- 1Cancer Institute, Longhua Hospital, Shanghai University of Traditional Chinese Medicine, Shanghai, China
- 2Department of Surgery, University of California, San Diego, La Jolla, CA, United States
- 3Anticancer Inc., San Diego, CA, United States
Lung adenocarcinoma is the most common pathological type of lung cancer with poor patient outcomes; therefore, developing novel therapeutic agents is critically needed. Andrographolide (AD), a major active component derived from the traditional Chinese medicine (TCM) Andrographis paniculate, is a potential antitumor drug, but the role of AD in lung adenocarcinoma remains poorly understood. In the present study, we demonstrated that AD inhibited the proliferation of broad-spectrum lung cancer cell lines in a dose-dependent manner. Meanwhile, we found that a high dose of AD induced Noxa-dependent apoptosis in human lung adenocarcinoma cells (A549 and H1299). Further studies revealed that Noxa was transcriptionally activated by activating transcription factor 4 (ATF4) in AD-induced apoptosis. Knockdown of ATF4 by small interfering RNA (siRNA) significantly diminished the transactivation of Noxa as well as the apoptotic population induced by AD. These results of the present study indicated that AD induced apoptosis of human lung adenocarcinoma cells by activating the ATF4/Noxa axis and supporting the development of AD as a promising candidate for the new era of chemotherapy.
Introduction
Lung adenocarcinoma is recalcitrant cancer with overall survival of less than 5 years (Denisenko et al., 2018). Intolerable side effects and multidrug resistance are still the main causes of the poor outcomes of patients with lung adenocarcinoma (Malhotra and Perry, 2003; Thomas et al., 2015; Jamal-Hanjani et al., 2017; Rotow and Bivona, 2017). Therefore, it is urgently needed to develop novel therapeutic agents with high efficiency and low toxicity to ameliorate patient outcomes.
Cell apoptosis is a process of programmed cell death, and inducing tumor cell apoptosis has become a strategy for cancer therapy (Gerl and Vaux, 2005; Hanahan and Weinberg, 2011). Among the apoptotic regulatory proteins, pro-apoptotic BH3-only (Bcl-2 homology domain 3) protein Noxa, a member of the Bcl-2 (B cell lymphoma-2) family proteins, has been defined as an antitumor drug target (Pérez-Galán et al., 2006; Albert et al., 2014; Guikema et al., 2017; Morsi et al., 2018). Inoue et al. reported that Noxa mediated HDAC (histone deacetylase) inhibitor-induced apoptosis and suggested that activated Noxa could be a potential clinical target for chronic lymphocytic leukemia and lymphoma therapy (Inoue et al., 2007). Shibue et al. reported that Noxa is necessary for irradiation-induced apoptosis and supported that upregulated Noxa may provide a new strategy for cancer therapy (Shibue et al., 2003). Activating transcription factor 4 (ATF4) is a universal stress-responsive gene and could transcriptionally activate Noxa in response to chemotherapy. Armstrong et al. reported that apoptosis induced by fenretinide and bortezomib via upregulating Noxa was dependent on ATF4 (Armstrong et al., 2010). Chen et al. found the same mechanism in a small molecule compound MLN4924-treated human esophageal cancer cells (Chen et al., 2016). In conclusion, targeting ATF4/Noxa axis could be a promising strategy for cancer treatment (Zhu et al., 2012; Nunez-Vazquez et al., 2020).
There is currently a great interest in developing traditional Chinese medicine (TCM) into first-line therapy for cancer (Tang et al., 1999; Efferth et al., 2007; Lu et al., 2019). Andrographolide (AD) is one of the major active components of the TCM Andrographis paniculate (Zhao et al., 2002). Previous studies have reported that AD exhibits a broad-spectrum antitumor efficacy in various cancer cells (Banerjee et al., 2016; Kumar et al., 2016; Islam et al., 2018; Chen et al., 2019). However, the antitumor efficacy and the underlying molecular mechanisms of AD on human lung adenocarcinoma cells remains poorly understood. In this study, we demonstrated that AD exhibits a broad-spectrum proliferation inhibitory effect in lung cancer cells, and firstly reported that AD induced lung adenocarcinoma cell apoptosis via activating ATF4/Noxa axis. The present study provides a basis to clinically develop AD for the new era of chemotherapy.
Materials and Methods
Cells and Reagents
Human lung adenocarcinoma cells (A549, H1299), human lung squamous cells (SK-MES-1), murine Lewis lung carcinoma cells (LLC), and normal human bronchial epithelial cells (BEAS-2B) were obtained from the American Type Culture Collection (Manassas, VA, United States). These cells were cultured in Dulbecco’s modified Eagle’s medium (DMEM, BasalMedia, Shanghai, China) which contains 10% fetal bovine serum (FBS, BasalMedia, Shanghai, China) and 1% penicillin–streptomycin solution (BasalMedia, Shanghai, China) at 37°C with 5% CO2. Andrographolide (Sigma-Aldrich, Germany) was dissolved into a concentration of 100 mM with dimethyl sulfoxide (DMSO, Sigma-Aldrich, Germany) and stored at -20°C. Antibody against β-actin was obtained from HuaBio (Hangzhou, China); Antibodies against cleaved-PARP (c-PARP), PARP, cleaved-caspase-3 (c-Casp3), caspase3 (Casp3), ATF4, c-Myc, Noxa, Puma, Bid, Bim, Bik, Bax, and Bak were all obtained from Cell Signaling Technology (Beverly, MA, United States).
Cell Proliferation Assay
Cells in the exponential growth phase were seeded in ATPlite plates in triplicate, 2000 cells per well, cultured overnight, and treated with 1‰ DMSO or AD at indicated concentrations for 24 h and 48 h, followed by the ATPlite luminescence assay (BD Pharmingen, Franklin Lakes, New Jersey, United States).
