- 1National Engineering Laboratory for AIDS Vaccine, Key Laboratory for Molecular Enzymology and Engineering, School of Life Sciences, Jilin University, Changchun, China
- 2Jilin Ginseng Academy, Changchun University of Chinese Medicine, Changchun, China
- 3Research Center of Traditional Chinese Medicine, The Affiliated Hospital of Changchun University of Chinese Medicine, Key Laboratory of Active Substances and Biological Mechanisms of Ginseng Efficacy, Changchun University of Chinese Medicine, Changchun, China
Panax ginseng C.A. Mey (ginseng) is a classic medicinal plant which is well known for enhancing immune capacity. Polysaccharides are one of the main active components of ginseng. We isolated water-soluble ginseng polysaccharides (WGP) and analyzed the physicochemical properties of WGP including molecular weight, monosaccharide composition, and structural characteristics. WGP had minimal effect on the growth of hepatocytes. Interestingly, WGP significantly increased the mRNA and protein levels of complement component 4 (C4), one of the core components of the complement system. Promoter reporter gene assays revealed that WGP significantly enhanced activity of the C4 gene promoter. Deletion analyses determined that the E-box1 and Sp1 regions play key roles in WGP-induced C4 transcription. Taken together, our results suggest that WGP promotes C4 biosynthesis through upregulation of transcription. These results provide new explanation for the intrinsic mechanism by which ginseng boosts human immune capacity.
Introduction
For Panax ginseng C.A. Mey (ginseng), a perennial plant belonging to genus Panax, is one of the most appreciated medicinal plants (Yun, 2001). Regulation of the immune response is one of the main biological activities of ginseng extracts (Wang et al., 2001; Senchina et al., 2009; Kang and Min, 2012; Kachur and Suntres, 2016; Yun and Yi, 2020). The reason for long-lasting usage of ginseng is that it contains numerous natural regulatory compounds, such as polysaccharides, ginsenoside, phytosterols, and peptides (Dai et al., 2017).
As an important active component of ginseng, water-soluble ginseng polysaccharides (WGP) have been proved playing an important role in the modulation of immunity (Lim et al., 2004; Kim et al., 2007; Sun, 2011; Yu et al., 2017; Li et al., 2019). Human immune system is an elaborate and layered defense system against infections through gradual increase of specificity to invading organisms (Medzhitov, 2007). Among the components of immune response, the complement system plays an important role in organismal defense. Complements can lower the B-cell activating threshold and promote antigen retention on the surface of dendritic cells (Janssen et al., 2006; Gros et al., 2008; Carter and Fearon, 2010; Carroll and Isenman, 2012). Interaction between the effector components and the complement receptors can alter the secretion of cytokines and regulate the direction of T cell differentiation by influencing the microenvironment, thereby affecting the outcome of inflammation (Lalli et al., 2008; Amsen et al., 2009; Kolev et al., 2013).
The C4 gene product, fourth component of human complement (C4), is an important component of the complement system (Galibert et al., 1997). C4 together with C2 forms the classical complement which activates C3 convertase (Dodds and Law, 1990). C4 is an important effector for both innate and adaptive immune systems among vertebrate animals. C4 is mainly expressed in the liver and induced during acute inflammation or tissue injury (Zhang et al., 2009).
Until now, little is known about the effects of ginseng on the production of complement components. In this study, we investigated the effect of WGP on C4 biosynthesis and explored the underlying molecular mechanism. We demonstrate that WGP enhances C4 production by promoting C4 gene transcription via the E-box1 and Sp1 regions in the promoter. Our results provide more clues to fully understand the mechanism by which ginseng enhances human immunity.
Materials and Methods
Preparation of Water-Soluble Ginseng Polysaccharides
Ginseng powder (400 g) was boiled in deionized water (4 L) for 3 h. After filtration, the solid phase was boiled in deionized water at the same ratio (g/ml) two more times. The filtrate was combined, centrifuged, and concentrated. The concentrated solution was mixed with anhydrous ethanol at a volume ratio of 1:3 and left overnight at 4°C. Discard the supernatant by centrifugation and the precipitate was successively washed with 75% ethanol, 95% ethanol, anhydrous ethanol, and ether. The crude ginseng polysaccharides were obtained after drying and subsequently redissolved in deionized water. After removal of proteins using a Sevage reagent, a 3 KD molecular sieve was used to remove small molecules to obtain WGP. The content of sugar was tracked and monitored by the phenol sulfuric acid method (Wang et al., 2020).
Molecular Weight Analysis of Water-Soluble Ginseng Polysaccharides
WGP (5 mg/ml) was filtered via 0.45 μm microfiltration membrane. The filtered sample (20 μL) was loaded into a TSK-Gel G4000PWXL column (Tosoh, Shanghai branch, China) controlled by LC-10Avp system (Shimadzu, Shanghai branch, China). High performance gel permeation chromatography (HPGPC) was performed using 0.2 M NaCl as mobile phase at flow rate of 0.5 ml/min. T-series Dextran standards were used for reference standards.
Monosaccharide Composition Analysis of Water-Soluble Ginseng Polysaccharides
Monosaccharide composition analysis was performed as previously described (Wang et al., 2020). Briefly, WGP (200 g) was hydrolysed in anhydrous methanol solution (1 ml) containing hydrochloric acid in nitrogen. Then, the sample was dried and hydrolysed in 2 M trifluoroacetate acid. After dried, the sample was dissolved using 0.3 M sodium hydroxide and added an equal volume of 0.5 M PMP (1-phenyl-3-methyl-5-pyrazolone) with thoroughly blending using pipettor. Placed the mixture (0.2 ml) for 30 min at 70°C, added 0.1 ml hydrochloric acid and 0.7 ml dichloromethane for extraction. The aqueous phase was filtered via 0.22 μm organic membrane. Conversion of monosaccharides with PMP were detected via high-performance liquid chromatography (HPLC). The sample (10 μL) was injected into a 4.6 mm × 250 mm COSMOSIL 5C18-PAQ column (Nacalai Tesque, Shanghai branch, China) controlled by an LC-20AT system (Shimadzu, Shanghai branch, China). HPLC was performed using a mobile phase composed of 19.5% Acetonitrile and 80.5% 0.1 M PBS (pH 7.0) at flow rate of 1 ml/min. The absorbance values at wavelength 245 nm were compared with those of monosaccharide standards including arabinose, fucose, galactose, galacturonic acid, glucose, glucuronic acid, mannose, rhamnose, and xylose to determine the monosaccharide composition of WGP.
