- School of Life Sciences, Beijing University of Chinese Medicine, Beijing, China
Calcium, as a second messenger, plays an important role in the pathogenesis of cardiovascular diseases (CVDs). The malfunction of calcium signaling in endothelial cells and vascular smooth muscle cells promotes hypertension. In cardiomyocytes, calcium overload induces apoptosis, leading to myocardial infarction and arrhythmias. Moreover, the calcium–calcineurin–nuclear factor of activated T cells (NFAT) pathway is essential for expressing the cardiac pro-hypertrophic gene. Heart failure is also characterized by reduced calcium transient amplitude and enhanced sarcoplasmic reticulum (SR) calcium leakage. Traditional Chinese medicine (TCM) has been used to treat CVDs for thousands of years in China. Because of its multicomponent and multitarget characteristics, TCM's unique advantages in CVD treatment are closely related to the modulation of multiple calcium handling proteins and calcium signaling pathways in different types of cells involved in distinct CVDs. Thus, we systematically review the diverse mechanisms of TCM in regulating calcium pathways to treat various types of CVDs, ranging from hypertrophic cardiomyopathy to diabetic heart disease.
Introduction
Cardiovascular diseases (CVDs) have become common diseases and the main cause of death worldwide (Roth et al., 2017). In 2016, 56 million people died globally, and CVDs caused one-third of the deaths. It is also estimated that 23.6 million people will die from CVDs by 2030 (World Health Organization). The number of patients with CVDs in China is expanding rapidly, reaching more than 290 million, and CVDs are currently the leading cause of mortality (∼40%) (Hu Shengshou et al., 2019). With the increasing number of CVD patients, the cost of CVD treatment has also increased, prioritizing the prevention and treatment of CVDs (Tarride et al., 2009; Bansilal et al., 2015).
Calcium ions (Ca2+), as a second messenger, play an important role in different types of cells involved in various CVD conditions, such as hypertension, arrhythmias, and myocardial infarction (Firth et al., 2013; Xia et al., 2016; Mustroph et al., 2017; Wilson et al., 2019). Under physiological conditions, regulation of intracellular Ca2+ concentration ([Ca2+]i) can effectively change the activity of vasodilation/contraction in vascular smooth muscle cells (VSMCs) (Marchand et al., 2012; Hao et al., 2019). Endothelial cells participate in the modulation of vascular tone by releasing vasoactive agents, such as nitric oxide (NO), which in turn alters [Ca2+]i and maintains blood vessel homeostasis (Takeuchi et al., 2004; Filippini et al., 2019). In cardiomyocytes, calcium influx through voltage-dependent calcium channels (VDCCs) activates ryanodine receptors (RyRs) to release calcium from the sarcoplasmic reticulum (SR), which is called calcium-induced calcium release (CICR), and elevated calcium levels activate myofilaments to cause contraction (Bers, 2008; Louch et al., 2015; Han et al., 2019). Dysregulation of Ca2+ signaling in the heart is tightly correlated with pathogenesis of CVDs. For example, Ca2+ release from nuclear envelope activates CaMKII and promotes nuclear translocation of histone deacetylase 5 (HDAC5), initiating the cardiac hypertrophy (Nakayama et al., 2010). For an inherited arrhythmia called catecholaminergic polymorphic ventricular tachycardia (CPVT), RyRs mutation and β-adrenergic stimulation make SR Ca2+ content exceed a threshold, promoting the generation of calcium waves that lead to arrhythmias (Eisner, 2014). Furthermore, Ca2+ overload induces apoptosis in cardiomyocytes under pathological conditions, leading to myocardial infarction (Vassalle and Lin, 2004; Jiao et al., 2019), and increased SR Ca2+ leakage and reduced SR Ca2+ uptake can be normally observed during heart failure (HF) (Kalyanasundaram et al., 2013). Moreover, hypertension is related to the malfunction of calcium signaling in endothelial cells and VSMCs (Touyz et al., 2018; Wilson et al., 2019). Thus, calcium signaling is crucial during the pathogenesis of CVDs, and more detailed mechanisms of CVDs pathogenesis due to Ca2+ signaling malfunction are referred to other reviews (Cartwright et al., 2011; Eisner, 2014; Landstrom et al., 2017).
Traditional Chinese medicine (TCM) has been used to treat CVDs for thousands of years, with antioxidative, anti-inflammatory, and protective effects on vascular endothelial cells (Pan et al., 2011; Tao et al., 2012; Liu et al., 2015; Lam et al., 2016). For example, a multicenter clinical trial has revealed that Qiliqiangxin (QLQX) capsules, in addition to standard treatment, effectively decreases the levels of N-terminal pro-B-type natriuretic peptide in patients with chronic heart failure (Li et al., 2013). Furthermore, the clinical intravenous use of TCM for patients with acute myocardial infarction is increasing in China, and Salvia miltiorrhiza represents the most common prescription (Spatz et al., 2018). A meta-analysis including seventeen randomized controlled trials suggest the primary end points of patients with unstable angina are reduced by Panax notoginseng saponins, an important effective constituent in Panax notoginseng (Duan et al., 2018). Accumulating evidence suggests that calcium signaling plays a central role in the underlying mechanisms of TCM's therapeutic effects (Qi et al., 2008; Wang et al., 2012; Li et al., 2018a; Jiayi et al., 2019; Li et al., 2020). For instance, baicalein, the active ingredient of Scutellaria baicalensis, has been shown to reduce calcium levels in cardiomyocytes and prevent heart remodeling (Zhao et al., 2016). Ginseng extract has been reported to reduce the blood pressure of patients in clinical trials, which is beneficial for CVD prevention, and targeting calcium channels in vascular tissues may be one of the underlying mechanisms (Liu and Huang, 2016). Based on the current knowledge, we systematically review the diverse mechanisms of TCM in regulating calcium pathways to treat various types of CVDs.
Protective Effects of Traditional Chinese Medicine and Its Bioactive Components on Various Types of Cardiovascular Diseases
Hypertrophic Cardiomyopathy
Several risk factors such as hypertension can induce compensatory muscle tissue thickening and chamber enlargement in the heart, which is called cardiac hypertrophy (Drazner, 2011). For physiological hypertrophy, heart growth or hypertrophy (such as increased heart function caused by regular exercise), is reversible and harmless with no abnormality in the heart structure (Maillet et al., 2013). Pathological hypertrophy, in contrast to physiological hypertrophy, is often linked with interstitial fibrosis and dysregulation of cardiac function, thereby promoting heart failure (HF) and sudden death (Iemitsu et al., 2001; Bernardo et al., 2010; Aoyagi and Matsui, 2011). The characteristics of cardiac pathological hypertrophy at the cellular level include cell enlargement, protein synthesis enhancement, and fetal gene activation (Cox and Marsh, 2014; Tham et al., 2015). Multiple calcium-related signaling pathways are involved in cardiac hypertrophy (Mukherjee and Spinale, 1998; Wilkins and Molkentin, 2004). For example, after binding with Ca2+/calmodulin (CaM), calcineurin dephosphorylates nuclear factor of activated T cells (NFAT) in the cytosol and promotes NFAT translocation into the cell nucleus, which regulates gene expression during cardiac hypertrophy (Dewenter et al., 2017).
TCM and its active ingredients can treat myocardial hypertrophy through calcium signaling pathways (Table 1). For instance, Dracocephalum heterophyllum Benth flavonoid (DHBF) has been reported to alleviate myocardial hypertrophy induced by angiotensin II (Ang II), reflected by downregulation of cardiac hypertrophy markers and reduction of cell surface area. DHBF protected hypertrophic cardiomyocytes, presumably by blocking Ang II type 1 receptor and L-type calcium channels (LTCCs) to assist NO release and to reduce intracellular calcium levels (Jiang et al., 2018). Stachydrine (STA), the main biologically active ingredient of Leonurus heterophyllus, attenuated cardiac hypertrophy and protected the heart by reducing the amplitude of calcium transients and prolonging the decay constant of calcium transients. The underlying mechanism is to reduce cAMP levels, to inhibit cAMP-dependent protein kinase (PKA) activation, and to prevent phospholamban (PLN) phosphorylation (Zhang et al., 2014). Tanshinone IIA, a compound from the root of Salvia miltirrhiza, has been shown to alleviate isoprenaline (ISO)-induced cardiomyocyte hypertrophy because it could attenuate the ISO-mediated upregulation of atrial natriuretic peptide (ANP), brain natriuretic peptide (BNP), calcineurin, NFATc3, and β-myosin heavy chain (β-MHC) (Tan et al., 2011). Astragalus membranaceus contains an active component called Astragalus polysaccharide (APS), which has been shown to play an essential role in anti-myocardial hypertrophy by targeting the Ca2+-mediated signaling cascade, including prevention of hypertrophy-related NFATc3 nuclear translocation and downregulation of calmodulin-dependent protein kinase II (CaMKII) activity (Dai et al., 2014). Smilax glabra flavonoids (SGF) suppress calcium release from SR by blocking RyR2, thereby protecting myocardial cells from hypertrophy (Shou et al., 2013). Moreover, Cai et al. found that Tu-fu-ling flavonoids (TFLF) could reverse the induction of myocardial hypertrophy by Ang II, and its mechanism might be similarly related to RyR2 and junctophilin-2 (JP2) (Cai et al., 2015). Scutellarin is a bioactive component of Erigeron breviscapus, which is widely used in the treatment of CVDs. The cardiac hypertrophy model based on transverse aortic ligation has been applied to study its cardioprotective effect, and the results revealed that scutellarin was capable of treating hypertension and myocardial hypertrophy by modulating the Ca2+-mediated calcineurin pathway (Pan et al., 2010). Taken together, TCM could reduce [Ca2+]i by blocking either calcium entry through LTCC or calcium release from SR, thereby inhibiting the calcium–calcineurin–NFAT signaling pathway and preventing cardiac hypertrophy (Figure 1).
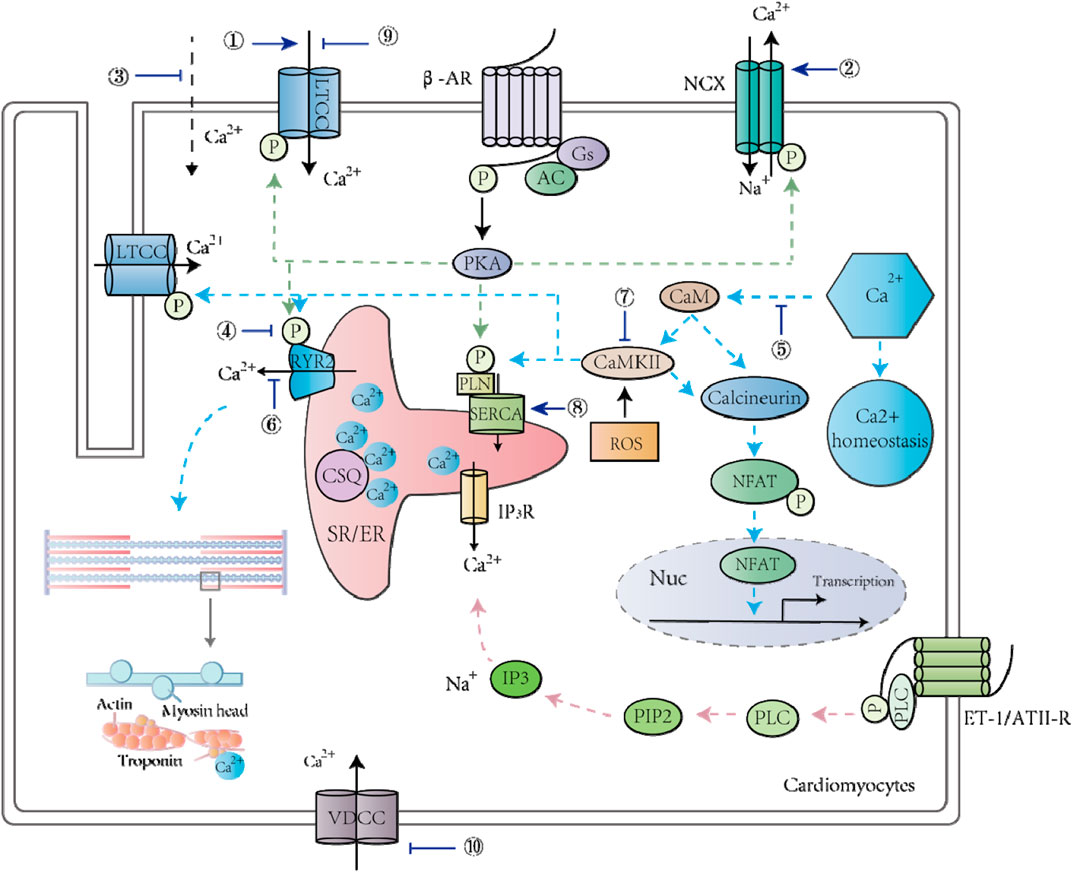
FIGURE 1. TCM and its active ingredients treat CVDs by regulating calcium signaling pathways in cardiomyocytes. ①Matrine; ②Matrine; ③Changrolin, Paeoniflorin monomer, Dehydroevodiamine alkaloid; ④Stachydrine; ⑤Scutellarin, Astragali Radix; ⑥Senkyunolide A, Smilax glabra flavonoids, Baicalein; ⑦Astragalus polysaccharide; ⑧Baicalein; ⑨6-Gin; Astragaloside IV; Wenxin Keli; Qiliqiangxin; Total flavones from AS; ⑩Senkyunolide A; Ligustrazine.
