- 1Department of Molecular Biology and Biotechnology, School of Sciences, Tezpur University, Tezpur, India
- 2Department of Biochemistry and Molecular Genetics, University of Colorado Anschutz Medical Campus, Aurora, CO, United States
- 3Institute of Advanced Study in Science and Technology, Guwahati, India
The Indian red scorpion (Mesobuthus tamulus) is one of the world’s deadliest scorpions, with stings representing a life-threatening medical emergency. This species is distributed throughout the Indian sub-continent, including eastern Pakistan, eastern Nepal, and Sri Lanka. In India, Indian red scorpions are broadly distributed in western Maharashtra, Saurashtra, Kerala, Andhra Pradesh, Tamil Nadu, and Karnataka; however, fatal envenomations have been recorded primarily in the Konkan region of Maharashtra. The Indian red scorpion venom proteome comprises 110 proteins belonging to 13 venom protein families. The significant pharmacological activity is predominantly caused by the low molecular mass non-enzymatic Na+ and K+ ion channel toxins. Other minor toxins comprise 15.6% of the total venom proteome. Indian red scorpion stings induce the release of catecholamine, which leads to pathophysiological abnormalities in the victim. A strong correlation has been observed between venom proteome composition and local (swelling, redness, heat, and regional lymph node involvement) and systemic (tachycardia, mydriasis, hyperglycemia, hypertension, toxic myocarditis, cardiac failure, and pulmonary edema) manifestations. Immediate administration of antivenom is the preferred treatment for Indian red scorpion stings. However, scorpion-specific antivenoms have exhibited poor immunorecognition and neutralization of the low molecular mass toxins. The proteomic analysis also suggests that Indian red scorpion venom is a rich source of pharmacologically active molecules that may be envisaged as drug prototypes. The following review summarizes the progress made towards understanding the venom proteome of the Indian red scorpion and addresses the current understanding of the pathophysiology associated with its sting.
A Brief Overview of Venomous Scorpions With Particular Reference to Indian Red Scorpion
Animal venoms are complex secretions consisting mainly of bioactive proteins and peptides (Fry et al., 2009; Casewell et al., 2013) that primarily serve as a chemical means of protection and subduing prey. Venom has evolved independently throughout the animal kingdom (Casewell et al., 2013; Suranse et al., 2018); consequently, it is present in all major animal lineages (Holford et al., 2018). All of the 1,500 extant scorpion species are venomous. While human envenomation by most results in only minor reactions, approximately 30 scorpions are considered medically significant (Table 1) (Bawaskar and Bawaskar, 2012). Severe and sometimes fatal envenomations have been documented from stings by Buthidae, Hemiscorpiidae, and Scorpionidae families (White, 2016) in Latin America, North Africa, the Middle East, and India (Reddy, 2013). Currently, 86 scorpion species have been described throughout India; however, only the Indian red scorpion (Mesobuthus tamulus), which belongs to the family Buthidae, and the Indian black scorpion (Heterometrus swammerdami, formerly Palamneus gravimanus) of the Scorpionidae family, pose a significant threat to humans– primarily young children, elderly, and immuno-compromised individuals (Tiwari and Deshpande, 1993; Badhe et al., 2007; Quintero-Hernandez et al., 2013; Reddy, 2013; Ortiz et al., 2015; Santos et al., 2016; Das et al., 2020). Limited clinical reports suggest that the venom of the Indian red scorpion exhibits higher toxicity compared to Indian black scorpion venom (Erfati, 1978; Bawaskar and Bawaskar, 1998; Madhavan, 2015; Senthilvelan et al., 2015), and as a consequence, urgent medical attention may be required following a sting. The potent toxicity of Indian red scorpion venom is attributed to the abundance of potassium channel toxins targeting the central nervous and cardiovascular systems (discussed below). This venom phenotype is also seen in other medically important scorpion species, such as the Iranian scorpion (Hemiscorpius lepturus) of the Hemiscorpiidae family (Prendini, 2000), and Pandinus imperator (Scorpionidae family), which is endemic to West Africa.
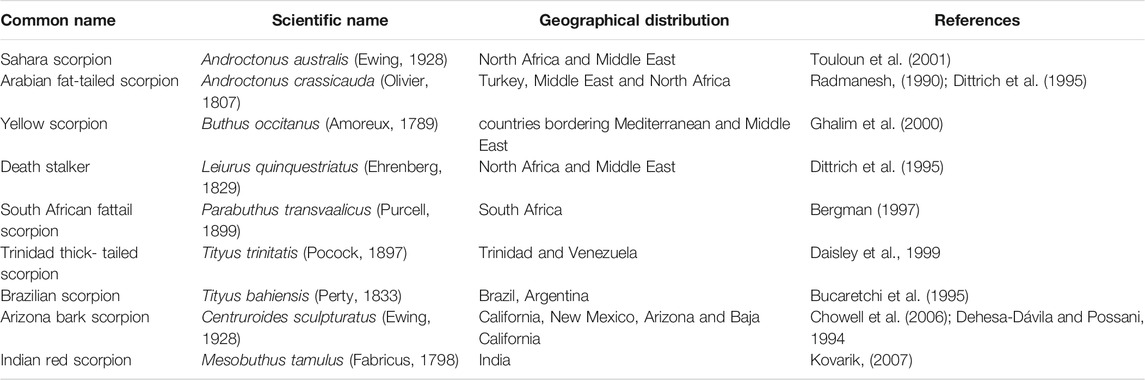
TABLE 1. List of some of the most dangerous and deadly scorpion species distributed across the world.
Given the medical threat of the Indian red scorpionand the recent characterization of its venom proteome (Das et al., 2020), the following review aims to highlight the current understanding of the venom proteome composition, the epidemiology of Indian red scorpion sting, and correlate venom phenotype to the pathophysiological symptoms observed following envenomation. Lastly, we address some of the different treatment regimens utilized by clinicians to treat scorpion stings. Searches of published reports were conducted with public databases (MEDLINE, Scopus) using the search engines-Science Direct (https://www.sciencedirect.com/), Google Scholar (https://scholar.google.com/), and PubMed (pubmed.ncbi.nlm.nih.gov). The different search words were “Indian red scorpion venom,” “Toxins and Indian red scorpion venom,” “epidemiology of Indian red scorpion sting,” “Proteomic analysis and Indian red scorpion venom,” “Pathophysiology of Indian red scorpion sting,” “Mesobuthus tamulus,” and “Treatment of scorpion sting.”
Geographical Distribution, Epidemiology, and Clinical Symptoms of Sting
The evolutionary history of scorpions is represented by a continuous fossil record that dates back to the Telychian Stage (Silurian, Llandovery) (Dunlop, 2010; Dunlop and Selden, 2013). Evidence suggests that scorpions have existed on earth for over 430 million years and first appeared as an aquatic organism during the Silurian period (Dunlop and Selden, 2013). The present-day Indian red scorpion ranges in size from 2 to 3.5 inches (5–9 cm) in length (Kovařík, 2007), has red pedipalps (claws), a tail, legs, and body covered in khaki-colored cuticles (Figure 1). These nocturnal predators are endemic to the Indian sub-continent. They are rarely found outside Eastern Nepal (Bhadani et al., 2006), Eastern Pakistan (Kovařík, 2007), or Sri Lanka (Kularatne et al., 2015).
In India, morbidity and mortality due to scorpion stings frequently occur in western Maharashtra, Saurashtra, Kerala, Andhra Pradesh, Tamil Nadu, and Karnataka. A case study involving 141 children admitted to Government Raja Mirasdhar Hospital (Thanjavur, southern India) with a real Indian red scorpion sting, demonstrated that childrenbetween 1–3 and 7–12 years of age exhibited the following: most adverse effects to envenomation. Eight patients displayed priapism and five patients up to 6 years. One patient older than 6 years exhibited pulmonary edema, a fatal and life-threatening sting effect (Yuvaraja et al., 2019). Records from a tertiary care and teaching hospital in southern India showed that 50 patients experiencing Indian red scorpion sting demonstrated dyspnoea (n = 13, 26%), chest pain (n = 9, 18%), vomiting (n = 6, 12%), sweating (n = 5, 10%), nausea (n = 3, 6%), priapism (n = 7, 14%) and piloerection (n = 6, 12%) (Madhavan, 2015).
An epidemiological study conducted in Mahad (200 km south of Mumbai, Western India) from 1984 to 1995 also showed that children <16 years tend to respond more poorly to Indian red scorpion sting (Bawaskar and Bawaskar, 1998); out of the 293 patients, six deaths were reported before hospital arrival. Patients were further divided into three broad groups based on the clinical symptoms- i) 111 (38%) patients exhibited hypertension within 1–10 h (mean 3.5 h), ii) 87 (30%) patients with tachycardia reported within 1–24 h (mean 6.7 h), and iii) 72 (24.5%) patients with pulmonary oedema reported within 6–24 h (mean 8 h) post scorpion sting (Bawaskar and Bawaskar, 1996; Bawaskar and Bawaskar, 1998).
A 14 year old healthy male from the Babaganj region of Northern India developed cardiac and gastrointestinal complications following an Indian red scorpion sting on the right big toe (Agrawal et al., 2015). Twenty-three Indian red scorpion stings have been documented in three localities of Jaffna, Sri Lanka, consisting of 13 (57%) males and 10 (43%) females. While the mean age was 30 years (Kularatne et al., 2015), 5 (22%) cases were children below 12. Upon admission to the hospital, all patients had evidence of either local or systemic manifestations envenoming (Kularatne et al., 2015; Ratnayake et al., 2016). Thirty-three scorpion stings were reported at Rims Teaching Hospital, Raichur, Karnataka, India, from 2009 to 2014, of which 22 were from the Indian black scorpion and 11 from the Indian red scorpion. The patients exhibited bradycardia, drowsiness, cutaneous manifestations, hypotension, and hypertension (Rajashekhar and Mudgal, 2017).
