- 1Department of Clinical and Administrative Pharmacy, University of Georgia College of Pharmacy, Augusta, GA, United States
- 2Department of Pharmacy, Augusta University Medical Center, Augusta, GA, United States
Catecholamine upregulation is a core pathophysiological feature in critical illness. Sustained catecholamine β-adrenergic induction produces adverse effects relevant to critical illness management. β-blockers (βB) have proposed roles in various critically ill disease states, including sepsis, trauma, burns, and cardiac arrest. Mounting evidence suggests βB improve hemodynamic and metabolic parameters culminating in decreased burn healing time, reduced mortality in traumatic brain injury, and improved neurologic outcomes following cardiac arrest. In sepsis, βB appear hemodynamically benign after acute resuscitation and may augment cardiac function. The emergence of ultra-rapid βB provides new territory for βB, and early data suggest significant improvements in mitigating atrial fibrillation in persistently tachycardic septic patients. This review summarizes the evidence regarding the pharmacotherapeutic role of βB on relevant pathophysiology and clinical outcomes in various types of critical illness.
Introduction
The catecholamine cascade is a defining element of critical illness (Preiser et al., 2014). The α- and β-adrenergic receptors form the response mechanism for endogenous catecholamines and exogenously administered catecholamine vasoactive agents (e.g., dobutamine, dopamine, norepinephrine, and epinephrine) (Preiser et al., 2014). These receptors elicit responses in nearly every major organ system and change their expression levels during the body’s stress response to critical illness (Belletti et al., 2020). Prolonged exposure to high levels of catecholamines in these altered states may evoke detrimental metabolic and hemodynamic effects. Higher levels of catecholamines appear in a myriad of critical illness etiologies and have been associated with higher mortality (Tripathi et al., 1981; Hamill et al., 1987; Benedict and Rose, 1992; Boldt et al., 1995; Dunser and Hasibeder, 2009).
β-blockers (βB) may be administered to manipulate the adrenergic response during critical illness. βB are mainstay medications for cardiovascular disease states, including post-myocardial infarction management (O'Gara et al., 2013), atrial fibrillation (AF) (January et al., 2014), and heart failure (HF); however, evaluation of the utility of βB extends beyond long-term cardiac management into acute management of critically ill patients (Writing et al., 2021). The purpose of this review is to critically evaluate available literature regarding βB therapy in critical illness and describe evidence-based βB use in presentations of critical illness including sepsis, severe burns, traumatic brain injury, and cardiac arrest.
Methods
A literature search was performed to identify studies including critically ill patients who received βB therapy. The PubMed database was searched for studies published between January 1970 and March 2021 using combinations of the search terms beta-blockers, cardiac arrest, critical illness, esmolol, intensive care unit, sepsis, septic shock, severe burns, and traumatic brain injury. Studies reporting on patients managed in the intensive care unit (ICU) setting treated with βB were included. No limits on study designs were made and included prospective, retrospective, observational, or interventional designs. References within original research articles, review articles, editorials, abstracts, meta-analyses, and systematic reviews were screened for inclusion. A summary of the included works outlining sample size, disease state, βB agent used, dosing, timing of initiation, and outcomes can be found in Table 1. Furthermore, a summary of frequently questions regarding βB in critical illness are summarized in Table 2.
Beta-Adrenergic Physiology and Rationale in Critical Illness
To appreciate the potential benefits of βB as a pharmacologicallyy supported intervention inhas several rationales for use during critical illness, conceptualization of β-adrenergic physiology during critical illness is necessary. Alterations in both as the dysregulated signaling molecules and receptorsadrenergic cascade provides intervenable pathways. Complexity further increases as the detrimental adrenergic susceptibilities differ among organ systems, withThese β-adrenergic effects in are pronounced in cardiac and pulmonary tissues most relevant to critically ill patientsduring a critically ill state (Dunser and Hasibeder, 2009). Figure 1 provides a visual representation ofillustrates the physiologic response to β receptor agonism and antagonism, emphasizing the negative effects of β receptor stimulation in critical illness.
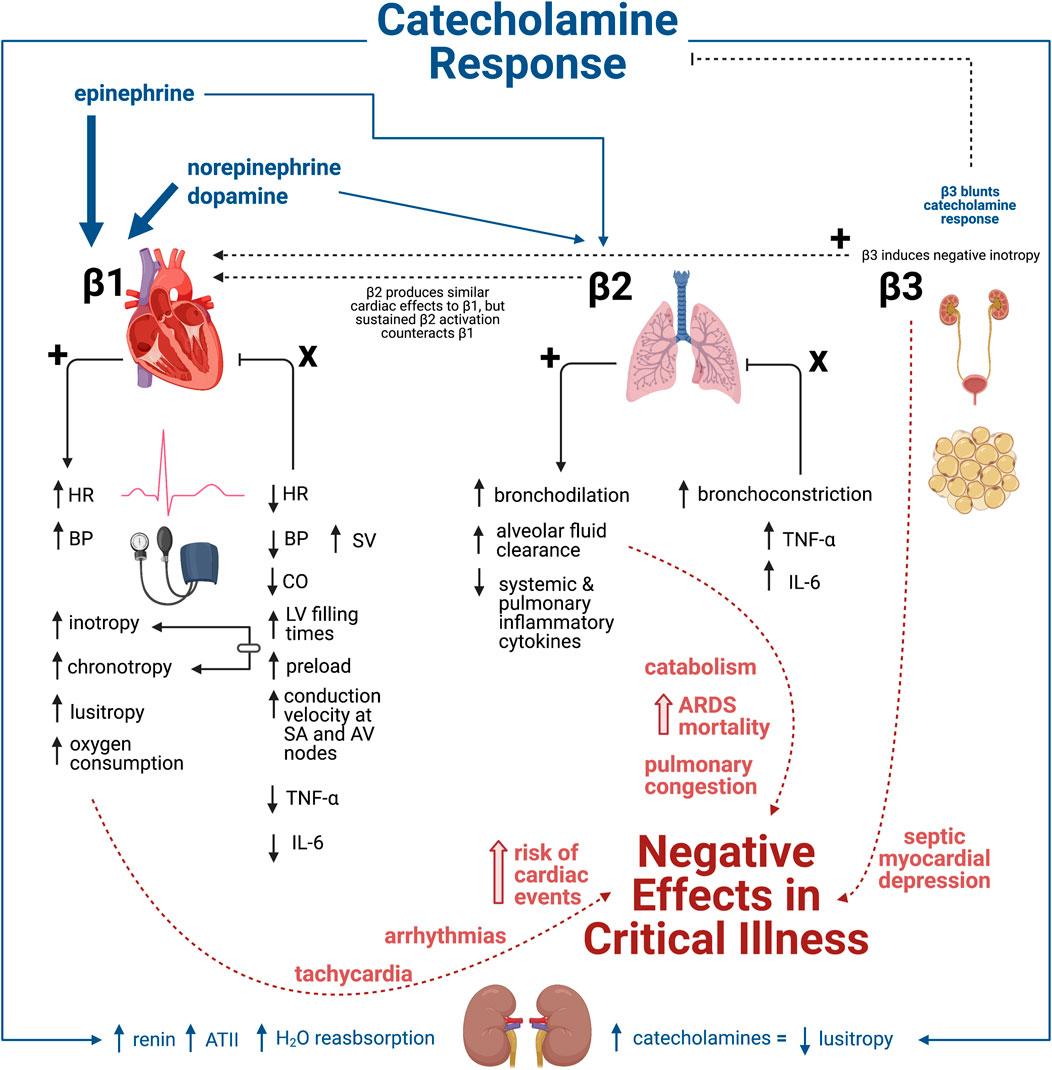
FIGURE 1. Physiologic response to β-adrenergic receptor agonism and antagonism in critical illness. The catecholamine response characterized by epinephrine, norepinephrine, and dopamine release result in stimulation of β1 (majorly) and β2 (minorly). In contrast, β3 agonism blunts the catecholamine response. The physiologic response to β1, β2, and β3 agonism culminates in numerous negative effects within critical illness that can ultimately lead to negative clinical outcomes including increased mortality. This introduces the beneficial physiologic response of βB antagonism as a way to mediate the detrimental effects of the hyperadrenergic state prominent in various types of critical illness. Illustration created with BioRender.com.
Catecholamine Up-Regulation
The principle sSympathetic nervous system (SNS) signaling hormones include the catecholamines norepinephrine, (agonist of α1, α2, β1, and minorly β2), epinephrine (agonist of α1, α2, β1, and β2), and dopamine, which increase during any state of stress (dose-dependent agonist of α1, α2, β1, and minorly β2) (Table 3). (Dunser and Hasibeder, 2009) The stress of Ccritical illness results in massive SNS signaling (Dunser and Hasibeder, 2009). Cardiac arrest and septic shock display profound increases in circulating epinephrine (up to 300 times baseline) and norepinephrine (14 times baseline) (Wortsman et al., 1984; Jones and Romano, 1989). High These higher circulating catecholamine levels are associated with increased mortality. and may potentially be used as an additional factor in predicting mortality These higher circulating catecholamines can predict mortality in the critically ill (Benedict and Rose, 1992; Boldt et al., 1995), but wWhether catecholamine upregulation represents treatable pathophysiology or a necessary compensation inevitably linked to disease severity and poorer outcomes remains debatedshould be targeted remains unclear, as it provides the physiologic adaptation to shock and critically ill states.
Cardiac β Effects
β-adrenergic pathways extensively regulate cardiac function and function and, specifically, hemodynamics due to extensive cardiac expression. β1 comprises 80% of cardiac β-receptors and mediates inotropy, chronotropy, lusitropy (i.e. relaxation rate), and dromotropy (i.e. conduction speed). However, at high concentrations of catecholamines, the lusitropic effect is overwhelmed by tachycardia and increased contractility (Dunser and Hasibeder, 2009; Wachter and Gilbert, 2012). β2 produces similar cardiac effects to β1 (Belletti et al., 2020), but sustained β2 activation leads to a counteracting of β1 effects (Lucia et al., 2018). Moreover, the cardiac-mediated epinephrine response appears independent of functional β2 and is mediated primarily by β1relies on β1 activation (Chruscinski et al., 1999). This Experimental evidence touts β1 activation appearspathways as proapoptotic to cardiac myocytes, while β2 may confer protection (Patterson et al., 2004). Although, interestingly, recent preclinical data in mice demonstrated prevention of cardiac mitochondrial dysfunction via ablation of β2 signaling after burns (El Ayadi et al., 2019). β1 predominates cardiac expression over β2 (4 to 1), but states such as HF can tip the balance nearly even through β1 downregulation by sustained adrenergic stimulation (Bristow et al., 1986). Increased β2 expression may provide benefits through increase contractility and angiogenesis, Research diverges in identifying cardioprotective vs. deleterious roles from the higher proportion of β2 expression as some reports indicate improvements in contractility, angiogenesis, and cardiac remodeling (Rengo et al., 2012). but In contrast, others implicatemay promote β2 as arrhythmiasmogenic (Nguyen et al., 2015). However, commonly used transgenic mice strains overexpressing β2-receptors may represent non-physiologic environments given that HF does not upregulate β2-receptors. This augmented receptor physiology may increase the rate of arrhythmogenicity attributed to β2-receptors in these animal studies (Bristow et al., 1986). Unlike β1 and β2, β3 induces negative inotropy and blunts the catecholamine response (Moniotte et al., 2001), and expression is upregulated in critical illness (Moniotte et al., 2007). IThe increased β3 expression may prime the heart for consequences like septic myocardial depression (Yang et al., 2018). Interestingly, Myagmar et al. recently described the absence of β2 and β3 in cardiac myocytes, while β1 was present in all myocytes. β2 and β3 are primarily in other cell types (e.g., endothelial cells) underscoring the reliance on β1 in cardiac muscle, which raise further concerns regarding the appropriateness of artificial β2 overexpression in cardiac myocytes (Myagmar et al., 2017).
Clinical evidence supporting harmful β-mediated harmful effects has been reported. β1 drives Ttachycardia in critical illness (primarily β1 driven) may increase the, increasing the risk of cardiac events in those with pre-existing heart disease (Sander et al., 2005). Additionally, left ventricular (LV) apical ballooning syndrome (i.e., Takotsubo syndrome) has a links to endogenous adrenergic stimulation (Wittstein et al., 2005) and specifically β-agonism (Hajsadeghi et al., 2018). In sepsis, despite elevated catecholamines, overall β-receptor downregulation contributes to septic myocardial dysfunction (Suzuki et al., 2017). Cumulatively, these cardiac effects support the rationale of study behindfor β1-selective βB (e.g., esmolol) in critical illness, as β2 and β3 are is potentially protective.
Cardiac β-Blockade Effects
Antagonism The antagonism of cardiac β-receptors produces negative inotropic and chronotropic effects asslows conduction velocity through sino-atrial and atrioventricular nodes and produces negative inotropic and chronotropic effects decreases. These effectsThis mechanism may decrease cardiac output and blood pressure, demonstrated by. Experimental studies have shown impairment ined right ventricular function and worsened perfusion with βB when useduse at the onset of septic shock (Coppola et al., 2015). Typically, these effects limit their use in critical illness application; h. However, experimental assessments are often limited by short observation times (several hours) compared to more extended follow-up in clinical studies that would evaluate judicious use of βB after acute hemodynamic stabilization. Preclinical studies have suggested positive effects as beta-blockade with agents selective for β1 agents may reduced tumor necrosis factor alpha (TNF-α) and interleukin 6 (IL-6) in the serum and myocardiumsystemic and cardiac inflammation (Suzuki et al., 2005; Hagiwara et al., 2009). HoweverIn contrast, antagonism of β2 increases TNF-α and IL-6inflammation and may exacerbate the physiologic changes seen inof sepsis (Lang et al., 2008), further supporting β1 selective benefits.
