- 1Department of Biochemistry, University of Nigeria, Nsukka, Nigeria
- 2Department of Science Laboratory Technology, University of Nigeria, Nsukka, Nigeria
- 3Natural Science Unit, School of General Studies, University of Nigeria, Nsukka, Nigeria
- 4School of Nutrition Sciences, University of Ottawa, Ottawa, ON, Canada
The health benefits and toxicity of plant products are largely dependent on their secondary metabolite contents. These compounds are biosynthesized by plants as protection mechanisms against environmental factors and infectious agents. This review discusses the traditional uses, phytochemical constituents and health benefits of plant species in genus Zanthoxylum with a focus on cancer, microbial and parasitic infections, and sickle cell disease as reported in articles published from 1970 to 2021 in peer-reviewed journals and indexed in major scientific databases. Generally, Z. species are widely distributed in Asia, America and Africa, where they are used as food and for disease treatment. Several compounds belonging to alkaloids, flavonoids, terpenoids, and lignans, among others have been isolated from Z. species. This review discusses the biological activities reported for the plant species and their phytochemicals, including anticancer, antibacterial, antifungal, antiviral, anti-trypanosomal, antimalarial and anti-sickling properties. The safety profiles and suggestions for conservation of the Z. species were also discussed. Taken together, this review demonstrates that Z. species are rich in a wide range of bioactive phytochemicals with multiple health benefits, but more research is needed towards their practical application in the development of functional foods, nutraceuticals and lead compounds for new drugs.
Introduction
Humans have continually depended on plants for food and medicine. Plants produce secondary metabolites in response to infective agents and environmental factors. Consequently, efforts have been made to isolate, characterize and investigate the beneficial effects of plant-derived secondary metabolites on human health. Notably, several bioactive compounds from plants have provided inspirations for the synthesis of chemical drugs, such as artesunates from artemisinins and quinolone antimalarials from quinine (Karunamoorthi et al., 2013; Lifongo et al., 2014; Pawar 2014; Numonov et al., 2019). Natural product chemists often rely on traditional knowledge on plants with medicinal potentials to produce crude extracts with biological activities. This process is followed by downstream processing to isolate the bioactive compounds, and structural characterization to identify them. In some cases, chemical modifications of the phytochemicals are used to produce more clinically effective and safer entities.
Zanthoxylum species, also known as Fagara species, have a long history of use as sources of food and drug by locals in different parts of Asia, America and Africa. In traditional medicine, many of the plant species are used in treating sickle cell anemia, trypanosomiasis, malaria and microbial infections, including tuberculosis and enteritis, with Z. zanthoxyloides Lam being the most reported species for these applications (Erichsen-Brown 1979; Burkill 1985). For example, fruits of Z. lepreurii Guill. and Perr. and Z. zanthoxyloides Lam are used in managing fever, malaria, tumors and sickle cell anemia (Tamdem 2019) while the stem bark, leaves, and roots are applied to suppress pain, and to treat arthritis, leprosy, stomachache and venereal diseases in Cameroon (Burkill 1998; Ngoumfo et al., 2010). Furthermore, different parts of Z. lepreurii are used to treat or manage tuberculosis, malaria, human immunodeficiency virus (HIV) and several types of bacterial infection in Uganda and other parts of Africa (Lamorde et al., 2010; Tabuti et al., 2010; Bunalema et al., 2014). In China and other parts of Eastern Asia, Z. bungeanum Maxim. (Syn. Z. piperitum Benn.) is widely used as a food condiment because of its perceived health benefits Hwang et al. (2008) and as cosmetics for maintaining skin quality (Hwang et al., 2020). In Chinese medicine, Z. bungeanum is used as spices and for treating infection and bone diseases (Lee and Lim 2008; Kim et al., 2017). The leaves, fruits and barks are used in treating bacterial and fungal infections, as spices, and for food preservation in Japan (Hatano et al., 2004). Similarly, different parts of Z. schinifolium Siebold and Zucc. are used as food condiments and for treating stomach pain, diarrhea, jaundice, and cold in Eastern Asia (Cui et al., 2009). Furthermore, herbal preparation from different parts of Z. americanum Mill. is traditionally used for treating tumors, fungal skin infections, respiratory, urinary, genital and gastrointestinal (GIT) diseases by herbal healers in Canada and United States (Moerman 1998). In Kanayatn Dayak Community, West Kalimantan, Indonesia, the stem and root of Z. bungeanum are consumed raw or after boiling in water to prevent alcohol intoxication and treat respiratory diseases (Sepsamli and Prihastanti 2019). Other traditional and ethnobotanical uses of Z. species have been discussed elsewhere (Patiño et al., 2012; Adewole 2020; Lu et al., 2020a; Obakiro et al., 2020; Okagu et al., 2021). The objectives of this review are to discuss (1) the potential of Z. species as sources of bioactive phytochemicals that can be applied in the management and treatment of cancer, microbial and parasitic infections, and sickle cell disease; (2) chemical constituents involved in these biological activities; and (3) safety issues and suggestions for conservation of the plant species.
Literature Search Strategy and Criteria for Selection of Articles
This study used a strategy similar to that reported by Nigussie et al. (2021). From repositories and search engines (PubMed, ScienceDirect, and Google Scholar), information related to the health benefits of Z. species, with emphasis on anticancer, anti-trypanosomal, antimicrobial, antiviral, antimalarial and anti-sickling properties, in peer-reviewed journals and ethnobotanical surveys published from 1970-July 3, 2021 were retrieved. The titles and abstracts of the studies were scanned using the inclusion criteria for this study. The search terms included cytotoxicity, anticancer, antimicrobial, antibacterial, anti-mycobacterial, antimalarial, antiviral, larvicidal, trypanocidal, anti-sickling and antiproliferative effect of Zanthoxylum species, Fagara species, and medicinal plants. In some cases, articles citing older papers and references of recent papers were used to obtain additional articles of interest. Studies reporting the biological activities of interest on different parts of Z. species including seeds, fruits, stem/stem bark, fruits, and root/root bark were included. Biological activities of crude extracts, their fractions and isolated compounds were also included. Where available, the mechanisms of action of the extract or isolated compounds were retrieved. Reviews, newspaper and other non-peer-reviewed articles were excluded. Similarly, studies reporting biological activities of Z. species other than those under consideration and in languages other than English were excluded. In this review, a test substance is considered bioactive when the outcome of the substance-treated group was substantial when determined qualitatively or quantitatively compared to controls (untreated group or group that received a standard drug).
The correctness of the scientific/botanical names of the plants reported in the included studies were confirmed with names available in botanical databases, including www.theplantlist.org, https://www.ipni.org/, https://www.ncbi.nlm.nih.gov/Taxonomy/Browser/, and https://www.tropicos.org. In cases where the plant name in the article was not the acceptable taxonomical nomenclature, the name in the botanical databases was used. A number of reviews have records of plant species in genus Zanthoxylum, including Z. armatum DC (Brijwal et al., 2013; Mukhtar and Kalsi 2018; Paul et al., 2018; Verma et al., 2021), Z. limonella (Supabphol and Tangjitjareonkun 2014), Z. nitidum (Roxb.) DC (Lu et al., 2020a), Z. rhetsa (Roxb.) DC (Maduka and Ikpa, 2021), and Zanthoxylum bungeanum Maxim (Zhang M. et al., 2017). Some of these reviews are not comprehensive, while others focused on health benefits related to metabolic diseases (Okagu et al., 2021) or the phytoconstituents such as alkaloids (Yuan et al., 2015; Wei et al., 2021), or were published in non-English languages (Zhang M. et al., 2017). In some previous reviews on traditional uses, only selected species were discussed with respect to a particular disease condition, e.g., Imaga (2010) on sickle cell anemia, Ochwang’i et al. (2014) on cancer, Sinan et al. (2019) on malaria, and Obakiro et al. (2020) on tuberculosis. These reviews were carefully analyzed and most of the reviewed studies were excluded from the present review. Hence, this review covers information on the phytochemistry and biological activities of interest for 25 plants species in Genus Zanthoxylum, namely Z. leprieurii Guill. and Perr., Z. bungeanum Maxim. (Syn. Z. nitidum Bunge; Z. piperitum Benn.; Z. bungeanum var. bungeanum; Z. simulans Hance); Z. schinifolium Siebold and Zucc., Z. clava-herculis L., Z. heitzii (Aubrév. and Pellegr.) P.G.Waterman, Z. chalybeum Engl., Z. ailanthoides Siebold and Zucc., Z. acanthopodium DC., Z. zanthoxyloides (Lam.) Zepern. and Timler, Z. paracanthum Kokwaro, Z. riedelianum Engl., Z. americanum Mill, Z. armatum DC. (Syn. Z. alatum Roxb.), Z. rhetsa DC., Z. buesgenii (Engl.) P.G.Waterman, Z. madagascariense Baker, Z. austrosinense C.C. Huang, Z. schreberi (J.F.Gmel.) Reynel ex C. Nelson (Syn. Z. monophyllum (Lam.) P. Wilson), Z. rhoifolium Lam., Z. davyi Waterm., Z. ovalifolium Tutcher, Z. fagara (L.) Sarg., Z. tingoassuiba A. St.-Hil., Z. gilletii (De Wild.) P.G.Waterman, and Z. poggei (Engl.) P.G.Waterman.
Zanthoxylum Species as Potential Sources of Anticancer Agents
Cancer is a disease that is characterized by uncontrolled cell division and loss of contact inhibition, leading to formation of tumors. Cancers are resistant to apoptosis and develop angiogenic and metastatic potentials. Prevalence of cancer is rising worldwide, even in developing countries where the rise is partly due to adoption of the Western diet and sedentary lifestyle, and increase in the aging population, among other factors (Morounke et al., 2017). Cancer-related deaths are higher in economically poor countries due to late detection and poor access to treatment and support (India State-Level Disease Burden Initiative Cancer Collaborators, 2018). The number of cancer cases and cancer deaths globally are projected to increase geometrically in the future (Smittenaar et al., 2016), thus placing cancer as a major global health issue. A number of approaches, such as radiation therapies, chemotherapies and surgeries, or their combination, are available for cancer management and treatment. Radiation therapies and surgeries are effective but present cancer patients with discomfort. In most cases, chemotherapies are linked with side effects and some cancers are resistant to chemotherapy. Development of clinically potent cancer drugs that are selectively toxic to cancer cells without harming normal cells has become a public health priority. One promising strategy is to search for natural products with cancer cell-specific cytotoxicity. Some natural compounds from plants and marine organisms have promising applications as anticancer agents (Lichota and Gwozdzinski 2018). Traditionally, medicinal plants have been used for treating cancer in different parts of the world (Abubakar et al., 2020; Adewole 2020). Medicinal plants belonging to Z. species and their phytochemicals with anticancer properties are discussed below.
The potential of Z. species in the treatment of cancer has been assessed using both drug-sensitive and drug-resistant cancer cells. For example, antiproliferative activities have been demonstrated using cell culture studies for extracts of Z. clava-herculis L. stem bark against lung cancer (A549) (Wansi et al., 2009), Z. ailanthoides Seibold. and Zucc. stem against human colon cancer cell line (Colo 205) (Chou et al., 2011), Z. heitzii (Aubrév. and Pellegr.) P.G.Waterman fruits and barks against cervical cancer (HeLa), breast cancer (MCF-7), acute monocytic leukemia (THP-1) and human Caucasian prostate cancer (PC-3) (Dzoyem et al., 2013), Z. leprieuii and Z. zanthoxyloides fruits against PC-3, MCF-7, liver (WRL-68), and colon (Caco-2) (Misra et al., 2013), Z. rhetsa DC. stem bark and root bark against human stomach-cancer cell lines, SCL, SCL-6, SCL-3706, SCL-9, Kato-3, and NUGC-4 (Ahsan et al., 2014), Z. chalybeum Engl. and Z. parachanthum Kowaro stem bark against drug-sensitive and multidrug-resistant leukemia cell lines (CCRF-CEM and CEM/ADR5000) (Omosa et al., 2019), Z. acanthopodium DC. seed against MCF-7 cell line (Arsita et al., 2019), Z. zanthoxyloides roots against liver cancer (HCC), larynx cancer (HEp2) and breast cancer (BT549) (Andima et al., 2020), and Z. paracanthum root bark against human breast cancer (HCC 1395) and human prostate cancer (DU 145) cell lines (Kaigongi et al., 2020). Most of these reports are inspired by the traditional uses of the plant in managing health conditions, including cancer. Specifically, decoctions of different parts of Zanthoxylum poggei (Engl.) P. G. Waterman are ingested to treat tumors, among other health issues, in Cameroon and Congo (Wouatsa et al., 2013). Using a combination of chromatographic and spectrophotometric techniques, acridone and indoloquinazoline alkaloids, poggeicridone and 2-methoxy-7,8-dehydroruteacarpine, respectively were isolated from stem bark of the plant. On exposure to cultured PC-3 cells, the two alkaloids elicited significant cytotoxic effects with IC50 values of 15.8 and 22.1 μM, respectively compared to IC50 value of 0.9 μM for doxorubicin, the reference anticancer drug (Wansi et al., 2016).
These reports have provided support to the traditional uses of decoctions of Z. species alone or as a cocktail with other plant species for managing cancer. Most anticancer natural products act by blocking different mechanisms through which cancer cells grow, multiply and invade other cells, as well as resist the immune system. These mechanisms include the induction of cell cycle arrest, apoptosis and oxidative stress, as well as inhibition of angiogenesis, metastasis and growth signaling pathways in cancer cells (Ahmad et al., 2003; Michalkova et al., 2021). As shown in Figure 1, extracts of Z. species have been demonstrated to elicit anticancer properties via inhibition of angiogenesis Harahap et al. (2018) and DNA synthesis, induction of apoptosis Alam et al. (2017) and cell cycle arrest at Go/G1 Pieme et al. (2014) and G2-M phases, and suppression of cyclooxygenase (COX)-2 and vascular endothelial growth factor receptor type-2 (VEGFR-2) expression (Harahap et al., 2018); Table 1 presents the anticancer properties of crude extracts of Z. species whose anticancer constituents are unknown.
