- 1College of Traditional Chinese Medicine, Jinan University, Guangzhou, China
- 2Medical College, Sun Yat-sen University, Guangzhou, China
Coronavirus Disease 19 (COVID-19) is a respiratory disease caused by severe acute respiratory syndrome coronavirus 2 (SARS-CoV-2), which has grown to a worldwide pandemic with substantial mortality. The symptoms of COVID-19 range from mild flu-like symptoms, including cough and fever, to life threatening complications. There are still quite a number of patients with COVID-19 showed enteric symptoms including nausea, vomiting, and diarrhea. The gastrointestinal tract may be one of the target organs of SARS-CoV-2. Angiotensin converting enzyme 2 (ACE2) is the main receptor of SARS-CoV-2 virus, which is significantly expressed in intestinal cells. ACE2 links amino acid malnutrition to microbial ecology and intestinal inflammation. Intestinal flora imbalance and endotoxemia may accelerate the progression of COVID-19. Many herbs have demonstrated properties relevant to the treatment of COVID-19, by supporting organs and systems of the body affected by the virus. Herbs can restore the structure of the intestinal flora, which may further modulate the immune function after SARS-CoV-2 infection. Regulation of intestinal flora by herbal medicine may be helpful for the treatment and recovery of the disease. Understanding the role of herbs that regulate intestinal flora in fighting respiratory virus infections and maintaining intestinal flora balance can provide new ideas for preventing and treating COVID-19.
Introduction
Coronaviruses are mainly divided into four genera, alpha (α), beta (β), gamma (γ), and delta (δ), which can infect humans and a variety of animals. The seven human coronaviruses (HCoV) that have been discovered are HCoV-229E, HCoV-NL63, HCoV-OC43, HCoV-HKU1, Severe acute respiratory syndrome coronavirus (SARS-CoV), Middle East respiratory syndrome coronavirus (MERS-CoV), and SARS-CoV-2. Coronaviruses can cause respiratory as well as gastrointestinal infections in humans and animals (Su et al., 2016). In addition to the respiratory system, the digestive tract is most commonly affected by coronavirus infections. The symptoms mainly include abdominal pain, nausea, vomiting, and diarrhea. Human gastrointestinal cells were highly susceptible to MERS-CoV, and the virus was able to maintain their replication robustly in small intestine cells (Xiong et al., 2020). About 1/3 of patients with Middle East respiratory syndrome (MERS) have gastrointestinal symptoms (Matoba et al., 2015; Corman et al., 2018). SARS-CoV-2 is an enveloped, positively charged single-stranded RNA virus belonging to the genus Coronavirus. SARS-CoV-2 is highly homologous to SARS coronavirus, and its nucleic acid sequence similarity reaches 70%. Similar to SARS virus infection, respiratory tract infection symptoms such as fever and cough are one of the most common clinical manifestations of COVID-19 patients (Zhou et al., 2020). Still, a considerable number of patients will also have gastrointestinal symptoms such as diarrhea (Zhang et al., 2020a).
Angiotensin-converting enzyme 2 (ACE2) is the primary receptor of the SARS-Cov-2 virus. ACE2 is significantly expressed in alveolar type II cells and intestinal cells (Hashimoto et al., 2012). Current studies believe that the gastrointestinal tract is one of the target organs of SARS-CoV-2 (Jin et al., 2020a). The positive viral RNA test in the stool of COVID-19 patients also confirms this to a certain extent and suggests SARS-CoV-2 transmission through the fecal-oral route. It undoubtedly poses a further challenge to the prevention and control of the COVID-19 epidemic. Although the respiratory symptoms related to COVID-19 have attracted significant attention, gastrointestinal symptoms are often overlooked, which can easily lead to a missed diagnosis. This review summarizes COVID-19, SARS, and MERS's clinical features associated with gastrointestinal symptoms, the related mechanisms of changes in the intestinal flora, and related herbs that can regulate intestinal flora. Understanding the gastrointestinal symptoms and possible mechanisms of COVID-19 is of great clinical significance for the early diagnosis, treatment, and control of the disease.
Clinical Features of Coronavirus Disease 19, Severe Acute Respiratory Syndrome, and Middle East Respiratory Syndrome With Gastrointestinal Symptoms
COVID-19 is mainly manifested by fever, fatigue, and dry cough, but some patients have abdominal pain, nausea, vomiting, diarrhea, and other symptoms. According to relevant reports on COVID-19 symptoms, the number, range, and severity of COVID-19-related symptoms may vary from person to person. Overall, our symptom analysis of independently published studies (Booth et al., 2003; Chan et al., 2003; Choi et al., 2003; Lee et al., 2003; Peiris et al., 2003a; Peiris et al., 2003b; Poutanen et al., 2003; Rainer et al., 2003; Tsang et al., 2003; Zaki et al., 2012; Assiri et al., 2013a; Assiri et al., 2013b; Memish et al., 2013; Al-Tawfiq et al., 2014; Saad et al., 2014; Noorwali et al., 2015; Oboho et al., 2015; Yin and Wunderink, 2018; Cattelan et al., 2020; Chen et al., 2020; Cummings et al., 2020; Guan et al., 2020a; Guan et al., 2020b; Huang C. et al., 2020; Li et al., 2020a; Qian et al., 2020; Shi S. et al., 2020; Suleyman et al., 2020; Wang D. et al., 2020; Wang Z. et al., 2020; Xu X. et al., 2020; Zhang et al., 2020a; Zhou et al., 2020) involving thousands of people shows that a small proportion but large number of patients experience gastrointestinal problems (Figure 1).
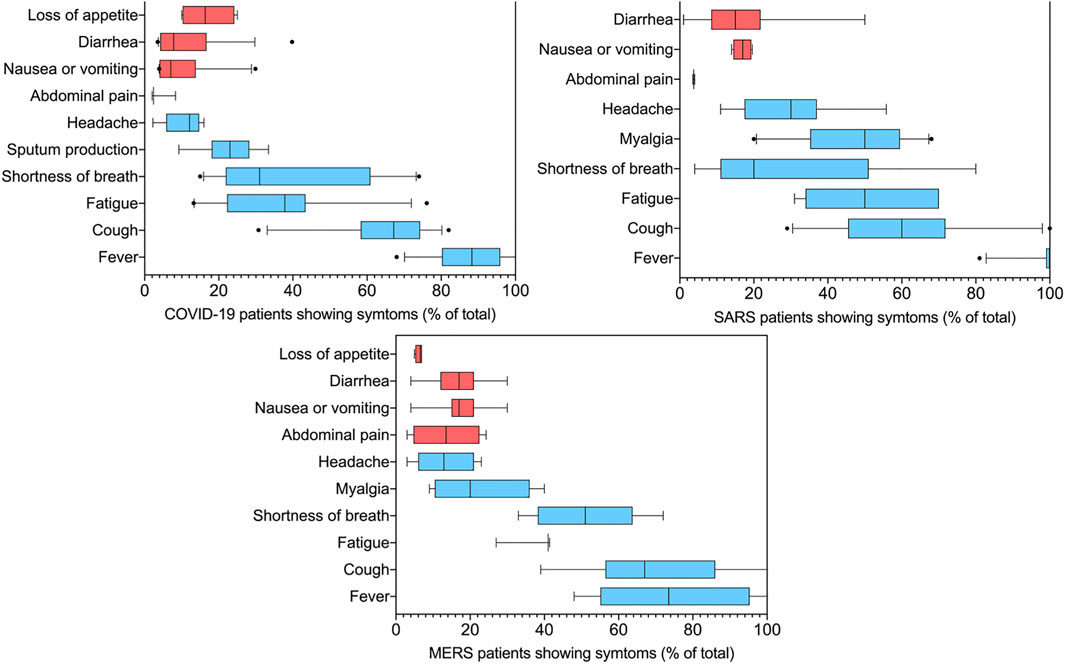
FIGURE 1. Summary of symptoms feature of COVID-19, SARS, and MERS. The data is the summary of 16 independent reports involving a total of 7322 COVID-19 patients, 10 independent reports involving a total of 1299 SARS patients, nine independent reports involving a total of 783 MERS patients. The red bars are those associated with gastrointestinal problems. In the box plots, the boundary of the box closest to zero indicates the 25th percentile, a black line within the box marks the median, and the boundary of the box farthest from zero indicates the 75th percentile. Whiskers above and below the box indicate the 10th and 90th percentiles. Points above and below the whiskers indicate outliers outside the 10th and 90th percentiles.
Among the 1,299 hospitalized patients with SARS included in the statistics, 514 (39%) developed gastrointestinal symptoms, included diarrhea (7–20%) (Booth et al., 2003; Chan et al., 2003; Peiris et al., 2003b; Tsang et al., 2003), there are also reports that a 50% (Poutanen et al., 2003) probability of appearing, nausea or vomiting (12–20%) (Lee et al., 2003; Yin and Wunderink, 2018). Similarly, in every two of the MERS inpatients, a gastrointestinal symptom occurs. Including loss of appetite (4–6%) (Assiri et al., 2013b; Oboho et al., 2015), nausea or vomiting (7–28%) (Assiri et al., 2013a; Oboho et al., 2015; Yin and Wunderink, 2018), abdominal pain (3–24%) (Assiri et al., 2013b; Memish et al., 2013) and diarrhea (5–30%) (Al-Tawfiq et al., 2014; Saad et al., 2014), the incidence of diarrhea was also reported as 75% (Memish et al., 2013). Consistent with SARS-CoV-1 and MERS-CoV, among a total of 7,322 COVID-19 patients, 1,104 patients suffered gastrointestinal problems, as high as 15% of cases. According to the report, 9–26% (Qian et al., 2020; Suleyman et al., 2020) patients loss their appetite (median 17%), 2–31% (Chen et al., 2020; Huang C. et al., 2020) had diarrhea (median 9.8%) with an outlier of the 40% (Zhang et al., 2020a) and 4–30% (Li et al., 2020b; Xu X. et al., 2020; Zhou et al., 2020)suffered from nausea or vomiting (median 6%). Incidence of gastrointestinal complaints, vomiting, and diarrhea caused by SARS-CoV-2 is similar to SARS-CoV-1 and MERS-CoV. Patients in severe or critical condition have the highest incidence of diarrhea (Guan et al., 2020b). In rare cases (Lee et al., 2020; Pan et al., 2020), diarrhea is the only symptom of COVID-19. The autopsy study of patients who died of COVID-19 also found that the small intestine showed segmental dilation and narrow changes (Wichmann et al., 2020). It is shown that the SARS-CoV-2 attacks not only the lungs but also the GI track. Theoretically, SARS-CoV-2 can bind to the angiotensin-converting enzyme 2 (ACE2) receptors in the intestinal cells, thus causing gastrointestinal disease and gastrointestinal symptoms such as abdominal pain and diarrhea.
Effect of Severe Acute Respiratory Syndrome Coronavirus 2 on Human Intestinal Tract
Severe Acute Respiratory Syndrome Coronavirus 2 Infection Association With Intestinal Flora Dysbiosis and Intestinal Barrier Disruption
At present, SARS-CoV-2 has been found and isolated in stool samples of patients with COVID-19 (Tang et al., 2020; Mohan et al., 2021), and changes in intestinal flora have been found in COVID-19 patients with gastrointestinal symptoms (Dhar and Mohanty, 2020). It is suggested that SARS-CoV-2 may cause intestinal flora imbalance while causing lung infection. The intestinal flora is composed of bacteria, viruses, fungi, and archaea. Viral infections can cause changes in the composition of intestinal flora, thereby causing intestinal barrier disfunction (Eckburg et al., 2005; Qin et al., 2015; Thaiss et al., 2016; Deng et al., 2020). Intestinal flora has a closely relationship with the expression of tight junction proteins in the intestinal epithelial cells. The dysbiosis of intestinal flora is accompanied by decreased expression of intestinal tight junction proteins such as Claudin-1, Occludin and ZO-1, leading to the disruption of intestinal barrier. The intestinal barrier function requires the complexity of epithelial, which relies on the differentiation of intestinal stem cells (Yu et al., 2020). ACE2 may dictate the stemness of intestinal stem cells by orchestrating calcium perturbation (Yu et al., 2020). For patients in severe or critical condition, the disordered intestinal flora leads to abnormal intestinal inflammation, which affects the gut-lung axis and aggravates the degree of systemic inflammation during the disease (Schuijt et al., 2016; Zhu et al., 2018). Unstable gut mycobiomes and prolonged dysbiosis persisted in patients with COVID-19 after nasopharyngeal clearance of SARS-CoV-2 (Zuo et al., 2020). A. flavus and Aspergillus niger, were detected in fecal samples from patients with COVID-19, even after clearance of SARS-CoV-2 from nasopharyngeal samples and resolution of respiratory symptoms (Zuo et al., 2020). In patients with SARS-CoV-2 infection accompanied by abdominal pain, diarrhea and other gastrointestinal symptoms, the probiotics such as Lactobacillus or Bifidobacterium in the intestines are significantly reduced (Xu K. et al., 2020). Nutritional support, and supplementation of probiotics can reduce bacterial translocation and secondary intestinal infections (Dhar and Mohanty, 2020). China’s Guidelines for the diagnosis and treatment of novel coronavirus pneumonia (version sixth) mentioned that herbal medicine with prebiotic effect could be used to maintain the intestinal flora homeostasis and prevent secondary bacterial infections.
Severe Acute Respiratory Syndrome Coronavirus 2’s Mechanism of Effect on Intestinal Mucosa-Associated Immune System
The SARS-CoV-2 Spike Glycoprotein can bind to the ACE2 receptor on intestinal epithelial cells’ surface (Walls et al., 2020). ACE2 is expressed in lung cells and intestinal epithelial cells of the esophagus, ileum, and colon. According to the combined data from human protein atlas (http://www.proteinatlas.org), genotype tissue expression and mammalian genome function annotations, the top three tissues with the highest expression levels belong to intestinal tissues (Figure 2). Therefore, SARS-CoV-2 not only infects the respiratory system but may also directly affect the GI system. ACE2 controls the functional expression in the intestines of one of the transport proteins, B0AT1, which acts specifically on neutral amino acids. (Scalise and Indiveri, 2020). The ACE2-B0AT1 exists as a dimer of heterodimers.
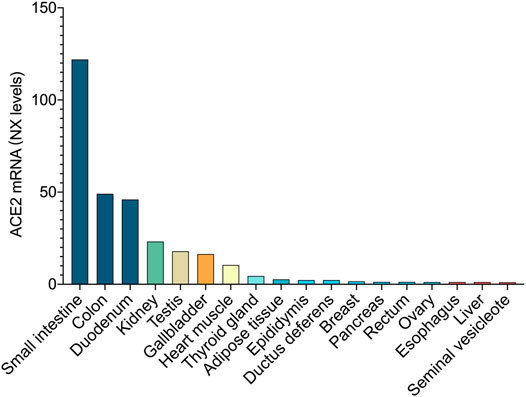
FIGURE 2. ACE2 expression at the mRNA level. In combined data from Human Protein Atlas, Genotype Tissue Expression, and Functional Annotation of The Mammalian Genome, the top three tissues with the highest expression belong to the intestinal tissue.
Deficiency of ACE2 can also causes a critical impairment of local tryptophan homeostasis which alters the susceptibility to intestinal inflammation (Vuille-dit-Bille et al., 2015). Dietary tryptophan is primarily absorbed via the B0AT1/ACE2 transport pathway on the luminal surface of small intestinal epithelial cells. These differences were reduced when the diet of the ACE2 mutant mice was supplemented with a source of tryptophan (Izcue and Powrie, 2012). Tryptophan has major effects on the host and notably on immunity and metabolism, gut microbiota, intestinal barrier, and transit (Gao et al., 2018; Taleb, 2019). Under normal physiological conditions, the barrier function of the intestinal mucosa is relatively complete, which can effectively prevent the invasion of harmful substances and maintain the stability of the body environment. The intestinal mucosal immune barrier is composed of gut associated lymphoid tissue (GALT) and diffuse immune cells (mainly refers to lamina propria lymphocytes and intraepithelial lymphocytes). GALT can bind to the antigen to produce secretory immunoglobulin A (SIgA). Intestinal mucosal intraepithelial lymphocytes (IEL) and lamina propria lymphocytes (LPL) is the efferent effect and regulatory site of the mucosal immune system (Qiao et al., 1991; Abuzakouk et al., 1998). The stimulated IEL (mainly T lymphocytes) can proliferate rapidly and release a variety of cytokines such as IL-2, IFN-γ, TNF-α. It has the functions of defense against intestinal pathogen invasion, anti-mucosal allergic reaction, suppression of immune response, elimination of damaged epithelial cells and promotion the production of SIgA (Viney and MacDonald, 1990; Olivares-Villagomez and Van Kaer, 2018). LPL (mainly T lymphocytes, B lymphocytes, macrophages, dendritic cells) can secrete a variety of Th2-type cytokines such as TNF, IL-4, IL-6, and IL-10. It contains a large number of plasma cells, which can promote the secretion of Ig A antibodies, neutralize and eliminate antigens. SIgA dimer exists on the surface of the gastrointestinal mucosa and is the main effector molecule of the intestinal mucosal immune response. It can prevent the invasion and adhesion of pathogens, and can bind the corresponding antigen to inhibit bacterial proliferation and neutralize toxins, and can resist proteolytic enzymes. Protect the intestinal mucosa from being digested, and exert local anti-infection and anti-allergic effects (Pal et al., 2013; Zhang J. et al., 2020).
In the absence of ACE2, the expression of the mTOR signaling pathway in the small intestine is reduced, resulting in a decrease in the expression of antimicrobial peptides in the Paneth cells of the small intestine (Hashimoto et al., 2012). The antimicrobial peptides in Paneth cells in the small intestine can change the composition of the intestinal flora and increase intestinal inflammation. Inhibition of the expression of antimicrobial peptides will lead to a sharp increase in the probability of endotoxin and endogenous infection, thereby producing and promoting inflammatory mediators. The cascade reaction caused by the inhibition of the expression of antimicrobial peptides leads to extensive tissue damage, gastrointestinal symptoms, and even multiple organ failure (MOF), leading to a poor prognosis for COVID-19 patients.