The hemodynamic benefits of βB may improve cardiac function in critical illness through may occur through increased left ventricula LVr filling times by reducing heart rateas heart rate (HR) lowers and there is enhanced ventricular-arterial (V-A) coupling (Mathieu et al., 2016). Patients with septic shock experience a V-A decoupling associated with poor LV function (Guarracino et al., 2014). Morelli et al. demonstrated β1 selective esmolol reduced arterial elastance, and increased stroke volume, reduced with esmolol (a β1 selective βB) heart rate in septic shock reduction, suggesting improved V-A coupling (Morelli et al., 2016). Further, esmolol increased stroke volume (SV) in septic shock despite decreases in cardiac output (CO). Figure 2 describes the hemodynamic effects of sepsis and concomitant βB. βBThese effects are attributable to the reductions in heart rateHR reductions, enhancing end-diastolic filling of the left ventricleLV and thus increasingto increase preload. No differences in oxygenation and tissue perfusion were noted despite decreased CO (Du et al., 2016). Experimentally, esmolol protects myocardial function in sepsis, likely through mitigating apoptotic pathways in the myocardium that are associated with elevated β1 stimulation (Herndon et al., 2001; Wang et al., 2017). Indeed, esmolol added to cardioplegic solutions for cardiac surgery reduced post-surgery troponins suggesting cardiac tissue preservation (Bignami et al., 2017). In acute decompensated HF, continuation of chronic βB appears to prevent death (Prins et al., 2015; Jones et al., 2020). While it may seem logical to stop negative inotropes in patients hospitalized with a failing heart, discontinuation of βB did not significantly affect hemodynamics in these patients (Butler et al., 2006). A meta-analysis of βB effects in septic shock trials supports neutral hemodynamic effects after initial resuscitation despite vasopressor support requirements after initial resuscitation (Lee et al., 2019). Taken together, these The extrapolation of preclinicalpreclinical and clinical data support beneficial, or at least safe, cardiac and hemodynamic effects cardiac βB during critical illness data. to clinical settings support beneficial effects, although clinical studies lack details discerning if these suggested mechanisms are the drivers of clinical benefit.
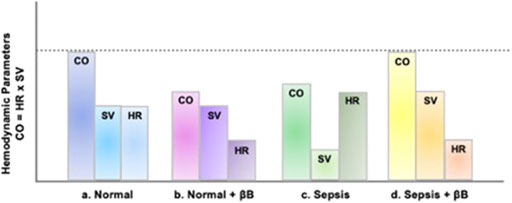
FIGURE 2. Hemodynamic effects of sepsis and β-Blockade. In panel A, the stroke volume (SV) and cardiac output (CO), stroke volume (SV), and heart rate (HR) of a normal, healthy individual are presented. In panel B, due to the negative inotropy associated with βB, which causes reduced HR, the overall CO is reduced despite normal SV. In panel C, sepsis results in tachycardia, due to excessive sympathetic activation. This increase in HR does not allow for adequate ventricular filling causing a decrease in CO secondary to a decrease in SV. In panel D, given that venous return (i.e., preload) is adequate, then βB-induced HR reduction allows for more left ventricular filling time, subsequently decreasing afterload and increasing SV enough to overcome decreased HR and improved CO.
Pulmonary β Effects
The pulmonary vasculature has modest concentrations of β-receptors. Within the lungs, β2 -receptors are the most consequential in the lungs as they outnumber β1 three to one in most pulmonary tissues and are the exclusive β-receptor present on pulmonary vascular smooth muscle (Carstairs et al., 1985). β2-receptors in the epithelium contribute to alveolar fluid clearance, while those in smooth muscle promote bronchodilation (Mutlu and Factor, 2008). β1-receptors present onof the alveolar wall and submucosal glands (Carstairs et al., 1985) and contributes to alveolar fluid clearance (Sakuma et al., 2001), although not to the extent of β2 (Mutlu et al., 2004).
Adrenergic overstimulation has several pulmonary effects germane to critical illness concerns, including pulmonary edema and elevated pulmonary pressures with right heart dysfunction, most notably through α-receptor-mediated vasoconstriction (Dunser and Hasibeder, 2009). α-receptor-mediated vasoconstriction increases the displaced blood volume into the pulmonary circulation, increasing congestion and capillary wall stress. Pressure increase and fluid retention readily shift fluid into the pulmonary interstitium and the alveoli, especially when inflammation disrupts the capillary barrier. Although less influential than α stimulation, β1 stimulation can augment right ventricular output, further increasing pulmonary blood volume and pulmonary capillary pressures (Rassler, 2012). However, β2-agonism is often associated with improvements in mechanisms thatmay mitigate prevent edema throughsuch as alveolar fluid clearance (Maron et al., 2009). β2-agonism may produce other protective pulmonary effects such as reductions in systemic and pulmonary inflammatory cytokines (Maris et al., 2005; Bosmann et al., 2012) and prevent capillary permeability (de Prost et al., 2008). Clinical trials failed to translate pre-clinical evidence into positive outcomes as β2-agonism increased acute respiratory distress syndrome mortalitylinical trials in critical illness have failed to show positive outcomes as β2-agonism increased mortality from acute respiratory distress syndrome (Gao Smith et al., 2012). The lack of benefit may occur secondary to dysfunctional β2-receptors during prolonged inflammatory states (Giembycz and Newton, 2006; Belletti et al., 2020). Notably, β2-mediated vasodilation may detrimentally affect blood shunting in cardiopulmonary resuscitation leading to the distribution of blood from well to unventilated alveoli (Thrush et al., 1997). The mode of critical illness likely influences the degree of pulmonary pathophysiology with β-receptor stimulation, with insufficient evidence to malignCurrently, insufficient evidence exists to support a role for β2 stimulation as helpful or harmful in critical illness and possible harm in long-term pulmonary dysfunction like acute respiratory distress syndrome.
Pulmonary β-Blockade Effects
βB provides a potential strategy to improve pulmonary adrenergic response. Prescribing of βB typically warrants caution in pulmonary pathologies, most notably chronic obstructive pulmonary disease (COPD) and asthma, as βB can reverse the benefits of β2-mediated bronchodilation, although cardioselectivity β1 blocking agents eliminates some concern (MacNee, 2019). Nevertheless, Aa recent large clinical trial determined metoprolol use in COPD patients without cardiac indications for a βB resulted in increased exacerbations (Dransfield et al., 2019). However, in critically ill patients with acute respiratory failure and COPD, β1-selective βB did not affect ICU length of stay (Kargin et al., 2014). Additionally, continuing cardioselective βB for patients with underlying cardiac indications hospitalized for COPD exacerbations appears safe (Stefan et al., 2012). In asthma, several clinical and database studies have suggested that βB use does not worsen airway hyperresponsiveness or asthma exacerbations (Short et al., 2013; Morales et al., 2017). A network meta-analysis of 24 clinical trials concluded that non-selective βB (specifically oral timolol and propranolol infusions) were associated with a higher incidence of asthma attacks than β1-selective βB. MoreoverAdditionally, antecedent cardioselective βB therapy has been associated with lower mortality in ICU patients with acute respiratory failure, and βB withdrawal may worsen mortality (Noveanu et al., 2010). In a retrospective assessment of βB commencement after about 7 days of ICU admission, no alterations in pulmonary function occurred (Van Herpen et al., 2019). Given the current evidence, compelling cardiac indications (e.g., atrial fibrillation, ischemic heart disease) should drive βB use in critically ill patients, and COPD and asthma should not restrict βB use. given the current evidence.
Some preclinical evidence suggests possible protective mechanisms of βB germane to critical illness. Maccari et al. reported various selective and non-selective βB prevented catecholamine-induced β2 downregulation in-vitro (Maccari et al., 2020). Other pre-clinical studies demonstrate βB lung-protective effects in sepsis-induced acute lung injury. The ultra-rapid βB, landiolol, suppressed lung injury and reduced lung injury associated protein, high-mobility group box 1 (HMGB-1), in a rat lipopolysaccharide sepsis model (Hagiwara et al., 2009). The mechanism of pulmonary benefit of βB in these settings remains a conjecture, although when applied in clinical settings, the effects do not appear detrimental to pulmonary physiology.
Disease-specific Evidence for β-Blockade
Sepsis
Dysregulated inflammatory response and catecholamine upregulation affect nearly every organ system in sepsis. Two specific derangements include hemodynamic compromise and metabolic alterations, which may open a role for βB (Plank et al., 1998; O'Dwyer et al., 2006; Furian et al., 2012). Sepsis leads to elevated serum pro-inflammatory cytokines (e.g.,TNF-α, IL-1β, and IL-6). Cytokine up-regulation has multiple deleterious effects, possibly mitigated by βB (Cain et al., 1999; Hsueh and Law, 2003). Indeed, βB have been proposed to reduce sepsis-induced cardiac dysfunction, improve the sepsis-induced hypermetabolic state, and play a role in immunomodulation by preventing lymphocyte apoptosis prevalent within inflammatory mechanisms of sepsis (Suzuki et al., 2017). Additionally, sepsis precipitates tachycardia, which reduces filling time and increases the risk of arrhythmias, potentially exacerbating the poor hemodynamics of impaired systemic vascular resistance (SVR) (Jacobi, 2002; Suzuki et al., 2017). Heart rate reduction via β1 blockade in the setting of adequate preload can decrease myocardial oxygen consumption, increase diastolic filling time, and increase coronary perfusion time, all potentially reducing the risk of myocardial ischemia and improvement in end-organ perfusion. β1 blockade may result in hemodynamically significant hypotension in patients without adequate preload and should therefore be used cautiously or avoided. The 2016 Surviving Sepsis Guidelines do not make recommendations regarding βB continuation or initiation in septic patients (Rhodes et al., 2017).
The hemodynamic improving effects of acute βB use in sepsis remain controversial; however, case series and small retrospective and prospective studies have established a plausible safety profile. As early as 1972, a case series in refractory septic shock patients documented the hemodynamic effects of propranolol (Berk et al., 1972). The cases conceptualized hyperdynamic vs. hypodynamic shock, given the observation that three patients dying after propranolol use had severely reduced CO compared to those who survived. Analysis of hemodynamic parameters continued in retrospective reviews of septic patients; however, unlike Berk et al., a study conducted by Schmittinger et al (Schmittinger et al., 2008) found no change in cardiac index (CI) following milrinone infusion with enteral metoprolol initiated after hemodynamic stabilization. HR control (65–95 bpm) was achieved in 39 out of 40 patients in addition to a significant increase in stroke volume index (SVI) (p = 0.002), and central venous pressure (CVP) along with dosages of norepinephrine, vasopressin, and milrinone all decreased (p < 0.001). Other small retrospective studies of βB in sepsis have not shown increases in mortality through acute βB use (Gutierrez et al., 2009).
Subsequent small prospective observational studies continued to analyze hemodynamic parameters following βB, specifically esmolol, in sepsis. Some studies demonstrated significantly decreased CO proportional to the decreases in HR (Gore and Wolfe, 2006) while others showed unchanged CO (Morelli et al., 2016) or insignificant reductions in CO (Balik et al., 2012). A more consistent trend was seen with regard to SV with evidence of significant (Du et al., 2016; Morelli et al., 2016) or negligible increases documented (Balik et al., 2012). In a subgroup analysis, Du et al. demonstrated that in patients with increased SV, esmolol therapy had a lower risk for decreased CO (Du et al., 2016). Similarly to Schmittinger et al. (Schmittinger et al., 2008), Morelli et al.(Morelli et al., 2016) observed reduced norepinephrine requirements after esmolol, although not all studies uniformly observed this difference (Balik et al., 2012). Measures of tissue perfusion, including lactate levels, were conflicting amongst studies, with some showing significant decreases in the esmolol group (Du et al., 2016) while others had more substantial reductions in the control group (Shang et al., 2016). The prospective studies did not analyze the risk or incidence of mortality associated with esmolol therapy, but Shang et al.(Shang et al., 2016) concluded a significantly shorter mechanical ventilation duration with esmolol compared to control (p < 0.05). Concerning the timing for the initiation of esmolol, these prospective studies were relatively consistent by attempting to correct preload through fluid resuscitation before administration of an esmolol loading dose. Nevertheless, timing, thresholds, and parameters for hemodynamic stabilization varied. These retrospective and prospective data are collectively limited by small sample sizes and lack relevant clinical outcomes, establishing impetus for larger randomized trials.
The seminal Morelli et al. (Morelli et al., 2013) phase 2 study of esmolol in septic shock patients requiring high-dose vasopressors revived discussion of βB in sepsis. Esmolol achieved the target HR (80–94 bpm) in all patients (−28 bpm; IQR = −37 to −21) compared to standard of care (−6 bpm; 95% CI = −14 to 0) and resulted in a mean reduction of 18 bpm (p < 0.001). The esmolol group exhibited improvements in SV and left ventricularLV stroke work index and decreases in norepinephrine and fluid requirements. Esmolol also improved pH, base excess, and arterial lactate. Several other randomized controlled trials (RCTs) evaluated βB in sepsis (Orbegozo Cortes et al., 2014; Yang et al., 2014; Wang et al., 2015; Xinqiang et al., 2015; Wang et al., 2017; Liu et al., 2019; Kakihana et al., 2020). Cumulatively, these trials have recently been assessed in systematic reviews and meta-analyses.
