- 1Department of Critical Care Medicine, the Second Affiliated Hospital of Xi’an Jiaotong University, Xi’an, China
- 2Department of Critical Care Medicine, the Second Affiliated Hospital of Xi’an Medical University, Xi’an, China
Background: It remains unclear whether the mean vancomycin trough concentration (VTC) derived from the entire course of therapy is of potential benefit for critically ill patients. This study was conducted to explore the association between mean serum VTC and mortality in intensive care units (ICUs).
Methods: 3,603 adult patients with two or more VTC records after receiving vancomycin treatment in the eICU Collaborative Research Database were included in this multicenter retrospective cohort study. Mean VTC was estimated using all measured VTCs and investigated as a continuous and categorical variable. Patients were categorised into four groups according to mean VTC: <10, 10–15, 15–20, and >20 mg/L. Multivariable logistic regression and subgroup analyses were performed to investigate the relationship of mean VTC with mortality.
Results: After adjusting for a series of covariates, logistic regression analyses indicated that mean VTC, as a continuous variable, was positively correlated with ICU (odds ratio, 1.038, 95% confidence interval, [1.014–1.063]) and hospital (1.025 [1.005–1.046]) mortalities. As a categorical variable, mean VTC of 10–15 mg/L was not associated with reduced ICU (1.705 [0.975–2.981]) and hospital (1.235 [0.829–1.841]) mortalities. Mean VTC of 15–20 mg/L was not correlated with a lower risk of hospital mortality (1.370 [0.924–2.029]). Moreover, mean VTCs of 15–20 and >20 mg/L were significantly associated with higher ICU mortality (1.924 [1.111–3.332]; 2.428 [1.385–4.258]), and mean VTC of >20 mg/L with higher hospital mortality (1.585 [1.053–2.387]) than mean VTC of <10 mg/L. Similar results were observed in patients with different Acute Physiology and Chronic Health Evaluation IV score, creatinine clearance, age, and body mass index subgroups.
Conclusion: Mean VTC was not associated with reduced ICU/hospital related mortality. Our results suggested that VTC monitoring might not guarantee vancomycin efficacy for ICU patients.
Introduction
Vancomycin, a glycolpeptide bactericide that acts by obstructing the synthesis of the bacterial cell wall, was isolated from streptomycin approximately half a century ago (Moellering, 2006). It is widely used to treat Gram-positive bacterial infections, including methicillin-resistant Staphylococcus aureus (MRSA) and exhibits time-dependent bactericidal activity with a long post-antibiotic effect (Álvarez et al., 2016; Liu et al., 2011; Rybak, 2006). Therapeutic drug monitoring (TDM) is an adjuvant and practical method used for vancomycin dosing adjustment in intensive care units (ICUs) because of its narrow therapeutic window (Tabah et al., 2015). Based on infection models and clinical pharmacokinetic/pharmacodynamic (PK/PD) studies, the area under the concentration-time curve over 24 h/minimum inhibitory concentration (AUC0–24 h/MIC) has been established as the most predictive index to reflect the clinical and microbiological efficacies of vancomycin (Jung et al., 2014; Moise-Broder et al., 2004). Bacterial clearance, along with improvements in clinical signs and symptoms, has been suggested to be associated with AUC0–24h/MIC ≥ 400 (Makmor-Bakry et al., 2019; Rybak et al., 2020). However, it is difficult to precisely determine multiple serum/tissue vancomycin concentrations during the same dosing interval to calculate the AUC in clinical practice (Rybak et al., 2009).
Serum vancomycin trough concentration (VTC) monitoring before the fourth dose has been suggested as the most practical method for TDM of vancomycin, as a VTC 15–20 mg/L may achieve an AUC0–24h/MIC ≥400 if the MIC is ≤ 1 mg/L (Rybak et al., 2009). The Infectious Diseases Society of America has recommended that the VTC should be maintained above 10 mg/L to avoid the development of resistance, and 15–20 mg/L to improve clinical outcomes (Rybak et al., 2009). The Chinese Pharmacological Society recommended 10–15 mg/L as the target VTC for adult patients, and 10–20 mg/L for adult patients with severe MRSA infections (Ye et al., 2016). However, plenty of studies have demonstrated that VTC did not have a considerable effect on treatment outcomes (Albur et al., 2012; Jung et al., 2014; Liang et al., 2018; Makmor-Bakry et al., 2019) and highlighted a high incidence of inappropriate VTC leading to increased healthcare costs (Seng et al., 2018). As a result, the latest consensus in 2020 advocated vancomycin area under the concentration-time curve (AUC) values obtained by Bayesian modelling as the most accurate approach for managing vancomycin dosing (Rybak et al., 2020). Nevertheless, to our knowledge, those most retrospective and prospective studies only focused on the initial VTC after vancomycin therapy. It remains unclear whether the mean VTC, estimated using all measured VTCs during the entire course of treatment, is beneficial for critically ill patients. Therefore, this multicenter observational study with a large sample was further performed to investigate the association of mean VTC with mortality in critically ill patients.
