- 1Institute of Neuroscience and Department of Neurology of the Second Affiliated Hospital of Guangzhou Medical University; Key Laboratory of Neurogenetics and Channelopathies of Guangdong Province and the Ministry of Education of China, Guangzhou, China
- 2Department of Neurology, Guangzhou First People’s Hospital, Guangzhou, China
- 3BGI-Shenzhen, Shenzhen, China
- 4Epilepsy Center and Department of Neurosurgery, The First Affiliated Hospital, Jinan University, Guangzhou, China
- 5Department of Neurology, Guangdong 999 Brain Hospital, Guangzhou, China
- 6The First People’s Hospital of Shunde, Foshan, China
- 7The Third People’s Hospital of Mianyang, Mianyang, China
- 8Department of Neurology, Nanhua Hospital Affiliated to South China University, Hengyang, China
- 9Department of Neurology, The Affiliated Yuebei People’s Hospital of Shantou University Medical College, Shaoguan, China
- 10The Affiliated Hospital of Xiangnan University, Chenzhou, China
- 11Department of Neurology, The Seventh Affiliated Hospital, Sun Yat-Set University, Guangzhou, China
- 12West China Hospital, Sichuan University, Chengdu, China
- 13Department of Neuroscience, Central Clinical School, Monash University, Alfred Hospital, Melbourne, VIC, Australia
To characterize human leukocyte antigen (HLA) loci as risk factors in aromatic antiepileptic drug-induced maculopapular exanthema (AED-MPE). A case-control study was performed to investigate HLA loci involved in AED-MPE in a southern Han Chinese population. Between January 2007 and June 2019, 267 patients with carbamazepine (CBZ), oxcarbazepine (OXC), or lamotrigine (LTG) associated MPE and 387 matched drug-tolerant controls from six centers were enrolled. HLA-A/B/C/DRB1 genotypes were determined using sequence-based typing. Potential risk alleles were validated by meta-analysis using data from different populations and in silico analysis of protein-drug interactions. HLA-DRB1*04:06 was significantly associated with OXC-MPE (p = 0.002, pc = 0.04). HLA-B*38:02 was associated with CBZ-MPE (p = 0.03). When pooled, HLA-A*24:02, HLA-A*30:01, and HLA-B*35:01 additionally revealed significant association with AED-MPE. Logistic regression analysis showed a multiplicative interaction between HLA-A*24:02 and HLA-B*38:02 in CBZ-MPE. Meta-analysis of data from different populations revealed that HLA-24*:02 and HLA-A*30:01 were associated with AED-MPE (p = 0.02 and p = 0.04, respectively). In silico analysis of protein-drug interaction demonstrated that HLA-A*24:02 and HLA-A*30:01 had higher affinities with the three aromatic AEDs than the risk-free HLA-A allele. HLA-DRB1*04:06 showed relatively specific high affinity with S-monohydroxy derivative of OXC. HLA-DRB1*04:06 is a specific risk allele for OXC-induced MPE in the Southern Han Chinese. HLA-A*24:02, possibly HLA-A*30:01, are common risk factors for AED-MPE. The multiplicative risk potential between HLA-A*24:02 and HLA-B*38:02 suggests that patients with two risk alleles are at greater risk than those with one risk allele. Inclusion of these HLA alleles in pre-treatment screening would help estimating the risk of AED-MPE.
Introduction
Aromatic antiepileptic drugs (AEDs), including carbamazepine (CBZ), lamotrigine (LTG), and oxcarbazepine (OXC), are commonly prescribed in clinical practice. However, these medications are also the most common causes of cutaneous adverse drug reactions (cADRs) (Arif et al., 2007; Mockenhaupt et al., 2008). The cADRs range from mild maculopapular exanthema (MPE), with increasing severity, to hypersensitivity syndrome (HSS), Stevens-Johnson syndrome (SJS), and toxic epidermal necrolysis (TEN) (Toledano and Gil-Nagel, 2008). MPE is relatively mild but generally requires medication discontinuation, disrupting AED treatment (Arif et al., 2007; Mockenhaupt et al., 2008). The overall incidence of MPE induced by AEDs (AED-MPE) is estimated to be 2.8%, and is higher at 3.7, 4.8, and 9% with CBZ, LTG, and OXC (Arif et al., 2007; Wang et al., 2012), respectively. CBZ, LTG, and OXC also have a 20–30% chance of cross-reactivity in MPE with other AEDs (Hirsch et al., 2008). MPE may evolve to severe SJS/TEN, which has high mortality of up to 30% (Roujeau, 1994). Several human leukocyte antigen (HLA) alleles have been demonstrated to be risk factors for CBZ-induced SJS/TEN, including HLA-B*15:02 in the Han Chinese and Southeast Asian populations (Chung et al., 2004; Tassaneeyakul et al., 2010; Shi et al., 2017), and HLA-A*31:01 in Japanese and European populations (McCormack et al., 2011; Ozeki et al., 2011), while HLA-A*24:02 is a common risk factor for AEDs-SJS/TEN (Shi et al., 2017). These findings have resulted in breakthroughs in the prevention of CBZ-induced SJS/TEN (Chen et al., 2011). Previously, several studies demonstrated that HLA alleles are possibly associated with AED-MPE, such as HLA-A*31:01 with CBZ-induced MPE (McCormack et al., 2011) and HLA-A*24:02 with LTG-induced MPE (Moon et al., 2015). However, the genetic HLA risk alleles of AED-MPE were largely undetermined.
To examine the HLA risk alleles in AED-MPE, we performed a multicenter case-control study with a cohort of 267 MPE cases from southern China. Significant findings were validated by meta-analyses of data from independent studies in other populations and computer simulation on protein-drug interactions1.
Materials and Methods
Recruitment of Cases and Drug-Tolerant Controls
The diagnoses of MPE were on the basis of the clinical morphology of the skin damage, which is characterized by cutaneous fine pink macules and papules, and lesions without mucosal or systemic involvement. A dermatologist confirmed the diagnosis. Cases with AED-MPE were determined according to the Naranjo algorithm (Naranjo. et al., 1981) and were recruited from six hospitals in three provinces in southern China, including Guangdong, Hunan, and Sichuan, between January 2007 and June 2019. These patients developed MPE within 8 weeks after receiving an aromatic AED. As a part of follow-up after AEDs were prescribed, the patients came to hospital when MPE were developed, and no other causes for the MPE were found. No cases were taking other medicines associated with cADRs such as abacavir and allopurinol. The tolerant controls were patients with epilepsy who received aromatic AEDs for at least 3 months without evidence of cutaneous adverse reactions. The controls were matched cases of Han Chinese from the same region who took the same AED, with a matching ratio of at least 1:1 (controls: cases). All individuals enrolled were unrelated ethnic southern Han Chinese. None of the four biological grandparents were from other races.
Standard Protocol Approvals, Registrations, and Patient Consents
This study adhered to the guidelines of the International Committee of Medical Journal Editors with regard to patient consent for research or participation and received approvals from local ethics committees of the participating hospitals.