Arrhythmia
Arrhythmia is a common heart disease characterized by abnormal heart rhythm, which can be either too fast, i.e., tachycardia, such as atrial fibrillation (AF), or too slow, i.e., bradycardia, which could occur in sick sinus node syndrome (Wang et al., 2017a; Zaza et al., 2018). There are many predisposing factors for arrhythmia. For example, hypoxia and oxidative stress mediate Ca2+ overload in myocardial cells and generate heart rhythm irregularities and other myocardial dysfunctions (Guan et al., 2015).
Several studies have evaluated and explored the therapeutic effects of common Chinese medicines and their active ingredients on arrhythmia through modulating cardiac calcium handling (Liu et al., 2017a; Yang et al., 2020a; Tian et al., 2018) (Table 2). The antiarrhythmic effect of matrine in Sophora tonkinensis was revealed in a rat arrhythmia model induced by coronary artery ligation. Matrine ameliorated ischemia-mediated abnormal reduction of intracellular Ca2+, presumably by enhancing the activity of LTCCs (Li et al., 2009). These results collectively indicated that long-term use of matrine resisted arrhythmia and myocardial infarction and protected heart function (Li et al., 2009). Changrolin, an active component of Dichroa febrifuga, could protect against arrhythmia because of its role in inhibiting multiple ion currents, such as the sodium current (INa) and calcium currents (ICa), and changing the electromechanical properties of isolated rat cardiomyocytes (Chen et al., 2010). As examined by the whole cell patch-clamp technique, paeoniflorin protected against arrhythmias by inhibiting L-type calcium current (ICaL) to reduce oscillatory afterpotentials or extrasystoles (Wang et al., 2011). Gan et al. found that isorhynchophylline, an alkaloid from Uncaria rhynchophylla, had various effects in treating hypertension, arrhythmia, and other diseases (Gan et al., 2011). More specifically, isorhynchophylline alleviated arrhythmia in rats and guinea pigs by inhibiting the calcium current and reducing the action potential duration in cardiomyocytes, leading to a decreased rate of arrhythmia occurrence and mortality (Gan et al., 2011).
One of the critical targets of numerous Chinese patent medicines in treating arrhythmia is calcium signaling. For example, Wenxin Keli (WXKL), a widely used TCM in China, prepared from five ingredients: Polygonatum sibiricum, Codonopsis, Notoginseng, amber, Nardostachys jatamansi DC. WXKL improved calcium homeostasis by modulating the LTCC, late sodium current (INaL), transient outward potassium current (Ito), and the downstream Ca2+/CaMKII pathway (Wang et al., 2017a; Xing et al., 2013; Wang et al., 2013; Chen et al., 2013; Xue et al., 2013). Ischemia and reperfusion injury, as modeled by hypoxia and reoxygenation at the cellular level, induce arrhythmia and cell death, which is mainly due to Ca2+ overload (Luo et al., 2017). WXKL prevented dysregulation of the INaL, sodium-calcium exchanger current (INCX) and Ca2+ overload induced by ischemia-reperfusion and protected cardiomyocytes from arrhythmia and death (Luo et al., 2017). Some clinical studies have found that WXKL can reduce heart rate, improve heart failure, and relieve heart failure complications (Zheng et al., 2018; Chen et al., 2014). Shensong Yangxin (SSYX) is composed of extracts from 12 herbs, including Panax ginseng, Ophiopogon japonicus, Cornus officinalis, etc. The whole cell patch-clamp technique has been used to examine the cardiac protection mechanism of SSYX, and the results suggested that SSYX alleviated arrhythmia by inhibiting various ion channels (ICa, INaL, Ik, Ito, and IK1) (Li et al., 2007). QLQX capsule is composed of 11 herbal medicines such as Astragali Radix, Panax ginseng, Alismatis Rhizoma, and Carthami Flos. QLQX capsule has been used in the clinical treatment of arrhythmia (Wei et al., 2012), and experiments in a rabbit model of electrical stimulation-induced AF showed that QLQX effectively rescued the pacing-mediated downregulation of the L-type dihydropyridine receptor (DHPR) and reduced AF inducibility (Tingting et al., 2019). In addition, QLQX effectively blocked ICaL in ventricular cardiomyocytes isolated from rats and modulated cardiac function (Wei et al., 2012). Thus, TCM systematically regulated multiple ion currents such as ICaL to prevent Ca2+ overload and to modulate the electrophysiological properties of cardiomyocytes, which is beneficial for arrhythmia treatment (Figure 1). Indeed, clinical trials showed that western medication plus QLQX reduced the levels of NT-proBNP and could improve the quality of life of patients with heart failure (Li et al., 2013; Wang et al., 2017b).
Hypertensive Heart Disease
Hypertension is a multifactorial disease and a known risk factor for CVDs (Kokubo and Matsumoto, 2016). The morbidity, mortality, and economic burden attributable to hypertension are high (Bernardes et al., 2013). Hypertension causes blood vessels to become too tight, resulting in atherosclerosis (Zaheer et al., 2016). With plaque formation and blood vessel narrowing, blood flow to the heart muscle will be reduced or interrupted, depriving the heart muscle of oxygen and nutrition, leading to a series of diseases, such as myocardial ischemia and myocardial infarction, coronary heart disease, and myocardial hypertrophy (Bentzon et al., 2014). At present, the pathogenesis of hypertension is mainly related to vascular endothelium malfunction (Spieker et al., 2006; Dharmashankar and Widlansky, 2010).
Several studies have revealed that common clinical prescriptions of Chinese medicines, such as Qianhu, Schisandrae, and Salvia miltirrhiza (Wenjie et al., 2014; Ye et al., 2018; Hu et al., 2012), treat hypertension by regulating calcium signaling (Table 3). The hypotensive effects and related mechanisms of MTE, the water-soluble portion of Marsdenia tenacissima, were evaluated by the isometric vessel tension study, and the results suggested that MTE induced vasodilation by reducing calcium influx and promoting endothelial NO release (Hao et al., 2019). Bottino Pontes et al. studied Pimpinella seed aqueous extract (AE) by evaluating its effects on arterial blood pressure, and the authors found that AE could lower blood pressure and promote bradycardia. This protective cardiovascular effect was attributed to calcium entry blockage in cardiomyocytes and VSMCs (Pontes et al., 2019). Schisandra chinensis contains an active substance called α-Iso-cubebene (ICB), which could reduce vascular tension by inhibiting the phosphorylation of the myosin light chain (MLC) and regulate the receptor-operated channel-mediated cytosolic calcium flux induced by phenylephrine (PE) or norepinephrine (NE). Therefore, ICB can potentially be used to treat vascular hypertension (Ye et al., 2018). Praeruptorin c (Pra-c) is an active ingredient extracted from Peucedanum praeruptorum. Persistent hypertension promotes cardiac hypertrophy and myocardial fibrosis, and experiments in spontaneously hypertensive rats showed that Pra-c lowered blood pressure, restored calcium homeostasis, and reversed ventricular remodeling by upregulating PLN, which regulated SR Ca2+ uptake by complexing with sarco/endoplasmic reticulum Ca2+-ATPase (SERCA) (Wenjie et al., 2014; Fernández-de Gortari and Espinoza-Fonseca, 2018). Berberine, an active alkaloid found in many TCMs, dilates blood vessels and reduces blood pressure. In mice, berberine exerted its antihypertensive and antiheart failure effects by inhibiting transient receptor potential channel 4 (TRPV4) and LTCCs to reduce intracellular calcium levels and to weaken CaM/MLC activity, thereby promoting VSMC relaxation (Wang et al., 2015). Tetrahydropalmatine (THP) is a bioactive ingredient derived from Corydalis yanhusuo. THP has been known to ameliorate hypertension by targeting the endothelium-dependent phosphatidylinositol 3-kinase (PI3K)/Akt signaling pathway, and Ca2+ and K+ channels are also involved (Zhou et al., 2019a). Studies have been conducted to investigate the effects of Danshen and Gegen (DG) on isolated coronary arteries and their potential mechanisms in treating hypertensive heart diseases. The researchers found that danshensu and salvianolic acid B, the active compound of Danshen, reduced the calcium flux of the left anterior descending artery in rats, and lithospermic acid similarly reduced the calcium level in cardiomyocytes (Hu et al., 2012). The influx of calcium in VSMCs caused cell contraction, and DG reduced [Ca2+]i by blocking LTCCs and partially opening the inward rectifier potassium channel (Kir), thereby relaxing blood vessels and relieving subsequent angina (Hu et al., 2012). Therefore, reducing [Ca2+]i and the activity of MLC in VSMCs as well as promoting NO production in endothelial cells served as the major mechanisms of TCM in treating hypertensive heart disease (Figure 2).
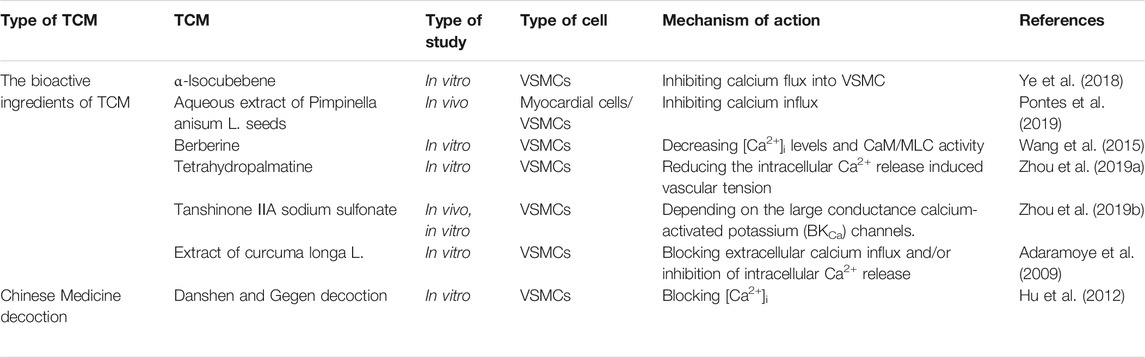
TABLE 3. The mechanism of TCM in the treatment of hypertensive heart disease through calcium signaling.
