- 1Department of Pharmacy, The Children's Hospital, Zhejiang University School of Medicine, National Clinical Research Center for Child Health, Hangzhou, China
- 2Department of Nephrology, The Children's Hospital, Zhejiang University School of Medicine, National Clinical Research Center for Child Health, Hangzhou, China
- 3Department of Pharmacy, Cancer Hospital of the University of Chinese Academy of Sciences (Zhejiang Cancer Hospital), Institute of Basic Medicine and Cancer (IBMC), Chinese Academy of Sciences, Hangzhou, China
Background: Tacrolimus (TAC) is an important immunosuppressant for children with primary nephrotic syndrome (PNS). The relationship between sampling time variability in TAC therapeutic drug monitoring and dosage regimen in such children is unknown.
Methods: In this single-center, prospective, observational study, we evaluated the sampling time variability, concentration error (CE), relative CE (RCE), and the impact of the sampling time on TAC dosage regimens in 112 PNS children with 188 blood samples. Nominal concentration (Cnom) at 12-h after last TAC dose was simulated based on observed concentration (Cobs) via previously published pharmacokinetic models, then CE and RCE were calculated. Inappropriate dosing adjustments resulting from deviated sampling time were evaluated based on a target Cnom of 5–10 ng/ml.
Results: We found that 32 and 68% of samples were respectively collected early (2–180 min) and delayed (4–315 min). Furthermore, 24, 22, 22, and 32% of blood samples were drawn within deviations of ≤0.5, 0.5–1, 1–2, and >2 h, respectively, and 0.3 ng/ml of CE and 6% RCE per hour of deviation occurred. Within a deviation of >2 h, 25% of Cobs might result in inappropriate dosing adjustments. Early and delayed sampling might result in inappropriate dose holding or unnecessary dose increments, respectively, in patients with Cobs ∼ 5 ng/ml.
Conclusions: Variable sampling time might lead to inappropriate dosing adjustment in a minority of children with PNS, particularly those with TAC Cobs ∼ 5 ng/ml collected with a deviation of >2 h.
Introduction
Tacrolimus (TAC) is a cornerstone of immunosuppression after organ transplantation (Kidney Disease Improving Global Outcomes KDIGO Transplant Work Group, 2009;Ericson et al., 2017). It is now administered to children with primary nephrotic syndrome (PNS), a renal disease classically characterized by massive proteinuria, hypoalbuminemia, edema, and hypercholesterolemia (Lombel et al., 2013; Kaku et al., 2015; Subspecialty Group of Nephrology Society of Pediatrics Chinese Medical Association, 2017; Trautmann et al., 2020). Owing to the substantial individual variability of its pharmacokinetic profile, TAC doses are usually adjusted based on trough concentrations (C0) determined by therapeutic drug monitoring (TDM) (Brunet et al., 2019). Up to now, the consensus target C0 of TAC in pediatric PNS is 5–10 ng/ml during the first few months of treatment (usually first 6 months), recommended by several professional societies, including the organization of Kidney Disease: Improving Global Outcomes, and Chinese Medical Association (Lombel et al., 2013; Subspecialty Group of Nephrology Society of Pediatrics Chinese Medical Association, 2017).
An accurate C0 is crucial for precise TAC dosing; however, it is greatly dependent on appropriate sampling. Ideally, TAC should be administered twice daily at 12-h intervals. Thus, TAC dose is commonly adjusted to maintain the 12-h trough levels in the target range. However, in real clinical practice, the observed C0 (Cobs) was always not sampled exactly at 12-h after TAC administrating. For example, in posttransplant patients, sampling time deviation (STD) varies in the clinical setting from to 8–14 h, or even 2–25 h after the last dose (Karaalp et al., 2004; Dasari et al., 2016; Strohbehn et al., 2018). Furthermore, the potential bias of clinical decisions caused by inaccurate C0 is considered financially inefficient and harmful (Wong et al., 2016; Strohbehn et al., 2018). Therefore, in pediatric PNS, TAC dose should be tailored based on the nominal C0 (Cnom) of 12-h target C0 of 5–10 ng/ml.
Little is known about the variability of TAC STD in children with PNS. We previously simulated a STD-related concentration error (CE) and an inappropriate decision of dose tailoring in silico (Wang et al., 2020). An approximate 7% relative CE (RCE) per hour of STD occurred. Compared to delayed sampling, early sampling was associated with a larger RCE and higher risk of inappropriate dosing. However, STD induced inappropriate clinical dose decision is necessary to be clarified in real-world practice.