As a carboxypeptidase, ACE2 can catalyze Ang Ⅱ to Ang (1-7), which further binds to the cell surface receptor MAS to establish a second axis through ACE2/ANG-(1-7)/MAS, whose end point is the metabolite ANG-(1-7). The downstream ERK, P38, and JNK signaling pathways are regulated by Mas, which plays a protective role in inhibiting the inflammatory response (Passos-Silva et al., 2013). Besides, Ang (1-7) can also directly inhibit or promote the release of nitric oxide from intestinal smooth muscle cells through Mas receptors (Santos et al., 2013), thereby intervening in the activation Ang (1-7) of NF-κB signaling pathways and reducing intestinal inflammatory damage (Souza Santos et al., 2018).
Restoring Intestinal Flora May Help Prevent and Treat Severe Acute Respiratory Syndrome Coronavirus 2 Infections
Restore the intestinal flora and reduce the intestinal barrier disruption may be of great value in preventing and treating SARS-CoV-2 infection. The intestinal flora can affect the occurrence of respiratory diseases through the production of metabolites (Saint-Georges-Chaumet and Edeas, 2016). A variety of specific microorganisms in the intestine can ferment undigested carbohydrates in the small intestine, produce short-chain fatty acids, and participate in energy metabolism (den Besten et al., 2013; Koeth et al., 2013; Ridaura et al., 2013; Koh et al., 2016). The specific microorganisms can enhance the intestinal epithelial barrier function, immune tolerance, maintain intestinal homeostasis, and reduce the occurrence of infection by down-regulating the expression of inflammatory factors. The intestinal flora can also maintain the ratio of helper T lymphocytes (Th) and reduce airway inflammation (Kao et al., 2020; Kreft et al., 2020). The intestinal flora can promotes the preferential differentiation of anti-inflammatory Treg/Th2 cells while suppressing the relative differentiation of pro-inflammatory Th1/Th17 cells (Li K. et al., 2020). Intestinal flora disorders can cause the dominant Th2 response. In general, the administration of targeted microecological preparations such as non-starch polysaccharides or related herbal medicines may have a specific preventive effect on SARS-CoV-2 infection (Li Y. et al., 2020).
For viral infections, though, overuse of antibiotics is ineffective and increases the patient's exposure to antibiotics in the short term. However, for patients with SARS-CoV-2 infection, especially patients with sepsis, empirical antibacterial treatment can be given within 1 h of the initial evaluation according to the China’s Guidelines for the diagnosis and treatment of novel coronavirus pneumonia (version sixth). However, the colonization resistance of the intestine would be destroyed as soon as antibiotics treatment for pathogenic bacterial infections, caused profound and lasting changes in the intestinal flora. Antibiotics can cause changes in the intestinal flora, which can also lead to an increase in opportunistic pathogens (such as Clostridium difficile) and more likely to cause infections. The intestinal flora contains probiotics, which can prevent the colonization of pathogens. Antibiotic treatment destroys the structure of the intestinal flora and reduces resistance to pathogenic bacteria (Knight and Girling, 2003; Li L. et al., 2021). The metabolites of the intestinal flora can also affect the permeability of the host intestinal mucosa barrier (Arpaia et al., 2013). The intestinal mucosal barrier not only participates in the intake of food nutrition and flora metabolites but also has a critical barrier function to prevent microbial invasion and inhibit the inflammatory response to the intestinal contents (Turner, 2009). The intestinal mucosal barrier includes continuous monolayer intestinal epithelial cells and the mucus they secrete. As the recognition site of intestinal microbes, intestinal epithelial cells often interact with microbes and their metabolites to promote the development of the intestinal immune system which participate in immune response and maintain the homeostasis of the intestinal flora (Cario and Podolsky, 2000; Eckburg et al., 2005). The intestinal mucosal immune system is composed of the intestinal epithelium and various secretions on its surface, scattered immune cells, intestinal microbiota, and intestinal-related lymphoid tissues (Hooper et al., 2012). In sepsis, the dysbiosis of intestinal flora will lead to changes in the physiological and anatomical structure of the intestinal mucosal barrier, the proliferation of intestinal cells is significantly reduced, and the apoptosis of intestinal villi and crypt cells is increased dramatically (Weinstein et al., 1975). Intestinal flora and its metabolites play a significant role in maintaining the health of the body.
In patients with SARS-CoV-2 infection, early administration of microecological preparations and prebiotics can restore the balance of intestinal flora and strengthen the intestinal barrier, which is a crucial measure to avoid the aggravation of COVID-19 (Hu et al., 2021). In addition to damages the lungs, SARS-CoV-2 infection cause damage to multiple organs such as the gastrointestinal tract. The change of the intestinal flora is one of its essential pathophysiological mechanisms (Olaimat et al., 2020). The use of prebiotics and herbal medicines with prebiotic effects regulate the intestinal flora, maintain the microecological balance of the gastrointestinal tract, improve the body's immunity, and reduce virus damage to the lungs (Heidari et al., 2021). Prebiotics and herbs with prebiotic effects may help control the progression of severe COVID-19 patients and speed up the recovery process of patients infected with SARS-CoV-2.
Herbal Medicine, Gut Microbiota and Coronavirus Disease 19
Herbal Medicines as Angiotensin-Converting Enzyme 2-Blockers in Intestinal Tract
Angiotensin-converting enzyme 2 (ACE2) efficiently binds the S1 domain of the SARS-CoV S protein. ACE2 is a functional receptor for SARS-CoV. SARS-CoV-2 uses the SARS-CoV receptor ACE2 for entry and the serine protease TMPRSS2 for S protein priming. Several retrospective studies conducted on COVID-19 patients infected with SARS-CoV-2 pointed out that the combination of herbal medicine and Western medicine can significantly improve the clinical symptoms of COVID-19 patients and shorten the treatment time for patients in severe or critical condition (He J. et al., 2020; Lee et al., 2021; Luo et al., 2021). As show in Figure 2, ACE2 is highly expressed in GI tract. Herbs are taken orally and absorbed through the intestine. It is important to understand which herbs and their active ingredients have potential inhibitory effects on ACE2. Here, we list the commonly used herbal prescriptions (Perez-Roses et al., 2015; Saranya et al., 2017; Chuan et al., 2020; Feng et al., 2020; Huang L. et al., 2020; Kim and Kim, 2020; Kim M. et al., 2020; Lin et al., 2020; Liu and Zhang, 2020; Ma J. et al., 2020; Ma Q. et al., 2020; Niu et al., 2020; Qu et al., 2020; Sen et al., 2020; Song et al., 2020; Sun K. et al., 2020; Tao et al., 2020; Wang S. et al., 2020; Wang et al., 2020b; Ya et al., 2020; Yao et al., 2020; Zhang et al., 2020b; Hong et al., 2021; Jia et al., 2021; Li Y. et al., 2021; Lin et al., 2021) in the “China Novel Coronavirus Pneumonia Diagnosis and Treatment Program.” Among them, some herbs with potential ACE2-blockers and their related active ingredients are sorted out in Figure 3. Research results show that the herbs as mentioned above may maintain the balance of RAS-related pathways (Saranya et al., 2017), inhibit the activation of the complement system (Perez-Roses et al., 2015), blunt inflammation (Kim M. et al., 2020; Kim and Kim, 2020), participate in immune regulation (Qu et al., 2020), inhibit the activity of SARS-CoV-2 3C-like protease (Chuan et al., 2020; Feng et al., 2020; Huang L. et al., 2020; Lin et al., 2020; Sen et al., 2020; Tao et al., 2020; Ya et al., 2020), and inhibit the binding process of ACE2 (Hoffmann et al., 2020; Ma Q. et al., 2020; Niu et al., 2020; Song et al., 2020; Wang et al., 2020b).
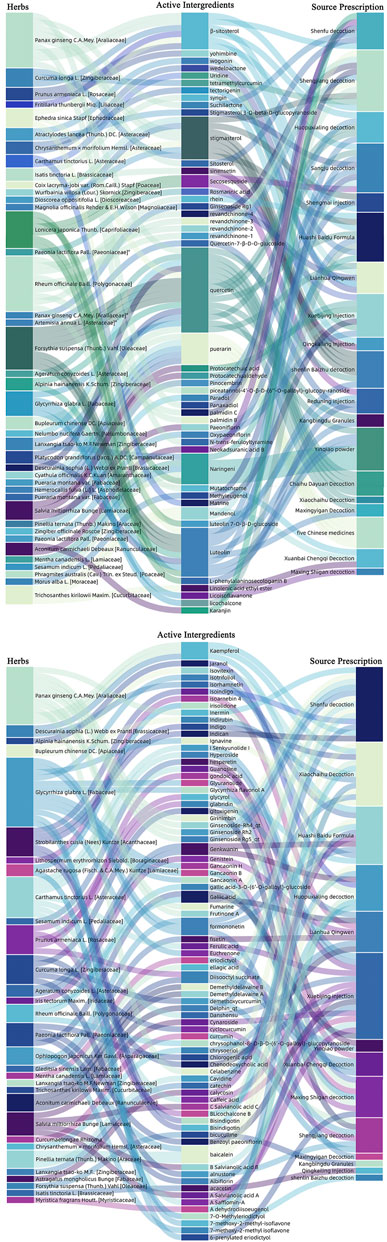
FIGURE 3. Herbs with potential ACE2 receptor blockers, as well as related active ingredients and derivative prescriptions. We analyzed the most commonly used Chinese medicine prescriptions in the “Diagnosis and Treatment Protocol for Novel Coronavirus Pneumonia.” Herbal medicines with potential ACE2 receptor blockers and related active ingredients have been sorted out.
Herbal Medicines Restore the Intestinal Flora
As mentioned above, restoring intestinal flora may help prevent and treat SARS-CoV-2 infections. In clinical practice, herbal medicines are usually extracted by water or ethanol and are mainly taken orally. An intestine is an important place for oral drugs to be metabolized in the body (Feng et al., 2019). After the oral medication, they interact with a large number of microorganisms in the intestine (Hamasaki et al., 2000; Feng et al., 2019). Some types of herbal medicines can regulate the intestinal flora’s composition and metabolism, thereby improving the body's dysfunction and pathological conditions. The intestinal flora can participate in the metabolic transformation of herbs, and at the same time, can transform herbal compounds to improve bioavailability (Zuo et al., 2002).
There are five main categories of herbal ingredients that can affect the intestinal flora, including glycoside, flavonoids, alkaloids, phenylpropanoids, and organic acids. The intestinal flora secretes glycoside hydrolases, lyases and esterases to cut sugar chains to obtain energy. An important type of product produced by polyglycolysis is short-chain fatty acids, including acetic acid, propionic acid and butyric acid. Short-chain fatty acids have a wide range of physiological function (Yang et al., 1996; Abdel-Hafez et al., 1998; Bae et al., 2004; Guo et al., 2012; Feng et al., 2015; Xia et al., 2016; Liu et al., 2019; Shao et al., 2019; Wang et al., 2021). Most flavonoids (except flavanols) naturally combine with sugars to form β-glycosides, and only a small part of flavonoids is absorbed by the small intestine. Most of the glycosylated flavonoids will reach the colon and will be broken down into phenolic acid or other metabolites that can be absorbed by the body under the action of the colonic microflora. Flavonoids existing in the colon have a certain regulatory effect on the intestinal flora, and flavonoids catabolized by microorganisms can change their bioavailability and activity (Lee et al., 2004; Xiuwei et al., 2005; Taiming et al., 2006; Knaup et al., 2007; Shi et al., 2009; Trinh et al., 2010; Zhou et al., 2014; Zhou et al., 2015; Xin et al., 2019; Jin et al., 2020b; Wang et al., 2020a). Alkaloids are a type of nitrogen-containing organic compounds derived from the biological world. They have significant physiological activities and are one of the important components of Chinese medicine. Some alkaloids have a certain degree of hydrophilicity and are also soluble in organic solvents. The structural characteristics of these alkaloids are often small molecules, ether bonds, coordination bonds, etc., which are prone to hydrolysis and dehydration reactions under the action of the intestinal flora (Ying et al., 2002; Weiming, 2005; Huaixia et al., 2006; Yufeng et al., 2008). With a lactone structure, phenylpropanoids is easy to undergo biotransformation such as lactone hydrolysis or demethylation under the action of the intestinal flora (Jan et al., 2009; Zhao et al., 2009). A small amount of organic acids is absorbed in the stomach and small intestine as a prototype, and then hydrolyzed under the action of the esterase of the intestinal mucosa and the intestinal flora for further metabolism. Intestinal bacteria can metabolize polyphenols or carbohydrates in the diet to produce organic acids (Weikao et al., 2008; Kim et al., 2013). The production of organic acids is related to Clostridium, Escherichia coli, and Lactobacillus. Lactic acid can regulate intestinal peristalsis and inhibit the reproduction of harmful bacteria.
Here, we sort out some representative herbs that interact with the intestinal flora according to the types of active ingredients, as well as the possible mechanisms of the interaction of these herbs and effective ingredients with the intestinal flora. The interaction between the effective ingredients of traditional Chinese medicine and the intestinal flora are summarized in Table 1.
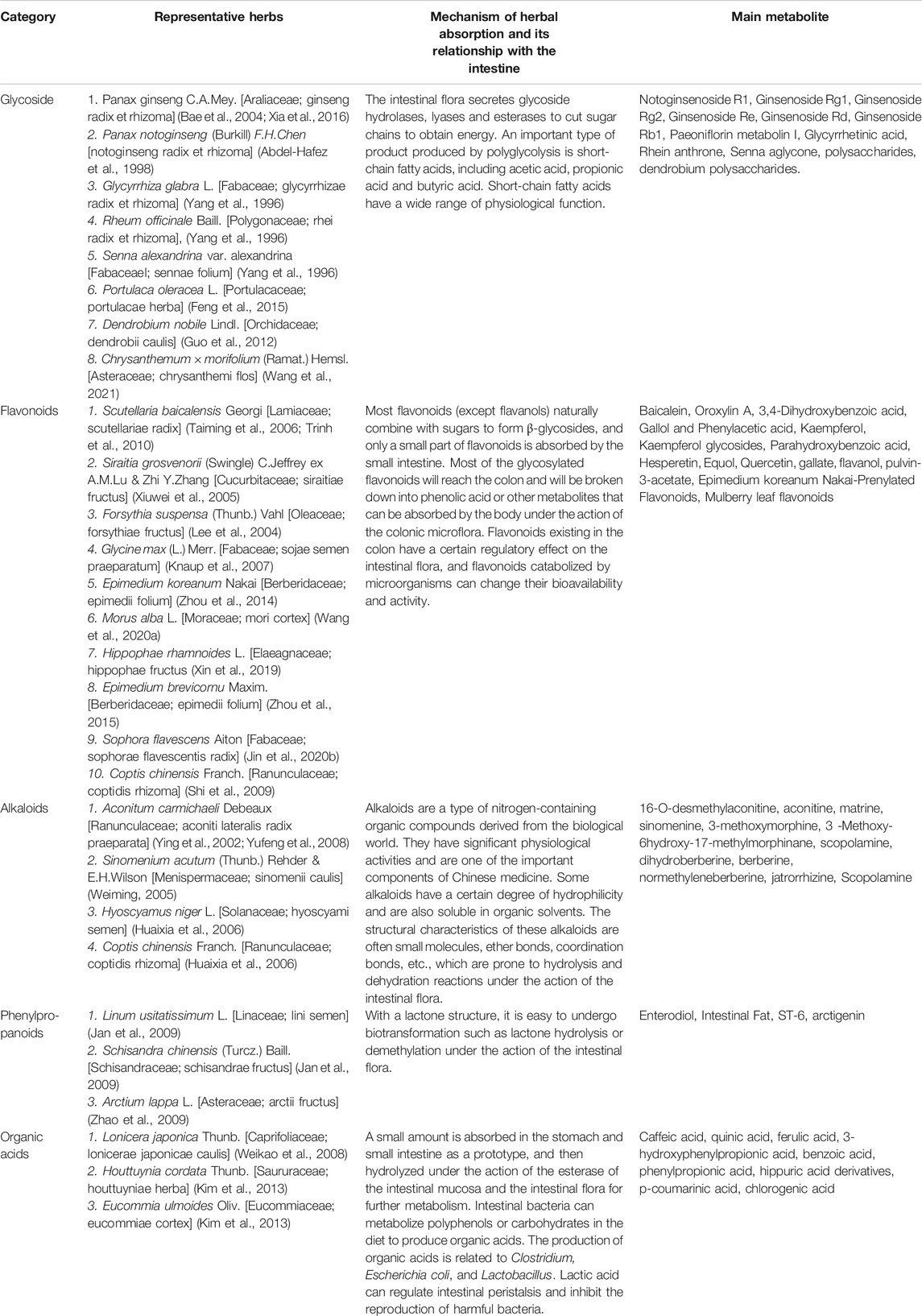
TABLE 1. The interaction between the effective ingredients of traditional Chinese medicine and the intestinal flora
Herbal Medicine That Regulates the Intestinal Mucosal Barrier
Stable intestinal tight junction function is an important prerequisite for the stability of the intestinal mucosal barrier. It is closely related to tight junction protein including Occludin, ZO-1, and Claudin, and plays a key role in maintaining intestinal permeability. Tight junction protein is a critical protein that connects the gaps between cells and regulates the intestinal mucosa's permeability. Tight junctions have two main functions: 1) Maintain the polarity of cells, prevent the top and bottom sides of integral membrane proteins from spreading from the side; 2) Prevent ions and molecules from passing through Intercellular space. Under normal circumstances, the tightly connected structure is complete and the function is normal, but the structure and function will be destroyed under pathological conditions. Herbs relieve the destruction of intestinal epithelial cell tight junctions by proinflammatory cytokines (such as LPS, TNF-α, IFN-γ, IL), up-regulating tight expression junction proteins, and enhance the effect of mechanical barriers (Kim et al., 2009; Shah et al., 2010; Li et al., 2017). There are quite a few herbs that can up-regulate Tight junction protein expression and reduce intestinal mucosal permeability.
The active ingredients in the herbal medicine increase the expression level of tight junction protein, improve the ultrastructure of intestinal epithelial cells, up-regulate the ultrastructure of intestinal epithelial cells ZO-1 and Occludin expression, and then repair the colonic epithelial barrier and reduce Intestinal permeability (Lina et al., 2014). Herbs can protect the intestinal mucosal immune barrier by regulating the levels of related cytokines (Pan et al., 2011; Liu et al., 2016). The mechanism may be to regulate lymphocytes, reduce the level of inflammatory factor TNF-α, and improve the intestinal barrier damage (Dai et al., 2009; Zhang et al., 2012). In China, many herbs have been clinically used to treat COVID-19. We searched for herbs that affected TJ protein from the “China Novel Coronavirus Pneumonia Diagnosis and Treatment Program” and the relevant data were shown in Table 2.