HLA High-Resolution Genotyping
Genomic DNA was obtained from peripheral blood using a QIAamp blood mini kit (Qiagen, Hilden, Germany). High-resolution sequence-based HLA-A, HLA-B, HLA-C, and HLA-DRB1 typing was performed by Shanghai Tissuebank Biotechnology (Shanghai, China), as described previously (Shi et al., 2017).
Meta-Analysis
To validate the potential risk alleles identified from the present cohort, we performed meta-analyses on data from other populations. We conducted a thorough biomedical literature search using PubMed, Embase, and the Cochrane Library. The following terms were used in our searches: “antiepileptic drugs” or “AEDs”, “HLA”, or “human leukocyte antigen”, “cutaneous adverse drug reactions” or “cADRs”, and “maculopapular eruption” or “MPE”. The last search was conducted on December 31, 2020.
Criteria for the selection of studies were: 1) the report was a case-control study on association between HLA and AED-cADRs; 2) the genotyping method and ethnicity were provided; 3) the carrier rate of HLA-A*24:02, HLA-A*30:01, HLA-B*35:01, HLA-B*38:02, and HLA-DRB1*04:06 in the cases and the tolerant controls was reported; 4) the most recent publication with the largest samples was selected when duplicate publications were identified. Exclusion criteria were: 1) case reports or case series, review articles, and basic genetic research; 2) repeat studies; 3) non-human studies.
Data managements and analyses were performed using RevMan 5.0.24 software (Cochrane Collaboration, Copenhagen, Denmark). A p-value of less than 0.1 and I2-value of higher than 50% were defined study heterogeneity, under which the association was assessed using the random model, or not using the fixed model.
Computer Simulation on Protein-Drug Docking
HLA molecules play a role in the development of cADRs potentially via binding with drugs (Illing et al., 2012; Norcross et al., 2012; Ostrov et al., 2012). Hence, the binding affinity between possible risk HLA molecules and AEDs were performed by in silico analysis. The three-dimensional (3D) structure of CBZ, LTG, OXC and its R enantiomer of monohydroxy derivative (R-MHD) and S-MHD, was downloaded from the PubChem database (https://pubchem.ncbi.nlm.nih.gov). The experimentally determined 3D protein structures included HLA-A*11:01, HLA-A*24:02, HLA-DRB1*01:01, and HLA-DRB1*04:01 (Protein Data Bank ID 6ID4.pdb (Gu et al., 2019), 2BCK.pdb (Cole et al., 2006), 3PDO.pdb (Gunther et al., 2010), and 4MCY.pdb (Scally et al., 2013), respectively). The structures of other HLA molecules were predicted by I-TASSER (Yang et al., 2015) based on the templates from the PDB database (https://www.rcsb.org/). A homology model of HLA-A molecule and a heterodimer model of HLA-DR complex (consists of HLA-DRA1 and HLA-DRB1 molecules) were used for docking by Rosseta docking_local_refine protocol (Lyskov and Gray, 2008). PyMOL align command was used for alignment. To set the same docking parameters, we aligned the 3D structural files of HLA-A molecules with HLA-A*24:02 as reference, and the files of HLA-DRB1 molecules with DRB1*04:01 as reference. We set the binding pocket of HLA-A in the condition of box center point at 25.453 Å (Å, x-axis), 20.524 Å (y-axis), and 13.601 Å (z-axis) with the box size of x = 16 Å, y = 30 Å, and z = 30 Å. The binding pocket of HLA-DRB1 was 43.798 Å (x-axis), 19.478 Å (y-axis), and −2.022 Å (z-axis) with a box size of x = 16 Å, y = 24 Å, and z = 23 Å. The search parameter exhaustiveness was set to 15. The Autodock Vina was used to construct the binding model and calculate the binding affinity between the drug and the target (Trott and Olson, 2010). The binding poses between drugs and HLA targets were shown by PyMOL 1.7.
Statistical Analysis
Statistical analysis was performed using SPSS version 19.0 (SPSS Inc., Chicago, IL, United States). A 2-by-2 χ2 test was used to compare the allele carrier rates between groups. An independent t-test was performed to compare the sex ratio, mean age, and initial dosages between groups. p-values less than 0.05 (two-sided) were considered statistically significant. To achieve sufficient power, the corrected p values (pc) were estimated by using Bonferroni’s correction for multiple comparisons (n = 24 for HLA-A, 51 for HLA-B, 25 for HLA-C, and 26 for HLA-DRB1 in the CBZ-MPE group; n = 19 for HLA-A, 46 for HLA-B, 23 for HLA-C, and 26 for HLA-DRB1 in the LTG-MPE group; n = 16 for HLA-A, 37 for HLA-B, 18 for HLA-C, and 19 for HLA-DRB1 in the OXC-MPE group; n = 24 for HLA-A, 51 for HLA-B, 27 for HLA-C, and 26 for HLA-DRB1 in the pooled group). Logistic regression was used to analyze the possible interaction between two risk alleles.
Results
Characteristics of Cases with MPE and Tolerant Controls
A total of 267 cases with aromatic AED-MPE (146 CBZ-induced MPE, 67 LTG-induced MPE, and 54 OXC-induced MPE) and 387 tolerant controls (180 exposed to CBZ, 102 to LTG, and 133 to OXC, overlapped in some cases due to use of more than one AED in the same individual) were included. All cases were taking aromatic AEDs for treatment of epilepsy. Their clinical characteristics are summarized in Table 1. There was no significant difference between the cases and tolerant controls in age and sex ratio. The CBZ and LTG controls showed significantly higher exposure doses than the cases (p = 1.22 × 10−9 and p = 2.87 × 10−5, respectively). No significant difference in OXC dose was found between cases with OXC-induced MPE and OXC tolerant controls. The median latency to MPE after CBZ, LTG, and OXC administration was 11.59 days (range: 1–60), 13.51 days (range: 1–60), and 12 days (range: 1–40), respectively. The majority of cases (132/141, 93.6%) developed MPE within 30 days after drug treatment.
As shown in Table 2, in CBZ-induced MPE, the carrier rate of HLA-B*38:02 was higher in the case group (18/145, 12.41%) than in the tolerant group (10/179, 5.59%; p = 0.03; OR: 2.4; 95% CI: 1.07–5.37). In LTG-induced MPE, no significant allele was found when compared to the tolerant-control group.
In OXC-induced MPE, the carrier rate of HLA-DRB1*04:06 was significantly higher in the case group than in the tolerant group (8/51, 15.69% vs. 1/94, 1.06%; p = 0.002; OR: 17.30; 95% CI: 2.10–142.72). After Bonferroni’s correction, the significant difference remained (pc = 0.038). Among the patients taking OXC, 8 (88.9%) of nine patients with HLA-DRB1*04:06 developed OXC-induced MPE. HLA-DRB1*04:06 has a sensitivity of 15.69% and a specificity of 98.94% for OXC-induced MPE.
Considering the shared aromatic ring, we performed a pooled analysis on the three antiepileptic drugs induced MPE (Table 2). HLA-B*38:02 and HLA-DRB1*04:06 remained to be significantly associated with AED-MPE (p = 0.03 and p = 0.02, respectively). In addition, HLA-A*24:02, HLA-A*30:01, and HLA-B*35:01 were demonstrated to be weakly associated alleles. HLA-A*24:02 was presented in 22.13% (56/253) of cases and 15.58% (48/308) of tolerant controls (p = 0.047); HLA-A*30:01 was present in 5.53% (14/253) of cases and 2.27% (7/308) of tolerant controls (p = 0.043); and HLA-B*35:01 was present in 4.21% (11/261) of cases and 1.16% (4/344) of tolerant controls (p = 0.020).
