- 1Department of Cardiology, Guangdong Second Provincial General Hospital, Guangzhou, China
- 2School of Public Health, Guangzhou Medical University, Guangzhou, China
Heart failure (HF), the leading cause of death among men and women world-wide, causes great health and economic burdens. HF can be triggered by many factors, such as coronary artery disease, heart attack, cardiomyopathy, hypertension, obesity, etc., all of which have close relations with calcium signal and the level of reactive oxygen species (ROS). Calcium is an essential second messenger in signaling pathways, playing a pivotal role in regulating the life and death of cardiomyocytes via the calcium-apoptosis link mediated by the cellular level of calcium. Meanwhile, calcium can also control the rate of energy production in mitochondria that are the major resources of ROS whose overproduction can lead to cell death. More importantly, there are bidirectional interactions between calcium and ROS, and such interactions may have therapeutic implications in treating HF through finely tuning the balance between these two by certain drugs. Many naturally derived products, e.g., flavones and isoflavones, have been shown to possess activities in regulating calcium and ROS simultaneously, thereby leading to a balanced microenvironment in heart tissues to exert therapeutic efficacies in HF. In this mini review, we aimed to provide an updated knowledge of the interplay between calcium and ROS in the development of HF. In addition, we summarized the recent studies (in vitro, in vivo and in clinical trials) using natural isolated flavones and isoflavones in treating HF. Critical challenges are also discussed. The information collected may help to evoke multidisciplinary efforts in developing novel agents for the potential prevention and treatment of HF.
Introduction
Heart diseases, including cardiovascular diseases, the world’s leading cause of death, are composed with a class of chronic, progressive, and/or lethal diseases, such as high blood pressure, high blood cholesterol, abnormal heart rhythms, coronary artery disease, ischemic heart disease, stroke, heart attacks, etc. (Virani et al., 2021). Heart failure (HF), the late stage of heart diseases, is a condition in which there is a dramatically reduced supply of blood pumped by the muscle of the heart (Dryer et al., 2021). While HF is a chronic and progressive disease, its onset and consequence are acute and prominent (Weintraub et al., 2010). In China, there are approximately 14 million patients suffering from HF, with a prevalence rate of 1.3% (0.9% in 2000). As in the United States, approximately 1.5 million people experience HF every year, causing over 690,000 deaths in 2019 (Ahmad and Anderson, 2021), indicating a great burden to health and finance.
While varied therapeutics for heart diseases that may lead to HF are available, such as nitrates, calcium channel blockers, angiotensin-converting enzyme (ACE) inhibitors, angiotensin II receptor blockers, and 3-hydroxy-3-methylglutaryl coenzyme A (HMG-CoA) reductase inhibitors, etc., novel agents, including those effective in preventing HF, are in urgent need to reduce the high morbidity, and mortality. Growing evidence has suggested that the prevention of HF is quite imperative (Horwich and Fonarow, 2017; Wang, 2019). Among all the newly developed regimens, nature-derived products can exert huge potential because of their unique properties and multiple functions such as regulating calcium and reactive oxygen species (ROS) levels in cells.
Here, we attempted to summarize the interactions between calcium cation (Ca2+) signaling and ROS level, both of which contribute to the progression of heart disease as well as HF (Bertero and Maack, 2018). Calcium can work as a direct signaling transductor or a second messenger in regulating neuronal transmission, electrical excitation and contractile function of myocytes (Landstrom et al., 2017; Terrar, 2020), or in promoting the growth, life or death of cells such as proliferation and apoptosis (Rizzuto et al., 2003; Lemos and Ehrlich, 2018) which has an intimate connection with the level of cellular ROS (Orrenius et al., 2003). Meanwhile, similar to high levels of ROS, high levels of cytoplasmic calcium, a term called calcium overload, can also induce cell death (Zhivotovsky and Orrenius, 2011). Therefore, it is feasible that the close interaction between ROS and calcium in inducing cell death can be endowed with therapeutic implications (Münzel et al., 2017). Interestingly, there are many natural products that can simultaneously reduce calcium overload and ROS over-production, exerting cardiovascular protective, and HF-preventing effects (Jiang et al., 2016; Mohiuddin, 2019). Flavones including isoflavones, which are one of the most abundant components in plants and fruits, have been intensely studied and applied in markets as a dietary supplement to prevent the incidence of heart diseases including HF (Dixon and Pasinetti, 2010; McCullough et al., 2012; Zamora-Ros et al., 2013). Therefore, we also attempted to summarize the current status (those studies conducted in the past decade) and challenges in using flavones as therapeutic agents in HF via the dual-regulation of calcium and ROS. The information gained may serve as a foundation for further in-depth study, including pharmacological and chemical modification research, and the development of flavones in clinical use.
The Interplay Between Calcium and ROS in Inducing Cardiomyocytes Death
ROS over-production and calcium accumulation in acute myocardial ischemic injury can be attributed to be the major causes of damage to the heart (Shen and Jennings, 1972). Calcium plays key roles in multiple aspects of heart tissue and cell biology. In this review, we highlighted its role in inducing cell death. Calcium concentrations in the outside and inside of cells, in endoplasmic reticulum (ER), and mitochondria are pivotal for maintaining cell functions, and its alterations could lead to cell death (Bagur and Hajnóczky, 2017). Calcium overload, especially in the mitochondria of cardiomyocytes, can cause HF as shown in cell-based models and mouse models (Luo and Anderson, 2013; Santulli et al., 2015; Mora et al., 2018). The malfunctional mitochondria due to calcium overload can further produce more ROS, which may also finally contribute to HF (Luo and Anderson, 2013; Santulli et al., 2015; Bertero and Maack, 2018).
ROS are one of the main inducers of cell death (Ryter et al., 2007). Normally, the ROS level remains in a controllable condition mediated by the producing systems and the active antioxidant enzymes (eliminating systems) in cells, and they, when working as signal transductors, can closely participate in almost every aspect of cell biology (Cui et al., 2018a; Bock and Tait, 2020). Under stress and malfunctioning conditions, the ROS level in cells can be increased due to varied reasons, leading to the apoptosis initiation (Cui et al., 2018a). Oxidative stress due to over-produced ROS is one of the hallmarks of cardiovascular disease, which has close connections with the progression of ischemia-reperfusion damage and atherosclerosis, both of which can eventually lead to HF (Panth et al., 2016).
There are bidirectional interactions between calcium and ROS as shown in Figure 1 (Görlach et al., 2015). Briefly, calcium can modulate the formation and production of ROS. First, ER and mitochondria are two major producers of various ROS; and calcium can induce ER stress, and enhance ATP production in mitochondria that requires oxygen, resulting in over-produced ROS. Second, NADPH oxidases (NOXs) that are calcium dependent, are another major source of ROS such as H2O2 and O2− (Rastogi et al., 2016; Burtenshaw et al., 2017). Calcium can either activate NOXs via directly binding to NOXs at certain domains or in an indirect way through signal transduction, leading to ROS over-production (Bánfi et al., 2004). Endothelial nitric oxide synthase (eNOS), one of three isoforms that synthesize nitric oxide (NO) (Cui et al., 2019), is another enzyme that is calcium dependent (Aoyagi et al., 2003; Devika and Jaffar Ali, 2013). Calcium can activate calmodulin, which then binds to eNOS, leading to its efficient NO production (Sessa, 2004). Furthermore, calcium can also induce ROS generation by impacting other key ROS-maintaining enzymes such as voltage dependent anion channels (VDAC) (Feno et al., 20192019), or certain complexes form the electron transporting chain (ETC) located in the inner mitochondrial membrane (Adam-Vizi and Starkov, 2010), etc.