Diversity of Indian Red Scorpion Venom
Geographical variation in sting severity has been reported in India (Reddy, 2013; Suranse et al., 2019) (Figure 2) and is a likely consequence of variation in population genetic structure, which drives phenotypic differences in venom composition (Newton et al., 2007). Several populations of Indian red scorpions collected from eight locations in Maharashtra (Bhate plateau, Sangameshwar, Jejuri, Shindavane, Pashan, Alandi, Kalyan, and Jalna) exhibited moderate genetic variation, with regression analysis suggesting that the genetic distance of subspecies increases by 0.006% (95%CI: 0.003–0.010%) per Kilometre of geographical separation (Suranse et al., 2017). It has also been suggested that genetic structure correlates to climatic differences in precipitation, specifically high, moderate, and low rainfall areas (Suranse et al., 2017), associated with differences in venom phenotype. For example, significant variation in the expression of venom peptides was observed between Indian red scorpions collected from the Konkan region of Maharashtra and the semi arid Deccan plateau (Newton et al., 2007). In addition, anecdotal reports suggest that stings from Indian red scorpions of the Konkan region on the western side of the Western Ghats are more severe than stings from populations on the eastern side of the Western Ghats. These differences are likely due to variations in venom peptide composition between the two populations (Newton et al., 2007). While other factors may contribute to the pathophysiology of the sting, these have not been addressed in the literature (Bawaskar and Bawaskar, 1992; Kankonkar et al., 1998; Murthy and Zare, 1998; Newton et al., 2007). Intra-specific venom variation has also been demonstrated between Indian red scorpions from Western India (Ratnagiri, Chiplun, and Ahmednagar) and Southern India Chennai by sodium dodecyl polyacrylamide gel electrophoresis (SDS-PAGE) (Badhe et al., 2006). Mice injected with equal concentrations of Indian red scorpion venom obtained from the above geographical regions showed significant variation in their blood sodium levels (Badhe et al., 2007). While in-depth analyses of Indian red scorpion venoms from different areas of the Indian sub-continent are currently lacking, this data would help uncover the geographical impact onvenom composition. Although venom variation has been shown to result in differences in sting severity and symptoms for scorpions from different regions of the world (Abroug et al., 2020), a detailed description of this topicis beyond the scope of the current review.
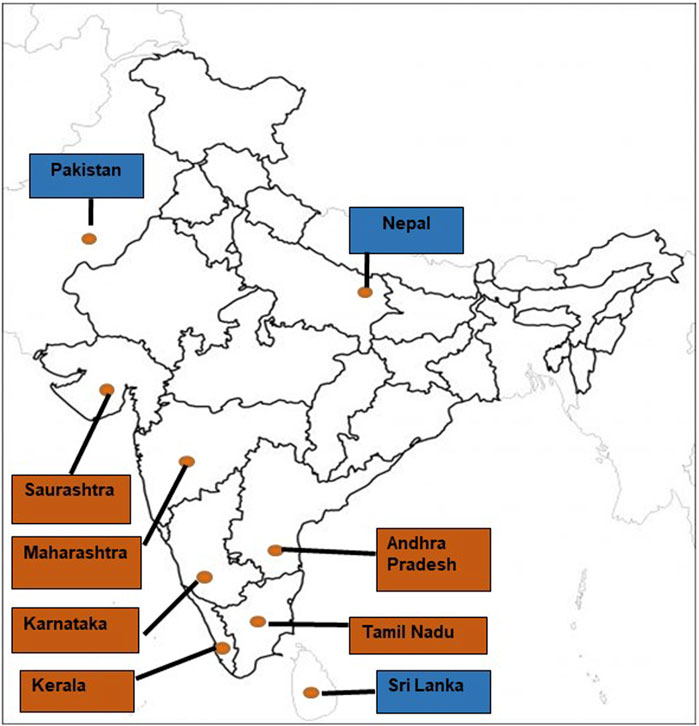
FIGURE 2. The geographical distribution of the Indian red scorpion throughout the Indian sub-continent [Brown fill: Indian states; Blue fill: neighbouring countries of India].
Characterization of Venom and Analyses of Sequence-Structure-Functional Impacts
Biochemical and Proteomic Characterization
Scorpion venomis a cocktail of enzymatic and non-enzymatic proteins with the latter classified into two additional categories based on their number of amino acids; i) short toxins are comprised of 30–40 amino acids; and ii) long toxins have 60–70 amino acids (Srairi-Abid et al., 2019). Non-enzymatic toxins can be divided into four groups based on their biological functions and pharmacological activity, namely Na+, K+, Ca2+, and Cl− channel toxins (Possani et al., 1999; Tytgat et al., 1999; de la Vega and Possani, 2004). The last decade has seen an expansion of research techniques utilized to identify, characterize, and quantify the venom composition of venomous animals. Traditional approaches have relied on biochemical analyses of venom enzymes and venom profiling by SDS-PAGE and gel filtration chromatography. However, more recently, these techniques have been coupled with high-throughput genomic, transcriptomic, and proteomics approaches to provide a more profound and more comprehensive analyses of a species venom (Gutiérrez et al., 1995; Calvete et al., 2009; de la Vega et al., 2010; Abdel-Rahman et al., 2013; Mukherjee et al., 2016; Santibáñez-López et al., 2016; Calvete, 2017; Kalita et al., 2018; Saviola et al., 2020). Several studies have also drawn a good correlation between venom composition with toxicity and pathophysiology of sting (Zelanis and Tashima, 2014; Chanda and Mukherjee, 2020; Manuwar et al., 2020).
Recently, our laboratory utilized liquid chromatography-mass spectrometry (LC-MS/MS)-based proteomics combined with biochemical and in vitro pharmacological activity assays to characterize the venom composition of the Indian red scorpion (Das et al., 2020). Proteomic analysis identified 110 proteins and polypeptides belonging to 13 protein families. The venom had a preponderance of ion channel toxins (Na+ and K+ channels affecting toxins). Other minor venom components are serine protease-like protein, serine protease inhibitor, antimicrobial peptide, hyaluronidase, makatoxin, lypolysis potentiating peptides, neurotoxin affecting Cl− channels, parabutoporin, Ca2+ channel toxins, bradykinin potentiating peptides, HMG CoA reductase inhibitor, and several other toxins with unknown pharmacological activity (Das et al., 2020, Supplementary Table S1). Further, the low molecular weight insect-selective toxins BtTx3 (3,796 Da) and ButaIT (3,856.7 Da) were identified. These toxins can be developed as insecticidal agents against lepidopteran insect species (Wudayagiri et al., 2001; Dhawan et al., 2002).
Indian red scorpion venom did not show activity for any of the tested enzymes (phospholipase A2, L-amino acid oxidase, adenosine tri-, di-, and monophosphatase, hyaluronidase, metalloproteinase, and fibrinogenolytic), was devoid of in vitro hemolytic activity, and also failed to interfere with blood coagulation and platelet modulation (activation or deaggregation) under in vitro conditions.The 3D structure of some of the toxins deposited in UniProt is shown in Supplementary Table S2. The occurrence of several other venom toxins from different Mesobuthus and Heterometrus species also found throughout the Indian subcontinent are shown in Supplementary Table S3.
Sturture-Function Analysis of Scorpion Toxins
Scorpion venoms contain numerous polypeptides cross-linked via three to four disulfide bridges that exert various physiological and pharmacological activities by targeting ion channel (s) function (Rochat et al., 1979; Zlotkin et al., 1991; Gordon et al., 1992). Regardless of the diverse primary structures, the majority of scorpion toxins have an identical Csαβ (cysteine-stabilized α/β motif) fold (Fontecilla-Camps et al., 1988). Further, Na+ channel toxins have been divided into mammalian and insect toxins, with the former sub-divided into α- and β- toxins (Jover et al., 1980; Couraud et al., 1982) and the latter sub-classified into depressant, excitatory, and α- insect toxins (Zlotkin et al., 1995). Several scorpion toxins that exclusively target Na+ and K+ channels have been studied extensively concerning their structure, mode of action, and pharmacological properties (Miller et al., 1985; Zlotkin et al., 1991; Delepierre et al., 1997). Little attention has been paid to identifying and purifying toxins from Indian red scorpion venom to develop different drug prototypes. However, the sequences of a few purified toxins have been determined. For example, ButaIT, is a novel short lepidopteran-selective toxin with 37 amino acids cross-linked by disulfide bridges and eight cysteine residues; it shares sequence homology with other fast toxins Peptide I, neurotoxin P2, Lqh-8/6, chlorotoxin, insectotoxin I5A, insect toxin 15, and insectotoxin I1. Three-dimensional structural modelling of this toxin revealed that similar to other scorpion toxins, ButaIT contains an α-helix and a β-sheet. Moreover, this toxin showed high target specificity towards Heliothis virescens, a notorious budworm on the cotton crop (Wudayagiri et al., 2001). Notably, the proteomic analysis demonstrated that Bukatoxin and Makatoxin represent approximately 2.3% of M. tamulus venom proteome (Das et al., 2020).
Although the structure-function relationship of these toxins from Indian red scorpion venom has not been elucidated; the functional site of Bukatoxin from Buthus martensii karch venom activates the Na+ channel in nitrergic inhibitory fibers, resulting in the neuronal release of nitric oxide (NO) (Gibson and McFadzean, 2001; Srinivasan et al., 2001; Reis et al., 2019). Bukatoxin shares 78 and 72% structural similarity with neurotoxin X from Mesobuthus eupeus venom (Grishin et al., 1979) and neurotoxin IV from Leiurus quinquestriatus quinquestriatus venom (Kopeyan et al., 1985), respectively. Similarly, Makatoxin I from venom of Buthus martensii karch contains 64 amino acids with eight half cystein residues, has a short J loop (cys- 16 to cys-22) and a long B loop (cys-36 to cys-46) (Gong et al., 1997) and exhibits 78 and 81% structural similarity with Bot I (Vargas et al., 1982) and Bot II (Gregoire and Rochat, 1983) toxins (both from Buthus occictanus tunetanus venom), respectively. Further, Makatoxin I shows 55–77% similarity with Lqq IV and Lqq III from Leiurus quinquestriatus quinquestriatus venom (Kopeyan et al., 1985; Kopeyan et al., 1993). The nitrergic action of Makatoxin I causes a release of NO that mediates a relaxant response in rat precontracted anococcygeus muscle (ACM) (Gong et al., 1997).
Pharmacological Targets of Indian Red Scorpion Toxins
Indian red scorpion venom is quite toxic towards mammals. The median lethal dose (LD50, s.c injection) against the juvenile and adult rats has been estimated at 1.3 ± 0.14 and 2.2 ± 0.21 mg/kg, respectively (Tiwari and Deshpande, 1993). Consequently, the Indian red scorpion sting represents a significant medical threat throughout its geographical range, including in India. The potent toxicity towards mammals is due to a predominance of neurotoxins (Das et al., 2020) that target the functionality of voltage-gated Na+ and K+ channels, including calcium-activated K+ media, which ultimately leads to the various clinical symptoms in different regions of India and Sri Lanka (Table 2).
