- 1Department of Pharmacology, Faculty of Medicine, Universiti Kebangsaan Malaysia, Kuala Lumpur, Malaysia
- 2Unit of Pharmacology, Department of Basic Medical Sciences, Faculty of Medicine, Universiti Sultan Zainal Abidin, Kuala Terengganu, Malaysia
- 3Drug and Herbal Research Centre, Faculty of Pharmacy, Universiti Kebangsaan Malaysia, Kuala Lumpur, Malaysia
- 4Unit of Pharmacology, Faculty of Medicine and Defense Health, Universiti Pertahanan Nasional Malaysia, Kuala Lumpur, Malaysia
- 5Cardiovacular Health Research Group, Faculty of Medicine, Universiti Kebangsaan Malaysia, Kuala Lumpur, Malaysia
Cardiac hypertrophy is characteristic of heart failure in patients who have experienced cardiac remodeling. Many medicinal plants, including Parkia speciosa Hassk., have documented cardioprotective effects against such pathologies. This study investigated the activity of P. speciosa empty pod extract against cardiomyocyte hypertrophy in H9c2 cardiomyocytes exposed to angiotensin II (Ang II). In particular, its role in modulating the Ang II/reactive oxygen species/nitric oxide (Ang II/ROS/NO) axis and mitogen-activated protein kinase (MAPK) pathway was examined. Treatment with the extract (12.5, 25, and 50 μg/ml) prevented Ang II-induced increases in cell size, NADPH oxidase activity, B-type natriuretic peptide levels, and reactive oxygen species and reductions in superoxide dismutase activity. These were comparable to the effects of the valsartan positive control. However, the extract did not significantly ameliorate the effects of Ang II on inducible nitric oxide synthase activity and nitric oxide levels, while valsartan did confer such protection. Although the extract decreased the levels of phosphorylated extracellular signal-related kinase, p38, and c-Jun N-terminal kinase, valsartan only decreased phosphorylated c-Jun N-terminal kinase expression. Phytochemical screening identified the flavonoids rutin (1) and quercetin (2) in the extract. These findings suggest that P. speciosa empty pod extract protects against Ang II-induced cardiomyocyte hypertrophy, possibly by modulating the Ang II/ROS/NO axis and MAPK signaling pathway via a mechanism distinct from valsartan.
Introduction
Cardiac hypertrophy initially develops as an adaptive response to compensate for reduced cardiac function (Bernardo and McMullen, 2016). Unfortunately, the sustained effects of pathological stimuli promote pathophysiological changes that lead to cardiac remodeling and, ultimately, heart failure (Wang et al., 2016). Angiotensin II (Ang II), a potent stimulus of cardiac myocyte growth factors, has been found to be elevated in cardiac failure (Zucker et al., 2015). Ang II can be used to mimic pressure-overload-induced cardiac hypertrophy (Ying et al., 2014) and has been widely employed as a hypertrophic stimulus in various in vitro cardiac disease models (Ding et al., 2019).
Exposure to Ang II stimulates the development of cardiac hypertrophy by activating G-protein-coupled receptors, which, in turn, activate several cascades, including the Ang II/reactive oxygen species/nitric oxide (Ang II/ROS/NO) axis as well as signaling kinases and phosphatases (Takano et al., 2003). Substantial evidence has linked Ang II-stimulated pathways to the activation of NADPH oxidase (NOX), which is a significant source of ROS in cardiovascular cells (Nguyen Dinh Cat et al., 2013). ROS have been implicated in the activation of mitogen-activated protein kinase (MAPK) and nuclear factor kappa B (NF-κB) pathways in Ang II-induced cardiac hypertrophy (Chen et al., 2020; Zhu et al., 2020). MAPK has three subfamilies—extracellular signal-related kinase (ERK1/2), c-Jun N-terminal kinase (JNK), and p38 kinase (p38)—that have been reported to play a role in cardiac hypertrophy (Zhang et al., 2003; Muslin, 2008; Cheng et al., 2017). Ang II also causes cardiac inflammation by promoting inducible nitric oxide synthase (iNOS) activity (Huang et al., 2017). These overlapping pathways eventually lead to cardiac remodeling and hypertrophy.
A potential therapeutic target for halting the progression of cardiac failure involves the prevention of pathological cardiac hypertrophy, for which numerous studies have attempted to identify novel therapies. While no drugs directly or specifically targeting pathological cardiac hypertrophy have been identified (Tran et al., 2016), neurohormonal blockers have been found to reduce cardiac hypertrophy indirectly. Ethnopharmacology is a promising screening tool in drug discovery, and many medicinal plants, including Eriobotrya japonica (Thunb.) Lindl (Chiang et al., 2018) and Nelumbo nucifera Gaertn. (Cho et al., 2019), have been shown to display cardioprotective activity against Ang II-induced cardiomyocyte hypertrophy.
Parkia speciosa Hassk., a leguminous plant in the family Fabaceae, grows indigenously in Southeast Asia and has traditionally been used to manage hypertension (Azliza et al., 2012) and heart problems (Yullia, 2008). The plant’s empty pods have been reported to display various pharmacological activities, including anti-inflammatory (Mustafa et al., 2018; Gui et al., 2019a), antioxidant (Gui et al., 2019b), and α-glucosidase-inhibiting (Saleh et al., 2021) properties. Extracts from its pods contain a higher antioxidant capacity than its seeds (Kamisah et al., 2013), likely associated with the pod’s flavonoid and phenolic components, including gallic acid, quercetin, gossypetin, and catechin (Ko et al., 2014; Saleh et al., 2021). Experiments in hypertensive rats support the pods’ hypotensive and cardioprotective properties (Kamisah et al., 2017). However, their effects on cardiomyocyte hypertrophy have yet to be investigated. As plant extracts with high flavonoid content have been shown to protect against cardiomyocyte hypertrophy (Sun et al., 2018; Cho et al., 2019), this study aimed to investigate the effects of P. speciosa empty pod extract on the Ang II/ROS/NO axis and MAPK signaling pathway in Ang II-treated cardiomyocytes.
Materials and Methods
Materials
P. speciosa pods (Figure 1) were purchased from a local trader at Slim River, Perak, Malaysia (3°49′31.0ʺN 101°29′12.1ʺE) in January 2018. A voucher specimen (UKMB40383) was deposited at the Universiti Kebangsaan Malaysia Herbarium. H9c2 cardiomyocytes were obtained commercially (American Type Culture Collection, Rockville, MD, United States). All chemicals were purchased from Sigma-Aldrich (St. Louis, MO, United States), and all antibodies for Western blotting were purchased from Cell Signaling Technology (Danvers, MA, United States) unless otherwise noted.
Empty Pod Extraction
The pods were cleaned, deseeded, and dried at room temperature. The dried, empty pods were ground and extracted in 95% ethanol in a 100 g:1 L ratio at room temperature for 9 days (Ko et al., 2014), with the ethanol changed every 3 days to improve yield. The extract was then filtered through cotton wool, and the filtrate was concentrated with a rotary vacuum evaporator (Buchi Rotavapor R-200 System, Marshall Scientific, Hampton, NH, United States). After freeze-drying (Labconco, Kansas City, MO, United States) for 5 days, the powder was stored at 4°C.
Phytochemical Screening
Phytochemical screening was conducted using high-performance liquid chromatography (HPLC) following the method of Tuszyńska (2014) with some modifications. Briefly, P. speciosa extract powder was dissolved in 100% aqueous methanol (10 mg/ml) before filtering through a nylon membrane (0.45 µm) (#PP013045; Membrane Solutions, Auburn, WA, United States). Since hydrolyzed glycosides are not applicable for in vitro experiments, the extract was not subjected to acid hydrolysis. HPLC was performed on a C18 column (150 × 4.6 mm, 5 μm; Phenomenex, Torrance, CA) using a Waters Series 600 (Waters, Milford, MA) fitted with a photodiode array detector and an autosampler with an injection volume of 20 μl. The samples were isocratically eluted using 0.2% orthophosphoric acid in methanol/water (60/40) at 0.75 ml/min with detection at 370 nm. This procedure was repeated on three separate days (interday) with at least three replicates/day (intraday) to determine precision.
Peaks in the extract samples were compared to catechin (K4512), rutin (R5143), quercetin (Q4951), kaempferol (K0133), ellagic acid (E2250), gallic acid (27,645), and caffeic acid (C0625) standards. The area under the curve (AUC) was calculated for five concentrations (62.5–1,000 μg/ml) of each standard run in triplicate and was used to prepare calibration curves. Fitted equations for the calibration curves were used to calculate the concentration of the compounds in P. speciosa extract.
H9c2 Cell Culture
H9c2 cells were cultured in Dulbecco’s Modified Eagle Medium (DMEM; Gibco BRL Life Technologies, Grand Island, NY, United States) supplemented with 10% fetal bovine serum (FBS), 100 U/ml penicillin G, and 100 μg/ml streptomycin at 37°C in a humidified atmosphere containing 5% CO2. The medium was changed every 2 d. The cells were grown to 60–70% confluency and serum-starved for 24 h prior to the experiment (Yan et al., 2013). Cells passaged 5–7 times and grown to a density of 1.6 × 104 cells/ml were used for the experiments.
Cytotoxicity Study
Cells were seeded in a 96-well plate and incubated with various concentrations of P. speciosa extract (3.125–400 μg/ml) for 24 h. Cell viability was assayed using 3-(4,5-dimethylthiazol-2-yl)-5-(3-carboxymethoxyphenyl)-2-(4-sulfophenyl)-2H-tetrazolium (MTS; Cat No: 197010, Abcam, Cambridge, United Kingdom) with detection at 490 nm. Dimethyl sulfoxide (DMSO) (<0.1%) was used as the vehicle for P. speciosa extract. A minimum of three biological replicates was performed in triplicate (n = 3).
