- 1Department of Chinese Medicine Authentication, School of Pharmacy, Second Military Medical University, Shanghai, China
- 2Department of Anesthesiology, Shanghai Eastern Hepatobiliary Surgery Hospital, Shanghai, China
- 3Department of Biological Science, College of Life Science, Fujian Agriculture and Forestry University, Fuzhou, China
- 4Department of Biochemical Pharmacy, School of Pharmacy, Second Military Medical University, Shanghai, China
Prostate cancer is the second most common malignant cancer in males. It involves a complex process driven by diverse molecular pathways that closely related to the survival, apoptosis, metabolic and metastatic characteristics of aggressive cancer. Prostate cancer can be categorized into androgen dependent prostate cancer and castration-resistant prostate cancer and cure remains elusive due to the developed resistance of the disease. Natural compounds represent an extraordinary resource of structural scaffolds with high diversity that can offer promising chemical agents for making prostate cancer less devastating and curable. Herein, those natural compounds of different origins and structures with potential cytotoxicity and/or in vivo anti-tumor activities against prostate cancer are critically reviewed and summarized according to the cellular signaling pathways they interfere. Moreover, the anti-prostate cancer efficacy of many nutrients, medicinal plant extracts and Chinese medical formulations were presented, and the future prospects for the application of these compounds and extracts were discussed. Although the failure of conventional chemotherapy as well as involved serious side effects makes natural products ideal candidates for the treatment of prostate cancer, more investigations of preclinical and even clinical studies are necessary to make use of these medical substances reasonably. Therefore, the elucidation of structure-activity relationship and precise mechanism of action, identification of novel potential molecular targets, and optimization of drug combination are essential in natural medicine research and development.
Introduction
It is nowadays evident that prostate cancer (PCa) is recognized as the second most common cancer and the fifth cause of cancer death in males (Torre et al., 2015)Initially, the usual therapy for prostate cancer is prostatectomy or radiation, which aims to remove or kill the malignant cells that have not spread or metastasized (Feldman and Feldman, 2001). However, numerous patients cannot be cured thoroughly by this treatment, and then followed by cancer recurrence and/or metastasis. The majority of prostate cancer growth is androgen dependent. Androgen deprivation therapy (ADT) such as surgery or gonadotropin-releasing hormone (GnRH) analog treatment, is the main therapeutic and dramatically effective intervention for the treatment of androgen dependent prostate cancer (ADPC) in putting patients with tumors in remission, as documented by the work on castration of Huggins, who was awarded the Nobel prize in 1966 (Huggins, 1978). Nevertheless, after this therapy, most of these prostate cancer patients gradually become androgen independent, go on to progress, metastasize and resist to ADT within 13–24 months accompanied by increased levels of prostate-specific antigen (PSA). Siegel et al. reported that failure of ADT is responsible for the ∼27,000 metastatic prostate cancer deaths in the United States annually (Siegel et al., 2017). This stage of prostate cancer is called castration-resistant prostate cancer (CRPC), which has poor prognosis (Small et al., 2004). At present, there is no effective therapy for CRPC besides docetaxel, which has been demonstrated to prolong overall survival in this patient population. However, the efficacy of docetaxel is not satisfactory and there are many severe adverse effects such as anemia, neutropenia, diarrhea, and sensory neuropathy. Although, therapeutic options have expanded rapidly since 2011, including AR inhibitors (enzalutamide, abiraterone), immunotherapy (sipuleucel-T), bone seeking radionuclides (radium-223), and second-line chemotherapy (cabazitaxel), all of these agents or interventions only have shown a median survival benefit of 2–5 months (Ritch and Cookson, 2016). So searching for more effective anti-prostate cancer drugs, especially with high efficacy and low toxicity, remains an urgent problem that needs to be resolved. Natural compounds represent an irreplaceable resource of structural scaffolds that can offer chemical agents for making prostate cancer less devastating and curable. In recent years, many natural products and extracts have been scientifically investigated in vitro and/or in vivo and proved as potential anti-prostate cancer agents, which are currently scattered across various publications. So a systematic summary and knowledge of future prospects are necessary to facilitate further chemical and pharmacological studies for anti-prostate cancer agents.
Herein, we reviewed the detailed molecular causes of prostate cancer and critically summarized the natural compounds (or extracts and Chinese herb preparations) that have been reported to inhibit prostate cancer cells proliferation/tumor growth, induce prostate cancer cells apoptosis or exhibit effects on specific signaling pathways involved in prostate cancer in vivo and in vitro. In addition, we also provided possible novel targets for screening natural compounds (or extracts or Chinese herb preparations) with anti-prostate cancer activity and discuss the future prospects for the application of these compounds and extracts and the novel available approaches and technological improvements that should be explored to treat prostate cancer.
Overview of the Molecular Basis of Prostate Cancer
Molecular Basis of Androgen Dependent Prostate Cancer
Androgens, principally testosterone (T) and dihydrotestosterone (DHT), are synthesized from cholesterol as the initial 27-carbon substrate via multiple enzymatic steps (Wadosky and Koochekpour, 2016). As a member of the ligand-activated nuclear hormone receptors superfamily, androgen receptor (AR) is a modular protein with four functional domains: an N-terminal regulatory domain (NTD), a DNA-binding domain (DBD), a small hinge region (H) and a ligand-binding domain (LBD) (Ho and Dehm, 2017). Upon binding to androgens, AR undergoes a conformational change, leading to nuclear translocation, phosphorylation, homodimer, and interaction with DNA (Li and Al-Azzawi, 2009). Subsequently, AR dimer binds to androgen-response elements (AREs), recruits essential co-factors and regulates the expression of androgen-regulated genes (Ho and Dehm, 2017).
The development and maintenance of the prostate is inseparable from androgen acting through the AR. Since Huggins and Hodges first demonstrated the responsiveness of prostate cancer to androgen deprivation, it has been clear that prostate cancer is dependent on androgen and AR activation for growth and survival (Huggins and Hodges, 1941). From then, hundreds of studies have demonstrated that androgen withdrawal results in initial regression of essentially all prostate cancers, albeit for a finite period, with the ultimate development of castration-resistant disease. Androgen deprivation therapy, via either orchidectomy or use of a gonadotropin-releasing hormone (GnRH) agonist has become the cornerstone of therapy in the treatment of prostate cancers. Newer agents, such as abiraterone, which block androgen synthetic pathways, have added clinical benefit in disseminated disease, demonstrating that even in “castration resistant disease” androgens may still be supporting prostate cancer growth (Morgentaler, 2009). These data support the notion that prostate cancer, in most cases, is a hormone (androgen) sensitive disease.
Overview of the Mechanisms of Castration-Resistant Prostate Cancer
Historically, there are much debate about the mechanisms of castration resistance, which are mainly summarized as the following by recent studies: canonical AR signaling, relying on AR nuclear translocation and AR-DNA binding, and non-nuclear AR signaling which requires neither AR nuclear translocation nor AR-DNA binding(Qin and Bin, 2019; Pisano et al., 2021).
Canonical AR Signaling
The potential mechanisms of canonical AR signaling that lead to CRPC can be categorized into three parts. 1) common alterations in AR, which can lead to AR increase its sensitivity to very low levels of androgens or constitutive activation of AR signaling; 2) AR activation by androgens converted from adrenalandrogens or synthesized intratumorally via the de novo route; 3) alterations in cofactors of the AR pathway (Figure 1).
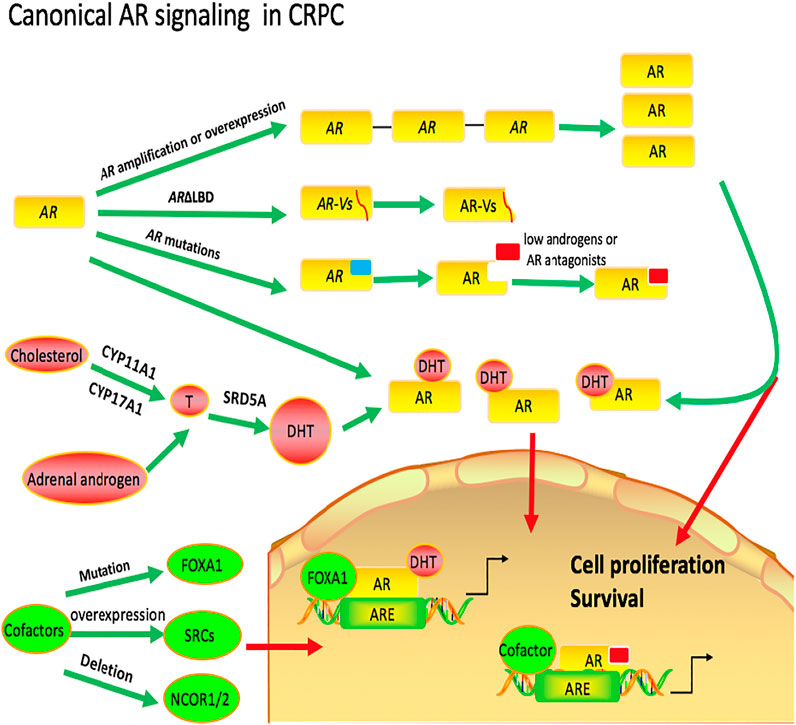
FIGURE 1. Canonical AR in castration-resistant prostate cancer. Common alterations in AR, including AR amplification or overexpression, AR mutations and truncated AR lacking ligand-binding domains (ARΔLBD), can increase its sensitivity to very low levels of androgens or lead to constitutive activation of AR signaling. There are two possible ways for the initial substrates to convert to intratumoral DHT in CRPC. The first way is that androgens are synthesized intratumorally via the de novo route and the second is that androgens are converted from adrenal androgens. Genomic and transcriptomic alterations in AR pathway coregulators are also associated with resistance to AR-targeted therapies in CRPC.
Common Alterations in AR
One possible mechanism by which the prostate cancer becomes resistant to androgen deprivation therapy is alterations in AR, including AR amplification or overexpression, AR mutations and truncated AR lacking ligand-binding domains (ARΔLBD). Thus, these changes in AR increase its sensitivity to very low levels of androgens or lead to constitutive activation of AR signaling. Strictly speaking, this mechanism of prostate cancers is not androgen-independent and the responses still depend on AR and androgen.
AR Amplification or Overexpression
Despite low circulating androgens in the CRPC patients, one potential mechanism that would allow tumor cell proliferation is by promoting the expression of the AR itself, which increases ligand-occupied receptor content (Feldman and Feldman, 2001). Plenty of studies have shown that approximately 50% of tumors that become castration resistant after ADT have amplified the AR gene, the most frequent genetic alteration reported for CRPC tumors, whereas none of the untreated primary tumors before androgen ablation had an AR gene amplification (Robinson et al., 2015; Djusberg et al., 2017).
Numerous studies provide the simplest explanation of how increased androgen receptor expression leads to resistance to anti-androgen therapy. According to one study, a three-to-five-fold increase in receptor levels could compensate for low ligand levels and restore androgen receptor signaling in xenotransplantation models. (Chen et al., 2004). Although tumors with AR amplification have increased levels of AR, the signal for cell proliferation presumably continues to require androgen (Visakorpi et al., 1995). Maybe this can explain why tumors with castration resistance have increased sensitivity to androgens in a low androgen environment.
AR Mutations
In CRPC, the frequency of AR mutations in pre-treated tumors is 5–30% (Grasso et al., 2012; Robinson et al., 2015; Kumar et al., 2016). Most mutations identified in CRPC were located in the AR-LBD. These alterations could facilitate AR signaling in CRPC by offering: 1) ligand facilitation, thereby inducing AR activation even in the presence of low or absent levels of androgens and 2) agonist properties to AR antagonists (Coutinho et al., 2016). In addition, mutations can also occur in the AR-NTD that account for about a third of all mutations described in AR. And mutations can usually cause alterations that contribute to AR transactivation, such as facilitated recruitment of co-factors and other components of the transcriptional machinery, promoted N/C interaction, increased response to DHT activation and enhanced protein stability and nuclear retention (Network C. G. A., 2015; Coutinho et al., 2016).
Truncated AR Lacking Ligand-Binding Domains (ARΔLBD)
Latest RNA sequencing data from big data sets, strongly suggests that constitutively active ARΔLBD may play a role in 40–50% of patients with CRPC (Robinson et al., 2015). Compared with hormone naïve PCa, ARΔLBDs are frequently upregulated in CRPC, and may serve as an adaptive response to therapies targeting the androgen/AR-signaling axis (Guo et al., 2009; Li et al., 2013). The recent genomic data on unique exon junctions reveals that at least 12 distinct AR-V mRNA species are detectable in primary PCa and 23 in CRPC (Abeshouse et al., 2015). However, among these variants, AR-V3/AR-V7 appears to be one of the most abundantly and ubiquitously expressed isoforms in our screening of a panel of human prostate cancer cell lines and tissues (Guo et al., 2009; Schweizer and Plymate, 2016). In addition, nonsense mutations leading to premature chain termination (Q641X, formerly Q640X) as well as enzymatic cleavage (tr-AR) were also shown to induce AR△LBDs (Haile and Sadar, 2011). F. Zengerling et al. reported that inhibition of IGF-1R resulted in a down-regulation of AR, Q641X and AR-V7 signaling in PCa cells (Zengerling et al., 2016), which suggests that IGF-1/IGF-1R axis is a modulator of the AR△LBD signaling, providing a rationale by targeting growth factor receptor for CRPC treatment.
AR Activation by DHT Synthesized Intratumorally via the de novo Route or Converted From Adrenal Androgens
There are two possible ways for the initial substrates to convert to intratumoral DHT in CRPC. The first way is that androgens are synthesized intratumorally via the de novo route and the second is that androgens are converted from adrenal androgens.
DHT Intratumorally via the de novo Route
The use of cholesterol for de novo steroidogenesis requires the components of the steroidogenic machinery present in the adrenals and gonads, including steroidogenic acute regulatory proteins, CYP11A1 and CYP17A1 (Miller and Auchus, 2011), which may play important roles in prostate cancer (Locke et al., 2008). Comparisons between primary prostate cancer and CRPC demonstrate that the transcription levels of these proteins are upregulated in CRPC (Montgomery et al., 2008) and CYP17A1 protein is detectable in a subset of metastatic CRPC cases (Efstathiou et al., 2012). In contrast to steroidogenesis in the adrenals and gonads, CRPC expresses steroid-5α-reductase (SRD5A1) with obvious 5α-reductase activity (Chang et al., 2011). One of the functions of robust SRD5A enzyme activity is that any de novo steroidogenesis would likely occur through the back door pathway that bypasses the requirement for T and involves 5-reduction of a 21-carbon steroid (progesterone or 17α-hydroxyprogesterone) instead of a 19-carbon androgen (Shaw et al., 2000; Auchus, 2004). Although this biochemical pathway may be engaged in CRPC, the relatively lengthy eight enzymatic steps required for conversion from cholesterol to DHT, the abundance of adrenal precursors present in serum, and the much closer pathway proximity of adrenal precursors to DHT, together suggest that adrenal precursors serve as the major substrate pool.
DHT Converted From Adrenal Androgens
There are two possible pathways from adrenal precursor steroids to DHT (Luu-The et al., 2008; Chang and Sharifi, 2012). The canonical adrenal pathway is the route that results in T synthesis as the penultimate metabolite, which undergoes 5-reduction to DHT (Scher and Sawyers, 2005; Ryan and Tindall, 2011; Hofland et al., 2012; Stein et al., 2012). This pathway is probably favored in the field because of the general notion that T must be the precursor to DHT and T is frequently detectable at concentrations greater than DHT in CRPC, as occurs with gonadal androgen physiology (Titus et al., 2005; Montgomery et al., 2008). In this pathway, DHEA is converted by 3β-hydroxysteroid dehydrogenase (3βHSD) to androstenedione (AD), which is then 17-keto reduced by aldo-keto reductase 1C3 (AKR1C3) or 17βHSD3 to T, the immediate precursor to DHT. The second possible pathway is that AD, like T, a 3-keto, △4-steroid, is also a potential substrate for SRD5A (Tomkins, 1957). AD is reduced to 5α-androstanedione (5α-dione), which then becomes the immediate precursor to DHT. The 5α-dione pathway is the major pathway for the synthesis of DHT in CRPC (Chang et al., 2011).
Alterations in Cofactors of the AR Pathway
Resistance to AR-targeted therapies in CRPC was also associated with genomic and transcriptomic alterations in coregulators of the AR pathway. Expression of 50 of the ∼200 AR-associated coregulators is aberrant in clinical CRPC specimens (Liu et al., 2017). For example, a higher frequency of mutations in FOXA1, the gene encoding a pioneer factor that facilitates AR chromatin binding and transcriptional activation, was found in CRPC (12%) than in primary prostate cancer (4%) (Watson et al., 2013; Abeshouse et al., 2015). In addition, as one class of coregulators, steroid receptor coactivators (SRC-1, SRC-2 and SRC-3) play a key role in facilitating aberrant AR signaling in CRPC e. There have been studies reported that all 3 SRCs is elevated in CRPC (Taylor et al., 2010; Grasso et al., 2012; Abeshouse et al., 2015; Beltran et al., 2016; Bernemann et al., 2016). GATA2, another AR pioneer factor in the AR signaling axis, is aberrant expressed in CRPC and associated with poor outcome (He et al., 2014; Yan et al., 2014). Gupta et al. detected genomic copy number changes of circulating tumor cells from 16 patients with CRPC resistant to abiraterone or enzalutamide and revealed that multiple genes encoding AR coregulators had copy number alterations, including copy number gains BRD4 (43.75%) (Gupta et al., 2017). Moreover, changes of AR corepressors also play a key role in CRPC. For example, loss of activity of the key nuclear receptor corepressors NCOR1 and NCOR2, is prevalent in CRPC due to mutation and/or deletion (Grasso et al., 2012; Abeshouse et al., 2015; Kumar et al., 2016).
Non-nuclear AR Signaling
Trafficking from the nucleus into the cytoplasm, AR may have unexpected consequences because AR has known functions in the cytoplasm, which is called non-genomic signaling (Foradori et al., 2008). One of the main characteristics of non-nuclear signaling is its rapidity with which it occurs. When steroid receptors stay in the cytosol, they can undergo several protein–protein interactions within seconds to minutes after steroid stimulation, which activates a variety of signaling pathways to promote the development of CRPC (Figure 2).
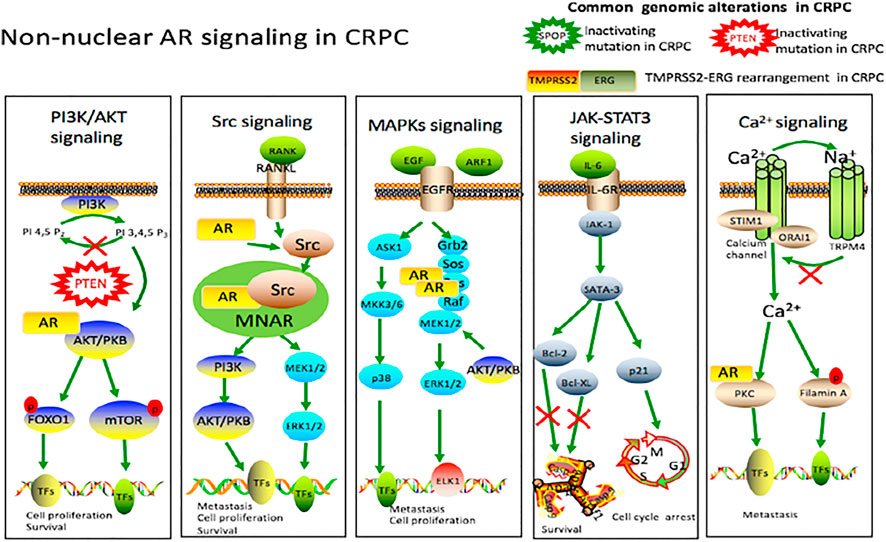
FIGURE 2. Non-nuclear AR signaling in castration-resistant prostate cancer. Cytokines, interleukins and the growth factors secreted by the prostate cancer cells activate various signaling cascades like PI3K/AKT, Src, MAPKs and JAK/STAT3 pathways involved in castration-resistant prostate cancer, leading to cell proliferation, survival and tumor metastasis. Intracellular Ca2+ centration can be modulated through Calcium channel. This increase in intracellular Ca2+ can lead to activation of PKC and filamin A, ultimately influencing gene transcription through phosphorylation. TRPM4 is also activated by a rise in intracellular Ca2+ in prostate cancer cells. Upon activation, a Na+ influx via TRPM4 depolarizes the membrane potential, which decreases the driving force for Ca2+, and thus contributes to migration of androgen-insensitive prostate cancer cells. There are other genomic alterations in castration-resistant prostate cancer, including PTEN mutation, SPOP mutation and TMPRSS2-ERG rearrangement.
