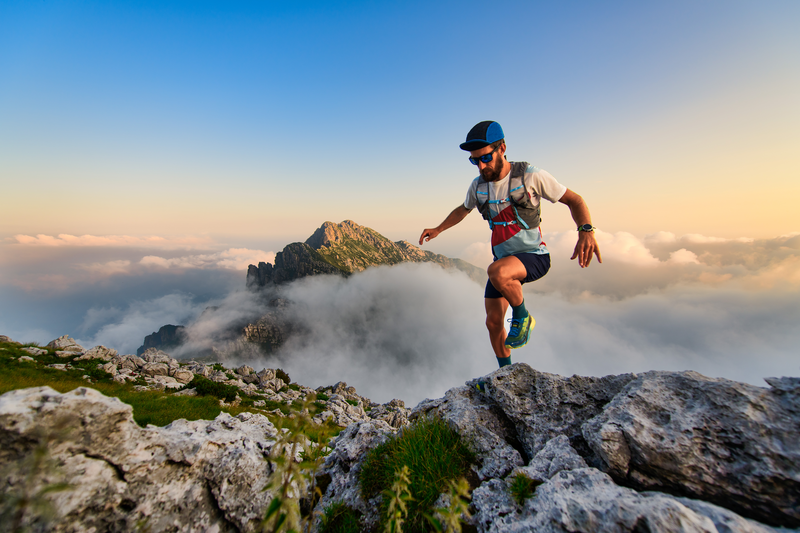
94% of researchers rate our articles as excellent or good
Learn more about the work of our research integrity team to safeguard the quality of each article we publish.
Find out more
ORIGINAL RESEARCH article
Front. Pharmacol. , 27 September 2021
Sec. Inflammation Pharmacology
Volume 12 - 2021 | https://doi.org/10.3389/fphar.2021.697912
This article is part of the Research Topic The Role of Neuroinflammation in Chronic Pain Development and Maintenance View all 11 articles
The mechanisms involved in the development and maintenance of cancer pain remain largely unidentified. Recently, it has been reported that β-adrenergic receptors (β-ARs), mainly β2-and β3-ARs, contribute to tumor proliferation and progression and may favor cancer-associated pain and neuroinflammation. However, the mechanism underlying β-ARs in cancer pain is still unknown. Here, we investigated the role of β1-, β2-and β3-ARs in a mouse model of cancer pain generated by the para-tibial injection of K7M2 osteosarcoma cells. Results showed a rapid tumor growth in the soft tissue associated with the development of mechanical allodynia in the hind paw ipsilateral to the injected site. In addition to reduce tumor growth, both propranolol and SR59230A, β1-/β2-and β3-AR antagonists, respectively, attenuated mechanical allodynia, the number of macrophages and an oxidative stress by-product accumulated in the ipsilateral tibial nerve. The selective β1-AR antagonist atenolol was able to slightly reduce the tumor growth but showed no effect in reducing the development of mechanical allodynia. Results suggest that the development of the mechanical allodynia in K7M2 osteosarcoma-bearing mice is mediated by oxidative stress associated with the recruitment of neural macrophages, and that antagonism of β2-and β3-ARs contribute not solely to the reduction of tumor growth, but also in cancer pain. Thus, the targeting of the β2-and β3-ARs signaling may be a promising therapeutic strategy against both tumor progression and the development of cancer-evoke pain in osteosarcoma.
Pain is one of the most common invalidating symptoms of cancer, affecting approximately 70% of cancer patients worldwide every year (van den Beuken-van Everdingen et al., 2007). Despite the significant advances in understanding, early detection, and treatment of cancer, progress in the knowledge of pain related to cancer and improvement in the use of analgesics have been limited. Causes of cancer pain are multifactorial and complex and are likely connected with an array of tumor- and host-related factors (Lam and Schmidt, 2010). Numerous receptors and their activators have been studied for a better understanding of the cancer pain mechanisms. These comprise the transient receptor potential (TRP) channels, including the vanilloid 1 (TRPV1) and ankyrin 1 (TRPA1) subtypes which are involved in bone (Ghilardi et al., 2005), and melanoma (Antoniazzi et al., 2019; De Logu et al., 2021) cancer pain models, respectively. The contribution of the acid-sensing ion channels (ASICs) has been reported in an osteolysis-induced bone cancer pain (Nagae et al., 2007) and the protease-activator receptor 2 (PAR2) in peripheral neurons is the target of serine proteases and tryptase released from cancer cells to promote the prolonged mechanical allodynia in mouse cancer nociceptive models (Lam and Schmidt, 2010).
β-ARs are G protein coupled receptors constituted of seven transmembrane domains (Hein and Kobilka, 1995; Hein, 2006), which mediate catecholamine-induced activation of adenylate cyclase. Three β-AR subtypes have been identified, the β1-, β2-and β3-ARs, which are mostly known for their role in the regulation of cardiovascular, airway, uterine, and other peripheral functions (Hein, 2006). β-ARs have also been implicated in functions of the central nervous system, including the regulation of sympathetic tone, as well as different disorders, including those associated with learning and memory, mood and food intake (Hein and Kobilka, 1995; Hein, 2006). Given their expression in peripheral nervous systems (Hein, 2006), emerging evidence also suggests a contribution of β-ARs in modulating different chronic pain conditions (Yalcin et al., 2010; Bunevicius et al., 2013; Zhang et al., 2018). β-ARs seem to be involved in pain processes mainly through activation of immunoregulatory cells, including T-cells (Laukova et al., 2012; Slota et al., 2015), mast cells (Chi et al., 2004), and macrophages (Chiarella et al., 2014; Kim et al., 2014) with associated increases in pro-inflammatory cytokines and nociceptors sensitization (Binshtok et al., 2008; Czeschik et al., 2008). Expression of β2-ARs within the nociceptive system suggested their potential implication in nociception and pain (Nicholson et al., 2005; Kanno et al., 2010; Hartung et al., 2014; Ciszek et al., 2016; Zhang et al., 2018), and human genetic studies also confirmed the contribution of β2-ARs to chronic pain disorders (Diatchenko et al., 2006). However, the recruitment of β2-ARs in the participation to pro- or antinociceptive mechanisms might depend on the considered stimulus since analgesic actions were observed with an agonist in a visceral pain model (Bentley and Starr, 1986) and with an antagonist in inflammatory pain models (Parada et al., 2003; Nackley et al., 2007). Conversely, either the stimulation or inhibition of the β1-AR subtype did not directly participate to the antinociceptive mechanisms in models of neuropathic pain (Yalcin et al., 2009a; Yalcin et al., 2009b). Instead, the β3-AR subtype has been primarily associated with metabolic regulation both in physiological and pathological conditions (Schena and Caplan, 2019).
