- 1State Key Laboratory of Organ Failure Research, National Clinical Research Center of Kidney Disease, Guangdong Provincial Key Laboratory of Renal Failure Research, Division of Nephrology, Nanfang Hospital, Southern Medical University, Guangzhou, China
- 2The First Medical College, Southern Medical University, Guangzhou, China
Ethnopharmacological Relevance: Triptolide (TP), the primary biologically active ingredient of Tripterygium wilfordii Hook F (TWHF), possesses the potential to solve the shortcomings of TWHF in treating diabetic kidney disease (DKD) in the clinic.
Aim of the Study: We conducted a meta-analysis to evaluate the efficacy of TP in treating DKD and offer solid evidence for further clinical applications of TP.
Materials and Methods: Eight databases (CNKI, VIP, CBM, WanFang, PubMed, Web of Science, EMBASE, and Cochrane library) were electronically searched for eligible studies until October 17, 2020. We selected animal experimental studies using TP versus renin–angiotensin system inhibitors or nonfunctional liquids to treat DKD by following the inclusion and exclusion criteria. Two researchers independently extracted data from the included studies and assessed the risk of bias with the Systematic Review Centre for Laboratory Animal Experimentation Risk of Bias tool. Fixed-effects meta-analyses, subgroup analyses, and meta-regression were conducted using RevMan 5.3 software. Inplasy registration number: INPLASY2020100042.
Results: Twenty-six studies were included. Meta-analysis showed that TP significantly reduced albuminuria (14 studies; standardized mean difference SMD: −1.44 [−1.65, −1.23], I2 = 87%), urine albumin/urine creatinine ratio (UACR) (8 studies; SMD: –5.03 [–5.74, −4.33], I2 = 84%), total proteinuria (4 studies; SMD: –3.12 [–3.75, −2.49], I2 = 0%), serum creatinine (18 studies; SMD: –0.30 [–0.49, −0.12], I2 = 76%), and blood urea nitrogen (12 studies; SMD: –0.40 [–0.60, −0.20], I2 value = 55%) in DKD animals, compared to the vehicle control. However, on comparing TP to the renin–angiotensin system (RAS) inhibitors in DKD treatment, there was no marked difference in ameliorating albuminuria (3 studies; SMD: –0.35 [–0.72, 0.02], I2 = 41%), serum creatinine (3 studies; SMD: –0.07 [–0.62, 0.48], I2 = 10%), and blood urea nitrogen (2 studies; SMD: –0.35 [–0.97, 0.28], I2 = 0%). Of note, TP exhibited higher capacities in reducing UACR (2 studies; SMD: –0.66 [–1.31, −0.01], I2 = 0%) and total proteinuria (2 studies; SMD: –1.18 [–1.86, −2049], I2 = 0%). Meta-regression implicated that the efficacy of TP in reducing DKD albuminuria was associated with applied dosages. In addition, publication bias has not been detected on attenuating albuminuria between TP and RAS inhibitors after the diagnosis of DKD.
Systematic Review Registration:https://clinicaltrials.gov/, identifier INPLASY2020100042
Introduction
Diabetic kidney disease (DKD) is a chronic clinical condition characterized by micro- or macro-albuminuria followed by a progressive decline in kidney functions (Fu et al., 2019; Kopel et al., 2019). Over the past years, the incidence of DKD and its mortality has been largely underestimated (Rao et al., 2012). As a major driver of excess mortality in diabetes (Koye et al., 2018), DKD places growing financial burdens on diabetes management on a global scale (Slabaugh et al., 2015), especially in emerging and developing economies (Thomas et al., 2016). At present, antidiabetic medications and renin–angiotensin system (RAS) inhibitors are routinely used to prevent DKD from entering end-stage renal disease (ESRD) (Hostetter, 2001). However, this first-line therapy for DKD has been considered unsatisfying because of its potential side effects, such as diabetic ketoacidosis (Fadini et al., 2017) and reversible AKI (Onuigbo, 2011). Therefore, a systemic evaluation of the efficacy and safety of the current therapeutic strategies for DKD is urgently needed.
Tripterygium wilfordii Hook F (TWHF), a well-known Chinese herb, has been intensely developed and widely applied in treating nephritis or DKD in the clinic (Li et al., 2014;Huang et al., 2020; Xu et al., 2020; Guo Y et al., 2021). However, restricted by its adverse reactions and complex pharmacology (Hong et al., 2016; Ren et al., 2019; Huang et al., 2020), the extracts from TWHF have become a new focus in the field. The primary biologically active ingredient of TWHF, triptolide (TP), was discovered in 1972 (Kupchan et al., 1972). It is a striking target for total synthesis because of its intriguing structural features and promising biological activities (Zhang et al., 2019). TP can suppress inflammation and enhance cytoprotection by inhibiting the secretion of proinflammatory cytochemokines (Zhao et al., 2000; Zhou et al., 2003; Krakauer et al., 2005; Lu et al., 2005; Liu et al., 2006; Hoyle et al., 2010; Hou et al., 2019). Similar to TWHF, TP also induces organ or tissue damages (Xi et al., 2017), including hepatotoxicity (Li et al., 2014), nephrotoxicity (Yang et al., 2011), and reproductive toxicity (Ni et al., 2008). Along with advances in technology, TP exhibits great capacities in enhancing its efficacy, reducing side effects, and improving bioavailability through the nanostructured TP delivery system (Ren et al., 2021). Furthermore, the newly designed and synthesized water-soluble TP derivatives also demonstrated their safety and efficacy (Liu, 2011). These innovations significantly increased the application of TP in treating DKD. In this study, in contrast to RAS inhibitors and nonfunctional liquids, the primary objective is to systemically evaluate the efficacy of TP in animal models with DKD. Our analyses provide confidence for clinicians to design personalized therapeutic strategies for DKD under the current precision medicine model.
Methods
This systematic review adhered to the preferred reporting items for systematic reviews and meta-analysis guidelines (Moher et al., 2009). The review protocol was registered in INPLASY before the beginning of the experiment (registration number: INPLASY2020100042).
Publication Searching
We followed the Systematic Review Center for Laboratory Animal Experimentation (SYRCLE) step-by-step guide (Leenaars et al., 2012) to draw up the search strategy. Animal experimental studies of “TP treats DKD” were electronically searched in China National Knowledge Infrastructure (CNKI), Chinese Science and Technology Journal Database (VIP), Chinese Biomedical Database (CBM), WanFang, PubMed, Web of Science, EMBASE, and Cochrane library published from database inception to October 17, 2020. The search strategy consists of following three search components: triptolide AND DKD/diabetic nephropathy AND animals, using the Medical Subject Heading (MeSH) terms and keywords to perform searching. A pre-published animal filter (Hooijmans et al., 2010) was applied to limit the range for animal studies. All references of eligible articles were screened carefully for additional analyses.
Inclusion and Exclusion Criteria
Included criteria: 1. Population: All animal models with DKD, regardless of species, age, or sex. 2. Intervention: The experimental groups used TP as monotherapy in any dosage. Comparison: The control groups received equal volumes of a nonfunctional liquid (normal saline) or did not receive treatment or recommended therapies according to clinical practice guidelines, including RAS inhibitors (angiotensin-converting enzyme inhibitors, ACEIs, or angiotensin AT (1)-receptor blockers, ARBs). 3. Study design: TP prepared in various forms, including extracts, granules, and injections, was eligible. 4. Outcome: The outcomes were changed in albuminuria, proteinuria excretion, and kidney function (serum creatinine, SCr, and blood urea nitrogen, BUN). 5. The literature is published either in Chinese or English.
Excluded criteria: 1. No in vivo studies (in vitro studies, clinical trials, review articles, case reports, comments, editorials, and abstracts). 2. Additional hypoglycemics were used during treatment. 3. Assessing TP combined with other herbal ingredients or complementary therapies. 4. Repeated literature. 5. Full text was not found.
