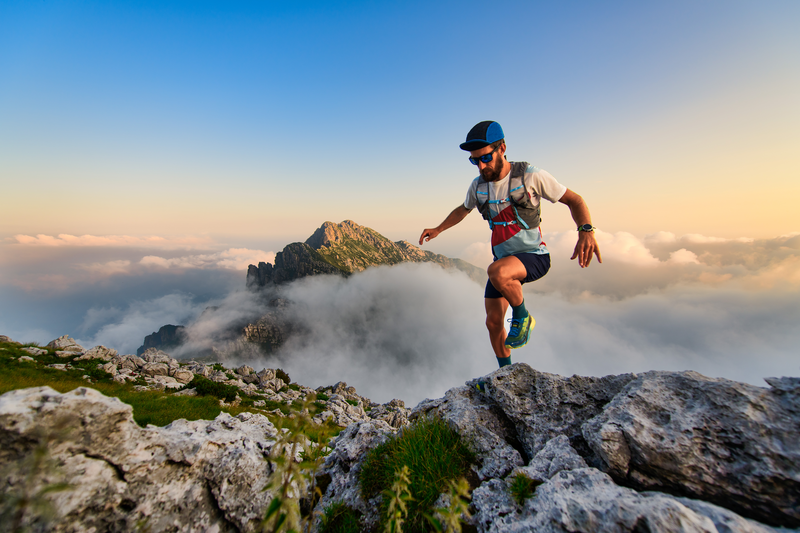
94% of researchers rate our articles as excellent or good
Learn more about the work of our research integrity team to safeguard the quality of each article we publish.
Find out more
REVIEW article
Front. Pharmacol. , 13 April 2021
Sec. Ethnopharmacology
Volume 12 - 2021 | https://doi.org/10.3389/fphar.2021.660083
Allergic rhinitis (AR) is a common inflammatory condition of the nasal mucosa and it is an immunoglobulin E–mediated disease. The incidence and prevalence of AR globally have been escalating over recent years. Antihistamines, intranasal corticosteroids, decongestants, intranasal anticholinergics, intranasal cromolyn, leukotriene receptor antagonists and immunotherapy have been used in the treatment of AR. However, there is a need to search for more effective and safer remedies as many of the current treatments have reported side effects. Medicinal plants have been used traditionally to relief symptoms of AR but their efficacy and safety have not been scientifically proven. In this review, up-to-date reports of studies on the anti-allergic rhinitis of several medicinal plants and their bioactive metabolites through suppression of the immune system are compiled and critically analyzed. The plant samples were reported to suppress the productions of immunoglobulin E, cytokines and eosinophils and inhibit histamine release. The suppression of cytokines production was found to be the main mechanistic effect of the plants to give symptomatic relief. The prospect of these medicinal plants as sources of lead molecules for development of therapeutic agents to treat AR is highlighted. Several bioactive metabolites of the plants including shikonin, okicamelliaside, warifteine, methylwarifteine, luteolin-7-O-rutinoside, tussilagone, petasin, and mangiferin have been identified as potential candidates for development into anti-allergic rhinitis agents. The data collection was mainly from English language articles published in journals, or studies from EBSCOHOST, Medline and Ovid, Scopus, Springer, and Google Scholar databases from the year 1985–2020. The terms or keywords used to find relevant studies were allergic rhinitis OR pollinosis OR hay fever, AND medicinal plant OR single plant OR single herb OR phytotherapy. This comprehensive review serves as a useful resource for medicinal plants with anti-allergic rhinitis potential, understanding the underlying mechanisms of action and for future exploration to find natural product candidates in the development of novel anti-allergic rhinitis agents.
Allergic rhinitis (AR) is one of the common allergic diseases affecting 30% of the world population (Tohidinik et al., 2019). It is also known as hay fever, typically causes symptoms of runny nose, clear nasal discharge, sneezing nasal pruritus and airflow obstruction caused by immunoglobulin E (IgE)-mediated reactions. AR is triggered by potential allergens and involves mucosal inflammation driven by type 2 helper T (Th2) cells (Wheatley and Togias, 2015). AR is one of the types of allergy that manifest an abnormal regulation of the immune system. Epidemiological studies have revealed that the prevalence of AR has increased progressively in more developed countries, and currently affects 10–40% of adults and 2–25% of children (Zhang and Zhang, 2019). It is one of the common types of allergic condition which might not appear to be serious as it is not associated with severe morbidity and mortality but affects the quality of life. The allergic disease may also substantially cause an economic burden to the patient due to inadequate management of the allergic disease (Zuberbier et al., 2014). Thus, the needs on medication and alternative therapy to alleviate the symptoms are very crucial. There are some diseases particularly allergic reactions that have been found to be associated with AR. The prevalence of AR has shown an increasing pattern in a similar fashion to that of asthma (Asher et al., 2006; Tohidinik et al., 2019). It was found that eczema in infancy might have a causal effect on AR in children with and perhaps without asthma (Hopper et al., 2012). There is a close epidemiologic relationship of allergic conjunctivitis with AR (Bielory, 2010). Genetic factor might be one of the etiologies of AR as a study has shown that having a parent with AR may double the risk (Westman et al., 2013). Seeing that it might be leading to development of other diseases, this health issue requires great involvement of patients and healthcare professionals.
The pathophysiology of AR disease is quite complex as it involves several chemical mediators or antibodies in immunological cascades. Therefore, these different mediators or antibodies involved in the pathophysiology of the disease might be the potential targets in treating AR. It is very crucial to understand the pathophysiology of this disease in developing a potential remedy and alternative therapy for a wide population of AR patients. Avoidance of potential allergens that might trigger an allergic reaction is the best way to prevent AR occurrence (Okubo et al., 2017). Allergens that could trigger the symptoms in AR include animal dander, dust mite, seafood as well as cigarette smoke. Heavy maternal cigarette smoking may increase the risk of having this disease in children in the first year of life (Skoner, 2001). Treating the symptoms by using conventional or alternative therapy may be efficient but it is important to identify the triggers or allergens to avoid repetitive occurrence. It may be difficult to avoid the potential allergen exposure and therefore the use of medicine is needed to provide fast relief. The use of conventional medicine has an increasing trend as the number of people having this disease has also increased.
Current treatment to treat symptoms of AR include the use of antihistamines, intranasal corticosteroids, decongestants, intranasal cromolyn, intranasal anticholinergics, leukotriene receptor antagonists and immunotherapy. Antihistamines inhibit receptor activity, and some can additionally stabilize the H1-receptor inverse agonists or mast cells (Leurs et al., 2002; Bousquet et al., 2008). The use of first-generation antihistamines has reported several side effects such as mental impairment, sedation and anticholinergic side effects (Bousquet et al., 2008). Antihistamine monotherapy has been reported to provide satisfactory relief of symptoms in some patients. However, for the relief of nasal congestion, antihistamines use is less effective and usually need to be combined with a decongestant or intranasal corticosteroid (May and Dolen, 2017). Intranasal corticosteroids decrease the inflammatory cytokines and mediators release, which help to reduce nasal mucosal inflammation that can reach maximum benefit within two weeks (Weiner et al., 1998). Corticosteroids administered by any route, including the intranasal route can cause adverse effects on the eyes, bones, the hypothalamic-pituitary-adrenal axis, and also local side effects such as candidiasis (Bielory, 2010). The side effects of the use of conventional medicines have led to many studies to find alternative ways to help to reduce the symptoms of this disease. Some natural products such as garlic and Andrographis paniculata (Burm.f.) Nees have been used to provide symptom relief for nasal congestion which is also one of the symptoms in AR. Various treatment options are targeting different key components to help to relieve AR symptoms. However, the scientific or evidence-based research on the efficacy and safety of the plants have not yet been proven in many studies upon AR models or subjects. Therefore, many studies have been conducted in search of medicinal plants that have a promising potential of being a better alternative to provide symptoms relief in AR.
The search for new therapeutic agents from medicinal plants to treat AR is definitely needed especially plants which have shown positive outcomes in exerting anti-allergic properties, particularly toward AR animal models. Medicinal plants and their bioactive metabolites possess different mechanisms of action in combating the mediators or immune system involved in AR inflammatory cascades or allergic reaction pathways. Some of these phytochemicals have anti-allergic properties as well as anti-inflammatory properties which may be useful to treat different types of allergic diseases and symptoms. Numerous studies have been performed to determine the anti-inflammatory and anti-allergic properties of medicinal plants particularly in treating AR through in vivo studies and clinical trials by using AR-induced model or AR patients, respectively. There are numerous number of mediators involved in the pathophysiology of AR. These different targeted cells or mediators are important key components for different types of phytochemicals found in medicinal plants. AR is one of the types of allergy that manifest an abnormal regulation of the immune system.
The databases employed for data collection are mainly from EBSCOHOST, Medline and Ovid, Scopus, Springer, and Google Scholar databases from 1985 to 2020. The keywords used during searching include allergic rhinitis OR pollinosis OR hay fever, AND medicinal plant OR single plant OR single herb OR phytotherapy. Studies included in this mechanistic review were in vivo, in vitro, and clinical studies on medicinal plants that showed significant suppressive effect on AR. The sources of medicinal plants were verified, and only studies on single medicinal plant were considered. Table 1 shows a list of medicinal plants and phytochemicals which exhibited anti-allergic rhinitis effect in vivo and in vitro studies while Table 2 is a list of plants that have been investigated for anti-allergic rhinitis activity in clinical trials. Studies that were excluded from the searching and data extraction were polyherbal formulations, used as an adjunct therapy or as supplementation only and studies that were not using AR-induced model in animal studies or AR patients for its clinical studies. In addition, toxicological data on the medicinal plants that showed strong anti-allergic rhinitis effect were also gathered to discuss their safety levels for possible use in treating AR.
RA is a type 1 hypersensitivity reaction which occurs when there are specific triggers from allergens’ exposure including pests, dust mites, some molds and pets. This will bring about the subsequent flow of biochemical and immunological activities leading to the clinical manifestation of the disease. Exposure to allergens may induce IgE antibodies to bind to the high-affinity receptor (FcεRI) on the surface of mast cells, basophils, and antigen-presenting cells and causes sensitization (Gould and Sutton, 2008). The most common symptoms in AR are sneezing, pruritus, and reflex secretory responses. These might be due to the release of histamine which activates H1 and H2 receptors on mucosal blood vessels and H1 receptors on sensory nerve endings leading to vascular engorgement (Togias, 2003). IgE expression and histamine release are the important key features in its pathophysiology as they occur during the early phase of the immunological pathway (Okubo et al., 2017). Pathways involved in the pathophysiology of AR will eventually lead to the manifestations of various common symptoms in AR triggered by the allergen in which the patient is sensitive. Understanding the pathophysiology of AR might in a way help to explain the pathways involved in eliciting the symptoms and thus explained the rational treatment approach indicated.