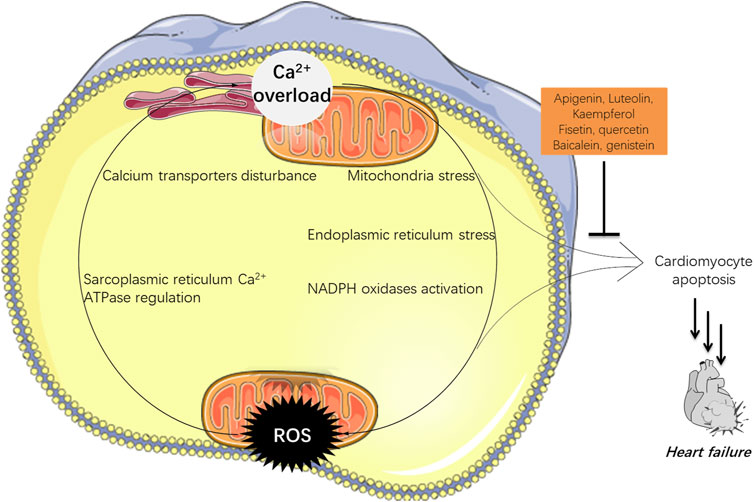
FIGURE 1. The interactions between calcium and ROS in HF. Over-loaded calcium could not only induce apoptosis, but also stimulate the production of ROS which may initiate cell death. Flavones hold potential in navigating both of them which can be adopted in HF treatment.
Meanwhile, ROS can also negatively influence myocardial calcium handling, causing arrhythmia, and augmenting cardiac remodeling by inducing hypertrophic signaling and apoptosis, which later contributes to HF (Senoner and Dichtl, 2019). ROS are ready to attack cellular biomolecules including calcium transporters on cell membranes or organelles’ membranes including ER and mitochondria, therefore affecting the calcium homeostasis (Zimmerman et al., 2011). Free radical H2O2 can bind to the residue of Cys674 at the sarcoplasmic reticulum Ca2+ ATPase (SERCA), leading to disturbed cardiac myocyte in a rat heart (Qin et al., 2013).
In a word, high levels of ROS can increase the uptake of calcium in cells; meanwhile the calcium level in cells can also stimulate the production of ROS. These two events working together can finally induce cardiomyocyte death and eventually HF.
Natural Flavones Exhibit Potential in Treating HF via Dual Regulation of Calcium and ROS
Flavones, including isoflavones, are a class of natural products categorized as flavonoids, sharing a common backbone of 2-phenylchromen-4-one (flavone) or 3-phenylchromen-4-one (isoflavone) (Figure 2) (Hostetler et al., 2017). Natural flavones are rich in fruits, vegetables, soybean, herbal plants, honey, and they have been used as herbal medicines for over 1,000 years. Importantly, the isolated/purified components have been used as supplemental nutrients for decades (Singh et al., 2014). Currently, dozens of flavones are under clinical trials for the treatment of diseases associated with cardiovascular dysfunction and other diseases including neurodegenerative diseases, diabetes mellitus, cancers, etc., suggesting their huge potentials (Hostetler et al., 2017; Cui et al., 2018b). Flavones are known as multi-targeting or multi-functional compounds since they can regulate/target multiple enzymes in vivo (Qiu et al., 2018; Ye et al., 2019), such as silent mating type information regulation 2 homolog (SIRT) (Kang et al., 2018), ABC transporters (Li and Paxton, 2013), cyclin-dependent kinases (CDKs) (Khuntawee et al., 2012), and certain microRNA (Lin et al., 2018), etc. In addition to their multi-functional property, flavones also exert medical efficacies via multi-mechanisms including the regulation of both ROS and calcium that contribute significantly to HF. A retrospective clinical meta-analysis of 23 years among 56,048 Danish people has indicated that the consumption of certain flavonoids (500 mg/day) can reduce the incidence and mortality of cardiovascular diseases (Bondonno et al., 2019), and such efficacies have been validated by other studies as well (Ponzo et al., 2015; Dalgaard et al., 2019), suggesting the beneficial effects of flavonoids in treating HF. Indeed, growing in vitro and in vivo studies have proven such effects (Mozaffarian and Wu, 2018). Here, we focus on those flavones and isoflavones (Figure 2) that exert their heart protective effects via dual regulating ROS and calcium signal.
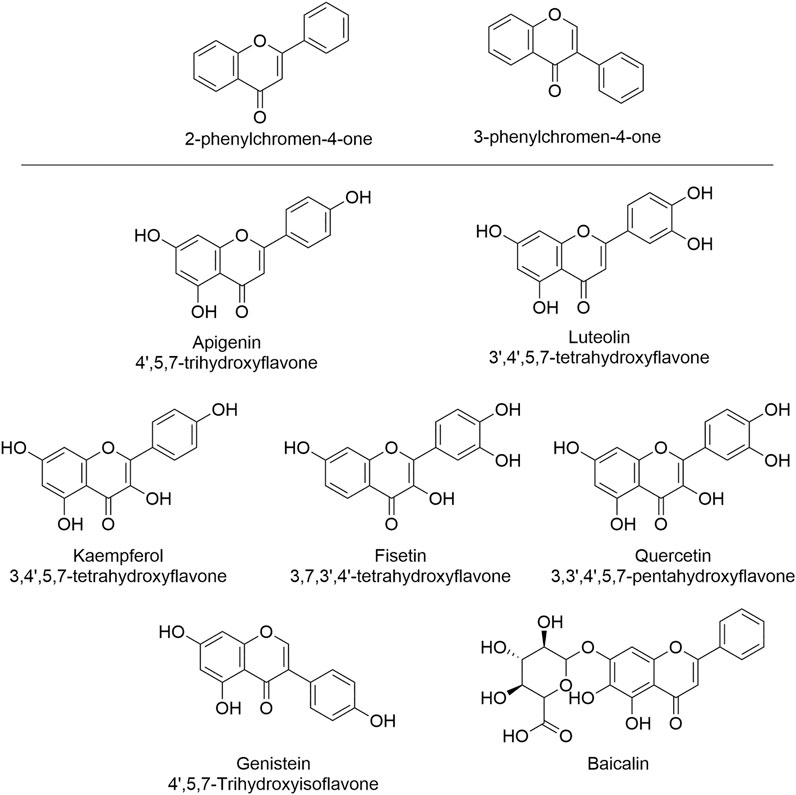
FIGURE 2. The structures of flavones and isoflavone that show HF-treating/preventing effects via the regulation of both ROS and calcium signal. These natural products can serve as leading compounds that can undergo structural modification to achieve the selective regulation of ROS and calcium.
Apigenin (4′,5,7-trihydroxyflavone), a dietary supplement that has demonstrated the ability to regulate both ROS and calcium (Maher and Hanneken, 2005; Wu et al., 2021), suggesting its potential in treating HF. Li et al. (2017) reported that apigenin (50 mg/kg) could relieve myocardial injury induced by endotoxin and decrease the death rate of cardiomyocytes in mice, suggesting a cardioprotective effect (Li et al., 2017). Apigenin worked via reducing oxidative stress as confirmed by increased cardiac glutathione (GSH) level, oxidative stress markers, and pro-inflammatory cytokines including tumor necrosis factor (TNF-α), interleukin 1β (IL-1β), macrophage inflammatory protein-2 (MIP-2) which have intimate networks with Ca2+-associated signals (Hendy and Canaff, 2016; Li et al., 2017).
Luteolin (3′,4′,5,7-tetrahydroxyflavone) is a flavone that has been serving as a supplemental nutrient for decades for improving memory and brain health (Swaminathan et al., 2019; Wang et al., 2021). Luteolin can protect heart from damage caused by over-produced ROS and over-loaded calcium (Wang et al., 2012; Yan et al., 2018). Wang et al. (2012) found that in an animal model of type I diabetic cardiomyopathy, luteolin (200 mg/kg) maintained certain cardiac functions as measured by the left ventricular systolic pressure, left ventricular developed pressure, left ventricular end diastolic pressure, and maximal rate of rise/fall left ventricle pressure development (Wang et al., 2012). They also found that luteolin worked by reducing oxidative stress as confirmed by decreased ROS-producing proteins and signal pathways (Wang et al., 2012). Madhesh and Vaiyapuri (2005 and 2012) found that luteolin (0.3 mg/kg/day) could protect cardiac function and prevent myocardial infarction by reducing mitochondrial lipid peroxidation (a route that produce ROS in vivo) and increasing the mitochondrial antioxidant levels as shown in isoproterenol induced myocardial infarction model in rats (Manju et al., 2005; Madhesh and Vaiyapuri, 2012). Luteolin appears to be an inhibitor of L-type calcium channels as confirmed by Yan et al. (2018), Yan et al. (2019). Luteolin (7.5, 15, or 30 µM) ameliorated calcium overload in freshly isolated cardiomyocytes, accompanied by suppressed Protein Kinase A (PKA) activity and enhanced Ca2+-Mg2+-ATPase activity (Yan et al., 2018). Luteolin’s regulatory role in calcium was also confirmed by Li et al.’s studies in 2015 and 2017 (Nai et al., 2015; Hu et al., 2017a).