Data Extraction and Quality Assessment
Studies according to the inclusion criteria were considered eligible for the analyses. Data of each included study were extracted by two authors independently and cross-checked in terms of the author, publication year, animal models (species, sex, weight, method of modeling, and the criteria for successful modeling), TP intervention (the type of intervention, dosage, and the duration of intervention), and outcomes. When results were only reported graphically, the graph data were measured using GetData, software downloaded on the website (http://getdata.com.ru/). The total quality assessment of each study was evaluated based on the Systematic Review Centre for Laboratory Animal Experimentation Risk of Bias tool (Hooijmans et al., 2014). According to its guidance, each domain of individual studies was graded as low, unclear, or high risk of bias.
Statistical Analysis
RevMan 5.3 software and Stata 15.1 software were used for data analysis. Studies were divided into two compilations to assess the differences between the TP and control groups and the differences between the TP and the RAS inhibitor groups. Continuous variables were expressed as standardized mean differences (SMDs) with a 95% confidence interval. A fixed effect was used in meta-analysis. Heterogeneity among the included studies was checked by using the chi-square test and I2 test. Substantial heterogeneity was considered to exist when I2 > 50%. Subgroup analyses and meta-regression were carried out to explore the possible sources of heterogeneity. High-risk studies were removed one by one from the group of the synthesized studies, and the remaining studies were re-analyzed to estimate the robustness of the results. Publication bias was evaluated by Begg’s test, Egger’s test, and the visual inspection of funnel plots for asymmetry.
Results
Search Results
As illustrated in Figure 1, we identified 252 articles throughout the database. After removing duplications and screening the articles based on the titles and abstracts, the full texts of 70 studies were assessed for eligibility. Forty-four additional studies were excluded with the reasons being unclear intervention details and no predetermined outcomes, combining other extracts from TWHF or additional hypoglycemics for treatment, unavailable full texts, presenting in vitro experiments, and no relevance to diseases or herbs. Therefore, 26 studies were ultimately included in the analyses.
Characteristics of the Included Studies
The details of the study characteristics are presented in Table 1. Twenty-six studies were published between 2008 and 2020. Five articles (Ma et al., 2013; Guo et al., 2016; Dong et al., 2017; Han et al., 2017; Li et al., 2017) were published in English, and the remaining 21 articles were published in Chinese. Four articles (Ma, 2009; Zhu, 2013; Han, 2018; Xue, 2018) were exhibited as dissertations, and the others were published in peer-reviewed journals. All research works tested the effects of TP on DKD, reporting at least one clinical parameter, including albuminuria, proteinuria, UACR, or kidney function. Among them, seven studies (Ma, 2009; Ma et al., 2009; Qao et al., 2009; Li et al., 2013; An et al., 2017; Dong et al., 2017; Ren et al., 2020) performed comparisons between TP and ACEI or ARB in treating DKD. Twenty-three studies used male Sprague–Dawley (SD) and Wistar rats, and the remaining three studies (Qao et al., 2009; Fan et al., 2018; Ren et al., 2020) used mutant or transgenic mice. The methods of modeling and the types of interventions are stated above. The duration of intervention ranged from 4 to 12 weeks. The dosage of TP varied from 0.2 ug/kg/d to 1.8 g/kg/d; 0.2 mg/kg/d was the commonly administered dose.
Risk of Bias of the Studies Included
According to SYRCLE’s risk of bias tool for animal studies, two researchers (MH and RF) independently evaluated all included studies. The detailed results of the analyses of bias are shown in Figures 2A,B. No studies fulfilled all methodological criteria that were analyzed. Regarding selection bias, random sequence generation processes were not reported clearly in 76.92% of studies (n = 20). In terms of the animals’ baseline characteristics, 23 studies (88.46%) did not report this information. The unclear risks of the bias found in the studies were related to allocation concealment, blinding of caregivers, and/or investigators or outcome assessors. Nine studies (34.62%) showed incomplete information regarding random housing. Three studies (11.54%) reported unclear arbitrary outcome assessment of detection bias for relevant outcome measures. Two studies (7.70%) presented a high risk for incomplete outcome data, while three (11.54%) for selective outcome reporting. Only one research (3.85%) suggests other potential sources of bias.
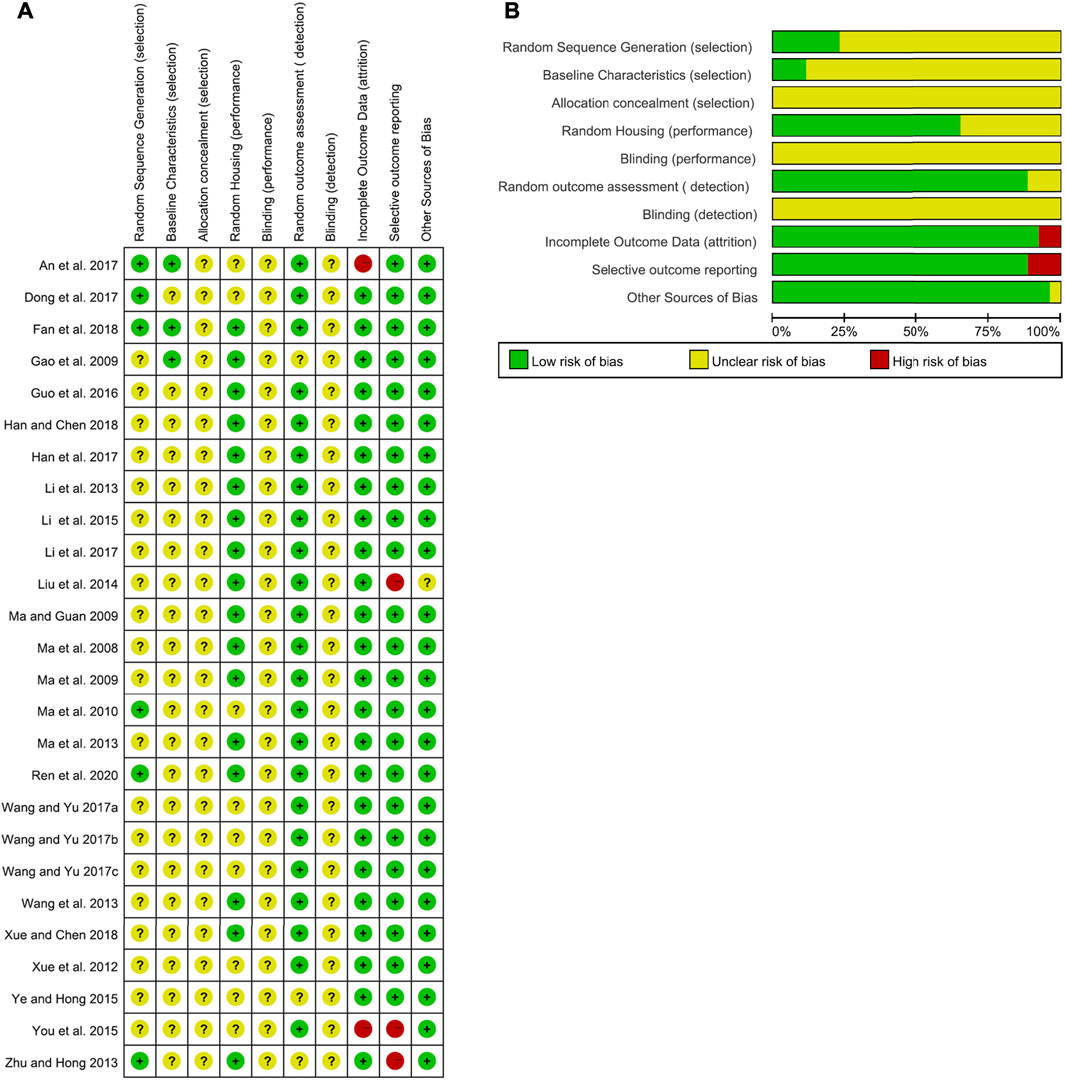
FIGURE 2. Risk of bias of the included studies. (A) Summary of the risk of bias. (B) Summary of the risk-of-bias assessment.