Nasal responsiveness occurs with regards to our body normal function or termed as homeostasis in which the body reacts toward an occurrence of abnormal changes with normal response and intensity. Hyperresponsiveness of AR disease might be due to alteration toward the normal responsiveness as a result of genetical or pathological factors affecting the structural or functional elements of the nasal mucosa (Sin and Togias, 2011). AR begins with the allergen sensitization as showed in Figure 1 which leads to the obvious clinical features present in AR patients. Sensitization is a process by which the immune system will produce the antibody or more specifically the IgE antibody, in response to certain types of particles or allergens it considered abnormal. The process begins in the nasal tissues where allergens will be engulfed by antigen-presenting cells (APCs), broken into allergenic peptides and migrate to lymph nodes (Godthelp et al., 1996).
APCs such as immature dendritic cells (DC) capture allergens, mature, and migrate to the draining lymph nodes. The processed allergen will then be presented to naive T cells (Eifan and Durham, 2016). Activation of epithelial cells simultaneously by non-antigenic pathways such as proteases may lead to epithelial cytokines release of which polarizes the action into Th2 response by inducing the expression of genes that promote Th2 survival (Georas et al., 2005). Therefore, Th2 cytokine inhibitors such as suplatast tosilate can be used in managing mild symptoms induced by Th2 response (Tomaki et al., 2000). The release of interleukin-4 (IL-4) may generate Th2 cell production that leads to B-cells activation (Shirakawa et al., 2000). It will become allergen-specific IgE-producing plasma cells that will then bind to mast cells and basophils. This occurrence will eventually lead to repeated AR events as the IgE binding to mast cell and basophils will recognize the presence of allergens invading the body when re-exposure occurred (Wheatley and Togias, 2015).
DC form a network that is localized within the submucosa and epithelium of the entire respiratory mucosa which as well includes the nasal mucosa (Hartmann et al., 2006). In AR patients, there is an elevation in the levels of both T-cells and DC (Sin and Togias, 2011). Processed antigens and the tissue microenvironment may send signals to DC that will then polarize naive T cells into either Th1 or Th2 cells and their respective cytokines (Liu, 2005). The epithelial cells release thymic stromal lymphopoietin and prostaglandin E2 which may also send signals to DC and cause such polarization (Bharadwaj et al., 2007). In both nonallergic and allergic individuals there are actually different proportions of allergen specific IL-10–producing Tr1 cells, IL-4–producing effector T cells and CD251 Tregs (Akdis et al., 2004; Maggi et al., 2007). Clinical allergy will develop based on the balance between certain Treg populations and Th2. Cytokines such as IL-3 and IL-4 provides the first essential signal that causes IgE production via B cells activation. Besides, these cytokines may as well induce clonal expansion in a case of IgE expressing memory B cells. In the second signal, costimulatory interaction between CD40 ligand on both B-cell surface and T-cell surface will eventually promote activation of B-cell and switch recombination for the production of IgE (Geha, 1992). A cascade of events including early and late phase responses may be triggered by re-exposure to allergens, leading to symptoms of AR characterized by sudden episodes of nasal itching, sneezing, rhinorrhea and nasal congestion (Sih and Mion, 2010). In the late-phase response, fatigue, congestion, malaise, and irritability occur at 6–24 h after exposure to an allergen that involves the influx of inflammatory cells into the nasal mucosa (Rondón et al., 2009; Haenuki et al., 2012). The pathophysiology of AR is diagrammatically presented in Figure 1.
Medicinal plants and their bioactive metabolites possess different mechanisms of action in combating the mediators of immune system involved in AR inflammatory cascades or allergic reaction pathways. Some of these phytochemicals have anti-allergic properties as well as anti-inflammatory properties which may be useful to treat different types of allergic diseases and symptoms. Several cellular, animal and clinical studies have been performed to evaluate the anti-allergic and anti-inflammatory properties of medicinal plants particularly in treating AR by using AR-induced model or AR patients. There are numerous number of mediators involved in the pathophysiology of AR. These different targeted cells or mediators are important key components for different types of phytochemicals found in many different medicinal plants. The phytochemicals may be effectively acting against the important key components involved and thus alleviating the symptoms in the patient with AR. Anti-allergic rhinitis effects of medicinal plants and their bioactive metabolites may be by suppression of the immune system through suppression of IgE, inhibition of cytokines production, inhibition of histamine release and suppression of eosinophil production.
IgE is identified as the key molecule that triggers type 1 hypersensitivity reactions such as atopic dermatitis and AR (Platts-Mills, 2001; Johansson et al., 2004). Production of IgE antibodies may be enhanced by the interleukins, particularly IL-4 and IL-3, produced subsequently after the activation of the Th2 cells. This will eventually increase the regulation of eosinophil functions and promote the growth of mast cells (Busse et al., 1995). IgE is an important potential key component targeted at the early stage of hypersensitive reaction as it leads to the activation of other chemical or immunological cascades in later stages. These antibodies, in turn, attached to specific receptors on other resident cells by binding to high-affinity (cεRI) surface receptors on basophils, mast cells or CD23 on B lymphocytes and eosinophils (Presta et al., 1994). IgE has a short half-life and thus having lower concentration compared to other immunoglobulins in the circulation. However, it is extremely bioactive as it binds to high-affinity receptors on the surface of basophils and mast cells. The basophils and mast cells may be highly sensitive to allergens even when the concentration of IgE is very low in the circulation. IgE production and mast cell degranulation is the significant type I allergic responses which cause the subsequent release of histamine and other mediators in an allergic reaction. Following the early phase of allergic reactions, cytokines release including IL-4, IL-5, IL-6, and TNF-α, enhances IgE synthesis. In contrast, IFN-γ, IFN-α and transforming factor (TGF)-β might also inhibit IgE production (Ayoub et al., 2003).
Several cellular and animal studies have been performed to investigate the suppressive effect of medicinal plants on IgE in AR-induced model. The effect of Bupleurum chinense DC. extract on allergic inflammatory responses was conducted in ovalbumin (OVA)-induced AR mouse model by determining the anti-OVA specific IgE, IgG1 and IgG2a in the serum (Bui et al., 2019c). Results showed that at doses of 100 and 200 mg/kg of B. chinense, there was a significant (<0.05 and <0.01, respectively) suppression of anti-OVA specific IgE in a dose-dependent pattern. The anti-OVA specific IgG1 in serum was also decreased in a dose-dependent manner which resulted in the inhibition of histamine release. This data indicated that oral administration of B. chinense might ameliorate allergic inflammation responses in AR early phase as this particular action mediated by the IgE antibody is the crucial key component that initiates hypersensitivity reaction in AR.
Cinnamomum verum J. Presl bark extract was investigated for its potential effect in suppressing IgE levels in an OVA-induced animal model (Aswar et al., 2015). At 10 and 30 μg/kg concentrations, the plant sample significantly prevented elevation of serum IgE levels as compared with AR control mice (p < 0.01 and <0.001, respectively). In comparison with the effect of IgE suppression by the plant extract, a positive control treated by xylometazoline showed statistically significant (p < 0.001) inhibition of serum IgE level as compared with AR control mice. This study concluded that the extracts from the bark of C. verum showed prophylactic potential against OVA-induced model through suppression of IgE as well as histamine release. This potential medicinal plant might be useful in managing AR. IgE may activate the mast cells via the high-affinity receptor FcεRI. Therefore, prevention of IgE serum elevation by the extract of C. verum indicated stabilization of mast cells as the mechanism of eosinophil recruitment without affecting level of nitric acid.
An in vivo study conducted in OVA-induced AR treated with a methanol extract of Ostericum grosseserratum (Maxim.) Kitag. roots showed significant inhibition of OVA-specific IgE production in the OVA-sensitized mice IgE (p < 0.001) as compared to control mice given OVA alone (Jung et al., 2011). As the release of cytokines IL-4 and IFN-γ has a significant relation with the production of IgE elicited after exposure to an allergen, 100 mg/kg of the methanol extract of O. grosseserratum significantly inhibited IL-4 production (p < 0.001), compared with OVA-sensitized mice, and enhanced IFN-γ production (p < 0.01). These results demonstrated that O. grosseserratum extract could inhibit IgE production by the regulation of IL- 4/IFN-γ ratio in allergic responses.
Shikonin (1), a derivative of naphthoquinone, isolated from the roots of Symphytum officinale L. was evaluated for its curative effect on IgE production during allergic reaction in an OVA-induced AR rat model. The findings indicated that in three shikonin groups (intraperitoneal injection with 200, 400 and 600 μg/kg of shikonin), the OVA-specific IgE and IL-4 serum levels were significantly decreased and the serum IFN-γ level was markedly increased as compared with the model group. In addition, the shikonin groups also decreased the GATA-3 protein expression level and increased the T-bet protein in nasal mucosa tissue. There were increase of GSH-Px and SOD levels but a decrease in the MDA level as compared to the model group (Wang et al., 2017). The findings suggested that shikonin could alleviate AR in the rat model through suppression of IgE level besides its regulation of GATA-3 and T-bet protein expression in nasal mucosa tissue and anti-oxidative stress effects.
Several clinical investigations have been conducted to verify whether the medicinal plants may target IgE, to suppress its release in patients with AR. Most of the clinical studies conducted were mainly focused on observing the relief of AR symptoms upon their administration. Measurement of IgE serum level was not commonly conducted in clinical studies. One of the clinical trials conducted to observe significant changes in IgE serum level was a double-blind and prospective study on the effect of the oil of Nigella sativa L. in 66 AR patients (Nikakhlagh et al., 2011). High level of IgE that was presented in 57.5% of the volunteers (>100 IU/ml) was not always accompanied by allergy symptoms. In fact, severe allergy symptoms have shown in patients with low IgE levels. Allergen-induced rhinitis that causes an up-regulation in the expression of high-affinity receptors reflects more of its true activity rather than the initial concentration of circulating IgE levels in the serum (Yamaguchi et al., 1997). The mean difference of total serum IgE before and after the treatment and placebo groups was statistically significant (p = 0.0708) while the differences in IgE before and after a nasal wash in the N. sativa-treatment and placebo groups were also statistically significant at p = 0.0065 and 0.2800, respectively. However, the average of IgE from the nasal wash in the N. sativa-treatment group before and after the treatment in between both study (p = 0.0017) and placebo group (p = 0.455) was insignificant (Nikakhlagh et al., 2011).
Okicamelliaside (2), an ellagic acid glucoside has been isolated from Camellia japonica L. It is a highly potent anti-degranulation and has potential to suppress allergic reaction in vivo (Onodera et al., 2010). In an in vivo study the compound was investigated for its ability to inhibit AR in male BALB/c mice which were stimulated with a Japanese cedar pollen extract and challenged by nasal instillation of the antigen. Intraperitoneal administration of okicamelliaside at 0.2 mg/kg for 24 days resulted in a decrease in the sneezing frequency during the 10 min immediately after the challenge. Okicamelliaside exhibited 12,000 times potency in suppressing sneezing effect compared to ketotifen fumarate, an anti-allergic drug used as control. The sneezing frequency varied widely between individuals in the control, ketotifen fumarate and okicamelliaside groups. However, there was no significant difference in IgE levels, suggesting that KF and okicamelliaside might exert anti-allergic activity by suppressing degranulation instead (Kuba et al., 2008). Okicamelliaside might have a poor activity against AR model but further investigation is needed to help to understand its anti-allergic effect through different cascades involving different key components particularly in AR.