PI3K/AKT Signaling Pathway
The PI3K/AKT pathway is one of the most frequently activated signal transduction pathways in human cancer, including prostate cancer(Hoxhaj and Manning, 2020; Park et al., 2018; Braglia et al., 2020). Alterations of the PI3K/AKT pathway, including altered expression, mutation, and copy number alterations, have been reported in 42% of primary prostate tumors and 100% of metastatic tumors (Taylor et al., 2010). Androgens induce the accumulation of TORC2 complex, rapamycin insensitive chaperone of mTOR and stress activated protein kinase interacting protein 1 in the nucleus, thus stimulating TORC2 to activate Akt (Fang et al., 2012). Activated AKT can stimulate many downstream functions via its kinase activity, including glycogen synthase kinase 3 (GSK3), tuberous sclerosis complex (TSC), FOXO transcription factors, NF-kappa-B and Bcl-2 family members BAD, which regulate a range of cellular processes (Liu et al., 2009; Courtney et al., 2010). It is estimated that genomic phosphatase and tensin homolog gene (PTEN) alterations, which is a negative inhibitor of PI3K/AKT pathway, occur in 9–45% of high-grade prostate intra-epithelial neoplasia (HG-PIN), 20–60% of localized prostate cancer, and up to 100% of cases of metastatic prostate cancer (Taylor et al., 2010; Jia et al., 2013).
Src Signaling Pathway
Preclinical studies have confirmed that non receptor tyrosine kinase c-Src and Src family kinase (SFK) regulate a complex signal network, driving the development of castration-resistance prostate cancer and bone metastasis. (Cai et al., 2011). After the establishment of bone metastasis, prostate cancer cells destroy the balance of osteoclasts and osteoblasts by secreting a variety of molecules, such as growth factors and cytokines that disrupt the normal process of bone maintenance and reconstruction (Yang et al., 2001; Mundy, 2002). The balance is in favor of osteoblastogenesis, which explains the usual condensing aspect of PCa-derived bone metastases. Src activity specifically affects ruffled borders of osteoclasts (essential for bone resorption), through dynamic regulating the interactions of actin cytoskeleton and formation of podosomes (Horne et al., 2005; Destaing et al., 2011). Src and other SFKs also play important roles in the antiapoptotic signal transduction of RANKL and other tumor necrosis factor family members in osteoclasts (Xing et al., 2001). One essential role for Src in osteoblasts has also been demonstrated that reduction of Src expression decreases osteoblast(responsible for bone formation) proliferation and increases differentiation (Marilena et al., 2000).
Recently, a large number of studies have shown that the activation of SRC is an important mediator of AR signaling. (Asim et al., 2008). AR can form a tertiary complex with the scaffold protein modulator of non-genomic actions of the estrogen receptor (MNAR/PELP1) and Src (Unni et al., 2004). Initially, Src is inactive within this complex. However, when AR binds to Src, this results in the activation of Src in this complex (AR/MNAR/Src) and the subsequent activation of a downstream effector, MEK (Unni et al., 2004). Subsequent studies have shown that AR-induced Src activation can promote cell proliferation through cell cycle progression from G1 phase to S phase (Migliaccio et al., 2002).
MAPKs Signaling Pathway
The MAPKs signaling cascade play important roles in regulating diverse biological functions including cell proliferation, motility and survival, which are essential to prostate carcinogenesis (Rossomando et al., 1989; Armenia et al., 2018; Abida et al., 2019). Studies of DHT-responsiveness in prostate cancer cells show that DHT treatment induces phosphorylation of ERK-1/2 within 1–2 min and peak levels of phosphorylation within 5–10 min (Liao et al., 2013). Activated ERK-1/2 then translocate to the nucleus and directly interact with and phosphorylate transcription factors (TFs), such as nuclear ETS domain-containing Elk1 (Marais et al., 1993; Gille et al., 1995; Yang et al., 1998). Elk1 transcriptionally regulates immediate early genes (IEGs) such as c-fos (Gille et al., 1995; Unni et al., 2004), and regulates the expression of several genes related to cell proliferation (Marais et al., 1993; Unni et al., 2004). In addition, recent studies showed that other molecules induce prostate cancer via MAPK signaling. Jason et al. reported that ADP-ribosylation factor 1 (ARF1), a crucial regulator in vesicle-mediated membrane trafficking and involved in the activation of signaling molecules, promotes the occurrence of prostate cancer via targeting oncogenic MAPK signaling (Davis et al., 2016). Gonzalo et al. reported that epidermal growth factor (EGF) could stimulate G0/G1-S transition via p38 MAPK to overcome the growth restriction of androgen deprivation in prostate cancer cells (Rodriguez-Berriguete et al., 2016).
JAK-STAT3 Signaling Pathway
Janus kinases (JAK) signal transducers and activator of transcription (STAT) pathway play an important role in differentiation, hematopoiesis, immune function and cell growth (Bolli et al., 2003). Recently, accumulating evidence indicated that IL-6 is indispensable for activation of JAK/STAT pathway, which is involved in the oncogenesis of prostate cancer (Liu X. et al., 2012). Compared with men with normal prostates, benign prostatic hyperplasia, prostatitis and localized disease, approximately 50% of patients with advanced prostate cancer have increased levels of serum IL-6 (Twillie et al., 1995). Upon the binding of IL-6 to the IL-6 receptor, JAK-1 and STAT-3 become activated in sequence (C Schindler and Jr, 1995). L Tam et al. reported that cytoplasmic expression of IL-6 receptor and pSTAT3 Tyr705 are associated with the shortened biochemical recurrence time and death time from hormone relapse, respectively. Therefore, it is reasonable to target this pathway in hormone-refractory prostate cancer treatments (Tam et al., 2007).
Ca2+ Signaling Pathway
Ca2+signaling is also involved in prostate cancer progression (Figiel et al., 2019; Chalmers and Monteith et al., 2018). Increased calcium intake from dairy products has been considered as a risk factor for prostate cancer (Foradori et al., 2007; Flourakis and Prevarskaya, 2009). As a primary signaling molecule, extracellular Ca2+ works through the Ca2+-sensing receptor (CaR, a G protein coupled receptor) which directly regulates cell signal transduction and the Ca2+ channels (Vaz et al., 2015). Depletion of intracellular Ca2+ stores serves as a signal for the activation of Ca2+ influx across the plasma membrane. The proteins STIM1 and ORAI1 were identified as the key components of store-operated Ca2+ entry (SOCE). When Ca2+ is released from intracellular Ca2+ pool, Ca2+ dissociates from a luminal EF hand motif of STIM1. As a consequence, STIM1 proteins aggregate and recruit Orai1 Ca2+channels, which then mediate SOCE (Kilch et al., 2016).
Recently, Huang et al. found that Ca2+ via CaR-mediated signaling induces filamin A cleavage, which is an actin-binding protein, and promotes the migration of AR-deficient and highly metastatic prostate cancer cells (Huang et al., 2016). In one additional study, Christian demonstrated that transient receptor potential melastatin 4 channel (TRPM4) is activated by a rise in intracellular Ca2+ in prostate cancer cells. Upon activation, a Na+ influx via TRPM4 depolarizes the membrane potential, which reduces the driving force for Ca2+ and limits SOCE, and thus promotes migration of androgen-insensitive prostate cancer cells (Christian et al., 2015).
Other Genomic Alterations in Castration-Resistant Prostate Cancer
Prostate cancer is characterized by a high genetic heterogeneity due to genomic alterations and instabilities associated with diverse PCa risk factors (Squire et al., 2011; Yap et al., 2016; Ciccarese et al., 2017; Rodrigues et al., 2017), which was evidenced by extensive genomic profiling analysis conducted on primary tumors (Network T. C. G. A., 2015) and on metastatic samples (Dan et al., 2015).
Speckle-type POZ protein (SPOP) is the substrate-binding subunit of a cullin-3 (CUL3)-based E3 ubiquitin ligase complex, which mediates the ubiquitylation and degradation of many target proteins. SPOP binds to the substrates through its N-terminal meprin and traf homology (MATH) domain, whereas it interacts with cullin-3 via BTB domain on its C terminal (Pintard et al., 2003; Xu et al., 2003; Zhuang et al., 2009). Recent cancer whole-genome and exome sequencing studies have shown that SPOP is the most frequently mutated gene (in up to 15% of cases) in primary prostate cancer (Barbieri et al., 2012; Network T. C. G. A., 2015). Interestingly, all SPOP somatic mutations identified in prostate cancer are clustered in its substrate binding MATH domain, thus having a dominant-negative effect on substrate binding and degradation (Theurillat et al., 2014). Recent studies have unanimously reported that SPOP interacts with bromodomain and extraterminal (BET) proteins that largely act as transcriptional coactivators and play vital roles in cell cycle, apoptosis, migration and invasion in physiological conditions. In addition, SPOP also promotes the ubiquitylation and proteasomal degradation of bromodomain-containing protein 2 (BRD2), BRD3 and BRD4, (Dai et al., 2017; Janouskova et al., 2017; Zhang et al., 2017). Pathologically, BET proteins are frequently overexpressed and are clinically linked to various types of human cancer (French et al., 2003; Crawford et al., 2008; Belkina and Denis, 2012). Recently, Janouskova et al. reported prostate cancer–associated SPOP mutants impaired its binding to BET proteins, leading to the reduced proteasomal degradation and accumulation of these proteins in prostate cancer cell lines and patient specimens, which subsequently causes resistance to BET inhibitors (Janouskova et al., 2017). Similar study has also demonstrated that SPOP-mutated prostate cancer cell lines and patient-derived organoids were intrinsically resistant to BET inhibitor-induced growth arrest and apoptosis (Dai et al., 2017). Furthermore, Dai et al. provided that stabilization of BRD4 may be a molecular mechanism for resistance to BET inhibitors in patients with prostate cancer bearing SPOP mutations (Dai et al., 2017). Taken together, these findings offer mechanistic insights into how SPOP mutations influence prostate cancer.
The TMPRSS2-ERG fusion gene arising from genetic rearrangement (fusion of encoding transmembrane protease serine 2, TMPRSS2 gene, and EST-related gene, ERG) has also been a central focus in prostate cancer, which leads to aberrant expression of the ETS transcription factor ERG (Tomlins et al., 2005; Kandoth, 2013). TMPRSS2-ERG is the most common gene rearrangement in prostate cancer and is present in approximately 50% of prostate cancer tissues in Western countries (Cary and Cooperberg, 2013). Previous studies indicated that ERG overexpression was driven by hijacking of androgen-responsive elements within the TMPRSS2 promoter (Tomlins et al., 2005; Wang et al., 2007; Thangapazham et al., 2014). However, Kron et al. found that the molecular process is more complex. Their study indicated that the frequent deletion allows a cluster of regulatory elements (CORE) in the TMPRSS2 promoter to expand into the rearranged ERG allele. This expanded CORE contains some CREs within the ERG locus that can promote ERG overexpression. Studies also revealed that overexpressed ERG co-opts prostate-specific master regulatory transcription factors, including AR, HOXB13 and FOXA1, in a process facilitated by their physical interaction with ERG and actives NOTCH signaling in primary prostate cancer (Kron et al., 2017). ERG overexpression is now an instrumental indicator in the diagnosis of prostate cancer. In addition, Graff et al. recently found that obesity and height might be correlated with the development of TMPRSS2-ERG-positive prostate cancer (Graff et al., 2018). Collectively, the functions and mechanisms of TMPRSS2-ERG increase the opportunities for finding new therapeutic targets for prostate cancer(Wang et al., 2017; Kong et al., 2020).
Natural Compounds That Exert Anti-Prostate Effects
Natural compounds that have been found to inhibit prostate cancer cells proliferation/tumor growth, promote prostate cancer cells apoptosis, or modulate specific signaling pathways involved in prostate cancer in vivo and in vitro are categorized and presented according to their source of isolation (marine organisms, microorganisms and plants) and the structural scaffolds. Besides the effects on prostate cancer cells growth or apoptosis, special emphasis was given to the mechanism of action of a compound interfering specific signaling pathways involved in prostate cancer.
Natural Compounds Obtained From Marine Organisms or Microorganisms
As is well known, marine organisms or microorganisms possess the capacity to produce a large amount of diverse secondary metabolites with unique structural features and biological properties. Thus, marine and microbial organisms represent interesting and important sources of single molecules with promising skeletons and significant anti-prostate cancer activity. Up to now, a total of 24 natural compounds (Figures 3) isolated from marine organisms have been found to exhibit significant anti-prostate cancer activity either in vivo or in vitro. Detailed information about the compounds origin, activity and mechanism of action is listed in Table 1. Most of them exhibit antiproliferative, apoptosis inducing or metastasis inhibitory activities, with various acting mechanisms such as induction of autophagy, inhibition of AR activation, PI-3K/AKT/mTOR or JAK/STAT signaling pathways (Senderowicz et al., 1995; Liu et al., 2006; Wang WL. et al., 2008; Hellsten et al., 2008; Gantar et al., 2012; Meimetis et al., 2012; Shin et al., 2013; Liberio et al., 2015; Liu et al., 2016). Especially, frondoside A not only caused cell type specific cell cycle arrest and induction of caspase-dependent or -independent apoptosis in vivo but also significantly inhibited the cell growth of PC-3 and DU145 with a notable reduction of lung metastasis and decrease of circulating tumor cells in the peripheral blood (Dyshlovoy et al., 2016). In addition, gliotoxin, chaetocin and chetomin exhibited antiangiogenic effects in vitro and attenuated tumor growth mainly by disrupting the HIF-1α/p300 complex, which makes them attractive molecules for the design of future chemotherapeutic agents (Cook et al., 2009).
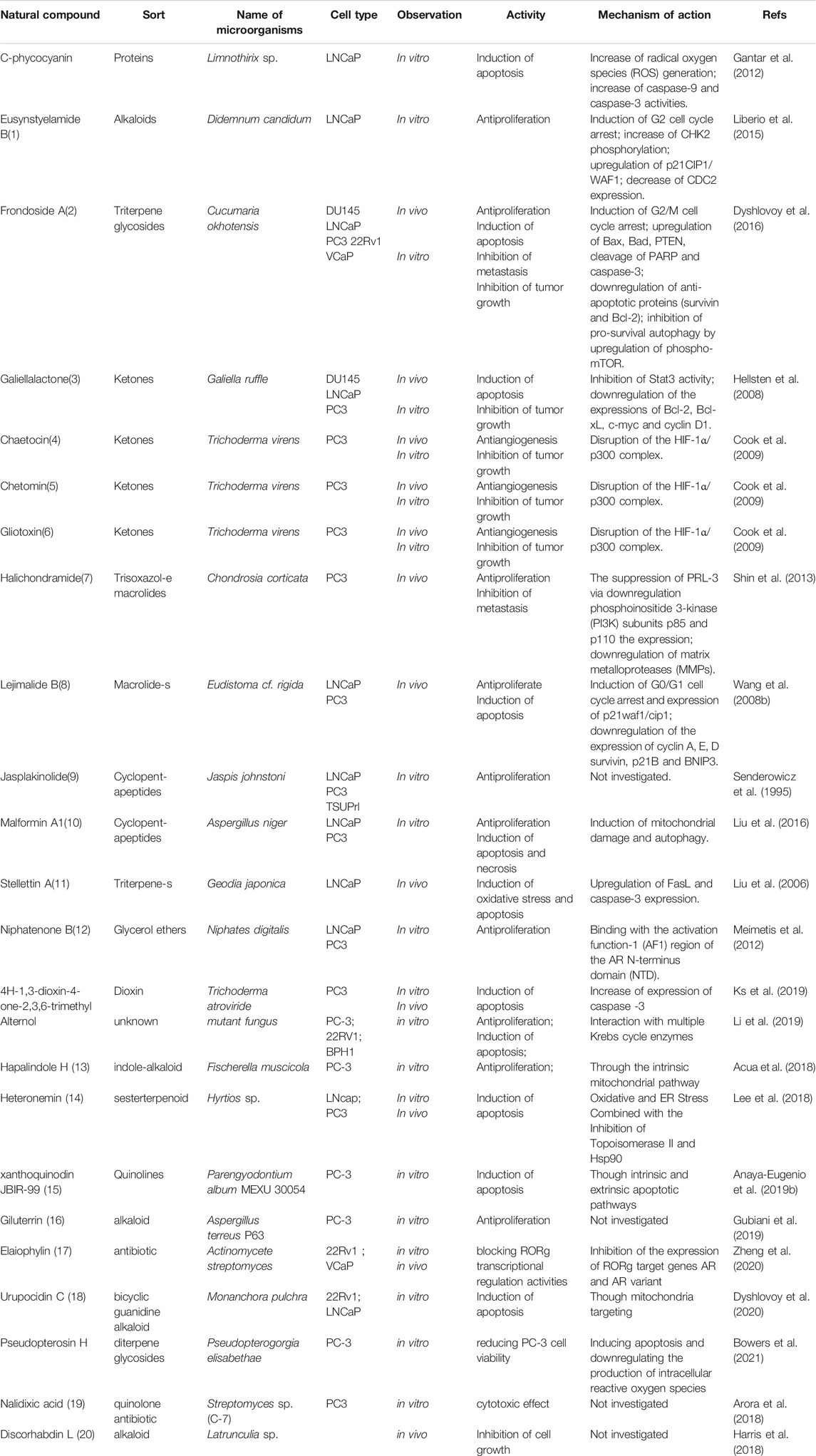
TABLE 1. Natural compounds obtained from marine organisms or microorganisms with anti-prostate cancer activities.
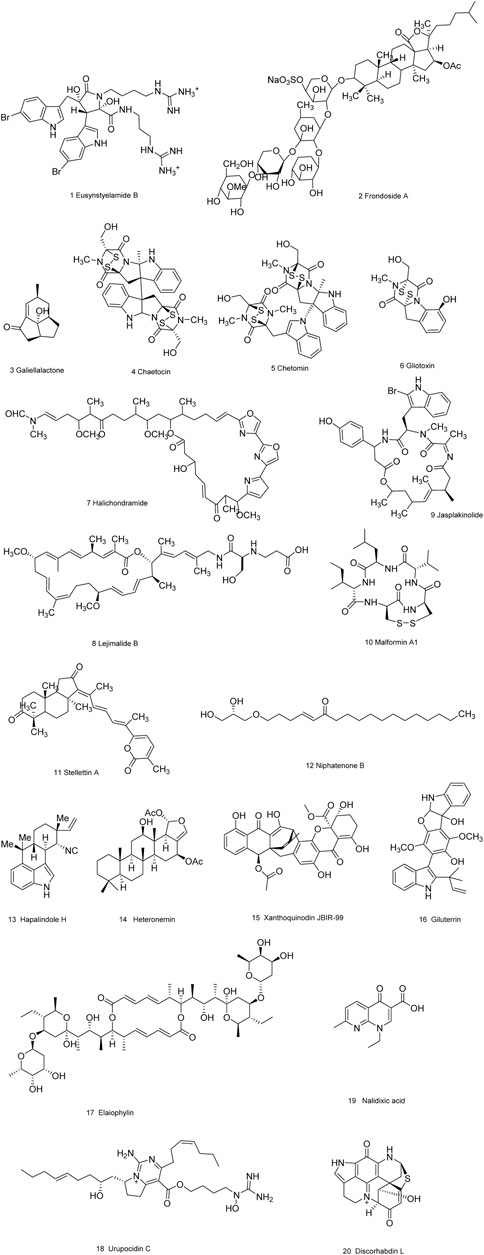
FIGURE 3. Natural compounds obtained from marine organisms or microorganisms with anti-prostate cancer activities.
