- 1Department of Oncology, Shanghai East Hospital, Tongji University School of Medicine, Shanghai, China
- 2Department of Medicine, Jinggangshan University, Ji’an, China
- 3International Education College, Jiangxi University of Traditional Chinese Medicine, Nanchang, China
- 4Department of Hepatobiliary Diseases, Affiliated Hospital of Jiangxi University of Traditional Chinese Medicine, Nanchang, China
- 5Department of Gastroenterology, The Second Affiliated Hospital of Nanchang University, Nanchang, China
Background: The etiology and carcinogenesis of hepatocellular carcinoma (HCC) are associated with various risk factors. Saponins extracted from Dioscorea zingiberensis C. H. Wright exhibit antitumor activity against HCC. This study aimed to investigate the effect and the underlying mechanism of Dioscorea Zingiberensis new saponin (ZnS) on HCC.
Methods: Human HCC cell lines, Huh7 and SMMC-7721, were treated with different concentrations of ZnS. Cell apoptosis was determined via flow cytometry assay. Differentially expressed lncRNAs (DElncRNAs) in ZnS-treated SMMC-7721 cells were determined through RNA-sequence. The role of lncRNA TCONS-00026762 in HCC was investigated gain of function analysis, along with cell proliferation, apoptosis, and invasion in HCC cells. A subcutaneous xenograft of SMMC-7721 cell lines was established to study the effects of TCONS-00026762 in vivo. The expression of apoptosis-related proteins was detected in vivo and in vitro via western blotting.
Results: ZnS inhibited the proliferation of HCC cell in a dose-dependent manner. ZnS could induce apoptosis in HCC cells. Illumina sequencing results showed that 493 DElncRNAs were identified in ZnS-treated SMMC-7721 cells. TCONS-00026762 expression was down-regulated in the ZnS-treated SMMC-7721 cells. TCONS-00026762 inhibited the effect of ZnS on the proliferation, apoptosis, and invasion of HCC cells. ZnS inhibited the tumor growth, while, TCONS-00026762 promoted tumor growth in vivo. Furthermore, ZnS and TCONS-00026762 regulated cell apoptotic pathways.
Conclusion: ZnS significantly inhibits the viability, apoptosis, invasion, and tumorigenicity of HCC cells by regulating the expression of TCONS-00026,762. Our findings provide novel insights into the potential role of lncRNA in HCC therapy.
Introduction
Hepatocellular carcinoma (HCC) is among top 10 cancers that exhibit high mortality rates worldwide. Over 840,000 new cases of HCC are reported and 780,000 people die of HCC annually (Ferlay et al., 2015; Bray et al., 2018; Yu et al., 2021). Around the world, the leading cause of liver cancer is chronic viral hepatitis, namely hepatitis B and C. However, due to the increase in hepatitis B vaccination, studies have predicted that the rising incidence of alcohol consumption and obesity worldwide will overtake viral hepatitis as the most common cause of HCC (Akinyemiju et al., 2017). And alcohol (ethanol) is already the most common cause of liver cancer in men (Akinyemiju et al., 2017), recognized as a Group 1 carcinogen by the International Agency for Research on Cancer (IARC) (Byrne and Targher, 2015). Alcohol is mainly detoxified and metabolized by the liver, and the intermediate product of metabolism, acetaldehyde, has a direct toxic effect on liver cells. Long-term intake of large amounts will undoubtedly increase the burden on the liver and accelerate liver damage, and alcohol can stimulate the secretion of the pituitary gland, accelerate the speed of cell division, increase the susceptibility to cancer, and thus easily induce alcoholic liver, alcoholic cirrhosis, and even liver cancer (Dunn and Shah, 2016). Due to the difficulty of early diagnosis, rapid disease development, and lack of targeted medicines, the survival rate of hepatocellular carcinoma is very limited (Fu and Wang, 2018). Most patients presenting with very advanced HCCs are untreatable and die within 3–6 months (Ferlay et al., 2015). Hence, it is important to explore new biomarkers and evaluate effective agents for diagnosis and treatment of HCC.
Long non-coding RNA (lncRNA) is a non-coding RNAs exceeding 200 nucleotides (nt) in length. Recent studies have confirmed that lncRNA plays a potentially complex and diverse role in the etiology, carcinogenesis, invasion, and metastasis of human cancers, including HCC (Yuan et al., 2014; Wang et al., 2015; Li et al., 2016). LncRNA regulates the expression of tumor suppressor/anticancer genes, mainly by sponging miRNAs and regulating mRNAs (Wang et al., 2015; Li et al., 2016; Lei et al., 2019). For example, Li et al., reported that long non-coding RNA HULC (highly up-regulating in liver cancer) expression activated ZEB1 (Zinc finger E-box binding homeobox 1)-induced epithelial-mesenchymal transition (EMT) by sponging miR-200a-3p (Bray et al., 2018). Long noncoding RNA NR2F1-AS1 (nuclear receptor subfamily 2 group F member 1 antisense RNA 1) and ARSR (Activated in RCC with sunitinib Resistance) are associated with drug resistance in HCC cells via modulation of signaling pathways and miRNA or mRNAs (Li et al., 2017; Huang et al., 2018). Accordingly, the dysregulation of lncRNA in HCC is associated with diverse biological processes in HCC cells, such as cell differentiation, proliferation, invasion, metastasis, and apoptosis (Li et al., 2015; Yang et al., 2017).
Some signal transductions mediated by lncRNA are closely related to the anticancer mechanism of various drugs. It has been reported that saponins extracted from plants belongs to the family Dioscoreaceae, including Dioscorea zingiberensis C. H. Wright, exhibit antitumor activity against HCC (Zhou et al., 2009; Zhao et al., 2018). D. zingiberensis C. H. Wright is rich in saponins, including protodioscin, dioscin, scutellaria, trifoliate, glucose triglucoside, glucose diglucoside and trillium glucoside (Zhu et al., 2010; Lei et al., 2011; Zhang et al., 2015). Saponins induce apoptosis and cell cycle arrest via the extracellular regulated protein kinase (ERK)/nuclear factor κB (NF-κB) signaling pathway in human HCC HepG2 cells (Auyeung et al., 2009; Long et al., 2015). Following, Qiu et al. confirmed that Rhizoma Paridis saponins through inhibiting cancer cellular metabolism to suppress proliferation in hepatoma H22 tumor murine (Qiu et al., 2016). In addition, Rhizoma Paridis saponin treatment reversed sorafenib-resistance in HCC cells (Yao et al., 2018). D. zingiberensis C. H. Wright, also known as turmeric, is a perennial herbaceous plant of the genus Dioscorea and a unique species cultivated in China. The main active ingredient in the rhizome of D. zingiberensis is a saponin, which displays antitussive, antiasthmatic, antithrombostic, and cholesterol lowering activities, in addition to preventing arteriosclerosis (Gong et al., 2011; Li et al., 2011; Du et al., 2016). The results of our previous study indicated that HCC cell proliferation was significantly reduced when treated with Zingiberensis new saponin (ZnS) from D. zingiberensis C. H. Wright. However, the mechanism underlying of antitumor activity of ZnS against HCC cells remains unclear.
In this study, we demonstrated that ZnS suppresses proliferation of HCC cells by inhibiting the expression of lncRNA TCONS-00026,762. The chemical structure of ZnS is shown in Figure 1A. We determined the differentially expressed lncRNAs by identifying the lncRNA profiles in HCC cells treated with ZnS. Overexpression of TCONS-00026762, via transfection into HCC cells, was assessed in order to investigate mechanisms underlying in HCC. ZnS and TCONS-00026762 regulated the proliferation of HCC cells and tumor growth by inducing cell apoptosis. Moreover, the expression of apoptosis-related proteins was examined, and it was demonstrated that ZnS, a naturally occurring phytochemical agent, inhibited HCC by regulating TCONS-00026762.