Previous studies on Cissampelos sympodialis Eichler has led to the discovery of several alkaloids and their bioactivities (Barbosa-Filho et al., 2000). It was discovered that warifteine (3) was the compound responsible for the immunomodulatory and anti-inflammatory effects of the plant on models of inflammation (Costa et al., 2008). Considering the urgency in developing a monotherapy to treat AR, an in vivo study has been conducted to analyze the therapeutic potential of warifteine (3) and methylwarifteine (4) on the combined AR and asthma syndrome model (CARAS) (Cavalcanti et al., 2020). In this study, the treatment with both compounds has shown a significant decrease of OVA-specific IgE at p < 0.01. Nuclear factor-kappa B (NF-κB) activation is related to the production of several inflammatory mediators, including cytokines in the inflamed site while the cytokines such as 1L-4,1L-5, and IL-13 are responsible for the allergic-specific IgE production promoting AR symptoms (Schuliga, 2015). In a similar study, the effect of these two compounds was conducted on NF-κB and both compounds have shown a significant decrease in NF-κB (p65) at p < 0.05 (Cavalcanti et al., 2020). The isolated compounds found in C. sympodialis may have a promising potential as a phytopharmaceutical prototype for allergies but a further study may have to be conducted for its efficacy and safety in a mammal or human model.
Allergen exposure against which they are sensitized, may results in nasal symptoms within minutes as there is cross-linking by the allergen of IgE bound to mucosal mast cells (Wheatley and Togias, 2015). The subsequent cascades are the release of histamine, cysteinyl leukotrienes and prostaglandin D2 (Barnes, 2011). Th2 inflammation develops in the nasal mucosa of the AR patient during the next hours, with the participation of a wide array of cytokines and chemokines. This is due to the complex interaction of mast cells, dendritic cells, eosinophils, T cells, epithelial cells, innate lymphoid cells, as well as the basophils (Barnes, 2011; Sin and Togias, 2011). Histamine irritation of the sensory nerve in the nasal mucosa, transmitted to the sneezing center in the brain particularly the medulla oblongata which then leads to sneezing in AR patients (Okubo et al., 2017). It may also cause plasma leakage through its secretion from the nasal glands or acting directly on the nasal mucosa vessels and leads to watery rhinorrhea (Ichikawa et al., 1991; Okubo et al., 2017). As histamine is one of the most important key components in an early phase of AR pathophysiology and gives immediate symptoms within few minutes of allergen exposure, it has been the potential target in managing AR disease in human. This might provide a fast relieve in a patient with AR stimulated by a certain type of allergens.
In vivo study on wild grape or Ampelopsis glandulosa var. brevipedunculata (Maxim.) Momiy. hot water extract was conducted on phorbol myristate acetate (PMA)-induced or with histamine-induced upregulation of H1 receptor messenger ribonucleic acid (mRNA) expression in human epithelial cells (HeLa) (Islam et al., 2018). Stimulation of HeLa cells with PMA or histamine induced significant and transient increase in H1R mRNA with a maximum of 3 h prior to stimulation (Das et al., 2007). There were significant differences at p < 0.01 in three different doses of wild grape extract (WGE) (10, 20, 30 μg/ml) compared to PMA-induced HeLa. Besides, there were significant differences at p < 0.05; p < 0.01 between 20 and 40 μg/ml of WGE, respectively, compared to histamine-induced HeLa. WGE suppressed histamine signaling through the inhibition of histamine-induced upregulation of H1R gene expression. They reported that PKCδ signaling was involved in the expression of the H1R gene. Western-blot analysis suggested that inhibition of phosphorylation of Tyr311 was the underlying mechanism of the suppressive effect of WGE on the up-regulation of H1R gene expression as Tyr311 was crucial for the activation of PKCδ (Islam et al., 2018). WGE showed a strong activity in inhibiting histamine release conducted against AR-induced model and thus might be helpful in alleviating the symptoms in AR. Clinical studies should be conducted in order to assess its safety and efficacy in a human model.
Extract of Wurfbainia villosa var. xanthioides (Wall. ex Baker) Skornick. and A.D.Poulsen from Zingiberaceae family significantly inhibited systemic allergic reaction and histamine release (Kim et al., 2007; Kim H.-G. et al., 2015). An in vivo study showed significant differences in the reduction of histamine release (p < 0.05) at the dose of 10–1000 mg/kg compared to 48/80-induced rat peritoneal mast cell (RPMC) histamine release. W. villosa extract has shown several actions that were beneficial in alleviating the symptoms in immediate hypersensitivity reaction. The activity of W. villosa in inhibiting histamine release was moderate since there was not so much of significant difference when a higher dose of its extract was used. Apart from its anti-allergic rhinitis effect, the ethyl acetate extract of W. villosa modulated tumor growth factor-β (TGF-β) related signaling, primarily via the Smad2/3 and Smad7 (gene chromosomes) signaling pathways, in bile duct ligation-induced liver fibrosis subject, suggesting that it has antifibrotic effects against cholestatic liver injury (Kim et al., 2019). Other mechanisms suggested that the ethyl acetate extract of W. villosa regulated fibrogenic cytokines, especially TGF-β (Lee et al., 2016).
Hydroalcoholic extract of Cinnamomum verum J. Presl standardized to type-A procynidines polyphenols (CZ-TAPP) was evaluated for its anti-allergic effect at the dose of 3, 10 and 30 μg/kg in OVA-induced AR in BALB/c mice. At 10 and 30 μg/kg treatment the animals showed significant prevention of elevated serum histamine levels as compared with AR control mice (p < 0.01 and p < 0.001, respectively). There were induction of rubbing reflex and sneezing in AR control mice as compared to normal mice upon histamine challenge. These elevation in histamine release may results in increased mucus secretion, vascular permeability, edema and contraction of smooth muscle. The treatment with C. verum extracts significantly reduced these histamine-induced symptoms. CZ-TAPP has showed a strong inhibition in IgE serum and histamine release as compared with AR control mice while showing reduction of symptoms as well such as sneezing and rubbing (Aswar et al., 2015).
OVA sensitization and challenge in an in vivo model led to an increase in histamine and OVA-specific IgE titers in sera, increased IL-4 release in nasal lavage and infiltration of inflammatory cells in the epithelium and sub epithelium of the nasal mucosa (Bahekar et al., 2008). Oral administration of Ostericum grosseserratum (Maxim.) Kitag. methanol extract at doses of 0.5 and 1.0 mg/ml significantly inhibited OVA-specific IgE production in the OVA-sensitized mice (p < 0.001) as compared to control mice given OVA alone (Jung et al., 2011). Based on this study, O. grosseserratum has shown a strong activity in AR-induced model. Another plant that has exhibited histamine suppression effect was Artemisia abrotanum L., prepared as a nasal spray preparation. The use of nasal spray preparation has shown a rapid onset of action and relief of nasal symptoms such as congestion, rhinorrhea, and sneezing within a few minutes of application (Remberg et al., 2004). The overall tolerability in all of the twelve patients dealing with a mild to moderate locally appearing stinging sensation was good although it was reported to happen immediately after applying the nasal spray preparation (Remberg et al., 2004). The histamine action was proved by the reduction of early AR symptoms deemed to be strongly related to the early phase cycle of AR pathophysiology. The flavonoids were likely the important compounds in this species that inhibited the effects mediated by histamine (Bergendorff and Sterner, 1995). This study might have shown a good response on its effect in alleviating the symptoms after its application. However, there was no evidence of histamine inhibition that was clearly explained or well conducted in this particular study.
The 50% ethanol extract of peppermint or Mentha × piperita L. has been reported to inhibit histamine release from peritoneal mast cells of actively sensitized rats (Inoue et al., 2002). The identify the compounds contained in the peppermint extract which leads to such a remarkable result was determined. There were six flavonoid glycosides isolated from the extract and luteolin-7-O-rutinoside 5) was the one that showed a potent inhibitory effect on histamine release from rat peritoneal mast cells. This compound caused a dose-related inhibition of histamine release with a significant effect at 30 and 100 Mµ (p < 0.05 and p < 0.01, respectively) as well as having inhibitory effects on sneezing and nasal rubbing at the dose of 300 mg/kg (p < 0.01). Another study has also been conducted to thoroughly study the structure of this compound and it was found that the catechol structure in the Bring and the C2–C3 double bond in the C ring were essential for the inhibition of the histamine release (Amellal et al., 1985).
Cytokines particularly cysteinyl-leukotrienes were released by mast cells activated by IgE-mediated mechanism in AR (Pawankar and Ra, 1996). In the early phase of IgE-mediated allergic reaction, some types of cytokines such as IL-4 and IL-13 will be released by activated T lymphocytes and will interact with B lymphocytes to induce the synthesis of allergen-specific IgE (Pawankar et al., 2011). Besides histamine, cytokines is also one of the major vasoactive mediators. Some examples of cytokines such as IL-5 and granulocyte/macrophage colony stimulating factor (GM-SCF) may help to overcome programmed cell death of eosinophils by keeping eosinophils alive for several days or even weeks (Plager et al., 1998). Eosinophils synthesize and release cytokines such as IL-3, IL-5 and GM-CSF that play crucial roles in the late phase and on-going allergic inflammation (Yang et al., 1995).
The nasal mucosal tissues of AR has illustrated an elevation in the levels of pro-inflammatory cytokines such as the IL-1, thymic stromal lymphopoietin (TSLP), and TNF-α (Kim H.-Y. et al., 2015). The administration of 10 mg/kg of Allium hookeri Thwaites ethanol extract significantly reduced the increased rubs scores and IgE and IL 4 levels in OVA-sensitized mice with significant values at p < 0.05. Being a specific inducer of IgE, IL 4 modulates a variety of inflammatory mediators release from immune cells, resulting in increased vascular permeability, increased mucus secretion in the nasal mucosa and inflammatory cells infiltration (Chai et al., 2017). Therefore, cytokine IL-4 is an important key component in alleviating AR symptoms through a downstream of its level in AR patients. The methanol extract of A. hookeri at various concentrations of 100, 200, 300 μg/mL has been shown to exhibit anti-inflammatory activity by inhibition of NO and ROS productions (Jang et al., 2017). Besides, it also downregulated NF-κB signaling pathways that consequently reduced proinflammatory mediators. Moreover, it could inhibit adipogenesis by promoting lipolysis by suppressing adipogenic factors expression including controlled amino acid therapy (CAAT)/enhancer binding protein (C/EBP) and lipoprotein lipase (LPL) in 3T3-L1(mouse cell line) adipocytes (Kim et al., 2019).
As discussed earlier, besides decreasing the serum levels of OVA-specific IgE, shikonin (1) was also observed to significantly decreased (p < 0.05) the serum levels of IL-4 and significantly increased (p < 0.05) the serum IFN-γ level (Wang et al., 2017). Previous study has reported that increasing the IFN-γ level and reducing the IL-4 level in the blood could alleviate the pathological lesion of AR in rats (Li et al., 1996). These findings indicated that the mechanism of shikonin attenuated AR may also involved reducing the level of IL-4 and increasing the level of IFN-γ in the body (Wang et al., 2017).
