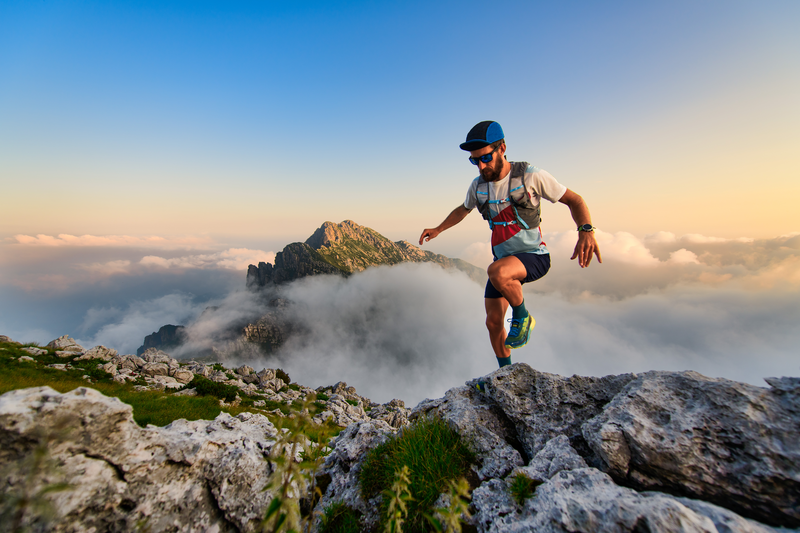
95% of researchers rate our articles as excellent or good
Learn more about the work of our research integrity team to safeguard the quality of each article we publish.
Find out more
REVIEW article
Front. Pharmacol. , 02 August 2021
Sec. Respiratory Pharmacology
Volume 12 - 2021 | https://doi.org/10.3389/fphar.2021.709538
This article is part of the Research Topic Advances in Cell-Based and Gene-Based Therapies for Respiratory Diseases View all 21 articles
Acute lung injury (ALI), a common and critical illness with high morbidity and mortality, is caused by multiple causes. It has been confirmed that oxidative stress plays an important role in the development of ALI. Ferroptosis, a newly discovered programmed cell death in 2012, is characterized by iron-dependent lipid peroxidation and involved in many diseases. To date, compelling evidence reveals the emerging role of ferroptosis in the pathophysiological process of ALI. Here, we review the role of ferroptosis in the pathogenesis of ALI and its therapeutic potential in ALI.
Acute lung injury (ALI), a common and critical illness with high morbidity and mortality, is caused by a variety of factors, including pulmonary and extrapulmonary factors (Ware et al., 2000; Mutlu et al., 2006; Matthay et al., 2019). The pathogenesis of ALI is not fully understood, and there is still no effective targeted intervention. Therefore, it is of great significance to study the pathogenesis and treatment of ALI. The pathogenesis of ALI was previously believed to involve inflammation, coagulation, oxidative stress, repair and so on (Matthay et al., 2019). In 2012, Dixon et al. proposed ferroptosis, a new concept of cell death. Studies have shown that ferroptosis is closely related to tumor, nervous system disease, infection, ischemia/reperfusion (I/R) injury, kidney injury and other diseases (Friedmann et al., 2014; Linkermann et al., 2014; Stockwell et al., 2017; Dar et al., 2018; Amaral et al., 2019; Hu et al., 2019; Mou et al., 2019; Han et al., 2020; Mao et al., 2020). In recent years, ferroptosis has also been confirmed to contribute to lipopolysaccharide (LPS)-induced ALI, intestinal I/R-induced ALI, oleic acid-induced ALI, and acute radiation-induced lung injury (RILI) (Li et al., 2019; Liu et al., 2020; Dong et al., 2020; Li et al., 2020; Zhou et al., 2019). In addition, ferroptosis inhibitors ferrostatin-1 and lipoxstatin-1, as well as inhibitor of apoptosis-stimulating protein of p53 (iASPP), can mediate protective effects against ALI by inhibiting ferroptosis (Liu et al., 2020; Li et al., 2020). This review summarizes the research progress, regulatory mechanism and therapeutic potential of ferroptosis in ALI (Figure 1).
FIGURE 1. The molecular mechanism and regulation of ferroptosis in ALI. Note: ALI: acute lung injury; GSH: glutathione peroxidase 4; ROS: reactive oxygen species; Nrf2: nuclear factor erythroid 2-related factor 2; HO-1: heme oxygenase-1; ARE: antioxidant response element; TFR1: transferrin receptor 1; DFO: deferoxamine; iASSP: inhibitor of apoptosis-stimulating protein of p53.
Ferroptosis was first discovered as a unique form of cell death by Dixon et al. when studying the mechanism of erastin killing tumor cells with RAS mutation in 2012 (Dixon et al., 2012). As a programmed cell death, ferroptosis is quite different from apoptosis and autophagy in morphology (Table 1). The characteristics of ferroptosis include decreased mitochondrial crista, increased mitochondrial membrane density, along with ruptured mitochondrial outer membrane as observed under electron microscopy, but the integrity of nucleus remains (Kazan et al., 2019). Besides mitochondria, other organelles such as golgi, endoplasmic reticulum and lysosome, are also involved in ferroptosis. Golgi stress-related lipid peroxidation, endoplasmic reticulum-related oxidative stress, and lysosome dysfunction can induce ferroptosis (Wu et al., 2020). The biological properties of ferroptosis are characterized by a large amount of iron accumulation and membrane lipid peroxidation products in the process of cell death. At present, the mechanism of ferroptosis mainly focuses on oxidative damage and iron metabolism (Stockwell et al., 2017; Li et al., 2020). Lipid peroxidation and iron metabolism signaling are recognized as the central mediators of ferroptosis (Xie et al., 2016).