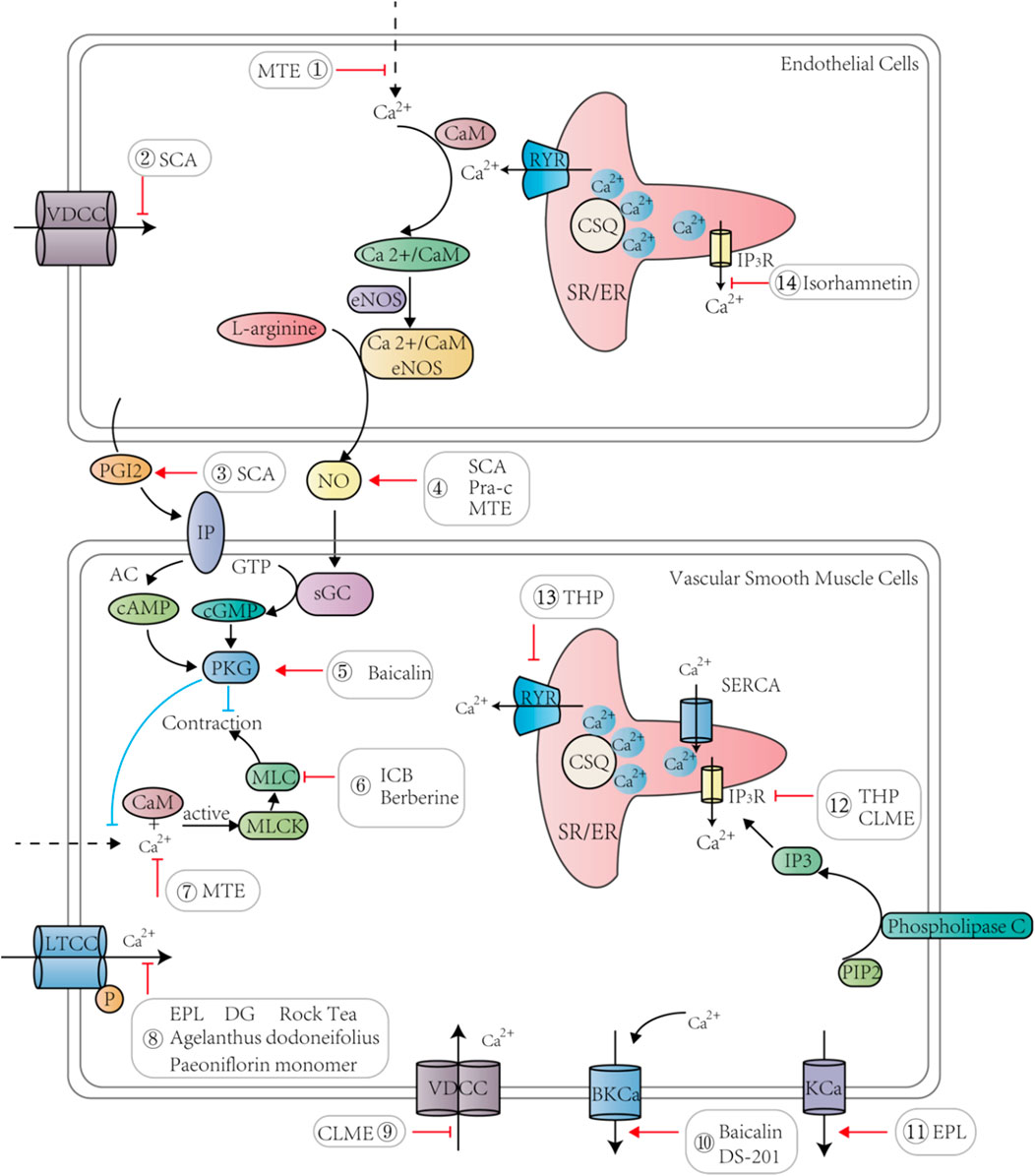
FIGURE 2. TCM and its active ingredients promote vasodilation and lower blood pressure by regulating calcium signaling pathways in endothelial cells and vascular smooth muscle cells.
Heart Failure
Congestive heart failure is a complex disease featuring impaired heart function, during which cardiac output is insufficient to accommodate venous return and cannot provide or maintain the required basic energy or metabolism (Li et al., 2017; Smith and Eisner, 2019). In a failing heart, the Ca2+ transient amplitude of cardiomyocytes is decreased, together with enhanced SR Ca2+ leakage and reduced SR Ca2+ uptake (Balke and Shorofsky, 1998; Luo and Anderson, 2013). In the early stages of HF, CaMKII promotes the phosphorylation of RyR2 at serine 2814/2815, leading to abnormal Ca2+ signals, such as a high level of calcium sparks (Chen et al., 2020).
Many active ingredients of TCM have played an important role in anti-heart failure by inhibiting calcium signal disorder and reducing Ca2+ overload (Li et al., 2018a; Cheng et al., 2018; Liu et al., 2016) (Table 4). Stachydrine hydrochloride alleviated phenylephrine-induced elevated levels of sarcomere contraction, calcium transients, and calcium sparks. Moreover, among a series of proteins in the calcium signaling cascade, the hyperphosphorylation of CaMKII, RyR2, and PLN was blocked by stachydrine hydrochloride, which facilitated the binding of FKBP12.6 to RyR2 (Chen et al., 2020). Stachydrine hydrochloride also decreased the level of SR calcium leakage into the cytoplasm, and the underlying mechanism might be related to the reduction of transverse aorta constriction (TAC)/PE-induced hyperphosphorylation of CaMKII (Chen et al., 2020). An in vivo model of abdominal aortic constriction in rats and an in vitro model of ISO-treated H9C2 cells have been used to study the beneficial effect of baicalein in Scutellaria baicalensis on myocardial function (Zhao et al., 2016), and the results indicated that baicalein regulated the expression and activity of SERCA and RyR2 to maintain calcium balance in the heart. Additionally, baicalin inhibited the elevated expression of sodium-calcium exchanger 1 (NCX1) and phospho-CaMKII (P-CaMKII) during HF, which played an essential role in regulating [Ca2+]i, thereby protecting myocardial structure and contractile function (Zhao et al., 2016). Sophora flavescens contains various biologically active components with pharmacological activities, such as sophoridine (Zhao et al., 2016), which protects against HF by increasing the activity of DHPR to improve CICR (Hu et al., 2016). Astragaloside IV (AS-IV), extracted from Astragalus membrananceus (Huangqi), also has the potential to treat HF because it inhibits LTCCs and protects against myocardial damage caused by Ca2+ overload (Zhao et al., 2017). In summary, TCM modulated the expression and activities of numerous calcium handling proteins to rescue the abnormal calcium homeostasis observed in failing cardiomyocytes, such as enhanced SR calcium leakage and Ca2+ overload (Figure 1).
Coronary Heart Disease and Myocardial Infarction
Coronary heart disease includes a series of clinical conditions, such as myocardial infarction and arrhythmia, caused by atherosclerosis, which leads to narrowing of the coronary artery lumen and insufficient blood supply to the heart muscle (Lei et al., 2018; Liu et al., 2018). Coronary heart disease is the main cause of sudden cardiac death worldwide, accompanied by apoptosis and myocardial fibrosis, leading to HF (Yang et al., 2018a; Yang et al., 2018b).
Xin-Ke-Shu (XKS), Guanxinshutong capsule (GXSTC), modified Yiqi decoction (MYQ), and other Chinese herbal compound preparations are involved in the regulation of calcium signaling during the treatment of coronary heart disease and myocardial infarction (Yang et al., 2018a; Liu et al., 2017b; Yu et al., 2017) (Table 5). Xin-Ke-Shu tablet (XKS) is mainly composed of Salvia miltiorrhiza, Radix Puerariae, Hawthorn, Panax Notoginseng and Radix Aucklandiae. XKS improved LAD-induced myocardial infarction in rats, which was attributed to its effect on inhibition of the overexpression of CaMKII, thereby alleviating Ca2+ overload. Moreover, XKS also regulates lysophosphatidylcholine and long-chain fatty acid expression and maintains cell membrane stability (Yang et al., 2018a). GXSTC is commonly used in the clinical treatment of coronary heart disease, angina pectoris, and other CVDs. Systematic pharmacology studies revealed that GXSTC ameliorated myocardial injury of ischemia-reperfusion through twelve pathways (including the calcium signaling pathway) and eight calcium-related targets. Furthermore, the four main active ingredients (FMAI) of GXSTC (protocatechuic acid, borneol, cryptotanshinone, and eugenol) prevented Ca2+ overload and protected cardiomyocytes (Liu et al., 2018). Studies have been conducted to examine the effect of MYQ on cardiac function and infarct size in the coronary artery blockage-reperfusion model, and the results suggested that long-term MYQ treatment alleviated ischemia/reperfusion (I/R) injury by regulating Ca2+-handling proteins (increasing NCX1 and SERCA2a expression) and the signaling pathways of apoptosis and autophagy (Yu et al., 2017).

TABLE 5. The mechanism of TCM in the treatment of coronary heart disease and myocardial infarction through calcium signaling.
Diabetic Heart Disease
Diabetes is a common metabolic disease that often causes various complications such as diabetic cardiomyopathy, which is usually due to the generation of advanced glycation end products, which directly damage myocardial cells (Poirier and Eckel, 2000; Athithan et al., 2019). Excessive endothelin 1 (ET-1) produced during diabetes further damages heart cells, resulting in weak cardiac contractility (Qi et al., 2008; Sena et al., 2013). During this process, the activity of calcium handling proteins in the SR is impaired, leading to the dysregulation of Ca2+ processing (Qi et al., 2008; Pereira et al., 2014).
The therapeutic effects of TCM on diabetic cardiomyopathy have also been investigated (Jiang et al., 2020; Liu et al., 2017c). In diabetes models established by streptozotocin injection, total triterpene acids (TTAs), an active chemical substance extracted from Cornus officinalis, played a beneficial role in diabetic cardiomyopathy by targeting calcium handling proteins (FKBP12.6, SERCA2a, and PLN) and normalizing calcium release from SR (Qi et al., 2008). Similarly, in streptozotocin-induced diabetic rats, total aralosides of aralia elata seem (TASAES) improved myocardial contractility and protected myocardial function, the possible mechanism of which was through increasing ICaL and inhibiting connective tissue growth factor (Xi et al., 2009).
Inflammation-Associated Heart Disease
Inflammation has been associated with numerous cardiovascular conditions, ranging from coronary heart disease to valvular heart diseases, and one of its well-known effects is promoting atherosclerosis (Wolf and Ley, 2019; Roifman et al., 2011). Thus, inflammation is considered an emerging target for the treatment of both inflammatory and commonplace heart diseases (Sethwala et al., 2021; Thackeray and Taqueti, 2020; Danesh et al., 2004). TCM’s anti-inflammatory properties may contribute to its therapeutic effects on CVDs (Hao et al., 2017; Li et al., 2019). For instance, a randomized clinical trial with a large sample size suggested the beneficial effect of QLQX in chronic HF treatment, and the possible mechanisms included the regulation of inflammation-related cytokines (Hao et al., 2017). Both total flavonoids from Glycyrrhizae radix et rhizoma (FGR) and total flavonoids from Spatholobi caulis (FSC) showed anti-inflammatory properties (Zhang et al., 2017). Total coumarins from Peucedani radix (CPR), as an anti-inflammatory herb, blocked the receptor-operated calcium channel (ROCC) and VDCC to inhibit calcium influx, thereby promoting vasodilation (Zhang et al., 2017).
Discussion
Overwhelming evidence suggests that TCM’s unique advantage in CVDs treatment is closely related to multiple calcium signaling pathways (Qi et al., 2008; Wang et al., 2019a; Siu and Ko, 2010). Because of the multicomponent and multitarget characteristics of TCM, diverse calcium handling proteins and signaling pathways in various types of cells involved in distinct CVDs can be regulated (Wheeler-Jones, 2005; Wang et al., 2019b; Wang et al., 2018) (Figures 3, 4). It is important to note that the structures of chemicals in TCM are highly related to their functions. For instance, the hydroxyl group in gingerol leads to a shortened aliphatic tail and decreased Van der Waals (VDW) interactions with the hydrophobic pocket of its ligand, which affects the potency of gingerol in activating Ca2+ permeable TRPV1 channels (Yin et al., 2019). In this review, we summarized the current research progress on the therapeutic effects of TCM on CVDs through modulation of calcium signaling.
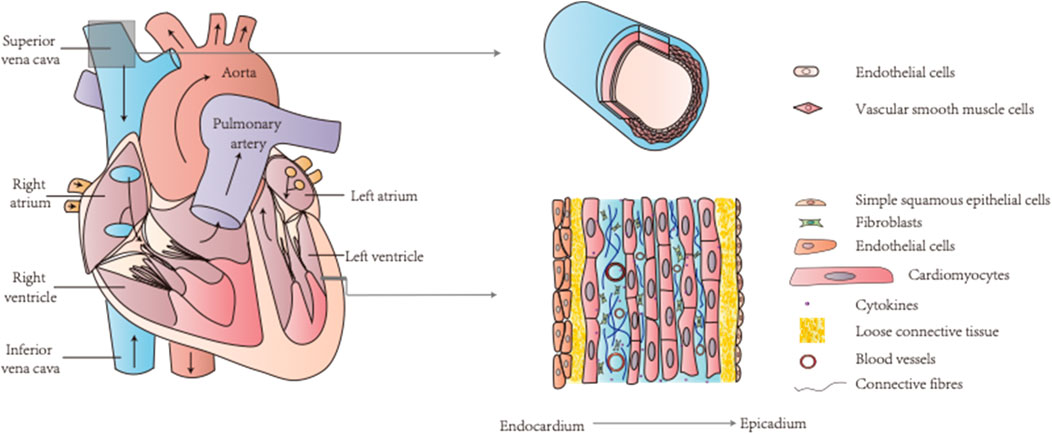
FIGURE 4. The heart and blood vessels are two key components of the circulatory system containing various types of cells. TCM can target calcium signaling in endothelial cells, vascular smooth muscle cells, and cardiomyocytes for CVDs treatment.