Neuropathic pain resulting from nerve injury may be attributable to an excessive immune response leading to the development of neuroinflammation in the peripheral (Trevisan et al., 2016; De Logu et al., 2017) or central nervous system (Tsuda et al., 2003). Neuroinflammation is an established cause of neuropathic pain in the sciatic nerve ligation mouse model (De Logu et al., 2017), in the reperfusion ischemia mouse model (De Logu et al., 2020a), and in the trigeminal nerve ligation mouse model (Trevisan et al., 2016). Moreover, inflammatory processes are involved in different pathologies, including cancer. It is widely recognized that the immune system interacts with the sensory nervous system contributing to maintenance of chronic pain (De Logu et al., 2017; De Logu et al., 2020a).
In model of sciatic nerve injury in rat, noradrenergic perivascular axons germinate in the dorsal root ganglia (DRG) to form unusual structures around axotomized sensory neurons of large diameter able to mediate pain signaling (McLachlan et al., 1993). In addition, increased mRNA levels of β2-AR in ipsilateral DRGs after sciatic nerve injury has been observed (Maruo et al., 2006). Moreover, the neuropathy produced by the axotomy of DRG neurons was relieved by manipulation of adrenergic receptors (ARs) (Chung and Chung, 2002), and noradrenaline, by the β3-AR stimulation, has been reported to mediate the release of adenosine-triphosphate (ATP) from DRG neurons, thus causing mechanical allodynia in rat model of peripheral nerve injury (Kanno et al., 2010). Immune cells respond by activation of ARs, primarily the β2-ARs, which regulate a variety of functions ranging from cellular migration to cytokine secretion (Wieduwild et al., 2020). However, little is known about the involvement of β-ARs in cancer evoked-pain.
Here, we investigated whether β1-, β2-and β3-ARs contribute to pain in a mouse model of osteosarcoma generated by the para-tibial injection of K7M2 cells, using different β-ARs blockers. Although the selectivity of the most of β-ARs blockers is questionable, based on previously reported data (Dal Monte et al., 2013; Calvani et al., 2019) we used atenolol, propranolol and SR59230A as blockers to modulate β1-, β2-and β3-ARs downstream effects, respectively. We observed that pharmacological antagonism of β2-and β3-ARs, reduced tumor growth and the ensuing mechanical allodynia caused by K7M2 osteosarcoma cells inoculation. Tumor growth was associated with an increase in the number of F4/80+ cells inside the tibial nerve that was attenuated by β2-and β3-ARs antagonism. These results corroborate the role of neuroinflammation in cancer pain and introduce the role of β-ARs as crucial players in the development and maintenance of chronic pain and in the modulation of neuroinflammation.
BALB/c mice (male, 4 weeks old, Envigo RMS) were used. Mice were housed in a temperature- and humidity-controlled vivarium (12 h dark/light cycle, free access to food and water). Behavioral experiments were done in a quiet, temperature-controlled (20–22°C) room between 9 am and 5 pm and were performed by an operator blinded to the drug treatment. Animals were anesthetized with a mixture of ketamine and xylazine (90 mg/kg and 3 mg/kg, respectively, i.p.) and euthanized with inhaled CO2 plus 10–50% O2.
Osteosarcoma K7M2 (CRL-2836, American Type Culture Collection, ATCC) murine cells were grown in Dulbecco′s Modified Eagle′s Medium (DMEM) supplemented with 10% fetal bovine serum (FBS), penicillin/streptomycin solution (1x) and glutamine (2 mM) at 37°C, 5% CO2 atmosphere, and 21% O2.
For cells inoculation, 50 µl of K7M2 (7 × 105) cells were suspended in phosphate buffer saline (PBS) and injected para-tibial. Control groups (sham) were injected with 50 µl of PBS. K7M2 cell lines are isogenic with the BALB/c mouse strain. Tumor growth rate was evaluated by measuring tumor mass with a caliber, and tumor mass volume calculated as volume = [(length x width)2/2]. Mice were sacrificed at day 20 after K7M2 cells inoculation or sham. SR59230A (Tocris Bioscence), propranolol (Merck Life Science) and atenolol (Merck Life Science) (10 mg/kg i.p.) or vehicle (NaCl 0.9%) were administered twice a day (every 8 h) starting from day 10 after K7M2 cells inoculation, when a palpable tumor was present, or sham. Phenyl-alpha-tert-butyl nitrone (PBN, 100 mg/kg, i.p.) or vehicle (4% dimethyl sulfoxide, DMSO, 4% tween 80 in 0.9% NaCl) was given at day 14 after K7M2 cells inoculation.
The measurement of mechanical paw withdrawal threshold (PWT) was carried out using von Frey filaments of increasing stiffness (0.02–2 g) applied to the plantar surface of the mouse hindpaw, according to the up-and-down paradigm (Chaplan et al., 1994). The 50% mechanical paw-withdrawal threshold (g) response was then calculated from the resulting scores.
Tibial nerves were dissected from euthanized mice and mechanically dissociated to obtain a single cell suspension. Briefly, nerves were dissociated in a solution containing HEPES (25 mM), Hanks’ Balanced Salt solution (HBSS, 1x), FBS 10% and dnase (10 µM) by using the gentleMACS Octo Dissociator (Miltenyi Biotec). Cells were then stained with anti-CD45-VioBlue conjugated antibody (130-110-664, Miltenyi Biotec), anti-F4/80-PE conjugated antibody (130-116-449, Miltenyi Biotec), and anti-CD64-APC conjugated antibody (130-126-950, Miltenyi Biotec). After staining, cells were subjected to flow cytometry by using a Miltenyi Biotec MACSQuant Analyzer 10. Results were analyzed by using FlowlogicTM Software. Gating strategy is reported in Figure 1A.
