- 1Department of Biochemistry and Physiology, Faculty of Pharmacy and Food Sciences, Universitat de Barcelona, Barcelona, Spain
- 2Department of Pharmacy, Faculty of Health Sciences, Universidad San Jorge, Zaragoza, Spain
- 3School of Pharmacy, Università di Camerino, Camerino, Italy
- 4Instituto Agroalimentario de Aragón-IA2, CITA-Universidad de Zaragoza, Zaragoza, Spain
Pteridophytes, represented by ferns and allies, are an important phytogenetic bridge between lower and higher plants. Ferns have evolved independently of any other species in the plant kingdom being its secondary metabolism a reservoir of phytochemicals characteristic of this taxon. The study of the potential uses of Polypodium vulgare L. (Polypodiaceae) as medicinal plant has increased in recent years particularly when in 2008 the European Medicines Agency published a monograph about the rhizome of this species. Our objective is to provide scientific knowledge on the polar constituents extracted from the fronds of P. vulgare, one of the main ferns of European distribution, to contribute to the validation of certain traditional uses. Specifically, we have characterized the methanolic extract of P. vulgare fronds (PVM) by HPLC-DAD and investigated its potential cytotoxicity, phototoxicity, ROS production and protective effects against oxidative stress by using in vitro methods. The 3T3, HaCaT, HeLa, HepG2, MCF-7 and A549 were the cell lines used to evaluate the possible cytotoxic behaviour of the PVM. HPLC-DAD was utilized to validate the polyphenolic profile of the extract. H2O2 and UVA were the prooxidant agents to induce oxidative stress by different conditions in 3T3 and HaCaT cell lines. Antioxidant activity of in vitro PVM in 3T3 and HaCaT cell lines was evaluated by ROS assay. Our results demonstrate that PVM contains significant amounts of shikimic acid together with caffeoylquinic acid derivatives and flavonoids such as epicatechin and catechin; PVM is not cytotoxic at physiological concentrations against the different cell lines, showing cytoprotective and cellular repair activity in 3T3 fibroblast cells. This biological activity could be attributed to the high content of polyphenolic compounds. The fronds of the P. vulgare are a source of polyphenolic compounds, which can be responsible for certain traditional uses like wound healing properties. In the present work, fronds of the common polypody are positioned as a candidate for pharmaceutical applications based on traditional medicine uses but also as potential food ingredients due to lack of toxicity at physiological concentrations.
Introduction
Oxidative stress is characterized by an imbalance between pro-oxidant agents and the antioxidant defence system. According to its origin, this antioxidant system is classified as endogenous or exogenous (Addor, 2017). The inability to maintain an adequate redox state, either due to excess production of free radicals or an alteration of the antioxidant system, triggers oxidative damage that affects fundamental biological structures (Willcox et al., 2004). In this sense, studies have associated oxidative stress with the development of different metabolic diseases (Cásedas et al., 2016; He et al., 2017). Nowadays, antioxidant is defined as “any substance that delays, prevents, or removes oxidative damage to a target molecule” (Burton and Ingold, 2015).
Numerous investigations have also established the link between sun exposure and skin alterations (Evans and Johnson, 2010; Kimlin and Guo, 2012). Today it is well documented that ultraviolet radiation affects animals in different causes, among which oxidative stress, inflammation, erythema, breakdown of the extracellular matrix, wrinkling and skin cancer. But the main effect of this ultraviolet irradiation is the increase in oxidative stress caused by the increase in ROS, which can lead to an imbalance in the endogenous antioxidant system (Gegotek et al., 2020). Ultraviolet irradiation is also the main etiologic factor in the development of skin cancers (Rundle et al., 2020). Phytochemicals can modulate the behaviour of tumour cells by acting on different pathways of molecular signalling such as exogenous antioxidant system. As examples of these pathways are the topoisomerase inhibition (genistein), kinase inhibition (apigenin) and modulation of multidrug resistance (2′,4′,6′-triOH-chalcone), among others (Ren et al., 2003). The doxorubicin, paclitaxel, vinblastine, etoposide, irinotecan, gemcitabine, and methotrexate are medically successful in anticancer therapy, for their security and efficacy, which are part of the list of anticancer agents provided or inspired by nature in recent years (Newman and Cragg, 2012; Silva et al., 2019). In addition, polyphenols, as flavonoids for their safety and accessibility, can also be key dietary molecules for cancer treatment and prevention respectively (Asensi et al., 2011; Shukla et al., 2014; Alvarado-Sansininea et al., 2018).
Ethnopharmacological investigations on traditional Chinese medicine have reported the therapeutic uses of ferns in current medicine. For that purpose, different bioassays were performed as for example antioxidant (Dryoathyrium boryanum (Willd.) Ching (Athyriaceae family) (Cao et al., 2013)), acetycholinesterase inhibition (Stenochlaena palustres (Burm. f.) Bedd. (Blechnaceae family) (Chear et al., 2016)), tyrosinase inhibition (Asplenium adiantum-nigrum L. (Aspleniaceae family) (Farràs et al., 2019)) and anti-tumour activity (Davallia cylindrica Ching (Davalliaceae family) (Cao et al., 2014)) attributed to some ferns.
On the other hand, investigations of ferns in recent years have put forward the idea of these species as a potential source of bioactive compounds with food interest. In certain Eastern cultures of the European continent fronds of ferns are used as a food source (Langhansova et al., 2021). The young fronds of ferns (named fiddleheads), which generally exhibit a higher total phenol content than the corresponding mature fronds, are a source of nutrients and phytochemicals with a high potential to reduce oxidative stress of diseases associated with ageing (Dvorakova et al., 2021).
Polypodium vulgare L. (The Plant List, 2021), commonly known as polypody in English or as polipodio in Spain for the shape of its fronds as feet (poly: many and podos: foot), as represented in Image 1, is a fern of the leptosporangiate class belonging to the Polypodiaceae family (Bolòs and Vigo, 1984; Khare, 2007; Quer, 2016). P. vulgare has been used as medicinal plant in Europe since ancient times. As example, in the middle of the last century the use of P. vulgare rhizome infusion as expectorant or diuretic in traditional Polish medicine is reported (Glensk et al., 2019b). Moreover, the fronds of P. vulgare have an ethnoveterinary use for treatment variolous, jaundice and parasitic diseases in Spain (Bonet and Vallès, 2007). The use of P. vulgare as food is restricted as sweetener in the case of its rhizome (Glensk et al., 2019a). Since 2008, the rhizome of P. vulgare has been accepted by European Medicines Agency (EMA) for its use as expectorant herbal medicine in cough and cold and in cases of occasional constipation (EMA, 2008).
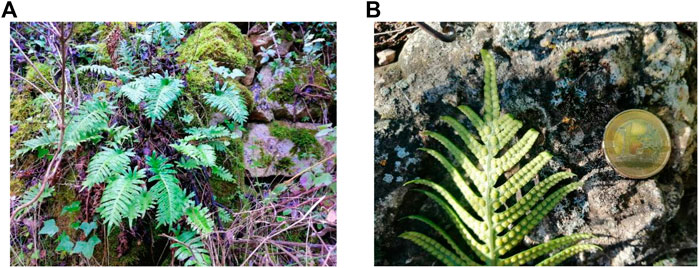
IMAGE 1. Photography of the face fronds (A) and underside frond (B) of fresh Polypodium vulgare L. (Polypodiaceae). Pictures were taken by Adrià Farràs at Prades mountains. The euro coin reflects the dimension of the frond (image 1B).
In the present study we want to highlight the insufficient number of studies dealing with ferns in comparison with angiosperms to support their potential uses (Chen and Kong, 2005; Cao et al., 2014). Hopefully, these studies will be a turning point for the promotion of traditional and local uses of ferns in Europe and particularly in Spain (Villar and Bonet, 2018).
Materials and Methods
Chemicals and Reagents Equations
All reagents were of analytical grade. Trypan blue (0.4%) dye, hydrogen peroxide (H2O2) 30% w/w, 2,5-diphenyl-3-(4,5-dimethyl-2-thiazolyl) tetrazolium bromide (MTT), dimethylsulfoxide (DMSO), 2,7-dichlorodihydrofluorescein diacetate (DCF) and chlorpromazine hydrochloride (CPZ, CAS No. 69–09-0) were supplied from Sigma-Aldrich (Madrid, Spain). Dulbecco’s modified Eagle’s medium (DMEM) with and without phenol red, fetal bovine serum (FBS), phosphate buffered saline (PBS), l-glutamine solution (200 mM), trypsin-ethylenediaminetetraacetic acid (EDTA) solution (170,000 U/L trypsin and 0.2 g/L EDTA) and penicillin-streptomycin solution (10,000 U/mL penicillin and 10 mg/mL streptomycin) were acquired from Lonza (Verviers, Belgium). All analytical standards used for liquid chromatography analysis shikimic acid, gallic acid, 5-O-caffeoylquinic acid, 3-O-caffeoylquinic acid, (+)-catechin hydrate, (−)-epicatechin, rutin, hyperoside, naringin, quercitrin, 3,5-di-O-caffeoylquinic acid, rosmarinic acid, cinnamic acid, eugenol and trans-cinnamaldheyde were purchased from Sigma-Aldrich (Milan, Italy). The 75 cm2 culture flasks and 96-well plates were obtained from TPP (Trasadingen, Switzerland). HyClone fetal bovine serum (FBS) was purchased from Thermo Scientific (Northumberland, United Kingdom).
Plant Material
The fronds of Polypodium vulgare L. were collected from the Prades mountains 41°17′34″N 1°02′42″E geographical coordinates (Tarragona, Spain). Previously we verified that this species was reported in the selected area by Banco de Datos de Biodiversidad de Cataluña (BDBC, 2015). When the fronds were dried, a sample voucher was stored at Herbarium of Universidad San Jorge (Zaragoza, Spain), Polypodium vulgare L.: voucher no. 003-2016.
Preparation of Methanolic Extract With the Fronds of Polypodium vulgare L.
