- 1Department of Nephrology, Shaanxi Traditional Chinese Medicine Hospital, Xi’an, China
- 2The First School of Clinical Medicine, Shaanxi University of Traditional Chinese Medicine, Xianyang, China
- 3Faculty of Life Science and Medicine, Northwest University, Xi’an, China
- 4Department of Clinical Pharmacy, Affiliated Hospital of Chengdu University, Chengdu, China
The Wnt/β-catenin signaling pathway plays important roles in embryonic development and tissue homeostasis. Wnt signaling is induced, and β-catenin is activated, associated with the development and progression of renal fibrosis. Wnt/β-catenin controls the expression of various downstream mediators such as snail1, twist, matrix metalloproteinase-7, plasminogen activator inhibitor-1, transient receptor potential canonical 6, and renin-angiotensin system components in epithelial cells, fibroblast, and macrophages. In addition, Wnt/β-catenin is usually intertwined with other signaling pathways to promote renal interstitial fibrosis. Actually, given the crucial of Wnt/β-catenin signaling in renal fibrogenesis, blocking this signaling may benefit renal interstitial fibrosis. There are several antagonists of Wnt signaling that negatively control Wnt activation, and these include soluble Fzd-related proteins, the family of Dickkopf 1 proteins, Klotho and Wnt inhibitory factor-1. Furthermore, numerous emerging small-molecule β-catenin inhibitors cannot be ignored to prevent and treat renal fibrosis. Moreover, we reviewed the knowledge focusing on anti-fibrotic effects of natural products commonly used in kidney disease by inhibiting the Wnt/β-catenin signaling pathway. Therefore, in this review, we summarize recent advances in the regulation, downstream targets, role, and mechanisms of Wnt/β-catenin signaling in renal fibrosis pathogenesis. We also discuss the therapeutic potential of targeting this pathway to treat renal fibrosis; this may shed new insights into effective treatment strategies to prevent and treat renal fibrosis.
Introduction
Chronic kidney disease (CKD) is an increasingly serious public health problem due to its high prevalence and mortality and greatly increases the risk of end-stage renal disease (ESRD), and cardiovascular disease (Webster et al., 2017). Renal fibrosis is the final pathological, dynamic, progressive, and irreversible process common to any ongoing CKD or maladaptive repair (Djudjaj and Boor, 2019). Renal fibrosis is the accumulation of scars in the parenchyma that is a pathological expansion of the normal wound healing process, characterized by inflammation, myofibroblast activation, migration, and matrix deposition and remodeling, leading to the replacement of functional parenchyma by fibrotic tissues (Humphreys, 2018; Distler et al., 2019). Renal interstitial fibrosis is mainly driven by various pro-fibrotic growth factors, forming a fibrotic micro-environment in the interstitial space (Chen et al., 2018c; Tang et al., 2019). In other words, the major pathological events of renal fibrosis include inflammatory cell infiltration, fibroblast activation and proliferation, and abnormal increase and excessive deposition of extracellular matrix (ECM) components, mainly composed of collagen, fibronectin, and proteoglycans (Liu et al., 2021). With the ECM continuous deposition, scar tissue replaces normal tissue, tubules, and peritubular capillaries are lost, resulting in disruption of tissue architecture and loss of renal function (Xing et al., 2021). Additionally, there is growing evidence that ECM-derived components could be used as danger-associated molecular patterns (DAMPs). As an important promoter of fibrogenesis, as long as the inflammatory stimulation persists, these DAMPs are generated and release signals during the phase cell activation and damage, ultimately promoting inflammation to fibrosis and kidney disease (Nastase et al., 2018).
The Wnt/β-catenin signaling pathway is an evolutionarily conserved developmental signaling pathway, playing an extremely important role in organogenesis, tissue homeostasis, and disease progression of multicellular organisms (Schunk et al., 2021). There are 19 identified encoding Wnt genes in the mammalian genome, all of which are cysteine-rich proteins (Langton et al., 2016). Wnt protein induces β-catenin-dependent signaling through Wnt receptor coiled Frizzled (FZD) and co-receptors low-density lipoprotein receptor-related protein-5/protein-6 (LRP5/6) (Janda et al., 2017). In addition, there are other receptors and co-receptors, including the tyrosine kinase receptors RYK, single transmembrane receptor tyrosine kinase, G-protein coupled receptor, etc., that trigger various downstream signaling pathways (Foulquier et al., 2018). Continuous accumulation of intracellular β-catenin signaling plays a crucial role in developing renal fibrosis, podocyte injury, proteinuria, persistent tissue damage during acute kidney injury, and cystic kidney diseases (Miao et al., 2019; Schunk et al., 2021). The latest research shows that given the crucial role of Wnt/β-catenin signaling in renal fibrogenesis, blocking this signaling may be beneficial to alleviate renal fibrosis (Xie et al., 2021; Yiu et al., 2021). Recent studies have shown that apigenin (API) could effectively relieve renal fibrosis via co-inhibiting uric acid (UA) reabsorption and the Wnt/β-catenin signaling pathway (Li et al., 2021). In addition, ischemia-reperfusion injury (IRI) could increase indoleamine-2,3-dioxygenase (IDO) expression to activate the Wnt/β-catenin pathway leading to renal fibrosis. Prostaglandin E2 (PGE2) could ameliorate kidney fibrosis via inhibiting IDO expression and reducing β-catenin resulting in lower expressions of α-smooth muscle actin (α-SMA), fibronectin (Pan et al., 2021). In vivo and in vitro, it has proved that the abnormally expressed cannabinoid receptor type 2 (CB2) is closely related to renal fibrosis via β-arrestin 1-induced β-catenin activation, and β-catenin could promote the activation and expression of CB2, and finally forms the vicious circle in the CB2/β-catenin pathway (Zhou et al., 2021). Therefore, it is of great significance to clarify the cellular and molecular mechanisms of the Wnt/β-catenin signaling pathway in tubulointerstitial fibrosis and provide a new treatment strategy for antifibrosis and delaying CKD progression. In this review, we summarize recent advances on the involvement of Wnt/β-catenin in the pathogenesis of tubulointerstitial fibrosis and the intervention effect of natural products targeting the Wnt/β-catenin signaling pathway.
Wnt/β-Catenin Signaling Pathways
The mechanisms of Wnt signaling consist of two main branches: the canonical and non-canonical pathways (Schunk et al., 2021). The canonical pathway is also known as the Wnt/β-catenin pathway. Furthermore, two master non-canonical pathways are the Wnt/planar cell polarity pathway (Wnt/PCP pathway) and the Wnt/calcium pathway (Wnt/Ca2+ pathway) (Hu et al., 2020). According to downstream effects, all Wnt ligands are divided into two categories: one is canonical, Wnt ligands that induce β-catenin-dependent pathway, including Wnt1, 2, 3, 8a, 8b, 10a, and 10b, and the other is non-canonical, Wnt ligands that mediate β-catenin-independent pathway, including Wnt4, 5a, 5b, 6, 7a, 7b, and 11 (Acebron and Niehrs, 2016). In the canonical Wnt/β-catenin signaling pathway, Wnt molecules transmit the intracellular signal in the intracellular matrix through interacting with FZD receptors and the co-receptor LRP5/6 (Figure 1). FZD proteins have seven transmembrane receptors, with a cysteine-rich domain responsible for the binding of Wnt proteins (Schunk et al., 2021). Since the activation of LRP5/6 depends on the binding of the canonical Wnt ligands to the FZD receptors, they are considered to be the key co-receptors of the canonical Wnt signaling pathway (Huang et al., 2019). After that, the interaction triggers the intracellular signal cascade and promotes the accumulation of non-phosphorylated β-catenin, which translocates into the nucleus, and cooperates with the transcription factors T cell factor (TCF)/lymphatic enhancer-binding factor (LEF) to trigger the transcription of Wnt target genes (Tessa et al., 2020). β-Catenin is an important co-factor that binds multiple transcriptional molecules and mediates fibrogenic signaling pathways (Yang et al., 2021). In a steady-state, β-catenin is inactivated by a “destruction complex,” which includes the proteins glycogen synthase kinase 3β (GSK3β), adenomatous polyposis coli (APC), casein kinase 1 (CK1), and axin (Wang et al., 2020a). The protein complex mediates the phosphorylation, ubiquitinylation, and degradation of β-catenin, and through continuous ubiquitination and degradation, the cytoplasmic levels are kept at a low level (Schunk et al., 2021). However, once Wnt ligands bind to co-receptors on cytomembrane, the combination of FZD and Dishevelled (DVL) could provide a recruitment platform for the β-catenin destruction (Gammons et al., 2016). DVL protein is recruited, and the ‘destruction complex’ is disrupted, protecting β-catenin from inactivation and degradation, thus leading to the stabilization, accumulation, and nuclear translocation of β-catenin (Nusse and Clevers, 2017). An increasing number of studies have demonstrated that fibroblast signaling pathways all merge on β-catenin to promote the β-catenin/TCF complex and mediate fibrogenesis (Yang et al., 2021). β-Catenin integrates the inputs of transforming growth factor β (TGF-β)/Smad, integrin/ILK, the Wnt/β-catenin pathway and renin-angiotensin system (RAS), which are activated in fibrotic primary and allograft kidney diseases (Huang et al., 2019).
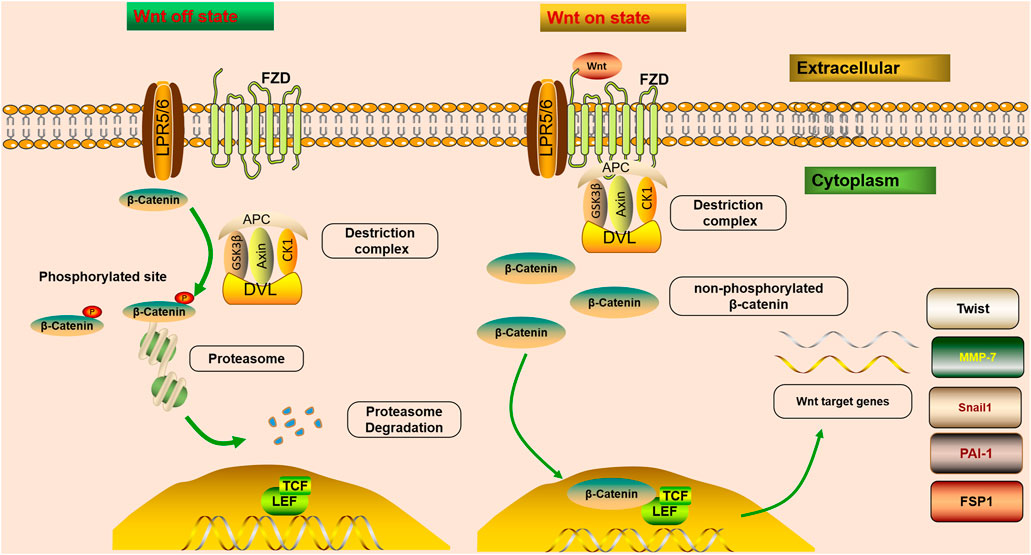
FIGURE 1. Wnt/β-catenin signaling is off or on state. In steady-state, β-catenin is inactivated by a “destruction complex,” and phosphorylation, ubiquitinylated, and degraded of β-catenin is mediated by the protein complex. Wnt on state; Wnt molecules transmit the intracellular signal in the intracellular matrix through interacting with FZD receptors and LRP5/6. After that, the interaction triggers the intracellular signal cascade and promotes the accumulation of non-phosphorylated β-catenin, which translocates into the nucleus, and cooperates with TCF/LEF to trigger the transcription of Wnt target genes. Once Wnt ligands bind to co-receptors on cytomembrane, the combination of FZD and DVL could provide a recruitment platform for the β-catenin destruction. Wnt/β-catenin controls the expression of various downstream mediators implicated in renal fibrosis, such as Snail1, MMP-7, PAI-1, Twist, and FSP1.
Wnt/β-Catenin Signaling Pathway in Renal Fibrosis
The Wnt/β-catenin pathway is one of the crucial signaling pathways resulting in kidney disease. An increasing number of studies have demonstrated that the activation of the Wnt/β-catenin signaling pathway serves a key role in promoting renal fibrosis by controlling the expression of various downstream mediators implicated in renal fibrosis (Chen et al., 2017b; Feng et al., 2019a; Schunk et al., 2021) (Figure 1). The transient activation of many signaling pathways has a beneficial effect on repairing damaged tissues. However, their sustained activation promotes fibrosis (Edeling et al., 2016). It has been confirmed that severe ischemia/reperfusion injury leads to sustained and excessive activation of Wnt/β-catenin, accompanied by interstitial myofibroblast activation and ECM deposition, characteristics of renal fibrotic lesions development (Xiao et al., 2016). Therefore, sustained and exaggerated Wnt/β-catenin activation mediates fibroblast activation. Although transient Wnt/β-catenin activation promotes tissue regeneration and repair after kidney injury, sustained or uncontrolled Wnt/β-catenin signaling stimulates podocyte injury and proteinuria, ultimately leading to irreversible renal fibrosis (Schunk et al., 2021).
Fibroblast
Fibroblasts are the main driving force for scar formation after kidney injury (Miao et al., 2021). The continuous activation of fibroblasts leads to the secretion of ECM components, such as collagen, proteoglycan, and fibronectin, leading to the development of renal fibrosis (Feng et al., 2019b). As an ECM glycoprotein, fibronectin serves a key role in wound healing and fibrosis by regulating the deposition of collagen and other ECM molecules. A study confirmed that upregulated Wnt/β-catenin signaling is related to the response of epithelial cells to wound, renal tubular cell damage, fibrous collagen, and immunoglobulin transcript expression (Venner et al., 2016). Thus, Wnt protein derived from renal tubules may play a critical role in fibroblast activation and renal fibrosis (Zhou et al., 2017). There is a complex regulatory network between renal tubular epithelial cells and fibroblasts, regulated by autocrine and paracrine Wnt/β-catenin signaling (Maarouf et al., 2016). The activated Wnt/β-catenin pathway can promote the fibroblast proliferation and differentiation of fibroblasts towards myofibroblasts; myofibroblasts are critical contributors to renal fibrosis. Their characteristics are secreting fibronectin and increasing the expression of α-SMA (Zhou et al., 2017). In a high glucose environment, activating the Wnt/β-catenin pathway could promote renal mesangial cell proliferation and fibronectin production. Interestingly, fibronectin is an important target gene of Wnt/β-catenin (Zhang et al., 2014). Therefore, inhibiting the activity of Wnt/β-catenin signaling in fibroblasts may help alleviate the progression of renal fibrosis (Duan et al., 2020).