Fourier Transform-Infrared Spectroscopy Analysis
Fourier transform-infrared spectroscopy (FT-IR) spectra of WGP was acquired via Tenor 27 spectrophotometer (Shimadzu, Shanghai branch, China). WGP was ground with KBr powder at a mass ratio of 3:1 and compressed into a pellet. The FT-IR spectra were recorded in range of 400–4,000 cm−1.
Nuclear Magnetic Resonance Analysis
The lyophilized WGP was dissolved in D2O. The 1H and 13C nuclear magnetic resonance (NMR) spectrum were performed on AV-500, 600, and 800 instruments (Bruker, Germany) using tetramethylsilane as the internal standard.
Cell Culture
Human hepatic cell line L-O2 was purchased from the American Type Culture Collection (Manassas, VA, United States). Cells were cultured using DMEM media containing 100 U/ml penicillin, 100 μg/ml streptomycin, 2 mM L-glutamine (Thermo Fisher Scientific, Shanghai branch, China) and 10% (V/V) fetal bovine serum (Abwbio, Guangzhou, China) and incubated in HERAcell150i incubator (Thermo Fisher Scientific) set to 5%CO2/95% air and 37°C. Cells were tested for mycoplasma monthly by the PCR method described by Uphoff and Drexler (Uphoff and Drexler, 2005).
In Vitro Cytotoxicity Assays
The L-O2 cells were treated with a series of equal ratio gradient concentrations of WGP for 72 h in a 96-well plate. MTT [3-(4, 5-dimethyl-thiazol-2yl)-2, 5-diphenyl tetrazolium bromide] (Sigma-Aldrich, Shanghai branch, China) was added at the final concentration of 0.5 mg/ml and incubated for 4 h in incubator. Then, the cells were lysed in 10% SDS containing 10 mM HCl overnight. The absorbance values at wavelength 590 nm were measured via a microplate reader.
Western Blotting
Whole cell lysates were obtained via ultrasonic cell disruption and subjected to SDS-polyacrylamide gel electrophoresis. Proteins were electrophoretically transferred onto PVDF (polyvinylidene difluoride) membrane (Thermo Fisher Scientific), and subsequently immunoblotted utilizing anti-β-actin (Proteintech, Rosemont, IL, United States) and -C4 (Abcam, Cambridge, MA, United States) antibodies. Visualization of immunoreactive proteins was conducted using the Odyssey Infrared Imaging System (LiCor, Lincoln, NE, United States). Densitometry measurements were performed using Odyssey V3.0 software (LiCor).
Real-Time PCR
Total RNA was extracted by TRIZOL method and used to make cDNAs via reverse transcription PCR Kit (Thermo Fisher Scientific), as described previously (Edwards et al., 2009). The LightCycler 480 real-time PCR meter (Roche, Indianapolis, IN, United States) and TaqMan probe Hs00246758_m1 (Thermo Fisher Scientific) were used to quantify C4 transcripts. Comparative Ct method was used to calculate the fold changes (Livak and Schmittgen, 2001). C4 transcripts were normalized to GAPDH transcripts measured by TaqMan probe (Hs02786624_g1).
Construction of C4 Promoter-Luciferase Reporter Plasmids
The C4 promoter region was PCR amplified from human genomic DNA via full length-forward and reverse primers (Table 1). Then, the PCR product was cloned into pGEM-T-Easy vector (Promega, Madison, WI, United States). A single clone of C4 promoter was identified and digested by XhoI and HindIII (Promega). The C4 promoter was then subcloned into reporter gene vector pGL4.19 basic (Promega) at the XhoI and HindIII restriction sites to generate pC4-1,007/+44. To generate the 5’-deletion constructs, pC4-119/+44, pC4-102/+44, pC4-92/+44, pC4-72/+44, and pC4-48/+44, genomic DNA fragments were PCR amplified from the pGL4.19 plasmid using forward (segment 1 to segment 5) and reverse primers (Table 1). Then, the PCR product was digested by HindIII and XhoI, and subcloned into pGL4.19 basic.
Statistical Analysis
Statistical analyses were performed using GraphPad Prism (GraphPad Software, San Diego, CA, United States). Differences were compared via the non-pair-wised two-sample t-test.
Results
Isolation and Physicochemical Characterization of Water-Soluble Ginseng Polysaccharides
WGP were obtained via hot-water extraction and alcohol precipitation from ginseng powder. The yield of WGP was 0.85%. Carbohydrate content of WGP was over 98% and protein impurity was less than 0.1%. HPGPC was used for molecular weight analysis of WGP. We obtained two overlapping peaks and a single symmetric peak indicating that the molecular weight range of the WGP was from 1 kD to 79.4 kD (Figure 1A). The peak at 1 kD is due to incomplete interception of molecular sieve. Then, monosaccharide compositions analysis of WGP hydrolysate was performed using HPLC and nine monosaccharide reference standards. Monosaccharide profile was obtained by comparing retention times of nine standards under the same analytical conditions (Figures 1B,C). Chromatographic results demonstrated that WGP was composed of galacturonic acid, galactose, glucose, arabinose, rhamnose, glucuronic acid, and mannose in molar proportions of 28.9: 24.4: 23.0: 13.9: 6.7: 2.6: 0.6, respectively. Xylose and fucose were not detected. Consistent with published studies, glucose, galacturonic acid, and galactose are the most common monosaccharide compositions of WGP (Shi et al., 2017; Shin et al., 2017; Chen et al., 2018; Song et al., 2018; Zhao et al., 2019; Park et al., 2020). However, the specific proportion of these monosaccharides has varied widely across these studies, which may be due to the different origins of ginseng.