Materials and Methods
Data Source and Study Design
This multicenter observational study was performed using the eICU Collaborative Research Database (eICU-CRD, version 2.0), which is a public de-identified ICU database comprising 200,859 patient unit encounters for 139,367 unique patients admitted between 2014 and 2015. The eICU database is available from https://physionet.org/content/eicu-crd/. Patients were admitted to 1 of 335 units at 208 hospitals located throughout the United States (Pollard et al., 2018). The eICU-CRD includes data on vital signs, laboratory measurements, medications, Acute Physiology and Chronic Health Evaluation (APACHE) components, care plan information, admission diagnoses, time-stamped diagnoses, and treatments. All researchers of this study received the necessary training and obtained permission to access the database.
Patient Selection
Adult patients (≥18 years) receiving vancomycin therapy with a single hospital admission and two or more TDM records on the first ICU stay were included in this study. The exclusion criteria were as follows: 1) patients with an ICU length of stay ≤24 h, 2) patients without records of their ICU discharge status, 3) patients with missing or unqualified covariates for multivariable adjustment, and 4) patients with an outlier value of VTC. The upper and lower fences represented values more and less than Q3 and Q1, respectively, by 1.5 times the interquartile range (IQR). The outlier value of VTC was defined as the value above or below the upper (mean VTC >30.70 mg/L) or lower (mean VTC <1.82 mg/L) fences (IQR was calculated using the formula: Q3—Q1).
Outcomes and Covariates
The outcomes of this study were ICU and hospital mortalities. The variables, mean VTC or serum creatinine (Scr), were calculated by dividing the sum of all collected VTC or Scr by the frequency of monitoring. Mean creatinine clearance (CCl) was determined by the Cockcroft-Gault equation: ([140—age in years] × weight in kg/[72 × mean Scr concentration in mg/dl]) × 0.85 if female (Cockcroft and Gault, 1976). Related treatments (such as dialysis, ventilation, vasodepressor, and vasopressor administration) were included, as these may reflect illness severity and/or affect VTC throughout ICU stay. Variables such as demographic data (e.g., age, sex, and ethnicity), initial body mass index (BMI), APACHE IV score, and comorbidities that could influence mortality (e.g., sepsis, burns, pancreatitis, gastrointestinal bleed, diabetes, heart failure, chronic obstructive pulmonary disease [COPD], hepatic failure, tumour, pneumonia, and renal failure) were also assessed during the first 24 h of ICU admission.
Statistical Analysis
Based on the recommended VTC in a series of guidelines (Rybak et al., 2009; Matsumoto et al., 2013; Ye et al., 2016), we divided the mean VTC into four categories: <10, 10–15, 15–20, and >20 mg/L. Continuous variables were presented as the medians (IQR) and compared using the Kruskal-Wallis H test. Categorical variables were presented as frequencies (percentages) and compared using χ2 or Fisher’s exact tests. A Chord diagram showed the connection between the initial VTC and mean VTC for each patient. Logistic regression models were used to investigate the association of the mean VTC, as a continuous and categorical variable, with ICU and hospital mortalities. To flexibly model and visualise the relationship between mean VTC and mortality, we also used restricted cubic splines with four knots at the fifth, 35th, 65th, and 95th percentiles. Interaction and subgroup analyses were conducted to determine whether the relationship between mean VTC and mortality persisted when the severity of the clinical status (≤64, or >64), CCl (≤80, or >80 ml/min), age (≤60, or >60 years), or BMI (≤30, or >30 kg/m2) changed. In addition, the subpopulation with records of vancomycin dose and duration was abstracted for another analysis. All tests were two-sided, and a p value < 0.05 was considered statistically significant. Data were extracted using the SAS version 9.4 software (SAS Institute, Cary, NC, United States), and all statistical analyses were performed using SPSS 22.0 (SPSS, Inc., Chicago, IL, United States) and Stata 14.0 (Stata Corp., College Station, TX, United States).
Results
Individual Selection and Clinical Characteristics
A total of 4,539 adult patients with a single hospital stay and at least two VTC records at the first ICU admission were extracted from the eICU-CRD. The followings were excluded: 718 patients with a length of ICU stay ≤24 h, 1 patient without a record of ICU discharge status, 142 patients with missing or unqualified covariates for multivariable adjustment, and 75 patients with a discrete VTC. Finally, 3,603 patients were included in this study (Figure 1). The patients were divided into the following four groups according to the mean VTC during ICU stay: <10 mg/L (n = 372, 10.3%), 10–15 mg/L (n = 1,165, 32.3%), 15–20 mg/L (n = 1,261 35.0%), and >20 mg/L (n = 805, 22.3%). Age, BMI, mean VTC, number of VTC, APACHE IV score, Scr, CCl, and use of vasodepressor, vasopressor and dialysis were significantly different among the four groups (p < 0.05). Additionally, there was a significant difference in the prevalence of COPD, heart failure, and renal failure (p < 0.05). Groups with a higher mean VTC had higher ICU (4.6, 8.2, 11.6, and 15.4%, respectively; p < 0.001) and hospital (10.5, 14.1, 18.5, and 22.0%, respectively; p < 0.001) mortalities (Table 1). The Chord diagram presented the connection between the mean VTC and initial VTC for each patient (Figure 2). Among all patients, there were 1,622 (45.0%) patients with the mean VTC and the initial VTC in the same groups. 250 (19.7%) patients with initial VTC <10 mg/L had a mean VTC 15–20 mg/L. Among patients with initial VTC 10–15 mg/L, 458 (44.3%) patients reached a mean VTC within 15–20 mg/L. And 182 (27.8%) patients with initial VTC >20 mg/L had a mean VTC 15–20 mg/L.