Based on the prevalence of MPE in previous reports (estimated to be 3.7, 9, and 2.8% for CBZ, OXC, and AEDs, respectively, (Arif et al., 2007; Wang et al., 2012) the positive prediction values (PPVs) for the individual tests ranged from 3.93 to 59.41%, and the negative prediction values (NPVs) were higher than 92.2%. The number need to test (NNT) using HLA-B*38:02 to prevent one case with CBZ-induced MPE was 218, using HLA-DRB1*04:06 to prevent one case with OXC-induced MPE was 71, using HLA-A*24:02, A*30:01, B*35:01, and B*38:02 to prevent one case with AED-induced MPE was 162, 646, 848, and 333, respectively, (Table 2).
HLA-B*15:02 is a strong and specific risk factor for CBZ-induced SJS/TEN (Chung et al., 2004; Tassaneeyakul et al., 2010; Shi et al., 2017), but we did not find any significant association between HLA-B*15:02 and CBZ-induced MPE or AED-MPE in the present study (Supplementary Table S2).
HLA-A*31:01 was previously reported to be associated with CBZ-induced cADRs in the European population (McCormack et al., 2011). Our previous studies did not find such association in CBZ-induced SJS (Shi et al., 2017). In the present study, whenever using separate or pooled analysis, HLA-A*31:01 did not show significant association with MPE (Supplementary Table S2). The difference may be due to the HLA-A*31:01 associated with AED-cADRs having ethnic specificity. Conversely, the carrier rate of HLA-B*40:01 and HLA-C*12:03 were lower in the CBZ-induced MPE group than in the tolerant group (p = 1.91 × 10−4, OR: 0.37; 95% CI: 0.21–0.62; and p = 0.001, OR: 0.13; 95% CI: 0.03–0.55, respectively, Supplementary Table S2), suggesting negative correlations.
Interaction Between Any Two HLA Alleles Located in Different Locus
The five potentially associated alleles are located in the HLA-A, HLA-B, or HLA-DRB1 separately and do not cluster as a haplotype (http://www.allelefrequencies.net/). We did not find any cluster from the data of this cohort, either (Supplementary Table S1). We then analyzed the potential interaction between any two alleles at different locus using logistic regression. In CBZ-induced MPE, the risk in patients who were positive for both HLA-A*24:02 and HLA-B*38:02 were significantly higher than in those who were positive for either HLA-A*24:02 or HLA-B*38:02 alone. A potential interaction between HLA-A*24:02 and HLA-B*38:02 was suggested (OR 7.29, Table 3). When combining the HLA-A*24:02 and HLA-B*38:02 into a single risk group (i.e., carriers of HLA-A*24:02 and/or HLA-B*38:02), the sensitivity increased to 31.43% for CBZ-induced MPE, with a specificity of 79.10%. The PPV and NPV for the individual combined HLA-A*24:02 and HLA-B*38:02 tests were 5.46 and 96.78%, and the NNT to prevent one case was 86 (Table 2). We did not find any other potential multiplicative interactions between the risk HLA alleles for the antiepileptic drugs-induced MPE.
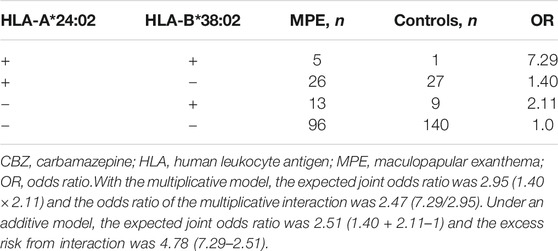
TABLE 3. Excess risk from interaction between HLA-A*24:02 and HLA-B*38:02 alleles in CBZ-Induced MPE (logistic regression).
Meta-Analysis
To test the significant association between these five alleles and AED-MPE, we employed meta-analysis of data from different studies and the data of the present study. A total of 152 published studies, and two unpublished data provided by the corresponding author (Supplementary Tables S3, S4) were collected. After exclusion, ten studies relevant to the association between HLA-A*24:02 and AED-MPE met the inclusion criteria, comprising of 488 cases with AED-MPE and 1277 AED-tolerant controls (Wang et al., 2010; Fricke-Galindo et al., 2014; Hsiao et al., 2014; Moon et al., 2015; Chen et al., 2017; Koomdee et al., 2017; Wang et al., 2019; Xu et al., 2019; Supplementary Table S4). The association between HLA-A*24:02 and AED-MPE was verified across different populations of China, Korea, Malaysia, Mexican and Thai (134/488 vs. 321/1277, p = 0.02, Figure 1A). HLA-A*30:01 was verified to be associated with AED-MPE based on data from eight studies including 458 cases and 855 tolerant controls (30/458 vs. 39/855, p = 0.04, Figure 1B). The other three alleles did not show significant association with AED-MPE (Supplementary Figure S1), but HLA-B*38:02 showed a tendency toward significant difference (33/357 vs. 37/644, p = 0.06).
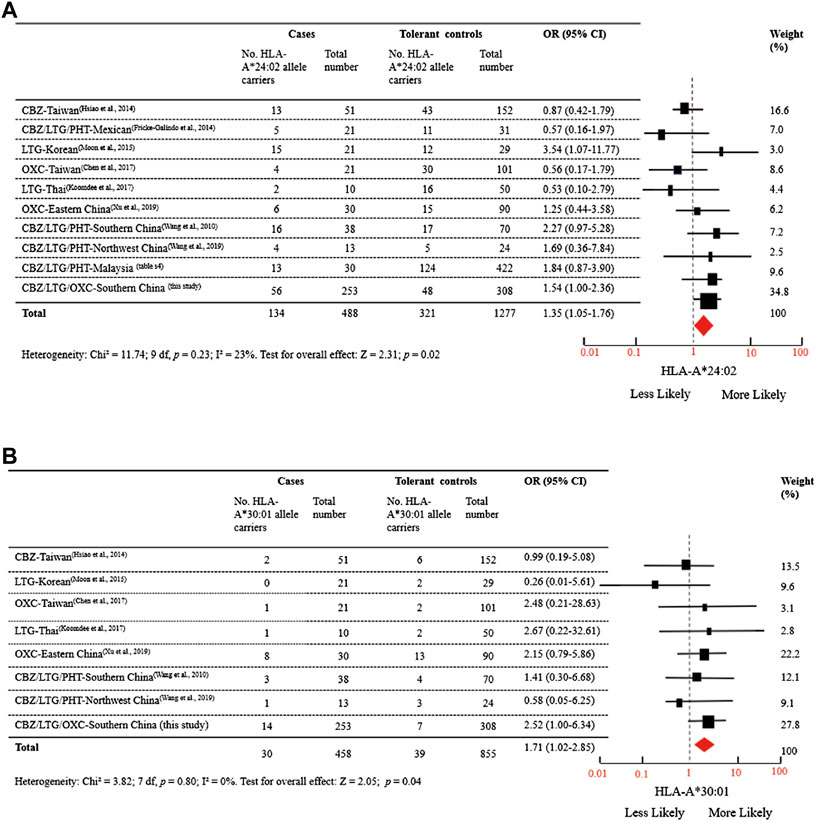
FIGURE 1. Distribution of HLA-A*24:02 and HLA-A*30:01 Alleles in MPE Induced by Aromatic Antiepileptic Drugs. (A) Data were from 10 studies relevant to the association between HLA-A*24:02 and AED-MPE, comprising of 488 cases with AED-MPE and 1277 AED-tolerant controls from China, Korea, Malaysia, Mexican, and Thai. (B) Data were from 8 studies relevant to the association between HLA-A*30:01 and AED-MPE, comprising of 458 cases with AED-MPE and 855 AED-tolerant controls from China, Korean, and Thai. I2 represent measures of heterogeneity. Study weighting (indicated by different sizes of squares) refers to the proportion of participants who were recruited from each study. The horizontal lines represent 95% confidence intervals (CIs). Diamonds indicate pooled odds ratios. CBZ, carbamazepine; LTG, lamotrigine; MPE, maculopapular exanthema; OR, odds ratio, OXC, oxcarbazepine; PHT, phenytoin.