Apart from interleukins, several other types of cytokines that might be involved in the cascades of allergic reaction in AR are interferon-gamma (IFN-ƴ) and retinoid-related orphan receptor gamma t (RORc). An in vivo study investigated the possible chemical mediators or key components involved in the pathophysiology of AR such as Th1 related cytokines as well as Th17 related cytokines (Yang et al., 2008). The experiment conducted on OVA-induced mice showed a significant decrease (p < 0.05) in the level of Th1 related cytokines such as IL-12 when treated with 100 mg/kg/day of Piper nigrum L. extract with a significant increase in IFN-ƴ (p < 0.05). The level of IL-12 showed a significant decrease at p < 0.001 when treated with a higher dose of P. nigrum at 200 mg/kg/day while there was a significant decrease with p< 0.01 in IFN-ƴ level given with the same dose when compared with OVA group. Besides, P. nigrum also showed a significant effect on Th17 related cytokines such as IL-5, IL-13, and RORc. The lowest dose of P. nigrum (50 mg/kg/day) given to the subject has also shown a significant decrease on IL-5 and IL-13 levels (p < 0.01 and p < 0.05, respectively). At the dose of 100 mg/kg/day, P. nigrum has shown a significant decrease in the levels of IL-5 with p < 0.01 and p < 0.05 in IL-13 and RORc levels. There’s a significant decrease at p < 0.001, p < 0.01, p < 0.05 in IL-5, RORc, IL-13 levels respectively compared with the OVA group when 200 mg/kg/ml of P. nigrum was given to the subject. This shows that P. nigrum has a strong activity in reducing IL-5 levels compared to other types of cytokines at 100 mg/kg/day. P. nigrum may provide a promising strategy for immunotherapy in airway diseases such as AR as it exhibits several different mechanisms that act against the cascades in AR model to alleviate the symptoms present through a subsequent series of a pathway in the pathophysiology of AR. RORc was shown to regulate Th17 differentiation, but it’s a deficiency, did not completely abolish TH17 related cytokine expression (Yang et al., 2008).
A syrup formulation of Zataria multiflora Boiss. was prepared and investigated in a randomized clinical trial conducted in 43 individuals with a history of seasonal AR. A 20% Z. multiflora hydroalcoholic extract was diluted to achieve the final concentration of thymol and carvacrol of 20.5 and 2.85 mg/100 ml, respectively. The study investigated the effect of this formulation on the expression of Treg related cytokines IL-17, IL-4 as well as other types of cytokines and TH17 cells. Z. multiflora syrup formulation decreased IL-17 expression with a significant difference at (p< 0.05) compared to the control group (Ariaee et al., 2018). IL-7 is a cytokine which produces TH17 cell that plays a major role in fighting extracellular pathogens (Kleinewietfeld and Hafler, 2013). This pro-inflammatory cytokine can upregulate T cell-triggered inflammation and hematopoiesis by stimulating stromal cells to secret other cytokines and growth factors (Wong et al., 2001). IL-17 may play a great role in reducing AR symptoms (Ariaee et al., 2018). The clinical study that has been carried out against Z. multiflora has shown a moderate activity in reducing IL-17 against patient with AR. Apart from its anti-allergic rhinitis effect, the erial parts of Z. multiflora has an anti-amnesic effect and might improve memory deficit through anticholinesterase activity. Multiple-dose injection of Z. multiflora extract could dose-dependently inhibit acetylcholinesterase (AchE) in the brain hippocampus of scopolamine-induced amnesia rats (Sheibani et al., 2019).
Tussilagone (6), a sesquiterpene compound isolated from the dried flower buds of Tussilago farfara L. has been identified as the major bioactive component of the plant (Jin et al., 2020). IL-6 is a type of cytokine that is also an important key component in the pathophysiology of AR. The aggravation of the inflammatory symptoms may be induced by the mast cells. This is caused by the recruitment of various inflammatory cells to the nasal mucosa by cytokines including IL-6 and TNF-α (Bernstein et al., 2002). Apart from reducing the levels of histamine and OVA-specific IgE in an in vivo study, tussilagone administered intraperitoneally at the dose of 50 and 25 mg/kg has shown a significant decrease (p< 0.01) in cytokine IL-6 levels OVA-sensitized guinea pigs. The inhibitory effect of tussilagone at 50 mg/kg was as good as clarityne, the positive control, at the dose of 10 mg/kg (Jin et al., 2020). Another study has also shown a significant decrease in LPS-stimulated production of IL-1β, and IL-6 mRNA through the inhibition of MAPK and NF-ƙB pathways (Choi et al., 2018). The phosphorylation of MAPKs may upregulate the gene expression of multiple inflammatory cytokines by transcription factor activation (Passante and Frankish, 2009). An in vitro study conducted on RBL-2H3 cells, a histamine-releasing cell has also shown an obvious suppression of p38 MAPK in a concentration-dependent manner after the treatment with tussilagone (6) at the doses of 1, 10, 100, 500 µM with a significant value of p< 0.01 given at the highest dose (500 µM). The inhibition of the MAPK pathway in the mast cells has been identified to be one of the mechanisms to alleviate AR symptoms in this study (Jin et al., 2020).
Petasol butenoate complex, Ze339, a herbal extract from Petasites hybridus (L.) G. Gaertn., B. Mey. and Schreb. leaves is known to be effective in treating AR. The use of this medicinal plant has led to decreased local production of IL-8 and LTB4 measured in nasal lining fluid and a faster recovery from nasal obstruction in allergic patients in a placebo-controlled double blind randomized clinical trial (Dumitru et al., 2011). The study has been conducted in 18 subjects with AR to grass pollen has shown a significant decrease in IL-8 and LTB4 expression compared to desloratadine (p = 0.025 and 0.014, respectively). Petasin (7) is a racemic mixture consisting of the isoforms of isopetasin (7a) and neopetasin (7b). They have been found to be the active components of the extract of this medicinal plant in an in vitro study on human nasal epithelial cells (Steiert et al., 2017). This study concluded that petasin decreased the PolyIC-induced IL-8 expression and neutrophil chemotaxis at the dose of 10 μg/ml with significant value of p < 0.01. Thus, Ze339 and its constituents, isopetasin and neopetasin are potential candidates for the development of agents for the treatment of immune deviations associated with continuous cytokine-induced inflammation.
Eosinophils and mass cells are the key inflammatory cells in allergic inflammation. Eosinophil infiltration has been considered as the major attribute of AR mucosal inflammation as it is (Minai-Fleminger and Levi-Schaffer, 2009; Wang et al., 2013). In fact eosinophils infiltration is the best marker of allergic inflammation that results in severe inflammation and causes the most severe symptoms (Ciprandi et al., 2005; Gelardi et al., 2008). IgE production is a proinflammatory process driven by cytokines that act against allergens’ exposure through the mucosal infiltration and actions of plasma cells, mast cells, and eosinophils (Skoner, 2001). Production of eosinophil is induced by Th2 cells in the early phase but it might be released during the latent recruitment phase through the release of cytokines and activation of endothelial cells (Baraniuk, 1997). Eosinophils produce several important cytokines such as IL-5 that act to promote eosinophil survival and activation (Tomaki et al., 2000). The eosinophil may as well degranulates and participate in the response to nasal challenge with allergen apart from triggering nasal provocation evidenced by the recovery of major basic protein in nasal lavage fluids (Naclerio et al., 1994).
An overall state of allergic conditions may be determined by the increase in eosinophils in the blood. Eosinophils level was increased in toluene 2,4-diisocyanate (TDI)-induced allergic mice model showing that this is one of the most important key components in understanding AR. Results from this study have shown that the treatment with an oral dose of Acanthus ilicifolius L. ethanolic extract (500 mg/kg) and cetrizine (20 mg/kg) could decrease the count of these inflammatory cells as compared to TDI-control (p< 0.05) (Sardar et al., 2018). Symptoms wise, A. ilicifolius ethanol extracts significantly suppressed sneezing and nasal score with medium activity with a significant value at p< 0.05 for both responding variables. It may be well explained with the significant suppression of related key components that play important roles in this disease such as eosinophil.
There is an association between the levels of eosinophil and Th17. Both components have been investigated in an in vivo study of Piper nigrum L. on OVA-induced subject which has a significant increase in eosinophil level with p < 0.001. Treatment with ethanol extract of P. nigrum at a dose of 50 mg/kg showed a significant decrease (p < 0.05) while at the doses of 100 and 200 mg/kg (p < 0.01), the extract showed a medium activity against eosinophil suppression compared with the OVA group (Bui et al., 2019a). This study has also been conducted on the effect of dexamethasone in suppressing eosinophil levels with a significant decrease in eosinophil levels at p < 0.001 compared to OVA-group. The study emphasized the important key components of eosinophil which are very crucial in most allergic reactions including AR. Thus, suppression of eosinophil may result in the alleviation of AR symptoms.
In another in vivo study, eosinophil suppression was found to be significant when treated with a standardized extract of Rosa multiflora Thunb. (Bui et al., 2019b). Different doses (100, 200 and 400 mg/kg) of standardized extract of R. multiflora were used in this study. At 100 mg/kg the extract showed medium activity with a significant decrease in eosinophil levels (p < 0.01) but stronger activity in eosinophil suppression was observed at the doses of 200 and 400 mg/kg (p < 0.001) when compared with OVA group. Dexamethasone, used as a positive control also showed a significant difference similar to 200 and 400 mg/kg of R. multiflorae extracts compared with the OVA-induced model. Hence, the standardized R. multiflorae extracts at the stated doses might have nearly the same efficacy as the present conventional drug, dexamethasone (Bui et al., 2019b). The relative symptomatic effect might be explained by observing the epithelial swelling of OVA-induced subject as eosinophils-derived mediators induced epithelial damage leading to nasal mucosal swelling (Skoner, 2001). A significant difference in amelioration of epithelial swelling was observed in OVA subject treated with 200 and 400 mg/kg of standardized R. multiflorae extracts with significant value at p < 0.01 while epithelial swelling was ameliorated with a significant decrease of p < 0.001 when treated with dexamethasone. Dexamethasone might have a better effect on alleviating the symptoms by ameliorating the epithelial swelling. However, a clinical trial on its effect on human subjects is needed to investigate its effects on the human subject. Yet, this medicinal plant may have a promising future as an alternative treatment for AR.
There was a statistically significant reduction in mean nasal smear eosinophil count observed in a patient administered with Urtidin F. C tablet containing 150 mg of Urtica dioica L. The treatment showed medium activity with significant value at p < 0.01 compared to pre-treatment while there are no significant differences in control and placebo group (p < 0.001 and p < 0.1, respectively) (Bakhshaee, 2017). This study was conducted in a randomized double-blind study clinical trial to identify the significant effects of the tablet formulation among AR patients. However, similar effects were demonstrated between the placebo group compared with the treated group based on the Sino-Nasal Outcome Test 22 (SNOT-22) of stinging nettle controlling the symptoms of AR. Therefore, the outcomes of this study showed a weak association between the effect of eosinophil suppression with its symptomatic relief outcomes.