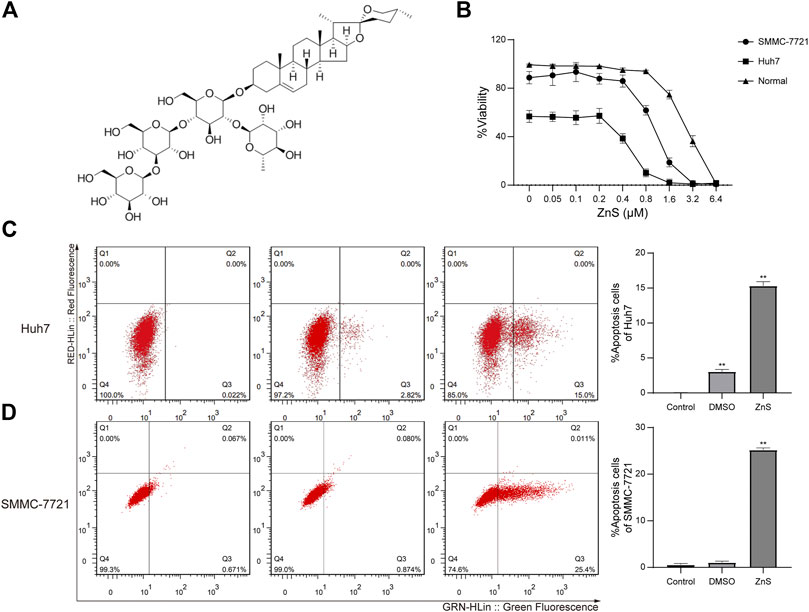
FIGURE 1. (A) The chemical structure of ZnS. (B) The viability rates of human normal liver cell line, HL-7702, and human HCC cell lines, Huh7 and SMMC-7721, treated with ZnS, were examined using CCK-8. Detection of apoptosis rates in two cell lines, Huh7 (C) and SMMC-7721 (D), after ZnS treatment by flow cytometry. Each trial was replicated at least three times. Data are expressed as mean ± SD, **, p < 0.01.
Material and Methods
Cell Lines, Culture Conditions, Plasmids, and Reagents
Human normal liver cell line, HL-7702, and human HCC cell lines, Huh7 and SMMC-7721, were purchased from the Chinese Academy of Sciences (Beijing, China). All cells were maintained in Dulbecco’s modified Eagle’s medium (DMEM, Hyclone, Thermo Scientific, Epsom, United Kingdom) supplemented with 10% fetal bovine serum (FBS, Hyclone) and 1% penicillin-streptomycin (Hyclone) at 37°C in 5% CO2. Full-length lncRNA TCONS-00026762 was amplified via PCR and then subcloned into the vector, pCDH-CMV-MCS-EF1-copGFP-T2A-Puro.
Cell Treatment
Cells were grown in 96-well plates (5 × 103 cells/well) and treated with ZnS (S12537, HPLC ≥98%, C51H82O22, Shanghai Hewu Biotechnology Co., Ltd., Shanghai, China) at concentrations of 0.05, 0.1, 0.2, 0.4, 0.8, 1.6 3.2, and 6.4 μM for 48 h. Cells treated with DMSO were regarded as the control. IC50 values of ZnS in the two HCC cell lines were detected using a cell viability assay.
Cell Viability Assay
After being treated with 48 h ZnS, cells were harvested, and the cell viability was assessed using a Cell Counting Kit 8 (CCK-8, Dojindo Laboratories, Kumamoto, Japan), according to the manufacturer’s instructions. Optical density at 450 nm absorbance was detected using a microplate spectrophotometer (Multiskan GO, Thermo Scientific). Six replicates were performed for each experiment. The IC50 values of ZnS in the two cell lines were also calculated.
Cell Apoptosis Assay
Cell apoptosis was analyzed by Annexin V-fluorescein isothiocyanate (FITC) and PI (Beyotime Institute of Biotechnology). Cells treated with ZnS for 48 h were harvested via centrifugation at 1,200 rpm/min for 1 min, and suspended in 1× binding buffer. The cells were then incubated with Annexin V-FITC solution in the dark at 4°C for 10–15 min, followed by a second harvesting (centrifugation at 1,200 rpm/min and suspension). Cells were then subjected to PI staining for observation via a BD FacScanto II flow cytometer (BD Biosciences, San Jose, CA, United States).
RNA-Sequencing and Computational Analysis
Total RNA was extracted using TRIzol (Invitrogen, Carlsbad, CA, United States). RNA qualification and quantification were conducted using a NanoDrop ND-2100 spectrophotometer (NanoDrop Technologies, Wilmington, DE, United States) and an Agilent 2,100 Bioanalyzer (Agilent Technologies, Palo Alto, CA, United States). Certified samples (RNA Integrity Number >7.0 and OD260/OD280 > 1.8) were subjected to elimination of ribosomal RNA (rRNA), using a Ribo-Minus kit (Thermo Fish, Waltham, MA, United States) and RNA fragmentation, via using fragmentation buffer (Ambion, Austin, TX, United States).
First strand cDNA and double strand DNA (dsDNA) were subsequently synthetized using reverse transcriptase (SuperScript III, Invitrogen), random primers, and dNTPs. Thereafter, cDNA samples were purified and ligated to adenylate 3′-ends and sequencing adaptors, followed by purification (XP beads, Beckman Coulter, Brea, CA, United States). Next, dsDNA containing uracil deoxyribonucleotides (U base) was degraded. Finally, PCR enrichment followed by purification was performed to obtain sequencing libraries. An Illumina HiSeq 4,000 pair-end sequencing (2 × 150 bp) platform was used.
LncRNA Profile Analysis
Reference-based assemblies of three samples in each group were merged using Cuffmerge (version 2.2.1, http://cole-trapnell-lab.github.io/cufflinks/cuffmerge/). Known lncRNAs were identified using the NONCODE database (http://www.bioinfo.org/noncode/). Novel lncRNAs in the assemblies were detected using the following criteria: ≥200 nt in length, Coding Potential Calculator (CPC) score ≤ 0, Coding Potential Assessment Tool (CPAT) probability ≤0.364 and phyloCSF score ≤ −20. Fragment perkilobase of transcript per million fragments mapped (FPKM) values were used to calculate the expression levels of the transcripts. Differentially expressed lncRNAs (DElncRNAs) were identified based on FPKM values under conditions of p < 0.05 and |log2FC| >1.
Quantitative Real-Time PCR (qRT-PCR)
SMMC-7721 cells grown in six-well plates were transfected with plasmids. After 24h, the cells were either treated with 1 μM ZnS for 24 h or left untreated and were then harvested. Total RNA was extracted using TRIzol reagent (Invitrogen, United States) in accordance with the manufacturer’s instructions. Thereafter, cDNA was synthesized from total RNA using a PrimeScript RT reagent kit (Tiangen, China). GAPDH mRNA was used as an internal control. The primer sequences used were as follows. TCONS-00026762 forward primer: 5′-AAT GAG GAG CAG GTT GGA CT-3′, TCONS-00026762 reverse primer: 5′-GAT CAC TTC CTC ACC TGG CT-3'; GAPDH forward primer: 5′-GGT GAA GGT CGG AGT CAA CG-3′, GAPDH reverse primer: 5′-CAA AGT TGT CAT GGA TGA ACC-3'. Finally, the expression was determined using the SYBR@ Green reagent kit (Roche, Indianapolis, IN, United States).