TABLE 1. Morphological comparison between ferroptosis, apoptosis, necrosis, pyroptosis and autophagy (Kazan et al., 2019); (Dixon et al., 2012); (Wu et al., 2020); (Stockwell et al., 2017); (Li et al., 2020).
Cystine/glutamate transporter (system Xc-) is an important antioxidant, composed of two subunits, SLC7A11 and SLC3A2L. It can transfer cystine into cells and excrete glutamate out of cells. Cystine and glutamate are exchanged by system Xc-in a ratio of 1:1. Cystine is reduced to cysteine by system Xc- and participates in the synthesis of glutathione (GSH) (Chen et al., 2015). Then GSH is reduced to corresponding alcohols under the action of glutathione peroxidase 4 (Gpx4) (Bridges et al., 2012). Therefore, inhibition of system Xc- and Gpx4 can reduce Cystine uptake and GSH synthesis, leading to oxidative damage and even cell death. This process is also different from apoptosis and autophagy.
As a substrate for the synthesis of lipid signal mediators, the amount and location of polyunsaturated fatty acids (PUFA) determine the degree of lipid peroxidation in cells. The ferroptosis signal transmitted by PUFA depends on the esterification of membrane-forming phospholipids and oxidation (D'Herde et al., 2017). Acyl CoA synthase long chain family member 4 (ACSL4) and Lysophosphatidylcholine acyltransferase 3 (LPCAT3) are involved in the biosynthesis and remodeling of polyunsaturated fatty acid PE (PUFA-PE) in cell membrane. With the activation of ACSL4, the free PUFA could be esterified with the help of LPCAT3, and then bound to the membrane phospholipid to form PUFA-PE. Therefore, the up-regulation of ACSL4 is considered as a biomarker and contributor of ferroptosis. PUFA-PE can promote lipoxygenase (LOXs)-mediated enzymatic reaction to form lipid hydroperoxides. Therefore, the depletion of LOXs in cells can prevent ferroptosis induced by erastin (Yang et al., 2016).
Circulating iron exists in the form of ferric iron (Fe3+) by binding to transferrin. Fe3+ iron is imported into the cell via the membrane protein transferrin receptor 1 (TFR1) and then locates in the endosome. Then the Fe3+ iron is reduced to ferrous iron (Fe2+) by reductase in the endosome. The release of Fe2+ can be mediated by divalent metal transporter1 (DMT1) from the endosome into the cytoplasm that is a labile iron pool. Ferritin is an iron storage protein complex, where excessive iron is stored in. It includes ferritin light chain (FTL) and ferritin heavy chain1 (FTH1) (Harrison et al., 1996). FTH1 catalyzes the conversion of Fe2+ form into the Fe3+ form and then the Fe3+ iron is bound to the ferritin shell, thus reducing the level of free iron. Excessive iron can lead to ferroptosis by producing ROS through Fenton reaction (Wang et al., 2018).
There are eight subtypes of glutathione peroxidase (Gpx) in mammals, among which Gpx4 is the key regulator of ferroptosis (Brigelius-Flohe et al., 2013; Conrad et al., 2015). Gpx4, a single copy gene located on chromosome 19, was isolated and purified from pig liver by Ursini and colleagues in 1982 (Forcina et al., 2019; Ursini et al., 1982; Hirschhorn et al., 2019). It is a selenoprotein that can repair oxidative damage of lipid cells. Gpx4 is unique among Gpx subtypes as it is the only enzyme capable of reducing the esterified oxidized fatty acids and cholesterol hydroperoxides (Conrad et al., 2015). Gpx4 can convert intracellular toxic lipid hydrogen peroxide (L-OOH) into nontoxic lipid alcohol (L-OH), and promote the decomposition of hydrogen peroxide (H2O2), which can protect cell membrane from oxidative damage (Forcina et al., 2019). Therefore, Gpx4 is essential for preventing cell damage and maintaining tissue homeostasis (Wortmann et al., 2013). It was found that a large number of ferroptosis occurred in renal tubular cells when Gpx4 gene was knocked out (Friedmann et al., 2014). Specific blockade of Gpx4 can lead to destruction of muscles, neurons, and other cells, suggesting that Gpx4 is essential for survival of adult cells. In conclusion, inactivation of Gpx4 can lead to accumulation of lipid peroxide and ferroptosis, and Gpx4 plays a negative regulatory role in the process of ferroptosis (Forcina et al., 2019; Kinowaki et al., 2018).
System Xc− is an important intracellular antioxidant system. It is an amino acid transporter expressed on mammalian cell membrane, composed of two subunits, SLC7A11 and SLC3A2L. Intracellular glutamate is exchanged with extracellular cystine via system Xc− by the ratio of 1:1. Cystine is involved in the synthesis of GSH, an important intracellular free radical scavenger. Inhibition of system Xc− can lead to a rapid decrease in intracellular GSH level and rapid ferroptosis (Bridges et al., 2012; Dixon et al., 2012). Studies have also found that tumor suppressor p53 can inhibit the uptake of cystine by inhibiting SLC7A11, thus inducing ferroptosis (Jiang et al., 2015).
Nuclear factor erythroid 2-related factor 2 (Nrf2) is an important transcription factor regulating cellular oxidative stress response, and antioxidant response element (ARE) is a downstream signal molecule of Nrf2(Canning et al., 2015; Xie et al., 2016; Krajka-Kuźniak et al., 2017). After activation of Nrf2/ARE signaling pathway, a classical signaling pathway, a series of cell protective genes can be induced, such as heme oxygenase−1 (HO−1), nicotinamide adenine dinucleotide phosphate quinone oxidoreductase (NQO1), glutathione peroxidase (GSH−Px) and so on (Chen et al., 2018). Nrf2 plays a very important role in ferroptosis by regulating iron homeostasis and lipid peroxidation (Sun et al., 2016; Kerins et al., 2018; Dodson et al., 2019). Studies show that Nrf2/HO-1 signaling pathway can regulate anti-inflammatory, antioxidant stress, and ferroptosis, which plays a multi-organ protective role (Zhang et al., 2019; Jiang et al., 2020). Nrf2-keap1 pathway can change tumor microenvironment and affect tumor growth by up regulating xCT (SLC7A11 or system Xc−). Nrf2 overexpression or Keap1 knockout can inhibit ferroptosis, accelerate the proliferation of glioma cells, and reduce the survival rate of tumor patients, suggesting a potential target for tumor treatment (Sartori et al., 2010).
