- 1Department of Biology, Georgia State University, Atlanta, GA, United States
- 2Division of Pulmonary and Critical Care, Department of Medicine, Massachusetts General Hospital, Harvard Medical School, Boston, MA, United States
- 3Department of Nutrition, Georgia State University, Atlanta, GA, United States
- 4Division of Biology and Medicine, Department of Molecular Biology, Cell Biology and Biochemistry, Brown University, Providence, RI, United States
- 5Department of Chemistry, Georgia State University, Atlanta, GA, United States
- 6Center for Molecular and Translational Medicine, Georgia State University, Atlanta, GA, United States
Although a few studies show that the use of electronic nicotine delivery systems (ENDS) may ameliorate objective and subjective outcomes in COPD smokers who switched to electronic cigarettes, it is unclear whether e-cigarette exposure alters lung pathological features and inflammatory response in COPD. Here, we employed βENaC-overexpressing mice bearing COPD-like pulmonary abnormality, and exposed them to ENDS. We found that ENDS exposure aggravated airspace enlargement and mucus production in βENaC-overexpressing mice, which was associated with increased MMP12 and Muc5ac, respectively. ENDS exposure to mice significantly increased the numbers of macrophages, particularly in M2 macrophages in bronchoalveolar lavage (BAL) fluid, despite ENDS did not induce M2 macrophage polarization in a cultured murine macrophage cell line (RAW264.7). There were no changes in neutrophils in BAL fluid by ENDS exposure. Multiple cytokine productions were increased including M-CSF, IL-1r
Introduction
Electronic nicotine delivery systems (ENDS), also referred to as e-cigarettes, are electronic devices that produce an aerosol by heating a liquid that contains nicotine predominantly (Breland et al., 2017). ENDS is generated for stopping traditional cigarette smoking that is a major risk factor for chronic obstructive pulmonary disease (COPD). Some studies show that ENDS use may ameliorate objective and subjective outcomes in COPD smokers who switched to e-cigarettes, which is associated with abstinence and conventional smoking reduction (Polosa et al., 2016; Polosa et al., 2020). However, ENDS is not safe. Emerging evidence shows that ENDS trigger severe lung injuries and lead to the development of various lung diseases (Shields et al., 2017; Gilpin, 2019; Bhatt et al., 2020; Bhatta and Glantz, 2020; Mcalinden et al., 2020; Traboulsi et al., 2020). In 2019, an epidemic of E-cigarette-associated lung injury affected 49 states of the United States with over 2,600 cases and 60 deaths reported (Belok et al., 2020; Cherian et al., 2020). Moreover, growing evidence reveals that ENDS use is directly associated with the diagnosis of COPD in humans in recent years, and the exposure to ENDS is reported to induce COPD-like phenotype in mice (Garcia-Arcos et al., 2016; Bowler et al., 2017; Perez et al., 2019; Xie et al., 2020). However, the mechanisms have not been fully uncovered yet.
COPD is a primary leading cause of death affecting an estimated 328 million worldwide (Eisner et al., 2010; Quaderi and Hurst, 2018). It is characterized by progressive and irreversible airflow obstruction caused by chronic lung inflammation, in which innate immune cells play a pivotal role (Rovina et al., 2013; Barnes, 2016). Especially, macrophages are key effector cells in COPD which orchestrate immune responses (Kapellos et al., 2018). Macrophages are observed remarkedly increased in lung tissues and bronchoalveolar lavage (BAL) fluid of COPD patients and are associated with COPD severity (Hiemstra, 2013). In general, macrophages consist of two major phenotypes (Mosser and Edwards, 2008). M1 macrophages, referred to as classically activated macrophages, have been described as cytotoxic and pro-inflammatory, and are characterized by secretion of pro-inflammatory cytokines such as interferon (IFN)-γ, IL-6, and IL-12 (Mantovani et al., 2004; Mantovani et al., 2013). M2 macrophages, referred to as alternatively activated macrophages, are considered anti-inflammatory and are related to tissue repair and fibrosis, producing majorly anti-inflammatory cytokines including transforming growth factor β1 (TGF-β1), IL-4, IL-10, M-CSF (Gordon and Martinez, 2010; Martinez and Gordon, 2014; Chávez-Galán et al., 2015). Two phenotypes can switch between each other by different stimuli (Das et al., 2015; Funes et al., 2018). Multiply research groups have reported an alteration of immunophenotypes of macrophages towards M2 phenotype in COPD subjects, with cytokine production skew towards an M2 profile in blood and BAL samples from patients, suggesting M2 macrophages phenotype is a critical player in COPD disease progression (Eapen et al., 2017a; Eapen et al., 2017b; Da Silva et al., 2020). However, it is unclear whether macrophages undergo phenotype alterations after ENDS exposure in COPD, understanding of which would be of great importance to uncover the impact of ENDS on COPD.
It has been shown that βENaC-overexpressing mice have COPD-like pulmonary abnormalities, including mucous hypersecretion, inflammatory and emphysematous phenotypes and pulmonary dysfunction (Johannesson et al., 2012; Seys et al., 2015; Shuto et al., 2016). In this study, we employed βENaC-overexpressing mice and exposed them to ENDS to determine whether ENDS aggravate or exacerbate COPD-like pathological changes. We also measured macrophage immunophenotype and lung fibrogenesis in these mice exposed to ENDS. We observed a significant exacerbation of COPD features in these mice, along with a remarkable change in multiple cytokine production in COPD mice after exposure to ENDS. Further investigations elucidated that the ENDS challenge increased the total macrophage population but did not change neutrophil numbers. Macrophage phenotype was altered with an increase in M2 phenotype after ENDS exposure. In addition, fibrotic phenotypes were enhanced in ENDS-exposed mice, evidenced by a dramatic increase in the level of pro-fibrotic cytokine TGF-β1 and an augment in collagen production. For the first time, our study shows that the ENDS affect macrophage phenotype alteration during COPD development in mice, which leads to an enhancement of lung fibrosis.
Materials and Methods
Animal Model
βENaC mice with C57BL/6J background were obtained from the Jackson Laboratory. All animal procedures described were approved by the Institutional Animal Care and Use Committee (IACUC) at Georgia State University. βENaC mice were bred with wild-type C57BL/6J mice to produce hemizygous βENaC for our studies. Animals were genotyped to determine the genetic overexpression of Scnn1b. Briefly, tail snips were harvested and lysed in Proteinase K at 56°C water bath overnight. DNA was extracted by Dneasy Blood and Tissue kit (Qiagen, Foster City, CA). The overexpression of the mouse Scnn1b gene was identified by PCR (Forward: CCTCCAAGAGTTCAACTACCG; Reverse: TCTACCAGCTCAGCCACAGTG) (Mall et al., 2004). After PCR, samples were run on a 4% agarose gel containing ethidium bromide. Mice carrying Scnn1b overexpression were tagged as βENaC mice for future studies.
Electronic Nicotine Delivery Systems Exposure to βENaC Mice
At 12-week of age, mice (n = 10/group) were randomized into either the ENDS exposure group or the control group. Mice in the ENDS group were exposed to e-cigarette vapor for 30 min daily at a frequency of 5 times per week for a total duration of 8 weeks as shown in Figure 1A. Mice were placed in a smoking chamber attached to an inExpose Smoking Robot (SCIREQ, Montreal, QC, Canada) with an attached e-cigarette accessory (ECX JoyeTech E-Vic Mini, SCIREQ, Canada) for the administration of vaporized S brand e-cigarette liquid containing nicotine at 50 mg/ml with caramel flavoring. After 8 weeks of e-cigarette vapor exposure, animals were euthanized within 24 h after the last e-cigarette vapor exposure using CO2. Then, bronchoalveolar lavage (BAL) fluid, and lung tissues were collected for analysis as described below.