Meanwhile, due to the retrospective nature of the previously published study (Wang et al., 2020), some factors partly decided blood level of TAC, such as genotype of cytochrome P-450 system (CYP3A) family (Huang et al., 2020) and co-therapy medications of cost-saving agents (CYP3A inhibitors such as fluconazole, diltiazem, and Schisandra extract) prolonged TAC elimination (Zhao et al., 2016; Vanhove et al., 2017; Huang et al., 2019; Wang et al., 2021), were not involved. Furthermore, age (ontogeny) sometimes dominates the enzymatic functions (phenotype) in pediatric patients (Sun, et al., 2018), and the age-related pattern in CYP3A expression and activity is worth exploring.
Therefore, this single-center, prospective, observational study aimed to evaluated the variability of TAC STD, STD-related CE, and its potential adverse impact on clinical decisions regarding TAC dosage regimens involved factors of age, CYP3A5 genotype, and CYP3A inhibitors.
Methods and Design
Patients and Ethics
This prospective observational study was conducted between April 2019 and March 2020 at a tertiary children’s teaching hospital in Hangzhou, Zhejiang Province, China.
We included consecutive patients who were diagnosed with PNS, aged <18 years, and then treated with a constant TAC dose for ≥3 days twice daily. Patients were excluded if they were unwilling to participate in the study, co-treated with traditional Chinese medicine except Wuzhi capsules [extract of Schisandra sphenanthera, which increased the TAC concentration (Chen et al., 2020)], or had serious infections or multiple organ injuries.
The Ethics Committee of the Children’s Hospital, Zhejiang University School of Medicine, approved the study (2019-IRB-039), which was registered in the Chinese Clinical Trial Registry (ChiCTR1900022724). All parents provided written informed consent before participation. All patient information was rendered anonymously before analysis.
TDM, Genotyping, and Data Collection
Whole blood (2 ml) anticoagulated with EDTA was sampled for TDM, and the sample time was extracted from electronic medical records (EMR). Concentrations of TAC were determined using ARCHITECT i1000 chemiluminescent microparticle immunoassay kits (Abbott Laboratories, Chicago, IL, United States) as described by the manufacturer. The detection range was 2.0–30.0 ng/ml, and the inter- and intra-coefficients of variation were ≤10%. Total DNA was extracted, and the CYP3A5*3 genotype was assessed using the polymerase chain reaction as we previously described (Huang et al., 2020).
Data about TAC dosages, frequency, and intake times were analyzed. Inpatient information was extracted from the EMR. Data were collected from outpatients using social networking applications; a special two-dimensional code (QR code) was automatically sent to every patient, and related complete information were acquired by investigators. Information including therapeutic regimens, concomitant drugs (CYP3A4/5 inducers/inhibitors and Wuzhi capsules), and subsequent dose regimens by clinicians after TDM was also collected.
The interval between blood sampling and the last TAC dose was calculated. Early and delayed sampling was defined as blood drawn before and after the 12-h dosing intervals, respectively.
Simulation of nominal TAC C0 and evaluation of concentration error of all measurable samples (Analysis set 1)
In this process, observed C0 (Cobs) ≤ 2.0 ng/ml (the lower limit of quantitation) by TDM was excluded for its unmeasurable value. Then nominal C0 (Cnom) at 12 h after the last TAC dose was simulated based on Cobs. A nonlinear mixed-effects model program, NONMEM®, version 7.4 (Icon, Inc., Lake Winola, PA, United States) and published population pharmacokinetic models (Huang et al., 2020; Chen et al., 2020) of Chinese children with PNS were applied for simulation (Supplementary Table S1).
The difference between the Cobs and Cnom was defined as the CE and calculated according to Eq. 1. The RCE was calculated using Eq. 2.
Evaluation of TAC dosing decision of subjects within the first 6 months of TAC treatment (Analysis set 2)
Because the consensus target C0 (5–10 ng/ml) only established for the first 6 months of TAC treatment in pediatric PNS (Lombel et al., 2013), the impact of STD on clinical dosing regimens was conducted among patients within 6 months after TAC treatment in this process.
We identified the following scenarios of inappropriate dosing decisions associated with STD: 1) inappropriate dose adjusting: when Cnom was within the target range (5–10 ng/ml) but Cobs was outside the target range and clinicians altered the TAC treatment; 2) inappropriate dose holding: when Cnom was outside the target range (<5 or >10 ng/ml) and Cobs was within it, but dosing was not altered. Inappropriate decisions were recorded, and subsequent therapy regimens and PNS management were followed up. The management of PNS was estimated as relapse and complete, partial, and or no remission.