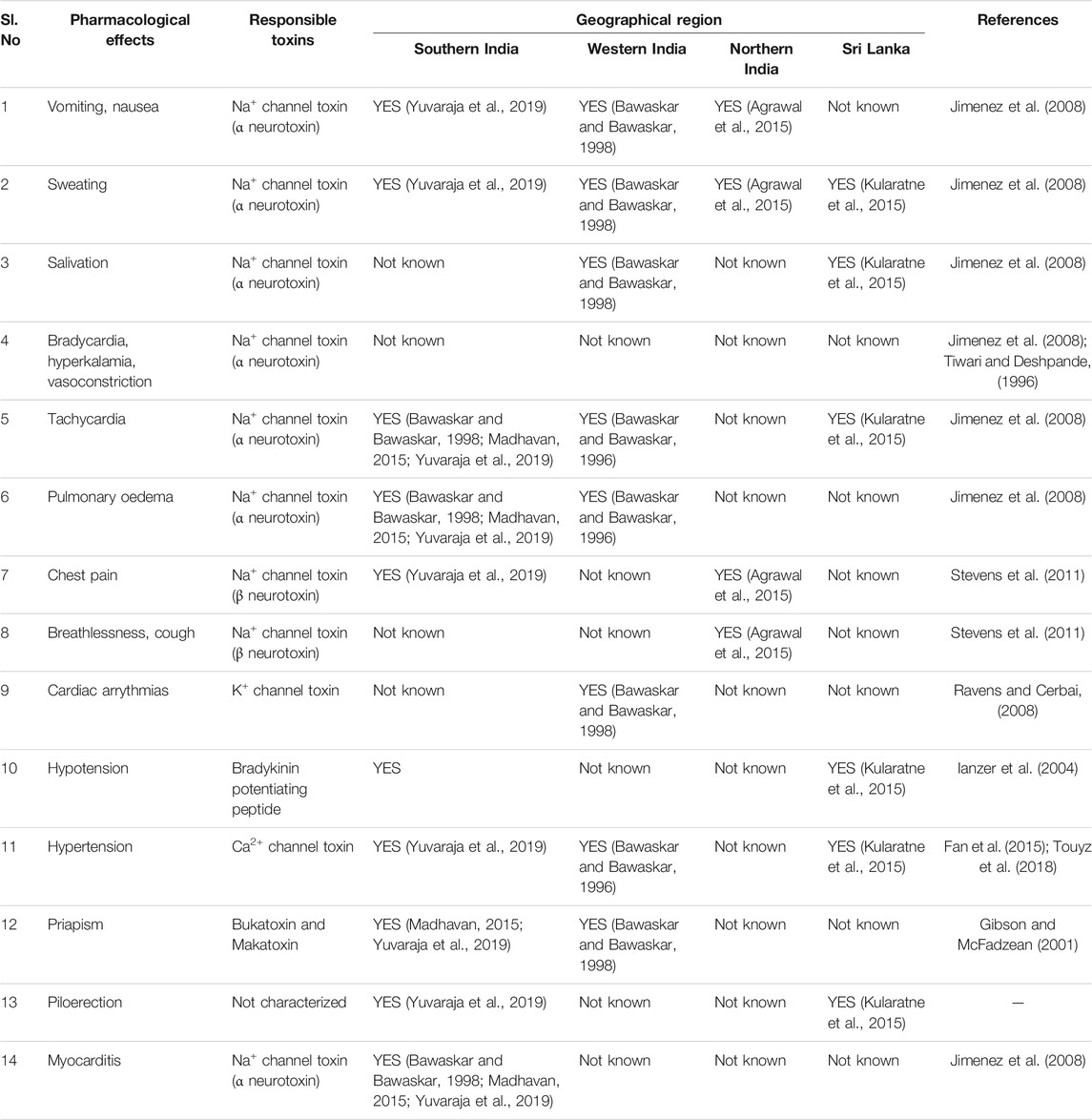
TABLE 2. Comparative list of the pharmacological effects induced by toxins from Indian red scorpion venoms of different geographical regions.
Proteomic analysis revealed that Na+ and K+ channel toxins are prominent in Indian red scorpion venom. These toxins are likely responsible for enhancing the release of neurotransmitters, either by slowing the inactivation of Na+ channels or blocking the K+ channels (Narahashi et al., 1972; Rowan et al., 1992; Vatanpour et al., 1993). Na+ channel toxins are further classified as α- and β-neurotoxins and can interfere with the function of the nervous system by modulating Na+ channel activity in nerve cells (Stevens et al., 2011). The α-toxin binds to site 3 receptors of Na+ channel, where it blocks the inactivation and prolongs the action potential (Catterall, 1976; Catterall, 1986; Couraud et al., 1982). On the other hand, the β-toxin binds to site 4 of Na+ channel and shifts the voltage activation towards more negative potentials, leading to spontaneous and repetitive firing (Couraud et al., 1982; Pinter et al., 1999).
Tamapin, a 3,459.1 Da toxin purified from Indian red scorpion venom, selectively blocks small conductance Ca2+-activated K+ (SK) channels expressed in the central nervous system. Tamapin has 31 amino acids and exhibits moderate sequence similarity to scyllatoxin (77%) and PO5 (74%), two SK channel blockers from Leiurus quinquestriatus, and Androctonus mauretanicus venoms, respectively. Further, the C-terminal tyrosine residue of tamapin is amidated, which likely impacts the pharmacological properties and potency of the toxin (Pedarzani et al., 2002). Tamulustoxin, another peptide toxin from Indian red scorpion venom, exhibits a slow time-dependent inactivation of the K+ channel that produces a functional effect on the prolonged depolarization or repetitive firing of action potentials (Strong et al., 2001). Notably, scorpion venom toxins that block Na+ and K+ channels mediate the synergistic effects responsible for the intense and persistent depolarization of the autonomic nerves, causing a massive release of autonomic neurotransmitters that evokes the “autonomic storm” response (Gwee et al., 2002). Scorpion venom toxins that target Ca2+ channels inhibit the contraction of pulmonary artery smooth muscle cells by decreasing intracellular calcium and causing pulmonary hypertension (Fan et al., 2015; Touyz et al., 2018).
Interestingly, Indian red scorpion venom lacked enzymatic activity, even though mass spectrometry identified several enzymes (Das et al., 2020). Proteomic analysis identified a serine protease-like protein and a hyaluronidase enzyme comprising 2.9 and 2.2% of venom, respectively. However, crude venom did not show serine protease activity or influence clotting time of platelet poor plasma; hyaluronidase activity was also absent (Das et al., 2020). Interestingly, hyaluronidase activity was demonstrated by some other scorpion species such (Heterometrus swammerdami/Palamneus gravimanus (Morey et al., 2006), Hemiscorpius lepturus (Seyedian et al., 2010), and Heterometrus fuvipes (Ramanaiah et al., 1990), and Strong et al. (2001) also reported enzymatic activities from scorpion venoms. It is also possible that the functionality of these enzymes differs from the conventional snake venom serine proteases and hyaluronidases, resulting in differences in substrate specificity between some snake and scorpion venom enzymes (Thakur and Mukherjee, 2015; Cid-Uribe et al., 2020; Das et al., 2020). Another possibility is that the serine protease-like protein and the hyaluronidase enzyme do not show in vitro enzyme activity due to their relatively low abundance in Indian red scorpion venom (Das et al., 2020). It is noteworthy that while hyaluronidase is a non-toxic enzyme, it enhances the diffusion rate of venom into the victim’s tissue and thus enhances the local systemic envenomation (Morey et al., 2006). Moreover, the presence of phospholipase A2 (MtPLA2,19 kDa) in Indian red scorpion venom was reported by Hariprasad et al. (2009). However, the activity of this enzyme was not detected, possibly due to its low abundance in the venom proteome (Das et al., 2020). PLA2 has been reported in the venom of other scorpions such as Anuroctonus phaiodactylus (Phaiodactylipin, 19.1 kDa) (Valdez-Cruz et al., 2004), Hemiscorpius lepturus (Hemipilin 1 and 2, 15 kDa) (Jridi et al., 2015, 2017), Heterometrus fulvipes (HfPLA2, 16 kDa) (Ramanaiah et al., 1990), Heterometrus laoticus (HmTx, 14 kDa) (Incamnoi et al., 2013), Pandinus imperator (IpTxi, 15 kDa) (Zamudio et al., 1997), Scorpio maurus (phospholipin and Sm-PLGV, 14.8 and 15.15 kDa, respectively) (Conde et al., 1999; Louati et al., 2013; Krayem et al., 2018). PLA2s exhibit a broad spectrum of pharmacological activities, ranging from myotoxicity, neurotoxicity, inflammatory, hemolytic, anticoagulant, anti-microbial to anti-tumoral activities (Krayem and Gargouri, 2020).
Elucidation of Mechanism of Toxicity
The mechanism of scorpion venom-mediated toxicity in an envenomed human is briefly discussed below.
Myocarditis, Bradycardia, and Hypotension
Indian red scorpion sting is often characterized by myocarditis, bradycardia, and hypotension (Bawaskar, 1982). The binding of scorpion venom neurotoxins to Na+ ion channels promotes membrane depolarization which triggers the release of catecholamines (Rowan et al., 1992; Jimenez et al., 2008; Stevens et al., 2011). Continuous elevation of these adrenalin hormones can downregulate β-adrenergic receptors, which are critical for the overall regulation of cardiac function (Wachter and Gilbert, 2012). This effect can diminish myofibrils’ role and cause myocarditis and bradycardia by lowering the number of contracting units, reducing the heart’s pumping ability (Singh and Deshpande, 2005; Kassim et al., 2008).
Tachycardia and Hypertension
Tachycardia is correlated with hypertension and may induce cardiovascular risk. As mentioned above, catecholamines released following scorpion envenomation can play a crucial role in initiating cardiac disorder by activating the β-receptors in the heart. The continuous release of endogenous and exogenous catecholamines post Indian scorpion sting causes an increase in spontaneous diastolic depolarization on cardiac fibers, which elevates the heart rate that leads to tachycardia or tachyarrhythmia (Reddy et al., 2017).
Pulmonary Edema
The fluid movement across the pulmonary capillary membrane can be described through a general transport equation named Starling’s equation (Starling, 1896). The elevated release of catecholamines as a consequence of scorpion sting can produce a profound shift influid movement, resulting in a collection of fluid in the extravascular tissue of the lung (pulmonary oedema) (Erdmann et al., 1975). Pulmonary oedema is a common clinical symptom of Indian red scorpion sting (Bagchi, and Deshpande, 1998).
