- 1Department of Neurology, Shanghai Fifth People’s Hospital, Fudan University, Shanghai, China
- 2Department of Neurology, Shidong Hospital Affiliated to University of Shanghai for Science and Technology, Shanghai, China
Background and Purpose: CSF therapy is considered a promising therapeutic approach for stroke. We performed a meta-analysis to explore the safety and efficacy of CSF in published clinical stroke studies.
Methods: We searched articles online and manually. Two reviewers selected studies independently, selecting data based on study quality, characteristics of intervention (administration time, observation time, type, dose, and injection approach of CSF), and the baseline characteristics of patients (age, sex, hypertension, diabetes, smoker, and lipids) were extracted. Main prognosis outcomes were measured as all-cause death in severe adverse events (SAE) and recurrent stroke in SAE. Secondary outcomes were measured as CD34+ cell counts in periphery blood at day 5, National Institutes of Health Stroke Scale (NIHSS), and Barthel index (BI), Side effects of CSF were taken as the indicator of safety. STATA13 software was used to perform the meta-analysis.Keywords: Stroke, Colony-stimulating factor, Meta-analysis, therapy, Neurological Diseases
Results: This meta-analysis involved 485 patients from eight studies. Among them, 475 patients from seven studies were gauged SAE (all-cause death), 393 patients from six studies were checked SAE (recurrent stroke); 137 patients from three studies underwent CD34+ measurement, 389 patients from six studies were tested NIHSS and 307 patients from five studies accessed BI. Compared with the control group, both all-causes death (RR= 1.73, 95%CI= (0.61, 4.92), P=0.735, I2=0.0%) and recurrent stroke (RR= 0.43, 95%CI= (0.14, 1.32), P=0.214, I2=33.1%) present no statistical differences, indicating that the application of CSF does not statistically alter the prognosis of patients with stroke. The application of CSF effectively enhanced CD34+ cell counts in periphery blood at day 5 (SMD= 1.23, 95%CI= (0.54, 1.92), P=0.04, I2=69.0%) but did not statistically impact NIHSS (SMD= −0.40, 95%CI= (−0.93, 0.13), P ≤ 0.001, I2=79.7%) or BI (SMD= 0.04, 95%CI= (−0.38, 0.46), P=0.068, I2=54.3%).
Conclusion: Our study consolidates the security of CSF administration for its exerting no effect on detrimental outcomes. It has proven to be effective in elevating CD34+ cell counts in periphery blood at day 5, indicating CSF may participate in stroke recovery, but its efficacy in stroke recovery remains detected.
Systematic Review Registration: PROSPERO, CRD42020166216.
Introduction
Stroke causes great burdens to society worldwide, especially in low-income and middle-income countries (Krishnamurthi et al., 2013). In China, stroke is the leading cause of death and disability-adjusted life-years (DALYs) (Zhou et al., 2019). Although emergency treatments like thrombolysis and mechanical embolectomy can save the lives of patients and promote neurological recovery, the use is limited by the strict administration conditions and severe side effects of these treatments (Bravata, 2005; Adams et al., 2007; Thomalla et al., 2007; Lambrinos et al., 2016). Thus, new therapeutic approaches are necessary for better recovery.
CSF is a group of glycoprotein, including granulocyte colony-stimulating factor (G-CSF), erythropoietin (EPO), macrophage colony-stimulating factor (M-CSF), granulocyte-macrophage colony-stimulating factor (GM-CSF), and interleukin-3 (IL-3), etc. (Wakefield et al., 1990) They were first discovered in bone marrow cells that had been cultured agar (Ichikawa et al., 1966; Landau and Sachs, 1971) and since then, increasing works have been down on CSFs. They have been demonstrated to enhance the proliferation and differentiation of stem cells, stimulate angiogenesis and neurogenesis as well as produce antiapoptotic function (Jung et al., 2006; Komine-Kobayashi et al., 2006; Greenbaum and Link, 2011; Kimáková et al., 2017), serving as promising candidates after stroke. Schäbitz and his colleagues found that G-CSF directly protects cells from glutamate-induced excitotoxicity in vitro and diminishes infarct volume after experimental stroke in vivo by targeting G-CSF receptors on neuron membrane (Schäbitz et al., 2003). Another study also reported the protective function of G-CSF in increasing survival rate and decreasing infarct volume, but they assumed that this function may due to the mobilization function of G-CSF on bone marrow stem cells. The mobilized bone marrow stem cells may differentiate into nervous and endothelial cells and repair the injured zone (Six et al., 2003). This assumption has been corroborated in later studies. One study verified that G-CSF mobilized peripheral blood progenitor cells leading to a significant reduction of stroke-induced hyperactivity (Willing et al., 2003), and compared with stem cells not pre-treated, pre-treated stem cells manifest stronger proliferation and improve motor function quicker (Hokari et al., 2009). Another study found that with G-CSF injection, more stem cells honed in on the subventricular area of injured brain tissue (Shyu et al., 2004). Not only bone marrow (BM) derived stem cells are mobilized by CSF. Administration of G-CSF and stem cell factor (SCF) in the subacute phase after focal cerebral ischemia contributes to neuronal generation from both BM-derived cells and intrinsic neural stem/progenitor cells (Kawada et al., 2006). Though CSF mobilized stem cells may exert protective function in stroke, we lack direct proof supporting the fact that the protections are due to stem cells differentiating into neurons and repairing the injured brain. Toth et al. found that most cells entering the brain maintained bone marrow identity and did not transdifferentiate into neural cells, but G-CSF combined with SCF administration and resulted in a 1.5-fold increase in vessel formation, thus the neuronal survival was attributed to the consequence of CSF-induced angiogenesis (Toth et al., 2008). Endogenous CSFs are also essential for brain recovery after stroke (Sevimli et al., 2009) that G-CSF-deficient mice manifested larger infarct volume, while G-CSF substitution rescues these detrimental effects (Strecker et al., 2010). Though it seems that CSFs are hopeful for stroke recovery, inconsistent outcomes from clinical trials challenge whether CSFs are tolerable and efficient for improving human neurological function after stroke. This meta-analysis aims to obtain the overall expression of the function of CSF on stroke and for better guiding research in the future. In this study, we include randomized controlled trials (RCT) targeting adult patients with acute, subacute, or chronic stroke and analyze if CSF administration makes differences in SAE, stem cell mobilization (CD34 + cell counts), and neurological prognosis (NIHSS and BI). Considering the heterogeneity that exists in both interventions and the baseline characteristic data of patients, we investigated the combined effects of the time of injection (administrationNo), the time of observing outcome (observationNo), the dose of CSF (doseNo), type of CSF (interventionNo), and baseline characteristics and for this purpose, we used the meta-regression technique (Thompson and Higgins, 2002).