Computer Simulation on Binding of HLA Molecules to AEDs
The genotyping-comparison and meta-analysis indicated that HLA-A*24:02 and HLA-A*30:01 were risk alleles for AEDs-MPE and HLA-DRB1*04:06 was risk factor for OXC-induced MPE. We then investigated the docking situation between the risk molecules and antiepileptic drugs by computer simulation.
HLA-A*11:01 is the most frequent allele in general population but not a risk allele for AED-MPE. The protein structure of HLA-A*11:01 has been identified experimentally (Gu et al., 2019). We then analyzed the docking model of HLA-A*24:02 and HLA-A*30:01 with a comparison to HLA-A*11:01. For HLA-A*24:02 and HLA-A*30:01, the three antiepileptic drugs were docked well into the groove region. For HLA-A*11:01, only OXC was docked into the groove region, while CBZ and LTG were docked into a region near the groove region (Figure 2A). HLA-A*24:02 have generally higher binding affinity scores with CBZ, LTG, and OXC (−8.0, −8.4, and −6.9 kal/mol, respectively). HLA-A*30:01 also showed relatively higher binding affinity with CBZ and OXC (−7.3 and −7.5 kal/mol, respectively).
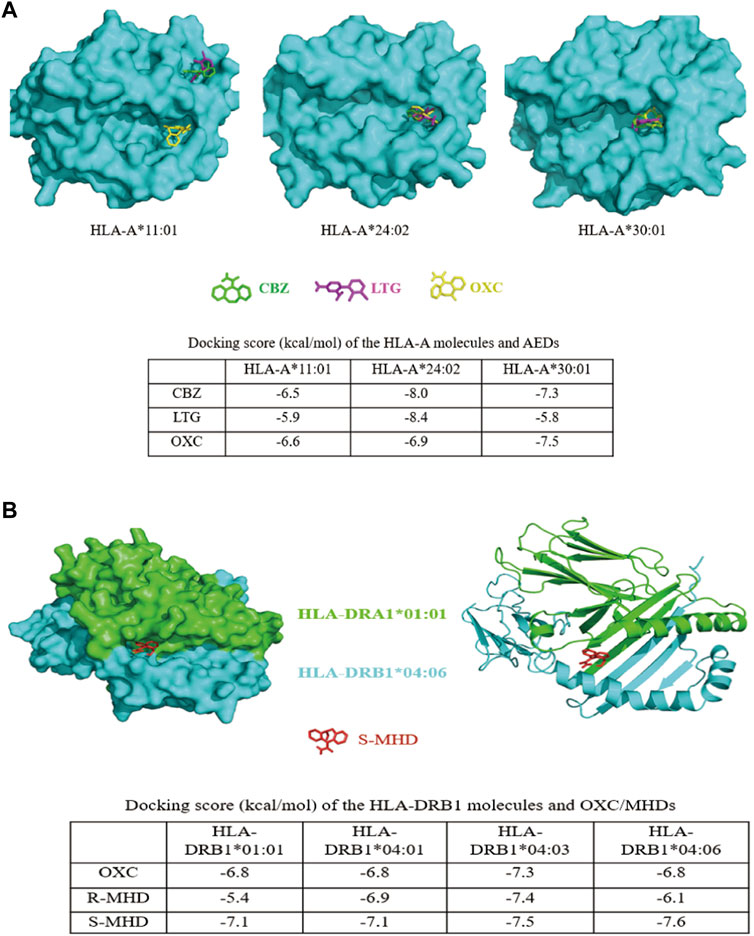
FIGURE 2. Three-dimensional models of interactions between HLA molecules and AEDs. (A)In silico modeling of interactions between the homology HLA-A molecules and AEDs. CBZ, LTG, and OXC were docked into the groove region of HLA-A*24:02 and HLA-A*30:01. For HLA-A*11:01, only OXC was docked into the groove region, while CBZ and LTG were docked into a region near the groove. The binding scores of HLA-A*24:02 with CBZ, LTG, and OXC, and HLA-A*30:01 with CBZ and OXC, were much higher than that of HLA-A*11:01 with the corresponding drugs (shown in the table). (B)In silico modeling of the interaction between the heterodimer of HLA-DRA1*01:01 and HLA-DRB1*04:06 and S-MHD. Similar to the model, OXC, R-MHD, and S-MHD were identically docked into the groove region of the HLA-DR molecules. The binding affinity of HLA-DRB1*04:06 and S-MHD (−7.6 kcal/mol) was the highest among 12 protein-drug interactions. HLA-DRB1*04:03 showed generally higher binding affinities with OXC, R-MHD, and S-MHD. All dock scores were listed in the table. CBZ, carbamazepine; LTG, lamotrigine; OXC, oxcarbazepine; R-MHD, R enantiomer of monohydroxy derivative; S-MHD, S enantiomer of monohydroxy derivative.
For HLA-DR, the protein structure of HLA-DRB1*01:01 and HLA-DRB1*04:01 have been identified experimentally (Gunther et al., 2010; Scally et al., 2013) and are risk-free molecules. Previously, HLA-DRB1*04:03 was showed to be a risk molecule for OXC-induced MPE in Korean (Moon et al., 2016). We therefore compared the docking models of HLA-DRB1*01:01, HLA-DRB1*04:01, HLA-DRB1*04:03, and HLA-DRB1*04:06. Considering OXC is rapidly metabolized to monohydroxylated derivatives (MHD) after oral administration in human (Flesch, 2004), we performed computer simulation on the docking of OXC and its metabolites R-MHD and S-MHD with the four heterodimer HLA-DR molecules. It was demonstrated that OXC, R-MHD, and S-MHD were identically docked into the groove region of the four molecules (Figure 2B and Supplementary Figure S2 (3-D protein-drug modeling figures provided, Supplementary Figure S2 is available on https://figshare.com/s/dae756a6f226a668db28), the docking model of S-MHD and HLA-DRB1*04:06 as a representative). HLA-DRB1*04:03 molecule showed generally higher binding affinities with OXC, R-MHD, and S-MHD (−7.3, −7.4, and −7.5 kcal/mol, respectively). However, HLA-DRB1*04:06 revealed the highest affinity with S-MHD (−7.6 kcal/mol), which is the main compound after oral taking of OXC in human (Flesch, 2004).
