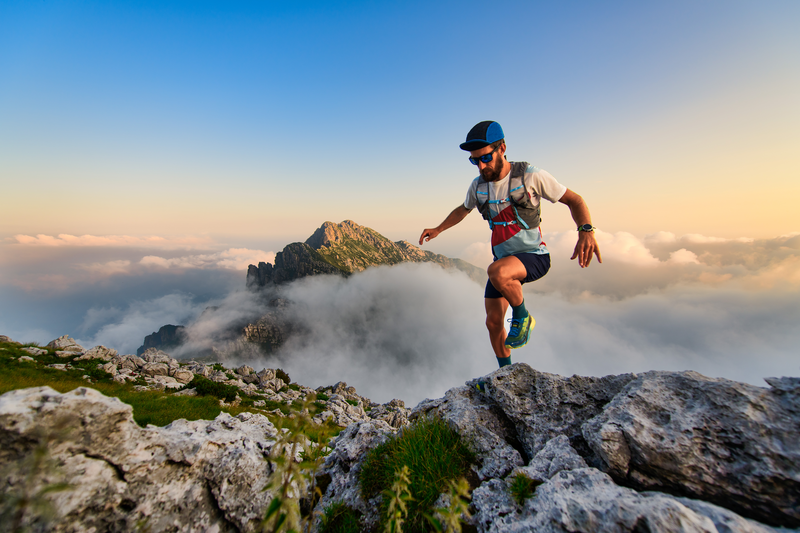
94% of researchers rate our articles as excellent or good
Learn more about the work of our research integrity team to safeguard the quality of each article we publish.
Find out more
SYSTEMATIC REVIEW article
Front. Pharmacol. , 21 May 2021
Sec. Ethnopharmacology
Volume 12 - 2021 | https://doi.org/10.3389/fphar.2021.663266
This article is part of the Research Topic Medicinal Plants in the Treatment of Myocardial Injury and Vascular Diseases View all 16 articles
Objective: Hydroxytyrosol (HT), a polyphenol of olive plant is well known for its antioxidant, anti-inflammatory and anti-atherogenic properties. The aim of this systematic search is to highlight the scientific evidence evaluating molecular efficiency of HT in halting the progression of intimal hyperplasia (IH), which is a clinical condition arises from endothelial inflammation.
Methods: A systematic search was performed through PubMed, Web of Science and Scopus, based on pre-set keywords which are Hydroxytyrosol OR 3,4-dihydroxyphenylethanol, AND Intimal hyperplasia OR Neointimal hyperplasia OR Endothelial OR Smooth muscles. Eighteen in vitro and three in vitro and in vivo studies were selected based on a pre-set inclusion and exclusion criteria.
Results: Based on evidence gathered, HT was found to upregulate PI3K/AKT/mTOR pathways and supresses inflammatory factors and mediators such as IL-1β, IL-6, E-selectin, P-selectin, VCAM-1, and ICAM-1 in endothelial vascularization and functioning. Two studies revealed HT disrupted vascular smooth muscle cells (SMC) cell cycle by dephosphorylating ERK1/2 and AKT pathways. Therefore, HT was proven to promote endothelization and inhibit vascular SMCs migration thus hampering IH development. However, none of these studies described the effect of HT collectively in both vascular endothelial cells (EC) and SMCs in IH ex vivo model.
Conclusions: Evidence from this concise review provides an insight on HT regulation of molecular pathways in reendothelization and inhibition of VSMCs migration. Henceforth, we propose effect of HT on IH prevention could be further elucidated through in vivo and ex vivo model.
Annually, millions of coronary artery bypass surgery (CABG) and percutaneous coronary interventions (PCI) are performed to treat ischemic heart disease. However, the development of intimal hyperplasia (IH) limits the long-term efficacy of these cardiovascular interventions (Mylonaki et al., 2018). Intimal hyperplasia is defined by thickening of the intimal layer of a blood vessel as a response to endothelial injury, which occurs during or post-surgical procedures such as PCI or CABG (Gellman et al., 1991). Endothelial injury triggers inflammation and platelet activation which subsequently stimulates the proliferation and migration of smooth muscle cells (SMCs) from media toward the intimal layer. SMCs migration is highly assisted by the secretion of inflammatory factors and mediators and degradation of multiple extracellular matrix (ECM) components in the media and adventitia (Jennette and Stone, 2014). This cascade reaction eventually leads to atherosclerosis where the blood vessel narrowed, and surrounding tissues falls into ischemic condition. Unfortunately, the formation of IH decreases the patency of bypass grafted veins to 40% after 10–20 years following surgery (de Vries and Quax, 2018).
Despite cutting edge therapies, IH remains as the main risk after CABG with no known remedy to reduce or relinquish the ever-progressing condition. Antithrombotic drugs are the classic approach to prevent IH (Hillis et al., 2012; Anderson et al., 2013). However, prolonged dual-antiplatelet therapy post angioplasty and stent implantation increases the risk of internal bleeding (Costa et al., 2015; Urban et al., 2019). First-generation drug-eluting stent (DES) incorporated with antiproliferative drugs like Sirolimus and Paclitaxel, were used to replace bare-metal stent (BMS) (Stone et al., 2007) has significantly reduced the recurrence of occlusion (Stettler et al., 2007; Jennette and Stone, 2014). Unfortunately, increased late stent thrombosis were also reported (Stone et al., 2007). DES efficiently prevent the migration of SMCs by disrupting SMCs cell cycle but with the price of delayed re-endothelization due to the antiproliferative effect of the drug on endothelial cells (ECs) (Camenzind et al., 2007; Joner et al., 2008).
Moving forward, bioresorbable stent (BRS) technology were introduced where the stents could be completely resolved after six months of implantation (Luo et al., 2014) leaving zero traces of stents material. This ultimately reduces future complications like stent migration, endothelial dysfunction, and restenosis (Gonzalo and Macaya, 2012). Unfortunately, BRS mechanical properties i.e. strut thickness, causes vessel injury and subsequently leads to platelet recruitment and thrombosis (Lee and Hernandez, 2018). In addition to that, concern about the degradation and disintegration of BRS into its by-products and its elimination in the coronary artery adds more challenges to the use of BRS. Large and small randomized trials of BRS implantation, unveiled thrombosis and intimal proliferation at one year follow up (Jinnouchi et al., 2019). Moreover, Optical Coherence Tomography (OCT) of an implanted Bioresorbable Novolimus-Eluting Coronary in patient revealed that the implanted scaffold collapsed and increased of neointimal proliferation in the artery (Alfonso and García-Guimaraes, 2017); Braun et al., 2016). Absorb Bioresorbable Vascular Scaffold (BVS; Abbott Vascular) is the first FDA-approved BRS, but it failed to ensure sustained success with increased late thrombosis events reported that leads to its withdrawal from the market due to low demand (Jinnouchi et al., 2019). BRSs are being redeveloped by taking into consideration several issues that include the strut thickness, degradation efficiency, scaffold thrombosis, and currently waiting to be evaluated in large-scale clinical trials (Regazzoli et al., 2017).
Various herbal plant-based components were studied for their cardiovascular protection effect (Barnard et al., 2019; Kim et al., 2019; Tuso et al., 2015). Xu et al. compiled a list of natural plant derived compounds such as flavonoids, polyphenols, alkaloids, and terpenes that were found to efficiently suppress VSMCs migration and proliferation (Xu et al., 2018). They further elucidated the involvement of typical cell regulatory and inflammatory pathways including MAPKs, PI3K/Akt, JAK-STAT, FAK, and NF-κB in VSMCs migration. However, they focused solely on activity of plant base compounds on VSMCs and not collectively with endothelial cells which is also an essential cell in pathophysiology of IH.
Polyphenol such as resveratrol is the most studied compound in IH prevention. Balloon catheters coated with resveratrol effectively deliver resveratrol to the targeted site and successfully reduce IH development in rabbit models (Tolva et al., 2016). In addition to that, a series of in vivo animal studies showed that resveratrol promoted re-endothelization and vascular healing post-surgical anastomosis (Yurdagul et al., 2014; Karaarslan et al., 2015; Kamann et al., 2019). Kamann et al. reported that resveratrol increases ECs proliferation via activating extracellular signal-regulated kinase (ERK) and estrogen receptor-dependent pathway under laminar shear stress (Yurdagul et al., 2014). Interestingly, curcumin also ameliorated IH by increasing endothelial angiogenesis and proliferation in an artery injured rat (Chen et al., 2015).
