- 1Department of Head and Neck Surgery, Hubei Cancer Hospital, Tongji Medical College, Huazhong University of Science and Technology, Wuhan, China
- 2Laboratory of Drug Discovery and Molecular Engineering, Department of Medicinal Plants, College of Plant Science and Technology, Huazhong Agricultural University, Wuhan, China
- 3National and Local Joint Engineering Research Center for Medicinal Plant Breeding and Cultivation, Wuhan, China
- 4Hubei Provincial Engineering Research Center for Medicinal Plants, Wuhan, China
- 5Ethnobotany and Pharmacognosy Lab, Department of Botany, University of Chittagong, Chittagong, Bangladesh
- 6ROII Office, University of the Punjab, Lahore, Pakistan
- 7Saint-Petersburg State Chemical Pharmaceutical University, Saint-Petersburg, Russia
The roots of Glycyrrhiza spp. have been utilized in Traditional Chinese medicine (TCM) for thousands of years. Non-traditional (aerial) parts constitute a large portion of the biomass of Glycyrrhiza plants and are mostly discarded after harvesting the roots and rhizomes. Through comparative phytochemical and anti-inflammatory activity analyses, this study explored the potential benefits of the aerial parts of Glycyrrhiza uralensis Fisch. ex DC. as medicinal materials. First, a combined approach based on GC/MS and UHPLC-ESI-QTof MS analysis was adopted for the identification and quantitative examination of medicinally important compounds from G. uralensis. Additionally, a bioassay-guided fractioning of ethanolic extracts of G. uralensis leaf material was performed and its anti-inflammatory activity was tested. The aerial portion of G. uralensis was rich in medicinally important compounds. Two compounds (henicosane-1 and decahydroisoquinoline-2) were found to exert a significant anti-inflammatory effect, inhibiting the release of pro-inflammatory mediators (NO and PGE2) and cytokines (IL-1β, IL6, and TNF-α), without exerting cytotoxic effects. Moreover, both compounds down-regulated iNOS and COX-2 mRNA expression. These results suggest that non-traditional parts of G. uralensis are suitable sources of bioactive metabolites that can be explored for medicinal purposes.
Introduction
Glycyrrhiza uralensis Fisch. ex DC. as well as Glycyrrhiza glabra L. (Fabaceae), commonly known as licorice, are traditional plants recognized through ages for their multiple health benefits and medicinal uses. Particularly, G. uralensis is mentioned in the pharmacopoeia of China, Russia, and other countries (Wang et al., 2020). “Licorice” is obtained from the underground parts of G. uralensis and related species. Different compounds including triterpenoid saponins, flavanones, chalcones, and coumarins have been isolated from the roots of Glycyrrhiza spp. (Wang et al., 2020). Glycyrrhiza spp. are widely cultivated, since these contain most of the bioactive compounds that are responsible for their medicinal and culinary attributes as a flavoring agent and spice (Bell et al., 2011; Dong et al., 2014). Currently licorice is used at different stages of processing grains and oil products, meat products, beverages, candies, jellies, dried fruits, seeds, and soy sauce etc. (Montoro et al., 2011). The roots of this plant are used to treat influenza, coughs, and liver damage in traditional medicinal formulations (Zarubaev et al., 2016).
Previous studies have shown that the extracts of the roots of G. uralensis contain antioxidant, anti-inflammatory, antiviral, cytotoxic, antidiabetic, inhibitors of angiotensin-converting enzyme 2 and transmembrane protease, serine 2, skin-whitening, hepatoprotective, and cholinergic properties (Ahn et al., 2010; Gou et al., 2020; Isbrucker and Burdock 2006; Wu et al., 2020). However, the aerial portion of this plant is of lesser importance to cultivators and usually constitutes an agro-industrial waste after the harvest of the roots or rhizomes that corresponds to merely one fourth of the whole biomass of the plant (Figure 1). It is worth mentioning that the aerial parts of G. uralensis also contains liquiritin and some other medicinally important compounds (Table 1). While previous studies are mostly limited to some major compounds identified from the roots such as glycyrrhizin, liquiritin, liquiritigenin, and isoliquiritigenin (Ji et al., 2016), the information related to the medicinal importance of many other compounds present in the foliar portion of this plant is scarce. Therefore, in this study we examined the leaves of G. uralensis for the presence of anti-inflammatory compounds. To the best of our knowledge, we described for the first time the presence of two bioactive compounds in the leaves of this plant, and reported the effect of the isolated compounds on the production of important pro-inflammatory mediators.
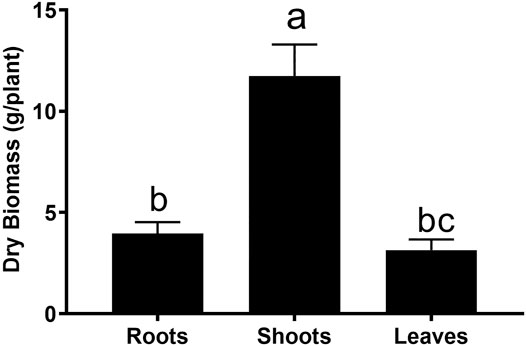
FIGURE 1. Comparative dry biomass of different parts of G. uralensis. Small letters represent level of significance among different treatments as inferred by DNMRT at p = 0.05.
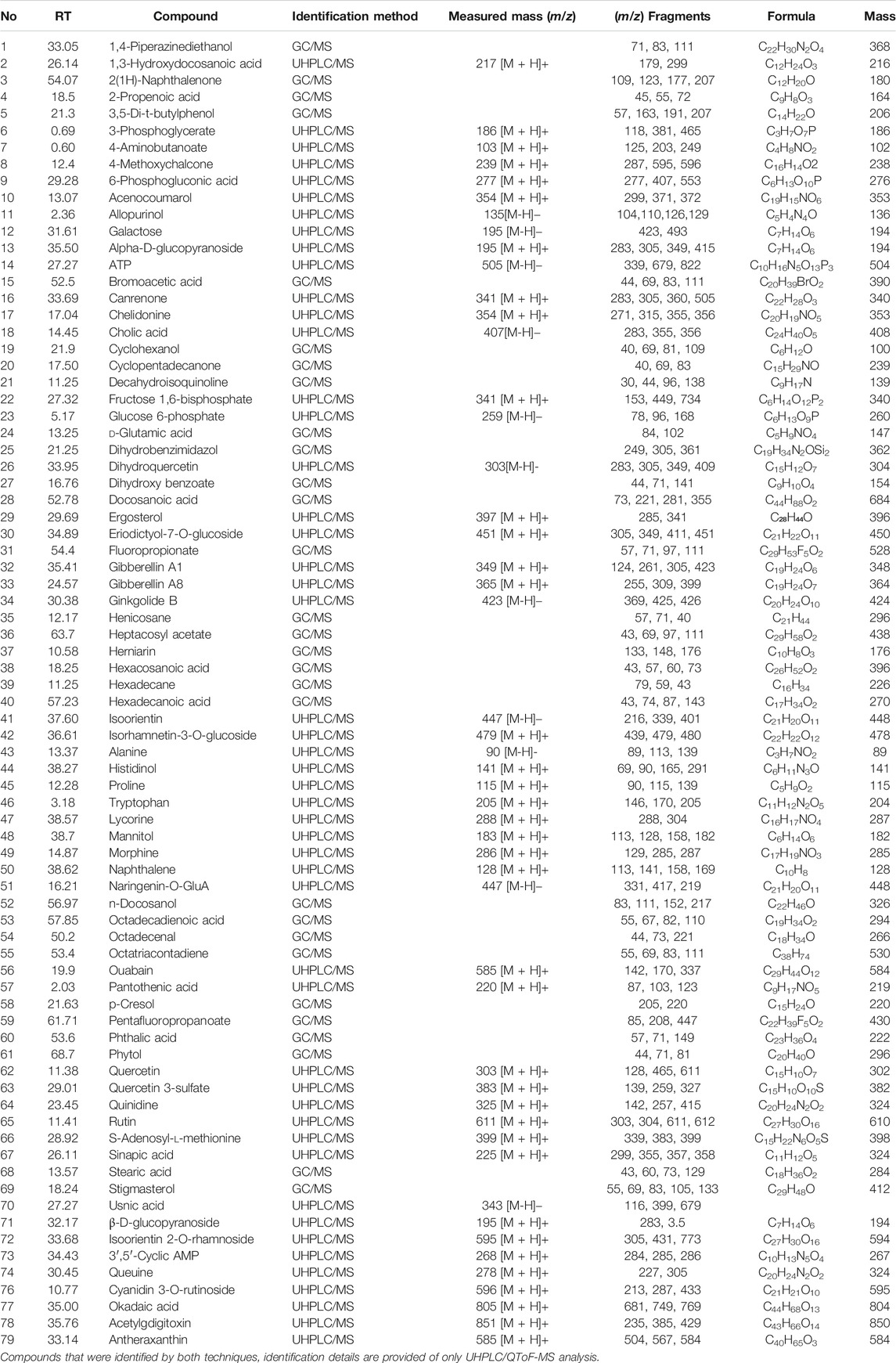
TABLE 1. Details of different compounds detected in leaf material of G. uralensis by performing GC/MS and LC/MS analysis.
