- 1Department of Clinical Pharmacology, Basil Hetzel Institute for Translational Health Research, The Queen Elizabeth Hospital, Woodville South, SA, Australia
- 2Discipline of Pharmacology, School of Medicine, University of Adelaide, Adelaide, SA, Australia
Tacrolimus is an essential immunosuppressant for the prevention of rejection in solid organ transplantation. Its low therapeutic index and high pharmacokinetic variability necessitates therapeutic drug monitoring (TDM) to individualise dose. However, rejection and toxicity still occur in transplant recipients with blood tacrolimus trough concentrations (C0) within the target ranges. Peripheral blood mononuclear cells (PBMC) have been investigated as surrogates for tacrolimus’s site of action (lymphocytes) and measuring allograft tacrolimus concentrations has also been explored for predicting rejection or nephrotoxicity. There are relatively weak correlations between blood and PBMC or graft tacrolimus concentrations. Haematocrit is the only consistent significant (albeit weak) determinant of tacrolimus distribution between blood and PBMC in both liver and renal transplant recipients. In contrast, the role of ABCB1 pharmacogenetics is contradictory. With respect to distribution into allograft tissue, studies report no, or poor, correlations between blood and graft tacrolimus concentrations. Two studies observed no effect of donor ABCB1 or CYP3A5 pharmacogenetics on the relationship between blood and renal graft tacrolimus concentrations and only one group has reported an association between donor ABCB1 polymorphisms and hepatic graft tacrolimus concentrations. Several studies describe significant correlations between in vivo PBMC tacrolimus concentrations and ex vivo T-cell activation or calcineurin activity. Older studies provide evidence of a strong predictive value of PBMC C0 and allograft tacrolimus C0 (but not blood C0) with respect to rejection in liver transplant recipients administered tacrolimus with/without a steroid. However, these results have not been independently replicated in liver or other transplants using current triple maintenance immunosuppression. Only one study has reported a possible association between renal graft tacrolimus concentrations and acute tacrolimus nephrotoxicity. Thus, well-designed and powered prospective clinical studies are still required to determine whether measuring tacrolimus PBMC or graft concentrations offers a significant benefit compared to current TDM.
Introduction
The first calcineurin inhibitor (CNI), ciclosporin, revolutionised solid organ transplantation in the early 1980s providing, for the first time, immunosuppression that selectively targeted T-cell mediated rejection. Calcineurin is a serine-threonine phosphatase that dephosphorylates nuclear factor of activated T-cells (NFAT) allowing the translocation of this nuclear receptor into the nucleus, initiating T-cell activation via upregulation of interleukin-2 expression (Brunet et al., 2019). The second CNI, tacrolimus entered clinical use in the early 1990s, further reducing the incidence of rejection, and rapidly becoming the cornerstone of maintenance immunosuppression in solid organ transplantation. Tacrolimus inhibits calcineurin by binding to its cytosolic receptor, FKBP12. Both ciclosporin and tacrolimus have low therapeutic indices and, due to their central CNI mechanism of action, have overlapping spectra of adverse effects including nephrotoxicity, one of the major dose-limiting toxicities (Brunet et al., 2019).
Tacrolimus pharmacokinetics display significant inter-individual variability due to differences in the hepatic and intestinal expression/activity of cytochrome P450 (CYP) 3A4, CYP3A5 and P-glycoprotein, arising from the effects of genetic polymorphisms, drug- and environmental-interactions (Christians et al., 2002; Staatz and Tett, 2004; Brunet et al., 2019). The latter two also contributing to intra-individual pharmacokinetic variability. Pharmacokinetic variability together with tacrolimu’s low therapeutic index has led to dosage individualisation using therapeutic drug monitoring (TDM) (Staatz and Tett, 2004). During its early clinical use blood tacrolimus concentration-response relationships with respect to rejection and/or adverse effects were described in clinical studies of renal and hepatic transplant recipients administered tacrolimus alone or with a steroid (with or without azathioprine) (Kershner and Fitzsimmons, 1996). However, despite relatively high therapeutic ranges, a continuing significant incidence of rejection and nephrotoxicity spurred the development of induction therapy and the establishment of triple maintenance immunosuppression consisting primarily of tacrolimus co-administered with a corticosteroid and mycophenolic acid (Wallemacq et al., 2009). This has allowed a significant decrease in the targeted therapeutic range of whole blood trough tacrolimus concentrations (C0Blood), minimising long-term nephrotoxicity (Brunet et al., 2019) whilst still maintaining an acceptably low risk of rejection. However, the effectiveness of the therapeutic range is controversial, and rejection still occurs in patients with C0Blood within the current therapeutic ranges (Wallemacq et al., 2009; Hu et al., 2019).
In whole blood tacrolimus distributes primarily within erythrocytes (approximately 85%), another 14% is distributed in plasma and only a small proportion (< 1%) is in the mononuclear cell fraction that contains lymphocytes (Figure 1) (Zahir et al., 2001; Zahir et al., 2004a; Zahir et al., 2004b). In plasma tacrolimus binds to soluble proteins and, to a lesser extent, lipoproteins, resulting in a low plasma unbound fraction (approximately 1%) (Zahir et al., 2001; Zahir et al., 2004a; Zahir et al., 2004b). Haematocrit and erythrocyte numbers significantly affect the distribution of tacrolimus into plasma (Zahir et al., 2004a), as does concentration-dependent binding within erythrocytes (Zahir et al., 2001). Although plasma protein binding is not concentration-dependent, it is significantly affected by plasma α1-acid glycoprotein and HDL-cholesterol concentrations (Zahir et al., 2004a). Since only unbound tacrolimus is available for distribution into lymphocytes and other tissues where it exerts pharmacological activity, the use of blood concentrations for tacrolimus TDM is problematic as changes or differences in blood concentrations may reflect alterations in binding to erythrocytes or plasma proteins, rather than any differences in unbound tacrolimus concentrations. This has led to considerable effort to measure tacrolimus concentrations directly at its sites of immunosuppression (lymphocytes) and of toxicity. Peripheral blood mononuclear cells (PBMC) are a readily accessible matrix that has been investigated as a convenient surrogate for lymphocyte tacrolimus concentrations. Allograft tissue biopsy samples have similarly been investigated as a potential adjunct to tacrolimus TDM. In 2016 Capron et al. (2016) reviewed the potential of monitoring intra-cellular immunosuppressant drug concentrations in transplantation, and Lemaitre et al. (2020) recently published an expert consensus on requirements for measuring PBMC tacrolimus concentrations. This review will update the evidence for tacrolimus concentration-effect relationships using either PBMC or graft tissue; the pharmacokinetics of tacrolimus in these biological matrices; and the relationship between blood, PBMC and graft tacrolimus concentrations.
