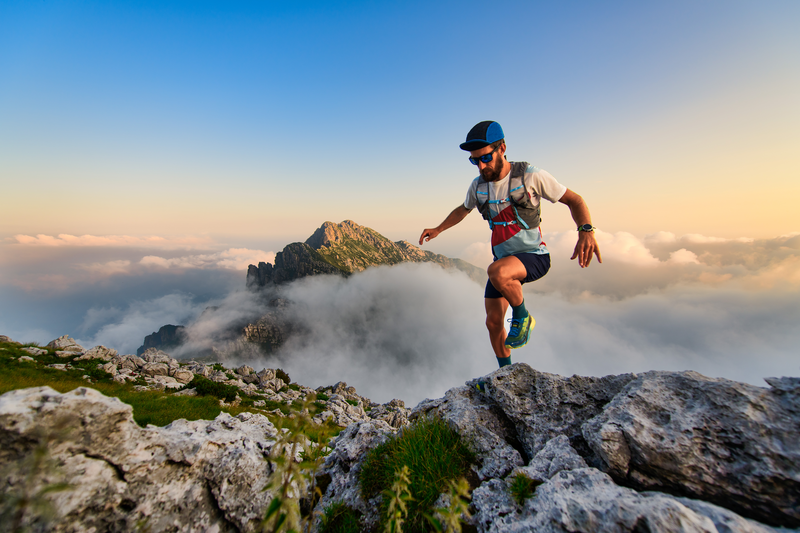
95% of researchers rate our articles as excellent or good
Learn more about the work of our research integrity team to safeguard the quality of each article we publish.
Find out more
MINI REVIEW article
Front. Pharmacol. , 25 August 2021
Sec. Ethnopharmacology
Volume 12 - 2021 | https://doi.org/10.3389/fphar.2021.703837
This article is part of the Research Topic Plant Products for Antiviral Therapeutics View all 16 articles
Introduction: The worldwide burden of viral infections has triggered a resurgence in the search for new and more efficient antiviral drugs. Scientists are also repurposing existing natural compounds such as the antimalarial drug artemisinin from Artemesia annua L. as potential drug candidates for some of the emerging and re-emerging viral infections such as covid-19
Aim: The aim of this review was to analyse the existing literature to explore the actual or potential natural antiviral compounds from African and Asian medicinal plants as lead compounds in the drug discovery process.
Methods: We searched the literature on African and Asian medicinal plant species as antiviral agents for HIV-1 and the novel coronavirus (SARS-CoV-2) in various databases and search engines such as Web of Science, Google Scholar and PubMed. The search was limited to in vitro, in vivo, and clinical studies and excluded in silico studies.
Results: We present 16 plant species with actual or potential antiviral activity against HIV-1 and SARS-CoV-2. These plant species span the continents of Africa and Asia where they are widely used for treating several other ailments.
Conclusion: Natural compounds from plants can play a significant role in the clinical management of HIV/AIDS and the covid-19 pandemic. More research needs to be conducted to investigate the potential toxicities of the various compounds and their efficacies in clinical settings.
The emergence and re-emergence of new infectious diseases especially of viral origin are of great concern to global public health (Louten, 2016; Mourya et al., 2019; Abrahão and de Arruda, 2020). This has reignited the search for natural compounds especially, from plants as potential sources of new drug candidates to add to the current armamentarium (Jans et al., 2020). This review focusses on HIV-1 and SARS-CoV-2, the viruses that cause AIDs and covid-19 respectively because over the last 50 years, these two viruses have caused huge pandemics globally: the HIV/AIDS pandemic and covid-19 pandemic (Illanes-Álvarez, et al., 2021). Since the start of the HIV/AIDS pandemic in the early 80’s, about 77.5 million people have become infected with HIV and over 34.7 million have succumbed to the diseases (UNAIDS, 2021). For the more recent covid-19 pandemic which begun in 2019, there have been 206,958,371 confirmed cases as of August 18, 2021 globally with 4,361,996 confirmed deaths, as of July 28, 2021 (WHO, 2021). The novel SARS-CoV-2 virus causes severe acute and fatal respiratory syndrome after gaining access to the host cells through the angiotensin-converting enzyme 2 (ACE2) co-receptors for the virus. The virus can also damage vital body organs by causing a cytokine storm (Ding et al., 2003; Hashemifesharaki and Gharibzahedi, 2020; Xu et al., 2020). Another similarity both pandemics share is causation by RNA viruses which have animal reservoirs and infections characterized by the increased synthesis of proinflammatory cytokines (Illanes-Álvarez et al., 2021).
Medicinal plants have a long history of healing many diseases. Natural compounds from plants are a rich reservoir of bioactive antiviral compounds. Recent scientific and technological advances have made it possible to easily isolate compounds from such plants with a wide range of biological activities (Martin and Ernst, 2003). Some natural products from plants contain metabolites that are key in preventing virus replication without affecting host metabolism. Such compounds are ideal candidates for the development of antiviral medicinal products with limited side effects. For instance, compounds such as 3β-friedelanol, friedelin, and epitaraxerol isolated from the ethanolic extract of Euphorbia neriifolia had potent anti-human coronavirus activity on human fibroblasts (MRC-5) infected cells (Chang et al., 2012). These natural plant products may additionally modify or enhance host immune responses against viruses; thus, alleviating symptoms and reducing mortality of viral infections (Kurokawa et al., 2010).
Fewer antiviral drugs are considered safe for human use than antibacterial agents mainly because viral pathogenesis is not as well understood at molecular level. Some viruses multiply and induce host cell lysis, while viruses incorporate themselves into the host chromosome and may remain dormant for years. Numerous viruses survive and thrive by hijacking the machinery of the host cell which includes different enzyme systems. Therefore, their inhibition is key to controlling viral diseases (Kurokawa et al., 2010).
Another approach that is vigorously being pursued is the repurposing of existing compounds and exploring their synergistic activity as potential drug candidates for diseases such as covid-19 (Prasad et al., 2020). Such compounds are often considered cheaper, safer, and efficacious alternatives to some of the currently available conventional drugs (Perera and Efferth, 2012). For instance, A. annua tea infusion is highly active against HIV-1 50% inhibitory concentration (IC50 = 2.0 μg/ml) but was originally used for treating malaria and fevers. Artemisinin, the main component from A. annua is currently the main stay for malaria treatment (Lubbe et al., 2012). Other studies are investigating the potential of already known compounds as potential candidates against SARS-CoV 2 and HIV-1. This mini-review reports on medicinal plant species and their metabolites as actual or potential antiviral candidates for HIV/AIDS and covid-19.