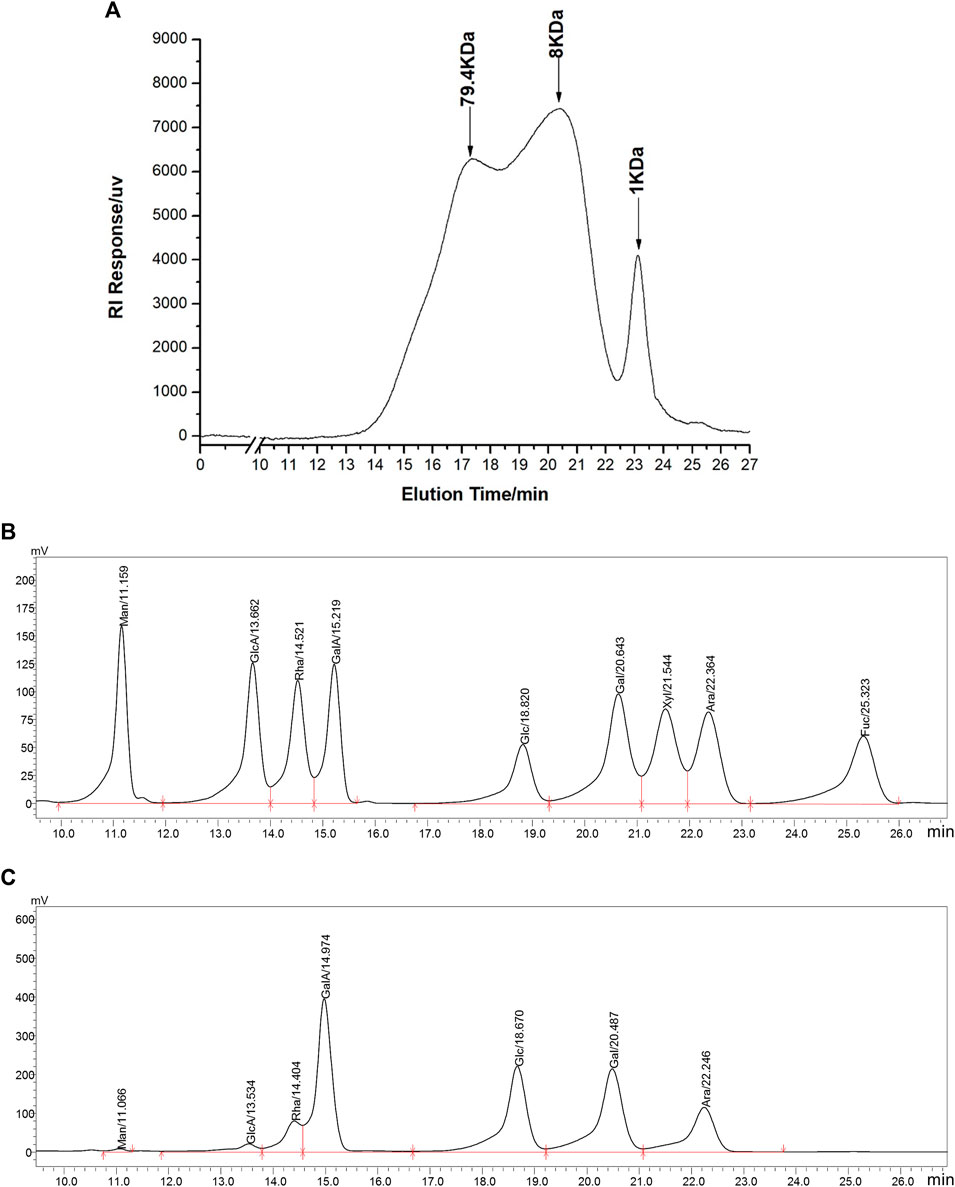
FIGURE 1. Chromatographic analysis of WGP. HPGPC was used to determine WGP molecular weight distribution (A). HPLC was used to separate monosaccharide standards (B) and analysis the monosaccharides composition in WGP (C). Man, GlcA, Rha, GalA, Glc, Gal, Xyl, Ara, and Fuc represent mannose, glucuronic acid, rhamnose, galacturonic acid, glucose, galactose, xylose, arabinose, and fucose, respectively.
The FT-IR spectrum of WGP showed characteristic hydroxyl and C-H stretching vibration peak at 3,416.51 and 2,929.93 cm−1, respectively. The absorption peaks at 1,625.99 and 1735.68 cm−1 were caused by bound water and C=O stretching vibration of uronic acid, respectively. The absorption peaks at 1,414.96 and 1,241.87 cm−1 represented C-H angular vibrations of carbohydrate. In addition, 1,153.18, 1,080.52, and 1,023.91 cm−1 were assigned to C-O-H and C-O-C stretching vibration peaks of pyran, indicated that WGP was connected by α-pyranoside bond. The absorption peaks at 936.61 and 851.02 cm−1 were the characteristic absorption peaks of α-Glcp (Figure 2A).
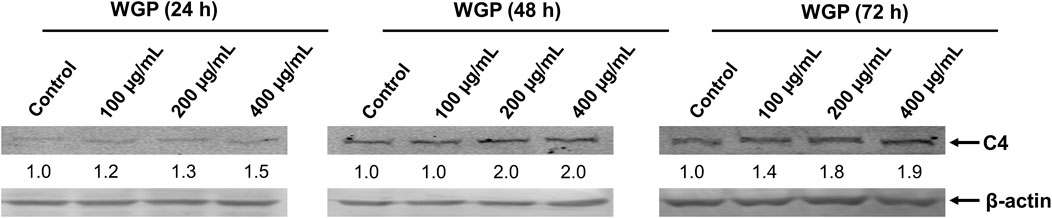
FIGURE 2. Structural characterization of WGP. FT-IR spectrum with the range of 4,000–400 cm−1(A) and 1H NMR (B), 13C NMR (C) spectra were used to characterize WGP structure.