Natural Compounds Isolated From Plants
Medicinal plants have always been a very good source of drugs, which could produce plenty of secondary metabolites with high structural diversity and versatile bioactivities. Many candidates with promising anti-prostate activity have been reported, including 7 alkaloids, 23 flavanoids, 25 terpenoids, 13 polyphenols, 10 lignans and 48 other compounds (Figures 4–9). Almost all these candidates show anti-prostate cancer activities via anti-proliferation, apoptosis induction or metastasis and invasion inhibition, involved in canonical AR signaling and non-AR signaling like caspase cascades, AKT/mTOR pathway, MAPKs pathway, NF-κB pathway, Ca2+ pathway and JAK/STATs pathway. Additionally, there exist other acting mechanisms, for example, anibamine exhibited anti-prostate cancer activity by binding to the chemokine receptor CCR5; fisetin inhibited tumor growth by downregulating the expression of NudC protein, MMP-2 and MMP-9; lycopene showed anti-prostate cancer effects by inhibiting androgen receptor element and signaling of insulin-like growth factor-1 (Afaq et al., 2008; Khan et al., 2008; Bureyko et al., 2009; Wertz, 2009; Zhang et al., 2010b; Chien et al., 2010; Suh et al., 2010; Tang et al., 2011; Holzapfel et al., 2013; Mukhtar et al., 2015). Detailed information is provided in Tables 2–7.
Extracts With Anti-Prostate Cancer Activity
Extracts consist of a group of bioactive natural compounds, which may exert and possess the advantages of synergistic effects against diseases. Recently, nutraceuticals have also received increasing attention as the agents or dietary supplements for cancer prevention and treatment, as well as some extracts derived from edible sources. Thus in this section we will respectively review those extracts and nutraceuticals that have the potential effects against prostate cancer either in vitro or in prostate cancer mice models. Chinese herbal compound preparations of more than one medicinal plants that have been reported to inhibit prostate cancer are also presented in this review.
Herbal Extracts
Traditional and folk herbal medicines from medicinal plants offer great potential for the discovery of novel anti-prostate cancer drugs. The plant extracts listed in Table 8 are complex mixtures, which need further investigations to reveal their bioative constituents through bioguided isolation and to clarify the roles of these different compounds in agaisnt prostate cancer when used alone or in combination. Also, the synergistic effect of the individual active components of these extracts and molecular mechanisms involved need further elucidation in order to evaluate the potential of these compounds as antineoplastic agents.
Chinese Herbal Compound Preparations
There are four traditional Chinese medical formulations reported to display significant anti-prostate cancer properties, that is, Zyflamend, PC-SPES and LCS101, which are composed of different medicinal plants (Table 9; Bemis et al., 2005; Hsieh et al., 1997; Cohen et al., 2015). Especially, PC-SPES significantly inhibited prostate tumor growth in tumor-bearing mouse model, mainly through cell cycle arrest and apoptosis induction, which is already clinically utilized for the treatment of clincal patients with prostate cancer (Hsieh et al., 1997).
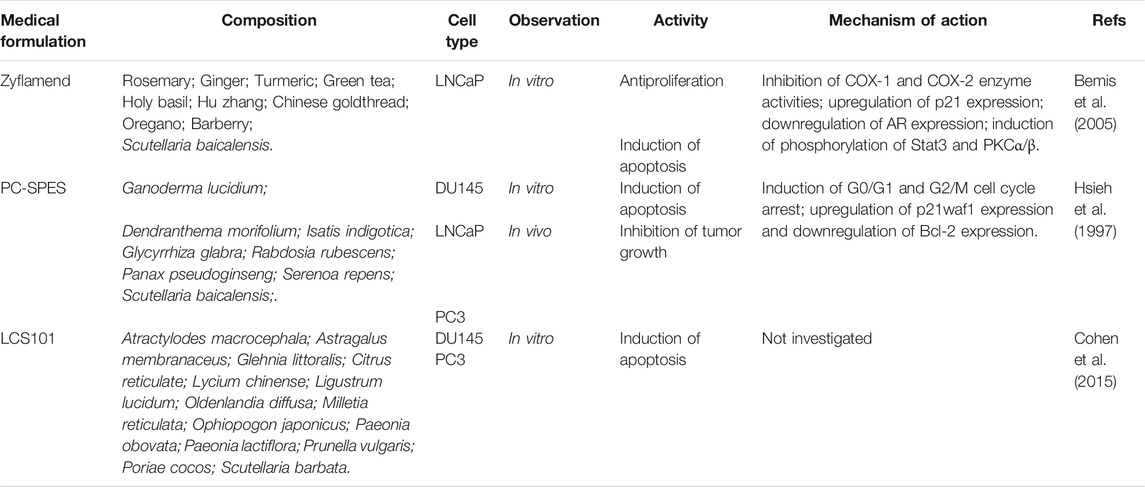
TABLE 9. Chinese herbal compound preparations obtained from plants with anti-prostate cancer activities.
Nutraceuticals and Extracts Derived From Edible Sources
Nowadays, dietary factors play an increasingly important role in the chemopreventive and/or therapeutic management of cancer (Table 10). The study of dietary agents (nutraceuticals or extracts derived from edible sources) in prostate cancer prevention is an important area of research since about 43–80% patients with prostate cancer are on alternative therapy based on dietary modification (Lippert et al., 1999; Nam et al., 1999). There are strong evidences that nutraceuticals and extracts derived from edible spices, vegetables or fruits such as vitamin D, pomegranate and tea polyphenols have demonstrated significant anti-prostate cancer activity when tested either in vitro and/or in vivo (Kasimsetty et al., 2009; Gregory et al., 2010; Koyama et al., 2010; Mordanmccombs et al., 2010; Hsu et al., 2011; Xiao et al., 2011; Turan et al., 2017). Especially, dietary phytochemicals that can selectively interfere cellular pathways involved in prostate cancer cells have attracted research interest of scientists in prostate cancer therapies in recent years.
Conclusion and Perspectives
Prostate cancer is the second most frequently diagnosed tumor and the fifth leading cause of cancer-related deaths in men in the worldwide (McEleny et al., 2002). And the mortality of prostate cancer mainly occurs as a result of the castrate resistant ones. Up to date, different kinds of drugs have been employed to improve the treatment condition, mainly including LHRH antagonists, antiandrogen (androgen receptor antagonists and androgen synthesis inhibitors), tyrosine kinase inhibitors, angiogenesis inhibitors, endothelin antagonists, matrix metalloproteinase inhibitors, antioxidants, and cell cycle inhibitors. However, as mentioned above, there is no effective therapy for CRPC at present, except for docetaxel, which is the only chemotherapeutic agent that has been proven to prolong the overall survival in CRPC patient population though with many adverse effects reported (Eyben et al., 2015). Hence, it is urgent for us to explore an effective treatment for prostate cancer, especially for CRPC. In recent years, many natural products and extracts have been scientifically investigated in vitro and/or in vivo and proved as potential anti-prostate cancer agents, which are currently scattered across various publications. So a systematic summary and knowledge of future prospects are necessary to facilitate further chemical and pharmacological studies for anti-prostate cancer agents.
In our review, we provided a comprehensive overview of the molecular basis of the incidence and development of prostate cancer, especially for castration-resistant prostate cancer (CRPC), which mainly including canonical AR signaling (AR amplification, over-expression, mutation, and unconventional activation), and non-nuclear AR signaling (PI3K/AKT, Src, MAPKs, JAK-STAT3, and Ca2+ signaling pathways). So most components involved in above-mentioned pathways represent potential targets for screening natural compounds and/or extracts with anti-prostate cancer activity. And natural compounds or extracts that could function as modulators of canonical AR or non-nuclear AR signalling pathways thus can be regarded as promising candidates for anti-prostate interventions.
So far, a great amount of natural products isolated from diverse sources have been found to significantly inhibit prostate cancer cell proliferation/tumor growth or affected cellular signaling pathways in prostate cancer. As shown in our paper, the majority of natural compounds with direct relevance to prostate cancer are primarily derived from plants, with comparatively few molecules from marine and microbial sources. For these reported bioactive constituents, there is still plenty of room for improvement regarding the studies focused on efficacy enhancement and side effects amelioration by semi-synthetic modifications based on quantitative structural activity relationship elucidation. Since marine and microbial organisms represent important sources for single molecules exploit, more available and improved approaches should be included in finding novel natural products with significant anti-prostate cancer activity from these resource. Especially, engineering bacteria or fungus with novel gene clusters, currently used mainly for the identification of antibiotics or anti-tumor drugs, would be another promising approach for discovering natural compounds with anti-prostate activity. Extracts are another applicable option for anti-prostate purposes, in which case the chemical profile should be further elucidated, possibly affording a pure bioactive compound with precise mechanism of action. Also clinically used Chinese herb preparations should be profiled using techniques such as HPLC–MS to standardize the complex system to make it more controllable, stable, and reproducible in prostate cancer treatment. Furthermore, drug combination of these reported natural compounds with conventional chemotherapeutic agents may also be a promising way in finding solution for prostate cancer treatment. Finally, safty large-scale studies are needed to evaluate promising compounds or extracts and determing non-toxic doses for treating prostate cancer in mammals.
In conclusion, tackling prostate cancer (especially CRPC) is a much needed task that requires not only the great progress in understanding the genetic basis of prostate cancer, but also the significant technological improvements in tracking of bioactive natural compounds and structural characterization, which will facilitate the identification of novel natural compounds with significant anti-prostate cancer properties for drug development and therefore can be translated into significant health benefits for humans.
Author Contributions
CZ, WX, and QY conceived and designed the outline of the manuscript; BB, QC, and RJ collated and summarized the literatures; XQ, HW and YB analyzed the data; RJ, BB, and CZ wrote the manuscript, QC, WX, and QY revised the manuscript and provided technical assistance to RJ and BB.
Funding
The work was supported by the National Nature Science Foundation of China (No. 82174081, 81770604), the Program of Shanghai Health System Subject Chief Scientist (2017BR004), and Shanghai Natural Science Fund (21ZR1478000).
Conflict of Interest
The authors declare that the research was conducted in the absence of any commercial or financial relationships that could be construed as a potential conflict of interest.
Publisher’s Note
All claims expressed in this article are solely those of the authors and do not necessarily represent those of their affiliated organizations, or those of the publisher, the editors and the reviewers. Any product that may be evaluated in this article, or claim that may be made by its manufacturer, is not guaranteed or endorsed by the publisher.
References
Aalinkeel, R., Bindukumar, B., Reynolds, J. L., Sykes, D. E., Mahajan, S. D., Chadha, K. C., et al. (2008). The Dietary Bioflavonoid, Quercetin, Selectively Induces Apoptosis of Prostate Cancer Cells by Down-Regulating the Expression of Heat Shock Protein 90. Prostate 68 (16), 1773–1789. doi:10.1002/pros.20845
Abeshouse, A., Ahn, J., Akbani, R., Ally, A., Amin, S., Andry, C. D., et al. (2015). The Molecular Taxonomy of Primary Prostate Cancer. Cell 163 (4), 1011–1025. doi:10.1016/j.cell.2015.10.025
Abou-Hashem, M. M. M., Abo-Elmatty, D. M., Mesbah, N. M., and Abd El-Mawgoud, A. M. (2019). Induction of Sub-G0 Arrest and Apoptosis by Seed Extract of Moringa Peregrina (Forssk.) Fiori in Cervical and Prostate Cancer Cell Lines. J. Integr. Med. 17 (6), 410–422. doi:10.1016/j.joim.2019.09.004
Acquaviva, R., Di Giacomo, C., Sorrenti, V., Galvano, F., Santangelo, R., Cardile, V., et al. (2012). Antiproliferative Effect of Oleuropein in Prostate Cell Lines. Int. J. Oncol. 41 (1), 31–38. doi:10.3892/ijo.2012.1428
Acuña, U. M., Mo, S., Zi, J., Orjala, J., and DE Blanco, E. J. C. (2018). Hapalindole H Induces Apoptosis as an Inhibitor of NF-ĸb and Affects the Intrinsic Mitochondrial Pathway in PC-3 Androgen-Insensitive Prostate Cancer Cells. Anticancer Res. 38 (6), 3299–3307. doi:10.21873/anticanres.12595
Afaq, F., Zaman, N., Khan, N., Syed, D. N., Sarfaraz, S., Zaid, M. A., et al. (2008). Inhibition of Epidermal Growth Factor Receptor Signaling Pathway by Delphinidin, an Anthocyanidin in Pigmented Fruits and Vegetables. Int. J. Cancer 123 (7), 1508–1515. doi:10.1002/ijc.23675
Al-Oqail, M. M., El-Shaibany, A., Al-Jassas, E., Al-Sheddi, E. S., Al-Massarani, S. M., and Farshori, N. N. (2016). In Vitro anti-proliferative Activities of Aloe Perryi Flowers Extract on Human Liver, colon, Breast, Lung, Prostate and Epithelial Cancer Cell Lines. Pak J. Pharm. Sci. 29 (2 Suppl. l), 723–729.
Anaya-Eugenio, G. D., Addo, E. M., Ezzone, N., Henkin, J. M., Ninh, T. N., Ren, Y., et al. (2019a). Caspase-Dependent Apoptosis in Prostate Cancer Cells and Zebrafish by Corchorusoside C from Streptocaulon Juventas. J. Nat. Prod. 82 (6), 1645–1655. doi:10.1021/acs.jnatprod.9b00140
Anaya-Eugenio, G. D., Rebollar-Ramos, D., González, M. D. C., Raja, H., Mata, R., and Carcache de Blanco, E. J. (2019b). Apoptotic Activity of Xanthoquinodin JBIR-99, from Parengyodontium Album MEXU 30054, in PC-3 Human Prostate Cancer Cells. Chem. Biol. Interact 311, 108798. doi:10.1016/j.cbi.2019.108798
Arias-González, I., García-Carrancá, A. M., Cornejo-Garrido, J., and Ordaz-Pichardo, C. (2018). Cytotoxic Effect of Kalanchoe Flammea and Induction of Intrinsic Mitochondrial Apoptotic Signaling in Prostate Cancer Cells. J. Ethnopharmacol 222, 133–147. doi:10.1016/j.jep.2018.04.038
Arjsri, P., Phetcharaburanin, J., Suksawat, M., Mapoung, S., Subkamkaew, C., Semmarath, W., et al. (2021). Spirogyra Neglecta (Hassall) Kützing Attenuates Metastasis of Castration-Resistant Human Prostate Cancer via the Blockage of AKT Signaling Pathway. South Afr. J. Bot. 139 (Suppl. 10), 26–37. doi:10.1016/j.sajb.2021.02.005
Arora, N., Kumar, S., Satti, N. K., Ali, A., Gupta, P., and Katoch, M. (2018). A Strain of Streptomyces Sp. Isolated from Rhizospheric Soil of Crataegus Oxycantha Producing Nalidixic Acid, a Synthetic Antibiotic. J. Appl. Microbiol. 124 (6), 1393–1400. doi:10.1111/jam.13736
Arulselvan, P., Ju, J., Gothai, S., Hasanpourghadi, M., Nasser, A., Aziz Ibrahim, I., et al. (2018). Anticancer Potential of Moringa Oleifera Flower Extract in Human Prostate Cancer PC-3 Cells via Induction of Apoptosis and Downregulation of AKT Pathway. Phcog Mag. 14 (58), 477. doi:10.4103/pm.pm_516_17
Asadi-Samani, M., Rafieian-Kopaei, M., Lorigooini, Z., and Shirzad, H. (2018). A Screening of Growth Inhibitory Activity of Iranian Medicinal Plants on Prostate Cancer Cell Lines. Biomedicine (Taipei) 8 (2), 8. doi:10.1051/bmdcn/2018080208
Asea, A., Ara, G., Teicher, B. A., Stevenson, M. A., and Calderwood, S. K. (2001). Effects of the Flavonoid Drug Quercetin on the Response of Human Prostate Tumours to Hyperthermia In Vitro and In Vivo. Int. J. Hyperthermia 17 (4), 347–356. doi:10.1080/02656730110053146
Asim, M., Siddiqui, I. A., Hafeez, B. B., Baniahmad, A., and Mukhtar, H. (2008). Src Kinase Potentiates Androgen Receptor Transactivation Function and Invasion of Androgen-independent Prostate Cancer C4-2 Cells. Oncogene 27 (25), 3596–3604. doi:10.1038/sj.onc.1211016
Auchus, R. J. (2004). The Backdoor Pathway to Dihydrotestosterone. Trends Endocrinol. Metab. 15 (9), 432–438. doi:10.1016/j.tem.2004.09.004
Azémar, M., Hildenbrand, B., Haering, B., Heim, M. E., and Unger, C. (2007). Clinical Benefit in Patients with Advanced Solid Tumors Treated with Modified Citrus Pectin: A Prospective Pilot Study. Clin. Med. Oncol. 1 (1), CMO.S285–80. doi:10.4137/CMO.S285
Bae, W. J., Choi, J. B., Kim, K. S., Ha, U. S., Hong, S. H., Lee, J. Y., et al. (2020). Inhibition of Proliferation of Prostate Cancer Cell Line DU-145 In Vitro and In Vivo Using Salvia Miltiorrhiza Bunge. Chin. J. Integr. Med. 26 (7), 533–538. doi:10.1007/s11655-017-2801-5