We searched the literature on medicinal plant species used in Africa and Asia with scientific evidence of antiviral activity against either HIV-1 or SARS-CoV-2 or both in various databases and search engines such as Web of Science, Google Scholar and PubMed. We selected the most important plant species basing on scientific evidence from one or more studies confirming their antiviral activities. The search was limited to in vitro, in vivo, and clinical studies but excluded in silico studies. The search focused on English only studies conducted within the last 50 years. The selected plant species are presented in detail as follows:
All the plant species selected are used in traditional medicine for treating various diseases including viral infections. Of the 16 medicinal plant species analysed, 11 were considered major because they had a larger body of scientific evidence to support their bioactivity or efficacy than the rest which were categoried as “other medicinal plant species with limited studies.”Table 1 shows a detailed breakdown of ten plant species with proven activity against SARS-CoV-2 and or HIV-1.
A. catechu is a moderate size deciduous tree with a dark grayish or brown rough bark (Chopra et al., 1956). It is indigenous to India and other Asian countries, as well as East Africa (Patel et al., 2009; Sunil et al., 2019). The stem bark, aqueous and ethanol extracts of A. catechu caused the suppression of HIV-1 infection partly through their inhibitory effect on HIV-1 protease and partly due to the interference in interaction of viral Trans-Activator of Transcription (Tat) protein. The n-butanol fraction of A. catechu exhibited strong inhibitory activity against HIV proteases (IC50 = 12.9 μg/ml). A. catechu acts through various other mechanisms such as inhibition of fusion of infected cells and blocking RNA synthesis (Nutan et al., 2013). The n-butanol fraction showed a dose-dependent inhibition against HIV-1NL4.3 infection of peripheral blood mononuclear cells (PBMC), and against HIV-1BaL(R-5-tropic) as well as two different primary viral isolates of HIV-1 (Nutan et al., 2013). A. catechu contains various compounds such as kaempferol, quercetin, catechin, rutin, isorhamnetin, epicatechin, afzelechin, epiafzelechin, mesquitol, ophioglonin, aromadendrin and phenols (Li et al., 2010). However, the specific bioactive compounds responsible for the anti-HIV activity were not established.
A. sativum (garlic) is a member of the onion family. It is a hardy perennial herb likely to have originated in central Asia where it was used as a food and medicine. Traditionally, it was used for preventing infections and the treatment of colds, influenza, bronchitis, whooping cough, gastroenteritis, dysentery and skin problems (Rivlin, 2001; Lissiman et al., 2014) and to boost immunity in people living with HIV/AIDS (Anywar et al., 2020a). A. sativum has a range of biological activities, including antiviral properties. It inhibits viral-cell attachment, viral reverse transcriptase, and further destruction of CD4T cells (Tatarintsev et al., 1992; Walder et al., 1997). Ajoene, a compound isolated from garlic showed dose-dependent HIV inhibition (EC50 = 0.35 μM). The lectins (ASA and ASA1) from garlic inhibited both HIV-1 replication and viral attachment in the later stages of infectious virus cycle of SARS-CoV (Keyaerts et al., 2007). Garlic extract inhibited angiotensin converting enzyme (ACE)2, a functional receptor for SARS-CoV Li et al. (2003) by 39.57% at a dose of 10 mg/ml Chaudhary et al. (2020) indicating its potential antiviral activity against SARS-CoV. A. sativum contains various other bioactive compounds including alliin, allicin, vinyldithis and flavonoids (Chavanet al., 2016; Kim et al., 2018; Baek et al., 2019; Phan et al., 2019; El-Saber Batiha et al., 2020).
A. korupensis is a West African liana with the only known population limited within Korup National Park and its vicinity, in Cameroon. A. korupensis has antiviral activity against HIV-1 and HIV-2. Different compounds with anti-HIV activity have been isolated from its bark and leaves such as michellamine B Manfredi et al. (1991), Foster and Sork (1997) and korundamine A (Hallock et al., 1998). Michellamine B is a naturally occurring naphthylisoquinoline alkaloid that inhibits the replication of both HIV-1 and HIV-2, and their associated cytopathic effects (CPE) on cultured human T-lymphoblastoid cells (CEM-SS and MT-2), with a 50% effective concentration (CC50) near 18 μg/ml (Manfredi et al., 1991). Korundamine A is also a naphthylisoquinoline alkaloid which is heterodimeric. Korundamine A exhibited anticytopathic activity against HIV-1 (EC50 = 2 μM) (Hallock et al., 1998). The anti-HIV activity of korundamine A is comparable to the anti-HIV activity of the michellamines. Korundamine A is also active against several resistant strains of HIV, with EC50 values of 8, l0 and 6 μM for the CEM-SS/OClOO, MT2/A17 and MT2/G9106 host cell/virus strain combinations, respectively (Hallock et al., 1998). Five michellamine-type dimeric naphthylisoquinoline alkaloids: michellamines A2, A3, A4, B2, and B3, were isolated from the root bark of a related species, the Central African liana Ancistrocladus congolensis, along with their two known parent compounds, the michellamines A and B (Bringmann et al., 2016).
A. annua is a shrub, often growing over 2 m high (Ferreira et al., 1997). It is native to China and has traditionally been used to treat malaria and fevers Willcox et al. (2004), Anywar et al. (2020b) and to boost immunity in people living with HIV/AIDS (Anywar et al., 2020a). A. annua extracts inhibit various viruses (Efferth et al., 2008). The ethanol extracts of A. annua significantly inhibited the effects on virus-induced CPE in SARS-CoV. The ethanol leaf extract of A. annua had antiviral activity against SARS-CoV-1 BJ-001 strain (EC50 = 34.5) and BJ-006 strain (EC50 = 39.2). A. annua was proposed as one of the potential candidates for the development of new anti-SARS-CoV drug (Li et al., 2005). In addition, A. annua tea infusion is highly active against HIV (IC50 = 2.0 μg/ml) but the main component, artemisinin was inactive at 25 μg/ml (Lubbe et al., 2012). The specific phytochemical compounds responsible for its anti-HIV-1 and anti-SARS-CoV activity have not established although it contains bioactive compounds such as quercetin, polyphenols, saponins, sterols, dicaffeoylquinic acid polyaccharides (Lin et al., 2014).
C. corylifolium Syn: P. corylifolia is a is a popular traditional medicinal herb that occurs mainly in Indonesia, Malaysia, Bangladesh, India, China, and Sri Lanka. P. corylifolia has well known antiviral, antioxidant, antibacterial and antidepressant activities (Chopra et al., 2013; Alam et al., 2018). P. corylifolia seed extract exhibited high activity against SARS-CoV-papain-like protease (PLpro) (IC50 = 15 μg/ml). SARS-CoV Plpro is an important enzyme in SARS virus replication (Kim et al., 2014). P. corylifolia contains various bioactive components (Schneiderová et al., 2013; Kim et al., 2014). All the six isolated bioactive flavonoids from P. corylifolia: bavachinin, neobavaisoflavone, isobavachalcone, 4′-O-methylbavachalcone, psoralidin and corylifol produced a dose-dependent inhibition of PLpro (IC50 = 4.2–38.4 µM) (Kim et al., 2014).
