- 1Pharmaceutical Sciences Research Center, Department of Pharmacy, Children’s Hospital of Nanjing Medical University, Nanjing, China
- 2School of Basic Medical Sciences and Clinical Pharmacy, China Pharmaceutical University, Nanjing, China
- 3Neonatal Intensive Care Unit, Children’s Hospital of Nanjing Medical University, Nanjing, China
Caffeine citrate is the drug of choice for the pharmacological treatment of apnea of prematurity. Factors such as maturity and genetic variation contribute to the interindividual variability in the clinical response to caffeine therapy in preterm infants, making the optimal dose administered controversial. Moreover, the necessity for therapeutic drug monitoring (TDM) of caffeine is still worth discussing due to the need to achieve the desired target concentrations as well as concerns about the safety of higher doses. Therefore, we reviewed the pharmacokinetic profile of caffeine in preterm infants, evidence of the safety and efficacy of different doses of caffeine, therapeutic concentration ranges of caffeine and impact of genetic variability on caffeine therapy. Whereas the safety and efficacy of standard-dose caffeine have been demonstrated, evidence for the safety of higher administered doses is insufficient. Thus, preterm infants who lack clinical response to standard-dose caffeine therapy are of interest for TDM when dose optimization is performed. Polymorphisms in pharmacodynamics-related genes, but not in pharmacokinetics-related genes, have a significant impact on the interindividual variability in clinical response to caffeine therapy. For preterm infants lacking clinical response, how to develop individualized medication regimens for caffeine remains to be explored.
Introduction
Apnea of prematurity (AOP), classified as central, obstructive, or mixed, is usually defined as a cessation of breathing in a premature infant for 20 s or longer, or a shorter pause accompanied by bradycardia (<100 bpm), cyanosis, or pallor (Eichenwald, 2016). It is a common problem among preterm infants, particularly extremely preterm infants (Saroha and Patel, 2020). The reported incidence of AOP varies, but it is clearly inversely related to gestational age. Its incidence is 10% in neonates born beyond 34 weeks gestation. However, in newborns who are at 30–34 weeks gestation at birth, the incidence ranges from 20 to 85%. Ninety percent of the extremely low birth weight (less than 1,000 g) newborn population are reported to have AOP (Eichenwald, 2016; Erickson et al., 2021). Observational studies have demonstrated associations between apneic events and deficits in cerebral oxygenation (Schmid et al., 2015; Horne et al., 2017), increased risk for retinopathy of prematurity (ROP) (Di Fiore et al., 2010), neurodevelopmental impairment (Janvier et al., 2004; Martin et al., 2011), and even death or disability (Lodha et al., 2015).
Several interventions decrease apneic event frequency and duration. These include respiratory interventions including continuous positive airway pressure and pharmacologic therapies, such as methylxanthines, which have been used for over 40 years (Gentle et al., 2018). Caffeine is the first choice among all methylxanthines because of its efficacy, better tolerability and wider therapeutic index as well as longer half-life (Dobson and Hunt, 2013). Researchers of the international Caffeine for Apnea of Prematurity (CAP) trial confirmed the short- and long-term benefits and safety of neonatal caffeine therapy, including reduced rates of bronchopulmonary dysplasia (BPD), patent ductus arteriosus (PDA), and of severe ROP (Schmidt et al., 2006), and improved survival rates without neurodevelopmental disability at 18–21 months of age (Schmidt et al., 2007). Five- and 11 years follow-up studies confirmed that neonatal caffeine therapy appeared to have lasting beneficial effects on motor function and is effective and safe even into middle school age (Schmidt et al., 2012; Schmidt et al., 2017; Murner-Lavanchy et al., 2018). Therefore, caffeine has now become one of the most preferred drugs worldwide for AOP treatment and has been named the “silver” or “magic” bullet (Aranda et al., 2010; Bancalari, 2014).
Despite caffeine’s frequent use in routine neonatal practice, there are controversies surrounding this medicine, which future researches may resolve, including the optimal dose of caffeine administration (Moschino et al., 2020) and therapeutic drug monitoring (TDM) (Shrestha and Jawa, 2017; Saroha and Patel, 2020). Of note, neonatal caffeine therapy results in significant intersubject variability, and it remains unclear why apneic episodes persist in some preterm infants but not in others (He et al., 2020). Therefore, we summarize pharmacokinetic studies of caffeine in a population of preterm infants, as well as evidence of the safety, efficacy and therapeutic concentration ranges at different doses. We also discuss the dose optimization and the necessity for TDM of caffeine, and provide the first review of the impact of genetic variability on the clinical response to caffeine therapy.
Pharmacokinetics of Caffeine in Preterm Infants
Most of the pharmacokinetic (PK) studies for caffeine were performed in premature neonates (Table 1 and Table 2). Due to the difficulty of adequate sampling in preterm babies, most studies have been population pharmacokinetic (PPK) studies using nonlinear mixed effects models (NONMEM) or P-pharm approaches (Table 2). The pharmacokinetics of caffeine is largely independent of the route of administration. Oral caffeine is almost completely bioavailable and is rapidly and completely absorbed from the gastrointestinal tract, reaching peak plasma concentrations in 30 min to 2 h after administration (Aranda et al., 1979a; Bonati et al., 1982; Blanchard and Sawers, 1983). Caffeine is hydrophilic and distributed evenly in all body fluids without tissue accumulation (Arnaud, 1976; Arnaud, 2011). It is also highly lipid-soluble to cross all biological membranes, including the blood-brain barrier, leading to a similar caffeine concentration between the plasma and cerebrospinal fluid of neonates (Turmen et al., 1979; Tanaka et al., 1984; Arnaud, 1987). The volume of distribution in preterm infants is mainly affected by the current body weight and gestational age, and its value is slightly greater than that in healthy adults, possibly due to the increased residence time of caffeine in the extracellular fluid (Aranda et al., 1979a; Bonati et al., 1982; Gorodischer and Karplus, 1982; Lelo et al., 1986; Thomson et al., 1996; Falcão et al., 1997; Lee et al., 1997; Lee et al., 2002; Kearns et al., 2003; Charles et al., 2008; Gao et al., 2020; Guo et al., 2020).
The metabolism of caffeine occurs primarily in the liver. In adults, with the catalysis by CYP2A1 and CYP2E1, caffeine undergoes 1-, 3-, and 7-demethylation to generate the biologically active metabolites theophylline, theobromine, and paraxanthine, which can then be further demethylated to monomethylxanthine (Gu et al., 1992; Thorn et al., 2012). Dimethylxanthine or monomethylxanthine is converted to methyluric acid by xanthine oxidase, whereas paraxanthine can also undergo 8-hydroxylation or generate 5-acetylamino-6-formylamino-3-methyluracil catalyzed by CYP2A6 or N-acetyltransferase-2, respectively (Begas et al., 2007; Thorn et al., 2012). However, in neonates, approximately 85% of caffeine is excreted unchanged in the urine, whereas this proportion in adults is less than 2% (Arnaud, 2011; Aldridge et al., 1979). CYP1A2 is the cytochrome P450 enzyme responsible for more than 90% of caffeine metabolism, studies have shown that CYP1A2 expression is not evident within the first 30 days of newborns’ life due to delayed ontogeny, and CYP1A2 content in liver microsomes of infants aged 1–3 months is only 10–15% of that in adults (Arnaud, 2011; Song et al., 2017; Sonnier and Cresteil, 1998). Correspondingly, the main metabolite in newborns during the first trimester of life is caffeine, whereas 8-hydroxylation appears early and matures approximately 1 month after birth, demethylation metabolism gradually matures with postnatal age, and acetylation is immature until at least 1 year of age (Aldridge et al., 1979; Carrier et al., 1988; Pons et al., 1989; Cazeneuve et al., 1994; al-Alaiyan et al., 2001; Blake et al., 2006). In addition, theophylline can be converted back to caffeine in premature infants by active methylation (Bory et al., 1978; Bory et al., 1979).
The serum half-life of caffeine in preterm infants is prolonged more than ten times that of adults because of immature hepatic metabolism and renal excretion (Aranda et al., 1979b; Bonati et al., 1982; Gorodischer and Karplus, 1982; Lelo et al., 1986; Pearlman et al., 1989; De Carolis et al., 1991). Caffeine’s clearance in preterm infants is influenced by various factors such as the current weight, postnatal age, gestational age, parenteral nutrition, and serum creatinine concentration, with values of approximately one-tenth of those in adults (Bonati et al., 1984; Lelo et al., 1986; Thomson et al., 1996; Falcão et al., 1997; Lee et al., 1997; Lee et al., 2002; Charles et al., 2008; Gao et al., 2020; Guo et al., 2020). For example, caffeine clearance shows a rapid maturation with postnatal age in a very recent study (Engbers et al., 2021). Earlier studies found that the elimination half-life and clearance of caffeine can reach adult levels at approximately 5–6 months after birth (Aranda et al., 1979b; Pons et al., 1988). However, a re-evaluation and validation of ontogeny functions for CYP1A2 describes an increase in relative intrisic metabolic clearance from birth to 3 years followed by a decrease to adult values (Salem et al., 2014). Therefore, the PK process of caffeine in neonates is variable and continues to mature with development, which needs to be taken into consideration when administered.