The circulatory system includes the heart and blood vessels as two key components, and diseases of the circulatory system, i.e., CVDs, are complex and involve various cell types, including endothelial cells, VSMCs, and cardiomyocytes (Wang et al., 2019b; Li et al., 2018b; Braile et al., 2020; Rohde et al., 2015) (Figure 4). An increase in calcium levels in endothelial cells promotes NO and other endothelium-derived factors that can be released to regulate vascular tone (Hao et al., 2019). The calcium signaling pathway of endothelial cells is a common target of TCM. For example, MTE promoted vasodilation by reducing calcium influx and inducing endothelial NO release (Hao et al., 2019). Moreover, isorhamnetin inhibited IP3-sensitive calcium pools from releasing calcium and protected endothelial cells (Jiayi et al., 2019). Schisantherin A (SCA) regulates NO and prostacyclin (PGI2) to induce endothelium-dependent vasodilation and obstructs VDCC to promote endothelium-independent vasodilation (Yang et al., 2020b). Thus, TCM can treat certain CVD conditions such as hypertensive heart disease by maintaining proper endothelial cell function through regulation of the calcium signaling pathway (Xiong et al., 2013; Yu et al., 2013; Zhang et al., 2020) (Figure 2).
The rapid spread of cellular signals between endothelial cells and neighboring VSMCs in blood vessels is due to their tight connections (Iaizzo, 2020). NO released by endothelial cells plays a crucial role in vasodilation, mainly by activating the soluble guanosine cyclase in VSMCs and inducing the production of cGMP that further decreases [Ca2+]i and promotes relaxation in VSMCs (Filippini et al., 2019; Hu et al., 2012; Vanhoutte et al., 2017). For vasoconstriction, VSMCs are first stimulated by extracellular signals such as vasoconstrictor agonists (Wang et al., 2018; Félétou, 2011), which results in a rapid increase and accumulation of intracellular calcium. This elevated level of calcium binds to CaM in the cytoplasm to activate downstream myosin light chain kinase (MLCK), which further phosphorylates MLC to change its conformation and promotes vasoconstriction (Hao et al., 2019; Kamm and Stull, 1985). Contraction of VSMCs regulates blood vessel tension and blood flow distribution and is closely related to atherosclerosis and coronary heart disease because of the phenotypic plasticity of VSMCs (Gomez and Owens, 2012). Some TCMs can regulate the function of VSMCs by acting on LTCCs and other calcium channels to reduce calcium levels (Figure 2). For instance, ICB promoted VSMC relaxation by blocking calcium entry and MLC phosphorylation (Ye et al., 2018). Berberine inhibited TRPV4 to reduce calcium influx and to decrease CaM/MLC activity, thereby regulating vascular tightness (Wang et al., 2015). The mechanism of tanshinone IIA sodium sulfonate (DS-201) vasodilation might be related to the activation of large-conductance calcium-dependent potassium channels (BKca), making DS-201 a combination therapy candidate with a BK Ca2+ agonist to treat hypertension (Zhou et al., 2019b). Baicalin has also been shown to activate the BKca current, cGMP-dependent protein kinase (PKG), and PKA pathways, which increase potassium outflow and promote hyperpolarization of the myocardial cell membrane, resulting in vasodilation (Lin et al., 2010). EPL promoted vasodilation by activating calcium-activated potassium channels (KCa) and ATP-sensitive K+ channel (KATP) channels and suppressing LTCCs to enhance the Akt- and NO-cGMP signaling pathways (Jin et al., 2012). Certain TCMs can also act on intracellular Ca2+ release to facilitate VSMC relaxation. For instance, the methanolic extract of Curcuma longa (CLME) prevented calcium release from the IP3-sensitive calcium store to promote vasodilation (Adaramoye et al., 2009). Therefore, TCM directly targets the calcium signaling pathway in VSMCs to promote vasodilation.
The heart is responsible for pumping blood to provide nutrition for various organs and tissues, which depends on the cardiomyocytes' rhythmic contraction and relaxation (Woodcock and Matkovich, 2005). After being excited, the membrane of cardiomyocytes will have action potentials caused by sequential opening and closure of multiple ion channels (Balse et al., 2012). In this process, activation of voltage-gated LTCCs promotes Ca2+ influx from the external environment, and the elevated calcium level in the cytoplasm activates SR to release more calcium ions (Woodcock and Matkovich, 2005; Zhu et al., 2019). Ca2+ then binds to troponin to induce cardiac contraction (Park et al., 2017). Thus, the proper regulation of intracellular calcium ions is crucial for maintaining heart functions (Marks, 2003). Indeed, the disturbance of calcium homeostasis has been observed in numerous types of CVDs, such as cardiac arrhythmias (Landstrom et al., 2017). Various studies have shown that Chinese herbal medicines and their active ingredients can improve cardiomyocyte function and treat CVDs by regulating the expression and activity of calcium channels and receptors (Hu et al., 2012; Yang et al., 2020b; Liu et al., 2013) (Figure 1). For example, senkyunolide A and ligustrazinecan hindered the opening of VDCC, rendering a calcium antagonistic effect (Lei et al., 2018). Magnesium lithospermate B (MLB) could be used to treat angina pectoris and coronary heart disease by reversibly inhibiting L-type Ca2+ currents (Wang et al., 2006). In addition to regulating LTCCs, which are responsible for the Ca2+ influx in cardiomyocytes, RyRs that mediate intracellular Ca2+ release from SR are also valid targets for TCM (Lei et al., 2018). FMAI from Guanxin Shutong capsule increased the expression of RyR2 and PLN in cardiomyocytes (Liu et al., 2017b). As a crucial calcium removal mechanism, NCX, in its forward mode, pumps 1 Ca2+ out of cells in exchange for 3 Na+ to reduce [Ca2+]i (Pott et al., 2011). Ginkgo biloba extract 50 (GBE50) reduced NCX abnormal expression to improve myocardial contractility (Liu et al., 2013). On the other hand, ginseng–aconite decoction (GAD) exerted a positive inotropic effect on cardiomyocytes by activating NCX’s reverse mode to induce Ca2+ entry (Cui et al., 2013). As a result of modulating multiple calcium handling proteins, TCM’s common effect is preventing Ca2+ overload, which is detrimental for cardiomyocytes (Vassalle and Lin, 2004). For example, the combination of ginsenoside Rb1, ruscogenin, and schisandrin, three representative ingredients in Sheng-Mai-San, inhibited Ca2+ overload and maintained cardiac histological characteristics during myocardial ischemic injury (Li et al., 2018a). Ophiopogonin reduced myocardial cell apoptosis induced by intracellular Ca2+ overload and endoplasmic reticulum stress (Yang et al., 2017). TCM can also regulate calcium-related signaling pathways. Ginsenoside reduced Ca2+ overload and altered the calcineurin signaling pathway, which improved the metabolism of ischemic myocardial tissue and protected myocardial structure (Yang et al., 2017). FMAI also reduced [Ca2+]i and prevented apoptosis by modulating the Ca2+/CaM/CaMK signaling pathway and changing gene expression in cardiomyocytes (Liu et al., 2017b). In summary, accumulating evidence suggests that TCM plays an important role in CVD treatments by reducing apoptosis, protecting cardiac structure and enhancing the contractile function of cardiomyocytes through modulation of Ca2+ homeostasis.
Conclusion
The pathophysiology of CVDs is multifactorial and complex, involving calcium signaling as a key player. With multicomponent and multitarget characteristics, Chinese herbal medicine has shown unique advantages in CVD treatment with few side effects. Accumulating evidence suggests that TCM plays an important role in maintaining calcium homeostasis and improving calcium signaling to alleviate the symptoms of CVDs. Thus, we reviewed the positive effects of TCM and its active ingredients on a wide range of CVDs and highlighted the central role of calcium signaling in their mechanism of action in endothelial cells, VSMCs and cardiomyocytes, which may provide basic guidance for follow-up research.
Author Contributions
SL designed the study. YL and ZZ prepared the figures and drafted the manuscript. TY and ZJ critically reviewed the manuscript. All authors read and approved the final manuscript.
Funding
This study is supported by the National Natural Science Foundation of China (Grant No. 81703942 and 81973698), Young Elite Scientists Sponsorship Program by CACM (Grant No. 2019-QNRC2-B08), Science Fund for Distinguished Young Scholars in BUCM (Grant No. BUCM-2019-JCRC004) and BUCM research start-up fund (to SL).
Conflict of Interest
The authors declare that the research was conducted in the absence of any commercial or financial relationships that could be construed as a potential conflict of interest.