E. neriifolia is a spiny herb native to Southeast Asia with a native range from Iran to Myanmar (Hieh et al., 1993; POWO, 2020). The herb is currently cultivated in southern Taiwan (Hieh et al., 1993). Terpenoids isolated from E. neriifolia exhibited a structure-activity relationship against the human coronavirus (HCoV). Twenty-three compounds (22 triterpenoids and 1 flavonoid glycoside) have been isolated from the ethanolic leaf extract of E. neriifolia leaves. Specifically, 3β-friedelanol exhibited more potent anti-viral activity against the corona virus (HCoV, strain 229E) than the positive control, actinomycin D (0.02 μg/ml) Chang et al. (2012) in MRC-5 cells (human fibroblasts cells).
M. indica is a medicinal and fruit tree widely used in the traditional medicine in India, and Africa (Makare et al., 2001; Anywar et al., 2020a). M. indica possess antiviral activities which have been attributed to the compound mangiferin, a naturally occurring gucosylxanthone (Guha et al., 1996; Wang et al., 2011). Mangiferin inhibited HIV-1ⅢB induced syncytium formation at non-cytotoxic concentrations, with a 50% effective concentration (EC50) of 16.9 μM. Mangiferin also demonstrated antiviral activity against various laboratory-derived, clinically isolated and resistant HIV-1 strains of HIV. Mangiferin acts by inhibiting HIV proteases (Wang et al., 2011). Mangiferin reduced the CPE in MT-2 cells and prevented cell death at > 10 μ/ml with a CC50 of 3.95 μ/ml and a lower IC50 of 125 μ/ml than the standard drug zidovuline (IC50 of 3.5 μ/ml). Mangiferin was thus not toxic at such high concentrations compared to the standard drug (Guha et al., 1996).
Panax ginseng is a highly valued medicinal plant that has been used for thousands of years. It is mostly cultivated in China, Korea, and Japan (Shehzad et al., 2012; 2013; Bai et al., 2018). Ginseng is a perennial herb whose rhizome is an important determinant of its quality (Choi, 2008). The consumption of Korean ginseng was shown to slow the depletion of CD4 T cells and serum CD8 levels in HIV-1-infected patients (Sung et al., 2005). A retrospective study that analyzed 252 HIV-1 patients diagnosed from 1986 to 2013 prior to the initiation of antiretroviral therapy showed that P. ginseng prolonged survival in HIV-1 patients. The study also showed significant correlations between the total amount of P. ginseng consumed and survival time (r = 0.64, p < 0.0001) and between total amount of P. ginseng and mean annual decrease in CD4+ T-cell count in all 252 patients (r = −0.17, p < 0.01) (Cho and Kim, 2017). The ginsenoside-Rb1, which is one of the active components of P. ginseng (Shehzad et al., 2012), showed antiviral activity against FP-21399, a bis-azo derivative with HIV inhibition activity by preventing viral entry at 100 μM (Zhang et al., 1998). The major pharmacologically active components of ginseng root are ginsenosides which make up 2–3% of the root (Jeong et al., 2003; Shehzad et al., 2012).
P. sidoides is a popular herb whose roots are widely used in traditional medicine in South Africa for different ailments such as diarrhoea, dysentery, ear, nose, throat disorders and respiratory tract infections (Kolodziej, 2002; Brendler and van Wyk, 2008; Michaelis et al., 2011). P. sidoides has potent anti-HIV-1 activity. It protected PBMC and macrophages from infection with various X4 and R5 tropic HIV-1 strains, and clinical isolates by directly interfering with viral infectivity and blocking viral attachment and entry of HIV-1 particles to target cells. P. sidoides contains active polyphenolic compounds with low cytotoxicity (Helfer et al., 2014). However, the specific phytochemical compounds responsible for its anti-HIV-1 activity were not established.
Phyllanthus amarus (Syn P. niruri) is a small shrub that is widespread in the tropics. The aqueous or alcohol extracts of P. amarus extracts inhibited HIV replication both in vivo and in vitro by blocking HIV-1 attachment to cells. P. amarus also inhibited the HIV-1 enzymes: integrase by 0.48–0.16 μg/ml, reverse transcriptase by 8.17–2.53 μg/ml, and protease by 21.80–6.28 μg/ml. The extracts blocked the interaction of HIV-1 gp120 and the CD4 receptor with 50% inhibitory concentrations of 2.65 μg/ml for the aqueous/alcohol extract and 0.48 μg/ml for geraniin, an isolated egallotanin from the plant. Additionally, sera from human volunteers reduced HIV replication by more than 30% at a concentration of 5% in vivo (Notka et al., 2004). The alkaloidal extract of P. niruri selectively inhibited the cytopathic effects of HIV-1 on human MT-4 cells at the tested concentrations (CC50 = 279.85 μg/ml; EC50 = 20.98 μg/ml). The selectivity index (SI) (defined as CC50/EC50) of the extract for the viral cells was 13.34 (Naik & Juvekar, 2003). P. amarus contains gallotannins, and the ellagitannins: geraniin and corilagin were shown to be the most potent mediators of the anti HIV-1 activities (Notka et al., 2004).
T. Cacao is a small evergreen tree native to South America (Baliga et al., 2014). The seeds of T. cacao are often used in the food industry especially for chocolate making (Wickramasuriya and Dunwell, 2018). T. cacao has anti-HIV activity. A sodium hydroxide cocoa husk extract inhibited the CPE of HIV-1 against HTLV-1-transformed T-cell lines; MT-2 and MT-4. Furthermore, the extract also inhibited syncytium formation between HIV-infected and uninfected lymphoblastoid T-cell line, MOLT-4. The extract is thought to act by inhibiting the adsorption of the virus (Unten et al., 1991). Cacao lignin-carbohydrate complex (LCC) has also shown strong antiviral and macrophage stimulatory activity (Sakagami, et al., 2010). However, cacao mass LCC demonstrated higher anti-HIV activity than cacao husk (Sakagami et al., 2011). The husk extract is made up of condensed or polymerized flavonoids such as catechin and anthocyanidin which are sometimes complexed with glucose (Kimura, 1979). T. cacao contains several bioactive compounds such theobromine, and polyphenols (Goya et al., 2016; Oyeleke, et al., 2018). However, the specific phytochemical compounds responsible for its anti-HIV-1 activity were not established.
Other plant species with promising as antiviral but with limited research are presented below.