Concentration-Response Study of P. speciosa Extract on H9c2 Cell Size
Cells were treated concurrently with Ang II (600 nM) (Siti et al., 2021) and various concentrations of P. speciosa extract (3.125–100 μg/ml) for 24 h in eight-well chamber slides. Cell size was measured using immunofluorescence staining. The best three extract concentrations for protecting against Ang II-induced cardiomyocyte hypertrophy were selected for further study. At least three biological replicates were performed in triplicate (n = 3).
Experimental Groups
H9c2 cells were randomly assigned to seven groups: 1) control (vehicle), 2) 50 μg/ml P. speciosa extract, 3) Ang II (600 nM; Siti et al., 2021), 4) Ang II and 12.5 μg/ml extract, 5) Ang II and 25 μg/ml extract, 6) Ang II and 50 μg/ml extract, and 7) Ang II and 20 μM valsartan (Al-Mazroua et al., 2013). Valsartan served as the positive control. Cells were treated concurrently with the extract and Ang II for 24 h.
Cell Size Quantification
Cell size was measured following the method of Jeong et al. (2015) with slight modifications described by Siti et al. (2021). Cells were stained with a primary antibody against α-actinin (1:200 dilution; ab9465, Abcam, Cambridge, MA, United States) followed by an Alexa Fluor 488-conjugated anti-mouse secondary antibody (1:200 dilution; A-11059, Invitrogen, Waltham, MA, United States) and visualized via fluorescence microscopy (Olympus Optical, Tokyo, Japan). A blinded assessor quantified the cardiomyocytes’ surface areas (>60 cells) using ImageJ software (U. S. National Institutes of Health, Bethesda, MD, United States) and compared them to control cells. A minimum of three biological replicates was performed in triplicate (n = 3).
Cellular B-Type Natriuretic Peptide and iNOS Levels
The cellular levels of B-type natriuretic peptide (BNP) and iNOS were estimated from cell lysates using commercial kits (Elabscience, Houston, TX, United States). Briefly, the biotinylated detection antibody and samples were incubated in micro-ELISA wells precoated with BNP or rat NOS2/iNOS antibodies, excess conjugates were removed, and an avidin-horseradish peroxidase (HRP) conjugate was added to develop a blue color. Upon addition of a stop solution, a yellow color change occurred, which was measured at 450 nm. BNP and iNOS levels were estimated against standard curves. A minimum of three biological replicates was performed in triplicate (n = 3).
Cellular Nitrite and Intracellular ROS Detection
Nitrite, a stable metabolite of NO, was measured following the method described by Siti et al. (2019). Cardiomyocytes were seeded in 96-well plates. Sample cell lysate (50 μl) was reacted with an equal volume of modified Griess reagent for 15 min at room temperature in the dark. The absorbance was measured at 540 nm (EnSpire® Multimode Plate Reader, PerkinElmer, Inc., MA, United States) and compared to a sodium nitrite standard curve to determine nitrite concentrations.
Global levels of ROS, including peroxynitrite and superoxide, were assessed in living cells using a commercial kit (ROS-ID® Total ROS/Superoxide Detection Kit, ENZ-51010, Enzo, NY, United States) according to the manufacturer’s protocol. Fluorescence signals were measured at 488 nm using a microplate reader (EnSpire® Multimode Plate Reader, PerkinElmer, Inc., MA, United States).
At least three biological replicates were performed in triplicate (n = 3) for all experiments.
NOX and Superoxide Dismutase Activities
NOX activity was measured according to the method described by Mustapha et al. (2010). Briefly, cell lysate (50 μg protein/sample), cytochrome c (250 μg/L), and NADPH (100 μM) were incubated at 37°C for 2 h with or without diphenyleneiodonium (DPI, 100 μM). The absorbance of the mixture was quantified at 550 nm. NOX activity was calculated using an extinction coefficient of 21 mMcm−1.
Superoxide dismutase (SOD) activity (U/mg of protein) was measured according to the procedure of Beyer and Fridovich (1987). Sample cell lysate (20 μl) and riboflavin (10 μl, 50 μM) were added into an assay mixture containing 27 ml of phosphate buffer (pH 7.8, 50 mM), EDTA (50 μM), 1.5 ml of L-methionine (20 mM), and 1 ml of nitroblue tetrazolium (1.5 mM). The mixture was illuminated for 7 min in an aluminum foil-coated box equipped with a 40 W fluorescent bulb, and absorbance was measured at 550 nm.
At least three biological replicates were performed in triplicate (n = 3) for all experiments.
Western Blot Analysis
Protein expression was measured by Western blot as previously described (Siti et al., 2021). Anti-phospho-ERK1/2 rabbit polyclonal (1:1,000) (#4377), anti-phospho-JNK1/2 rabbit monoclonal (1:1,000) (#4668), and anti-phospho-p38 mouse monoclonal (1:500) (sc-166182; Santa Cruz Biotechnology, Dallas, TX, United States) were the primary antibodies used in this study. β-Actin mouse monoclonal antibodies (1:500) (sc-47778; Santa Cruz Biotechnology, Dallas, TX, United States) served as the loading control. HRP-conjugated IgG anti-mouse (1:2000) (sc-516102; Santa Cruz Biotechnology, Dallas, TX, United States) was used as the secondary antibody. Blots were visualized on a gel doc system and analyzed with ImageJ software (U. S. National Institutes of Health, Bethesda, MD, United States). A minimum of three biological replicates was performed in triplicate (n = 3).
Statistical Analysis
All data are reported as mean ± standard error of the mean (SEM) from a minimum of three biological replicates performed in triplicate. The Shapiro-Wilk test was used to test for normality. Results were analyzed using one-way analysis of variance (ANOVA) followed by Tukey’s post hoc test in SPSS version 24.0 software (IBM Corp., Armonk, NY, United States), with p < 0.05 considered significant.
Results
Phytochemical Screening of the Extract
There were 11 peaks detected in the sample extract chromatogram (Figure 2A). Two peaks were identified as rutin (1) and quercetin (2) (Figure 3) when compared against the peaks of the standards (Figure 2B). The remaining compounds could not be unequivocally identified owing to peak shape (blunted or multiple peaks). The retention time (tR) for rutin (1) and quercetin (2) in the extract resembled that of the standards (Table 1). Based on the rutin and quercetin calibration curves (Figure 4), the P. speciosa empty pod crude ethanolic extract contained 15.5 μg rutin/mg extract (1) and 0.11 μg quercetin/mg extract (2) (Table 2).
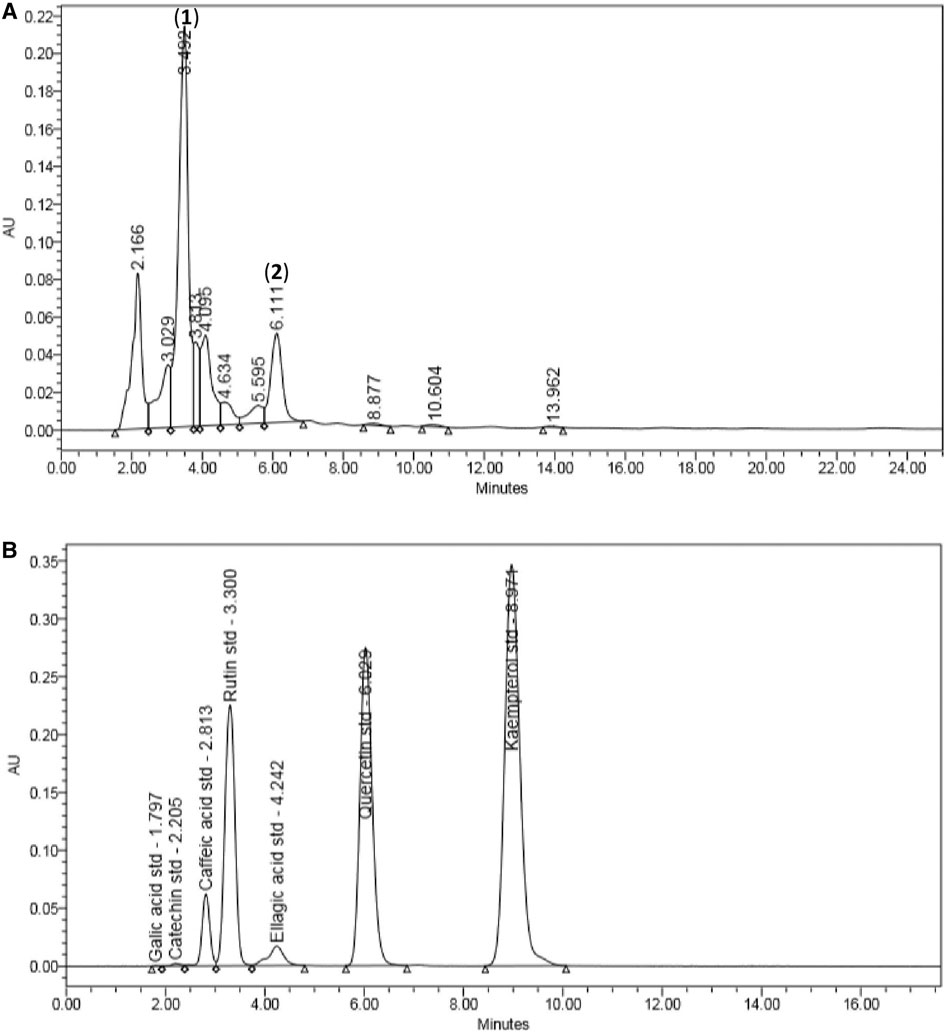
FIGURE 2. Chromatographic analysis of (A) Parkia speciosa empty pod crude extract with detected rutin (1) and quercetin (2), and (B) the standard known compounds.
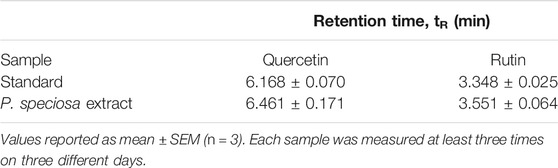
TABLE 1. Retention time (tR) of quercetin and rutin in 10 mg Parkia speciosa crude extract compared to standard compounds.