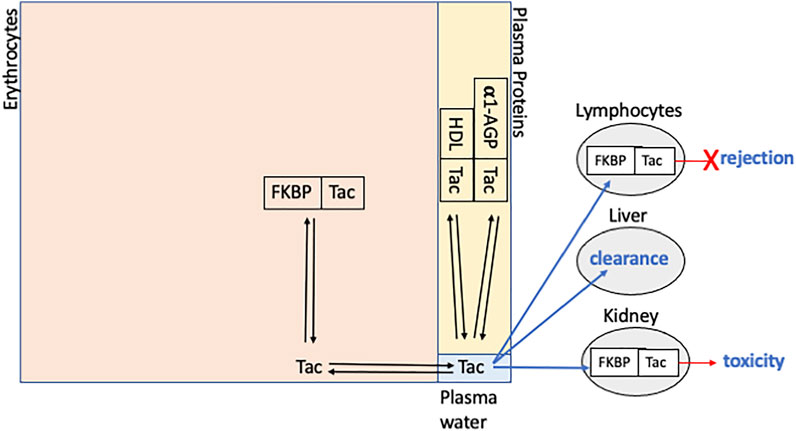
FIGURE 1. Schematic representation of tacrolimus (Tac) distribution within whole blood showing binding to FKBP12 (FKBP), high density lipoproteins (HDL) and alpha-1 acid glycoprotein (α1-AGP). The large rectangle is divided into sections whose area approximates relative distribution between erythrocytes, plasma proteins and plasma water (unbound tacrolimus). The PBMC compartment is not shown and represents < 1% of tacrolimus in whole blood. Blue arrows indicate distribution into: lymphocytes, where biding with FKBP12 results in inhibition of calcineurin and prevention of rejection; liver, the primary site of clearance; and kidneys, where binding with FKBP12 and inhibition of calcineurin may be associated with nephrotoxicity.
PBMC Tacrolimus Concentrations
Rejection and Nephrotoxicity
Zahir et al. provided the first evidence for a possible clinical benefit of measuring PBMC tacrolimus concentrations in a study of 40 adult liver transplant recipients. They reported that a lower proportion of total blood tacrolimus was associated with the leucocyte fraction in patients with rejection compared to those without (Zahir et al., 2004a; Zahir et al., 2004b). In 90 adult liver transplant recipients studied 7 days post transplantation, Capron et al. (2012) later observed that whilst there was no relationship between C0Blood and rejection, trough PBMC tacrolimus concentrations (C0PBMC) were significantly lower in patients with rejection compared to those without, regardless of whether rejection was classified histologically or clinically (Table 1A). Importantly, C0PBMC measured on days 3 and 5 were also significantly different between recipients who would be classified as rejectors or non-rejectors on day-7, suggesting the potential to predict early rejection, In addition, day-7 C0PBMC correlated with the histological grading of rejection. To date, this is the only study that has directly and prospectively compared prediction of rejection by C0Blood and matched C0PBMC taken on the same day as the protocol liver biopsy used to classify rejection. However, patients received only tacrolimus monotherapy for maintenance immunosuppression, with or without anti-lymphocytic induction therapy, and there was a high incidence (41%) of moderate/severe histological rejection.
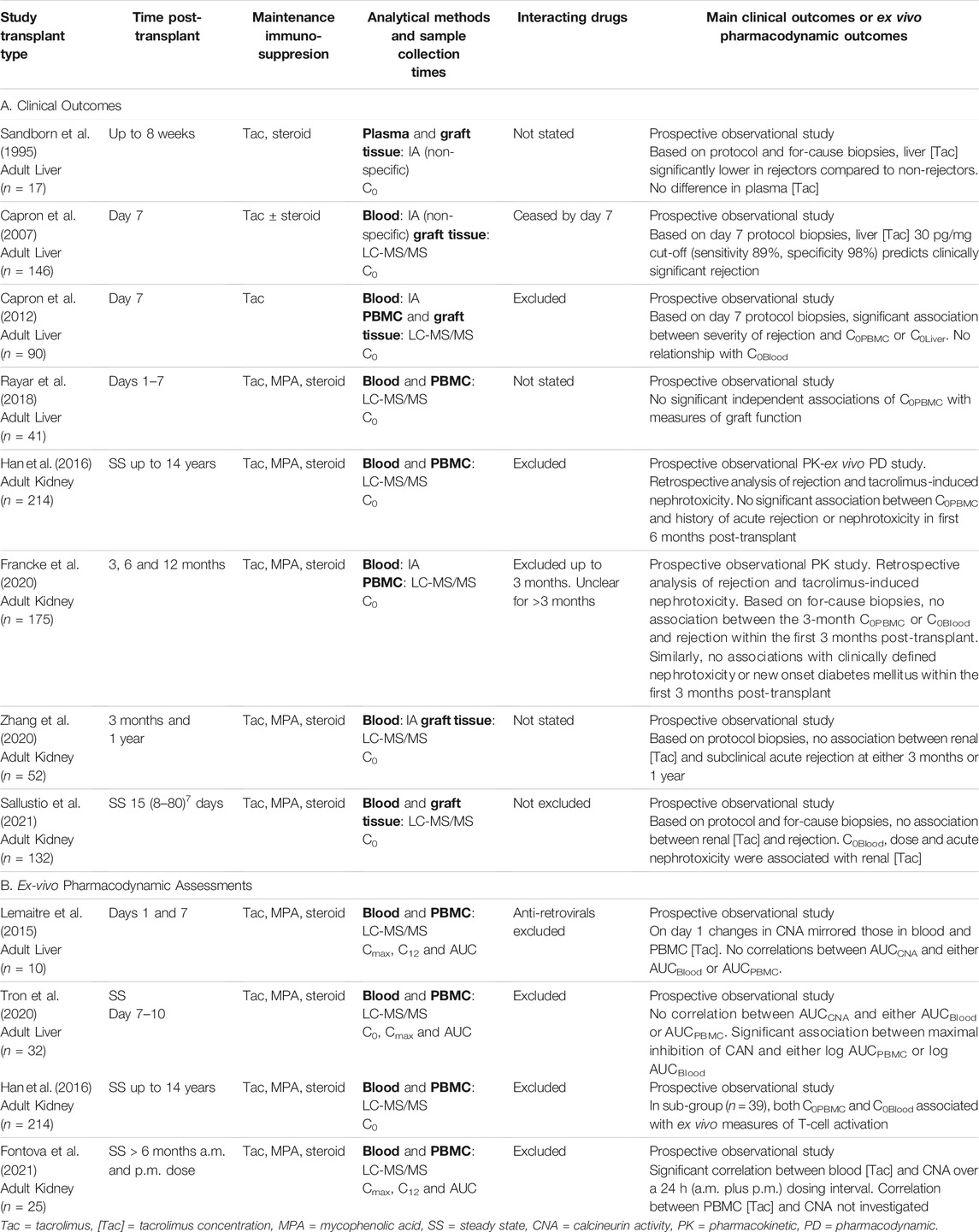
TABLE 1. Summary of clinical studies investigating blood, PBMC and allograft tacrolimus concentrations and their associations with clinical outcomes or ex-vivo pharmacodynamic assessments.
Two other clinical studies that measured PBMC tacrolimus concentrations in liver transplants have also included clinical outcome data. These more recent studies recruited patients receiving triple maintenance immunosuppression with tacrolimus, mycophenolic acid and a corticosteroid (Lemaitre et al., 2015; Rayar et al., 2018). In 10 transplant recipients recruited at steady state (Table 1B), only one case of acute rejection was observed precluding any investigation of the relationship between rejection and tacrolimus concentrations in PBMC (Lemaitre et al., 2015). A study of 41 patients (Table 1A) recruited in the first week post transplantation did not collect data on rejection but found no association between C0PBMC and measures of graft function (Rayar et al., 2018).
