- 1Department of Biomedical Science, Kulliyyah of Allied Health Sciences, International Islamic University Malaysia, Kuantan, Malaysia
- 2Pharmacognosy Research Group, Department of Pharmaceutical Chemistry, Kulliyyah of Pharmacy, International Islamic University Malaysia, Kuantan, Malaysia
- 3Faculty of Pharmacy, Airlangga University, Surabaya, Indonesia
- 4Department of Biomedical Sciences, Faculty of Medicine and Health Sciences, Universiti Malaysia Sabah, Kota Kinabalu, Malaysia
- 5Laboratory of Halal Science Research, Halal Products Research Institute, Universiti Putra Malaysia, Serdang, Malaysia
The leaves of Neolamarckia cadamba (NC) (Roxb.) Bosser (family: Rubiaceae) are traditionally used to treat breast cancer in Malaysia; however, this traditional claim is yet to be scientifically verified. Hence, this study was aimed to evaluate the anticancer effect of NC leaves’ ethanol extract against breast cancer cell line (MCF-7 cells) using an in vitro cell viability, cytotoxicity, and gene expression assays followed by the gas chromatography analysis to further confirm active principles. Results revealed 0.2 mg/ml as the half maximal inhibitory concentration (IC50) against MCF-7. The extract exerted anticancer effect against MCF-7 cells in a dose- and time-dependent manner. The cell cycle assay showed that the extract arrested MCF-7 cells in the G0/G1 phase, and apoptosis was observed after 72 h by the Annexin-V assay. The gene expression assay revealed that the cell cycle arrest was associated with the downregulation of CDK2 and subsequent upregulation of p21 and cyclin E. The extract induced apoptosis via the mediation of the mitochondrial cell death pathways. A chromatography analysis revealed the contribution of D-pinitol and myo-inositol as the two major bioactive compounds to the activity observed. Overall, the study demonstrated that NC leaves’ ethanol extract exerts anticancer effect against MCF-7 human breast cancer cells through the induction of apoptosis and cell cycle arrest, thereby justifying its traditional use for the treatment of breast cancer in Malaysia.
Introduction
Breast cancer is the most commonly occurring cancer in women and the second most common cancer overall in terms of fatality after lung cancer (Bray et al., 2018). The rate of breast cancer patients’ cases is increasing every year, both in developed and developing countries (Torre et al., 2015). It is estimated that 26 million new cancer cases and 17 million cancer deaths are likely to occur per year globally by 2030 (Thun et al., 2010). In Asia alone, the incident from 2008 to 2030 is estimated to increase from 6.1 to 10.7 million cancer cases and 4.1 to 7.5 million deaths (Sankaranarayanan et al., 2014). Malaysia is one of the Asian countries with an increased prevalence of breast cancer cases (Azizah et al., 2016), affecting both genders but increased exponentially with age in women and thus accounted for the leading cause of cancer-related deaths among women in Malaysia (Lukong, 2017). Surgery, radiotherapy, chemotherapy, hormonal therapy, and gene-targeted therapy have been greatly used in treating breast cancer patients. The advances of current treatments and detecting methods have contributed to the increased survival rate (Blumen et al., 2016). Unfortunately, these treatments cause short-term and long-term side effects for the patients. Moreover, studies have demonstrated that the burden of the cost related to cancer treatment is uncharacteristically rising (Blumen et al., 2016; Trogdon et al., 2017). Therefore, using natural sources as alternative, effective, and non-invasive entities to treat cancer is warranted.
According to the World Health Organization (WHO), many countries, including developing countries, still use plants and natural source–related products for therapeutic purposes (Rayan et al., 2017). About 60% of anticancer agents have been originated from the natural sources globally (Newman and Cragg, 2016). The nature-derived compounds are readily available, generally more tolerated, and considered non-toxic to normal human cells (Shah et al., 2013).
Neolamarckia cadamba (Roxb.) Bosser (family: Rubiaceae) is a tree that is traditionally used for the treatment of various illnesses. This underexplored evergreen tropical plant has been widely used in the Ayurvedic medicine system of India in treating eye infection, skin diseases, dyspepsia, gum-related troubles, stomatitis, cough, fever, anemia, blood disorders, and stomach ache (Dubey et al., 2011; Pandey and Negi, 2016). In addition, the leaves of this plant have been shown to possess several pharmacological properties, including antioxidant (Kaur and Kumar, 2011; Chandel et al., 2012), antidiabetic (Ahmed et al., 2011), antitumor (Dolai et al., 2012), anti-inflammatory, antipyretic, analgesic (Mondal et al., 2009), antimicrobial (Rafshanjani et al., 2014), and anticancer effects (Singh et al., 2013). Furthermore, the N. cadamba leaves have also been used as a topical application to treat breast cancer. However, this traditional application has never been scientifically investigated to verify its anticancer effect. Therefore, the main purpose of the study was to evaluate the anticancer effects of N. cadamba leaves’ extract on breast cancer cell line (MCF-7) with emphasis on the mechanism of action, that is, apoptosis induction and cell cycle arrest. The characterization of N. cadamba leaves’ extract was also carried out using a gas chromatography–mass spectrometry (GCMS) approach to identify bioactive compounds.
Novelty
1. It is the pioneer study highlighting the anticancer effect of Neolamarckia cadamba (Roxb.) Bosser leaves’ ethanol extract on breast cancer cells for the first time.
2. Neolamarckia cadamba leaves’ ethanol extract showed dose- and time-dependent anticancer effects.
3. Treated cells were arrested in the G1 phase and underwent apoptosis via the mitochondria pathway.
4. Findings of this study further support the traditional use of Neolamarckia cadamba (Roxb.) Bosser leaves as an anticancer agent in the treatment of breast cancer.
Materials and Methods
Materials and Chemicals
The GCMS reagents were purchased from Sigma-Aldrich, United States (Pyridine and N-Methyl-N-(trimethylsilyl)tri-fluoroacetamide-MSTFA) and Supelco, United States (methoxyamine HCl). The normal human fibroblast (HDF) and human breast adenocarcinoma (MCF-7) cells were obtained from American Type Culture Collection (ATCC), United States. All cells were maintained in complete growth medium (CGM) consisting of Dulbecco’s modified Eagle medium (DMEM) (Nacalei Tesque, Japan), 10% fetal bovine serum (Nacalei Tesque, Japan), and 1% of penicillin–streptomycin (Nacalei Tesque, Japan). The phosphate-buffered saline (PBS) from Gibco, United States, was used for cell-washing purposes. Reagents for apoptosis (Nexin reagent) and a cell cycle reagent were purchased from Merck Millipore, United States. Kits used for the mRNA analysis were purchased from Analytik Jena, Germany (InnuPREP DNA/RNA mini kit), BIOLINE, United Kingdom (SensiFAST cDNA synthesis and SensiFAST SYBR® No-ROX kits), and Integrated DNA technologies, Singapore (primer).
Preparation of Neolamarckia cadamba Leaves’ 80% Ethanol Extract (NC)
Fresh leaves of N. cadamba were collected from Balok, 25200 Kuantan, Pahang DM, Malaysia, and deposited (voucher specimen #: PIIUM 0266) in the Herbarium, Kulliyyah of Pharmacy, IIUM, Malaysia. The leaves were prepared according to the previous method (Liu et al., 2017) with slight modification. The leaves were washed and air-dried. The dried leaves were pulverized and soaked in 80% ethanol three times using an ultrasonic sonicator. The extract was filtered, concentrated by a rotary evaporator, and freeze-dried. It was stored at −20°C until further usage. The extract was dissolved in 80% ethanol to obtain a stock solution of 100 mg/ml. Working solutions were prepared fresh from ethanol stock in the DMEM medium by serial dilutions. The solutions were then filtered with a sterilized 0.22 µl syringe filter before being used in an in vitro assay.