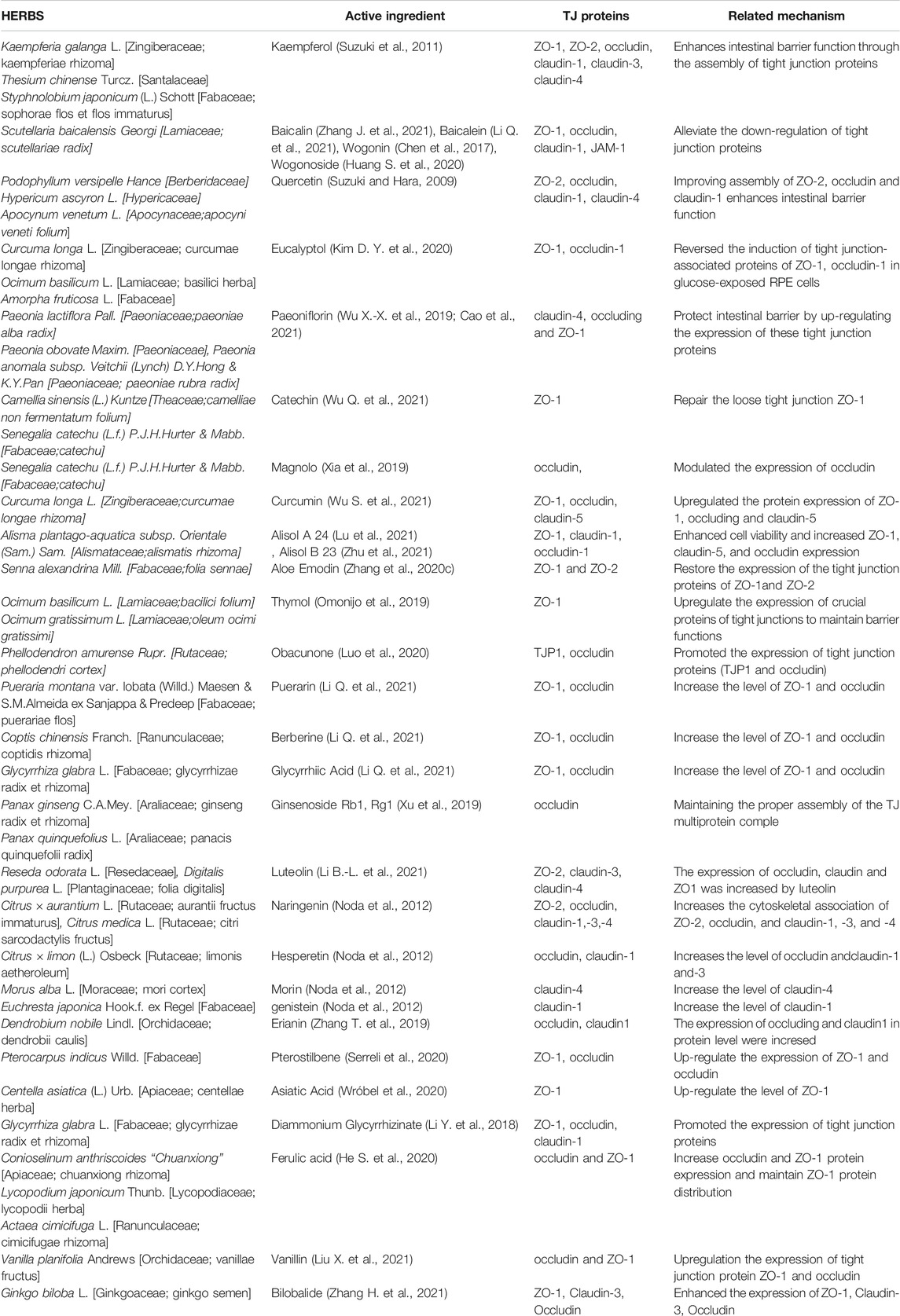
TABLE 2. Herbs for the treatment of COVID-19 that can affect the expression of tight junction proteins
Herbal Medicines Beneficial to the Intestinal Mucosa-Associated Immune System
In addition to protecting the integrity of the intestinal mucosa, herbal medicine also has a regulatory effect on the intestinal mucosa's immune function with a wide range of immunomodulatory effects. In recent years, extensive research on herbs’ intestinal mucosal immunity has helped to reveal its mechanism of action (Yan et al., 2009; Cai et al., 2018). Many herbal medicines, especially those that can restore intestinal flora, can increase the number of M cells in epithelial cells related to Peel's node follicles, promote the proliferation and activation of lymphocytes of Peel's node, and induce local mucosal immune response (Xu and Du, 2020). In addition, herbal medicine can also increase the content of SIgA, IL-2, and IL-4 cytokines in intestinal mucosal proliferating immune cells, improve the body's immunity (Liu et al., 2003; Allam et al., 2015; Chen et al., 2016), and improve the oxidative stress state of intestinal mucosa (Ghaffari et al., 2018; Ghaffari et al., 2019). The presence of a large number of lymphocytes and cytokines near the intestinal mucosal epithelium is one of the important targets of herbal medicine (Tang and Li, 2014). Lymphocytes are an important part of the immune system. Different lymphocytes have different functions. T lymphocytes participate in cellular immunity, among which regulatory T lymphocytes mainly maintain the homeostasis of intestinal mucosal immunity, and Th17 mainly defends against extracellular bacterial infections and mediates chronic inflammation. B lymphocytes participate in humoral immunity and can differentiate into plasma cells under the stimulation of antigens. NK lymphocytes can directly kill certain target cells. There are a large number of cytokines near the intestinal mucosal epithelium, including lymphokines, interleukin (IL), tumor necrosis factor (TNF), interferon (IFN) and so on (Pitman and Blumberg, 2000). Herbal medicine has a good regulatory effect on intestinal immunity, and can regulate pro-inflammatory cytokines (IL-2, IFN-γ, TNF-α) and anti-inflammatory cytokines (IL-4, IL-5, IL-6, IL-10) Expression (Wu et al., 2003; Yasui and Irahara, 2007).
The secretion balance of pro-inflammatory cytokines and anti-inflammatory cytokines is the guarantee for the body to produce a correct immune response. The immunomodulatory effect of herbal medicine on the body is one of the important mechanisms to prevent and treat intestinal mucosal damage. Herbs can affect the expression of IL-2, IL-4, IFN-γ, and SIg A in the intestinal mucosa, can increase the proliferation of mucosal cells and maintain the integrity of the intestinal mucosa (Zhang et al., 2010; Deng et al., 2018; Zhang M.-X. et al., 2019; Pu et al., 2020; Fang et al., 2021). Herbal medicine, rich in a variety of biologically active ingredients and nutrients, achieves its immunomodulatory effect by activating macrophages, T lymphocytes, B lymphocytes and blood complement proteins. Herbs can reduce the intestinal inflammatory response, reduce intestinal inflammatory factors, and reduce the damage of intestinal epithelial cells by reducing the levels of TNF-α, IL-6 and other inflammatory factors (Zhang, 1984; Ou et al., 2017; Liu et al., 2018; Gao et al., 2019; Zhang H.-Y. et al., 2021; Pang et al., 2021).
Herbal Medicines Restore Intestinal Flora Which Might Be Effective on Alleviating Specific Complications Caused by Coronavirus Disease 19
Acute Respiratory Distress Syndrome and Multiple Organ Dysfunction Syndrome
MODS refers to the occurrence of two or more system or organ dysfunctions or failures at the same time or sequentially after 24 h of acute damage to the body. It is a clinical syndrome in which multiple organ function changes in patients with acute injury cannot maintain a stable internal environment. MODS is dangerous and has a high mortality rate. Among the patients in severe or critical condition after infected by SARS-CoV-2, about two out of three patients will develop severely life-threatening ARDS (Chiumello et al., 2020; Chivato Martin-Falquina et al., 2021), manifested as sudden, stubborn, and rapidly progressing hypoxemia. The mortality rate is much higher. The occurrence of ARDS or MODS may involve the imbalance of intestinal flora (Li et al., 2014; Dickson et al., 2016). The intestines and lungs are closely connected and affect each other. Under stress conditions such as trauma and infection in the body, the intestinal flora is unbalanced, releasing a large amount of active oxygen, and the intestinal barrier function is impaired (Lyte and Bailey, 1997; Souza et al., 2004). The bacteria enter the blood and spread through the blood to other tissues or organs of the host, and finally cause a series of A cascade of inflammatory factors broke out and developed into severe sepsis, leading to ARDS (Anders et al., 2013; Dickson et al., 2016). After the bacterial translocation, the lung is the first organ to be injured, indicating that intestinal infection is the inducement of acute lung injury. Studies have shown that mouse lung microbes transformed from Firmicutes and Proteus to pseudo-nematode community structure (Yajima et al., 2001). In clinical trials, patients with acute respiratory distress syndrome (ARDS) have higher intestinal bacteria levels in bronchoalveolar lavage fluid, which is correlated with the degree of systemic inflammation (Dickson et al., 2016). The primary source of pulmonary flora in sepsis patients may be the lower digestive tract, indicating that the intestinal flora is the bridge between the lungs and the intestines (Dickson et al., 2016). However, the discovery that intestinal flora can cause lung infections may provide new ideas for preventing acute respiratory distress syndrome caused by SARS-CoV-2 infections.
The pathogenesis of MODS is currently unclear. However, the intestine is an important source of bacteria and endotoxins in patients in severe or critical condition, the intestine is one of the important sites for inflammatory cell activation and release of inflammatory mediators (Deng et al., 2001; van der Voort, 2006). For the treatment of MODS, attention should be paid to the prevention and treatment of intestinal dysfunction. Herbal medicine has the following functions in preventing MODS. 1) Herbal medicine can strengthen the effect of enteral nutrition, enhance the body's immune protein synthesis, and more effectively improve the patient's serum protein, muscle, fat and other nutritional indicators (Yongbing et al., 2008; Hu et al., 2011; Pang et al., 2012; Zhang Q. et al., 2020). Early enteral nutrition, especially nutrients with immunomodulatory components, can prevent intestinal mucosal atrophy and reduce the occurrence of bacterial translocation and sepsis. 2) Herbs can regulate the intestinal flora, which can prevent the overgrowth and reproduction of Gram-negative bacteria (Wu Y.-R. et al., 2019; Liu Y.-T. et al., 2021). 3) Herbal medicine has an antioxidant effect and can effectively reduce the damage of oxygen free radicals to cell membranes (Wu Y.-R. et al., 2019; Liu Y.-T. et al., 2021). Herbs can also protect intestinal endothelial cells from oxygen free radical damage, prevent intestinal toxins from entering the blood circulation (Xiyu et al., 2006; Li M. et al., 2018). 4) As mentioned above, herbal medicine can enhance the intestinal barrier function and prevent bacterial translocation.
As the most extensive and crucial functional organ of the GI tract, the intestinal flora is bound to participate in specific complications' occurrence and development. In particular, some abnormal changes in the small intestine found on autopsy suggest the correlation between the SARS-CoV-2 infection and the intestinal flora. Of course, this correlation needs more research to confirm.
Sepsis
Sepsis is one of the main causes of death of patients in severe or critical condition, and its pathophysiological mechanism is more complicated. SARS-CoV-2 invades the body, produces pro-inflammatory and anti-inflammatory reactions, and releases a large number of inflammatory mediators to cause sepsis (Shi et al., 1999; Lin et al., 2000). The intestine, as the largest “reservoir of bacteria” in the body, is considered to be the “priming” organ for sepsis-induced multiple organ dysfunction syndrome (Haseeb and Salwen, 2005). The diversity and stability of the intestinal flora can enhance the host's defense capabilities. When sepsis occurs, the intestinal microenvironment changes, leading to pathological changes such as the destruction of intestinal epithelial cells, inflammatory reactions, and the invasion of pathogenic bacteria, which cause local and remote organ damage (Muller-Werdan and Werdan, 2003). After an autopsy, it was found that the intestinal mucosal barrier of patients who died of COVID-19 was damaged (Varga et al., 2020).
Most of the nutrient supply of intestinal epithelial cells comes from direct absorption from the intestinal lumen. The intestinal flora mainly depends on the intestinal nutrients to survive and participate in the metabolism of nutrients (Guarner and Malagelada, 2003). Some polysaccharides and other ingredients in herbal medicine can be metabolized by bacteria into short-chain fatty acids (SFCA)(Rechkemmer et al., 1988; Huo et al., 2020), including butyric acid, acetic acid, propionic acid, etc. Among them, butyric acid is helpful for the repair of intestinal mucosa and the prognosis of sepsis. SFCA can also be combined with G protein-coupled receptor (GPCR) to play a key role in promoting the stability of the intestinal environment and regulating inflammation. It also affects the function of dendritic cells and regulatory T lymphocytes and the secretion of IgA antibodies. Play an anti-inflammatory effect, thereby maintaining intestinal homeostasis. In conclusion, herbal medicine can maintain the intestinal microecological balance, prevent the migration of flora, and avoid the induction of endotoxemia, which is extremely important for the prevention and treatment of sepsis (Varon, 2009; Varon and Varon, 2015; Fan et al., 2020).
Herbal Medicines Regulate the Immune Function of Other Respiratory Diseases by Restore Intestinal Flora
The gastrointestinal tract is considered the largest immunological organ in the body having a central role in regulating immune homeostasis (Takiishi et al., 2017). The intestinal flora plays a vital role in the function regulation, immune defense, and material metabolism of the human body (Eckburg et al., 2005; Thaiss et al., 2016). The intestinal mucosa has the function of producing immune tolerance and resisting pathogen invasion. Studies have shown that the intestinal flora can activate the TLR2, TLR3, TLR4, TLR7, and TLR9 signaling pathway (Cario and Podolsky, 2000; Szebeni et al., 2008; Heimesaat et al., 2010) and induce regulatory T cells (Treg). Tregs can negatively regulate the intensity and time of immune responses, and their abnormalities can lead to immune imbalance. The intestinal flora can also regulate helper T cells (Th) to induce the activation of neutrophils and intestinal epithelial cells (Wu et al., 2009; Wu et al., 2010; Geuking et al., 2011). Intestinal flora can also stimulate B lymphocytes to produce inhibitory cytokines, thereby inhibiting the occurrence of inflammation. Therefore, intestinal flora can initiate the body’s active immune response to invading microorganisms while maintaining its immune balance.
Gut-Lung Axis intestinal immunity participates in the regulation of lung immunity and systemic immunity. The “gut-lung axis” refers to the fact that the intestinal flora can affect and regulate the lungs’ immunity and function. Intestinal flora can induce inflammation in mice’s lungs through Toll-Like receptor and promote the infiltration of neutrophils (Sato et al., 2020; Liao et al., 2021). T helper 17 cells’ intestinal induction is a critical step in mucosal protection (Gaboriau-Routhiau et al., 2009), which can recruit neutrophils and promote the secretion of antibacterial factors by bronchial epithelium. Immunization of rat intestines by inactivated atypical Haemophilus influenzae can simultaneously increase the number of specific Th17 cells in mesenteric lymph nodes and airways (Essilfie et al., 2011; Olliver et al., 2011). The production of specific antibodies in the respiratory tract coincides with the intestinal response to antigen exposure. Therefore, intestinal flora plays a significant role in the lung defense against microbial invasion (Jung et al., 1995; Hooper and Macpherson, 2010). The immune transmission of the gastrointestinal and respiratory tracts can be achieved through mucosal immunity (Akbari et al., 2001; Aujla et al., 2008). It means that in the treatment of COVID-19, the crucial role of intestinal flora in the regulation of the gut-lung axis should not be ignored.
Pneumonia
Infectious lung diseases may cause pathological changes through the following two aspects. One is that immune disorders destroy the intestinal microecological disorders, leading to inflammation. As the intestinal cavity is exposed to many exogenous antigens, the immune system must be strictly controlled to maintain a symbiotic relationship with symbiotic bacteria. The host can distinguish beneficial microorganisms from harmful pathogens and establish a healthy microbial community. The mucosal immune system is responsible for removing pathogens. An inappropriate immune response in this process will destroy the intestines' homeostasis, cause microecological disorders, and lead to metabolic dysfunction and local or systemic inflammation (Williams, 2003; van Wijk and Cheroutre, 2010). After mice are infected with the influenza virus, the intestinal flora becomes unbalanced and adaptive immune suppression, further aggravating lung inflammation (Deng et al., 2020). Also, the administration of antibiotics will cause the disturbance of the intestinal microflora, which will last for a long time. Changes in disease status are mainly caused by changing the susceptibility to infection, the colonization of antibiotic-resistant strains in the intestine, and resistance genes (Sartor, 2004; Buffie and Pamer, 2013). The intestinal microecological imbalance can cause the conditional pathogenic bacteria in the intestine to move up to the oropharynx or lower respiratory tract, causing lower respiratory tract infection (Khalmatova, 2006).
Chronic Obstructive Pulmonary Disease
The microecological imbalance of the intestinal flora directly or indirectly promotes the occurrence and development, and severity of COPD. A large increase in Gram-negative bacilli will release endotoxin into the blood, and then return to the right atrium via the inferior vena cava, and perfused into the lungs via the pulmonary artery and capillaries. Endotoxin damage promotes the occurrence and development of COPD (Zhou et al., 2019; Hu et al., 2020). The gut microbiota components, especially Gram-negative bacilli, are also the main species of the lung microbiota of COPD patients, and these bacteria can cause acute exacerbations of COPD (Sun Z. et al., 2020). Herbs have beneficial effects in improving symptoms in stable COPD patients over a 3 month treatment period. The potential underlying mechanism may be attributable to the difference in gut microbiota among patients (Hu et al., 2020) and by inhibiting Th17/Treg’s ratio via restore gut microbiota (Peng et al., 2019).