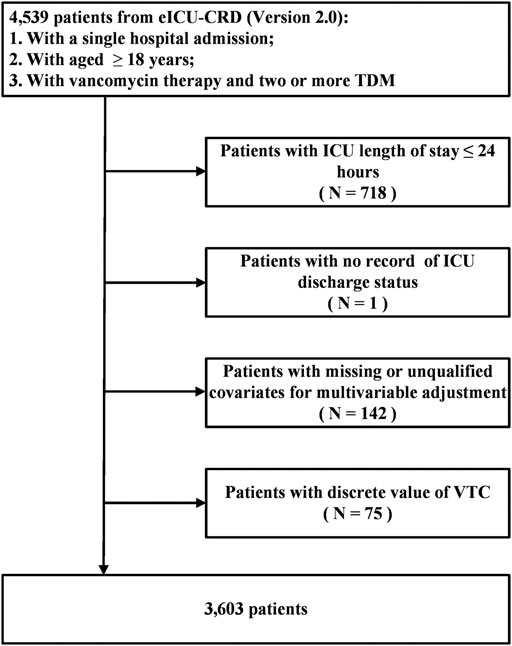
FIGURE 1. Flow Chart of Participant Selection. Cohort selection and criteria for exclusion, a total of 3,603 patients were included in the analysis. eICU-CRD, eICU Collaborative Research Database; TDM, therapeutic drug monitoring; ICU, intensive care unit; VTC, vancomycin trough concentration.
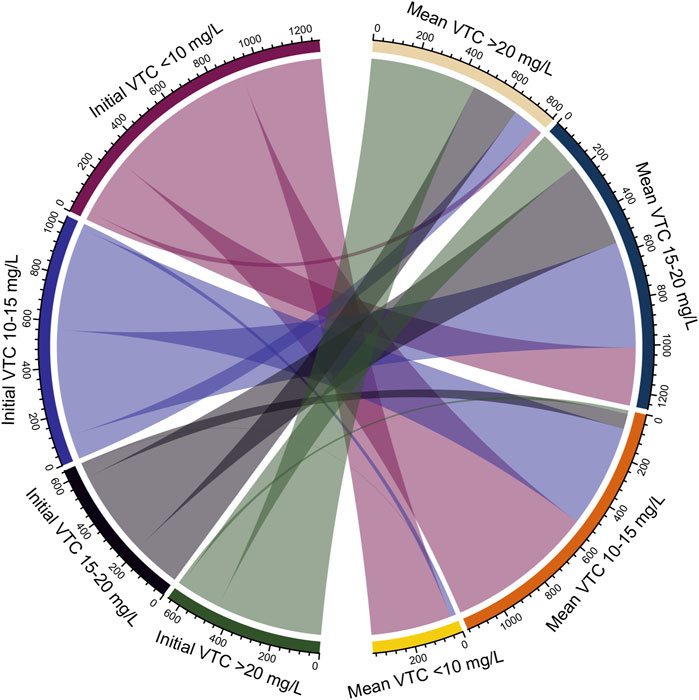
FIGURE 2. Connection Between the Mean VTC and Initial VTC. A chord diagram presented the difference of the mean VTC with initial VTC for each patient. VTC, vancomycin trough concentration.
Association of Mean VTC With Mortality
The univariable logistic regression model revealed that the mean VTC, as a continuous variable, was positively correlated with ICU (odds ratio, 1.073, 95% confidence interval, [1.051–1.095]) and hospital (1.054 [1.036–1.072]) mortalities. This association was still robust (1.038 [1.014–1.063]; 1.025 [1.005–1.046], respectively) after adjusting for age, sex, ethnicity, BMI, APACHE IV score, CCl, the use of ventilation, dialysis, vasodepressor and vasopressor, and diagnoses at ICU admission (Table 2). When mean VTC was considered as a categorical variable, patients with mean VTCs of 10–15, 15–20, and >20 mg/L were associated with higher ICU mortality (1.854 [1.091–3.150]; 2.734 [1.632–4.582]; 3.802 [2.254–6.414]), and those with mean VTCs of 15–20 and >20 mg/L showed a significant increase for hospital mortality (1.935 [1.349–2.776]; 2.407 [1.660–3.489]) compared with those with mean VTC <10 mg/L in the univariable logistic regression analyses. After multivariable adjustment, mean VTC of 10–15 mg/L was not associated with reduced ICU (1.705 [0.975–2.981]) and hospital (1.235 [0.829–1.841]) mortalities. Mean VTC of 15–20 mg/L was not correlated with a lower risk of hospital mortality (1.370 [0.924–2.029]). Moreover, mean VTCs of 15–20 and >20 mg/L were significantly associated with higher ICU mortality (1.924 [1.111–3.332]; 2.428 [1.385–4.258]), and mean VTC of >20 mg/L with increased hospital mortality (1.585 [1.053–2.387]) compared with mean VTC of <10 mg/L (Table 2). Restricted cubic splines visually showed that the risks of ICU (A) and hospital (B) mortalities increased with an increasing mean VTC (Supplementary Figure 1).