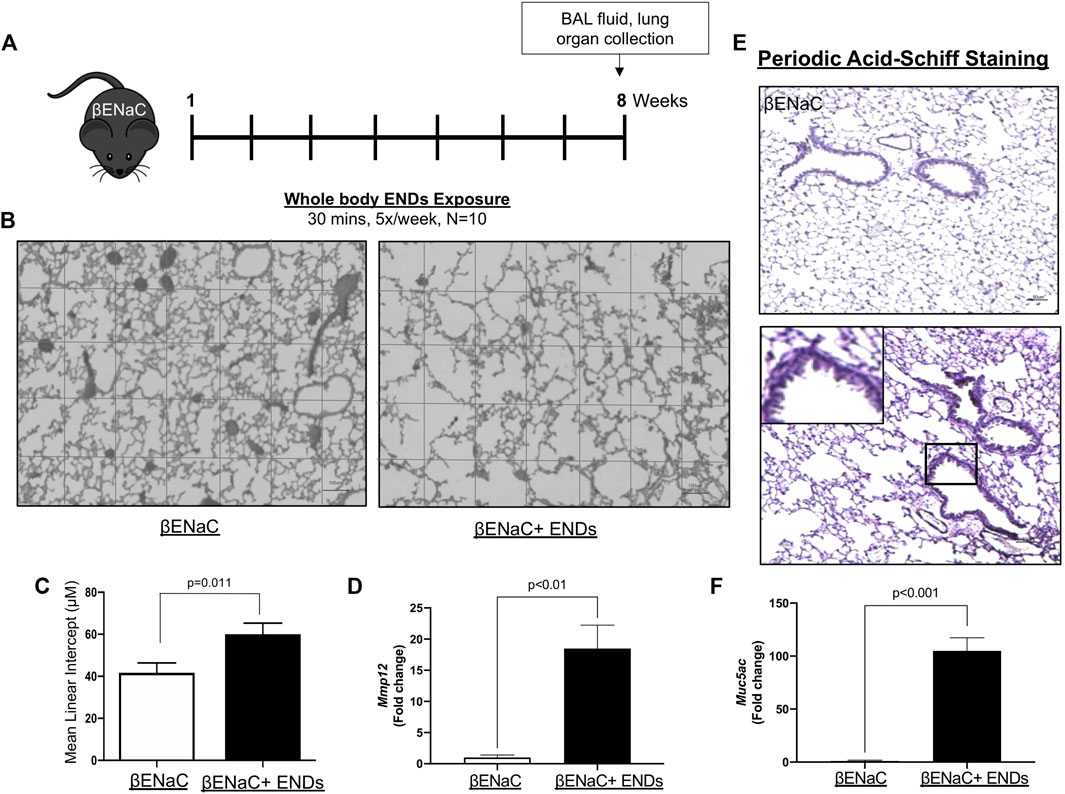
FIGURE 1. E-cigarette exposure aggravates COPD in βENaC mice. (A) Experimental schematic of whole body ENDS exposure in βENaC mice. (B) ENDS exposure caused emphysematous phenotypes changes in βENaC mice. Representative data of H&E staining images using lung sections of sham air (left) (n = 4) or ENDS exposed (right) (n = 4) mice. (C) Quantification of the free distance between gas exchange surfaces in lungs of βENaC mice with or without ENDS exposure. (D,F) Determination of mRNA levels of COPD-related proteins MMP12 (D) and Muc5ac (F) in lung tissues from βENaC mice with or without ENDS exposure by qPCR. (E) Representative images of Periodic Acid-Schiff staining in lung sections of βENaC mice with or without ENDS exposure. The inset indicates the enlarged image of the boxed area showing positive staining. All data presented at mean
Cell Counts and Cytokine Measurements in Bronchoalveolar Lavage Fluid
After the mice were euthanized, tracheostomy was performed, and lungs were immediately flushed with 1 ml ice-cold phosphate-buffered saline (PBS) twice for collection of BAL fluid. Total cells from the BAL were centrifuged and counted using Countess™ II Automated Cell Counter (Invitrogen, Carlsbad, CA) as previously described (Mall et al., 2004). Total immune cell count (5,000 cells/slide) was performed on Shandon cytospin slides (Thermo Shandon, Pittsburgh, PA) stained with Diff-Quik (Dade Bering, Newark, DE). The BAL supernatants were stored at −80°C until analysis.
Morphometric Assessment
In order to determine the effects of ENDS exposure on the development of emphysema, we did a morphometric assessment according to the previous protocol (Bartalesi et al., 2005; Mall et al., 2008). Briefly, the mouse lungs were inflated with 1% low melting-point agarose to 25 cm of H2O pressure. After 48 h of fixation, lungs were paraffin-embedded, sectioned, and stained with hematoxylin and eosin (H&E). The paraffin slides were baked at 60°C for 2 h, then deparaffinized with xylene and rehydrated with graded ethanol solutions. Next, the slides were incubated with Mayer’s Hematoxylin for 10 min and washed under tap water for 10 min. Slides were then immersed in Eosin for 30 s and washed under tap water, after which, the slides were dehydrated and mounted with a mounting medium. Mean linear intercepts were determined and calculated (Dunnill, 1962).
Immunohistochemistry
The mouse lungs were inflated with 1% low melting-point agarose to 25 cm of fixative pressure and then fixed with 4% neutral buffered formalin for 48 h. Later, these tissue samples were embedded in paraffin, and sectioned into 4 µm sections using a rotary microtome. For immunohistochemical analysis, tissue sections were deparaffinized with xylene and rehydrated with graded concentrations of ethanol, followed by antigen retrieval in 10 mM citric acid solution (pH = 6) for 20 min using a pressure cooker. Endogenous peroxidase activity was blocked by 3% hydrogen peroxide. After blocking with 5% BSA for 30 min, the slides were incubated with the primary antibodies against the following proteins at 4°C overnight: α-smooth muscle actin (α-SMA) 1:500 (Ab5694; Abcam); Arginase I 1:1,000 (sc-20150; Santa Cruz). After washing, the sections were incubated with the appropriate HRP polymers and developed with 3–3′ diaminobenzidine solution (DAB substrate kit; Vector Laboratories). After counterstaining with hematoxylin, the slides were dehydrated and mounted with a mounting medium.
Periodic-Acid Schiff Staining
Periodic-acid Schiff (PAS; Sigma Aldrich, Saint Louis, MO) staining was used to evaluate mucus accumulation and immune cell infiltration. The Periodic-acid Schiff staining was performed following the manufacturer’s instructions. All images were taken at 20x magnification using Keyence Fluorescent Microscope (Keyence, Itasca, IL).
Sirius Red Staining
Sirius Red staining was performed with Novaultra Sirius Red Stain Kit (IW-3012, IHC world) following the instructions of the manufacturer. In brief, the paraffin-embedded slides were deparaffinized in xylene and hydrated in gradient concentrations of ethanol. The slides were stained with Weigert’s hematoxylin for 8 min, followed by picro-sirius red for 1 h. After two washes in acidified water, slides were dehydrated, mounted in a resinous medium, and covered by cover slides.
Cell Culture
Raw264.7 cells were purchased from ATCC and cultured in DMEM media supplied with 10% fetal bovine serum. Raw264.7 cells were maintained in a cell culture incubator at 37°C and 5% CO2. To test whether ENDS can induce macrophage polarization, Raw264.7 were incubated with DMSO or ENDS with nicotine (50 mg/ml) or IL-4+IL-13 (IL-4 20 ng/ml; IL-13 20 ng/ml) for 48 h and harvested for analysis of protein expression by western blot.
Flow Cytometry
Freshly harvested lung tissues were minced and dissociated using collagenase D (Sigma 11088858001, 0.5 mg/ml) and DNase I for 20 min at 37°C on a shaker. Cell suspensions were subsequently passed through a 40 μm cell strainer to get a single cell suspension. Non-specific Fc-mediated interactions were blocked by incubating cells with CD16/CD32 antibodies (Thermofisher, 14–0161–82). Cells were labeled with fluorophore-conjugated antibodies, including CD45-FITC (BioLegend 103,108), F4/80-PE/Cy5 (BioLegend, 123111), CD11b-APC (Thermofisher, 17–0112–82), Ly6G-BV421(BioLegend, 127627), CD206-e450 (Thermofisher, 48–2061–82). At the last step, Cells were resuspended in FACS staining buffer and acquired using LSRFortessa flow cytometer (BD Biosciences). Data were analyzed by FlowJo software (Tree Star).