Construction of Stable Cell Lines
SMMC-7721 cells were infected with lentivirus carrying TCONS-00026,762 or control lentivirus (Igebiotech, Guangzhou, China). The efficacy of TCONS-00026762 overexpression was detected via fluorescence analysis.
Matrigel Invasion Assay
First, 4 × 105 cells were seeded into the upper chamber coated with Matrigel (BD Biosciences, San Diego, CA, United States) and incubated in 200 μL serum-free media. The lower chambers were filled with 600 μL complete medium for 24 h. After removing cells on the upper surface, transwell filters were fixed with paraformaldehyde and stained with crystal violet (RiboBio, Guangzhou, China). The experiment was performed in triplicate. Invading cells were captured using a Motic inverted microscope (AE30, Motic, Hong Kong, China), and the average number of invading cells in three randomly selected fields was calculated.
Tumor Formation in Nude Mice
This animal study and all animal experiments were approved by the Medical Experimental Animal Care Commission of Shanghai East Hospital (Approval number: 2019-52). Animal experiments were performed in accordance with the National Institutes of Health Guide for the Care and Use of Laboratory Animals. Twenty 4-week-old male BALB/c nude mice were randomly assigned to control group (SMMC-7721 cells), ZnS group (SMMC-7721 cells), ZnS + NC group (SMMC-7721 cells with LV-control) and ZnS + TCONS-00026762 (SMMC-7721 with overexpression-TCONS-00026762). The mice were orally administered ZnS at 60 mg/kg/day. Three weeks later, all mice were sacrificed, and the weight of each tumor was measured. Tumor tissues were integrally stripped out.
Immunohistochemistry
Paraffin-embedded slides of xenograft tumors from the nude mice were dewaxed with xylene I, II, and III for 10 min each, and 100, 90, 70, and 50% alcohol for 2 min each, followed by washing with PBS three times. Samples were subsequently heated in 0.01 M citrate buffer (pH 6.0), incubated in serum for 30 min at 37°C and finally incubated overnight at 4°C with anti-ki67 antibodies. The slides were then incubated with secondary antibodies for 2 h at 37°C, and washed with PBS. After development with DAB-H2O2 for 10 min at room temperature, slides were observed for staining under a light microscope.
Western Blotting
Lysis buffer, containing 10% glycerol, 2.3% SDS, 62.5 mM Tris, pH 6.8 150 mM NaCl, 10 mM EDTA, 1 μg/ml eupeptic, 1 μg/ml pepstatin, 5 μg/ml chymostatin, 1 μg/ml aprotinin, and 1 mM phenylmethylsulphonyl fluoride, was used to collect samples. Thereafter, 8% SDS- PAGE and polyvinylidene difluoride (PVDF) membranes were used to separate and transfer the protein, respectively. Blots were incubated with anti-AKT, anti-p-AKT, anti-ERK1/2, anti-p-ERK1/2, anti-Bcl-2, anti-pro-caspase 8, or anti- PARP-cleaved, respectively. Proteins were then washed three times with TBST for 5 min. Finally, the membranes were incubated with secondary antibodies for 1 h. The results were analyzed using an ECL detection system. GAPDH was used as the reference protein.
Statistical Analysis
All experimental data were expressed as mean ± SD. Statistical analyses were performed using GraphPad Prism. Differences between groups were analyzed using Student’s unpaired t-test. IC50 values of ZnS in cell lines were calculated via nonlinear regression (curve fit). The 95 confidence intervals were calculated. Differences in the IC50 values of ZnS in the two cell lines were compared using the unpaired t-test. Statistically significance was set at p < 0.05.
Results
ZnS Inhibited the Proliferation of HCC Cells
The inhibitive effect of ZnS on HCC cell growth was studied by performing cell viability assays with different concentrations of ZnS (0–6.4 μM). The viability rate of the two HCC cell lines was inhibited by ZnS in a dose-dependent manner (Figure 1B), while the viability rate of normal cells remained stable. The IC50 value of ZnS in Huh7 cells was determined to be 0.51 μM, and that in SMMC-7721 cells was 1.0 μM. The IC50 values of ZnS in the two cell lines were significantly different. Thus, ZnS was able to effectively inhibit the growth of HCC cells but had less influence at low and medium concentrations.
ZnS Promoted Apoptosis in HCC Cells
In order to determine the inhibitory effect of ZnS on HCC cells, we investigated the effect of ZnS on the apoptosis of HCC cells. Huh7 and SMMC-7721 cells were treated for 48 h with 0.51 μM ZnS and 1.0 μM ZnS, respectively. The cell apoptosis percentages of Huh7 and SMMC-7721 cells were increased significantly by ZnS treatment compared with those of the blank controls (Figures 1C,D). In addition, we found that the apoptosis percentage of the Huh7 cells was increased by DMSO treatment compared with blank control. These results suggested that the administration of ZnS to HCC cells significantly increased cell apoptosis. Because of the stronger inhibitive effect of ZnS in SMMC-7721, SMMC-7721 cell line was selected for further sequencing analysis.
LncRNA Profiling and Validation
SMMC-7721 cells were treated with 1.0 μM ZnS or DMSO for 48 h before total cellular RNA was isolation. High-throughput sequencing was performed to detect lncRNAs. A total of 493 DElncRNAs, including 267 up-regulated and 226 down-regulated lncRNAs, were identified as differentially expressed lncRNAs in ZnS-treated SMMC-7721 cells (Figure 2A). To find lncRNA that might be related to the development and progression of hepatocellular carcinoma, we confirmed the location of top 30 differentially expressed lncRNAs and predicted their adjacent/localized genes through Ensemble BLAST. The results showed that the TCONS-00026762 is located on chromosome 10p15.1 and largely overlaps aldo-keto reductase family 1 member C1 (AKR1C1). AKR1C1 is reportedly involved in the initiation and progression of cancer (Chang et al., 2019; Huebbers et al., 2019; Wang et al., 2019; Zhao et al., 2019). So, lncRNA TCONS-00026762 was selected for further investigation. The result of qRT-PCR showed that the expression of TCONS-00026762 was markedly down-regulated in the ZnS group (Figure 2B).
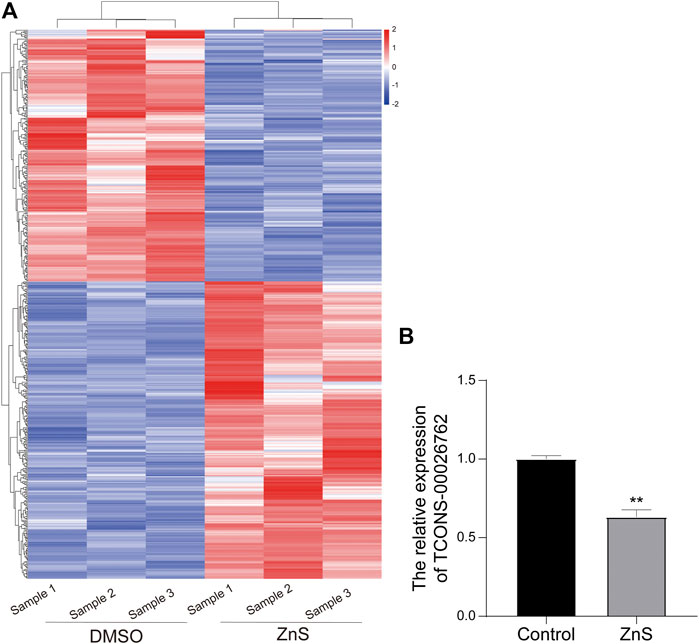
FIGURE 2. Analysis of differentially expressed lncRNAs treated with ZnS. (A) The heatmap of differentially expressed lncRNAs between DMSO and ZnS groups. (B) The qRT-PCR analysis of TCONS-00026,762 expression. Each trial was replicated at least three times. Data are expressed as mean ± SD, **, p < 0.01.