References
Adaramoye, O. A., Anjos, R. M., Almeida, M. M., Veras, R. C., Silvia, D. F., Oliveira, F. A., et al. (2009). Hypotensive and Endothelium-independent Vasorelaxant Effects of Methanolic Extract from Curcuma Longa L. In Rats. J. Ethnopharmacology 124 (3), 457–462. doi:10.1016/j.jep.2009.05.021
Aoyagi, T., and Matsui, T. (2011). Phosphoinositide-3 Kinase Signaling in Cardiac Hypertrophy and Heart Failure. Cpd 17 (18), 1818–1824. doi:10.2174/138161211796390976
Athithan, L., Gulsin, G. S., McCann, G. P., and Levelt, E. (2019). Diabetic Cardiomyopathy: Pathophysiology, Theories and Evidence to Date. Wjd 10 (10), 490–510. doi:10.4239/wjd.v10.i10.490
Balke, C., and Shorofsky, S. R. (1998). Alterations in Calcium Handling in Cardiac Hypertrophy and Heart Failure. Cardiovasc. Res. 37 (2), 290–299. doi:10.1016/s0008-6363(97)00272-1
Balse, E., Steele, D. F., Abriel, H., Coulombe, A., Fedida, D., and Hatem, S. N. (2012). Dynamic of Ion Channel Expression at the Plasma Membrane of Cardiomyocytes. Physiol. Rev. 92 (3), 1317–1358. doi:10.1152/physrev.00041.2011
Bansilal, S., Castellano, J. M., and Fuster, V. (2015). Global burden of CVD: Focus on Secondary Prevention of Cardiovascular Disease. Int. J. Cardiol. 201 (Suppl. 1), S1–S7. doi:10.1016/s0167-5273(15)31026-3
Bentzon, J. F., Otsuka, F., Virmani, R., and Falk, E. (2014). Mechanisms of Plaque Formation and Rupture. Circ. Res. 114 (12), 1852–1866. doi:10.1161/circresaha.114.302721
Bernardes, M. J. C., Carvalho, F. S. d., Lima Silveira, L., Paula, J. R. d., Bara, M. T. F., Garrote, C. F., et al. (2013). Hypotensive Effect of Aspidosperma Subincanum Mart. In Rats and its Mechanism of Vasorelaxation in Isolated Arteries. J. Ethnopharmacology 145 (1), 227–232. doi:10.1016/j.jep.2012.10.057
Bernardo, B. C., Weeks, K. L., Pretorius, L., and McMullen, J. R. (2010). Molecular Distinction between Physiological and Pathological Cardiac Hypertrophy: Experimental Findings and Therapeutic Strategies. Pharmacol. Ther. 128 (1), 191–227. doi:10.1016/j.pharmthera.2010.04.005
Bers, D. M. (2008). Calcium Cycling and Signaling in Cardiac Myocytes. Annu. Rev. Physiol. 70, 23–49. doi:10.1146/annurev.physiol.70.113006.100455
Braile, M., Marcella, S., Cristinziano, L., Galdiero, M. R., Modestino, L., Ferrara, A. L., et al. (2020). VEGF-A in Cardiomyocytes and Heart Diseases. Ijms 21 (15), 5294. doi:10.3390/ijms21155294
Cai, Y., Tu, J., Pan, S., Jiang, J., Shou, Q., Ling, Y., et al. (2015). Medicinal Effect and its JP2/RyR2-Based Mechanism of Smilax Glabra Flavonoids on Angiotensin II-Induced Hypertrophy Model of Cardiomyocytes. J. Ethnopharmacol. 169, 435–440. doi:10.1016/j.jep.2015.04.026
Cartwright, E. J., Mohamed, T., Oceandy, D., and Neyses, L. (2011). Calcium Signaling Dysfunction in Heart Disease. Biofactors 37 (3), 175–181. doi:10.1002/biof.149
Chen, W.-h., Yang, D., Wang, W.-y., Zhang, J., and Wang, Y.-p. (2010). Cellular Electrophysiological Effects of Changrolin in Isolated Rat Cardiac Myocytes. Eur. J. Pharmacol. 647 (1-3), 139–146. doi:10.1016/j.ejphar.2010.08.024
Chen, Y., Li, Y., Guo, L., Chen, W., Zhao, M., Gao, Y., et al. (2013). Effects of Wenxin Keli on the Action Potential and L-type Calcium Current in Rats with Transverse Aortic Constriction-Induced Heart Failure. Evidence-Based Complement. Altern. Med. 2013, 1–12. doi:10.1155/2013/572078
Chen, Y., Xiong, X., Wang, C., Wang, C., Zhang, Y., Zhang, X., et al. (2014). The Effects of Wenxin Keli on Left Ventricular Ejection Fraction and Brain Natriuretic Peptide in Patients with Heart Failure: a Meta-Analysis of Randomized Controlled Trials. Evidence-Based Complement. Altern. Med. 2014, 1–9. doi:10.1155/2014/242589
Chen, H.-H., Wang, S.-N., Cao, T.-T., Zheng, J.-L., Tian, J., Shan, X.-L., et al. (2020). Stachydrine Hydrochloride Alleviates Pressure Overload-Induced Heart Failure and Calcium Mishandling on Mice. J. Ethnopharmacol. 248, 112306. doi:10.1016/j.jep.2019.112306
Cheng, Y., Song, J., Hu, P., and Zhu, Y. (2018). Tetrahydroxystilbene Glucoside (TSG) Restores the Effect of Transient Hypoxia on Reperfusion Injury in Senescent H9c2 Cells by Regulating Mitochondrial Energy Metabolism. Evidence-Based Complement. Altern. Med. 2018, 1–7. doi:10.1155/2018/2545024
Cox, E. J., and Marsh, S. A. (2014). A Systematic Review of Fetal Genes as Biomarkers of Cardiac Hypertrophy in Rodent Models of Diabetes. PLoS One 9 (3), e92903. doi:10.1371/journal.pone.0092903
Cui, H. Z., Kim, H. Y., Kang, D. G., and Lee, H. S. (2013). Ginseng-Aconite Decoction Elicits a Positive Inotropic Effect via the Reverse Mode Na+/Ca2+ Exchanger in Beating Rabbit Atria. J. Ethnopharmacol. 148 (2), 624–631. doi:10.1016/j.jep.2013.05.014
Dai, H., Jia, G., Liu, X., Liu, Z., and Wang, H. (2014). Astragalus Polysaccharide Inhibits Isoprenaline-Induced Cardiac Hypertrophy via Suppressing Ca2+-Mediated calcineurin/NFATc3 and CaMKII Signaling Cascades. Environ. Toxicol. Pharmacol. 38 (1), 263–271. doi:10.1016/j.etap.2014.05.008
Danesh, J., Wheeler, J. G., Hirschfield, G. M., Eda, S., Eiriksdottir, G., Rumley, A., et al. (2004). C-reactive Protein and Other Circulating Markers of Inflammation in the Prediction of Coronary Heart Disease. N. Engl. J. Med. 350 (14), 1387–1397. doi:10.1056/NEJMoa032804
Dewenter, M., von der Lieth, A., Katus, H. A., and Backs, J. (2017). Calcium Signaling and Transcriptional Regulation in Cardiomyocytes. Circ. Res. 121 (8), 1000–1020. doi:10.1161/circresaha.117.310355
Dharmashankar, K., and Widlansky, M. E. (2010). Vascular Endothelial Function and Hypertension: Insights and Directions. Curr. Hypertens. Rep. 12 (6), 448–455. doi:10.1007/s11906-010-0150-2
Drazner, M. H. (2011). The Progression of Hypertensive Heart Disease. Circulation 123 (3), 327–334. doi:10.1161/circulationaha.108.845792
Duan, L., Xiong, X., Hu, J., Liu, Y., and Wang, J. (2018). Efficacy and Safety of Oral Panax Notoginseng Saponins for Unstable Angina Patients: A Meta-Analysis and Systematic Review. Phytomedicine 47, 23–33. doi:10.1016/j.phymed.2018.04.044
Eisner, D. (2014). Calcium in the Heart: from Physiology to Disease. Exp. Physiol. 99 (10), 1273–1282. doi:10.1113/expphysiol.2013.077305
Félétou, M. (2011). The Endothelium:Part 1: Multiple Functions of the Endothelial Cells—Focus on Endothelium-Derived Vasoactive Mediators. France: Morgan & Claypool Life Sciences.
Fernández-de Gortari, E., and Espinoza-Fonseca, L. M. (2018). Structural Basis for Relief of Phospholamban-Mediated Inhibition of the Sarcoplasmic Reticulum Ca2+-ATPase at Saturating Ca2+ Conditions. J. Biol. Chem. 293 (32), 12405–12414. doi:10.1074/jbc.RA118.003752
Filippini, A., D’Amore, A., and D’Alessio, A. (2019). Calcium Mobilization in Endothelial Cell Functions. Int. J. Mol. Sci. 20 (18), 4525. doi:10.3390/ijms20184525
Firth, A. L., Won, J. Y., and Park, W. S. (2013). Regulation of Ca2+Signaling in Pulmonary Hypertension. Korean J. Physiol. Pharmacol. 17 (1), 1–8. doi:10.4196/kjpp.2013.17.1.1
Gan, R., Dong, G., Yu, J., Wang, X., Fu, S., and Yang, S. (2011). Protective Effects of Isorhynchophylline on Cardiac Arrhythmias in Rats and guinea Pigs. Planta Med. 77 (13), 1477–1481. doi:10.1055/s-0030-1270742
Gomez, D., and Owens, G. K. (2012). Smooth Muscle Cell Phenotypic Switching in Atherosclerosis. Cardiovasc. Res. 95 (2), 156–164. doi:10.1093/cvr/cvs115
Guan, S., Ma, J., Chu, X., Gao, Y., Zhang, Y., Zhang, X., et al. (2015). Effects of Total Flavones fromAcanthopanax Senticosuson L-type Calcium Channels, Calcium Transient and Contractility in Rat Ventricular Myocytes. Phytother. Res. 29 (4), 533–539. doi:10.1002/ptr.5278
Han, X., Zhang, Y., Liang, Y., Zhang, J., Li, M., Zhao, Z., et al. (2019). 6‐Gingerol, an Active Pungent Component of Ginger, Inhibits L‐type Ca 2+ Current, Contractility, and Ca 2+ Transients in Isolated Rat Ventricular Myocytes. Food Sci. Nutr. 7 (4), 1344–1352. doi:10.1002/fsn3.968
Hao, P., Jiang, F., Cheng, J., Ma, L., Zhang, Y., and Zhao, Y. (2017). Traditional Chinese Medicine for Cardiovascular Disease. J. Am. Coll. Cardiol. 69 (24), 2952–2966. doi:10.1016/j.jacc.2017.04.041
Hao, H., Tian, W., Pan, C., Jiao, Y., Deng, X., Fan, J., et al. (2019). Marsdenia Tenacissima Extract Dilated Small Mesenteric Arteries via Stimulating Endothelial Nitric Oxide Synthase and Inhibiting Calcium Influx. J. Ethnopharmacol. 238, 111847. doi:10.1016/j.jep.2019.111847
Hu, F., Koon, C. M., Chan, J. Y. W., Lau, K. M., Kwan, Y. W., and Fung, K. P. (2012). Involvements of Calcium Channel and Potassium Channel in Danshen and Gegen Decoction Induced Vasodilation in Porcine Coronary LAD Artery. Phytomedicine 19 (12), 1051–1058. doi:10.1016/j.phymed.2012.07.007
Hu, S.-T., Shen, Y.-F., Gong, J.-M., and Yang, Y.-J. (2016). Effect of Sophoridine on Ca2+ Induced Ca2+ Release during Heart Failure. Physiol. Res. 65 (1), 43–52. doi:10.33549/physiolres.933052
Hu Shengshou, G. R., Liu, L., Zhu, M., Wang, W., Wang, Y., Wu, Z., et al. (2019). Summary of the 2018 Report on Cardiovascular Diseases in China.
Iaizzo, P. A. Gap Junctions (Cell-To-Cell Conduction),”Altas of Human CARDIAC Anatomy. Available at: http://www.vhlab.umn.edu/atlas/conduction-system-tutorial/gap-junctions.shtml (accessed November 23, 2020).
Iemitsu, M., Miyauchi, T., Maeda, S., Sakai, S., Kobayashi, T., Fujii, N., et al. (2001). Physiological and Pathological Cardiac Hypertrophy Induce Different Molecular Phenotypes in the Rat. Am. J. Physiology-Regulatory, Integr. Comp. Physiol. 281 (6), R2029–R2036. doi:10.1152/ajpregu.2001.281.6.R2029
Jiang, H., Zhang, C., and He, W. (2018). The Effects of Dracocephalum Heterophyllum Benth Flavonoid on Hypertrophic Cardiomyocytes Induced by Angiotensin II in Rats. Med. Sci. Monit. 24, 6322–6330. doi:10.12659/MSM.908912
Jiang, Z., Fu, L., Xu, Y., Hu, X., Yang, H., Zhang, Y., et al. (2020). Cyclovirobuxine D Protects against Diabetic Cardiomyopathy by Activating Nrf2-Mediated Antioxidant Responses. Sci. Rep. 10 (1), 6427. doi:10.1038/s41598-020-63498-3
Jiao, L., Li, M., Shao, Y., Zhang, Y., Gong, M., Yang, X., et al. (2019). lncRNA-ZFAS1 Induces Mitochondria-Mediated Apoptosis by Causing Cytosolic Ca2+ Overload in Myocardial Infarction Mice Model. Cell Death Dis 10 (12), 942. doi:10.1038/s41419-019-2136-6
Jiayi, C., Tianyi, N., Dan, T., Tingguo, K., Qingfeng, W., and Qianqian, Z. (2019). Isorhamnetin Protects Endothelial Cells Model CRL1730 from Oxidative Injury by Hydrogen Peroxide. Pak J. Pharm. Sci. 32 (1), 131–136.