RNA Isolation and RT-qPCR Analysis
The expressions of inflammatory cytokines, proteins, and growth factors were assessed with qPCR using lung tissues. The total RNA was isolated using the RNeasy Plus mini kit (Qiagen) according to the manufacturer’s instructions. For RT-qPCR, 0.5 μg of total RNA was reverse-transcribed to cDNA using Maxima First Strand cDNA Synthesis Kit (Thermo Fisher K1641). Real-time qPCR was then performed using 2 μL of cDNA using Luna Universal qPCR Master Mix (NEB M3003) with SYBR® Green as the fluorescent dye and the 7,500 Fast Real-Time PCR System (Life Technologies). The primer pairs are used at a final concentration of 250 nM. Primer sequences are listed in the box below (Table 1). Cyclophilin was used as the reference gene. The relative transcript abundance of target genes compared with the reference gene was expressed in the cycle threshold (ΔCt) as ΔCt = Ct(target)—Ct(reference). The relative difference in transcript levels of the treated group compared with the control group was expressed as ΔΔCt = ΔCt(treated)—ΔCt(control). The relative fold changes in the transcript level were represented as 2−ΔΔCt.
Data Analysis
In the case of only two groups, two-tail t-tests were performed to assess the differences. All analyses were carried out using Prism7.0 software (GraphPad Software Inc., San Diego, CA). Values are represented as mean ± SEM. Levels of significance designated as p < 0.05 unless otherwise indicated.
Results
E-Cigarette Exposure Aggravates Chronic Obstructive Pulmonary Disease in βENaC Mice
In our study, the
E-Cigarette Exposure Elevates the Inflammatory Response
COPD is characterized by lung inflammation, leading to progressive and irreversible airflow obstruction (King, 2015). Next, we determined the cytokine production in mice with or without exposure to ENDS. We measured the production of major cytokines by real-time qPCRs using lungs from mice of different groups. The results showed that the ENDS exposure significantly increased the production of multiple cytokines, including M-CSF, IL-1r
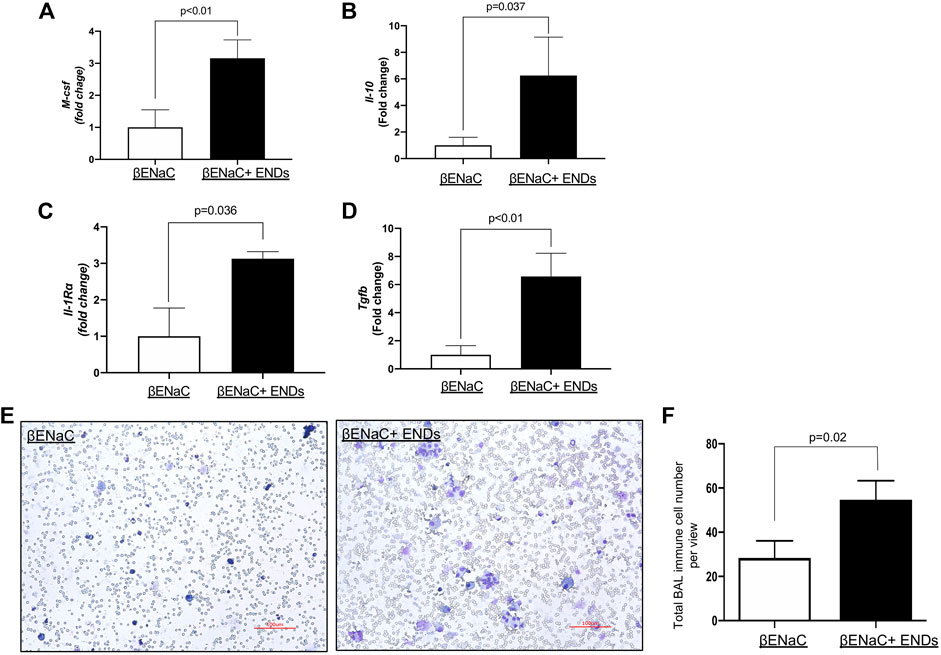
FIGURE 2. E-cigarette exposure elevates inflammatory response. (A–D) Determination of mRNA levels of inflammatory cytokines in lung tissues from βENaC mice with or without ENDS exposure by qPCR. (E) Representative images of Diff-Quik staining of BAL from βENaC mice with or without ENDS exposure (n = 4 per group). (F) Quantification of Diff-Quik staining as in (E) (n = 4 per group). All data presented at mean
E-Cigarette Increases Macrophage Numbers in Lungs
Since ENDS exposure significantly augmented levels of cytokines, which are predominantly secreted by immune cells, for instance, macrophages, so our next question was asked as to whether ENDS exposure modulated populations of immune cells. We then assessed the numbers of macrophages and neutrophils with FACS and found that ENDS exposure increased the numbers of macrophages (Figures 3A,B), but did not change that of neutrophils (Figures 3C,D).
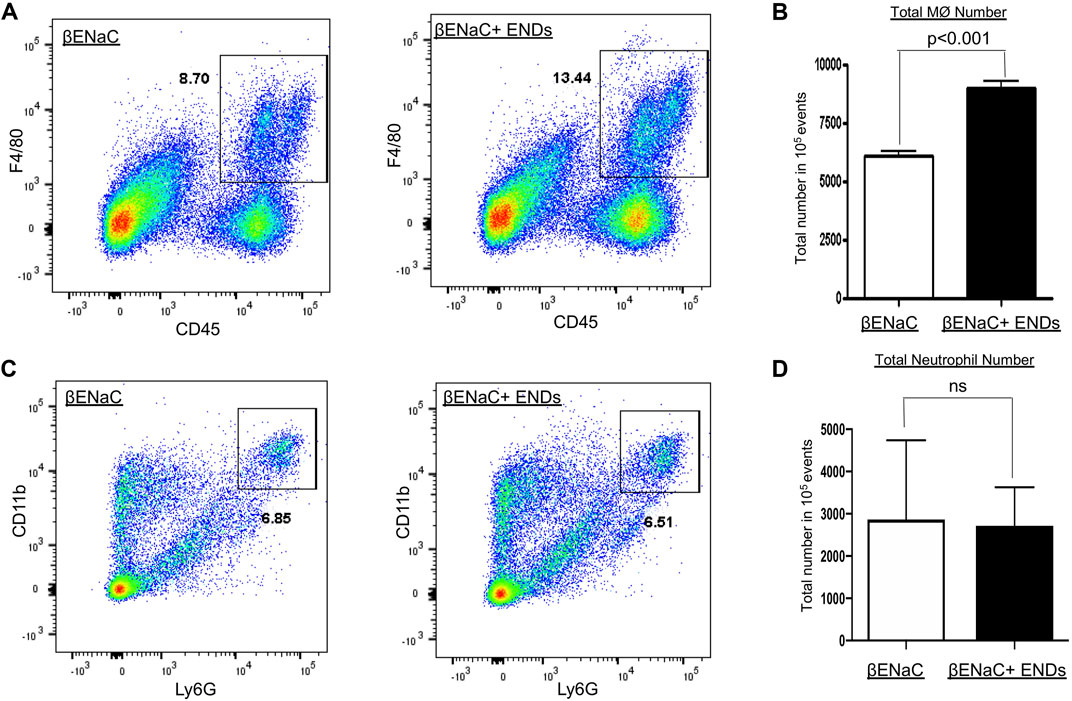
FIGURE 3. E-cigarette increases macrophage numbers in lungs. (A,C) FACS analysis of cell population of CD45+F4/80+ macrophages and CD11b+Ly6G+ neutrophils in lung tissues of βENaC mice with or without ENDS exposure (n = 3 per group). (B,D) Quantification of FCAS analysis of macrophage and neutrophil population as in (A) and (C), respectively. All data presented at mean
E-Cigarette Increases the Population of M2 Phenotype of Macrophages
Macrophages have two subtypes, M1 and M2. M1 and M2 macrophages have different functions and distinct cytokine profiles. The qPCR results showed ENDS promoted the productions of multiple cytokines including M-CSF, IL-1r
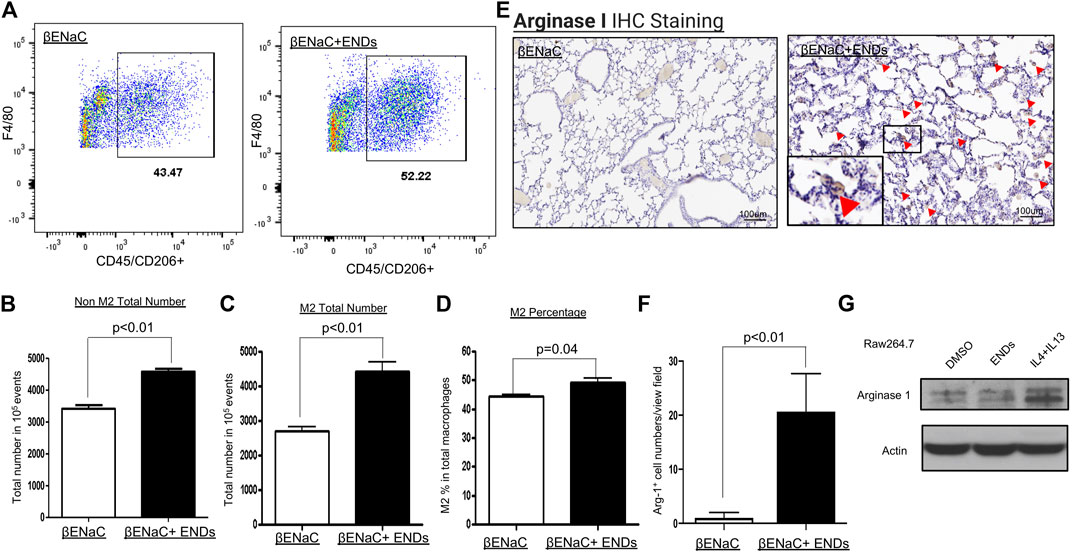
