- 1School of Pharmacy and State Key Laboratory of Quality Research in Chinese Medicine, Macau University of Science and Technology, Macau, China
- 2Department of Cardiothoracic Surgery, Zhuhai People’s Hospital, Jinan University Medical School, Guangzhou, China
Perivascular adipose tissue (PVAT) is a unique fat depot with local and systemic impacts. PVATs are anatomically, developmentally, and functionally different from classical adipose tissues and they are also different from each other. PVAT adipocytes originate from different progenitors and precursors. They can produce and secrete a wide range of autocrine and paracrine factors, many of which are vasoactive modulators. In the context of obesity-associated low-grade inflammation, these phenotypic and functional differences become more evident. In this review, we focus on the recent findings of PVAT’s heterogeneity by comparing commonly studied adipose tissues around the thoracic aorta (tPVAT), abdominal aorta (aPVAT), and mesenteric artery (mPVAT). Distinct origins and developmental trajectory of PVAT adipocyte potentially contribute to regional heterogeneity. Regional differences also exist in ways how PVAT communicates with its neighboring vasculature by producing specific adipokines, vascular tone regulators, and extracellular vesicles in a given microenvironment. These insights may inspire new therapeutic strategies targeting the PVAT.
Introduction
Obesity is becoming a substantial public health concern since it gives rise to a wide range of disorders (Olshansky et al., 2005). Obesity prevalence has tripled since 1975 and it is now one of the WHO’s priorities to cease the quick rise in obesity (Jaacks et al., 2019). The burgeoning global epidemic of obesity, consequently leading to type 2 diabetes, dyslipidemias, cardiovascular disease, and even some cancers will soon be devastating if actions are not taken (Alberti et al., 2009; Grundy, 2012; Scheen and Van Gaal, 2014). Obesity occurs due to excess energy intake, dramatic fat mass accumulation caused by increased adipocyte size (hypertrophy), or increased adipocyte number (hyperplasia), or both. Given the systemic impacts, obesity cannot be seen simply as a fat accumulation, and it goes hand-in-hand with many metabolic complications. People often experienced obesity as a chronic affliction characterized by low-grade inflammation. Despite the absence of the four cardinal signs of typical inflammation, the unresolved inflammation, entwined with fibrosis and defected angiogenesis-induced hypoxia, is a common pathway in the development of various cardiometabolic diseases.
Excessive fat accumulation can happen in various adipose depots, including a kind of unique adipose tissue known as perivascular adipose tissue (PVAT). PVAT surrounds most blood vessels except capillaries, pulmonary and cerebral blood vessels. PVAT plays wide-ranging physiological roles far beyond supporting connective tissue. It is now considered a metabolically active organ that regulates vascular function in both autocrine and paracrine fashions by producing various adipokines (Rajsheker et al., 2010; Van de Voorde et al., 2013). Over the decades, our understanding of PVAT biology has increased simultaneously with a rising prevalence of obesity and related metabolic complications. Not only does PVAT actively maintain vascular homeostasis, but it also markedly modulates various inflammation-related cardiovascular diseases. Due to the proximity of PVAT to the associated blood vessels, PAVT can be a unique target to improve arterial function in the setting of obesity.
PVATs are not only different from classical adipose tissues, they vary from location to location developmentally and functionally (Chang et al., 2020). Most intriguingly, PVAT’s origin largely remains unknown, despite the development of cellular fate mapping and lineage tracing in other fat depots (Sanchez-Gurmaches et al., 2016; Guimaraes-Camboa et al., 2017; Schwalie et al., 2018; Merrick et al., 2019; Shao et al., 2019; Cattaneo et al., 2020). Numerous publications have presented us with a bulk of mixed information, given the fact that these publications always include more than one PVAT, which challenges readers outside of the field. The general information on the pathophysiological functions of PVAT has been the subject of many recent comprehensive reviews (Nosalski and Guzik, 2017; Hildebrand et al., 2018; Chang et al., 2020). In this review, we aim to form a conceptual picture of PVAT heterogeneity, mainly focusing on histology, developmental origin, and briefly on secretome.
Depot-Specific and Polychromatic Adipose Tissue
Nomenclature of Perivascular Adipose Tissues
A PVAT is generally named after its adjacent vessel’s name, such as pericoronary adipose tissue, referring to the fat tissue around the coronary artery. Over the years, various non-standard names were used for the same PVAT, which can be merely confusing or even misleading. For instance, “cardiac” PVAT surrounding the coronary artery or epicardial adipose tissue was abbreviated as either “C-PVAT” (Drosos et al., 2016), or “PVAT-CA” (Lu et al., 2017), or even “epi” for shorter (Mazurek et al., 2003). It is not uncommon that these names are mistakenly referred to, for example, in the case of pericardial and epicardial fat (Iacobellis, 2009). These facts reason the need to standardize nomenclature for PVATs. Some research articles have used a combined format to define these concepts (Tran et al., 2018; Ye et al., 2019). Throughout this review, we will use their traditional names according to their locations but with some specifications. In particular, we “standardized” these names by introducing a common name, “PVAT,” following a lowercase letter, indicating where it is localized. For example, pericoronary adipose tissue is termed as “cPVAT,” thoracic periaortic adipose tissue is abbreviated as “tPVAT,” and abdominal periaortic adipose tissue as “aPVAT.” Adipose tissue around the mesenteric artery is called “mPVAT.” A comparison among the four most commonly studied PVATs is presented in Table 1 and Figure 1.
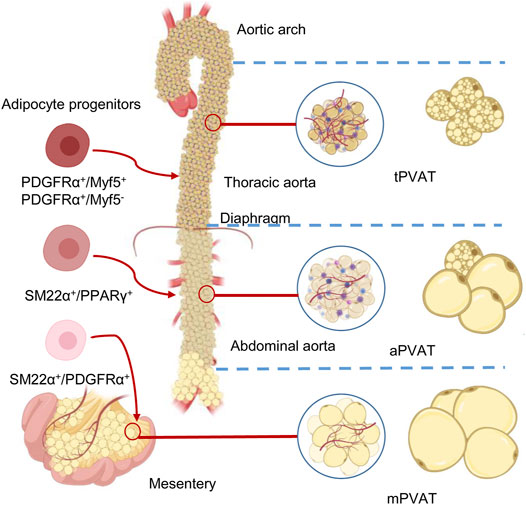
FIGURE 1. Anatomical, morphological, and developmental differences of the perivascular adipose tissues (PVATs). The color differences reflect the different proportions of brown adipocytes in the adipose tissue. Adipose tissues around thoracic aorta (tPVAT), abdominal aorta (aPVAT), and mesenteric artery (mPVAT) have distinct fat cell populations that are derived from different adipocyte progenitors. These progenitors could be identified by highly expressed cell markers, such as platelet-derived growth factor receptor-α (PGDFRα), myogenic factor 5 (Myf5), smooth muscle protein 22-α (SM22α), and peroxisome proliferator-activated receptor-γ (PPARγ).
Multicolored Adipose Tissue
Adipose tissues are empirically color-coded as white (WAT), brown (BAT), and beige/brown-in-white (brite) adipose tissues. The color explicitly reflects the main adipocyte population and the amount of iron-containing mitochondria in each adipocyte. The more mitochondria, the darker the color is. In addition to major depots, there are other location-specific adipose tissues, including mammary “pink” adipose tissue and bone marrow adipose tissue, which is primarily red in young individuals and turns yellow during aging. It would undoubtedly be oversimplified if we classify adipose tissues based solely on the appearing color and neglect their complexity and heterogeneity. Classical inter-scapular BAT has been previously assumed to contain a homogeneous population of brown adipocytes. A recent study has revealed a new brown adipocyte subpopulation with low thermogenic activity coexists with the classical high-thermogenic brown adipocytes (Song et al., 2020). More interestingly, these two brown adipocyte subpopulations may interconvert dynamically in response to the environmental temperature (Song et al., 2020). Using AdipoChaser mouse models, Wang et al. also demonstrated that “pink” mammary adipose tissue can undergo reversible dedifferentiation during lactation, where de-differentiated fibroblasts proliferate and re-differentiate into adipocytes upon weaning (Wang et al., 2018).
PVAT’s color is not visually presented. Instead, it is determined by the subcellular composition and enrichment of specific genetic biomarkers. For example, tPVAT, characterized as brown adipose tissue, is labeled with highly expressed uncoupling protein-1 (UCP-1) and cell death activator CIDE-A (Cidea) (Tran et al., 2018). Conversely, aPVAT is seemingly white but contains a mixture of beige and white adipocytes (Padilla et al., 2013). Therefore, when we talk about the color of a PVAT, we mainly use generalized terminology to reflect their cellular characteristics and corresponding genetic profiles. Generally speaking, white PVAT adipocytes share a lot of similarities with their visceral counterparts, which are morphologically classified by the appearance of large unilocular lipid droplets, fewer mitochondria, and small cytoplasmic volumes. Brown PVAT adipocytes, on the other hand, are distinguished by multilocular lipid droplets and high density of mitochondria. In rodents, arteries including the mesenteric, carotid, and femoral are surrounded by WAT, while the thoracic aorta is encircled by BAT and the abdominal aorta by beige PVAT (a mixture of white and brown adipocytes) (Brown et al., 2014).
Histological Distinctions of Perivascular Adipose Tissues
Although the majority of adipose tissue, either by volume or by function, is adipocytes, white adipocytes comprise only one-third of the total cell number in WAT. The remaining 70% of cells are a heterogeneous collection of largely undefined stromal and immune cells (Sarantopoulos et al., 2018). Understandably, PVAT is composed of adipocytes, nerves, and a stromal vascular fraction consisting of monocytes, endothelial cells, pericytes, macrophages, T cells, and mesenchymal stem cells (Chatterjee et al., 2009). Large vessels are separated from their PVATs by a layer of adventitia composed of elastic bundles, fibroblasts, nerves, and vasa vasorum. On the other hand, PVATs around small vessels and microvessels are seen as a seamless continuum from their associated blood vessels. Different PVATs are dominated by diverse adipocytes, indicating regional phenotypic heterogeneity in a given vasculature (Fitzgibbons et al., 2011).
Histology of Pericoronary Adipose Tissue
Broadly, cardiac fat depots include epicardial and pericardial adipose tissues. A big portion of epicardial fat surrounding the coronary arteries is called pericoronary adipose tissue (cPVAT) (Fernandez-Alfonso et al., 2017). Human cPVAT was firstly considered as a unique subtype of WAT since the cellular morphology and gene profile were more consistent with WAT than BAT (Chatterjee et al., 2009). Differentiated pericoronary adipocytes are irregularly shaped, smaller in size, and lower in lipid accumulation relative to subcutaneous and perirenal counterparts (Chatterjee et al., 2009). Meanwhile, human cPVAT exhibits an increased capacity to attract immune cells and induce angiogenesis, contributing to coronary atherosclerosis development (Chatterjee et al., 2009). Notably, these features were observed in differentiated preadipocytes in vitro, which might differ from in vivo physiological settings.
