- 1Department of Laboratory Diagnostics and Clinical Immunology of Developmental Age, Medical University of Warsaw, Warsaw, Poland
- 2Department of Methodology, Centre for Preclinical Research, Medical University of Warsaw, Warsaw, Poland
- 3Doctoral School, Medical University of Warsaw, Warsaw, Poland
- 4Department of Internal Medicine and Endocrinology, Medical University of Warsaw, Warsaw, Poland
Neutrophils are crucial elements of innate immune system, which assure host defense via a range of effector functions, such as phagocytosis, degranulation, and NET formation. The latest literature clearly indicates that modulation of effector functions of neutrophils may affect the treatment efficacy. Pharmacological modulation may affect molecular mechanisms activating or suppressing phagocytosis, degranulation or NET formation. In this review, we describe the role of neutrophils in physiology and in the course of bacterial and viral infections, illustrating the versatility and plasticity of those cells. This review also focus on the action of plant extracts, plant-derived compounds and synthetic drugs on effector functions of neutrophils. These recent advances in the knowledge can help to devise novel therapeutic approaches via pharmacological modulation of the described processes.
Neutrophils in Health and Disease
Role in Health
Neutrophils are produced in the venous sinuses of the bone marrow, where they derive from a common myeloid progenitor cells (Figure 1; Nerlov and Graf, 1998). Recently, a novel classification of bone marrow neutrophil-lineage cells emerged. Progenitor stem cells evaluate into a pool of preneutrophils, further differentiating into non-proliferative immature neutrophils and, eventually, mature neutrophils, equipped with numerous machineries to combat pathogens. Maturation of this lineage is orchestrated by transcription factors such as GFI1 and Pu.1 (Evrard et al., 2018). Neutrophils are continually generated in the bone marrow (daily production may reach up to 2 × 1011 cells), where also begin the maturation process (Borregaard, 2010). Granulocyte-colony stimulating factor (G-CSF) is the main agent responsible for their development, production and release (Lieschke et al., 1994). In vivo studies showed that neutrophils may circulate in human blood for ∼10 h (Athens et al., 1961a; McMillan and Scott, 1968) to 5 days (Pillay et al., 2010). Nonetheless, in the course of the development of inflammatory reaction, neutrophils become active, and their lifespan is extended. This is a result of interactions with various cytokines, (e.g. IL-1 beta, tumor necrosis factor (TNF), IFN-gamma), as well as bacterial products, such as lipopolysaccharide (LPS) (Colotta et al., 1992; Summers et al., 2010; Kim et al., 2011). Mature neutrophils (with segmented nuclei and fully formed granules) may also proliferate outside of the bone marrow—in the spleen after infection (Deniset et al., 2017) or when they come in contact with serum amyloid A (De Santo et al., 2010). Longer lifespan allows them to shape many processes: tissue healing, inflammation resolution and modulation of adaptive immune responses, but also may cause adverse effects, including tissue damage (Liew and Kubes, 2019). Neutrophils’ maturation and aging goes in parallel with natural drift in their phenotype and function in the absence of inflammation. These cells undergo daily modifications (Casanova-Acebes et al., 2013), such as: expression of adhesion molecules affecting tissue trafficking, (e.g. CD62L, CD11b, and CD49 days), expression of chemokines receptors, (e.g. CXCR2 and CXCR4), pathogen receptors (Toll-like and NOD-like receptors), activity of pathways related to cell activation (such as NF-kB and MAPK signaling), and cell death (as revised in (Adrover et al., 2016)). This process is influenced by the microbiome, as its depletion with broad-spectrum antibiotics reduces neutrophil number and aging, which can be reversed by LPS stimulation. Similar results were observed in germ-free and TLR2, TLR4, and Myd88 knock-out mice. Neutrophils not stimulated by microbiota are less responsive to pathogens, e.g., their NET formation is decreased (Zhang et al., 2015). The circadian cycle affects the function of neutrophils, possibly as a result of cyclic release of newly formed cells from the bone marrow (Ella et al., 2018). Such a regulation has an impact on the neutrophils’ functions in immunity and inflammation (He et al., 2018).
Over 50 years ago, study of Mauer et al. showed that granulocytes rapidly disappear from circulation when injected into healthy volunteers (Mauer et al., 1960), with further conclusion that total blood granulocyte pool is made up of two compartments, i.e., circulating granulocyte pool and marginal granulocyte pool. They are in an equilibrium with each other and it is possible to move cells from the latter to the former compartment by stimuli like epinephrine or physical exercise (Athens et al., 1961b). Further studies revealed that in absence of inflammation neutrophils can be found in other compartments, including bone marrow (where they inhibit hematopoietic niches and trigger the release of hematopoietic progenitor cells into the bloodstream), spleen (mainly in the marginal zone and red pulp; supporting B cell maturation and antibody production), liver, lung (where they prevent formation of melanoma metastases and affect lung transcriptome in diurnal pattern), intestine (where phagocytosis of incoming neutrophils by resident macrophages regulates cytokine production and participates in the remote regulation of bone marrow niches), white adipose tissue, skin, skeletal muscle, peripheral lymph nodes, intestine (as isolated patches in the intestine) kidneys, and heart. Only immune-privileged organs, i.e., ovaries, testes, and brain are free from neutrophils. Apoptotic neutrophils located in skin, muscle, and intestine are phagocyted by dendritic cells and macrophages, which inhibits IL-23 expression and thus controls granulopoiesis by regulating G-CSF production (Casanova-Acebes et al., 2013). How neutrophils persist in these organs is not fully elucidated yet. Intravascular transit time in the liver is around 2 min (Peters et al., 1985), in the spleen and bone marrow—10 min (Peters et al., 1985; Ussov et al., 1995). Recently, two pools of neutrophils were identified in the spleen. Mobile neutrophils were roaming around the red pulp, while the stationary ones remained in the perivascular area, both populations differ in terms of Ly6G expression. Mature, (i.e. Ly6Ghigh) splenic neutrophils were necessary to eradicate Staphylococci during systemic infection. This process takes place mainly in the red pulp, whereas immature neutrophils, (i.e. Ly6Gint), residing perivascular area, undergo emergency proliferation and mobilization (Deniset et al., 2017) Using intravital microscopy large quantities of neutrophils resident in the lung under normal conditions were visualized (Kreisel et al., 2010). Other study showed that neutrophils in this location are not simply “stuck” in the capillary bed, but rather are on the move–tethering, crawling or adhered to cell surfaces (Yipp et al., 2017). Lung is an organ with a high number of neutrophils in marginated pool, maintained due to expression of adhesion molecules on neutrophils themselves and on endothelial cells. After stimulation, such as infection, profile of adhesion molecules evolve quickly, allowing transmigration (Sibille and Marchandise, 1993). Moreover, the persistence time in this organ is way longer than the mean neutrophil intravascular transit time estimated for other organs (Peters et al., 1985; Lien et al., 1987; Ussov et al., 1995).
Granulocytes’ clearance from the circulation takes place mainly in the liver, spleen, and bone marrow (Shi et al., 2001; Hong et al., 2012). As they grow older, the expression of CXC-chemokine receptor 4 (CXCR4) increases, which probably leads them back to the bone marrow, where they are eliminated (Casanova-Acebes et al., 2013). The same receptor also negatively regulates release of the newly formed neutrophils from the bone marrow (Eash et al., 2009; Summers et al., 2010). CXCR4 is not the only factor responsible for the destruction of neutrophils as cells that lack this receptor have the same half-life as the wild type neutrophils (Eash et al., 2009). Granulocytes may also die in the blood vessels and are later removed by the Kupffer cells (liver-resident macrophages) (Shi et al., 2001). The neutrophils clearance by Kupffer or dendritic cells is regulated, in part, by the IL-23, IL-17 and granulocyte colony-stimulating factor (G-CSF) cytokine axis. These mediators stimulate granulocyte production in the bone marrow as well (Stark et al., 2005). There are still significant gaps in the knowledge about the neutrophil clearance by other cells. Neutrophils die primarily by an intrinsic mechanism of apoptosis, regulated by Bcl-2 protein family. MCL-1 is the key anti-apoptotic agent, antagonizing the pro-apoptotic effects of remaining Bcl-2 family members. Severe neutropenia without affecting other cells circulating in the blood was observed in MCL-1 knock-out mice (Csepregi et al., 2018). During the resolution of inflammation, macrophages dispose apoptotic neutrophils by phagocytosis, releasing lipid mediators. These molecules play a role in re-establishing homeostasis and their absence likely leads to chronic inflammation (Buckley et al., 2014). Some neutrophils sacrifice themselves, releasing neutrophil extracellular traps (NETs) to fight pathogens (Brinkmann et al., 2004; Stark et al., 2005). Neutrophils are also capable of vital netosis–i.e., releasing NETs by a living cell (Yipp and Kubes, 2013).
Significant heterogeneity and functional versatility are observed among neutrophils (Scapini et al., 2016; Silvestre-Roig et al., 2016; Deniset and Kubes, 2018). Both, in steady state and in the course of inflammation, these cells undertake various roles, not always beneficial. It is still unclear whether this variety results from different programs of neutrophils’ maturation and activation or is somehow influenced by external factors. In patients with autoimmune disorders, low density neutrophils/granulocytes were more frequent, though their functions are not precisely defined (Hacbarth and Kajdacsy-Balla, 1986; Scapini et al., 2016). Polymorphonuclear myeloid-derived suppressor cells (PMN-MDSCs) are responsible for the failure of many cancer therapies and poor clinical outcomes. They derive from pathologically activated neutrophils, however, the exact mechanism is of their action is unclear (Gabrilovich, 2017). Two distinct fractions of tumor-associated neutrophils are identified in cancer patients. N1—a pro-inflammatory and anti-tumor subset, induced by TGF-β blockade and N2–a protumor group, increasing in number, following stimulation by TGF-β (Fridlender et al., 2009). The concept of neutrophils’ heterogeneity cannot be universally summarized. We still see the vast gaps in the knowledge that preclude a succinct explanation for this phenomenon. Nevertheless, this heterogeneity may have a significant impact on choosing potential targets for future therapeutic agents (Nemeth et al., 2020).
To exert their effects, neutrophils must first reach the target tissue. The recruitment of granulocytes into the inflamed site involves tethering, rolling, adhesion, crawling and transmigration (Kolaczkowska and Kubes, 2013). The entire process is initiated via activated endothelium that exposes adhesion molecules, that enable leukocytes recruitment. Activation occurs via stimulation by mediators such as histamine, cysteinyl-leukotrienes and cytokines, usually released by resident leukocytes, when the pathogens are present (Ley et al., 2007). Endothelial cells may also be activated directly. If pattern-recognition receptor (PRR) connects with its ligand, the number of adhesion molecules increases on the endothelium surface. P-selectin and E-selectin are responsible for further neutrophil recruitment steps (Petri et al., 2008). They bind to their ligands, including P-selectin glycoprotein ligand 1 (PSGL1), capturing free-flowing neutrophils to the endothelium surface and promote subsequent granulocytes’ rolling along the vessel. Adhesion, crawling and transmigration depend, to a large extent, on integrin interactions with cell adhesion molecules (CAMs) (Phillipson et al., 2006). Luminal surface of endothelium exposes chemokines, which activate rolling neutrophils, thus inducing conformational changes and completing the extravasation process. Neutrophils are capable of returning to the bloodstream via a process called reverse transendothelial cell migration (rTEM). Neutrophils that underwent rTEM are characterized by high CD54 and low CXCR1 expression and are identified more frequently in case of systemic inflammation than in healthy donors (Buckley et al., 2006). Other forms of neutrophils’ motility are: 1) reverse luminal crawling—moving along blood vessel against blood flow; 2) reverse abluminal crawling—occurs while seeking essential directional cues to fully breach venular walls and 3) reverse interstitial migration–a movement directed away from inflammation site within interstitium, which may result in remote organ damage, as documented in ischemic injury (Nourshargh et al., 2016).
Role in Disease
Neutrophils play key roles in many diseases, protecting against pathogens and regulating innate and adaptive immunity. On the other hand, when hyperactive or abnormally stimulated, they may lead to tissue damage and exacerbate existing pathology.
During sepsis, neutrophils show enhanced responsiveness to chemokines, resulting in their accumulation at the infection site (Angus et al., 2001; Martin et al., 2003; Sônego et al., 2016). Some hypotheses suggest that sepsis is associated with an early overwhelming innate immune response, characterized by dysregulation of the overproduction of cytokines (TNF-α, IL-1β, IL-6, IL-8) (Benjamim et al., 2004; Faix, 2013; Chousterman et al., 2017). Concentrations of circulating pro-inflammatory cytokines are low or undetectable in healthy individuals but their production is stimulated during invasion by pathogenic microorganisms. In human and experimental animal models of sepsis, cytokines are released in a sequential manner resulting in a “cytokine cascade” (Steinhauser et al., 1999). It is initiated when a stimulus, such as Gram-negative bacterial endotoxin, (e.g. lipopolysaccharides released by E. coli), induces production of the “early inflammatory cytokines,” like TNF-α and IL-1β. TNF-α was shown to be a central factor of immune regulation and mediator of the pathophysiological changes associated with bacteremia and sepsis syndrome (Benjamim et al., 2004). Overproduction of TNF-α correlates with enhanced properties of phagocytes. In contrast, IL-1β serum levels are only slightly increased during sepsis. The release of “early inflammatory cytokines,” intensifies the production of the “late inflammatory cytokines”—IL-6 and IL-8. IL-6 was recognized as a marker of sepsis with high specificity (Kuster et al., 1998). The increased plasma IL-8 concentration in adult sepsis-occurring patients may correlate with mortality (Marty et al., 1994). Although various pro-inflammatory cytokines contribute to the inflammatory cascade, other cytokines also display anti-inflammatory properties, serving to counterbalance a potentially inadequate pro-inflammatory state. In sepsis, interleukin 10 has been shown to act as the primary endogenous modulator of inflammatory response.
The dysregulation of “cytokine balance” influences of the immune cells functions including phagocytosis and NETosis. The question is: how may cytokine profile affect those processes? Where is the balance between “too much” and “not enough?” A similar question was asked by Garner and colleagues (Garner et al., 1996), who showed that the increasing concentration of TNF-α and IL-1β may suddenly upregulate Fc receptor (FcγR)-mediated phagocytosis by human polymorphonuclear neutrophils (PMN). Nevertheless, the mechanisms of this enhanced phagocytosis remain unknown. This same issue was investigated by Erwig and colleagues. They exposed macrophages on single cytokine and cytokine mixture (IFN-γ, TNF-α, TGF-β, IL-4, IL-6, and IL-10). After 48 h their function was evaluated for nitric oxide (NO) generation, uptake of apoptotic neutrophils, and β-glucuronidase expression. The phagocytic properties of leukocytes were augmented by TNF-α (40 vs 29% controls) and decreased by IFN-γ, IL-10, and IL-4 (Erwig et al., 1998).
Similarly to phagocytosis, NET formation (Galluzzi et al., 2018) may be effective anti-microbial mechanism in the course of sepsis, however with time it may contribute to tissue and endothelial damage, finally leading to disseminated intravascular coagulation (DIC), with high mortality rate. Interestingly, both SOFA (Sequential Organ Failure Assessment) score and acute kidney injury (AKI) correlates with cell-free DNA, measured in septic patient serum (Klopf et al., 2021).
Some studies highlight neutrophils contribution to antiviral immunity (Saitoh et al., 2012; Jenne et al., 2013). In the course of COVID-19, neutrophils-to-lymphocytes ratio was established as an independent prognostic factor (Wang et al., 2020). Recent study showed that increased amounts of reactive oxygen species (ROS) and the release of NETs are associated with intensive thrombi formation–one of the most prevalent and serious COVID-19 complications (Arcanjo et al., 2020). In COVID-19 patients, cell-free DNA, MPO-DNA complexes, and citrullinated histone H3 have been assessed and appeared to be elevated. Interestingly, cell-free DNA and MPO-DNA complexes were significantly higher in the blood from mechanically ventilated vs. non-ventilated patients. It was suggested that vascular damage, resulting in acute respiratory distress syndrome (ARDS) and multiorgan dysfunction, may be due to NET formation (Klopf et al., 2021). The abovementioned findings may link cytokine storm (as discussed in (Ragab et al., 2020)), microangiopathic occlusions and ARDS, and justifies use of steroids, (i.e. dexamethasone, as shown in RECOVERY trial (Horby et al., 2021)) and tocilizumab (Zhang et al., 2020b) in COVID-19 treatment.