Materials and Methods
Chemicals and Reagents
All solvents and standards used for GC/MS and UHPLC ESI-QTof MS analysis were of chromatography grade and obtained from Sigma-Aldrich (St. Louis, MO, United States). Dulbecco’s modified Eagle’s medium (DMEM), penicillin, streptomycin and fetal bovine serum (FBS) were purchased from Thermo Scientific (Logan, UT, United States). Griess reagent, acetylsalicylic acid (aspirin), dimethylsulfoxide (DMSO), lipopolysaccharide (LPS), pyridine, ribitol were obtained from Sigma-Aldrich (St. Louis, MO, United States). MOX and MSTFA mixtures were purchased from Thermo Fisher (TX, United States). 13C-ribitol was obtained from Omicron Biochemicals Inc, (IN, United States). ELISA kits were obtained from Bio-Rad (CA, United States) and Sigma-Aldrich (St. Louis, MO, United States). The RAW 264.7 cell lines at sixth passage were used that were originally purchased from Sigma-Aldrich (St. Louis, MO, United States). Deionized water (Milli-Q) was used in the whole study (Millipore, Billerica, MA, United States).
Comparative Metabolomics of the Roots and Aerial Parts of G. uralensis
Plant Material and Preparation of the Extracts
Different parts of G. uralensis were obtained from Qinghaihu Pharmaceutical, Co. Ltd. (Qinghai, China). Prof. Dr Xuebo Hu, from College of Plant Sciences and Technology, Huazhong Agricultural University, China, verified the identity of plant material (The specimens were kept at Institute for Medicinal Plants, Huazhong Agricultural University with voucher number 2017-Gu-0001, 2017-Gu-0002 and 2017-Gu-0003 for the roots, stems and shoots). In order to obtain an extract, the air-dried powdered (500 mg) material of roots and shoots of G. uralensis was macerated separately in 25 ml of MeOH/CHCl3/H2O (2.5:1:0.5, v/v) solution overnight under continuous stirring (Weckwerth et al., 2004). The whole process was performed twice. The material was filtered through a Whatman No. 1 filter paper. The solvent was evaporated using rotary evaporator under low-pressure to obtain a semi-solid consistency.
Extract Derivatization and GC/MS Analysis
Gas chromatography coupled with mass spectrometry (GC/MS) analysis was performed as described by Weckwerth et al. (2004) with some modifications. Here, 13C-ribitol (0.02 μg/μL) was used as an internal standard. Dried samples were derivatized using standard MOX and MSTFA mixtures as described by Mari et al. (2013). The clear supernatant was obtained after centrifugation and poured into clean GC-vials for analysis. The sample (1 µL) was injected in a Shimadzu GC/MS-QP2010 SE (Shimadzu, Japan) instrument at the constant temperature of 230°C in splitless mode. Chromatographic separation was performed using HP-5MS capillary column (30 m × 0.25 mm × 0.25 µm) and helium as carrier gas at a constant flow rate of 1.0 ml/min. The GC/MS temperature gradient used during analysis was same as adopted by Mari et al. (2013). Mass analyzer was set at full scan mode (40–800 m/z) and the ion source temperature was maintained at 250°C, with EI ionization at 70 eV.
UHPLC ESI-QTof MS analysis
Previously prepared plant extracts were dissolved in pure methanol following sonication for 5 min. The obtained solution was centrifuged, and the supernatant was passed through cellulose filters (0.2 µm pore size). Afterward, the sample (0.2 μL) was injected in an ultra-performance liquid chromatography coupled with electrospray ionization-quadrupole time-of-flight mass spectrometry (UHPLC-ESI-QTof MS/MS) instrument. The chromatographic separation (Figure 2) was performed on a Waters ACQUITY UHPLC I-class system (Waters Corporation, Dublin, Ireland) fitted with a Waters ACQUITY UHPLC BEH C18 column. The mobile phases were deionized H2O containing 0.1% of formic acid (A) and MeOH containing 0.1% of formic acid (B) at constant flow rate of 0.7 ml/min. The gradient was set as follows: 5% B at 0 min, linearly increasing from 5 to 10% B within 5 min, from 10 to 100% B within 22.5 min, and held at 100% B for 2.5 min. The chromatographic system was coupled with Waters Xevo QTof-MS system via an electrospray ionization (ESI) interface operating in full scan mode. The ESI source and MS parameters were set as adopted by (Muema et al., 2017).
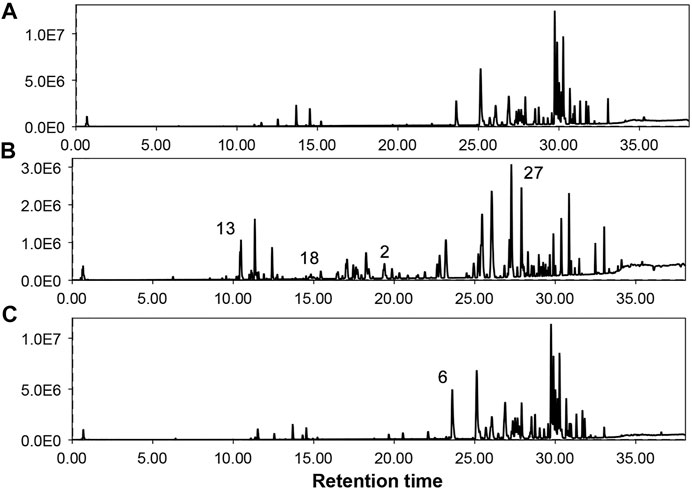
FIGURE 2. Total ion chromatograms of different parts of G. uralensis obtained from UHPLC-QTOF/MS analysis. (A) = Shoots, (B) = Leaves, (C) = Roots. Numbers over peaks represents different compounds as mentioned in Table 2.
Compound Identification and Data Analysis
MzMine version 2.30 (mzmine.github.io) was used for both qualitative and quantitative analysis of both GC/MS and LC/MS data. The alignment was carried out as a function of retention time, using a tolerance window of 0.2 min and 10 ppm mass accuracy (Molina-Calle et al., 2017). Metabolites were identified by comparing mass spectra with spectral libraries (NIST and Wiley), online database MassBank (http://www.massbank.jp/) and previously published literature (Zhang and Ye 2009; Siracusa et al., 2011; Farag et al., 2012; Ammar et al., 2017; Yu et al., 2017; Hefny Gad et al., 2018). Metabolites were identified with a spectral match factor higher than 800. The resulting data matrix based on the relative abundance of metabolites of different parts of G. uralensis was exported into the online tool ClustVis (https://biit.cs.ut.ee/clustvis/) to create heat maps and principal component analysis (PCA) plots. All samples were analyzed three times and mean data was used to perform statistical analysis. Furthermore, comparative quantifications of different medicinally important compounds were performed for their prevalence in aerial and below ground parts of G. uralensis.
Isolation and Identification of Anti-inflammatory Compound/s from the Leaves of G. uralensis
Compound Extraction and Isolation
An overview of the purification process of compounds is shown in Supplementary Figure S1. Briefly, dried leaves of G. uralensis were ground into a fine powder prior to use. Leaf powder (∼1 kg) was first extracted with ∼20 L of EtOH at ∼ 77°C for 5 h. Afterward, the solvent was removed by rotary evaporator and lyophilized to obtain a dry material. This dried material was further extracted using an EtOH-H2O (v/v) based solvent system and a stepwise elution method with increasing EtOH concentration (from 20 to 100%) to yield five fractions (F1-F5). Subsequently, the selected bioactive sub-fractions, eluted at 60% EtOH (F 4.6) and 70% EtOH (F 4.7), were processed with column chromatography on silica gel to yield five sub-fractions (A–E). Parent fractions were separated into sub-fractions based on thin layer chromatography (TLC) to obtain pure compounds. Sub-fraction 4.6°C was passed through a silica gel column to obtain four sub-fractions, 4.6Ca–4.6Cd. Then, sub-fraction 4.6 Cb was purified on silica gel eluted with EtOH-H2O (3:1 v/v) to obtain compound 1 (6.8 mg). Similarly, compound 2 (826.5 mg) was purified using EtOH-H2O (4:1 v/v) from sub-fraction 4.7Bc. Purified compounds were identified by comparing spectral data with previously published data and authentic pure reference compounds.
