in SBP, cardiac and aortic TBARS content, serum BNP level, and cardiac ACE activities in the l-NAME-induced hypertensive rat model. The effects of captopril were comparable to that of C. asiatica. The results were supported by other studies showing that daily administration of captopril (5 mg/kg) could reduce hypertension and cardiac remodeling in rats with long-term exposure to l-NAME via inhibition on ACE (Maneesai et al., 2016b; Bunbupha et al., 2019). The mechanism of l-NAME leads to raising the blood pressure by inhibiting the eNOS activity, thus diminishes NO production. Hence, a drug like sodium nitroprusside that promotes NO release could be a more suitable choice as the positive control. However, it must be administered parenterally, making its daily administration a difficult routine (Hottinger et al., 2014).
We acknowledged other limitations in the current study. We only used a single dose of ethanolic extract of C. asiatica, guided from the results of our pilot study using three different doses; 300, 500 and 1,000 mg/kg (Supplementary Material). At the dose of 300 mg/kg, C. asiatica was reported to have high anti-oxidant properties (Muchtaromah et al., 2016) and at the dose of 500 mg/kg, this extract possessed significant diuretic property (Roopesh et al., 2011) which had the potential as an anti-hypertensive agent. Meanwhile, C. asiatica at the dose of 1,000 mg/kg exhibited a significant reduction in the ischemic area of cardiac tissues in rat model with post-myocardial infarction event (Pragada et al., 2004). Our pilot study showed that 500 and 1,000 mg/kg of ethanolic extract of C. asiatica significantly prevented the systolic blood pressure in l-NAME-induced hypertensive rats after 3 weeks of treatment and the lower dose was chosen for our main study. The rationale of using only a single dose of the extract is to minimize the number of animals needed in animal experimentation to comply with 3Rs (refinement, reduction and replacement) for best practice using animals. Future studies should be considered to establish a detailed dose-response relationship and to investigate different routes/timing of administration of the extract. Another limitation in this study is the assessment of vascular responsiveness, serial echocardiography, and histomorphology for cardiac and aortic tissues were not performed. The vascular reactivity test and serial echocardiography demonstrate the vascular and cardiac function changes. In contrast, a histomorphology test is a useful tool for assessing tissue remodeling development in hypertension and cardiac failure. Hence, additional studies are required to obtain these results. Nevertheless, this is the first study that investigated the role of C. asiatica in preventing hypertension and cardiac remodeling in the chronic NO deficient state. The present findings proposed that the amelioration of oxidative status and RAAS activity are the most likely mechanisms contributing to these protective effects. Given the protective potentials of this herbal plant, C. asiatica is highly advocated as a promising preventive approach in hypertension and its cardiac complication. Further studies are recommended to investigate the effect of C. asiatica in other hypertensive rat models or more severe stages of hypertension.
Data Availability Statement
The datasets presented in this study can be found in online repositories. The names of the repository/repositories and accession number(s) can be found in the article/Supplementary Material.
Ethics Statement
The animal study was reviewed and approved by Universiti Kebangsaan Malaysia Animal Ethic Committee (UKMAEC), Universiti Kebangsaan Malaysia.
Author Contributions
MB designed all the experiments. MB and MM performed all experiments under the supervision of NM. MB collected and analyzed data and wrote the manuscript. MM, JSFZ and AHJ contributed to data collection. YK and NM revised the first copy of the manuscript and which was approved by all authors. NM supervised the work from designing to finalizing the manuscript for journal submission.
Funding
The authors would like to acknowledge the financial funding provided by the Faculty of Medicine, Universiti Kebangsaan Malaysia (Grant no. FF-2020-015).
Conflict of Interest
The authors declare that the research was conducted in the absence of any commercial or financial relationships that could be construed as a potential conflict of interest.
Publisher’s Note
All claims expressed in this article are solely those of the authors and do not necessarily represent those of their affiliated organizations, or those of the publisher, the editors and the reviewers. Any product that may be evaluated in this article, or claim that may be made by its manufacturer, is not guaranteed or endorsed by the publisher.