Effects of TP in Treating DKD
Effects on the Changes of Albuminuria, Urine Albumin/Urine Creatinine Ratio (UACR), or Proteinuria
The change in albuminuria was measured in 14 studies (Qao et al., 2009; Zhu, 2013; Liu et al., 2014; Li et al., 2015; Ye and Hong et al., 2015; You et al., 2015; Guo et al., 2016; An et al., 2017; Han et al., 2017; Li et al., 2017; Fan et al., 2018; Han, 2018; Xue, 2018; Ren et al., 2020). The pooled estimation indicated that TP reduced albuminuria significantly (SMD: −1.44 [−1.65, −1.23], I2 = 87%), albeit with substantial heterogeneity (Figure 3).
Because of the heterogeneity associated with these studies, we performed the subgroup analyses of treatment duration (p < 0.00001), the dosage of triptolide (p = 0.002), method of modeling (p = 0.0003), and species of modeling (p = 0.85). Moreover, the meta-regression results showed that there was a linear relationship between the effect and low doses of TP. The studies in which dosage was more significant than 400 μg/kg/d were considered the high-dosage subgroup, whereas others were considered the low-dosage subgroup. The sensitivity analysis found no significant changes.
Eight studies (Li et al., 2013; Ma et al., 2008; Ma, 2009; Ma et al., 2009; Ma et al., 2010; Ma et al., 2013; Wang et al., 2013; Xue et al., 2012) examined the UACR. The outcome was −5.03 mg/mg (95% CI [−5.74, −4.33]), though heterogeneity was significant (I2 = 84%, Figure 4). Through sensitivity analysis, it is found that heterogeneity was significantly reduced when Wang’s study (Wang et al., 2013) was eliminated (the I2 value reduced from 84% to 0) (Figure 5).
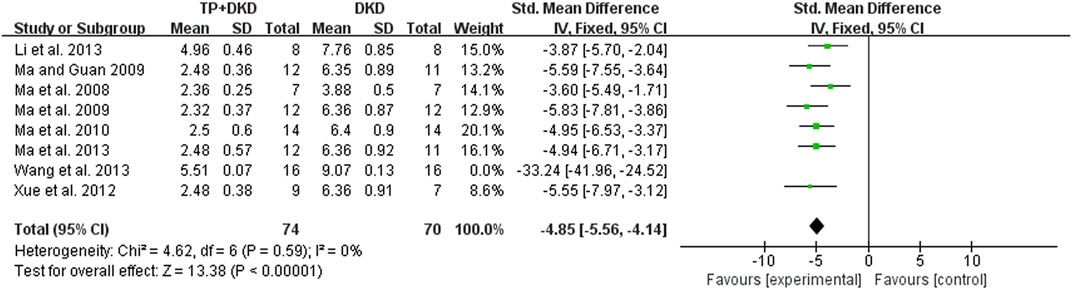
FIGURE 5. Forest plot of UACR outcome (not included by Wang et al., 2013).
As for proteinuria, the meta-analysis result of the four studies (Dong et al., 2017; Wang and Yu, 2017a; Wang and Yu, 2017b; Wang and Yu, 2017c) also suggested that it lowered the level of proteinuria. The I2 value was less than 50% (Figure 6). Since the study (Dong et al., 2017) contained two groups, we divided it into Dong et al., 2017-A and -B in this meta-analysis.
Effects on Kidney Function Changes
The kidney function was reflected by measuring the concentration of serum creatinine (SCr) and blood urea nitrogen (BUN) in the included studies. The pooled result of 18 studies (Fan et al., 2018; Guo et al., 2016; Han et al., 2017; Han, 2018; Liu et al., 2014; Li et al., 2013; Li et al., 2017; Ma et al., 2008; Ma, 2009; Ma et al., 2010; Ren et al., 2020; Wang et al., 2013; Wang and Yu, 2017a, 2017b, 2017c; Xue et al., 2012; Xue, 2018; Zhu, 2013) showed that TP had a positive effect on reducing SCr levels, with an SMD (and 95% CI) of −0.30 [−0.49, −0.12](Figure 7). The study (Fan et al., 2018) contained three groups. We then divided it into Fan et al., 2018-A, and -B, and -C in this meta-analysis. Although these results showed high heterogeneity (I2 value= 76%), no outliers were identified by using the sensitivity analysis. Neither treatment duration nor the dosage of TP in subgroup analyses showed differences in SCr levels.
The BUN levels were examined in 12 studies (Ma, 2009; Li et al., 2013; Ma et al., 2013; Wang et al., 2013; Zhu, 2013; Liu et al., 2014; Guo et al., 2016; Han et al., 2017; Li et al., 2017; Fan et al., 2018; Han, 2018; Xue, 2018) (Figure 8). The meta-analysis results showed that the performance of TP was excellent in reducing BUN levels (SMD: −0.40 [−0.60, −0.20], I2 = 55%), compared to the control group.
Comparison of TP and ACEI or ARB in Treating DKD
Effects on the Changes of Albuminuria, Urinary Albumin/Urine Creatinine Ratio (UACR), or Proteinuria
We found three studies (An et al., 2017; Qao et al., 2009; Ren et al., 2020) which analyzed albuminuria (Figure 9A), two studies (Li et al., 2013, Ma et al., 2009) which analyzed the urine albumin/urine creatinine ratio (UACR) (Figure 9B), and one (Dong et al., 2017) which analyzed proteinuria (Figure 9C).
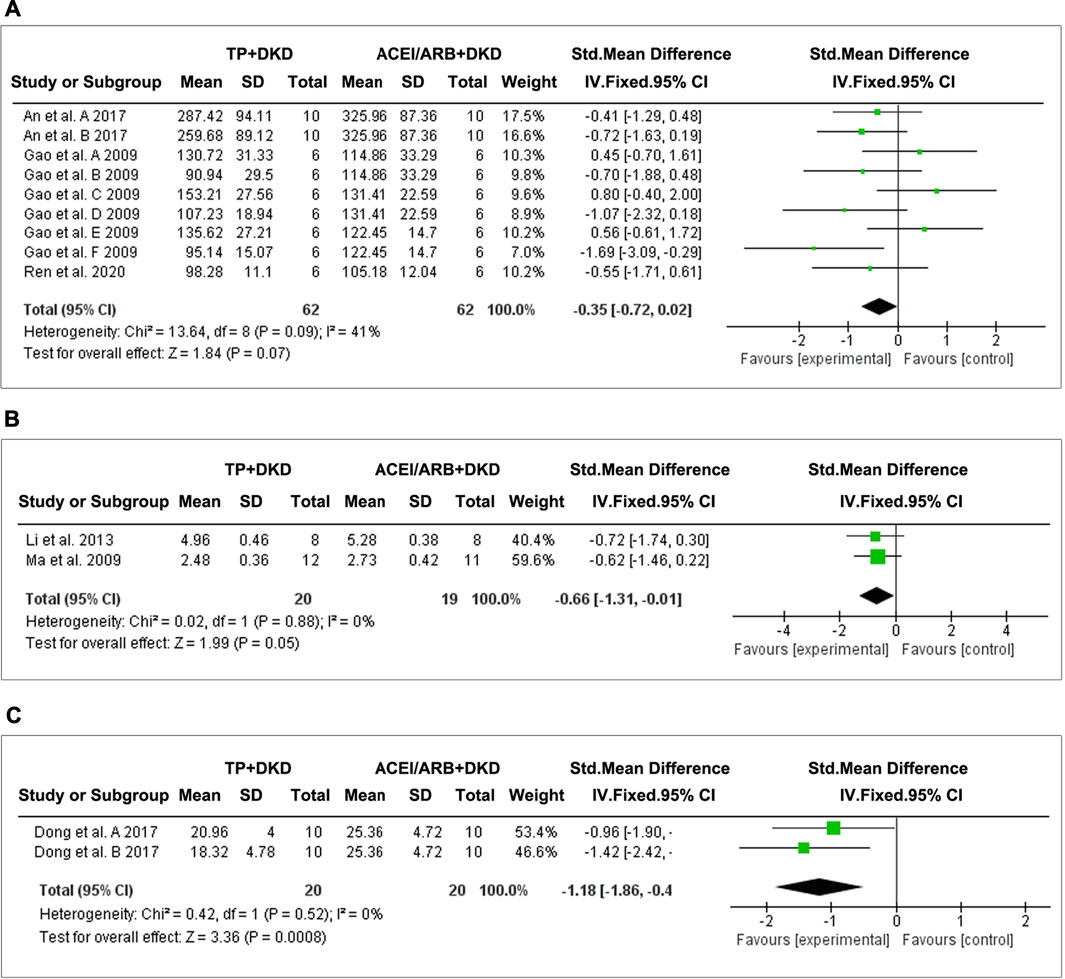
FIGURE 9. Forest plot of (A) albuminuria, (B) UACR, and (C) total proteinuria outcome comparing with RAS inhibitor.