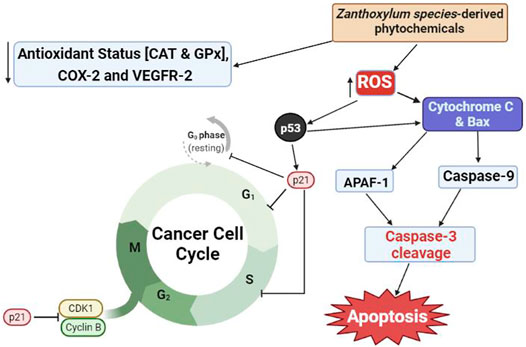
FIGURE 1. Proposed mechanism of anticancer activities of natural products from Zanthoxylum species. CAT, catalase; GPx, glutathione peroxidase; COX-2, cyclooxygenase-2; VEGFR-2, vascular endothelial growth factor receptor-2; CDK1, cyclin-dependent kinase-1; ROS, reactive oxygen species; Bax, Bcl-2-associated X protein; APAF-1, apoptosis protein activating factor-1.
Several studies have isolated the Z. species phytochemicals that may be responsible for their in vitro anticancer properties. Based on their classes, chemical compounds derived from Z. species with substantial inhibitory activities against cultured cancer cells are discussed below. An orbitide, [1-8-NαC]-zanriorb A1, isolated from Z. riedelianum Engl. leaves inhibited the proliferation of Jurkat leukemia T cells (IC50 218 nM) by inducing apoptosis (Beirigo et al., 2016). In addition, phenolic compounds isolated from Z. ailanthoides stem, chlorogenic acid, flavone and isoflaxidin, were shown to suppress Colo 205 viability and induce apoptosis and cell cycle arrest at the G2/M-phase via upregulation of the expression of apoptosis-inducing factor, endonuclease G, and caspases 3, 7, and 9 while suppressing fatty acid synthase (FAS) (Chou et al., 2011). FAS is a multifunctional enzyme complex that is gaining attention as a target for cancer management. The inhibition of FAS activity in many cancer cells induce restimulation-induced cell death, one of the notable apoptotic pathways (Fhu and Ali 2020). Similarly, an alkamide, 4-(isoprenyloxy)-3-methoxy-3,4-deoxymethylenedioxyfagaramide, isolated from Z. chalybeum stem bark was moderately cytotoxic against CCRF-CEM and CEM/ADR5000 cells with IC50 values of 29.13 and 31 μM, respectively (Omosa et al., 2019), although the bioactivity mechanism is unknown. Such mechanistic information may facilitate the identification of specific molecular targets and derivatives of the compound with enhanced potency.
Furthermore, Z. species have been reported to contain several coumarins with broad-spectrum anticancer activities. For example, coumarins from Z. schinifolium stem, collinin, 8-methoxyanisocoumarin H and acetoxyschinifolin, significantly halted the proliferation of PC-3, HL-60, and colorectal (SNUC5) cancer cells with respective IC50 values of 4.62, 4.39 and 6.26 μM by collinin, IC50 of 5.02, 12.22 and 33.5 by 8-methoxyanisocoumarin H, and IC50 values of 5.12, 33.81 and 35.11 μM by acetoxyschinifolin. The coumarins acted by inducing apoptosis and suppression of the expression of genes (p-ERK1/2 MAPK, p-AKT, and c-myc) involved in cancer development and progression (Li et al., 2013). Furthermore, a pyranocoumarin from Z. ailanthoides stem bark, luvangetin exhibited weaker cytotoxic activity against human lung cancer (A-549) cells with an IC50 value of 4.28 μg/ ml compared to 5-fluorouracil, a known anticancer agent (IC50 of 0.6 μg/ ml) (Cao et al., 2013). Nonetheless, the potential of luvangetin can be further explored by structural modification to possibly obtain more potent anticancer derivatives.
Zanthoxylum-isolated lignans have also been reported to have anticancer activities. Sesamin from Z. parachanthum demonstrated cytotoxic activity against CCRF-CEM and CEM/ADR5000 cancer cells with IC50 values of 40.74 and 30.70 µM, respectively (Omosa et al., 2019). In addition, (-)-xanthoxylol-3,3-dimethylallyl ether from Z. bungeanum Maxim stem bark was cytotoxic against MCF-7 cancer cells with an IC50 of 18.65 μg/ ml (Yang et al., 2009) while asarinin from Z. americanum stem suppressed the proliferation of HL-60 cancer cells with IC50 of 11.64 μM (Ju Y et al., 2001). Interestingly, the cytotoxicity of kobusin from Z. rhetsa bark against mouse melanoma (B16-F10) cells was weaker (IC50 values of 112.2 μg/ ml) Santhanam et al. (2016) than the activity of kobusin from Z. armatum bark against human lung (A549) and pancreatic (MIA-PaCa) cancer cells (IC50 values of 34.71 and 32.86 μg/ ml, respectively) (Mukhija et al., 2014). This demonstrates that activity is dependent on the cancer cell type, possibly because of differences in bioaccessibility of the compounds, their molecular targets and anticancer mechanisms. In general, the lignans acted by inducing apoptosis and cell cycle arrest, and inhibiting DNA synthesis in the cancer cells. In addition to the broad-spectrum antiproliferative properties, the potential of these lignans as anticancer agents is strengthened by the absence of cytotoxicity to human dermal fibroblasts and peripheral blood mononuclear cells.
In many studies, Zanthoxylum alkaloids were reported to exhibit cytotoxic activity against cancer cells in vitro (Wei et al., 2021). Specifically, a furoquinoline alkaloid, skimmianine, demonstrated cytotoxic activity against MCF-7 cancer cells with an IC50 value of 8.03 μg/ ml while an aporphine alkaloid, liriodenine, was cytotoxic against MCF-7, NCI-H460, and SF-268 cancer cells with IC50 values of 3.19, 2.38 and 2.19 μg/ ml, respectively (Andima et al., 2020). Recently, acridone alkaloids, fabiocinine and arborinine, and skimmianine from Z. leprieurii Guill. and Perr. root bark were reported to exhibit selective cytotoxicity against HeLa cells with IC50 values of 28.49, 62.71 and 12.8 μg/ ml, respectively, which were lower than the activity of anticancer agent, emetine (IC50 values of 0.026 μg/ ml against HeLa cells) (Eze et al., 2020). Similarly, an indole alkaloid, canthin-6-one from Z. parachanthum inhibited CCRF-CEM and CEM/ADR5000 cancer cell proliferation with IC50 values of 15.82 and 10.52 µM, respectively (Omosa et al., 2019). Unlike doxorubicin, canthin-6-one demonstrated selective cytotoxicity against the drug-resistant cell line without affecting normal human peripheral blood mononuclear cells (Omosa et al., 2019). In other cancer cells (HCC 1395 and DU 145), canthin-6-one and its derivative, 10-methoxycanthin-6-one, from the same plant were strongly cytotoxic with IC50 values of 8.12, and 9.43 μg/ ml, and 14.70 and 1.58 μg/ ml, respectively (Kaigongi et al., 2020). Despite its lower cytotoxicity against cancer cells compared to doxorubicin, the better selectivity/nontoxicity to normal cells positions canthin-6-one as a promising candidate with a broad-spectrum anticancer activity.
Furthermore, benzophenanthridine alkaloid, 1-methoxy-12-methyl-12,13-dihydro-(1,3) dioxolo (4′,5′:4,5) benzo (1,2-c) phenanthridine-2,13-diol, from the aerial parts of Z. buesgenii (Engl.) P.G.Waterman showed moderate to strong cytotoxicity against sensitive and multidrug resistant cancer cells (CCRF-CEM, CEM/ADR5000, MDA-MB231, MDA-MB231/BCRP, HCT116 (p53+/+), HCT116 (p53−/−), U87MG, U87MG.ΔEGFR, and HepG2) with IC50 values of 0.24, 31.58, 30.14, 65.01, 42.46, 62.34, 60.55, 61.84, and 22.37, respectively, while sparing normal human liver (AML12) cells (Sandjo et al., 2014). Due to the broad-spectrum anticancer activity of the benzophenanthridine and aporphine alkaloids, further studies are required to understand the molecular mechanism of action against the cancer cells. Benzophenanthridine alkaloid from another species (Z. madagascariense Baker), rutaceline, showed inhibitory activity against Caco-2 cells by inducing apoptosis, cell cycle arrest at the G0/G1 phase and DNA fragmentation, and by inhibiting DNA synthesis (Pachón et al., 2007). Acting via similar mechanisms (induction of apoptosis and cell cycle arrest by strong binding to cyclin-dependent kinases (CDK2 and CDK6) and caspases 3 and 8), the ability of a benzophenanthridine alkaloid from Z. zanthoxyloides roots, dihydrochelerythrine to exhibit significant cytotoxicity against HCC and BT549 cancer cells Andima et al. (2020) demonstrates the strong anticancer potential of the benzophenanthridine alkaloids from Z. species. Through unknown mechanisms, other alkaloids such as isoquinoline alkaloids (e.g. nitidine, fagaronine chelerythridine and sanguinarine) from Z. bungeanum elicited selective DNA damage and cytotoxicity against mouse lymphocytic leukemia cells in vitro (Kaminskyy et al., 2008; Liao et al., 2013; Tian et al., 2017). Similarly, Z. austrosinense C.C. Huang root-derived carbazole alkaloids, zanthoaustrones A–C, exhibited strong antiproliferative activities against human leukemia (HL-60), liver (SMMC-7721), lung (A-549), breast (MCF-7) and colon (SW480) cancer cell lines (Fu et al., 2020). Despite the promising in vitro anticancer activities reported, the experimental designs often did not include appropriate positive controls. This is needed for validation of anticancer activities prior to in vivo studies, considering potential differences in assay conditions that may influence cellular activities.
Redox imbalance in cancer cells caused by reduction in antioxidant status and elevation of ROS production and lipid peroxidation has been targeted as a major mechanism through which some plant-derived compounds induce apoptosis (Redza-Dutordoir and Averill-Bates 2016). Other mechanisms include caspase-mediated signaling, which induces apoptosis, and p53-mediated cell cycle arrest (Zhang Y. et al., 2017). For example, an acridone alkaloid derivative (2-aminoacetamido-10-(3,5-dimethoxy)-benzyl-9(10H)-acridone hydrochloride) was shown to kill leukemia cells by decreasing mitochondrial transmembrane potential while increasing the expression of Bax, cytochrome C and apoptosis protein activating factor-1 to form an apoptosome (Wang et al., 2013). Formation of apoptosome activates caspase-9 with concomitant activation of caspase-3, the final inducer of apoptosis. The increase in intracellular ROS production induced by natural products also alters membrane phospholipid composition and integrity, all of which contribute to cancer cell death (Rahman et al., 2021).
Taken together, Z. species contain a repertoire of phytochemicals with promising application in the treatment of cancer. However, the reviewed studies were conducted in cell cultures in vitro, without validation of physiological anticancer effects of the extracts or isolated compounds using model organisms or in humans. This is a major limitation of the studies because of pharmacokinetic and pharmacodymanic considerations, which influence the bioaccessibility, bioavailability and target binding/sensitivity of the compounds. Furthermore, some cancer cells have developed mechanisms for resisting the cytotoxic actions of some anticancer agents, such as reduced expression of drug targets while upregulating the expression of alternative survival pathways (Pistritto et al., 2016; Bukowski et al., 2020). Consequently, the multiple target mechanisms of anticancer activities identified in vitro for some Z. species-derived compounds make them strong candidates for in vivo studies and human clinical trials, and further development as anticancer agents. Chemical compounds isolated from Z. species with anticancer properties and their molecular mechanisms are presented in Table 2; Figure 1.
Zanthoxylum Species as Potential Sources of Antimicrobial Agents
Microorganisms play many roles essential for human survival and are used as sources of drugs such as antibiotics. However, many strains of bacteria and fungi, such as Streptococcus mutans, S. aereus, Mycobacterium tuberculosis, K. pneumoniae, Candida species, and Escherichia coli are causative agents of many diseases of clinical importance. Several classes of antimicrobial drugs are used to control microbial infections, by suppressing microbial growth or killing them. However, survival pressure has led to the emergence and spread of antibiotics-resistant strains of many microorganisms, including those that are resistant to multiple drugs of the same or different classes (multi-drug resistant strains). These drug resistant strains have led to prolonged treatment duration, frequent hospitalization, increased healthcare cost and mortality from treatable microbial infections (Naylor et al., 2018). This necessitates the urgent search for clinically effective antimicrobial agents against these “superbugs”.
Antimicrobial agents from herbs that are traditionally used in treating microbial infections are being isolated and assessed for activities against drug-resistant microbial strains. Many natural products derived from genus Zanthoxylum show promising antimicrobial activities against bacteria and fungi of public health importance. Investigations for antimicrobial activities are mostly guided by traditional uses of the plant species in the treatment of infectious diseases. Among the Z. species, Z. zanthoxyloides is well known for its use in the management of microbial infections in China and Korea and other parts of Asia as well as in Uganda, Nigeria and Ghana (Anokbonggo et al., 1990; Ngono-Ngane et al., 2000; Imaga 2010; Ynalvez et al., 2012; Ouédraogo et al., 2019; Adeeyo et al., 2020). Additionally, Z. zanthoxyloides is used as chewing stick and to treat oral infections and toothaches (Anyanwu and Okoye 2017), thus suggesting that the plant species may have antimicrobial activities against oral pathogens. Similarly, Z. rhetsa has been used against urogenital microbial infections and in disinfection of contaminated surfaces in Bangladesh (Yusuf et al., 1994), while Z. lemairei (De Wild) P.G. Waterman is used against malaria and diarrhea (Adesina et al., 1997), Z. chalybeum stem bark against malaria in Rwanda and Ivory Coast (Kamanzi Atindehou et al., 2002), and Z. schreberi (J.F.Gmel.) Reynel ex C. Nelson in treating eye infections in the Caribbean, Venezuela, Colombia and Costa Rica (Rodríguez-Guzmán et al., 2011).
