- 1Department of Respiratory, Changchun University of Chinese Medicine, Changchun, China
- 2State Key Laboratory of Quality Research in Chinese Medicines, Faculty of Chinese Medicine, Macau University of Science and Technology, Macau, China
- 3College of Integrated Traditional Chinese and Western Medicine, Changchun University of Chinese Medicine, Changchun, China
- 4Jilin Ginseng Academy, Key Laboratory of Active Substances and Biological Mechanisms of Ginseng Efficacy, Ministry of Education, Jilin Provincial Key Laboratory of Bio-Macromolecules of Chinese Medicine, Changchun University of Chinese Medicine, Changchun, China
- 5Department of Scientific Research, Changchun University of Chinese Medicine, Changchun, China
Respiratory diseases, especially the pandemic of respiratory infectious diseases and refractory chronic lung diseases, remain a key clinical issue and research hot spot due to their high prevalence rates and poor prognosis. In this review, we aimed to summarize the recent advances in the therapeutic effects and molecular mechanisms of key common bioactive compounds from Chinese herbal medicine. Based on the theories of traditional Chinese medicine related to lung diseases, we searched several electronic databases to determine the high-frequency Chinese medicines in clinical application. The active compounds and metabolites from the selected medicines were identified using the Traditional Chinese Medicine Systems Pharmacology Database (TCMSP) by analyzing oral bioavailability and drug similarity index. Then, the pharmacological effects and molecular mechanisms of the selected bioactive compounds in the viral and bacterial infections, inflammation, acute lung injury (ALI), chronic obstructive pulmonary disease (COPD), pulmonary fibrosis, asthma, and lung cancer were summarized. We found that 31 bioactive compounds from the selected 10 common Chinese herbs, such as epigallocatechin-3-gallate (EGCG), kaempferol, isorhamnetin, quercetin, and β-sitosterol, can mainly regulate NF-κB, Nrf2/HO-1, NLRP3, TGF-β/Smad, MAPK, and PI3K/Akt/mTOR pathways to inhibit infection, inflammation, extracellular matrix deposition, and tumor growth in a series of lung-related diseases. This review provides novel perspectives on the preclinical study and clinical application of Chinese herbal medicines and their bioactive compounds against respiratory diseases.
Introduction
Respiratory diseases include respiratory infectious diseases, asthma, chronic obstructive pulmonary disease (COPD), interstitial pulmonary disease (ILD), and lung cancer. These diseases are characterized by the injuries of bronchial and alveolar tissue to cause respiratory dysfunction and even respiratory failure. Respiratory infectious diseases mainly caused by viruses or bacteria and often contagious, remain a major global public health problem. For example, since the outbreak of coronavirus disease (COVID-19) at the end of 2019, there have been nearly 180 million confirmed cases, including 3.9 million deaths by June 25, 2021 (Mortality et al., 2019; Berlin et al., 2020). Pneumonia is another common respiratory infection, it can lead to hospitalization and death in all age group, and the annual costs exceed $10 billion in the United States and Europe (Global, 2018; Stets et al., 2019). Chronic respiratory diseases such as COPD, ILD, pulmonary fibrosis (PF), and lung cancer, seriously affect human health, these diseases were associated with more than 4 million deaths (7% of all deaths) worldwide in 2017 (Global, 2018). They are induced by long-term exposure to airborne pollutants, tobacco, or kitchen smoke, and their mortality by 18.0% in the last 30 years (Li et al., 2020a). COPD has become the fourth leading cause of death worldwide (Ferkol and Schraufnagel, 2014). Moreover, the quality of life in patients with ILD and IPF is severely affected due to progressive scarring of the lung parenchyma and impairment of pulmonary function (Wollin et al., 2019; Spagnolo et al., 2021). In addition, lung cancer has poor survival and high mortality, and it is the most common cause of cancer-related death worldwide (Siegel et al., 2021). The concern due to the global burden of respiratory diseases, such as the ongoing global pandemic of COVID-19, COPD, and lung cancer, has stimulated research on the treatment and prevention of respiratory diseases. Therefore, the therapeutic effects and molecular mechanisms of potential intervention strategies have become a hot spot for multidisciplinary research.
Traditional Chinese medicine (TCM) has a history of more than 3,000 years and has been used for the prevention and treatment of many respiratory diseases. The ancient medicine books named “Shennong Ben Cao Jing” and “Shanghan Lun” clearly recorded the theories of traditional Chinese medicine, such as reducing phlegm and relieving cough and asthma, and many prescriptions for the prevention and treatment of lung-related diseases. Based on thousands of years of clinical application and the modernization of TCM research, hundreds of Chinese medicines have been shown to be effective in the current clinical applications for treating respiratory infections, asthma, chronic lung diseases, and lung cancer; these effects are based on the therapeutic and improved effects for acute respiratory symptoms and lung dysfunction (Ren et al., 2020; Zhang et al., 2021). Importantly, bioactive compounds or their metabolites from these medicines with high-frequency use, such as saponins, flavonoids, alkaloids, and phenolic acids, are critical for the prevention and treatment of respiratory diseases (Shahidi and Yeo, 2018; Ory et al., 2019; Russo et al., 2020). Currently, the roles of different medicines are summarized in most review, not for potential active components of these medicines for fighting the diseases of respiratory systems. It is necessary to summarize the recent findings regarding the therapeutic effects and molecular mechanisms of bioactive compounds from commonly used Chinese medicines for preventing and treating a series of lung-related diseases. In this review, we first searched English or Chinese electronic databases for clinical studies of TCM against respiratory diseases to identify the Chinese medicines with high-frequency use in the clinical setting. The active components and their metabolites from the selected medicines were identified using the Traditional Chinese Medicine Systems Pharmacology Database (TCMSP) by analyzing oral bioavailability and drug similarity index. Then, the published studies for advanced research of those bioactive compounds after screening in multiple disorders of respiratory system were collected. Finally, we summarized the pharmacological effects and molecular mechanisms of the selected bioactive compounds in the viral and bacterial infections, inflammation, acute lung injury (ALI), COPD, PF, and lung cancer. This review provides new insights into the clinical use of medicinal herbs for the prevention and treatment of respiratory diseases.
High-Frequency Use of Chinese Medicine and Literature Collection
According to the theories of TCM involving lung-related diseases, we searched Chinese or English electronic databases including CNKI database, Wanfang Data Knowledge Service Platform, VIP Chinese Science and Technology Journal database, PubMed database, and Web of Science database with keywords such as “traditional Chinese medicine,” “Chinese medicine,” or “respiratory diseases.” After the literature retrieval, the Chinese medicines widely used in clinical applications for reducing phlegm (Morus alba L., Moraceae family, Chinese name: Sangbaipi, peel; Ginkgo biloba L., Ginkgoaceae family, Chinese name: Baiguo, seed; Aster tataricus L.f., Compositae family, Chinese name: Ziwan, root) and relieving cough and asthma (Perilla frutescens (L.) Britton, Lamiaceae family, Chinese name: Suzi, seed; Tussilago farfara L., Compositae family, Chinese name: Kuandonghua, flower; Datura metel L., Solanaceae family, Chinese name: Yangjinhua, flower; Ardisia japonica (Thunb.) Blume, Primulaceae family, Chinese name: Aidicha, leaf; Lepidium apetalum Willd., Brassicaceae family, Chinese name: Tinglizi, seed; Eriobotrya japonica (Thunb.) Lindl., Rosaceae family, Chinese name: Pipaye leaf; Prunus mandshurica (Maxim.) Koehne., Rosaceae family, Chinese name: Kuxingren, seed) were selected.
The effective components and their metabolites of the selected 10 medical plants were searched in the Traditional Chinese Medicine Systems Pharmacology Database (TCMSP, https://old.tcmsp-e.com/index.php, version 2.3). The active compounds of each herb were sorted out by the screening criteria with (oral bioavailability ≥30% and drug-likeness ≥0.18) for the ADME (absorption, distribution, metabolism, and excretion) evaluation system. After sorting, we identified 165 bioactive compounds from these 10 herbs, such as epigallocatechin-3-gallate (EGCG), kaempferol, apigenin, ellagic acid and resveratrol for further analysis. Then, we searched the databases (PubMed, EMBASE, or Web of Science) using the keywords for one of the ingredients from the TCMSP and a type of disease, such as respiratory infection, COVID-19, inflammation, ALI, PF, COPD, asthma, or lung cancer to obtain articles published from January 2000 to May 2021.
Articles that included both components and disease terms, excluding review articles were identified as reference lists (4,519 articles). Titles and abstracts of all the records were screened to exclude irrelevant studies (duplicates: n = 3,276; publication before 2000: n = 171, non-English: n = 20). We further excluded the irrelevant records for the subject (n = 416), target herbs (n = 216), Chinese medicinal formulae/mixture compounds (n = 60), targeting drug delivery system (n = 25), or computational study without experimental validation (n = 60). Moreover, 74 reports for component analysis were added to obtain 349 full-text articles for eligibility assessment. Finally, 129 articles for the therapeutic effects and molecular mechanisms of 31 bioactive compounds from the selected 10 herbs were enrolled in the final analysis, after excluding similar studies or those not relevant to our topic of this review (n = 234). The detailed flow chart of the published articles collection is shown in Figure 1.
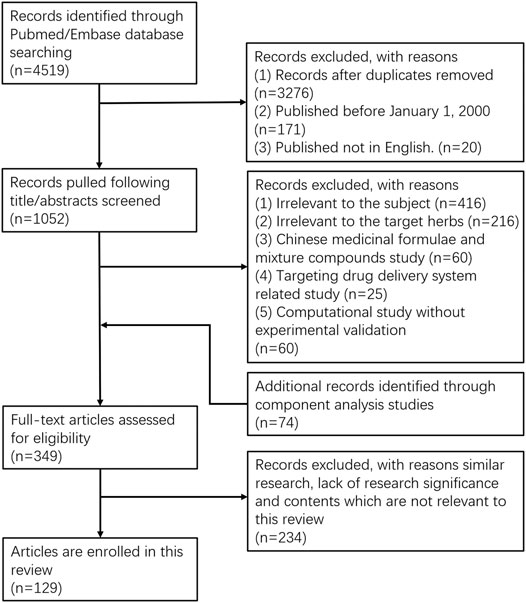
FIGURE 1. Flow diagram of literature collection methods for bioactive compounds from commonly used of traditional Chinese medicine in clinical applications.
Therapeutic Effects and Molecular Mechanisms of Bioactive Compounds Against Respiratory Diseases
Viral and Bacterial Infections
Bacterial and viral infections account for up to 70% of all pathogenic diseases in humans (Smith et al., 2014). Influenza is one of the most prevalent respiratory diseases, and accounts for nearly 5–15% of people all respiratory infections. Although most patients recover, about 0.5 million people die of influenza each year (Petrova and Russell, 2018). The outbreak of COVID-19 has become a global health emergency on a pandemic scale, which has given rise to various studies and developments of antiviral drugs and vaccines. Coronaviruses identify the angiotensin-converting enzyme 2 (ACE2) as the main entry point into the respiratory epithelial cells of the host (Zhou et al., 2020a). Potential targets, including retinoic acid-inducible gene I (RIG-I)/melanoma differentiation-associated gene 5/mitochondrial antiviral signaling/TNF receptor-associated factor 3/interferon regulatory factor 3 (IRF3)/IRF7, and Toll-like receptors (TLRs)/TIR-domain-containing adapter-inducing interferon-β/nuclear factor kappa B (NF-κB)/mitogen-activated protein kinase (MAPK)/activating protein-1 (AP-1) pathways as intercellular sensors have been detected to study translation and budding process of SARS-CoV-1 and MERS viruses infection with SARS-CoV-1 using in vitro and in vivo models (Stertz et al., 2007), which may cause cellular death, hyperinflammation, and cytokine storm during viral infections (Azkur et al., 2020).
TCM could be a great potential resource for the development of innovative pharmacotherapies against infections. It has been reported that Lianhuaqingwen granules (active ingredients including Forsythia suspensa (Thunb.) Vahl, Lonicera japonica Thunb., and Prunus mandshurica (Maxim.) Koehne., Rosaceae) (Jia et al., 2015), Shufeng Jiedu capsule (active ingredients including Forsythia suspensa (Thunb.) Vahl, Strobilanthes cusia (Nees) Kuntze, and Bupleurum chinense DC.) (Liu et al., 2019a), Huoxiang Zhengqi dropping pills (active ingredients including Pogostemon cablin (Blanco) Benth., Platycodon grandiflorus (Jacq.) A.DC., and Pinellia ternata (Thunb.) Makino) (Li et al., 2006) and Haishiyi formula (active ingredients including Ephedra sinica Stapf, Prunus mandshurica (Maxim.) Koehne., and Atractylodes macrocephala Koidz.) can improve clinical symptoms, such as fatigue, cough, and fever, reduce the usage rate of antibiotics, and prevent the progression to severe COVID-19 (Xiao et al., 2020a; Tian et al., 2020; Xia et al., 2021). Currently, canti-COVID-19 agents mainly target SARS-CoV-2 spike receptor-binding domain or ACE2 enzyme activity to block the entry of COVID-19 to the cells. EGCG from Eriobotrya japonica (Thunb.) Lindl., and Ginkgo biloba L., and isorhamnetin found in Lepidium apetalum Willd., Eriobotrya japonica (Thunb.) Lindl., Ginkgo biloba L., and Aster tataricus L.f. exhibit the ability to prevent SARS-CoV-2 from entering into ACE2+ cells (Henss et al., 2021; Maiti and Banerjee, 2021; Zhan et al., 2021). Neochlorogenic acid from Tussilago farfara L. and Lianhuaqingwen granules inhibit the ACE2 enzyme activity (Chen et al., 2021a). Hesperidin and hyperoside from Eriobotrya japonica (Thunb.) Lindl. show antiviral and anti-inflammatory effects against H1N1 virus (Ding et al., 2018; Ling et al., 2020). In H9N2 virus-induced pneumonia, kaempferol inhibits TLR4/Myeloid differentiation factor 88 (MyD88)/NF-κB signaling pathways to reduce the production of inflammatory factors and enhance antioxidant ability (Zhang et al., 2017a). β-sitosterol from eight herbs, such as Morus alba L., and Datura metel L., inhibits RIG-I and signal transducer and activator of transcription 1 (STAT1) signaling pathway to improve interferon sensitization (Zhou et al., 2020b). As for bacterial infections, it has been reported that benzaldehyde has a good inhibitory effect on a variety of bacteria (Lee et al., 2014a). SARS-CoV-2 spike receptor-binding domain, ACE2, and inflammatory response are essential targets of these bioactive compounds, which may be related to Toll-like receptor and MAPK signaling pathways. Overall, the antiviral and antibacterial effects of these active compounds mentioned above are shown in Table 1.