Priapism
Bukatoxin and makatoxin are two α-neurotoxins from Indian red scorpion venom (Das et al., 2020) that cause a persistent activation of Na+ channel in nitrergic inhibitory fibers resulting in the release of NO (Gibson and McFadzean, 2001; Gwee et al., 2002; Reis et al., 2019). Upon entering the sting victim’s body, these neurotoxins stimulate the production of acetylcholine, which binds to endothelial cell receptors following activation of NO synthase to produce NO, which then passes through the smooth muscle cell to activate guanylate cyclase leading to the synthesis of cGMP. After that, cGMP activates protein kinase G, which acts as a vasodilator of smooth muscle causing priapism (unwanted, prolonged, painful penile erection), a common clinical symptom primarily observed in children post scorpion sting (Bawaskar, 1982; Hofmann et al., 1992; Lincoln and Cornwell, 1993; Lohmann et al., 1997). According to Bawaskar (1982), the chances of developing cardiac manifestations at a later stage of priapism are very high.
A Brief Account on Treatment of Scorpion Sting in India
As Indian red scorpion sting can be lethal, significant effort must be directed towards understanding the associated pathophysiological symptoms and adequately treating the envenomated victim. Despite advances in understanding pathophysiology and therapy, mortality remains high in many rural areas, mainly due to inefficient access to medical facilities (Reddy, 2013). Following a scorpion sting, patients should be observed for 24 h irrespective of the species involved. For the cases of severe envenomation, therapeutic efforts should be directed towards treating the over stimulated autonomic nervous system and correcting hypovolemia. Different treatment strategies utilized for Indian red scorpion sting are summarized in Table 3. Depending upon the severity of the sting, antivenom availability, and access to proper medical facilities, a single or a combination of treatment methods may be administered (Bawaskar and Bawaskar, 2011).
Indian red scorpion venom contains an abundance of low molecular mass toxins, which are poor immunogens (Das et al., 2020), and as a consequence, it can be challenging to raise toxin-specific antibodies in the horse (El Ayeb and Delori, 1984; Kankonkar et al., 1998; Das et al., 2020). Kankonkar et al. (1998) demonstrated that the use of an adjuvant and extending the immunization period resulted in the production of a potent equine antiserum capable of neutralizing the major lethal factors of scorpion venom. However, early administration of antivenom is necessary to prevent the release of catecholamine and minimize the intoxication following scorpion envenomation (Chippaux, 2012).
Conclusion on Future Directions for Research and Augmentation of Clinical Treatment on Scorpion Sting
Indian red scorpion sting is a typical medical emergency in many Indian sub-tropical countries. Sting severity is more significant in children, the elderly, and immuno-compromised adults and can be lethal if not properly treated. The geographical variation in Indian red scorpion venom toxicity and its pharmacological effects often leads to differences in local and systemic symptoms. In-depth proteomic analyses are necessary to correlate the geographical variation in Indian red scorpion venom composition with sting severity. While various treatments are available for the clinical management of scorpion sting, early administration of scorpion antivenom is the preferred choice, even though the poor immunogenicity of scorpion antivenom might present additional clinical challenges. The adjuvant may be an excellent choice to enhance the antigenicity of low molecular mass toxins of scorpion venom (Kankonkar et al., 1998; Bermúdez-Méndez et al., 2018). Additional studies on scorpion venom composition are warranted to produce highly effective antivenom that will target a broad range of species.
Further, the Indian red scorpion venom is proving to be an essential source of biologically active compounds which could have immense medical and pharmacological value (Strong et al., 2001). Proteomic profiling of the Indian red scorpion venom can provide a catalogue of novel molecules with robust pharmacological characteristics that may be explored as potential life-threatening therapeutics.
While proteomics has identified an abundance of low molecular mass ion channel toxins in Indian red scorpion venom, many proteins or peptides of low abundance may not be identified due to the limited number of sequences in protein reference databases. Therefore, additional –omic analyses, such as genomics and transcriptomics (Zhijian et al., 2006; Ma et al., 2009; Rokyta and Ward, 2017; Ward et al., 2018), are encouraged to promote the discovery of novel scorpion venom toxins. This research will increase our understanding of Indian red scorpion venom (Schendel et al., 2019), its toxicity mechanism, and identify novel drug prototypes from this venom (Uzair et al., 2018).
In addition, venom proteins (toxins) of low abundance can be challenging to detect without optimizing standard mass spectrometric methods or applying alternative techniques, such as western blotting with a particular antibody. Most mass spectrometry-based proteomic experiments are conducted in data-dependent acquisition (DDA) mode, where the stochastic nature of precursor ion selection for tandem MS (MS/MS) creates a bias by omitting low abundance or poorly ionizing peptides (Bateman et al., 2014; Lee et al., 2019). Alternative strategies such as data-independent acquisition (DIA) can enhance the identification and quantification of low abundant proteins (Hu et al., 2016) and could assist in the discovery of novel proteins and peptides from scorpion venoms. Alternatively, sample decomplexing via offline liquid chromatography or gel electrophoresis before mass spectrometry can also promote the identification of low abundant proteins.
Lastly, antibodies raised explicitly against low molecular mass toxins using toxicovenomics, antivenomics, and affinity purification can supplement commercially available scorpion antivenoms and would be an ideal approach for better in-patient management of Indian red scorpion sting. Repurposed drugs can also be explored as possible antidotes to treat scorpion stings.
Author Contributions
AM conceived the idea. AM, BD, and AS wrote the manuscript, and AM and AS edited the manuscript. All authors approved the final version of the manuscript.
Conflict of Interest
The authors declare that the research was conducted in the absence of any commercial or financial relationships that could be construed as a potential conflict of interest.
Publisher’s Note
All claims expressed in this article are solely those of the authors and do not necessarily represent those of their affiliated organizations, or those of the publisher, the editors and the reviewers. Any product that may be evaluated in this article, or claim that may be made by its manufacturer, is not guaranteed or endorsed by the publisher.
Acknowledgments
BD is recipient of Junior Research Fellowship from CSIR, New Delhi.
Supplementary Material
The Supplementary Material for this article can be found online at: https://www.frontiersin.org/articles/10.3389/fphar.2021.710680/full#supplementary-material
References
Abdel-Rahman, M. A., Quintero-Hernandez, V., and Possani, L. D. (2013). Venom Proteomic and Venomous Glands Transcriptomic Analysis of the Egyptian Scorpion Scorpio Maurus Palmatus (Arachnida: Scorpionidae). Toxicon 74, 193–207. doi:10.1016/j.toxicon.2013.08.064
Abroug, F., Nouira, S., Haguiga, H., Elatrous, S., Belghith, M., Boujdaria, R., et al. (1997). High-dose Hydrocortisone Hemisuccinate in Scorpion Envenomation. Ann. Emerg. Med. 30, 23–27. doi:10.1016/S0196-0644(97)70105-7
Abroug, F., Ouanes-Besbes, L., Tilouche, N., and Elatrous, S. (2020). Scorpion Envenomation: State of the Art. Intensive Care Med. 46, 401–410. doi:10.1007/s00134-020-05924-8
Agrawal, A., Kumar, A., Consul, S., and Yadav, A. (2015). Scorpion Bite, a Sting to the Heart!. Indian J. Crit. Care Med. 19, 233–236. doi:10.4103/0972-5229.154570
Akella, A., Tiwari, A. K., Rai, O. P., and Deshpande, S. B. (2016). Morphine Blocks the Mesobuthus Tamulus Venom-Induced Augmentation of Phenyldiguanide Reflex and Pulmonary Edema in Anesthetized Rats. Indian J. Pharmacol. 48, 74–77. doi:10.4103/0253-7613.174560
Amoreux, P. J. (1789). Description méthodique d’une espèce de scorpion roux commune à Souvignargues en Languedoc et détails historiques à ce sujet. Observations sur la Physique, sur l’Histoire naturelle et sur les Arts 35, 9–16.
Badhe, R. V., Thomas, A. B., Deshpande, A. D., Salvi, N., and Waghmare, A. (2007). The Action of Red Scorpion (Mesobuthus Tamulus Coconsis, Pocock) Venom and its Isolated Protein Fractions on Blood Sodium Levels. J. Venom. Anim. Toxins Incl. Trop. Dis. 13, 82–93. doi:10.1590/S1678-91992007000100006
Badhe, R. V., Thomas, A. B., Harer, S. L., Deshpande, A. D., Salvi, N., and Waghmare, A. (2006). Intraspecific Variation in Protein Pattern of Red Scorpion (Mesobuthus Tamulus, Coconsis, Pocock) Venoms from Western and Southern India. J. Venom. Anim. Toxins Incl. Trop. Dis. 12, 612–619. doi:10.1590/S1678-91992006000400008
Bagchi, S., and Deshpande, S. B. (1998). Indian Red Scorpion (Buthus Tamulus) Venom-Induced Augmentation of Cardiac Reflexes Is Mediated through the Mechanisms Involving Kinins in Urethane Anaesthetized Rats. Toxicon 36, 309–320. doi:10.1016/S0041-0101(97)00121-9
Bahloul, M., Chaari, A., Dammak, H., Samet, M., Chtara, K., Chelly, H., et al. (2013). Pulmonary Edema Following Scorpion Envenomation: Mechanisms, Clinical Manifestations, Diagnosis and Treatment. Int. J. Cardiol. 162, 86–91. doi:10.1016/j.ijcard.2011.10.013
Bateman, N. W., Goulding, S. P., Shulman, N. J., Gadok, A. K., Szumlinski, K. K., MacCoss, M. J., et al. (2014). Maximizing Peptide Identification Events in Proteomic Workflows Using Data-dependent Acquisition (DDA). Mol. Cell Proteomics 13, 329–338. doi:10.1074/mcp.M112.026500
Bawaskar, H. S., and Bawaskar, P. H. (2011). Efficacy and Safety of Scorpion Antivenom Plus Prazosin Compared with Prazosin Alone for Venomous Scorpion (Mesobuthus Tamulus) Sting: Randomised Open Label Clinical Trial. BMJ 342, c7136. doi:10.1136/bmj.c7136
Bawaskar, H. S., and Bawaskar, P. H. (1998). Indian Red Scorpion Envenoming. Indian J. Pediatr. 65, 383–391. doi:10.1007/BF02761131
Bawaskar, H. S., and Bawaskar, P. H. (1992). Management of the Cardiovascular Manifestations of Poisoning by the Indian Red Scorpion (Mesobuthus Tamulus). Br. Heart J. 68, 478–480. doi:10.1136/hrt.68.11.478
Bawaskar, H. S., and Bawaskar, P. H. (2012). Scorpion Sting: Update. J. Assoc. Physicians India 60, 46–55.