Alternatively, quercetin (Khandelwal et al., 2012) and salvianolic acid A (SAA) (Sun et al., 2012) were also found to inhibit proliferation of VSMCs too. Intriguingly, a green tea polyphenol, epigallocatechin-3-gallate (EGCg), suppressed neointimal hyperplasia (NIH) in rabbit model by inhibiting the proliferation of VSMCs via inactivation of MAPKs pathway In a recent study, Wei delivered mesoporous silica nanoparticles encapsulated honokiol (HNK), a small molecule polyphenol after balloon injury and HNK greatly suppressed intimal thickening by reducing phosphorylation of Smad3 (Wei et al., 2020).
Olive oil is the primary source of fat and polyphenols in Mediterranean Diet (MD) (Widmer et al., 2015). In 2013, the United Nations Educational, Scientific and Cultural Organization (UNESCO) include MD in the “Representative List of the Intangible Cultural Heritage of Humanity”. MD was also classified in the 2015–2020 Dietary Guidelines for Americans as a healthy diet (Romagnolo and Selmin, 2017). Phytochemicals from olive plant showed positive correlation with the reduction of cardiovascular diseases symptoms and risk factors (Tejada et al., 2016; Guasch-ferré et al., 2019).
Hydroxytyrosol (HT) is a most potent antioxidant, with 154.16 g/mol M mass found in the olive plant (Granados-Principal et al., 2010). HT is naturally derived from the hydrolysis of oleuropein (Tagliafierro et al., 2015) and alternatively, from dopamine metabolism in humans (Rodríguez-Morató et al., 2016). In nature, HT is hydrophilic hence readily absorb in a dose-dependent manner in animals and humans and are excreted in the urine as glucuronide conjugates (Kamil et al., 2020). HT is a well-studied phytochemical for its vascular protection (Hernáez et al., 2017; Nemzer et al., 2019), antioxidant (Adawiyah Razali et al., 2019; Soler-Cantero et al., 2012; Tejada et al., 2016), anti-inflammatory (Chin and Pang, 2017; Ng et al., 2017; Vilaplana-Pérez et al., 2014; Li et al., 2017), anti-atherogenic properties including the inhibition of LDL oxidation (Storniolo et al., 2019); and anti-platelet aggregation (De Roos et al., 2011). A couple of independent research elucidated HT potential in the attenuation of IH development (Xu et al., 2018; Man et al., 2020) However, HT has not been employed in any in vivo model to treat IH. Therefore, we aim to collect the scientific evidence of HT in the suppression of IH. This systematic review collate in vitro and in vivo studies that elucidate the underlying molecular action of HT in the attenuation of IH.
The selection and screening process were carried out based on PRISMA guideline as presented in Figure 1. A systematic screening through three databases (PubMed, Scopus and Web of Science) were performed. Original articles related to the molecular action of Hydroxytyrosol in intimal hyperplasia were searched using the following keywords: Hydroxytyrosol OR 3,4-dihydroxyphenylethanol AND Intimal hyperplasia OR Neointimal hyperplasia OR Endothelial OR Smooth muscle cells.
Full-text articles published between 2011–2020 in English were included. Only research/original articles were selected while review articles, proceeding abstract, and case studies were excluded. The search included all in vitro and in vivo studies. Titles and abstracts were meticulously screened and only articles that correlate to the molecular and cellular mechanism of action of Hydroxytyrosol in intimal hyperplasia were selected.
Two independent reviewers thoroughly screen the selected research articles. All related articles from the database searches were combined and duplicates were removed. The rest of the articles were screened further to meet the selection criteria. The title was first screened, follow by the abstracts for relevance to the selected topic. Unrelated articles that do not fall into the inclusion criteria were removed. The extracted data are tabulated concisely as follows: 1) Reference 2) Aim 3) Cells and Treatment 4) Test(s) 5) Finding(s) 6) Signaling molecules/Pathways 7) Conclusion/correlation with IH.
The quality of the selected studies was validated using a modified version of Office of Health Assessment and Translation (OHAT). The checklist is designed as presented in Table 3 to evaluate the potential risk of bias of both in vivo and in vitro studies by assessing 1) reporting bias, 2) performance bias, 3) detection bias, and 4) selection bias.
Initially, a total of 335 articles were identified from all database search and 216 articles remained after the removal of duplicates. The reviewers validate selected articles independently according to designed inclusion and exclusion criteria to minimize bias. Screening of title and abstract were done twice and a total of 35 papers were identified. During the final screening of the full text, 11 non-related articles, and 3 articles that used nonvascular cells were excluded. In the end, a total of 19 in vitro and 4 both in vitro and in vivo studies were selected for the review. Figure 1 shows the selection and screening process based on PRISMA guidelines.
Three electronic database searches identified 19 in vitro (Table 1) and 4 studies that include both in vivo and in vitro (Table 2) analysis related to the action of HT in enhancing endothelial function and inhibiting proliferation of VMSCs which are involved in the suppression of intimal hyperplasia development. Data extracted from the selected articles is presented in Table 1. Most experiments were conducted utilizing human umbilical vein endothelial cells (HUVECs), human vascular endothelial cells (HVEC) and porcine pulmonary artery endothelial cells (PAECs). However, monocyte cell lines such as U937 and Jurkat were also used in 3 studies. Bovine vascular smooth muscle cells (BVSMVs) and human microvascular endothelial cells (HMVECs) were utilized in 2 studies. Human microvascular cell line, bovine aorta endothelial cells (BAECs), human peripheral blood cells, myoblast, rat vascular smooth muscle cells, and vascular adventitia fibroblast were also utilized. Two in vivo studies were conducted on mice while one was done in a rat model.
Risk bias analysis was conducted using modified version of Office of Health Assessment and Translation (OHAT). Overall, twenty-one out of twenty-three studies showed low risk bias. Two in vitro, two in vivo and one in vitro and ex vivo studies showed low risk of bias when they fulfill the selection criteria and reported all outcomes. In contrast, two studies showed high substantial risk of bias due to insufficient sample number and unclear adverse event reporting. A summary of risk bias analysis presented in Table 3.
Antioxidant potential of HT comes from its chemical presence of hydroxyl (OH) groups in the ortho position. These OH groups are responsible in forming stable hydrogen bonds by scavenging reactive oxygen species (ROS) such as hydrogen peroxide (H2O2), superoxide ion (O2−), hydroxyl radical (OH−), and reactive nitrogen species (RNS) (Napolitano et al., 2010). HT regulates vascular homeostasis by balancing cellular oxidation stress and in addition to that, treatment with HT increase the production of nitric oxide (NO) which directly plays a crucial role in endothelial cells (ECs) functioning (Sandoo et al., 2010) such as inhibition of inflammation, cell adhesion (Reglero-Real et al., 2016), platelets interactions (Hamilos et al., 2018) as well as maintaining vessel tone (Norton and Popel, 2016).
Imbalance cellular redox reactions in ECs arise from vascular complications like thrombosis (Yang et al., 2017), intimal growth (Nedeljkovic et al., 2003), inflammation, and infarction (Pober and Sessa, 2007). These events are likely activate transcription factors which mediate the secretion of inflammatory factors and cells to the site of inflammation which eventually, delays the healing process (Martinon, 2010; Yang et al., 2017). Interestingly, Pi et al. showed that organic compound extracted from plant i.e. apocynin reduces endogenous ROS level in mice with carotid injury that subsequently suppressed the secretion of pro-inflammatory molecules and VSMC proliferation (Pi et al., 2013). Similarly, heart failure drug like simvastatin and Ivabradine reduces the generation of ROS in IH progression in hyperlipidaemic rabbits (Koniari et al., 2016).
These findings strongly support the correlation between oxidation machinery and the prevention of IH. From our database search, 9 studies reported that HT efficiently prevented ROS production (Nakbi et al., 2011; Zrelli et al., 2011a; Zrelli et al., 2011b; Scoditti et al., 2012; Zrelli et al., 2013; Kouka et al., 2017; Torul et al., 2020). HT was also reported to be able to phosphorylate endothelial nitric oxide synthase (eNOS) which increases nitric oxide (NO) synthesis that essentially needed for vascular integrity and protection (Tousoulis et al., 2011; Zhao et al., 2015; Loscalzo and Jin, 2010). This effect could potentially promote reendothelization in IH repair.