Gas Chromatography–Mass Spectrometry (GCMS) Analysis of NC
Derivatization of the samples was performed for the GCMS analysis following the method described (Liu et al., 2017). Briefly, about 50 µl pyridine, 100 µl of methoxyamine HCl (20 mg/ml in pyridine), and 300 µl of MSTFA were added to the extract. The extract solutions were filtered and covered with aluminum foil to be left overnight at room temperature before the analysis. The GCMS analysis was carried out using GCMS-TQ8040 (Shimadzu, Japan), where the analysis was carried out using a DB-5MS 5% phenyl methyl siloxane (30 mm × 0.25 mm, i.d. × 0.25 µm thickness). The initial oven temperature used was set to 50°C for 5 min and raised to 200°C at the rate of 5°C/min and held for 5 min before reaching the target temperature of 300°C at the same rate. The carrier gas applied was helium with a flow rate of 1.0 ml/min, while the injector and detector temperature were set up to 250 and 280°C, respectively. Full-scan mass spectra were retrieved by setting parameters ranging from 50 to 550 m/z. The chromatograms of the compounds were compared to the database of the National Institute of Standards and Technology (NIST) 2014.
Effect of NC on Cell Viability of MCF-7 and HDF Cell Growth
All cells were maintained in a sterilized environment set to 37°C with 5% CO2 humidified atmosphere. The cell viability of NC-treated cells was measured using the trypan blue exclusion (TBE) method as previously described (Hsieh et al., 2005). In determining the median concentration of NC on MCF-7 cells, the cells were seeded at 4.7 × 104 cells/ml in 6-well plates. After overnight, different concentrations of NC prepared by serial dilution (500.0, 250.0, 125.0, 62.5, 31.3, 15.6, 7.8, 3.9, 1.95, and 0.9 μg/ml) were added to MCF-7 cells and kept for 72 h before performing cell density evaluation using TBE. MCF-7 cells were exposed to NC for 24, 48, 72, and 96 h prior evaluation with the TBE assay to determine the MCF-7 growth exposure with NC. The cytotoxicity of NC against normal HDF cells, which were cultured at 5.0 × 104 cells/ml in 6-well plates, was determined by examining the IC50 value of NC after 72 h. The untreated cells were not exposed to NC and were used as a negative control.
Cell Cycle Analysis
MCF-7 cells collected from the well were prepared in triplicate prior to staining with the cell cycle reagent (Guava Cell Cycle Reagent), following the manufacturer’s instructions. The PI-stained cells were observed using a Guava EasyCyte flow cytometer system (Hsieh et al., 2005).
Apoptosis Analysis Using Annexin V
Briefly, MCF-7 cells were exposed to NC for 72 h. The collected cells were treated with the FITC Annexin-V Apoptosis Detection Kit I, following the manufacturer’s instructions. The cells were treated with 1x binding buffer, FITC Annexin-V, and propidium iodide (PI) before their application to the Guava easyCyte flow cytometer system (EMD Millipore, Germany) (Hsieh et al., 2005).
Real-Time Quantitative PCR Analysis of Target Genes
The quantification of the mRNA expression of the targeted primer mRNA corresponding to apoptosis, cell cycle, and metastasis was carried out following our previously published study (Strober, 2015). Briefly, the RNA extraction kit was used to extract the total RNA from the plant treated and non-treated MCF-7 cells after 72 h. The RNA’s purity and integrity were evaluated prior to complementary DNA (cDNA) synthesis. The cDNA was prepared using a cDNA synthesis kit with 200 ng of RNA template following the manufacturer’s instructions. Primers were selected from the National Centre for Biotechnology Information (NCBI) database based on the criteria listed below.
i. Melting temperature, Tm (59–65°C)
ii. Amplicon size (70–150 bases)
iii. Forward and reverse primers (spanning exon–exon junctions)
iv. Primer’s 3′ end with a C or G residue
v. GC content (40–60%)
vi. Primer’s sequence (18–25 nucleotides)
The PCR amplifications were conducted using SYBR green in CFX96 Touch™ Real-Time PCR (Bio-Rad, United States). The efficiency of the primer PCR was evaluated using 1:10 serial dilutions of the cDNA sample. The standard curve’s amplification efficiency (E) range was set between 93.5 and 115.4%, while the correlation coefficients (R2) ranged from 0.984 to 0.998. The optimized parameters of the amplifications include 2 min at 50°C, 10 min at 95°C, 45 cycles of 15 s at 95°C, and 1 min at 60°C. The CFX Manager Software was used to analyze the results obtained by applying threshold cycle (CT) values. The reference genes used were glyceraldehyde 3-phosphate dehydrogenase (GAPDH) and β-actin (ACTB). The primers’ detailed information is displayed in Supplementary Table S1.
Statistical Analysis
Statistical and the IC50 values’ (half maximal inhibitory concentration) calculations were carried out using GraphPad Prism software version 6. The cytotoxicity data were analyzed using multiple t-tests. The flow cytometry analysis and gene expression data were determined using multiple t-tests (Holm–Sidak method). All data were displayed as mean ± SD (standard deviation). * Signifies the significant difference when p < 0.05.
Results
Gas Chromatography–Mass Spectrometry Analysis of NC
Table 1 presents the putative compounds detected in the NC leaves’ 80% ethanol extract. The metabolites with the similarity index (SI) of more than 85% based on the National Institute of Standards and Technology (NIST) 14 database can be regarded as the putative compounds. The chromatogram showed the highest peak area corresponding to D-pinitol, followed by myo-inositol, oleic acid, hexadecanoic acid, and octadecanoic acid.
Breast Cancer Cells (MCF-7) Viability After NC Treatment
The inhibitory activity of NC leaves’ 80% ethanol extract was investigated against MCF-7 cells. The cell viability was directly tested using the trypan blue exclusion assay method where the dead cells were stained blue. Figure 1A shows the antiproliferative activity of NC leaves’ 80% ethanol extract on MCF-7 cells after 72 h of incubation. The finding revealed an IC50 of 206.0 ± 3.4 μg/ml. Cell viability was found to decrease with the increasing concentration of the extract used. Figure 1B demonstrates the IC50 value of NC to inhibit MCF-7 growth with cell viability percentage difference between NC and UT by 6.3, 12.2, 23.1, and 35.4% at 24, 48, 72, and 96 h, respectively. From the findings, it can be seen that the NC inhibition effect on MCF-7 growth increased over time. Figure 1C depicts the image of MCF-7 cells treated with NC in comparison to the untreated as a control. The image shows the cells exhibiting a distinguished plasma membrane with intact nuclei, and cells were seen to grow adjacent to the neighboring cells in a monolayer. Most of the cells were attached to the tissue culture plate. In contrast, cells treated with NC extract for 72 h showed apoptotic-like features including cell rounding, loss of contact with the adjacent cells, cell shrinkage, and formation of apoptotic bodies. Figure 1D presents the viability of normal HDF cells exposed to NC leaves’ 80% ethanol extract for 72 h. The graph reveals that NC leaves’ 80% ethanol extract had slight toxicity on HDF cells by inhibiting cell growth by 19.5% when compared to UT. Overall, the NC ethanol extract exhibited a concentration and time inhibition pattern related to the MCF-7 cell growth and slight toxicity on normal HDF cells. IC50 obtained was used for further investigation in this study.