Discussion
In the present study, we performed a multicenter case-control study and identified HLA-DRB1*04:06 as a specific risk factor for OXC-induced MPE and potentially HLA-B*38:02 as a risk allele for CBZ-induced MPE in Southern Han Chinese. Further studies suggest a multiplicative interaction between HLA-A*24:02 and HLA-B*38:02 in CBZ-induced MPE and that patients with two risk alleles are at greater risk than those with one risk allele alone. Meta-analysis on data from different populations revealed that HLA-A*24:02 and HLA-A*30:01 alleles were possibly common risk factors for AED-MPE. These genetic associations were supported by evidence from computer simulation on interactions between HLA molecules and AEDs.
OXC, a 10-keto analog of CBZ with less frequent and severe cADRs than CBZ (He et al., 2012; Chen et al., 2017), is commonly used as an alternative to CBZ in clinics. However, several studies reported that the incidence of OXC-induced cADRs was as high as 5–9% (Alvestad et al., 2007; Hirsch et al., 2008; Wang et al., 2012), resulting in drug discontinuation in almost all cases (Shorvon, 2000; Kalis and Huff, 2001; Wang et al., 2012). To date, the risk factors for OXC-induced MPE are largely unknown. In this multicenter case-control study in southern Han Chinese, we found that HLA-DRB1*04:06 was associated with OXC-induced MPE with high OR value (17.30), which remained significant difference after Bonferroni’s correction. Among the 145 epilepsy patients treated with OXC, eight (88.89%) of nine patients with HLA-DRB1*04:06 developed MPE. The high specificity (98.94%) and positive predictive value (88.89%) suggest potential implication of HLA-DRB1*04:06 detection in clinical practice. Therefore, the patients who were positive for HLA-DRB1*04:06 should be carefully looked when OXC was prescribed. However, the sensitivity of HLA-DRB1*04:06 for OXC-induced MPE is 15.96%, which may be due to the relatively low frequency (2.1%) of HLA-DRB1*04:06 in the Southern Han Chinese. HLA-DRB1*04:06 presents at frequencies of 2.1–3.5% in Chinese and 0–6.8% in other populations (www.allelefrequencies.net/default.asp). Given that the incidence of OXC-induced MPE is of up to 9% (Wang et al., 2012), the PPV and NPV of this allele are 59.41 and 92.25%, respectively. The NNT using HLA-DRB1*04:06 to prevent one case with OXC-induced MPE is 71.
AED-MPE is mainly mediated by CD4+ T cells recognized by HLA class II molecules (Pichler, 2002), which include the molecules encoded by HLA-DR, HLA-DQ, or HLA-DP alleles. HLA-DRB1*04:06 was the first risk HLA-DR molecule identified for OXC-induced MPE in the Southern Han Chinese. Similarly, HLA-DRB1*04:03 has been identified as a potential risk factor for OXC-induced MPE in the Korean population with a specificity of 98.57% and a sensitivity of 17.50% (Moon et al., 2016). HLA-DRB1*04:03 is also a less frequent allele in the Korean population (2.96%, www.allelefrequencies.net/default.asp). Generally, TCRs monitor the universe of antigens to which an individual is exposed by surveying the ligands (or peptides) presented on an antigen-presenting cell membrane. Different HLA molecules have different binding specificities, resulting in a specific profile of presented peptides. When T cells encounter an unknown peptide, an immune response is triggered. In case of cutaneous adverse drug reactions (cADRs), HLA molecule, drug, peptide, and T cell receptor (TCRs) are potentially involved. Previously, three models have been proposed to explain the underlying mechanism of immune-mediated cADRs: 1) a peptide, which is uniquely presented by the HLA allele, is modified by the drug; 2) the HLA molecule itself is modified by the drug in a region and then exposed to the TCR; 3) the binding specificity of the HLA molecule with peptide is altered by the presence of drug. In the latter two models, the drug needs to bind HLA molecules. Several studies have revealed that abacavir could bind inside the peptide-binding groove of HLA-B*57:01 to alter the repertoire of endogenous peptides, resulted in affecting TCR interface to trigger HLA-associated drug hypersensitivity, provided experimental evidence supporting the third model (Illing et al., 2012; Norcross et al., 2012; Ostrov et al., 2012). Based on these previous studies, we analyzed the binding of culprit AEDs with HLA molecules. Among of 12 drug-protein interactions, the docking score of S-MHD binding with HLA-DRB1*04:06 was the highest (Figure 2B). After oral administration of OXC in human, only 2% of the total doses is present as OXC, approximately 70% as MHDs, and the others are rapidly eliminated. Of the MHDs, the S-MHD over R-MHD ratio is 4:1 (Flesch, 2004). Therefore, the strong interaction between HLA-DRB1*04:06 and S-MHD potentially explained HLA-DRB1*04:06 as a risk allele in OXC-induced MPE in the Southern Han Chinese. However, it is uncertain whether AEDs binds with the risk HLA allele to change the shape and chemistry of the antigen-binding cleft, thereby altering the repertoire of endogenous peptides that can bind the allele. In addition, the dock scores between HLA-DRB1*04:03 and OXC, R-MHD, and S-MHD were relatively high, potentially explaining the association between HLA-DRB1*04:03 and OXC-MPE in the Korean population. The mechanisms underlying the tiny difference of risk allele between the two populations are unknown, but ethnic specificity may be one of explanations (Lee et al., 2010), such as endogenous peptides and T cell receptors (Geursen et al., 1993; Ko et al., 2011).
We previously reported that HLA-A*24:02 was a common risk factor for AED-SJS/TEN with a tendency of risk for MPE (Shi et al., 2017). In this study, we compared a relatively large cohort comprising of CBZ, LTG, and OXC induced-MPE to tolerant controls (Table 2) and performed meta-analysis of the data from different populations (Figure 1A) to demonstrate that HLA-A*24:02 was a potentially common risk allele for AED-MPE. The binding scores of CBZ, LTG, and OXC with HLA-A*24:02 molecule were much higher than that of the risk-free HLA-A*11:01 molecule (Figure 2A), providing additional evidence of HLA-A*24:02 as a common risk for cADRs. Similarly, HLA-A*30:01 was suggested to be a possibly common risk factor for AED-MPE (Figure 1A and Figure 2A).
Previous studies indicated that some HLA alleles appeared to be a specific risk for AED-cADRs, such as HLA-B*15:02 for CBZ-induced SJS/TEN (Chung et al., 2004), whereas other HLA alleles showed common risk potential, such as HLA-A*24:02 for AED-SJS/TEN (Shi et al., 2017). In the present study, HLA-DRB1*04:06 was demonstrated as a specific risk factor for OXC-induced MPE, while HLA-A*24:02 and HLA-A*30:01 as potentially common risk factors for AED-MPE (Table 1). These findings may be explained by highly polymorphic HLA alleles and the highly heterogeneous binding affinities of the risk HLA molecules with AEDs.