In addition to that, HT also protect cells from H2O2 induced cytotoxicity and apoptosis by decreasing superoxide release (Nakbi et al., 2011; Torul et al., 2020) while activating JNK and p38 MAPKs pathways (Kitsati et al., 2016). Interestingly, a particular study by Zrelli found that HT activate the AMPK-FOXO3 pathway by enhancing catalase activity to reduce oxidative stress (Zrelli et al., 2011b). Expression of FOXO3 appears to protect cells from oxidative injury by regulating the expression of the antioxidant enzyme such as catalase and peroxiredoxin (Hou et al., 2010; Olmos et al., 2009). Similarly, another set of studies stated AMPK directly activates FOXO3 transcriptional activity to provide cellular resistance toward oxidative stress (Greer et al., 2007; Li et al., 2009).
Endothelial injury is a precursor for intimal hyperplasia (Garg and Hassid, 1989; de Vries and Quax, 2018). Inflammatory cytokines, chemokines, immune cells, and platelets are recruited to the site of injury to initiate repair mechanism which starts off with vascular inflammation and followed with healing process that are regulated by the immune system to maintain vascular health (D’Angelo et al., 2020). However, prolonged exposure to inflammatory molecules has a detrimental effect on vascular cells. Especially, during vascular injury, the secretion of ICAM-1 and MCP-1 attract platelet and leukocyte to the injured site. Gradually, the activated platelets trigger Thromboxane A2 and PDGF release which causes the VSMC to proliferate and migrate (Davies and Hagen, 1989; Huang et al., 2002). Thus, downregulating inflammatory factors and mediators potentially could prevent further progression of IH. Olive oil extracts have been shown to decrease the inflammatory activation in endothelial cells (Burja et al., 2019).
In ECs inflammation, nuclear factor-kappa B (NFκB) transcription factor regulates inflammatory mediators such as MCP-1, VCAM-1, ICAM-1, and E-selectin which recruits leukocytes, IL-6, and IL-8. (Pamukcu et al., 2011). From our systematic search, Scoditti et al. found that HT treatment decrease the expression of MMP-9, ICAM-1, IL-1b, TNF-α, and COX-2 by inactivating NF-κβ, PKCβ1, and PKCα in PMA activated human monocytes (Scoditti et al., 2014). Upon consumption, HT metabolized into glucuronide, sulfate methyl and methyl–sulphate conjugates (Kotronoulas et al., 2013; Rubió et al., 2014). It is crucial to test biological activity of HT metabolites together with HT assessing in vascular protection ability of HT. Catalan and colleagues synthesized physiological HT metabolites using Caco-2 cells. They reported that HT with its metabolites decrease inflammatory mediatorssuch as E-selectin, P-selectin, ICAM-1, and VCAM-1 but HT metabolite alone could only decrease MCP-1 level (Catalán et al., 2015). They further elucidate HT and HT metabolites potential in rat and endothelial cell model where they reported that HT and HT derivate supplemented aorta, stained less for E-selectin, MCP-1, and ICAM-1. Furthermore, they found that HT and HT metabolites provide endothelial protection through regulation of ERK, JNK, and MAPK interrelated pathways (Catalán et al., 2018). Moreover, Hydroxytyrosol acetate (HT-Ac), were also found to be able to suppress inflammatory response by upregulating SIRT-6 expression in hypercholesterolemic mice and TNF-α treated HUVECs. These studies shed light on the activation of TNFRSF1A and PKM2 pathways which are responsible for anti-inflammatory activity (Yao et al., 2019) thus proves HT inhibits inflammatory angiogenesis.
Inflammatory angiogenesis contribute immensely in the formation of tumor vasculature. Tumor angiogenesis produces new blood vessels from existing vessels to supply nutrients and oxygen to tumor cells (Aguilar-Cazares et al., 2019). HT successfully inhibited inflammatory angiogenesis in phorbol myristate acetate (PMA) stimulated endothelial cells through inhibition of proinflammatory enzyme cyclooxygenase (COX)-2 and matrix degrading enzymes matrix metalloproteinases (MMPs) which are proinflammatory mediators in cancer and atherosclerosis (Fortes et al., 2012; Scoditti et al., 2012).
Re-endothelization is a prime event in IH repair. Delay in re-endothelization results in non-successful vascular interventions. Abate et al. reported that HT promote angiogenesis and wound healing in HUVECs cells via activating PI3K/AKT/mTOR pathways while upregulating the migration and adhesion-related protein expression (Abate et al., 2020). In another study, HT combined with pulsed electromagnetic field treatment, enhanced HUVECs migration and proliferation via regulation of Akt, mTOR, and TGF-β pathways (Cheng et al., 2017). Besides, two independent research by Zrelli et al. (2011b, 2015) demonstrates HT action of vascular healing through heme oxygenase-1 (HO-1) activation. High HO-1 expression protects cells from endothelial injury (Marcantoni et al., 2012; Kim et al., 2013). Additionally, they also reported that HT promotes vascular healing by stimulating the Nrf2 pathway which upregulates expression of HO-1 that is supported by PI3K, Akt, Erk ½. Lamy and colleagues, revealed that HT prevent endothelial apoptosis by suppressing ERK-1/2, SAPK and JNK pathways (Lamy et al., 2014).
Proliferation and migration of VMSCs are huge contributors to intimal thickening. Naturally, VSMCs exist in both contractile and synthetic phenotypes which are responsible to maintain vascular homeostasis (Michel et al., 2012; Basatemur et al., 2019). Endothelial injury tends to trigger generation of inflammatory factors such as platelet-derived growth factor (PDGF), fibroblast growth factor (FGF), and transforming growth factor-beta (TGFβ), which accelerate the migration of VMSCs into the intima layer (Lindqvist et al., 2001). HT promote VMSCs apoptosis via the production of NO and subsequent inactivation of Akt mediated by PP2A pathway in PDGF induced rat VMSCs (Zrelli et al., 2011a).
Regulation of VSMCs proliferation determines by MAPKs family members such as c-Jun N terminal kinase (JNK), extracellular signal-regulated kinase ½ (ERK), and p38 (Xu et al., 2018). MAPK chains also promote PDGF-stimulated VSMCs migration in the vascular injury model (Zhan et al., 2003). In a study by Liu et al., sulphur dioxide prevented VSMCs proliferation by inactivating Erk/MAP kinase pathway (Liu et al., 2014). Therefore, HT successfully inhibit bovine VMSCs proliferation in the same manner by disrupting the cell cycle regulated by ERK ½ (Abe et al., 2012).
On another hand, Matrix Metalloproteinases (MMP) are crucial extracellular matrix (ECM) components in maintaining vessel integrity and angiogenesis (Raffetto and Khalil, 2008). Amongst the different type of MMPs, MMP-2 were shown to enhanced VMSCs migration by disrupting the ECM in an in vitro model (Belo et al., 2015). Therefore, HT's ability to inhibit MMP-2 expression (Fortes et al., 2012) could therefore suppress VSMCs migration. Just as important, expression of MMP-9 that breaks the barrier between VSMCs and ECs were found to be downregulated by HT treatment (Nakbi et al., 2011; Scoditti et al., 2012; Scoditti et al., 2014). Phenotype switching of VSMCs from contractile to synthetic, marks the beginning of VSMCs remodeling (Wadey et al., 2018). In a past study, Resveratrol stimulate differentiation of VSMCs and inhibit migration by activating SIRT1 and AMPK (Thompson et al., 2014). In the same way, HT regulate the expression of SIRT1 in TNF-α stimulated vascular adventitia fibroblast (VAFs). HT and SIRT1 were shown to have good compatibility (Wang et al., 2018). These findings thus support HT ability in prevention of excessive vascular remodeling.
Ethnopharmacology has been an ever-growing field especially in the discovery of new compound in treatments of various diseases. Linking our ancestor knowledge in medicinal plants and giving it a scientific prove are both exciting and beneficial in future medical treatment. The association of plant derived antioxidants, specifically Hydroxytyrosol (HT) with lower risk factor and mortality in cardiovascular disease patients that consume olives products are well recognized. HT were found to exerted cardioprotective and anti-atherosclerotic effects in a randomized, double-blinded, placebo-controlled, crossover trial that were performed for 20 weeks (Quirós-Fernández et al., 2019). However, until now HT has not been investigated in attenuating intimal hyperplasia (IH) which if found beneficial could change the treatment of CVD patients significantly.