Dosage of Caffeine in Preterm Infants
Standard Dose of Caffeine and Its History
Caffeine is often available as caffeine citrate, which comes in both oral and injectable formulations, and the dose of caffeine base is half that of caffeine citrate (Shrestha and Jawa, 2017). As early as in 1977, Aranda et al. published the first study of caffeine used to treat AOP (Aranda et al., 1977). In that study, 18 preterm infants received an intravenous loading dose of 20 mg/kg caffeine citrate followed by a maintenance dose of 5–10 mg/kg once or twice daily for 2–3 days, and a marked reduction in apnea spells was observed. In the next 10 years, the same dose regimen was tested in several studies with small sample sizes (n = 16 to n = 23), and the therapeutic effect of caffeine on AOP was observed by comparison with placebo or theophylline (Murat et al., 1981; Brouard et al., 1985; Anwar et al., 1986; Bairam et al., 1987). In 1999, a multicenter, double-blind, randomized trial of caffeine citrate was performed using the above dose regimen. In this trial, eighty-five infants who were 28–32 weeks post-conception and 24 h or more after birth were randomized to caffeine or placebo for up to 10 days, and the results showed that this dose regimen was safe and effective for those recruited neonates (Erenberg et al., 2000). Based partly on such data, the U.S. Food and Drug Administration approved the dose regimen of caffeine citrate as a loading dose of 20 mg/kg followed by an intravenous or oral maintenance dose of 5 mg/kg/day, which is similar to what was approved by the European Medicines Agency (Erenberg et al., 2000; NDA 20-793/S-001, 2000; European Medicines Agency, 2009). Therefore, in this review, we refer to this as the “standard dose” regimen for caffeine.
In 2006, a large, multicenter, randomized, placebo-controlled trial, called the CAP trial, revealed the short- and long-term efficacy and safety of the standard dose regimen of caffeine (Schmidt et al., 2006; Schmidt et al., 2007). In the CAP trial, preterm infants with very low birth weight (VLBW, 500–1,250 g) were randomized to placebo or caffeine citrate at a loading dose of 20 mg/kg, followed by a maintenance dose of 5 mg/kg/24 h, which could be increased to 10 mg/kg/24 h for persistent apnea. This trial demonstrated several well-known beneficial short-terms effects of caffeine (Schmidt et al., 2006). Regarding the long-term effects, preterm infants had a higher rate of survival without neurodevelopmental disability and a lower incidence of severe ROP, cerebral palsy and cognitive delay at a corrected age of 18–21 months (Schmidt et al., 2007), with an improvement in gross motor function at 5 years (Schmidt et al., 2012). In addition, they also revealed that neonatal caffeine therapy at the doses used in CAP trial is effective and safe into miiddle school age (Doyle et al., 2017; Schmidt et al., 2017; Murner-Lavanchy et al., 2018; Schmidt et al., 2019). Due to the CAP trial, the standard-dose caffeine regimen has been widely used (Table 3). However, variable clinical outcomes do exist after standard-dose caffeine treatment.
Higher Doses of Caffeine
Many studies have shown higher doses of caffeine to be more effective with negligible adverse effects (Table 4). Multiple studies have reported that higher doses of caffeine are more effective in reducing episodes of apnea and reducing extubation failure rates (Scanlon et al., 1992; Mohammed et al., 2015; Zhao et al., 2016; Wan et al., 2020). Among them, Mohammad et al. compared a higher dose (loading 40 mg/kg and maintenance of 20 mg/kg/day) with standard-dose caffeine citrate in 120 preterm infants < 32 weeks gestation with AOP within the first 10 days of life (Mohammed et al., 2015). In this trial, the higher dose of caffeine, in addition to being observed to have a better therapeutic effect, was also associated with a significant increase in tachycardia episodes. However, the clinical findings in this trial had no significant impact on physicians’ decision to withhold caffeine.
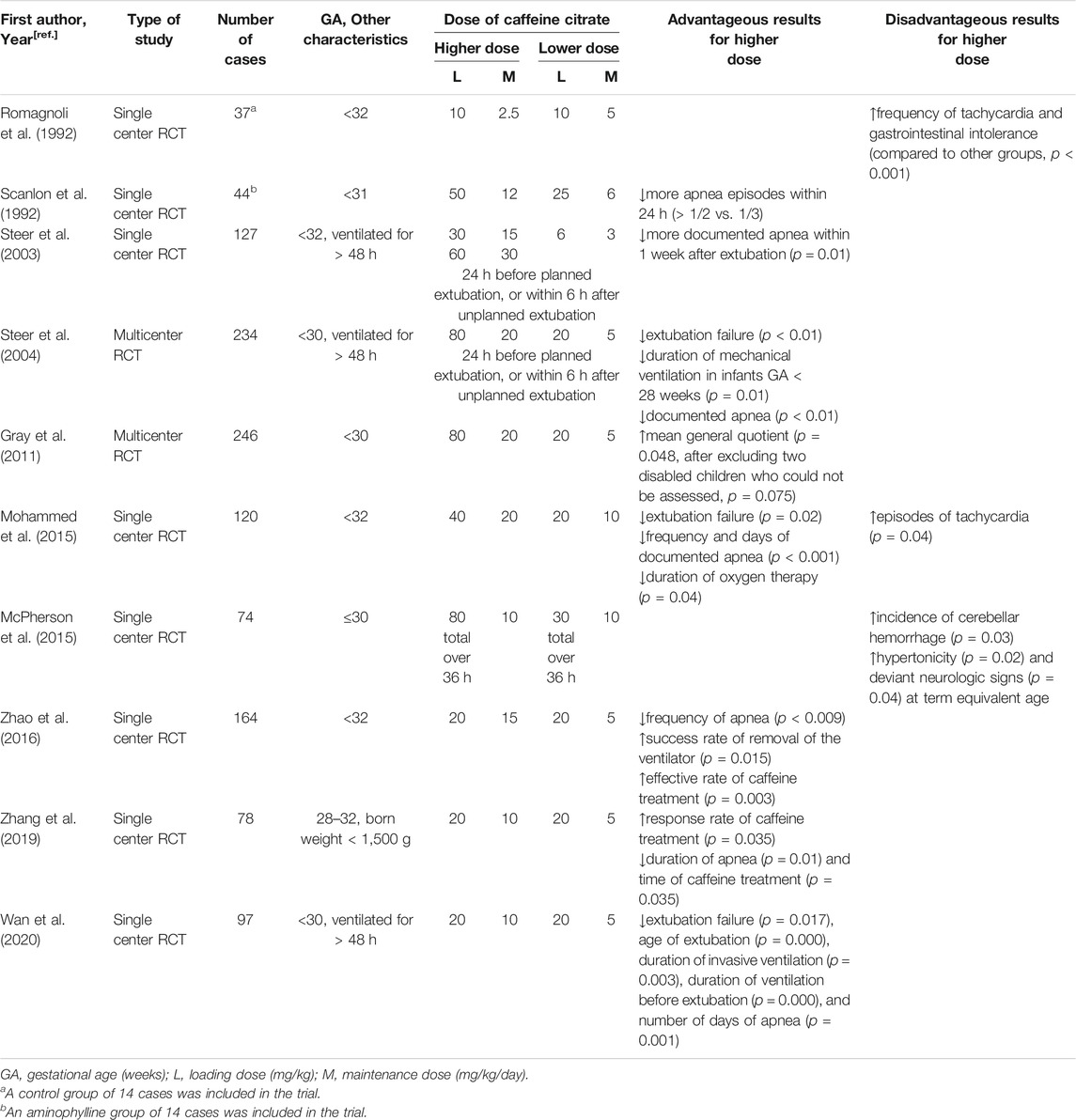
TABLE 4. Advantageous and Disadvantageous Results for Higher vs. Lower Doses of Caffeine in Randomized Controlled Trials.
Other RCTs examined different dosing regimens of caffeine citrate for periextubation management of ventilated preterm infants. In 2003, Steer et al. compared three dose regimens of caffeine citrate (3, 15 and 30 mg/kg) for periextubation management of 127 preterm infants < 32 weeks gestation who were ventilated for > 48 h and found that there was no statistically significant difference in the incidence of extubation failure between different dosing groups (Steer et al., 2003). However, in a subsequent multicenter, double-blind RCT, the same authors found that a dose of 20 mg/kg was given 24 h before a planned extubation or within 6 h of an unplanned extubation reduced the rate of extubation failure within 48 h compared to a lower dose of 5 mg/kg, without evidence of harm in the first year of life (Steer et al., 2004). With the inclusion of additional subjects in the above-mentioned study, Gray compared the long-term effects of the two dose regimens used in Steer’s study (Gray et al., 2011). In this trial, 20 mg/kg/day caffeine citrate resulted in neither adverse outcomes in cognitive development, temperament, morbidity, mortality or disability at 1 year nor in behavior at 2 years.
Several findings also revealed the benefits of higher doses of caffeine for VLBW preterm infants. A retrospective analysis suggested that a higher average daily dose of caffeine citrate was associated with better neurodevelopmental outcomes in VLBW infants (Ravichandran et al., 2019). Another RCT trial found that a maintenance dose as high as 10 mg/kg better reduced the duration of apnea and caffeine treatment in this population (Zhang et al., 2019).