Macrophages
According to the activation mechanism and cell function, macrophages are divided into classically activated macrophages (M1) and alternatively activated macrophages (M2) (Wang et al., 2014a). M1, a pro-inflammatory phenotype, releases cytokines that inhibit the proliferation of surrounding cells and damage contiguous tissue, while M2, an anti-inflammatory phenotype, releases cytokines that promote the proliferation of contiguous cells and tissue repair (Wang et al., 2014a; Cosin-Roger et al., 2019). If macrophages fail to acquire a tissue-healing phenotype, dysregulated signals can be drivers of disease processes, such as sustained, exuberant inflammation and fibrosis (Smigiel and Parks, 2018). Thus, macrophages serve a key role in immune surveillance and in the maintenance of renal homeostasis. Macrophages recruited from the bone marrow can transition directly into myofibroblasts during renal injury. This process is defined as macrophage-to-myofibroblast transition, which may play a crucial role in the progression of chronic inflammation to pathogenic fibrosis (Tang et al., 2019). Sustained accumulation and activation of macrophages in kidney tissue could lead to the production of multiple pro-fibrotic cytokines and ultimately induce renal fibrosis. Additionally, as key inflammatory cells, macrophages could promote ECM synthesis and deposition, resulting in renal fibrosis by releasing inflammatory cytokines, TGF-β, and matrix-degrading enzyme inhibitors (Yang et al., 2019). Activation of Wnt/β-catenin signaling stimulates renal inflammation, comprising macrophages infiltration, pro-inflammatory cytokines release, and cell adhesion molecules expression in renal injury. Moreover, tubular cell-derived Wnt ligands mediate pro-inflammatory activation of renal macrophages during fibrosis (Wong et al., 2018). Previous studies have shown that the hyperactive of Wnt/β-catenin signaling could promote renal fibrosis by stimulating macrophage M2 polarization and promoting the proliferation and accumulation of macrophages. The continuous accumulation and activation of macrophages may lead to various fibrotic cytokines and ultimately lead to kidney fibrosis (Feng et al., 2018). Therefore, as an important source of Wnt protein in adult tissues, macrophages proliferate and accumulate in kidney tissues through the activation of the Wnt/β-catenin signal, considered a key factor in renal fibrosis (Cosin-Roger et al., 2019).
Snail1
Snail family zinc finger 1 (Snail1) is a transcription factor expressed during embryonic renal development and is widely expressed in various kidney injury models, including unilateral ureteral obstruction (UUO), 5/6 nephrectomy, and hypoxia. It is involved in regulating fatty acid metabolism, cell cycle arrest, and inflammatory response, major biological processes responsible for renal fibrogenesis (Simon-Tillaux and Hertig, 2017). Snail1 is a key transcription factor driving epithelial-mesenchymal transition (EMT); the stabilized β-catenin enters the cell nucleus, forms a complex with TCF, and activates the transcription of Snail1 to drive EMT. Moreover, the up-regulation of TGF-β1 promotes Snail1-mediated EMT of renal tubular epithelial cells during renal fibrosis. Not only that, but also Snail1 is a critical transcription target of β-catenin that upregulates β-catenin transcriptional activity; as such, both β-catenin and Snail1 may be activated simultaneously to produce an additive or synergistic effect in promoting EMT (García de Herreros and Baulida, 2012). Thus, the Snail1-induced EMT process is a key mechanism that initiates the reaction cascade leading to fibrosis (Bai et al., 2016). Additionally, it has been found in a modern study that the Snail1/β-catenin signaling pathway may be involved in promoting renal fibrosis related to diabetes (Kim et al., 2017). Furthermore, it has been demonstrated that Snail1s could interact with β-catenin functionally, thereby increasing the expression of Wnt-dependent target genes (Stemmer et al., 2008).
Matrix Metalloproteinase-7
MMP-7, also known as matrilysin, is a secreted zinc- and calcium-dependent endopeptidase, a transcriptional target of classic Wnt/β-catenin signaling, a pathological mediator, and therapeutic target of renal fibrosis (Wozniak et al., 2021). Under normal physiologic conditions, MMP-7 is almost not expressed in adult kidneys but upregulated in various renal diseases, including AKI and CKD (Wozniak et al., 2021). MMP-7 can degrade ECM components and cleave various substrates, such as E-cadherin, Fas ligand, and nephrin. Therefore, it plays a key role in regulating various biological processes, such as cell proliferation, apoptosis, EMT, and podocyte damage (Tan et al., 2019). Furthermore, MMP-7, via its proteolytic activity, mediates proteolytic degradation of E-cadherin, resulting in β-catenin liberation and activation, leading to renal fibrosis in a Wnt-independent fashion. It is worth noting that the release of β-catenin mediated by MMP-7 further induces the expression of MMP-7, eventually forming a vicious circle (Liu et al., 2020). In other words, on the one hand, activation of Wnt/β-catenin promotes the occurrence of fibrosis by upregulating pro-fibrotic mediators, including MMP-7, PAI-1. On the other hand, MMP-7, as the most powerful β-catenin downstream target, can activate the Wnt/β-catenin signaling pathway after renal injury (Tan et al., 2019). In summary, renal MMP-7 levels correlate with Wnt/β-catenin activity, and urinary MMP-7 may be a noninvasive biomarker of pro-fibrotic signaling in the kidney. However, in several AKI animal models (IRI, cisplatin administration, and folic-acid induced AKI), MMP7 exerts protective effects on the kidney as an adaptive response. Therefore, the role of MMP-7 as a therapeutic target for kidney disease needs further study.
Plasminogen Activator Inhibitor-1
PAI-1, as a member of the serine protease inhibitor family, interferes with ECM and fibrin degradation, mediated by urokinase-type plasminogen activator (uPA) and tissue-type plasminogen activator (tPA) to suppress fibrinolysis and contribute to interstitial fibrosis in the kidney injury (Flevaris and Vaughan, 2017). Increasing evidence shows the role of PAI1 in renal fibrosis (Zhou et al., 2015a). PAI-1 promotes fibrosis by participating in various cellular processes, such as inflammation, cell adhesion, and migration. Conversely, some specific factors that promote fibrosis, such as oxidative stress and hypoxia, could also affect the expression of the PAI-1 gene (Rabieian et al., 2018). And depleting PAI-1 can alleviate interstitial fibrosis by decreasing fibroblast activation and proliferation in the renal interstitium (Yao et al., 2019). Therefore, PAI-1, a key molecule in renal fibrosis progression, has increased expression in various kidney disease models (Hamasaki et al., 2013). In addition, a recent study indicated that PAI-1 could promote cell migration through LRP1-dependent β-catenin activation (Kozlova et al., 2015). Since the promoter region of PAI-1 contains a TCF/LEF binding site, PAI-1 is an important target gene of β-catenin signaling in renal injury (Malik et al., 2020).
Components of the Renin-Angiotensin System
The RAS serves a vital role in maintaining renal hemodynamics and the occurrence of hypertension and kidney disease (Navar, 2014). Unanimously, renal tissue RAS has various pathophysiological functions in regulating blood pressure, growth of kidney cells, and glomerular sclerosis, leading to renal fibrosis development (Urushihara et al., 2012). The (pro)renin receptor ((P)RR), consisting of 350 amino acids, has been considered as a single-transmembrane protein encoded by ATP6AP2, an X chromosome-located gene, and the transmembrane receptors enhance the tissue RAS by binding to their ligands renin and/or prorenin; therefore, it is initially considered to be an important part of the RAS and is ubiquitously expressed in the human body (Ramkumar and Kohan, 2016; Ichihara and Yatabe, 2019). The receptor plays crucial roles in various pathways, involved in extensive physiological and pathological processes, such as the cell cycle, autophagy, acid-base balance, energy metabolism, T cell homeostasis, blood pressure regulation, cardiac remodeling, and maintaining podocyte structure (Ichihara and Yatabe, 2019; Wang et al., 2020a). The Wnt-RAS signaling serves a vital role in the development and progression of CKD (Zhou et al., 2020). There are putative TCF/LEF binding sites in the RAS promoter region through bioinformatics analyses, and β-catenin could trigger LEF-1 to bind to these sites in renal tubular cells (Zhou et al., 2015b). In addition, (P)RR is necessary for signal transduction through FZD-LRP5/6 (Li et al., 2017). Moreover, accumulating evidence has demonstrated that (P)RR is a downstream target and a crucial element in Wnt signal transmission, promoting kidney damage and fibrosis through amplifying Wnt/β-catenin signaling transduction. In addition, Wnt/β-catenin, as the main upstream regulator, controls the expression of multiple RAS genes. In other words, the overactivity of β-catenin or different Wnt ligands leads to the expression of all RAS genes (Zhou and Liu, 2015; Zhao et al., 2018). Hence, targeting Wnt/β-catenin would concurrently inhibit all RAS genes, accordingly suppressing inflammation and alleviating renal fibrosis (Zhou and Liu, 2016). Studies have demonstrated that the fibrogenic action of Wnt/β-catenin is dependent on RAS activation, and Wnt/β-catenin regulates multiple RAS genes. At the same time, RAS can induce the expression of multiple Wnt genes in vivo and in vitro; as such, the Wnt/β-catenin-RAS axis can be known as a vicious circle in aggravating the renal injury (Xiao et al., 2019).
Transient Receptor Potential Canonical 6
TRPC6 has been implicated in the pathogenesis of kidney diseases, including focal segmental glomerulosclerosis (FSGS), diabetic nephropathy (DN), immune-mediated kidney disease, and renal fibrosis. As a result, TRPC6 has become a critical target of therapeutic agents to prevent and treat various kidney diseases (Hall et al., 2019). TRPC6 is another transcriptional target of the Wnt/β-catenin signaling cascade, and the Wnt/β-catenin signaling pathway may potentially be active in the pathogenesis of TRPC6-mediated diabetic podocyte injury (Zhang et al., 2013; Kim and Dryer, 2021). TRPC6 knockout shows protection on UUO-triggered kidney tubulointerstitial injury, interstitial fibrosis, and α-SMA expression (Gu et al., 2020). Mutations and over-activation in TRPC6 channel activity play an important role in podocyte damage in DN(Staruschenko et al., 2019; Wang et al., 2020c), However its role in renal fibrosis and the interaction with the Wnt signaling pathway in renal fibrosis still need further study.
In summary, the Wnt/β-catenin signaling pathway could activate renal fibrosis-related cytokines and up-regulate the expression of downstream target genes, eventually inhibit the main pathological process in the fibrosis process, and improve renal fibrosis. Therefore, we can further explore the downstream targets of the Wnt/β-catenin signaling pathway on this basis to further improve the mechanism of the Wnt/β-catenin signaling pathway in renal fibrosis.
The Crosstalk Between Wnt/β-Catenin and Other Signaling Pathways on Renal Interstitial Fibrosis
Various pathways intersect and regulate each other to induce appropriate responses to a series of complex stimuli. Therefore, the synergy between other pathological signaling pathways and Wnt may play an important role in promoting renal fibrosis. RNA sequencing showed that deleting TGF-β receptors in proximal renal tubular cells regulated many growth factor pathways, but Wnt/β-catenin signaling is the most affected pathway due to the activity of β-catenin that is impaired in vivo and in vitro (Nlandu-Khodo et al., 2017). The participation of the Wnt signaling pathway enhances the pro-fibrotic effect of the TGF-β signaling pathway (Yang et al., 2020). In hypoxic pathological damage of organs, overactivation hypoxia-inducible factor-1α (HIF-1α) activates the Wnt/β-catenin signaling pathway, thereby aggravating renal interstitial fibrosis development (Qi et al., 2017). Although the Hedgehog (HH) signaling pathway is considered upstream of the Wnt/β-catenin signaling pathway, there is overlap between the two phenotypic results, suggesting a synergistic effect. Wnt and Notch interact mostly synergistically in the stem cell and epithelial cell compartment to trigger fibrosis development via suppressing epithelial differentiation; Notch, as a negative regulator of the Wnt/β-catenin signaling pathway, promotes β-catenin degradation by establishing a complex with β-catenin (Edeling et al., 2016; Chatterjee and Sil, 2019). However, the mechanism of the interaction between the signaling pathways is still unclear, and the studies on the mechanism of their synergy with the Wnt/β-catenin signaling pathway should be further explored.
Targeting Wnt/β-Catenin Signaling as a Therapeutic Potential for Renal Fibrosis
Accumulating evidence has demonstrated that inhibiting the Wnt signaling pathway could alleviate renal interstitial fibrosis by attenuating apoptosis and expression of fibrosis-associated markers in renal cells (Ren et al., 2019; Cai et al., 2020; Huang et al., 2020). Endogenous Wnt inhibitors can negatively regulate the Wnt signaling pathway by binding to Wnt ligands competitively with Wnt receptors or co-receptors, such as Dickkopf1 (DKK1), secreted frizzled protein 1 (Sfrp1), Wnt inhibitor 1 (Wif-1), Klotho (Kawazoe et al., 2021). In addition, exogenous Wnt signaling inhibitors accompanied by natural products cannot be ignored to prevent and treat renal fibrosis. Collectively, the Wnt/β-catenin signaling pathway may serve as a potential treatment strategy for renal fibrotic disorders.