Powdered fronds of the plant material were macerated with methanol for 24 h. After this, the methanolic extract was filtered using a Whatman no. 4 filter paper and to evaporate the solvent, a rotatory evaporator with a thermostatic bath at 30°C was used. This process was repeated three times to obtain the correspondence exhaustion extract as described by ourselves (Farràs et al., 2019). Finally, extracts were conserved at −20°C until we need. Homogenization of the plant extract with the corresponding culture medium was obtained by sonication.
Phytochemical Characterization by Liquid Chromatography With Diode-Array Detection (HPLC-DAD)
HPLC-DAD studies were performed using a Hewlett-Packard HP-1090 Series II (Palo Alto, CA, United States), equipped with a vacuum degasser, a binary pump, an autosampler and a model 1046A HP photodiode array detector (DAD) following a previous developed method with some modifications (Caprioli et al., 2016). The chromatographic separation was accomplished on a Synergi Polar-RP C18 (4.6 mm × 250 mm, 4 µm) analytical column from Phenomenex (Chesire, United Kingdom). The column was preceded by a security cartridge. The mobile phase for HPLC-DAD (diode array detector) analyses was a mixture of (A) water with 0.1% formic acid (v/v) and (B) acetonitrile with 0.1% formic acid, flowing at 0.8 mL/min in gradient conditions: 0 min, 20% B; 0–15 min, 60% B; 15–20 min, 60% B; 20–25 min, 20% B, 25–30 min, 20% B. The column temperature was set at 30°C and the injection volume was 5 µL. UV spectra were recorded in the range 230–350 nm, where 230 nm was used for quantification of shikimic acid, 256 nm for rutin and hyperoside, 272 nm for gallic acid, 280 nm for (+)-catechin hydrate and (−)-epicatechin, 325 nm for 3-O-caffeoylquinic acid, 5-O-caffeoylquinic acid and 3,5-di-O-caffeoylquinic acid.
Calibration curves of the analysed compounds (Supplementary Material S1) were constructed injecting standard solutions at six different concentrations, i.e., 0.5, 1, 5, 10, 50 and 100 mg/mL, in HPLC-DAD. All the calibration curves of the analysed compounds showed a correlation coefficient greater than 0.9930. The limits of detection and the limits of quantification of the analyzed compounds, expressed in µg/mL were estimated on the basis of 3:1 and 10:1 S/Ns (signal to noise ratio). LODs and LOQs were in the range of 0.03–0.15 and 0.1–0.5 μg/mL, respectively. Retention time stability was utilized to demonstrate the specificity of the method. Reproducibility of the chromatographic retention time for each compound in was examined five times per day over a 5-day period (n = 25). The retention times using this method were stable with a percent RSD value of ≤1.33%.
Cell Culture
The mouse fibroblast cell line, NIH 3T3, and the spontaneously immortalized human keratinocyte cell line, HaCaT, were used in all in vitro experiments. In the case of cytotoxic assay, cell viability also was evaluated by the cervical cancer cell line HeLa, the liver cancer cell line HepG2, the breast cancer cell line MCF-7 and lung cancer cell line A549. 3T3 and A549 were purchased from Sigma-Aldrich as a worldwide provider of European Collection of Authenticated Cell Cultures (ECACC), whereas HaCaT, HeLa and MCF-7 were obtained from Eucellbank (Celltec-Universitat de Barcelona, Spain). HepG2 cell line was kindly donated by Dr. Borràs of Experimental Toxicology and Ecotoxicology Platform (UTOX-CERETOX) of Parc Científic of Universitat de Barcelona.
Cell maintenance and culture were performed in Dulbecco’s Modified Eagle’s medium (DMEM) supplemented with 10% heat-inactivated fetal bovine serum (FBS), 2 mM l-glutamine and 100 U/mL:100 U/mL streptomycin-penicillin mixture (10% FBS-DMEM) at 37°C in a 5% carbon dioxide (CO2)-humidified incubator. Cells were routinely subcultured in 75 cm2 flasks.
Experimental treatments were performed when cells reached 80% of confluence, culture medium was removed, cells were rinsed with PBS and then detached by trypsinization (trypsin-EDTA). From the cellular suspension obtained and after adjusting cell density at 1 x 105 cells/mL, 100 were seeded in 96 well microplates and incubated overnight (37°C and 5% CO2). Cell density was adjusted by counting the number of viable cells with the trypan blue (0.4%) dye exclusion.
Cytotoxicity Activity of Methanolic Extract of Polypodium vulgare L. in Non-Tumoral and Tumoral Cells Lines
Non-tumoral (3T3 and HaCaT) and tumoral cells lines (HeLa, HepG2, MCF-7 and A549) were treated for 24 h (37°C and 5% CO2) with increasing concentrations of methanolic extract 0.01, 0.1, 1 and 2 mg/mL P. vulgare in 5% FBS-DMEM. For each independent experiment and plate, untreated cells (maintained with culture medium) were included as negative controls. Cytotoxicity of PVM was determined by the NRU and MTT assays.
Determination of Cell Viability by Neutral Red Uptake and 2,5-Diphenyl-3-(4,5-Dimethyl-2-Thiazolyl) Tetrazolium Bromide Assays
Cell viability were determined by the NRU and MTT methods after treatments.
The Borenfreund and Puerner protocol for the determination of cell viability by NRU has been followed with some described adaptations (Borenfreund and Puerner, 1985). Once the incubation time of the cells with the treatments had elapsed, the supernatant was extracted from each well and 100 μl of NR solution was applied (0.05 mg/mL in serum-free DMEM without phenol red). After 3 hours, the supernatant was removed by inversion from the plate and 100 µl of the developer NR solution was added. In the developer solution, the formaldehyde was replaced by an acidic ethanol solution (Riddell et al., 1986). The quantification of the remnant NR, which corresponds to the NRU bound to the lysosomes, is proportional to the viable cells (Weyermann et al., 2005). After 5–10 min of shaking the plate, the absorbance was obtained at 550 nm, by means of the Tecan Sunrise microplate reader (Männedorf, Switzerland).
The MTT assay based on the experimental protocol of Mosmann (Mosmann, 1983) was used with the some previous adaptations (Zanette et al., 2011); 100 µL of an MTT solution (0.5 mg/mL in serum-free DMEM without phenol red) was added in each well following incubation of the plates for at least 3 h in cell culture incubation conditions (37°C and 5% CO2). At the end of incubation, supernatant was removed and 100 µL of the organic dissolvent dimethyl sulfoxide (DMSO) was added to each well to dissolve the formazan crystals (Präbst, 2017). The amount of soluble formazan is proportional to the number of cells with optimal mitochondrial activity (Fotakis and Timbrell, 2006). Absorbance was measured at 550 nm using a Tecan Sunrise microplate reader (Männedorf, Switzerland), previous homogenization of the well content by gently shaking each microplate during 5 min at 100 rpm/min.
Cell viability for NRU and MTT assays were calculated using the following equation:
where Acontrol and Asample are the absorbance of the control and each sample, respectively.
Cytoprotective Activity in 3T3 and HaCaT Cell Lines
Potential protective effect of the extract was then explored in the non-tumoral cell lines against oxidative stress induced by hydrogen peroxide (Cásedas et al., 2020). Cells were pre-treated with 0.01, 0.1, 1 and 2 mg/mL PVM (100 μL) dissolved by 5% FBS-DMEM for 24 h following addition of H2O2 (in 5% FBS-DMEM) at a final concentration 2 mM for 2.5 h. Finally, cell viability was determined by NRU and MTT assay. In each microplate negative and positive controls were included. In this case, positive controls consist of cells treated by H2O2 at 2 mM during 2.5 h without previous pre-treatment with the extracts.
Cytoprotective activity was calculated as follows:
where CV is the cell viability for each condition described in the formula.
Cellular Repair Activity in 3T3 Cells
Cellular repair properties were evaluated using 100 μL H2O2 at 2 mM during 2.5 h before applying PVM at different concentrations (0.01, 0.1, 1 and 2 mg/mL). Cell viability was assessed by NRU and MTT assays 24 h after incubation with the treatments.
Cellular repair activity was calculated as follows:
where CV is the cell viability for each condition described in the formula.
Phototoxicity Activity of Methanolic Extract of Polypodium vulgare L. in 3T3 and HaCaT Cell Lines
In parallel with the study of the potential cytoprotective protection of the extract, we have explored the potential phototoxic activity of PVM. For this purpose we followed the Organization for Economic Cooperation and Development (OECD) TG 432 (2019) (OECD, 2019) with some adaptions.
Briefly, 3T3 and HaCaT cells were plated at a density of 1 x 105 cells/mL (100 μL) in a 96 well microplate in 10% FBS-DMEM for 24 h. Cells were treated with PVM samples and incubated for 1 h (37°C, 5% CO2) before being irradiated with 1.8 J/cm2 of ultraviolet A (UVA) light. To avoid as much as possible protein interferences with protein and light absorbing components, PVM samples were solubilized in serum-free DMEM without phenol red. Moreover, for comparative purposes and to correctly interpret the data, negative controls consisted in non-treated cells whereas positive controls consisted in cells treated with the well-known phototoxic chemical chloropromazine.
After irradiation, cell media was replaced for 100 μL of fresh medium (10% FBS-DMEM) and cell viability was determined after 24 h of incubation by the NRU and MTT colorimetric assays.
Light exposure was performed in a photostability UV chamber (58 × 34 × 28 cm) equipped with three UVA lamps Actinic BL TL/TL-D/T5 (Philips, 43 V, 352 nm, 15 W) as described by our research group (Martínez et al., 2013). Dosage and time exposition of cells to UVA light was regularly settled thanks to a photoradiometer Delta OHM provided with a UVA probe (HD2302 - Italy). We followed the equation:
where E stands for ultraviolet dose, t represents the time expressed in seconds and, finally, P is the lamp potency.