Supplementary Material
The Supplementary Material for this article can be found online at: https://www.frontiersin.org/articles/10.3389/fphar.2021.742562/full#supplementary-material
References
Adamcova, M., Ruzickova, S., and Simko, F. (2013). Multiplexed Immunoassays for Simultaneous Quantification of Cardiovascular Biomarkers in the Model of H(G)-nitro-L-arginine Methylester (L-NAME) Hypertensive Rat. J. Physiol. Pharmacol. 64 (2), 211–217.
Ahmadinejad, F., Geir Møller, S., Hashemzadeh-Chaleshtori, M., Bidkhori, G., and Jami, M. S. (2017). Molecular Mechanisms behind Free Radical Scavengers Function against Oxidative Stress. Antioxidants (Basel) 6 (3), 51. doi:10.3390/antiox6030051
Aldubayan, M. A., Ahmed, A. S., Emara, A. M., Ahmed, A. A., and Elgharabawy, R. M. (2020). Sinapic Acid Attenuates Cardiovascular Disorders in Rats by Modulating Reactive Oxygen Species and Angiotensin Receptor Expression. Oxid Med. Cel Longev 2020, 1436858. doi:10.1155/2020/1436858
Aluko, E. O., Adejumobi, O. A., and Fasanmade, A. A. (2019). Peristrophe Roxburghiana Leaf Extracts Exhibited Anti-hypertensive and Anti-lipidemic Properties in L-NAME Hypertensive Rats. Life Sci. 234, 116753. doi:10.1016/j.lfs.2019.116753
Andarwulan, N., Batari, R., Sandrasari, D. A., Bolling, B., and Wijaya, H. (2010). Flavonoid Content and Antioxidant Activity of Vegetables from Indonesia. Food Chem. 121 (4), 1231–1235. doi:10.1016/j.foodchem.2010.01.033
Azerad, R. (2016). Chemical Structures, Production and Enzymatic Transformations of Sapogenins and Saponins from Centella asiatica (L.) Urban. Fitoterapia 114, 168–187. doi:10.1016/j.fitote.2016.07.011
Bakogiannis, C., Theofilogiannakos, E., Papadopoulos, C., Lazaridis, C., Bikakis, I., Tzikas, S., et al. (2019). A Translational Approach to the Renin-Angiotensin-Aldosterone System in Heart Failure. Ann. Res. Hospitals 3, 1–11. doi:10.21037/arh.2019.05.01
Balasuriya, N., and Rupasinghe, H. P. (2012). Antihypertensive Properties of Flavonoid-Rich Apple Peel Extract. Food Chem. 135 (4), 2320–2325. doi:10.1016/j.foodchem.2012.07.023
Bartosz, M., Kedziora, J., and Bartosz, G. (1997). Antioxidant and Prooxidant Properties of Captopril and Enalapril. Free Radic. Biol. Med. 23 (5), 729–735. doi:10.1016/s0891-5849(97)00014-2
Benzie, I. F., and Tomlinson, B. (1998). Antioxidant Power of Angiotensin-Converting Enzyme Inhibitors In Vitro. Br. J. Clin. Pharmacol. 45 (2), 168–169. doi:10.1046/j.1365-2125.1998.00664.x
Berkban, T., Boonprom, P., Bunbupha, S., Welbat, J. U., Kukongviriyapan, U., Kukongviriyapan, V., et al. (2015). Ellagic Acid Prevents L-NAME-Induced Hypertension via Restoration of eNOS and P47phox Expression in Rats. Nutrients 7 (7), 5265–5280. doi:10.3390/nu7075222
Bradford, M. M. (1976). A Rapid and Sensitive Method for the Quantitation of Microgram Quantities of Protein Utilizing the Principle of Protein-Dye Binding. Anal. Biochem. 72 (1), 248–254. doi:10.1006/abio.1976.9999
Brinkhaus, B., Lindner, M., Schuppan, D., and Hahn, E. G. (2000). Chemical, Pharmacological and Clinical Profile of the East Asian Medical Plant Centella asiatica. Phytomedicine 7 (5), 427–448. doi:10.1016/s0944-7113(00)80065-3
Bunbupha, S., Pakdeechote, P., Kukongviriyapan, U., Prachaney, P., and Kukongviriyapan, V. (2014). Asiatic Acid Reduces Blood Pressure by Enhancing Nitric Oxide Bioavailability with Modulation of eNOS and P47phox Expression in L-NAME-Induced Hypertensive Rats. Phytother Res. 28 (10), 1506–1512. doi:10.1002/ptr.5156