Materials and Methods
We did this meta-analysis in accordance with the Preferred Reporting Items for Systematic Reviews and Meta-Analyses (PRISMA) reporting guidelines (Moher et al., 2009).
Retrieving the Literature
We searched the PUBMED, EMBASE, and Cochrane library databases. The PUBMED strategy was depicted as follows: (((((((“Stroke” [Mesh]) OR (cerebrovascular accident*[Title/Abstract])) OR (cerebrovascular apoplexy [Title/Abstract])) OR (accident*, cerebrovascular [Title/Abstract])) OR (brain vascular accident*[Title/Abstract])) OR (stroke [Title/Abstract])) AND (((((((((((((((“Colony-Stimulating Factors” [Mesh]) OR (CSF [Title/Abstract])) OR (Granulocyte colony-stimulating factor [Title/Abstract])) OR (G-CSF [Title/Abstract])) OR (erythropoietin [Title/Abstract])) OR (EPO [Title/Abstract])) OR (macrophage colony-stimulating factor [Title/Abstract])) OR (M-CSF [Title/Abstract])) OR (granulocyte-macrophage colony-stimulating factor [Title/Abstract])) OR (GM-CSF [Title/Abstract])) OR (interleukin-3 [Title/Abstract])) OR (IL-3 [Title/Abstract])) OR (Thrombopoietin [Title/Abstract])) OR (TPO [Title/Abstract])) OR (Colony-stimulating factor*[Title/Abstract]))) AND ((((((randomized controlled trial) OR (randomized)) OR (placebo)) OR (clinical trials, randomized)) OR (RCT)) OR (trials, randomized controlled)). We included articles in English. The bibliographies were cross checked respectively by two investigators. A third investigator resolved issues when divisions arose.
Selection of Studies and Data Extraction
Inclusion criteria: 1) the design of the included study was an RCT on stroke with adult population; 2) the intervention involves the administration of one kind of CSF in stroke; 3) the research reported one or more outcome measures including NIHSS, BI scores, SAE, and laboratory values for CD34+; 4) the subjects were diagnosed with stroke by clinical manifestation, laboratory tests and magnetic resonance imaging (MRI) or computerized tomography (CT), despite ischemic or hemorrhagic, acute, subacute or chronic.
SAE (all-cause death) and SAE (recurrent stroke) were considered as the main outcome. CD34+ cell counts in periphery blood at day 5, NIHSS (an inventory reflecting the severity of the stroke, with higher scores indicating more severe stroke), and BI scores (an inventory reflecting the ability to live independently, with lower scores indicating more severe functional disorders) as efficacy outcomes were also recorded.
Quality Assessment
The methodological quality of included RCTs was assessed critically by two reviewers according to Cochrane Handbook 5.1. Each study was rigorously appraised in accordance with the following seven scales: 1) random sequence generation; 2) allocation concealment; 3) blinding of participants and personnel; 4) blinding of outcome assessment; 5) incomplete outcome data; 6) selective reporting; 7) other bias. The quality of the evidence in individual studies was graded according to the GRADE workgroup recommendation by two researchers (Atkins et al., 2004).
Statistical Analysis
We calculated the standardized mean difference (SMD) and the relative ratio (RR) and their respective 95% confidence interval (CI) as measures of the relationship between CSF and outcomes. RR was calculated for dichotomous variables and SMD for continuous variables. I2fn2 statistic was used to determine heterogeneity. We performed meta-analysis using a fixed effects model to achieve the pooled RR and SMD within the included studies, a random-effects model was used when statistically significant heterogeneity was existent (I2>50%, p < 0.05) or I2>50% but proves no statistical significance (p > 0.05). If the heterogeneity was still not eliminated, the sensitivity analysis and meta-regression would be applied. All analyses were conducted by Stata 13.0. A p value of less than 0.05 was considered statistically significant.
Results
Study Inclusion and Study Characteristics
From online searches, nine abstracts that met the inclusion criteria were chosen. One research reported that their data did not obey normal distribution, and the sample size was quite small (three experimental groups to three matched groups). It is not appropriate to transform the interquartile range into mean and standard deviation. Therefore, this article was excluded (Alasheev et al., 2011). Manual searching did not identify any further eligible data. (see Figure 1). Thus, the meta-analysis is based on the data from eight articles (Table1). Outcomes were assessed in a total of 475 patients for SAE (all-cause death) and 393 patients for SAE (recurrent stroke), 137 patients for CD34+ measurements, 389 patients for NIHSS, and 307 patients for Barthel Index. A combination of administration time, observing time, CSF dose, CSF type, and baseline characteristics (age, hypertension, diabetes, abnormal plasma lipid condition, sex, smoker) in the studies were presented separately for comparisons of NIHSS and Barthel Index (see Supplementary Figures S1, S2).