TCONS-00026762 Regulated the Movability of HCC Cells
TCONS-00026762 over-expression was established in Hun-7 and SMMC-7721 cell lines via lentiviral infection. ZnS significantly inhibited the proliferation of HCC cells. However, TCONS-00026762 could enhance the viability of HCC cells (Figure 3A). The flow cytometry analysis results were consistent with the above findings. ZnS treatment significantly induce apoptosis in HCC cells than in the blank control. TCONS-00026762 protected HCC cells from apoptosis (Figure 3B). Furthermore, transwell assays showed that ZnS significantly inhibited HCC cell invasion, while TCONS-00026762 promoted HCC cell invasion (Figure 3C).
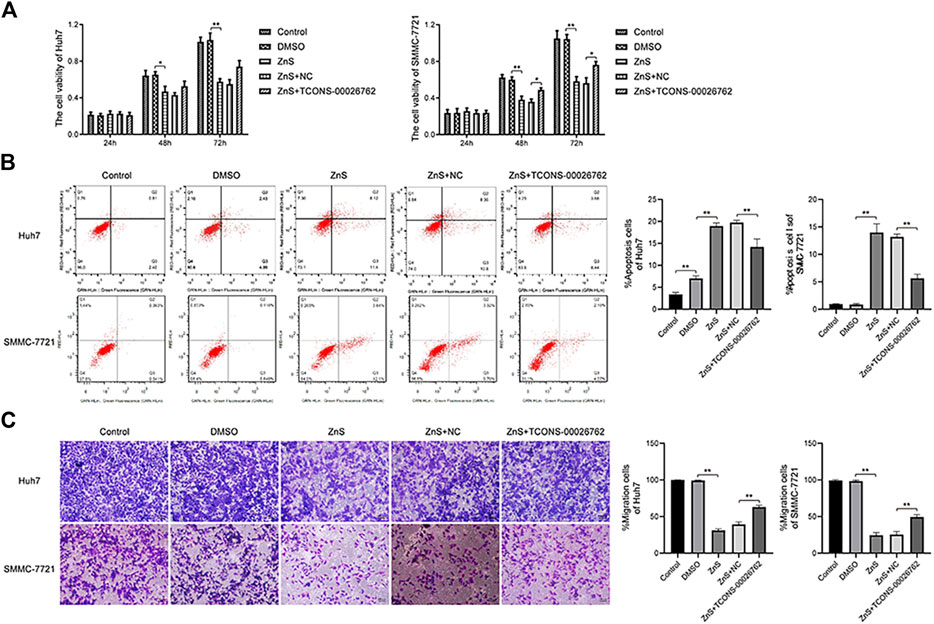
FIGURE 3. TCONS-00026,762 regulated the movability of HCC cells. (A) The viability rates of Huh7 and SMMC-7721 cells. (B) The flow cytometry analysis results and apoptosis rates of Huh7 and SMMC-7721 cells. (C) The invasion of Huh7 and SMMC-7721 cells were detected by transwell. Scale bar, 100 μm. Each trial was replicated at least three times. Data are expressed as mean ± SD, *, p < 0.05, **, p < 0.01.
TCONS-00026762 Regulated SMMC-7721-Derived Tumor Progression
We constructed a xenograft model by subcutaneously implanting SMMC-7721 cells in nude mice. Following 28 days of culturing, ZnS significantly inhibited tumor growth, while TCONS-00026762 caused significantly tumor formation (Figures 4A–F).
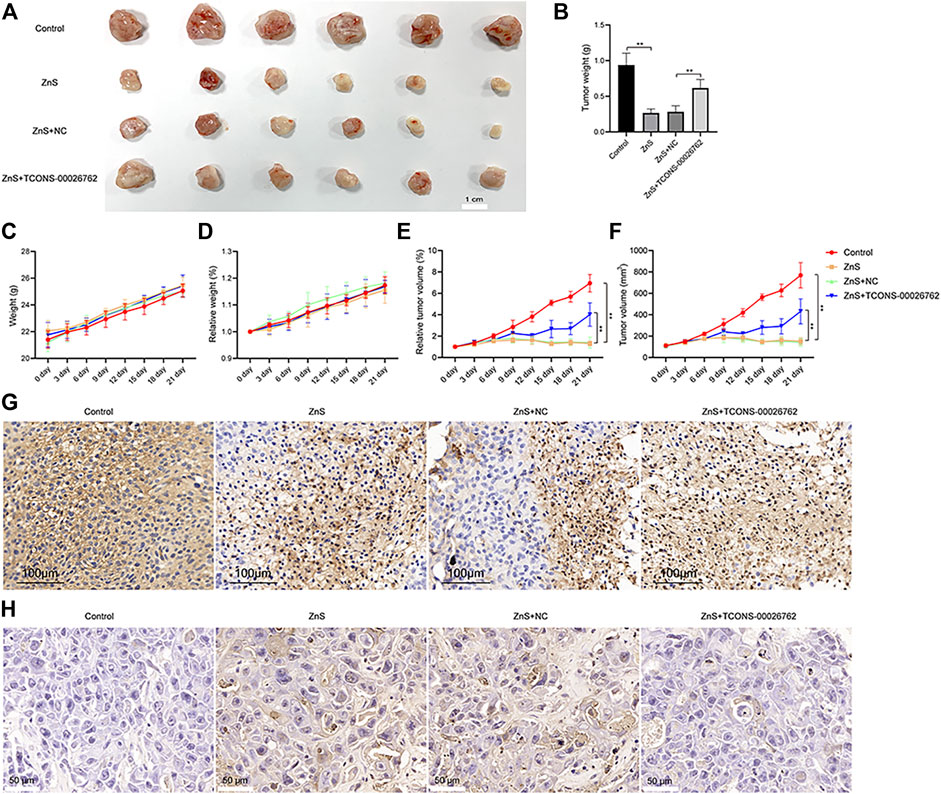
FIGURE 4. The inhibitory effect of ZnS and TCONS-00026762. (A) SMMC-7721 cells were subcutaneously injected into the right flank of nude mice to establish tumors. Scale bar, 1 cm. (B) The tumor weight measured (C and D) The weight and relative weight of nude mice were measured (E and F) The tumor volume and tumor relative weight were measured (G) Immunohistochemical detection of ki67 expression in tumor tissue. Scale bar, 100 μm (H) The apoptosis of tumor tissue was analyzed by TUNNEL. Scale bar, 50 μm. Error bars represents mean ± SD, **, p < 0.01.
Ki67 protein is a widely used cellular marker of proliferation present during all active phases of the cell cycle (Zhao et al., 2017). We examined the expression of ki67 in tumors via immunohistochemistry (Figure 4G). The results indicated that ZnS suppressed the expression of ki67, whereas TCONS-00026762 improved ki67 expression. Furthermore, we detected cell apoptosis in tumors via TUNNEL. The results showed that ZnS induced apoptosis in tumor tissues, whereas TCONS-00026762 could reduce apoptosis (Figure 4H). The results above suggested that ZnS induced apoptosis in SMMC-7721-derived tumor progression by regulating the expression of TCONS-00026762.