Instead of the whole plant parts, solvent extracts of different parts of Z. species have been investigated for antimicrobial activities. As shown in Table 3, the potency of antimicrobial activity [expressed as minimum inhibitory concentration (MIC) or inhibition zone diameter (IZD)] varies with the different plant species, part used (fruits, leaves, root bark, stem bark), microorganism tested, solvent used for extraction, or type of assay used in the studies. Moderately polar solvents appear to be the best medium for extraction of the antimicrobial compounds compared with highly polar and non-polar solvents (Gonçalves et al., 2019). It is also worth noting that most of the microorganisms studied are drug-sensitive species and only a few studies tested the extracts on drug-resistant microorganisms. Thus, it is challenging to assess and conclude on the potential of individual plant extracts as presented in the literature. It is apparent that the Z. species crude extracts contain antimicrobial principles, which may need to be purified to enhance the activity or for elucidating molecular mechanisms. Nonetheless, the combination of several potentially active principles in the crude extracts may present an opportunity. For instance, aqueous-methanol extract of Z. zanthoxyloides root bark showed antibacterial activity against Streptococcus mutans, Sarcina lutea, and Lactobacillus sp. at IZD of 20, 32, and 56 mm, respectively, at 100 mg/ ml compared to the stronger but limited effect of antibiotic drug, amoxicillin, which was active (IZD of 22 mm at 10 μg/ ml) only against Lactobacillus sp. (Okafor et al., 2017). Multi-component extracts with such promising antimicrobial activity can be further investigated for safety and pharmacological effects as low-cost alternatives to purified compounds or drugs. Aside from solvent extracts, the antimicrobial activities of multi-component essential oils derived from Z. species have been reported (Table 3). In one study, bioautophagy-directed fractionation of essential oil from Z. armatum leaves led to the isolation of β-fenchol and linalool, which had antifungal activities against A. alternata and C. lunata (Guleria et al., 2013).
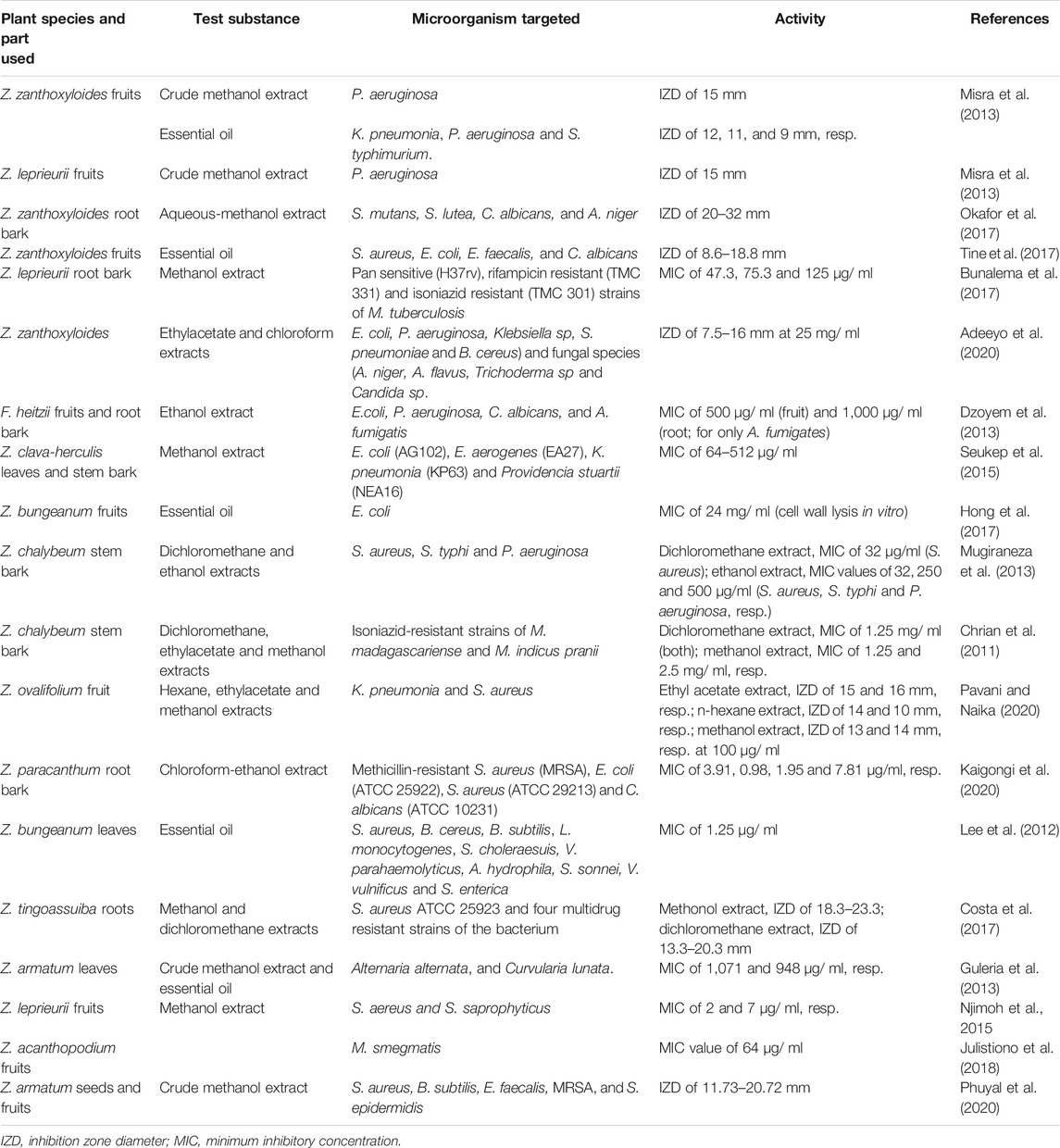
TABLE 3. Summary of the antimicrobial activities of solvent extracts and essential oils from different part of Zanthoxylum species.
In general, studies on the Z. species crude extracts and essential oils reported antimicrobial activity as IZD or MIC, and seldom investigated their molecular mechanisms. In one study, n-hexane extract of Z. acanthopodium fruits that was active against M. smegmatis was reported to induce loss of intracellular sodium and potassium ion concentration, suggesting that the extract acted by damaging the bacterial cell wall (Julistiono et al., 2018). Hong et al. (2017) also reported that essential oil from Z. bungeanum fruits, containing 6,9,12,15-hexadeca-tetraenoic acid-methyl ester, 4-terpinenylacetate, D-limonene, eucalyptol, α-terpineol, β-linalool, δ-cadinene and β-pinene, caused lysis of cultured E. coli membrane. This mechanism was supported by the high amount of bacterial intracellular (nucleic acids and proteins) and cell membrane components in the culture medium. In vivo evaluation in a mouse model of enteritis demonstrated that Z. bungeanum fruit-derived essential oil downregulated the expression of pro-inflammatory cytokines (Hong et al., 2017); this indicates that anti-inflammatory mechanism played a role in host protection by the essential oil against E. coli infection. Indeed, elucidation of molecular mechanisms would be more logical for isolated compounds with defined molecular targets in the microorganisms or host. Nonetheless, knowledge of the molecular basis of antimicrobial effect would enhance the direct utilization of crude extracts of Z. species for pharmacological applications.
Efforts have been made to isolate compounds that are responsible for the reported antimicrobial activities of Z. species extracts. Most of the compounds are alkaloids and some possess antimicrobial activity against both drug-sensitive and drug-resistant species of public health importance. For example, alkaloids (6-acetonyldihydronitidine, 6-acetonyldihydroavicine and 6-acetonyldihydrochelerythrine) from the stem bark of Z. rhoifolium strongly inhibited the growth of S. aureus, S. epidermidis, K. pneumonie, S. Setubal, and E. coli with respective MIC values of 1.06, 1.06, 3.12, 3.12, and 1.06 μg/ ml (6-acetonyldihydronitidine), 1.06, 3.12, 1.06, 3.12, and 3.12 μg/ ml (6-acetonyldihydroavicine), and 12.5, 6.25, 6.25, 6.25, and 12.5 μg/ ml (6-acetonyldihydrochelerythrine) (Gonzaga et al., 2003). Other antimicrobial alkaloids isolated from Z. species include dihydrochelerythrine from Z. rhetsa roots and stem (Tantapakul et al., 2012); bis-[6-(5,6-dihydro-chelerythrinyl)] ether, 6-ethoxy-chelerythrine and 4-methoxy-N-methyl-2-quinolone from Z. schreberi leaves and bark (Rodríguez-Guzmán et al., 2011); β-carboline alkaloids (10-methoxycanthin-6-one and canthin-6-one) and phenanthridine alkaloids (8-acetonyldihydrochelerythrine and 8-oxochelerythrine) from Z. paracanthum root bark (Kaigongi et al., 2020); N-methylcanadine from Z. tingoassuiba A. St.-Hil. roots (Costa et al., 2017); and acridone alkaloids (hydroxy-1,3-dimethoxy-10-methyl-9-acridone and 3-hydroxy-1,5,6-trimethoxy-9-acridone) from Z. leprieurii stem bark (Bunalema et al., 2017). Similar to its broad-spectrum anticancer activity, canthin-6-one was strongly active against several bacteria (S. aureus, E. coli, Proteus vulgaris and Klebsiella aerogenes) and fungi (A. niger and C. albicans), with corresponding MIC values of 0.227, 0.114, 0.114, 0.227, and 0.114 µM, respectively. Furthermore, the acridone alkaloids strongly inhibited first line drug-resistant (H37rv), rifampicin-resistant (TMC 331) and isoniazid-resistant (TMC 301) strains of M. tuberculosis with MIC values of 1.5–8.3 μg/ ml (Bunalema et al., 2017), which positions Z. leprieurii as a potential source of anti-tuberculosis agents. Structure-activity relationship studies are needed to identify the pharmacophores of the alkaloids against specific microorganisms or their molecular targets. Likewise, antimicrobial mechanisms of the alkaloids are largely unknown, although Wouatsa et al. (2013) reported that acridone alkaloids, 3-hydroxy-1,5,6-trimethoxy-9-acridone and 2,4′-hydroxyzanthacridone oxide, from Z. leprieurii fruit extract acted by inhibition of aromatase and glycosyltransferase, which are involved in biosynthesis of bacterial lipopolysaccharides and cell wall.
Apart from alkaloids, other antimicrobial compounds isolated from different Z. species include lignans (e.g., sesamin and syringaresinol) (Rahman et al., 2008), tetraflavonoids (e.g., lemairones A and B) (Bitchagno et al., 2015), and phytosterol (e.g., stigmasterol) (Kaigongi et al., 2020). Lemairones A and B from Z. lemairei leaves moderately inhibited multidrug-resistant bacteria, K pneumoniae KP55 and E. coli AG100 with MIC values of 128 mg/ ml and 64 mg/ ml, respectively against E. coli AG100, and MIC value of 128 mg/ ml against K. pneumoniae KP55 (Bitchagno et al., 2015). Furthermore, a polymeric procyanidin from Z. bungeanum fruit exhibited cytotoxicity against drug resistant strains of S. aureus with an MIC value of 128 μg/ ml; the compound acted by inhibiting β-lactamase activity and by inducing cell wall damage (Kusuda et al., 2006). This study also showed that the isolated compound has potential for further development as an adjuvant of antibacterial drugs for mitigating the burden of drug-resistant microbial infections. Taken together, it is recommended that future studies should use drug resistant microorganisms since the ultimate goal is to overcome antimicrobial resistance. Findings from the studies reviewed suggest that some of the compounds isolated from Z. species have promising future as source of new antibiotics. Figure 2 shows representative Z. species-derived compounds with anticancer, antiparasitic, antimicrobial or anti-sickling activities.
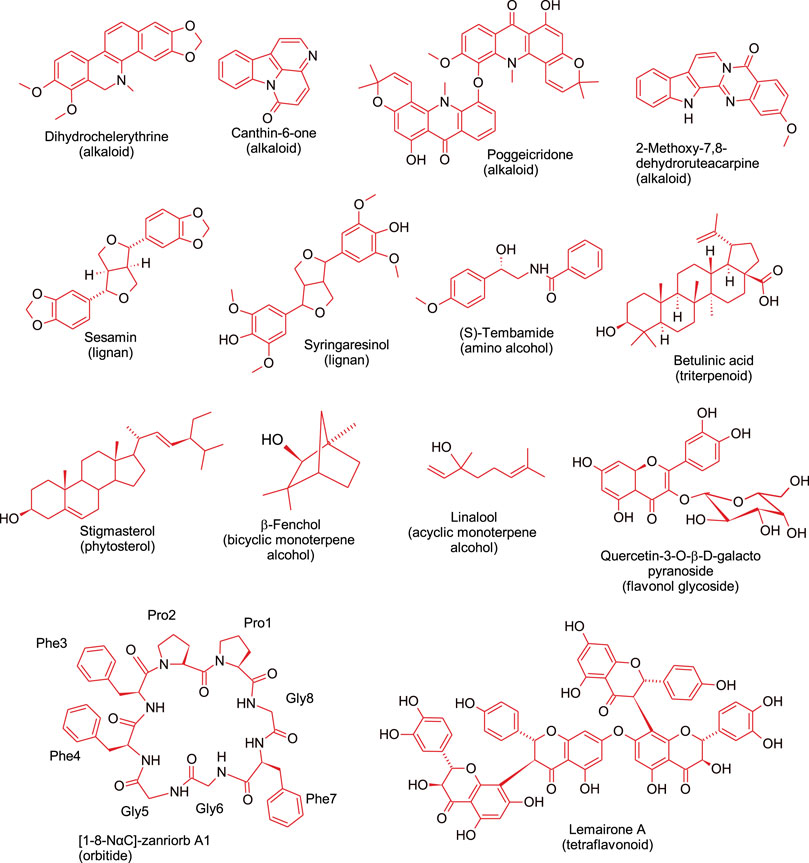
FIGURE 2. Representative bioactive compounds, from different phytochemical classes, isolated from Zanthoxylum species.
Antiviral Potentials of Zanthoxylum Species
The emergence and spread of viruses such as SARS-CoV-2, HIV and hepatitis B have led to heightened efforts in search for effective remedies. These efforts include repurposing of drugs developed for other viral diseases as well as exploring for new drug candidates in medicinal plants used in treating viral infections by traditional medicine practitioners. A few studies have reported antiviral activities for extracts from Z. species. Following the folkloric use in treating oral pathogens and symptoms related to picornaviridae infection, Choi (2016) found that the methanol extract of Z. piperitum leaves were cytotoxic to human rhinoviruses - HRVs (HRV2 and HRV3) and enteroviruses (coxsackie A16, B3, and B4 viruses, and human enterovirus71) of picornaviridae virus family with IC50 values of 59, 39, 45, 68, 93, and 4.4 μg/ml, respectively. As the extract had low toxicity against human cells (Hela and Vero cells), the active ingredients, if isolated, can serve as bioactive candidates against viral diseases caused by members of the picornaviridae family. Moreover, leaves of Z. bungeanum are used in Korea and Japan for treating respiratory diseases. To support this use, Ha et al. (2014) reported the anti-influenza virus A/NWS/33 (H1N1) effects of flavonol glycosides, quercetin-3-O-β-D-galactopyranoside, quercetin-3-O-α-L-rhamnopyranoside and kaempferol-3-O-α-L-rhamnopyranoside isolated from the Z. bungeanum leaves; the flavonol glycosides also inhibited influenza A virus neuraminidase activity with IC50 values of 434, 211, and 273 μg/ ml, respectively. Influenza A virus neuraminidase is involved in the release of newly made virus particle from infected cells, making it a good target for reducing the spread in host cells. Considering the multiple molecular targets of polyphenols, it is also possible that the antiviral activity was mediated via additional unknown mechanisms.