Bawaskar, H. S., and Bawaskar, P. H. (1996). Severe Envenoming by the Indian Red Scorpion Mesobuthus Tamulus: the Use of Prazosin Therapy. QJM 89, 701–704. doi:10.1093/qjmed/89.9.701
Bawaskar, H. S., and Bawaskar, P. H. (1994). Vasodilators: Scorpion Envenoming and the Heart (An Indian Experience). Toxicon 32, 1031–1040. doi:10.1016/0041-0101(94)90386-7
Bawaskar, H. S. (1982). Diagnostic Cardiac Premonitory Signs and Symptoms of Red Scorpion Sting. Lancet 1, 552–554. doi:10.1016/S0140-6736(82)92057-8
Bergman, N. J. (1997). Clinical description of Parabuthus transvaalicus scorpionism in Zimbabwe. Toxicon 35, 759–771. doi:10.1016/S0041-0101(96)00041-4
Bermúdez-Méndez, E., Fuglsang-Madsen, A., Føns, S., Lomonte, B., Gutiérrez, J., and Laustsen, A. (2018). Innovative Immunization Strategies for Antivenom Development. Toxins 10, 452. doi:10.3390/toxins10110452
Bhadani, U. K., Tripathi, M., Sharma, S., and Pandey, R. (2006). Scorpion Sting Envenomation Presenting with Pulmonary Edema in Adults: a Report of Seven Cases from Nepal. Indian J. Med. Sci. 60, 19–23. doi:10.4103/0019-5359.19672
Bhoite, R. R., Bhoite, G. R., Bagdure, D. N., and Bawaskar, H. S. (2015). Anaphylaxis to Scorpion Antivenin and its Management Following Envenomation by Indian Red Scorpion, Mesobuthus Tamulus. Indian J. Crit. Care Med. 19, 547–549. doi:10.4103/0972-5229.164807
Birdsall, H. H. (2015). “Adaptive immunity: antibodies and immunodeficiencies”, in principles and practice of infectious diseases. Editors J.E. Bennett, R. Dolin, M.J. Bliser, and Amsterdam (Elsevier), 34–49. doi:10.1016/C2012-1-00075-6
Bucaretchi, F., Baracat, E. C., Nogueira, R. J., Chaves, A., Zambrone, F. A., Fonseca, M. R., et al. (1995). A Comparative Study of Severe Scorpion Envenomation in Children Caused by Tityus Bahiensis and Tityus Serrulatus. Rev. Inst. Med. Trop. Sao Paulo 37, 331–336. doi:10.1590/S0036-46651995000400008
Calvete, J. J., Sanz, L., Angulo, Y., Lomonte, B., and Gutiérrez, J. M. (2009). Venoms, Venomics, Antivenomics. FEBS Lett. 583, 1736–1743. doi:10.1016/j.febslet.2009.03.029
Calvete, J. J. (2017). Venomics: Integrative Venom Proteomics and beyond. Biochem. J. 474, 611–634. doi:10.1042/BCJ20160577
Casewell, N. R., Wüster, W., Vonk, F. J., Harrison, R. A., and Fry, B. G. (2013). Complex Cocktails: the Evolutionary novelty of Venoms. Trends Ecol. Evol. 28, 219–229. doi:10.1016/j.tree.2012.10.020
Catterall, W. A. (1986). Molecular Properties of Voltage-Sensitive Sodium Channels. Annu. Rev. Biochem. 55, 953–985. doi:10.1146/annurev.bi.55.070186.004513
Catterall, W. A. (1976). Purification of a Toxic Protein from Scorpion Venom Which Activates the Action Potential Na+ Ionophore. J. Biol. Chem. 251, 5528–5536. doi:10.1016/S0021-9258(17)33092-2
Chanda, A., and Mukherjee, A. K. (2020). Mass Spectrometric Analysis to Unravel the Venom Proteome Composition of Indian Snakes: Opening New Avenues in Clinical Research. Expert Rev. Proteomics 17, 411–423. doi:10.1080/14789450.2020.1778471
Chippaux, J. P. (2012). Emerging Options for the Management of Scorpion Stings. Drug Des. Devel. Ther. 6, 165–173. doi:10.2147/DDDT.S24754
Chowell, G., Díaz-Dueñas, P., Bustos-Saldaña, R., Mireles, A. A., and Fet, V. (2006). Epidemiological and Clinical Characteristics of Scorpionism in Colima, Mexico (2000-2001). Toxicon 47, 753–758. doi:10.1016/j.toxicon.2006.02.004
Cid-Uribe, J. I., Veytia-Bucheli, J. I., Romero-Gutierrez, T., Ortiz, E., and Possani, L. D. (2020). Scorpion Venomics: a 2019 Overview. Expert Rev. Proteomics 17, 67–83. doi:10.1080/14789450.2020.1705158
Conde, R., Zamudio, F. Z., Becerril, B., and Possani, L. D. (1999). Phospholipin, a Novel Heterodimeric Phospholipase A2 from Pandinus Imperator Scp6pion Venom. FEBS Lett. 460, 447–450. doi:10.1016/S0014-5793(99)01392-7
Couraud, F., Jover, E., Dubois, J. M., and Rochat, H. (1982). Two Types of Scorpion Receptor Sites, One Related to the Activation, the Other to the Inactivation of the Action Potential Sodium Channel. Toxicon 20, 9–16. doi:10.1016/0041-0101(82)90138-6
Das, B., Patra, A., and Mukherjee, A. K. (2020). Correlation of Venom Toxinome Composition of Indian Red Scorpion (Mesobuthus Tamulus) with Clinical Manifestations of Scorpion Stings: Failure of Commercial Antivenom to Immune-Recognize the Abundance of Low Molecular Mass Toxins of This Venom. J. Proteome Res. 19, 1847–1856. doi:10.1021/acs.jproteome.0c00120
Daisley, H., Alexander, D., and Pitt-Miller, P. (1999). Acute myocarditis following Tityus trinitatis envenoming: morphological and pathophysiological characteristics. Toxicon 37, 159–165. doi:10.1016/S0041-0101(98)00174-3
Dehesa-Dávila, M., and Possani, L. D. (1994). Scorpionism and serotherapy in Mexico. Toxicon 32, 1015–1018. doi:10.1016/0041-0101(94)90383-2
de la Vega, R. C., and Possani, L. D. (2004). Current Views on Scorpion Toxins Specific for K+-channels. Toxicon 43, 865–875. doi:10.1016/j.toxicon.2004.03.022
de la Vega, R. C., Schwartz, E. F., and Possani, L. D. (2010). Mining on Scorpion Venom Biodiversity. Toxicon 56, 1155–1161. doi:10.1016/j.toxicon.2009.11.010
Delepierre, M., Prochnicka-Chalufour, A., and Possani, L. D. (1997). A Novel Potassium Channel Blocking Toxin from the Scorpion Pandinus Imperator: A 1H NMR Analysis Using a Nano-NMR Probe. Biochemistry 36, 2649–2658. doi:10.1021/bi9617116
Devi, C. S., Reddy, C. N., Devi, S. L., Subrahmanyam, Y. R., Bhatt, H. V., Suvarnakumari, G., et al. (1970). Defibrination Syndrome Due to Scorpion Venom Poisoning. Br. Med. J. 1, 345–347. doi:10.1136/bmj.1.5692.345
Dhawan, R. D., Joseph, S., Sethi, A., and Lala, A. K. (2002). Purification and Characterization of a Short Insect Toxin from the Venom of the Scorpion Buthus Tamulus. FEBS Lett. 528, 261–266. doi:10.1016/S0014-5793(02)03326-4
Dittrich, K., Power, A. P., and Smith, N. A. (1995). Scorpion Sting Sydrome - a Ten Year Experience. Ann. Saudi Med. 15, 148–155. doi:10.5144/0256-4947.1995.148
Dunlop, J. A. (2010). Geological History and Phylogeny of Chelicerata. Arthropod Struct. Dev. 39, 124–142. doi:10.1016/j.asd.2010.01.003
Dunlop, J. A., and Selden, P. A. (2013). Scorpion Fragments from the Silurian of Powys, Wales. Arachnology 16, 27–32. doi:10.13156/arac.2013.16.1.27
Ehrenberg, C. G. (1829). Coccus manniparus H. et E.[2 pages unnumbered. Klug, F. and Ehrenberg, CG Symbolae physicae seu icones et descriptiones insectorum quae ex itinere per Africam borealem et Asiam occidentalem. Dccas Prima, Berolini ex officina Academica.
El Ayeb, M., and Delori, P. (1984). “Immunology and Immunochemistry of Scorpion Neurotoxins,” in Handbook of Natural Toxins (Rome: CRC Press).
Erdmann, A. J., Vaughan, T. R., Brigham, K. L., Woolverton, W. C., and Staub, N. C. (1975). Effect of Increased Vascular Pressure on Lung Fluid Balance in Unanesthetized Sheep. Circ. Res. 37, 271–284. doi:10.1161/01.RES.37.3.271
Erfati, P. (1978). “Epidemiology, Symptomatology and Treatment of Buthidae Stings,” in Arthopod Venoms, Handbook of Experimental Pharmacology. Editor S. Bettini (New York: Springer-Verlag), 312–315.
Ewing, H. E. (1928). The scorpions of the western part of the United States, with notes on those occurring in northern Mexico. Proc. U. S. Natl. Mus.