Intracellular Reactive Oxygen Species (ROS) Induced by H2O2 of Methanolic Extract of Polypodium vulgare L. in 3T3 and HaCaT Cell Lines
ROS production was tested accordingly (Ferreira et al., 2018). After the incubation of the cells with the different concentrations of the extract for 24 h as reported in previous sections for in vitro assays, cells were washed twice with PBS and DCF (100 µM) was applied to each well for 45 min (37°C and 5% CO2). DCF that has not penetrated cells was removed by washing twice with cell culture medium and then H2O2 (1 and 2 mM) was added to induce oxidative stress. The fluorescence intensity of the oxidized product of DCF was registered (λexcitation 480 nm; λemision 530 nm) at 0, 1, 2 and 3 h by a plate reader ThermoFisher SCIENTIFIC VARIOSKAN LUX (ThermoFisher SCIENTIFIC, Waltham, Massachusetts, United States). Results were expressed as Fluorescence Intensity (FI) which have adimensional units. The FIz h Vs 0 h were calculated as follows:
where FIz h is the intensity fluorescence at z h (z as 1, 2 or 3 h) of incubation and FI0 h the amount fluorescence intensity at 0 h.
The FI for each specific time was calculated using this formula:
The ∆ROS, which have adimensional units for FI, was obtained using the following formula:
Statistical Analysis
All experiments were carried out in triplicates and almost three independent experiments were assayed, on different days, except for the cytoprotection PVM HaCaT against 2 mM H2O2 (2.5 h) MTT for which the results correspond to n = 2 experiments. Statistical significance for MTT cell viability and fluorescence intensity was analysed by using GraphPad Prism version 7, San Diego, CA, United States. Data are presented as mean ± standard error. Activities have been compared using a two-way analysis of variance (ANOVA) by Bonferroni. Statistical differences were considered as follows: p ≤ 0.05 (*), p ≤ 0.01 (**), p ≤ 0.001 (***) and p ≤ 0.0001 (****).
Results
Phytochemical Characterization by Liquid Chromatography With Diode-Array Detection (HPLC-DAD)
Different types of polyphenols were monitored in the extract. The extract proved to contain different types of phenolic acids and flavonoids, as observed in Table 1 (77,823.7 mg/kg). However, naringin, quercitrin, rosmarinic acid, cinnamic acid, eugenol and trans-cinnamaldheyde were not detected.
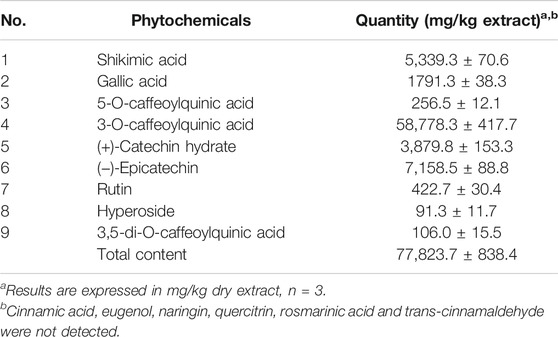
TABLE 1. Quantitative determination of metabolites in the methanolic extract Polypodium vulgare L. by HPLC-DAD reported at 272 nm.
The major constituents in the extract, as seen in Figure 1, were 3-O-caffeoylquinic acid (58,778.3 mg/kg), epicatechin (7,158.5 mg/kg), shikimic acid (5,339.3 mg/kg) and catechin (3,879.8 mg/kg) which were phenol acids. The peculiar secondary metabolites found were hyperoside and 3,5-di-O-caffeoylquinic acid, with low concentrations (91.3 and 106.0 mg/kg respectively).
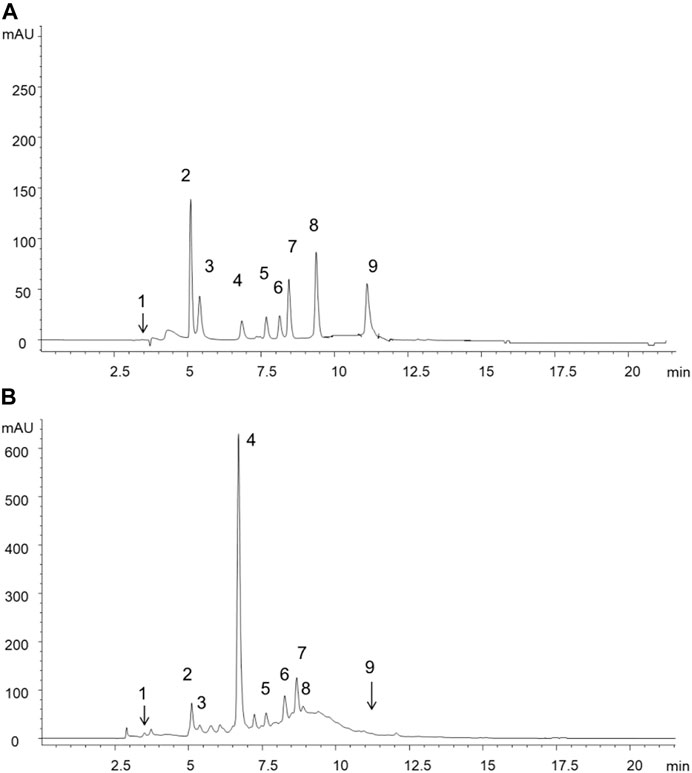
FIGURE 1. HPLC-DAD chromatograms reported only at 272 nm for sake of clarity and corresponding to (A) standard mixture solution (B) extract of methanolic fronds extract of Polypodium vulgare L. List of compounds: 1 = shikimic acid, 2 = gallic acid, 3 = 5-O-caffeoylquinic acid, 4 = 3-O-caffeoylquinic acid, 5 = catechin, 6 = epicatechin, 7 = rutin, 8 = hyperoside, 9 = 3,5-di-O-caffeoylquinic acid.
Cytotoxic Activity in Non-Tumoral and Tumoral Cell Lines
A set of cytotoxic assays was carried out to determine the cytotoxic potential; however, data on NRU method were not shown.
Figure 2 shows cell viability obtained by the MTT assay for the different cell lines described here. First, we evaluated the cytotoxic activity of the PVM in 3T3 and HaCaT as a representation of non-tumoral cell lines. In both cell lines a marked increase in cytotoxicity was observed, at concentrations of 1 and 2 mg/mL PVM compared to 0.01 and 0.1 mg/mL PVM but with a slightly higher cytotoxicity activity of PVM in HaCaT (35.3%) than in 3T3 (46.4%) at 1 mg/mL PVM.
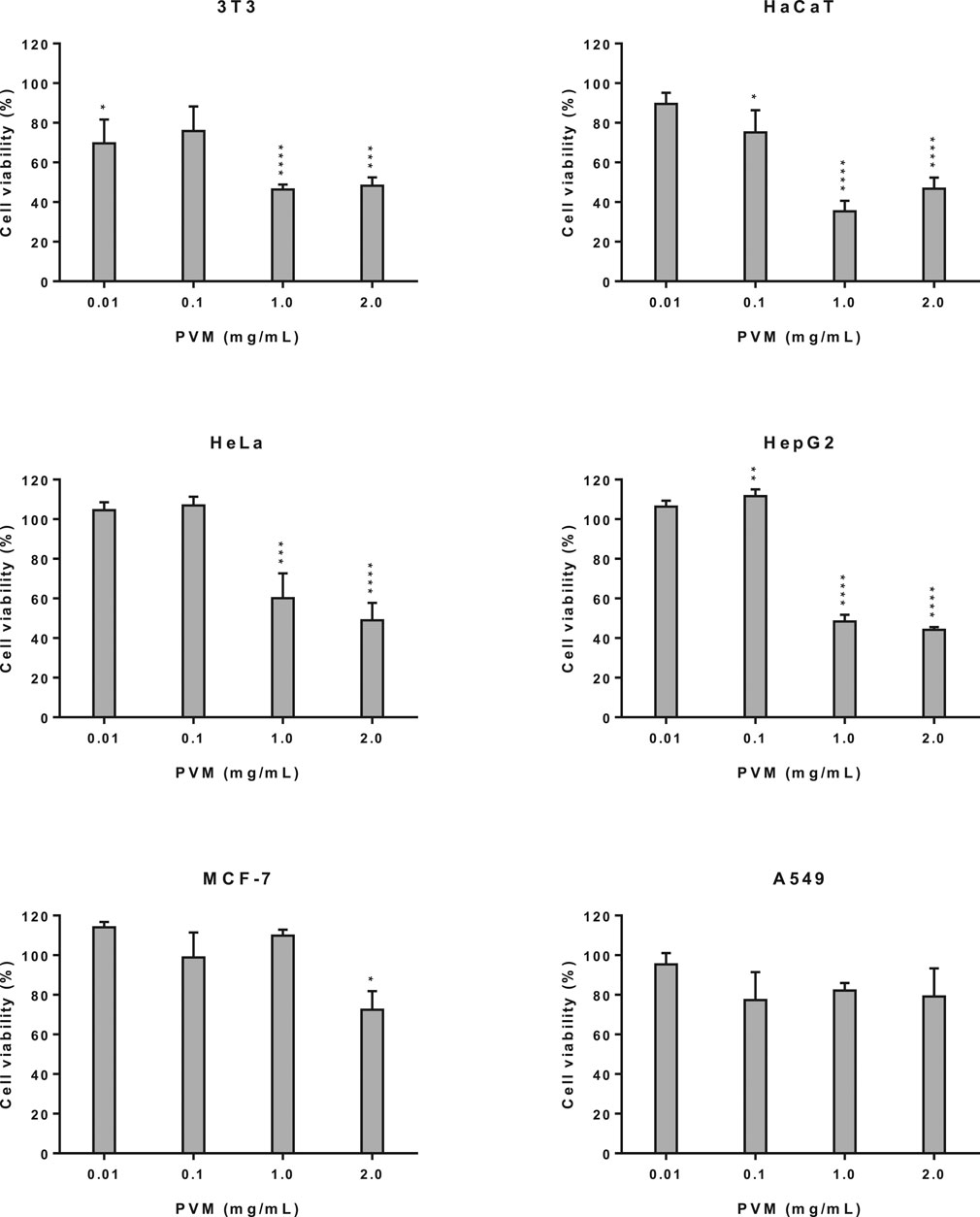
FIGURE 2. Cytotoxicity activity of PVM in 3T3, HaCaT, HeLa, HepG2, MCF-7 and A549 cell lines by MTT assay and expressed as percentage of cell viability respect to control cells. Results are expressed as mean ± standard error of n = 3. Control cells were maintained only with culture medium. A two-way analysis of variance (ANOVA) and a Bonferroni post hoc assay have been performed. Statistical differences were considered as follows: *p ≤ 0.05, **p ≤ 0.01, ***p ≤ 0.001 and ****p ≤ 0.0001 compared with no treated cells (negative control).