In addition to influenza virus, the antiviral potentials of Z. species-derived phytochemicals have also been reported against hepatitis B virus. A coumarin, collinin, from chloroform extract of Z. schionifolium bark exhibited anti-hepatitis B virus activity (ED50 of 68.3 μg/ ml) and also inhibited HBV-DNA replication (IC50 of 17.1 μg/ ml) (Chang et al., 1997). Using a different assay, two alkaloids, 5,6-dihydro-6-methoxynitidine and 5-methoxydictamnine, from Z. bungeanum Maxim roots at 0.2 µM showed higher anti-HBV activities by respectively inhibiting 43.3 and 49.3% of viral multiplication than 10 µM of standard antiviral drug, lamivudine with 29.6% inhibition (Yang and Chen 2008). In addition, a benzophenanthridine alkaloid (decarine), a furoquinoline alkaloid (γ-fagarine), and an amino alcohol derivative ( + )-tembamide) from Z. ailanthoides root bark showed anti-HIV activities (EC50 values <0.05 μg/ ml) with no cytotoxicity against normal H9 lymphocyte cells (Cheng et al., 2005). It is challenging to compare the antiviral potential of the Zanthoxylum compounds because of differences in the structural type of the compounds, target virus, and assay method used in these studies. Nonetheless, due to their selected toxicity to viruses, the Z. species-derived alkaloids warrant further investigation for potential use in treating viral infections.
Zanthoxylum Species as Potential Source of Anti-parasitic Agents
Human African trypanosomiasis (sleeping sickness), a neglected tropical disease, is a Trypanosoma species-caused parasitic disease that is endemic in sub-Saharan Africa, where majority of the victims are poor rural dwellers. Trypanosoma species is transmitted by tsetse flies and cause a fatal disease if not properly treated. A few T. species, such as T. brucei gambiense, is responsible for the vast majority of Trypanosoma infection in West and Central Africa while T. rhodesiense is mostly responsible for infections in East Africa where less than 10% of the infection exists. The initial (hematolymphatic) stage of infection is asymptomatic and, if detected early, is treatable with pentamidine or suramin while the second (meningo-encephalitic) stage, which is characterized by the invasion of the central nervous system by the parasite, is treatable with melarsoprol or eflornithine (Simarro et al., 2011). Unfortunately, these drugs do not guarantee total recovery as the success rate in most cases is less than 90%. In addition to the growing drug resistance of the parasites, some devastating toxicities accompy the use of these drugs (Shaw et al., 2010). This presents the need for safer and potent alternatives, especially from natural products and medicinal plants that have a history of application in treating the disease. Medicinal plants are used for treating trypanosomiasis in Nigeria (Atawodi et al., 2003; Bulus et al., 2016; Okwor et al., 2020); thus, they have strong prospects for use as sources of clinically relevant anti-trypanosoma agents. In Nigeria and Ghana, roots, stem and leaves of Z. zanthoxyloides are used in treating trypanosomiasis (Mann et al., 2011). When cultured with the root extract, the viability of T. brucei was demonstrated to be suppressed (IC50 = 3.41 μg/ ml) by induction of apoptosis and cell cycle arrest at G0/G1 phase (Dofuor et al., 2019). Subsequent investigation by the same group resulted in the isolation of an alkaloid, skimmianine, and an oxylipin, 9-oxo-10,12-octadecadienoic acid, from Z. zanthoxyloides root as the cytotoxic principles against T. brucei (GUTat 3.1 strains; EC50 values of 1.7 and 1.2 µM, respectively) (Dofuor et al., 2020). Although less active than diminazene aceturate, an antitrypanosomal drug (EC50 of 0.5 µM), the alkaloids acted by inducing cell cycle arrest at G0-G1 and (G2-M) phases and by inhibiting DNA synthesis of the parasite. Similarly, an acridone alkaloid, arborinine, derived from Z. leprieurii stem bark exhibited anti-trypanosomal activity in cultured Trypanosoma brucei (s427) cells with an IC50 of 13.2 μg/ ml by unknown mechanisms (Eze et al., 2020). There is a need to test the clinical effectiveness of these isolated compounds in trypanosoma infection to clarify if their in vitro activities can translate into clinically relevant in vivo effects. This is because some therapeutic agents that are active in culture studies are not biostable in the gastrointestinal tract or may face transport barriers during transepithelial transport when orally ingested (Udenigwe et al., 2021).
Apart from trypanosomiasis, malaria is another parasitic disease targeted with some Z. species. Malaria is caused by Plasmodium species and is transmitted by Anopheles species through the blood of an infected human. An estimate of over 200 million people die from malaria-related events and a vast majority of these deaths occur in sub-Saharan Africa with Nigeria bearing the highest burden (WHO, 2019). Majority of those who contract and die from malaria are poor rural dwellers who resort to cheap and ineffective drugs that relieve the symptoms, leading to relapses and increase in the development of resistant strains of the parasite (Karunamoorthi et al., 2013). In addition, some individuals are sensitive to some prescription antimalarial drugs (Haakenstad et al., 2019). Consequently, traditional medicine practitioners harness the therapeutic potentials of medicinal plants to treat malaria. Many Zanthoxylum species have been investigated as sources of antimalarial agents. For example, an in vitro study by Mofor et al. (2017) reported that extract of Z. clava-herculis stem bark inhibited multidrug resistant strain of P. species with IC50 of 4.94 μg/ ml and with low toxicity against monkey kidney epithelial cell line. Despite the prospects, the phytochemicals responsible for the antimalarial activity and their mechanism of action are unknown. Other studies have attempted to isolate some antimalarial principles from Z. species. Among the compounds, alkaloids, lignans and amides dominated as active compounds. For instance, sesamine from Z. gilletii stem bark showed significant anti-plasmodial activities against chloroquine-sensitive Sierra Leone (D6), chloroquine-resistant Indochina (W2), and artemisinin-resistant strain (3D7) of P. falciparum with IC50 of 1.92, 3.23, and 2.94 μg/ ml, respectively (Masinde 2014). Secondary metabolites such as syncarpamide and decarine from Z. syncarpum stem also significantly inhibited both chloroquine-sensitive and chloroquine-resistant strains of malaria parasite; IC50 values of 2.04 and 1.44 µM were recorded against P. falciparum D6 strain and 3.06 and 0.88 µM against P. falciparum W2 strain (Ross et al., 2004). Syncarpamide was cytotoxic against African green monkey kidney (VERO) fibroblast cell line only at high concentration of 56 μM, outside the range of bioactivity concentrations. This suggests that the Z. syncarpum compounds can potentially exhibit anti-plasmodial effect with low toxicity to the host.
Based on the use of different Z. zanthoxyloides parts for treating malaria (Adesina 2005; Enechi et al., 2019), Goodman et al. (2019) that four alkaloids, bis-dihydrochelerythrinyl ether, skimmianine, buesgenine and chelerythrine, isolated from roots, root-bark and stem-bark exhibited anti-plasmodial activity against chloroquine-sensitive (3D7) strains of P. falciparum (IC50 values of 4.3, 0.7, 2.0, and 0.4 μg/ ml, respectively). Previous studies on other alkaloids showed that nitidine from Z. gilletii stem bark exhibited anti-plasmodial activity against P. falciparum strain FcB1 with IC50 < 5 μg/ml by halting DNA synthesis in the parasite (Zirihi et al., 2005; Zirihi et al., 2009). Moreover, 8-acetonyldihydrochelerythrine from Z. gilletii stem bark inhibited D6, W2, and 3D7 strains of P. falciparum with IC50 values of 4.06, 4.02, and 3.37 μg/ ml, respectively through unknown mechanisms (Masinde, 2014). Apart from alkaloids, an amide, fagaramide, isolated from Z. gilletii stem bark was moderately active (IC50 of 7.73, 15.15, and 7.72 μg/ ml) against D6, W2, and 3D7 strains of P. falciparum, respectively (Masinde, 2014). Another amide, pellitorine, and a furanoquinolines, γ-fagarine, from Z. zanthoxyloides roots, root-bark and stem-bark also inhibited 3D7 strains of P. falciparum with IC50 values of 2.2 and 2.0 μg/ ml, respectively (Goodman et al., 2019). It is possible that the anti-plasmodial compounds may have acted alone or together if present in the plant extracts used in treating malaria (Enechi et al., 2019; Amah et al., 2021). Despite the promising results, the mechanism of action of the isolated compounds are unknown and the research design in some cases did not include reference antimalarial agents for comparison. Future studies need to evaluate the clinical efficacy of the isolated anti-plasmodial compounds in susceptible populations as a treatment option for combatting resistant species of the malarial parasites.
Potential Application of Zanthoxylum Species in Sickle Cell Disease
Sickle cell disease (SSD) is a group of genetic diseases resulting from inheritance of two abnormal copies of hemoglobin genes. The most common among them is sickle cell anemia. This disease is characterized by hemolytic anemia and occlusion of the blood vessels that reoccurs often. This occlusion is the cause of the excruciating crisis in the joints, a common occurrence in people with SSD. Upon hemolysis, hemoglobin in the erythrocytes is released as free heme (which is pro-inflammatory) and free iron (which by Fenton-type reaction interacts with hydrogen peroxide to form reactive oxygen species). These two components collectively worsen the complications associated with SSD. Consequently, there has been increased and continuous awareness on the prevention of SSD, and improvement in treatment regimen and other intervention strategies. People who cannot afford anti-sickling drugs like hydroxyurea, nitric oxide, purified poloxamer 118 and piracetam resort to medicinal plants with history of use in subsiding crisis associated with SSD (Okpuzor et al., 2008; Amujoyegbe et al., 2016). Medicinal plants have been investigated for anti-sickling activities and several have shown promising results. Notably, some members of genus Zanthoxylum (e.g., Z. zanthoxyloides, Z. leprieuri and Z. gilletii) are among the plants with history of traditional use in managing SSD (Akakpo-Akue et al., 2020). Some studies have reported the anti-sickling activities of Z. species and some anti-sickling compounds in these plant species have been isolated and characterized.
Among the species, Z. zanthoxyloides, Z. lemairei, Z. leprieurii, Z. tessmannii and Z. gilletii have been investigated for anti-sickling activity in vitro (Egunyomi et al., 2009; Ouattara et al., 2009). Moreover, the specific compounds responsible for anti-sickling activity of the plant extracts were scarcely reported. Particularly, three divallinoylquinic acids (burkinabins A, B, and C) isolated from Z. zanthoxyloides root bark at 1.964 mg/ ml inhibited sickling of deoxygenated erythrocytes by 77, 78.6 and 82.5% for burkinabins A, B, and C, respectively, which were similar to the effect of sodium chromoglycate, a reference anti-sickling agent (Ouattara et al., 2009). In addition, phenolic acids, such as syringic acid, vanillic acid, proto-catechuic acid, and p-hydroxy-benzoic acid, have been documented to play major roles in the anti-sickling activities of Z. zanthoxyloides (Nurain et al., 2017). However, this conclusion was only based on their identification in high amount in the active plant root extracts. Hence, bioassay-guided fractionation studies are needed to confirm the bioactivity of the phenolic acids, and to isolate other active compounds in the Z. species that showed anti-sickling activities. Generally, the ability of burkinabins A-C to demonstrate good anti-sickling properties has positioned the Z. species as promising sources of therapeutic agents for managing SSD. Clinical trials with the isolated anti-sickling compounds from Z. species are recommended and further studies are needed to modify the compounds to more potent and safer derivatives.
Safety of Zanthoxylum Species
Knowing that not all things natural is safe, there is a need to be cautious in the use of natural products for food and drug. Many natural products have exhibited different levels of toxicity, including lethality at high doses (Al-Nuaimi 2018). For instance, Z. chalybeum root bark extract at 4,000 mg/ kg elevated serum creatinine, sodium and potassium levels in rats, as well induced histomorphological deterioration of the intestine in a manner consistent with tumor formation (Engeu et al., 2008). In addition, in vivo and in vitro studies showed that Z. chalybeum leaves, stem bark and root bark (2000 mg/ kg) caused mortality of mice and elicited toxicity against normal human renal epithelium cells. However, after solvent fractionation, no sign of toxicity was recorded at a maximum dose of 5,000 mg/ kg, suggesting that the toxic compounds might be acting in synergy. Furthermore, Z. gilletii stem bark applied in treating erectile dysfunction in South Africa and Peru was also reported to elicit histological changes in the reproductive system of male rat after oral administration daily for 14 days. Similarly, at high concentrations, Z. zanthoxyloides stem bark extract was genotoxic and cytotoxic against human leukocytes (Ogunbolude et al., 2014), while Z. lepreurii and Z. zanthoxyloides roots extracts were cytotoxic against normal human prostate epithelium cells (Tamdem 2019). Furthermore, Z. zanthoxyloides root bark induced seizure and substantial damage to the liver and kidney, resulting in mortality in mice that received large doses of the herbal materials; LD50 was recorded to be 5 g/ kg (Ogwal-Okeng et al., 2003). Z. zanthoxyloides stem bark was also reported to decrease bile release, affecting negatively the serum lipid levels of rats fed the extract (Umaru et al., 2019). In Ugandan folkloric practice, overdose of Z. zanthoxyloides has been recorded to cause short-duration and self-healing stomach disturbances (Anokbonggo et al., 1990). Lastly, Z. heitzii stem bark at doses higher than 6 g/ kg elicited toxicity and organ damage in rats (Ntchapda et al., 2015). Collectively, toxicities of the Z. species vary based on species, plant part, extraction solvent, dosage, level of phytochemical fractionation, and animal model studied. It is worth noting that the doses of the plant extracts that caused toxicity far exceed those that led to the desirable bioactivity. Nonetheless, caution should be taken to avoid consumption of high doses of the plant preparations to avoid deleterious effects. Furthermore, safe doses of some Z. species are yet to be reported and this information is necessary for traditional medicine practitioners to properly administer the natural products and for the safety of consumers.