Evidence indeed showed cPVAT was composed of small unilocular adipocytes positively stained with brown fat marker UCP-1 (Chatterjee et al., 2013; Sacks et al., 2013). Sacks et al. further proposed that it would be more reasonable that cPVAT might closely resemble beige adipocytes (Sacks et al., 2013; Sacks and Symonds, 2013). Consistent expression of UCP-1 in cPVAT has been repeatedly reported since then (Chechi et al., 2017), but less expression of adiponectin, an anti-inflammatory adipokine was also reported (Numaguchi et al., 2019). Thus cPVAT can be classified as beige adipose tissue, with a large proportion of white adipocytes, which may contribute to coronary atherosclerosis development.
Histology of Thoracic Periaortic Adipose Tissue
Thoracic periaortic PVAT (tPVAT) expands from the aortic arch at the T4 vertebra to the diaphragm’s aortic hiatus at the T10–T11 vertebrae. The comparison of adipocyte size, inflammation, and macrophage polarization indicated that tPVAT was close to subcutaneous adipose tissue (Fitzgibbons et al., 2011). It is now widely accepted that tPVAT is morphologically and functionally like BAT (Figure 2A). Multilocular brown adipocytes and abundant mitochondria were observed under light or electron microscopy (Fitzgibbons et al., 2011; Padilla et al., 2013), which might contribute to the decreased atherosclerotic plaque burden (Fitzgibbons et al., 2011).
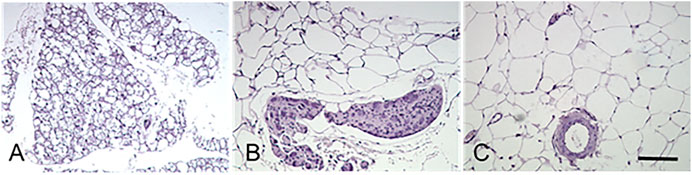
FIGURE 2. Histological characteristics of the perivascular adipose tissues. Murine adipose tissues around thoracic aorta (tPVAT, panel (A)), abdominal aorta (aPVAT, panel (B)), and mesenteric artery (mPVAT, panel (C)) were stained by hematoxylin and eosin. Brown adipocytes in tPVAT are characterized by small-sized, multilocular lipid droplets, whereas white adipocytes in mPVAT are classified by the appearance of a larger unilocular lipid droplet and small cytoplasmic space. aPVAT has mixed adipocyte populations. Scale bar, 100 µm.
In tPVAT, the adipocyte phenotypic switching via specific signaling pathways is evident in both whitening and browning directions. In a diet-induced obesity mouse model, tPVAT adipocytes become “whiter”, with a primarily unilocular appearance and larger lipid (Galvez-Prieto et al., 2008; Sacks and Symonds, 2013). Aging together with miR-146b-3p downregulation could decrease browning (Pan et al., 2018). Reduced brown adipogenic differentiation of resident stromal cells via loss of peroxisome proliferator-activated receptor-γ (PPARγ) coactivator-1 α (PGC-1α) was also observed in aged animals (Pan et al., 2019). Cold is a capable inducer for perivascular adipocyte to re-brown and to restore protective effects on metabolism and vascular function (Boa et al., 2017; Bussey et al., 2018). This relationship between tPVAT and adiposity, employed as a predictor for cardiovascular diseases, warrants further investigation in a clinical setting.
Histology of Abdominal Periaortic Adipose Tissue
Abdominal periaortic adipose tissue (aPVAT) is the adipose segments surrounding the abdominal aorta from below the diaphragm to the femoral bifurcation. As a continuum of thoracic aorta, the abdominal aorta is encircled by 4- to 10-fold more adipose tissue than the adipose tissue around the aortic arch in rats (Henrichot et al., 2005). aPVAT is more prone to accumulation when exposed to a high-fat diet and shows significant adipocyte hyperplasia and hypertrophy (Henrichot et al., 2005). A comparison with tPVAT demonstrates that aPVAT contains a mixture of cells, with a predominant proportion of unilocular white adipocytes and a small portion of brown adipocytes (Police et al., 2009). Moreover, the average aPVAT adipocyte size is much bigger than that of tPVAT (Figure 2B). Electron microscopy demonstrated that aPVAT was composed of white adipocytes with fewer mitochondria (Padilla et al., 2013). Upon a 4 month high-fat diet feeding, mouse aPVAT showed higher gene expression of inflammatory cytokines than tPVAT. Leptin coincided with the inflammatory cytokines in aPAVT, whereas UCP-1 was elevated in tPVAT but suppressed in aPVAT throughout diet-induced obesity (Police et al., 2009). Further studies provided evidence that only aPVAT-, but not tPVAT-conditioned medium could promote inflammatory cytokine MCP-1 generation and macrophage migration (Police et al., 2009). aPVAT was found to express a higher level of inflammatory receptors, including CD11c, IL-6R, and TNFR1/2 relative to tPVAT and inter-scapular brown adipose tissues (Padilla et al., 2013). These convincing pieces of evidence differentiate two segments of PVAT, although they wrap along the same artery. These intriguing features remain enigmatic due to the lack of solid advances in the developmental origin, transdifferentiation, and cellular fate mapping of PVAT.
Histology of Mesenteric Perivascular Adipose Tissue
In humans and mice, the fat tissue around the resistance mesenteric arteries forms mesenteric perivascular adipose tissue (mPVAT) (Figure 2C). Among adipose tissues collected from different locations, mPVAT was traditionally categorized as visceral WAT (Walden et al., 2012) with slightly smaller adipocyte size than classical visceral adipocytes (Caesar et al., 2010). However, mPVAT adipocytes are four times larger than periaortic adipocytes, and mPVAT expresses much less brown adipocyte marker of UCP-1 and PR domaining containing 16 (PRDM16) compared with brown adipocytes (Galvez-Prieto et al., 2008; Walden et al., 2012). The enlarged cross-sectional area of mPVAT adipocytes during obesity could be reduced but not completely reversed after caloric restriction (Bussey et al., 2016).
mPVAT expressed WAT specific marker transcription factor 21 (Tcf21), beige specific marker T-box protein 1 (Tbx1), and transcription factor Pat2. Besides, treatment with a β3-agonist, CL-316,243, could increase beige markers in mPVAT (Friederich-Persson et al., 2017). Other brown adipocyte gene markers, including myosin regulatory light chain (Mylpf), lim homebox 8 (Lhx8), zinc fingers in the cerebellum1 (Zic1), and T-box 15 (Tbx15) are barely seen in mPVAT, whereas a series of white adipose genes such as homeobox C8 (Hoxc8), transcription factor 21 (Tcf21), and dermatopontin (Dpt) are highly expressed in mPVAT (Walden et al., 2012). These findings adequately help classify mPVAT as a WAT.
Different Origin of Perivascular Adipose Tissues
Typical mature adipocytes originate from progenitor cells, which are committed preadipocytes derived from stem cells of multiple sources (Rodeheffer et al., 2008). The distinction between WAT and BAT reasonably leads to a simple classification of two precursor populations, giving rise to white and brown adipocytes, respectively. A commonly known classifier is myf5, which encodes myogenic factor 5 (Myf5) (Timmons et al., 2007), whereas PRDM16 may be a controller for brown adipocyte generation (Seale et al., 2008). However, using mTmG reporter, a study revealed that white adipocytes in the subcutaneous and retroperitoneal WAT also originate from Myf5-expressing precursors. This approach also demonstrated that Myf5 expression could not track many brown adipocytes (Sanchez-Gurmaches and Guertin, 2014a). The adipogenic capacity of vasculature-residing mural cells (e.g., pericytes) in the adipose tissue was well documented in many papers (Tang et al., 2008; Gupta et al., 2012; Tran et al., 2012; Berry and Rodeheffer, 2013). This statement is challenged by a study where pericytes do not contribute to adipocytes’ generation, although they seem to act as progenitors in vitro (Guimaraes-Camboa et al., 2017). Merrick et al. further indicated that reticular interstitium rather than the vasculature is the residing site for interstitial progenitor cells, which give rise to the preadipocytes expressing intercellular adhesion molecule–1 and another group of cells expressing protein CD142 (Merrick et al., 2019). Most recent results further revealed that only fibroblasts, neither mural nor endothelial cells, are cells of the vascular wall with significant adipogenic potential in vivo in both WAT and BAT (Cattaneo et al., 2020). Thus developmental origins of adipose tissue and the mechanisms controlling its expansion are just beginning and more intriguing findings are expected to come soon. Adipocytes of different PVATs may originate from distinct precursors (Hepler et al., 2017; Tran et al., 2018). Despite the aforementioned development of cellular fate mapping and lineage tracing in other adipose depots, the origins of PVAT adipocytes, in general, are barely known. This session presents distinct adipocyte development in Table 1 and Figure 1. A clear definition of adipocyte origin can reveal the determined precursors and the regulatory mechanisms.
Origin of Epicardial and Periaortic Arch Perivascular Adipose Tissue
Epicardial fat originates from the splanchnic mesoderm in human (Sacks et al., 2013) and in mouse (Walden et al., 2012). Periaortic adipose tissue is potentially derived from Myf5+ progenitors (Sanchez-Gurmaches and Guertin, 2014a). A more recent study showed that periaortic adipocyte progenitors expressed smooth muscle protein 22-alpha (SM22α) during development. Besides, knockout of PPARγ in neural crest cells leads to developmental delay of the periaortic arch PVAT (Fu et al., 2019). This evidence indicates that periaortic arch PVAT adipocytes have multiple lineages, mainly from ectoderm-derived neural crest cells, rather than the mesoderm-derived Myf5+ progenitors (Fu et al., 2019). A possible explanation is that ectoderm-derived neural crest cells have a broad differentiation potential and give rise to a diverse range of cell types. For example, neural crest cells were once identified as one of the progenitors of white adipocytes (Sanchez-Gurmaches and Guertin, 2014b). Another study revealed that neural crest-derived cells resided along the vessels within the subcutaneous adipose tissue. These results demonstrate that neural crest-derived adipocyte-committed progenitors contribute to adipogenesis (Sowa et al., 2013).