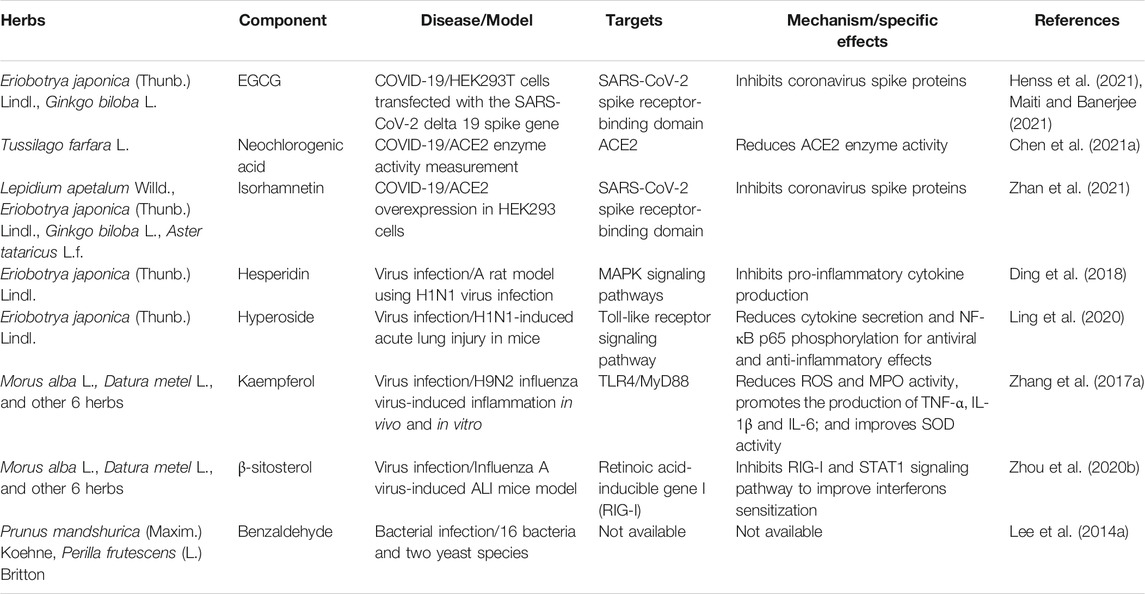
TABLE 1. Summary of effects and mechanisms of bioactive compounds against bacterial and viral infections.
Inflammation and ALI
ALI is common in pulmonary infection, lung contusion, pulmonary embolism, and near-drowning, it can lead to acute respiratory distress syndrome (ARDS) (Suresh et al., 2000). The mortality of ARDS ranges from 35 to 46%, which is higher than mortality of breast cancer or HIV infection (Fan et al., 2018). Patients recovered from ARDS may experience physical, neuropsychiatric, and neurocognitive morbidity that persistently impair their quality of life (Fan et al., 2014). Inflammation, bacterial and viral infections are the most common causes of ALI (Fan and Fan, 2018). The pathogenesis of ALI is believed to be related to inflammation, oxidative stress, cell apoptosis, and hypoxia, involving major cytokines such as tumor necrosis factor-α (TNF-α), interleukin (IL)-6, and IL-1β, IL-9, and IL-8, as well as the chemokines, such as chemokine-2 (CCL-2), monocyte chemotactic factors (MIP), and macrophage chemoattractant protein (MCP). The critical signaling pathways mainly include NF-κB, MAPK, nucleotide-binding oligomerization domain, NOD-like receptor family pyrin domain containing 3 (NLRP3), TLRs, adrenergic receptors, the Janus kinase (JAK)/STAT, and AMP-activated protein kinase (AMPK)- anti-thymocyte globulin (ATG7) signaling pathways (Chang et al., 2018a; Nadeem et al., 2018). The potential targets include of superoxide dismutase (SOD), glutamate-cysteine ligase catalytic subunit (GCLC), NAD(P)H, quinone-1 (NQO1), catalase (CAT), glutathione peroxidase (GSH-Px), and heme oxygenase-1 (HO-1) (Sun et al., 2018; Zhou et al., 2020a). Collectively, intrapulmonary oxidants derived from either activated lung macrophages or oxidant-generating enzymes delivered into the lung are two main pathways of oxidative stress, which can induce ALI and, more seriously, ARDS (Ward, 2010).
The model of ALI is mainly based on the induction by lipopolysaccharide (LPS) in in vivo and in vitro experiments. Other inflammatory substances and harmful chemicals such as N-methyl-d-aspartate, methamphetamine, and paraquat (PQ) are also used in ALI studies. TNF-α and other cytokines are commonly used in the in vitro model construction of ALI. The changes in pulmonary function, lung wet/dry ratios, the morphology of lung tissue, and inflammatory factors in alveolar lavage fluid and serum are generally used to evaluate the inflammatory response. Canonical NF-κB pathway directly induces proinflammatory cytokines such as TNF-α, IL-1β, and IL-6. Subsequently, the activation of IL-1R1 and TNFR1 can make a positive feedback to activate the crucial pathway of inflammation via the NF-κB pathway (Yu et al., 2020a). Ellagic acid, apigenin, EGb761, galangin, isorhamnetin, and kaempferol from Ginkogo biloba, Aster tataricus L.f., Eriobotrya japonica (Thunb.) Lindl., and Lepidium apetalum Willd. can reduce the production of inflammatory cytokines and oxidative stress to prevent LPS-induced ALI in mice through the NF-κB pathway (Cornélio Favarin et al., 2013; Huang et al., 2013; Lee et al., 2014b; Shu et al., 2014; Wang et al., 2014; Chi et al., 2016; Li et al., 2016; Luan et al., 2016; Liu et al., 2018a; Qian et al., 2019; Júlio de Souza et al., 2020; Ren et al., 2021). TLR4/MYD88, an upstream player of the NF-κB pathway, mediates the inflammation and ALI. Both processes are ameliorated by ferulic acid and hesperidin from Aster tataricus L.f. and Eriobotrya japonica (Thunb.) Lindl., which have anti-inflammatory activities and protective effects against ALI by downregulating cytokines and chemokines (Ma et al., 2015; Wu et al., 2021). Furthermore, myeloid differentiation 2 (MD2) and high-mobility group box 1 (HMGB1) are the key targets of hesperidin, through which it can effectively inhibit inflammation during ALI (Liu et al., 2015; Ma et al., 2015). Rutin and moracin M from Eriobotrya japonica (Thunb.) Lindl. and Morus alba L. improve ALI through a crosstalk of the MAPK and the NF-kB signaling pathways (Yeh et al., 2014; Liu et al., 2015; Ma et al., 2015; Huang et al., 2016; Lee et al., 2016; Ye et al., 2019). The NLRP3 inflammasome processes the interleukin precursors into their mature forms, such as IL-1β and IL-18, which results in inflammation (Afonina et al., 2017). The bioactive components from Prunus mandshurica (Maxim.) Koehne, Eriobotrya japonica (Thunb.) Lindl., and Morus alba L. amygdalin and resveratrol suppress NF-κB activity and ROS production via inhibiting NLRP3 inflammasome (Jiang et al., 2016a; Zhang et al., 2017b). SIRT1, the NAD+-dependent protein deacetylase, provides “stop signals” for inflammatory and oxidative stress (Jiang et al., 2016a; de Oliveira et al., 2019; Tsai et al., 2019). Resveratrol and oleanolic acid from Perilla frutescens (L.) Britton, Eriobotrya japonica (Thunb.) Lindl. and Morus alba L. reduce PTEN and NF-κB acetylation through the activation of SIRT1 (Peng et al., 2019; Wang et al., 2020a). Quercetin and formononetin from most of the 10 herbs enhance Nrf2/HO-1-mediated cytoprotective effects and prevent LPS-induced lung inflammation (Ma et al., 2013; Takashima et al., 2014; Wang et al., 2018a; Chen et al., 2021b). Luteolin downregulates cytokine and oxidative stress, ICAM-1 through the NF-κB pathway and induces Treg differentiation against ALI (Rungsung et al., 2018; Xie et al., 2021). According to the role of miRNAs in lung inflammation, it has been shown that resveratrol downregulates miR-193a to target transforming growth factor-β2 (TGF-β2), TGFβ receptor (TGFβR3), and death receptor-6 (Alghetaa et al., 2018). Liquiritin from Prunus mandshurica (Maxim.) Koehne inhibits the expression of TRPV1 and TRPA1 thereby providing anti-inflammatory and anticough effects (Liu et al., 2020). Taken together, 16 active compounds in 10 herbs have potential roles in inhibiting lung inflammation and injury through NF-κB, MAPK, NLRP3, PI3K/Akt, SIRT1, and HO-1 pathways. More details for the therapeutic effects and molecular mechanism of these compounds against inflammation and ALI are shown in Table 2.
Chronic Obstructive Pulmonary Disease
The pathogenesis of COPD is related to chronic inflammation, oxidative stress, cellular senescence, corticosteroid resistance, cell apoptosis, and changes in pulmonary histology and functions. The proinflammatory cytokines and chemokines (TNF-α, IL-1, IL-6, and IL-8), the signaling pathways (NF-κB and MAPK pathways), and various stress-related molecules (SOD, MDA, GSH) participate in the different pathological stages of COPD (Yang et al., 2020). IL-8 recruits neutrophils and secretes several neutrophil elastases and metalloproteases, e.g., MMP-9, which results in alveolar destruction. GM-CSF and IL-6 contribute to the increase in airway smooth muscle mass and proliferation, leading to bronchial obstruction (Knobloch et al., 2018; Jamal Jameel et al., 2021). Human airway smooth muscle cells (HASMCs) contributing to the secretion of cytokines and chemokines are related to non-type 2 airway inflammation and remodeling processes in COPD (Knobloch et al., 2013; Knobloch et al., 2016; Knobloch et al., 2019). Exposure to gases from cigarette smoking and inhaled particles such as PM2.5 are two archetypical inducing factors of COPD, which means that cigarette smoke and PM2.5 are commonly used for establishing in vivo and in vitro models of COPD (Rabe and Watz, 2017). Many studies have shown that multiple herbs, such as Tussilago farfara L., Eriobotrya japonica (Thunb.) Lindl., and Morus alba L. can inhibit the progression of COPD. Tussilagone and EGCG from the herbs mentioned above enhance the antiproliferative activity through the inhibition of the NF-κB pathway (Choi et al., 2018; Lakshmi et al., 2020). Amygdalin ameliorates the process of epithelial-mesenchymal transition (EMT) through the TGF-β/Smad pathway in cigarette smoke-exposed BEAS-2B cell line and mice model (Wang et al., 2019). Ursolic acid attenuates emphysema and enhances airway remodeling via unfolded protein response (UPR) signaling pathways (Lin et al., 2019; Li et al., 2020b). Liquiritin can reduce pulmonary inflammation by targeting the TGF-β pathway (Guan et al., 2012). Resveratrol inhibits the autophagic process and decreases IL-1β production by inactivation of NLRP3 inflammasome (Ding et al., 2019) or regulation of p53 destabilization (Navarro et al., 2017). Alveolar macrophages are important immune and inflammatory regulatory cells in the lung tissue (Gerlach et al., 2015). Resveratrol reduces the expression of MMP-9, GM-CSF and inflammatory mediators including IL-6, IL-8, and MCP-1 in alveolar macrophages under the stimulation of different harmful substances (Culpitt et al., 2003; Knobloch et al., 2011). Other reports have shown that resveratrol inhibits cytokines and chemokines (CCL-2, IL-6, IL-8) and ameliorates bronchial obstruction-related secretory proteins (GM-CSF and VEGF) in HASMCs from smokers and COPD patients. Similar to the findings against inflammation and ALI, SIRT1 and p38 MAPK are regarded as therapeutic targets of resveratrol in lipoteichoic acid (LTA)- or TNF-α-stimulated HASMC models (Knobloch et al., 2010; Knobloch et al., 2014). The abovementioned therapeutic effects and mechanisms of resveratrol have also been demonstrated in animal models (Chen et al., 2016; Wang et al., 2017a). Together, these findings suggest that six main compounds can regulate NF-κB, UPR, TGF-β, MAPK and SIRT1 pathways to inhibit COPD in different cell and animal models (Table 3).