Asthma
Changes in microbial composition accompany bacterial and viral respiratory tract infections. Changes in the intestinal flora may promote the immune response dominated by respiratory allergies, thus playing an essential role in respiratory diseases' pathogenesis (Bjorksten et al., 2001). Also, the increase in asthma risk is related to the rise in the number and abundance of Bacteroides fragilis and total anaerobes in the intestine (Shi H.-L. et al., 2020). The intestinal composition flora of asthma patients has undergone significant changes. Herbal medicine can regulate the intestinal flora, thereby improving asthmatic airway inflammation (Fang et al., 2019; Le et al., 2020; Xueren et al., 2020). Herbs can also regulate immune function through intestinal flora and are used to treat acute exacerbations of bronchial asthma (Huiyang et al., 2020). By increasing the number of probiotics, reducing the number of harmful flora and restoring the flora’s diversity, herbal medicine has certain advantages and effects in the treatment of intestinal microflora disorders and allergic asthma.
Conclusion
In many parts of the world, herbal medicine can be used to regulate and maintain the intestinal flora balance, thereby reducing the incidence of secondary bacterial infections. Due to hypoxia, inflammatory factors, and the use of antibacterial drugs, patients in severe or critical condition will have severe disturbances in the intestinal microenvironment, and critically ill patients are more likely to die from secondary bacterial infections. The “Diagnosis and Treatment Protocol for Novel Coronavirus Pneumonia” (fourth trial edition, trial fifth revised edition, sixth trial edition) [40–42] also mentioned the use of intestinal microecological regulators to maintain the intestinal flora Balance and prevent secondary bacterial infections. Many patients with mild COVID-19 chose to self-isolate at home to enhance their immunity and achieve self-healing. The immune function of patients is essential in defeating and clearing the virus. The gut microbiota in particular plays important roles in host metabolism, immunity and anti-inflammation. Herbs can restore the structure of the intestinal flora, which may further modulate the immune function after SARS-CoV-2 infection. Regulation of intestinal flora by herbal medicine may be helpful for the treatment and recovery of the disease (Figure 4). Understanding the role of herbs that regulate intestinal flora in fighting respiratory virus infections and maintaining intestinal flora balance can provide new ideas for preventing and treating COVID-19.
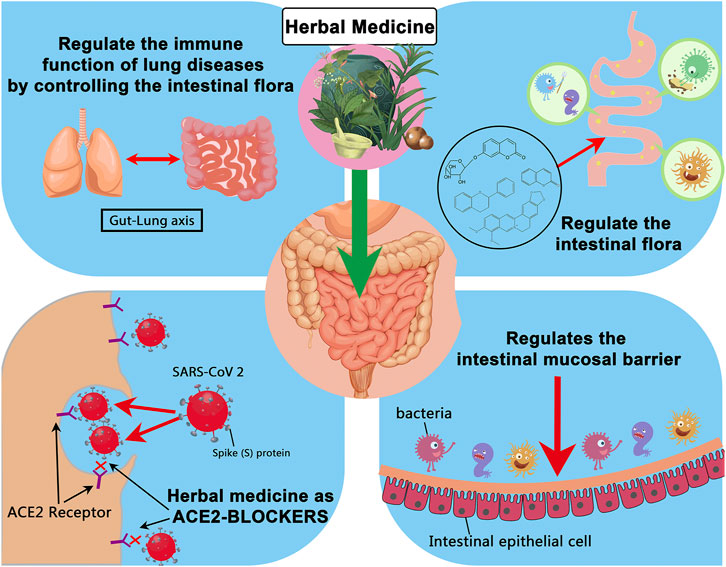
FIGURE 4. Summary of possible ways in which herbal medicines can affect the prognosis of COVID-19 by regulating the intestinal flora.
Author Contributions
LD and ZC conceived the paper. LD and YL wrote the manuscript. HX and YL contributed to the manuscript revision.
Funding
This work was supported by the National Natural Science Foundation of China (No. 81830114 and No. 81774164 and No.82004232); Natural Science Foundation of Guangdong, China (No. 2020A1515010756); Project of Administration of Traditional Chinese Medicine of Guangdong Province (No. 20201074); Keypoint research and invention program of Guangdong Province (No. 2020B1111100010).
Conflict of Interest
The authors declare that the research was conducted in the absence of any commercial or financial relationships that could be construed as a potential conflict of interest.
Acknowledgments
We thank Ray Liu for the helpful comments on the manuscript.
References
Abdel-Hafez, A. A., Meselhy, M. R., Nakamura, N., Hattori, M., Watanabe, H., Mohamed, T. A., et al. (1998). Potent Anticonvulsant Paeonimetabolin-I Derivatives Obtained by Incubation of Paeoniflorin and Thiol Compounds with Lactobacillus Brevis. Chem. Pharm. Bull. 46 (9), 1486–1487. doi:10.1248/cpb.46.1486
Abuzakouk, M., Carton, J., Feighery, C., OʼDonoghue, D. P., Weir, D. G., and OʼFarrelly, C. (1998). CD4+CD8+ and CD8α+β- T Lymphocytes in Human Small Intestinal Lamina Propria. Eur. J. Gastroenterol. Hepatol. 10 (4), 325–330. doi:10.1097/00042737-199804000-00009
Akbari, O., DeKruyff, R. H., and Umetsu, D. T. (2001). Pulmonary Dendritic Cells Producing IL-10 Mediate Tolerance Induced by Respiratory Exposure to Antigen. Nat. Immunol. 2 (8), 725–731. doi:10.1038/90667
Al-Tawfiq, J. A., Hinedi, K., Ghandour, J., Khairalla, H., Musleh, S., Ujayli, A., et al. (2014). Middle East Respiratory Syndrome Coronavirus: a Case-Control Study of Hospitalized Patients. Clin. Infect. Dis. 59 (2), 160–165. doi:10.1093/cid/ciu226
Allam, S., Krueger, D., Demir, I. E., Ceyhan, G., Zeller, F., and Schemann, M. (2015). Extracts from Peppermint Leaves, Lemon Balm Leaves and in Particular Angelica Roots Mimic the Pro-Secretory Action of the Herbal Preparation STW 5 in the Human Intestine. Phytomedicine 22 (12), 1063–1070. doi:10.1016/j.phymed.2015.08.008
Anders, H.-J., Andersen, K., and Stecher, B. (2013). The Intestinal Microbiota, a Leaky Gut, and Abnormal Immunity in Kidney Disease. Kidney Int. 83 (6), 1010–1016. doi:10.1038/ki.2012.440
Arpaia, N., Campbell, C., Fan, X., Dikiy, S., van der Veeken, J., deRoos, P., et al. (2013). Metabolites Produced by Commensal Bacteria Promote Peripheral Regulatory T-Cell Generation. Nature 504 (7480), 451–455. doi:10.1038/nature12726
Assiri, A., Al-Tawfiq, J. A., Al-Rabeeah, A. A., Al-Rabiah, F. A., Al-Hajjar, S., Al-Barrak, A., et al. (2013a). Epidemiological, Demographic, and Clinical Characteristics of 47 Cases of Middle East Respiratory Syndrome Coronavirus Disease from Saudi Arabia: A Descriptive Study. Lancet Infect. Dis. 13 (9), 752–761. doi:10.1016/S1473-3099(13)70204-4
Assiri, A., McGeer, A., Perl, T. M., Price, C. S., Al Rabeeah, A. A., Cummings, D. A. T., et al. (2013b). Hospital Outbreak of Middle East Respiratory Syndrome Coronavirus. N. Engl. J. Med. 369 (5), 407–416. doi:10.1056/NEJMoa1306742
Aujla, S. J., Chan, Y. R., Zheng, M., Fei, M., Askew, D. J., Pociask, D. A., et al. (2008). IL-22 Mediates Mucosal Host Defense Against Gram-Negative Bacterial Pneumonia. Nat. Med. 14 (3), 275–281. doi:10.1038/nm1710
Bae, E. A., Han, M. J., Kim, E. J., and Kim, D. H. (2004). Transformation of Ginseng Saponins to Ginsenoside Rh2 by Acids and Human Intestinal Bacteria and Biological Activities of Their Transformants. Arch. Pharm. Res. 27 (1), 61–67. doi:10.1007/bf02980048
Björkstén, B., Sepp, E., Julge, K., Voor, T., and Mikelsaar, M. (2001). Allergy Development and the Intestinal Microflora during the First Year of Life. J. Allergy Clin. Immunol. 108 (4), 516–520. doi:10.1067/mai.2001.118130
Booth, C. M., Matukas, L. M., Tomlinson, G. A., Rachlis, A. R., Rose, D. B., Dwosh, H. A., et al. (2003). Clinical Features and Short-Term Outcomes of 144 Patients with SARS in the Greater Toronto Area. JAMA 289 (21), 2801–2809. doi:10.1001/jama.289.21.joc30885
Buffie, C. G., and Pamer, E. G. (2013). Microbiota-Mediated Colonization Resistance Against Intestinal Pathogens. Nat. Rev. Immunol. 13 (11), 790–801. doi:10.1038/nri3535
Cai, B., Wan, P., Sun, H., Chen, D., Chen, H., Chen, X., et al. (2018). Protective Effects of Enteral Nutrition Supplemented withCrassostrea hongkongensisPolysaccharides Against 5-Fluorouracil-Induced Intestinal Mucosal Damage in Rats. J. Med. Food 21 (4), 348–355. doi:10.1089/jmf.2017.4025
Cao, X. Y., Ni, J. H., Wang, X., Feng, G. Z., Li, H. D., Bao, W. L., et al. (2021). Total Glucosides of Paeony Restores Intestinal Barrier Function Through Inhibiting Lyn/Snail Signaling Pathway in Colitis Mice. Phytomedicine 87, 153590. doi:10.1016/j.phymed.2021.153590
Cario, E., and Podolsky, D. K. (2000). Differential Alteration in Intestinal Epithelial Cell Expression of Toll-Like Receptor 3 (TLR3) and TLR4 in Inflammatory Bowel Disease. Infect. Immun. 68 (12), 7010–7017. doi:10.1128/iai.68.12.7010-7017.2000
Cattelan, A. M., Di Meco, E., Trevenzoli, M., Frater, A., Ferrari, A., Villano, M., et al. (2020). Clinical Characteristics and Laboratory Biomarkers Changes in COVID-19 Patients Requiring or Not Intensive or Sub-intensive Care: A Comparative Study. BMC Infect. Dis. 20 (1), 934. doi:10.1186/s12879-020-05647-7
Chan, J. W. M., Ng, C. K., Chan, Y. H., Mok, T. Y. W., Lee, S., Chu, S. Y. Y., et al. (2003). Short Term Outcome and Risk Factors for Adverse Clinical Outcomes in Adults With Severe Acute Respiratory Syndrome (SARS). Thorax 58 (8), 686–689. doi:10.1136/thorax.58.8.686
Chen, C., Guo, D., and Lu, G. (2017). Wogonin Protects Human Retinal Pigment Epithelium Cells from LPS-Induced Barrier Dysfunction and Inflammatory Responses by Regulating the TLR4/NF-Κb Signaling Pathway. Mol. Med. Rep. 15 (4), 2289–2295. doi:10.3892/mmr.2017.6252
Chen, J., Pang, W., Shi, W., Yang, B., Kan, Y., He, Z., et al. (2016). Structural Elucidation of a Novel Polysaccharide from Pseudostellaria Heterophylla and Stimulating Glucose Uptake in Cells and Distributing in Rats by Oral. Molecules 21 (9), 1233. doi:10.3390/molecules21091233
Chen, N., Zhou, M., Dong, X., Qu, J., Gong, F., Han, Y., et al. (2020). Epidemiological and Clinical Characteristics of 99 Cases of 2019 Novel Coronavirus Pneumonia in Wuhan, China: A Descriptive Study. Lancet 395 (10223), 507–513. doi:10.1016/s0140-6736(20)30211-7
Chiumello, D., Pozzi, T., Storti, E., Caccioppola, A., Pontiroli, A. E., and Coppola, S. (2020). Body Mass index and Acute Respiratory Distress Severity in Patients With and Without SARS-CoV-2 Infection. Br. J. Anaesth. 125 (4), E376–E377. doi:10.1016/j.bja.2020.07.006
Chivato Martín-Falquina, I., García-Morán, S., and Jiménez Moreno, M. A. (2021). Acute Pancreatitis in SARS-CoV-2 Infection. Beyond Respiratory Distress. Rev. Esp. Enferm Dig. 113 (5), 388–389. doi:10.17235/reed.2021.7781/2020
Choi, K. W., Chau, T. N., Tsang, O., Tso, E., Chiu, M. C., Tong, W. L., et al. (2003). Outcomes and Prognostic Factors in 267 Patients with Severe Acute Respiratory Syndrome in Hong Kong. Ann. Intern. Med. 139 (9), 715–723. doi:10.7326/0003-4819-139-9-200311040-00005
Chuan, W., Min, L. H., Yan, W., Pan, Z., Ping, L. J., Bin, W., et al. (2020). Mechanism Study of Matrine against SARS-CoV-2 Based on Network Pharmacology and Molecular Docking. Pharmacol. Clin. Chin. Materia Med. 36 (4), 23–29. doi:10.13412/j.cnki.zyyl.20200629.001
Corman, V. M., Muth, D., Niemeyer, D., and Drosten, C. (2018). Hosts and Sources of Endemic Human Coronaviruses. Adv. Virus Resin. 100, 163–188. doi:10.1016/bs.aivir.2018.01.001
Cummings, M. J., Baldwin, M. R., Abrams, D., Jacobson, S. D., Meyer, B. J., Balough, E. M., et al. (2020). Epidemiology, Clinical Course, and Outcomes of Critically Ill Adults with COVID-19 in New York City: A Prospective Cohort Study. Lancet 395 (10239), 1763–1770. doi:10.1016/s0140-6736(20)31189-2
Dai, Y. C., Tang, Z. P., Ma, G. T., Yin, P., Gong, Y. P., Liu, W., et al. (2009). Treatment with Parenteral Nutrition Support and Chinese Herbs in One Case of Primary Small Intestinal Lymphangiectasia. Chin. Med. Sci. J. 24 (4), 248–251. doi:10.1016/s1001-9294(10)60011-9
den Besten, G., van Eunen, K., Groen, A. K., Venema, K., Reijngoud, D. J., and Bakker, B. M. (2013). The Role of Short-Chain Fatty Acids in the Interplay between Diet, Gut Microbiota, and Host Energy Metabolism. J. Lipid Res. 54 (9), 2325–2340. doi:10.1194/jlr.R036012
Deng, L., Xu, H., Liu, P., Wu, S., Shi, Y., Lv, Y., et al. (2020). Prolonged Exposure to High Humidity and High Temperature Environment Can Aggravate Influenza Virus Infection through Intestinal flora and Nod/RIP2/NF-Κb Signaling Pathway. Vet. Microbiol. 251, 108896. doi:10.1016/j.vetmic.2020.108896
Deng, X., Wang, X., Lasson, Å., Sun, Z., Soltesz, V., and Andersson, R. (2001). The Involvement of Multiple Protease-Antiprotease Systems and Gut Origin Sepsis in Zymosan-Associated Endothelial Barrier Injury and Multiple Organ Dysfunction in Rats. Shock 16 (4), 298–303. doi:10.1097/00024382-200116040-00012
Deng, Y., Han, X., Tang, S., Li, C., Xiao, W., and Tan, Z. (2018). Magnolol and Honokiol Attenuate Apoptosis of Enterotoxigenic Escherichia Coli-Induced Intestinal Epithelium by Maintaining Secretion and Absorption Homeostasis and Protecting Mucosal Integrity. Med. Sci. Monit. 24, 3348–3356. doi:10.12659/msm.910350
Dhar, D., and Mohanty, A. (2020). Gut Microbiota and Covid-19- Possible Link and Implications. Virus. Res. 285, 198018. doi:10.1016/j.virusres.2020.198018
Dickson, R. P., Singer, B. H., Newstead, M. W., Falkowski, N. R., Erb-Downward, J. R., Standiford, T. J., et al. (2016). Enrichment of the Lung Microbiome with Gut Bacteria in Sepsis and the Acute Respiratory Distress Syndrome. Nat. Microbiol. 1 (10), 16113. doi:10.1038/nmicrobiol.2016.113
Eckburg, P. B., Bik, E. M., Bernstein, C. N., Purdom, E., Dethlefsen, L., Sargent, M., et al. (2005). Diversity of the Human Intestinal Microbial flora. Science 308 (5728), 1635–1638. doi:10.1126/science.1110591
Essilfie, A. T., Simpson, J. L., Horvat, J. C., Preston, J. A., Dunkley, M. L., Foster, P. S., et al. (2011). Haemophilus Influenzae Infection Drives IL-17-Mediated Neutrophilic Allergic Airways Disease. Plos Pathog. 7 (10), e1002244. doi:10.1371/journal.ppat.1002244
Fan, T. T., Cheng, B. L., Fang, X. M., Chen, Y. C., and Su, F. (2020). Application of Chinese Medicine in the Management of Critical Conditions: A Review on Sepsis. Am. J. Chin. Med. 48 (6), 1315–1330. doi:10.1142/s0192415x20500640
Fang, C., Chunli, S., Mei, Y., Xiaoping, G., Liying, W., Bin, H., et al. (2019). Effect of Lycium Barbarum Polysaccharide on Gut Microbiota in Allergic Asthmatic Mice. Mod. Food Sci. Technol. 35 (09), 67–73. doi:10.13982/j.mfst.1673-9078.2019.9.007
Fang, W., Zhao, P., Shen, A., Liu, L., Chen, H., Chen, Y., et al. (2021). Effects of Qing Hua Chang Yin on Lipopolysaccharide induced Intestinal Epithelial Tight junction Injury in Caco2 Cells. Mol. Med. Rep. 23 (3), 205. doi:10.3892/mmr.2021.11844
Feng, L., Li, S., Dai, L., Li, C., Li, Y., Wu, F., et al. (2015). Effects of Polysaccharides from Portulaca Oleracea L. On Intestinal Mucosal Cytokines and Intestinal flora in Mice with Ulcerative Colitis. Chin. J. Microecology 27 (2), 139–142.