The clinical manifestations of ALI are characterized by diffuse pulmonary infiltration, refractory hypoxemia and respiratory distress. The pathological manifestations are injury of pulmonary capillary endothelial cells and alveolar epithelial cells, and diffuse alveolar and interstitial edema. As is well known, ALI can be caused by various extrapulmonary factors (such as sepsis, surgery, burns, fluid resuscitation, severe pancreatitis,etc.) and pulmonary factors (such as pulmonary inflammation, pulmonary contusion, aspiration,etc.). However, the confirmed pathogenesis of ALI is very complex, mainly involving the uncontrolled inflammatory reaction, the regulation of aquaporin, the imbalance of coagulation/fibrinolysis system, apoptosis, autophagy, pyrosis and so on (Sartori et al., 2010; Kovarova et al., 2012; Matthay et al., 2019).
Under normal circumstances, the lung relies on the phagocytosis of macrophages, transferrin in secretion, antioxidant molecules on the surface of respiratory tract epithelium, and respiratory ciliary expectoration system to maintain iron homeostasis. Once the protective mechanisms are destroyed by endogenous or exogenous factors, oxidative stress injury occurs in the lung (Turi et al., 2004). It was found that there was iron excess in the lower respiratory tract of ALI patients (Stites et al., 1999; Ghio et al., 2003). Iron accumulation can lead to inflammatory reaction, oxidative stress and mitochondrial dysfunction, and eventually cause lung damage through ferroptosis (Yoshida et al., 2019). Iron excess can also induce or aggravate hyperoxia-induced lung injury in patients with mechanical ventilation or diving operators, while intravenous deferoxamine can attenuate lung injury (Sha et al., 2019). Iron also plays an important role in hypoxia-induced lung injury. Iron supplementation can promote inflammatory response and oxidative stress, and aggravates lung injury induced by high altitude in rats (Salama et al., 2014). In addition, it has been found that the total GSH decreased and oxidized glutathione (GSSG) increased in alveolar epithelial lining fluid of ALI patients and animal models (Schmidt et al., 2004; Britt et al., 2014). In conclusion, in the pathological process of ALI, the release of various reactive oxygen species and the generation of free radicals can damage alveolar epithelial cells, and iron overload can further promote the conversion of hydrogen peroxide into free radicals through Fenton reaction, which increases the cytotoxicity, thus promoting the occurrence and development of ALI (Zhang et al., 2019). In conclusion, these studies suggest that iron metabolism and oxidative stress may involve in the pathogenesisof ALI. With the development of ferroptosis research, more and more studies have found that ferroptosis is involved in the pathogenesis of ALI. It has been confirmed that ferroptosis exists in some animal models or cell models of ALI.
Sepsis is a systemic inflammatory response syndrome caused by severe infection, with rapid progression and poor prognosis. ALI often occurs in the early stage of sepsis, but no effective treatments are currently available for it (Rubenfeld et al., 2005). Sepsis-induced lung injury is essentially an acute pathological injury of lung tissue caused by uncontrolled inflammatory reaction. In vitro (Liu et al., 2020), it was found that the expression of ferroptosis markers, SLC7A11, and GPx4, were down-regulated, while the levels of malondialdehyde (MDA) and total iron were significantly increased in a dose-dependent manner after lipopolysaccharide (LPS) intervention on the human bronchial epithelial cell line, BEAS-2B. Ferrostatin-1, an inhibitor of ferroptosis, could reverse the above effects, suggesting that ferroptosis played a very important role in the pathogenesis of LPS-induced ALI. In vivo (Yu et al., 2014; Liu et al., 2020), similar conclusions were drawn in ALI models established by intratracheal or intravenous injection of LPS. Ferroptosis may participate in LPS-induced ALI through Nrf2/ARE signaling pathway (Yu et al., 2014; Liu et al., 2020). Another study showed that HO−1 played a protective role in the pathogenesis of sepsis-induced lung injury, and artesunate could improve sepsis-induced lung injury by activating Nrf2 and promoting HO−1 expression (Luo et al., 2014; Cao et al., 2016; see Table 2). In conclusion, ferroptosis may be a potential therapeutic target for sepsis-induced lung injury, thus indicating the therapeutic potential of ferroptosis inhibitors for it.
Intestinal I/R injury can be caused by severe trauma, extensive burns, severe infection, shock, intestinal obstruction, cardiac surgery, etc. Once intestinal I/R injury occurs, intestinal mucosal barrier is destroyed, intestinal bacteria and toxins are translocated, and then a large number of cytokines and inflammatory mediators are released into blood circulation, leading to systemic inflammatory response and injury of distant organs. The lung is the earliest and most vulnerable organ, known as intestinal I/R-induced ALI, which plays a vital role in the development of multiple organ dysfunction syndrome (MODS) (de Perrot et al., 2003; Marco et al., 2007; Mörs et al., 2017; Li et al., 2020). So far, the pathogenesis of intestinal I/R-induced ALI has not been fully elucidated, and there is no specific medicine.