L. aggregata is a common herb in China and Japan. Its roots are mainly utilized for the treatment of pain, inflammation, indigestion, cold and hernia (Xiao et al., 2011; Wei et al., 2017). L. aggregata exhibited significant antiviral activity by inhibiting virus-induced CPE against the SARS-CoV strain BJ001 (EC50 = 88.2 ± 7.7 μg/ml) (Li et al., 2005). The specific phytochemical compounds responsible for its anti-SARS-CoV-2 activity were not established.
L. radiata is a herb native to China, Korea, Japan, and Nepal with various biological activities including: antiviral, and anti-inflammatory (Cedrón et al., 2010; Lamoral-Theys et al., 2010; Kretzing et al., 2011). The ethanolic stem extract of L. radiata exhibited inhibitory effects against SARS- CoV-2, which have been attributed to lycorine Li et al. (2005), the main active component (Yang et al., 2019).
O. acanthium is a herbaceous plant native to Europe, Xinjiang and W. Himalaya in Asia, and NW. Africa (POWO, 2020). O. acanthium has anti-inflammatory, anti-cancer, antiviral, and antioxidant properties. O. acanthium was effective at inhibiting ACE-2 activity by more 80% (Sharifi et al., 2013), which could potentially be effective against SARS-CoV-2. Although the specific compounds responsible for its anti-SARS-CoV-2 activity were not established, extracts of O. acanthium have a wide range of bio-active components including flavonoids, triterpenoids, phenylpropanoids, sesquiterpene lactones and sterols (Csupor-Löffler et al., 2014; Abusamra et al., 2015).
P. lingua is an epiphytic fern that mainly occurs in Korea, Japan, China, and other Asian states. P. lingua has antiviral, antioxidant, antibacterial and anti-cancer activities (Zheng, 1990; Fan et al., 2020). The leaves of P. lingua have traditionally been used to treat various viral infections. The chloroform leaf extract of P. lingua had antiviral activity against SARS-CoV-1 BJ-001 strain (EC50 = 43.2) and BJ-006 strain (EC50 = 40.5). P. lingua was proposed as one of the potential candidates for the development of new anti-SARS-CoV drugs (Li et al., 2005). Although the specific compounds responsible for its anti SARS-CoV activity were not established, P. lingua is known to contain several bioactive compounds such as flavonoids, chlorogenic acid, mangiferin, isomangiferin, astragaline and trifoline (Xiao et al., 2017). In separate studies, mangiferin has been shown to have potent anti-HIV-1 activities (Guha et al., 1996; Wang et al., 2011) as discussed under M. indica.
T. kirilowii is a Chinese medicinal herb with anti-HIV-1 activity (Lo et al., 2017). Trichobitacin from T. kirilowii suppresses HIV-1 induced formation of cell syncytia. Trichobitacin is a novel ribosome-inactivating protein that has been isolated from the roots T. kirilowii (Zheng et al., 2000). Trichobitacin also reduced the expression of HIV-1 p24 antigen and the number of HIV antigen positive cells in acutely HIV-1 infected culture (IC50 = 5 μg) (Zheng et al., 2000). According to Byers et al. (1994), clinical studies involving the use of trichosanthin to treat AIDS patients failing treatment with antiretroviral agents such as zidovudine may help to prevent loss of CD4+ cells.
This review shows that 16 plant species used traditionally in Africa and Asia for treating various ailments possess in vitro and in vivo anti-HIV-1 and anti-SARS-CoV-2 activity. This affirms the potential of medicinal plants as a reservoir of potential antiviral compounds. These compounds need further investigation as potential drug candidates for HIV-1, SARS-CoV-1 and other pathogenic agents. Most of the plant species lack clinical studies to support their efficacy despite showing in vitro bioactivity. The potential therapeutic effects of these medicinal plants and others against SARS-CoV-2 and HIV-1 should be explored through further research, on their efficacy and safety, herbal-drug interactions, clinical trials and product development.
Only two plant species; A. sativum and A. annua showed biological activity against both HIV-1 and SARS-CoV-2. Eight of the 16 plant species plant had been tested for activity against SARS-CoV-2 whereas 10 had been tested against HIV-1. Only P. ginseng and T. kirilowii were subjected clinical trials. The rest of the plant species had only been evaluated in cellular based-assays in vivo.
Although the general phytochemistry of the plant species reviewed is known, it is only in a handful of cases where the specific compounds responsible for the antiviral activity was determined. The assays conducted involved the use of various extracts including crude and pure compounds as well as fractions of the extracts. The commonest extracts or solvents used were ethanol and water. The isolated pure compounds tested were geraniin from P. amarus, ginsenoside-Rb1 from P. ginseng, mangiferin from M. indica, six isolated bioactive flavonoids from C. corylifolium, michellamine B and korundamine A from Ancistrocladus korupensis and lectins and ajoene from A. sativum.
The cytotoxicity of most of the plant species was generally low where determined. However, the cytotoxicity data need to be backed by in vitro or in vivo toxicity data. This is important because be toxic and need to be thoroughly investigated to rule out or mitigate any possible harmful effects before use (Anywar et al., 2021). Medicinal plants could potentially be toxic and need to be thoroughly investigated to rule out or mitigate any possible harmful effects before use. This can be seen from the compound trichosanthin from T. kirilowii used to treat AIDS patients. Even though clinical studies involving the use of trichosanthin to treat AIDS patients failing treatment with antiretroviral drugs such as zidovudine may help to prevent loss of CD4+ cells (Byers et al. (1994). Trichosanthin has undesirable side effects which have greatly restricted its clinical application (Zhao et al., 1999).
The plant extracts reviewed work mainly as inhibitors of the various viral enzymes involved in the virus life cycle. Although the mechanisms of action of most of the major medicinal plants were well understood, there were cases such as Cullen corylifolium against SARS-CoV-2, Korundamine A from Ancistrocladus korupensis in the different strains of HIV-1 and the water infusion Artemisia annua against HIV-1 where the mode of action was no determined.
Although the aspect of herb-drug interactions between the medicinal plants and conventional medicines are largely unknown, potential threats exist and need to be investigated. For instance, P. ginseng was found to interact with some anti-HIV drugs and change their pharmacokinetic properties. Ginsenoside Rh2, a compound from P. ginseng increased the accumulation and decreased the efflux of ritonavir through P-glycoprotein (P-gp) in Caco-2 cells and MDCK-MDR1 cells (Shi et al., 2013).
The potential therapeutic effects of T. cacao against SARS-CoV-2 should be explored through further research.
AG conceptualised the research idea. All the authors participated in drafting, reviewing and reading the manuscript.
The authors declare that the research was conducted in the absence of any commercial or financial relationships that could be construed as a potential conflict of interest.
All claims expressed in this article are solely those of the authors and do not necessarily represent those of their affiliated organizations, or those of the publisher, the editors and the reviewers. Any product that may be evaluated in this article, or claim that may be made by its manufacturer, is not guaranteed or endorsed by the publisher.