Cell Cycle Analysis of NC Against MCF-7 Cells
To understand the growth inhibition found earlier, NC leaves’ 80% ethanol extract–treated MCF-7 cells were subjected to cell cycle analysis with UT serving as control. Figure 2A demonstrates MCF-7 cells treated with NC leaves’ 80% ethanol extract and UT DNA histogram at 72 h of incubation, which indicates that NC leaves’ 80% ethanol extract exerted MCF-7 arrest in G1/G0 compared to UT. Figure 2B shows the proportion of cells according to cell cycle phase from Figure 2A. The graph reveals that NC leaves’ 80% ethanol extract significantly induced G1/G0 arrest in MCF-7 cells evidenced by the increase of cell population by 22.9% in G1/G0 and simultaneous decrease of G2/M by 21.9% compared to UT.
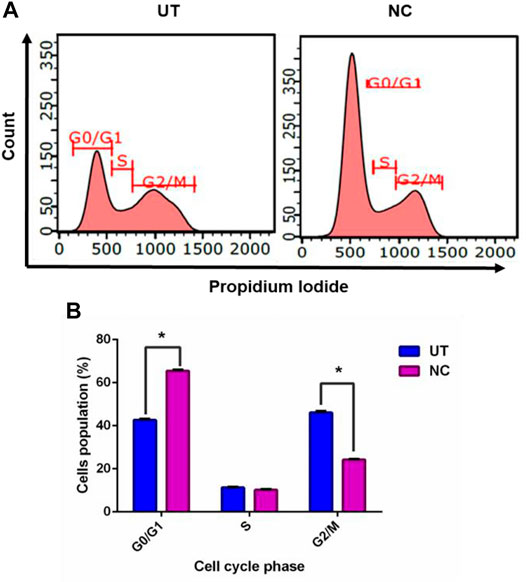
FIGURE 1. Effect of NC leaves’ 80% ethanol extract on MCF-7 cell viability. (A) The median concentration of MCF-7 treated with NC leaves’ 80% ethanol extract for 72 h. (B) Antiproliferative effect of NC leaves’ 80% ethanol extract at 24, 28, 72, and 96 h with untreated cells (UT) as controls. (C) Image of MCF-7 cells with NC leaves’ 80% ethanol extract treated and untreated at 72 h. (D) Cytotoxic effect of NC leaves’ 80% ethanol extract median concentration on normal human dermal fibroblast (HDF) cells at 72 h incubation. The results presented as mean ± SD (n = 3) (bar graph = 25 μm). * indicates p < 0.05.
Apoptosis Analysis of NC Leaves 80% Ethanol Extract Against MCF-7 Cells
As evidenced earlier, NC leaves’ 80% ethanol extract showed growth inhibition and cell death; hence, further investigation was conducted to evaluate the possibility of cells to undergo apoptosis using flow cytometer analysis. The Annexin-V assay helped to differentiate individual cells in live, early apoptotic, late apoptotic, and necrotic quadrants following 72 h of NC leaves’ 80% ethanol extract treatment to MCF-7 cells. Figure 3A shows MCF-7 cells, NC leaves’ 80% ethanol extract-treated cells, and UT dot plot distribution in quadrants. The Annexin-V assay revealed that NC leaves’ 80% ethanol extract–treated cells induces early and late apoptosis by 12.0 and 32.1% compared to UT. On the contrary, UT cells are majorly located in the live quadrant. Figure 3B demonstrates the proportion percentages of apoptotic (early and late apoptosis) and live cells of NC leaves’ 80% ethanol extract–treated and UT MCF-7 cells. The proportion of apoptotic percentage from NC leaves’ 80% of ethanol extract–treated cells was significantly higher by 44.3% compared to UT 8.6%, while the proportion of live cells percentage was higher in UT MCF-7 by 91.5% than in NC-treated cells by 65.7%.
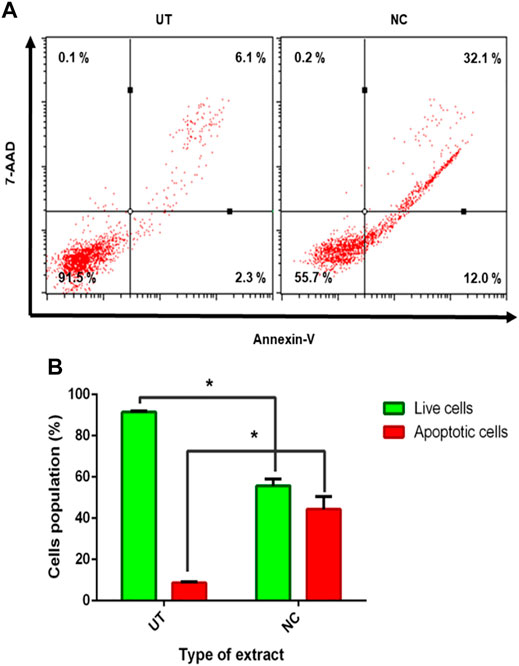
FIGURE 2. Effect of NC leaves’ 80% ethanol extract on the MCF-7 cell cycle. (A) Representation of DNA histogram of NC leaves’ 80% ethanol extract–treated cells, with UT as control at 72 h of incubation. DNA histograms display cell cycle phases of treated cells, namely, G1/G0, S, and G2/M (n = 3). (B) The proportion of cells according to the cell cycle phase are presented in percentage mean ± SD (n = 3). * indicates p < 0.05 concerning untreated.
Effects of NC Leaves’ 80% Ethanol Extract on Gene Expression of MCF-7
A deeper investigation was further performed to investigate gene expression of NC leaves’ 80% ethanol extract supplement to MCF-7 cells with regards to cell cycle arrest and apoptosis induction. Five genes related to apoptosis were measured for their expressions through the qPCR assay viz. Bax, Bcl-2, caspase-9, caspase-7, and cytochrome c. The expression of cell cycle–related genes on MCF-7–treated cells with a growth arrest at the G0/G1 phase, cyclin E, CDK2, and p21 were studied. Figure 4 shows that upon NC leaves’ 80% ethanol extract treatment, the expression of cyclin E and p21 were found to be upregulated with 1.6 ± 0.54 and 1.7 ± 0.02 folds, respectively. Meanwhile, CDK2 was downregulated with 0.9 ± 0.07 folds. From this result, the upregulated expression of p21 in NC-treated cells indicates that the p21 inhibits the activity of CDK2 and cyclin E catalytic complex formation which blocks the progression to the next stage of the cell cycle.
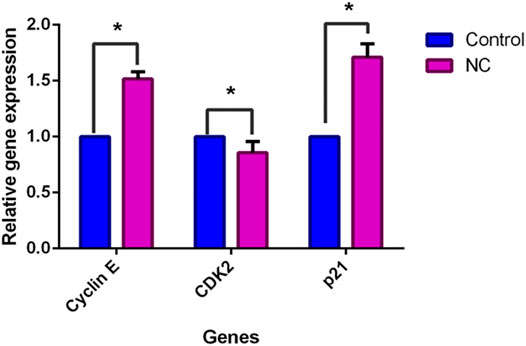
FIGURE 3. Effect of NC leaves 80% ethanol extract on MCF-7 cells. (A) Representation of dot plot distribution of cells in living, death, and early and late apoptosis quadrants of MCF-7 cells treated with NC leaves 80% ethanol extract at 72 h with UT as control (n = 3). (B) The proportion percentages of apoptotic (early and late apoptosis) and live cells are presented as percentage mean ± SD (n = 3). * indicates p < 0.05 concerning untreated.