Association of Mean VTC With Mortality in Different Subgroups
We further analyzed the association between mean VTC and mortality in different predefined subgroups: APACHE IV score ≤64 (n = 1,841) and APACHE IV score >64 (n = 1,762); CCl ≤80 ml/min (n = 1,393) and CCl >80 ml/min (n = 2,210); age ≤60 years (n = 1,674) and age>60 years (n = 1,929); BMI ≤30 kg/m2 (n = 2,245) and BMI >30 kg/m2 (n = 1,358). There was no significant interaction stratified by APACHE IV score (ICU mortality, pinteraction = 0.545; hospital mortality, pinteraction = 0.058), CCl (ICU mortality, pinteraction = 0.807; hospital mortality, pinteraction = 0.769), age (ICU mortality, pinteraction = 0.469; hospital mortality, pinteraction = 0.314) and BMI (ICU mortality, pinteraction = 0.636; hospital mortality, pinteraction = 0.627). Notably, mean VTC, as a continuous variable, was significantly correlated with both ICU and hospital mortalities in APACHE IV score >64, CCl ≤80 ml/min, age ≤60 years and BMI ≤30 kg/m2 subgroups. When mean VTC was used as a categorical variable, similar results were observed across the different subgroups. Compared with patients with mean VTC of <10 mg/L, those with mean VTCs of 10–15 and 15–20 mg/L were not associated with reduced ICU and hospital mortalities in all subgroups (Table 3; Supplementary Tables 1, 2, 3).
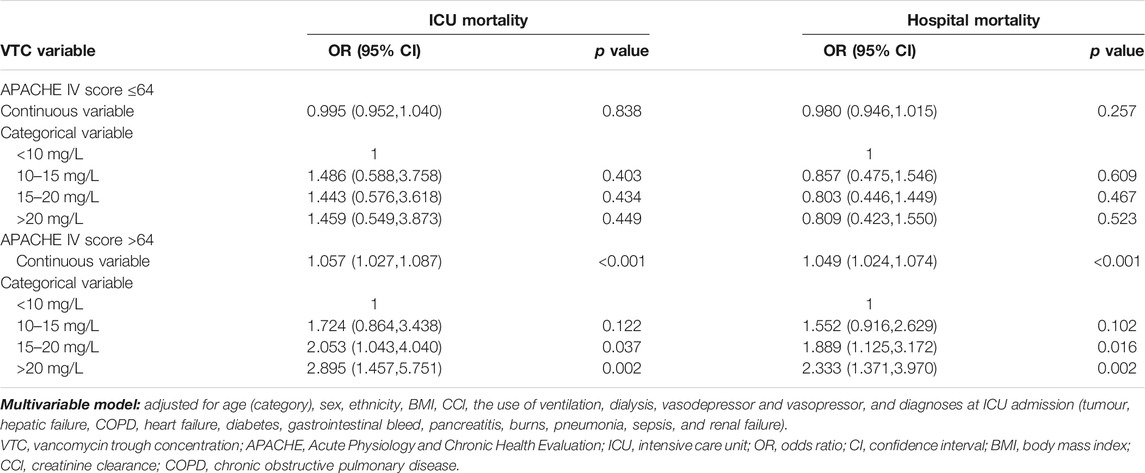
TABLE 3. Multivariable analysis for the association of mean VTC with mortality in Apache IV score subgroups.
We collected 1,553 patients with the records of vancomycin dose and duration to exclude the impact of vancomycin administration on the prognosis of patients. There were significantly statistical differences in total vancomycin dose, average daily dose, and duration of vancomycin exposure across the four groups (p < 0.05). The multivariable logistic regression model was built to adjust a series of covariates, including average daily dose and duration of vancomycin exposure, which demonstrated that the relationship between mean VTC and mortality still persisted (Supplementary Table 4).
Discussion
In this retrospective multicenter cohort study, we recruited a total of 3,603 critically ill patients with two or more VTC monitoring records after vancomycin treatment from 335 different ICUs at 208 hospitals in the eICU-CRD. Mean VTC was calculated by dividing the sum of all collected VTC by the frequency of monitoring for each patient. Our study showed that mean VTCs of 10–15, 15–20, and >20 mg/L were not associated with reduced ICU and hospital mortalities for critically ill patients. Patients with mean VTCs of 15–20 and >20 mg/L were found to be exposed to even higher risks of ICU or hospital mortality compared with those with mean VTC of <10 mg/L. The results indicated that maintaining therapeutic serum VTC between 15 mg/L and 20 mg/L throughout the entire course of vancomycin treatment failed to demonstrate a benefit for ICU and hospital mortalities in ICU patients, and implied that VTC might not be a suitable monitoring indicator to ensure vancomycin efficacy for critically ill patients.