Only three studies in kidney transplant recipients provide any data on clinical outcomes and tacrolimus concentrations in blood and PBMC (Francke et al., 2020; Han et al., 2016; Klaasen et al., 2018). Again, transplant recipients received triple maintenance immunosuppression as above. In a small study of 29 patients (Supplementary Table S1), the incidence of rejection 1 week post-transplant was too low to adequately investigate any relationship with PBMC tacrolimus concentrations (Klaasen et al., 2018). Two larger studies (Francke et al., 2020; Han et al., 2016) carried out at steady state assessed clinical outcomes retrospectively (Table 1A). Han et al. reported 15.6% of kidney transplant recipients experienced rejection within the first 6 months post-transplant (Han et al., 2016). However, the authors did not state how rejection was defined and found no association between rejection and C0PBMC measured in 214 patients up to 14 years post-transplant. Using only for-cause biopsies in 175 recipients, Francke et al. reported 8% developed biopsy proven rejection in the first 3 months of renal transplantation, with no difference in the 3-month C0PBMC, C0Blood or their ratio between patients who had and hadn’t experienced rejection (Francke et al., 2020). Both studies (Han et al., 2016; Francke et al., 2020) also investigated tacrolimus-induced nephrotoxicity and found no difference between C0PBMC in patients who had or hadn’t experienced nephrotoxicity over the first 6 or 3 months of renal transplantation, respectively.
Overall, there is very little evidence for clinical utility of C0PBMC in the prediction of rejection or tacrolimus-induced nephrotoxicity. This may mostly be due to small sample sizes, the low incidence of rejection with modern triple maintenance immunosuppression, and the often retrospective assessment of rejection and nephrotoxicity. The only clinical evidence for prediction of rejection is from a single well-designed study in liver transplant patients receiving tacrolimus monotherapy, which may not be directly translatable to patients on modern maintenance immunosuppression or to other transplant types.
Ex vivo Calcineurin Activity and T-Cell Activation
In the absence of sufficient clinical outcome data, several groups have investigated the relationship between blood or PBMC tacrolimus concentrations and ex vivo calcineurin activity (CNA) or T-cell activation (Table 1B). In 10 de novo liver transplant recipients studied on days 1 and 7 following commencement of tacrolimus therapy Lemaitre et al. (2015) reported that, over a 12-h dosing interval, inhibition of PBMC CNA on day 1 mirrored tacrolimus concentrations in both blood and PBMC, with an average maximum inhibition of 38% occurring slightly after attainment of maximum tacrolimus concentrations in blood (CmaxBlood) and PBMC (CmaxPBMC). On day 7, no change in CNA was observed over the dosing interval, and less peak to trough variability in the tacrolimus concentration vs time curves was apparent in both blood and PBMC compared to day 1. There was no correlation between the 12-h area under the CNA vs time curve (AUCCNA) on days 1 or 7 and the corresponding 0–12 h areas under the tacrolimus concentration vs time curves in whole blood (AUCBlood) or PBMC (AUCPBMC). In this study, although PBMC tacrolimus concentrations and CNA were measured in the whole PBMC fraction, the authors accounted for granulocyte contamination of PBMC by expressing the tacrolimus concentrations per 106 leukocytes, rather than the conventional use of total cells. However, tacrolimus would likely also distribute into granulocytes, potentially biasing the relationship between measured intracellular tacrolimus concentrations and CNA.
In 32 de novo liver transplant recipients studied between day 7–10 post-transplant, Tron et al. (2020) confirmed that maximal inhibition of CNA (CNAImax) occurred 2 h post-dose, slightly after attainment of CmaxBlood and CmaxPBMC (1.6 h post-dose). CNAImax was correlated with both log-CmaxPBMC and log-CmaxBlood with a median 37% maximal inhibition compared to baseline CNA measured before administration of the first post-transplant tacrolimus dose. The authors calculated CmaxBlood and CmaxPBMC producing 50% inhibition of CNA (IC50) of 18 μg/L and 100 pg/106 cells, respectively. Similar to an in vitro IC50 of 160 pg/106 cells calculated using PBMC isolated from healthy volunteers (Tron et al., 2019). Using a population pharmacokinetic model developed in the same study (discussed below in Whole blood and PBMC tacrolimus pharmacokinetics), Tron et al. (2020) estimated that in recipients with C0Blood of <4, 4-6 or 6–10 μg/L only 13, 39, and 42%, respectively, were likely to attain CmaxPBMC greater than the IC50. In comparison, Capron et al. reported mean (s.d.) C0PBMC of 90.9 (41.2) vs 33.8 (16.7) pg/106 cells in liver transplant patients with no/mild vs moderate/severe biopsy graded rejection; and 48.7 (11.9) vs 22.0 (6.1) pg/106 cells in patients without vs with clinically significant rejection (Capron et al., 2012). This may suggest that in vivo prevention of rejection potentially occurs at tacrolimus PBMC concentrations lower than those required for ex vivo inhibition of PBMC CNA. Interestingly, Tron et al. also reported significant inter-individual variability in baseline CNA (coefficient of variation (CV) = 66%), indicating a considerable component of inter-individual pharmacodynamic variability. Most recently, Fontova et al. (2021) also reported an average 29% maximum inhibition of CNA within a 12 h dosing interval and a significant inverse correlation between AUCBlood and the area under the percentage inhibition of CNA versus time curve in renal transplant recipients. Although they also measured AUCPBMC a similar analysis was not performed.
Han et al. (2016) investigated C0Blood and C0PBMC in 213 stable renal transplant recipients, and quantitated interferon-γ (IFN-γ) and interleukin-2 (IL-2) expressing T-cells in a subset of 39 recipients grouped according to C0PBMC. They reported that, following ex vivo activation with phorbol-12-myristate 13-acetate and ionomycin, the proportion of CD3+CD4+IFN-γ+, CD3+CD4+IL-2+ and CD3+CD8+IL-2+ T-cells was significantly greater in the low C0PBMC group. However, a significantly higher proportion of CD3+CD4+IFN-γ+ and CD3+CD4+IL-2+ cells was also observed when the comparisons were based on low versus high C0Blood.
In general, these studies show that both blood and PBMC tacrolimus concentrations correlate with inhibition of CNA or measures of lymphocyte activation, although the correlations appear stronger using PBMC concentrations. The reports are also consistent with other studies indicating that tacrolimus does not completely inhibit lymphocyte CNA (Fukudo et al., 2005) and support the role of triple maintenance immunosuppression in allowing lower tacrolimus exposures whilst still maintaining a relatively low risk of rejection.
Whole Blood and PBMC Tacrolimus Pharmacokinetics
Tacrolimus PBMC concentrations have been measured in recipients of liver, kidney and heart transplants, with mean C0PBMC ranging from 22.5–266 pg/106 cells and corresponding mean C0Blood between 3.4–10.5 μg/L (Capron et al., 2010; Capron et al., 2012; Lemaitre et al., 2013; Lemaitre et al., 2015; Han et al., 2016; Klaasen et al., 2018; Romano et al., 2018; Francke et al., 2020; Tron et al., 2020; Fontova et al., 2021) (Supplementary Table S1). Most studies report greater inter-individual variability in PBMC compared to blood tacrolimus concentrations, with CVs for C0PBMC and C0Blood ranging from 40 to 110% and 20–57%, respectively (Capron et al., 2010; Capron et al., 2012; Lemaitre et al., 2013; Lemaitre et al., 2015; Han et al., 2016; Klaasen et al., 2018; Romano et al., 2018; Tron et al., 2020). Whether this reflects greater physiological variability or analytical variability is unclear. Only one study has assessed variability of the PBMC preparation step and reported a CV of 7.3%, which was greater than the intra-assay imprecision but similar to inter-assay imprecision (Klaasen et al., 2018). This provides some confidence that inter-individual variability in tacrolimus PBMC pharmacokinetics may indeed be greater than in blood, supporting a potential benefit of measuring concentrations in PBMC.