Four meta-analyses also synthesized the findings from the trials comparing higher and lower doses of caffeine citrate (Table 5). Among of them, three papers reported that higher caffeine dosage regimens might be better in reducing the risk of BPD and extubation failure (Chen et al., 2018; Pakvasa et al., 2018; Vliegenthart et al., 2018; Brattström et al., 2019), two reported a decrease in apnea frequency (Chen et al., 2018; Brattström et al., 2019) and one reported a shortened duration of mechanical ventilation (Brattström et al., 2019). Regarding safety concerns, three meta-analyses concluded a higher risk of tachycardia with higher dose of caffeine (Chen et al., 2018; Pakvasa et al., 2018), but no other adverse outcomes were increased.
However, a pilot RCT found an increased incidence of cerebellar hemorrhage (CBH) in infants < 31 weeks’ gestation who were randomized to a higher-dose caffeine citrate (loading 80 mg/kg) (McPherson et al., 2015). Further analysis of this trial demonstrated that early high-dose caffeine therapy was associated with a trend toward an increase in seizure incidence (40 vs 58%, p = 0.1) and burden (48.9 vs 170.9, p = 0.1) (Vesoulis et al., 2016). These results discouraged a larger RCT. More recently, a retrospective study of 218 preterm infants < 28 weeks’ gestation who received a loading dose of caffeine citrate within the first 36 h of life was conducted (Firman et al., 2019). The use of early high loading dose caffeine citrate (a median dose of 80 mg/kg) was not shown to be associated with CBH. Although the two studies obtained different short-term outcomes, they both found that at 2 years of age, the Bayley-III scores used to assess neurodevelopment were not significantly different between the two dose groups.
Collectively, most previous RCTs had small sample sizes, and only two of them have reported 2 years clincal outcomes, which, although positive, need to be treated with caution. Thus, whether to use higher caffeine dosage regimens and how to optimize the caffeine dose are still questionable.
Therapeutic Drug Monitoring of Caffeine
Therapeutic Concentration of Standard Dose of Caffeine
The role of TDM for the control of therapeutic ranges of caffeine has often been challenged due to its benign safety profile when standard dosing is used. As early as in 1977, Aranda et al. revealed that the plasma concentration of standard dose caffeine needed to be monitored and the effective therapeutic concentration was established at 5–20 mg/L by referring to the use of theophylline (Aranda et al., 1977). Subsequently, the same authors also noted that the minimum effective plasma concentration of caffeine was 3–4 mg/L, but an optimal ventilatory response was observed at greater than 8 mg/L, and slight toxicity manifesting as temporary jitteriness was not detected until 50–84 mg/L (Aranda et al., 1979a; Aranda and Turmen, 1979). Therefore, Aranda et al. concluded that the optimal therapeutic concentration of caffeine is 8–20 mg/L, which both produces an adequate response to control apnea and avoids the risk of toxic effects (Aranda and Turmen, 1979).
Blood caffeine levels in preterm infants were almost within this conventional target range in other studies using similar standard dose regimens. In an RCT, 37 preterm infants rapidly achieved the therapeutic concentration within 24 h after starting treatment with a significant reduction in apneic episodes (Romagnoli et al., 1992). A study of 18 Asian preterm infants reported mean serum caffeine concentrations of 10–20 mg/L, and concluded that conventional caffeine therapeutic concentrations should be adhered to in order to ensure safety and efficacy (Lee et al., 2002). Leon et al. found that when the maintenance dose was 6 mg/kg, the 25th to 75th percentile range of mean serum caffeine concentrations in 108 preterm infants was comparable between two different loading dose groups (20 or 25 mg/kg), ranging from 18 to 23 mg/L (Leon et al., 2007). Another study found that the majority of preterm infants achieved target plasma caffeine levels of 5–20 mg/L when treated with a median dose of 5.0 mg/kg (range 2.5–10.9 mg/kg), with 95% of measures within this range in a cohort of 101 preterm infants with 23–32 weeks gestation, including those with renal or hepatic dysfunction (Natarajan et al., 2007a).
Therefore, blood caffeine concentrations of 5–20 or 8–20 mg/L have been commonly recognized as effective therapeutic concentrations for AOP treatment. Routine monitoring of caffeine levels is not recommended by the American Academy of Pediatrics Committee on Fetus and Newborn in their statement on AOP (Eichenwald, 2016). However, when we traced back to the origin, we recognized that the study by Aranda et al. was the fisrt study to determine the therapeutic concentration range of caffeine only based on 18 premature infants’ data (Aranda et al., 1977). Surprisingly, the blood caffeine concentrations were not measured in the well-known CAP trial and the drug was monitored according to its clinical effect only (Schmidt et al., 2006). Of note, the study by Natarajan et al. included a group of preterm neonates (n = 94) who lacked clinical response and had median to 75th quartile of plasma caffeine concentrations of 10.2–14.1 mg/L, suggesting that some neonates may need higher targets of caffeine to control apnea (Natarajan et al., 2007a). Collectively, whether to monitor the level of caffeine in preterm neonates using standard doses still needs to be explored.
Therapeutic Concentration of Higher Dose of Caffeine
Many studies have shown that using higher dose of caffeine was more effective with negligible adverse effects than the standard-dose regimen and explored different effective therapeutic ranges of caffeine. A caffeine PK study including 13 premature infants found that the blood caffeine level varied widely from 12 to 36 mg/L when the single dose regimen of 15 mg/kg was used (Gorodischer and Karplus, 1982). Another RCT reported that 73% of the plasma caffeine concentration measurements in the high-dose group ranged from 26 to 40 mg/L, and apnea episodes were reduced more rapidly within 8 and 24 h without serious adverse effects compared to the standard-dose group (Scanlon et al., 1992). In a PK study conducted by Lee et al., in which no undesired consequences occurred when the mean serum caffeine concentrations were 35.8 or 69.0 mg/L (Lee et al., 1997), a therapeutic concentration > 35 mg/L was proposed to effectively prevent apnea after extubation. Similarly, Steer et al. reported that two higher dose groups with mean serum caffeine concentrations of 31.4 and 59.9 mg/L had short-term benefits and safety during peri-extubation among 127 infants < 32 weeks gestation (Steer et al., 2003). Subsequently, a commentary by Dr. Gal in 2007 questioned the traditional therapeutic concentration (Gal, 2007). According to his findings, higher serum caffeine concentrations produced more significant clinical responses including the reduced incidence of apnea, bradycardia, and of oxygen desaturation, which affirmed a target range of 8–40 mg/L, proposed by Natarajan et al. in another review (Natarajan et al., 2007b). In addition, a retrospective chart review of 198 infants born ≤ 29 weeks gestation showed that serum concentrations of caffeine > 14.5 mg/L were correlated with a reduction in the incidence of chronic lung disease (Chavez Valdez et al., 2011).
However, a small observational prospective study found that serum caffeine levels ≥ 20 mg/L were associated with increased proinflammatory cytokines in preterm infants during the first week of life (Alur et al., 2015). In another study of 115 preterm infants, there was no association between episodes of apnea and serum caffeine concentrations, although there was a significant but weak correlation between caffeine concentration and heart rate (Yu et al., 2016). Meanwhile, some cases reported acute intoxication due to overdose. A case report in 1980 presented two full-term infants with acute caffeine overdose who still had seizure activity when caffeine levels decreased to 31.9 mg/L and 10 mg/L, respectively, although the effect of perinatal asphyxia could not be ruled out (Banner and Czajka, 1980). Another 31 weeks gestational neonate experienced toxic reactions, including hypertonia, sweating, tachycardia, heart failure, pulmonary edema, metabolic disturbances and gastric dilatation, due to the blood caffeine level’s reaching 217.5 mg/L at 36.5 h after dosing, but these symptoms disappeared on day 7 at plasma concentrations of 60–70 mg/L (Anderson et al., 1999). Neurological symptoms, such as uninterrupted tremors, hypertonia, persistent reflex posture, crying, and digestive disorders were reported in a 33 weeks preterm newborn with a serum caffeine level of 160 mg/L at 66 h after administration, whereas his psychomotor development returned to normal after 3 months of age (Perrin et al., 1987). In addition, it is unfortunate that blood caffeine concentrations of subjects were not provided in most RCTs investigating doses of caffeine for AOP (Gray et al., 2011; McPherson et al., 2015; Mohammed et al., 2015; Zhao et al., 2016; Zhang et al., 2019; Wan et al., 2020). Due to lack of high-quality evidence for the long-term safety of high levels of caffeine, further determination of the therapeutic concentration range is difficult.
Therapeutic Drug Monitoring and Dose Optimization of Caffeine
In the aforementioned studies, thetherapeutic concentration of caffeine was commonly recognized as 5–20 or 8–20 mg/L when using the standard dose regimen. However, some preterm neonates lacked a positive clinical response, although their caffeine levels were within the therapeutic concentration range, suggesting that these neonates may need to use higher doses to control apnea episodes. But using high doses may induce adverse reactions, and how to determine therapeutic doses for neonates who lack a clinical response still needs to be investigated. Therefore, is it feasible to guide dose optimization based on the monitoring caffeine levels?