Pulmonary Fibrosis
It is now clear that many elements of the innate and adaptive immune response participate in the differentiation and activation of fibroblasts. The pathogenesis of PF is related to adaptive and innate immune activation, inflammation, epithelial/endothelial damage, EMT and cell apoptosis. Specifically, the activation of TGF-β or NF-κB pathway is the primary factor driving the progression of PF (Kitani et al., 2003; Wynn and Ramalingam, 2012). Some natural products, such as β-sitosterol, quercetin, ferulic acid, hesperidin, and EGb761 from various herbs, inhibit PF by downregulating TGF-β. β-sitosterol and ferulic acid suppress EMT and reduce extracellular matrix (ECM) through the TGF-β/Smad-dependent signaling pathways (Park et al., 2019; Ali et al., 2021). Quercetin suppresses Akt/mammalian target of rapamycin (mTOR) pathway in TGF-β-mediated responses and reduces fibrotic factors, such as collagen I, collagen III, and IL-6 (Xiao et al., 2020b). Another study has reported that quercetin enhances the expression of caveolin1 (CAV1), the cell membrane lipid raft and a protective factor for PF, to inhibit ligand-induced apoptosis in fibroblasts (Hohmann et al., 2019). For other bioactive compounds, hesperidin and EGb761 improve the progression of PF by mediating the proinflammatory cytokines and apoptosis-related proteins via the crosstalk of NF-κB and TGF-β pathways (Zhou et al., 2019; Pan et al., 2020).
Hyperoside inhibits the EMT via the regulation of the Akt/GSK3β pathway (Huang et al., 2020). Ellagic acid suppresses ECM accumulation by regulating the Wnt pathway (Li et al., 2021). EGCG reduces the production of cytokines through the Nrf-2/HO-1 pathway (Sriram et al., 2009; You et al., 2014). Galangin and isorhamnetin attenuate EMT and inflammatory damage in bleomycin or TGF-β-induced PF models (Zheng et al., 2019; Wang et al., 2020b). Kaempferol promotes autophagy in the therapeutic effects on PF (Liu et al., 2019b). Resveratrol regulates miR-21/Smad7 to alleviate serious PF symptoms (Wang et al., 2018b). Rosmarinic acid targets miR-19b-3p/MYPT1 to relieve the pulmonary fibrosis caused by radiotherapy (Zhang et al., 2020). Collectively, these results indicate that these bioactive compounds can reduce EMT and ECM deposition to inhibit progressive lung fibrosis by regulating TGF-β, Akt/GSK3β, Nrf-2/HO-1, or microRNA-mediated pathways (Table 4).
Asthma
Asthma is associated with the activation of IgE-mediated mast cells and eosinophilic inflammation. Inhaled corticosteroids which have a therapeutic effect on allergic reactions and sensitivity of type 2 inflammation, are the cornerstone treatment for asthma. Airway inflammation and remodeling, and airway hyperresponsiveness (AHR) promote the pathogenesis of asthma (Mishra et al., 2018). Naïve CD4 T cells are exposed to antigens and differentiate into various T helper (Th) cell types (e.g., Th1, Th2, Th17). Th2 cells play an important role in disease pathogenesis and progression (Chen and Kolls, 2013; Gaurav and Agrawal, 2013). However, neutrophilic inflammation has also been observed during asthma exacerbations as well asc in severe asthma patients (Ray and Kolls, 2017). Through the literature search, natural products from 10 different medical plants have a good inhibitory effect on the inflammation based on eosinophils and neutrophils in asthma. Unsurprisingly, the dysregulation of the NF-κB and MAPK signaling pathways associated with inflammation and immune response, plays a major role in asthma (Freund-Michel and Frossard, 2008; Zhang et al., 2013). Rosmarinic acid, tussilagone, formononetin, galangin, ellagic acid, and ginkgolide B can downregulate the levels of histamine, ovalbumin (OVA)-specific IgE, Th2 cytokines, and chemokines (IL-4, IL-5, IL-13, CCL5, and CCL11) in serum and bronchial alveolar lavage fluid through the suppression of the NF-κB and MAPK signaling pathways (Chu et al., 2011; Alves et al., 2013; Kim et al., 2013; Zha et al., 2013; Zhou et al., 2014; Liang et al., 2016a; Liang et al., 2016b; Henry et al., 2020; Jin et al., 2020; Yi et al., 2020). EGCG inhibits MMP-9 production, ROS generation, and EMT to reduce airway remodeling by upregulating PTEN (Kim et al., 2006; Yang et al., 2018). Kaempferol ameliorates airway hyperplasia and hypertrophy via the Syk-PLCγ and PKCμ-ERK-cPLA2-COX2 and NF-κB signaling pathways (Gong et al., 2012; Shin et al., 2015; Molitorisova et al., 2021). Glabridin, β-sitosterol and quercetin can suppress the level of serum IgE, TNF-α, IL-4, and IL-5, but the mechanism has not been thoroughly explored (Rogerio et al., 2007; Mahajan and Mehta, 2011; Dogan et al., 2020). Luteolin inhibits the inflammatory responses and autophagy via the PI3K/Akt/mTOR pathway (Jang et al., 2017; Wang et al., 2021). Taken together, in asthma, these 10 bioactive compounds can inhibit inflammatory reactions and airway remodeling through the MAPK and NF-κB pathways in OVA-induced animal models (Table 5).
Lung Cancer
Lung cancer is the malignant tumor with the highest mortality rate. It causes 1.6 million deaths every year, but treatment can effectively prolong survival and quality of life (Siegel et al., 2021). TCM treatment can effectively improve the quality of life and survival time of patients with advanced lung cancer with or without conventional therapy (Sun et al., 2019; Jiang et al., 2016b; Xu et al., 2011). Active components of TCM participate in the treatment of lung cancer through the regulation of multiple pathways (Table 6). Ursolic acid and β-sitosterol show a good lung cancer-inhibiting effect via the TGF-β/Smad pathway (Ruan et al., 2019; Sundarraj et al., 2012; Wang et al., 2017b). Caffeic acid and sanguinarine enhance the antiproliferative effect of paclitaxel in lung cancer A549 and H1299 cells (Lin et al., 2012). Sanguinarine can target NF-κB pathway-mediated autophagy and mitophagy to block lung cancer progression (Yu et al., 2020b). Meanwhile, the p53 protein is a transcription factor that inhibits cell proliferation or survival, acting as a key tumor suppressor protein (Skoulidis and Heymach, 2019). Loss or mutant of p53 induces lung cancer with shortened latency and increases rapid progression and poor prognosis (Donehower et al., 2019). Natural products such as hyperoside, resveratrol, liquiritin, and formononetin have a good effect on improving the antitumor function of p53 and inducing the apoptosis of tumor cells. Hyperoside increases Caspase-9/Caspase-3 activation to induce apoptosis in in vitro and in vivo models of A549 and H1975 cells (Liu et al., 2016; Lü, 2016). Resveratrol decreases antiapoptotic factors, Bcl-2 and Bcl-xl and the levels of MMP2, and MMP9 by upregulating the p53/HO-1 pathways against lung cancer (Liu et al., 2010; Rasheduzzaman et al., 2018; Li et al., 2019). Liquiritin decreases the expression levels of PCNA, p-PTEN, caspase family, and PARP (Zhou and Ho, 2014). Formononetin promotes Mcl-1 ubiquitination and degradation via Fbw7 to enhance the EGFR-TKI sensitivity (Yang et al., 2014; Yu et al., 2020c). The PI3K/Akt signaling pathway is an important dysregulated pathway in tumorigenesis, which controls lung cancer growth, metabolism, motility, and other key cellular processes (Janku et al., 2018). Isorhamnetin and apigenin inhibit EMT and decrease invasion by inhibiting Akt activation (Chang et al., 2018b; Luo et al., 2019). Moracin N induces autophagy mTOR signaling pathway (Gao et al., 2020). Furthermore, isorhamnetin as a potential application in adjuvant radiotherapy inhibits the activation of NF-κB and increases the expression of IL-13 (Du et al., 2020). Resveratrol and ellagic acid promote lung cancer cell apoptosis via the PI3K/Akt signaling pathway (Liu et al., 2018b; Li et al., 2019). Amygdalin downregulates the phosphorylation of Akt to inhibit invasion and migration of H1299 and PA cells (Qian et al., 2015). Inactivation of STAT3 is a target for increasing cisplatin sensitivity in lung cancer treatment, galangin, and laricitrin are STAT3 inhibitors in adjuvant chemotherapy (Chang et al., 2016a; Chang et al., 2016b; Yu et al., 2018). Oleanolic acid enhances mitophagy through the PINK1/Parkin axis in A549 cells (Castrejón-Jiménez et al., 2019). Rosmarinic acid could reverse the cisplatin resistance by inhibiting the expression of P-gp, MDR1, and MAPK pathways and plays a key role in the treatment of non-small cell lung cancer (NSCLC) (Liao et al., 2020). EGCG from Eriobotrya japonica (Thunb.) Lindl. and Ginkgo biloba L. can suppress the levels of Axl and Tyro three to reduce the resistance to platinum (Kim and Lee, 2014). Ginkgolide B and glabridin from Ginkgo biloba L. have inhibitory effects on autophagy and angiogenesis, mediated by Beclin-1 or FAK/Src complex, respectively (Tsai et al., 2011; Wang et al., 2020c). In H1975 cell model, ursolic acid inhibits the Wnt/β-catenin pathway to suppress proliferation and induce apoptosis (Yang et al., 2019). As a cisplatin sensitizing agent, ginkgetin enhances the ferroptosis-mediated disruption of the Nrf2/HO-1 axis (Lou et al., 2021). Kaempferol downregulates Nrf2 and upregulates miR-340 to induce apoptosis and autophagy (Han et al., 2018; Fouzder et al., 2021). As for quercetin, it can target aurora B or miR-16-5p/WEE1 pathways to inhibit lung cancer progression and enhance the radiosensitivity of NSCLC cells (Xingyu et al., 2016; Wang et al., 2020d). Hesperidin exhibits antiproliferative and apoptosis induction effects by regulating the miR-132/ZEB2 signaling pathway (Birsu Cincin et al., 2015; Tan et al., 2020). Luteolin inhibits cell proliferation and induces apoptosis via miR-34a-5p targeting MDM4 and RhoA (Jiang et al., 2018; Masraksa et al., 2020). Taken together, these results demonstrate that these bioactive compounds have anticancer effects by targeting multiple pathways, including NF-κB, p53, TGF-β, or miRNAs (Table 6).
Collectively, the network of bioactive compounds, targets, signal pathways, and different pulmonary diseases is visualized in Figure 2. These bioactive compounds, such as isorhamnetin, formononetin, resveratrol, and galangin are active substances of types of saponins, flavonoids, and alkaloids, which can regulate different key targets (NF-κB, PI3K/Akt, Nrf-2, NLRP3) to regulate cytokine production, oxidative stress or chemotherapy sensitivity against a series of lung-related diseases.
Discussion
According to the theories of TCM and commonly used Chinese medicines in the clinical application against respiratory diseases, this review summarizes the pharmacological effects and molecular mechanisms of 31 active compounds of 10 Chinese herbal medicines in six main lung-related diseases, including pulmonary infection, ALI, PF, COPD, asthma, and lung cancer. Many studies have demonstrated that bioactive compounds can ameliorate bacterial, virus, and LPS-induced pulmonary infection by targeting the NF-κB, MAPK, Nrf2/HO-1, and NLRP3 pathways, reducing the release of cytokines and chemokines, and suppressing inflammation by pathological reaction, oxidative stress, and ROS production. Eriobotrya japonica (Thunb.) Lindl. and its compounds (EGCG, isorhamnetin, hesperidin, hyperoside, kaempferol, β-sitosterol) may be considered an effective Chinese herbal medicine for the treatment of viral infections. Flavonoids, including apigenin, galangin, isorhamnetin, rutin, moracin M, amygdalin, hesperidin, quercetin, formononetin, luteolin, and liquiritin, exhibit good bioactivity against ALI. As for inflammation, bioactive compounds from Aster tataricus L.f. and Eriobotrya japonica (Thunb.) Lindl. have potential anti-inflammatory activity, suggesting that apigenin, quercetin, luteolin, and isorhamnetin are effective anti-inflammatory compounds. In the studies of COPD, bioactive compounds have mainly attenuated cigarette smoke-induced emphysema, airway remodeling, and inflammation through the NF-κB, MAPK, and TGF-β/Smad pathways, and resveratrol is one of the important and effective bioactive substances against COPD. Multiple components, including β-sitosterol, ferulic acid, quercetin, hesperidin, EGb761, and resveratrol, are directly or indirectly related to TGF-β/Smad, which is a crucial target for PF. These components can effectively suppress biological process of EMT and ECM. In asthma, rosmarinic acid, tussilagone, formononetin, and galangin targeting the MAPK and NF-κB pathways to reduce OVA-specific IgE, and ameliorate airway hyperplasia and hypertrophy. Importantly, these active components such as organic acids and flavonoids can inhibit the proliferation and migration of lung cancer and increase its sensitivity to radiotherapy and chemotherapy. Hyperoside, resveratrol, glabridin, luteolin, and kaempferol are considered potential candidates for the treatment of lung cancer based on a large number of studies. Collectively, ECCG, kaempferol, isorhamnetin, quercetin, and β-sitosterol are important bioactive compounds for prevention and treatment of ALI, PF, and lung cancer. Taken together, multiple bioactive compounds from the 10 different herbs have potential therapeutic effects against respiratory diseases by regulating various molecular pathways (Figures 2, 3).