Therefore, we compile studies that utilize HT in vascular remodeling and critically review the mechanism that were elucidated. Endothelial functioning and healing are a crucial point in preventing further progression of IH, as endothelial injury triggers migration of SMCs. HT antioxidant property provides an oxidative stress defense friendly environment that prevents endothelial dysfunction and apoptosis. This is facilitated by the activation of AMPK-FOXO3 (Zrelli et al., 2011b). The molecular action of HT downregulates NFκB pathway which improves NO production. HT also promote cellular survival from ROS induction (Torul et al., 2020). These series of evidence, allow us to proposed HT that could promote reendothelization in the site of endothelial injury.
Migration of smooth muscle cell (SMCs) is the direct causal effect following EC disruption in IH. Overall, direct effect of HT on SMCs were inhibition of proliferation and migration. HT inhibited SMCs migration and proliferation via blocking cell cycle regulated by ERK1/2 phosphorylation (Abate et al., 2012). Zrelli proved that NO production and Akt dephosphorylation could prevent VSMCs proliferation. He also reported these events triggered by activation of PP2A that leads to cell apoptosis (Zrelli et al., 2011a). Correspondingly, HT directly effect MMP 9 and MMP 2 reduction which indirectly inhibits migration of SMCs (Nakbi et al., 2011; Scoditti et al., 2012; Fortes et al., 2012; Scoditti et al., 2014).
With regards to dosage, up to 160 μM, HT promotes endothelial proliferation and functioning endothelium. HT efficiently reduced SMCs proliferation at a dosage of 100 µM. These findings strongly support our theory for the use HT as treatment for intimal hyperplasia where with further research, a perfect dosage that enables HT enhance reendothelization while inhibits SMCs migration. Therefore, we hope this evidence compilation will encourage researchers to investigate the use of HT in ex vivo intimal hyperplasia organ culture models in future.
This systematic review collect evidences on molecular action of HT in the attenuation of IH in both in vitro and in vivo models. Supporting study on HT activity at the molecular level is presented in Tables 1 and 2 and further simplified in Figure 2. These consolidated findings uncovered the underlying pathways influenced by HT in IH suppression. HT promotes reendothelization by activating cell regulation pathways including AMPK/FOXO3, PI3K/AKT/mTOR and supressing VSMCs migration by disrupting cell cycle via inactivation of ERK1/2 and AKT. These findings can be further be applied in the treatment of IH by delivery of HT in future translational studies.
FIGURE 2. Hydroxytyrosol regulating key genes, inflammatory molecules and pathways in reendotelisation and inhibition of VSMCs
The original contributions presented in the study are included in the article/Supplementary Material, further inquiries can be directed to the corresponding author.
NS, MY, RI, and MR designed the research topic and questions of the systematic review. UV, NS, MY, and RI designed the study. UV, NS, and MY performed article selection and screening. UV, and NS carried out data collection, extraction, manuscript writing and data analysis. MY, RI, and MR performed final proofread of the manuscript.
This research is fully funded under the Fundamental Research Grant Scheme (FRGS) FRGS/1/2019/SKK08/UKM/03/3 by the Ministry of Education Malaysia. The funder does not have any contribution and decision on publishing or preparation of the manuscript.
The authors declare that the research was conducted in the absence of any commercial or financial relationships that could be construed as a potential conflict of interest.
All the authors would like to thank the Faculty of Medicine, UKM, for the resources use to complete this review.
Abate, M., Pisanti, S., Caputo, M., Citro, M., Vecchione, C., and Martinelli, R. (2020). 3-Hydroxytyrosol Promotes Angiogenesis In Vitro by Stimulating Endothelial Cell Migration. Int. J. Mol. Sci. 21 (10), 3657. doi:10.3390/ijms21103657
Abe, R., Beckett, J., Abe, R., Nixon, A., Rochier, A., Yamashita, N., et al. (2012). Olive Oil Polyphenols Differentially Inhibit Smooth Muscle Cell Proliferation through a G1/S Cell Cycle Block Regulated by ERK1/2. Int. J. Angiol. 21 (2), 069–076. doi:10.1055/s-0032-1315630
Adawiyah Razali, R., Lokanathan, Y., Yazid, M. D., Ansari, A. S., Bin Saim, A., and Bt Hj Idrus, R. (2019). Modulation of Epithelial to Mesenchymal Transition Signaling Pathways by Olea Europaea and its Active Compounds. Int. J. Mol. Sci. 20 (14), 3492. doi:10.3390/ijms20143492
Aguilar-Cazares, D., Chavez-Dominguez, R., Carlos-Reyes, A., Lopez-Camarillo, C., Hernadez de la Cruz, O. N., and Lopez-Gonzalez, J. S. (2019). Contribution of Angiogenesis to Inflammation and Cancer. Front. Oncol. 9, 1399. doi:10.3389/fonc.2019.01399
Alfonso, F., and García-Guimaraes, M. (2017). Restenosis of Coronary Bioresorbable Vascular Scaffolds. Revista Española de Cardiología (English Edition) 70 (7), 527–531. doi:10.1016/j.rec.2017.02.024
Anderson, J. L., Halperin, J. L., Albert, N. M., Bozkurt, B., Brindis, R. G., Curtis, L. H., et al. (2013). Management of Patients with Peripheral Artery Disease (Compilation of 2005 and 2011 ACCF/AHA Guideline Recommendations). Circulation 127 (13), 1425–1443. doi:10.1161/cir.0b013e31828b82aa
Barnard, N. D., Goldman, D. M., Loomis, J. F., Kahleova, H., Levin, S. M., Neabore, S., et al. (2019). Plant-based Diets for Cardiovascular Safety and Performance in Endurance Sports. Nutrients 11 (1), 1–10. doi:10.3390/nu11010130
Basatemur, G. L., Jørgensen, H. F., Clarke, M. C. H., Bennett, M. R., and Mallat, Z. (2019). Vascular Smooth Muscle Cells in Atherosclerosis. Nat. Rev. Cardiol. 16 (12), 727–744. doi:10.1038/s41569-019-0227-9
Belo, V. A., Guimarães, D. A., and Castro, M. M. (2015). Matrix Metalloproteinase 2 as a Potential Mediator of Vascular Smooth Muscle Cell Migration and Chronic Vascular Remodeling in Hypertension. J. Vasc. Res. 52 (4), 221–231. doi:10.1159/000441621
Braun, D., Baquet, M., Massberg, S., Mehilli, J., and Hausleiter, J. (2016). Collapse of a Bioresorbable Novolimus-Eluting Coronary Scaffold. JACC: Cardiovasc. Interventions 9 (1), e13–e14. doi:10.1016/j.jcin.2015.10.019
Burja, B., Kuret, T., Janko, T., Topalović, D., Živković, L., Mrak-Poljšak, K., et al. (2019). Olive Leaf Extract Attenuates Inflammatory Activation and DNA Damage in Human Arterial Endothelial Cells. Front. Cardiovasc. Med. 6, 56. doi:10.3389/fcvm.2019.00056