Chacko et al. evaluated 9 studies in a systematic review and found benefit from most studies with regards to heart rate control, decreased mortality, and acid-base parameters although strength of evidence is limited due to heterogeneity and inclusion of only one RCT (Chacko and Gopal, 2015). Sanfilippo et al. (Sanfilippo et al., 2015) was the next systematic review published that included two RCTs with the additional evidence from Yang et al. (Yang et al., 2014) At this time, the sizeable differences in sample size and trial design did not allow for a meta-analysis, but this systematic review further affirmed that βB use in septic and septic shock patients conferred decreased HR without significant adverse effects (Sanfilippo et al., 2015). The previous systematic reviews include a range of trial designs, but a meta-analysis conducted in 2018 evaluated the use of esmolol on septic shock and sepsis from five RCTs (Liu et al., 2018). The three trials that reported survival rate showed that esmolol use when compared to control was associated with a significantly increased rate of survival (RR = 2.06; 95% CI = 1.52–2.79; p = 0.006). With regard to hemodynamics, esmolol use showed no influence on MAP, CVP, or central venous oxygen saturation (ScVO2) but did reduce HR and cardiac biomarker troponin I. In 2019, Lee et al. (Lee et al., 2019) published a systematic review of 14 studies which included 5 RCTs, although only three of these RCTs were the same as those included in the Liu et al. meta-analysis. Six of the studies assessed βB use and mortality, which despite possible publication bias, demonstrated average odds ratio of 0.4072 (95% CI = 0.2602–0.6373) in favor of βB use.
Since the publication of these systematic reviews and meta-analyses there has been an increased focus on the treatment of tachyarrhythmias in sepsis. Initial evidence garnering support for βB use in septic patients with atrial fibrillation resulted from a 2016 propensity-matched cohort study. This analysis concluded that βB were associated with lower hospital mortality when compared to calcium channel blockers (CCBs), digoxin, and amiodarone (Walkey et al., 2016). With regard to rate control, Bosch et al. found that in comparison to CCBs, amiodarone, and digoxin, βB improved HR control to <110 bpm at 1 hour for the treatment of sepsis-associated atrial fibrillation, although this effect did not persist to show meaningful difference at 6 h (Bosch et al., 2020). While these studies included a variety of βB agents, newer evidence has shifted to solely focus on the use of ultra-short-acting βB, esmolol and landiolol. Of note, landiolol is not available for use in the United States. Kakihana et al. (Kakihana et al., 2020) analyzed the safety and efficacy of landiolol in a multicenter, open-label RCT in Japan that showed significant improvements in HR control and decreased incidence of new-onset arrhythmias. This trial specifically focused on a patient population with HR ≥ 100 bpm maintained for at least 10 min without a change in catecholamine dose and with a diagnosis of atrial fibrillation, atrial flutter, or sinus tachycardia. The most common adverse effect was hypotension, which quickly resolved in all instances given the ultra-short-acting nature of the drug. Hasegawa et al. performed a systematic review and meta-analysis of seven RCTs associated with esmolol and landiolol use in patients with persistent tachycardia (defined as HR > 95 bpm) despite initial resuscitation.(Hasegawa et al., 2021). Six of the RCTs included reported 28-days mortality. The use of ultra-short-acting βB in this patient population of 572 patients was found to be associated with significantly lower 28-days mortality (RR = 0.68; 95% CI = 0.54–0.85; p < 0.001) with an absolute risk reduction of 18.2% conferring a number needed to treat of 6 to prevent one patient death.
The use of beta-blockade in septic patients remains controversial especially with regard to timing of initiation. Tachycardia in the early stages of un-resuscitated sepsis is a major compensatory mechanism to ensure cardiac output, oxygen delivery, and perfusion. The use of beta-blockade, specifically with esmolol and landiolol, has been shown to reduce heart rate in the septic patients without deleterious effects on end-organ perfusion and may be associated with improved survival rates. Despite some dosing and timing variation within the RCTs that have been conducted, there is a general consensus that βB should not be initiated until at least 6 h, and in some trials 24 h, after the initial fluid resuscitation and vasopressor use. With this in mind, βB therapy may be initiated while patients are still requiring vasopressors. In fact, many studies described potential for decreased norepinephrine requirements with βB, instigating hypotheses of βB as vasopressor-sparing with potential to avoid deleterious effects of prolonged, high catecholamine requirements. Therefore, use of esmolol should be based on patient specific factors and likely should be considered only after initial resuscitation and once hemodynamic stabilization with vasopressors is achieved. Without large randomized controlled trials evaluating and elucidating the optimal dosing regimen and initiation timing considerations, the cost of esmolol infusion course should be considered as many hospital formularies restrict its use due to the extensive significant cost of the drug.
There are numerous retrospective studies that have investigated premorbid βB exposure prior to admission to the ICU with a diagnosis for sepsis that have conferred mortality benefit. Macchia et al. performed a retrospective observational study in 9,465 septic patients that concluded lower 28-days mortality in patients previously prescribed βB (Macchia et al., 2012). As part of a national cohort of Medicare beneficiaries, Singer et al. determined outpatient βB prescription was associated with a significantly reduced in-hospital and 30-days mortality, with no difference in regards to cardioselective compared to non-selective βB (Singer et al., 2017). In contrast, a recent observational cohort study by Guz et al. found that antecedent cardioselective βB were associated with a stronger protective effect on 30-days mortality rate reduction than noncardioselective βB for patients admitted with sepsis (Guz et al., 2021). Based on additional subgroup analyses according to tachycardia stratification, both patients with absolute and relative tachycardia on presentation exhibited reduced 30-days mortality rates with βB use.
Beyond initiation of βB in sepsis or premorbid βB use, continuation of chronic βB in patients admitted with sepsis and septic shock remains controversial, with common practice being to discontinue anti-hypertensive therapy upon admission. A prospective, observational study evaluated 296 patients admitted with severe sepsis or septic shock who were on chronic beta-blocker therapy (Fuchs et al., 2017). Chronic beta-blocker therapy was continued in 167 patients and was associated with significant decreases in hospital, 28-days, and 90-days mortality (p < 0.05) compared to βB cessation. Continuation of beta-blockade therapy was also associated with decreased crystalloid requirements during the first 24 h (p = 0.049) without increases in need for vasopressor, inotropic support, or low-dose steroids. To build on these results, a systematic review including a total of nine studies and over 6,500 patients found that premorbid beta-blocker exposure, regardless of continuation, in patients with sepsis was associated with reduced mortality (Tan et al., 2019). Although the precise mechanism of benefit in these settings is unknown, potential explanations beyond the mechanisms mentioned previously in this section include the prevention of rebound effects of tachycardia, hypertension, and arrhythmias caused by abrupt βB withdrawal.
In summary, Tthe hemodynamic evidence for βB use in sepsis has been proven as there are numerous studies demonstrating decreased HR without significant change in MAP, CVP, or ScVO2. Further, the recent evidence for ultra-short-acting βB, esmolol and landiolol, especially with regard to decreased incidence of arrhythmias and 28-days mortality benefit is clinically significant. In fact, some are realizing a need to stratify subgroups within septic cohorts based on the potential benefit of cardiovascular intervention to decrease the negative consequences of tachyarrhythmias (Morelli et al., 2020). The inconsistencies in terms of dosing and timing of initiation within the existing evidence require subsequent investigation in robust randomized controlled trials. Overall, esmolol was the most studied βB in sepsis, and initial doses varied over a wide range of either weight based dosing (most commonly 0.05 mg/kg/min) or standard dosing (most commonly 25 mg/h) with doses titrated to heart rate reductions of 20% or a heart rate goal of 70–100 bpm. Additionally, there is ample evidence to show antecedent βB use confers mortality benefit, but there is only one RCT evaluating the continuation of chronic βB therapy in acute sepsis, which warrants supplementation.
Burns
Severe burns lead to catecholamine release and a hypermetabolic state characterized by increased cardiac output, increased energy requirements, muscle breakdown, and general catabolism (e.g., reduced bone density, etc) (Wilmore et al., 1974; Herndon et al., 2001). This response lasts for at least 9 months and up to 2 years and is associated with a hypercatabolic state leading to muscle and bone loss (Hart et al., 2000; Przkora et al., 2006). β1 receptor mediated lipolysis and agonism of β2 receptors can cause glycogenolysis and gluconeogenesis within hepatocytes due to catecholamine stimulation (Novotny et al., 2009). Hypermetabolism can negatively impact the function of skeletal muscle, skin, and the immune system, ultimately resulting in multiorgan dysfunction and even death (Núñez-Villaveirán et al., 2015).
As such, βB are an attractive intervention to prevent the hyperadrenergic cascade that follows burn injury. Preclinical animal studies examining propranolol to improve wound healing following burn injury have noted enhanced wound healing and reduced activity of local inflammatory pathways (Romana-Souza et al., 2008; Zhang et al., 2009). Nearly all studies investigating βB in burn injuries have been conducted in pediatric patients using propranolol (Núñez-Villaveirán et al., 2015). Propranolol has been associated with a decrease in HR and oxygen consumption and the reversal of catabolism, evidenced by significant reductions in resting energy expenditure (REE) and prevention of lean body mass loss (Herndon et al., 2001; Jeschke et al., 2007; Williams et al., 2011; Herndon et al., 2012).
Baron et al. (Baron et al., 1997) deemed propranolol use safe and effective for ≥10 days following severe burns (≥40% of total body surface area [TBSA]) in 22 children aged 1–10 years old. In this population, propranolol use demonstrated significantly decreased HR and rate pressure product (RPP), defined as MAP multiplied by HR, without adverse effects. Herndon et al. (Herndon et al., 2001) extended this time frame to at least 2 weeks by evaluating propranolol in 25 pediatric burn patients (>40% of TBSA). Propranolol showed successful attenuation of the hypermetabolic response by decreasing REE, oxygen consumption, and muscle catabolism. Additionally, lean mass loss at 2 weeks was prevented by propranolol (9% loss vs 1% loss; p = 0.003). Similarly, Jeschke et al. (Jeschke et al., 2007) found improvements in REE with propranolol in 245 severely burned children. In a separate trial, Herndon et al. (Herndon et al., 2012) investigated propranolol given within 96 h from admission and continued for a year compared to control in 179 pediatric burn patients with burns >30% of TBSA. While there was no significant difference in mortality (p = 0.72), propranolol use did result in reduced cardiac work and improved lean body mass and bone density without adverse events. In patients receiving propranolol, the percent of predicted HR was significantly lower and persisted up to a year postburn; however, significant reductions in REE and RPP were only sustained through 6 months, while no difference was seen at 1 year.
A large clinical trial evaluated propranolol’s effects on cardiac function when started 24–72 h after admission versus control in 406 children with burns >30% of TBSA (Williams et al., 2011). CO decreased after 2 weeks of starting propranolol and the reduction continued throughout the trial. SV, when compared to non-burned children of the same age, was higher in patients receiving propranolol versus control (112 ± 8% vs. 94 ± 5%; p < 0.02), likely a function of the reduced HR allowing for longer ventricular filling times. RPP decreased in the group receiving propranolol, indicating lower myocardial oxygen consumption. These results suggest that propranolol has a significant hemodynamic impact on pediatric burn patients.
Data for βB use in burned adults are limited, but the available evidence supports conclusions comparable to these pediatric studies. Arbabi et al. (Arbabi et al., 2004) compared three cohorts: preexisting βB use continued during hospitalization, new hospital βB use, and no βB use in adult burn patients. Unlike the pediatric studies, βB selection varied with most receiving metoprolol, atenolol, and esmolol, and few receiving propranolol. Preexisting βB was associated with a significantly lower rate of mortality than βB initiation during hospitalization and no βB (5 vs. 27% and 13%, respectively). The higher mortality rate in the hospital-initiated βB group may be due to the presence of tachyarrhythmias treated with βB and more severe underlying disease, which was supported by prolonged ICU and hospital stays in the group. Preexisting βB was associated with a shorter mean healing time of 21 days when compared to control (p = 0.02). These data suggest antecedent βB use may confer a lower risk of mortality and accelerated healing time, which complements the data for improved outcomes in other adrenergic stress states like sepsis.
In 2009, Mohammadi et al. (Mohammadi et al., 2009) randomized 79 adult burn patients to propranolol or control and assessed wound healing dynamics. Patients receiving propranolol had more rapid healing times and reductions in required graft size (13.75 vs 18.75%; p = 0.006) in addition to shorter hospital length of stay (24.41 vs 30.95 days; p = 0.05). To build on these results, Ali et al. (Ali et al., 2015) evaluated the effect of propranolol on wound healing and blood loss in a cohort of 69 adult burn patients. Patients receiving propranolol initiated within 48 h of admission had a shorter recovery time with an average of 10 ± 5 days in between skin grafting procedures, whereas patients in the control group had an average of 17 ± 12 days in between procedures (p = 0.02). When hematocrit levels were drawn perioperatively, patients receiving propranolol showed a 5–7% increase compared to control (p = 0.002). Notably, the propranolol patients required larger grafts, but no differences in blood transfusions were observed, thus concluding the utility of propranolol for diminishing blood loss during skin graft procedures and improving wound healing. Further investigation in a recent Pakistani clinical trial of propranolol in 70 patients started day three post-burn demonstrated similar reductions in healing time (about a 1 week reduction) and time to graft readiness (10 days reduction) (Cheema et al., 2020). Propranolol also resulted in shorter hospitalization (26.7 vs. 33.6 days; p < 0.001).
Overall, the evidence suggest βB are effective in improving burn recovery in both pediatric and adult patients. By mitigating the adrenergic response at early time points after burns, βB can lessen the negative effects of the hyperadrenergic burn state. The 2012 American Burn Association (ABA) Burn Quality Consensus Conference Summary agreed that βB use is beneficial in pediatric and adult burn patients but recommended further research due to the lack of level one evidence at that time (Gibran et al., 2013). The International Society for Burn Injuries (ISBI) released the Practice Guidelines for Burn Care, Part 2 in 2018 with a recommendation to use a nonselective βB in burn patients ≤18 years old with the goal of reducing HR to 75% of the admission HR (Allorto et al., 2018). Since the publication of the ISBI guidelines in 2018, there has been no new evidence in pediatric burn patients; however, the Cheema et al. trial in Pakistan provides additional, robust evidence in adult burn patients which may lead to increased guidance in this population. While these guideline statements do make recommendations for βB use and monitoring including HR and weight loss, they do not specify timing or dosing. Based on the studies evaluated, propranolol initiated within one to 3 days after burn injury has the strongest evidence in both children and adults.