Bunbupha, S., Pakdeechote, P., Maneesai, P., Prachaney, P., and Boonprom, P. (2019). Carthamus Tinctorius L. Extract Attenuates Cardiac Remodeling in L-NAME-Induced Hypertensive Rats by Inhibiting the NADPH Oxidase-Mediated TGF-Β1 and MMP-9 Pathway. Ann. Anat. 222, 120–128. doi:10.1016/j.aanat.2018.12.006
Bunbupha, S., Prachaney, P., Kukongviriyapan, U., Kukongviriyapan, V., Welbat, J. U., and Pakdeechote, P. (2015). Asiatic Acid Alleviates Cardiovascular Remodelling in Rats with L-NAME-Induced Hypertension. Clin. Exp. Pharmacol. Physiol. 42 (11), 1189–1197. doi:10.1111/1440-1681.12472
Capettini, L. S., Cortes, S. F., and Lemos, V. S. (2010). Relative Contribution of eNOS and nNOS to Endothelium-dependent Vasodilation in the Mouse Aorta. Eur. J. Pharmacol. 643 (2), 260–266. doi:10.1016/j.ejphar.2010.06.066
Casadei, B., and Sears, C. E. (2003). Nitric-oxide-mediated Regulation of Cardiac Contractility and Stretch Responses. Prog. Biophys. Mol. Biol. 82 (1-3), 67–80. doi:10.1016/s0079-6107(03)00006-3
Conen, D., Zeller, A., Pfisterer, M., and Martina, B. (2006). Usefulness of B-type Natriuretic Peptide and C-Reactive Protein in Predicting the Presence or Absence of Left Ventricular Hypertrophy in Patients with Systemic Hypertension. Am. J. Cardiol. 97 (2), 249–252. doi:10.1016/j.amjcard.2005.08.028
D'Souza, S. P., and Baxter, G. F., (2003). B Type Natriuretic Peptide: a Good Omen in Myocardial Ischaemia. Heart 89 (7), 707–709. doi:10.1136/heart.89.7.707
Dai, D. F., Johnson, S. C., Villarin, J. J., Chin, M. T., Nieves-Cintrón, M., Chen, T., et al. (2011). Mitochondrial Oxidative Stress Mediates Angiotensin II-Induced Cardiac Hypertrophy and Galphaq Overexpression-Induced Heart Failure. Circ. Res. 108 (7), 837–846. doi:10.1161/CIRCRESAHA.110.232306
De Giusti, V. C., Caldiz, C. I., Ennis, I. L., Pérez, N. G., Cingolani, H. E., and Aiello, E. A. (2013). Mitochondrial Reactive Oxygen Species (ROS) as Signaling Molecules of Intracellular Pathways Triggered by the Cardiac Renin-Angiotensin II-Aldosterone System (RAAS). Front. Physiol. 4 (126), 126. doi:10.3389/fphys.2013.00126
De Giusti, V. C., Garciarena, C. D., and Aiello, E. A. (2009). Role of Reactive Oxygen Species (ROS) in Angiotensin II-Induced Stimulation of the Cardiac Na+/HCO3- Cotransport. J. Mol. Cel Cardiol 47 (5), 716–722. doi:10.1016/j.yjmcc.2009.07.023
Drazner, M. H. (2011). The Progression of Hypertensive Heart Disease. Circulation 123 (3), 327–334. doi:10.1161/CIRCULATIONAHA.108.845792
Eirin, A., Lerman, A., and Lerman, L. O. (2014). Mitochondrial Injury and Dysfunction in Hypertension-Induced Cardiac Damage. Eur. Heart J. 35 (46), 3258–3266. doi:10.1093/eurheartj/ehu436
Förstermann, U., and Sessa, W. C. (2012). Nitric Oxide Synthases: Regulation and Function. Eur. Heart J. 33 (7), 829–837d. doi:10.1093/eurheartj/ehr304
Giani, J. F., Janjulia, T., Kamat, N., Seth, D. M., Blackwell, W. L., Shah, K. H., et al. (2014). Renal Angiotensin-Converting Enzyme Is Essential for the Hypertension Induced by Nitric Oxide Synthesis Inhibition. J. Am. Soc. Nephrol. 25 (12), 2752–2763. doi:10.1681/ASN.2013091030