HLA alleles are co-expressed on the cell surface. Our previous study demonstrated that patients with two risk alleles are at greater risk for developing AED-SJS/TEN than those with one risk allele (Shi et al., 2017). In the present study, we found the multiplicative risk potential between HLA-A*24:02 and HLA-B*38:02 (Table 3). The patients with the two alleles increased about 3–5 times the risk for CBZ-induced MPE when compared to the patients with one allele alone. In addition, the NNT using the two risk alleles to prevent one case with CBZ-induced MPE is decreased to 86 (Table 2). Therefore, attentions should be paid to the multiplicative risk potential of HLA alleles.
A recent study reported that HLA-B*15:02 and HLA-B*58:01 were associated with CBZ-induced MPE in the Thai population (Sukasem et al., 2018). Our previous study found that HLA-B*15:02 was associated with CBZ-induced SJS/TEN (Shi et al., 2017). In this study, we did not find its association with CBZ-induced MPE in the Han Chinese population. Another risk allele HLA-B*58:01 is worthy of verifying in more cases with CBZ-induced MPE. Similarly, HLA-A*31:01 was reported to be a risk factor for CBZ-induced cADRs in the European population (McCormack et al., 2011), we did not find such association in the Han Chinese population, in our previous study (Shi et al., 2017), as well as in the present study. The differences may be explained by the different ethnicity. According to the Clinical Pharmacogenetics Implementation Consortium (CPIC) guidelines, HLA-A*31:01 and HLA-B*15:02 should be tested before taking CBZ and CBZ/OXC in patients firstly prescribed, respectively, (Leckband et al., 2013; Phillips et al., 2018). From our findings, the efficacy of HLA-A*31:01 testing for the Han Chinese population should be evaluated further.
The present study had some limitations. We found several HLA alleles to be associated with AED-MPE, but their risk potential was not as strong as that of HLA alleles associated with AED-SJS/TEN (Chung et al., 2004; Shi et al., 2017). MPE is a milder phenotype of cADRs, which would generally be affected by other factors (He et al., 2012; McCormack et al., 2018) that we did not consider in this study. Further efforts are required to identify novel HLA or other genetic risk alleles of cADRs, including that for specific populations. We only analyzed the binding of culprit AEDs with HLA molecules, not considering the complicated neo-self peptides specifically in the presence of AEDs and the complexity of TCRs, which should be investigated further. In addition, HLA-B*40:01 and HLA-C*12:03 were found to be negatively associated with AED-MPE (Supplementary Table S2). However, the precise role of these alleles in the developing of cADRs remains unknown.
In summary, our data suggest that HLA-DRB1*04:06 is a highly specific risk factor for OXC-induced MPE in the Southern Han Chinese and that HLA-A*24:02, possibly HLA-A*30:01 are common risk factors for AED-MPE. Inclusion of these HLA alleles in pre-treatment screening would help estimate the risk of AED-MPE in individuals.
Data Availability Statement
The original contributions presented in the study are included in the article/Supplementary Material, further inquiries can be directed to the corresponding author.
Ethics Statement
The studies involving human participants were reviewed and approved by the Ethics Committee of the Second Affiliated Hospital of Guangzhou Medical University (2017-hs-30). Written informed consent to participate in this study was provided by the participants’ legal guardian/next of kin.
Author Contributions
WL and YS designed and conceptualized the study, analyzed and interpreted data, and drafted and revised the manuscript. WL and YS had full access to all of the data in the study and take responsibility for the integrity of the data and the accuracy of the data analysis. FM and BM contributed to the clinical data collection, meta-analysis and statistical analysis. YM and HL contributed to in silico analysis of protein-drug interactions. All authors collected data, revised the manuscript and contributed to the writing.
All authors listed have made a substantial, direct, and intellectual contribution to the work and approved it for publication.
Funding
This work was funded by the National Natural Science Foundation of China (grant no. 81601136), Science and Technology Project of Guangdong Province (grant nos. 2017B090904036 and 2017B030314159), Science and Technology Project of Guangzhou (grant nos. 201904010275 and 201904020028), Foundation for High-level Talents in Higher Education of Guangdong (grant no. 2013-167), Health Science and Technology Project of Guangzhou (grant no. 20191A011076), and Multi-center Clinical Research Fund Project of the Second Affiliated Hospital of Guangzhou Medical University (grant no. DZX-002). The funders had no role in study design, data collection and analysis, decision to publish or preparation of the manuscript.
Conflict of Interest
Authors YM and HL were employed by the company BGI-Shenzhen.
The remaining authors declares that the research was conducted in the absence of any commercial or financial relationships that could be construed as a potential conflict of interest.
Acknowledgments
We are grateful to He Shanheng Charity Foundation for contributing to the development of our institute.
Supplementary Material
The Supplementary Material for this article can be found online at: https://www.frontiersin.org/articles/10.3389/fphar.2021.671572/full#supplementary-material
Footnotes
1For the China Epilepsy Gene 1.0 Project: www.epg1.cn
References
Alvestad, S., Lydersen, S., and Brodtkorb, E. (2007). Rash From Antiepileptic Drugs: Influence by Gender, Age, and Learning Disability. Epilepsia. 48 (7), 1360–1365. doi:10.1111/j.1528-1167.2007.01109.x