Overall, the evidence suggest βB are effective in improving burn recovery in both pediatric and adult patients. By mitigating the adrenergic response at early time points after burns, βB can lessen the negative effects of the hyperadrenergic burn state. Dosing evaluated in these studies with the strongest evidence in both children and adults was propranolol 1–3 mg/kg/day within one to 3 days after burn injury and titrated based on hemodynamic effects. Adults maintained on another βB agent may be better served continuing their current βB instead of switching to propranolol; however, no evidence has addressed head-to-head comparisons of βB providing an area for future research.
Traumatic Brain Injury
Following traumatic brain injury (TBI), a systemic hyperadrenergic state develops characterized by adrenal release of catecholamines and sympathetic activation (Clifton et al., 1981). The surge in catecholamine levels causes vasoconstriction, worsened cerebral ischemia, increased intracranial pressure, all leading to ongoing secondary injury to brain tissue (Lazaridis, 2017; Rizoli et al., 2017). βB can theoretically inhibit the catecholamine interaction with beta adrenergic receptors thus obstructing the detrimental sympathetic nervous system hyperactivity associated with severe TBI. Benefit may also be seen from βB by decreasing the cerebral oxygen demand, thus improving relative ischemia (Clifton et al., 1981).
Substantial pre-clinical evidence has collectively found that βB reduce cerebral ischemia and increase cerebral perfusion following traumatic insult (Goyagi et al., 2006; Ley et al., 2009; Goyagi et al., 2010; Iwata et al., 2010; Ley et al., 2010; Umehara et al., 2010; Goyagi et al., 2012; Song et al., 2014). Neurological deficit scores and infarct volumes were decreased in rats or mice that were treated with βB. Differences in the route of administration, agent chosen, dose, and timing varied but globally use of βB appears to confer benefit. Propranolol (Goyagi et al., 2006; Ley et al., 2009; Iwata et al., 2010; Ley et al., 2010), esmolol (Goyagi et al., 2006; Goyagi et al., 2010; Iwata et al., 2010; Umehara et al., 2010; Goyagi et al., 2012), landiolol (Goyagi et al., 2006; Goyagi et al., 2010; Iwata et al., 2010; Umehara et al., 2010; Goyagi et al., 2012), carvedilol (Goyagi et al., 2006), and betaxalol (Song et al., 2014) have all been investigated. Goyagi et al. (Goyagi et al., 2006) found no difference between intravascular versus intrathecal administration, Song et al. (Song et al., 2014) only investigated intraventricular administration, and all other studies used intravascular administration. Iwata et al. was the only study to indicate medication preference where esmolol and landiolol showed superior neuroprotection compared to propranolol in postischemic treatment. (Iwata et al., 2010). Higher doses of propranolol (4 mg/kg) were preferred to lower doses (1 mg/kg) (Ley et al., 2010), while no difference was observed amongst varying doses of esmolol and landiolol (Goyagi et al., 2012). Conflicting evidence exists for the timing of βB administration, where Ley et al. (Ley et al., 2010) observed that initiation of βB treatment pre-TBI was equivalent to post-TBI while Iwata (Iwata et al., 2010) found only post-TBI initiation benefit.
To date, only one RCT regarding beta-blocker use in TBI has been conducted by Cruickshank et al. (Cruickshank et al., 1987) Secondary to unclear randomization and allocation concealment method in addition to incomplete outcome data reported, the trial has largely been discounted due to a high risk of bias; however, it did show a positive correlation between arterial noradrenaline concentration and cardiac damage. (Cruickshank et al., 1987) (Alali et al., 2017) Additionally, fewer βB-group patients experienced supraventricular tachycardia (6 vs. 28; p < 0.0001) and ST-segment and T-wave changes (15 vs. 26; p = 0.062). βB use also inhibited further increases in myocardial isoenzyme of creatine kinase (CKMB) and abolished focal myocardial necrotic lesions compared to placebo. The remainder of the clinical evidence regarding βB use in TBI is from one multi-institutional, prospective, observational study and nine observational cohort studies, but overwhelmingly, this evidence concludes a mortality benefit for use of βB in TBI (Alali et al., 2017; Chen et al., 2017).
Within the nine retrospective cohort studies conducted, eight analyzed a primary outcome of in-hospital mortality (Arbabi et al., 2007; Cotton et al., 2007; Inaba et al., 2008; Schroeppel et al., 2010; Schroeppel et al., 2014; Mohseni et al., 2015; Ko et al., 2016; Murry et al., 2016; Zangbar et al., 2016). After adjustments, βB use after TBI was associated with statistically significant lower mortality in seven out of the eight studies with primary outcomes of in-hospital mortality (Arbabi et al., 2007; Cotton et al., 2007; Inaba et al., 2008; Schroeppel et al., 2010; Mohseni et al., 2015; Ko et al., 2016; Zangbar et al., 2016). Schroeppel et al. (Schroeppel et al., 2014) showed similar adjusted odds of mortality amongst all subjects, but subgroup analysis revealed lower odds of mortality in patients who received propranolol. The βB cohorts typically were comprised of older subjects (Arbabi et al., 2007; Inaba et al., 2008; Schroeppel et al., 2010; Mohseni et al., 2015) with more severe head injuries (Arbabi et al., 2007; Inaba et al., 2008; Schroeppel et al., 2010; Mohseni et al., 2015) as indicated by lower GCS levels (Arbabi et al., 2007; Ko et al., 2016) and therefore investigators adjusted for potential confounding factors. In-hospital mortality was a secondary outcome in Murry et al. (Murry et al., 2016) where no difference was observed, although no adjustments were made. A meta-analysis of all nine cohort studies, which included 8,245 patients, revealed a statistically significant mortality reduction when patients were exposed to beta-blockers after TBI (pooled OR = 0.39; p < 0.00001) (Alali et al., 2017). In all of the cohort studies, βB were initiated during hospital stay after the TBI had occurred and continued for varied durations. Two of the more recent studies from 2016 made an effort to administer propranolol earlier in the time course (within twelve (Murry et al., 2016) or twenty-four (Ko et al., 2016) hours of admission). Various βB were used amongst the studies with no preference cited between agents except in the aforementioned Schroeppel et al. study where propranolol reduced mortality compared to atenolol, carvedilol, esmolol, labetalol, metoprolol, and sotalol (Schroeppel et al., 2014). In 2017, based on a meta-analysis of these observational cohort studies, the Eastern Association of Surgery and Trauma (EAST), made a conditional recommendation for in-hospital use of βB in adults admitted to the ICU with severe, acute TBI and no contraindications to βB (Alali et al., 2017). The recommendation requires that hypotension (systolic blood pressure [SBP] < 90 mmHg) and symptomatic bradycardia (HR < 50 bpm) are avoided, but there is no formal recommendation on when to initiate βB, which βB to use, and how to titrate the βB therapy (Alali et al., 2017). In general, hypotension should warrant βB discontinuation or dose reduction.
In 2018, to build on the optimistic findings of these small single-center trials, the American Association for the Surgery of Trauma (AAST) conducted a multi-institutional, prospective observational trial (Ley et al., 2018). After analysis of 2,252 patients, the trial concluded that patients who received βB after TBI had a significantly lower adjusted (adjusted OR = 0.35; p < 0.001) and unadjusted mortality rate (13.8 vs. 17.7%; p = 0.013) in congruence with the 2017 EAST guideline recommendation. Further investigation revealed a reduction in mortality associated with propranolol use compared to all other βB (9.3 vs. 15.9%; p = 0.003). This study revealed no difference in neurological outcomes associated with βB use and patients who received propranolol had increased length of stay despite the aforementioned survival advantage.
NCT02957331, a randomized, open-label interventional trial, released study results on June 4, 2020 investigating the use of propranolol after TBI (Rizoli et al., 2017). The results show a difference of 7.7% propranolol arm versus 33.33% non-propranolol arm for all-cause 30-days mortality, although no analysis has been published. Propranolol was dosed to target a HR < 100 bpm and was held if the patient became hypotensive (SBP <100 mmHg) or bradycardic (HR < 60 bpm). The DASH After TBI trial (NCT01322048) is an ongoing, randomized, double-blind trial comparing propranolol and clonidine use to placebo (Patel et al., 2012). The primary outcome is ventilator-free days supplemented by multiple secondary outcomes, including all-cause mortality and neuropsychological outcomes. Interim data demonstrates decreased ventilator-free days and decreased percentage of all-cause mortality associated with adrenergic blockade (propranolol and clonidine) (Ley et al., 2009). No neuropsychological outcomes have been reported at this time. Only one propensity-matched case control study has addressed neuropsychological outcomes thus far, where beta-blockade was associated with shorter length of hospital stay and reduced risk of poor long-term functional outcome (Ahl et al., 2017).
In summary, βB use after TBI has been associated with decreased in-hospital mortality in one multi-institutional, prospective, observational trial, and nine retrospective cohort studies. Only one RCT has been conducted where mortality was not investigated; however, existing evidence supports the most recent 2017 EAST guideline recommendations of using βB following TBI. Studies evaluated a variety of both selective and nonselective βB in patients with a TBI; however, dosing was not reported in a majority of cases. Continued investigation in more robust trial designs may aid with clarification of preferred agent, dosing, titration, timing for initiation.
Cardiac Arrest
Epinephrine is part of the algorithm to treat pulseless ventricular tachycardia (VT) and ventricular fibrillation (VF) (Panchal et al., 2020); however, epinephrine, itself a catecholamine, can increase oxygen requirement of an already strained heart and may potentiate VF risk (Monroe and French, 1960). Thus, in addition to endogenous catecholamine release that can occur during ischemia, the cycle of catecholamine administration during VF may lead to refractory VF (RVF) or electrical storm (Nademanee et al., 2000; Lee et al., 2016). βB have been hypothesized to improve outcomes in VF by inhibiting elevated catecholamine levels to decrease myocardial oxygen demand and lower the threshold for VF (Lee et al., 2016). Animal studies have shown that βB have improved rates of resuscitation when used in cardiac arrest (Ditchey et al., 1994; Cammarata et al., 2004; Huang et al., 2004; Killingsworth et al., 2004). Several small trials evaluated the use of βB in refractory VF and electrical storm treatment and concluded that their use increases the rates of ROSC and overall survival (Nademanee et al., 2000; Skrifvars et al., 2003; Driver et al., 2014; Lee et al., 2016).
A small study evaluated the use of esmolol versus control on the incidence of sustained ROSC in patients with RVF (Lee et al., 2016). Sustained ROSC was greater in patients who received esmolol compared with those in the control group (56 vs. 16%; p = 0.007). Although there were significantly more patients who received esmolol that survived to ICU admission, survival and neurological outcome at 30 days, 3 months, and 6 months was not significant (p = 0.36). Similarly, Driver et al. (Driver et al., 2014) assessed the outcomes of 6 patients receiving esmolol versus 19 control patients who had RVF that started as VT or VF either outside of the hospital or in the emergency department. Patients in the esmolol group had greater incidence of sustained ROSC (67 vs. 32%) and survival to ICU admission (66 vs. 32%). Differing from the previous trial, patients receiving esmolol in this study had increased frequencies of survival to hospital discharge (50 vs. 16%) and discharge with fair neurologic outcome (50 vs. 11%), although these results were not statistically significant due to small sample size.
Nademanee et al. (Nademanee et al., 2000) studied the effects of sympathetic blockade in 27 patients vs. anti-arrhythmic agents in 22 patients with electrical storm. These patients had a myocardial infarction between 72 h and 3 months prior to developing electrical storm. Patients in the sympathetic blockade group received either propranolol, esmolol, or left stellate ganglionic blockade (LSGB). Because patients receiving beta blockers were analyzed in a combined group with those receiving LSGB, this limits our interpretation of the statistical analyses from the trial. No subgroup analysis of βB use alone was presented. Patients in the control group received lidocaine, procainamide, and/or bretylium tosylate as the anti-arrhythmic agent. Patients receiving a sympathetic blocker had a significantly higher survival rate at 1 week than patients who received an anti-arrhythmic (22 vs. 82%; p < 0.0001). Survival rate at 1 year was also greater in patients who received a sympathetic blocker versus an anti-arrhythmic (67 vs. 5%; p < 0.0001).
These three studies by Lee et al. (Lee et al., 2016), Driver et al. (Driver et al., 2014), and Nademanee et al. (Nademanee et al., 2000) were recently analyzed in a systematic review and meta-analysis by Gottlieb et al. (Gottlieb et al., 2020) Cumulatively, 115 patients were included with similar results to the individual studies of beta-blockade association with improved outcomes ranging from ROSC to survival with favorable neurologic outcome. The risk of bias was considered moderate to severe given the influence of confounding factors and selection of participants.
Propranolol was compared to metoprolol for electrical storm in combination with amiodarone for patients who had congestive heart failure and an implantable cardioverter-defibrillator (ICD) to assess the last VT or VF event that required the ICD for arrhythmia termination (Chatzidou et al., 2018). Patients receiving propranolol had 2.67 times fewer events of VT or VF (p = 0.001), as well as 2.34 times less incidences of ICD firings (p = 0.004). After 24 h, more patients receiving propranolol than metoprolol had not had an arrhythmia (p = 0.03). Propranolol was associated with higher likelihood of arrhythmia termination (p < 0.001), faster arrhythmia termination (p < 0.05), and decreased hospital length of stay when compared to metoprolol (p < 0.05). As such, non-selective β1 and β2 blockade appeared to result in more significant decreases in catecholamines and cardiac norepinephrine spillover leading to improvements in electrical storm control, whereas β1-selective blockers have been associated with increased cardiac norepinephrine spillover.