Extract Cytotoxicity
Treatment with 0.1% DMSO alone had no effect on cell viability as compared to the control (data not shown), indicating that its use as a vehicle for P. speciosa extract did not contribute to cytotoxicity. The median inhibitory concentration (IC50) of the extract was approximately 108.35 μg/ml (Figure 5). Subsequent experiments used sub-IC50 extract concentrations.
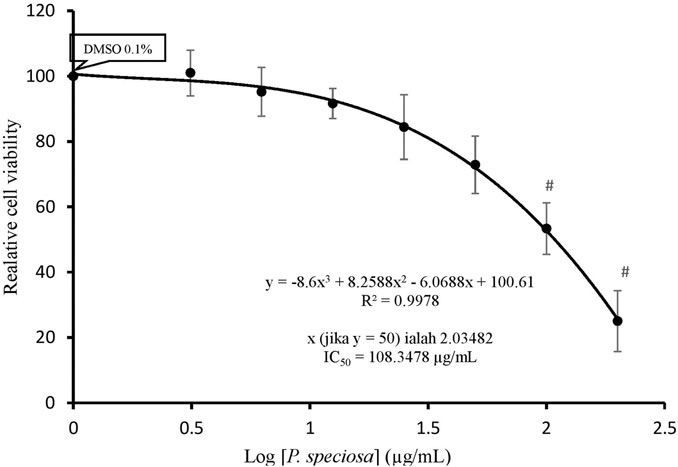
FIGURE 5. Cell viability of H9c2 cells after 24 h exposure to Parkia speciosa empty pod extract at increasing concentrations. *p < 0.05 vs. control. Results are presented as the mean ± SEM (n = 3).
Optimizing Extract Concentration for Antihypertrophic Activity
Ang II-induced cardiomyocyte hypertrophy was significantly alleviated with 6.25, 12.5, 25, and 50 μg/ml extract (Figure 6). Cell size was significantly reduced at 100 μg/ml extract compared to both the control and Ang II treatments (p < 0.05). Based on these findings, 12.5, 25, and 50 μg/ml extract were used in the subsequent experiments.
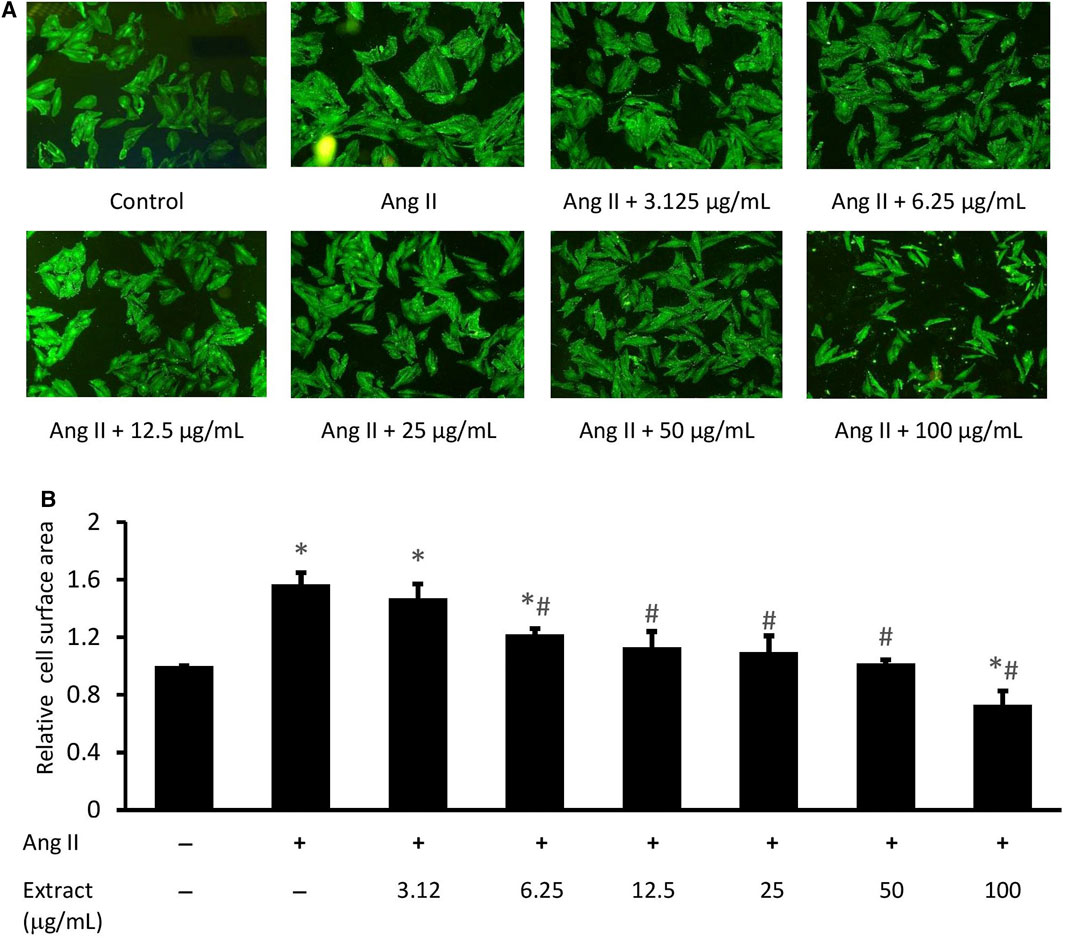
FIGURE 6. Effect of various concentrations of Parkia speciosa empty pod extract on cell size in H9c2 cells exposed to Ang II (600 nM) for 24 h. (A) Representative immunofluorescent image (magnification × 100) and (B) quantitative analysis of immunofluorescent cells. *p < 0.05 vs. control (no treatment) group. #p < 0.05 vs. Ang II group. Bars represent the mean ± SEM (n = 3).
Cell Size and BNP Levels
Ang II-treated cells showed a significant increase in cell size (1.52 ± 0.04 times) and cellular BNP levels (50.49 ± 1.16 ng/mg protein) compared to the control (27.29 ± 2.08 ng/mg protein) (Figure 7). Treatment with valsartan or the selected extract concentrations significantly reduced Ang II-induced changes in cell size and BNP levels (p < 0.05). There were no significant differences in these effects across the three extract concentrations or valsartan treatments. Treatment with 50 μg/ml extract alone did not significantly affect cell size or BNP levels.
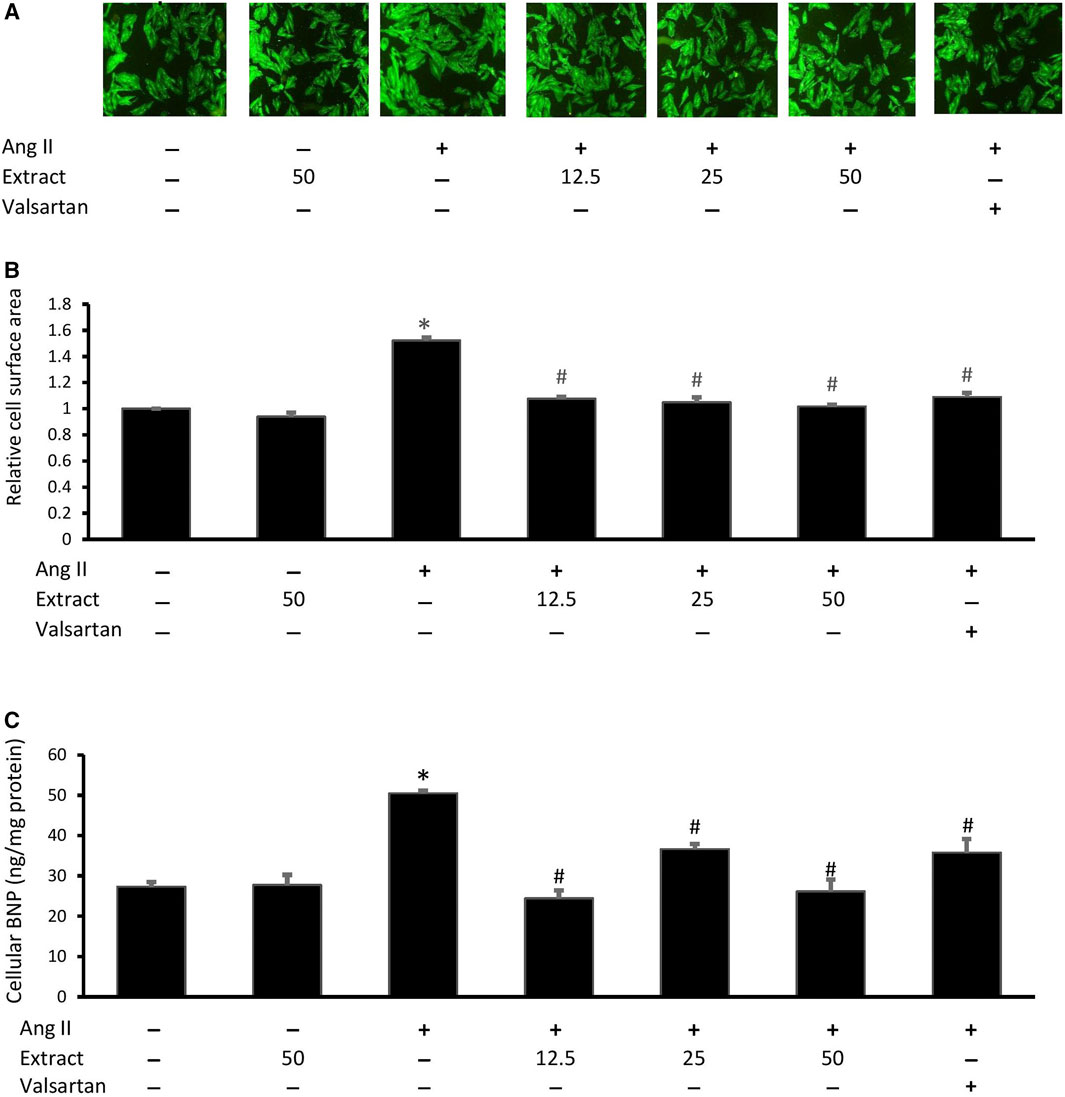
FIGURE 7. Effect of Parkia speciosa empty pod extract (μg/ml) or valsartan (20 μM, positive control) co-incubation on Ang II (600 nM)-induced H9c2 cell hypertrophy seen in (A) representative immunofluorescent-stained cells (magnification × 100), (B) cell surface area, and (C) B-type natriuretic peptide (BNP) after 24 h *p < 0.05 vs. control (no treatment) group. #p < 0.05 vs. Ang II group. Results are presented as the mean ± SEM (n = 3).