Western Blotting
Cells were harvested and lysed in RIPA buffer (Beyotime, Shanghai, China). Protein concentrations were determined by the protein assay kit (Epizyme, Shanghai, China). Gel electrophoresis (SDS–PAGE) was used to separate the total proteins of the samples. Then, the proteins were transferred onto a polyvinylidene fluoride membrane (PVDF). 5% nonfat milk in TBST was used to block the PVDF membrane for 1 h at room temperature. The membranes with a primary antibody were co-incubated at 4°C and washed three times with TBST overnight, and then co-incubated with a secondary antibody at room temperature for 1 h. After then, washed three times with TBST and visualized with the ECL kit (Share Bio, Shanghai, China) and film (Tanon, Shanghai, China).
Real-Time Polymerase Chain Reaction Analyses (RT-PCR)
Ultrapure RNA kit (ComWin Biotech, Beijing, China) was used to isolate total RNA, and 1 μg total RNA was reversed to cDNA by using the PrimerScript reverse transcription reagent kit (Vazyme Biotech, Nanjing, China). Then, the cDNA was quantified with RT-PCR by using the Power SYBR Green PCR MasterMix (Vazyme Biotech, Nanjing, China) on the ABI 7500 thermocycler (Applied Biosystems, Foster City, CA, United States). The mRNA abundance of each sample was normalized to β-actin. Primers were designed and synthesized by BioSune (Shanghai, China). The sequences of the primers were as follows: human β-actin: forward 5′-CGTGCGTGACATTAAGGAGAAG-3′ and reverse 5′-AAGGAAGGCTGGAAGAGTGC-3′; human ATF4: forward 5′-ATGACCGAAATGAGCTTCCTG-3′ and reverse 5′-GCTGGAGAACCCATGAGGT-3′; human Noxa: forward 5′-ACCAAGCCGGATTTGCGATT-3′ and reverse 5′-ACTTGCACTTGTTCCTCGTGG-3′; and human c-Myc: forward 5′-GGCTCCTGGCAAAAGGTCA-3′ and reverse 5′-CTGCGTAGTTGTGCTGATGT-3′.
Apoptosis Assay
Cells were harvested after treatment with different concentrations of AD for 24 h and then stained by using Annexin V-FITC/PI stain kit following the manufacturer’s instructions (BD Pharmingen, New Jersey, United States). The stained cells were analyzed with flow cytometry (BD, New Jersey, United States).
Gene Silencing Using Small Interfering RNA
The siRNA oligonucleotides were transfected into cells by using Lipofectamine 2000. Briefly, Opti-MEM (Invitrogen) was used to incubate with siRNA and Lipofectamine 2000 separately for 5 min at room temperature and mixed for 20 min, and then the mixture together with the serum-free medium were applied to the cells (final concentration of siRNA is 20 nM). All siRNAs were synthesized by GenePharma (Shanghai, China). The sequences of the siRNA were as follows: negative control: 5′-UUCUCCGAACGUGUCACGUTT-3′; siATF4: 5′-GCCUAGGUCUCUUAGAUGA-3′; siNoxa#1: 5′-GUAAUUAUUGACACAUUUC-3′ (Alves et al., 2006); and siNoxa#2: 5′-GGUGCACGUUUCAUCAAUUUG-3′ (Yao et al., 2014).
Statistical Analysis
Data were displayed as mean ± standard deviation. All data represented three independent experiments. Significant differences between groups were assessed by the two-tailed unpaired Student’s t-test of GraphPad Prism software. Four levels of significance were used for all tests (*p ≤ 0.05, **p ≤ 0.01, ***p≤ 0.001, and ***p≤ 0.0001).
Results
Andrographolide Inhibited the Proliferation of Lung Cancer Cells
The chemical structure of AD is shown in Figure 1A. To evaluate the inhibitory efficacy of AD on the proliferation of lung cancer cells, we determined the half-maximal inhibitory concentration IC50 of AD in five cell lines including squamous carcinoma cells (SK-MES-1), adenocarcinoma cells (A549, H1299), murine Lewis lung cancer cells (LLC), and normal human bronchial epithelial cells (BEAS-2B) at 48 h. As shown in Figure 1B, the IC50 values were approximately 8.72, 3.69, 10.99, 5.2, and 52.10 μM in A549, H1299, SK-MES-1, LLC, and BEAS-2B cells, respectively. The results indicated that AD has a broad-spectrum inhibitory effect on lung cancer cells but has a weaker inhibitory effect on the normal human bronchial epithelial cells.
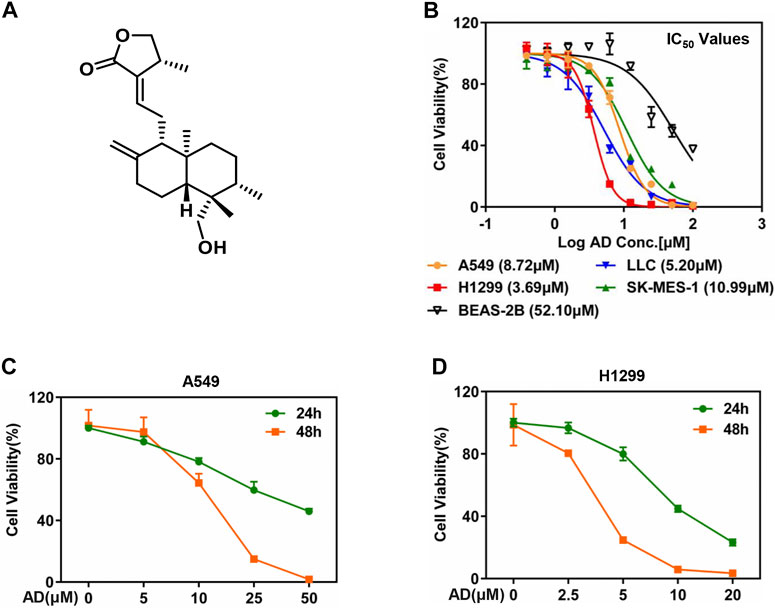
FIGURE 1. Andrographolide inhibited the proliferation of lung cancer cells. (A) The chemical structure of AD. (B) Cells were seeded in ATPlite plates in triplicate, 2000 cells per well, cultured overnight, and treated with 1‰ DMSO or various concentrations of AD (100, 50, 25, 12.5, 6.25, 3.13, 1.56, and 0.78 μM) for 48 h. The ATPlite cell viability assay was used to determine the half-maximal inhibitory concentrations IC50 of A549, H1299, SK-MES-1, LLC, and BEAS-2B cells, respectively. (C–D) Cells were seeded in ATPlite plates in triplicate, 2000 cells per well, cultured overnight, and treated with 1‰ DMSO or indicated concentrations of AD for 24 h and 48 h, followed by the ATPlite cell viability assay.