The cytotoxicity study was extended to tumoral cells such us HeLa, HepG2, MCF-7 and A549 cells. As we can observe in Figure 2, PVM on HeLa and HepG2 cells presents a similar cytotoxic behaviour, presenting a significant decrease in cell viability at the highest concentration assessed, being this decrease slightly higher in HepG2 (44.2%) than HeLa (49.0%). No cytotoxic effects have been determined at 0.01 and 0.1 mg/mL of the extract.
For MCF-7 and A549 cells (Figure 2) no statistical differences among cell viability is observed at the different concentrations studied of PVM, although values show a slight decrease to 72.5% in MCF-7 at 2 mg/mL and 77.3% in A549 at 0.1 mg/mL.
The present results exhibited that, cytotoxicity effects only appear at 1 and 2 mg/mL PVM in 3T3, HaCaT, HeLa and HepG2.
Cytoprotective Activity in 3T3 and HaCaT Cell Lines
Before the potential cytoprotective activity were studied, the deleterious effects of H2O2 in the cells were initially determined. From this previous assay (data not shown), we have established that cell viability obtained at 2 mM of H2O2 for 2.5 h (30.5 and 41.0% for 3T3 and HaCaT respectively) allows us to evaluate potential beneficial effects of PVM.
As observed in Figure 3, cell viability in 3T3 increases mainly in parallel to PVM concentrations indicating some cytoprotective effect although not statistically significant, being this cytoprotective activity of 18.9 and 26.5% at 0.1 and 2 mg/mL, respectively. In the case of HaCaT, no cytoprotective effect has been observed in any of the concentrations tested.
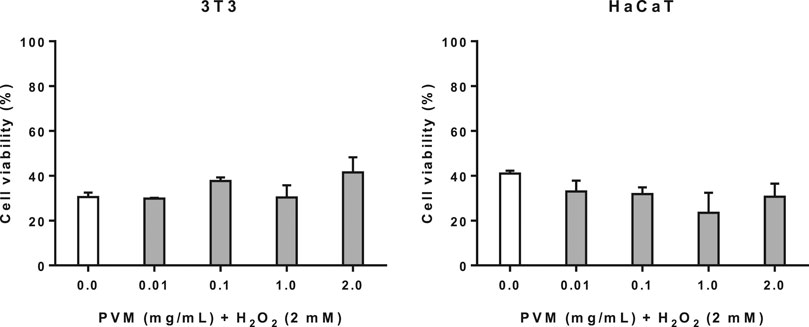
FIGURE 3. Cytoprotective activity of PVM in 3T3 and HaCaT cell lines for 2 mM H2O2 during 2.5 h by MTT assay and expressed as percentage of cell viability respect to untreated cells control. H2O2 cell viability was used as positive control. Results are expressed as mean ± standard error of n = 3 and n = 2 respectively. A two-way analysis of variance (ANOVA) and a Bonferroni post hoc assay have been performed. No statistically significant differences were found.
Cellular Repair Activity in 3T3 Cells
In the cellular repair assay, we have used the same conditions of H2O2 as in cytoprotection assay (2 mM H2O2 for 2.5 h). As we can observe in Figure 4, there is an increase in cell viability as PVM concentration rises; however, this discrete reparation effect is proportional to the concentration of the extract.
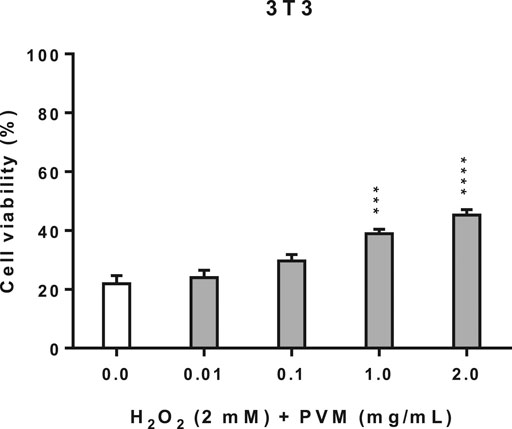
FIGURE 4. Cellular repair activity of PVM in 3T3 cell line for 2 mM H2O2 during 2.5 h by MTT assay and expressed as percentage of cell viability respect to untreated cells control. H2O2 cell viability was used as positive control. Results are expressed as mean ± standard error of n = 3. A two-way analysis of variance (ANOVA) and a Bonferroni post hoc assay have been performed. Statistical differences were considered as follows: ***p ≤ 0.001 and ****p ≤ 0.0001 compared with positive control.
Phototoxicity Activity of Methanolic Extract of Polypodium vulgare L. in 3T3 and HaCaT Cell Lines
The validity of the assay has been determined by calculating the ratio of cell viability in irradiated respect to non-irradiated conditions of both negative and positive control cells. Doses of 1.8 J/cm2 of UVA light affects the viability of non-treated cells that decrease in both cell lines. In the phototoxicity assay is important to consider that cell viability of control cells not treated but irradiated present a cell viability of about 63.3 and 75.0% respect from the non-irradiated ones for 3T3 and HaCaT respectively. Indicating that 3T3 are much sensitive to light than HaCaT and that interpretation of data should be interpreted carefully, as seen in Figure 5. However, the effect of the photosensitizer CPZ in 3T3 and HaCaT is confirmed by the important drop of cell viability when exposed to UVA respect not exposed to UVA in both cell lines, 26.9 and 13.7% cell viability respectively. Considering these ratios, the viability obtained when cells were exposed to UVA in the presence of PVM is considered.
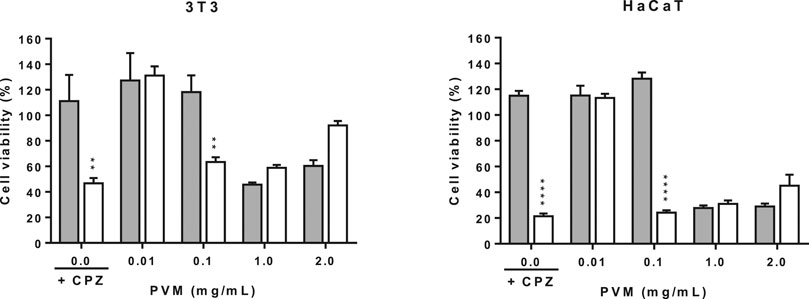
FIGURE 5. Phototoxicity activity of PVM in 3T3 and HaCaT cell lines by MTT assay and expressed as percentage of cell viability respect to the correspondent control cells. Chloropromazine cell viability was used as positive control. Gray columns correspond to cells non exposed to UVA light and white columns correspond to cells exposed to 1.8 J/cm2 of UVA light. Results are expressed as mean ± standard error of n = 3. A two-way analysis of variance (ANOVA) and a Bonferroni post hoc assay have been performed. Statistical differences were considered as follows: **p ≤ 0.01 and ****p ≤ 0.0001 compared with correspondence no irradiated/irradiated positive control.
In general, PVM did not show phototoxic behaviour in the assayed conditions, except at 0.1 mg/mL. At this concentration, there is a decrease in viability when cells are exposed to light.
Intracellular Reactive Oxygen Species (ROS) Induced by H2O2 of Methanolic Extract of Polypodium vulgare L. in 3T3 and HaCaT Cell Lines
The production of ROS was explored by the fluorescence intensity with the DCF probe. As shown in Figure 6, for each cell line was obtained the same tendency of ROS production at 2 h, with similar pattern recorded at 1 and 3 h (data not shown).
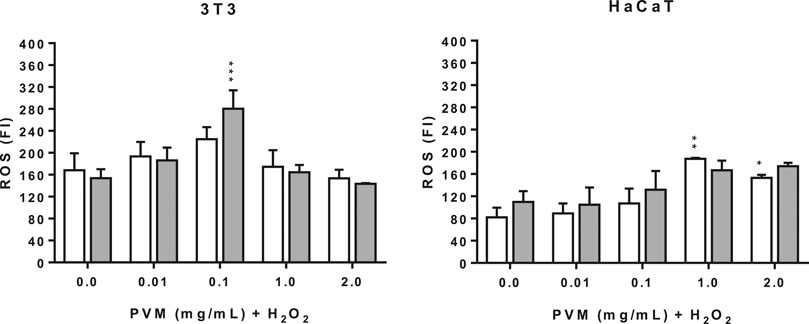
FIGURE 6. Intracellular ROS induced by 1 and 2 mM H2O2 for 2 h treatment with PVM in 3T3 and HaCaT cells. H2O2: positive control. White columns correspond to 1 mM H2O2 and gray columns correspond to 2 mM H2O2. Results are expressed as mean ± standard error of n = 3. A two-way analysis of variance (ANOVA) and a Bonferroni post hoc assay have been performed. Statistical differences were considered as follows: *p ≤ 0.05, **p ≤ 0.01 and ***p ≤ 0.001 compared with the correspondent positive control.
The production of ROS in the conditions tested here are significantly different for both cell lines. In the case of 3T3, positive controls show similar values of ROS production at the two concentrations of H2O2 but higher than those obtained in the case of HaCaT. This observation suggests that keratinocytes are less sensitive than 3T3.
In the case of 3T3 there is a peak of intracellular ROS production in the presence of PVM at 0.1 mg/mL, which is more pronounced in cells treated with 2 mM H2O2, followed by an important reduction at 1 mg/mL and, finally, reverted at 2 mg/mL. This pattern is independent of the final concentration of H2O2. In contrast, the production of ROS is dose dependent in the case of HaCaT, except at 2 mg/mL PVM at 1 mM H2O2. One explanation to this different behavior between the two cell lines can be attributed to the presence of different protective antioxidant systems and that can also explain the ROS production showed by the positive controls.