Intracellular ROS Levels and NOX and SOD Activities
While Ang II significantly increased the intracellular ROS levels and NOX activity and decreased the SOD activity in H9c2 cells compared to the control (Figures 8A–C), co-treatment with valsartan or the selected extract concentrations prevented these effects. There were no significant differences in intracellular ROS levels across the three extract concentrations. SOD activity was rescued similarly across all treatments. Treatment with 50 μg/ml extract alone did not significantly affect these parameters.
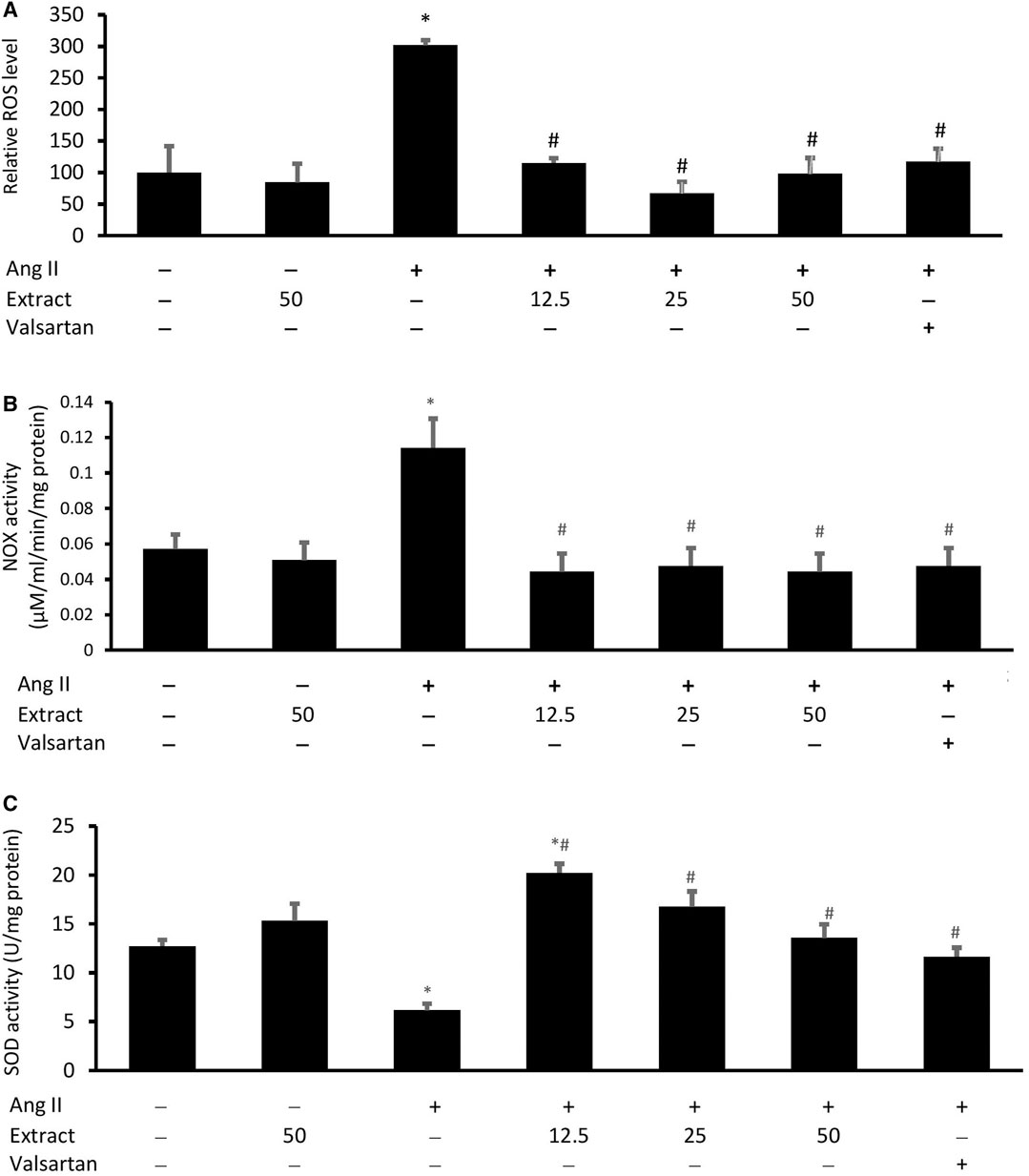
FIGURE 8. (A) Cellular reactive oxygen species (ROS) level, (B) NADPH oxidase (NOX) activities, and (C) superoxide dismutase (SOD) activities in cells co-treated with Ang II (600 nM) and three concentrations of Parkia speciosa extract (μg/ml) or valsartan (20 μM, positive control) for 24 h *p < 0.05 vs. control (no treatment) group. #p < 0.05 vs. Ang II group. Bars represent means ± SEM (n = 3).
Cellular iNOS and Nitrite Levels
Ang II significantly increased H9c2 cellular iNOS levels (0.016 ± 0.002 pg/mg protein, p < 0.05) compared to the control (0.008 ± 0.001 pg/mg protein) (Figure 9A). While co-treatment with P. speciosa empty pod extract did not prevent this change, iNOS levels were rescued with valsartan (p < 0.05). Exposure to Ang II significantly reduced H9c2 cellular nitrite levels (18.96 ± 3.49 mM/mg protein, p < 0.05) compared to the control (31.79 ± 4.29 mM/mg protein) (Figure 9B). Neither valsartan nor the selected extract concentrations significantly prevented this change (p > 0.05). Treatment with 50 μg/ml extract alone did not significantly affect these parameters.
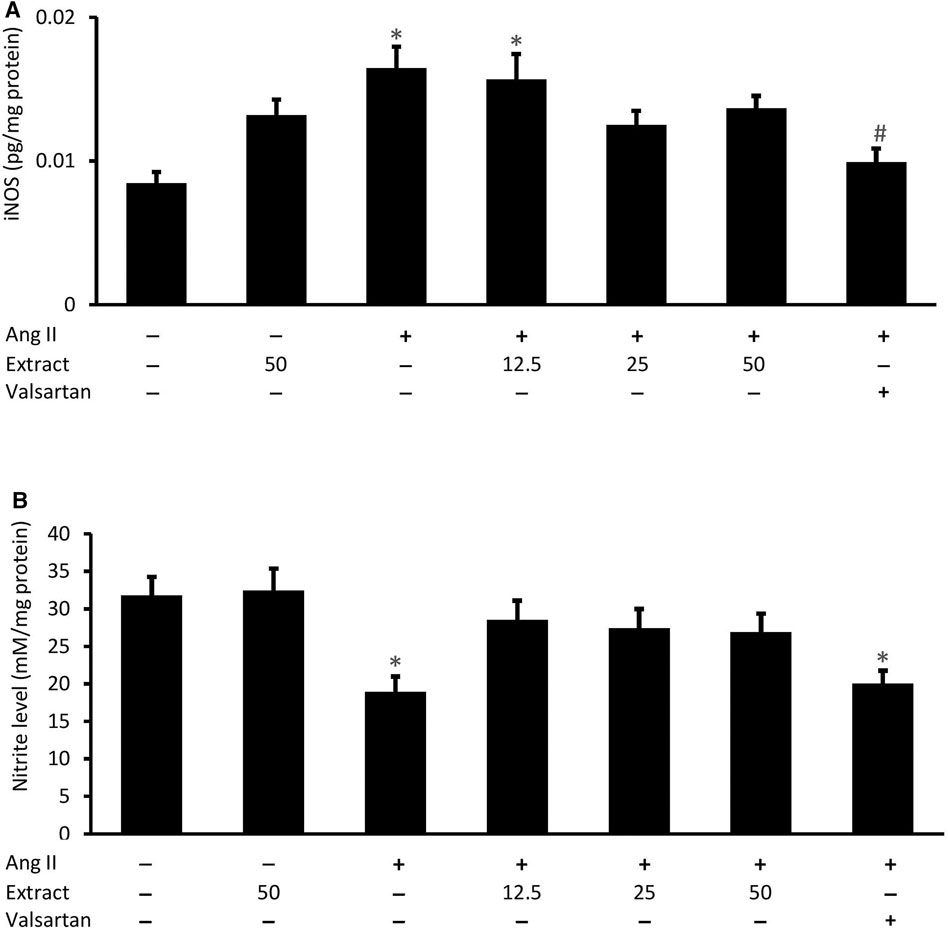
FIGURE 9. Cellular (A) inducible nitric oxide synthase (iNOS) activity and (B) nitrite level in groups co-treated with Ang II (600 nM) and three concentrations of Parkia speciosa empty pod extract or valsartan (20 μM, positive control) for 24 h *p < 0.05 vs. control (no treatment) group. #p < 0.05 vs. Ang II cells. Bars represent means ± SEM (n = 3).