The authors acknowledge their individual research institutions.
Abrahão, J. S., and de Arruda, L. B. (2020). Special Issue "Emerging Viruses: Surveillance, Prevention, Evolution, and Control". Viruses 12 (3), 306. doi:10.3390/v12030306
Abusamra, Y. A., Scuruchi, M., Habibatni, S., Maammeri, Z., Benayache, S., D'Ascola, A., et al. (2015). Evaluation of putative cytotoxic activity of crude extracts from Onopordum acanthium leaves and Spartium junceum flowers against the U-373 glioblastoma cell line. Pak J. Pharm. Sci. 28 (4), 1225–1232.
Alam, F., Khan, G. N., and Asad, M. H. H. B. (2018). Psoralea corylifolia L: Ethnobotanical, biological, and chemical aspects: A review. Phytother Res. 32 (4), 597–615. doi:10.1002/ptr.6006
Anywar, G., Kakudidi, E., Byamukama, R., Mukonzo, J., Schubert, A., and Oryem-Origa, H. (2020a). Indigenous traditional knowledge of medicinal plants used by herbalists in treating opportunistic infections among people living with HIV/AIDS in Uganda. J. Ethnopharmacol, 246, 112205. doi:10.1016/j.jep.2019.112205
Anywar, G., Kakudidi, E., Byamukama, R., Mukonzo, J., Schubert, A., and Oryem-Origa, H. (2020b). Medicinal plants used by traditional medicine practitioners to boost the immune system in people living with HIV/AIDS in Uganda. Eur. J. Integr. Med. 35, 101011. doi:10.1016/j.eujim.2019.101011
Anywar, G. U., Kakudidi, E. K., Byamukama, R., Mukonzo, J. K., Schubert, A., Oryem-Origa, H., et al. (2021). A review of the toxicity and phytochemistry of medicinal plant species used by herbalists in treating people living with HIV/AIDS in Uganda. Front. Pharmacol. 12, 435. doi:10.3389/fphar.2021.615147
Baek, S. C., Nam, K. H., Yi, S. A., Jo, M. S., Lee, K. H., Lee, Y. H., et al. (2019). Anti-adipogenic Effect of β-Carboline Alkaloids from Garlic (Allium sativum). Foods 8 (12), 673. doi:10.3390/foods8120673
Bai, L., Gao, J., Wei, F., Zhao, J., Wang, D., and Wei, J. (2018). Therapeutic Potential of Ginsenosides as an Adjuvant Treatment for Diabetes. Front. Pharmacol. 9, 423. doi:10.3389/fphar.2018.00423
Baliga, M. S., Saxena, A., Kaur, K., Kalekhan, F., Chacko, A., Venkatesh, P., et al. (2014). “Polyphenols in the Prevention of Ulcerative Colitis,” in Polyphenols in Human Health and Disease (Massachusetts, United States: Academic Press), 655–663. doi:10.1016/b978-0-12-398456-2.00050-5
Brendler, T., and van Wyk, B. E. (2008). A historical, scientific and commercial perspective on the medicinal use of Pelargonium sidoides (Geraniaceae). J. Ethnopharmacol, 119(3), 420–433. doi:10.1016/j.jep.2008.07.037
Bringmann, G., Steinert, C., Feineis, D., Mudogo, V., Betzin, J., and Scheller, C. (2016). HIV-inhibitory michellamine-type dimeric naphthylisoquinoline alkaloids from the Central African liana Ancistrocladus congolensis. Phytochemistry, 128, 71–81. doi:10.1016/j.phytochem.2016.04.005
Byers, V. S., Levin, A. S., Malvino, A., Waites, L., Robins, R. A., and Baldwin, R. W. (1994). A phase II study of effect of addition of trichosanthin to zidovudine in patients with HIV disease and failing antiretroviral agents. AIDS Res. Hum. Retroviruses 10 (4), 413–420. doi:10.1089/aid.1994.10.413
Cedrón, J. C., Gutiérrez, D., Flores, N., Ravelo, A. G., and Estévez-Braun, A. (2010). Synthesis and antiplasmodial activity of lycorine derivatives. Bioorg. Med. Chem., 18(13), 4694–4701. doi:10.1016/j.bmc.2010.05.023
Chang, F. R., Yen, C. T., Ei-Shazly, M., Lin, W. H., Yen, M. H., Lin, K. H., et al. (2012). Anti-human coronavirus (anti-HCoV) triterpenoids from the leaves of Euphorbia neriifolia. Nat. Prod. Commun. 7 (11), 1415–1417. doi:10.1177/1934578x1200701103
Chaudhary, N., Sabikhi, L., Hussain, S. A., and Kumar, M. H. S. (2020). A comparative study of the antioxidant and ACE inhibitory activities of selected herbal extracts. J. Herbal Med., 22, 100343. doi:10.1016/j.hermed.2020.100343
Chavan, R. D., Shinde, P., Girkar, K., Madage, R., and Chowdhary, A. (2016). Assessment of anti-influenza activity and hemagglutination inhibition of Plumbago indica and Allium sativum Extracts. Pharmacognosy Res. 8 (2), 105–111. doi:10.4103/0974-8490.172562
Cho, Y. K., and Kim, J. E. (2017). Effect of Korean Red Ginseng intake on the survival duration of human immunodeficiency virus type 1 patients. J. Ginseng Res. 41 (2), 222–226. doi:10.1016/j.jgr.2016.12.006
Choi, K. T. (2008). Botanical characteristics, pharmacological effects and medicinal components of Korean Panax ginseng C A Meyer. Acta Pharmacol. Sin 29 (9), 1109–1118. doi:10.1111/j.1745-7254.2008.00869.x
Chopra, B., Dhingra, A. K., and Dhar, K. L. (2013). Psoralea corylifolia L. (Buguchi) - folklore to modern evidence: review. Fitoterapia 90, 44–56. doi:10.1016/j.fitote.2013.06.016
Chopra, R. N., Nayar, S. L., and Chopra, I. C. (1956). Glossary of Indian medicinal plants, Vol. 1. New Delhi: Council of Scientific & Industrial Research.
Csupor-Löffler, B., Zupkó, I., Molnár, J., Forgo, P., and Hohmann, J. (2014). Bioactivity-guided isolation of antiproliferative compounds from the roots of Onopordum acanthium. Nat. Product. Commun. 9 (3), 337–340.
Ding, Y., Wang, H., Shen, H., Li, Z., Geng, J., Han, H., et al. (2003). The clinical pathology of severe acute respiratory syndrome (SARS): a report from China. The J. Pathol. A J. Pathological Soc. Great Britain Ireland 200 (3), 282–289.