Endogenous Wnt Inhibitors
Secreted Frizzled-Related Protein1
Humans have five secreted frizzled-related proteins (Sfrp1-5) with cysteine-rich domains (CRD), and these Sfrps have a strong homology with FZD receptors, therefore compete with FZD receptors for Wnt binding. In other words, Sfrps act as a Wnt inhibitor. The Sfrp family consists of secreted glycoproteins that can competitively bind to Wnt, inhibiting the canonical and non-canonical Wnt signaling pathways (Cruciat and Niehrs, 2013). Studies have confirmed that Sfrp1 regulates cell proliferation and differentiation by regulating Wnt/β-catenin signaling, showing low expression in various tumor tissues (Qiao et al., 2017). Thus, Sfrp1 is a Wnt antagonist that acts as a negative regulator of Wnt/β-catenin signaling and serves a key role in fibrotic diseases. In the mouse model of UUO, knockout of Sfrp1 significantly increases the expression of α-SMA and the protein level of vimentin; meanwhile, it decreases the protein level of E-cadherin, which enhances the epithelial to mesenchymal transition (Matsuyama et al., 2014). Additionally, down-regulation of Sfrp1 activates the Wnt/β-catenin signaling pathway, increased ECM deposition, eventually lead to renal fibrosis. Therefore, Sfrp1 acts as a negative regulator of the Wnt signaling pathway and suppresses renal fibrosis via inhibiting the Wnt/β-catenin signaling pathway.
Klotho
Klotho, an anti-aging protein, reduces renal fibrosis after AKI. Klotho serves a key role in regulating various cellular processes by interacting with multiple signaling molecules, including oxidative stress, fibrosis, inflammation, autophagy, and apoptosis (Hu et al., 2013). Therefore, Klotho is a critical gene, controlling aging and kidney homeostasis, and an ideal intervention target for various kidney diseases and even extrarenal complications (Xia and Cao, 2021). Furthermore, Klotho plays an important role in anti-fibrotic activities by inhibiting oxidative stress and excessive inflammation. Hence, Klotho deficiency enhances renal fibrosis (Lindberg et al., 2014). It has been shown that the extracellular domain of Klotho inhibits Wnt signaling via binding to multiple Wnt ligands (Muñoz-Castañeda et al., 2020). Thus, Klotho is a critical negative regulator of canonical Wnt signaling and suppresses renal fibrosis in the obstructed kidney model by simultaneously suppressing multiple growth factor signaling pathways such as fibroblast growth factor-2 (FGF-2), Wnt, and TGF-β1 (Guan et al., 2014). A study has found that Klotho represses the Wnt/β-catenin pathway in renal tubular epithelial cells (TECs) to exert a stronger anti-fibrotic effect (Zhang et al., 2018a). Wnt/β-catenin activation is considered to be the key factor resulting in Klotho downregulation (Muñoz-Castañeda et al., 2017). In addition, the upregulation of Klotho prevents Wnt activation, thereby inhibiting the deposition of ECM and reducing the transcription of cytokines, ultimately improving renal fibrosis (Zhou L. et al., 2013). Therefore, Klotho is termed an antagonist of endogenous Wnt/β-catenin activity, and increasing Klotho levels could be a strategy to reduce the morbidity and mortality of kidney-related diseases (Muñoz-Castañeda et al., 2020).
Dickkopf 1
DKK1 is an important member of the DKK family (DKK1, DKK2, DKK3, DKK4) and is widely expressed in many fields. It has been considered a secreted protein that can suppress the Wnt signaling transduction pathway (Huang et al., 2018). DKK1 may play a crucial role in the fibrotic process in several organs such as the liver, lungs, and kidneys (Klavdianou et al., 2017). Gene therapy using DKK1 significantly suppresses fibroblast-specific protein 1(fsp1), a marker for fibroblasts and myofibroblasts, type I collagen, and fibronectin mRNA in the model of obstructive nephropathy, thereby repressing the activation of myofibroblast and improving renal fibrosis (He et al., 2009). In vivo, DKK1 effectively inhibited inflammation and fibrosis associated with ureteral obstruction (Johnson et al., 2017). DKK1 can inhibit the canonical Wnt signaling pathway through binding to LRP5/6, as well as interrupting the formation of the LRP and Wnt protein complex. (Hou et al., 2021), Therefore, DKK1 is termed an inhibitor of canonical Wnt/β-catenin signaling (Lipphardt et al., 2019). As a Wnt antagonist, DKK1 blocks Wnt-mediated fibrosis and also down-regulates its expression under fibrotic conditions. Therefore, it is termed a comprehensive regulator of the Wnt signaling pathway and has been proven to participate in renal fibrosis, glucose metabolism, and inflammation (Huang et al., 2018). Overall, there is no doubt that the outlook for DKK1 target therapy is promising. DKK1, DKK2, and DKK4 act as Wnt antagonists by directly binding to LRP5/6, thereby inhibiting Wnt/β-catenin-dependent signaling (Joiner et al., 2013). However, in UUO rat models and adenine-induced nephropathy, DKK3 is a major driver of renal fibrosis (Federico et al., 2016), and the exact role of DKK3 on Wnt/β-catenin signaling remains poorly unclear.
Wnt Inhibitor Factor 1
Wif-1 is an antagonist of the Wnt signaling pathway, inhibiting the Wnt signaling pathway by binding to the Wnt ligand. Hypermethylation of the Wif-1 promoter leads to down-regulation of Wif-1 expression, which activates the Wnt signaling pathway, further promotes cell proliferation, and induces cell apoptosis (Lin et al., 2017). On the contrary, the recovered Wif-1 expression level inhibits the Wnt signaling pathway. In fibroblasts from Systemic sclerosis (SSc) patients, an autoimmune disease characterized by extensive visceral organ and skin fibrosis, expression of Wif-1 is decreased. And knockdown of Wif-1 in normal fibroblasts induces Wnt signaling and collagen production (Svegliati et al., 2014). In the prevention and treatment of various diseases, Wif-1 plays an important role in inhibiting cell proliferation and migration by inhibiting the Wnt/β-catenin signaling pathway, but its role in renal fibrosis needs further study.
Exogenous Wnt Inhibitors
The Wnt/β-catenin signaling pathway can be therapeutically targeted at several steps (Figure 2). Firstly, blocking the production of all active Wnts. Porcupine (PORCN) is a membrane-bound O-acyltransferase required for Wnt palmitoylation, secretion, and biologic activity, and PORCN inhibitors prevent the release of Wnt ligands, such as Wnt-c59, LGK974(Wnt-974), IWP2, and IWP-L6 (Moon et al., 2017). Secondly, inhibitors of GSK3β, axin, DVL, and CK1 interfere with Wnt/β-catenin downstream signaling. Numerous studies have shown that GSK-3β activation plays a wide spectrum of important roles in tissue fibrosis (Zhuang et al., 2018; Zeng et al., 2019). SB-216763, a GSK-3β inhibitor, protects against Aldo-induced renal injury by activating autophagy and might be a therapeutic option for renal fibrosis (Zhang et al., 2018b). Substrate competitive inhibitors (SCIs), a novel small molecule GSK-3 inhibitor, is considered to be highly selective and more suitable for clinical practice (Rippin et al., 2020). In addition, CHIR99021 (Ng-Blichfeldt et al., 2019), L807mts, (Licht-Murava et al., 2016) and LY2090314 (Tu et al., 2017) have been produced as emerging small molecule inhibitors of GSK-3β. Tankyrase (Tnks) is a transferase that targets axin for proteasome degradation, and loss of Tnks activity leads to accelerated β-catenin destruction. XAV939 and inhibitors of Wnt response (IWR) act as Tankyrase inhibitors, which ultimately promote β-catenin degradation and inhibit Wnt/β-catenin signaling transcription (Kulak et al., 2015). In addition, E-7449 (Plummer et al., 2020) and K-476 (Kinosada et al., 2021), as new inhibitors of Tnks, have been widely used in anti-tumor therapy, and their anti-fibrotic mechanism needs to be further studied. BMD4722 specifically inhibits DVL by inhibiting the protein-protein interaction approach (Ma et al., 2018). IC261 is discovered as an ATP-competitive inhibitor of CK1 (Xian et al., 2021). Finally, inhibitors of TCF/LEF prevent Wnt/β-catenin-dependent gene transcription. CREB-binding protein (CBP) acts as a co-activator of multiple transcription factors for Wnt signal transduction formed with TCF. ICG-001, a small-molecule β-catenin inhibitor, inhibits the canonical Wnt/β-catenin signaling pathway by binding to CBP and blocking the interaction between β-catenin and CBP (Akcora et al., 2018). It inhibits renal tubular EMT by suppressing the transcription of a series of β-catenin-driven genes, such as Snail1, PAI-1, collagen I, fibronectin, and RAS, thereby ameliorates interstitial myofibroblast activation, represses matrix deposition, relieves proteinuria, ameliorates kidney inflammation, and alleviates fibrosis and exerts renal protection (Xiao et al., 2019). In a UUO murine model, ICG-001 could reduce the macrophage-to-myofibroblast transition of bone marrow-derived macrophages in renal fibrosis by inhibiting the β-catenin/TCF interaction (Yang et al., 2019). Therefore, ICG-001 not only prevents but reverses established fibrosis (Henderson et al., 2010). Moreover, PRI-724, as a selective inhibitor of CBP/β-catenin interaction, specifically destroys the interaction between β-catenin and CBP and has an encouraging effect in anti-liver fibrosis (Nishikawa et al., 2018).
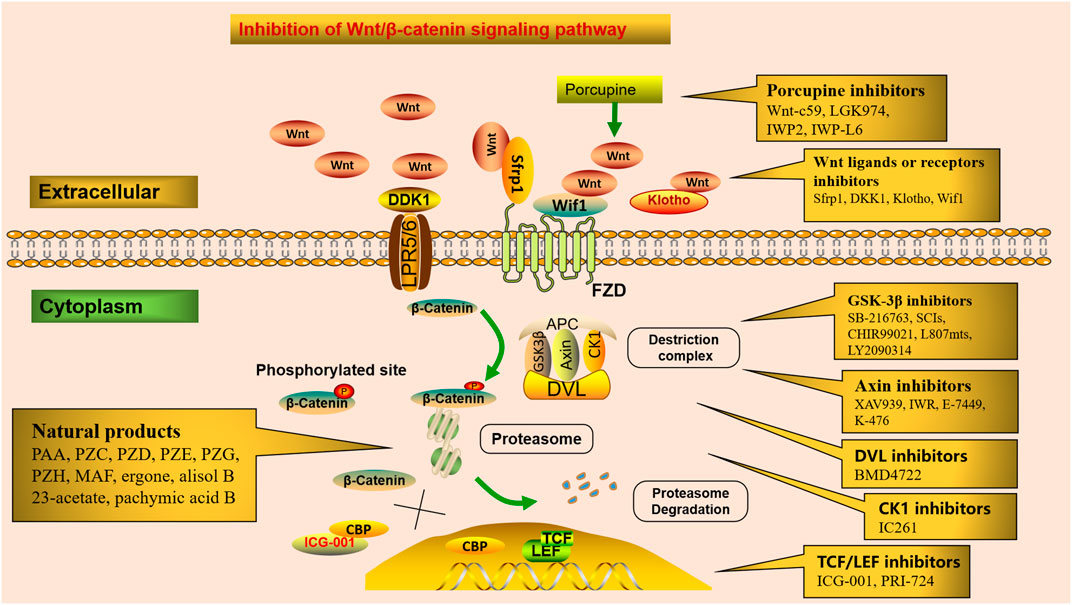
FIGURE 2. Potential therapeutic targets in the Wnt/β-catenin signaling. Numerous small molecules inhibit Wnt/β-catenin signaling at different steps of the pathway. Porcupine inhibitors prevent Wnt ligands secretion. Receptor or co-receptor inhibitors prevent the receptor actions of Wnt ligands. Inhibitors of GSK3β, axin, DVL, and CK1 interfere with Wnt/β-catenin downstream signaling. Inhibitors of TCF/LEF suppress Wnt/β-catenin-dependent gene transcription. Several natural products alleviate renal fibrosis by regulating the Wnt/β-catenin signaling pathway.
Specific Inhibition of Wnt/β-Catenin Signaling by Natural Products
Modern clinical pharmacological studies have confirmed that Chinese herbal medicines (CHMs) have a wide range of biological activities and play a broad and important role in regulating immune function by exerting their anti-cancer, anti-inflammatory, and anti-fibrosis effects (Zhao, 2013; Chen et al., 2016; Chen et al., 2018a; Wu et al., 2021). Clinical trials and experimental studies have shown that CHMs have great advantages in reducing proteinuria and improving renal function, by focusing on the anti-inflammatory, anti-oxidative, anti-apoptotic and anti-fibrotic effects (Chen et al., 2019a; Miao et al., 2020; Wang et al., 2020b; Wang et al., 2021a; Wang et al., 2021b). In addition, CHMs play a vital role in alleviating renal fibrosis by regulating the Wnt/β-catenin signaling pathway (Liu et al., 2019; Dai et al., 2020).
“Concept of holism” and “treatment based on syndrome differentiation” are the basic principles of traditional Chinese medicine (TCM) throughout the treatment of diseases. A study confirmed that Qishen Yiqi dripping pill (QYDP) reduces the renal Wnt1, β-catenin, TGF-β1, and Smad2 gene expression and downregulates collagen I, α-SMA, and fibronectin expression significantly in diabetic rats. The study results showed that QYDP ameliorates kidney function and renal fibrosis in diabetic rats by repressing the Wnt/β-catenin and TGF-β/Smad2 signaling pathways (Zhang et al., 2020). Qingshen Buyang Formula significantly reduces the expression of collagen I and fibronectin, the main components of ECM. Furthermore, by inhibiting EMT and Wnt/β-catenin signaling pathway, it improves renal injury and relieves renal fibrosis (Zhang et al., 2019). Zhen-Wu-tang alleviates adenine-induced chronic renal failure (CRF) by regulating the canonical Wnt4/β-catenin signaling, associated with improvement of renal fibrosis because it suppresses the overexpression of collagen IV and fibronectin, two key components of fibrosis (La et al., 2018). Huang Gan Formula is a new prescription developed and simplified based on uremia clearing granule and the theoretical basis of TCM. HGF inhibits the Wnt/β-catenin signaling pathway, significantly reducing glomerulosclerosis and tubular interstitial fibrosis and improving residual renal function (Mo et al., 2015).