Discussion
Despite the significant biological activities attributed to the Polypodiaceae family reported in various studies, such as antidiabetic (Phymatopteris triloba (Houtt.) Pic. Serm.) (Chai et al., 2013), anticancer (Polypodium leucotomos) (Gonzalez et al., 2010) and anti-inflammatory activities (Polypodium leucotomos) (Choudhry et al., 2014), there are many other Polypodiaceae ferns not yet characterized or studied specifically for their potential food or medical uses. This is the case of Polypodium vulgare L. Probably, the most studied Polypodiaceae fern is Polypodium leucotomos because of the commercialization of its standardized aqueous extract fronds (PLE) (known as Fernblock and formulated in cosmetic products and food supplements used to protect from sun exposition) (Palomino, 2015) and its standardized ethanolic dry extract rhizome (known as the oral medicine Difur for skin inflammatory disorders) (AEMPS, 2013). In addition, the aqueous extract fronds of P. leucotomos has been reported as a natural product for the treatment of skin alterations such as photodermatosis, adjunctive treatment of melasma (as chemopreventive), vitiligo, psoriasis vulgaris and atopic dermatitis, among others (Choudhry et al., 2014; Parrado et al., 2018; Thompson and Kim, 2020).
As stated by Messeguer (Messeguer et al., 1998), the two major drugs used of P. vulgare are rhizomes and fronds as reported for other species of Polypodium (Liu et al., 1998). In the present study we have obtained a methanol extract from the fronds of the fern. Some studies reported the isolated phytochemical composition of the rhizome, describing the different types of phytochemicals namely flavonoids as flavan-3-ol derivatives (Glensk et al., 2019a), triterpenoids hydrocarbons, triterpenoids alcohols of the cycloartane group, saponin glycosides (Arai et al., 1989; Arai et al., 1991), phytoecdysteroids (Messeguer et al., 1998) and others (Dar et al., 2012). However, to date no published work or study has dealt with the composition of the fronds of P. vulgare except for the one of Messeguer describing the presence of some phytoecdysteroids (Messeguer et al., 1998). Among the few articles reporting the composition and bioactivity of this fern, Sofiane et al. (2015) describes antioxidant, antimicrobial and anti-inflammatory activities attributing these activities to different groups of phytochemicals but unspecifying the part of the plant used (drug plant). Also, Glensk et al. (2019b) reports the antimicrobial activity of the rhizome attributed to osladin. It is widely known that the production of secondary metabolites is a response to environmental conditions (environmental stress, period of the year, among other variables) to which the plant is exposed (Wu et al., 2017). To eliminate this variable, the fronds from which the extract was obtained in the present study were collected at the same time of the year (November 2016). Using HPLC-DAD, we have determined a high number of phenolic related compounds and a small fraction of flavonoids (less than 15% of the total polyphenolic species) represented by (+)-catechin hydrate, (−)-epicatechin, rutin and hyperoside. This observation agrees with our previous study using thin layer chromatography (TLC) (Farràs et al., 2019). It is well known that flavonoids, due to their radical scavenging ability provided by its chemical structure described elsewhere (Rice-Evans et al., 1996; Prochazkova et al., 2011; Wen et al., 2014), have a greater antioxidant capacity than certain phenolic acids (Leopoldini et al., 2011); however, flavonoids are residual components of our extract. Other phenolics such as p-coumaric, ferulic, caffeic, vanillic and chlorogenic acids, were reported as the major polyphenol phytochemicals in P. leucotomos (Gombau et al., 2006; García et al., 2006). Another important aspect to consider is the synergy that the different phytochemicals present in an extract against the oxidative damage (Yen et al., 2013; Naik and Sellappan, 2020).
In the Asian continent, a variety of ferns have been used as remedies as the case of the Gusuibu ferns (Chang et al., 2007). Nevertheless, other fern species, such as Pteridium aquilinum (L.) Kuhn (Dennstaedtiaceae family), contain ptaquiloside, a toxic compound that can cause cancer (da Costa et al., 2012; O’Connor et al., 2019). For this reason, the objective of the present work is to study the bioactivity of the polar constituents of P. vulgare including its cytotoxic potential in non-tumoral (3T3 and HaCaT) and tumoral cells (HeLa, HepG2, MCF-7 and A549). The protective activity of the extract against oxidative stress is also studied by different assays. Four concentrations of the extract (0.01, 0.1, 1 and 2 mg/mL) were selected in the present study considering physiological and non physiological concentrations for a better understanding.
There are many viability assays used to evaluate cytotoxic activity of different substances and products. NRU has been proven to be a sensitive assay to study the cytotoxic activity and potential protection of procyanidin fractions from grape and pine against the H2O2 insult (Ugartondo et al., 2007; Mitjans et al., 2011). In addition, MTT is regarded as a gold standard of cytotoxicity assays as it is highly sensitive and a high-throughput screening assay. However, recently Karakas have described an interfering effect of the methanolic extract of different Turkish’s plant extracts resulting in false-positive viability (Karakas et al., 2017). In this sense, potential interferences of our fern extracts with the MTT assay were performed previously to study their cytotoxic activity. Taken together these aspects, we considered studying the biological activity of our extracts by these two assays. In our case, NRU failed to be sensitive according to our MTT data. However, our results can indicate that mechanism of cytotoxic behaviour of PVM does not include lysosomal damage.
The potential use of ferns to prevent or treat tumoral processes, as the case of some Asiatic fern species, has been demonstrated by the cytotoxic, pro-apoptotic or cell cycle-arresting effects of non-characterized plant extracts (Tomsik, 2014). In our case, no relevant cytotoxic effects have been reported for the extract in the different cell lines, except in the case of the HaCaT cells, but only at the very high concentrations. The phytochemical characterization by HPLC-DAD indicates that epicatechin is the second major compound of the flavonoid components of the extract. Moreover (Cao et al., 2014), a strong cytotoxic behaviour of an ethanol extract of the whole fern Davallia cylindrica Ching in A549 cells has been described and attributed to the high content of quercitrin and some of its derivatives. Using HPLC-DAD we failed to detect quercitrin (a glycosylated derivative of quercetin), which can explain the absence of relevant cytotoxicity (Farràs et al., 2019). Results obtained with 3T3 and HaCaT cell lines open the possibility to validate the traditional use of this species in the Sobrarbe region as disinfectant and wound healing (Huesca) (Villar and Bonet, 2018), mostly considering that fibroblasts, the most common cells in connective tissue, play a critical role in wound healing and keratinocytes form epidermis, which is a biological and physical barrier against injuries. These results are in line with the fact that the pteridophytes presents an antibiotic properties (Banerjee and Sen, 1980).
The protective effect of the extract was assessed in this study against hydrogen peroxide in 3T3 and HaCaT cells. Our results show that H2O2 causes slightly higher mortality in 3T3 (30.5% cell viability) than in HaCaT (41.0% cell viability), which can be explained by a higher antioxidant defence system on keratinocytes than fibroblasts (Pérez et al., 1995). This minor mortality in HaCaT can justify that the extract failed to present cytoprotective capacity in such cell line. We have observed a discrete cytoprotective effect of PVM in the 3T3 cells being the first report that deals with this kind of assays using a fern extract from the Polypodiaceae family. However, Gomes et al. (2001) and Gombau et al. (2006) have described the potential antioxidant activity of P. leucotomos, by different in vitro methods.
H2O2 is recognized as a pleiotropic compound in the induction of oxidative stress (Sies and Jones, 2020). However, its effect on the induction of oxidative stress differs in whether a pre-treatment (cytoprotective activity), co-treatment or post-treatment (cellular repair activity) trial is performed (Siddiqui et al., 2011). In the post-treatment test, as result of an oxidative stress that triggers severe cellular damage, cells are sometimes unable to regain redox homeostasis despite being subsequently treated with an antioxidant agent. Considering our results of cytoprotection, the cellular repair test was only performed in the 3T3 cell line. This assay showed that there is a significant increase in cellular viability directly proportional to PVM concentration suggesting the capacity of the extract to induce cellular repair mechanisms.
Other authors have reported that P. leucotomos was able to protect human fibroblast from cytoskeletal disarrangements induced by UVA light (1 J/cm2) (Alonso-Lebrero et al., 2003). Moreover, Philips et al. (2003) reported that a concentration lower than 0.1% improves cellular membrane integrity and inhibits MMP-1 on fibroblast and keratinocytes thus suggesting its potential use in prevention on skin photoaging. However, before studying the potential photoprotective activity of the extract, we should discard any phototoxic reactions. In the present study, the determination of phototoxicity is based on the OECD TG 432 (OECD, 2019), where the BALB/c 3T3 cell line has been replaced by NIH 3T3 and we included the HaCaT cell line and the determination of cell viability by MTT as previously reported (Baccarin et al., 2015). In general, we can conclude that PVM is not phototoxic although the decrease in cell viability at 0.1 mg/mL in both cell lines needs to be clarified. One interpretation could arise from the direct toxic effects of UVA light over the cells that can be reverted by the presence of the extract at high concentrations but not at moderate ones as 0.1 mg/mL PVM. Further investigation should be conducted to explore the cellular mechanisms that are activated. Contact time of the extract also should be considered, thus in this phototoxicity test is 1 h plus the time of UVA exposition, whereas in the rest of assays the extract remains approximately 24 h in contact with cells.
It is known that UV damages mitochondrial DNA (Hseu et al., 2015), for this reason it would be interesting to also evaluate the potential phototoxic or photoprotective activity of the PVM by other assays such as the comet assay in a similar way as previously described for pomegranate seed oil nanoemulsion in HaCaT (Baccarin et al., 2015). Currently, the mechanisms by which P. leucotomos protects against UV-induced DNA damage, such as overexpression of the p53 gene, have already been described (Parrado et al., 2020).
Hydrogen peroxide is an oxidative agent that promote the endogenous generation of ROS in diverse cell lines (Uguz et al., 2016). If high ROS concentrations trigger cell death, the loss of mitochondrial functionality begins with the consequent apoptosis (Moloney and Cotter, 2018). In our case, there is an increase of ROS production in both cell lines except at 2 mg/mL. This increase of ROS production observed here and, particularly in HaCaT cells apart from the lowest concentration extract tested (0.01 mg/mL PVM), may be explained by the pro-oxidant effect of polyphenols (Santos et al., 2018). The mechanism why this ROS can diminish cell viability needs to be clarified and further explored in other tumoral cell lines as a first step to better characterize the chemotherapeutic potential of PVM (Skibola and Smith, 2000).