Study Quality
Two researchers assessed the quality of the included studies independently by using ReVman (see Figures 2, 3). Three out of the eight articles are open-labeled, thus presenting high risk in terms of “allocation concealment” and “blinding of participants and personnel”. In these three open-labeled studies, Zhou depicted that the outcomes were all analyzed by researchers blinded to patient groupings. Prasad (Prasad et al., 2011) did not refer to the blinding of outcome assessment. Gorthi showed that patients groupings were transparent to all participants. Thus, in the part of “Blinding of outcome assessment”, Zhou was ranked as “low risk”, Prasad was graded by “unclear risk” while Gorthi presented “high risk”. All studies presented low risks in terms of “attrition bias” and most studies present low or unclear risks in “selection bias”, “reporting bias” and “other bias”. The quality of the evidence in individual studies was graded according to the GRADE workgroup recommendation by two researchers (See Figure 4).
Efficacy, Safety and Meta-Regression Analysis
Efficacy
No significant differences were found in the heterogeneity test in all-causes deaths (I2 = 0.0%) and recurrent stroke (I2 = 33.1%). Both of the I2 are lower than 50%, thus a fixed effect model was employed to combine effect size. Even though the CSF administration seems to have no effects on prognosis (see Figures 5, 6) that all-causes death (RR = 1.73, 95%CI= (0.61, 4.92), p = 0.735, I2 = 0.0%) and recurrent stroke (RR = 0.43, 95%CI= (0.14, 1.32), p = 0.214, I2 = 33.1%) present no statistical differences, SAE (all-causes death) was prone to increase while SAE (recurrent stroke) tended to decrease.
The heterogeneity test presents significant differences in CD34+ (I2 = 69%), NIHSS (I2 = 79.7%) and BI (I2 = 54.3%), a random effects model was chosen here. CSF administration elevates CD34 + level at day 5 (SMD = 1.23, 95%CI= (0.54, 1.92), p = 0.04, I2 = 69.0%) significantly, but does not impact NIHSS (SMD = -0.40, 95%CI= (-0.93, 0.13), p ≤ 0.001, I2 = 79.7%) nor BI (SMD = 0.04, 95%CI= (-0.38, 0.46), p = 0.068, I2 = 54.3%) (see Figures 7–9). It indicates that even if the CSF application mobilizes CD34+, the neurological tests do not show significant changes.
Safety
Shyu (Shyu et al., 2006) reported that three participants in the experimental group claimed headache, bone pain, and transient liver function abnormality. Prasad (Prasad et al., 2011) reported one case of bone pain and one case of deep venous thrombosis in the experimental group. Sprigg (Sprigg et al., 2016) reported that in the experimental group, six people claimed bone pain, three people outlined that they had headache, and no one had been infected while in the controlled group. Three people claimed bone pain, four persons claimed headache, and two got infected. Zhou et al., 2019 had reported that gastrointestinal reactions had been found in 19 people, and bone pain was found in 15 people, fever was found in 12 people and deep venous thrombosis was found in one person in the experimental group, while no other side effects had been found in the control group. Overall, they found the application of CSF to be safe.
Meta-Regression Analysis
We conducted meta-regression to find out the cause of high heterogeneities. However, neither administration factors (the time of injection, the time of observing outcome, dose of CSF, and type of CSF) nor baseline characteristics of the patients altered the effects of CSF treatment (see Supplementary Figures S1, S2) on NIHSS or BI.
Sensitivity analysis demonstrates that the overall result is not impacted by dismissing research in each turn, and our outcomes are reliable (see Figures 10, 11).
Publication Bias
No publication bias is found in the funnel plot (see Figure 12).
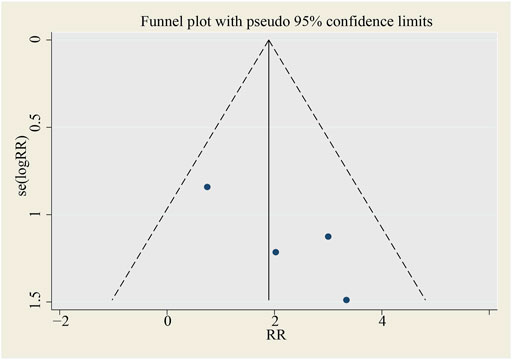
FIGURE 12. Funnel plot for SAE (all‐cause death). SMD, standardized mean difference, SE, standard error.
Discussion
Safety and Efficacy of Colony-Stimulating Factors on Stroke
This meta-analysis includes the newest research and demonstrates the safety of CSF administration on stroke recovery. Interestingly, though no statistical significance has been found, CSFs tend to increase the onset of SAE (all-cause death) and decrease SAE (recurrent stroke), implying that CSF treatment is a promising therapeutic approach for patients with stroke. Though there is a trend that CSF may be of benefit for neurological improvement (NIHSS and BI), no significant outcomes have been drawn. To confirm its safety and effectiveness, a larger scale of RCTs will be needed in the future.