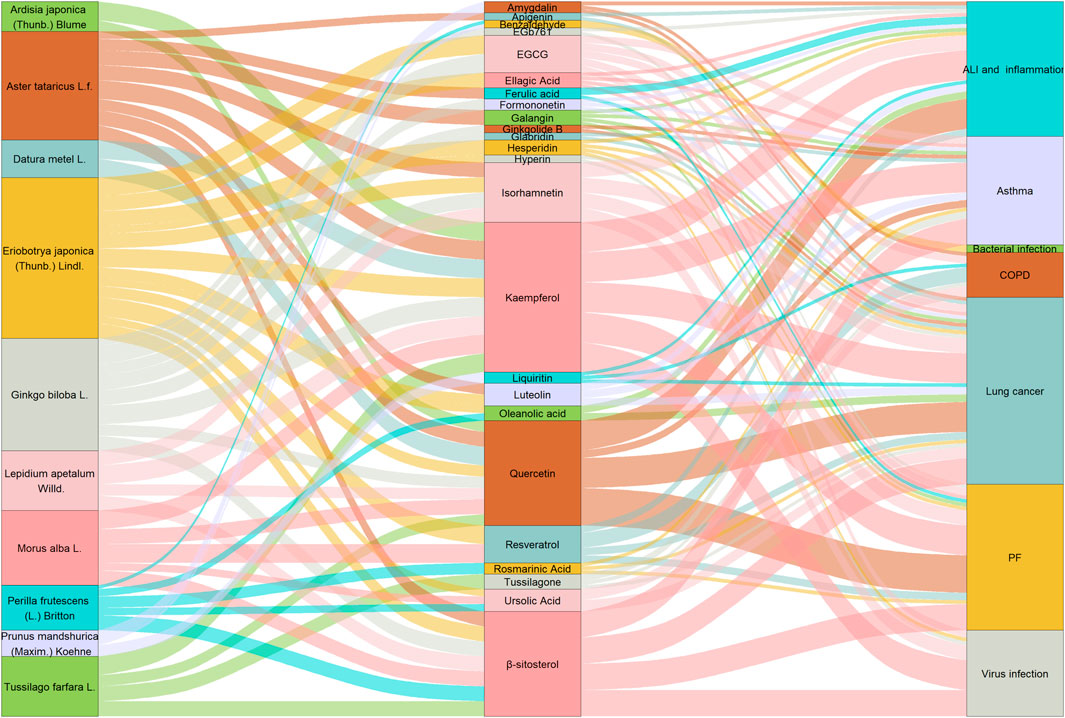
FIGURE 3. Network of different herbs, main bioactive compounds, and a series of lung-related diseases.
However, in the studies of different bioactive compounds on respiratory diseases, three important aspects should be considered. Firstly, only key and common active ingredients in each herb are summarized, which might not be fully representative of the herb. More active components should be further identified to explore their pharmacological effects against respiratory diseases. Secondly, multiple compounds In a herb can target similar or different signaling pathways to play the potential roles in those diseases. The network of various targets of different components might be used to explain the combined effect of the formula. Thirdly, different signaling pathways or pathological procedures in infection, inflammation, COPD, or lung cancer are potential targets for these active ingredients. However, the potential targets and the binding role of these active compounds still remain unclear. It should be a future direction for most researchers to confirm specific targets of those potential drug candidates using multiple modern techniques. Finally, the quantitative analysis for the biological activity, toxicity and selectivity of 31 bioactive compounds should be performed in a kind of respiratory diseases to predict the promising candidates for drug development using systematic review and meta-analysis. Overall, this review provides novel perspectives on the preclinical study and clinical application of herbal medicines and their bioactive compounds against respiratory diseases.
Conclusion
In summary, 10 Chinese herbal medicines were selected based on the theories of TCM and high-frequency use of Chinese medicines in clinical application. The pharmacological effects and molecular mechanisms of 31 bioactive compounds from these 10 Chinese herbs in infection, ALI, PF, COPD, asthma, and lung cancer were summarized. The bioactive compounds, such as epigallocatechin-3-gallate, kaempferol, isorhamnetin, quercetin, and β-sitosterol, can mainly regulate the NF-κB, Nrf2/HO-1, NLRP3, TGF-β/Smad, MAPK, and PI3K/Akt/mTOR pathways to inhibit infection, inflammation, extracellular matrix deposition, and tumor growth in a series of lung-related diseases. This review provides novel perspectives on the preclinical study and clinical application of Chinese herbal medicines and their bioactive compounds against respiratory diseases.
Author Contributions
JW and QW collected, analyzed, and reviewed the literatures and wrote the draft manuscript; JW, QW, LD, SS, and YL added/checked references and assembled figures/tables; LS, TW, and DZ supervised the manuscript; ZW and XL designed and revised the whole manuscript. All authors have read and agreed to the published version of the manuscript.
Funding
This study was supported by the Science and Technology Development Plan Project of Jilin Province (2020122235JC, 20200404057YY, 20200901003SF), National Natural Science Foundation of China (81804013), and Science and Technology Project of Education Department of Jilin Province (JJKH20210964KJ).
Conflict of Interest
The authors declare that the research was conducted in the absence of any commercial or financial relationships that could be construed as a potential conflict of interest.
Publisher’s Note
All claims expressed in this article are solely those of the authors and do not necessarily represent those of their affiliated organizations, or those of the publisher, the editors and the reviewers. Any product that may be evaluated in this article, or claim that may be made by its manufacturer, is not guaranteed or endorsed by the publisher.
Acknowledgments
We thank LetPub (www.letpub.com) for its linguistic assistance during the preparation of this manuscript.
References
Afonina, I. S., Zhong, Z., Karin, M., and Beyaert, R. (2017). Limiting Inflammation-The Negative Regulation of NF-Κb and the NLRP3 Inflammasome. Nat. Immunol. 18 (8), 861–869. doi:10.1038/ni.3772
Alghetaa, H., Mohammed, A., Sultan, M., Busbee, P., Murphy, A., Chatterjee, S., et al. (2018). Resveratrol Protects Mice against SEB-Induced Acute Lung Injury and Mortality by miR-193a Modulation that Targets TGF-β Signalling. J. Cel Mol. Med. 22 (5), 2644–2655. doi:10.1111/jcmm.13542
Ali, S. A., Saifi, M. A., Pulivendala, G., Godugu, C., and Talla, V. (2021). Ferulic Acid Ameliorates the Progression of Pulmonary Fibrosis via Inhibition of TGF-Β/smad Signalling. Food Chem. Toxicol. 149, 111980. doi:10.1016/j.fct.2021.111980
Alves, Cde. F., Angeli, G. N., Favarin, D. C., de Andrade, E. L., Chica, J. E., Faccioli, L. H., et al. (2013). The Effects of Proresolution of Ellagic Acid in an Experimental Model of Allergic Airway Inflammation. Mediators Inflamm. 2013, 863198. doi:10.1155/2013/863198
Azkur, A. K., Akdis, M., Azkur, D., Sokolowska, M., van de Veen, W., Brüggen, M. C., et al. (2020). Immune Response to SARS-CoV-2 and Mechanisms of Immunopathological Changes in COVID-19. Allergy 75 (7), 1564–1581. doi:10.1111/all.14364
Berlin, D. A., Gulick, R. M., and Martinez, F. J. (2020). Severe Covid-19. N. Engl. J. Med. 383 (25), 2451–2460. doi:10.1056/NEJMcp2009575
Birsu Cincin, Z., Unlu, M., Kiran, B., Sinem Bireller, E., Baran, Y., and Cakmakoglu, B. (2015). Anti-proliferative, Apoptotic and Signal Transduction Effects of Hesperidin in Non-small Cell Lung Cancer Cells. Cel Oncol (Dordr) 38 (3), 195–204. doi:10.1007/s13402-015-0222-z
Castrejón-Jiménez, N. S., Leyva-Paredes, K., Baltierra-Uribe, S. L., Castillo-Cruz, J., Campillo-Navarro, M., Hernández-Pérez, A. D., et al. (2019). Ursolic and Oleanolic Acids Induce Mitophagy in A549 Human Lung Cancer Cells. Molecules 24 (19). doi:10.3390/molecules24193444
Chang, H. Y., Chen, Y. C., Lin, J. G., Lin, I. H., Huang, H. F., Yeh, C. C., et al. (2018). Asatone Prevents Acute Lung Injury by Reducing Expressions of NF-[Formula: See text]B, MAPK and Inflammatory Cytokines. Am. J. Chin. Med. 46 (3), 651–671. doi:10.1142/s0192415x18500349
Chang, J. H., Cheng, C. W., Yang, Y. C., Chen, W. S., Hung, W. Y., Chow, J. M., et al. (2018). Downregulating CD26/DPPIV by Apigenin Modulates the Interplay between Akt and Snail/Slug Signaling to Restrain Metastasis of Lung Cancer with Multiple EGFR Statuses. J. Exp. Clin. Cancer Res. 37 (1), 199. doi:10.1186/s13046-018-0869-1
Chang, W. A., Hung, J. Y., Jian, S. F., Lin, Y. S., Wu, C. Y., Hsu, Y. L., et al. (2016). Laricitrin Ameliorates Lung Cancer-Mediated Dendritic Cell Suppression by Inhibiting Signal Transducer and Activator of Transcription 3. Oncotarget 7 (51), 85220–85234. doi:10.18632/oncotarget.13240
Chang, W. A., Hung, J. Y., Tsai, Y. M., Hsu, Y. L., Chiang, H. H., Chou, S. H., et al. (2016). Laricitrin Suppresses Increased Benzo(a)pyrene-Induced Lung Tumor-Associated Monocyte-Derived Dendritic Cell Cancer Progression. Oncol. Lett. 11 (3), 1783–1790. doi:10.3892/ol.2016.4153
Chen, J., Yang, X., Zhang, W., Peng, D., Xia, Y., Lu, Y., et al. (2016). Therapeutic Effects of Resveratrol in a Mouse Model of LPS and Cigarette Smoke-Induced COPD. Inflammation 39 (6), 1949–1959. doi:10.1007/s10753-016-0430-3
Chen, K., and Kolls, J. K. (2013). T Cell-Mediated Host Immune Defenses in the Lung. Annu. Rev. Immunol. 31, 605–633. doi:10.1146/annurev-immunol-032712-100019
Chen, X., Wu, Y., Chen, C., Gu, Y., Zhu, C., Wang, S., et al. (2021). Identifying Potential Anti-COVID-19 Pharmacological Components of Traditional Chinese Medicine Lianhuaqingwen Capsule Based on Human Exposure and ACE2 Biochromatography Screening. Acta Pharm. Sin B 11 (1), 222–236. doi:10.1016/j.apsb.2020.10.002
Chen, Y., Wei, D., Zhao, J., Xu, X., and Chen, J. (2021). Reduction of Hyperoxic Acute Lung Injury in Mice by Formononetin. PloS one 16 (1), e0245050. doi:10.1371/journal.pone.0245050
Chi, G., Zhong, W., Liu, Y., Lu, G., Lü, H., Wang, D., et al. (2016). Isorhamnetin Protects Mice from Lipopolysaccharide-Induced Acute Lung Injury via the Inhibition of Inflammatory Responses. Inflamm. Res. 65 (1), 33–41. doi:10.1007/s00011-015-0887-9
Choi, B. S., Kim, Y. J., Yoon, Y. P., Lee, H. J., and Lee, C. J. (2018). Tussilagone Suppressed the Production and Gene Expression of MUC5AC Mucin via Regulating Nuclear Factor-Kappa B Signaling Pathway in Airway Epithelial Cells. Korean J. Physiol. Pharmacol. 22 (6), 671–677. doi:10.4196/kjpp.2018.22.6.671
Chu, X., Ci, X., He, J., Wei, M., Yang, X., Cao, Q., et al. (2011). A Novel Anti-inflammatory Role for Ginkgolide B in Asthma via Inhibition of the ERK/MAPK Signaling Pathway. Molecules 16 (9), 7634–7648. doi:10.3390/molecules16097634
Cornélio Favarin, D., Martins Teixeira, M., Lemos de Andrade, E., de Freitas Alves, C., Lazo Chica, J. E., Artério Sorgi, C., et al. (2013). Anti-inflammatory Effects of Ellagic Acid on Acute Lung Injury Induced by Acid in Mice. Mediators Inflamm. 2013, 164202. doi:10.1155/2013/164202
Culpitt, S. V., Rogers, D. F., Fenwick, P. S., Shah, P., De Matos, C., Russell, R. E., et al. (2003). Inhibition by Red Wine Extract, Resveratrol, of Cytokine Release by Alveolar Macrophages in COPD. Thorax 58 (11), 942–946. doi:10.1136/thorax.58.11.942
de Oliveira, M. T. P., de Sá Coutinho, D., Tenório de Souza, É., Stanisçuaski Guterres, S., Pohlmann, A. R., Silva, P. M. R., et al. (2019). Orally Delivered Resveratrol-Loaded Lipid-Core Nanocapsules Ameliorate LPS-Induced Acute Lung Injury via the ERK and PI3K/Akt Pathways. Int. J. Nanomedicine 14, 5215–5228. doi:10.2147/ijn.S200666
Ding, S., Wang, H., Wang, M., Bai, L., Yu, P., and Wu, W. (2019). Resveratrol Alleviates Chronic "Real-World" Ambient Particulate Matter-Induced Lung Inflammation and Fibrosis by Inhibiting NLRP3 Inflammasome Activation in Mice. Ecotoxicol Environ. Saf. 182, 109425. doi:10.1016/j.ecoenv.2019.109425
Ding, Z., Sun, G., and Zhu, Z. (2018). Hesperidin Attenuates Influenza A Virus (H1N1) Induced Lung Injury in Rats through its Anti-inflammatory Effect. Antivir. Ther. 23 (7), 611–615. doi:10.3851/imp3235
Dogan, M. F., Parlar, A., Cam, S. A., Tosun, E. M., Uysal, F., and Arslan, S. O. (2020). Glabridin Attenuates Airway Inflammation and Hyperresponsiveness in a Mice Model of Ovalbumin-Induced Asthma. Pulm. Pharmacol. Ther. 63, 101936. doi:10.1016/j.pupt.2020.101936
Donehower, L. A., Soussi, T., Korkut, A., Liu, Y., Schultz, A., Cardenas, M., et al. (2019). Integrated Analysis of TP53 Gene and Pathway Alterations in the Cancer Genome Atlas. Cell Rep. 28 (11), 1370–e5. doi:10.1016/j.celrep.2019.08.06110.1016/j.celrep.2019.07.001
Du, Y., Jia, C., Liu, Y., Li, Y., Wang, J., and Sun, K. (2020). Isorhamnetin Enhances the Radiosensitivity of A549 Cells through Interleukin-13 and the NF-Κb Signaling Pathway. Front. Pharmacol. 11, 610772. doi:10.3389/fphar.2020.610772