FIGURE 4. E-cigarette increases the population of M2 phenotype of macrophages. (A) FACS analysis of the cell population of F4/80+CD206+ M2 macrophages in lung tissues of βENaC mice with or without ENDS exposure (n = 3 per group). The M2 macrophages were pre-gated on live CD45+ cells. (B) Quantification of FACS analysis of CD45+F4/80+CD206- non-M2 macrophage cell number in lung tissues of βENaC mice with or without ENDS exposure. (C) Quantification of FACS analysis of CD45+F4/80+CD206+ M2 macrophage cell number in lung tissues of βENaC mice with or without ENDS exposure. (D) Quantification of FACS analysis of CD206+CD45+F4/80+ M2 phenotype percentage in CD45+F4/80+ total macrophages in lung tissues of βENaC mice with or without ENDS exposure. (E) Representative images of immunohistochemical (IHC) staining of Arginase I in lung tissues of βENaC mice with or without ENDS exposure. The inset indicates the enlarged image of the boxed area showing positive staining. (F) Quantification of IHC staining of Arginase I as in (E). The positive staining areas were calculated as percentages of total area, using five randomly selected sections per mouse, a total of four mice in each group. (G) Representative blots showing the expression level of Arginase I after indicated treatments. Actin was used as an internal control. Raw264.7 were treated with DMSO or ENDS (50 mg/ml) or IL-4+IL-13 (IL-4 20 ng/ml; IL-13 20 ng/ml) for 48 h and harvested for analysis of protein expression by western blot. Columns and error bars represent means
Since we observed that ENDS increased the M2 macrophage population, then we asked whether ENDS directly induced M2 macrophages polarization. We tested our hypothesis using murine macrophage cell line Raw264.7. We treated Raw264.7 with DMSO, ENDS, or IL-4+IL-13 and then harvested cells for immunoblots of M2 marker Arginase 1. IL-4+IL-13, which are well-known to induce M2 macrophage polarization, are used as a positive control. Immunoblot results demonstrated that ENDS treatment did not increase Arginase 1 expression compared to DMSO treatment, while IL-4+IL-13 upregulated expression of Arginase 1 in Raw264.7 (Figure 4G). Our results suggest that ENDS do not induce M2 macrophage polarization directly in the cultured murine macrophage cell line.
E-Cigarette Exposure Enhances Fibrotic Phenotypes in the Lungs
Substantial studies have shown that M2 macrophages promote lung fibrosis (Song et al., 2000; Bargagli et al., 2011; Hou et al., 2018); and ENDS elevate extracellular matrix accumulation and profibrotic progress (Chapman et al., 2019; Shi et al., 2019; Wang et al., 2019; Wang et al., 2020), we next questioned whether fibrotic phenotypes were enhanced in the lungs after exposure to ENDS. We assessed collagen content using Sirius Red staining and found that ENDS exposure dramatically increased collagen levels in mice (Figures 5A,B). The collagen is predominantly produced by myofibroblasts, a group of key effector cells in fibrosis progression which is considered as a hallmark of fibrosis (Hinz, 2007; Hinz et al., 2007; Kendall and Feghali-Bostwick, 2014). We then stained myofibroblasts in lung tissues from mice with or without ENDS delivery, using a commonly used marker
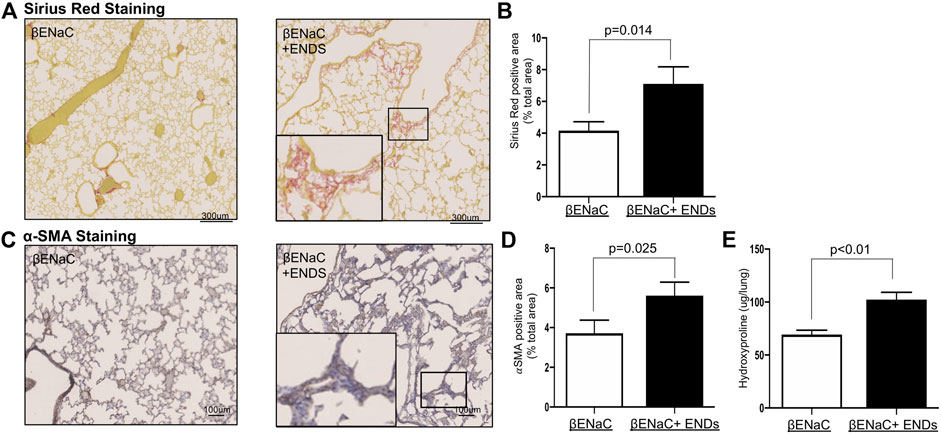
FIGURE 5. E-cigarette exposure enhances fibrosis in the lungs. (A) Representative images of Pico-Sirius Red staining in lung sections from βENaC mice with or without ENDS exposure (n = 4 per group). The inset indicates the enlarged image of the boxed area showing positive staining. (B) Quantitative analysis of Pico-Sirius Red staining as in (A). The positive staining areas were calculated as percentages of total area, using five randomly selected sections per mouse, a total of four mice in each group. (C) Representative images of
Discussion
Smoking is the leading cause of COPD, which accounts for as much as 90% of COPD risk. ENDS are considered as a safe replacement for cigarette smoking. However, increasing concerns have been raised in recent years as ENDS use shows a high correlation with COPD occurrence (Bowler et al., 2017; Perez et al., 2019), the underneath mechanisms of which have not been fully understood. Here we report that ENDS rewired cytokine production in lungs of COPD mice caused by ENDS exposure. Increasing evidence indicates that chronic inflammatory and immune responses play key roles in the development and progression of COPD (Rovina et al., 2013). Our study is the first one to elucidate that ENDS shaped an anti-inflammatory environment by increasing levels of several important anti-inflammatory cytokines and augmenting the population of the anti-inflammatory M2 phenotype of macrophages. We also found that ENDS increased fibrosis in COPD mice. It has been reported that the development of fibrosis in COPD results in a reduction in lung elasticity, which further worsens COPD (Rovina et al., 2013).