Camenzind, E., Steg, P. G., and Wijns, W. (2007). Response to Camenzind et al: Commentary. Circulation 115 (11), 1455. doi:10.1161/circulationaha.106.666800
Catalán, Ú., López de las Hazas, M.-C., Piñol, C., Rubió, L., Motilva, M.-J., Fernandez-Castillejo, S., et al. (2018). Hydroxytyrosol and its Main Plasma Circulating Metabolites Attenuate the Initial Steps of Atherosclerosis through Inhibition of the MAPK Pathway. J. Funct. Foods 40, 280–291. doi:10.1016/j.jff.2017.11.007
Catalán, Ú., López de las Hazas, M.-C., Rubió, L., Fernández-Castillejo, S., Pedret, A., de la Torre, R., et al. (2015). Protective Effect of Hydroxytyrosol and its Predominant Plasmatic Human Metabolites against Endothelial Dysfunction in Human Aortic Endothelial Cells. Mol. Nutr. Food Res. 59 (12), 2523–2536. doi:10.1002/mnfr.201500361
Chen, D., Tao, X., Wang, Y., Tian, F., Wei, Y., Chen, G., et al. (2015). Curcumin Accelerates Reendothelialization and Ameliorates Intimal Hyperplasia in Balloon-Injured Rat Carotid Artery via the Upregulation of Endothelial Cell Autophagy. Int. J. Mol. Med. 36 (6), 1563–1571. doi:10.3892/ijmm.2015.2365
Cheng, Y., Qu, Z., Fu, X., Jiang, Q., and Fei, J. (2017). Hydroxytyrosol Contributes to Cell Proliferation and Inhibits Apoptosis in Pulsed Electromagnetic Fields Treated Human Umbilical Vein Endothelial Cells In Vitro. Mol. Med. Rep. 16 (6), 8826–8832. doi:10.3892/mmr.2017.7701
Chin, K. Y., and Pang, K. L. (2017). Therapeutic Effects of Olive and its Derivatives on Osteoarthritis: From Bench to Bedside. Nutrients 9 (10), 1–18. doi:10.3390/nu9101060
Costa, F., Vranckx, P., Leonardi, S., Moscarella, E., Ando, G., Calabro, P., et al. (2015). Impact of Clinical Presentation on Ischaemic and Bleeding Outcomes in Patients Receiving 6- or 24-month Duration of Dual-Antiplatelet Therapy after Stent Implantation: a Pre-specified Analysis from the PRODIGY (Prolonging Dual-Antiplatelet Treatment after Grading Stent-Induced Intimal Hyperplasia) Trial. Eur. Heart J. 36 (20), 1242–1251. doi:10.1093/eurheartj/ehv038
D'Angelo, C., Goldeck, D., Pawelec, G., Gaspari, L., Di Iorio, A., and Paganelli, R. (2020). Exploratory Study on Immune Phenotypes in Alzheimer's Disease and Vascular Dementia. Eur. J. Neurol. 27 (10), 1887–1894. doi:10.1111/ene.14360
Davies, M. G., and Hagen, P.-0. (1989). Pathobiology of Intimal Hyperplasia. Br. J. Surg., 81(9), 1254–1269. doi:10.1002/bjs.1800810904
De Roos, B., Zhang, X., Rodriguez Gutierrez, G., Wood, S., Rucklidge, G. J., Reid, M. D., et al. (2011). Anti-platelet Effects of Olive Oil Extract: In vitro Functional and Proteomic Studies. Eur. J. Nutr. 50 (7), 553–562. doi:10.1007/s00394-010-0162-3
de Vries, M. R., and Quax, P. H. A. (2018). Inflammation in Vein Graft Disease. Front. Cardiovasc. Med. 5, 1–13. doi:10.3389/fcvm.2018.00003
Fortes, C., García-Vilas, J. A., Quesada, A. R., and Medina, M. Á. (2012). Evaluation of the Anti-angiogenic Potential of Hydroxytyrosol and Tyrosol, Two Bio-Active Phenolic Compounds of Extra Virgin Olive Oil, in Endothelial Cell Cultures. Food Chem. 134 (1), 134–140. doi:10.1016/j.foodchem.2012.02.079
Fuccelli, R., Fabiani, R., and Rosignoli, P. (2018). Hydroxytyrosol exerts anti-inflammatory and anti-oxidant activities in a mouse model of systemic inflammation. Molecules 23 (12). doi:10.3390/molecules23123212
García-Vilas, J. A., Quesada, A. R., and Medina, M. Á. (2017). Hydroxytyrosol Targets Extracellular Matrix Remodeling by Endothelial Cells and Inhibits Both Ex Vivo and In Vivo Angiogenesis. Food Chem. 221, 1741–1746. doi:10.1016/j.foodchem.2016.10.111
Garg, U. C., and Hassid, A. (1989). Nitric Oxide-Generating Vasodilators and 8-Bromo-Cyclic Guanosine Monophosphate Inhibit Mitogenesis and Proliferation of Cultured Rat Vascular Smooth Muscle Cells. J. Clin. Invest. 83 (5), 1774–1777. doi:10.1172/jci114081
Gellman, J., Ezekowitz, M. D., Sarembock, I. J., Azrin, M. A., Nochomowitz, L. E., Lerner, E., et al. (1991). Effect of Lovastatin on Intimal Hyperplasia after Balloon Angioplasty: A Study in an Atherosclerotic Hypercholesterolemic Rabbit. J. Am. Coll. Cardiol. 17 (1), 251–259. doi:10.1016/0735-1097(91)90735-r
Gonzalo, N., Gonzalo, N., and Macaya, C. (2012). Absorbable Stent: Focus on Clinical Applications and Benefits. Vhrm 8 (1), 125–132. doi:10.2147/vhrm.s22551
Granados-Principal, S., Quiles, J. L., Ramirez-Tortosa, C. L., Sanchez-Rovira, P., and Ramirez-Tortosa, M. C. (2010). Hydroxytyrosol: From Laboratory Investigations to Future Clinical Trials. Nutr. Rev. 68 (4), 191–206. doi:10.1111/j.1753-4887.2010.00278.x
Greer, E. L., Oskoui, P. R., Banko, M. R., Maniar, J. M., Gygi, M. P., Gygi, S. P., et al. (2007). The Energy Sensor AMP-Activated Protein Kinase Directly Regulates the Mammalian FOXO3 Transcription Factor. J. Biol. Chem. 282 (41), 30107–30119. doi:10.1074/jbc.m705325200
Guasch-ferré, M., Merino, J., Sun, Q., Fitó, M., Salas-salvadó, J., Fern, D., et al. (2019). Supplementation in a Physically Active Population . Sports Med. - Open 20 (1), 1–17. doi:10.3390/ijms20184567
Hamilos, M., Petousis, S., and Parthenakis, F. (2018). Interaction between Platelets and Endothelium: From Pathophysiology to New Therapeutic Options. Cardiovasc. Diagn. Ther. 8 (5), 568–580. doi:10.21037/cdt.2018.07.01
Hernáez, Á., Castañer, O., Elosua, R., Pintó, X., Estruch, R., Salas-Salvadó, J., et al. (2017). Mediterranean Diet Improves High-Density Lipoprotein Function in High-Cardiovascular-Risk Individuals. Circulation 135 (7), 633–643. doi:10.1161/circulationaha.116.023712
Hillis, L. D., Smith, P. K., Anderson, J. L., Bittl, J. A., Bridges, C. R., Byrne, J. G., et al. (2012). 2011 ACCF/AHA guideline for coronary artery bypass graft surgery: executive summary: a report of the American College of Cardiology Foundation/American Heart Association Task Force on Practice Guidelines. J. Thorac. Cardiovasc. Surg. 143 (1), 4–34. doi:10.1016/j.jtcvs.2011.10.015
Hou, X., Song, J., Li, X.-N., Zhang, L., Wang, X., Chen, L., et al. (2010). Metformin Reduces Intracellular Reactive Oxygen Species Levels by Upregulating Expression of the Antioxidant Thioredoxin via the AMPK-FOXO3 Pathway. Biochem. Biophysical Res. Commun. 396 (2), 199–205. doi:10.1016/j.bbrc.2010.04.017
Huang, B., Dreyer, T., Heidt, M., Yu, J. C. M., Philipp, M., Hehrlein, F. W., et al. (2002). Insulin and Local Growth Factor PDGF Induce Intimal Hyperplasia in Bypass Graft Culture Models of Saphenous Vein and Internal Mammary Artery. Eur. J. Cardio-Thoracic Surg. 21 (6), 1002–1008. doi:10.1016/s1010-7940(02)00111-2