Bao, X., Zhu, J., Ren, C., Zhao, A., Zhang, M., Zhu, Z., et al. (2021). β-Elemonic Acid Inhibits Growth and Triggers Apoptosis in Human Castration-Resistant Prostate Cancer Cells through the Suppression of JAK2/STAT3/MCL-1 and NF-ĸb Signal Pathways. Chem. Biol. Interact 342, 109477. doi:10.1016/j.cbi.2021.109477
Barbieri, C. E., Baca, S. C., Lawrence, M. S., Demichelis, F., Blattner, M., Theurillat, J. P., et al. (2012). Exome Sequencing Identifies Recurrent SPOP, FOXA1 and MED12 Mutations in Prostate Cancer. Nat. Genet. 44 (6), 685–689. doi:10.1038/ng.2279
Baron, A., Mancini, M., Caldwell, E., Cabrelle, A., Bernardi, P., and Pagano, F. (2009). Serenoa Repens Extract Targets Mitochondria and Activates the Intrinsic Apoptotic Pathway in Human Prostate Cancer Cells. BJU Int. 103 (9), 1275–1283. doi:10.1111/j.1464-410X.2008.08266.x
Belkina, A. C., and Denis, G. V. (2012). BET Domain Co-regulators in Obesity, Inflammation and Cancer. Nat. Rev. Cancer 12 (7), 465–477. doi:10.1038/nrc3256
Beltran, H., Prandi, D., Mosquera, J. M., Benelli, M., Puca, L., Cyrta, J., et al. (2016). Divergent Clonal Evolution of Castration-Resistant Neuroendocrine Prostate Cancer. Nat. Med. 22 (3), 298–305. doi:10.1038/nm.4045
Bemis, D. L., Capodice, J. L., Anastasiadis, A. G., Katz, A. E., and Buttyan, R. (2005). Zyflamend, a Unique Herbal Preparation with Nonselective COX Inhibitory Activity, Induces Apoptosis of Prostate Cancer Cells that Lack COX-2 Expression. Nutr. Cancer 52 (2), 202–212. doi:10.1207/s15327914nc5202_10
Ben-Eltriki, M., Deb, S., Adomat, H., and Tomlinson Guns, E. S. (2016). Calcitriol and 20(S)-protopanaxadiol Synergistically Inhibit Growth and Induce Apoptosis in Human Prostate Cancer Cells. J. Steroid Biochem. Mol. Biol. 158, 207–219. doi:10.1016/j.jsbmb.2015.12.002
Bernemann, C., Schnoeller, T. J., Luedeke, M., Steinestel, K., Boegemann, M., Schrader, A. J., et al. (2017). Expression of AR-V7 in Circulating Tumour Cells Does Not Preclude Response to Next Generation Androgen Deprivation Therapy in Patients with Castration Resistant Prostate Cancer. Eur. Urol. 71, 1–3. doi:10.1016/j.eururo.2016.07.021
Bodle, C. R., Mackie, D. I., Hayes, M. P., Schamp, J. H., Miller, M. R., Henry, M. D., et al. (2017). Natural Products Discovered in a High-Throughput Screen Identified as Inhibitors of RGS17 and as Cytostatic and Cytotoxic Agents for Lung and Prostate Cancer Cell Lines. J. Nat. Prod. 80 (7), 1992–2000. doi:10.1021/acs.jnatprod.7b00112
Bolli, R., Dawn, B., and Xuan, Y. T. (2003). Role of the JAK-STAT Pathway in protection against Myocardial Ischemia/reperfusion Injury. Trends Cardiovasc. Med. 13 (2), 72–79. doi:10.1016/s1050-1738(02)00230-x
Bonham, M., Posakony, J., Coleman, I., Montgomery, B., Simon, J., and Nelson, P. S. (2005). Characterization of Chemical Constituents in Scutellaria Baicalensis with Antiandrogenic and Growth-Inhibitory Activities toward Prostate Carcinoma. Clin. Cancer Res. 11 (10), 3905–3914. doi:10.1158/1078-0432.CCR-04-1974
Bowers, Z., Caraballo, D., Jebelli, J., Famuyiwa, T., and Kumi-Diaka, J. (2021). Therapeutic Potential of Pseudopterosin H on a Prostate Cancer Cell Line. FASEB 35. doi:10.1096/fasebj.2021.35.S1.04601
Bureyko, T., Hurdle, H., Metcalfe, J. B., Clandinin, M. T., and Mazurak, V. C. (2009). Reduced Growth and Integrin Expression of Prostate Cells Cultured with Lycopene, Vitamin E and Fish Oil In Vitro. Br. J. Nutr. 101 (7), 990–997. doi:10.1017/S0007114508051684
Burton, L. J., Smith, B. A., Smith, B. N., Loyd, Q., Nagappan, P., McKeithen, D., et al. (2015). Muscadine Grape Skin Extract Can Antagonize Snail-Cathepsin L-Mediated Invasion, Migration and Osteoclastogenesis in Prostate and Breast Cancer Cells. Carcinogenesis 36 (9), 1019–1027. doi:10.1093/carcin/bgv084
Cai, H., Smith, D. A., Memarzadeh, S., Lowell, C. A., Cooper, J. A., and Witte, O. N. (2011). Differential Transformation Capacity of Src Family Kinases during the Initiation of Prostate Cancer. Proc. Natl. Acad. Sci. U S A. 108 (16), 6579–6584. doi:10.1073/pnas.1103904108
Cary, K. C., and Cooperberg, M. R. (2013). Biomarkers in Prostate Cancer Surveillance and Screening: Past, Present, and Future. Ther. Adv. Urol. 5 (6), 318–329. doi:10.1177/1756287213495915
Castro, M. J., Careaga, V. P., Sacca, P. A., Faraoni, M. B., Murray, A. P., and Calvo, J. C. (2019). Lupane Triterpenoids and New Derivatives as Antiproliferative Agents against Prostate Cancer Cells. Anticancer Res. 39 (7), 3835–3845. doi:10.21873/anticanres.13533
Chan, M. L., Liang, J. W., Hsu, L. C., Chang, W. L., Lee, S. S., and Guh, J. H. (2015). Zerumbone, a Ginger Sesquiterpene, Induces Apoptosis and Autophagy in Human Hormone-Refractory Prostate Cancers through Tubulin Binding and Crosstalk between Endoplasmic Reticulum Stress and Mitochondrial Insult. Naunyn Schmiedebergs Arch. Pharmacol. 388 (11), 1223–1236. doi:10.1007/s00210-015-1152-z
Chang, H. T., Chou, C. T., Chen, I. L., Liang, W. Z., Kuo, D. H., Huang, J. K., et al. (2013). Mechanisms of Resveratrol-Induced Changes in [Ca(2+)]i and Cell Viability in PC3 Human Prostate Cancer Cells. J. Recept Signal. Transduct Res. 33 (5), 298–303. doi:10.3109/10799893.2013.822886
Chang, K. H., Li, R., Papari-Zareei, M., Watumull, L., Zhao, Y. D., Auchus, R. J., et al. (2011). Dihydrotestosterone Synthesis Bypasses Testosterone to Drive Castration-Resistant Prostate Cancer. Proc. Natl. Acad. Sci. U S A. 108 (33), 13728–13733. doi:10.1073/pnas.1107898108
Chang, K. H., and Sharifi, N. (2012). Prostate Cancer-From Steroid Transformations to Clinical Translation. Nat. Rev. Urol. 9 (12), 721–724. doi:10.1038/nrurol.2012.175
Chaudhary, A., Pechan, T., and Willett, K. L. (2007). Differential Protein Expression of Peroxiredoxin I and II by Benzo(a)pyrene and Quercetin Treatment in 22Rv1 and PrEC Prostate Cell Lines. Toxicol. Appl. Pharmacol. 220 (2), 197–210. doi:10.1016/j.taap.2006.12.030
Chen, C. D., Welsbie, D. S., Tran, C., Baek, S. H., Chen, R., Vessella, R., et al. (2004). Molecular Determinants of Resistance to Antiandrogen Therapy. Nat. Med. 10 (1), 33–39. doi:10.1038/nm972
Chen, L., Yan, F., Chen, W., Zhao, L., Zhang, J., Lu, Q., et al. (2018). Procyanidin from Peanut Skin Induces Antiproliferative Effect in Human Prostate Carcinoma Cells DU145. Chem. Biol. Interact 288, 12–23. doi:10.1016/j.cbi.2018.04.008
Chen, Y. C., Kung, F. L., Tsai, I. L., Chou, T. H., Chen, I. S., and Guh, J. H. (2010). Cryptocaryone, a Natural Dihydrochalcone, Induces Apoptosis in Human Androgen Independent Prostate Cancer Cells by Death Receptor Clustering in Lipid Raft and Nonraft Compartments. J. Urol. 183 (6), 2409–2418. doi:10.1016/j.juro.2010.01.065
Chiang, Y. T., Wang, K., Fazli, L., Qi, R. Z., Gleave, M. E., Collins, C. C., et al. (2014). GATA2 as a Potential Metastasis-Driving Gene in Prostate Cancer. Oncotarget 5 (2), 451–461. doi:10.18632/oncotarget.1296
Chien, C. S., Shen, K. H., Huang, J. S., Ko, S. C., and Shih, Y. W. (2010). Antimetastatic Potential of Fisetin Involves Inactivation of the PI3K/Akt and JNK Signaling Pathways with Downregulation of MMP-2/9 Expressions in Prostate Cancer PC-3 Cells. Mol. Cel Biochem 333 (1-2), 169–180. doi:10.1007/s11010-009-0217-z
Chintharlapalli, S., Papineni, S., Ramaiah, S. K., and Safe, S. (2007). Betulinic Acid Inhibits Prostate Cancer Growth through Inhibition of Specificity Protein Transcription Factors. Cancer Res. 67 (6), 2816–2823. doi:10.1158/0008-5472.Can-06-3735
Cho, H. D., Lee, J. H., Moon, K. D., Park, K. H., Lee, M. K., and Seo, K. I. (2018). Auriculasin-induced ROS Causes Prostate Cancer Cell Death via Induction of Apoptosis. Food Chem. Toxicol. 111, 660–669. doi:10.1016/j.fct.2017.12.007
Ciccarese, C., Massari, F., Iacovelli, R., Fiorentino, M., Montironi, R., Di Nunno, V., et al. (2017). Prostate Cancer Heterogeneity: Discovering Novel Molecular Targets for Therapy. Cancer Treat. Rev. 54, 68–73. doi:10.1016/j.ctrv.2017.02.001
Cohen, Z., Maimon, Y., Yoeli-Lerner, M., Yang, P., Samuels, N., and Berger, R. (2015). Selective Anticancer Effects and protection from Chemotherapy by the Botanical Compound LCS101: Implications for Cancer Treatment. Int. J. Oncol. 46 (1), 308–316. doi:10.3892/ijo.2014.2711
Colgate, E. C., Miranda, C. L., Stevens, J. F., Bray, T. M., and Ho, E. (2007). Xanthohumol, a Prenylflavonoid Derived from Hops Induces Apoptosis and Inhibits NF-kappaB Activation in Prostate Epithelial Cells. Cancer Lett. 246 (1-2), 201–209. doi:10.1016/j.canlet.2006.02.015
Cook, K. M., Hilton, S. T., Mecinovic, J., Motherwell, W. B., Figg, W. D., and Schofield, C. J. (2009). Epidithiodiketopiperazines Block the Interaction between Hypoxia-Inducible Factor-1alpha (HIF-1alpha) and P300 by a Zinc Ejection Mechanism. J. Biol. Chem. 284 (39), 26831–26838. doi:10.1074/jbc.M109.009498
Courtney, K. D., Corcoran, R. B., and Engelman, J. A. (2010). The PI3K Pathway as Drug Target in Human Cancer. J. Clin. Oncol. 28 (6), 1075–1083. doi:10.1200/Jco.2009.25.3641
Coutinho, I., Day, T. K., Tilley, W. D., and Selth, L. A. (2016). Androgen Receptor Signaling in Castration-Resistant Prostate Cancer: a Lesson in Persistence. Endocr. Relat. Cancer 23 (12), T179–T197. doi:10.1530/ERC-16-0422
Crawford, N. P., Alsarraj, J., Lukes, L., Walker, R. C., Officewala, J. S., Yang, H. H., et al. (2008). Bromodomain 4 Activation Predicts Breast Cancer Survival. Proc. Natl. Acad. Sci. U S A. 105 (17), 6380–6385. doi:10.1073/pnas.0710331105
Dai, W., Sun, C., Huang, S., and Zhou, Q. (2016). Carvacrol Suppresses Proliferation and Invasion in Human Oral Squamous Cell Carcinoma. Onco Targets Ther. 9, 2297–2304. doi:10.2147/ott.S98875
Dai, X., Gan, W., Li, X., Wang, S., Zhang, W., Huang, L., et al. (2017). Prostate Cancer-Associated SPOP Mutations Confer Resistance to BET Inhibitors through Stabilization of BRD4. Nat. Med. 23 (9), 1063–1071. doi:10.1038/nm.4378
Dan, R., Vanallen, E., Wu, Y. M., Schultz, N., Lonigro, R., Mosquera, J. M., et al. (2015). Integrative Clinical Genomics of Advanced Prostate Cancer. Cell 161 (5), 1215. doi:10.1016/j.cell.2015.05.001
Dasari, S., Samy, A. L. P. A., Kajdacsy-Balla, A., Bosland, M. C., and Munirathinam, G. (2018). Vitamin K2, a Menaquinone Present in Dairy Products Targets Castration-Resistant Prostate Cancer Cell-Line by Activating Apoptosis Signaling. Food Chem. Toxicol. 115, 218–227. doi:10.1016/j.fct.2018.02.018
Davis, J. E., Xie, X., Guo, J., Huang, W., Chu, W. M., Huang, S., et al. (2016). ARF1 Promotes Prostate Tumorigenesis via Targeting Oncogenic MAPK Signaling. Oncotarget 7 (26), 39834–39845. doi:10.18632/oncotarget.9405
Deepak, M., and Handa, S. S. (2000). Antiinflammatory Activity and Chemical Composition of Extracts of Verbena Officinalis. Phytother Res. 14 (6), 463–465. doi:10.1002/1099-1573(200009)14:6<463::aid-ptr611>3.0.co;2-g
Deng, Y., Li, Y., Yang, F., Zeng, A., Yang, S., Luo, Y., et al. (2017). The Extract from Punica Granatum (Pomegranate) Peel Induces Apoptosis and Impairs Metastasis in Prostate Cancer Cells. Biomed. Pharmacother. 93, 976–984. doi:10.1016/j.biopha.2017.07.008
Destaing, O., Block, M. R., Planus, E., and Albiges-Rizo, C. (2011). Invadosome Regulation by Adhesion Signaling. Curr. Opin. Cel Biol 23 (5), 597–606. doi:10.1016/j.ceb.2011.04.002
Ding, W., Hu, Z., Zhang, Z., Ma, Q., Tang, H., and Ma, Z. (2015). Physapubescin B Exhibits Potent Activity against Human Prostate Cancer In Vitro and In Vivo. J. Agric. Food Chem. 63 (43), 9504–9512. doi:10.1021/acs.jafc.5b03045
Djusberg, E., Jernberg, E., Thysell, E., Golovleva, I., Lundberg, P., Crnalic, S., et al. (2017). High Levels of the AR-V7 Splice Variant and Co-amplification of the Golgi Protein Coding YIPF6 in AR Amplified Prostate Cancer Bone Metastases. Prostate 77 (6), 625–638. doi:10.1002/pros.23307
Dyshlovoy, S. A., Kudryashova, E. K., Kaune, M., Makarieva, T. N., Shubina, L. K., Busenbender, T., et al. (2020). Urupocidin C: a New marine Guanidine Alkaloid Which Selectively Kills Prostate Cancer Cells via Mitochondria Targeting. Sci. Rep. 10 (1), 9764. doi:10.1038/s41598-020-66428-5
Dyshlovoy, S. A., Menchinskaya, E. S., Venz, S., Rast, S., Amann, K., Hauschild, J., et al. (2016). The marine Triterpene Glycoside Frondoside A Exhibits Activity In Vitro and In Vivo in Prostate Cancer. Int. J. Cancer 138 (10), 2450–2465. doi:10.1002/ijc.29977
Efstathiou, E., Titus, M., Tsavachidou, D., Tzelepi, V., Wen, S., Hoang, A., et al. (2012). Effects of Abiraterone Acetate on Androgen Signaling in Castrate-Resistant Prostate Cancer in Bone. J. Clin. Oncol. 30 (6), 637–643. doi:10.1200/JCO.2010.33.7675
Erdogan, S., and Turkekul, K. (2020). Neferine Inhibits Proliferation and Migration of Human Prostate Cancer Stem Cells through P38 MAPK/JNK Activation. J. Food Biochem. 44 (6). doi:10.1111/jfbc.13253
Eroğlu, C., Avcı, E., Vural, H., and Kurar, E. (2018). Anticancer Mechanism of Sinapic Acid in PC-3 and LNCaP Human Prostate Cancer Cell Lines. Gene 671, 127–134. doi:10.1016/j.gene.2018.05.049
Estrada, A. C., Syrovets, T., Pitterle, K., Lunov, O., Büchele, B., Schimana-Pfeifer, J., et al. (2010). Tirucallic Acids Are Novel Pleckstrin Homology Domain-dependent Akt Inhibitors Inducing Apoptosis in Prostate Cancer Cells. Mol. Pharmacol. 77 (3), 378–387. doi:10.1124/mol.109.060475
Fang, Z., Zhang, T., Dizeyi, N., Chen, S., Wang, H., Swanson, K. D., et al. (2012). Androgen Receptor Enhances P27 Degradation in Prostate Cancer Cells through Rapid and Selective TORC2 Activation. J. Biol. Chem. 287 (3), 2090–2098. doi:10.1074/jbc.M111.323303
Feldman, B. J., and Feldman, D. (2001). The Development of Androgen-independent Prostate Cancer. Nat. Rev. Cancer 1 (1), 34–45. doi:10.1038/35094009
Fernández-Pomares, C., Juárez-Aguilar, E., Domínguez-Ortiz, M. Á., Gallegos-Estudillo, J., Herrera-Covarrubias, D., Sánchez-Medina, A., et al. (2018). Hydroalcoholic Extract of the Widely Used Mexican Plant Justicia Spicigera Schltdl. Exerts a Cytostatic Effect on LNCaP Prostate Cancer Cells. J. Herbal Med. 12, 66–72. doi:10.1016/j.hermed.2017.09.003
Flourakis, M., and Prevarskaya, N. (2009). Insights into Ca2+ Homeostasis of Advanced Prostate Cancer Cells. Biochim. Biophys. Acta 1793 (6), 1105–1109. doi:10.1016/j.bbamcr.2009.01.009
Foradori, C. D., Weiser, M. J., and Handa, R. J. (2008). Non-genomic Actions of Androgens. Front. Neuroendocrinol 29 (2), 169–181. doi:10.1016/j.yfrne.2007.10.005
Foradori, C. D., Werner, S. B., Sandau, U. S., Clapp, T. R., and Handa, R. J. (2007). Activation of the Androgen Receptor Alters the Intracellular Calcium Response to Glutamate in Primary Hippocampal Neurons and Modulates Sarco/endoplasmic Reticulum Calcium ATPase 2 Transcription. Neuroscience 149 (1), 155–164. doi:10.1016/j.neuroscience.2007.06.054
French, C. A., Miyoshi, I., Kubonishi, I., Grier, H. E., Perez-Atayde, A. R., and Fletcher, J. A. (2003). BRD4-NUT Fusion Oncogene: a Novel Mechanism in Aggressive Carcinoma. Cancer Res. 63 (2), 304–307. doi:10.1016/S0165-4608(02)00679-9
Gantar, M., Dhandayuthapani, S., and Rathinavelu, A. (2012). Phycocyanin Induces Apoptosis and Enhances the Effect of Topotecan on Prostate Cell Line LNCaP. J. Med. Food 15 (12), 1091–1095. doi:10.1089/jmf.2012.0123
Gapter, L., Wang, Z., Glinski, J., and Ng, K. Y. (2005). Induction of Apoptosis in Prostate Cancer Cells by Pachymic Acid from Poria Cocos. Biochem. Biophys. Res. Commun. 332 (4), 1153–1161. doi:10.1016/j.bbrc.2005.05.044