We demonstrated that ENDS exposure elevated inflammation response and enhanced lung fibrosis. Lung inflammation and fibrosis are both essentially implicated in the development and progression of COPD; and they also are closed correlated (Ward and Hunninghake, 1998; Rao et al., 2020). Observations that lung tissues from patients with lung fibrosis display significant inflammation are widely documented, suggesting that the inflammatory process could result in lung fibrosis (Ward and Hunninghake, 1998; Bringardner et al., 2008). Intensive investigations reveal that fibrosis seems to be the end outcome of unresolved chronic inflammatory reactions induced by a variety of stimuli and tissue injury (Wynn, 2008). Specifically, in COPD, the pathogenesis usually starts with an inflammatory process (Cornwell et al., 2010). Under certain circumstances, inflammation reactions are not resolved. Such a chronic, persistent inflammatory response results in extensive fibrosis, and consequently, causes an exacerbation of COPD conditions (Barnes, 2014; Barnes, 2019). In the current study, we found that ENDS exposure elevated inflammation response by increasing multiple cytokine production and enhanced pro-fibrotic activity by augmenting M2 macrophage numbers, both of which worsen the COPD symptoms in mice.
We observed ENDS exposure remarkedly elevated the population of M2 macrophages. The balance of M1/M2 macrophages is well-recognized to control the fate of an organ in inflammation or fibrosis (Patel et al., 2017; Chen et al., 2020). M1 macrophages are known as a pro-inflammatory or anti-fibrotic phenotype as they trigger inflammatory responses by producing proinflammatory cytokines and contribute to tissue destruction (Byrne et al., 2016). Conversely, M2 macrophages are known as an anti-inflammatory or pro-fibrotic phenotype, which are implicated in the aberrant wound-healing process during fibrosis by producing pro-fibrotic cytokines (Byrne et al., 2016; Shapouri-Moghaddam et al., 2018). M2 macrophage numbers are reported to be elevated during fibrotic disease; and excessive M2 macrophages contribute to a pathological fibroproliferative response and consequently promote lung fibrosis found in the later phase of acute lung injury and acute respiratory distress syndrome (Janssen et al., 2011; Xiang et al., 2016). Our finding showing that ENDS elevated M2 macrophage numbers suggests ENDS could lead to a fibrotic amplification which may exacerbate COPD in patients.
We further intended to uncover the underlying mechanisms of how ENDS promoted the alteration of macrophage phenotype. We asked whether ENDS directly regulated the M2 phenotype switch. ENDS is commonly made up of four basic ingredients: water, nicotine, flavorings, and propylene glycol (PG) and/or vegetable glycerin base (VG). Nicotine is usually thought to be the major toxic component in ENDS. It has been shown that cigarette smoke extract (CSE) and pure nicotine can induce M2 phenotype change (Lu et al., 2017; Alqasrawi et al., 2020), so we hypothesized that ENDS may directly induce macrophage M2 polarization via nicotine. However, murine macrophage Raw264.7 did not display an increase in Arginase 1 expression level after the treatment of nicotine-containing ENDS juice, which means the ENDS might not directly induce macrophage M2 polarization. This suggests the importance of vaping smoking in causing the M2 macrophage phenotype. Our results elucidated that anti-inflammatory cytokines were increased after ENDS delivery. Such change in cytokines profile is reported as a stimulus to induce macrophage polarization in murine models of emphysema (Kohler et al., 2019). So, the change in cytokine profile, instead of being a consequence of M2 macrophage polarization, might be the cause of M2 macrophage phenotype change.
Myofibroblasts are major effector cells during lung injury and repair. Our results showed that ENDS exposure increased the number of
Besides, a typical feature of widespread peribronchiolar fibrosis with collagen deposition in COPD patients suggests fibrosis is probably the earliest and an important mechanism for COPD progression, which however has been long neglected with very few studies (Mcdonough et al., 2011; Hogg et al., 2017; Koo et al., 2018; Barnes, 2019). In this study, we have also investigated fibrotic phenotypes in mice after ENDS exposure. We observed a significant increase in collagen accumulation and α-SMA positive myofibroblast population, indicating ENDS enhanced fibrotic phenotypes in COPD mice. It is plausible that the increase in M2 macrophages induced by ENDS results in the augment of fibrotic phenotypes.
In addition, a notable phenomenon in our study is that ENDS remarkably enriched the myofibroblast population, suggesting ENDS might induce the activation of myofibroblasts. The myofibroblast is the predominant cell type that is responsible for the deposition of extracellular matrix and is considered as a hallmark of fibrotic diseases (Hinz et al., 2007; Hinz, 2016; Pakshir et al., 2020). And we observed lung fibrosis was enhanced in mice who received ENDS exposure. It would be interesting to understand whether ENDS can stimulate the differentiation of fibroblasts to myofibroblasts. This might uncover another potential risk of ENDS as it might promote lung fibrosis development via inducing myofibroblasts differentiation.
In conclusion, our study elucidates that ENDS exposure elevates COPD features and causes inflammatory response alteration with an increase in M2 macrophage number. The changes in inflammatory response resulted from ENDS further lead to an enhancement in lung fibrosis. Our study provides us a deeper understanding of the mechanism how ENDS affects COPD. Lastly, though βENaC-overexpressing mice serve as a good species exposed to ENDS for induction of animal model of COPD, the effect of ENDS on wild type mice still requires further investigations.
Data Availability Statement
The original contributions presented in the study are included in the article/Supplementary Material, further inquiries can be directed to the corresponding author.
Ethics Statement
The animal study was reviewed and approved by IACUC at Georgia State University.
Author Contributions
HH, GP, MM, and XJ planned and designed experiments and wrote the manuscript. HH, GP, and MM carried out the animal studies performed molecular and cell biology experiments. HH and GP acquired histology analysis. GP performed flow cytometry and FACs analysis. HY, JY, M-HZ, Z-RL, and XJ provided intellectual oversight and reviewed the manuscript. HY provided pathological support and statistical analyses.
Funding
This work was supported by FAMRI foundation YFEL141014 to XJ. This work was also supported by R42AA112713 from NIH to JY, an Institutional Development Award from the NIGMS of NIH under grant #P20GM103652 to HY and Dr. Ralph and Marian Falk Medical Research Trust Awards to HY.
Conflict of Interest
The authors declare that the research was conducted in the absence of any commercial or financial relationships that could be construed as a potential conflict of interest.
Publisher’s Note
All claims expressed in this article are solely those of the authors and do not necessarily represent those of their affiliated organizations, or those of the publisher, the editors and the reviewers. Any product that may be evaluated in this article, or claim that may be made by its manufacturer, is not guaranteed or endorsed by the publisher.
Acknowledgments
We thank Jian-dong Li (Georgia State University) for his useful discussions.