In Figure 5A, Bax (proapoptotic) and cytochrome c were downregulated by NC leaves’ 80% ethanol extract by 2.1 ± 0.07-fold and 5.1 ± 0.42-fold, respectively, whereas Bcl-2 was downregulated by 0.9 ± 0.04-fold. Meanwhile, in Figure 5B caspase family (caspase-9 and caspase-7) expressions were upregulated with NC leaves’ 80% ethanol extract treatment by 3.1 ± 0.65 and 1.8 ± 0.19-fold, respectively. The upregulation of Bax, cytochrome c, and caspases family corresponded with the downregulation of Bcl-2 gene, suggesting that NC extract treatment lowered cell survival and induced apoptosis via the intrinsic mitochondrial pathway.
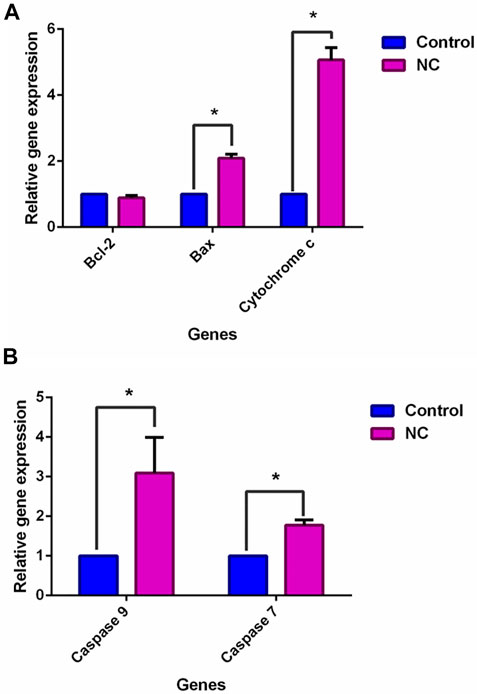
FIGURE 4. Effect of NC leaves 80% ethanol extract on cell cycle-related gene expression upon treatment with IC50 for 72 h. Relative expression for cyclin E, CDK2, and p21 were calculated by measuring the fold change with GAPDH and β-actin as reference genes in untreated cells (the ratio was equal to 1). Data presented in mean ± standard deviation of triplicates of the experiment. * indicates a significant difference when p < 0.05.
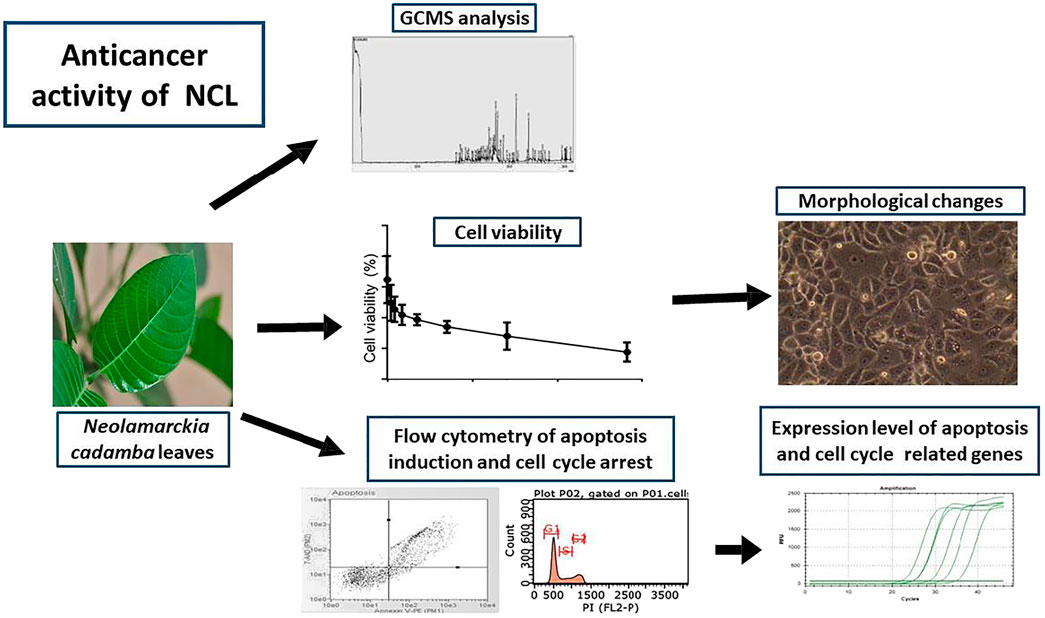
FIGURE 5. Effect of NC leaves 80% ethanol extract on apoptosis-related gene expression upon treatment with IC50 for 72 h. Relative expression for (A) Bcl-2, Bax, and cytochrome c, and (B) caspase-9 and caspase-7 were calculated by measuring the fold change with GAPDH and β-actin as a reference gene in untreated cells, whose ratio was equal to 1. Data presented in mean ± standard deviation of triplicates of the experiment. * indicates a significant difference when p < 0.05.
Discussion
Throughout the whole world, breast cancer is affecting women the most compared to other types of cancers with a high incidence and mortality rate (Harbeck et al., 2019; Lin et al., 2019). Additionally, there are some undocumented and unverified claims for N. cadamba leaves that it is used to treat breast cancer in different parts of Malaysia; however, no scientific study using breast cancer cell lines has ever been carried out to confirm its potential in the management of breast cancer. Hence, this study was performed to verify N. cadamba leaves’ extract as a potential anticancer agent with minimal toxicities. In this study, NC leaves’ 80% ethanol extract was initially characterized with GCMS and then assessed for its in vitro anticancer properties and mechanism of action using MCF-7 cells. Phytochemical characterization of NC leaves s 80% ethanol extract through the GCMS analysis identified eight putative bioactive compounds including D-pinitol, myo-inositol, oleic acid, hexadecanoic acid, octadecanoic acid, β-D-(+)-talopyranose, levoglucosan, and phenol, 2, 4-bis(1,1-dimethylethyl).
Among the compounds, D-pinitol (6-methoxycyclohexane-1,2,3,4,5-pentol) was found to be the most abundant one. D-pinitol is a natural, sugar-like cyclitol, a cyclic polyol found in a variety of plants including soybean and has been reported to exert diverse biological activities particularly antioxidant, antiviral, antidiabetic, anti-inflammatory, and anticancer. D-pinitol has been reported to inhibit prostate cancer metastasis through the inhibition of αVβ3 integrin by modulating FAK, c-Src, and NF-κB pathways (Lin et al., 2013). Furthermore, a study revealed that d-pinitol was able to induce apoptosis in MCF-7 by upregulating Bax and downregulating Bcl2 expressions. It was also demonstrated that D-pinitol promoted apoptosis in MCF-7 cells via induction of p53 and Bax and inhibition of Bcl-2 and NF-κB (Rengarajan et al., 2014). Myo-inositol, the next most abundant bioactive compound in N. cadamba leaves’ extract, has been earlier isolated from fresh samples of Cosmos caudatus Kunth (Javadi et al., 2015) and Sapindus mukorossi Gaertn (Liu et al., 2018). Myo-inositol has been currently used clinically to treat polycystic ovary syndrome (PCOS) (Artini et al., 2013).
Hexadecanoic acid was identified in our study as another bioactive compound, and identified earlier from methanol (44.88%) and hexane (17.96%) extracts of N. cadamba leaves. Additionally, octadecanoic acid was also found in methanol, ethyl acetate, chloroform, and hexane extract (Zayed et al., 2014). N-hexadecanoic acid found in Kigelia africana subsp. africana (syn. Kigelia pinnata) leaves had caused cytotoxicity in HCT-116 cell lines (Ravi and Krishnan, 2017). Moreover, octadecanoic acid has induced apoptosis in breast cancer cells (Evans et al., 2009). Oleic acid found in almond oil (Prunus dulcis (Mill.) D. A.Webb) has displayed antiproliferative and anticancer effects on colon carcinoma cells (Mericli et al., 2017). Meanwhile, the ability of phenol, 2,4-bis(1,1-dimethylethyl) compound docked with Bcl-2 showing low binding affinity (−7.4 kcal/mol) after caryophyllene compound (−7.5 kcal/mol) indicated the potential of Solanum trilobatum L. extract compounds as a potent anticancer agent (Xavier et al., 2013).
