- 1Department of Pharmacology, School of Clinical Medicine, Faculty of Health Sciences, University of the Free State, Bloemfontein, South Africa
- 2Department of Biochemistry, School of Life Sciences, University of KwaZulu-Natal, (Westville Campus), Durban, South Africa
- 3Department of Pharmacology, School of Science and Technology, Nottingham Trent University, Nottingham, UK
- 4Department of Pure and Industrial Chemistry, Faculty of Physical Sciences, University of Nigeria, Nsukka, Nigeria
Cannabis sativa L. is a crop utilized globally for recreational, therapeutic, and religious purposes. Although considered as an illicit drug in most countries, C. sativa until recently started gaining attention for its medicinal application. This study sought to investigate the hepatoprotective effect of C. sativa on iron-mediated oxidative hepatic injury. Hepatic injury was induced ex vivo by incubating hepatic tissues with Fe2+, which led to depleted levels of reduced glutathione, superoxide dismutase, catalase and ENTPDase activities, triglyceride, and high-density lipoprotein–cholesterol (HDL-C). Induction of hepatic injury also caused significant elevation of malondialdehyde, nitric oxide, cholesterol, and low-density lipoprotein–cholesterol (LDL-C) levels while concomitantly elevating the activities of ATPase, glycogen phosphorylase, glucose-6-phosphatase, fructose-1,6-bisphosphatase, amylase, and lipase. Treatment with the hexane, dichloromethane (DCM), and ethanol extracts of C. sativa leaves significantly (p < 0.05) reversed these levels and activities to almost near normal. However, there was no significant effect on the HDL-C level. The extracts also improved the utilization of glucose in Chang liver cells. High-performance liquid chromatography (HPLC) analysis showed the presence of phenolics in all extracts, with the ethanol extract having the highest constituents. Cannabidiol (CBD) was identified in all the extracts, while Δ-9-tetrahydrocannabinol (Δ-9-THC) was identified in the hexane and DCM extracts only. Molecular docking studies revealed strong interactions between CBD and Δ-9-THC with the β2 adrenergic receptor of the adrenergic system. The results demonstrate the potential of C. sativa to protect against oxidative-mediated hepatic injury by stalling oxidative stress, gluconeogenesis, and hepatic lipid accumulation while modulating cholinergic and purinergic activities. These activities may be associated with the synergistic effect of the compounds identified and possible interactions with the adrenergic system.
Introduction
The roles of the liver in the metabolic functions of the body are well documented (Abdel-Salam et al., 2014; Seif, 2016). The liver is among the prominent organs in the body required for maintaining and regulating the body’s homeostasis, thus playing a key role in the detoxification and excretion of endogenous and exogenous compounds as well as carbohydrate, protein, and fat metabolism (Pandit et al., 2012; Seif, 2016). The liver has also been reported to play a principal role in iron homeostasis as well as storing excess iron (Anderson and Frazer, 2005; Rishi and Subramaniam, 2017).
Iron is regarded as one of the essential elements owing to its roles in the body leading to optimal health. It is involved in several metabolic reactions and is a cofactor for enzymes involved in most physiological functions due to its ability to assume two different ionic states [ferrous (Fe2+) and ferric (Fe3+)] (Rishi and Subramaniam, 2017). This ability also makes it an influential regulator of the cell’s redox homeostatic state (Andrews, 1999; Erukainure et al., 2017). However, dysregulation of iron homeostasis leading to excessive increase in hepatic (liver) iron storage has been implicated in exacerbated production of free radicals (Anderson and Shah, 2013). This increased production causes an imbalance in the redox state owing to suppression of the tissues’ intrinsic antioxidant defense system, which consequently leads to oxidative hepatic injury. Oxidative injury has been implicated as an important mechanism in the pathogenesis and progression of several hepatic diseases such as cirrhosis, hepatitis, hepatocellular carcinoma, and fibrosis (Anderson and Shah, 2013; Milic et al., 2016).
Medicinal plants have been utilized in ancient history in the folkloric treatment and management of liver diseases and several ailments such as diabetes, cancer, and malaria. Folkloric medicine and its method of treatment are often correlated with the tradition and culture of the community where the plants are indigenous, thus contributing to the cultural heritage and health system of the community. The efficacies of these plants can be associated with their phytochemical and nutritional components (Chukwuma et al., 2019). Among such plants is Cannabis sativa L.
A member of the Cannabis genus and the Cannabaceae family, C. sativa popularly referred to as marijuana, Indian hemp, and weed is an annual herbaceous plant utilized for recreational, therapeutic, and religious purposes as well as for food (Kuddus et al., 2013; Bonini et al., 2018). It is globally widespread, with Africa reported for its highest production, as it accounts for over 25% of the global production (UNODC, 2012). Its major phytochemical constituents consist of phytocannabinoids, which are a class of C21–C22 terpenophenolic compounds, with tetrahydrocannabinol (THC), cannabinol (CBN), and cannabidiol (CBD) being the most common (Andre et al., 2016). Its use in traditional medicine has been reported for treating a number of ailments including diabetes, liver diseases, cancer, and neurological disorders particularly in Chinese and Indian traditional medicine (Russo, 2005; Brand and Zhao, 2017; Ryz et al., 2017). These are supported by a number of studies that have shown the therapeutic properties of C. sativa and its constituents, which include anticancer activity (Guzman, 2003), antidiabetic activity (Ren et al., 2016), pain suppression (Whiting et al., 2015), anti-neurodegeneration activity (Aso and Ferrer, 2016), sleep management (Ramar et al., 2018), protection from liver diseases (Musa et al., 2012; Hussein et al., 2014), anti-colitis activity, and anti-epilepsy activity (Fusar-Poli et al., 2009). Despite its medicinal properties, C. sativa is considered an illicit drug in most countries but has recently started gaining attention for its medicinal application.
This study sought out to investigate the hepatoprotective potential of C. sativa leaves by determining their ability to suppress oxidative stress and hepatic lipid level, as well as their effect on carbohydrate metabolism, purinergic activities, and cholinergic dysfunction in ferric-mediated oxidative hepatic injury.
Materials and Methods
Plant Material
This research has been undertaken with the approval (Permit No. POS 248/2019/2020) of the South African Health Products Regulatory Authority to conduct, collect, possess, transport, and store cannabis plant, plant parts, and products for research purposes. The study was also conducted to collect cannabis plants in Lesotho under the permit (Permit #: 01/LS/2019/10/02-01).
The leaves of C. sativa were obtained from Mohale’s Hoek District, Lesotho (GPS coordinates: 30.333,776″S and 27.651,201″E). The plant was authenticated by the Geo Potts Herbarium at the University of the Free State, Bloemfontein 9300, South Africa, and assigned the voucher number BLFU MGM 0018. This was further verified in the plant list online database (http://www.theplantlist.org/tpl1.1/search?q=Cannabis+Sativa+L).
The leaves were air-dried and pulverized to dry powder before undergoing sequential extraction using solvents of increasing polarity, namely, hexane, dichloromethane (DCM), and ethanol, for 48 h with gentle agitation of 100 rpm at room temperature. Each solvent was decanted and concentrated in vacuo using an R-215 rotary evaporator (Buchi, Flawil, Switzerland). The extracts were collected in glass vials and stored in the dark at ambient room temperature.
Animals
Five male albino rats (Sprague Dawley strain) that weighed between 180 and 200 g were obtained from the Biomedical Research Unit (BRU), University of KwaZulu-Natal, Durban, South Africa. The animals were housed in plastic cages, fed on pelletized chows, and provided with water ad libitum, all under a 12-h light-dark cycle of natural photoperiod.
Rats were deprived of food (overnight fasting) 12 h prior to sacrifice. They were humanely sacrificed by euthanizing with isoform. Their livers were harvested, and bloodstains were removed by rinsing in 0.9% NaCl solution and then homogenized in 50 mM of sodium phosphate buffer, pH 7.5 (with 1% Triton X-100). The homogenized samples were then centrifuged at 15,000 rpm for 10 min at 4°C. The supernatant was collected and used immediately for ex vivo studies.
Rats were maintained under the approved guidelines of the Animal Ethics Committee of the University of KwaZulu-Natal, Durban, South Africa, and the study received ethical approval from the University of KwaZulu-Natal Animal Ethics Committee (Protocol approval number: AREC/22/019D).