In the respiratory system, neutrophils act like a double-edged sword. On the one hand, they defend organism against pathogens, but on the other they cause extensive tissue damage in e.g., acute lung injury, chronic obstructive pulmonary disease, or neutrophilic asthma (Grommes and Soehnlein, 2011; Meijer et al., 2013; Krishnamoorthy et al., 2018). Neutrophils also contribute to formation and destabilization of atherosclerotic plaques (Soehnlein, 2012). The accumulation of these cells is associated with a greater risk of plaque rupture (Ionita et al., 2010). Moreover, neutrophils accumulated at the plaque site may release NETs, which leads to smooth muscle cells damage, prompting destabilization (Silvestre-Roig et al., 2019).
In ischemic diseases, such as myocardial infarction or stroke, inflammatory processes cause severe tissue damage, especially after reperfusion (Eltzschig and Eckle, 2011). In myocardial infarction model, Ly6Chigh monocytes and neutrophils contributed comparably to proteolysis, which may result in left ventricle rupture (Anzai et al., 2017). As mentioned above, neutrophils may undergo rTEM, stimulated by LTB4, from area of ischemia-reperfusion injury and contribute to distant organ damage (Colom et al., 2015).
The role of NET formation in autoimmune disease was reviewed by Mitsios et al. (Mitsios et al., 2016) and Lee et al. (Lee et al., 2017). Some authors suggest that the anti-DNA immunization in systemic lupus erythematosus may be initiated by NETs. Released nucleic acid activates the dendritic cells, which in turn prompt antibodies production by B cells, causing systemic autoimmunity (Garcia-Romo et al., 2011; Lande et al., 2011). Consistently, citrullinated histones, present in NETs were suggested as the trigger activating anti-citrullinated peptide autoantibodies (ACPAs) production, prevalent in rheumatoid arthritis (Khandpur et al., 2013). Release of proteinase 3 (PR3) and myeloperoxidase (MPO) may initiate antineutrophil cytoplasmic antibody-associated vasculitis (Kessenbrock et al., 2009).
Cancer biology is influences by neutrophils’ behavior. As mentioned before, neutrophils may exert pro-inflammatory or pro-tumoral effects (Fridlender et al., 2009). This phenotypic switch and overall plasticity may explain the vast spectrum of neutrophil activities, both harmful and beneficial to the host (Sagiv et al., 2015). Some of the undesirable effects include suppression of T cell-mediated antitumor immunity (Coffelt et al., 2015; Steele et al., 2016) or elastase-mediated degradation of IRS1 (Houghton et al., 2010), enhancing cancer cell proliferation. On the other hand, some studies reported that neutrophils prevent metastasis formation (Granot et al., 2011) or inhibit early cancer growth (Blaisdell et al., 2015). In solid tumors, increased neutrophils number in tumor microenvironment is a negative outcome predictor (Templeton et al., 2014; Gentles et al., 2015).
TO Phagocytose, to Degranulate or to Make Extracellular Traps?
Neutrophils are crucial elements of innate immune system, which assure host defense via a range of effector functions, such as phagocytosis, degranulation, and NET formation (Figure 2; Kolaczkowska and Kubes, 2013).
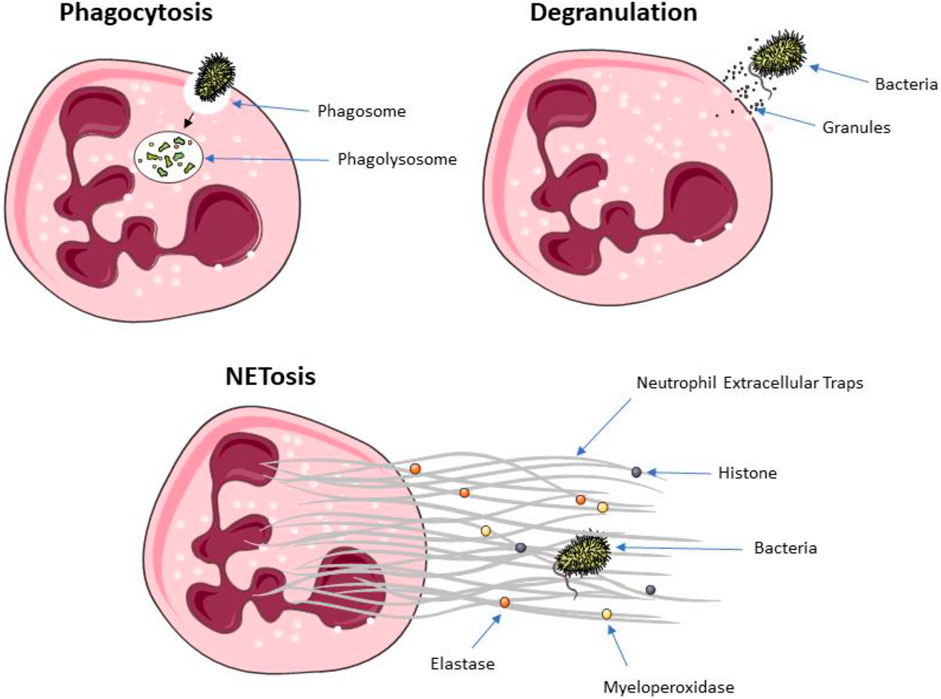
FIGURE 2. The killing mechanisms of neutrophils: phagocytosis, degranulation, and extracellular traps release.
Phagocytosis is a fundamental mechanism for the effective elimination of disease-causing agents (Schumann, 2016). It enables clearance of microbes, but also dead cells and tissue debris (Jaumouillé and Waterman, 2020). Thus, phagocytosis is a fundamental component responsible for tissue homeostasis and the innate immune response (Jaumouillé and Waterman, 2020). This process is initialized by internalization of targeted organism/particle. Two different mechanisms have been identified for the internalization of diverse particulate material: 1) the trigger mechanism where discreet signaling initiates formation of plasma membrane protrusions, shaped by actin, that surround nearby material, and 2) the zipper mechanism where cell surface receptors sequentially bind to ligands on the target particle, which leads to a complete wrapping of the particle by the plasma membrane (Nordenfelt and Tapper, 2011). Only a small number of pathogens, (e.g., Salmonella or Shigella) is able to initiate the process, whereas the zipper mechanism, involving a wide spectrum of phagocytic receptors, allows to successfully bind many species of pathogen/particles (Jaumouillé and Waterman, 2020). The essential phagocytic receptors are Fcγ class receptors, which recognize IgG, the complement receptor 3 (αMβ2 integrin). While TLRs and NOD receptors are not classified as phagocytic receptors, their activation may increase phagocytosis (Nordenfelt and Tapper, 2011). Receptor activation initiates signaling cascades that re-model lipids in the cell membrane and leads to rearrangement of actin cytoskeleton in order to extend the cell membrane around the particle. After reconstructing the plasma membrane of the phagocyte, bacteria are ultimately internalized in phagosome (Fairn and Grinstein, 2012). The dynamics of phagosome maturation is highly dependent on the several regulators like Rab proteins synthesis. Among known Rab proteins, Rab5 and Rab7 are directly involved in regulation of phagosome functions (Mottola, 2014).
Unlike macrophages, maturation of neutrophilic phagosomes is dependent on fusion with preformed granules (Nordenfelt and Tapper, 2011; Gierlikowska et al., 2020b). Membrane proteins and granule contents are directed to distinct locations by precise trafficking and fusion/fission processes (Nordenfelt and Tapper, 2011). Activation of granules is assisted by recruitment of NADPH oxidase (Johansson et al., 1995). It is suggested that an early alkalization of neutrophil phagosomes leads to the oxidative burst in the phagosomes (Segal et al., 1981).
Besides ROS production, neutrophils eliminate pathogens via production of microbicidal molecules following degranulation (Mortaz et al., 2018).
Degranulation is important for pathogen killing, but also modulates the immune response during infectious or non-infectious diseases (Mortaz et al., 2018). Different granule types are released sequentially (Kolaczkowska and Kubes, 2013). At the beginning, tertiary granules are released, (e.g. cathepsin, gelatinase, known as metalloprotease 9), then secondary granules, (e.g. lactoferrin, alkaline phosphatase, lysozyme, cathelicidin, NADPH oxidase and collagenase). At the end, primary granules are secreted containing the most pro-inflammatory and antimicrobial proteins, such as elastase, MPO, cathepsin G, defensins (HNP-1, HNP-2 and HNP-3 peptides) and bacterial permeability increasing protein (BPI) (Faurschou and Borregaard, 2003). Normal neutrophil degranulation involves the release of primary and secondary granules mainly into the phagosome, which prevents host tissue damage (Sengeløv et al., 1995). Bacteria are able to disrupt, dysregulate, or induce excessive neutrophil degranulation, in order to diminish the protective effects of neutrophil degranulation in a way that ultimately benefits the pathogen and extend the disease course, thus pharmacological modulation may potentially bring promising results for recovering (Eichelberger and Goldman, 2020).
Neutrophils use a few strategies to counteract infections. Two of those strategies have been thoroughly studied and are described above: phagocytosis and degranulation. They can also utilize NADPH oxidase to form ROS which have antimicrobial potential (Mantovani et al., 2011). A new mechanism that combats infections—NET formation–has been described in 2004 (Brinkmann et al., 2004). Once the process is initiated, the chromatin in neutrophils’ DNA loosens up and it forms complexes with numerous granular and cytoplasmic proteins, which are subsequently released into the extracellular space. Depending on the origin of proteins, they could by classified as histones (H1, H2A, H2B, H3, and H4), azurophilic granules (MPO, elastase, defensins, cathepsin G), specific granules (lactoferrin, alkaline phosphatase, NADPH oxidase, lysozyme, collagenase), tertiary granules (gelatinase, cathepsin) and cytosol proteins (LL-37, proteinase 3, neutrophil serine protease 4 and tryptase) (Brinkmann et al., 2004). The biological function of extracellular traps is to sequester a specific space, enabling accumulation of high concentration of antimicrobial agents, preferably at the site of infection. Thus extracellular traps immobilize and kill microorganisms, which prevent them from dissemination (Manda et al., 2014).
Modulations of killing mechanisms (like phagocytosis, degranulation, or NET formation) could be of interest, as it may potentially boost pathogen killing or protect host from own tissue damage.
Pharmacological Modulation of Phagocytosis via Medical Plants
Therapies targeted at innate immune response modulation via medicinal plants and metabolites isolated from them experience a renaissance in recent years (Ríos, 2010; Licciardi and Underwood, 2011; Grigore et al., 2020; Behl et al., 2021).
After performing a literature search using relevant MeSH terms and keywords, we identified 53 plant extracts, which were tested on neutrophil model and their influence on phagocytic properties were evaluated (Table 1). Selected plants belonged to diverse families: Anacardiaceae, Araceae, Araliaceae, Arecaceae, Apocynaceae, Asphodelaceae, Asteraceae, Boraginaceae, Capparaceae, Celastraceae, Convolvulaceae, Cucurbitaceae. Elaeocarpaceae, Euphorbiaceae, Fabaceae, Geraniaceae, Hypericaceae, Lamiaceae, Lythraceae, Malvaceae, Melastomataceae, Meliaceae, Menispermaceae, Moraceae, Moringaceae, Phyllanthaceae, Poaceae, Ranunculaceae, Rosaceae, Rubiaceae, Santalaceae, Sapindaceae, Saxifragaceae, Theaceae, Zingiberaceae, and Zosteraceae. Plant materials were collected in Europe, Asia, Southern and Northern America and Africa. The geographic location directly determines the phytochemical composition of plants and their pharmacological activity (Czerwińska and Melzig, 2018; Kiss et al., 2020). Thus, evaluation of the medical plants collected from different locations, may highlight future directions for searching other plants (with similar chemical composition), and then select them for compounds isolation (Gierlikowska et al., 2020a). We noticed that 33 of the selected plant extracts stimulated phagocytic activity of neutrophils, 18 decreased phagocytic activity and 2 extracts did not affect the process.
Pharmacological modulation of phagocytosis may affect the uptake of pathogens, biochemical changes inside of phago- and lysosomes, phagolysosomes formation and modulation of intracellular killing via oxygen-dependent and oxygen-independent degradation (Table 1).
Among selected plant extracts, two of them, obtained from Tinospora crispa (L.) and Heliotropium sarmentosum (Lam.), modulated expression of surface receptors. The neutrophils treated with T. crispa as well as H. sarmentosum extracts overexpressed MAC-1 cell surface receptor leading to increased phagocytic activity (Chen et al., 2014; Ahmad et al., 2015). MAC-1 is a complement receptor (“CR3”) consisting of CD11b (integrin αM) and CD18 (integrin β2) (Todd, 1996). The integrin CD11b is responsible for direct binding to intercellular adhesion molecule-1 (ICAM-1) leading to firm adhesion to endothelium and transmigration of phagocytes to infected tissues (Ross and Vĕtvicka, 1993). Considering that T. crispa has been used traditionally in the treatment of rheumatoic arthritis, urinary tract infections, fever, inflammation, fracture, and hypertension (Ahmad et al., 2015), the modulation of phagocytic properties of neutrophils creates new research perspectives for further evaluation. Based on the use in traditional medicine, H. sarmentosum has demonstrated anti-inflammatory, antinociceptive and antipyretic activities (Chen et al., 2014), thus similarly to T. crispa modulation of phagocytosis may point to unknown immunomodulatory properties of this extract.
Pharmacological stimulation of C3b and Fcγ expression on neutrophil surface was proved for Echinacea purpurea (L.) Moench (Melchart et al., 1995; Isaykina et al., 2008). E. purpurea has a well-documented biological effect in a variety of diseases, particularly bacterial and viral infections. Recently, many studies are focused on immunomodulatory effects of echinacea. The phytochemical analysis confirmed that alkamides, flavonoids, derivatives of quercetin, kaempferol and caffeic acid, are considered important for biological activity (Barnes et al., 2005).
Popov and colleagues showed that Bergenia crassifolia (L.) treatment resulted in stimulation of neutrophilic uptake capacity (Popov et al., 2005). This observation justifies the traditional use of B. crassifolia as a treatment of bacterial infections (Shikov et al., 2014). The plant presents a multitude of bioactive agents, including tannins (pedunculagin, tellimagrandin I), polysaccharides, flavonoids (bergenin, kaempferol, and quercetin derivatives) aldehydes (2,4-heptadienal, benzaldehyde), terpenes (thymol, geraniol), phenolics (arbutin, ellagic acid, hydroquinone), phenolic acids (caffeoyl quinic acid, gallic acid, quinic acid) and other compounds (bergenan) (Shikov et al., 2014). The selected compounds are typical for Bergenia species and exerts anti-inflammatory, antimicrobial, antioxidant, and immunomodulating properties (Shikov et al., 2014). A well-studied compound is bergenin, which stimulates the uptake of apoptotic human neutrophils and then intensifies the ROS production by mouse macrophages (Shikov et al., 2014).
Tinospora cordifolia (Willd.), Ocimum tenuiflorum L. and Semecarpus anacardium L. stimulated lysosomal enzymes activity increasing phagocytic properties of neutrophils (Mukherjee et al., 2005; Ramprasath et al., 2006; Sharma et al., 2012). T. cordifolia extract was tested on Escherichia coli-induced peritonitis in a mice model—both phagocytic and intracellular bactericidal capacities of neutrophils were enhanced by extract treatment (Thatte et al., 1992). The reduction of bacterial colonization in mice model was also observed after O. tenuiflorum treatment (Saini et al., 2009).
Ipomoea batatas (L.) was identified as an extract capable of stimulating phagolysosome formation inside of neutrophils (Miyazaki et al., 2005).
Inside of newly formed phagolysosomes, ROS are produced more intensively (Nathan, 2006; Urban et al., 2006). One of the major functions of phagocytes is to produce ROS during the oxidative burst (Brungs et al., 2015). Thus, stimulating this process may have beneficial effects during bacterial infections. Echium amoenum Fisch (Asadollahi et al., 2015), Grewia asiatica L. (Mesaik et al., 2013), Saccharum officinarum L. (El-Abasy et al., 2002) and Acer pictum subsp. mono (Maxim.) stimulated ROS production and enhanced phagocytosis efficiency. These plants have documented antioxidant and immunomodulatory properties (Abed et al., 2012; Akram et al., 2014; Mehmood et al., 2020).
The signaling pathway leading to ROS release is highly dependent on spleen tyrosine kinase (Syk) activation (Brungs et al., 2015). Three plant extracts (Cuscuta epithymum (L.), Ipomoea batatas (L.) and Euphorbia hirta L.) stimulated Syk phosphorylation resulting in a more pronounced ROS production and an increased phagocytic activity (Sudam et al., 2017).