Refer to Therapeutic Concentration of Standard Dose of Caffeine, routine monitoring of blood caffeine levels is generally not recommended. Leon et al. found that when a caffeine dose regimen close to standard (loading 20 or 25 mg/kg and maintenance of 6 mg/kg/day) was used, the serum drug concentrations were maintained in a safe therapeutic range and were independent of corrected gestational age, weight, and postnatal age within the first 2 weeks of life (Leon et al., 2007). Nevertheless, a PPK study found that the day-to-day variability in caffeine clearance of preterm neonates was twice the interindividual variability, implying that adjusting maintenance doses in light of previous serum concentrations is futile (Charles et al., 2008). However, some studies reported that higher levels of caffeine resulted in a greater response, and caffeine concentration monitoring was essential to ensure reaching the expected drug levels (Gal, 2007; Kahn and Godin, 2016). The 2019 guidelines of the National Institute for Health and Care Excellence recommend that caffeine levels should be monitored using reference ranges from the local laboratories to ensure safety when the daily maintenance dose is higher than 20 mg/kg (NICE, 2019). Combined with clinical practice, a growing body of research has endorsed the view that therapeutic monitoring of caffeine is of interest when therapeutic response is lacking or toxicity is suspected (Natarajan et al., 2007a; Gal, 2007; Leon et al., 2007; Gal, 2009; Kahn and Godin, 2016; Yu et al., 2016).
Naturally, the dose optimization of caffeine cannot be generalized. On the one hand, the change in caffeine clearance in preterm infants is a postnatal maturational progression (Aranda and Beharry, 2020). For routine use of caffeine, Koch et al. developed a simulated PK model and proposed an adjustment strategy based on postnatal age to maintain stable caffeine concentrations, with steps of increasing the caffeine maintenance daily dose by 1 mg/kg every 1 to 2 postnatal weeks, 6 mg/kg in the second week, 7 mg/kg in the third to fourth weeks, and 8 mg/kg in the fifth to eighth weeks (Koch et al., 2017). Recently, it has also been proposed that individualized caffeine medication can be administered with the help of a physiologic based pharmacokinetics (PBPK) model (Abduljalil et al., 2020; Aranda and Beharry, 2020; Verscheijden et al., 2020). On the other hand, the clinical response is specific to each individual and influenced by many factors such as gestational age, birth weight and genetic variability (Gal, 2007; Bloch-Salisbury et al., 2010; Francart et al., 2013; Ravichandran et al., 2019; He et al., 2020). Although increasing evidence has proven that the higher dose of caffeine is beneficial for newborns, there are also potential toxic risks and unknown long-term safety problems. In addition, the reported therapeutic concentration ranges of caffeine may not be simply combined together because of the differences such as the population, sample size, biological matrix, as well as assay methods in each study. This highlights the need to tailor the most appropriate range of individual therapeutic concentration according to blood caffeine levels, and the development of minimally invasive sampling techniques and noninvasive sampling of caffeine may contribute to achieving this requirement (Patel et al., 2013; Bruschettini et al., 2016; Chaabane et al., 2017).
Impact of Genetic Variability on the Clinical Response to Caffeine Therapy
Earlier studies have found that heritability impacts the incidence of AOP, which raised interest in elucidating the effects of genetic factors on AOP as well as caffeine therapy (Tamim et al., 2003; Bloch-Salisbury et al., 2010). The therapeutic effect of caffeine depends on the disposition process of caffeine in vivo, that is, PK, and the interaction with target receptors, that is, pharmacodynamics (PD). Researches to date are precisely based on these two aspects.
In terms of PK, a recent retrospective study found that there were no significant differences in caffeine systemic exposure levels between apneic and apnea-free groups, as well as no significant association between the C0/D ratio and genetic variations in CYP1A2 genes (rs2472299 and rs762551) (He et al., 2020). Correspondingly, in another PPK study of Chinese preterm neonates, the investigators found no significant association between several genetic variants in CYP1A2 (rs2069514, rs2069521, rs2069526, rs2470890, rs35694136, rs3743484, rs56107638 and rs762551) and PK parameters (Gao et al., 2020). These findings echo delayed CYP1A2 ontogenesis and immature metabolism in premature infants, indicating that the contribution of genetic polymorphisms in caffeine-metabolizing enzymes to the variability in treatment response is limited. Notably, however, it has also been reported that the distribution of the aryl hydrocarbon receptor (AHR) CC genotype (rs4410790) differed significantly between the two groups with different responses to caffeine treatment in Chinese preterm neonates (He et al., 2020). Although AHR is normally a transcription factor that can regulate CYP1A2 expression, the authors stated that this finding may not be explained by the AHR-CYP1A2 metabolic pathway mechanisms.
In contrast, several studies have reported the effect of genetic polymorphisms associated with caffeine PD on treatment response. Adenosine receptor (AR) gene polymorphisms are the most described genetic factors in those current studies. AR is a class of G protein-coupled receptors with four known subtypes, A1, A2A, A2B and A3, which is encoded by the ADORA1, ADORA2A, ADORA2B, and ADORA3 genes, respectively (Chen et al., 2013; Borea et al., 2018). With a molecular structure similar to that of adenosine, caffeine acts as a nonspecific antagonist of A1AR and A2AAR to exert pharmacological effects at physiological concentrations (McLellan et al., 2016; Kumar and Lipshultz, 2019). In some studies, AR gene polymorphisms have already been found to be associated with intersubject variability in sensitivity to caffeine-induced anxiety (Childs et al., 2008; Rogers et al., 2010). Referring to these findings, Kumral et al. conducted a retrospective case-control study and found that ADORA1 (rs16851030) CC genotype carriers had better responsiveness to caffeine than CT or TT genotype carriers.They also revealed that the correlation between ADORA2A (rs35320474, rs5751876, rs3761422) CT or TT genotypes and vulnerability to AOP as well as the correlation between ADORA2A (rs35320474) CT or TT genotypes and greater risk of BPD (Kumral et al., 2012). A significantly increased frequency of ADORA2A (rs5751876) CT, TT genotypes and T allele in caffeine nonresponders compared to caffeine responders was also reported in another prospective case-control study of Egyptian preterm neonates (Mokhtar et al., 2018). Moreover, a most recent retrospective study of Chinese preterm infants found that carriers of ADORA1 T > G (rs10920568), G > T (rs12744240) and ADORA3 C > A (rs10776727) as well as T > C (rs2298191) mutant genotypes did not respond to caffeine treatment, whereas ADORA2A T > A (rs34923252) and A > C (rs5996696) mutation genotype carriers responded better (He et al., 2020). In addition, this study also showed that a variant (rs521704, C > A) in the coding gene of adenosine dehydrogenase (ADA), which catalyzes adenosine metabolism, was associated with the response of premature infants to caffeine therapy (He et al., 2020). Phosphodiesterase (PDE), one of the targets of caffeine at nonphysiological concentrations, was also correlated, as carriers of the homozygous mutant genotype of PDE4D (rs10075508, C > T) responded poorly to standard-dose caffeine treatment (McLellan et al., 2016; Kumar and Lipshultz, 2019; He et al., 2020).
Collectively, although the sample size number of these studies is small, several genetic polymorphisms have been revealed to be associated with individual variances in response to caffeine therapy. Therefore, studies with larger sample sizes are needed to confirm these findings and further researches are warranted to explain how genetic variants play a critical role in the response to caffeine therapy in premature infants.
Conclusion
Caffeine is effective in reducing apnea frequency in preterm neonates. The available evidence has confirmed the efficacy and safety of standard doses of caffeine, and routine TDM seems unnecessary in neonates who respond positively to caffeine treatment. However, the well-known CAP trial only started caffeine treatment when apnea occurred, and when to start standard-dose caffeine therapy is also a quite controversial issue that requires long-term safety studies. For developmental premature infants, a dosing adjustment strategy based on postnatal age was proposed to maintain stable caffeine concentrations, and individualized caffeine medication may be administered with the help of PPK and PBPK models. For neonates lacking a positive clinical response, as the evidence for the use of higher doses of caffeine is insufficient, and TDM should be performed to achieve the desired blood caffeine level and ensure safety. The long-term results of larger trials of higher doses of caffeine are expected and would be more reasonable if corresponding blood caffeine concentrations could be provided. In addition, the study of genetic factors has preliminarily revealed the association between genetic polymorphisms and clinical response to caffeine therapy. Further studies are required to explain how genetic variants play a role in the response to caffeine therapy in premature infants. And how to establish an approach to individualize medication regimens for infants with poor clinical response by integrating tools such as TDM, genetic testing, PPK and PBPK models is also a direction for future exploration.
Author Contributions
JL, HG, FC, JX: Wrote the manuscript and prepared the tables. XH, YH, YX: Revised the manuscript. RC, XD: Contributed to the language polish. HG, FC: Provided financial support. All the authors reviewed and agreed the final manuscript.
Funding
This research was supported by the Specially Appointed Medical Expert Project of the Jiangsu Commission of Health (2019) and Special Fund for Clinical Research of the Wu Jieping Medical Foundation (320.6750.2020-04-07). This study was also supported by the Scientific Research Support Foundation for Top Young Scholars at the Children’s Hospital of Nanjing Medical University (2020).
Conflict of Interest
The authors declare that the research was conducted in the absence of any commercial or financial relationships that could be construed as a potential conflict of interest.
Publisher’s Note
All claims expressed in this article are solely those of the authors and do not necessarily represent those of their affiliated organizations, or those of the publisher, the editors and the reviewers. Any product that may be evaluated in this article, or claim that may be made by its manufacturer, is not guaranteed or endorsed by the publisher.