Metoprolol was also studied in patients resuscitated from out-of-hospital VF in a forward multiple logistic regression analysis to predict survival conducted by Skrifvars et al.(Skrifvars et al., 2003) Out of 102 patients total, 79 received beta-blocking agents (80%) which included the use of either metoprolol (intravenous or oral) or bisoprolol (oral). βB use during the first 72 h of post-resuscitation care was associated with survival at 6 months from the event in both the univariate (p < 0.001) and multiple logistic regression analyses (p = 0.002).
The 2017 AHA/ACC/HRS Guideline for the Management of Patients with Ventricular Arrythmias and Prevention of Sudden Cardiac Death (SCD) support the use of βB as first-line antiarrhythmic therapy for the treatment of ventricular arrhythmias and reducing the risk of SCD (Al-Khatib et al., 2018). Additionally, βB use is associated with a significant reduction in mortality in the setting of acute myocardial infarction (AMI) in addition to suppressing recurrent VF in patients with recent MI. The 2018 AHA Focused Update on ACLS Use of Antiarrhythmic Drugs During and Immediately After Cardiac Arrest does not recommend βB use immediately following cardiac arrest given limited evidence (Panchal et al., 2018). Upon review, esmolol 300–500 μg/kg loading dose followed by 0–100 μg/kg/min infusion was the most evaluated βB in the cardiac arrest studies, but propranolol, bisoprolol and metoprolol at variable doses were additionally studied. There is also some controversy as one study used a loading dose of esmolol 300–500 μg/kg while another studied esmolol 300–500 mg/kg accounting for a substantial one-thousandfold difference.
In summary, Tthe demonstration of improved rates of ROSC and sustained outcomes in addition to increased survival from βB (most notably with esmolol) use in patients with RVF is promising; however, larger studies are necessary to offer increased guidance on βB use during and after cardiac arrest in the coming years. Furthermore, additional research is needed to compare specific βB agents in cardiac arrest to build on existing evidence that non-selective agents may lead to fewer arrhythmias, improved arrhythmia termination, and decreased hospital length of stay when compared to β1-selective βB. However, once hemodynamic stabilization is achieved, current evidence is in line with guideline recommendations to initiate βB therapy to reduce risk of repeated VF (Al-Khatib et al., 2018).
Conclusion
Although negative inotropes appear counterintuitive in hemodynamically compromised critical illness, dampening catecholamine signaling may confer a wide range of benefits, dependent on etiology. In Sepsis, immediately post fluid resuscitation and initial stabilization, re-initiation of home βB therapy should be strongly considered. Additionally, existing evidence suggests βB use may improve recovery following burn injury, reduce mortality rate in TBI, and increase achievement of ROSC in RVF cardiac arrest. Further, promising new data in sepsis suggest a potential role as well as further inquiry.
Author Contributions
All authors participated in drafting and critical revisions. TJ/AN participated in conceptualization and planning.
Funding
This work was supported by the National Center for Advancing Translational Sciences (NCATS) of the National Institutes of Health (NIH) under Award Numbers UL1TR002378 and KL2TR002381 awarded to AN and the UL1TR002378 and TL1TR002382 awarded to TJ.
Conflict of Interest
The authors declare that the research was conducted in the absence of any commercial or financial relationships that could be construed as a potential conflict of interest.
Publisher’s Note
All claims expressed in this article are solely those of the authors and do not necessarily represent those of their affiliated organizations, or those of the publisher, the editors and the reviewers. Any product that may be evaluated in this article, or claim that may be made by its manufacturer, is not guaranteed or endorsed by the publisher.
References
Ahl, R., Thelin, E. P., Sjölin, G., Bellander, B. M., Riddez, L., Talving, P., et al. (2017). β-Blocker after Severe Traumatic Brain Injury Is Associated with Better Long-Term Functional Outcome: a Matched Case Control Study. Eur. J. Trauma Emerg. Surg. 43 (6), 783–789. doi:10.1007/s00068-017-0779-5
Al-Khatib, S. M., Stevenson, W. G., Ackerman, M. J., Bryant, W. J., Callans, D. J., Curtis, A. B., et al. (2018). 2017 AHA/ACC/HRS Guideline for Management of Patients with Ventricular Arrhythmias and the Prevention of Sudden Cardiac Death: A Report of the American College of Cardiology/American Heart Association Task Force on Clinical Practice Guidelines and the Heart Rhythm Society. Circulation 138(13), e272-e391. doi: doi:doi:10.1161/CIR.0000000000000549
Alali, A. S., Mukherjee, K., McCredie, V. A., Golan, E., Shah, P. S., Bardes, J. M., et al. (2017). Beta-blockers and Traumatic Brain Injury: A Systematic Review, Meta-Analysis, and Eastern Association for the Surgery of Trauma Guideline. Ann. Surg. 266 (6), 952–961. doi:10.1097/SLA.0000000000002286
Ali, A., Herndon, D. N., Mamachen, A., Hasan, S., Andersen, C. R., Grogans, R. J., et al. (2015). Propranolol Attenuates Hemorrhage and Accelerates Wound Healing in Severely Burned Adults. Crit. Care 19, 217. doi:10.1186/s13054-015-0913-x
Allorto, N., Atieh, B., Bolgiani, A., Chatterjee, P., Cioffi, W., Dziewulski, P., et al. (2018). ISBI Practice Guidelines for Burn Care, Part 2. Burns 44 (7), 1617–1706. doi:10.1016/j.burns.2018.09.012
Arbabi, S., Ahrns, K. S., Wahl, W. L., Hemmila, M. R., Wang, S. C., Brandt, M. M., et al. (2004). Beta-blocker Use Is Associated with Improved Outcomes in Adult Burn Patients. J. Trauma 56 (2), 265–271. discussion 269-271. doi:10.1097/01.TA.0000109859.91202.C8
Arbabi, S., Campion, E. M., Hemmila, M. R., Barker, M., Dimo, M., Ahrns, K. S., et al. (2007). Beta-blocker Use Is Associated with Improved Outcomes in Adult Trauma Patients. J. Trauma 62 (1), 56–62. doi:10.1097/TA.0b013e31802d972b
Balik, M., Rulisek, J., Leden, P., Zakharchenko, M., Otahal, M., Bartakova, H., et al. (2012). Concomitant Use of Beta-1 Adrenoreceptor Blocker and Norepinephrine in Patients with Septic Shock. Wien Klin Wochenschr 124 (15-16), 552–556. doi:10.1007/s00508-012-0209-y
Baron, P. W., Barrow, R. E., Pierre, E. J., and Herndon, D. N. (1997). Prolonged Use of Propranolol Safely Decreases Cardiac Work in Burned Children. J. Burn Care Rehabil. 18 (3), 223–227. doi:10.1097/00004630-199705000-00008
Belletti, A., Landoni, G., Lomivorotov, V. V., Oriani, A., and Ajello, S. (2020). Adrenergic Downregulation in Critical Care: Molecular Mechanisms and Therapeutic Evidence. J. Cardiothorac. Vasc. Anesth. 34 (4), 1023–1041. doi:10.1053/j.jvca.2019.10.017
Benedict, C. R., and Rose, J. A. (1992). Arterial Norepinephrine Changes in Patients with Septic Shock. Circ. Shock 38 (3), 165–172.
Berk, J. L., Hagen, J. F., Maly, G., and Koo, R. (1972). The Treatment of Shock with Beta Adrenergic Blockade. Arch. Surg. 104 (1), 46–51. doi:10.1001/archsurg.1972.04180010040011
Bignami, E., Guarnieri, M., Franco, A., Gerli, C., De Luca, M., Monaco, F., et al. (2017). Esmolol before cardioplegia and as cardioplegia adjuvant reduces cardiac troponin release after cardiac surgery. A randomized trial. Perfusion 34 (2), 313–320. doi:10.1177/0267659116681437
Boldt, J., Menges, T., Kuhn, D., Diridis, C., and Hempelmann, G. (1995). Alterations in Circulating Vasoactive Substances in the Critically Ill-Aa Comparison between Survivors and Non-survivors. Intensive Care Med. 21 (3), 218–225. doi:10.1007/BF01701475
Bosch, N. A., Rucci, J. M., Massaro, J. M., Winter, M. R., Quinn, E. K., Chon, K. H., et al. (2021). Comparative Effectiveness of Heart Rate Control Medications for the Treatment of Sepsis-Associated Atrial Fibrillation. Chest 159, 1452–1459. doi:10.1016/j.chest.2020.10.049
Bosmann, M., Grailer, J. J., Zhu, K., Matthay, M. A., Sarma, J. V., Zetoune, F. S., et al. (2012). Anti-inflammatory Effects of β2 Adrenergic Receptor Agonists in Experimental Acute Lung Injury. Faseb j 26 (5), 2137–2144. doi:10.1096/fj.11-201640
Bristow, M. R., Ginsburg, R., Umans, V., Fowler, M., Minobe, W., Rasmussen, R., et al. (1986). Beta 1- and Beta 2-Adrenergic-Receptor Subpopulations in Nonfailing and Failing Human Ventricular Myocardium: Coupling of Both Receptor Subtypes to Muscle Contraction and Selective Beta 1-receptor Down-Regulation in Heart Failure. Circ. Res. 59 (3), 297–309. doi:10.1161/01.res.59.3.297
Butler, J., Young, J. B., Abraham, W. T., Bourge, R. C., Adams, K. F., Clare, R., et al. (2006). Beta-Blocker Use and Outcomes Among Hospitalized Heart Failure Patients. J. Amer. College Cardiol. 47 (12), 2462–2469. doi:10.1016/j.jacc.2006.03.030
Cain, B. S., Meldrum, D. R., Dinarello, C. A., Meng, X., Joo, K. S., Banerjee, A., et al. (1999). Tumor Necrosis Factor-Alpha and Interleukin-1beta Synergistically Depress Human Myocardial Function. Crit. Care Med. 27 (7), 1309–1318. doi:10.1097/00003246-199907000-00018
Cammarata, G., Weil, M. H., Sun, S., Tang, W., Wang, J., and Huang, L. (2004). Beta1-adrenergic Blockade during Cardiopulmonary Resuscitation Improves Survival. Crit. Care Med. 32 (9 Suppl. l), S440–S443. doi:10.1097/01.ccm.0000134263.32657.34
Carstairs, J. R., Nimmo, A. J., and Barnes, P. J. (1985). Autoradiographic Visualization of Beta-Adrenoceptor Subtypes in Human Lung. Am. Rev. Respir. Dis. 132 (3), 541–547. doi:10.1164/arrd.1985.132.3.541
Chacko, C. J., and Gopal, S. (2015). Systematic Review of Use of β-blockers in Sepsis. J. Anaesthesiol Clin. Pharmacol. 31 (4), 460–465. doi:10.4103/0970-9185.169063
Chatzidou, S., Kontogiannis, C., Tsilimigras, D. I., Georgiopoulos, G., Kosmopoulos, M., Papadopoulou, E., et al. (2018). Propranolol versus Metoprolol for Treatment of Electrical Storm in Patients with Implantable Cardioverter-Defibrillator. J. Am. Coll. Cardiol. 71 (17), 1897–1906. doi:10.1016/j.jacc.2018.02.056