Besides the plant extracts that stimulated phagocytosis, we also presented plant materials that inhibited this process or had no effect on it (Table 1). As mentioned above, 18 plant extracts decreased phagocytic activity of neutrophils and 2 extracts did not affect this process. Only few molecular mechanisms responsible for suppression of phagocytosis were identified. They were dependent on the inhibition of MAC-1 cell surface expression (Phyllanthus amarus, Camellia sinensis (L.)) (Ilangkovan et al., 2015; Albuquerque et al., 2016), suppression of CR1, CR3, CR4, and Fcγ receptors expression (Areca catechu L.) (Hung et al., 2006) and inhibition of ROS production (Cucumis maderaspatanus L. Ajuga reptans L. Ajuga genevensis L. Osbeckia octandra DC, Cedrela fissilis Vell, Trichilia glabra L. Phyllanthus debilis, Crataegus laevigata (Poir.), Ixora coccinea L. Zingiber zerumbet (L.)) (t Hart et al., 1990; Benencia et al., 1995; Benencia et al., 2000; Dalli et al., 2008; Wickramasinghe et al., 2014; Akhtar et al., 2019; Toiu et al., 2019).
Two plant extracts: Punica granatum L. (Oliveira et al., 2010) and Melia azedarach L. (Benencia et al., 1994) did not affect phagocytosis. P. granatum, is known as a medical plant used for hypertension, diabetes, as well as a few types of cancer, but not for bacteria-induced diseases (Ge et al., 2021). M. azedarach was also used for the cancer-related disease treatment (Ervina et al., 2020; Shrestha et al., 2021).
We identified a few 9) plant-derived compounds regulating the neutrophils’ phagocytosis (Table 2). Seven compounds stimulated this process (gingerol, arecoline, 11-hydroxymustakone, N-methyl-2-pyrrolidone, N-formylannonain, magnoflorine, and tinocordiside) (Hung et al., 2006; Sharma et al., 2012; Jin et al., 2016) and two did not affect phagocytosis (cordifolioside A, syringin) (Sharma et al., 2012). Gingerol was isolated from Pinellia pedatisecta Schott, arecoline from Areca catechu L. and the rest of the selected compounds were isolated from Tinospora cordifolia (Willd.). P. pedatisecta as well as A. catechu are well-known in Chinese medicine as anti-inflammatory agents (Li et al., 2017; Wang et al., 2019). T. cordifolia is also known in Asian traditional medicine as an anti-inflammatory agent (Yates et al., 2021).
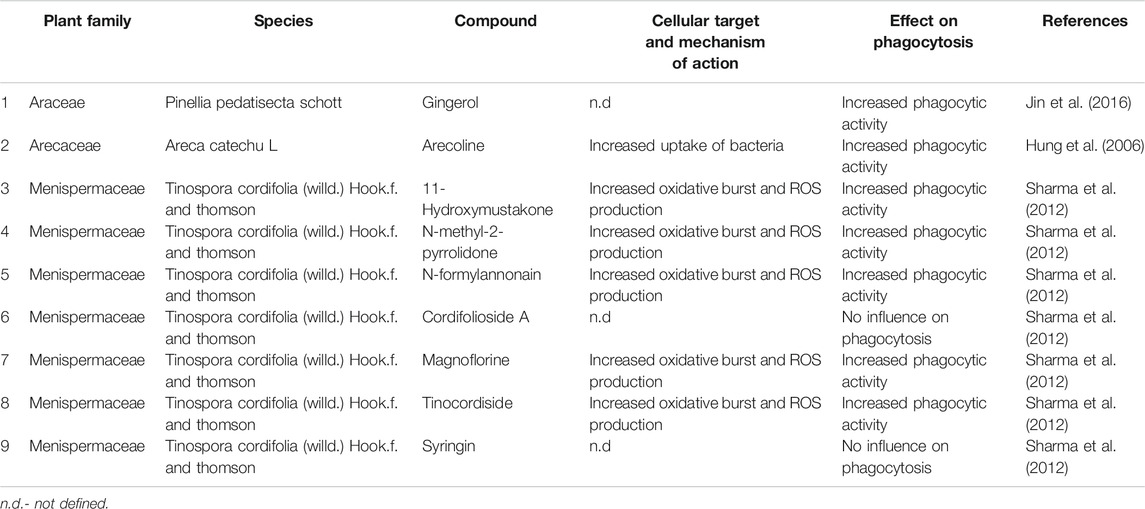
TABLE 2. List of selected compounds isolated from plant material with documented impact on phagocytosis.
Pharmacological Modulation of Phagocytosis by Selected Drugs
Some papers reported the effects of synthetic drugs on phagocytic function of neutrophils, but the data are scarce (Table 3). Numerous macrolide antibiotics stimulate phagocytosis performed by macrophages (Hodge et al., 2006; Xu et al., 2019), at least for some of the compounds using phosphatidylserine receptor-dependent pathway (Yamaryo et al., 2003). These results encouraged researchers to test influence of erythromycin (Cuffini et al., 2009) and azithromycin (Pohl et al., 2020) on neutrophil’s phagocytosis, which revealed that the former indeed stimulates phagocytosis, whereas the latter does not affect it. Fosfomycin was shown to enhance intracellular killing of S. aureus in both macrophages and neutrophils (Shen et al., 2016). Among antibiotics, chloramphenicol was also tested showing no significant results (Bystrzycka et al., 2017). Clinical significance of those findings remains uncertain, nevertheless testing the influence of other antibiotics, especially macrolides, on phagocytic function should be encouraged.
The interaction between melatonin and immune system is being widely explored, as discussed previously (Carrillo-Vico et al., 2013). Among others, its influence on neutrophil-mediated phagocytosis was tested, showing its inhibitory role in this phenomenon (Xu et al., 2019). The data on beneficial role of melatonin against polymicrobial sepsis also exist (Xu et al., 2019), thus further studies on the exact role of melatonin are needed.
Pharmacological Modulation of Degranulation via Medical Plants
We have identified 14 plant extracts, whose impact on degranulation was tested (Table 4). All the extracts inhibited the degranulation, but only in 5 cases the mechanism of action was explained. Decreased degranulation resulted from inhibiting the elastase and MPO synthesis and release. An elastase-inhibitory effect was observed after Typhonium roxburghii treatment (Korinek et al., 2016), as well as Panax notoginseng (Jin et al., 2007), Lobelia chinensis Lour (Kuo et al., 2011) and Tamarindus indica L. (Paula et al., 2009). Selected plants were widely used to treat cancer and inflammatory-related diseases (Jin et al., 2007; Kuo et al., 2011; Korinek et al., 2016).
Anti-MPO activity was observed for Anvillea garcinii subsp. radiate (Boukemara et al., 2016). This plant was used for symptomatic treatment of cold (Perveen et al., 2020), but the successful treatment was related to the presence of flavonoids and sesquiterpene lactones, which show significant antibacterial and antifungal properties (Perveen et al., 2020). Thus, based on traditional use of A. garcinii, further evaluation of phagocytosis seems to be worth exploring.
For the rest of tested extracts (Cnidium monnieri (L.), Angelica gigas Nakai, Carthamus tinctorius L. Cinnamomum cassia (L.), Guarea kunthiana A. Juss. Prunus persica (L.), Rehmannia glutinosa (Gaertn.), Eugenia aurata, and Eugenia punicifolia) authors documented only final effect on degranulation without identifying the molecular basis of the observed effect (Park et al., 2004; Costa et al., 2016; Jerjomiceva et al., 2016).
Chung and colleagues identified Hypericum geminiflorum Hemsl. as a source of compounds modulating degranulation (Table 5). Gemichalcone A and gemichalcone B. isolated from H. geminiflorum decreased the degranulation of neutrophils (Chung et al., 2002). Plants belonging to the Hypericum species were used as diuretics, analgetics, antiphlogistics and antidepressant agents (Zhang et al., 2020a). Immunomodulatory, anti-inflammatory or antimicrobial properties of Hypericium species are unknown. Another example of a plant-derived compound decreasing degranulation of neutrophils is quercetin (Kanashiro et al., 2007; Pečivová et al., 2012). Although quercetin is widespread in many plant species, in both mentioned articles quercetin was used as a synthesized compound. Quercetin is a plant molecule that has shown many pharmacological activities, such as antimicrobial, antiviral, anticancer, having potential for treating metabolic, allergic, and inflammatory disorders, cardiovascular diseases, and arthritis (Batiha et al., 2020). The documented pharmacological effects encouraged to introduction of quercetin to the pharmaceutical market, however, low solubility in water, which is a key factor in drug absorption and its bioavailability, limits its use (Batiha et al., 2020). Further attempts to increase the quercetin solubility are urgently needed. Interestingly, quercetin, as well as described above gemichalcone A and gemichalcone B belong to flavonoids (Panche et al., 2016), which may indicate a specific chemical group of plant-derived compounds that modulate neutrophil degranulation.

TABLE 5. List of selected compounds isolated from plant material with documented impact on degranulation of neutrophils.
Another source of compounds modulating degranulation is Salvia officinalis L.–it contains salvianolic acid capable to stimulate degranulation (Wang et al., 2010). Although mentioned data refer to mast cells the observed pharmacological effect is noteworthy, and in our opinion further studies on neutrophil model may also bring promising results S. officinalis was used for the treatment of inflammation, gout, paralysis, ulcers, rheumatoid arthritis, dizziness, tremor, diarrhea, and hyperglycemia (Ghorbani and Esmaeilizadeh, 2017). Salvianolic acid is considered a chemo-preventive agent which suppresses oxidative stress and apoptosis (Wang et al., 2021). It was also documented that salvianolic acid may also be an antioxidant and anti-inflammatory agent which modulates PI3K/Akt/mTOR signaling pathway (Jin et al., 2021).
Pharmacological Modulation of Degranulation via Drugs
Number of synthetic drugs known to regulate neutrophils degranulation is even lower than that affecting phagocytosis (Table 6). Similarly, two macrolide antibiotics were tested, and it was shown that erythromycin stimulates degranulation (Abdelghaffar et al., 1997), whereas azithromycin does not affect this process (Pohl et al., 2020). Chloramphenicol was also investigated, but exerted no effect on this function (Bystrzycka et al., 2017). Again, whether these effects are of clinical significance, remains unclear.
Pharmacological Modulation of NETosis via Medical Plants
A few plant extracts were tested for their influence on NETosis (Table 7), showing that extracts obtained from Anacardium occidentale L. (Hollands et al., 2016), Verbesina erstediana Benth (Yaseen et al., 2017), and Guarea kunthiana A. Juss (Jerjomiceva et al., 2016) increased it, whereas Salvia miltiorrhiza Bunge (Tao et al., 2018), Eugenia aurata (Costa et al., 2016), and Eugenia punicifolia (Costa et al., 2016) decreased it.
A. occidentale has shown significant antibacterial activity and might have potential applications as new antibacterial drug (Shabeeba and Rathinasamy, 2018). A. occidentale extract has been tested in mice and rat models as a therapy of gastric-related conditions (Konan and Bacchi, 2007; Morais et al., 2010; Goulart da Silva et al., 2021). Thus, further evaluation of its immunomodulatory properties may open novel prospects. V. erstediana and G. kunthianawere were used in the folk medicine in treatment of bacterial infections, especially S. aureus (Jerjomiceva et al., 2016; Yaseen et al., 2017). Thus, documented antimicrobial properties justify the traditional use.
It is unknown why E. aurata and E. punicifolia extracts decreased NET release. For S. miltiorrhiza it has been speculated that decreased NET formation may results from the inhibition of MPO and NADPH oxidase (NOX) activity (Tao et al., 2018).
Six plant-derived compounds were investigated (Table 8). Anacardic acid, as the only substance, was able to increase NET production (Hollands et al., 2016), whereas catechin hydrate, epicatechin, rutin trihydrate (Kirchner et al., 2013), celastrol (Yu et al., 2015), and polydatin (Liao et al., 2018) decreased it. Anacardic acid belongs to phenolic lipids (Hollands et al., 2016), a diversified group of compounds derived from mono and dihydroxyphenols (Stasiuk and Kozubek, 2010). Anacardic acid shows antioxidant capacity, what may be related with suppression of superoxide generation (Trevisan et al., 2006). According to Hollands et al. inhibition of ROS production (inter alia superoxide generation) directly affects NET formation (Hollands et al., 2016).
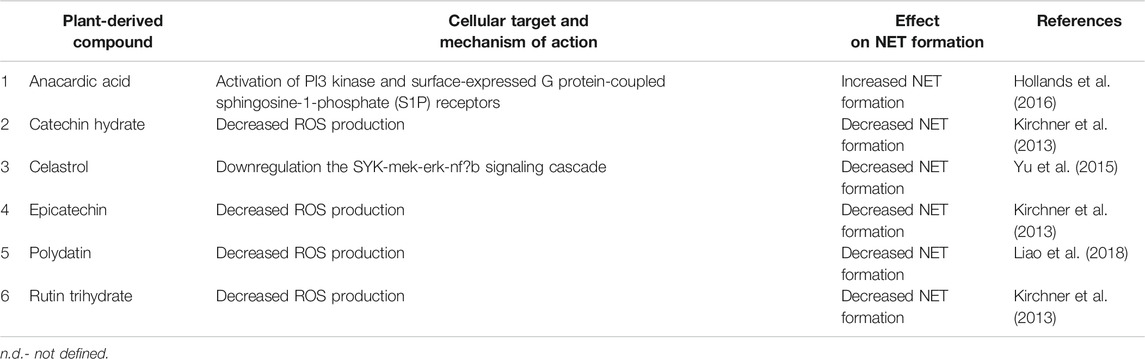
TABLE 8. List of selected compounds isolated from plant material with documented impact on NET formation.
Other compounds (catechin hydrate, epicatechin, rutin trihydrate, celastrol, and polydatin) classified as NET formation inhibitors belong to different chemical groups, thus an unambiguous assignment of a chemical structure to a biological function is not possible. Interestingly, excluding celastrol, compounds decreased NET formation via modulation of ROS production. Although anacardic acid also decreased ROS production, the biological effect was opposite to the one observed for other compounds. Because ROS production is crucial for efficient pathogen killing and NET formation, the dependency between chemical structure and pharmacological effect, as well as potential cytotoxicity need to be evaluated in the future.
Pharmacological Modulation of NET Formation via Drugs
We identified 19 drugs, whose impact on NET formation was tested, mostly antibiotics and antimycotics (Table 9). β-lactams belong to the most widely used class of antibiotics (Bush and Bradford, 2016), but only two drugs from this group were tested. Amoxicillin increased NET formation (Bystrzycka et al., 2016), whereas no such effect was observed for cefotaxime (Manda-Handzlik et al., 2017). Three representatives of macrolides were investigated with different results. Clarithromycin increased NETosis (Konstantinidis et al., 2016; Arampatzioglou et al., 2018), erythromycin decreased it (Zhang et al., 2019), whereas azithromycin exerted no effect (Manda-Handzlik et al., 2017). Among other groups single particles were tested. Enrofloxacin (fluoroquinolone used in veterinary medicine (López-Cadenas et al., 2013)) increased NET formation (Jerjomiceva et al., 2014), chloramphenicol (Bystrzycka et al., 2017) and gentamicin (Manda-Handzlik et al., 2017) decreased it, whereas minocycline (tetracycline used in acne vulgaris, but with some safety issues, as revised in (Garner et al., 2012)) did not affect this effect (Irizarry-Caro et al., 2018).
Neutrophils play an essential role in counteracting mycoses, including NET release, as recently revised (Urban and Nett, 2019). Both amphotericin B, usually used in severe cases (Hamill, 2013), and voriconazole, a drug of choice in invasive aspergillosis (Malani et al., 2015), decreased NET formation (Decker et al., 2018).
Above-mentioned data clearly reveal the complexity of the issue. It obvious that stimulation of NET production during an infection is beneficial, as it increases pathogen clearance and thus infection control, on the other hand it can participate in host tissue’s damage, as it is observed in acute respiratory distress syndrome (Grégoire et al., 2018), or even promote bacteria proliferation (Czaikoski et al., 2016). The effects of antimicrobial drugs are not easy to interpret due to difficulties in separating direct antimicrobial and immune-modulating properties. To further investigate this matter, in vivo studies on combinations of antibiotics or antimycotics with NET-modulating particles are needed. The substances discussed below can be used as such particles.
It was demonstrated that acidification inhibits ROS-dependent NET release (Behnen et al., 2017), whereas more alkaline pH stimulates NET formation mediated by both NOX2-independent (Naffah de Souza et al., 2017) and NOX2-dependent (Khan et al., 2018) pathways. These results are of interest since hypoperfusion-induced lactic acidemia is common in sepsis and septic shock and should be managed accordingly (Rhodes et al., 2017). It is worth verifying whether increasing pH affects NET release during the treatment.