FIGURE 1. Tumor growth and mechanical allodynia induced by K7M2 osteosarcoma cells in BALB/c mice (A and B) Time dependent increase in tumor size and mechanical allodynia after para-tibial K7M2 osteosarcoma cell inoculation or sham in BALB/c mice (C) Representative gating strategy of flow cytometry analysis and relative quantification of F4/80+/CD64+ monocytes/macrophages in tibial nerve, and (D) typical images and pooled data of F4/80+ cells in the ipsilateral tibial nerve (stained with the neural marker PGP9.5) after para-tibial K7M2 osteosarcoma cell inoculation or sham in BALB/c mice. BL, baseline. PWT, paw withdrawal threshold. N = 6 mice. *p < 0.05, ***p < 0.001 K7M2 vs Sham. Data are presented as mean ± SEM, data points overlaid (C, D). Two-way ANOVA and Bonferroni post hoc test (A, B), unpaired student t-test (C, D).
The tumor and tibial nerve were dissected from anesthetized and transcardially perfused with PBS, followed by 4% paraformaldehyde, mice. The tibial nerve and the hindlimb were postfixed for 24 h, and cryoprotected in sucrose 30%. Immunofluorescence staining was performed according to standard procedures. Briefly, after the antibody retrieval with citrate buffer pH 6, tissue sections (10 µm) were incubated with the following primary antibodies: F4/80 [1:50, MA516624, rat monoclonal (Cl:A3-1), RRID:AB_2538120 Thermo Fisher Scientific], PGP9.5 (1:250, ab108986, rabbit monoclonal, RRID:AB_ 10891773 Abcam), β1 receptor (1:400, ab3442, rabbit polyclonal, RRID:AB_ 10890808 Abcam), β2 receptor (1:100, ab182136, rabbit monoclonal, RRID:AB_ 2747383 Abcam), β3 receptor (1:100, ab94506, rabbit monoclonal, RRID:AB_ 10863818 Abcam) diluted in block solution (5% normal goat serum and normal donkey serum, in PBS and triton-x100, PBST) 1 h at room temperature. Sections were then incubated for 2 h in the dark with the fluorescent secondary antibody polyclonal, Alexa Fluor® 488 (1:600, Thermo Fisher Scientific) or Alexa Fluor® 594 (1:600, Thermo Fisher Scientific). Sections were coverslipped using a water-based mounting medium with 4′6′-diamidino-2-phenylindole (DAPI, Abcam). The analysis of negative controls (non-immune serum) was simultaneously performed to exclude the presence of non-specific immunofluorescent staining, cross-immunostaining, or fluorescence bleed-through. For histological evaluation, sections were stained with hematoxylin/eosin and based on the morphology, the boundaries of the nerve trunk corresponding to the epineurium were identified and reported in adjacent immunofluorescence images with dashed lines. The number of F4/80+ cells was counted in 104 μm2 boxes in the tibial nerve trunk.
H2O2 level was assessed by using the Amplex Red® assay (Thermo Fisher Scientific). Tibial nerve tissue was rapidly removed and placed into modified Krebs/HEPES buffer [composition in mmol/l: 99.01 NaCl, 4.69 KCl, 2.50 CaCl2, 1.20 MgSO4, 1.03 KH2PO4, 25.0 NaHCO3, 20.0 Na-HEPES, and 5.6 glucose (pH 7.4)]. Samples were minced and incubated with Amplex red (100 μM) and HRP (1 U/ml) (1 h, 37°C) in modified Krebs/HEPES buffer protected from light. Fluorescence excitation and emission were at 540 and 590 nm, respectively. H2O2 production was calculated using H2O2 standard and expressed as μmol/l of mg of dry tissue.
RAW 264.7 cells were seeded in 96-well plates (30.000 cells/well) and grown in phenol red-free Roswell Park Memorial Institute (RPMI). The cultured medium was replaced with Krebs-Ringer phosphate (KRP, composition in mM: 2 CaCl2; 5.4 KCl; 0.4 MgSO4; 135 NaCl; 10 d-glucose; 10 HEPES [pH 7.4]) added with SR59230A, propranolol, atenolol (all, 100 nM) or vehicle (0.01% DMSO in KRP) for 20 min at room temperature. Cells were activated by the addition of phorbol-myristate-acetate (PMA, 16 μM), along with Amplex Red (50 μM) and HRP (1 U/ml) at a total volume of 100 μl. Signal was detected 60 min after exposure to the stimuli, at 560 nm H2O2 release was calculated using H2O2 standards and expressed as nmol/1 (Idelman et al., 2015).
The group size of n = 6 animals for behavioral experiments was determined by sample size estimation using G*Power (v3.1) (Faul et al., 2007) to detect size effect in a post-hoc test with type 1 and 2 error rates of 5 and 20%, respectively. Mice were allocated to vehicle or treatment groups using a randomization procedure (http://www.randomizer.org/). Investigators were blinded to the treatments, which were revealed only after data collection. No animals were excluded from experiments.
Results are expressed as mean ± standard error of the mean (SEM). For multiple comparisons, a one-way analysis of variance (ANOVA) followed by the post-hoc Bonferroni’s test or Dunnett’s test was used. Two groups were compared using Student’s t-test. For behavioral experiments with repeated measures, the two-way mixed model ANOVA followed by the post-hoc Bonferroni’s test was used. Statistical analyses were performed on raw data using Graph Pad Prism 8 (GraphPad Software Inc.). p values less than 0.05 (p < 0.05) were considered significant. Statistical tests used and the sample size for each analysis are listed in the figure legends.
Para-tibial inoculation of K7M2 osteosarcoma cells into the hindlimb of BALB/c mice induced a time-dependent (0–20 days) increase in hindlimb thickness, mainly due to tumor growth (Figure 1A). Tumor growth was associated with a parallel increase in mechanical allodynia (Figure 1B) and in the number of F4/80+/CD64+ monocytes/macrophages inside the tibial nerve trunk ipsilateral to the inoculated hindlimb, as shown by flow cytometric analysis and immunofluorescence staining (Figures 1C, D). Control (sham) mice did not develop either the increase in the hindlimb volume or mechanical allodynia or in the number of monocytes/macrophages inside the tibial nerve ipsilateral to the injected hindlimb (Figures 1A–D). These data show a time-dependent association between the tumor mass growth and the development of the mechanical allodynia and for the first time that the tumor growth in K7M2 osteosarcoma-bearing mice is associated with an increased neuroinflammation in the tibial nerve, revealed by the increased number of macrophages (F4/80+/CD64+ cells).