González, A., Ravassa, S., López, B., Moreno, M. U., Beaumont, J., San José, G., et al. (2018). Myocardial Remodeling in Hypertension. Hypertension 72 (3), 549–558. doi:10.1161/HYPERTENSIONAHA.118.11125
Grocott-Mason, R., Fort, S., Lewis, M. J., and Shah, A. M. (1994). Myocardial Relaxant Effect of Exogenous Nitric Oxide in Isolated Ejecting Hearts. Am. J. Physiol. 266 (5 Pt 2), H1699–H1705. doi:10.1152/ajpheart.1994.266.5.H1699
Harrison, C. B., Drummond, G. R., Sobey, C. G., and Selemidis, S. (2010). Evidence that Nitric Oxide Inhibits Vascular Inflammation and Superoxide Production via a P47phox-dependent Mechanism in Mice. Clin. Exp. Pharmacol. Physiol. 37 (4), 429–434. doi:10.1111/j.1440-1681.2009.05317.x
Harwoko, P. S., and Nugroho, A. E. (2014). Triterpenoid-rich Fraction of centella Asiatica Leaves and In Vivo Antihypertensive Activity. Int. Food Res. J. 21 (1), 149–154.
Hashim, P., Sidek, H., Helan, M. H., Sabery, A., Palanisamy, U. D., and Ilham, M. (2011). Triterpene Composition and Bioactivities of Centella asiatica. Molecules 16 (2), 1310–1322. doi:10.3390/molecules16021310
Hettihewa, S. K., Hemar, Y., and Rupasinghe, H. P. V. (2018). Flavonoid-Rich Extract of Actinidia Macrosperma (A Wild Kiwifruit) Inhibits Angiotensin-Converting Enzyme In Vitro. Foods 7 (146), 1–8. doi:10.3390/foods7090146
Hottinger, D. G., Beebe, D. S., Kozhimannil, T., Prielipp, R. C., and Belani, K. G. (2014). Sodium Nitroprusside in 2014: A Clinical Concepts Review. J. Anaesthesiol Clin. Pharmacol. 30 (4), 462–471. doi:10.4103/0970-9185.142799
Jackson, G., Gibbs, C. R., Davies, M. K., and Lip, G. Y. (2000). ABC of Heart Failure. Pathophysiology. BMJ 320 (7228), 167–170. doi:10.1136/bmj.320.7228.167
James, J. T., and Dubery, I. A. (2009). Pentacyclic Triterpenoids from the Medicinal Herb, Centella asiatica (L.) Urban. Molecules 14 (10), 3922–3941. doi:10.3390/molecules14103922
Jia, N., Dong, P., Ye, Y., Qian, C., and Dai, Q. (2012). Allopurinol Attenuates Oxidative Stress and Cardiac Fibrosis in Angiotensin II-Induced Cardiac Diastolic Dysfunction. Cardiovasc. Ther. 30 (2), 117–123. doi:10.1111/j.1755-5922.2010.00243.x
Jin, S., Teng, X., Xiao, L., Xue, H., Guo, Q., Duan, X., et al. (2017). Hydrogen Sulfide Ameliorated L-NAME-Induced Hypertensive Heart Disease by the Akt/eNOS/NO Pathway. Exp. Biol. Med. (Maywood) 242 (18), 1831–1841. doi:10.1177/1535370217732325
Kakishita, M., Nakamura, K., Asanuma, M., Morita, H., Saito, H., Kusano, K., et al. (2003). Direct Evidence for Increased Hydroxyl Radicals in Angiotensin II-Induced Cardiac Hypertrophy through Angiotensin II Type 1a Receptor. J. Cardiovasc. Pharmacol. 42 (Suppl. 1), S67–S70. doi:10.1097/00005344-200312001-00015
Kamisah, Y., Zuhair, J. S. F., Juliana, A. H., and Jaarin, K. (2017). Parkia Speciosa Empty Pod Prevents Hypertension and Cardiac Damage in Rats Given N(G)-nitro-l-arginine Methyl Ester. Biomed. Pharmacother. 96, 291–298. doi:10.1016/j.biopha.2017.09.095
Ke, J. Y., Kliewer, K. L., Hamad, E. M., Cole, R. M., Powell, K. A., Andridge, R. R., et al. (2015). The Flavonoid, Naringenin, Decreases Adipose Tissue Mass and Attenuates Ovariectomy-Associated Metabolic Disturbances in Mice. Nutr. Metab. (Lond) 12 (1), 1. doi:10.1186/1743-7075-12-1
Kementerian Kesihatan Malaysia (2018). Clinical Practise Guidelines Management of Hypertension. 5th Edn, 30. Available at http://www.acadmed.org.my/index.cfm?&menuid=67 (Accessed November 11, 2021).