Feng, W., Ao, H., Peng, C., and Yan, D. (2019). Gut Microbiota, a New Frontier to Understand Traditional Chinese Medicines. Pharmacol. Res. 142, 176–191. doi:10.1016/j.phrs.2019.02.024
Feng, W. L., Ting, L. H., Yao, W., Li, L. X., Yao, C. Q., Jie, X., et al. (2020). Study on Mechanism of Shengmai Injection against Novel Coronavirus Pneumonia Based on Network Pharmacology and Molecular Docking Technology. Chin. Traditional Herbal Drugs 51 (11), 2977–2987. doi:10.7501/j.issn.0253-2670.2020.11.016
Gaboriau-Routhiau, V., Rakotobe, S., Lécuyer, E., Mulder, I., Lan, A., Bridonneau, C., et al. (2009). The Key Role of Segmented Filamentous Bacteria in the Coordinated Maturation of Gut Helper T Cell Responses. Immunity 31 (4), 677–689. doi:10.1016/j.immuni.2009.08.020
Gao, C., Liu, L., Zhou, Y., Bian, Z., Wang, S., and Wang, Y. (2019). Novel Drug Delivery Systems of Chinese Medicine for the Treatment of Inflammatory Bowel Disease. Chin. Med. 14 (17), 23. doi:10.1186/s13020-019-0245-x
Gao, J., Xu, K., Liu, H., Liu, G., Bai, M., Peng, C., et al. (2018). Impact of the Gut Microbiota on Intestinal Immunity Mediated by Tryptophan Metabolism. Front. Cell Infect. Microbiol. 8 (6), 13. doi:10.3389/fcimb.2018.00013
Geuking, M. B., Cahenzli, J., Lawson, M. A. E., Ng, D. C. K., Slack, E., Hapfelmeier, S., et al. (2011). Intestinal Bacterial Colonization Induces Mutualistic Regulatory T Cell Responses. Immunity 34 (5), 794–806. doi:10.1016/j.immuni.2011.03.021
Ghaffari, A., Rafraf, M., Navekar, R., and Asghari-Jafarabadi, M. (2018). Effects of Turmeric and Chicory Seed Supplementation on Antioxidant and Inflammatory Biomarkers in Patients with Non-alcoholic Fatty Liver Disease (NAFLD). Adv. Integr. Med. 5 (3), 89–95. doi:10.1016/j.aimed.2018.01.002
Ghaffari, A., Rafraf, M., Navekar, R., Sepehri, B., Asghari-Jafarabadi, M., and Ghavami, S.-M. (2019). Turmeric and Chicory Seed Have Beneficial Effects on Obesity Markers and Lipid Profile in Non-alcoholic Fatty Liver Disease (NAFLD). Int. J. Vitamin Nutr. Res. 89 (5-6), 293–302. doi:10.1024/0300-9831/a000568
Guan, W.-J., Ni, Z.-Y., Hu, Y., Liang, W.-H., Ou, C.-Q., He, J.-X., et al. (2020b). Clinical Characteristics of Coronavirus Disease 2019 in China. N. Engl. J. Med. 382 (18), 1708–1720. doi:10.1056/NEJMoa2002032
Guan, W.-J., Liang, W.-H., Zhao, Y., Liang, H.-R., Chen, Z.-S., Li, Y.-M., et al. (2020a). Comorbidity and its Impact on 1590 Patients with COVID-19 in China: A Nationwide Analysis. Eur. Respir. J. 55 (5), 2000547. doi:10.1183/13993003.00547-2020
Guarner, F., and Malagelada, J.-R. (2003). Gut flora in Health and Disease. Lancet 361 (9356), 512–519. doi:10.1016/s0140-6736(03)12489-0
Guo, J., Cheng, Z., Qi, D., Ma, S., Du, Y., Zhao, H., et al. (2012). Regulatory Effects of Dendrobium Polysaccharides on Intestinal Dysbiosis: an Evaluation by PCR-DGGE. Chin. J. Microecol. 24 (10), 873–875. doi:10.13381/j.cnki.cjm.2012.10.009
Hamasaki, N., Ishii, E., Tominaga, K., Tezuka, Y., Nagaoka, T., Kadota, S., et al. (2000). Highly Selective Antibacterial Activity of Novel Alkyl Quinolone Alkaloids from a Chinese Herbal Medicine, Gosyuyu (Wu-Chu-Yu), against Helicobacter Pylori In Vitro. Microbiol. Immunol. 44 (1), 9–15. doi:10.1111/j.1348-0421.2000.tb01240.x
Haseeb, M. A., and Salwen, M. J. (2005). Collateral Damage: Sepsis-Induced Gut Injury. Crit. Care Med. 33 (10), 2439–2440. doi:10.1097/01.Ccm.0000182999.95340.C3
Hashimoto, T., Perlot, T., Rehman, A., Trichereau, J., Ishiguro, H., Paolino, M., et al. (2012). ACE2 Links Amino Acid Malnutrition to Microbial Ecology and Intestinal Inflammation. Nature 487 (7408), 477–481. doi:10.1038/nature11228
He, J., Chen, G., Jiang, Y., Jin, R., Shortridge, A., Agusti, S., et al. (2020). Comparative Infection Modeling and Control of COVID-19 Transmission Patterns in China, South Korea, Italy and Iran. Sci. Total Environ. 747 (19), 141447. doi:10.1016/j.scitotenv.2020.141447
He, S., Guo, Y., Zhao, J., Xu, X., Wang, N., and Liu, Q. (2020). Ferulic Acid Ameliorates Lipopolysaccharide-Induced Barrier Dysfunction via MicroRNA-200c-3p-Mediated Activation of PI3K/AKT Pathway in Caco-2 Cells. Front. Pharmacol. 11, 376. doi:10.3389/fphar.2020.00376
Heidari, Z., Tajbakhsh, A., Gheibi-Hayat, S. M., Moattari, A., Razban, V., Berenjian, A., et al. (2021). Probiotics/ Prebiotics in Viral Respiratory Infections: Implication for Emerging Pathogens. Recent Pat. Biotechnol. [Epub ahead of print]. doi:10.2174/1872208315666210419103742
Heimesaat, M. M., Nogai, A., Bereswill, S., Plickert, R., Fischer, A., Loddenkemper, C., et al. (2010). MyD88/TLR9 Mediated Immunopathology and Gut Microbiota Dynamics in a Novel Murine Model of Intestinal Graft-Versus-Host Disease. Gut 59 (8), 1079–1087. doi:10.1136/gut.2009.197434
Hoffmann, M., Kleine-Weber, H., Schroeder, S., Krüger, N., Herrler, T., Erichsen, S., et al. (2020). SARS-CoV-2 Cell Entry Depends on ACE2 and TMPRSS2 and is Blocked by a Clinically Proven Protease Inhibitor. Cell 181 (2), 271–280. doi:10.1016/j.cell.2020.02.052
Hong, Z. H., Hong, Z. X., Zhen, M., Sheng, G. D., Xian, H. G., Liu, Y., et al. (2021). Discussion on the Network Pharmacological Mechanism and Preliminary Evidence of Huopo Xialing Decoction for the Treatment of 2019-nCoV Inflammatory Storm by Integrating“Pathway-Target-Active Component”. Modernization Traditional Chin. Med. Materia Medica-World Sci. Technol. 23 (2), 536–551.
Hooper, L. V., Littman, D. R., and Macpherson, A. J. (2012). Interactions between the Microbiota and the Immune System. Science 336 (6086), 1268–1273. doi:10.1126/science.1223490
Hooper, L. V., and Macpherson, A. J. (2010). Immune Adaptations that Maintain Homeostasis with the Intestinal Microbiota. Nat. Rev. Immunol. 10 (3), 159–169. doi:10.1038/nri2710
Hu, J., Zhang, L., Lin, W., Tang, W., Chan, F. K. L., and Ng, S. C. (2021). Review Article: Probiotics, Prebiotics and Dietary Approaches during COVID-19 Pandemic. Trends Food Sci. Technol. 108, 187–196. doi:10.1016/j.tifs.2020.12.009
Hu, Y., Ma, Y., Wang, J., and Zhu, Z. H. (2011). Early Enteral Infusion of Traditional Chinese Medicine Preparation Can Effectively Promote the Recovery of Gastrointestinal Function after Esophageal Cancer Surgery. J. Thorac. Dis. 3 (4), 249–254. doi:10.3978/j.issn.2072-1439.2011.09.08
Hu, Y., Shi, Q., Ying, S., Zhu, D., Chen, H., Yang, X., et al. (2020). Effects of Compound Caoshi Silkworm Granules on Stable COPD Patients and Their Relationship with Gut Microbiota. Medicine 99 (22), e20511. doi:10.1097/md.0000000000020511
Huaixia, C., Peng, D., Fengmei, H., and Yong, C. (2006). Study on the Metabolism of Scopolamine in Rat Intestinal flora. J. Hubei Univ. 4, 414–416.
Huang, C., Wang, Y., Li, X., Ren, L., Zhao, J., Hu, Y., et al. (2020). Clinical Features of Patients Infected with 2019 Novel Coronavirus in Wuhan, China. Lancet 395 (10223), 497–506. doi:10.1016/s0140-6736(20)30183-5
Huang, L., Xu, R., Liu, Y., and Liu, Z. (2020). Study on Active Compounds of Yupingfeng San for Preventing and Treating COVID-19 Based on Network Pharmacology and Molecular Docking Technology. Pharmacol. Clin. Chin. Mater. Med. 36 (2), 116–121. doi:10.13412/j.cnki.zyyl.20200407.005
Huang, S., Fu, Y., Xu, B., Liu, C., Wang, Q., Luo, S., et al. (2020). Wogonoside Alleviates Colitis by Improving Intestinal Epithelial Barrier Function via the MLCK/pMLC2 Pathway. Phytomedicine 68, 153179. doi:10.1016/j.phymed.2020.153179
Huiyang, S., Li, X., and Oumei, H. (2020). Effect of Modified Maxing Shigan Decoction on T Lymphocyte Subsets,inflammatory Factors and Intestinal flora in Children with Acute Exacerbation of Bronchial Asthma. Hebei J. Traditional Chin. Med. 42 (4), 567–571. doi:10.3969/j.issn.1002-2619.2020.04.019
Huo, W., Qi, P., Cui, L., Zhang, L., Dai, L., Liu, Y., et al. (2020). Polysaccharide from Wild Morels Alters the Spatial Structure of Gut Microbiota and the Production of Short-Chain Fatty Acids in Mice. Biosci. Microb. Food Health 39 (4), 219–226. doi:10.12938/bmfh.2020-018
Izcue, A., and Powrie, F. (2012). Malnutrition Promotes Rogue Bacteria. Nature 487 (7408), 437–439. doi:10.1038/487437a
Jan, K. C., Hwang, L. S., and Ho, C. T. (2009). Biotransformation of Sesaminol Triglucoside to Mammalian Lignans by Intestinal Microbiota. J. Agric. Food Chem. 57 (14), 6101–6106. doi:10.1021/jf901215j
Jia, S., Luo, H., Liu, X., Fan, X., Huang, Z., Lu, S., et al. (2021). Dissecting the Novel Mechanism of Reduning Injection in Treating Coronavirus Disease 2019 (COVID-19) Based on Network Pharmacology and Experimental Verification. J. Ethnopharmacol. 273 (12), 113871. doi:10.1016/j.jep.2021.113871
Jin, X., Lian, J. S., Hu, J. H., Gao, J., Zheng, L., Zhang, Y. M., et al. (2020a). Epidemiological, Clinical and Virological Characteristics of 74 Cases of Coronavirus-Infected Disease 2019 (COVID-19) with Gastrointestinal Symptoms. Gut 69 (6), 1002–1009. doi:10.1136/gutjnl-2020-320926
Jin, X., Lu, Y., Chen, S., and Chen, D. (2020b). UPLC-MS Identification and Anticomplement Activity of the Metabolites of Sophora Tonkinensis Flavonoids Treated with Human Intestinal Bacteria. J. Pharm. Biomed. Anal. 184, 113176. doi:10.1016/j.jpba.2020.113176
Jung, H. C., Eckmann, L., Yang, S. K., Panja, A., Fierer, J., Morzycka-Wroblewska, E., et al. (1995). A Distinct Array of Proinflammatory Cytokines Is Expressed in Human colon Epithelial Cells in Response to Bacterial Invasion. J. Clin. Invest. 95 (1), 55–65. doi:10.1172/jci117676
Kao, H.-F., Wang, Y.-C., Tseng, H.-Y., Wu, L. S.-H., Tsai, H.-J., Hsieh, M.-H., et al. (2020). Goat Milk Consumption Enhances Innate and Adaptive Immunities and Alleviates Allergen-Induced Airway Inflammation in Offspring Mice. Front. Immunol. 11 (18), 184. doi:10.3389/fimmu.2020.00184
Khalmatova, B. T. (2006). Intestinal Microbiocenosis in Children with Thymomegaly and Acute Lower Respiratory Tract Infections and Regimen of its Correction. Zh Mikrobiol. Epidemiol. Immunobiol. 1 (7), 97–99. doi:10.16438/j.0513-4870.2020-0126
Kim, Y.-H., Cho, K.-H., and Moon, B.-S. (2009). Banhabaikchulcheunma-tang Down-Regulates LPS-Induced Production of Pro-inflammatory Cytokines. J. Physiol. Pathol. Korean Med. 23 (1), 192–198.
Kim, B.-G., Jung, W. D., Mok, H., and Ahn, J.-H. (2013). Production of Hydroxycinnamoyl-Shikimates and Chlorogenic Acid in Escherichia coli: Production of Hydroxycinnamic Acid Conjugates. Microb. Cell Fact 12 (5), 15. doi:10.1186/1475-2859-12-15
Kim, D. Y., Kang, M.-K., Lee, E.-J., Kim, Y.-H., Oh, H., Kim, S.-I., et al. (2020). Eucalyptol Inhibits Amyloid-β-Induced Barrier Dysfunction in Glucose-Exposed Retinal Pigment Epithelial Cells and Diabetic Eyes. Antioxidants 9 (10), 1000. doi:10.3390/antiox9101000
Kim, M., and Kim, Y. B. (2020). A Network-Based Pharmacology Study of Active Compounds and Targets of Fritillaria Thunbergii against Influenza. Comput. Biol. Chem. 89, 107375. doi:10.1016/j.compbiolchem.2020.107375
Kim, M., Park, K. H., and Kim, Y. B. (2020). Identifying Active Compounds and Targets of Fritillariae Thunbergii against Influenza-Associated Inflammation by Network Pharmacology Analysis and Molecular Docking. Molecules 25 (17), 3853. doi:10.3390/molecules25173853
Knaup, B., Kahle, K., Erk, T., Valotis, A., Scheppach, W., Schreier, P., et al. (2007). Human Intestinal Hydrolysis of Phenol Glycosides - a Study with Quercetin Andp-Nitrophenol Glycosides Using Ileostomy Fluid. Mol. Nutr. Food Res. 51 (11), 1423–1429. doi:10.1002/mnfr.200700036
Knight, D., and Girling, K. (2003). Gut flora in Health and Disease. Lancet 361 (9371), 1831. doi:10.1016/s0140-6736(03)13438-1
Koeth, R. A., Wang, Z., Levison, B. S., Buffa, J. A., Org, E., Sheehy, B. T., et al. (2013). Intestinal Microbiota Metabolism of L-Carnitine, a Nutrient in Red Meat, Promotes Atherosclerosis. Nat. Med. 19 (5), 576–585. doi:10.1038/nm.3145
Koh, A., De Vadder, F., Kovatcheva-Datchary, P., and Bäckhed, F. (2016). From Dietary Fiber to Host Physiology: Short-Chain Fatty Acids as Key Bacterial Metabolites. Cell 165 (6), 1332–1345. doi:10.1016/j.cell.2016.05.041
Kreft, L., Hoffmann, C., and Ohnmacht, C. (2020). Therapeutic Potential of the Intestinal Microbiota for Immunomodulation of Food Allergies. Front. Immunol. 11 (14), 1853. doi:10.3389/fimmu.2020.01853
Le, S., Xiaomei, C., Chongming, W., and Shunxing, G. (2020). Advances and prospects of pharmacological activities of Dendrobium officinale Kimura et Migo polysaccharides. Acta Pharmaceut. Sin. 55 (10), 2322–2329. doi:10.16438/j.0513-4870.2020-0126
Lee, D. Y. W., Li, Q. Y., Liu, J., and Efferth, T. (2021). Traditional Chinese Herbal Medicine at the Forefront Battle against COVID-19: Clinical Experience and Scientific Basis. Phytomedicine 80, 153337. doi:10.1016/j.phymed.2020.153337
Lee, I.-C., Huo, T.-I., and Huang, Y.-H. (2020). Gastrointestinal and Liver Manifestations in Patients with COVID-19. J. Chin. Med. Assoc. 83 (6), 521–523. doi:10.1097/jcma.0000000000000319
Lee, N.-K., Choi, S.-H., Park, S.-H., Park, E.-K., and Kim, D.-H. (2004). Antiallergic Activity of Hesperidin Is Activated by Intestinal Microflora. Pharmacology 71 (4), 174–180. doi:10.1159/000078083
Lee, N., Hui, D., Wu, A., Chan, P., Cameron, P., Joynt, G. M., et al. (2003). A Major Outbreak of Severe Acute Respiratory Syndrome in Hong Kong. N. Engl. J. Med. 348 (20), 1986–1994. doi:10.1056/nejmoa030685
Li, B.-L., Zhao, D.-Y., Du, P.-L., Wang, X.-T., Yang, Q., and Cai, Y.-R. (2021). Luteolin Alleviates Ulcerative Colitis through SHP-1/STAT3 Pathway. Inflamm. Res. 70 (6), 705–717. doi:10.1007/s00011-021-01468-9
Li, J., Wei, Y., Li, X., Zhu, D., Nie, B., Zhou, J., et al. (2017). Herbal Formula Xian-Fang-Huo-Ming-Yin Regulates Differentiation of Lymphocytes and Production of Pro-inflammatory Cytokines in Collagen-Induced Arthritis Mice. BMC Comp. Altern. Med. 17 (1), 12. doi:10.1186/s12906-016-1526-x