The combined results suggested no differences in albuminuria (SMD: −0.35 [−0.72, 0.02], I2 = 41%) between TP and ACEI or ARB. Interestingly, TP significantly reduced proteinuria (SMD: −1.18 [−1.86, −0.49], I2 = 0%) and the UACR (SMD: −0.66 [−1.31, −0.01], I2 = 0%). Due to data limitations, the reliability of this result was reduced.
Effects on Kidney Function Changes
Three studies evaluated the effects of TP on SCr, and two studies assessed BUN. The pooled results implicated that, when comparing TP and ACEI or ARB, no significant differences were shown on the changes of SCr (SMD: −0.07 [−0.62, 0.48], I2 = 10%) (Figure 10A) and BUN (SMD: −0.35 [−0.97, 0.28], I2 = 0%) (Figure 10B).
Publication Bias
Begg’s and Egger’s tests were used to analyze albuminuria changes after treating DKD with TP. The funnel plot of the tests was asymmetric, and the outcome of p-value was less than 0.05, both of which indicated that there was publication bias (Figure 11A). The potential publication bias might be due to the high percentage of positive results being published. There was no publication bias for the effects on albuminuria between TP and ACEI or ARB in treating DKD (Figure 11B).
Discussion
Currently, there are no effective treatments to halt DKD in the clinic which is a global health concern. The data on comparing the efficacy and safety of the current DKD therapeutic interventions remain lacking. Herein, we performed a systematic review by including 26 studies to analyze the effects of TP, an extract from a traditional Chinese herb, in treating DKD. We collected the majority of therapeutic parameters used in DKD diagnosis or clinical response evaluation, including albuminuria, proteinuria, UACR, SCr, and BUN. Serving as a characteristic indicator of the constant deterioration of DKD (de Boer et al., 2011), albuminuria and proteinuria play a key role in renal disease progression and cardiovascular events (Lin et al., 2018). It is also a sensitive biomarker for the lesions caused by DKD (Guh, 2010). As for early DKD screening (McGrath and Edi, 2019), UACR, a preferred measure of albuminuria (Sumida et al., 2020), is recommended to be detected routinely in diabetic patients who have the potential risk of renal impair (Association, 2019). In addition, to maximize the sensitivity of screening tests, SCr (Kramer, 2004) and BUN (Xie et al., 2018) are recommended as effective indicators, associating closely in renal function assessment (Zhuang et al., 2020). Under the intervention of TP, the assessment of its efficacy will be best done with the comprehensive analyzation toward the floating of the parameters above.
Our study found that TP markedly decreases proteinuria and albuminuria in DKD animal models, consistent with the previous report (Yuan et al., 2019). Since the 24-hr urinary albumin showed a high heterogeneity (χ2 = 283.65, I2 = 87%, Figure 3), we further analyzed TP effects by setting up different subgroups according to the dosages and the duration of TP treatment. Although the heterogeneity remains high, the result did exhibit significant differences (χ2 = 42.98, p < 0.00001, I2 = 93.0%, Figure 3) among the subgroups. According to meta-regression analysis in the low-dose subgroups (TP ≤ 400 ug/kg/d), the anti-albuminuric effects were enhanced with increasing dosage. In contrast, such effects declined in the high-dose subgroups (TP > 400 ug/kg/d). Similar results were not observed in the duration subgroup during TP treatment. It might be due to the functional “working window” of TP being relatively narrow. In other words, the therapeutic dose of TP is close to its toxic dose (Li et al., 2014; Fan et al., 2018).
A key finding of our analyses is that the effects of TP on reducing albuminuria have no differences compared to RAS inhibitors in DKD models. It is well known that RAS inhibitors are the cornerstone therapy of DKD. We speculate that such equivalent effects of TP and RAS inhibitors should attribute to the following mechanisms: 1) TP protects podocytes by inactivating the Toll-like receptor/NF-κB signaling pathway in diseased glomeruli which maintains the integrity of slit-diaphragm proteins such as nephrin and podocin (Ma et al., 2010; Wang et al., 2013; Ren et al., 2020). 2) TP ameliorates inflammation by regulating the balance of T-helper cells and repressing macrophage infiltration (Zhu, 2013; Guo et al., 2016). 3) TP alleviates oxidative stress by downregulating the renal cortex oxidative carbonyl protein and nitrotyrosine (Dong et al., 2017). 4) TP reduces glomerular mesangial cell proliferation by inactivating the PDK1/AKT/mTOR pathway (Han et al., 2017). 5) TP ameliorates glomerulosclerosis by suppressing the Notch1 pathway and regulating the content of Glut-1 and Glut-4 (You et al., 2015; Han, 2018). Of note, Li reported that a combination of TP and RAS inhibitors (irbesartan) reduced albuminuria synergistically (Li et al., 2013). The involved mechanism is unclear.
TP is the major effective monomer of the mixture, tripterygium glycosides (TGs). TG is the commercialized and commonly used extract from the TWHF herb in treating primary nephritis. In contrast to TG, TP has a similar effect on decreasing proteinuria and albuminuria induced by nephritis and DKD (Li et al., 2019). Specific to DKD intervention, the advantages of TP lies in its precision. However, a major concern regarding TP in clinical use is its multi-organ toxicity and the narrow therapeutic window (Li et al., 2014; Liu et al., 2019; Xu et al., 2019; Li et al., 2020). TP is the most important ingredient that leads to toxicity (Li et al., 2015). Primarily, triptolide is eliminated through hepatic and renal pathways. It has been revealed that the induction or inhibition of CYP3A played an important role in TP-induced hepatotoxicity (Shen et al., 2014). In addition to CYP-mediated metabolism, P-glycoprotein also played an important role in the disposition of TP and TP-induced hepatotoxicity (Xiao-Mei et al., 2013). Furthermore, members of the cytochrome P450 protein family that are involved in fatty acid (FA) metabolism, such as CYP2E1, showed the correlation between TP and its damage in kidneys. The proteomics data further suggested that FAs were involved in TP-induced toxicity (Menglin et al., 2017). Along with the advances in technology, this shortcoming of TP has been partially solved through building innovative drug delivery systems, developing water-soluble analogs, designing combinational strategies, and inventing derivatives based on structure–activity relationships (Chen et al., 2018; Liu et al., 2019). For instance, TP-encapsulated mesoscale nanoparticles (TP-MNPs) could be delivered explicitly to diseased organs to exert their therapeutic effects (Deng et al., 2019). Impressively, triptolide aminoglycoside (TPAG) is also able to protect against renal ischemia/reperfusion injury with lower toxicity to the kidney, liver, genital system, and immune system (Qi et al., 2015). A new medication developed based on TP, 14-succinate triptolide-fragment peptide (TPS-PF-A299-585), attenuates the thickening of the glomerular basement membrane in a membranous nephropathic rodent model. In vitro, TPS-PF-A299-585 presents anti-inflammatory activities equivalent to those of TP in the cultured kidney epithelial cells after incubation with lipopolysaccharides (Yuan et al., 2015). Intriguingly, a low-dose 14-succinyl triptolide-lysozyme (TPS-LZM) significantly hampered the progression of renal ischemia/reperfusion, whereas triptolide or lysozyme could not functionally work individually (Zhang et al., 2009). These renovations are promising and likely to be translated into DKD treatment in the clinical setting. In addition, a combinational prescription of TP, catalpol, and Panax notoginseng saponins markedly attenuates hepatotoxicity induced by TP (Zhou et al., 2018). It should be pointed out that it is a principle used to improve herbal effects and decrease its toxicity by regulating the formula in Chinese medicine. Therefore, the concrete drug–drug interactions need to be further explored.