WGP 1H NMR spectrum exhibited seven anomeric proton signals at δ5.32, 5.31, 5.14, 5.13, 5.06, 5.02, and 5.00 ppm, suggesting that the analyte was made up of seven monosaccharides. Intense signals within δ3.10–4.20 ppm represented CH-O and CH2-O groups of carbohydrate. The chemical shift from δ3.15–4.13 ppm was contributed by H-2 to H-6 protons. Meanwhile, no signal was observed at δ5.50 ppm indicating that WGP contained glucopyranose, consistent with the FT-IR result (Figure 2B). The structure of WGP was further analyzed by 13C NMR spectroscopy. β-1,4-Linked Gal residues exhibited six signals at δ105.15, 70.70, 73.05, 75.44, 74.05, and 61.58 ppm, corresponding to their C-1 to C-6. Signals at δ105.13 and 82.84 ppm were attributed to C-1 and C-3 of β-1,3-Gal, respectively. Furthermore, δ101.42 ppm was the heterocephalic carbon position of α-Glcp. Peak at δ78.04 ppm indicated that C-4 of α-Glcp had been replaced. δ73.07, 74.81, 72.54, and 62.83 ppm were the positions of C-2, C-3, C-5, and C-6 of α-1,4-Glcp, respectively. The anomeric signals at δ108.58 and 83.72 ppm were due to C-1 and C-3 carbons of α-1,3,5-Ara (Figure 2C). These results were consistent with the monosaccharide composition analysis and FT-IR spectrum of WGP.
Water-Soluble Ginseng Polysaccharides Induce C4 Expression in Hepatocytes
C4 is mostly synthesized in the liver (Galibert et al., 1997). Therefore, we chose human normal hepatocyte L-O2 cells as model. First, we investigated the cytotoxic effect of WGP on L-O2 cells by treating the L-O2 cells with WGP for 72 h. The results obtained from MTT assays showed that WGP treatment had minimal effect on viable cells, with the inhibition rate of viable cells less than 14% (Figure 3). Then, we determined the effect of WGP on the protein levels of C4 by western blotting. As shown in Figure 4, WGP increased C4 protein levels as early as 24 h and lasted for 72 h in a dose dependent way. These results show that WGP increase C4 production in hepatocytes.
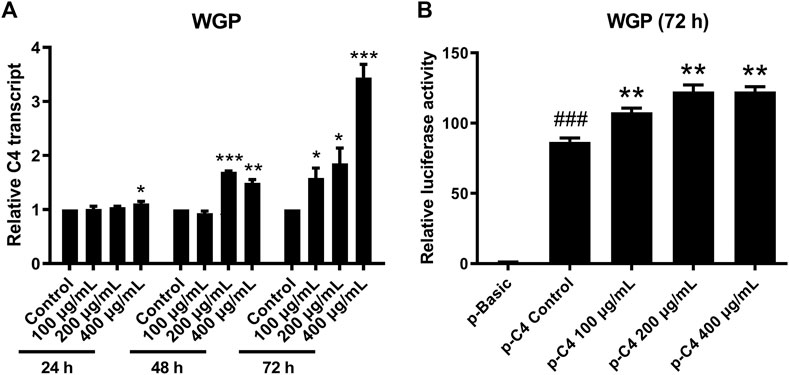
FIGURE 3. WGP have minimal effect on the growth of hepatocytes. L-O2 cells were treated with WGP at a series of equal ratio gradient concentrations for 72 h and then subjected to MTT assay. Data are presented as mean ± standard errors of the mean (SEM) from 3 independent experiments.
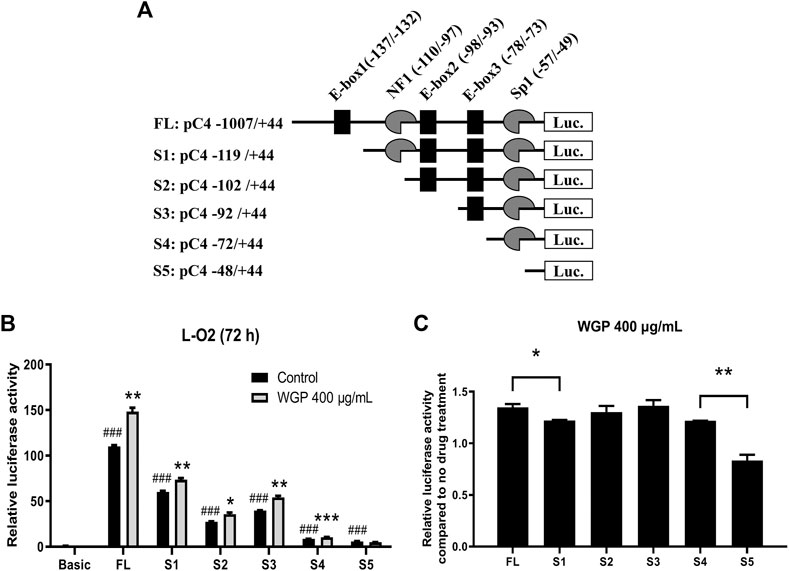
FIGURE 4. WGP increase C4 production in hepatocytes. L-O2 cells were treated with WGP at a series of equal ratio gradient concentrations for 24, 48 or 72 h. Protein levels of C4 were determined using western blotting. The fold changes of C4 densitometry measurements were compared to β-actin and then normalized to the vehicle control.
Effect of Water-Soluble Ginseng Polysaccharides on C4 Gene Transcription
To determine if WGP enhance C4 production through transcriptional mechanisms, we treated L-O2 cells with WGP for 24, 48, and 72 h, and then measured C4 mRNA levels by real-time PCR. Treatment of L-O2 cells with WGP for 48 and 72 h significantly increased levels of C4 mRNA, indicating WGP enhances C4 gene transcription (Figure 5A). To determine if WGP truly enhances C4 gene transcription, pGL4.19 reporter plasmid consisting of −1,007 to +44 region of the C4 promoter (designated FL) was used to confirm the effect of WGP on C4 transcription. Transient transfection of pC4-1,007/+44 into L-O2 cells was performed first, then the cells were treated with WGP for 72 h. The p-C4-1,007/+44 construct showed significant reporter activity compared to pGL4.19 basic in the absence or presence of WGP. Interestingly, treatment of the cells with WGP for 72 h significantly enhanced the C4 promoter reporter gene activity (Figure 5B). Taken together, these results demonstrate that WGP increase the production of C4 by promoting C4 gene transcription.