In keeping with a greater variability of tacrolimus PBMC pharmacokinetics, a lack of statistically significant correlation between C0Blood and C0PBMC has been reported in some studies (Capron et al., 2010; Capron et al., 2012; Lemaitre et al., 2013; Romano et al., 2018). However, others have found significant, albeit weak, correlations between blood and PBMC tacrolimus concentrations at C0 (Pensi et al., 2015; Han et al., 2016; Klaasen et al., 2018; Francke et al., 2020; Tron et al., 2020), 1.5 h post dose (Klaasen et al., 2018) and Cmax (Tron et al., 2020), and between AUCBlood and AUCPBMC (Lemaitre et al., 2015; Tron et al., 2020; Fontova et al., 2021). Tron et al. (2020) also reported that C0Blood correlated with both CmaxPBMC and AUCPBMC. Fontova et al. recently demonstrated circadian variability in both blood and PBMC tacrolimus pharmacokinetics, with higher blood and PBMC tacrolimus exposures following the morning (compared to evening) dose; and stronger correlations between the 0–12 h tacrolimus AUC in blood or PBMC and the corresponding 12 h (C12), rather than the pre-dose (C0) trough concentrations (Fontova et al., 2021). Previous studies have also reported better correlations between C12Blood and AUCBlood (Barraclough et al., 2011; Marquet et al., 2018).
Since T-cells can comprise between 12–92% of total cell numbers in PBMC preparations, one study has compared tacrolimus C0 in whole blood, PBMC, purified CD4+ T-cells and purified CD19+ B-cells of kidney transplant recipients (Romano et al., 2018). In this study, tacrolimus C0 were higher in T- and B-cells compared to PBMC, and there was a significant correlation between C0 in blood and T-cells, but not between C0 values in any of the other matrices. A study in healthy volunteers administered tacrolimus also found a significant correlation between tacrolimus concentrations in blood and T-cells (but not PBMC) (In 't Veld et al., 2019), and in contrast to the study in transplant recipients, tacrolimus concentrations in T-cells were lower than those in PBMC. Taken together, all studies indicate that across liver, renal and heart transplant groups, the relationship between blood and PBMC tacrolimus concentrations is relatively modest and may be affected by the composition of the PBMC fraction. This may, in part, contribute to the poor correlation between blood and PBMC tacrolimus concentrations, as rejection and inflammation are likely to alter the cellular composition of this matrix.
As previously discussed, only unbound tacrolimus distributes from plasma into erythrocytes and the other cells contained in whole blood. Thus, changes in haematocrit, red cell number and plasma proteins may impact the proportion of tacrolimus in whole blood that is distributed within the PBMC fraction. In addition, leucocyte uptake and efflux of tacrolimus may involve carrier mediated processes which may be subject to saturability, induction, inhibition and genetic polymorphisms. All these processes may also affect the rate and extent of tacrolimus distribution within the different compartments in whole blood. The C0PBMC/C0Blood ratio is an indication of the proportion of whole blood tacrolimus that is distributed within PBMC. Identifying covariates that determine this ratio may assist in the prediction of tacrolimus C0PBMC from C0Blood.
In kidney transplant recipients, Capron et al. (2010) used multiple linear regression to assess pharmacogenetic and other clinical variables (Table 2A) as predictors of C0PBMC, C0PBMC/dose and C0PBMC/C0Blood. They reported that recipient ABCB1 SNPs (1199GA, 3435TT), CYP3A5 non-expressor genotype, a CYP3A5*3—ABCB1 1199GA interaction, and the log of mean corpuscular volume (MCV) were independent determinants of C0PBMC/Dose one week after renal transplantation, whilst at steady-state ABCB1 1199GA was no longer significant. The effect of CYP3A5 most likely reflected the TDM guided lower doses of tacrolimus in non-expressors. In contrast, ABCB1 SNPs (1199GA, 3435CT, 3435TT) and total plasma protein concentrations were independent determinants of C0PBMC/C0Blood one week post-transplantation, whilst at steady-state ABCB1 3435TT was no longer significant (Table 2A). In this study the variants of ABCB1 (the gene coding for P-glycoprotien) were independent predictors of a higher C0PBMC/C0Blood ratio (Table 2A), consistent with reduced efflux of tacrolimus from PBMC. In addition, high total plasma protein was an independent predictor of a lower ratio (Table 2A), consistent with increased binding of tacrolimus to plasma proteins and therefore less unbound tacrolimus available for distribution into PBMC. Unfortunately, haematocrit does not appear to have been tested as a covariate in this analysis.
In contrast, in stable renal transplants, Han et al. (2016) found no association between recipient ABCB1 SNPs and C0PBMC/C0Blood, but did find a significant association with sex, haematocrit and transplant duration using analysis of covariance (Table 2A). In addition, haematocrit and transplant duration were also significantly associated with C0PBMC. Unfortunately, tacrolimus dose was not investigated. More recently, using multiple linear regression in kidney transplants, Francke et al. (2020) also found no effect of recipient ABCB1 1199/3435 variant alleles, CYP3A5*3 or CYP3A4*22 on C0PBMC/C0Blood ratio but age, albumin and haematocrit were independent predictors of the ratio at 3 months post-transplant (Table 2A), similar to (Han et al., 2016).
Tron et al. (2020) developed a 2-compartment population pharmacokinetic model describing the relationship between blood and PBMC tacrolimus concentrations approximately 1 week after liver transplantation. Although they did not find any demographic or pharmacogenetic covariates that significantly improved their model, univariate analyses of model-derived AUCs and observed C0 and Cmax revealed a significant but weak inverse correlation between haematocrit and AUCPBMC and between haematocrit and the ratio of AUCPBMC/AUCBlood (Table 2B), similar to the studies in renal transplants. High haematocrit is consistent with a larger erythrocyte binding compartment and therefore less unbound tacrolimus available for distribution into PBMC. In the above renal and hepatic transplant studies, haematocrit was the only consistent determinant of the proportion of whole blood tacrolimus that is present within PBMC. Haematocrit is also a significant covariate in many solid organ transplant population models of whole blood tacrolimus pharmacokinetics (Brooks et al., 2016).
Tron et al. also found that recipient (but not donor) 2677TT and 1236/2677/3435 homozygous triple variant ABCB1 SNPs were associated with a lower AUCPBMC/AUCBlood ratio, whilst the recipient ABCB1 1199A variant allele had no effect (Table 2B) (Tron et al., 2020). In this study the lower AUCPBMC/AUCBlood ratio in carriers of the ABCB1 3435T variant contradicts its association with higher C0PBMC/C0Blood ratios reported by Capron et al. (2010) 1 week after renal transplantation. In addition, the association between recipient ABCB1 1199A variant and C0PBMC/C0Blood ratios reported by Capron et al. (2010) 1 week after renal transplantation was not observed for the AUC ratio reported by Tron et al. (2020). In kidney transplants, recipient genotypes would relate to hepatic, intestinal and PBMC enzyme/transporter activities (Capron et al., 2010), whilst in liver transplants (Tron et al., 2020) recipient genotypes would relate to intestinal (not hepatic) and PBMC activities, potentially explaining some of the pharmacogenetic discordance between studies. Additionally, relatively small sample sizes and very small numbers of patients who were carriers of variant alleles may also have contributed to discordant observations.