A major issue in our current study is the high heterogeneity of the effect size of 24-h urinary albumin in DKD. At the subgroup layer, a remarkable difference (χ2 = 16.07, p = 0.0003, I2 = 87.6%) was present among subgroups of modeling. At present, there are no ideal experimental models that show all characteristics of DKD in humans. Different animal models or backgrounds could easily cause heterogeneity. Interestingly, no statistical difference (χ2 = 0.04, p = 0.85, I2 = 0%) was shown among the subgroups of strains (groups of genetic DN animal models and a group of Sprague–Dawley rats). Furthermore, our study has difficulties evaluating the risk of bias because the experiment information is lacking in some studies. Moreover, potential publication bias is shown (Figure 11). It suggests that negative outcomes are published rarely. Also, the data of certain indexes are inadequate, and the side effect profiles and adverse events are absent. With regard to this, the effects of TP might be overestimated. We should be cautious in interpreting the results.
Specific to the sensitivity analysis of UACR (Figures 4, 5), Wang’s study is considered as the main factor causing high heterogeneity. Based on the full text of the article, the composition and duration of high-fat diets, streptozotocin (STZ) administration, the timing of TP treatment, and weight of animals were similar to others except Ma et al., 2008. A significant difference was that SD rats were used by Li et al., 2013 and Wang et al., 2013, whereas Wistar rats were used by others. Marques et al. found that most metabolic effects, such as hyperleptinemia and decreased oral glucose tolerance, created by a high-fat diet revealed themselves earlier or more prominently in Wistar rats rather than SD rats, although the influence caused by the high-fat diet were generally alike in both strains (Marques et al., 2016). In comparison, the studies conducted by Li et al., 2013 and Wang et al., 2013, which utilized SD rats, have a slightly higher UACR in DKD groups than other studies. It is unclear whether the impact of STZ injection or the treatment of TP is different in those two strains. However, the remaining studies seemed to be a homogeneous group after removing the study conducted by Wang et al., 2013. Compared to others, Wang’s study had a larger sample size and presented a more advanced level of UACR with a minor standard deviation in both DKD and TP groups. Accordingly, it had a larger standardized mean difference effect size and confidence interval. Consequently, we ascribed the prominent effects of the study by Wang et al., 2013 to the better administration or careful treatment in the entire experiment. In addition, the standards of successfully established DKD models were different among studies included. The factors mentioned above result in multiple metabolic conditions and kidney lesions causing heterogeneity. Pooled effect sizes showed that TP decreased SCr and BUN slightly with lower heterogeneity.
There are some limitations in this review. First, as only two studies creating the group of TP combined with an RAS inhibitor, we did not have enough data to appraise the effects of this medication—co-administration in DKD models. In addition, the high heterogeneity of various factors resulted in subgroup analysis on dosage, and the treatment duration of triptolide failed to reduce it. Significant differences were caused by discordance in strains, methodologies, and criteria for modeling, stages of disease, dosages and durations of intervention, and even experiment management in each trial. It is hard to avoid it now, since there is no agreement on the establishment of animal models of DKD yet. Therefore, differences in dose, formula, and duration remain existent in the included studies in which DKD was totally induced by high-fat dietary intervention plus streptocizin injection. Furthermore, the potential bias and comparatively small studies resulted in overrated curative powers. Thus, the conclusion should be interpreted and generalized carefully. More preclinical trials with rigorous designing need to be performed to strengthen the evidence in the future.
Conclusion
Triptolide exhibits similar pharmacological effects to RAS inhibitors on reducing albuminuria and preserving renal function after the onset of DKD. Although there was data heterogeneity, this meta-analysis result provides the clinicians with potential options in designing interventional strategies for DKD patients. Meanwhile, the renovations on TP derivatives and drug-delivering systems are believed to be promising to shed light on DKD prevention in the future.
Data Availability Statement
The original contributions presented in the study are included in the article/Supplementary Material; further inquiries can be directed to the corresponding author.
Author Contributions
DL and HF conceived the project. DL, HM, and FR wrote and revised the manuscript. DL, FR, and HM constructed the mathematical model. DL, HM, and FR edited the manuscript. HF supervised the entire project.
Funding
This work was supported by the National Natural Science Foundation of China (Grants 81970587 and 81770737) and the Guangzhou Regenerative Medicine and Health Guangdong Laboratory (grants 2018GZR110104001 and 2018GZR0201003), and College Students’ Innovative Entrepreneurial Training Plan Program (S201912121084). Fu H is the recipient of the Outstanding Youths Development Scheme of Nanfang Hospital (2015J006) and Southern Medical University (2020JQPY004).
Conflict of Interest
The authors declare that the research was conducted in the absence of any commercial or financial relationships that could be construed as a potential conflict of interest.
Publisher’s Note
All claims expressed in this article are solely those of the authors and do not necessarily represent those of their affiliated organizations, or those of the publisher, the editors and the reviewers. Any product that may be evaluated in this article, or claim that may be made by its manufacturer, is not guaranteed or endorsed by the publisher.
References
An, Z., Dong, X., Guo, Y., Zhou, J., and Qin, T. (2017). Effect of Triptolide on Urinary Albumin Excretion in Rats with Diabetic Nephropathy. J. Nanchang Univ. Sci. 57, 27–29. doi:10.13764/j.cnki.ncdm.2017.01.007
Association, A. D. (2019). 11. Microvascular Complications and Foot Care: Standards of Medical Care in Diabetes-2019. Diabetes Care 42 (Suppl. 1), S124–s138. doi:10.2337/dc19-S011
Chen, S. R., Dai, Y., Zhao, J., Lin, L., Wang, Y., and Wang, Y. (2018). A Mechanistic Overview of Triptolide and Celastrol, Natural Products from Tripterygium Wilfordii Hook F. Front. Pharmacol. 9, 104–113. doi:10.3389/fphar.2018.00104
de Boer, I. H., Rue, T. C., Cleary, P. A., Lachin, J. M., Molitch, M. E., Steffes, M. W., et al. (2011). Long-term Renal Outcomes of Patients with Type 1 Diabetes Mellitus and Microalbuminuria: an Analysis of the Diabetes Control and Complications Trial/Epidemiology of Diabetes Interventions and Complications Cohort. Arch. Intern. Med. 171, 412–420. doi:10.1001/archinternmed.2011.16
Deng, X., Zeng, T., Li, J., Huang, C., Yu, M., Wang, X., et al. (2019). Kidney-targeted Triptolide-Encapsulated Mesoscale Nanoparticles for High-Efficiency Treatment of Kidney Injury. Biomater. Sci. 7, 5312–5323. doi:10.1039/c9bm01290g
Dong, X. G., An, Z. M., Guo, Y., Zhou, J. L., and Qin, T. (2017). Effect of Triptolide on Expression of Oxidative Carbonyl Protein in Renal Cortex of Rats with Diabetic Nephropathy. J. Huazhong Univ. Sci. Technolog Med. Sci. 37, 25–29. doi:10.1007/s11596-017-1689-9
Fadini, G. P., Bonora, B. M., and Avogaro, A. (2017). SGLT2 Inhibitors and Diabetic Ketoacidosis: Data from the FDA Adverse Event Reporting System. Diabetologia 60, 1385–1389. doi:10.1007/s00125-017-4301-8
Fan, H., Yang, J., Wang, M., Du, J., and Fang, F. (2018). Experimental Study of Tripterygium Glycosides in Delaying the Progression of Diabetic Nephropathy. Chin. J. Clin. Healthc. 21, 377–382. doi:10.3969/J.issn.16726790.2018.03.024
Fu, H., Liu, S., Bastacky, S. I., Wang, X., Tian, X. J., and Zhou, D. (2019). Diabetic Kidney Diseases Revisited: A New Perspective for a new era. Mol. Metab. 30, 250–263. doi:10.1016/j.molmet.2019.10.005
Gao, Q., Liu, Z., Qin, W., Zheng, C., Zhang, M., Zeng, C., et al. (2009). Triptolide Ameliorates Proteinuria and Improves Renal Lesion in Diabetic Db/db Mice. Chin. J. Nephrol. Dial. Transpl. 18, 519–528. doi:10.3969/j.issn.1006-298X.2009.06.004
Guh, J. Y. (2010). Proteinuria versus Albuminuria in Chronic Kidney Disease. Nephrology (Carlton) 15 Suppl 2 (Suppl. 2), 53–56. doi:10.1111/j.1440-1797.2010.01314.x
Guo, H., Pan, C., Chang, B., Wu, X., Guo, J., Zhou, Y., et al. (2016). Triptolide Improves Diabetic Nephropathy by Regulating Th Cell Balance and Macrophage Infiltration in Rat Models of Diabetic Nephropathy. Exp. Clin. Endocrinol. Diabetes 124, 389–398. doi:10.1055/s-0042-106083
Guo, Y., Guo, N., Wang, J., Wang, R., and Tang, L. (2021). Retrospective Analysis of Tripterygium Wilfordii Polyglycoside Combined with Angiotensin Receptor Blockers for the Treatment of Primary Membranous Nephropathy with Sub-nephrotic Proteinuria. Ren. Fail. 43, 729–736. doi:10.1080/0886022X.2021.1918555
Han, F., Xue, M., Chang, Y., Li, X., Yang, Y., Sun, B., et al. (2017). Triptolide Suppresses Glomerular Mesangial Cell Proliferation in Diabetic Nephropathy Is Associated with Inhibition of PDK1/Akt/mTOR Pathway. Int. J. Biol. Sci. 13, 1266–1275. doi:10.7150/ijbs.20485
Han, F. (2018). Study on the Mechanism of Triptolide in Improving Diabetic Nephropathy via PDK1/Akt and miR-137/Notch1 Pathway. Dissertation. Tianjin (China). Tianjin Medicial university.