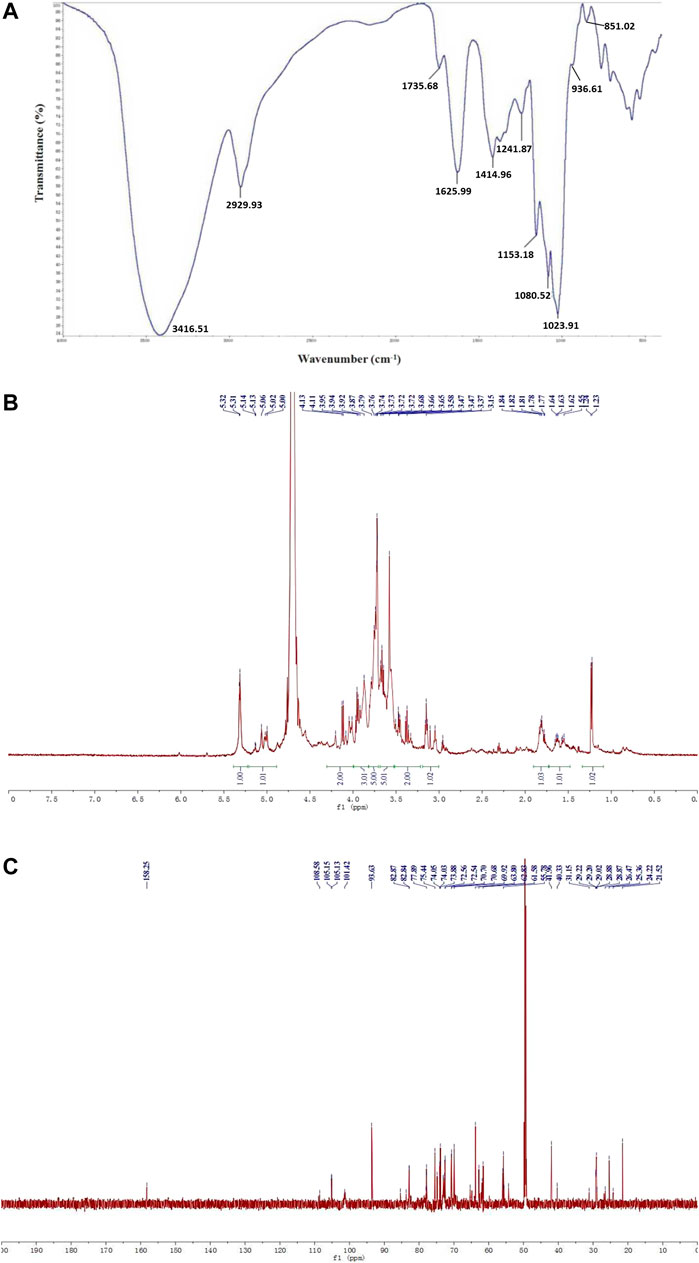
FIGURE 5. WGP promote C4 gene transcription. L-O2 cells were treated with WGP at a series of equal ratio gradient concentrations for up to 72 h. Real-time PCR method was used to detect the levels of C4 transcripts (A). L-O2 cells was transiently transfected with the full-length pC4 -1,007/+75 (p-C4) plasmid and then treated with WGP or vehicle control for 72 h. Luciferase assays were performed to determine the C4 promoter activity (B). Data are presented as mean of triplicates ±SEM from one representative experiment. * indicates p < 0.05, ** indicates p < 0.005, and *** indicates p < 0.001 compared to vehicle control. ### indicates p < 0.001 compared to the basic vector control.
Water-Soluble Ginseng Polysaccharides Enhance C4 Transcription Potentially via the E-box1 and Sp1 Elements
C4 promoter contains three E-boxes (positions −137 to −132, −98 to −93, and −78 to −73; designated E-box1, E-box2, and E-box3), one NF1 binding site (positions −110 to −97), and one Sp1 (positions −57 to −49) binding element (Figure 6A). To determine which Cis-acting element(s) is (are) vital to the enhancing effect of WGP, a series of 5’ deletion constructs (designated S1-S5; Figure 6A) were generated by using the −1,007 to +144 region of the C4 promoter as the template. As shown in Figures 6B,C, deletion from −1,007 to −119 significantly decreased C4 promoter activity and significantly decreased the enhancing effect of WGP on C4 promoter activity compared to the FL construct, indicating the E-box1 element in this region plays an important role in mediating the enhancing effect of WGP on C4 promoter. In contrast, further deletions from −119 to −102, −102 to −92, and −92 to −72 did not significantly affect the enhancing effect of WGP on C4 promoter. Interestingly, deletion from −72 to −48 completely abolished the enhancing effect of WGP, indicating the Sp1 element in this region also plays an important role in mediating the WGP effect on C4 promoter. Taken together, results from our deletion analyses suggest that the E-box1 and Sp1 cis-elements play vital roles in mediating the enhancing effect of WGP on C4 gene transcription.