Li, K., Hao, Z., Du, J., Gao, Y., Yang, S., and Zhou, Y. (2020). Bacteroides Thetaiotaomicron Relieves colon Inflammation by Activating Aryl Hydrocarbon Receptor and Modulating CD4+T Cell Homeostasis. Int. Immunopharmacol. 90, 107183. doi:10.1016/j.intimp.2020.107183
Li, L. Q., Huang, T., Wang, Y. Q., Wang, Z. P., Liang, Y., Huang, T. B., et al. (2020b). Response to Char’s Comment: Comment on Li et al: COVID‐19 Patients’ Clinical Characteristics, Discharge Rate, and Fatality Rate of Meta‐Analysis. J. Med. Virol. 92 (9), 1433. doi:10.1002/jmv.25924
Li, L. Q., Huang, T., Wang, Y. q., Wang, Z. p., Liang, Y., Huang, T. b., et al. (2020a). COVID‐19 Patients' Clinical Characteristics, Discharge Rate, and Fatality Rate of Meta‐analysis. J. Med. Virol. 92 (6), 577–583. doi:10.1002/jmv.25757
Li, L., Wang, Q., Gao, Y., Liu, L., Duan, Y., Mao, D., et al. (2021). Colistin and Amoxicillin Combinatorial Exposure Alters the Human Intestinal Microbiota and Antibiotic Resistome in the Simulated Human Intestinal Microbiota. Sci. Total Environ. 750, 141415. doi:10.1016/j.scitotenv.2020.141415
Li, M., Pare, P. W., Zhang, J., Kang, T., Zhang, Z., Yang, D., et al. (2018). Antioxidant Capacity Connection with Phenolic and Flavonoid Content in Chinese Medicinal Herbs. Rec.Nat.Prod. 12 (3), 239–250. doi:10.25135/rnp.24.17.08.138
Li, Q., Cui, Y., Xu, B., Wang, Y., Lv, F., Li, Z., et al. (2021). Main Active Components of Jiawei Gegen Qinlian Decoction Protects against Ulcerative Colitis under Different Dietary Environments in a Gut Microbiota-Dependent Manner. Pharmacol. Res. 170, 105694. doi:10.1016/j.phrs.2021.105694
Li, Y., Chu, F., Li, P., Johnson, N., Li, T., Wang, Y., et al. (2021). Potential Effect of Maxing Shigan Decoction against Coronavirus Disease 2019 (COVID-19) Revealed by Network Pharmacology and Experimental Verification. J. Ethnopharmacol. 271, 113854. doi:10.1016/j.jep.2021.113854
Li, Y., Liu, T., Yan, C., Xie, R., Guo, Z., Wang, S., et al. (2018). Diammonium Glycyrrhizinate Protects against Nonalcoholic Fatty Liver Disease in Mice through Modulation of Gut Microbiota and Restoration of Intestinal Barrier. Mol. Pharm. 15 (9), 3860–3870. doi:10.1021/acs.molpharmaceut.8b00347
Li, Y., Liu, X.-Y., Ma, M.-M., Qi, Z.-J., Zhang, X.-Q., Li, Z., et al. (2014). Changes in Intestinal Microflora in Rats with Acute Respiratory Distress Syndrome. World J. Gastroenterol. 20 (19), 5849–5858. doi:10.3748/wjg.v20.i19.5849
Li, Y., Liu, X., Guo, L., Li, J., Zhong, D., Zhang, Y., et al. (2020). Traditional Chinese Herbal Medicine for Treating Novel Coronavirus (COVID-19) Pneumonia: Protocol for a Systematic Review and Meta-Analysis. Syst. Rev. 9 (1), 75. doi:10.1186/s13643-020-01343-4
Liao, S., Liu, S., Zhang, Y., Bahi, J., and Oualha, N. (2021). Preparation of Anti Toll-like Receptor-4 Nano-Antibody and its Effect on Gram Negative Sepsis. J. Nanosci. Nanotechnol. 21 (2), 1048–1053. doi:10.1166/jnn.2021.18664
Lin, C.-C., Yen, F.-L., Hsu, F.-F., and Lin, J.-M. (2010). Anti-hypercholesterolaemia, Antioxidant Activity and Free Radical Scavenger Effects of Traditional Chinese Medicine Prescriptions Used for Stroke. J. Pharm. Pharmacol. 52 (11), 1387–1393. doi:10.1211/0022357001777388
Lin, H., Wang, X., Liu, M., Huang, M., Shen, Z., Feng, J., et al. (2021). Exploring the Treatment of COVID ‐19 with Yinqiao Powder Based on Network Pharmacology. Phytotherapy Res. 35 (5), 2651–2664. doi:10.1002/ptr.7012
Lin, Y. X., Liang, Y. Y., Jie, Z., Feng, W. R., and Qiang, N. L. (2020). Exploring Potential Effect of Shengjiang San on SARS-CoV-2 Based on Network Pharmacology and Molecular Docking. Chin. Traditional Herbal Drugs 51 (07), 1795–1803. doi:10.7501/J.ISSN.0253-2670.2020.07.013
Lina, L., Zhiguang, S., and Jing, Y. (2014). Research Progress in Intervention of Intestinal Epithelial Function of Irritable Bowel Syndrome Patients by Herbal Medicine. Res. Prog. Intervention Intestinal Epithelial Funct. Irritable Bowel Syndr. Patients by Herbal Med. 25 (4), 519–522. doi:10.3969/j.issn.1003-9783.2014.04.032
Liu, B., Liu, T., Wang, X., Zheng, X., Wang, H., and Ma, L. (2016). Effects of Guchang Capsule on Dextran Sulphate Sodium-Induced Experimental Ulcerative Colitis in Mice. Evid. Based Comp. Altern. Med. 2016, 1–12. doi:10.1155/2016/3150651
Liu, C.-M., Chen, J., Yang, S., Mao, L.-G., Jiang, T.-T., Tu, H.-H., et al. (2018). The Chinese Herbal Formula Zhibai Dihuang Granule Treat Yin-Deficiency-Heat Syndrome Rats by Regulating the Immune Responses. J. Ethnopharmacol. 225, 271–278. doi:10.1016/j.jep.2018.05.001
Liu, C.-S., Liang, X., Wei, X.-H., Jin, Z., Chen, F.-L., Tang, Q.-F., et al. (2019). Gegen Qinlian Decoction Treats Diarrhea in Piglets by Modulating Gut Microbiota and Short-Chain Fatty Acids. Front. Microbiol. 10, 825. doi:10.3389/fmicb.2019.00825
Liu, G., and Zhang, T. (2020). Feasibility of Chaihu Dayuan Decoction in the Treatment of COVID-19 Based on Network Pharmacology and Molecular Docking Technology. Pharmacol. Clin. Chin. Materia Med. 36 (2), 102–108. doi:10.13412/j.cnki.zyyl.20200409.001
Liu, S.-P., Dong, W. G., Wu, D. F., Luo, H. S., and Yu, J. P. (2003). Protective Effect Ofangelica Sinensispolysaccharide on Experimental Immunological colon Injury in Rats. World J. Gastroenterol. 9 (12), 2786–2790. doi:10.3748/wjg.v9.i12.2786
Liu, X., Yang, J., Li, J., Xu, C., and Jiang, W. (2021). Vanillin Attenuates Cadmium-Induced Lung Injury through Inhibition of Inflammation and Lung Barrier Dysfunction through Activating AhR. Inflammation 44. doi:10.1007/s10753-021-01492-1
Liu, Y.-T., Qi, S.-L., and Sun, K.-W. (2021). Traditional Chinese Medicine, Liver Fibrosis, Intestinal flora: Is There Any Connection?-A Narrative Review. Ann. Palliat. Med. 10 (4), 4846–4857. doi:10.21037/apm-20-2129
Lu, L., Lu, T., Shen, J., Lv, X., Wei, W., Wang, H., et al. (2021). Alisol A 24-acetate Protects against Brain Microvascular Endothelial Cells Injury through Inhibiting miR-92a-3p/Tight Junctions axis. Aging 13. doi:10.18632/aging.203094
Luo, H., Yang, M., Tang, Q.-L., Hu, X.-Y., Willcox, M. L., and Liu, J.-P. (2021). Characteristics of Registered Clinical Trials on Traditional Chinese Medicine for Coronavirus Disease 2019 (COVID-19): A Scoping Review. Eur. J. Integr. Med. 41, 101251. doi:10.1016/j.eujim.2020.101251
Luo, X., Yue, B., Yu, Z., Ren, Y., Zhang, J., Ren, J., et al. (2020). Obacunone Protects against Ulcerative Colitis in Mice by Modulating Gut Microbiota, Attenuating TLR4/NF-Κb Signaling Cascades, and Improving Disrupted Epithelial Barriers. Front. Microbiol. 11, 497. doi:10.3389/fmicb.2020.00497
Lyte, M., and Bailey, M. T. (1997). Neuroendocrine-bacterial Interactions in a Neurotoxin-Induced Model of Trauma. J. Surg. Res. 70 (2), 195–201. doi:10.1006/jsre.1997.5130
Ma, J., J., Huo, X., Chen, X., Zhu, W., Yao, M., Qiao, Y., et al. (2020). Study on Screening Chinese Traditional Medicine against 2019-nCoV Based on Mpro and PLP. China J. Chin. Materia. Med. 45 (6), 1219–1224.
Ma, Q., Li, R., Pan, W., Huang, W., Liu, B., Xie, Y., et al. (2020). Phillyrin (KD-1) Exerts Anti-viral and Anti-inflammatory Activities against Novel Coronavirus (SARS-CoV-2) and Human Coronavirus 229E (HCoV-229E) by Suppressing the Nuclear Factor Kappa B (NF-Κb) Signaling Pathway. Phytomedicine 78, 153296. doi:10.1016/j.phymed.2020.153296
Matoba, Y., Abiko, C., Ikeda, T., Aoki, Y., Suzuki, Y., Yahagi, K., et al. (2015). Detection of the Human Coronavirus 229E, HKU1, NL63, and OC43 between 2010 and 2013 in Yamagata, Japan. Jpn. J. Infect. Dis. 68 (2), 138–141. doi:10.7883/yoken.JJID.2014.266
Memish, Z. A., Zumla, A. I., Al-Hakeem, R. F., Al-Rabeeah, A. A., and Stephens, G. M. (2013). Family Cluster of Middle East Respiratory Syndrome Coronavirus Infections. N. Engl. J. Med. 368 (26), 2487–2494. doi:10.1056/NEJMoa1303729
Mohan, S. V., Hemalatha, M., Kopperi, H., Ranjith, I., and Kumar, A. K. (2021). SARS-CoV-2 in Environmental Perspective: Occurrence, Persistence, Surveillance, Inactivation and Challenges. Chem. Eng. J. 405, 126893. doi:10.1016/j.cej.2020.126893
Müller-Werdan, U., and Werdan, K. (2003). End-organ Damage in Inflammation and Sepsis. Internist 44 (7), 864–871. doi:10.1007/s00108-003-0956-y
Niu, W., Wu, F., Cui, H., Cao, W., Chao, Y., Wu, Z., et al. (2020). Network Pharmacology Analysis to Identify Phytochemicals in Traditional Chinese Medicines that May Regulate ACE2 for the Treatment of COVID-19. Evid. Based Complement. Alternat. Med. 2020, 7493281. doi:10.1155/2020/7493281
Noda, S., Tanabe, S., and Suzuki, T. (2012). Differential Effects of Flavonoids on Barrier Integrity in Human Intestinal Caco-2 Cells. J. Agric. Food Chem. 60 (18), 4628–4633. doi:10.1021/jf300382h
Noorwali, A. A., Turkistani, A. M., Asiri, S. I., Trabulsi, F. A., Alwafi, O. M., Alzahrani, S. H., et al. (2015). Descriptive Epidemiology and Characteristics of Confirmed Cases of Middle East Respiratory Syndrome Coronavirus Infection in the Makkah Region of Saudi Arabia, March to June 2014. Ann. Saudi Med. 35 (3), 203–209. doi:10.5144/0256-4947.2015.203
Oboho, I. K., Tomczyk, S. M., Al-Asmari, A. M., Banjar, A. A., Al-Mugti, H., Aloraini, M. S., et al. (2015). 2014 MERS-CoV Outbreak in Jeddah - A Link to Health Care Facilities. N. Engl. J. Med. 372 (9), 846–854. doi:10.1056/NEJMoa1408636
Olaimat, A. N., Aolymat, I., Al-Holy, M., Ayyash, M., Abu Ghoush, M., Al-Nabulsi, A. A., et al. (2020). The Potential Application of Probiotics and Prebiotics for the Prevention and Treatment of COVID-19. Npj Sci. Food 4 (5), 17. doi:10.1038/s41538-020-00078-9
Olivares-Villagómez, D., and Van Kaer, L. (2018). Intestinal Intraepithelial Lymphocytes: Sentinels of the Mucosal Barrier. Trends Immunol. 39 (4), 264–275. doi:10.1016/j.it.2017.11.003
Olliver, M., Hiew, J., Mellroth, P., Henriques-Normark, B., and Bergman, P. (2011). Human Monocytes Promote Th1 and Th17 Responses to Streptococcus Pneumoniae. Infect. Immun. 79 (10), 4210–4217. doi:10.1128/iai.05286-11
Omonijo, F. A., Liu, S., Hui, Q., Zhang, H., Lahaye, L., Bodin, J.-C., et al. (2019). Thymol Improves Barrier Function and Attenuates Inflammatory Responses in Porcine Intestinal Epithelial Cells during Lipopolysaccharide (LPS)-Induced Inflammation. J. Agric. Food Chem. 67 (2), 615–624. doi:10.1021/acs.jafc.8b05480
Ou, Y.-y., Jiang, Y., Li, H., Zhang, Y.-y., Lu, Y., and Chen, D.-f. (2017). Polysaccharides from Arnebia Euchroma Ameliorated Endotoxic Fever and Acute Lung Injury in Rats through Inhibiting Complement System. Inflammation 40 (1), 275–284. doi:10.1007/s10753-016-0478-0
Pal, S., Mitra, M., Mishra, J., Saha, S., and Bhattacharya, B. (2013). Correlation of Total Salivary Secretory Immunoglobulin A (SIgA) and Mutans Specific SIgA in Children Having Different Caries Status. J. Indian Soc. Pedod Prev. Dent 31 (4), 270–274. doi:10.4103/0970-4388.121831
Pan, L., Mu, M., Yang, P., Sun, Y., Wang, R., Yan, J., et al. (2020). Clinical Characteristics of COVID-19 Patients with Digestive Symptoms in Hubei, China: A Descriptive, Cross-Sectional, Multicenter Study. Am. J. Gastroenterol. 115 (5), 766–773. doi:10.14309/ajg.0000000000000620
Pan, M.-H., Chiou, Y.-S., Tsai, M.-L., and Ho, C.-T. (2011). Anti-inflammatory Activity of Traditional Chinese Medicinal Herbs. J. Tradit. Complement. Med. 1 (1), 8–24. doi:10.1016/s2225-4110(16)30052-9
Pang, J., Ma, S., Xu, X., Zhang, B., and Cai, Q. (2021). Effects of Rhizome of Atractylodes Koreana (Nakai) Kitam on Intestinal flora and Metabolites in Rats with Rheumatoid Arthritis. J. Ethnopharmacol. 279, 114026. doi:10.1016/j.jep.2021.114026
Pang, X., He, X., Su, F., and Wu, Q. (2012). Study on the Therapeutic Effects of Chinese Medicinal Formulae for Strengthening Spleen Combined with Enteral Nutrition Treatment. Chin. J. Hosp. Pharm. 32 (16), 1282–1284. doi:10.1016/s0254-6272(13)60069-2
Passos-Silva, D. G., Verano-Braga, T., and Santos, R. A. S. (2013). Angiotensin-(1-7): Beyond the Cardio-Renal Actions. Clin. Sci. 124 (7–8), 443–456. doi:10.1042/cs20120461
Peiris, J., Chu, C., Cheng, V., Chan, K., Hung, I., Poon, L., et al. (2003a). Clinical Progression and Viral Load in a Community Outbreak of Coronavirus-Associated SARS Pneumonia: a Prospective Study. Lancet 361 (9371), 1767–1772. doi:10.1016/s0140-6736(03)13412-5
Peiris, J., Lai, S., Poon, L., Guan, Y., Yam, L., Lim, W., et al. (2003b). Coronavirus as a Possible Cause of Severe Acute Respiratory Syndrome. Lancet 361 (9366), 1319–1325. doi:10.1016/s0140-6736(03)13077-2
Peng, G., Wang, Y., Liu, Z., Kong, J., and Li, Y. (2019). Xuanbai Chengqi Decoction Alleviates Pulmonary Inflammation in Mouse Model of Chronic Obstructive Pulmonary Disease through Inhibiting the Ratio of Th17/Treg via Gut Commensal Microbiota. Eur. J. Immunol. 49, 1890. doi:10.1002/eji.201970400
Pérez-Rosés, R., Risco, E., Vila, R., Peñalver, P., and Cañigueral, S. (2015). Effect of Some Essential Oils on Phagocytosis and Complement System Activity. J. Agric. Food Chem. 63 (5), 1496–1504. doi:10.1021/jf504761m
Pitman, R. S., and Blumberg, R. S. (2000). First Line of Defense: the Role of the Intestinal Epithelium as an Active Component of the Mucosal Immune System. J. Gastroenterol. 35 (11), 805–814. doi:10.1007/s005350070017
Poutanen, S. M., Low, D. E., Henry, B., Finkelstein, S., Rose, D., Green, K., et al. (2003). Identification of Severe Acute Respiratory Syndrome in Canada. N. Engl. J. Med. 348 (20), 1995–2005. doi:10.1056/nejmoa030634
Pu, J., Chen, D., Tian, G., He, J., Zheng, P., Mao, X., et al. (2020). Effects of Benzoic Acid, Bacillus Coagulans and Oregano Oil Combined Supplementation on Growth Performance, Immune Status and Intestinal Barrier Integrity of Weaned Piglets. Anim. Nutr. 6 (2), 152–159. doi:10.1016/j.aninu.2020.02.004
Qian, G.-Q., Yang, N.-B., Ding, F., Ma, A. H. Y., Wang, Z.-Y., Shen, Y.-F., et al. (2020). Epidemiologic and Clinical Characteristics of 91 Hospitalized Patients with COVID-19 in Zhejiang, China: A Retrospective, Multi-Centre Case Series. QJM 113 (7), 474–481. doi:10.1093/qjmed/hcaa089