Cheema, S. A., Ahmed, U. T., Nasir, H., Dogar, S. R., and Mustafa, Z. (2020). Effects of Propranolol in Accelerating Wound Healing and Attenuation of Hypermetabolism in Adult Burn Patients. J. Coll. Physicians Surg. Pak 30 (1), 46–50. doi:10.29271/jcpsp.2020.01.46
Chen, Z., Tang, L., Xu, X., Wei, X., Wen, L., and Xie, Q. (2017). Therapeutic Effect of Beta-Blocker in Patients with Traumatic Brain Injury: A Systematic Review and Meta-Analysis. J. Crit. Care 41, 240–246. doi:10.1016/j.jcrc.2017.05.035
Chruscinski, A. J., Rohrer, D. K., Schauble, E., Desai, K. H., Bernstein, D., and Kobilka, B. K. (1999). Targeted Disruption of the Beta2 Adrenergic Receptor Gene. J. Biol. Chem. 274 (24), 16694–16700. doi:10.1074/jbc.274.24.16694
Clifton, G. L., Ziegler, M. G., and Grossman, R. G. (1981). Circulating Catecholamines and Sympathetic Activity after Head Injury. Neurosurgery 8 (1), 10–14. doi:10.1227/00006123-198101000-00003
Coppola, S., Froio, S., and Chiumello, D. (2015). β-Blockers in Critically Ill Patients: from Physiology to Clinical Evidence. Crit. Care 19 (1), 119. doi:10.1186/s13054-015-0803-2
Cotton, B. A., Snodgrass, K. B., Fleming, S. B., Carpenter, R. O., Kemp, C. D., Arbogast, P. G., et al. (2007). Beta-blocker Exposure Is Associated with Improved Survival after Severe Traumatic Brain Injury. J. Trauma 62 (1), 26–35. doi:10.1097/TA.0b013e31802d02d0
Cruickshank, J. M., Neil-Dwyer, G., Degaute, J. P., Hayes, Y., Kuurne, T., Kytta, J., et al. (1987). Reduction of Stress/catecholamine-Induced Cardiac Necrosis by Beta 1-selective Blockade. Lancet 2 (8559), 585–589. doi:10.1016/s0140-6736(87)92984-9
de Lucia, C., Eguchi, A., and Koch, W. J. (2018). New Insights in Cardiac β-Adrenergic Signaling during Heart Failure and Aging. Front. Pharmacol. 9, 904. doi:10.3389/fphar.2018.00904
de Prost, N., Dreyfuss, D., Ricard, J. D., and Saumon, G. (2008). Terbutaline Lessens Protein Fluxes across the Alveolo-Capillary Barrier during High-Volume Ventilation. Intensive Care Med. 34 (4), 763–770. doi:10.1007/s00134-007-0954-y
Ditchey, R. V., Rubio-Perez, A., and Slinker, B. K. (1994). Beta-adrenergic Blockade Reduces Myocardial Injury during Experimental Cardiopulmonary Resuscitation. J. Am. Coll. Cardiol. 24 (3), 804–812. doi:10.1016/0735-1097(94)90032-9
Dransfield, M. T., Voelker, H., Bhatt, S. P., Brenner, K., Casaburi, R., Come, C. E., et al. (2019). Metoprolol for the Prevention of Acute Exacerbations of COPD. N. Engl. J. Med. 381 (24), 2304–2314. doi:10.1056/NEJMoa1908142
Driver, B. E., Debaty, G., Plummer, D. W., and Smith, S. W. (2014). Use of Esmolol after Failure of Standard Cardiopulmonary Resuscitation to Treat Patients with Refractory Ventricular Fibrillation. Resuscitation 85 (10), 1337–1341. doi:10.1016/j.resuscitation.2014.06.032
Du, W., Wang, X. T., Long, Y., and Liu, D. W. (2016). Efficacy and Safety of Esmolol in Treatment of Patients with Septic Shock. Chin. Med. J. (Engl) 129 (14), 1658–1665. doi:10.4103/0366-6999.185856
Dünser, M. W., and Hasibeder, W. R. (2009). Sympathetic Overstimulation during Critical Illness: Adverse Effects of Adrenergic Stress. J. Intensive Care Med. 24 (5), 293–316. doi:10.1177/0885066609340519
El Ayadi, A., Prasai, A., Jay, J., Bhattari, N., Guilory, A., Herndon, D., et al. (2019). The Role of Beta-2 Adrenergic Receptors in Cardiac Bioenergetics Following Severe Burns. FASEB J. 33 (S1), lb281. doi:10.1096/fasebj.2019.33.1_supplement.lb281
Fuchs, C., Wauschkuhn, S., Scheer, C., Vollmer, M., Meissner, K., Kuhn, S. O., et al. (2017). Continuing Chronic Beta-Blockade in the Acute Phase of Severe Sepsis and Septic Shock Is Associated with Decreased Mortality Rates up to 90 Days. Br. J. Anaesth. 119 (4), 616–625. doi:10.1093/bja/aex231
Furian, T., Aguiar, C., Prado, K., Ribeiro, R. V., Becker, L., Martinelli, N., et al. (2012). Ventricular Dysfunction and Dilation in Severe Sepsis and Septic Shock: Relation to Endothelial Function and Mortality. J. Crit. Care 27 (3), 319.e9–15. doi:10.1016/j.jcrc.2011.06.017
Gao Smith, F., Perkins, G. D., Gates, S., Young, D., McAuley, D. F., Tunnicliffe, W., et al. (2012). Effect of Intravenous β-2 Agonist Treatment on Clinical Outcomes in Acute Respiratory Distress Syndrome (Balti-2): a Multicentre, Randomised Controlled Trial. Lancet 379 (9812), 229–235. doi:10.1016/s0140-6736(11)61623-1
Gibran, N. S., Wiechman, S., Meyer, W., Edelman, L., Fauerbach, J., Gibbons, L., et al. (2013). Summary of the 2012 ABA Burn Quality Consensus Conference. J. Burn Care Res. 34 (4), 361–385. doi:10.1097/BCR.0b013e31828cb249
Giembycz, M. A., and Newton, R. (2006). Beyond the dogma: novel β2-adrenoceptor signalling in the airways. European Respiratory J. 27 (6), 1286–1306. doi:10.1183/09031936.06.00112605
Gore, D. C., and Wolfe, R. R. (2006). Hemodynamic and Metabolic Effects of Selective Beta1 Adrenergic Blockade during Sepsis. Surgery 139 (5), 686–694. doi:10.1016/j.surg.2005.10.010
Gottlieb, M., Dyer, S., and Peksa, G. (2020). Reply to: Beta-Blockade for the Treatment of Cardiac Arrest Due to Ventricular Fibrillation or Pulseless Ventricular Tachycardia: A Systematic Review and Meta-Analysis. Resuscitation 150, 193–125. doi:10.1016/j.resuscitation.2019.11.01910.1016/j.resuscitation.2020.02.020
Goyagi, T., Horiguchi, T., Nishikawa, T., Tobe, Y., and Masaki, Y. (2012). Neuroprotective Effects of Selective β-1 Adrenoceptor Antagonists, Landiolol and Esmolol, on Transient Forebrain Ischemia in Rats; a Dose-Response Study. Brain Res. 1461, 96–101. doi:10.1016/j.brainres.2012.04.040
Goyagi, T., Horiguchi, T., Nishikawa, T., and Tobe, Y. (2010). Post-treatment with Selective β1 Adrenoceptor Antagonists Provides Neuroprotection against Transient Focal Ischemia in Rats. Brain Res. 1343, 213–217. doi:10.1016/j.brainres.2010.04.079
Goyagi, T., Kimura, T., Nishikawa, T., Tobe, Y., and Masaki, Y. (2006). Beta-adrenoreceptor Antagonists Attenuate Brain Injury after Transient Focal Ischemia in Rats. Anesth. Analg 103 (3), 658–663. doi:10.1213/01.ane.0000228859.95126.69
Guarracino, F., Ferro, B., Morelli, A., Bertini, P., Baldassarri, R., and Pinsky, M. R. (2014). Ventriculoarterial Decoupling in Human Septic Shock. Crit. Care 18 (2), R80. doi:10.1186/cc13842
Gutierrez, J., Hossam, A., Lazarezcu, R., Kay, E., and Rundek, T. (2009). Effect of Beta Blockers on Sepsis Outcome. Med. Sci. Monit. 15 (10), CR499–503.
Guz, D., Buchritz, S., Guz, A., Ikan, A., Babich, T., Daitch, V., et al. (2021). β-Blockers, Tachycardia, and Survival Following Sepsis: An Observational Cohort Study. Clin. Infect. Dis. 73, e921–e926. doi:10.1093/cid/ciab034
Hagiwara, S., Iwasaka, H., Maeda, H., and Noguchi, T. (2009). Landiolol, an Ultrashort-Acting Beta1-Adrenoceptor Antagonist, Has Protective Effects in an LPS-Induced Systemic Inflammation Model. Shock 31 (5), 515–520. doi:10.1097/SHK.0b013e3181863689
Hajsadeghi, S., Rahbar, M. H., Iranpour, A., Salehi, A., Asadi, O., and Jafarian, S. R. (2018). Dobutamine-induced Takotsubo Cardiomyopathy: A Systematic Review of the Literature and Case Report. Anatol J. Cardiol. 19 (6), 412–416. doi:10.14744/AnatolJCardiol.2018.78642
Hamill, R. W., Woolf, P. D., McDonald, J. V., Lee, L. A., and Kelly, M. (1987). Catecholamines Predict Outcome in Traumatic Brain Injury. Ann. Neurol. 21 (5), 438–443. doi:10.1002/ana.410210504
Hart, D. W., Wolf, S. E., Mlcak, R., Chinkes, D. L., Ramzy, P. I., Obeng, M. K., et al. (2000). Persistence of Muscle Catabolism after Severe Burn. Surgery 128 (2), 312–319. doi:10.1067/msy.2000.108059
Hasegawa, D., Sato, R., Prasitlumkum, N., Nishida, K., Takahashi, K., Yatabe, T., et al. (2021). Effect of Ultrashort-Acting β-Blockers on Mortality in Patients with Sepsis with Persistent Tachycardia Despite Initial Resuscitation. Chest 159, 2289–2300. doi:10.1016/j.chest.2021.01.009
Herndon, D. N., Hart, D. W., Wolf, S. E., Chinkes, D. L., and Wolfe, R. R. (2001). Reversal of Catabolism by Beta-Blockade after Severe Burns. N. Engl. J. Med. 345 (17), 1223–1229. doi:10.1056/NEJMoa010342
Herndon, D. N., Rodriguez, N. A., Diaz, E. C., Hegde, S., Jennings, K., Mlcak, R. P., et al. (2012). Long-term Propranolol Use in Severely Burned Pediatric Patients: a Randomized Controlled Study. Ann. Surg. 256 (3), 402–411. doi:10.1097/SLA.0b013e318265427e
Hsueh, W. A., and Law, R. (2003). The central Role of Fat and Effect of Peroxisome Proliferator-Activated Receptor-Gamma on Progression of Insulin Resistance and Cardiovascular Disease. Am. J. Cardiol. 92 (4), 3J–9J. doi:10.1016/s0002-9149(03)00610-6
Huang, L., Weil, M. H., Cammarata, G., Sun, S., and Tang, W. (2004). Nonselective Beta-Blocking Agent Improves the Outcome of Cardiopulmonary Resuscitation in a Rat Model. Crit. Care Med. 32 (9 Suppl. l), S378–S380. doi:10.1097/01.ccm.0000134266.65164.7c
Inaba, K., Teixeira, P. G., David, J. S., Chan, L. S., Salim, A., Brown, C., et al. (2008). Beta-blockers in Isolated blunt Head Injury. J. Am. Coll. Surg. 206 (3), 432–438. doi:10.1016/j.jamcollsurg.2007.10.005
Iwata, M., Inoue, S., Kawaguchi, M., Nakamura, M., Konishi, N., and Furuya, H. (2010). Posttreatment but Not Pretreatment with Selective Beta-Adrenoreceptor 1 Antagonists Provides Neuroprotection in the hippocampus in Rats Subjected to Transient Forebrain Ischemia. Anesth. Analg 110 (4), 1126–1132. doi:10.1213/ANE.0b013e3181d278f7
Jacobi, J. (2002). Pathophysiology of Sepsis. Am. J. Health Syst. Pharm. 59 Suppl 1 (Suppl. 1), S3–S8. doi:10.1093/ajhp/59.suppl_1.S3
January, C. T., Wann, L. S., Alpert, J. S., Calkins, H., Cigarroa, J. E., Cleveland, J. C., et al. (2014). 2014 AHA/ACC/HRS Guideline for the Management of Patients with Atrial Fibrillation: Executive Summary. J. Am. Coll. Cardiol. 64 (21), 2246–2280. doi:10.1016/j.jacc.2014.03.021
Jeschke, M. G., Norbury, W. B., Finnerty, C. C., Branski, L. K., and Herndon, D. N. (2007). Propranolol Does Not Increase Inflammation, Sepsis, or Infectious Episodes in Severely Burned Children. J. Trauma 62 (3), 676–681. doi:10.1097/TA.0b013e318031afd3
Jones, S. B., and Romano, F. D. (1989). Dose- and Time-dependent Changes in Plasma Catecholamines in Response to Endotoxin in Conscious Rats. Circ. Shock 28 (1), 59–68.