Origin of Thoracic Periaortic Adipose Tissue
Unlike periaortic arch PVAT, neural crest cells do not contribute to tPVAT development (Ye et al., 2019). Only about 10–30% of the brown adipocytes in tPVAT derive from Myf5+ sources (Ye et al., 2019). A lineage-tracing study further elaborates that anterior tPVAT adipocytes can be traced to SM22α+ progenitors, whereas left lateral tPVAT presents both SM22α+ and Myf5+ features (Ye et al., 2019). However, recent cell differentiation assays and genetic fate mapping studies show that fibroblastic progenitor cells but not vascular smooth muscle cells (VSMCs) are responsible for tPVAT adipogenesis (Angueira et al., 2021), which contradicts the previous findings (Chang et al., 2012; Ye et al., 2019). Progenitor cells for tPVAT are from a fibroblastic lineage, including (Pdgfra+; Ly6a+; Pparg−) and preadipocytes (Pdgfra+; Ly6a−; Pparg+). Bona fide VSMCs were not found to contribute to adipocyte formation in tPVAT (Angueira et al., 2021). Single-cell transcriptomic analyses both at embryonic (E18) and perinatal (P3, after birth) stages identified transcription factor early B cell factor-2 (EBF2) as a critical modulator of BAT (Angueira et al., 2020) and tPVAT development (Angueira et al., 2021).
Origin of Abdominal Periaortic Adipose Tissue
Abdominal PVAT preadipocytes demonstrate lower brown adipocyte developmental transcription factors relative to tPVAT (Tran et al., 2018). Abdominal PVAT lacks Zic1 gene, encoding zinc finger proteins that are critical for early BAT development (Contreras et al., 2016). In the absence of the adipogenic transcription factor PPARγ in the VSMCs, failure of aPVAT development was observed (Chang et al., 2012). These findings demonstrate that aPVAT shares, at least to some extent, similar developmental origins with SM22α+ and PPARγ+ VSMCs.
Origin of Mesenteric Perivascular Adipose Tissue
Few studies have focused on the developmental origin of mPVAT, and some comparative data may provide limited clues. Given the proximity, mPVAT’s developmental origins are thought to be similar to the visceral adipose tissue. Indeed, both mPVAT and perigonadal adipose tissue expressed comparable levels of white adipocyte signature gene Tcf21 and the brite adipocyte-specific genes Tbx1 and Tmem26 (Contreras et al., 2016). Mesenteric and perigonadal adipose tissue are also found to share the same mesothelial origin in lineage tracing experiments, and preadipocytes of mPVAT have a transcriptional profile closer to that of subcutaneous, but not omental preadipocytes (Chau et al., 2014). Also, in this cell lineage analysis, 28% of mature mesenteric adipocytes were Wt1+ positive, suggesting the source of mesenchymal stem cells in mesenteric adipocytes is different from that of BAT, where Wt1 expression is undetectable (Chau et al., 2014). Chang et al. have suggested that mPVAT shares a developmental origin with VSMCs because the deletion of the PPAR γ in VSMCs resulted in a dramatic loss of mPVAT (Chang et al., 2012).
Different Secretome of Perivascular Adipose Tissues
Adipose tissue is capable to synthesize and secrete various substances just as endocrine cells do. In this sense, adipose tissue is the largest endocrine organ, and more than 600 hundred identified factors produced by adipocytes are collectively termed adipokines or adipocytokines (Halberg et al., 2008; Lehr et al., 2012). Paracrine crosstalk between PVAT and its neighboring artery, also known as “vasocrine” communication, actively regulates vascular inflammation and arterial remodeling (Yudkin et al., 2005). Anatomically distinct PVAT depots can release a quite different range of adipokines. Previous literatures have deeply explored and frequently revisited this topic (Omar et al., 2014; Owen et al., 2014; Akoumianakis et al., 2017; Nosalski and Guzik, 2017; Xia and Li, 2017; Oikonomou and Antoniades, 2019; Chang et al., 2020; Kim et al., 2020). We briefly summarized PVAT-derived adipokines, vascular tone regulators, and newly discovered exosomes/extracellular vesicles in this part. Unlike previous reviews, these factors are categorized by specific PVAT depots where detectable levels are reported (e.g. PCR, Western-blot, or immunostaining).
Pro- and Anti-Inflammatory Adipokines of Perivascular Adipose Tissues
Most PVAT-generated cytokines/chemokines such as tumor necrosis factor (TNF)-α, interleukin-6 (IL-6), plasminogen activator inhibitor-1 (PAI-1), and monocyte chemoattractant protein-1 (MCP-1), are pro-inflammatory and pro-atherosclerotic. Adiponectin is one of the few anti-inflammatory factors that possess multiple salutary effects for cardiovascular disease prevention (Xu and Vanhoutte, 2012). Phenotypic differences between tPVAT and aPVAT are evident that tPVAT generates much less pro-inflammatory cytokines, and is thus resistant to diet-induced inflammation (Police et al., 2009; Fitzgibbons et al., 2011). mPVAT and aPVAT are similarly prone to the expansion of adipocytes and diet-induced inflammation (Li et al., 2019). However, mPVAT is more sensitive to the high-fat diet challenge, where the adipose “browning” genes are dramatically down-regulated (Hou et al., 2016). Previous reviews in this field have elaborated on PVAT-derived adipokines and their interactions with other vascular cells (Brown et al., 2014; Gil-Ortega et al., 2015; Akoumianakis et al., 2017; Kim et al., 2019; Chang et al., 2020). The PVAT-generated representative pro- and anti-inflammatory factors are briefly updated in Table 2. Note that one type of adipokine can be generated from multiple sites and at the same time one PVAT can release a variety of adipokines. Members of the same adipokine family (i.e., pro-inflammatory or constricting) all share the same predicted vascular function.
Perivascular Adipose Tissue-Derived Vasodilators and Contracting Factors
Many PVAT-derived factors are also vascular tone regulators. Since the discovery of adipocyte-derived relaxing factor (ADRF) in 2002 (Lohn et al., 2002), understanding of the mechanisms by which PVAT maintains vascular homeostasis has been sought. A wide range of PVAT-derived relaxing factors have been proposed, such as adiponectin (Lynch et al., 2013), prostacyclin (Chang et al., 2012), gaseous molecules (e.g. NO and H2S) (Szasz and Webb, 2012), methyl palmitate (Lee et al., 2011), angiotensin 1–7 (Lee et al., 2009), and omentin (Yamawaki et al., 2010). Potential contracting candidates include angiotensin II (Lu et al., 2010), endothelin-1 (Almabrouk et al., 2014; Tano et al., 2014), and resistin (Small et al., 2019) released by adipocytes. Depending on PVAT location and different circumstances, some factors including H2S (Lucchesi et al., 2005), leptin, TNF-α, IL-6, and apelin may act as either vasorelaxant or constricting factors (Maenhaut and Van de Voorde, 2011). For example, leptin could induce vasodilation to modulate blood pressure homeostasis (Fruhbeck, 1999; Lembo et al., 2000); however, obesity-induced hyperleptinemia resulted in an increase of endothelin-1, which then leads to vasoconstriction (Quehenberger et al., 2002). Some reports have demonstrated that TNF-α causes vascular dilation mediated by NO (Maenhaut and Van de Voorde, 2011) or hydrogen peroxide (Cheranov and Jaggar, 2006) production. On the other hand, TNF-α can also constrict blood vessels by increasing endothelin-1 (Wort et al., 2009) and angiotensinogen levels (Brasier et al., 1996). Based on their principle functions and originating depots, these factors are summarized in Table 3.
Perivascular Adipose Tissue-Derived Extracellular Vesicle
Inter-cell and inter-organ signaling within PVAT remain a mystery. Recently, many studies, including our own, have delved deep to identify the messengers conveying the communication between a blood vessel and its surrounding PVAT (Li et al., 2019). Apart from the secretory cytokines and chemokines factors, adipocytes also secrete many types of extracellular vesicles (EVs) (Deng et al., 2009; Ogawa et al., 2010), typically including exosomes and microvesicles. EVs play important roles in intercellular communication by selective packaging of lipids, proteins, and microRNAs (miRNAs) (Valadi et al., 2007; van Niel et al., 2018). Adipose tissue was proved to constitute an essential source of circulating exosomal miRNAs, as a form of adipokine that acts locally or distantly (Thomou et al., 2017). In addition, these EVs could be taken up by neighboring or distant cells to modulate these recipient’s functions (Bang et al., 2014; Sun et al., 2016). For instance, adipocyte-derived exosomal miRNAs enable the metabolic regulation of neighboring macrophages (Ogawa et al., 2010; Zhang et al., 2016). Vice versa, macrophages can secrete miRNA-containing exosomes to modulate local adipocyte function (Ying et al., 2017). Similarly, the endothelial-adipocyte interplay was the result of EV-mediated reciprocal trafficking of caveolin 1 (Crewe et al., 2018).
These observations led us to hypothesize that whether PVAT-adipocytes secrete exosomal miRNAs, if so, how they regulate vascular function in the context of obesity. Indeed, we have demonstrated that perivascular adipocytes produce and secrete miRNA-containing EVs, which can be taken up in neighboring VSMCs (Li et al., 2019). One of the most enriched miRNAs in PVAT and PVAT-derived EVs, miR-221-3p, is transported into adjacent VSMCs. The study further provided an EV-miR-221-3p-mediated mechanism by which PVAT triggers an early vascular remodeling in vascular inflammation (Li et al., 2019). In another study, increased miR-221/222 in the arteries promoted neointimal hyperplasia in the femoral artery following wire injury (Lightell et al., 2018).
Conclusion
Anatomically distinct PVATs vary in developmental origin, cellular composition, and secretome. The farther away a PVAT is from the heart, the more white-like the adipocytes are. This is true even in the same stem aorta but wrapped with distinct PVATs in the chest cage and the abdominal cavity. PVAT progenitor cells include but may not be limited to mural cells (pericyte or smooth muscle cells) and fibroblasts. These potential progenitors give rise to committed preadipocytes and contribute to adipogenesis. Adipogenesis and angiogenesis appear to co-exist, and preadipocytes and pericytes may co-develop as well, which should be further studied. The developmental trajectory of PVAT adipocytes is somehow still a “bloody mess” (Rosen and Spiegelman, 2014).
Adipocytes and their neighboring vascular cells constitute a perivascular microenvironment. In an inflammatory setting, a family of intercellular message-conveying machinery is involved in these cells’ interplay. Perivascular adipocytes, partially via alteration of their secretome, modulate the nearby VSMCs (Miao and Li, 2012) and endothelial cells (Sena et al., 2017). For obesity, secretion of anti-inflammatory adiponectin is markedly reduced, whereas the generation of pro-inflammatory cytokines is dramatically elevated. Besides, the contiguity with adventitia makes it plausible that paracrine or vasocrine crosstalks between PVAT and the encircled blood vessel are reciprocal and bidirectional.