To investigate a possible role of β-ARs in the modulation of tumor growth and mechanical allodynia induced by para-tibial inoculation of K7M2 osteosarcoma cells, 10 days after K7M2 osteosarcoma cells inoculation, when allodynia was already present, mice were treated with the β1-AR antagonist atenolol, the β1-/β2-AR antagonist propranolol, the β3-AR antagonist SR59230A, or vehicle. As already reported in other cancer mouse models (Calvani et al., 2019; Bruno et al., 2020; Calvani et al., 2020), the treatment with β2-and β3-AR antagonists induced a significant reduction in tumor size (Figure 2A), thus confirming a pivotal role of β2-and β3-ARs in tumor growth. Conversely, the treatment with atenolol induced a slight, but not significant, reduction in the tumor size, denoting a minor role of the β1-AR subtype in controlling tumor growth (Figure 2A). Surprisingly, K7M2 osteosarcoma-bearing mice treated with propranolol and SR59230A, but not with atenolol exhibited a marked reduction in the mechanical allodynia (Figure 2B).
FIGURE 2. β2-and β3-, but not β1-ARs antagonism reduce K7M2 osteosarcoma growth and mechanical allodynia in BALB/c mice (A) Time dependent increase in tumor size and (B) mechanical allodynia after para-tibial K7M2 osteosarcoma cell inoculation or sham in BALB/c mice treated (daily, starting from day 10) with atenolol (10 mg/kg, i.p.), propranolol (10 mg/kg, i.p.), SR59230A (10 mg/kg, i.p.), or vehicle (Veh) (C and D) Representative immunofluorescence images of β1-, β2-and β3-ARs expression in (C) tumor cells and (D) macrophages (F4/80+ cells) in tibial nerve, 20 days after para-tibial inoculation of K7M2 osteosarcoma cells. BL, baseline. PWT, paw withdrawal threshold. N = 6 mice. **p < 0.01, ***p < 0.001 vs Sham; §p < 0.05, §§p < 0.01, §§§p < 0.001 vs K7M2 + Veh. Data are presented as mean ± SEM. Two-way ANOVA and Bonferroni post hoc test.
To investigate the role played by the β-ARs in tumor and in macrophages accumulating in the neural space, we first evaluated the expression of the three β-AR subtypes in tumor cells and in macrophages located in the tibial nerve. Immunofluorescence staining showed the expression of all three β-AR subtypes in the tumor cells (Figure 2C). Macrophages only expressed β2-and β3-but not β1-AR (Figure 2D).
Overall, these data showed that while β-ARs blockade, mainly β2-and β3-ARs and to a lesser extent β1-AR, is able to significantly influence the osteosarcoma tumor growth, the targeting of β2-and β3-ARs, but not of β1-AR, mitigated the established mechanical allodynia resulting from osteosarcoma tumor growth.
We previously observed that the development of mechanical allodynia in several neuropathic pain models (De Logu et al., 2017; De Logu et al., 2020a; De Logu et al., 2020b; De Logu et al., 2021) was associated with the increase in monocytes/macrophages inside the sciatic nerve ipsilateral to the injury. Thus, based on results showing the efficacy of β-AR antagonism to reduce the mechanical allodynia (Figure 2B), we then investigated whether the number of macrophages recruited in tibial nerve of osteosarcoma-bearing mice, could be modulated by β-AR antagonists administration.
We observed that the repeated treatment with the β1/2- and β3-AR antagonists (propranolol and SR59230A), but not with the β1-AR antagonist (atenolol), showed a significant reduction in the number of macrophages in tibial nerve compared to vehicle-treated mice (Figures 3A, B).
FIGURE 3. β2-and β3-AR antagonists reduce neuroinflammation in tibial nerve of K7M2 osteosarcoma-bearing mice (A) Representative flow cytometry analysis and relative quantification of F4/80+/CD64+ macrophages (B) typical immunofluorescence images and pooled data of F4/80+ cells and (C) H2O2 content in the tibial nerve after para-tibial K7M2 osteosarcoma cell inoculation or sham in BALB/c mice treated (daily, starting from day 10) with atenolol (10 mg/kg, i.p.) propranolol (10 mg/kg, i.p.), SR59230A (10 mg/kg, i.p.) or vehicle (Veh) (D) H2O2 level in RAW 264.7 stimulated with PMA (16 μM) in presence of SR59230A, propranolol, atenolol (all, 100 nM) or Veh (n = 3 independent experiments) (E) Mechanical allodynia at day 14 after para-tibial K7M2 osteosarcoma cell inoculation or sham in BALB/c mice treated with PBN (100 mg/kg, i.p.) or Veh. BL, baseline. PWT, paw withdrawal threshold. N = 6 mice. *p < 0.05, ***p < 0.001 vs Sham, Veh-Veh; §p < 0.05, §§§p < 0.001 vs K7M2 + Veh, Veh-PMA, K7M2 + Veh PBN. Data are presented as mean ± SEM. One-way (A–C) or two-way (D–E) ANOVA and Bonferroni post hoc test.
It has been known that the increase in macrophages number evokes an increase in oxidative stress at the tissue level, leading to the development of pain (De Logu et al., 2017; De Logu et al., 2020a; De Logu et al., 2020b; De Logu et al., 2021). Accordingly, in the tibial nerve of K7M2 osteosarcoma-bearing mice, we observed an increase in H2O2 levels compared to sham mice (Figure 3C), and the repeated treatment with propranolol and SR59230A, but not with atenolol, significantly reduced the increase in H2O2 levels in tibial nerve (Figure 3C). Given the absence of the β1-AR expression in macrophages, we speculated that the analgesic effect observed in K7M2 osteosarcoma-bearing mice following the repeated treatment with propranolol and SR59230A, was mainly due to a modulation of neuroinflammation (H2O2 release) dependent on β2-and β3-ARs blockade on tibial macrophages. To corroborate our hypothesis, we stimulated a murine macrophage cell line (RAW 264.7) with the pro-oxidant agent phorbol 12-myristate 13-acetate (PMA) alone or in combination with the different β-AR antagonists. Results showed that PMA induced a release of H2O2 from RAW 264.7 macrophages which was reduced in the presence of β2-and β3-AR but not with β1-AR antagonists (Figure 3D).