Induction of Oxidative Injury
Oxidative injury was induced in vitro using earlier established protocols with slight modifications (Akomolafe et al., 2013; Kizil et al., 2010). Briefly, 100 µl of the hepatic tissue supernatant was incubated with 15–240 μg/ml each of hexane, DCM, and ethanol extracts in the presence of 0.1 mM of FeSO4 at 37°C for 30 min. CBD was used as a reference drug, as it is the main non-psychoactive compound in C. sativa. Reactions without the extract or reference drug represented negative control (untreated). The normal control consisted of hepatic tissue homogenates without extracts, reference drugs, and FeSO4. The choice of doses was based on previous reports on the hepatoprotective properties of medicinal plants (Sanni et al., 2018).
Antioxidative Activities
The hepatic tissue was analyzed for levels of reduced glutathione (GSH) (Ellman, 1959), catalase and superoxide dismutase (SOD) activities (Chance and Maehly, 1955; Kakkar et al., 1984), and malondialdehyde (MDA) level (Chowdhury and Soulsby, 2002).
Nitric Oxide Level
The level of nitric oxide (NO) in the hepatic tissues was determined using the Griess method (Tsikas, 2005; Erukainure et al., 2019).
Cholinergic Enzyme Activities
The cholinergic status of the hepatic tissues was estimated using Ellman’s procedure to analyze the activity of acetylcholinesterase (Ellman et al., 1961).
Purinergic Enzyme Activities
The purinergic activities of the hepatic tissues were analyzed by determining the adenylpyrophosphatase (ATPase) (Adewoye et al., 2000; Erukainure et al., 2017) and ecto-nucleoside triphosphate diphosphohydrolase (ENTPDase) (Akomolafe et al., 2017) activities.
Gluconeogenic Enzyme Activities
The hepatic tissues were examined for gluconeogenic activities by determining the activities of glycogen phosphorylase (Cornblath et al., 1963; Balogun and Ashafa, 2017), glucose-6-phosphatase (Mahato et al., 2011; Erukainure et al., 2017), and fructose-1,6-bisphosphatase (Gancedo and Gancedo, 1971; Balogun and Ashafa, 2017) in the supernatants.
Hepatic Amylase Activity
The α-amylase activity of the hepatic tissues was determined by employing an earlier established method (Oboh et al., 2017).
Hepatic Lipase Activity
The lipase activity of the hepatic tissues was determined by employing a previously modified protocol (Kim et al., 2010; Erukainure et al., 2020).
Hepatic Lipid Profile
The lipid profile of the hepatic tissues was analyzed using a modified method described earlier (Erukainure et al., 2020). Briefly, hepatic tissues were incubated alongside 0.1 mM of FeSO4 and 240 μg/ml of the plant extracts or reference drug at 37°C overnight. The samples were thereafter centrifuged at 15,000 rpm for 10 min at 4°C. The tissue supernatants were decanted and used immediately to determine the total cholesterol, triglycerides (TGs), and high-density lipoprotein–cholesterol (HDL-C) of the hepatic tissues via an Automated Chemistry Analyzer (Labmax Plenno, Labtest Co. Ltd., Lagoa Santa, Brazil) with commercial assay kits according to the manufacturer’s manual.
Glucose Utilization in Chang Liver Cells
Chang liver cells (ATCC® CCL-13™) were obtained from the American Type Culture Collection (ATCC®), Manassas, VA, United States. The cells were seeded into flat-bottom 96-well culture plates (NUNC, Roskilde, Denmark) at a density of 6,000 cells/well in a volume of 200 µl/well of growth medium. After 72 h, 10 µl of the plant extracts (250 μg/ml) or metformin (20 µM) in Roswell Park Memorial Institute (RPMI) 1640 media (Helm AG, Hamburg, Germany) was added to the 200 µl medium already in the well to give a final concentration of 12.5 μg/ml and 1 µM for the plant extracts and metformin, respectively. They were thereafter subjected to induction for 48 h. After incubation, the growth medium was aspirated from the wells and replaced with 100 µl of freshly prepared incubation buffer (RPMI 1640 diluted with phosphate-buffered saline (PBS) to 8 mM of glucose with 0.1% (w/v) of bovine serum albumin (BSA; Roche Diagnostics, Mannheim, Germany)) with and/or without metformin or the extracts. The cells were further incubated for 3 h at 37°C. A volume of 50 μl of the reaction mixture was mixed with 200 μl of glucose oxidase reagent (SERA-PAK Plus, Bayer, Leverkusen, Germany) in a 96-well plate and incubated for 15 min at 37°C. Absorbance was read at 510 nm using a microplate reader. Glucose utilization was calculated by subtracting the amount of glucose left in the medium from the amount given at time 0 (Oyedemi et al., 2013).
High-Performance Liquid Chromatography of Plant Extracts
High-performance liquid chromatography (HPLC)–diode array detection analysis of the extracts (hexane, DCM, and ethanol) was carried out with an Agilent 1100 series (Agilent, Waldbronn, Germany) instrument equipped with a photodiode array, an autosampler, a column thermostat, and a degasser. The stationary phase consisted of A Phenomenex: Luna 5 µm C18 2) (150 × 4.6 mm; 5 μm particle size) column. Water containing 0.1% of formic acid (A) and acetonitrile (B) served as mobile phases at a flow rate of 1 ml/min. The gradient elution was as follows: initial ratio 95% A:5% B, maintained for 10 min, changed to 90% A:10% B in 10 min, changed to 70% A:30% B in 10 min, to 50% A:50% B in 10 min, maintained for 0.5 min, and back to initial ratio in 0.5 min. The temperature was set to 30°C, injection volume was 20.0 μl, and chromatograms were recorded at 254 nm (Erukainure et al., 2020).
Molecular Docking Studies
Molecular docking was performed to determine the binding energy of THC and CBD with cannabinoid receptors. The 3D structure was retrieved from the cholesterol-bound form of human β2 adrenergic receptor (PDB access code: 3D4S (Hanson et al., 2008)) with a resolution of 2.8 Å. The suitable grid box was determined using AutoDock tools (Sanner, 1999). The structures of the ligands were retrieved from PUBMED and optimized using Gaussian 09 (Frisch et al., 2009); this was done to obtain minimized conformation. The determined dimension was X = 30 Y = 30 Z = 30 with 1.00 Å as the grid spacing. The optimum binding site for the ligand (Yang et al., 2013) was determined using the Lamarckian genetic algorithm method. Gasteiger charges were computed using the AutoDock Tools graphical user interface supplied by MGL Tools (Morris et al., 2009).
Statistical Analysis
Data were analyzed by one-way ANOVA and presented as mean ± SD. Statistical significance was established at p < 0.05 using Tukey’s honestly significant difference (HSD)–multiple range post-hoc test. Statistical analyses were done using IBM Statistical Package for the Social Sciences (SPSS) for Windows, version 23.0 (IBM Corp., Armonk, NY, USA).
Results and Discussion
In the present study, the induction of oxidative injury in isolated rat hepatic tissues was established by incubating with FeSO4 in the presence of C. sativa extracts (hexane, DCM, and ethanol) and CBD. Induction of oxidative injury significantly depleted GSH level, SOD, and catalase activities, while concomitantly elevating MDA and NO levels as shown in Figure 1A–D; Figure 2, thus signifying an occurrence of oxidative stress and proinflammation on incubation of hepatic tissues with FeSO4. Oxidative stress has been reported in the pathogenesis and progression of hepatotoxicity and liver diseases (Zhu et al., 2012; Cichoż-Lach and Michalak, 2014), with a clear-cut in studies that reported increased oxidative stress markers in alcoholic hepatitis (Lu et al., 2008; Zhu et al., 2012). Treatment with C. sativa significantly reversed these activities and levels and was comparable with the active compound CBD, which served as a standard drug. These effect reversals indicate an ameliorative effect of C. sativa on hepatic oxidative stress. This is in tune with earlier reports on the protective properties of C. sativa on liver diseases (Musa et al., 2012; Hussein et al., 2014).