Kementerian Kesihatan Malaysia (2019). Clinical Practise Guidelines on Management of Heart Failure. 4th EdnAvailable at https://www.malaysianheart.org/?p=cpg (Accessed November 11, 2021).
Kinnunen, P., Vuolteenaho, O., and Ruskoaho, H. (1993). Mechanisms of Atrial and Brain Natriuretic Peptide Release from Rat Ventricular Myocardium: Effect of Stretching. Endocrinology 132 (5), 1961–1970. doi:10.1210/endo.132.5.8477647
Kumar, S., Prahalathan, P., and Raja, B. (2014). Vanillic Acid: a Potential Inhibitor of Cardiac and Aortic wall Remodeling in L-NAME Induced Hypertension through Upregulation of Endothelial Nitric Oxide Synthase. Environ. Toxicol. Pharmacol. 38 (2), 643–652. doi:10.1016/j.etap.2014.07.011
Kumari, S., Deori, M., Elancheran, R., Kotoky, J., and Devi, R. (2016). In Vitro and In Vivo Antioxidant, Anti-hyperlipidemic Properties and Chemical Characterization of Centella asiatica (L.) Extract. Front. Pharmacol. 7 (400), 400–412. doi:10.3389/fphar.2016.00400
Ledwozyw, A., Michalak, J., Stepień, A., and Kadziołka, A. (1986). The Relationship between Plasma Triglycerides, Cholesterol, Total Lipids and Lipid Peroxidation Products during Human Atherosclerosis. Clin. Chim. Acta 155 (3), 275–283. doi:10.1016/0009-8981(86)90247-0
Lüscher, T. F., and Vanhoutte, P. M. (1986). Endothelium-dependent Contractions to Acetylcholine in the Aorta of the Spontaneously Hypertensive Rat. Hypertension 8 (4), 344–348. doi:10.1161/01.hyp.8.4.344
Maneesai, P., Bunbupha, S., Kukongviriyapan, U., Prachaney, P., Tangsucharit, P., Kukongviriyapan, V., et al. (2016). Asiatic Acid Attenuates Renin-Angiotensin System Activation and Improves Vascular Function in High-Carbohydrate, High-Fat Diet Fed Rats. BMC Complement. Altern. Med. 16 (123), 123. doi:10.1186/s12906-016-1100-6
Maneesai, P., Bunbupha, S., Kukongviriyapan, U., Senggunprai, L., Kukongviriyapan, V., Prachaney, P., et al. (2017). Effect of Asiatic Acid on the Ang II-At1r-NADPH Oxidase-NF-Κb Pathway in Renovascular Hypertensive Rats. Naunyn Schmiedebergs Arch. Pharmacol. 390 (10), 1073–1083. doi:10.1007/s00210-017-1408-x
Maneesai, P., Prasarttong, P., Bunbupha, S., Kukongviriyapan, U., Kukongviriyapan, V., Tangsucharit, P., et al. (2016). Synergistic Antihypertensive Effect of Carthamus tinctorius L. Extract and Captopril in L-NAME-Induced Hypertensive Rats via Restoration of eNOS and AT₁R Expression. Nutrients 8 (3), 122. doi:10.3390/nu8030122
Muchtaromah, B., Ahmad, M., Suyono, S., Romaidi, R., Bahri, S., and Kumalasari, H. P. (2016). Dosage and Administration Length of Centella asiatica (L.) Urban Decrease the Level of Sod and Mda and Improve Brain Histological Condition of Rats. Jurnal Teknologi (Sciences Engineering) 78 (5), 57–61. doi:10.11113/jt.v78.8238
Münzel, T., Camici, G. G., Maack, C., Bonetti, N. R., Fuster, V., and Kovacic, J. C. (2017). Impact of Oxidative Stress on the Heart and Vasculature: Part 2 of a 3-Part Series. J. Am. Coll. Cardiol. 70 (2), 212–229. doi:10.1016/j.jacc.2017.05.035
Nadruz, W. (2015). Myocardial Remodeling in Hypertension. J. Hum. Hypertens. 29 (1), 1–6. doi:10.1038/jhh.2014.36
Nansy, E., Pramono, S., and Nugroho, A. (2015). Total Flavonoid Content and In Vivo Hypotensive Effect of Chloroform Insoluble Fraction of Centella asiatica Leaf Extract. Int. Food Res. J. 22 (5), 2119.