Most preclinical studies corroborate the efficacy of CSFs for stroke recovery. CSFs achieved a neuroprotective function by directly binding CSF receptor (Schäbitz et al., 2003; Villa et al., 2003) or by indirectly mobilizing BM-derived stem cells (Six et al., 2003; Willing et al., 2003) and intrinsic neural stem/progenitor cells (Kawada et al., 2006) to the subventricular zone (SVZ). This protection remains when administered in the acute, subacute phase (Kawada et al., 2006) and chronic phase (Schneider et al., 2006; Zhao et al., 2007; Piao et al., 2009; Larpthaveesarp et al., 2016) of stroke. G-CSF is also reported to have a survival-enhancing capacity and a beneficial effect on the functional outcome for aged rats (Popa-Wagner et al., 2010). The beneficial effects of CSFs are via anti-apoptosis (Kretz et al., 2005; Schneider et al., 2005; Li et al., 2007; Mengozzi et al., 2012; Souvenir et al., 2014; Komnig et al., 2018), anti-inflammation (Villa et al., 2003; Dietel et al., 2012; Mengozzi et al., 2012; Yuen et al., 2017) angiogenesis (Li et al., 2007; Liu et al., 2010), neurogenesis (Kretz et al., 2005; Tsai et al., 2006; Gonzalez et al., 2013), oligodendrogenesis (Zhang et al., 2010; Gonzalez et al., 2013; Wang et al., 2017), and the protection for synaptic functions and axonal myelination (Schneider et al., 2005; Liu et al., 2010; Zhang et al., 2010; Mengozzi et al., 2012). It is also reported that CSFs can protect the brain after stroke by ameliorating astrocyte swelling and maintaining the brain liquid equilibrium (Gunnarson et al., 2009) or remodeling the neuron-vascular network (Cui et al., 2015). Besides direct interventions, some research corroborates that CSFs are effective in extending the therapeutic time window of t-PA and thereby indirectly enhancing the possibility of stroke recovery (dela Peña et al., 2015; Dela Peña et al., 2018). Moreover, some analogues of EPO are studied as well (Villa et al., 2007; Gan et al., 2012). They are proven to produce fewer side effects but generate similar protective effects, and are currently regarded as a brand-new and hopeful therapy for stroke (Gan et al., 2012).
Though CSFs seemed promising, not all preclinical research draws positive conclusions about CSFs on stroke. One article reported that EPO did not protect brain tissue after stroke, and if the plasma level of EPO is too high, it is detrimental for elevating hematocrit and increases accompanying blood viscosity (Wiessner et al., 2001). Another study demonstrated that G-CSF might lead to negative outcomes in stroke by exaggerating inflammatory responses (Taguchi et al., 2007). The fact that CSFs are detrimental under certain circumstances partly accounts for the tendency that CSF increases the onset of SAE and indicates that all factors that influence CSFs plasma level (including the dose and application approach) may affect the outcome, and this is the reason we select the dose of CSF and other details of interventions to detect the origins of heterogeneity. Age and comorbidities like diabetes have also been reported to influence the function of G-CSF on stroke in preclinical studies (Lan et al., 2008; Popa-Wagner et al., 2010; Buga et al., 2013). Thus, we explore whether these factors will impact the function of CSFs on stroke in this meta-analysis (see Supplementary Figures S1, S2). However, none of them have been proven to make a significant difference. This may be in part due to the limited number of enrolled trials and missing data, partly owing to some undetected factors. For example, though several studies have corroborated that CSFs rescue ischemic tissue through anti-inflammation function (Villa et al., 2003; Dietel et al., 2012; Mengozzi et al., 2012; Yuen et al., 2017), another study claims that G-CSF will induce an exaggerated inflammatory response, thus leading to cortical atrophy and impaired behavioral function (Taguchi et al., 2007). Some conditions causing the transformation of the role of CSFs in inflammation do exist, and some other stroke-related phenotypes have also been identified and need further investigation.
Methodological Considerations
This meta-analysis only collects RCTs to guarantee the reliability of its conclusions. There is no evidence of publication bias and statistical significance in the results of sensitivity analysis to ensure stability and creditability.
There exist several disadvantages. First, this meta-analysis does not exclude research that uses CSF except G-CSF, however, only one study takes EPO as an intervention, the other seven studies subcutaneously inject G-CSF, which makes subdivision and analysis impossible. If omitted, the sample size will be greatly impacted, thus decreasing the power of the results. Secondly, Sprigg (Sprigg et al., 2006) had conducted a concentration gradient of G-CSF, for fully utilizing all data the research provided, we synthesized the outcome, which means the omission of the details. Even though most trials have taken subcutaneous injection of 10 μg/kg G-CSF as an intervention, there are exceptions (Sprigg et al., 2006; Yip et al., 2011). CSF is proven to be safe on the whole, but it is not clear which kind of CSF at which maximum concentration is safe. Last but not least, in terms of quality assessment, only three out of the eight studies are ranked as having a low bias in all scales. The studies by Gorthi, Prasad, and Zhou are open-label, and thus ranked as having a high bias in the scales of “Selection bias” and “Performance bias”. The fact that patients and their families know exactly what kind of treatment they are undergoing could trigger placebo effects, which impact the real effect of CSFs. Though the study of Yip et al. (Yip et al., 2011) claims that their article is randomized, they do not describe how they randomize participants into different groups, which may also shake the reliability of our study.
Implications for Further Studies
According to the quality assessment by GRADE. The qualities of CD34+, SAE (all-cause death), and SAE (recurrent stroke) are high while NIHSS and BI are low. Our analysis showed that only one study investigated the dose of G-CSF and its efficacy in humans with stroke (Sprigg et al., 2006), whereas no studies investigated CSF in stroke with other conditions such as stroke complications, CSF administration, and baseline characteristics of patients (e.g. age, smoke, alcohol abuse). Rigorously designed randomized controlled studies are needed to explore how these factors will impact the function of CSF in stroke further. Furthermore, the studies included in this meta-analysis do not investigate whether the subtypes of ischemic stroke will influence the function of CSF, while most animal studies that focus on CSF and stroke use middle cerebral artery occlusion (MCAO) models, which only mimic strokes that have happened in the middle cerebral artery territory. This may partly explain the failure of the transformation from preclinical studies to clinical studies, which should also be paid attention to in future studies.