To finally confirm that the increase in H2O2 level was associated to mechanical allodynia in our model, K7M2 osteosarcoma-bearing mice were treated with the antioxidant PBN, at day 14 after cells inoculation, when mechanical allodynia was already established. Data showed that a single injection of PBN was able to transiently revert mechanical allodynia (Figure 3E).
Overall, our data suggest that macrophages accumulating in tibial nerve of K7M2 osteosarcoma-bearing mice induce the development of mechanical allodynia by releasing oxidative stress, and that this process is finely regulated by β2-and β3-but not by β1-ARs modulation.
Cancer-induced pain represents a high-priority complication related to tumor growth, not only in primary bone cancer, but also in bone cancer metastasis. Despite the growing interest in the research of new treatments for cancer pain, opioids remain the leading therapeutic option, which, however, shows marked limitations. Poor understanding of molecular mechanisms implicated in the development and maintenance of cancer-induced pain limits the identification of novel therapeutic targets for this condition. Previous studies highlighted the role of β-ARs modulation in models of various neuropathic pain (Kanno et al., 2010; Fávaro-Moreira et al., 2012; Zhang et al., 2018). However, the mechanism by which these receptors promote the development and maintenance of pain is poorly understood. Our data show that β-ARs, mainly β2-and β3-ARs sustain both tumor growth and cancer pain in a syngeneic osteosarcoma murine model. Indeed, the systemic treatment with the β1-AR antagonist atenolol did not affect osteosarcoma tumor growth, while the β1/2- and β3-ARs antagonists, propranolol and SR59230A respectively, reduced osteosarcoma tumor growth and were also able to modulate the mechanical allodynia developed with the tumor growth. As these receptors are expressed both on tumor cells and inflammatory cells, including macrophages, administration of β-ARs antagonists is able to target both of these pathological processes.
First, we showed that the osteosarcoma-bearing mice developed a sustained mechanical allodynia, starting from day 10 after K7M2 osteosarcoma cells inoculation, that was absent in sham mice. Then, based on previous data that showed the pivotal role of peripheral nerve macrophages in the development of pain in mouse models of neuropathic and cancer pain (De Logu et al., 2017; De Logu et al., 2020a; De Logu et al., 2021), we wondered whether also in this cancer model, macrophages in the peripheral (tibial) nerve, which runs ipsilateral to the tumor, played a role in the development and maintenance of mechanical allodynia. Data showed that the number of neural macrophages in the tibial nerve of osteosarcoma-bearing mice were clearly increased compared to sham mice.
To test the involvement of the β-ARs in the maintenance of cancer-related pain in osteosarcoma-bearing mice, we treated the mice (daily) with the β1-AR antagonist atenolol, the β1/2-AR antagonist propranolol and the β3-AR antagonist SR59230A. Results showed that atenolol treatment had no effect in reducing mechanical allodynia, while propranolol and SR59230A treatment significantly reduced the mechanical allodynia. Moreover, propranolol and SR59230A were also able to reduce the tumor growth, confirming the crucial role of the β2-and β3-AR subtypes in sustaining pro-tumoral signaling in agreement with previous data obtained in other mouse models of cancer (Lamkin et al., 2012; Magnon et al., 2013; Calvani et al., 2019; Bruno et al., 2020; Calvani et al., 2020).
Since the development of mechanical allodynia was associated with an increase in neural macrophages and that β-ARs antagonism was able to reduce osteosarcoma-induced pain, we wondered if there was a correlation between β-ARs activity and the modulation of neuroinflammation. Surprisingly, β2-and β3-ARs antagonists were able to significantly reduce the number of macrophages in the tibial nerve compared to vehicle treated mice. According to previously reported data showing that peripheral β2-and β3-ARs drive functional pain via increased activation of immune cells which promote neuroinflammation and neuropathic pain (Kanno et al., 2010; Zhang et al., 2018), and in the light of our previous data (De Logu et al., 2017; De Logu et al., 2020a; De Logu et al., 2021), our results suggested that the reduction in mechanical allodynia observed in propranolol- and SR59230A-treated mice was partially due to the reduced inflammatory cells (macrophages) in tibial nerve associated with the decreased tumor size following drug treatment. Furthermore, the evaluation of β-ARs expression in osteosarcoma tumor and in neural (tibial nerve) macrophages, showed that while all the three β-ARs were expressed in the tumor, only the β2-and β3-AR subtypes were expressed on neural macrophages. These results, together with data showing that both β1/2-AR and β3-AR but not β1-AR antagonism was able to affect cancer-evoked mechanical allodynia, supported the hypothesis that β2-and β3-AR signaling on neural macrophages sustained the mechanical allodynia developed in osteosarcoma-bearing mice.
To corroborate this hypothesis, we focused our attention to the increase in the oxidative stress as biological process correlated to the macrophages activity in the tibial nerves, and responsible for the nociceptor activation and the ensuing pain condition (De Logu et al., 2017). We observed that, in tibial nerves of osteosarcoma-bearing mice, the amount of H2O2, a reactive oxygen species (ROS) known to be responsible of nociception activation in several pain model (Antoniazzi et al., 2019; Brusco et al., 2020; De Logu et al., 2021), resulted increased compared to sham mice, and that treatment with propranolol and SR59230A, but not with atenolol, abrogated this effect in vivo. Accordingly, ROS (H2O2) production in murine macrophages was reverted by the treatment with propranolol and SR59230A, but not with atenolol. Finally, we reported that the treatment with an antioxidant transiently abrogated the established mechanical allodynia in vivo, thus confirming a leading role of oxidative burst in maintaining pain condition.
To date, there are no clinical studies aiming at investigating whether the use of β-AR antagonists could be effective in counteract cancer-pain in humans. However, a phase 2 study (NCT01222091) aimed at exploring whether propranolol treatment improved thermal and mechanical hypersensitivity after administration of an opioid (remifentanil), showed that concomitant infusion of propranolol with remifentanil prevented the remifentanil-induced post infusion hyperalgesia (Chu et al., 2012). These data highlight the great significance of investigating the role of the β-adrenergic system as pharmacological target for preventing different type of pain.
Overall, our data suggest a role for the β-ARs signaling, mainly through β2-and β3-ARs, in sustaining both tumor growth and cancer-induced pain in a syngeneic osteosarcoma murine model and identify macrophages recruited in the peripheral nerve and oxidative stress generation, as cellular and molecular mediators for the development of such pain. Furthermore, our study highlights the ability of β-ARs antagonists in modulating both tumor growth and the induction of cancer-evoked pain, giving to these receptors unique features as promising therapeutic targets for cancer therapy and associated pain.