Considering the morbidity and prognosis of lung adenocarcinoma, we next focused on lung adenocarcinoma cells to explore the antitumor property of AD. First, we selected four concentrations of AD for further studies according to the IC50 values. Then, we examined the inhibitory effect of these concentrations of AD on the proliferation of the two lung adenocarcinoma cells. As shown in Figures 1C,D, AD significantly suppressed the cell proliferation of the two lung adenocarcinoma cells in a dose- and time-dependent manner.
Andrographolide Triggered Apoptosis of Human Lung Adenocarcinoma Cells
To further investigate the underlying mechanisms of AD-induced cell growth inhibition, we determined cell apoptosis by Annexin V-FITC/PI stain and FACS in both A549 and H1299 cells after AD treatment for 24 h. As shown in Figure 2A, a significant dose-dependent increased apoptosis was detected in the two cells, especially in high concentration groups. Then, we examined c-PARP and c-Casp3 by immunoblotting to further confirm the induction of apoptosis by AD in A549 and H1299 cells. The results indicated that the expression levels of c-Casp3 and c-PARP were greatly increased in a dose- and time-dependent manner (Figures 2B,C). These findings demonstrated that AD induced apoptosis of the human lung adenocarcinoma cells.
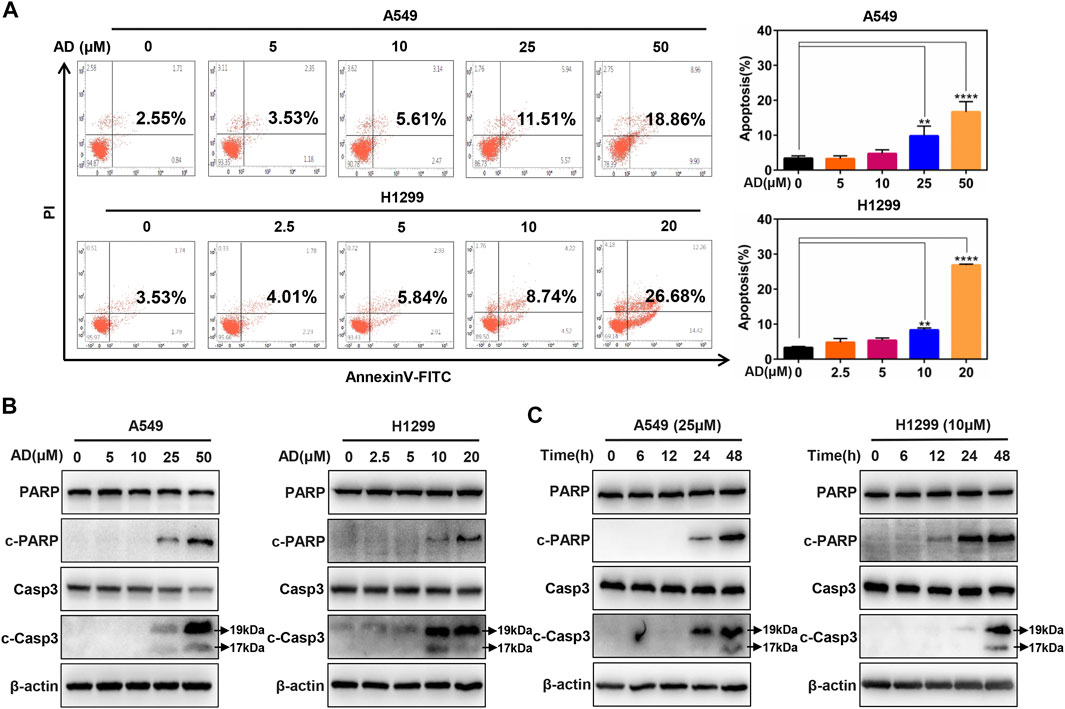
FIGURE 2. Andrographolide triggered apoptosis in human lung adenocarcinoma cells (A) Cells were treated with 1‰ DMSO or indicated concentrations of AD for 24 h, then subjected to Annexin V-FITC/PI staining, followed by the flow cytometric analysis. (B–C) After being treated with 1‰ DMSO or AD at indicated concentrations and different times, cell protein was extracted and detected by Western blotting with antibodies against PARP, c-PARP, Casp3 and c-Casp3, and β-actin (** p ≤ 0.01; **** p ≤ 0.0001).
The BH3-Only Protein Noxa Was Upregulated by Andrographolide Treatment
Given that the role of the classical pro-apoptotic Bcl-2 family proteins in regulating apoptotic cell death, we next determined the expression of the pro-apoptotic proteins, including BH3-only proteins Puma, Bim, Bik, Bid, and Noxa, as well as their downstream pro-apoptotic proteins Bak and Bax in A549 and H1299 cells. These results indicated that only Noxa was significantly upregulated in the protein level in a dose-dependent manner (Figure 3A). Moreover, the mRNA level of Noxa was also substantially elevated by treatment of AD (Figure 3B). These findings indicated that Noxa was transcriptionally activated by AD treatment and suggested that Noxa plays a crucial role in AD-induced apoptosis.