Mannitol and hypertonic saline, agents administered to patients with intracranial edema (Burgess et al., 2016) may suppress of NOX2-dependent NET production (Nadesalingam et al., 2018). Again, the clinical significance of this observation is not clear.
Metformin remains the first line of pharmacological treatment in type 2 diabetes mellitus (Baker et al., 2021) and may be beneficial in numerous other conditions (Lv and Guo, 2020). Studies showed that it decreases NET release through inhibition of elastase, proteinase-3 and histones (Menegazzo et al., 2018). Clinical significance of this phenomenon is not clear, nevertheless it should be noted, that in sepsis and septic shock accompanied with hyperglycemia insulin is a drug of choice (Rhodes et al., 2017). As mentioned, NET formation seems to play a role in atherosclerosis (Soehnlein, 2012), which may be prevented by a long-term metformin treatment (as revised in (Luo et al., 2019)).
Melatonin was also tested for its effect on NET production and increased it (Xu et al., 2019). Among other hormones only 17-β-estradiol was tested–it also increased NET formation (Yasuda et al., 2019). Since women have overall stronger immune responses, as revised in (Moulton, 2018), further studies are of interest.
Memantine is a drug approved for Alzheimer’s disease and tested for other applications (Lu and Nasrallah, 2018). It was shown that through stimulation of MPO production, it increases NET formation (Peng et al., 2020). Clozapine, the first atypical antipsychotic drug, whose side effects involve agranulocytosis (Khokhar et al., 2018) was also tested and was shown to exert no influence on NET formation (Irizarry-Caro et al., 2018).
Drug-induced lupus is a condition similar to systemic lupus erythematosus and triggered by initiation of a certain drug, usually resolving after the medication’s withdrawal (Borchers et al., 2007). Carmona-Rivera et al. demonstrated a role of NETosis in this pathology (Carmona-Rivera et al., 2017). In this context, an interesting approach was proposed by (Irizarry-Caro et al., 2018), who focused on drugs related to drug-induced lupus. They investigated hydralazin, a direct-acting vasodilator (Kandler et al., 2011) and procainamide, and showed that both drugs indeed increase NET production, which may contribute to autoimmunity.
Conclusion and Prospects
The Chinese and European Pharmacopeia convincingly report possible modulations of the immune response via medical plants. So far, natural products classified as immunomodulators were cursorily explored, without comprehensive identification of molecular mechanisms responsible for the observed effects. Firstly, there is a need to perform detailed phytochemical analyses of plant extracts, then select pure compounds for further biological evaluation. A specific assessment of purified compounds could help to identify cellular targets, determine bioactive dosage, biodistribution and kinetics. The knowledge of molecular mechanisms regulated by plant-derived compounds may potentially help to identify therapeutic targets, as well as potentially limit the spread of an infection. Moreover, the chemical structures of selected secondary metabolites can serve as lead structures for synthesis of new substances. Similar conclusions could be drawn for synthetic drugs. Only few synthetic drugs were tested for their influence on immunomodulatory neutrophil functions and most of the data brought interesting results, thus further studies are urgently needed. For synthetic drugs, two areas seem to be the most important: identifying new substances, which affect effector functions of neutrophils, and testing approved drugs, especially antibiotics, antimycotics and these responsible for autoimmune reactions re, for their impact on immunomodulatory functions of neutrophils.
Author Contributions
All authors listed have made substantial, direct, and intellectual contribution to the work and approved the final version for publication.
Funding
BG was supported by the National Science Center, Poland, grant number Preludium 2020/37/N/NZ6/02355.
Conflict of Interest
The authors declare that the research was conducted in the absence of any commercial or financial relationships that could be construed as a potential conflict of interest.
References
Abdelghaffar, H., Vazifeh, D., and Labro, M. T. (1997). Erythromycin A-Derived Macrolides Modify the Functional Activities of Human Neutrophils by Altering the Phospholipase D-Phosphatidate Phosphohydrolase Transduction Pathway: L-Cladinose Is Involved Both in Alterations of Neutrophil Functions and Modulation of This Transductional Pathway. J. Immunol. 159, 3995–4005.
Abed, A., Minaiyan, M., Ghannadi, A., Mahzouni, P., and Babavalian, M. R. (2012). Effect of Echium amoenum Fisch. et Mey a Traditional Iranian Herbal Remedy in an Experimental Model of Acute Pancreatitis. ISRN Gastroenterol. 2012, 141548. doi:10.5402/2012/141548
Adrover, J. M., Nicolás-Ávila, J. A., and Hidalgo, A. (2016). Aging: A Temporal Dimension for Neutrophils. Trends Immunol. 37, 334–345. doi:10.1016/j.it.2016.03.005
Ahmad, W., Jantan, I., Kumolosasi, E., and Bukhari, S. N. (2015). Immunostimulatory Effects of the Standardized Extract of Tinospora Crispa on Innate Immune Responses in Wistar Kyoto Rats. Drug Des. Devel Ther. 9, 2961–2973. doi:10.2147/DDDT.S85405
Akhtar, N. M. Y., Jantan, I., Arshad, L., and Haque, M. A. (2019). Standardized Ethanol Extract, Essential Oil and Zerumbone of Zingiber Zerumbet Rhizome Suppress Phagocytic Activity of Human Neutrophils. BMC Complement. Altern. Med. 19, 331. doi:10.1186/s12906-019-2748-5
Akram, M., Hamid, A., Khalil, A., Ghaffar, A., Tayyaba, N., Saeed, A., et al. (2014). Review on Medicinal Uses, Pharmacological, Phytochemistry and Immunomodulatory Activity of Plants. Int. J. Immunopathol Pharmacol. 27, 313–319. doi:10.1177/039463201402700301
Albuquerque, K. F. F. S., Marinovic, M. P., Morandi, A. C., Bolin, A. P., and Otton, R. (2016). Green Tea Polyphenol Extract In Vivo Attenuates Inflammatory Features of Neutrophils from Obese Rats. Eur. J. Nutr. 55, 1261–1274. doi:10.1007/s00394-015-0940-z
An, B.-S., Kang, J.-H., Yang, H., Yang, M.-P., and Jeung, E.-B. (2013). Effects of Acer Okamotoanum Sap on the Function of Polymorphonuclear Neutrophilic Leukocytes In Vitro and In Vivo. Mol. Med. Rep. 7, 654–658. doi:10.3892/mmr.2012.1190
Angus, D. C., Linde-Zwirble, W. T., Lidicker, J., Clermont, G., Carcillo, J., and Pinsky, M. R. (2001). Epidemiology of Severe Sepsis in the United States: Analysis of Incidence, Outcome, and Associated Costs of Care. Crit. Care Med. 29, 1303–1310. doi:10.1097/00003246-200107000-00002
Anzai, A., Choi, J. L., He, S., Fenn, A. M., Nairz, M., Rattik, S., et al. (2017). The Infarcted Myocardium Solicits GM-CSF for the Detrimental Oversupply of Inflammatory Leukocytes. J. Exp. Med. 214, 3293–3310. doi:10.1084/jem.20170689
Arampatzioglou, A., Papazoglou, D., Konstantinidis, T., Chrysanthopoulou, A., Mitsios, A., Angelidou, I., et al. (2018). Clarithromycin Enhances the Antibacterial Activity and Wound Healing Capacity in Type 2 Diabetes Mellitus by Increasing LL-37 Load on Neutrophil Extracellular Traps. Front. Immunol. 9, 2064. doi:10.3389/fimmu.2018.02064
Arcanjo, A., Logullo, J., Menezes, C. C. B., De Souza Carvalho Giangiarulo, T. C., Dos Reis, M. C., De Castro, G. M. M., et al. (2020). The Emerging Role of Neutrophil Extracellular Traps in Severe Acute Respiratory Syndrome Coronavirus 2 (COVID-19). Sci. Rep. 10, 19630. doi:10.1038/s41598-020-76781-0
Asadollahi, F., Mehrzad, J., Chaichi, M. J., and Taghavi Razavizadeh, A. (2015). Photoimmunological Properties of Borage in Bovine Neutrophil In Vitro Model. J. Photochem. Photobiol. B: Biol. 151, 270–275. doi:10.1016/j.jphotobiol.2015.08.023
Athens, J. W., Haab, O. P., Raab, S. O., Mauer, A. M., Ashenbrucker, H., Cartwright, G. E., et al. (1961a). Leukokinetic Studies. Iv. The Total Blood, Circulating and Marginal Granulocyte Pools and the Granulocyte Turnover Rate in Normal Subjects*. J. Clin. Invest. 40, 989–995. doi:10.1172/jci104338
Athens, J. W., Raab, S. O., Haab, O. P., Mauer, A. M., Ashenbrucker, H., Cartwright, G. E., et al. (1961b). Leukokinetic Studies. Iii. The Distribution of Granulocytes in the Blood of Normal Subjects*. J. Clin. Invest. 40, 159–164. doi:10.1172/jci104230
Baker, C., Retzik-Stahr, C., Singh, V., Plomondon, R., Anderson, V., and Rasouli, N. (2021). Should Metformin Remain the First-Line Therapy for Treatment of Type 2 Diabetes?. Ther. Adv. Endocrinol. Metab. 12, 2042018820980225. doi:10.1177/2042018820980225
Barnes, J., Anderson, L. A., Gibbons, S., and Phillipson, J. D. (2005). Echinacea Species (Echinacea Angustifolia (DC.) Hell., Echinacea Pallida (Nutt.) Nutt.,Echinacea Purpurea (L.) Moench): a Review of Their Chemistry, Pharmacology and Clinical Properties. J. Pharm. Pharmacol. 57, 929–954. doi:10.1211/0022357056127
Batiha, G. E., Beshbishy, A. M., Ikram, M., Mulla, Z. S., El-Hack, M. E. A., Taha, A. E., et al. (2020). The Pharmacological Activity, Biochemical Properties, and Pharmacokinetics of the Major Natural Polyphenolic Flavonoid: Quercetin. Foods 9, 374. doi:10.3390/foods9030374
Behl, T., Kumar, K., Brisc, C., Rus, M., Nistor-Cseppento, D. C., Bustea, C., et al. (2021). Exploring the Multifocal Role of Phytochemicals as Immunomodulators. Biomed. Pharmacother. 133, 110959. doi:10.1016/j.biopha.2020.110959
Behnen, M., Möller, S., Brozek, A., Klinger, M., and Laskay, T. (2017). Extracellular Acidification Inhibits the ROS-dependent Formation of Neutrophil Extracellular Traps. Front. Immunol. 8, 184. doi:10.3389/fimmu.2017.00184
Benencia, F., Courrèges, M. C., Massouh, E. J., and Coulombié, F. C. (1994). Effect of Melia Azedarach L. Leaf Extracts on Human Complement and Polymorphonuclear Leukocytes. J. Ethnopharmacol. 41, 53–57. doi:10.1016/0378-8741(94)90057-4
Benencia, F., Courrèges, M. C., Nores, M. M., and Coulombié, F. C. (1995). Immunomodulatory Activities of Cedrela Tubiflora Leaf Aqueous Extracts. J. Ethnopharmacol. 49, 133–139. doi:10.1016/0378-8741(95)01311-3
Benencia, F., Courrèges, M. C., and Coulombié, F. C. (2000). Anti-inflammatory Activities of Trichilia Glabra Aqueous Leaf Extract. J. Ethnopharmacol. 71, 293–300. doi:10.1016/s0378-8741(00)00192-6
Benjamim, C. F., Hogaboam, C. M., and Kunkel, S. L. (2004). The Chronic Consequences of Severe Sepsis. J. Leukoc. Biol. 75, 408–412. doi:10.1189/jlb.0503214
Blaisdell, A., Crequer, A., Columbus, D., Daikoku, T., Mittal, K., Dey, S. K., et al. (2015). Neutrophils Oppose Uterine Epithelial Carcinogenesis via Debridement of Hypoxic Tumor Cells. Cancer Cell 28, 785–799. doi:10.1016/j.ccell.2015.11.005
Borchers, A. T., Keen, C. L., and Gershwin, M. E. (2007). Drug-induced Lupus. Ann. N Y Acad. Sci. 1108, 166–182. doi:10.1196/annals.1422.019
Borregaard, N. (2010). Neutrophils, from Marrow to Microbes. Immunity 33, 657–670. doi:10.1016/j.immuni.2010.11.011
Boudoukha, C., Bouriche, H., Ortega, E., and Senator, A. (2016). Immunomodulatory Effects ofSantolina Chamaecyparissusleaf Extracts on Human Neutrophil Functions. Pharm. Biol. 54, 667–673. doi:10.3109/13880209.2015.1071853
Boukemara, H., Hurtado-Nedelec, M., Marzaioli, V., Bendjeddou, D., El Benna, J., and Marie, J. C. (2016). Anvillea Garcinii Extract Inhibits the Oxidative Burst of Primary Human Neutrophils. BMC Complement. Altern. Med. 16, 433. doi:10.1186/s12906-016-1411-7
Brinkmann, V., Reichard, U., Goosmann, C., Fauler, B., Uhlemann, Y., Weiss, D. S., et al. (2004). Neutrophil Extracellular Traps Kill Bacteria. Science 303, 1532–1535. doi:10.1126/science.1092385
Brungs, S., Kolanus, W., and Hemmersbach, R. (2015). Syk Phosphorylation—a Gravisensitive Step in Macrophage Signalling. Cell Commun. Signaling 13, 9. doi:10.1186/s12964-015-0088-8
Buckley, C. D., Ross, E. A., Mcgettrick, H. M., Osborne, C. E., Haworth, O., Schmutz, C., et al. (2006). Identification of a Phenotypically and Functionally Distinct Population of Long-Lived Neutrophils in a Model of Reverse Endothelial Migration. J. Leukoc. Biol. 79, 303–311. doi:10.1189/jlb.0905496
Buckley, C. D., Gilroy, D. W., and Serhan, C. N. (2014). Proresolving Lipid Mediators and Mechanisms in the Resolution of Acute Inflammation. Immunity 40, 315–327. doi:10.1016/j.immuni.2014.02.009
Burgess, S., Abu-Laban, R. B., Slavik, R. S., Vu, E. N., and Zed, P. J. (2016). A Systematic Review of Randomized Controlled Trials Comparing Hypertonic Sodium Solutions and Mannitol for Traumatic Brain Injury. Ann. Pharmacother. 50, 291–300. doi:10.1177/1060028016628893
Bush, K., and Bradford, P. A. (2016). β-Lactams and β-Lactamase Inhibitors: An Overview. Cold Spring Harb Perspect. Med. 6. doi:10.1101/cshperspect.a025247
Bystrzycka, W., Moskalik, A., Sieczkowska, S., Manda-Handzlik, A., Demkow, U., and Ciepiela, O. (2016). The Effect of Clindamycin and Amoxicillin on Neutrophil Extracellular Trap (NET) Release. cejoi 1, 1–5. doi:10.5114/ceji.2016.58811
Bystrzycka, W., Manda-Handzlik, A., Sieczkowska, S., Moskalik, A., Demkow, U., and Ciepiela, O. (2017). Azithromycin and Chloramphenicol Diminish Neutrophil Extracellular Traps (NETs) Release. Int. J. Mol. Sci. 18, 2666. doi:10.3390/ijms18122666
Carmona-Rivera, C., Purmalek, M. M., Moore, E., Waldman, M., Walter, P. J., Garraffo, H. M., et al. (2017). A Role for Muscarinic Receptors in Neutrophil Extracellular Trap Formation and Levamisole-Induced Autoimmunity. JCI Insight 2, e89780. doi:10.1172/jci.insight.89780
Carrillo-Vico, A., Lardone, P., Álvarez-Sánchez, N., Rodríguez-Rodríguez, A., and Guerrero, J. (2013). Melatonin: Buffering the Immune System. Ijms 14, 8638–8683. doi:10.3390/ijms14048638
Casanova-Acebes, M., Pitaval, C., Weiss, L. A., Nombela-Arrieta, C., Chèvre, R., A-González, N., et al. (2013). Rhythmic Modulation of the Hematopoietic Niche through Neutrophil Clearance. Cell 153, 1025–1035. doi:10.1016/j.cell.2013.04.040
Chang, D. M., Chang, W. Y., Kuo, S. Y., and Chang, M. L. (1997). The Effects of Traditional Antirheumatic Herbal Medicines on Immune Response Cells. J. Rheumatol. 24, 436–441.