Cell Line and Cell Culture
The cell line RAW 264.7 was maintained in DMEM medium supplemented with 10% FBS, 100 U/ml penicillin, and 100 mg/ml streptomycin. The cells were grown at 37°C and 5% CO2 in humidified incubator (ABI 371, Thermo Fisher Scientific Inc, United States).
Determination of NO Production
Nitric oxide (NO) production was measured with the Griess reagent (Sigma-Aldrich, Darmstadt, Germany). Briefly, RAW264.7 cells (1 × 105 cells/well) were incubated in a 48-well plate with different concentrations of test materials for 1 h. Cells were stimulated with lipopolysaccharide (LPS, 1 μg/ml) for 24 h. Supernatants (100 μl) were collected, mixed with an equal volume of the Griess reagent, and incubated at 37°C for 10 min. Afterward, optical density (OD) was measured at 540 nm using a microplate reader (xMark, BIO-RAD, CA, United States). Acetylsalicylic acid (aspirin) (100 µM) was used as positive control in all subsequent assays (Gao et al., 2015). Each experiment was repeated twice, and measurements were taken in triplicate.
Measurement of Pro-Inflammatory Cytokine (PGE2, TNF-α, IL-1β, and IL-6) Production
The production of pro-inflammatory cytokine was determined by commercially available ELISA kits (Bio-Rad, CA, United States; Sigma-Aldrich, Darmstadt, Germany). RAW 264.7 cells (1 × 105 cells/well) were plated in 48-well plates and incubated with test material for 1 h prior to LPS (1 μg/ml) stimulation. Cell-free culture supernatants were collected for the determination of PGE2, IL-1β, IL-6, and TNF-α concentration according to the manufacturer’s instructions.
RT-qPCR Analysis
Total RNA from RAW 264.7 cells was extracted using TRIzol reagent (Invitrogen, United States) according to the manufacturer’s instructions. First strand complementary DNA (cDNA) was synthesized by using MMLV based reverse transcriptase kit (Invitrogen, United States). Afterward, cDNA was amplified with gene-specific primers using 2Xn-Taq polymerase mixture (Enzynomics, Korea). The primer sequences are listed in Supplementary Table S1.
Statistical Analysis
Data were analyzed statistically by performing one-way analysis of variance (ANOVA) followed by Duncan’s New Multiple Range Test using SPSS version 21 (Chicago, IL).
Results
Comparative Metabolomics of Roots and Aerial Parts of G. uralensis
Roots of G. uralensis are mostly used in Chinese traditional medicine. Since the aerial parts are normally discarded, we wonder if these parts could also be utilized. In an analysis of the biomass distribution, it was found that roots accounted about 30% of the whole plant biomass (Figure 1). Therefore, medicinal evaluation of the aerial parts must be performed.
Considering the lack of research dealing with the use of the non-traditional (aerial) parts of G. uralensis for the exploration of medicinally valuable compounds, a preliminary study was performed focusing on the comparative metabolomics of the roots and aerial parts of this plant. The extracts of aerial and below ground parts of G. uralensis were analyzed by GC/MS and UHPLC-ESI-QTof MS/MS analysis (Figure 2). Analysis of the mass spectrum data led to the identification of 79 compounds from the leaves of G. uralensis. The identified compounds can be seen in Tables 1 and 2, along with the main identification parameters obtained from the existing databases. Based on our comparative study, both traditional (roots) and non-traditional (aerial) parts showed a varying profile of different compounds like phenolic, saponins, flavonoids, flavonoid glycosides, coumarins, chalcones, and tannins. To provide a global overview, the relative abundance of the compounds in different parts of the plant is shown in a heatmap (Figure 3). The most abundant group of compounds included phenolic acids and their derivatives, which were identified in both positive and negative ionization mode that generated [M + H] and [M-H] precursor ions (Table 1). Another group identified in the leaf samples was the medicinally valued flavanones and glycoside compounds, which are characteristic of Glycyrrhiza spp. Other important groups of compounds putatively identified in the leaf samples were saccharides, tannins, and sulfoxides. In addition, lipids and their derivatives were identified in the samples in [M + H] and [M-H] modes. Overall, the results show that the leaves of G. uralensis contain a high diversity of all the examined classes of compounds when compared with the roots and shoots (Figure 2).
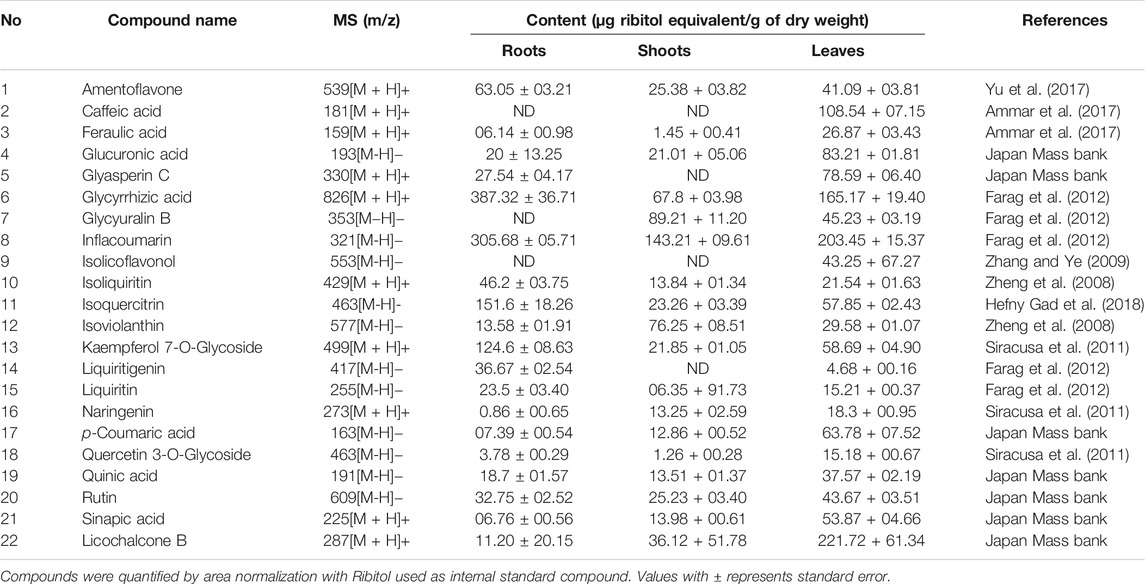
TABLE 2. Comparative quantifications of some major bioactive compounds presented in different parts of G. uralensis.
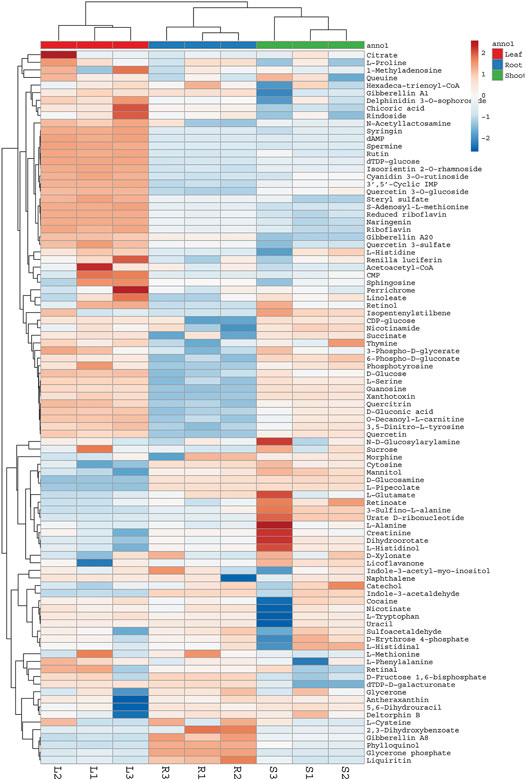
FIGURE 3. Heat map showing relative abundance of different compounds detected in aerial parts and roots of G. uralensis. Heat map was constructed using web based ClustVis tool. L = Leaves, R = Roots, S = Shoots.