Kaempferol is another widely used dietary supplement with a chemical name of 3,4′,5,7-tetrahydroxyflavone, and it can protect heart via the navigation of both ROS and calcium (An and Kim, 2015; Guo et al., 2015). An in vitro study by Guo et al. (2015) showed that in A/R-induced injury model, kaempferol (10, 20, or 40 μM) inhibited mitochondria-mediated apoptosis and increased the cell viability of cardiomyocytes via reducing ROS production mediated by activating SIRT1 (Guo et al., 2015). Calmodulin kinase II (CaMKII), a key player in calcium signaling pathways, can be activated by higher levels of ROS, thereby resulting in abnormally slow heart rhythm or cardiomyocytes death (Di Carlo et al., 2014; Santalla et al., 2014). Kaempferol (15 mM) significantly reduced the CaMKII oxidization and sinus nodal cell death, warranting further in vivo verification (An and Kim, 2015). It is also worth noting that the concentration used in this study is 15 mM, which is much higher than the other studies that fall in submicromolar concentrations. Such high concentrations may cause problems in clinical trials when translating the in vitro doses into those in humans, requiring an in-depth pharmacokinetic study.
Fisetin (3,7,3′,4′-tetrahydroxyflavone) is an abundant flavone existing in strawberry, apple, persimmon, grape, onion, and cucumber. Fisetin demonstrated multiple health benefits including preventing HF via regulating ROS and calcium (Rodius et al., 2020). Shanmugam et al. (2018) confirmed the cardiovascular protective effect of fisetin using a Langendorff isolated heart perfusion system (Shanmugam et al., 2018). Fisetin pretreatment (20 mg/kg) showed a strong protective effect against the damage induced by myocardial ischemia reperfusion in the isolated rat heart. Fisetin improved mitochondrial physiology, biogenesis, and functions including maintaining the ETC and reducing superoxide (O2−) generated in mitochondria, suggesting a mitochondria-mediated mechanism (Shanmugam et al., 2018). The further in silico analysis and computer-aided docking study showed that fisetin might be a potent glycogen synthase kinase 3β (GSK3β) inhibitor (Shanmugam et al., 2018), warranting further study.
Quercetin (3,3′,4′,5,7-pentahydroxyflavone) is an important dietary flavone in fruits and vegetables, and it possesses anti-inflammatory and anti-oxidative properties which may benefit the patients with cardiovascular diseases (Patel et al., 2018). Jing et al. (2016) found that quercetin pretreatment (20 mg/kg) reversed cardiomyocytes apoptosis induced by posttraumatic stress and it restored cardiac function as shown in a rat model (Jing et al., 2016). In vitro study of pretreatment with quercetin at 20 μM showed that it can obviously maintain the cell viability, decreased TNF-α, ROS level and calcium overload in H9c2 cells, suggesting the beneficial effects of quercetin in treating cardiac injury (Jing et al., 2016). Quercetin can also protect the heart from myocardial ischemia reperfusion injury via the dual regulation of ROS level and calcium overload (Zhang et al., 2020). Furthermore, a meta-analysis of clinical trials showed that the consumption of quercetin (at the dose of more than 500 mg/day) exhibited significant reduction of blood pressure, suggesting a beneficial effect of quercetin in HF (Serban et al., 2016).
Baicalein, enriched in natural products and herbal medicines, is a glycosylated flavone that regulates ROS and calcium in cells (Xin et al., 2020), showing promising therapeutic effects in treating and preventing HF (Zhao et al., 2016). Zhao et al. (2016) found that in HF in vivo model established by abdominal aorta constriction in rats and in vitro isoproterenol-induced H9C2 cells, baicalein (50, 100, and 200 mg/kg in vivo or 5, 10, 20 μM in vitro) significantly alleviated HF syndromes by improving heart function as confirmed by hematoxylin-eosin and ELISA measuring the pathomorphological changes and down-regulated TNF-α, angiotensin II, and BNP in peripheral blood (Zhao et al., 2016). Baicalein reduced myocardial fibrosis in vivo through inhibiting the expression and activities of matrix metalloproteinase-2 and -9 (MMP-2/9). Furthermore, baicalein was found to suppress isoproterenol-induced cardiomyocytes hypertrophy and apoptosis in vivo and in vitro, probably via regulating calcium related proteins such as the phosphorylated Ca2+/calmodulin-dependent protein kinase II (CaMKII), Na+/Ca2+-exchangers (NCX1) and sarcoplasmic reticulum Ca2+ ATPase 2 (SERCA2) (Zhao et al., 2016).
Genistein (4′,5,7-trihydroxyisoflavone) is an isoflavone that is found in soy-based products, being widely used as a supplemental nutrient for years (Williamson-Hughes et al., 2006; Mamagkaki et al., 2021). Genistein is also a dual regulator of ROS and calcium (Uddin and Kabir, 2019). Matori et al. (2012) found that genistein (1 mg/kg/day for 9 days) could restore cardiopulmonary structure and function, and reverse the loss of capillaries induced by pulmonary hypertension in the rat model, demonstrating its potential in preventing HF (Matori et al., 2012). In addition, a randomized double-blind case-control study conducted among postmenopausal women with metabolic syndrome showed that genistein (54 mg/day) significantly improved heart functions measured by the left ventricular ejection fraction and remodeling, suggesting a favorable outcome when applied in human with cardiovascular diseases (De Gregorio et al., 2017).
Other potential flavones such as rutin, quercetin-3-O-rutinoside which is the glycosylated quercetin (Chu et al., 2014; Lv et al., 2018), chrysin (5,7-Dihydroxyflavone) (Farkhondeh et al., 2019; Xingyue et al., 2021), wogonin (5,7-Dihydroxy-8-methoxyflavone) (Khan et al., 2016; Khan and Kamal, 2019), also possess cardiovascular protective and HF-preventing efficacies via an ROS-calcium associated mechanism, rendering them as attractive drug candidates or dietary supplements.
Discussion and Future Perspective
The information discussed above has indicated that 1) there is a vicious cycle between overloaded calcium and over-produced ROS, and both contribute to HF; 2) certain flavones can protect cardiovascular via down-regulating both intracellular calcium content and ROS level, thereby demonstrating potentials in preventing/treating HF as summarized in Table 1. As shown in the original studies, luteolin, kaempferol, and baicalein, demonstrated a dose-dependent mode of action; while the other four including apigenin, fisetin, quercetin, and genistein, were tested with one dose/concentration to exert the HF-treating/preventing effects, warranting further pharmacological study in vivo. In addition, combinational strategies of certain flavones and conventional drugs can also be developed and applied in HF treatment via synergistic effects (Guerrero et al., 2012; Zeka et al., 2017).
Meanwhile, cautions should also be made. Firstly, these flavones are not specific regulators of ROS or calcium, undermining their potential as drug candidates which require the selective targeting of certain pathogenic mechanisms/proteins. As for these small-molecule flavones, it appears to be true that none of them has a selective bio-target in vivo, and it is well accepted that most of them might exert their bioactivities via interacting with membrane proteins (Cyboran et al., 2012; Ingólfsson et al., 2014; Phan et al., 2014), requiring more studies such as medicinal chemical modification to improve the selectivity and druglikeness (Boniface and Elizabeth, 2019). As far as the authors concerned, it seems to be more reasonable to develop them as supplemental nutrients in preventing HF.