Conservation of Zanthoxylum Species for Future Applications
Considering the wide use of plants in the genus Zanthoxylum and the risk of extinction, conservationists have been advocating for measures to minimize overexploitation, especially for the species whose roots are the most commonly used part (Mbinile et al., 2020). For example, Ouédraogo et al. (2019) showed that the chemical constituents of the stem bark and root bark of Z. zanthoxyloides are similar; hence, extracts from these parts are likely to have similar biological activities. This observation needs to be confirmed by comparative phytochemomics and assessment of biological activities. If the relatedness is established, scientists and traditional practitioners would have sustainable alternatives and thus minimize overharvesting of the plant root. In addition, reforestation of medicinal plants and maintenance of their cell cultures for reuse should be emphasized (Li et al., 2020). Furthermore, genetic modification of medicinal plants to become more resilient to environmental threats, such as drought, will also help to make the species more sustainable.
It is worth noting that the names of some of the plants used in the studies reviewed were the “synonyms” as shown in plant databases. For example, some studies like Wu et al. (2007) and Fan et al. (2019) reported on Zanthoxylum simulans while Yang and Chen (2008), Yang et al. (2009), Chakthong et al. (2019), Sepsamli and Prihastanti, (2019),Lu et al. (2020a) and Lu et al. (2020b) reported on Zanthoxylum nitidum instead of the accepted nomenclature, “Zanthoxylum bungeanum Maxim”. This issue was noted in several other papers, such as Chrian et al. (2011), Rodriguez-Guzman et al. (2011), Okafor et al. (2017), and Wansi et al. (2009). To address this issue, a recent study applied DNA barcoding for correct identification of plant species that were incorrectly named in previous studies (Veldmana et al., 2020). The authors noted that some medicinal plant researchers do not consult plant taxonomy experts and others do not confirm the plant identity by comparing their features with those available in reputable plant databases. Another possible source of this confusion is the local assignment of arbitrary names to many plant species by herbalists. This could also be associated with intraspecies and interspecies genetic diversity, and variance development because of changes in the environment (Feng et al., 2020). The issue could also be linked to the dependence of researchers in some countries on traditional medicine practitioners to provide and identify therapeutic plants. This system is not reliable. In one instance, some Zanthoxylum species with different chemical constituents and biological activities (Z. bungeanum, Z. schinifolium, and Z. piperitum) were distributed and intermixed as “Zanthoxyli pericarpium” by traditional medicine practitioners in Korea (Jang et al., 2020). Without proper identification, the outcome of research conducted with incorrectly labelled plant samples will be misleading. It is recommended that experts in phytotaxonomy should validate the identity of the plant species prior to further research and development. In addition, molecular characterization of the plant and the use of specific biomarkers may be helpful in ensuring that names given to plants used for phytopharmacological research are credible.
Conclusion
Zanthoxylum species are reservoir of phytochemicals with health-promoting properties, such as anti-sickling, anticancer and anti-infectious disease activities (Figure 3). The majority of the biological properties reported for Z. species were inspired by their traditional uses as therapeutic agents. Considering that the roots of Z. species are the most sourced parts in trado-medicinal uses, reforestation of the plants is highly recommended to avoid overharvesting. Similarly, the plant culture can be utilized instead of the freshly harvested plants. In many studies, drug-sensitive strains of infectious agents and cancer cells were used to assess bioactivity. Hence, future research should target the activity of Z. species against drug-resistant species or strains. This is important because one of the ultimate goals of new drug development is to curb drug resistance. Furthermore, several Z. species phytochemicals with strong bioactivities against infectious microorganisms, especially against drug-resistant strains of malarial parasites, viruses and other microbes, as well as drug-resistant cancer cells should be subjected to clinical trials as potential natural alternatives to synthetic drugs. In addition, since many studies were conducted in vitro, there is a dearth of information on the intestinal transport, biostability, bioaccessibility, and bioavailability of many of the active compounds. Additional research is needed to clarify the specific chemical compounds responsible for the promising biological activities of some of the plant extracts as well as the bioactivity and mechanisms of action of some of the isolated compounds. Future research should also confirm bioactivities and safety of the compounds in vivo using animal models and humans. Finally, researchers should endeavor to mimic the traditional methods used in preparation of the plant extract to ensure the preservation of the bioactive principles of interest.
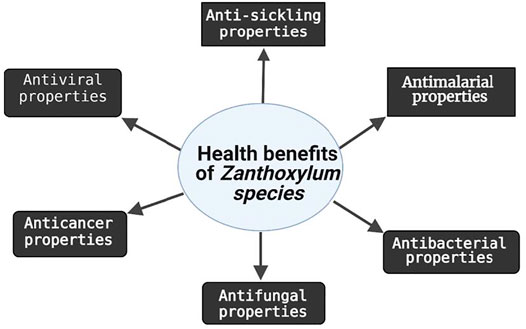
FIGURE 3. Summary of biological activities of extracts or compounds from different parts of Zanthoxylum species.
Author Contributions
IOU, JCN, and CCU contributed to conception and design of the study. EA conducted the literature search. IOU, JCN, and ECA wrote sections of the first draft of the manuscript. IOU and CCU revised the manuscript. All authors read and approved the submitted version.
Conflict of Interest
The authors declare that the research was conducted in the absence of any commercial or financial relationships that could be construed as a potential conflict of interest.
Publisher’s Note
All claims expressed in this article are solely those of the authors and do not necessarily represent those of their affiliated organizations, or those of the publisher, the editors and the reviewers. Any product that may be evaluated in this article, or claim that may be made by its manufacturer, is not guaranteed or endorsed by the publisher.
References
Abubakar, I. B., Ukwuani-Kwaja, A. N., Garba, A. D., Singh, D., Malami, I., Salihu, T. S., et al. (2020). Ethnobotanical Study of Medicinal Plants Used for Cancer Treatment in Kebbi State, North-west Nigeria. Acta Ecologica Sinica 40, 306–314. doi:10.1016/j.chnaes.2020.02.007
Adeeyo, A. O., Odelade, K. A., Msagati, T. A. M., and Odiyo, J. O. (2020). Antimicrobial Potencies of Selected Native African Herbs against Water Microbes. J. King Saud Univ. - Sci. 32, 2349–2357. doi:10.1016/j.jksus.2020.03.013
Adesina, S. K., Olugbade, T. A., Akinwusi, D. D., and Bergenthal, D. (1997). Extractives from Zanthoxylum Lemairei Root and Stem. Pharmazie 52, 720–724.
Adesina, S. (2005). The Nigerian Zanthoxylum: Chemical and Biological Values. Afr. J. Trad. Compl. Alt. Med. 2 (3), 282–301. doi:10.4314/ajtcam.v2i3.31128
Adewole, K. E. (2020). Nigerian Antimalarial Plants and Their Anticancer Potential: A Review. J. Integr. Med. 18, 92–113. doi:10.1016/j.joim.2020.01.001
Ahmad, M. U., Rahman, M. A., Huq, E., and Chowdhury, R. (2003). Alkaloids of Zanthoxylum Budrunga. Fitoterapia 74, 191–193. doi:10.1016/s0367-326x(02)00286-1
Ahsan, M., Haque, M. R., Hossain, M. B., Islam, S. N., Gray, A. I., and Hasan, C. M. (2014). Cytotoxic Dimeric Quinolone-Terpene Alkaloids from the Root Bark of Zanthoxylum Rhetsa. Phytochemistry 103, 8–12. doi:10.1016/j.phytochem.2014.03.008
Ahsan, M., Zaman, T. A., Hasan, C. M., Ito, C., and Islam, S. K. (2000). Constituents and Cytotoxicity of Zanthoxylum Rhesta Stem Bark. Fitoterapia 71, 697–700. doi:10.1016/s0367-326x(00)00214-8
Al-Nuaimi, A. A. H. D. (2018). Extracts of Plants Used as Traditional Medicines Have Toxic Effect on the Liver and Kidney. Mojap 5 (1), 32‒41. doi:10.15406/mojap.2018.05.00161
Alam, F., Najum Us Saqib, Q., and Waheed, A. (2017). Cytotoxic Activity of Extracts and Crude Saponins from Zanthoxylum Armatum DC. Against Human Breast (MCF-7, MDA-MB-468) and Colorectal (Caco-2) Cancer Cell Lines. BMC Complement. Altern. Med. 17, 368. doi:10.1186/s12906-017-1882-1
Amah, C. C., Enechi, O. C., Ekpo, D. E., Okagu, I. U., Ononiwu, C. P., and Joshua, P. E. (2021). Safety Assessment and Antimalarial Property of Methanol Extract of Fagara Zanthoxyloides Root-Bark on Plasmodium Berghei-infected Mice. Comp. Clin. Pathol. 30 (1), 217–228. doi:10.1007/s00580-021-03202-7
Ameh, S. J., Tarfa, F. D., and Ebeshi, B. U. (2012). Traditional Herbal Management of Sickle Cell Anemia: Lessons from Nigeria. Anemia. 2012, 1–9. doi:10.1155/2012/607436
Amujoyegbe, O. O., Idu, M., Agbedahunsi, J. M., and Erhabor, J. O. (2016). Ethnomedicinal Survey of Medicinal Plants Used in the Management of Sickle Cell Disorder in Southern Nigeria. J. Ethnopharmacol 185, 347–360. doi:10.1016/j.jep.2016.03.042
Andima, M., Coghi, P., Yang, L. J., Wai Wong, V. K., Mutuku Ngule, C., Heydenreich, M., et al. (2019). Antiproliferative Activity of Secondary Metabolites from Zanthoxylum Zanthoxyloides Lam: In Vitro and In Silico Studies. Pc 10 (1), 44–51. doi:10.5530/pc.2020.1.8
Anokbonggo, W. W., Odoi-Adome, R., and Oluju, P. M. (1990). Traditional Methods in Management of Diarrhoeal Diseases in Uganda. Bull. World Health Organ. 68 (3), 359–363.
Anyanwu, M. U., and Okoye, R. C. (2017). Antimicrobial Activity of Nigerian Medicinal Plants. J. Intercult Ethnopharmacol 6 (2), 240–259. doi:10.5455/jice.20170106073231
Arsita, E. V., Saragih, D. E., and Aldrin, K. (2019). Anticancer Potential from Ethanol Extract of Zanthoxylum Acanthopodium DC. Seed to against MCF-7 Cell Line. IOP Conf. Ser. Earth Environ. Sci. 293, 012016. doi:10.1088/1755-1315/293/1/012016
Atawodi, S. E., Bulus, T., Ibrahim, S., Ameh, D. A., Nok, A. J., Mamman, M., et al. (2003). In Vitro trypanocidal Effect of Methanolic Extract of Some Nigerian savannah Plants. Afr. J. Biotech. 2 (9), 317–321.
Bitchagno, G. T. M., Tankeo, S. B., Tsopmo, A., Mpetga, J. D. S., Tchinda, A. T., Fobofou, S. A. T., et al. (2015). Lemairones A and B: Two New Antibacterial Tetraflavonoids from the Leaves of Zanthoxylum Lemairei (Rutaceae). Phytochemistry Lett. 14, 1–7. doi:10.1016/j.phytol.2015.08.012
Brijwal, L., Pandey, A., and Tamta, S. (2013). An Overview on Phytomedicinal Approaches of Zanthoxylum Armatum DC.: An Important Magical Medicinal Plant. J. Med. Plants Res. 7 (8), 366–370. doi:10.5897/JMPR12.743
Bukowski, K., Kciuk, M., and Kontek, R. (2020). Mechanisms of Multidrug Resistance in Cancer Chemotherapy. Int. J. Mol. Sci. 21 (9), 3233. doi:10.3390/ijms21093233
Bulus, T., Ahmed, A. B., Aboi, T. Y., and Danbaki, D. A. (2016). Determination of IC50 and IC90 = Ethanolic Extracts of Some Medicinal Plants against Trypanosoma Brucei Brucei. Arch. Clin. Microbiol. 7, 3. doi:10.4172/1989-8436.100048
Bunalema, L., Fotso, G. W., Waako, P., Tabuti, J., and Yeboah, S. O. (2017). Potential of Zanthoxylum Leprieurii as a Source of Active Compounds against Drug Resistant Mycobacterium tuberculosis. BMC Complement. Altern. Med. 17, 89. doi:10.1186/s12906-017-1602-x
Bunalema, L., Obakiro, S., Tabuti, J. R., and Waako, P. (2014). Knowledge on Plants Used Traditionally in the Treatment of Tuberculosis in Uganda. J. Ethnopharmacol 151, 999–1004. doi:10.1016/j.jep.2013.12.020
Burkill, H. M. (1998). The Useful Plants of West Tropical Africa, Families M-R. 2nd edition, Vol. 4. Kew: The Royal Botanic Garden, 1–969.
Burkill, H. M. (1985). The Useful Plant of West Tropical Africa. Kew-UK: Royal Botanic Gardens, 4–6.
Cao, X. L., Xu, J., Bai, G., Zhang, H., Liu, Y., Xiang, J. F., et al. (2013). Isolation of Anti-tumor Compounds from the Stem Bark of Zanthoxylum Ailanthoides Sieb. & Zucc. By Silica Gel Column and Counter-current Chromatography. J. Chromatogr. B Analyt Technol. Biomed. Life Sci. 929, 6–10. doi:10.1016/j.jchromb.2013.04.006
Chakthong, S., Ampaprom, R., Inparn, S., Phetkul, U., Chusri, S., Limsuwan, S., et al. (2019). New Alkylamide from the Stems of Zanthoxylum Nitidum. Nat. Prod. Res. 33 (2), 153–161. doi:10.1080/14786419.2018.1440218
Chang, C.-T., Doong, S.-L., Tsai, I.-L., and Chen, I.-S. (1997). Coumarins and Anti-HBV Constituents from Zanthoxylum Schinifolium. Phytochemistry 45, 1419–1422. doi:10.1016/S0031-9422(97)89023-1
Cheng, M. J., Lee, K. H., Tsai, I. L., and Chen, I. S. (2005). Two New Sesquiterpenoids and Anti-HIV Principles from the Root Bark of Zanthoxylum Ailanthoides. Bioorg. Med. Chem. 13 (21), 5915–5920. doi:10.1016/j.bmc.2005.07.050
Choi, H. J. (2016). Evaluation of Antiviral Activity of Zanthoxylum Species against Picornaviruses. Osong Public Health Res. Perspect. 7 (6), 400–403. doi:10.1016/j.phrp.2016.11.003
Chou, S. T., Peng, H. Y., Chang, C. T., Yang, J. S., Chung, H. K., Yang, S. T., et al. (2011). Zanthoxylum Ailanthoides Sieb And Zucc. Extract Inhibits Growth and Induces Cell Death through G2/M-phase Arrest and Activation of Apoptotic Signals in Colo 205 Human colon Adenocarcinoma Cells. Anticancer Res. 31, 1667–1676.