Although ABCB1 genetic polymorphisms are not major determinants of tacrolimus blood clearance, the ABCB1 1199G>A SNP has been shown to increase in vitro intra-cellular accumulation of tacrolimus in HEK293 and K562 recombinant cell lines (Dessilly et al., 2014). However, its relative role in the net efflux of tacrolimus from PBMC is unknown and currently its effects on the PBMC:blood concentration ratio is contradictory. Unfortunately, direct comparison of these studies is difficult due to differences in the preparation of PBMC; cellular composition of PBMC, sample size; ethnicity of transplant recipients; exclusion of drugs that interact with CYP3A and P-glycoprotein; transplant duration; covariates investigated; statistical analyses and corrections for multiple comparisons.
Most of the above studies have addressed inter-individual variability in the C0PBMC/C0Blood ratio but not intra-individual variability. Han et al. (2016) measured C0PBMC/C0Blood on two occasions in a small subset of renal transplant recipients and reported that the ratio measured >1 year post-transplant was significantly lower than that measured in the first year. In contrast, two later studies with larger sample sizes found no effect of time post-transplant on C0PBMC/C0Blood when subjects were repeatedly sampled at different time-points after transplantation (Klaasen et al., 2018; Francke et al., 2020). They reported median or mean intra-individual CVs of 45% (range 5.9–88%) (Klaasen et al., 2018) and 39.0% (range 3.5–173.2%) (Francke et al., 2020), which were lower than the CVs for inter-individual variability (Francke et al., 2020). Interestingly, in the patients with the greatest intra-individual variability, the variability could not be explained by changes in haematocrit (Francke et al., 2020).
One important determinant of tacrolimus distribution (and activity), which has not been addressed, is intra-cellular binding capacity (Figure 1). FKBP12 is the major erythrocyte cytoplasmic protein to which tacrolimus binds (Nagase et al., 1994). Whilst haematocrit is an estimate erythrocyte numbers, it does not address variability in erythrocyte expression of FKBP12. Although inhibition of calcineurin in lymphocytes is mediated by the tacrolimus-FKBP12 complex, tacrolimus also binds to other FKBPs whose expression differs between tissues and cell types (Baughman et al., 1997). Thus, differences or changes in the expression of FKBPs are likely to affect both inter- and intra-individual variability in whole blood and PBMC tacrolimus pharmacokinetics. In addition, variability in FKBP expression within lymphocytes may also affect the degree of calcineurin inhibition by tacrolimus (Kung and Halloran, 2000).
Allograft Tacrolimus Concentrations
Rejection
In 1992 Sandborn et al. reported that liver transplant recipients with cellular rejection had lower graft tissue ciclosporin concentrations than those without (Sandborn et al., 1992). They later expanded these observations to tacrolimus in a group of 17 de novo liver transplant recipients administered maintenance immunosuppression consisting of tacrolimus and prednisolone (Sandborn et al., 1995). Seven of the 17 patients developed nine episodes of histological rejection and had graft tacrolimus concentrations (measured using clinical biopsy samples) that were significantly lower than those in the patients without rejection. However, they found no difference in plasma tacrolimus concentrations between patients with and without rejection. These early reports (Table 1A) provided much of the impetus for better understanding the role of graft tacrolimus concentrations in determining risk of rejection. In these early studies both plasma and tissue tacrolimus concentrations were measured by an immunoassay with significant cross-reactivity to tacrolimus metabolites (Wallemacq et al., 2009). Since the metabolite/parent tacrolimus concentration ratio changes over a dosing interval and may also differ between plasma and other tissues, these early observations may have been subject to significant analytical bias. Capron et al. next investigated the relationship between graft tacrolimus concentrations and rejection in 146 de novo liver transplant recipients administered tacrolimus and corticosteroid maintenance immunosuppression (Table 1A) (Capron et al., 2007). They reported that day 7 graft tacrolimus concentrations (measured by a specific LC-MS/MS method) were significantly lower in patients with moderate/severe histological rejection compared to those with no (or mild) rejection, and that there was a strong first-order exponential correlation between Banff histology score and hepatic tacrolimus concentrations (r2 = 0.98 p = 0.002). A cut-off hepatic tacrolimus concentration of 30 pg/mg of tissue predicted clinically significant rejection with 89% sensitivity and 98% specificity. In comparison, there was no difference in C0Blood in patients with or without mild/moderate rejection. However, C0Blood were measured with an immunoassay also associated with significant metabolite cross-reactivity (Wallemacq et al., 2009). This was followed by another study (also discussed in Rejection and nephrotoxicity) in liver transplant recipients (Table 1A) again reporting significantly lower liver tacrolimus concentrations (and C0PBMC) in patients with moderate/severe histological rejection compared to those with no/mild rejection, and a significant relationship between liver tacrolimus concentrations (and C0PBMC) and Banff scores (Capron et al., 2012). Even though C0Blood were measured by a relatively specific immunoassay there was still no association between C0Blood and rejection.
Two studies (Table 1A) have recently investigated potential relationships between renal graft tacrolimus concentrations and clinical outcomes (Zhang et al., 2020; Sallustio et al., 2021). In 52 renal transplant recipients there was no difference in renal tacrolimus concentrations between patients with or without histologically classified subclinical acute rejection at 3 months or 1 year post transplantation (Zhang et al., 2020). In a larger study of 132 renal transplant recipients, biopsy-proven rejection was similarly not associated with renal tacrolimus concentrations (Sallustio et al., 2021). In both studies, patients received triple maintenance immunosuppression with tacrolimus, mycophenolic acid and prednisolone and rejection episodes were observed in 21% of patients (Zhang et al., 2020) and 44% of biopsy samples (Sallustio et al., 2021). These later studies contrast with the earlier results in hepatic transplantation. However, the renal transplant recipients were administered current triple maintenance immunosuppression, whereas the hepatic transplant recipients were administered maintenance immunosuppression of tacrolimus monotherapy or tacrolimus and a steroid (Table 1A). Therefore, the utility of hepatic tacrolimus concentrations as predictors of rejection with modern triple therapy is yet to be determined.
Nephrotoxicity
Despite a reduction in the target C0Blood therapeutic range, evidence of chronic tacrolimus induced nephrotoxicity is still present in 34 and 72% of renal allograft biopsies by 5 and 10 years post-transplantation (Nankivell et al., 2016). Although high C0Blood are associated with increased risk of nephrotoxicity, it is unclear whether renal CNI concentrations may be better predictors. Of the two studies that have measured intra-renal tacrolimus concentrations in clinical allograft biopsies (Table 1A), only one has investigated nephrotoxicity, reporting that the relationship between blood and renal tacrolimus concentrations may be different (steeper) in patients with acute nephrotoxicity compared to those without (Sallustio et al., 2021). However, these results were based on a very small incidence of acute nephrotoxicity and require confirmation.