As serum VTC has been suggested as a surrogate marker for the AUC/MIC index to monitor vancomycin efficacy, several studies have been designed to verify the association of VTC with clinical and microbiological outcomes in critically ill patients (Rybak et al., 2009). Surprisingly, most studies found no statistical difference in treatment outcomes according to the VTC level. Two retrospective studies have shown that VTC alone is not a good indicator for vancomycin treatment success among patients with MRSA bacteremia (Jung et al., 2014; Makmor-Bakry et al., 2019). Two other retrospective cohort studies have demonstrated that VTC >15 mg/L fails to improve the outcomes of patients with MRSA infections (Clemens et al., 2011; Hall et al., 2014). Additionally, two prospective, multicenter, observational studies have demonstrated no significant association between VTC level and vancomycin treatment response in a Chinese population diagnosed with gram-positive bacterial infections (Liang et al., 2018; Shen et al., 2018). Moreover, the latest consensus suggests that there are minimal to no data to support the safety and efficacy of a target VTC of 15–20 mg/L in patients with serious MRSA infections (Rybak et al., 2020). However, those aforementioned studies only explored the relationship between single steady-state VTC and outcomes. In a retrospective study of 76 critically ill patients confirmed MRSA infections, Cheong reported that the initial VTC was not associated with treatment response, which was consistent with the results of the other studies. Surprisingly, a corrected VTC, calculated as dividing the sum of each measured VTC multiplied by the number of days at that level by the total number of days under treatment, was higher among patients with improved clinical presentation and laboratory results than among those with poor clinical outcomes (Cheong et al., 2012). Because of the lack of large-scale population studies on multiple VTC records after receiving vancomycin therapy, which may represent personalised PK/PD profiles of vancomycin, whether the mean VTC derived from the entire course of therapy is of potential benefit for critically ill patients remains unclear.
In this study, the mean VTC was estimated using all collected VTCs throughout the therapy course, providing an overall level of VTC during the ICU stay. The chord diagram showed 19.7% patients with initial VTC < 10 mg/L, 44.3% patients with initial VTC 10–15 mg/L, and 27.8% patients with initial VTC > 20 mg/L had reached a mean VTC 15–20 mg/L eventually, which indicated that initial sub-therapeutic or excessive VTC had been adjusted to achieve suggested VTC. Therefore, mean VTC could reflect exposure dosage of vancomycin after adjustment for those with lower or higher initial VTC, to some extent. We further found that mean VTCs of 15–20 and >20 mg/L were significantly correlated with higher ICU mortality (1.924-fold and 2.428-fold), mean VTC of >20 mg/L with higher hospital mortality (1.585-fold) than a mean VTC of <10 mg/L. Moreover, mean VTCs of 10–15 and 15–20 mg/L were not associated with reduced ICU/hospital related mortality. Therefore, this present study is one in a growing number of studies demonstrating that maintaining therapeutic VTC might not ensure vancomycin efficacy for critically ill patients. For clinics, our findings suggested that the interpretation of VTC results should be considered cautiously in clinical practice.
The APACHE IV score is useful for assessing the severity of illness and predicting outcomes in ICU patients (Ko et al., 2018; Zimmerman et al., 2006). To diminish the influence of disease severity itself, all individuals were divided into two subgroups for further investigations based on the median first APACHE IV score (≤64 or >64). Vancomycin is eliminated primarily via the renal route, with >80–90% recovered unchanged in urine within 24 h after administration of a single dose (Rybak, 2006). A decrease in the glomerular filtration rate for any cause would increase the VTC, making the association between mortality and VTC difficult to assess (Cantú et al., 1994). To exclude the influence of renal function on outcomes, we classified the patients into two groups according to CCl (≤80 or >80 ml/min) to assess this relationship. Meanwhile, the age and BMI of patient associated with mean VTC can be very significant for mortality (Haeseker et al., 2016; Tsai et al., 2018). Sensitivity analyses were also performed in patients of different ages (≤60 or >60 years) and BMIs (≤30 or >30 kg/m2). There is no interaction between mean VTC and those variables for ICU as well as hospital mortalities, which indicated that those variables did not impact the association between mean VTC and mortality. Subgroup analyses also found similar results about the relationships of mean VTC with mortality in all predefined subgroups. Therefore, mean VTC was not associated with improved outcomes regardless of the severity of illness, the degree of renal function, age, or BMI. Based on these results, The AUC/MIC or Bayesian AUC-only estimation may be considered as possible alternative methods for vancomycin administration.
A few underlying mechanisms may explain our results. First, Patel et al. reported that patients with a VTC of 15–20 mg/L could achieve an AUC0-24/MIC ratio of ≥400 when the MIC value is ≤ 1 (Patel et al., 2011). However, several studies have demonstrated a poor correlation between VTC and AUC0–24 because of high inter-individual variability (Bakke et al., 2017; Haeseker et al., 2016; Neely et al., 2014; Tsai et al., 2018). A 3-years, prospective study indicated that 68% of adults who were administered vancomycin with an AUC0-24 ≥ 400 mg h/L had a trough concentration of <15 mg/L (Neely et al., 2018). Additionally, a prospective study of Chinese patients suggested that Cmax, AUC0–24, and AUC0–24 h/MIC are not significantly associated with clinical and microbiological outcomes based on multivariable logistic regression analysis (Shen et al., 2018). Second, vancomycin has been associated with nephrotoxicity, which is the most serious common side effect of vancomycin linked to a higher risk of mortality (Jeffres, 2017). A multicenter prospective clinical trial including 288 adult patients indicated that a VTC of >15 mg/L is associated with a 3-fold increased risk of nephrotoxicity (Bosso et al., 2011). Another retrospective study in the 315 vancomycin-treated patients observed that vancomycin nephrotoxicity is independently correlated with higher VTC of >20 mg/L (Park et al., 2018). The result is similar to those of our study, in which mean VTC >15 mg/L was associated with increased ICU or hospital mortality. Therefore, the optimal TDM of vancomycin in ICU patients warrants further investigation.