Abbreviations
ADA, adenosine dehydrogenase; AHR, aryl hydrocarbon receptor; AOP, apnea of prematurity; AR, adenosine receptor; BPD, bronchopulmonary dysplasia; CAP, Caffeine for Apnea of Prematurity; CBH, cerebellar hemorrhage; NONMEM, nonlinear mixed effects models; PBPK, physiologic based pharmacokinetics; PD, pharmacodynamics; PDA, patent ductus arteriosus; PDE, phosphodiesterase; PK, pharmacokinetics; PPK, population pharmacokinetics; RCT, randomized controlled trial; ROP, retinopathy of prematurity; TDM, therapeutic drug monitoring; VLBW, very low birth weight.
References
Abduljalil, K., Pan, X., Pansari, A., Jamei, M., and Johnson, T. N. (2020). Preterm Physiologically Based Pharmacokinetic Model. Part II: Applications of the Model to Predict Drug Pharmacokinetics in the Preterm Population. Clin. Pharmacokinet. 59 (4), 501–518. doi:10.1007/s40262-019-00827-4
al-Alaiyan, S., al-Rawithi, S., and Raines, D. (2001). Caffeine Metabolism in Premature Infants. J. Clin. Pharmacol. 41 (6), 620–627. doi:10.1177/00912700122010500
Aldridge, A., Aranda, J. V., and Neims, A. H. (1979). Caffeine Metabolism in the Newborn. Clin. Pharmacol. Ther. 25 (4), 447–453. doi:10.1002/cpt1979254447
Alur, P., Bollampalli, V., Bell, T., Hussain, N., and Liss, J. (2015). Serum Caffeine Concentrations and Short-Term Outcomes in Premature Infants of ⩽29 Weeks of Gestation. J. Perinatol 35 (6), 434–438. doi:10.1038/jp.2014.226
Anderson, B. J., Gunn, T. R., Holford, N. H. G., and Johnson, R. (1999). Caffeine Overdose in a Premature Infant: Clinical Course and Pharmacokinetics. Anaesth. Intensive Care 27 (3), 307–311. doi:10.1177/0310057x9902700316
Anwar, M., Mondestin, H., Mojica, N., Novo, R., Graff, M., Hiatt, M., et al. (1986). Effect of Caffeine on Pneumogram and Apnoea of Infancy. Arch. Dis. Child. 61 (9), 891–895. doi:10.1136/adc.61.9.891
Aranda, J. V., and Beharry, K. D. (2020). Pharmacokinetics, Pharmacodynamics and Metabolism of Caffeine in Newborns. Semin. Fetal Neonatal Med. 25 (6), 101183. doi:10.1016/j.siny.2020.101183
Aranda, J. V., and Turmen, T. (1979). Methylxanthines in Apnea of Prematurity. Clin. Perinatol. 6 (1), 87–108. doi:10.1016/s0095-5108(18)31165-5
Aranda, J. V., Gorman, W., Bergsteinsson, H., and Gunn, T. (1977). Efficacy of caffeine in treatment of apnea in the low-birth-weight infant. J. Pediatr. 90 (3), 467–472. doi:10.1016/s0022-3476(77)80718-x
Aranda, J. V., Cook, C. E., Gorman, W., Collinge, J. M., Loughnan, P. M., Outerbridge, E. W., Aldridge, A., and Neims, A. H. (1979). Pharmacokinetic Profile of Caffeine in the Premature Newborn Infant With Apnea. J. Pediatr. 94 (4), 663–668. doi:10.1016/s0022-3476(79)80047-5
Aranda, J. V., Collinge, J. M., Zinman, R., and Watters, G. (1979). Maturation of Caffeine Elimination in Infancy. Arch. Dis. Child. 54 (12), 946–949. doi:10.1136/adc.54.12.946
Aranda, J. V., Beharry, K., Valencia, G. B., Natarajan, G., and Davis, J. (2010). Caffeine Impact on Neonatal Morbidities. J. Matern. Fetal. Neonatal Med. 23 (Suppl 3), 20–23. doi:10.3109/14767058.2010.517704
Arnaud, M. J. (1976). Identification, Kinetic and Quantitative Study of [2-14C] and [1-Me-14C]Caffeine Metabolites in Rat's Urine by Chromatographic Separations. Biochemical Medicine 16 (1), 67–76. doi:10.1016/0006-2944(76)90010-7
Arnaud, M. J. (1987). The Pharmacology of Caffeine. Prog Drug Res 31, 273–313. doi:10.1007/978-3-0348-9289-6_9
Arnaud, MJ (2011). Pharmacokinetics and Metabolism of Natural Methylxanthines in Animal and Man. Handb Exp Pharmacol 2011, 33–91. doi:10.1007/978-3-642-13443-2_3
Bairam, A., Boutroy, M.-J., Badonnel, Y., and Vert, P. (1987). Theophylline Versus caffeine: Comparative Effects in Treatment of Idiopathic Apnea in the Preterm Infant. J. Pediatr. 110 (4), 636–639. doi:10.1016/s0022-3476(87)80569-3
Bancalari, E. (2014). Current Management of Apnea in Premature Infants: Is Caffeine the Magic Bullet? Early Hum. Develop. 90, S1–S2. doi:10.1016/s0378-3782(14)50001-1
Banner, W., and Czajka, P. A. (1980). Acute Caffeine Overdose in the Neonate. Arch. Pediatr. Adolesc. Med. 134 (5), 495–498. doi:10.1001/archpedi.1980.02130170045015
Begas, E., Kouvaras, E., Tsakalof, A., Papakosta, S., and Asprodini, E. K. (2007). In Vivo evaluation of CYP1A2, CYP2A6, NAT-2 and Xanthine Oxidase Activities in a Greek Population Sample by the RP-HPLC Monitoring of Caffeine Metabolic Ratios. Biomed. Chromatogr. 21 (2), 190–200. doi:10.1002/bmc.736
Blake, M. J., Abdel-Rahman, S. M., Pearce, R. E., Leeder, J. S., and Kearns, G. L. (2006). Effect of Diet on the Development of Drug Metabolism by Cytochrome P-450 Enzymes in Healthy Infants. Pediatr. Res. 60 (6), 717–723. doi:10.1203/01.pdr.0000245909.74166.00
Blanchard, J., and Sawers, S. J. A. (1983). The Absolute Bioavailability of Caffeine in Man. Eur. J. Clin. Pharmacol. 24 (1), 93–98. doi:10.1007/bf00613933
Bloch-Salisbury, E., Hall, M. H., Sharma, P., Boyd, T., Bednarek, F., and Paydarfar, D. (2010). Heritability of Apnea of Prematurity: a Retrospective Twin Study. Pediatrics 126 (4), e779–e787. doi:10.1542/peds.2010-0084
Bonati, M., Latini, R., Galletti, F., Young, J. F., Tognoni, G., and Garattini, S. (1982). Caffeine Disposition After Oral Doses. Clin. Pharmacol. Ther. 32 (1), 98–106. doi:10.1038/clpt.1982.132
Bonati, M., Latini, R., Tognoni, G., Young, J. F., and Garattini, S. (1984). Interspecies Comparison of In Vivo Caffeine Pharmacokinetics in Man, Monkey, Rabbit, Rat and Mouse. Drug Metab. Rev. 15 (7), 1355–1383. doi:10.3109/03602538409029964
Borea, P. A., Gessi, S., Merighi, S., Vincenzi, F., and Varani, K. (2018). Pharmacology of Adenosine Receptors: The State of the Art. Physiol. Rev. 98 (3), 1591–1625. doi:10.1152/physrev.00049.2017
Bory, C., Baltassat, P., Porthault, M., Bethenod, M., Frederich, A., and Aranda, J. (1978). Biotransformation of Theophylline to Caffeine in Premature Newborn. Lancet 312 (8101), 1204–1205. doi:10.1016/s0140-6736(78)92188-8
Bory, C., Baltassat, P., Porthault, M., Bethenod, M., Frederich, A., and Aranda, J. V. (1979). Metabolism of Theophylline to Caffeine in Premature Newborn Infants. J. Pediatr. 94 (6), 988–993. doi:10.1016/s0022-3476(79)80246-2