Several compounds isolated from natural products promote urination and eliminate edema, which greatly benefits renal disease and fibrosis (Zhao et al., 2009; Zhao et al., 2012a; Zhao L. et al., 2013; Zhao Y.-Y. et al., 2013; Tian et al., 2014; Zhao et al., 2014). As an edible mushroom, Poria Cocos is widely used for diuretic, anti-inflammatory, antioxidant, lipid-lowering, and anti-fibrotic effects (Zhao et al., 2012b; Feng et al., 2013; Wang et al., 2013b; Miao et al., 2016; Chen et al., 2019b; Feng et al., 2019c). Poricoic acid A (PAA), as the main triterpenoid compound of Poria Cocos, exhibits renoprotective effects, (Chen et al., 2019c; Feng et al., 2019a; Chen et al., 2020a) and PAA showed anti-fibrotic effects via regulating the Wnt/β-catenin pathway (Chen et al., 2019d). In the HK-2 cell and UUO model, poricoic acid ZC (PZC), poricoic acid ZD (PZD), and poricoic acid ZE (PZE) could alleviate renal fibrosis by effectively blocking RAS by simultaneously targeting multiple RAS components, correlated with activation of Wnt/β-catenin pathways (Wang et al., 2018a). Additionally, poricoic acid ZG (PZG) and poricoic acid ZH (PZH) significantly suppress the activation of Wnt/β-catenin signaling and relieve renal fibrosis (Wang et al., 2018b). 25-O-methylalisol F is a new tetracyclic triterpenoid compound isolated from the Alismatis rhizome that exhibits renoprotective effects (Chen et al., 2014; Feng et al., 2014; Tian et al., 2014; Dou et al., 2018), and is a novel RAS inhibitor by simultaneously targeting multiple RAS components (Chen et al., 2018b). Ergone, alisol B 23-acetate, and pachymic acid B inhibit ECM accumulation, suppress oxidative stress and inflammation, and regulates the Wnt/β-catenin signaling pathway (Zhao et al., 2011; Chen et al., 2017a; Chen et al., 2019e; Chen et al., 2020b).
Curcumin alleviated ECM accumulation in diabetic nephropathy by down-regulating Wnt/β-catenin signaling and rescued diabetic renal injury (Ho et al., 2016). Salvia miltiorrhiza extracts relieve renal injury by suppressing the relative expression levels of wnt4, β-catenin, and TGF-β in renal tissue (Xiang et al., 2019). Salidroside protects against T1DM-induced kidney injury and renal fibrosis by ameliorating TGF-β1 and the Wnt1/3a/β-catenin signaling pathway (Shati and Alfaifi, 2020). It has been demonstrated that Tripterygium wilfordii treatment inhibits the upregulation of Wnt1 and β-catenin expression in hyperglycemia-induced kidney tissue and attenuates the renal injury in rats caused by diabetes (Chang et al., 2018). Triptonide can effectively inhibit canonical Wnt/β-catenin signaling by targeting the downstream C-terminal transcription domain of β-catenin or a nuclear component associated with β-catenin (Chinison et al., 2016). Astragaloside IV has been shown to have possible inhibitory effects on renal interstitial fibrosis by effectively inhibiting the upregulation of proteins in the Wnt/β-catenin signaling pathway in UUO model rats (Wang et al., 2014b). Quercetin inhibits β-catenin signaling transduction, thereby inhibiting the activation of fibroblasts and renal fibrosis (Ren et al., 2016).
In short, based on the findings described above, the Wnt/β-catenin signaling pathway can be therapeutically targeted at several steps. Lots of emerging small molecule inhibitors that target Wnt and/or β-catenin are under development (Table 1). Additionally, the natural products, active ingredients, crude extracts, and traditional Chinese medicine formulas play an important role in anti-kidney fibrosis by inhibiting the Wnt/β-catenin signaling pathway. However, the specific molecular mechanisms of numerous inhibitors still need in-depth study, and more inhibitors need to be further developed.
Concluding Remarks
In summary, Wnt/β-catenin signaling plays a role in the pathogenesis of renal interstitial fibrosis, and targeting this pathway to treat renal fibrosis could yield positive results. Accumulating evidence has demonstrated that the role of Wnt/β-catenin signaling in the process of kidney repair and regeneration after AKI cannot be ignored (Kuure et al., 2007; Lasagni et al., 2015; Zhou et al., 2016; Jiao et al., 2017), and activating Wnt/β-catenin signaling could alleviate AKI (Li et al., 2020). A moderate increase in Wnt/β-catenin signaling is beneficial, but excessive activation of this pathway could trigger renal fibrosis (Guo et al., 2019; Sun et al., 2020). In addition, early intervention, but not late with β-catenin inhibitor significantly attenuates the apoptosis and inflammation induced by aristolochic acid (AA) (Kuang et al., 2021). Therefore, the dual role of Wnt/β-catenin signaling in CKD needs further study. And more researches are required to determine whether this pathway should be augmented in AKI to CKD. If so, the optimal treatment duration and the safe and effective dose also need to be determined.
At present, there are many studies on the Wnt/β-catenin signaling pathway. However, the mechanism of the non-canonical Wnt pathway in renal fibrosis still needs further investigation; Additionally, there are few clinical studies targeting Wnt/β-catenin signaling pathway to treat renal fibrosis, which is also the direction of our future efforts. since an FZD receptor can interact with different Wnt ligands to activate the Wnt pathway, coupled with the presence of several Wnt ligands. Therefore, understanding the selective binding of each Wnt protein to a specific FZD receptor and the resulting cascade reaction requires further research; Many signaling pathways play an important role in the process of renal fibrosis. However, the studies on the mechanism of their synergy with the Wnt/β-catenin signaling pathway should be further explored. In addition, a better understanding of the interactions between these pathways is needed to identify key molecules that regulate their interactions, which may serve as potential therapeutic targets; More small molecules that inhibit Wnt/β-catenin signaling are in development, which will explore more strategies to regulate this signaling pathway and provide more options for the effective therapies of renal fibrosis. The traditional Chinese medicine compound formula for the effective treatment of renal fibrosis is complex, and the active ingredients of Chinese medicine monomers or extracts are unknown. Therefore, the advantages of emerging natural products, CHMs, or new drugs in anti-renal fibrosis still need long-term research. Furthermore, to fully clarify the therapeutic effect of natural products in renal fibrosis, there is an urgent need to conduct studies that pay attention to identifying active ingredients, exploring action mechanisms, and rigorous pharmacological evaluation to ensure safety and accord the standards for clinical use. In conclusion, Wnt/β-catenin provides a broad prospect for treating renal interstitial fibrosis, but the understanding of Wnt/β-catenin remains a significant challenge.
Author Contributions
Y-YZ, X-YY, and J-RC were responsible for the conception. S-SL wrote the manuscript. S-SL and QS prepared the figures and tables. M-RH and PS are responsible for revising. Y-YZ and X-YY checked the article. S-SL were responsible for the final approval of the version to be submitted. All authors read and approved the final manuscript.
Funding
This study was supported by the Shaanxi Key Science and Technology Plan Project (No. 2019ZDLSF04-04-02), National Natural Science Foundation of China (Nos. 82074002, 81673578, 81872985), and National Key Research and Development Project of China (No. 2019YFC1709405).
Conflict of Interest
The authors declare that the research was conducted in the absence of any commercial or financial relationships that could be construed as a potential conflict of interest.
Publisher’s Note
All claims expressed in this article are solely those of the authors and do not necessarily represent those of their affiliated organizations, or those of the publisher, the editors and the reviewers. Any product that may be evaluated in this article, or claim that may be made by its manufacturer, is not guaranteed or endorsed by the publisher.
References
Acebron, S. P., and Niehrs, C. (2016). β-Catenin-Independent Roles of Wnt/LRP6 Signaling. Trends Cel Biol. 26 (12), 956–967. doi:10.1016/j.tcb.2016.07.009
Akcora, B. Ö., Storm, G., and Bansal, R. (2018). Inhibition of Canonical WNT Signaling Pathway by β-catenin/CBP Inhibitor ICG-001 Ameliorates Liver Fibrosis In Vivo through Suppression of Stromal CXCL12. Biochim. Biophys. Acta (Bba) - Mol. Basis Dis. 1864 (3), 804–818. doi:10.1016/j.bbadis.2017.12.001
Bai, X., Geng, J., Zhou, Z., Tian, J., and Li, X. (2016). MicroRNA-130b Improves Renal Tubulointerstitial Fibrosis via Repression of Snail-Induced Epithelial-Mesenchymal Transition in Diabetic Nephropathy. Sci. Rep. 6, 20475. doi:10.1038/srep20475
Cai, J., Liu, Z., Huang, X., Shu, S., Hu, X., Zheng, M., et al. (2020). The Deacetylase Sirtuin 6 Protects against Kidney Fibrosis by Epigenetically Blocking β-catenin Target Gene Expression. Kidney Int. 97 (1), 106–118. doi:10.1016/j.kint.2019.08.028
Chang, B., Chen, W., Zhang, Y., Yang, P., and Liu, L. (2018). Tripterygium Wilfordii Mitigates Hyperglycemia-Induced Upregulated Wnt/β-Catenin Expression and Kidney Injury in Diabetic Rats. Exp. Ther. Med. 15 (4), 3874–3882. doi:10.3892/etm.2018.5901
Chatterjee, S., and Sil, P. C. (2019). Targeting the Crosstalks of Wnt Pathway with Hedgehog and Notch for Cancer Therapy. Pharmacol. Res. 142, 251–261. doi:10.1016/j.phrs.2019.02.027
Chen, D.-Q., Cao, G., Chen, H., Argyopoulos, C. P., Yu, H., Su, W., et al. (2019c). Identification of Serum Metabolites Associating with Chronic Kidney Disease Progression and Anti-fibrotic Effect of 5-methoxytryptophan. Nat. Commun. 10 (1), 1476. doi:10.1038/s41467-019-09329-0
Chen, D.-Q., Cao, G., Chen, H., Liu, D., Su, W., Yu, X.-Y., et al. (2017a). Gene and Protein Expressions and Metabolomics Exhibit Activated Redox Signaling and Wnt/β-Catenin Pathway Are Associated with Metabolite Dysfunction in Patients with Chronic Kidney Disease. Redox Biol. 12, 505–521. doi:10.1016/j.redox.2017.03.017
Chen, D.-Q., Cao, G., Zhao, H., Chen, L., Yang, T., Wang, M., et al. (2019b). Combined Melatonin and Poricoic Acid A Inhibits Renal Fibrosis through Modulating the Interaction of Smad3 and β-catenin Pathway in AKI-To-CKD Continuum. Ther. Adv. Chronic Dis. 10, 204062231986911. doi:10.1177/2040622319869116
Chen, D.-Q., Feng, Y.-L., Cao, G., and Zhao, Y.-Y. (2018a). Natural Products as a Source for Antifibrosis Therapy. Trends Pharmacol. Sci. 39 (11), 937–952. doi:10.1016/j.tips.2018.09.002
Chen, D.-Q., Feng, Y.-L., Chen, L., Liu, J.-R., Wang, M., Vaziri, N. D., et al. (2019a). Poricoic Acid A Enhances Melatonin Inhibition of AKI-To-CKD Transition by Regulating Gas6/Axl NF κB/Nrf2 axis. Free Radic. Biol. Med. 134, 484–497. doi:10.1016/j.freeradbiomed.2019.01.046
Chen, D.-Q., Feng, Y.-L., Tian, T., Chen, H., Yin, L., Zhao, Y.-Y., et al. (2014). Diuretic and Anti-diuretic Activities of Fractions of Alismatis Rhizoma. J. Ethnopharmacology 157, 114–118. doi:10.1016/j.jep.2014.09.022
Chen, D.-Q., Wang, Y.-N., Vaziri, N. D., Chen, L., Hu, H.-H., and Zhao, Y.-Y. (2020a). Poricoic Acid A Activates AMPK to Attenuate Fibroblast Activation and Abnormal Extracellular Matrix Remodelling in Renal Fibrosis. Phytomedicine 72, 153232. doi:10.1016/j.phymed.2020.153232
Chen, H., Tian, T., Miao, H., and Zhao, Y.-Y. (2016). Traditional Uses, Fermentation, Phytochemistry and Pharmacology of Phellinus Linteus: A Review. Fitoterapia 113, 6–26. doi:10.1016/j.fitote.2016.06.009
Chen, H., Wang, M.-C., Chen, Y.-Y., Chen, L., Wang, Y.-N., Vaziri, N. D., et al. (2020b). Alisol B 23-acetate Attenuates CKD Progression by Regulating the Renin-Angiotensin System and Gut-Kidney axis. Ther. Adv. Chronic Dis. 11, 204062232092002. doi:10.1177/2040622320920025