Mangiferin 8) isolated from Mangifera indica L. has showed a significant decrease in eosinophil level compared to the OVA group at p < 0.01 given at the dose of 5 and 20 mg/kg. The result showed a similar significant difference in eosinophil level in the animal models treated with 2.5 mg/kg of dexamethasone (Piao et al., 2020). The number of eosinophils, goblet cells, and mast cells infiltrating the nasal mucosa were estimated quantitatively in the histologic sections. It was shown that mangiferin (8) at the dose of 20 mg/kg produced a better protective effect on inflammatory cell production compared to 5 mg/kg mangiferin (8) (Piao et al., 2020). Mangiferin (8) may have the potential to be a better alternative to alleviate AR symptoms than conventional medicines with less side effects. However, the safety and efficacy of this compound may need further research.
The medicinal plants discussed in this review might have potential to be developed as alternatives to treat symptoms of AR. However, further studies should be carried out to determine their safety level for human use specifically in treating AR. Safety is the most important aspect in developing medicinal or health products. Thus, toxicity studies of the medicinal plants and their bioactive metabolites should be performed and reviewed to identify their potential acute or chronic toxicity that might be encountered upon using them as therapeutic agents for treating allergic diseases such as AR. Some toxicity studies have been conducted for some of these potential medicinal plants.
An acute and chronic toxicity studies on the 95% ethanol extract of Cinnamomum verum J. Presl have been conducted by Shah et al. (1998). Thirty-five mice were randomly divided into six C. verum-treatment groups and a control and the mice were observed for signs of toxicity and mortality after 24 h. There was some decreased locomotor activity at 3 g/kg dose, suggesting that it might possess narcotic effects at high doses. In a chronic toxicity study, the mice were treated with 100 mg/kg of C. verum extract for three weeks. Mortality in the C. verum treatment group was statistically insignificant (p > 0.05) compared to the control. It was observed that only one male mouse developed inflammation of hind limb which was cleared up during the next few weeks. The condition of the viscera and vital organs weight in the treated animals were normal and comparable to the control except for a decrease in the weight of the liver. There was a decrease in hemoglobin contents base on hematological studies.
The fixed oil of Nigella sativa L. was evaluated for its acute toxicity in mice. Oral dose administration of the oil in the mice showed a LD50 value of 28.8 ml/kg, while intraperitoneally administration exhibited a LD50 value of 2.06 ml/kg (Zaoui et al., 2002). It was observed that oral and intraperitoneal administration of the oil at all doses resulted in behavioral perturbations with immediate agitation, temporary writhing, followed by a quiet attitude period and sedation. Diarrhea was generally observed and the animals died 12 h after oil administration. In a chronic toxicity study, the rats were administered a daily woral dose of 2 ml/kg body wt. for 12 weeks. The leukocyte and platelet counts were observed and showed that there was a significant difference as compared to the control (p < 0.01). Besides, the platelet and leukocyte counts were significantly reduced as compared to the control (p < 0.01). The compound believed to cause toxicity in Petasites hybridus (L.) G. Gaertn., B. Mey. and Schreb. is pyrrolizidine alkaloids (Dreger et al., 2009). The secondary metabolite in the plants known with its hepatotoxic, cardiotoxic, pneumotoxic and nephrotoxic properties provides a defense mechanism against herbivores. Acute toxicity study of P. hybridus extract in Wistar rats revealed oral LD50 value of ≥2500 mg/kg body weight with 833–1250-fold, and intraperitoneal LD50 value of approximately 1000 mg/kg body weight with 333–500-fold higher than the recommended human doses of common butterbur extract (Danesch and Rittinghausen, 2003). Hemorrhagic necrosis, hepatomegaly, and ascites were found to be the signs of acute toxicity. Moreover, hepatic veins obstruction that results in venous-occlusive disease while in chronic poisoning, liver failure due to necrosis, fibrosis, and cirrhosis were some of the effects observed in subacute toxicity testing (Aydın et al., 2013).
The acute toxicity test of aqueous and hexane extracts of Urtica dioica L. at the concentrations of 250, 500, 1000, and 2000 mg/kg administered orally in Wistar rats have shown no mortality during 24 h period. No stereotypical toxic symptoms such as ataxia, convulsion, increased diuresis or diarrhea were observed, except the fourth group (2000 mg/kg) which exhibited symptoms like diuresis and diarrhea (Dar et al., 2013). Acute toxicity effects of aqueous and ethanolic extracts of erial parts of Zataria multiflora Boiss extracts in mice were conducted. The animals were injected intraperitoneally with various doses of the extracts and the mortality was determined at 48 h after treatment. Based on the LD50 values, the ethanolic extract (3.47 g/kg) was more toxic than the aqueous extract (3.85 g/kg) and these extracts are relatively toxic. The maximum non-fatal doses for the aqueous and ethanol extracts were 2.2 and 2 g/kg, respectively (Hosseinzadeh et al., 2000).
The most common potential target in treating AR is the suppression of histamine release as histamine is one of the most important key components in an early phase of AR pathophysiology and gives immediate symptoms within few minutes of allergen exposure. Current conventional treatment administers antihistamine drugs as first-line therapy. However, other mechanistic effects such as suppression of IgE, inhibition of cytokines production and suppression of eosinophil production have also been used as targets in efforts to search for bioactive principles from medicinal plants with strong anti-allergic rhinitis activity. Various in vivo, in vitro and clinical studies on medicinal plants and their bioactive metabolites have been carried out to evaluate their anti-allergic and anti-inflammatory properties particularly in treating AR by using various immune cells, AR-induced models or AR patients. There was remarkable amount of experimental data that have been generated and they can be further developed as potential source of new anti-allergic rhinitis agents. However, most studies on the medicinal plants including clinical trials were carried out using the crude extracts of the plants as the extracts were not standardized or chemically characterized and the active chemical markers were mostly not identified. The bioactive metabolites contributing to the anti-allergic rhinitis effect have not been well determined. For future studies sufficient preclinical testing should be generated using standardized extracts, which include bioavailability, pharmacokinetic and toxicological studies, before they can be subjected to clinical studies. Based on in vitro and in vivo studies several bioactive metabolites of the plant extracts including shikonin (1), okicamelliaside (2), warifteine (3), methylwarifteine (4), luteolin-7-O-rutinoside (5), tussilagone (6), petasin (7), and mangiferin (8) (Figure 2) have been identified as potential candidates for development into anti-allergic rhinitis agents. These bioactive compounds have to be further subjected to systematic and operationally thorough controlled randomized trials to prove its safety and efficacy for human use in treating AR.
NAR gathered the literature and drafted the manuscript. KH and IJ participated in the design and concept of the manuscript. All authors were involved in editing and KH gave the approval of the final version to be submitted for publication.
The authors would like to thank Universiti Kebangsaan Malaysia (UKM) for the financial support under grant number FRGS/1/2019/STG01/UKM/02/10.
The authors declare that the research was conducted in the absence of any commercial or financial relationships that could be construed as a potential conflict of interest.
Akdis, M., Verhagen, J., Taylor, A., Karamloo, F., Karagiannidis, C., Crameri, R., et al. (2004). Immune responses in healthy and allergic individuals are characterized by a fine balance between allergen-specific T Regulatory 1 and T Helper 2 cells. J. Exp. Med. 199, 1567–1575. doi:10.1084/jem.20032058
Amellal, M., Bronner, C., Briancon, F., Haag, M., Anton, R., and Landry, Y. (1985). Inhibition of mast cell histamine release by flavonoids and biflavonoids. Planta Med. 51, 16–20. doi:10.1055/s-2007-969381
Ariaee, N., Ghorbani, J., Panahi, M., Mohamadi, M., Asili, J., Ranjbar, A., et al. (2018). Oral administration of Zataria multiflora extract decreases IL-17 expression in perennial allergic rhinitis. Rep. Biochem. Mol. Biol. 6, 203–207.
Asher, M. I., Montefort, S., Björkstén, B., Lai, C. K., Strachan, D. P., Weiland, S. K., et al. (2006). Worldwide time trends in the prevalence of symptoms of asthma, allergic rhinoconjunctivitis, and eczema in childhood: isaac phases one and three repeat multicountry cross-sectional surveys. The Lancet 368, 733–743. doi:10.1016/s0140-6736(06)69283-0
Aswar, U. M., Kandhare, A. D., Mohan, V., Thakurdesai, P. A., and Thakurdesai, P. A. (2015). Anti-allergic effect of intranasal administration of type-a procyanidin polyphenols based standardized extract of Cinnamon bark in ovalbumin sensitized BALB/c Mice. Phytother. Res. 29, 423–433. doi:10.1002/ptr.5269
Aydın, A. A., Zerbes, V., Parlar, H., and Letzel, T. (2013). The medical plant butterbur (Petasites): analytical and physiological (re)view. J. Pharm. Biomed. Anal. 75, 220–229. doi:10.1016/j.jpba.2012.11.028
Ayoub, M., Lallouette, P., Sütterlin, B. W., Bessler, W. G., Huber, M., and Mittenbühler, K. (2003). Modulation of the Th1/Th2 bias by an immunoglobulin histamine complex in the ovalbumin allergy mouse model. Int. Immunopharmacol. 3, 523–539. doi:10.1016/s1567-5769(03)00031-6
Bahekar, P. C., Shah, J. H., Ayer, U. B., Mandhane, S. N., and Thennati, R. (2008). Validation of Guinea pig model of allergic rhinitis by oral and topical drugs. Int. Immunopharmacol. 8, 1540–1551. doi:10.1016/j.intimp.2008.06.010
Bai, X., Chai, Y., Shi, W., Li, Y., Zhang, T., and Liu, P. (2020). Lonicera japonica polysaccharides attenuate ovalbumin-induced allergic rhinitis by regulation of Th17 cells in BALB/c mice. J. Funct. Foods 65, 103758. doi:10.1016/j.jff.2019.103758
Bakhshaee, M., Mohammad Pour, A. H., Esmaeili, M., Jabbari Azad, F., Alipour Talesh, G., Salehi, M., et al. (2017). Efficacy of supportive therapy of allergic rhinitis by stinging nettle (Urtica dioica) root extract: a randomized, double-blind, placebo- controlled, clinical trial. Iran J. Pharm. Res. 16, 112.