Secondly, the dual-regulation of ROS and calcium might not be the mere mechanism that leads to cardio-protective effects by flavones (Najjar and Feresin, 2021). HF, the late stage of heart diseases, can be triggered by various factors; consequently, flavones can also exert HF-preventing efficacies via multiple mechanisms which have been intensively studied over the past decade (Grassi et al., 2013; Choy et al., 2019; Ciumărnean et al., 2020a; Ciumărnean et al., 2020b; Fusi et al., 2020; Yamagata and Yamori, 2020; Jiang et al., 2021). This fact can further support the strategy of developing flavones as supplemental nutrients.
Last, in spite of the fact that the aforementioned flavones can generally reduce the level of ROS in cardiomyocytes, a significant proportion of them (at varied concentrations) can also induce the production, leading to cell death which can be applied in cancer treatment (Lu et al., 2007; Lin et al., 2011; Shih et al., 2017; Souza et al., 20172017; Cui et al., 2018b; Cataneo et al., 2019; Korga et al., 2019). Thus, the therapeutic windows of each flavone should be determined before their application (or trials) in humans.
Multiple clinical trials are ongoing and several conducted previously have been completed as shown in the Supplemental Table S1. It is worth noting that quercetin, whose name has been used since 1857, has been widely tested in clinical trials for the treatment of different diseases including heart diseases. As one of the most abundant, and widely studied and applied as nutritional supplement (Jing et al., 2016; Patel et al., 2018), it is the authors’ opinion that quercetin has a greater potential in treating/preventing HF among all the others. However, by far, using flavones as drug candidates in HF treatment/prevention is still in its early stage. One of the major obstacles that refrain the effects in vivo and in clinical trials is that the stability, selectivity, and overall poor bioavailability that fails to reach consistent exposure levels, etc. (Ross and Kasum, 2002; Wu et al., 2011; Thilakarathna and Rupasinghe, 2013; Hu et al., 2017b). Bioavailability of certain flavones has been tested in human, and the results indicated that only a small proportion can be absorbed (Meyer et al., 2006; Kanaze et al., 2007), such as15–24% of genistein (Lu and Anderson, 1998). Such low bioavailability may require high doses in humans, and a typical dose is 500 mg/day, and doses below this may not benefit patients with heart diseases/conditions (Kirienko and Radak, 2016; Serban et al., 2016; Bondonno et al., 2019). Therefore, to achieve the full potential in HF, further in vitro and in vivo studies are required to determine the dose, administration methods, safety, and pharmacokinetic and pharmacodynamics profiles.
Conclusion
Overload of calcium and elevated ROS production can form a vicious cycle to induce cardiomyocytes death that may finally lead to HF. A number of flavones show the dual-regulation of calcium and ROS, demonstrating their therapeutic potential in HF.
Author Contributions
Conceptualization: TY, DH, and QC. Writing: TY, DH, HC, SC, and HW. Review and editing: TY and DH. All authors contributed to the article and approved the submitted version.
Conflict of Interest
The authors declare that the research was conducted in the absence of any commercial or financial relationships that could be construed as a potential conflict of interest.
Publisher’s Note
All claims expressed in this article are solely those of the authors and do not necessarily represent those of their affiliated organizations, or those of the publisher, the editors and the reviewers. Any product that may be evaluated in this article, or claim that may be made by its manufacturer, is not guaranteed or endorsed by the publisher.
Supplementary Material
The Supplementary Material for this article can be found online at: https://www.frontiersin.org/articles/10.3389/fphar.2021.718496/full#supplementary-material
References
Adam-Vizi, V., and Starkov, A. A. (2010). Calcium and Mitochondrial Reactive Oxygen Species Generation: How to Read the Facts. J. Alzheimers Dis. 20 (Suppl. 2), S413–S426. doi:10.3233/JAD-2010-100465
Ahmad, F. B., and Anderson, R. N. (2021). The Leading Causes of Death in the US for 2020. JAMA 325 (18), 1829–1830. doi:10.1001/jama.2021.5469
An, M., and Kim, M. (2015). Protective Effects of Kaempferol against Cardiac Sinus Node Dysfunction via CaMKII Deoxidization. Anat. Cel. Biol. 48 (4), 235–243. doi:10.5115/acb.2015.48.4.235
Aoyagi, M., Arvai, A. S., Tainer, J. A., and Getzoff, E. D. (2003). Structural Basis for Endothelial Nitric Oxide Synthase Binding to Calmodulin. EMBO J. 22 (4), 766–775. doi:10.1093/emboj/cdg078
Bagur, R., and Hajnóczky, G. (2017). Intracellular Ca2+ Sensing: Its Role in Calcium Homeostasis and Signaling. Mol. Cel. 66 (6), 780–788. doi:10.1016/j.molcel.2017.05.028
Bánfi, B., Tirone, F., Durussel, I., Knisz, J., Moskwa, P., Molnár, G. Z., et al. (2004). Mechanism of Ca2+ Activation of the NADPH Oxidase 5 (NOX5). J. Biol. Chem. 279 (18), 18583–18591. doi:10.1074/jbc.M310268200
Bertero, E., and Maack, C. (2018). Calcium Signaling and Reactive Oxygen Species in Mitochondria. Circ. Res. 122 (10), 1460–1478. doi:10.1161/CIRCRESAHA.118.310082
Bock, F. J., and Tait, S. W. G. (2020). Mitochondria as Multifaceted Regulators of Cell Death. Nat. Rev. Mol. Cel. Biol. 21 (2), 85–100. doi:10.1038/s41580-019-0173-8
Bondonno, N. P., Dalgaard, F., Kyrø, C., Murray, K., Bondonno, C. P., Lewis, J. R., et al. (2019). Flavonoid Intake Is Associated with Lower Mortality in the Danish Diet Cancer and Health Cohort. Nat. Commun. 10 (1), 3651. doi:10.1038/s41467-019-11622-x
Boniface, P. K., and Elizabeth, F. I. (2019). Flavones as a Privileged Scaffold in Drug Discovery: Current Developments. Curr. Org. Synth. 16 (7), 968–1001. doi:10.2174/1570179416666190719125730
Burtenshaw, D., Hakimjavadi, R., Redmond, E. M., and Cahill, P. A. (2017). Nox, Reactive Oxygen Species and Regulation of Vascular Cell Fate. Antioxidants (Basel) 6 (4). doi:10.3390/antiox6040090
Cataneo, A. H. D., Tomiotto-Pellissier, F., Miranda-Sapla, M. M., Assolini, J. P., Panis, C., Kian, D., et al. (2019). Quercetin Promotes Antipromastigote Effect by Increasing the ROS Production and Anti-amastigote by Upregulating Nrf2/HO-1 Expression, Affecting Iron Availability. Biomed. Pharmacother. 113, 108745. doi:10.1016/j.biopha.2019.108745
Choy, K. W., Murugan, D., Leong, X. F., Abas, R., Alias, A., and Mustafa, M. R. (2019). Flavonoids as Natural Anti-inflammatory Agents Targeting Nuclear Factor-Kappa B (NFκB) Signaling in Cardiovascular Diseases: A Mini Review. Front. Pharmacol. 10, 1295. doi:10.3389/fphar.2019.01295
Chu, J. X., Li, G. M., Gao, X. J., Wang, J. X., and Han, S. Y. (2014). Buckwheat Rutin Inhibits AngII-Induced Cardiomyocyte Hypertrophy via Blockade of CaN-dependent Signal Pathway. Iran. J. Pharm. Res. 13 (4), 1347–1355.