Statistical Analysis
Data were statistically analyzed using SPSS version 24.0 (IBM Corp, Armonk, NY, United States) and GraphPad Prism version 8.0 (GraphPad Software Inc., San Diego, CA, United States). Continuous data are presented as mean value ± standard deviation (SD) and median value with interquartile range (IQR) for normally and non-normally distributed variables, and analyzed using t-test and Mann-Whitney U test, respectively. Categorical data are presented as frequencies and proportions and were analyzed using chi-square tests. Correlations between STD and CE or RCE in total patients or subgroups of 0–3, 3–6, 6–9, 9–12, and 12–18 years old were investigated using linear regression. Differences were considered statistically significant at p < 0.05.
Results
We collected 188 blood samples from 112 patients with PNS, and then STD were estimated. Furthermore, CE and inappropriate clinical decision were estimated based on 160 measurable blood samples from 101 patients, and 93 samples of 59 patients within 6 months after initial treatment, respectively (Figure 1; Table 1).
Sampling Time Deviations of TAC TDM
Among all samples, 128 (68.1%) were delayed while 60 (31.9%) were early sampled, and 76.6% were collected from outpatients (dark blue in Figure 2). Approximately a quarter (23.9%) and a half (46.3%) samples were collected within STD ≤0.5 h and ≤1 h, respectively. Meanwhile, the STD was longer in delayed than in early samplings (median, 70; range, 4–315; IQR, 30–180 min vs median, 45; range, 2–180; IQR, 26–90 min; p < 0.05).
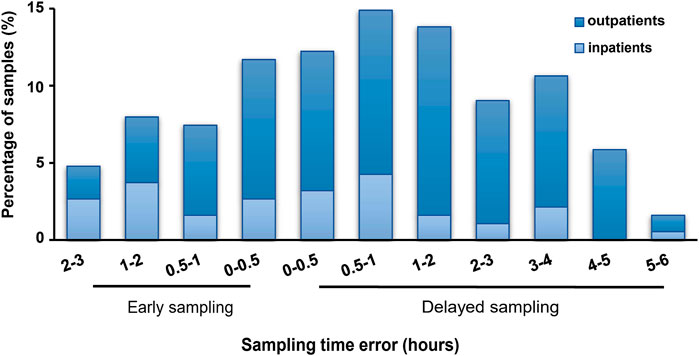
FIGURE 2. Sampling time deviations of tacrolimus therapeutic drug monitoring in pediatric primary nephrotic syndrome. All samples (n = 188); dark blue: samples from outpatients (n = 144); light blue: samples from inpatients (n = 44).
Impact of Sampling Time on TAC Concentration Error
The 160 samples with measured Cobs were used for Cnom simulation via NONMEM. Mean Cobs and Cnom were 5.5 ± 3.0 and 5.8 ± 3.0 ng/ml, respectively (Table 1, “Analysis set 1”). The CE and RCE ranges in early samples were 0–1.1 ng/ml (Figure 3A) and 0.2–22.4% (Figure 3C), respectively, and in delayed samples were 0–2.2 ng/ml (Figure 3A), and 0.3–32.9% (Figure 3C), respectively. Linear relationships were strong between STD and CE as well as RCE (correlation coefficients for CE and RCE, 0.860 and 0.956, respectively; p < 0.001 for both). Approximately, 0.3 ng/ml of CE (Figure 3B) and 5.9% of RCE (Figure 3D) occurred for every hour of STD.
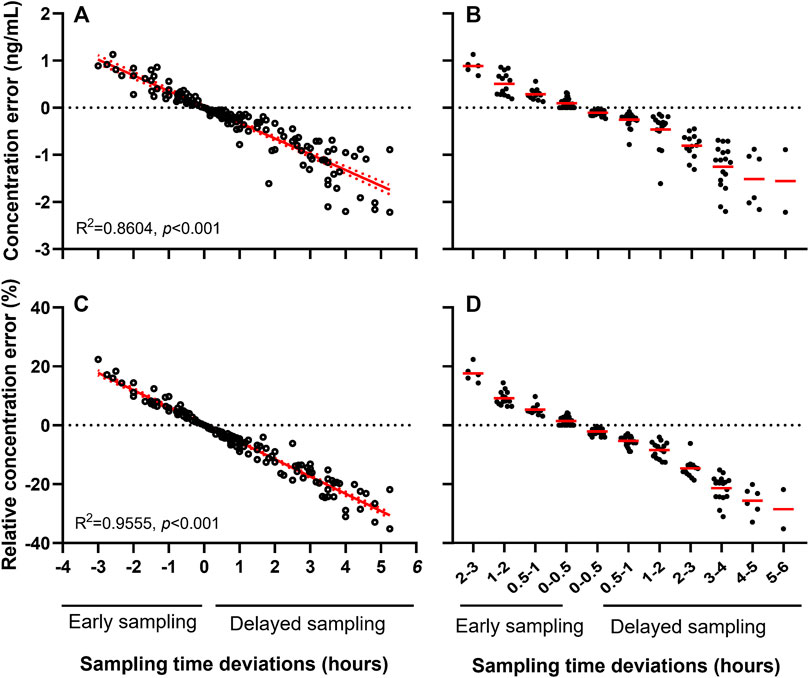
FIGURE 3. Sampling time deviation induced concentration error (A, B) and relative concentration error (C, D) of tacrolimus.