Jones, T. W., Smith, S. E., Van Tuyl, J. S., and Newsome, A. S. (2020). Sepsis With Preexisting Heart Failure: Management of Confounding Clinical Features. J. Intensive Care Med., 885066620928299. doi:10.1177/0885066620928299
Kakihana, Y., Nishida, O., Taniguchi, T., Okajima, M., Morimatsu, H., Ogura, H., et al. (2020). Efficacy and Safety of Landiolol, an Ultra-short-acting β1-selective Antagonist, for Treatment of Sepsis-Related Tachyarrhythmia (J-Land 3S): a Multicentre, Open-Label, Randomised Controlled Trial. Lancet Respir. Med. 8 (9), 863–872. doi:10.1016/S2213-2600(20)30037-0
Kargin, F., Takir, H. B., Salturk, C., Goksenoglu, N. C., Karabay, C. Y., Mocin, O. Y., et al. (2014). The Safety of Beta-Blocker Use in Chronic Obstructive Pulmonary Disease Patients with Respiratory Failure in the Intensive Care Unit. Multidiscip Respir. Med. 9 (1), 8. doi:10.1186/2049-6958-9-8
Killingsworth, C. R., Wei, C. C., Dell'Italia, L. J., Ardell, J. L., Kingsley, M. A., Smith, W. M., et al. (2004). Short-acting Beta-Adrenergic Antagonist Esmolol Given at Reperfusion Improves Survival after Prolonged Ventricular Fibrillation. Circulation 109 (20), 2469–2474. doi:10.1161/01.CIR.0000128040.43933.D3
Ko, A., Harada, M. Y., Barmparas, G., Thomsen, G. M., Alban, R. F., Bloom, M. B., et al. (2016). Early Propranolol after Traumatic Brain Injury Is Associated with Lower Mortality. J. Trauma Acute Care Surg. 80 (4), 637–642. doi:10.1097/TA.0000000000000959
Lang, C. H., Nystrom, G., and Frost, R. A. (2008). β-Adrenergic Blockade Exacerbates Sepsis-Induced Changes in Tumor Necrosis Factor α and Interleukin-6 in Skeletal Muscle and Is Associated with Impaired Translation Initiation. J. Trauma Acute Care Surg. 64 (2), 477–486. doi:10.1097/01.TA.0000249375.43015.01
Lazaridis, C. (2017). Cerebral Oxidative Metabolism Failure in Traumatic Brain Injury: "Brain Shock". J. Crit. Care 37, 230–233. doi:10.1016/j.jcrc.2016.09.027
Lee, Y. H., Lee, K. J., Min, Y. H., Ahn, H. C., Sohn, Y. D., Lee, W. W., et al. (2016). Refractory Ventricular Fibrillation Treated with Esmolol. Resuscitation 107, 150–155. doi:10.1016/j.resuscitation.2016.07.243
Lee, Y. R., Seth, M. S., Soney, D., and Dai, H. (2019). Benefits of Beta-Blockade in Sepsis and Septic Shock: A Systematic Review. Clin. Drug Investig. 39 (5), 429–440. doi:10.1007/s40261-019-00762-z
Ley, E. J., Leonard, S. D., Barmparas, G., Dhillon, N. K., Inaba, K., Salim, A., et al. (2018). Beta Blockers in Critically Ill Patients with Traumatic Brain Injury: Results from a Multicenter, Prospective, Observational American Association for the Surgery of Trauma Study. J. Trauma Acute Care Surg. 84 (2), 234–244. doi:10.1097/TA.0000000000001747
Ley, E. J., Park, R., Dagliyan, G., Palestrant, D., Miller, C. M., Conti, P. S., et al. (2010). In Vivo effect of Propranolol Dose and Timing on Cerebral Perfusion after Traumatic Brain Injury. J. Trauma 68 (2), 353–356. doi:10.1097/TA.0b013e3181c8269a
Ley, E. J., Scehnet, J., Park, R., Schroff, S., Dagliyan, G., Conti, P. S., et al. (2009). The In Vivo Effect of Propranolol on Cerebral Perfusion and Hypoxia after Traumatic Brain Injury. J. Trauma 66 (1), 154–161. discussion 159-161. doi:10.1097/TA.0b013e31819388be
Liu, H., Ding, X. F., Zhang, S. G., Wang, H. X., Luo, Y. G., Duan, X. G., et al. (2019). [Effect of Esmolol in Septic Shock Patients with Tachycardia: a Randomized Clinical Trial]. Zhonghua Yi Xue Za Zhi 99 (17), 1317–1322. doi:10.3760/cma.j.issn.0376-2491.2019.17.009
Liu, P., Wu, Q., Tang, Y., Zhou, Z., and Feng, M. (2018). The Influence of Esmolol on Septic Shock and Sepsis: A Meta-Analysis of Randomized Controlled Studies. Am. J. Emerg. Med. 36 (3), 470–474. doi:10.1016/j.ajem.2017.11.013
Maccari, S., Vezzi, V., Barbagallo, F., Stati, T., Ascione, B., Grò, M. C., et al. (2020). β-blockers Reverse Agonist-Induced β(2)-AR Downregulation Regardless of Their Signaling Profile. Int. J. Mol. Sci. 21 (2), 512. doi:10.3390/ijms21020512
Macchia, A., Romero, M., Comignani, P. D., Mariani, J., D'Ettorre, A., Prini, N., et al. (2012). Previous Prescription of β-blockers Is Associated with Reduced Mortality Among Patients Hospitalized in Intensive Care Units for Sepsis. Crit. Care Med. 40 (10), 2768–2772. doi:10.1097/CCM.0b013e31825b9509
MacNee, W. (2019). Beta-Blockers in COPD - A Controversy Resolved? N. Engl. J. Med. 381 (24), 2367–2368. doi:10.1056/NEJMe1912664
Maris, N. A., de Vos, A. F., Dessing, M. C., Spek, C. A., Lutter, R., Jansen, H. M., et al. (2005). Antiinflammatory Effects of Salmeterol after Inhalation of Lipopolysaccharide by Healthy Volunteers. Am. J. Respir. Crit. Care Med. 172 (7), 878–884. doi:10.1164/rccm.200503-451OC
Maron, M. B., Luther, D. J., Pilati, C. F., Ohanyan, V., Li, T., Koshy, S., et al. (2009). Beta-adrenoceptor Stimulation of Alveolar Fluid Clearance Is Increased in Rats with Heart Failure. Am. J. Physiol. Lung Cel Mol Physiol 297 (3), L487–L495. doi:10.1152/ajplung.90629.2008
Mathieu, C., Zieleskiewicz, L., and Leone, M. (2016). Beta-blockers in Septic Shock: a Magnifying Glass on the Relation Heart Vessel. J. Thorac. Dis. 8 (8), E802–E804. doi:10.21037/jtd.2016.07.12
Mohammadi, A. A., Bakhshaeekia, A., Alibeigi, P., Hasheminasab, M. J., Tolide-ei, H. R., Tavakkolian, A. R., et al. (2009). Efficacy of Propranolol in Wound Healing for Hospitalized Burn Patients. J. Burn Care Res. 30 (6), 1013–1017. doi:10.1097/BCR.0b013e3181b48600
Mohseni, S., Talving, P., Thelin, E. P., Wallin, G., Ljungqvist, O., and Riddez, L. (2015). The Effect of β-blockade on Survival after Isolated Severe Traumatic Brain Injury. World J. Surg. 39 (8), 2076–2083. doi:10.1007/s00268-015-3039-z
Moniotte, S., Belge, C., Sekkali, B., Massion, P. B., Rozec, B., Dessy, C., et al. (2007). Sepsis Is Associated with an Upregulation of Functional Beta3 Adrenoceptors in the Myocardium. Eur. J. Heart Fail. 9 (12), 1163–1171. doi:10.1016/j.ejheart.2007.10.006
Moniotte, S., Kobzik, L., Feron, O., Trochu, J.-N., Gauthier, C., and Balligand, J.-L. (2001). Upregulation of β3-Adrenoceptors and Altered Contractile Response to Inotropic Amines in Human Failing Myocardium. Circulation 103 (12), 1649–1655. doi:10.1161/01.CIR.103.12.1649
Monroe, R. G., and French, G. (1960). Ventricular Pressure-Volume Relationships and Oxygen Consumption in Fibrillation and Arrest. Circ. Res. 8, 260–266. doi:10.1161/01.res.8.1.260
Morales, D. R., Lipworth, B. J., Donnan, P. T., Jackson, C., and Guthrie, B. (2017). Respiratory Effect of Beta-Blockers in People with Asthma and Cardiovascular Disease: Population-Based Nested Case Control Study. BMC Med. 15 (1), 18. doi:10.1186/s12916-017-0781-0
Morelli, A., Ertmer, C., Westphal, M., Rehberg, S., Kampmeier, T., Ligges, S., et al. (2013). Effect of Heart Rate Control with Esmolol on Hemodynamic and Clinical Outcomes in Patients with Septic Shock: a Randomized Clinical Trial. Jama 310 (16), 1683–1691. doi:10.1001/jama.2013.278477
Morelli, A., Singer, M., Ranieri, V. M., D'Egidio, A., Mascia, L., Orecchioni, A., et al. (2016). Heart Rate Reduction with Esmolol Is Associated with Improved Arterial Elastance in Patients with Septic Shock: a Prospective Observational Study. Intensive Care Med. 42 (10), 1528–1534. doi:10.1007/s00134-016-4351-2
Morelli, A., Whitehouse, T., and Rehberg, S. (2020). β-Blockade in Sepsis: Regulation of Persisting Sepsis-Related Tachycardia. Lancet Respir. Med. 8 (9), 833–834. doi:10.1016/S2213-2600(20)30063-1
Murry, J. S., Hoang, D. M., Barmparas, G., Harada, M. Y., Bukur, M., Bloom, M. B., et al. (2016). Prospective Evaluation of Early Propranolol after Traumatic Brain Injury. J. Surg. Res. 200 (1), 221–226. doi:10.1016/j.jss.2015.06.045
Mutlu, G. M., Dumasius, V., Burhop, J., McShane, P. J., Meng, F. J., Welch, L., et al. (2004). Upregulation of Alveolar Epithelial Active Na+ Transport Is Dependent on Beta2-Adrenergic Receptor Signaling. Circ. Res. 94 (8), 1091–1100. doi:10.1161/01.Res.0000125623.56442.20
Mutlu, G. M., and Factor, P. (2008). Alveolar Epithelial Beta2-Adrenergic Receptors. Am. J. Respir. Cel Mol Biol 38 (2), 127–134. doi:10.1165/rcmb.2007-0198TR
Myagmar, B. E., Flynn, J. M., Cowley, P. M., Swigart, P. M., Montgomery, M. D., Thai, K., et al. (2017). Adrenergic Receptors in Individual Ventricular Myocytes. Circulation Res. 120 (7), 1103–1115. doi:10.1161/CIRCRESAHA.117.310520
Nademanee, K., Taylor, R., Bailey, W. E., Rieders, D. E., and Kosar, E. M. (2000). Treating Electrical Storm : Sympathetic Blockade versus Advanced Cardiac Life Support-Guided Therapy. Circulation 102 (7), 742–747. doi:10.1161/01.cir.102.7.742
Nguyen, M. N., Kiriazis, H., Ruggiero, D., Gao, X. M., Su, Y., Jian, A., et al. (2015). Spontaneous Ventricular Tachyarrhythmias in β2-adrenoceptor Transgenic Mice in Relation to Cardiac Interstitial Fibrosis. Am. J. Physiol. Heart Circ. Physiol. 309 (5), H946–H957. doi:10.1152/ajpheart.00405.2015
Noveanu, M., Breidthardt, T., Reichlin, T., Gayat, E., Potocki, M., Pargger, H., et al. (2010). Effect of Oral β-blocker on Short and Long-Term Mortality in Patients with Acute Respiratory Failure: Results from the BASEL-II-ICU Study. Crit. Care 14 (6), R198. doi:10.1186/cc9317
Novotny, N. M., Lahm, T., Markel, T. A., Crisostomo, P. R., Wang, M., Wang, Y., et al. (2009). Beta-Blockers in Sepsis: Reexamining the Evidence. Shock 31 (2), 113–119. doi:10.1097/SHK.0b013e318180ffb6
Núñez-Villaveirán, T., Sánchez, M., Millán, P., and García-de-Lorenzo, A. (2015). Systematic Review of the Effect of Propanolol on Hypermetabolism in Burn Injuries. Med. Intensiva 39 (2), 101–113. doi:10.1016/j.medin.2014.08.002
O'Dwyer, M. J., Mankan, A. K., Stordeur, P., O'Connell, B., Duggan, E., White, M., et al. (2006). The Occurrence of Severe Sepsis and Septic Shock Are Related to Distinct Patterns of Cytokine Gene Expression. Shock 26 (6), 544–550. doi:10.1097/01.shk.0000235091.38174.8d
O'Gara, P. T., Kushner, F. G., Ascheim, D. D., Casey, D. E., Chung, M. K., de Lemos, J. A., et al. (2013). 2013 ACCF/AHA Guideline for the Management of ST-Elevation Myocardial Infarction. A Rep. Am. Coll. Cardiol. Foundation/American Heart Assoc. Task Force Pract. Guidel. 61 (4), e78–e140. doi:10.1016/j.jacc.2012.11.019
Orbegozo Cortes, D., Njimi, H., Dell'Anna, A. M., and Taccone, F. S. (2014). Esmolol for Septic Shock: More Than Just Heart Rate Control? Minerva Anestesiol 80 (2), 254–258.