Chrian, M., Erasto, P., and Otieno, J. (2011). Antimycobacterial Activity and Cytotoxicity Effect of Extracts of Hallea Rubrostipulata and Zanthoxylum Chalybeum. Spatula DD 1, 147–152. doi:10.5455/spatula.20110811025348
Costa, R. S., Lins, M. O., Le Hyaric, M., Barros, T. F., and Velozo, E. S. (2017). In Vitro antibacterial Effects of Zanthoxylum Tingoassuiba Root Bark Extracts and Two of its Alkaloids against Multiresistant Staphylococcus aureus. Revista Brasileira de Farmacognosia 27, 195–198. doi:10.1016/j.bjp.2016.11.001
Cui, H. Z., Choi, H. R., Choi, D. H., Cho, K. W., Kang, D. G., and Lee, H. S. (2009). Aqueous Extract of Zanthoxylum Schinifolium Elicits Contractile and Secretory Responses via Beta1-Adrenoceptor Activation in Beating Rabbit Atria. J. Ethnopharmacol 126, 300–307. doi:10.1016/j.jep.2009.08.025
de A Gonzaga, W., Weber, A. D., Giacomelli, S. R., Dalcol, , Hoelzel, S. C., and Morel, A. F. (2003). Antibacterial Alkaloids from Zanthoxylum Rhoifolium. Planta Med. 69, 371–374. doi:10.1055/s-2003-38882
Dofuor, A. K., Djameh, G. I., Ayertey, F., Bolah, P., Amoa-Bosompem, M., Kyeremeh, K., et al. (2019). Antitrypanosomal Effects of Zanthoxylum Zanthoxyloides (Lam.) Zepern. & Timler Extracts on African Trypanosomes. Evid. Based Complement. Alternat Med. 2019, 1730452. doi:10.1155/2019/1730452
Dofuor, A. K., Ayertey, F., Bolah, P., Djameh, G. I., Kyeremeh, K., Ohashi, M., et al. (2020). Isolation and Antitrypanosomal Characterization of Furoquinoline and Oxylipin from Zanthoxylum Zanthoxyloides. Biomolecules 10, 1670. doi:10.3390/biom10121670
Dos S Beirigo, P. J., Torquato, H. F., dos Santos, C. H., Carvalho, M. G., Castro, R. N., Paredes-Gamero, E. J., et al. (2016). [1-8-NαC]-Zanriorb A1, a Proapoptotic Orbitide from Leaves of Zanthoxylum Riedelianum. J. Nat. Prod. 79 (5), 1454–1458. doi:10.1021/acs.jnatprod.5b00177
Dzoyem, J. P., Guru, S. K., Pieme, C. A., Kuete, V., Sharma, A., Khan, I. A., et al. (2013). Cytotoxic and Antimicrobial Activity of Selected Cameroonian Edible Plants. BMC Complement. Altern. Med. 13, 78. doi:10.1186/1472-6882-13-78
Egunyomi, A., Moody, J. O., and Eletu, O. M. (2009). Antisickling Activities of Two Ethnomedicinal Plant Recipes Used for the Management of Sickle Cell Anaemia in Ibadan, Nigeria. Afr. J. Biotech. 8 (1), 20–25.
Enechi, O. C., Amah, C. C., Okagu, I. U., Ononiwu, C. P., Azidiegwu, V. C., Ugwuoke, E. O., et al. (2019). Methanol Extracts of Fagara Zanthoxyloides Leaves Possess Antimalarial Effects and Normalizes Haematological and Biochemical Status of Plasmodium Berghei-Passaged Mice. Pharm. Biol. 57 (1), 577–585. doi:10.1080/13880209.2019.1656753
Engeu, P. E., Tumusiime, R., Agwaya, M., Mugisha, G., Grace, N., Galiwango, B., et al. (2008). Repeat-dose Effects of Zanthoxylum Chalybeum Root Bark Extract: A Traditional Medicinal Plant Used for Various Diseases in Uganda. Afr. J. Pharm. Pharmacol. 2 (6), 101–105.
Eze, F. I., Siwe-Noundou, X., Isaacs, M., Patnala, S., Osadebe, P. O., and Krause, R. W. M. (2020). Anti-cancer and Anti-trypanosomal Properties of Alkaloids from the Root Bark of Zanthoxylum Leprieurii Guill and Perr. Trop. J. Pharm. Res. 19 (11), 2377–2383. doi:10.4314/tjpr.v19i11.19
Fan, M., Tian, Y., Chen, G., Sarker, S. D., Nahar, L., Wu, J., et al. (2019). Enrichment and Analysis of Quaternary Alkaloids from Zanthoxylum Simulans Using Weak Cation Exchange Solid-phase Extraction Coupled with LC-MS. Phytochem. Anal. 30, 727–734. doi:10.1002/pca.28602019
Feng, S., Liu, Z., Hu, Y., Tian, J., Yang, T., and Wei, A. (2020). Genomic Analysis Reveals the Genetic Diversity, Population Structure, Evolutionary History and Relationships of Chinese Pepper. Hortic. Res. 7, 158. doi:10.1038/s41438-020-00376-z
Fhu, C. W., and Ali, A. (2020). Fatty Acid Synthase: An Emerging Target in Cancer. Molecules 25 (17), 3935. doi:10.3390/molecules25173935
Fu, Y. H., Guo, J. M., Xie, Y. T., Hua, J., Dai, Y. Y., Zhang, W., et al. (2020). Structural Characterization, Antiproliferative and Anti-inflammatory Activities of Alkaloids from the Roots of Zanthoxylum Austrosinense. Bioorg. Chem. 102, 104101. doi:10.1016/j.bioorg.2020.104101
Gbadamosi, I. T. (2015). An Inventory of Ethnobotanicals Used in the Management of Sickle Cell Disease in Oyo State, Nigeria. Bot. Res Int 8 (4), 65–72.
Gonçalves, M., Margarida Madureira, A., Duarte, A., Catarino, L., Monteiro, A., and Teixeira, G. (2019). Zanthoxylum Zanthoxyloides and Zanthoxylum Leprieurii: a Micromorphological, Phytochemical and Antibacterial Evaluation. Ann. Med. 51 (Suppl. 1), 81. doi:10.1080/07853890.2018.1561716
Goodman, C. D., Hoang, A. T., Diallo, D., Malterud, K. E., McFadden, G. I., and Wangensteen, H. (2019). Anti-plasmodial Effects of Zanthoxylum Zanthoxyloides. Planta Med. 85, 1073–1079. doi:10.1055/a-0973-0067
Guleria, S., Tiku, A. K., Koul, A., Gupta, S., Singh, G., and Razdan, V. K. (2013). Antioxidant and Antimicrobial Properties of the Essential Oil and Extracts of Zanthoxylum Alatum Grown in North-Western Himalaya. ScientificWorldJournal 2013, 790580. doi:10.1155/2013/7905802013
Ha, S. Y., Youn, H., Song, C. S., Kang, S. C., Bae, J. J., Kim, H. T., et al. (2014). Antiviral Effect of Flavonol Glycosides Isolated from the Leaf of Zanthoxylum Piperitum on Influenza Virus. J. Microbiol. 52 (4), 340–344. doi:10.1007/s12275-014-4073-5
Haakenstad, A., Harle, A. C., Tsakalos, G., Micah, A. E., Tao, T., Anjomshoa, M., et al. (2019). Tracking Spending on Malaria by Source in 106 Countries, 2000-16: an Economic Modelling Study. Lancet Infect. Dis. 19 (7), 703–716. doi:10.1016/S1473-3099(19)30165-3
Harahap, U., Hasibuan, P. A. Z., Sitorus, P., Arfian, N., and Satria, D. (2018). Antimigration Activity of an Ethylacetate Fraction of Zanthoxylum Acanthopodium DC. Fruits in 4T1 Breast Cancer Cells. Asian Pac. J. Cancer Prev. 19, 565–569. doi:10.22034/APJCP.2018.19.2.565
Hatano, T., Inada, K., Ogawa, T. O., Ito, H., and Yoshida, T. (2004). Aliphatic Acid Amides of the Fruits of Zanthoxylum Piperitum. Phytochemistry 65, 2599–2604. doi:10.1016/j.phytochem.2004.08.018
Hong, L., Jing, W., Qing, W., Anxiang, S., Mei, X., Qin, L., et al. (2017). Inhibitory Effect of Zanthoxylum Bungeanum Essential Oil (ZBEO) on Escherichia coli and Intestinal Dysfunction. Food Funct. 8, 1569–1576. doi:10.1039/C6FO01739H
Hwang, K. A., Kwon, J. E., Noh, Y., Park, B., Jeong, Y. J., Lee, S. M., et al. (2008). Effects of Zanthoxylum Piperitum Ethanol Extract on Osteoarthritis Inflammation and Pain. Biomed. Pharmacother. 105, 481–490. doi:10.1016/j.biopha.2018.05.109
Hwang, W., Kim, D., Kwon, O. S., Kim, Y. S., Ahn, B., and Kang, N. G. (2020). Topical Application of Zanthoxylum Piperitum Extract Improves Lateral Canthal Rhytides by Inhibiting Muscle Contractions. Sci. Rep. 10 (1), 21514. doi:10.1038/s41598-020-78610-w
Imaga, N. O. A., Shaire, E. A., Ogbeide, S., and Samuel, K. A. (2011). In Vitro biochemical Investigations of the Effects of Carica Papaya and Fagara Zanthoxyloides on Antioxidant Status and Sickle Erythrocytes. Afr. J. Biochem. Res. 5 (8), 226–236.
Imaga, N. O. A. (2010). The Use of Phytomedicines as Effective Therapeutic Agents in Sickle Cell Anemia. Sci. Res. Essays 5 (24), 3803–3807.
India State-Level Disease Burden Initiative Cancer Collaborators (2018). The burden of Cancers and Their Variations across the States of India: the Global Burden of Disease Study 1990-2016. Lancet Oncol. 19, 1289–1306. doi:10.1016/S1470-2045(18)30447-9
J, A.-A., T.K.M, K., Kiyinlma, C., Gnamien Marcel, A., Yvette, F., A, Y.-C., et al. (2020). Ethnobotanical Study of Medicinal Plants Used against Sickle Cell Anaemia in the Eastern Part of the Côte d'Ivoire. J.Anim.Plant Sci. 45 (1), 7839–7852. doi:10.35759/JAnmPlSci.v45-1.7
Jang, H. S., Jeong, B., Choi, S. Y., Lee, J., Kwon, Y. S., and Yang, H. (2020). The Rapid Discrimination and Quality Assessment of Three Zanthoxylum Species Using 1H NMR Spectrometry. Int. J. Anal. Chem. 2020, 1–7. doi:10.1155/2020/3830258
Ju, Y., Still, C. C., Sacalis, J. N., Li, J., and Ho, C. T. (2001). Cytotoxic Coumarins and Lignans from Extracts of the Northern Prickly Ash (Zanthoxylum Americanum). Phytother Res. 15 (5), 441–443. doi:10.1002/ptr.686
Julistiono, H., Lestari, F. G., Iryanto, R., and Lotulung, P. D. (2018). Antimycobacterial Activity of Fruit of Zanthoxylum Acanthopodium DC against M Ycobacterium Smegmatis. Avicenna J. Phytomed 8 (5), 432–438.
Kaigongi, M. M., Lukhoba, C. W., Yaouba, S., Makunga, N. P., Githiomi, J., and Yenesew, A. (2020). In Vitro antimicrobial and Antiproliferative Activities of the Root Bark Extract and Isolated Chemical Constituents of Zanthoxylum Paracanthum Kokwaro (Rutaceae). Plants (Basel) 9, 920. doi:10.3390/plants9070920
Kaminskyy, V., Lin, K. W., Filyak, Y., and Stoika, R. (2008). Differential Effect of Sanguinarine, Chelerythrine and Chelidonine on DNA Damage and Cell Viability in Primary Mouse Spleen Cells and Mouse Leukemic Cells. Cell Biol Int 32 (2), 271–277. doi:10.1016/j.cellbi.2007.09.004
Kamanzi Atindehou, K., Kone, M., Terreaux, C., and Traore, D. (2002). Evaluation of the Antimicrobial Potential of Medicinal Plants From the Ivory Coast. Phytother. Res. 16 (5), 497–502. doi:10.1002/ptr.970
Karunamoorthi, K., Sabesan, S., Jegajeevanram, K., and Vijayalakshmi, J. (2013). Role of Traditional Antimalarial Plants in the Battle against the Global Malaria burden. Vector Borne Zoonotic Dis. 13 (8), 521–544. doi:10.1089/vbz.2011.0946
Kim, M. H., Lee, H. J., Park, J. C., Hong, J., and Yang, W. M. (2017). Zanthoxylum Piperitum Reversed Alveolar Bone Loss of Periodontitis via Regulation of Bone Remodeling-Related Factors. J. Ethnopharmacol 195, 137–142. doi:10.1016/j.jep.2016.10.057
Kusuda, M., Inada, K., Ogawa, T. O., Yoshida, T., Shiota, S., Tsuchiya, T., et al. (2006). Polyphenolic Constituent Structures of Zanthoxylum Piperitum Fruit and the Antibacterial Effects of its Polymeric Procyanidin on Methicillin-Resistant Staphylococcus aureus. Biosci. Biotechnol. Biochem. 70 (6), 1423–1431. doi:10.1271/bbb.50669
Lamorde, M., Tabuti, J. R., Obua, C., Kukunda-Byobona, C., Lanyero, H., Byakika-Kibwika, P., et al. (2010). Medicinal Plants Used by Traditional Medicine Practitioners for the Treatment of HIV/AIDS and Related Conditions in Uganda. J. Ethnopharmacol 130, 43–53. doi:10.1016/j.jep.2010.04.004
Lassané, O., Aminata, P. N., Moussa, C., Latifou, L., and Martin, K. (2019). Stem Bark of Zanthoxylum Zanthoxylodes a Possible Substitute of Root Bark for the Conservation of the Species in Burkina Faso. Afr. J. Biotechnol. 18 (9), 197–205. doi:10.5897/AJB2019.16743
Lee, J.-H., Jang, M., Seo, J., and Kim, G. H. (2012). Antibacterial Effects of Natural Volatile Essential Oil Fromzanthoxylum Piperitum A.P. Dc. Against Foodborne Pathogens. J. Food Biochem. 36 (6), 667–674. doi:10.1111/j.1745-4514.2011.00581.x
Lee, S. J., and Lim, K. T. (2008). Glycoprotein of Zanthoxylum Piperitum DC Has a Hepatoprotective Effect via Anti-oxidative Character In Vivo and In Vitro. Toxicol. Vitro 22, 376–385. doi:10.1016/j.tiv.2007.10.002
Li, W., Sun, Y. N., Yan, X. T., Yang, S. Y., Kim, E. J., Kang, H. K., et al. (2013). Coumarins and Lignans from Zanthoxylum Schinifolium and Their Anticancer Activities. J. Agric. Food Chem. 61, 10730–10740. doi:10.1021/jf403479c
Li, Z., Tariq, A., Pan, K., Graciano, C., Sun, F., Song, D., et al. (2020). Role of glycine max in Improving Drought Tolerance in Zanthoxylum Bungeanum. Peer J. 8, e9040. doi:10.7717/peerj.9040
Liao, J., Xu, T., Zheng, J. X., Lin, J. M., Cai, Q. Y., Yu, D. B., et al. (2013). Nitidine Chloride Inhibits Hepatocellular Carcinoma Cell Growth In Vivo through the Suppression of the JAK1/STAT3 Signaling Pathway. Int. J. Mol. Med. 32 (1), 79–84. doi:10.3892/ijmm.2013.1358
Lichota, A., and Gwozdzinski, K. (2018). Anticancer Activity of Natural Compounds from Plant and marine Environment. Int. J. Mol. Sci. 19, 3533. doi:10.3390/ijms19113533
Lifongo, L. L., Simoben, C. V., Ntie-Kang, F., Babiaka, S. B., and Judson, P. N. (2014). A Bioactivity versus Ethnobotanical Survey of Medicinal Plants from Nigeria, West Africa. Nat. Prod. Bioprospect 4, 1–19. doi:10.1007/s13659-014-0005-7
Lu, Q., Li, C., and Wu, G. (2020b). Insight into the Inhibitory Effects of Zanthoxylum Nitidum against Helicobacter pylori Urease and jack Bean Urease: Kinetics and Mechanism. J. Ethnopharmacol 249, 112419. doi:10.1016/j.jep.2019.112419
Lu, Q., Ma, R., Yang, Y., Mo, Z., Pu, X., and Li, C. (2020a). Zanthoxylum Nitidum (Roxb.) DC: Traditional Uses, Phytochemistry, Pharmacological Activities and Toxicology. J. Ethnopharmacol. 260, 112946. doi:10.1016/j.jep.2020.112946
Maduka, T. O., and Ikpa, C. B. C. (2021). Zanthoxylum Rhetsa (Roxb.) DC.: a Systemic Review of its Ethnomedicinal Properties, Phytochemistry and Pharmacology. WNOFNS 37, 41–57.