Additionally, in recent years, phenolic compounds are positioning themselves as the reference antioxidant substances of natural origin (Virgili and Marino, 2008; Piccolella et al., 2019). Thus, certain foods and plant extracts with high antioxidant properties are positioned as a powerful adjuvant treatment to counteract the adverse drug reactions (ADR) associated with the chemotherapy (Oyenihi and Smith, 2019).
Conclusion
The absence of cytotoxicity at physiological concentrations determined in the six cell lines of the present study, together with the high concentration of phenolics in the fronds of P. vulgare, is decisive to confirm this fern as a source of bioactive and antioxidant compounds with pharmaceutical applications. Some traditional uses of P. vulgare, such as the wound healing benefits, have still not been proved but this is the first time that the fronds are positioned as potential bioactive agents. This article could also be an inflexion point to justify further research of the fronds of P. vulgare.
Data Availability Statement
The raw data supporting the conclusions of this article will be made available by the authors, without undue reservation.
Author Contributions
Conceptualization: MM and VL; methodology: AF, MM and FM; analysis: AF, FM, GC and MM; investigation: AF, MM and VL; resources: MM, MPV and VL; writing-original draft preparation: AF; writing-review and editing: MM and VL; supervision: MM and VL; funding acquisition: MM and MPV. All authors have read and agreed to the published version of the manuscript.
Funding
This work was financially supported by project 307,629 of Fundació Bosch & Gimpera - Universitat de Barcelona.
Conflict of Interest
The authors declare that the research was conducted in the absence of any commercial or financial relationships that could be construed as a potential conflict of interest.
The handling editor declared a past co-authorship with several of the authors FM, GC.
Publisher’s Note
All claims expressed in this article are solely those of the authors and do not necessarily represent those of their affiliated organizations, or those of the publisher, the editors and the reviewers. Any product that may be evaluated in this article, or claim that may be made by its manufacturer, is not guaranteed or endorsed by the publisher.
Acknowledgments
We acknowledge the technical support of Universitat de Barcelona and Universidad San Jorge.
Supplementary Material
The Supplementary Material for this article can be found online at: https://www.frontiersin.org/articles/10.3389/fphar.2021.727528/full#supplementary-material
References
Addor, F. A. S. (2017). Antioxidants in Dermatology. An Bras Dermatol. 92, 356–362. doi:10.1590/abd1806-4841.20175697
AEMPS (2013). PROSPECTO: INFORMACIÓN PARA EL PACIENTE Difur 120 Mg Cápsulas Duras. Spain: Agencia Española de Medicamentos y Productos Sanitarios (AEMPS), 4.
Alonso-Lebrero, J. L., Domínguez-Jiménez, C., Tejedor, R., Brieva, A., and Pivel, J. P. (2003). Photoprotective Properties of a Hydrophilic Extract of the Fern Polypodium leucotomos on Human Skin Cells. J. Photochem. Photobiol. B 70, 31–37. doi:10.1016/s1011-1344(03)00051-4
Alvarado-Sansininea, J. J., Sánchez-Sánchez, L., López-Muñoz, H., Escobar, M. L., Flores-Guzmán, F., Tavera-Hernández, R., et al. (2018). Quercetagetin and Patuletin: Antiproliferative, Necrotic and Apoptotic Activity in Tumor Cell Lines. Molecules 23, 18. doi:10.3390/molecules23102579
Arai, Y., Shiojima, K., and Ageta, H. (1989). Fern Constituents: Cyclopodmenyl Acetate, a Cycloartanoid Having a New 33-carbon Skeleton, Isolated from Polypodium vulgare. Chem. Pharm. Bull. 37, 560–562. doi:10.1248/cpb.37.560
Arai, Y., Yamaide, M., Yamazaki, S., and Ageta, H. (1991). Fern Constituents: Triterpenoids Isolated from Polypodium vulgare, P. fauriei and P. virginianum. Phytochemistry 30, 3369–3377. doi:10.1016/0031-9422(91)83212-4
Asensi, M., Ortega, A., Mena, S., Feddi, F., and Estrela, J. M. (2011). Natural Polyphenols in Cancer Therapy. Crit. Rev. Clin. Lab. Sci. 48, 197–216. doi:10.3109/10408363.2011.631268
Baccarin, T., Mitjans, M., Ramos, D., Lemos-Senna, E., and Vinardell, M. P. (2015). Photoprotection by Punica granatum Seed Oil Nanoemulsion Entrapping Polyphenol-Rich Ethyl Acetate Fraction against UVB-Induced DNA Damage in Human Keratinocyte (HaCaT) Cell Line. J. Photochem. Photobiol. B 153, 127–136. doi:10.1016/j.jphotobiol.2015.09.005
Banerjee, R. D., and Sen, S. P. (1980). Antibiotic Activity of pteridophytes. Econ. Bot. 34, 284–298. doi:10.1007/bf02858649
BDBC (2015). Barcelona: Generalidad de Cataluña. Available at: http://biodiver.bio.ub.es/biocat/index.jsp (Accessed December 15, 2015).
Bolòs, O., and Vigo, J. (1984). “Flora Dels Països Catalans I,” in Polipodiàcies: Polypodium vulgare L. Editorial Barcino, Barcelona: Editorial Barcino, 188–190.
Bonet, M. A., and Vallès, J. (2007). Ethnobotany of Montseny Biosphere reserve (Catalonia, Iberian Peninsula): Plants Used in Veterinary Medicine. J. Ethnopharmacol. 110, 30–47. doi:10.1016/j.jep.2006.09.016
Borenfreund, E., and Puerner, J. A. (1985). Toxicity Determined In Vitro by Morphological Alterations and Neutral Red Absorption. Toxicol. Lett. 24, 119–124. doi:10.1016/0378-4274(85)90046-3
Burton, G., and Ingold, K. (2015). “Antioxidant Defences Synthesized In Vivo,” in Free Radicals in Biology and Medicine. 5 ed. (Oxford: Oxford University Press), 77–152.
Cao, J., Xia, X., Chen, X., Xiao, J., and Wang, Q. (2013). Characterization of Flavonoids from Dryopteris erythrosora and Evaluation of Their Antioxidant, Anticancer and Acetylcholinesterase Inhibition Activities. Food Chem. Toxicol. 51, 242–250. doi:10.1016/j.fct.2012.09.039
Cao, J., Xia, X., Dai, X., Wang, Q., and Xiao, J. (2014). Chemical Composition and Bioactivities of Flavonoids-Rich Extract from Davallia cylindrica Ching. Environ. Toxicol. Pharmacol. 37, 571–579. doi:10.1016/j.etap.2014.01.011
Caprioli, G., Alunno, A., Beghelli, D., Bianco, A., Bramucci, M., Frezza, C., et al. (2016). Polar Constituents and Biological Activity of the Berry-Like Fruits from Hypericum androsaemum L. Front. Plant Sci. 7, 232. doi:10.3389/fpls.2016.00232
Cásedas, G., Les, F., Choya-Foces, C., Hugo, M., and López, V. (2020). The Metabolite Urolithin-A Ameliorates Oxidative Stress in Neuro-2a Cells, Becoming a Potential Neuroprotective Agent. Antioxidants (Basel) 9, 16. doi:10.3390/antiox9020177
Cásedas, G., Les, F., Gómez-Serranillos, M. P., Smith, C., and López, V. (2016). Bioactive and Functional Properties of Sour Cherry Juice (Prunus cerasus). Food Funct. 7, 4675–4682. doi:10.1039/c6fo01295g
Chai, T. T., Elamparuthi, S., Yong, A. L., Quah, Y., Ong, H. C., and Wong, F. C. (2013). Antibacterial, Anti-glucosidase, and Antioxidant Activities of Selected highland Ferns of Malaysia. Bot. Stud. 54, 55. doi:10.1186/1999-3110-54-55
Chang, H-C., Huang, G-J., Agrawal, D. C., Kuo, C-L., Wu, C-R., and Tsay, H-S. (2007). Antioxidant Activities and Polyphenol Contents of Six Folk Medicinal Ferns Used as “Gusuibu”. Bot. Stud. 48, 397–406.
Chear, N. J., Khaw, K. Y., Murugaiyah, V., and Lai, C. S. (2016). Cholinesterase Inhibitory Activity and Chemical Constituents of Stenochlaena palustris Fronds at Two Different Stages of Maturity. J. Food Drug Anal. 24, 358–366. doi:10.1016/j.jfda.2015.12.005
Chen, C., and Kong, A. N. (2005). Dietary Cancer-Chemopreventive Compounds: from Signaling and Gene Expression to Pharmacological Effects. Trends Pharmacol. Sci. 26, 318–326. doi:10.1016/j.tips.2005.04.004
Choudhry, S. Z., Bhatia, N., Ceilley, R., Hougeir, F., Lieberman, R., Hamzavi, I., et al. (2014). Role of Oral Polypodium Leucotomos Extract in Dermatologic Diseases: a Review of the Literature. J. Drugs Dermatol. 13, 148–153.
Dar, P. A., Sofi, G., and Jafri, M. A. (2012). Polypodium vulgare Linn. A Versatile Herbal Medicine: a Review. Int. J. Pharm. Sci. Res. 3, 1616–1620.
Dvorakova, M., Pumprova, K., Antonínová, Ž., Rezek, J., Haisel, D., Ekrt, L., et al. (2021). Nutritional and Antioxidant Potential of Fiddleheads from European Ferns. Foods 10, 15. doi:10.3390/foods10020460
EMA (2008). Assesment Report on Polypodium vulgare L., Rhizoma. London: EMEA/HMPC/600669/2007 European Medicines Agency (EMA).