TCONS-00026762 Regulated the Expression of Apoptosis-Related Proteins
Based on above results, we examined the expression of apoptosis-related proteins in vitro and in vivo. The results of the western blotting assay showed that the expressions of p-AKT, p-ERK1/2, Bcl-2 and pro-caspase8 were down-regulated in cells and tumor tissues treated with ZnS, while the expression of PARP-cleaved was up-regulated (Figure 5). In addition, TCONS-00026762 could up-regulated the phosphorylation of AKT and ERK1/2 and the expression of Bcl-2 and caspase8, and down-regulated the expression of PARP-cleaved (Figure 5). Overall, these results indicate that ZnS regulated the expression of apoptosis-related proteins by reducing the expression of TCONS-00026,762, there by inducing cell apoptosis.
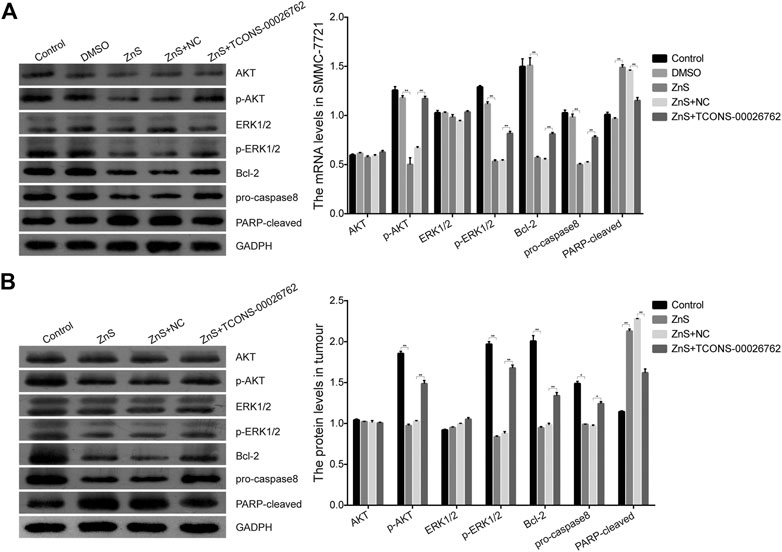
FIGURE 5. The expression levels of apoptosis-related proteins in SMMC-7721 cells (A) and tumor tissue (B). Each treatment was replicated three times. Error bars represents mean ± SD, *, p < 0.05, **, p < 0.01.
Discussion
HCC, which is widely considered as one of the most aggressive and lethal malignant tumors, is a great threat to human health (Ferlay et al., 2015; Bray et al., 2018). Therefore, it is crucial to identify effective drugs or treatments that can reduce the risk of metastasis and recurrence of HCC. Traditional Chinese medicines or natural extracts, which display high levels of efficacy and low toxicity compared to other drugs, have shown much promise in this direction (Karimi et al., 2013; Giordani et al., 2015). ZnS is used in the treatment of various cancers and therefore may be an effective drug against HCC. We investigated the inhibitory effect of ZnS on HCC as well as the regulation mechanisms underlying lncRNA expression.
Reportedly, ZnS exerts cytotoxic activity against many cancers, such as colon carcinoma, lung carcinoma and melanoma (Tong et al., 2012). ZnS inhibited proliferation and induced apoptosis in C26 cells (colon carcinoma), HepG2 (HCC), A549 (lung carcinoma) and B16 (melanoma) (Tong et al., 2012). Zhao et al., reported that the zingiberenesis saponin I increased the expression levels of cleaved PARP, caspase-3/9, and Bax, and reduced Bcl-2 in C26 cells. Administering zingiberenesis saponin I promoted DNA fragmentation in C26 cells was (Qingbing et al., 2010). Our present study suggested that administering ZnS to Huh7 and SMMC-7721 cells promoted cell apoptosis and inhibited proliferation in a dose-dependent manner. These results suggested that ZnS exerts an antitumor effect on HCC cells by inhibiting cell proliferation and promoting apoptosis.
The anticancer mechanism of ZnS may be closely related to lncRNA. It has been reported that ingredients of D. zingiberensis Wright, such as saponin and dioscin, inhibited the growth of gastric cancer cells (HGC-27, MGC803 and SGC7901) by decreasing the expression of lncRNA HOTAIR (Ma et al., 2016). Our Illumina sequencing analysis showed that ZnS induced dysregulation of lncRNA in SMMC-7721 cells. These results suggested that these DElncRNAs play important roles in ZnS-mediated antitumor effects in HCC cells. The novel lncRNA TCONS-00026762 attracted our attention due to its largely unknown role in HCC progression. Blast in NCBI, TCONS-00026762 largely overlaps with the promoter and exon of AKR1C1. AKR1C1 is a member of the human aldo-keto reductase family, which catalyze NADPH-dependent reductions (Dhagat et al., 2008; Rizner and Penning, 2014; Zhu et al., 2018). Mounting evidence reveals that AKR1C1 is volved in a variety of cancers, including hepatocellular carcinoma, lung cancer, gastric cancer, and cervical cancer, and the over-expression of AKR1C1 has been found to be associated with carcinogenesis (Hsu et al., 2001; Ji et al., 2004; Fukumoto et al., 2005; Woenckhaus et al., 2006; Seo et al., 2007; Chang et al., 2009; Chien et al., 2009; Rizner, 2012; Matsunaga et al., 2014). In addition, the low expression of AKR1C1 may slow down the progression of cancer (Klippel et al., 2012). All these results suggest that AKR1C1 plays an important role in carcinogenesis. Therefore, TCONS-00026762 was selected for further experiments.
In order to investigate the function of TCONS-00026762 in HCC progression, TCONS-00026762 was over-expressed in the SMMC-7721 cell line via lentivirus packaging. The results showed that TCONS-00026762 could reduce the effect of ZnS on HCC cells, suggesting that ZnS inhibits cell proliferation and invasion and induces cell apoptosis by suppressing the expression of TCONS-00026762. To determine the effect of TCONS-00026762 on tumorigenesis in vivo, we constructed mouse transplanted tumor models, using in nude mice transplanted with SMMC-7721 cells. ZnS dramatically suppressed the tumor growth and promoted cell apoptosis, as expected. Furthermore, ZnS significantly inhibited the effect of TCONS-00026762 on tumor progression.
Cell apoptosis is a form of programmed cell death, which is regulated by multiple genes and it is an effective cellular mechanism against cancer (Renault and Chipuk, 2014; Song et al., 2018). According to a previous study, PI3K/AKT signaling pathway plays an important role in cell apoptosis. Inhibition of PI3K/Akt signaling pathway could inhibit tumor growth and promote apoptosis (Fang et al., 2007; Zhou et al., 2020). ERK1 and ERK2, collectively referred to as ERK 1/2, regulate multiple biological processes including development, apoptosis, and inflammation. (Joshi, 2014). Bcl-2, an apoptosis inhibitory protein, play an important role in the regulation of apoptosis. Inhibition of Bcl-2 promoted the apoptosis (Shimizu et al., 1999). Pro-caspase8 is reportedly activated by autocatalytic cleavage, and activated caspase-8 induces cell death (Muzio et al., 1998). PARP is a key enzyme in the detection of DNA damage and occurrence. PARP is cleaved to 89-kDa fragment form, which could induce apoptosis through the access of endonucleases to chromatin. In the present study, we observed that ZnS significantly inhibit the activation of the p-Akt, p-ERK, Bcl-2 and pro-caspase8 and up-regulated expression and cleavage of PARP. And TCONS-00026762 offset the effects of ZnS, indicating that ZnS promotes the apoptosis of HCC cells by suppressing the expression of TCONS-00026762.