References
Alqasrawi, D., Abdelli, L. S., and Naser, S. A. (2020). Mystery Solved: Why Smoke Extract Worsens Disease in Smokers with Crohn's Disease and Not Ulcerative Colitis? Gut MAP!. Microorganisms 8, 666. doi:10.3390/microorganisms8050666
An, Y., Liu, F., Chen, Y., and Yang, Q. (2020). Crosstalk between Cancer‐associated Fibroblasts and Immune Cells in Cancer. J. Cel Mol Med 24, 13–24. doi:10.1111/jcmm.14745
Bagalad, B., Mohan Kumar, K., and Puneeth, H. (2017). Myofibroblasts: Master of Disguise. J. Oral Maxillofac. Pathol. 21, 462–463. doi:10.4103/jomfp.jomfp_146_15
Bargagli, E., Prasse, A., Olivieri, C., Muller-Quernheim, J., and Rottoli, P. (2011). Macrophage-Derived Biomarkers of Idiopathic Pulmonary Fibrosis. Pulm. Med. 2011, 717130. doi:10.1155/2011/717130
Barnes, P. J. (2014). Cellular and Molecular Mechanisms of Chronic Obstructive Pulmonary Disease. Clin. Chest Med. 35, 71–86. doi:10.1016/j.ccm.2013.10.004
Barnes, P. J. (2016). Inflammatory Mechanisms in Patients with Chronic Obstructive Pulmonary Disease. J. Allergy Clin. Immunol. 138, 16–27. doi:10.1016/j.jaci.2016.05.011
Barnes, P. J. (2019). Small Airway Fibrosis in COPD. Int. J. Biochem. Cel Biol. 116, 105598. doi:10.1016/j.biocel.2019.105598
Bartalesi, B., Cavarra, E., Fineschi, S., Lucattelli, M., Lunghi, B., Martorana, P. A., et al. (2005). Different Lung Responses to Cigarette Smoke in Two Strains of Mice Sensitive to Oxidants. Eur. Respir. J. 25, 15–22. doi:10.1183/09031936.04.00067204
Belok, S. H., Parikh, R., Bernardo, J., and Kathuria, H. (2020). E-cigarette, or Vaping, Product Use-Associated Lung Injury: a Review. Pneumonia 12, 1–8. doi:10.1186/s41479-020-00075-2
Bhatt, J. M., Ramphul, M., and Bush, A. (2020). An Update on Controversies in E-Cigarettes. Paediatric Respir. Rev. 36, 75. doi:10.1016/j.prrv.2020.09.003
Bhatta, D. N., and Glantz, S. A. (2020). Association of E-Cigarette Use with Respiratory Disease Among Adults: a Longitudinal Analysis. Am. J. Prev. Med. 58, 182–190. doi:10.1016/j.amepre.2019.07.028
Bowler, R. P., Hansel, N. N., Hansel, N. N., Jacobson, S., Graham Barr, R., Make, B. J., et al. (2017). Electronic Cigarette Use in US Adults at Risk for or with COPD: Analysis from Two Observational Cohorts. J. Gen. Intern. Med. 32, 1315–1322. doi:10.1007/s11606-017-4150-7
Breland, A., Soule, E., Lopez, A., Ramôa, C., El-Hellani, A., and Eissenberg, T. (2017). Electronic Cigarettes: what Are They and what Do They Do?. Ann. N.Y. Acad. Sci. 1394, 5–30. doi:10.1111/nyas.12977
Bringardner, B. D., Baran, C. P., Eubank, T. D., and Marsh, C. B. (2008). The Role of Inflammation in the Pathogenesis of Idiopathic Pulmonary Fibrosis. Antioxid. Redox Signaling 10, 287–302. doi:10.1089/ars.2007.1897
Byrne, A. J., Maher, T. M., and Lloyd, C. M. (2016). Pulmonary Macrophages: A New Therapeutic Pathway in Fibrosing Lung Disease?. Trends Mol. Med. 22, 303–316. doi:10.1016/j.molmed.2016.02.004
Caramori, G., Casolari, P., Di Gregorio, C., Saetta, M., Baraldo, S., Boschetto, P., et al. (2009). MUC5AC Expression Is Increased in Bronchial Submucosal Glands of Stable COPD Patients. Histopathology 55, 321–331. doi:10.1111/j.1365-2559.2009.03377.x
Chapman, D. G., Casey, D. T., Ather, J. L., Aliyeva, M., Daphtary, N., Lahue, K. G., et al. (2019). The Effect of Flavored E-Cigarettes on Murine Allergic Airways Disease. Scientific Rep. 9, 13671. doi:10.1038/s41598-019-50223-y
Chávez-Galán, L., Olleros, M. L., Vesin, D., and Garcia, I. (2015). Much More Than M1 and M2 Macrophages, There Are Also CD169(+) and TCR(+) Macrophages. Front. Immunol. 6, 263. doi:10.3389/fimmu.2015.00263
Chen, X., Tang, J., Shuai, W., Meng, J., Feng, J., and Han, Z. (2020). Macrophage Polarization and its Role in the Pathogenesis of Acute Lung Injury/acute Respiratory Distress Syndrome. Inflamm. Res. 69, 883–895. doi:10.1007/s00011-020-01378-2
Cherian, S. V., Kumar, A., and Estrada-Y-Martin, R. M. (2020). E-cigarette or Vaping Product-Associated Lung Injury: a Review. Am. J. Med. 133, 657–663. doi:10.1016/j.amjmed.2020.02.004
Churg, A., Zhou, S., and Wright, J. L. (2012). Matrix Metalloproteinases in COPD. Eur. Respir. J. 39, 197–209. doi:10.1183/09031936.00121611
Comito, G., Giannoni, E., Segura, C. P., Barcellos-De-Souza, P., Raspollini, M. R., Baroni, G., et al. (2014). Cancer-associated Fibroblasts and M2-Polarized Macrophages Synergize during Prostate Carcinoma Progression. Oncogene 33, 2423–2431. doi:10.1038/onc.2013.191
Cornwell, W., Kim, V., Song, C., and Rogers, T. (2010). Pathogenesis of Inflammation and Repair in Advanced COPD. Semin. Respir. Crit. Care Med. 31, 257–266. doi:10.1055/s-0030-1254066
Da Silva, C. O., Gicquel, T., Daniel, Y., Bartholo, T., Vene, E., Loyer, P., et al. (2020). Alteration of Immunophenotype of Human Macrophages and Monocytes after Exposure to Cigarette Smoke. Scientific Rep. 10, 1–13. doi:10.1038/s41598-020-68753-1
Da Silva, M. D., Bobinski, F., Sato, K. L., Kolker, S. J., Sluka, K. A., and Santos, A. R. S. (2015). IL-10 Cytokine Released from M2 Macrophages Is Crucial for Analgesic and Anti-inflammatory Effects of Acupuncture in a Model of Inflammatory Muscle Pain. Mol. Neurobiol. 51, 19–31. doi:10.1007/s12035-014-8790-x
Das, A., Sinha, M., Datta, S., Abas, M., Chaffee, S., Sen, C. K., et al. (2015). Monocyte and Macrophage Plasticity in Tissue Repair and Regeneration. Am. J. Pathol. 185, 2596–2606. doi:10.1016/j.ajpath.2015.06.001
Dunnill, M. S. (1962). Quantitative Methods in the Study of Pulmonary Pathology. Thorax 17, 320–328. doi:10.1136/thx.17.4.320
Eapen, M. S., Hansbro, P. M., Mcalinden, K., Kim, R. Y., Ward, C., Hackett, T. L., et al. (2017a). Abnormal M1/M2 Macrophage Phenotype Profiles in the Small Airway wall and Lumen in Smokers and Chronic Obstructive Pulmonary Disease (COPD). Sci. Rep. 7, 13392. doi:10.1038/s41598-017-13888-x
Eapen, M. S., Myers, S., Walters, E. H., and Sohal, S. S. (2017b). Airway Inflammation in Chronic Obstructive Pulmonary Disease (COPD): a True Paradox. Expert Rev. Respir. Med. 11, 827–839. doi:10.1080/17476348.2017.1360769
Eisner, M. D., Anthonisen, N., Coultas, D., Kuenzli, N., Perez-Padilla, R., Postma, D., et al. (2010). An Official American Thoracic Society Public Policy Statement: Novel Risk Factors and the Global burden of Chronic Obstructive Pulmonary Disease. Am. J. Respir. Crit. Care Med. 182, 693–718. doi:10.1164/rccm.200811-1757st