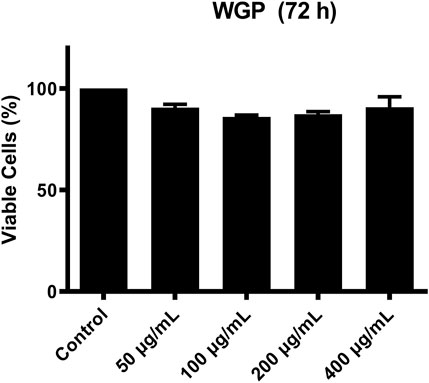
FIGURE 6. WGP enhance C4 transcription via the E-box1 and Sp1 elements. (A) The critical cis-regulatory elements (NF-1, E-box1∼3, Sp1) on the plus (+) and minus (-) DNA strand are shown. Numbering is relative to the C4 translation start site. A series of 5’-deletion constructs were generated by PCR amplification and subcloning into the pGL4.19 basic vector. (B) The C4 promoter constructs were transiently transfected into L-O2 cells. Luciferase assay was used to measure the activity of C4 promoter. * indicates p < 0.05, ** indicates p < 0.005, and *** indicates p < 0.001 compared to no drug treatment control. ### indicates p < 0.001 compared to basic vector control. (C) Fold changes of WGP treatment compared to vehicle control are graphed. * indicates p < 0.05 and ** indicates p < 0.005. Data are presented as mean of triplicates ±SEM from one representative experiment.
Discussion
Immunomodulatory activity is one of the main functions of ginseng polysaccharides (Guo et al., 2021). The immune system orchestrated by immune organs, immune cells, and immunoreactive substances has the functions of surveillance, defense, and regulation. In the modern world, increasing stress has shifted humans’ lifestyle leading to various health issues, such as cardiovascular disorders, hypertension, diabetes, and hypoimmunity (Parkin and Cohen, 2001). Hypoimmunity predisposes people to infection and cancer. In this study, we explored the immunomodulatory effect of ginseng polysaccharides from the perspective of C4, an important component of the complement system. Here, we demonstrate that WGP possess a strong and significant inductive effect on both protein and mRNA levels of C4 in hepatocyte. Complement deficiency including C4 has been associated with susceptibility to infection. C4 has been shown to inhibit adenoviral infections by inactivating viral capsids (Schröder-Braunstein and Kirschfink, 2019). Reduced levels of complement system components can cause autoimmune diseases due to the lacking of clearance of immunocomplexes (Conigliaro et al., 2019). In these scenarios, taking ginseng polysaccharide or ginseng extract is a potential adjuvant therapy.
C4 is composed of two isotypes, C4A and C4B. Both of them are encoded 10 kb apart in the major histocompatibility complex (MHC) class III area on the sixth human chromosome. C4A and C4B genes have similarly sequences located separately and closely (Carroll et al., 1984a; Carroll et al., 1984b). C4 promoter lacks a canonical TATA-box which is commonly found at promoters of genes transcribed by RNA polymerase II (Roeder, 1991). C4 promoter contains a nuclear factor 1 (NF-1) site at −110 to −97, an Sp1 site at −57 to −49, and three basic helix-loop-helix -like transcription factor sites at −137 to −132, −98 to −93, and −78 to −73, respectively referred to as E-box1, E-box2, and E-box3. These regions appear to be critical for the transcript activity of C4 gene (Vaishnaw et al., 1998). Thus, we performed deletion analyses within the C4 gene promoter. We found that E-box1 and Sp1 elements play key roles in WGP-regulated C4 transcription. A previous study has shown that the E-box motif of C4 promoter can be recognized by one of the many basic helix-loop-helix (bHLH) or basic helix-loop-helix-leucine zipper (bHLH-LZ) transcription factor family members (Littlewood and Evan, 1995). Moreover, IFN-γ could increase transcription of C4 via the E-box sequence at −78 to −73 (Banerjee et al., 2011). Thus, additional studies are warranted to determine the factors modulated by WGP in hepatocytes. However, these studies are not in the scope of this paper.
In conclusion, our results demonstrate that polysaccharides derived from ginseng can significantly increase production of C4 through transcriptional mechanisms. These results provide a molecular explanation for the immunity enhancing function of ginseng extracts.
Data Availability Statement
The original contributions presented in the study are included in the article/Supplementary Material, further inquiries can be directed to the corresponding authors.
Author Contributions
GW: conceptualization, project administration, investigation, funding acquisition, and writing the original draft; LS: conceptualization, project administration, and supervision; SL, FL, JL, TW, and CH: investigation and data curation.
Funding
This work was supported by a grant from National Key Research and Development Program of China (2017YFC1702103). This study was supported by the Key Laboratory for Molecular Enzymology and Engineering, the Ministry of Education, National Engineering Laboratory for AIDS Vaccine, School of Life Sciences, Jilin University, and Jilin Ginseng Academy, Research Center of Traditional Chinese Medicine, the Affiliated Hospital of Changchun University of Chinese Medicine, Key Laboratory of Active Substances and Biological Mechanisms of Ginseng Efficacy, the Ministry of Education, Changchun University of Chinese Medicine.
Conflict of Interest
The authors declare that the research was conducted in the absence of any commercial or financial relationships that could be construed as a potential conflict of interest.
Publisher’s Note
All claims expressed in this article are solely those of the authors and do not necessarily represent those of their affiliated organizations, or those of the publisher, the editors and the reviewers. Any product that may be evaluated in this article, or claim that may be made by its manufacturer, is not guaranteed or endorsed by the publisher.
References
Amsen, D., Antov, A., and Flavell, R. A. (2009). The Different Faces of Notch in T-Helper-Cell Differentiation. Nat. Rev. Immunol. 9 (2), 116–124. doi:10.1038/nri2488
Banerjee, A., Mazumdar, B., Meyer, K., Di Bisceglie, A. M., Ray, R. B., and Ray, R. (2011). Transcriptional Repression of C4 Complement by Hepatitis C Virus Proteins. J. Virol. 85 (9), 4157–4166. doi:10.1128/JVI.02449-10
Carroll, M. C., Belt, T., Palsdottir, A., and Porter, R. R. (1984a). Structure and Organization of the C4 Genes. Philos. Trans. R. Soc. Lond. B Biol. Sci. 306 (1129), 379–388. doi:10.1098/rstb.1984.0098
Carroll, M. C., Campbell, R. D., Bentley, D. R., and Porter, R. R. (1984b). A Molecular Map of the Human Major Histocompatibility Complex Class III Region Linking Complement Genes C4, C2 and Factor B. Nature 307 (5948), 237–241. doi:10.1038/307237a0
Carroll, M. C., and Isenman, D. E. (2012). Regulation of Humoral Immunity by Complement. Immunity 37 (2), 199–207. doi:10.1016/j.immuni.2012.08.002
Carter, R. H., and Fearon, D. T. (2010). Pillars Article: CD19: Lowering the Threshold for Antigen Receptor Stimulation of B Lymphocytes. Science, 1992. 256: 105-107. J. Immunol. 184 (5), 2233–2235.