Evans, J. A., and Johnson, E. J. (2010). The Role of Phytonutrients in Skin Health. Nutrients 2, 903–928. doi:10.3390/nu2080903
Farràs, A., Cásedas, G., Les, F., Terrado, E. M., Mitjans, M., and López, V. (2019). Evaluation of Anti-Tyrosinase and Antioxidant Properties of Four Fern Species for Potential Cosmetic Applications. Forests 10, 14. doi:10.3390/f10020179
Ferreira, I., Silva, A., Martins, J. D., Neves, B. M., and Cruz, M. T. (2018). Nature and Kinetics of Redox Imbalance Triggered by Respiratory and Skin Chemical Sensitizers on the Human Monocytic Cell Line THP-1. Redox Biol. 16, 75–86. doi:10.1016/j.redox.2018.02.002
Fotakis, G., and Timbrell, J. A. (2006). In Vitro Cytotoxicity Assays: Comparison of LDH, Neutral Red, MTT and Protein Assay in Hepatoma Cell Lines Following Exposure to Cadmium Chloride. Toxicol. Lett. 160, 171–177. doi:10.1016/j.toxlet.2005.07.001
García, F., Pivel, J. P., Guerrero, A., Brieva, A., Martínez-Alcázar, M. P., Caamaño-Somoza, M., et al. (2006). Phenolic Components and Antioxidant Activity of Fernblock, an Aqueous Extract of the Aerial Parts of the Fern Polypodium leucotomos. Methods Find Exp. Clin. Pharmacol. 28, 157–160. doi:10.1358/mf.2006.28.3.985227
Gegotek, A., Domingues, P., and Skrzydlewska, E. (2020). Natural Exogenous Antioxidant Defense against Changes in Human Skin Fibroblast Proteome Disturbed by UVA Radiation. Oxid. Med. Cell. Longev. 2020, 1–12. doi:10.1155/2020/3216415
Gil da Costa, R. M., Bastos, M. M., Oliveira, P. A., and Lopes, C. (2012). Bracken-associated Human and Animal Health Hazards: Chemical, Biological and Pathological Evidence. J. Hazard. Mater. 203-204, 1–12. doi:10.1016/j.jhazmat.2011.12.046
Glensk, M., Dudek, M. K., Ciach, M., and Wlodarczyk, M. (2019a). Isolation and Structural Determination of Flavan-3-ol Derivatives from the Polypodium vulgare L. Rhizomes Water Extract. Nat. Product. Res. 10, 1–10. doi:10.1080/14786419.2019.1655302
Glensk, M., Tichaczek-Goska, D., Sroda-Pomianek, K., Wlodarczyk, M., Wesolowski, C. A., and Wojnicz, D. (2019b). Differing Antibacterial and Antibiofilm Properties of Polypodium vulgare L. Rhizome Aqueous Extract and One of its Purified Active Ingredients-Osladin. J. Herbal Med. 17-18, 100261. doi:10.1016/j.hermed.2019.100261
Gombau, L., García, F., Lahoz, A., Fabre, M., Roda-Navarro, P., Majano, P., et al. (2006). Polypodium leucotomos Extract: Antioxidant Activity and Disposition. Toxicol. Vitro 20, 464–471. doi:10.1016/j.tiv.2005.09.008
Gomes, A. J., Lunardi, C. N., Gonzalez, S., and Tedesco, A. C. (2001). The Antioxidant Action of Polypodium leucotomos Extract and Kojic Acid: Reactions with Reactive Oxygen Species. Braz. J. Med. Biol. Res. 34, 1487–1494. doi:10.1590/s0100-879x2001001100018
Gonzalez, S., Gilaberte, Y., and Philips, N. (2010). Mechanistic Insights in the Use of a Polypodium leucotomos Extract as an Oral and Topical Photoprotective Agent. Photochem. Photobiol. Sci. 9, 559–563. doi:10.1039/b9pp00156e
He, L., He, T., Farrar, S., Ji, L., Liu, T., and Ma, X. (2017). Antioxidants Maintain Cellular Redox Homeostasis by Elimination of Reactive Oxygen Species. Cell Physiol. Biochem. 44, 532–553. doi:10.1159/000485089
Hseu, Y. C., Lo, H. W., Korivi, M., Tsai, Y. C., Tang, M. J., and Yang, H. L. (2015). Dermato-protective Properties of Ergothioneine through Induction of Nrf2/ARE-Mediated Antioxidant Genes in UVA-Irradiated Human Keratinocytes. Free Radic. Biol. Med. 86, 102–117. doi:10.1016/j.freeradbiomed.2015.05.026
Karakas, D., Ari, F., and Ulukaya, E. (2017). The MTT Viability Assay Yields Strikingly False-Positive Viabilities Although the Cells Are Killed by Some Plant Extracts. Turkish J. Biol. 41, 919–925. doi:10.3906/biy-1703-104
Khare, C. (2007). “Polypodium vulgare Linn.,” in Indian Medicinal Plants. Editor C. Khare (New York, NY: Springer). doi:10.1007/978-0-387-70638-2_1262
Kimlin, M. G., and Guo, Y. (2012). Assessing the Impacts of Lifetime Sun Exposure on Skin Damage and Skin Aging Using a Non-invasive Method. Sci. Total Environ. 425, 35–41. doi:10.1016/j.scitotenv.2012.02.080
Langhansova, L., Pumprova, K., Haisel, D., Ekrt, L., Pavicic, A., Zajíčková, M., et al. (2021). European Ferns as Rich Sources of Antioxidants in the Human Diet. Food Chem. 356, 129637. doi:10.1016/j.foodchem.2021.129637
Leopoldini, M., Russo, N., and Toscano, M. (2011). The Molecular Basis of Working Mechanism of Natural Polyphenolic Antioxidants. Food Chem. 125, 288–306. doi:10.1016/j.foodchem.2010.08.012
Liu, B., Diaz, F., Bohlin, L., and Vasänge, M. (1998). Quantitative Determination of Antiinflammatory Principles in Some Polypodium Species as a Basis for Standardization. Phytomedicine 5, 187–194. doi:10.1016/S0944-7113(98)80026-3
Martínez, V., Galbiati, V., Corsini, E., Martín-Venegas, R., Vinardell, M. P., and Mitjans, M. (2013). Establishment of an In Vitro Photoassay Using THP-1 Cells and IL-8 to Discriminate Photoirritants from Photoallergens. Toxicol. Vitro 27, 1920–1927. doi:10.1016/j.tiv.2013.06.013
Messeguer, J., Melé, E., Reixach, N., Irurre-Santilari, J., and Casas, J. (1998). “Polypodium vulgare L. (Wood Fern): In Vitro Cultures and the Production of Phytoecdysteroids,” in Medicinal and Aromatic Plants X (Berlin, Heidelberg: Springer), 333–348.
Mitjans, M., Ugartondo, V., Martínez, V., Touriño, S., Torres, J. L., and Vinardell, M. P. (2011). Role of Galloylation and Polymerization in Cytoprotective Effects of Polyphenolic Fractions against Hydrogen Peroxide Insult. J. Agric. Food Chem. 59, 2113–2119. doi:10.1021/jf1025532
Moloney, J. N., and Cotter, T. G. (2018). ROS Signalling in the Biology of Cancer. Semin. Cel Dev. Biol. 80, 50–64. doi:10.1016/j.semcdb.2017.05.023
Mosmann, T. (1983). Rapid Colorimetric Assay for Cellular Growth and Survival: Application to Proliferation and Cytotoxicity Assays. J. Immunol. Methods 65, 55–63. doi:10.1016/0022-1759(83)90303-4
Naik, A. V., and Sellappan, K. (2020). In Vitro evaluation of Annona muricata L. (Soursop) Leaf Methanol Extracts on Inhibition of Tumorigenicity and Metastasis of Breast Cancer Cells. Biomarkers 25, 701–710. doi:10.1080/1354750X.2020.1836025
Newman, D. J., and Cragg, G. M. (2012). Natural Products as Sources of New Drugs over the 30 Years from 1981 to 2010. J. Nat. Prod. 75, 311–335. doi:10.1021/np200906s
O'Connor, P. J., Alonso-Amelot, M. E., Roberts, S. A., and Povey, A. C. (2019). The Role of Bracken Fern Illudanes in Bracken Fern-Induced Toxicities. Rev. Mutat. Res. 782, 39. doi:10.1016/j.mrrev.2019.05.001
OECD (2019). “OECD Test No. 432: In Vitro 3T3 NRU Phototoxicity Test,” in OECD Guidelines for the Testing of Chemicals, Section 4 (Organization for Economic Cooperation and Development (OECD)).
Oyenihi, A. B., and Smith, C. (2019). Are Polyphenol Antioxidants at the Root of Medicinal Plant Anti-cancer success? J. Ethnopharmacol. 229, 54–72. doi:10.1016/j.jep.2018.09.037
Palomino, O. M. (2015). Current Knowledge in Polypodium leucotomos Effect on Skin protection. Arch. Dermatol. Res. 307, 199–209. doi:10.1007/s00403-014-1535-x
Parrado, C., Nicolas, J., Juarranz, A., and Gonzalez, S. (2020). The Role of the Aqueous Extract Polypodium leucotomos in Photoprotection. Photochem. Photobiol. Sci. 19, 831–843. doi:10.1039/d0pp00124d
Parrado, C., Philips, N., Gilaberte, Y., Juarranz, A., and González, S. (2018). Oral Photoprotection: Effective Agents and Potential Candidates. Front. Med. (Lausanne) 5, 188. doi:10.3389/fmed.2018.00188
Pérez, S., Sergent, O., Morel, P., Chevanne, M., Dubos, M. P., Cillard, P., et al. (1995). Kinetics of Lipid Peroxidation Induced by UVB on Epidermal Cell Cultures. Comptes Rendus des Seances de la Societe de Biologie et de ses Filiales 189, 453–465.
Philips, N., Smith, J., Keller, T., and Gonzalez, S. (2003). Predominant Effects of Polypodium leucotomos on Membrane Integrity, Lipid Peroxidation, and Expression of Elastin and Matrixmetalloproteinase-1 in Ultraviolet Radiation Exposed Fibroblasts, and Keratinocytes. J. Dermatol. Sci. 32, 1–9. doi:10.1016/s0923-1811(03)00042-2
Piccolella, S., Crescente, G., Candela, L., and Pacifico, S. (2019). Nutraceutical Polyphenols: New Analytical Challenges and Opportunities. J. Pharm. Biomed. Anal. 175, 112774. doi:10.1016/j.jpba.2019.07.022
Präbst, K., Engelhardt, H., Ringgeler, S., and Hübner, H. (2017). “Basic Colorimetric Proliferation Assays: MTT, WST, and Resazurin,” in Basic Colorimetric Proliferation Assays: MTT, WST, and Resazurin. First ed. (New York: Springer), 1–17. doi:10.1007/978-1-4939-6960-9_1
Procházková, D., Boušová, I., and Wilhelmová, N. (2011). Antioxidant and Prooxidant Properties of Flavonoids. Fitoterapia 82, 513–523. doi:10.1016/j.fitote.2011.01.018
Quer, P. F. (2016). “Plantas Medicinales. El Dioscórides Renovado” in PTERIDÓFITOS: POLIPODIO (Polypodium vulgare L.) (Barcelona: Ediciones Península), 73–75.