Fernando, M. R., Giembycz, M. A., and Mckay, D. M. (2016). Bidirectional Crosstalk via IL-6, PGE2 and PGD2 between Murine Myofibroblasts and Alternatively Activated Macrophages Enhances Anti-inflammatory Phenotype in Both Cells. Br. J. Pharmacol. 173, 899–912. doi:10.1111/bph.13409
Funes, S. C., Rios, M., Escobar-Vera, J., and Kalergis, A. M. (2018). Implications of Macrophage Polarization in Autoimmunity. Immunology 154, 186–195. doi:10.1111/imm.12910
Garcia-Arcos, I., Geraghty, P., Baumlin, N., Campos, M., Dabo, A. J., Jundi, B., et al. (2016). Chronic Electronic Cigarette Exposure in Mice Induces Features of COPD in a Nicotine-dependent Manner. Thorax 71, 1119–1129. doi:10.1136/thoraxjnl-2015-208039
Gilpin, W. (2019). Cellular Automata as Convolutional Neural Networks. Phys. Rev. E 100, 032402. doi:10.1103/physreve.100.032402
Gordon, S., and Martinez, F. O. (2010). Alternative Activation of Macrophages: Mechanism and Functions. Immunity 32, 593–604. doi:10.1016/j.immuni.2010.05.007
Hiemstra, P. S. (2013). Altered Macrophage Function in Chronic Obstructive Pulmonary Disease. Ann. ATS 10, S180–S185. doi:10.1513/annalsats.201305-123aw
Hinz, B. (2007). Formation and Function of the Myofibroblast during Tissue Repair. J. Invest. Dermatol. 127, 526–537. doi:10.1038/sj.jid.5700613
Hinz, B., Phan, S. H., Thannickal, V. J., Galli, A., Bochaton-Piallat, M.-L., and Gabbiani, G. (2007). The Myofibroblast. Am. J. Pathol. 170, 1807–1816. doi:10.2353/ajpath.2007.070112
Hogg, J. C., Paré, P. D., and Hackett, T.-L. (2017). The Contribution of Small Airway Obstruction to the Pathogenesis of Chronic Obstructive Pulmonary Disease. Physiol. Rev. 97, 529. doi:10.1152/physrev.00025.2015
Hou, J., Shi, J., Chen, L., Lv, Z., Chen, X., Cao, H., et al. (2018). M2 Macrophages Promote Myofibroblast Differentiation of LR-MSCs and Are Associated with Pulmonary Fibrogenesis. Cell Commun. Signaling 16, 89. doi:10.1186/s12964-018-0300-8
Hunninghake, G. M., Cho, M. H., Tesfaigzi, Y., Soto-Quiros, M. E., Avila, L., Lasky-Su, J., et al. (2009). MMP12,Lung Function, and COPD in High-Risk Populations. N. Engl. J. Med. 361, 2599–2608. doi:10.1056/nejmoa0904006
Janssen, W. J., Barthel, L., Muldrow, A., Oberley-Deegan, R. E., Kearns, M. T., Jakubzick, C., et al. (2011). Fas Determines Differential Fates of Resident and Recruited Macrophages during Resolution of Acute Lung Injury. Am. J. Respir. Crit. Care Med. 184, 547–560. doi:10.1164/rccm.201011-1891oc
Johannesson, B., Hirtz, S., Schatterny, J., Schultz, C., and Mall, M. A. (2012). CFTR Regulates Early Pathogenesis of Chronic Obstructive Lung Disease in βENaC-overexpressing Mice. PloS one 7, e44059. doi:10.1371/journal.pone.0044059
Johnson, Z. I., Lopresti, S., Lantonio, B., Wells, A., Ismail, N., Brown, B. N., et al. (2018). Bi-directional Macrophage-Fibroblast Crosstalk Directs Wound Resolution Factors. FASEB J. 32, 412–414. doi:10.1096/fasebj.2018.32.1_supplement.414.2
Kapellos, T. S., Bassler, K., Aschenbrenner, A. C., Fujii, W., and Schultze, J. L. (2018). Dysregulated Functions of Lung Macrophage Populations in COPD. J. Immunol. Res. 2018, 2349045. doi:10.1155/2018/2349045
Kendall, R. T., and Feghali-Bostwick, C. A. (2014). Fibroblasts in Fibrosis: Novel Roles and Mediators. Front. Pharmacol. 5, 123. doi:10.3389/fphar.2014.00123
King, P. T. (2015). Inflammation in Chronic Obstructive Pulmonary Disease and its Role in Cardiovascular Disease and Lung Cancer. Clin. translational Med. 4, 68. doi:10.1186/s40169-015-0068-z
Kohler, J. B., Cervilha, D. A. B., Riani Moreira, A., Santana, F. R., Farias, T. M., Alonso Vale, M. I. C., et al. (2019). Microenvironmental Stimuli Induce Different Macrophage Polarizations in Experimental Models of Emphysema. Biol. Open 8, bio040808. doi:10.1242/bio.040808
Koo, H.-K., Vasilescu, D. M., Booth, S., Hsieh, A., Katsamenis, O. L., Fishbane, N., et al. (2018). Small Airways Disease in Mild and Moderate Chronic Obstructive Pulmonary Disease: a Cross-Sectional Study. Lancet Respir. Med. 6, 591–602. doi:10.1016/s2213-2600(18)30196-6
Li, J., and Ye, Z. (2020). The Potential Role and Regulatory Mechanisms of MUC5AC in Chronic Obstructive Pulmonary Disease. Molecules 25, 4437. doi:10.3390/molecules25194437
Lu, J., Xie, L., Liu, C., Zhang, Q., and Sun, S. (2017). PTEN/PI3k/AKT Regulates Macrophage Polarization in Emphysematous Mice. Scand. J. Immunol. 85, 395–405. doi:10.1111/sji.12545
Mall, M. A., Harkema, J. R., Trojanek, J. B., Treis, D., Livraghi, A., Schubert, S., et al. (2008). Development of Chronic Bronchitis and Emphysema in β-Epithelial Na+Channel-Overexpressing Mice. Am. J. Respir. Crit. Care Med. 177, 730–742. doi:10.1164/rccm.200708-1233oc
Mall, M., Grubb, B. R., Harkema, J. R., O'neal, W. K., and Boucher, R. C. (2004). Increased Airway Epithelial Na+ Absorption Produces Cystic Fibrosis-like Lung Disease in Mice. Nat. Med. 10, 487–493. doi:10.1038/nm1028
Mantovani, A., Biswas, S. K., Galdiero, M. R., Sica, A., and Locati, M. (2013). Macrophage Plasticity and Polarization in Tissue Repair and Remodelling. J. Pathol. 229, 176–185. doi:10.1002/path.4133
Mantovani, A., Sica, A., Sozzani, S., Allavena, P., Vecchi, A., and Locati, M. (2004). The Chemokine System in Diverse Forms of Macrophage Activation and Polarization. Trends Immunology 25, 677–686. doi:10.1016/j.it.2004.09.015
Martinez, F. O., and Gordon, S. (2014). The M1 and M2 Paradigm of Macrophage Activation: Time for Reassessment. F1000prime Rep. 6, 13. doi:10.12703/p6-13
Mcalinden, K. D., Eapen, M. S., Lu, W., Sharma, P., and Sohal, S. S. (2020). The Rise of Electronic Nicotine Delivery Systems and the Emergence of Electronic-Cigarette-Driven Disease. Am. J. Physiol. Lung Cel Mol Physiol 319, L585–L595. doi:10.1152/ajplung.00160.2020
Mcdonough, J. E., Yuan, R., Suzuki, M., Seyednejad, N., Elliott, W. M., Sanchez, P. G., et al. (2011). Small-airway Obstruction and Emphysema in Chronic Obstructive Pulmonary Disease. N. Engl. J. Med. 365, 1567–1575. doi:10.1056/nejmoa1106955
Monteran, L., and Erez, N. (2019). The Dark Side of Fibroblasts: Cancer-Associated Fibroblasts as Mediators of Immunosuppression in the Tumor Microenvironment. Front. Immunol. 10, 1835. doi:10.3389/fimmu.2019.01835