In the past, apoptosis was considered to be the main regulatory cell death mode in various ischemic injury models. However, in recent years, more and more studies have found that ferroptosis is the main driving factor of ischemic injury (Tonnus et al., 2017). Studies in vivo and in vitro have confirmed that ferroptosis occurs in type II alveolar epithelial cells of mice with intestinal I/R injury, and ferroptosis is involved in intestinal I/R-induced ALI (Dong et al., 2020; Li et al., 2020). Ferroptosis inhibitor ferrostatin-1 can improve intestinal I/R-induced ALI by alleviating pulmonary edema and inhibiting lipid peroxidation, while iron can reverse the above effects. It has been found that Nrf2 regulates ferroptosis by promoting the expression of HO−1 and SLC7A11, which plays a protective role in ferroptosis. Nrf2 may be a key regulator of intestinal I/R-induced ALI (Meng et al., 2016; Dong et al., 2020). Further research shows that iASPP can alleviate intestinal I/R-induced ALI and ferroptosis through Nrf2/HIF-1α/TF signaling pathway (Li et al., 2020; see Table 2). At present, the mechanism research is still in its infancy.
Intravenous injection of oleic acid is one of the methods to establish ALI models (Zhou et al., 2014). Once oleic acid microbubbles enter pulmonary capillaries, it results in pulmonary vascular congestion, increased capillary permeability and pulmonary interstitial edema, which is consistent with the pathological changes of ALI (Lee et al., 2014). In mice injected with oleic acid, iron overload, decrease in glutathione levels, GPx4 (a marker of ferroptosis) and ferritin, and increase in MDA content, were observed in lung tissues, accompanied with morphological changes of ferroptosis such as mitochondrial wrinkle and mitochondrial membrane rupture (Zhou et al., 2019; see Table 2). However, the specific molecular signaling pathways remain unclear.
The incidence of RILI was 16.7–50.3%, which increased the mortality and disability of lung cancer patients (S N et al., 2019). Oxidative damage of lung tissue, induced by a large amount of Reactive Oxygen Species (ROS) produced by radiation, is a key factor in the pathogenesis of RILI(Li et al., 2019). It has been found that ferroptosis played an important role in RILI, and ferroptosis inhibitor significantly reduced ROS in lung tissue and inflammatory factors in serum (Li et al., 2019; see Table 2).
Ferroptosis is characterized by a large amount of iron accumulation and lipid peroxidation. Accordingly, the therapeutic targets for ferroptosis should focus on inhibiting iron metabolism and lipid peroxidation. Iron metabolism inhibitors and iron chelators, such as deferoxamine (DFO), can inhibit ferroptosis by inhibiting iron uptake (Wang et al., 2020). Inhibitors of lipid metabolism inhibit polyunsaturated fatty acids (PUFA) incorporation into phospholipid membranes, such as thiazolidinediones and knockdown of long-chain acyl-CoA synthetases (ACSL4) (Dixon et al., 2015; Doll et al., 2017). Ferroptosis inhibitor ferrostatin-1 can improve intestinal I/R-induced ALI by inhibiting lipid peroxidation and alleviating pulmonary edema (Dong et al., 2020). It can also alleviate sepsis-induced ALI by promoting the expression of SLC7A11 and GPx4 and reducing the levels of MDA and iron in lung tissue (Liu et al., 2020). Another ferroptosis inhibitor, lipoxstatin-1, can promote the expression of GSH and GPx4, and reduce the content of MDA, iron and transferrin. In addition, lipoxstatin-1 can reverse the effect of erastin on promoting ferroptosis, and alleviate intestinal I/R-induced ALI, suggesting the therapeutic potential of lipoxstatin-1 (Li et al., 2020). IASPP, which is a known inhibitor of p53 transcriptional activity, mainly exists in the cytoplasm. IASPP inhibits ferroptosis through Nrf2/HIF-1α/TF signaling pathway and plays a protective role in intestinal I/R-induced ALI, as demonstrated in MLE-2 cells (Li et al., 2020). Although these ferroptosis inhibitors have been proved to have the effect of improving ALI, they are still in animal models and/or in vitro studies, lack of clinical evidence (see Table 3).
As a new form of programmed cell death, ferroptosis is known to mainly involve in tumor, nervous system diseases, I/R injury and acute kidney injury. In recent years, more and more studies have found that ferroptosis is also involved in the pathogenesis of ALI. Ferroptosis has been confirmed in many ALI animal models or cell models, but its specific mechanism has not been fully elucidated. Ferroptosis may be a target for the treatment of ALI, focusing on the inhibition of iron metabolism and lipid peroxidation. Ferrostatin-1, lipoxstatin-1 and iASPP have been proved to have the effects on inhibiting ferroptosis and protecting ALI. However, they only stay in animal models and/or in vitro studies, lack of clinical evidence. In the future, more comprehensive and in-depth scientific researches are needed to further explore the relationship between ferroptosis and ALI, to provide more theoretical basis for clinical work.
All authors listed have made a substantial, direct, and intellectual contribution to the work and approved it for publication.
The authors declare that the research was conducted in the absence of any commercial or financial relationships that could be construed as a potential conflict of interest.
All claims expressed in this article are solely those of the authors and do not necessarily represent those of their affiliated organizations, or those of the publisher, the editors and the reviewers. Any product that may be evaluated in this article, or claim that may be made by its manufacturer, is not guaranteed or endorsed by the publisher.