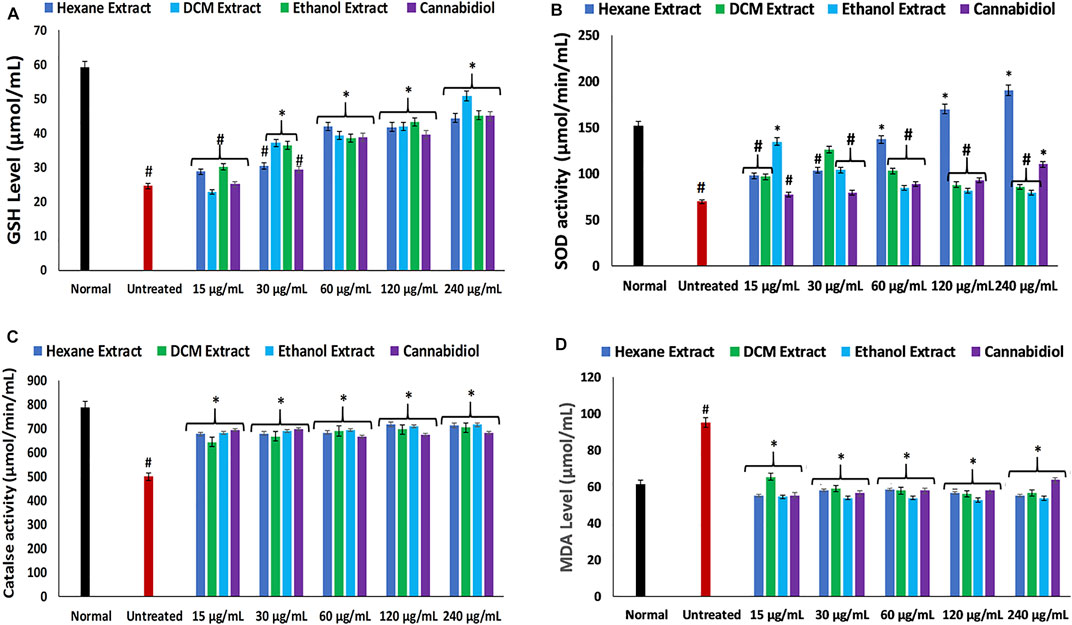
FIGURE 1. Effect of Cannabis sativa on (A) GSH level, (B) SOD, (C) catalase, and (D) MDA level in oxidative hepatic injury. Data = mean ± SD; n = 3. *Statistically significant (p < 0.05) compared with untreated tissues; #statistically significant (p < 0.05) compared with normal tissues. Normal: liver tissues not treated with FeSO4 and/or C. sativa. Untreated: liver tissues treated with FeSO4 only. GSH, reduced glutathione; SOD, superoxide dismutase; MDA, malondialdehyde.
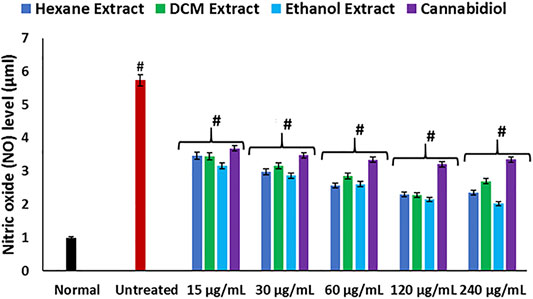
FIGURE 2. Effect of Cannabis sativa on NO level in oxidative hepatic injury. Data = mean ± SD; n = 3. *Statistically significant (p < 0.05) compared with untreated tissues; #statistically significant (p < 0.05) compared with the normal tissues. Normal: liver tissues not treated with FeSO4 and/or C. sativa. Untreated: liver tissues treated with FeSO4 only.
Hepatic cholinergic dysfunction characterized by increased acetylcholinesterase activity has been associated with the pathogenesis of hepatotoxicity, as it triggers inflammation of the hepatocytes (García-Ayllón et al., 2012; García-Ayllón et al., 2006). The increased acetylcholinesterase activity occurrence on induction of oxidative injury (Figure 3) corroborates the occurrence of oxidative stress (Figures 1A–D) and elevated NO level (Figure 2), as a rise in oxidative stress and proinflammation has been implicated in increased acetylcholinesterase activity (Melo et al., 2003; Shaked et al., 2009). The reduced activity on treatment with C. sativa therefore indicates a protective effect of the extracts against cholinergic dysfunction in oxidative hepatotoxicity.
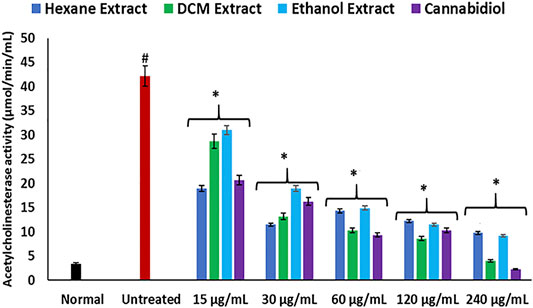
FIGURE 3. Effect of Cannabis sativa on acetylcholinesterase activity in oxidative hepatic injury. Data = mean ± SD; n = 3. *Statistically significant (p < 0.05) compared with untreated tissues; #statistically significant (p < 0.05) compared with normal tissues. Normal: liver tissues not treated with FeSO4 and/or C. sativa. Untreated: liver tissues treated with FeSO4 only.
Disturbances in hepatic purinergic enzyme activities have been implicated in hepatotoxicity (Sun et al., 2013). These enzymes catalyze phospho-hydrolysis of adenosine triphosphate (ATP) and adenosine monophosphate (AMP) to release the endogenous signaling nucleotide (Akinyemi et al., 2017; Bagatini et al., 2018). In this study, induction of oxidative injury led to a significant elevation of ATPase activity, with concomitant depletion of ENTPDase activity as depicted in Figures 4A,B. The increased ATPase activity portrays a depleted hepatic ATP activity, while the depleted ENTPDase activity depicts depleted levels of adenosine (Salau et al., 2019). There was a significant reversal of these activities on treatment with C. sativa extracts, thus indicating a modulatory effect of the extracts on purinergic activities in oxidative-mediated hepatotoxicity.
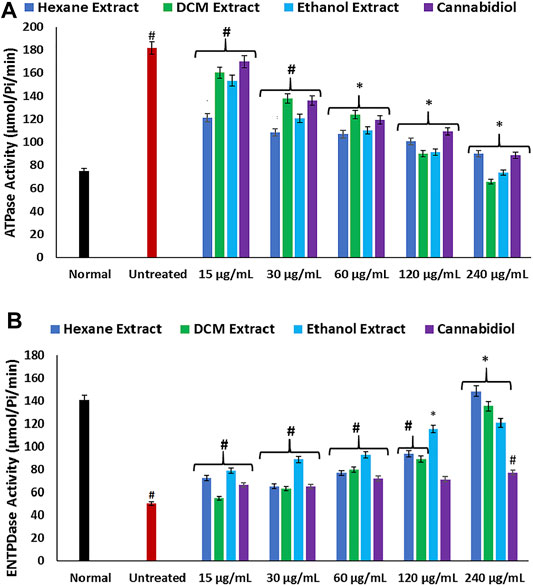
FIGURE 4. Effect of Cannabis sativa on (A) ATPase and (B) ENTPDase activities in oxidative hepatic injury. Data = mean ± SD; n = 3. *Statistically significant (p < 0.05) compared with untreated tissues; #statistically significant (p < 0.05) compared with normal tissues. Normal: liver tissues not treated with FeSO4 and/or C. sativa. Untreated: liver tissues treated with FeSO4 only. ATPase, adenylpyrophosphatase; ENTPDase, ecto-nucleoside triphosphate diphosphohydrolase.
FeSO4-induced oxidative injury significantly elevated the activities of glycogen phosphorylase, glucose-6-phosphatase, and fructose-1,6-bisphosphatase as shown in Figures 5A–C. Elevation of these carbohydrate metabolizing enzymes indicates increased gluconeogenesis, which insinuates elevated hepatic concentration of glucose (Erukainure et al., 2019). Increased gluconeogenesis has been implicated in oxidative injury and recognized as one of the main mechanisms of liver diseases (Teimouri et al., 2006; Sunny et al., 2011). Elevated hepatic glucose levels portray a toxic effect, as surplus glucose can be channeled to the polyol pathway, advanced glycation end-product (AGE) formation, the hexosamine pathway, and protein kinase C (PKC) isoforms, where they serve as fuel (Erukainure, Ochuko L et al., 2019; Luo et al., 2016). Excessive glucose can also undergo oxidation to produce the enediol radical in the presence of transition metal, which breaks down to reactive ketoaldehydes and superoxide anion (O2.−) radicals (Maritim et al., 2003). Treatment with C. sativa extracts led to significantly (p < 0.05) reverse these enzyme activities, thus indicating a glycogenic effect. The glycolytic effect of the extracts insinuates the reduced availability of glucose for oxidation and the aforementioned oxidative pathways.