Whole Blood and Allograft Tacrolimus Pharmacokinetics
Measurement of allograft tacrolimus concentrations is ethically limited to the use of biopsies collected for clinical assessment of graft dysfunction or as part of established routine clinical monitoring protocols. Thus, there are no clinical data on graft tacrolimus AUCs. However, similar to the studies of PBMC tacrolimus exposures, Capron et al. found no correlation between C0Blood and graft tacrolimus concentrations in liver transplant recipients, using a relatively non-specific immunoassay to measure C0Blood (Capron et al., 2007) and also in a later publication in which C0Blood were measured with a more specific immunoassay (Capron et al., 2012). In contrast, the latter study reported a good correlation (r2 = 0.55, p = 0.001) between C0PBMC and hepatic tacrolimus concentrations (Capron et al., 2012), possibly indicating that both PBMC and hepatic tacrolimus concentrations are more closely related to unbound plasma tacrolimus concentrations than those in whole blood. In renal transplant recipients, weak correlations have been reported between C0Blood and graft tacrolimus concentrations with r2 values of 0.13 (p = 0.01) (Zhang et al., 2020) and 0.19 (p = 7.4 × 10−10) (Sallustio et al., 2021). In addition, a better correlation between dose and renal tacrolimus concentrations than between dose and C0Blood (Sallustio et al., 2021) has also been reported, again potentially indicating that renal tacrolimus concentrations better reflect unbound plasma tacrolimus concentrations, hence, dose.
Similar to PBMC, there appears to be greater inter-individual variability in intra-graft tacrolimus concentrations than C0Blood (Supplementary Table S1), with mean (s.d.) concentrations of 91.3 (52.2) pg/mg of tissue and 8.9 (3.0) μg/L, respectively, and CVs of 57 and 34%, respectively in liver transplant recipients (Capron et al., 2012). In renal transplantation, C0Blood and graft tacrolimus concentrations ranged from 2.6 to 52.3 μg/L and 33–828 pg/mg of tissue, respectively (Sallustio et al., 2021). Analytical variability in measurement of intra-renal tacrolimus concentrations appears relatively small, with one study reporting intra- and inter-assay CVs between 5.9 and 14.1% for replicate analyses of in vivo renal cortical tissue tacrolimus concentrations (Noll et al., 2013).
Only one group (Elens et al., 2007) has investigated the effect of ABCB1 and CYP3A5 genetic polymorphisms on hepatic tacrolimus concentrations in liver transplantation (post hoc analysis of (Capron et al., 2007) in Table 1A). Using multiple linear regression analysis, donor ABCB1 2677 G/T, T/T and G/A, 1199G/A and day 7 log plasma bilirubin concentrations were independent predictors of day 7 hepatic tacrolimus concentrations, whist the same genotypes (but not bilirubin) were independent predictors of dose-corrected hepatic tacrolimus concentrations (Elens et al., 2007). In renal transplants, donor or recipient ABCB1 and CYP3A5 genetic polymorphisms had no effect on renal tacrolimus concentrations (Zhang et al., 2020; Sallustio et al., 2021), but C0Blood (p = 1 × 10−8), dose (p = 0.02) and acute nephrotoxicity (main effect p = 0.01 and first-order interaction with C0Blood p = 0.002) were independent predictors of renal tacrolimus concentrations (Sallustio et al., 2021). Interestingly, a greater role of P-glycoprotein in determining hepatic versus renal tacrolimus concentrations is supported by animal work showing that knockout of P-glycoprotein expression in mice results in increased tissue/blood tacrolimus concentration ratios in liver but not in kidneys (Yokogawa et al., 1999).
Conclusion
Although investigation of PBMC and allograft tissue as alternate matrices for tacrolimus TDM has been conducted for more than 20 years, there is little consistent evidence for a clinical benefit with respect to the prediction of rejection. Most studies are limited by their retrospective or post-hoc design, small sample sizes and insufficient statistical power. The only evidence of a strong predictive value of PBMC and allograft tacrolimus C0 with respect to rejection was in liver transplant recipients administered immunosuppression regimens that are no longer used clinically (Capron et al., 2012). The results have not been independently replicated in liver or other transplant groups using current immunosuppressant regimens. Only one study has reported an association between renal tacrolimus concentrations and histological evidence of acute nephrotoxicity (Sallustio et al., 2021). Thus, well-designed and powered prospective clinical studies are still required to determine whether TDM of tacrolimus using PBMC or graft concentrations offers a significant clinical benefit compared to current TDM based on blood tacrolimus concentrations. Harmonisation of analytical methods may be an important initial step to significantly facilitate comparisons between laboratories and generalisation of results (Lemaitre et al., 2020).
Population pharmacokinetic modelling could provide a robust sparse sampling strategy with which to investigate the relationship between tacrolimus concentrations in whole blood and PBMC (or allograft tissue) and pharmacodynamics (e.g., rejection), and could also allow for the assessment significant covariates, including the effects of drugs that may interfere with tacrolimus distribution (e.g., inhibitors/inducers of efflux or uptake proteins such as P-glycoprotein or SLCO1B proteins (Elens et al., 2007; Boivin et al., 2013)). Validated models may facilitate prediction of PBMC or allograft tacrolimus concentrations without the need to carry our frequent actual analysis in patients. However, like whole blood, PBMC are a heterogenous collection of cells and their use may have limitations similar to the use of whole blood.
Author Contributions
The author confirms being the sole contributor of this work and has approved it for publication.
Conflict of Interest
The author declares that the research was conducted in the absence of any commercial or financial relationships that could be construed as a potential conflict of interest.
Publisher’s Note
All claims expressed in this article are solely those of the authors and do not necessarily represent those of their affiliated organizations, or those of the publisher, the editors, and the reviewers. Any product that may be evaluated in this article, or claim that may be made by its manufacturer, is not guaranteed or endorsed by the publisher.