The root extract contained higher concentrations of most of the medicinally important compounds (Table 2). Moreover, these compounds showed different abundance among roots shoots and leaves. For instance, roots contained approximately ten times more flavanones (13.64% of the total mass extract) compared with the leaves (4.07% of the total mass extract). Similarly, compounds like liquiritin, glycyrrhetic acid, feraulic acid, and isoquercitrin were found abundantly in the roots when compared with the leaves and shoot. Alternatively, some compounds like caffeic acid, glyasperin C, isolicoflavonol, and isolicoflavonol were found in the leaves but not in the shoots. Quantitative data showed that the leaves of G. uralensis contained some phenolic acids (sinapic acid and p-coumaric acid), isoviolanthin, and glycyuralin B in higher concentrations in comparison with the roots and shoots. Indeed, phenolic content in 1 g of leaf extract were equivalent to 9.63% of the total mass extract, which is approximately three times lower as compared to the root extracts (2.87% of the total mass extract).
Furthermore, a quantitative data set was created to compare metabolic profile of different plant parts by performing PCA analysis. It showed great extent of variability in the chemical composition of extracts obtained from the roots, shoots, and leaves of G. uralensis. PCA plot showed three distinct groups corresponding to different plant parts (Figure 4).
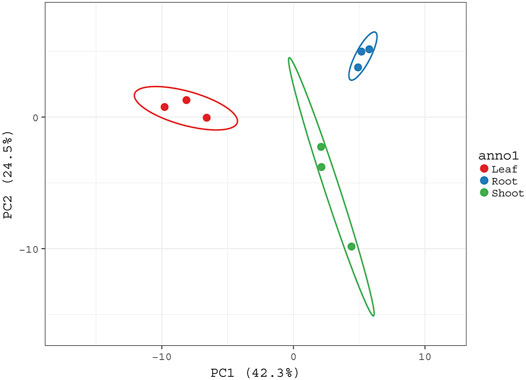
FIGURE 4. PCA score plot sowing the variability of metabolic profiles of aerial rats and roots of G. uralensis. PCA plot was constructed based on abundance of different metabolites present in aerial and roots of G. uralensis. All three groups corresponding to different plant parts are well separated from each other based on the variability of their metabolic profiles.
Isolation and Identification of Anti-Inflammatory Compounds From the Leaves of G. uralensis
Effect of G. uralensis Leaf Extracts on LPS-induced NO Production
To determine the anti-inflammatory effects of G. uralensis leaf extracts, we initially investigated the inhibitory effects of crude extracts (at concentration of 25,50,75 and 100 μg/ml) and fractionated leaf extracts (at concentration of 2.5,5.7.5 and 10 μg/ml) against NO production using LPS-induced RAW 264.7 cells. Among the tested fractions, F4 showed the highest inhibitory effect against NO release (Figure 5).
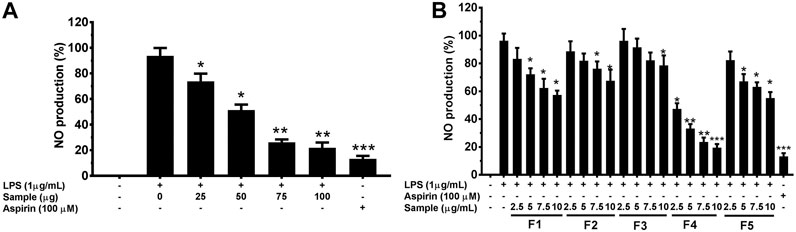
FIGURE 5. Effects of crude extracts (A) and fractions (B) EtOH leaf extracts of G. uralensis on nitric oxide in RAW 264.7 cells. Cells (1.0 × 105 cells/ml) were stimulated by LPS (1 μg/ml) for 24 h in the presence of rude extracts and fractions at varying concentrations. Culture media were collected in order to measure NO by the Griess reaction. Values are the mean ± standard error of triplicate experiments. *p < 0.05, **p < 0.01 and **p < 0.001 for the comparison with the LPS-stimulated group.
Identification of the Active Compounds and Their Inhibitory Effect in LPS-Induced NO and PGE2 Production
Firstly, the compounds were putatively identified with MS analysis (Figure 6). Afterward, the identification was confirmed by comparing retention and molecular indices with pure authentic internal standards. Compounds belonging to fraction four, were identified as henicosane (1) and decahydroisoquinoline (2) also known as perhydroisoquinoline.
To assess the inhibitory effect of purified compound 1 and 2 in LPS stimulated RAW 264.7 cells, the production of NO was measured by the Griess reaction and PGE2 by ELISA, respectively. As shown in Figures 7A,B the production of NO and PGE2 was markedly increased by stimulation with LPS. In contrast, treatment with compound 1 and 2 inhibited the production of both mediators in a concentration-dependent manner (Figures 7A,B). This remarkable effect was not related to the nonspecific cytotoxicity, since both compounds showed non-significant effects on RAW 264.7 cell viability, as determined by the methyltetrazolium (MTT) assay (Figure 7C). Hence, the inhibition of NO and PGE2 was due to a direct inhibitory effect of the test compounds.
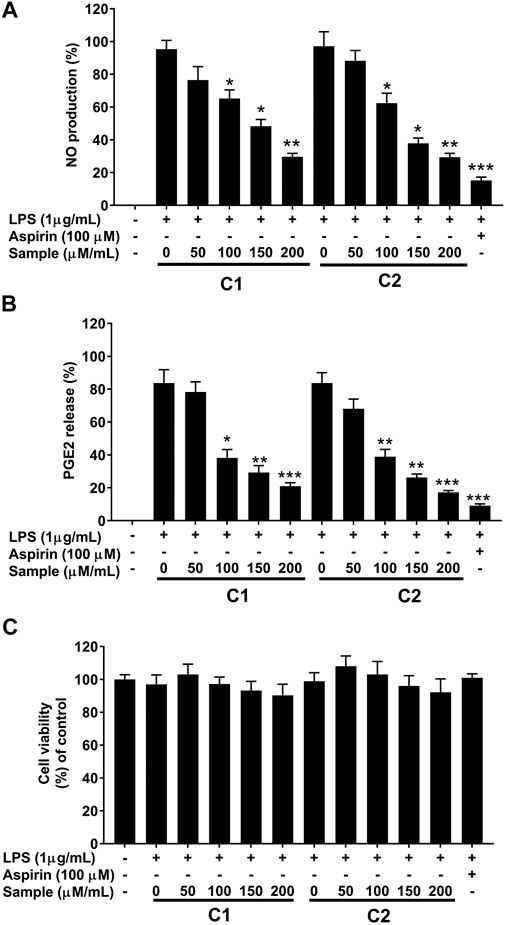
FIGURE 7. Effects of purified compounds on production of nitric oxide and prostaglandin E2 and cytotoxicity in RAW 264.7 cells. Cells (1.0 × 105 cells/ml) were stimulated by LPS (1 μg/ml) for 24 h in the presence of compounds (50, 100, 150, and 200 µ/ml). Culture media were collected in order to measure (A) NO and (B) PGE2 production by the Griess reaction and ELISA assay, respectively. (C) Cytotoxicity was determined using the MTT method. Values are the mean ± standard error of triplicate experiments. *p < 0.05, **p < 0.01 and **p < 0.001 for the comparison with the LPS-stimulated group.
Effect of Purified Compounds in LPS-induced iNOS and COX-2 Expression
Furthermore, the anti-inflammatory effect of the purified compounds was correlated with the expression levels of inducible nitric oxide synthase (iNOS) and cyclooxygenase-2 (COX-2), as revealed by RT-qPCR analysis. LPS simulation significantly increased the expression of these inflammation related genes (Figure 8), whereas, the presence of compound 1 and 2 significantly attenuated their induction in a concentration-dependent manner (Figure 8).