Qiao, L., Schürmann, G., Betzler, M., and Meuer, S. C. (1991). Activation and Signaling Status of Human Lamina Propria T Lymphocytes. Gastroenterology 101 (6), 1529–1536. doi:10.1016/0016-5085(91)90388-2
Qin, N., Zheng, B., Yao, J., Guo, L., Zuo, J., Wu, L., et al. (2015). Influence of H7N9 Virus Infection and Associated Treatment on Human Gut Microbiota. Sci. Rep. 5, 14771. doi:10.1038/srep14771
Qu, L., Xu, Y., Cao, G., Xu, K., Tu, J., Lin, X., et al. (2020). Effects of Atractylodes Oil on Inflammatory Response and Serum Metabolites in Adjuvant Arthritis Rats. Biomed. Pharmacother. 127, 110130. doi:10.1016/j.biopha.2020.110130
Rainer, T. H., Cameron, P. A., Smit, D., Ong, K. L., Hung, A. N. W., Nin, D. C. P., et al. (2003). Evaluation of WHO Criteria for Identifying Patients with Severe Acute Respiratory Syndrome Out of Hospital: Prospective Observational Study. BMJ 326 (7403), 1354–1358. doi:10.1136/bmj.326.7403.1354
Rechkemmer, G., Rönnau, K., and Engelhardt, W. V. (1988). Fermentation of Polysaccharides and Absorption of Short Chain Fatty Acids in the Mammalian Hindgut. Comp. Biochem. Physiol. A: Physiol. 90 (4), 563–568. doi:10.1016/0300-9629(88)90668-8
Ridaura, V. K., Faith, J. J., Rey, F. E., Cheng, J., Duncan, A. E., Kau, A. L., et al. (2013). Gut Microbiota from Twins Discordant for Obesity Modulate Metabolism in Mice. Science 341 (6150), 1241214. doi:10.1126/science.1241214
Saad, M., Omrani, A. S., Baig, K., Bahloul, A., Elzein, F., Matin, M. A., et al. (2014). Clinical Aspects and Outcomes of 70 Patients with Middle East Respiratory Syndrome Coronavirus Infection: a Single-center Experience in Saudi Arabia. Int. J. Infect. Dis. 29, 301–306. doi:10.1016/j.ijid.2014.09.003
Saint-Georges-Chaumet, Y., and Edeas, M. (2016). Microbiota-mitochondria Inter-talk: Consequence for Microbiota-Host Interaction. Pathog. Dis. 74 (1), ftv096. doi:10.1093/femspd/ftv096
Santos, R. A. S., Ferreira, A. J., Verano-Braga, T., and Bader, M. (2013). Angiotensin-Converting Enzyme 2, Angiotensin-(1-7) and Mas: New Players of the Renin-Angiotensin System. J. Endocrinol. 216 (2), R1–R17. doi:10.1530/joe-12-0341
Santos, R. A. S., Sampaio, W. O., Alzamora, A. C., Motta-Santos, D., Alenina, N., Bader, M., et al. (2018). The Ace2/Angiotensin-(1-7)/Mas Axis of the Renin-Angiotensin System: Focus on Angiotensin-(1-7). Physiol. Rev. 98 (1), 505–553. doi:10.1152/physrev.00023.2016
Saranya, J., Shilpa, G., Raghu, K. G., and Priya, S. (2017). Morus alba Leaf Lectin (MLL) Sensitizes MCF-7 Cells to Anoikis by Inhibiting Fibronectin Mediated Integrin-FAK Signaling through Ras and Activation of P38 MAPK. Front. Pharmacol. 8 (7), 34. doi:10.3389/fphar.2017.00034
Sartor, R. B. (2004). Therapeutic Manipulation of the Enteric Microflora in Inflammatory Bowel Diseases: Antibiotics, Probiotics, and Prebiotics. Gastroenterology 126 (6), 1620–1633. doi:10.1053/j.gastro.2004.03.024
Sato, R., Reuter, T., Hiranuma, R., Shibata, T., Fukui, R., Motoi, Y., et al. (2020). The Impact of Cell Maturation and Tissue Microenvironments on the Expression of Endosomal Toll-like Receptors in Monocytes and Macrophages. Int. Immunol. 32 (12), 785–798. doi:10.1093/intimm/dxaa055
Scalise, M., and Indiveri, C. (2020). Repurposing Nimesulide, a Potent Inhibitor of the B0AT1 Subunit of the SARS-CoV-2 Receptor, as a Therapeutic Adjuvant of COVID-19. SLAS Discov. 25 (10), 1171–1173. doi:10.1177/2472555220934421
Schuijt, T. J., Lankelma, J. M., Scicluna, B. P., de Sousa e Melo, F., Roelofs, J. J. T. H., de Boer, J. D., et al. (2016). The Gut Microbiota Plays a Protective Role in the Host Defence against Pneumococcal Pneumonia. Gut 65 (4), 575–583. doi:10.1136/gutjnl-2015-309728
Sen, D., Debnath, P., Debnath, B., Bhaumik, S., and Debnath, S. (2020). Identification of Potential Inhibitors of SARS-CoV-2 Main Protease and Spike Receptor from 10 Important Spices through Structure-Based Virtual Screening and Molecular Dynamic Study. J. Biomol. Struct. Dyn. 39, 1–22. doi:10.1080/07391102.2020.1819883
Serreli, G., Melis, M. P., Zodio, S., Naitza, M. R., Casula, E., Peñalver, P., et al. (2020). Altered Paracellular Permeability in Intestinal Cell Monolayer Challenged with Lipopolysaccharide: Modulatory Effects of Pterostilbene Metabolites. Food Chem. Toxicol. 145, 111729. doi:10.1016/j.fct.2020.111729
Shah, V. O., Ferguson, J. E., Hunsaker, L. A., Deck, L. M., and Vander Jagt, D. L. (2010). Natural Products Inhibit LPS-Induced Activation of Pro-Inflammatory Cytokines in Peripheral Blood Mononuclear Cells. Nat. Product. Res. 24 (12), 1177–1188. doi:10.1080/14786410903112680
Shao, S., Wang, D., Zheng, W., Li, X., Zhang, H., Zhao, D., et al. (2019). A Unique Polysaccharide from Hericium erinaceus Mycelium Ameliorates Acetic Acid-Induced Ulcerative Colitis Rats by Modulating the Composition of the Gut Microbiota, Short Chain Fatty Acids Levels and GPR41/43 Respectors. Int. Immunopharmacol. 71, 411–422. doi:10.1016/j.intimp.2019.02.038
Shi, H.-L., Lan, Y.-H., Hu, Z.-C., Yan, Z.-N., Liu, Z.-Z., Kadier, X., et al. (2020). Microecology Research: A New Target for the Prevention of Asthma. Chin. Med. J. 133 (22), 2712–2720. doi:10.1097/cm9.0000000000001127
Shi, R., Zhou, H., Liu, Z., Ma, Y., Wang, T., Liu, Y., et al. (2009). Influence ofCoptis Chinensison Pharmacokinetics of Flavonoids after Oral Administration ofRadix Scutellariaein Rats. Biopharm. Drug Dispos. 30 (7), 398–410. doi:10.1002/bdd.674
Shi, S., Qin, M., Shen, B., Cai, Y., Liu, T., Yang, F., et al. (2020). Association of Cardiac Injury with Mortality in Hospitalized Patients with COVID-19 in Wuhan, China. JAMA Cardiol. 5 (7), 802–810. doi:10.1001/jamacardio.2020.0950
Shi, Y., Lin, W., Fan, B., Jia, Z., Yao, S., Kang, J., et al. (1999). Fast Repair of Hydroxy Radical Purine Deoxynucleotide Adducts by Phenylpropanoid Glycosides and Their Derivatives from Chinese Herbs. Biochim. Biophys. Acta 1472 (1–2), 115–127. doi:10.1016/s0304-4165(99)00111-7
Song, L., Bi, S., Gu, J., Wu, T., and He, L. (2020). Screening Novel Inhibitors Targeting SARS-CoV-2 S Protein-ACE2 Interaction Based on Molecular Docking. Chin. Traditional Herbal Drugs 51 (9), 2361–2367. doi:10.7501/j.issn.0253-2670.2020.09.010
Souza, D. G., Vieira, A. T., Soares, A. C., Pinho, V., Nicoli, J. R., Vieira, L. Q., et al. (2004). The Essential Role of the Intestinal Microbiota in Facilitating Acute Inflammatory Responses. J. Immunol. 173 (6), 4137–4146. doi:10.4049/jimmunol.173.6.4137
Su, S., Wong, G., Shi, W., Liu, J., Lai, A. C. K., Zhou, J., et al. (2016). Epidemiology, Genetic Recombination, and Pathogenesis of Coronaviruses. Trends Microbiol. 24 (6), 490–502. doi:10.1016/j.tim.2016.03.003
Suleyman, G., Fadel, R. A., Malette, K. M., Hammond, C., Abdulla, H., Entz, A., et al. (2020). Clinical Characteristics and Morbidity Associated with Coronavirus Disease 2019 in a Series of Patients in Metropolitan Detroit. JAMA Netw. Open 3 (6), e2012270. doi:10.1001/jamanetworkopen.2020.12270
Sun, K., Zhang, X., Liu, J., and Sun, R. (2020). Network Pharmacological Analysis and Mechanism Prediction of Xiaochaihu Decoction in Treatment of COVID-19 with Syndrome of Pathogenic Itieat Lingering in Lung and Obstructive Cardinalate. Chin. Tradit. Herbal Drugs 51 (7), 1750–1760. doi:10.7501/J.ISSN.0253-2670.2020.07.009
Sun, Z., Zhu, Q. L., Shen, Y., Yan, T., and Zhou, X. (2020). Dynamic Changes of Gut and Lung Microorganisms during Chronic Obstructive Pulmonary Disease Exacerbations. Kaohsiung J. Med. Sci. 36 (2), 107–113. doi:10.1002/kjm2.12147
Suzuki, T., and Hara, H. (2009). Quercetin Enhances Intestinal Barrier Function through the Assembly of Zonnula Occludens-2, Occludin, and Claudin-1 and the Expression of Claudin-4 in Caco-2 Cells. J. Nutr. 139 (5), 965–974. doi:10.3945/jn.108.100867
Suzuki, T., Tanabe, S., and Hara, H. (2011). Kaempferol Enhances Intestinal Barrier Function through the Cytoskeletal Association and Expression of Tight junction Proteins in Caco-2 Cells. J. Nutr. 141 (1), 87–94. doi:10.3945/jn.110.125633
Szebeni, B., Veres, G., Dezsõfi, A., Rusai, K., Vannay, Á., Mraz, M., et al. (2008). Increased Expression of Toll-like Receptor (TLR) 2 and TLR4 in the Colonic Mucosa of Children with Inflammatory Bowel Disease. Clin. Exp. Immunol. 151 (1), 34–41. doi:10.1111/j.1365-2249.2007.03531.x
Taiming, L., Xuehua, J., Meijuan, Z., Sijian, L., and Zhuo, C. (2006). Absorption Characteristics of Baicalin and Baicalein in Rat Small Intestine. Chin. Pharm. J. 23, 1784–1787.
Takiishi, T., Fenero, C. I. M., and Câmara, N. O. S. (2017). Intestinal Barrier and Gut Microbiota: Shaping Our Immune Responses throughout Life. Tissue Barriers 5 (4), e1373208. doi:10.1080/21688370.2017.1373208
Taleb, S. (2019). Tryptophan Dietary Impacts Gut Barrier and Metabolic Diseases. Front. Immunol. 10, 2113. doi:10.3389/fimmu.2019.02113
Tang, A., Tong, Z.-d., Wang, H.-l., Dai, Y.-x., Li, K.-f., Liu, J.-n., et al. (2020). Detection of Novel Coronavirus by RT-PCR in Stool Specimen from Asymptomatic Child, China. Emerg. Infect. Dis. 26 (6), 1337–1339. doi:10.3201/eid2606.200301
Tang, X.-s., and Li, N.-h. (2014). Future View and Development of Immunology: Exploring the Immunology Based on Chinese Medicine and Culture. Chin. J. Integr. Med. 20 (8), 563–569. doi:10.1007/s11655-014-1757-y
Tao, X., Ke, Z., Zhang, X., Deng, Y., Cao, Z., Cao, L., et al. (2020). Investigate Mechanism of Jinzhen Oral Liquid for Prevention COVID-19 Based on Network Pharmacology and Molecular Docking Technology. Chin. Traditional Herbal Drugs 51 (9), 2326–2333.
Thaiss, C. A., Zmora, N., Levy, M., and Elinav, E. (2016). The Microbiome and Innate Immunity. Nature 535 (7610), 65–74. doi:10.1038/nature18847
Trinh, H.-t., Joh, E.-h., Kwak, H.-y., Baek, N.-i., and Kim, D.-h. (2010). Anti-pruritic Effect of Baicalin and its Metabolites, Baicalein and Oroxylin A, in Mice. Acta Pharmacol. Sin 31 (6), 718–724. doi:10.1038/aps.2010.42
Tsang, K. W., Ho, P. L., Ooi, G. C., Yee, W. K., Wang, T., Chan-Yeung, M., et al. (2003). A Cluster of Cases of Severe Acute Respiratory Syndrome in Hong Kong. N. Engl. J. Med. 348 (20), 1977–1985. doi:10.1056/nejmoa030666
Turner, J. R. (2009). Intestinal Mucosal Barrier Function in Health and Disease. Nat. Rev. Immunol. 9 (11), 799–809. doi:10.1038/nri2653
van der Voort, P. H. J. (2006). Linking Gut-Associated Lymphoid Tissue to Multiple Organ Dysfunction Syndrome and Infection. Crit. Care Med. 34 (1), 257–258. doi:10.1097/01.Ccm.0000196087.29912.A0
van Wijk, F., and Cheroutre, H. (2010). Mucosal T Cells in Gut Homeostasis and Inflammation. Expert Rev. Clin. Immunol. 6 (4), 559–566. doi:10.1586/eci.10.34
Varga, Z., Flammer, A. J., Steiger, P., Haberecker, M., Andermatt, R., Zinkernagel, A. S., et al. (2020). Endothelial Cell Infection and Endotheliitis in COVID-19. Lancet 395 (10234), 1417–1418. doi:10.1016/s0140-6736(20)30937-5
Varon, A., and Varon, J. (2015). Shenfu, Traditional Chinese Medicine in Sepsis: Are We Ready for it?. Am. J. Emerg. Med. 33 (9), 1244–1245. doi:10.1016/j.ajem.2015.03.047
Varon, J. (2009). Herbal Medicine in Acute Care Medicine: Past, Present, and Future?. Am. J. Emerg. Med. 27 (1), 113–114. doi:10.1016/j.ajem.2008.09.006
Viney, J. L., and MacDonald, T. T. (1990). Selective Death of T Cell Receptor γ/δ+ Intraepithelial Lymphocytes by Apoptosis. Eur. J. Immunol. 20 (12), 2809–2812. doi:10.1002/eji.1830201242
Vuille-dit-Bille, R. N., Camargo, S. M., Emmenegger, L., Sasse, T., Kummer, E., Jando, J., et al. (2015). Human Intestine Luminal ACE2 and Amino Acid Transporter Expression Increased by ACE-Inhibitors. Amino Acids 47 (4), 693–705. doi:10.1007/s00726-014-1889-6
Walls, A. C., Park, Y.-J., Tortorici, M. A., Wall, A., McGuire, A. T., and Veesler, D. (2020). Structure, Function, and Antigenicity of the SARS-CoV-2 Spike Glycoprotein. Cell 181 (2), 281–292. doi:10.1016/j.cell.2020.02.058
Wang, D., Hu, B., Hu, C., Zhu, F., Liu, X., Zhang, J., et al. (2020). Clinical Characteristics of 138 Hospitalized Patients with 2019 Novel Coronavirus-Infected Pneumonia in Wuhan, China. JAMA 323 (11), 1061–1069. doi:10.1001/jama.2020.1585
Wang, J., Liang, Q., Zhao, Q., Tang, Q., Ahmed, A. F., Zhang, Y., et al. (2021). The Effect of Microbial Composition and Proteomic on Improvement of Functional Constipation by Chrysanthemum Morifolium Polysaccharide. Food Chem. Toxicol. 153, 112305. doi:10.1016/j.fct.2021.112305
Wang, S., Li, C., and Wang, Z. (2020). Mechanism of Xuanbai Chengqi Decoction in the Treatment of Severe COVID-19 Case Based on Network Pharmacology and Literature Screening. Modern. Traditional Chin. Med. Mater. 22 (10), 3472–3484. doi:10.11842/wst.20200720002
Wang, Y., Chen, B., Cao, J., Huang, Y., Wang, G., Peng, K., et al. (2020a). Effects of Mulberry Leaf Flavonoids on Intestinal Mucosal Morphology and Intestinal Flora of Litopenaeus Vannamei. Chin. J. Anim. Nutr. 32 (4), 1817–1825. doi:10.3969/j.issn.1006-267x.2020.04.040
Wang, Y., Pan, B., Fang, S., Zhang, J., Pan, Z., and Liu, L. (2020b). Discussion of Pharmacological Mechanism and Component Compatibility of Five Chinese Medicines Targeting ACE2 for Treatment of COVID-19 Using Network Pharmacology. Chin. Traditional Herbal Drugs 51 (18), 4691–4697. doi:10.7501/j.issn.0253-2670.2020.18.012
Wang, Z., Yang, B., Li, Q., Wen, L., and Zhang, R. (2020). Clinical Features of 69 Cases with Coronavirus Disease 2019 in Wuhan, China. Clin. Infect. Dis. 71 (15), 769–777. doi:10.1093/cid/ciaa272
Weikao, C., Wenzheng, J., and Hengshan, T. (2008). The In Vivo Process of Chlorogenic Acid and Drug Interaction. Pharmacol. Clin. Chin. Materia Med. 03, 118–120. doi:10.13412/j.cnki.zyyl.2008.03.051
Weiming, C. (2005). Study on the Chemical Constituents of Sinomenium Chinensis and the Metabolism of Sinomenine in Rats. PhD. (Shenyang, China: Shenyang Pharmaceutical University).