Supplementary Material
The Supplementary Material for this article can be found online at: https://www.frontiersin.org/articles/10.3389/fphar.2021.733285/full#supplementary-material
References
Barraclough, K. A., Isbel, N. M., Kirkpatrick, C. M., Lee, K. J., Taylor, P. J., Johnson, D. W., et al. (2011). Evaluation of Limited Sampling Methods for Estimation of Tacrolimus Exposure in Adult Kidney Transplant Recipients. Br. J. Clin. Pharmacol. 71, 207–223. doi:10.1111/j.1365-2125.2010.03815.x
Baughman, G., Wiederrecht, G. J., Chang, F., Martin, M. M., and Bourgeois, S. (1997). Tissue Distribution and Abundance of Human FKBP51, and FK506-Binding Protein that Can Mediate Calcineurin Inhibition. Biochem. Biophys. Res. Commun. 232, 437–443. doi:10.1006/bbrc.1997.6307
Boivin, A. A., Cardinal, H., Barama, A., Naud, J., Pichette, V., Hébert, M. J., et al. (2013). Influence of SLCO1B3 Genetic Variations on Tacrolimus Pharmacokinetics in Renal Transplant Recipients. Drug Metab. Pharmacokinet. 28, 274–277. doi:10.2133/dmpk.dmpk-12-sh-093
Brooks, E., Tett, S. E., Isbel, N. M., and Staatz, C. E. (2016). Population Pharmacokinetic Modelling and Bayesian Estimation of Tacrolimus Exposure: Is This Clinically Useful for Dosage Prediction Yet. Clin. Pharmacokinet. 55, 1295–1335. doi:10.1007/s40262-016-0396-1
Brunet, M., van Gelder, T., Åsberg, A., Haufroid, V., Hesselink, D. A., Langman, L., et al. (2019). Therapeutic Drug Monitoring of Tacrolimus-Personalized Therapy: Second Consensus Report. Ther. Drug Monit. 41, 261–307. doi:10.1097/FTD.0000000000000640
Capron, A., Haufroid, V., and Wallemacq, P. (2016). Intra-Cellular Immunosuppressive Drugs Monitoring: A Step Forward towards Better Therapeutic Efficacy after Organ Transplantation. Pharmacol. Res. 111, 610–618. doi:10.1016/j.phrs.2016.07.027
Capron, A., Lerut, J., Latinne, D., Rahier, J., Haufroid, V., and Wallemacq, P. (2012). Correlation of Tacrolimus Levels in Peripheral Blood Mononuclear Cells with Histological Staging of Rejection after Liver Transplantation: Preliminary Results of a Prospective Study. Transpl. Int. 25, 41–47. doi:10.1111/j.1432-2277.2011.01365.x
Capron, A., Lerut, J., Verbaandert, C., Mathys, J., Ciccarelli, O., Vanbinst, R., et al. (2007). Validation of a Liquid Chromatography-Mass Spectrometric Assay for Tacrolimus in Liver Biopsies after Hepatic Transplantation: Correlation with Histopathologic Staging of Rejection. Ther. Drug Monit. 29, 340–348. doi:10.1097/FTD.0b013e31805c73f1
Capron, A., Mourad, M., De Meyer, M., De Pauw, L., Eddour, D. C., Latinne, D., et al. (2010). CYP3A5 and ABCB1 Polymorphisms Influence Tacrolimus Concentrations in Peripheral Blood Mononuclear Cells after Renal Transplantation. Pharmacogenomics 11, 703–714. doi:10.2217/pgs.10.43
Christians, U., Jacobsen, W., Benet, L. Z., and Lampen, A. (2002). Mechanisms of Clinically Relevant Drug Interactions Associated with Tacrolimus. Clin. Pharmacokinet. 41, 813–851. doi:10.2165/00003088-200241110-00003
Dessilly, G., Elens, L., Panin, N., Capron, A., Decottignies, A., Demoulin, J. B., et al. (2014). ABCB1 1199G>A Genetic Polymorphism (Rs2229109) Influences the Intracellular Accumulation of Tacrolimus in HEK293 and K562 Recombinant Cell Lines. PLoS One 9, e91555. doi:10.1371/journal.pone.0091555
Elens, L., Capron, A., Kerckhove, V. V., Lerut, J., Mourad, M., Lison, D., et al. (2007). 1199G>A and 2677G>T/A Polymorphisms of ABCB1 Independently Affect Tacrolimus Concentration in Hepatic Tissue after Liver Transplantation. Pharmacogenet Genomics 17, 873–883. doi:10.1097/FPC.0b013e3282e9a533
Fontova, P., Colom, H., Rigo-Bonnin, R., van Merendonk, L. N., Vidal-Alabró, A., Montero, N., et al. (2021). Influence of the Circadian Timing System on Tacrolimus Pharmacokinetics and Pharmacodynamics after Kidney Transplantation. Front. Pharmacol. 12, 636048. doi:10.3389/fphar.2021.636048
Francke, M. I., Hesselink, D. A., Li, Y., Koch, B. C. P., de Wit, L. E. A., van Schaik, R. H. N., et al. (2020). Monitoring the Tacrolimus Concentration in Peripheral Blood Mononuclear Cells of Kidney Transplant Recipients. Br. J. Clin. Pharmacol. 87 (4), 1918–1929. doi:10.1111/bcp.14585
Fukudo, M., Yano, I., Masuda, S., Fukatsu, S., Katsura, T., Ogura, Y., et al. (2005). Pharmacodynamic Analysis of Tacrolimus and Cyclosporine in Living-Donor Liver Transplant Patients. Clin. Pharmacol. Ther. 78, 168–181. doi:10.1016/j.clpt.2005.04.008
Han, S. S., Yang, S. H., Kim, M. C., Cho, J. Y., Min, S. I., Lee, J. P., et al. (2016). Monitoring the Intracellular Tacrolimus Concentration in Kidney Transplant Recipients with Stable Graft Function. PLoS One 11, e0153491. doi:10.1371/journal.pone.0153491
Hu, R., Barratt, D. T., Coller, J. K., Sallustio, B. C., and Somogyi, A. A. (2019). Is There a Temporal Relationship between Trough Whole Blood Tacrolimus Concentration and Acute Rejection in the First 14 Days After Kidney Transplantation. Ther. Drug Monit. 41, 528–532. doi:10.1097/FTD.0000000000000656
In 't Veld, A. E., Grievink, H. W., Saghari, M., Stuurman, F. E., de Kam, M. L., and de Vries, A. P. J. (2019). Immunomonitoring of Tacrolimus in Healthy Volunteers: The First Step from PK- to PD-Based Therapeutic Drug Monitoring. Int. J. Mol. Sci. 20, 4710. doi:10.3390/ijms20194710
Kershner, R. P., and Fitzsimmons, W. E. (1996). Relationship of FK506 Whole Blood Concentrations and Efficacy and Toxicity after Liver and Kidney Transplantation. Transplantation 62, 920–926. doi:10.1097/00007890-199610150-00009
Klaasen, R. A., Bergan, S., Bremer, S., Daleq, L., Andersen, A. M., Midtvedt, K., et al. (2018). Longitudinal Study of Tacrolimus in Lymphocytes During the First Year After Kidney Transplantation. Ther. Drug Monit. 40, 558–566. doi:10.1097/FTD.0000000000000539
Kung, L., and Halloran, P. F. (2000). Immunophilins May Limit Calcineurin Inhibition by Cyclosporine and Tacrolimus at High Drug Concentrations. Transplantation 70, 327–335. doi:10.1097/00007890-200007270-00017
Lemaitre, F., Antignac, M., and Fernandez, C. (2013). Monitoring of Tacrolimus Concentrations in Peripheral Blood Mononuclear Cells: Application to Cardiac Transplant Recipients. Clin. Biochem. 46, 1538–1541. doi:10.1016/j.clinbiochem.2013.02.011
Lemaitre, F., Blanchet, B., Latournerie, M., Antignac, M., Houssel-Debry, P., Verdier, M. C., et al. (2015). Pharmacokinetics and Pharmacodynamics of Tacrolimus in Liver Transplant Recipients: Inside the white Blood Cells. Clin. Biochem. 48, 406–411. doi:10.1016/j.clinbiochem.2014.12.018