Pakdeechote, P., Bunbupha, S., Kukongviriyapan, U., Prachaney, P., Khrisanapant, W., and Kukongviriyapan, V. (2014). Asiatic Acid Alleviates Hemodynamic and Metabolic Alterations via Restoring eNOS/iNOS Expression, Oxidative Stress, and Inflammation in Diet-Induced Metabolic Syndrome Rats. Nutrients 6 (1), 355–370. doi:10.3390/nu6010355
Panza, J. A., Quyyumi, A. A., Brush, J. E., and Epstein, S. E. (1990). Abnormal Endothelium-dependent Vascular Relaxation in Patients with Essential Hypertension. N. Engl. J. Med. 323 (1), 22–27. doi:10.1056/NEJM199007053230105
Pittella, F., Dutra, R. C., Junior, D. D., Lopes, M. T., and Barbosa, N. R. (2009). Antioxidant and Cytotoxic Activities of Centella asiatica (L) Urb. Int. J. Mol. Sci. 10 (9), 3713–3721. doi:10.3390/ijms10093713
Pragada, R. R., Veeravalli, K. K., Chowdary, K. P., and Routhu, K. V. (2004). Cardioprotective Activity of Hydrocotyle Asiatica L. In Ischemia-Reperfusion Induced Myocardial Infarction in Rats. J. Ethnopharmacol 93 (1), 105–108. doi:10.1016/j.jep.2004.03.025
Pryor, W. A., and Squadrito, G. L. (1995). The Chemistry of Peroxynitrite: a Product from the Reaction of Nitric Oxide with Superoxide. Am. J. Physiol. 268 (5 Pt 1), L699–L722. doi:10.1152/ajplung.1995.268.5.L699
Rababa'h, A. M., Guillory, A. N., Mustafa, R., and Hijjawi, T. (2018). Oxidative Stress and Cardiac Remodeling: An Updated Edge. Curr. Cardiol. Rev. 14 (1), 53–59. doi:10.2174/1573403X14666180111145207
Rajeshwari, T., Raja, B., Manivannan, J., and Silambarasan, T. (2014). Valproic Acid Attenuates Blood Pressure, Vascular Remodeling and Modulates ET-1 Expression in L-NAME Induced Hypertensive Rats. Biomed. Prev. Nutr. 4 (2), 195–202. doi:10.1016/j.bionut.2013.09.002
Robaczewska, J., Kedziora-Kornatowska, K., Kozakiewicz, M., Zary-Sikorska, E., Pawluk, H., Pawliszak, W., et al. (2016). Role of Glutathione Metabolism and Glutathione-Related Antioxidant Defense Systems in Hypertension. J. Physiol. Pharmacol. 67 (3), 331–337.
Roopesh, C., Salomi, K. R., Nagarjuna, S., and Reddy, Y. P. (2011). Diuretic Activity of Methanolic and Ethanolic Extracts of Centella asiatica Leaves in Rats. Int. Res. J. Pharm. 2 (11), 163–165.