The results showed that the population of MCF-7 cell lines was reduced to 50% from 100% of viable cells. NC extract exhibited a growth inhibitory effect toward MCF-7 in a concentration- and time-dependent manner in which the percentage of viable cells decreased in correspondence with the increase of NC extract concentration. Different bioactive compounds were reported in N. cadamba leaves using different solvents and associated with various biological activities, including antioxidant, anticancer, or antitumor activity (Zayed et al., 2014). Sun and Hai Liu (2006) described that the existence of different components in the natural sources may produce additive and synergistic effects rather than a single effect. The biochemical and different morphological changes such as cell shrinkage, cell blebbing, the formation of apoptotic bodies, nuclear condensation, and DNA fragmentation are the hallmarks of apoptosis (Ouyang et al., 2012). The apoptotic-like features of MCF-7 cells were observed under a phase-contrast inverted microscope. Cell rounding, cell shrinkage, membrane blebs, loose contact with adjacent cells, the formation of apoptotic bodies, and cell vacuolization were seen in the treated MCF-7 cells. The appearance of apoptotic cell features has also been reported in MCF-7 cells treated with rapamycin under inverted microscope observation (Khairi et al., 2014). Alteration in the morphology of MCF-7 cells following the NC extract treatment indicated that the cells experienced apoptosis (Wen et al., 2017). One of the main challenges with the current treatments is the capacity to hit cancer cells without killing normal cells surrounding the cancer cells. However, the findings in this study revealed that NC extract was slightly toxic toward normal cells.
Inhibition of proliferation and/or induction of apoptosis in cancer cells is considered one of the main important criteria for many anticancer agents (Barhoi et al., 2021; Orabi et al., 2021). The flow cytometric analysis in the present study revealed that MCF-7 cells underwent apoptosis upon treatment with NC, abundantly in late apoptosis. A similar finding was found on MCF-7 cells after the treatment with rapamycin in which late apoptosis up to 23.2% had been reported (Khairi et al., 2014). Early apoptosis was detected in the present study indicating the externalization of negatively charged phosphatidylserine (PS) (Riedl et al., 2011; Jamali et al., 2018), which is mainly localized in the inner layer of the membrane. Late apoptosis is also known as secondary necrosis, causing loss of membrane integrity (Poon et al., 2010).
The cell cycle consists of consecutive four phases that are important in cell division and cell proliferation. The unscheduled proliferation and genomic instability give rise to the accumulation of tumor cells (Hanahan and Weinberg, 2000). The finding in this study suggests that NC leaves 80% ethanol extract caused cell cycle arrest at the G0/G1 phase in MCF-7 cells after 72 h of exposure. The results clearly showed a significant increase of cell distribution in the G0/G1 phase accompanied by a decrease of cell distribution in phase S. Accumulation of cells in the G0/G1 phase has been reported in MCF-7 cancer cells (Sun and Hai Liu, 2006; Choi and Kim, 2008) and other cancer cell lines (Fan et al., 2015; Karade and Jadhav, 2018) after plant extract treatment. A similar finding was also reported in a study in which epirubicin caused the G0/G1 cell cycle arrest on MCF-7 and T47D cells (Xiong et al., 2016). Upon detecting the DNA damage, cell cycle checkpoints stop the progression of the cell cycle from one phase to the next phase to ensure the fidelity of genetic information (Vermeulen et al., 2003). The arrest of MCF-7 cells at the G0/G1 phase prevents the cells from entering the next phases of the cell cycle, the S and G2/M phases, which eventually inhibits the synthesis of DNA and suppresses proliferation of the cells. To find out the possible mechanism of the extract to induce apoptosis and cell cycle arrest in MCF-7 cells, the qPCR assay was performed. The upregulation of cytochrome c links with the upregulation of Bax expression and downregulation of Bcl-2 (Ilhan, 2020). Bax executes its action on mitochondria by promoting the release of cytochrome c, via increasing the permeability of mitochondria membrane, into the intracellular space (Kilbride and Prehn, 2013). The inhibition of Bcl-2 activity involves with the heterodimer formation on Bcl-2 by Bax gene to counteract the antiapoptotic effects of Bcl-2 (Adams and Cory, 2007). The liberation of cytochrome c would activate apoptosome, which then activates caspase-9 and the effector caspases, caspase-3 and caspase-7 leading to cell death (Olsson & Zhivotovsky, 2011; Westphal et al., 2011; Looi et al., 2013). The morphological and biochemical changes are associated with caspase activation upon apoptotic stimuli (Duclos et al., 2017). The upregulation of caspase-9 and caspase-7 expressions was detected upon NC treatment on MCF-7 cells. Activation of the effector caspases via proteolytic cleavage by caspase-9 and cytochrome c is believed to play a crucial role in the execution of apoptosis (Hassan et al., 2014). These results might suggest that NC extract exerts apoptosis through the intrinsic pathway. The expression of p21 (tumor suppressor gene) stops the cell cycle progression from dividing damaged cells. The binding of p21 on CDKs or cyclin-CDK complexes disrupts these interactions and inhibits cell cycle progression (Karimian et al., 2016; Kreis et al., 2019). Upregulated p21 and downregulated CDK2 support the G0/G1 cell cycle arrest in the previous data. Interestingly, cyclin E in the present study was found to be upregulated, which suggested that cyclin E independently binds to CDK2 for halting the cell cycle. It was suggested that inhibition of breast cancer cell MCF-7 proliferation may be linked to the upregulation of cyclin E and p21 as well as downregulation of CDK2 upon NC extract treatment. In addition, p21 was found to be associated with apoptosis. A study found the cytochrome c blockage and caspase-3 inactivation in HCT116 p21−/− but not in HCT p53−/− and HCT116 Bax−/− of colon cancer cells after treatment with curcumin. The study also found a reduced expression of Apaf-1 in the HCT116 p21−/− cells, whereas it was not expressed in wild type (Gogada et al., 2011). Cytochrome c release and Apaf-1 are required for caspase-9 activation of the intrinsic apoptosis pathway (Zhou et al., 2015), which in turn activates executioner caspases, including caspase-3 and caspase-7. Based on the present findings, it is suggested that p21 upregulated expressions may have been involved in apoptosis activities by promoting the cytochrome c release to inhibit the proliferation of MCF-7 cells upon treatment.
Conclusion
The present research data suggest that NC leaves ethanol extract inhibits cellular growth in MCF-7 cells by inducing apoptosis and cell cycle arrest. As supported by the flow cytometric analysis, upregulation of Bax, cytochrome c, and caspases (caspase-9 and -7) with downregulation of Bcl-2 in MCF-7 cells suggest that NC leaves ethanol extract promotes apoptosis via the intrinsic pathway. In addition, upregulation of p21 and cyclin E, and downregulation of CDK2 expressions suggest that cell cycle arrest occurs in MCF-7 cells upon NC leaves’ ethanol extract treatment. Through this finding, the upregulation of cytochrome c may be associated with the upregulation of p21, which ultimately leads to apoptosis induction. Other than that, upregulation of cyclin E alone may have caused cell cycle arrest, in which overexpression may reduce the proliferation of MCF-7 cells. The GCMS analysis revealed that D-pinitol, myo-inositol, hexadecanoic acid (palmitic acid), octadecanoic acid (stearic acid), oleic acid, and phenol, 2,4-bis(1,1-dimethylethyl) compounds may have contributed to the aforementioned anticancer effects of the NC leaves ethanol extract. Nevertheless, other compounds that could not be identified in the GCMS library might have also played some roles in the anticancer effects of the NC leaves ethanol extract. Therefore, further research is still required to identify other possible bioactive compounds possessing anticancer effects and evaluate all the possible bioactive anticancer candidates. Additionally, studies involving an in vivo model are still necessary to further analyze NC anticancer effects and the mechanism of action.