Jin, S. N., Wen, J. F., Wang, T. T., Kang, D. G., Lee, H. S., and Cho, K. W. (2012). Vasodilatory Effects of Ethanol Extract of Radix Paeoniae Rubra and its Mechanism of Action in the Rat Aorta. J. Ethnopharmacol. 142 (1), 188–193. doi:10.1016/j.jep.2012.04.035
Kalyanasundaram, A., Lacombe, V. A., Belevych, A. E., Brunello, L., Carnes, C. A., Janssen, P. M. L., et al. (2013). Up-regulation of Sarcoplasmic Reticulum Ca2+ Uptake Leads to Cardiac Hypertrophy, Contractile Dysfunction and Early Mortality in Mice Deficient in CASQ2. Cardiovasc. Res. 98 (2), 297–306. doi:10.1093/cvr/cvs334
Kamm, K. E., and Stull, J. T. (1985). The Function of Myosin and Myosin Light Chain Kinase Phosphorylation in Smooth Muscle. Annu. Rev. Pharmacol. Toxicol. 25, 593–620. doi:10.1146/annurev.pa.25.040185.003113
Kokubo, Y., and Matsumoto, C. (2016). Hypertension Is a Risk Factor for Several Types of Heart Disease: Review of Prospective Studies. Adv. Exp. Med. Biol. 956, 419–426. doi:10.1007/5584_2016_99
Lam, P., Cheung, F., Tan, H., Wang, N., Yuen, M., and Feng, Y. (2016). Hepatoprotective Effects of Chinese Medicinal Herbs: A Focus on Anti-Inflammatory and Anti-Oxidative Activities. Int. J. Mol. Sci. 17 (4), 465. doi:10.3390/ijms17040465
Landstrom, A. P., Dobrev, D., and Wehrens, X. H. T. (2017). Calcium Signaling and Cardiac Arrhythmias. Circ. Res. 120 (12), 1969–1993. doi:10.1161/circresaha.117.310083
Lei, W., Ni, J., Xia, X., Jiang, M., and Bai, G. (2018). Searching for Synergistic Calcium Antagonists and Novel Therapeutic Regimens for Coronary Heart Disease Therapy from a Traditional Chinese Medicine, Suxiao Jiuxin Pill. J. Chromatogr. B 1092, 220–227. doi:10.1016/j.jchromb.2018.06.015
Li, N., Ma, K.-j., Wu, X.-f., Sun, Q., Zhang, Y.-h., and Pu, J.-l. (2007). Effects of Chinese Herbs on Multiple Ion Channels in Isolated Ventricular Myocytes. Chin. Med. J. 120 (12), 1068–1074. doi:10.1097/00029330-200706020-00008
Li, X., Chu, W., Liu, J., Xue, X., Lu, Y., Shan, H., et al. (2009). Antiarrhythmic Properties of Long-Term Treatment with Matrine in Arrhythmic Rat Induced by Coronary Ligation. Biol. Pharm. Bull. 32 (9), 1521–1526. doi:10.1248/bpb.32.1521
Li, X., Zhang, J., Huang, J., Ma, A., Yang, J., Li, W., et al. (2013). A Multicenter, Randomized, Double-Blind, Parallel-Group, Placebo-Controlled Study of the Effects of Qili Qiangxin Capsules in Patients with Chronic Heart Failure. J. Am. Coll. Cardiol. 62 (12), 1065–1072. doi:10.1016/j.jacc.2013.05.035
Li, Z., Li, S., Hu, L., Li, F., Cheung, A. C., shao, W., et al. (2017). Mechanisms Underlying Action of Xinmailong Injection, a Traditional Chinese Medicine, in Cardiac Function Improvement. Ajtcam 14 (2), 241–252. doi:10.21010/ajtcam.v14i2.26
Li, F., Fan, X.-X., Chu, C., Zhang, Y., Kou, J.-P., and Yu, B.-Y. (2018a). A Strategy for Optimizing the Combination of Active Components Based on Chinese Medicinal Formula Sheng-Mai-San for Myocardial Ischemia. Cell Physiol. Biochem. 45 (4), 1455–1471. doi:10.1159/000487572
Li, M., Qian, M., Kyler, K., and Xu, J. (2018b). Endothelial-Vascular Smooth Muscle Cells Interactions in Atherosclerosis. Front. Cardiovasc. Med. 5, 151. doi:10.3389/fcvm.2018.00151
Li, S.-m., Li, J.-g., and Xu, H. (2019). A New Perspective for Chinese Medicine Intervention for Coronary Artery Disease: Targeting Inflammation. Chin. J. Integr. Med. 25 (1), 3–8. doi:10.1007/s11655-018-2995-1
Li, M., Xie, X., Chen, H., Xiong, Q., Tong, R., Peng, C., et al. (2020). Aconitine Induces Cardiotoxicity through Regulation of Calcium Signaling Pathway in Zebrafish Embryos and in H9c2 Cells. J. Appl. Toxicol. 40 (6), 780–793. doi:10.1002/jat.3943
Lin, Y.-L., Dai, Z.-K., Lin, R.-J., Chu, K.-S., Chen, I.-J., Wu, J.-R., et al. (2010). Baicalin, a Flavonoid from Scutellaria Baicalensis Georgi, Activates Large-Conductance Ca2+-Activated K+ Channels via Cyclic Nucleotide-dependent Protein Kinases in Mesenteric Artery. Phytomedicine 17 (10), 760–770. doi:10.1016/j.phymed.2010.01.003
Liu, C., and Huang, Y. (2016). Chinese Herbal Medicine on Cardiovascular Diseases and the Mechanisms of Action. Front. Pharmacol. 7, 469. doi:10.3389/fphar.2016.00469
Liu, A.-H., Bao, Y.-M., Wang, X.-Y., and Zhang, Z.-X. (2013). Cardio-Protection by Ginkgo Biloba Extract 50 in Rats with Acute Myocardial Infarction Is Related to Na+-Ca2+ Exchanger. Am. J. Chin. Med. 41 (4), 789–800. doi:10.1142/s0192415x13500535
Liu, Q., Li, J., Hartstone-Rose, A., Wang, J., Li, J., Janicki, J. S., et al. (2015). Chinese Herbal Compounds for the Prevention and Treatment of Atherosclerosis: Experimental Evidence and Mechanisms. Evidence-Based Complement. Altern. Med. 2015, 1–15. doi:10.1155/2015/752610
Liu, Y.-T., Zhou, C., Jia, H.-M., Chang, X., and Zou, Z.-M. (2016). Standardized Chinese Formula Xin-Ke-Shu Inhibits the Myocardium Ca2+ Overloading and Metabolic Alternations in Isoproterenol-Induced Myocardial Infarction Rats. Sci. Rep. 6, 30208. doi:10.1038/srep30208
Liu, Z. Y., Huang, J., Liu, N. N., Zheng, M., Zhao, T., Zhao, B. C., et al. (2017a). Molecular Mechanisms of Increased Heart Rate in Shenxianshengmai-Treated Bradycardia Rabbits. Chin. Med. J. (Engl) 130 (2), 179–186. doi:10.4103/0366-6999.197999
Liu, F., Huang, Z.-Z., Sun, Y.-H., Li, T., Yang, D.-H., Xu, G., et al. (2017b). Four Main Active Ingredients Derived from a Traditional Chinese Medicine Guanxin Shutong Capsule Cause Cardioprotection during Myocardial Ischemia Injury Calcium Overload Suppression. Phytother. Res. 31 (3), 507–515. doi:10.1002/ptr.5787
Liu, Y., Zhao, Y.-B., Wang, S.-W., Zhou, Y., Tang, Z.-S., and Li, F. (2017c). Mulberry Granules Protect against Diabetic Cardiomyopathy through the AMPK/Nrf2 Pathway. Int. J. Mol. Med. Sep. 40 (3), 913–921. doi:10.3892/ijmm.2017.3050
Liu, F., Du, X., Liu, P.-R., Sun, Y.-H., and Zhang, Y.-M. (2018). Screening and Analysis of Key Active Constituents in Guanxinshutong Capsule Using Mass Spectrum and Integrative Network Pharmacology. Chin. J. Nat. Medicines 16 (4), 302–312. doi:10.1016/s1875-5364(18)30060-8
Louch, W. E., Koivumäki, J. T., and Tavi, P. (2015). Calcium Signalling in Developing Cardiomyocytes: Implications for Model Systems and Disease. J. Physiol. 593 (5), 1047–1063. doi:10.1113/jphysiol.2014.274712
Luo, M., and Anderson, M. E. (2013). Mechanisms of Altered Ca 2+ Handling in Heart Failure. Circ. Res. 113 (6), 690–708. doi:10.1161/circresaha.113.301651
Luo, A., Liu, Z., Cao, Z., Hao, J., Wu, L., Fu, C., et al. (2017). Wenxin Keli Diminishes Ca2+ Overload Induced by Hypoxia/reoxygenation in Cardiomyocytes through Inhibiting INaL and ICaL. Pacing Clin. Electrophysiol. 40 (12), 1412–1425. doi:10.1111/pace.13206
Maillet, M., van Berlo, J. H., and Molkentin, J. D. (2013). Molecular Basis of Physiological Heart Growth: Fundamental Concepts and New Players. Nat. Rev. Mol. Cel Biol 14 (1), 38–48. doi:10.1038/nrm3495
Marchand, A., Abi-Gerges, A., Saliba, Y., Merlet, E., and Lompré, A.-M. (2012). Calcium Signaling in Vascular Smooth Muscle Cells: from Physiology to Pathology. Adv. Exp. Med. Biol. 740, 795–810. doi:10.1007/978-94-007-2888-2_35
Marks, A. R. (2003). Calcium and the Heart: a Question of Life and Death. J. Clin. Invest. 111 (5), 597–600. doi:10.1172/jci18067
Mukherjee, R., and Spinale, F. G. (1998). L-type Calcium Channel Abundance and Function with Cardiac Hypertrophy and Failure: a Review. J. Mol. Cell Cardiol. 30 (10), 1899–1916. doi:10.1006/jmcc.1998.0755
Mustroph, J., Neef, S., and Maier, L. S. (2017). CaMKII as a Target for Arrhythmia Suppression. Pharmacol. Ther. 176, 22–31. doi:10.1016/j.pharmthera.2016.10.006
Nakayama, H., Bodi, I., Maillet, M., DeSantiago, J., Domeier, T. L., Mikoshiba, K., et al. (2010). The IP 3 Receptor Regulates Cardiac Hypertrophy in Response to Select Stimuli. Circ. Res. 107 (5), 659–666. doi:10.1161/circresaha.110.220038
Pan, Z.-W., Zhang, Y., Mei, D.-H., Zhang, R., Wang, J.-H., Zhang, X.-Y., et al. (2010). Scutellarin Exerts its Anti-hypertrophic Effects via Suppressing the Ca2+-Mediated Calcineurin and CaMKII Signaling Pathways. Naunyn-schmied Arch. Pharmacol. 381 (2), 137–145. doi:10.1007/s00210-009-0484-y
Pan, M.-H., Chiou, Y.-S., Tsai, M.-L., and Ho, C.-T. (2011). Anti-inflammatory Activity of Traditional Chinese Medicinal Herbs. J. Traditional Complement. Med. 1 (1), 8–24. doi:10.1016/s2225-4110(16)30052-9
Park, K. C., Gaze, D. C., Collinson, P. O., and Marber, M. S. (2017). Cardiac Troponins: from Myocardial Infarction to Chronic Disease. Cardiovasc. Res. 113 (14), 1708–1718. doi:10.1093/cvr/cvx183
Pereira, L., Ruiz-Hurtado, G., Rueda, A., Mercadier, J.-J., Benitah, J.-P., and Gómez, A. M. (2014). Calcium Signaling in Diabetic Cardiomyocytes. Cell Calcium 56 (5), 372–380. doi:10.1016/j.ceca.2014.08.004
Poirier, P., and Eckel, R. H. (2000). Advanced Glycation End Products and Coronary Heart Disease in Type 2 Diabetes. Diabetes Care 23 (9), 1441–1442. doi:10.2337/diacare.23.9.1441
Pontes, V. C. B., Rodrigues, D. P., Caetano, A., and Gamberini, M. T. (2019). Preclinical Investigation of the Cardiovascular Actions Induced by Aqueous Extract of Pimpinella Anisum L. Seeds in Rats. J. Ethnopharmacol. 237, 74–80. doi:10.1016/j.jep.2019.03.050
Pott, C., Eckardt, L., and I. Goldhaber, J. (2011). Triple Threat: the Na+/Ca2+ Exchanger in the Pathophysiology of Cardiac Arrhythmia, Ischemia and Heart Failure. Cdt 12 (5), 737–747. doi:10.2174/138945011795378559
Qi, M.-Y., Liu, H.-R., Dai, D.-Z., Li, N., and Dai, Y. (2008). Total Triterpene Acids, Active Ingredients from Fructus Corni, Attenuate Diabetic Cardiomyopathy by Normalizing ET Pathway and Expression of FKBP12.6 and SERCA2a in Streptozotocin-Rats. J Pharm. Pharmacol. 60 (12), 1687–1694. doi:10.1211/jpp/60.12.0016