Samhan-Arias, A. K., Tyurina, Y. Y., and Kagan, V. E. (2011). Lipid Antioxidants: Free Radical Scavenging versus Regulation of Enzymatic Lipid Peroxidation. J. Clin. Biochem. Nutr. 48 (1), 91–95. doi:10.3164/jcbn.11-009FR
Schulz, E., Gori, T., and Münzel, T. (2011). Oxidative Stress and Endothelial Dysfunction in Hypertension. Hypertens. Res. 34 (6), 665–673. doi:10.1038/hr.2011.39
Sedeek, M., Hébert, R. L., Kennedy, C. R., Burns, K. D., and Touyz, R. M. (2009). Molecular Mechanisms of Hypertension: Role of Nox Family NADPH Oxidases. Curr. Opin. Nephrol. Hypertens. 18 (2), 122–127. doi:10.1097/MNH.0b013e32832923c3
Silambarasan, T., Manivannan, J., Krishna Priya, M., Suganya, N., Chatterjee, S., and Raja, B. (2014). Sinapic Acid Prevents Hypertension and Cardiovascular Remodeling in Pharmacological Model of Nitric Oxide Inhibited Rats. PloS one 9 (12), e115682. doi:10.1371/journal.pone.0115682
Singh, V. P., Le, B., Khode, R., Baker, K. M., and Kumar, R. (2008). Intracellular Angiotensin II Production in Diabetic Rats Is Correlated with Cardiomyocyte Apoptosis, Oxidative Stress, and Cardiac Fibrosis. Diabetes 57 (12), 3297–3306. doi:10.2337/db08-0805
Sorriento, D., De Luca, N., Trimarco, B., and Iaccarino, G. (2018). The Antioxidant Therapy: New Insights in the Treatment of Hypertension. Front. Physiol. 9, 258. doi:10.3389/fphys.2018.00258
Sung, J. H., Jo, Y. S., Kim, S. J., Ryu, J. S., Kim, M. C., Ko, H. J., et al. (2013). Effect of Lutein on L-NAME-Induced Hypertensive Rats. Korean J. Physiol. Pharmacol. 17 (4), 339–345. doi:10.4196/kjpp.2013.17.4.339
Tabassum, R., Vaibhav, K., Shrivastava, P., Khan, A., Ejaz Ahmed, M., Javed, H., et al. (2013). Centella asiatica Attenuates the Neurobehavioral, Neurochemical and Histological Changes in Transient Focal Middle Cerebral Artery Occlusion Rats. Neurol. Sci. 34 (6), 925–933. doi:10.1007/s10072-012-1163-1
Takemoto, M., Egashira, K., Usui, M., Numaguchi, K., Tomita, H., Tsutsui, H., et al. (1997). Important Role of Tissue Angiotensin-Converting Enzyme Activity in the Pathogenesis of Coronary Vascular and Myocardial Structural Changes Induced by Long-Term Blockade of Nitric Oxide Synthesis in Rats. J. Clin. Invest. 99 (2), 278–287. doi:10.1172/JCI119156
Tóth, M., Vuorinen, K. H., Vuolteenaho, O., Hassinen, I. E., Uusimaa, P. A., Leppäluoto, J., et al. (1994). Hypoxia Stimulates Release of ANP and BNP from Perfused Rat Ventricular Myocardium. Am. J. Physiol. 266 (4 Pt 2), H1572–H1580. doi:10.1152/ajpheart.1994.266.4.H1572
Touyz, R. M., and Briones, A. M. (2011). Reactive Oxygen Species and Vascular Biology: Implications in Human Hypertension. Hypertens. Res. 34 (1), 5–14. doi:10.1038/hr.2010.201
Touyz, R. M., and Schiffrin, E. L. (1999). Ang II-Stimulated Superoxide Production Is Mediated via Phospholipase D in Human Vascular Smooth Muscle Cells. Hypertension 34 (4 Pt 2), 976–982. doi:10.1161/01.hyp.34.4.976
Tsuchiya, K., Sakai, H., Suzuki, N., Iwashima, F., Yoshimoto, T., Shichiri, M., et al. (2007). Chronic Blockade of Nitric Oxide Synthesis Reduces Adiposity and Improves Insulin Resistance in High Fat-Induced Obese Mice. Endocrinology 148 (10), 4548–4556. doi:10.1210/en.2006-1371