Conclusion
In summary, though the safety of CSF therapy has been corroborated, our meta-analysis does not provide evidence that CSF administration statistically significantly improves the neurological deficits in patients with stroke. Larger, higher-quality RCTs are necessary for fully addressing the problem, and the administration of CSFs should be undertaken with more caution.
Data Availability Statement
The original contributions presented in the study are included in the article/Supplementary Material, further inquiries can be directed to the corresponding authors.
Author Contributions
XC collected and analyzed data and finally wrote the whole manuscript, WS assisted in assessing the reliability of the included studies, PZ and DW critically read and edited the manuscript.
Funding
This study was supported by Shanghai Minhang District Health and Family Planning Commission (great discipline of Shanghai Minhang District No. 2020MWDXK01) and Shanghai Fifth People’s Hospital Construction Project for Key Specialty (Grant No. 2020WYZDZK04).
Conflict of Interest
The authors declare that the research was conducted in the absence of any commercial or financial relationships that could be construed as a potential conflict of interest.
Supplementary Material
The Supplementary Material for this article can be found online at: https://www.frontiersin.org/articles/10.3389/fphar.2021.704509/full#supplementary-material
SUPPLEMENTARY FIGURE 1. Meta-regression for NIHSS.
SUPPLEMENTARY FIGURE 2. Meta-regression for Barthel index.
References
Adams, H. P., del Zoppo, G., Alberts, M. J., Bhatt, D. L., Brass, L., Furlan, A., et al. (2007). Guidelines for the Early Management of Adults with Ischemic Stroke. Stroke 38 (5), 1655–1711. doi:10.1161/strokeaha.107.181486
Alasheev, A. M., Belkin, A. A., Leiderman, I. N., Ivanov, R. A., and Isakova, T. M. (2011). Granulocyte-colony-stimulating Factor for Acute Ischemic Stroke: a Randomized Controlled Trial (STEMTHER). Transl. Stroke Res. 2 (3), 358–365. doi:10.1007/s12975-011-0091-3
Atkins, D., Eccles, M., Eccles, M., Flottorp, S., Guyatt, G. H., Henry, D., et al. (2004). Systems for Grading the Quality of Evidence and the Strength of Recommendations I: Critical Appraisal of Existing Approaches the GRADE Working Group. BMC Health Serv. Res. 4 (1), 38. doi:10.1186/1472-6963-4-38
Bravata, D. M. (2005). Intravenous Thrombolysis in Acute Ischaemic Stroke. CNS Drugs 19 (4), 295–302. doi:10.2165/00023210-200519040-00002
Buga, A.-M., Di Napoli, M., and Popa-Wagner, A. (2013). Preclinical Models of Stroke in Aged Animals with or without Comorbidities: Role of Neuroinflammation. Biogerontology 14 (6), 651–662. doi:10.1007/s10522-013-9465-0
Cui, L., Duchamp, N. S., Boston, D. J., Ren, X., Zhang, X., Hu, H., et al. (2015). NF-κB Is Involved in Brain Repair by Stem Cell Factor and Granulocyte-colony Stimulating Factor in Chronic Stroke. Exp. Neurol. 263, 17–27. doi:10.1016/j.expneurol.2014.08.026
dela Peña, I. C., Yoo, A., Tajiri, N., Acosta, S. A., Ji, X., Kaneko, Y., et al. (2015). Granulocyte colony-stimulating Factor Attenuates Delayed tPA-Induced Hemorrhagic Transformation in Ischemic Stroke Rats by Enhancing Angiogenesis and Vasculogenesis. J. Cereb. Blood Flow Metab. 35 (2), 338–346. doi:10.1038/jcbfm.2014.208
dela Peña, I., Yang, S., Shen, G., Fang Liang, H., Solak, S., and Borlongan, C. (2018). Extension of Tissue Plasminogen Activator Treatment Window by Granulocyte-Colony Stimulating Factor in a Thromboembolic Rat Model of Stroke. Ijms 19 (6), 1635. doi:10.3390/ijms19061635
Dietel, B., Cicha, I., Kallmünzer, B., Tauchi, M., Yilmaz, A., Daniel, W. G., et al. (2012). Suppression of Dendritic Cell Functions Contributes to the Anti-inflammatory Action of Granulocyte-colony Stimulating Factor in Experimental Stroke. Exp. Neurol. 237 (2), 379–387. doi:10.1016/j.expneurol.2012.06.019
Gan, Y., Xing, J., Jing, Z., Stetler, R. A., Zhang, F., Luo, Y., et al. (2012). Mutant Erythropoietin without Erythropoietic Activity Is Neuroprotective against Ischemic Brain Injury. Stroke 43 (11), 3071–3077. doi:10.1161/strokeaha.112.663120
Gonzalez, F. F., Larpthaveesarp, A., McQuillen, P., Derugin, N., Wendland, M., Spadafora, R., et al. (2013). Erythropoietin Increases Neurogenesis and Oligodendrogliosis of Subventricular Zone Precursor Cells after Neonatal Stroke. Stroke 44 (3), 753–758. doi:10.1161/strokeaha.111.000104
Greenbaum, A. M., and Link, D. C. (2011). Mechanisms of G-CSF-Mediated Hematopoietic Stem and Progenitor Mobilization. Leukemia 25 (2), 211–217. doi:10.1038/leu.2010.248