Mosser, D. M., and Edwards, J. P. (2008). Exploring the Full Spectrum of Macrophage Activation. Nat. Rev. Immunol. 8, 958–969. doi:10.1038/nri2448
Pakshir, P., Noskovicova, N., Lodyga, M., Son, D. O., Schuster, R., Goodwin, A., et al. (2020). The Myofibroblast at a Glance. J. Cel Sci. 133, jcs227900. doi:10.1242/jcs.227900
Patel, U., Rajasingh, S., Samanta, S., Cao, T., Dawn, B., and Rajasingh, J. (2017). Macrophage Polarization in Response to Epigenetic Modifiers during Infection and Inflammation. Drug Discov. Today 22, 186–193. doi:10.1016/j.drudis.2016.08.006
Perez, M. F., Atuegwu, N. C., Mead, E. L., Oncken, C., and Mortensen, E. M. (2019). Adult E-Cigarettes Use Associated with a Self-Reported Diagnosis of COPD. Int. J. Environ. Res. Public Health 16, 3938. doi:10.3390/ijerph16203938
Polosa, R., Morjaria, J. B., Caponnetto, P., Prosperini, U., Russo, C., Pennisi, A., et al. (2016). Evidence for Harm Reduction in COPD Smokers Who Switch to Electronic Cigarettes. Respir. Res. 17, 166. doi:10.1186/s12931-016-0481-x
Polosa, R., Morjaria, J. B., Prosperini, U., Busà, B., Pennisi, A., Malerba, M., et al. (2020). COPD Smokers Who Switched to E-Cigarettes: Health Outcomes at 5-year Follow up. Ther. Adv. Chronic Dis. 11, 2040622320961617. doi:10.1177/2040622320961617
Quaderi, S., and Hurst, J. (2018). The Unmet Global burden of COPD. Glob. Health Epidemiol. Genom 3, e4. doi:10.1017/gheg.2018.1
Rao, W., Wang, S., Duleba, M., Niroula, S., Goller, K., Xie, J., et al. (2020). Regenerative Metaplastic Clones in COPD Lung Drive Inflammation and Fibrosis. Cell 181, 848–864.e818. doi:10.1016/j.cell.2020.03.047
Rovina, N., Koutsoukou, A., and Koulouris, N. G. (2013). Inflammation and Immune Response in COPD: where Do We Stand?. Mediators Inflamm. 2013, 413735. doi:10.1155/2013/413735
Rőszer, T. (2015). Understanding the Mysterious M2 Macrophage through Activation Markers and Effector Mechanisms. Mediators Inflamm. 2015, 816460. doi:10.1155/2015/816460
Seys, L. J., Verhamme, F. M., Dupont, L. L., Desauter, E., Duerr, J., Agircan, A. S., et al. (2015). Airway Surface Dehydration Aggravates Cigarette Smoke-Induced Hallmarks of COPD in Mice. PLoS One 10, e0129897. doi:10.1371/journal.pone.0129897
Shapouri-Moghaddam, A., Mohammadian, S., Vazini, H., Taghadosi, M., Esmaeili, S. A., Mardani, F., et al. (2018). Macrophage Plasticity, Polarization, and Function in Health and Disease. J. Cel Physiol 233, 6425–6440. doi:10.1002/jcp.26429
Shi, H., Fan, X., Horton, A., Haller, S. T., Kennedy, D. J., Schiefer, I. T., et al. (2019). The Effect of Electronic-Cigarette Vaping on Cardiac Function and Angiogenesis in Mice. Scientific Rep. 9, 4085. doi:10.1038/s41598-019-40847-5
Shields, P. G., Berman, M., Brasky, T. M., Freudenheim, J. L., Mathe, E., Mcelroy, J. P., et al. (2017). A Review of Pulmonary Toxicity of Electronic Cigarettes in the Context of Smoking: a Focus on Inflammation. Cancer Epidemiol. Biomarkers Prev. 26, 1175–1191. doi:10.1158/1055-9965.epi-17-0358
Shuto, T., Kamei, S., Nohara, H., Fujikawa, H., Tasaki, Y., Sugahara, T., et al. (2016). Pharmacological and Genetic Reappraisals of Protease and Oxidative Stress Pathways in a Mouse Model of Obstructive Lung Diseases. Scientific Rep. 6, 1–15. doi:10.1038/srep39305
Song, E., Ouyang, N., Hörbelt, M., Antus, B., Wang, M., and Exton, M. S. (2000). Influence of Alternatively and Classically Activated Macrophages on Fibrogenic Activities of Human Fibroblasts. Cell Immunol. 204, 19–28. doi:10.1006/cimm.2000.1687
Traboulsi, H., Cherian, M., Abou Rjeili, M., Preteroti, M., Bourbeau, J., Smith, B. M., et al. (2020). Inhalation Toxicology of Vaping Products and Implications for Pulmonary Health. Int. J. Mol. Sci. 21, 3495. doi:10.3390/ijms21103495
Van Linthout, S., Miteva, K., and Tschöpe, C. (2014). Crosstalk between Fibroblasts and Inflammatory Cells. Cardiovasc. Res. 102, 258–269. doi:10.1093/cvr/cvu062
Wang, Q., Ahmad Khan, N., Muthumalage, T., Lawyer, G. R., Mcdonough, S. R., Chuang, T. D., et al. (2019). Dysregulated Repair and Inflammatory Responses by E‐cigarette‐derived Inhaled Nicotine and Humectant Propylene Glycol in a Sex‐dependent Manner in Mouse Lung. FASEB BioAdvances 1, 609–623. doi:10.1096/fba.2019-00048
Wang, Q., Lucas, J. H., Sundar, I., and Rahman, I. (2020). Electronic-Cigarette Induces Dysregulated Repair Response and Extracellular Matrix Remodeling in Mouse Lung via ±7 Nicotinic Acetylcholine Receptor. 21 (1), 154. doi:10.21203/rs.2.23829/v1
Ward, P. A., and Hunninghake, G. W. (1998). Lung Inflammation and Fibrosis. Am. J. Respir. Crit. Care Med. 157, S123–S129. doi:10.1164/ajrccm.157.4.nhlbi-10
Wynn, T. (2008). Cellular and Molecular Mechanisms of Fibrosis. J. Pathol. 214, 199–210. doi:10.1002/path.2277
Xiang, J., Cheng, S., Feng, T., Wu, Y., Xie, W., Zhang, M., et al. (2016). Neotuberostemonine Attenuates Bleomycin-Induced Pulmonary Fibrosis by Suppressing the Recruitment and Activation of Macrophages. Int. Immunopharmacology 36, 158–164. doi:10.1016/j.intimp.2016.04.016
Keywords: ENDS, COPD, M2 macrophage, lung fibrosis, inflammation
Citation: Han H, Peng G, Meister M, Yao H, Yang JJ, Zou M-H, Liu Z-R and Ji X (2021) Electronic Cigarette Exposure Enhances Lung Inflammatory and Fibrotic Responses in COPD Mice. Front. Pharmacol. 12:726586. doi: 10.3389/fphar.2021.726586
Received: 17 June 2021; Accepted: 14 July 2021;
Published: 28 July 2021.
Edited by:
Jian Gao, Second Affiliated Hospital of Dalian Medical University, ChinaReviewed by:
Hongguang Nie, China Medical University, ChinaQixin Wang, University of Rochester, United States
Copyright © 2021 Han, Peng, Meister, Yao, Yang, Zou, Liu and Ji. This is an open-access article distributed under the terms of the Creative Commons Attribution License (CC BY). The use, distribution or reproduction in other forums is permitted, provided the original author(s) and the copyright owner(s) are credited and that the original publication in this journal is cited, in accordance with accepted academic practice. No use, distribution or reproduction is permitted which does not comply with these terms.
*Correspondence: Xiangming Ji, xji4@gsu.edu