Jennette, J. C., and Stone, J. R. (2014). “Diseases of Medium-Sized and Small Vessels,” in Cellular and Molecular Pathobiology of Cardiovascular Disease, 197–219. doi:10.1016/B978-0-12-405206-2.00011-9
Jinnouchi, H., Torii, S., Sakamoto, A., Kolodgie, F. D., Virmani, R., and Finn, A. V. (2019). Fully Bioresorbable Vascular Scaffolds: Lessons Learned and Future Directions. Nat. Rev. Cardiol. 16 (5), 286–304. doi:10.1038/s41569-018-0124-7
Joner, M., Nakazawa, G., Finn, A. V., Quee, S. C., Coleman, L., and Acampado, E. (2018). Endothelial cell recovery between comparator polymer-based drug-eluting stents. J. Am. Coll. Cardiol. 52 (5), 333–342. doi:10.1016/j.jacc.2008.04.030
Kamann, S., Haase, T., Stolzenburg, N., Löchel, M., Peters, D., and Schnorr, J. (2019). Resveratrol- Coated Balloon Catheters in Porcine Coronary and Peripheral Arteries. Int. J. Mol. Sci. 20 (9), 2285. doi:10.3390/ijms20092285
Kamil, K., Yazid, M. D., Idrus, R. B. H., and Kumar, J. (2020). Hydroxytyrosol Promotes Proliferation of Human Schwann Cells: An In Vitro Study. Int. J. Environ. Res. Public Health 17 (12), 1–11. doi:10.3390/ijerph17124404
Karaarslan, K., Abud, B., Albayrak, G., Aykut, K., Ergür, B. U., and Silistreli, E. (2015). The Effect of Resveratrol on Intimal Hyperplasia and Endothelial Proliferation of Rabbit Carotid Artery Anastomosis. Interactive Cardiovasc. Thorac. Surg. 20 (1), 15–20. doi:10.1093/icvts/ivu316
Khandelwal, A. R., Hebert, V. Y., Kleinedler, J. J., Rogers, L. K., Ullevig, S. L., Asmis, R., et al. (2012). Resveratrol and Quercetin Interact to Inhibit Neointimal Hyperplasia in Mice with a Carotid Injury. J. Nutr. 142 (8), 1487–1494. doi:10.3945/jn.112.162628
Kim, H., Caulfield, L. E., Garcia-Larsen, V., Steffen, L. M., Coresh, J., and Rebholz, C. M. (2019). Plant-Based Diets Are Associated with a Lower Risk of Incident Cardiovascular Disease, Cardiovascular Disease Mortality, and All-Cause Mortality in a General Population of Middle- Aged Adults. J. Am. Heart Assoc. 8 (16), e012865. doi:10.1161/jaha.119.012865
Kim, N., Hwangbo, C., Lee, S., and Lee, J.-H. (2013). Eupatolide Inhibits PDGF-Induced Proliferation and Migration of Aortic Smooth Muscle Cells through ROS-dependent Heme Oxygenase-1 Induction. Phytother. Res. 27 (11), 1700–1707. doi:10.1002/ptr.4924
Kitsati, N., Mantzaris, M. D., and Galaris, D. (2016). Hydroxytyrosol Inhibits Hydrogen Peroxide-Induced Apoptotic Signaling via Labile Iron Chelation. Redox Biol. 10 , 233–242. doi:10.1016/j.redox.2016.10.006
Koniari, I., Mavrilas, D., Apostolakis, E., Papadimitriou, E., Papadaki, H., Papalois, A., et al. (2016). Inhibition of Atherosclerosis Progression, Intimal Hyperplasia, and Oxidative Stress by Simvastatin and Ivabradine May Reduce Thoracic Aorta's Stiffness in Hypercholesterolemic Rabbits. J. Cardiovasc. Pharmacol. Ther. 21 (4), 412–422. doi:10.1177/1074248415617289
Kotronoulas, A., Pizarro, N., Serra, A., Robledo, P., Joglar, J., Rubió, L., et al. (2013). Dose-dependent Metabolic Disposition of Hydroxytyrosol and Formation of Mercapturates in Rats. Pharmacol. Res. 77, 47–56. doi:10.1016/j.phrs.2013.09.001
Kouka, P., Priftis, A., Stagos, D., Angelis, A., Stathopoulos, P., Xinos, N., et al. (2017). Assessment of the Antioxidant Activity of an Olive Oil Total Polyphenolic Fraction and Hydroxytyrosol from a Greek Olea Europea Variety in Endothelial Cells and Myoblasts. Int. J. Mol. Med. 40 (3), 703–712. doi:10.3892/ijmm.2017.3078
Lamy, S., Ouanouki, A., Béliveau, R., and Desrosiers, R. R. (2014). Olive Oil Compounds Inhibit Vascular Endothelial Growth Factor Receptor-2 Phosphorylation. Exp. Cel Res. 322 (1), 89–98. doi:10.1016/j.yexcr.2013.11.022
Lee, D. H., and Hernandez, J. M. d. la. T. (2018). The Newest Generation of Drug-Eluting Stents and beyond. Eur. Cardiol. Rev. 13 (1), 54–59. doi:10.15420/ecr.2018:8:2
Li, Q., Wu, X., Liu, Y., Zhang, M., Bai, X., and Chen, C. (2017). The Effect of Anagliptin on Intimal Hyperplasia of Rat Carotid Artery after Balloon Injury. Mol. Med. Rep. 16 (6), 8003–8010. doi:10.3892/mmr.2017.7667
Li, X. N., Song, J., Zhang, L., LeMaire, S. A., Hou, X., Zhang, C., et al. (2009). Activation of the AMPK-FOXO3 Pathway Reduces Fatty Acid-Induced Increase in Intracellular Reactive Oxygen Species by Upregulating Thioredoxin. Diabetes 58 (10), 2246–2257. doi:10.2337/db08-1512
Lindqvist, A., Nilsson, B. O., Ekblad, E., and Hellstrand, P. (2001). Platelet-derived Growth Factor Receptors Expressed in Response to Injury of Differentiated Vascular Smooth Muscle In Vitro: Effects on Ca2+ and Growth Signals. Acta Physiol. Scand. 173 (2), 175–184. doi:10.1046/j.1365-201x.2001.00873.x
Liu, D., Huang, Y., Bu, D., Liu, A. D., Holmberg, L., Jia, Y., et al. (2014). Sulfur Dioxide Inhibits Vascular Smooth Muscle Cell Proliferation via Suppressing the Erk/MAP Kinase Pathway Mediated by cAMP/PKA Signaling. Cel Death Dis. 5. doi:10.1038/cddis.2014.229
Loscalzo, J., and Jin, R. C. (2010). Vascular Nitric Oxide: Formation and Function. J. Blood Med. 2010 (1), 147–162. doi:10.2147/JBM.S7000
Luo, Q., Liu, X., Li, Z., Huang, C., Zhang, W., Meng, J., et al. (2014). Degradation Model of Bioabsorbable Cardiovascular Stents. PLoS ONE 9 (11), 1–9. doi:10.1371/journal.pone.0110278
Man, R. C., Sulaiman, N., Ishak, M. F., Idrus, R. B. H., Rahman, M. R. A., and Yazid, M. D. (2020). The Effects of Pro-inflammatory and Anti-inflammatory Agents for the Suppression of Intimal Hyperplasia: An Evidence-Based Review. Int. J. Environ. Res. Public Health 17 (21), 1–22. doi:10.3390/ijerph17217825
Marcantoni, E., Di Francesco, L., Dovizio, M., Bruno, A., and Patrignani, P. (2012). Novel Insights into the Vasoprotective Role of Heme Oxygenase-1. Int. J. Hypertens. 2012, 127910. doi:10.1155/2012/127910
Martinon, F. (2010). Signaling by ROS Drives Inflammasome Activation. Eur. J. Immunol. 40 (3), 616–619. doi:10.1002/eji.200940168
Michel, J. B., Li, Z., and Lacolley, P. (2012). Smooth Muscle Cells and Vascular Diseases. Cardiovasc. Res. 95 (2), 135–137. doi:10.1093/cvr/cvs172
Mylonaki, I., Allain, E., Strano, F., Allémann, E., Corpataux, J. M., Meda, P., et al. (2018). Evaluating Intimal Hyperplasia under Clinical Conditions. Interactive Cardiovasc. Thorac. Surg. 27 (3), 427–436. doi:10.1093/icvts/ivy101