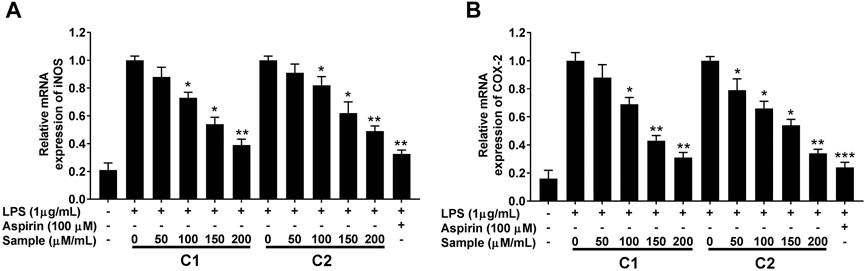
FIGURE 8. Effects of purified compounds on iNOS (A) and COX-2 (B) genes expression in RAW264.7 cells. Cells were pretreated with the indicated concentrations of purified compounds for 30 min and simulated with LPS (1 μg/ml) for 24 h. Gene expression was analyzed by quantitative RT-PCR analysis using gene specific primers in a concentration-dependent manner. Values are the mean ± standard error of triplicate experiments. Values are the mean + S.E. of triplicate experiments. *p < 0.05, **p < 0.01 and ***p < 0.001 for the comparison with the LPS-stimulated group. iNOS; nitric oxide synthase, COX-2; cyclooxygenase-2.
Effect of Purified Compounds on LPS-Induced Pro-inflammatory Cytokines Production
The inhibitory effect of purified compounds on LPS-simulated RAW 264.7 cell was further analyzed by measuring the changes in the release and the transcription levels of pro-inflammatory cytokines (IL-1β, IL-6, and TNF-α) using ELISA and RT-qPCR analysis, respectively. As can be seen in Figure 9, treatment with compound 1 and 2 lowered the expression levels of all tested LPS-induced pro-inflammatory cytokines at both mRNA and protein levels in a concentration-dependent manner.
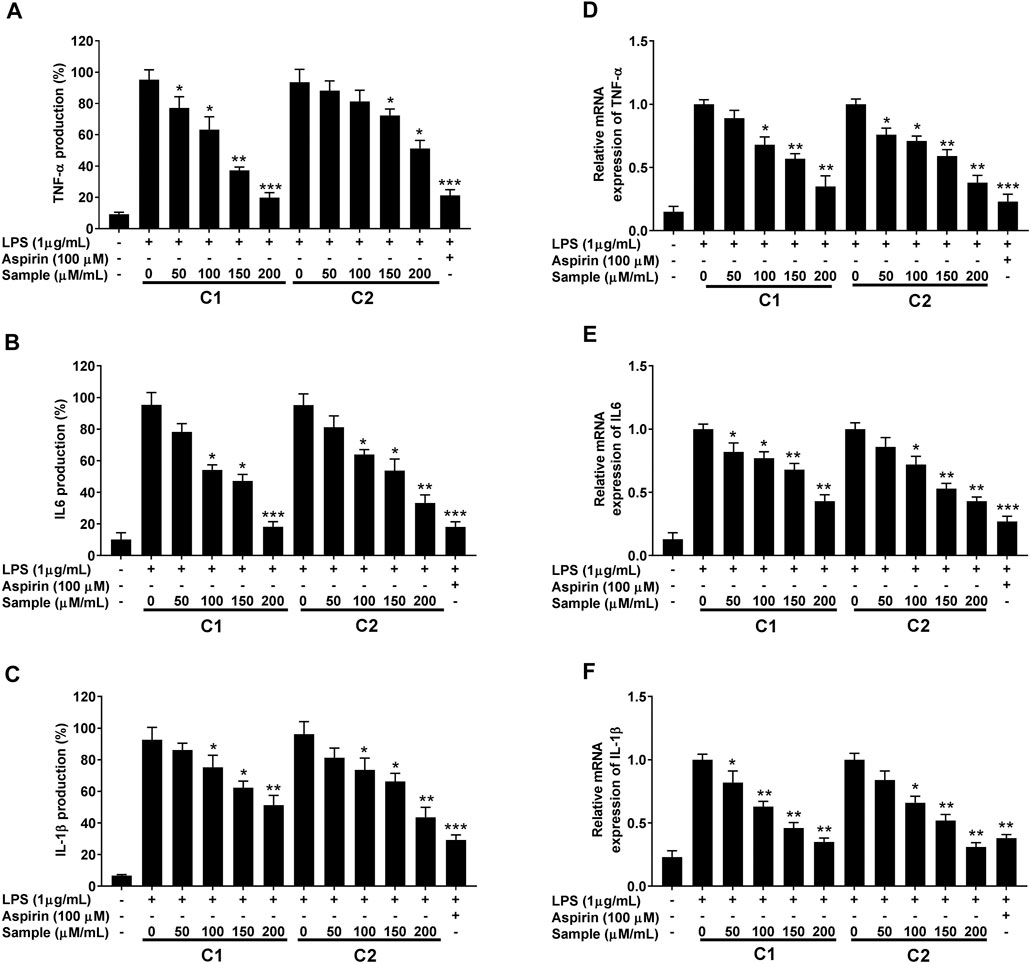
FIGURE 9. Inhibitory effect of purified compounds on pro-inflammatory cytokine production in RAW 264.7 cells. Cells (1.0 × 105 cells/ml) were stimulated by LPS (1 μg/ml) for 24 h in the presence of compounds (2.5, 5, and 10 µ/ml). Supernatants were collected, the TNF-α, IL-6 and IL-1β production in the supernatants was determined by ELISA (A–C). Gene expression was analyzed by quantitative RT-PCR analysis using gene specific primers (D–F). Values are the mean ± standard error of triplicate experiments. Values are the mean ± standard error of triplicate experiments. *p < 0.05, **p < 0.01 and ***p < 0.001 for the comparison with the LPS-stimulated group.
Discussion
Glycyrrhiza spp. plays an important role in many prescriptions used in complementary and alternative medicines (Ayeka et al., 2017). These plants are used in traditional Chinese medicine to treat many diseases and act as ingredients in the confectionary industry in Japan. To obtain a chemical profile of the roots and aerial parts of G. uralensis, an analytical method based on GC/MS and UHPLC/MS was developed. Simultaneously acquired UHPLC/MS total ion chromatograms for the roots, shoots, and leaves extracts of G. uralensis are show in Figure 2; while the identities, retention times, and observed molecular and fragment ions for metabolites are presented in Tables 1 and 2. Metabolites were identified by matching their m/z attributes with those reported in the literature, standard compound libraries (NIST and Wiley), and the “Japan Mass Bank” (Horai et al., 2010). When possible, the identification was confirmed with standard compounds available in-house.
In this study, the overall chemical profile of G. uralensis in terms of the types and contents is in agreement to previous studies (Bai et al., 2020; Kitagawa et al., 1993; Song et al., 2017; Yu et al., 2021). The roots and aerial parts showed the presence of varying abundance of different classes of phytochemicals such as total phenolics, flavonoids, tannins, and saccharides. As shown in Table 2, some of these medicinally valuable compounds were quantified by the normalization of peak areas with authentic internal standards. Remarkably, more than 40 phenolic compounds were identified in the leaves of G. uralensis, suggesting that their abundance is related to the medicinal usefulness of the aerial parts when compared with the roots (Abureidah et al., 2014). Consistently, the UHPLC/ESI/MS total ion chromatogram of the leaf extract of G. uralensis showed the presence of several medicinally valuable phenolic acids: sinapic acid with m/z [M + H]+ of 225, p-coumaric acid with m/z [M + H]+ of 163, and ferulic acid with m/z [M + H]+ of 159; as well as flavonoids: isoliquiritin showing m/z [M + H]+ at 429 and isoquercitrin with m/z [M + H]+ at 463 (Table 1). Some previous studies have also reported presence of same types of medicinally important flavonoids (Fukai et al., 1991; Yuldashev 1998) and phenolics (Nomura et al., 2002) in aerial and belowground parts of G. uralensis.
Moreover, O- and C-glycosylated forms were also identified from the aerial parts of G. uralensis. The C-glucosides entities were proposed based on their fragmentation pattern, which involved the sugar moiety by losses of 2, 3, or 4 (HCHO) (De et al., 2012). However, further stereochemical differentiation of the isomers was not possible by UHPLC/ESI/QTof/MS [20]. Some dominant glycosides in the aerial parts and roots of the plant were: kaempferol 7-O-glucoside characterized by its main fragment at m/z [M + H]+ 499 (relative intensity 100%); isoorientin 2-O-rhamnoside with m/z [M + H]+ at 595; and quercetin 3-O-glycoside, with a main fragment at m/z [M + H]+ 463 (relative intensity 100%). The concentration of quercetin 3-O-glycoside was higher in the leaves than in the roots, whereas the opposite was seen for kaempferol 7-O-glucoside that was present in higher quantities in the root extracts (Table 1). The same types of glycosides have been reported in aerial parts of G. uralensis (Jia et al., 1992).