The continuing worldwide upsurge in obesity prompts us to unveil PVAT’s significant role in vascular function. A better understanding of regional heterogeneity among PVATs is just a start. PVAT’s function and dysfunction in vascular homeostasis and cardiovascular pathogeny remain our long-term tasks to pursue. Developmental fate mapping is an essential technique for answering some of these questions. Single-cell techniques have empowered this process by helping draw sophisticated cellular atlas. Some researchers have explored the heterogeneity of PVAT at a single-cell level and uncovered distinct clusters with specific signature markers and signaling pathways (Angueira et al., 2021). Creating a more precise map of such a complex tissue from single-cell sequencing data is, therefore, a challenging task, which on the other hand, opens an opportunity for us to dive into this unknown.
Author Contributions
XL and YZ conceived the design and concepts. XL and YZ wrote the manuscript. ZM contributed key information for tables and figures. All authors contributed to the article and approved the submitted version.
Funding
This work was supported by the Macau FDCT grants (0123/2020/A to XL) and (0007/2019/AKP, 033/2017/AMJ, and 0067/2018/A2 to YZ).
Conflict of Interest
The authors declare that the research was conducted in the absence of any commercial or financial relationships that could be construed as a potential conflict of interest.
References
Akoumianakis, I., Tarun, A., and Antoniades, C. (2017). Perivascular Adipose Tissue as a Regulator of Vascular Disease Pathogenesis: Identifying Novel Therapeutic Targets. Br. J. Pharmacol. 174, 3411–3424. doi:10.1111/bph.13666
Alberti, K. G. M. M., Eckel, R. H., Grundy, S. M., Zimmet, P. Z., Cleeman, J. I., Donato, K. A., et al. (2009). Harmonizing the Metabolic Syndrome: A Joint Interim Statement of the International Diabetes Federation Task Force on Epidemiology and Prevention; National Heart, Lung, and Blood Institute; American Heart Association; World Heart Federation; International Atherosclerosis Society; and Interna. Circulation 120, 1640–1645. doi:10.1161/circulationaha.109.192644
Almabrouk, T. A. M., Ewart, M. A., Salt, I. P., and Kennedy, S. (2014). Perivascular Fat, AMP ‐Activated Protein Kinase and Vascular Diseases. Br. J. Pharmacol. 171, 595–617. doi:10.1111/bph.12479
Angueira, A. R., Sakers, A. P., Holman, C. D., Cheng, L., Arbocco, M. N., Shamsi, F., et al. (2021). Defining the Lineage of Thermogenic Perivascular Adipose Tissue. Nat. Metab. 3, 469–484. doi:10.1038/s42255-021-00380-0
Angueira, A. R., Shapira, S. N., Ishibashi, J., Sampat, S., Sostre-Colón, J., Emmett, M. J., et al. (2020). Early B Cell Factor Activity Controls Developmental and Adaptive Thermogenic Gene Programming in Adipocytes. Cell Rep. 30, 2869–2878. doi:10.1016/j.celrep.2020.02.023
Awata, W. M. C., Gonzaga, N. A., Borges, V. F., Silva, C. B. P., Tanus-Santos, J. E., Cunha, F. Q., et al. (2019). Perivascular Adipose Tissue Contributes to Lethal Sepsis-Induced Vasoplegia in Rats. Eur. J. Pharmacol. 863, 172706. doi:10.1016/j.ejphar.2019.172706
Ayala-Lopez, N., Martini, M., Jackson, W. F., Darios, E., Burnett, R., Seitz, B., et al. (2014). Perivascular Adipose Tissue Contains Functional Catecholamines. Pharmacol. Res. Perspect. 2, e00041. doi:10.1002/prp2.41
Bang, C., Batkai, S., Dangwal, S., Gupta, S. K., Foinquinos, A., Holzmann, A., et al. (2014). Cardiac Fibroblast-Derived microRNA Passenger Strand-Enriched Exosomes Mediate Cardiomyocyte Hypertrophy. J. Clin. Invest. 124, 2136–2146. doi:10.1172/jci70577
Berry, R., and Rodeheffer, M. S. (2013). Characterization of the Adipocyte Cellular Lineage In Vivo. Nat. Cel Biol 15, 302–308. doi:10.1038/ncb2696
Boa, B. C. S., Yudkin, J. S., van Hinsbergh, V. W. M., Bouskela, E., and Eringa, E. C. (2017). Exercise Effects on Perivascular Adipose Tissue: Endocrine and Paracrine Determinants of Vascular Function. Br. J. Pharmacol. 174, 3466–3481. doi:10.1111/bph.13732
Brasier, A. R., Li, J., and Wimbish, K. A. (1996). Tumor Necrosis Factor Activates Angiotensinogen Gene Expression by the Rel A Transactivator. Hypertension 27, 1009–1017. doi:10.1161/01.hyp.27.4.1009
Britton, K. A., Pedley, A., Massaro, J. M., Corsini, E. M., Murabito, J. M., Hoffmann, U., et al. (2012). Prevalence, Distribution, and Risk Factor Correlates of High Thoracic Periaortic Fat in the Framingham Heart Study. J. Am. Heart Assoc. 1, e004200. doi:10.1161/jaha.112.004200
Brown, N. K., Zhou, Z., Zhang, J., Zeng, R., Wu, J., Eitzman, D. T., et al. (2014). Perivascular Adipose Tissue in Vascular Function and Disease. Arterioscler Thromb. Vasc. Biol. 34, 1621–1630. doi:10.1161/atvbaha.114.303029
Bussey, C. E., Withers, S. B., Aldous, R. G., Edwards, G., and Heagerty, A. M. (2016). Obesity-Related Perivascular Adipose Tissue Damage is Reversed by Sustained Weight Loss in the Rat.Arterioscler. Thromb. Vasc. Biol. 36, 1377–1385. doi:10.1161/atvbaha.116.307210
Bussey, C. E., Withers, S. B., Saxton, S. N., Bodagh, N., Aldous, R. G., and Heagerty, A. M. (2018). β3 -Adrenoceptor Stimulation of Perivascular Adipocytes Leads to Increased Fat Cell-Derived NO and Vascular Relaxation in Small Arteries. Br. J. Pharmacol. 175, 3685–3698. doi:10.1111/bph.14433
Caesar, R., Manieri, M., Kelder, T., Boekschoten, M., Evelo, C., Müller, M., et al. (2010). A Combined Transcriptomics and Lipidomics Analysis of Subcutaneous, Epididymal and Mesenteric Adipose Tissue Reveals Marked Functional Differences. PLoS One 5, e11525. doi:10.1371/journal.pone.0011525
Cattaneo, P., Mukherjee, D., Spinozzi, S., Zhang, L., Larcher, V., Stallcup, W. B., et al. (2020). Parallel Lineage-Tracing Studies Establish Fibroblasts as the Prevailing In Vivo Adipocyte Progenitor. Cell Rep. 30, 571–582. doi:10.1016/j.celrep.2019.12.046
Chang, L., Garcia-Barrio, M. T., and Chen, Y. E. (2020). Perivascular Adipose Tissue Regulates Vascular Function by Targeting Vascular Smooth Muscle Cells. Arterioscler. Thromb. Vasc. Biol. 40, 1094–1109. doi:10.1161/atvbaha.120.312464
Chang, L., Villacorta, L., Li, R., Hamblin, M., Xu, W., Dou, C., et al. (2012). Loss of Perivascular Adipose Tissue on Peroxisome Proliferator-Activated Receptor-γ Deletion in Smooth Muscle Cells Impairs Intravascular Thermoregulation and Enhances Atherosclerosis. Circulation 126, 1067–1078. doi:10.1161/circulationaha.112.104489
Chatterjee, T. K., Aronow, B. J., Tong, W. S., Manka, D., Tang, Y., Bogdanov, V. Y., et al. (2013). Human Coronary Artery Perivascular Adipocytes Overexpress Genes Responsible for Regulating Vascular Morphology, Inflammation, and Hemostasis. Physiol. Genomics 45, 697–709. doi:10.1152/physiolgenomics.00042.2013
Chatterjee, T. K., Stoll, L. L., Denning, G. M., Harrelson, A., Blomkalns, A. L., Idelman, G., et al. (2009). Proinflammatory Phenotype of Perivascular Adipocytes. Circ. Res. 104, 541–549. doi:10.1161/circresaha.108.182998
Chau, Y.-Y., Bandiera, R., Serrels, A., Martínez-Estrada, O. M., Qing, W., Lee, M., et al. (2014). Visceral and Subcutaneous Fat Have Different Origins and Evidence Supports a Mesothelial Source. Nat. Cell Biol. 16, 367–375. doi:10.1038/ncb2922
Chechi, K., Voisine, P., Mathieu, P., Laplante, M., Bonnet, S., Picard, F., et al. (2017). Functional Characterization of the Ucp1-Associated Oxidative Phenotype of Human Epicardial Adipose Tissue. Sci. Rep. 7, 15566. doi:10.1038/s41598-017-15501-7
Cheng, K.-H., Chu, C.-S., Lee, K.-T., Lin, T.-H., Hsieh, C.-C., Chiu, C.-C., et al. (2008). Adipocytokines and Proinflammatory Mediators From Abdominal and Epicardial Adipose Tissue in Patients With Coronary Artery Disease. Int. J. Obes. 32, 268–274. doi:10.1038/sj.ijo.0803726
Cheranov, S. Y., and Jaggar, J. H. (2006). TNF-alpha Dilates Cerebral Arteries via NAD(P)H Oxidase-Dependent Ca2+ Spark Activation. Am. J. Physiol. Cell Physiol. 290, C964–C971. doi:10.1152/ajpcell.00499.2005
Contreras, G. A., Thelen, K., Ayala-Lopez, N., and Watts, S. W. (2016). The Distribution and Adipogenic Potential of Perivascular Adipose Tissue Adipocyte Progenitors is Dependent on Sexual Dimorphism and Vessel Location. Physiol. Rep. 4, e12993. doi:10.14814/phy2.12993
Crewe, C., Joffin, N., Rutkowski, J. M., Kim, M., Zhang, F., Towler, D. A., et al. (2018). An Endothelial-to-Adipocyte Extracellular Vesicle Axis Governed by Metabolic State. Cell 175, 695–708. doi:10.1016/j.cell.2018.09.005