Gille, H., Kortenjann, M., Thomae, O., Moomaw, C., Slaughter, C., Cobb, M. H., et al. (1995). ERK Phosphorylation Potentiates Elk-1-mediated Ternary Complex Formation and Transactivation. EMBO J. 14 (5), 951–962. doi:10.1002/j.1460-2075.1995.tb07076.x
Gioti, K., Papachristodoulou, A., Benaki, D., Beloukas, A., Vontzalidou, A., Aligiannis, N., et al. (2020). Glycyrrhiza Glabra-Enhanced Extract and Adriamycin Antiproliferative Effect on PC-3 Prostate Cancer Cells. Nutr. Cancer 72 (2), 320–332. doi:10.1080/01635581.2019.1632357
Graff, R. E., Ahearn, T. U., Pettersson, A., Ebot, E. M., Gerke, T., Penney, K. L., et al. (2018). Height, Obesity, and the Risk of TMPRSS2:ERG-Defined Prostate Cancer. Cancer Epidemiol. Biomarkers Prev. 27 (2), 193–200. doi:10.1158/1055-9965.EPI-17-0547
Grasso, C. S., Wu, Y. M., Robinson, D. R., Cao, X., Dhanasekaran, S. M., Khan, A. P., et al. (2012). The Mutational Landscape of Lethal Castration-Resistant Prostate Cancer. Nature 487 (7406), 239–243. doi:10.1038/nature11125
Gregory, K. J., Zhao, B., Bielenberg, D. R., Dridi, S., Wu, J., Jiang, W., et al. (2010). Vitamin D Binding Protein-Macrophage Activating Factor Directly Inhibits Proliferation, Migration, and uPAR Expression of Prostate Cancer Cells. PLoS One 5 (10), e13428. doi:10.1371/journal.pone.0013428
Gubiani, J. R., Oliveira, M. C. S., Neponuceno, R. A. R., Camargo, M. J., Garcez, W. S., Biz, A. R., et al. (2019). Cytotoxic Prenylated Indole Alkaloid Produced by the Endophytic Fungus Aspergillus terreus P63. Phytochemistry Lett. 32, 162–167. doi:10.1016/j.phytol.2019.06.003
Gumushan Aktas, H., and Akgun, T. (2018). Naringenin Inhibits Prostate Cancer Metastasis by Blocking Voltage-Gated Sodium Channels. Biomed. Pharmacother. 106, 770–775. doi:10.1016/j.biopha.2018.07.008
Guo, Z., Yang, X., Sun, F., Jiang, R., Linn, D. E., Chen, H., et al. (2009). A Novel Androgen Receptor Splice Variant Is Up-Regulated during Prostate Cancer Progression and Promotes Androgen Depletion-Resistant Growth. Cancer Res. 69 (6), 2305–2313. doi:10.1158/0008-5472.Can-08-3795
Gupta, S., Li, J., Kemeny, G., Bitting, R. L., Beaver, J., Somarelli, J. A., et al. (2017). Whole Genomic Copy Number Alterations in Circulating Tumor Cells from Men with Abiraterone or Enzalutamide-Resistant Metastatic Castration-Resistant Prostate Cancer. Clin. Cancer Res. 23 (5), 1346–1357. doi:10.1158/1078-0432.CCR-16-1211
Haile, S., and Sadar, M. D. (2011). Androgen Receptor and its Splice Variants in Prostate Cancer. Cell Mol Life Sci 68 (24), 3971–3981. doi:10.1007/s00018-011-0766-7
Hamidullah, , Kumar, R., Singh Saini, K., Kumar, A., Kumar, S., Ramakrishna, E., et al. (2015). Quercetin-6-C-β-D-glucopyranoside, Natural Analog of Quercetin Exhibits Anti-prostate Cancer Activity by Inhibiting Akt-mTOR Pathway via Aryl Hydrocarbon Receptor. Biochimie 119, 68–79. doi:10.1016/j.biochi.2015.10.012
Han, H., Qiu, L., Wang, X., Qiu, F., Wong, Y., and Yao, X. (2011). Physalins A and B Inhibit Androgen-independent Prostate Cancer Cell Growth through Activation of Cell Apoptosis and Downregulation of Androgen Receptor Expression. Biol. Pharm. Bull. 34(10), 1584–1588. doi:10.1248/bpb.34.1584
Harris, E. M., Strope, J. D., Beedie, S. L., Huang, P. A., Goey, A. K. L., Cook, K. M., et al. (2018). Preclinical Evaluation of Discorhabdins in Antiangiogenic and Antitumor Models. Mar. Drugs 16 (7). doi:10.3390/md16070241
Hastak, K., Gupta, S., Ahmad, N., Agarwal, M. K., Agarwal, M. L., and Mukhtar, H. (2003). Role of P53 and NF-kappaB in Epigallocatechin-3-Gallate-Induced Apoptosis of LNCaP Cells. Oncogene 22 (31), 4851–4859. doi:10.1038/sj.onc.1206708
He, B., Lanz, R. B., Fiskus, W., Geng, C., Yi, P., Hartig, S. M., et al. (2014). GATA2 Facilitates Steroid Receptor Coactivator Recruitment to the Androgen Receptor Complex. Proc. Natl. Acad. Sci. U S A. 111 (51), 18261–18266. doi:10.1073/pnas.1421415111
Hellsten, R., Johansson, M., Dahlman, A., Dizeyi, N., Sterner, O., and Bjartell, A. (2008). Galiellalactone Is a Novel Therapeutic Candidate against Hormone-Refractory Prostate Cancer Expressing Activated Stat3. Prostate 68 (3), 269–280. doi:10.1002/pros.20699
Ho, Y., and Dehm, S. M. (2017). Androgen Receptor Rearrangement and Splicing Variants in Resistance to Endocrine Therapies in Prostate Cancer. Endocrinology 158 (6), 1533–1542. doi:10.1210/en.2017-00109
Hofland, J., van Weerden, W. M., Steenbergen, J., Dits, N. F., Jenster, G., and de Jong, F. H. (2012). Activin A Stimulates AKR1C3 Expression and Growth in Human Prostate Cancer. Endocrinology 153 (12), 5726–5734. doi:10.1210/en.2011-2065
Holzapfel, N. P., Holzapfel, B. M., Champ, S., Feldthusen, J., Clements, J., and Hutmacher, D. W. (2013). The Potential Role of Lycopene for the Prevention and Therapy of Prostate Cancer: from Molecular Mechanisms to Clinical Evidence. Int. J. Mol. Sci. 14 (7), 14620–14646. doi:10.3390/ijms140714620
Holzmann, C., Kappel, S., Kilch, T., Jochum, M. M., Urban, S. K., Jung, V., et al. (2015). Transient Receptor Potential Melastatin 4 Channel Contributes to Migration of Androgen-Insensitive Prostate Cancer Cells. Oncotarget 6 (39), 41783–41793. doi:10.18632/oncotarget.6157
Hong, M. Y., Seeram, N. P., and Heber, D. (2008). Pomegranate Polyphenols Down-Regulate Expression of Androgen-Synthesizing Genes in Human Prostate Cancer Cells Overexpressing the Androgen Receptor. J. Nutr. Biochem. 19 (12), 848–855. doi:10.1016/j.jnutbio.2007.11.006
Horne, W. C., Sanjay, A., Bruzzaniti, A., and Baron, R. (2005). The Role(s) of Src Kinase and Cbl Proteins in the Regulation of Osteoclast Differentiation and Function. Immunol. Rev. 208 (1), 106–125. doi:10.1111/j.0105-2896.2005.00335.x
Hsieh, T., Chen, S. S., Wang, X., and Wu, J. M. (1997). Regulation of Androgen Receptor (AR) and Prostate Specific Antigen (PSA) Expression in the Androgen-Responsive Human Prostate LNCaP Cells by Ethanolic Extracts of the Chinese Herbal Preparation, PC-SPES. Biochem. Mol. Biol. Int. 42 (3), 535–544. doi:10.1080/15216549700202941
Hsu, A., Bray, T. M., Helferich, W. G., Doerge, D. R., and Ho, E. (2010). Differential Effects of Whole Soy Extract and Soy Isoflavones on Apoptosis in Prostate Cancer Cells. Exp. Biol. Med. (Maywood) 235 (1), 90–97. doi:10.1258/ebm.2009.009128
Hsu, J. C., Zhang, J., Dev, A., Wing, A., Bjeldanes, L. F., and Firestone, G. L. (2005). Indole-3-carbinol Inhibition of Androgen Receptor Expression and Downregulation of Androgen Responsiveness in Human Prostate Cancer Cells. Carcinogenesis 26 (11), 1896–1904. doi:10.1093/carcin/bgi155
Hsu, J. L., Lee, Y. J., Leu, W. J., Dong, Y. S., Pan, S. L., Uang, B. J., et al. (2014). Moniliformediquinone Induces In Vitro and In Vivo Antitumor Activity through Glutathione Involved DNA Damage Response and Mitochondrial Stress in Human Hormone Refractory Prostate Cancer. J. Urol. 191 (5), 1429–1438. doi:10.1016/j.juro.2013.11.102
Hsu, J. W., Yasmin-Karim, S., King, M. R., Wojciechowski, J. C., Mickelsen, D., Blair, M. L., et al. (2011). Suppression of Prostate Cancer Cell Rolling and Adhesion to Endothelium by 1α,25-Dihydroxyvitamin D3. Am. J. Pathol. 178 (2), 872–880. doi:10.1016/j.ajpath.2010.10.036
Huang, C., Miller, R. T., and Freter, C. E. (2016). Signaling Regulation and Role of Filamin A Cleavage in Ca2+-Stimulated Migration of Androgen Receptor-Deficient Prostate Cancer Cells. Oncotarget 8 (3), 3840–3853. doi:10.18632/oncotarget.9472
Huang, D. M., Shen, Y. C., Wu, C., Huang, Y. T., Kung, F. L., Teng, C. M., et al. (2004). Investigation of Extrinsic and Intrinsic Apoptosis Pathways of New Clerodane Diterpenoids in Human Prostate Cancer PC-3 Cells. Eur. J. Pharmacol. 503 (1-3), 17–24. doi:10.1016/j.ejphar.2004.09.040
Huang, W. J., Bi, L. Y., Li, Z. Z., Zhang, X., and Ye, Y. (2013). Formononetin Induces the Mitochondrial Apoptosis Pathway in Prostate Cancer Cells via Downregulation of the IGF-1/IGF-1R Signaling Pathway. Pharm. Biol. 52 (4), 466–470. doi:10.3109/13880209.2013.842600
Huang, Y. S., Mao, J. X., Zhang, L., Guo, H. W., Yan, C., and Chen, M. (2021). Antiprostate Cancer Activity of Ineupatolide Isolated from Carpesium Cernuum L. Biomed. Res. Int. 2021, 5515961. doi:10.1155/2021/5515961
Huang, Y. W., Wang, L. S., Chang, H. L., Ye, W., Dowd, M. K., Wan, P. J., et al. (2006). Molecular Mechanisms of (-)-Gossypol-Induced Apoptosis in Human Prostate Cancer Cells. Anticancer Res. 26 (3A), 1925–1933.
Huggins, C. (1978). Endocrine-induced Regression of Cancers. Am. J. Surg. 136 (2), 233–238. doi:10.1016/0002-9610(78)90235-0
Huggins, C., and Hodges, C. (1941). Studies in Prostate Cancer: the Effect of Castration, of Estrogen and of Androgen Injection on Serum Phosphatases in Metastatic Carcinoma of Prostate.
Janouskova, H., El Tekle, G., Bellini, E., Udeshi, N. D., Rinaldi, A., Ulbricht, A., et al. (2017). Opposing Effects of Cancer-type-specific SPOP Mutants on BET Protein Degradation and Sensitivity to BET Inhibitors. Nat. Med. 23 (9), 1046–1054. doi:10.1038/nm.4372
Jeon, Y. J., Jung, S. N., Chang, H., Yun, J., Lee, C. W., Lee, J., et al. (2015a). Artocarpus Altilis (Parkinson) Fosberg Extracts and Geranyl Dihydrochalcone Inhibit STAT3 Activity in Prostate Cancer DU145 Cells. Phytother Res. 29 (5), 749–756. doi:10.1002/ptr.5311
Jeon, Y. J., Jung, S. N., Yun, J., Lee, C. W., Choi, J., Lee, Y. J., et al. (2015b). Ginkgetin Inhibits the Growth of DU-145 Prostate Cancer Cells through Inhibition of Signal Transducer and Activator of Transcription 3 Activity. Cancer Sci. 106 (4), 413–420. doi:10.1111/cas.12608
Jia, S., Gao, X., Lee, S. H., Maira, S. M., Wu, X., Stack, E. C., et al. (2013). Opposing Effects of Androgen Deprivation and Targeted Therapy on Prostate Cancer Prevention. Cancer Discov. 3 (1), 44–51. doi:10.1158/2159-8290.CD-12-0262
Jiang, H., Sun, J., Xu, Q., Liu, Y., Wei, J., Young, C. Y., et al. (2013). Marchantin M: a Novel Inhibitor of Proteasome Induces Autophagic Cell Death in Prostate Cancer Cells. Cell Death Dis 4 (8), e761. doi:10.1038/cddis.2013.285
Jiang, J., Eliaz, I., and Sliva, D. (2011). Suppression of Growth and Invasive Behavior of Human Prostate Cancer Cells by ProstaCaidâ„¢: Mechanism of Activity. Int. J. Oncol. 38 (6), 1675–1682. doi:10.3892/ijo.2011.996
Jiang, S., Zu, Y., Fu, Y., Zhang, Y., and Efferth, T. (2008). Activation of the Mitochondria-Driven Pathway of Apoptosis in Human PC-3 Prostate Cancer Cells by a Novel Hydrophilic Paclitaxel Derivative, 7-Xylosyl-10-Deacetylpaclitaxel. Int. J. Oncol. 33 (1), 103–111. doi:10.3892/ijo.33.1.103
Jin, Y., Kim, Y. H., Park, J. Y., Lee, Y. J., Oh, H. M., Choi, S. K., et al. (2018). Methyllucidone Inhibits STAT3 Activity by Regulating the Expression of the Protein Tyrosine Phosphatase MEG2 in DU145 Prostate Carcinoma Cells. Bioorg. Med. Chem. Lett. 28 (5), 853–857. doi:10.1016/j.bmcl.2018.02.012
Jongsomchai, K., Leardkamolkarn, V., and Mahatheeranont, S. (2020). A rice Bran Phytochemical, Cyanidin 3-glucoside, Inhibits the Progression of PC3 Prostate Cancer Cell. Anat. Cel Biol 53 (4), 481–492. doi:10.5115/acb.20.085
Jung, S. N., Shin, D. S., Kim, H. N., Jeon, Y. J., Yun, J., Lee, Y. J., et al. (2015). Sugiol Inhibits STAT3 Activity via Regulation of Transketolase and ROS-Mediated ERK Activation in DU145 Prostate Carcinoma Cells. Biochem. Pharmacol. 97 (1), 38–50. doi:10.1016/j.bcp.2015.06.033
Kasimsetty, S. G., Bialonska, D., Reddy, M. K., Thornton, C., Willett, K. L., and Ferreira, D. (2009). Effects of Pomegranate Chemical Constituents/intestinal Microbial Metabolites on CYP1B1 in 22Rv1 Prostate Cancer Cells. J. Agric. Food Chem. 57 (22), 10636–10644. doi:10.1021/jf902716r
Kaur, M., Velmurugan, B., Rajamanickam, S., Agarwal, R., and Agarwal, C. (2009). Gallic Acid, an Active Constituent of Grape Seed Extract, Exhibits Anti-proliferative, Pro-apoptotic and Anti-tumorigenic Effects against Prostate Carcinoma Xenograft Growth in Nude Mice. Pharm. Res. 26 (9), 2133–2140. doi:10.1007/s11095-009-9926-y
Khan, F., Pandey, P., Ahmad, V., and Upadhyay, T. K. (2020a). Moringa Oleifera Methanolic Leaves Extract Induces Apoptosis and G0/G1 Cell Cycle Arrest via Downregulation of Hedgehog Signaling Pathway in Human Prostate PC-3 Cancer Cells. J. Food Biochem. 44 (8), e13338. doi:10.1111/jfbc.13338
Khan, F., Pandey, P., Jha, N. K., Jafri, A., and Khan, I. (2020b). Antiproliferative Effect of Moringa Oleifera Methanolic Leaf Extract by Down-Regulation of Notch Signaling in DU145 Prostate Cancer Cells. Gene Rep. 19, 100619. doi:10.1016/j.genrep.2020.100619
Khan, N., Asim, M., Afaq, F., Abu Zaid, M., and Mukhtar, H. (2008). A Novel Dietary Flavonoid Fisetin Inhibits Androgen Receptor Signaling and Tumor Growth in Athymic Nude Mice. Cancer Res. 68 (20), 8555–8563. doi:10.1158/0008-5472.CAN-08-0240
Kilch, T., Kappel, S., and Peinelt, C. (2016). Regulation of Ca(2+) Signaling in Prostate Cancer Cells. Channels (Austin) 10 (4), 170–171. doi:10.1080/19336950.2015.1137176
Kim, K. Y., Cho, H. J., Yu, S. N., Kim, S. H., Yu, H. S., Park, Y. M., et al. (2013). Interplay of Reactive Oxygen Species, Intracellular Ca2+ and Mitochondrial Homeostasis in the Apoptosis of Prostate Cancer Cells by Deoxypodophyllotoxin. J. Cel Biochem 114 (5), 1124–1134. doi:10.1002/jcb.24455
Kim, S. H., Bommareddy, A., and Singh, S. V. (2011). Garlic Constituent Diallyl Trisulfide Suppresses X-Linked Inhibitor of Apoptosis Protein in Prostate Cancer Cells in Culture and In Vivo. Cancer Prev. Res. (Phila) 4 (6), 897–906. doi:10.1158/1940-6207.CAPR-10-0323
Kimira, M., Arai, Y., Shimoi, K., and Watanabe, S. (1998). Japanese Intake of Flavonoids and Isoflavonoids from Foods. J. Epidemiol. 8 (3), 168–175. doi:10.2188/jea.8.168
Koka, P. S., Mondal, D., Schultz, M., Abdel-Mageed, A. B., and Agrawal, K. C. (2010). Studies on Molecular Mechanisms of Growth Inhibitory Effects of Thymoquinone against Prostate Cancer Cells: Role of Reactive Oxygen Species. Exp. Biol. Med. (Maywood) 235 (6), 751–760. doi:10.1258/ebm.2010.009369
Kong, E. H., Kim, Y. J., Kim, Y. J., Cho, H. J., Yu, S. N., Kim, K. Y., et al. (2008). Piplartine Induces Caspase-Mediated Apoptosis in PC-3 Human Prostate Cancer Cells. Oncol. Rep. 20 (4), 785–792. doi:10.3892/or_00000075
Koyama, S., Cobb, L. J., Mehta, H. H., Seeram, N. P., Heber, D., Pantuck, A. J., et al. (2010). Pomegranate Extract Induces Apoptosis in Human Prostate Cancer Cells by Modulation of the IGF-IGFBP axis. Growth Horm. IGF Res. 20 (1), 55–62. doi:10.1016/j.ghir.2009.09.003
Kron, K. J., Murison, A., Zhou, S., Huang, V., Yamaguchi, T. N., Shiah, Y. J., et al. (2017). TMPRSS2-ERG Fusion Co-opts Master Transcription Factors and Activates NOTCH Signaling in Primary Prostate Cancer. Nat. Genet. 49 (9), 1336–1345. doi:10.1038/ng.3930
Kumar, A., Coleman, I., Morrissey, C., Zhang, X., True, L. D., Gulati, R., et al. (2016). Substantial Interindividual and Limited Intraindividual Genomic Diversity Among Tumors from Men with Metastatic Prostate Cancer. Nat. Med. 22 (4), 369–378. doi:10.1038/nm.4053
Labow, R. S., and Layne, D. S. (1972). The Formation of Glucosides of Isoflavones and of Some Other Phenols by Rabbit Liver Microsomal Fractions. Biochem. J. 128 (3), 491–497. doi:10.1042/bj1280491
Lee, D. H., Szczepanski, M. J., and Lee, Y. J. (2009). Magnolol Induces Apoptosis via Inhibiting the EGFR/PI3K/Akt Signaling Pathway in Human Prostate Cancer Cells. J. Cel Biochem 106 (6), 1113–1122. doi:10.1002/jcb.22098