Chen, M.-H., Lo, D.-Y., Liao, J.-W., Hsuan, S.-L., Chien, M.-S., Lin, C.-C., et al. (2012). Immunostimulation of Sugar Cane Extract on Neutrophils to Salmonella typhimurium Infection in Mice. Phytother. Res. 26, 1062–1067. doi:10.1002/ptr.3676
Chen, M.-L., Wu, S., Tsai, T.-C., Wang, L.-K., Chou, W.-M., and Tsai, F.-M. (2014). The Caffeic Acid in Aqueous Extract ofTournefortia Sarmentosaenhances Neutrophil Phagocytosis ofEscherichia Coli. Immunopharmacol. Immunotoxicol. 36, 390–396. doi:10.3109/08923973.2014.956753
Chousterman, B. G., Swirski, F. K., and Weber, G. F. (2017). Cytokine Storm and Sepsis Disease Pathogenesis. Semin. Immunopathol 39, 517–528. doi:10.1007/s00281-017-0639-8
Chung, M.-I., Weng, J.-R., Wang, J.-P., Teng, C.-M., and Lin, C.-N. (2002). Antiplatelet and Anti-inflammatory Constituents and New Oxygenated Xanthones from Hypericum Geminiflorum. Planta Med. 68, 25–29. doi:10.1055/s-2002-19871
Coffelt, S. B., Kersten, K., Doornebal, C. W., Weiden, J., Vrijland, K., Hau, C.-S., et al. (2015). IL-17-producing γδ T Cells and Neutrophils Conspire to Promote Breast Cancer Metastasis. Nature 522, 345–348. doi:10.1038/nature14282
Colom, B., Bodkin, J. V., Beyrau, M., Woodfin, A., Ody, C., Rourke, C., et al. (2015). Leukotriene B4-Neutrophil Elastase Axis Drives Neutrophil Reverse Transendothelial Cell Migration In Vivo. Immunity 42, 1075–1086. doi:10.1016/j.immuni.2015.05.010
Colotta, F., Re, F., Polentarutti, N., Sozzani, S., and Mantovani, A. (1992). Modulation of Granulocyte Survival and Programmed Cell Death by Cytokines and Bacterial Products. Blood 80, 2012–2020. doi:10.1182/blood.v80.8.2012.2012
Costa, M. F., Jesus, T. I., Lopes, B. R., Angolini, C. F., Montagnolli, A., Gomes, L. P., et al. (2016). Eugenia Aurata and Eugenia Punicifolia HBK Inhibit Inflammatory Response by Reducing Neutrophil Adhesion, Degranulation and NET Release. BMC Complement. Altern. Med. 16, 403. doi:10.1186/s12906-016-1375-7
Csepregi, J. Z., Orosz, A., Zajta, E., Kása, O., Németh, T., Simon, E., et al. (2018). Myeloid-Specific Deletion of Mcl-1 Yields Severely Neutropenic Mice that Survive and Breed in Homozygous Form. J.I. 201, 3793–3803. doi:10.4049/jimmunol.1701803
Cuffini, A. M., Tullio, V., Banche, G., Allizond, V., Mandras, N., Roana, J., et al. (2009). The Erythromycin-Resistance inS. PyogenesDoes Not Limit the Human Polymorphonuclear Cell Antimicrobial Activity. Int. J. Immunopathol Pharmacol. 22, 239–242. doi:10.1177/039463200902200127
Czaikoski, P. G., Mota, J. M., Nascimento, D. C., Sônego, F., Castanheira, F. V., Melo, P. H., et al. (2016). Neutrophil Extracellular Traps Induce Organ Damage during Experimental and Clinical Sepsis. PLoS One 11, e0148142. doi:10.1371/journal.pone.0148142
Czerwińska, M. E., and Melzig, M. F. (2018). Cornus Mas and Cornus Officinalis-Analogies and Differences of Two Medicinal Plants Traditionally Used. Front. Pharmacol. 9, 894. doi:10.3389/fphar.2018.00894
Dalli, E., Milara, J., Cortijo, J., Morcillo, E., Cosinsales, J., and Sotillo, J. (2008). Hawthorn Extract Inhibits Human Isolated Neutrophil Functions. Pharmacol. Res. 57, 445–450. doi:10.1016/j.phrs.2008.05.001
De Santo, C., Arscott, R., Booth, S., Karydis, I., Jones, M., Asher, R., et al. (2010). Invariant NKT Cells Modulate the Suppressive Activity of IL-10-secreting Neutrophils Differentiated with Serum Amyloid A. Nat. Immunol. 11, 1039–1046. doi:10.1038/ni.1942
Decker, C., Wurster, S., Lazariotou, M., Hellmann, A.-M., Einsele, H., Ullmann, A. J., et al. (2018). Analysis of the In Vitro Activity of Human Neutrophils against Aspergillus fumigatus in Presence of Antifungal and Immunosuppressive Agents. Med. Mycol. 56, 514–519. doi:10.1093/mmy/myx069
Deniset, J. F., and Kubes, P. (2018). Neutrophil Heterogeneity: Bona Fide Subsets or Polarization States? J. Leukoc. Biol. 103, 829–838. doi:10.1002/jlb.3ri0917-361r
Deniset, J. F., Surewaard, B. G., Lee, W.-Y., and Kubes, P. (2017). Splenic Ly6Ghigh Mature and Ly6Gint Immature Neutrophils Contribute to Eradication of S. Pneumoniae. J. Exp. Med. 214, 1333–1350. doi:10.1084/jem.20161621
Eash, K. J., Means, J. M., White, D. W., and Link, D. C. (2009). CXCR4 Is a Key Regulator of Neutrophil Release from the Bone Marrow under Basal and Stress Granulopoiesis Conditions. Blood 113, 4711–4719. doi:10.1182/blood-2008-09-177287
Eichelberger, K. R., and Goldman, W. E. (2020). Manipulating Neutrophil Degranulation as a Bacterial Virulence Strategy. Plos Pathog. 16, e1009054. doi:10.1371/journal.ppat.1009054
El-Abasy, M., Motobu, M., Shimura, K., Na, K.-J., Kang, C.-B., Koge, K., et al. (2002). Immunostimulating and Growth-Promoting Effects of Sugar Cane Extract (SCE) in Chickens. J. Vet. Med. Sci. 64, 1061–1063. doi:10.1292/jvms.64.1061
Ella, K., Mócsai, A., and Káldi, K. (2018). Circadian Regulation of Neutrophils: Control by a Cell-Autonomous Clock or Systemic Factors? Eur. J. Clin. Invest. 48 (Suppl. 2), e12965. doi:10.1111/eci.12965
Eltzschig, H. K., and Eckle, T. (2011). Ischemia and Reperfusion-From Mechanism to Translation. Nat. Med. 17, 1391–1401. doi:10.1038/nm.2507
Ervina, M., Poerwono, H., Widyowati, R., Matsunami, K., and Sukardiman, (2020). Bio-selective Hormonal Breast Cancer Cytotoxic and Antioxidant Potencies of Melia Azedarach L. Wild Type Leaves. Biotechnol. Rep. (Amst) 25, e00437. doi:10.1016/j.btre.2020.e00437
Erwig, L. P., Kluth, D. C., Walsh, G. M., and Rees, A. J. (1998). Initial Cytokine Exposure Determines Function of Macrophages and Renders Them Unresponsive to Other Cytokines. J. Immunol. 161, 1983–1988.
Evrard, M., Kwok, I. W. H., Chong, S. Z., Teng, K. W. W., Becht, E., Chen, J., et al. (2018). Developmental Analysis of Bone Marrow Neutrophils Reveals Populations Specialized in Expansion, Trafficking, and Effector Functions. Immunity 48, 364–379. doi:10.1016/j.immuni.2018.02.002
Fairn, G. D., and Grinstein, S. (2012). How Nascent Phagosomes Mature to Become Phagolysosomes. Trends Immunol. 33, 397–405. doi:10.1016/j.it.2012.03.003
Faix, J. D. (2013). Biomarkers of Sepsis. Crit. Rev. Clin. Lab. Sci. 50, 23–36. doi:10.3109/10408363.2013.764490
Faurschou, M., and Borregaard, N. (2003). Neutrophil Granules and Secretory Vesicles in Inflammation. Microbes Infect. 5, 1317–1327. doi:10.1016/j.micinf.2003.09.008
Fidan, I., Ozkan, S., Gurbuz, I., Yesilyurt, E., Erdal, B., Yolbakan, S., et al. (2008). The Efficiency ofViscum Album Ssp. albumandHypericum Perforatumon Human Immune CellsIn Vitro. Immunopharmacol. Immunotoxicol. 30, 519–528. doi:10.1080/08923970802135286
Figueiredo-Rinhel, A. S. G., De Melo, L. L., Bortot, L. O., Santos, E. O. L., Andrade, M. F., Azzolini, A. E. C. S., et al. (2017). Baccharis Dracunculifolia DC (Asteraceae) Selectively Modulates the Effector Functions of Human Neutrophils. J. Pharm. Pharmacol. 69, 1829–1845. doi:10.1111/jphp.12822
Fridlender, Z. G., Sun, J., Kim, S., Kapoor, V., Cheng, G., Ling, L., et al. (2009). Polarization of Tumor-Associated Neutrophil Phenotype by TGF-β: "N1" versus "N2" TAN. Cancer Cell 16, 183–194. doi:10.1016/j.ccr.2009.06.017
Gabrilovich, D. I. (2017). Myeloid-Derived Suppressor Cells. Cancer Immunol. Res. 5, 3–8. doi:10.1158/2326-6066.cir-16-0297
Galluzzi, L., Vitale, I., Aaronson, S. A., Abrams, J. M., Adam, D., Agostinis, P., et al. (2018). Molecular Mechanisms of Cell Death: Recommendations of the Nomenclature Committee on Cell Death 2018. Cell Death Differ 25, 486–541. doi:10.1038/s41418-017-0012-4
Garcia-Romo, G. S., Caielli, S., Vega, B., Connolly, J., Allantaz, F., Xu, Z., et al. (2011). Netting Neutrophils Are Major Inducers of Type I IFN Production in Pediatric Systemic Lupus Erythematosus. Sci. Transl Med. 3, 73ra20. doi:10.1126/scitranslmed.3001201
Garner, C. V., D'amico, R., and Simms, H. H. (1996). Cytokine-mediated Human Polymorphonuclear Neutrophil Phagocytosis: Evidence of Differential Sensitivities to Manipulation of Intracellular Mechanisms. J. Surg. Res. 60, 84–90. doi:10.1006/jsre.1996.0015
Garner, S. E., Eady, A., Bennett, C., Newton, J. N., Thomas, K., and Popescu, C. M. (2012). Minocycline for Acne Vulgaris: Efficacy and Safety. Cochrane Database Syst. Rev. 15. Cd002086. doi:10.1002/14651858.cd002086.pub2
Ge, S., Duo, L., Wang, J., Gegen, Z., Yang, J., Li, Z., et al. (2021). A Unique Understanding of Traditional Medicine of Pomegranate, Punica Granatum L. And its Current Research Status. J. Ethnopharmacol 10, 113877. doi:10.1016/j.jep.2021.113877
Gentles, A. J., Newman, A. M., Liu, C. L., Bratman, S. V., Feng, W., Kim, D., et al. (2015). The Prognostic Landscape of Genes and Infiltrating Immune Cells across Human Cancers. Nat. Med. 21, 938–945. doi:10.1038/nm.3909
Ghorbani, A., and Esmaeilizadeh, M. (2017). Pharmacological Properties of Salvia Officinalis and its Components. J. Traditional Complement. Med. 7, 433–440. doi:10.1016/j.jtcme.2016.12.014
Ghule, B. V., Murugananthan, G., Nakhat, P. D., and Yeole, P. G. (2006). Immunostimulant Effects of Capparis Zeylanica Linn. Leaves. J. Ethnopharmacol. 108, 311–315. doi:10.1016/j.jep.2006.03.041
Gierlikowska, B., Gierlikowski, W., Bekier, K., Skalicka-Woźniak, K., Czerwińska, M. E., and Kiss, A. K. (2020a). Inula Helenium and Grindelia Squarrosa as a Source of Compounds with Anti-inflammatory Activity in Human Neutrophils and Cultured Human Respiratory Epithelium. J. Ethnopharmacol, 249, 112311. doi:10.1016/j.jep.2019.112311
Gierlikowska, B., Gierlikowski, W., and Demkow, U. (2020b). Alantolactone Enhances the Phagocytic Properties of Human Macrophages and Modulates Their Proinflammatory Functions. Front. Pharmacol. 11, 1339. doi:10.3389/fphar.2020.01339
Goulart Da Silva, G., De Oliveira Braga, L. E., Souza De Oliveira, E. C., Valério Tinti, S., De Carvalho, J. E., Goldoni Lazarini, J., et al. (2021). Cashew Apple Byproduct: Gastroprotective Effects of Standardized Extract. J. Ethnopharmacol. 269, 113744. doi:10.1016/j.jep.2020.113744
Granot, Z., Henke, E., Comen, E. A., King, T. A., Norton, L., and Benezra, R. (2011). Tumor Entrained Neutrophils Inhibit Seeding in the Premetastatic Lung. Cancer Cell 20, 300–314. doi:10.1016/j.ccr.2011.08.012
Grégoire, M., Uhel, F., Lesouhaitier, M., Gacouin, A., Guirriec, M., Mourcin, F., et al. (2018). Impaired Efferocytosis and Neutrophil Extracellular Trap Clearance by Macrophages in ARDS. Eur. Respir. J. 52, 1702590. doi:10.1183/13993003.02590-2017
Grigore, A., Cord, D., Tanase, C., and Albulescu, R. (2020). Herbal Medicine, a Reliable Support in COVID Therapy. J. Immunoassay Immunochemistry 41, 976–999. doi:10.1080/15321819.2020.1862867
Grommes, J., and Soehnlein, O. (2011). Contribution of Neutrophils to Acute Lung Injury. Mol. Med. 17, 293–307. doi:10.2119/molmed.2010.00138
Gupta, A., Gautam, M. K., Singh, R. K., Kumar, M. V., Rao Ch, V., Goel, R. K., et al. (2010). Immunomodulatory Effect of Moringa Oleifera Lam. Extract on Cyclophosphamide Induced Toxicity in Mice. Indian J. Exp. Biol. 48, 1157–1160.
Hacbarth, E., and Kajdacsy-Balla, A. (1986). Low Density Neutrophils in Patients with Systemic Lupus Erythematosus, Rheumatoid Arthritis, and Acute Rheumatic Fever. Arthritis Rheum. 29, 1334–1342. doi:10.1002/art.1780291105
Hajto, T., Hostanska, K., and Gabius, H. J. (1989). Modulatory Potency of the Beta-galactoside-specific Lectin from Mistletoe Extract (Iscador) on the Host Defense System In Vivo in Rabbits and Patients. Cancer Res. 49, 4803–4808.
Hamill, R. J. (2013). Amphotericin B Formulations: a Comparative Review of Efficacy and Toxicity. Drugs 73, 919–934. doi:10.1007/s40265-013-0069-4
Haq, A., Abdullatif, M., Lobo, P. I., Khabar, K. S. A., Sheth, K. V., and Al-Sedairy, S. T. (1995). Nigella Sativa: Effect on Human Lymphocytes and Polymorphonuclear Leukocyte Phagocytic Activity. Immunopharmacol, 30, 147–155. doi:10.1016/0162-3109(95)00016-m
He, W., Holtkamp, S., Hergenhan, S. M., Kraus, K., De Juan, A., Weber, J., et al. (2018). Circadian Expression of Migratory Factors Establishes Lineage-specific Signatures that Guide the Homing of Leukocyte Subsets to Tissues. Immunity 49, 1175–1190. doi:10.1016/j.immuni.2018.10.007
Heroor, S., Beknal, A., and Mahurkar, N. (2013). Immunomodulatory Activity of Methanolic Extracts of Fruits and Bark of Ficus Glomerata Roxb. In Mice and on Human Neutrophils. Indian J. Pharmacol. 45, 130–135. doi:10.4103/0253-7613.108287
Hodge, S., Hodge, G., Brozyna, S., Jersmann, H., Holmes, M., and Reynolds, P. N. (2006). Azithromycin Increases Phagocytosis of Apoptotic Bronchial Epithelial Cells by Alveolar Macrophages. Eur. Respir. J. 28, 486–495. doi:10.1183/09031936.06.00001506
Hollands, A., Corriden, R., Gysler, G., Dahesh, S., Olson, J., Raza Ali, S., et al. (2016). Natural Product Anacardic Acid from Cashew Nut Shells Stimulates Neutrophil Extracellular Trap Production and Bactericidal Activity. J. Biol. Chem. 291, 13964–13973. doi:10.1074/jbc.m115.695866
Hong, C., Kidani, Y., A-Gonzalez, N., Phung, T., Ito, A., Rong, X., et al. (2012). Coordinate Regulation of Neutrophil Homeostasis by Liver X Receptors in Mice. J. Clin. Invest. 122, 337–347. doi:10.1172/jci58393
Horby, P., Horby, P., Lim, W. S., Emberson, J. R., Mafham, M., Bell, J. L., et al. (2021). Dexamethasone in Hospitalized Patients with Covid-19. N. Engl. J. Med. 384, 693–704. doi:10.1056/NEJMoa2021436
Houghton, A. M., Rzymkiewicz, D. M., Ji, H., Gregory, A. D., Egea, E. E., Metz, H. E., et al. (2010). Neutrophil Elastase-Mediated Degradation of IRS-1 Accelerates Lung Tumor Growth. Nat. Med. 16, 219–223. doi:10.1038/nm.2084
Hu, S., Concha, C., Cooray, R., and Holmberg, O. (1995). Ginseng-enhanced Oxidative and Phagocytic Activities of Polymorphonuclear Leucocytes from Bovine Peripheral Blood and Stripping Milk. Vet. Res. 26, 155–161.