Ren, W., Qiao, Z., Wang, H., Zhu, L., and Zhang, L. (2003). Flavonoids: Promising Anticancer Agents. Med. Res. Rev. 23, 519–534. doi:10.1002/med.10033
Rice-Evans, C. A., Miller, N. J., and Paganga, G. (1996). Structure-Antioxidant Activity Relationships of Flavonoids and Phenolic Acids. Free Radic. Biol. Med. 20, 933–956. doi:10.1016/0891-5849(95)02227-9
Riddell, R. J., Clothier, R. H., and Balls, M. (1986). An Evaluation of Three In Vitro Cytotoxicity Assays. Food Chem. Toxicol. 24, 469–471. doi:10.1016/0278-6915(86)90095-5
Rundle, C. W., Militello, M., Barber, C., Presley, C. L., Rietcheck, H. R., and Dellavalle, R. P. (2020). Epidemiologic Burden of Skin Cancer in the US and Worldwide. Curr. Derm Rep. 9, 309–322. doi:10.1007/s13671-020-00311-4
Santos, J. S., Deolindo, C. T. P., Hoffmann, J. F., Chaves, F. C., do Prado-Silva, L., Sant'Ana, A. S., et al. (2018). Optimized Camellia sinensis var. sinensis, Ilex paraguariensis, and Aspalathus linearis Blend Presents High Antioxidant and Antiproliferative Activities in a Beverage Model. Food Chem. 254, 348–358. doi:10.1016/j.foodchem.2018.02.021
Shukla, S., Meeran, S. M., and Katiyar, S. K. (2014). Epigenetic Regulation by Selected Dietary Phytochemicals in Cancer Chemoprevention. Cancer Lett. 355, 9–17. doi:10.1016/j.canlet.2014.09.017
Siddiqui, M. A., Kashyap, M. P., Kumar, V., Tripathi, V. K., Khanna, V. K., Yadav, S., et al. (2011). Differential protection of Pre-, Co- and post-treatment of Curcumin against Hydrogen Peroxide in PC12 Cells. Hum. Exp. Toxicol. 30, 192–198. doi:10.1177/0960327110371696
Sies, H., and Jones, D. P. (2020). Reactive Oxygen Species (ROS) as Pleiotropic Physiological Signalling Agents. Nat. Rev. Mol. Cel Biol. 21, 363–383. doi:10.1038/s41580-020-0230-3
Silva, V. A. O., Alves, A. L. V., Rosa, M. N., Silva, L. R. V., Melendez, M. E., Cury, F. P., et al. (2019). Hexane Partition from Annona crassiflora Mart. Promotes Cytotoxity and Apoptosis on Human Cervical Cancer Cell Lines. Invest. New Drugs 37, 602–615. doi:10.1007/s10637-018-0657-y
Skibola, C. F., and Smith, M. T. (2000). Potential Health Impacts of Excessive Flavonoid Intake. Free Radic. Biol. Med. 29, 375–383. doi:10.1016/s0891-5849(00)00304-x
Sofiane, G., Wafa, N., and Ouarda, D. (2015). Antioxidant, Antimicrobial and Anti-inflammatory Activities of Flavonoids and Tannins Extracted from Polypodium vulgare L. Asian J. Biochem. Pharm. Res. 5, 114–122.
The Plant List. 2021. Polypodium vulgare L.. Available at: http://www.theplantlist.org/tpl1.1/record/tro-26600283 (Accessed January 20, 2021)
Thompson, K. G., and Kim, N. (2020). Dietary Supplements in Dermatology: A review of the Evidence for Zinc, Biotin, Vitamin D, Nicotinamide, and Polypodium. J. Am. Acad. Dermatol. doi:10.1016/j.jaad.2020.04.123
Tomsik, P. (2014). Ferns and Lycopods-A Potential Treasury of Anticancer Agents but Also a Carcinogenic Hazard. Phytotherapy Res. 28, 798–810. doi:10.1002/ptr.v28.5
Ugartondo, V., Mitjans, M., Touriño, S., Torres, J. L., and Vinardell, M. P. (2007). Comparative Antioxidant and Cytotoxic Effect of Procyanidin Fractions from Grape and Pine. Chem. Res. Toxicol. 20, 1543–1548. doi:10.1021/tx700253y
Uguz, A. C., Oz, A., and Naziroglu, M. (2016). Curcumin Inhibits Apoptosis by Regulating Intracellular Calcium Release, Reactive Oxygen Species and Mitochondrial Depolarization Levels in SH-SY5Y Neuronal Cells. J. Recept. Signal Transduct. 36, 395–401. doi:10.3109/10799893.2015.1108337
Villar, L., and Bonet, M. À. (2018). “Polypodium L.” in Inventario Español de los Conocimientos Tradicionales relativos a la Biodiversidad. 2 ed. (Madrid: Ministerio de Agricultura y Pesca, Alimentación y Medio Ambiente de España), 51–55.
Virgili, F., and Marino, M. (2008). Regulation of Cellular Signals from Nutritional Molecules: a Specific Role for Phytochemicals, beyond Antioxidant Activity. Free Radic. Biol. Med. 45, 1205–1216. doi:10.1016/j.freeradbiomed.2008.08.001
Wen, L., He, J., Wu, D., Jiang, Y., Prasad, K. N., Zhao, M., et al. (2014). Identification of Sesquilignans in Litchi (Litchi chinensis Sonn.) Leaf and Their Anticancer Activities. J. Funct. Foods 8, 26–34. doi:10.1016/j.jff.2014.02.017
Weyermann, J., Lochmann, D., and Zimmer, A. (2005). A Practical Note on the Use of Cytotoxicity Assays. Int. J. Pharm. 288, 369–376. doi:10.1016/j.ijpharm.2004.09.018
Willcox, J. K., Ash, S. L., and Catignani, G. L. (2004). Antioxidants and Prevention of Chronic Disease. Crit. Rev. Food Sci. Nutr. 44, 275–295. doi:10.1080/10408690490468489
Wu, S., Li, J., Wang, Q., Cao, H., Cao, J., and Xiao, J. (2017). Seasonal Dynamics of the Phytochemical Constituents and Bioactivities of Extracts from Stenoloma chusanum (L.) Ching. Food Chem. Toxicol. 108, 458–466. doi:10.1016/j.fct.2016.10.003
Yen, W. J., Chyau, C. C., Lee, C. P., Chu, H. L., Chang, L. W., and Duh, P. D. (2013). Cytoprotective Effect of white tea against H2O2-Induced Oxidative Stress In Vitro. Food Chem. 141, 4107–4114. doi:10.1016/j.foodchem.2013.06.106
Zanette, C., Pelin, M., Crosera, M., Adami, G., Bovenzi, M., Larese, F. F., et al. (2011). Silver Nanoparticles Exert a Long-Lasting Antiproliferative Effect on Human Keratinocyte HaCaT Cell Line. Toxicol. Vitro 25, 1053–1060. doi:10.1016/j.tiv.2011.04.005
Glossary
3T3 = NIH 3T3 mouse fibroblast cell line
A549 lung cancer cell line
ADR Adverse Drug Reactions
CO2 carbon dioxide
CV Cell Viability
CPZ chlorpromazine hydrochloride
E ultraviolet dose
EMA European Medicines Agency
DCF 2,7-dichlorodihydrofluorescein diacetate
DMEM Dulbecco’s Modified Eagle’s Medium
DMSO dimethyl sulfoxide
EDTA ethylenediaminetetraacetic acid
ECACC European Collection of Authenticated Cell Cultures
FBS Fetal Bovine Serum
FI Fluorescence Intensity
HaCaT spontaneously immortalized human keratinocyte cell line
HeLa cervical cancer cell line
HepG2 liver cancer cell line
HPLC-DAD liquid chromatography with diode-array detection
H2O2 hydrogen peroxide
MCF-7 breast cancer cell line
MTT 2,5-diphenyl-3-(4,5-dimethyl-2-thiazolyl) tetrazolium bromide
NR Neutral Red
NRU Neutral Red Uptake
OECD Organisation for Economic Cooperation and Development
PBS Phosphate Buffered Saline
PL Polypodium leucotomos
PLE aqueous extract of Polypodium leucotomos
PVM methanolic extract of Polypodium vulgare L. fronds
ROS Reactive Oxygen Species
RNS Reactive Nitrogen Species
TLC Thin Layer Chromatography
UV ultraviolet
UVA ultraviolet A
Keywords: cytoprotection, cytotoxicity, polyphenols, polypody, ferns, medicinal plants
Citation: Farràs A, Mitjans M, Maggi F, Caprioli G, Vinardell MP and López V (2021) Polypodium vulgare L. (Polypodiaceae) as a Source of Bioactive Compounds: Polyphenolic Profile, Cytotoxicity and Cytoprotective Properties in Different Cell Lines. Front. Pharmacol. 12:727528. doi: 10.3389/fphar.2021.727528
Received: 18 June 2021; Accepted: 26 August 2021;
Published: 16 September 2021.
Edited by:
Gokhan Zengin, Selcuk University, TurkeyReviewed by:
Sengul Uysal, Erciyes University, TurkeyPaula C. Castilho, Universidade da Madeira, Portugal
Copyright © 2021 Farràs, Mitjans, Maggi, Caprioli, Vinardell and López. This is an open-access article distributed under the terms of the Creative Commons Attribution License (CC BY). The use, distribution or reproduction in other forums is permitted, provided the original author(s) and the copyright owner(s) are credited and that the original publication in this journal is cited, in accordance with accepted academic practice. No use, distribution or reproduction is permitted which does not comply with these terms.
*Correspondence: Montserrat Mitjans, bW9udHNlbWl0amFuc0B1Yi5lZHU=; Víctor López, aWxvcGV6QHVzai5lcw==