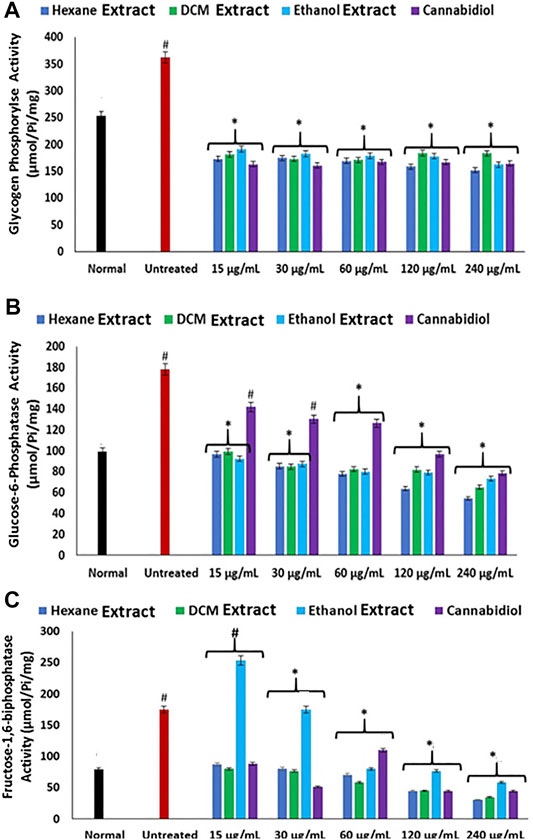
FIGURE 5. Effect of Cannabis sativa on (A) glycogen phosphorylase, (B) glucose-6-phosphatase, and (C) fructose-1,6-bisphosphatase activities in oxidative hepatic injury. Data = mean ± SD; n = 3. *Statistically significant (p < 0.05) compared with untreated tissues; #statistically significant (p < 0.05) compared with normal tissues. Normal: liver tissues not treated with FeSO4 and/or C. sativa. Untreated: liver tissues treated with FeSO4 only.
The increased hepatic amylase activity in inducing oxidative injury as depicted in Figure 6A further corroborates an elevated hepatic concentration of glucose in oxidative hepatotoxicity. Amylase catalyzes the disintegration of dietary carbohydrates to glucose and has been implicated in hyperglycemia (Oyebode et al., 2019; Sanni et al., 2019). Induction of oxidative injury also led to elevated activity of hepatic lipase (Figure 6B). Elevated hepatic lipase activity has been implicated in hepatotoxicity as it regulates TG levels, leading to a buildup of lipids in the liver (Bersot et al., 1999; Mahley et al., 2000). Its elevation on induction of oxidative injury therefore insinuates a dysregulated TG metabolism, thus contributing to liver steatosis, which is characterized by increased hepatic lipid accumulation (Kawano and Cohen, 2013). Treatment with C. sativa significantly inhibited hepatic amylase and lipase activities, which indicates the ability of the extracts to modulate glucose and lipid accumulations in oxidative hepatotoxicity.
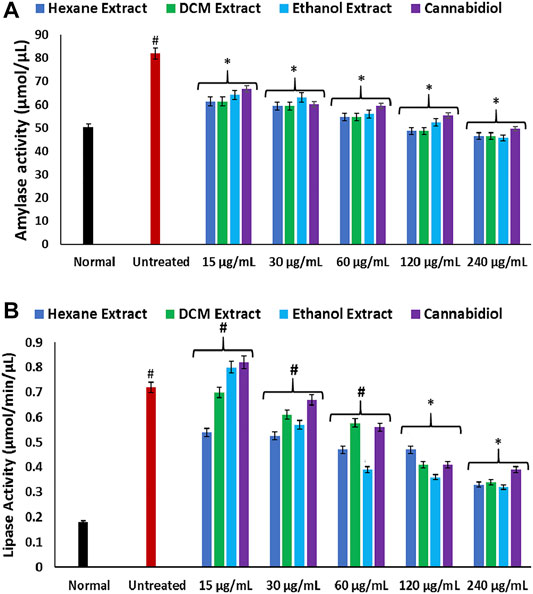
FIGURE 6. Effect of Cannabis sativa on (A) amylase and (B) lipase activities in oxidative hepatic injury. Data = mean ± SD; n = 3. *Statistically significant (p < 0.05) compared with untreated tissues; #statistically significant (p < 0.05) compared with normal tissues. Normal: liver tissues not treated with FeSO4 and/or C. sativa. Untreated: liver tissues treated with FeSO4 only.
As shown in Figure 7, induction of oxidative injury instigated significant (p < 0.05) elevation of hepatic levels of cholesterol, low-density lipoprotein–cholesterol (LDL-C), with concomitant depleted levels of TG and HDL-C, thereby depicting a dysregulated lipid profile, which is a hallmark of most liver diseases such as hepatitis and cirrhosis (Cheung and Sanyal, 2008; Min et al., 2012). These levels correlate with the increased hepatic lipase activity on induction of oxidative injury (Figure 6B) and insinuate an accumulation of lipids. Treatment with C. sativa significantly (p < 0.05) reduced hepatic levels of cholesterol and LDL-C but had no effect on the TG and HDL-C levels. The ability of the extracts to deplete hepatic levels of cholesterol and LDL-C may further portray a modulatory effect on hepatic lipid metabolism in oxidative-mediated hepatotoxicity.
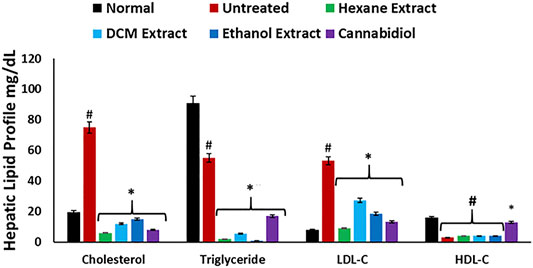
FIGURE 7. Effect of Cannabis sativa on lipid profile in oxidative hepatic injury. Data = mean ± SD; n = 3. *Statistically significant (p < 0.05) compared with untreated tissues; #statistically significant (p < 0.05) compared with normal tissues. Normal: liver tissues not treated with FeSO4 and/or C. sativa. Untreated: liver tissues treated with FeSO4 only.
To further ascertain the effect of C. sativa extracts on hepatic glucose metabolism, their ability to stimulate glucose utilization in the liver was determined in Chang liver cells. Impaired hepatic glucose utilization characterized by hepatic glucose intolerance leading to accumulation of glucose has been implicated in the pathogenesis of hepatotoxicity (Riggio et al., 1982; Imano et al., 1999; Merli et al., 1999). All extracts stimulated liver glucose utilization at the highest concentration and were compared favorably with metformin as shown in Figure 8, thus further insinuating the ability of the extracts to improve glucose metabolism, which corroborates decreased gluconeogenic activity (Figures 5A–C).
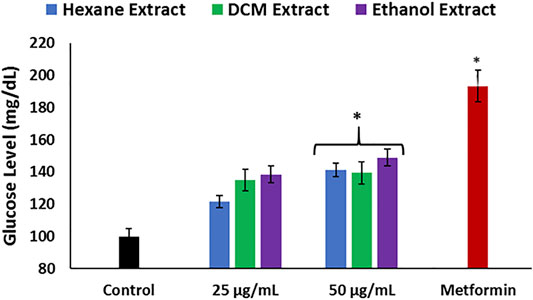
FIGURE 8. Effect of Cannabis sativa on glucose utilization in Chang liver cells. Data = mean ± SD; n = 3. *Statistically significant (p < 0.05) compared with control.
HPLC analysis of the extract revealed the presence of phenolics in all extracts, with the ethanol extract having the highest number of identified compounds as shown in Table 1. The HPLC fingerprints are presented in Supplementary Figures S1A–C. The presence of CBD was also detected in all the extracts, while Δ-9-THC was identified in the hexane and DCM extracts only. The antioxidative and hepatoprotective properties of phenolics are well established (Madrigal-Santillán et al., 2014; Erukainure et al., 2018). CBD and Δ-9-THC have been recognized as the main phytoconstituent of C. sativa and have been reported for their hepatoprotective properties (Dibba et al., 2018; Ismail et al., 2018). Therefore, the studied activities of C. sativa extracts may be associated with the synergistic effect of the identified compounds.
To understand the role of C. sativa extracts in modulating hepatotoxicity, CBD and Δ-9-THC were molecularly docked with the human β2 adrenergic receptor. Δ-9-THC showed the best binding potential as revealed by its binding energy of −9.0 kcal/mol as shown in Table 2. It binds to the TYR 316 residue of the human β2 adrenergic receptor as depicted in Table 2 and Figure 9, thus insinuating a potent molecular interaction with the β2 adrenergic receptor. CBD also showed a potent interaction with a binding energy of −8.7 kcal/mol and bound to TYR 308 and THR 110 residues of the human β2 adrenergic receptor (Table 2; Supplementary Figure S2). The β2 adrenergic receptor has been implicated in hepatotoxicity, as its activation has been linked to exacerbated hepatic TG, lipogenesis, and dysregulated fatty acid metabolism (Shi et al., 2021). The molecular interactions between the phytocannabinoids and the receptor may therefore indicate the potentials of CBD and Δ-9-THC to inactivate the β2 adrenergic receptor, thus modulating the adrenergic system. This may explain the ability of C. sativa extracts to inhibit hepatic lipase activity (Figure 6B) and suppress hepatic TG level (Figure 7) in oxidative hepatic injury.