This study was beset by some limitations. TCONS-00026762 largely overlaps with AKR1C1, indicating a possible association between TCONS-00026762 and AKR1C1. This relationship was not validated in this study. Therefore, the combined effect of TCONS-00026762 and AKR1C1 on HCC progression requires further investigation. Additionally, due to the enhanced tissue specificity of lncRNAs and the ability of lncRNAs to function as competing endogenous RNAs (ceRNAs) to regulate microRNA/mRNA, therapeutic targeting of lncRNAs may result in fewer off-target effects than other therapeutic strategies (Ballantyne et al., 2016). These characteristics highlight the idea that therapeutic approaches that target lncRNAs may be faster and more efficient than methods that target proteins. Accordingly, we suggest that lncRNAs could be utilized as effective therapeutic targets for HCC in future.
In conclusion, ZnS, as a traditional Chinese medicine, not only inhibited the growth and invasion capability of HCC cells, but also promoted the apoptosis of HCC cells and tumors by suppressing lncRNA TCONS-00026762 expression. These findings suggest that ZnS could be an effective drug for the treatment of HCC in the future.
Data Availability Statement
The data presented in the study are deposited in the BioProject database, accession number: PRJNA718909.
Ethics Statement
The animal study was reviewed and approved by the Medical Experimental Animal Care Commission of Shanghai East Hospital.
Author Contributions
Conception and design of the research: ZW; Acquisition of data: XL; Analysis and interpretation of data: PZ; Statistical analysis: KH; Obtaining funding: YG; Drafting the manuscript: XL; Revision of manuscript for important intellectual content: YG. All authors contributed to manuscript revision, read, and approved the submitted version.
Funding
This work was supported by grants from the National Natural Science Foundation of China (Grant No. 81472576).
Conflict of Interest
The authors declare that the research was conducted in the absence of any commercial or financial relationships that could be construed as a potential conflict of interest.
Supplementary Material
The Supplementary Material for this article can be found online at: https://www.frontiersin.org/articles/10.3389/fphar.2021.678620/full#supplementary-material.
References
Akinyemiju, T., Akinyemiju, T., Abera, S., Ahmed, M., Alam, N., Alemayohu, M. A., et al. (2017). The Burden of Primary Liver Cancer and Underlying Etiologies from 1990 to 2015 at the Global, Regional, and National Level: Results from the Global Burden of Disease Study 2015. JAMA Oncol. 3 (12), 1683–1691. doi:10.1001/jamaoncol.2017.3055
Auyeung, K. K., Law, P. C., and Ko, J. K. (2009). Astragalus Saponins Induce Apoptosis via an ERK-independent NF-kappaB Signaling Pathway in the Human Hepatocellular HepG2 Cell Line. Int. J. Mol. Med. 23 (2), 189–196. doi:10.3892/ijmm_00000116
Ballantyne, M., McDonald, R., and Baker, A. (2016). lncRNA/MicroRNA Interactions in the Vasculature. Clin. Pharmacol. Ther. 99 (5), 494–501. doi:10.1002/cpt.355
Bray, F., Ferlay, J., Soerjomataram, I., Siegel, R. L., Torre, L. A., and Jemal, A. (2018). Global Cancer Statistics 2018: GLOBOCAN Estimates of Incidence and Mortality Worldwide for 36 Cancers in 185 Countries. CA: A Cancer J. Clinicians 68 (6), 394–424. doi:10.3322/caac.21492
Byrne, C. D., and Targher, G. (2015). NAFLD: a Multisystem Disease. J. Hepatol. 62 (1 Suppl. l), S47–S64. doi:10.1016/j.jhep.2014.12.012
Chang, H.-C., Chen, Y.-L., Chan, C.-P., Yeh, K.-T., Kuo, S.-J., Ko, C.-J., et al. (2009). Overexpression of Dihydrodiol Dehydrogenase as a Prognostic Marker in Resected Gastric Cancer Patients. Dig. Dis. Sci. 54 (2), 342–347. doi:10.1007/s10620-008-0350-7
Chang, W.-M., Chang, Y.-C., Yang, Y.-C., Lin, S.-K., Chang, P. M.-H., and Hsiao, M. (2019). AKR1C1 Controls Cisplatin-Resistance in Head and Neck Squamous Cell Carcinoma through Cross-Talk with the STAT1/3 Signaling Pathway. J. Exp. Clin. Cancer Res. 38 (1), 245. doi:10.1186/s13046-019-1256-2
Chien, C.-W., Ho, I.-C., and Lee, T.-C. (2009). Induction of Neoplastic Transformation by Ectopic Expression of Human Aldo-Keto Reductase 1C Isoforms in NIH3T3 Cells. Carcinogenesis 30 (10), 1813–1820. doi:10.1093/carcin/bgp195
Dhagat, U., Endo, S., Sumii, R., Hara, A., and El-Kabbani, O. (2008). Selectivity Determinants of Inhibitor Binding to Human 20α-Hydroxysteroid Dehydrogenase: Crystal Structure of the Enzyme in Ternary Complex with Coenzyme and the Potent Inhibitor 3,5-Dichlorosalicylic Acid†. J. Med. Chem. 51 (15), 4844–4848. doi:10.1021/jm8003575
Du, D., Zhang, R., Xing, Z., Liang, Y., Li, S., Jin, T., et al. (2016). 9,10-Dihydrophenanthrene Derivatives and One 1,4-anthraquinone Firstly Isolated from Dioscorea Zingiberensis C. H. Wright and Their Biological Activities. Fitoterapia 109, 20–24. doi:10.1016/j.fitote.2015.11.022
Dunn, W., and Shah, V. H. (2016). Pathogenesis of Alcoholic Liver Disease. Clin. Liver Dis. 20 (3), 445–456. doi:10.1016/j.cld.2016.02.004
Fang, J., Ding, M., Yang, L., Liu, L.-Z., and Jiang, B.-H. (2007). PI3K/PTEN/AKT Signaling Regulates Prostate Tumor Angiogenesis. Cell Signal. 19 (12), 2487–2497. doi:10.1016/j.cellsig.2007.07.025
Ferlay, J., Soerjomataram, I., Dikshit, R., Eser, S., Mathers, C., Rebelo, M., et al. (2015). Cancer Incidence and Mortality Worldwide: Sources, Methods and Major Patterns in GLOBOCAN 2012. Int. J. Cancer 136 (5), E359–E386. doi:10.1002/ijc.29210