Baraniuk, J. (1997). Pathogenesis of allergic rhinitis. J. Allergy Clin. Immunol. 99, S763–S772. doi:10.1016/s0091-6749(97)70125-8
Barbosafilho, J., Vasconcelosleitaodacunha, E., and Gray, A. (2000). Alkaloids of the menispermaceae. Alkaloids: Chem. Biol. 54, 1–190. doi:10.1016/s0099-9598(00)54002-4
Barnes, P. J. (2011). Pathophysiology of allergic inflammation. Immunological Rev. 242, 31–50. doi:10.1111/j.1600-065x.2011.01020.x
Bergendorff, O., and Sterner, O. (1995). Spasmolytic flavonols fromArtemisia abrotanum. Planta Med. 61 (04), 370–371. doi:10.1055/s-2006-958106
Bernstein, D. I., Bernstein, C. K., Deng, C., Murphy, K. J., Bernstein, I. L., Bernstein, J. A., et al. (2002). Evaluation of the clinical efficacy and safety of grapeseed extract in the treatment of fall seasonal allergic rhinitis: a pilot study. Ann. Allergy Asthma Immunol. 88, 272–278. doi:10.1016/s1081-1206(10)62008-x
Bharadwaj, A. S., Bewtra, A. K., and Agrawal, D. K. (2007). Dendritic cells in allergic airway inflammationThis article is one of a selection of papers published in the Special Issue on Recent Advances in Asthma Research. Can. J. Physiol. Pharmacol. 85, 686–699. doi:10.1139/y07-062
Bielory, L. (2010). Allergic conjunctivitis and the impact of allergic rhinitis. Curr. Allergy Asthma Rep. 10, 122–134. doi:10.1007/s11882-010-0087-1
Bousquet, J., Khaltaev, N., Cruz, A. A., Denburg, J., Fokkens, W. J., Togias, A., et al. (2008). Allergic rhinitis and its impact on asthma (aria) 2008 update (in collaboration with the world health organization, GA(2)len and AllerGen). Allergy 63 (Suppl 86), 8–160. doi:10.1111/j.1398-9995.2007.01620.x
Bui, T. T., Fan, Y., Piao, C. H., Nguyen, T. V., Shin, D.-u., Jung, S. Y., et al. (2020). Piper nigrum extract improves OVA-induced nasal epithelial barrier dysfunction via activating Nrf2/HO-1 signaling. Cell Immunol. 351, 104035. doi:10.1016/j.cellimm.2019.104035
Bui, T. T., Kwon, D.-A., Choi, D. W., Jung, S. Y., Lee, S.-Y., Piao, C. H., et al. (2019b). Rosae multiflorae fructus extract and its four active components alleviate ovalbumin-induced allergic inflammatory responses via regulation of Th1/Th2 imbalance in BALB/c rhinitis mice. Phytomedicine 55, 238–248. doi:10.1016/j.phymed.2018.06.044
Bui, T. T., Piao, C. H., Hyeon, E., Fan, Y., Choi, D. W., Jung, S. Y., et al. (2019c). Preventive effect of Bupleurum chinense on nasal inflammation via suppressing T helper type 2, eosinophil and mast cell activation. Am. J. Chin. Med. 47, 405–421. doi:10.1142/s0192415x19500204
Bui, T. T., Piao, C. H., Hyeon, E., Fan, Y., Van Nguyen, T., Jung, S. Y., et al. (2019a). The protective role of Piper nigrum fruit extract in an ovalbumin-induced allergic rhinitis by targeting of NFκBp65 and STAT3 signalings. Biomed. Pharmacother. 109, 1915–1923. doi:10.1016/j.biopha.2018.11.073
Busse, W. W., Coffman, R. L., Gelfand, E. W., Kay, A. B., and Rosenwasser, L. J. (1995). Mechanisms of persistent airway inflammation in asthma. A role for T cells and T-cell products. Am. J. Respir. Crit. Care Med. 152, 388–393. doi:10.1164/ajrccm.152.1.7599853
Cavalcanti, R. F. P., Gadelha, F. A. A. F., de Jesus, T. G., Cavalcante-Silva, L. H. A., Paiva Ferreira, L. K. D., Paiva Ferreira, L. A. M., et al. (2020). Warifteine and methylwarifteine inhibited the type 2 immune response on combined allergic rhinitis and asthma syndrome (CARAS) experimental model through NF-κB pathway. Int. Immunopharmacol. 85, 106616. doi:10.1016/j.intimp.2020.106616
Chai, R., Liu, B., and Qi, F. (2017). The Significance of the levels of Il-4, Il-31 and Tlsp in patients with asthma and/or rhinitis. Immunotherapy 9, 331–337. doi:10.2217/imt-2016-0131
Choi, B.-S., Kim, Y.-j., Yoon, Y. P., Lee, H. J., and Lee, C. J. (2018). Tussilagone suppressed the production and gene expression of MUC5AC mucin via regulating nuclear factor-kappa B signaling pathway in airway epithelial cells. Korean J. Physiol. Pharmacol. 22, 671–677. doi:10.4196/kjpp.2018.22.6.671
Ciprandi, G., Vizzaccaro, A., Cirillo, I., Tosca, M., Massolo, A., and Passalacqua, G. (2005). Nasal eosinophils display the best correlation with symptoms, pulmonary function and inflammation in allergic rhinitis. Int. Arch. Allergy Immunol. 136, 266–272. doi:10.1159/000083953
Costa, H. F., Bezerra-Santos, C. R., Barbosa Filho, J. M., Martins, M. A., and Piuvezam, M. R. (2008). Warifteine, a bisbenzylisoquinoline alkaloid, decreases immediate allergic and thermal hyperalgesic reactions in sensitized animals. Int. Immunopharmacology 8, 519–525. doi:10.1016/j.intimp.2007.11.009
Danesch, U., and Rittinghausen, R. (2003). Safety of a patented special butterbur root extract for migraine prevention. Headache 43, 76–78. doi:10.1046/j.1526-4610.2003.03015.x
Dar, S. A., Ganai, F. A., Yousuf, A. R., Balkhi, M.-u. -H., Bhat, T. M., and Sharma, P. (2013). Pharmacological and toxicological evaluation ofUrtica dioica. Pharm. Biol. 51, 170–180. doi:10.3109/13880209.2012.715172
Das, A. K., Yoshimura, S., Mishima, R., Fujimoto, K., Mizuguchi, H., Dev, S., et al. (2007). Stimulation of histamine H1 receptor up-regulates histamine H1 receptor itself through activation of receptor gene transcription. J. Pharmacol. Sci. 103, 374–382. doi:10.1254/jphs.fp0061411
Dreger, M., Stanisławska, M., Krajewska-Patan, A., Mielcarek, S., Mikołajczak, P. Ł., and Buchwald, W. (2009). Pyrrolizidine alkaloids–chemistry, biosynthesis, pathway, toxicity, safety and perspectives of medicinal usage. Herba Pol. 55, 127–147.
Dumitru, A. F., Shamji, M., Wagenmann, M., Hindersin, S., Scheckenbach, K., Greve, J., et al. (2011). Petasol butenoate complex (Ze 339) relieves allergic rhinitis-induced nasal obstruction more effectively than desloratadine. J. Allergy Clin. Immunol. 127, 1515–1521. doi:10.1016/j.jaci.2011.02.045
Eifan, A. O., and Durham, S. R. (2016). Pathogenesis of rhinitis. Clin. Exp. Allergy 46, 1139–1151. doi:10.1111/cea.12780
Geha, R. (1992). Regulation of IgE synthesis in humans. J. Allergy Clin. Immunol. 90, 143–150. doi:10.1016/0091-6749(92)90064-9
Gelardi, M., Del Giudice, A. M., Fiorella, M. L., Fiorella, R., Russo, C., Soleti, P., et al. (2008). Non-allergic rhinitis with eosinophils and mast cells constitutes a new severe nasal disorder. Int. J. Immunopathol Pharmacol. 21, 325–331. doi:10.1177/039463200802100209
Georas, S. N., Guo, J., De Fanis, U., and Casolaro, V. (2005). T-helper cell type-2 regulation in allergic disease. Eur. Respir. J. 26, 1119–1137. doi:10.1183/09031936.05.00006005
Godthelp, T., Fokkens, W. J., Kleinjan, A., Holm, A. F., Mulder, P. G. H., Prens, E. P., et al. (1996). Antigen presenting cells in the nasal mucosa of patients with allergic rhinitis during allergen provocation. Clin. Exp. Allergy 26, 677–688. doi:10.1046/j.1365-2222.1996.1072451.x
Gould, H. J., and Sutton, B. J. (2008). IgE in allergy and asthma today. Nat. Rev. Immunol. 8, 205–217. doi:10.1038/nri2273
Haenuki, Y., Matsushita, K., Futatsugi-Yumikura, S., Ishii, K. J., Kawagoe, T., Imoto, Y., et al. (2012). A critical role of IL-33 in experimental allergic rhinitis. J. Allergy Clin. Immunol. 130, 184–194. doi:10.1016/j.jaci.2012.02.013
Hajiheydari, M. R., Yarmohammadi, M. E., Izadi, P., Jafari, F., Emadi, F., Emaratkar, E., et al. (2017). Effect of Nepeta bracteata Benth. on allergic rhinitis symptoms: a randomized double-blind clinical trial. J. Res. Med. Sci. 22, 128. doi:10.4103/jrms.JRMS_316_17
Hartmann, E., Graefe, H., Hopert, A., Pries, R., Rothenfusser, S., Poeck, H., et al. (2006). Analysis of plasmacytoid and myeloid dendritic cells in nasal epithelium. Cvi 13, 1278–1286. doi:10.1128/cvi.00172-06
Hopper, J. L., Bui, Q. M., Erbas, B., Matheson, M. C., Gurrin, L. C., Burgess, J. A., et al. (2012). Does eczema in infancy cause hay fever, asthma, or both in childhood? insights from a novel regression model of sibling data. J. Allergy Clin. Immunol. 130, 1117–1122. doi:10.1016/j.jaci.2012.08.003
Hosseinzadeh, H., Ramezani, M., and Salmani, G.-A. (2000). Antinociceptive, anti-inflammatory and acute toxicity effects of Zataria multiflora Boiss extracts in mice and rats. J. Ethnopharmacology 73, 379–385. doi:10.1016/s0378-8741(00)00238-5
Ichikawa, K., Okuda, M., Naka, F., and Yago, H. (1991). The sources of chemical substances in allergic nasal fluid. Rhinology 29, 143–149.
Inoue, T., Sugimoto, Y., Masuda, H., and Kamei, C. (2002). Antiallergic effect of flavonoid glycosides obtained from Mentha piperita L. Biol. Pharm. Bull. 25, 256–259. doi:10.1248/bpb.25.256
Islam, R., Mizuguchi, H., Shaha, A., Nishida, K., Yabumoto, M., Ikeda, H., et al. (2018). Effect of wild grape on the signaling of histamine H1 receptor gene expression responsible for the pathogenesis of allergic rhinitis. J. Med. Invest. 65, 242–250. doi:10.2152/jmi.65.242
Jang, J.-Y., Lee, M.-J., You, B.-R., Jin, J.-S., Lee, S.-H., Yun, Y.-R., et al. (2017). Allium hookeri root extract exerts anti-inflammatory effects by nuclear factor-Κb down-regulation in lipopolysaccharide-induced raw 264.7 Cells. BMC Complement. Altern. Med. 17, 126. doi:10.1186/s12906-017-1709-0
Jin, C., Ye, K., Luan, H., Liu, L., Zhang, R., Yang, S., et al. (2020). Tussilagone inhibits allergic responses in OVA-induced allergic rhinitis Guinea pigs and IgE-stimulated RBL-2H3 cells. Fitoterapia 144, 1–7. doi:10.1016/j.fitote.2020.104496
Johansson, S. G. O., Bieber, T., Dahl, R., Friedmann, P. S., Lanier, B. Q., Lockey, R. F., et al. (2004). Revised nomenclature for allergy for global use: report of the nomenclature review committee of the world allergy organization, October 2003. J. Allergy Clin. Immunol. 113, 832–836. doi:10.1016/j.jaci.2003.12.591
Jung, H. W., Jung, J. K., and Park, Y. K. (2011). Antiallergic effect of Ostericum koreanum root extract on ovalbumin-induced allergic rhinitis mouse model and mast cells. Asian Pac. J. Allergy Immunol. 29, 338–348.