Data Availability Statement
The raw data supporting the conclusions of this article will be made available by the authors, without undue reservation.
Author Contributions
AK and RW conceptualized the idea and outlined the research project. SR and AK performed all preliminary tests, experiments, and wrote the research manuscript. ZZ and QA provided research ideas and laboratory facilities, and edited the research manuscript. All authors equally participated in the preparation of the final research manuscript and approved it for publication without any conflict of interest.
Funding
This research was funded by the Malaysian Ministry of Education, grant number FRGS 16-046-0545 (Granted to AK) and IIUM Publication Research Initiative Grant fund, grant number PRIGS18-027-0027 (Granted to AK).
Conflict of Interest
The authors declare that the research was conducted in the absence of any commercial or financial relationships that could be construed as a potential conflict of interest.
Reviewer MA declared a past coauthorship with one of the author ZZ to the handling editor.
Publisher’s Note
All claims expressed in this article are solely those of the authors and do not necessarily represent those of their affiliated organizations, or those of the publisher, the editors, and the reviewers. Any product that may be evaluated in this article, or claim that may be made by its manufacturer, is not guaranteed or endorsed by the publisher.
Acknowledgments
We truly acknowledge technical assistance and equipment by the Molecular Laboratory staff of Kulliyyah of Medicine, Central Research and Animal Facility Cream (CREAM), and the Department of Pharmaceutical Chemistry, Kulliyyah of Pharmacy in International Islamic University Malaysia (IIUM). Thanks to Airlangga University for the invitation in Adjunct Professorship Program 2019.
Supplementary Material
The Supplementary Material for this article can be found online at: https://www.frontiersin.org/articles/10.3389/fphar.2021.741683/full#supplementary-material
References
Adams, J. M., and Cory, S. (2007). Bcl-2-Regulated Apoptosis: Mechanism and Therapeutic Potential. Curr. Opin. Immunol. 19, 488–496. doi:10.1016/j.coi.2007.05.004
Ahmed, F., Rahman, S., Ahmed, N., Hossain, M., Biswas, A., Sarkar, S., et al. (2011). Evaluation of Neolamarckia Cadamba (Roxb.) Bosser Leaf Extract on Glucose Tolerance in Glucose-Induced Hyperglycemic Mice. Afr. J. Tradit Complement. Altern. Med. 8, 79–81. doi:10.4314/ajtcam.v8i1.60549
Artini, P. G., Di Berardino, O. M., Papini, F., Genazzani, A. D., Simi, G., Ruggiero, M., et al. (2013). Endocrine and Clinical Effects of Myo-Inositol Administration in Polycystic Ovary Syndrome. A Randomized Study. Gynecol. Endocrinol. 29, 375–379. doi:10.3109/09513590.2012.743020
Azizah, A. M., Nor Saleha, I. T., Noor Hashimah, A., Asmah, Z. A., and Mastulu, W. (2016). Malaysian National Cancer Registry Report 2007-2011: Malaysia Cancer Statistic, Data and Figure. Malaysia: Malaysia Natl. Cancer Inst.
Barhoi, D., Upadhaya, P., Barbhuiya, S. N., Giri, A., and Giri, S. (2021). Aqueous Extract of Moringa Oleifera Exhibit Potential Anticancer Activity and Can Be Used as a Possible Cancer Therapeutic Agent: A Study Involving In Vitro and In Vivo Approach. J. Am. Coll. Nutr. 40, 70–85. doi:10.1080/07315724.2020.1735572
Blumen, H., Fitch, K., and Polkus, V. (2016). Comparison of Treatment Costs for Breast Cancer, by Tumor Stage and Type of Service. Am. Health Drug Benefits 9, 23–32.
Bray, F., Ferlay, J., Soerjomataram, I., Siegel, R. L., Torre, L. A., and Jemal, A. (2018). Global Cancer Statistics 2018: GLOBOCAN Estimates of Incidence and Mortality Worldwide for 36 Cancers in 185 Countries. CA. Cancer J. Clin. 68, 394–424. doi:10.3322/caac.21492
Chandel, M., Sharma, U., Kumar, N., Singh, B., and Kaur, S. (2012). Antioxidant Activity and Identification of Bioactive Compounds from Leaves of Anthocephalus Cadamba by Ultra-performance Liquid Chromatography/electrospray Ionization Quadrupole Time of Flight Mass Spectrometry. Asian Pac. J. Trop. Med. 5, 977–985. doi:10.1016/S1995-7645(12)60186-2
Choi, E. J., and Kim, G. H. (2008). Daidzein Causes Cell Cycle Arrest at the G1 and G2/M Phases in Human Breast Cancer MCF-7 and MDA-MB-453 Cells. Phytomedicine 15, 683–690. doi:10.1016/j.phymed.2008.04.006
Dolai, N., Karmakar, I., Suresh Kumar, R. B., Kar, B., Bala, A., and Haldar, P. K. (2012). Evaluation of Antitumor Activity and In Vivo Antioxidant Status of Anthocephalus Cadamba on Ehrlich Ascites Carcinoma Treated Mice. J. Ethnopharmacol. 142, 865–870. doi:10.1016/j.jep.2012.05.050
Dubey, A., Nayak, S., and Goupale, D. C. (2011). A Review on Phytochemical, Pharmacological and Toxicological Studies on Neolamarckia Cadamba. Der. Pharm. Let. 3, 45–54.