Fu, J., and Wang, H. (2018). Precision Diagnosis and Treatment of Liver Cancer in China. Cancer Lett. 412, 283–288. doi:10.1016/j.canlet.2017.10.008
Fukumoto, S.-i., Yamauchi, N., Moriguchi, H., Hippo, Y., Watanabe, A., Shibahara, J., et al. (2005). Overexpression of the Aldo-Keto Reductase Family Protein AKR1B10 Is Highly Correlated with Smokers' Non-small Cell Lung Carcinomas. Clin. Cancer Res. 11 (5), 1776–1785. doi:10.1158/1078-0432.CCR-04-1238
Giordani, M. A., Collicchio, T. C. M., Ascêncio, S. D., de Oliveira Martins, D. T., Balogun, S. O., Bieski, I. G. C., et al. (2015). Hydroethanolic Extract of the Inner Stem Bark of Cedrela Odorata Has Low Toxicity and Reduces Hyperglycemia Induced by an Overload of Sucrose and Glucose. J. Ethnopharmacology 162, 352–361. doi:10.1016/j.jep.2014.12.059
Gong, G., Qin, Y., and Huang, W. (2011). Anti-thrombosis Effect of Diosgenin Extract from Dioscorea Zingiberensis C.H. Wright In Vitro and In Vivo. Phytomedicine 18 (6), 458–463. doi:10.1016/j.phymed.2010.08.015
Hsu, N. Y., Ho, H. C., Chow, K. C., Lin, T. Y., Shih, C. S., Wang, L. S., et al. (2001). Overexpression of Dihydrodiol Dehydrogenase as a Prognostic Marker of Non-small Cell Lung Cancer. Cancer Res. 61 (6), 2727–2731. doi:10.1002/1097-0142(20010315)91:6<1185::AID-CNCR1116>3.0.CO;2
Huang, H., Chen, J., Ding, C. M., Jin, X., Jia, Z. M., and Peng, J. (2018). Lnc RNA NR 2F1‐ AS 1 Regulates Hepatocellular Carcinoma Oxaliplatin Resistance by Targeting ABCC 1 via miR‐363. J. Cel Mol Med. 22 (6), 3238–3245. doi:10.1111/jcmm.13605
Huebbers, C. U., Verhees, F., Poluschkin, L., Olthof, N. C., Kolligs, J., Siefer, O. G., et al. (2019). Upregulation of AKR1C1 and AKR1C3 Expression in OPSCC with Integrated HPV16 and HPV‐negative Tumors Is an Indicator of Poor Prognosis. Int. J. Cancer 144 (10), 2465–2477. doi:10.1002/ijc.31954
Ji, Q., Aoyama, C., Nien, Y.-D., Liu, P. I., Chen, P. K., Chang, L., et al. (2004). Selective Loss of AKR1C1 and AKR1C2 in Breast Cancer and Their Potential Effect on Progesterone Signaling. Cancer Res. 64 (20), 7610–7617. doi:10.1158/0008-5472.CAN-04-1608
Joshi, S. (2014). Mnk Kinase Pathway: Cellular Functions and Biological Outcomes. Wjbc 5 (3), 321–333. doi:10.4331/wjbc.v5.i3.321
Karimi, A., Moradi, M. T., Saeedi, M., Asgari, S., and Rafieian-Kopaei, M. (2013). Antiviral Activity of Quercus Persica L.: High Efficacy and Low Toxicity. Adv. Biomed. Res. 2, 36. doi:10.4103/2277-9175.109722
Klippel, S., Jakubikova, J., Delmore, J., Ooi, M., McMillin, D., Kastritis, E., et al. (2012). Methyljasmonate Displaysin Vitroandin Vivoactivity against Multiple Myeloma Cells. Br. J. Haematol. 159 (3), 340–351. doi:10.1111/j.1365-2141.2012.09253.x
Lei, J., Niu, H., Li, T., and Huang, W. (2011). A Novel β-glucosidase from Aspergillus Fumigates Releases Diosgenin from Spirostanosides of Dioscorea Zingiberensis C. H. Wright (DZW). World J. Microbiol. Biotechnol. 28 (3), 1309–1314. doi:10.1007/s11274-011-0907-z
Lei, T., Zhu, X., Zhu, K., Jia, F., and Li, S. (2019). EGR1-induced Upregulation of lncRNA FOXD2-AS1 Promotes the Progression of Hepatocellular Carcinoma via Epigenetically Silencing DKK1 and Activating Wnt/β-Catenin Signaling Pathway. Cancer Biol. Ther. 20 (7), 1007–1016. doi:10.1080/15384047.2019.1595276
Li, C., Chen, J., Zhang, K., Feng, B., Wang, R., and Chen, L. (2015). Progress and Prospects of Long Noncoding RNAs (lncRNAs) in Hepatocellular Carcinoma. Cell Physiol Biochem. 36 (2), 423–434. doi:10.1159/000430109
Li, P., Mao, Z., Lou, J., Li, Y., Mou, Y., Lu, S., et al. (2011). Enhancement of Diosgenin Production in Dioscorea Zingiberensis Cell Cultures by Oligosaccharides from its Endophytic Fungus Fusarium Oxysporum Dzf17. Molecules 16 (12), 10631–10644. doi:10.3390/molecules161210631
Li, S.-P., Xu, H.-X., Yu, Y., He, J.-D., Wang, Z., Xu, Y.-J., et al. (2016). LncRNA HULC Enhances Epithelial-Mesenchymal Transition to Promote Tumorigenesis and Metastasis of Hepatocellular Carcinoma via the miR-200a-3p/ZEB1 Signaling Pathway. Oncotarget 7 (27), 42431–42446. doi:10.18632/oncotarget.9883
Li, Y., Ye, Y., Feng, B., and Qi, Y. (2017). Long Noncoding RNA lncARSR Promotes Doxorubicin Resistance in Hepatocellular Carcinoma via Modulating PTEN-PI3K/Akt Pathway. J. Cel. Biochem. 118 (12), 4498–4507. doi:10.1002/jcb.26107
Long, F.-Y., Chen, Y.-S., Zhang, L., Kuang, X., Yu, Y., Wang, L.-F., et al. (2015). Pennogenyl Saponins Induce Cell Cycle Arrest and Apoptosis in Human Hepatocellular Carcinoma HepG2 Cells. J. Ethnopharmacology 162, 112–120. doi:10.1016/j.jep.2014.12.065
Ma, T., Wang, R.-p., and Zou, X. (2016). Dioscin Inhibits Gastric Tumor Growth through Regulating the Expression Level of lncRNA HOTAIR. BMC Complement. Altern. Med. 16 (1), 383. doi:10.1186/s12906-016-1360-1
Matsunaga, T., Yamaguchi, A., Morikawa, Y., Kezuka, C., Takazawa, H., Endo, S., et al. (2014). Induction of Aldo-Keto Reductases (AKR1C1 and AKR1C3) Abolishes the Efficacy of Daunorubicin Chemotherapy for Leukemic U937 Cells. Anticancer Drugs 25 (8), 868–877. doi:10.1097/CAD.0000000000000112
Muzio, M., Stockwell, B. R., Stennicke, H. R., Salvesen, G. S., and Dixit, V. M. (1998). An Induced Proximity Model for Caspase-8 Activation. J. Biol. Chem. 273 (5), 2926–2930. doi:10.1074/jbc.273.5.2926
Qingbing, Z., Wen, H., Wenfang, W., Dan, S., Hua, L., Qingyi, T., et al. (2010).Study on the Water-Insoluble Steroidal Saponins from Dioscorea Zingiberensis and Cell Growth Inhibitive Effects of Steroidal Saponins against Human Hepatocellular Carcinoma hepG2 Cells West China J. Pharm. Ences. 5, 527–529.