Our study has some limitations. First, given the retrospective nature of the study, the risk of unmeasured confounding effects and the introduction of bias were unavoidable. However, this multicenter study of VTC included the largest sample size to date, allowing the findings to be generalised. Second, there is no available information on microbiological outcomes and acute kidney injury after vancomycin treatment in eICU-CRD. Nevertheless, mortality may represent clinical outcomes to confirm the prognostic value of VTC, as critically ill patients with gram-positive bacterial infection have high mortality, particularly owing to MRSA infections (Calfee et al., 2014). Third, hospital protocols for the time of VTC monitoring, target VTC, and adjustments of vancomycin were not available due to the retrospective design. However, the sensitivity analysis found the association was persisted in less than half of the patients with records on vancomycin dose and duration. Fourth, we can provide only the association between mean VTC and mortality rather than causality. In the future, a well-designed randomised controlled trial with more treatment information should be considered to evaluate causality between VTC and mortality.
Conclusion
In conclusion, mean VTC was not associated with reduced ICU/hospital related mortality, independent of the degree of disease severity, renal function, age, and BMI. Our results indicated that VTC monitoring might not ensure vancomycin efficacy for ICU patients. Therefore, the interpretation of VTC results should be considered cautiously for clinics in clinical practice. The AUC/MIC or Bayesian AUC-only estimation may be considered as possible alternative methods for vancomycin administration, and warrant further investigations in future studies.
Data Availability Statement
Publicly available datasets were analyzed in this study. This data can be found here: https://eicu-crd.mit.edu.
Ethics Staement
As all protected patient health-related information in the eICU had been deleted, the requirement for individual patient consent was waived. The database is released under the Health Insurance Portability and Accountability Act (HIPAA) safe harbor provision. The re-identification risk was certified as meeting safe harbor standards by Privacert (Cambridge, MA) (HIPAA Certification no. 1031219-2).
Author Contributions
GW, YH, and JR conceived of the study. GW provided funding for the study. GW, XL and XW provided critical appraisal and revision. JL, RL, YG, XJ, JZ, and JR extracted and collected data from eICU-CRD. YH, XJ, and JL performed the statistical analysis. YH and JR wrote the manuscript. YH, JR, JL, XJ, YG, RL, and JZ revised the manuscript. All authors read and approved the final manuscript.
Funding
This study was supported by the National Natural Science Foundation of China (81770057).
Conflict of Interest
The authors declare that the research was conducted in the absence of any commercial or financial relationships that could be construed as a potential conflict of interest.
Acknowledgments
We appreciate the researchers at the MIT Laboratory for Computational Physiology and collaborating research groups to keep eICU-CRD databases available.
Supplementary Material
The Supplementary Material for this article can be found online at: https://www.frontiersin.org/articles/10.3389/fphar.2021.690157/full#supplementary-material
References
Albur, M. S., Bowker, K., Weir, I., and MacGowan, A. (2012). Factors Influencing the Clinical Outcome of Methicillin-Resistant Staphylococcus aureus Bacteraemia. Eur. J. Clin. Microbiol. Infect. Dis. 31 (3), 295–301. doi:10.1007/s10096-011-1310-2
Álvarez, R., López Cortés, L. E., Molina, J., Cisneros, J. M., and Pachón, J. (2016). Optimizing the Clinical Use of Vancomycin. Antimicrob. Agents Chemother. 60 (5), 2601–2609. doi:10.1128/aac.03147-14
Bakke, V., Sporsem, H., Von der Lippe, E., Nordøy, I., Lao, Y., Nyrerød, H. C., et al. (2017). Vancomycin Levels Are Frequently Subtherapeutic in Critically Ill Patients: a Prospective Observational Study. Acta Anaesthesiol Scand. 61 (6), 627–635. doi:10.1111/aas.12897
Bosso, J. A., Nappi, J., Rudisill, C., Wellein, M., Bookstaver, P. B., Swindler, J., et al. (2011). Relationship between Vancomycin Trough Concentrations and Nephrotoxicity: a Prospective Multicenter Trial. Antimicrob. Agents Chemother. 55 (12), 5475–5479. doi:10.1128/aac.00168-11