Darios, E. S., Winner, B. M., Charvat, T., Krasinksi, A., Punna, S., and Watts, S. W. (2016). The Adipokine Chemerin Amplifies Electrical Field-Stimulated Contraction in the Isolated Rat superior Mesenteric Artery. Am. J. Physiol. Heart Circ. Physiol. 311, H498–H507. doi:10.1152/ajpheart.00998.2015
Deng, Z.-b., Poliakov, A., Hardy, R. W., Clements, R., Liu, C., Liu, Y., et al. (2009). Adipose Tissue Exosome-Like Vesicles Mediate Activation of Macrophage-Induced Insulin Resistance. Diabetes 58, 2498–2505. doi:10.2337/db09-0216
Dobrian, A. D., Hatcher, M. A., Brotman, J. J., Galkina, E. V., Taghavie-Moghadam, P., Pei, H., et al. (2015). STAT4 Contributes to Adipose Tissue Inflammation and Atherosclerosis. J. Endocrinol. 227, 13–24. doi:10.1530/joe-15-0098
Drosos, I., Chalikias, G., Pavlaki, M., Kareli, D., Epitropou, G., Bougioukas, G., et al. (2016). Differences Between Perivascular Adipose Tissue Surrounding the Heart and the Internal Mammary Artery: Possible Role for the Leptin-Inflammation-Fibrosis-Hypoxia axis. Clin. Res. Cardiol. 105, 887–900. doi:10.1007/s00392-016-0996-7
Fang, L., Zhao, J., Chen, Y., Ma, T., Xu, G., Tang, C., et al. (2009). Hydrogen Sulfide Derived From Periadventitial Adipose Tissue is a Vasodilator. J. Hypertens. 27, 2174–2185. doi:10.1097/hjh.0b013e328330a900
Fernández-Alfonso, M. S., Gil-Ortega, M., Aranguez, I., Souza, D., Dreifaldt, M., Somoza, B., et al. (2017). Role of PVAT in Coronary Atherosclerosis and Vein Graft Patency: Friend or Foe? Br. J. Pharmacol. 174, 3561–3572. doi:10.1111/bph.13734
Fitzgibbons, T. P., Kogan, S., Aouadi, M., Hendricks, G. M., Straubhaar, J., and Czech, M. P. (2011). Similarity of Mouse Perivascular and Brown Adipose Tissues and Their Resistance to Diet-Induced Inflammation. Am. J. Physiol. Heart Circ. Physiol. 301, H1425–H1437. doi:10.1152/ajpheart.00376.2011
Fortuño, A., Rodríguez, A., Gómez-Ambrosi, J., Muñiz, P., Salvador, J., Díez, J., et al. (2002). Leptin Inhibits Angiotensin II-Induced Intracellular Calcium Increase and Vasoconstriction in the Rat Aorta. Endocrinology 143, 3555–3560. doi:10.1210/en.2002-220075
Friederich-Persson, M., Nguyen Dinh Cat, A., Persson, P., Montezano, A. C., and Touyz, R. M. (2017). Brown Adipose Tissue Regulates Small Artery Function through NADPH Oxidase 4-Derived Hydrogen Peroxide and Redox-Sensitive Protein Kinase G-1α. Arterioscler Thromb. Vasc. Biol. 37, 455–465. doi:10.1161/atvbaha.116.308659
Fruhbeck, G. (1999). Pivotal Role of Nitric Oxide in the Control of Blood Pressure after Leptin Administration. Diabetes 48, 903–908. doi:10.2337/diabetes.48.4.903
Fu, M., Xu, L., Chen, X., Han, W., Ruan, C., Li, J., et al. (2019). Neural Crest Cells Differentiate into Brown Adipocytes and Contribute to Periaortic Arch Adipose Tissue Formation. Arterioscler. Thromb. Vasc. Biol. 39, 1629–1644. doi:10.1161/atvbaha.119.312838
Gaborit, B., Venteclef, N., Ancel, P., Pelloux, V., Gariboldi, V., Leprince, P., et al. (2015). Human Epicardial Adipose Tissue Has a Specific Transcriptomic Signature Depending on its Anatomical Peri-Atrial, Peri-Ventricular, or Peri-Coronary Location. Cardiovasc. Res. 108, 62–73. doi:10.1093/cvr/cvv208
Gálvez, B., de Castro, J., Herold, D., Dubrovska, G., Arribas, S., González, M. C., et al. (2006). Perivascular Adipose Tissue and Mesenteric Vascular Function in Spontaneously Hypertensive Rats. Arterioscler Thromb. Vasc. Biol. 26, 1297–1302. doi:10.1161/01.ATV.0000220381.40739.dd
Gálvez-Prieto, B., Bolbrinker, J., Stucchi, P., de Las Heras, A. I., Merino, B., Arribas, S., et al. (2008). Comparative Expression Analysis of the Renin-Angiotensin System Components Between White and Brown Perivascular Adipose Tissue. J. Endocrinol. 197, 55–64. doi:10.1677/joe-07-0284
Gao, Y.-J., Lu, C., Su, L.-Y., Sharma, A. M., and Lee, R. M. K. W. (2007). Modulation of Vascular Function by Perivascular Adipose Tissue: the Role of Endothelium and Hydrogen Peroxide. Br. J. Pharmacol. 151, 323–331. doi:10.1038/sj.bjp.0707228
Gao, Y., Takemori, K., Su, L., An, W., Lu, C., Sharma, A., et al. (2006). Perivascular Adipose Tissue Promotes Vasoconstriction: the Role of Superoxide Anion. Cardiovasc. Res. 71, 363–373. doi:10.1016/j.cardiores.2006.03.013
Gil-Ortega, M., Somoza, B., Huang, Y., Gollasch, M., and Fernández-Alfonso, M. S. (2015). Regional Differences in Perivascular Adipose Tissue Impacting Vascular Homeostasis. Trends Endocrinol. Metab. 26, 367–375. doi:10.1016/j.tem.2015.04.003
Gil-Ortega, M., Stucchi, P., Guzmán-Ruiz, R., Cano, V., Arribas, S., González, M. C., et al. (2010). Adaptative Nitric Oxide Overproduction in Perivascular Adipose Tissue During Early Diet-Induced Obesity. Endocrinology 151, 3299–3306. doi:10.1210/en.2009-1464
Greulich, S., Chen, W. J., Maxhera, B., Rijzewijk, L. J., van der Meer, R. W., Jonker, J. T., et al. (2013). Cardioprotective Properties of Omentin-1 in Type 2 Diabetes: Evidence from Clinical and In Vitro Studies. PloS one 8, e59697. doi:10.1371/journal.pone.0059697
Grundy, S. M. (2012). Pre-Diabetes, Metabolic Syndrome, and Cardiovascular Risk. J. Am. Coll. Cardiol. 59, 635–643. doi:10.1016/j.jacc.2011.08.080
Gruzdeva, O., Uchasova, E., Dyleva, Y., Borodkina, D., Akbasheva, O., Antonova, L., et al. (2019). Adipocytes Directly Affect Coronary Artery Disease Pathogenesis via Induction of Adipokine and Cytokine Imbalances. Front. Immunol. 10, 2163. doi:10.3389/fimmu.2019.02163
Guimarães-Camboa, N., Cattaneo, P., Sun, Y., Moore-Morris, T., Gu, Y., Dalton, N. D., et al. (2017). Pericytes of Multiple Organs do not Behave as Mesenchymal Stem Cells In Vivo. Cell Stem Cell 20, 345–359. doi:10.1016/j.stem.2016.12.006
Gupta, R. K., Mepani, R. J., Kleiner, S., Lo, J. C., Khandekar, M. J., Cohen, P., et al. (2012). Zfp423 Expression Identifies Committed Preadipocytes and Localizes to Adipose Endothelial and Perivascular Cells. Cell Metab. 15, 230–239. doi:10.1016/j.cmet.2012.01.010
Halberg, N., Wernstedt-Asterholm, I., and Scherer, P. E. (2008). The Adipocyte as an Endocrine Cell. Endocrinol. Metab. Clin. North America 37, 753–768. doi:10.1016/j.ecl.2008.07.002
Han, F., Zhang, Y., Shao, M., Mu, Q., Jiao, X., Hou, N., et al. (2018). C1q/TNF-related Protein 9 Improves the Anti-contractile Effects of Perivascular Adipose Tissue via the AMPK-eNOS Pathway in Diet-Induced Obese Mice. Clin. Exp. Pharmacol. Physiol. 45, 50–57. doi:10.1111/1440-1681.12851
Henrichot, E., Juge-Aubry, C. E., Pernin, A., Pache, J.-C., Velebit, V., Dayer, J.-M., et al. (2005). Production of Chemokines by Perivascular Adipose Tissue. Arterioscler. Thromb. Vasc. Biol. 25, 2594–2599. doi:10.1161/01.atv.0000188508.40052.35
Hepler, C., Vishvanath, L., and Gupta, R. K. (2017). Sorting Out Adipocyte Precursors and Their Role in Physiology and Disease. Genes Dev. 31, 127–140. doi:10.1101/gad.293704.116
Hildebrand, S., Stumer, J., and Pfeifer, A. (2018). PVAT and its Relation to Brown, Beige, and White Adipose Tissue in Development and Function. Front. Physiol. 9, 70. doi:10.3389/fphys.2018.00070
Horimatsu, T., Patel, A. S., Prasad, R., Reid, L. E., Benson, T. W., Zarzour, A., et al. (2018). Remote Effects of Transplanted Perivascular Adipose Tissue on Endothelial Function and Atherosclerosis. Cardiovasc. Drugs Ther. 32, 503–510. doi:10.1007/s10557-018-6821-y
Hou, N., Liu, Y., Han, F., Wang, D., Hou, X., Hou, S., et al. (2016). Irisin Improves Perivascular Adipose Tissue Dysfunction via Regulation of the Heme Oxygenase-1/Adiponectin Axis in Diet-Induced Obese Mice. J. Mol. Cell. Cardiol. 99, 188–196. doi:10.1016/j.yjmcc.2016.09.005
Iacobellis, G., Pistilli, D., Gucciardo, M., Leonetti, F., Miraldi, F., Brancaccio, G., et al. (2005). Adiponectin Expression in Human Epicardial Adipose Tissue In Vivo is Lower in Patients With Coronary Artery Disease. Cytokine 29, 251–255. doi:10.1016/j.cyto.2004.11.002
Iacobellis, G., di Gioia, C. R., Di Vito, M., Petramala, L., Cotesta, D., De Santis, V., et al. (2009). Epicardial Adipose Tissue and Intracoronary Adrenomedullin Levels in Coronary Artery Disease. Horm. Metab. Res. 41, 855–860. doi:10.1055/s-0029-1231081
Iacobellis, G. (2009). Epicardial and Pericardial Fat: Close, But Very Different. Obesity (Silver Spring) 17, 625. doi:10.1038/oby.2008.575