Wang, X., Cai, X., Wang, W., Jin, Y., Chen, M., Huang, X., et al. (2018). Effect of Asiaticoside on Endothelial Cells in Hypoxia-induced P-ulmonary H-ypertension. Mol. Med. Rep. 17 (2), 2893–2900. doi:10.3892/mmr.2017.8254
Weber, M., and Hamm, C. (2006). Role of B-type Natriuretic Peptide (BNP) and NT-proBNP in Clinical Routine. Heart 92 (6), 843–849. doi:10.1136/hrt.2005.071233
Wei, J., Huang, Q., Huang, R., Chen, Y., Lv, S., Wei, L., et al. (2013). Asiatic Acid from Potentilla Chinensis Attenuate Ethanol-Induced Hepatic Injury via Suppression of Oxidative Stress and Kupffer Cell Activation. Biol. Pharm. Bull. 36 (12), 1980–1989. doi:10.1248/bpb.b13-00634
Wiemer, G., Linz, W., Hatrik, S., Schölkens, B. A., and Malinski, T. (1997). Angiotensin-converting Enzyme Inhibition Alters Nitric Oxide and Superoxide Release in Normotensive and Hypertensive Rats. Hypertension. 1979 30 (5), 1183–1190. doi:10.1161/01.hyp.30.5.1183
World Health Organization (2017). Cardiovascular Diseases (CVDs). Available at https://www.who.int/en/news-room/fact-sheets/detail/cardiovascular-diseases-(cvds) (Accessed November 11, 2021).
Wunpathe, C., Maneesai, P., Rattanakanokchai, S., Bunbupha, S., Kukongviriyapan, U., Tong-Un, T., et al. (2020). Tangeretin Mitigates L-NAME-Induced Ventricular Dysfunction and Remodeling through the AT1R/pERK1/2/pJNK Signaling Pathway in Rats. Food Funct. 11 (2), 1322–1333. doi:10.1039/c9fo02365h
Yu, B. P. (1994). Cellular Defenses against Damage from Reactive Oxygen Species. Physiol. Rev. 74 (1), 139–162. doi:10.1152/physrev.1994.74.1.139
Zainol, M. K., Abd-Hamid, A., Yusof, S., and Muse, R. (2003). Antioxidative Activity and Total Phenolic Compounds of Leaf, Root and Petiole of Four Accessions of Centella asiatica (L.) Urban. Food Chem. 81 (4), 575–581. doi:10.1016/s0308-8146(02)00498-3
Keywords: Centella asiatica, cardiac damage, hypertension, nitro-l-arginine methyl ester, nitric oxide
Citation: Bunaim MK, Kamisah Y, Mohd Mustazil MN, Fadhlullah Zuhair JS, Juliana AH and Muhammad N (2021) Centella asiatica (L.) Urb. Prevents Hypertension and Protects the Heart in Chronic Nitric Oxide Deficiency Rat Model. Front. Pharmacol. 12:742562. doi: 10.3389/fphar.2021.742562
Received: 16 July 2021; Accepted: 03 November 2021;
Published: 03 December 2021.
Edited by:
Li-Tung Huang, Kaohsiung Chang Gung Memorial Hospital, TaiwanReviewed by:
Seyed Zachariah Moradi, Kermanshah University of Medical Sciences, IranSongül Karakaya, Atatürk University, Turkey
Copyright © 2021 Bunaim, Kamisah, Mohd Mustazil, Fadhlullah Zuhair, Juliana and Muhammad. This is an open-access article distributed under the terms of the Creative Commons Attribution License (CC BY). The use, distribution or reproduction in other forums is permitted, provided the original author(s) and the copyright owner(s) are credited and that the original publication in this journal is cited, in accordance with accepted academic practice. No use, distribution or reproduction is permitted which does not comply with these terms.
*Correspondence: Norliza Muhammad, bm9ybGl6YV9zc3BAcHB1a20udWttLmVkdS5teQ==