Ciumărnean, L., Milaciu, M. V., Runcan, O., Vesa, Ș. C., Răchișan, A. L., Negrean, V., et al. (2020). The Effects of Flavonoids in Cardiovascular Diseases. Molecules 25 (18). doi:10.3390/molecules25184320
Ciumărnean, L., Milaciu, M. V., Runcan, O., Vesa, Ș. C., Răchișan, A. L., Negrean, V., et al. (2020). The Effects of Flavonoids in Cardiovascular Diseases. Molecules 25 (18). doi:10.3390/molecules25184320
Cui, Q., Wang, J. Q., Assaraf, Y. G., Ren, L., Gupta, P., Wei, L., et al. (2018). Modulating ROS to Overcome Multidrug Resistance in Cancer. Drug Resist. Updat. 41, 1–25. doi:10.1016/j.drup.2018.11.001
Cui, Q., Yang, D. H., and Chen, Z. S. (2018). Special Issue: Natural Products: Anticancer and beyond. Molecules 23 (6), 1246–1249. doi:10.3390/molecules23061246
Cui, Q., Yang, Y., Ji, N., Wang, J. Q., Ren, L., Yang, D. H., et al. (2019). Gaseous Signaling Molecules and Their Application in Resistant Cancer Treatment: from Invisible to Visible. Future Med. Chem. 11 (4), 323–336. doi:10.4155/fmc-2018-0403
Cyboran, S., Oszmiański, J., and Kleszczyńska, H. (2012). Interaction between Plant Polyphenols and the Erythrocyte Membrane. Cell. Mol. Biol. Lett. 17 (1), 77–88. doi:10.2478/s11658-011-0038-4
Dalgaard, F., Bondonno, N. P., Murray, K., Bondonno, C. P., Lewis, J. R., Croft, K. D., et al. (2019). Associations between Habitual Flavonoid Intake and Hospital Admissions for Atherosclerotic Cardiovascular Disease: a Prospective Cohort Study. Lancet Planet. Health 3 (11), e450–59. doi:10.1016/S2542-5196(19)30212-8
De Gregorio, C., Marini, H., Alibrandi, A., Di Benedetto, A., Bitto, A., Adamo, E. B., et al. (2017). Genistein Supplementation and Cardiac Function in Postmenopausal Women with Metabolic Syndrome: Results from a Pilot Strain-Echo Study. Nutrients 9 (6). doi:10.3390/nu9060584
Devika, N. T., and Jaffar Ali, B. M. (2013). Analysing Calcium Dependent and Independent Regulation of eNOS in Endothelium Triggered by Extracellular Signalling Events. Mol. Biosyst. 9 (11), 2653–2664. doi:10.1039/c3mb70258h
Di Carlo, M. N., Said, M., Ling, H., Valverde, C. A., De Giusti, V. C., Sommese, L., et al. (2014). CaMKII-dependent Phosphorylation of Cardiac Ryanodine Receptors Regulates Cell Death in Cardiac Ischemia/reperfusion Injury. J. Mol. Cel. Cardiol. 74, 274–283. doi:10.1016/j.yjmcc.2014.06.004
Dixon, R. A., and Pasinetti, G. M. (2010). Flavonoids and Isoflavonoids: from Plant Biology to Agriculture and Neuroscience. Plant Physiol. 154 (2), 453–457. doi:10.1104/pp.110.161430
Dryer, C., Cotter, E. K., and Flynn, B. (2021). Overview of the 2021 Update to the 2017 ACC Expert Consensus Decision Pathway for Optimization of Heart Failure with Reduced Ejection Fraction. J. Cardiothorac. Vasc. Anesth. doi:10.1053/j.jvca.2021.03.041
Farkhondeh, T., Samarghandian, S., and Bafandeh, F. (2019). The Cardiovascular Protective Effects of Chrysin: A Narrative Review on Experimental Researches. Cardiovasc. Hematol. Agents Med. Chem. 17 (1), 17–27. doi:10.2174/1871525717666190114145137
Feno, S., Butera, G., Vecellio Reane, D., Rizzuto, R., and Raffaello, A. (20192019). Crosstalk between Calcium and ROS in Pathophysiological Conditions. Oxid. Med. Cel. Longev. 2019, 9324018. doi:10.1155/2019/9324018
Fusi, F., Trezza, A., Tramaglino, M., Sgaragli, G., Saponara, S., and Spiga, O. (2020). The Beneficial Health Effects of Flavonoids on the Cardiovascular System: Focus on K+ Channels. Pharmacol. Res. 152, 104625. doi:10.1016/j.phrs.2019.104625
Görlach, A., Bertram, K., Hudecova, S., and Krizanova, O. (2015). Calcium and ROS: A Mutual Interplay. Redox Biol. 6, 260–271. doi:10.1016/j.redox.2015.08.010
Grassi, D., Desideri, G., Di Giosia, P., De Feo, M., Fellini, E., Cheli, P., et al. (2013). Tea, Flavonoids, and Cardiovascular Health: Endothelial protection. Am. J. Clin. Nutr. 98 (6 Suppl. l), 1660S–1666S. doi:10.3945/ajcn.113.058313
Guerrero, L., Castillo, J., Quiñones, M., Garcia-Vallvé, S., Arola, L., Pujadas, G., et al. (2012). Inhibition of Angiotensin-Converting Enzyme Activity by Flavonoids: Structure-Activity Relationship Studies. PLoS One 7 (11), e49493. doi:10.1371/journal.pone.0049493
Guo, Z., Liao, Z., Huang, L., Liu, D., Yin, D., and He, M. (2015). Kaempferol Protects Cardiomyocytes against Anoxia/reoxygenation Injury via Mitochondrial Pathway Mediated by SIRT1. Eur. J. Pharmacol. 761, 245–253. doi:10.1016/j.ejphar.2015.05.056
Hendy, G. N., and Canaff, L. (2016). Calcium-sensing Receptor, Proinflammatory Cytokines and Calcium Homeostasis. Semin. Cel. Dev. Biol. 49, 37–43. doi:10.1016/j.semcdb.2015.11.006
Horwich, T. B., and Fonarow, G. C. (2017). Prevention of Heart Failure. JAMA Cardiol. 2 (1), 116. doi:10.1001/jamacardio.2016.3394
Hostetler, G. L., Ralston, R. A., and Schwartz, S. J. (2017). Flavones: Food Sources, Bioavailability, Metabolism, and Bioactivity. Adv. Nutr. 8 (3), 423–435. doi:10.3945/an.116.012948
Hu, M., Wu, B., and Liu, Z. (2017). Bioavailability of Polyphenols and Flavonoids in the Era of Precision Medicine. Mol. Pharm. 14 (9), 2861–2863. doi:10.1021/acs.molpharmaceut.7b00545
Hu, W., Xu, T., Wu, P., Pan, D., Chen, J., Chen, J., et al. (2017). Luteolin Improves Cardiac Dysfunction in Heart Failure Rats by Regulating Sarcoplasmic Reticulum Ca2+-ATPase 2a. Sci. Rep. 7, 41017. doi:10.1038/srep41017
Ingólfsson, H. I., Thakur, P., Herold, K. F., Hobart, E. A., Ramsey, N. B., Periole, X., et al. (2014). Phytochemicals Perturb Membranes and Promiscuously Alter Protein Function. ACS Chem. Biol. 9 (8), 1788–1798. doi:10.1021/cb500086e
Jiang, Y. Q., Chang, G. L., Wang, Y., Zhang, D. Y., Cao, L., and Liu, J. (2016). Geniposide Prevents Hypoxia/Reoxygenation-Induced Apoptosis in H9c2 Cells: Improvement of Mitochondrial Dysfunction and Activation of GLP-1R and the PI3K/AKT Signaling Pathway. Cell. Physiol. Biochem. 39 (1), 407–421. doi:10.1159/000445634
Jiang, Y., Sun-Waterhouse, D., Chen, Y., Li, F., and Li, D. (2021). Epigenetic Mechanisms Underlying the Benefits of Flavonoids in Cardiovascular Health and Diseases: Are Long Non-coding RNAs Rising Stars? Crit. Rev. Food Sci. Nutr., 1–19. doi:10.1080/10408398.2020.1870926
Jing, Z., Wang, Z., Li, X., Li, X., Cao, T., Bi, Y., et al. (2016). Protective Effect of Quercetin on Posttraumatic Cardiac Injury. Sci. Rep. 6, 30812. doi:10.1038/srep30812
Kanaze, F. I., Bounartzi, M. I., Georgarakis, M., and Niopas, I. (2007). Pharmacokinetics of the Citrus Flavanone Aglycones Hesperetin and Naringenin after Single Oral Administration in Human Subjects. Eur. J. Clin. Nutr. 61 (4), 472–477. doi:10.1038/sj.ejcn.1602543
Kang, H. W., Lee, S. G., Otieno, D., and Ha, K. (2018). Flavonoids, Potential Bioactive Compounds, and Non-shivering Thermogenesis. Nutrients 10 (9). doi:10.3390/nu10091168
Khan, S., and Kamal, M. A. (2019). Can Wogonin Be Used in Controlling Diabetic Cardiomyopathy? Curr. Pharm. Des. 25 (19), 2171–2177. doi:10.2174/1381612825666190708173108
Khan, S., Zhang, D., Zhang, Y., Li, M., and Wang, C. (2016). Wogonin Attenuates Diabetic Cardiomyopathy through its Anti-inflammatory and Anti-oxidative Properties. Mol. Cel. Endocrinol. 428, 101–108. doi:10.1016/j.mce.2016.03.025
Khuntawee, W., Rungrotmongkol, T., and Hannongbua, S. (2012). Molecular Dynamic Behavior and Binding Affinity of Flavonoid Analogues to the Cyclin Dependent Kinase 6/cyclin D Complex. J. Chem. Inf. Model. 52 (1), 76–83. doi:10.1021/ci200304v
Kirienko, A., and Radak, D. (2016). Clinical Acceptability Study of Once-Daily versus Twice-Daily Micronized Purified Flavonoid Fraction in Patients with Symptomatic Chronic Venous Disease: a Randomized Controlled Trial. Int. Angiol. 35 (4), 399–405.