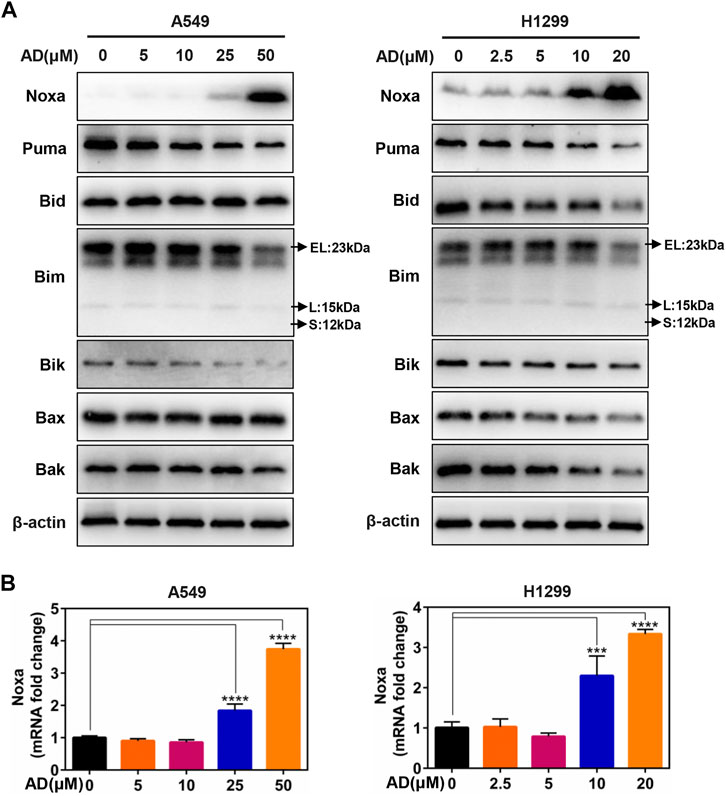
FIGURE 3. The BH3-only protein Noxa mediated andrographolide-induced apoptosis. (A) Cells were treated with 1‰ DMSO or the indicated concentrations of AD for 24 h. Cell protein was extracted and detected by Western blotting with antibodies against Noxa, Puma, Bid, Bim, Bik, Bax, Bak, and β-actin. (B) The mRNA level of Noxa was quantified by RT-PCR (normalized to β-actin) (*** p ≤ 0.001; **** p ≤ 0.0001).
Noxa Knockdown Inhibited Andrographolide-Induced Apoptosis
To confirm the role of Noxa in AD-induced apoptosis in A549 and H1299 cells, Noxa was knocked down by small interfering RNA (siRNA). As shown in Figure 4A and supplementary Figure S1A, Noxa knockdown significantly diminished AD-induced apoptosis as well as the expression levels of c-Casp3 and c-PARP (Figure 4B and Supplementary Figure S1B). These findings demonstrated that AD induced Noxa-dependent apoptosis of human lung adenocarcinoma cells.
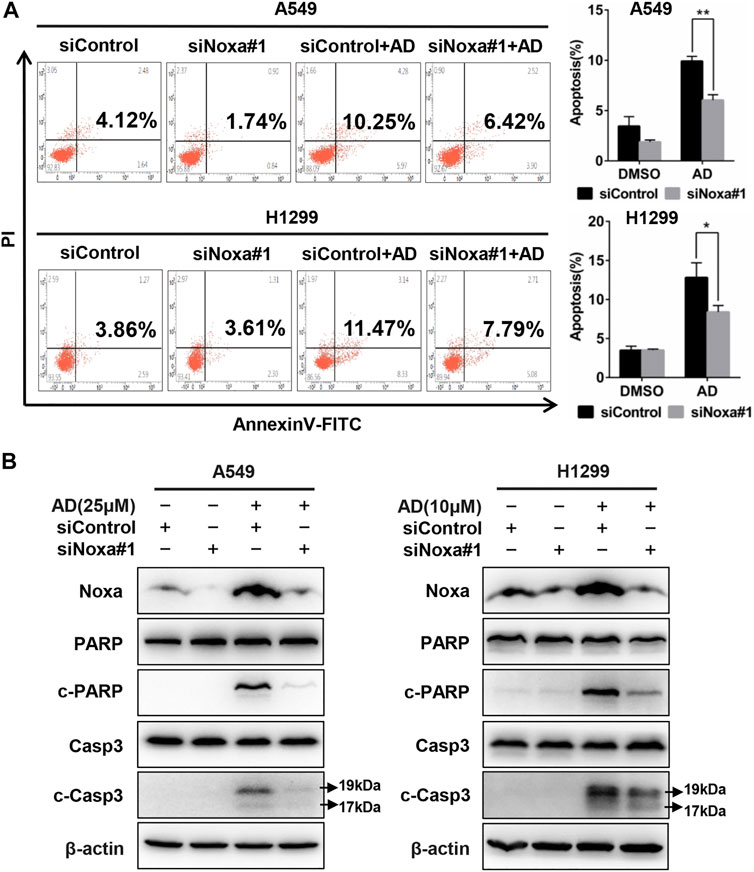
FIGURE 4. Noxa knockdown significantly decreased andrographolide-induced apoptosis in human lung adenocarcinoma cells. (A) Cells were transfected with siControl or siNoxa#1 and treated with 1‰ DMSO and AD (A549 25 μM and H1299 10 μM) for 24 h. Apoptosis was determined and quantified with Annexin V-FITC/PI staining analysis. (B) Cell protein was extracted and detected by western blotting with antibodies against Noxa, PARP, c-PARP, Casp3, c-Casp3, and β-actin (* p ≤ 0.05; ** p ≤ 0.01).
Andrographolide-Induced Apoptosis in Human Lung Adenocarcinoma Cells via ATF4/Noxa Axis
Previous studies in our laboratory unveiled that ATF4 transcriptionally activated Noxa in esophageal squamous cell carcinoma cells after treated by small-molecule compound MLN4924 (Chen et al., 2016). In contrast, it was c-Myc but not ATF4 that transactivated Noxa to trigger cell apoptosis in head and neck squamous cell carcinoma cells after treated by MLN4924 (Zhang et al., 2019). To confirm the transcription factors responsible for Noxa induction upon AD treatment in both A549 and H1299 cells, we examined the protein and mRNA expression of c-Myc and ATF4. The results indicated that AD treatment strongly induced the upregulation of ATF4, but not c-Myc at both protein and mRNA levels (Figures 5A,B). Furthermore, ATF4 knockdown suppressed the transactivation of Noxa as well as the cleavage of PARP and caspase 3 in AD-treated A549 and H1299 cells (Figure 5C). Consistently, the apoptotic population of AD-treated cells was significantly diminished in both two ATF4 knockdown cells (Figure 5D). Taken together, AD transcriptionally activated ATF4, which induced the transcriptional activation of the pro-apoptotic protein Noxa to trigger apoptosis in both A549 and H1299 cells.