Jaacks, L. M., Vandevijvere, S., Pan, A., McGowan, C. J., Wallace, C., Imamura, F., et al. (2019). The Obesity Transition: Stages of the Global Epidemic. Lancet Diabetes Endocrinol. 7, 231–240. doi:10.1016/s2213-8587(19)30026-9
Jung, H., Park, K., Cho, Y., Chung, S., Cho, H., Cho, S., et al. (2006). Resistin is Secreted From Macrophages in Atheromas and Promotes Atherosclerosis. Cardiovasc. Res. 69, 76–85. doi:10.1016/j.cardiores.2005.09.015
Kassan, M., Galan, M., Partyka, M., Trebak, M., and Matrougui, K. (2011). Interleukin-10 Released by CD4 + CD25 + Natural Regulatory T Cells Improves Microvascular Endothelial Function Through Inhibition of NADPH Oxidase Activity in Hypertensive Mice. Arterioscler Thromb. Vasc. Biol. 31, 2534–2542. doi:10.1161/atvbaha.111.233262
Kim, H. W., Belin de Chantemèle, E. J., and Weintraub, N. L. (2019). Perivascular Adipocytes in Vascular Disease. Arterioscler. Thromb. Vasc. Biol. 39, 2220–2227. doi:10.1161/atvbaha.119.312304
Kim, H. W., Shi, H., Winkler, M. A., Lee, R., and Weintraub, N. L. (2020). Perivascular Adipose Tissue and Vascular Perturbation/Atherosclerosis. Arterioscler. Thromb. Vasc. Biol. 40, 2569–2576. doi:10.1161/atvbaha.120.312470
Kohn, C., Schleifenbaum, J., Szijarto, I. A., Marko, L., Dubrovska, G., Huang, Y., et al. (2012). Differential Effects of Cystathionine-Gamma-Lyase-Dependent Vasodilatory H2S in Periadventitial Vasoregulation of Rat and Mouse Aortas. PLoS One 7, e41951. doi:10.1371/journal.pone.0041951
Kostopoulos, C. G., Spiroglou, S. G., Varakis, J. N., Apostolakis, E., and Papadaki, H. H. (2014). Adiponectin/T-Cadherin and Apelin/APJ Expression in Human Arteries and Periadventitial Fat: Implication of Local Adipokine Signaling in Atherosclerosis? Cardiovasc. Pathol. 23, 131–138. doi:10.1016/j.carpath.2014.02.003
Langheim, S., Dreas, L., Veschini, L., Maisano, F., Foglieni, C., Ferrarello, S., et al. (2010). Increased Expression and Secretion of Resistin in Epicardial Adipose Tissue of Patients With Acute Coronary Syndrome. Am. J. Physiol. Heart Circul. Physiol. 298, H746–H753. doi:10.1152/ajpheart.00617.2009
Lee, R. M., Lu, C., Su, L.-Y., and Gao, Y.-J. (2009). Endothelium-Dependent Relaxation Factor Released by Perivascular Adipose Tissue. J. Hypertens. 27, 782–790. doi:10.1097/hjh.0b013e328324ed86
Lee, Y.-C., Chang, H.-H., Chiang, C.-L., Liu, C.-H., Yeh, J.-I., Chen, M.-F., et al. (2011). Role of Perivascular Adipose Tissue-Derived Methyl Palmitate in Vascular Tone Regulation and Pathogenesis of Hypertension. Circulation 124, 1160–1171. doi:10.1161/circulationaha.111.027375
Lehman, S. J., Massaro, J. M., Schlett, C. L., O’Donnell, C. J., Hoffmann, U., and Fox, C. S. (2010). Peri-aortic Fat, Cardiovascular Disease Risk Factors, and Aortic Calcification: the Framingham Heart Study. Atherosclerosis 210, 656–661. doi:10.1016/j.atherosclerosis.2010.01.007
Lehr, S., Hartwig, S., Lamers, D., Famulla, S., Müller, S., Hanisch, F.-G., et al. (2012). Identification and Validation of Novel Adipokines Released from Primary Human Adipocytes. Mol. Cell Proteomics 11, 010504. doi:10.1074/mcp.m111.010504
Lembo, G., Vecchione, C., Fratta, L., Marino, G., Trimarco, V., d’Amati, G., et al. (2000). Leptin Induces Direct Vasodilation Through Distinct Endothelial Mechanisms. Diabetes 49, 293–297. doi:10.2337/diabetes.49.2.293
Li, X., Ballantyne, L. L., Yu, Y., and Funk, C. D. (2019). Perivascular Adipose Tissue-Derived Extracellular Vesicle miR‐221‐3p Mediates Vascular Remodeling. FASEB J. 33, 12704–12722. doi:10.1096/fj.201901548r
Lightell, D. J., Moss, S. C., and Woods, T. C. (2018). Upregulation of miR-221 and -222 in Response to Increased Extracellular Signal-Regulated Kinases 1/2 Activity Exacerbates Neointimal Hyperplasia in Diabetes Mellitus. Atherosclerosis 269, 71–78. doi:10.1016/j.atherosclerosis.2017.12.016
Lohmann, C., Schäfer, N., von Lukowicz, T., Sokrates Stein, M. A., Borén, J., Rütti, S., et al. (2009). Atherosclerotic Mice Exhibit Systemic Inflammation in Periadventitial and Visceral Adipose Tissue, Liver, and Pancreatic Islets. Atherosclerosis 207, 360–367. doi:10.1016/j.atherosclerosis.2009.05.004
Löhn, M., Dubrovska, G., Lauterbach, B., Luft, F. C., Gollasch, M., and Sharma, A. M. (2002). Periadventitial Fat Releases a Vascular Relaxing Factor. FASEB J. 16, 1057–1063. doi:10.1096/fj.02-0024com
Lu, C., Su, L.-Y., Lee, R. M. K. W., and Gao, Y.-J. (2010). Mechanisms for Perivascular Adipose Tissue-Mediated Potentiation of Vascular Contraction to Perivascular Neuronal Stimulation: the Role of Adipocyte-Derived Angiotensin II. Eur. J. Pharmacol. 634, 107–112. doi:10.1016/j.ejphar.2010.02.006
Lu, D., Wang, W., Xia, L., Xia, P., and Yan, Y. (2017). Gene Expression Profiling Reveals Heterogeneity of Perivascular Adipose Tissues Surrounding Coronary and Internal Thoracic Arteries. Acta Biochim. Biophys. Sin (Shanghai) 49, 1075–1082. doi:10.1093/abbs/gmx113
Lucchesi, P. A., Belmadani, S., and Matrougui, K. (2005). Hydrogen Peroxide Acts as Both Vasodilator and Vasoconstrictor in the Control of Perfused Mouse Mesenteric Resistance Arteries. J. Hypertens. 23, 571–579. doi:10.1097/01.hjh.0000160214.40855.79
Lynch, F. M., Withers, S. B., Yao, Z., Werner, M. E., Edwards, G., Weston, A. H., et al. (2013). Perivascular Adipose Tissue-Derived Adiponectin Activates BK(Ca) Channels to Induce Anticontractile Responses. Am. J. Physiol. Heart Circ. Physiol. 304, H786–H795. doi:10.1152/ajpheart.00697.2012
Maenhaut, N., and Van de Voorde, J. (2011). Regulation of Vascular Tone by Adipocytes. BMC Med. 9, 25. doi:10.1186/1741-7015-9-25
Manka, D., Chatterjee, T. K., Stoll, L. L., Basford, J. E., Konaniah, E. S., Srinivasan, R., et al. (2014). Transplanted Perivascular Adipose Tissue Accelerates Injury-Induced Neointimal Hyperplasia. Arterioscler. Thromb. Vasc. Biol. 34, 1723–1730. doi:10.1161/atvbaha.114.303983
Mazurek, T., Zhang, L., Zalewski, A., Mannion, J. D., Diehl, J. T., Arafat, H., et al. (2003). Human Epicardial Adipose Tissue is a Source of Inflammatory Mediators. Circulation 108, 2460–2466. doi:10.1161/01.cir.0000099542.57313.c5
Merrick, D., Sakers, A., Irgebay, Z., Okada, C., Calvert, C., Morley, M. P., et al. (2019). Identification of a Mesenchymal Progenitor Cell Hierarchy in Adipose Tissue. Science 364, eaav2501. doi:10.1126/science.aav2501
Meyer, M. R., Fredette, N. C., Barton, M., and Prossnitz, E. R. (2013). Regulation of Vascular Smooth Muscle Tone by Adipose-Derived Contracting Factor. PLoS One 8, e79245. doi:10.1371/journal.pone.0079245
Miao, C.-Y., and Li, Z.-Y. (2012). The Role of Perivascular Adipose Tissue in Vascular Smooth Muscle Cell Growth. Br. J. Pharmacol. 165, 643–658. doi:10.1111/j.1476-5381.2011.01404.x
Moos, M. P. W., John, N., Gräbner, R., Nossmann, S., Günther, B., Vollandt, R., et al. (2005). The Lamina Adventitia is the Major Site of Immune Cell Accumulation in Standard Chow-Fed Apolipoprotein E-Deficient Mice. Arterioscler. Thromb. Vasc. Biol. 25, 2386–2391. doi:10.1161/01.atv.0000187470.31662.fe
Nosalski, R., and Guzik, T. J. (2017). Perivascular Adipose Tissue Inflammation in Vascular Disease. Br. J. Pharmacol. 174, 3496–3513. doi:10.1111/bph.13705
Numaguchi, R., Furuhashi, M., Matsumoto, M., Sato, H., Yanase, Y., Kuroda, Y., et al. (2019). Differential Phenotypes in Perivascular Adipose Tissue Surrounding the Internal Thoracic Artery and Diseased Coronary Artery. J. Am. Heart Assoc. 8, e011147. doi:10.1161/jaha.118.011147
Ogawa, R., Tanaka, C., Sato, M., Nagasaki, H., Sugimura, K., Okumura, K., et al. (2010). Adipocyte-derived Microvesicles Contain RNA that Is Transported Into Macrophages and Might be Secreted into Blood Circulation. Biochem. Biophys. Res. Commun. 398, 723–729. doi:10.1016/j.bbrc.2010.07.008
Oikonomou, E. K., and Antoniades, C. (2019). The Role of Adipose Tissue in Cardiovascular Health and Disease. Nat. Rev. Cardiol. 16, 83–99. doi:10.1038/s41569-018-0097-6
Olshansky, S. J., Passaro, D. J., Hershow, R. C., Layden, J., Carnes, B. A., Brody, J., et al. (2005). A Potential Decline in Life Expectancy in the United States in the 21st Century. N. Engl. J. Med. 352, 1138–1145. doi:10.1056/nejmsr043743
Omar, A., Chatterjee, T. K., Tang, Y., Hui, D. Y., and Weintraub, N. L. (2014). Proinflammatory Phenotype of Perivascular Adipocytes. Arterioscler. Thromb. Vasc. Biol. 34, 1631–1636. doi:10.1161/atvbaha.114.303030