Amaral, E. P., Costa, D. L., Namasivayam, S., Riteau, N., Kamenyeva, O., Mittereder, L., et al. (2019). A Major Role for Ferroptosis in Mycobacterium Tuberculosis-Induced Cell Death and Tissue Necrosis. J. Exp. Med. 216 (3), 556–570. doi:10.1084/jem.20181776
Bridges, R. J., Natale, N. R., and Patel, S. A. (2012). System Xc- Cystine/glutamate Antiporter: an Update on Molecular Pharmacology and Roles within the CNS. Br. J. Pharmacol. 165 (1), 20–34. doi:10.1111/j.1476-5381.2011.01480.x
Brigelius-Flohé, R., and Maiorino, M. (2013). Glutathione Peroxidases. Biochim. Biophys. Acta (Bba) - Gen. Subjects 1830 (5), 3289–3303. doi:10.1016/j.bbagen.2012.11.020
Britt, R. D., Velten, M., Locy, M. L., Rogers, L. K., and Tipple, T. E. (2014). The Thioredoxin Reductase-1 Inhibitor Aurothioglucose Attenuates Lung Injury and Improves Survival in a Murine Model of Acute Respiratory Distress Syndrome. Antioxid. Redox Signaling 20 (17), 2681–2691. doi:10.1089/ars.2013.5332
Canning, P., Sorrell, F. J., and Bullock, A. N. (2015). Structural Basis of Keap1 Interactions with Nrf2. Free Radic. Biol. Med. 88 (Pt B), 101–107. doi:10.1016/j.freeradbiomed.2015.05.034
Cao, T.-h., Jin, S.-g., Fei, D.-s., Kang, K., Jiang, L., Lian, Z.-y., et al. (2016). Artesunate Protects against Sepsis-Induced Lung Injury via Heme Oxygenase-1 Modulation. Inflammation 39 (2), 651–662. doi:10.1007/s10753-015-0290-2
Chen, L., Li, X., Liu, L., Yu, B., Xue, Y., and Liu, Y. (2015). Erastin Sensitizes Glioblastoma Cells to Temozolomide by Restraining xCT and Cystathionine-γ-Lyase Function. Oncol. Rep. 33 (3), 1465–1474. doi:10.3892/or.2015.3712
Chen, Q. M., and Maltagliati, A. J. (2018). Nrf2 at the Heart of Oxidative Stress and Cardiac protection. Physiol. Genomics 50 (2), 77–97. doi:10.1152/physiolgenomics.00041.2017
Conrad, M., and Friedmann Angeli, J. P. (2015). Glutathione Peroxidase 4 (Gpx4) and Ferroptosis: What's So Special about it? Mol. Cell Oncol. 2 (3), e995047. doi:10.4161/23723556.2014.995047
D'Herde, K., and Krysko, D. V. (2017). Oxidized PEs Trigger Death. Nat. Chem. Biol. 13 (1), 4–5. doi:10.1038/nchembio.2261
Dar, H. H., Tyurina, Y. Y., Mikulska-Ruminska, K., Shrivastava, I., Ting, H.-C., Tyurin, V. A., et al. (2018). Pseudomonas aeruginosa Utilizes Host Polyunsaturated Phosphatidylethanolamines to Trigger Theft-Ferroptosis in Bronchial Epithelium. J. Clin. Invest. 128 (10), 4639–4653. doi:10.1172/JCI99490
de Perrot, M., Liu, M., Waddell, T. K., and Keshavjee, S. (2003). Ischemia-reperfusion-induced Lung Injury. Am. J. Respir. Crit. Care Med. 167 (4), 490–511. doi:10.1164/rccm.200207-670so
Dixon, S. J., Lemberg, K. M., Lamprecht, M. R., Skouta, R., Zaitsev, E. M., Gleason, C. E., et al. (2012). Ferroptosis: an Iron-dependent Form of Nonapoptotic Cell Death. Cell 149 (5), 1060–1072. doi:10.1016/j.cell.2012.03.042
Dixon, S. J., Winter, G. E., Musavi, L. S., Lee, E. D., Snijder, B., Rebsamen, M., et al. (2015). Human Haploid Cell Genetics Reveals Roles for Lipid Metabolism Genes in Nonapoptotic Cell Death. ACS Chem. Biol. 10 (7), 1604–1609. doi:10.1021/acschembio.5b00245
Dodson, M., Castro-Portuguez, R., and Zhang, D. D. (2019). NRF2 Plays a Critical Role in Mitigating Lipid Peroxidation and Ferroptosis. Redox Biol. 23, 101107. doi:10.1016/j.redox.2019.101107
Doll, S., Proneth, B., Tyurina, Y. Y., Panzilius, E., Kobayashi, S., Ingold, I., et al. (2017). ACSL4 Dictates Ferroptosis Sensitivity by Shaping Cellular Lipid Composition. Nat. Chem. Biol. 13 (1), 91–98. doi:10.1038/nchembio.2239
Dong, H., Qiang, Z., Chai, D., Peng, J., Xia, Y., Hu, R., et al. (2020). Nrf2 Inhibits Ferroptosis and Protects against Acute Lung Injury Due to Intestinal Ischemia Reperfusion via Regulating SLC7A11 and HO-1. Aging 12 (13), 12943–12959. doi:10.18632/aging.103378
Fan, Z., Wirth, A.-K., Chen, D., Wruck, C. J., Rauh, M., Buchfelder, M., et al. (2017). Nrf2-Keap1 Pathway Promotes Cell Proliferation and Diminishes Ferroptosis. Oncogenesis 6 (8), e371. doi:10.1038/oncsis.2017.65
Forcina, G. C., and Dixon, S. J. (2019). GPX4 at the Crossroads of Lipid Homeostasis and Ferroptosis. Proteomics 19 (18), 1800311. doi:10.1002/pmic.201800311
Friedmann Angeli, J. P., Schneider, M., Proneth, B., Tyurina, Y. Y., Tyurin, V. A., Hammond, V. J., et al. (2014). Inactivation of the Ferroptosis Regulator Gpx4 Triggers Acute Renal Failure in Mice. Nat. Cel Biol 16 (12), 1180–1191. doi:10.1038/ncb3064
Ghio, A. J., Carter, J. D., Richards, J. H., Richer, L. D., Grissom, C. K., and Elstad, M. R. (2003). Iron and Iron-Related Proteins in the Lower Respiratory Tract of Patients with Acute Respiratory Distress Syndrome. Crit. Care Med. 31 (2), 395–400. doi:10.1097/01.ccm.0000050284.35609.97