Beside polyphenolic compounds, other polar compounds were identified including sugars, amino acids, and organic acids. Monosaccharides were detected at m/z 195 (galactose), m/z 259 (glucose 6-phosphate), and m/z 341 (fructose 1,6-bisphosphate). Amino acids eluted between 1 and 14 min corresponding to alanine (m/z 90), proline (m/z 115), and tryptophan (m/z 205). The known organic acids were identified as sinapic acid, stearic acid, usnic acid, and cholic acid, among others (Table 1).
The PCA was performed to highlight the varying metabolic profiles of the aerial and underground parts of G. uralensis. Pre-processed metabolomics data sets from different plant parts were analyzed to generate a PCA plot in which three different groups could be discriminated, thus indicating the varying distribution of components in the leaves, roots, and shoots of G. uralensis (Figure 4). In our study, the integration of data obtained from GC/MS and UHPLC/QTof/MS into a single matrix for PCA allowed the clear separation of extracts from the different plant parts, thereby highlighting the importance of both techniques for sample classification.
In our effort to screen bioactive compounds from the aerial parts of G. uralensis, a bio-guided fractioning allowed the isolation of two phytochemicals (henicosane-1 and decahydroisoquinoline-2) that exhibited significant anti-inflammatory effects. Furthermore, the mechanisms mediating this effect were investigated using RAW 264.7 cells. The results revealed that both the compounds significantly reduced the production of NO and PGE2, as well as the mRNA levels of iNOS and COX-2 in LPS-stimulated RAW 264.7 macrophages (Figures 5, 7).
Henicosane belongs to alkanes that are found in various eukaryotic organisms (Coates et al., 2014). These compounds are considered among the main constituents in the most of the plants (Mathis and Ourisson, 1964). Long chain alkanes have been widely isolated from plant fractions possessing medicinal properties (Aiello et al., 2000; Bush and McInerney 2013; Ghasemi Pirbalouti et al., 2014). Methane, a simplest alkane has shown the protective effect to inhibit some inflammatory signals caused by LPS in macrophages and suppress immune response in mice by intensifying IL-10 expression through PI3K/AKT/GSK-3β pathway (Zhang et al., 2016). Second bioactive compound (decahydroisoquinoline) purified in study is an isoquinoline alkaloid. The phytochemical and biological investigation of different plants have led to the isolation of several isoquinoline alkaloids with medicinal properties (Iranshahy et al., 2014; Khan and Kumar 2015; Haider et al., 2018; Bala et al., 2019). An increasing number of recent studies have reported that alkaloids are effective for treating inflammatory disorders and bring good ground for hope of drug development (Peng et al., 2019).
Macrophages play a key role in the immunopathological phenomena during inflammation and infection owing to their phagocytic and cytotoxic capacities (Laskin and Pendino 1995; Mosser and Edwards 2008; Decano et al., 2016). Pro-inflammatory mediators (NO and PGE2) and cytokines (IL-1β, IL-6, and TNF-α) are overproduced by macrophages under inflammation (Fujiwara and Kobayashi 2005; Jin et al., 2008). Lipopolysaccharides (LPS)s are the main components of the cell wall of Gram-negative bacteria, which upon recognition by murine macrophages, elicit their activation with a distinctive up-regulation of iNOS expression (Nathan and Xie 1994). In fact, high levels of NO production are of crucial importance in the process of macrophage response (MacMicking et al., 1997). Therefore, the suppression of NO is considered an important therapeutic target to treat inflammation (Batkhuu et al., 2002; Lee et al., 2008; Yoshitake et al., 2008). In our study, we successfully established that the compounds C1 and C2 isolated from the leaves of G. uralensis are strong suppressors of NO production by LPS-stimulated RAW 264.7 murine macrophages. This was accompanied by the inhibition of PGE2 and inflammatory cytokines (IL-1β, IL-6, and TNF-α), as assessed by ELISA and qRT-PCR analysis (Figures 8, 9). As both test compounds showed an effect in all the evaluated downstream targets, including iNOS and COX-2 enzymes, our findings suggest that the anti-inflammatory effect of compound 1 and 2 from G. uralensis, may be due to the targeting of upstream signaling such as that related to the mitogen-activated protein (MAP) kinase or the nuclear factor (NF)-κB signal pathways.
Conclusion
Our findings suggest that henicosane 1) and decahydroisoquinoline 2) isolated from the leaves of G. uralensis are valuable anti-inflammatory metabolites. This study supports the pharmacological importance of the non-traditional aerial parts of G. uralensis as potential sources of new natural compounds for the treatment of inflammation. Secondly, these aerial parts, which are currently considered an agro-industrial waste, can be used to recover liquiritin and some other medicinally valuable components.
Data Availability Statement
The datasets presented in this study can be found in online repositories. The names of the repository/repositories and accession number(s) can be found in the article/Supplementary Material.
Author Contributions
Validation, BL; Investigation, WA, AA, SA, XH; Resources, NY, WK; Data Curation, SA, TA, BL; Visualization, XH; Project Administration, XH; Funding Acquisition, XH; manuscript writing and editing XH, WA, MF, AS.
Funding
This research was supported by Hubei Technology Innovation Center for Agricultural Sciences-‘2020 key technology research and demonstration project of safe and efficient production of genuine medicinal materials’ (No. 2020-620-000-002-04), National Key R&D Program of China (No. 2017YFD0501500), and the Fundamental Research Funds for the Central Universities program (No. 2662017PY110 and 2662015PY181) to XH. The project was supported by Health Commission of Hubei Province (ZY 2019M032) to JC. The research was also supported by National Science and Technology Fundamental Resources Investigation Program of China (No. 2018FY100704 to XL).
Conflict of Interest
The authors declare that the research was conducted in the absence of any commercial or financial relationships that could be construed as a potential conflict of interest.
Supplementary Material
The Supplementary Material for this article can be found online at: https://www.frontiersin.org/articles/10.3389/fphar.2021.658670/full#supplementary-material
References
Abu-Reidah, I. M., del Mar Contreras, M., Arráez-Román, D., Fernández-Gutiérrez, A., and Segura-Carretero, A. (2014). UHPLC-ESI-QTOF-MS-based Metabolic Profiling ofVicia fabaL. (Fabaceae) Seeds as a Key Strategy for Characterization in Foodomics. Electrophoresis 35, 1571–1581. doi:10.1002/elps.201300646
Ahn, J. Y., Kim, S., Jung, S. E., and Ha, T. Y. (2010). Effect of Licorice (Glycyrrhiza Uralensis Fisch) on Amyloid-β-Induced Neurotoxicity in PC12 Cells. Food Sci. Biotechnol. 19, 1391–1395. doi:10.1007/s10068-010-0198-4
Aiello, A., Carbonelli, S., Esposito, G., Fattorusso, E., Iuvone, T., and Menna, M. (2000). Novel Bioactive Sulfated Alkene and Alkanes from the Mediterranean AscidianHalocynthia Papillosa. J. Nat. Prod. 63, 1590–1592. doi:10.1021/np000281o
Ammar, S., Contreras, M. d. M., Gargouri, B., Segura-Carretero, A., and Bouaziz, M. (2017). RP-HPLC-DAD-ESI-QTOF-MS Based Metabolic Profiling of the Potential Olea Europaea By-Product "wood" and its Comparison with Leaf Counterpart. Phytochem. Anal. 28, 217–229. doi:10.1002/pca.2664
Ayeka, P. A., Bian, Y. H., Githaiga, P. M., and Zhao, Y. (2017). The Immunomodulatory Activities of Licorice Polysaccharides (Glycyrrhiza Uralensis Fisch.) in CT 26 Tumor-Bearing Mice. Bmc Complement. Altern. Med. 17, 536. doi:10.1186/s12906-017-2030-7
Bai, H., Bao, F., Fan, X., Han, S., Zheng, W., Sun, L., et al. (2020). Metabolomics Study of Different Parts of Licorice from Different Geographical Origins and Their Anti‐inflammatory Activities. J. Sep. Sci. 43, 1593–1602. doi:10.1002/jssc.201901013
Bala, M., Kumar, S., Pratap, K., Verma, P. K., Padwad, Y., and Singh, B. (2019). Bioactive Isoquinoline Alkaloids from Cissampelos Pareira. Nat. Prod. Res. 33, 622–627. doi:10.1080/14786419.2017.1402319
Batkhuu, J., Hattori, K., Takano, F., Fushiya, S., Oshiman, K.-i., and Fujimiya, Y. (2002). Suppression of NO Production in Activated Macrophages In Vitro and Ex Vivo by Neoandrographolide Isolated from Andrographis Paniculata. Biol. Pharm. Bull. 25, 1169–1174. doi:10.1248/bpb.25.1169
Bell, L. W., Bennett, R. G., Ryan, M. H., and Clarke, H. (2011). The Potential of Herbaceous Native Australian Legumes as Grain Crops: a Review. Renew. Agric. Food Syst. 26, 72–91. doi:10.1017/s1742170510000347
Bush, R. T., and McInerney, F. A. (2013). Leaf Wax N-Alkane Distributions in and across Modern Plants: Implications for Paleoecology and Chemotaxonomy. Geochimica et Cosmochimica Acta 117, 161–179. doi:10.1016/j.gca.2013.04.016
Coates, R. C., Podell, S., Korobeynikov, A., Lapidus, A., Pevzner, P., Sherman, D. H., et al. (2014). Characterization of Cyanobacterial Hydrocarbon Composition and Distribution of Biosynthetic Pathways. PLOS one 9, e85140. doi:10.1371/journal.pone.0085140
De, B. D., Schulze, A. E., Joubert, E., De, V. A., Malherbe, C. J., and Stander, M. A. (2012). Food Ingredient Extracts of Cyclopia Subternata (Honeybush): Variation in Phenolic Composition and Antioxidant Capacity. Molecules 17, 14602–14624.