Chen, H., Yang, T., Wang, M.-C., Chen, D.-Q., Yang, Y., and Zhao, Y.-Y. (2018b). Novel RAS Inhibitor 25-O-Methylalisol F Attenuates Epithelial-To-Mesenchymal Transition and Tubulo-Interstitial Fibrosis by Selectively Inhibiting TGF-β-Mediated Smad3 Phosphorylation. Phytomedicine 42, 207–218. doi:10.1016/j.phymed.2018.03.034
Chen, L., Cao, G., Wang, M., Feng, Y. L., Chen, D. Q., Vaziri, N. D., et al. (2019d). The Matrix Metalloproteinase‐13 Inhibitor Poricoic Acid ZI Ameliorates Renal Fibrosis by Mitigating Epithelial‐Mesenchymal Transition. Mol. Nutr. Food Res. 63 (13), e1900132. doi:10.1002/mnfr.201900132
Chen, L., Chen, D.-Q., Liu, J.-R., Zhang, J., Vaziri, N. D., Zhuang, S., et al. (2019e). Unilateral Ureteral Obstruction Causes Gut Microbial Dysbiosis and Metabolome Disorders Contributing to Tubulointerstitial Fibrosis. Exp. Mol. Med. 51 (3), 1–18. doi:10.1038/s12276-019-0234-2
Chen, L., Chen, D.-Q., Wang, M., Liu, D., Chen, H., Dou, F., et al. (2017b). Role of RAS/Wnt/β-catenin axis Activation in the Pathogenesis of Podocyte Injury and Tubulo-Interstitial Nephropathy. Chem. Biol. Interact. 273, 56–72. doi:10.1016/j.cbi.2017.05.025
Chen, L., Yang, T., Lu, D.-W., Zhao, H., Feng, Y.-L., Chen, H., et al. (2018c). Central Role of Dysregulation of TGF-β/Smad in CKD Progression and Potential Targets of its Treatment. Biomed. Pharmacother. 101, 670–681. doi:10.1016/j.biopha.2018.02.090
Chinison, J., Aguilar, J. S., Avalos, A., Huang, Y., Wang, Z., Cameron, D. J., et al. (2016). Triptonide Effectively Inhibits Wnt/β-Catenin Signaling via C-Terminal Transactivation Domain of β-catenin. Sci. Rep. 6, 32779. doi:10.1038/srep32779
Cosin-Roger, J., Ortiz-Masià, M. D., and Barrachina, M. D. (2019). Macrophages as an Emerging Source of Wnt Ligands: Relevance in Mucosal Integrity. Front. Immunol. 10, 2297. doi:10.3389/fimmu.2019.02297
Cruciat, C.-M., and Niehrs, C. (2013). Secreted and Transmembrane Wnt Inhibitors and Activators. Cold Spring Harbor Perspect. Biol. 5 (3), a015081. doi:10.1101/cshperspect.a015081
Dai, H., Liu, F., Qiu, X., Liu, W., Dong, Z., Jia, Y., et al. (2020). Alleviation by Mahuang Fuzi and Shenzhuo Decoction in High Glucose-Induced Podocyte Injury by Inhibiting the Activation of Wnt/β-Catenin Signaling Pathway, Resulting in Activation of Podocyte Autophagy. Evid. Based Complement. Alternat. Med. 2020, 1–11. doi:10.1155/2020/7809427
Distler, J. H. W., Györfi, A.-H., Ramanujam, M., Whitfield, M. L., Königshoff, M., and Lafyatis, R. (2019). Shared and Distinct Mechanisms of Fibrosis. Nat. Rev. Rheumatol. 15 (12), 705–730. doi:10.1038/s41584-019-0322-7
Djudjaj, S., and Boor, P. (2019). Cellular and Molecular Mechanisms of Kidney Fibrosis. Mol. Aspects Med. 65, 16–36. doi:10.1016/j.mam.2018.06.002
Dou, F., Miao, H., Wang, J. W., Chen, L., Wang, M., Chen, H., et al. (2018). An Integrated Lipidomics and Phenotype Study Reveals Protective Effect and Biochemical Mechanism of Traditionally Used Alisma Orientale Juzepzuk in Chronic Renal Disease. Front. Pharmacol. 9, 53. doi:10.3389/fphar.2018.00053
Duan, Y., Qiu, Y., Huang, X., Dai, C., Yang, J., and He, W. (2020). Deletion of FHL2 in Fibroblasts Attenuates Fibroblasts Activation and Kidney Fibrosis via Restraining TGF-Β1-Induced Wnt/β-Catenin Signaling. J. Mol. Med. 98 (2), 291–307. doi:10.1007/s00109-019-01870-1
Edeling, M., Ragi, G., Huang, S., Pavenstädt, H., and Susztak, K. (2016). Developmental Signalling Pathways in Renal Fibrosis: the Roles of Notch, Wnt and Hedgehog. Nat. Rev. Nephrol. 12 (7), 426–439. doi:10.1038/nrneph.2016.54
Federico, G., Meister, M., Mathow, D., Heine, G. H., Moldenhauer, G., Popovic, Z. V., et al. (2016). Tubular Dickkopf-3 Promotes the Development of Renal Atrophy and Fibrosis. JCI Insight 1 (1), e84916. doi:10.1172/jci.insight.84916
Feng, Y.-L., Cao, G., Chen, D.-Q., Vaziri, N. D., Chen, L., Zhang, J., et al. (2019b). Microbiome-metabolomics Reveals Gut Microbiota Associated with Glycine-Conjugated Metabolites and Polyamine Metabolism in Chronic Kidney Disease. Cell. Mol. Life Sci. 76 (24), 4961–4978. doi:10.1007/s00018-019-03155-9
Feng, Y.-L., Chen, H., Chen, D.-Q., Vaziri, N. D., Su, W., Ma, S.-X., et al. (2019c). Activated NF-κB/Nrf2 and Wnt/β-Catenin Pathways Are Associated with Lipid Metabolism in CKD Patients with Microalbuminuria and Macroalbuminuria. Biochim. Biophys. Acta (Bba) - Mol. Basis Dis. 1865 (9), 2317–2332. doi:10.1016/j.bbadis.2019.05.010
Feng, Y.-L., Chen, H., Tian, T., Chen, D.-Q., Zhao, Y.-Y., and Lin, R.-C. (2014). Diuretic and Anti-diuretic Activities of the Ethanol and Aqueous Extracts of Alismatis Rhizoma. J. Ethnopharmacol. 154 (2), 386–390. doi:10.1016/j.jep.2014.04.017
Feng, Y.-L., Lei, P., Tian, T., Yin, L., Chen, D.-Q., Chen, H., et al. (2013). Diuretic Activity of Some Fractions of the Epidermis of Poria Cocos. J. Ethnopharmacol. 150 (3), 1114–1118. doi:10.1016/j.jep.2013.10.043
Feng, Y. L., Chen, D. Q., Vaziri, N. D., Guo, Y., and Zhao, Y. Y. (2019a). Small Molecule Inhibitors of Epithelial‐Mesenchymal Transition for the Treatment of Cancer and Fibrosis. Med. Res. Rev. 40, 54–78. doi:10.1002/med.21596
Feng, Y., Ren, J., Gui, Y., Wei, W., Shu, B., Lu, Q., et al. (2018). Wnt/β-Catenin-Promoted Macrophage Alternative Activation Contributes to Kidney Fibrosis. J. Am. Soc. Nephrol. 29 (1), 182–193. doi:10.1681/asn.2017040391
Flevaris, P., and Vaughan, D. (2017). The Role of Plasminogen Activator Inhibitor Type-1 in Fibrosis. Semin. Thromb. Hemost. 43 (2), 169–177. doi:10.1055/s-0036-1586228
Foulquier, S., Daskalopoulos, E. P., Lluri, G., Hermans, K. C. M., Deb, A., and Blankesteijn, W. M. (2018). WNT Signaling in Cardiac and Vascular Disease. Pharmacol. Rev. 70 (1), 68–141. doi:10.1124/pr.117.013896
Gammons, M. V., Rutherford, T. J., Steinhart, Z., Angers, S., and Bienz, M. (2016). Essential Role of the Dishevelled DEP Domain in a Wnt-dependent Human-Cell-Based Complementation Assay. J. Cell Sci. 129 (20), 3892–3902. doi:10.1242/jcs.195685
García de Herreros, A., and Baulida, J. (2012). Cooperation, Amplification, and Feed-Back in Epithelial-Mesenchymal Transition. Biochim. Biophys. Acta (Bba) - Rev. Cancer 1825 (2), 223–228. doi:10.1016/j.bbcan.2012.01.003
Gu, L.-f., Ge, H.-t., Zhao, L., Wang, Y.-j., Zhang, F., Tang, H.-t., et al. (2020). Huangkui Capsule Ameliorates Renal Fibrosis in a Unilateral Ureteral Obstruction Mouse Model through TRPC6 Dependent Signaling Pathways. Front. Pharmacol. 11, 996. doi:10.3389/fphar.2020.00996
Guan, X., Nie, L., He, T., Yang, K., Xiao, T., Wang, S., et al. (2014). Klotho Suppresses Renal Tubulo-Interstitial Fibrosis by Controlling Basic Fibroblast Growth Factor-2 Signalling. J. Pathol. 234 (4), 560–572. doi:10.1002/path.4420
Guo, Q., Zhong, W., Duan, A., Sun, G., Cui, W., Zhuang, X., et al. (2019). Protective or Deleterious Role of Wnt/beta-Catenin Signaling in Diabetic Nephropathy: An Unresolved Issue. Pharmacol. Res. 144, 151–157. doi:10.1016/j.phrs.2019.03.022
Hall, G., Wang, L., and Spurney, R. F. (2019). TRPC Channels in Proteinuric Kidney Diseases. Cells 9 (1), 44. doi:10.3390/cells9010044
Hamasaki, Y., Doi, K., Maeda-Mamiya, R., Ogasawara, E., Katagiri, D., Tanaka, T., et al. (2013). A 5-hydroxytryptamine Receptor Antagonist, Sarpogrelate, Reduces Renal Tubulointerstitial Fibrosis by Suppressing PAI-1. Am. J. Physiol. Renal Physiol. 305 (12), F1796–F1803. doi:10.1152/ajprenal.00151.2013
He, W., Dai, C., Li, Y., Zeng, G., Monga, S. P., and Liu, Y. (2009). Wnt/β-Catenin Signaling Promotes Renal Interstitial Fibrosis. J. Am. Soc. Nephrol. 20 (4), 765–776. doi:10.1681/asn.2008060566
Henderson, J., Wilkinson, S., Przyborski, S., Stratton, R., and O’Reilly, S. (2020). microRNA27a-3p Mediates Reduction of the Wnt Antagonist sFRP-1 in Systemic Sclerosis. Epigenetics 16, 808–817. doi:10.1080/15592294.2020.1827715
Henderson, W. R., Chi, E. Y., Ye, X., Nguyen, C., Tien, Y.-t., Zhou, B., et al. (2010). Inhibition of Wnt/ -catenin/CREB Binding Protein (CBP) Signaling Reverses Pulmonary Fibrosis. Proc. Natl. Acad. Sci. 107 (32), 14309–14314. doi:10.1073/pnas.1001520107
Ho, C., Hsu, Y.-C., Lei, C.-C., Mau, S.-C., Shih, Y.-H., and Lin, C.-L. (2016). Curcumin Rescues Diabetic Renal Fibrosis by Targeting Superoxide-Mediated Wnt Signaling Pathways. Am. J. Med. Sci. 351 (3), 286–295. doi:10.1016/j.amjms.2015.12.017
Hou, N.-N., Kan, C.-X., Huang, N., Liu, Y.-P., Mao, E.-W., Ma, Y.-T., et al. (2021). Relationship between Serum Dickkopf-1 and Albuminuria in Patients with Type 2 Diabetes. World J. Diabetes 12 (1), 47–55. doi:10.4239/wjd.v12.i1.47
Hu, H.-H., Cao, G., Wu, X.-Q., Vaziri, N. D., and Zhao, Y.-Y. (2020). Wnt Signaling Pathway in Aging-Related Tissue Fibrosis and Therapies. Ageing Res. Rev. 60, 101063. doi:10.1016/j.arr.2020.101063
Hu, M. C., Kuro-o, M., and Moe, O. W. (2013). Klotho and Chronic Kidney Disease. Contrib. Nephrol. 180, 47–63. doi:10.1159/000346778
Huang, H., Huang, X., Luo, S., Zhang, H., Hu, F., Chen, R., et al. (2020). The MicroRNA MiR-29c Alleviates Renal Fibrosis via TPM1-Mediated Suppression of the Wnt/β-Catenin Pathway. Front. Physiol. 11, 331. doi:10.3389/fphys.2020.00331
Huang, P., Yan, R., Zhang, X., Wang, L., Ke, X., and Qu, Y. (2019). Activating Wnt/β-Catenin Signaling Pathway for Disease Therapy: Challenges and Opportunities. Pharmacol. Ther. 196, 79–90. doi:10.1016/j.pharmthera.2018.11.008
Huang, Y., Liu, L., and Liu, A. (2018). Dickkopf-1: Current Knowledge and Related Diseases. Life Sci. 209, 249–254. doi:10.1016/j.lfs.2018.08.019
Humphreys, B. D. (2018). Mechanisms of Renal Fibrosis. Annu. Rev. Physiol. 80, 309–326. doi:10.1146/annurev-physiol-022516-034227
Ichihara, A., and Yatabe, M. S. (2019). The (Pro)renin Receptor in Health and Disease. Nat. Rev. Nephrol. 15 (11), 693–712. doi:10.1038/s41581-019-0160-5
Janda, C. Y., Dang, L. T., You, C., Chang, J., de Lau, W., Zhong, Z. A., et al. (2017). Surrogate Wnt Agonists that Phenocopy Canonical Wnt and β-catenin Signalling. Nature 545 (7653), 234–237. doi:10.1038/nature22306
Jiao, X., Cai, J., Yu, X., and Ding, X. (2017). Paracrine Activation of the Wnt/β-Catenin Pathway by Bone Marrow Stem Cell Attenuates Cisplatin-Induced Kidney Injury. Cell Physiol. Biochem. 44 (5), 1980–1994. doi:10.1159/000485904
Johnson, B. G., Ren, S., Karaca, G., Gomez, I. G., Fligny, C., Smith, B., et al. (2017). Connective Tissue Growth Factor Domain 4 Amplifies Fibrotic Kidney Disease through Activation of LDL Receptor-Related Protein 6. J. Am. Soc. Nephrol. 28 (6), 1769–1782. doi:10.1681/asn.2016080826
Joiner, D. M., Ke, J., Zhong, Z., Xu, H. E., and Williams, B. O. (2013). LRP5 and LRP6 in Development and Disease. Trends Endocrinol. Metab. 24 (1), 31–39. doi:10.1016/j.tem.2012.10.003
Kawazoe, M., Kaneko, K., and Nanki, T. (2021). Glucocorticoid Therapy Suppresses Wnt Signaling by Reducing the Ratio of Serum Wnt3a to Wnt Inhibitors, sFRP-1 and Wif-1. Clin. Rheumatol. 40, 2947–2954. doi:10.1007/s10067-020-05554-x
Kim, D. Y., Kang, M.-K., Park, S.-H., Lee, E.-J., Kim, Y.-H., Oh, H., et al. (2017). Eucalyptol Ameliorates Snail1/β-catenin-dependent Diabetic Disjunction of Renal Tubular Epithelial Cells and Tubulointerstitial Fibrosis. Oncotarget 8 (63), 106190–106205. doi:10.18632/oncotarget.22311
Kim, E. Y., and Dryer, S. E. (2021). Effects of TRPC6 Inactivation on Glomerulosclerosis and Renal Fibrosis in Aging Rats. Cells 10 (4), 856. doi:10.3390/cells10040856
Kinosada, H., Okada-Iwasaki, R., Kunieda, K., Suzuki-Imaizumi, M., Takahashi, Y., Miyagi, H., et al. (2021). The Dual Pocket Binding Novel Tankyrase Inhibitor K-476 Enhances the Efficacy of Immune Checkpoint Inhibitor by Attracting CD8+ T Cells to Tumors. Am. J. Cancer Res. 11 (1), 264–276.