Han, C., Liu, Y., Dai, R., Ismail, N., Su, W., and Li, B. (2020). Ferroptosis and its Potential Role in Human Diseases. Front. Pharmacol. 11, 239. doi:10.3389/fphar.2020.00239
Harrison, P. M., and Arosio, P. (1996). The Ferritins: Molecular Properties, Iron Storage Function and Cellular Regulation. Biochim. Biophys. Acta (Bba) - Bioenerg. 1275 (3), 161–203. doi:10.1016/0005-2728(96)00022-9
Hirschhorn, T., and Stockwell, B. R. (2019). The Development of the Concept of Ferroptosis. Free Radic. Biol. Med. 133, 130–143. doi:10.1016/j.freeradbiomed.2018.09.043
Hu, Z., Zhang, H., Yang, S.-k., Wu, X., He, D., Cao, K., et al. (2019). Emerging Role of Ferroptosis in Acute Kidney Injury. Oxidative Med. Cell Longevity 2019, 1–8. doi:10.1155/2019/8010614
Jiang, L., Kon, N., Li, T., Wang, S.-J., Su, T., Hibshoosh, H., et al. (2015). Ferroptosis as a P53-Mediated Activity during Tumour Suppression. Nature 520 (7545), 57–62. doi:10.1038/nature14344
Jiang, T., Cheng, H., Su, J., Wang, X., Wang, Q., Chu, J., et al. (2020). Gastrodin Protects against Glutamate-Induced Ferroptosis in HT-22 Cells through Nrf2/HO-1 Signaling Pathway. Toxicol. Vitro 62, 104715. doi:10.1016/j.tiv.2019.104715
Kazan, K., and Kalaipandian, S. (2019). Ferroptosis: Yet Another Way to Die. Trends Plant Sci. 24 (6), 479–481. doi:10.1016/j.tplants.2019.03.005
Kerins, M. J., and Ooi, A. (2018). The Roles of NRF2 in Modulating Cellular Iron Homeostasis. Antioxid. Redox Signaling 29 (17), 1756–1773. doi:10.1089/ars.2017.7176
Kinowaki, Y., Kurata, M., Ishibashi, S., Ikeda, M., Tatsuzawa, A., Yamamoto, M., et al. (2018). Glutathione Peroxidase 4 Overexpression Inhibits ROS-Induced Cell Death in Diffuse Large B-Cell Lymphoma. Lab. Invest. 98 (5), 609–619. doi:10.1038/s41374-017-0008-1
Kovarova, M., Hesker, P. R., Jania, L., Nguyen, M., Snouwaert, J. N., Xiang, Z., et al. (2012). NLRP1-dependent Pyroptosis Leads to Acute Lung Injury and Morbidity in Mice. J.I. 189 (4), 2006–2016. doi:10.4049/jimmunol.1201065
Krajka-Kuźniak, V., Paluszczak, J., and Baer-Dubowska, W. (2017). The Nrf2-ARE Signaling Pathway: an Update on its Regulation and Possible Role in Cancer Prevention and Treatment. Pharmacol. Rep. 69 (3), 393–402. doi:10.1016/j.pharep.2016.12.011
Lee, S. M., Choi, H., Yang, G., Park, K. C., Jeong, S., and Hong, S. (2014). MicroRNAs Mediate Oleic Acid-Induced Acute Lung Injury in Rats Using an Alternative Injury Mechanism. Mol. Med. Rep. 10 (1), 292–300. doi:10.3892/mmr.2014.2155
Li, J., Cao, F., Yin, H.-l., Huang, Z.-j., Lin, Z.-t., Mao, N., et al. (2020). Ferroptosis: Past, Present and Future. Cell Death Dis 11 (2), 88. doi:10.1038/s41419-020-2298-2
Li, J., Lu, K., Sun, F., Tan, S., Zhang, X., Sheng, W., et al. (2021). Panaxydol Attenuates Ferroptosis against LPS-Induced Acute Lung Injury in Mice by Keap1-Nrf2/HO-1 Pathway. J. Transl Med. 19 (1), 96. doi:10.1186/s12967-021-02745-1
Li, X., Zhuang, X., and Qiao, T. (2019). Role of Ferroptosis in the Process of Acute Radiation-Induced Lung Injury in Mice. Biochem. Biophysical Res. Commun. 519 (2), 240–245. doi:10.1016/j.bbrc.2019.08.165
Li, Y., Cao, Y., Xiao, J., Shang, J., Tan, Q., Ping, F., et al. (2020). Inhibitor of Apoptosis-Stimulating Protein of P53 Inhibits Ferroptosis and Alleviates Intestinal Ischemia/reperfusion-Induced Acute Lung Injury. Cell Death Differ 27 (9), 2635–2650. doi:10.1038/s41418-020-0528-x
Linkermann, A., Skouta, R., Himmerkus, N., Mulay, S. R., Dewitz, C., De Zen, F., et al. (2014). Synchronized Renal Tubular Cell Death Involves Ferroptosis. Proc. Natl. Acad. Sci. USA 111 (47), 16836–16841. doi:10.1073/pnas.1415518111
Liu, P., Feng, Y., Li, H., Chen, X., Wang, G., Xu, S., et al. (2020). Ferrostatin-1 Alleviates Lipopolysaccharide-Induced Acute Lung Injury via Inhibiting Ferroptosis. Cell Mol Biol Lett 25, 10. doi:10.1186/s11658-020-00205-0
Liu, X., Wang, L., Xing, Q., Li, K., SiMa, J. X., Ma, X., et al. (2021). Sevoflurane Inhibits Ferroptosis: A New Mechanism to Explain its Protective Role against Lipopolysaccharide-Induced Acute Lung Injury. Life Sci. 275, 119391. doi:10.1016/j.lfs.2021.119391
Luo, Y.-p., Jiang, L., Kang, K., Fei, D.-s., Meng, X.-l., Nan, C.-c., et al. (2014). Hemin Inhibits NLRP3 Inflammasome Activation in Sepsis-Induced Acute Lung Injury, Involving Heme Oxygenase-1. Int. Immunopharmacology 20 (1), 24–32. doi:10.1016/j.intimp.2014.02.017
Mao, H., Zhao, Y., Li, H., and Lei, L. (2020). Ferroptosis as an Emerging Target in Inflammatory Diseases. Prog. Biophys. Mol. Biol. 155, 20–28. doi:10.1016/j.pbiomolbio.2020.04.001