Korga, A., Ostrowska, M., Jozefczyk, A., Iwan, M., Wojcik, R., Zgorka, G., et al. (2019). Apigenin and Hesperidin Augment the Toxic Effect of Doxorubicin against HepG2 Cells. BMC Pharmacol. Toxicol. 20 (1), 22. doi:10.1186/s40360-019-0301-2
Landstrom, A. P., Dobrev, D., and Wehrens, X. H. T. (2017). Calcium Signaling and Cardiac Arrhythmias. Circ. Res. 120 (12), 1969–1993. doi:10.1161/CIRCRESAHA.117.310083
Lemos, F. O., and Ehrlich, B. E. (2018). Polycystin and Calcium Signaling in Cell Death and Survival. Cell Calcium 69, 37–45. doi:10.1016/j.ceca.2017.05.011
Li, F., Lang, F., Zhang, H., Xu, L., Wang, Y., Zhai, C., et al. (2017). Apigenin Alleviates Endotoxin-Induced Myocardial Toxicity by Modulating Inflammation, Oxidative Stress, and Autophagy. Oxid. Med. Cel. Longev. 2017, 2302896. doi:10.1155/2017/2302896
Li, Y., and Paxton, J. W. (2013). The Effects of Flavonoids on the ABC Transporters: Consequences for the Pharmacokinetics of Substrate Drugs. Expert Opin. Drug Metab. Toxicol. 9 (3), 267–285. doi:10.1517/17425255.2013.749858
Lin, C. C., Kuo, C. L., Lee, M. H., Lai, K. C., Lin, J. P., Yang, J. S., et al. (2011). Wogonin Triggers Apoptosis in Human Osteosarcoma U-2 OS Cells through the Endoplasmic Reticulum Stress, Mitochondrial Dysfunction and Caspase-3-dependent Signaling Pathways. Int. J. Oncol. 39 (1), 217–224. doi:10.3892/ijo.2011.1027
Lin, C. M., Wang, B. W., Pan, C. M., Fang, W. J., Chua, S. K., Hou, S. W., et al. (2018). Effects of Flavonoids on MicroRNA 145 Regulation through Klf4 and Myocardin in Neointimal Formation In Vitro and In Vivo. J. Nutr. Biochem. 52, 27–35. doi:10.1016/j.jnutbio.2017.08.016
Lu, H. F., Hsueh, S. C., Ho, Y. T., Kao, M. C., Yang, J. S., Chiu, T. H., et al. (2007). ROS Mediates Baicalin-Induced Apoptosis in Human Promyelocytic Leukemia HL-60 Cells through the Expression of the Gadd153 and Mitochondrial-dependent Pathway. Anticancer Res. 27 (1A), 117–125.
Lu, L. J., and Anderson, K. E. (1998). Sex and Long-Term Soy Diets Affect the Metabolism and Excretion of Soy Isoflavones in Humans. Am. J. Clin. Nutr. 68 (6 Suppl. l), 1500S–1504S. doi:10.1093/ajcn/68.6.1500S
Luo, M., and Anderson, M. E. (2013). Mechanisms of Altered Ca²⁺ Handling in Heart Failure. Circ. Res. 113 (6), 690–708. doi:10.1161/CIRCRESAHA.113.301651
Lv, L., Yao, Y., Zhao, G., and Zhu, G. (2018). Rutin Inhibits Coronary Heart Disease through ERK1/2 and Akt Signaling in a Porcine Model. Exp. Ther. Med. 15 (1), 506–512. doi:10.3892/etm.2017.5365
Madhesh, M., and Vaiyapuri, M. (2012). Effect of Luteolin on Lipid Peroxidation and Antioxidants in Acute and Chronic Periods of Isoproterenol Induced Myocardial Infarction in Rats. J. Acute Med. 2 (3), 70–76. doi:10.1016/j.jacme.2012.06.001
Maher, P., and Hanneken, A. (2005). Flavonoids Protect Retinal Ganglion Cells from Oxidative Stress-Induced Death. Invest. Ophthalmol. Vis. Sci. 46 (12), 4796–4803. doi:10.1167/iovs.05-0397
Mamagkaki, A., Bouris, I., Parsonidis, P., Vlachou, I., Gougousi, M., and Papasotiriou, I. (2021). Genistein as a Dietary Supplement; Formulation, Analysis and Pharmacokinetics Study. PLoS One 16 (4), e0250599. doi:10.1371/journal.pone.0250599
Manju, V., Balasubramaniyan, V., and Nalini, N. (2005). Rat Colonic Lipid Peroxidation and Antioxidant Status: the Effects of Dietary Luteolin on 1,2-dimethylhydrazine challenge. Cel. Mol. Biol. Lett. 10 (3), 535–551.
Matori, H., Umar, S., Nadadur, R. D., Sharma, S., Partow-Navid, R., Afkhami, M., et al. (2012). Genistein, a Soy Phytoestrogen, Reverses Severe Pulmonary Hypertension and Prevents Right Heart Failure in Rats. Hypertension 60 (2), 425–430. doi:10.1161/HYPERTENSIONAHA.112.191445
McCullough, M. L., Peterson, J. J., Patel, R., Jacques, P. F., Shah, R., and Dwyer, J. T. (2012). Flavonoid Intake and Cardiovascular Disease Mortality in a Prospective Cohort of US Adults. Am. J. Clin. Nutr. 95 (2), 454–464. doi:10.3945/ajcn.111.016634
Meyer, H., Bolarinwa, A., Wolfram, G., and Linseisen, J. (2006). Bioavailability of Apigenin from Apiin-Rich Parsley in Humans. Ann. Nutr. Metab. 50 (3), 167–172. doi:10.1159/000090736
Mohiuddin, A. (2019). Natural Foods and Indian Herbs of Cardiovascular Interest. Pharm. Pharmacol. Int. J. 7 (2), 60–84. doi:10.15406/ppij.2019.07.00235
Mora, M. T., Ferrero, J. M., Gomez, J. F., Sobie, E. A., and Trenor, B. (2018). Ca2+ Cycling Impairment in Heart Failure Is Exacerbated by Fibrosis: Insights Gained from Mechanistic Simulations. Front. Physiol. 9, 1194. doi:10.3389/fphys.2018.01194
Mozaffarian, D., and Wu, J. H. Y. (2018). Flavonoids, Dairy Foods, and Cardiovascular and Metabolic Health: A Review of Emerging Biologic Pathways. Circ. Res. 122 (2), 369–384. doi:10.1161/CIRCRESAHA.117.309008
Münzel, T., Camici, G. G., Maack, C., Bonetti, N. R., Fuster, V., and Kovacic, J. C. (2017). Impact of Oxidative Stress on the Heart and Vasculature: Part 2 of a 3-Part Series. J. Am. Coll. Cardiol. 70 (2), 212–229. doi:10.1016/j.jacc.2017.05.035
Nai, C., Xuan, H., Zhang, Y., Shen, M., Xu, T., Pan, D., et al. (2015). Luteolin Exerts Cardioprotective Effects through Improving Sarcoplasmic Reticulum Ca(2+)-ATPase Activity in Rats during Ischemia/Reperfusion In Vivo. Evid. Based Complement. Alternat Med. 2015, 365854.