Rohde, D., Busch, M., Volkert, A., Ritterhoff, J., Katus, H. A., Peppel, K., et al. (2015). Cardiomyocytes, Endothelial Cells and Cardiac Fibroblasts: S100A1's Triple Action in Cardiovascular Pathophysiology. Future Cardiol. 11 (3), 309–321. doi:10.2217/fca.15.18
Roifman, I., Beck, P. L., Anderson, T. J., Eisenberg, M. J., and Genest, J. (2011). Chronic Inflammatory Diseases and Cardiovascular Risk: a Systematic Review. Can. J. Cardiol. 27 (2), 174–182. doi:10.1016/j.cjca.2010.12.040
Roth, G. A., Johnson, C., Abajobir, A., Abd-Allah, F., Abera, S. F., Abyu, G., et al. (2017). Global, Regional, and National Burden of Cardiovascular Diseases for 10 Causes, 1990 to 2015. J. Am. Coll. Cardiol. 70 (1), 1–25. doi:10.1016/j.jacc.2017.04.052
Sena, C. M., Pereira, A. M., and Seiça, R. (2013). Endothelial Dysfunction - a Major Mediator of Diabetic Vascular Disease. Biochim. Biophys. Acta (BBA) - Mol. Basis Dis. 1832 (12), 2216–2231. doi:10.1016/j.bbadis.2013.08.006
Sethwala, A. M., Goh, I., and Amerena, J. V. (2021). Combating Inflammation in Cardiovascular Disease. Heart Lung Circ. 30, 197–206. doi:10.1016/j.hlc.2020.09.003
Shou, Q., Pan, S., Tu, J., Jiang, J., Ling, Y., Cai, Y., et al. (2013). Modulation Effect of Smilax Glabra Flavonoids on Ryanodine Receptor Mediated Intracellular Ca2+ Release in Cardiomyoblast Cells. J. Ethnopharmacol. 150 (1), 389–392. doi:10.1016/j.jep.2013.08.009
Siu, A. H.-L., and Ko, K. M. (2010). Herba Cistanche Extract Enhances Mitochondrial Glutathione Status and Respiration in Rat Hearts, with Possible Induction of Uncoupling Proteins. Pharm. Biol. 48 (5), 512–517. doi:10.3109/13880200903190985
Smith, G. L., and Eisner, D. A. (2019). Calcium Buffering in the Heart in Health and Disease. Circulation 139 (20), 2358–2371. doi:10.1161/circulationaha.118.039329
Spatz, E. S., Wang, Y., Beckman, A. L., Wu, X., Lu, Y., Du, X., et al. (2018). Traditional Chinese Medicine for Acute Myocardial Infarction in Western Medicine Hospitals in China. Circ. Cardiovasc. Qual. Outcomes 11 (3), e004190. doi:10.1161/circoutcomes.117.004190
Spieker, L. E., Flammer, A. J., and Lüscher, T. F. (2006). The Vascular Endothelium in Hypertension. Handb Exp. Pharmacol. 176 (Pt 2), 249–283. doi:10.1007/3-540-36028-x_8
Takeuchi, K., Watanabe, H., Tran, Q. K., Ozeki, M., Sumi, D., Hayashi, T., et al. (2004). Nitric Oxide: Inhibitory Effects on Endothelial Cell Calcium Signaling, Prostaglandin I2 Production and Nitric Oxide Synthase Expression. Cardiovasc. Res. 62 (1), 194–201. doi:10.1016/j.cardiores.2003.12.028
Tan, X., Li, J., Wang, X., Chen, N., Cai, B., Wang, G., et al. (2011). Tanshinone IIA Protects against Cardiac Hypertrophy via Inhibiting calcineurin/NFATc3 Pathway. Int. J. Biol. Sci. 7 (3), 383–389. doi:10.7150/ijbs.7.383
Tao, X., Jing-Bo, P., Wen-Tong, Z., Xin, Z., Tao-tao, Z., Shi-jun, Y., et al. (2012). Antiatherogenic and Anti-Ischemic Properties of Traditional Chinese Medicine Xinkeshu via Endothelial Protecting Function. Evidence-Based Complement. Altern. Med. 2012, 1–9. doi:10.1155/2012/302137
Tarride, J.-E., Lim, M., DesMeules, M., Luo, W., Burke, N., O’Reilly, D., et al. (2009). A Review of the Cost of Cardiovascular Disease. Can. J. Cardiol. 25 (6), e195–e202. doi:10.1016/s0828-282x(09)70098-4
Thackeray, J. T., and Taqueti, V. R. (2020). Imaging Inflammation in Cardiovascular Disease: Translational Perspective and Overview. Q. J. Nucl. Med. Mol. Imaging 64 (1), 1–3. doi:10.23736/s1824-4785.20.03247-1
Tham, Y. K., Bernardo, B. C., Ooi, J. Y. Y., Weeks, K. L., and McMullen, J. R. (2015). Pathophysiology of Cardiac Hypertrophy and Heart Failure: Signaling Pathways and Novel Therapeutic Targets. Arch. Toxicol. 89 (9), 1401–1438. doi:10.1007/s00204-015-1477-x
Tian, G., Sun, Y., Liu, S., Li, C., Chen, S., Qiu, R., et al. (2018). Therapeutic Effects of Wenxin Keli in Cardiovascular Diseases: An Experimental and Mechanism Overview. Front. Pharmacol. 9, 1005. doi:10.3389/fphar.2018.01005
Tingting, H., Guangzhong, L., Yanxiang, Z., DongDong, Y., Li, S., and Li, W. (2019). Qiliqiangxin Attenuates Atrial Structural Remodeling in Prolonged Pacing-Induced Atrial Fibrillation in Rabbits. Naunyn-schmiedeberg's Arch. Pharmacol. 392 (5), 585–592. doi:10.1007/s00210-018-01611-0
Touyz, R. M., Alves-Lopes, R., Rios, F. J., Camargo, L. L., Anagnostopoulou, A., Arner, A., et al. (2018). Vascular Smooth Muscle Contraction in Hypertension. Cardiovasc. Res. 114 (4), 529–539. doi:10.1093/cvr/cvy023
Vanhoutte, P. M., Shimokawa, H., Feletou, M., and Tang, E. H. C. (2017). Endothelial Dysfunction and Vascular Disease - a 30th Anniversary Update. Acta Physiol. 219 (1), 22–96. doi:10.1111/apha.12646
Vassalle, M., and Lin, C.-I. (2004). Calcium Overload and Cardiac Function. J. Biomed. Sci. 11 (5), 542–565. doi:10.1007/bf02256119
Wang, W., Hu, G.-Y., and Wang, Y.-P. (2006). Selective Modulation of L-type Calcium Current by Magnesium Lithospermate B in guinea-pig Ventricular Myocytes. Life Sci. 78 (26), 2989–2997. doi:10.1016/j.lfs.2005.11.024
Wang, R. R., Li, N., Zhang, Y. H., Ran, Y. Q., and Pu, J. L. (2011). The Effects of Paeoniflorin Monomer of a Chinese Herb on Cardiac Ion Channels. Chin. Med. J. (Engl) 124 (19), 3105–3111. doi:10.3760/cma.j.issn.0366-6999.2011.19.028
Wang, Y., Liu, Z., Li, C., Li, D., Ouyang, Y., Yu, J., et al. (2012). Drug Target Prediction Based on the Herbs Components: the Study on the Multitargets Pharmacological Mechanism of Qishenkeli Acting on the Coronary Heart Disease. Evidence-Based Complement. Altern. Med. 2012, 1–10. doi:10.1155/2012/698531
Wang, X., Wang, X., Gu, Y., Wang, T., and Huang, C. (2013). Wenxin Keli Attenuates Ischemia-Induced Ventricular Arrhythmias in Rats: Involvement of L-type Calcium and Transient Outward Potassium Currents. Mol. Med. Rep. 7 (2), 519–524. doi:10.3892/mmr.2012.1195
Wang, M., Xu, X., Xu, H., Wen, F., Zhang, X., Sun, H., et al. (2014). Effect of the Total Saponins of Aralia Elata (Miq) Seem on Cardiac Contractile Function and Intracellular Calcium Cycling Regulation. J. Ethnopharmacol. 155 (1), 240–247. doi:10.1016/j.jep.2014.05.024
Wang, J., Guo, T., Peng, Q. S., Yue, S. W., and Wang, S. X. (2015). Berberine via Suppression of Transient Receptor Potential Vanilloid 4 Channel Improves Vascular Stiffness in Mice. J. Cel. Mol. Med. 19 (11), 2607–2616. doi:10.1111/jcmm.12645
Wang, T., Lu, M., Du, Q., Yao, X., Zhang, P., Chen, X., et al. (2017a). An Integrated Anti-arrhythmic Target Network of a Chinese Medicine Compound, Wenxin Keli, Revealed by Combined Machine Learning and Molecular Pathway Analysis. Mol. Biosyst. 13 (5), 1018–1030. doi:10.1039/c7mb00003k
Wang, X., Hou, Y., Mao, J., Zhang, Y., Li, Z., Zhao, Y., et al. (2017b). Western Medication Plus Traditional Chinese Medicine Preparations in Patients with Chronic Heart Failure: a Prospective, Single-Blind, Randomized, Controlled, and Multicenter Clinical Trial. J. Tradit Chin. Med. 37 (6), 756–766. doi:10.1016/S0254-6272(18)30038-4
Wang, D., Uhrin, P., Mocan, A., Waltenberger, B., Breuss, J. M., Tewari, D., et al. (2018). Vascular Smooth Muscle Cell Proliferation as a Therapeutic Target. Part 1: Molecular Targets and Pathways. Biotechnol. Adv. 36 (6), 1586–1607. doi:10.1016/j.biotechadv.2018.04.006
Wang, R., Wang, M., Wang, S., Yang, K., Zhou, P., Xie, X., et al. (2019a). An Integrated Characterization of Contractile, Electrophysiological, and Structural Cardiotoxicity of Sophora Tonkinensis Gapnep. In Human Pluripotent Stem Cell-Derived Cardiomyocytes. Stem Cel Res Ther 10 (1), 20. doi:10.1186/s13287-018-1126-4
Wang, H., Li, H., Sun, Q., Yao, Y., Yuan, C., Liu, G., et al. (2019b). Factors Regulating the Change of Vascular Smooth Muscle Cells in Cardiovascular Diseases: A Mini Review. Transl Surg. 4 (2), 22. doi:10.4103/ts.ts_4_19
Wei, Y., Liu, X., Hou, L., Che, W., The, E., and Jhummon, M. V. (2012). Qiliqiangxin Affects L Type Current in the Normal and Hypertrophied Rat Heart. Evidence-Based Complement. Altern. Med. 2012, 1–4. doi:10.1155/2012/131830
Wenjie, W., Houqing, L., Liming, S., Ping, Z., and Gengyun, S. (2014). Effects of Praeruptorin C on Blood Pressure and Expression of Phospholamban in Spontaneously Hypertensive Rats. Phytomedicine 21 (3), 195–198. doi:10.1016/j.phymed.2013.08.028
Wheeler-Jones, C. P. D. (2005). Cell Signalling in the Cardiovascular System: an Overview. Heart 91 (10), 1366–1374. doi:10.1136/hrt.2005.072280
Wilkins, B. J., and Molkentin, J. D. (2004). Calcium-calcineurin Signaling in the Regulation of Cardiac Hypertrophy. Biochem. Biophysical Res. Commun. 322 (4), 1178–1191. doi:10.1016/j.bbrc.2004.07.121
Wilson, C., Zhang, X., Buckley, C., Heathcote, H. R., Lee, M. D., and McCarron, J. G. (2019). Increased Vascular Contractility in Hypertension Results From Impaired Endothelial Calcium Signaling. Hypertension 74 (5), 1200–1214. doi:10.1161/hypertensionaha.119.13791
Wolf, D., and Ley, K. (2019). Immunity and Inflammation in Atherosclerosis. Circ. Res. 124 (2), 315–327. doi:10.1161/circresaha.118.313591
Woodcock, E. A., and Matkovich, S. J. (2005). Cardiomyocytes Structure, Function and Associated Pathologies. Int. J. Biochem. Cel Biol. 37 (9), 1746–1751. doi:10.1016/j.biocel.2005.04.011
Xi, S., Zhou, G., Zhang, X., Zhang, W., Cai, L., and Zhao, C. (2009). Protective Effect of Total Aralosides of Aralia Elata (Miq) Seem (TASAES) against Diabetic Cardiomyopathy in Rats during the Early Stage, and Possible Mechanisms. Exp. Mol. Med. 41 (8), 538–547. doi:10.3858/emm.2009.41.8.059
Xia, P., Liu, Y., and Cheng, Z. (2016). Signaling Pathways in Cardiac Myocyte Apoptosis. Biomed. Res. Int. 2016, 1–22. doi:10.1155/2016/9583268