Fan, E., Brodie, D., and Slutsky, A. S. (2018). Acute Respiratory Distress Syndrome: Advances in Diagnosis and Treatment. Jama 319 (7), 698–710. doi:10.1001/jama.2017.21907
Fan, E., Dowdy, D. W., Colantuoni, E., Mendez-Tellez, P. A., Sevransky, J. E., Shanholtz, C., et al. (2014). Physical Complications in Acute Lung Injury Survivors: a Two-Year Longitudinal Prospective Study. Crit. Care Med. 42 (4), 849–859. doi:10.1097/ccm.0000000000000040
Fan, E. K. Y., and Fan, J. (2018). Regulation of Alveolar Macrophage Death in Acute Lung Inflammation. Respir. Res. 19 (1), 50. doi:10.1186/s12931-018-0756-5
Ferkol, T., and Schraufnagel, D. (2014). The Global burden of Respiratory Disease. Ann. Am. Thorac. Soc. 11 (3), 404–406. doi:10.1513/AnnalsATS.201311-405PS
Fouzder, C., Mukhuty, A., and Kundu, R. (2021). Kaempferol Inhibits Nrf2 Signalling Pathway via Downregulation of Nrf2 mRNA and Induces Apoptosis in NSCLC Cells. Arch. Biochem. Biophys. 697, 108700. doi:10.1016/j.abb.2020.108700
Freund-Michel, V., and Frossard, N. (2008). The Nerve Growth Factor and its Receptors in Airway Inflammatory Diseases. Pharmacol. Ther. 117 (1), 52–76. doi:10.1016/j.pharmthera.2007.07.003
Gao, C., Sun, X., Wu, Z., Yuan, H., Han, H., Huang, H., et al. (2020). A Novel Benzofuran Derivative Moracin N Induces Autophagy and Apoptosis through ROS Generation in Lung Cancer. Front. Pharmacol. 11, 391. doi:10.3389/fphar.2020.00391
Gaurav, R., and Agrawal, D. K. (2013). Clinical View on the Importance of Dendritic Cells in Asthma. Expert Rev. Clin. Immunol. 9 (10), 899–919. doi:10.1586/1744666x.2013.837260
Gerlach, K., Köhler-Bachmann, S., Jungck, D., Körber, S., Yanik, S., Knoop, H., et al. (2015). Endothelin Receptor-Antagonists Suppress Lipopolysaccharide-Induced Cytokine Release from Alveolar Macrophages of Non-smokers, Smokers and COPD Subjects. Eur. J. Pharmacol. 768, 123–130. doi:10.1016/j.ejphar.2015.10.040
Global, regional. (2018). Global, Regional, and National Incidence, Prevalence, and Years Lived with Disability for 354 Diseases and Injuries for 195 Countries and Territories, 1990-2017: a Systematic Analysis for the Global Burden of Disease Study 2017. Lancet 392 (10159), 1789–1858. doi:10.1016/s0140-6736(18)32279-7
Gong, J. H., Shin, D., Han, S. Y., Kim, J. L., and Kang, Y. H. (2012). Kaempferol Suppresses Eosionphil Infiltration and Airway Inflammation in Airway Epithelial Cells and in Mice with Allergic Asthma. J. Nutr. 142 (1), 47–56. doi:10.3945/jn.111.150748
Guan, Y., Li, F. F., Hong, L., Yan, X. F., Tan, G. L., He, J. S., et al. (2012). Protective Effects of Liquiritin Apioside on Cigarette Smoke-Induced Lung Epithelial Cell Injury. Fundam. Clin. Pharmacol. 26 (4), 473–483. doi:10.1111/j.1472-8206.2011.00956.x
Han, X., Liu, C. F., Gao, N., Zhao, J., and Xu, J. (2018). Kaempferol Suppresses Proliferation but Increases Apoptosis and Autophagy by Up-Regulating microRNA-340 in Human Lung Cancer Cells. Biomed. Pharmacother. 108, 809–816. doi:10.1016/j.biopha.2018.09.087
Henry, L. J. K., Ramar, M. K., Palanisamy, S., Natesan, S., and Kandasamy, R. (2020). Mechanistic Investigation of PPARγ-Facilitated Anti-asthmatic Effects of Galangin (Norizalpinin): Insights from In Silico and In vivo Analyses. Biochem. Biophys. Res. Commun. 526 (3), 833–840. doi:10.1016/j.bbrc.2020.03.158
Henss, L., Auste, A., Schürmann, C., Schmidt, C., von Rhein, C., Mühlebach, M. D., et al. (2021). The green tea Catechin Epigallocatechin Gallate Inhibits SARS-CoV-2 Infection. J. Gen. Virol. 102 (4). doi:10.1099/jgv.0.001574
Hohmann, M. S., Habiel, D. M., Coelho, A. L., Verri, W. A., and Hogaboam, C. M. (2019). Quercetin Enhances Ligand-Induced Apoptosis in Senescent Idiopathic Pulmonary Fibrosis Fibroblasts and Reduces Lung Fibrosis In Vivo. Am. J. Respir. Cel Mol. Biol. 60 (1), 28–40. doi:10.1165/rcmb.2017-0289OC
Huang, C. H., Yang, M. L., Tsai, C. H., Li, Y. C., Lin, Y. J., and Kuan, Y. H. (2013). Ginkgo Biloba Leaves Extract (EGb 761) Attenuates Lipopolysaccharide-Induced Acute Lung Injury via Inhibition of Oxidative Stress and NF-κb-dependent Matrix Metalloproteinase-9 Pathway. Phytomedicine 20 (3-4), 303–309. doi:10.1016/j.phymed.2012.11.004
Huang, J., Tong, X., Zhang, L., Zhang, Y., Wang, L., Wang, D., et al. (2020). Hyperoside Attenuates Bleomycin-Induced Pulmonary Fibrosis Development in Mice. Front. Pharmacol. 11, 550955. doi:10.3389/fphar.2020.550955
Huang, Y. C., Horng, C. T., Chen, S. T., Lee, S. S., Yang, M. L., Lee, C. Y., et al. (2016). Rutin Improves Endotoxin-Induced Acute Lung Injury via Inhibition of iNOS and VCAM-1 Expression. Environ. Toxicol. 31 (2), 185–191. doi:10.1002/tox.22033
Jamal Jameel, K., Gallert, W. J., Yanik, S. D., Panek, S., Kronsbein, J., Jungck, D., et al. (2021). Biomarkers for Comorbidities Modulate the Activity of T-Cells in COPD. Int. J. Mol. Sci. 22 (13). doi:10.3390/ijms22137187
Jang, T. Y., Jung, A. Y., Kyung, T. S., Kim, D. Y., Hwang, J. H., and Kim, Y. H. (2017). Anti-allergic Effect of Luteolin in Mice with Allergic Asthma and Rhinitis. Cent. Eur. J. Immunol. 42 (1), 24–29. doi:10.5114/ceji.2017.67315
Janku, F., Yap, T. A., and Meric-Bernstam, F. (2018). Targeting the PI3K Pathway in Cancer: Are We Making Headway? Nat. Rev. Clin. Oncol. 15 (5), 273–291. doi:10.1038/nrclinonc.2018.28
Jia, W., Wang, C., Wang, Y., Pan, G., Jiang, M., Li, Z., et al. (2015). Qualitative and Quantitative Analysis of the Major Constituents in Chinese Medical Preparation Lianhua-Qingwen Capsule by UPLC-DAD-QTOF-MS. ScientificWorldJournal 2015, 731765. doi:10.1155/2015/731765
Jiang, L., Zhang, L., Kang, K., Fei, D., Gong, R., Cao, Y., et al. (2016). Resveratrol Ameliorates LPS-Induced Acute Lung Injury via NLRP3 Inflammasome Modulation. Biomed. Pharmacother. 84, 130–138. doi:10.1016/j.biopha.2016.09.020
Jiang, Y., Liu, L. S., Shen, L. P., Han, Z. F., Jian, H., Liu, J. X., et al. (2016). Traditional Chinese Medicine Treatment as Maintenance Therapy in Advanced Non-small-cell Lung Cancer: A Randomized Controlled Trial. Complement. Ther. Med. 24, 55–62. doi:10.1016/j.ctim.2015.12.006
Jiang, Z. Q., Li, M. H., Qin, Y. M., Jiang, H. Y., Zhang, X., and Wu, M. H. (2018). Luteolin Inhibits Tumorigenesis and Induces Apoptosis of Non-small Cell Lung Cancer Cells via Regulation of MicroRNA-34a-5p. Int. J. Mol. Sci. 19 (2). doi:10.3390/ijms19020447
Jin, C., Ye, K., Luan, H., Liu, L., Zhang, R., Yang, S., et al. (2020). Tussilagone Inhibits Allergic Responses in OVA-Induced Allergic Rhinitis guinea Pigs and IgE-Stimulated RBL-2H3 Cells. Fitoterapia 144, 104496. doi:10.1016/j.fitote.2020.104496
Júlio de Souza, A. L., Beatriz Mahler Pereira, A., Robison de Oliveira, J., Santos Ramalho, L., Ismarsi de Souza, H., Lacerda Nascimento, A., et al. (2020). Dermatophagoides Pteronyssinus-Induced Pro-inflammatory Responses Mediated via STAT3 and NF-kappaB Signaling Pathways in Human Bronchial Epithelial Cells - Inhibitory Effects of Lafoensia Pacari and Ellagic Acid. J. Pharmacol. Sci. 142 (4), 157–164. doi:10.1016/j.jphs.2020.01.004
Kim, H. H., Bae, Y., and Kim, S. H. (2013). Galangin Attenuates Mast Cell-Mediated Allergic Inflammation. Food Chem. Toxicol. 57, 209–216. doi:10.1016/j.fct.2013.03.015
Kim, K. C., and Lee, C. (2014). Reversal of Cisplatin Resistance by Epigallocatechin Gallate Is Mediated by Downregulation of Axl and Tyro 3 Expression in Human Lung Cancer Cells. Korean J. Physiol. Pharmacol. 18 (1), 61–66. doi:10.4196/kjpp.2014.18.1.61
Kim, S. H., Park, H. J., Lee, C. M., Choi, I. W., Moon, D. O., Roh, H. J., et al. (2006). Epigallocatechin-3-gallate Protects Toluene Diisocyanate-Induced Airway Inflammation in a Murine Model of Asthma. FEBS Lett. 580 (7), 1883–1890. doi:10.1016/j.febslet.2006.02.052
Kitani, A., Fuss, I., Nakamura, K., Kumaki, F., Usui, T., and Strober, W. (2003). Transforming Growth Factor (TGF)-beta1-producing Regulatory T Cells Induce Smad-Mediated Interleukin 10 Secretion that Facilitates Coordinated Immunoregulatory Activity and Amelioration of TGF-Beta1-Mediated Fibrosis. J. Exp. Med. 198 (8), 1179–1188. doi:10.1084/jem.20030917
Knobloch, J., Hag, H., Jungck, D., Urban, K., and Koch, A. (2011). Resveratrol Impairs the Release of Steroid-Resistant Cytokines from Bacterial Endotoxin-Exposed Alveolar Macrophages in Chronic Obstructive Pulmonary Disease. Basic Clin. Pharmacol. Toxicol. 109 (2), 138–143. doi:10.1111/j.1742-7843.2011.00707.x
Knobloch, J., Jungck, D., Charron, C., Stoelben, E., Ito, K., and Koch, A. (2018). Superior Anti-inflammatory Effects of Narrow-Spectrum Kinase Inhibitors in Airway Smooth Muscle Cells from Subjects with Chronic Obstructive Pulmonary Disease. J. Allergy Clin. Immunol. 141 (3), 1122–1124. doi:10.1016/j.jaci.2017.09.026
Knobloch, J., Jungck, D., Kronsbein, J., Stoelben, E., Ito, K., and Koch, A. (2019). LABAs and p38MAPK Inhibitors Reverse the Corticosteroid-Insensitivity of IL-8 in Airway Smooth Muscle Cells of COPD. J. Clin. Med. 8 (12). doi:10.3390/jcm8122058
Knobloch, J., Lin, Y., Konradi, J., Jungck, D., Behr, J., Strauch, J., et al. (2013). Inflammatory Responses of Airway Smooth Muscle Cells and Effects of Endothelin Receptor Antagonism. Am. J. Respir. Cel Mol. Biol. 49 (1), 114–127. doi:10.1165/rcmb.2012-0287OC
Knobloch, J., Sibbing, B., Jungck, D., Lin, Y., Urban, K., Stoelben, E., et al. (2010). Resveratrol Impairs the Release of Steroid-Resistant Inflammatory Cytokines from Human Airway Smooth Muscle Cells in Chronic Obstructive Pulmonary Disease. J. Pharmacol. Exp. Ther. 335 (3), 788–798. doi:10.1124/jpet.110.166843
Knobloch, J., Wahl, C., Feldmann, M., Jungck, D., Strauch, J., Stoelben, E., et al. (2014). Resveratrol Attenuates the Release of Inflammatory Cytokines from Human Bronchial Smooth Muscle Cells Exposed to Lipoteichoic Acid in Chronic Obstructive Pulmonary Disease. Basic Clin. Pharmacol. Toxicol. 114 (2), 202–209. doi:10.1111/bcpt.12129
Knobloch, J., Yanik, S. D., Körber, S., Stoelben, E., Jungck, D., and Koch, A. (2016). Tnfα-induced Airway Smooth Muscle Cell Proliferation Depends on Endothelin Receptor Signaling, GM-CSF and IL-6. Biochem. Pharmacol. 116, 188–199. doi:10.1016/j.bcp.2016.07.008
Lakshmi, S. P., Reddy, A. T., Kodidhela, L. D., and Varadacharyulu, N. C. (2020). Epigallocatechin Gallate Diminishes Cigarette Smoke-Induced Oxidative Stress, Lipid Peroxidation, and Inflammation in Human Bronchial Epithelial Cells. Life Sci. 259, 118260. doi:10.1016/j.lfs.2020.118260
Lee, C. Y., Yang, J. J., Lee, S. S., Chen, C. J., Huang, Y. C., Huang, K. H., et al. (2014). Protective Effect of Ginkgo Biloba Leaves Extract, EGb761, on Endotoxin-Induced Acute Lung Injury via a JNK- and Akt-dependent NFκB Pathway. J. Agric. Food Chem. 62 (27), 6337–6344. doi:10.1021/jf501913b
Lee, H. H., Ahn, J. H., Kwon, A. R., Lee, E. S., Kwak, J. H., and Min, Y. H. (2014). Chemical Composition and Antimicrobial Activity of the Essential Oil of Apricot Seed. Phytother Res. 28 (12), 1867–1872. doi:10.1002/ptr.5219