Najjar, R. S., and Feresin, R. G. (2021). Protective Role of Polyphenols in Heart Failure: Molecular Targets and Cellular Mechanisms Underlying Their Therapeutic Potential. Int. J. Mol. Sci. 22 (4). doi:10.3390/ijms22041668
Orrenius, S., Zhivotovsky, B., and Nicotera, P. (2003). Regulation of Cell Death: the Calcium-Apoptosis Link. Nat. Rev. Mol. Cel. Biol. 4 (7), 552–565. doi:10.1038/nrm1150
Panth, N., Paudel, K. R., and Parajuli, K. (2016). Reactive Oxygen Species: A Key Hallmark of Cardiovascular Disease. Adv. Med. 2016, 9152732. doi:10.1155/2016/9152732
Patel, R. V., Mistry, B. M., Shinde, S. K., Syed, R., Singh, V., and Shin, H. S. (2018). Therapeutic Potential of Quercetin as a Cardiovascular Agent. Eur. J. Med. Chem. 155, 889–904. doi:10.1016/j.ejmech.2018.06.053
Phan, H. T., Yoda, T., Chahal, B., Morita, M., Takagi, M., and Vestergaard, M. C. (2014). Structure-dependent Interactions of Polyphenols with a Biomimetic Membrane System. Biochim. Biophys. Acta 1838 (10), 2670–2677. doi:10.1016/j.bbamem.2014.07.001
Ponzo, V., Goitre, I., Fadda, M., Gambino, R., De Francesco, A., Soldati, L., et al. (2015). Dietary Flavonoid Intake and Cardiovascular Risk: a Population-Based Cohort Study. J. Transl. Med. 13, 218. doi:10.1186/s12967-015-0573-2
Qin, F., Siwik, D. A., Lancel, S., Zhang, J., Kuster, G. M., Luptak, I., et al. (2013). Hydrogen Peroxide-Mediated SERCA Cysteine 674 Oxidation Contributes to Impaired Cardiac Myocyte Relaxation in Senescent Mouse Heart. J. Am. Heart Assoc. 2 (4), e000184. doi:10.1161/JAHA.113.000184
Qiu, T., Wu, D., Yang, L., Ye, H., Wang, Q., Cao, Z., et al. (2018). Exploring the Mechanism of Flavonoids through Systematic Bioinformatics Analysis. Front. Pharmacol. 9, 918. doi:10.3389/fphar.2018.00918
Rastogi, R., Geng, X., Li, F., and Ding, Y. (2016). NOX Activation by Subunit Interaction and Underlying Mechanisms in Disease. Front. Cel. Neurosci. 10, 301. doi:10.3389/fncel.2016.00301
Rizzuto, R., Pinton, P., Ferrari, D., Chami, M., Szabadkai, G., Magalhães, P. J., et al. (2003). Calcium and Apoptosis: Facts and Hypotheses. Oncogene 22 (53), 8619–8627. doi:10.1038/sj.onc.1207105
Rodius, S., de Klein, N., Jeanty, C., Sánchez-Iranzo, H., Crespo, I., Ibberson, M., et al. (2020). Fisetin Protects against Cardiac Cell Death through Reduction of ROS Production and Caspases Activity. Sci. Rep. 10 (1), 2896. doi:10.1038/s41598-020-59894-4
Ross, J. A., and Kasum, C. M. (2002). Dietary Flavonoids: Bioavailability, Metabolic Effects, and Safety. Annu. Rev. Nutr. 22, 19–34. doi:10.1146/annurev.nutr.22.111401.144957
Ryter, S. W., Kim, H. P., Hoetzel, A., Park, J. W., Nakahira, K., Wang, X., et al. (2007). Mechanisms of Cell Death in Oxidative Stress. Antioxid. Redox Signal. 9 (1), 49–89. doi:10.1089/ars.2007.9.49
Santalla, M., Valverde, C. A., Harnichar, E., Lacunza, E., Aguilar-Fuentes, J., Mattiazzi, A., et al. (2014). Aging and CaMKII Alter Intracellular Ca2+ Transients and Heart Rhythm in Drosophila melanogaster. PLoS One 9 (7), e101871. doi:10.1371/journal.pone.0101871
Santulli, G., Xie, W., Reiken, S. R., and Marks, A. R. (2015). Mitochondrial Calcium Overload Is a Key Determinant in Heart Failure. Proc. Natl. Acad. Sci. U S A. 112 (36), 11389–11394. doi:10.1073/pnas.1513047112
Senoner, T., and Dichtl, W. (2019). Oxidative Stress in Cardiovascular Diseases: Still a Therapeutic Target? Nutrients 11 (9), 11. doi:10.3390/nu11092090
Serban, M. C., Sahebkar, A., Zanchetti, A., Mikhailidis, D. P., Howard, G., Antal, D., et al. (2016). Effects of Quercetin on Blood Pressure: A Systematic Review and Meta-Analysis of Randomized Controlled Trials. J. Am. Heart Assoc. 5 (7). doi:10.1161/JAHA.115.002713
Shanmugam, K., Ravindran, S., Kurian, G. A., and Rajesh, M. (2018). Fisetin Confers Cardioprotection against Myocardial Ischemia Reperfusion Injury by Suppressing Mitochondrial Oxidative Stress and Mitochondrial Dysfunction and Inhibiting Glycogen Synthase Kinase 3β Activity. Oxid. Med. Cel. Longev. 2018, 9173436. doi:10.1155/2018/9173436
Shen, A. C., and Jennings, R. B. (1972). Kinetics of Calcium Accumulation in Acute Myocardial Ischemic Injury. Am. J. Pathol. 67 (3), 441–452.