Lee, E., Son, J. E., Byun, S., Lee, S. J., Kim, Y. A., Liu, K., et al. (2013a). CDK2 and mTOR Are Direct Molecular Targets of Isoangustone A in the Suppression of Human Prostate Cancer Cell Growth. Toxicol. Appl. Pharmacol. 272 (1), 12–20. doi:10.1016/j.taap.2013.04.030
Lee, M. G., Liu, Y. C., Lee, Y. L., El-Shazly, M., Lai, K. H., Shih, S. P., et al. (2018). Heteronemin, a Marine Sesterterpenoid-type Metabolite, Induces Apoptosis in Prostate LNcap Cells via Oxidative and ER Stress Combined with the Inhibition of Topoisomerase II and Hsp90. Mar. Drugs 16 (6). doi:10.3390/md16060204
Lee, S.-E., Sivtseva, S., Lim, C., Okhlopkova, Z., and Cho, S. (2021). Artemisia Kruhsiana Leaf Extract Induces Autophagic Cell Death in Human Prostate Cancer Cells. Chin. J. Nat. Medicines 19 (2), 134–142. doi:10.1016/s1875-5364(21)60014-6
Lee, S. E., Okhlopkova, Z., Lim, C., and Cho, S. (2020). Dracocephalum Palmatum Stephan Extract Induces Apoptosis in Human Prostate Cancer Cells via the Caspase-8-Mediated Extrinsic Pathway. Chin. J. Nat. Med. 18 (10), 793–800. doi:10.1016/s1875-5364(20)60019-x
Lee, S. Y., Cho, J. S., Yuk, D. Y., Moon, D. C., Jung, J. K., Yoo, H. S., et al. (2009). Obovatol Enhances Docetaxel-Induced Prostate and Colon Cancer Cell Death through Inactivation of Nuclear Transcription Factor-Κb. J. Pharmacol. Sci. 111 (2), 124–136. doi:10.1254/jphs.09048fp
Lee, W., Kim, K. Y., Yu, S. N., Kim, S. H., Chun, S. S., Ji, J. H., et al. (2013b). Pipernonaline from Piper Longum Linn. Induces ROS-Mediated Apoptosis in Human Prostate Cancer PC-3 Cells. Biochem. Biophys. Res. Commun. 430 (1), 406–412. doi:10.1016/j.bbrc.2012.11.030
Leu, W. J., Chang, H. S., Chan, S. H., Hsu, J. L., Yu, C. C., Hsu, L. C., et al. (2014). Reevesioside A, a Cardenolide Glycoside, Induces Anticancer Activity against Human Hormone-Refractory Prostate Cancers through Suppression of C-Myc Expression and Induction of G1 Arrest of the Cell Cycle. Plos One 9 (1), e87323. doi:10.1371/journal.pone.0087323
Levrier, C., Sadowski, M. C., Rockstroh, A., Gabrielli, B., Kavallaris, M., Lehman, M., et al. (2016). 6α-Acetoxyanopterine: A Novel Structure Class of Mitotic Inhibitor Disrupting Microtubule Dynamics in Prostate Cancer Cells. Mol. Cancer Ther. 16 (1), 3–15. doi:10.1158/1535-7163.mct-16-0325
Lewandowska, U., Szewczyk, K., Owczarek, K., Hrabec, Z., Podsędek, A., Koziołkiewicz, M., et al. (2013). Flavanols from Japanese Quince (Chaenomeles Japonica) Fruit Inhibit Human Prostate and Breast Cancer Cell Line Invasiveness and Cause Favorable Changes in Bax/Bcl-2 mRNA Ratio. Nutr. Cancer 65 (2), 273–285. doi:10.1080/01635581.2013.749292
Li, C., Han, X., Zhang, H., Wu, J., and Li, B. (2015a). Effect of Scopoletin on Apoptosis and Cell Cycle Arrest in Human Prostate Cancer Cells In Vitro. Trop. J. Pharm. Res. 14 (4), 611–617. doi:10.4314/tjpr.v14i4.8
Li, C., He, C., Xu, Y., Xu, H., Tang, Y., Chavan, H., et al. (2019). Alternol Eliminates Excessive ATP Production by Disturbing Krebs Cycle in Prostate Cancer. Prostate 79, 628–639. doi:10.1002/pros.23767
Li, J., and Al-Azzawi, F. (2009). Mechanism of Androgen Receptor Action. Maturitas 63 (2), 142–148. doi:10.1016/j.maturitas.2009.03.008
Li, Q. Q., Wang, G., Huang, F., Banda, M., and Reed, E. (2010). Antineoplastic Effect of Beta-Elemene on Prostate Cancer Cells and Other Types of Solid Tumour Cells. J. Pharm. Pharmacol. 62 (8), 1018–1027. doi:10.1111/j.2042-7158.2010.01135.x
Li, T., Zhao, X., Mo, Z., Huang, W., Yan, H., Ling, Z., et al. (2014). Formononetin Promotes Cell Cycle Arrest via Downregulation of Akt/Cyclin D1/CDK4 in Human Prostate Cancer Cells. Cell Physiol Biochem 34 (4), 1351–1358. doi:10.1159/000366342
Li, W. (2020). Isolobetyol, a New Polyacetylene Derivative from Platycodon Grandiflorum Root. Nat. Prod. Res., 1–4. doi:10.1080/14786419.2020.1779269
Li, W., Li, D.-Y., Wang, H.-D., Zheng, Z.-J., Hu, J., and Li, Z.-Z. (2015b). Juglans Regia Hexane Extract Exerts Antitumor Effect, Apoptosis Induction and Cell Circle Arrest in Prostate Cancer Cells In Vitro. Trop. J. Pharm. Res. 14 (3), 399. doi:10.4314/tjpr.v14i3.7
Li, X., Fan, S., Pan, X., Xiaokaiti, Y., Duan, J., Shi, Y., et al. (2016). Nordihydroguaiaretic Acid Impairs Prostate Cancer Cell Migration and Tumor Metastasis by Suppressing Neuropilin 1. Oncotarget 7 (52), 86225–86238. doi:10.18632/oncotarget.13368
Li, X., Lao, Y., Zhang, H., Wang, X., Tan, H., Lin, Z., et al. (2015c). The Natural Compound Guttiferone F Sensitizes Prostate Cancer to Starvation Induced Apoptosis via Calcium and JNK Elevation. BMC Cancer 15 (1), 254. doi:10.1186/s12885-015-1292-z
Li, X., Li, X., Wang, J., Ye, Z., and Li, J. C. (2012). Oridonin Up-Regulates Expression of P21 and Induces Autophagy and Apoptosis in Human Prostate Cancer Cells. Int. J. Biol. Sci. 8 (6), 901–912. doi:10.7150/ijbs.4554
Li, Y., Chan, S. C., Brand, L. J., Hwang, T. H., Silverstein, K. A., and Dehm, S. M. (2013). Androgen Receptor Splice Variants Mediate Enzalutamide Resistance in Castration-Resistant Prostate Cancer Cell Lines. Cancer Res. 73 (2), 483–489. doi:10.1158/0008-5472.CAN-12-3630
Li, Y., and Sarkar, F. H. (2002). Inhibition of Nuclear Factor kappaB Activation in PC3 Cells by Genistein Is Mediated via Akt Signaling Pathway. Clin. Cancer Res. 8 (7), 2369–2377. doi:10.1159/000064924
Liao, R. S., Ma, S., Miao, L., Li, R., Yin, Y., and Raj, G. V. (2013). Androgen Receptor-Mediated Non-genomic Regulation of Prostate Cancer Cell Proliferation. Transl Androl. Urol. 2 (3), 187–196. doi:10.3978/j.issn.2223-4683.2013.09.07
Liberio, M. S., Sadowski, M. C., Davis, R. A., Rockstroh, A., Vasireddy, R., Lehman, M. L., et al. (2015). The Ascidian Natural Product Eusynstyelamide B Is a Novel Topoisomerase II Poison that Induces DNA Damage and Growth Arrest in Prostate and Breast Cancer Cells. Oncotarget 6 (41), 43944–43963. doi:10.18632/oncotarget.6267
Lin, C. C., Chen, K. B., Tsai, C. H., Tsai, F. J., Huang, C. Y., Tang, C. H., et al. (2019). Casticin Inhibits Human Prostate Cancer DU 145 Cell Migration and Invasion via Ras/Akt/NF‐κB Signaling Pathways. J. Food Biochem. 43. doi:10.1111/jfbc.12902
Lippert, M. C., Mcclain, R., Boyd, J. C., and Theodorescu, D. (1999). Alternative Medicine Use in Patients with Localized Prostate Carcinoma Treated with Curative Intent. Cancer 86 (12), 2642–2648. doi:10.1002/(SICI)1097-0142(19991215)86:123.0.CO;2-4
Liu, K., Park, C., Li, S., Lee, K. W., Liu, H., He, L., et al. (2012a). Aloe-emodin Suppresses Prostate Cancer by Targeting the mTOR Complex 2. Carcinogenesis 33 (7), 1406–1411. doi:10.1093/carcin/bgs156
Liu, P., Cheng, H., Roberts, T. M., and Zhao, J. J. (2009). Targeting the Phosphoinositide 3-kinase Pathway in Cancer. Nat. Rev. Drug Discov. 8 (8), 627–644. doi:10.1038/nrd2926
Liu, S., Kumari, S., Hu, Q., Senapati, D., Venkadakrishnan, V. B., Wang, D., et al. (2017). A Comprehensive Analysis of Coregulator Recruitment, Androgen Receptor Function and Gene Expression in Prostate Cancer. Elife 6, e28482. doi:10.7554/eLife.28482
Liu, W. K., Cheung, F. W., and Che, C. T. (2006). Stellettin A Induces Oxidative Stress and Apoptosis in HL-60 Human Leukemia and LNCaP Prostate Cancer Cell Lines. J. Nat. Prod. 69 (6), 934–937. doi:10.1021/np058122y
Liu, X., He, Z., Li, C. H., Huang, G., Ding, C., and Liu, H. (2012b). Correlation Analysis of JAK-STAT Pathway Components on Prognosis of Patients with Prostate Cancer. Pathol. Oncol. Res. 18 (1), 17–23. doi:10.1007/s12253-011-9410-y
Liu, Y., Wang, M., Wang, D., Li, X., Wang, W., Lou, H., et al. (2016). Malformin A1 Promotes Cell Death through Induction of Apoptosis, Necrosis and Autophagy in Prostate Cancer Cells. Cancer Chemother. Pharmacol. 77 (1), 63–75. doi:10.1007/s00280-015-2915-4
Locke, J. A., Guns, E. S., Lubik, A. A., Adomat, H. H., Hendy, S. C., Wood, C. A., et al. (2008). Androgen Levels Increase by Intratumoral De Novo Steroidogenesis during Progression of Castration-Resistant Prostate Cancer. Cancer Res. 68 (15), 6407–6415. doi:10.1158/0008-5472.CAN-07-5997
Lu, Z., Wang, H., Zhu, M., Song, W., Wang, J., Wu, C., et al. (2018). Ophiopogonin D', a Natural Product from Radix Ophiopogonis, Induces In Vitro and In Vivo RIPK1-dependent and Caspase-independent Apoptotic Death in Androgen-independent Human Prostate Cancer Cells. Front. Pharmacol. 9, 432. doi:10.3389/fphar.2018.00432
Luu-The, V., Bélanger, A., and Labrie, F. (2008). Androgen Biosynthetic Pathways in the Human Prostate. Best Pract. Res. Clin. Endocrinol. Metab. 22 (2), 207–221. doi:10.1016/j.beem.2008.01.008
Ma, B., Zhang, H., Wang, Y., Zhao, A., Zhu, Z., Bao, X., et al. (2018). Corosolic Acid, a Natural Triterpenoid, Induces ER Stress-dependent Apoptosis in Human Castration Resistant Prostate Cancer Cells via Activation of IRE-1/JNK, PERK/CHOP and TRIB3. J. Exp. Clin. Cancer Res. 37 (1), 210. doi:10.1186/s13046-018-0889-x
Malik, A., Afaq, F., Sarfaraz, S., Adhami, V. M., Syed, D. N., and Mukhtar, H. (2005). Pomegranate Fruit Juice for Chemoprevention and Chemotherapy of Prostate Cancer. Proc. Natl. Acad. Sci. U S A. 102 (41), 14813–14818. doi:10.1073/pnas.0505870102
Marais, R., Wynne, J., and Treisman, R. (1993). The SRF Accessory Protein Elk-1 Contains a Growth Factor-Regulated Transcriptional Activation Domain. Cell 73 (2), 381–393. doi:10.1016/0092-8674(93)90237-k
Marilena, M., Sims, N. A., Susanne, V., Silvia, M., Anna, T., Silvia, B., et al. (2000). Decreased C-Src Expression Enhances Osteoblast Differentiation and Bone Formation. J. Cel Biol. 151 (2), 311–320.
Masood, M., Rasul, A., Sarfraz, I., Jabeen, F., Liu, S., Liu, X., et al. (2019).Hispolon Induces Apoptosis against Prostate DU145 Cancer Cells via Modulation of Mitochondrial and STAT3 PathwaysPak J. Pharm. Sci., 32, 2237–2243.
Masoodi, K. Z., Wani, W., Dar, Z. A., Mansoor, S., Anam-ul-Haq, S., Farooq, I., et al. (2020). Sea Buckthorn (Hippophae Rhamnoides L.) Inhibits Cellular Proliferation, Wound Healing and Decreases Expression of Prostate Specific Antigen in Prostate Cancer Cells In Vitro. J. Funct. Foods 73, 104102. doi:10.1016/j.jff.2020.104102
McEleny, K. R., Watson, R. W., Coffey, R. N., O'Neill, A. J., and Fitzpatrick, J. M. (2002). Inhibitors of Apoptosis Proteins in Prostate Cancer Cell Lines. Prostate 51 (2), 133–140. doi:10.1002/pros.10061
Meimetis, L. G., Williams, D. E., Mawji, N. R., Banuelos, C. A., Lal, A. A., Park, J. J., et al. (2012). Niphatenones, Glycerol Ethers from the Sponge Niphates Digitalis Block Androgen Receptor Transcriptional Activity in Prostate Cancer Cells: Structure Elucidation, Synthesis, and Biological Activity. J. Med. Chem. 55 (1), 503–514. doi:10.1021/jm2014056
Meng, F. M., Yang, J. B., Yang, C. H., Jiang, Y., Zhou, Y. F., Yu, B., et al. (2012). Vitexicarpin Induces Apoptosis in Human Prostate Carcinoma PC-3 Cells through G2/M Phase Arrest. Asian Pac. J. Cancer Prev. 13 (12), 6369–6374. doi:10.7314/Apjcp.2012.13.12.6369
Meng, Y., Lin, Z. M., Ge, N., Zhang, D. L., Huang, J., and Kong, F. (2015). Ursolic Acid Induces Apoptosis of Prostate Cancer Cells via the PI3K/Akt/mTOR Pathway. Am. J. Chin. Med. 43 (7), 1471–1486. doi:10.1142/S0192415X15500834
Miao, L., Yun, X., Tao, R., Wang, Y., Fan, G., Zhu, Y., et al. (2018). Bakuchiol Exhibits Anti-metastasis Activity through NF-Κb Cross-Talk Signaling with AR and ERβ in Androgen-independent Prostate Cancer Cells PC-3. J. Pharmacol. Sci. 138, 1–8. doi:10.1016/j.jphs.2017.04.004
Migliaccio, A., Castoria, G., Di Domenico, M., de Falco, A., Bilancio, A., Lombardi, M., et al. (2002). Sex Steroid Hormones Act as Growth Factors. J. Steroid Biochem. Mol. Biol. 83 (1), 31–35. doi:10.1016/s0960-0760(02)00264-9
Miller, W. L., and Auchus, R. J. (2011). The Molecular Biology, Biochemistry, and Physiology of Human Steroidogenesis and its Disorders. Endocr. Rev. 32 (1), 81–151. doi:10.1210/er.2010-0013
Ming, M., Sun, F. Y., Zhang, W. T., and Liu, J. K. (2016). Therapeutic Effect of Oridonin on Mice with Prostate Cancer. Asian Pac. J. Trop. Med. 9 (2), 184–187. doi:10.1016/j.apjtm.2016.01.007
Mirza, M. B., Elkady, A. I., Al-Attar, A. M., Syed, F. Q., Mohammed, F. A., and Hakeem, K. R. (2018). Induction of Apoptosis and Cell Cycle Arrest by Ethyl Acetate Fraction of Phoenix Dactylifera L. (Ajwa Dates) in Prostate Cancer Cells. J. Ethnopharmacol 218, 35–44. doi:10.1016/j.jep.2018.02.030
Montgomery, R. B., Mostaghel, E. A., Vessella, R., Hess, D. L., Kalhorn, T. F., Higano, C. S., et al. (2008). Maintenance of Intratumoral Androgens in Metastatic Prostate Cancer: a Mechanism for Castration-Resistant Tumor Growth. Cancer Res. 68 (11), 4447–4454. doi:10.1158/0008-5472.CAN-08-0249
Mordan-McCombs, S., Brown, T., Wang, W. L., Gaupel, A. C., Welsh, J., Tenniswood, M., et al. (2010). Tumor Progression in the LPB-Tag Transgenic Model of Prostate Cancer Is Altered by Vitamin D Receptor and Serum Testosterone Status. J. Steroid Biochem. Mol. Biol. 121 (2), 368–371. doi:10.1016/j.jsbmb.2010.03.062
Morgentaler, A. (2009). Testosterone Therapy in Men with Prostate Cancer: Scientific and Ethical Considerations. J. Urol. 181 (3), 972–979. doi:10.1016/j.juro.2008.11.031
Mukhtar, E., Adhami, V. M., Sechi, M., and Mukhtar, H. (2015). Dietary Flavonoid Fisetin Binds to β-tubulin and Disrupts Microtubule Dynamics in Prostate Cancer Cells. Cancer Lett. 367 (2), 173–183. doi:10.1016/j.canlet.2015.07.030
Mundy, G. R. (2002). Metastasis to Bone: Causes, Consequences and Therapeutic Opportunities. Nat. Rev. Cancer 2 (8), 584–593. doi:10.1038/nrc867
Nakase, I., Gallis, B., Takatani-Nakase, T., Oh, S., Lacoste, E., Singh, N. P., et al. (2009). Transferrin Receptor-dependent Cytotoxicity of Artemisinin-Transferrin Conjugates on Prostate Cancer Cells and Induction of Apoptosis. Cancer Lett. 274 (2), 290–298. doi:10.1016/j.canlet.2008.09.023
Nam, R. K., Fleshner, N., Rakovitch, E., Klotz, L., Trachtenberg, J., Choo, R., et al. (1999). Prevalence and Patterns of the Use of Complementary Therapies Among Prostate Cancer Patients: an Epidemiological Analysis. J. Urol. 161 (5), 1521–1524. doi:10.1108/1359854091097007210.1097/00005392-199905000-00028
Network, C. G. A. (2015a). The Molecular Taxonomy of Primary Prostate Cancer. Cell 163 (4), 1011–1025. doi:10.1016/j.cell.2015.10.025
Network, T. C. G. A. (2015b). The Molecular Taxonomy of Primary Prostate Cancer. Cell 163 (4), 1011–1025. doi:10.1016/j.cell.2015.10.025
Nguyen, C., Mehaidli, A., Baskaran, K., Grewal, S., Pupulin, A., Ruvinov, I., et al. (2019). Dandelion Root and Lemongrass Extracts Induce Apoptosis, Enhance Chemotherapeutic Efficacy, and Reduce Tumour Xenograft Growth In Vivo in Prostate Cancer. Evid. Based Complement. Alternat Med. 2019, 2951428. doi:10.1155/2019/2951428
Nishimura, M., Fuchino, H., Takayanagi, K., Kawakami, H., Nakayama, H., Kawahara, N., et al. (2021). Toxicity of Jegosaponins A and B from Styrax japonica Siebold Zuccarini in Prostate Cancer Cells and Zebrafish Embryos Resulting from Increased Membrane Permeability. Ijms 22 (12), 6354. doi:10.3390/ijms22126354
Pan, X. Y., Guo, H., Han, J., Hao, F., An, Y., Xu, Y., et al. (2012). Ginsenoside Rg3 Attenuates Cell Migration via Inhibition of Aquaporin 1 Expression in PC-3M Prostate Cancer Cells. Eur. J. Pharmacol. 683 (1–3), 27–34. doi:10.1016/j.ejphar.2012.02.040
Pintard, L., Willis, J. H., Willems, A., Johnson, J. L., Srayko, M., Kurz, T., et al. (2003). The BTB Protein MEL-26 Is a Substrate-specific Adaptor of the CUL-3 Ubiquitin-Ligase. Nature 425 (6955), 311–316. doi:10.1038/nature01959