Calfee, D. P., Salgado, C. D., Milstone, A. M., Harris, A. D., Kuhar, D. T., Moody, J., et al. (2014). Strategies to Prevent Methicillin-Resistant Staphylococcus aureus Transmission and Infection in Acute Care Hospitals: 2014 Update. Infect. Control. Hosp. Epidemiol. 35 (Suppl. 2), S108–S132. doi:10.1017/s0899823x00193882
Cantú, T. G., Yamanaka-Yuen, N. A., and Lietman, P. S. (1994). Serum Vancomycin Concentrations: Reappraisal of Their Clinical Value. Clin. Infect. Dis. 18 (4), 533–543. doi:10.1093/clinids/18.4.533
Cheong, J. Y., Makmor-Bakry, M., Lau, C. L., and Abdul Rahman, R. (2012). The Relationship between Trough Concentration of Vancomycin and Effect on Methicillin-Resistant Staphylococcus aureus in Critically Ill Patients. S Afr. Med. J. 102 (7), 616–619. doi:10.7196/samj.5343
Clemens, E. C., Chan, J. D., Lynch, J. B., and Dellit, T. H. (2011). Relationships between Vancomycin Minimum Inhibitory Concentration, Dosing Strategies, and Outcomes in Methicillin-Resistant Staphylococcus aureus Bacteremia. Diagn. Microbiol. Infect. Dis. 71 (4), 408–414. doi:10.1016/j.diagmicrobio.2011.08.002
Cockcroft, D. W., and Gault, M. H. (1976). Prediction of Creatinine Clearance from Serum Creatinine. Nephron 16 (1), 31–41. doi:10.1159/000180580
Haeseker, M., Croes, S., Neef, C., Bruggeman, C., Stolk, L., and Verbon, A. (2016). Evaluation of Vancomycin Prediction Methods Based on Estimated Creatinine Clearance or Trough Levels. Ther. Drug Monit. 38 (1), 120–126. doi:10.1097/ftd.0000000000000250
Hall, R. G., Blaszczyk, A. T., Thompson, K. A., Brouse, S. D., Giuliano, C. A., Frei, C. R., et al. (2014). Impact of Empiric Weight-Based Vancomycin Dosing on Nephrotoxicity and Mortality in Geriatric Patients with Methicillin-Resistant Staphylococcus aureus Bacteraemia. J. Clin. Pharm. Ther. 39 (6), 653–657. doi:10.1111/jcpt.12203
Jeffres, M. N. (2017). The Whole Price of Vancomycin: Toxicities, Troughs, and Time. Drugs 77 (11), 1143–1154. doi:10.1007/s40265-017-0764-7
Jung, Y., Song, K. H., Cho, Je., Kim, H. S., Kim, N. H., Kim, T. S., et al. (2014). Area under the Concentration-Time Curve to Minimum Inhibitory Concentration Ratio as a Predictor of Vancomycin Treatment Outcome in Methicillin-Resistant Staphylococcus aureus Bacteraemia. Int. J. Antimicrob. Agents 43 (2), 179–183. doi:10.1016/j.ijantimicag.2013.10.017
Ko, M., Shim, M., Lee, S. M., Kim, Y., and Yoon, S. (2018). Performance of Apache IV in Medical Intensive Care Unit Patients: Comparisons with Apache II, SAPS 3, and MPM0 III. Acute Crit. Care 33 (4), 216–221. doi:10.4266/acc.2018.00178
Liang, X., Fan, Y., Yang, M., Zhang, J., Wu, J., Yu, J., et al. (2018). A Prospective Multicenter Clinical Observational Study on Vancomycin Efficiency and Safety with Therapeutic Drug Monitoring. Clin. Infect. Dis. 67 (Suppl. l_2), S249–s255. doi:10.1093/cid/ciy680
Liu, C., Bayer, A., Cosgrove, S. E., Daum, R. S., Fridkin, S. K., Gorwitz, R. J., et al. (2011). Clinical Practice Guidelines by the Infectious Diseases Society of america for the Treatment of Methicillin-Resistant Staphylococcus aureus Infections in Adults and Children. Clin. Infect. Dis. 52 (3), e18–55. doi:10.1093/cid/ciq146
Makmor-Bakry, M., Ahmat, A., Shamsuddin, A., Lau, C. L., and Ramli, R. (2019). Association between Single Trough-Based Area under the Curve Estimation of Vancomycin and Treatment Outcome Among Methicillin-Resistant Staphylococcus aureus Bacteremia Patients. Anaesthesiol Intensive Ther. 51 (3), 218–223. doi:10.5114/ait.2019.87362
Matsumoto, K., Takesue, Y., Ohmagari, N., Mochizuki, T., Mikamo, H., Seki, M., et al. (2013). Practice Guidelines for Therapeutic Drug Monitoring of Vancomycin: a Consensus Review of the Japanese Society of Chemotherapy and the Japanese Society of Therapeutic Drug Monitoring. J. Infect. Chemother. 19 (3), 365–380. doi:10.1007/s10156-013-0599-4
Moellering, R. C. (2006). Vancomycin: a 50-year Reassessment. Clin. Infect. Dis. 42 (Suppl. 1), S3–S4. doi:10.1086/491708
Moise-Broder, P. A., Forrest, A., Birmingham, M. C., and Schentag, J. J. (2004). Pharmacodynamics of Vancomycin and Other Antimicrobials in Patients with Staphylococcus aureus Lower Respiratory Tract Infections. Clin. Pharmacokinet. 43 (13), 925–942. doi:10.2165/00003088-200443130-00005
Neely, M. N., Kato, L., Youn, G., Kraler, L., Bayard, D., van Guilder, M., et al. (2018). Prospective Trial on the Use of Trough Concentration versus Area under the Curve to Determine Therapeutic Vancomycin Dosing. Antimicrob. Agents Chemother. 62 (2). doi:10.1128/aac.02042-17
Neely, M. N., Youn, G., Jones, B., Jelliffe, R. W., Drusano, G. L., Rodvold, K. A., et al. (2014). Are Vancomycin Trough Concentrations Adequate for Optimal Dosing? Antimicrob. Agents Chemother. 58 (1), 309–316. doi:10.1128/aac.01653-13