Decano, J. L., Mattson, P. C., and Aikawa, M. (2016). Macrophages in Vascular Inflammation: Origins and Functions. Curr. Atheroscler. Rep. 18, 34. doi:10.1007/s11883-016-0585-2
Dong, Y., Zhao, M., Zhao, T., Feng, M., Chen, H., Zhuang, M., et al. (2014). Bioactive Profiles, Antioxidant Activities, Nitrite Scavenging Capacities and Protective Effects on H2O2-Injured PC12 Cells of Glycyrrhiza Glabra L. Leaf and Root Extracts. Molecules 19, 9101–9113. doi:10.3390/molecules19079101
Farag, M. A., Porzel, A., and Wessjohann, L. A. (2012). Comparative Metabolite Profiling and Fingerprinting of Medicinal Licorice Roots Using a Multiplex Approach of GC-MS, LC-MS and 1D NMR Techniques. Phytochemistry 76, 60–72. doi:10.1016/j.phytochem.2011.12.010
Fujiwara, N., and Kobayashi, K. (2005). Macrophages in Inflammation. Cdtia 4, 281–286. doi:10.2174/1568010054022024
Fukai, T., Qing-Hua, W., and Nomura, T. (1991). Six Prenylated Phenols from Glycyrrhiza Uralensis. Phytochemistry 30, 1245–1250. doi:10.1016/s0031-9422(00)95210-5
Gao, L-N., Zhou, X., Zhang, Y., Cui, Y-L., Yu, C-Q., and Gao, S. (2015). The Anti-inflammatory Activities of Ethanol Extract from Dan-Lou Prescription In Vivo and In Vitro. BMC Complement. Altern. Med. 15, 317. doi:10.1186/s12906-015-0848-4
Ghasemi Pirbalouti, A., Fatahi-Vanani, M., Craker, L., and Shirmardi, H. (2014). Chemical Composition and Bioactivity of Essential Oils ofHypericum Helianthemoides. Hypericum perforatumandHypericum Scabrum. Pharm. Biol. 52, 175–181. doi:10.3109/13880209.2013.821663
Gou, S.-h., He, M., Li, B.-b., Zhu, N.-y., and Ni, J.-m. (2020). Hepatoprotective Effect of Total Flavonoids from Glycyrrhiza Uralensis Fisch in Liver Injury Mice. Nat. Product. Res., 1–5. doi:10.1080/14786419.2020.1824223
Haider, S., Chittiboyina, A. G., and Khan, I. A. (2018). Effective Synthetic Strategies for the Construction of Isoquinoline Scaffold Found in Biologically Active Natural Products. Coc 22, 148–164. doi:10.2174/1385272821666171005150423
Hefny Gad, M., Tuenter, E., El-Sawi, N., Younes, S., El-Ghadban, E.-M., Demeyer, K., et al. (2018). Identification of Some Bioactive Metabolites in a Fractionated Methanol Extract fromIpomoea aquatica(Aerial Parts) through TLC, HPLC, UPLC-ESI-QTOF-MS and LC-SPE-NMR Fingerprints Analyses. Phytochem. Anal. 29, 5–15. doi:10.1002/pca.2709
Horai, H., Arita, M., Kanaya, S., Nihei, Y., Ikeda, T., Suwa, K., et al. (2010). MassBank: a Public Repository for Sharing Mass Spectral Data for Life Sciences. J. Mass. Spectrom. 45, 703–714. doi:10.1002/jms.1777
Iranshahy, M., Quinn, R. J., and Iranshahi, M. (2014). Biologically Active Isoquinoline Alkaloids with Drug-like Properties from the Genus Corydalis. RSC Adv. 4, 15900–15913. doi:10.1039/c3ra47944g
Isbrucker, R. A., and Burdock, G. A. (2006). Risk and Safety Assessment on the Consumption of Licorice Root (Glycyrrhiza sp.), its Extract and Powder as a Food Ingredient, with Emphasis on the Pharmacology and Toxicology of Glycyrrhizin. Regul. Toxicol. Pharmacol. 46, 167–192. doi:10.1016/j.yrtph.2006.06.002
Ji, S., Li, Z., Song, W., Wang, Y., Liang, W., Li, K., et al. (2016). Bioactive Constituents of Glycyrrhiza uralensis(Licorice): Discovery of the Effective Components of a Traditional Herbal Medicine. J. Nat. Prod. 79, 281–292. doi:10.1021/acs.jnatprod.5b00877
Jia, S. S., Ma, C. M., Li, Y. H., and Hao, J. H. (1992). [Glycosides of Phenolic Acid and Flavonoids from the Leaves of Glycyrrhiza Uralensis Ficsh]. Yao Xue Xue Bao 27, 441–444.