Hung, S.-L., Lee, Y.-Y., Liu, T.-Y., Peng, J.-L., Cheng, Y.-Y., and Chen, Y.-T. (2006). Modulation of Phagocytosis, Chemotaxis, and Adhesion of Neutrophils by Areca Nut Extracts. J. Periodontol. 77, 579–585. doi:10.1902/jop.2006.050217
Ilangkovan, M., Jantan, I., Mesaik, M. A., and Bukhari, S. N. (2015). Immunosuppressive Effects of the Standardized Extract of Phyllanthus Amarus on Cellular Immune Responses in Wistar-Kyoto Rats. Drug Des. Devel Ther. 9, 4917–4930. doi:10.2147/DDDT.S88189
Ionita, M. G., Van Den Borne, P., Catanzariti, L. M., Moll, F. L., De Vries, J.-P. P. M., Pasterkamp, G., et al. (2010). High Neutrophil Numbers in Human Carotid Atherosclerotic Plaques Are Associated with Characteristics of Rupture-Prone Lesions. Atvb 30, 1842–1848. doi:10.1161/atvbaha.110.209296
Irizarry-Caro, J. A., Carmona-Rivera, C., Schwartz, D. M., Khaznadar, S. S., Kaplan, M. J., and Grayson, P. C. (2018). Brief Report: Drugs Implicated in Systemic Autoimmunity Modulate Neutrophil Extracellular Trap Formation. Arthritis Rheumatol. 70, 468–474. doi:10.1002/art.40372
Isaykina, N. V., Perevozchicova, T. V., and Kalinkina, G. I. (2008). Immunotropic Activity of Plant Extract Echinosol. Bull. Exp. Biol. Med. 146, 223–225. doi:10.1007/s10517-008-0257-5
Jaumouillé, V., and Waterman, C. M. (2020). Physical Constraints and Forces Involved in Phagocytosis. Front. Immunol. 11, 1097. doi:10.3389/fimmu.2020.01097
Jenne, C. N., Wong, C. H. Y., Zemp, F. J., Mcdonald, B., Rahman, M. M., Forsyth, P. A., et al. (2013). Neutrophils Recruited to Sites of Infection Protect from Virus Challenge by Releasing Neutrophil Extracellular Traps. Cell Host & Microbe 13, 169–180. doi:10.1016/j.chom.2013.01.005
Jerjomiceva, N., Seri, H., Völlger, L., Wang, Y., Zeitouni, N., Naim, H. Y., et al. (2014). Enrofloxacin Enhances the Formation of Neutrophil Extracellular Traps in Bovine Granulocytes. J. Innate Immun. 6, 706–712. doi:10.1159/000358881
Jerjomiceva, N., Seri, H., Yaseen, R., De Buhr, N., Setzer, W. N., Naim, H. Y., et al. (2016). Guarea Kunthiana Bark Extract Enhances the Antimicrobial Activities of Human and Bovine Neutrophils. Nat. Prod. Commun. 11, 767–770. doi:10.1177/1934578x1601100617
Jin, U.-H., Park, S.-G., Suh, S.-J., Kim, J.-K., Kim, D.-S., Moon, S.-K., et al. (2007). Inhibitory Effect ofPanax Notoginseng on Nitric Oxide Synthase, Cyclo-Oxygenase-2 and Neutrophil Functions. Phytother. Res. 21, 142–148. doi:10.1002/ptr.2018
Jin, Y. P., Wu, H., Yu, H. L., Pan, Y. Z., Chen, Y. Q., Wang, K. L., et al. (2016). [Antagonism Mechanism of Gingerols against Inflammatory Effect of Toxic Raphides from Pinella Pedatisecta]. Zhongguo Zhong Yao Za Zhi. 41, 1087–1092. doi:10.4268/cjcmm20160619
Jin, L., Chen, C., Huang, L., Bu, L., Zhang, L., and Yang, Q. (2021). Salvianolic Acid A Blocks Vasculogenic Mimicry Formation in Human Non-small Cell Lung Cancer via PI3K/Akt/mTOR Signalling. Clin. Exp. Pharmacol. Physiol. 48, 508–514. doi:10.1111/1440-1681.13464
Johansson, A., Jesaitis, A. J., Lundqvist, H., Magnusson, K.-E., Sjölin, C., Karlsson, A., et al. (1995). Different Subcellular Localization of Cytochrome B and the Dormant NADPH-Oxidase in Neutrophils and Macrophages: Effect on the Production of Reactive Oxygen Species during Phagocytosis. Cell Immunol. 161, 61–71. doi:10.1006/cimm.1995.1009
Kakalij, R. M., Alla, C. P., Kshirsagar, R. P., Kumar, B. H., Mutha, S. S., and Diwan, P. V. (2014). Ameliorative Effect of Elaeocarpus Ganitrus on Gentamicin-Induced Nephrotoxicity in Rats. Indian J. Pharmacol. 46, 298–302. doi:10.4103/0253-7613.132163
Kanashiro, A., Souza, J. G., Kabeya, L. M., C. S. Azzolini, A. E., and Lucisano-Valim, Y. M. (2007). Elastase Release by Stimulated Neutrophils Inhibited by Flavonoids: Importance of the Catechol Group. Z. Naturforsch C J. Biosci. 62, 357–361. doi:10.1515/znc-2007-5-607
Kandler, M. R., Mah, G. T., Tejani, A. M., Stabler, S. N., and Salzwedel, D. M. (2011). Hydralazine for Essential Hypertension. Cochrane Database Syst. Rev. 9, Cd004934. doi:10.1002/14651858.cd004934.pub4
Kessenbrock, K., Krumbholz, M., Schönermarck, U., Back, W., Gross, W. L., Werb, Z., et al. (2009). Netting Neutrophils in Autoimmune Small-Vessel Vasculitis. Nat. Med. 15, 623–625. doi:10.1038/nm.1959
Khan, M. A., Philip, L. M., Cheung, G., Vadakepeedika, S., Grasemann, H., Sweezey, N., et al. (2018). Regulating NETosis: Increasing pH Promotes NADPH Oxidase-dependent NETosis. Front. Med. (Lausanne) 5, 19. doi:10.3389/fmed.2018.00019
Khandpur, R., Carmona-Rivera, C., Vivekanandan-Giri, A., Gizinski, A., Yalavarthi, S., Knight, J. S., et al. (2013). NETs Are a Source of Citrullinated Autoantigens and Stimulate Inflammatory Responses in Rheumatoid Arthritis. Sci. Transl Med. 5, 178ra140. doi:10.1126/scitranslmed.3005580
Khokhar, J. Y., Henricks, A. M., Sullivan, E. D. K., and Green, A. I. (2018). Unique Effects of Clozapine: A Pharmacological Perspective. Adv. Pharmacol. 82, 137–162. doi:10.1016/bs.apha.2017.09.009
Kim, M.-H., Granick, J. L., Kwok, C., Walker, N. J., Borjesson, D. L., Curry, F.-R. E., et al. (2011). Neutrophil Survival and C-Kit+-Progenitor Proliferation in Staphylococcus Aureus-Infected Skin Wounds Promote Resolution. Blood 117, 3343–3352. doi:10.1182/blood-2010-07-296970
Kirchner, T., Hermann, E., Möller, S., Klinger, M., Solbach, W., Laskay, T., et al. (2013). Flavonoids and 5-aminosalicylic Acid Inhibit the Formation of Neutrophil Extracellular Traps. Mediators Inflamm. 2013, 710239. doi:10.1155/2013/710239
Kiss, A. K., Michalak, B., Patyra, A., and Majdan, M. (2020). UHPLC‐DAD‐ESI‐MS/MS and HPTLC Profiling of Ash Leaf Samples from Different Commercial and Natural Sources and Their In Vitro Effects on Mediators of Inflammation. Phytochem. Anal. 31, 57–67. doi:10.1002/pca.2866
Klopf, J., Brostjan, C., Eilenberg, W., and Neumayer, C. (2021). Neutrophil Extracellular Traps and Their Implications in Cardiovascular and Inflammatory Disease. Int. J. Mol. Sci. 22, 559. doi:10.3390/ijms22020559
Kolaczkowska, E., and Kubes, P. (2013). Neutrophil Recruitment and Function in Health and Inflammation. Nat. Rev. Immunol. 13, 159–175. doi:10.1038/nri3399
Konan, N. A., and Bacchi, E. M. (2007). Antiulcerogenic Effect and Acute Toxicity of a Hydroethanolic Extract from the Cashew (Anacardium Occidentale L.) Leaves. J. Ethnopharmacol 112, 237–242. doi:10.1016/j.jep.2007.03.003
Konstantinidis, T., Kambas, K., Mitsios, A., Panopoulou, M., Tsironidou, V., Dellaporta, E., et al. (2016). Immunomodulatory Role of Clarithromycin in Acinetobacter Baumannii Infection via Formation of Neutrophil Extracellular Traps. Antimicrob. Agents Chemother. 60, 1040–1048. doi:10.1128/aac.02063-15
Korinek, M., Chen, K.-M., Jiang, Y.-H., El-Shazly, M., Stocker, J., Chou, C.-K., et al. (2016). Anti-allergic Potential of Typhonium Blumei: Inhibition of Degranulation via Suppression of PI3K/PLCγ2 Phosphorylation and Calcium Influx. Phytomedicine 23, 1706–1715. doi:10.1016/j.phymed.2016.10.011
Kreisel, D., Nava, R. G., Li, W., Zinselmeyer, B. H., Wang, B., Lai, J., et al. (2010). In vivo two-photon Imaging Reveals Monocyte-dependent Neutrophil Extravasation during Pulmonary Inflammation. Proc. Natl. Acad. Sci. 107, 18073–18078. doi:10.1073/pnas.1008737107
Krishnamoorthy, N., Douda, D. N., Brüggemann, T. R., Ricklefs, I., Duvall, M. G., Abdulnour, R. E., et al. (2018). Neutrophil Cytoplasts Induce T(H)17 Differentiation and Skew Inflammation toward Neutrophilia in Severe Asthma. Sci. Immunol. 3. doi:10.1126/sciimmunol.aao4747
Kuo, P.-C., Hwang, T.-L., Lin, Y.-T., Kuo, Y.-C., and Leu, Y.-L. (2011). Chemical Constituents from Lobelia Chinensis and Their Anti-virus and Anti-inflammatory Bioactivities. Arch. Pharm. Res. 34, 715–722. doi:10.1007/s12272-011-0503-7
Küster, H., Weiss, M., Willeitner, A. E., Detlefsen, S., Jeremias, I., Zbojan, J., et al. (1998). Interleukin-1 Receptor Antagonist and Interleukin-6 for Early Diagnosis of Neonatal Sepsis 2 Days before Clinical Manifestation. Lancet. 352, 1271–1277. doi:10.1016/s0140-6736(98)08148-3
Lande, R., Ganguly, D., Facchinetti, V., Frasca, L., Conrad, C., Gregorio, J., et al. (2011). Neutrophils Activate Plasmacytoid Dendritic Cells by Releasing Self-DNA-Peptide Complexes in Systemic Lupus Erythematosus. Sci. Translational Med. 3, 73ra19. doi:10.1126/scitranslmed.3001180
Lee, Y.-Y., Lin, M.-B., Cheng, C.-F., Chang, L.-Y., Liu, T.-Y., and Hung, S.-L. (2014). Inhibitory Effects of Areca Nut Extract on Expression of Complement Receptors and Fc Receptors in Human Neutrophils. J. Periodontol. 85, 1096–1106. doi:10.1902/jop.2013.130498
Lee, K. H., Kronbichler, A., Park, D. D.-Y., Park, Y., Moon, H., Kim, H., et al. (2017). Neutrophil Extracellular Traps (NETs) in Autoimmune Diseases: A Comprehensive Review. Autoimmun. Rev. 16, 1160–1173. doi:10.1016/j.autrev.2017.09.012
Ley, K., Laudanna, C., Cybulsky, M. I., and Nourshargh, S. (2007). Getting to the Site of Inflammation: the Leukocyte Adhesion Cascade Updated. Nat. Rev. Immunol. 7, 678–689. doi:10.1038/nri2156
Li, L., Luo, Z., Liu, Y., Wang, H., Liu, A., Yu, G., et al. (2017). Screening and Identification of the Metabolites in Rat Plasma and Urine after Oral Administration of Areca Catechu L. Nut Extract by Ultra-high-pressure Liquid Chromatography Coupled with Linear Ion Trap-Orbitrap Tandem Mass Spectrometry. Molecules 22, 1026. doi:10.3390/molecules22061026
Liao, P., He, Y., Yang, F., Luo, G., Zhuang, J., Zhai, Z., et al. (2018). Polydatin Effectively Attenuates Disease Activity in Lupus-Prone Mouse Models by Blocking ROS-Mediated NET Formation. Arthritis Res. Ther. 20, 254. doi:10.1186/s13075-018-1749-y
Licciardi, P. V., and Underwood, J. R. (2011). Plant-derived Medicines: a Novel Class of Immunological Adjuvants. Int. Immunopharmacology 11, 390–398. doi:10.1016/j.intimp.2010.10.014
Lien, D. C., Wagner, W. W., Capen, R. L., Haslett, C., Hanson, W. L., Hofmeister, S. E., et al. (1987). Physiological Neutrophil Sequestration in the Lung: Visual Evidence for Localization in Capillaries. J. Appl. Physiol. 62, 1236–1243. doi:10.1152/jappl.1987.62.3.1236
Lieschke, G., Grail, D., Hodgson, G., Metcalf, D., Stanley, E., Cheers, C., et al. (1994). Mice Lacking Granulocyte Colony-Stimulating Factor Have Chronic Neutropenia, Granulocyte and Macrophage Progenitor Cell Deficiency, and Impaired Neutrophil Mobilization. Blood 84, 1737–1746. doi:10.1182/blood.v84.6.1737.bloodjournal8461737
Liew, P. X., and Kubes, P. (2019). The Neutrophil's Role during Health and Disease. Physiol. Rev. 99, 1223–1248. doi:10.1152/physrev.00012.2018
Lo, D.-Y., Chen, T.-H., Chien, M.-S., Koge, K., Hosono, A., Kaminogawa, S., et al. (2005). Effects of Sugar Cane Extract on the Modulation of Immunity in Pigs. J. Vet. Med. Sci. 67, 591–597. doi:10.1292/jvms.67.591
López-Cadenas, C., Sierra-Vega, M., García-Vieitez, J., Diez-Liébana, M., Sahagún-Prieto, A., and Fernández- Martínez, N. (2013). Enrofloxacin: Pharmacokinetics and Metabolism in Domestic Animal Species. Cdm 14, 1042–1058. doi:10.2174/1389200214666131118234935
Lu, S., and Nasrallah, H. A. (2018). The Use of Memantine in Neuropsychiatric Disorders: An Overview. Ann. Clin. Psychiatry 30, 234–248.