The raw data supporting the conclusions of this article will be made available by the authors, without undue reservation.
The animal study was reviewed and approved by University of Florence research permit n° 936/2017-PR.
GB, FL, RN, MC, CF conceived and supervised the project, interpreted the results and finalized the manuscript. GB, FL, DA, RN contributed to experimental designs, performed experiments, interpreted the results, generated figures and wrote the manuscript. GB, DA, AS, FL performed experiments, interpreted the results. All authors discussed the results and revision of the manuscript and approved the manuscript.
The research leading to these results has received funding from AIRC under IG 2020-ID. 24503 project–P.I. RN.
The authors declare that the research was conducted in the absence of any commercial or financial relationships that could be construed as a potential conflict of interest.
The reviewer PB declared a shared affiliation with one of the authors, FL, to the handling editor at time of review.
All claims expressed in this article are solely those of the authors and do not necessarily represent those of their affiliated organizations, or those of the publisher, the editors and the reviewers. Any product that may be evaluated in this article, or claim that may be made by its manufacturer, is not guaranteed or endorsed by the publisher.
Antoniazzi, C. T. D., Nassini, R., Rigo, F. K., Milioli, A. M., Bellinaso, F., Camponogara, C., et al. (2019). Transient Receptor Potential Ankyrin 1 (TRPA1) Plays a Critical Role in a Mouse Model of Cancer Pain. Int. J. Cancer 144, 355–365. doi:10.1002/ijc.31911
Bentley, G. A., and Starr, J. (1986). The Antinociceptive Action of Some Beta-Adrenoceptor Agonists in Mice. Br. J. Pharmacol. 88, 515–521. doi:10.1111/j.1476-5381.1986.tb10231.x
Binshtok, A. M., Wang, H., Zimmermann, K., Amaya, F., Vardeh, D., Shi, L., et al. (2008). Nociceptors Are Interleukin-1beta Sensors. J. Neurosci. 28, 14062–14073. doi:10.1523/JNEUROSCI.3795-08.2008
Bruno, G., Cencetti, F., Pini, A., Tondo, A., Cuzzubbo, D., Fontani, F., et al. (2020). β3-Adrenoreceptor Blockade Reduces Tumor Growth and Increases Neuronal Differentiation in Neuroblastoma via SK2/S1P2 Modulation. Oncogene 39, 368–384. doi:10.1038/s41388-019-0993-1
Brusco, I., Li Puma, S., Chiepe, K. B., da Silva Brum, E., de David Antoniazzi, C. T., de Almeida, A. S., et al. (2020). Dacarbazine Alone or Associated with Melanoma-Bearing Cancer Pain Model Induces Painful Hypersensitivity by TRPA1 Activation in Mice. Int. J. Cancer 146, 2797–2809. doi:10.1002/ijc.32648
Bunevicius, A., Hinderliter, A., Klatzkin, R., Patel, A., Arizmendi, C., and Girdler, S. S. (2013). Beta-Adrenergic Receptor Mechanisms and Pain Sensitivity in Women with Menstrually Related Mood Disorders. J. Pain 14, 1349–1360. doi:10.1016/j.jpain.2013.05.014
Calvani, M., Bruno, G., Dabraio, A., Subbiani, A., Bianchini, F., Fontani, F., et al. (2020). β3-Adrenoreceptor Blockade Induces Stem Cells Differentiation in Melanoma Microenvironment. Int. J. Mol. Sci. 21, 1420. doi:10.3390/ijms21041420
Calvani, M., Bruno, G., Dal Monte, M., Nassini, R., Fontani, F., Casini, A., et al. (2019). β3 -Adrenoceptor as a Potential Immuno-Suppressor Agent in Melanoma. Br. J. Pharmacol. 176, 2509–2524. doi:10.1111/bph.14660
Chaplan, S. R., Bach, F. W., Pogrel, J. W., Chung, J. M., and Yaksh, T. L. (1994). Quantitative Assessment of Tactile Allodynia in the Rat Paw. J. Neurosci. Methods 53, 55–63. doi:10.1016/0165-0270(94)90144-9
Chi, D. S., Fitzgerald, S. M., Pitts, S., Cantor, K., King, E., Lee, S. A., et al. (2004). MAPK-Dependent Regulation of IL-1- and Beta-Adrenoreceptor-Induced Inflammatory Cytokine Production from Mast Cells: Implications for the Stress Response. BMC Immunol. 5, 22. doi:10.1186/1471-2172-5-22
Chiarella, S. E., Soberanes, S., Urich, D., Morales-Nebreda, L., Nigdelioglu, R., Green, D., et al. (2014). β₂-Adrenergic Agonists Augment Air Pollution-Induced IL-6 Release and Thrombosis. J. Clin. Invest. 124, 2935–2946. doi:10.1172/JCI75157
Chu, L. F., Cun, T., Ngai, L. K., Kim, J. E., Zamora, A. K., Young, C. A., et al. (2012). Modulation of Remifentanil-Induced Postinfusion Hyperalgesia by the β-Blocker Propranolol in Humans. Pain 153, 974–981. doi:10.1016/j.pain.2012.01.014
Chung, J. M., and Chung, K. (2002). Importance of Hyperexcitability of DRG Neurons in Neuropathic Pain. Pain Pract. 2, 87–97. doi:10.1046/j.1533-2500.2002.02011.x
Ciszek, B. P., O'Buckley, S. C., and Nackley, A. G. (2016). Persistent Catechol-O-Methyltransferase-Dependent Pain Is Initiated by Peripheral β-Adrenergic Receptors. Anesthesiology 124, 1122–1135. doi:10.1097/ALN.0000000000001070
Czeschik, J. C., Hagenacker, T., Schäfers, M., and Büsselberg, D. (2008). TNF-Alpha Differentially Modulates Ion Channels of Nociceptive Neurons. Neurosci. Lett. 434, 293–298. doi:10.1016/j.neulet.2008.01.070