Pinto, J. T., Sinha, R., Papp, K., Facompre, N. D., Desai, D., and El-Bayoumy, K. (2007). Differential Effects of Naturally Occurring and Synthetic Organoselenium Compounds on Biomarkers in Androgen Responsive and Androgen Independent Human Prostate Carcinoma Cells. Int. J. Cancer 120 (7), 1410–1417. doi:10.1002/ijc.22500
Pradhan, N., Parbin, S., Kausar, C., Kar, S., Mawatwal, S., Das, L., et al. (2019). Paederia Foetida Induces Anticancer Activity by Modulating Chromatin Modification Enzymes and Altering Pro-inflammatory Cytokine Gene Expression in Human Prostate Cancer Cells. Food Chem. Toxicol. 130, 161–173. doi:10.1016/j.fct.2019.05.016
Rabiau, N., Kossaï, M., Braud, M., Chalabi, N., Satih, S., Bignon, Y. J., et al. (2010). Genistein and Daidzein Act on a Panel of Genes Implicated in Cell Cycle and Angiogenesis by Polymerase Chain Reaction Arrays in Human Prostate Cancer Cell Lines. Cancer Epidemiol. 34 (2), 200–206. doi:10.1016/j.canep.2009.12.018
Ritch, C. R., and Cookson, M. S. (2016). Advances in the Management of Castration Resistant Prostate Cancer. BMJ 355, i4405. doi:10.1136/bmj.i4405
Robinson, D., Van Allen, E. M., Wu, Y. M., Schultz, N., Lonigro, R. J., Mosquera, J. M., et al. (2015). Integrative Clinical Genomics of Advanced Prostate Cancer. Cell 161 (5), 1215–1228. doi:10.1016/j.cell.2015.05.001
Rodrigues, D. N., Boysen, G., Sumanasuriya, S., Seed, G., Marzo, A. M., and de Bono, J. (2017). The Molecular Underpinnings of Prostate Cancer: Impacts on Management and Pathology Practice. J. Pathol. 241 (2), 173–182. doi:10.1002/path.4826
Rodríguez-Berriguete, G., Torrealba, N., Fraile, B., Paniagua, R., and Royuela, M. (2016). Epidermal Growth Factor Induces P38 MAPK-dependent G0/G1-To-S Transition in Prostate Cancer Cells upon Androgen Deprivation Conditions. Growth Factors 34 (1-2), 5–10. doi:10.3109/08977194.2015.1132712
Rosa, H. H., Carvalho, P., Ortmann, C. F., Schneider, N. F. Z., Reginatto, F. H., Simões, C. M. O., et al. (2020). Cytotoxic Effects of a Triterpene-Enriched Fraction of Cecropia Pachystachya on the Human Hormone-Refractory Prostate Cancer PC3 Cell Line. Biomed. Pharmacother. 130, 110551. doi:10.1016/j.biopha.2020.110551
Russo, A., Piovano, M., Clericuzio, M., Lombardo, L., Tabasso, S., Chamy, M. C., et al. (2007). Putrescine-1,4-dicinnamide from Pholiota Spumosa (Basidiomycetes) Inhibits Cell Growth of Human Prostate Cancer Cells. Phytomedicine 14 (2-3), 185–191. doi:10.1016/j.phymed.2006.09.010
Ryan, C. J., and Tindall, D. J. (2011). Androgen Receptor Rediscovered: the New Biology and Targeting the Androgen Receptor Therapeutically. J. Clin. Oncol. 29 (27), 3651–3658. doi:10.1200/JCO.2011.35.2005
Sachan, R., Kundu, A., Jeon, Y., Choi, W. S., Yoon, K., Kim, I. S., et al. (2018). Afrocyclamin A, a Triterpene Saponin, Induces Apoptosis and Autophagic Cell Death via the PI3K/Akt/mTOR Pathway in Human Prostate Cancer Cells. Phytomedicine 51, 139–150. doi:10.1016/j.phymed.2018.10.012
Sajjadi, S.-E., Ghanadian, M., Aghaei, M., and Salehi, A. (2018). Two New Dammarane Triterpenes Isolated from Cleome Khorassanica Bunge & Bien with Cytotoxicity against DU-145 and LNCaP Prostate Cancer Cell Lines. J. Asian Nat. Prod. Res. 22, 38–46. doi:10.1080/10286020.2018.1538211
Salmani, J. M. M., Zhang, X. P., Jacob, J. A., and Chen, B. A. (2017). Apigenin's Anticancer Properties and Molecular Mechanisms of Action: Recent Advances and Future Prospectives. Chin. J. Nat. Med. 15 (5), 321–329. doi:10.1016/S1875-5364(17)30052-3
Saravanakumar, K., Mandava, S., Chellia, R., Jeevithan, E., Babu Yelamanchi, R. S., Mandava, D., et al. (2019). Novel Metabolites from Trichoderma Atroviride against Human Prostate Cancer Cells and Their Inhibitory Effect on Helicobacter pylori and Shigella Toxin Producing Escherichia coli. Microb. Pathogenesis 126, 19–26. doi:10.1016/j.micpath.2018.10.011
Sarveswaran, S., Gautam, S. C., and Ghosh, J. (2012). Wedelolactone, a Medicinal Plant-Derived Coumestan, Induces Caspase-dependent Apoptosis in Prostate Cancer Cells via Downregulation of PKCε without Inhibiting Akt. Int. J. Oncol. 41 (6), 2191–2199. doi:10.3892/ijo.2012.1664
Sarveswaran, S., Liroff, J., Zhou, Z., Nikitin, A. Y., and Ghosh, J. (2010). Selenite Triggers Rapid Transcriptional Activation of P53, and P53-Mediated Apoptosis in Prostate Cancer Cells: Implication for the Treatment of Early-Stage Prostate Cancer. Int. J. Oncol. 36 (6), 1419–1428. doi:10.3892/ijo_00000627
Scher, H. I., and Sawyers, C. L. (2005). Biology of Progressive, Castration-Resistant Prostate Cancer: Directed Therapies Targeting the Androgen-Receptor Signaling axis. J. Clin. Oncol. 23 (32), 8253–8261. doi:10.1200/JCO.2005.03.4777
Schindler, C., and Darnell, J. E. (1995). Transcriptional Responses to Polypeptide Ligands: the JAK-STAT Pathway. Annu. Rev. Biochem. 64 (64), 621–651. doi:10.1146/annurev.bi.64.070195.003201
Schleich, S., Papaioannou, M., Baniahmad, A., and Matusch, R. (2006). Activity-guided Isolation of an Antiandrogenic Compound of Pygeum Africanum. Planta Med. 72 (6), 547–551. doi:10.1055/s-2006-941472
Schweizer, M. T., and Plymate, S. R. (2016). Targeting Constitutively Active Androgen Receptor Splice Variants in Castration Resistant Prostate Cancer. Expert Opin. Ther. Targets 20 (8), 903–906. doi:10.1517/14728222.2016.1159676
Senderowicz, A. M., Kaur, G., Sainz, E., Laing, C., Inman, W. D., Rodríguez, J., et al. (1995). Jasplakinolide's Inhibition of the Growth of Prostate Carcinoma Cells In Vitro with Disruption of the Actin Cytoskeleton. J. Natl. Cancer Inst. 87 (1), 46–51. doi:10.1093/jnci/87.1.46
Seo, Y., Ryu, K., Park, J., Jeon, D. K., Jo, S., Lee, H. K., et al. (2017). Inhibition of ANO1 by Luteolin and its Cytotoxicity in Human Prostate Cancer PC-3 Cells. PLoS One 12 (3), e0174935. doi:10.1371/journal.pone.0174935
Shanmugam, M. K., Rajendran, P., Li, F., Nema, T., Vali, S., Abbasi, T., et al. (2011). Ursolic Acid Inhibits Multiple Cell Survival Pathways Leading to Suppression of Growth of Prostate Cancer Xenograft in Nude Mice. J. Mol. Med. (Berl) 89 (7), 713–727. doi:10.1007/s00109-011-0746-2
Shaw, G., Renfree, M. B., Leihy, M. W., Shackleton, C. H. L., Roitman, E., and Wilson, J. D. (2000). Prostate Formation in a Marsupial Is Mediated by the Testicular Androgen 5alpha -androstane-3alpha ,17beta -diol. Proc. Natl. Acad. Sci. 97 (22), 12256–12259. doi:10.1073/pnas.220412297
Sheng, Y., Zou, M., Wang, Y., and Li, Q. (2015). 2',4'-dihydroxychalcone, a Flavonoid Isolated from Herba Oxytropis, Suppresses PC-3 Human Prostate Cancer Cell Growth by Induction of Apoptosis. Oncol. Lett. 10 (6), 3737–3741. doi:10.3892/ol.2015.3795
Shigemura, K., Arbiser, J. L., Sun, S. Y., Zayzafoon, M., Johnstone, P. A., Fujisawa, M., et al. (2007). Honokiol, a Natural Plant Product, Inhibits the Bone Metastatic Growth of Human Prostate Cancer Cells. Cancer 109 (7), 1279–1289. doi:10.1002/cncr.22551
Shin, Y., Kim, G. D., Jeon, J. E., Shin, J., and Lee, S. K. (2013). Antimetastatic Effect of Halichondramide, a Trisoxazole Macrolide from the marine Sponge Chondrosia Corticata, on Human Prostate Cancer Cells via Modulation of Epithelial-To-Mesenchymal Transition. Mar. Drugs 11 (7), 2472–2485. doi:10.3390/md11072472
Siegel, R. L., Miller, K. D., Fedewa, S. A., Ahnen, D. J., Meester, R. G. S., Barzi, A., et al. (2017). Colorectal Cancer Statistics, 2017. CA Cancer J. Clin. 67 (3), 177–193. doi:10.3322/caac.21395
Sigstedt, S. C., Hooten, C. J., Callewaert, M. C., Jenkins, A. R., Romero, A. E., Pullin, M. J., et al. (2008). Evaluation of Aqueous Extracts of Taraxacum officinale on Growth and Invasion of Breast and Prostate Cancer Cells. Int. J. Oncol. 32 (5), 1085–1090. doi:10.3892/ijo.32.5.1085
Singh, S. V., Zeng, Y., Xiao, D., Vogel, V. G., Nelson, J. B., Dhir, R., et al. (2005). Caspase-dependent Apoptosis Induction by Guggulsterone, a Constituent of Ayurvedic Medicinal Plant Commiphora Mukul, in PC-3 Human Prostate Cancer Cells Is Mediated by Bax and Bak. Mol. Cancer Ther. 4 (11), 1747–1754. doi:10.1158/1535-7163.MCT-05-0223
Small, E. J., Halabi, S., Dawson, N. A., Stadler, W. M., Rini, B. I., Picus, J., et al. (2004). Antiandrogen Withdrawal Alone or in Combination with Ketoconazole in Androgen-independent Prostate Cancer Patients: a Phase III Trial (CALGB 9583). J. Clin. Oncol. 22 (6), 1025–1033. doi:10.1200/JCO.2004.06.037
Squire, J. A., Park, P. C., Yoshimoto, M., Alami, J., Williams, J. L., Evans, A., et al. (2011). Prostate Cancer as a Model System for Genetic Diversity in Tumors. Adv. Cancer Res. 112, 183–216. doi:10.1016/B978-0-12-387688-1.00007-7
Steigerová, J., Rárová, L., Oklešt'ková, J., Křížová, K., Levková, M., Sváchová, M., et al. (2012). Mechanisms of Natural Brassinosteroid-Induced Apoptosis of Prostate Cancer Cells. Food Chem. Toxicol. 50 (11), 4068–4076. doi:10.1016/j.fct.2012.08.031
Stein, M. N., Goodin, S., and Dipaola, R. S. (2012). Abiraterone in Prostate Cancer: a New Angle to an Old Problem. Clin. Cancer Res. 18 (7), 1848–1854. doi:10.1158/1078-0432.CCR-11-1805
Su, X., Li, T., Liu, Z., Huang, Q., Liao, K., Ren, R., et al. (2018). Licochalcone A Activates Keap1-Nrf2 Signaling to Suppress Arthritis via Phosphorylation of P62 at Serine 349. Free Radic. Biol. Med. 115, 471–483. doi:10.1016/j.freeradbiomed.2017.12.004
Su, Z. Y., Khor, T. O., Shu, L., Lee, J. H., Saw, C. L., Wu, T. Y., et al. (2013). Epigenetic Reactivation of Nrf2 in Murine Prostate Cancer TRAMP C1 Cells by Natural Phytochemicals Z-Ligustilide and Radix Angelica Sinensis via Promoter CpG Demethylation. Chem. Res. Toxicol. 26 (3), 477–485. doi:10.1021/tx300524p
Suh, Y., Afaq, F., Khan, N., Johnson, J. J., Khusro, F. H., and Mukhtar, H. (2010). Fisetin Induces Autophagic Cell Death through Suppression of mTOR Signaling Pathway in Prostate Cancer Cells. Carcinogenesis 31 (8), 1424–1433. doi:10.1093/carcin/bgq115
Sun, B. L., Cai, E. B., Zhao, Y., Wang, Y., Yang, L. M., and Wang, J. Y. (2021). Arctigenin Triggers Apoptosis and Autophagy via PI3K/Akt/mTOR Inhibition in PC-3M Cells. Chem. Pharm. Bull. (Tokyo) 69 (5), 472–480. doi:10.1248/cpb.c21-00021
Sun, H., Zhang, A. H., Liu, S. B., Qiu, S., Li, X. N., Zhang, T. L., et al. (2018). Cell Metabolomics Identify Regulatory Pathways and Targets of Magnoline against Prostate Cancer. J. Chromatogr. B Analyt Technol. Biomed. Life Sci. 1102–1103, 143–151. doi:10.1016/j.jchromb.2018.10.017
Swami, S., Krishnan, A. V., Moreno, J., Bhattacharyya, R. S., Gardner, C., Brooks, J. D., et al. (2009). Inhibition of Prostaglandin Synthesis and Actions by Genistein in Human Prostate Cancer Cells and by Soy Isoflavones in Prostate Cancer Patients. Int. J. Cancer 124 (9), 2050–2059. doi:10.1002/ijc.24161
Tam, L., Mcglynn, L. M., Traynor, P., Mukherjee, R., Bartlett, J. M., and Edwards, J. (2007). Expression Levels of the JAK/STAT Pathway in the Transition from Hormone-Sensitive to Hormone-Refractory Prostate Cancer. Br. J. Cancer 97 (3), 378–383. doi:10.1038/sj.bjc.6603871
Tang, Y., Li, X., Liu, Z., Simoneau, A. R., Xie, J., and Zi, X. (2010). Flavokawain B, a Kava Chalcone, Induces Apoptosis via Up-Regulation of Death-Receptor 5 and Bim Expression in Androgen Receptor Negative, Hormonal Refractory Prostate Cancer Cell Lines and Reduces Tumor Growth. Int. J. Cancer 127 (8), 1758–1768. doi:10.1002/ijc.25210
Tang, Y., Parmakhtiar, B., Simoneau, A. R., Xie, J., Fruehauf, J., Lilly, M., et al. (2011). Lycopene Enhances Docetaxel's Effect in Castration-Resistant Prostate Cancer Associated with Insulin-like Growth Factor I Receptor Levels. Neoplasia 13 (2), 108–119. doi:10.1593/neo.101092
Taylor, B. S., Schultz, N., Hieronymus, H., Gopalan, A., Xiao, Y., Carver, B. S., et al. (2010). Integrative Genomic Profiling of Human Prostate Cancer. Cancer Cell 18 (1), 11–22. doi:10.1016/j.ccr.2010.05.026
Thangapazham, R., Saenz, F., Katta, S., Mohamed, A. A., Tan, S. H., Petrovics, G., et al. (2014). Loss of the NKX3.1 Tumorsuppressor Promotes the TMPRSS2-ERG Fusion Gene Expression in Prostate Cancer. BMC Cancer 14 (1), 16. doi:10.1186/1471-2407-14-16
Theurillat, J. P., Udeshi, N. D., Errington, W. J., Svinkina, T., Baca, S. C., Pop, M., et al. (2014). Prostate Cancer. Ubiquitylome Analysis Identifies Dysregulation of Effector Substrates in SPOP-Mutant Prostate Cancer. Science 346 (6205), 85–89. doi:10.1126/science.1250255
Thirugnanam, S., Xu, L., Ramaswamy, K., and Gnanasekar, M. (2008). Glycyrrhizin Induces Apoptosis in Prostate Cancer Cell Lines DU-145 and LNCaP. Oncol. Rep. 20 (6), 1387–1392. doi:10.3892/or_00000157
Ting, H., Deep, G., Kumar, S., Jain, A. K., Agarwal, C., and Agarwal, R. (2016). Beneficial Effects of the Naturally Occurring Flavonoid Silibinin on the Prostate Cancer Microenvironment: Role of Monocyte Chemotactic Protein-1 and Immune Cell Recruitment. Carcinogenesis 37 (6), 589–599. doi:10.1093/carcin/bgw039
Titus, M. A., Schell, M. J., Lih, F. B., Tomer, K. B., and Mohler, J. L. (2005). Testosterone and Dihydrotestosterone Tissue Levels in Recurrent Prostate Cancer. Clin. Cancer Res. 11 (13), 4653–4657. doi:10.1158/1078-0432.CCR-05-0525
Tomkins, G. M. (1957). The Enzymatic Reduction of delta 4-3-ketosteroids. J. Biol. Chem. 225 (1), 13–24. doi:10.1016/S0002-9610(34)90209-910.1016/s0021-9258(18)64906-3
Tomlins, S. A., Rhodes, D. R., Perner, S., Dhanasekaran, S. M., Mehra, R., Sun, X. W., et al. (2005). Recurrent Fusion of TMPRSS2 and ETS Transcription Factor Genes in Prostate Cancer. Science 310 (5748), 644–648. doi:10.1126/science.1117679
Torre, L. A., Bray, F., Siegel, R. L., Ferlay, J., Lortet-Tieulent, J., and Jemal, A. (2015). Global Cancer Statistics, 2012. CA Cancer J. Clin. 65 (2), 87–108. doi:10.3322/caac.21262
Trang, N. T., Wanner, M. J., Phuong, le. V. N., Koomen, G. J., and Dung, N. X. (1993). Thymoquinone from Eupatorium Ayapana. Planta Med. 59 (1), 99. doi:10.1055/s-2006-959619
Turan, I., Demir, S., Kilinc, K., Burnaz, N. A., Yaman, S. O., Akbulut, K., et al. (2017). Antiproliferative and Apoptotic Effect of Morus Nigra Extract on Human Prostate Cancer Cells. Saudi Pharm. J. 25 (2), 241–248. doi:10.1016/j.jsps.2016.06.002
Twillie, D. A., Eisenberger, M. A., Carducci, M. A., Hseih, W. S., Kim, W. Y., and Simons, J. W. (1995). Interleukin-6: a Candidate Mediator of Human Prostate Cancer Morbidity. Urology 45 (3), 542–549. doi:10.1016/S0090-4295(99)80034-X
Unni, E., Sun, S., Nan, B., McPhaul, M. J., Cheskis, B., Mancini, M. A., et al. (2004). Changes in Androgen Receptor Nongenotropic Signaling Correlate with Transition of LNCaP Cells to Androgen independence. Cancer Res. 64 (19), 7156–7168. doi:10.1158/0008-5472.CAN-04-1121
Vanella, L., Di Giacomo, C., Acquaviva, R., Barbagallo, I., Cardile, V., Kim, D. H., et al. (2013). Apoptotic Markers in a Prostate Cancer Cell Line: Effect of Ellagic Acid. Oncol. Rep. 30 (6), 2804–2810. doi:10.3892/or.2013.2757
Vaz, C. V., Rodrigues, D. B., Socorro, S., and Maia, C. J. (2015). Effect of Extracellular Calcium on Regucalcin Expression and Cell Viability in Neoplastic and Non-neoplastic Human Prostate Cells. Biochim. Biophys. Acta 1853 (10 Pt A), 2621–2628. doi:10.1016/j.bbamcr.2015.07.006
Visakorpi, T., Hyytinen, E., Koivisto, P., Tanner, M., Keinänen, R., Palmberg, C., et al. (1995). In Vivo amplification of the Androgen Receptor Gene and Progression of Human Prostate Cancer. Nat. Genet. 9 (4), 401–406. doi:10.1038/ng0495-401