Efferth, T., Romero, M. R., Wolf, D. G., Stamminger, T., Marin, J. J., and Marschall, M. (2008). The antiviral activities of artemisinin and artesunate. Clin. Infect. Dis. 47, 804–811. doi:10.1086/591195
El-Saber Batiha, G., Magdy Beshbishy, A., G Wasef, L., Elewa, Y. H. A., A Al-Sagan, A., Abd El-Hack, M. E., et al. (2020). Chemical constituents and pharmacological activities of garlic (Allium sativum L.): A Review. Nutrients 12 (3), 872. doi:10.3390/nu12030872
Fan, Y., Feng, H., Liu, L., Zhang, Y., Xin, X., and Gao, D. (2020). Chemical components and antibacterial activity of the essential oil of six Pyrrosia species. Chem. Biodivers, 17(10), e2000526. doi: doi: doi:10.1002/cbdv.202000526
Ferreira, J. F. S., Simon, J. E., and Janick, J. (1997). Artemisia annua: botany, horticulture, pharmacology. Horticult. Rev. 19, 319–371.
Foster, P., and Sork, V. (1997). Population and genetic structure of the West African rain forest liana Ancistrocladus korupensis (Ancistrocladaceae). Am. J. Bot., 84(8), 1078–1091. doi: doi: doi:10.2307/2446151
Guha, S., Ghosal, S., and Chattopadhyay, U. (1996). Antitumor, immunomodulatory and anti-HIV effect of mangiferin, a naturally occurring glucosylxanthone. Chemotherapy 42 (6), 443–451. doi:10.1159/000239478
Hallock, Y. F., Cardellina, J. H., Schäffer, M., Bringmann, G., François, G., and Boyd, M. R. (1998). Korundamine A, a novel HIV-inhibitory and antimalarial "hybrid" naphthylisoquinoline alkaloid heterodimer from Ancistrocladus korupensis. Bioorg. Med. Chem. Lett., 8(13), 1729–1734. doi:10.1016/S0960-894X(98)00304-7
Hashemifesharaki, R., and Gharibzahedi, S. M. T. (2020). Future nutrient-dense diets rich in vitamin D: a new insight toward the reduction of adverse impacts of viral infections similar to COVID-19. Nutrire 45 (2), 19. doi:10.1186/s41110-020-00122-4
Helfer, M., Koppensteiner, H., Schneider, M., Rebensburg, S., Forcisi, S., Müller, C., et al. (2014). The root extract of the medicinal plant Pelargonium sidoides is a potent HIV-1 attachment inhibitor. PloS one 9 (1), e87487. doi:10.1371/journal.pone.0087487
Hieh, C. F., Chaw, S. M., and Wang, J. C. (1993). Composite: Flora of Taiwan. 2n Edn. (Taipei: Editorial Committee of the Flora of Taiwan), 3, 414–504.
Illanes-Álvarez, F., Márquez-Ruiz, D., Márquez-Coello, M., Cuesta-Sancho, S., and Girón-González, J. A. (2021). Similarities and differences between HIV and SARS-CoV-2. Int. J. Med. Sci. 18 (3), 846–851. doi:10.7150/ijms.50133
Jeong, C. S., Hyun, J. E., Kim, Y. S., and Lee, E. S. (2003). Ginsenoside Rb1: the anti-ulcer constituent from the head of Panax ginseng. Arch. Pharm. Res. 26 (11), 906–911. doi:10.1007/BF02980198
Keyaerts, E., Vijgen, L., Pannecouque, C., Van Damme, E., Peumans, W., Egberink, H., et al. (2007). Plant lectins are potent inhibitors of coronaviruses by interfering with two targets in the viral replication cycle. Antivir. Res, 75(3), 179–187. doi:10.1016/j.antiviral.2007.03.003
Kim, D. W., Seo, K. H., Curtis-Long, M. J., Oh, K. Y., Oh, J. W., Cho, J. K., et al. (2014). Phenolic phytochemical displaying SARS-CoV papain-like protease inhibition from the seeds of Psoralea corylifolia. J. Enzyme Inhib. Med. Chem. 29 (1), 59–63. doi:10.3109/14756366.2012.753591
Kim, S., Kim, D. B., Jin, W., Park, J., Yoon, W., Lee, Y., et al. (2018). Comparative studies of bioactive organosulphur compounds and antioxidant activities in garlic (Allium sativum L.), elephant garlic (Allium ampeloprasum L.) and onion (Allium cepa L.). Nat. Prod. Res. 32 (10), 1193–1197. doi:10.1080/14786419.2017.1323211
Kimura, K. (1979). Manufacturing procedure of natural pigment from cacao bean. Jpn. Patent No. Showa 54, 10567.
Kolodziej, H. (2002). Pelargonium reniforme and Pelargonium sidoides: their botany, chemistry and medicinal use. Geranium and Pelargonium. London: Taylor & Francis, 262–290.
Kretzing, S., Abraham, G., Seiwert, B., Ungemach, F. R., Krügel, U., and Regenthal, R. (2011). Dose-dependent emetic effects of the Amaryllidaceous alkaloid lycorine in beagle dogs. Toxicon, 57(1), 117–124. doi:10.1016/j.toxicon.2010.10.012
Kurokawa, M., Shimizu, T., Watanabe, W., and Shiraki, K. (2010). Development of new antiviral agents from natural products. Open Antimicrob. Agents J. 2 (1), 49–57. doi:10.2174/1876518101002020049
Lamoral-Theys, D., Decaestecker, C., Mathieu, V., Dubois, J., Kornienko, A., Kiss, R., et al. (2010). Lycorine and its derivatives for anticancer drug design. Mini Rev. Med. Chem. 10 (1), 41–50. doi:10.2174/138955710791112604
Li, S. Y., Chen, C., Zhang, H. Q., Guo, H. Y., Wang, H., Wang, L., et al. (2005). Identification of natural compounds with antiviral activities against SARS-associated coronavirus. Antivir. Res 67 (1), 18–23. doi:10.1016/j.antiviral.2005.02.007Li
Li, W., Moore, M. J., Vasilieva, N., Sui, J., Wong, S. K., Berne, M. A., et al. (2003). Angiotensin-converting enzyme 2 is a functional receptor for the SARS coronavirus. Nature 426 (6965), 450–454. doi:10.1038/nature02145
Li, X., Wang, H., Liu, C., and Chen, R. (2010). Chemical constituents of Acacia catechu. Zhongguo Zhong Yao Za Zhi 35 (11), 1425–1427.