Luo, F., Das, A., Chen, J., Wu, P., Li, X., and Fang, Z. (2019). Metformin in Patients with and without Diabetes: a Paradigm Shift in Cardiovascular Disease Management. Cardiovasc. Diabetol. 18, 54. doi:10.1186/s12933-019-0860-y
Lv, Z., and Guo, Y. (2020). Metformin and its Benefits for Various Diseases. Front. Endocrinol. (Lausanne) 11, 191. doi:10.3389/fendo.2020.00191
Malani, A. N., Kerr, L. E., and Kauffman, C. A. (2015). Voriconazole: How to Use This Antifungal Agent and what to Expect. Semin. Respir. Crit. Care Med. 36, 786–795. doi:10.1055/s-0035-1562903
Manda, A., Pruchniak, M. P., Araźna, M., and Demkow, U. A. (2014). Neutrophil Extracellular Traps in Physiology and Pathology. cejoi 1, 116–121. doi:10.5114/ceji.2014.42136
Manda-Handzlik, A., Bystrzycka, W., Sieczkowska, S., Demkow, U., and Ciepiela, O. (2017). Antibiotics Modulate the Ability of Neutrophils to Release Neutrophil Extracellular Traps. Adv. Exp. Med. Biol. 944, 47–52. doi:10.1007/978-3-319-44488-8_59
Mantovani, A., Cassatella, M. A., Costantini, C., and Jaillon, S. (2011). Neutrophils in the Activation and Regulation of Innate and Adaptive Immunity. Nat. Rev. Immunol. 11, 519–531. doi:10.1038/nri3024
Martin, G. S., Mannino, D. M., Eaton, S., and Moss, M. (2003). The Epidemiology of Sepsis in the United States from 1979 through 2000. N. Engl. J. Med. 348, 1546–1554. doi:10.1056/nejmoa022139
Marty, C., Misset, B., Tamion, F., Fitting, C., Carlet, J., and Cavaillon, J.-M. (1994). Circulating Interleukin-8 Concentrations in Patients with Multiple Organ Failure of Septic and Nonseptic Origin. Crit. Care Med. 22, 673–679. doi:10.1097/00003246-199404000-00025
Mauer, A. M., Athens, J. W., Ashenbrucker, H., Cartwright, G. E., and Wintrobe, M. M. (1960). Leukokinetic Studies. Ii. A Method for Labeling Granulocytes In Vitro with Radioactive Diisopropylfluorophosphate (Dfp32)*. J. Clin. Invest. 39, 1481–1486. doi:10.1172/jci104167
Mcmillan, R., and Scott, J. L. (1968). Leukocyte Labeling with 51Chromium. Blood 32, 738–754. doi:10.1182/blood.v32.5.738.738
Mehmood, A., Ishaq, M., Usman, M., Zhao, L., Ullah, A., and Wang, C. (2020). Nutraceutical Perspectives and Value Addition of Phalsa ( Grewia Asiatica L.): A Review. J. Food Biochem. 44, e13228. doi:10.1111/jfbc.13228
Meijer, M., Rijkers, G. T., and Van Overveld, F. J. (2013). Neutrophils and Emerging Targets for Treatment in Chronic Obstructive Pulmonary Disease. Expert Rev. Clin. Immunol. 9, 1055–1068. doi:10.1586/1744666x.2013.851347
Melchart, D., Linde, K., Worku, F., Sarkady, L., Holzmann, M., Jurcic, K., et al. (1995). Results of Five Randomized Studies on the Immunomodulatory Activity of Preparations of Echinacea. J. Altern. Complement. Med. 1, 145–160. doi:10.1089/acm.1995.1.145
Menegazzo, L., Scattolini, V., Cappellari, R., Bonora, B. M., Albiero, M., Bortolozzi, M., et al. (2018). The Antidiabetic Drug Metformin Blunts NETosis In Vitro and Reduces Circulating NETosis Biomarkers In Vivo. Acta Diabetol. 55, 593–601. doi:10.1007/s00592-018-1129-8
Mesaik, M. A., Ahmed, A., Khalid, A. S., Jan, S., Siddiqui, A. A., Perveen, S., et al. (2013). Effect of Grewia Asiatica Fruit on Glycemic Index and Phagocytosis Tested in Healthy Human Subjects. Pak J. Pharm. Sci. 26, 85–89.
Mitsios, A., Arampatzioglou, A., Arelaki, S., Mitroulis, I., and Ritis, K. (2016). NETopathies? Unraveling the Dark Side of Old Diseases through Neutrophils. Front. Immunol. 7, 678. doi:10.3389/fimmu.2016.00678
Miyazaki, Y., Kusano, S., Doi, H., and Aki, O. (2005). Effects on Immune Response of Antidiabetic Ingredients from White-Skinned Sweet Potato (Ipomoea Batatas L.). Nutrition 21, 358–362. doi:10.1016/j.nut.2004.11.001
Morais, T. C., Pinto, N. B., Carvalho, K. M. M. B., Rios, J. B., Ricardo, N. M. P. S., Trevisan, M. T. S., et al. (2010). Protective Effect of Anacardic Acids from Cashew (Anacardium Occidentale) on Ethanol-Induced Gastric Damage in Mice. Chem. Biol. Interact. 183, 264–269. doi:10.1016/j.cbi.2009.10.008
Mortaz, E., Alipoor, S. D., Adcock, I. M., Mumby, S., and Koenderman, L. (2018). Update on Neutrophil Function in Severe Inflammation. Front. Immunol. 9, 2171. doi:10.3389/fimmu.2018.02171
Mostafa, E., Fayed, M. A. A., Radwan, R. A., and Bakr, R. O. (2019). Centaurea Pumilio L. Extract and Nanoparticles: A Candidate for Healthy Skin. Colloids Surf. B: Biointerfaces 182, 110350. doi:10.1016/j.colsurfb.2019.110350
Mottola, G. (2014). The Complexity of Rab5 to Rab7 Transition Guarantees Specificity of Pathogen Subversion Mechanisms. Front Cel Infect Microbiol. 4, 180. doi:10.3389/fcimb.2014.00180
Moulton, V. R. (2018). Sex Hormones in Acquired Immunity and Autoimmune Disease. Front. Immunol. 9, 2279. doi:10.3389/fimmu.2018.02279
Mukherjee, R., Dash, P. K., and Ram, G. C. (2005). Immunotherapeutic Potential of Ocimum Sanctum (L) in Bovine Subclinical Mastitis. Res. Vet. Sci. 79, 37–43. doi:10.1016/j.rvsc.2004.11.001
Mukherjee, R., De, U. K., and Ram, G. C. (2010). Evaluation of Mammary Gland Immunity and Therapeutic Potential of Tinospora Cordifolia against Bovine Subclinical Mastitis. Trop. Anim. Health Prod. 42, 645–651. doi:10.1007/s11250-009-9471-z
Nadesalingam, A., Chen, J. H. K., Farahvash, A., and Khan, M. A. (2018). Hypertonic Saline Suppresses NADPH Oxidase-dependent Neutrophil Extracellular Trap Formation and Promotes Apoptosis. Front. Immunol. 9, 359. doi:10.3389/fimmu.2018.00359
Naffah de Souza, C., Breda, L. C. D., Khan, M. A., De Almeida, S. R., Câmara, N. O. S., Sweezey, N., et al. (2017). Alkaline pH Promotes NADPH Oxidase-independent Neutrophil Extracellular Trap Formation: A Matter of Mitochondrial Reactive Oxygen Species Generation and Citrullination and Cleavage of Histone. Front. Immunol. 8, 1849. doi:10.3389/fimmu.2017.01849
Nathan, C. (2006). Neutrophils and Immunity: Challenges and Opportunities. Nat. Rev. Immunol. 6, 173–182. doi:10.1038/nri1785
Németh, T., Sperandio, M., and Mócsai, A. (2020). Neutrophils as Emerging Therapeutic Targets. Nat. Rev. Drug Discov. 19, 253–275. doi:10.1038/s41573-019-0054-z
Nerlov, C., and Graf, T. (1998). PU.1 Induces Myeloid Lineage Commitment in Multipotent Hematopoietic Progenitors. Genes Dev. 12, 2403–2412. doi:10.1101/gad.12.15.2403
Nordenfelt, P., and Tapper, H. (2011). Phagosome Dynamics during Phagocytosis by Neutrophils. J. Leukoc. Biol. 90, 271–284. doi:10.1189/jlb.0810457
Nourshargh, S., Renshaw, S. A., and Imhof, B. A. (2016). Reverse Migration of Neutrophils: where, when, How, and Why? Trends Immunol. 37, 273–286. doi:10.1016/j.it.2016.03.006
Oliveira, R. A., Narciso, C. D., Bisinotto, R. S., Perdomo, M. C., Ballou, M. A., Dreher, M., et al. (2010). Effects of Feeding Polyphenols from Pomegranate Extract on Health, Growth, Nutrient Digestion, and Immunocompetence of Calves. J. Dairy Sci. 93, 4280–4291. doi:10.3168/jds.2010-3314
Ou, J. G. (1991). [Effect of Chinese Medicine "gu Chi Wan" on Chemotaxis and Phagocytosis of Polymorphonuclear Leukocytes from Peripheral Blood in Juvenile Periodontitis Patients]. Zhonghua Kou Qiang Yi Xue Za Zhi. 26, 51–53.
Panche, A. N., Diwan, A. D., and Chandra, S. R. (2016). Flavonoids: an Overview. J. Nutr. Sci. 5, e47. doi:10.1017/jns.2016.41
Park, W. H., Park, S. Y., Kim, H. M., and Kim, C. H. (2004). Effect of a Korean Traditional Formulation, Hwaotang, on Superoxide Generation in Human Neutrophils, Platelet Aggregation in Human Blood, and Nitric Oxide, Prostaglandin E2 Production and Paw Oedema Induced by Carrageenan in Mice. Immunopharmacol. Immunotoxicol. 26, 53–73. doi:10.1081/iph-120029945
Paula, F. S., Kabeya, L. M., Kanashiro, A., De Figueiredo, A. S. G., Azzolini, A. E. C. S., Uyemura, S. A., et al. (2009). Modulation of Human Neutrophil Oxidative Metabolism and Degranulation by Extract of Tamarindus indica L. Fruit Pulp. Food Chem. Toxicol. 47, 163–170. doi:10.1016/j.fct.2008.10.023
Pečivová, J., Mačičková, T., Sviteková, K., and Nosáľ, R. (2012). Quercetin Inhibits Degranulation and Superoxide Generation in PMA Stimulated Neutrophils. Interdiscip. Toxicol. 5, 81–83.
Peng, L., Li, L., He, X. L., Yu, J. Y., Zeng, Z. J., Yang, W. J., et al. (2020). Memantine Displays Antimicrobial Activity by Enhancing Escherichia coli Pathogen-Induced Formation of Neutrophil Extracellular Traps. Front. Cel Infect Microbiol. 10, 47. doi:10.3389/fcimb.2020.00047
Perveen, S., Alqahtani, J., Orfali, R., Aati, H. Y., Al-Taweel, A. M., Ibrahim, T. A., et al. (2020). Antibacterial and Antifungal Sesquiterpenoids from Aerial Parts of Anvillea Garcinii. Molecules 25. 1730. doi:10.3390/molecules25071730
Peters, A. M., Saverymuttu, S. H., Keshavarzian, A., Bell, R. N., and Lavender, J. P. (1985). Splenic Pooling of Granulocytes. Clin. Sci. (Lond) 68, 283–289. doi:10.1042/cs0680283
Petri, B., Phillipson, M., and Kubes, P. (2008). The Physiology of Leukocyte Recruitment: an In Vivo Perspective. J. Immunol. 180, 6439–6446. doi:10.4049/jimmunol.180.10.6439
Phillipson, M., Heit, B., Colarusso, P., Liu, L., Ballantyne, C. M., and Kubes, P. (2006). Intraluminal Crawling of Neutrophils to Emigration Sites: a Molecularly Distinct Process from Adhesion in the Recruitment Cascade. J. Exp. Med. 203, 2569–2575. doi:10.1084/jem.20060925
Pillay, J., Den Braber, I., Vrisekoop, N., Kwast, L. M., De Boer, R. J., Borghans, J. A. M., et al. (2010). In vivo labeling with 2H2O Reveals a Human Neutrophil Lifespan of 5.4 Days. Blood 116, 625–627. doi:10.1182/blood-2010-01-259028
Pohl, K., Grimm, X. A., Caceres, S. M., Poch, K. R., Rysavy, N., Saavedra, M., et al. (2020). Mycobacterium Abscessus Clearance by Neutrophils Is Independent of Autophagy. Infect. Immun. 88, e00024–20. doi:10.1128/iai.00024-20
Popov, S. V., Popova, G. Y., Nikolaeva, S. Y., Golovchenko, V. V., and Ovodova, R. G. (2005). Immunostimulating Activity of Pectic Polysaccharide fromBergenia Crassifolia (l.) Fritsch. Phytother. Res. 19, 1052–1056. doi:10.1002/ptr.1789
Ragab, D., Salah Eldin, H., Taeimah, M., Khattab, R., and Salem, R. (2020). The COVID-19 Cytokine Storm; what We Know So Far. Front. Immunol. 11, 1446. doi:10.3389/fimmu.2020.01446
Ramprasath, V. R., Shanthi, P., and Sachdanandam, P. (2006). Effect ofSemecarpus Anacardium Linn. Nut Milk Extract on Rat Neutrophil Functions in Adjuvant Arthritis. Cell Biochem. Funct. 24, 333–340. doi:10.1002/cbf.1243
Rasheed, H. M. F., Rasheed, F., Qureshi, A. W., and Jabeen, Q. (2016). Immunostimulant Activities of the Aqueous Methanolic Extract of Leptadenia Pyrotechnica , a Plant from Cholistan Desert. J. Ethnopharmacology 186, 244–250. doi:10.1016/j.jep.2016.03.039
Rege, N., Bapat, R. D., Koti, R., Desai, N. K., and Dahanukar, S. (1993). Immunotherapy with Tinospora Cordifolia: a New Lead in the Management of Obstructive Jaundice. Indian J. Gastroenterol. 12, 5–8.
Rhodes, A., Evans, L. E., Alhazzani, W., Levy, M. M., Antonelli, M., Ferrer, R., et al. (2017). Surviving Sepsis Campaign: International Guidelines for Management of Sepsis and Septic Shock: 2016. Intensive Care Med. 43, 304–377. doi:10.1007/s00134-017-4683-6
Ríos, J.-L. (2010). Effects of Triterpenes on the Immune System. J. Ethnopharmacol. 128, 1–14. doi:10.1016/j.jep.2009.12.045
Ross, G. D., and Vĕtvicka, V. (1993). CR3 (CD11b, CD18): a Phagocyte and NK Cell Membrane Receptor with Multiple Ligand Specificities and Functions. Clin. Exp. Immunol. 92, 181–184. doi:10.1111/j.1365-2249.1993.tb03377.x
Sagiv, J. Y., Michaeli, J., Assi, S., Mishalian, I., Kisos, H., Levy, L., et al. (2015). Phenotypic Diversity and Plasticity in Circulating Neutrophil Subpopulations in Cancer. Cel Rep. 10, 562–573. doi:10.1016/j.celrep.2014.12.039
Saini, A., Sharma, S., and Chhibber, S. (2009). Induction of Resistance to Respiratory Tract Infection with Klebsiella pneumoniae in Mice Fed on a Diet Supplemented with Tulsi (Ocimum Sanctum) and Clove (Syzgium Aromaticum) Oils. J. Microbiol. Immunol. Infect. 42, 107–113.
Saitoh, T., Komano, J., Saitoh, Y., Misawa, T., Takahama, M., Kozaki, T., et al. (2012). Neutrophil Extracellular Traps Mediate a Host Defense Response to Human Immunodeficiency Virus-1. Cell Host & Microbe 12, 109–116. doi:10.1016/j.chom.2012.05.015
Scapini, P., Marini, O., Tecchio, C., and Cassatella, M. A. (2016). Human Neutrophils in the Saga of Cellular Heterogeneity: Insights and Open Questions. Immunol. Rev. 273, 48–60. doi:10.1111/imr.12448
Schumann, J. (2016). It Is All about Fluidity: Fatty Acids and Macrophage Phagocytosis. Eur. J. Pharmacol. 785, 18–23. doi:10.1016/j.ejphar.2015.04.057
Segal, A. W., Geisow, M., Garcia, R., Harper, A., and Miller, R. (1981). The Respiratory Burst of Phagocytic Cells Is Associated with a Rise in Vacuolar pH. Nature 290, 406–409. doi:10.1038/290406a0
Sengeløv, H., Follin, P., Kjeldsen, L., Lollike, K., Dahlgren, C., and Borregaard, N. (1995). Mobilization of Granules and Secretory Vesicles during In Vivo Exudation of Human Neutrophils. J. Immunol. 154, 4157–4165.
Shabeeba, M. A., and Rathinasamy, K. (2018). Antibacterial and Anticancer Activity of the Purified Cashew Nut Shell Liquid: Implications in Cancer Chemotherapy and Wound Healing. Nat. Prod. Res. 32, 2856–2860.