Vitali, F., Pennisi, G., Attaguile, G., Savoca, F., and Tita, B. (2011). Antiproliferative and Cytotoxic Activity of Extracts from Cistus Incanus L. And Cistus Monspeliensis L. on Human Prostate Cell Lines. Nat. Prod. Res. 25 (3), 188–202. doi:10.1080/14786410802583148
von Eyben, F., Kiljunen, T., Kangasmaki, A., Kairemo, K., von Eyben, R., and Joensuu, T. (2015). Management of Advanced Prostate Cancer. Ann. Oncol. 26 (11), 178–182. doi:10.1093/annonc/mdv327
Wadosky, K. M., and Koochekpour, a. S. (2016). Molecular Mechanisms Underlying Resistance to Androgen Deprivation Therapy in Prostate Cancer. Oncotarget 27, 64447–64470. doi:10.18632/oncotarget.10901
Wang, J., Eltoum, I. E., and Lamartiniere, C. A. (2004). Genistein Alters Growth Factor Signaling in Transgenic Prostate Model (TRAMP). Mol. Cel Endocrinol 219 (1–2), 171–180. doi:10.1016/j.mce.2003.12.018
Wang, J. L., Chou, C. T., Liang, W. Z., Yeh, J. H., Kuo, C. C., Lee, C. Y., et al. (2016a). Effect of 2,5-dimethylphenol on Ca(2+) Movement and Viability in PC3 Human Prostate Cancer Cells. Toxicol. Mech. Methods 26 (5), 327–333. doi:10.3109/15376516.2016.1158893
Wang, L. G., Liu, X. M., and Chiao, J. W. (2006). Repression of Androgen Receptor in Prostate Cancer Cells by Phenethyl Isothiocyanate. Carcinogenesis 27 (10), 2124–2132. doi:10.1093/carcin/bgl075
Wang, P., Henning, S. M., Magyar, C. E., Elshimali, Y., Heber, D., and Vadgama, J. V. (2016b). Green tea and Quercetin Sensitize PC-3 Xenograft Prostate Tumors to Docetaxel Chemotherapy. J. Exp. Clin. Cancer Res. 35 (1), 73. doi:10.1186/s13046-016-0351-x
Wang, Q., Grkovic, T., Font, J., Bonham, S., Pouwer, R. H., Bailey, C. G., et al. (2014). Monoterpene Glycoside ESK246 from Pittosporum Targets LAT3 Amino Acid Transport and Prostate Cancer Cell Growth. ACS Chem. Biol. 9 (6), 1369–1376. doi:10.1021/cb500120x
Wang, Q., Li, W., Liu, X. S., Carroll, J. S., Jänne, O. A., Keeton, E. K., et al. (2007). A Hierarchical Network of Transcription Factors Governs Androgen Receptor-dependent Prostate Cancer Growth. Mol. Cel 27 (3), 380–392. doi:10.1016/j.molcel.2007.05.041
Wang, W., Rayburn, E. R., Hao, M., Zhao, Y., Hill, D. L., Zhang, R., et al. (2008a). Experimental Therapy of Prostate Cancer with Novel Natural Product Anti-cancer Ginsenosides. Prostate 68 (8), 809–819. doi:10.1002/pros.20742
Wang, W. L., McHenry, P., Jeffrey, R., Schweitzer, D., Helquist, P., and Tenniswood, M. (2008b). Effects of Iejimalide B, a marine Macrolide, on Growth and Apoptosis in Prostate Cancer Cell Lines. J. Cel Biochem 105 (4), 998–1007. doi:10.1002/jcb.21898
Wang, X., Wang, B., Zhou, L., Wang, X., Veeraraghavan, V. P., Mohan, S. K., et al. (2020). Ganoderma Lucidum Put Forth Anti-tumor Activity against PC-3 Prostate Cancer Cells via Inhibition of Jak-1/STAT-3 Activity. Saudi J. Biol. Sci. 27 (10), 2632–2637. doi:10.1016/j.sjbs.2020.05.044
Wang, Y., Liu, Q., Liu, Z., Li, B., Sun, Z., Zhou, H., et al. (2012). Berberine, a Genotoxic Alkaloid, Induces ATM-Chk1 Mediated G2 Arrest in Prostate Cancer Cells. Mutat. Res. 734 (1-2), 20–29. doi:10.1016/j.mrfmmm.2012.04.005
Watson, I. R., Takahashi, K., Futreal, P. A., and Chin, L. (2013). Emerging Patterns of Somatic Mutations in Cancer. Nat. Rev. Genet. 14 (10), 703–718. doi:10.1038/nrg3539
Wei, R., Ma, Q., Zhong, G., He, J., and Sang, Z. (2020). Isolation and Characterization of Flavonoid Derivatives with Anti-prostate Cancer and Hepatoprotective Activities from the Flowers of Hosta Plantaginea (Lam.) Aschers. J. Ethnopharmacol 253, 112685. doi:10.1016/j.jep.2020.112685
Wertz, K. (2009). Lycopene Effects Contributing to Prostate Health. Nutr. Cancer 61 (6), 775–783. doi:10.1080/01635580903285023
Wolfram, J., Suri, K., Huang, Y., Molinaro, R., Borsoi, C., Scott, B., et al. (2014). Evaluation of Anticancer Activity of Celastrol Liposomes in Prostate Cancer Cells. J. Microencapsul 31 (5), 501–507. doi:10.3109/02652048.2013.879932
Wu, Q., Kohli, M., Bergen, H. R., Cheville, J. C., Karnes, R. J., Cao, H., et al. (2014). Preclinical Evaluation of the Supercritical Extract of Azadirachta Indica (Neem) Leaves In Vitro and In Vivo on Inhibition of Prostate Cancer Tumor Growth. Mol. Cancer Ther. 13 (5), 1067–1077. doi:10.1158/1535-7163.MCT-13-0699
Xiang, N., Zhao, R., and Zhong, W. (2009). Sodium Selenite Induces Apoptosis by Generation of Superoxide via the Mitochondrial-dependent Pathway in Human Prostate Cancer Cells. Cancer Chemother. Pharmacol. 63 (2), 351–362. doi:10.1007/s00280-008-0745-3
Xiao, D., Zeng, Y., Prakash, L., Badmaev, V., Majeed, M., and Singh, S. V. (2011). Reactive Oxygen Species-dependent Apoptosis by Gugulipid Extract of Ayurvedic Medicine Plant Commiphora Mukul in Human Prostate Cancer Cells Is Regulated by C-Jun N-Terminal Kinase. Mol. Pharmacol. 79 (3), 499–507. doi:10.1124/mol.110.068551
Xing, L., Venegas, A. M., Chen, A., Garrett-Beal, L., Boyce, B. F., Varmus, H. E., et al. (2001). Genetic Evidence for a Role for Src Family Kinases in TNF Family Receptor Signaling and Cell Survival. Genes Dev. 15 (2), 241–253. doi:10.1101/gad.840301
Xu, L., Wei, Y., Reboul, J., Vaglio, P., Shin, T. H., Vidal, M., et al. (2003). BTB Proteins Are Substrate-specific Adaptors in an SCF-like Modular Ubiquitin Ligase Containing CUL-3. Nature 425 (6955), 316–321. doi:10.1038/nature01985
Xu, T., Li, T., Zhang, Y., Huan, S., Cui, T., and Sun, L. (2017). Inhibition of Akt/NF-κB/survivin Pathway by Embelin on Castration-Resistant Prostate Cancer Cells. Oncotarget 34, 3700–3710. doi:10.1038/onc.2014.302
Xu, Y. M., Liu, M. X., Grunow, N., Wijeratne, E. M., Paine-Murrieta, G., Felder, S., et al. (2015). Discovery of Potent 17β-Hydroxywithanolides for Castration-Resistant Prostate Cancer by High-Throughput Screening of a Natural Products Library for Androgen-Induced Gene Expression Inhibitors. J. Med. Chem. 58 (17), 6984–6993. doi:10.1021/acs.jmedchem.5b00867
Yaacob, N. S., Hamzah, N., Nik Mohamed Kamal, N. N., Zainal Abidin, S. A., Lai, C. S., Navaratnam, V., et al. (2010). Anticancer Activity of a Sub-fraction of Dichloromethane Extract of Strobilanthes Crispus on Human Breast and Prostate Cancer Cells In Vitro. BMC Complement. Altern. Med. 10 (1), 1–14. doi:10.1186/1472-6882-10-42
Yan, J., and Katz, A. (2010). PectaSol-C Modified Citrus Pectin Induces Apoptosis and Inhibition of Proliferation in Human and Mouse Androgen-dependent and- Independent Prostate Cancer Cells. Integr. Cancer Ther. 9 (2), 197–203. doi:10.1177/1534735410369672
Yang, C., Gundala, S. R., Mukkavilli, R., Vangala, S., Reid, M. D., and Aneja, R. (2015). Synergistic Interactions Among Flavonoids and Acetogenins in Graviola (Annona Muricata) Leaves Confer protection against Prostate Cancer. Carcinogenesis 36 (6), 656–665. doi:10.1093/carcin/bgv046
Yang, H., Landis-Piwowar, K. R., Lu, D., Yuan, P., Li, L., Reddy, G. P., et al. (2008). Pristimerin Induces Apoptosis by Targeting the Proteasome in Prostate Cancer Cells. J. Cel Biochem 103 (1), 234–244. doi:10.1002/jcb.21399
Yang, J., Fizazi, K., Peleg, S., Sikes, C. R., Raymond, A. K., Jamal, N., et al. (2001). Prostate Cancer Cells Induce Osteoblast Differentiation through a Cbfa1-dependent Pathway. Cancer Res. 61 (14), 5652–5659. doi:10.1016/S0165-4608(01)00481-2
Yang, J., Wang, C., Zhang, Z., Chen, X., Jia, Y., Wang, B., et al. (2017). Curcumin Inhibits the Survival and Metastasis of Prostate Cancer Cells via the Notch-1 Signaling Pathway. Apmis 125 (2), 134–140. doi:10.1111/apm.12650
Yang, S. H., Yates, P. R., Whitmarsh, A. J., Davis, R. J., and Sharrocks, A. D. (1998). The Elk-1 ETS-Domain Transcription Factor Contains a Mitogen-Activated Protein Kinase Targeting Motif. Mol. Cel Biol 18 (2), 710–720. doi:10.1128/MCB.18.2.710
Yap, T. A., Smith, A. D., Ferraldeschi, R., Al-Lazikani, B., Workman, P., and de Bono, J. S. (2016). Drug Discovery in Advanced Prostate Cancer: Translating Biology into Therapy. Nat. Rev. Drug Discov. 15 (10), 699–718. doi:10.1038/nrd.2016.120
Yin, P., Kawamura, T., He, M., Vanaja, D. K., and Young, C. Y. (2009). Phenethyl Isothiocyanate Induces Cell Cycle Arrest and Reduction of Alpha- and Beta-Tubulin Isotypes in Human Prostate Cancer Cells. Cell Biol Int 33 (1), 57–64. doi:10.1016/j.cellbi.2008.09.013
Yoon, Y. J., Kim, Y. H., Lee, Y. J., Choi, J., Kim, C. H., Han, D. C., et al. (2019). 2'-Hydroxycinnamaldehyde Inhibits Proliferation and Induces Apoptosis via Signal Transducer and Activator of Transcription 3 Inactivation and Reactive Oxygen Species Generation. Cancer Sci. 110 (1), 366–378. doi:10.1111/cas.13852
Yu, Z., Xu, J., Shao, M., and Zou, J. (2020). Germacrone Induces Apoptosis as Well as Protective Autophagy in Human Prostate Cancer Cells. Cancer Manag. Res. 12, 4009–4016. doi:10.2147/cmar.S250522
Yuan, H., Pan, Y., and Young, C. Y. (2004). Overexpression of C-Jun Induced by Quercetin and Resverol Inhibits the Expression and Function of the Androgen Receptor in Human Prostate Cancer Cells. Cancer Lett. 213 (2), 155–163. doi:10.1016/j.canlet.2004.04.003
Zabaiou, N., Fouache, A., Trousson, A., Buñay-Noboa, J., Marceau, G., Sapin, V., et al. (2019). Ethanolic Extract of Algerian Propolis Decreases Androgen Receptor Transcriptional Activity in Cultured LNCaP Cells. J. Steroid Biochem. Mol. Biol. 189, 108–115. doi:10.1016/j.jsbmb.2019.02.016
Zeng, Q., Zeng, Y., Nie, X., Guo, Y., and Zhan, Y. (2020). Britanin Exhibits Potential Inhibitory Activity on Human Prostate Cancer Cell Lines through PI3K/Akt/NF-Κb Signaling Pathways. Planta Med. 86 (18), 1401–1410. doi:10.1055/a-1211-4656
Zeng, Y., and Yang, Y. (2018). Piperine Depresses the Migration Progression via Downregulating the Akt/mTOR/MMP-9 S-ignaling P-athway in DU145 C-ells. Mol. Med. Rep. 17 (5). doi:10.3892/mmr.2018.8653
Zengerling, F., Azoitei, A., Herweg, A., Jentzmik, F., and Cronauer, M. V. (2016). Inhibition of IGF-1R Diminishes Transcriptional Activity of the Androgen Receptor and its Constitutively Active, C-Terminally Truncated Counterparts Q640X and AR-V7. World J. Urol. 34 (5), 633–639. doi:10.1007/s00345-015-1674-5
Zhang, C., Shu, L., Kim, H., Khor, T. O., Wu, R., Li, W., et al. (2016). Phenethyl Isothiocyanate (PEITC) Suppresses Prostate Cancer Cell Invasion Epigenetically through Regulating microRNA-194. Mol. Nutr. Food Res. 60 (6), 1427–1436. doi:10.1002/mnfr.201500918
Zhang, C., Su, Z. Y., Khor, T. O., Shu, L., and Kong, A. N. (2013). Sulforaphane Enhances Nrf2 Expression in Prostate Cancer TRAMP C1 Cells through Epigenetic Regulation. Biochem. Pharmacol. 85 (9), 1398–1404. doi:10.1016/j.bcp.2013.02.010
Zhang, L. Y., Wu, Y. L., Gao, X. H., and Guo, F. (2014). Mitochondrial Protein Cyclophilin-D-Mediated Programmed Necrosis Attributes to Berberine-Induced Cytotoxicity in Cultured Prostate Cancer Cells. Biochem. Biophys. Res. Commun. 450 (1), 697–703. doi:10.1016/j.bbrc.2014.06.039
Zhang, P., Wang, D., Zhao, Y., Ren, S., Gao, K., Ye, Z., et al. (2017). Intrinsic BET Inhibitor Resistance in SPOP-Mutated Prostate Cancer Is Mediated by BET Protein Stabilization and AKT-mTORC1 Activation. Nat. Med. 23(9), 1055–1062. doi:10.1038/nm.4379
Zhang, X., Haney, K. M., Richardson, A. C., Wilson, E., Gewirtz, D. A., Ware, J. L., et al. (2010b). Anibamine, a Natural Product CCR5 Antagonist, as a Novel lead for the Development of Anti-prostate Cancer Agents. Bioorg. Med. Chem. Lett. 20 (15), 4627–4630. doi:10.1016/j.bmcl.2010.06.003
Zhang, X., Yeung, E. D., Wang, J., Panzhinskiy, E. E., Tong, C., Li, W., et al. (2010a). Isoliquiritigenin, a Natural Anti-oxidant, Selectively Inhibits the Proliferation of Prostate Cancer Cells. Clin. Exp. Pharmacol. Physiol. 37 (8), 841–847. doi:10.1111/j.1440-1681.2010.05395.x
Zhang, X., Bi, L., Ye, Y., and Chen, J. (2014). Formononetin Induces Apoptosis in PC-3 Prostate Cancer Cells through Enhancing the Bax/Bcl-2 Ratios and Regulating the p38/Akt Pathway. Nutr. Cancer 66 (4), 656–661. doi:10.1080/01635581.2014.894098
Zhao, D., Lin, F., Wu, X., Zhao, Q., Zhao, B., Lin, P., et al. (2012). Pseudolaric Acid B Induces Apoptosis via Proteasome-Mediated Bcl-2 Degradation in Hormone-Refractory Prostate Cancer DU145 Cells. Toxicol. Vitro 26 (4), 595–602. doi:10.1016/j.tiv.2012.02.004
Zhao, F., Huang, W., Zhang, Z., Mao, L., Han, Y., Yan, J., et al. (2016). Triptolide Induces Protective Autophagy through Activation of the CaMKKβ-AMPK Signaling Pathway in Prostate Cancer Cells. Oncotarget 7 (5), 5366–5382. doi:10.18632/oncotarget.6783
Zhao, Y., Cheng, X., Wang, G., Liao, Y., and Qing, C. (2020). Linalool Inhibits 22Rv1 Prostate Cancer Cell Proliferation and Induces Apoptosis. Oncol. Lett. 20 (6), 289. doi:10.3892/ol.2020.12152
Zheng, J., Wang, J., Wang, Q., Zou, H., Wang, H., Zhang, Z., et al. (2020). Targeting Castration-Resistant Prostate Cancer with a Novel RORγ Antagonist Elaiophylin. Acta Pharmaceutica Sinica B 10, 2313–2322. doi:10.1016/j.apsb.2020.07.001
Zhou, Y., Liu, Y. E., Cao, J., Zeng, G., Shen, C., Li, Y., et al. (2009). Vitexins, Nature-Derived Lignan Compounds, Induce Apoptosis and Suppress Tumor Growth. Clin. Cancer Res. 15 (16), 5161–5169. doi:10.1158/1078-0432.CCR-09-0661
Zhu, Y., Liu, C., Nadiminty, N., Lou, W., Tummala, R., Evans, C. P., et al. (2013). Inhibition of ABCB1 Expression Overcomes Acquired Docetaxel Resistance in Prostate Cancer. Mol. Cancer Ther. 12 (9), 1829–1836. doi:10.1158/1535-7163.MCT-13-0208
Zhuang, M., Calabrese, M. F., Liu, J., Waddell, M. B., Nourse, A., Hammel, M., et al. (2009). Structures of SPOP-Substrate Complexes: Insights into Molecular Architectures of BTB-Cul3 Ubiquitin Ligases. Mol. Cel 36 (1), 39–50. doi:10.1016/j.molcel.2009.09.022
Zingue, S., Gbaweng Yaya, A. J., Michel, T., Ndinteh, D. T., Rutz, J., Auberon, F., et al. (2019). Bioguided Identification of Daucosterol, a Compound that Contributes to the Cytotoxicity Effects of Crateva Adansonii DC (Capparaceae) to Prostate Cancer Cells. J. Ethnopharmacol 247, 112251. doi:10.1016/j.jep.2019.112251
Zingue, S., Rutz, J., Maxeiner, S., Ndinteh, D. T., Chun, F. K.-H., Jüngel, E., et al. (2021). In Vitro pro-apoptotic and Anti-migratory Effects of Treculia Africana Decne. (Moraceae) and Entandrophragma Angolense Welw (Meliaceae) Extracts on Prostate Cancer Cells. J. Herbal Med. 28, 100443. doi:10.1016/j.hermed.2021.100443
Keywords: prostate cancer, natural compounds, apoptosis, androgen receptor, mechanism
Citation: Bai B, Chen Q, Jing R, He X, Wang H, Ban Y, Ye Q, Xu W and Zheng C (2021) Molecular Basis of Prostate Cancer and Natural Products as Potential Chemotherapeutic and Chemopreventive Agents. Front. Pharmacol. 12:738235. doi: 10.3389/fphar.2021.738235
Received: 08 July 2021; Accepted: 06 September 2021;
Published: 23 September 2021.
Edited by:
Wei Peng, Chengdu University of Traditional Chinese Medicine, ChinaReviewed by:
Tao Guo, Henan University of Traditional Chinese Medicine, ChinaLoh Teng Hern Tan, Monash University Malaysia, Malaysia
Copyright © 2021 Bai, Chen, Jing, He, Wang, Ban, Ye, Xu and Zheng. This is an open-access article distributed under the terms of the Creative Commons Attribution License (CC BY). The use, distribution or reproduction in other forums is permitted, provided the original author(s) and the copyright owner(s) are credited and that the original publication in this journal is cited, in accordance with accepted academic practice. No use, distribution or reproduction is permitted which does not comply with these terms.
*Correspondence: Qi Ye, cWlwaGFybUAxMjYuY29t; Weiheng Xu, eHV3ZWloZW5nNzExNEAxNjMuY29t; Chengjian Zheng, Y2p6aGVuZzE5ODRAMTI2LmNvbQ==
†These authors have contributed equally to this work