Gunnarson, E., Song, Y., Kowalewski, J. M., Brismar, H., Brines, M., Cerami, A., et al. (2009). Erythropoietin Modulation of Astrocyte Water Permeability as a Component of Neuroprotection. Proc. Natl. Acad. Sci. 106 (5), 1602–1607. doi:10.1073/pnas.0812708106
Hokari, M., Kuroda, S., Chiba, Y., Maruichi, K., and Iwasaki, Y. (2009). Synergistic Effects of Granulocyte-colony Stimulating Factor on Bone Marrow Stromal Cell Transplantation for Mice Cerebral Infarct. Cytokine 46 (2), 260–266. doi:10.1016/j.cyto.2009.02.008
Ichikawa, Y., Pluznik, D. H., and Sachs, L. (1966). In Vitro control of the Development of Macrophage and Granulocyte Colonies. Proc. Natl. Acad. Sci. 56 (2), 488–495. doi:10.1073/pnas.56.2.488
Jung, K.-H., Chu, K., Lee, S.-T., Kim, S.-J., Sinn, D.-I., Kim, S. U., et al. (2006). Granulocyte colony-stimulating Factor Stimulates Neurogenesis via Vascular Endothelial Growth Factor with STAT Activation. Brain Res. 1073-1074 (1), 190–201. doi:10.1016/j.brainres.2005.12.037
Kawada, H., Takizawa, S., Takanashi, T., Morita, Y., Fujita, J., Fukuda, K., et al. (2006). Administration of Hematopoietic Cytokines in the Subacute Phase after Cerebral Infarction Is Effective for Functional Recovery Facilitating Proliferation of Intrinsic Neural Stem/progenitor Cells and Transition of Bone Marrow-Derived Neuronal Cells. Circulation 113 (5), 701–710. doi:10.1161/circulationaha.105.563668
Kimáková, P., Solár, P., Solárová, Z., Komel, R., and Debeljak, N. (2017). Erythropoietin and its Angiogenic Activity. Ijms 18 (7), 1519. doi:10.3390/ijms18071519
Komine-Kobayashi, M., Zhang, N., Liu, M., Tanaka, R., Hara, H., Osaka, A., et al. (2006). Neuroprotective Effect of Recombinant Human Granulocyte colony-stimulating Factor in Transient Focal Ischemia of Mice. J. Cereb. Blood Flow Metab. 26 (3), 402–413. doi:10.1038/sj.jcbfm.9600195
Komnig, D., Gertz, K., Habib, P., Nolte, K. W., Meyer, T., Brockmann, M. A., et al. (2018). Faim2 Contributes to Neuroprotection by Erythropoietin in Transient Brain Ischemia. J. Neurochem. 145 (3), 258–270. doi:10.1111/jnc.14296
Kretz, A., Happold, C. J., Marticke, J. K., and Isenmann, S. (2005). Erythropoietin Promotes Regeneration of Adult CNS Neurons via Jak2/Stat3 and PI3K/AKT Pathway Activation. Mol. Cell Neurosci. 29 (4), 569–579. doi:10.1016/j.mcn.2005.04.009
Krishnamurthi, R. V., Feigin, V. L., Forouzanfar, M. H., Mensah, G. A., Connor, M., Bennett, D. A., et al. (2013). Global and Regional burden of First-Ever Ischaemic and Haemorrhagic Stroke during 1990-2010: Findings from the Global Burden of Disease Study 2010. Lancet Glob. Health 1 (5), e259–e281. doi:10.1016/s2214-109x(13)70089-5
Lambrinos, A., Schaink, A. K., Dhalla, I., Krings, T., Casaubon, L. K., Sikich, N., et al. (2016). Mechanical Thrombectomy in Acute Ischemic Stroke: A Systematic Review. Can. J. Neurol. Sci. 43 (4), 455–460. doi:10.1017/cjn.2016.30
Lan, X., Qu, H., Yao, W., and Zhang, C. (2008). Granulocyte-colony Stimulating Factor Inhibits Neuronal Apoptosis in a Rat Model of Diabetic Cerebral Ischemia. Tohoku J. Exp. Med. 216 (2), 117–126. doi:10.1620/tjem.216.117
Landau, T., and Sachs, L. (1971). Characterization of the Inducer Required for the Development of Macrophage and Granulocyte Colonies. Proc. Natl. Acad. Sci. 68 (10), 2540–2544. doi:10.1073/pnas.68.10.2540
Larpthaveesarp, A., Georgevits, M., Ferriero, D. M., and Gonzalez, F. F. (2016). Delayed Erythropoietin Therapy Improves Histological and Behavioral Outcomes after Transient Neonatal Stroke. Neurobiol. Dis. 93, 57–63. doi:10.1016/j.nbd.2016.04.006
Li, Y., Lu, Z., Keogh, C. L., Yu, S. P., and Wei, L. (2007). Erythropoietin-induced Neurovascular protection, Angiogenesis, and Cerebral Blood Flow Restoration after Focal Ischemia in Mice. J. Cereb. Blood Flow Metab. 27 (5), 1043–1054. doi:10.1038/sj.jcbfm.9600417
Liu, S.-P., Lee, S.-D., Lee, H.-T., Liu, D. D., Wang, H.-J., Liu, R.-S., et al. (2010). Granulocyte Colony-Stimulating Factor Activating HIF-1α Acts Synergistically with Erythropoietin to Promote Tissue Plasticity. PLoS One 5 (4), e10093. doi:10.1371/journal.pone.0010093
Mengozzi, M., Cervellini, I., Villa, P., Erbayraktar, Z., Gökmen, N., Yilmaz, O., et al. (2012). Erythropoietin-induced Changes in Brain Gene Expression Reveal Induction of Synaptic Plasticity Genes in Experimental Stroke. Proc. Natl. Acad. Sci. 109 (24), 9617–9622. doi:10.1073/pnas.1200554109