Chen, Q. L., Chen, Y. J., Zhou, S. S., Yip, K. M., Xu, J., Chen, H. B., et al. (2018). Laser Microdissection Hyphenated with High Performance Gel Permeation Chromatography-Charged Aerosol Detector and Ultra Performance Liquid Chromatography-Triple Quadrupole Mass Spectrometry for Histochemical Analysis of Polysaccharides in Herbal Medicine: Ginseng, a Case Study. Int. J. Biol. Macromol 107, 332–342. doi:10.1016/j.ijbiomac.2017.08.162
Conigliaro, P., Triggianese, P., Ballanti, E., Perricone, C., Perricone, R., and Chimenti, M. S. (2019). Complement, Infection, and Autoimmunity. Curr. Opin. Rheumatol. 31 (5), 532–541. doi:10.1097/bor.0000000000000633
Dai, C., Heemers, H., and Sharifi, N. (2017). Androgen Signaling in Prostate Cancer. Cold Spring Harb Perspect. Med. 7 (9). doi:10.1101/cshperspect.a030452
Dodds, A. W., and Law, S. K. (1990). The Complement Component C4 of Mammals. Biochem. J. 265 (2), 495–502. doi:10.1042/bj2650495
Edwards, H., Xie, C., LaFiura, K. M., Dombkowski, A. A., Buck, S. A., Boerner, J. L., et al. (2009). RUNX1 Regulates Phosphoinositide 3-kinase/AKT Pathway: Role in Chemotherapy Sensitivity in Acute Megakaryocytic Leukemia. Blood 114 (13), 2744–2752. doi:10.1182/blood-2008-09-179812
Galibert, M. D., Boucontet, L., Goding, C. R., and Meo, T. (1997). Recognition of the E-C4 Element from the C4 Complement Gene Promoter by the Upstream Stimulatory Factor-1 Transcription Factor. J. Immunol. 159 (12), 6176–6183.
Gros, P., Milder, F. J., and Janssen, B. J. (2008). Complement Driven by Conformational Changes. Nat. Rev. Immunol. 8 (1), 48–58. doi:10.1038/nri2231
Guo, M., Shao, S., Wang, D., Zhao, D., and Wang, M. (2021). Recent Progress in Polysaccharides from Panax Ginseng C. A. Meyer. Food Funct. 12 (2), 494–518. doi:10.1039/d0fo01896a
Janssen, B. J., Christodoulidou, A., McCarthy, A., Lambris, J. D., and Gros, P. (2006). Structure of C3b Reveals Conformational Changes that Underlie Complement Activity. Nature 444 (7116), 213–216. doi:10.1038/nature05172
Kachur, K., and Suntres, Z. E. (2016). The Antimicrobial Properties of Ginseng and Ginseng Extracts. Expert Rev. Anti Infect. Ther. 14 (1), 81–94. doi:10.1586/14787210.2016.1118345
Kang, S., and Min, H. (2012). Ginseng, the 'Immunity Boost': The Effects of Panax Ginseng on Immune System. J. Ginseng Res. 36 (4), 354–368. doi:10.5142/jgr.2012.36.4.354
Kim, H. J., Kim, M. H., Byon, Y. Y., Park, J. W., Jee, Y., and Joo, H. G. (2007). Radioprotective Effects of an Acidic Polysaccharide of Panax Ginseng on Bone Marrow Cells. J. Vet. Sci. 8 (1), 39–44. doi:10.4142/jvs.2007.8.1.39
Kolev, M., Le Friec, G., and Kemper, C. (2013). The Role of Complement in CD4⁺ T Cell Homeostasis and Effector Functions. Semin. Immunol. 25 (1), 12–19. doi:10.1016/j.smim.2013.04.012
Lalli, P. N., Strainic, M. G., Yang, M., Lin, F., Medof, M. E., and Heeger, P. S. (2008). Locally Produced C5a Binds to T Cell-Expressed C5aR to Enhance Effector T-Cell Expansion by Limiting Antigen-Induced Apoptosis. Blood 112 (5), 1759–1766. doi:10.1182/blood-2008-04-151068
Li, B., Zhang, N., Feng, Q., Li, H., Wang, D., Ma, L., et al. (2019). The Core Structure Characterization and of Ginseng Neutral Polysaccharide with the Immune-Enhancing Activity. Int. J. Biol. Macromol 123, 713–722. doi:10.1016/j.ijbiomac.2018.11.140
Lim, T. S., Na, K., Choi, E. M., Chung, J. Y., and Hwang, J. K. (2004). Immunomodulating Activities of Polysaccharides Isolated from Panax Ginseng. J. Med. Food 7 (1), 1–6. doi:10.1089/109662004322984626
Littlewood, T. D., and Evan, G. I. (1995). Transcription Factors 2: helix-loop-helix. Protein Profile 2 (6), 621–702.