Panchal, A. R., Bartos, J. A., Cabañas, J. G., Donnino, M. W., Drennan, I. R., Hirsch, K. G., et al. (2020). Part 3: Adult Basic and Advanced Life Support: 2020 American Heart Association Guidelines for Cardiopulmonary Resuscitation and Emergency Cardiovascular Care. Circulation 142(16_Suppl. l_2), S366–S468. doi: doi:doi:10.1161/CIR.0000000000000916
Panchal, A. R., Berg, K. M., Kudenchuk, P. J., Del Rios, M., Hirsch, K. G., Link, M. S., et al. (2018). 2018 American Heart Association Focused Update on Advanced Cardiovascular Life Support Use of Antiarrhythmic Drugs during and Immediately after Cardiac Arrest: An Update to the American Heart Association Guidelines for Cardiopulmonary Resuscitation and Emergency Cardiovascular Care. Circulation 138 (23), e740–e749. doi:10.1161/CIR.0000000000000613
Patel, M. B., McKenna, J. W., Alvarez, J. M., Sugiura, A., Jenkins, J. M., Guillamondegui, O. D., et al. (2012). Decreasing Adrenergic or Sympathetic Hyperactivity after Severe Traumatic Brain Injury Using Propranolol and Clonidine (DASH after TBI Study): Study Protocol for a Randomized Controlled Trial. Trials 13, 177. doi:10.1186/1745-6215-13-177
Patterson, A. J., Zhu, W., Chow, A., Agrawal, R., Kosek, J., Xiao, R. P., et al. (2004). Protecting the Myocardium: a Role for the Beta2 Adrenergic Receptor in the Heart. Crit. Care Med. 32 (4), 1041–1048. doi:10.1097/01.ccm.0000120049.43113.90
Plank, L. D., Connolly, A. B., and Hill, G. L. (1998). Sequential Changes in the Metabolic Response in Severely Septic Patients during the First 23 Days after the Onset of Peritonitis. Ann. Surg. 228 (2), 146–158. doi:10.1097/00000658-199808000-00002
Preiser, J. C., Ichai, C., Orban, J. C., and Groeneveld, A. B. (2014). Metabolic Response to the Stress of Critical Illness. Br. J. Anaesth. 113 (6), 945–954. doi:10.1093/bja/aeu187
Prins, K. W., Neill, J. M., Tyler, J. O., Eckman, P. M., and Duval, S. (2015). Effects of Beta-Blocker Withdrawal in Acute Decompensated Heart Failure: A Systematic Review and Meta-Analysis. JACC Heart Fail. 3 (8), 647–653. doi:10.1016/j.jchf.2015.03.008
Przkora, R., Barrow, R. E., Jeschke, M. G., Suman, O. E., Celis, M., Sanford, A. P., et al. (2006). Body Composition Changes with Time in Pediatric Burn Patients. J. Trauma 60 (5), 968–971. doi:10.1097/01.ta.0000214580.27501.19
Rassler, B. (2012). Contribution of α - and β -Adrenergic Mechanisms to the Development of Pulmonary Edema. Scientifica (Cairo) 2012, 829504. doi:10.6064/2012/829504
Rengo, G., Zincarelli, C., Femminella, G. D., Liccardo, D., Pagano, G., de Lucia, C., et al. (2012). Myocardial β(2) -adrenoceptor Gene Delivery Promotes Coordinated Cardiac Adaptive Remodelling and Angiogenesis in Heart Failure. Br. J. Pharmacol. 166 (8), 2348–2361. doi:10.1111/j.1476-5381.2012.01954.x
Rhodes, A., Evans, L. E., Alhazzani, W., Levy, M. M., Antonelli, M., Ferrer, R., et al. (2017). Surviving Sepsis Campaign: International Guidelines for Management of Sepsis and Septic Shock: 2016. Intensive Care Med. 43 (3), 304–377. doi:10.1097/CCM.000000000000225510.1007/s00134-017-4683-6
Rizoli, S. B., Jaja, B. N., Di Battista, A. P., Rhind, S. G., Neto, A. C., da Costa, L., et al. (2017). Catecholamines as Outcome Markers in Isolated Traumatic Brain Injury: the COMA-TBI Study. Crit. Care 21 (1), 37. doi:10.1186/s13054-017-1620-6
Romana-Souza, B., Nascimento, A. P., and Monte-Alto-Costa, A. (2008). Low-dose Propranolol Improves Cutaneous Wound Healing of Burn-Injured Rats. Plast. Reconstr. Surg. 122 (6), 1690–1699. doi:10.1097/PRS.0b013e31818cbf67
Sakuma, T., Tuchihara, C., Ishigaki, M., Osanai, K., Nambu, Y., Toga, H., et al. (2001). Denopamine, a Beta(1)-Adrenergic Agonist, Increases Alveolar Fluid Clearance in Ex Vivo Rat and guinea Pig Lungs. J. Appl. Physiol. (1985) 90 (1), 10–16. doi:10.1152/jappl.2001.90.1.101985
Sander, O., Welters, I. D., Foëx, P., and Sear, J. W. (2005). Impact of Prolonged Elevated Heart Rate on Incidence of Major Cardiac Events in Critically Ill Patients with a High Risk of Cardiac Complications. Crit. Care Med. 33 (1), 81–82. doi:10.1097/01.Ccm.0000150028.64264.14
Sanfilippo, F., Santonocito, C., Morelli, A., and Foex, P. (2015). Beta-blocker Use in Severe Sepsis and Septic Shock: a Systematic Review. Curr. Med. Res. Opin. 31 (10), 1817–1825. doi:10.1185/03007995.2015.1062357
Schmittinger, C. A., Dünser, M. W., Haller, M., Ulmer, H., Luckner, G., Torgersen, C., et al. (2008). Combined Milrinone and Enteral Metoprolol Therapy in Patients with Septic Myocardial Depression. Crit. Care 12 (4), R99. doi:10.1186/cc6976
Schroeppel, T. J., Fischer, P. E., Zarzaur, B. L., Magnotti, L. J., Clement, L. P., Fabian, T. C., et al. (2010). Beta-adrenergic Blockade and Traumatic Brain Injury: Protective? J. Trauma 69 (4), 776–782. doi:10.1097/TA.0b013e3181e981b8
Schroeppel, T. J., Sharpe, J. P., Magnotti, L. J., Weinberg, J. A., Clement, L. P., Croce, M. A., et al. (2014). Traumatic Brain Injury and β-blockers: Not All Drugs Are Created Equal. J. Trauma Acute Care Surg. 76 (2), 504–509. discussion 509. doi:10.1097/TA.0000000000000104
Shang, X., Wang, K., Xu, J., Gong, S., Ye, Y., Chen, K., et al. (2016). The Effect of Esmolol on Tissue Perfusion and Clinical Prognosis of Patients with Severe Sepsis: A Prospective Cohort Study. Biomed. Res. Int. 2016, 1038034. doi:10.1155/2016/1038034
Short, P. M., Williamson, P. A., Anderson, W. J., and Lipworth, B. J. (2013). Randomized Placebo-Controlled Trial to Evaluate Chronic Dosing Effects of Propranolol in Asthma. Am. J. Respir. Crit. Care Med. 187 (12), 1308–1314. doi:10.1164/rccm.201212-2206OC
Singer, K. E., Collins, C. E., Flahive, J. M., Wyman, A. S., Ayturk, M. D., and Santry, H. P. (2017). Outpatient Beta-Blockers and Survival from Sepsis: Results from a National Cohort of Medicare Beneficiaries. Am. J. Surg. 214 (4), 577–582. doi:10.1016/j.amjsurg.2017.06.007
Skrifvars, M. B., Pettilä, V., Rosenberg, P. H., and Castrén, M. (2003). A Multiple Logistic Regression Analysis of In-Hospital Factors Related to Survival at Six Months in Patients Resuscitated from Out-Of-Hospital Ventricular Fibrillation. Resuscitation 59 (3), 319–328. doi:10.1016/s0300-9572(03)00238-7
Song, D., Xu, J., Du, T., Yan, E., Hertz, L., Walz, W., et al. (2014). Inhibition of Brain Swelling after Ischemia-Reperfusion by β-adrenergic Antagonists: Correlation with Increased K+ and Decreased Ca2+ Concentrations in Extracellular Fluid. Biomed. Res. Int. 2014, 873590. doi:10.1155/2014/873590
Stefan, M. S., Rothberg, M. B., Priya, A., Pekow, P. S., Au, D. H., and Lindenauer, P. K. (2012). Association between β-blocker Therapy and Outcomes in Patients Hospitalised with Acute Exacerbations of Chronic Obstructive Lung Disease with Underlying Ischaemic Heart Disease, Heart Failure or Hypertension. Thorax 67 (11), 977–984. doi:10.1136/thoraxjnl-2012-201945
Suzuki, T., Morisaki, H., Serita, R., Yamamoto, M., Kotake, Y., Ishizaka, A., et al. (2005). Infusion of the Beta-Adrenergic Blocker Esmolol Attenuates Myocardial Dysfunction in Septic Rats. Crit. Care Med. 33 (10), 2294–2301. doi:10.1097/01.ccm.0000182796.11329.3b
Suzuki, T., Suzuki, Y., Okuda, J., Kurazumi, T., Suhara, T., Ueda, T., et al. (2017). Sepsis-induced Cardiac Dysfunction and β-adrenergic Blockade Therapy for Sepsis. J. Intensive Care 5, 22. doi:10.1186/s40560-017-0215-2
Tan, K., Harazim, M., Tang, B., Mclean, A., and Nalos, M. (2019). The Association between Premorbid Beta Blocker Exposure and Mortality in Sepsis-A Systematic Review. Crit. Care 23 (1), 298. doi:10.1186/s13054-019-2562-y
Tripathi, F. M., Guha, S., Khanna, N. N., Chansoria, J. P. N., Sinha, J. K., and Udupa, K. N. (1981). Plasma Catecholamines in thermal burns. Burns 8 (2), 99–101. doi:10.1016/0305-4179(81)90029-2
Thrush, D. N., Downs, J. B., and Smith, R. A. (1997). Is Epinephrine Contraindicated During Cardiopulmonary Resuscitation? Circulation 96 (8), 2709–2714. doi:10.1161/01.CIR.96.8.2709
Umehara, S., Goyagi, T., Nishikawa, T., Tobe, Y., and Masaki, Y. (2010). Esmolol and Landiolol, Selective Beta1-Adrenoreceptor Antagonists, Provide Neuroprotection against Spinal Cord Ischemia and Reperfusion in Rats. Anesth. Analg 110 (4), 1133–1137. doi:10.1213/ANE.0b013e3181cdb06b
Van Herpen, C. H., van Blokland, D. A., and van Zanten, A. R. H. (2019). Metabolic effects of beta-blockers in critically ill patients: A retrospective cohort study. Heart Lung 48 (4), 278–286. doi:10.1016/j.hrtlng.2019.02.004
Wachter, S. B., and Gilbert, E. M. (2012). Beta-adrenergic Receptors, from Their Discovery and Characterization through Their Manipulation to Beneficial Clinical Application. Cardiology 122 (2), 104–112. doi:10.1159/000339271
Walkey, A. J., Evans, S. R., Winter, M. R., and Benjamin, E. J. (2016). Practice Patterns and Outcomes of Treatments for Atrial Fibrillation during Sepsis: A Propensity-Matched Cohort Study. Chest 149 (1), 74–83. doi:10.1378/chest.15-0959
Wang, S., Li, M., Duan, J., Yi, L., Huang, X., Chen, D., et al. (2017). [Effect of Esmolol on Hemodynamics and Clinical Outcomes in Patients with Septic Shock]. Zhonghua Wei Zhong Bing Ji Jiu Yi Xue 29 (5), 390–395. doi:10.3760/cma.j.issn.2095-4352.2017.05.002
Wang, Z., Wu, Q., Nie, X., Guo, J., and Yang, C. (2015). Combination Therapy with Milrinone and Esmolol for Heart protection in Patients with Severe Sepsis: a Prospective, Randomized Trial. Clin. Drug Investig. 35 (11), 707–716. doi:10.1007/s40261-015-0325-3
Williams, F. N., Herndon, D. N., Kulp, G. A., and Jeschke, M. G. (2011). Propranolol Decreases Cardiac Work in a Dose-dependent Manner in Severely Burned Children. Surgery 149 (2), 231–239. doi:10.1016/j.surg.2010.05.015
Wilmore, D. W., Long, J. M., Mason, A. D., Skreen, R. W., and Pruitt, B. A. (1974). Catecholamines: Mediator of the Hypermetabolic Response to Thermal Injury. Ann. Surg. 180 (4), 653–669. doi:10.1097/00000658-197410000-00031
Wittstein, I. S., Thiemann, D. R., Lima, J. A., Baughman, K. L., Schulman, S. P., Gerstenblith, G., et al. (2005). Neurohumoral Features of Myocardial Stunning Due to Sudden Emotional Stress. N. Engl. J. Med. 352 (6), 539–548. doi:10.1056/NEJMoa043046
Wortsman, J., Frank, S., and Cryer, P. E. (1984). Adrenomedullary Response to Maximal Stress in Humans. Am. J. Med. 77 (5), 779–784. doi:10.1016/0002-9343(84)90512-6
Writing, C., Maddox, T. M., Januzzi, J. L., Allen, L. A., Breathett, K., Butler, J., et al. (2021). 2021 Update to the 2017 ACC Expert Consensus Decision Pathway for Optimization of Heart Failure Treatment: Answers to 10 Pivotal Issues about Heart Failure with Reduced Ejection Fraction: A Report of the American College of Cardiology Solution Set Oversight Committee. J. Am. Coll. Cardiol. 77 (6), 772–810. doi:10.1016/j.jacc.2020.11.022
Xinqiang, L., Weiping, H., Miaoyun, W., Wenxin, Z., Wenqiang, J., Shenglong, C., et al. (2015). [Esmolol Improves Clinical Outcome and Tissue Oxygen Metabolism in Patients with Septic Shock through Controlling Heart Rate]. Zhonghua Wei Zhong Bing Ji Jiu Yi Xue 27 (9), 759–763.
Yang, N., Shi, X. L., Zhang, B. L., Rong, J., Zhang, T. N., Xu, W., et al. (2018). The Trend of β3-Adrenergic Receptor in the Development of Septic Myocardial Depression: A Lipopolysaccharide-Induced Rat Septic Shock Model. Cardiology 139 (4), 234–244. doi:10.1159/000487126
Yang, S., Liu, Z., Yang, W., Zhang, G., Hou, B., Liu, J., et al. (2014). [Effects of the β-blockers on Cardiac protection and Hemodynamics in Patients with Septic Shock: a Prospective Study]. Zhonghua Wei Zhong Bing Ji Jiu Yi Xue 26 (10), 714–717. doi:10.3760/cma.j.issn.2095-4352.2014.10.007
Zangbar, B., Khalil, M., Rhee, P., Joseph, B., Kulvatunyou, N., Tang, A., et al. (2016). Metoprolol Improves Survival in Severe Traumatic Brain Injury Independent of Heart Rate Control. J. Surg. Res. 200 (2), 586–592. doi:10.1016/j.jss.2015.08.020
Keywords: beta-blockers, critical illness, sepsis, esmolol, tachyarrhythmia, hemodynamics
Citation: Bruning R, Dykes H, Jones TW, Wayne NB and Sikora Newsome A (2021) Beta-Adrenergic Blockade in Critical Illness. Front. Pharmacol. 12:735841. doi: 10.3389/fphar.2021.735841
Received: 03 July 2021; Accepted: 27 September 2021;
Published: 15 October 2021.
Edited by:
Mahmoud El-Mas, Alexandria University, EgyptReviewed by:
Folke Bror Sjoberg, Linköping University Hospital, SwedenSamuel Tisherman, University of Maryland, United States
Copyright © 2021 Bruning, Dykes, Jones, Wayne and Sikora Newsome. This is an open-access article distributed under the terms of the Creative Commons Attribution License (CC BY). The use, distribution or reproduction in other forums is permitted, provided the original author(s) and the copyright owner(s) are credited and that the original publication in this journal is cited, in accordance with accepted academic practice. No use, distribution or reproduction is permitted which does not comply with these terms.
*Correspondence: Andrea Sikora Newsome, sikora@uga.edu