Meanwhile, considering the potential influence of different age on the pharmacokinetics of TAC, correlation of RCE and STD was further analyzed in various subpopulation with 0–3, 3–6, 6–9, 9–12, and 12–18 years old. RCE by 1-h STD was increased from 5.35% of 0–3 aged patients to 6.16% of 12–18 aged patients (Supplementary Figure S1A–E). Moreover, it was disclosed that RCE per hour of STD was significantly linked to age (p = 0.0134, r = 0.950) (Supplementary Figure S1F).
Impact of Sampling Time on TAC Dosing Decision
TAC dosing decision after TDM in 93 samples collected within 6 months after initial treatment (Table 1, “Analysis set 2”) were evaluated in 59 patients. Among 93 samples, 9 paired Cobs and Cnom failed to consistently within target range, and occurred at scenarios with STD of 1–2 h (2 samples), 2–3 h (4 samples), 3–4 h (2 samples), and 4–5 h (1 sample). Furthermore, 6 of the 9 TAC dose decisions were inappropriate (Table 2). The respective CE and RCE ranges of −2.2–1.0 ng/ml and −33.3–25.0% in these incidents indicated a high incidence of inevitable inaccurate clinical decisions based on TAC dose adjustments.
Two scenarios, inappropriate TAC dose holdings for early sampling and unnecessary dose increases for delayed sampling, were observed. Doses were inappropriately withheld in three children (Table 2, Nos. 1–3) with overestimated Cobs, and this led to unsatisfactory clinical efficacy. PNS relapsed in one child at 15 days after TDM, and the other two children were partial remission, and the regimens were subsequently modified by combination with Wuzhi capsules or rituximab. Three children with inappropriately increased instead of being maintained with under-estimated Cobs, although the clinical outcomes were as expected (Table 2, Nos. 4–6).
Notably, all these samples had Cobs ∼5 ng/ml and were collected with STD >2 h; that is, the probability of inappropriate incidents was much higher when samples were collected with STD >2 h (25.0%, 6 in 24 samples) than ≤2 h (0 in 69 samples). As this study included only Chinese children, among whom, only one was a CYP3A5*1/*3 carrier; therefore, the influence of genetic polymorphisms was not statistically comparable. Similarly, only one child received Wuzhi capsules, and the effect of co-administered drugs was not estimated.
Discussion
TAC dosage regimens should be optimized based on TDM. However, obtaining accurate TAC concentrations in clinical practice is challenging owing to unsatisfactory patient compliance, measurement uncertainty, circadian rhythms, and other factors (Ertas et al., 2002;Eaton et al., 2018; Fontova et al., 2021). An important and prevalent cause of measurement uncertainty is STD (Saint-Marcoux et al., 2003; Choi et al., 2013; Moeller et al., 2014;Jakobsen et al., 2017). The present study is the first to determine the prevalence of TAC sampling time variability in pediatric patients with PNS and to elucidate possible STD-related concentration errors and inappropriate dose adjustments.
As disclosed in the present study, about half and one third of blood samples, respectively, were collected with STD of > 1 h and > 2 h. STD was common in the TDM of TAC. It was similar to the findings of adult organ transplantation (Karaalp et al., 2004; Dasari et al., 2016;Strohbehn et al., 2018). However, a specific time frame for collecting blood samples of TAC C0, has not been defined owing to the lack of evidence regarding a relationship between STD, and adverse events. Generally, a “clinically ideal” state that allows for a tolerance of 1 h around each TDM event has gained experiential acceptance (Karaalp et al., 2004). Notably, in children with PNS, a slight bias (CE 0.3 ng/ml and RCE 6%) within this interval did not result in subsequent inappropriate dose adjustment. Therefore, this “ideal” time frame of ±1 h might be clinically applicable.