Moher, D., Liberati, A., Tetzlaff, J., and Altman, D. G. (2009). Preferred Reporting Items for Systematic Reviews and Meta-Analyses: the PRISMA Statement. Plos Med. 6 (7), e1000097. doi:10.1371/journal.pmed.1000097
Piao, C.-S., Gonzalez-Toledo, M. E., Xue, Y.-Q., Duan, W.-M., Terao, S., Granger, D. N., et al. (2009). The Role of Stem Cell Factor and Granulocyte-colony Stimulating Factor in Brain Repair during Chronic Stroke. J. Cereb. Blood Flow Metab. 29 (4), 759–770. doi:10.1038/jcbfm.2008.168
Popa-Wagner, A., Stöcker, K., Balseanu, A. T., Rogalewski, A., Diederich, K., Minnerup, J., et al. (2010). Effects of Granulocyte-colony Stimulating Factor after Stroke in Aged Rats. Stroke 41 (5), 1027–1031. doi:10.1161/strokeaha.109.575621
Prasad, K., Kumar, A., Sahu, J. K., Srivastava, M. V. P., Mohanty, S., Bhatia, R., et al. (2011). Mobilization of Stem Cells Using G-CSF for Acute Ischemic Stroke: A Randomized Controlled, Pilot Study. Stroke Res. Treat. 2011, 1–7. doi:10.4061/2011/283473
Schäbitz, W. R., Kollmar, R., Schwaninger, M., Juettler, E., Bardutzky, J., Schölzke, M. N., et al. (2003). Neuroprotective Effect of Granulocyte colony-stimulating Factor after Focal Cerebral Ischemia. Stroke 34 (3), 745–751. doi:10.1161/01.str.0000057814.70180.17
Schneider, A., Krüger, C., Steigleder, T., Weber, D., Pitzer, C., Laage, R., et al. (2005). The Hematopoietic Factor G-CSF Is a Neuronal Ligand that Counteracts Programmed Cell Death and Drives Neurogenesis. J. Clin. Invest. 115 (8), 2083–2098. doi:10.1172/jci23559
Schneider, A., Wysocki, R., Pitzer, C., Krüger, C., Laage, R., Schwab, S., et al. (2006). An Extended Window of Opportunity for G-CSF Treatment in Cerebral Ischemia. BMC Biol. 4, 36. doi:10.1186/1741-7007-4-36
Sevimli, S., Diederich, K., Strecker, J.-K., Schilling, M., Klocke, R., Nikol, S., et al. (2009). Endogenous Brain protection by Granulocyte-colony Stimulating Factor after Ischemic Stroke. Exp. Neurol. 217 (2), 328–335. doi:10.1016/j.expneurol.2009.03.018
Shyu, W.-C., Lin, S.-Z., Yang, H.-I., Tzeng, Y.-S., Pang, C.-Y., Yen, P.-S., et al. (2004). Functional Recovery of Stroke Rats Induced by Granulocyte colony-stimulating Factor-Stimulated Stem Cells. Circulation 110 (13), 1847–1854. doi:10.1161/01.cir.0000142616.07367.66
Shyu, W.-C., Lin, S. Z., Lee, C. C., Liu, D. D., and Li, H. (2006). Granulocyte colony-stimulating Factor for Acute Ischemic Stroke: a Randomized Controlled Trial. Can. Med. Assoc. J. 174 (7), 927–933. doi:10.1503/cmaj.051322
Six, I., Gasan, G., Mura, E., and Bordet, R. (2003). Beneficial Effect of Pharmacological Mobilization of Bone Marrow in Experimental Cerebral Ischemia. Eur. J. Pharmacol. 458 (3), 327–328. doi:10.1016/s0014-2999(02)02785-1
Souvenir, R., Flores, J. J., Ostrowski, R. P., Manaenko, A., Duris, K., and Tang, J. (2014). Erythropoietin Inhibits HIF-1α Expression via Upregulation of PHD-2 Transcription and Translation in an In Vitro Model of Hypoxia-Ischemia. Transl. Stroke Res. 5 (1), 118–127. doi:10.1007/s12975-013-0312-z
Sprigg, N., Bath, P. M., Zhao, L., Willmot, M. R., Gray, L. J., Walker, M. F., et al. (2006). Granulocyte-Colony-Stimulating Factor Mobilizes Bone Marrow Stem Cells in Patients with Subacute Ischemic Stroke. Stroke 37 (12), 2979–2983. doi:10.1161/01.STR.0000248763.49831.c3
Sprigg, N., O’Connor, R., Woodhouse, L., Krishnan, K., England, T. J., Connell, L. A., et al. (2016). Granulocyte Colony Stimulating Factor and Physiotherapy after Stroke: Results of a Feasibility Randomised Controlled Trial: Stem Cell Trial of Recovery EnhanceMent after Stroke-3 (STEMS-3 ISRCTN16714730). PLoS One 11 (9), e0161359. doi:10.1371/journal.pone.0161359
Strecker, J.-K., Sevimli, S., Schilling, M., Klocke, R., Nikol, S., Schneider, A., et al. (2010). Effects of G-CSF Treatment on Neutrophil Mobilization and Neurological Outcome after Transient Focal Ischemia. Exp. Neurol. 222 (1), 108–113. doi:10.1016/j.expneurol.2009.12.012
Taguchi, A., Wen, Z., Myojin, K., Yoshihara, T., Nakagomi, T., Nakayama, D., et al. (2007). Granulocyte colony-stimulating Factor Has a Negative Effect on Stroke Outcome in a Murine Model. Eur. J. Neurosci. 26 (1), 126–133. doi:10.1111/j.1460-9568.2007.05640.x
Thomalla, G., Sobesky, J., Köhrmann, M., Fiebach, J. B., Fiehler, J., Zaro Weber, O., et al. (2007). Two Tales: Hemorrhagic Transformation but Not Parenchymal Hemorrhage after Thrombolysis Is Related to Severity and Duration of Ischemia. Stroke 38 (2), 313–318. doi:10.1161/01.str.0000254565.51807.22