Hong, Y., Gui, Z., Cai, X., and Lan, L. (2016). Clinical Efficacy and Safety of Tripterygium Glycosides in Treatment of Stage IV Diabetic Nephropathy: A Meta-Analysis. Open Med. (Wars) 11, 611–617. doi:10.1515/med-2016-0099
Hooijmans, C. R., Rovers, M. M., de Vries, R. B., Leenaars, M., Ritskes-Hoitinga, M., and Langendam, M. W. (2014). SYRCLE's Risk of Bias Tool for Animal Studies. BMC Med. Res. Methodol. 14, 43. doi:10.1186/1471-2288-14-43
Hooijmans, C. R., Tillema, A., Leenaars, M., and Ritskes-Hoitinga, M. (2010). Enhancing Search Efficiency by Means of a Search Filter for Finding All Studies on Animal Experimentation in PubMed. Lab. Anim. 44, 170–175. doi:10.1258/la.2010.009117
Hostetter, T. H. (2001). Prevention of End-Stage Renal Disease Due to Type 2 Diabetes. N. Engl. J. Med. 345, 910–912. doi:10.1056/NEJM200109203451209
Hou, W., Liu, B., and Xu, H. (2019). Triptolide: Medicinal Chemistry, Chemical Biology and Clinical Progress. Eur. J. Med. Chem. 176, 378–392. doi:10.1016/j.ejmech.2019.05.032
Hoyle, G. W., Hoyle, C. I., Chen, J., Chang, W., Williams, R. W., and Rando, R. J. (2010). Identification of Triptolide, a Natural Diterpenoid Compound, as an Inhibitor of Lung Inflammation. Am. J. Physiol. Lung Cel. Mol. Physiol. 298, L830–L836. doi:10.1152/ajplung.00014.2010
Huang, W. J., Liu, W. J., Xiao, Y. H., Zheng, H. J., Xiao, Y., Jia, Q., et al. (2020). Tripterygium and its Extracts for Diabetic Nephropathy: Efficacy and Pharmacological Mechanisms. Biomed. Pharmacother. 121, 109599. doi:10.1016/j.biopha.2019.109599
Kopel, J., Pena-Hernandez, C., and Nugent, K. (2019). Evolving Spectrum of Diabetic Nephropathy. World J. Diabetes 10, 269–279. doi:10.4239/wjd.v10.i5.269
Koye, D. N., Magliano, D. J., Nelson, R. G., and Pavkov, M. E. (2018). The Global Epidemiology of Diabetes and Kidney Disease. Adv. Chronic Kidney Dis. 25, 121–132. doi:10.1053/j.ackd.2017.10.011
Krakauer, T., Chen, X., Howard, O. M., and Young, H. A. (2005). Triptolide Attenuates Endotoxin- and Staphylococcal Exotoxin-Induced T-Cell Proliferation and Production of Cytokines and Chemokines. Immunopharmacol. Immunotoxicol. 27, 53–66. doi:10.1081/iph-51294
Kramer, H. (2004). Screening for Kidney Disease in Adults with Diabetes Mellitus: Don't Forget Serum Creatinine. Am. J. Kidney Dis. 44, 921–923. doi:10.1053/j.ajkd.2004.09.00210.1016/s0272-6386(04)01277-6
Kupchan, S. M., Court, W. A., Dailey, R. G., Gilmore, C. J., and Bryan, R. F. (1972). Triptolide and Tripdiolide, Novel Antileukemic Diterpenoid Triepoxides from Tripterygium Wilfordii. J. Am. Chem. Soc. 94, 7194–7195. doi:10.1021/ja00775a078
Leenaars, M., Hooijmans, C. R., van Veggel, N., ter Riet, G., Leeflang, M., Hooft, L., et al. (2012). A Step-by-step Guide to Systematically Identify All Relevant Animal Studies. Lab. Anim. 46, 24–31. doi:10.1258/la.2011.011087
Li, J., Shen, F., Guan, C., Wang, W., Sun, X., Fu, X., et al. (2014). Activation of Nrf2 Protects against Triptolide-Induced Hepatotoxicity. PLoS One 9, e100685. doi:10.1371/journal.pone.0100685
Li, K., Luan, J., Sui, A., Ma, R., Zhou, L., and Wang, Y. (2013). Influence of Irbesrtan Combined with Triptolide on ANGPTL2 and VEGF in the Kidney of 2 Type Diabetes Rat Models. Chin. J. Integr. Tradit. West. 14, 959–962. https://kns.cnki.net/kcms/detail/detail.aspx?FileName=JXSB201311009&DbName=CJFQ2013.