Inappropriate decisions on TAC dose adjustment induced by STD within deviations of ≤2 h were not generally remarkable. However, clinicians should be aware of patients with Cobs around the lower limit of the C0 target, particularly those with Cobs ∼ 5 ng/ml, and sampled within STD >2 h, for the excessive risk of inappropriate dose-holding induced unsatisfactory TAC effect in early sampled patients, and inappropriate dose-increase induced unnecessary costs in delayed sampled patients, respectively. Therefore, a repeat TDM at an accurate sampling time might be necessary. Moreover, educating patients and their family caregivers about sampling adherence is crucial (Shi et al., 2020). Medical workers also require education about pharmacokinetics and TDM, and laboratory technicians and nurses should be reminded about correct sampling times and their importance (Schiff et al., 2003;Chen et al., 2003).
To preliminarily disclosed the cause of individual variate of CE, the role of CYP3A5, the most efficient metabolizing enzyme of TAC, and was evaluated. CYP3A5*3 genetic polymorphism is widely regarded as an essential factor to the high individual variability of TAC (Woillard et al., 2017; Brunet et al., 2019; Mendrinou et al., 2020; van Gelder et al., 2020), and pediatric PNS patients with the CYP3A5*1 allele (rapid metabolizer) have lower TAC exposure than those who do not express CYP3A5 (CYP3A5*3/*3, slow metabolizer) (Huang et al., 2019). Meanwhile, the metabolism of TAC is inhibited by CYP inhibitors. However, the hypothesized greater effect of STD on a TAC rapid metabolizer without a combination of CYP inhibitors was not observed, and the long-term outcomes did not seem remarkably impaired. It might partly be attributed to most Chinese are slow metabolizers, and the negative influence of STD might be moderate in Chinese pediatric patients with PNS, despite limited data.
Besides CYP3A5, other metabolic CYP enzymes of TAC, such as CYP3A4 and CYP3A7 (Barbarino et al., 2013), can be involved in the further study to fully disclose the individual genetic variate of STD caused CE. Moreover, we found increased RCE along with aging in pediatric PNS patients. It was consistent with our previous finding in silico (Wang et al., 2020). This phenomenon may be particularly due to the age-related pattern in CYP3A protein expression and activity, and age (ontogeny) sometimes dominate the enzymatic functions (phenotype) in pediatric patients (Sun, et al., 2018). Activity of CYP3A4 is very low before birth but increases rapidly, whereas CYP3A7 displays a significant decline in postnatal periods. For CYP3A5, expression and function stay stable from gestation through adulthood. However, the ontogeny was not enough to explain it, for other factors as high body weight, daily dose, and corticosteroid dose, etc. may also related to increased RCE (Wang et al., 2020). But, nevertheless, the potential influence of different age on RCE should be valued.
This study has some limitations. First, we simulated Cnom based on previous population pharmacokinetic models, and bias towards real Cnom should be considered. Second, the impact of STD on TAC toxicity and adverse events was not investigated because of having few samples with TAC concentrations >10 ng/ml. Third, most Cobs below limit of quantitation were in delayed samples and were excluded during Cnom simulation because of the lack of exact values, which might weaken their clinical implications. Fourth, we included only patients within 6 months after TAC treatment who reached the target C0 of 5–10 ng/ml, because a specific C0 target for PNS after 6 months has not been recommended. Moreover, whether inaccurate TAC values could affect the long-term outcomes of pediatric PNS remains undefined, nonetheless, we linked them to the closely followed dosage decision; thus, these mysteries need to be resolved in studies with multi-center and large-sample clinical studies.
Conclusions
The present study found that the time of collecting blood samples from children with PNS to measure TAC varied in the clinical setting, and could result in a TAC concentration error as high as 30%. Although the impact of inappropriate sampling times on clinical decisions might have been moderate, STD >2 h associated with inaccurate C0 exposure should be worthy of attention.
Data Availability Statement
The original contributions presented in the study are included in the article/Supplementary Material, further inquiries can be directed to the corresponding authors.
Ethics Statement
The studies involving human participants were reviewed and approved by The Ethics Committee of the Children’s Hospital, Zhejiang University School of Medicine. Written informed consent to participate in this study was provided by the participants’ legal guardian/next of kin. Written informed consent was obtained from the minor(s)’ legal guardian/next of kin for the publication of any potentially identifiable images or data included in this article.
Author Contributions
All authors have contributed significantly to the work. LH, JM, and LF designed the research. LH, JW, JY, and HZ participated in data collection. JW and YH analyzed the data. LH, JW, MJ, and LF participated in manuscript preparation.
Funding
This project is supported by the National Natural Science Foundation of China (81973396), Natural Science Foundation of Zhejiang Province (LSY19H300001), and Hospital pharmacy Research Funding Project of Zhejiang Pharmaceutical Association (2014ZYY05).
Conflict of Interest
The authors declare that the research was conducted in the absence of any commercial or financial relationships that could be construed as a potential conflict of interest.