Brattström, P., Russo, C., Ley, D., and Bruschettini, M. (2019). High-versus Low-Dose Caffeine in Preterm Infants: a Systematic Review and Meta-Analysis. Acta Paediatr. 108 (3), 401–410. doi:10.1111/apa.14586
Brouard, C., Moriette, G., Murat, I., Flouvat, B., Pajot, N., Walti, H., et al. (1985). Comparative Efficacy of Theophylline and Caffeine in the Treatment of Idiopathic Apnea in Premature Infants. Arch. Pediatr. Adolesc. Med. 139 (7), 698–700. doi:10.1001/archpedi.1985.02140090060028
Bruschettini, M., Barco, S., Romantsik, O., Risso, F., Gennai, I., Chinea, B., et al. (2016). DBS-LC-MS/MS Assay for Caffeine: Validation and Neonatal Application. Bioanalysis 8 (18), 1893–1902. doi:10.4155/bio-2016-0127
Carrier, O., Pons, G., Rey, E., Richard, M.-O., Moran, C., Badoual, J., et al. (1988). Maturation of Caffeine Metabolic Pathways in Infancy. Clin. Pharmacol. Ther. 44 (2), 145–151. doi:10.1038/clpt.1988.129
Cazeneuve, C., Pons, G., Rey, E., Treluyer, J., Cresteil, T., Thiroux, G., et al. (1994). Biotransformation of Caffeine in Human Liver Microsomes from Foetuses, Neonates, Infants and Adults. Br. J. Clin. Pharmacol. 37 (5), 405–412. doi:10.1111/j.1365-2125.1994.tb05706.x
Chaabane, A., Chioukh, F. Z., Chadli, Z., Ben Fredj, N., Ben Ameur, K., Ben Hmida, H., et al. (2017). Therapeutic Drug Monitoring of Caffeine in Preterm Infants: Could Saliva Be an Alternative to Serum? Therapies 72 (6), 685–689. doi:10.1016/j.therap.2017.06.004
Charles, B. G., Townsend, S. R., Steer, P. A., Flenady, V. J., Gray, P. H., and Shearman, A. (2008). Caffeine Citrate Treatment for Extremely Premature Infants with Apnea: Population Pharmacokinetics, Absolute Bioavailability, and Implications for Therapeutic Drug Monitoring. Ther. Drug Monit. 30 (6), 709–716. doi:10.1097/ftd.0b013e3181898b6f
Chavez Valdez, R., Ahlawat, R., Wills-Karp, M., Nathan, A., Ezell, T., and Gauda, E. B. (2011). Correlation Between Serum Caffeine Levels and Changes in Cytokine Profile in a Cohort of Preterm Infants. J. Pediatr. 158 (1), 57–64.e1. doi:10.1016/j.jpeds.2010.06.051
Chen, J.-F., Eltzschig, H. K., and Fredholm, B. B. (2013). Adenosine Receptors as Drug Targets - What Are the Challenges? Nat. Rev. Drug Discov. 12 (4), 265–286. doi:10.1038/nrd3955
Chen, J., Jin, L., and Chen, X. (2018). Efficacy and Safety of Different Maintenance Doses of Caffeine Citrate for Treatment of Apnea in Premature Infants: A Systematic Review and Meta-Analysis. Biomed. Res. Int. 2018, 9061234. doi:10.1155/2018/9061234
Childs, E., Hohoff, C., Deckert, J., Xu, K., Badner, J., and de Wit, H. (2008). Association between ADORA2A and DRD2 Polymorphisms and Caffeine-Induced Anxiety. Neuropsychopharmacol 33 (12), 2791–2800. doi:10.1038/npp.2008.17
De Carolis, M. P., Romagnoli, C., Muzii, U., Tortorolo, G., Chiarotti, M., De Giovanni, N., et al. (1991). Pharmacokinetic Aspects of Caffeine in Premature Infants. Dev. Pharmacol. Ther. 16 (3), 117–122. doi:10.1159/000480570
Di Fiore, J. M., Bloom, J. N., Orge, F., Schutt, A., Schluchter, M., Cheruvu, V. K., et al. (2010). A Higher Incidence of Intermittent Hypoxemic Episodes Is Associated with Severe Retinopathy of Prematurity. J. Pediatr. 157 (1), 69–73. doi:10.1016/j.jpeds.2010.01.046
Dobson, N. R., and Hunt, C. E. (2013). Pharmacology Review: Caffeine Use in Neonates: Indications, Pharmacokinetics, Clinical Effects, Outcomes. NeoReviews 14 (11), e540–e550. doi:10.1542/neo.14-11-e540
Doyle, L. W., Ranganathan, S., and Cheong, J. L. Y. (2017). Neonatal Caffeine Treatment and Respiratory Function at 11 Years in Children Under 1,251 G at Birth. Am. J. Respir. Crit. Care Med. 196 (10), 1318–1324. doi:10.1164/rccm.201704-0767oc
Eichenwald, E. C. (2016). Committee on Fetus and Newborn, American Academy of Pediatrics. Apnea of Prematurity. Pediatrics 137 (1), e20153757. doi:10.1542/peds.2015-3757
Engbers, A. G. J., Völler, S., Poets, C. F., Knibbe, C. A. J., Reiss, I. K. M., Koch, B. C. P., et al. (2021). The Pharmacokinetics of Caffeine in Preterm Newborns: No Influence of Doxapram but Important Maturation With Age. Neonatology 118 (1), 106–113. doi:10.1159/000513413
Erenberg, A., Leff, R. D., Haack, D. G., Mosdell, K. W., Hicks, G. M., Wynne, B. A., et al. (2000). Caffeine Citrate for the Treatment of Apnea of Prematurity: a Double-Blind, Placebo-Controlled Study. Pharmacotherapy 20 (6), 644–652. doi:10.1592/phco.20.7.644.35167
Erickson, G., Dobson, N. R., and Hunt, C. E. (2021). Immature Control of Breathing and Apnea of Prematurity: the Known and Unknown. J. Perinatol. doi:10.1038/s41372-021-01010-z
European Medicines Agency (2009). Peyona (previously Nymusa). Available at: https://www.ema.europa.eu/en/medicines/human/EPAR/peyona-previously-nymusa (Accessed January 10, 2021)
Falcão, A. C., de Gatta, M. M. F., Iribarnegaray, M. F. D., Buelga, D. S., García, M. J., Dominguez-Gil, A., et al. (1997). Population Pharmacokinetics of Caffeine in Premature Neonates. Eur. J. Clin. Pharmacol. 52 (3), 211–217. doi:10.1007/s002280050276
Firman, B., Molnar, A., and Gray, P. H. (2019). Early High‐dose Caffeine Citrate for Extremely Preterm Infants: Neonatal and Neurodevelopmental Outcomes. J. Paediatr. Child. Health 55 (12), 1451–1457. doi:10.1111/jpc.14446
Francart, S. J., Allen, M. K., and Stegall-Zanation, J. (2013). Apnea of Prematurity: Caffeine Dose Optimization. J. Pediatr. Pharmacol. Ther. 18 (1), 45–52. doi:10.5863/1551-6776-18.1.45
Gal, P. (2007). Caffeine Therapeutic Drug Monitoring Is Necessary and Cost-Effective. J. Pediatr. Pharmacol. Ther. 12 (4), 212–215. doi:10.5863/1551-6776-12.4.212
Gal, P. (2009). Optimum Use of Therapeutic Drug Monitoring and Pharmacokinetics-Pharmacodynamics in the NICU. J. Pediatr. Pharmacol. Ther. 14 (2), 66–74. doi:10.5863/1551-6776-14.2.66
Gao, X. B., Zheng, Y., Yang, F., Wang, C. H., Jiang, Z. H., Wu, Y. E., Jacqz-Aigrain, E., et al. (2020). Developmental Population Pharmacokinetics of Caffeine in Chinese Premature Infants With Apnoea of Prematurity: A post-marketing Study to Support Paediatric Labelling in China. Br. J. Clin. Pharmacol.