Li, M., Hu, T., Tie, C., Qu, L., Zheng, H., and Zhang, J. (2017). Quantitative Proteomics and Targeted Fatty Acids Analysis Reveal the Damage of Triptolide in Liver and Kidney. Proteomics 17, 1700001. doi:10.1002/pmic.201700001
Li, W., Bai, X., Wu, Y., Huang, W., Tian, X., and Men, H. (2015). Protective Effect of Triptolide on Kidney of Early Diabetes Mellitus (DM)Model Rats. J. Hebei North. Univ. Sci. Ed. 31, 77–80. doi:10.3969/j.issn.1673-1492.2015.05.017
Li, X. J., Jiang, Z. Z., and Zhang, L. Y. (2014). Triptolide: Progress on Research in Pharmacodynamics and Toxicology. J. Ethnopharmacol. 155, 67–79. doi:10.1016/j.jep.2014.06.006
Li, X. X., Du, F. Y., Liu, H. X., Ji, J. B., and Xing, J. (2015). Investigation of the Active Components in Tripterygium Wilfordii Leading to its Acute Hepatotoxicty and Nephrotoxicity. J. Ethnopharmacol. 162, 238–243. doi:10.1016/j.jep.2015.01.004
Li, X. Y., Wang, S. S., Han, Z., Han, F., Chang, Y. P., Yang, Y., et al. (2017). Triptolide Restores Autophagy to Alleviate Diabetic Renal Fibrosis through the miR-141-3p/PTEN/Akt/mTOR Pathway. Mol. Ther. Nucleic Acids 9, 48–56. doi:10.1016/j.omtn.2017.08.011
Li, Y., Hu, Q., Li, C., Liang, K., Xiang, Y., Hsiao, H., et al. (2019). PTEN-induced Partial Epithelial-Mesenchymal Transition Drives Diabetic Kidney Disease. J. Clin. Invest. 129, 1129–1151. doi:10.1172/JCI121987
Li, Y., Chen, S., Xia, X., Peng, Y., Yang, X., and Wang, G. (2020). Analysis on Adverse Reactions Caused by Preparations Containing Tripterygium Wilfordii in Henan Province from 2004 to 2019. Drugs Clin. 35, 988–994. doi:10.7501/j.issn.1674-5515.2020.05.036
Lin, Y. C., Chang, Y. H., Yang, S. Y., Wu, K. D., and Chu, T. S. (2018). Update of Pathophysiology and Management of Diabetic Kidney Disease. J. Formos. Med. Assoc. 117, 662–675. doi:10.1016/j.jfma.2018.02.007
Liu, B., Fan, D., Shu, H., He, X., Lyu, C., and Lyu, A. (2019). Side Effect and Attenuation of Triptolide. Chin. J. Exp. Tradit. Med. Formulae 25, 181–190. doi:10.13422/j.cnki.syfjx.20191429
Liu, Q., Chen, T., Chen, G., Li, N., Wang, J., Ma, P., et al. (2006). Immunosuppressant Triptolide Inhibits Dendritic Cell-Mediated Chemoattraction of Neutrophils and T Cells through Inhibiting Stat3 Phosphorylation and NF-kappaB Activation. Biochem. Biophys. Res. Commun. 345, 1122–1130. doi:10.1016/j.bbrc.2006.05.024
Liu, Q. (2011). Triptolide and its Expanding Multiple Pharmacological Functions. Int. Immunopharmacol. 11, 377–383. doi:10.1016/j.intimp.2011.01.012
Liu, Q., Chen, S., Liu, F., Wang, X., Shi, Z., and Chen, H. (2014). Effects of Triptolide on Expressions of NF-Κb,NOS and VEGF in Glomeruli of Diabetes Mellitus Rats. J. Clin. Exp. Med. 13, 1925–1929. doi:10.3969/j.issn.1671-4695.2014.23.002
Lu, Y., Fukuda, K., Nakamura, Y., Kimura, K., Kumagai, N., and Nishida, T. (2005). Inhibitory Effect of Triptolide on Chemokine Expression Induced by Proinflammatory Cytokines in Human Corneal Fibroblasts. Invest. Ophthalmol. Vis. Sci. 46, 2346–2352. doi:10.1167/iovs.05-0010
Ma, R., Liu, L., Liu, X., Wang, Y., Jiang, W., and Xu, L. (2013). Triptolide Markedly Attenuates Albuminuria and Podocyte Injury in an Animal Model of Diabetic Nephropathy. Exp. Ther. Med. 6, 649–656. doi:10.3892/etm.2013.1226
Ma, R., Liu, L., Xu, Y., and Jiang, W. (2008). Protective Effect of Riptolide on Renal Tissures in Type 2 Diabetic Rats. Chin. J. Hypertens. 16, 1120–1124. doi:10.3969/j.issn.1673-7245.2008.12.01710.11569/wcjd.v16.i33.3775
Ma, R., Liu, X., Liu, L., and Li, H. (2010). Effects of Triptolide on the Podocyte Protein Expression of Nephrin and Podocin and its Mechanism in Type 2 Diabetic Rats. Chin. J. Diabetes Mellit. 02, 291–296. doi:10.3760/cma.j.issn.1674-5809.2010.04.013
Ma, R. (2009). The Protective Effect of the Combination of Triptolide and Irbesartan on the Podocytes in Type 2 Diabetic Rat Model and its mechanismDissertation. Shandong (China): Shandong University.
Ma, R., Wang, Y., Wei, Z., Lu, H., Liu, L., and Guan, G. (2009). Effect of Triptolide on Podocyte Injury and its Mechanism in the Kidney of 2 Type Diabetic Rats. Chin. J. Immunol. 25, 404–408. https://kns.cnki.net/kcms/detail/detail.aspx?FileName=ZMXZ200905007&DbName=CJFQ2009.
McGrath, K., and Edi, R. (2019). Diabetic Kidney Disease: Diagnosis, Treatment, and Prevention. Am. Fam. Physician 99, 751–759. doi:10.1002/0470021616.ch2
Moher, D., Liberati, A., Tetzlaff, J., and Altman, D. G. (2009). Preferred Reporting Items for Systematic Reviews and Meta-Analyses: the PRISMA Statement. BMJ 339, b2535. doi:10.1136/bmj.b2535
Ni, B., Jiang, Z., Huang, X., Xu, F., Zhang, R., Zhang, Z., et al. (2008). Male Reproductive Toxicity and Toxicokinetics of Triptolide in Rats. Arzneimittelforschung 58, 673–680. doi:10.1055/s-0031-1296570
Onuigbo, M. A. (2011). Can ACE Inhibitors and Angiotensin Receptor Blockers Be Detrimental in CKD Patients? Nephron. Clin. Pract. 118, c407–19. doi:10.1159/000324164
Qi, B., Wang, X., Zhou, Y., Han, Q., He, L., Gong, T., et al. (2015). A Renal-Targeted Triptolide Aminoglycoside (TPAG) Conjugate for Lowering Systemic Toxicities of Triptolide. Fitoterapia 103, 242–251. doi:10.1016/j.fitote.2015.04.008
Rao, C., Adair, T., Bain, C., and Doi, S. A. (2012). Mortality from Diabetic Renal Disease: a Hidden Epidemic. Eur. J. Public Health 22, 280–284. doi:10.1093/eurpub/ckq205
Ren, D., Zuo, C., and Xu, G. (2019). Clinical Efficacy and Safety of Tripterygium Wilfordii Hook in the Treatment of Diabetic Kidney Disease Stage IV: A Meta-Analysis of Randomized Controlled Trials. Medicine (Baltimore) 98, e14604. doi:10.1097/MD.0000000000014604
Ren, L., Zhu, M., Zhu, H., Ding, M., Fei, D., Huo, L., et al. (2020). Effect of Triptolide on Podocytes in Mice with Diabetic Nephropathy by Regulating Toll-like Receptor (TLR)/Nuclear Factor-Kb (NF-Κb) Signal Pathway. Zhejiang J. Integr. Tradit. Chin. West. Med. 30, 191–195. doi:10.3969/j.issn.1005-4561.2020.03.007
Ren, Q., Li, M., Deng, Y., Lu, A., and Lu, J. (2021). Triptolide Delivery: Nanotechnology-Based Carrier Systems to Enhance Efficacy and Limit Toxicity. Pharmacol. Res. 165, 105377. doi:10.1016/j.phrs.2020.105377
Shen, G., Zhuang, X., Xiao, W., Kong, L., Tan, Y., and Li, H. (2014). Role of CYP3A in Regulating Hepatic Clearance and Hepatotoxicity of Triptolide in Rat Liver Microsomes and sandwich-cultured Hepatocytes. Food Chem. Toxicol. 71, 90–96. doi:10.1016/j.fct.2014.05.020
Slabaugh, S. L., Curtis, B. H., Clore, G., Fu, H., and Schuster, D. P. (2015). Factors Associated with Increased Healthcare Costs in Medicare Advantage Patients with Type 2 Diabetes Enrolled in a Large Representative Health Insurance Plan in the US. J. Med. Econ. 18, 106–112. doi:10.3111/13696998.2014.979292
Sumida, K., Nadkarni, G. N., Grams, M. E., Sang, Y., Ballew, S. H., Coresh, J., et al. (2020). Conversion of Urine Protein-Creatinine Ratio or Urine Dipstick Protein to Urine Albumin-Creatinine Ratio for Use in Chronic Kidney Disease Screening and Prognosis : An Individual Participant-Based Meta-Analysis. Ann. Intern. Med. 173, 426–435. doi:10.7326/m20-0529
Thomas, M. C., Cooper, M. E., and Zimmet, P. (2016). Changing Epidemiology of Type 2 Diabetes Mellitus and Associated Chronic Kidney Disease. Nat. Rev. Nephrol. 12, 73–81. doi:10.1038/nrneph.2015.173
Wang, D., and Yu, R. (2017c). Effects of Triptolide on Expressions of iNOS and COX-2 in Renal Tissue of Diabetic Nephropathy Rats. Prog. Anat. Sci. 23, 148–150. doi:10.16695/j.cnki.1006-2947.2017.02.011
Wang, D., and Yu, R. (2017a). Effects of Triptolide on Expressions of PGE2 and NF-Κb in Renal Tissue of Diabetic Nephropathy Rats. Prog. Anat. Sci. 23, 10–12. doi:10.16695/j.cnki.1006-2947.2017.01.003
Wang, D., and Yu, R. (2017b). Effects of Triptolide on Expressions of TNF-α and MCP-1 in Renal Tissue of Diabetic Nephropathy Rats. Prog. Anat. Sci. 23, 71–73. doi:10.16695/j.cnki.1006-2947.2017.01.020
Wang, Y., Li, K., Hu, M., and Luan, J. (2013). Influence of ANGPTL2 on the Podocytes in the Kidney of 2 Type Diabetes Rat Models and Effect of Triptolide. Chin. J. Clin. Ed. 7, 10746–10751. doi:10.3877/cma.j.issn.1674-0785.2013.23.082
Xi, C., Peng, S., Wu, Z., Zhou, Q., and Zhou, J. (2017). WITHDRAWN: Toxicity of Triptolide and the Molecular Mechanisms Involved. Environ. Toxicol. Pharmacol. 90, 531–541. doi:10.1016/j.biopha.2017.04.00310.1016/j.etap.2017.09.013
Xie, Y., Bowe, B., Li, T., Xian, H., Yan, Y., and Al-Aly, Z. (2018). Higher Blood Urea Nitrogen Is Associated with Increased Risk of Incident Diabetes Mellitus. Kidney Int. 93, 741–752. doi:10.1016/j.kint.2017.08.033
Xu, L., Zhao, B., Wang, H., Liu, L., Chen, A., Wang, H., et al. (2020). Tripterygium Wilfordii Hook F Treatment for Stage IV Diabetic Nephropathy: Protocol for a Prospective, Randomized Controlled Trial. Biomed. Res. Int. 2020, 9181037. doi:10.1155/2020/9181037
Xu, Y., Fan, Y. F., Zhao, Y., and Lin, N. (2019). [Overview of Reproductive Toxicity Studies on Tripterygium Wilfordii in Recent 40 years]. Zhongguo Zhong Yao Za Zhi 44, 3406–3414. doi:10.19540/j.cnki.cjcmm.20190524.401
Xue, M. (2018). Effect of Triptolide on Diabetic Renal Tubular Epithelial-Mesenchymal Transition via miR-188- 5p/PTEN Pathway Master’s Thesis. Tianjin (China): Tianjin Medical University.