Shih, Y. L., Hung, F. M., Lee, C. H., Yeh, M. Y., Lee, M. H., Lu, H. F., et al. (2017). Fisetin Induces Apoptosis of HSC3 Human Oral Cancer Cells through Endoplasmic Reticulum Stress and Dysfunction of Mitochondria-Mediated Signaling Pathways. In Vivo 31 (6), 1103–1114. doi:10.21873/invivo.11176
Singh, M., Kaur, M., and Silakari, O. (2014). Flavones: an Important Scaffold for Medicinal Chemistry. Eur. J. Med. Chem. 84, 206–239. doi:10.1016/j.ejmech.2014.07.013
Souza, R. P., Bonfim-Mendonça, P. S., Gimenes, F., Ratti, B. A., Kaplum, V., Bruschi, M. L., et al. (20172017). Oxidative Stress Triggered by Apigenin Induces Apoptosis in a Comprehensive Panel of Human Cervical Cancer-Derived Cell Lines. Oxid. Med. Cel. Longev. 2017, 1512745. doi:10.1155/2017/1512745
Swaminathan, A., Basu, M., Bekri, A., Drapeau, P., and Kundu, T. K. (2019). The Dietary Flavonoid, Luteolin, Negatively Affects Neuronal Differentiation. Front. Mol. Neurosci. 12, 41. doi:10.3389/fnmol.2019.00041
Terrar, D. A. (2020). Calcium Signaling in the Heart. Adv. Exp. Med. Biol. 1131, 395–443. doi:10.1007/978-3-030-12457-1_16
Thilakarathna, S. H., and Rupasinghe, H. P. (2013). Flavonoid Bioavailability and Attempts for Bioavailability Enhancement. Nutrients 5 (9), 3367–3387. doi:10.3390/nu5093367
Uddin, M. S., and Kabir, M. T. (2019). Emerging Signal Regulating Potential of Genistein against Alzheimer's Disease: A Promising Molecule of Interest. Front. Cel. Dev. Biol. 7, 197. doi:10.3389/fcell.2019.00197
Virani, S. S., Alonso, A., Aparicio, H. J., Benjamin, E. J., Bittencourt, M. S., Callaway, C. W., et al. (2021). Heart Disease and Stroke Statistics-2021 Update: A Report from the American Heart Association. Circulation 143 (8), e254–743. doi:10.1161/CIR.0000000000000950
Wang, D. D. (2019). Dietary Patterns and Precision Prevention of Heart Failure. J. Am. Coll. Cardiol. 73 (16), 2046–2048. doi:10.1016/j.jacc.2019.02.037
Wang, G., Li, W., Lu, X., Bao, P., and Zhao, X. (2012). Luteolin Ameliorates Cardiac Failure in Type I Diabetic Cardiomyopathy. J. Diabetes Complications 26 (4), 259–265. doi:10.1016/j.jdiacomp.2012.04.007
Wang, Z., Zeng, M., Wang, Z., Qin, F., Chen, J., and He, Z. (2021). Dietary Luteolin: A Narrative Review Focusing on its Pharmacokinetic Properties and Effects on Glycolipid Metabolism. J. Agric. Food Chem. 69 (5), 1441–1454. doi:10.1021/acs.jafc.0c08085
Weintraub, N. L., Collins, S. P., Pang, P. S., Levy, P. D., Anderson, A. S., Arslanian-Engoren, C., et al. (2010). Acute Heart Failure Syndromes: Emergency Department Presentation, Treatment, and Disposition: Current Approaches and Future Aims: a Scientific Statement from the American Heart Association. Circulation 122 (19), 1975–1996. doi:10.1161/CIR.0b013e3181f9a223
Williamson-Hughes, P. S., Flickinger, B. D., Messina, M. J., and Empie, M. W. (2006). Isoflavone Supplements Containing Predominantly Genistein Reduce Hot Flash Symptoms: a Critical Review of Published Studies. Menopause 13 (5), 831–839. doi:10.1097/01.gme.0000227330.49081.9e
Wu, B., Kulkarni, K., Basu, S., Zhang, S., and Hu, M. (2011). First-pass Metabolism via UDP-Glucuronosyltransferase: a Barrier to Oral Bioavailability of Phenolics. J. Pharm. Sci. 100 (9), 3655–3681. doi:10.1002/jps.22568
Wu, Q., Li, W., Zhao, J., Sun, W., Yang, Q., Chen, C., et al. (2021). Apigenin Ameliorates Doxorubicin-Induced Renal Injury via Inhibition of Oxidative Stress and Inflammation. Biomed. Pharmacother. 137, 111308. doi:10.1016/j.biopha.2021.111308
Xin, L., Gao, J., Lin, H., Qu, Y., Shang, C., Wang, Y., et al. (2020). Regulatory Mechanisms of Baicalin in Cardiovascular Diseases: A Review. Front. Pharmacol. 11, 583200. doi:10.3389/fphar.2020.583200
Xingyue, L., Shuang, L., Qiang, W., Jinjuan, F., and Yongjian, Y. (2021). Chrysin Ameliorates Sepsis-Induced Cardiac Dysfunction through Upregulating Nfr2/Heme Oxygenase 1 Pathway. J. Cardiovasc. Pharmacol. 77 (4), 491–500. doi:10.1097/FJC.0000000000000989
Yamagata, K., and Yamori, Y. (2020). Inhibition of Endothelial Dysfunction by Dietary Flavonoids and Preventive Effects against Cardiovascular Disease. J. Cardiovasc. Pharmacol. 75 (1), 1–9. doi:10.1097/FJC.0000000000000757
Yan, Q., Li, Y., Yan, J., Zhao, Y., Liu, Y., and Liu, S. (2018). Effects of Luteolin on Regulatory Proteins and Enzymes for Myocyte Calcium Circulation in Hypothermic Preserved Rat Heart. Exp. Ther. Med. 15 (2), 1433–1441. doi:10.3892/etm.2017.5514
Yan, Q., Li, Y., Yan, J., Zhao, Y., Liu, Y., and Liu, S. (2019). Luteolin Improves Heart Preservation through Inhibiting Hypoxia-dependent L-type Calcium Channels in Cardiomyocytes. Exp. Ther. Med. 17 (3), 2161–2171. doi:10.3892/etm.2019.7214
Ye, Q., Liu, K., Shen, Q., Li, Q., Hao, J., Han, F., et al. (2019). Reversal of Multidrug Resistance in Cancer by Multi-Functional Flavonoids. Front. Oncol. 9, 487. doi:10.3389/fonc.2019.00487
Zamora-Ros, R., Jiménez, C., Cleries, R., Agudo, A., Sánchez, M. J., Sánchez-Cantalejo, E., et al. (2013). Dietary Flavonoid and Lignan Intake and Mortality in a Spanish Cohort. Epidemiology 24 (5), 726–733. doi:10.1097/EDE.0b013e31829d5902
Zeka, K., Ruparelia, K., Arroo, R. R. J., Budriesi, R., and Micucci, M. (2017). Flavonoids and Their Metabolites: Prevention in Cardiovascular Diseases and Diabetes. Diseases 5 (3). doi:10.3390/diseases5030019
Zhang, Y. M., Zhang, Z. Y., and Wang, R. X. (2020). Protective Mechanisms of Quercetin against Myocardial Ischemia Reperfusion Injury. Front. Physiol. 11, 956. doi:10.3389/fphys.2020.00956
Zhao, F., Fu, L., Yang, W., Dong, Y., Yang, J., Sun, S., et al. (2016). Cardioprotective Effects of Baicalein on Heart Failure via Modulation of Ca(2+) Handling Proteins In Vivo and In Vitro. Life Sci. 145, 213–223. doi:10.1016/j.lfs.2015.12.036
Zhivotovsky, B., and Orrenius, S. (2011). Calcium and Cell Death Mechanisms: a Perspective from the Cell Death Community. Cell Calcium 50 (3), 211–221. doi:10.1016/j.ceca.2011.03.003
Keywords: heart failure, calcium overload, ROS, flavones, treatment
Citation: Yu T, Huang D, Wu H, Chen H, Chen S and Cui Q (2021) Navigating Calcium and Reactive Oxygen Species by Natural Flavones for the Treatment of Heart Failure. Front. Pharmacol. 12:718496. doi: 10.3389/fphar.2021.718496
Received: 24 June 2021; Accepted: 18 October 2021;
Published: 09 November 2021.
Edited by:
Alessandro Mugelli, University of Florence, ItalyReviewed by:
Elisabetta Bigagli, University of Florence, ItalyLaura Pucci, National Research Council (CNR), Italy
Copyright © 2021 Yu, Huang, Wu, Chen, Chen and Cui. This is an open-access article distributed under the terms of the Creative Commons Attribution License (CC BY). The use, distribution or reproduction in other forums is permitted, provided the original author(s) and the copyright owner(s) are credited and that the original publication in this journal is cited, in accordance with accepted academic practice. No use, distribution or reproduction is permitted which does not comply with these terms.
*Correspondence: Tianhao Yu, ZXNobGRyQDEyNi5jb20=
†These authors have contributed equally to this work