Owen, M. K., Noblet, J. N., Sassoon, D. J., Conteh, A. M., Goodwill, A. G., and Tune, J. D. (2014). Perivascular Adipose Tissue and Coronary Vascular Disease. Arterioscler. Thromb. Vasc. Biol. 34, 1643–1649. doi:10.1161/atvbaha.114.303033
Owen, M. K., Witzmann, F. A., McKenney, M. L., Lai, X., Berwick, Z. C., Moberly, S. P., et al. (2013). Perivascular Adipose Tissue Potentiates Contraction of Coronary Vascular Smooth Muscle. Circulation 128, 9–18. doi:10.1161/circulationaha.112.001238
Padilla, J., Jenkins, N. T., Vieira-Potter, V. J., and Laughlin, M. H. (2013). Divergent Phenotype of Rat Thoracic and Abdominal Perivascular Adipose Tissues. Am. J. Physiol. Regul. Integr. Comp. Physiol. 304, R543–R552. doi:10.1152/ajpregu.00567.2012
Pan, X. X., Cao, J.-M., Cai, F., Ruan, C. C., Wu, F., and Gao, P. J. (2018). Loss of miR-146b-3p Inhibits Perivascular Adipocyte Browning With Cold Exposure During Aging. Cardiovasc. Drugs Ther. 32, 511–518. doi:10.1007/s10557-018-6814-x
Pan, X. X., Ruan, C. C., Liu, X. Y., Kong, L. R., Ma, Y., Wu, Q. H., et al. (2019). Perivascular Adipose Tissue-Derived Stromal Cells Contribute to Vascular Remodeling during Aging. Aging Cell 18, e12969. doi:10.1111/acel.12969
Park, S. Y., Kim, K. H., Seo, K. W., Bae, J. U., Kim, Y. H., Lee, S. J., et al. (2014). Resistin Derived From Diabetic Perivascular Adipose Tissue Up‐regulates Vascular Expression of Osteopontin via the AP ‐1 Signalling Pathway. J. Pathol. 232, 87–97. doi:10.1002/path.4286
Patel, V. B., Basu, R., and Oudit, G. Y. (2016). ACE2/Ang 1-7 Axis: A Critical Regulator of Epicardial Adipose Tissue Inflammation and Cardiac Dysfunction in Obesity. Adipocyte 5, 306–311. doi:10.1080/21623945.2015.1131881
Police, S. B., Thatcher, S. E., Charnigo, R., Daugherty, A., and Cassis, L. A. (2009). Obesity Promotes Inflammation in Periaortic Adipose Tissue and Angiotensin II-Induced Abdominal Aortic Aneurysm Formation. Arterioscler. Thromb. Vasc Biol. 29, 1458–1464. doi:10.1161/atvbaha.109.192658
Quehenberger, P., Exner, M., Sunder-Plassmann, R., Ruzicka, K., Bieglmayer, C., Endler, G., et al. (2002). Leptin Induces Endothelin-1 in Endothelial Cells In Vitro. Circ. Res. 90, 711–718. doi:10.1161/01.res.0000014226.74709.90
Rajsheker, S., Manka, D., Blomkalns, A. L., Chatterjee, T. K., Stoll, L. L., and Weintraub, N. L. (2010). Crosstalk Between Perivascular Adipose Tissue and Blood Vessels. Curr. Opin. Pharmacol. 10, 191–196. doi:10.1016/j.coph.2009.11.005
Rodeheffer, M. S., Birsoy, K., and Friedman, J. M. (2008). Identification of White Adipocyte Progenitor Cells In Vivo. Cell 135, 240–249. doi:10.1016/j.cell.2008.09.036
Rosen, E. D., and Spiegelman, B. M. (2014). What we Talk about When we Talk About Fat. Cell 156, 20–44. doi:10.1016/j.cell.2013.12.012
Sacks, H. S., Fain, J. N., Bahouth, S. W., Ojha, S., Frontini, A., Budge, H., et al. (2013). Adult Epicardial Fat Exhibits Beige Features. J. Clin. Endocrinol. Metab. 98, E1448–E1455. doi:10.1210/jc.2013-1265
Sacks, H. S., Fain, J. N., Holman, B., Cheema, P., Chary, A., Parks, F., et al. (2009). Uncoupling Protein-1 and Related Messenger Ribonucleic Acids in Human Epicardial and Other Adipose Tissues: Epicardial Fat Functioning as Brown Fat. J. Clin. Endocrinol. Metab. 94, 3611–3615. doi:10.1210/jc.2009-0571
Sacks, H., and Symonds, M. E. (2013). Anatomical Locations of Human Brown Adipose Tissue: Functional Relevance and Implications in Obesity and Type 2 Diabetes. Diabetes 62, 1783–1790. doi:10.2337/db12-1430
Sakamoto, S., Tsuruda, T., Hatakeyama, K., Imamura, T., Asada, Y., and Kitamura, K. (2014). Impact of Age-dependent Adventitia Inflammation on Structural Alteration of Abdominal Aorta in Hyperlipidemic Mice. PLoS One 9, e105739. doi:10.1371/journal.pone.0105739
Sanchez-Gurmaches, J., and Guertin, D. A. (2014a). Adipocyte Lineages: Tracing Back the Origins of Fat. Biochim. Biophys. Acta 1842, 340–351. doi:10.1016/j.bbadis.2013.05.027
Sanchez-Gurmaches, J., and Guertin, D. A. (2014b). Adipocytes Arise From Multiple Lineages That are Heterogeneously and Dynamically Distributed. Nat. Commun. 5, 4099. doi:10.1038/ncomms5099
Sanchez-Gurmaches, J., Hung, C.-M., and Guertin, D. A. (2016). Emerging Complexities in Adipocyte Origins and Identity. Trends Cell Biol. 26, 313–326. doi:10.1016/j.tcb.2016.01.004
Sarantopoulos, C. N., Banyard, D. A., Ziegler, M. E., Sun, B., Shaterian, A., and Widgerow, A. D. (2018). Elucidating the Preadipocyte and its Role in Adipocyte Formation: A Comprehensive Review. Stem Cell Rev. Rep. 14, 27–42. doi:10.1007/s12015-017-9774-9
Saxton, S. N., Ryding, K. E., Aldous, R. G., Withers, S. B., Ohanian, J., and Heagerty, A. M. (2018). Role of Sympathetic Nerves and Adipocyte Catecholamine Uptake in the Vasorelaxant Function of Perivascular Adipose Tissue. Arterioscler. Thromb. Vasc. Biol. 38, 880–891. doi:10.1161/atvbaha.118.310777
Scheen, A. J., and Van Gaal, L. F. (2014). Combating the Dual burden: Therapeutic Targeting of Common Pathways in Obesity and Type 2 Diabetes. Lancet Diabetes Endocrinol. 2, 911–922. doi:10.1016/s2213-8587(14)70004-x
Schleifenbaum, J., Köhn, C., Voblova, N., Dubrovska, G., Zavarirskaya, O., Gloe, T., et al. (2010). Systemic Peripheral Artery Relaxation by KCNQ Channel Openers and Hydrogen Sulfide. J. Hypertens. 28, 1875–1882. doi:10.1097/hjh.0b013e32833c20d5
Schmid, P. M., Resch, M., Steege, A., Fredersdorf-Hahn, S., Stoelcker, B., Birner, C., et al. (2011). Globular and Full-Length Adiponectin Induce NO-dependent Vasodilation in Resistance Arteries of Zucker Lean But Not Zucker Diabetic Fatty Rats. Am. J. Hypertens. 24, 270–277. doi:10.1038/ajh.2010.239
Schwalie, P. C., Dong, H., Zachara, M., Russeil, J., Alpern, D., Akchiche, N., et al. (2018). A Stromal Cell Population That Inhibits Adipogenesis in Mammalian Fat Depots. Nature 559, 103–108. doi:10.1038/s41586-018-0226-8
Seale, P., Bjork, B., Yang, W., Kajimura, S., Chin, S., Kuang, S., et al. (2008). PRDM16 Controls a Brown Fat/Skeletal Muscle Switch. Nature 454, 961–967. doi:10.1038/nature07182
Sena, C. M., Pereira, A., Fernandes, R., Letra, L., and Seiça, R. M. (2017). Adiponectin Improves Endothelial Function in Mesenteric Arteries of Rats Fed a High-Fat Diet: Role of Perivascular Adipose Tissue. Br. J. Pharmacol. 174, 3514–3526. doi:10.1111/bph.13756
Shao, M., Wang, Q. A., Song, A., Vishvanath, L., Busbuso, N. C., Scherer, P. E., et al. (2019). Cellular Origins of Beige Fat Cells Revisited. Diabetes 68, 1874–1885. doi:10.2337/db19-0308
Silaghi, A., Achard, V., Paulmyer-Lacroix, O., Scridon, T., Tassistro, V., Duncea, I., et al. (2007). Expression of Adrenomedullin in Human Epicardial Adipose Tissue: Role of Coronary Status. Am. J. Physiol. Endocrinol. Metab. 293, E1443–E1450. doi:10.1152/ajpendo.00273.2007
Small, H. Y., McNeilly, S., Mary, S., Sheikh, A. M., and Delles, C. (2019). Resistin Mediates Sex-Dependent Effects of Perivascular Adipose Tissue on Vascular Function in the Shrsp. Sci. Rep. 9, 6897. doi:10.1038/s41598-019-43326-z
Smith, E., Prasad, K.-M. R., Butcher, M., Dobrian, A., Kolls, J. K., Ley, K., et al. (2010). Blockade of Interleukin-17A Results in Reduced Atherosclerosis in Apolipoprotein E-Deficient Mice. Circulation 121, 1746–1755. doi:10.1161/circulationaha.109.924886
Song, A., Dai, W., Jang, M. J., Medrano, L., Li, Z., Zhao, H., et al. (2020). Low- and High-Thermogenic Brown Adipocyte Subpopulations Coexist in Murine Adipose Tissue. J. Clin. Invest. 130, 247–257. doi:10.1172/JCI129167
Sowa, Y., Imura, T., Numajiri, T., Takeda, K., Mabuchi, Y., Matsuzaki, Y., et al. (2013). Adipose Stromal Cells Contain Phenotypically Distinct Adipogenic Progenitors Derived From Neural Crest. PLoS One 8, e84206. doi:10.1371/journal.pone.0084206
Spiroglou, S. G., Kostopoulos, C. G., Varakis, J. N., and Papadaki, H. H. (2010). Adipokines in Periaortic and Epicardial Adipose Tissue: Differential Expression and Relation to Atherosclerosis. J. Atheroscler. Thromb. 17, 115–130. doi:10.5551/jat.1735
Sun, X., Lin, J., Zhang, Y., Kang, S., Belkin, N., Wara, A. K., et al. (2016). MicroRNA-181b Improves Glucose Homeostasis and Insulin Sensitivity by Regulating Endothelial Function in White Adipose Tissue. Circ. Res. 118, 810–821. doi:10.1161/circresaha.115.308166