TABLE 2. Binding energies and core amino acid residue between residues and ligand from molecular docking.
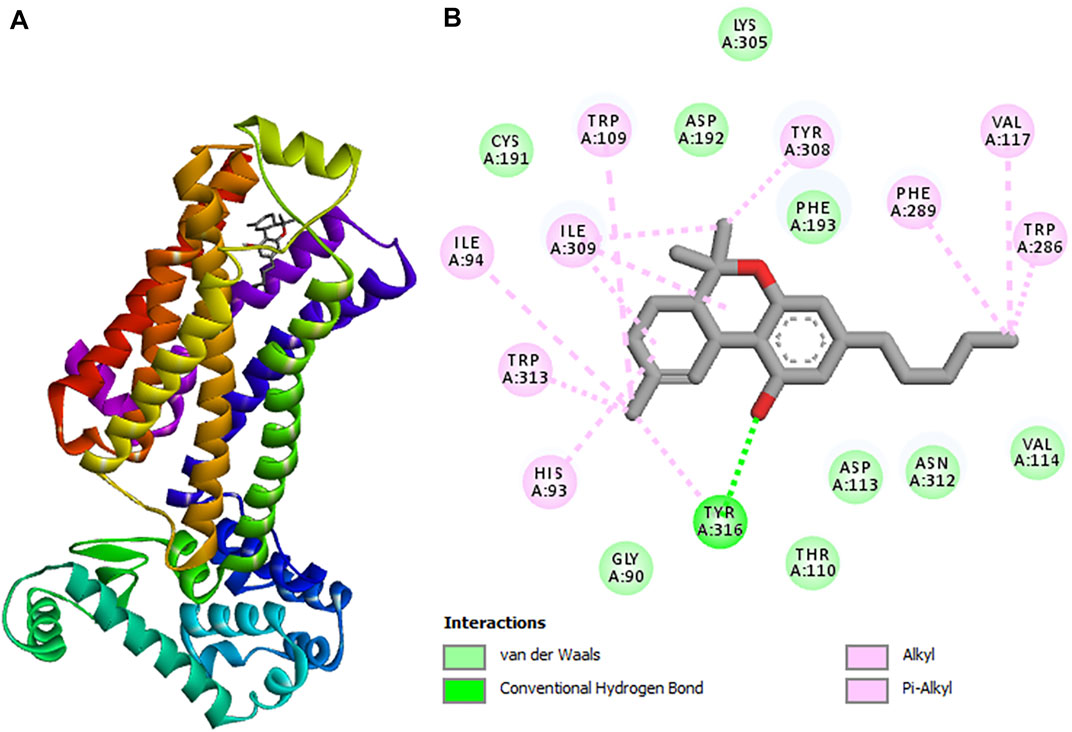
FIGURE 9. 3D structure of (A) tetrahydrocannabinol (highest binding affinity) in complex with cannabinoid receptor. (B) 2D representations displaying the interactions with amino acid residues.
These results indicate that the induction of oxidative hepatic injury led to oxidative stress (Figure 1), which has been implicated in proinflammation when O2.− arising from suppressed SOD activity (Figure 1B) reacts with high NO level (Figure 2) to form peroxynitrite radicals. Oxidative stress has also been reported to exacerbate acetylcholinesterase activity (Figure 3), with concomitant dysregulation of lipid metabolism as depicted by the increased lipase activity (Figure 6B) and exacerbated hepatic levels of cholesterol, TG, and LDL-C (Figure 7). Hysterical gluconeogenesis as depicted by the increased activities of glycogen phosphorylase, glucose-6-phosphatase, fructose-1,6-bisphosphatase, and amylase activity (Figures 5A, 6A) results in high hepatic levels of glucose and depleted levels of ATPs and adenosines (Figures 4A,B) in response to low hepatic glucose utilization (Figure 8). Excessive glucose levels arising from hysterical gluconeogenesis may contribute to oxidative stress (Figure 1) via oxidation to the enediol radical. Thus, a proposed hepatoprotective mechanism of C. sativa against oxidative hepatic injury involves its ability to mitigate oxidative stress (Figure 1), proinflammation (Figure 2), lipid dysmetabolism (Figures 6B, 7), glucose dysmetabolism (Figures 5, 6A), and cholinergic and purinergic dysfunction (Figures 3, 4) while concomitantly stimulating hepatic glucose uptake and utilization (Figure 8).
Conclusion
The data obtained in this study indicate the ability of C. sativa to protect against oxidative-mediated hepatic injury by stalling oxidative stress, gluconeogenesis, and hepatic lipid accumulation while modulating cholinergic and purinergic activities. These activities may be associated with the synergistic effect of the identified phenolics, CBD, and Δ-9-THC and possible interactions with the adrenergic system. However, the present study is the first of several studies we have outlined to investigate the therapeutic effect of C. sativa on oxidative-mediated hepatic injury. Further in vivo studies to decipher the molecular mechanism behind these activities are currently in place in our lab.
Data Availability Statement
The original contributions presented in the study are included in the article/supplementary material. Further inquiries can be directed to the corresponding author.
Ethics Statement
The animal study was reviewed and approved by the University of KwaZulu-Natal, Durban, South Africa.
Author Contributions
OE and MM conceptualized the project. OE, VS, SO, MI, and OO carried out the experiments. CI carried out the computational studies. OE, MM, and SO drafted the original manuscript. All authors read and approved the manuscript.
Conflict of Interest
The authors declare that the research was conducted in the absence of any commercial or financial relationships that could be construed as a potential conflict of interest.
Publisher’s Note
All claims expressed in this article are solely those of the authors and do not necessarily represent those of their affiliated organizations, or those of the publisher, the editors, and the reviewers. Any product that may be evaluated in this article, or claim that may be made by its manufacturer, is not guaranteed or endorsed by the publisher.
Acknowledgments
The authors are thankful to the University of the Free State for technical support and postdoctoral support for OE, SO, and OO. The authors are also thankful for the assistance and support from the Free State Provincial Police and the Police at Port St Johns and Lusikisiki in the Eastern Cape. The authors are further thankful to Ms. Mirranda Javu, senior community liaison officer, Department of Pharmacology UFS, for community engagement and negotiations on the cannabis collections. Similar appreciation goes to Mr. L. R. Mafura and Mr. S. E. Molomo, both from the Ministry of Forestry, Range, Soil and Water Conservation, Mafeteng, Lesotho, for the assistance with the cannabis project and cannabis wild collections. MM is thankful to the IKS Based Technology Innovation Unit of DSI South Africa for the financial support (Grant contracts: DST/CON 0162/201 and DST/CON 0206/2019/2020). OE is thankful to the University of the Free State, Bloemfontein, South Africa, for Incentives for Rated Researchers (2019060769) and the National Research Foundation (NRF) for Scarce Skills Postdoctoral Research Grant (UID: 132822).
Supplementary Material
The Supplementary Material for this article can be found online at: https://www.frontiersin.org/articles/10.3389/fphar.2021.705402/full#supplementary-material
Abbreviations
ATPase, adenylpyrophosphatase; CBD, cannabidiol; DCM, dichloromethane; ENTPDase, ecto-nucleoside triphosphate diphosphohydrolase; FeSO4, iron II sulfate; GSH, reduced glutathione; HDL-C, high-density lipoprotein-cholesterol; HPLC, high-performance liquid chromatography; LDL-C, low-density lipoprotein-cholesterol; MDA, malondialdehyde; NO, nitric oxide; SOD, superoxide dismutase; and Δ-9-THC, Δ-9-tetrahydrocannabinol.
References
Abdel-Salam, O. M. E., Sleem, A. A., and Shafee, N. (2014). Hepatoprotective Effects of Cynara Extract and Silymarin on Carbon Tetrachloride-Induced Hepatic Damage in Rats. Comp. Clin. Pathol. 23(3), 709–716. doi:10.1007/s00580-012-1675-3
Adewoye, O. E., Bolarinwa, A. F., and Olorunsogo, O. O. (2000). Ca++, Mg++-ATPase Activity in Insulin-dependent and Non-insulin Dependent Diabetic Nigerians. Afr. J. Med. Med. Sci. 29(3-4), 195–199 .