MAPK Protein Expression
After 24 h, Ang II-treated H9c2 cells expressed elevated levels of phosphorylated ERK1/2, p38, and JNK (p < 0.05) (Figure 10). Co-treatment with all three selected extract concentrations prevented these elevated levels to a similar extent, while valsartan only rescued p-JNK expression. Treatment with 50 μg/ml extract alone did not significantly affect MAPK protein expression.
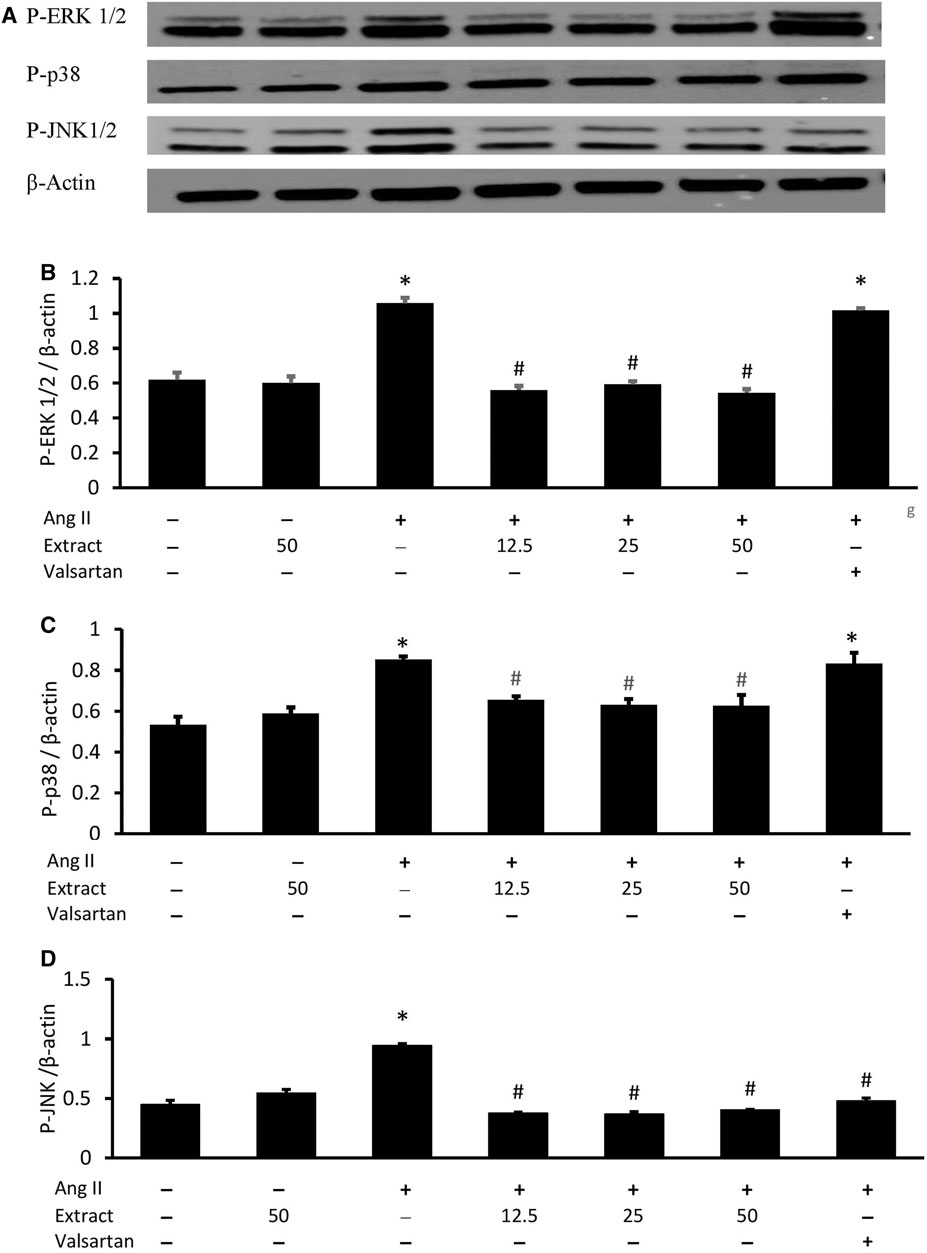
FIGURE 10. Effects of Parkia speciosa empty pod extract and valsartan (20 μM, positive control) on (A) representative immunoblots via Western blot analysis, quantitative analysis of phosphorylated (B) extracellular signal-related kinases (P-ERK1/2), (C) p38 kinase (P-p38), and (D) c-Jun N-terminal kinases (P-JNK) protein expressions in H9c2 cells that were exposed to Ang II (600 nM) for 24 h *p < 0.05 vs. control (no treatment) group. #p < 0.05 vs. Ang II cells. Bars represent means ± SEM (n = 3).
Discussion
Exposure to Ang II induced an ROS/NO axis imbalance, apparent in augmented intracellular superoxide/ROS (O2−•/ROS) levels, increased NOX and iNOS activities, and decreased SOD activity. This imbalance led to cardiomyocyte hypertrophy, which manifested in increased cell size and elevated BNP levels, indicative of ventricular dysfunction. Ang II is reported to promote cardiac hypertrophy by stimulating growth factors (Ding et al., 2019). The findings of this study confirm previous reports regarding the involvement of oxidative stress in the development of Ang II-induced cardiomyocyte hypertrophy (Guan et al., 2017; Hong et al., 2019). Binding of Ang II to the Ang II type 1 receptor (AT1R) enhances the activation of NOX (Masi et al., 2019), which is a substantial producer of ROS, including O2−• (Wen et al., 2019). The elevated levels of O2−• detected in the H9c2 cells depleted the antioxidant SOD, which functions as a first line of defense against cardiomyocyte hypertrophy by converting the radical anion into water and hydrogen peroxide (Campos-Shimada et al., 2020).
The detrimental effects of Ang II on the ROS/NO axis were prevented by co-treating with P. speciosa empty pod extract. Notably, the cardioprotective effects of the extract were not concentration-dependent. These findings highlight the extract’s antioxidant properties, in agreement with previous work (Kamisah et al., 2017; Gui et al., 2019b). The empty pod extract was employed in this study as it is reported to contain a higher antioxidant capacity than the seed extract (Kamisah et al., 2013; Zaini and Mustaffa 2017). The cardioprotective effects of the extract are likely associated with its flavonoid content, with rutin (1) and quercetin (2) identified among its primary metabolites in this work. Studies have reported the presence of other flavonoids (Ko et al., 2014; Ghasemzadeh et al., 2018) not detected in this work, likely due to differences in chromatographic analysis. HPLC analysis in this study was unable to identify the remaining contents in the extract unambiguously, which may have included other flavonoids and phenolic acids. As these other metabolites could play a role in the cardioprotective effects of P. speciosa extract, additional studies should focus on their identification. The purpose of identifying the metabolites in this study was to aid in understanding how the extract could provide its cardioprotective effects. To determine the specific mechanisms by which the extract prevents cardiomyocyte hypertrophy, studies should investigate the activity of the individual extract components. For example, previous work using commercial quercetin and rutin demonstrated their antioxidant and antihypertrophic activities in Ang II-treated cardiomyocytes (Siti et al., 2021). Flavonoids, such as quercetin and rutin, can exert their antioxidant effects by binding SOD and increasing its activity (Cos et al., 1998; Zhuang et al., 2016). Metabolites in the extract may also directly prevent the prooxidant effects of Ang II itself.
Another source of cellular ROS is iNOS, which is upregulated in response to increased microenvironmental inflammation (Cinelli et al., 2020). Ang II was found to activate iNOS activity in this work, consistent with previous findings (Restini et al., 2017). Ang II promotes inflammation via activation of the NF-κB signaling pathway and the release of tumor necrosis factor-α (TNFα) and interleukin 6 (Huang et al., 2017). However, this study found that despite increasing iNOS activity, exposure to Ang II decreased NO levels. NO can react with O2−• to generate peroxynitrite radicals (Radi, 2018), reducing its own level. Co-treatment with the extract did not prevent the harmful effects of Ang II on iNOS activity or NO levels. However, ethyl acetate fractions of the extract have been reported to reduce both parameters in cardiomyocytes and human umbilical vein endothelial cells exposed to TNFα (Mustafa et al., 2018; Gui JS. et al., 2019). The discrepancy between these findings could stem from differences in the models and the type of fraction used. Rutin (50 µM) and quercetin (331 µM) have been reported to reverse the effects of Ang II on iNOS activity and NO levels (Siti et al., 2021). The highest concentration of extract used in this work (50 μg/ml) contained much lower concentrations of the flavonoids [0.790 μg/ml rutin (1) and 0.105 μg/ml quercetin (2)], possibly rationalizing the poor protection.
Valsartan was used as the positive control in this work due to its ability to reduce cardiomyocyte hypertrophy and BNP levels (Xu et al., 2015; Wu et al., 2017), as well as its use in the clinical management of heart failure (Vaduganathan et al., 2020). The antioxidant activity of valsartan has manifested in suppressed ROS levels (Chen et al., 2014; Tian et al., 2018). Valsartan displayed similar protective effects against Ang II-induced cardiomyocyte hypertrophy and oxidative stress in this study. It acts as an AT1R blocker to prevent Ang II receptor activation and downstream pathological events. Valsartan demonstrated better anti-inflammatory properties than the extract in suppressing the negative effects of Ang II. Previous work reported similar beneficial effects of valsartan on iNOS expression (Mohammed et al., 2015).
Ang II treatment was found to increase the levels of phosphorylated ERK1/2, JNK1/2, and p38, consistent with previous studies (Sriramula and Francis, 2015; Yokota and Wang, 2016; Lu et al., 2020). Exposure to Ang II triggers signal transduction, which activates the MAPK cascade via phosphorylation of ERK1/2, JNK, and p38 prior to nuclear translocation. This leads to the activation of numerous transcription factors (Zhang et al., 2003), some of which regulate the expression of hypertrophic gene products, such as BNP (Nayer et al., 2014).