Xing, Y., Gao, Y., Chen, J., Zhu, H., Wu, A., Yang, Q., et al. (2013). Wenxin-Keli Regulates the Calcium/Calmodulin-dependent Protein Kinase II Signal Transduction Pathway and Inhibits Cardiac Arrhythmia in Rats with Myocardial Infarction. Evidence-Based Complement. Altern. Med. 2013, 1–15. doi:10.1155/2013/464508
Xiong, X., Yang, X., Liu, W., Chu, F., Wang, P., and Wang, J. (2013). Trends in the Treatment of Hypertension from the Perspective of Traditional Chinese Medicine. Evidence-Based Complement. Altern. Med. 2013, 1–13. doi:10.1155/2013/275279
Xue, X., Guo, D., Sun, H., Wang, D., Li, J., Liu, T., et al. (2013). Wenxin Keli Suppresses Ventricular Triggered Arrhythmias via Selective Inhibition of Late Sodium Current. Pacing Clin. Electrophysiol. 36 (6), 732–740. doi:10.1111/pace.12109
Yang, Y., Tian, Y., Hu, S., Bi, S., Li, S., Hu, Y., et al. (2017). Extract of Sheng-Mai-San Ameliorates Myocardial Ischemia-Induced Heart Failure by Modulating Ca2+-Calcineurin-Mediated Drp1 Signaling Pathways. Int. J. Mol. Sci. 18 (9), 1825. doi:10.3390/ijms18091825
Yang, Y., Jia, H., Yu, M., Zhou, C., Sun, L., Zhao, Y., et al. (2018a). Chinese Patent Medicine Xin-Ke-Shu Inhibits Ca 2+ Overload and Dysfunction of Fatty Acid β -oxidation in Rats with Myocardial Infarction Induced by LAD Ligation. J. Chromatogr. B 1079, 85–94. doi:10.1016/j.jchromb.2018.01.038
Yang, G., Min, D., Yan, J., Yang, M., and Lin, G. (2018b). Protective Role and Mechanism of Snakegourd Peel against Myocardial Infarction in Rats. Phytomedicine 42, 18–24. doi:10.1016/j.phymed.2018.03.014
Yang, H.-J., Kong, B., Shuai, W., Zhang, J.-j., and Huang, H. (2020a). Shensong Yangxin Protects Against Metabolic Syndrome-Induced Ventricular Arrhythmias by Inhibiting Electrical Remodeling. Front. Pharmacol. 11, 993. doi:10.3389/fphar.2020.00993
Yang, S., Xu, Z., Lin, C., Li, H., Sun, J., Chen, J., et al. (2020b). Schisantherin A Causes Endothelium-dependent and -independent Vasorelaxation in Isolated Rat Thoracic Aorta. Life Sci. 245, 117357. doi:10.1016/j.lfs.2020.117357
Ye, B. H., Kim, E. J., Baek, S. E., Choi, Y. W., Park, S. Y., and Kim, C. D. (2018). α-Isocubebene Modulates Vascular Tone by Inhibiting Myosin Light Chain Phosphorylation in Murine Thoracic Aorta. Korean J. Physiol. Pharmacol. 22 (4), 437–445. doi:10.4196/kjpp.2018.22.4.437
Yin, Y., Dong, Y., Vu, S., Yang, F., Yarov‐Yarovoy, V., Tian, Y., et al. (2019). Structural Mechanisms Underlying Activation of TRPV1 Channels by Pungent Compounds in Gingers. Br. J. Pharmacol. 176 (17), 3364–3377. doi:10.1111/bph.14766
Yu, T.-t., Guo, K., Chen, H.-c., Lan, C.-z., Wang, J., Huang, L.-l., et al. (2013). Effects of Traditional Chinese Medicine Xin-Ji-Er-Kang Formula on 2K1C Hypertensive Rats: Role of Oxidative Stress and Endothelial Dysfunction. BMC Complement. Altern. Med. 13, 173. doi:10.1186/1472-6882-13-173
Yu, X., Zhao, X.-D., Bao, R.-Q., Yu, J.-Y., Zhang, G.-X., and Chen, J.-W. (2017). The Modified Yi Qi Decoction Protects Cardiac Ischemia-Reperfusion Induced Injury in Rats. BMC Complement. Altern. Med. 17 (1), 330. doi:10.1186/s12906-017-1829-6
Zaheer, M., Chrysostomou, P., and Papademetriou, V. (2016). “Hypertension and Atherosclerosis: Pathophysiology, Mechanisms and Benefits of BP Control,” in Hypertension and Cardiovascular Disease. Editor E. A. Andreadis (Washington, DC, United States: Springer International Publishing), 201–216. doi:10.1007/978-3-319-39599-9_14
Zaza, A., Ronchi, C., and Malfatto, G. (2018). Arrhythmias and Heart Rate: Mechanisms and Significance of a Relationship. Arrhythm Electrophysiol. Rev. 7 (4), 1–237. doi:10.15420/aer.2018.12.3
Zhang, C., Shan, X.-L., Liao, Y.-L., Zhao, P., Guo, W., Wei, H.-C., et al. (2014). Effects of Stachydrine on Norepinephrine-Induced Neonatal Rat Cardiac Myocytes Hypertrophy and Intracellular Calcium Transients. BMC Complement. Altern. Med. 14, 474. doi:10.1186/1472-6882-14-474
Zhang, H. P., Zhang, D.-D., Ke, Y., and Bian, K. (2017). The Vasodilatory Effects of Anti-Inflammatory Herb Medications: A Comparison Study of Four Botanical Extracts. Evidence-Based Complement. Altern. Med. 2017, 1–15. doi:10.1155/2017/1021284
Zhang, D.-Y., Cheng, Y.-B., Guo, Q.-H., Shan, X.-L., Wei, F.-F., Lu, F., et al. (2020). Treatment of Masked Hypertension with a Chinese Herbal Formula. Circulation 142 (19), 1821–1830. doi:10.1161/circulationaha.120.046685
Zhao, F., Fu, L., Yang, W., Dong, Y., Yang, J., Sun, S., et al. (2016). Cardioprotective Effects of Baicalein on Heart Failure via Modulation of Ca2+ Handling Proteins In Vivo and In Vitro. Life Sci. 145, 213–223. doi:10.1016/j.lfs.2015.12.036
Zhao, M.-M., Lian, W.-W., Li, Z., Shao, D.-X., Chen, S.-C., Sun, X.-F., et al. (2017). Astragaloside IV Inhibits Membrane Ca2+ Current but Enhances Sarcoplasmic Reticulum Ca2+ Release. Am. J. Chin. Med. 45 (4), 863–877. doi:10.1142/s0192415x1750046x
Zheng, R., Tian, G., Zhang, Q., Wu, L., Xing, Y., and Shang, H. (2018). Clinical Safety and Efficacy of Wenxin Keli-Amiodarone Combination on Heart Failure Complicated by Ventricular Arrhythmia: A Systematic Review and Meta-Analysis. Front. Physiol. 9, 487. doi:10.3389/fphys.2018.00487
Zhou, Z.-Y., Zhao, W.-R., Shi, W.-T., Xiao, Y., Ma, Z.-L., Xue, J.-G., et al. (2019a). Endothelial-Dependent and Independent Vascular Relaxation Effect of Tetrahydropalmatine on Rat Aorta. Front. Pharmacol. 10, 336. doi:10.3389/fphar.2019.00336
Zhou, Y., Liu, X., Zhang, X., Wen, J., Cheng, J., Li, P., et al. (2019b). Decreased Vasodilatory Effect of Tanshinone ⅡA Sodium Sulfonate on Mesenteric Artery in Hypertension. Eur. J. Pharmacol. 854, 365–371. doi:10.1016/j.ejphar.2019.04.049
Zhu, J., Ye, Q., Xu, S., Chang, Y.-x., Liu, X., Ma, Y., et al. (2019). Shengmai Injection Alleviates H2O2 induced Oxidative Stress through Activation of AKT and Inhibition of ERK Pathways in Neonatal Rat Cardiomyocytes. J. Ethnopharmacol. 239, 111677. doi:10.1016/j.jep.2019.01.001
Glossary
AF atrial fibrillation
Ang II angiotensin II
ANP atrial natriuretic peptide
APS astragalus polysaccharide
AS-IV astragaloside IV
BNP brain natriuretic peptide
CaM calmodulin
CaMKII calmodulin-dependent protein kinase II
cAMP cyclic adenosine monophosphate
cGMP cyclic guanosine monophosphate;
CICR calcium-induced calcium release
CLME methanolic extract of curcuma longa
CPR total coumarins from Peucedani radix
CSQ calsequestrin
CVDs cardiovascular diseases
DeHE dehydroevodiamine alkaloid
DG danshen and gegen decoction
DHBF dracocephalum heterophyllum benth flavonoid
DHPR dihydropyridine receptor
DS-201 tanshinone IIA sodium sulfonate
eNOS endothelial nitric oxide synthase
EPL ethanol extract of paeonia lactiflora pall
ET-1 endothelin 1
FMAI four main active ingredients
FSC total flavonoids from spatholobi caulis
GAD ginseng–aconite decoction
GBE50 ginkgo biloba extract 50
GXSTC guanxinshutong capsule
HF congestive heart failure
I/R ischemia/reperfusion
ICa calcium currents
ICaL L-type calcium current
ICB α-Iso-cubebene
IK delayed rectifier current
IK1 inward rectifier potassium currents
INa sodium current
INaL the late sodium curren
INCX sodium-calcium exchanger current
IP3R Inositol trisphosphate receptor
ISO isoprenaline
Ito transient outward potassium current
JP2 junctophilin-2
KATP channel ATP-sensitive K+ channel
KCa channel calcium-activated potassium channel;
Kir channel inward rectifier potassium channel
LTCC L-type calcium channel
MLB magnesium lithospermate B
MLC myosin light chain
MLCK myosin light chain kinases
MTE marsdenia tenacissima extract
MYQ the modified yiqi decoction
NCX sodium-calcium exchanger
NE norepinephrine
NFAT nuclear factor of activated T cells
NO nitric oxide
P-CaMKII Phospho-CaMKII
PE phenylephrine
PGI2 prostacyclin
PI3K phosphatidylinositol 3-kinase
PKA cAMP-dependent protein kinase
PKG cGMP-dependent protein kinase;
PLN phospholamban
Pra-c praeruptorin c
QLQX qiliqiangxin
RCT randomized controlled trial
RyR ryanodine receptor
SCA schisantherin A;
SERCA sarco/endoplasmic reticulum Ca2+-ATPase
sGC soluble guanylate cyclase
SR sarcoplasmic reticulum
STA stachydrine
TAC transverse aorta constriction
TASAES total aralosides of aralia elata seem
TCM traditional Chinese medicine
TFAS total flavones from acanthopanax senticosusa
TFLF tu-fu-ling flavonoids;
THP tetrahydropalmatine
TRPV4 transient receptor potential channel 4
TTAs total triterpene acids
VDCC voltage-dependent calcium channel
VSMCs vascular smooth muscle cells
WXKL wenxin keli
XKS xin-ke-shu
β-AR β-adrenergic receptors
β-MHC β-myosin heavy chain
Keywords: traditional Chinese medicine, cardiovascular diseases, calcium signaling, cardiomyocytes, endothelial cells, vascular smooth muscle cells
Citation: Li Y, Zhang Z, Li S, Yu T and Jia Z (2021) Therapeutic Effects of Traditional Chinese Medicine on Cardiovascular Diseases: the Central Role of Calcium Signaling. Front. Pharmacol. 12:682273. doi: 10.3389/fphar.2021.682273
Received: 18 March 2021; Accepted: 01 June 2021;
Published: 09 July 2021.
Edited by:
Zhiyu Wang, Guangzhou University of Chinese Medicine, ChinaReviewed by:
Zijian Zhang, Baylor College of Medicine, United StatesYu Chiang Hung, Kaohsiung Chang Gung Memorial Hospital, Taiwan
Copyright © 2021 Li, Zhang, Li, Yu and Jia. This is an open-access article distributed under the terms of the Creative Commons Attribution License (CC BY). The use, distribution or reproduction in other forums is permitted, provided the original author(s) and the copyright owner(s) are credited and that the original publication in this journal is cited, in accordance with accepted academic practice. No use, distribution or reproduction is permitted which does not comply with these terms.
*Correspondence: Sen Li, senli@bucm.edu.cn
†These authors have contributed equally to this work and share first authorship