Fan, Z., Chen, Y., and Liu, H. (2015). “Calcium Channel Blockers for Pulmonary Arterial Hypertension,” in Cochrane Database of Systematic Reviews (United States: John Wiley & Sons), 1465–1858. doi:10.1002/14651858.cd010066.pub2
Fontecilla-Camps, J. C., Habersetzer-Rochat, C., and Rochat, H. (1988). Orthorhombic Crystals and Three-Dimensional Structure of the Potent Toxin II from the Scorpion Androctonus Australis Hector. Proc. Natl. Acad. Sci. U S A. 85, 7443–7447. doi:10.1073/pnas.85.20.7443
Freire-Maia, L., Campos, J. A., and Amaral, C. F. (1994). Approaches to the Treatment of Scorpion Envenoming. Toxicon 32, 1009–1014. doi:10.1016/0041-0101(94)90382-4
Fry, B. G., Roelants, K., Champagne, D. E., Scheib, H., Tyndall, J. D., King, G. F., et al. (2009). The Toxicogenomic Multiverse: Convergent Recruitment of Proteins into Animal Venoms. Annu. Rev. Genomics Hum. Genet. 10, 483–511. doi:10.1146/annurev.genom.9.081307.164356
Ghalim, N., El-Hafny, B., Sebti, F., Heikel, J., Lazar, N., Moustanir, R., et al. (2000). Scorpion Envenomation and Serotherapy in Morocco. Am. J. Trop. Med. Hyg. 62, 277–283. doi:10.4269/ajtmh.2000.62.277
Gibson, A., and McFadzean, L. (2001). Biology of the Anococcygeus Muscle. Int. Rev. Cytol. 205, 1–35. doi:10.1016/S0074-7696(01)05001-X
Gong, J., Kini, R. M., Gwee, M. C., Gopalakrishnakone, P., and Chung, M. C. (1997). Makatoxin I, a novel toxin isolated from the venom of the scorpion Buthus martensi Karsch, exhibits nitrergic actions. J. Biol. Chem. 272, 8320–8324. doi:10.1074/jbc.272.13.8320
Gordon, D., Moskowitz, H., Eitan, M., Warner, C., Catterall, W. A., and Zlotkin, E. (1992). Localization of Receptor Sites for Insect-Selective Toxins on Sodium Channels by Site-Directed Antibodies. Biochemistry 31, 7622–7628. doi:10.1021/bi00148a025
Gregoire, J., and Rochat, H. (1983). Covalent Structure of Toxins I and II from the Scorpion Buthus Occitanus Tunetanus. Toxicon 21, 153–162. doi:10.1016/0041-0101(83)90058-2
Grishin, E. V., Soldatov, N. M., Soldatova, L. N., and Ovchinnikov, Y. A. (1979). Studies of the Toxins from Buthus Eupeus Scorpion Venom. Toxicon 17, 60. doi:10.1016/0041-0101(79)90062-x
Gueron, M., Ilia, R., and Sofer, S. (1992). The Cardiovascular System after Scorpion Envenomation. A Review. J. Toxicol. Clin. Toxicol. 30, 245–258. doi:10.3109/15563659209038636
Gutiérrez, J. M., Romero, M., Díaz, C., Borkow, G., and Ovadia, M. (1995). Isolation and Characterization of a Metalloproteinase with Weak Hemorrhagic Activity from the Venom of the Snake Bothrops asper (Terciopelo). Toxicon 33, 19–29. doi:10.1016/0041-0101(94)00138-X
Gwee, M. C., Nirthanan, S., Khoo, H. E., Gopalakrishnakone, P., Kini, R. M., and Cheah, L. S. (2002). Autonomic Effects of Some Scorpion Venoms and Toxins. Clin. Exp. Pharmacol. Physiol. 29, 795–801. doi:10.1046/j.1440-1681.2002.03726.x
Hariprasad, G., Saravanan, K., Singh, S. B., Das, U., Sharma, S., and Kaur, P. (2009). Group III PLA2 from the Scorpion, Mesobuthus Tamulus: Cloning and Recombinant Expression in E. coli. Electron. J. Biotechnol. 12, 6–7. doi:10.2225/vol12-issue3-fulltext-7
Hofmann, F., Dostmann, W., Keilbach, A., Landgraf, W., and Ruth, P. (1992). Structure and Physiological Role of cGMP-dependent Protein Kinase. Biochim. Biophys. Acta 1135, 51–60. doi:10.1016/0167-4889(92)90165-8
Holford, M., Daly, M., King, G. F., and Norton, R. S. (2018). Venoms to the rescue. Science 361, 842–844. doi:10.1126/science.aau7761
Hu, A., Noble, W. S., and Wolf-Yadlin, A. (2016). Technical Advances in Proteomics: New Developments in Data-independent Acquisition. F1000Res 5, 1. doi:10.12688/f1000research.7042.1
Ianzer, D., Konno, K., Marques-Porto, R., Vieira Portaro, F. C., Stöcklin, R., Martins de Camargo, A. C., et al. (2004). Identification of Five New Bradykinin Potentiating Peptides (BPPs) from Bothrops jararaca Crude Venom by Using Electrospray Ionization Tandem Mass Spectrometry after a Two-step Liquid Chromatography. Peptides 25, 1085–1092. doi:10.1016/j.peptides.2004.04.006
Incamnoi, P., Patramanon, R., Thammasirirak, S., Chaveerach, A., Uawonggul, N., Sukprasert, S., et al. (2013). Heteromtoxin (HmTx), a Novel Heterodimeric Phospholipase A(2) from Heterometrus Laoticus Scorpion Venom. Toxicon 61, 62–71. doi:10.1016/j.toxicon.2012.10.012
Jimenez, E. C., Sasakawa, N., and Kumakura, K. (2008). Effects of Sodium Channel-Targeted Conotoxins on Catecholamine Release in Adrenal Chromaffin Cells. Philipp. J. Sci. 137, 127–132.
Jover, E., Couraud, F., and Rochat, H. (1980). Two Types of Scorpion Neurotoxins Characterized by Their Binding to Two Separate Receptor Sites on Rat Brain Synaptosomes. Biochem. Biophys. Res. Commun. 95, 1607–1614. doi:10.1016/S0006-291X(80)80082-9
Jridi, I., Catacchio, I., Majdoub, H., Shahbazeddah, D., El Ayeb, M., Frassanito, M. A., et al. (2015). Hemilipin, a Novel Hemiscorpius Lepturus Venom Heterodimeric Phospholipase A2, Which Inhibits Angiogenesis In Vitro and In Vivo. Toxicon 105, 34–44. doi:10.1016/j.toxicon.2015.08.022
Jridi, I., Catacchio, I., Majdoub, H., Shahbazzadeh, D., El Ayeb, M., Frassanito, M. A., et al. (2017). The Small Subunit of Hemilipin2, a New Heterodimeric Phospholipase A2 from Hemiscorpius Lepturus Scorpion Venom, Mediates the Antiangiogenic Effect of the Whole Protein. Toxicon 126, 38–46. doi:10.1016/j.toxicon.2016.12.001
Kalita, B., Singh, S., Patra, A., and Mukherjee, A. K. (2018). Quantitative Proteomic Analysis and Antivenom Study Revealing that Neurotoxic Phospholipase A2 Enzymes, the Major Toxin Class of Russell's viper Venom from Southern India, Shows the Least Immuno-Recognition and Neutralization by Commercial Polyvalent Antivenom. Int. J. Biol. Macromol. 118, 375–385. doi:10.1016/j.ijbiomac.2018.06.083
Kankonkar, R. C., Kulkurni, D. G., and Hulikavi, C. B. (1998). Preparation of a Potent Anti-scorpion-venom-serum against the Venom of Red Scorpion (Buthus Tamalus). J. Postgrad. Med. 44, 85–92.
Kassim, T. A., Clarke, D. D., Mai, V. Q., Clyde, P. W., and Mohamed Shakir, K. M. (2008). Catecholamine-induced Cardiomyopathy. Endocr. Pract. 14, 1137–1149. doi:10.4158/EP.14.9.1137
Kokki, H. (2003). Nonsteroidal Anti-inflammatory Drugs for Postoperative Pain: a Focus on Children. Paediatr. Drugs 5, 103–123. doi:10.2165/00128072-200305020-00004
Kopeyan, C., Mansuelle, P., Martin-Eauclaire, M. F., Rochat, H., and Miranda, F. (1993). Characterization of Toxin III of the Scorpion Leiurus Quinquestriatus Quinquestriatus: a New Type of Alpha-Toxin Highly Toxic Both to Mammals and Insects. Nat. Toxins 1, 308–312. doi:10.1002/nt.2620010510
Kopeyan, C., Martinez, G., and Rochat, H. (1985). Primary Structure of Toxin IV of Leiurus Quinquestriatus Quinquestriatus. FEBS Lett. 181, 211–217. doi:10.1016/0014-5793(85)80262-3
Kovařík, F. (2007). A Revision of the Genus Hottentotta Birula, 1908, with Descriptions of Four New Species (Scorpiones, Buthidae). Euscorpius, 1–107.
Krayem, N., and Gargouri, Y. (2020). Scorpion Venom Phospholipases A2: A Minireview. Toxicon 184, 48–54. doi:10.1016/j.toxicon.2020.05.020
Krayem, N., Parsiegla, G., Gaussier, H., Louati, H., Jallouli, R., Mansuelle, P., et al. (2018). Functional Characterization and FTIR-Based 3D Modeling of Full Length and Truncated Forms of Scorpio Maurus Venom Phospholipase A2. Biochim. Biophys. Acta Gen. Subj. 1862, 1247–1261. doi:10.1016/j.bbagen.2018.02.004
Kularatne, S. A., Dinamithra, N. P., Sivansuthan, S., Weerakoon, K. G., Thillaimpalam, B., Kalyanasundram, V., et al. (2015). Clinico-epidemiology of Stings and Envenoming of Hottentotta Tamulus (Scorpiones: Buthidae), the Indian Red Scorpion from Jaffna Peninsula in Northern Sri Lanka. Toxicon 93, 85–89. doi:10.1016/j.toxicon.2014.11.225
Lee, H. Y., Kim, E. G., Jung, H. R., Jung, J. W., Kim, H. B., Cho, J. W., et al. (2019). Refinements of LC-MS/MS Spectral Counting Statistics Improve Quantification of Low Abundance Proteins. Sci. Rep. 9, 13653–13710. doi:10.1038/s41598-019-49665-1
Leite Junior, J. B., de Mello Bastos, J. M., Samuels, R. I., Carey, R. J., and Carrera, M. P. (2019). Reversal of Morphine Conditioned Behavior by an Anti-dopaminergic post-trial Drug Treatment during Re-consolidation. Behav. Brain Res. 359, 771–782. doi:10.1016/j.bbr.2018.08.009
Lincoln, T. M., and Cornwell, T. L. (1993). Intracellular Cyclic GMP Receptor Proteins. Faseb. J. 7, 328–338. doi:10.1096/fasebj.7.2.7680013
Lohmann, S. M., Vaandrager, A. B., Smolenski, A., Walter, U., and De Jonge, H. R. (1997). Distinct and Specific Functions of cGMP-dependent Protein Kinases. Trends Biochem. Sci. 22, 307–312. doi:10.1016/s0968-0004(97)01086-4
Louati, H., Krayem, N., Fendri, A., Aissa, I., Sellami, M., Bezzine, S., et al. (2013). A Thermoactive Secreted Phospholipase A₂ Purified from the Venom Glands of Scorpio Maurus: Relation between the Kinetic Properties and the Hemolytic Activity. Toxicon 72, 133–142. doi:10.1016/j.toxicon.2013.06.017
Ma, Y., Zhao, R., He, Y., Li, S., Liu, J., Wu, Y., et al. (2009). Transcriptome Analysis of the Venom Gland of the Scorpion Scorpiops Jendeki: Implication for the Evolution of the Scorpion Venom Arsenal. BMC genomics 10, 290. doi:10.1186/1471-2164-10-290
Madhavan, J. (2015). A Study on Clinical Presentation and Outcome of Scorpion Sting in Children. Thanjavur: Doctoral dissertation, Thanjavur Medical College.