Sharma, V., Thakur, M., Chauhan, N. S., and Dixit, V. K. (2010). Immunomodulatory Activity of Petroleum Ether Extract ofAnacyclus Pyrethrum. Pharm. Biol. 48, 1247–1254. doi:10.3109/13880201003730642
Sharma, U., Bala, M., Kumar, N., Singh, B., Munshi, R. K., and Bhalerao, S. (2012). Immunomodulatory Active Compounds from Tinospora Cordifolia. J. Ethnopharmacol. 141, 918–926. doi:10.1016/j.jep.2012.03.027
Shen, F., Tang, X., Cheng, W., Wang, Y., Wang, C., Shi, X., et al. (2016). Fosfomycin Enhances Phagocyte-Mediated Killing of Staphylococcus aureus by Extracellular Traps and Reactive Oxygen Species. Sci. Rep. 6, 19262. doi:10.1038/srep19262
Shi, J., Gilbert, G. E., Kokubo, Y., and Ohashi, T. (2001). Role of the Liver in Regulating Numbers of Circulating Neutrophils. Blood 98, 1226–1230. doi:10.1182/blood.v98.4.1226
Shikov, A. N., Pozharitskaya, O. N., Makarova, M. N., Makarov, V. G., and Wagner, H. (2014). Bergenia Crassifolia (L.) Fritsch—Pharmacology and Phytochemistry. Phytomedicine 21, 1534–1542. doi:10.1016/j.phymed.2014.06.009
Shrestha, S. S., Ferrarese, I., Sut, S., Zengin, G., Grana, S., Ak, G., et al. (2021). Phytochemical Investigations and In Vitro Bioactivity Screening on Melia Azedarach L. Leaves Extract from Nepal. Chem. Biodivers 8, 1–18. doi:10.1002/cbdv.202001070
Shukla, S., Mehta, A., John, J., Mehta, P., Vyas, S. P., and Shukla, S. (2009). Immunomodulatory Activities of the Ethanolic Extract of Caesalpinia Bonducella Seeds. J. Ethnopharmacol. 125, 252–256. doi:10.1016/j.jep.2009.07.002
Sibille, Y., and Marchandise, F. X. (1993). Pulmonary Immune Cells in Health and Disease: Polymorphonuclear Neutrophils. Eur. Respir. J. 6, 1529–1543.
Silvestre-Roig, C., Hidalgo, A., and Soehnlein, O. (2016). Neutrophil Heterogeneity: Implications for Homeostasis and Pathogenesis. Blood 127, 2173–2181. doi:10.1182/blood-2016-01-688887
Silvestre-Roig, C., Braster, Q., Wichapong, K., Lee, E. Y., Teulon, J. M., Berrebeh, N., et al. (2019). Externalized Histone H4 Orchestrates Chronic Inflammation by Inducing Lytic Cell Death. Nature 569, 236–240. doi:10.1038/s41586-019-1167-6
Soehnlein, O. (2012). Multiple Roles for Neutrophils in Atherosclerosis. Circ. Res. 110, 875–888. doi:10.1161/circresaha.111.257535
Solanki, Y. B., and Jain, S. M. (2010). Immunostimolatory Activities ofVigna mungoL. Extract in Male Sprague-Dawley Rats. J. Immunotoxicol. 7, 213–218. doi:10.3109/15476911003792278
Sônego, F., Castanheira, F. V., Ferreira, R. G., Kanashiro, A., Leite, C. A., Nascimento, D. C., et al. (2016). Paradoxical Roles of the Neutrophil in Sepsis: Protective and Deleterious. Front. Immunol. 7, 155. doi:10.3389/fimmu.2016.00155
Stark, M. A., Huo, Y., Burcin, T. L., Morris, M. A., Olson, T. S., and Ley, K. (2005). Phagocytosis of Apoptotic Neutrophils Regulates Granulopoiesis via IL-23 and IL-17. Immunity 22, 285–294. doi:10.1016/j.immuni.2005.01.011
Stasiuk, M., and Kozubek, A. (2010). Biological Activity of Phenolic Lipids. Cell. Mol. Life Sci. 67, 841–860. doi:10.1007/s00018-009-0193-1
Steele, C. W., Karim, S. A., Leach, J. D. G., Bailey, P., Upstill-Goddard, R., Rishi, L., et al. (2016). CXCR2 Inhibition Profoundly Suppresses Metastases and Augments Immunotherapy in Pancreatic Ductal Adenocarcinoma. Cancer Cell 29, 832–845. doi:10.1016/j.ccell.2016.04.014
Steinhauser, M. L., Kunkel, S. L., and Hogaboam, C. M. (1999). New Frontiers in Cytokine Involvement during Experimental Sepsis. ILAR J. 40, 142–150. doi:10.1093/ilar.40.4.142
Sudam, V. S., Potnuri, A. G., and Subhashini, N. J. P. (2017). Syk—GTP RAC-1 Mediated Immune-Stimulatory Effect of Cuscuta Epithymum, Ipomoea Batata and Euphorbia Hirta Plant Extracts. Biomed. Pharmacother. 96, 742–749. doi:10.1016/j.biopha.2017.10.060
Sudha, P., Asdaq, S. M., Dhamingi, S. S., and Chandrakala, G. K. (2010). Immunomodulatory Activity of Methanolic Leaf Extract of Moringa Oleifera in Animals. Indian J. Physiol. Pharmacol. 54, 133–140.
Summers, C., Rankin, S. M., Condliffe, A. M., Singh, N., Peters, A. M., and Chilvers, E. R. (2010). Neutrophil Kinetics in Health and Disease. Trends Immunol. 31, 318–324. doi:10.1016/j.it.2010.05.006
T Hart, L. A., Nibbering, P. H., Van Den Barselaar, M. T., Van Dijk, H., Van Den Berg, A. J., and Labadie, R. P. (1990). Effects of Low Molecular Constituents from Aloe Vera Gel on Oxidative Metabolism and Cytotoxic and Bactericidal Activities of Human Neutrophils. Int. J. Immunopharmacol 12, 427–434. doi:10.1016/0192-0561(90)90026-j
Tao, L., Xu, M., Dai, X., Ni, T., Li, D., Jin, F., et al. (2018). Polypharmacological Profiles Underlying the Antitumor Property of Salvia Miltiorrhiza Root (Danshen) Interfering with NOX-dependent Neutrophil Extracellular Traps. Oxid Med. Cel Longev 2018, 4908328. doi:10.1155/2018/4908328
Templeton, A. J., Mcnamara, M. G., Šeruga, B., Vera-Badillo, F. E., Aneja, P., Ocaña, A., et al. (2014). Prognostic Role of Neutrophil-To-Lymphocyte Ratio in Solid Tumors: a Systematic Review and Meta-Analysis. J. Natl. Cancer Inst. 106, dju124. doi:10.1093/jnci/dju124
Thatte, U. M., Kulkarni, M. R., and Dahanukar, S. A. (1992). Immunotherapeutic Modification of Escherichia coli Peritonitis and Bacteremia by Tinospora Cordifolia. J. Postgrad. Med. 38, 13–15.
Todd, R. F., 3rd (1996). The Continuing Saga of Complement Receptor Type 3 (CR3). J. Clin. Invest. 98, 1–2. doi:10.1172/jci118752
Toiu, A., Mocan, A., Vlase, L., Pârvu, A. E., Vodnar, D. C., Gheldiu, A. M., et al. (2019). Comparative Phytochemical Profile, Antioxidant, Antimicrobial and In Vivo Anti-inflammatory Activity of Different Extracts of Traditionally Used Romanian Ajuga Genevensis L. And A. Reptans L. (Lamiaceae). Molecules 24, 1597. doi:10.3390/molecules24081597
Toshkova, R., Nikolova, N., Ivanova, E., Ivancheva, S., and Serkedjieva, J. (2004). In vitro investigation on the Effect of a Plant Preparation with Antiviral Activity on the Functions of Mice Phagocyte Cells. Pharmazie 59, 150–154.
Trevisan, M. T. S., Pfundstein, B., Haubner, R., Würtele, G., Spiegelhalder, B., Bartsch, H., et al. (2006). Characterization of Alkyl Phenols in Cashew (Anacardium Occidentale) Products and Assay of Their Antioxidant Capacity. Food Chem. Toxicol. 44, 188–197. doi:10.1016/j.fct.2005.06.012
Urban, C. F., and Nett, J. E. (2019). Neutrophil Extracellular Traps in Fungal Infection. Semin. Cel Dev. Biol. 89, 47–57. doi:10.1016/j.semcdb.2018.03.020
Urban, C. F., Lourido, S., and Zychlinsky, A. (2006). How Do Microbes Evade Neutrophil Killing? Cell Microbiol. 8, 1687–1696. doi:10.1111/j.1462-5822.2006.00792.x
Ussov, W. Y., Aktolun, C., Myers, M. J., Jamar, F., and Peters, A. M. (1995). Granulocyte Margination in Bone Marrow: Comparison with Margination in the Spleen and Liver. Scand. J. Clin. Lab. Invest. 55, 87–96. doi:10.3109/00365519509075382
Van Der Nat, J., Klerx, J., Van Dijk, H., De Silva, K., and Labadie, R. (1986). Immunomodulatory Activity of an Aqueous Extract of the Stem Bark of Azadirachta indica. Planta Med. 52, 428–429. doi:10.1055/s-2007-969236
Wang, M.-X., Liu, Y. Y., Hu, B. H., Wei, X. H., Chang, X., Sun, K., et al. (2010). Total Salvianolic Acid Improves Ischemia-Reperfusion-Induced Microcirculatory Disturbance in Rat Mesentery. Wjg 16, 5306–5316. doi:10.3748/wjg.v16.i42.5306
Wang, W., Mao, S., Yu, H., Wu, H., Shan, X., Zhang, X., et al. (2019). Pinellia Pedatisecta Lectin Exerts a Proinflammatory Activity Correlated with ROS-MAPKs/NF-Κb Pathways and the NLRP3 Inflammasome in RAW264.7 Cells Accompanied by Cell Pyroptosis. Int. Immunopharmacol. 66, 1–12. doi:10.1016/j.intimp.2018.11.002
Wang, S., Fu, L., Huang, K., Han, J., Zhang, R., and Fu, Z. (2020). Neutrophil-to-lymphocyte Ratio on Admission Is an Independent Risk Factor for the Severity and Mortality in Patients with Coronavirus Disease 2019. J. Infect. 82, e16-e18. doi:10.1016/j.jinf.2020.09.022
Wang, J., Ma, Y., Guo, M., Yang, H., and Guan, X. (2021). Salvianolic Acid B Suppresses EMT and Apoptosis to Lessen Drug Resistance through AKT/mTOR in Gastric Cancer Cells. Cytotechnology 73, 49–61. doi:10.1007/s10616-020-00441-4
Wickramasinghe, R., Kumara, R. R., De Silva, E. D., Ratnasooriya, W. D., and Handunnetti, S. (2014). Inhibition of Phagocytic and Intracellular Killing Activity of Human Neutrophils by Aqueous and Methanolic Leaf Extracts of Ixora Coccinea. J. Ethnopharmacol. 153, 900–907. doi:10.1016/j.jep.2014.03.064
Xu, L., Zhang, W., Kwak, M., Zhang, L., Lee, P. C. W., and Jin, J. O. (2019). Protective Effect of Melatonin against Polymicrobial Sepsis Is Mediated by the Anti-bacterial Effect of Neutrophils. Front. Immunol. 10, 1371. doi:10.3389/fimmu.2019.01371
Yamaryo, T., Oishi, K., Yoshimine, H., Tsuchihashi, Y., Matsushima, K., and Nagatake, T. (2003). Fourteen-member Macrolides Promote the Phosphatidylserine Receptor-dependent Phagocytosis of Apoptotic Neutrophils by Alveolar Macrophages. Aac 47, 48–53. doi:10.1128/aac.47.1.48-53.2003
Yang, S.-H., Hong, C.-Y., and Yu, C.-L. (2002). The Stimulatory Effects of Nasal Discharge from Patients with Perennial Allergic Rhinitis on Normal Human Neutrophils Are Normalized after Treatment with a New Mixed Formula of Chinese Herbs. Int. Immunopharmacol. 2, 1627–1639. doi:10.1016/s1567-5769(02)00133-9
Yaseen, R., Branitzki-Heinemann, K., Moubasher, H., Setzer, W. N., Naim, H. Y., and Von Köckritz-Blickwede, M. (2017). In Vitro Testing of Crude Natural Plant Extracts from Costa Rica for Their Ability to Boost Innate Immune Cells against Staphylococcus aureus. Biomedicines 5. doi:10.3390/biomedicines5030040
Yasuda, H., Sonoda, A., Yamamoto, M., Kawashima, Y., Takishita, Y., Morita, A., et al. (2019). 17-β-estradiol Enhances Neutrophil Extracellular Trap Formation by Interaction with Estrogen Membrane Receptor. Arch. Biochem. Biophys. 663, 64–70. doi:10.1016/j.abb.2018.12.028
Yates, C. R., Bruno, E. J., and Yates, M. E. D. (2021). Tinospora Cordifolia: A Review of its Immunomodulatory Properties. J. Diet. Suppl., 1–15. doi:10.1080/19390211.2021.1873214
Yipp, B. G., and Kubes, P. (2013). NETosis: How Vital Is it? Blood 122, 2784–2794. doi:10.1182/blood-2013-04-457671
Yipp, B. G., Kim, J. H., Lima, R., Zbytnuik, L. D., Petri, B., Swanlund, N., et al. (2017). The Lung Is a Host Defense Niche for Immediate Neutrophil-Mediated Vascular Protection. Sci. Immunol. 2, eaam8929. doi:10.1126/sciimmunol.aam8929
Yu, Y., Koehn, C. D., Yue, Y., Li, S., Thiele, G. M., Hearth-Holmes, M. P., et al. (2015). Celastrol Inhibits Inflammatory Stimuli-Induced Neutrophil Extracellular Trap Formation. Cmm 15, 401–410. doi:10.2174/1566524015666150505160743
Zaporozhets, T. S., Besednova, N. N., Liamkin, G. P., Loenko Iu, N., and Popov, A. M. (1991). [Immunomodulating Properties of Pectin from Seawater Grass Zostera]. Antibiot. Khimioter 36, 31–34.
Zhang, D., Chen, G., Manwani, D., Mortha, A., Xu, C., Faith, J. J., et al. (2015). Neutrophil Ageing Is Regulated by the Microbiome. Nature 525, 528–532. doi:10.1038/nature15367
Zhang, H., Qiu, S. L., Tang, Q. Y., Zhou, X., Zhang, J. Q., He, Z. Y., et al. (2019). Erythromycin Suppresses Neutrophil Extracellular Traps in Smoking-Related Chronic Pulmonary Inflammation. Cell Death Dis. 10, 678. doi:10.1038/s41419-019-1909-2
Zhang, R., Ji, Y., Zhang, X., Kennelly, E. J., and Long, C. (2020a). Ethnopharmacology of Hypericum Species in China: A Comprehensive Review on Ethnobotany, Phytochemistry and Pharmacology. J. Ethnopharmacol. 254, 112686. doi:10.1016/j.jep.2020.112686
Keywords: neutrophils, phagocytosis, degranulation, extracellular traps, inflammation
Citation: Gierlikowska B, Stachura A, Gierlikowski W and Demkow U (2021) Phagocytosis, Degranulation and Extracellular Traps Release by Neutrophils—The Current Knowledge, Pharmacological Modulation and Future Prospects. Front. Pharmacol. 12:666732. doi: 10.3389/fphar.2021.666732
Received: 10 February 2021; Accepted: 19 April 2021;
Published: 04 May 2021.
Edited by:
Alexey Victorovich Sokolov, Institute of Experimental Medicine (RAS), RussiaReviewed by:
Anderson Guimarães-Costa, Federal University of Rio de Janeiro, BrazilRoman A. Zinovkin, Lomonosov Moscow State University, Russia
Copyright © 2021 Gierlikowska, Stachura, Gierlikowski and Demkow. This is an open-access article distributed under the terms of the Creative Commons Attribution License (CC BY). The use, distribution or reproduction in other forums is permitted, provided the original author(s) and the copyright owner(s) are credited and that the original publication in this journal is cited, in accordance with accepted academic practice. No use, distribution or reproduction is permitted which does not comply with these terms.
*Correspondence: Barbara Gierlikowska, YmFyYmFyYS5naWVybGlrb3dza2FAd3VtLmVkdS5wbA==