Xue, X., Zhai, L., Liu, X., and Ma, R. (2012). The Effect and Significance of Triptolide on the Expression of Nephrin,Podocin in Type 2 Diabetic Rat Kidneys. J. Clin. Ration. Drug Use 05, 9–11. doi:10.3969/j.issn.1674-3296.2012.21.005
Yang, F., Zhuo, L., Ananda, S., Sun, T., Li, S., and Liu, L. (2011). Role of Reactive Oxygen Species in Triptolide-Induced Apoptosis of Renal Tubular Cells and Renal Injury in Rats. J. Huazhong Univ. Sci. Technolog Med. Sci. 31, 335–341. doi:10.1007/s11596-011-0377-4
Ye, X., and Hong, Y. (2015). Effect of Triptolide on the Expression of Regulated upon Activation normal T-Cell Expressed and Secreted in Renal Tissue of Diabetic Nephropathy Rats. Chin. J. Clin. Pharmacol. 31, 31–33. doi:10.13699/j.cnki.1001-6821.2015.01.010
You, L., Guo, H., and Li, Y. (2015). Influence of Triptolide on the Expression of Glut-1, Glut-4 in Kidney of Diabetic Ne-Phropathy Rats. Pract. Pharm. Clin. Remedies 18, 390–393. doi:10.14053/j.cnki.ppcr.201504004
Yuan, K., Li, X., Lu, Q., Zhu, Q., Jiang, H., Wang, T., et al. (2019). Application and Mechanisms of Triptolide in the Treatment of Inflammatory Diseases-A Review. Front. Pharmacol. 10, 1469–1512. doi:10.3389/fphar.2019.01469
Yuan, Z. X., Wu, X. J., Mo, J., Wang, Y. L., Xu, C. Q., and Lim, L. Y. (2015). Renal Targeted Delivery of Triptolide by Conjugation to the Fragment Peptide of Human Serum Albumin. Eur. J. Pharm. Biopharm. 94, 363–371. doi:10.1016/j.ejpb.2015.06.012
Zhang, X., Xiao, Z., and Xu, H. (2019). A Review of the Total Syntheses of Triptolide. Beilstein J. Org. Chem. 15, 1984–1995. doi:10.3762/bjoc.15.194
Zhang, Z., Zheng, Q., Han, J., Gao, G., Liu, J., Gong, T., et al. (2009). The Targeting of 14-succinate Triptolide-Lysozyme Conjugate to Proximal Renal Tubular Epithelial Cells. Biomaterials 30, 1372–1381. doi:10.1016/j.biomaterials.2008.11.035
Zhao, G., Vaszar, L. T., Qiu, D., Shi, L., and Kao, P. N. (2000). Anti-inflammatory Effects of Triptolide in Human Bronchial Epithelial Cells. Am. J. Physiol. Lung Cel. Mol. Physiol. 279, L958–L966. doi:10.1152/ajplung.2000.279.5.L958
Zhou, H. F., Niu, D. B., Xue, B., Li, F. Q., Liu, X. Y., He, Q. H., et al. (2003). Triptolide Inhibits TNF-Alpha, IL-1 Beta and NO Production in Primary Microglial Cultures. Neuroreport 14, 1091–1095. doi:10.1097/01.wnr.0000073682.00308.47
Zhou, L., Zhou, C., Feng, Z., Liu, Z., Zhu, H., and Zhou, X. (2018). Triptolide-induced Hepatotoxicity Can Be Alleviated when Combined with Panax Notoginseng Saponins and Catapol. J. Ethnopharmacol. 214, 232–239. doi:10.1016/j.jep.2017.12.033
Zhu, J. (2013). Effect of Triptolide on the Expression of RANTES in the Kidney of Diabetic Rats Master’s Thesis. Zhejiang (China). Zhejiang Chinese Medical University.
Zhuang, X.-M., Shen, G.-L., Xiao, W.-B., Tan, Y., Lu, C., and Li, H. (2013). Assessment of the Roles of P-Glycoprotein and Cytochrome P450 in Triptolide-Induced Liver Toxicity in sandwich-cultured Rat Hepatocyte Model. Drug Metab. Dispos 41, 2158–2165. doi:10.1124/dmd.113.054056
Zhuang, Z., Wang, Z. H., Huang, Y. Y., Zheng, Q., and Pan, X. D. (2020). Protective Effect and Possible Mechanisms of Ligustrazine Isolated from Ligusticum Wallichii on Nephropathy in Rats with Diabetes: A Preclinical Systematic Review and Meta-Analysis. J. Ethnopharmacol 252, 112568. doi:10.1016/j.jep.2020.112568
Keywords: triptolide, renin-angiotensin system inhibitor, diabetic kidney diseases, albuminuria, kidney function
Citation: Liang D, Mai H, Ruan F and Fu H (2021) The Efficacy of Triptolide in Preventing Diabetic Kidney Diseases: A Systematic Review and Meta-Analysis. Front. Pharmacol. 12:728758. doi: 10.3389/fphar.2021.728758
Received: 22 June 2021; Accepted: 12 August 2021;
Published: 01 October 2021.
Edited by:
Annalisa Chiavaroli, University of Studies G.d’Annunzio Chieti and Pescara, ItalyReviewed by:
Sofia Viana, University of Coimbra, PortugalGabino Garrido, Catholic University of the North, Chile
Copyright © 2021 Liang, Mai, Ruan and Fu. This is an open-access article distributed under the terms of the Creative Commons Attribution License (CC BY). The use, distribution or reproduction in other forums is permitted, provided the original author(s) and the copyright owner(s) are credited and that the original publication in this journal is cited, in accordance with accepted academic practice. No use, distribution or reproduction is permitted which does not comply with these terms.
*Correspondence: Haiyan Fu, aHlfZnU0MjZAMTI2LmNvbQ==