Khinchi, M. S., Poulsen, L. K., Carat, F., André, C., Hansen, A. B., Malling, H.-J., et al. (2004). Clinical efficacy of sublingual and subcutaneous birch pollen allergen-specific immunotherapy: a randomized, placebo-controlled, double-blind, double-dummy study. Allergy 59, 45–53. doi:10.1046/j.1398-9995.2003.00387.x
Kim, H.-G., , Han, J.-M., Lee, J.-S., Lee, J. S., and Son, C.-G. (2015). Ethyl acetate fraction of Amomum xanthioides improves bile duct ligation-induced liver fibrosis of rat model via modulation of pro-fibrogenic cytokines. Scientific Rep. 5, 14531. doi:10.1038/srep14531
Kim, H.-Y., , Nam, S.-Y., Hong, S.-W., Kim, M.-J., Jeong, H.-J., and Kim, H.-M. (2015). Protective effects of rutin through regulation of vascular endothelial growth factor in allergic rhinitis. Am. J. Rhinol. Allergy 29, e87–e94. doi:10.2500/ajra.2015.29.4195
Kim, H. J., Jang, M.-J., Lee, J.-Y., and Lee, S.-H. (2019). Allium hookeri root extract inhibits adipogenesis by promoting lipolysis in high fat diet-induced obese mice. Nutrients 11, 2262. doi:10.3390/nu11102262
Kim, S.-H., Lee, S., Kim, I. K., Kwon, T. K., Moon, J.-Y., Park, W.-H., et al. (2007). Suppression of mast cell-mediated allergic reaction by Amomum xanthiodes. Food Chem. Toxicol. 45, 2138–2144. doi:10.1016/j.fct.2007.05.011
Kleinewietfeld, M., and Hafler, D. A. (2013). The plasticity of human treg and Th17 cells and its role in autoimmunity. Semin. Immunol. 25, 305–312. doi:10.1016/j.smim.2013.10.009
Kobayashi, M., Matsushita, H., Shioya, I., Nagai, M., Tsukiyama, R.-I., Saito, M., et al. (2004a). Quality of life improvement with soy sauce ingredients, shoyu polysaccharides, in perennial allergic rhinitis: a double-blind placebo-controlled clinical study. Int. J. Mol. Med. 14, 885–889.
Kobayashi, M., Matsushita, H., Yoshida, K., Tsukiyama, R.-I., Sugimura, T., and Yamamoto, K. (2004b). In vitro and in vivo anti-allergic activity of soy sauce. Int. J. Mol. Med. 14, 879–884.
Kobayashi, S., and Tanabe, S. (2006). Evaluation of the anti-allergic activity of Citrus unshiu using rat basophilic leukemia RBL-2H3 cells as well as basophils of patients with seasonal allergic rhinitis to pollen. Int. J. Mol. Med. 17, 511–515.
Kuba, M., Tsuha, K., Tsuha, K., Matsuzaki, G., and Yasumoto, T. (2008). In vivo analysis of the anti-allergic activities of Camellia japonica extract and okicamelliaside, a degranulation inhibitor. J. Health Sci. 54, 584–588. doi:10.1248/jhs.54.584
Lee, S.-B., Kim, H.-G., Kim, H.-S., Lee, J.-S., Im, H.-J., Kim, W.-Y., et al. (2016). Ethyl acetate fraction of Amomum xanthioides exerts antihepatofibrotic actions via the regulation of fibrogenic cytokines in a dimethylnitrosamine-induced rat model. Evid.-Based Complement. Alternat. Med. 16, 1–14. doi:10.1155/2016/6014380
Leurs, R., Church, M. K., and Taglialatela, M. (2002). H1-antihistamines: inverse agonism, anti-inflammatory actions and cardiac effects. Clin. Exp. Allergy 32, 489–498. doi:10.1046/j.0954-7894.2002.01314.x
Li, Y., Simons, F. E. R., Jay, F. T., and Hayglass, K. T. (1996). Allergen-driven limiting dilution analysis of human Il-4 and Ifn-Γ production in allergic rhinitis and clinically tolerant individual. Int. Immunol. 8 (6), 897–904. doi:10.1093/intimm/8.6.897
Liu, Y. J. (2005). IPC: professional type 1 interferon-producing cells and plasmacytoid dendritic cell precursors. Annu. Rev. Immunol. 23, 275–306. doi:10.1146/annurev.immunol.23.021704.115633
Lou, H., Wang, X., Wei, Q., Zhao, C., Xing, Z., Zhang, Q., et al. (2020). Artemisia annua sublingual immunotherapy for seasonal allergic rhinitis: a multicenter, randomized trial. World Allergy Organ. J. 13, 100458. doi:10.1016/j.waojou.2020.100458
Maeda-Yamamoto, M., Ema, K., Monobe, M., Shibuichi, I., Shinoda, Y., Yamamoto, T., et al. (2009). The efficacy of early treatment of seasonal allergic rhinitis with benifuuki green tea containing O-methylated catechin before pollen exposure: an open randomized study. Allergol. Int. 58, 437–444. doi:10.2332/allergolint.08-oa-0066
Maeta, A., Uzaki, M., Nishi, H., Asano, M., and Takahashi, K. (2017). Anti-allergic effects of a hot water extract of Stephania tetrandra S. Moore in RBL-2H3 cells and an allergic rhinitis mouse model. Food Sci. Technology Res. 23, 575–582. doi:10.3136/fstr.23.575
Maggi, L., Santarlasci, V., Liotta, F., Frosali, F., Angeli, R., Cosmi, L., et al. (2007). Demonstration of circulating allergen-specific Cd4+ Cd25highfoxp3+ T-regulatory cells in both nonatopic and atopic individuals. J. Allergy Clin. Immunol. 120, 429–436. doi:10.1016/j.jaci.2007.05.002
Matkovic, Z., Zivkovic, V., Korica, M., Plavec, D., Pecanic, S., and Tudoric, N. (2010). Efficacy and safety of Astragalus membranaceus in the treatment of patients with seasonal allergic rhinitis. Phytotherapy Res. 24, 175–181. doi:10.1002/ptr.2877
May, J. R., and Dolen, W. K. (2017). Management of allergic rhinitis: a review for the community pharmacist. Clin. Ther. 39, 2410–2419. doi:10.1016/j.clinthera.2017.10.006
Michalak, B., Piwowarski, J. P., Granica, S., Waltenberger, B., Atanasov, A. G., Khan, S. Y., et al. (2019). Eupatoriopicrin inhibits pro-inflammatory functions of neutrophils via suppression of IL- 8 and TNF-alpha production and p38 and ERK 1/2 MAP kinases. J. Nat. Prod. 82, 375–385. doi:10.1021/acs.jnatprod.8b00939
Minai-Fleminger, Y., and Levi-Schaffer, F. (2009). Mast cells and eosinophils: the two key effector cells in allergic inflammation. Inflamm. Res. 58, 631–638. doi:10.1007/s00011-009-0042-6
Naclerio, R. M., Baroody, F. M., Kagey-Sobotka, A., and Lichtenstein, L. M. (1994). Basophils and eosinophils in allergic rhinitis. J. Allergy Clin. Immunol. 94, 1303–1309. doi:10.1016/0091-6749(94)90346-8
Nikakhlagh, S., Rahim, F., Aryani, F. H. N., Syahpoush, A., Brougerdnya, M. G., and Saki, N. (2011). Herbal treatment of allergic rhinitis: the use of Nigella sativa. Am. J. Otolaryngol. 32, 402–407. doi:10.1016/j.amjoto.2010.07.019
Okubo, K., Kurono, Y., Ichimura, K., Enomoto, T., Okamoto, Y., Kawauchi, H., et al. (2017). Japanese guidelines for allergic rhinitis 2017. Allergol. Int. 66, 205–219. doi:10.1016/j.alit.2016.11.001
Onodera, K.-I., Tsuha, K., Yasumoto-Hirose, M., Tsuha, K., Hanashiro, K., Naoki, H., et al. (2010). Okicamelliaside, an extraordinarily potent anti-degranulation glucoside isolated from leaves of Camellia japonica. Biosci. Biotechnol. Biochem. 74, 2532–2534. doi:10.1271/bbb.100630
Passante, E., and Frankish, N. (2009). The RBL-2H3 cell line: its provenance and suitability as a model for the mast cell. Inflamm. Res. 58, 737–745. doi:10.1007/s00011-009-0074-y
Pawankar, R., Mori, S., Ozu, C., and Kimura, S. (2011). Overview on the pathomechanisms of allergic rhinitis. Asia Pac. Allergy 1, 157–167. doi:10.5415/apallergy.2011.1.3.157
Pawankar, R., and Ra, C. (1996). Heterogeneity of mast cells and T cells in the nasal mucosa. J. Allergy Clin. Immunol. 98, S248–S262. doi:10.1016/s0091-6749(96)70073-8
Peng, W., Han, P., Yu, L., Chen, Y., Ye, B., Qin, L., et al. (2019). Anti-allergic rhinitis effects of caffeoylquinic acids from the fruits of Xanthium strumarium in rodent animals via alleviating allergic and inflammatory reactions. Revista Brasileira de Farmacognosia 29, 46–53. doi:10.1016/j.bjp.2018.10.004
Piao, C. H., Fan, Y. J., Nguyen, T. V., Song, C. H., and Chai, O. H. (2020). Mangiferin alleviates ovalbumin-induced allergic rhinitis via Nrf2/HO-1/NF-κB signaling pathways. Int. J. Mol. Sci. 21, 1–12. doi:10.3390/ijms21103415
Plager, D., Stuad, S., and Gleich, G. (1998). Human eosinophil granule major basic protein and its novel homolog. Allergy 53, 33–40. doi:10.1111/j.1398-9995.1998.tb04937.x
Platts-Mills, T. A. (2001). The role of immunoglobulin E in allergy and asthma. Am. J. Respir. Crit. Care Med. 164, S1–S5. doi:10.1164/ajrccm.164.supplement_1.2103024
Presta, L., Shields, R., O’connell, L., Lahr, S., Porter, J., Gorman, C., et al. (1994). The binding site on human immunoglobulin E for its high affinity receptor. J. Biol. Chem. 269, 26368–26373. doi:10.1016/s0021-9258(18)47203-1
Remberg, P., Björk, L., Hedner, T., and Sterner, O. (2004). Characteristics, clinical effect profile and tolerability of a nasal spray preparation of Artemisia abrotanum L. for allergic rhinitis. Phytomedicine 11, 36–42. doi:10.1078/0944-7113-00350
Rondón, C., Fernández, J., López, S., Campo, P., Doña, I., Torres, M. J., et al. (2009). Blanca nasal inflammatory mediators and specific IgE production after nasal challenge with grass pollen in local allergic rhinitis. J. Allergy Clin. Immunol. 124, 1005–1011. doi:10.1016/j.jaci.2009.07.018