Lissiman, E., Bhasale, A. L., and Cohen, M. (2014). Garlic for the common cold. Cochrane Database Syst. Rev. 2014 (11), CD006206. doi:10.1002/14651858.CD006206.pub4
Lo, H. Y., Li, T. C., Yang, T. Y., Li, C. C., Chiang, J. H., Hsiang, C. Y., et al. (2017). Hypoglycemic effects of Trichosanthes kirilowii and its protein constituent in diabetic mice: the involvement of insulin receptor pathway. BMC Complement. Altern. Med. 17, 53. doi:10.1186/s12906-017-1578-6
Louten, J. (2016). Emerging and reemerging viral diseases. Essent. Hum. Virol., 291–310. doi:10.1016/B978-0-12-800947-5.00016-8
Lubbe, A., Seibert, I., Klimkait, T., and van der Kooy, F. (2012). Ethnopharmacology in overdrive: The remarkable anti-HIV activity of Artemisia annua. J. Ethnopharmacol, 141(3), 854–859. doi:10.1016/j.jep.2012.03.024
Makare, N., Bodhankar, S., and Rangari, V. (2001). Immunomodulatory activity of alcoholic extract of Mangifera indica L. in mice. J. Ethnopharmacol 78 (2-3), 133–137. doi:10.1016/s0378-8741(01)00326-9
Manfredi, K. P., Blunt, J. W., Cardellina, J. H., McMahon, J. B., Pannell, L. L., Cragg, G. M., et al. (1991). Novel alkaloids from the tropical plant Ancistrocladus abbreviatus inhibit cell killing by HIV-1 and HIV-2. J. Med. Chem. 34 (12), 3402–3405. doi:10.1021/jm00116a011
Martin, K. W., and Ernst, E. (2003). Antiviral agents from plants and herbs: a systematic review. Antivir. Ther. 8 (2), 77–90.
Michaelis, M., Doerr, H. W., and Cinatl, J. (2011). Investigation of the influence of EPs® 7630, a herbal drug preparation from Pelargonium sidoides, on replication of a broad panel of respiratory viruses. Phytomedicine, 18(5), 384–386. doi:10.1016/j.phymed.2010.09.008Mourya
Mourya, D. T., Yadav, P. D., Ullas, P. T., Bhardwaj, S. D., Sahay, R. R., Chadha, M. S., et al. (2019). Emerging/re-emerging viral diseases & new viruses on the Indian horizon. Indian J. Med. Res. 149 (4), 447–467. doi:10.4103/ijmr.IJMR_1239_18
Naik, A. D., and Juvekar, A. R. (2003). Effects of alkaloidal extract of Phyllanthus niruri on HIV replication. Indian J. Med. Sci. 57 (9), 387–393.
Notka, F., Meier, G., and Wagner, R. (2004). Concerted inhibitory activities of Phyllanthus amarus on HIV replication in vitro and ex vivo. Antivir. Res 64 (2), 93–102. doi:10.1016/j.antiviral.2004.06.010
Nutan, M. M., Modi, M., Dezzutti, C. S., Kulshreshtha, S., Rawat, A. K., Srivastava, S. K., et al. (2013). Extracts from Acacia catechu suppress HIV-1 replication by inhibiting the activities of the viral protease and Tat. Virol. J. 10 (1), 309–317. doi:10.1186/1743-422x-10-309
Oyeleke, S. A., Ajayi, A. M., Umukoro, S., Aderibigbe, A. O., and Ademowo, O. G. (2018). Anti-inflammatory activity of Theobroma cacao L. stem bark ethanol extract and its fractions in experimental models. J. Ethnopharmacol 222, 239–248. doi:10.1016/j.jep.2018.04.050
Patel, J. D., Kumar, V., and Bhatt, S. A. (2009). Antimicrobial screening and phytochemical analysis of the resin part ofAcacia catechu. Pharm. Biol. 47 (1), 34–37. doi:10.1080/13880200802400527
Perera, C., and Efferth, T. (2012). Antiviral medicinal herbs and phytochemicals. J. Pharmacogn 3 (1), 45–48.
Phan, A. D. T., Netzel, G., Chhim, P., Netzel, M. E., and Sultanbawa, Y. (2019). Phytochemical characteristics and antimicrobial activity of Australian grown garlic (Allium Sativum L.) Cultivars. Foods 8 (9), 358. doi:10.3390/foods8090358
Plants of the world (POWO) (2020). http://powo.science.kew.org/taxon/urn:lsid:ipni.org:names:235234-1.
Prasad, A., Muthamilarasan, M., and Prasad, M. (2020). Synergistic antiviral effects against SARS-CoV-2 by plant-based molecules. Plant Cel Rep 39 (9), 1109–1114. doi:10.1007/s00299-020-02560-w
Rivlin, R. S. (2001). Historical perspective on the use of garlic. J. Nutr. 131 (3), 951S–4S. doi:10.1093/jn/131.3.951S
Sagaya Jansi, R., Khusro, A., Agastian, P., Alfarhan, A., Al-Dhabi, N. A., Arasu, M. V., et al. (2020). Emerging paradigms of viral diseases and paramount role of natural resources as antiviral agents. Sci. Total Environ., 143539. doi:10.1016/j.scitotenv.2020.143539
Sakagami, H., Kawano, M., Thet, M. M., Hashimoto, K., Satoh, K., Kanamoto, T., et al. (2011). Anti-HIV and immunomodulation activities of cacao mass lignin-carbohydrate complex. In Vivo 25 (2), 229–236.
Sakagami, H., Kushida, T., Oizumi, T., Nakashima, H., and Makino, T. (2010). Distribution of lignin-carbohydrate complex in plant kingdom and its functionality as alternative medicine. Pharmacol. Ther., 128(1), 91–105. doi: doi: doi:10.1016/j.pharmthera.2010.05.004
Schneiderová, K., Slapetová, T., Hrabal, R., Dvořáková, H., Procházková, P., Novotná, J., et al. (2013). Tomentomimulol and mimulone B: two new C-geranylated flavonoids from Paulownia tomentosa fruits. Nat. Prod. Res. 27 (7), 613–618. doi:10.1080/14786419.2012.683002
Sharifi, N., Souri, E., Ziai, S. A., Amin, G., and Amanlou, M. (2013). Discovery of new angiotensin converting enzyme (ACE) inhibitors from medicinal plants to treat hypertension using an in vitro assay. Daru 21 (1), 74. doi:10.1186/2008-2231-21-74
Shehzad, O., Khan, S., Ha, I. J., Park, Y., and Kim, Y. S. (2012). Rational development of a selection model for solvent gradients in single-step separation of ginsenosides from Panax ginseng using high-speed counter-current chromatography. J. Sep. Sci. 35, 1462–1469. doi:10.1002/jssc.201200135
Shehzad, O., Kim, H. P., and Kim, Y. S. (2013). State-of-the-art separation of ginsenosides from Korean white and red ginseng by countercurrent chromatography. Anal. Bioanal. Chem. 405, 4523–4530. doi:10.1007/s00216-012-6609-z
Shi, J., Cao, B., Zha, W. B., Wu, X. L., Liu, L. S., Xiao, W. J., et al. (2013). Pharmacokinetic interactions between 20(S)-ginsenoside Rh2 and the HIV protease inhibitor ritonavir in vitro and in vivo. Acta Pharmacol. Sin 34 (10), 1349–1358. doi:10.1038/aps.2013.69
Sung, H., Kang, S. M., Lee, M. S., Kim, T. G., and Cho, Y. K. (2005). Korean red ginseng slows depletion of CD4 T cells in human immunodeficiency virus type 1-infected patients. Clin. Diagn. Lab. Immunol. 12 (4), 497–501. doi:10.1128/CDLI.12.4.497-501.2005
Sunil, M. A., Sunitha, V. S., Radhakrishnan, E. K., and Jyothis, M. (2019). Immunomodulatory activities of Acacia catechu, a traditional thirst quencher of South India. J. Ayurveda Integr. Med., 10(3), 185–191. doi: doi: doi:10.1016/j.jaim.2017.10.010
Tatarintsev, A. V., Vrzhets, P. V., Ershov, D. E., Shchegolev, A. A., Turgiev, A. S., Karamov, E. V., et al. (1992). The ajoene blockade of integrin-dependent processes in an HIV-infected cell system. Vestn Ross Akad Med. Nauk (11–12), 6–10.