Jin, M., Moon, T. C., Quan, Z., Lee, E., Kim, Y. K., Yang, J. H., et al. (2008). The Naturally Occurring Flavolignan, Deoxypodophyllotoxin, Inhibits Lipopolysaccharide-Induced iNOS Expression through the NF-Κb Activation in RAW264.7 Macrophage Cells. Biol. Pharm. Bull. 31, 1312–1315. doi:10.1248/bpb.31.1312
Khan, A. Y., and Suresh Kumar, G. (2015). Natural Isoquinoline Alkaloids: Binding Aspects to Functional Proteins, Serum Albumins, Hemoglobin, and Lysozyme. Biophys. Rev. 7, 407–420. doi:10.1007/s12551-015-0183-5
Kitagawa, I., Hori, K., Sakagami, M., Zhou, J.-L., and Yoshikawa, M. (1993). Saponin and Sapogenol. XLVIII. On the Constituents of the Roots of Glycyrrhiza Uralensis FISCHER from Northeastern China. (2). Licorice-Saponins D3, E2, F3, G2, H2, J2, and K2Licorice-Saponins D3, E2, F3, G2, H2, J2, and K2. Chem. Pharm. Bull. 41, 1337–1345. doi:10.1248/cpb.41.1337
Laskin, D. L., and Pendino, K. J. (1995). Macrophages and Inflammatory Mediators in Tissue Injury. Annu. Rev. Pharmacol. Toxicol. 35, 655–677. doi:10.1146/annurev.pa.35.040195.003255
Lee, H. J., Jeong, H. S., Kim, D. J., Noh, Y. H., Yuk, D. Y., and Hong, J. T. (2008). Inhibitory Effect of Citral on NO Production by Suppression of iNOS Expression and NF-Κb Activation in RAW264.7 Cells. Arch. Pharm. Res. 31, 342–349. doi:10.1007/s12272-001-1162-0
MacMicking, J., Xie, Q.-w., and Nathan, C. (1997). Nitric Oxide and Macrophage Function. Annu. Rev. Immunol. 15, 323–350. doi:10.1146/annurev.immunol.15.1.323
Mari, A., Lyon, D., Fragner, L., Montoro, P., Piacente, S., Wienkoop, S., et al. (2013). Phytochemical Composition of Potentilla Anserina L. Analyzed by an Integrative GC-MS and LC-MS Metabolomics Platform. Metabolomics 9, 599–607. doi:10.1007/s11306-012-0473-x
Mathis, C., and Ourisson, G. (1964). Étude chimio-taxonomique du genre Hypericum. Phytochemistry 3, 115–131. doi:10.1016/s0031-9422(00)84003-0
Molina-Calle, M., de Medina, V. S., Priego-Capote, F., and de Castro, M. D. L. (2017). Establishing Compositional Differences between Fresh and Black Garlic by a Metabolomics Approach Based on LC-QTOF MS/MS Analysis. J. Food Compost. Anal. 62, 155–163. doi:10.1016/j.jfca.2017.05.004
Montoro, P., Maldini, M., Russo, M., Postorino, S., Piacente, S., and Pizza, C. (2011). Metabolic Profiling of Roots of Liquorice (Glycyrrhiza Glabra) from Different Geographical Areas by ESI/MS/MS and Determination of Major Metabolites by LC-ESI/MS and LC-ESI/MS/MS. J. Pharm. Biomed. Anal. 54, 535–544. doi:10.1016/j.jpba.2010.10.004
Mosser, D. M., and Edwards, J. P. (2008). Exploring the Full Spectrum of Macrophage Activation. Nat. Rev. Immunol. 8, 958–969. doi:10.1038/nri2448
Muema, J. M., Bargul, J. L., Nyanjom, S. G., Mutunga, J. M., and Njeru, S. N. (2017). Potential of green tea (Camellia sinensis) proanthocyanidins-rich fraction for controlling malaria mosquito populations through disruption of larval development. Proceedings of the Africa Health Agenda International Conference, Nairobi, 7–9 March 2017.
Nathan, C., and Xie, Q. W. (1994). Regulation of Biosynthesis of Nitric Oxide. J. Biol. Chem. 269, 13725–13728. doi:10.1016/s0021-9258(17)36703-0
Nomura, T., Fukai, T., and Akiyama, T. (2002). Chemistry of Phenolic Compounds of Licorice (Glycyrrhiza Species) and Their Estrogenic and Cytotoxic Activities. Pure Appl. Chem. 74, 1199–1206. doi:10.1351/pac200274071199
Peng, J., Zheng, T-T., Li, X., Liang, Y., Wang, L-J., Huang, Y-C., et al. (2019). Plant-derived Alkaloids: the Promising Disease-Modifying Agents for Inflammatory Bowel Disease. Front. Pharmacol. 10, 351. doi:10.3389/fphar.2019.00351
Siracusa, L., Saija, A., Cristani, M., Cimino, F., D'Arrigo, M., Trombetta, D., et al. (2011). Phytocomplexes from Liquorice (Glycyrrhiza Glabra L.) Leaves - Chemical Characterization and Evaluation of Their Antioxidant, Anti-genotoxic and Anti-inflammatory Activity. Fitoterapia 82, 546–556. doi:10.1016/j.fitote.2011.01.009
Song, W., Qiao, X., Chen, K., Wang, Y., Ji, S., Feng, J., et al. (2017). Biosynthesis-based Quantitative Analysis of 151 Secondary Metabolites of Licorice to Differentiate Medicinal glycyrrhiza Species and Their Hybrids. Anal. Chem. 89 (5), 3146–3153. doi:10.1021/acs.analchem.6b04919
Wang, C., Chen, L., Xu, C., Shi, J., Chen, S., Tan, M., et al. (2020). A Comprehensive Review for Phytochemical, Pharmacological, and Biosynthesis Studies onGlycyrrhizaspp. Am. J. Chin. Med. 48, 17–45. doi:10.1142/s0192415x20500020
Weckwerth, W., Wenzel, K., and Fiehn, O. (2004). Process for the Integrated Extraction, Identification and Quantification of Metabolites, Proteins and RNA to Reveal Their Co-regulation in Biochemical Networks. Proteomics 4, 78–83. doi:10.1002/pmic.200200500
Wu, C-Y., Lin, Y-S., Yang, Y-H., Shu, L-H., Cheng, Y-C., and Te Liu, H. (2020). Potential Simultaneous Inhibitors of Angiotensin-Converting Enzyme 2 and Transmembrane Protease, Serine 2. Front. Pharmacol. 11, 584158. doi:10.3389/fphar.2020.584158
Yoshitake, J., Kato, K., Yoshioka, D., Sueishi, Y., Sawa, T., Akaike, T., et al. (2008). Suppression of NO Production and 8-nitroguanosine Formation by Phenol-Containing Endocrine-Disrupting Chemicals in LPS-Stimulated Macrophages: Involvement of Estrogen Receptor-dependent or -independent Pathways. Nitric Oxide 18, 223–228. doi:10.1016/j.niox.2008.01.003
Yu, P., Li, Q., Feng, Y., Chen, Y., Ma, S., and Ding, X. (2021). Quantitative Analysis of Flavonoids in Glycyrrhiza Uralensis Fisch by 1H-qNMR. J. Anal. Methods Chem. 2021, 1–6. doi:10.1155/2021/6655572
Yu, S., Yan, H., Zhang, L., Shan, M., Chen, P., Ding, A., et al. (2017). A Review on the Phytochemistry, Pharmacology, and Pharmacokinetics of Amentoflavone, a Naturally-Occurring Biflavonoid. Molecules 22, 299. doi:10.3390/molecules22020299
Yuldashev, M. P. (1998). Flavonoids of the Epigeal Part ofGlycyrrhiza Uralensis. Chem. Nat. Compd. 34, 508–509. doi:10.1007/bf02329609
Zarubaev, V. V., Anikin, V. B., and Smirnov, V. S. (2016). Anti-viral Activity of Glycirrhetinic and Glycirrhizic Acids. iimm 6, 199–206. doi:10.15789/2220-7619-2016-3-199-206
Zhang, Q., and Ye, M. (2009). Chemical Analysis of the Chinese Herbal Medicine Gan-Cao (Licorice). J. Chromatogr. A 1216, 1954–1969. doi:10.1016/j.chroma.2008.07.072
Zhang, X., Li, N., Shao, H., Meng, Y., Wang, L., Wu, Q., et al. (2016). Methane Limit LPS-Induced NF-κB/MAPKs Signal in Macrophages and Suppress Immune Response in Mice by Enhancing PI3K/AKT/GSK-3β-mediated IL-10 Expression. Scientific Rep. 6, 1–14. doi:10.1038/srep29359
Keywords: licorice, inflammation, henicosane, pro-inflammatory cytokines, metabolomics
Citation: Jiang L, Akram W, Luo B, Hu S, Faruque MO, Ahmad S, Yasin NA, Khan WU, Ahmad A, Shikov AN, Chen J and Hu X (2021) Metabolomic and Pharmacologic Insights of Aerial and Underground Parts of Glycyrrhiza uralensis Fisch. ex DC. for Maximum Utilization of Medicinal Resources. Front. Pharmacol. 12:658670. doi: 10.3389/fphar.2021.658670
Received: 29 January 2021; Accepted: 12 May 2021;
Published: 01 June 2021.
Edited by:
Fawzi Mohamad Mahomoodally, University of Mauritius, MauritiusReviewed by:
Steven Dentali, Dentali Botanical Sciences, United StatesWei Sun, China Academy of Chinese Medical Sciences, China
Copyright © 2021 Jiang, Akram, Luo, Hu, Faruque, Ahmad, Yasin, Khan, Ahmad, Shikov, Chen and Hu. This is an open-access article distributed under the terms of the Creative Commons Attribution License (CC BY). The use, distribution or reproduction in other forums is permitted, provided the original author(s) and the copyright owner(s) are credited and that the original publication in this journal is cited, in accordance with accepted academic practice. No use, distribution or reproduction is permitted which does not comply with these terms.
*Correspondence: Jian Chen, Y2hlbmoyMDE1QDE2My5jb20=; Xuebo Hu, eHVlYm9odUBtYWlsLmh6YXUuZWR1LmNu