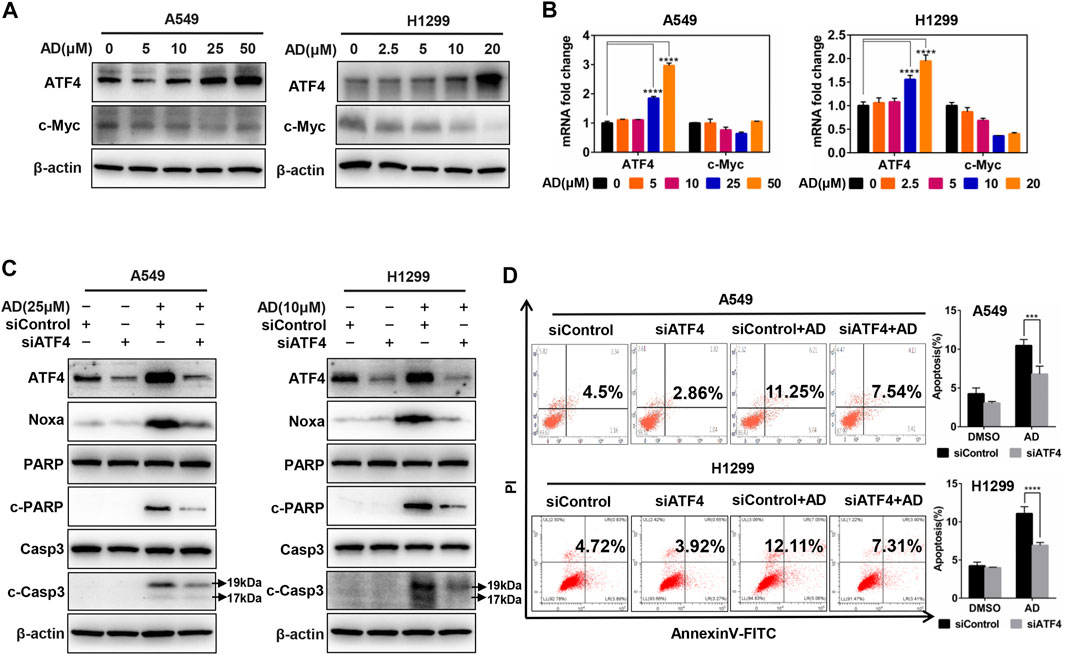
FIGURE 5. Andrographolide induced apoptosis in human lung adenocarcinoma cells via ATF4/Noxa axis. (A–B) Cells were treated with 1‰ DMSO or indicated concentrations of AD for 24 h; cell protein was extracted and detected by western blotting with antibodies against ATF4 and c-Myc. The mRNA level of ATF4 and c-Myc was quantified with RT-PCR (normalized to β-actin). (C–D) Cells were transfected with siControl or siATF4, then, treated with 1‰ DMSO or AD (A549 25 μM, H1299 10 μM) for 24 h, cell protein was extracted and detected by western blotting with antibodies against ATF4, Noxa, PARP, c-PARP, Casp3, c-Casp3, and β-actin. Apoptosis was determined and quantified by AnnexinV-FITC/PI staining analysis (* p ≤ 0.05; *** p ≤ 0.001; **** p ≤ 0.0001.).
Discussion
In the present study, we found that AD exhibits a broad-spectrum inhibition of proliferation in lung cancer cells, and firstly demonstrated that AD activated the ATF4/Noxa axis to induce apoptosis of human lung adenocarcinoma cells (Figure 6). In recent years, emerging evidence has indicated that AD could exert an antitumor effect by inducing apoptosis of diverse cancer cells. Yang et al. reported that AD induced apoptosis of T-cell acute lymphoblastic leukemia cell (Jurkat) via inhibition of the PI3K/Akt pathway (Yang et al., 2016). Wu et al. reported that AD activated the LKB1/AMPK signal pathways and induced apoptosis of human nasopharyngeal carcinoma cells (C666–1) (Wu et al., 2018). Peng et al. also reported that AD induced apoptosis of nasopharyngeal carcinoma cells (HK1 and CNE-1) via inhibiting the NF-κB signal pathway (Peng et al., 2015). These findings collectively indicated that the mechanisms of AD-induced apoptosis may be cancer-type dependent.
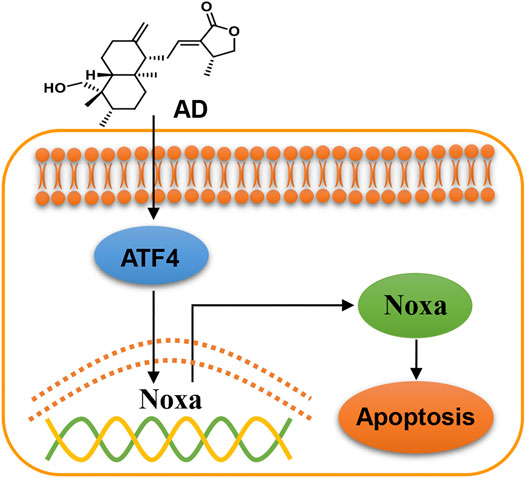
FIGURE 6. Working model of andrographolide-induced ATF4/Noxa-dependent apoptosis. AD triggered human lung adenocarcinoma cells apoptosis by transcriptionally activating ATF4 and subsequently transactivating the pro-apoptotic BH3-only protein Noxa.