Akinyemi, A. J., Onyebueke, N., Faboya, O. A., Onikanni, S. A., Fadaka, A., and Olayide, I. (2017). Curcumin Inhibits Adenosine Deaminase and Arginase Activities in Cadmium-Induced Renal Toxicity in Rat Kidney. J. Food Drug Anal. 25(2), 438–446. doi:10.1016/j.jfda.2016.06.004
Akomolafe, S. F., Akinyemi, A. J., Ogunsuyi, O. B., Oyeleye, S. I., Oboh, G., Adeoyo, O. O., et al. (2017). Effect of Caffeine, Caffeic Acid and Their Various Combinations on Enzymes of Cholinergic, Monoaminergic and Purinergic Systems Critical to Neurodegeneration in Rat Brain—In Vitro. NeuroToxicology. 62, 6–13. doi:10.1016/j.neuro.2017.04.008
Akomolafe, S. F., Oboh, G., Akindahunsi, A. A., Akinyemi, A. J., and Tade, O. G. (2013). Inhibitory Effect of Aqueous Extract of Stem Bark of Cissus Populnea on Ferrous Sulphate-And Sodium Nitroprusside-Induced Oxidative Stress in Rat’s Testes In Vitro. ISRN Pharmacol. 2013.
Anderson, E. R., and Shah, Y. M. (2013). Iron Homeostasis in the Liver. Compr. Physiol. 3(1), 315–330. doi:10.1002/cphy.c120016
Anderson, G. J., and Frazer, D. M. (2005). Hepatic Iron Metabolism. Thieme Medical Publishers, Inc.,New York.
Andre, C. M., Hausman, J.-F., and Guerriero, G. (2016). Cannabis Sativa: the Plant of the Thousand and One Molecules. Front. Plant Sci. 7, 19. doi:10.3389/fpls.2016.00019
Andrews, N. C. (1999). Disorders of Iron Metabolism. New Eng. J. Med. 341(26), 1986–1995. doi:10.1056/NEJM199912233412607
Aso, E., and Ferrer, I. (2016). CB2 Cannabinoid Receptor as Potential Target against Alzheimer's Disease. Front. Neurosci. 10, 243. doi:10.3389/fnins.2016.00243
Bagatini, M. D., dos Santos, A. A., Cardoso, A. M., Mânica, A., Reschke, C. R., and Carvalho, F. B. (2018). The Impact of Purinergic System Enzymes on Noncommunicable, Neurological, and Degenerative Diseases. J. Immunol. Res. 2018. doi:10.1155/2018/4892473
Balogun, F., and Ashafa, A. (2017). Aqueous Root Extracts of Dicoma Anomala (Sond.) Extenuates Postprandial Hyperglycaemia In Vitro and its Modulation on the Activities of Carbohydrate-Metabolizing Enzymes in Streptozotocin-Induced Diabetic Wistar Rats. S. Afr. J. Bot. 112, 102–111. doi:10.1016/j.sajb.2017.05.014
Bersot, T. P., Vega, G. L., Grundy, S. M., Palaoğlu, K. E., Atagündüz, P., Özbayrakçi, S., et al. (1999). Elevated Hepatic Lipase Activity and Low Levels of High Density Lipoprotein in a Normotriglyceridemic, Nonobese Turkish Population. J. Lipid. Res. 40(3), 432–438. doi:10.1016/s0022-2275(20)32447-0
Bonini, S. A., Premoli, M., Tambaro, S., Kumar, A., Maccarinelli, G., Memo, M., et al. (2018). Cannabis Sativa: A Comprehensive Ethnopharmacological Review of a Medicinal Plant with a Long History. J. Ethnopharmacol. 227, 300–315. doi:10.1016/j.jep.2018.09.004
Brand, E. J., and Zhao, Z. (2017). Cannabis in Chinese Medicine: Are Some Traditional Indications Referenced in Ancient Literature Related to Cannabinoids? Front. Pharmacol. 8, 108. doi:10.3389/fphar.2017.00108
Chance, B., and Maehly, A. (1955). Assay of Catalases and Peroxidases. Methods Enzymol. 2, 764–775. doi:10.1016/s0076-6879(55)02300-8
Cheung, O., and Sanyal, A. J. (2008). Abnormalities of Lipid Metabolism in Nonalcoholic Fatty Liver Disease, Semin. Liver Dis. 351–359. doi:10.1055/s-0028-1091979
Chowdhury, P., and Soulsby, M. (2002). Lipid Peroxidation in Rat Brain Is Increased by Simulated Weightlessness and Decreased by a Soy-Protein Diet. Ann. Clin. Lab. Sci. 32(2), 188–192.
Chukwuma, C. I., Matsabisa, M. G., Ibrahim, M. A., Erukainure, O. L., Chabalala, M. H., and Islam, M. S. (2019). Medicinal Plants with Concomitant Anti-diabetic and Anti-hypertensive Effects as Potential Sources of Dual Acting Therapies against Diabetes and Hypertension: A Review. J. Ethnopharmacol. 235 329–360. doi:10.1016/j.jep.2019.02.024
Cichoż-Lach, H., and Michalak, A. (2014). Oxidative Stress as a Crucial Factor in Liver Diseases. World J. Gastroenterol. 20(25), 8082–8091. doi:10.3748/wjg.v20.i25.8082
Cornblath, M., Randle, P., Parmeggiani, A., and Morgan, H. (1963). Regulation of Glycogenolysis in Muscle Effects of Glucagon and Anoxia on Lactate Production, Glycogen Content, and Phosphorylase Activity in the Perfused Isolated Rat Heart. J. Biol. Chem. 238(5), 1592–1597. doi:10.1016/s0021-9258(18)81105-x
Dibba, P., Li, A. A., Cholankeril, G., Iqbal, U., Gadiparthi, C., Khan, M. A., et al. (2018). The Role of Cannabinoids in the Setting of Cirrhosis. Medicines. 5(2), 52. doi:10.3390/medicines5020052
Ellman, G. L., Courtney, K. D., Andres, V., and Featherstone, R. M. (1961). A New and Rapid Colorimetric Determination of Acetylcholinesterase Activity. Biochem. Pharmacol. 7(2), 88–95. doi:10.1016/0006-2952(61)90145-9
Ellman, G. L. (1959). Tissue Sulfhydryl Groups. Arch. Biochem. Biophys. 82(1), 70–77. doi:10.1016/0003-9861(59)90090-6
Erukainure, O. L., Chukwuma, C. I., Matsabisa, M. G., Salau, V. F., Koorbanally, N. A., and Islam, M. S. (2020). Buddleja Saligna Willd (Loganiaceae) Inhibits Angiotensin-Converting Enzyme Activity in Oxidative Cardiopathy with Concomitant Modulation of Nucleotide Hydrolyzing Enzymatic Activities and Dysregulated Lipid Metabolic Pathways. J. Ethnopharmacol. 248, 112358. doi:10.1016/j.jep.2019.112358
Erukainure, O. L., Ijomone, O. M., Sanni, O., Aschner, M., and Islam, M. S. (2019). Type 2 Diabetes Induced Oxidative Brain Injury Involves Altered Cerebellar Neuronal Integrity and Elemental Distribution, and Exacerbated Nrf2 Expression: Therapeutic Potential of Raffia palm (Raphia Hookeri) Wine. Metab. Brain Dis. 34, 1385–1399. doi:10.1007/s11011-019-00444-x
Erukainure, O. L., Mopuri, R., Oyebode, O. A., Koorbanally, N. A., and Islam, M. S. (2017). Dacryodes Edulis Enhances Antioxidant Activities, Suppresses DNA Fragmentation in Oxidative Pancreatic and Hepatic Injuries; and Inhibits Carbohydrate Digestive Enzymes Linked to Type 2 Diabetes. Biomed. Pharmacother. 96, 37–47. doi:10.1016/j.biopha.2017.09.106
Erukainure, O. L., Oyebode, O. A., Ibeji, C. U., Koorbanally, N. A., and Islam, M. S. (2019). Vernonia Amygdalina Del. Stimulated Glucose Uptake in Brain Tissues Enhances Antioxidative Activities; and Modulates Functional Chemistry and Dysregulated Metabolic Pathways. Metab. Brain Dis. 34(3), 721–732. doi:10.1007/s11011-018-0363-7
Erukainure, O. L., Sanni, O., and Islam, M. S. (2018). Clerodendrum Volubile: Phenolics and Applications to Health, in: R. Watson, V. Preedy, and S. Zibadi (Eds.), Polyphenols: Mechanisms of Action in Human Health and Disease. Elsevier, Amsterdam, Netherlands. doi:10.1016/b978-0-12-813006-3.00006-4
Frisch, M., Trucks, G., Schlegel, H. B., Scuseria, G., Robb, M., Cheeseman, J., et al. (2009). Gaussian 09, Revision D. 01. Gaussian, Inc., Wallingford CT.