Lee, J. H., Ko, H. J., Woo, E. R., Lee, S. K., Moon, B. S., Lee, C. W., et al. (2016). Moracin M Inhibits Airway Inflammation by Interrupting the JNK/c-Jun and NF-Κb Pathways In Vitro and In Vivo. Eur. J. Pharmacol. 783, 64–72. doi:10.1016/j.ejphar.2016.04.055
Li, C., Chen, J., Yuan, W., Zhang, W., Chen, H., and Tan, H. (2020). Preventive Effect of Ursolic Acid Derivative on Particulate Matter 2.5-induced Chronic Obstructive Pulmonary Disease Involves Suppression of Lung Inflammation. IUBMB life 72 (4), 632–640. doi:10.1002/iub.2201
Li, K., Yuan, J., and Su, W. (2006). Determination of Liquiritin, Naringin, Hesperidin, Thymol, Imperatorin, Honokiol, Isoimperatorin, and Magnolol in the Traditional Chinese Medicinal Preparation Huoxiang-Zhengqi Liquid Using High-Performance Liquid Chromatography. Yakugaku Zasshi 126 (11), 1185–1190. doi:10.1248/yakushi.126.1185
Li, X., Huang, K., Liu, X., Ruan, H., Ma, L., Liang, J., et al. (2021). Ellagic Acid Attenuates BLM-Induced Pulmonary Fibrosis via Inhibiting Wnt Signaling Pathway. Front. Pharmacol. 12, 639574. doi:10.3389/fphar.2021.639574
Li, X., Cao, X., Guo, M., Xie, M., and Liu, X. (2020). Trends and Risk Factors of Mortality and Disability Adjusted Life Years for Chronic Respiratory Diseases from 1990 to 2017: Systematic Analysis for the Global Burden of Disease Study 2017. BMJ 368, m234. doi:10.1136/bmj.m234
Li, Y., Chi, G., Shen, B., Tian, Y., and Feng, H. (2016). Isorhamnetin Ameliorates LPS-Induced Inflammatory Response through Downregulation of NF-Κb Signaling. Inflammation 39 (4), 1291–1301. doi:10.1007/s10753-016-0361-z
Li, Y., Yang, Y., Liu, X., Long, Y., and Zheng, Y. (2019). PRMT5 Promotes Human Lung Cancer Cell Apoptosis via Akt/Gsk3β Signaling Induced by Resveratrol. Cel Transpl. 28 (12), 1664–1673. doi:10.1177/0963689719885083
Liang, Z., Nie, H., Xu, Y., Peng, J., Zeng, Y., Wei, Y., et al. (2016). Therapeutic Effects of Rosmarinic Acid on Airway Responses in a Murine Model of Asthma. Int. Immunopharmacol 41, 90–97. doi:10.1016/j.intimp.2016.10.010
Liang, Z., Xu, Y., Wen, X., Nie, H., Hu, T., Yang, X., et al. (2016). Rosmarinic Acid Attenuates Airway Inflammation and Hyperresponsiveness in a Murine Model of Asthma. Molecules 21 (6). doi:10.3390/molecules21060769
Liao, X. Z., Gao, Y., Sun, L. L., Liu, J. H., Chen, H. R., Yu, L., et al. (2020). Rosmarinic Acid Reverses Non-small Cell Lung Cancer Cisplatin Resistance by Activating the MAPK Signaling Pathway. Phytother Res. 34 (5), 1142–1153. doi:10.1002/ptr.6584
Lin, C. L., Chen, R. F., Chen, J. Y., Chu, Y. C., Wang, H. M., Chou, H. L., et al. (2012). Protective Effect of Caffeic Acid on Paclitaxel Induced Anti-proliferation and Apoptosis of Lung Cancer Cells Involves NF-Κb Pathway. Int. J. Mol. Sci. 13 (5), 6236–6245. doi:10.3390/ijms13056236
Lin, L., Hou, G., Han, D., Kang, J., and Wang, Q. (2019). Ursolic Acid Protected Lung of Rats from Damage Induced by Cigarette Smoke Extract. Front. Pharmacol. 10, 700. doi:10.3389/fphar.2019.00700
Ling, L. J., Lu, Y., Zhang, Y. Y., Zhu, H. Y., Tu, P., Li, H., et al. (2020). Flavonoids from Houttuynia Cordata Attenuate H1N1-Induced Acute Lung Injury in Mice via Inhibition of Influenza Virus and Toll-like Receptor Signalling. Phytomedicine 67, 153150. doi:10.1016/j.phymed.2019.153150
Liu, H., Yu, H., Cao, Z., Gu, J., Pei, L., Jia, M., et al. (2019). Kaempferol Modulates Autophagy and Alleviates Silica-Induced Pulmonary Fibrosis. DNA Cel Biol. 38 (12), 1418–1426. doi:10.1089/dna.2019.4941
Liu, P. L., Tsai, J. R., Charles, A. L., Hwang, J. J., Chou, S. H., Ping, Y. H., et al. (2010). Resveratrol Inhibits Human Lung Adenocarcinoma Cell Metastasis by Suppressing Heme Oxygenase 1-mediated Nuclear Factor-kappaB Pathway and Subsequently Downregulating Expression of Matrix Metalloproteinases. Mol. Nutr. Food Res. 54 (Suppl. 2), S196–S204. doi:10.1002/mnfr.200900550
Liu, Q., Liang, X., Niu, C., and Wang, X. (2018). Ellagic Acid Promotes A549 Cell Apoptosis via Regulating the Phosphoinositide 3-kinase/protein Kinase B Pathway. Exp. Ther. Med. 16 (1), 347–352. doi:10.3892/etm.2018.6193
Liu, X., Zhang, H., Xu, J., Gong, S., Han, Y., Zhang, T., et al. (2019). Identification of Absorbed Components and Their Metabolites in Rat Plasma after Oral Administration of Shufeng Jiedu Capsule Using Ultra-performance Liquid Chromatography/quadrupole Time-Of-Flight Mass Spectrometry. Rapid Commun. Mass. Spectrom. 33 (19), 1494–1501. doi:10.1002/rcm.8498
Liu, X. X., Yu, D. D., Chen, M. J., Sun, T., Li, G., Huang, W. J., et al. (2015). Hesperidin Ameliorates Lipopolysaccharide-Induced Acute Lung Injury in Mice by Inhibiting HMGB1 Release. Int. Immunopharmacol 25 (2), 370–376. doi:10.1016/j.intimp.2015.02.022
Liu, Y., Li, Z., Xue, X., Wang, Y., Zhang, Y., and Wang, J. (2018). Apigenin Reverses Lung Injury and Immunotoxicity in Paraquat-Treated Mice. Int. Immunopharmacol 65, 531–538. doi:10.1016/j.intimp.2018.10.046
Liu, Y. H., Liu, G. H., Mei, J. J., and Wang, J. (2016). The Preventive Effects of Hyperoside on Lung Cancer In Vitro by Inducing Apoptosis and Inhibiting Proliferation through Caspase-3 and P53 Signaling Pathway. Biomed. Pharmacother. 83, 381–391. doi:10.1016/j.biopha.2016.06.035
Liu, Z., Wang, P., Lu, S., Guo, R., Gao, W., Tong, H., et al. (2020). Liquiritin, a Novel Inhibitor of TRPV1 and TRPA1, Protects against LPS-Induced Acute Lung Injury. Cell calcium 88, 102198. doi:10.1016/j.ceca.2020.102198
Lou, J. S., Zhao, L. P., Huang, Z. H., Chen, X. Y., Xu, J. T., Tai, W. C., et al. (2021). Ginkgetin Derived from Ginkgo Biloba Leaves Enhances the Therapeutic Effect of Cisplatin via Ferroptosis-Mediated Disruption of the Nrf2/HO-1 axis in EGFR Wild-type Non-small-cell Lung Cancer. Phytomedicine 80, 153370. doi:10.1016/j.phymed.2020.153370
Lü, P. (2016). Inhibitory Effects of Hyperoside on Lung Cancer by Inducing Apoptosis and Suppressing Inflammatory Response via Caspase-3 and NF-Κb Signaling Pathway. Biomed. Pharmacother. 82, 216–225. doi:10.1016/j.biopha.2016.05.006
Luan, R. L., Meng, X. X., and Jiang, W. (2016). Protective Effects of Apigenin against Paraquat-Induced Acute Lung Injury in Mice. Inflammation 39 (2), 752–758. doi:10.1007/s10753-015-0302-2
Luo, W., Liu, Q., Jiang, N., Li, M., and Shi, L. (2019). Isorhamnetin Inhibited Migration and Invasion via Suppression of Akt/ERK-Mediated Epithelial-To-Mesenchymal Transition (EMT) in A549 Human Non-small-cell Lung Cancer Cells. Biosci. Rep. 39 (9). doi:10.1042/bsr20190159
Ma, H., Feng, X., and Ding, S. (2015). Hesperetin Attenuates Ventilator-Induced Acute Lung Injury through Inhibition of NF-Κb-Mediated Inflammation. Eur. J. Pharmacol. 769, 333–341. doi:10.1016/j.ejphar.2015.11.038
Ma, Z., Ji, W., Fu, Q., and Ma, S. (2013). Formononetin Inhibited the Inflammation of LPS-Induced Acute Lung Injury in Mice Associated with Induction of PPAR Gamma Expression. Inflammation 36 (6), 1560–1566. doi:10.1007/s10753-013-9700-5
Mahajan, S. G., and Mehta, A. A. (2011). Suppression of Ovalbumin-Induced Th2-Driven Airway Inflammation by β-sitosterol in a guinea Pig Model of Asthma. Eur. J. Pharmacol. 650 (1), 458–464. doi:10.1016/j.ejphar.2010.09.075
Maiti, S., and Banerjee, A. (2021). Epigallocatechin Gallate and Theaflavin Gallate Interaction in SARS-CoV-2 Spike-Protein central Channel with Reference to the Hydroxychloroquine Interaction: Bioinformatics and Molecular Docking Study. Drug Dev. Res. 82 (1), 86–96. doi:10.1002/ddr.21730
Masraksa, W., Tanasawet, S., Hutamekalin, P., Wongtawatchai, T., and Sukketsiri, W. (2020). Luteolin Attenuates Migration and Invasion of Lung Cancer Cells via Suppressing Focal Adhesion Kinase and Non-receptor Tyrosine Kinase Signaling Pathway. Nutr. Res. Pract. 14 (2), 127–133. doi:10.4162/nrp.2020.14.2.127
Mishra, V., Banga, J., and Silveyra, P. (2018). Oxidative Stress and Cellular Pathways of Asthma and Inflammation: Therapeutic Strategies and Pharmacological Targets. Pharmacol. Ther. 181, 169–182. doi:10.1016/j.pharmthera.2017.08.011
Molitorisova, M., Sutovska, M., Kazimierova, I., Barborikova, J., Joskova, M., Novakova, E., et al. (2021). The Anti-asthmatic Potential of Flavonol Kaempferol in an Experimental Model of Allergic Airway Inflammation. Eur. J. Pharmacol. 891, 173698. doi:10.1016/j.ejphar.2020.173698
Mortality, Morbidity, and Hospitalisations Due to Influenza Lower Respiratory Tract Infections, 2017: an Analysis for the Global Burden of Disease Study 2017. Lancet Respir. Med. (2019) 7(1):69–89. doi:10.1016/s2213-2600(18)30496-x
Nadeem, A., Al-Harbi, N. O., Ahmad, S. F., Ibrahim, K. E., Siddiqui, N., and Al-Harbi, M. M. (2018). Glucose-6-phosphate Dehydrogenase Inhibition Attenuates Acute Lung Injury through Reduction in NADPH Oxidase-Derived Reactive Oxygen Species. Clin. Exp. Immunol. 191 (3), 279–287. doi:10.1111/cei.13097
Navarro, S., Reddy, R., Lee, J., Warburton, D., and Driscoll, B. (2017). Inhaled Resveratrol Treatments Slow Ageing-Related Degenerative Changes in Mouse Lung. Thorax 72 (5), 451–459. doi:10.1136/thoraxjnl-2016-208964
Ory, L., Nazih, E. H., Daoud, S., Mocquard, J., Bourjot, M., Margueritte, L., et al. (2019). Targeting Bioactive Compounds in Natural Extracts - Development of a Comprehensive Workflow Combining Chemical and Biological Data. Anal. Chim. Acta 1070, 29–42. doi:10.1016/j.aca.2019.04.038
Pan, L., Lu, Y., Li, Z., Tan, Y., Yang, H., Ruan, P., et al. (2020). Ginkgo Biloba Extract EGb761 Attenuates Bleomycin-Induced Experimental Pulmonary Fibrosis in Mice by Regulating the Balance of M1/M2 Macrophages and Nuclear Factor Kappa B (NF-Κb)-Mediated Cellular Apoptosis. Med. Sci. Monit. 26, e922634. doi:10.12659/msm.922634
Park, Y. J., Bang, I. J., Jeong, M. H., Kim, H. R., Lee, D. E., Kwak, J. H., et al. (2019). Effects of β-Sitosterol from Corn Silk on TGF-Β1-Induced Epithelial-Mesenchymal Transition in Lung Alveolar Epithelial Cells. J. Agric. Food Chem. 67 (35), 9789–9795. doi:10.1021/acs.jafc.9b02730
Peng, X. P., Li, X. H., Li, Y., Huang, X. T., and Luo, Z. Q. (2019). The Protective Effect of Oleanolic Acid on NMDA-Induced MLE-12 Cells Apoptosis and Lung Injury in Mice by Activating SIRT1 and Reducing NF-Κb Acetylation. Int. Immunopharmacol 70, 520–529. doi:10.1016/j.intimp.2019.03.018
Petrova, V. N., and Russell, C. A. (2018). The Evolution of Seasonal Influenza Viruses. Nat. Rev. Microbiol. 16 (1), 47–60. doi:10.1038/nrmicro.2017.118
Qian, J., Chen, X., Chen, X., Sun, C., Jiang, Y., Qian, Y., et al. (2019). Kaempferol Reduces K63-Linked Polyubiquitination to Inhibit Nuclear Factor-Κb and Inflammatory Responses in Acute Lung Injury in Mice. Toxicol. Lett. 306, 53–60. doi:10.1016/j.toxlet.2019.02.005
Qian, L., Xie, B., Wang, Y., and Qian, J. (2015). Amygdalin-mediated Inhibition of Non-small Cell Lung Cancer Cell Invasion In Vitro. Int. J. Clin. Exp. Pathol. 8 (5), 5363–5370.