Manuwar, A., Dreyer, B., Böhmert, A., Ullah, A., Mughal, Z., Akrem, A., et al. (2020). Proteomic Investigations of Two Pakistani Naja Snake Venoms Species Unravel the Venom Complexity, Posttranslational Modifications, and Presence of Extracellular Vesicles. Toxins (Basel) 12, 669. doi:10.3390/toxins12110669
Miller, C., Moczydlowski, E., Latorre, R., and Phillips, M. (1985). Charybdotoxin, a Protein Inhibitor of Single Ca2+-Activated K+ Channels from Mammalian Skeletal Muscle. Nature 313, 316–318. doi:10.1038/313316a0
Morey, S. S., Kiran, K. M., and Gadag, J. R. (2006). Purification and Properties of Hyaluronidase from Palamneus Gravimanus (Indian Black Scorpion) Venom. Toxicon 47, 188–195. doi:10.1016/j.toxicon.2005.10.014
Mukherjee, A. K., Kalita, B., and Mackessy, S. P. (2016). A Proteomic Analysis of Pakistan Daboia russelii Russelii Venom and Assessment of Potency of Indian Polyvalent and Monovalent Antivenom. J. Proteomics 144, 73–86. doi:10.1016/j.jprot.2016.06.001
Murthy, K. R., and Zare, M. A. (1998). Effect of Indian Red Scorpion (Mesobuthus Tamulus Concanesis, Pocock) Venom on Thyroxine and Triiodothyronine in Experimental Acute Myocarditis and its Reversal by Species Specific Antivenom. Indian J. Exp. Biol. 36, 16–21. doi:10.1016/j.jprot.2016.06.001
Narahashi, T., Shapiro, B. I., Deguchi, T., Scuka, M., and Wang, C. M. (1972). Effects of Scorpion Venom on Squid Axon Membranes. Am. J. Physiol. -Legacy Content 222, 850–857. doi:10.1152/ajplegacy.1972.222.4.850
Newton, K. A., Clench, M. R., Deshmukh, R., Jeyaseelan, K., and Strong, P. N. (2007). Mass Fingerprinting of Toxic Fractions from the Venom of the Indian Red Scorpion, Mesobuthus Tamulus: Biotope-specific Variation in the Expression of Venom Peptides. Rapid Commun. Mass. Spectrom. 21, 3467–3476. doi:10.1002/rcm.3240
Olivier, A.G. (1807). Entomologie ou histoire naturelle des Insectes, avec leurs caracteres générique et spécifiques, leur description, leur synonymie, et leur figure enluminée, 612. Paris: Desray, 63. Coléoptères. Tome cinquième.
Ortiz, E., Gurrola, G. B., Schwartz, E. F., and Possani, L. D. (2015). Scorpion Venom Components as Potential Candidates for Drug Development. Toxicon 93, 125–135. doi:10.1016/j.toxicon.2014.11.233
Pandurang, K. S., Singh, J., Bijesh, S., and Singh, H. P. (2014). Effectiveness of Anti Scorpion Venom for Red Scorpion Envenomation. Indian Pediatr. 51, 131–133. doi:10.1007/s13312-014-0345-8
Pinter, M. M., Pogarell, O., and Oertel, W. H. (1999). Efficacy, safety, and tolerance of the non-ergoline dopamine agonist pramipexole in the treatment of advanced Parkinson’s disease: a double blind, placebo controlled, randomised, multicentre study. J. Neurol. Neurosurg. Psychiatry 66, 436–441. doi:10.1136/jnnp.66.4.436
Pedarzani, P., D'hoedt, D., Doorty, K. B., Wadsworth, J. D., Joseph, J. S., Jeyaseelan, K., et al. (2002). Tamapin, a Venom Peptide from the Indian Red Scorpion (Mesobuthus Tamulus) that Targets Small Conductance Ca2+-Activated K+ Channels and Afterhyperpolarization Currents in central Neurons. J. Biol. Chem. 277, 46101–46109. doi:10.1074/jbc.M206465200
Perty, M. (1833). “Brasilianische Spinnen”. inDelectus Animalium Articulatorum quae in itinere per razilian ann. 1817 et 1820 colligerunt. Editors J.B. de Spix (Monachii Martius), 191–209.
Pocock, R. I. (1897). LIII.—Descriptions of some new species of scorpions of the Genus Tityus, with notes upon some forms allied to T. americanus (Linn.). J. Nat. Hist 19, 510–521. doi:10.1080/00222939708680571
Possani, L. D., Becerril, B., Delepierre, M., and Tytgat, J. (1999). Scorpion Toxins Specific for Na+-Channels. Eur. J. Biochem. 264, 287–300. doi:10.1046/j.1432-1327.1999.00625.x
Prendini, L. (2000). Phylogeny and Classification of the Superfamily Scorpionoidea Latreille 1802 (Chelicerata, Scorpiones): An Exemplar Approach. Cladistics 16, 1–78. doi:10.1111/j.1096-0031.2000.tb00348.x
Purcell, W. F. (1899). On the South African species of Peripatidae in the collection of the South African Museum. Ann. S. Afr. Mus. 1, 331–5. doi:10.5962/bhl.part.13902
Quintero-Hernández, V., Jiménez-Vargas, J. M., Gurrola, G. B., Valdivia, H. H., and Possani, L. D. (2013). Scorpion Venom Components that Affect Ion-Channels Function. Toxicon 76, 328–342. doi:10.1016/j.toxicon.2013.07.012
Radha Krishna Murthy, K., Vakil, A. E., Yeolekar, M. E., and Vakil, Y. E. (1988). Reversal of Metabolic & Electrocardiographic Changes Induced by Indian Red Scorpion (Buthus Tamulus) Venom by Administration of Insulin, Alpha Blocker & Sodium Bicarbonate. Indian J. Med. Res. 88, 450–457.
Radmanesh, M. (1990). Androctonus Crassicauda Sting and its Clinical Study in Iran. J. Trop. Med. Hyg. 93, 323–326.
Rahimtoola, S. H., Raphael, M. J., Pitney, W. R., Olsen, E. J., and Webb-peploe, M. (1970). Therapeutic Defibrination and Heparin Therapy in the Prevention and Resolution of Experimental Venous Thrombosis. Circulation 42, 729–737. doi:10.1161/01.CIR.42.4.729
Rajashekhar, , and Mudgal, S. M. (2017). Epidemiological and Clinical Study of Scorpion Envenomation in Patients Admitted at Rims Teaching Hospital. Raichur. Int. J. Sci. Study 5 (3), 73–76.
Ramanaiah, M., Parthasarathy, P. R., and Venkaiah, B. (1990). Isolation and Characterization of Hyaluronidase from Scorpion (Heterometrus Fulvipes) Venom. Biochem. Int. 20, 301–310.
Rang, H. P. (2003). “Anthelmintic Drugs,” in Pharmacology. Editors H. P. Rang, M. M. Dale, J. M. Ritter, and P. K. Moore.
Ratnayake, R. M., Kumanan, T., and Selvaratnam, G. (2016). Acute Myocardial Injury after Scorpion (Hottentotta Tamulus) Sting. Ceylon Med. J. 61, 86–87. doi:10.4038/cmj.v61i2.8293
Ravens, U., and Cerbai, E. (2008). Role of Potassium Currents in Cardiac Arrhythmias. Europace 10, 1133–1137. doi:10.1093/europace/eun193
Reader, T. A., Brière, R., and Grondin, L. (1987). Alpha-1 and Alpha-2 Adrenoceptor Binding in Cerebral Cortex: Competition Studies with [3H]prazosin and [3H]idazoxan. J. Neural Transm. 68, 79–95. doi:10.1007/BF01244641
Reddy, B. R. C. (2013). Scorpion Envenomation: What Is New? Medicine Update. 23a ed. Nova Delhi, India: Jaypee Brothers Medical Publishers, 421–423.
Reddy C, R., Bompelli, N., Khardenavis, V., and Deshpande, A. (2017). Scorpion Bite-Induced Ischaemic Stroke. BMJ Case Rep. 2017, 1. doi:10.1136/bcr-2017-221606
Reis, M. B., Zoccal, K. F., Gardinassi, L. G., and Faccioli, L. H. (2019). Scorpion Envenomation and Inflammation: Beyond Neurotoxic Effects. Toxicon 167, 174–179. doi:10.1016/j.toxicon.2019.06.219
Rochat, H., Bernard, P., and Couraud, F. (1979). Scorpion Toxins: Chemistry and Mode of Action. Adv. Cytopharmacol 3, 325–334.
Rokyta, D. R., and Ward, M. J. (2017). Venom-gland Transcriptomics and Venom Proteomics of the Black-Back Scorpion (Hadrurus Spadix) Reveal Detectability Challenges and an Unexplored Realm of Animal Toxin Diversity. Toxicon 128, 23–37. doi:10.1016/j.toxicon.2017.01.014
Rowan, E. G., Vatanpour, H., Furman, B. L., Harvey, A. L., Tanira, M. O., and Gopalakrishnakone, P. (1992). The Effects of Indian Red Scorpion Buthus Tamulus Venom In Vivo and In Vitro. Toxicon 30, 1157–1164. doi:10.1016/0041-0101(92)90431-4
Santibáñez-López, C., Cid-Uribe, J., Batista, C., Ortiz, E., and Possani, L. (2016). Venom Gland Transcriptomic and Proteomic Analyses of the Enigmatic Scorpion Superstitionia Donensis (Scorpiones: Superstitioniidae), with Insights on the Evolution of its Venom Components. Toxins 8, 367. doi:10.3390/toxins8120367
Santos, M. S. V., Silva, C. G. L., Neto, B. S., Grangeiro Júnior, C. R. P., Lopes, V. H. G., Teixeira Júnior, A. G., et al. (2016). Clinical and Epidemiological Aspects of Scorpionism in the World: a Systematic Review. Wilderness Environ. Med. 27, 504–518. doi:10.1016/j.wem.2016.08.003
Saviola, A. J., Negrão, F., and Yates, J. R. (2020). Proteomics of Select Neglected Tropical Diseases. Annu. Rev. Anal. Chem. (Palo Alto Calif. 13, 315–336. doi:10.1146/annurev-anchem-091619-093003
Schendel, V., Rash, L. D., Jenner, R. A., and Undheim, E. A. B. (2019). The Diversity of Venom: the Importance of Behavior and Venom System Morphology in Understanding its Ecology and Evolution. Toxins (Basel) 11, 666. doi:10.3390/toxins11110666
Senthilvelan, M., Reddy, P. P. K., Resveen, H., Chandramohan, M., Santhosh, S. J., Abraham, D. J., et al. (2015). A Case Report of Severe Acute Myocarditis and Pulmonary Odema Following Scorpion Sting. Int. J. Modn. Res. Revs. 3, 815–817.