While addressing the mechanisms of apoptosis induced by AD in human lung adenocarcinoma cells, we defined that the BH3-only protein Noxa mediated AD-induced apoptosis of human lung adenocarcinoma cells (A549 and H1299). BH3-only proteins Puma, Bim, Bik, Bid, Noxa, and their downstream proteins Bak and Bax all play critical roles in pro-apoptotic cell death (Gross et al., 1999; Cory et al., 2003; Breckenridge and Xue, 2004). It has been reported that some of the BH3-only proteins could mediate AD-induced cell apoptosis. Zhou et al. reported that AD promoted the cleavage of the BH3-only protein Bid to induce apoptosis of breast cancer cells (MDA-MB-231), cervical cancer cells (HeLa), and hepatoma cells (HepG2) (Zhou et al., 2006). Yang et al. reported that Bax and Bak are necessary for AD-induced apoptosis of lymphoma cells (Yang et al., 2010). In our study, we found that Noxa was significantly upregulated by AD in human lung adenocarcinoma cells, and Noxa knockdown significantly decreased AD-induced apoptosis. These results demonstrated that AD induced Noxa-dependent apoptosis of human lung adenocarcinoma cells.
The present study demonstrated that ATF4 transcriptionally activated Noxa in AD-treated lung adenocarcinoma cells. As a universal stress-responsive gene, ATF4 can be activated by several stimulations, such as oxidative stress, endoplasmic reticulum (ER) stress, and oxygen deprivation (Harding et al., 2003; Blais et al., 2004). A large number of studies indicated that activated ATF4 could induce cancer cell apoptosis for the oncotherapy (Jiang et al., 2013; Nunez-Vazquez et al., 2020; Xu et al., 2020). Zong et al. reported that radiation could upregulate ATF4 expression to induce cell apoptosis, and the overexpression of ATF4 could increase cell sensitivity to apoptosis in response to radiation (Zong et al., 2017). Sharma et al. found that cisplatin induced Noxa-dependent apoptosis through the upregulation of ATF4 in head and neck squamous cell carcinoma cells (Sharma et al., 2018). In this study, we found that both protein and mRNA levels of Noxa were upregulated after AD treatment, which suggested that Noxa was transcriptionally activated. Further studies showed that both the protein and mRNA levels of ATF4 were upregulated after AD treatment. Moreover, ATF4 knockdown inhibited the upregulation of Noxa as well as AD-induced apoptosis in human lung adenocarcinoma cells. Therefore, we confirmed that ATF4 transcriptionally activated Noxa in AD-induced apoptosis of human lung adenocarcinoma cells.
In summary, the present study firstly demonstrated that AD induced Noxa-dependent apoptosis by transactivating ATF4 in human lung cancer cells. These findings provided a scientific basis for developing AD as a promising candidate for the new era of chemotherapy.
Data Availability Statement
The original contributions presented in the study are included in the article/Supplementary Material, and further inquiries can be directed to the corresponding author.
Author Contributions
LJ designed and supervised the project. JZ, CL, and LZ carried out the experiments and drafted the manuscript, LJ finalized the manuscript. YH, TX, and YZ performed statistical analyses. XC and RH coordinated the study over the entire time. All authors read and approved the final manuscript.
Funding
This work was supported by the National Natural Science Foundation of China (Grant Nos. 81625018, 81820108022, 82003297), Innovation Program of Shanghai Municipal Education Commission (2019-01-07-00-10-E00056), Program of Shanghai Academic/Technology Research Leader (18XD1403800), National Thirteenth Five-Year Science and Technology Major Special Project for New Drug and Development (2017ZX09304001), Special scientific research project of Postgraduate Innovative Training of Shanghai University of Traditional Chinese Medicine (Y2020004).
Conflict of Interest
RH was employed by Anticancer Inc.
The remaining authors declare that the research was conducted in the absence of any commercial or financial relationships that could be construed as a potential conflict of interest.
Acknowledgments
We are thankful to all authorities of the National Natural Science Foundation of China, Innovation Program of Shanghai Municipal Education Commission, Program of Shanghai Academic/Technology Research Leader, National Thirteenth Five-Year Science and Technology Major Special Project for New Drug and Development, and Shanghai University of Traditional Chinese Medicine for their support.
Supplementary Material
The Supplementary Material for this article can be found online at: https://www.frontiersin.org/articles/10.3389/fphar.2021.680589/full#supplementary-material
References
Albert, M.-C., Brinkmann, K., and Kashkar, H. (2014). Noxa and Cancer Therapy. Mol. Cell Oncol. 1 (1), e29906, doi:10.4161/mco.29906
Alves, N. L., Derks, I. A. M., Berk, E., Spijker, R., van Lier, R. A. W., and Eldering, E. (2006). The Noxa/Mcl-1 Axis Regulates Susceptibility to Apoptosis under Glucose Limitation in Dividing T Cells. Immunity 24 (6), 703–716. doi:10.1016/j.immuni.2006.03.018