Publisher’s Note
All claims expressed in this article are solely those of the authors and do not necessarily represent those of their affiliated organizations, or those of the publisher, the editors and the reviewers. Any product that may be evaluated in this article, or claim that may be made by its manufacturer, is not guaranteed or endorsed by the publisher.
Acknowledgments
The authors thank all the pediatric patients, families, and nursing and support staff for their contributions to this study. The authors would also like to thank Professor Zheng Jiao of Shanghai Chest Hospital, Shanghai Jiao Tong University for data editing and processing.
Supplementary material
The Supplementary Material for this article can be found online at: https://www.frontiersin.org/articles/10.3389/fphar.2021.726667/full#supplementary-material
References
Barbarino, J. M., Staatz, C. E., Venkataramanan, R., Klein, T. E., and Altman, R. B. (2013). PharmGKB Summary: Cyclosporine and Tacrolimus Pathways. Pharmacogenet Genomics 23, 563–585. doi:10.1097/FPC.0b013e328364db84
Brunet, M., van Gelder, T., Åsberg, A., Haufroid, V., Hesselink, D. A., Langman, L., et al. (2019). Therapeutic Drug Monitoring of Tacrolimus-Personalized Therapy: Second Consensus Report. Ther. Drug Monit. 41, 261–307. doi:10.1097/FTD.0000000000000640
Chen, P., Tanasijevic, M. J., Schoenenberger, R. A., Fiskio, J., Kuperman, G. J., and Bates, D. W. (2003). A Computer-Based Intervention for Improving the Appropriateness of Antiepileptic Drug Level Monitoring. Am. J. Clin. Pathol. 119, 432–438. doi:10.1309/a96xu9yku298hb2r
Chen, X., Wang, D. D., Xu, H., and Li, Z. P. (2020). Optimization of Initial Dosing Scheme of Tacrolimus in Pediatric Refractory Nephrotic Syndrome Patients Based on CYP3A5 Genotype and Coadministration with Wuzhi-Capsule. Xenobiotica 50, 606–613. doi:10.1080/00498254.2019.1669844
Choi, L., Crainiceanu, C. M., and Caffo, B. S. (2013). Practical Recommendations for Population PK Studies with Sampling Time Errors. Eur. J. Clin. Pharmacol. 69, 2055–2064. doi:10.1007/s00228-013-1576-7
Dasari, B. V., Hodson, J., Nassir, A., Widmer, J., Isaac, J., Mergentel, H., et al. (2016). Variations in Practice to Therapeutic Monitoring of Tacrolimus Following Primary Adult Liver Transplantation. Int. J. Organ. Transpl. Med 7, 1–8.
Eaton, C. K., Gutierrez-Colina, A. M., Quast, L. F., Liverman, R., Lee, J. L., Mee, L. L., et al. (2018). Multimethod Assessment of Medication Nonadherence and Barriers in Adolescents and Young Adults with Solid Organ Transplants. J. Pediatr. Psychol. 43, 789–799. doi:10.1093/jpepsy/jsy016
Ericson, J. E., Zimmerman, K. O., Gonzalez, D., Melloni, C., Guptill, J. T., Hill, K. D., et al. (2017). A Systematic Literature Review Approach to Estimate the Therapeutic Index of Selected Immunosuppressant Drugs after Renal Transplantation. Ther. Drug Monit. 39, 13–20. doi:10.1097/FTD.0000000000000364
Ertas, O. S., and Kayali, A. (2002). Estimation of Basic Uncertainties in Clinical Analysis. Eur. J. Drug Metab. Pharmacokinet. 27, 75–78. doi:10.1007/BF03190419
Fontova, P., Colom, H., Rigo-Bonnin, R., van Merendonk, L. N., Vidal-Alabró, A., Montero, N., et al. (2021). Influence of the Circadian Timing System on Tacrolimus Pharmacokinetics and Pharmacodynamics after Kidney Transplantation. Front. Pharmacol. 12 (12), 636048. doi:10.3389/fphar.2021.636048
Huang, L., Liu, Y., Jiao, Z., Wang, J., Fang, L., and Mao, J. (2020). Population Pharmacokinetic Study of Tacrolimus in Pediatric Patients with Primary Nephrotic Syndrome: A Comparison of Linear and Nonlinear Michaelis-Menten Pharmacokinetic Model. Eur. J. Pharm. Sci. 143, 105199. doi:10.1016/j.ejps.2019.105199