Rabe, K. F., and Watz, H. (2017). Chronic Obstructive Pulmonary Disease. Lancet 389 (10082), 1931–1940. doi:10.1016/s0140-6736(17)31222-9
Rasheduzzaman, M., Jeong, J. K., and Park, S. Y. (2018). Resveratrol Sensitizes Lung Cancer Cell to TRAIL by P53 Independent and Suppression of Akt/NF-Κb Signaling. Life Sci. 208, 208–220. doi:10.1016/j.lfs.2018.07.035
Ray, A., and Kolls, J. K. (2017). Neutrophilic Inflammation in Asthma and Association with Disease Severity. Trends Immunol. 38 (12), 942–954. doi:10.1016/j.it.2017.07.003
Ren, J. L., Zhang, A. H., and Wang, X. J. (2020). Traditional Chinese Medicine for COVID-19 Treatment. Pharmacol. Res. 155, 104743. doi:10.1016/j.phrs.2020.104743
Ren, X., Han, L., Li, Y., Zhao, H., Zhang, Z., Zhuang, Y., et al. (2021). Isorhamnetin Attenuates TNF ‐α‐induced Inflammation, Proliferation, and Migration in Human Bronchial Epithelial Cells via MAPK and NF‐κB Pathways. Anat. Rec. 304 (4), 901–913. doi:10.1002/ar.24506
Rogerio, A. P., Kanashiro, A., Fontanari, C., da Silva, E. V., Lucisano-Valim, Y. M., Soares, E. G., et al. (2007). Anti-inflammatory Activity of Quercetin and Isoquercitrin in Experimental Murine Allergic Asthma. Inflamm. Res. 56 (10), 402–408. doi:10.1007/s00011-007-7005-6
Ruan, J. S., Zhou, H., Yang, L., Wang, L., Jiang, Z. S., Sun, H., et al. (2019). Ursolic Acid Attenuates TGF-Β1-Induced Epithelial-Mesenchymal Transition in NSCLC by Targeting Integrin αVβ5/MMPs Signaling. Oncol. Res. 27 (5), 593–600. doi:10.3727/096504017x15051723858706
Rungsung, S., Singh, T. U., Rabha, D. J., Kumar, T., Cholenahalli Lingaraju, M., Parida, S., et al. (2018). Luteolin Attenuates Acute Lung Injury in Experimental Mouse Model of Sepsis. Cytokine 110, 333–343. doi:10.1016/j.cyto.2018.03.042
Russo, M., Moccia, S., Spagnuolo, C., Tedesco, I., and Russo, G. L. (2020). Roles of Flavonoids against Coronavirus Infection. Chem. Biol. Interact 328, 109211. doi:10.1016/j.cbi.2020.109211
Shahidi, F., and Yeo, J. (2018). Bioactivities of Phenolics by Focusing on Suppression of Chronic Diseases: A Review. Int. J. Mol. Sci. 19 (6). doi:10.3390/ijms19061573
Shin, D., Park, S. H., Choi, Y. J., Kim, Y. H., Antika, L. D., Habibah, N. U., et al. (2015). Dietary Compound Kaempferol Inhibits Airway Thickening Induced by Allergic Reaction in a Bovine Serum Albumin-Induced Model of Asthma. Int. J. Mol. Sci. 16 (12), 29980–29995. doi:10.3390/ijms161226218
Shu, Y. S., Tao, W., Miao, Q. B., Lu, S. C., and Zhu, Y. B. (2014). Galangin Dampens Mice Lipopolysaccharide-Induced Acute Lung Injury. Inflammation 37 (5), 1661–1668. doi:10.1007/s10753-014-9894-1
Siegel, R. L., Miller, K. D., Jemal, A., and Jemal, A. (2021). Cancer Statistics, 2017. CA Cancer J. Clin. 67 (1), 7–30. doi:10.3322/caac.2165410.3322/caac.21387
Skoulidis, F., and Heymach, J. V. (2019). Co-occurring Genomic Alterations in Non-small-cell Lung Cancer Biology and Therapy. Nat. Rev. Cancer 19 (9), 495–509. doi:10.1038/s41568-019-0179-8
Smith, K. F., Goldberg, M., Rosenthal, S., Carlson, L., Chen, J., Chen, C., et al. (2014). Global Rise in Human Infectious Disease Outbreaks. J. R. Soc. Interf. 11 (101), 20140950. doi:10.1098/rsif.2014.0950
Spagnolo, P., Kropski, J. A., Jones, M. G., Lee, J. S., Rossi, G., Karampitsakos, T., et al. (2021). Idiopathic Pulmonary Fibrosis: Disease Mechanisms and Drug Development. Pharmacol. Ther. 222, 107798. doi:10.1016/j.pharmthera.202010.1016/j.pharmthera.2020.107798107798
Sriram, N., Kalayarasan, S., and Sudhandiran, G. (2009). Epigallocatechin-3-gallate Exhibits Anti-fibrotic Effect by Attenuating Bleomycin-Induced Glycoconjugates, Lysosomal Hydrolases and Ultrastructural Changes in Rat Model Pulmonary Fibrosis. Chem. Biol. Interact 180 (2), 271–280. doi:10.1016/j.cbi.2009.02.017
Stertz, S., Reichelt, M., Spiegel, M., Kuri, T., Martínez-Sobrido, L., García-Sastre, A., et al. (2007). The Intracellular Sites of Early Replication and Budding of SARS-Coronavirus. Virology 361 (2), 304–315. doi:10.1016/j.virol.2006.11.027
Stets, R., Popescu, M., Gonong, J. R., Mitha, I., Nseir, W., Madej, A., et al. (2019). Omadacycline for Community-Acquired Bacterial Pneumonia. N. Engl. J. Med. 380 (6), 517–527. doi:10.1056/NEJMoa1800201
Sun, K., Huang, R., Yan, L., Li, D. T., Liu, Y. Y., Wei, X. H., et al. (2018). Schisandrin Attenuates Lipopolysaccharide-Induced Lung Injury by Regulating TLR-4 and Akt/FoxO1 Signaling Pathways. Front. Physiol. 9, 1104. doi:10.3389/fphys.2018.01104
Sun, L., Yim, W. S., Fahey, P., Wang, S., Zhu, X., Qiao, J., et al. (2019). Investigation on Advanced Non-small-cell Lung Cancer Among Elderly Patients Treated with Chinese Herbal Medicine versus Chemotherapy: A Pooled Analysis of Individual Data. Evid. Based Complement. Alternat Med. 2019, 1898345. doi:10.1155/2019/1898345
Sundarraj, S., Thangam, R., Sreevani, V., Kaveri, K., Gunasekaran, P., Achiraman, S., et al. (2012). γ-Sitosterol from Acacia Nilotica L. Induces G2/M Cell Cycle Arrest and Apoptosis through C-Myc Suppression in MCF-7 and A549 Cells. J. Ethnopharmacol 141 (3), 803–809. doi:10.1016/j.jep.2012.03.014
Suresh, R., Kupfer, Y., and Tessler, S. (2000). Acute Respiratory Distress Syndrome. N. Engl. J. Med. 343 (9), 660–661. doi:10.1056/nejm200008313430914
Takashima, K., Matsushima, M., Hashimoto, K., Nose, H., Sato, M., Hashimoto, N., et al. (2014). Protective Effects of Intratracheally Administered Quercetin on Lipopolysaccharide-Induced Acute Lung Injury. Respir. Res. 15 (1), 150. doi:10.1186/s12931-014-0150-x
Tan, S., Dai, L., Tan, P., Liu, W., Mu, Y., Wang, J., et al. (2020). Hesperidin Administration Suppresses the Proliferation of Lung Cancer Cells by Promoting Apoptosis Via targeting the miR-132/ZEB2 S-ignalling P-athway. Int. J. Mol. Med. 46 (6), 2069–2077. doi:10.3892/ijmm.2020.4756
Tian, J., Yan, S., Wang, H., Zhang, Y., Zheng, Y., Wu, H., et al. (2020). Hanshiyi Formula, a Medicine for Sars-CoV2 Infection in China, Reduced the Proportion of Mild and Moderate COVID-19 Patients Turning to Severe Status: A Cohort Study. Pharmacol. Res. 161, 105127. doi:10.1016/j.phrs.2020.105127
Tsai, Y. F., Chen, C. Y., Chang, W. Y., Syu, Y. T., and Hwang, T. L. (2019). Resveratrol Suppresses Neutrophil Activation via Inhibition of Src Family Kinases to Attenuate Lung Injury. Free Radic. Biol. Med. 145, 67–77. doi:10.1016/j.freeradbiomed.2019.09.021
Tsai, Y. M., Yang, C. J., Hsu, Y. L., Wu, L. Y., Tsai, Y. C., Hung, J. Y., et al. (2011). Glabridin Inhibits Migration, Invasion, and Angiogenesis of Human Non-small Cell Lung Cancer A549 Cells by Inhibiting the FAK/rho Signaling Pathway. Integr. Cancer Ther. 10 (4), 341–349. doi:10.1177/1534735410384860
Wang, J., He, F., Chen, L., Li, Q., Jin, S., Zheng, H., et al. (2018). Resveratrol Inhibits Pulmonary Fibrosis by Regulating miR-21 through MAPK/AP-1 Pathways. Biomed. Pharmacother. 105, 37–44. doi:10.1016/j.biopha.2018.05.104
Wang, J., Liu, Y. T., Xiao, L., Zhu, L., Wang, Q., and Yan, T. (2014). Anti-inflammatory Effects of Apigenin in Lipopolysaccharide-Induced Inflammatory in Acute Lung Injury by Suppressing COX-2 and NF-kB Pathway. Inflammation 37 (6), 2085–2090. doi:10.1007/s10753-014-9942-x
Wang, L., Liu, H., He, Q., Gan, C., Li, Y., Zhang, Q., et al. (2020). Galangin Ameliorated Pulmonary Fibrosis In Vivo and In Vitro by Regulating Epithelial-Mesenchymal Transition. Bioorg. Med. Chem. 28 (19), 115663. doi:10.1016/j.bmc.2020.115663
Wang, Q., Chen, Y., Lu, H., Wang, H., Feng, H., Xu, J., et al. (2020). Quercetin Radiosensitizes Non-small Cell Lung Cancer Cells through the Regulation of miR-16-5p/WEE1 axis. IUBMB life 72 (5), 1012–1022. doi:10.1002/iub.2242
Wang, S., Wuniqiemu, T., Tang, W., Teng, F., Bian, Q., Yi, L., et al. (2021). Luteolin Inhibits Autophagy in Allergic Asthma by Activating PI3K/Akt/mTOR Signaling and Inhibiting Beclin-1-Pi3kc3 Complex. Int. Immunopharmacol 94, 107460. doi:10.1016/j.intimp.2021.107460
Wang, X., Li, M., Hu, M., Wei, P., and Zhu, W. (2017). BAMBI Overexpression Together with β-sitosterol Ameliorates NSCLC via Inhibiting Autophagy and Inactivating TGF-β/Smad2/3 Pathway. Oncol. Rep. 37 (5), 3046–3054. doi:10.3892/or.2017.5508
Wang, X., Liu, M., Zhu, M. J., Shi, L., Liu, L., Zhao, Y. L., et al. (2020). Resveratrol Protects the Integrity of Alveolar Epithelial Barrier via SIRT1/PTEN/p-Akt Pathway in Methamphetamine-Induced Chronic Lung Injury. Cell Prolif 53 (3), e12773. doi:10.1111/cpr.12773
Wang, X., Shao, Q. H., Zhou, H., Wu, J. L., Quan, W. Q., Ji, P., et al. (2020). Ginkgolide B Inhibits Lung Cancer Cells Promotion via Beclin-1-dependent Autophagy. BMC Complement. Med. Ther. 20 (1), 194. doi:10.1186/s12906-020-02980-x
Wang, X. F., Song, S. D., Li, Y. J., Hu, Z. Q., Zhang, Z. W., Yan, C. G., et al. (2018). Protective Effect of Quercetin in LPS-Induced Murine Acute Lung Injury Mediated by cAMP-Epac Pathway. Inflammation 41 (3), 1093–1103. doi:10.1007/s10753-018-0761-3
Wang, X. L., Li, T., Li, J. H., Miao, S. Y., and Xiao, X. Z. (2017). The Effects of Resveratrol on Inflammation and Oxidative Stress in a Rat Model of Chronic Obstructive Pulmonary Disease. Molecules 22 (9). doi:10.3390/molecules22091529
Wang, Z., Fang, K., Wang, G., Guan, X., Pang, Z., Guo, Y., et al. (2019). Protective Effect of Amygdalin on Epithelial-Mesenchymal Transformation in Experimental Chronic Obstructive Pulmonary Disease Mice. Phytother Res. 33 (3), 808–817. doi:10.1002/ptr.6274
Ward, P. A. (2010). Oxidative Stress: Acute and Progressive Lung Injury. Ann. N. Y Acad. Sci. 1203, 53–59. doi:10.1111/j.1749-6632.2010.05552.x
Wollin, L., Distler, J. H. W., Redente, E. F., Riches, D. W. H., Stowasser, S., Schlenker-Herceg, R., et al. (2019). Potential of Nintedanib in Treatment of Progressive Fibrosing Interstitial Lung Diseases. Eur. Respir. J. 54 (3). doi:10.1183/13993003.00161-2019