Duclos, C., Lavoie, C., and Denault, J. B. (2017). Caspases Rule the Intracellular Trafficking Cartel. FEBS J. 284, 1394–1420. doi:10.1111/febs.14071
Evans, L. M., Cowey, S. L., Siegal, G. P., and Hardy, R. W. (2009). Stearate Preferentially Induces Apoptosis in Human Breast Cancer Cells. Nutr. Cancer 61, 746–753. doi:10.1080/01635580902825597
Fan, W., Sun, L., Zhou, J. Q., Zhang, C., Qin, S., Tang, Y., et al. (2015). Marsdenia Tenacissima Extract Induces G0/G1 Cell Cycle Arrest in Human Esophageal Carcinoma Cells by Inhibiting Mitogen-Activated Protein Kinase (Mapk) Signaling Pathway. Chin. J. Nat. Med. 13, 428–437. doi:10.1016/S1875-5364(15)30036-4
Gogada, R., Amadori, M., Zhang, H., Jones, A., Verone, A., Pitarresi, J., et al. (2011). Curcumin Induces Apaf-1-dependent, P21-Mediated Caspase Activation and Apoptosis. Cell Cycle 10, 4128–4137. doi:10.4161/cc.10.23.18292
Hanahan, D., and Weinberg, R. A. (2000). The Hallmarks of Cancer. Cell 100, 57–70. doi:10.1016/s0092-8674(00)81683-9
Harbeck, N., Penault-Llorca, F., Cortes, J., Gnant, M., Houssami, N., Poortmans, P., et al. (2019). Breast Cancer. Nat. Rev. Dis. Primers 5, 66. doi:10.1038/s41572-019-0111-2
Hassan, M., Watari, H., AbuAlmaaty, A., Ohba, Y., and Sakuragi, N. (20142014). Apoptosis and Molecular Targeting Therapy in Cancer. Biomed. Res. Int. 2014, 150845. doi:10.1155/2014/150845
Hsieh, T. C., Wijeratne, E. K., Liang, J. Y., Gunatilaka, A. L., and Wu, J. M. (2005). Differential Control of Growth, Cell Cycle Progression, and Expression of NF-kappaB in Human Breast Cancer Cells MCF-7, MCF-10A, and MDA-MB-231 by Ponicidin and Oridonin, Diterpenoids from the Chinese Herb Rabdosia Rubescens. Biochem. Biophys. Res. Commun. 337, 224–231. doi:10.1016/j.bbrc.2005.09.040
Ilhan, S. (2020). Essential Oils from Vitex Agnus Castus L. Leaves Induces Caspase-dependent Apoptosis of Human Multidrug-Resistant Lung Carcinoma Cells through Intrinsic and Extrinsic Pathways. Nutr. Cancer 73, 1–9. doi:10.1080/01635581.2020.1823439
Jamali, T., Kavoosi, G., Safavi, M., and Ardestani, S. K. (2018). In-vitro Evaluation of Apoptotic Effect of OEO and Thymol in 2D and 3D Cell Cultures and the Study of Their Interaction Mode with DNA. Sci. Rep. 8, 15787. doi:10.1038/s41598-018-34055-w
Javadi, N., Abas, F., Mediani, A., Abd Hamid, A., Khatib, A., Simoh, S., et al. (2015). Effect of Storage Time on Metabolite Profile and Alpha-Glucosidase Inhibitory Activity of Cosmos Caudatus Leaves - GCMS Based Metabolomics Approach. J. Food Drug Anal. 23, 433–441. doi:10.1016/j.jfda.2015.01.005
Karade, P. G., and Jadhav, N. R. (2018). In Vitro Studies of the Anticancer Action of Tectaria Cicutaria in Human Cancer Cell Lines: G0/G1 P53-Associated Cell Cycle Arrest-Part I. J. Tradit. Complement. Med. 8, 459–464. doi:10.1016/j.jtcme.2017.07.003
Karimian, A., Ahmadi, Y., and Yousefi, B. (2016). Multiple Functions of P21 in Cell Cycle, Apoptosis and Transcriptional Regulation after DNA Damage. DNA Repair (Amst) 42, 63–71. doi:10.1016/j.dnarep.2016.04.008
Kaur, S., and Kumar, S. (2011). Studies on the Genoprotective/Antioxidant Potential of Methanol Extract of Anthocephalus Cadamba (Roxb.). Miq. J. Med. Plants Res. 5, 4764–4770. doi:10.5897/JMPR.9001158
Khairi, M., Shamsuddin, S., and Jaafar, H. (2014). Effects of Rapamycin on Cell Apoptosis in MCF-7 Human Breast Cancer Cells. Asian Pac. J. Cancer Prev. 15, 10659–10663. doi:10.7314/apjcp.2014.15.24.10659
Kilbride, S. M., and Prehn, J. H. (2013). Central Roles of Apoptotic Proteins in Mitochondrial Function. Oncogene 32, 2703–2711. doi:10.1038/onc.2012.348
Kreis, N. N., Louwen, F., and Yuan, J. (2019). The Multifaceted P21 (Cip1/Waf1/CDKN1A) in Cell Differentiation, Migration and Cancer Therapy. Cancers (Basel) 11, 1220. doi:10.3390/cancers11091220
Lin, L., Yan, L., Liu, Y., Yuan, F., Li, H., and Ni, J. (2019). Incidence and Death in 29 Cancer Groups in 2017 and Trend Analysis from 1990 to 2017 from the Global Burden of Disease Study. J. Hematol. Oncol. 12, 96. doi:10.1186/s13045-019-0783-9
Lin, T. H., Tan, T. W., Tsai, T. H., Chen, C. C., Hsieh, T. F., Lee, S. S., et al. (2013). D-pinitol Inhibits Prostate Cancer Metastasis through Inhibition of αVβ3 Integrin by Modulating FAK, C-Src and NF-Κb Pathways. Int. J. Mol. Sci. 14, 9790–9802. doi:10.3390/ijms14059790
Liu, J., Liu, Y., Wang, Y., Abozeid, A., Zu, Y. G., and Tang, Z. H. (2017). The Integration of GC-MS and LC-MS to Assay the Metabolomics Profiling in Panax Ginseng and Panax Quinquefolius Reveals a Tissue- and Species-specific Connectivity of Primary Metabolites and Ginsenosides Accumulation. J. Pharm. Biomed. Anal. 135, 176–185. doi:10.1016/j.jpba.2016.12.026
Liu, M., Chen, Y. L., Kuo, Y. H., Lu, M. K., and Liao, C. C. (2018). Aqueous Extract of Sapindus Mukorossi Induced Cell Death of A549 Cells and Exhibited Antitumor Property In Vivo. Sci. Rep. 8, 4831. doi:10.1038/s41598-018-23096-w
Looi, C. Y., Arya, A., Cheah, F. K., Muharram, B., Leong, K. H., Mohamad, K., et al. (2013). Induction of Apoptosis in Human Breast Cancer Cells via Caspase Pathway by Vernodalin Isolated from Centratherum Anthelminticum (L.) Seeds. PloS one 8, e56643. doi:10.1371/journal.pone.0056643
Lukong, K. E. (2017). Understanding Breast Cancer - the Long and Winding Road. BBA Clin. 7, 64–77. doi:10.1016/j.bbacli.2017.01.001
Mericli, F., Becer, E., Kabadayı, H., Hanoglu, A., Yigit Hanoglu, D., Ozkum Yavuz, D., et al. (2017). Fatty Acid Composition and Anticancer Activity in Colon Carcinoma Cell Lines of Prunus Dulcis Seed Oil. Pharm. Biol. 55, 1239–1248. doi:10.1080/13880209.2017.1296003
Mondal, S., Dash, G. K., and Acharyya, S. (2009). Analgesic, Anti-inflammatory and Antipyretic Studies of Neolamarckia Cadamba Barks. J. Pharm. Res. 2, 1133–1136.