Lemaitre, F., Vethe, N. T., DʼAvolio, A., Tron, C., Robertsen, I., De Winter, B., et al. (2020). Measuring Intracellular Concentrations of Calcineurin Inhibitors: Expert Consensus from the International Association of Therapeutic Drug Monitoring and Clinical Toxicology Expert Panel. Ther. Drug Monit. 42, 665–670. doi:10.1097/FTD.0000000000000780
Marquet, P., Albano, L., Woillard, J. B., Rostaing, L., Kamar, N., Sakarovitch, C., et al. (2018). Comparative Clinical Trial of the Variability Factors of the Exposure Indices Used for the Drug Monitoring of Two Tacrolimus Formulations in Kidney Transplant Recipients. Pharmacol. Res. 129, 84–94. doi:10.1016/j.phrs.2017.12.005
Nagase, K., Iwasaki, K., Nozaki, K., and Noda, K. (1994). Distribution and Protein Binding of FK506, a Potent Immunosuppressive Macrolide Lactone, in Human Blood and its Uptake by Erythrocytes. J. Pharm. Pharmacol. 46, 113–117. doi:10.1111/j.2042-7158.1994.tb03752.x
Nankivell, B. J., PʼNg, C. H., OʼConnell, P. J., and Chapman, J. R. (2016). Calcineurin Inhibitor Nephrotoxicity through the Lens of Longitudinal Histology: Comparison of Cyclosporine and Tacrolimus Eras. Transplantation 100, 1723–1731. doi:10.1097/TP.0000000000001243
Noll, B. D., Coller, J. K., Somogyi, A. A., Morris, R. G., Russ, G. R., Hesselink, D. A., et al. (2013). Validation of an LC-MS/MS Method to Measure Tacrolimus in Rat Kidney and Liver Tissue and its Application to Human Kidney Biopsies. Ther. Drug Monit. 35, 617–623. doi:10.1097/FTD.0b013e31828e8162
Pensi, D., De Nicolò, A., Pinon, M., Calvo, P. L., Nonnato, A., Brunati, A., et al. (2015). An UPLC-MS/MS Method Coupled with Automated On-Line SPE for Quantification of Tacrolimus in Peripheral Blood Mononuclear Cells. J. Pharm. Biomed. Anal. 107, 512–517. doi:10.1016/j.jpba.2015.01.054
Rayar, M., Tron, C., Locher, C., Chebaro, A., Beaurepaire, J. M., Blondeau, M., et al. (2018). Tacrolimus Concentrations Measured in Excreted Bile in Liver Transplant Recipients: The STABILE Study. Clin. Ther. 40, 2088–2098. doi:10.1016/j.clinthera.2018.10.015
Romano, P., da Luz Fernandes, M., de Almeida Rezende Ebner, P., Duarte de Oliveira, N., Mitsue Okuda, L., Agena, F., et al. (2018). UPLC-MS/MS Assay Validation for Tacrolimus Quantitative Determination in Peripheral Blood T CD4+ and B CD19+ Lymphocytes. J. Pharm. Biomed. Anal. 152, 306–314. doi:10.1016/j.jpba.2018.01.002
Sallustio, B. C., Noll, B. D., Hu, R., Barratt, D. T., Tuke, J., Coller, J. K., et al. (2021). Tacrolimus Dose, Blood Concentrations and Acute Nephrotoxicity, but Not CYP3A5/ABCB1 Genetics, Are Associated with Allograft Tacrolimus Concentrations in Renal Transplant Recipients. Br. J. Clin. Pharmacol. 87 (10), 3901–3909. doi:10.1111/bcp.14806
Sandborn, W. J., Lawson, G. M., Cody, T. J., Porayko, M. K., Hay, J. E., Gores, G. J., et al. (1995). Early Cellular Rejection after Orthotopic Liver Transplantation Correlates with Low Concentrations of FK506 in Hepatic Tissue. Hepatology 21, 70–76. doi:10.1002/hep.1840210113
Sandborn, W. J., Lawson, G. M., Krom, R. A., and Wiesner, R. H. (1992). Hepatic Allograft Cyclosporine Concentration Is Independent of the Route of Cyclosporine Administration and Correlates with the Occurrence of Early Cellular Rejection. Hepatology 15, 1086–1091. doi:10.1002/hep.1840150619
Staatz, C. E., and Tett, S. E. (2004). Clinical Pharmacokinetics and Pharmacodynamics of Tacrolimus in Solid Organ Transplantation. Clin. Pharmacokinet. 43, 623–653. doi:10.2165/00003088-200443100-00001
Tron, C., Allard, M., Petitcollin, A., Ferrand-Sorre, M. J., Verdier, M. C., Querzerho-Raguideau, J., et al. (2019). Tacrolimus Diffusion across the Peripheral Mononuclear Blood Cell Membrane: Impact of Drug Transporters. Fundam. Clin. Pharmacol. 33, 113–121. doi:10.1111/fcp.12412
Tron, C., Woillard, J. B., Houssel-Debry, P., David, V., Jezequel, C., Rayar, M., et al. (2020). Pharmacogenetic-Whole Blood and Intracellular Pharmacokinetic-Pharmacodynamic (PG-PK2-PD) Relationship of Tacrolimus in Liver Transplant Recipients. PLoS One 15, e0230195. doi:10.1371/journal.pone.0230195
Wallemacq, P., Armstrong, V. W., Brunet, M., Haufroid, V., Holt, D. W., Johnston, A., et al. (2009). Opportunities to Optimize Tacrolimus Therapy in Solid Organ Transplantation: Report of the European Consensus Conference. Ther. Drug Monit. 31, 139–152. doi:10.1097/FTD.0b013e318198d092
Yokogawa, K., Takahashi, M., Tamai, I., Konishi, H., Nomura, M., Moritani, S., et al. (1999). P-Glycoprotein-Dependent Disposition Kinetics of Tacrolimus: Studies in Mdr1a Knockout Mice. Pharm. Res. 16, 1213–1218. doi:10.1023/a:1018993312773
Zahir, H., McCaughan, G., Gleeson, M., Nand, R. A., and McLachlan, A. J. (2004). Changes in Tacrolimus Distribution in Blood and Plasma Protein Binding Following Liver Transplantation. Ther. Drug Monit. 26, 506–515. doi:10.1097/00007691-200410000-00008
Zahir, H., McCaughan, G., Gleeson, M., Nand, R. A., and McLachlan, A. J. (2004). Factors Affecting Variability in Distribution of Tacrolimus in Liver Transplant Recipients. Br. J. Clin. Pharmacol. 57, 298–309. doi:10.1046/j.1365-2125.2003.02008.x
Zahir, H., Nand, R. A., Brown, K. F., Tattam, B. N., and McLachlan, A. J. (2001). Validation of Methods to Study the Distribution and Protein Binding of Tacrolimus in Human Blood. J. Pharmacol. Toxicol. Methods 46, 27–35. doi:10.1016/s1056-8719(02)00158-2
Keywords: tacrolimus, transplantatation, rejection, nephrotoxicity, therapeutic drug monitoring, intra-cellular concentrations
Citation: Sallustio BC (2021) Monitoring Intra-cellular Tacrolimus Concentrations in Solid Organ Transplantation: Use of Peripheral Blood Mononuclear Cells and Graft Biopsy Tissue. Front. Pharmacol. 12:733285. doi: 10.3389/fphar.2021.733285
Received: 01 July 2021; Accepted: 23 September 2021;
Published: 26 October 2021.
Edited by:
Ji-Young Park, Korea University, South KoreaReviewed by:
Salvador F Aliño, University of Valencia, SpainYuanbin Song, Sun Yat-sen University Cancer Center, China
Copyright © 2021 Sallustio. This is an open-access article distributed under the terms of the Creative Commons Attribution License (CC BY). The use, distribution or reproduction in other forums is permitted, provided the original author(s) and the copyright owner(s) are credited and that the original publication in this journal is cited, in accordance with accepted academic practice. No use, distribution or reproduction is permitted which does not comply with these terms.
*Correspondence: Benedetta C. Sallustio, benedetta.sallustio@sa.gov.au