Fusar-Poli, P., Crippa, J. A., Bhattacharyya, S., Borgwardt, S. J., Allen, P., Martin-Santos, R., et al. (2009). Distinct Effects of Δ9-tetrahydrocannabinol and Cannabidiol on Neural Activation during Emotional Processing. Arch. Gen. Psychiatr. 66(1), 95–105. doi:10.1001/archgenpsychiatry.2008.519
Gancedo, J. M., and Gancedo, C. (1971). Fructose-1, 6-diphosphatase, Phosphofructokinase and Glucose-6-Phosphate Dehydrogenase from Fermenting and Non Fermenting Yeasts. Arch. Mikrobiol. 76(2), 132–138. doi:10.1007/BF00411787
García‐Ayllón, M. S., Silveyra, M. X., Candela, A., Compañ, A., Clària, J., Jover, R., et al. (2006). Changes in Liver and Plasma Acetylcholinesterase in Rats with Cirrhosis Induced by Bile Duct Ligation. Hepatology. 43(3), 444–453.
García-Ayllón, M.-S., Millán, C., Serra-Basante, C., Bataller, R., and Sáez-Valero, J. (2012). Readthrough Acetylcholinesterase Is Increased in Human Liver Cirrhosis. PLoS One. 7(9), doi:e44598
Guzman, M. (2003). Cannabinoids: Potential Anticancer Agents. Nat. Rev. Cancer 3(10), 745. doi:10.1038/nrc1188
Hanson, M. A., Cherezov, V., Griffith, M. T., Roth, C. B., Jaakola, V.-P., Chien, E. Y., et al. (2008). A Specific Cholesterol Binding Site Is Established by the 2.8 Å Structure of the Human β2-adrenergic Receptor. Structure. 16(6), 897–905. doi:10.1016/j.str.2008.05.001
Hussein, N. A. E. M., El-Toukhy, M. A. E.-F., Kazem, A. H., Ali, M. E.-S., Ahmad, M. A. E.-R., Ghazy, H. M. R., et al. (2014). Protective and Therapeutic Effects of Cannabis Plant Extract on Liver Cancer Induced by Dimethylnitrosamine in Mice. Alex. J. Med. 50(3), 241–251. doi:10.1016/j.ajme.2014.02.003
Imano, E., Kanda, T., Nakatani, Y., Motomura, M., Arai, K., Matsuhisa, M., et al. (1999). Impaired Splanchnic and Peripheral Glucose Uptake in Liver Cirrhosis. J. Hepatol. 31(3), 469–473. doi:10.1016/s0168-8278(99)80039-7
Ismail, M., Hasan, H., El-Orfali, Y., Ismail, H., and Khawaja, G. (2018). Anti-inflammatory, Antioxidative, and Hepatoprotective Effects of Trans 9-tetrahydrocannabinol/sesame Oil on Adjuvant-Induced Arthritis in Rats. Evid-based. Comp. Altern. Med. 2018, 9365464. doi:10.1155/2018/9365464
Kakkar, P., Das, B., and Viswanathan, P. (1984). A Modified Spectrophotometric Assay of Superoxide Dismutase. Indian J. Biochem. Biophys. 21, 130–132.
Kawano, Y., and Cohen, D. E. (2013). Mechanisms of Hepatic Triglyceride Accumulation in Non-alcoholic Fatty Liver Disease. J. Gastroenterol. 48(4), 434–441. doi:10.1007/s00535-013-0758-5
Kim, Y. S., Lee, Y. M., Kim, H., Kim, J., Jang, D. S., Kim, J. H., et al. (2010). Anti-obesity Effect of Morus Bombycis Root Extract: Anti-lipase Activity and Lipolytic Effect. J. Ethnopharmacol. 130(3), 621–624. doi:10.1016/j.jep.2010.05.053
Kizil, M., Kizil, G., Yavuz, M., and Ceken, B. (2010). Protective Activity of Ethanol Extract of Three Achillea Species against Lipid Peroxidation, Protein Oxidation and DNA Damage In Vitro. Acta Aliment. 39(4), 457–470. doi:10.1556/aalim.39.2010.4.7
Kuddus, M., Ginawi, I. A., and Al-Hazimi, A. (2013). Cannabis Sativa: An Ancient Wild Edible Plant of India. Emir. J. Food Agric. 25(10), 736–745. doi:10.9755/ejfa.v25i10.16400
Lu, Y., Zhuge, J., Wang, X., Bai, J., and Cederbaum, A. I. (2008). Cytochrome P450 2E1 Contributes to Ethanol‐induced Fatty Liver in Mice. Hepatology. 47(5), 1483–1494. doi:10.1002/hep.22222
Luo, X., Wu, J., Jing, S., and Yan, L.-J. (2016). Hyperglycemic Stress and Carbon Stress in Diabetic Glucotoxicity. Aging Dis. 7(1), 90–110. doi:10.14336/AD.2015.0702
Madrigal-Santillán, E., Madrigal-Bujaidar, E., Álvarez-González, I., Sumaya-Martínez, M. T., Gutiérrez-Salinas, J., Bautista, M., et al. (2014). Review of Natural Products with Hepatoprotective Effects. World J. Gastroenterol. 20(40), 14787–14804.
Mahato, A. K., Bhattacharya, S., and Shanthi, N. (2011). Design, Synthesis and Glucose-6-Phosphatase Inhibitory Activity of Diaminoguanidine Analogues of 3-Guanidinopropionic Acid and Amino Substituted (Pyridin-2-Yl) Thiourea Derivatives. J. Pharm. Sci. Res. 3, 896–902.
Mahley, R. W., Pépin, J., Palaogğlu, K. E., Malloy, M. J., Kane, J. P., and Bersot, T. P. (2000). Low Levels of High Density Lipoproteins in Turks, a Population with Elevated Hepatic Lipase: High Density Lipoprotein Characterization and Gender-specific Effects of Apolipoprotein E Genotype. J. Lipid. Res. 41(8), 1290–1301. doi:10.1016/s0022-2275(20)33437-4
Maritim, A., Sanders, R., and Watkins, J. (2003). Diabetes, Oxidative Stress, and Antioxidants: a Review. J. Biochem. Mol. Toxicol. 17(1), 24–38. doi:10.1002/jbt.10058
Melo, J. B., Agostinho, P., and Oliveira, C. R. (2003). Involvement of Oxidative Stress in the Enhancement of Acetylcholinesterase Activity Induced by Amyloid Beta-Peptide. Neurosci. Res. 45(1), 117–127. doi:10.1016/s0168-0102(02)00201-8
Merli, M., Leonetti, F., Riggio, O., Valeriano, V., Ribaudo, M. C., Sprati, F., et al. (1999). Glucose Intolerance and Insulin Resistance in Cirrhosis Are Normalized after Liver Transplantation. Hepatology. 30(3), 649–654. doi:10.1002/hep.510300306
Milic, S., Mikolasevic, I., Orlic, L., Devcic, E., Starcevic-Cizmarevic, N., Stimac, D., et al. (2016). The Role of Iron and Iron Overload in Chronic Liver Disease. Med. Sci. Monit. 22, 2144. doi:10.12659/msm.896494
Min, H.-K., Kapoor, A., Fuchs, M., Mirshahi, F., Zhou, H., Maher, J., et al. (2012). Increased Hepatic Synthesis and Dysregulation of Cholesterol Metabolism Is Associated with the Severity of Nonalcoholic Fatty Liver Disease. Cell. Metab. 15(5), 665–674. doi:10.1016/j.cmet.2012.04.004
Morris, G. M., Huey, R., Lindstrom, W., Sanner, M. F., Belew, R. K., Goodsell, D. S., et al. (2009). AutoDock4 and AutoDockTools4: Automated Docking with Selective Receptor Flexibility. J. Comput. Chem. 30(16), 2785–2791. doi:10.1002/jcc.21256
Musa, E., Jah Elnabi, M., Osman, E., and Dahab, M. (2012). Hepatoprotective and Toxicity Assessment of Cannabis Sativa Seed Oil in Albino Rat. Int. J. Chem. Biochem. Sci. 1, 69–76.