Livak, K. J., and Schmittgen, T. D. (2001). Analysis of Relative Gene Expression Data Using Real-Time Quantitative PCR and the 2(-Delta Delta C(T)) Method. Methods 25 (4), 402–408. doi:10.1006/meth.2001.1262
Medzhitov, R. (2007). Recognition of Microorganisms and Activation of the Immune Response. Nature 449 (7164), 819–826. doi:10.1038/nature06246
Park, D. H., Han, B., Shin, M. S., and Hwang, G. S. (2020). Enhanced Intestinal Immune Response in Mice after Oral Administration of Korea Red Ginseng-Derived Polysaccharide. Polymers (Basel) 12 (10), 2186. doi:10.3390/polym12102186
Parkin, J., and Cohen, B. (2001). An Overview of the Immune System. Lancet 357 (9270), 1777–1789. doi:10.1016/S0140-6736(00)04904-7
Roeder, R. G. (1991). The Complexities of Eukaryotic Transcription Initiation: Regulation of Preinitiation Complex Assembly. Trends Biochem. Sci. 16 (11), 402–408. doi:10.1016/0968-0004(91)90164-q
Schröder-Braunstein, J., and Kirschfink, M. (2019). Complement Deficiencies and Dysregulation: Pathophysiological Consequences, Modern Analysis, and Clinical Management. Mol. Immunol. 114, 299–311. doi:10.1016/j.molimm.2019.08.002
Senchina, D. S., Shah, N. B., Doty, D. M., Sanderson, C. R., and Hallam, J. E. (2009). Herbal Supplements and Athlete Immune Function-Wwhat's Proven, Disproven, and Unproven? Exerc. Immunol. Rev. 15, 66–106.
Shi, H., Yu, L., Shi, Y., Lu, J., Teng, H., Zhou, Y., et al. (2017). Structural Characterization of a Rhamnogalacturonan I Domain from Ginseng and its Inhibitory Effect on Galectin-3. Molecules 22 (6), 1016. doi:10.3390/molecules22061016
Shin, M. S., Hwang, S. H., Yoon, T. J., Kim, S. H., and Shin, K. S. (2017). Polysaccharides from Ginseng Leaves Inhibit Tumor Metastasis via Macrophage and NK Cell Activation. Int. J. Biol. Macromol 103, 1327–1333. doi:10.1016/j.ijbiomac.2017.05.055
Song, Y. R., Sung, S. K., Jang, M., Lim, T. G., Cho, C. W., Han, C. J., et al. (2018). Enzyme-assisted Extraction, Chemical Characteristics, and Immunostimulatory Activity of Polysaccharides from Korean Ginseng (Panax Ginseng Meyer). Int. J. Biol. Macromol 116, 1089–1097. doi:10.1016/j.ijbiomac.2018.05.132
Sun, Y. (2011). Structure and Biological Activities of the Polysaccharides from the Leaves, Roots and Fruits of Panax Ginseng C.A. Meyer: An Overview. Carbohydr. Polym. 85 (3), 490–499. doi:10.1016/j.carbpol.2011.03.033
Uphoff, C. C., and Drexler, H. G. (2005). Detection of Mycoplasma Contaminations. Methods Mol. Biol. 290, 13–23. doi:10.1385/1-59259-838-2:013
Vaishnaw, A. K., Mitchell, T. J., Rose, S. J., Walport, M. J., and Morley, B. J. (1998). Regulation of Transcription of the TATA-Less Human Complement Component C4 Gene. J. Immunol. 160 (9), 4353–4360.
Wang, J., Zhang, Y., Xu, X., Jin, W., Jing, C., Leng, X., et al. (2020). ASP2-1, a Polysaccharide from Acorus Tatarinowii Schott, Inhibits Osteoclastogenesis via Modulation of NFATc1 and Attenuates LPS-Induced Bone Loss in Mice. Int. J. Biol. Macromol 165 (Pt B), 2219–2230. doi:10.1016/j.ijbiomac.2020.10.077
Wang, M., Guilbert, L. J., Ling, L., Li, J., Wu, Y., Xu, S., et al. (2001). Immunomodulating Activity of CVT-E002, a Proprietary Extract from North American Ginseng (Panax Quinquefolium). J. Pharm. Pharmacol. 53 (11), 1515–1523. doi:10.1211/0022357011777882
Yu, X. H., Liu, Y., Wu, X. L., Liu, L. Z., Fu, W., and Song, D. D. (2017). Isolation, Purification, Characterization and Immunostimulatory Activity of Polysaccharides Derived from American Ginseng. Carbohydr. Polym. 156, 9–18. doi:10.1016/j.carbpol.2016.08.092
Yun, M., and Yi, Y. S. (2020). Regulatory Roles of Ginseng on Inflammatory Caspases, Executioners of Inflammasome Activation. J. Ginseng Res. 44 (3), 373–385. doi:10.1016/j.jgr.2019.12.006
Yun, T. K. (2001). Brief Introduction of Panax Ginseng C.A. Meyer. J. Korean Med. Sci. 16, S3–S5. doi:10.3346/jkms.2001.16.S.S3
Zhang, J., Koh, J., Lu, J., Thiel, S., Leong, B. S., Sethi, S., et al. (2009). Local Inflammation Induces Complement Crosstalk Which Amplifies the Antimicrobial Response. Plos Pathog. 5 (1), e1000282. doi:10.1371/journal.ppat.1000282
Keywords: ginseng, water-soluble ginseng polysaccharides, complement component 4, C4 transcription, C4 promoter
Citation: Liu S, Liu F, Wang T, Liu J, Hu C, Sun L and Wang G (2021) Polysaccharides Extracted From Panax Ginseng C.A. Mey Enhance Complement Component 4 Biosynthesis in Human Hepatocytes. Front. Pharmacol. 12:734394. doi: 10.3389/fphar.2021.734394
Received: 01 July 2021; Accepted: 30 August 2021;
Published: 10 September 2021.
Edited by:
Jing Zhao, University of Macau, ChinaReviewed by:
Wang Lingchong, Nanjing University of Chinese Medicine, ChinaLin Sun, Northeast Normal University, China
Copyright © 2021 Liu, Liu, Wang, Liu, Hu, Sun and Wang. This is an open-access article distributed under the terms of the Creative Commons Attribution License (CC BY). The use, distribution or reproduction in other forums is permitted, provided the original author(s) and the copyright owner(s) are credited and that the original publication in this journal is cited, in accordance with accepted academic practice. No use, distribution or reproduction is permitted which does not comply with these terms.
*Correspondence: Liwei Sun, c3VubnlsaWx3ZWlAMTYzLmNvbQ==; Guan Wang, d2cxMEBqbHUuZWR1LmNu
†These authors have contributed equally to this work