Treatment with P. speciosa extract reduced the expression of P-ERK, P-p38, and P-JNK, suggesting that its antihypertrophic activity may function via modulation of the MAPK signaling pathway. The extract may prevent Ang II from binding AT1R, suppressing downstream events leading to hypertrophy, although this requires further investigation. Quercetin (2) has been shown to block activation of the MAPK signaling pathway. As rutin (1) lacks this property (Siti et al., 2021), the inhibitory effects of the extract on the MAPK signaling pathway are most likely due to quercetin and/or other unidentified phytochemical contents. Nevertheless, both rutin and quercetin have been reported to ameliorate cardiac hypertrophy via multiple routes, including apoptosis, autophagy, and prohypertrophic pathways (Siti et al., 2020a; Siti et al., 2020b). Therefore, rutin may confer protection via mechanisms other than inhibition of MAPK signaling.
Apart from reducing P-JNK levels, valsartan had no effect on MAPK signaling. Similar effects have been reported for losartan, another AT1R blocker, in a study on myocardial hypertrophy in hypertensive rats (Izumi et al., 2000). Valsartan has been shown to mitigate the Ang II-induced activation of p38, ERK1/2, and JNK in HL-1 cardiomyocytes (Liu et al., 2015). These contradictory findings could derive from differences in the types of cells or models used. The findings in this work suggest that the cardioprotective effect of valsartan on cardiomyocyte hypertrophy occurs via modulation of the Ang II/ROS/NO axis rather than regulation of the MAPK pathway.
Few studies apart from this one have investigated the cardioprotective potential of P. speciosa extract. This study demonstrated the protective effects of P. speciosa empty pod extract against Ang II-induced cardiomyocyte hypertrophy in H9c2 cells (Figure 11), and may support the traditional use of the plant in ameliorating cardiac problems. The antihypertrophic properties of the extract were investigated by cotreating cardiomyocytes with extract and Ang II. While this study presents promising findings, the antihypertrophic effects of the extract should be investigated as a post-treatment in future investigations. Results from this work suggest that the extract could be used as a supplement to ameliorate cardiac remodeling, although further studies are required before clinical use. Future research should also explore other possible mechanisms of action, such as the extract’s effects on calcium regulatory proteins or other pathways, including the specificity protein-1/GATA binding protein-4 (Sp1/GATA4) or phosphatidylinositol 3-kinase/protein kinase B/glycogen synthase kinase-3β (PI3K/Akt/GSK-3β) signaling pathways in hypertrophied cardiomyocytes.
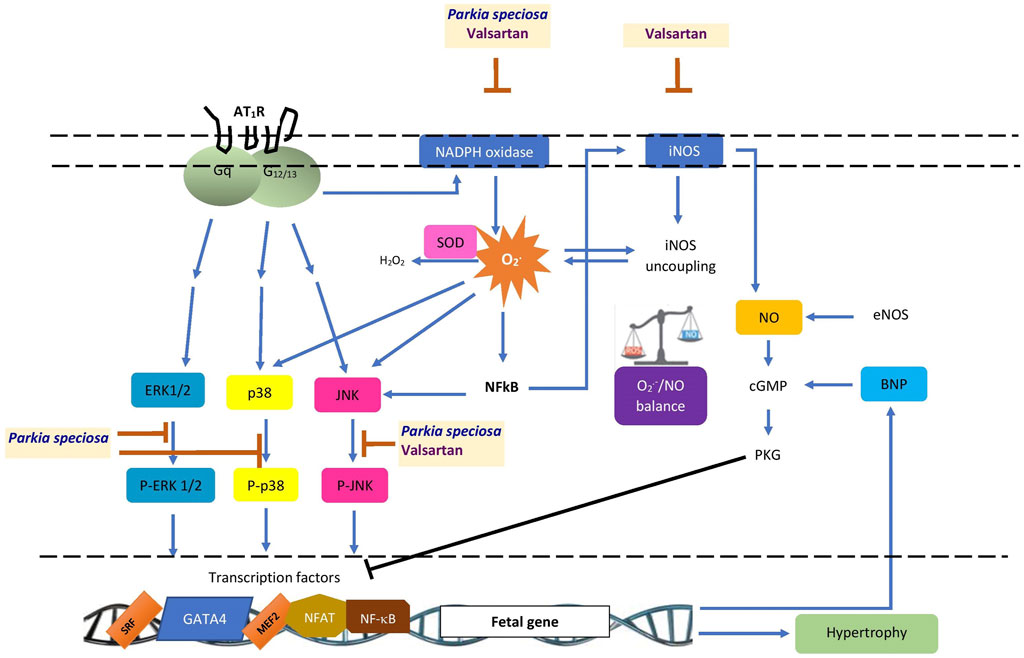
FIGURE 11. Schematic summary of the possible site of cardioprotective effects of Parkia speciosa empty pod extract. AT1R, angiotensin II type 1 receptor; BNP, B-type natriuretic peptide; cGMP, cyclic guanosine monophosphate; eNOS, endothelial nitric oxide synthase; ERK1/2, extracellular signal-related kinase; iNOS, inducible nitric oxide synthase; JNK, c-Jun N-terminal kinase; MEF2, myocyte enhancer factor-2; NFAT, nuclear factor of activated T-cells; NF-κB, nuclear factor kappa B; NO, nitric oxide; PKG, protein kinase G; p38, p38 kinase; P-ERK1/2, phosphorylated extracellular signal-related kinase (ERK1/2), P-JNK, phosphorylated c-Jun N-terminal kinase; P-p38, phosphorylated p38 kinase; SOD, superoxide dismutase; SRF, serum response factor; ⊥, inhibition.
Conclusion
P. speciosa empty pod extract afforded protection against Ang II-induced cardiomyocyte hypertrophy by mitigating oxidative stress and modulating the MAPK signaling pathway. These effects may be attributed to its rich rutin (1) and quercetin (2) content. Notably, the protective effects of the extract appear to occur via mechanisms distinct from valsartan.
Data Availability Statement
The raw data supporting the conclusions of this article will be made available by the authors, without undue reservation.
Author Contributions
HS performed the experiments, as well as wrote the article. JJ supervised the extraction and phytochemical screening. AA and YK supervised the study. All authors designed the study, revised the article and approved its submission.
Funding
This study received a funding from the Faculty of Medicine, Universiti Kebangsaan Malaysia (FF-2019-021).
Conflict of Interest
The authors declare that the research was conducted in the absence of any commercial or financial relationships that could be construed as a potential conflict of interest.
Publisher’s Note
All claims expressed in this article are solely those of the authors and do not necessarily represent those of their affiliated organizations, or those of the publisher, the editors and the reviewers. Any product that may be evaluated in this article, or claim that may be made by its manufacturer, is not guaranteed or endorsed by the publisher.
Acknowledgments
The authors would like to acknowledge technical assistance given by En Fadhlullah Zuhair Japar Sidik and Puan Juliana Abdul Hamid.
References
Al-Mazroua, H. A., Al-Rasheed, N. M., and Korashy, H. M. (2013). Downregulation of the Cardiotrophin-1 Gene Expression by Valsartan and Spironolactone in Hypertrophied Heart Rats In Vivo and Rat Cardiomyocyte H9c2 Cell Line In Vitro: A Novel Mechanism of Cardioprotection. J. Cardiovasc. Pharmacol. 61 (4), 337–344. doi:10.1097/FJC.0b013e318283a565
Azliza, M. A., Ong, H. C., Vikineswary, S., Noorlidah, A., and Haron, N. W. (2012). Ethno-medicinal Resources Used by the Temuan in Ulu Kuang Village. Stud. Ethno-Medicine 6 (1), 17–22. doi:10.1080/09735070.2012.11886415
Bernardo, B. C., and McMullen, J. R. (2016). Molecular Aspects of Exercise-Induced Cardiac Remodeling. Cardiol. Clin. 34 (4), 515–530. doi:10.1016/j.ccl.2016.06.002
Beyer, W. F., and Fridovich, I. (1987). Assaying for Superoxide Dismutase Activity: Some Large Consequences of Minor Changes in Conditions. Anal. Biochem. 161 (2), 559–566. doi:10.1016/0003-2697(87)90489-1
Campos-Shimada, L. B., Hideo Gilglioni, E., Fernandes Garcia, R., Rizato Martins-Maciel, E., Luiza Ishii-Iwamoto, E., and Luzia Salgueiro-Pagadigorria, C. (2020). Superoxide Dismutase: a Review and a Modified Protocol for Activities Measurements in Rat Livers. Arch. Physiol. Biochem. 126 (4), 292–299. doi:10.1080/13813455.2018.1520891
Chen, G., Pan, S. Q., Shen, C., Pan, S. F., Zhang, X. M., and He, Q. Y. (2014). Puerarin Inhibits Angiotensin II-Induced Cardiac Hypertrophy via the Redox-Sensitive ERK1/2, P38 and NF-Κb Pathways. Acta Pharmacol. Sin. 35 (4), 463–475. doi:10.1038/aps.2013.185
Chen, Y., Ge, Z., Huang, S., Zhou, L., Zhai, C., Chen, Y., et al. (2020). Delphinidin Attenuates Pathological Cardiac Hypertrophy via the AMPK/NOX/MAPK Signaling Pathway. Aging (Albany NY) 12 (6), 5362–5383. doi:10.18632/aging.102956
Cheng, C. I., Lee, Y. H., Chen, P. H., Lin, Y. C., Chou, M. H., and Kao, Y. H. (2017). Cobalt Chloride Induces RhoA/ROCK Activation and Remodeling Effect in H9c2 Cardiomyoblasts: Involvement of PI3K/Akt and MAPK Pathways. Cell Signal 36, 25–33. doi:10.1016/j.cellsig.2017.04.013
Chiang, J. T., Badrealam, K. F., Shibu, M. A., Kuo, C. H., Huang, C. Y., Chen, B. C., et al. (2018). Eriobotrya Japonica Ameliorates Cardiac Hypertrophy in H9c2 Cardiomyoblast and in Spontaneously Hypertensive Rats. Environ. Toxicol. 33 (11), 1113–1122. doi:10.1002/tox.22589