Ross, S. M. (2016). Allergic rhinitis: a proprietary extract of Pinus pinaster Aiton (pycnogenol) is found to improve the symptoms associated with allergic rhinitis. Holist. Nurs. Pract. 30, 301–304. doi:10.1097/hnp.0000000000000170
Saito, S., Takagi, H., Wakasa, Y., Ozawa, K., and Takaiwa, F. (2020). Safety and efficacy of rice seed-based oral allergy vaccine for Japanese cedar pollinosis in Japanese monkeys. Mol. Immunol. 125, 63–69. doi:10.1016/j.molimm.2020.06.019
Sardar, P. K., Dev, S., Al Bari, M. A., Paul, S., Yeasmin, M. S., Das, A. K., et al. (2018). Antiallergic, anthelmintic and cytotoxic potentials of dried aerial parts of Acanthus ilicifolius L. Clin. Phytoscience 4, 34. doi:10.1186/s40816-018-0094-7
Schuliga, M. (2015). NF-kappaB signaling in chronic inflammatory airway disease. Biomolecules 5, 1266–1283. doi:10.3390/biom5031266
Shah, A., Al-Shareef, A., Ageel, A., and Qureshi, S. (1998). Toxicity studies in mice of common spices, Cinnamomum zeylanicum bark and Piper longum fruits. Plant Foods Hum. Nutr. 52, 231–239. doi:10.1023/a:1008088323164
Sheibani, V., Mandegary, A., Vazifekhahan, E., Kasbzade, Z., Asadi, A., and Sharififar, F. (2019). Zataria multiflora Boiss. extract improves spatial memory and learning capacity in scopolamine-induced amnesic rats. Avicenna J. Phytomedicine 9, 587–596. doi:10.22038/AJP.2019.13540
Shin, S. -H., Ye, M. -K., Lee, D. -W., and Che, M. -H. (2020). Immunomodulative effects of Chamaecyparis obtusa essential oil in mouse model of allergic rhinitis. Molecules 25, 4517. doi:10.3390/molecules25194517
Shirakawa, T., Deichmann, K. A., Izuhara, K., Mao, X. Q., Adra, C. N., and Hopkin, J. M. (2000). Atopy and asthma: genetic variants of IL-4 and IL-13 signalling. Immunol. Today 21, 60–64. doi:10.1016/s0167-5699(99)01492-9
Sih, T., and Mion, O. (2010). Allergic rhinitis in the child and associated comorbidities. Pediatr. Allergy Immunol. 21, e107–e113. doi:10.1111/j.1399-3038.2009.00933.x
Sin, B., and Togias, A. (2011). Pathophysiology of allergic and nonallergic rhinitis. Proc. Am. Thorac. Soc. 8, 106–114. doi:10.1513/pats.201008-057rn
Skoner, D. P. (2001). Allergic rhinitis: definition, epidemiology, pathophysiology, detection, and diagnosis. J. Allergy Clin. Immunol. 108, S2–S8. doi:10.1067/mai.2001.115569
Steiert, S. A., Zissler, U. M., Chaker, A. M., Esser-von-Bieren, J., Daniela Dittlein, D., Guerth, F., et al. (2017). Anti-inflammatory effects of the petasin phyto drug Ze339 are mediated by inhibition of the STAT pathway. BioFactors 43, 388–399. doi:10.1002/biof.1349
Tanticharoenwiwat, P., Kulalert, P., Ayudhya, T. D. N., Koontongkaew, S., Jiratchariyakul, W., Soawakontha, R., et al. (2017). Inhibitory effect of Phlai capsules on skin test responses among allergic rhinitis patients: a randomized, three-way crossover study. J. Integr. Med. 15, 462–468. doi:10.1016/s2095-4964(17)60353-4
Thomet, O. A. R., Schapowal, A., Heinisch, I. V. W. M., Wiesmann, U. N., and Simon, H. U. (2002). Anti-inflammatory activity of an extract of Petasites hybridus in allergic rhinitis. Int. Immunopharmacol. 2, 997–1006. doi:10.1016/s1567-5769(02)00046-2
Till, S., Walker, S., Dickason, R., Huston, D. D., O’Brien, F., Lamb, J., et al. (1997). IL-5 production by allergen-stimulated T cells following grass pollen immunotherapy for seasonal allergic rhinitis. Clin. Exp. Immunol. 110, 114–121. doi:10.1111/j.1365-2249.1997.494-ce1392.x
Togias, A. (2003). Rhinitis and asthma: evidence for respiratory system integration. J. Allergy Clin. Immunol. 111, 1171–1183. doi:10.1067/mai.2003.1592
Tohidinik, H. R., Mallah, N., and Takkouche, B. (2019). History of allergic rhinitis and risk of asthma; a systematic review and meta-analysis. World Allergy Organ. J. 12, 100069. doi:10.1016/j.waojou.2019.100069
Tomaki, M., Zhao, L.-L., Lundahl, J., Sjöstrand, M., Jordana, M., Lindén, A., et al. (2000). Eosinophilopoiesis in a murine model of allergic airway eosinophilia: involvement of bone marrow Il-5 and Il-5 receptor Α. J. Immunol. 165, 4040–4050. doi:10.4049/jimmunol.165.7.4040
Wang, M., Liu, G., and Li, H. (2017). Curative effects of shikonin on allergic rhinitis in rats and the related mechanism. Biomed. Res. 28, 9253–9257.
Wang, M., Zhang, W., Shang, J., Yang, J., Zhang, L., and Bachert, C. (2013). Immunomodulatory effects of IL-23 and IL-17 in a mouse model of allergic rhinitis. Clin. Exp. Allergy 43, 956–966. doi:10.1111/cea.12123
Weiner, J. M., Abramson, M. J., and Puy, R. M. (1998). Intranasal corticosteroids versus oral H1 receptor antagonists in allergic rhinitis: systematic review of randomised controlled trials. Br. Med. J. 317, 1624–1629. doi:10.1136/bmj.317.7173.1624
Westman, M., Kull, I., Lind, T., Melen, E., Stjärne, P., Toskala, E., et al. (2013). The link between parental allergy and offspring allergic and nonallergic rhinitis. Allergy 68, 1571–1578. doi:10.1111/all.12267
Wheatley, L. M., and Togias, A. (2015). Allergic rhinitis. New Engl. J. Med. 372, 456–463. doi:10.1056/nejmcp1412282
Wong, C., Ho, C., Ko, F., Chan, C., Ho, A., Hui, D., et al. (2001). Proinflammatory cytokines (Il‐17, il‐6, il‐18 and il‐12) and Th cytokines (Ifn‐Γ, il‐4, il‐10 and il‐13) in patients with allergic asthma. Clin. Exp. Immunol. 125, 177–183. doi:10.1046/j.1365-2249.2001.01602.x
Yamaguchi, M., Lantz, C. S., Oettgen, H. C., Katona, I. M., Fleming, T., Miyajima, I., et al. (1997). Ige enhances mouse mast cell Fcεri expression in vitro and in vivo: evidence for a novel amplification mechanism in Ige-dependent reactions. J. Exp. Med. 185, 663–672. doi:10.1084/jem.185.4.663
Yang, P., Okuda, M., Pawankar, R., and Aihara, K. (1995). Electron microscopical studies of the cell population in nasal secretions. Rhinology 33, 70–77. doi:10.1016/0956-716x(95)00341-r
Yang, X. O., Pappu, B. P., Nurieva, R., Akimzhanov, A., Kang, H. S., Chung, Y., et al. (2008). T helper 17 lineage differentiation is programmed by orphan nuclear receptors Rorα and Rorγ. Immunity 28, 29–39. doi:10.1016/j.immuni.2007.11.016
Yonekura, S., Okamoto, Y., Yamasaki, K., Horiguchi, S., Hanazawa, T., Matsune, S., et al. (2011). A randomized, double-blind, placebo-controlled study of Ten-Cha (Rubus suavissimus) on house dust mite allergic rhinitis. Auris Nasus Larynx 38, 600–607. doi:10.1016/j.anl.2010.11.017
Zaoui, A., Cherrah, Y., Mahassini, N., Alaoui, K., Amarouch, H., and Hassar, M. (2002). Acute and chronic toxicity of Nigella sativa fixed oil. Phytomedicine 9, 69–74. doi:10.1078/0944-7113-00084
Zhang, Y., and Kang, H. (2020). Protective effect of Asarum sieboldii essential oil on ovalbumin induced allergic rhinitis in rat. Biosci. Rep. 40, 1–9. doi:10.1042/bsr20191370
Zhang, Y., and Zhang, L. (2019). Increasing prevalence of allergic rhinitis in China. Allergy Asthma Immunol. Res. 11, 156–169. doi:10.4168/aair.2019.11.2.156
Keywords: medicinal plants, anti-allergic rhinitis, immune system, immunoglobulin E, molecular mechanisms, toxicology
Citation: Rahim NA, Jantan I, Said MM, Jalil J, Abd Razak AF and Husain K (2021) Anti-Allergic Rhinitis Effects of Medicinal Plants and Their Bioactive Metabolites via Suppression of the Immune System: A Mechanistic Review. Front. Pharmacol. 12:660083. doi: 10.3389/fphar.2021.660083
Received: 28 January 2021; Accepted: 10 March 2021;
Published: 13 April 2021.
Edited by:
Luca Rastrelli, University of Salerno, ItalyReviewed by:
Kevin Spelman, Consultant, Ashland, OR, United StatesCopyright © 2021 Rahim, Jantan, Said, Jalil, Abd Razak and Husain. This is an open-access article distributed under the terms of the Creative Commons Attribution License (CC BY). The use, distribution or reproduction in other forums is permitted, provided the original author(s) and the copyright owner(s) are credited and that the original publication in this journal is cited, in accordance with accepted academic practice. No use, distribution or reproduction is permitted which does not comply with these terms.
*Correspondence: Khairana Husain, a2hhaXJhbmFAdWttLmVkdS5teQ==
Disclaimer: All claims expressed in this article are solely those of the authors and do not necessarily represent those of their affiliated organizations, or those of the publisher, the editors and the reviewers. Any product that may be evaluated in this article or claim that may be made by its manufacturer is not guaranteed or endorsed by the publisher.
Research integrity at Frontiers
Learn more about the work of our research integrity team to safeguard the quality of each article we publish.