Weinstein, W. M., Onderdonk, A. B., Bartlett, J. G., Louie, T. J., and Gorbach, S. L. (1975). Antimicrobial Therapy of Experimental Intraabdominal Sepsis. J. Infect. Dis. 132 (3), 282–286. doi:10.1093/infdis/132.3.282
Wichmann, D., Sperhake, J.-P., Lütgehetmann, M., Steurer, S., Edler, C., Heinemann, A., et al. (2020). Autopsy Findings and Venous Thromboembolism in Patients with COVID-19. Ann. Intern. Med. 173 (4), 268–277. doi:10.7326/M20-2003
Williams, J. E. (2003). Portal to the interior: Viral Pathogenesis and Natural Compounds that Restore Mucosal Immunity and Modulate Inflammation. Altern. Med. Rev. 8 (4), 395–409. doi:10.1136/ebm.8.2.59
Wróbel, A., Serefko, A., Szopa, A., and Poleszak, E. (2020). Asiatic Acid, a Natural Compound that Exerts Beneficial Effects on the Cystometric and Biochemical Parameters in the Retinyl Acetate-Induced Model of Detrusor Overactivity. Front. Pharmacol. 11, 574108. doi:10.3389/fphar.2020.574108
Wu, H.-J., Ivanov, I. I., Darce, J., Hattori, K., Shima, T., Umesaki, Y., et al. (2010). Gut-Residing Segmented Filamentous Bacteria Drive Autoimmune Arthritis via T Helper 17 Cells. Immunity 32 (6), 815–827. doi:10.1016/j.immuni.2010.06.001
Wu, Q., Chen, Y., Ouyang, Y., He, Y., Xiao, J., Zhang, L., et al. (2021). Effect of Catechin on Dietary AGEs Absorption and Cytotoxicity in Caco2 Cells. Food Chem. 355, 129574. doi:10.1016/j.foodchem.2021.129574
Wu, S., Guo, T., Qi, W., Li, Y., Gu, J., Liu, C., et al. (2021). Curcumin Ameliorates Ischemic Stroke Injury in Rats by Protecting the Integrity of the Blood brain Barrier. Exp. Ther. Med. 22 (1), 783. doi:10.3892/etm.2021.10215
Wu, S., Rhee, K.-J., Albesiano, E., Rabizadeh, S., Wu, X., Yen, H.-R., et al. (2009). A Human Colonic Commensal Promotes colon Tumorigenesis via Activation of T Helper Type 17 T Cell Responses. Nat. Med. 15 (9), 1016–1022. doi:10.1038/nm.2015
Wu, X.-X., Huang, X.-L., Chen, R.-R., Li, T., Ye, H.-J., Xie, W., et al. (2019). Paeoniflorin Prevents Intestinal Barrier Disruption and Inhibits Lipopolysaccharide (LPS)-Induced Inflammation in Caco-2 Cell Monolayers. Inflammation 42 (6), 2215–2225. doi:10.1007/s10753-019-01085-z
Wu, Y.-R., Y.-R., Kuang, G.-y., Lu, F.-g., Wang, H.-x., Lu, M., and Zhou, Q. (2019). Pathological Relationship between Intestinal Flora and Osteoarthritis and Intervention Mechanism of Chinese Medicine. Chin. J. Integr. Med. 25 (9), 716–720. doi:10.1007/s11655-019-3224-2
Wu, Z., Huang, X., and Qi, M. (2003). Study on Relationship between Traditional Chinese Medicine and Cytokines. Chin. Traditional Herbal Drugs 34 (9), 10–12.
Xia, G., Ting, G., Yang, M., Yanjing, L., Wenzhe, H., Zhenzhong, W., et al. (2016). Metabolism of Six Saponins by Rat Intestinal Bacteria In Vitro. China J. Chin. Materia Med. 41, 2329–2338. doi:10.4268/cjcmm20161226
Xia, T., Zhang, J., Han, L., Jin, Z., Wang, J., Li, X., et al. (2019). Protective Effect of Magnolol on Oxaliplatin‐induced Intestinal Injury in Mice. Phytotherapy Res. 33 (4), 1161–1172. doi:10.1002/ptr.6311
Xin, L., Liu, X.-H., Yang, J., Shen, H.-Y., Ji, G., Shi, X.-F., et al. (2019). The Intestinal Absorption Properties of Flavonoids in Hippophaë Rhamnoides Extracts by an In Situ Single-Pass Intestinal Perfusion Model. J. Asian Nat. Prod. Res. 21 (1), 62–75. doi:10.1080/10286020.2017.1396976
Xiong, L.-J., Zhou, M.-Y., He, X.-Q., Wu, Y., and Xie, X.-L. (2020). The Role of Human Coronavirus Infection in Pediatric Acute Gastroenteritis. Pediatr. Infect. Dis. J. 39 (7), 645–649. doi:10.1097/inf.0000000000002752
Xiuwei, Y., Jianye, Z., Wei, X., Jun, L., and Weiqing, Z. (2005). The Biotransformation of Kaempferitrin by Human Intestinal flora. Acta Pharmaceut. Sin. 08, 717–712. doi:10.16438/j.0513-4870.2005.08.009
Xiyu, C., Xin, C. U. I., Chunzhao, L. I. U., and Wen, N. I. (2006). Recent Advances of Antioxidant Activity of Chinese Herbal Medicines. Nat. Product. 18 (3), 514–518. doi:10.16333/j.1001-6880.2006.03.041
Xu, H., Liu, Y., Wang, D., and Zhang, Z. (2019). Shenmai Injection Maintains Blood-Brain Barrier Integrity Following Focal Cerebral Ischemia via Modulating the Expression and Trafficking of Occludin in Lipid Rafts. J. Ethnopharmacol. 237, 55–63. doi:10.1016/j.jep.2019.03.034
Xu, K., Cai, H., Shen, Y., Ni, Q., Chen, Y., Hu, S., et al. (2020). Management of Corona Virus Disease-19 (COVID-19): The Zhejiang Experience. Zhejiang Da Xue Xue Bao Yi Xue Ban 49 (1), 147–157. doi:10.3785/j.issn.1008-9292.2020.02.02
Xu, X., Han, M., Li, T., Sun, W., Wang, D., Fu, B., et al. (2020). Effective Treatment of Severe COVID-19 Patients with Tocilizumab. Proc. Natl. Acad. Sci. USA 117 (20), 10970–10975. doi:10.1073/pnas.2005615117
Xu, Y., and Du, Y. (2020). Effects of Lentinan on Endothelial Cell Activity, Inflammatory Response, Endoplasmic Reticulum Stress, and Apoptosis in Sepsis. Adv. Polym. Technology 2020, 1–9. doi:10.1155/2020/1640208
Xueren, O., Yanni, L., Xiaoling, L., and Hua, X. (2020). Effect of Shen Ling Baizhu Powder on Airway Inflammation and Regulation of Gut Microbiota in Asthmatic Mice. Tradit. Chin. Drug Res. Clin. Pharmacol. 31 (11), 1282–1288. doi:10.19378/j.issn.1003-9783.2020.11.004
Ya, Z., Qiang, L. Z., Qin, Z. X., and Long, W. B. (2020). To Investigation of the Mechanism of Xuebijing Injection in COVID-19 Treatment Based on Network Pharmacology and Molecular Docking. Chin. J. Comp. Med. 30 (07), 57–64. doi:10.3969/j.issn.1671-7856.2020.07.008
Yajima, M., Nakayama, M., Hatano, S., Yamazaki, K., Aoyama, Y., Yajima, T., et al. (2001). Bacterial Translocation in Neonatal Rats: The Relation between Intestinal flora, Translocated Bacteria, and Influence of Milk. J. Pediatr. Gastroenterol. Nutr. 33 (5), 592–601. doi:10.1097/00005176-200111000-00015
Yan, Q. I. U., Bao-an, C. U. I., Fa-ming, D., and Yuan-liang, H. U. (2009). Effects of Four Polysaccharides on Mucosal Immunity in Vaccinated Chickens. Chin. J. Prev. Vet. Med. 31 (12), 974–977.
Yang, L., Akao, T., Kobashi, K., and Hattori, M. (1996). Purification and Characterization of a Novel Sennoside-Hydrolyzing .BETA.-Glucosidase from Bifidobacterium Sp. Strain SEN, a Human Intestinal Anaerobe. Biol. Pharm. Bull. 19 (5), 705–709. doi:10.1248/bpb.19.705
Yao, Y., He, Z., Liu, X., He, Y., Lei, Y., Zhang, S., et al. (2020). Potential Material Basis of Kangbingdu Granules for Treatment of Coronavirus Disease 2019 (COVID-19) Based on Network Pharmacology and Molecular Docking Technology. Chin. Tradit. Herbal Drugs 51 (6), 1386–1396. doi:10.7501/j.issn.0253-2670.2020.06.003
Yasui, T., and Irahara, M. (2007). Effects of Traditional Chinese Medicine on Cytokines. J. Psychosomatic Obstet. Gynecol. 28, 25. doi:10.18977/jspog.12.1-2_82_1
Yin, Y., and Wunderink, R. G. (2018). MERS, SARS and Other Coronaviruses as Causes of Pneumonia. Respirology 23 (2), 130–137. doi:10.1111/resp.13196
Ying, S., Honggui, Z., Xiangguo, S., Mingyu, D., and Dafang, Z. (2002). Study on Metabolites of Aconitine in Rabbit Urine. Acta Pharm. Sin. 10, 781–783. doi:10.16438/j.0513-4870.2002.10.007
Yongbing, W., Yunbao, L. U. O., Chiran, H. A. N., Wei, Q. I. U., and Zhenggang, Z. H. U. (2008). Influences of Enteral Nutrition Combined with TCM Intervention on Inflammation and Intestinal Barrier Function in Severe Acute Pancreatitis in Rabbits. J. Shanghai Jiaotong Univ. Med. Sci. 28 (8), 978–982.
Yu, W., Ou, X., Liu, X., Zhang, S., Gao, X., Cheng, H., et al. (2020). ACE2 Contributes to the Maintenance of Mouse Epithelial Barrier Function. Biochem. Biophys. Res. Commun. 533 (4), 1276–1282. doi:10.1016/j.bbrc.2020.10.002
Yufeng, Z., Fengrui, S., Xinhua, G., and Shuying, L. (2008). Studies on the Biotransformation of Aconitine in Human Intestinal Bacteria Using Soft-Ionization Mass Spectrometry. Chem. J. Chin. Univ. 01, 55–59.
Zaki, A. M., van Boheemen, S., Bestebroer, T. M., Osterhaus, A. D. M. E., and Fouchier, R. A. M. (2012). Isolation of a Novel Coronavirus from a Man with Pneumonia in Saudi Arabia. N. Engl. J. Med. 367 (19), 1814–1820. doi:10.1056/NEJMoa1211721
Zhang, H.-Y., Tian, J.-X., Lian, F.-M., Li, M., Liu, W.-K., Zhen, Z., et al. (2021). Therapeutic Mechanisms of Traditional Chinese Medicine to Improve Metabolic Diseases via the Gut Microbiota. Biomed. Pharmacother. 133, 110857. doi:10.1016/j.biopha.2020.110857
Zhang, H., Wang, Y., Su, Y., Fang, X., and Guo, W. (2021). The Alleviating Effect and Mechanism of Bilobalide on Ulcerative Colitis. Food Funct. 12. doi:10.1039/d1fo01266e
Zhang, J.-J., Dong, X., Cao, Y.-y., Yuan, Y.-d., Yang, Y.-b., Yan, Y.-q., et al. (2020a). Clinical Characteristics of 140 Patients Infected with SARS‐CoV‐2 in Wuhan, China. Allergy 75 (7), 1730–1741. doi:10.1111/all.14238
Zhang, J., Mi, Y., Zhou, R., Liu, Z., Huang, B., Guo, R., et al. (2020). The TLR4-MyD88-NF-Κb Pathway is Involved in sIgA-Mediated IgA Nephropathy. J. Nephrol. 33 (6), 1251–1261. doi:10.1007/s40620-020-00722-3
Zhang, J., Zhang, Z., Xu, J., Ye, C., Fu, S., Hu, C.-A. A., et al. (2021). Protective Effects of Baicalin on Peritoneal Tight Junctions in Piglets Challenged with Glaesserella Parasuis. Molecules 26 (5), 1268. doi:10.3390/molecules26051268
Zhang., M.-X., Song, T.-Z., Zheng, H.-Y., Wang, X.-H., Lu, Y., Zhang, H.-D., et al. (2019). Superior Intestinal Integrity and Limited Microbial Translocation Are Associated with Lower Immune Activation in SIVmac239-Infected Northern Pig-Tailed Macaques (Macaca Leonina). Zoolog. Res. 40 (6), 522–531. doi:10.24272/j.issn.2095-8137.2019.047
Zhang, N., Guo, S., Li, H., Li, J., Xu, X., Wan, C., et al. (2012). Effects of Sijunzi Decoction on Small Intestinal T Lymphocyte Subsets Differentiation in Reserpine Induced Spleen Deficiency Rats. J. Anim. Vet. Adv. 11 (9), 1290–1298. doi:10.3923/javaa.2012.1290.1298
Zhang, Q., Yu, Q., and Zhu, Y. (2020). Clinical Effect of the Traditional Chinese Medicine for Invigorating the Spleen and Activating the Internal Circulation Combined with the Enteral Nutrition Support on the Postoperative Recovery of Gastric Cancer. Basic Clin. Pharmacol. Toxicol. 127, 218. doi:10.1111/bcpt.13455
Zhang, T., Ouyang, H., Mei, X., Lu, B., Yu, Z., Chen, K., et al. (2019). Erianin Alleviates Diabetic Retinopathy by Reducing Retinal Inflammation Initiated by Microglial Cells via Inhibiting Hyperglycemia‐mediated ERK1/2-Nf‐κb Signaling Pathway. FASEB J. 33 (11), 11776–11790. doi:10.1096/fj.201802614RRR
Zhang, X.-P., Jiang, J., Yu, Y.-P., Cheng, Q.-H., and Chen, B. (2010). Effect of Danshen on Apoptosis and NF-Kappa B Protein Expression of the Intestinal Mucosa of Rats with Severe Acute Pancreatitis or Obstructive Jaundice. Hepatobiliary Pancreat. Dis. Int. 9 (5), 537–546.
Zhang, Y., Gan, Z., Liu, Z., Luo, J., Tang, C., Liu, C., et al. (2020b). Exploring Mechanism of Qingkailing Injection in Treatment of Coronavirus Disease 2019 (COVID-19) Based on Network Pharmacology and Molecular Docking. Chin. Traditional Herbal Drugs 51 (12), 3201–3210. doi:10.7501/j.issn.0253-2670.2020.12.012
Zhang, Y., Song, Z., Huang, S., Zhu, L., Liu, T., Shu, H., et al. (2020c). Aloe Emodin Relieves Ang II‐induced Endothelial junction Dysfunction via Promoting Ubiquitination Mediated NLRP3 Inflammasome Inactivation. J. Leukoc. Biol. 108 (6), 1735–1746. doi:10.1002/jlb.3ma0520-582r
Zhang, Y. Z. (1984). Application of C3 Determination in the Treatment of Pulmonary Cancer by Traditional Chinese Medicine. Zhonghua Zhong Liu Za Zhi 6 (1), 41–43.
Zhao, Y., Song, F., Zhao, L., and Liu, S. (2009). Studies on the Biotransformation of Arctigenin Using Electrospray Ionization Mass Spectrometry. Acta Chim. Sinica 67 (10), 1123–1126.
Zhou, F., Yu, T., Du, R., Fan, G., Liu, Y., Liu, Z., et al. (2020). Clinical Course and Risk Factors for Mortality of Adult Inpatients with COVID-19 in Wuhan, China: a Retrospective Cohort Study. Lancet 395 (10229), 1054–1062. doi:10.1016/S0140-6736(20)30566-3
Zhou, J., Chen, Y., Wang, Y., Gao, X., Qu, D., and Liu, C. (2014). A Comparative Study on the Metabolism of Epimedium Koreanum Nakai-Prenylated Flavonoids in Rats by an Intestinal Enzyme (Lactase Phlorizin Hydrolase) and Intestinal Flora. Molecules 19 (1), 177–203. doi:10.3390/molecules19010177
Zhou, J., Ma, Y. H., Zhou, Z., Chen, Y., Wang, Y., and Gao, X. (2015). Intestinal Absorption and Metabolism of Epimedium Flavonoids in Osteoporosis Rats. Drug Metab. Dispos 43 (10), 1590–1600. doi:10.1124/dmd.115.064386
Zhou, Y., Ning, H., and Huang, R. (2019). The Influence of Intestinal flora on Asthma and COPD:Research advance. Chin. J. Microecology 31 (6), 740–744. doi:10.13381/j.cnki.cjm.201906029
Zhu, H.-C., Jia, X.-K., Fan, Y., Xu, S.-H., Li, X.-Y., Huang, M.-Q., et al. (2021). Alisol B 23-Acetate Ameliorates Azoxymethane/Dextran Sodium Sulfate-Induced Male Murine Colitis-Associated Colorectal Cancer via Modulating the Composition of Gut Microbiota and Improving Intestinal Barrier. Front. Cell Infect. Microbiol. 11, 640225. doi:10.3389/fcimb.2021.640225
Zhu, H., Lu, X., Ling, L., Li, H., Ou, Y., Shi, X., et al. (2018). Houttuynia Cordata Polysaccharides Ameliorate Pneumonia Severity and Intestinal Injury in Mice with Influenza Virus Infection. J. Ethnopharmacol. 218, 90–99. doi:10.1016/j.jep.2018.02.016
Zuo, F., Zhou, Z.-M., Yan, M.-Z., Liu, M.-L., Xiong, Y.-L., Zhang, Q., et al. (2002). Metabolism of Constituents in Huangqin-Tang, a Prescription in Traditional Chinese Medicine, by Human Intestinal flora. Biol. Pharm. Bull. 25 (5), 558–563. doi:10.1248/bpb.25.558
Keywords: herbal medicine, gut microbiota, COVID-19, SARS-CoV-2, intestinal mucosal barrier
Citation: Chen Z, Lv Y, Xu H and Deng L (2021) Herbal Medicine, Gut Microbiota, and COVID-19. Front. Pharmacol. 12:646560. doi: 10.3389/fphar.2021.646560
Received: 27 December 2020; Accepted: 25 June 2021;
Published: 07 July 2021.
Edited by:
Rudolf Bauer, University of Graz, AustriaReviewed by:
Shih-Jie Chou, Taipei Veterans General Hospital, TaiwanElizabeth Mary Williamson, University of Reading, United Kingdom
Thomas Efferth, Johannes Gutenberg University Mainz, Germany
Copyright © 2021 Chen, Lv, Xu and Deng. This is an open-access article distributed under the terms of the Creative Commons Attribution License (CC BY). The use, distribution or reproduction in other forums is permitted, provided the original author(s) and the copyright owner(s) are credited and that the original publication in this journal is cited, in accordance with accepted academic practice. No use, distribution or reproduction is permitted which does not comply with these terms.
*Correspondence: Li Deng, dengli@jnu.edu.cn
†These authors have contributed equally to this work