Matthay, M. A., Zemans, R. L., Zimmerman, G. A., Arabi, Y. M., Beitler, J. R., Mercat, A., et al. (2019). Acute Respiratory Distress Syndrome. Nat. Rev. Dis. Primers 5 (1), 18. doi:10.1038/s41572-019-0069-0
Meng, Q.-T., Cao, C., Wu, Y., Liu, H.-M., Li, W., Sun, Q., et al. (2016). Ischemic post-conditioning Attenuates Acute Lung Injury Induced by Intestinal Ischemia-Reperfusion in Mice: Role of Nrf2. Lab. Invest. 96 (10), 1087–1104. doi:10.1038/labinvest.2016.87
Mörs, K., Hörauf, J.-A., Kany, S., Wagner, N., Sturm, R., Woschek, M., et al. (2017). Ethanol Decreases Inflammatory Response in Human Lung Epithelial Cells by Inhibiting the Canonical NF-kB-Pathway. Cell Physiol Biochem 43 (1), 17–30. doi:10.1159/000480313
Mou, Y., Wang, J., Wu, J., He, D., Zhang, C., Duan, C., et al. (2019). Ferroptosis, a New Form of Cell Death: Opportunities and Challenges in Cancer. J. Hematol. Oncol. 12 (1), 34. doi:10.1186/s13045-019-0720-y
Marco, M., Andrade, C. F., Han, B., Seth, R., Zhang, Y., Bai, X. H., et al. (2007). Intestinal Ischemia-Reperfusion-Induced Acute Lung Injury and Oncotic Cell Death in Multiple Organs. Shock 28 (2), 227–238. doi:10.1097/01.shk.0000278497.47041.e3
Mutlu, G. M., and Budinger, G. R. (2006). Incidence and Outcomes of Acute Lung Injury. N. Engl. J. Med. 354 (4), 416–417. doi:10.1056/NEJMc053159
Rubenfeld, G. D., Caldwell, E., Peabody, E., Weaver, J., Martin, D. P., Neff, M., et al. (2005). Incidence and Outcomes of Acute Lung Injury. N. Engl. J. Med. 353 (16), 1685–1693. doi:10.1056/NEJMoa050333
Salama, S. A., Omar, H. A., Maghrabi, I. A., AlSaeed, M. S., and El-Tarras, A. E. (2014). Iron Supplementation at High Altitudes Induces Inflammation and Oxidative Injury to Lung Tissues in Rats. Toxicol. Appl. Pharmacol. 274 (1), 1–6. doi:10.1016/j.taap.2013.10.034
Sartori, C., Rimoldi, S. F., and Scherrer, U. (2010). Lung Fluid Movements in Hypoxia. Prog. Cardiovasc. Dis. 52 (6), 493–499. doi:10.1016/j.pcad.2010.02.005
Schmidt, R., Luboeinski, T., Markart, P., Ruppert, C., Daum, C., Grimminger, F., et al. (2004). Alveolar Antioxidant Status in Patients with Acute Respiratory Distress Syndrome. Eur. Respir. J. 24 (6), 994–999. doi:10.1183/09031936.04.00120703
Sha, X. L., Li, Y., Wang, X., Wang, J., and Chen, S. (2019). Effect of Deferoxamine on Pulmonary Surfactant Protein D and Antioxidant Enzymes in Rats Exposed to Hyperoxia Ventilation. J. Clin. Anesthesiol 35 (10), 1002–1005. doi:10.12089/jca.2019.10.016
S. N., S. G., Raviraj, R., Nagarajan, D., Zhao, W., and Zhao, W. (2019). Radiation-induced Lung Injury: Impact on Macrophage Dysregulation and Lipid Alteration - a Review. Immunopharmacology and Immunotoxicology 41 (3), 370–379. doi:10.1080/08923973.2018.1533025
Stites, S. W., Plautz, M. W., Bailey, K., O'Brien-Ladner, A. R., and Wesselius, L. J. (1999). Increased Concentrations of Iron and Isoferritins in the Lower Respiratory Tract of Patients with Stable Cystic Fibrosis. Am. J. Respir. Crit. Care Med. 160 (3), 796–801. doi:10.1164/ajrccm.160.3.9811018
Stockwell, B. R., Friedmann Angeli, J. P., Bayir, H., Bush, A. I., Conrad, M., Dixon, S. J., et al. (2017). Ferroptosis: a Regulated Cell Death Nexus Linking Metabolism, Redox Biology, and Disease. Cell 171 (2), 273–285. doi:10.1016/j.cell.2017.09.021
Sun, X., Ou, Z., Chen, R., Niu, X., Chen, D., Kang, R., et al. (2016). Activation of the P62-Keap1-NRF2 Pathway Protects against Ferroptosis in Hepatocellular Carcinoma Cells. Hepatology 63 (1), 173–184. doi:10.1002/hep.2825110.1002/hep.28251
Tonnus, W., and Linkermann, A. (2017). The In Vivo Evidence for Regulated Necrosis. Immunol. Rev. 277 (1), 128–149. doi:10.1111/imr.12551
Turi, J. L., Yang, F., Garrick, M. D., Piantadosi, C. A., and Ghio, A. J. (2004). The Iron Cycle and Oxidative Stress in the Lung. Free Radic. Biol. Med. 36 (7), 850–857. doi:10.1016/j.freeradbiomed.2003.12.008
Ursini, F., Maiorino, M., Valente, M., Ferri, L., and Gregolin, C. (1982). Purification from Pig Liver of a Protein Which Protects Liposomes and Biomembranes from Peroxidative Degradation and Exhibits Glutathione Peroxidase Activity on Phosphatidylcholine Hydroperoxides. Biochim. Biophys. Acta (Bba) - Lipids Lipid Metab. 710 (2), 197–211. doi:10.1016/0005-2760(82)90150-3
Wang, C., Yuan, W., Hu, A., Lin, J., Xia, Z., Yang, C., et al. (2020). Dexmedetomidine Alleviated Sepsis-induced M-yocardial F-erroptosis and S-eptic H-eart I-njury. Mol. Med. Rep. 22 (1), 175–184. doi:10.3892/mmr.2020.11114
Wang, S., Luo, J., Zhang, Z., Dong, D., Shen, Y., and Fang, Y. (2018). Iron and Magnetic: New Research Direction of the Ferroptosis-Based Cancer Therapy. Am. J. Cancer Res. 8 (10), 1933–1946.