Arif, H., Buchsbaum, R., Weintraub, D., Koyfman, S., Salas-Humara, C., Bazil, C. W., et al. (2007). Comparison and Predictors of Rash Associated with 15 Antiepileptic Drugs. Neurology. 68 (20), 1701–1709. doi:10.1212/01.wnl.0000261917.83337.db
Chen, C.-B., Hsiao, Y.-H., Wu, T., Hsih, M.-S., Tassaneeyakul, W., Jorns, T. P., et al. (2017). Risk and Association of HLA with Oxcarbazepine-Induced Cutaneous Adverse Reactions in Asians. Neurology. 88 (1), 78–86. doi:10.1212/WNL.0000000000003453
Chen, P., Lin, J.-J., Lu, C.-S., Ong, C.-T., Hsieh, P. F., Yang, C.-C., et al. (2011). Carbamazepine-induced Toxic Effects and HLA-B*1502 Screening in Taiwan. N. Engl. J. Med. 364 (12), 1126–1133. doi:10.1056/NEJMoa1009717
Chung, W.-H., Hung, S.-I., Hong, H.-S., Hsih, M.-S., Yang, L.-C., Ho, H.-C., et al. (2004). Medical Genetics: A Marker for Stevens-Johnson Syndrome. Nature. 428 (6982), 486. doi:10.1038/428486a
Cole, D. K., Rizkallah, P. J., Gao, F., Watson, N. I., Boulter, J. M., Bell, J. I., et al. (2006). Crystal Structure of HLA-A*2402 Complexed with a Telomerase Peptide. Eur. J. Immunol. 36 (1), 170–179. doi:10.1002/eji.200535424
Flesch, G. R. (2004). Overview of the Clinical Pharmacokinetics of Oxcarbazepine. Clin. Drug Invest. 24 (4), 185–203. doi:10.2165/00044011-200424040-00001
Fricke-Galindo, I., Martínez-Juárez, I. E., Monroy-Jaramillo, N., Jung-Cook, H., Falfán-Valencia, R., Ortega-Vázquez, A., et al. (2014). HLA-A*02:01:01/-B*35:01:01/-C*04:01:01 Haplotype Associated with Lamotrigine-Induced Maculopapular Exanthema in Mexican Mestizo Patients. Pharmacogenomics. 15 (15), 1881–1891. doi:10.2217/pgs.14.135
Geursen, A., Skinner, M. A., Townsend, L. A., Perko, L. K., Farmiloe, S. J., Peake, J. S., et al. (1993). Population Study of T Cell Receptor Vβ Gene Usage in Peripheral Blood Lymphocytes: Differences in Ethnic Groups. Clin. Exp. Immunol. 94 (1), 201–207. doi:10.1111/j.1365-2249.1993.tb06001.x
Gu, Y., Wong, Y. H., Liew, C. W., Chan, C. E. Z., Murali, T. M., Yap, J., et al. (2019). Defining the Structural Basis for Human Alloantibody Binding to Human Leukocyte Antigen Allele HLA-A*11:01. Nat. Commun. 10 (1), 893. doi:10.1038/s41467-019-08790-1
Gunther, S., Schlundt, A., Sticht, J., Roske, Y., Heinemann, U., Wiesmuller, K.-H., et al. (2010). Bidirectional Binding of Invariant Chain Peptides to an MHC Class II Molecule. Proc. Natl. Acad. Sci. 107 (51), 22219–22224. doi:10.1073/pnas.1014708107
He, N., Min, F.-L., Shi, Y.-W., Guo, J., Liu, X.-R., Li, B.-M., et al. (2012). Cutaneous Reactions Induced by Oxcarbazepine in Southern Han Chinese: Incidence, Features, Risk Factors and Relation to HLA-B Alleles. Seizure. 21 (8), 614–618. doi:10.1016/j.seizure.2012.06.014
Hirsch, L. J., Arif, H., Nahm, E. A., Buchsbaum, R., Resor, S. R., and Bazil, C. W. (2008). Cross-sensitivity of Skin Rashes with Antiepileptic Drug Use. Neurology. 71 (19), 1527–1534. doi:10.1212/01.wnl.0000334295.50403.4c
Hsiao, Y.-H., Hui, R. C.-Y., Wu, T., Chang, W.-C., Hsih, M.-S., Yang, C.-H., et al. (2014). Genotype-phenotype Association between HLA and Carbamazepine-Induced Hypersensitivity Reactions: Strength and Clinical Correlations. J. Dermatol. Sci. 73 (2), 101–109. doi:10.1016/j.jdermsci.2013.10.003
Illing, P. T., Vivian, J. P., Dudek, N. L., Kostenko, L., Chen, Z., Bharadwaj, M., et al. (2012). Immune Self-Reactivity Triggered by Drug-Modified HLA-Peptide Repertoire. Nature 486 (7404), 554–558. doi:10.1038/nature11147
Kalis, M., and Huff, N. A. (2001). Oxcarbazepine, an Antiepileptic Agent. Clin. Ther. 23 (5), 680–700. discussion 645. doi:10.1016/s0149-2918(01)80019-9
Ko, T.-M., Chung, W.-H., Wei, C.-Y., Shih, H.-Y., Chen, J.-K., Lin, C.-H., et al. (2011). Shared and Restricted T-Cell Receptor Use Is Crucial for Carbamazepine-Induced Stevens-Johnson Syndrome. J. Allergy Clin. Immunol. 128 (6), 1266–1276.e11. doi:10.1016/j.jaci.2011.08.013
Koomdee, N., Pratoomwun, J., Jantararoungtong, T., Theeramoke, V., Tassaneeyakul, W., Klaewsongkram, J., et al. (2017). Association of HLA-A and HLA-B Alleles with Lamotrigine-Induced Cutaneous Adverse Drug Reactions in the Thai Population. Front. Pharmacol. 8, 879. doi:10.3389/fphar.2017.00879
Leckband, S. G., Kelsoe, J. R., Dunnenberger, H. M., George, A. L., Tran, E., Berger, R., et al. (2013). Clinical Pharmacogenetics Implementation Consortium Guidelines for HLA-B Genotype and Carbamazepine Dosing. Clin. Pharmacol. Ther. 94 (3), 324–328. doi:10.1038/clpt.2013.103
Lee, M. T. M., Hung, S.-I., Wei, C.-Y., and Chen, Y.-T. (2010). Pharmacogenetics of Toxic Epidermal Necrolysis. Expert Opin. Pharmacother. 11 (13), 2153–2162. doi:10.1517/14656566.2010.495120
Lyskov, S., and Gray, J. J. (2008). The RosettaDock Server for Local Protein-Protein Docking. Nucleic Acids Res. 36 (Web Server issue), W233–W238. doi:10.1093/nar/gkn216
McCormack, M., Alfirevic, A., Bourgeois, S., Farrell, J. J., Kasperavičiūtė, D., Carrington, M., et al. (2011). HLA-A*3101 and Carbamazepine-Induced Hypersensitivity Reactions in Europeans. N. Engl. J. Med. 364 (12), 1134–1143. doi:10.1056/NEJMoa1013297
McCormack, M., Gui, H., Ingason, A., Speed, D., Wright, G. E. B., Zhang, E. J., et al. (2018). Genetic Variation in CFH Predicts Phenytoin-Induced Maculopapular Exanthema in European-descent Patients. Neurology 90 (4), e332–e341. doi:10.1212/WNL.0000000000004853
Mockenhaupt, M., Viboud, C., Dunant, A., Naldi, L., Halevy, S., Bavinck, J. N. B., et al. (2008). Stevens-Johnson Syndrome and Toxic Epidermal Necrolysis: Assessment of Medication Risks with Emphasis on Recently Marketed Drugs. The EuroSCAR-Study. J. Invest. Dermatol. 128 (1), 35–44. doi:10.1038/sj.jid.5701033
Moon, J., Kim, T.-J., Lim, J.-A., Sunwoo, J.-S., Byun, J.-I., Lee, S.-T., et al. (2016). HLA-B*40:02 and DRB1*04:03 Are Risk Factors for Oxcarbazepine-Induced Maculopapular Eruption. Epilepsia. 57 (11), 1879–1886. doi:10.1111/epi.13566