Szasz, T., and Webb, R. C. (2012). Perivascular Adipose Tissue: More Than Just Structural Support. Clin. Sci. 122, 1–12. doi:10.1042/cs20110151
Takaoka, M., Suzuki, H., Shioda, S., Sekikawa, K., Saito, Y., Nagai, R., et al. (2010). Endovascular Injury Induces Rapid Phenotypic Changes in Perivascular Adipose Tissue. Arterioscler Thromb. Vasc. Biol. 30, 1576–1582. doi:10.1161/atvbaha.110.207175
Tang, W., Zeve, D., Suh, J. M., Bosnakovski, D., Kyba, M., Hammer, R. E., et al. (2008). White Fat Progenitor Cells Reside in the Adipose Vasculature. Science 322, 583–586. doi:10.1126/science.1156232
Tano, J.-Y., Schleifenbaum, J., and Gollasch, M. (2014). Perivascular Adipose Tissue, Potassium Channels, and Vascular Dysfunction. Arterioscler. Thromb. Vasc. Biol. 34, 1827–1830. doi:10.1161/atvbaha.114.303032
Thomou, T., Mori, M. A., Dreyfuss, J. M., Konishi, M., Sakaguchi, M., Wolfrum, C., et al. (2017). Adipose-Derived Circulating miRNAs Regulate Gene Expression in Other Tissues. Nature 542, 450–455. doi:10.1038/nature21365
Timmons, J. A., Wennmalm, K., Larsson, O., Walden, T. B., Lassmann, T., Petrovic, N., et al. (2007). Myogenic Gene Expression Signature Establishes that Brown and white Adipocytes Originate from Distinct Cell Lineages. Proc. Natl. Acad. Sci. 104, 4401–4406. doi:10.1073/pnas.0610615104
Toczylowski, K., Hirnle, T., Harasiuk, D., Zabielski, P., Lewczuk, A., Dmitruk, I., et al. (2019). Plasma Concentration and Expression of Adipokines in Epicardial and Subcutaneous Adipose Tissue are Associated With Impaired Left Ventricular Filling Pattern. J. Transl. Med. 17, 310. doi:10.1186/s12967-019-2060-7
Tran, K.-V., Fitzgibbons, T., Min, S. Y., DeSouza, T., and Corvera, S. (2018). Distinct Adipocyte Progenitor Cells Are Associated with Regional Phenotypes of Perivascular Aortic Fat in Mice. Mol. Metab. 9, 199–206. doi:10.1016/j.molmet.2017.12.014
Tran, K.-V., Gealekman, O., Frontini, A., Zingaretti, M. C., Morroni, M., Giordano, A., et al. (2012). The Vascular Endothelium of the Adipose Tissue Gives Rise to Both White and Brown Fat Cells. Cell Metab. 15, 222–229. doi:10.1016/j.cmet.2012.01.008
Valadi, H., Ekström, K., Bossios, A., Sjöstrand, M., Lee, J. J., and Lötvall, J. O. (2007). Exosome-Mediated Transfer of mRNAs and microRNAs is a Novel Mechanism of Genetic Exchange Between Cells. Nat. Cell Biol. 9, 654–659. doi:10.1038/ncb1596
Van de Voorde, J., Pauwels, B., Boydens, C., and Decaluwé, K. (2013). Adipocytokines in Relation to Cardiovascular Disease. Metabolism 62, 1513–1521. doi:10.1016/j.metabol.2013.06.004
van Niel, G., D'Angelo, G., and Raposo, G. (2018). Shedding Light on the Cell Biology of Extracellular Vesicles. Nat. Rev. Mol. Cell Biol. 19, 213–228. doi:10.1038/nrm.2017.125
Vianello, E., Marrocco-Trischitta Massimiliano, M., Dozio, E., Bandera, F., Tacchini, L., Canciani, E., et al. (2019). Correlational Study on Altered Epicardial Adipose Tissue as a Stratification Risk Factor for Valve Disease Progression through IL-13 Signaling. J. Mol. Cell. Cardiol. 132, 210–218. doi:10.1016/j.yjmcc.2019.05.012
Waldén, T. B., Hansen, I. R., Timmons, J. A., Cannon, B., and Nedergaard, J. (2012). Recruited vs. Nonrecruited Molecular Signatures of Brown, "brite," and white Adipose Tissues. Am. J. Physiol. Endocrinol. Metab. 302, E19–E31. doi:10.1152/ajpendo.00249.2011
Wang, P., Xu, T.-Y., Guan, Y.-F., Su, D.-F., Fan, G.-R., and Miao, C.-Y. (2009). Perivascular Adipose Tissue-Derived Visfatin Is a Vascular Smooth Muscle Cell Growth Factor: Role of Nicotinamide Mononucleotide. Cardiovasc. Res. 81, 370–380. doi:10.1093/cvr/cvn288
Wang, Q. A., Song, A., Chen, W., Schwalie, P. C., Zhang, F., Vishvanath, L., et al. (2018). Reversible De-Differentiation of Mature White Adipocytes Into Preadipocyte-Like Precursors During Lactation. Cell Metab. 28, 282–288. doi:10.1016/j.cmet.2018.05.022
Weston, A. H., Egner, I., Dong, Y., Porter, E. L., Heagerty, A. M., and Edwards, G. (2013). Stimulated Release of a Hyperpolarizing Factor (ADHF) From Mesenteric Artery Perivascular Adipose Tissue: Involvement of Myocyte BKCa Channels and Adiponectin. Br. J. Pharmacol. 169, 1500–1509. doi:10.1111/bph.12157
Withers, S. B., Simpson, L., Fattah, S., Werner, M. E., and Heagerty, A. M. (2014). cGMP-Dependent Protein Kinase (PKG) Mediates the Anticontractile Capacity of Perivascular Adipose Tissue. Cardiovasc. Res. 101, 130–137. doi:10.1093/cvr/cvt229
Wort, S. J., Ito, M., Chou, P.-C., Mc Master, S. K., Badiger, R., Jazrawi, E., et al. (2009). Synergistic Induction of Endothelin-1 by Tumor Necrosis Factor α and Interferon γ Is Due to Enhanced NF-κB Binding and Histone Acetylation at Specific κB Sites. J. Biol. Chem. 284, 24297–24305. doi:10.1074/jbc.m109.032524
Xia, N., Horke, S., Habermeier, A., Closs, E. I., Reifenberg, G., Gericke, A., et al. (2016). Uncoupling of Endothelial Nitric Oxide Synthase in Perivascular Adipose Tissue of Diet-Induced Obese Mice. Arterioscler. Thromb. Vasc. Biol. 36, 78–85. doi:10.1161/atvbaha.115.306263
Xia, N., and Li, H. (2017). The Role of Perivascular Adipose Tissue in Obesity-Induced Vascular Dysfunction. Br. J. Pharmacol. 174, 3425–3442. doi:10.1111/bph.13650
Xiong, W., Zhao, X., Villacorta, L., Rom, O., Garcia-Barrio, M. T., Guo, Y., et al. (2018). Brown Adipocyte-Specific PPARγ (Peroxisome Proliferator-Activated Receptor γ) Deletion Impairs Perivascular Adipose Tissue Development and Enhances Atherosclerosis in Mice. Arterioscler. Thromb. Vasc. Biol. 38, 1738–1747. doi:10.1161/atvbaha.118.311367
Xu, A., and Vanhoutte, P. M. (2012). Adiponectin and Adipocyte Fatty Acid Binding Protein in the Pathogenesis of Cardiovascular Disease. Am. J. Physiol. Heart Circ. Physiol. 302, H1231–H1240. doi:10.1152/ajpheart.00765.2011
Yamawaki, H., Tsubaki, N., Mukohda, M., Okada, M., and Hara, Y. (2010). Omentin, a Novel Adipokine, Induces Vasodilation in Rat Isolated Blood Vessels. Biochem. Biophys. Res. Commun. 393, 668–672. doi:10.1016/j.bbrc.2010.02.053
Yasue, S., Masuzaki, H., Okada, S., Ishii, T., Kozuka, C., Tanaka, T., et al. (2010). Adipose Tissue-Specific Regulation of Angiotensinogen in Obese Humans and Mice: Impact of Nutritional Status and Adipocyte Hypertrophy. Am. J. Hypertens. 23, 425–431. doi:10.1038/ajh.2009.263
Ye, M., Ruan, C.-C., Fu, M., Xu, L., Chen, D., Zhu, M., et al. (2019). Developmental and Functional Characteristics of the Thoracic Aorta Perivascular Adipocyte. Cell. Mol. Life Sci. 76, 777–789. doi:10.1007/s00018-018-2970-1
Ying, W., Riopel, M., Bandyopadhyay, G., Dong, Y., Birmingham, A., Seo, J. B., et al. (2017). Adipose Tissue Macrophage-Derived Exosomal miRNAs Can Modulate In Vivo and In Vitro Insulin Sensitivity. Cell 171, 372–384. doi:10.1016/j.cell.2017.08.035
Yudkin, J. S., Eringa, E., and Stehouwer, C. D. (2005). “Vasocrine” Signalling from Perivascular Fat: a Mechanism Linking Insulin Resistance to Vascular Disease. Lancet 365, 1817–1820. doi:10.1016/s0140-6736(05)66585-3
Zhang, Y., Mei, H., Chang, X., Chen, F., Zhu, Y., and Han, X. (2016). Adipocyte-Derived Microvesicles From Obese Mice Induce M1 Macrophage Phenotype through Secreted miR-155. J. Mol. Cell Biol. 8, 505–517. doi:10.1093/jmcb/mjw040
Keywords: adipocyte, preadipocyte, adipokine, cellular heterogeneity, obesity, vascular inflammation
Citation: Li X, Ma Z and Zhu YZ (2021) Regional Heterogeneity of Perivascular Adipose Tissue: Morphology, Origin, and Secretome. Front. Pharmacol. 12:697720. doi: 10.3389/fphar.2021.697720
Received: 20 April 2021; Accepted: 10 June 2021;
Published: 22 June 2021.
Edited by:
Ana Paula Davel, State University of Campinas, BrazilReviewed by:
InKyeom Kim, Kyungpook National University, South KoreaQiongxin Wang, University of Alabama at Birmingham, United States
Copyright © 2021 Li, Ma and Zhu. This is an open-access article distributed under the terms of the Creative Commons Attribution License (CC BY). The use, distribution or reproduction in other forums is permitted, provided the original author(s) and the copyright owner(s) are credited and that the original publication in this journal is cited, in accordance with accepted academic practice. No use, distribution or reproduction is permitted which does not comply with these terms.
*Correspondence: Xinzhi Li, eGl6bGlAbXVzdC5lZHUubW8g; Yi Zhun Zhu, eXp6aHVAbXVzdC5lZHUubW8=