Newman, D. J., and Cragg, G. M. (2016). Natural Products as Sources of New Drugs from 1981 to 2014. J. Nat. Prod. 79, 629–661. doi:10.1021/acs.jnatprod.5b01055
Olsson, M., and Zhivotovsky, B. (2011). Caspases and Cancer. Cell Death Differ 18, 1441–1449. doi:10.1038/cdd.2011.30
Orabi, K. Y., Abaza, M. S., Luqmani, Y. A., and Al-Attiyah, R. (2021). Psiadin and Plectranthone Selectively Inhibit Colorectal Carcinoma Cells Proliferation via Modulating Cyclins Signaling and Apoptotic Pathways. PLoS ONE 16, e0252820. doi:10.1371/journal.pone.0252820
Ouyang, L., Shi, Z., Zhao, S., Wang, F. T., Zhou, T. T., Liu, B., et al. (2012). Programmed Cell Death Pathways in Cancer: A Review of Apoptosis, Autophagy and Programmed Necrosis. Cell Prolif 45, 487–498. doi:10.1111/j.1365-2184.2012.00845.x
Pandey, A., and Negi, P. S. (2016). Traditional Uses, Phytochemistry and Pharmacological Properties of Neolamarckia Cadamba: A Review. J. Ethnopharmacol. 181, 118–135. doi:10.1016/j.jep.2016.01.036
Poon, I. K., Hulett, M. D., and Parish, C. R. (2010). Molecular Mechanisms of Late Apoptotic/Necrotic Cell Clearance. Cel Death Differ 17, 381–397. doi:10.1038/cdd.2009.195
Rafshanjani, M., Shuaib, A., Parvin, S., and Kader, M. (2014). Antimicrobial and Preliminary Cytotoxic Effects of Ethanol Extract and its Fractions of Anthocephalus Cadamba (Roxb.) Miqstem Bark. Int. J. Pharm. Life Sci. 5, 4038–4044. doi:10.7897/2230-8407.0511175
Ravi, L., and Krishnan, K. (2017). Cytotoxic Potential of N-Hexadecanoic Acid Extracted from Kigelia Pinnata Leaves. Asian J. Cel Biol. 12, 20–27. doi:10.3923/ajcb.2017.20.27
Rayan, A., Raiyn, J., and Falah, M. (2017). Nature Is the Best Source of Anticancer Drugs: Indexing Natural Products for Their Anticancer Bioactivity. PLoS One 12, e0187925. doi:10.1371/journal.pone.0187925
Rengarajan, T., Nandakumar, N., Rajendran, P., Haribabu, L., Nishigaki, I., and Balasubramanian, M. P. (2014). D-pinitol Promotes Apoptosis in MCF-7 Cells via Induction of P53 and Bax and Inhibition of Bcl-2 and NF-Κb. Asian Pac. J. Cancer Prev. 15, 1757–1762. doi:10.7314/apjcp.2014.15.4.1757
Riedl, S., Rinner, B., Asslaber, M., Schaider, H., Walzer, S., Novak, A., et al. (20111808). In Search of a Novel Target - Phosphatidylserine Exposed by Non-apoptotic Tumor Cells and Metastases of Malignancies with Poor Treatment Efficacy. Biochim. Biophys. Acta 1808, 2638–2645. doi:10.1016/j.bbamem.2011.07.026
Sankaranarayanan, R., Ramadas, K., and Qiao, Y. L. (2014). Managing the Changing Burden of Cancer in Asia. BMC Med. 12, 3. doi:10.1186/1741-7015-12-3
Shah, U., Shah, R., Acharya, S., and Acharya, N. (2013). Novel Anticancer Agents from Plant Sources. Chin. J. Nat. Medicines 11, 16–23. doi:10.1016/s1875-5364(13)60002-3
Singh, S., Ishar, M. P. S., Saxena, A. K., and Kaur, A. (2013). Cytotoxic Effect of Anthocephalus Cadamba Miq. Leaves on Human Cancer Cell Lines. Pharmacognosy J. 5, 127–129. doi:10.1016/j.phcgj.2013.04.005
Strober, W. (2015). Trypan Blue Exclusion Test of Cell Viability. Curr. Protoc. Immunol. 111, A3. doi:10.1002/0471142735.ima03bs111
Sun, J., and Hai Liu, R. (2006). Cranberry Phytochemical Extracts Induce Cell Cycle Arrest and Apoptosis in Human MCF-7 Breast Cancer Cells. Cancer Lett. 241, 124–134. doi:10.1016/j.canlet.2005.10.027
Thun, M. J., DeLancey, J. O., Center, M. M., Jemal, A., and Ward, E. M. (2010). The Global Burden of Cancer: Priorities for Prevention. Carcinogenesis 31, 100–110. doi:10.1093/carcin/bgp263
Torre, L. A., Bray, F., Siegel, R. L., Ferlay, J., Tieulent, J. L., and Jemal, A. (2015). Global Cancer Statistics, 2012. CA Cancer J. Clin. 65, 87–108. doi:10.3322/caac.21262
Trogdon, J. G., Ekwueme, D. U., Poehler, D., Thomas, C. C., Hayes, K. R., and Allaire, B. T. (2017). Medical Costs of Treating Breast Cancer Among Younger Medicaid Beneficiaries by Stage at Diagnosis. Breast Cancer Res. Treat. 166, 207–215. doi:10.1007/s10549-017-4386-2
Vermeulen, K., Van Bockstaele, D. R., and Berneman, Z. N. (2003). The Cell Cycle: A Review of Regulation, Deregulation and Therapeutic Targets in Cancer. Cel Prolif 36, 131–149. doi:10.1046/j.1365-2184.2003.00266.x
Wen, Y., Chen, Z., Lu, J., Ables, E., Scemama, J. L., Yang, L. V., et al. (2017). Quantitative Analysis and Comparison of 3D Morphology between Viable and Apoptotic MCF-7 Breast Cancer Cells and Characterization of Nuclear Fragmentation. PLoS One 12, e0184726. doi:10.1371/journal.pone.0184726
Westphal, D., Dewson, G., Czabotar, P. E., and Kluck, R. M. (2011). Molecular Biology of Bax and Bak Activation and Action. Biochim. Biophys. Acta 1813, 521–531. doi:10.1016/j.bbamcr.2010.12.019
Xavier, A. P., Santharam, L., Panigrahi, S., Muthuraman, M. S., and Pemiah, B. (2013). Antitumor Potential of Ethanolic Extract of Solanum Trilobatum against Ehrlich’s Ascites Carcinoma. Int. J. Pharm. Tech. Res. 5, 1119–1125.
Xiong, J., Fan, F., Zhang, Y., Chen, W., and Mao, W. (2016). Epirubicin Inhibits Proliferation of Breast Cancer Cells through Upregulating P21 (Cip1) Expression. Int. J. Clin. Exp. Med. 9, 22764–22772.
Zayed, M. Z., Ahmad, F. B., Ho, W. S., and Pang, S. L. (2014). GC-MS Analysis of Phytochemical Constituents in Leaf Extracts of Neolamarckia Cadamba (Rubiaceae) from Malaysia. Int. J. Pharm. Pharm. Sci. 6, 123–127.
Keywords: Neolamarckia cadamba, breast cancer, gas chromatography, gene expression assay, mechanisms of action, in vitro anticancer
Citation: Razali S, Firus Khan A, Khatib A, Ahmed Q, Abdul Wahab R and Zakaria Z (2021) An In Vitro Anticancer Activity Evaluation of Neolamarckia cadamba (Roxb.) Bosser Leaves’ Extract and its Metabolite Profile. Front. Pharmacol. 12:741683. doi: 10.3389/fphar.2021.741683
Received: 15 July 2021; Accepted: 13 September 2021;
Published: 13 October 2021.
Edited by:
Alessandra Durazzo, Council for Agricultural Research and Economics, ItalyReviewed by:
Ali M. Alqahtani, King Khalid University, Saudi ArabiaMuhammad Nadeem Akhtar, Islamia University, Pakistan
Ahmed El-Sharkawy, Institute of Genetics and Biophysics (CNR), Italy
Harika Atmaca, Celal Bayar University, Turkey
Copyright © 2021 Razali, Firus Khan, Khatib, Ahmed, Abdul Wahab and Zakaria. This is an open-access article distributed under the terms of the Creative Commons Attribution License (CC BY). The use, distribution or reproduction in other forums is permitted, provided the original author(s) and the copyright owner(s) are credited and that the original publication in this journal is cited, in accordance with accepted academic practice. No use, distribution or reproduction is permitted which does not comply with these terms.
*Correspondence: Alfi Khatib, YWxmaWtoYXRpYkBpaXVtLmVkdS5teQ==; Zainul Amiruddin Zakaria, ZHJ6YXpha2FyaWFAZ21haWwuY29t