Moon, J., Park, H.-K., Chu, K., Sunwoo, J.-S., Byun, J.-I., Lim, J.-A., et al. (2015). The HLA-A*2402/Cw*0102 Haplotype Is Associated with Lamotrigine-Induced Maculopapular Eruption in the Korean Population. Epilepsia. 56 (10), e161–e167. doi:10.1111/epi.13087
Naranjo, C. A., Busto, U., Sellers, E. M., Sandor, P., Ruiz, I., Roberts, E. A., et al. (1981). A Method for Estimating the Probability of Adverse Drug Reactions. Clin. Pharmacol. Ther. 30 (2), 239–245. doi:10.1038/clpt.1981.154
Norcross, M. A., Luo, S., Lu, L., Boyne, M. T., Gomarteli, M., Rennels, A. D., et al. (2012). Abacavir Induces Loading of Novel Self-Peptides into HLA-B*57: 01: an Autoimmune Model for HLA-Associated Drug Hypersensitivity. AIDS. 26 (11), F21–F29. doi:10.1097/QAD.0b013e328355fe8f
Ostrov, D. A., Grant, B. J., Pompeu, Y. A., Sidney, J., Harndahl, M., Southwood, S., et al. (2012). Drug Hypersensitivity Caused by Alteration of the MHC-Presented Self-Peptide Repertoire. Proc. Natl. Acad. Sci. 109 (25), 9959–9964. doi:10.1073/pnas.1207934109
Ozeki, T., Mushiroda, T., Yowang, A., Takahashi, A., Kubo, M., Shirakata, Y., et al. (2011). Genome-wide Association Study Identifies HLA-A*3101 Allele as a Genetic Risk Factor for Carbamazepine-Induced Cutaneous Adverse Drug Reactions in Japanese Population. Hum. Mol. Genet. 20 (5), 1034–1041. doi:10.1093/hmg/ddq537
Phillips, E. J., Sukasem, C., Whirl‐Carrillo, M., Müller, D. J., Dunnenberger, H. M., Chantratita, W., et al. (2018). Clinical Pharmacogenetics Implementation Consortium Guideline for HLA Genotype and Use of Carbamazepine and Oxcarbazepine: 2017 Update. Clin. Pharmacol. Ther. 103 (4), 574–581. doi:10.1002/cpt.1004
Pichler, W. J. (2002). Pharmacological Interaction of Drugs with Antigen-specific Immune Receptors: the P-I Concept. Curr. Opin. Allergy Clin. Immunol. 2 (4), 301–305. doi:10.1097/00130832-200208000-00003
Roujeau, J.-C. (1994). The Spectrum of Stevens-Johnson Syndrome and Toxic Epidermal Necrolysis: a Clinical Classification. J. Invest. Dermatol. 102 (6), 28S–30S. doi:10.1111/1523-1747.ep12388434
Scally, S. W., Petersen, J., Law, S. C., Dudek, N. L., Nel, H. J., Loh, K. L., et al. (2013). A Molecular Basis for the Association of the HLA-DRB1 Locus, Citrullination, and Rheumatoid Arthritis. J. Exp. Med. 210 (12), 2569–2582. doi:10.1084/jem.20131241
Shi, Y.-W., Min, F.-L., Zhou, D., Qin, B., Wang, J., Hu, F.-Y., et al. (2017). HLA-A*24:02 as a Common Risk Factor for Antiepileptic Drug-Induced Cutaneous Adverse Reactions. Neurology. 88 (23), 2183–2191. doi:10.1212/wnl.0000000000004008
Sukasem, C., Chaichan, C., Nakkrut, T., Satapornpong, P., Jaruthamsophon, K., Jantararoungtong, T., et al. (2018). Association between HLA-B Alleles and Carbamazepine-Induced Maculopapular Exanthema and Severe Cutaneous Reactions in Thai Patients. J. Immunol. Res. 2018, 1–11. doi:10.1155/2018/2780272
Tassaneeyakul, W., Tiamkao, S., Jantararoungtong, T., Chen, P., Lin, S.-Y., Chen, W.-H., et al. (2010). Association between HLA-B*1502 and Carbamazepine-Induced Severe Cutaneous Adverse Drug Reactions in a Thai Population. Epilepsia. 51 (5), 926–930. doi:10.1111/j.1528-1167.2010.02533.x
Toledano, R., and Gil-Nagel, A. (2008). Adverse Effects of Antiepileptic Drugs. Semin. Neurol. 28 (3), 317–327. doi:10.1055/s-2008-1079336
Trott, O., and Olson, A. J. (2009). AutoDock Vina: Improving the Speed and Accuracy of Docking with a New Scoring Function, Efficient Optimization, and Multithreading. J. Comput. Chem. 31 (2), 455–461. doi:10.1002/jcc.21334
Wang, X.-Q., Lang, S.-Y., Shi, X.-B., Tian, H.-J., Wang, R.-F., and Yang, F. (2010). Cross-reactivity of Skin Rashes with Current Antiepileptic Drugs in Chinese Population. Seizure. 19 (9), 562–566. doi:10.1016/j.seizure.2010.09.003
Wang, X.-Q., Lang, S.-Y., Shi, X.-B., Tian, H.-J., Wang, R.-F., and Yang, F. (2012). Antiepileptic Drug-Induced Skin Reactions: a Retrospective Study and Analysis in 3793 Chinese Patients with Epilepsy. Clin. Neurol. Neurosurg. 114 (7), 862–865. doi:10.1016/j.clineuro.2012.01.019
Wang, X., Chao, L., Liu, X., Xu, X., and Zhang, Q. (2019). Association between HLA Genotype and Cutaneous Adverse Reactions to Antiepileptic Drugs Among Epilepsy Patients in Northwest China. Front. Neurol. 10, 1. doi:10.3389/fneur.2019.00001
Xu, J., Shi, X., Qiu, Y., Zhang, Y., Chen, S., Shi, Y., et al. (2019). Association between HLA-A*3201 Allele and Oxcarbazepine-Induced Cutaneous Adverse Reactions in Eastern Han Chinese Population. Seizure. 65, 25–30. doi:10.1016/j.seizure.2018.12.011
Keywords: antiepileptic drugs, oxcarbazepine, maculopapular exanthema, human leukocyte antigen, risk factor
Citation: Shi Y-W, Wang J, Min F-L, Bian W-J, Mao B-J, Mao Y, Qin B, Li B-M, Ou Y-M, Hou Y-Q, Zou X, Guan B-Z, He N, Chen Y-J, Li X-L, Wang J, Deng W-Y, Liu H-K, Shen N-X, Liu X-R, Yi Y-H, Zhou L-M, Zhou D, Kwan P and Liao W-P (2021) HLA Risk Alleles in Aromatic Antiepileptic Drug-Induced Maculopapular Exanthema. Front. Pharmacol. 12:671572. doi: 10.3389/fphar.2021.671572
Received: 26 February 2021; Accepted: 07 May 2021;
Published: 26 May 2021.
Edited by:
Chonlaphat Sukasem, Mahidol University, ThailandReviewed by:
Dean Naisbitt, University of Liverpool, United KingdomVincent Yip, University of Liverpool, United Kingdom
Copyright © 2021 Shi, Wang, Min, Bian, Mao, Mao, Qin, Li, Ou, Hou, Zou, Guan, He, Chen, Li, Wang, Deng, Liu, Shen, Liu, Yi, Zhou, Zhou, Kwan and Liao. This is an open-access article distributed under the terms of the Creative Commons Attribution License (CC BY). The use, distribution or reproduction in other forums is permitted, provided the original author(s) and the copyright owner(s) are credited and that the original publication in this journal is cited, in accordance with accepted academic practice. No use, distribution or reproduction is permitted which does not comply with these terms.
*Correspondence: Wei-Ping Liao, d3BsaWFvQDE2My5uZXQ=