Dal Monte, M., Casini, G., Filippi, L., Nicchia, G. P., Svelto, M., and Bagnoli, P. (2013). Functional Involvement of β3-Adrenergic Receptors in Melanoma Growth and Vascularization. J. Mol. Med. (Berl) 91, 1407–1419. doi:10.1007/s00109-013-1073-6
De Logu, F., De Prá, S. D., de David Antoniazzi, C. T., Kudsi, S. Q., Ferro, P. R., Landini, L., et al. (2020). Macrophages and Schwann Cell TRPA1 Mediate Chronic Allodynia in a Mouse Model of Complex Regional Pain Syndrome Type I. Brain Behav. Immun. 88, 535–546. doi:10.1016/j.bbi.2020.04.037
De Logu, F., Nassini, R., Materazzi, S., Carvalho Gonçalves, M., Nosi, D., Rossi Degl'Innocenti, D., et al. (2017). Schwann Cell TRPA1 Mediates Neuroinflammation that Sustains Macrophage-dependent Neuropathic Pain in Mice. Nat. Commun. 8, 1887. doi:10.1038/s41467-017-01739-2
De Logu, F., Trevisan, G., Marone, I. M., Coppi, E., Padilha Dalenogare, D., Titiz, M., et al. (2020). Oxidative Stress Mediates Thalidomide-Induced Pain by Targeting Peripheral TRPA1 and central TRPV4. BMC Biol. 18, 197. doi:10.1186/s12915-020-00935-9
De Logu, F., Marini, M., Landini, L., Souza Monteiro de Araujo, D., Bartalucci, N., Trevisan, G., et al. (2021). Peripheral Nerve Resident Macrophages and Schwann Cells Mediate Cancer-Induced Pain. Cancer Res. 81 (12), 3387–3401. doi:10.1158/0008-5472.can-20-3326
Diatchenko, L., Anderson, A. D., Slade, G. D., Fillingim, R. B., Shabalina, S. A., Higgins, T. J., et al. (2006). Three Major Haplotypes of the Beta2 Adrenergic Receptor Define Psychological Profile, Blood Pressure, and the Risk for Development of a Common Musculoskeletal Pain Disorder. Am. J. Med. Genet. B Neuropsychiatr. Genet. 141b, 449–462. doi:10.1002/ajmg.b.30324
Faul, F., Erdfelder, E., Lang, A. G., and Buchner, A. (2007). G*Power 3: A Flexible Statistical Power Analysis Program for the Social, Behavioral, and Biomedical Sciences. Behav. Res. Methods 39, 175–191. doi:10.3758/bf03193146
Fávaro-Moreira, N. C., Parada, C. A., and Tambeli, C. H. (2012). Blockade of β₁-, β₂- and β₃-Adrenoceptors in the Temporomandibular Joint Induces Antinociception Especially in Female Rats. Eur. J. Pain 16, 1302–1310. doi:10.1002/j.1532-2149.2012.00132.x
Ghilardi, J. R., Röhrich, H., Lindsay, T. H., Sevcik, M. A., Schwei, M. J., Kubota, K., et al. (2005). Selective Blockade of the Capsaicin Receptor TRPV1 Attenuates Bone Cancer Pain. J. Neurosci. 25, 3126–3131. doi:10.1523/JNEUROSCI.3815-04.2005
Hartung, J. E., Ciszek, B. P., and Nackley, A. G. (2014). β2- and β3-Adrenergic Receptors Drive COMT-Dependent Pain by Increasing Production of Nitric Oxide and Cytokines. Pain 155, 1346–1355. doi:10.1016/j.pain.2014.04.011
Hein, L. (2006). Adrenoceptors and Signal Transduction in Neurons. Cell Tissue Res. 326, 541–551. doi:10.1007/s00441-006-0285-2
Hein, L., and Kobilka, B. K. (1995). Adrenergic Receptor Signal Transduction and Regulation. Neuropharmacology 34, 357–366. doi:10.1016/0028-3908(95)00018-2
Idelman, G., Smith, D. L. H., and Zucker, S. D. (2015). Bilirubin Inhibits the Up-Regulation of Inducible Nitric Oxide Synthase by Scavenging Reactive Oxygen Species Generated by the Toll-Like Receptor 4-Dependent Activation of NADPH Oxidase. Redox Biol. 5, 398–408. doi:10.1016/j.redox.2015.06.008
Kanno, T., Yaguchi, T., and Nishizaki, T. (2010). Noradrenaline Stimulates ATP Release from DRG Neurons by Targeting Beta(3) Adrenoceptors as a Factor of Neuropathic Pain. J. Cel Physiol. 224, 345–351. doi:10.1002/jcp.22114
Kim, M. H., Gorouhi, F., Ramirez, S., Granick, J. L., Byrne, B. A., Soulika, A. M., et al. (2014). Catecholamine Stress Alters Neutrophil Trafficking and Impairs Wound Healing by β2-Adrenergic Receptor-Mediated Upregulation of IL-6. J. Invest. Dermatol. 134, 809–817. doi:10.1038/jid.2013.415
Lam, D. K., and Schmidt, B. L. (2010). Serine Proteases and Protease-Activated Receptor 2-Dependent Allodynia: A Novel Cancer Pain Pathway. Pain 149, 263–272. doi:10.1016/j.pain.2010.02.010
Lamkin, D. M., Sloan, E. K., Patel, A. J., Chiang, B. S., Pimentel, M. A., Ma, J. C., et al. (2012). Chronic Stress Enhances Progression of Acute Lymphoblastic Leukemia via β-Adrenergic Signaling. Brain Behav. Immun. 26, 635–641. doi:10.1016/j.bbi.2012.01.013
Laukova, M., Vargovic, P., Csaderova, L., Chovanova, L., Vlcek, M., Imrich, R., et al. (2012). Acute Stress Differently Modulates β1, β2 and β3 Adrenoceptors in T Cells, but Not in B Cells, from the Rat Spleen. Neuroimmunomodulation 19, 69–78. doi:10.1159/000329002
Magnon, C., Hall, S. J., Lin, J., Xue, X., Gerber, L., Freedland, S. J., et al. (2013). Autonomic Nerve Development Contributes to Prostate Cancer Progression. Science 341, 1236361. doi:10.1126/science.1236361
Maruo, K., Yamamoto, H., Yamamoto, S., Nagata, T., Fujikawa, H., Kanno, T., et al. (2006). Modulation of P2X Receptors via Adrenergic Pathways in Rat Dorsal Root Ganglion Neurons after Sciatic Nerve Injury. Pain 120, 106–112. doi:10.1016/j.pain.2005.10.016
McLachlan, E. M., Jänig, W., Devor, M., and Michaelis, M. (1993). Peripheral Nerve Injury Triggers Noradrenergic Sprouting within Dorsal Root Ganglia. Nature 363, 543–546. doi:10.1038/363543a0