Armstrong, J. L., Flockhart, R., Veal, G. J., Lovat, P. E., and Redfern, C. P. F. (2010). Regulation of Endoplasmic Reticulum Stress-Induced Cell Death by ATF4 in Neuroectodermal Tumor Cells. J. Biol. Chem. 285 (9), 6091–6100. doi:10.1074/jbc.M109.014092
Banerjee, M., Chattopadhyay, S., Choudhuri, T., Bera, R., Kumar, S., Chakraborty, B., et al. (2016). Cytotoxicity and Cell Cycle Arrest Induced by Andrographolide Lead to Programmed Cell Death of MDA-MB-231 Breast Cancer Cell Line. J. Biomed. Sci. 23, 40. doi:10.1186/s12929-016-0257-0
Blais, J. D., Filipenko, V., Bi, M., Harding, H. P., Ron, D., Koumenis, C., et al. (2004). Activating Transcription Factor 4 Is Translationally Regulated by Hypoxic Stress. Mcb 24 (17), 7469–7482. doi:10.1128/MCB.24.17.7469-7482.2004
Breckenridge, D. G., and Xue, D. (2004). Regulation of Mitochondrial Membrane Permeabilization by BCL-2 Family Proteins and Caspases. Curr. Opin. Cel Biol. 16 (6), 647–652. doi:10.1016/j.ceb.2004.09.009
Chen, P., Hu, T., Liang, Y., Li, P., Chen, X., Zhang, J., et al. (2016). Neddylation Inhibition Activates the Extrinsic Apoptosis Pathway through ATF4-CHOP-DR5 Axis in Human Esophageal Cancer Cells. Clin. Cancer Res. 22 (16), 4145–4157. doi:10.1158/1078-0432.Ccr-15-2254
Chen, Y.-P., Liu, Y.-W., Lee, D., Qiu, J. T., Lee, T.-Y., and Liu, S.-J. (2019). Biodegradable Andrographolide-Eluting Nanofibrous Membranes for the Treatment of Cervical Cancer. Ijn Vol. 14 421–429. doi:10.2147/ijn.S186714
Cory, S., Huang, D. C. S., and Adams, J. M. (2003). The Bcl-2 Family: Roles in Cell Survival and Oncogenesis. Oncogene 22 (53), 8590–8607. doi:10.1038/sj.onc.1207102
Denisenko, T. V., Budkevich, I. N., and Zhivotovsky, B. (2018). Cell Death-Based Treatment of Lung Adenocarcinoma. Cell Death Dis 9 (2), 117. doi:10.1038/s41419-017-0063-y
Efferth, T., Li, P. C. H., Konkimalla, V. S. B., and Kaina, B. (2007). From Traditional Chinese Medicine to Rational Cancer Therapy. Trends Mol. Med. 13 (8), 353–361. doi:10.1016/j.molmed.2007.07.001
Gerl, R., and Vaux, D. J. C. (2005). Apoptosis in the Development and Treatment of Cancer. Carcinogenesis 26 (2), 263–270. doi:10.1093/carcin/bgh283
Gross, A., McDonnell, J. M., and Korsmeyer, S. J. (1999). BCL-2 Family Members and the Mitochondria in Apoptosis. Genes Develop. 13 (15), 1899–1911. doi:10.1101/gad.13.15.1899
Guikema, J. E., Amiot, M., and Eldering, E. (2017). Exploiting the Pro-apoptotic Function of NOXA as a Therapeutic Modality in Cancer. Expert Opin. Ther. Targets 21 (8), 767–779. doi:10.1080/14728222.2017.1349754
Hanahan, D., and Weinberg, R. A. (2011). Hallmarks of Cancer: the Next Generation. Cell 144 (5), 646–674. doi:10.1016/j.cell.2011.02.013
Harding, H. P., Zhang, Y., Zeng, H., Novoa, I., Lu, P. D., Calfon, M., et al. (2003). An Integrated Stress Response Regulates Amino Acid Metabolism and Resistance to Oxidative Stress. Mol. Cel 11 (3), 619–633. doi:10.1016/s1097-2765(03)00105-9
Inoue, S., Riley, J., Gant, T. W., Dyer, M. J. S., and Cohen, G. M. (2007). Apoptosis Induced by Histone Deacetylase Inhibitors in Leukemic Cells Is Mediated by Bim and Noxa. Leukemia 21 (8), 1773–1782. doi:10.1038/sj.leu.2404760
Islam, M. T., Ali, E. S., Uddin, S. J., Islam, M. A., Shaw, S., Khan, I. N., et al. (2018). Andrographolide, a Diterpene Lactone from Andrographis Paniculata and its Therapeutic Promises in Cancer. Cancer Lett. 420, 129–145. doi:10.1016/j.canlet.2018.01.074
Jamal-Hanjani, M., Wilson, G., McGranahan, N., Birkbak, N., Watkins, T., Veeriah, S., et al. (2017). Tracking the Evolution of Non-small-cell Lung Cancer, N. Engl. J. Med., 376(22), 2109–2121. doi:10.1056/NEJMoa1616288
Jiang, Q., Li, F., Shi, K., Wu, P., An, J., Yang, Y., et al. (2013). ATF4 Activation by the p38MAPK-eIF4E axis Mediates Apoptosis and Autophagy Induced by Selenite in Jurkat Cells. FEBS Lett. 587 (15), 2420–2429. doi:10.1016/j.febslet.2013.06.011
Kumar, D., Kumar, S., Gorain, M., Tomar, D., Patil, H. S., Radharani, N. N. V., et al. (2016). Notch1-MAPK Signaling Axis Regulates CD133+ Cancer Stem Cell-Mediated Melanoma Growth and Angiogenesis. J. Invest. Dermatol. 136 (12), 2462–2474. doi:10.1016/j.jid.2016.07.024
Lu, C.-L., Li, X., Zhou, H.-M., Zhang, C., Yang, Y.-Y., Feng, R.-L., et al. (2019). Randomised Controlled Trials of Traditional Chinese Medicine in Cancer Care Published in Chinese: an Overview. The Lancet 394, S26. doi:10.1016/s0140-6736(19)32362-1
Malhotra, V., and Perry, M. J. C. b. (2003). Classical Chemotherapy: Mechanisms, Toxicities and the Therapeutic Window, Cancer Biol. Ther. 2, S2–S4. doi:10.4161/cbt.199
Morsi, R. Z., Hage-Sleiman, R., Kobeissy, H., and Dbaibo, G. (2018). Noxa: Role in Cancer Pathogenesis and Treatment. Ccdt 18 (10), 914–928. doi:10.2174/1568009618666180308105048
Núñez-Vázquez, S., Sánchez-Vera, I., Saura-Esteller, J., Cosialls, A. M., Noisier, A. F. M., Albericio, F., et al. (2020). NOXA Upregulation by the Prohibitin-Binding Compound Fluorizoline Is Transcriptionally Regulated by Integrated Stress Response-Induced ATF3 and ATF4. FEBS J. 288(4), 1271–1285. doi:10.1111/febs.15480