Klavdianou, K., Liossis, S.-N., and Daoussis, D. (2017). Dkk1: A Key Molecule in Joint Remodelling and Fibrosis. Mediterr. J. Rheumatol. 28 (4), 174–182. doi:10.31138/mjr.28.4.174
Kozlova, N., Jensen, J. K., Franklin Chi, T., Samoylenko, A., and Kietzmann, T. (2015). PAI-1 Modulates Cell Migration in a LRP1-dependent Manner via β-catenin and ERK1/2. Thromb. Haemost. 113 (5), 988–998. doi:10.1160/th14-08-0678
Kuang, Q., Wu, S., Xue, N., Wang, X., Ding, X., and Fang, Y. (2021). Selective Wnt/β-Catenin Pathway Activation Concomitant with Sustained Overexpression of miR-21 Is Responsible for Aristolochic Acid-Induced AKI-To-CKD Transition. Front. Pharmacol. 12, 667282. doi:10.3389/fphar.2021.667282
Kulak, O., Chen, H., Holohan, B., Wu, X., He, H., Borek, D., et al. (2015). Disruption of Wnt/β-Catenin Signaling and Telomeric Shortening Are Inextricable Consequences of Tankyrase Inhibition in Human Cells. Mol. Cel. Biol. 35 (14), 2425–2435. doi:10.1128/mcb.00392-15
Kuure, S., Popsueva, A., Jakobson, M., Sainio, K., and Sariola, H. (2007). Glycogen Synthase Kinase-3 Inactivation and Stabilization of β-Catenin Induce Nephron Differentiation in Isolated Mouse and Rat Kidney Mesenchymes. J. Am. Soc. Nephrol. 18 (4), 1130–1139. doi:10.1681/asn.2006111206
La, L., Wang, L., Qin, F., Jiang, J., He, S., Wang, C., et al. (2018). Zhen-wu-tang Ameliorates Adenine-Induced Chronic Renal Failure in Rats: Regulation of the Canonical Wnt4/beta-Catenin Signaling in the Kidneys. J. Ethnopharmacology 219, 81–90. doi:10.1016/j.jep.2017.12.013
Langton, P. F., Kakugawa, S., and Vincent, J.-P. (2016). Making, Exporting, and Modulating Wnts. Trends Cel Biol. 26 (10), 756–765. doi:10.1016/j.tcb.2016.05.011
Lasagni, L., Angelotti, M. L., Ronconi, E., Lombardi, D., Nardi, S., Peired, A., et al. (2015). Podocyte Regeneration Driven by Renal Progenitors Determines Glomerular Disease Remission and Can Be Pharmacologically Enhanced. Stem Cell Rep. 5 (2), 248–263. doi:10.1016/j.stemcr.2015.07.003
Li, X., Zheng, P., Ji, T., Tang, B., Wang, Y., and Bai, S. (2020). LINC00052 Ameliorates Acute Kidney Injury by Sponging miR-532-3p and Activating the Wnt Signaling Pathway. Aging 13 (1), 340–350. doi:10.18632/aging.104152
Li, Y., Zhao, Z., Luo, J., Jiang, Y., Li, L., Chen, Y., et al. (2021). Apigenin Ameliorates Hyperuricemic Nephropathy by Inhibiting URAT1 and GLUT9 and Relieving Renal Fibrosis via the Wnt/β-Catenin Pathway. Phytomedicine 87, 153585. doi:10.1016/j.phymed.2021.153585
Li, Z., Zhou, L., Wang, Y., Miao, J., Hong, X., Hou, F. F., et al. (2017). (Pro)renin Receptor is an Amplifier of Wnt/β-Catenin Signaling in Kidney Injury and Fibrosis. J. Am. Soc. Nephrol. 28 (8), 2393–2408. doi:10.1681/asn.2016070811
Licht-Murava, A., Paz, R., Vaks, L., Avrahami, L., Plotkin, B., Eisenstein, M., et al. (2016). A Unique Type of GSK-3 Inhibitor Brings New Opportunities to the Clinic. Sci. Signal. 9 (454), ra110. doi:10.1126/scisignal.aah7102
Lin, B., Hong, H., Jiang, X., Li, C., Zhu, S., Tang, N., et al. (2017). WNT Inhibitory Factor 1 Promoter Hypermethylation Is an Early Event during Gallbladder Cancer Tumorigenesis that Predicts Poor Survival. Gene 622, 42–49. doi:10.1016/j.gene.2017.04.034
Lindberg, K., Amin, R., Moe, O. W., Hu, M.-C., Erben, R. G., Östman Wernerson, A., et al. (2014). The Kidney Is the Principal Organ Mediating Klotho Effects. J. Am. Soc. Nephrol. 25 (10), 2169–2175. doi:10.1681/asn.2013111209
Lipphardt, M., Dihazi, H., Jeon, N. L., Dadafarin, S., Ratliff, B. B., Rowe, D. W., et al. (2019). Dickkopf-3 in Aberrant Endothelial Secretome Triggers Renal Fibroblast Activation and Endothelial-Mesenchymal Transition. Nephrol. Dial. Transpl. 34 (1), 49–62. doi:10.1093/ndt/gfy100
Liu, D., Chen, L., Zhao, H., Vaziri, N. D., Ma, S.-C., and Zhao, Y.-Y. (2019). Small Molecules from Natural Products Targeting the Wnt/β-Catenin Pathway as a Therapeutic Strategy. Biomed. Pharmacother. 117, 108990. doi:10.1016/j.biopha.2019.108990
Liu, Y., Su, Y.-Y., Yang, Q., and Zhou, T. (2021). Stem Cells in the Treatment of Renal Fibrosis: A Review of Preclinical and Clinical Studies of Renal Fibrosis Pathogenesis. Stem Cell Res. Ther. 12 (1), 333. doi:10.1186/s13287-021-02391-w
Liu, Z., Tan, R. J., and Liu, Y. (2020). The Many Faces of Matrix Metalloproteinase-7 in Kidney Diseases. Biomolecules 10 (6), 960. doi:10.3390/biom10060960
Ma, S., Choi, J., Jin, X., Kim, H.-Y., Yun, J.-H., Lee, W., et al. (2018). Discovery of a Small-Molecule Inhibitor of Dvl-CXXC5 Interaction by Computational Approaches. J. Comput. Aided Mol. Des. 32 (5), 643–655. doi:10.1007/s10822-018-0118-x
Maarouf, O. H., Aravamudhan, A., Rangarajan, D., Kusaba, T., Zhang, V., Welborn, J., et al. (2016). Paracrine Wnt1 Drives Interstitial Fibrosis Without Inflammation by Tubulointerstitial Cross-Talk. J. Am. Soc. Nephrol. 27 (3), 781–790. doi:10.1681/asn.2014121188
Malik, S. A., Modarage, K., and Goggolidou, P. (2020). The Role of Wnt Signalling in Chronic Kidney Disease (CKD). Genes 11 (5), 496. doi:10.3390/genes11050496
Matsuyama, M., Nomori, A., Nakakuni, K., Shimono, A., and Fukushima, M. (2014). Secreted Frizzled-Related Protein 1 (Sfrp1) Regulates the Progression of Renal Fibrosis in a Mouse Model of Obstructive Nephropathy. J. Biol. Chem. 289 (45), 31526–31533. doi:10.1074/jbc.M114.584565
Miao, H., Cao, G., Wu, X. Q., Chen, Y. Y., Chen, D. Q., Chen, L., et al. (2020). Identification of Endogenous 1‐aminopyrene as a Novel Mediator of Progressive Chronic Kidney Disease via Aryl Hydrocarbon Receptor Activation. Br. J. Pharmacol. 177 (15), 3415–3435. doi:10.1111/bph.15062
Miao, H., Wu, X.-Q., Zhang, D.-D., Wang, Y.-N., Guo, Y., Li, P., et al. (2021). Deciphering the Cellular Mechanisms Underlying Fibrosis-Associated Diseases and Therapeutic Avenues. Pharmacol. Res. 163, 105316. doi:10.1016/j.phrs.2020.105316
Miao, H., Zhao, Y.-H., Vaziri, N. D., Tang, D.-D., Chen, H., Chen, H., et al. (2016). Lipidomics Biomarkers of Diet-Induced Hyperlipidemia and its Treatment with Poria Cocos. J. Agric. Food Chem. 64 (4), 969–979. doi:10.1021/acs.jafc.5b05350
Miao, J., Liu, J., Niu, J., Zhang, Y., Shen, W., Luo, C., et al. (2019). Wnt/β‐catenin/RAS Signaling Mediates Age‐related Renal Fibrosis and Is Associated with Mitochondrial Dysfunction. Aging Cell 18 (5), e13004. doi:10.1111/acel.13004
Mo, L., Xiao, X., Song, S., Miao, H., Liu, S., Guo, D., et al. (2015). Protective Effect of Huang Gan Formula in 5/6 Nephrectomized Rats by Depressing the Wnt/β-Catenin Signaling Pathway. Drug Des. Devel. Ther. 9, 2867–2881. doi:10.2147/dddt.S81157
Moon, J., Zhou, H., Zhang, L.-s., Tan, W., Liu, Y., Zhang, S., et al. (2017). Blockade to Pathological Remodeling of Infarcted Heart Tissue Using a porcupine Antagonist. Proc. Natl. Acad. Sci. USA 114 (7), 1649–1654. doi:10.1073/pnas.1621346114
Muñoz-Castañeda, J. R., Herencia, C., Pendón-Ruiz de Mier, M. V., Rodriguez-Ortiz, M. E., Diaz-Tocados, J. M., Vergara, N., et al. (2017). Differential Regulation of Renal Klotho and FGFR1 in normal and Uremic Rats. Faseb j 31 (9), 3858–3867. doi:10.1096/fj.201700006R
Muñoz-Castañeda, J. R., Rodelo-Haad, C., Pendon-Ruiz de Mier, M. V., Martin-Malo, A., Santamaria, R., and Rodriguez, M. (2020). Klotho/FGF23 and Wnt Signaling as Important Players in the Comorbidities Associated With Chronic Kidney Disease. Toxins 12 (3), 185. doi:10.3390/toxins12030185
Nastase, M. V., Zeng-Brouwers, J., Wygrecka, M., and Schaefer, L. (2018). Targeting Renal Fibrosis: Mechanisms and Drug Delivery Systems. Adv. Drug Deliv. Rev. 129, 295–307. doi:10.1016/j.addr.2017.12.019
Navar, L. G. (2014). Intrarenal Renin-Angiotensin System in Regulation of Glomerular Function. Curr. Opin. Nephrol. Hypertens. 23 (1), 38–45. doi:10.1097/01.mnh.0000436544.86508.f1
Ng-Blichfeldt, J.-P., de Jong, T., Kortekaas, R. K., Wu, X., Lindner, M., Guryev, V., et al. (2019). TGF-β Activation Impairs Fibroblast Ability to Support Adult Lung Epithelial Progenitor Cell Organoid Formation. Am. J. Physiol. Lung Cell Mol. Physiol. 317 (1), L14–l28. doi:10.1152/ajplung.00400.2018
Nishikawa, K., Osawa, Y., and Kimura, K. (2018). Wnt/β-Catenin Signaling as a Potential Target for the Treatment of Liver Cirrhosis Using Antifibrotic Drugs. Int. J. Mol. Sci. 19 (10), 3103. doi:10.3390/ijms19103103
Nlandu-Khodo, S., Neelisetty, S., Phillips, M., Manolopoulou, M., Bhave, G., May, L., et al. (2017). Blocking TGF-β and β-Catenin Epithelial Crosstalk Exacerbates CKD. J. Am. Soc. Nephrol. 28 (12), 3490–3503. doi:10.1681/asn.2016121351
Nusse, R., and Clevers, H. (2017). Wnt/β-Catenin Signaling, Disease, and Emerging Therapeutic Modalities. Cell 169 (6), 985–999. doi:10.1016/j.cell.2017.05.016
Pan, B., Zhang, H., Hong, Y., Ma, M., Wan, X., and Cao, C. (2021). Indoleamine-2,3-Dioxygenase Activates Wnt/β-Catenin Inducing Kidney Fibrosis after Acute Kidney Injury. Gerontology, 1–9. doi:10.1159/00051504
Plummer, R., Dua, D., Cresti, N., Drew, Y., Stephens, P., Foegh, M., et al. (2020). First-in-Human Study of the PARP/Tankyrase Inhibitor E7449 in Patients with Advanced Solid Tumours and Evaluation of a Novel Drug-Response Predictor. Br. J. Cancer 123 (4), 525–533. doi:10.1038/s41416-020-0916-5
Proffitt, K. D., Madan, B., Ke, Z., Pendharkar, V., Ding, L., Lee, M. A., et al. (2013). Pharmacological Inhibition of the Wnt Acyltransferase PORCN Prevents Growth of WNT-Driven Mammary Cancer. Cancer Res. 73 (2), 502–507. doi:10.1158/0008-5472.Can-12-2258
Qi, C., Zhang, J., Chen, X., Wan, J., Wang, J., Zhang, P., et al. (2017). Hypoxia Stimulates Neural Stem Cell Proliferation by Increasing HIF-1α E-xpression and A-ctivating Wnt/β-C-atenin S-ignaling. Cell Mol. Biol. 63 (7), 12–19. doi:10.14715/cmb/2017.63.7.2
Qiao, B., He, B.-X., Cai, J.-H., Tao, Q., and King-Yin Lam, A. (2017). MicroRNA-27a-3p Modulates the Wnt/β-Catenin Signaling Pathway to Promote Epithelial-Mesenchymal Transition in Oral Squamous Carcinoma Stem Cells by Targeting SFRP1. Sci. Rep. 7, 44688. doi:10.1038/srep44688