Oboh, G., Olasehinde, T. A., and Ademosun, A. O. (2017). Inhibition of Enzymes Linked to Type-2 Diabetes and Hypertension by Essential Oils from Peels of orange and Lemon. Int. J. Food Prop. 20(Suppl. 1), S586–S594. doi:10.1080/10942912.2017.1303709
Oyebode, O. A., Erukainure, O. L., Ibeji, C. U., Koorbanally, N. A., and Islam, M. S. (2019). Phytochemical Constituents, Antioxidant and Antidiabetic Activities of Different Extracts of the Leaves, Stem and Root Barks of Alstonia Boonei: an In Vitro and In Silico Study. Bot. Lett. 166 (4), 444–456. doi:10.1080/23818107.2019.1624980
Oyedemi, S., Koekemoer, T., Bradley, G., van de Venter, M., and Afolayan, A. (2013). In Vitro anti-hyperglycemia Properties of the Aqueous Stem Bark Extract from Strychnos Henningsii (Gilg). Int. J. Diabetes Develop. Countries. 33(2), 120–127. doi:10.1007/s13410-013-0120-8
Pandit, A., Sachdeva, T., and Bafna, P. (2012). Drug-induced Hepatotoxicity: a Review. J. Appl. Pharm. Sci. 2(5), 233–243. doi:10.7324/japs.2012.2541
Ramar, K., Rosen, I., Kirsch, D., Chervin, R., Carden, K., Aurora, R., et al. (2018). American Academy of Sleep Medicine Board of Directors. Medical Cannabis and the Treatment of Obstructive Sleep Apnea: an American Academy of Sleep Medicine Position Statement. J. Clin. Sleep Med. 14(4), 679–681. doi:10.5664/jcsm.7070
Ren, Y., Liang, K., Jin, Y., Zhang, M., Chen, Y., Wu, H., et al. (2016). Identification and Characterization of Two Novel α-glucosidase Inhibitory Oligopeptides from Hemp (Cannabis Sativa L.) Seed Protein. J. Funct. Foods 26, 439–450. doi:10.1016/j.jff.2016.07.024
Riggio, O., Merli, M., Cangiano, C., Capocaccia, R., Cascino, A., Lala, A., et al. (1982). Glucose Intolerance in Liver Cirrhosis. Metabolism. 31(6), 627–634. doi:10.1016/0026-0495(82)90103-2
Rishi, G., and Subramaniam, V. N. (2017). The Liver in Regulation of Iron Homeostasis. Am. J. Physiol. Gastrointest. Liver Physiol. 313(3), G157–G165. doi:10.1152/ajpgi.00004.2017
Russo, E. (2005). Cannabis in India: Ancient Lore and Modern Medicine, in: R. Mechoulam (Ed.) Cannabinoids as Therapeutics. Springer, New York, US, pp. 1–22.
Ryz, N. R., Remillard, D. J., and Russo, E. B. (2017). Cannabis Roots: a Traditional Therapy with Future Potential for Treating Inflammation and Pain. Cannabis Cannabinoid Res. 2(1), 210–216. doi:10.1089/can.2017.0028
Salau, V. F., Erukainure, O. L., Ibeji, C. U., Olasehinde, T. A., Koorbanally, N. A., and Islam, M. S. (2019). Ferulic Acid Modulates Dysfunctional Metabolic Pathways and Purinergic Activities, while Stalling Redox Imbalance and Cholinergic Activities in Oxidative Brain Injury. Neurotox. Res., 37. doi:10.1007/s12640-019-00099-7
Sanner, M. F. (1999). Python: a Programming Language for Software Integration and Development. J. Mol. Graph. Model. 17(1), 57–61.
Sanni, O., Erukainure, O. L., Chukwuma, C. I., Koorbanally, N. A., Ibeji, C. U., and Islam, M. S. (2019). Azadirachta indica Inhibits Key Enzyme Linked to Type 2 Diabetes In Vitro, Abates Oxidative Hepatic Injury and Enhances Muscle Glucose Uptake Ex Vivo. Biomed. Pharmacother. 109, 734–743. doi:10.1016/j.biopha.2018.10.171
Sanni, O., Erukainure, O. L., Oyebode, O. A., Koorbanally, N. A., and Islam, M. S. (2018). Concentrated Hot Water-Infusion of Phragmanthera Incana Improves Muscle Glucose Uptake, Inhibits Carbohydrate Digesting Enzymes and Abates Fe2+-Induced Oxidative Stress in Hepatic Tissues. Biomed. Pharmacother. 108, 417–423. doi:10.1016/j.biopha.2018.09.014
Seif, H. S. A. (2016). Physiological Changes Due to Hepatotoxicity and the Protective Role of Some Medicinal Plants. Beni-Suef Univ. J. Basic Appl. Sci. 5(2), 134–146. doi:10.1016/j.bjbas.2016.03.004
Shaked, I., Meerson, A., Wolf, Y., Avni, R., Greenberg, D., Gilboa-Geffen, A., et al. (2009). MicroRNA-132 Potentiates Cholinergic Anti-inflammatory Signaling by Targeting Acetylcholinesterase. Immunity. 31(6), 965–973. doi:10.1016/j.immuni.2009.09.019
Shi, Y., Pizzini, J., Wang, H., Das, F., Abdul Azees, P. A., Ghosh Choudhury, G., et al. (2021). β2-Adrenergic Receptor Agonist Induced Hepatic Steatosis in Mice: Modeling Nonalcoholic Fatty Liver Disease in Hyperadrenergic States. Amer. J. Physiol. Endocrin. Metab. 321(1), E90–E104. doi:10.1152/ajpendo.00651.2020
Sun, X., Han, L., Seth, P., Bian, S., Li, L., Csizmadia, E., et al. (2013). Disordered Purinergic Signaling and Abnormal Cellular Metabolism Are Associated with Development of Liver Cancer in Cd39/ENTPD1 Null Mice. Hepatology. 57(1), 205–216. doi:10.1002/hep.25989
Sunny, N. E., Parks, E. J., Browning, J. D., and Burgess, S. C. (2011). Excessive Hepatic Mitochondrial TCA Cycle and Gluconeogenesis in Humans with Nonalcoholic Fatty Liver Disease. Cel. Metab. 14(6), 804–810. doi:10.1016/j.cmet.2011.11.004
Teimouri, F., Amirkabirian, N., Esmaily, H., Mohammadirad, A., Aliahmadi, A., and Abdollahi, M. (2006). Alteration of Hepatic Cells Glucose Metabolism as a Non-cholinergic Detoxication Mechanism in Counteracting Diazinon-Induced Oxidative Stress. Hum. Exp. Toxicol. 25(12), 697–703. doi:10.1177/0960327106075064
Tsikas, D. (2005). Review Methods of Quantitative Analysis of the Nitric Oxide Metabolites Nitrite and Nitrate in Human Biological Fluids. Free Rad. Res. 39(8), 797–815. doi:10.1080/10715760500053651
UNODC. (2012). Cannabis: A Short Review. https://www.unodc.org/documents/drug-prevention-and-treatment/cannabis_review.pdf. (Accessed November 12 2019).
Whiting, P. F., Wolff, R. F., Deshpande, S., Di Nisio, M., Duffy, S., Hernandez, A. V., et al. (2015). Cannabinoids for Medical Use: a Systematic Review and Meta-Analysis. JAMA 313(24), 2456–2473. doi:10.1001/jama.2015.6358
Yang, B., Hao, F., Li, J., Chen, D., and Liu, R. (2013). Binding of Chrysoidine to Catalase: Spectroscopy, Isothermal Titration Calorimetry and Molecular Docking Studies. J. Photochem. Photobiol. Biol. 128, 35–42. doi:10.1016/j.jphotobiol.2013.08.006
Keywords: cannabidiol, gluconeogenesis, hepatic injury, oxidative stress, Cannabis sativa L.
Citation: Erukainure OL, Matsabisa MG, Salau VF, Oyedemi SO, Oyenihi OR, Ibeji CU and Islam MS (2021) Cannabis sativa L. (var. indica) Exhibits Hepatoprotective Effects by Modulating Hepatic Lipid Profile and Mitigating Gluconeogenesis and Cholinergic Dysfunction in Oxidative Hepatic Injury. Front. Pharmacol. 12:705402. doi: 10.3389/fphar.2021.705402
Received: 05 May 2021; Accepted: 22 November 2021;
Published: 21 December 2021.
Edited by:
Gabriel A. Agbor, Institute of Medical Research and Studies of Medicinal Plants (IMPM), CameroonReviewed by:
Zeynep M. Coskun, Demiroglu Bilim University, TurkeyAlexandra Acco, Federal University of Paraná, Brazil
Copyright © 2021 Erukainure, Matsabisa, Salau, Oyedemi, Oyenihi, Ibeji and Islam. This is an open-access article distributed under the terms of the Creative Commons Attribution License (CC BY). The use, distribution or reproduction in other forums is permitted, provided the original author(s) and the copyright owner(s) are credited and that the original publication in this journal is cited, in accordance with accepted academic practice. No use, distribution or reproduction is permitted which does not comply with these terms.
*Correspondence: Motlalepula G. Matsabisa, bWF0c2FiaXNhbWdAdWZzLmFjLnph