Gentle, S. J., Travers, C. P., and Carlo, W. A. (2018). Caffeine Controversies. Curr. Opin. Pediatr. 30 (2), 177–181. doi:10.1097/mop.0000000000000588
Gorodischer, R., and Karplus, M. (1982). Pharmacokinetic Aspects of Caffeine in Premature Infants With Apnoea. Eur. J. Clin. Pharmacol. 22 (1), 47–52. doi:10.1007/bf00606424
Gray, P. H., Flenady, V. J., Charles, B. G., Steer, P. A., and Caffeine Collaborative Study, G. (2011). Caffeine Citrate for Very Preterm Infants: Effects on Development, Temperament and Behaviour. J. Paediatr. Child. Health 47 (4), 167–172. doi:10.1111/j.1440-1754.2010.01943.x
Gu, L., Gonzalez, F. J., Kalow, W., and Tang, B. K. (1992). Biotransformation of Caffeine, Paraxanthine, Theobromine and Theophylline by cDNA-Expressed Human CYP1A2 and CYP2E1. Pharmacogenetics 2 (2), 73–77. doi:10.1097/00008571-199204000-00004
Guo, A., Zhu, Z., Xue, J., Di, X., Fan, J., Huang, L., et al. (2020). Population Pharmacokinetic Study of Caffeine Citrate in Chinese Premature Infants With Apnea. J. Clin. Pharm. Ther. 45 (6), 1414–1421. doi:10.1111/jcpt.13240
He, X., Qiu, J. C., Lu, K. Y., Guo, H. L., Li, L., Jia, W. W., et al. (2020). Therapy for Apnoea of Prematurity: A Retrospective Study on Effects of Standard Dose and Genetic Variability on Clinical Response to Caffeine Citrate in Chinese Preterm Infants. Adv. Ther. 38 (1), 607–626. doi:10.1007/s12325-020-01544-2
Horne, R. S. C., Fung, A. C. H., NcNeil, S., Fyfe, K. L., Odoi, A., and Wong, F. Y. (2017). The Longitudinal Effects of Persistent Apnea on Cerebral Oxygenation in Infants Born Preterm. J. Pediatr. 182, 79–84. doi:10.1016/j.jpeds.2016.11.081
Janvier, A., Khairy, M., Kokkotis, A., Cormier, C., Messmer, D., and Barrington, K. J. (2004). Apnea Is Associated with Neurodevelopmental Impairment in Very Low Birth Weight Infants. J. Perinatol 24 (12), 763–768. doi:10.1038/sj.jp.7211182
Kahn, D. J., and Godin, R. (2016). Is it Time to Embrace the Caffeine Level? Pediatrics 137 (5), e20160403A. doi:10.1542/peds.2016-0403a
Kearns, G. L., Abdel-Rahman, S. M., Alander, S. W., Blowey, D. L., Leeder, J. S., and Kauffman, R. E. (2003). Developmental Pharmacology - Drug Disposition, Action, and Therapy in Infants and Children. N. Engl. J. Med. 349 (12), 1157–1167. doi:10.1056/nejmra035092
Koch, G., Datta, A. N., Jost, K., Schulzke, S. M., van den Anker, J., and Pfister, M. (2017). Caffeine Citrate Dosing Adjustments to Assure Stable Caffeine Concentrations in Preterm Neonates. J. Pediatr. 191, 50–56. doi:10.1016/j.jpeds.2017.08.064
Kumar, V. H. S., and Lipshultz, S. E. (2019). Caffeine and Clinical Outcomes in Premature Neonates. Children (Basel) 6 (11), 118. doi:10.3390/children6110118
Kumral, A., Tuzun, F., Yesilirmak, D. C., Duman, N., and Ozkan, H. (2012). Genetic Basis of Apnoea of Prematurity and Caffeine Treatment Response: Role of Adenosine Receptor Polymorphisms. Acta Paediatr. 101 (7), e299–e303. doi:10.1111/j.1651-2227.2012.02664.x
Lee, T. C., Charles, B., Steer, P., Flenady, V., and Shearman, A. (1997). Population Pharmacokinetics of Intravenous Caffeine in Neonates with Apnea of Prematurity*. Clin. Pharmacol. Ther. 61 (6), 628–640. doi:10.1016/s0009-9236(97)90097-7
Lee, H. S., Khoo, Y. M., Chirino-Barcelo, Y., Tan, K. L., and Ong, D. (2002). Caffeine in Apnoeic Asian Neonates: a Sparse Data Analysis. Br. J. Clin. Pharmacol. 54 (1), 31–37. doi:10.1046/j.1365-2125.2002.01589.x
Lelo, A., Birkett, D., Robson, R., and Miners, J. (1986). Comparative Pharmacokinetics of Caffeine and its Primary Demethylated Metabolites Paraxanthine, Theobromine and Theophylline in Man. Br. J. Clin. Pharmacol. 22 (2), 177–182. doi:10.1111/j.1365-2125.1986.tb05246.x
Leon, A. E., Michienzi, K., Ma, C. X., and Hutchison, A. A. (2007). Serum Caffeine Concentrations in Preterm Neonates. Am. J. Perinatol 24 (1), 39–47. doi:10.1055/s-2006-958163
Lodha, A., Seshia, M., McMillan, D. D., Barrington, K., Yang, J., Lee, S. K., et al. (2015). Association of Early Caffeine Administration and Neonatal Outcomes in Very Preterm Neonates. JAMA Pediatr. 169 (1), 33–38. doi:10.1001/jamapediatrics.2014.2223
Martin, R. J., Wang, K., Köroğlu, Ö., Di Fiore, J., and Kc, P. (2011). Intermittent Hypoxic Episodes in Preterm Infants: Do They Matter? Neonatology 100 (3), 303–310. doi:10.1159/000329922
McLellan, T. M., Caldwell, J. A., and Lieberman, H. R. (2016). A Review of Caffeine's Effects on Cognitive, Physical and Occupational Performance. Neurosci. Biobehav. Rev. 71, 294–312. doi:10.1016/j.neubiorev.2016.09.001
McPherson, C., Neil, J. J., Tjoeng, T. H., Pineda, R., and Inder, T. E. (2015). A Pilot Randomized Trial of High-Dose Caffeine Therapy in Preterm Infants. Pediatr. Res. 78 (2), 198–204. doi:10.1038/pr.2015.72
Mohammed, S., Nour, I., Shabaan, A. E., Shouman, B., Abdel-Hady, H., and Nasef, N. (2015). High versus Low-Dose Caffeine for Apnea of Prematurity: a Randomized Controlled Trial. Eur. J. Pediatr. 174 (7), 949–956. doi:10.1007/s00431-015-2494-8
Mokhtar, W. A., Fawzy, A., Allam, R. M., Zidan, N., and Hamed, M. S. (2018). Association between Adenosine Receptor Gene Polymorphism and Response to Caffeine Citrate Treatment in Apnea of Prematurity; an Egyptian Single-Center Study. Egypt. Pediatr. Assoc. Gaz. 66 (4), 115–120. doi:10.1016/j.epag.2018.09.001
Moschino, L., Zivanovic, S., Hartley, C., Trevisanuto, D., Baraldi, E., and Roehr, C. C. (2020). Caffeine in Preterm Infants: Where Are We in 2020? ERJ Open Res. 6 (1), 00330–2019. doi:10.1183/23120541.00330-2019
Murat, I., Moriette, G., Blin, M. C., Couchard, M., Flouvat, B., De Gamarra, E., et al. (1981). The Efficacy of Caffeine in the Treatment of Recurrent Idiopathic Apnea in Premature Infants. J. Pediatr. 99 (6), 984–989. doi:10.1016/s0022-3476(81)80038-8
Murner-Lavanchy, I. M., Doyle, L. W., Schmidt, B., Roberts, R. S., Asztalos, E. V., Costantini, B., et al. (2018). Neurobehavioral Outcomes 11 Years after Neonatal Caffeine Therapy for Apnea of Prematurity. Pediatrics 141 (5), e20174047. doi:10.1542/peds.2017-4047
Natarajan, G., Botica, M.-L., Thomas, R., and Aranda, J. V. (2007). Therapeutic Drug Monitoring for Caffeine in Preterm Neonates: an Unnecessary Exercise? Pediatrics 119 (5), 936–940. doi:10.1542/peds.2006-2986
Natarajan, G., Lulic-Botica, M., and Aranda, J. V. (2007). Pharmacology Review: Clinical Pharmacology of Caffeine in the Newborn. NeoReviews 8 (5), e214–e221. doi:10.1542/neo.8-5-e214
NDA 20-793/S-001 (2000). NDA 20-793/S-001. Available at: https://www.accessdata.fda.gov/drugsatfda_docs/label/2000/20793s1lbl.pdf (Accessed January 10, 2021).
NICE (2019). Specialist Neonatal Respiratory Care for Babies Born Preterm. Available at: https://www.nice.org.uk/guidance/ng124/chapter/Recommendations (Accessed December 20, 2020).
Pakvasa, M. A., Saroha, V., and Patel, R. M. (2018). Optimizing Caffeine Use and Risk of Bronchopulmonary Dysplasia in Preterm Infants. Clin. Perinatology 45 (2), 273–291. doi:10.1016/j.clp.2018.01.012
Patel, P., Mulla, H., Kairamkonda, V., Spooner, N., Gade, S., Della Pasqua, O., et al. (2013). Dried Blood Spots and Sparse Sampling: a Practical Approach to Estimating Pharmacokinetic Parameters of Caffeine in Preterm Infants. Br. J. Clin. Pharmacol. 75 (3), 805–813. doi:10.1111/j.1365-2125.2012.04392.x
Pearlman, S. A., Duran S., C., Wood, M. A., Maisels, M. J., and Berlin, Jr., C. M. (1989). Caffeine Pharmacokinetics in Preterm Infants Older Than 2 Weeks. Dev. Pharmacol. Ther. 12 (2), 65–69. doi:10.1159/000480966
Perrin, C., Debruyne, D., Lacotte, J., Laloum, D., Bonte, J.-B., and Moulin, M. (1987). Treatment of Caffeine Intoxication by Exchange Transfusion in a Newborn. Acta Paediatr. 76 (4), 679–681. doi:10.1111/j.1651-2227.1987.tb10546.x
Pons, G., Carrier, O., Richard, M.-O., Rey, E., d’Athis, P., Moran, C., et al. (1988). Developmental Changes of Caffeine Elimination in Infancy. Dev. Pharmacol. Ther. 11 (5), 258–264. doi:10.1159/000457700
Pons, G., Rey, E., Carrier, O., Richard, M. O., Moran, C., Badoual, J., et al. (1989). Maturation of AFMU Excretion in Infants. Fundam. Clin. Pharmacol. 3 (6), 589–595. doi:10.1111/j.1472-8206.1989.tb00461.x