Thompson, S. G., and Higgins, J. P. T. (2002). How Should Meta-Regression Analyses Be Undertaken and Interpreted?. Statist. Med. 21 (11), 1559–1573. doi:10.1002/sim.1187
Toth, Z. E., Leker, R. R., Shahar, T., Pastorino, S., Szalayova, I., Asemenew, B., et al. (2008). The Combination of Granulocyte colony-stimulating Factor and Stem Cell Factor Significantly Increases the Number of Bone Marrow-Derived Endothelial Cells in Brains of Mice Following Cerebral Ischemia. Blood 111 (12), 5544–5552. doi:10.1182/blood-2007-10-119073
Tsai, P. T., Ohab, J. J., Kertesz, N., Groszer, M., Matter, C., Gao, J., et al. (2006). A Critical Role of Erythropoietin Receptor in Neurogenesis and post-stroke Recovery. J. Neurosci. 26 (4), 1269–1274. doi:10.1523/jneurosci.4480-05.2006
Villa, P., Bigini, P., Mennini, T., Agnello, D., Laragione, T., Cagnotto, A., et al. (2003). Erythropoietin Selectively Attenuates Cytokine Production and Inflammation in Cerebral Ischemia by Targeting Neuronal Apoptosis. J. Exp. Med. 198 (6), 971–975. doi:10.1084/jem.20021067
Villa, P., van Beek, J., Larsen, A. K., Gerwien, J., Christensen, S., Cerami, A., et al. (2007). Reduced Functional Deficits, Neuroinflammation, and Secondary Tissue Damage after Treatment of Stroke by Nonerythropoietic Erythropoietin Derivatives. J. Cereb. Blood Flow Metab. 27 (3), 552–563. doi:10.1038/sj.jcbfm.9600370
Wakefield, P. E., James, W. D., Samlaska, C. P., and Meltzer, M. S. (1990). Colony-stimulating Factors. J. Am. Acad. Dermatol. 23 (5 Pt 1), 903–912. doi:10.1016/0190-9622(90)70313-7
Wang, R., Li, J., Duan, Y., Tao, Z., Zhao, H., and Luo, Y. (2017). Effects of Erythropoietin on Gliogenesis during Cerebral Ischemic/Reperfusion Recovery in Adult Mice. Aging Dis. 8 (4), 410–419. doi:10.14336/ad.2016.1209
Wiessner, C., Allegrini, P. R., Ekatodramis, D., Jewell, U. R., Stallmach, T., and Gassmann, M. (2001). Increased Cerebral Infarct Volumes in Polyglobulic Mice Overexpressing Erythropoietin. J. Cereb. Blood Flow Metab. 21 (7), 857–864. doi:10.1097/00004647-200107000-00011
Willing, A. E., Vendrame, M., Mallery, J., Cassady, C. J., Davis, C. D., Sanchez-Ramos, J., et al. (2003). Mobilized Peripheral Blood Cells Administered Intravenously Produce Functional Recovery in Stroke. Cel Transpl. 12 (4), 449–454. doi:10.3727/000000003108746885
Yip, H.-K., Tsai, T.-H., Lin, H.-S., Chen, S.-F., Sun, C.-K., Leu, S., et al. (2011). Effect of Erythropoietin on Level of Circulating Endothelial Progenitor Cells and Outcome in Patients after Acute Ischemic Stroke. Crit. Care 15 (1), R40. doi:10.1186/cc10002
Yuen, C. M., Yeh, K. H., Wallace, C. G., Chen, K. H., Lin, H. S., Sung, P. H., et al. (2017). EPO-cyclosporine Combination Therapy Reduced Brain Infarct Area in Rat after Acute Ischemic Stroke: Role of Innate Immune-Inflammatory Response, Micro-RNAs and MAPK Family Signaling Pathway. Am. J. Transl Res. 9 (4), 1651–1666.
Zhang, L., Chopp, M., Zhang, R. L., Wang, L., Zhang, J., Wang, Y., et al. (2010). Erythropoietin Amplifies Stroke-Induced Oligodendrogenesis in the Rat. PLoS One 5 (6), e11016. doi:10.1371/journal.pone.0011016
Zhao, L.-R., Berra, H. H., Duan, W.-M., Singhal, S., Mehta, J., Apkarian, A. V., et al. (2007). Beneficial Effects of Hematopoietic Growth Factor Therapy in Chronic Ischemic Stroke in Rats. Stroke 38 (10), 2804–2811. doi:10.1161/strokeaha.107.486217
Keywords: stroke, colony-stimulating factor, meta-analysis, therapy, neurological diseases
Citation: Chen X, Sun W, Zhong P and Wu D (2021) Colony-Stimulating Factors on Mobilizing CD34+ Cells and Improving Neurological Functions in Patients With Stroke: A Meta-Analysis and a Systematic Review. Front. Pharmacol. 12:704509. doi: 10.3389/fphar.2021.704509
Received: 03 May 2021; Accepted: 07 June 2021;
Published: 22 July 2021.
Edited by:
Francisco Lopez-Munoz, Camilo José Cela University, SpainReviewed by:
Amit Kumar, Laval University, CanadaEiichiro Nagata, Tokai University Isehara Hospital, Japan
Copyright © 2021 Chen, Sun, Zhong and Wu. This is an open-access article distributed under the terms of the Creative Commons Attribution License (CC BY). The use, distribution or reproduction in other forums is permitted, provided the original author(s) and the copyright owner(s) are credited and that the original publication in this journal is cited, in accordance with accepted academic practice. No use, distribution or reproduction is permitted which does not comply with these terms.
*Correspondence: Danhong Wu, ZGFuaG9uZ3d1QGZ1ZGFuLmVkdS5jbg==; Ping Zhong, enBoZ2xAMTYzLmNvbQ==