Cho, S., Cho, H. W., Woo, K. W., Jeong, J., Lim, J., Park, S., et al. (2019). Nelumbo nucifera Receptaculum Extract Suppresses Angiotensin II-Induced Cardiomyocyte Hypertrophy. Molecules 24 (9), 1647. doi:10.3390/molecules24091647
Cinelli, M. A., Do, H. T., Miley, G. P., and Silverman, R. B. (2020). Inducible Nitric Oxide Synthase: Regulation, Structure, and Inhibition. Med. Res. Rev. 40 (1), 158–189. doi:10.1002/med.21599
Cos, P., Ying, L., Calomme, M., Hu, J. P., Cimanga, K., Van Poel, B., et al. (1998). Structure-activity Relationship and Classification of Flavonoids as Inhibitors of Xanthine Oxidase and Superoxide Scavengers. J. Nat. Prod. 61 (1), 71–76. doi:10.1021/np970237h
Ding, J., Tang, Q., Luo, B., Zhang, L., Lin, L., Han, L., et al. (2019). Klotho Inhibits Angiotensin II-Induced Cardiac Hypertrophy, Fibrosis, and Dysfunction in Mice through Suppression of Transforming Growth Factor-Β1 Signaling Pathway. Eur. J. Pharmacol. 859, 172549. doi:10.1016/j.ejphar.2019.172549
Ghasemzadeh, A., Jaafar, H. Z. E., Bukhori, M. F. M., Rahmat, M. H., and Rahmat, A. (2018). Assessment and Comparison of Phytochemical Constituents and Biological Activities of Bitter Bean (Parkia Speciosa Hassk.) Collected from Different Locations in Malaysia. Chem. Cent. J. 12 (1), 12. doi:10.1186/s13065-018-0377-6
Guan, X. H., Hong, X., Zhao, N., Liu, X. H., Xiao, Y. F., Chen, T. T., et al. (2017). CD38 Promotes Angiotensin II-Induced Cardiac Hypertrophy. J. Cel. Mol. Med. 21 (8), 1492–1502. doi:10.1111/jcmm.13076
Gui, J. S., Jalil, J., Jubri, Z., and Kamisah, Y. (2019a). Parkia Speciosa Empty Pod Extract Exerts Anti-inflammatory Properties by Modulating NFκB and MAPK Pathways in Cardiomyocytes Exposed to Tumor Necrosis Factor-α. Cytotechnology 71, 79–89. doi:10.1007/s10616-018-0267-8
Gui, J. S., Mustafa, N., Jalil, J., Jubri, Z., and Kamisah, Y. (2019b). Modulation of NOX4 and MAPK Signalling Pathways by Parkia Speciosa Empty Pods in H9c2 Cardiomyocytes Exposed to H2O2. Indian J. Pharm. Sci. 81, 1029–1035. doi:10.36468/pharmaceutical-sciences.600
Hong, E. Y., Kim, T. Y., Hong, G. U., Kang, H., Lee, J. Y., Park, J. Y., et al. (2019). Inhibitory Effects of Roseoside and Icariside E4 Isolated from a Natural Product Mixture (No-Ap) on the Expression of Angiotensin Ii Receptor 1 and Oxidative Stress in Angiotensin II-Stimulated H9C2 Cells. Molecules 24 (3), 414. doi:10.3390/molecules24030414
Huang, X., Wang, Y., Zhang, Z., Wang, Y., Chen, X., Wang, Y., et al. (2017). Ophiopogonin D and EETs Ameliorate Ang II-Induced Inflammatory Responses via Activating PPARα in HUVECs. Biochem. Biophys. Res. Commun. 490 (2), 123–133. doi:10.1016/j.bbrc.2017.06.007
Izumi, Y., Kim, S., Zhan, Y., Namba, M., Yasumoto, H., and Iwao, H. (2000). Important Role of Angiotensin II-Mediated C-Jun NH(2)-terminal Kinase Activation in Cardiac Hypertrophy in Hypertensive Rats. Hypertension 36 (4), 511–516. doi:10.1161/01.hyp.36.4.511
Jeong, M. H., Kim, S. J., Kang, H., Park, K. W., Park, W. J., Yang, S. Y., et al. (2015). Cucurbitacin I Attenuates Cardiomyocyte Hypertrophy via Inhibition of Connective Tissue Growth Factor (CCN2) and TGF- β/Smads Signalings. PLoS One 10, e0136236. doi:10.1371/journal.pone.0136236
Kamisah, Y., Othman, F., Qodriyah, H. M., and Jaarin, K. (2013). Parkia Speciosa Hassk.: A Potential Phytomedicine. Evid. Based Complement. Alternat Med. 2013, 709028. doi:10.1155/2013/709028
Kamisah, Y., Zuhair, J. S. F., Juliana, A. H., and Jaarin, K. (2017). Parkia Speciosa Empty Pod Prevents Hypertension and Cardiac Damage in Rats Given N(G)-nitro-L-arginine Methyl Ester. Biomed. Pharmacother. 96, 291–298. doi:10.1016/j.biopha.2017.09.095
Ko, H.-J., Ang, L.-H., and Ng, L.-T. (2014). Antioxidant Activities and Polyphenolic Constituents of Bitter BeanParkia Speciosa. Int. J. Food Properties 17 (9), 1977–1986. doi:10.1080/10942912.2013.775152
Liu, L., Geng, J., Zhao, H., Yun, F., Wang, X., Yan, S., et al. (2015). Valsartan Reduced Atrial Fibrillation Susceptibility by Inhibiting Atrial Parasympathetic Remodeling through MAPKs/Neurturin Pathway. Cell. Physiol. Biochem. 36 (5), 2039–2050. doi:10.1159/000430171
Lu, Y., Sun, X., Peng, L., Jiang, W., Li, W., Yuan, H., et al. (2020). Angiotensin II-Induced Vascular Remodeling and Hypertension Involves Cathepsin L/V- MEK/ERK Mediated Mechanism. Int. J. Cardiol. 298, 98–106. doi:10.1016/j.ijcard.2019.09.070
Masi, S., Uliana, M., and Virdis, A. (2019). Angiotensin II and Vascular Damage in Hypertension: Role of Oxidative Stress and Sympathetic Activation. Vascul Pharmacol. 115, 13–17. doi:10.1016/j.vph.2019.01.004
Mohammed, H. E., Askar, M. E., Ali, S. I., and Fathy, O. M. (2015). Effect of Rennin Inhibitors and Sngiotensin II Receptor Antagonists on Left Ventricular Hypertrophy in Renovascular Hypertensive Rats. Int. J. Pharm. Pharm. Sci. 7 (9), 292–298.
Muslin, A. J. (2008). MAPK Signalling in Cardiovascular Health and Disease: Molecular Mechanisms and Therapeutic Targets. Clin. Sci. (Lond) 115 (7), 203–218. doi:10.1042/CS20070430
Mustafa, N. H., Ugusman, A., Jalil, J., and Kamisah, Y. (2018). Anti-inflammatory Property of Parkia Speciosa Empty Pod Extract in Human Umbilical Vein Endothelial Cells. J. App Pharm. Sci. 8, 152–158. doi:10.7324/JAPS.2018.8123
Mustapha, N. M., Tarr, J. M., Kohner, E. M., and Chibber, R. (2010). NADPH Oxidase versus Mitochondria-Derived ROS in Glucose-Induced Apoptosis of Pericytes in Early Diabetic Retinopathy. J. Ophthalmol. 2010, 746978. doi:10.1155/2010/746978
Nayer, J., Aggarwal, P., and Galwankar, S. (2014). Utility of point-of-care Testing of Natriuretic Peptides (Brain Natriuretic Peptide and N-Terminal Pro-brain Natriuretic Peptide) in the Emergency Department. Int. J. Crit. Illn. Inj. Sci. 4 (3), 209–215. doi:10.4103/2229-5151.141406
Nguyen Dinh Cat, A., Montezano, A. C., Burger, D., and Touyz, R. M. (2013). Angiotensin II, NADPH Oxidase, and Redox Signaling in the Vasculature. Antioxid. Redox Signal. 19 (10), 1110–1120. doi:10.1089/ars.2012.4641
Radi, R. (2018). Oxygen Radicals, Nitric Oxide, and Peroxynitrite: Redox Pathways in Molecular Medicine. Proc. Natl. Acad. Sci. U S A. 115, 5839–5848. doi:10.1073/pnas.1804932115
Restini, C. B. A., Garcia, A. F. E., Natalin, H. M., Natalin, G. M., and Rizzi, E. (2017). “Signaling Pathways of Cardiac Remodeling Related to Angiotensin II,” in Renin-Angiotensin System: Past, Present and Future. Editor A. N. Tolekova. Rijeka, Croatia IntechOpen, 51–68. doi:10.5772/66076
Saleh, M. S. M., Jalil, J., Mustafa, N. H., Ramli, F. F., Asmadi, A. Y., and Kamisah, Y. (2021). UPLC-MS-Based Metabolomics Profiling for α-Glucosidase Inhibiting Property of Parkia Speciosa Pods. Life 11 (2), 78. doi:10.3390/life11020078
Siti, H. N., Jalil, J., Asmadi, A. Y., and Kamisah, Y. (2021). Rutin Modulates MAPK Pathway Differently from Quercetin in Angiotensin II-Induced H9c2 Cardiomyocyte Hypertrophy. Int. J. Mol. Sci. 22 (10), 5063. doi:10.3390/ijms22105063
Siti, H. N., Kamisah, Y., Mohamed, S., and Jaarin, K. (2019). Effects of Citrus Leaf Extract on Aortic Vascular Reactivity in Hypertensive Rats Fed Repeatedly Heated Vegetable Oil. Appl. Physiol. Nutr. Metab. 44 (4), 373–380. doi:10.1139/apnm-2018-0175
Siti, H. N., Jalil, J., Asmadi, A. Y., and Kamisah, Y. (2020b). Effects of Quercetin on Cardiac Function in Pressure Overload and Postischemic Cardiac Injury in Rodents: a Systematic Review and Meta-Analysis. Cardiovasc. Drugs Ther doi:10.1007/s10557-020-07100-y