Huang, L., Wang, J., Yang, J., Zhang, H., Ni, Y., Zhu, Z., et al. (2019). Impact of CYP3A4/5 and ABCB1 Polymorphisms on Tacrolimus Exposure and Response in Pediatric Primary Nephrotic Syndrome. Pharmacogenomics 20, 1071–1083. doi:10.2217/pgs-2019-0090
Jakobsen, M. I., Larsen, J. R., Svensson, C. K., Johansen, S. S., Linnet, K., Nielsen, J., et al. (2017). The Significance of Sampling Time in Therapeutic Drug Monitoring of Clozapine. Acta Psychiatr. Scand. 135, 159–169. doi:10.1111/acps.12673
Kaku, Y., Ohtsuka, Y., Komatsu, Y., Ohta, T., Nagai, T., Kaito, H., et al. (2015). Clinical Practice Guideline for Pediatric Idiopathic Nephrotic Syndrome 2013: General Therapy. Clin. Exp. Nephrol. 19, 34–53. doi:10.1007/s10157-014-1031-9
Kamdem, L. K., Streit, F., Zanger, U. M., Brockmöller, J., Oellerich, M., Armstrong, V. W., et al. (2005). Contribution of CYP3A5 to the In Vitro Hepatic Clearance of Tacrolimus. Clin. Chem. 51, 1374–1381. doi:10.1373/clinchem.2005.050047
Karaalp, A., Demir, D., Gören, M. Z., Akc, A., ISkender, E., Yananl, H. R., et al. (2004). Therapeutic Drug Monitoring of Immunosuppressant Drugs in Marmara University Hospital. Ther. Drug Monit. 26, 263–266. doi:10.1097/00007691-200406000-00007
Kidney Disease Improving Global Outcomes KDIGO Transplant Work Group (2009). KDIGO Clinical Practice Guideline for the Care of Kidney Transplant Recipients. Am. J. Transpl. 9 Suppl 3, S1–S155. doi:10.1111/j.1600-6143.2009.02834.x
Lombel, R. M., Gipson, D. S., Hodson, E. M., and Kidney Disease, and Improving Global Outcomes, (2013). Treatment of Steroid-Sensitive Nephrotic Syndrome: New Guidelines from KDIGO. Pediatr. Nephrol. 28, 415–426. doi:10.1007/s00467-012-2304-810.1007/s00467-012-2310-x
Mendrinou, E., Mashaly, M. E., Al Okily, A. M., Mohamed, M. E., Refaie, A. F., Elsawy, E. M., et al. (2020). CYP3A5 Gene-Guided Tacrolimus Treatment of Living-Donor Egyptian Kidney Transplanted Patients. Front. Pharmacol. 11, 1218. doi:10.3389/fphar.2020.01218
Moeller, J., Lieb, R., Meyer, A. H., Quack Loetscher, K., Krastel, B., and Meinlschmidt, G. (2014). Nonadherence with Ambulatory Saliva Sampling Is Associated with Biased Salivary Testosterone Estimates. Psychoneuroendocrinology 44, 13–19. doi:10.1097/FTD.000000000000074210.1016/j.psyneuen.2014.02.012
Saint-Marcoux, F., Rousseau, A., Le Meur, Y., Estenne, M., Knoop, C., Debord, J., et al. (2003). Influence of Sampling-Time Error on Cyclosporine Measurements Nominally at 2 hours after Administration. Clin. Chem. 49, 813–815. doi:10.1373/49.5.813
Schiff, G. D., Klass, D., Peterson, J., Shah, G., and Bates, D. W. (2003). Linking Laboratory and Pharmacy: Opportunities for Reducing Errors and Improving Care. Arch. Intern. Med. 163 (8), 893–900. doi:10.1001/archinte.163.8.893
Shi, Y. X., Liu, C. X., Liu, F., Zhang, H. M., Yu, M. M., Jin, Y. H., et al. (2020). Efficacy of Adherence-Enhancing Interventions for Immunosuppressive Therapy in Solid Organ Transplant Recipients: A Systematic Review and Meta-Analysis Based on Randomized Controlled Trials. Front. Pharmacol. 11, 578887. doi:10.3389/fphar.2020.578887
Strohbehn, G. W., Pan, W. W., Petrilli, C. M., Heidemann, L., Larson, S., Aaronson, K. D., et al. (2018). Large-Scale Variability of Inpatient Tacrolimus Therapeutic Drug Monitoring at an Academic Transplant Center: A Retrospective Study. Ther. Drug Monit. 40, 394–400. doi:10.1097/FTD.0000000000000526
Subspecialty Group of Nephrology Society of Pediatrics Chinese Medical Association (2017). Evidence-based Guideline on Diagnosis and Treatment of Steroid-Resistant Nephrotic Syndrome. Chin. J. Pediatr. 55, 805–809. doi:10.3760/cma.j.issn.0578-1310.2017.11.002