Seyedian, R., Pipelzadeh, M. H., Jalali, A., Kim, E., Lee, H., Kang, C., et al. (2010). Enzymatic Analysis of Hemiscorpius Lepturus Scorpion Venom Using Zymography and Venom-specific Antivenin. Toxicon 56, 521–525. doi:10.1016/j.toxicon.2010.05.008
Sibley, D. R., Monsma, F. J., and Shen, Y. (1993). Molecular Neurobiology of Dopaminergic Receptors. Int. Rev. Neurobiol. 35, 391–415. doi:10.1016/S0074-7742(08)60573-5
Singh, S. K., and Deshpande, S. B. (2005). Intra-arterial Injection of Mesobuthus Tamulus Venom Elicits Cardiorespiratory Reflexes Involving Perivascular Afferents. Toxicon 46, 820–826. doi:10.1016/j.toxicon.2005.08.014
Srairi-Abid, N., Othman, H., Aissaoui, D., and BenAissa, R. (2019). Anti-tumoral Effect of Scorpion Peptides: Emerging New Cellular Targets and Signaling Pathways. Cell Calcium 80, 160–174. doi:10.1016/j.ceca.2019.05.003
Srinivasan, K. N., Nirthanan, S., Sasaki, T., Sato, K., Cheng, B., Gwee, M. C., et al. (2001). Functional Site of Bukatoxin, an Alpha-type Sodium Channel Neurotoxin from the Chinese Scorpion (Buthus Martensi Karsch) Venom: Probable Role of the (52)PDKVP(56) Loop. FEBS Lett. 494, 145–149. doi:10.1016/S0014-5793(01)02342-0
Starling, E. H. (1896). On the Absorption of Fluids from the Connective Tissue Spaces. J. Physiol. 19, 312–326. doi:10.1113/jphysiol.1896.sp000596
Stevens, M., Peigneur, S., and Tytgat, J. (2011). Neurotoxins and Their Binding Areas on Voltage-Gated Sodium Channels. Front. Pharmacol. 2, 71. doi:10.3389/fphar.2011.00071
Strong, P. N., Clark, G. S., Armugam, A., De-Allie, F. A., Joseph, J. S., Yemul, V., et al. (2001). Tamulustoxin: a Novel Potassium Channel Blocker from the Venom of the Indian Red Scorpion Mesobuthus Tamulus. Arch. Biochem. Biophys. 385, 138–144. doi:10.1006/abbi.2000.2135
Suranse, V., Sawant, N. S., Bastawade, D. B., and Dahanukar, N. (2019). Haplotype Diversity in Medically Important Red Scorpion (Scorpiones: Buthidae: Hottentotta Tamulus) from India. J. Genet. 98, 17. doi:10.1007/s12041-019-1058-5
Suranse, V., Sawant, N. S., Paripatyadar, S. V., Krutha, K., Paingankar, M. S., Padhye, A. D., et al. (2017). First Molecular Phylogeny of Scorpions of the Family Buthidae from India. Mitochondrial DNA A. DNA Mapp. Seq. Anal. 28, 606–611. doi:10.3109/24701394.2016.1149830
Suranse, V., Srikanthan, A., and Sunagar, K. (2018). Animal Venoms: Origin, Diversity and Evolution. Chichester: eLS, 1–20. doi:10.1002/9780470015902.a0000939.pub2
Thakur, R., and Mukherjee, A. K. (2015). A Brief Appraisal on Russell's Viper Venom (Daboia russelii Russelii) Proteinases. Singapore: Snake Venoms, 1–18. doi:10.1007/978-94-007-6648-8_19-1
Tiwari, A. K., and Deshpande, S. B. (1996). Augmentation of Phenyldiguanide-Induced Bradycardia by Buthus Tamulus Venom in Adult Rats. Indian J. Exp. Biol. 34, 667–670.
Tiwari, A. K., and Deshpande, S. B. (1993). Toxicity of Scorpion (Buthus Tamulus) Venom in Mammals Is Influenced by the Age and Species. Toxicon 31, 1619–1622. doi:10.1016/0041-0101(93)90346-K
Touloun, O., Slimani, T., and Boumezzough, A. (2001). Epidemiological Survey of Scorpion Envenomation in Southwestern Morocco. J. Venom. Anim. Toxins 7, 199–218. doi:10.1590/S0104-79302001000200006
Touyz, R. M., Alves-Lopes, R., Rios, F. J., Camargo, L. L., Anagnostopoulou, A., Arner, A., et al. (2018). Vascular Smooth Muscle Contraction in Hypertension. Cardiovasc. Res. 114, 529–539. doi:10.1093/cvr/cvy023
Tytgat, J., Chandy, K. G., Garcia, M. L., Gutman, G. A., Martin-Eauclaire, M. F., van der Walt, J. J., et al. (1999). A Unified Nomenclature for Short-Chain Peptides Isolated from Scorpion Venoms: Alpha-KTx Molecular Subfamilies. Trends Pharmacol. Sci. 20, 444–447. doi:10.1016/S0165-6147(99)01398-X
Uzair, B., Bint-e-Irshad, S., Khan, B. A., Azad, B., Mahmood, T., Rehman, M. U., et al. (2018). Scorpion Venom Peptides as a Potential Source for Human Drug Candidates. Protein Pept. Lett. 25, 702–708. doi:10.2174/0929866525666180614114307
Valdez-Cruz, N. A., Batista, C. V. F., and Possani, L. D. (2004). Phaiodactylipin, a Glycosylated Heterodimeric Phospholipase A2 from the Venom of the Scorpion Anuroctonus Phaiodactylus. Eur. J. Biochem. 271, 1453–1464. doi:10.1111/j.1432-1033.2004.04047.x
Vargas, O., Gregoire, J., Martin, M. F., Bechis, G., and Rochat, H. (1982). Neurotoxins from the Venoms of Two Scorpions: Buthus Occitanus Tunetanus and Buthus Occitanus Mardochei. Toxicon 20, 79.
Vatanpour, H., Rowan, E. G., and Harvey, A. L. (1993). Effects of Scorpion (Buthus Tamulus) Venom on Neuromuscular Transmission In Vitro. Toxicon 31, 1373–1384. doi:10.1016/0041-0101(93)90203-U
Wachter, S. B., and Gilbert, E. M. (2012). Beta-adrenergic Receptors, from Their Discovery and Characterization through Their Manipulation to Beneficial Clinical Application. Cardiology 122, 104–112. doi:10.1159/000339271
Ward, L. M., Shih, P. M., and Fischer, W. W. (2018). MetaPOAP: Presence or Absence of Metabolic Pathways in Metagenome-Assembled Genomes. Bioinformatics 34, 4284–4286. doi:10.1093/bioinformatics/bty510
White, J. (2016). “Overview of Scorpion Envenoming,” in Overview of Scorpion Envenoming. Critical Care Toxicology. Editor J. Brent (Switzerland: Springer International Publishing), 1–15. doi:10.1007/978-3-319-20790-2_147-1
Wudayagiri, R., Inceoglu, B., Herrmann, R., Derbel, M., Choudary, P. V., and Hammock, B. D. (2001). Isolation and Characterization of a Novel Lepidopteran-Selective Toxin from the Venom of South Indian Red Scorpion, Mesobuthus Tamulus. BMC Biochem. 2, 16. doi:10.1186/1471-2091-2-16
Yugandhar, B., Radha Krishna Murthy, K., and Sattar, S. A. (1999). Insulin Administration in Severe Scorpion Envenoming. J. Venom. Anim. Toxins 5, 200–219. doi:10.1590/s0104-79301999000200007
Yuvaraja, K., Chidambaram, N., Umarani, R., Bhargav, K. M., Kumar, S. P., Prabhu, T., et al. (2019). A Study on Clinical Features, Complications and Management of Scorpion Sting Envenomation at a Tertiary Care Hospital, in Rural South India. J. Clin. Sci. Res. 8, 140.
Zamudio, F. Z., Conde, R., Arévalo, C., Becerril, B., Martin, B. M., Valdivia, H. H., et al. (1997). The Mechanism of Inhibition of Ryanodine Receptor Channels by Imperatoxin I, a Heterodimeric Protein from the Scorpion Pandinus Imperator. J. Biol. Chem. 272, 11886–11894. doi:10.1074/jbc.272.18.11886
Zelanis, A., and Tashima, A. K. (2014). Unraveling Snake Venom Complexity with 'omics' Approaches: Challenges and Perspectives. Toxicon 87, 131–134. doi:10.1016/j.toxicon.2014.05.011
Zhijian, C., Feng, L., Yingliang, W., Xin, M., and Wenxin, L. (2006). Genetic Mechanisms of Scorpion Venom Peptide Diversification. Toxicon 47, 348–355. doi:10.1016/j.toxicon.2005.11.013
Zlotkin, E., Eitan, M., Bindokas, V. P., Adams, M. E., Moyer, M., Burkhart, W., et al. (1991). Functional Duality and Structural Uniqueness of Depressant Insect-Selective Neurotoxins. Biochemistry 30, 4814–4821. doi:10.1021/bi00233a025
Zlotkin, E., Moskowitz, H., Herrmann, R., Pelhate, M., and Gordon, D. (1995). “Insect Sodium Channel as the Target for Insect-Selective Neurotoxins from Scorpion Venom,” in Molecular Action of Insecticides on Ion Channels. Editor J. M. Dark (Washington: Springer) 56–85. doi:10.1021/bk-1995-0591.ch004
Keywords: Indian red scorpion, venom composition, pathophysiology of scorpion sting, catecholamines, therapy against Mesobuthus tamulus scorpion sting
Citation: Das B, Saviola AJ and Mukherjee AK (2021) Biochemical and Proteomic Characterization, and Pharmacological Insights of Indian Red Scorpion Venom Toxins. Front. Pharmacol. 12:710680. doi: 10.3389/fphar.2021.710680
Received: 17 May 2021; Accepted: 16 August 2021;
Published: 28 September 2021.
Edited by:
Choo Hock Tan, University of Malaya, MalaysiaReviewed by:
Juan J Calvete, Consejo Superior de Investigaciones Científicas (CSIC), SpainSakthivel Vaiyapuri, University of Reading, United Kingdom
Copyright © 2021 Das, Saviola and Mukherjee. This is an open-access article distributed under the terms of the Creative Commons Attribution License (CC BY). The use, distribution or reproduction in other forums is permitted, provided the original author(s) and the copyright owner(s) are credited and that the original publication in this journal is cited, in accordance with accepted academic practice. No use, distribution or reproduction is permitted which does not comply with these terms.
*Correspondence: Ashis K. Mukherjee, akm@tezu.ernet.in