Nakbi, A., Dabbou, S., Champion, S., Fouchier, F., Mehri, S., Attia, N., et al. (2011). Modulation of the Superoxide Anion Production and MMP-9 Expression in PMA Stimulated THP-1 Cells by Olive Oil Minor Components: Tyrosol and Hydroxytyrosol. Food Res. Int. 44 (2), 575–581. doi:10.1016/j.foodres.2010.12.010
Napolitano, A., De Lucia, M., Panzella, L., and d’Ischia, M. (2010). “The Chemistry of Tyrosol and Hydroxytyrosol: Implications for Oxidative Stress,” in Olives and Olive Oil in Health and Disease Prevention, 1225–1232. doi:10.1016/B978-0-12-374420-3.00134-0
Nedeljkovic, Z. S., Gokce, N., and Loscalzo, J. (2003). Mechanisms of Oxidative Stress and Vascular Dysfunction. Postgrad. Med. J. 79 (930), 195–200. doi:10.1136/pmj.79.930.195
Nemzer, B. V., Yashin, A. Y., Vedenin, A. N., Yashin, Y. I., Yashunsky, D. V., Nifantiev, N. E., et al. (2019). Selected Powerful Natural Antioxidants: Structure, Food Sources, Antioxidant Activities, and Important Health Benefits. J. Food Res. 8 (1), 60. doi:10.5539/jfr.v8n1p60
Ng, S. F., Tan, L. S., and Buang, F. (2017). Transdermal Anti-inflammatory Activity of Bilayer Film Containing Olive Compound Hydroxytyrosol: Physical Assessment, In Vivo Dermal Safety and Efficacy Study in Freund’s Adjuvant-Induced Arthritic Rat Model. Drug Dev. Ind. Pharm. 43 (1), 108–119. doi:10.1080/03639045.2016.1224893
Norton, K. A., and Popel, A. S. (2016). Effects of Endothelial Cell Proliferation and Migration Rates in a Computational Model of Sprouting Angiogenesis. Scientific Rep. 6, 1–10. doi:10.1038/srep36992
Olmos, Y., Valle, I., Borniquel, S., Tierrez, A., Soria, E., Lamas, S., et al. (2009). Mutual Dependence of Foxo3a and PGC-1α in the Induction of Oxidative Stress Genes. J. Biol. Chem. 284 (21), 14476–14484. doi:10.1074/jbc.m807397200
Pamukcu, B., Lip, G. Y. H., and Shantsila, E. (2011). The Nuclear Factor - Kappa B Pathway in Atherosclerosis: A Potential Therapeutic Target for Atherothrombotic Vascular Disease. Thromb. Res. 128 (2), 117–123. doi:10.1016/j.thromres.2011.03.025
Pi, Y., Zhang, L. L., Li, B. H., Guo, L., Cao, X. J., Gao, C. Y., et al. (2013). Inhibition of Reactive Oxygen Species Generation Attenuates TLR4-Mediated Proinflammatory and Proliferative Phenotype of Vascular Smooth Muscle Cells. Lab. Invest. 93 (8), 880–887. doi:10.1038/labinvest.2013.79
Pober, J. S., and Sessa, W. C. (2007). Evolving Functions of Endothelial Cells in Inflammation. Nat. Rev. Immunol. 7 (10), 803–815. doi:10.1038/nri2171
Quirós-Fernández, R., López-Plaza, B., Bermejo, L. M., Palma-Milla, S., and Gómez-Candela, C. (2019). Supplementation with Hydroxytyrosol and Punicalagin Improves Early Atherosclerosis Markers Involved in the Asymptomatic Phase of Atherosclerosis in the Adult Population: A Randomized, Placebo-Controlled, Crossover Trial. Nutrients 11 (3), 1–16. doi:10.3390/nu11030640
Raffetto, J. D., and Khalil, R. A. (2008). Matrix Metalloproteinases and Their Inhibitors in Vascular Remodeling and Vascular Disease. Biochem. Pharmacol. 75 (2), 347–359. doi:10.1016/j.bcp.2007.07.004
Regazzoli, D., Leone, P. P., Colombo, A., and Latib, A. (2017). New Generation Bioresorbable Scaffold Technologies: An Update on Novel Devices and Clinical Results. J. Thorac. Dis. 9 (Suppl. 9), S979–S985. doi:10.21037/jtd.2017.07.104
Reglero-Real, N., Colom, B., Bodkin, J. V., and Nourshargh, S. (2016). Endothelial Cell Junctional Adhesion Molecules. Arteriosclerosis, Thromb. Vasc. Biol. 36 (10), 2048–2057. doi:10.1161/atvbaha.116.307610
Rodríguez-Morató, J., Boronat, A., Kotronoulas, A., Pujadas, M., Pastor, A., Olesti, E., et al. (2016). Metabolic Disposition and Biological Significance of Simple Phenols of Dietary Origin: Hydroxytyrosol and Tyrosol. Drug Metab. Rev. 48 (2), 218–236. doi:10.1080/03602532.2016.1179754
Romagnolo, D. F., and Selmin, O. I. (2017). Mediterranean Diet and Prevention of Chronic Diseases. Nutr. Today 52 (5), 208–222. doi:10.1097/nt.0000000000000228
Rubió, L., Farràs, M., de La Torre, R., Macià, A., Romero, M. P., Valls, R. M., et al. (2014). Metabolite Profiling of Olive Oil and Thyme Phenols after a Sustained Intake of Two Phenol-Enriched Olive Oils by Humans: Identification of Compliance Markers. Food Res. Int. 65 59–68. doi:10.1016/j.foodres.2014.05.009
Sandoo, A., Veldhuijzen van Zanten, J. J. C. ., Metsios, G. S., Carroll, D., and Kitas, G. D. (2010). The Endothelium and its Role in Regulating Vascular Tone. Open Cardiovasc. Med. J. 4 (1), 302–312. doi:10.2174/1874192401004010302
Scoditti, E., Calabriso, N., Massaro, M., Pellegrino, M., Storelli, C., Martines, G., et al. (2012). Mediterranean Diet Polyphenols Reduce Inflammatory Angiogenesis through MMP-9 and COX-2 Inhibition in Human Vascular Endothelial Cells: A Potentially Protective Mechanism in Atherosclerotic Vascular Disease and Cancer. Arch. Biochem. Biophys. 527 (2), 81–89. doi:10.1016/j.abb.2012.05.003
Scoditti, E., Nestola, A., Massaro, M., Calabriso, N., Storelli, C., De Caterina, R., et al. (2014). Hydroxytyrosol Suppresses MMP-9 and COX-2 Activity and Expression in Activated Human Monocytes via PKCα and PKCβ1 Inhibition. Atherosclerosis 232 (1), 17–24. doi:10.1016/j.atherosclerosis.2013.10.017
Soler-Cantero, A., Jové, M., Cacabelos, D., Boada, J., Naudí, A., Romero, M. P., et al. (2012). Plant-Derived Phenolics Inhibit the Accrual of Structurally Characterised Protein and Lipid Oxidative Modifications. PLoS ONE 7 (8), e43308. doi:10.1371/journal.pone.0043308
Stettler, C., Wandel, S., Allemann, S., Kastrati, A., Morice, M. C., Schömig, A., et al. (2007). Outcomes Associated with Drug-Eluting and Bare-Metal Stents: a Collaborative Network Meta- Analysis. Lancet 370 (9591), 937–948. doi:10.1016/s0140-6736(07)61444-5
Stone, G. W., Moses, J. W., Ellis, S. G., Schofer, J., Dawkins, K. D., Morice, M.-C., et al. (2007). Safety and Efficacy of Sirolimus- and Paclitaxel-Eluting Coronary Stents. New Engl. J. Med. 356 (10), 998–1008. doi:10.1056/nejmoa067193
Storniolo, C. E., Sacanella, I., Mitjavila, M. T., Lamuela-Raventos, R. M., and Moreno, J. J. (2019). Bioactive Compounds of Cooked Tomato Sauce Modulate Oxidative Stress and Arachidonic Acid Cascade Induced by Oxidized LDL in Macrophage Cultures. Nutrients 11 (8), 1880. doi:10.3390/nu11081880
Sun, L., Zhao, R., Zhang, L., Zhang, T., Xin, W., Lan, X., et al. (2012). Salvianolic acid A inhibits PDGF-BB induced vascular smooth muscle cell migration and proliferation while does not constrain endothelial cell proliferation and nitric oxide biosynthesis. Molecules. 17 (3), 3333–3347.