Sun, J. Y., Xu, Z. J., Sun, F., Guo, H. L., Ding, X. S., Chen, F., et al. (2018). Individualized Tacrolimus Therapy for Pediatric Nephrotic Syndrome: Considerations for Ontogeny and Pharmacogenetics of CYP3A. Curr. Pharm. Des. 24, 2765–2773. doi:10.2174/1381612824666180829101836
Trautmann, A., Vivarelli, M., Vivarelli, M., Samuel, S., Gipson, D., Sinha, A., et al. (2020). International Pediatric Nephrology AssociationIPNA Clinical Practice Recommendations for the Diagnosis and Management of Children with Steroid-Resistant Nephrotic Syndrome. Pediatr. Nephrol. 35, 1529–1561. doi:10.1007/s00467-020-04519-1
van Gelder, T., Meziyerh, S., Swen, J. J., de Vries, A. P. J., and Moes, D. J. A. R. (2020). The Clinical Impact of the C0/D Ratio and the CYP3A5 Genotype on Outcome in Tacrolimus Treated Kidney Transplant Recipients. Front. Pharmacol. 11, 1142. doi:10.3389/fphar.2020.01142
Vanhove, T., Bouwsma, H., Hilbrands, L., Swen, J. J., Spriet, I., Annaert, P., et al. (2017). Determinants of the Magnitude of Interaction between Tacrolimus and Voriconazole/Posaconazole in Solid Organ Recipients. Am. J. Transpl. 17, 2372–2380. doi:10.1111/ajt.14232
Wang, J., Gao, P., Zhang, H., Hu, Y., Ni, Y., Zhu, Z., et al. (2020). Evaluation of Concentration Errors and Inappropriate Dose Tailoring of Tacrolimus Caused by Sampling-Time Deviations in Pediatric Patients with Primary Nephrotic Syndrome. Ther. Drug Monit. 42, 392–399. doi:10.1097/FTD.0000000000000717
Wang, J., Huang, L., Gao, P., Hu, Y., Ni, Y., Zhu, Z., et al. (2021). Diltiazem on Tacrolimus Exposure and Dose Sparing in Chinese Pediatric Primary Nephrotic Syndrome: Impact of CYP3A4, CYP3A5, ABCB1, and SLCO1B3 Polymorphisms. Eur. J. Clin. Pharmacol. 77, 71–77. doi:10.1007/s00228-020-02977-y
Woillard, J. B., Mourad, M., Neely, M., Capron, A., van Schaik, R. H., van Gelder, T., et al. (2017). Tacrolimus Updated Guidelines through popPK Modeling: How to Benefit More from CYP3A Pre-emptive Genotyping Prior to Kidney Transplantation. Front. Pharmacol. 8, 358. doi:10.3389/fphar.2017.00358
Wong, K. R., Nelson, L. A., Elliott, E. S. R., Liu, Y., Sommi, R. W., and Winans, E. A. (2016). Utilization of Antipsychotic Therapeutic Drug Monitoring at a State Psychiatric Hospital. Ment. Health Clin. 6, 1–7. doi:10.9740/mhc.2016.01.001
Keywords: sampling time, therapeutic drug monitoring, tacrolimus, nephrotic syndrome, pediatrics
Citation: Huang L, Wang J, Yang J, Zhang H, Hu Y, Miao J, Mao J and Fang L (2022) Impact of Sampling Time Variability on Tacrolimus Dosage Regimen in Pediatric Primary Nephrotic Syndrome: Single-Center, Prospective, Observational Study. Front. Pharmacol. 12:726667. doi: 10.3389/fphar.2021.726667
Received: 17 June 2021; Accepted: 16 December 2021;
Published: 07 January 2022.
Edited by:
Pavel Anzenbacher, Palacký University, Olomouc, CzechiaReviewed by:
Xiao Chen, Children’s Hospital of Fudan University, ChinaJoanna Sobiak, Poznan University of Medical Sciences, Poland
Copyright © 2022 Huang, Wang, Yang, Zhang, Hu, Miao, Mao and Fang. This is an open-access article distributed under the terms of the Creative Commons Attribution License (CC BY). The use, distribution or reproduction in other forums is permitted, provided the original author(s) and the copyright owner(s) are credited and that the original publication in this journal is cited, in accordance with accepted academic practice. No use, distribution or reproduction is permitted which does not comply with these terms.
*Correspondence: Luo Fang, ZmFuZ2x1b0B6amNjLm9yZy5jbg==; Jianhua Mao, bWFvamg4OEBnbWFpbC5jb20=
†LH and JW have contributed equally to this work