Qiu, P., Man, S., Yang, H., Fan, W., Yu, P., and Gao, W. (2016). Utilization of Metabonomics to Identify Serum Biomarkers in Murine H22 Hepatocarcinoma and Deduce Antitumor Mechanism of Rhizoma Paridis Saponins. Chemico-Biological Interactions 256, 55–63. doi:10.1016/j.cbi.2016.06.026
Renault, T. T., and Chipuk, J. E. (2014). Death upon a Kiss: Mitochondrial Outer Membrane Composition and Organelle Communication Govern Sensitivity to BAK/BAX-Dependent Apoptosis. Chem. Biol. 21 (1), 114–123. doi:10.1016/j.chembiol.2013.10.009
Rizner, T. L. (2012). Enzymes of the AKR1B and AKR1C Subfamilies and Uterine Diseases. Front. Pharmacol. 3, 34. doi:10.3389/fphar.2012.00034
Rizner, T. L., and Penning, T. M. (2014). Role of Aldo-Keto Reductase Family 1 (AKR1) Enzymes in Human Steroid Metabolism. Steroids 79, 49–63. doi:10.1016/j.steroids.2013.10.012
Seo, D.-C., Sung, J.-M., Cho, H.-J., Yi, H., Seo, K.-H., Choi, I.-S., et al. (2007). Gene Expression Profiling of Cancer Stem Cell in Human Lung Adenocarcinoma A549 Cells. Mol. Cancer 6, 75. doi:10.1186/1476-4598-6-75
Shimizu, S., Narita, M., Tsujimoto, Y., and Tsujimoto, Y. (1999). Bcl-2 Family Proteins Regulate the Release of Apoptogenic Cytochrome C by the Mitochondrial Channel VDAC. Nature 399 (6735), 483–487. doi:10.1038/20959
Song, D., Liang, H., Qu, B., Li, Y., Liu, J., Zhang, Y., et al. (2018). Ivermectin Inhibits the Growth of Glioma Cells by Inducing Cell Cycle Arrest and Apoptosis In Vitro and In Vivo. J. Cel Biochem. 120 (1), 622–633. doi:10.1002/jcb.27420
Tong, Q.-Y., He, Y., Zhao, Q.-B., Qing, Y., Huang, W., and Wu, X.-H. (2012). Cytotoxicity and Apoptosis-Inducing Effect of Steroidal Saponins from Dioscorea Zingiberensis Wright against Cancer Cells. Steroids 77 (12), 1219–1227. doi:10.1016/j.steroids.2012.04.019
Wang, F., Ying, H.-Q., He, B.-S., Pan, Y.-Q., Deng, Q.-W., Sun, H.-L., et al. (2015). Upregulated lncRNA-UCA1 Contributes to Progression of Hepatocellular Carcinoma through Inhibition of miR-216b and Activation of FGFR1/ERK Signaling Pathway. Oncotarget 6 (10), 7899–7917. doi:10.18632/oncotarget.3219
Wang, L., Liu, Y., and Yu, G. (2019). Avasimibe Inhibits Tumor Growth by Targeting FoxM1-AKR1C1 in Osteosarcoma. Ott Vol. 12, 815–823. doi:10.2147/OTT.S165647
Woenckhaus, M., Klein-Hitpass, L., Grepmeier, U., Merk, J., Pfeifer, M., Wild, P., et al. (2006). Smoking and Cancer-Related Gene Expression in Bronchial Epithelium and Non-small-cell Lung Cancers. J. Pathol. 210 (2), 192–204. doi:10.1002/path.2039
Yang, Y., Chen, L., Gu, J., Zhang, H., Yuan, J., Lian, Q., et al. (2017). Recurrently Deregulated lncRNAs in Hepatocellular Carcinoma. Nat. Commun. 8 (1), 14421. doi:10.1038/ncomms14421
Yao, J., Man, S., Dong, H., Yang, L., Ma, L., and Gao, W. (2018). Combinatorial Treatment of Rhizoma Paridis Saponins and Sorafenib Overcomes the Intolerance of Sorafenib. J. Steroid Biochem. Mol. Biol. 183, 159–166. doi:10.1016/j.jsbmb.2018.06.010
Yu, J., Chen, G. G., and Lai, P. B. S. (2021). Targeting Hepatocyte Growth Factor/c‐mesenchymal-Epithelial Transition Factor axis in Hepatocellular Carcinoma: Rationale and Therapeutic Strategies. Med. Res. Rev. 41 (1), 507–524. doi:10.1002/med.21738
Yuan, J.-h., Yang, F., Wang, F., Ma, J.-z., Guo, Y.-j., Tao, Q.-f., et al. (2014). A Long Noncoding RNA Activated by TGF-β Promotes the Invasion-Metastasis Cascade in Hepatocellular Carcinoma. Cancer Cell 25 (5), 666–681. doi:10.1016/j.ccr.2014.03.010
Zhang, X., Li, J., Ito, Y., and Sun, W. (2015). Simultaneous Quantification of Five Steroid Saponins from Dioscorea Zingiberensis C.H. Wright in Rat Plasma by HPLC-MS/MS and its Application to the Pharmacokinetic Studies. Steroids 93, 16–24. doi:10.1016/j.steroids.2014.08.023
Zhao, D., Zhang, C.-J., Yang, R., Chen, J.-P., Ma, L., Liu, G., et al. (2017). Effect of 1,25(OH2D3 on the Proliferation of Human Mesangial Cells and Their Expression of Ki67. Genet. Mol. Res. 16 (2). doi:10.4238/gmr16029191
Zhao, S. F., Wagn, S. G., Zhao, Z. Y., and Li, W. L. (2019). AKR1C13, Notably AKR1C3, Are Distinct Biomarkers for Liver Cancer Diagnosis and Prognosis: Database Mining in Malignancies. Oncol. Lett. 18 (5), 4515–4522. doi:10.3892/ol.2019.10802
Zhao, Y.-Z., Zhang, Y.-Y., Han, H., Fan, R.-P., Hu, Y., Zhong, L., et al. (2018). Advances in the Antitumor Activities and Mechanisms of Action of Steroidal Saponins. Chin. J. Nat. Medicines 16 (10), 732–748. doi:10.1016/s1875-5364(18)30113-4
Zhou, J., Jiang, Y. y., Chen, H., Wu, Y. c., and Zhang, L. (2020). Tanshinone I Attenuates the Malignant Biological Properties of Ovarian Cancer by Inducing Apoptosis and Autophagy via the Inactivation of PI3K/AKT/mTOR Pathway. Cell Prolif 53 (2), e12739. doi:10.1111/cpr.12739
Zhou, X.-y., Fu, F.-h., Li, Z., Dong, Q.-j., He, J., and Wang, C.-h. (2009). Escin, a Natural Mixture of Triterpene Saponins, Exhibits Antitumor Activity against Hepatocellular Carcinoma. Planta Med. 75 (15), 1580–1585. doi:10.1055/s-0029-1185838
Zhu, H., Chang, L.-L., Yan, F.-J., Hu, Y., Zeng, C.-M., Zhou, T.-Y., et al. (2018). AKR1C1 Activates STAT3 to Promote the Metastasis of Non-Small Cell Lung Cancer. Theranostics 8 (3), 676–692. doi:10.7150/thno.21463
Zhu, J., Guo, X., Fu, S., Zhang, X., and Liang, X. (2010). Characterization of Steroidal Saponins in Crude Extracts from Dioscorea Zingiberensis C. H. Wright by Ultra-performance Liquid Chromatography/electrospray Ionization Quadrupole Time-Of-Flight Tandem Mass Spectrometry. J. Pharm. Biomed. Anal. 53 (3), 462–474. doi:10.1016/j.jpba.2010.05.019
Keywords: Hepatocellular carcinoma (HCC), long non-coding RNA (lncRNA), dioscorea zingiberensis new saponin (ZnS), TCONS-00026762, apoptosis
Citation: Liu X, Zhou P, He K, Wen Z and Gao Y (2021) Dioscorea Zingiberensis New Saponin Inhibits the Growth of Hepatocellular Carcinoma by Suppressing the Expression of Long Non-coding RNA TCONS-00026762. Front. Pharmacol. 12:678620. doi: 10.3389/fphar.2021.678620
Received: 10 March 2021; Accepted: 01 April 2021;
Published: 03 May 2021.
Edited by:
Jiang-Jiang Qin, Institute of Cancer and Basic Medicine, Chinese Academy of Sciences, ChinaReviewed by:
Qiyang Shou, Zhejiang Chinese Medical University, ChinaQi Zeng, Xidian University, China
Copyright © 2021 Liu, Zhou, He, Wen and Gao. This is an open-access article distributed under the terms of the Creative Commons Attribution License (CC BY). The use, distribution or reproduction in other forums is permitted, provided the original author(s) and the copyright owner(s) are credited and that the original publication in this journal is cited, in accordance with accepted academic practice. No use, distribution or reproduction is permitted which does not comply with these terms.
*Correspondence: Zhili Wen, wenzhili2020@163.com