UNAIDS (2021). Global HIV & AIDS statistics — Fact sheet. https://www.unaids.org/sites/default/files/media_asset/UNAIDS_FactSheet_en.pdf.
Unten, S., Ushijima, H., Shimizu, H., Tsuchie, H., Kitamura, T., Moritome, N., et al. (1991). Effect of cacao husk extract on human immunodeficiency virus infection. Lett. Appl. Microbiol., 13(6), 251–254. doi: doi: doi:10.1111/j.1472-765X.1991.tb00621.x
Walder, R., Kalvatchev, Z., Garzaro, D., Barrios, M., and Apitz-Castro, R. (1997). In vitro suppression of HIV-1 replication by ajoene [(e)-(z)-4,5,9-trithiadodeca-1,6,11-triene-9 oxide]. Biomed. Pharmacother., 51(9), 397–403. doi: doi: doi:10.1016/S0753-3322(97)89433-4
Wang, R. R., Gao, Y. D., Ma, C. H., Zhang, X. J., Huang, C. G., Huang, J. F., et al. (2011). Mangiferin, an anti-HIV-1 agent targeting protease and effective against resistant strains. Molecules 16 (5), 4264–4277. doi:10.3390/molecules16054264
Wei, G., Chen, H., Nie, F., Ma, X., and Jiang, H. (2017). 1, 3, 6-Trihydroxy-7-Methyl-9, 10-Anthracenedione Isolated from genus Lindera with Anti-cancer Activity. Anticancer Agents Med. Chem. 17 (11), 1604–1607. doi:10.2174/1871520615666150914114649
WHO (2021). WHO Coronavirus (COVID-19) Dashboard. https://covid19.who.int.
Wickramasuriya, A. M., and Dunwell, J. M. (2018). Cacao biotechnology: current status and future prospects. Plant Biotechnol. J. 16 (1), 4–17. doi:10.1111/pbi.12848
Willcox, M., Bodeker, G., Bourdy, G., Dhingra, V., Falquet, J., Ferreira, J. F., et al. (2004). Artemisia annua as a traditional herbal antimalarial. Traditional Med. plants Malar. 4, 43–59. doi:10.1201/9780203502327-14
Xiao, M., Cao, N., Fan, J. J., Shen, Y., and Xu, Q. (2011). Studies on flavonoids from the leaves of Lindera aggregata. Zhong Yao Cai 34 (1), 62–64.
Xiao, W., Peng, Y., Tan, Z., Lv, Q., Chan, C. O., Yang, J., et al. (2017). Comparative evaluation of chemical profiles of Pyrrosiae folium originating from three pyrrosia species by HPLC-DAD combined with multivariate statistical analysis. Molecules 22 (12), 2122. doi:10.3390/molecules22122122
Xu, X., Chen, P., Wang, J., Feng, J., Zhou, H., Li, X., et al. (2020). Evolution of the novel coronavirus from the ongoing Wuhan outbreak and modeling of its spike protein for risk of human transmission. Sci. China Life Sci. 63 (3), 457–460. doi:10.1007/s11427-020-1637-5
Yang, L., Zhang, J. H., Zhang, X. L., Lao, G. J., Su, G. M., Wang, L., et al. (2019). Tandem mass tag-based quantitative proteomic analysis of lycorine treatment in highly pathogenic avian influenza H5N1 virus infection. PeerJ 7, e7697. doi:10.7717/peerj.7697
Zhao, J., Ben, L. H., Wu, Y. L., Hu, W., Ling, K., Xin, S. M., et al. (1999). Anti-HIV agent trichosanthin enhances the capabilities of chemokines to stimulate chemotaxis and G protein activation, and this is mediated through interaction of trichosanthin and chemokine receptors. J. Exp. Med. 190 (1), 101–111. doi:10.1084/jem.190.1.101
Zheng, M. (1990). Experimental study of 472 herbs with antiviral action against the herpes simplex virus. Zhong Xi Yi Jie He Za Zhi 10 (1), 39–41.
Keywords: medicinal plants, antiviral, COVID-19, HIV/aids, immune system, infectious diseases
Citation: Anywar G, Akram M and Chishti MA (2021) African and Asian Medicinal Plants as a Repository for Prospective Antiviral Metabolites Against HIV-1 and SARS CoV-2: A Mini Review. Front. Pharmacol. 12:703837. doi: 10.3389/fphar.2021.703837
Received: 30 April 2021; Accepted: 10 August 2021;
Published: 25 August 2021.
Edited by:
Banasri Hazra, Jadavpur University, IndiaReviewed by:
Roodabeh Bahramsoltani, Tehran University of Medical Sciences, IranCopyright © 2021 Anywar, Akram and Chishti. This is an open-access article distributed under the terms of the Creative Commons Attribution License (CC BY). The use, distribution or reproduction in other forums is permitted, provided the original author(s) and the copyright owner(s) are credited and that the original publication in this journal is cited, in accordance with accepted academic practice. No use, distribution or reproduction is permitted which does not comply with these terms.
*Correspondence: Godwin Anywar, Z29kd2luYW55d2FyQGdtYWlsLmNvbQ==
Disclaimer: All claims expressed in this article are solely those of the authors and do not necessarily represent those of their affiliated organizations, or those of the publisher, the editors and the reviewers. Any product that may be evaluated in this article or claim that may be made by its manufacturer is not guaranteed or endorsed by the publisher.
Research integrity at Frontiers
Learn more about the work of our research integrity team to safeguard the quality of each article we publish.