Ware, L. B., and Matthay, M. A. (2000). The Acute Respiratory Distress Syndrome. N. Engl. J. Med. 342 (18), 1334–1349. doi:10.1056/NEJM200005043421806
Wortmann, M., Schneider, M., Pircher, J., Hellfritsch, J., Aichler, M., Vegi, N., et al. (2013). Combined Deficiency in Glutathione Peroxidase 4 and Vitamin E Causes Multiorgan Thrombus Formation and Early Death in Mice. Circ. Res. 113 (4), 408–417. doi:10.1161/CIRCRESAHA.113.279984
Wu, Y., Zhang, S., Gong, X., Tam, S., Xiao, D., Liu, S., et al. (2020). The Epigenetic Regulators and Metabolic Changes in Ferroptosis-Associated Cancer Progression. Mol. Cancer 19 (1), 39. doi:10.1186/s12943-020-01157-x
Xie, Y., Hou, W., Song, X., Yu, Y., Huang, J., Sun, X., et al. (2016). Ferroptosis: Process and Function. Cel Death Differ 23 (3), 369–379. doi:10.1038/cdd.2015.158
Yang, W. S., Kim, K. J., Gaschler, M. M., Patel, M., Shchepinov, M. S., and Stockwell, B. R. (2016). Peroxidation of Polyunsaturated Fatty Acids by Lipoxygenases Drives Ferroptosis. Proc. Natl. Acad. Sci. USA 113 (34), E4966–E4975. doi:10.1073/pnas.1603244113
Yoshida, M., Minagawa, S., Araya, J., Sakamoto, T., Hara, H., Tsubouchi, K., et al. (2019). Involvement of Cigarette Smoke-Induced Epithelial Cell Ferroptosis in COPD Pathogenesis. Nat. Commun. 10 (1), 3145. doi:10.1038/s41467-019-10991-7
Yu, J. B., Shi, J., Gong, L. R., Dong, S. A., Xu, Y., Zhang, Y., et al. (2014). Role of Nrf2/ARE Pathway in Protective Effect of Electroacupuncture against Endotoxic Shock-Induced Acute Lung Injury in Rabbits. PLoS One 9 (8), e104924. doi:10.1371/journal.pone.0104924
Zhang, V., Nemeth, E., and Kim, A. (2019). Iron in Lung Pathology. Pharmaceuticals 12 (1), 30. doi:10.3390/ph12010030
Zhang, X., Ding, M., Zhu, P., Huang, H., Zhuang, Q., Shen, J., et al. (2019). New Insights into the Nrf-2/HO-1 Signaling Axis and its Application in Pediatric Respiratory Diseases. Oxidative Med. Cell Longevity 2019, 1–9. doi:10.1155/2019/3214196
Zhou, H., Li, F., Niu, J. Y., Zhong, W. Y., Tang, M. Y., and Lin, D. (2019). Ferroptosis Was Involved in the Oleic Acid-Induced Acute Lung Injury in Mice. Sheng Li Xue Bao. 71 (5), 689–697.
Keywords: ferroptosis, acute lung injury, iron metabolism, lipid peroxidation, ferrostatin-1, lipoxstatin-1, iASPP
Citation: Yin X, Zhu G, Wang Q, Fu YD, Wang J and Xu B (2021) Ferroptosis, a New Insight Into Acute Lung Injury. Front. Pharmacol. 12:709538. doi: 10.3389/fphar.2021.709538
Received: 14 May 2021; Accepted: 22 July 2021;
Published: 02 August 2021.
Edited by:
Johnatas Dutra Silva, Queen’s University Belfast, United KingdomReviewed by:
Chunbin Zou, University of Pittsburgh, United StatesCopyright © 2021 Yin, Zhu, Wang, Fu, Wang and Xu. This is an open-access article distributed under the terms of the Creative Commons Attribution License (CC BY). The use, distribution or reproduction in other forums is permitted, provided the original author(s) and the copyright owner(s) are credited and that the original publication in this journal is cited, in accordance with accepted academic practice. No use, distribution or reproduction is permitted which does not comply with these terms.
*Correspondence: Biao Xu, MjAxODUwMzFAbmp1Y20uZWR1LmNu
†These authors have contributed equally to this work
Disclaimer: All claims expressed in this article are solely those of the authors and do not necessarily represent those of their affiliated organizations, or those of the publisher, the editors and the reviewers. Any product that may be evaluated in this article or claim that may be made by its manufacturer is not guaranteed or endorsed by the publisher.
Research integrity at Frontiers
Learn more about the work of our research integrity team to safeguard the quality of each article we publish.