Rabieian, R., Boshtam, M., Zareei, M., Kouhpayeh, S., Masoudifar, A., and Mirzaei, H. (2018). Plasminogen Activator Inhibitor Type‐1 as a Regulator of Fibrosis. J. Cell Biochem. 119 (1), 17–27. doi:10.1002/jcb.26146
Ramkumar, N., and Kohan, D. E. (2016). The Nephron (Pro)renin Receptor: Function and Significance. Am. J. Physiol. Renal Physiol. 311 (6), F1145–f1148. doi:10.1152/ajprenal.00476.2016
Ren, J., Li, J., Liu, X., Feng, Y., Gui, Y., Yang, J., et al. (2016). Quercetin Inhibits Fibroblast Activation and Kidney Fibrosis Involving the Suppression of Mammalian Target of Rapamycin and β-catenin Signaling. Sci. Rep. 6, 23968. doi:10.1038/srep23968
Ren, X., Zhu, R., Liu, G., Xue, F., Wang, Y., Xu, J., et al. (2019). Effect of Sitagliptin on Tubulointerstitial Wnt/β‐Catenin Signalling in Diabetic Nephropathy. Nephrology 24 (11), 1189–1197. doi:10.1111/nep.13641
Rippin, I., Khazanov, N., Ben Joseph, S., Kudinov, T., Berent, E., Arciniegas Ruiz, S. M., et al. (2020). Discovery and Design of Novel Small Molecule GSK-3 Inhibitors Targeting the Substrate Binding Site. Int. J. Mol. Sci. 21 (22), 8709. doi:10.3390/ijms21228709
Schunk, S. J., Floege, J., Fliser, D., and Speer, T. (2021). WNT-β-catenin Signalling - A Versatile Player in Kidney Injury and Repair. Nat. Rev. Nephrol. 17 (3), 172–184. doi:10.1038/s41581-020-00343-w
Shati, A. A., and Alfaifi, M. Y. (2020). Salidroside Protects against Diabetes Mellitus‐Induced Kidney Injury and Renal Fibrosis by Attenuating TGF‐β1 and Wnt1/3a/β‐Catenin Signalling. Clin. Exp. Pharmacol. Physiol. 47 (10), 1692–1704. doi:10.1111/1440-1681.13355
Simon-Tillaux, N., and Hertig, A. (2017). Snail and Kidney Fibrosis. Nephrol. Dial. Transpl. 32 (2), 224–233. doi:10.1093/ndt/gfw333
Smigiel, K. S., and Parks, W. C. (2018). Macrophages, Wound Healing, and Fibrosis: Recent Insights. Curr. Rheumatol. Rep. 20 (4), 17. doi:10.1007/s11926-018-0725-5
Staruschenko, A., Spires, D., and Palygin, O. (2019). Role of TRPC6 in Progression of Diabetic Kidney Disease. Curr. Hypertens. Rep. 21 (7), 48. doi:10.1007/s11906-019-0960-9
Stemmer, V., de Craene, B., Berx, G., and Behrens, J. (2008). Snail Promotes Wnt Target Gene Expression and Interacts with β-catenin. Oncogene 27 (37), 5075–5080. doi:10.1038/onc.2008.140
Sun, Z., Xu, S., Cai, Q., Zhou, W., Jiao, X., Bao, M., et al. (2020). Wnt/β-Catenin Agonist BIO Alleviates Cisplatin-Induced Nephrotoxicity without Compromising its Efficacy of Anti-proliferation in Ovarian Cancer. Life Sci. 263, 118672. doi:10.1016/j.lfs.2020.118672
Svegliati, S., Marrone, G., Pezone, A., Spadoni, T., Grieco, A., Moroncini, G., et al. (2014). Oxidative DNA Damage Induces the ATM-Mediated Transcriptional Suppression of the Wnt Inhibitor WIF-1 in Systemic Sclerosis and Fibrosis. Sci. Signal. 7 (341), ra84. doi:10.1126/scisignal.2004592
Tan, R. J., Li, Y., Rush, B. M., Cerqueira, D. M., Zhou, D., Fu, H., et al. (2019). Tubular Injury Triggers Podocyte Dysfunction by β-Catenin-Driven Release of MMP-7. JCI Insight 4 (24). doi:10.1172/jci.insight.122399
Tang, P. M.-K., Nikolic-Paterson, D. J., and Lan, H.-Y. (2019). Macrophages: Versatile Players in Renal Inflammation and Fibrosis. Nat. Rev. Nephrol. 15 (3), 144–158. doi:10.1038/s41581-019-0110-2
Tessa, H., David, M. W., and S, G. L. (2020). Wnt/β-Catenin in Acute Kidney Injury and Progression to Chronic Kidney Disease. Semin. Nephrol. 40 (2), 126–137. doi:10.1016/j.semnephrol.2020.01.004
Tian, T., Chen, H., and Zhao, Y.-Y. (2014). Traditional Uses, Phytochemistry, Pharmacology, Toxicology and Quality Control of Alisma Orientale (Sam.) Juzep: A Review. J. Ethnopharmacol. 158, 373–387. doi:10.1016/j.jep.2014.10.061
Tu, C., Xu, R., Koleti, M., and Zoldan, J. (2017). Glycogen Synthase Kinase-3 Inhibition Sensitizes Human Induced Pluripotent Stem Cells to Thiol-Containing Antioxidants Induced Apoptosis. Stem Cell Res. 23, 182–187. doi:10.1016/j.scr.2017.07.019
Urushihara, M., Kinoshita, Y., Kondo, S., and Kagami, S. (2012). Involvement of the Intrarenal Renin-Angiotensin System in Experimental Models of Glomerulonephritis. J. Biomed. Biotechnol. 2012, 1–6. doi:10.1155/2012/601786
Venner, J. M., Famulski, K. S., Reeve, J., Chang, J., and Halloran, P. F. (2016). Relationships Among Injury, Fibrosis, and Time in Human Kidney Transplants. JCI Insight 1 (1), e85323. doi:10.1172/jci.insight.85323
Wang, J., Nishiyama, A., Matsuyama, M., Wang, Z., and Yuan, Y. (2020a). The (Pro)renin Receptor: a Novel Biomarker and Potential Therapeutic Target for Various Cancers. Cell Commun. Signal 18 (1), 39. doi:10.1186/s12964-020-0531-3
Wang, L., Chi, Y.-F., Yuan, Z.-T., Zhou, W.-C., Yin, P.-H., Zhang, X.-M., et al. (2014a). Astragaloside IV Inhibits the Up-Regulation of Wnt/β-Catenin Signaling in Rats with Unilateral Ureteral Obstruction. Cell Physiol. Biochem. 33 (5), 1316–1328. doi:10.1159/000358699
Wang, M., Chen, D.-Q., Chen, L., Cao, G., Zhao, H., Liu, D., et al. (2018a). Novel Inhibitors of the Cellular Renin-Angiotensin System Components, Poricoic Acids, Target Smad3 Phosphorylation and Wnt/β-Catenin Pathway against Renal Fibrosis. Br. J. Pharmacol. 175 (13), 2689–2708. doi:10.1111/bph.14333
Wang, M., Chen, D.-Q., Chen, L., Liu, D., Zhao, H., Zhang, Z.-H., et al. (2018b). Novel RAS Inhibitors Poricoic Acid ZG and Poricoic Acid ZH Attenuate Renal Fibrosis via a Wnt/β-Catenin Pathway and Targeted Phosphorylation of Smad3 Signaling. J. Agric. Food Chem. 66 (8), 1828–1842. doi:10.1021/acs.jafc.8b00099
Wang, M., Hu, H.-H., Chen, Y.-Y., Chen, L., Wu, X.-Q., and Zhao, Y.-Y. (2020b). Novel Poricoic Acids Attenuate Renal Fibrosis through Regulating Redox Signalling and Aryl Hydrocarbon Receptor Activation. Phytomedicine 79, 153323. doi:10.1016/j.phymed.2020.153323
Wang, N., Liang, H., and Zen, K. (2014b). Molecular Mechanisms that Influence the Macrophage m1-m2 Polarization Balance. Front. Immunol. 5, 614. doi:10.3389/fimmu.2014.00614
Wang, Q., Tian, X., Wang, Y., Wang, Y., Li, J., Zhao, T., et al. (2020c). Role of Transient Receptor Potential Canonical Channel 6 (TRPC6) in Diabetic Kidney Disease by Regulating Podocyte Actin Cytoskeleton Rearrangement. J. Diabetes Res. 2020, 1–11. doi:10.1155/2020/6897390
Wang, X., Moon, J., Dodge, M. E., Pan, X., Zhang, L., Hanson, J. M., et al. (2013a). The Development of Highly Potent Inhibitors for Porcupine. J. Med. Chem. 56 (6), 2700–2704. doi:10.1021/jm400159c
Wang, Y.-N., Hu, H.-H., Zhang, D.-D., Wu, X.-Q., Liu, J.-L., Guo, Y., et al. (2021a). The Dysregulation of Eicosanoids and Bile Acids Correlates with Impaired Kidney Function and Renal Fibrosis in Chronic Renal Failure. Metabolites 11 (2), 127. doi:10.3390/metabo11020127
Wang, Y.-N., Wu, X.-Q., Zhang, D.-D., Hu, H.-H., Liu, J.-L., Vaziri, N. D., et al. (2021b). Polyporus Umbellatus Protects Against Renal Fibrosis by Regulating Intrarenal Fatty Acyl Metabolites. Front. Pharmacol. 12, 633566. doi:10.3389/fphar.2021.633566
Wang, Y.-Z., Zhang, J., Zhao, Y.-L., Li, T., Shen, T., Li, J.-Q., et al. (2013b). Mycology, Cultivation, Traditional Uses, Phytochemistry and Pharmacology of Wolfiporia cocos (Schwein.) Ryvarden et Gilb.: A review. J. Ethnopharmacol. 147 (2), 265–276. doi:10.1016/j.jep.2013.03.027
Webster, A. C., Nagler, E. V., Morton, R. L., and Masson, P. (2017). Chronic Kidney Disease. Lancet 389 (10075), 1238–1252. doi:10.1016/s0140-6736(16)32064-5
Wong, D. W. L., Yiu, W. H., Chan, K. W., Li, Y., Li, B., Lok, S. W. Y., et al. (2018). Activated Renal Tubular Wnt/β-Catenin Signaling Triggers Renal Inflammation during Overload Proteinuria. Kidney Int. 93 (6), 1367–1383. doi:10.1016/j.kint.2017.12.017
Wozniak, J., Floege, J., Ostendorf, T., and Ludwig, A. (2021). Key Metalloproteinase-Mediated Pathways in the Kidney. Nat. Rev. Nephrol. 17, 513–527. doi:10.1038/s41581-021-00415-5
Wu, X.-Q., Zhang, D.-D., Wang, Y.-N., Tan, Y.-Q., Yu, X.-Y., and Zhao, Y.-Y. (2021). AGE/RAGE in Diabetic Kidney Disease and Ageing Kidney. Free Radic. Biol. Med. 171, 260–271. doi:10.1016/j.freeradbiomed.2021.05.025
Xia, J., and Cao, W. (2021). Epigenetic Modifications of Klotho Expression in Kidney Diseases. J. Mol. Med. 99 (5), 581–592. doi:10.1007/s00109-021-02044-8
Xian, J., Bu, F., Wang, Y., Long, F., Zhang, Z., Wu, C., et al. (2021). A Rationale for Drug Design provided by Co-Crystal Structure of IC261 in Complex with Tubulin. Molecules 26 (4), 946. doi:10.3390/molecules26040946
Xiang, X., Cai, H.-D., Su, S.-l., Dai, X.-x., Zhu, Y., Guo, J.-m., et al. (2019). Salvia Miltiorrhiza Protects against Diabetic Nephropathy through Metabolome Regulation and Wnt/β-Catenin and TGF-β Signaling Inhibition. Pharmacol. Res. 139, 26–40. doi:10.1016/j.phrs.2018.10.030
Xiao, L., Xu, B., Zhou, L., Tan, R. J., Zhou, D., Fu, H., et al. (2019). Wnt/β-catenin Regulates Blood Pressure and Kidney Injury in Rats. Biochim. Biophys. Acta (Bba) - Mol. Basis Dis. 1865 (6), 1313–1322. doi:10.1016/j.bbadis.2019.01.027
Xiao, L., Zhou, D., Tan, R. J., Fu, H., Zhou, L., Hou, F. F., et al. (2016). Sustained Activation of Wnt/β-Catenin Signaling Drives AKI to CKD Progression. J. Am. Soc. Nephrol. 27 (6), 1727–1740. doi:10.1681/asn.2015040449
Xie, H., Miao, N., Xu, D., Zhou, Z., Ni, J., Yin, F., et al. (2021). FoxM1 Promotes Wnt/β‐Catenin Pathway Activation and Renal Fibrosis via Transcriptionally Regulating Multi‐Wnts Expressions. J. Cell Mol. Med. 25 (4), 1958–1971. doi:10.1111/jcmm.15948
Xing, L., Chang, X., Shen, L., Zhang, C., Fan, Y., Cho, C., et al. (2021). Progress in Drug Delivery System for Fibrosis Therapy. Asian J. Pharm. Sci. 16 (1), 47–61. doi:10.1016/j.ajps.2020.06.005
Yang, X., Wang, H., Tu, Y., Li, Y., Zou, Y., Li, G., et al. (2020). WNT1‐Inducible Signaling Protein‐1 Mediates TGF‐β1‐Induced Renal Fibrosis in Tubular Epithelial Cells and Unilateral Ureteral Obstruction Mouse Models via Autophagy. J. Cell Physiol. 235 (3), 2009–2022. doi:10.1002/jcp.29187
Yang, Y., Feng, X., Liu, X., Wang, Y., Hu, M., Cao, Q., et al. (2019). Fate Alteration of Bone Marrow-Derived Macrophages Ameliorates Kidney Fibrosis in Murine Model of Unilateral Ureteral Obstruction. Nephrol. Dial. Transpl. 34 (10), 1657–1668. doi:10.1093/ndt/gfy381