Park, S. J., Lim, N. R., Park, H. J., Yang, J. W., Kim, M. J., Kim, K., et al. (2018). Evaluation of Risk Factors for Vancomycin-Induced Nephrotoxicity. Int. J. Clin. Pharm. 40 (5), 1328–1334. doi:10.1007/s11096-018-0634-8
Patel, N., Pai, M. P., Rodvold, K. A., Lomaestro, B., Drusano, G. L., and Lodise, T. P. (2011). Vancomycin: We Can't Get There from Here. Clin. Infect. Dis. 52 (8), 969–974. doi:10.1093/cid/cir078
Pollard, T. J., Johnson, A. E. W., Raffa, J. D., Celi, L. A., Mark, R. G., and Badawi, O. (2018). The eICU Collaborative Research Database, a Freely Available Multi-center Database for Critical Care Research. Sci. Data 5, 180178. doi:10.1038/sdata.2018.178
Rybak, M., Lomaestro, B., Rotschafer, J. C., Moellering, R., Craig, W., Billeter, M., et al. (2009). Therapeutic Monitoring of Vancomycin in Adult Patients: a Consensus Review of the American Society of Health-System Pharmacists, the Infectious Diseases Society of America, and the Society of Infectious Diseases Pharmacists. Am. J. Health Syst. Pharm. 66 (1), 82–98. doi:10.2146/ajhp080434
Rybak, M. J., Le, J., Lodise, T. P., Levine, D. P., Bradley, J. S., Liu, C., et al. (2020). Therapeutic Monitoring of Vancomycin for Serious Methicillin-Resistant Staphylococcus aureus Infections: A Revised Consensus Guideline and Review by the American Society of Health-System Pharmacists, the Infectious Diseases Society of America, the Pediatric Infectious Diseases Society, and the Society of Infectious Diseases Pharmacists. Am. J. Health Syst. Pharm. 77 (11), 835–864. doi:10.1093/ajhp/zxaa036
Rybak, M. J. (2006). The Pharmacokinetic and Pharmacodynamic Properties of Vancomycin. Clin. Infect. Dis. 42 (Suppl. 1), S35–S39. doi:10.1086/491712
Seng, J. J. B., Yong, M. H. A., Peh, Z. X., Soong, J. L., and Tan, M. H. (2018). Appropriateness of Vancomycin Therapeutic Drug Monitoring and its Outcomes Among Non-dialysis Patients in a Tertiary Hospital in Singapore. Int. J. Clin. Pharm. 40 (5), 977–981. doi:10.1007/s11096-018-0670-4
Shen, K., Yang, M., Fan, Y., Liang, X., Chen, Y., Wu, J., et al. (2018). Model-based Evaluation of the Clinical and Microbiological Efficacy of Vancomycin: A Prospective Study of Chinese Adult In-House Patients. Clin. Infect. Dis. 67 (Suppl. l_2), S256–s262. doi:10.1093/cid/ciy667
Tabah, A., De Waele, J., Lipman, J., Zahar, J. R., Cotta, M. O., Barton, G., et al. (2015). The ADMIN-ICU Survey: a Survey on Antimicrobial Dosing and Monitoring in ICUs. J. Antimicrob. Chemother. 70 (9), 2671–2677. doi:10.1093/jac/dkv165
Tsai, D., Stewart, P. C., Hewagama, S., Krishnaswamy, S., Wallis, S. C., Lipman, J., et al. (2018). Optimised Dosing of Vancomycin in Critically Ill Indigenous Australian Patients with Severe Sepsis. Anaesth. Intensive Care 46 (4), 374–380. doi:10.1177/0310057x1804600405
Ye, Z. K., Chen, Y. L., Chen, K., Zhang, X. L., Du, G. H., He, B., et al. (2016). Therapeutic Drug Monitoring of Vancomycin: a Guideline of the Division of Therapeutic Drug Monitoring, Chinese Pharmacological Society. J. Antimicrob. Chemother. 71 (11), 3020–3025. doi:10.1093/jac/dkw254
Keywords: vancomycin trough concentration, intensive care unit, mortality, eICU collaborative research database, observational study
Citation: Hou Y, Ren J, Li J, Jin X, Gao Y, Li R, Zhang J, Wang X, Li X and Wang G (2021) Relationship Between Mean Vancomycin Trough Concentration and Mortality in Critically Ill Patients: A Multicenter Retrospective Study. Front. Pharmacol. 12:690157. doi: 10.3389/fphar.2021.690157
Received: 02 April 2021; Accepted: 06 July 2021;
Published: 19 July 2021.
Edited by:
Elena Ramírez, University Hospital La Paz, SpainReviewed by:
Muhammad Usman, University of Veterinary and Animal Sciences, PakistanJun Jie Benjamin Seng, Ministry of Health, Singapore
Copyright © 2021 Hou, Ren, Li, Jin, Gao, Li, Zhang, Wang, Li and Wang. This is an open-access article distributed under the terms of the Creative Commons Attribution License (CC BY). The use, distribution or reproduction in other forums is permitted, provided the original author(s) and the copyright owner(s) are credited and that the original publication in this journal is cited, in accordance with accepted academic practice. No use, distribution or reproduction is permitted which does not comply with these terms.
*Correspondence: Xinyu Li, bHh5X2ljdUAxNjMuY29t; Gang Wang, Z2FuZ193YW5nQHhqdHUuZWR1LmNu
†These authors have contributed equally to this work