Mallya, R., Malim, F., Naik, A., and Bhitre, M. (2019). Evaluation of Anthelmintic Potential of Leaves and Fruits of Zanthoxylum Rhetsa. Pj 11 (3), 475–478. doi:10.5530/pj.2019.11.75
Mann, A., Ifarajimi, O. R., Adewoye, A. T., Ukam, C., Udeme, E. E., Okorie, , et al. (2011). In Vivo antitrypanosomal Effects of Some Ethnomedicinal Plants from Nupeland of North Central Nigeria. Afr. J. Tradit Complement. Altern. Med. 8 (1), 15–21. doi:10.4314/ajtcam.v8i1.60486
Masinde, W. R. G. (2014). Phytochemical Investigation of Zanthoxylum Gilletii (Rutaceae) for Antiplasmodial Biomolecules. Kenya: University of Nairobi.
Mbinile, S. D., Munishi, L. K., Ngondya, I. B., and Ndakidemi, P. A. (2020). Conservation and Management Challenges Facing a Medicinal Plant Zanthoxylum Chalybeum in Simanjiro Area, Northern Tanzania. Sustainability 12, 4140. doi:10.3390/su12104140
Michalkova, R., Mirossay, L., Gazdova, M., Kello, M., and Mojzis, J. (2021). Molecular Mechanisms of Antiproliferative Effects of Natural Chalcones. Cancers (Basel) 13, 2730. doi:10.3390/cancers13112730
Misra, L. N., Wouatsa, N. A., Kumar, S., Venkatesh Kumar, R., and Tchoumbougnang, F. (2013). Antibacterial, Cytotoxic Activities and Chemical Composition of Fruits of Two Cameroonian Zanthoxylum Species. J. Ethnopharmacol. 148, 74–80. doi:10.1016/j.jep.2013.03.069
Mofor, C. T., Zofou, D., Nangmo, L., Boyom, F. F., Fokou, E., Titanji, V. P. K., et al. (2017). Phytochemical Screening, Antiplasmodial Activity against Multi-Drug Resistant Parasites, Cytotoxicity and Antioxidant Profiling of Extracts from Fagara Macrophylla and Eremomastax Speciosa. Int. J. Ind. Herbs Drugs 2 (2), 1–8.
Morounke, S. G., Ayorinde, J. B., Benedict, A. O., Adedayo, F. F., Adewale, F. O., Oluwadamilare, I., et al. (2017). Epidemiology and Incidence of Common Cancers in Nigeria. J. Cancer Biol. Res. 5 (3), 1105.
Mugiraneza, P. J., Munyabuhoro, S., and Bajyana, S. E. (2013). In Vitro antimicrobial Investigation of Zanthoxylum Chalybeum Stem Bark. J. Med. Plants Res. 7 (21), 1577–1579. doi:10.5897/JMPR12.1231
Mukhija, M., Lal Dhar, K., and Nath Kalia, A. (2014). Bioactive Lignans from Zanthoxylum Alatum Roxb. Stem Bark with Cytotoxic Potential. J. Ethnopharmacol 152 (1), 106–112. doi:10.1016/j.jep.2013.12.039
Mukhtar, H. M., and Kalsi, V. (2018). A Review on Medicinal Properties of Zanthoxylum Armatum DC. Rese. Jour. Pharm. Technol. 11 (5), 2131–2138. doi:10.5958/0974-360X.2018.00395.5
Naylor, N. R., Atun, R., Zhu, N., Kulasabanathan, K., Silva, S., Chatterjee, A., et al. (2018). Estimating the burden of Antimicrobial Resistance: a Systematic Literature Review. Antimicrob. Resist. Infect. Control. 7, 58. doi:10.1186/s13756-018-0336-y
Ngane, A. N., Biyiti, L., Zollo, P. H., and Bouchet, P. (2000). Evaluation of antifungal activity of extracts of two Cameroonian Rutaceae: Zanthoxylum leprieurii Guill. et Perr. and Zanthoxylum xanthoxyloides Waterm. J. Ethnopharmacol 70 (3), 335–342. doi:10.1016/S0378-8741(99)00188-9
Ngoumfo, R. M., Jouda, J. B., Mouafo, F. T., Komguem, J., Mbazoa, C. D., Shiao, T. C., et al. (2010). In Vitro cytotoxic activity of isolated acridones alkaloids from Zanthoxylum leprieurii Guill. et Perr. Bioorg. Med. Chem. 18, 3601–3605. doi:10.1016/j.bmc.2010.03.040
Nigussie, D., Davey, G., Tufa, T. B., Brewster, M., Legesse, B. A., Fekadu, A., et al. (2021). Antibacterial and Antifungal Activities of Ethiopian Medicinal Plants: a Systematic Review. Front. Pharmacol. 12, 633921. doi:10.3389/fphar.2021.633921
Nogueira, J., Vinturelle, R., Mattos, C., Tietbohl, L. A., Santos, M. G., Junior, I. S., et al. (2014b). Acaricidal Properties of the Essential Oil from Zanthoxylum Caribaeum against Rhipicephalus Microplus. J. Med. Entomol. 51 (5), 971–975. doi:10.1603/me13236
Ntchapda, F., Maguirgue, K., Kemeta, A. D. R., Jean, M., Djedouboum, A., and Dimo, T. (2015). Acute Toxicity Studies of the Stem Bark Extract of Zanthoxylum Heitzii A. & P. (Rutaceae) on Haematological Parameters, and Body Temperature. Int. J. Pharma Res. Health Sci. 3 (2), 630–642.
Numonov, S., Sharopov, F., Salimov, A., Sukhrobov, P., Atolikshoeva, S., Safarzoda, R., et al. (2019). Assessment of Artemisinin Contents in Selected Artemisia Species from Tajikistan (Central Asia). Medicines (Basel) 6, 23. doi:10.3390/medicines6010023
Nurain, I. O., Bewaji, C. O., Johnson, J. S., Davenport, R. D., and Zhang, Y. (2017). Potential of Three Ethnomedicinal Plants as Antisickling Agents. Mol. Pharm. 14 (1), 172–182. doi:10.1021/acs.molpharmaceut.6b00767
Obakiro, S. B., Kiprop, A., Kowino, I., Kigondu, E., Odero, M. P., Omara, T., et al. (2020). Ethnobotany, Ethnopharmacology, and Phytochemistry of Traditional Medicinal Plants Used in the Management of Symptoms of Tuberculosis in East Africa: A Systematic Review. Trop. Med. Health 48, 68. doi:10.1186/s41182-020-00256-1
Ochwang'i, D. O., Kimwele, C. N., Oduma, J. A., Gathumbi, P. K., Mbaria, J. M., and Kiama, S. G. (2014). Medicinal Plants Used in Treatment and Management of Cancer in Kakamega County, Kenya. J. Ethnopharmacol 151 (3), 1040–1055. doi:10.1016/j.jep.2013.11.051
Ogunbolude, Y., Ibrahim, M., Elekofehinti, O. O., Adeniran, A., Abolaji, A. O., Rocha, J. B., et al. (2014). Effects of Tapinanthus Globiferus and Zanthoxylum Zanthoxyloides Extracts on Human Leukocytes In Vitro. J. Intercult Ethnopharmacol 3 (4), 167–172. doi:10.5455/jice.20140826110059
Ogwal-Okeng, J. W., Obua, C., and Anokbonggo, W. W. (2003). Acute Toxicity Effects of the Methanolic Extract of Fagara Zanthoxyloides (Lam.) Root-Bark. Afr. Health Sci. 3 (3), 124–126.
Okafor, S. N., Chibueze, G. C., Obonga, W. O., Ezeokonkwo, M. A., and Muogbo, C. C. (2017). Aqueous Methanol Extract of the Root Bark of Zanthoxylum Zanthoxyloides Provides Natural Remedy for Dental Caries and Toothache. World J. Pharm. Res. 6 (7), 336–349.
Okagu, I. U., Ndefo, J. C., Aham, E. C., and Udenigwe, C. C. (2021). Zanthoxylum Species: a Comprehensive Review of Traditional Uses, Phytochemistry, Pharmacological and Nutraceutical Applications. Molecules 26 (13), 4023. doi:10.3390/molecules26134023
Okpuzor, J., Adebesin, O., Ogbunugafor, H., and Amadi, I. (2008). The Potential of Medicinal Plants in Sickle Cell Disease Control: a Review. Int. J. Biomed. Health Sci. 4 (2), 47–55.
Okwor, O. H., Ogugua, V. N., and Okagu, I. U. (2020). Therapeutic Evaluation of Anti-trypanosoma Activity of Ethanol Extracts of Jatropha Curcas Roots in Comparison with Diminazene Aceturate in Trypanosoma Brucei Brucei-Parasitized Rats. Comp. Clin. Pathol. 29 (6), 1189–1198. doi:10.1007/s00580-020-03171-3
Omara, T. (2020). Antimalarial Plants Used across Kenyan Communities. Evid. Based Complement. Alternat Med. 2020, 4538602. doi:10.1155/2020/4538602
Omosa, L. K., Mbogo, G. M., Korir, E., Omole, R., Seo, E.-J., Yenesew, A., et al. (2019). Cytotoxicity of Fagaramide Derivative and Canthin-6-One from Zanthoxylum (Rutaceae) Species against Multidrug Resistant Leukemia Cells. Nat. Product. Res. 35, 579–586. doi:10.1080/14786419.2019.1587424
Ouattara, B., Jansen, O., Angenot, L., Guissou, I. P., Frédérich, M., Fondu, P., et al. (2009). Antisickling Properties of Divanilloylquinic Acids Isolated from Fagara Zanthoxyloides Lam. (Rutaceae). Phytomedicine 16 (2-3), 125–129. doi:10.1016/j.phymed.2008.10.013
Pachón, G., Rasoanaivo, H., Azqueta, A., Rakotozafy, J. C., Raharisololalao, A., de Cerain, A. L., et al. (2007). Anticancer Effect of a New Benzophenanthridine Isolated from Zanthoxylum Madagascariense (Rutaceline). In Vivo 21, 417–422.
Patiño, L. O. J., Prieto, R. J. A., and Cuca, S. L. E. (2012). “Zanthoxylum Genus as Potential Source of Bioactive Compounds,” in Bioactive Compounds in Phytomedicine. Rasooli I (Croatia: InTech Open), 185–218. doi:10.5772/26037
Paul, A., Kumar, A., Singh, G., and Choudhary, A. (2018). Medicinal, Pharmaceutical and Pharmacological Properties of Zanthoxylum Armatum: a Review. J. Pharmacogn Phytochem. 7 (4), 892–900.
Pavani, P., and Naika, R. (2020). Evalution of Anti-bacterial Activity of Zanthoxylum Ovalifolium Wight (Rutaceae) against Selected Pathogenic Bacteria. Plant Arch. 20 (1), 2591–2594.