Pérez-Galán, P., Roué, G., Villamor, N., Montserrat, E., and Colomer, D. J. B. (2006). The Proteasome Inhibitor Bortezomib Induces Apoptosis in Mantle-Cell Lymphoma through Generation of ROS and Noxa Activation Independent of P53 Status. Blood. 107(1), 257–264. doi:10.1182/blood-2005-05-2091
Peng, T., Hu, M., Wu, T., Zhang, C., Chen, Z., Huang, S., et al. (2015). Andrographolide Suppresses Proliferation of Nasopharyngeal Carcinoma Cells via Attenuating NF-Κb Pathway, Biomed. Res. Int., 2015, 735056. doi:10.1155/2015/735056
Rotow, J., and Bivona, T. G. (2017). Understanding and Targeting Resistance Mechanisms in NSCLC. Nat. Rev. Cancer 17 (11), 637–658. doi:10.1038/nrc.2017.84
Sharma, K., Vu, T. T., Cook, W., Naseri, M., Zhan, K., Nakajima, W., et al. (2018). p53‐independent Noxa Induction by Cisplatin Is Regulated by ATF3/ATF4 in Head and Neck Squamous Cell Carcinoma Cells. Mol. Oncol. 12 (6), 788–798. doi:10.1002/1878-0261.12172
Shibue, T., Takeda, K., Oda, E., Tanaka, H., Murasawa, H., Takaoka, A., et al. (2003). Integral Role of Noxa in P53-Mediated Apoptotic Response. Genes Develop. 17 (18), 2233–2238. doi:10.1101/gad.1103603
Tang, J. L., Zhan, S. Y., and Ernst, E. (1999). Review of Randomized Controlled Trials of Traditional Chinese Medicine, BMJ 319(7203):160–161.doi:10.1136/bmj.319.7203.160
Thomas, A., Liu, S. V., Subramaniam, D. S., and Giaccone, G. (2015). Refining the Treatment of NSCLC According to Histological and Molecular Subtypes. Nat. Rev. Clin. Oncol. 12 (9), 511–526. doi:10.1038/nrclinonc.2015.90
Wu, B., Chen, X., Zhou, Y., Hu, P., Wu, D., Zheng, G., et al. (2018). Andrographolide Inhibits Proliferation and Induces Apoptosis of Nasopharyngeal Carcinoma Cell Line C666-1 through LKB1-AMPK-dependent Signaling Pathways. Pharmazie 73 (10), 594–597. doi:10.1691/ph.2018/8583
Xu, L., Jiao, J., Sun, X., Sang, W., Gao, X., Yang, P., et al. (2020). Cladribine Induces ATF4 Mediated Apoptosis and Synergizes with SAHA in Diffuse Large B-Cell Lymphoma Cells. Int. J. Med. Sci. 17 (10), 1375–1384. doi:10.7150/ijms.41793
Yang, S., Evens, A. M., Prachand, S., Singh, A. T. K., Bhalla, S., David, K., et al. (2010). Mitochondrial-Mediated Apoptosis in Lymphoma Cells by the Diterpenoid Lactone Andrographolide, the Active Component of Andrographis Paniculata. Clin. Cancer Res. 16 (19), 4755–4768. doi:10.1158/1078-0432.Ccr-10-0883
Yang, T., Yao, S., Zhang, X., and Guo, Y. (2016). Development, and therapyAndrographolide Inhibits Growth of Human T-Cell Acute Lymphoblastic Leukemia Jurkat Cells by Downregulation of PI3K/AKT and Upregulation of P38 MAPK Pathways. Dddt 10, 1389–1397. doi:10.2147/dddt.S94983
Yao, W.-T., Wu, J.-F., Yu, G.-Y., Wang, R., Wang, K., Li, L.-H., et al. (2014). Suppression of Tumor Angiogenesis by Targeting the Protein Neddylation Pathway. Cel Death Dis 5 (2), e1059. doi:10.1038/cddis.2014.21
Zhang, W., Liang, Y., Li, L., Wang, X., Yan, Z., Dong, C., et al. (2019). The Nedd8‐activating Enzyme Inhibitor MLN 4924 ( TAK ‐924/Pevonedistat) Induces Apoptosis via c‐Myc‐Noxa axis in Head and Neck Squamous Cell Carcinoma. Cell Prolif 52 (2), e12536, doi:10.1111/cpr.12536
Zhao, J., Yang, G., Liu, H., Wang, D., Song, X., and Chen, Y. (2002). Determination of Andrographolide, Deoxyandrographolide and Neoandrographolide in the Chinese Herb Andrographis Paniculata by Micellar Electrokinetic Capillary Chromatography. Phytochem. Anal. 13 (4), 222–227. doi:10.1002/pca.644
Zhou, J., Zhang, S., Choon-Nam, O., and Shen, H.-M. (2006). Critical Role of Pro-apoptotic Bcl-2 Family Members in Andrographolide-Induced Apoptosis in Human Cancer Cells. Biochem. Pharmacol. 72 (2), 132–144. doi:10.1016/j.bcp.2006.04.019
Zhu, H., Yang, W., He, L.-j., Ding, W.-j., Zheng, L., Liao, S.-d., et al. (2012). Upregulating Noxa by ER Stress, Celastrol Exerts Synergistic Anti-cancer Activity in Combination with ABT-737 in Human Hepatocellular Carcinoma Cells. PLoS One 7 (12), e52333, doi:10.1371/journal.pone.0052333
Keywords: TCM, andrographolide, lung adenocarcinoma, apoptosis, ATF4, noxa
Citation: Zhang J, Li C, Zhang L, Heng Y, Xu T, Zhang Y, Chen X, Hoffman RM and Jia L (2021) Andrographolide Induces Noxa-Dependent Apoptosis by Transactivating ATF4 in Human Lung Adenocarcinoma Cells. Front. Pharmacol. 12:680589. doi: 10.3389/fphar.2021.680589
Received: 15 March 2021; Accepted: 09 April 2021;
Published: 29 April 2021.
Edited by:
Haiyang Yu, Tianjin University of Traditional Chinese Medicine, ChinaReviewed by:
Ping Chen, Zhengzhou University, ChinaZhen W Ang, Chinese Academy of Medical Sciences, China
Copyright © 2021 Zhang, Li, Zhang, Heng, Xu, Zhang, Chen, Hoffman and Jia. This is an open-access article distributed under the terms of the Creative Commons Attribution License (CC BY). The use, distribution or reproduction in other forums is permitted, provided the original author(s) and the copyright owner(s) are credited and that the original publication in this journal is cited, in accordance with accepted academic practice. No use, distribution or reproduction is permitted which does not comply with these terms.
*Correspondence: Lijun Jia, ljjia@shutcm.edu.cn
†These authors have contributed equally to this work and share first authorship