Yang, Y., Nankivell, B. J., Hua, W., Rao, P., Ren, X., Yu, H., et al. (2021). Renal Tubular Cell Binding of β‐Catenin to TCF1 versus FoxO1 is Associated with Chronic Interstitial Fibrosis in Transplanted Kidneys. Am. J. Transpl. 21 (2), 727–739. doi:10.1111/ajt.16287
Yao, L., Wright, M. F., Farmer, B. C., Peterson, L. S., Khan, A. M., Zhong, J., et al. (2019). Fibroblast-Specific Plasminogen Activator Inhibitor-1 Depletion Ameliorates Renal Interstitial Fibrosis after Unilateral Ureteral Obstruction. Nephrol. Dial. Transpl. 34 (12), 2042–2050. doi:10.1093/ndt/gfz050
Yiu, W. H., Li, Y., Lok, S. W. Y., Chan, K. W., Chan, L. Y. Y., Leung, J. C. K., et al. (2021). Protective Role of Kallistatin in Renal Fibrosis via Modulation of Wnt/β-Catenin Signaling. Clin. Sci. 135 (3), 429–446. doi:10.1042/cs20201161
Zeng, Z., Wang, Q., Yang, X., Ren, Y., Jiao, S., Zhu, Q., et al. (2019). Qishen Granule Attenuates Cardiac Fibrosis by Regulating TGF-β /Smad3 and GSK-3β Pathway. Phytomedicine 62, 152949. doi:10.1016/j.phymed.2019.152949
Zhang, F., Wan, X., Cao, Y.-Z., Sun, D., and Cao, C.-C. (2018a). Klotho Gene-Modified BMSCs Showed Elevated Antifibrotic Effects by Inhibiting the Wnt/β-Catenin Pathway in Kidneys After Acute Injury. Cell Biol. Int. 42 (12), 1670–1679. doi:10.1002/cbin.11068
Zhang, Q., Xiao, X., Zheng, J., Li, M., Yu, M., Ping, F., et al. (2020). Qishen Yiqi Dripping Pill Protects against Diabetic Nephropathy by Inhibiting the Wnt/β-Catenin and Transforming Growth Factor-β/Smad Signaling Pathways in Rats. Front. Physiol. 11, 613324. doi:10.3389/fphys.2020.613324
Zhang, X., Fang, J., Chen, Z., Zhao, B., Wu, S., and Pan, Y. (2019). Qingshen Buyang Formula Attenuates Renal Fibrosis in 5/6 Nephrectomized Rats via Inhibiting EMT and Wnt/β-Catenin Pathway. Evid. Based Complement. Alternat. Med. 2019, 5370847. doi:10.1155/2019/5370847
Zhang, Y. D., Ding, X. J., Dai, H. Y., Peng, W. S., Guo, N. F., Zhang, Y., et al. (2018b). SB‐216763, A GSK‐3β Inhibitor, Protects against Aldosterone‐Induced Cardiac, and Renal Injury by Activating Autophagy. J. Cell Biochem. 119 (7), 5934–5943. doi:10.1002/jcb.26788
Zhang, Z.-H., Zhao, Y.-Y., Cheng, X.-l., Dai, Z., Zhou, C., Bai, X., et al. (2013). General Toxicity of Pinellia Ternata (Thunb.) Berit. In Rat: a Metabonomic Method for Profiling of Serum Metabolic Changes. J. Ethnopharmacol. 149 (1), 303–310. doi:10.1016/j.jep.2013.06.039
Zhang, Z.-Y., Mai, Y., Yang, H., Dong, P.-Y., Zheng, X.-L., and Yang, G.-S. (2014). CTSB Promotes Porcine Preadipocytes Differentiation by Degrading Fibronectin and Attenuating the Wnt/β-Catenin Signaling Pathway. Mol. Cell Biochem. 395 (1-2), 53–64. doi:10.1007/s11010-014-2111-6
Zhao, Y.-Y., Cheng, X.-L., Cui, J.-H., Yan, X.-R., Wei, F., Bai, X., et al. (2012a). Effect of Ergosta-4,6,8(14),22-Tetraen-3-One (Ergone) on Adenine-Induced Chronic Renal Failure Rat: A Serum Metabonomic Study Based on Ultra Performance Liquid Chromatography/High-Sensitivity Mass Spectrometry Coupled with MassLynx I-FIT Algorithm. Clin. Chim. Acta 413 (19–20), 1438–1445. doi:10.1016/j.cca.2012.06.005
Zhao, Y.-Y., Feng, Y.-L., Du, X., Xi, Z.-H., Cheng, X.-L., and Wei, F. (2012b). Diuretic Activity of the Ethanol and Aqueous Extracts of the Surface Layer of Poria Cocos in Rat. J. Ethnopharmacol. 144 (3), 775–778. doi:10.1016/j.jep.2012.09.033
Zhao, Y.-Y., Li, H.-T., Feng, Y.-L., Bai, X., and Lin, R.-C. (2013). Urinary Metabonomic Study of the Surface Layer of Poria Cocos as an Effective Treatment for Chronic Renal Injury in Rats. J. Ethnopharmacol. 148 (2), 403–410. doi:10.1016/j.jep.2013.04.018
Zhao, Y.-Y. (2013). Traditional Uses, Phytochemistry, Pharmacology, Pharmacokinetics and Quality Control of Polyporus Umbellatus (Pers.) Fries: A Review. J. Ethnopharmacol. 149 (1), 35–48. doi:10.1016/j.jep.2013.06.031
Zhao, Y.-y., Xie, R.-m., Chao, X., Zhang, Y., Lin, R.-c., and Sun, W.-j. (2009). Bioactivity-Directed Isolation, Identification of Diuretic Compounds from Polyporus umbellatus. J. Ethnopharmacol. 126 (1), 184–187. doi:10.1016/j.jep.2009.07.033
Zhao, Y.-Y., Zhang, L., Mao, J.-R., Cheng, X.-H., Lin, R.-C., Zhang, Y., et al. (2011). Ergosta-4,6,8(14),22-Tetraen-3-One Isolated from Polyporus Umbellatus Prevents Early Renal Injury in Aristolochic Acid-Induced Nephropathy Rats. J. Pharm. Pharmacol. 63 (12), 1581–1586. doi:10.1111/j.2042-7158.2011.01361.x
Zhao, Y., Wang, C., Wang, C., Hong, X., Miao, J., Liao, Y., et al. (2018). An Essential Role for Wnt/β-Catenin Signaling in Mediating Hypertensive Heart Disease. Sci. Rep. 8 (1), 8996. doi:10.1038/s41598-018-27064-2
Zhao, Y. Y., Chen, H., Tian, T., Chen, D. Q., Bai, X., and Wei, F. (2014). A Pharmaco-Metabonomic Study on Chronic Kidney Disease and Therapeutic Effect of Ergone by UPLC-QTOF/HDMS. PLoS One 9 (9), e115467. doi:10.1371/journal.pone.0115467
Zhou, D., Fu, H., Zhang, L., Zhang, K., Min, Y., Xiao, L., et al. (2017). Tubule-Derived Wnts are Required for Fibroblast Activation and Kidney Fibrosis. J. Am. Soc. Nephrol. 28 (8), 2322–2336. doi:10.1681/asn.2016080902
Zhou, D., Tan, R. J., Fu, H., and Liu, Y. (2016). Wnt/β-Catenin Signaling in Kidney Injury and Repair: A Double-Edged Sword. Lab. Invest. 96 (2), 156–167. doi:10.1038/labinvest.2015.153
Zhou, G., Li, J., Zeng, T., Yang, P., and Li, A. (2020). The Regulation Effect of WNT-RAS Signaling in Hypothalamic Paraventricular Nucleus on Renal Fibrosis. J. Nephrol. 33 (2), 289–297. doi:10.1007/s40620-019-00637-8
Zhou, L., Li, Y., Hao, S., Zhou, D., Tan, R. J., Nie, J., et al. (2015b). Multiple Genes of the Renin-Angiotensin System Are Novel Targets of Wnt/β-Catenin Signaling. J. Am. Soc. Nephrol. 26 (1), 107–120. doi:10.1681/asn.2014010085
Zhou, L., Li, Y., Zhou, D., Tan, R. J., and Liu, Y. (2013). Loss of Klotho Contributes to Kidney Injury by Derepression of Wnt/β-Catenin Signaling. J. Am. Soc. Nephrol. 24 (5), 771–785. doi:10.1681/asn.2012080865
Zhou, L., and Liu, Y. (2016). Wnt/β-Catenin Signaling and Renin-Angiotensin System in Chronic Kidney Disease. Curr. Opin. Nephrol. Hypertens. 25 (2), 100–106. doi:10.1097/mnh.0000000000000205
Zhou, L., and Liu, Y. (2015). Wnt/β-Catenin Signalling and Podocyte Dysfunction in Proteinuric Kidney Disease. Nat. Rev. Nephrol. 11 (9), 535–545. doi:10.1038/nrneph.2015.88
Zhou, L., Mo, H., Miao, J., Zhou, D., Tan, R. J., Hou, F. F., et al. (2015a). Klotho Ameliorates Kidney Injury and Fibrosis and Normalizes Blood Pressure by Targeting the Renin-Angiotensin System. Am. J. Pathol. 185 (12), 3211–3223. doi:10.1016/j.ajpath.2015.08.004
Zhou, S., Wu, Q., Lin, X., Ling, X., Miao, J., Liu, X., et al. (2021). Cannabinoid Receptor Type 2 Promotes Kidney Fibrosis Through Orchestrating β-catenin Signaling. Kidney Int. 99 (2), 364–381. doi:10.1016/j.kint.2020.09.025
Zhuang, S., Hua, X., He, K., Zhou, T., Zhang, J., Wu, H., et al. (2018). Inhibition of GSK‐3β Induces AP‐1‐mediated Osteopontin Expression to Promote Cholestatic Liver Fibrosis. FASEB J. 32 (8), 4494–4503. doi:10.1096/fj.201701137R
Glossary
α-SMA α-smooth muscle actin
AA aristolochic acid
AKI acute kidney injury
APC adenomatous polyposis coli
API apigenin
CB2 cannabinoid receptor type 2
CBP CREB-binding protein
CHMs Chinese herbal medicines
CK1 casein kinase 1
CKD Chronic kidney disease
CRD cysteine‐rich domains
CRF chronic renal failure
DAMPs danger-associated molecular patterns
DKK1 Dickkopf1
DN diabetic nephropathy
DVL Dishevelled
ECM extracellular matrix
EMT epithelial-mesenchymal transition
ESRD end-stage renal disease
fsp1 fibroblast-specific protein 1
FGF-2 fibroblast growth factor-2
FSGS glomerulosclerosis
FZD Frizzled
GSK3β glycogen synthase kinase 3β
HH Hedgehog
HIF-1α hypoxia inducible factor-1α
IDO indoleamine-2,3-dioxygenase
IRI ischemia-reperfusion injury
IWR inhibitors of Wnt response
LEF lymphatic enhancer-binding factor
LRP5/6 low-density lipoprotein receptor-related protein-5/protein-6
MMP-7 matrix metalloproteinase-7
PAA Poricoic acid A
PAI-1 plasminogen activator inhibitor-1
PGE2 prostaglandin E2
PORCN Porcupine
(P)RR (pro)renin receptor
PZC poricoic acid ZC
PZD poricoic acid ZD
PZE poricoic acid ZE
PZG poricoic acid ZG
PZH poricoic acid ZH
RAS renin-angiotensin system
SCIs Substrate competitive inhibitors
Sfrp1 secreted frizzled protein 1
Snail1 Snail family zinc finger 1
SSc Systemic sclerosis
tPA tissue-type plasminogen activator
TCF transcription factors T cell factor
TCM traditional Chinese medicine
TECs tubular epithelial cells
TGF-β transforming growth factor β
Tnks Tankyrase
TRPC6 transient receptor potential canonical 6
UA uric acid
Upa urokinase-type plasminogen activator
UUO unilateral ureteral obstruction
Wif-1 Wnt inhibitor 1
Wnt/Ca2+ pathway Wnt/calcium pathway
Wnt/PCP pathway Wnt/planar cell polarity pathway
Keywords: Wnt/β-catenin, chronic kidney disease, renal fibrosis, traditional Chinese medicine, natural product
Citation: Li S-S, Sun Q, Hua M-R, Suo P, Chen J-R, Yu X-Y and Zhao Y-Y (2021) Targeting the Wnt/β-Catenin Signaling Pathway as a Potential Therapeutic Strategy in Renal Tubulointerstitial Fibrosis. Front. Pharmacol. 12:719880. doi: 10.3389/fphar.2021.719880
Received: 03 June 2021; Accepted: 03 August 2021;
Published: 16 August 2021.
Edited by:
Zhiyong Guo, Second Military Medical University, ChinaReviewed by:
Songyan Gao, Shanghai University, ChinaDong Wang, Anhui University of Chinese Medicine, China
Copyright © 2021 Li, Sun, Hua, Suo, Chen, Yu and Zhao. This is an open-access article distributed under the terms of the Creative Commons Attribution License (CC BY). The use, distribution or reproduction in other forums is permitted, provided the original author(s) and the copyright owner(s) are credited and that the original publication in this journal is cited, in accordance with accepted academic practice. No use, distribution or reproduction is permitted which does not comply with these terms.
*Correspondence: Xiao-Yong Yu, Z3ViNzA3MjVAMTI2LmNvbQ==; Ying-Yong Zhao, enl5QG53dS5lZHUuY24=