Wu, X., Lin, L., and Wu, H. (2021). Ferulic Acid Alleviates Lipopolysaccharide‐induced Acute Lung Injury through Inhibiting TLR4/NF‐κB Signaling Pathway. J. Biochem. Mol. Toxicol. 35 (3), e22664. doi:10.1002/jbt.22664
Wynn, T. A., and Ramalingam, T. R. (2012). Mechanisms of Fibrosis: Therapeutic Translation for Fibrotic Disease. Nat. Med. 18 (7), 1028–1040. doi:10.1038/nm.2807
Xia, L., Shi, Y., Su, J., Friedemann, T., Tao, Z., Lu, Y., et al. (2021). Shufeng Jiedu, a Promising Herbal Therapy for Moderate COVID-19:Antiviral and Anti-inflammatory Properties, Pathways of Bioactive Compounds, and a Clinical Real-World Pragmatic Study. Phytomedicine 85, 153390. doi:10.1016/j.phymed.2020.153390
Xiao, M., Tian, J., Zhou, Y., Xu, X., Min, X., Lv, Y., et al. (2020). Efficacy of Huoxiang Zhengqi Dropping Pills and Lianhua Qingwen Granules in Treatment of COVID-19: A Randomized Controlled Trial. Pharmacol. Res. 161, 105126. doi:10.1016/j.phrs.2020.105126
Xiao, Y., Zhou, L., Zhang, T., Qin, C., Wei, P., Luo, L., et al. (2020). Anti-fibrosis Activity of Quercetin Attenuates Rabbit Tracheal Stenosis via the TGF-β/AKT/mTOR Signaling Pathway. Life Sci. 250, 117552. doi:10.1016/j.lfs.2020.117552
Xie, K., Chai, Y.-s., Lin, S.-h., Xu, F., and Wang, C.-j. (2021). Luteolin Regulates the Differentiation of Regulatory T Cells and Activates IL-10-Dependent Macrophage Polarization against Acute Lung Injury. J. Immunol. Res. 2021, 1–12. doi:10.1155/2021/8883962
Xingyu, Z., Peijie, M., Dan, P., Youg, W., Daojun, W., Xinzheng, C., et al. (2016). Quercetin Suppresses Lung Cancer Growth by Targeting Aurora B Kinase. Cancer Med. 5 (11), 3156–3165. doi:10.1002/cam4.891
Xu, Z. Y., Jin, C. J., Zhou, C. C., Wang, Z. Q., Zhou, W. D., Deng, H. B., et al. (2011). Treatment of Advanced Non-small-cell Lung Cancer with Chinese Herbal Medicine by Stages Combined with Chemotherapy. J. Cancer Res. Clin. Oncol. 137 (7), 1117–1122. doi:10.1007/s00432-011-0975-3
Yang, K., Chen, Y., Zhou, J., Ma, L., Shan, Y., Cheng, X., et al. (2019). Ursolic Acid Promotes Apoptosis and Mediates Transcriptional Suppression of CT45A2 Gene Expression in Non-small-cell Lung Carcinoma Harbouring EGFR T790M Mutations. Br. J. Pharmacol. 176 (24), 4609–4624. doi:10.1111/bph.14793
Yang, N., Zhang, H., Cai, X., and Shang, Y. (2018). Epigallocatechin-3-gallate Inhibits Inflammation and Epithelial-mesenchymal T-ransition through the PI3K/AKT P-athway via U-pregulation of PTEN in A-sthma. Int. J. Mol. Med. 41 (2), 818–828. doi:10.3892/ijmm.2017.3292
Yang, Y., Zhao, Y., Ai, X., Cheng, B., and Lu, S. (2014). Formononetin Suppresses the Proliferation of Human Non-small Cell Lung Cancer through Induction of Cell Cycle Arrest and Apoptosis. Int. J. Clin. Exp. Pathol. 7 (12), 8453–8461.
Yang, Y., Jin, X., Jiao, X., Li, J., Liang, L., Ma, Y., et al. (2020). Advances in Pharmacological Actions and Mechanisms of Flavonoids from Traditional Chinese Medicine in Treating Chronic Obstructive Pulmonary Disease. Evidence-Based Complement. Altern. Med. 2020, 1–10. doi:10.1155/2020/8871105
Ye, J., Guan, M., Lu, Y., Zhang, D., Li, C., Li, Y., et al. (2019). Protective Effects of Hesperetin on Lipopolysaccharide-Induced Acute Lung Injury by Targeting MD2. Eur. J. Pharmacol. 852, 151–158. doi:10.1016/j.ejphar.2019.02.042
Yeh, C. H., Yang, J. J., Yang, M. L., Li, Y. C., and Kuan, Y. H. (2014). Rutin Decreases Lipopolysaccharide-Induced Acute Lung Injury via Inhibition of Oxidative Stress and the MAPK-NF-Κb Pathway. Free Radic. Biol. Med. 69, 249–257. doi:10.1016/j.freeradbiomed.2014.01.028
Yi, L., Cui, J., Wang, W., Tang, W., Teng, F., Zhu, X., et al. (2020). Formononetin Attenuates Airway Inflammation and Oxidative Stress in Murine Allergic Asthmaflammation and Oxidative Stress in Murine Allergic Asthma. Front. Pharmacol. 11, 533841. doi:10.3389/fphar.2020.533841
You, H., Wei, L., Sun, W. L., Wang, L., Yang, Z. L., Liu, Y., et al. (2014). The green tea Extract Epigallocatechin-3-Gallate Inhibits Irradiation-Induced Pulmonary Fibrosis in Adult Rats. Int. J. Mol. Med. 34 (1), 92–102. doi:10.3892/ijmm.2014.1745
Yu, H., Lin, L., Zhang, Z., Zhang, H., and Hu, H. (2020). Targeting NF-Κb Pathway for the Therapy of Diseases: Mechanism and Clinical Study. Signal. Transduct Target. Ther. 5 (1), 209. doi:10.1038/s41392-020-00312-6
Yu, S., Gong, L. S., Li, N. F., Pan, Y. F., and Zhang, L. (2018). Galangin (GG) Combined with Cisplatin (DDP) to Suppress Human Lung Cancer by Inhibition of STAT3-Regulated NF-Κb and Bcl-2/Bax Signaling Pathways. Biomed. Pharmacother. 97, 213–224. doi:10.1016/j.biopha.2017.10.059
Yu, X., Gao, F., Li, W., Zhou, L., Liu, W., and Li, M. (2020). Formononetin Inhibits Tumor Growth by Suppression of EGFR-Akt-Mcl-1 axis in Non-small Cell Lung Cancer. J. Exp. Clin. Cancer Res. 39 (1), 62. doi:10.1186/s13046-020-01566-2
Yu, Y., Luo, Y., Fang, Z., Teng, W., Yu, Y., Tian, J., et al. (2020). Mechanism of Sanguinarine in Inhibiting Macrophages to Promote Metastasis and Proliferation of Lung Cancer via Modulating the Exosomes in A549 Cells. Onco Targets Ther. 13, 8989–9003. doi:10.2147/ott.S261054
Zha, W.-J., Qian, Y., Shen, Y., Du, Q., Chen, F.-F., Wu, Z.-Z., et al. (2013). Galangin Abrogates Ovalbumin-Induced Airway Inflammation via Negative Regulation of NF-Κb. Evidence-Based Complement. Altern. Med. 2013, 1–14. doi:10.1155/2013/767689
Zhan, Y., Ta, W., Tang, W., Hua, R., Wang, J., Wang, C., et al. (2021). Potential Antiviral Activity of Isorhamnetin against SARS‐CoV ‐2 Spike Pseudotyped Virus In Vitro. Drug Dev. Res. doi:10.1002/ddr.21815
Zhang, A., Pan, W., Lv, J., and Wu, H. (2017). Protective Effect of Amygdalin on LPS-Induced Acute Lung Injury by Inhibiting NF-Κb and NLRP3 Signaling Pathways. Inflammation 40 (3), 745–751. doi:10.1007/s10753-017-0518-4
Zhang, R., Ai, X., Duan, Y., Xue, M., He, W., Wang, C., et al. (2017). Kaempferol Ameliorates H9N2 Swine Influenza Virus-Induced Acute Lung Injury by Inactivation of TLR4/MyD88-Mediated NF-Κb and MAPK Signaling Pathways. Biomed. Pharmacother. 89, 660–672. doi:10.1016/j.biopha.2017.02.081
Zhang, T., Ma, S., Liu, C., Hu, K., Xu, M., and Wang, R. (2020). Rosmarinic Acid Prevents Radiation-Induced Pulmonary Fibrosis through Attenuation of ROS/MYPT1/TGFβ1 Signaling via miR-19b-3p. Dose Response 18 (4), 1559325820968413. doi:10.1177/1559325820968413
Zhang, Y., Cardell, L. O., Edvinsson, L., and Xu, C. B. (2013). MAPK/NF-κB-dependent Upregulation of Kinin Receptors Mediates Airway Hyperreactivity: a New Perspective for the Treatment. Pharmacol. Res. 71, 9–18. doi:10.1016/j.phrs.2013.02.004
Zhang, Y., Lu, P., Qin, H., Zhang, Y., Sun, X., Song, X., et al. (2021). Traditional Chinese Medicine Combined with Pulmonary Drug Delivery System and Idiopathic Pulmonary Fibrosis: Rationale and Therapeutic Potential. Biomed. Pharmacother. 133, 111072. doi:10.1016/j.biopha.2020.111072
Zheng, Q., Tong, M., Ou, B., Liu, C., Hu, C., and Yang, Y. (2019). Isorhamnetin Protects against Bleomycin-Induced Pulmonary Fibrosis by Inhibiting Endoplasmic Reticulum Stress and Epithelial-Mesenchymal Transition. Int. J. Mol. Med. 43 (1), 117–126. doi:10.3892/ijmm.2018.3965
Zhou, B. X., Li, J., Liang, X. L., Pan, X. P., Hao, Y. B., Xie, P. F., et al. (2020). β-Sitosterol Ameliorates Influenza A Virus-Induced Proinflammatory Response and Acute Lung Injury in Mice by Disrupting the Cross-Talk between RIG-I and IFN/STAT Signaling. Acta Pharmacol. Sin 41 (9), 1178–1196. doi:10.1038/s41401-020-0403-9
Zhou, E., Fu, Y., Wei, Z., and Yang, Z. (2014). Inhibition of Allergic Airway Inflammation through the Blockage of NF-Κb Activation by Ellagic Acid in an Ovalbumin-Induced Mouse Asthma Model. Food Funct. 5 (9), 2106–2112. doi:10.1039/c4fo00384e
Zhou, P., Yang, X. L., Wang, X. G., Hu, B., Zhang, L., Zhang, W., et al. (2020). A Pneumonia Outbreak Associated with a New Coronavirus of Probable Bat Origin. Nature 579 (7798), 270–273. doi:10.1038/s41586-020-2012-7
Zhou, Y., and Ho, W. S. (2014). Combination of Liquiritin, Isoliquiritin and Isoliquirigenin Induce Apoptotic Cell Death through Upregulating P53 and P21 in the A549 Non-small Cell Lung Cancer Cells. Oncol. Rep. 31 (1), 298–304. doi:10.3892/or.2013.2849
Keywords: Chinese herbal medicines, bioactive compounds, respiratory diseases, therapeutic use, molecular mechanisms of pharmacological action
Citation: Wang J, Wu Q, Ding L, Song S, Li Y, Shi L, Wang T, Zhao D, Wang Z and Li X (2021) Therapeutic Effects and Molecular Mechanisms of Bioactive Compounds Against Respiratory Diseases: Traditional Chinese Medicine Theory and High-Frequency Use. Front. Pharmacol. 12:734450. doi: 10.3389/fphar.2021.734450
Received: 01 July 2021; Accepted: 16 August 2021;
Published: 27 August 2021.
Edited by:
Wenzhi Yang, Tianjin University of Traditional Chinese Medicine, ChinaReviewed by:
Roodabeh Bahramsoltani, Tehran University of Medical Sciences, IranRosario Rojas, Universidad Peruana Cayetano Heredia, Peru
Copyright © 2021 Wang, Wu, Ding, Song, Li, Shi, Wang, Zhao, Wang and Li. This is an open-access article distributed under the terms of the Creative Commons Attribution License (CC BY). The use, distribution or reproduction in other forums is permitted, provided the original author(s) and the copyright owner(s) are credited and that the original publication in this journal is cited, in accordance with accepted academic practice. No use, distribution or reproduction is permitted which does not comply with these terms.
*Correspondence: Zeyu Wang, zeyu781022@163.com; Xiangyan Li, xiangyan_li1981@163.com
†These authors have contributed equally to this work