Nackley, A. G., Tan, K. S., Fecho, K., Flood, P., Diatchenko, L., and Maixner, W. (2007). Catechol-O-Methyltransferase Inhibition Increases Pain Sensitivity through Activation of Both Beta2- and Beta3-Adrenergic Receptors. Pain 128, 199–208. doi:10.1016/j.pain.2006.09.022
Nagae, M., Hiraga, T., and Yoneda, T. (2007). Acidic Microenvironment Created by Osteoclasts Causes Bone Pain Associated with Tumor Colonization. J. Bone Miner Metab. 25, 99–104. doi:10.1007/s00774-006-0734-8
Nicholson, R., Dixon, A. K., Spanswick, D., and Lee, K. (2005). Noradrenergic Receptor mRNA Expression in Adult Rat Superficial Dorsal Horn and Dorsal Root Ganglion Neurons. Neurosci. Lett. 380, 316–321. doi:10.1016/j.neulet.2005.01.079
Parada, C. A., Yeh, J. J., Joseph, E. K., and Levine, J. D. (2003). Tumor Necrosis Factor Receptor Type-1 in Sensory Neurons Contributes to Induction of Chronic Enhancement of Inflammatory Hyperalgesia in Rat. Eur. J. Neurosci. 17, 1847–1852. doi:10.1046/j.1460-9568.2003.02626.x
Schena, G., and Caplan, M. J. (2019). Everything You Always Wanted to Know about β3-AR * (* but Were Afraid to Ask). Cells 8, 357. doi:10.3390/cells8040357
Slota, C., Shi, A., Chen, G., Bevans, M., and Weng, N. P. (2015). Norepinephrine Preferentially Modulates Memory CD8 T Cell Function Inducing Inflammatory Cytokine Production and Reducing Proliferation in Response to Activation. Brain Behav. Immun. 46, 168–179. doi:10.1016/j.bbi.2015.01.015
Trevisan, G., Benemei, S., Materazzi, S., De Logu, F., De Siena, G., Fusi, C., et al. (2016). TRPA1 Mediates Trigeminal Neuropathic Pain in Mice Downstream of Monocytes/Macrophages and Oxidative Stress. Brain 139, 1361–1377. doi:10.1093/brain/aww038
Tsuda, M., Shigemoto-Mogami, Y., Koizumi, S., Mizokoshi, A., Kohsaka, S., Salter, M. W., et al. (2003). P2X4 Receptors Induced in Spinal Microglia Gate Tactile Allodynia after Nerve Injury. Nature 424, 778–783. doi:10.1038/nature01786
van den Beuken-van Everdingen, M. H., de Rijke, J. M., Kessels, A. G., Schouten, H. C., van Kleef, M., and Patijn, J. (2007). Prevalence of Pain in Patients with Cancer: A Systematic Review of the Past 40 Years. Ann. Oncol. 18, 1437–1449. doi:10.1093/annonc/mdm056
Wieduwild, E., Girard-Madoux, M. J., Quatrini, L., Laprie, C., Chasson, L., Rossignol, R., et al. (2020). β2-Adrenergic Signals Downregulate the Innate Immune Response and Reduce Host Resistance to Viral Infection. J. Exp. Med. 217, e20190554. doi:10.1084/jem.20190554
Yalcin, I., Choucair-Jaafar, N., Benbouzid, M., Tessier, L. H., Muller, A., Hein, L., et al. (2009). Beta(2)-Adrenoceptors Are Critical for Antidepressant Treatment of Neuropathic Pain. Ann. Neurol. 65, 218–225. doi:10.1002/ana.21542
Yalcin, I., Tessier, L. H., Petit-Demoulière, N., Doridot, S., Hein, L., Freund-Mercier, M. J., et al. (2009). Beta2-Adrenoceptors Are Essential for Desipramine, Venlafaxine or Reboxetine Action in Neuropathic Pain. Neurobiol. Dis. 33, 386–394. doi:10.1016/j.nbd.2008.11.003
Yalcin, I., Tessier, L. H., Petit-Demoulière, N., Waltisperger, E., Hein, L., Freund-Mercier, M. J., et al. (2010). Chronic Treatment with Agonists of Beta(2)-Adrenergic Receptors in Neuropathic Pain. Exp. Neurol. 221, 115–121. doi:10.1016/j.expneurol.2009.10.008
Keywords: β-adrenergic receptors, cancer pain, osteosarcoma, neuroinflammation, macrophages
Citation: Bruno G, De Logu F, Souza Monteiro de Araujo D, Subbiani A, Lunardi F, Rettori S, Nassini R, Favre C and Calvani M (2021) β2-and β3-Adrenergic Receptors Contribute to Cancer-Evoked Pain in a Mouse Model of Osteosarcoma via Modulation of Neural Macrophages. Front. Pharmacol. 12:697912. doi: 10.3389/fphar.2021.697912
Received: 20 April 2021; Accepted: 15 September 2021;
Published: 27 September 2021.
Edited by:
Pallavi R. Devchand, University of Calgary, CanadaReviewed by:
Paola Bagnoli, University of Pisa, ItalyCopyright © 2021 Bruno, De Logu, Souza Monteiro de Araujo, Subbiani, Lunardi, Rettori, Nassini, Favre and Calvani. This is an open-access article distributed under the terms of the Creative Commons Attribution License (CC BY). The use, distribution or reproduction in other forums is permitted, provided the original author(s) and the copyright owner(s) are credited and that the original publication in this journal is cited, in accordance with accepted academic practice. No use, distribution or reproduction is permitted which does not comply with these terms.
*Correspondence: Romina Nassini, cm9taW5hLm5hc3NpbmlAdW5pZmkuaXQ=
†These authors have contributed equally to this work
Disclaimer: All claims expressed in this article are solely those of the authors and do not necessarily represent those of their affiliated organizations, or those of the publisher, the editors and the reviewers. Any product that may be evaluated in this article or claim that may be made by its manufacturer is not guaranteed or endorsed by the publisher.
Research integrity at Frontiers
Learn more about the work of our research integrity team to safeguard the quality of each article we publish.