Siti, H. N., Jalil, J., Asmadi, A. Y., and Kamisah, Y. (2020a). Roles of Rutin in Cardiac Remodeling. J. Funct. Foods 64, 103606. doi:10.1016/j.jff.2019.103606
Sriramula, S., and Francis, J. (2015). Tumor Necrosis Factor - Alpha Is Essential for Angiotensin II-Induced Ventricular Remodeling: Role for Oxidative Stress. PLoS One 10 (9), e0138372. doi:10.1371/journal.pone.0138372
Sun, S., Li, T., Jin, L., Piao, Z. H., Liu, B., Ryu, Y., et al. (2018). Dendropanax Morbifera Prevents Cardiomyocyte Hypertrophy by Inhibiting the Sp1/GATA4 Pathway. Am. J. Chin. Med. 46 (5), 1021–1044. doi:10.1142/S0192415X18500532
Takano, H., Zou, Y., Hasegawa, H., Akazawa, H., Nagai, T., and Komuro, I. (2003). Oxidative Stress-Induced Signal Transduction Pathways in Cardiac Myocytes: Involvement of ROS in Heart Diseases. Antioxid. Redox Signal. 5 (6), 789–794. doi:10.1089/152308603770380098
Tian, H., Yu, D., Hu, Y., Zhang, P., Yang, Y., Hu, Q., et al. (2018). Angiotensin II Upregulates Cyclophilin A by Enhancing ROS Production in Rat Cardiomyocytes. Mol. Med. Rep. 18 (5), 4349–4355. doi:10.3892/mmr.2018.9448
Tran, H. A., Lin, F., and Greenberg, B. H. (2016). Potential New Drug Treatments for Congestive Heart Failure. Expert Opin. Investig. Drugs 25 (7), 811–826. doi:10.1080/13543784.2016.1181749
Tuszyńska, M. (2014). Validation of the Analytical Method for the Determination of Flavonoids in Broccoli. J. Hortic. Res. 22 (1), 131–140. doi:10.2478/johr-2014-0016
Vaduganathan, M., Claggett, B. L., Desai, A. S., Anker, S. D., Perrone, S. V., Janssens, S., et al. (2020). Prior Heart Failure Hospitalization, Clinical Outcomes, and Response to Sacubitril/valsartan Compared with Valsartan in HFpEF. J. Am. Coll. Cardiol. 75 (3), 245–254. doi:10.1016/j.jacc.2019.11.003
Wang, K., Long, B., Liu, F., Wang, J. X., Liu, C. Y., Zhao, B., et al. (2016). A Circular RNA Protects the Heart from Pathological Hypertrophy and Heart Failure by Targeting miR-223. Eur. Heart J. 37 (33), 2602–2611. doi:10.1093/eurheartj/ehv713
Wen, Y., Liu, R., Lin, N., Luo, H., Tang, J., Huang, Q., et al. (2019). NADPH Oxidase Hyperactivity Contributes to Cardiac Dysfunction and Apoptosis in Rats with Severe Experimental Pancreatitis through ROS-Mediated MAPK Signaling Pathway. Oxid. Med. Cel. Longev. 2019, 4578175. doi:10.1155/2019/4578175
Xu, W. P., Yao, T. Q., Jiang, Y. B., Zhang, M. Z., Wang, Y. P., Yu, Y., et al. (2015). Effect of the Angiotensin II Receptor Blocker Valsartan on Cardiac Hypertrophy and Myocardial Histone Deacetylase Expression in Rats with Aortic Constriction. Exp. Ther. Med. 9 (6), 2225–2228. doi:10.3892/etm.2015.2374
Yan, L., Zhang, J. D., Wang, B., Lv, Y. J., Jiang, H., Liu, G. L., et al. (2013). Quercetin Inhibits Left Ventricular Hypertrophy in Spontaneously Hypertensive Rats and Inhibits Angiotensin II-Induced H9C2 Cells Hypertrophy by Enhancing PPAR-γ Expression and Suppressing AP-1 Activity. PLoS One 8 (9), e72548. doi:10.1371/journal.pone.0072548
Ying, H., Xu, M. C., Tan, J. H., Shen, J. H., Wang, H., and Zhang, D. F. (2014). Pressure Overload-Induced Cardiac Hypertrophy Response Requires Janus Kinase 2-histone Deacetylase 2 Signaling. Int. J. Mol. Sci. 15 (11), 20240–20253. doi:10.3390/ijms151120240
Yokota, T., and Wang, Y. (2016). p38 MAP Kinases in the Heart. Gene 575 (2 Pt 2), 369–376. doi:10.1016/j.gene.2015.09.030
Yullia, T. (2008). “Prakata,” in Variasi Masakan Petai & Jengkol, Tim Dapur DeMedia (Jakarta Selatan, DeMedia Pustaka). Jakarta Selatan and DeMedia, 2.
Zaini, N., and Mustaffa, F. (2017). Review: Parkia Speciosa as Valuable, Miracle of Nature. Ajmah 2 (3), 1–9. doi:10.9734/AJMAH/2017/30997
Zhang, W., Elimban, V., Nijjar, M. S., Gupta, S. K., and Dhalla, N. S. (2003). Role of Mitogen-Activated Protein Kinase in Cardiac Hypertrophy and Heart Failure. Exp. Clin. Cardiol. 8 (4), 173–183.
Zhu, W., Wu, R. D., Lv, Y. G., Liu, Y. M., Huang, H., and Xu, J. Q. (2020). BRD4 Blockage Alleviates Pathological Cardiac Hypertrophy through the Suppression of Fibrosis and Inflammation via Reducing ROS Generation. Biomed. Pharmacother. 121, 109368. doi:10.1016/j.biopha.2019.109368
Zhuang, X., Zhao, B., Liu, S., Song, F., Cui, F., Liu, Z., et al. (2016). Noncovalent Interactions between Superoxide Dismutase and Flavonoids Studied by Native Mass Spectrometry Combined with Molecular Simulations. Anal. Chem. 88 (23), 11720–11726. doi:10.1021/acs.analchem.6b03359
Zucker, I. H., Schultz, H. D., Patel, K. P., and Wang, H. (2015). Modulation of Angiotensin II Signaling Following Exercise Training in Heart Failure. Am. J. Physiol. Heart Circ. Physiol. 308 (8), H781–H791. doi:10.1152/ajpheart.00026.2015
Glossary
Ang II angiotensin II
ANOVA one-way analysis of variance
AT1R Ang II type 1 receptor
AUC area under the curve
BNP B-type natriuretic peptide
cGMP cyclic guanosine monophosphate
DMEM Dulbecco’s Modified Eagle Medium
DMSO dimethyl sulfoxide
DPI diphenyleneiodonium
EDTA ethylenediaminetetraacetic acid
ELISA enzyme-linked immunosorbent assay
eNOS endothelial nitric oxide synthase
ERK1/2 extracellular signal-related kinase
FBS fetal bovine serum
HPLC high-performance liquid chromatography
HRP horseradish peroxidase
IC50 median inhibitory concentration
iNOS inducible nitric oxide synthase
JNK c-Jun N-terminal kinase
MAPK mitogen-activated protein kinase
MEF2 myocyte enhancer factor-2
MTS 3-(4,5-dimethylthiazol-2-yl)-5-(3-carboxymethoxyphenyl)-2-(4-sulfophenyl)-2H-tetrazolium
NADPH nicotinamide adenine dinucleotide phosphate
NF-κB nuclear factor kappa-B
NO nitric oxide
NOX NADPH oxidase
NFAT nuclear factor of activated T-cells
NOS2 nitric oxide synthase 2
O2−• superoxide anion
P-ERK1/2 phosphorylated ERK1/2
p38 p38 kinase
PI3K/Akt/GSK-3β phosphatidylinositol 3-kinase/protein kinase B/glycogen synthase kinase-3β
P-p38 phosphorylated p38
P-JNK phosphorylated JNK
PKG protein kinase G
ROS reactive oxygen species
SEM standard error of the mean
SOD superoxide dismutase
Sp1/GATA4 specificity protein-1/GATA binding protein-4
SPSS Statistical Product and Service Solutions
SRF serum response factor
TNFα tumor necrosis factor-α
tR retention time
Keywords: Parkia speciosa, angiotensin II, NADPH oxidase, iNOS, ERK, p38, JNK
Citation: Siti H, Jalil J, Asmadi A and Kamisah Y (2021) Parkia speciosa Hassk. Empty Pod Extract Alleviates Angiotensin II-Induced Cardiomyocyte Hypertrophy in H9c2 Cells by Modulating the Ang II/ROS/NO Axis and MAPK Pathway. Front. Pharmacol. 12:741623. doi: 10.3389/fphar.2021.741623
Received: 15 July 2021; Accepted: 28 September 2021;
Published: 14 October 2021.
Edited by:
Uraiwan Panich, Mahidol University, ThailandReviewed by:
Raquel Bridi, Pontificia Universidad Católica de Chile, ChileLei Chen, Guangdong Ocean University, China
Copyright © 2021 Siti, Jalil, Asmadi and Kamisah. This is an open-access article distributed under the terms of the Creative Commons Attribution License (CC BY). The use, distribution or reproduction in other forums is permitted, provided the original author(s) and the copyright owner(s) are credited and that the original publication in this journal is cited, in accordance with accepted academic practice. No use, distribution or reproduction is permitted which does not comply with these terms.
*Correspondence: Yusof Kamisah, a2FtaXNhaF95QHlhaG9vLmNvbQ==