Pawar, H. A. (2014). Natural Product as a Source of lead to the Design of New Drugs. Nat. Prod. Chem. Res. 2014 2, 156. doi:10.4172/2329-6836.1000156
Phuyal, N., Jha, P. K., Raturi, P. P., and Rajbhandary, S. (2020). In Vitro antibacterial Activities of Methanolic Extracts of Fruits, Seeds, and Bark of Zanthoxylum Armatum DC. J. Trop. Med. 2020, 2803063. doi:10.1155/2020/2803063
Pieme, C. A., Santosh, G. K., Tekwu, E. M., Askun, T., Aydeniz, H., Ngogang, J. Y., et al. (2014). Fruits and Barks Extracts of Zanthozyllum Heitzii a Spice from Cameroon Induce Mitochondrial Dependent Apoptosis and Go/G1 Phase Arrest in Human Leukemia HL-60 Cells. Biol. Res. 47, 54. doi:10.1186/0717-6287-47-54
Pistritto, G., Trisciuoglio, D., Ceci, C., Garufi, A., and D'Orazi, G. (2016). Apoptosis as Anticancer Mechanism: Function and Dysfunction of its Modulators and Targeted Therapeutic Strategies. Aging (Albany NY) 8 (4), 603–619. doi:10.18632/aging.100934
Rahman, M. A., Hannan, M. A., Dash, R., Rahman, M. H., Islam, R., Uddin, M. J., et al. (2021). Phytochemicals as a Complement to Cancer Chemotherapy: Pharmacological Modulation of the Autophagy-Apoptosis Pathway. Front. Pharmacol. 12, 639628. doi:10.3389/fphar.2021.639628
Rahman, M. M., Gray, A. I., Khondkar, P., and Islam, M. A. (2008). Antimicrobial Activities of Alkaloids and Lignans from Zanthoxylum Budrunga. Nat. Prod. Comm. 3 (1), 45–47. doi:10.1177/1934578x0800300110
Redza-Dutordoir, M., and Averill-Bates, D. A. (2016). Activation of Apoptosis Signalling Pathways by Reactive Oxygen Species. Biochim. Biophys. Acta 1863 (12), 2977–2992. doi:10.1016/j.bbamcr.2016.09.012
Rodríguez-Guzmán, R., Fulks, L. C., Radwan, M. M., Burandt, C. L., and Ross, S. A. (2011). Chemical Constituents, Antimicrobial and Antimalarial Activities of Zanthoxylum Monophyllum. Planta Med. 77, 1542–1544. doi:10.1055/s-0030-1270782
Ross, S. A., Sultana, G. N., Burandt, C. L., ElSohly, M. A., Marais, J. P., and Ferreira, D. (2004). Syncarpamide, a New Antiplasmodial (+)-norepinephrine Derivative from Zanthoxylum Syncarpum. J. Nat. Prod. 67, 88–90. doi:10.1021/np030417t
Sandjo, L. P., Kuete, V., Tchangna, R. S., Efferth, T., and Ngadjui, B. T. (2014). Cytotoxic Benzophenanthridine and Furoquinoline Alkaloids from Zanthoxylum Buesgenii (Rutaceae). Chem. Cent. J. 8, 61. doi:10.1186/s13065-014-0061-4
Santhanam, R. K., Ahmad, S., Abas, F., Safinar Ismail, I., Rukayadi, Y., Tayyab Akhtar, M., et al. (2016). Bioactive Constituents of Zanthoxylum Rhetsa Bark and its Cytotoxic Potential against B16-F10 Melanoma Cancer and normal Human Dermal Fibroblast (HDF) Cell Lines. Molecules 21, 652. doi:10.3390/molecules21060652
Sepsamli, L., and Prihastanti, J. E. (2019). Ethnobotany of Balimo (Zanthoxylum Nitidum) in the Kanayatn Dayak Community in Tapakng, West Kalimantan. Biosaintifika 11 (3), 318–324. doi:10.15294/biosaintifika.v11i3.20688
Seukep, J. A., Ngadjui, B., and Kuete, V. (2015). Antibacterial Activities of Fagara Macrophylla, Canarium Schweinfurthii, Myrianthus Arboreus, Dischistocalyx Grandifolius and Tragia Benthamii against Multi-Drug Resistant Gram-Negative Bacteria. SpringerPlus 4, 567. doi:10.1186/s40064-015-1375-y
Shaw, A., Robays, J., Fèvre, E. M., Lutumba, P., and Boelaert, M. (2010). “The Burden of Human African Trypanosomiasis,” in The Burden of Human African Trypanosomiasis. Handbook of Disease Burdens and Quality of Life Measures. Editors V. R. Preedy, and R. R. Watson (New York, NY: Springer), 1433–1442. doi:10.1007/978-0-78665-0_8310.1007/978-0-387-78665-0_83
Simarro, P. P., Diarra, A., Ruiz Postigo, J. A., Franco, J. R., and Jannin, J. G. (2011). The Human African Trypanosomiasis Control and Surveillance Programme of the World Health Organization 2000-2009: the Way Forward. Plos Negl. Trop. Dis. 5 (2), e1007. doi:10.1371/journal.pntd.0001007
Sinan, K. I., Zengin, G., Bene, K., and Mahomoodally, M. F. (2019). Chemistry and Pharmacology of Three Antiplasmodial Traditional Medicinal Plants from Tropical Africa - A Review. South Afr. J. Bot. 126, 265–276. doi:10.1016/j.sajb.2019.04.024
Smittenaar, C. R., Petersen, K. A., Stewart, K., and Moitt, N. (2016). Cancer Incidence and Mortality Projections in the UK until 2035. Br. J. Cancer 115, 1147–1155. doi:10.1038/bjc.2016.304
Supabphol, R., and Tangjitjareonkun, J. (2014). Chemical Constituents and Biological Activities of Zanthoxylum Limonella (Rutaceae): A Review. Trop. J. Pharm. Res. 13 (12), 2119–2130. doi:10.4314/tjpr.v13i12.25
Tabuti, J. R., Kukunda, C. B., and Waako, P. J. (2010). Medicinal Plants Used by Traditional Medicine Practitioners in the Treatment of Tuberculosis and Related Ailments in Uganda. J. Ethnopharmacol 127, 130–136. doi:10.1016/j.jep.2009.09.035
Tamdem, S. B. G. (2019). Phytochemical, Chemopreventive and Antimalarial Activity Evaluation of Five Selected Medicinal Plants from the Cameroonian flora. UK: Liverpool John Moores University.
Tantapakul, C., Phakhodee, W., Ritthiwigrom, T., Yossathera, K., Deachathai, S., and Laphookhieo, S. (2012). Antibacterial Compounds from Zanthoxylum Rhetsa. Arch. Pharm. Res. 35 (7), 1139–1142. doi:10.1007/s12272-012-0703-9
Tian, Y., Zhang, C., and Guo, M. (2017). Comparative Study on Alkaloids and Their Anti-proliferative Activities from Three Zanthoxylum Species. BMC Complement. Altern. Med. 17 (1), 460. doi:10.1186/s12906-017-1966-y
Tine, Y., Diop, A., Diatta, W., Desjobert, J. M., Boye, C. S., Costa, J., et al. (2017). Chemical Diversity and Antimicrobial Activity of Volatile Compounds from Zanthoxylum Zanthoxyloides Lam. According to Compound Classes, Plant Organs and Senegalese Sample Locations. Chem. Biodivers 14 (1), e1600125. doi:10.1002/cbdv.201600125
Udenigwe, C. C., Abioye, R. O., Okagu, I. U., and Obeme-Nmom, J. I. (2021). Bioaccessibility of Bioactive Peptides: Recent Advances and Perspectives. Curr. Opin. Food Sci. 39, 182–189. doi:10.1016/j.cofs.2021.03.005
Umaru, H. A., Clarkson, W. P., and Christopher, K. (2019). Effect of Stem Bark Extract of Fagara Zanthoxyloides on Bile Secretion in Rats. Am. J. Res. Comm. 7 (7), 1–9.
Veldman, S., Ju, Y., Otieno, J. N., Abihudi, S., Posthouwer, C., Gravendeel, B., et al. (2020). DNA Barcoding Augments Conventional Methods for Identification of Medicinal Plant Species Traded at Tanzanian Markets. J. Ethnopharmacol 250, 112495. doi:10.1016/j.jep.2019.112495
Verma, K. K., Kumar, B., Raj, H., and Sharma, A. (2021). A Review on Chemical Constituents, Traditional Uses, Pharmacological Studies of Zanthoxylum Armatum (Rutaceae). J. Drug Deliv. Ther. 11 (2-S), 136–142. doi:10.22270/jddt.v11i2-S.4786
Wang, Y., Gao, D., Chen, Z., Li, S., Gao, C., Cao, D., et al. (2013). Acridone Derivative 8a Induces Oxidative Stress-Mediated Apoptosis in CCRF-CEM Leukemia Cells: Application of Metabolomics in Mechanistic Studies of Antitumor Agents. PLoS One 8 (5), e63572. doi:10.1371/journal.pone.0063572
Wang, Y., Zhao, C. M., Guo, T., Zhu, Y. L., and Zhao, P. (2015). Preliminary Screening of 44 Plant Extracts for Anti-tyrosinase and Antioxidant Activities. Pak J. Pharm. Sci. 28 (5), 1737–1744.
Wansi, J. D., Nwozo, S. O., Mbaze, L. M., Devkota, K. P., Donkwe Moladje, S. M., Fomum, Z. T., et al. (2009). Amides from the Stem Bark of Fagara Macrophylla. Planta Med. 75, 517–521. doi:10.1055/s-0029-1185327
Wansi, J. D., Tadjong Tcho, A., Toze, F. A. A., Nahar, L., Martin, C., and Sarker, S. D. (2016). Cytotoxic Acridone and Indoloquinazoline Alkaloids from Zanthoxylum Poggei. Phytochemistry Lett. 17, 293–298. doi:10.1016/j.phytol.2016.08.010
Wei, W. J., Chen, X. H., Guo, T., Liu, X. Q., Zhao, Y., Wang, L. L., et al. (2021). A Review on Classification and Biological Activities of Alkaloids from the Genus Zanthoxylum Species. Mini Rev. Med. Chem. 21 (3), 336–361. doi:10.2174/1389557520666200910091905
World Health Organization (Who), (2019). World Malaria Report 2019 Availableat: https://www.who.int/publications/i/item/world-malaria-report-2019/ Accessedon.October 2, 2020 .
Wouatsa, V. N., Misra, L., Kumar, S., Prakash, O., Khan, F., Tchoumbougnang, F., et al. (2013). Aromatase and Glycosyl Transferase Inhibiting Acridone Alkaloids from Fruits of Cameroonian Zanthoxylum Species. Chem. Cent. J. 7, 125. doi:10.1186/1752-153X-7-125
Wu, J., Mei, W. L., and Dai, H. F. (2007). A New Monoterpenoid Glycoside from Roots of Zanthoxylum Simulans. Chin. Tradit Herb Drug 38 (4), 488–490. doi:10.1002/chin.200713185
Yang, C. H., Cheng, M. J., Lee, S. J., Yang, C. W., Chang, H. S., and Chen, I. S. (2009). Secondary Metabolites and Cytotoxic Activities from the Stem Bark of Zanthoxylum Nitidum. Chem. Biodivers 6, 846–857. doi:10.1002/cbdv.200800107
Yang, G., and Chen, D. (2008). Alkaloids from the Roots of Zanthoxylum Nitidum and Their Antiviral and Antifungal Effects. Chem. Biodivers 5, 1718–1722. doi:10.1002/cbdv.200890160
Ynalvez, R. A., Cardenas, C., Addo, J. K., Adukpo, G. E., Dadson, B. A., and Addo-Mensa, A. (2012). Evaluation of the Antimicrobial Activity of Zanthoxylum Zanthoxyloides Root Bark Extracts. Res. J. Med. Plant 6 (2), 149–159. doi:10.3923/rjmp.2012.149.159
Yuan, H. M., Qiu, L., Xie, Z. J., Zou, L., Zheng, J., and Fu, Q. (2015). Research Progress on Alkaloids Constituents from Zanthoxylum and Their Pharmacological Activities. Zhongguo Zhong Yao Za Zhi 40 (23), 4573–4584. doi:10.4268/cjcmm20152309
Yusuf, M., Chowdhury, J. U., Wahab, M. A., and Begum, J. (1994). Medicinal Plants of Bangladesh. Chittagong, Bangladesh: BCSIR Laboratories.
Zhang, M., Wang, J., Zhu, L., Li, T., Jiang, W., Zhou, J., et al. (2017a). Zanthoxylum Bungeanum Maxim. (Rutaceae): A Systematic Review of its Traditional Uses, Botany, Phytochemistry, Pharmacology, Pharmacokinetics, and Toxicology. Int. J. Mol. Sci. 18 (10), 2172. doi:10.3390/ijms18102172
Zhang, Y., Dong, H., Zhang, J., and Zhang, L. (2017b). Inhibitory Effect of Hyperoside Isolated from Zanthoxylum Bungeanum Leaves on SW620 Human Colorectal Cancer Cells via Induction of the P53 Signaling Pathway and Apoptosis. Mol. Med. Rep. 16 (2), 1125–1132. doi:10.3892/mmr.2017.6710
Zirihi, G. N., Mambu, L., Guédé-Guina, F., Bodo, B., and Grellier, P. (2005). In Vitro antiplasmodial Activity and Cytotoxicity of 33 West African Plants Used for Treatment of Malaria. J. Ethnopharmacol 98, 281–285. doi:10.1016/j.jep.2005.01.004
Zirihi, G. N., N'guessan, K., Etien, D. T., and Serikouassi, B. P. H. (2009). Evaluation In Vitro of Antiplasmodial Activity of Ethanolic Extracts of Funtumia Elastica, Rauvolfia Vomitoria and Zanthoxylum Gilletii on Plasmodium Falciparum Isolates from Côted’Ivoire. J. Anim. Plant Sci. 5 (1), 406–413.
Keywords: Zanthoxylum, Ethnobotany, health benefits, phytochemicals, functional foods, Nutraceutials
Citation: Okagu IU, Ndefo JC, Aham EC and Udenigwe CC (2021) Zanthoxylum Species: A Review of Traditional Uses, Phytochemistry and Pharmacology in Relation to Cancer, Infectious Diseases and Sickle Cell Anemia. Front. Pharmacol. 12:713090. doi: 10.3389/fphar.2021.713090
Received: 21 May 2021; Accepted: 31 August 2021;
Published: 15 September 2021.
Edited by:
Michael Heinrich, UCL School of Pharmacy, United KingdomReviewed by:
Eugenia Teodor, National Institute of Research and Development for Biological Sciences (NIRDBS), RomaniaYan-Hui Fu, Hainan Normal University, China
Copyright © 2021 Okagu, Ndefo, Aham and Udenigwe. This is an open-access article distributed under the terms of the Creative Commons Attribution License (CC BY). The use, distribution or reproduction in other forums is permitted, provided the original author(s) and the copyright owner(s) are credited and that the original publication in this journal is cited, in accordance with accepted academic practice. No use, distribution or reproduction is permitted which does not comply with these terms.
*Correspondence: Joseph Chinedum Ndefo, am9zZXBoLm5kZWZvQHVubi5lZHUubmc=; Chibuike. C. Udenigwe, Y3VkZW5pZ3dAdW90dGF3YS5jYQ==