Tagliafierro, L., Officioso, A., Sorbo, S., Basile, A., and Manna, C. (2015). The Protective Role of Olive Oil Hydroxytyrosol against Oxidative Alterations Induced by Mercury in Human Erythrocytes. Food Chem. Toxicol. 82, 59–63. doi:10.1016/j.fct.2015.04.029
Tejada, S., Pinya, S., Mar Bibiloni, M. del., Tur, J. A., Pons, A., and Sureda, A. (2016). Cardioprotective Effects of the Polyphenol Hydroxytyrosol from Olive Oil. Curr. Drug Targets 18 (13), 1477–1486. doi:10.2174/1389450117666161005150650
Terzuoli, E., Nannelli, G., Giachetti, A., Morbidelli, L., Ziche, M., and Donnini, S. (2020). Targeting endothelial‐to‐mesenchymal transition: the protective role of hydroxytyrosol sulfate metabolite. Eur. J. Nutrit. 59. doi:10.1007/s00394-019-01920-x
Thompson, A. M., Martin, K. A., and Rzucidlo, E. M. (2014). Resveratrol Induces Vascular Smooth Muscle Cell Differentiation through Stimulation of SirT1 and AMPK. PLoS ONE 9 (1), 1–10. doi:10.1371/journal.pone.0085495
Tolva, V., Mazzola, S., Zerbi, P., Casana, R., Albertini, M., Calvillo, L., et al. (2016). A Successful Experimental Model for Intimal Hyperplasia Prevention Using a Resveratrol- Delivering Balloon. J. Vasc. Surg. 63 (3), 788–794. doi:10.1016/j.jvs.2014.09.035
Torul, H., Küçükboyaci, N., Tamer, U., and Karasu, Ç. (2020). Evaluation of Phenolic Compounds and Protective Effects of Olive (Olea Europaea L.) Leaf Extracts on Endothelial Cells against Hydrogen Peroxide-Induced Toxicity. J. Res. Pharm. 24 (4), 497–507. doi:10.35333/jrp.2020.198
Tousoulis, D., Kampoli, A.-M., Tentolouris Nikolaos Papageorgiou, C., and Stefanadis, C. (2011). The Role of Nitric Oxide on Endothelial Function. Curr. Vasc. Pharmacol. 10 (1), 4–18. doi:10.2174/157016112798829760
Tuso, P., Stoll, S. R., and William, W. Li. (2015). “A Plant-Based Diet, Atherogenesis, and Coronary Artery Disease Prevention,” in Ethnicity, 19, 62–67. doi:10.7812/TPP/14-0361
Urban, P., Mehran, R., Colleran, R., Angiolillo, D. J., Byrne, R. A., Capodanno, D., et al. (2019). Defining High Bleeding Risk in Patients Undergoing Percutaneous Coronary Intervention. Circulation 140 (3), 240–261. doi:10.1161/circulationaha.119.040167
Vilaplana-Pérez, C., Auñón, D., García-Flores, L. A., and Gil-Izquierdo, A. (2014). Hydroxytyrosol and Potential Uses in Cardiovascular Diseases, Cancer, and AIDS. Front. Nutr. 1, 1–11. doi:10.3389/fnut.2014.00018
Wadey, K., Lopes, J., Bendeck, M., and George, S. (2018). Role of Smooth Muscle Cells in Coronary Artery Bypass Grafting Failure. Cardiovasc. Res. 114 (4), 601–610. doi:10.1093/cvr/cvy021
Wang, W., Jing, T., Yang, X., He, Y., Wang, B., Xiao, Y., et al. (2018). Hydroxytyrosol Regulates the Autophagy of Vascular Adventitial Fibroblasts through the SIRT1-Mediated Signaling Pathway. Can. J. Physiol. Pharmacol. 96, 88–96. doi:10.1139/cjpp-2016-0676
Wei, X., Fang, Z., Sheng, J., Wang, Y., and Lu, P. (2020). Honokiol-mesoporous Silica Nanoparticles Inhibit Vascular Restenosis via the Suppression of TGF-β Signaling Pathway. Int. J. Nanomedicine 15, 5239–5252. doi:10.2147/ijn.s250911
Widmer, R. J., Flammer, A. J., Lerman, L. O., and Lerman, A. (2015). The Mediterranean diet, its components, and cardiovascular disease. Am. J. Med. 128 (3), 229–238. doi:10.1016/j.amjmed.2014.10.014
Xu, K., Al-Ani, M. K., Pan, X., Chi, Q., Dong, N., and Qiu, X. (2018). Plant-derived Products for Treatment of Vascular Intima Hyperplasia Selectively Inhibit Vascular Smooth Muscle Cell Functions. Evidence-Based Complement. Altern. Med. 2018, 3549312. doi:10.1155/2018/3549312
Yang, X., Li, Y., Li, Y., Ren, X., Zhang, X., Hu, D., et al. (2017). Oxidative Stress-Mediated Atherosclerosis: Mechanisms and Therapies. Front. Physiol. 8, 1–16. doi:10.3389/fphys.2017.00600
Yao, F., Yang, G., Xian, Y., Wang, G., Zheng, Z., Jin, Z., et al. (2019). The Protective Effect of Hydroxytyrosol Acetate against Inflammation of Vascular Endothelial Cells Partly through the SIRT6-Mediated PKM2 Signaling Pathway. Food Funct. 10 (9), 5789–5803. doi:10.1039/c9fo00586b
Yurdagul, A., Kleinedler, J. J., McInnis, M. C., Khandelwal, A. R., Spence, A. L., Orr, A. W., et al. (2014). Resveratrol Promotes Endothelial Cell Wound Healing under Laminar Shear Stress through an Estrogen Receptor-α-dependent Pathway. Am. J. Physiol. - Heart Circulatory Physiol. 306 (6), 797–806. doi:10.1152/ajpheart.00892.2013
Zhan, Y., Kim, S., Izumi, Y., Izumiya, Y., Nakao, T., Miyazaki, H., et al. (2003). Role of JNK, P38, and ERK in Platelet-Derived Growth Factor-Induced Vascular Proliferation, Migration, and Gene Expression. Arteriosclerosis, Thromb. Vasc. Biol. 23 (5), 795–801. doi:10.1161/01.ATV.0000066132.32063.F2
Zhao, Y., Vanhoutte, P. M., and Leung, S. W. S. (2015). Vascular Nitric Oxide: Beyond eNOS. J. Pharmacol. Sci. 129 (2), 83–94. doi:10.1016/j.jphs.2015.09.002
Zrelli, H., Kusunoki, M., and Miyazaki, H. (2015). Role of Hydroxytyrosol-dependent Regulation of HO-1 Expression in Promoting Wound Healing of Vascular Endothelial Cells via Nrf2 De Novo Synthesis and Stabilization. Phytotherapy Res. 29 (7), 1011–1018. doi:10.1002/ptr.5339
Zrelli, H., Matsuka, M., Araki, M., Zarrouk, M., and Miyazaki, H. (2011a). Hydroxytyrosol Induces Vascular Smooth Muscle Cells Apoptosis through No Production and PP2A Activation with Subsequent Inactivation of Akt. Planta Med. 77 (15), 1680–1686. doi:10.1055/s-0030-1271073
Zrelli, H., Matsuoka, M., Kitazaki, S., Zarrouk, M., and Miyazaki, H. (2011b). Hydroxytyrosol Reduces Intracellular Reactive Oxygen Species Levels in Vascular Endothelial Cells by Upregulating Catalase Expression through the AMPK-FOXO3a Pathway. Eur. J. Pharmacol. 660 (2–3), 275–282. doi:10.1016/j.ejphar.2011.03.045
Zrelli, H., Wei Wu, C., Zghonda, N., Shimizu, H., and Miyazaki, H. (2013). Combined Treatment of Hydroxytyrosol with Carbon Monoxide-Releasing Molecule-2 Prevents TNF α -induced Vascular Endothelial Cell Dysfunction through No Production with Subsequent NF B Inactivation. Biomed. Res. Int. 2013, 912431. doi:10.1155/2013/912431
Keywords: hydroxytyrosol, intimal hyperplasia, neointimal hyperplasia, smooth muscle cell, endothelial cell
Citation: Vijakumaran U, Yazid MD, Hj Idrus RB, Abdul Rahman MR and Sulaiman N (2021) Molecular Action of Hydroxytyrosol in Attenuation of Intimal Hyperplasia: A Scoping Review. Front. Pharmacol. 12:663266. doi: 10.3389/fphar.2021.663266
Received: 02 February 2021; Accepted: 29 April 2021;
Published: 21 May 2021.
Edited by:
Masliza Mahmod, University of Oxford, United KingdomReviewed by:
Jian Yang, The First People's Hospital of Yichang, ChinaCopyright © 2021 Vijakumaran, Yazid, Hj Idrus, Abdul Rahman and Sulaiman. This is an open-access article distributed under the terms of the Creative Commons Attribution License (CC BY). The use, distribution or reproduction in other forums is permitted, provided the original author(s) and the copyright owner(s) are credited and that the original publication in this journal is cited, in accordance with accepted academic practice. No use, distribution or reproduction is permitted which does not comply with these terms.
*Correspondence: Nadiah Sulaiman, bmFkaWFoc3VsYWltYW5AdWttLmVkdS5teQ==
Disclaimer: All claims expressed in this article are solely those of the authors and do not necessarily represent those of their affiliated organizations, or those of the publisher, the editors and the reviewers. Any product that may be evaluated in this article or claim that may be made by its manufacturer is not guaranteed or endorsed by the publisher.
Research integrity at Frontiers
Learn more about the work of our research integrity team to safeguard the quality of each article we publish.