Ravichandran, S., Chouthai, N. S., Patel, B., Sharma, A., Gupte, A., Ma, M. M., et al. (2019). Higher Daily Doses of Caffeine Lowered the Incidence of Moderate to Severe Neurodevelopmental Disabilities in Very Low Birth Weight Infants. Acta Paediatr. 108 (3), 430–435. doi:10.1111/apa.14465
Rogers, P. J., Hohoff, C., Heatherley, S. V., Mullings, E. L., Maxfield, P. J., Evershed, R. P., et al. (2010). Association of the Anxiogenic and Alerting Effects of Caffeine with ADORA2A and ADORA1 Polymorphisms and Habitual Level of Caffeine Consumption. Neuropsychopharmacol 35 (9), 1973–1983. doi:10.1038/npp.2010.71
Romagnoli, C., De Carolis, M. P., Muzii, U., Zecca, E., Tortorolo, G., Chiarotti, M., et al. (1992). Effectiveness and Side Effects of Two Different Doses of Caffeine in Preventing Apnea in Premature Infants. Ther. Drug Monit. 14 (1), 14–19. doi:10.1097/00007691-199202000-00003
Salem, F., Johnson, T. N., Abduljalil, K., Tucker, G. T., and Rostami-Hodjegan, A. (2014). A Re-evaluation and Validation of Ontogeny Functions for Cytochrome P450 1A2 and 3A4 Based on In Vivo Data. Clin. Pharmacokinet. 53 (7), 625–636. doi:10.1007/s40262-014-0140-7
Saroha, V., and Patel, R. M. (2020). Caffeine for Preterm Infants: Fixed Standard Dose, Adjustments for Age or High Dose? Semin. Fetal Neonatal Med. 25, 101178. doi:10.1016/j.siny.2020.101178
Scanlon, J. E., Chin, K. C., Morgan, M. E., Durbin, G. M., Hale, K. A., and Brown, S. S. (1992). Caffeine or Theophylline for Neonatal Apnoea? Arch. Dis. Child. 67 (4 Spec No), 425–428. doi:10.1136/adc.67.4_spec_no.425
Schmid, M. B., Hopfner, R. J., Lenhof, S., Hummler, H. D., and Fuchs, H. (2015). Cerebral Oxygenation during Intermittent Hypoxemia and Bradycardia in Preterm Infants. Neonatology 107 (2), 137–146. doi:10.1159/000368294
Schmidt, B., Roberts, R. S., Davis, P., Doyle, L. W., Barrington, K. J., Ohlsson, A., et al. (2006). Caffeine Therapy for Apnea of Prematurity. N. Engl. J. Med. 354 (20), 2112–2121. doi:10.1056/nejmoa054065
Schmidt, B., Roberts, R. S., Davis, P., Doyle, L. W., Barrington, K. J., Ohlsson, A., et al. (2007). Long-term Effects of Caffeine Therapy for Apnea of Prematurity. N. Engl. J. Med. 357 (19), 1893–1902. doi:10.1056/nejmoa073679
Schmidt, B., Anderson, P. J., Doyle, L. W., Dewey, D., Grunau, R. E., Asztalos, E. V., et al. (2012). Survival without Disability to Age 5 Years after Neonatal Caffeine Therapy for Apnea of Prematurity. JAMA 307 (3), 275–282. doi:10.1001/jama.2011.2024
Schmidt, B., Roberts, R. S., Anderson, P. J., Asztalos, E. V., Costantini, L., Davis, P. G., et al. (2017). Academic Performance, Motor Function, and Behavior 11 Years after Neonatal Caffeine Citrate Therapy for Apnea of Prematurity. JAMA Pediatr. 171 (6), 564–572. doi:10.1001/jamapediatrics.2017.0238
Schmidt, B., Anderson, P. J., Asztalos, E. V., Doyle, L. W., Grunau, R. E., Moddemann, D., et al. (2019). Self-reported Quality of Life at Middle School Age in Survivors of Very Preterm Birth. JAMA Pediatr. 173 (5), 487–489. doi:10.1001/jamapediatrics.2018.4853
Shrestha, B., and Jawa, G. (2017). Caffeine Citrate - Is it a Silver Bullet in Neonatology? Pediatr. Neonatal. 58 (5), 391–397. doi:10.1016/j.pedneo.2016.10.003
Song, G., Sun, X., Hines, R. N., McCarver, D. G., Lake, B. G., Osimitz, T. G., et al. (2017). Determination of Human Hepatic CYP2C8 and CYP1A2 Age-dependent Expression to Support Human Health Risk Assessment for Early Ages. Drug Metab. Dispos 45 (5), 468–475. doi:10.1124/dmd.116.074583
Sonnier, M., and Cresteil, T. (1998). Delayed Ontogenesis of CYP1A2 in the Human Liver. Eur. J. Biochem. 251 (3), 893–898. doi:10.1046/j.1432-1327.1998.2510893.x
Steer, P., Flenady, V., Shearman, A., Lee, T., Tudehope, D., and Charles, B. (2003). Periextubation Caffeine in Preterm Neonates: a Randomized Dose Response Trial. J. Paediatr. Child. Health 39 (7), 511–515. doi:10.1046/j.1440-1754.2003.00207.x
Steer, P., Flenady, V., Shearman, A., Charles, B., Gray, P. H., Henderson-Smart, D., et al. (2004). High Dose Caffeine Citrate for Extubation of Preterm Infants: a Randomised Controlled Trial. Arch. Dis. Child. - Fetal Neonatal Ed. 89 (6), F499–F503. doi:10.1136/adc.2002.023432
Tamim, H., Khogali, M., Beydoun, H., Melki, I., Yunis, K., and National Collaborative Perinatal Neonatal, N. (2003). Consanguinity and Apnea of Prematurity. Am. J. Epidemiol. 158 (10), 942–946. doi:10.1093/aje/kwg226
Tanaka, H., Nakazawa, K., Arima, M., and Iwasaki, S. (1984). Caffeine and its Dimethylxanthines and Fetal Cerebral Development in Rat. Brain Develop. 6 (4), 355–361. doi:10.1016/s0387-7604(84)80111-4
Thomson, A. H., Kerr, S., and Wright, S. (1996). Population Pharmacokinetics of Caffeine in Neonates and Young Infants. Ther. Drug Monit. 18 (3), 245–253. doi:10.1097/00007691-199606000-00005
Thorn, C. F., Aklillu, E., McDonagh, E. M., Klein, T. E., and Altman, R. B. (2012). PharmGKB Summary. Pharmacogenet Genomics 22 (5), 389–395. doi:10.1097/fpc.0b013e3283505d5e
Turmen, T., Louridas, T. A., and Aranda, J. V. (1979). Relationship of Plasma and CSF Concentrations of Caffeine in Neonates With Apnea. J. Pediatr. 95 (4), 644–646. doi:10.1016/s0022-3476(79)80788-x
Verscheijden, L. F. M., Koenderink, J. B., Johnson, T. N., de Wildt, S. N., and Russel, F. G. M. (2020). Physiologically-based Pharmacokinetic Models for Children: Starting to Reach Maturation? Pharmacol. Ther. 211, 107541. doi:10.1016/j.pharmthera.2020.107541
Vesoulis, Z. A., McPherson, C., Neil, J. J., Mathur, A. M., and Inder, T. E. (2016). Early High-Dose Caffeine Increases Seizure Burden in Extremely Preterm Neonates: A Preliminary Study. J. Caffeine Res. 6 (3), 101–107. doi:10.1089/jcr.2016.0012
Vliegenthart, R., Miedema, M., Hutten, G. J., van Kaam, A. H., and Onland, W. (2018). High versus standard dose caffeine for apnoea: a systematic review. Arch Dis Child. Fetal Neonatal Ed 103 (6), F523–F529. doi:10.1136/archdischild-2017-313556
Wan, L, Huang, L, and Chen, P (2020). Caffeine Citrate Maintenance Doses Effect on Extubation and Apnea Postventilation in Preterm Infants. Pediatr Pulmonol. 55, 2635–2640. doi:10.1002/ppul.24948
Yu, T, Balch, AH, Ward, RM, Korgenski, EK, and Sherwin, CM (2016). Incorporating Pharmacodynamic Considerations into Caffeine Therapeutic Drug Monitoring in Preterm Neonates. BMC Pharmacol Toxicol 17 (1), 22. doi:10.1186/s40360-016-0065-x
Zhang, X, Zhang, HT, Lyu, Y, Wang, LF, and Yang, ZY (2019). [Clinical effect and safety of different maintenance doses of caffeine citrate in treatment of apnea in Very Low Birth Weight Preterm Infants: a Prospective Randomized Controlled Trial]. Zhongguo Dang Dai Er Ke Za Zhi 21 (6), 558–561.
Keywords: preterm infant, apnea of prematurity, caffeine, pharmacokinetics, therapeutic drug monitoring, polymorphism
Citation: Long J-Y, Guo H-L, He X, Hu Y-H, Xia Y, Cheng R, Ding X-S, Chen F and Xu J (2021) Caffeine for the Pharmacological Treatment of Apnea of Prematurity in the NICU: Dose Selection Conundrum, Therapeutic Drug Monitoring and Genetic Factors. Front. Pharmacol. 12:681842. doi: 10.3389/fphar.2021.681842
Received: 17 March 2021; Accepted: 12 July 2021;
Published: 26 July 2021.
Edited by:
Raffaele Simeoli, Bambino Gesù Children Hospital (IRCCS), ItalyReviewed by:
Carl Hunt, Uniformed Services University of the Health Sciences, United StatesSinno Simons, Erasmus Medical Center, Netherlands
Copyright © 2021 Long, Guo, He, Hu, Xia, Cheng, Ding, Chen and Xu. This is an open-access article distributed under the terms of the Creative Commons Attribution License (CC BY). The use, distribution or reproduction in other forums is permitted, provided the original author(s) and the copyright owner(s) are credited and that the original publication in this journal is cited, in accordance with accepted academic practice. No use, distribution or reproduction is permitted which does not comply with these terms.
*Correspondence: Feng Chen, Y3kuY2hlbjUwOEBnbWFpbC5jb20=; Jing Xu, bmp4dWppbmdAMTYzLmNvbQ==
‡Visiting graduate student from China Pharmaceutical University.
†These authors have contributed equally to this work.